- Department of Environmental Science and Ecology, School of Environmental Science and Engineering, Shaanxi University of Science and Technology, Xi’an, China
Drought and salinity are two abiotic stresses that affect plant productivity. We exposed 2-year-old Platycladus orientalis saplings to single and combined stress of drought and salinity. Subsequently, the responses of physiological traits and soil properties were investigated. Biochemical traits such as leaf and root phytohormone content significantly increased under most stress conditions. Single drought stress resulted in significantly decreased nonstructural carbohydrate (NSC) content in stems and roots, while single salt stress and combined stress resulted in diverse response of NSC content. Xylem water potential of P. orientalis decreased significantly under both single drought and single salt stress, as well as the combined stress. Under the combined stress of drought and severe salt, xylem hydraulic conductivity significantly decreased while NSC content was unaffected, demonstrating that the risk of xylem hydraulic failure may be greater than carbon starvation. The tracheid lumen diameter and the tracheid double wall thickness of root and stem xylem was hardly affected by any stress, except for the stem tracheid lumen diameter, which was significantly increased under the combined stress. Soil ammonium nitrogen, nitrate nitrogen and available potassium content was only significantly affected by single salt stress, while soil available phosphorus content was not affected by any stress. Single drought stress had a stronger effect on the alpha diversity of rhizobacteria communities, and single salt stress had a stronger effect on soil nutrient availability, while combined stress showed relatively limited effect on these soil properties. Regarding physiological traits, responses of P. orientalis saplings under single and combined stress of drought and salt were diverse, and effects of combined stress could not be directly extrapolated from any single stress. Compared to single stress, the effect of combined stress on phytohormone content and hydraulic traits was negative to P. orientalis saplings, while the combined stress offset the negative effects of single drought stress on NSC content. Our study provided more comprehensive information on the response of the physiological traits and soil properties of P. orientalis saplings under single and combined stress of drought and salt, which would be helpful to understand the adapting mechanism of woody plants to abiotic stress.
1 Introduction
Forests provide great economic and ecological value for human beings, however, are facing a severe threat of the increasing impacts of climate change. Drought conditions, exacerbated by climate change, are expected to become more frequent and intense, leading to large-scale tree mortality (Bradford et al., 2022). Meanwhile, approximately 1 billion hectares of land worldwide are affected by salinity, particularly in arid and semi-arid regions, posing additional challenges to plant survival (Wicke et al., 2011; Hopmans et al., 2021). Woody plants often face the dual stress of drought and salinity in natural conditions (Liu et al., 2022a). Water deficit results in reduced xylem water potential, prohibited xylem water transport and carbon metabolism processes, and ultimately tree death (Cui et al., 2019; Mantova et al., 2022). Similarly, soil salinity inhibits water absorption due to osmotic stress and can lead to ion toxicity, further affecting plant health (Lambers et al., 2008).
In response to drought and salt stress, woody plants show instant biochemical adjustment (Li et al., 2023), such as hormone regulation, e.g., abscisic acid (ABA), gibberellic acid (GA3), jasmonic acid (JA) and its methyl ester methyl jasmonate (JA-Me), and indole acetic acid (IAA) (Ryu and Cho, 2015). For example, ABA induces leaf stomatal closure to reduce water loss by transpiration and thus maintains higher xylem water potential under drought or salt stress (Martin-StPaul et al., 2017; Ouyang et al., 2017). GAs play an important role in woody plants’ resistance to salt stress (Colebrook et al., 2014), and their content changes under salt stress may be species-specific, e.g., reduced content was found in Oryza sativa, Phaseolus acutifolius, whereas increased content was found in P. vulgaris (Yurekli et al., 2004; Farooq et al., 2022). Similarly, under drought and salt stress, JA and JA-Me also have a positive effect on stress tolerance (Kang et al., 2008; Daszkowska-Golec and Szarejko, 2013), and the changes in its content were also species-specific (Kang et al., 2005; de Ollas et al., 2013). IAA regulates soluble sugar content, promotes root development and maintains chlorophyll content, which plays a crucial role in plant growth, development, and health (Talebzadeh and Valeo, 2022; Verma et al., 2022). Under drought and salt stress, biochemical traits such as leaf chlorophyll content may increase, decrease, or remain unchanged, depending on the species, and the duration and intensity of the stress (Anjum et al., 2011; Acosta-Motos et al., 2017). Non-structural carbohydrates (NSC), including soluble sugars and starch, represent the balance between carbon supply and carbon assimilation in woody plants, and it has been shown that both drought and salt stress can lead to a decrease in NSC content in woody plants (Sevanto et al., 2014; Lima et al., 2021). NSC content predicts the process of carbon starvation, which occurs when a plant is unable to supply enough carbohydrates for the metabolic processes necessary for survival, leading to plant mortality (Weber et al., 2019; McDowell et al., 2022).
Xylem is the essential tissue responsible for long-distance water transport in woody plants, besides the above-mentioned biochemical traits, the response of xylem hydraulic and anatomical traits under drought and salt stress has been investigated intensively over the last few decades (Polle and Chen, 2015; Lens et al., 2022). Specifically, woody plants could adjust their xylem anatomical traits to balance xylem hydraulic efficiency and safety, hence to avoid the potential risk on its growth and development under drought and salt stress (Liu et al., 2022b; Li et al., 2024). For instance, woody plants construct narrower xylem conduits with thicker conduit cell walls under drought stress (Li et al., 2022b), which show lower hydraulic efficiency but higher safety (Cai and Tyree, 2010; Hacke et al., 2017). Under salt stress, woody plants may also show xylem hydraulic and anatomical changes. For instance, under salt stress, poplar shows decreased vessel lumen and increased vessel wall thickness, although the xylem hydraulic conductivity of salt-tolerant poplar was unchanged, whereas that of salt-sensitive poplar decreased (Junghans et al., 2006).
Drought and salt stress negatively affect soil properties, plant growth and productivity (Ali et al., 2017). Soil microbial communities and nutrient availability, both crucial for maintaining soil fertility, exhibit dynamic responses to environmental stresses, with consequential effects on plant growth (Dai et al., 2019; Xu et al., 2020). The rhizobacterial communities are sensitive to changes in soil moisture and salinity (Rath et al., 2019), with drought conditions known to induce shifts in their composition and function (Naylor and Coleman-Derr, 2018). Salinity stress can also alter microbial diversity and abundance, which is evaluated by alpha diversity (Willis, 2019), and further affecting plant growth indirectly (Ma et al., 2020). Meanwhile, both drought and salt can affect soil nutrient content and effectiveness in ways that alter nutrient uptake by plants and microbes, or the cycling of nitrogen, phosphorus, and potassium (e.g., by affecting nitrogen mineralization, soil phosphorus adsorption-desorption behavior, and mobility of mineral elements) (Chang, 2023).
Previous work has been done on investigating the effects of single stress such as drought and salt on soil physicochemical properties, microbial communities, and physiological traits of woody plants (Daliakopoulos et al., 2016; Li et al., 2023), however, studies on combined drought and salt stresses are still rare. Combined stress may result in very similar or completely different response of plant physiological traits, compared with single stress (Suzuki et al., 2014). Due to the frequent tree mortality events under combined abiotic stress (Niinemets, 2010; Teshome et al., 2020), it is crucial to understand the response of plant physiological traits and soil properties under combined stress, as well as its difference compared with single stress. P. orientalis is widely used for ecological restoration in western China and is a major silvicultural species, however, its response to drought, salt, and their combined stress remains unclear. In this study, we aim to investigate the effects of drought, salinity, and their combined stress on the physiological traits of P. orientalis, as well as its rhizobacterial communities and soil nutrient (N, P, K) content. We hypothesize that more significant responses would be observed under combined stress compared with single stress, and drought and salt stress may have different effects on different traits. Our detailed investigation on the responses of physiological traits and soil properties under drought and salt stress would be helpful to understand the adaptive mechanisms of this species with abiotic stress.
2 Materials and methods
2.1 Plant material and experimental design
The experiment was performed in a growth chamber with a light period of 16 h at day/8 h at night, a temperature of 26 °C at day/20 °C at night, and a relative humidity of 50-60%. We determined two salt stress levels by preliminary experiments (150 mmol NaCl, moderate salinity, and 300 mmol NaCl, severe salinity, per kg of soil) and set up two watering patterns (well-watered and slight drought, which corresponded to the soil water concentration of 70-80% of the maximum water-holding capacity of the soil, and the soil water concentration of 40-50% of the maximum water-holding capacity of the soil, respectively).
In April 2023, thirty P. orientalis saplings (one per pot) were planted using approximately 2 L pots. All saplings were two years old and about 30 cm tall. The soil used was commercial nutrient soil containing mainly peat, coir and perlite, and each pot was filled with 500 g of soil. After micro-acclimation for one month, the saplings were treated with drought and/or salt stress. Twenty saplings were irrigated with a quantity of 600 mM solution of NaCl to reach the set soil salinity. Subsequently, irrigation was stopped for half of the saplings in each soil salinity (0, 150, 300 mmol/kg) until the soil water content was reduced to 40-50% of the maximum soil water content, which took 15 days. Therefore, there were in total six groups with five saplings in each group, namely control (well-watered, W), well-watered + moderate salinity (WMS), well-watered + severe salinity (WSS), drought (D), drought + moderate salinity (DMS), and drought + severe salinity (DSS). Eventually, the single drought stress, the single salt stress, and the combined drought and salt stress lasted for 30, 45, and 30 days, respectively. The stresses started from May 15th, 2023, and ended on July 1st, 2023. In each group, 3-5 saplings were used for determination of biochemical traits, xylem hydraulic and anatomical traits, with at least two technical replications included in each sapling for determination of biochemical traits and xylem hydraulic traits.
At the end of the stress experiment, the hydraulic conductivity of the xylem was first measured. Subsequently, we cut each sapling, separating the remaining roots, stems, and leaves for biochemical and anatomical measurements. All sampled leaves were in a mature state at the time of collection, and the leaves used for biochemical index determination were not differentiated by the age.
2.2 Determination of plant hormone content
Fine roots and leaves of 3-5 saplings per group were collected for determination of phytohormone content (ABA, GA3, JA-Me, IAA) using enzyme linked immunosorbent assay (ELISA) as described by Yang et al. (2001). Briefly, 0.5 g of fresh plant material was weighed, snap-frozen in liquid nitrogen, and ground to a powder, which was subsequently extracted with cold 80% (v/v) methanol with butylated hydroxytoluene at 4°C overnight. After centrifugation and extraction of the supernatant, the extracts were passed through a C18 Sep-Pak cartridge (Waters, Milford, MA), dried in N2, and dilution with PBS (phosphate buffered saline). Subsequently, 50 μL of standard samples and diluted samples to be tested were added to a 96-well enzyme plate, followed by 50 μL of diluted samples containing a certain amount of antibody. After incubation at 37°C for 0.5 h, the enzyme plate was subsequently washed with a washing solution containing PBS and Tween-20. The appropriate amount of enzyme secondary antibody was added to the diluted sample, and 100 μL was added to each well of the enzyme plate and incubated at 37°C for 0.5 h, and then the plate was washed again. After washing the plates again with washing solution, o-phenylenediamine was added to develop the color, followed by termination of the reaction with sulfuric acid and measurement of the absorbance values of individual samples at 490 nm.
2.3 Determination of leaf chlorophyll content
To determine leaf chlorophyll content, 0.1 g of fresh leaves were collected from each sapling and extracted in 95% ethanol for 24 h. The chlorophyll extract was centrifuged at 8000 r/min for 10 min and the supernatant was taken. The absorbance of the extract was measured at 665 nm, 649 nm and 470 nm, and the chlorophyll A, chlorophyll B and carotenoid contents were calculated according to Equations 1–3 (Zhao and Cang, 2016).
Where Ca is the content of the chlorophyll A, Cb is the content of the chlorophyll B, Cc is the content of carotenoid, A665 is the absorbance at the wavelength of 665 nm, A649 is the absorbance at wavelength of 649 nm, and A470 is the absorbance at wavelength of 470 nm.
2.4 Determination of non-structural carbohydrates content
NSC content was determined as described in Li et al. (2008). Leaves, stems, and roots of 3-5 saplings per group were collected and immediately dried in an oven at 105°C for 30 min to terminate the enzyme activity, followed by drying at 65°C for 48 h to constant weight. To determine the soluble sugar content, 0.1 g of dried sample from each sapling was weighed and added to 10 mL of 80% ethanol solution, then centrifuged and the supernatant was extracted in a water bath at 80°C for 30 min. To extract starch, the residue was mixed with 10 mL of distilled water and after boiling water bath for 30min, 2 mL of 9.2 M perchloric acid was added, and the supernatant was extracted by centrifugation, and the extraction was repeated for a total of three times. 2 mL of the diluted extract was taken in a test tube, followed by the sequential addition of 0.5 mL of anthrone-ethyl acetate solution (20 g/L) and 5 mL of sulfuric acid (18.4 mol/L). The test tubes were cooled in cold water after a water bath at 100°C for 10 min and the absorbance at 630 nm was determined.
2.5 Determination of xylem anatomical traits
To prepare microscopic sections, a 2 cm long sample was selected from the middle of the main stem of 3-5 saplings per group. These samples were then stored in 70% alcohol and later rehydrated in pure water for 1.0 hours. After the rehydration process, the samples were slightly dried at room temperature and fixed on a microtome (Leica RM2126RT, Germany). Sections with a thickness of 20μm were sliced and afterward stained with 1% safranin. After staining for 2 min, the sections were rinsed with pure water, and then dehydrated into 50%, 75%, 95%, and 100% ethanol for several seconds (Li et al., 2022b). For the observation and collection of microscopic images, a light microscope (Olympus CX43, Japan) was utilized. Under 200× magnification, a pie-shaped region of the section with at least 200 consecutive tracheids included was selected from the outermost annual rings of the xylem, and the tracheid double wall thickness and tracheid lumen diameter were measured by the software Image J. For each image, the measurement of the diameter of the lumen and the double wall thickness of the tracheid was selected from the outermost 5 layers of xylem, which were inferred as newly developed xylem during the stress period, due to the great overlap between the xylem growing period of this species and the stress period of our experiment.
2.6 Determination of xylem hydraulic traits
Every three days, the midday leaf water potential of each sapling was monitored using a pressure chamber (PMS Instruments, Albany, OR, USA). The leaves for xylem water potential measurements were enclosed in plastic bags that were surrounded by aluminum foil to stop transpiration and balance the xylem water potential of stems and leaves (Begg and Turner, 1970). For xylem hydraulic conductivity measurement, a 2 cm long stem segment was collected underwater from the middle part of 3-5 saplings per group (McCulloh et al., 2014; Maruta et al., 2022), and the xylem specific hydraulic conductivity (Kh) was measured by using the Sperry apparatus and calculated according to Equation 4, where the pressure drop of degassed KCl solution (0.01 mol/L) generates a certain water flow in the stem (Sperry et al., 1988).
Where F is the velocity of water flow through the stem, L (m) is the length of the stem, ΔP (MPa) is the pressure difference generating water flow in the stem segment, and A is the xylem area of the cross-section of the stem segment. The influence of resin on the determination of xylem hydraulic conductivity was excluded because there were no resin canals in the xylem of P. orientalis, and no influence of resin on the determination of xylem hydraulic conductivity was found in the preliminary experiments.
2.7 Determination of soil nutrient (N, P, K) content
Non-rhizosphere soil samples used for soil nutrient content were collected and determined with reference to NY/T 1121-2006 soil testing standards (MOA, 2006). Briefly, the ammonium nitrogen (NH4-N) content was determined by the potassium chloride extraction-indigo phenol blue colorimetric method; the nitrate nitrogen (NO3-N) content was determined by the phenol disulfonic acid colorimetric method. Available phosphorus (AP) content was determined by sodium bicarbonate extraction-molybdenum antimony colorimetry, and available potassium (AK) content was determined by flame spectrophotometry.
2.8 Structure of rhizobacterial community
Soil DNA was extracted from 0.5 g low-temperature lyophilized rhizosphere soil of 3 saplings according to the user manual of the FastDNA™ SPIN Kit for Soil (MP Biomedicals LLC, Santa Ana, CA). The concentration and quality of the extracted DNA were determined by NanoDropOne (NanoDrop Technologies, Wilmington, DE, USA). Qualified DNA samples were stored at −80 °CC for subsequent experiments. For bacteria, primers barcoded-338F (ACTCCTACGGGAGGCAGCA) and barcoded-06R (GGACTACHVGGGTWTCTAAT), targeting a broad diversity of bacteria with few biases against a particular group, were used to amplify the V4 region of the bacterial 16S rRNA gene (Bergmann et al., 2011). The PCRs (50 μL) contained 25 μL Premix Ex Taq (Takara Biotechnology), 13 μL sterilized water, 5 μL of each primer (2 μM), and 2 μL of diluted template DNA (1–10 ng). The PCR conditions were as follows: (i) initial denaturation at 94 °CC for 5 min; (ii) denaturation at 94 °CC for 40 s, annealing at 56 °CC for 60 s, and extension at 72 °CC for 60 s. The second step was repeated 30 times: (iii) elongation at 72 °CC for 10 min. The length and concentration of the PCR products were determined using 1% agarose gel electrophoresis. PCR products were mixed in equal density ratios according to GeneTools Analysis Software (Version 4.03.05.0, SynGene). Then, a mixture of PCR products was purified with an E.Z.N.A. Gel Extraction Kit (Omega, USA) before sequencing on an Illumina Nova6000 platform, and 250 bp paired-end reads were generated (Guangdong Magigene Biotechnology Co., Ltd. Guangzhou, China).
The structure of the rhizobacterial community of P. orientalis was analyzed under different water and salinity conditions. The effective reads were merged and grouped into operational taxonomic units (OTUs) in UPARSE at a 97% similarity. Fastp (version 0.14.1) was used to control the quality of the raw data. The primers were removed using Cutadapt software to obtain clean paired-end reads. Paired-end clean reads were merged using usearch-fastq_mergepairs (V10), and the spliced sequences were called raw tags. Fastp was used to control the quality of the raw data to obtain clean paired-end tags. Sequences were clustered into operational taxonomic units (OTUs) at a 97% identity threshold using UPARSE. Taxonomic assignment was carried out using OTUs with SILVA (16 S) and Unite (ITS). Bacterial community abundance indices including Chao1 and ACE and diversity indices including Shannon and Simpson were comprehensively analyzed.
2.9 Statistical analysis
Statistical analyses were performed using IBM SPSS Statistics 26 (IBM Corp., USA). Three-way analyses of variance were performed to analyze the effects of time, drought, salinity, and their interactions on xylem water potential. Two-way analyses of variance were performed to analyze the effects of drought, salinity, and their interactions on phytohormone content, leaf chlorophyll content, NSC content, xylem hydraulic and anatomical traits, as well as soil nutrient (N, P, K) content and alpha diversity of rhizobacterial community. The differences between mean values were compared by Duncan’s tests at a significance level of P< 0.05. The software Origin 2023 (OriginLab Corp., USA) was used for generating graphs.
3 Results
3.1 Plant hormone content
The ABA contents in leaves increased significantly (P< 0.05; Figure 1A) in the WSS, D, DMS and DSS groups, however, did not change significantly in the WMS group. Root ABA content significantly increased (P< 0.05) in the D and DMS groups, significantly decreased (P< 0.05) in the WMS group, however, did not show significant change in the WSS and DSS groups (P > 0.05) compared with the control group. Leaf GA3 contents were significantly higher (P< 0.05; Figure 1B) in the WMS, D, DMS, and DSS groups than in the control group, and increased more under the combined stress than under the single stress. Root GA3 content was significantly higher (P< 0.05) than the control under both drought and salt as well as combined stress. Leaf JA-Me content was significantly higher (P< 0.05; Figure 1C) in the WMS, DMS, and DSS groups than in the control group, but was not significantly different (P > 0.05) from the control in the WSS and D groups. The JA-Me content in roots was significantly increased (P< 0.05) in WSS and D groups and significantly decreased (P< 0.05) in DSS group, whereas in WMS and DMS groups, it was not significantly different (P > 0.05) from the control group. Leaf IAA content increased significantly (P< 0.05; Figure 1D) under both drought and salt stress, whereas root growth hormone increased significantly (P< 0.05) in WSS, D, and DMS groups, decreased significantly (P< 0.05) in DSS group, and did not change significantly (P< 0.05) in WMS group. Overall, drought and salt and their interactions had significant effects (P< 0.05) on all four hormones in P. orientalis leaves, whereas single drought stress had no significant effect on root JA-Me and single drought or salt stress had no significant effect on root IAA (P > 0.05; Table 1).
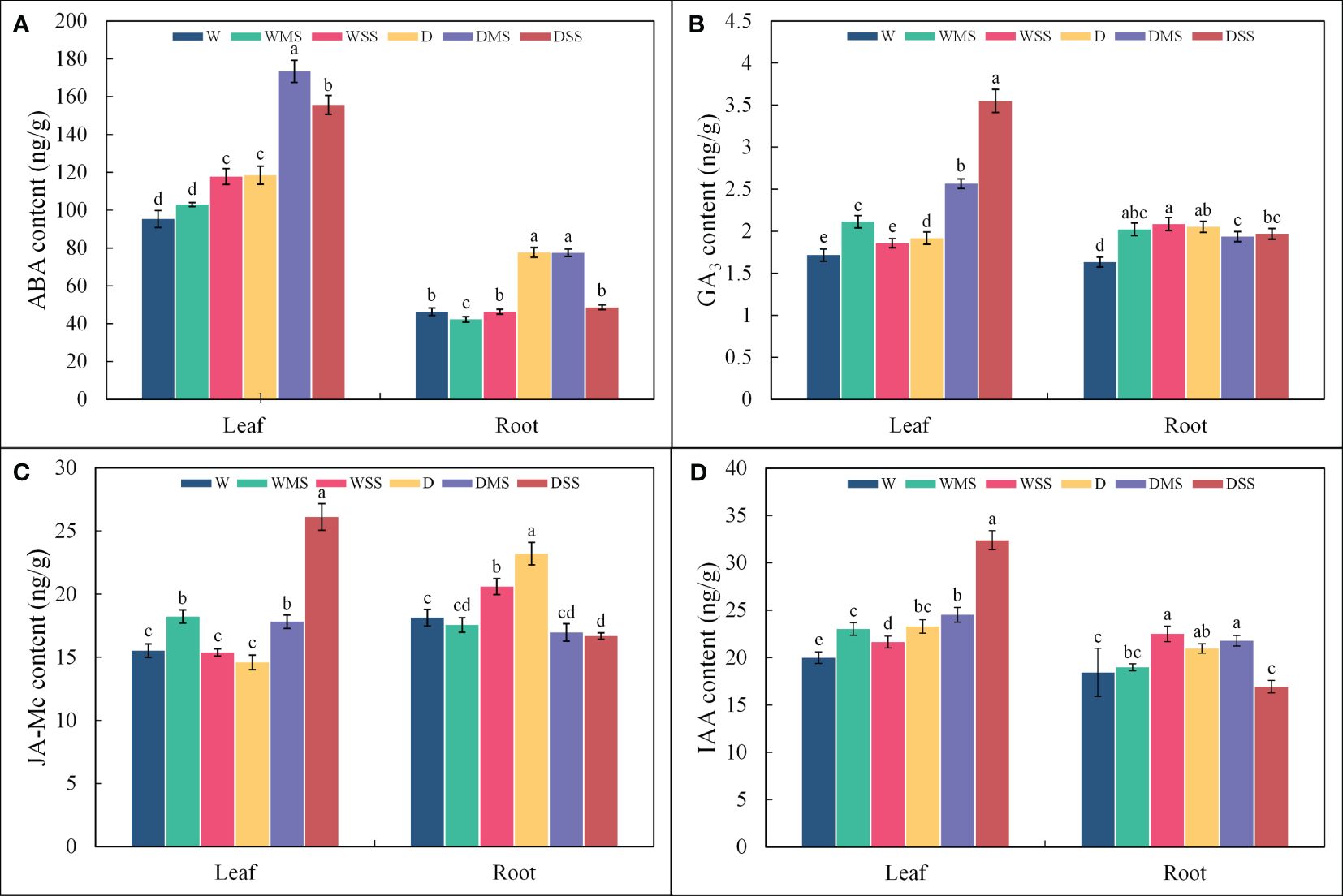
Figure 1 ABA, GA3, JA-Me and IAA contents in roots and leaves of P. orientalis from different groups. (A) ABA; (B) GA3; (C) JA-Me; (D) IAA, where different lower-case letters represent significant differences between groups. The phytohormone contents were calculated from at least three samples in each group.

Table 1 Significance of the effects of the factors and their interactions on ABA, GA3, JA-Me and IAA, where the bolded number representing the p-values less than 0.05.
3.2 Leaf chlorophyll content
There were no significant changes in chlorophyll A and chlorophyll B contents under drought and salt stress. The carotenoid content was significantly decreased in WMS, WSS, and DSS group (P > 0.05; Figure 2). In addition, leaf dehydration was visually observed.
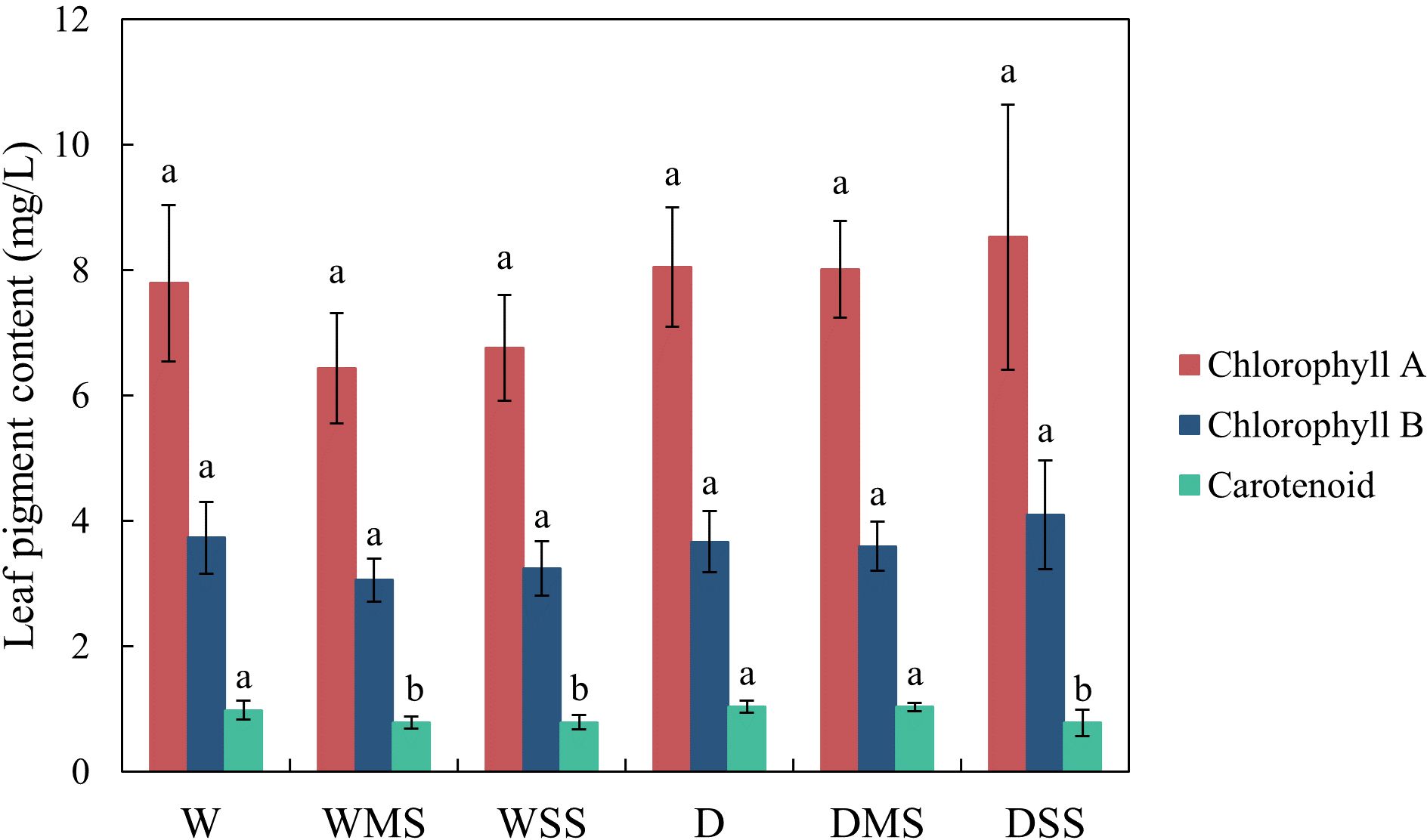
Figure 2 Leaf chlorophyll A, chlorophyll B and carotenoid contents in the different groups. The chlorophyll contents were calculated from at least three samples in each group.
3.3 NSC content
There were no significant differences in the content of soluble sugars in leaves, stems, and roots among the different groups (P > 0.05), and the contents of soluble sugars, starch and NSC in leaves were not significantly different among the groups (P > 0.05). However, for stems and roots, the starch concentration significantly decreased in the D group compared with the W group (P< 0.05), while the starch and NSC concentration in the DMS and DSS group are significantly higher than the D group (P< 0.05). In addition, the results of the interaction analysis showed that both the starch and NSC content of roots and stems were significantly affected by salinity and drought × salinity interaction (Figure 3, Table 2).
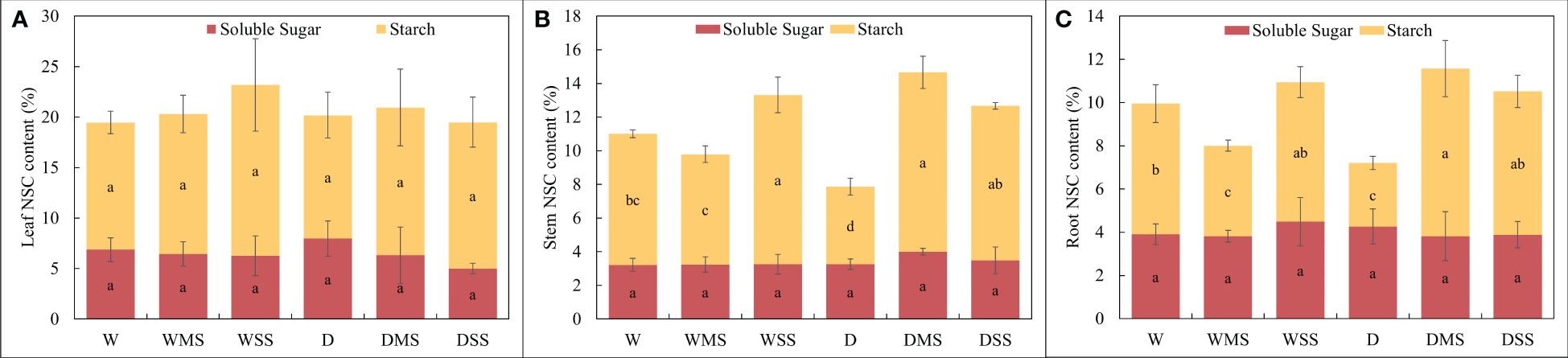
Figure 3 Soluble sugar, starch and NSC concentration in different groups. (A) leaf; (B) stem; (C) root. The soluble sugar, starch and NSC concentrations were calculated from at least three samples in each group.

Table 2 Significance of the effects of the factors and their interactions on soluble sugar, starch, and NSC, where the bolded number representing the p-values less than 0.05.
3.4 Xylem hydraulic and anatomical traits
Neither drought nor salt stress significantly affected tracheid lumen diameter and tracheid double-wall thickness in roots and stems (P > 0.05; Table 3). However, the interaction between drought and salt resulted in a significant increase (P< 0.05; Table 3) in stem tracheid lumen diameter. Under drought and salt stresses, midday leaf water potential of P. orientalis saplings decreased significantly with stress duration (P< 0.01; Figure 4, Table 4). There is a significant interaction between drought and salinity with time, drought and salt lead to a progressive decrease in water potential over time, which became statistically significant after 15 days of stress. Therefore, from the 15th day of stress, the water potentials of the D, DMS, and DSS groups were significantly lower (P< 0.05) than those of the W, WMS, and WSS groups. There was a negative and significant correlation between xylem water potential and leaf ABA content at the time of sampling (day 30 of stress) (Figure 1, Figure 4, P< 0.05). There was no statistically significant difference between the xylem hydraulic conductivity of the control group and W, WMS, and WSS groups (P > 0.05). The mean xylem hydraulic conductivity decreased under the combined stress of drought and salt but was only significantly different between the D and DSS group (P< 0.05; Figure 5). The results of the interaction analysis showed that both drought and salt significantly affected the xylem hydraulic conductivity of P. orientalis saplings, however, there is no interaction between drought and salinity.
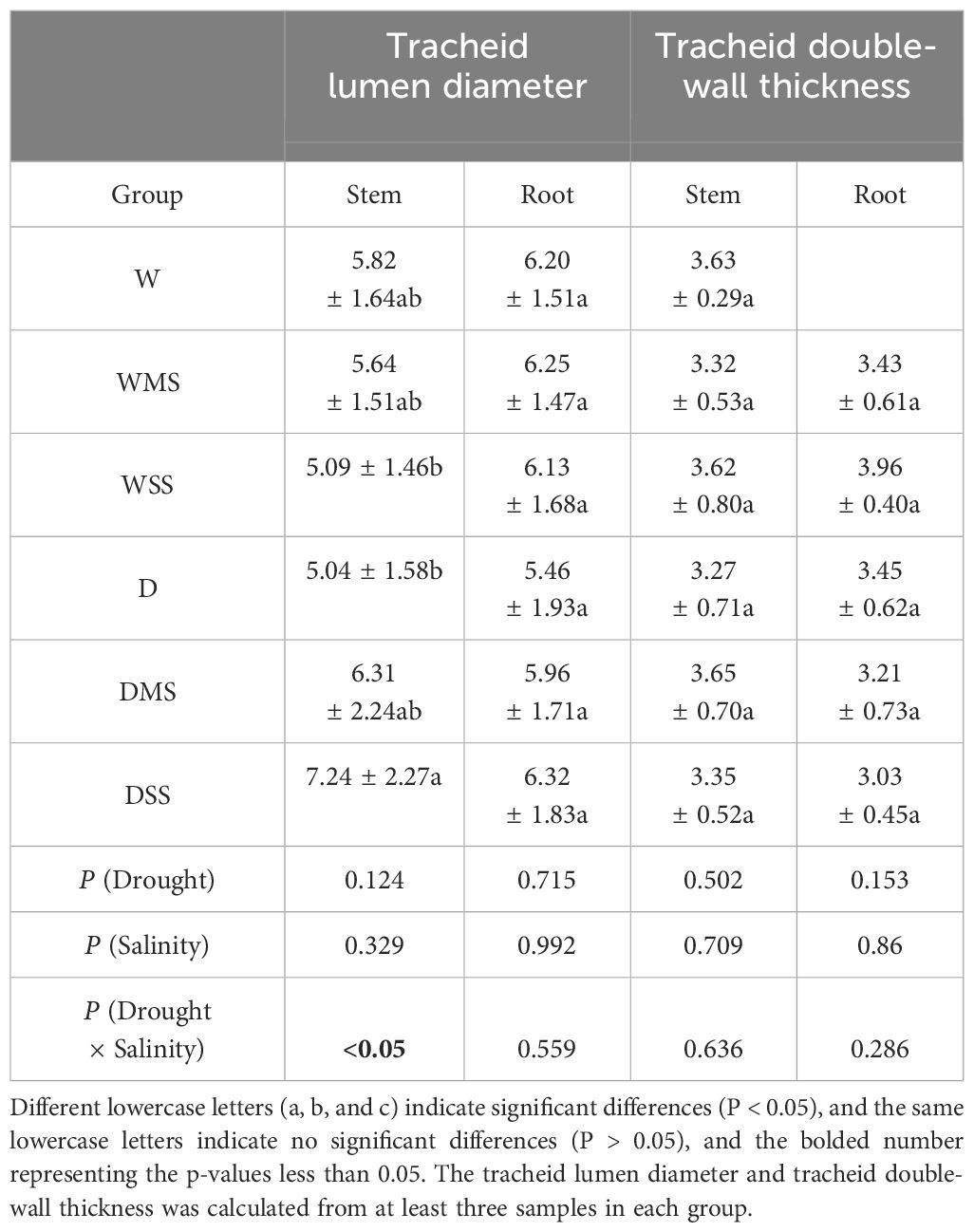
Table 3 Xylem anatomical traits of different groups of P. orientalis saplings and significance of the effects of the factors and their interactions on anatomical traits.
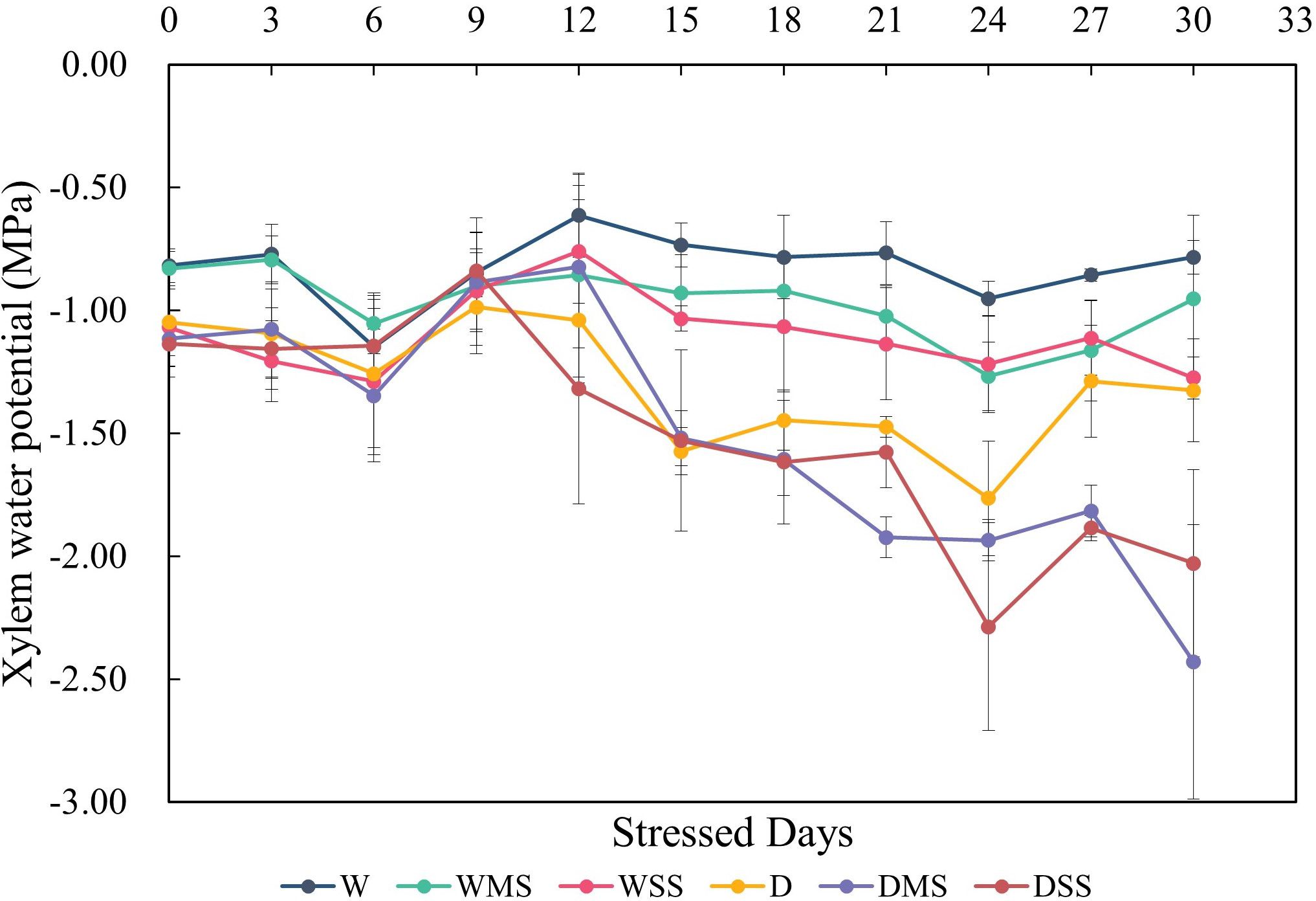
Figure 4 Xylem water potential variations in different groups of P. orientalis saplings during stresses. The water potential was calculated from three samples in each group.

Table 4 Significance of the effects of the factors and their interactions on xylem water potential and hydraulic conductivity, where the bolded number representing the p-values less than 0.05.
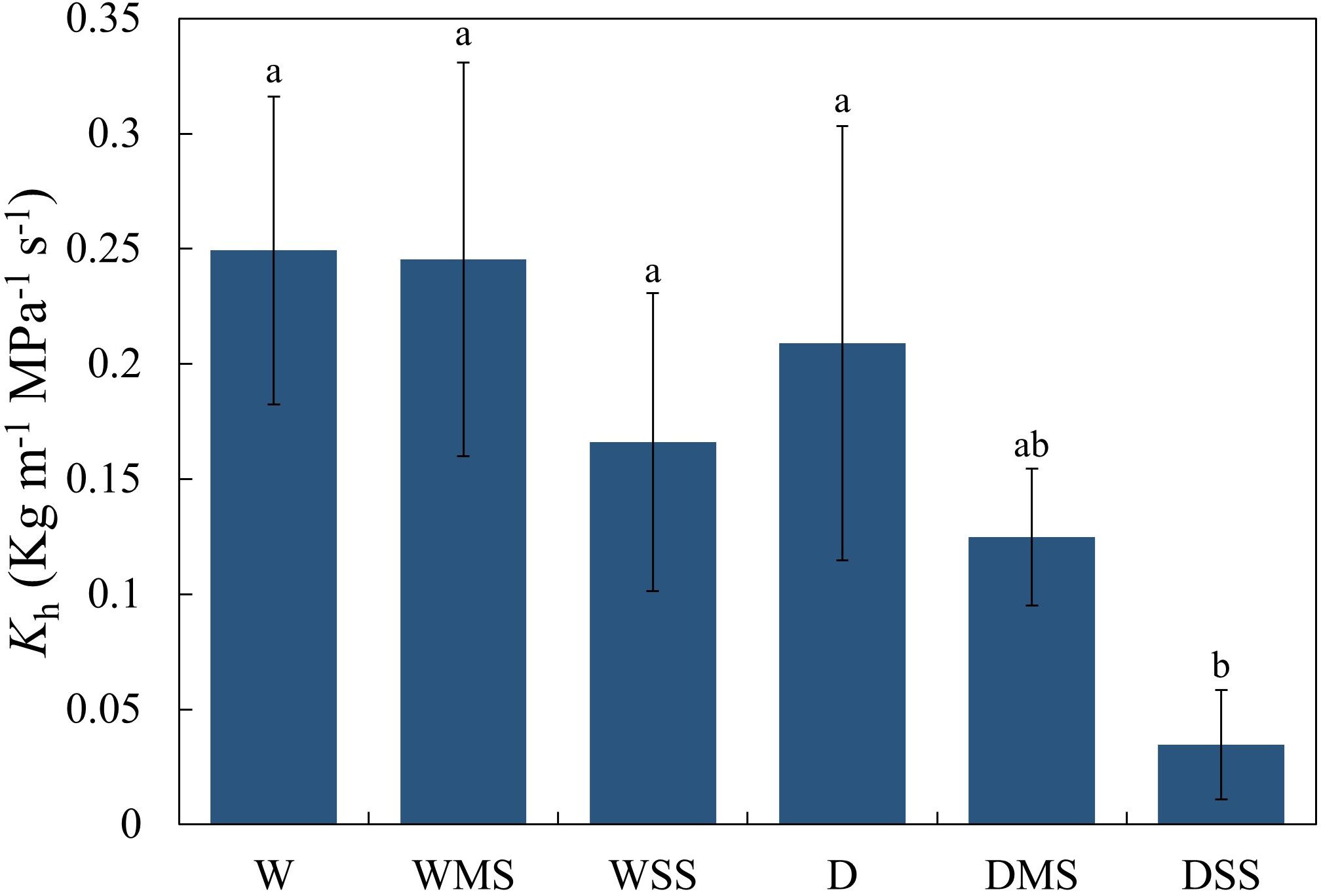
Figure 5 Xylem hydraulic conductivity of different groups. Different lowercase letters indicate significant differences (P< 0.05), and the same lowercase letters indicate no significant differences (P > 0.05). The xylem hydraulic conductivity was calculated from at least three samples in each group.
3.5 Soil nutrient (N, P, K) content
Soil nitrate nitrogen contents significantly decreased in the WSS, DMS, and DSS group (P< 0.05; Table 5). The results of the interaction analysis showed that drought had no significant effect on soil nitrate nitrogen content, while salinity and the interaction of drought × salinity had significant effects on nitrate nitrogen content (P< 0.05; Table 5). Ammonium nitrogen content was significantly increased in the WMS and DMS group; however, ammonium nitrogen in the D, WSS, and DSS group showed no significant difference from control group (Table 5). The results of the interaction analysis showed that only salt stress significantly affected soil ammonium nitrogen (P< 0.05; Table 5). Drought stress, salt stress, and the combination of the two did not significantly affect soil available phosphorus (P > 0.05; Table 5). Soil available potassium content increased significantly under single stresses of drought or salt (P< 0.05; Table 5) but decreased significantly in the DSS group (P< 0.05; Table 5). Besides, the soil available potassium content followed the pattern that WSS > WMS > W and D > DMS > DSS. The results of the interaction analysis showed that drought did not significantly affect soil available potassium content, while salinity and drought × salinity interaction significantly affected soil available potassium content (Table 5).
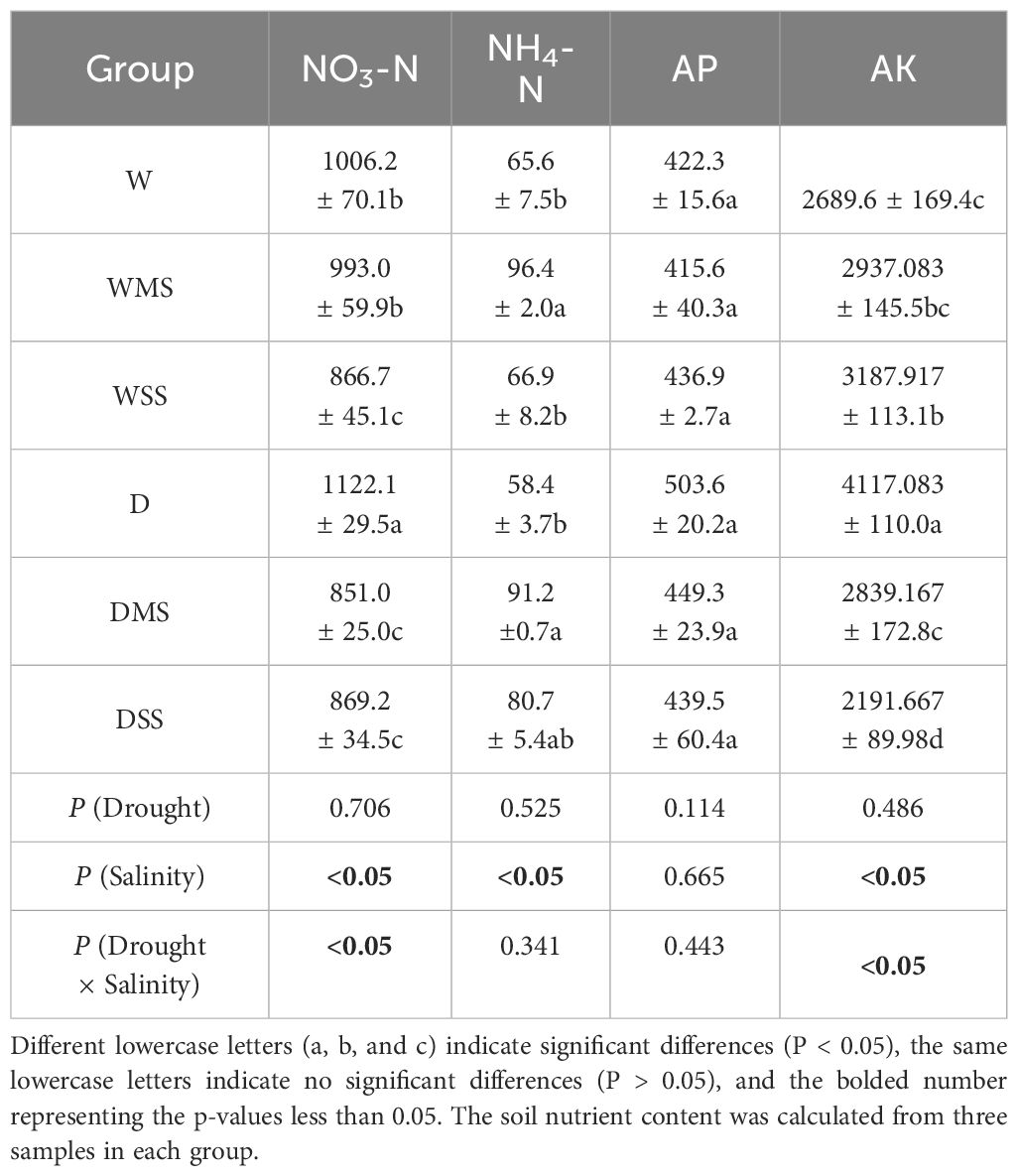
Table 5 Soil nutrient content of different groups and significance of the effects of the factors and their interactions on soil nutrient content.
3.6 Rhizobacteria community
In total, 2,685 OTUs were present in the different groups, and the number of OTUs unique to the different groups ranged from 704 to 1,310 (Figure 6). For the 18 samples in the six groups, the coverage index for each replicate reached 0.98 or more, indicating that most of the bacterial sequences could be covered by sequencing. Drought stress significantly affected the alpha diversity of rhizobacteria community. Specifically, single drought stress reduced ACE, chao1, and Shannon indices, as well as increased Simpson index, suggesting a decrease in rhizobacterial abundance and diversity. Nevertheless, single salt stress and the combined stress of drought and salinity did not significantly affect rhizobacterial alpha diversity (P > 0.05; Table 6; Figure 7).
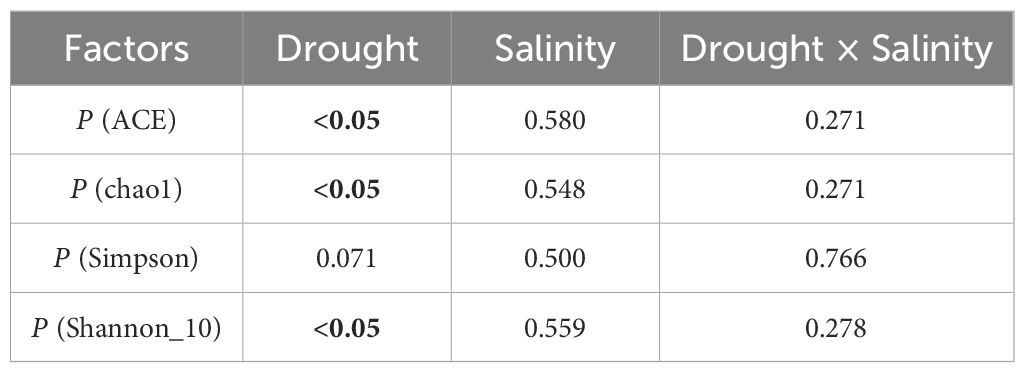
Table 6 Significance of the effects of the factors and their interactions on abundance and diversity of rhizobacteria community, where the bolded number representing the p-values less than 0.05.
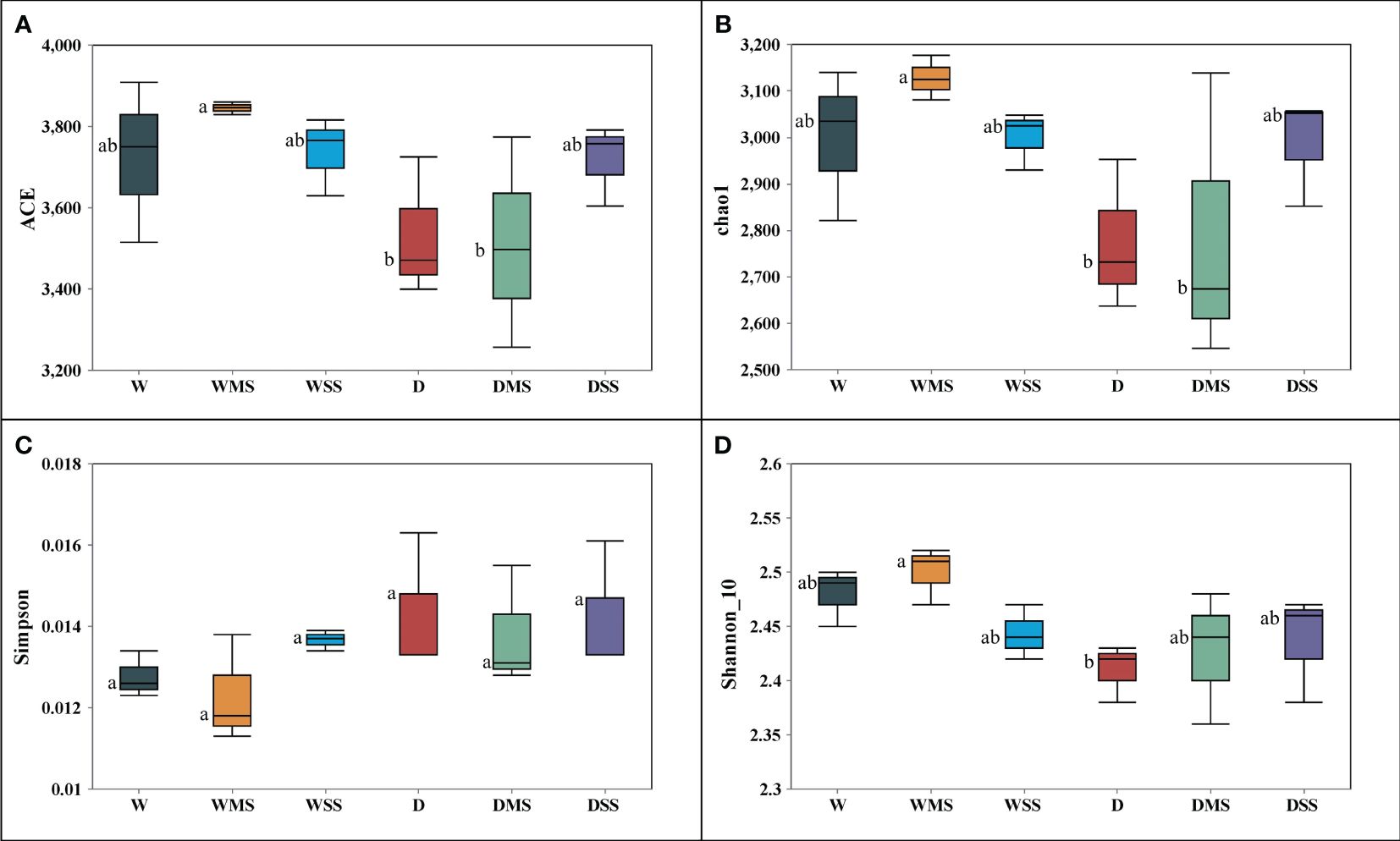
Figure 7 Indices reflecting alpha diversity of rhizobacteria among different groups. (A) ACE index; (B) chao1 index; (C) Simpson index; (D) Shannon_10. The alpha diversity was calculated from three samples in each group.
4 Discussion
4.1 Effects of single and combined stress of drought and salinity on the biochemical traits
In our study, compared with the control group, leaf ABA content in P. orientalis increased significantly under drought and salt stress. ABA induces stomatal closure to decrease water loss and ion transport from root to shoot (Li et al., 2023). Therefore, the increase of ABA is one of the responses to drought and salt stress in P. orientalis saplings. Single drought stress and combined stress of drought and moderate salt stress resulted in significantly higher root ABA content, while root ABA content under combined stress of drought and severe salt stress was not significantly different from the control. Previous studies have shown that Cupressaceae tree species exhibit p-type ABA dynamics under severe drought, i.e., ABA levels increase early in the drought and decrease as the water potential further decreases (Brodribb et al., 2014; Mercado-Reyes et al., 2023). Similar reasons may explain the lower root ABA in DSS group compared with DMS group in our study. Roots are the primary site of ABA synthesis, and the decrease in root ABA content may result from inhibited synthesis under stress (Mercado-Reyes et al., 2023). We observed significantly lower leaf ABA in the DSS group than in the DMS group and hypothesized that p-type ABA dynamics also applied in the leaves of P. orientalis saplings.
The gibberellin content of both roots and leaves increased under drought and salt stress. Gibberellins have positive effects on plant growth and tolerance under drought and salt stress (Moula et al., 2020; Gaion and Carvalho, 2021). JA and JA-Me are endogenous growth regulators, exhibit phytohormones crosstalk with ABA in the pathway for stomatal closure (Xing et al., 2022), and can also improve plant tolerance to drought and salt (Seo et al., 2005; Daszkowska-Golec and Szarejko, 2013). Under abiotic stresses such as drought and salinity, the JA signaling factor PeJAZ2 in Populus euphratica interacts with PeOST1, which is involved in stomatal regulation. The overexpression of PeJAZ2 activates the expression of ABA signal transduction genes, leading to the induction of stomatal closure and an increase in water use efficiency (Rao et al., 2023). The significant increase in leaf JA-Me content under the stresses may improve the tolerance of P. orientalis to salt stress in the form of induced stomatal closure. However, the role of JA in plant salt tolerance mechanism varies among species. For instance, both exogenous JA-Me and the accumulation of endogenous JA enhance the salt tolerance of Abelmoschus esculentus (Wang et al., 2023). Conversely, in Oryza sativa, the accumulation of endogenous JA and its signal transduction negatively affected salt tolerance (Ndecky et al., 2023). In this study, there was no significant increase in roots in JA-Me in the DMS and DSS group. We hypothesize that the ability of P. orientalis to improve stress tolerance through hormonal regulation is limited, and the combined stress of drought and salt exceeds this limitation, leading to dysfunction starting from the roots. IAA contributes to the development of plant vascular tissues and plays an important role in plant growth and development (Ryu and Cho, 2015), and its content in leaves increased under drought and salt stress. IAA can regulate xylem size (Junghans et al., 2006), and an increase in the IAA content may have contributed to the increase in tubular lumen diameter in the DSS group. There is a synergistic effect between IAA and GAs, with IAA promoting the biosynthesis of GAs (Zhu et al., 2022) and GAs promoting the transport of IAA (Li et al., 2015). In our study, both drought and salt stress resulted in a significant increase in leaf GA3 and IAA content, which may be due to the synergistic effect of these two. In addition, exogenous application of IAA with GAs increased superoxide dismutase (SOD) and peroxidase (POD) activities of Solanum tuberosum under salt stress and had a positive effect on its growth (Khalid and Aftab, 2020).
Overall, under single stress, the content of ABA, JA-Me and GA3 in P. orientalis saplings significantly increased, which can enhance the resistance to abiotic stresses (Colebrook et al., 2014; Sah et al., 2016; Wang et al., 2021b). However, under combined stress of drought and severe salt, the content of ABA and JA-Me in roots did not significantly increase, indicating that hormone regulation ability of P. orientalis saplings was limited.
Chlorophyll A and B are the main pigments involved in light harvesting and energy transfer in photosynthesis, while carotenoids are accessory pigments that protect the photosynthetic apparatus from excess light and oxidative damage (Sonobe et al., 2020). Drought stress can reduce the chlorophyll content of plants by affecting the biosynthesis and degradation of chlorophyll, while salt stress can also decrease the chlorophyll content of plants by impairing the chloroplast structure and function (Hamani et al., 2020; Song et al., 2021). In addition, the increase of ABA, GAs, JA-Me and IAA may inhibit chlorophyll synthesis or promote its degradation (Liu et al., 2017; Zhu et al., 2017). However, in our study, chlorophyll A and chlorophyll B of P. orientalis saplings were not significantly affected by drought, salt, and their combined stresses, which is consistent with Acosta-Motos et al. (2015). In this study, chlorophyll content was expressed as the ratio of chlorophyll extract content to leaf fresh weight. Under drought and salt stress, plant leaves are usually dehydrated (Chaves et al., 2009; Kuromori et al., 2022), which is consistent with our findings. Therefore, we hypothesize that the decrease in fresh weight due to leaf dehydration and the decrease in chlorophyll extract content may occur at the same time, which in turn leads to a lack of significance in the changes in chlorophyll content that we calculated (Hou et al., 2015). In addition, species that are tolerant to both drought and salt may not show significant change on the chlorophyll content (Anjum et al., 2011; Acosta-Motos et al., 2017), and P. orientalis is tolerant to both drought and salt (Ou et al., 2023), therefore its chlorophyll content didn’t change under drought and salt stress, which may help to maintain its photosynthetic capacity. This further leads to unchanged leaf NSC content in our study.
Under single drought stress, the NSC content of stem and root was decreased, which was in agreement with other studies (He et al., 2020; Ji et al., 2020). Besides, the NSC content of different organs responded differently to drought and salt stress. Leaf NSC contents did not change significantly in any group, while the NSC contents in stems and roots were significantly increased in the DMS group and significantly decreased in the D group, indicating that the effects of combined stress on NSC content are not additive. The NSC content of root and stem was significantly higher in the DSS and DMS group than the D group, however, the pattern does not apply to the WSS, WMS and W group (Figure 3). This suggested that the effect of salinity on NSC content varied depending on soil moisture content, i.e., there was an interaction between drought and salt stress, which was positive on the NSC content in our study. NSC content significantly decreased in roots under single drought stress but increased or remained unchanged under combined stress, indicating that the effects of combined stress on NSC content are not additive. Previous studies have shown that salt addition can mitigate the negative effects of drought on NSC content in Robinia pseudoacacia, which is consistent with our results (Fan et al., 2024). Likewise, salt addition increased water use efficiency and protected dry matter production of Atriplex lentiformis under drought stress (Glenn et al., 2012). In our study, root NSC content significantly increased in the DMS group, reflecting the strong carbon storage capacity of P. orientalis under combined stress, as well as the positive interaction between drought and salt stress on NSC content. However, the interaction between drought and salt is complex and its effects may be species-specific. For example, under drought, salt and their combined stress, the starch and NSC content of Juglans regia L. and Tamarix chinensis was significantly decreased (Wang et al., 2021a; Mao et al., 2023), while the NSC content in the roots of Triticum aestivum under combined drought and salt stress was significantly increased (Fu et al., 2023).
In summary, our results suggest that the NSC contents of roots and stems in P. orientalis are more responsive to salt stress than leaves. Also, starch content is more sensitive to the stresses than soluble sugar content. Fluctuations in starch and NSC contents reflect the osmotic adjustment ability under drought and salt stresses, while stable soluble sugar levels suggest P. orientalis can maintain carbon supply ability under stresses (Thalmann et al., 2016). Further studies are needed to elucidate the molecular mechanisms of these metabolic changes to clarify the different patterns of changes in NSC content under different stresses.
4.2 Effects of single and combined stress of drought and salinity on the xylem anatomical and hydraulic traits
Our study showed that drought and salt stress affected the lumen diameter of xylem tracheids in P. orientalis. Under well-watered conditions, the lumen diameter decreased with increasing salt concentration, although it is insignificant, which is consistent with Rajput et al. (2015), indicating that salt stress slightly reduced the water transport capacity of the stem xylem. This could be due to the osmotic stress and ion toxicity caused by salt stress, which may impair the expansion of xylem tracheids (Li et al., 2023). Surprisingly, tracheid lumen diameter of stems increased under combined drought and salt stress, which may be related to the significant increase in IAA content (Junghans et al., 2006). The variation in vessel diameter of poplar conduits under salt stress were related to IAA content (Ryu and Cho, 2015), although the mechanisms regulating the variation of xylem anatomical traits under salt stress are still unknown (Eckert et al., 2019). However, no significant changes in root and stem tracheid double-wall thickness were observed under drought and salt stresses. Overall, the anatomical structure of P. orientalis showed limited changes under drought and salt stress, and the combined stress of drought and salt on tracheid diameter resulted in effect that is different from any single stress.
Drought and salt stress could result in reduced xylem water potential and hydraulic conductivity (Li et al., 2023). The results of this study also show that drought and salt stresses affect the xylem water potential. The decrease in leaf water potential was more pronounced in the D, DMS, DSS group than in the W, WMS, WSS group, suggesting that drought stress had a stronger effect on xylem hydraulic traits than salt stress. ABA is an important phytohormone for the regulation of xylem water potential under drought and salt stress (Zhang et al., 2006). In our study, the negative and significant relationship between ABA content and xylem water potential confirmed the regulation of ABA on xylem water potential in P. orientalis saplings (Figure 1, Figure 4). The xylem hydraulic conductivity did not change significantly in the WMS and WSS group, suggesting that the P. orientalis saplings were able to maintain their water transport capacity under single salt stress. The xylem hydraulic conductivity in the D group also showed no significant difference from the W group. This may be due to the mild degree of drought in this study, which did not induce xylem embolism in P. orientalis saplings. However, although the xylem tracheid lumen diameter increased in the DSS group, the xylem hydraulic conductivity decreased significantly in the DSS group, which may be related to the occurrence of xylem embolism and the inhibition of aquaporins activity (Shekoofa and Sinclair, 2018). In addition, a lower ratio of GA3/ABA was found to inhibit leaf growth and reduce water loss (Zhu, 2023). In our study, the ratio of GA3/ABA was significantly reduced in WSS, D, and DMS groups (P< 0.05), which may maintain the xylem hydraulic conductivity. However, the ratio of GA3/ABA was significantly increased in the DSS group (P< 0.05), which may have increased water loss, leading to a significant decrease in xylem hydraulic conductivity and demonstrated the limit of hormone regulation in P. orientalis seedlings. Overall, combined stress leads to a more severe loss of xylem hydraulic conductivity compared with single stress.
Previous studies have shown that both single or combined stress of drought and salt can lead to a decrease in hydraulic conductivity and NSC content (Wang et al., 2021a; Cao et al., 2023), resulting in hydraulic failure and carbon starvation, which is recognized as the main mechanisms of tree mortality (McDowell, 2011; McDowell et al., 2022). In our study, NSC content in stems and roots was significantly decreased under single drought stress, whereas the NSC content of all organs was not decreased under the combined stress, suggesting that combined stress of drought and moderate salt can offset the negative effects of single drought stress on NSC content in P. orientalis saplings. However, when drought was combined with severe salt stress, there was no significant change in NSC content, while the hydraulic conductivity had been reduced by approximately 86%. Therefore, when drought is combined with severe salinity, the risk of hydraulic failure occurs much earlier than carbon starvation under combined stress, and further research is needed on investigating the mechanisms of tree mortality under combined stress.
4.3 Effects of single and combined stress of drought and salinity on the soil properties
The results of this study showed that drought and salinity have different effects on soil nitrogen and phosphorus availability. Nitrogen and phosphorus are essential nutrients for plant growth and development, and their uptake and metabolism are influenced by soil water and salt conditions. Salt stress may inhibit the decomposition of soil organic matter to reduce potential mineralized N, as well as inhibit nitrification and denitrification by soil microorganisms, and ultimately significantly affect soil nitrate and ammonium N levels. Drought stress may not affect soil nitrate and ammonium nitrogen contents significantly, but it may reduce the plant uptake and translocation of these nutrients due to the low soil water potential (Tian et al., 2021). Soil available phosphorus comes mainly from organic matter decomposition and mineral dissolution. Phosphorus is one of the most immobile nutrients in soils and increased soil salinity reduces the rate of phosphorus uptake by plants, so soil available phosphorus levels may not change significantly under drought and salt stress (Miura, 2012; Su et al., 2021). These findings imply that salinity stress has a greater impact on soil nitrogen cycling than drought stress, and that nitrogen management is more critical than phosphorus management under saline and dry conditions. In our study, drought led to an increase in soil available potassium content, which is consistent with Shi et al. (2010). Plants can only take up potassium from the soil solution (Ashley et al., 2005); therefore, under drought stress, the uptake of potassium by plants decreases (Wang et al., 2018), resulting in higher potassium content remaining in the soil. The decrease in soil available potassium due to the combined drought and salt stress may be due to the competition between Na and K in the soil for the exchange places (Wang et al., 2013; Stavi et al., 2021), resulting in a decrease in K availability. However, soil effective potassium content increased under salt stress, probably due to salt-induced K efflux and reduced K uptake in plants (Wu et al., 2018). Considering that our study results were based on the pot experiment, further investigations could be done to examine the response of natural soil nutrient content under the combined stresses of drought and salt.
Physiological changes in plants under drought or salt stress, such as changes in root exudates, affect the rhizobacteria community (Balestrini et al., 2024). Our results showed that drought and salt stress have different effects on the rhizobacteria alpha diversity. Soil bacterial abundance and diversity are important indicators of soil health and function, and they are influenced by various environmental factors, such as soil pH, moisture, nutrients, and salinity (Lelana et al., 2022; Li et al., 2022a). In this study, drought significantly decreased the ACE, Chao1 and Simpson indices (Table 6), indicating that drought reduced the abundance and diversity of rhizobacteria community. This could be due to the water loss, nutrient variation, and osmotic imbalance caused by drought stress (Kim et al., 2017). However, salt stress did not significantly affect all the indices shown, indicating that soil bacterial abundance and diversity was not affected by salinity. Similar to the our study, salinity did not significantly affect the alpha diversity of rhizosphere microbial community of Spinacia oleracea (Ibekwe et al., 2017). Our results suggest that drought stress is more detrimental to alpha diversity of rhizobacteria community than salt stress, and that there is no interaction between drought and salinity. In addition, the use of commercial nutrient soils may result in soil microbial responses that differ from the natural soil. More studies in natural soil are needed to further elucidate the mechanisms by which drought and salinity affect rhizosphere microbial communities.
5 Conclusions
Our study showed that single drought and salt stress and their combined stress affected various physiological traits in P. orientalis saplings. The responses of P. orientalis saplings under single and combined stress of drought and salt were diverse, and effects of combined stress could not be directly extrapolated from any single stress. ABA, GA3, JA-Me and IAA contents were significantly increased in leaves and roots under most stress conditions. Drought stress reduced the starch storage in stems and roots, while the combined stress of drought and salt stress did not decrease it. The combined stress of drought and salinity resulted in higher starch and NSC contents in stems and roots than single drought stress. The content of soluble sugars in different organs was not affected by any stress. NSC content significantly decreased in roots under single drought stress but increased or remained unchanged under combined stress, indicating that the effects of combined stress on NSC content are not additive. Xylem water potential of P. orientalis saplings were significantly reduced under both drought and salt stress. Xylem hydraulic conductivity was only significantly decreased under the combined stress, while NSC content was unaffected, demonstrating that the risk of xylem hydraulic failure due to combined stress is greater than carbon starvation. The anatomical structure of root and stem xylem was hardly affected, except for the stem xylem tracheid lumen diameter which was significantly increased under the combined stress of drought and severe salt. Drought stress affected rhizobacteria alpha diversity, however, under any of the stresses, the alpha diversity of rhizobacteria communities was not significantly different from the control. Salt stress significantly reduced nitrate nitrogen content and increased ammonium nitrogen content. Available phosphorus content did not change significantly, and soil available potassium was significantly affected by salinity. Overall, salt stress has a greater impact on soil nutrient availability, whereas drought stress more significantly affects the alpha diversity of rhizobacteria communities, and the interaction of drought and salt has limited effects on soil properties. Regarding physiological traits, responses of P. orientalis saplings under single and combined stress of drought and salt exhibited variability, indicating that the effects of combined stress cannot be directly extrapolated from any single stress. Notably, compared to single stress, the effect of combined stress on phytohormone content and xylem hydraulic traits was negative to P. orientalis saplings, while the negative effects of single drought stress on NSC content were offset under the combined stress. Our results suggest that P. orientalis may have different strategies to cope with drought and salinity stress, however, the mechanisms leading to the different responses to drought and salt as well as combined stresses remain unclear. An intensive study of the response mechanisms of this species to single and combined stress of drought and salt is essential to improve our understanding of it. Therefore, it is necessary to investigate the similarities, differences, and interactions between the molecular mechanisms of drought and salt-induced plant responses in future studies.
Data availability statement
The datasets presented in this study can be found in online repositories. The names of the repository/repositories and accession number(s) can be found below: NCBI SRA, SRR27143335-SRR27143352.
Author contributions
ShL: Conceptualization, Funding acquisition, Methodology, Project administration, Resources, Supervision, Writing – review & editing. SeL: Data curation, Formal analysis, Investigation, Methodology, Validation, Visualization, Writing – original draft. JW: Investigation, Writing – review & editing. ZL: Investigation, Writing – review & editing. CY: Investigation, Writing – review & editing. MW: Investigation, Writing – review & editing. JG: Resources, Supervision, Writing – review & editing.
Funding
The author(s) declare financial support was received for the research, authorship, and/or publication of this article. This research was funded by National Natural Science Foundation of China, grant number 32001291, Key Research and Development Program of Shaanxi, grant number 2024SF-YBXM-540, and Talent Project of Shaanxi University of Science and Technology, grant number 126022037.
Conflict of interest
The authors declare that the research was conducted in the absence of any commercial or financial relationships that could be construed as a potential conflict of interest.
Publisher’s note
All claims expressed in this article are solely those of the authors and do not necessarily represent those of their affiliated organizations, or those of the publisher, the editors and the reviewers. Any product that may be evaluated in this article, or claim that may be made by its manufacturer, is not guaranteed or endorsed by the publisher.
References
Acosta-Motos, J. R., Diaz-Vivancos, P., Álvarez, S., Fernández-García, N., Sánchez-Blanco, M. J., Hernández, J. A. (2015). NaCl-induced physiological and biochemical adaptative mechanisms in the ornamental Myrtus communis L. plants. J. Plant Physiol. 183, 41–51. doi: 10.1016/j.jplph.2015.05.005
Acosta-Motos, J., Ortuño, M., Bernal-Vicente, A., Diaz-Vivancos, P., Sanchez-Blanco, M., Hernandez, J. (2017). Plant responses to salt stress: adaptive mechanisms. Agronomy 7, 18. doi: 10.3390/agronomy7010018
Ali, S., Rizwan, M., Qayyum, M. F., Ok, Y. S., Ibrahim, M., Riaz, M., et al. (2017). Biochar soil amendment on alleviation of drought and salt stress in plants: a critical review. Environ. Sci. pollut. Res. 24, 12700–12712. doi: 10.1007/s11356-017-8904-x
Anjum, S. A., Xie, X., Wang, L. C., Saleem, M. F., Man, C., Lei, W. (2011). Morphological, physiological and biochemical responses of plants to drought stress. Afr. J. Agric. Res. 6, 2026–2032. doi: 10.5897/AJAR10.027
Ashley, M. K., Grant, M., Grabov, A. (2005). Plant responses to potassium deficiencies: role potassium transport proteins. J. Exp. Bot. 57, 425–436. doi: 10.1093/jxb/erj034
Balestrini, R., Sillo, F., Boussageon, R., Wipf, D., Courty, P. E. (2024). The hidden side of interaction: microbes and roots get together to improve plant resilience. J. Plant Interact. 19, 2323991. doi: 10.1080/17429145.2024.2323991
Begg, J. E., Turner, N. C. (1970). Water potential gradients in field tobacco. Plant Physiol. 46, 343–346. doi: 10.1104/pp.46.2.343
Bergmann, G. T., Bates, S. T., Eilers, K. G., Lauber, C. L., Caporaso, J. G., Walters, W. A., et al. (2011). The under-recognized dominance of Verrucomicrobia in soil bacterial communities. Soil Biol. Biochem. 43, 1450–1455. doi: 10.1016/j.soilbio.2011.03.012
Bradford, J. B., Shriver, R. K., Robles, M. D., McCauley, L. A., Woolley, T. J., Andrews, C. A., et al. (2022). Tree mortality response to drought—density interactions suggests opportunities to enhance drought resistance. J. Appl. Ecol. 59, 549–559. doi: 10.1111/1365-2664.14073
Brodribb, T. J., McAdam, S. A., Jordan, G. J., Martins, S. C. (2014). Conifer species adapt to low-rainfall climates by following one of two divergent pathways. Proc. Natl. Acad. Sci. U. S. A. 111, 14489–14493. doi: 10.1073/pnas.1407930111
Cai, J., Tyree, M. T. (2010). The impact of vessel size on vulnerability curves: data and models for within-species variability in saplings of aspen, Populus tremuloides Michx. Plant Cell Environ. 33, 1059–1069. doi: 10.1111/j.1365-3040.2010.02127.x
Cao, H., Ding, R., Kang, S., Du, T., Tong, L., Zhang, Y., et al. (2023). Drought, salt, and combined stresses in plants: Effects, tolerance mechanisms, and strategies. Adv. Agron. 178, 107–163. doi: 10.1016/bs.agron.2022.11.004
Chang, X. (2023). Effects of arbuscular mycorrhizal fungi on the rhizosphere soil microenvironment of plants under drought and salt stress. Master’s Thesis. Xianyang: Northwest A&F University. (in Chinese with English abstract)
Chaves, M. M., Flexas, J., Pinheiro, C. (2009). Photosynthesis under drought and salt stress: regulation mechanisms from whole plant to cell. Ann. Bot. 103, 551–560. doi: 10.1093/aob/mcn125
Colebrook, E. H., Thomas, S. G., Phillips, A. L., Hedden, P. (2014). The role of gibberellin signalling in plant responses to abiotic stress. J. Exp. Biol. 217, 67–75. doi: 10.1242/jeb.089938
Cui, G., Zhang, Y., Zhang, W., Lang, D., Zhang, X., Li, Z., et al. (2019). Response of carbon and nitrogen metabolism and secondary metabolites to drought stress and salt stress in plants. J. Plant Biol. 62, 387–399. doi: 10.1007/s12374-019-0257-1
Dai, L., Zhang, G., Yu, Z., Ding, H., Xu, Y., Zhang, Z. (2019). Effect of drought stress and developmental stages on microbial community structure and diversity in peanut rhizosphere soil. Int. J. Mol. Sci. 20, 2265. doi: 10.3390/ijms20092265
Daliakopoulos, I., Tsanis, I., Koutroulis, A., Kourgialas, N., Varouchakis, A., Karatzas, G., et al. (2016). The threat of soil salinity: A European scale review. Sci. Total Environ. 573, 727–739. doi: 10.1016/j.scitotenv.2016.08.177
Daszkowska-Golec, A., Szarejko, I. (2013). Open or close the gate - stomata action under the control of phytohormones in drought stress conditions. Front. Plant Sci. 4. doi: 10.3389/fpls.2013.00138
de Ollas, C., Hernando, B., Arbona, V., Gomez-Cadenas, A. (2013). Jasmonic acid transient accumulation is needed for abscisic acid increase in citrus roots under drought stress conditions. Physiol. Plant 147, 296–306. doi: 10.1111/j.1399-3054.2012.01659.x
Eckert, C., Sharmin, S., Kogel, A., Yu, D., Kins, L., Strijkstra, G.-J., et al. (2019). What makes the wood? Exploring the molecular mechanisms of xylem acclimation in hardwoods to an ever-changing environment. Forests 10, 358. doi: 10.3390/f10040358
Fan, Y., Wang, J., Yan, M., Wang, X., Du, G., Li, H., et al. (2024). Salt addition mitigate mortality risk and prolong survival of robinia pseudoacacia subjected to drought stress. Agronomy 14, 439. doi: 10.3390/agronomy14030439
Farooq, M., Khan, M. A., Zhao, D. D., Asif, S., Kim, E. G., Jang, Y. H., et al. (2022). Extrinsic role of gibberellin mitigating salinity effect in different rice genotypes. Front. Plant Sci. 13. doi: 10.3389/fpls.2022.1041181
Fu, Y., Li, P., Mounkaila Hamani, A. K., Wan, S., Gao, Y., Wang, X. (2023). Effects of single and combined drought and salinity stress on the root morphological characteristics and root hydraulic conductivity of different winter wheat varieties. Plants 12, 2694. doi: 10.3390/plants12142694
Gaion, L. A., Carvalho, R. F. (2021). Stomatal response to drought is modulated by gibberellin in tomato. Acta Physiol. Plant 43, 129. doi: 10.1007/s11738-021-03286-2
Glenn, E. P., Nelson, S. G., Ambrose, B., Martinez, R., Soliz, D., Pabendinskas, V., et al. (2012). Comparison of salinity tolerance of three Atriplex spp. well-watered drying soils. Environ. Exp. Bot. 83, 62–72. doi: 10.1016/j.envexpbot.2012.04.010
Hacke, U. G., Spicer, R., Schreiber, S. G., Plavcová, L. (2017). An ecophysiological and developmental perspective on variation in vessel diameter. Plant Cell Environ. 40, 831–845. doi: 10.1111/pce.12777
Hamani, A. K. M., Wang, G., Soothar, M. K., Shen, X., Gao, Y., Qiu, R., et al. (2020). Responses of leaf gas exchange attributes, photosynthetic pigments and antioxidant enzymes in NaCl-stressed cotton (Gossypium hirsutum L.) seedlings to exogenous glycine betaine and salicylic acid. BMC Plant Biol. 20, 1–14. doi: 10.1186/s12870-020-02624-9
He, W., Liu, H., Qi, Y., Liu, F., Zhu, X. (2020). Patterns in nonstructural carbohydrate contents at the tree organ level in response to drought duration. Global Change Biol. 26, 3627–3638. doi: 10.1111/gcb.15078
Hopmans, J. W., Qureshi, A., Kisekka, I., Munns, R., Grattan, S., Rengasamy, P., et al. (2021). Critical knowledge gaps and research priorities in global soil salinity. Adv. Agron. 169, 1–191. doi: 10.1016/bs.agron.2021.03.001
Hou, S., Zhang, Q., Liu, S., Zeng, L., Wu, Y., Liu, G. (2015). Growth and physiological responses of Melaleuca bracteata cv.’Revolution Gold’to water stress. Acta Bot. Boreal.-Occident. Sin. 34, 2491–2499. doi: 10.7606/j.issn.1000-4025.2014.12.2491
Ibekwe, A. M., Ors, S., Ferreira, J. F., Liu, X., Suarez, D. L. (2017). Seasonal induced changes in spinach rhizosphere microbial community structure with varying salinity and drought. Sci. Total Environ. 579, 1485–1495. doi: 10.1016/j.scitotenv.2016.11.151
Ji, L., Attaullah, K., Wang, J., Yu, D., Yang, Y., Yang, L., et al. (2020). Root traits determine variation in nonstructural carbohydrates (NSCs) under different drought intensities and soil substrates in three temperate tree species. Forests 11, 415. doi: 10.3390/f11040415
Junghans, U., Polle, A., Duchting, P., Weiler, E., Kuhlman, B., Gruber, F., et al. (2006). Adaptation to high salinity in poplar involves changes in xylem anatomy and auxin physiology. Plant Cell Environ. 29, 1519–1531. doi: 10.1111/j.1365-3040.2006.01529.x
Kang, J. S., Frank, J., Kang, C. H., Kajiura, H., Vikram, M., Ueda, A., et al. (2008). Salt tolerance of Arabidopsis thaliana requires maturation of N-glycosylated proteins in the Golgi apparatus. Proc. Natl. Acad. Sci. U. S. A. 105, 5933–5938. doi: 10.1073/pnas.0800237105
Kang, D. J., Seo, Y. J., Lee, J. D., Ishii, R., Kim, K., Shin, D., et al. (2005). Jasmonic acid differentially affects growth, ion uptake and abscisic acid concentration in salt-tolerant and salt-sensitive rice cultivars. J. Agron. Crop Sci. 191, 273–282. doi: 10.1111/j.1439-037X.2005.00153.x
Khalid, A., Aftab, F. (2020). Effect of exogenous application of IAA and GA 3 on growth, protein content, and antioxidant enzymes of Solanum tuberosum L. grown in vitro under salt stress. In Vitro Cell. Dev. Biol.-Plant 56, 377–389. doi: 10.1007/s11627-019-10047-x
Kim, B. R., Shin, J., Guevarra, R., Lee, J. H., Kim, D. W., Seol, K. H., et al. (2017). Deciphering diversity indices for a better understanding of microbial communities. J. Microbiol. Biotechnol. 27, 2089–2093. doi: 10.4014/jmb.1709.09027
Kuromori, T., Fujita, M., Takahashi, F., Yamaguchi-Shinozaki, K., Shinozaki, K. (2022). Inter-tissue and inter-organ signaling in drought stress response and phenotyping of drought tolerance. Plant J. 109, 342–358. doi: 10.1111/tpj.15619
Lambers, H., Chapin, F. S., Pons, T. L. (2008). Plant physiological ecology (New York: Springer). doi: 10.1007/978-0-387-78341-3
Lelana, N. E., Utami, S., Widyati, E., Puspitaningtyas, D. M., Supriadi, B., Oktarina, S., et al. (2022). The bacterial composition and diversity in a eucalyptus pellita plantation in south sumatra, Indonesia. Diversity 14, 442. doi: 10.3390/d14060442
Lens, F., Gleason, S. M., Bortolami, G., Brodersen, C., Delzon, S., Jansen, S. (2022). Functional xylem characteristics associated with drought-induced embolism in angiosperms. New Phytol. 236, 2019–2036. doi: 10.1111/nph.18447
Li, M., Dai, G., Mu, L. (2022a). Composition and diversity of soil bacterial communities under identical vegetation along an elevational gradient in Changbai Mountains, China. Front. Microbiol. 13, 1065412. doi: 10.3389/fmicb.2022.1065412
Li, S., Huang, X., Zheng, R., Zhang, M., Zou, Z., Heal, K. V., et al. (2024). Xylem plasticity of root, stem, and branch in Cunninghamia lanceolata under drought stress: implications for whole-plant hydraulic integrity. Front. Plant Sci. 15. doi: 10.3389/fpls.2024.1308360
Li, S., Li, X., Wang, J., Chen, Z., Lu, S., Wan, X., et al. (2022b). Hydraulic traits are coupled with plant anatomical traits under drought–rewatering cycles in Ginkgo biloba L. Tree Physiol. 42, 1216–1227. doi: 10.1093/treephys/tpab174
Li, S., Lu, S., Wang, J., Chen, Z., Zhang, Y., Duan, J., et al. (2023). Responses of physiological, morphological and anatomical traits to abiotic stress in woody plants. Forests 14, 1784. doi: 10.3390/f14091784
Li, M. H., Xiao, W. F., Shi, P., Wang, S. G., Zhong, Y. D., Liu, X. L., et al. (2008). Nitrogen and carbon source-sink relationships in trees at the Himalayan treelines compared with lower elevations. Plant Cell Environ. 31, 1377–1387. doi: 10.1111/j.1365-3040.2008.01848.x
Li, G., Zhu, C., Gan, L., Ng, D., Xia, K. (2015). ). GA(3) enhances root responsiveness to exogenous IAA by modulating auxin transport and signalling in Arabidopsis. Plant Cell Rep. 34, 483–494. doi: 10.1007/s00299-014-1728-y
Lima, L. L., Frosi, G., Lopes, R., Santos, M. G. (2021). Remobilization of leaf Na(+) content and use of nonstructural carbohydrates vary depending on the time when salt stress begins in woody species. Plant Physiol. Biochem. 158, 385–395. doi: 10.1016/j.plaphy.2020.11.026
Liu, X., Li, Y., Zhong, S. (2017). Interplay between light and plant hormones in the control of arabidopsis seedling chlorophyll biosynthesis. Front. Plant Sci. 8. doi: 10.3389/fpls.2017.01433
Liu, M., Liu, X., Zhao, Y., Korpelainen, H., Li, C. (2022a). Sex-specific nitrogen allocation tradeoffs in the leaves of Populus cathayana cuttings under salt and drought stress. Plant Physiol. Biochem. 172, 101–110. doi: 10.1016/j.plaphy.2022.01.009
Liu, Y., Su, M., Han, Z. (2022b). Effects of NaCl stress on the growth, physiological characteristics and anatomical structures of Populus talassica× Populus euphratica seedlings. Plants 11, 3025. doi: 10.3390/plants11223025
Ma, Y., Dias, M. C., Freitas, H. (2020). Drought and salinity stress responses and microbe-induced tolerance in plants. Front. Plant Sci. 11,591911. doi: 10.3389/fpls.2020.591911
Mantova, M., Herbette, S., Cochard, H., Torres-Ruiz, J. M. (2022). Hydraulic failure and tree mortality: from correlation to causation. Trends Plant Sci. 27, 335–345. doi: 10.1016/j.tplants.2021.10.003
Mao, P., Lin, Q., Pang, Y., Wang, K., Ni, R., Han, X., et al. (2023). Eco-physiological response mechanism of Tamarix chinensis to soil water changes in coastal wetlands of the Yellow River Delta. Front. Mar. Sci. 10. doi: 10.3389/fmars.2023.1231928
Martin-StPaul, N., Delzon, S., Cochard, H. (2017). Plant resistance to drought depends on timely stomatal closure. Ecol. Lett. 20, 1437–1447. doi: 10.1111/ele.12851
Maruta, E., Yazaki, K., Ogasa, M. Y., Taneda, H. (2022). Pit aspiration causes an apparent loss of xylem hydraulic conductivity in a subalpine fir (Abies mariesii Mast.) overwintering at the alpine timberline. Tree Physiol. 42, 1228–1238. doi: 10.1093/treephys/tpab173
McCulloh, K. A., Johnson, D. M., Meinzer, F. C., Woodruff, D. R. (2014). The dynamic pipeline: hydraulic capacitance and xylem hydraulic safety in four tall conifer species. Plant Cell Environ. 37, 1171–1183. doi: 10.1111/pce.12225
McDowell, N. G. (2011). Mechanisms linking drought, hydraulics, carbon metabolism, and vegetation mortality. Plant Physiol. 155, 1051–1059. doi: 10.1104/pp.110.170704
McDowell, N. G., Ball, M., Bond-Lamberty, B., Kirwan, M. L., Krauss, K. W., Megonigal, J. P., et al. (2022). Processes and mechanisms of coastal woody-plant mortality. Global Change Biol. 28, 5881–5900. doi: 10.1111/gcb.16297
Mercado-Reyes, J. A., Pereira, T. S., Manandhar, A., Rimer, I. M., McAdam, S. A. M. (2023). Extreme drought can deactivate ABA biosynthesis in embolism-resistant species. Plant Cell Environ 47, 497–510. doi: 10.1111/pce.14754
Miura, K. (2012). “Nitrogen and phosphorus nutrition under salinity stress,”. Ecophysiology responses Plants under salt stress. (New York NY: Springer), 425–441. doi: 10.1007/978-1-4614-4747-4_16
MOA (2006). Agricultural standard of people’s republic of China: soil testing (NY/T 1121-2006) (Beijing: Ministry of Agriculture of the People’s Republic of China).
Moula, I., Boussadia, O., Koubouris, G., Hassine, M. B., Boussetta, W., Van Labeke, M. C., et al. (2020). Ecophysiological and biochemical aspects of olive tree (Olea europaea L.) in response to salt stress and gibberellic acid-induced alleviation. S. Afr. J. Bot. 132, 38–44. doi: 10.1016/j.sajb.2020.04.022
Naylor, D., Coleman-Derr, D. (2018). Drought stress and root-associated bacterial communities. Front. Plant Sci. 8, 303756.doi: 10.3389/fpls.2017.02223
Ndecky, S., Nguyen, T. H., Eiche, E., Cognat, V., Pflieger, D., Pawar, N., et al. (2023). Jasmonate signaling controls negative and positive effectors of salt stress tolerance in rice. J. Exp. Bot. 74, 3220–3239. doi: 10.1093/jxb/erad086
Niinemets, Ü. (2010). Responses of forest trees to single and multiple environmental stresses from seedlings to mature plants: past stress history, stress interactions, tolerance and acclimation. For. Ecol. Manage. 260, 1623–1639. doi: 10.1016/j.foreco.2010.07.054
Ou, C., Cheng, W., Wang, Z., Yao, X., Yang, S. (2023). Exogenous melatonin enhances Cd stress tolerance in Platycladus orientalis seedlings by improving mineral nutrient uptake and oxidative stress. Ecotoxicol. Environ. Saf. 252, 114619. doi: 10.1016/j.ecoenv.2023.114619
Ouyang, W., Struik, P. C., Yin, X., Yang, J. (2017). Stomatal conductance, mesophyll conductance, and transpiration efficiency in relation to leaf anatomy in rice and wheat genotypes under drought. J. Exp. Bot. 68, 5191–5205. doi: 10.1093/jxb/erx314
Polle, A., Chen, S. (2015). On the salty side of life: molecular, physiological and anatomical adaptation and acclimation of trees to extreme habitats. Plant Cell Environ. 38, 1794–1816. doi: 10.1111/pce.12440
Rajput, V. D., Chen, Y., Ayup, M. (2015). Effects of high salinity on physiological and anatomical indices in the early stages of Populus euphratica growth. Russ. J. Plant Physiol. 62, 229–236. doi: 10.1134/S1021443715020168
Rao, S., Tian, Y., Zhang, C., Qin, Y., Liu, M., Niu, S., et al. (2023). The JASMONATE ZIM-domain–OPEN STOMATA1 cascade integrates jasmonic acid and abscisic acid signaling to regulate drought tolerance by mediating stomatal closure in poplar. J. Exp. Bot. 74, 443–457. doi: 10.1093/jxb/erac418
Rath, K. M., Fierer, N., Murphy, D. V., Rousk, J. (2019). Linking bacterial community composition to soil salinity along environmental gradients. ISME J. 13, 836–846. doi: 10.1038/s41396-018-0313-8
Ryu, H., Cho, Y.-G. (2015). Plant hormones in salt stress tolerance. J. Plant Biol. 58, 147–155. doi: 10.1007/s12374-015-0103-z
Sah, S. K., Reddy, K. R., Li, J. (2016). Abscisic acid and abiotic stress tolerance in crop plants. Front. Plant Sci. 7, 190245. doi: 10.3389/fpls.2016.00571
Seo, H., Kim, S., Jang, S., Choo, Y., Sohn, E., Lee, I. (2005). Effect of jasmonic acid on endogenous gibberellins and abscisic acid in rice under NaCl stress. Biol. Plant 49, 447–450. doi: 10.1007/s10535-005-0026-5
Sevanto, S., Mcdowell, N. G., Dickman, L. T., Pangle, R., Pockman, W. T. (2014). How do trees die? A test of the hydraulic failure and carbon starvation hypotheses. Plant Cell Environ. 37, 153–161. doi: 10.1111/pce.12141
Shekoofa, A., Sinclair, T. R. (2018). Aquaporin activity to improve crop drought tolerance. Cells 7, 123. doi: 10.3390/cells7090123
Shi, Y., Li, J., Shao, J., Deng, S., Wang, R., Li, N., et al. (2010). Effects of Stockosorb and Luquasorb polymers on salt and drought tolerance of Populus popularis. Sci. Hortic. 124, 268–273. doi: 10.1016/j.scienta.2009.12.031
Song, Y., Feng, L., Alyafei, M. A. M., Jaleel, A., Ren, M. (2021). Function of chloroplasts in plant stress responses. Int. J. Mol. Sci. 22, 13464. doi: 10.3390/ijms222413464
Sonobe, R., Yamashita, H., Mihara, H., Morita, A., Ikka, T. (2020). Estimation of leaf chlorophyll a, b and carotenoid contents and their ratios using hyperspectral reflectance. Remote Sens. 12, 3265. doi: 10.3390/rs12193265
Sperry, J., Donnelly, J., Tyree, M. (1988). A method for measuring hydraulic conductivity and embolism in xylem. Plant, Cell Environ. 11, 35–40. doi: 10.3390/rs12193265
Stavi, I., Thevs, N., Priori, S. (2021). Soil salinity and sodicity in drylands: A review of causes, effects, monitoring, and restoration measures. Front. Environ. Sci. 330. doi: 10.3389/fenvs.2021.712831
Su, R., Zhang, Z., Chang, C., Peng, Q., Cheng, X., Pang, J., et al. (2021). Interactive effects of phosphorus fertilization and salinity on plant growth, phosphorus and sodium status, and tartrate exudation by roots of two alfalfa cultivars. Ann. Bot. 129, 53–64. doi: 10.1093/aob/mcab124
Suzuki, N., Rivero, R.M., Shulaev, V., Blumwald, E., Mittler, R. (2014). Abiotic and biotic stress combinations. New Phytol. 203 (1), 32–43. doi: 10.1111/nph.12797
Talebzadeh, F., Valeo, C. (2022). Evaluating the effects of environmental stress on leaf chlorophyll content as an index for tree health. IOP Conference Series: Earth and Environmental Science 1006. 012007. doi: 10.1088/1755-1315/1006/1/012007
Teshome, D. T., Zharare, G. E., Naidoo, S. (2020). The threat of the combined effect of biotic and abiotic stress factors in forestry under a changing climate. Front. Plant Sci. 11, 601009. doi: 10.3389/fpls.2020.601009
Thalmann, M., Pazmino, D., Seung, D., Horrer, D., Nigro, A., Meier, T., et al. (2016). Regulation of leaf starch degradation by abscisic acid is important for osmotic stress tolerance in plants. Plant Cell 28, 1860–1878. doi: 10.1105/tpc.16.00143
Tian, J., Pang, Y., Zhao, Z. (2021). Drought, salinity, and low nitrogen differentially affect the growth and nitrogen metabolism of sophora japonica (L.) in a semi-hydroponic phenotyping platform. Front. Plant Sci. 12. doi: 10.3389/fpls.2021.715456
Verma, S., Negi, N. P., Pareek, S., Mudgal, G., Kumar, D. (2022). Auxin response factors in plant adaptation to drought and salinity stress. Physiol. Plant 174, e13714. doi: 10.1111/ppl.13714
Wang, X., Mohamed, I., Ali, M., Abbas, M. H. H., Shah, G. M., Chen, F. (2018). Potassium distribution in root and non-root zones of two cotton genotypes and its accumulation in their organs as affected by drought and potassium stress conditions. J. Plant Nutr. Soil Sci. 182, 72–81. doi: 10.1002/jpln.201800026
Wang, Y., Mostafa, S., Zeng, W., Jin, B. (2021b). Function and mechanism of jasmonic acid in plant responses to abiotic and biotic stresses. Int. J. Mol. Sci. 22, 8568. doi: 10.3390/ijms22168568
Wang, F., Wan, C., Wu, W., Zhang, Y., Pan, Y., Chen, X., et al. (2023). Methyl jasmonate (MeJA) enhances salt tolerance of okra (Abelmoschus esculentus L.) plants by regulating ABA signaling, osmotic adjustment substances, photosynthesis and ROS metabolism. Sci. Hortic. 319, 112145.
Wang, B., Zhang, J., Pei, D., Yu, L. (2021a). Combined effects of water stress and salinity on growth, physiological, and biochemical traits in two walnut genotypes. Physiol. Plant 172, 176–187. doi: 10.1111/ppl.13316
Wang, M., Zheng, Q., Shen, Q., Guo, S. (2013). The critical role of potassium in plant stress response. Int. J. Mol. Sci. 14, 7370–7390. doi: 10.3390/ijms14047370
Weber, R., Gessler, A., Hoch, G. (2019). High carbon storage in carbon-limited trees. New Phytol. 222, 171–182. doi: 10.1111/nph.15599
Wicke, B., Smeets, E., Dornburg, V., Vashev, B., Gaiser, T., Turkenburg, W., et al. (2011). The global technical and economic potential of bioenergy from salt-affected soils. Energy Environ. Sci. 4, 2669–2681. doi: 10.1039/C1EE01029H
Willis, A. D. (2019). Rarefaction, alpha diversity, and statistics. Front. Microbiol. 10, 492464. doi: 10.3389/fmicb.2019.02407
Wu, H., Zhang, X., Giraldo, J. P., Shabala, S. (2018). It is not all about sodium: revealing tissue specificity and signalling roles of potassium in plant responses to salt stress. Plant Soil 431, 1–17. doi: 10.1007/s11104-018-3770-y
Xing, J., Li, M., Li, J., Shen, W., Li, P., Zhao, J., et al. (2022). Stem canker pathogen Botryosphaeria dothidea inhibits poplar leaf photosynthesis in the early stage of inoculation. Front. Plant Sci. 13, 1008834. doi: 10.3389/fpls.2022.1008834
Xu, Y., Zhang, G., Ding, H., Ci, D., Dai, L., Zhang, Z. (2020). Influence of salt stress on the rhizosphere soil bacterial community structure and growth performance of groundnut (Arachis hypogaea L.). Int. Microbiol. 23, 453–465. doi: 10.1007/s10123-020-00118-0
Yang, Y., Xu, C., Wang, B., Jia, J. (2001). Effects of plant growth regulators on secondary wall thickening of cotton fibres. Plant Growth Regul. 35, 233–237. doi: 10.1023/A:1014442015872
Yurekli, F., Porgali, Z. B., Turkan, I. (2004). Variations in abscisic acid, indole-3-acetic acid, gibberellic acid and zeatin concentrations in two bean species subjected to salt stress. Acta Biol. Cracov. Bot. 46, 201–212.
Zhang, J., Jia, W., Yang, J., Ismail, A. M. (2006). ). Role of ABA in integrating plant responses to drought and salt stresses. Field Crops Res. 97, 111–119. doi: 10.1016/j.fcr.2005.08.018
Zhao, S., Cang, J. (2016). Plant physiology lab instruction (Beijing: China: Agricultural Science and Technology Press).
Zhu, Q. (2023). The study on the response of endogenous hormones to ecological factors and influence of biosynthesis of main flavor components in tea plant. [dissertation]. Fuzhou: Fujian Agriculture and Forestry University. (2008). (in Chinese with English abstract).
Zhu, X., Chen, J., Qiu, K., Kuai, B. (2017). Phytohormone and light regulation of chlorophyll degradation. Front. Plant Sci. 8. doi: 10.3389/fpls.2017.01911
Keywords: salt, drought, xylem hydraulics, physiological traits, soil properties
Citation: Li S, Lu S, Wang J, Liu Z, Yuan C, Wang M and Guo J (2024) Divergent effects of single and combined stress of drought and salinity on the physiological traits and soil properties of Platycladus orientalis saplings. Front. Plant Sci. 15:1351438. doi: 10.3389/fpls.2024.1351438
Received: 07 December 2023; Accepted: 22 May 2024;
Published: 06 June 2024.
Edited by:
Arun K. Shanker, Central Research Institute for Dryland Agriculture (ICAR), IndiaReviewed by:
Abdenour Kheloufi, University of Batna 2, AlgeriaUsman Khalid Chaudhry, Ömer Halisdemir University, Türkiye
Roman Gebauer, Mendel University in Brno, Czechia
Copyright © 2024 Li, Lu, Wang, Liu, Yuan, Wang and Guo. This is an open-access article distributed under the terms of the Creative Commons Attribution License (CC BY). The use, distribution or reproduction in other forums is permitted, provided the original author(s) and the copyright owner(s) are credited and that the original publication in this journal is cited, in accordance with accepted academic practice. No use, distribution or reproduction is permitted which does not comply with these terms.
*Correspondence: Shan Li, lishan@sust.edu.cn