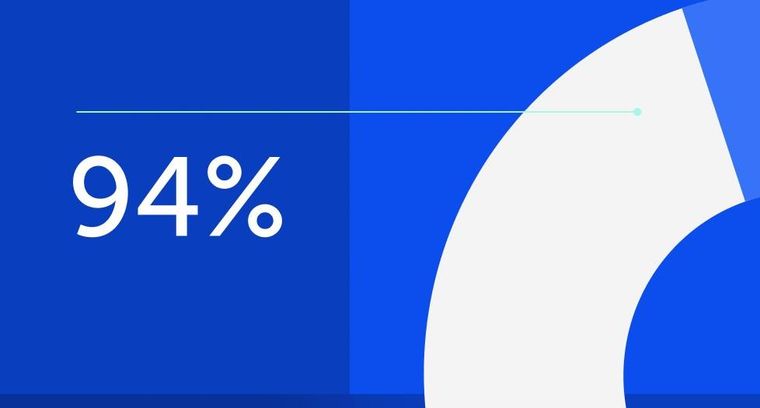
94% of researchers rate our articles as excellent or good
Learn more about the work of our research integrity team to safeguard the quality of each article we publish.
Find out more
ORIGINAL RESEARCH article
Front. Plant Sci., 04 March 2024
Sec. Plant Symbiotic Interactions
Volume 15 - 2024 | https://doi.org/10.3389/fpls.2024.1364807
This article is part of the Research TopicThe Role of the Microbiome in Plant and Soil Health in a Changing ClimateView all 13 articles
Introduction: Microbial biofertilizers or biocontrol agents are potential sustainable approaches to overcome the limitations of conventional agricultural practice. However, the limited catalog of microbial candidates for diversified crops creates hurdles in successfully implementing sustainable agriculture for increasing global/local populations. The present study aimed to explore the wheat rhizosphere microbiota for microbial strains with a biofertilizer and biocontrol potential.
Methods: Using a microbial culturing-based approach, 12 unique microbial isolates were identified and screened for biofertilizer/biocontrol potential using genomics and physiological experimentations.
Results and discussion: Molecular, physiological, and phylogenetic characterization identified Stenotrophomonas maltophilia BCM as a potential microbial candidate for sustainable agriculture. Stenotrophomonas maltophilia BCM was identified as a coccus-shaped gram-negative microbe having optimal growth at 37°C in a partially alkaline environment (pH 8.0) with a proliferation time of ~67 minutes. The stress response physiology of Stenotrophomonas maltophilia BCM indicates its successful survival in dynamic environmental conditions. It significantly increased (P <0.05) the wheat seed germination percentage in the presence of phytopathogens and saline conditions. Genomic characterization decoded the presence of genes involved in plant growth promotion, nutrient assimilation, and antimicrobial activity. Experimental evidence also correlates with genomic insights to explain the potential of Stenotrophomonas maltophilia BCM as a potential biofertilizer and biocontrol agent. With these properties, Stenotrophomonas maltophilia BCM could sustainably promote wheat production to ensure food security for the increasing population, especially in native wheat-consuming areas.
Wheat is a principal source of calories and nutritional sustenance for most of the world’s population. The escalating global population poses a formidable challenge to food production systems, necessitating a heightened focus on augmenting wheat production by at least 1.5% per year by 2050 to meet burgeoning dietary demands. We have easily achieved it using fertilizers, hybrid seeds, and pesticides. However, their continuous use has adversely affected soil quality, which limits us from using another chemical-based green revolution. The disruption of soil ecology highlights the urgency for sustainable and integrated interventions to meet requirements without affecting soil ecology. The development of biofertilizers and their integration into agricultural practices pave the way toward achieving growth targets sustainability. Biofertilizer microbial strains are pivotal in enhancing plant growth and productivity through their intricate interactions with the rhizosphere. These strains, often comprising beneficial bacteria or mycorrhizal fungi, contribute to sustainable agriculture by promoting nutrient availability, facilitating nutrient uptake, and inducing plant systemic resistance. Symbiotic microbes like Rhizobium, Sinorhizobium, Azoarcus, Mesorhizobium, Frankia, Allorhizobium, Bradyrhizobium, Burkholderia, Azorhizobium, and Achromobacter strains (Nosheen et al., 2021) and free-living microbes like Azospirillum, Azotobacter, Azoarcus, Gluconacetobacter, and Herbaspirillum (Steenhoudt and Vanderleyden, 2000) have proven their potential to meet plant nitrogen requirements (Shah et al., 2021). The phosphate solubilization potential of Agrobacterium sp., Azotobacter sp., Bacillus sp., Burkholderia sp., Enterobacter sp., Erwinia sp., Pseudomonas sp., etc. (Shah et al., 2021) can be employed to meet our plant phosphate requirement. The potassium-solubilizing microbe like Bacillus edaphicus (Sheng and He, 2006), Bacillus megaterium, Arthrobacter sp (Keshavarz Zarjani et al., 2013), and Paenibacillus glucanolyticus (Sangeeth et al., 2012) enhances plant production. Various microbes were characterized for their plant growth-promoting potential by plant hormone secretion (Kumar et al., 2023), siderophores generation (Kumar et al., 2023), nutrient assimilation (Fatima and Senthil-Kumar, 2015), and biotic and abiotic stress resistance (Tsukanova et al., 2017). Employment of these microbial biofertilizers could fulfill the plant growth requirements for increased crop production. The wheat yield enhancement also requires employing biocontrol agents to overcome phytopathogen infestation. Wheat phytopathogens Rhizoctonia solani and Fusarium oxysporum severely affect seed germination and seedling growth (Harris and Moen, 1985; El Chami et al., 2023). It ultimately results in bare patches in crop areas up to 20% (Anees et al., 2010). These pathogens have significantly reduced the production of wheat. Australia’s southern and western cropping regions have documented annual losses of $59 million and $166 million, respectively (Murray and Brennan, 2009). Also, this pathogen has a wide host range (Cook et al., 2002), and thus, it is much more difficult to control. Crop rotation strategy implementation may reduce the fungal infestation to some extent. However, even this strategy reduced grain production (Volsi et al., 2022). Various biocontrol agents like Trichoderma sp., Mycorrhizal fungi, and Pseudomonas fluorescens demonstrated antagonism toward Rhizoctonia solani and Fusarium oxysporum. The applicability of these control agents in wheat cultivation lies in their ability to enhance plant defense mechanisms, induce systemic resistance, and compete for resources with pathogenic fungi. Biocontrol agents primarily excel in mitigating phytopathogens; however, their role in directly promoting plant growth in wheat may be comparatively less pronounced.
A limited number of strains are known to exert the dual effect of biocontrol and biofertilization. Bacillus subtilis and Bacillus amyloliquefaciens represent a group with dual functionality, producing antimicrobial compounds such as polyketides, ribosomal peptides, and bacteriocins for biocontrol and contributing to plant growth promotion through the production of siderophores (Caulier et al., 2019). Pseudomonas fluorescens is recognized for biocontrol against various pathogens such as soil-borne Fusarium solani and Sclerotinea rolscii (Ganeshan and Manoj Kumar, 2005) and is known to stimulate plant growth by producing growth-promoting substances such as Indole-3-acetic acid and siderophores (Sah et al., 2021). However, their poor survivability in dynamic soil ecosystems, host specificity, etc. (Shah et al., 2021) requires enriching a catalog of plant growth-promoting strains. Therefore, identifying dual players from the host native niches will bestow the advantage of natural colonization and prepare a stage for its utilization up to its full potential. Hereby, the present study was designed to explore/elucidate the wheat rhizosphere microbial world to identify potential biocontrol agents with plant growth-promoting potential to increase wheat crop yield.
Rhizospheric soil was collected from wheat plants grown in an experimental field at Maharshi Dayanand University Rohtak (28° 52’ 44’’ NL and 76° 37’ 19’’ EL), Haryana, India. A measurement of 5.0g of soil was suspended in 20 ml ultrapure sterile water to perform physiochemical analysis. Furthermore, soil suspension was serially diluted up to 10-8. A measurement of 0.1ml of each dilution was spread evenly on self-devised minimal media A (pH 7.2) [Urea (200mg), calcium phosphate (250mg), Ferrous sulfate (20mg), Synthetic Sea salt (200mg), Pectin (50mg), Inulin (50mg), Starch (50mg), Sorbitol (50mg), Carboxyl methyl cellulose (50mg), and Ammonium sulfate (50mg) dissolved in 100ml distilled water]. Culture plates were incubated at 16°C, 25°C, and 37°C to isolate diverse microbes. The bacterial growth was observed for 48 hours, and morphologically diverse microbial colonies were sub-cultured at 37°C to achieve their pure cultures.
Isolated bacterial cultures were screened to assess their antifungal activity using a disc diffusion assay (Balouiri et al., 2016). Wheat rhizosphere microbes, Rhizoctonia solani, and Fusarium oxysporum were grown in LB and YEPD broth, respectively, with continuous shaking at 200 rpm for 24 hours at 28°C. A measurement of 0.1 ml of 1.0 OD600nm overnight-grown fungal culture was spread evenly on PDA plates in sterile conditions, discs were placed at the center of the plates, and 50µl of the overnight-grown bacterial culture (A600nm: 1.0) was applied to the disc, followed by incubation at 28°C for 48 hours. The fungal growth inhibition was checked by observing the presence of the growth inhibition zone (Balouiri et al., 2016).
Gram staining of biocontrol microbe was performed with a gram staining kit (K001-1KT, Himedia). Growth of biocontrol microbe was observed at different pHs (3, 4, 5, 7, 8, 9, 10, 11, and 12) and temperatures (10°C, 15°C, 20°C, 25°C, 30°C, 35°C, 40°C, 45°C, 50°C, 55°C, and 60°C) to identify its optimal growth conditions. Its growth pattern was observed in LB broth for 48 hrs at 37°C with constant shaking at 200rpm to check its doubling time (Wang et al., 2015). Substrate utilization preference of the identified microbe was assessed with a Hi-carbo kit (Himedia, KB009A-1KT, KB009B-1KT, and KB009C-1KT). Biochemical properties of the identified strain were performed using assays for amylase (Swain et al., 2006), catalase (Iwase et al., 2013), pectinase (Oumer and Abate, 2018), cellulase (Kasana et al., 2008), esterase (Ramnath et al., 2017), and protease (Vijayaraghavan et al., 2017). The antibiotic susceptibility of the microbial isolate was assessed using a Combi IV kit (Himedia, OD023) and G-VI-plus (Himedia, OD034). The stress response physiology of the identified strain was assessed by performing assays for salt stress tolerance, metal stress tolerance, and oxidative stress (Yadav et al., 2023). DNA was extracted from the microbial isolate using the alkali lysis method (Chauhan et al., 2009). The qualitative and quantitative analysis of the DNA was performed with agarose gel electrophoresis and Qubit HS DNA estimation kits (Invitrogen, USA), respectively. The 16S rRNA gene was amplified and sequenced to decode its taxonomic affiliation using a standardized methodology (Yadav et al., 2023).
Stenotrophomonas maltophilia BCM was sequenced using Illumina MiSeq using Nextera XT DNA Library Prep kit. Raw reads were quality checked using FASTQC v0.11.9 (http://www.bioinformatics.babraham.ac.uk/projects/fastqc) and fastQ Validator v0.1.1 (https://github.com/statgen/fastQValidator). Contaminated reads were removed to get the corrected reads. The SPAdes v3.15.1 assembler was used for the de-novo assembly. Further, BUSCO v5.0.0 assessment tools were used with the latest bacterial orthologue catalog (bacteria_odb10) for analyzing the completeness of a set of predicted genes in bacterial genome assemblies. Assembled contigs were used for functional annotation via PROKKA. SSU rRNA gene was extracted, and the BLASTn was performed to identify the taxonomic affiliation of BCM. Database homologs with more than 97% 16S rRNA gene similarity were chosen for comparative analysis. Genomes were downloaded from the NCBI web server and annotated via PROKKA (Seemann, 2014). J-species software (http://jspecies.ribohost.com/jspeciesws/) assessed the genome level similarity using average nucleotide identity and tetra-correlation values. CRISPR/Casin genome was identified using the CRISPR identifier. Antibiotic resistance genes using CARD identifier were assembled and contigs were used to draw a circular genomic map via the Proksee tool (https://proksee.ca/). The antibiotic resistance, metal/metalloid resistance, and oxidative stress resistance protein features were identified using rapid annotation using a subsystem technology (RAST) server (https://rast.nmpdr.org/rast.cgi?page=Jobs). The genome was checked for pathogenesis with the Island Viewer 4 with the default parameters. Phylogenomic characterization of Stenotrophomonas maltophilia BCM and shortlisted strains were plotted using roary_plots.py v0.1.0 (https://github.com/sanger-pathogens/Roary/blob/master/contrib/roary_plots/roary_plots.py). The core multiple sequence alignments were used to infer the phylogenomic tree using FastTree v2.1.10 (Price et al., 2010).
The biocontrol potential of Stenotrophomonas maltophilia BCM was assessed against Rhizoctonia solani and Fusarium oxysporum in terms of their effect on seed germination efficiency, root and shoot length of wheat plantlets (Moraes et al., 2014), and alpha-amylase activity (Singh and Kayastha, 2014). Biofilm inhibition activity of Stenotrophomonas maltophilia BCM was checked against Chromobacterium violaceum. Anti-biofilm activity was calculated by estimating the amount of violacein production by Chromobacterium violaceum in the presence of Stenotrophomonas maltophilia BCM (Adeyemo et al., 2022).
Wheat seed germination assay was performed in the presence of Stenotrophomonas maltophilia BCM. Seeds were initially soaked in overnight-grown microbial culture corresponding to 1011 cells/ml containing different concentrations of NaCl ranging from 0.0 M to 1.0 M for 16 hours at 37°C, while control seeds were soaked directly at different concentrations of NaCl ranging from 0 to 1M for 16 hours at 37°C. Seeds were finally wrapped in germination sheets, inserted in 50 ml culture tubes containing 5 ml Hoagland solution, and incubated for 7 days in the dark at room temperature. Seed germination percentage, alpha-amylase activity, and root and shoot length were measured after the incubation (Singh and Kayastha, 2014).
Stenotrophomonas maltophilia BCM were screened for nitrate reductase activity (Kim and Seo, 2018), auxin production (Ehmann, 1977), ammonia production (Bhattacharyya et al., 2020), and siderophore biosynthesis (Himpsl and Mobley, 2019) for the assessment of their bio-fertilization potential.
The wheat rhizospheric soil from where the microbe was isolated has been collected and identified to have 7.3 pH, 22.6˚C temperature, and 11.5 ± 1.10% moisture content. Twelve morphologically diverse microbes were purified from the wheat rhizosphere, of which three showed antifungal activity against at least one fungal strain. Only the BCM strain was found to show activity against both fungal strains and was used for further study. Antifungal activity assay showed that the microbial isolate BCM led to a growth inhibition zone of 17 ± 0.57 mm and 15 ± 0.57735 mm against Rhizoctonia solani and Fusarium oxysporum, respectively. These results indicate that microbial isolate BCM harbors the potential to develop an efficient biocontrol agent.
The 16S rRNA gene of the microbial isolate BCM shared 99.48% homology with Stenotrophomonas maltophilia LMG 25348 in the NCBI 16S rRNA gene database, indicating it as a species of Stenotrophomonas maltophilia. The16S rRNA gene-based phylogenetic analysis also confirms similar observations (Figure 1). Based on taxonomic and phylogenetic observations, microbial isolate BCM was labeled Stenotrophomonas maltophilia BCM for downstream analysis. Microscopic investigation indicated Stenotrophomonas maltophilia BCM as a gram-negative, rod-shaped, and motile bacterium. Stenotrophomonas maltophilia BCM showed optimum growth at pH 7.0 and 35˚C (Figure 2). Growth pattern analysis indicates that it attains a log phase of growth after 15 hours and has a doubling time of around 67.8 minutes (Supplementary Figure SF1). Stenotrophomonas maltophilia BCM showed growth of 0.493 O.D. at 600nm when grown anaerobically for 24 hrs at 37°C, indicating its facultative anaerobic nature. Stenotrophomonas maltophilia BCM was positive for amylase, esterase, lipase, protease, and catalase activity. Substrate utilization assay of Stenotrophomonas maltophilia BCM indicates that its substrate utilization profile is similar to other Stenotrophomonas maltophilia species (Supplementary Table S1). Antibiotic susceptibility assay suggests it is resistant toward bacitracin, cephalothin, erythromycin, novobiocin, oxytetracycline, ceftazidime, cefotaxime, and ofloxacin antibiotics while showing sensitivity toward nation, lincomycin, claxon, and amikacin. The antibiotic resistance profile of Stenotrophomonas maltophilia BCM was similar to other Stenotrophomonas maltophilia species while overlapping with Stenotrophomonas maltophilia smyn44 (Supplementary Table S2). Similar biochemical, substrate utilization, and antibiotic resistance profiles of Stenotrophomonas maltophilia BCM to other Stenotrophomonas maltophilia species strengthen the 16S rRNA gene-based taxonomic observations. Stress response physiology assays indicate that it can successfully grow in the presence of salts [up to 5.85% NaCl (w/v), 8.9% KCl (w/v), and 4.2% LiCl (w/v)] (Figure 3), metals [up to 0.1% Na3AsO4 (w/v), 0.12% NaAsO2 (w/v), and 0.54% CdCl2(w/v)] (Figure 4), oxidizing agents [up to 5.96% (v/v) H2O2] (Figure 5), as observed for other Stenotrophomonas sp. (Supplementary Table S3).
Figure 1 Phylogenetic affiliation of Stenotrophomonas maltophilia BCM with the other Stenotrophomonas species. The phylogenetic tree was constructed with the Neighbor-joining method of phylogenetics with 1000 bootstrap replications using MEGA-X software. The 16S rRNA gene sequence of Stenotrophomonas ginsengisoli DCY01 was represented as out-group.
Figure 2 Growth profile of Stenotrophomonas metophilia BCM under diverse temperatures (10°C to 60°C with an interval of 5°C) and pH (from 3 to 12 with an interval of one pH unit) conditions. Stenotrophomonas maltophilia BCM growth was observed in LB broth after 16 hrs of uninterrupted growth at respective temperature and pH conditions with constant shaking at 200 rotations per minute (rpm). The experiments were carried out in triplicates, and growth was observed by reading the absorbance of the culture at 600nm. Plotted values are the mean of triplicates along with the observed standard deviation.
Figure 3 Growth profile of Stenotrophomonas maltophilia BCM in the presence of NaCl, KCl, and LiCl. Stenotrophomonas maltophilia BCM growth was observed in salt-supplemented LB broth after 16 hrs of uninterrupted growth at 37°C with constant shaking at 200 rpm. The experiments were carried out in triplicates, and growth was observed by reading the absorbance of the culture at 600nm. Values plotted here are the triplicates’ mean and the observed standard deviation.
Figure 4 Growth profile of Stenotrophomonas maltophilia in the presence of cadmium chloride (A), sodium arsenite (B), and sodium arsenate (C). Stenotrophomonas maltophilia BCM growth was observed in metal/metalloid-supplemented LB broth after 16 hrs of uninterrupted growth at 37°C with constant shaking at 200 rpm. The experiments were carried out in triplicates, and growth was observed by reading the absorbance of the culture at 600nm. Values plotted here are the triplicates’ mean and the observed standard deviation.
Figure 5 Growth profile of Stenotrophomonas maltophilia BCM in the presence of NaCl , KCl, and LiCl. Stenotrophomonas maltophilia BCM growth was observed in salt-supplemented LB broth after 16 hrs of uninterrupted growth at 37°C with constant shaking at 200 rpm. The experiments were carried out in triplicates, and growth was observed by reading the absorbance of the culture at 600nm. Values plotted here are the triplicates' mean and the observed standard deviation.
Genome sequencing of Stenotrophomonas maltophilia BCM resulted in the generation of 551495 paired-end raw reads. Stenotrophomonas maltophilia BCM genome was assembled into 447 contigs, accounting for a total 4519592 bp size, with 66.5% GC content and N50 length of 139100bp (Supplementary Table S4). Functional annotation of the genome identified 3949 protein-coding, 7 rRNA, 74 tRNA, and 1 tmRNA gene (Figure 6A). Average nucleotide identity (ANI) was performed to check the relationship of microbe at the genomic level. The average ANI among different species of Stenotrophomonas was 77-99% toward the lower end of the 77-100% spectrum, suggesting significant interspecific genomic variations. Furthermore, the ANI score of Stenotrophomonas maltophilia BCM with Stenotrophomonas maltophilia smyn44 was 99.57, while it was comparatively higher compared with other species members (Supplementary Table S5). The affiliation of Stenotrophomonas maltophilia BCM as a member of Stenotrophomonas maltophilia species was further confirmed using terra correlation. Stenotrophomonas maltophilia BCM has been awarded a 0.9996 z-score against Stenotrophomonas maltophilia AU12-09 during terra-correlation, confirming its similarity with Stenotrophomonas maltophilia. Other Stenotrophomonas species exhibited good similarity (z-score ∼ 0.93-0.99) (Supplementary Table S6). The matrix generated using the Roary tool showed the comprehensive nature of the genome in which the microbial isolate showed the highest similarity with Stenotrophomonas maltophilia smyn44 (Figure 6B). The genomic matrix also revealed that all Stenotrophomonas genomes share only a few numbers of genes as their core genome. Shell and cloud genome collectively forms the central part of the genomes. Stenotrophomonas maltophilia BCM genome has neither a pathogenic gene/island nor any virulence-related genes, indicating its non-pathogenic nature. In addition to the genes for antimicrobial activity (Table 1), Stenotrophomonas maltophilia BCM genome harbors genes encoding proteins for plant growth promotion activities like auxin biosynthesis, nitrogen assimilation, siderophore biosynthesis, and phosphate solubilization (Table 2). Stenotrophomonas maltophilia BCM genome also harbors genes responsible for arsenic resistance, oxidative stress tolerance, metal stress tolerance, and salt tolerance (Supplementary Table S7), explaining its stress response physiology. The 24 CAZymes clusters within its genome also justify its diverse carbohydrate utilization profile (Supplementary Table S8). Additionally, its genome encodes various hydrolases, some of which might extend anti-pathogenic behavior to the host (Supplementary Table S9).
Figure 6 Genome map (A) and phylogenomic profile (B) of the Stenotrophomonas maltophilia BCM. The circular genome map (A) was drawn using the Proksee online tool (https://proksee.ca. The phylogenomic tree (B) was constructed with the FastTree v2.1.10 tool via Roary.
Table 1 Genetic features involved in Stenotrophomonas maltophilia BCM genome involved in biocontrolling activity.
Table 2 Genetic features identified within Stenotrophomonas maltophilia BCM genome encoding various proteins involved in nutrient assimilation and solubilization.
Several proteins were identified as essential for effective colonization in plant rhizosphere (Kumar et al., 2023). An in-depth analysis of the Stenotrophomonas maltophilia BCM genome identifies the presence of genes encoding proteins for the synthesis of Type 1 and IV pili, exopolysaccharide (Table 3) essential for plant surface adhesion, auto-aggregation, and early biofilm formation (Kumar et al., 2023).
Table 3 Genetic features within the genome encoding proteins for Stenotrophomonas maltophilia BCM colonization in wheat rhizosphere.
The protective effect of Stenotrophomonas maltophilia BCM on wheat seed germination was assessed. A 10% ± 1 and 5% ± 0.57735 seed germination was observed in the presence of Rhizoctonia solani and Fusarium oxysporum, respectively. Seeds’ pre-treatment with Stenotrophomonas maltophilia BCM showed a germination efficiency of 75.33 ± 0.57735% and 87.66 ± 0.57705% in the presence of Rhizoctonia solani and Fusarium oxysporum, respectively (Figure 7). Stenotrophomonas maltophilia BCM was observed to increase ~750 and ~1753-fold seed germination in the presence of Rhizoctonia solani and Fusarium oxysporum, respectively. These results strongly indicate the potential biocontrol behavior of Stenotrophomonas maltophilia BCM. Stenotrophomonas maltophilia BCM enhanced seed germination in the presence of phytopathogens and significantly improved seed germination in reference to the control (P=0.024) (Figure 7). Rhizoctonia solani and Fusarium oxysporum were also found to significantly reduce (P=0.0001 and P=0.0018) alpha-amylase activity in wheat seeds to 0.42 IU and 0.22 IU, respectively. Seeds’ pre-treatment with Stenotrophomonas maltophilia BCM significantly increased alpha-amylase activity (P=0.000136 and P=0.0000262) in the presence of Rhizoctonia solani and Fusarium oxysporum, respectively (Figure 8). The significant improvement of alpha-amylase activity in wheat seeds after pre-treatment with Stenotrophomonas maltophilia BCM could be a possible reason for enhanced seed germination.
Figure 7 Impact of Stenotrophomonas maltophilia BCM on the wheat seed germination percentage under biotic stress. Seeds were pre-inoculated with 2× 108 CFU/ml of test organisms (S. maltophilia BCM and phytopathogenic fungal strains as per experimental conditions) for 16 hours before seed germination experiments. All assays were carried out in triplicates.
Figure 8 Impact of Stenotrophomonas maltophilia BCM on the alpha-amylase activity in wheat seeds during germination under biotic stress. Seeds were pre-inoculated with 2× 108 CFU/ml of test organisms (S. maltophilia BCM and phytopathogenic fungal strains as per experimental conditions) for 16 hours before alpha-amylase activity assays. Plotted values are the mean of triplicates along with the observed standard deviation.
Pre-treatment of seeds with Stenotrophomonas maltophilia BCM not only enhanced seed germination but also improved the growth of wheat plantlets. Wheat seeds pre-treated with Stenotrophomonas maltophilia BCM showed a significantly enhanced shoot length (P=0.027) and root length (P=0.010) compared to the untreated seeds (Figure 9). Stenotrophomonas maltophilia BCM was also found to significantly improve the root (P=0.017 and 0.039) and shoot length (P=0.020 and 0.136) of wheat plantlets infected with Rhizoctonia solani and Fusarium oxysporum, respectively. The average shoot and root length of wheat plantlets treated with Stenotrophomonas maltophilia BCM were significantly higher (P=0.0297 and 0.0023) compared to the control even after Rhizoctonia solani and Fusarium oxysporum exposure, indicating its biocontrol behavior. Stenotrophomonas maltophilia BCM also interacted with Chromobacterium violaceum and significantly reduced violacein production (P=0.001). This indicates the potential of Stenotrophomonas maltophilia BCM as a quorum-sensing inhibitor and antibiofilm activity, which is good for a bio-controlling agent.
Figure 9 Impact of Stenotrophomonas maltophilia BCM on root and shoot length of WC-306 plantlets under biotic stress. Seeds were pre-inoculated with 2× 108 CFU/ml of test organisms (S. maltophilia BCM and phytopathogenic fungal strains as per experimental conditions) for 16 hours before seedling growth experiments. Plotted values are the mean of triplicates along with the observed standard deviation.
Stenotrophomonas maltophilia BCM’s role in wheat seed germination in abiotic stress like high salinity (a key bottleneck in wheat germination and crop production) was also assessed. Increased salt concentration significantly reduced seed germination (Figure 10A). Seeds’ pre-treatment with Stenotrophomonas maltophilia BCM showed enhanced seed germination at high salinity conditions (Figure 10B). Pre-treatment of seeds with Stenotrophomonas maltophilia BCM not only enhanced seed germination but also improved the growth of wheat plantlets. Wheat seeds pre-treated with Stenotrophomonas maltophilia BCM showed a significantly enhanced shoot length (P=0.001) and root length (P=00.0018) compared to the untreated in high salinity conditions.
Figure 10 Impact of Stenotrophomonas maltophilia BCM on root and shoot length of WC-306 plantlets under saline stress (NaCl (A) and KCl (B)). Seeds were pre-inoculated with 2× 108 CFU/ml of test organisms (S. maltophilia BCM and phytopathogenic fungal strains as per experimental conditions) for 16 hours before seedling growth experiments. Plotted values are the mean of triplicates along with the observed standard deviation.
Stenotrophomonas maltophilia BCM genome harbors key genes responsible for plant growth promotion activities like auxin biosynthesis, nitrogen assimilation, siderophore biosynthesis, and phosphate solubilization. Stenotrophomonas maltophilia BCM showed nitrate reductase activity (14IU), extracellular alkaline (0.22IU), and acid phosphatase activity (0.1 IU). It was also found to produce and secrete plant growth-promoting hormones in the surrounding environment. The presence of genes responsible for plant growth promotion and their bioactivity indicate biocontrol behavior. Stenotrophomonas maltophilia BCM also harbors plant growth-promotion activity.
A boost in crop production of cereals is required to ensure food security for the expanding global population (Tilman et al., 2011). In the past, applying agrochemicals has boosted agricultural yield and helped to fulfill food requirements. Continuous applications of agrochemicals negatively impact the environment and human health. These agrochemicals severely affect soil fertility, creating hurdles in enhancing crop production to ensure food security (Gamage et al., 2023). In addition, the evolution of plant pathogens for pesticide resistance, higher infectivity, and broad host range are significant challenges (Newman and Derbyshire, 2020). Sustainable agricultural practices offer solutions to ensure healthy soil ecology, limit infections, and enhance crop production (Mehmet Tuğrul, 2020). Plants harbor several microbial companions on underground and above-ground surfaces (Van Dijk et al., 2022). These micro-residents improve the host plant’s growth by enhancing nutrient assimilations, cell division, and plant reproduction and preventing the invasion of pathogens (Koza et al., 2022). Sustainable agricultural practices advocate the employment of such micro-residents to improve crop production. Identification and characterization of such candidate microbes became a quest of researchers around the globe.
Wheat is one of the prime food sources for fulfilling the hunger of most of the global population. Increases in soil salinity, reduced soil fertility, emergence of phytopathogens, and climate change threaten wheat production (Srinivas et al., 2019). There is an emergent need to identify potential microbial agents that can improve resistance toward both biotic (phytopathogens) and abiotic (salinity) stress in wheat for better crop production. In the present study, an effort was made to explore the wheat rhizosphere microbiota to identify potential microbial candidates to enhance plant growth by inhabiting phytopathogens infection, improving nutrient assimilation, and extending resistance toward various stressors.
Culture-dependent exploration of wheat rhizosphere microbiota identified 12 morphologically different bacterial isolates. Antifungal assays indicate that the isolate BCM showed effective growth inhibition of Rhizoctonia solani and Fusarium oxysporum phytopathogens. The 16S rRNA gene-based phylogenetic characterization of BCM isolate reveals suitable homology with Stenotrophomonas maltophilia, accordingly labeled as Stenotrophomonas maltophilia BCM. Stenotrophomonas maltophilia is a group of diazotrophic bacteria isolated from diverse habitats, predominantly from plant-associated ecosystems (Pinski et al., 2020). Rhizospheric Stenotrophomonas maltophilia species have the potential as biofertilizers, biocontrol agents, pesticide remediation (Kumar et al., 2023), and stress resistance (Singh and Jha, 2017). These studies strengthen the candidature of Stenotrophomonas maltophilia BCM as a biofertilizer and biocontrol agent. Most of this information was drawn based on experimentation with diverse plant species (Kumar et al., 2023). As a result, it is essential to understand its physiological, genomic, and plant-associated properties to understand its efficiency.
The morphological, physiological, and chemotaxonomic profile of BCM was similar to other Stenotrophomonas maltophilia strains, confirming 16S rRNA gene-based phylogenetic observations. Likewise, other Stenotrophomonas sp., Stenotrophomonas maltophilia BCM, was observed to successfully thrive under diverse physicochemical (pH and temperature) and stress conditions (saline, exposure to metal/metalloid, and antibiotic). Survival in diverse environments extended the ubiquitous nature of Stenotrophomonas sp (Kumar et al., 2023). Stenotrophomonas maltophilia BCM harbors a G+C-rich genome of 4519592bps encoding a diverse array of proteins, extending metabolic robustness to the host. Average Nucleotide Identity and phylogenomic observations further validate its taxonomic affiliation. Stenotrophomonas maltophilia BCM encodes a diverse array of hydrolytic enzymes (CAzymes, proteases, chitinase, glucanases, and lipases), phytohormones production (IAA), nutrients (Phosphate) solubilization, and phenazine production. The presence of protein-encoding features for phosphate solubilization, siderophore production, nitrogen fixation, and phytohormone production could significantly boost plant growth (Souza et al., 2015) to act as a biofertilizer. Various Stenotrophomonas sp. were already characterized for the presence of these features and were placed under the category of PGPRs (Majeed et al., 2015). Chitinolytic and proteolytic enzymes could effectively hydrolyze fungal cell walls and inhibit fungal growth (Hamid et al., 2013). Phenazine is another potent antifungal compound, and phenazine-producing microbes can effectively protect plants against fungal phytopathogens (Karmegham et al., 2020). Stenotrophomonas maltophilia BCM genome harbors genes encoding chitinase, protease, and proteins involved in phenazine production, indicating its potential as a biocontrol agent. Type I and IV pili encoding genes are essential for adhesion, autoaggregation, and biofilm formation (Elvers et al., 2001); these proteins allow the PGPR Stenotrophomonas sp. to associate with plant host in the rhizosphere (Kumar et al., 2023). The presence of these genes in the Stenotrophomonas maltophilia BCM genome further confirms its strong interaction with wheat rhizosphere. Despite the enormous potential of Stenotrophomonas sp., their application for crop improvement is challenged by the pathogenic nature of Stenotrophomonas maltophilia (Ryan et al., 2009). Surprisingly, the Stenotrophomonas maltophilia BCM genome lacks gene clusters for inducing pathogenicity in animals and plants, confirming its safe application. Stenotrophomonas maltophilia BCM genome indicates its application as a biocontrol agent and biofertilizer; however, these properties must be validated experimentally.
In-vitro experiments confirm phosphate solubilization, nitrate reduction, and auxin synthesis properties of Stenotrophomonas maltophilia BCM. These experimental observations confirm genomic observations based on the biofertilizer potential of Stenotrophomonas maltophilia BCM. Additionally, in-vitro antifungal experiments confirm its potential as a biocontrol agent. PGPR and biocontrol behavior of Stenotrophomonas maltophilia BCM were further confirmed in in-vivo studies. Wheat seed germination experiments in the presence of phytopathogens, Fusarium oxysporum, and Rhizoctonia solani indicated that Stenotrophomona smaltophilia BCM effectively protects the host seedling from fungal infection. These observations support the genome-based observations indicting Stenotrophomonas maltophilia BCM as a biocontrol agent. Stenotrophomonas maltophilia BCM not only protects the wheat seedlings from fungal infection but also significantly improves seed germination percentage and plant growth in the presence and absence of phytopathogens. Stenotrophomonas maltophilia BCM also improved wheat seed germination percentage and seedling growth under saline conditions, indicating its potential to overcome salty conditions. Conclusively, wheat rhizosphere isolates Stenotrophomonas maltophilia BCM showed good PGPR and biocontrol potential under a diverse range of stresses in the study, projecting its potential application for sustainable agriculture. However, long-term analysis under field conditions is required to validate the outcomes. These analyses are essential for implanting the isolate in agricultural practices.
The whole genome sequence of Stenotrophomonas maltophilia BCM has been uploaded to the NCBI server with an SRA accession number SRX23009035 and Bio project accession ID PRJNA1056133.
PS: Data curation, Formal analysis, Investigation, Methodology, Validation, Visualization, Writing – original draft, Writing – review & editing. RP: Writing – original draft, Writing – review & editing. NC: Data curation, Methodology, Project administration, Validation, Writing – original draft, Writing – review & editing.
The author(s) declare that no financial support was received for the research, authorship, and/or publication of this article.
The authors acknowledge CSIR-Institute of Genomics and Integrative Biology, New Delhi, India, for DNA sequencing facility.
The authors declare that the research was conducted in the absence of any commercial or financial relationships that could be construed as a potential conflict of interest.
All claims expressed in this article are solely those of the authors and do not necessarily represent those of their affiliated organizations, or those of the publisher, the editors and the reviewers. Any product that may be evaluated in this article, or claim that may be made by its manufacturer, is not guaranteed or endorsed by the publisher.
The Supplementary Material for this article can be found online at: https://www.frontiersin.org/articles/10.3389/fpls.2024.1364807/full#supplementary-material
Adeyemo, R. O., Famuyide, I. M., Dzoyem, J. P., Lyndy Joy, M. (2022). Anti-biofilm, antibacterial, and anti-quorum sensing activities of selected South African plants traditionally used to treat diarrhoea. Evidence-Based Complement. Altern. Med. 2022, 1–12. doi: 10.1155/2022/1307801
Anees, M., Edel-Hermann, V., Steinberg, C. (2010). Build up of patches caused by Rhizoctonia solani. Soil Biol. Biochem. 42, 1661–1672. doi: 10.1016/j.soilbio.2010.05.013
Balouiri, M., Sadiki, M., Ibnsouda, S. K. (2016). Methods for in vitro evaluating antimicrobial activity: A review. J. Pharm. Anal. 6, 71–79. doi: 10.1016/j.jpha.2015.11.005
Bhattacharyya, C., Banerjee, S., Acharya, U., Mitra, A., Mallick, I., Haldar, A., et al. (2020). Evaluation of plant growth promotion properties and induction of antioxidative defense mechanism by tea rhizobacteria of Darjeeling, India. Sci. Rep. 10, 15536. doi: 10.1038/s41598-020-72439-z
Caulier, S., Nannan, C., Gillis, A., Licciardi, F., Bragard, C., Mahillon, J. (2019). Overview of the antimicrobial compounds produced by members of the bacillus subtilis group. Front. Microbiol. 10. doi: 10.3389/fmicb.2019.00302
Chauhan, N. S., Ranjan, R., Purohit, H. J., Kalia, V. C., Sharma, R. (2009). Identification of genes conferring arsenic resistance to Escherichia coli from an effluent treatment plant sludge metagenomic library: Arsenic resistance genes from sludge metagenome. FEMS Microbiol. Ecol. 67, 130–139. doi: 10.1111/j.1574-6941.2008.00613.x
Cook, R. J., Weller, D. M., El-Banna, A. Y., Vakoch, D., Zhang, H. (2002). Yield responses of direct-seeded wheat to rhizobacteria and fungicide seed treatments. Plant Dis. 86, 780–784. doi: 10.1094/PDIS.2002.86.7.780
Ehmann, A. (1977). The van URK-Salkowski reagent — a sensitive and specific chromogenic reagent for silica gel thin-layer chromatographic detection and identification of indole derivatives. J. Chromatogr. A. 132, 267–276. doi: 10.1016/S0021-9673(00)89300-0
El Chami, J., El Chami, E., Tarnawa, Á., Kassai, K. M., Kende, Z., Jolánkai, M. (2023). Effect of Fusarium infection on wheat quality parameters. Cereal Res. Commun. 51, 179–187. doi: 10.1007/s42976-022-00295-w
Elvers, K. T., Leeming, K., Lappin-Scott, H. M. (2001). Binary culture biofilm formation by Stenotrophomonas maltophilia and Fusarium oxysporum. J. Ind. Microbiol. Biotechnol. 26, 178–183. doi: 10.1038/sj.jim.7000100
Fatima, U., Senthil-Kumar, M. (2015). Plant and pathogen nutrient acquisition strategies. Front. Plant Sci. 6, 750. doi: 10.3389/fpls.2015.00750
Gamage, A., Gangahagedara, R., Gamage, J., Jayasinghe, N., Kodikara, N., Suraweera, P., et al. (2023). Role of organic farming for achieving sustainability in agriculture. Farming. System. 1, 100005. doi: 10.1016/j.farsys.2023.100005
Ganeshan, G., Manoj Kumar, A. (2005). Pseudomonas fluorescens, a potential bacterial antagonist to control plant diseases. J. Plant Interact. 1, 123–134. doi: 10.1080/17429140600907043
Hamid, R., Khan, M. A., Ahmad, M., Ahmad, M. M., Abdin, M. Z., Musarrat, J., et al. (2013). Chitinases: an update. J. Pharm. Bioallied. Sci. 5, 21–29. doi: 10.4103/0975-7406.106559
Harris, J. R., Moen, R. (1985). Replacement of Rhizoctonia solani on wheat seedlings by a succession of root-rot fungi. Trans. Br. Mycological. Soc. 84, 11–20. doi: 10.1016/S0007-1536(85)80214-X
Himpsl, S. D., Mobley, H. L. T. (2019). “Siderophore detection using chrome azurol S and cross-feeding assays,” in Proteus mirabilis Methods in Molecular Biology. Ed. Pearson, M. M. (Springer New York, New York, NY), 97–108. doi: 10.1007/978-1-4939-9601-8_10
Iwase, T., Tajima, A., Sugimoto, S., Okuda, K., Hironaka, I., Kamata, Y., et al. (2013). A Simple Assay for measuring catalase activity: A visual approach. Sci. Rep. 3, 3081. doi: 10.1038/srep03081
Karmegham, N., Vellasamy, S., Natesan, B., Sharma, M. P., Al Farraj, D. A., Elshikh, M. S. (2020). Characterization of antifungal metabolite phenazine from rice rhizosphere fluorescent pseudomonads (FPs) and their effect on sheath blight of rice. Saudi. J. Biol. Sci. 27, 3313–3326. doi: 10.1016/j.sjbs.2020.10.007
Kasana, R. C., Salwan, R., Dhar, H., Dutt, S., Gulati, A. (2008). A rapid and easy method for the detection of microbial cellulases on agar plates using gram’s iodine. Curr. Microbiol. 57, 503–507. doi: 10.1007/s00284-008-9276-8
Keshavarz Zarjani, J., Aliasgharzad, N., Oustan, S., Emadi, M., Ahmadi, A. (2013). Isolation and characterization of potassium solubilizing bacteria in some Iranian soils. Arch. Agron. Soil Sci. 59, 1713–1723. doi: 10.1080/03650340.2012.756977
Kim, J., Seo, H. (2018). In vitro nitrate reductase activity assay from arabidopsis crude extracts. BIO-PROTOCOL 8, 1–6. doi: 10.21769/BioProtoc.2785
Koza, N. A., Adedayo, A. A., Babalola, O. O., Kappo, A. P. (2022). Microorganisms in plant growth and development: roles in abiotic stress tolerance and secondary metabolites secretion. Microorganisms 10, 1528. doi: 10.3390/microorganisms10081528
Kumar, A., Rithesh, L., Kumar, V., Raghuvanshi, N., Chaudhary, K., Abhineet, et al. (2023). Stenotrophomonas in diversified cropping systems: friend or foe? Front. Microbiol. 14. doi: 10.3389/fmicb.2023.1214680
Majeed, A., Abbasi, M. K., Hameed, S., Imran, A., Rahim, N. (2015). Isolation and characterization of plant growth-promoting rhizobacteria from wheat rhizosphere and their effect on plant growth promotion. Front. Microbiol. 6. doi: 10.3389/fmicb.2015.00198
Mehmet Tuğrul, K. (2020). “Soil management in Sustainable Agriculture,” in Sustainable Crop Production. Eds. Hasanuzzaman, M., Carvalho Minhoto Teixeira Filho, M., Fujita, M., Assis Rodrigues Nogueira, T. (London, United Kingdom: IntechOpen Limited). doi: 10.5772/intechopen.88319
Moraes, A. P. R., Videira, S. S., Bittencourt, V. R. E. P., Bittencourt, A. J. (2014). Antifungal activity of Stenotrophomonas maltophilia in Stomoxys calcitranslarvae. Rev. Bras. Parasitol. Vet. 23, 194–199. doi: 10.1590/S1984-29612014037
Murray, G. M., Brennan, J. P. (2009). Estimating disease losses to the Australian wheat industry. Austral. Plant Pathol. 38, 558. doi: 10.1071/AP09053
Newman, T. E., Derbyshire, M. C. (2020). The evolutionary and molecular features of broad host-range necrotrophy in plant pathogenic fungi. Front. Plant Sci. 11. doi: 10.3389/fpls.2020.591733
Nosheen, S., Ajmal, I., Song, Y. (2021). Microbes as biofertilizers, a potential approach for sustainable crop production. Sustainability 13, 1868. doi: 10.3390/su13041868
Oumer, O. J., Abate, D. (2018). Screening and molecular identification of pectinase producing microbes from coffee pulp. BioMed. Res. Int. 2018, 1–7. doi: 10.1155/2018/2961767
Pinski, A., Zur, J., Hasterok, R., Hupert-Kocurek, K. (2020). Comparative Genomics of Stenotrophomonas maltophilia and Stenotrophomonas rhizophila Revealed Characteristic Features of Both Species. IJMS 21, 4922. doi: 10.3390/ijms21144922
Price, M. N., Dehal, P. S., Arkin, A. P. (2010). FastTree 2 – approximately maximum-likelihood trees for large alignments. PloS One 5, e9490. doi: 10.1371/journal.pone.0009490
Ramnath, L., Sithole, B., Govinden, R. (2017). Identification of lipolytic enzymes isolated from bacteria indigenous to Eucalyptus wood species for application in the pulping industry. Biotechnol. Rep. 15, 114–124. doi: 10.1016/j.btre.2017.07.004
Ryan, R. P., Monchy, S., Cardinale, M., Taghavi, S., Crossman, L., Avison, M. B., et al. (2009). The versatility and adaptation of bacteria from the genus Stenotrophomonas. Nat. Rev. Microbiol. 7, 514–525. doi: 10.1038/nrmicro2163
Sah, S., Krishnani, S., Singh, R. (2021). Pseudomonas mediated nutritional and growth promotional activities for sustainable food security. Curr. Res. Microb. Sci. 2, 100084. doi: 10.1016/j.crmicr.2021.100084
Sangeeth, K. P., Bhai, ,.S., Srinivasan, V. (2012). Paenibacillus glucanolyticus, a promising potassium solubilizing bacterium isolated from black pepper (Piper nigrum L.) rhizosphere. J. Spic. Aromat. Crops. 21, 118–124.
Seemann, T. (2014). Prokka: rapid prokaryotic genome annotation. Bioinformatics 30, 2068–2069. doi: 10.1093/bioinformatics/btu153
Shah, A., Nazari, M., Antar, M., Msimbira, L. A., Naamala, J., Lyu, D., et al. (2021). PGPR in agriculture: A sustainable approach to increasing climate change resilience. Front. Sustain. Food Syst. 5. doi: 10.3389/fsufs.2021.667546
Sheng, X. F., He, L. Y. (2006). Solubilization of potassium-bearing minerals by a wild-type strain of Bacillus edaphicus and its mutants and increased potassium uptake by wheat. Can. J. Microbiol. 52, 66–72. doi: 10.1139/w05-117
Singh, K., Kayastha, A. M. (2014). α-Amylase from wheat (Triticum aestivum) seeds: Its purification, biochemical attributes and active site studies. Food Chem. 162, 1–9. doi: 10.1016/j.foodchem.2014.04.043
Singh, R. P., Jha, P. N. (2017). The PGPR Stenotrophomonas maltophilia SBP-9 Augments Resistance against Biotic and Abiotic Stress in Wheat Plants. Front. Microbiol. 8. doi: 10.3389/fmicb.2017.01945
Souza, R., Ambrosini, A., Passaglia, L. M. P. (2015). Plant growth-promoting bacteria as inoculants in agricultural soils. Genet. Mol. Biol. 38, 401–419. doi: 10.1590/S1415-475738420150053
Srinivas, C., Nirmala Devi, D., Narasimha Murthy, K., Mohan, C. D., Lakshmeesha, T. R., Singh, B., et al. (2019). Fusarium oxysporum f. sp. lycopersici causal agent of vascular wilt disease of tomato: Biology to diversity– A review. Saudi. J. Biol. Sci. 26, 1315–1324. doi: 10.1016/j.sjbs.2019.06.002
Steenhoudt, O., Vanderleyden, J. (2000). Azospirillum, a free-living nitrogen-fixing bacterium closely associated with grasses: genetic, biochemical and ecological aspects. FEMS Microbiol. Rev. 24, 487–506. doi: 10.1111/j.1574-6976.2000.tb00552.x
Swain, M. R., Kar, S., Padmaja, G., Ray, R. C. (2006). Partial characterization and optimization of production of extracellular alpha-amylase from Bacillus subtilis isolated from culturable cow dung microflora. Pol. J. Microbiol. 55, 289–296.
Tilman, D., Balzer, C., Hill, J., Befort, B. L. (2011). Global food demand and the sustainable intensification of agriculture. Proc. Natl. Acad. Sci. U.S.A. 108, 20260–20264. doi: 10.1073/pnas.1116437108
Tsukanova, K. A., Сhеbоtаr, V.К., Meyer, J. J. M., Bibikova, T. N. (2017). Effect of plant growth-promoting Rhizobacteria on plant hormone homeostasis. South Afr. J. Bot. 113, 91–102. doi: 10.1016/j.sajb.2017.07.007
Van Dijk, L. J. A., Abdelfattah, A., Ehrlén, J., Tack, A. J. M. (2022). Soil microbiomes drive aboveground plant–pathogen–insect interactions. Oikos 2022, e09366. doi: 10.1111/oik.09366
Vijayaraghavan, P., Rajendran, P., Prakash Vincent, S. G., Arun, A., Abdullah Al-Dhabi, N., Valan Arasu, M., et al. (2017). Novel sequential screening and enhanced production of fibrinolytic enzyme by bacillus sp. IND12 using response surface methodology in solid-state fermentation. BioMed. Res. Int. 2017, 1–13. doi: 10.1155/2017/3909657
Volsi, B., Higashi, G. E., Bordin, I., Telles, T. S. (2022). The diversification of species in crop rotation increases the profitability of grain production systems. Sci. Rep. 12, 19849. doi: 10.1038/s41598-022-23718-4
Wang, L., Fan, D., Chen, W., Terentjev, E. M. (2015). Bacterial growth, detachment and cell size control on polyethylene terephthalate surfaces. Sci. Rep. 5, 15159. doi: 10.1038/srep15159
Keywords: biofertilizer, biocontrol agent, wheat rhizosphere, plant growth promotion, genome characterization, sustainable agriculture, comparative genomics
Citation: Sharma P, Pandey R and Chauhan NS (2024) Biofertilizer and biocontrol properties of Stenotrophomonas maltophilia BCM emphasize its potential application for sustainable agriculture. Front. Plant Sci. 15:1364807. doi: 10.3389/fpls.2024.1364807
Received: 03 January 2024; Accepted: 09 February 2024;
Published: 04 March 2024.
Edited by:
Anoop Kumar Srivastava, Central Citrus Research Institute (ICAR), IndiaReviewed by:
Skaidre Suproniene, Lithuanian Research Centre for Agriculture and Forestry, LithuaniaCopyright © 2024 Sharma, Pandey and Chauhan. This is an open-access article distributed under the terms of the Creative Commons Attribution License (CC BY). The use, distribution or reproduction in other forums is permitted, provided the original author(s) and the copyright owner(s) are credited and that the original publication in this journal is cited, in accordance with accepted academic practice. No use, distribution or reproduction is permitted which does not comply with these terms.
*Correspondence: Nar Singh Chauhan, bnNjaGF1aGFuQG1kdXJvaHRhay5hYy5pbg==
Disclaimer: All claims expressed in this article are solely those of the authors and do not necessarily represent those of their affiliated organizations, or those of the publisher, the editors and the reviewers. Any product that may be evaluated in this article or claim that may be made by its manufacturer is not guaranteed or endorsed by the publisher.
Research integrity at Frontiers
Learn more about the work of our research integrity team to safeguard the quality of each article we publish.