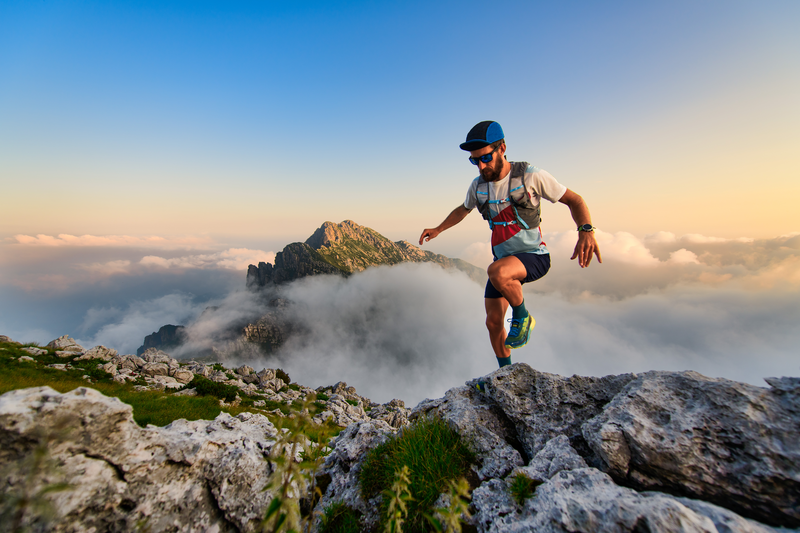
95% of researchers rate our articles as excellent or good
Learn more about the work of our research integrity team to safeguard the quality of each article we publish.
Find out more
ORIGINAL RESEARCH article
Front. Plant Sci. , 01 March 2024
Sec. Plant Abiotic Stress
Volume 15 - 2024 | https://doi.org/10.3389/fpls.2024.1369397
Ultraviolet (UV)-B radiation is considered one of the major detrimental rays coming from the Sun. UV-B radiation has a harmful impact on plant growth and development. The effect of UV-B radiation was studied on 64 rice (Oryza sativa L.) genotypes during the vegetative season. An equal number of genotypes from the japonica (50%) and indica (50%) subspecies were phenotyped using the Soil-Plant-Atmosphere-Research (SPAR) units. The 10 kJ UV-B was imposed 12 days after planting (DAP) and continued for three weeks (21 d). Based on the combined ultraviolet-B radiation response index (CUVBRI) for each genotype, the 64 rice genotypes were classified into sensitive, moderately sensitive, moderately tolerant, and tolerant. Various shoot traits, such as plant height, tiller, and leaf numbers, were measured. We also studied critical root phenological traits like root volume, diameter, tips, and forks. Out of all the studied shoot traits, leaf area showed maximum reduction for both indica (54%) and japonica (48%). Among the root traits, root length decreased by negligible (1%) for indica as compared to japonica (5%), while root crossing and forks showed a maximum decline for japonica (37 and 42%), respectively. This study is timely, meaningful, and required because it will help breeders select a tolerant or sensitive rice line for better yield and production under abiotic stresses.
Sun rays are the principal source of energy received by the Earth. Out of all the radiation reaching the planet Earth, nearly 7-9% of the radiation is ultraviolet (UV) radiation (100-400 nm) (Frohnmeyer and Staiger, 2003). However, the ratio is mere and lethal for the entire living system. UV radiation is divided into three sub-radiations: UV-A (315-400 nm), UV-B (280-315 nm), and UV-C (100-280 nm). UV-C is the most hazardous of the three radiations to reach the Earth’s surface. Still, it is less critical for plant health because the atmosphere absorbs much of this radiation. The ozone layer absorbs approximately 95% of UV-B radiation and reaches the Earth’s surface with an average intensity of 1 Wm−2 (Cejka et al., 2011). Anthropogenic factors are a significant cause of the depletion of the ozone layer, leading to UV-B radiations on the Earth’s surface (UNEP, 2014). The amount of UV-B radiation has increased due to stratospheric ozone (O3) depletion caused by anthropogenic chlorofluorocarbons (Sah et al., 2022). In the future, changes in UV radiation reaching the Earth’s surface are expected to be influenced more by climate factors (cloud cover, aerosols, and surface reflectivity) than by changes in stratospheric ozone, assuming full compliance with the Montreal Protocol (Bernhard et al., 2020; Barnes et al., 2023; Bernhard et al., 2023). However, uncertainties persist. A recent study suggests a potential 3–8% increase in the UV Index over the tropics and mid-latitudes by 2100, depending on greenhouse gas scenarios, cloud cover, and aerosol concentrations. Additional factors affecting UV exposure include increased wildfires, which generate aerosols and harm the ozone layer, land-use practices (e.g., deforestation), melting of snow and ice, and shifts in species distribution due to climate change (Barnes et al., 2023). UV-B radiation is difficult to separate from the Sun’s radiation. Plants are sensitive to UV-B during crop growth and development (Kakani et al., 2003a). UV-B can affect plant’s morphology, anatomy, and physiology (Hollosy, 2002; Reddy et al., 2003; Kakani et al., 2003a; Kakani et al., 2003b; Mathur and Jajoo, 2015; Mathur et al., 2018; Ramamoorthy et al., 2022). Effects of UV-B have been studied in the field (Sullivan et al., 2007) and in horticulture crops (Craver et al., 2014). Studies on maize reported that UV-B radiations decrease the number of chloroplasts in the leaves (Santos et al., 1993; Fagerberg and Bornman, 1997), reduce rubisco activity, decrease stomata numbers and leaf structure (Lingakumar and Kulandaivelu, 1993). Likewise, studies in cotton crops showed adverse effects of UV-B on pigments, including leaf necrosis, chlorosis, and early leaf senescence (Reddy et al., 2003; Kakani et al., 2003b). Such inhibitory effects of UV-B led to a decrease in photosynthesis and protein biosynthesis in many crops such as cotton (Kakani et al., 2003a), rice (Kumagai et al., 2001), sugarcane (Yuan et al., 2011), buckwheat (Yao et al., 2008), corn (Singh et al., 2014; Wijewardana et al., 2016), wheat (Mathur and Jajoo, 2015; Mathur et al., 2018), sweet potato (Chen et al., 2020; Ramamoorthy et al., 2022).
Rice (Oryza sativa L.) is one of the most important crops, extensively cultivated around the globe. Irrigated rice accounts for approximately 55% of the global harvested area and contributes 75% of global rice production, with 25% use of global agricultural freshwater (Thanawong et al., 2014). Rice provides ~27% of dietary energy needs, ~20% of nutritional protein, and ~3% dietary fiber (Kennedy et al., 2003). Rice also contains bioactive compounds such as anthocyanins, flavonoids, and polyphenols (Fukagawa and Ziska, 2019). Therefore, rice cultivation has been under tremendous pressure to keep its productivity with population growth and demands.
UV-B can affect the morphological and physiological processes in rice. Teramura et al. (1991) reported that rice sensitivity is latitude-based. Asian rice genotypes have different sensitivity as compared to other rice genotypes. Rice exposed to UV-B at the vegetative stage negatively affects coleoptile growth (Idris et al., 2021), biomass-related traits, and yield-determining traits (Kumagai et al., 2001). In response to UV-B, rice leaves accumulate higher malondialdehyde (MDA) content (Du et al., 2011) and anthocyanin (in purple rice strain) (Hada et al., 2003). Rice genotypes were also screened for UV-B tolerance based on the various phenolic concentrations and biomass weight (Teramura et al., 1991; Dai et al., 1994; Cassi-Lit et al., 1997).
Few studies (Jumaa et al., 2020) focused on phenotyping rice genotypes for vegetative stages. A knowledge gap exists regarding the impact of UV-B stress on rice genotypes during the early vegetative stage, particularly concerning shoot and root physiology. Previous studies did not consider the phenotypic variability in shoot and root traits across various rice genotypes in response to UV-B stress during the vegetative stage. This study addresses this gap and enhances our understanding of the intricate interactions between UV-B stress and rice genotypes at the critical early vegetative stage. The specific objectives of the study were to (i) quantify UV-B induced variability in morpho-physiological at the early growth stage, (ii) determine the correlation between physiology and vigor under UV-B, and (iii) identify rice genotypes tolerant to UV-B stress at early vegetative stage. The study will be helpful in the future in identifying and selecting tolerant and susceptible rice lines or varieties for various abiotic stresses.
A panel (64 genotypes) consisting of an equal number of genotypes from japonica (50%) and indica (50%) (Supplementary Table 1) were phenotyped using the sunlit controlled environment facility, known as Soil-Plant-Atmosphere-Research (SPAR) units at the Rodney Foil Plant Science Research Center, Mississippi State University, Mississippi State, MS, USA. Four uniform seeds of each genotype were sown in the 384 PVC plastic pots filled with gravel (300 g) at the bottom and a mixture of sand and topsoil with a 3:1 (v/v) ratio. Pots were arranged in a randomized complete block design (RCBD) with three replications, and each genotype was placed randomly. For irrigation, Hoagland’s nutrient solution (Hewitt, 1952) was provided three times based on 120% of the evapotranspiration measured in each SPAR unit as described in Reddy et al. (2001) using an automated and computer-controlled drip irrigation system with one dripper per pot.
This experiment used 384 pots (64 rice genotypes x two treatments x three replications) to examine variability among rice genotypes for UV-B radiations. The ten kJ UV-B was imposed 12 days after planting (DAP) and continued for three weeks (21 d). A square-wave supplementation system (constant UV-B supplements) was used to provide desired UV-B radiation dosages, which were delivered from 0.5 m above the plant canopy for eight h each day, from 08:00 to 16:00 h by eight fluorescent UV-B-313 lamps (Q-Panel Company, Cleveland, OH, USA) mounted horizontally on a metal frame inside each SPAR chamber, driven by 40 W dimming ballasts. The UV-B radiation delivered at the top of the plant canopy was monitored at ten different locations in each SPAR chamber daily at 10:00 h with a UVX digital radiometer (UVP Inc., San Gabriel, CA, USA), which was calibrated against an Optronic Laboratory (Orlando FL, USA) Model 754 Spectroradiometer that was being used initially to quantify the lamp output. As needed, the lamp output was adjusted to maintain the desired UV-B level, as described by Reddy et al (2003, 2013). Across treatments, typical temperatures (30/22°C; day/night) were maintained during the experiments. Air temperature in each SPAR unit was monitored and adjusted every 10 s throughout the day and night. The mean temperature (day/night) and relative humidity (day) were 26.0 ± 0.8°C and 46.0 ± 13%, respectively. The (CO2) in each SPAR unit was monitored and adjusted every 10 s throughout the day and maintained within set points ± 10 µmol mol-1 as 420 µmol mol–1.
Physiological traits such as chlorophyll content, flavonoid, anthocyanin, and nitrogen balance index (NBI) were measured using a handheld Dualex Scientific instrument (Force A DX16641, Paris, France) at 33 DAP or 21 days after stress (DAS). The nitrogen balance index (NBI) was automatically calculated as a ratio of the chlorophyll content (ChI) to the flavonol index (FLAV), i.e., NBI = ChI/FLAV.
Chlorophyll fluorescence was measured using the FluorPen FP 100 (Photo System Instruments, Kolackova, Czech Republic). We followed the instrument protocol described by Reddy et al. (2021). To determine the maximum potential quantum efficiency of Photosystem II, fluorescence parameters such as maximal fluorescence intensity (Fm), maximal variable fluorescence (Fv), and the ratio of Fv to Fm (Fv/Fm) were measured (Reddy et al., 2021).
Plant height, tiller numbers, and leaf number on the main axis were measured manually. Plant height was measured using the metric yard rule. The total leaf area was measured using the LI-3100 leaf-area meter (LI-COR, Inc., USA) 33 DAP or 21 DAS.
Individual plant’s roots and shoots were separated. The soil adhered to the roots and was washed thoroughly with a mild-speed water stream. Harvested roots were scanned using an Epson Expression 11000XL scanner at a resolution of 800 dots per inch, and the digital images were analyzed using WinRHIZO Pro 2009C software (Regent Instruments, Québec, Canada). Information extracted from the WinRHIZO software was used to determine the UV-B effect on root traits such as the longest root length, total root length, root surface area, root volume, average root diameter, root tips, root forks, and root crossings. Finally, we estimated the whole plant’s dry weight (leaf +stem +root) across treatments.
Statistical analyses were carried out using RStudio 3.6.1 (https://rstudio.com/). Two-way ANOVA was performed on the phenotypic data to estimate the source of variation in genotype (G), treatment (T), and their interaction (G x T) using the library (“Agricolae”), in R, a language and environment (https://www.R-project.org/, R Core). Genotype or treatment means were compared with the Least Significant Difference (LSD) test, and the probability level of p< 0.05 was considered statistically significant. Sigma Plot 13.0 (Systat Software, Inc., San Jose, CA, USA) was used to plot all graphs.
Further, rice genotypes were classified into UV-B tolerant and sensitive groups based on the genotype response index (Reddy et al., 2021). Initially, the Individual UV-B response index (IUV-BRI) for each parameter was calculated as the value of a parameter (Pl) at the 10 kJ of UV-B of a given genotype divided by the value for the same parameter (Po) at the optimum 0 kJ of UV-B (Equation 1).
Then, each genotype’s Combined UV-B response index (CUV-BRI) (Equation 2) was calculated as the sum of all IUV-BRIs derived from plant height (PH), tiller number (TN), leaf number (LN), leaf area (LA), leaf dry weight (LWT), shoot dry weight (SWT), root dry weight (RWT), stem dry weight (StWT), root/shoot (RS), total dry mass (TDM), longest root length (LRL), total root length (TRL), root surface area (RSA), root diameter (RD), root volume (RV), Tips, Forks, crossings (Cr), quantum efficiency of Photosystem II (Fv/Fm), chlorophyll (Chl) content, flavonoid (Flav), anthocyanin (Anth), and nitrogen balance index (NBI).
All the measured and derived phenotypic traits of 64 rice genotypes decreased (3 to 53%) in response to UV-B stress (Supplementary Tables 2A, B). Morphological characters such as plant height, tiller numbers, and leaves on the main axis varied between indica and japonica genotypes in response to UV-B.
In response to UV-B, plant height (PH) decreased by 24% in indica and 21% in japonica (Figure 1A). Genotype RU1504122 (japonica) showed the maximum decrease (10 cm) in plant height. In contrast, we recorded the highest PH (17 cm) for genotype RU1504114 (japonica). In indica, the tiller number (TN) was reduced by 10% and by 20% in japonica under UV-B (Figure 1B). Individually, the maximum decrease in TN was recorded for UV-B sensitive genotypes RU1204156 (japonica, 3 no. plant-1). Maximum values for TN were obtained for COLOMBIA XXI (indica, 5 no. plant-1). Under UV-B, leaf number on the main axis decreased to 7% and 2% for indica and japonica, respectively (Figure 1C). Maximum decrease in leaf number was observed for CT18247-12-8-1-4-2-2 (indica, 3 no. main axis-1), while the maximum increase in N-22 (Aus, 4 no. main axis-1) and NIPPONBARE (japonica, 4 no. main axis-1). Among all the 64 genotypes, RU1204156 (japonica) showed the lowest values for leaf area (57.2 cm2 plant-1) and recorded the highest value for MERMENTAU (japonica, 97 cm2 plant-1) (Figure 1D). As compared to the control, leaf weight, stem weight, root weight, and shoot weight were negatively affected under UV-B (Figures 2A–D). Compared to the control, the leaf weight of the japonica genotype (RU1204156) showed the least value (0.32 g plant-1), while RU1404156 recorded the maximum value. Stem weight ranged from 0.23 g plant-1 (indica, CT18244-9-4-4-2-1-2) to 0.47 g plant-1 (japonica, RU1404156). Likewise, for root weight, the indica genotype (CT18244-9-4-4-2-1-2) showed minimum values under UV-B.
Figure 1 Ultraviolet-B radiation effects on shoot growth traits, (A) plant height, (B) tillers, (C) leaves on the main axis and (D) total leaf area of 64 indica and japonica rice genotypes. Measurements were taken 33 days after sowing and 21 days after UV-B treatment. The middle line indicates the median, and the box shows the range of the 25th to 75th percentiles of the total data. The whiskers indicate the interquartile range and the outer circle lines with high or low scores in each category.
Figure 2 Ultraviolet-B radiation effects on shoot growth traits, (A) leaf weight, (B) stem weight, (C) root weight, and (D) shoot weight of 64 indica and japonica rice genotypes. Measurements were taken 33 days after sowing and 21 days after UV-B treatment. The middle line indicates the median, and the box shows the range of the 25th to 75th percentiles of the total data. The whiskers indicate the interquartile range, and the outer circle lines have high or low scores in each category.
Figure 3 represents various root parameters for all indica and japonica rice lines. The highest decrease in root surface area was recorded for CL111 (238 cm2 plant-1, japonica), while a maximum increase was observed for the japonica genotype (RU1404156). Compared to control plants, the total root length decreased by around 31% for all, 28% for indica, and 34% for japonica, respectively (Figure 3). The total root length (TRL) and the longest root length (LRL) decreased maximum for a UV-B moderately tolerant genotype, NIPPONBARE. The highest values for TRL and LRL were recorded for IR09L179 (indica) and RU1304154 (japonica). The root surface area (RSA) values declined in response to UV-B for both indica and japonica, 32% and 35%, respectively (Figure 3C). Root volume (RV) decreased significantly for japonica (36%) and indica (34%) (Figure 3D).
Figure 3 Ultraviolet-B radiation effects on root growth traits, (A) longest root length, (B) total root length, (C) root surface area, and (D) root volume of 64 indica and japonica rice genotypes. Measurements were taken at 33 days after sowing and 21 days after UV-B treatment. The middle line indicates the median, and the box shows the range of the 25th to 75th percentiles of the total data. The whiskers indicate the interquartile range and the outer circle lines with high or low scores in each category.
As compared to other root parameters, the average root diameter (RD) showed a lesser decrease (5%) for indica and (2%) japonica (Figure 4A), while recorded the highest value for RD for NIPPONBARE (0.32 cm root-1) and lowest for N-22 (Aus, 0.25 cm root-1). For root tips, indica genotype (CT18233-15-6-6-4-8-1) showed a maximum decrease (~21,939 no. plant-1), while recorded the highest values for japonica RU1404156 (23,137 no. plant-1). The relative decline in root tips for indica and japonica was around 32% and 33%, respectively. Maximum reduction in root fork and root crossing was observed for the UV-B japonica genotype (RU1504083), and RU1404156 (japonica) showed the highest value for both traits. The reduction in indica for root fork was around 37%, while for japonica, it was 42%. Root crossing decreased more for japonica (42%) as compared to indica (33%) genotypes (Figures 4A–D). Whole plant weight decreased maximum in an indica genotype (CT18244-9-4-4-2-1-2), followed by a japonica genotype (RU1204156). On average, 23% and 19% increases in root-to-shoot ratio were observed for indica and japonica (Figures 5A, B). The maximum root-to-shoot ratio was recorded in japonica Bowman, followed by the Cheniere genotype.
Figure 4 Ultraviolet-B radiation effects on root growth and developmental traits, (A) average root diameter, (B) root tips, (C) root forks, and (D) root crossings of 64 indica and japonica rice genotypes. Measurements were taken 33 days after sowing and 21 days after UV-B treatment. The middle line indicates the median, and the box shows the range of the 25th to 75th percentiles of the total data. The whiskers indicate the interquartile range and the outer circle lines with high or low scores in each category.
Figure 5 Ultraviolet-B radiation effects on growth traits, (A) whole plant weight, and (B) root and shoot ratio of 64 indica and japonica rice genotypes. Measurements were taken 33 days after sowing and 21 days after UV-B treatment. The middle line indicates the median, and the box shows the range of the 25th to 75th percentiles of the total data. The whiskers indicate the interquartile range and the outer circle lines with high or low scores in each category.
The quantum efficiency of PSII (Fv/Fm) (Figure 6A) showed an 8% and 6% decrease in indica and japonica. The lowest value was recorded for the indica genotype (CT19561-3-57-2P-2-1-2-M) (Table 1). FEDEARROZ 21 (indica) (Table 1) showed the highest value for Fv/Fm. We also measured Chlorophyll (Chl) content for control and UV-B radiations for indica and japonica (Figure 6B). We observed a slight difference (7% for indica and 6% for japonica) for Chl content for both the sub-species (Figure 6B). An indica genotype (CT18247-12-8-1-4-2-2) showed maximum decrease followed by UV-B sensitive japonica genotype (CL111), respectively. We recorded the highest decrease in the nitrogen balance index for the japonica genotype CL111. Out of the two, indica showed a better nitrogen balance (26%) than japonica (24%) when exposed to UV-B radiation (Figure 6C).
Figure 6 Ultraviolet-B radiation effects on (A) chlorophyll fluorescence, (B) chlorophyll index, and (C) nitrogen balance index of 64 indica and japonica rice genotypes. Measurements were made 33 days after sowing and 21 days after UV-B treatment. The middle line indicates the median, and the box shows the range of the 25th to 75th percentiles of the total data. The whiskers indicate the interquartile range, and the outer circle lines have high or low scores in each category.
Table 1 Classification of 64 indica and japonica rice genotypes into various ultraviolet (UV) B radiation tolerant groups based on cumulative UV-B response indices.
We recorded the highest value for the flavonoid index for indica genotype (CT18593-1-7-2-2-5), followed by the japonica genotype (CL111). Overall, in response to UV-B radiations, flavonoid content increased significantly, around (29%) in indica and (24%) in japonica (Figure 7A). Maximum values for anthocyanin were recorded for indica genotype (IR65600-81-5-2-3); overall changes in anthocyanin for indica and japonica were 4% and 2% under UV-B treatment (Figure 7B).
Figure 7 Ultraviolet-B radiation effects on (A) flavonoid index and (B) anthocyanin index of 64 indica and japonica rice genotypes. Measurements were made 33 days after sowing and 21 days after UV-B treatment. The middle line indicates the median, and the box shows the range of the 25th to 75th percentiles of the total data. The whiskers indicate the interquartile range and the outer circle lines with high or low scores in each category.
A combined UV-B response index (CUV-BRI) was calculated to categorize the genotypes into UV-B sensitive, moderately sensitive, moderately tolerant, and tolerant. A strong correlation was observed for root and shoot traits (r2 = 0.92). In contrast, we observed a weaker correlation for physiology traits (Figure 8). The correlation indicated that root and shoot traits are more important and should be used as a criterion for genotype selection under UV-B conditions.
Figure 8 Correlation between combined cumulative shoot, root, or physiology UV-B-response index and total UV-B response index of all 64 indica and japonica rice genotypes traits.
The results unveiled that UV-B has detrimental effects on rice growth and development. Dai et al. (1994) reported that rice’s sensitivity to UV-B’s plant height was much lower than leaf area and plant dry weight, which is corroborated by our study. Decreased plant height under UV-B could be due to one of the following reasons: (i) reduced carbohydrate content (Zhao et al., 2003), (ii) damaged cell components, and interaction of growth regulators (Ensminger and Schafer, 1992; Mohammed and Tarpley, 2013), (iii) shorter internodes rather than fewer nodes, (iv) decreased levels of growth hormone (indole acetic acid) in plants (Kakani et al., 2003b). A general growth delay has been documented to protect from UV-B radiation as it directly affects cell division (Kakani et al., 2003b).
In the present study, leaf area significantly decreased for both indica and japonica under UV-B. Reduction in leaf area due to UV-B is associated with damage to photosynthetic pigments. Thus, leaf area can be considered one of the critical parameters in selecting the rice genotype tolerant to UV-B. The decrease in light capture and photosynthetic activity due to UV-B was also supported by stomatal closure in plants (He et al., 2013; Martínez-Lüscher et al., 2013; Tossi et al., 2014). This stomata closure will decrease photosynthesis in situ and potentially decrease biomass production, as evident in our study (reduced plant, stem, and root weight). The decrease in chlorophyll pigments and photosynthesis reduced the plant height of most crop plants, including rice (Sah et al., 2022). Our study supports that the decreased stem and root weight in the present work could probably be through a reduction in cell division rather than reduced cell size, as reported in previous studies (Kakani et al., 2003b; Meng et al., 2019; Sah et al., 2022).
Roots are essential for growth and development, anchoring and structural support to plants for their growth substrate, facilitating water and nutrient uptake from the soil and sensing and responding to environmental signals such as biotic and abiotic stresses (Meng et al., 2019). In response to environmental stresses, root systems are continuously reshaped by the initiation and elongation of new roots throughout the growth period, facilitating the plant’s adaptation to biotic and abiotic stresses (Fukai and Cooper, 1995; Gowda et al., 2011; Meng et al., 2019). We observed a decrease in the root parameters as compared to the control. The probable reason could be the downregulation of photosynthesis and cell division in response to UV-B, which negatively affected rice genotypes’ root diameter and length (Meng et al., 2019; Yang et al., 2020; Sah et al., 2022).
Root length is an essential component of root architecture to survive in complex soil conditions. Root elongation is essentially driven by root apical meristems localized in the apical meristems of roots (Meng et al., 2019). We observed a slight negative impact on root length in response to UV-B supplementation. Root biomass decreased in response to UV-B. A potential reason for the decrease in root tips and the number of root crossings might be a modification in auxin transport within the roots under UV-B exposure (Yamamoto et al., 2007; Cho et al., 2014; Wang et al., 2014; Qin et al., 2017; Roychoudhry and Kepinski, 2022).
UV-B radiation directly impacts the DNA, proteins, and lipids (Sah et al., 2022). We observed a reduction in total chlorophyll content under UV-B. A previous study by Sah et al. (2022) also reported decreased chlorophyll a/b binding protein expression in some rice cultivars under UV-B. UV-B decreases the function of photosynthetic pigments, which can lead to a loss of photosynthetic capacity. Further, UV-B is also known to interfere with the light-harvesting complex (LHCII) of PSII and quantum efficiency in rice (Sah et al., 2022). In our study, a decline in quantum efficiency (Fv/Fm) was observed, which could present a reduction in the rate of primary charge separation and a decrease in the stabilization of charge separation and disconnection of some minor antennae from PSII (Mathur and Jajoo, 2015). The reduction in quantum efficiency of PSII can also be due to the downregulation of CP43 protein under UV-B (Sah et al., 2022). Decreased photosynthetic efficiency was also associated with reduced nitrogen balance, leading to lower leaf chlorophyll content.
Further, nitrogen is a fundamental constituent of many leaf cell components, particularly those associated with the photosynthetic apparatus, including carboxylation enzymes and chlorophyll. For these reasons, leaf nitrogen concentration strongly regulates maximum photosynthetic capacity (Rousseaux et al., 1998; Kakani et al., 2003b). Nitrogen balance decreased in both genotypes, indicating that UV-B has impacted the plant’s chlorophyll content, photosynthesis, and proteins. Hidema et al. (1996) also reported a decreased nitrogen content in rice plants, which is consistent with the present study.
Environmental conditions during early developmental stages appear to be essential to the development of plants as far as subsequent sensitivity to UV-B damage (Sullivan et al., 2007; Mathur et al., 2018). In response to the damaging effects of UV-B radiation, plants have developed some defense strategies to overcome or compensate for these detrimental effects. These defense mechanisms include an accumulation of UV-B absorbing pigments and UV-A photons to repair most UV-B-induced DNA damage (Britt, 1996). Carotenoids are yet other pigments that indirectly protect plants against the harmful impact of UV-B radiations by protecting photosynthetic apparatus.
Accumulating certain phenolic compounds such as anthocyanin and flavonoids plays a significant role in the UV-B repair mechanism in plants. Our results depict that flavonoid content increased in the indica rice genotype compared to japonica, indicating that the indica genotype may have a better UV-B repair mechanism. Markham et al. (1998) reported that in UV-tolerant rice cultivar M202, flavonoids play a more elusive role in the plant’s UV-B protection and screening. In plants, the synthesis of UV-absorbing flavonoids constitutes an effective non-enzymatic mechanism to mitigate photoinhibition and photooxidative damage caused by UV stress. This mechanism includes either reducing the penetration of incident UV radiation or acting as a quencher of reactive oxygen species (ROS). Increased flavonoid concentration under UV-B in the current study implies that plants maintain protective mechanisms to protect the underlying tissues against harmful radiation. The anthocyanin index did not show a significant change, but slight alterations in the anthocyanin index indicated that these are protective mechanisms in plants. Aggregating flavonoids, anthocyanin, and other UV-absorbing metabolites in tissues reduce epidermal UV transmittance and are crucial mechanisms in plant’s acclimation to changing UV environments.
An extensive set of 64 rice genotypes, including indica and japonica sub-species, were screened and categorized as UV-B sensitive, moderately sensitive, moderately tolerant, and tolerant. UV-B had a detrimental effect on both rice ecotypes, but the damage differed for indica and japonica. Based on CUVBRI, we hypothesized that leaf area could be used as one of the early-stage indicators for UV-B stress tolerance in rice genotypes. The genotypes studied and identified will give breeders a more comprehensive range of genotype selection under UV-B treatments. The study can be further helpful in determining and selecting a better tolerant or susceptible rice line for better yield and production under abiotic stresses.
The original contributions presented in the study are included in the article/Supplementary Material. Further inquiries can be directed to the corresponding authors.
SM: Writing – original draft, Writing – review & editing. RB: Writing – review & editing. SJ: Data curation, Methodology, Writing – review & editing. NK: Data curation, Writing – review & editing. VR: Funding acquisition, Writing – review & editing. WG: Writing – review & editing. KR: Conceptualization, Data curation, Software, Supervision, Visualization, Writing – review & editing.
The author(s) declare financial support was received for the research, authorship, and/or publication of this article. This research was partly supported by the National Institute for Food and Agriculture, NIFA 2019-30552, 2022 3463, and MIS 043050.
SM would like to acknowledge the Agricultural Research Service (ARS) Research Participation Program administered by the Oak Ridge Institute for Science and Education (ORISE) through an interagency agreement between the US. Department of Energy (DOE) and the US. Department of Agriculture (USDA). ORISE is managed by ORAU under DOE contract number DE-SC0014664. We thank David Brand for his technical support during the experiment and Dr. Ed. Redona for providing the rice seed.
The authors declare that research was conducted in the absence of any commercial or financial relationships that could be construed as a potential conflict of interest.
All claims expressed in this article are solely those of the authors and do not necessarily represent those of their affiliated organizations, or those of the publisher, the editors and the reviewers. Any product that may be evaluated in this article, or claim that may be made by its manufacturer, is not guaranteed or endorsed by the publisher.
The Supplementary Material for this article can be found online at: https://www.frontiersin.org/articles/10.3389/fpls.2024.1369397/full#supplementary-material
Supplementary Table 2 | (A) Descriptive statistics of physiological and dry weight traits of 64 rice genotypes under control (CNT) and ultraviolet (UV)-B. *P< 0.05, **P< 0.01, ***P< 0.001. (B) Descriptive statistics of physiological and dry weight traits of 64 rice genotypes under control (CNT) and ultraviolet (UV)-B. *P< 0.05, **P< 0.01, ***P< 0.001.
Anth, Anthocyanin; Chl, Chlorophyll; Cro, Crossings, Forks, Tips; CUV-BRI, Combined UV-B response index; DAP, Days after planting; Flav, Flavonoids; Fv/Fm, Quantum efficiency of Photosystem II; IUV-BRI, Individual UV-B response index; LA, Leaf area; LN, Leaf number; LRL, Longest root length; LWT, Leaf dry weight; NBI, Nitrogen balance index; OsMOGS, mannosyl-oligosaccharide glucosidase; PH, Plant height; RD, Root diameter; RV, Root volume; RWT, Root dry weight, RSA, Root surface area; RS, root/shoot; SHWT, Shoot dry weight; SPAR, Soil-Plant-Atmosphere-Research; StWT, Stem dry weight; TN, Tillers number; TRL, Total root length; TDM, Total dry mass; UV, Ultraviolet.
Barnes, P. W., Robson, T. M., Zepp, R. G., Bornman, J. F., Jansen, M. A. K., Ossola, R., et al. (2023). Interactive effects of changes in UV radiation and climate on terrestrial ecosystems, biogeochemical cycles, and feedbacks to the climate System. Photochem. Photobiol. Sci. 22, 1049–1091. doi: 10.1007/s43630-023-00376-7
Bernhard, G. H., Neale, R. E., Barnes, P. W., Neale, P. J., Zepp, R. G., Wilson, S. R.. (2020). Environmental effects of stratospheric ozone depletion, UV radiation and interactions with climate change: UNEP Environmental Effects Assessment Panel, update 2019. Photochem. Photobiol. Sci. 19, 542–584. doi: 10.1039/d0pp90011g
Bernhard, G. H., Bais, A. F., Aucamp, P. J., Klekociuk, A. R., Liley, J. B., McKenzie, R. L. (2023). Stratospheric ozone, UV radiation, and climate interactions. Photochem. Photobiol. Sci. 22, 991–1009. doi: 10.1007/s43630-023-00373-w
Britt, A. B. (1996). DNA damage and repair in plants. Annual Review. Plant Physiol. Plant Mol. Biol. 4, 75–100. doi: 10.1146/annurev.arplant.47.1.75
Cassi-Lit, M., Whitecross, M. J., Nayudu, M., Tanner, G. J. (1997). UV-B irradiation induces differential leaf damage, ultrastructural changes and accumulation of specific phenolic compounds in rice cultivars. Aust. J. Plant Physiol. 24, 261–274. doi: 10.1071/PP96080
Cejka, C., Ardan, T., Sirc, J., Michálek, J., Beneš, J., Brůnová, B., et al. (2011). Hydration and transparency of the rabbit cornea irradiated with UV-B-doses of 0.25 J/cm2 and 0.5 J/cm2 compared with equivalent UVB radiation exposure reaching the human cornea from sunlight. Curr. Eye. Res. 36, 607–613. doi: 10.3109/02713683.2011.574332
Chen, Z., Gao, W., Reddy, K. R., Chen, M., Taduri, S., Meyers, S. L., et al. (2020). Ultraviolet (UV)-B effects on growth and yield of three contrasting sweet potato cultivars. Photosynthetica. 58 1), 37–44. doi: 10.32615/ps.2019.137
Cho, S. H., Yoo, S. C., Zhang, H., Lim, J. H., Paek, N. C. (2014). Rice narrow leaf regulates leaf and adventitious root development. Plant Mol. Biol. Rep. 32, 270–281. doi: 10.1007/s11105-013-0675-z
Craver, J. K., Miller, C. T., Williams, K. A. (2014). Ultraviolet radiation affects intumescence development in ornamental sweet potato (Ipomoea batatas). Hortscience 49, 1277–1283. doi: 10.21273/HORTSCI.49.10.1277
Dai, Q., Peng, S., Chavez, A. Q., Vergara, B. S. (1994). Intraspecific response of 188 rice cultivars to enhanced ultraviolet-B radiation. Environ. Exp. Bot. 34, 433–442. doi: 10.1016/0098-8472(94)90026-4
Du, H., Liang, Y., Pei, K., Ma, K. (2011). UV radiation-responsive proteins in rice leaves: a proteomic analysis. Plant Cell Physiol. 52, 306–316. doi: 10.1093/pcp/pcq186
Ensminger, P. A., Schafer, E. (1992). Blue and ultraviolet-B light photoreceptors in parsley cells. J. Photochem. Photobiol. 55, 437–447. doi: 10.1111/j.1751-1097.1992.tb04259.x
Fagerberg, W. R., Bornman, J. F. (1997). Ultraviolet-B radiation caused shade type ultrastructural changes in Brassica napus. Physiol. Plant 101, 833–844. doi: 10.1111/j.1399-3054.1997.tb01071.x
Frohnmeyer, H., Staiger, D. (2003). Ultraviolet-B radiation-mediated responses in plants balancing damage and protection. Plant Physiol. 133, 1420–1428. doi: 10.1104/pp.103.030049
Fukagawa, N. K., Ziska, L. H. (2019). Rice: importance for global nutrition. J. Nutr. Sci. Vitaminol. 65, S2–S3. doi: 10.3177/jnsv.65.S2
Fukai, S., Cooper, M. (1995). Development of drought resistant cultivars using physio-morphological traits in rice. Field Crops Res. 40, 67–87. doi: 10.1016/0378-4290(94)00096-U
Gowda, V. R. P., Henry, A., Yamauchi, A., Shashidha, H. E., Serraj, R. (2011). Root biology and genetic improvement for drought avoidance in rice. Field Crop Res. 122, 1–13. doi: 10.1016/j.fcr.2011.03.001
Hada, H., Hidema, J., Maekawa, M., Kumagai, T. (2003). Higher amounts of anthocyanins and UV-absorbing compounds effectively lowered CPD photorepair in purple rice (Oryza sativa L.). Plant Cell Environ. 26, 1691–1701. doi: 10.1046/j.1365-3040.2003.01087.x
He, J. M., Ma, X. G., Zhang, Y., Sun, T. F., Xu, F. F., Chen, Y. P., et al. (2013). Role and interrelationship of Gα protein, hydrogen peroxide, and nitric oxide in ultraviolet B-induced stomatal closure in Arabidopsis leaves. Plant Physiol. 161, 1570–1583. doi: 10.1104/pp.112.211623
Hewitt, E. J. (1952). Sand and water culture methods used in the study of plant nutrition. Technical Communication No. 22 (Commonwealth Bureau of Horticulture and Plantation Crops, Commonwealth Agricultural Bureaux, Farnham Royal, Buckinghamshire), 187–190.
Hidema, J., Kang, H. S., Kumagai, T. (1996). Differences in the sensitivity to UVB radiation of two cultivars of rice (Oryza sativa L.). Plant Cell Physiol. 37, 742–747. doi: 10.1093/oxfordjournals.pcp.a029008
Hollosy, F. (2002). Effect of UV radiation on plant cells. Micron. 33, 179–197. doi: 10.1016/s0968-4328(01)00011-7
Idris, M., Seo, N., Jiang, L., Kiyota, S., Hidema, J., Iino, M. (2021). UV-B signalling in rice: Response identification, gene expression profiling and mutant isolation. Plant Cell Environ. 44, 1468–1485. doi: 10.1111/pce.13988
Jumaa, S. H., Kakar, N., Redona, E. D., Lone, A. (2020). Assessing the early-season vigor of a diverse rice population by using morphophysiological traits. SABRAO. J. Breed. Genet. 52, 248–270.
Kakani, V. G., Reddy, K. R., Zhao, D., Mohammed, A. R. (2003a). Effects of ultraviolet-B radiation on cotton (Gossypium hirsutum L.) morphology and anatomy. Ann. Bot. (Lond) 91, 817–826. doi: 10.1093/aob/mcg086
Kakani, V. G., Reddy, K. R., Zhao, D., Sailaja, K. (2003b). Field crop responses to ultraviolet- B radiation: A review. Agric. For. Meteorol. 120, 191–218. doi: 10.1016/j.agrformet.2003.08.015
Kennedy, G., Burlingame, B., Nguyen, V. N. (2003). "Nutritional contribution of rice and impact of biotechnology and biodiversity in rice-consuming countries.," in Proceedings of the 20th session of the International Rice Commission, Bangkok, Thailand, 23-26 July 2002., 59–69.
Kumagai, T., Hidema, J., Kang, H. S., Sato, T. (2001). Effects of supplemental UV-B radiation on the growth and yield of two cultivars of Japanese lowland rice (Oryza sativa L.) under the field in a cool rice-growing region of Japan. Agric. Ecosyst. Environ. 83, 201–208. doi: 10.1016/S0167-8809(00)00180-8
Lingakumar, K., Kulandaivelu, G. (1993). Changes induced by ultraviolet-B radiation in vegetative growth, foliar characteristics and photosynthetic activities in Vigna ungiculata. Aust. J. Plant Physiol. 20, 299–308. doi: 10.1071/PP9930299
Markham, K. R., Tanner, G. J., Caasi, M. L., Whitecross, M. I., Nayudu, M., Mitchell, K. A. (1998). Possible protective role for 3', 4’-dihydroxyflavones induced by enhanced UV-B in a UV tolerant rice cultivar. Phytochemistry 49, 1913–1919. doi: 10.1016/S0031-9422(98)00438-5
Martínez-Lüscher, J., Morales, F., Delrot, S., Sánchez-Díaz, M., Gomés, E., Aguirreolea, J., et al. (2013). Short- and long-term physiological responses of grapevine leaves to UV-B radiation. Plant Sci. 213, 114–122. doi: 10.1016/j.plantsci.2013.08.010
Mathur, S., Jajoo, A. (2015). Investigating deleterious effects of ultraviolet (UV) radiations on wheat by a quick method. Acta Physiol. Plant 37, 121. doi: 10.1007/s11738-015-1874-z
Mathur, S., Tripathi, V., Jajoo, A. (2018). “Damaging effects of ultraviolet radiations (UVR) on photosynthetic apparatus,” in Format Environment and Photosynthesis a Future Prospect. Eds. Singh, V. P., Singh, S. (Studium Press New Delhi, (India) Pvt. Ltd.), 333–351.
Meng, F., Xiang, D., Zhu, J., Li, Y., Mao, C. (2019). Molecular mechanisms of root development in rice. Rice 12, 1. doi: 10.1186/s12284-018-0262-x
Mohammed, A. R., Tarpley, L. (2013). Effects of enhanced ultraviolet-b (UV-B) radiation and antioxidative-type plant growth regulators on rice (Oryza sativa L.) leaf photosynthetic rate, photochemistry and physiology. J. Agricult. Sci. 5, 5. doi: 10.5539/jas.v5n5p115
Qin, H., Zhang, Z., Wang, J., Chen, X., Wei, P., Huang, R. (2017). The activation of OsEIL1 on YUC8 transcription and auxin biosynthesis is required for ethylene-inhibited root elongation in rice early seedling development. PloS Genet. 13, e1006955. doi: 10.1371/journal.pgen.1006955
Ramamoorthy, P., Bheemanahalli, R., Meyers, S. L., Shankle, M. W., Reddy, K. R. (2022). Drought, low nitrogen stress, and ultraviolet-b radiation effects on growth, development, and physiology of sweet potato cultivars during early season. Genes 13, 156. doi: 10.3390/genes13010156
Reddy, K. R., Kakani, V. G., Zhao, D., Gao, W. (2003). Cotton responses to ultraviolet-B radiation: experimentation and algorithm development. Agric. For. Meteorol. 120, 249–265. doi: 10.1016/j.agrformet.2003.08.029
Reddy, K. R., Read, J., McKinion, J. M. (2001). Soil-plant–atmosphere–research (SPAR) facility: A tool for plant research and modeling. Biotronics 30, 27–50.
Reddy, K. R., Seghal, A., Jumaa, S., Bheemanahalli, R., Kakar, N., Redoña, E. D., et al. (2021). Morpho-physiological characterization of diverse rice genotypes for seedling stage high- and low-temperature tolerance. 11(1), 112. doi: 10.3390/agronomy11010112
Reddy, K. R., Singh, S. K., Koti, S., Kakani, V. K., Zhao, D., Gao, W., et al. (2013). Quantifying corn growth and physiological responses to ultraviolet-b radiation for modelling. Agron. J. 105, 1367–1377. doi: 10.2134/agronj2013.0113
Rousseaux, M. C., Ballare, C. L., Scopel, A. L., Searles, P. S., Caldwell, M. M. (1998). Solar ultraviolet-B radiation affects plant-insect interactions in a natural ecosystem of Tierra del Fuego. Oecologia 116, 528–535. doi: 10.1007/s004420050618
Roychoudhry, S., Kepinski, S. (2022). Auxin in root development. Cold Spring Harb. Perspect. Biol 14(4), a039933. doi: 10.1101/cshperspect.a039933
Sah, S. K., Reddy, K. R., Li, J. (2022). Silicon enhances plant vegetative growth and soil water retention of soybean (Glycine max) plants under water-limiting conditions. Plants 11 (13), 1687. doi: 10.3390/plants11131687
Santos, I., Almeida, J. M., Salema, R. (1993). Plants of Zea mays L. developed under enhanced UV-B radiation. I. Some ultrastructural and biochemical aspects. J. Plant Physiol. 141, 450–456. doi: 10.1016/S0176-1617(11)80193-8
Singh, S. K., Reddy, K. R., Reddy, V. R., Gao, W. (2014). Maize growth and developmental responses to temperature and ultraviolet-B radiation interaction. Photosynthetica 52, 262–271. doi: 10.1007/s11099-014-0029-6
Sullivan, J. H., Gitz, D. C., III, Liu-Gitz, L., Xu, C., Gao, W., Slusser, J. (2007). Coupling short-term changes in ambient UV-B levels with induction of UV-screening compounds. Photoch. Photobio. 83, 863–870. doi: 10.1111/j.1751-1097.2007.00138.x
Teramura, A. H., Ziska, L. H., Sztein, A. E. (1991). Changes in growth and photosynthetic capacity of rice with increased UVB radiation. Physiol. Plant 83, 373–380. doi: 10.1111/j.1399-3054.1991.tb00108.x
Thanawong, K., Perret, S. R., Basset-Mens, C. (2014). Eco-efficiency of paddy rice production in Northeastern Tailand: a comparison of rain-fed and irrigated cropping systems. J. Cle. Prod. 73, 204–217. doi: 10.1016/j.jclepro.2013.12.067
Tossi, V., Lamattina, L., Jenkins, G. I., Cassia, R. O. (2014). Ultraviolet-B induced stomatal closure in Arabidopsis is regulated by the UV RESISTANCE LOCUS8 photoreceptor in a nitric oxide-dependent mechanism. Plant Physiol. 164, 2220–2230. doi: 10.1104/pp.113.231753
UNEP, (United Nations Environment Programme) (2014). Environmental effects of ozone depletion and its interactions with climate change, ISBN: 978-9966-076-04-5 ISBN, 978-9966-076-04-5. (Secretariat for The Vienna Convention for the Protection of the Ozone Layer and The Montreal Protocol on Substances that Deplete the Ozone Layer: United Nations Environment Programme (UNEP)).
Wang, S., Xu, Y., Li, Z., Zhang, S., Lim, J. M., Lee, K. O., et al. (2014). OsMOGS is required for N-glycan formation and auxin-mediated root development in rice (Oryza sativa L.). Plant J. 78, 632–645. doi: 10.1111/tpj.12497
Wijewardana, C., Henry, W. B., Gao, W., Reddy, K. R. (2016). Interactive effects on CO2, drought, and ultraviolet-B radiation on maize growth and development. J. Photochem. Photobiol. B.: Biol. 160, 198–209. doi: 10.1016/j.jphotobiol.2016.04.004
Yamamoto, Y., Kamiya, N., Morinaka, Y., Matsuoka, M., Sazuka, T. (2007). Auxin biosynthesis by the YUCCA genes in rice. Plant Physiol. 143, 1362–1371. doi: 10.1104/pp.106.091561
Yang, J., Li, M. R., Qin, L., Feng, S. H., Zhan, F. D., Li, Y., et al. (2020). The effects of enhanced UV-B radiation on root morphology and secretion content of rice (Oryza sativa Linn.). IOP. Conf. Series. Earth Environ. Sci. 446, 32073. doi: 10.1088/1755-1315/446/3/032073
Yao, Y. A., Yang, Y. Q., Li, Y., Lutts, S. (2008). Intraspecific responses of Fagopyrum esculentum to enhanced ultraviolet B radiation. Plant Growth Regul. 56, 297–306. doi: 10.1007/s10725-008-9309-0
Yuan, L., Yongmei, H., Yanqun, Z., Fangdong, Z. (2011). Identification and cloning of molecular markers for UV-B tolerant gene in wild sugarcane (Saccharum spontaneum L.). J. Photochem. Photobiol. B.: Biol. 105, 119–125. doi: 10.1016/j.jphotobiol.2011.07.002
Zhao, D., Reddy, K. R., Kakani, V. G., Read, J. J., Sullivan, J. H. (2003). Growth and physiological responses of cotton (Gossypium hirsutum L.) to elevated carbon dioxide and ultraviolet-B radiation under controlled environmental conditions. Plant Cell Environ. 26, 771–778. doi: 10.1046/j.1365-3040.2003.01019.x
Keywords: combined UV response index, genetic variability, individual UV response index, root, shoot
Citation: Mathur S, Bheemanahalli R, Jumaa SH, Kakar N, Reddy VR, Gao W and Reddy KR (2024) Impact of ultraviolet-B radiation on early-season morpho-physiological traits of indica and japonica rice genotypes. Front. Plant Sci. 15:1369397. doi: 10.3389/fpls.2024.1369397
Received: 12 January 2024; Accepted: 12 February 2024;
Published: 01 March 2024.
Edited by:
Anket Sharma, Texas Tech University, United StatesReviewed by:
Rupal Singh Tomar, Saint Louis University, United StatesCopyright © 2024 Mathur, Bheemanahalli, Jumaa, Kakar, Reddy, Gao and Reddy. This is an open-access article distributed under the terms of the Creative Commons Attribution License (CC BY). The use, distribution or reproduction in other forums is permitted, provided the original author(s) and the copyright owner(s) are credited and that the original publication in this journal is cited, in accordance with accepted academic practice. No use, distribution or reproduction is permitted which does not comply with these terms.
*Correspondence: Kambham Raja Reddy, a3JyZWRkeUBwc3MubXNzdGF0ZS5lZHU=; Sonal Mathur, bWF0aHVya3NvbmFsQGdtYWlsLmNvbQ==
†Present address: Salah Hameed Jumaa, Department of Field Crop Science, Tikrit University, Salah Al-Deen Governorate, Tikrit, Iraq
Naqeebullah Kakar, Agronomy Department, Kansas State University, Manhattan, KS, United States
Disclaimer: All claims expressed in this article are solely those of the authors and do not necessarily represent those of their affiliated organizations, or those of the publisher, the editors and the reviewers. Any product that may be evaluated in this article or claim that may be made by its manufacturer is not guaranteed or endorsed by the publisher.
Research integrity at Frontiers
Learn more about the work of our research integrity team to safeguard the quality of each article we publish.