- 1College of Agriculture, Henan University of Science and Technology, Luoyang, China
- 2Key Laboratory of Huang-Huai-Hai Smart Agricultural Technology, Ministry of Agriculture and Rural Affairs, Zhengzhou, China
- 3Institute of Agricultural Information Technology, Henan Academy of Agricultural Sciences, Zhengzhou, China
The judicious management of water and nitrogen (N) is pivotal for augmenting crop productivity and N use efficiency, while also mitigating environmental concerns. With the advent of the High−Farmland Construction Program in China, one−off irrigation has become feasible for most dryland fields, presenting a novel opportunity to explore the synergistic strategies of water and N management. This study delves into the impact of one−off alternate furrow irrigation (AFI) and topdressing N fertilizer (TN) on soil nitrate−N distribution, and N productivity—including plant N accumulation, translocation, and allocation, and grain yield, protein content, N use efficiency of winter wheat (Triticum aestivum L.) in 2018−2019 and 2019−2020. Experimental treatments administered at the jointing stage comprised of two irrigation methods—every (EFI) and alternative (AFI) furrow irrigation at 75 mm, and two topdressing N rates—0 (NTN) and 60 (TN) kg N ha−1. Additionally, a conventional local farmer practice featuring no irrigation and no topdressing N (NINTN) was served as control. Compared to NINTN, EFINTN substantially increased aboveground N accumulation, grain yield, and protein yield, albeit with a reduction in grain protein content by 8.1%−10.6%. AFI, in turn, led to higher nitrate−N accumulation in the 60−160 cm soil depth at booting and anthesis, but diminished levels at maturity, resulting in a significant surge in N accumulation from anthesis to maturity and its contribution to grain, N fertilizer partial factor productivity (PFPN), and N uptake efficiency (NUPE), thereby promoting grain yield by 9.9% and preserving grain protein content. Likewise, TN enhanced soil nitrate−N at key growth stages, reflected in marked improvements in N accumulation both from booting to anthesis and from anthesis to maturity, as well as in grain yield, protein content, and protein yield. The combination of AFI and TN (AFITN) yielded the highest grain yield, protein content, with PFPN, NUPE, and N internal efficiency outstripping those of EFINTN, but not AFINTN. In essence, one−off AFI coupled with TN at the jointing stage is a promising strategy for optimizing soil nitrate−N and enhancing wheat N productivity in dryland where one−off irrigation is assured.
1 Introduction
Wheat stands as the cornerstone of nutrition and vegetable protein in the human diet, occupying over 20% of the world’s arable territory and feeds about 30% of the world population (Zhang et al., 2019; Liu et al., 2020). Despite this, around 75% of wheat production emanates from drylands area in world—regions mainly characterized by aridity, semi−aridity, and semi−humid drought−prone (Khan et al., 2017; Zhang et al., 2019). Water scarcity and nutrient shortfalls impose significant constraints on the pursuit of enhanced and consistent wheat yields in these regions (Luo et al., 2021). Soil moisture deficits not only curtail nutrient uptake efficiency but also compromise the productivity of both water and nutrients, leading to low and unstable wheat yields (Gan et al., 2013; Zhang et al., 2020). Nonetheless, the swift progression of the High−Standard Farmland Construction Program in China, culminating in 66.7 million hectares of high−caliber farmland by 2022 and plans for an additional 13.3 million hectares during the 14th Five−Year Plan, has cemented the provision of one−off irrigation for wheat growth across numerous dryland areas—locales previously bereft of irrigation (Wang et al., 2022; Zhao et al., 2023b). However, management strategies that leverage this one−off irrigation are still underdeveloped, it is a urgent necessity to refine water and nutrient management tactics to grasp this one-off irrigation opportunity to bolster wheat productivity.
Nitrogen (N), a linchpin for crop physiological and agronomic health, is crucial for robust plant growth and development (Wang et al., 2014). An adequate water regimen enhances grain N uptake and overall crop productivity (Kumari et al., 2017); conversely, water imbalances can precipitate N inefficiencies (Sarker et al., 2020; Zhao et al., 2023b). Research by Jia et al. (2021) acknowledged that alternate furrow irrigation (AFI) targets the root zone with precision, thereby optimizing water provisioning, bolstering shoot N accumulation, and augmenting N use efficiency (NUE), ultimately yielding richer wheat harvests. However, instances under low rainfall condition have shown AFI to be detrimental to N uptake, leading to decreased crop yields when contrasted with conventional every furrow irrigation (EFI) due to diminished irrigation volumes (Benjamin et al., 1997; Sepaskhah and Hosseini, 2008). Yet, combining AFI with other approaches, such as N management and planting modes, has been demonstrated to enhance NUE in wheat crops (Ghasemi-Aghbolaghi and Sepaskhah, 2018). Topdressing nitrogen (TN), a strategic intervention for crop yield improvement, furnishes N nutrition vital for subsequent growth stages, particularly when soil N content is suboptimal (Li et al., 2020; Ji et al., 2021; Fu et al., 2022). Zain et al. (2021) discovered that TN applied at the jointing stage syncs with wheat’s N demands, optimizing NUE in China North Plain. In drought−prone zones, a one-off irrigation based on 0−40 cm soil moisture levels at the regreening stage and coupled with a 50% N fertilizer topdressing, has proven to increase shoot N accumulation, NUE, and grain yield while minimizing soil nitrate-N residue at harvest (Zhao et al., 2023b). These insights suggest that judicious irrigation paired with TN at the proper stage are pivotal for elevating grain yield and NUE, enabling an environmentally congenial wheat production system. However, farmers usually apply all N fertilizers before sowing due to irrigation and labor constraints, which lead to a misalignment between the wheat’s water and N requirements and result in some environmental issues (Wang and Li, 2019). Thus, this condition reinforce the need for AFI and TN protocols in enhancing winter wheat productivity in drylands.
The presence of nitrate−N in soil is integral for plant N uptake; however, if it exceeds safety thresholds, it is at risk of leaching, denitrification, and emissions in winter wheat production systems (Zhou and Buterbach-Bahl, 2014; Dai et al., 2015; Huang et al., 2017; Zhao et al., 2023a). Notably, dryland farms in China often exhibit substantial soil nitrate−N concentrations, such as 601 kg ha−1 in 100−180 cm (Dai et al., 2015), 1065 kg ha−1 in 0−300 cm (Guo et al., 2010), and a staggering 708−1500 kg ha−1 within a 0−380 cm soil profile (Zhao et al., 2023a)—figures that underscore the urgency for agro−operations focused on optimizing soil nitrate−N to favor crop uptake and minimize environmental risks (Adel et al., 2019; Wang and Li, 2019).
The furrow-seeded (FS) framework, is widely applied in drylands for the purpose to increase crop yields and N use efficiency (Sharma et al., 2012; Gan et al., 2013; Mehdi et al., 2017; Ali et al., 2018; Zhang et al., 2020; Yang et al., 2021). In FS system, the ridges in the field alternate with the corresponding furrows, which made irrigation more conveniently and precisely (Jia et al., 2021). Previous studies have demonstrated that, under RF, the winter wheat yield in dryland could be significantly increased via irrigation (Ali et al., 2018; Li et al., 2019). For example, Li et al. (2019) found that a total amount of irrigation of 165 mm during the growth stage increased wheat yield by 46.1%. Luo et al. (2020) reported that the irrigation of 7.8–11.8 mm at wintering and jointing increased wheat yield by 10.0–27.1%. However, these researches mainly focus on optimizing amount and/or its technology of irrigation, but not pay attention in one-off irrigation. Moreover, the influence of coupled FI and TN techniques on soil nitrate−N and N productivity in FS winter wheat remains elusive. This gap inhibits the full utilization of water resources, which come from High−Standard Farmland Construction efforts, to boost wheat productivity.
Within this context, our study proposed that coupled AFI with TN could optimize soil nitrate−N and maximize N productivity in winter wheat. Employing AFI and TN at the jointing stage of winter wheat in a semi−humid and drought−prone region, this research aims to: (1) elucidate the effects of FI, AFI, and TN, as well as their interactions, on soil nitrate−N accumulation; (2) evaluate their impact on aboveground N accumulation, translocation, allocation, grain yield, grain protein content, protein yield, and N use efficiency; and (3) identify an optimal agronomic strategy that synergizes soil nitrate−N, crop yield, quality, and efficiency within the FS system in drylands where one−off irrigation is assured.
2 Materials and methods
2.1 Study site description
From October 2018 and June 2020, a two−year field experiment was carried out at Nandasu village, located in Xiaolangdi town, Mengjin district, Luoyang, Henan province—one of typical dryland winter wheat producing regions in China. The study site was characterized by an average annual air temperature of 13.7°C and a mean annual frost−free period of 210 days, the area enjoys an average annual sunshine duration of 2196 hours and receives an average annual precipitation of 650 mm. Notably, around 60% of this rainfall occurs from June to September, which marginally overlaps with the winter wheat year. The predominant cropping system in this locale is the winter wheat−summer maize rotation, with winter wheat typically sown in early to mid−October and harvested at the beginning of June the following year.
During the two experimental years, the recorded annual precipitation was 602.1 mm in 2018−2019 and 692.7 mm in 2019−2020, with 16.5% and 38.0% of this, respectively, occurring during the winter wheat season (Figure 1). The experimental site’s soil, formed from cinnamon−colored parent material and classified as a calcareous Eum−Orthic Anthrosol according to Chinese soil taxonomy, displayed consistent baseline properties at the initiation of the experiment for both years. Within the upper 0−20 cm soil layer, assessments revealed a soil field capacity ranging from 27.3% to 27.4%, bulk density between 1.35 and 1.36 g cm-3, pH of 8.2, organic matter content averaging 13.1−13.2 g kg−1, total N content fixed at 0.81 g kg−1, available phosphorus contents ranging from 12.1 to 13.2 mg kg−1, and available potassium levels between 121.6 and 125.4 mg kg−1.
2.2 Experimental design and field management
Our study incorporated a two−factor experimental design beyond the control treatment, focusing on furrow irrigation (FI) techniques and topdressing nitrogen (TN) rates. The primary treatments involved two FI techniques: every furrow irrigation (EFI) and alternate furrow irrigation (AFI), each with 75 mm of water applied at the jointing stage. For the secondary treatments, we employed two TN rates: a Zero−N topdressing (NTN) at 0 kg ha−1, and a N topdressing (TN) at 60 kg ha−1, administered concurrently with the irrigation. Additionally, the traditional local farmer planting practice featuring no irrigation and no topdressing N (NINTN) was served as control. Consequently, the experimental framework encompassed five distinct treatments:
1. Conventional no irrigation and no topdressing N (NINTN).
2. Every furrow irrigation without topdressing N (EFINTN).
3. Alternate furrow irrigation without topdressing N (AFINTN).
4. Every furrow irrigation with topdressing N (EFITN).
5. Alternate furrow irrigation with topdressing N (AFITN).
The application amount of basal fertilizer is set according to local farmer’ practice, and both the application amount of irrigation and N fertilizer topdressing are recommended by local agricultural experts. The specific amounts for irrigation and fertilizer application are detailed in Table 1.
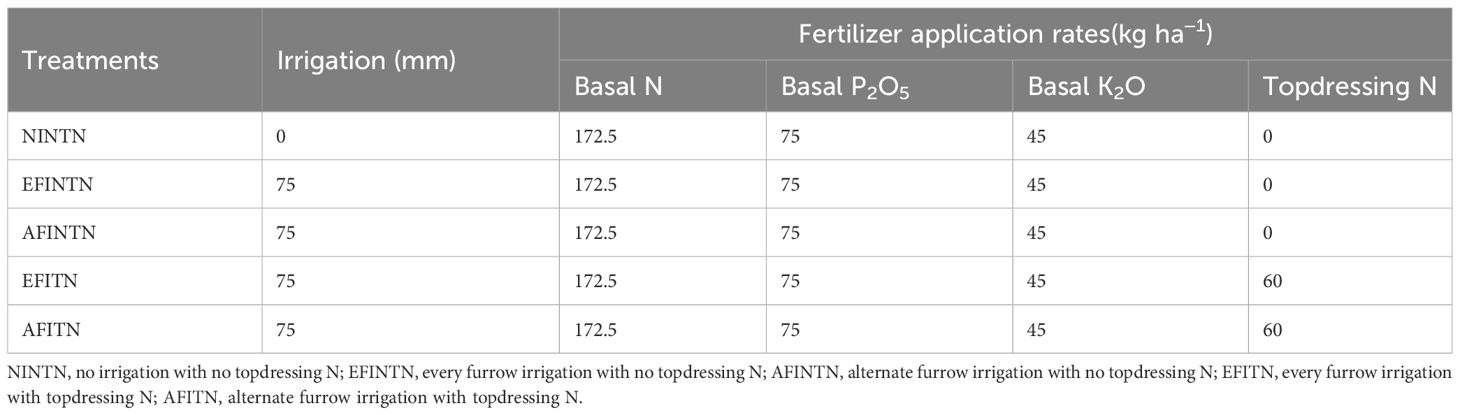
Table 1 The irrigation amount and fertilizer application rates in different treatments in 2018–2019 and 2019–2020.
Our study utilized a completely randomized plot design with three replications for each treatment. Each plot measured 20 meters in length and 6.12 meters in width, and all were situated within the same field with a 1−meter buffer between each. Upon sowing, we utilized a no−till fertilizer seeder (model 2BMQF−6/12A, produced by Luoyang Xinle Machinery Co., Ltd) to apply compound fertilizers (N:P2O5:K2O ratio of 23:10:6) at a rate of 750 kg per hectare as the base fertilizer.
The 2BMQF−6/12A no−till seeder is designed to perform multiple tasks—including furrowing, ridging, fertilizing, sowing, and soil repacking—simultaneously. Post−sowing, the equipment was adjusted to create ridges 20 cm wide and 10 cm high, with furrows that were 14 cm wide. Consequently, the spacing for wheat plants in the wider rows was 20 cm, compared to 14 cm in the narrower rows, averaging to 17 cm overall (Figure 2).
Winter wheat variety Zhoumai36 was sown using a seeding rate of 187.5 kg per hectare, at a depth of 3−5 cm within the furrows. The base fertilizers were precisely drilled between two seed rows at a depth of 10 cm. Sowing dates were October 13, 2018, and October 15, 2019, with respective harvest dates of May 30, 2019, and June 2, 2020. The previous crop in the both two years were summer maize (Zhengdan958), which harvest at early-October. Irrigation conducted at the jointing stage (Zadoks 31) on March 19, 2019, and March 22, 2020, with both the AFI and EFI treatments receiving 75 mm, calibrated for the entire plot area and regulated using a mechanical water meter (with 0.01m³ accuracy and operating at outlet valve pressures between 0.10−0.12 MPa). Specifically, each plot received 9.18 m³ of water, and the irrigation space per furrow was 2.8 m² (20 m × 0.14 m). Under EFI treatment, the total irrigated area per plot was 50.4 m² (20 m × 2.52 m), with 0.51 m³ of water for each irrigated furrow. Conversely, under AFI treatment, the irrigated area per plot was halved to 25.2 m² (20 m × 1.26 m), with 1.02 m³ of water for each irrigated furrow—effectively doubling the irrigation volume per furrow compared to EFI (Figure 2).
For TN treatments, a uniform rate of 60 kg N per hectare was hand−broadcast in the watering furrows just before the irrigation event. To manage weeds, pests, and diseases, we employed the same herbicides and pesticides used by local farmers.
2.3 Measurements and methods
2.3.1 Soil nitrate−N
During the 2018−2019 and 2019−2020 years, soil core samples were collected randomly at key phenological stages: booting (Zadoks 43), anthesis (Zadoks 65), and maturity (Zadoks 94). We sampled these using a soil auger with an internal diameter of 4.0 cm, extracting cores from 0 to 200 cm soil depths in 20 cm intervals. For NINTN and EFI treatments, we randomly took three soil cores from the center between two plant rows within a furrow. In the case of AFI treatments, we collected three soil cores from the center of irrigated furrows and another three from the center of non−irrigated furrows. The individual soil samples from identical depths and the same plot were combined, yielding round 300 grams after a homogeneous mix. This composite sample was immediately sealed in a labeled plastic bag to preserve it for further laboratory analysis. In the laboratory, the nitrate-N was quantified with the method described by Huang et al. (2017), fresh soil samples weighing 5.0 g were extracted with 50 mL of a 1.0 mol L-1 KCl after shaking it continuously for 1h. We then filtered the resultant mixture and promptly measured the nitrate−N concentration within the filtrate using a high−resolution digital colorimeter, the AutoAnalyzer 3 (AA3) from SEAL Company in Germany.
The soil nitrate−N accumulation (SNA, kg N ha–1) in the 0−200 cm soil profile was calculated as follows (Dai et al., 2015; Huang et al., 2017):
where Ti is the soil layer thickness (cm), Di is the soil bulk density (g cm−3), Ci is the soil nitrate concentration (mg kg−1), i.e., i = 20, 40, 60, 80, 100, 120, 140, 160, 180, 200. The soil bulk density of 1.32, 1.34 and 1.38 g cm−3 was used in 0−20, 20−40, and 40−200 cm according to the average value of local field, and 0.1 is the conversion coefficient.
2.3.2 Plant N
At the jointing (Zadoks 31) stage, we collected four 0.5 m−long samples of winter wheat from random locations within the experimental field. Additional samples were harvested from each plot at anthesis (Zadoks 65) and maturity (Zadoks 94) stages. The process involved counting the tillers and removing the roots using scissors where the stem intersected with the root system. The aboveground biomass at both anthesis and maturity stages was categorized into stem, leaves, sheath, and ear components. Specifically, at maturity, the ear was further divided into grain and chaff (glume + rachis). Subsequently, the biomass was oven−dried at 105°C for 30 minutes, followed by drying at 65°C for 24 hours to establish the dry weight. The oven−dried samples, encompassing grain, straw, and glume, were finely ground in preparation for chemical analysis. Utilizing a mixture of sulfuric acid and hydrogen peroxide (H2SO4−H2O2), the samples were digested, allowing us to determine N concentrations in the digestion solution with an AutoAnalyzer 3 (AA3, SEAL Company, Germany) using the method prescribed by Huang et al. (2017). The N accumulation in each plant organ was calculated by multiplying the organ’s dry weight (expressed in kg per hectare) by its respective total N concentration (in g kg−1). The total aboveground N accumulation (NA, in kg ha−1) was then computed by aggregating the N accumulation figures for each organ. Further, we calculated various N−related parameters including accumulation, translocation, and allocation based on the Equations 2-7 (Huang et al. 2021).
2.3.3 Grain yield, protein content and protein yield
At the maturity stage, four representative sampling areas, each measuring 2 m by 1.36 m, were randomly selected within each plot. The wheat plants within these areas were manually harvested to assess grain yield. Following harvest, the plants were air−dried, threshed, and the grain obtained was weighed. To accurately determine grain moisture content and dry weight, 100 g samples of the air−dried grain were further oven−dried at 90°C for 30 minutes and then at a reduced temperature of 65°C for a duration of 24 hours. Grain yield calculations for each plot were standardized to a uniform moisture content of 12.5%, using the air−dried grain weight and its determined water content to adjust the figures accordingly. The grain protein content was then calculated by multiplying the grain’s total N content, expressed in g kg−1, by the factor 0.57—which is specific to cereal grains. Lastly,the protein yield, expressed in kilograms per hectare (kg ha−1), was calculated using the Equation 8, as documented by Huang et al. (2021):
2.3.4 N use efficiency
The N fertilizer partial factor productivity, N internal efficiency, N uptake efficiency and N harvest index were calculated using the Equations 9-12, respectively (Huang et al. 2017):
where Yg is the grain yield (kg ha−1); and FN is the fertilizer rate for N (kg N ha−1); NAM is the total N accumulation in aboveground parts at maturity (kg ha−1); NAg is the N accumulation in grain at maturity (kg ha–1).
2.4 Statistical analysis
The means of the data for each treatment were computed by averaging the values across all plots. Differences between these means were assessed using analysis of variance (ANOVA) followed by the Least Significant Difference (LSD) test at a significance level of P = 0.05. These statistical analyses were conducted using the SPSS statistical software (version 18, IBM Corp., Chicago, IL, USA). Graphical representations of the data were created with Microsoft Excel 2010.
3 Results
3.1 Soil nitrate−N
Significant differences in soil nitrate−N accumulation were observed among the treatments at the booting, anthesis, and maturity stages in both years, as shown in Table 2 and Figure 3. Compared to NINTN treatment, the EFINTN treatment did not alter the soil nitrate−N accumulation at the booting stage. However, it resulted in a notable reduction in soil nitrate−N accumulation by 7.0−9.4% at the anthesis stage and 7.3−12.1% at the maturity stage, respectively. These reductions suggest that furrow irrigation at the jointing stage enhanced the uptake of soil nitrate−N by the wheat crop. In contrast, when compared to the every furrow irrigation (EFI) treatments, the alternate furrow irrigation (AFI) treatments showed increased soil nitrate−N accumulation levels at booting and anthesis stages, with a marked decrease in the upper soil layer but a significant increase in the 80−140 cm soil layer. Nevertheless, at the maturity stage, AFI treatments exhibited a significant reduction in soil nitrate−N accumulation, ranging from 6.8−8.1%. Irrespective of the irrigation method, the topdressing nitrogen (TN) treatments exhibited a significant increase in soil nitrate−N accumulation at booting (28.3%) and anthesis (24.6%) stages when compared to the no topdressing nitrogen (NTN) treatments. However, the TN treatments did not show a significant effect on the soil nitrate−N accumulation at maturity. The findings suggest that employing both AFI and TN techniques at the jointing stage could enhance soil N availability during the middle growth stages of winter wheat but had no beneficial impact at maturity. Across the two years, the soil nitrate−N accumulation at the maturity stage was observed to be AFITN = AFINTN< EFITN = EFINNT< NINTN and the AFITN, AFINTN, EFITN, and EFINNT treatments reduced the soil nitrate−N accumulation by 9.7%, 17.1%, 8.5%, and 14.6%, respectively, compared to the NINTN treatment. These results indicated that implementing the AFINTN technique effectively decreased the risk of nitrate−N accumulation, even with an application of 60 kg N ha−1 at the jointing stage.
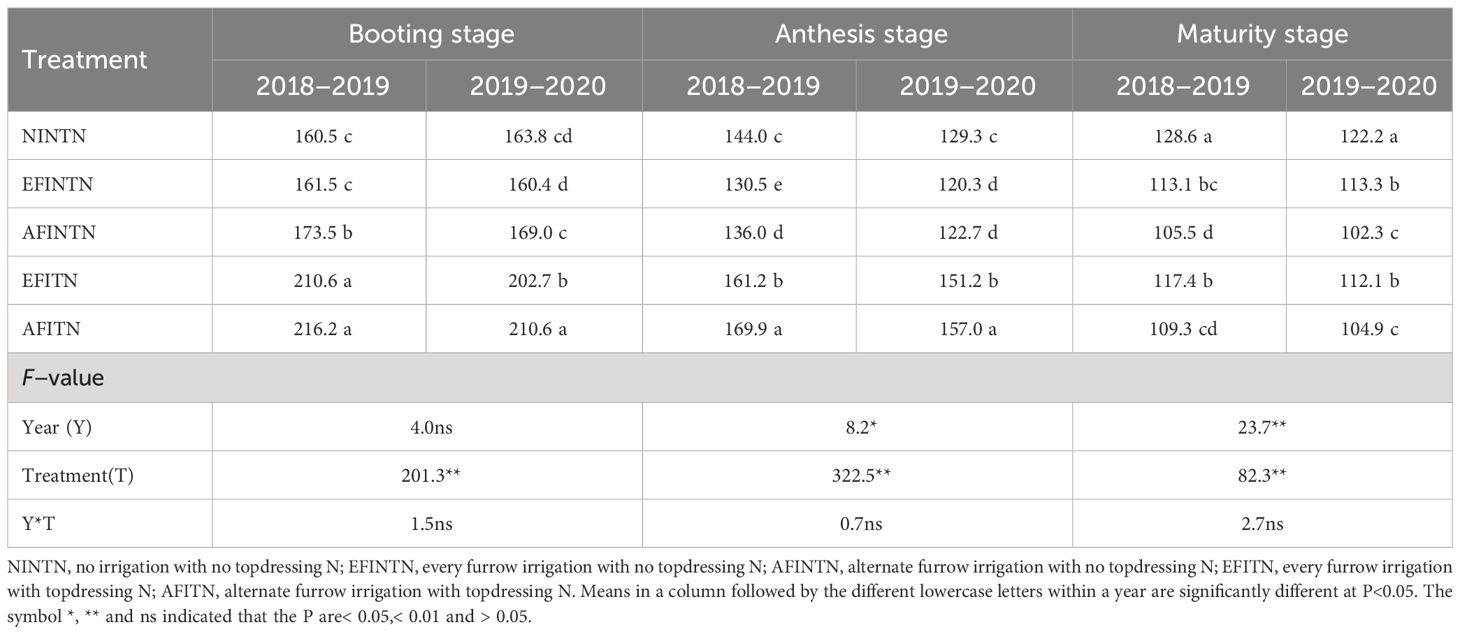
Table 2 Soil nitrate−N accumulation kg N ha-1) at booting, anthesis and maturity stages affected by the FI, AFI and TN techniques in 2018−2019 and 2019−2020.
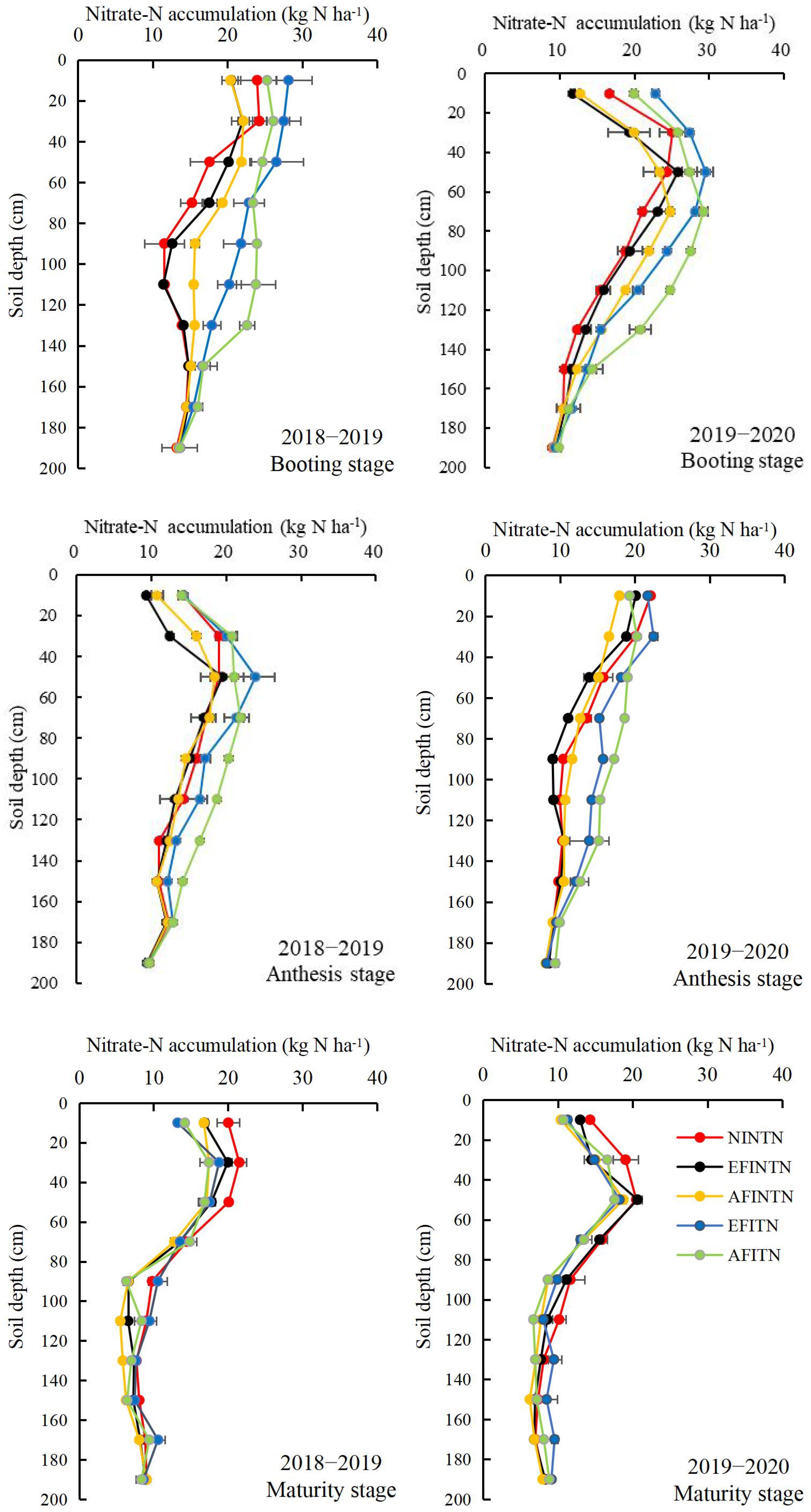
Figure 3 Soil nitrate−N accumulation at the different soil depths affected by the FI, AFI and TN techniques in 2018−2019 and 2019−2020. NINTN, no irrigation with no topdressing N; EFINTN, every furrow irrigation with no topdressing N; AFINTN, alternate furrow irrigation with no topdressing N; EFITN, every furrow irrigation with topdressing N; AFITN, alternate furrow irrigation with topdressing N. Bars indicated standard deviation.
3.2 Plant N accumulation, translocation, and allocation
3.2.1 Periodical N accumulation during various growth stages and its rate to aboveground N at maturity
Aboveground N accumulation during different growth stages, as well as its proportion of aboveground total N accumulation at maturity, were influenced by the furrow irrigation (FI), AFI, and TN techniques over the two years, as detailed in Table 3. Compared to the NINTN treatment, the EFINTN treatment significantly boosted both the overall N accumulation, specifically the N accumulation from jointing to anthesis and from anthesis to maturity. Consequentially, the N accumulation at anthesis was increased by 14.8% and the aboveground N accumulation at maturity by 22.8%. Compared to the EFI treatments, AFI treatments under the same TN rates decreased both the N accumulation from jointing to anthesis and its rate to aboveground N accumulation at maturity. Nevertheless, they significantly raised N accumulation from anthesis to maturity by 63.2% and its rate to aboveground total N accumulation at maturity by 48.9%, culminating in a 9.4−10.1% increase in aboveground N accumulation at maturity across the two years. Additionally, within the same FI technique, TN treatments markedly raised the N accumulation from jointing to anthesis by 42.9−50.0% and the rate of periodical N accumulation from jointing to anthesis to aboveground N accumulation at maturity by 12.9−18.0%, as well as the N accumulation from anthesis to maturity by 63.5−97.9% and the rate of periodical N accumulation from anthesis to maturity to aboveground N accumulation at maturity by 29.5−56.1%, ultimately enhancing the aboveground N accumulation at maturity by 23.1−29.5%, averaged across the two years. These findings demonstrate that both AFI and TN techniques have the potential to promote aboveground N accumulation during the mid and late growth periods and its rate to aboveground total N accumulation at maturity in dryland winter wheat.
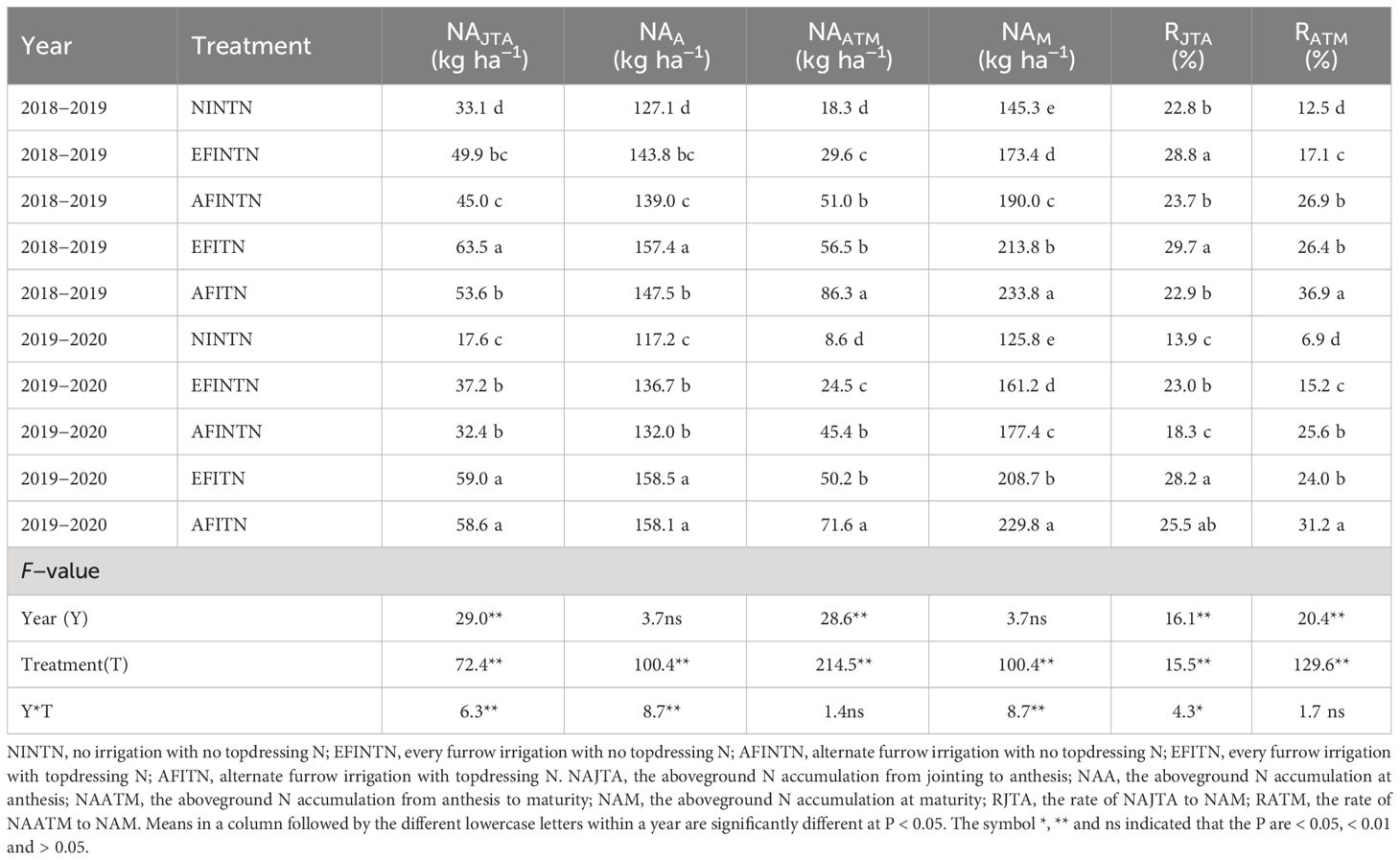
Table 3 Periodical N accumulation amount and its rate to aboveground N accumulation at maturity affected by the FI, AFI and TN techniques in 2018−2019 and 2019−2020.
3.2.2 Pre-anthesis N translocation and its contribution to grain
Table 4 illustrates the translocation amount and translocation rate of pre-anthesis N from vegetative organs to grains, which can be ranked as follows: the TN treatments exhibited higher values than NTN treatments, and the EFI treatments showed higher values than AFI treatments. Conversely, the contribution rates of pre-anthesis N to grain N were higher for the NTN treatments than TN treatments, and for the AFI treatments than EFI treatments. Consequently, the optimized values for pre-anthesis N translocation amount and its rate were achieved under the EFITN treatment. However, the contribution rates of pre-anthesis N translocation to grain N was most substantially recorded under the NINTN treatment over the two years.
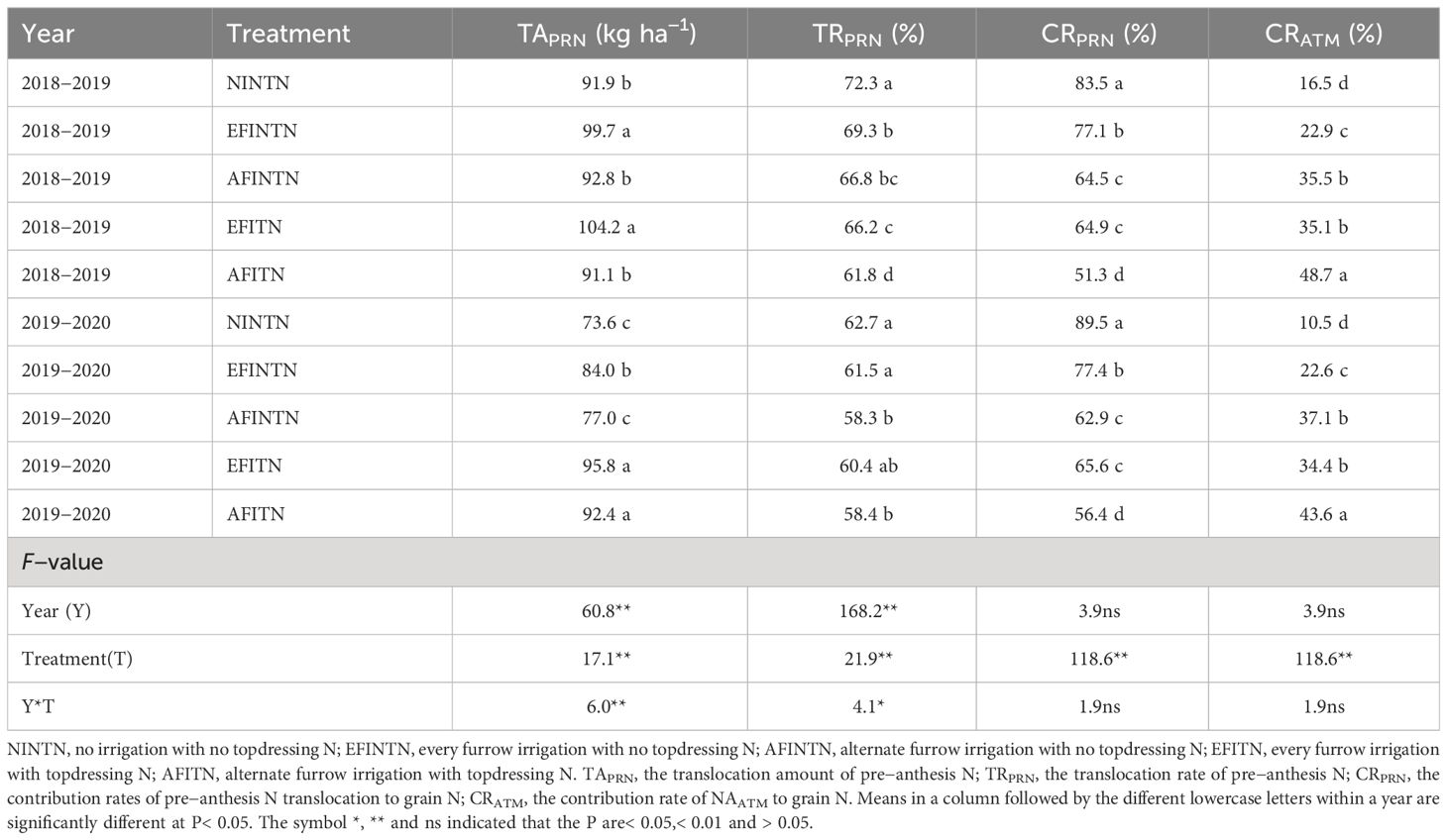
Table 4 N translocation from vegetative organ to grain, and contribution rate of post-anthesis N accumulation to grain of winter wheat affected by the FI, AFI and TN techniques in 2018−2019 and 2019−2020.
Both AFI and TN treatments, when compared within the same TN rate or FI technique, significantly enhanced the contribution rate of aboveground N accumulation from anthesis to maturity to grain N. The highest values for aboveground N accumulation from anthesis to maturity to grain N were observed under the AFITN treatment, followed by EFITN or AFINTN, then enhanced compared to EFINTN, and, finally, NINTN. This descending order of effectiveness was consistent in the two years. Notably, the differences in aboveground N accumulation from anthesis to maturity to grain N among treatments were statistically significant, with the exception of no significant difference discerned between EFITN and AFINTN.
3.2.3 N allocation in various organs at maturity
Table 5 presents data indicating that the EFINTN treatment significantly increased N allocation in different winter wheat organs in both years when compared with the NINTN treatment, with the exception of the glume + rachis in 2018−2019. Furthermore, when compared to the EFI treatments, the AFI treatments boosted grain N allocation by 11.6% averaged across the years and TN techniques. In comparison to the NTN treatments, the TN treatments significantly increased N allocation: 28.5% in grain, 21.2% in the glume + rachis, and 19.8% in the stem + leaf + sheath, averaged across the years and FI techniques. Finally, the AFITN treatment yielded the greatest N allocation in grain, which increased by 80.2%, 44.1%, 28.6%, and 11.4% when compared to the NINTN, EFINTN, AFINTN, and EFITN respectively, averaged across the two years. These findings underscore that N allocation in various organs of winter wheat at maturity is significantly influenced by both FI and AFI, as well as TN techniques. Moreover, the data suggest that the synergistic interaction between AFI and TN is a major determinant of N distribution patterns in winter wheat.
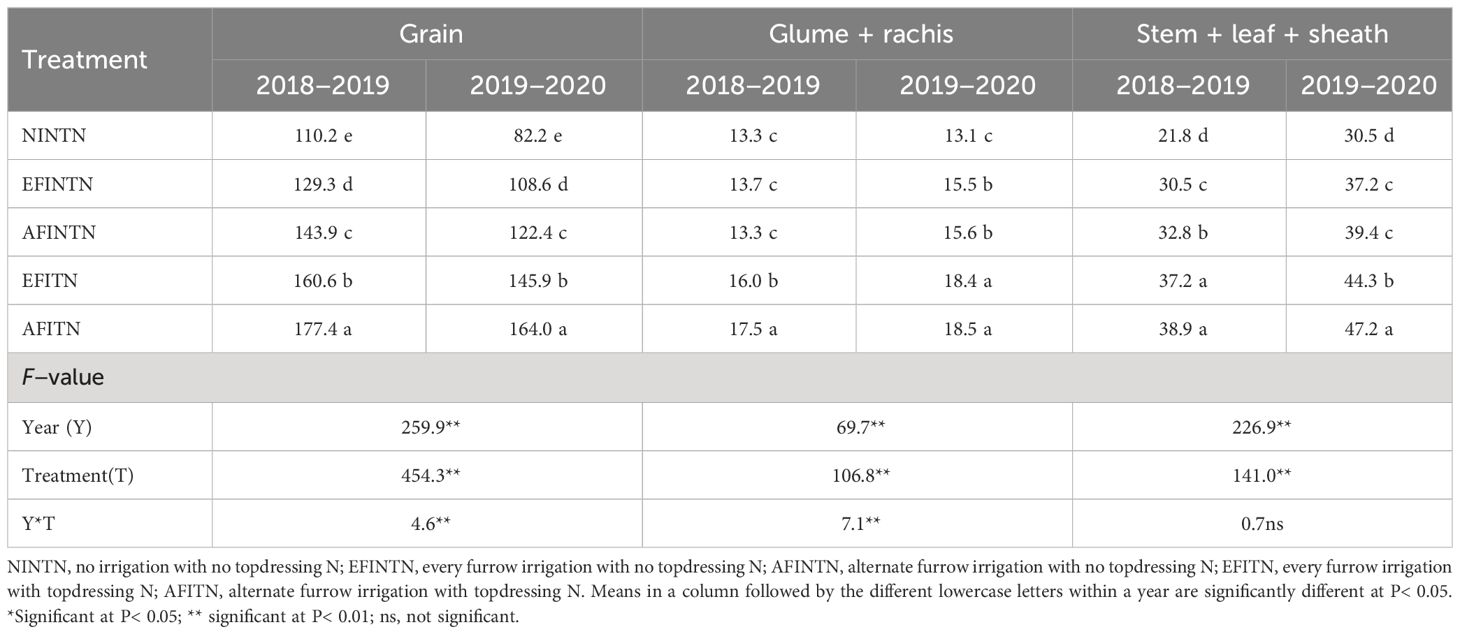
Table 5 N allocation (kg ha−1) in various organs of wheat at maturity affected by the FI, AFI and TN techniques in 2018−2019 and 2019−2020.
3.3 Grain yield, protein content, and protein yield
Table 6 reveals significant variances among treatments in terms of grain yield, protein content, and protein yield. The AFITN treatment achieved the highest grain and protein yields, followed by EFITN, AFINTN, EFINTN, and lastly, NINTN, all in descending order with statistical significance (P< 0.05) over the two years. Regarding grain protein content, there was no statistical difference was detected between EFINTN and AFINTN treatments; but there was a respective decrease of 8.8% and 10.7% when compared to the NINTN treatment, averaged across the two seasons. TN treatments under same furrow irrigation (FI) technique exhibited significant increases in grain protein content—ranging from 4.4% to 14.9%—when compared to the NTN treatments. Of note, the enhancements under AFI were higher than those under EFI. Furthermore, in comparison to the NINTN treatment, the grain protein content under the EFITN treatment diminished significantly by 2.7% to 4.1%, whereas it did not decrease under the AFITN treatment and in fact exhibited an increase of 2.7% in 2019−2020. These results conclusively indicates that the strategic coupled application of AFI and TN techqiue at the jointing stage can substantially enhance grain yield and either sustain or potentially increase the protein content, thereby significantly elevating the protein yield of dryland winter wheat.
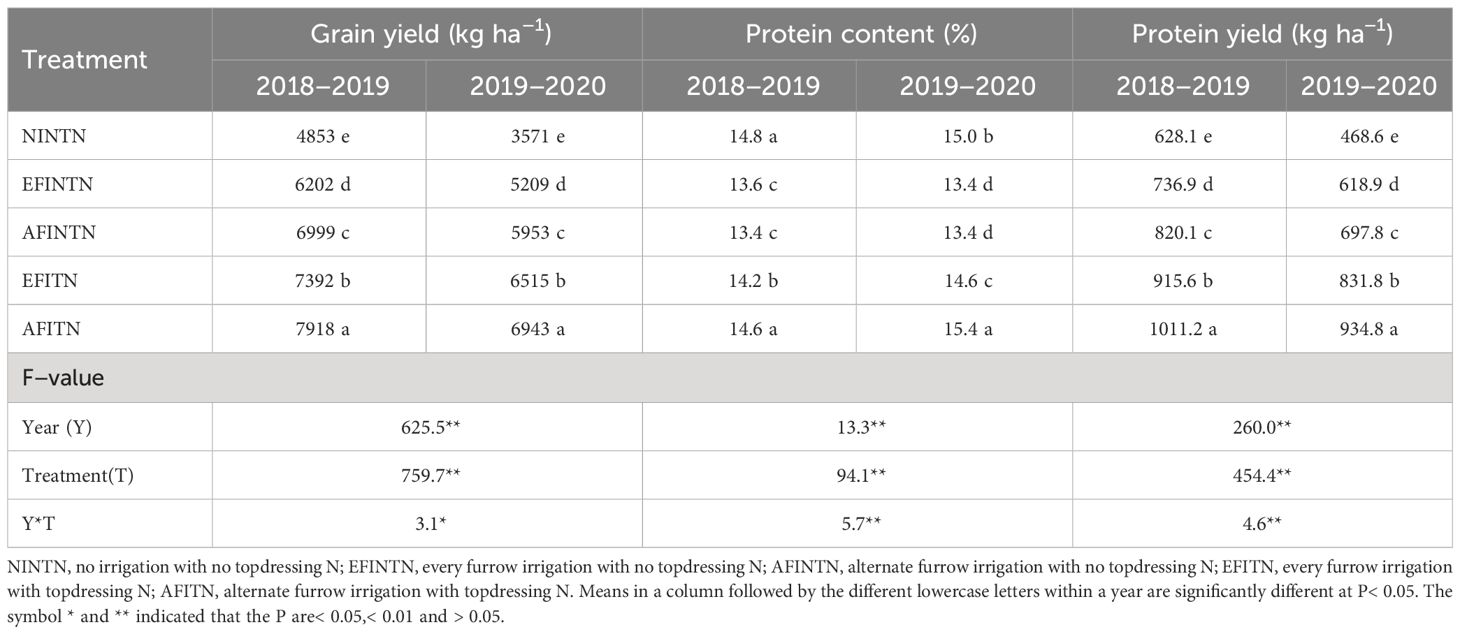
Table 6 Grain yield, protein content, protein yield of winter wheat affected by the FI, AFI and TN techniques in 2018−2019 and 2019−2020.
3.4 N use efficiency
Across both years, a significant divergence in N fertilizer partial factor productivity (PFPN), N uptake efficiency (NUPE), and N internal efficiency (NIE) was observed among the different treatments, generally ordered as AFINTN > EFINTN > AFITN > EFITN > NINTN (Table 7). Specifically, the PFPN under the AFINTN treatment surpassed EFINTN, AFITN, EFITN, and NINTN by 13.4%, 17.3%, 25.6%, and 53.9%, respectively, as well as NUPE by 9.8%, 6.5%, 17.0%, and 35.7%, while NIE showed improvements of 3.4%, 9.8%, 7.0%, and 13.9%, averaged across the two years. These results suggest that both FI and AFI techniques bolster N use efficiency, whereas the TN technique had a diminishing effect. In 2018–2019 year, the differences in the N harvest index (NHI) among treatments were not statistically significant. Nonetheless, in 2019–2020, the NHI was ranked as AFITN > EFITN > AFINTN > EFINTN > NINTN, with significant differences among all treatments exception between EFITN and AFINTN or AFITN.
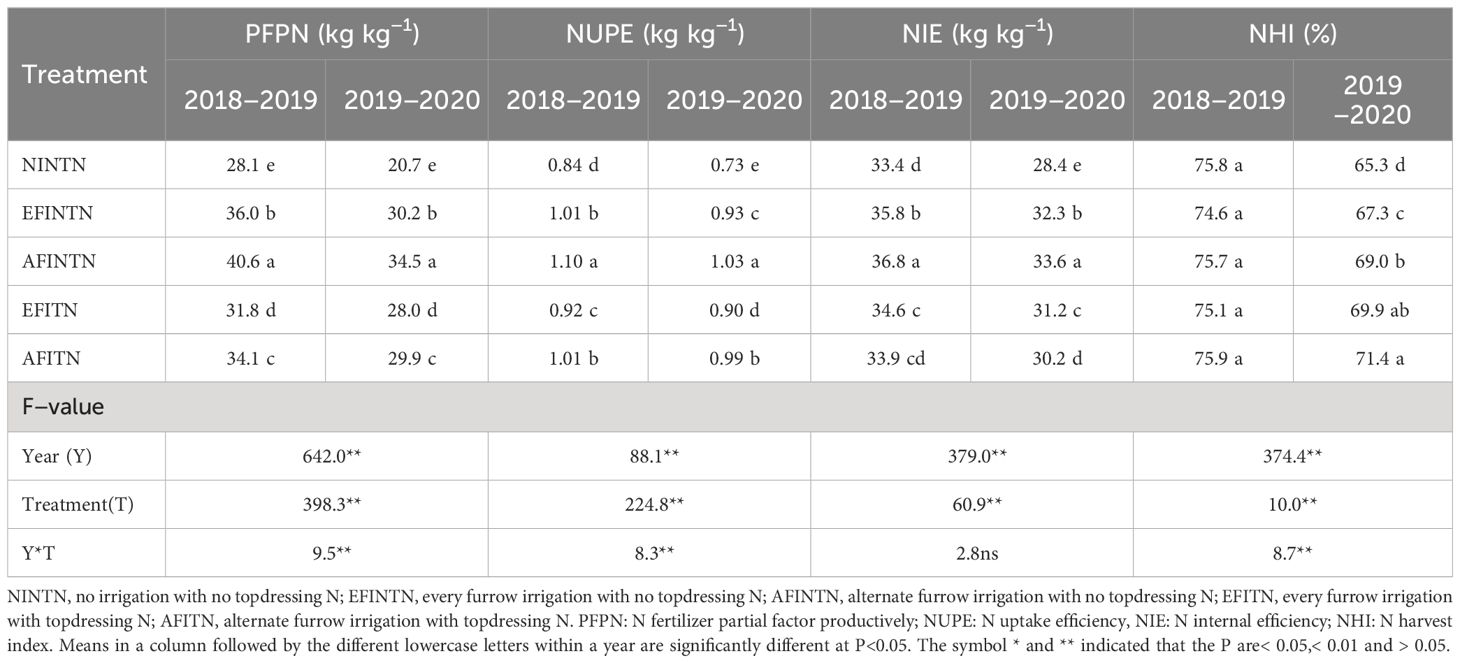
Table 7 N use efficiency of winter wheat affected by the FI, AFI and TN techniques in 2018−2019 and 2019−2020.
4 Discussion
4.1 Impact of FI, AFI, and TN at jointing on soil nitrate−N
Soil nitrate−N, the predominant form of N absorbed by crops in dry farming regions, is influenced by a myriad of factors including N fertilizer application, plant uptake and utilization, soil water penetration, and leaching processes (Zhou and Buterbach-Bahl, 2014; Wang and Li, 2019). Our study found that compared to the NINTN treatment, the EFINTN treatment resulted in lower soil nitrate−N accumulation at both the anthesis and maturity stages, suggesting that furrow irrigation (FI) reduced soil nitrate−N accumulation in dryland winter wheat farming systems. This reduction was primarily attributed to the improved N uptake by the crop due to better soil moisture from irrigation (Table 2, Adel et al., 2019). When comparing irrigation methods, alternate furrow irrigation (AFI) elevated soil nitrate−N accumulation at the booting and anthesis stages, particularly within the 80−140 cm soil layer, compared to every furrow irrigation (EFI). This enhancement, however, diminished progressively with wheat growth, especially from anthesis to maturity, leading to lower soil nitrate−N accumulation under AFI than under EFI at the maturity stage (Table 2, Figure 3). These findings suggest that AFI enhances subsoil nitrate−N accumulation in the mid−growth stages but reduces it at maturity stage of wheat, potentially due to the dual role of water movement and plant N uptake. Firstly, soil nitrate−N is prone to download leaching with irrigation water, more irrigation amount in the irrigated furrow resulted in less nitrate−N in the upper and more nitrate−N in the deeper soil layers (Khan et al., 2020). Secondly, AFI’s moist environment is known to improve plant N requirement (Jia et al., 2021) and the distribution, density, and activity of crop root (Liang et al., 2000; Wang et al., 2014), all of these would help to increase soil nitrate-N absorption by wheat (Jia et al., 2021).
Previous research demonstrated that N fertilizer topdressing can modulate soil nitrate−N accumulation, and its effects influenced by water management strategies (Zhao and Yu, 2006; Shi et al., 2012; Li et al., 2020). Han et al. (2014) compared AFI and N topdressing paired to conventional irrigation and fertilization, noting that the former increased nitrate−N in the upper soil (0–60 cm) by 30−60% while reducing it in the deeper layers (60–200 cm) by 8−44% in summer maize farmland, mainly due to the enhancement of N requirement and root morphology from the optimized moisture introduced by AFI. In our investigation, TN treatments with 60 kg N ha−1 topdressing at the jointing stage consistently improved the soil nitrate−N accumulation in most soil layers at the booting and anthesis stages, regardless of the irrigation techqiue employed, although the differences waned as the wheat matured. Notably, the combination of AFI and TN synergistically increased the soil nitrate−N accumulation, leading to the AFITN treatment exhibiting the highest soil nitrate−N accumulation during the booting and anthesis stages (Table 2, Figure 3). This implies that integrating AFI with TN at the jointing stage can bolster the soil nitrate−N accumulation and providing a favorable N supply in wheat’s middle growth phase. This soil nitrate−N accumulation enhancement mainly ascribed the N from topdressing and soil N mineralization. The greater moisture in the irrigated furrow and the partially dry soils under AFI system has strong air permeability, which generate favorable conditions for microbial activity, thus enhancing soil N mineralization and affecting the nitrate-N distribution in soil (Tian et al., 2016; Pu et al., 2022).
In terms of environmental risk, less residual nitrate−N at wheat maturity is preferable for reducing N loss and water pollution (Huang et al., 2017; Lu et al., 2019). Researches have indicated that FI technique can contribute significantly to nitrate−N leaching and groundwater contamination within agricultural systems, potentially leaching up to 40% of the nitrate−N in the root development zone (Siyal et al., 2012; Iqbal et al., 2019). Luo et al. (2020) observed that supplemental irrigation under rainfed systems could substantially increase soil nitrate−N concentration. However, studies like those by Skinner et al. (1999) have shown that AFI may lessen the risk of nitrate−N leaching when irrigation water is weekly applied, similar to EFI techniques throughout crop growth stages. Optimal irrigation and fertilization under furrow irrigation system shown by Adel et al. (2019) was also found to increase root water and N uptake while reducing nitrate−N losses, compared to conventional practices. Variations in experimental conditions may account for differences in study outcomes. In our case, FI treatments exhibited a substantial reduction in the soil nitrate−N accumulation at the maturity stage of wheat, especially under AFI treatments, meaning that AFI at jointing is prone to reduce the soil nitrate−N accumulation at maturity in wheat cropping system (Table 2, Figure 3). This aligns with Jia et al. (2021), who found that AFI with 180 mm of water reduced nitrate−N leaching by an average of 8.3% and 16.6% compared to flood irrigation with 180 mm and 270 mm, respectively. In turn, TN treatments did not show a discernible effect on the soil nitrate−N accumulation at maturity stage of winter wheat (Table 2, Figure 3). This showed that the 60 kg N ha-1 topdressing under one-off furrow irrigation did not increase the environment risk from nitrate−N. Moreover, all combinations of FI and TN techniques decreased soil nitrate−N accumulation at the maturity stage of winter wheat when compared to NINTN, particularly highlighting the effectiveness of AFITN in minimizing the risk of nitrate−N leaching (Table 2, Figure 3). These results indicated that at the maturity stage of winter wheat, the effectiveness of TN-introduced soil nitrate−N accumulation increase was lower than the FI-introduced soil nitrate−N accumulation decrease, leading to a marked decrease of soil nitrate−N accumulation, and thus alleviating the environmental risk.
4.2 Influence of FI, AFI and TN at jointing on winter wheat grain yield and protein content
Boosting grain yield remains a primary driver in wheat production, particularly in dryland regions where the potential for yield increases eclipses that of more infertile areas (Wang and Li, 2019). Our research reveals that the techniques of furrow irrigation (FI), alternative furrow irrigation (AFI), and topdressing nitrogen (TN) all contributed to a significant upsurge in winter wheat’s grain yield. This mainly ascribe to the improvement of soil moisture (Wu et al., 2023) and N supply (Table 2, Figure 3). Notably, the AFITN treatment outperformed the NINTN by 63.2–94.4% in the two years, indicating a substantial margin for yield enhancement in dryland furrow−seeded (FS) wheat systems provided by a coupled application of one-off AFI and TN at the jointing stage.
Evolving living standards have ramped up demand for high−quality grain and wheat flour (Lin et al., 2015). Grain protein content is a critical quality parameter for winter wheat, vital for both food and non−food applications (Luo et al., 2018; Hu et al., 2021). In our study, compared to the NINTN treatment, the FI technique under NTN (EFINTN and AFINTN) treatments decreased grain protein content by 8.1–10.6%. This reduction is often linked to the dilutive effect on N concentration due to the increased grain yield (Sissons et al., 2014; Hu et al., 2021), as supported by Luo et al. (2020), who documented a 5.4% drop in protein content in FI−treated winter wheat. Similarly, Sarker et al. (2020) found that AFI maintained yield and grain N content in maize despite a 37.0% reduction in irrigation water in a sub−tropical South Asian climate.
The interplay of plant N requirement, accumulation, translocation, and allocation, and the N supply-demand relationships in various wheat growth stages greatly dictates variations in wheat yield and grain protein content, all of which can be refined through judicious irrigation and N management (Aziz et al., 2018; Jia et al., 2021; Damme et al., 2022). An Iranian field experiment illustrated AFI’s superiority over EFI in bolstering shoot N uptake and grain protein content in the FS barley system (Ghasemi-Aghbolaghi and Sepaskhah, 2018). Similarly, in China’s Huang−Huai−Hai Plain, AFI distributed at wintering and grain filling stage outpaced EFI treatments at anthesis stage with the same irrigation amount, improving both grain yield and grain N accumulation (Jia et al., 2021). Our investigation further corroborates that, AFI did not significantly alter wheat grain protein content under NTN while increased by 2.8–5.5% under TN (Table 6), indicating the TN treatments could minimized N dilution under AFI system despite the augmented yield. The reason being, although AFI did not noticeably alter the N accumulation at anthesis and the translocation amount of pre-anthesis N, it considerably enhanced the N accumulation from anthesis to maturity, and its contribution to grain N in winter wheat, supporting the significant increase of grain N accumulation at maturity (Table 4). Four potential reasons are discernible: (1) AFITN’s promotion of robust root systems (Wang et al., 2012) and supply-demand status (Zhang et al., 2012), leading to heightened N uptake and assimilation (Jia et al., 2021; Yang et al., 2021); (2) the improved soil moisture and soil nitrate−N accumulation enhancing the N accumulation from anthesis to maturity and its contribution to grain N, resulting in the enhancement of grain protein content (Table 6); (3) the 2−folds irrigation volume within the irrigated furrow under AFI allowing the deep soil deposition of fertilizer N, which eluded fertilizer N loss via volatilization, favored root development and N uptake during later growth phases (Wang et al., 2014). (4) the moderate moisture levels under AFI assist N cycle phases, improving N availability and leading to amplified grain N storage (Ashraf et al., 2016).
Optimized N supply at key growth stages is often used to improve plant N characteristics, increase crop yield and grain protein content (Aziz et al., 2018; Damme et al., 2022). Wang et al. (2021) found that employing topdressing N technique at the jointing stage significantly or extremely significantly enhanced the N accumulation, yield, protein content of all the tested wheat. Similar results were also obtained in this study, employed the 60 kg N kg-1 topdressing at the jointing stage show significant advantages in N accumulation at anthesis, N transloctaion amout of pre-anthesis N, N accumulation from anthesis to maturity and its contribution to grain N (Tables 3, 4), grain N allocation (Table 5) and consequently boosting grain yield and protein content (Table 6), thereby addressing the grain protein content reduction caused by FI and AFI. As a result, the AFITN combination yielded top levels in grain yield, and protein content, culminating in an optimal protein yield. These results illustrated that, the topdressing N may employ to balance the N supply-demand for simultaneously increasing grain protein quality and the FI-introduced increase of N requirement.
4.3 Enhancement of N use efficiency by FI, AFI, and TN at jointing
Enhancing N use efficiency (NUE) is crucial for sustainable and environmentally friendly wheat production (Zhang et al., 2020; Zhao et al., 2023b). Studies, such as Jia et al. (2021), found that alternate furrow irrigation (AFI), with an equivalent water volume of 150 mm, significantly increased winter wheat’s N uptake efficiency (NUPE) by 8.0% compared to the flood irrigation. Han et al. (2014) also demonstrated that AFI combined with separated N fertilizer applications, improved N agronomic efficiency by 36−56% in summer maize compared to conventional irrigation methods. Comparable enhancements in N use efficiency (NUE) of AFI under the same irrigation quantities were recorded with sugar beets in Turkey (Mon et al., 2016) and safflower in Iran (Shahrokhnia and Sepaskhah, 2016). Conducted under semi−humid, drought−prone conditions, our study shows that implementing furrow irrigation (FI) with 75 mm at the jointing stage significantly enhances the partial factor productivity of nitrogen (PFPN), NUPE, and N internal efficiency (NIE) by 24.2−55.6%, 16.4−36.0%, and 3.9−14.2% respectively across the two years. Herein, the gains under the AFI treatments surpassed those under the EFI treatments, whereas improvements under the TN treatments fell short of the NTN treatments. These outcomes suggest that NUE in winter wheat can be markedly augmented through FI and AFI, particularly for AFI under both NTN and TN conditions. The enhancement results chiefly from FI and AFI’s significant increase in soil moisture content (Wu et al., 2023) and the optimized nitrate−N distribution from booting to maturity (Table 2, Figure 3), thus balancing the plant N requirement and soil N supply, finally elevating the plant N absorption, accumulation, and utilization proficiency (Chen et al., 2020; Jia et al., 2021). Contrastingly, there have been instances where AFI was associated with reduced NUE in winter wheat (Sepaskhah and Hosseini, 2008) and plant N uptake for sugar beets in Iran (Mehdi et al., 2017). Ghasemi-Aghbolaghi and Sepaskhah (2018) also reported a decrease in both N internal efficiency (NIE) and NAE for barley using AFI in Iran. Variations in experimental conditions such as climate, soil properties, and cultivation managements may account for differences in study outcomes. In this study, although the precipitation amount in whole year and wheat growing season is higher, the N uptake efficiency in 2019−2020 was obviously lower than 2018−2019, which mainly because the consecutively extreme high temperature (from2 May to 4 May, over 40°C) occurred during early grain filling stage in 2020−2021, thus resulting in a marked decrease in grains per spike and 1000-grain weight (Wu et al., 2023), and finally decreasing the grain yield (Table 6) and grain N accumulation (Table 5). Ru et al. (2022) also reported that short-term extreme heat stress after anthesis resulted in a pronounced decrease in yield and NUE by reducing grain number per spike and thousand kernel weight. Hence, the effects of climate such as temperature should be considered in wheat production to enhance wheat yield and nutrient use efficiency.
N management remains a pivotal influence on N use efficiency in winter wheat, and the effectiveness relates to water conditions (Ji et al., 2021; Zain et al., 2021; Zhao et al., 2023b). In our study, compared to NTN, TN treatments of 60 kg ha−1 topdressing at the jointing stage has a dampening impact on PFPN, NUE, and NIE under both EFI and AFI, but these efficiencies still register significantly higher than that under the traditional NINTN treatment. This maybe ascribe to FI’s promotion of N uptake and assimilation (Jia et al., 2021; Yang et al., 2021). In our case, the decrease of soil nitrate−N accumulation (Figure 3, Table 2) at different growth stages among treatments can also provide an evidence for the marked difference of soil nitrate−N uptake by wheat This reveals that the potential negative influence of TN on N use efficiency can be mitigated by FI and AFI strategies, especially in dryland where at least one-off irrigation is assured.
5 Conclusion
The obtained results indicate that under the furrow seeding system, when implemented the furrow irrigation (FI), alternate furrow irrigation (AFI), and topdressing nitrogen (TN) techniques, significantly enhances nitrate−N levels at the booting and anthesis stages. This elevation leads to increased aboveground N accumulation, particularly post-anthesis, which contributes considerably to grain yield and protein yield. Concurrently, TN treatment notably enhances grain protein content, while AFI markedly improves N use efficiency. Therefore, the coupled application of AFI and TN not only boosts yield, quality, and efficiency but also mitigates soil nitrate−N residues at the maturity stage of winter wheat production. This coupled strategy holds great promise for dryland regions where a one−off irrigation event is assured during the growth stages of winter wheat.
Data availability statement
The raw data supporting the conclusions of this article will be made available by the authors, without undue reservation.
Author contributions
MH: Data curation, Formal analysis, Investigation, Writing – original draft, Writing – review & editing, Software. WL: Data curation, Formal analysis, Writing – review & editing. CH: Data curation, Formal analysis, Writing – review & editing. JW: Conceptualization, Investigation, Project administration, Writing – review & editing. HW: Conceptualization, Investigation, Project administration, Writing – review & editing. GF: Conceptualization, Project administration, Writing – review & editing. MS: Writing – review & editing. YL: Conceptualization, Funding acquisition, Project administration, Writing – review & editing, Investigation. GL: Conceptualization, Funding acquisition, Project administration, Software, Writing – review & editing.
Funding
The author(s) declare financial support was received for the research, authorship, and/or publication of this article. This study was financially supported by National Key Research and Development Program of China (under Grant No. 2022YFD2300800; 2022YFD2001005), the Science and Technology Research Project of Henan, China (under Grant No. 222102110087; 232102111009; 232102110051).
Acknowledgments
The author would like to thank the reviewers for their valuable comments and suggestions for this work.
Conflict of interest
The authors declare that the research was conducted in the absence of any commercial or financial relationships that could be construed as a potential conflict of interest.
Publisher’s note
All claims expressed in this article are solely those of the authors and do not necessarily represent those of their affiliated organizations, or those of the publisher, the editors and the reviewers. Any product that may be evaluated in this article, or claim that may be made by its manufacturer, is not guaranteed or endorsed by the publisher.
References
Adel, M., Sina, B., Fariborz, A. (2019). Effects of irrigation and fertilization management on reducing nitrogen losses and increasing corn yield under furrow irrigation. Agric. Water Manage. 213, 1116–1129. doi: 10.1016/j.agwat.2018.11.007
Ali, S., Xu, Y. Y., Jia, Q., Irshad, A., Wei, T., Ren, X. L., et al. (2018). Cultivation techniques combined with deficit irrigation improves winter wheat photosynthetic characteristics, dry matter translocation and water use efficiency under simulated rainfall conditions. Agric. Water Manage. 201, 207–218. doi: 10.1016/j.agwat.2018.01.017
Ashraf, U., Salim, M. N., Sher, A., Sabir, S. U. R., Khan, A., Pan, S. G., et al. (2016). Maize growth, yield formation and water-nitrogen usage in response to varied irrigation and nitrogen supply under semi-arid climate. Turk. J. Field Crops 21, 88–96. doi: 10.17557/tjfc.93898
Aziz, O., Hussain, S., Rizwan, M., Riaz, M., Bashir, S., Lin, L. R., et al. (2018). Increasing water productivity, nitrogen economy, and grain yield of rice by water saving irrigation and fertilizer–N management. Environ. Sci. pollut. Res. 25, 16601–16615. doi: 10.1007/s11356-018-1855-z
Benjamin, J. G., Porter, L. K., Duke, H. R., Ahuja, L. R. (1997). Corn growth and nitrogen uptake with furrow irrigation and fertilizer bands. Agron. J. 89, 609–612. doi: 10.2134/agronj1997.00021962008900040012x
Chen, X. W., Zhao, H. B., Liu, J. F., Mao, A. R. (2020). Winter wheat nitrogen utilization under different mulching techniques on the Loess Plateau. Agron. J. 112, 1391–1405. doi: 10.1002/agj2.20105
Dai, J., Wang, Z. H., Li, F. C., He, G., Wang, S., Li, Q., et al. (2015). Optimizing nitrogen input by balancing winter wheat yield and residual nitrate in soil in a long–term dryland field experiment in the Loess Plateau of China. Field Crops Res. 181, 32–41. doi: 10.1016/j.fcr.2015.06.014
Damme, L., Jing, S. X., Montcalm, A. M., Jepson, M., Andersen, M. N., Hansen, E. M. (2022). Proper management of irrigation and nitrogen–application increases crop N–uptake efficiency and reduces nitrate leaching. Acta Agric. Scand. B Soil Plant Sci. 72, 913–922. doi: 10.1080/09064710.2022.2122864
Fu, X., Wang, J., Peng, Z. P., Yang, X. N., Zhang, S. H. (2022). Late topdressing can sustain wheat grain yield under straw mulching in the Loess Plateau of China. Agron. J. 114, 3487–3497. doi: 10.1002/agj2.21200
Gan, Y. T., Siddique, K. H. M., Turner, N. C., Li, X. G., Niu, J. Y., Yang, C., et al. (2013). Ridge furrow mulching systems—an innovative technique for boosting crop productivity in semiarid rain–fed environments. Adv. Agron. 125, 429–476. doi: 10.1016/B978-0-12-405942-9.00007-4
Ghasemi-Aghbolaghi, S., Sepaskhah, A. R. (2018). Barley (Hordeum vulgare L.) response to partial root drying irrigation, planting method and nitrogen application rates. Int. J. Plant Prod. 12 (1), 13–24. doi: 10.1007/s42106–017-0002-y
Guo, S. L., Wu, J. S., Dang, T. H., Liu, W. Z., Li, Y., Wei, W. X., et al. (2010). Impacts of fertilizer practices on environmental risk of nitrate in semiarid farmlands in the Loess Plateau of China. Plant Soil. 330, 1–13. doi: 10.1007/s11104-009-0204-x
Han, K., Yang, Y., Zhou, C. J., Shangguan, Y. X., Zhang, L., Li, N., et al. (2014). Management of furrow irrigation and nitrogen application on summer maize. Agronomy 106, 1402–1410. doi: 10.2134/agronj13.0367
Hu, C. L., Sadras, V. O., Lu, G. Y., Zhang, P. X., Han, Y., Liu, L., et al. (2021). A global meta–analysis of split nitrogen application for improved wheat yield and grain protein content. Soil Till Res. 213, 105111. doi: 10.1016/j.still.2021.105111
Huang, M., Wang, Z. H., Luo, L. C., Wang, S., Hui, X. L., He, G., et al. (2017). Soil testing at harvest to enhance productivity and reduce nitrate residues in dryland wheat production. Field Crops Res. 212, 153–164. doi: 10.1016/j.fcr.2017.07.011
Huang, M., Wu, J. Z., Li, Y. J., Fu, G. Z., Zhao, K. N., Zhang, Z. W., et al. (2021). Effects of tillage practices and nitrogen fertilizer application rates on grain yield, protein content in winter wheat and soil nitrate residue in dryland. Sci. Agric. Sin. 54, 5206–5219. doi: 10.3864/j.issn.0578–1752.2021.24.004
Iqbal, S., Arif, M., Thierfelder, C., Yasmeen, T., Li, T. (2019). Reducing nitrogen losses and increasing maize productivity in organic manures amending soils by increasing the ridge to furrow production. Exp. Agric. 55, 428–442. doi: 10.1017/S0014479718000091
Ji, J. C., Liu, J. L., Chen, J. J., Niu, Y. J., Xuan, K. F., Jiang, Y. F., et al. (2021). Optimization of topdressing for winter wheat by accurate growth monitoring and improved production estimation. Remote Sens. 13, 2349. doi: 10.3390/rs13122349
Jia, D. Y., Dai, X. L., Xie, Y. Y., He, M. R. (2021). Alternate furrow irrigation improves grain yield and nitrogen use efficiency in winter wheat. Agric. Water Manage. 244, 106606. doi: 10.1016/j.agwat.2020.106606
Khan, S., Abbas, S. K., Irshad, S., Mazhar, S. A., Batool, S. (2020). Impact of various irrigation practices on nitrate movement in soil profile and wheat productivity. Appl. Water Sci. 10, 6. doi: 10.1007/s13201-020-01238-8
Khan, Z. I., Ahmad, K., Rehman, S., Siddique, S., Bashir, H., Zafar, A., et al. (2017). Health risk assessment of heavy metals in wheat using different water qualities: implication for human health. Environ. Sci. Pollut. Res. 24, 947e955. doi: 10.1007/s11356-016-7865-9
Kumari, K., Dass, A., Sudhishri, S., Kaur, D., Rani, A. (2017). Yield components, yield and nutrient uptake pattern in maize (Zea mays) under varying irrigation and nitrogen levels. Indian J. Agron. 62, 104–107. doi: 10.59797/ija.v62i1.4265
Li, W. W., Xiong, L., Wang, C. J., Liao, Y. C., Wu, W. (2019). Optimized ridge-furrow with plastic film mulching system to use precipitation efficiently for winter wheat production in dry semi-humid areas. Agric. Water Manage. 218, 211–221. doi: 10.1016/j.agwat.2019.03.048
Li, X. X., Shi, Z. L., Wang, J. C., Wang, F., Jiang, R. F. (2020). Economical nitrogen application rate of winter wheat under rice–wheat rotation in the Yangtze River basin of China. Chin. J. Appl. Ecol. 31, 3015–3022. doi: 10.13287/j.1001–9332.202009.027
Liang, Z. S., Kang, S. Z., Shi, P. Z., Pan, Y. H., He, L. J. (2000). Effect of alternate furrow irrigation on maize production, root density and water–saving benefit. Sci. Agric. Sin. 33, 26–32.
Lin, Z., Chang, X. H., Wang, D. M., Zhao, G. C., Zhao, B. Q. (2015). Long–term fertilization effects on processing quality of wheat grain in the North China Plain. Field Crops Res. 174, 55–60. doi: 10.1016/j.fcr.2015.01.008
Liu, Y., Zhang, X. L., Xi, L. Y., Liao, Y. C., Han, J. (2020). Ridge–furrow planting promotes wheat grain yield and water productivity in the irrigated sub–humid region of China. Agric. Water Manage. 231, 105935. doi: 10.1016/j.agwat.2019.105935
Lu, J., Bai, Z. H., Velthof, G. L., Wu, Z. G., Chadwick, D., Ma, L. (2019). Accumulation and leaching of nitrate in soils in wheat–maize production in China. Agric. Water Manage. 212, 407–415. doi: 10.1016/j.agwat.2018.08.039
Luo, J., Liang, Z. M., Xi, L. Y., Liao, Y. C., Liu, Y. (2020). Plastic–covered ridge–furrow planting combined with supplemental irrigation based on measuring soil moisture promotes wheat grain yield and irrigation water use efficiency in irrigated fields on the loess plateau, China. Agronomy 10 (7), 1010. doi: 10.3390/agronomy10071010
Luo, L. C., Wang, Z. H., Huang, M., Hui, X. L., Wang, S., Zhao, Y., et al. (2018). Plastic film mulch increased winter wheat grain yield but reduced its protein content in dryland of Northwest China. Field Crops Res. 218, 69–77. doi: 10.1016/j.fcr.2018.01.005
Luo, C. L., Zhang, X. F., Duan, H. X., Zhou, R., Mo, F., Mburu, D. M., et al. (2021). Responses of rainfed wheat productivity to varying ridge–furrow size and rate in semiarid eastern African Plateau. Agric. Water Manage. 249, 106813. doi: 10.1016/j.agwat.2021.106813
Mehdi, B., Ali, R. S., Seyed, H. A. (2017). Irrigation and nitrogen managements affect nitrogen leaching and root yield of sugar beet. Nutr. Cycl. Agroecosystems 108, 211–230. doi: 10.1007/s10705-017-9853-y
Mon, J., Bronson, K. F., Hunsaker, D. J., Thorp, K. R., White, J. W., French, A. N. (2016). Interactive effects of nitrogen fertilization and irrigation on grain yield, canopy temperature, and nitrogen use efficiency in overhead sprinkler–irrigated durum wheat. Field Crops Res 191, 54–65. doi: 10.1016/j.fcr.2016.02.011
Pu, J. X., Fan, Y. Q., Feng, X. Y., Liu, Y. H., Qiao, S. T., Liu, R. H. (2022). Effects of alternate partial root−zone irrigation on soil moisture and nutrient conditions and crop growth. China Soils Fert. 9, 209−215. doi: 10.11838/sfsc.1673−6257.21376
Ru, C., Hu, X. T., Chen, D. Y., Song, T. Y., Wang, W. N., Lv, M. W., et al. (2022). Nitrogen modulates the effects of short-term heat, drought and combined stresses after anthesis on photosynthesis, nitrogen metabolism, yield, and water and nitrogen use efficiency of wheat. Water 14, 1407. doi: 10.3390/w14091407
Sarker, K. K., Hossain, A., Timsina, J., Biswas, S. K., Malone, S. L., Alam, M. K., et al. (2020). Alternate furrow irrigation can maintain grain yield and nutrient content, and increase crop water productivity in dry season maize in subtropical climate of South Asia. Agric. Water Manage. 238, 106229. doi: 10.1016/j.agwat.2020.106229
Sepaskhah, A. R., Hosseini, S. N. (2008). Effects of alternate furrow irrigation and nitrogen application rates on yield and water–and nitrogen–use efficiency of winter wheat (Triticum aestivum L.). Plant Prod. Sci. 11, 250–259. doi: 10.1626/pps.11.250
Shahrokhnia, M. H., Sepaskhah, A. R. (2016). Effects of irrigation strategies, planting methods and nitrogen fertilization on yield, water and nitrogen efficiencies of safflower. Agri Water Manage. 172, 18–30. doi: 10.1016/j.agwat.2016.04.010
Sharma, P., Shukla, M. K., Sammis, T. W., Steiner, R. L., Mexal, J. G. (2012). Nitrate-nitrogen leaching from three specialty crops of New Mexico under furrow irrigation system. Agri Water Manage. 109, 71–80. doi: 10.1016/j.agwat.2012.02.008
Shi, Z. L., Yang, S. J., Zhang, C. H., Gu, K. J. (2012). Effect of nitrogen application on soil nitrate nitrogen content, root growth and nitrogen utilization of winter wheat in rice–wheat rotation. J. Soil Water Conserv. 26, 118–122. doi: 10.13870/j.cnki.stbcxb.2012.05.054
Sissons, M., Ovenden, B., Adorada, D., Milgate, A. (2014). Durum wheat quality in high input irrigation systems in south–eastern Australia. Crop Pasture Sci. 65, 411–422. doi: 10.1071/CP13431
Siyal, A. A., Bristow, K. L., Simunek, J. (2012). Minimizing nitrogen leaching from furrow irrigation through novel fertilizer placement and soil surface management strategies. Agric. Water Manage. 115, 242–251. doi: 10.1016/j.agwat.2012.09.008
Skinner, H., Hanson, J., Benjamin, J. G. (1999). Nitrogen uptake and partitioning under alternate–and every–furrow irrigation. Plant Soil. 210, 11–20. doi: 10.1023/A:1004695301778
Tian, D., Gao, M., Xue, C. (2016). Effects of soil moisture and nitrogen addition on nitrogen mineralization and soil pH in purple soil of three different textures. J. Soil Water Conserv. 30, 256–261. doi: 10.13870/j.cnki.stbcxb.2016.01.046
Wang, Z. H., Li, S. X. (2019). Nitrate N loss by leaching and surface runoff in agricultural land: a global issue (a review). Adv. Agron. 156, 159–217. doi: 10.1016/bs.agron.2019.01.007
Wang, Y., Li, G. Q., Wang, S. W., Zhang, Y. G., Li, D. H., Zhou, H., et al. (2022). A comprehensive evaluation of benefit of high-standard farmland development in China. Sustainablity 14, 10361.doi:10.3390/su141610361. doi: 10.3390/su141610361
Wang, Z. C., Liu, F. L., Kang, S. Z., Jensen, C. R. (2012). Alternate partial root–zone drying irrigation improves nitrogen nutrition in maize (Zea mays L.) leaves. Environ. Exp. Bot. 75, 36–40. doi: 10.1016/j.envexpbot.2011.08.015
Wang, C. Y., Liu, W. X., Li, Q. X., Ma, D. Y., Lu, H. F., Feng, W., et al. (2014). Effects of different irrigation and nitrogen regimes on root growth and its correlation with above–ground plant parts in high-yielding wheat under field conditions. Field Crops Res. 165, 138–114. doi: 10.1016/j.fcr.2014.04.011
Wang, Y. J., Wang, D. M., Wang, Y. J., Yang, Y. S., Chang, X. H., Shi, S. B. (2021). Response of yield and quality of winter wheat materials from different ecological regions to nitrogen topdressing rate. J. Plant Nutri. Ferti. 27, 719–727. doi: 10.11674/zwyf.20541
Wu, J. Z., Guan, H. Y., Wang, Z. M., Li, Y. J., Fu, G. Z., Huang, M., et al. (2023). Alternative furrow irrigation combined with topdressing nitrogen at jointing help yield formation and water use of winter wheat under no–till ridge furrow planting system in semi–humid drought–prone areas of China. Agronomy 13, 1390. doi: 10.3390/agronomy13051390
Yang, Z. S., Wu, J. Z., Huang, M., Li, Y. J., Fu, G. Z., Zhao, K. N., et al. (2021). Effects of furrow planting and fertilization optimization on wheat yield, water and fertilizer use efficiency in dryland. Acta Agric. Boreali–Sin. 36, 157–166. doi: 10.7668/hbnxb.20192122
Zain, M., Si, Z. Y., Li, S., Gao, Y., Mehmood, F., Rahman, S. U., et al. (2021). The coupled effects of irrigation scheduling and nitrogen fertilization mode on growth, yield and water use efficiency in drip–irrigated winter wheat. Sustainability 13, 1–18. doi: 10.3390/su13052742
Zhang, L. D., Gao, L. H., Zhang, L. X., Wang, S. ,. Z., Sui, X. ,. L., Zhang, Z. ,. X. (2012). Alternate furrow irrigation and nitrogen level effects on migration of water and nitrate-nitrogen in soil and root growth of cucumber in solar-greenhouse. Sci. Hortic. 138, 43–49. doi: 10.1016/j.scienta.2012.02.003
Zhang, X. D., Kamran, M., Li, F. J., Xue, X. K., Jia, Z. K., Han, Q. F. (2020). Optimizing fertilization under ridge–furrow rainfall harvesting system to improve foxtail millet yield and water use in a semiarid region, China. Agric. Water Manage. 227, 105852. doi: 10.1016/j.agwat.2019.105852
Zhang, X., Li, X. H., Luo, L. C., Ma, Q. X., Ma, Q., Hui, X. L., et al. (2019). Monitoring wheat nitrogen requirement and top soil nitrate for nitrate residue controlling in drylands. J. Clean. Prod. 241, 118372. doi: 10.1016/j.jclepro.2019.118372
Zhao, K. N., Huang, M., Li, Y. J., Wu, J. Z., Tian, W. Z., Li, J. H., et al. (2023a). Combined organic fertilizer and straw return enhanced summer maize productivity and optimized soil nitrate–N distribution in rainfed summer maize–winter wheat rotation on the Southeast Loess Plateau. Soil Sci. Plant Nutr. 23, 938–952. doi: 10.1007/s42729-022-01094-2
Zhao, K. N., Wang, H. T., Wu, J. Z., Liu, A. K., Huang, X. L., Li, G. Q., et al. (2023b). One–off irrigation improves water and nitrogen use efficiency and productivity of wheat as mediated by nitrogen rate and tillage in drought–prone areas. Field Crops Res. 295, 108898. doi: 10.1016/j.fcr.2023.108898
Zhao, J. Y., Yu, Z. W. (2006). Effects of nitrogen rate on nitrogen fertilizer use of winter wheat and content of soil nitrate–N under different fertility condition. Acta Ecol. Sin. 26, 815–822.
Keywords: one-off alternate furrow irrigation, topdressing N, dryland winter wheat, soil nitrate-N, grain yield, N use efficiency
Citation: Huang M, Li W, Hu C, Wu J, Wang H, Fu G, Shaaban M, Li Y and Li G (2024) Coupled one-off alternate furrow irrigation with nitrogen topdressing at jointing optimizes soil nitrate-N distribution and wheat nitrogen productivity in dryland. Front. Plant Sci. 15:1372385. doi: 10.3389/fpls.2024.1372385
Received: 18 January 2024; Accepted: 09 May 2024;
Published: 28 May 2024.
Edited by:
Xiaoli Hui, Northwest A&F University, ChinaReviewed by:
Jiameng Guo, Henan Agricultural University, ChinaLv Xiaokang, Hebei Agricultural University, China
Lina Jiang, Henan Normal University, China
Hafeez Ur Rehman, University of Agriculture Faisalabad, Pakistan
Copyright © 2024 Huang, Li, Hu, Wu, Wang, Fu, Shaaban, Li and Li. This is an open-access article distributed under the terms of the Creative Commons Attribution License (CC BY). The use, distribution or reproduction in other forums is permitted, provided the original author(s) and the copyright owner(s) are credited and that the original publication in this journal is cited, in accordance with accepted academic practice. No use, distribution or reproduction is permitted which does not comply with these terms.
*Correspondence: Youjun Li, bHlqQGhhdXN0LmVkdS5jbg==; Guoqiang Li, Z3FsaUBobmFncmkub3JnLmNu