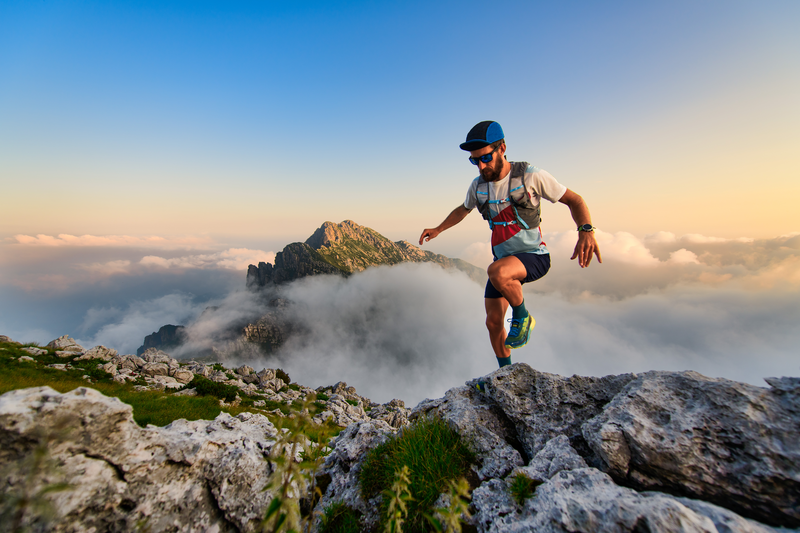
95% of researchers rate our articles as excellent or good
Learn more about the work of our research integrity team to safeguard the quality of each article we publish.
Find out more
ORIGINAL RESEARCH article
Front. Plant Sci. , 28 February 2024
Sec. Crop and Product Physiology
Volume 15 - 2024 | https://doi.org/10.3389/fpls.2024.1351843
This article is part of the Research Topic Meeting the Triple-H Challenge: Advanced Crop-Soil-Fertilizer Management Strategies to Maximize Crop Yield, Quality, and Nutrient Efficiency View all 15 articles
Intercropping has the potential to enhance yields and nutrient availability in resource-limited agricultural systems. However, the effects on crop yield nutrients and soil properties can vary considerably depending on the specific plant combinations and intercropping ratios used. In this study, the advantages and impacts of intercropping C. esculentus with legumes were investigated by measuring their biomass, nutrient content, and soil properties. The experiment included five intercropping treatments: monoculture of C. esculentus (MC), intercropping of C. esculentus with Medicago sativa L. (alfalfa) at row spacing ratios of 4:4 (4:4CM) and 8:4 (8:4CM), and intercropping of C. esculentus with Glycine max (L.) Merr. (soybean), also at row spacing ratios of 4:4 (4:4CG) and 8:4 (8:4CG). Our results demonstrated that all four intercropping treatments (4:4CM, 4:4CG, 8:4CM, and 8:4CG) significantly increased the biomass of C. esculentus by approximately 41.05%, 41.73%, 16.08%, and 18.43%, respectively, compared with monoculture cultivation alone, among which the 4:4CG treatment was optimum. However, no significant differences were observed in alfalfa or soybean biomass across different intercropping ratios. A notable increase was found in the total nitrogen (TN) and total phosphorus (TP) contents in the leaves, roots, and tubers of C. esculentus under intercropping, along with increased soil organic carbon (SOC), alkaline-hydrolyzed nitrogen (AN), available phosphorus (AP), microbial biomass carbon (MBC), microbial biomass nitrogen (MBN), and soil water content (SWC), and significantly reduced the soil pH. Among the intercropping treatments, the 4:4CG treatment also exhibited the most favorable soil properties. In particular, compared with MC, the 4:4CG treatment resulted in significant increases of 163.8%, 394.6%, and 716.8% in SOC, AN, and AP contents, respectively. The same treatment also led to significant increases of 48.34%, 46.40%, and 208.65% in MBC, MBN, and SWC, respectively. Overall, the findings suggest that the use of 4:4CG intercropping is an effective approach for sustainable farming management in Xinjiang.
Approximately 45% of the Earth’s land area comprises drylands (Prăvălie, 2016), and with the ongoing climate warming, these global drylands are projected to expand in the forthcoming decades (Zhang et al., 2021). However, such an expansion poses a remarkable threat to food security, thus underscoring the urgent need to identify suitable crops and cultivation methods for these dry regions. C. esculentus, commonly known as tiger nut and belonging to the family Cyperaceae Juss., is indigenous to the Mediterranean region of Africa (Follak et al., 2016). It is widely distributed in many northern temperate regions and is becoming increasingly popular as an energy crop in China, India, Egypt, and the United States (Ayeni, 2022). C. esculentus possesses exceptional traits, such as drought resistance and salinity tolerance (Duan et al., 2022; Zhang et al., 2023; Zhu et al., 2023). The tubers of C. esculentus are rich in fats, proteins, sugars, other nutrients, and a wide range of active compounds, making it a high-quality, high-yield, and high-value crop (Coskuner et al., 2002). In addition, the above-ground leafy part of C. esculentus, which has a growing period of around 120 days, is usually harvested for animal fodder, while the underground tuber part can be used as high-quality fodder, making it an ecologically vital cash crop (Kizzie-Hayford et al., 2015).
Intercropping, which refers to the concurrent cultivation of two or more crops on the same plot (Willey, 1990), offers heightened crop yield and stability compared with monocropping (Lan et al., 2023; Parvin et al., 2023). This agricultural practice mitigates the adverse environmental impacts associated with modern farming methods while simultaneously optimizing soil nutrient, water, and resource utilization (Chen et al., 2017). Various common species can be utilized for intercropping (Qu et al., 2023), and studies have demonstrated notable advantages when legumes are included—for instance, Qu et al. (2022) revealed that rape with common vetch significantly increased biomass, reduced soil pH, and increased soil organic carbon (SOC) and soil available phosphorus (AP). Similarly, Huang et al. (2022) demonstrated that the intercropping of tea plant with both soybean and zoysia significantly increased SOC, total nitrogen (TN), and tea quality. These findings can be attributed to the ability of legumes to alleviate nutrient stress and enhance nutrient availability through nitrogen (N) fixation or transfer to non-leguminous plants (Hobbie and Högberg, 2012; Shao et al., 2020). In addition, legumes serve as nutrient suppliers by delivering nutrients to non-leguminous plants via root secretions or arbuscular mycorrhizal fungal networks (Hauggaard-Nielsen and Jensen, 2005; Li et al., 2008; Adomako et al., 2022). Together with the root exudates, the legume-based intercropping system also promotes a beneficial rhizobacterial community, thereby improving soil quality and the rhizosphere soil environment for better resource uptake (Duchene et al., 2017; Chamkhi et al., 2022; Zhao et al., 2022). This synergistic effect on nutrient acquisition contributes to the growth of both intercropped species, ultimately leading to increased crop yield (Lu et al., 2024).
In addition, Black and Ong (2000) reported that, compared with monocropping, intercropping reduced crop water consumption (WC) without affecting total biomass production. Rezig et al. (2013) also showed that intercropping led to WC reduction in potato and sulla, resulting in increased water use efficiency of both crops. This phenomenon can be attributed to two factors. First, intercropping systems can improve water use efficiency and land occupancy by significantly developing canopy and reducing soil evapotranspiration. Second, the proportion of evapotranspiration flow is higher in intercropping than in monoculture, resulting in an expansion of the vegetation cover area and a subsequent increase in evapotranspiration. In turn, this reduces soil evapotranspiration in intercropping systems and increases soil water content (SWC). Therefore, it is important to study different intercropping treatments to increase crop yield and plant nutrients, improve soil nutrients, reduce soil pH, and increase SWC in arid areas.
In intercropping systems, the growth and resource capture of crops are determined by both intraspecific and interspecific competition among plants (Vandermeer, 1989). Therefore, maintaining appropriate ratios in intercropping systems is crucial to mitigate plant competition and optimize environmental resource uptake (Koocheki et al., 2016). Typically, a higher proportion of legumes can enhance atmospheric N fixation through symbiotic interactions with rhizobia, thus mitigating competition with non-leguminous plants for soil N resources; however, increasing proportions of intercropped plants may lead to reduced yields from the main crop (Andersen et al., 2005; Viaud et al., 2023). In addition, legumes possess the ability to enhance N availability in intercropping systems by fixing N, minimizing N leaching, and supplying N to neighboring crops (Vrignon-Brenas et al., 2016), yet whether this increased N availability offsets the competitive effects of higher proportions remains unclear from existing findings. Therefore, different intercropping proportions were also explored in the current study to investigate whether increased N availability from intercropping with legumes could counteract the competitive nature of high proportions of C. esculentus and whether it could improve C. esculentus yields and nutrients.
The southern fringe of the Taklamakan Desert in Xinjiang is located within the arid and semiarid belt of Asia and Europe, which features a typical temperate continental arid climate (Mazdiyasni and AghaKouchak, 2015). Xinjiang’s predominant sandy soil, coupled with the snakeberry’s robust root system and vigorous tillering capacity, contributes to sand fixation, soil stabilization, and wind protection (Aydar et al., 2020). Medicago sativa L. (alfalfa) and Glycine max (L.) Merr. (soybean) are both major legume crops in this region, which can significantly enhance the fertility and physicochemical properties of soil, thus establishing a mutually beneficial relationship between utilization and conservation (Rau et al., 2023). Alfalfa and soybean are sensitive to drought, with limited growth and lower yields in dry areas. However, some studies have demonstrated that intercropping with other crops can reduce the drought sensitivity of alfalfa and soybean, reduce water consumption, and increase yields (Ouda et al., 2007; Mao et al., 2012; Zhang et al., 2021; Jamali et al., 2023). In view of the limited research on intercropping C. esculentus, an emerging oilseed crop, the present study presents an innovative approach to intercropping C. esculentus with alfalfa and soybean in different proportions to assess the effects on their yield, nutrients, and soil properties. The goal of the current work is to fully utilize the economic and ecological potential of C. esculentus and to understand the key methods of growing oil salsa bean in such drylands. Furthermore, this study aims to determine whether intercropping two legumes can alleviate the competitive effects caused by the high rates of C. esculentus. To this end, two hypotheses were formulated: (1) intercropping significantly enhances the yield and nutrients of C. esculentus and enriches soil nutrient content and (2) legumes effectively counteract the competitive effects among C. esculentus when intercropped with high proportions of C. esculentus.
This experiment was conducted in June 2021 in the C. esculentus planting area (81°06′18″ E, 37°25′99″ N) located in Tuan Jie New Village in the Hotan Prefecture of the Xinjiang Uygur Autonomous Region, China (Figure 1). The area is a newly reclaimed sandy land located in the Taklamakan Desert that features a temperate continental desert climate, with an average annual temperature of approximately 13.1°C, an average annual precipitation of 43.8 mm, and a total evapotranspiration of 2,624.4 mm. The initial soil indicators were collected from the area at depths of 0–20 cm, air-dried, and sieved through a 2-mm sieve to remove rhizomes and debris. Next, the physical and chemical properties of the soils were determined with three replicates for all measurements, thereby yielding the following results: soil capacity (1.54 g·cm−3), pH (8.72), SOC (2.11 g·kg−1), AN (1.86 mg·kg−1), AP (1.23 mg·kg−1), MBC (23.09 mg·kg−1), and MBN (2.16 mg·kg−1).
Figure 1 Location of the C. esculentus planting demonstration area in Hotan Prefecture, Xinjiang, China.
The experimental variety of C. esculentus used in this study was “XinKe No. 1.” The experiment followed a one-way randomized design, including the following five treatments: C. esculentus monoculture (MC), C. esculentus and alfalfa according to a 4:4 intercropping (4:4CM), C. esculentus and soybean according to a 4:4 intercropping (4:4CG), C. esculentus and alfalfa according to an 8:4 intercropping (8:4CM), and C. esculentus and soybean according to an 8:4 intercropping (8:4CG). All rows were uniformly spaced at intervals of 30 cm, and each row strip measured 60 cm in width (Figure 2). Each treatment plant was planted in a one-hectare experimental plot. A base fertilizer comprising 300 kg·hm−2 urea (N ≥ 18%), 300 kg·hm−2 diammonium phosphate (P2O ≥ 48%), and 300 kg·hm−2 humic acid was applied, while water application amounted to 2,025 m3·hm-2.
Figure 2 Diagram of different intercropping treatments of C. esculentus with alfalfa and soybean (the numbers indicate the distance in centimeters). MC, 4:4CM, 4:4CG, 8:4CM, and 8:4CG represent monocropping C. esculentus, 4:4 C. esculentus/Medicago sativa L. intercropping, 4:4 C. esculentus/Glycine max (L.) Merr., 8:4 C. esculentus/Medicago sativa L., and 8:4 C. esculentus L./Glycine max (L.) Merr. intercropping, respectively.
The sowing process took place on June 10, 2021 using mechanically opened furrows and strip planting techniques. The sowing rate for the planting treatments remained constant at 300 kg·hm−2. Throughout the growing season, fertilizers and water were administered via drip irrigation, with each drip irrigation strip spaced at intervals of 30 cm apart. In the early growth stage of C. esculentus (July), water was dripped four times, with a total volume of approximately 9.38 m3·hm-2, along with an application of approximately 1.69 m3·hm-2 urea and 0.75 kg·hm-2 potassium sulfate (K ≥ 52%). During the middle stage of irrigation (from August to the end of September), water was applied 12 times, followed by additional four applications during the late stage of irrigation (from the end of September to the beginning of October), using identical quantities for both water and fertilizer inputs. Using appropriate herbicides and pesticides, weed and pest control measures were implemented at regular monthly intervals.
The resulting plants were harvested on October 10, 2021. Three 10 × 5-m plots were set up at 5 m apart. Three designated sampling points were selected in each plot, and plant samples (leaves, roots, and tubers) were collected from the central areas (0.5 m × 0.5 m) of these points, with 10 plants randomly selected from each point to record above- and below-ground biomass. Next, soil samples were collected from three random points in each plot using a soil sampler; each point had a 2-cm diameter and a depth range of 0–20 cm. All soil samples were thoroughly mixed to form a uniform sample and then passed through a 2-mm sieve to remove roots and debris.
The plant samples were washed with water, purified, and dried at 75°C for 48 h until the biomass was constant and then subjected to the determination of indicators. Subsequently, the dry matter was ground using a vibrating disc mill (RS200, Retsch GmbH Inc., Haan, Germany). After passing through a 1-mm sieve, the nutrient content and quality of the different organs were assessed. Organic carbon (OC) and total nitrogen (TN) concentrations were measured using a CN autoanalyzer (Eurovector, Milan, Italy), and total phosphorus (TP) concentrations were analyzed by using Mo–Sb colorimetric method after persulphate oxidation (Lu et al., 2015).
The soil samples were passed through a 2-mm sieve to eliminate rhizomes and debris. Subsequently, the samples were air-dried at 105°C for 48 h. The soil pH was determined using a pH meter (PHS-3C, China), while soil organic carbon (SOC) content was assessed using the potassium dichromate oxidation-heating method (Jiang et al., 2017). Soil alkali-hydrolyzable nitrogen (AN) concentration was quantified through the Kjeldahl method (Bremner and Mulvaney, 1982), while AP content was measured using the ammonium molybdate method after completing the H2SO4 digestion of the samples (Liu et al., 2021). Soil microbial biomass carbon (MBC) and microbial biomass nitrogen (MBN) were quantified via fumigation extraction (Vance et al., 1987). The soil samples were weighed and measured both before and after being air-dried in an oven at 105°C to determine the SWC and soil bulk density (Chehab et al., 2020). The particle size distribution of the soil was analyzed using the pipette method (Chen et al., 1991). Following the guidelines of the International System of Soil Classification (ISSC), the particle sizes were classified into four categories: clay (0–0.002 mm), silt (0.002–0.02 mm), very fine sands (Vfs, 0.02–0.2 mm), and medium and coarse sands (Macs, 0.2–2 mm).
The effects of intercropping on the nutrients, above- and below-ground biomass, and soil properties of C. esculentus were tested using one-way analysis of variance (ANOVA). Tukey’s test was employed to compare differences among intercropping treatments. All statistical analyses were performed using R v4.1.0 (R Core Team, 2018) and SPSS 23.0 software (SPSS Inc., Chicago, IL, USA), with the significance level set at P < 0.05. Pearson correlation analysis with the corrplot software package was utilized to investigate and visualize plant–soil relationships.
The biomass obtained from the 4:4CM and 4:4CG treatments of oilseed bean was significantly higher (P < 0.05) than that obtained via the MC treatment, with increases of 41.05% and 41.73%, respectively (Table 1). The biomass of oilseed bean in the 8:4CM and 8:4CG treatments was not significantly different (P > 0.05) from that of the MC treatment. Furthermore, the biomass of alfalfa and soybean did not differ significantly (P > 0.05) among different intercropping treatments. The C. esculentus leaf biomass, produced by the 4:4CM treatment, was significantly higher than that produced by MC at 56.85% (P < 0.05), while those produced by the 4:4CG, 8:4CM, and 8:4CG treatments were not significantly different from that produced by the MC treatment (P > 0.05) (Table 2). Regarding the root biomass of C. esculentus, the 4:4CG treatment yielded significantly higher biomass by 78.41% (P < 0.05) than MC. In addition, for tuber biomass, both 4:4CM and 4:4CG treatments yielded significantly higher tuber biomass than the MC treatment by 29.77% and 39.01% (P < 0.05), respectively, while the 8:4CM and 8:4CG treatments did not have any significant (P > 0.05) difference from MC.
Table 1 Total biomass of C. esculentus, alfalfa, and soybean under different intercropping treatments (kg·acre-2/g).
Table 2 Biomass of the separate components of C. esculentus under various intercropping treatments (kg·acre-2/g).
The OC contents in leaves, roots, and tubers of C. esculentus were not significantly different (P > 0.05) in all treatments (Figure 3A). In comparison, the TN contents in the leaves and roots of C. esculentus were significantly higher (P < 0.05) in all the intercropping treatments compared with MC (Figure 3B), although there were no significant differences (P > 0.05) among the intercropping treatments. The highest TN contents were found in the leaves and roots of aubergine under the 8:4CM treatment, which increased by 149.08% and 295.03%, respectively, compared with the MC treatment. The TP content of C. esculentus leaves and roots was significantly higher in the 8:4CM treatment than in MC (Figure 3C), with increases of 258.3% and 258.3%, respectively. However, there were no significant differences (P > 0.05) among the various intercropping treatments. Furthermore, the TN and TP contents of C. esculentus tubers did not show significant differences (P > 0.05) among all treatments.
Figure 3 Effect of different intercropping treatments on (A) organic carbon content, (B) total nitrogen content, and (C) total phosphorus content of the leaves, roots, and tubers of C. esculentus. The different small letters indicate significant differences among the four treatments (P < 0.05). MC, 4:4CM, 4:4CG, 8:4CM, and 8:4CG represent monocropping C. esculentus, 4:4 C. esculentus/Medicago sativa L. intercropping, 4:4 C. esculentus/Glycine max (L.) Merr., 8:4 C. esculentus/Medicago sativa L., and 8:4 C. esculentus/Glycine max (L.) Merr. intercropping, respectively.
All intercropping treatments showed a significant increase in the SOC, with the 4:4CG treatment being the most significant (P < 0.05) (Figure 4A). In particular, the 4:4CG treatment increased the SOC by 163.89% compared with the MC treatment. In terms of the AN content, the 4:4CM, 4:4CG, and 8:4CG treatments showed significant (P < 0.05) increases compared with MC (Figure 4B), with the 4:4CG treatment showing the most significant increase of 163.89% compared with the MC. Meanwhile, there was no significant difference (P > 0.05) between the 8:4CM treatment and MC. For the AP, all intercropping treatments showed significant (P < 0.05) increases compared with MC (Figure 4C), with the 4:4CG treatment being the most significant, increasing the AP by 163.89% compared with the MC treatment.
Figure 4 Effects of different intercropping treatments on (A) soil organic carbon content, (B) soil alkali-hydrolyzable nitrogen content, and (C) soil available phosphorus content. The different small letters indicate significant differences among the five treatments (P < 0.05). MC, 4:4CM, 4:4CG, 8:4CM, and 8:4CG represent monocropping C. esculentus, 4:4 C. esculentus/Medicago sativa L. intercropping, 4:4 C. esculentus/Glycine max (L.) Merr., 8:4 C. esculentus/Medicago sativa L., and 8:4 C. esculentus/Glycine max (L.) Merr. intercropping, respectively.
The soil pH values of all four intercropping patterns were significantly lower than those of the monocropping treatments (Figure 5A), with the lowest pH value in the 4:4CG treatment (P < 0.05), which was reduced by 5.78%, 3.18%, 4.51%, and 4.01% compared with the MC, 4:4CM, 8:4CM, and 8:4CG treatments, respectively. In addition, SWC was significantly higher (P < 0.05) in the intercropping treatments than in the monocropping treatments, with 4:4CG averaging the highest with an increase of 212.07% compared with the MC treatment (Figure 5B). MBC was significantly higher (P < 0.05) in both the 4:4CM and 4:4CG treatments than MC (Figure 5C), with the former being the highest on average, showing an increase of 93.57% compared with MC. However, there was no significant difference between the 8:4CM and 8:4CG intercrop treatments and MC (P > 0.05). Moreover, MBN was significantly higher (P < 0.05) in the 4:4CM, 4:4CG, 8:4CM, and 8:4CG treatments than in the MC treatment (Figure 5D), with increases of 75.09%, 86.57%, 61.25%, and 74.68%, respectively. There were no significant differences (P > 0.05) among the intercrop treatments.
Figure 5 Effects of different intercropping treatments on (A) soil pH, (B) soil water content (SWC), (C) soil microbiomass carbon, and (D) soil microbiomass nitrogen content. The different small letters indicate significant differences among the four treatments (P < 0.05). MC, 4:4CM, 4:4CG, 8:4CM, and 8:4CG represent monocropping C. esculentus, 4:4 C. esculentus/Medicago sativa L. intercropping, 4:4 C. esculentus/Glycine max (L.) Merr., 8:4 C. esculentus/Medicago sativa L., and 8:4 C. esculentus/Glycine max (L.) Merr. intercropping, respectively.
The MC and intercropping treatments significantly altered the proportion of grain size in the initial soil (IS, Figure 6). In the IS, the largest proportion of Vfs was 45%, while the smallest proportion of clay was 9%. However, after the MC and intercropping treatments, the percentage of grain size in the soil changed significantly (P < 0.05). There was also a significant decrease in Macs and a significant increase in the percentage of clay particles in the MC and all intercropping treatment soils (P < 0.05), with Macs having the smallest percentage of soil particle size, while the percentages of clay, silt, and Vfs in the soils were roughly similar.
Figure 6 A comparison of original soil samples, monocropping, and intercropping treatments was analyzed to characterize soil particle size distribution. The different small letters indicate significant differences among the four treatments (P < 0.05). Vfs and Macs represent very fine sand and medium and coarse sand, respectively. IS, MC, 4:4CM, 4:4CG, 8:4CM, and 8:4CG represent initial soil, monocropping C. esculentus, 4:4 C. esculentus/Medicago sativa L. intercropping, 4:4 C. esculentus/Glycine max (L.) Merr., 8:4 C. esculentus/Medicago sativa L., and 8:4 C. esculentus/Glycine max (L.) Merr. intercropping, respectively.
The SOC, AN, and AP were significantly and positively correlated with the total biomass of C. esculentus (P < 0.05, Figure 7). The MBC and MBN were significantly and positively correlated with the total biomass, TN and TP in leaves, TN and TP in roots, and SOC in C. esculentus (P < 0.05). Similarly, the SWC was significantly and positively correlated with the TN and TP in leaves and roots, soil nutrients, the MBC, and MBN (P < 0.05). At the same time, significant negative correlations were observed between pH and total biomass and between soil nutrients and SWC (P < 0.05). In terms of soil particle size, a significant negative correlation was found between clay and Vfs, Macs, and the SOC and between AN and AP (P < 0.05).
Intercropping, especially with legumes, confers substantial advantages in crop yield compared with monocropping (Qu et al., 2022; Lan et al., 2023; Parvin et al., 2023). This finding aligns with that of the present study, demonstrating higher C. esculentus biomass in intercropping treatments than in MC. This increase can be attributed to the legumes’ ability to achieve biological N fixation, thus alleviating N limitations in crops and fostering enhanced plant growth and development (Zhao et al., 2022). Moreover, intercropping optimizes soil nutrient management and fortifies crop nutrient supply, ultimately augmenting plant biomass (Zhang et al., 2016; Eusun et al., 2022; Lu et al., 2024). Conventionally, a higher proportion of the main crop correlates with increased yield (Tehulie and Nigatie, 2023). However, the present study revealed no significant differences in C. esculentus, alfalfa, and soybean yields among treatments with varying intercropping proportions. This finding suggests that the competitive effect of C. esculentus may outweigh the promoting effect of legumes in high-proportion cropping practices.
Beyond yield enhancements, intercropping with legumes increases the essential nutrient content in plants, particularly N and P (Schwerdtner and Spohn, 2022; Zhao et al., 2022). Furthermore, our results showed that the TN and TP contents of C. esculentus leaves and roots were significantly increased compared with the MC treatment. This study thus presents findings that are consistent with previous results—for example, the research of Li et al. (2022) on corn–soybean intercropping demonstrated significantly higher TN and TP contents in corn leaves compared with MC or corn–millet intercropping. Similarly, Li et al. (2010) observed a 9%–30% increase in maize’s N uptake in intercropping scenarios compared with MC. This increased N content in C. esculentus may be attributed to the process of legumes transferring nutrients to non-legumes (Chu et al., 2004; Rau et al., 2023). At the same time, the improvement in P nutrition in C. esculentus through intercropping may be attributed to P uptake released during legume root residue decomposition (Li et al., 2003). Furthermore, the enhanced N uptake promotes root growth and spatial distribution while delaying root senescence at the same time. Thus, the resulting boost in root uptake capacity contributes to the increased N content in C. esculentus (Zheng et al., 2021). These factors collectively underscore the multifaceted benefits of intercropping strategies involving legumes.
Intercropping increases soil nutrients, thereby boosting crop yield and nutrient uptake capacity (Liu et al., 2014; Chamkhi et al., 2022). In particular, Lu et al. (2023) demonstrated that intercropping maize and soya bean increased AN and AP in inter-root soils. Similarly, Cuartero et al. (2022) concluded that the intercropping of melon with cowpea significantly increased the TC, TN, and AP levels in the soil compared with monocropping. Consistent with previous studies, our study revealed that intercropping C. esculentus with legumes significantly increased SOC, AN, and AP, thus enriching soil nutrients. This enhancement may be attributed to the increased populations of bacteria involved in soil nutrient cycling, such as rhizobia, phosphate-solubilizing, and potassium-solubilizing bacteria (Duchene et al., 2017; Zhang et al., 2018).
Moreover, distinct differences were observed among various intercropping practices (Qu et al., 2023), with the SOC, AN, and AP contents being significantly higher in C. esculentus and soybean intercropping treatments than in C. esculentus and alfalfa intercropping treatments. This finding may be attributed to the fact that the intercropping of C. esculentus with soybean increases the relative abundance of proteobacteria, which may potentially enhance N2 fixation (Rahav et al., 2016; Li et al., 2018; Huang et al., 2022; Jamali et al., 2023) and promote soil fertility. Among the intercropping of C. esculentus and soybean, soil nutrients were again significantly higher in the 4:4 intercropping treatment than in the 8:4 treatment. This is because the reduced competition intensity stemming from the low-proportion planting density of C. esculentus (Edmeades and Daynard, 1979; Tetio-Kagho and Gardner, 1988) decreases the competition for soil nutrient resources (Hara, 1986; Weiner, 1990; Schwinn and Weiner, 1998; Hara, 2005). In addition, the competition between C. esculentus and soybean may be lower than that with alfalfa, thus appearing to be significantly highest in the SOC, AN, and AP levels under the 4:4CG treatment.
The results of our study also showed a significant decrease in the pH level and a significant increase in the SWC of soil when C. esculentus was intercropped with legumes. These results are supported by previous studies, such as that of Wang et al. (2023), who observed a considerable decrease in soil pH after intercropping tea with legumes compared with monocropping. Shen et al. (2023) reported increased SWC when intercropping maize and soybean. These findings can be explained by the fact that intercropping with legumes can increase N fixation and decrease pH by increasing N accumulation (Chen et al., 2023; Liu et al., 2023). In addition, acidic metabolites are produced by intercropping plants with alfalfa and soybeans, resulting in a decrease in pH. These acids act as main osmotic pressure regulators, contributing to the increased SWC in intercropping (Rezig et al., 2013). Our results also showed that intercropping with legumes increases soil SWC, which is supported by previous studies indicating that intercropping systems can significantly improve soil physicohydraulic properties and optimize soil structure (Chen et al., 2019; Shen et al., 2023). This finding suggests that the intercropping of oilseed bean with soybean significantly improves adaptation to drought and provides a basis for promoting soybean cultivation in arid regions. The current study also revealed that, unlike the grain size of the IS, monocropping and intercropping both increased the clay percentage and reduced the percentage of Macs compared with the original soil’s grain size. However, no significant difference was observed in soil particle size between the MC and intercropping treatments, possibly due to the study’s short duration. Thus, additional experiments with extended durations must be conducted to further delve into this aspect.
Intercropping with legumes notably increased the concentrations of microbial C and N in the soil. This finding aligns with that of Qu et al. (2022), who revealed significant increases in MBC and MBN under intercropping, directly proving the notion that enhanced stimulation of the microbial community can be achieved through intercropping. This enhancement may stem from the strong correlation observed between total microbial biomass and soil C concentration (Goyal et al., 1993; Elfstrand et al., 2007). Notably, 4:4CG intercropping exhibited the highest MBC and MBN contents, which are likely due to the increased soil nutrient concentration that encourages microbial colonization and accumulation, subsequently boosting soil microbial activity (Wu et al., 2016). These alterations in soil properties and the subsequent increase in soil microbial population may have exerted a positive feedback on plant growth and productivity (Hauggaard-Nielsen and Jensen, 2005; Adomako et al., 2022). Therefore, further studies are needed to assess the feedback effects with microorganisms under C. esculentus intercropping.
The evaluation of C. esculentus yield, nutrient content, and soil properties within a C. esculentus and legume intercropping system encompassed various intercropping methods and ratios. The study results showed that intercropping significantly increased the yield and nutrients of C. esculentus compared with monocropping, although there were no significant differences between the intercropping treatments. This finding suggests that increasing the proportion of C. esculentus in intercropping does not increase the yield and nutrient content of the former. Furthermore, intercropping with legumes was not effective in counteracting the competitive effects of high proportions of C. esculentus. Meanwhile compared with monocropping, intercropping had significant positive effects on soil nutrients, with the 4:4CG treatment being the most effective. Therefore, the use of 4:4CG intercropping treatment is important to improve yield, plant nutrients, and soil quality, especially in arid and nutrient-deficient lands, such as Xinjiang. In addition, designing long-term field observations to understand the interaction mechanism of intercropping between oilseed rape and legumes is an important direction for future research.
The original contributions presented in the study are included in the article/supplementary material. Further inquiries can be directed to the corresponding authors.
XS: Writing – original draft, Writing – review & editing. YL: Writing – review & editing. XL: Writing – review & editing. LL: Writing – review & editing.
The author(s) declare that financial support was received for the research, authorship, and/or publication of this article. This research was supported funding from the Xinjiang Key Research and Development Programme Project (2022B02040-2).
The authors sincerely acknowledge the National Field Scientific Observation and Experimental Station of Celle Desert Grassland Ecosystem, Xinjiang Institute of Ecology and Geography, Chinese Academy of Sciences and the researchers for their assistance.
The authors declare that the research was conducted in the absence of any commercial or financial relationships that could be construed as a potential conflict of interest.
All claims expressed in this article are solely those of the authors and do not necessarily represent those of their affiliated organizations, or those of the publisher, the editors and the reviewers. Any product that may be evaluated in this article, or claim that may be made by its manufacturer, is not guaranteed or endorsed by the publisher.
Adomako, M. O., Roiloa, S., Yu, F. H. (2022). Potential roles of soil microorganisms in regulating the effect of soil nutrient heterogeneity on plant performance. Microorganisms 10 (12), 2399. doi: 10.3390/microorganisms10122399
Andersen, M. K., Hauggaard-Nielsen, H., Ambus, P., Jensen, E. S. (2005). Biomass production, symbiotic nitrogen fixation and inorganic N use in dual and tri-component annual intercrops. Plant Soil 266, 273–287. doi: 10.1007/s11104-005-0997-1
Aydar, E. F., Tutuncu, S., Ozcelik, B. (2020). Plant-based milk substitutes: Bioactive compounds, conventional and novel processes, bioavailability studies, and health effects. J. Funct. Food. 70, 103975. doi: 10.1016/j.jff.2020.103975
Ayeni, A. O. (2022). Hoop house and field evaluation of tigernut (Cyperus esculentus L. var. sativus boeck) selections in new Jersey, USA. Plants 11, 897. doi: 10.3390/plants11070897
Black, C. R., Ong, C. K. (2000). Utilisation of light and water in tropical agriculture. Agric. For. Meteorol. 104, 25–47. doi: 10.1016/S0168-1923(00)00145-3
Bremner, J. M., Mulvaney, C. S. (1982). Nitrogen-total. Methods of soil analysis. Part 2. Chemical and microbiological properties. Methodsofsoilan 2, 595–624.
Chamkhi, I., Cheto, S., Geistlinger, J., Zeroual, Y., Kouisni, L., Bargaz, A., et al. (2022). Legume-based intercropping systems promote beneficial rhizobacterial community and crop yield under stressing conditions. Ind. Crops Prod. 183, 114985. doi: 10.1016/j.indcrop.2022.114958
Chehab, H., Tekaya, M., Hajlaoui, H., Abdelhamid, S., Gouiaa, M., Sfina, H., et al. (2020). Complementary irrigation with saline water and soil organic amendments modified soil salinity, leaf Na+, productivity and oil phenols of olive trees (cv. Chemlali) grown under semiarid conditions. Agric. Water Manage. 237, 106183. doi: 10.1016/j.agwat.2020.106183
Chen, C., Liu, W., Wu, J., Jiang, X., Zhu, X. (2019). Can intercropping with the cash crop help improve the soil physico-chemical properties of rubber plantations? Geoderma 335, 149–160. doi: 10.1016/j.geoderma.2018.08.023
Chen, C., Xiao, W., Chen, H. Y. H. (2023). Mapping global soil acidification under N deposition. Glob. Change Biol. 29, 4652–4661. doi: 10.1111/gcb.16813
Chen, J., Wei, F., Zheng, C., Wu, Y. Y., Adriano, D. C. (1991). Background concentrations of elements in soils of China. Water Air Soil pollut. 57, 699–712. doi: 10.1007/BF00282934
Chen, X., Tian, Y., Guo, F., Chen, G., He, H., Li, H. (2017). The effect of monocropping peanut and cassava/peanut intercropping on physical and chemical properties in peanut rhizosphere soil under the biochar application and straw mulching. IOP Conf. Ser. Earth Environ. Sci. 59, 12021. doi: 10.1088/1755-1315/59/1/012021
Chu, G., Shen, Q., Cao, J. (2004). Nitrogen fixation and N transfer from peanut to rice cultivated in aerobic soil in an intercropping system and its effect on soil N fertility. Plant Soil 263, 17–27. doi: 10.1023/B:PLSO.0000047722.49160.9e
Coskuner, Y., Ercan, R., Karababa, E., Nazlican, A. N. (2002). Physical and chemical properties of chufa (Cyperus esculentus L.) tubers grown in the C, ukurova region of Turkey. J. Sci. Food Agric. 82, 625–631. doi: 10.1002/jsfa.1091
Cuartero, J., Pascual, J. A., Vivo, J. M., Özbolat, O., Sánchez-Navarro, V., Egea-Cortines, M., et al. (2022). A first-year melon/cowpea intercropping system improves soil nutrients and changes the soil microbial community. Agriculture Ecosyst. Environ. 328, 107856. doi: 10.1016/j.agee.2022.107856
Duan, Y., Ren, W., Zhao, J., Luo, C., Liu, Y. (2022). Planting Cyperus esculentus augments soil microbial biomass and diversity, but not enzymatic activities. PeerJ 10, e14199. doi: 10.7717/peerj.14199
Duchene, O., Vian, J.-F., Celette, F. (2017). Intercropping with legume for agroecological cropping systems: Complementarity and facilitation processes and the importance of soil microorganisms. A review. Agric. Ecosyst. Environ. 240, 148–161. doi: 10.1016/j.agee.2017.02.019
Edmeades, G., Daynard, T. (1979). The development of plant to-plant variability in maize at different planting densities. Can. J. Plant Sci. 59 (3), 561–576. doi: 10.4141/cjps79-095
Elfstrand, S., Hedlund, K., Mårtensson, A. (2007). Soil enzyme activities, microbial community composition and function after 47 years of continuous green manuring. Appl. Soil Ecol. 35, 610–621. doi: 10.1016/j.apsoil.2006.09.011
Eusun, H., Weronika, C., Dorte Bodin, D., Kristian, T. K. (2022). Exploitation of neighbouring subsoil for nutrient acquisition under annual-perennial strip intercropping systems. bioRxiv. Plant Biol. 109, 475–506. doi: 10.1016/j.agee.2022.108106
Follak, S., Belz, R., Bohren, C., De Castro, O., Del Guacchio, E., Pascual-Seva, N., et al. (2016). Biological flora of Central Europe: Cyperus esculentus L. Perspect. Plant Ecol. Evol. Syst. 23, 33–51. doi: 10.1016/j.ppees.2016.09.003
Goyal, S., Mishra, M., Dhankar, S., Kapoor, K., Batra, R. (1993). Microbial biomass turnover and enzyme activities following the application of farmyard manure to field soils with and without previous long-term applications. Biol. Fertil. Soils 15, 60–64. doi: 10.1007/BF00336290
Hara, T. (1986). Growth of individuals in plant populations. Ann. Bot. 57, 55–68. doi: 10.1093/oxfordjournals.aob.a087094
Hara, T. (2005). Mode of competition and size-structure dynamics in plant communities. Plant Spec. Biol. 8 (2-3), 75–84. doi: 10.1111/j.1442-1984.1993.tb00059.x
Hauggaard-Nielsen, H., Jensen, E. S. (2005). Facilitative root interactions in intercrops. Plant Soil 274, 237–250. doi: 10.1007/s11104-004-1305-1
Hobbie, E. A., Högberg, P. (2012). Nitrogen isotopes link mycorrhizal fungi and plants to nitrogen dynamics. New Phytol. 196, 367–382. doi: 10.1111/j.1469-8137.2012.04300.x
Huang, Z., Cui, C., Cao, Y., Dai, J., Cheng, X., Hua, S., et al. (2022). Tea plant–legume intercropping simultaneously improves soil fertility and tea quality by changing Bacillus species composition. Hortic. Res.-England. 9, uhac046. doi: 10.1093/hr/uhac046
Jamali, Z. H., Ali, S., Qasim, M., Song, C., Anwar, M., Du, J., et al. (2023). Assessment of molybdenum application on soybean physiological characteristics in maize-soybean intercropping. Front. Plant Sci. 14. doi: 10.3389/fpls.2023.1240146
Jiang, Y. F., Ye, Y. C., Guo, X., Rao, L., Sun, K., Li, W. F. (2017). Spatial variability of ecological stoichiometry of soil nitrogen and phosphorus in farmlands of Jiangxi province and its influencing factors. Acta Pedol. Sin. 54, 1527–1539.
Kizzie-Hayford, N., Jaros, D., Schneider, Y., Rohm, H. (2015). Physico-chemical properties of globular tiger nut proteins. Eur. Food. Res. Technol. 241, 835–841. doi: 10.1007/s00217-015-2508-9
Koocheki, A., Seyyedi, S. M., Gharaei, S. (2016). Evaluation of the effects of saffron–cumin intercropping on growth, quality and land equivalent ratio under semi-arid conditions. Sci. Hortic. 201, 190–198. doi: 10.1016/j.scienta.2016.02.005
Lan, Y., Zhang, H., He, Y., Jiang, C., Yang, M., Ye, S. (2023). Legume-bacteria-soil interaction networks linked to improved plant productivity and soil fertility in intercropping systems. Ind. Crop Prod. 196, 116504. doi: 10.1016/j.indcrop.2023.116504
Li, L., Duan, R., Li, R., Zou, Y., Liu, J., Chen, F., et al. (2022). Impacts of corn intercropping with soybean, peanut and millet through different planting patterns on population dynamics and community diversity of insects under fertilizer reduction. Front. Plant Sci. 13. doi: 10.3389/fpls.2022.936039
Li, L., Zhang, F., Li, X., Christie, P., Sun, J., Yang, S., et al. (2003). Interspecific facilitation of nutrient uptake by intercropped maize and faba bean. Nutr. Cycl. Agroecosyst. 65, 61–71. doi: 10.1023/A:1021885032241
Li, Q. Z., Sun, J. H., Wei, X. J., Christie, P., Zhang, F. S., Li, L. (2010). Overyielding and interspecific interactions mediated by nitrogen fertilization in strip intercropping of maize with faba bean, wheat and barley. Plant Soil 339 (1-2), 147–161. doi: 10.1007/s11104-010-0561-5
Li, Y., Pan, F., Yao, H. (2018). Response of symbiotic and asymbiotic nitrogen-fixing microorganisms to nitrogen fertilizer application. J. Soils Sediments 19, 1948–1958. doi: 10.1007/s11368-018-2192-z
Li, Y., Ran, W., Zhang, R., Sun, S., Xu, G. (2008). Facilitated legume nodulation, phosphate uptake and nitrogen transfer by arbuscular inoculation in an upland rice and mung bean intercropping system. Plant Soil 315, 285–296. doi: 10.1007/s11104-008-9751-9
Liu, T., Cheng, Z., Meng, H., Ahmad, I., Zhao, H. (2014). Growth, yield and quality of spring tomato and physicochemical properties of medium in a tomato/garlic intercropping system under plastic tunnel organic medium cultivation. Sci. Hortic. 170, 159–168. doi: 10.1016/j.scienta.2014.02.039
Liu, H. Y., Huang, N., Zhao, C. M., Li, J. H. (2023). Responses of carbon cycling and soil organic carbon content to nitrogen addition in grasslands globally. Soil Biol. Biochem. 186, 109164. doi: 10.1016/j.soilbio.2023.109164
Liu, Q., Yin, R., Tan, B., You, C., Zhang, L., Zhang, J., et al. (2021). Nitrogen addition and plant functional type independently modify soil mesofauna effects on litter decomposition. Soil Biol. Biochem. 160, 108340. doi: 10.1016/j.soilbio.2021.108340
Lu, J., Liu, Y., Zou, X., Zhang, X., Yu, X., Wang, Y., et al. (2024). Rotational strip peanut/cotton intercropping improves agricultural production through modulating plant growth, root exudates, and soil microbial communities. Agric. Ecosyst. Environ. 359, 108767. doi: 10.1016/j.agee.2023.108767
Lu, X., Yan, Y., Sun, J., Zhang, X., Chen, Y., Wang, X., et al. (2015). Carbon, nitrogen, and phosphorus storage in alpine grassland ecosystems of Tibet: Effects of grazing exclusion. Ecol. Evol. 19, 4492–4504. doi: 10.1002/ece3.1732
Lu, M., Zhao, J., Lu, Z., Li, M., Yang, J., Fullen, M., et al. (2023). Maize–soybean intercropping increases soil nutrient availability and aggregate stability. Plant Soil. doi: 10.1007/s11104-023-06282-2
Mao, L., Zhang, L., Li, W., van der Werf, W., Sun, J., Spiertz, H., et al. (2012). Yield advantage and water saving in maize/pea intercrop. Field Crops Res. 138, 11–20. doi: 10.1016/j.fcr.2012.09.019
Mazdiyasni, O., AghaKouchak, A. (2015). Substantial increase in concurrent droughts and heatwaves in the United States. Proc. Natl. Acad. Sci. U.S.A. 112, 11484–11489. doi: 10.1073/pnas.1422945112
Ouda, S. ,. A., Mesiry, T. ,. E., Adballah, E. ,. F., Gaballah, M. ,. S. (2007). Effect of water stress on the yield of soybean and maize grown under different intercropping patterns. Austral. J. basic Appl. Sci. 1, 578–585.
Parvin, S., Bajwa, A., Uddin, S., Sandral, G., Rose, M. T., Van Zwieten, L., et al. (2023). Impact of wheat-vetch temporary intercropping on soil functions and grain yield in a dryland semi-arid environment. Plant Soil. doi: 10.1007/s11104-023-05914-x
Prăvălie, R. (2016). Drylands extent and environmental issues. A global approach. Earth-Sci. Rev. 161, 259–278. doi: 10.1016/j.earscirev.2016.08.003
Qu, J., Li, L., Wang, Y, Yang, J., Zhao, X. (2022). Effects of rape/common vetch intercropping on biomass, soil characteristics, and microbial community diversity. Front. Environ. Sci. 10, 947014. doi: 10.3389/fenvs.2022.947014
Qu, J., Li, L., Zhao, P., Zhang, T., Chen, G., Yang, J., et al. (2023). Effects of intercropping oat and common vetch on plant biomass yield and soil nitrogen and phosphorus availability in different soil characteristics. J. Soil Sci. Plant Nutr. 23, 3258–3270. doi: 10.1007/s42729-023-01211-9
Rahav, E., Giannetto, M. J., Bar-Zeev, E. (2016). Contribution of mono and polysaccharides to heterotrophic N2 fixation at the eastern Mediterranean coastline. Sci. Rep. 6, (1). doi: 10.1038/srep27858
Rau, A., Zhu, K., Nurlan, B., Mirobit, M., Yessenkul, K., Bek, M. H., et al. (2023). Agronomic and reclamation strategies to enhance soil fertility, productivity and water accessibility. Front. Sustain. Food Syst. 7. doi: 10.3389/fsufs.2023.1288481
Rezig, M., Sahli, A., Ben jeddi, F., Harbaoui, Y. (2013). Potato (Solanum tuberosum L.) and Sulla (Hedysarum coronarium L.) Intercropping in Tunisia: Effects in Water Consumption and Water Use Efficiency. J. Aric. Sci. 5. doi: 10.5539/jas.v5n10p123
Schwerdtner, U., Spohn, M. (2022). Plant species interactions in the rhizosphere increase maize N and P acquisition and maize yields in intercropping. J. Soil Sci. Plant Nutr. 22, 3868–3884. doi: 10.1007/s42729-022-00936-3
Schwinn, S., Weiner, J. (1998). Mechanisms determining the degree of size asymmetry in competition among plants. Oecologia 113, 447–455. doi: 10.1007/s004420050397
Shao, Z., Wang, X., Gao, Q., Zhang, H., Yu, H., Wang, Y., et al. (2020). Root contact between maize and alfalfa facilitates nitrogen transfer and uptake using techniques of foliar 15N-labeling. Agronomy 10 (3), 360. doi: 10.3390/agronomy10030360
Shen, L., Wang, X., Liu, T., Wei, W., Zhang, S., Keyhani, A., et al. (2023). Border row effects on the distribution of root and soil resources in maize–soybean strip intercropping systems. Soil Tillage Res. 233, 105812. doi: 10.1016/j.still.2023.105812
Tehulie, N. S., Nigatie, T. Z. (2023). Response of intercropping coffee (Coffea arabica L.) with banana (Musa spp.) on yield, yield components, and quality of coffee. Crop Sci. 63, 888–898. doi: 10.1002/csc2.20862
Tetio-Kagho, F., Gardner, F. P. (1988). Responses of maize to plant population density. II. Reproductive development, yield, and yield adjustments. Agron. J. 80, 935–940. doi: 10.2134/agronj1988.00021962008000060019x
Vance, E. D., Brookes, P. C., Jenkinson, D. S. (1987). An extraction method for measuring soil microbial biomass C. Soil Biol. Biochem. 19, 703–707. doi: 10.1016/0038-0717(87)90052-6
Vandermeer, J. H. (1989). The ecology of intercropping (Cambridge: Cambridge University Press). doi: 10.1017/CBO9780511623523.
Viaud, P., Heuclin, B., Letourmy, P., Christina, M., Versini, A., Mansuy, A., et al. (2023). Sugarcane yield response to legume intercropped: A meta-analysis. Field Crop Res. 295, 108882. doi: 10.1016/j.fcr.2023.108882
Vrignon-Brenas, S., Celette, F., Piquet-Pissaloux, A., Jeuffroy, M. H., David, C. (2016). Early assessment of ecological services provided by forage legumes in relay intercropping. Eur. J. Agron. 75, 89–98. doi: 10.1016/j.eja.2016.01.011
Weiner, J. (1990). Asymmetric competition in plant populations. Trends Ecol. Evol. 5, 360–364. doi: 10.1016/0169-5347(90)90095-U
Willey, R. W. (1990). Resource use in intercropping systems. Agric. Water Manage. 17, 215–231. doi: 10.1016/0378-3774(90)90069-B
Wu, G. L., Liu, Y., Tian, F. P., Shi, Z. H. (2016). Legumes functional group promotes soil organic carbon and nitrogen storage by increasing plant diversity. Land Degrad. Dev. 28, 1336–1344. doi: 10.1002/ldr.2570
Zhang, R. Y., Liu, C., Chen, P. X., Jiang, M. M., Zhu, W. X., Liu, H. M. (2023). Sequential extraction of oligosaccharide and polysaccharides from defatted tiger nut (Cyperus esculentus) meal for its comprehensive utilization. J. Food Meas. Charact. 17, 4357–4370. doi: 10.1007/s11694-023-01978-6
Zhang, W. P., Liu, G. C., Sun, J. H., Fornara, D., Zhang, L. Z., Zhang, F. F., et al. (2016). Temporal dynamics of nutrient uptake by neighbouring plant species: evidence from intercropping. Funct. Ecol. 31, 469–479. doi: 10.1111/1365-2435.12732
Zhang, M. M., Wang, N., Hu, Y. B., Sun, G. Y. (2018). Changes in soil physicochemical properties and soil bacterial community in mulberry (Morus alba L.)/alfalfa (Medicago sativa L.) intercropping system. Microbiol. Open 7 (2), e00555. doi: 10.1002/mbo3.555
Zhang, C., Yang, Y., Yang, D., Wu, X. (2021). Multidimensional assessment of global dryland changes under future warming in climate projections. J. Hydrol. 592. doi: 10.1016/j.jhydrol.2020.125618
Zhao, J., Chen, J., Beillouin, D., Lambers, H., Yang, Y., Smith, P., et al. (2022). Global systematic review with meta-analysis reveals yield advantage of legume-based rotations and its drivers. Nat. Commun. 13, 4926. doi: 10.1038/s41467-022-32464-0
Zheng, B., Zhang, X., Chen, P., Du, Q., Zhou, Y., Yang, H., et al. (2021). Improving maize’s N uptake and N use efficiency by strengthening roots’ absorption capacity when intercropped with legumes. PeerJ 9, e11658. doi: 10.7717/peerj.11658
Keywords: Cyperus esculentus L., legumes, intercropping, biomass, plant nutrients, soil properties
Citation: Shen X, Liu Y, Li X and Li L (2024) Effect of intercropping with legumes at different rates on the yield and soil physicochemical properties of Cyperus esculentus L. in arid land. Front. Plant Sci. 15:1351843. doi: 10.3389/fpls.2024.1351843
Received: 07 December 2023; Accepted: 29 January 2024;
Published: 28 February 2024.
Edited by:
Xiaoli Hui, Northwest A&F University, ChinaReviewed by:
Esmaeil Rezaei-Chiyaneh, Urmia University, IranCopyright © 2024 Shen, Liu, Li and Li. This is an open-access article distributed under the terms of the Creative Commons Attribution License (CC BY). The use, distribution or reproduction in other forums is permitted, provided the original author(s) and the copyright owner(s) are credited and that the original publication in this journal is cited, in accordance with accepted academic practice. No use, distribution or reproduction is permitted which does not comply with these terms.
*Correspondence: Xiangyi Li, bGl4eUBtcy54amIuYWMuY24=; Lei Li, bGlsZWlAbXMueGpiLmFjLmNu
Disclaimer: All claims expressed in this article are solely those of the authors and do not necessarily represent those of their affiliated organizations, or those of the publisher, the editors and the reviewers. Any product that may be evaluated in this article or claim that may be made by its manufacturer is not guaranteed or endorsed by the publisher.
Research integrity at Frontiers
Learn more about the work of our research integrity team to safeguard the quality of each article we publish.