- 1College of Life Sciences, Zhejiang Normal University, Jinhua, China
- 2Jinhua Mountain Observation and Research Station for Subtropical Forest Ecosystems, Jinhua, China
Pollen analysis, a crucial tool in botany and ecology for examining historical biotic dynamics, has elicited debate owing to its complex link with vegetation. The challenge lies in discerning the ecological significance of pollen data. In this study, we conducted detailed quadrat surveys on Jinhua Mountain, subtropical China, analyzing topsoil pollen to determine whether pollen signals accurately reflect key ecological components in the forests and shrublands. We performed direct comparisons between pollen and plant compositions and calculated pollen percentages and plant Important Values (IVs) for each quadrat. The results indicate greater homogeneity in pollen composition across the study area compared to plant composition, particularly in the high percentage of Pinus pollen. However, distinct plant communities exhibited significantly different pollen compositions, as evidenced by the multi-response permutation test. This divergence aligns with variations in the dominant plant species across different communities. There were significant correlations between pollen percentages and plant IVs, with correlation coefficients of 0.55 (p < 0.001) at the quadrat level and 0.78 (p < 0.001) at the taxon level. These results support the utility of pollen analysis for representing ecologically significant values in subtropical Chinese forests and shrublands. Such correlations might also be extrapolated to pollen-based paleoecological studies.
1 Introduction
Pollen serves as a robust indicator for discerning spatial and temporal variations in species assemblages and interspecific interactions, rendering it well-suited for high-throughput evaluation of global ecological shifts (Hornick et al., 2021). Pollen analysis remains the predominant method for recovering historical data regarding vegetation, climate, and the environment (Prentice et al., 1998; Xu et al., 2007; Zhao et al., 2015; Birks, 2019; Chevalier et al., 2020; Zheng et al., 2021). The majority of investigations in this domain hinge upon the ecological significance of pollen, specifically its representation of vegetation (Davis, 1963; Parsons and Prentice, 1981; Sugita, 1994).
Understanding the complex relationship between pollen and vegetation is a fundamental aspect of pollen analysis. Influenced by various processes, including pollen production, transport, and deposition, this relationship is complex (Xu et al., 2007; Xu et al., 2016). To elucidate the pollen-plant connection and enhance the pollen-vegetation calibration, several approaches have been introduced and applied, including pollen representation value (R value), extended pollen representation value (ERV), relative pollen productivity (RPP), and relevant source area of pollen (RSAP) estimation (Davis, 1963; Andersen, 1970; Prentice and Parsons, 1983; Prentice, 1985; Prentice and Webb, 1986; Jackson and Kearsley, 1998; Sugita, 2007a, b). With the increasing accessibility of data related to pollen R values and RPP for pollen-vegetation calibration (Li et al., 2018; Wieczorek and Herzschuh, 2020; Xia and Ni, 2024), the pollen-vegetation relationship has gained substantial recognition. Chinese palynologists have also been dedicated to investigating this relationship, including R value, RPP estimation, and quantitative aspects of the pollen-plant connection (Yu et al., 2001; Xiao et al., 2011; Zheng et al., 2015; Xu et al., 2016; Li et al., 2017; Cui et al., 2020). Although uncertainties persist, regression correlations tend toward a linear curve in specific regions following calibration with R values and RPP (Xu et al., 2016). However, limited attention has been directed toward whether pollen can adequately reflect the role of a plant within a community. This represents a minor barrier between community ecology rooted in investigative approaches and pollen-based paleoecology (Seddon et al., 2014).
Pollen percentage constitutes the most commonly utilized data type in calculating pollen representation, RPP, and pollen source areas, whereas the type of plant data employed varies across different studies. For example, Andersen (1970) considered plant frequency, tree crown area, or tree basal area within a given plot, whereas subsequent forest-related studies frequently incorporated tree percentage (abundance) (Prentice and Webb, 1986; Jackson and Kearsley, 1998). In the context of steppe vegetation, coverage has been the prevailing metric for studying pollen-vegetation relationships (Xu et al., 2007). This choice is justified because counting individual plants is more challenging than estimating coverage. From a community ecology perspective, both abundance and coverage are crucial, as they provide quantitative insights into individual plants and the spatial extent they occupy (Song, 2016). However, abundance and/or coverage sometimes fall short of depicting a taxon’s functional role within the community. Instead, dominants or edificators play a pivotal role in shaping the community structure and maintaining its stability and ecosystem services. In vegetation ecology, dominants or edificators typically exhibit high importance values, amalgamating several measurements such as abundance, frequency, dominance, and height (Curtis and Mclntosh, 1951). Whether the pollen percentage aligns with numeric measures of taxon importance remains an open question.
To address these issues, we conducted extensive fieldwork on Jinhua Mountain, located in eastern subtropical China. Furthermore, pollen analysis was performed on the soil samples obtained from the quadrats. A vegetation map of Jinhua Mountain was compiled in 2017. Over the course of 2017-2018, plant quadrats from different vegetation types and plant communities were investigated to study the quantitively relationship between soil pollen percentage and plant community. Soil samples collected before the investigation were subjected to pollen identification. Our primary objectives were to compare discrepancies between pollen signals and the contemporary vegetation community in Jinhua Mountain and to determine whether pollen signals could effectively reflect significant ecological components within the subtropical Chinese plant community.
2 Materials and methods
2.1 Quadrat investigation and soil sample collection
Jinhua Mountain (E 119.48°–119.77°, N 29.15°–29.32°, 1312 m above sea level) is situated to the north of Jinhua City in Zhejiang Province in the middle-eastern region of China. This mountain predominantly consists of rhyolite with localized limestone occurrences. Influenced by a subtropical monsoonal climate, the mean annual temperature in the Jinhua region stands at 17.7°C. Notably, January recorded an average temperature of 5.2°C, while July experienced an average temperature of 29.5°C. The mean annual precipitation is 1436 mm, with 70% of the precipitation occurring between March and August. The vegetation on Jinhua Mountain is characterized by evergreen broad-leaved forests located at the northern boundary of the central subtropical zone. The region has abundant plant germplasm resources and a diverse array of plant species (Fan et al., 2019). As elevation increases, the vegetation transitions through various types, including evergreen broadleaf forests (Castanopsis sclerophylla (Lindl.) Schottky, Schima superba Gardner & Champ), evergreen deciduous broadleaved forests (Liquidambar formosana Hance, Eurya muricata Dunn, Castanea seguinii Dode), coniferous broadleaf mixed forests (Pinus massoniana Lamb., Castanea henryi (Skan) Rehder & E. H. Wilson, Dalbergia hupeana Hance), coniferous forests (Pinus taiwanensis Hayata, Pin. massoniana), and ultimately culminate in mountaintop shrublands dominated by Rhododendron simsii Planch. and Rhododendron molle (Blume) G. Don. Additionally, cultivated cedar (Cunninghamia lanceolata (Lamb.) Hook.) and hairy bamboo (Phyllostachys edulis (Carrière) J. Houz.) forests are also present in this region.
A comprehensive vegetation survey was conducted during 2017 and 2018. In total, 24 quadrats were rigorously selected, including five distinct vegetation types: coniferous forest, evergreen broad-leaved forest, mixed evergreen and deciduous broad-leaved forest, mixed coniferous and broad-leaved forest, and shrubland (Figure 1; Table 1). The dimensions of the quadrats were 30 × 30 m for forested areas and 30 × 10 m for shrubland regions. Each forested quadrat was further subdivided into nine 10 × 10 m sub-quadrats. Within these sub-quadrats, five 4 × 4 m plots were designated in the upper-left corner to scrutinize the shrub layer. Similarly, within the upper-left corner of each 4 × 4 m sub-quadrat, a 1 × 1 m quadrat was established to examine the herbaceous layer.
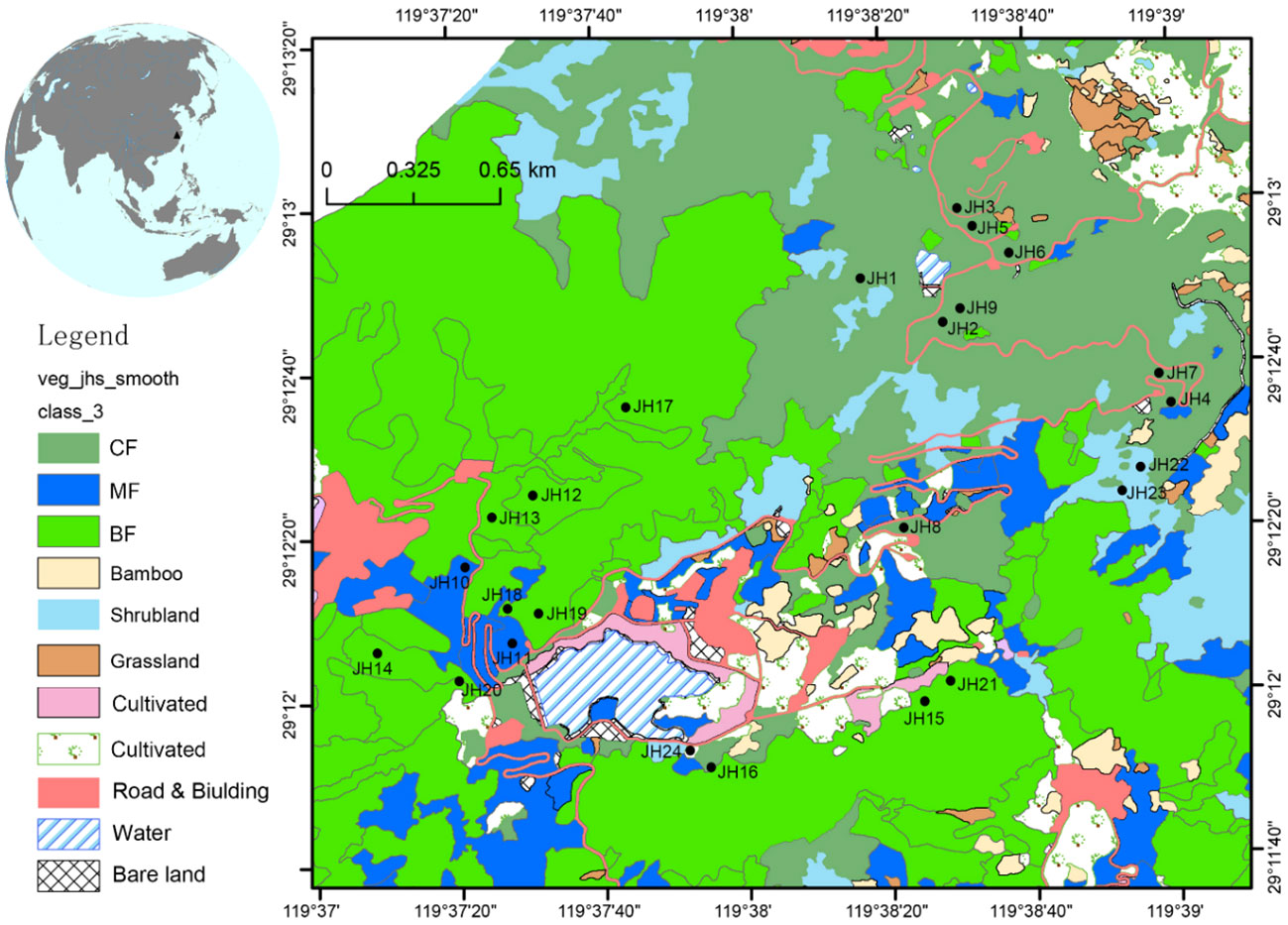
Figure 1 Map showing locations of Jinhua Mountain (top-left) and 24 quadrats in Jinhua Mountain (JH1-24). Vegetation map was cited from Fan (2020). CF, subtropical coniferous forest. MF, mixed coniferous and broad-leaved forest. BF, broad-leaved forest.
All standing woody species with a diameter at breast height (DBH) equal to or exceeding 1 cm underwent rigorous examination, and their species name, plant count, DBH, height, crown width, and coordinates were duly recorded. For the shrub and herbaceous layers, species name, plant count, height, and coverage were documented. Within the shrubland quadrats, the species name, plant count, DBH, height, crown width, and coordinates of woody plants with a DBH of ≥ 1 cm were rigorously measured and recorded. For woody plants with a DBH < 1 cm, the species name, plant count, base diameter, height, and coordinates were documented for all trees with a height ≥ 10 cm.
Furthermore, within each 10 × 10 m sub-quadrat, a 1 × 1 m herbaceous quadrat located in the upper-left corner was designated to record the species name, plant count, height, and coverage within the herbaceous layer. Notably, at the commencement of the quadrat investigation, surface soil samples from to 0-1 cm depth were methodically collected within each 10 × 10 m sub-quadrat.
2.2 Laboratory works
The soil samples designated for pollen analysis underwent a series of rigorous treatments. Initially, they were subjected to heavy liquid flotation, which included treatment with HCl (10%) and KOH (10%) to eliminate carbonate and humic acid residues, respectively. Subsequently, the samples were passed through a 200 μm sieve and subjected to centrifugation. To process the samples further, we introduced 30 mL of ZnBr2 and performed centrifugation twice as a part of this procedure. Subsequently, the samples underwent two rounds of centrifugation with deionized water to remove the heavy liquid.
The final step of the process involved adding 5 mL of mixed acetic anhydride and concentrated sulfuric acid (9:1) to the samples, which were subsequently heated in a water bath at 80°C for 3 min. Subsequently, the samples were centrifuged and washed until the solution reached a neutral pH. To facilitate the quantification of pollen concentrations, tablets containing a known quantity of Lycopodium L. spores were introduced into each sample prior to the aforementioned treatments. Pollen was identified using a Zeiss Scope A1 at Zhejiang Normal University, with a minimum count of 500 terrestrial pollen grains per sample.
2.3 Numeric analysis
Numeric characteristics of vegetation communities, including abundance, coverage, frequency, and important values (IVs), are widely employed as valuable metrics in the field of community ecology (Song, 2016). IVs, in particular, serve as a crucial and commonly used index that reflects the comprehensive significance of individual taxa within a plant community (Curtis and Mclntosh, 1951). We computed the average IVs for each taxon based on the relative frequency, height, and dominance derived from the quadrat data.
Frequency was calculated as the number of plots in which a species was observed divided by the total number of survey plots. Relative frequency was computed by dividing the frequency by the sum of the frequencies of all species. Height was determined as the total height of individuals of a species, whereas the relative height was obtained by dividing the height by the sum of the heights of all species. Dominance was quantified as the total breast area of a species, and relative dominance was established by dividing dominance by the sum of the dominance of all species. Given the substantial importance of woody taxa compared to fern and herbaceous taxa in forest and shrub quadrats, we focused solely on calculating the IVs for woody taxa investigated within forest quadrats and shrub sub-quadrats. To facilitate comparisons with pollen results from the same quadrats, we summed the IVs of taxa with the same genus or family, aligning with the taxon names in the Chinese Pollen Database (Chen et al., 2021).
The percentage of pollen was initially computed for each surface soil sample. The percentage of woody taxa was derived from the total tree and shrub pollen counts, whereas the herbaceous and fern taxa were based on the overall pollen counts. The pollen percentage was subsequently calculated as the average percentage within each quadrat for the numerical analysis. To create vegetation and pollen stratigraphic diagrams, we utilized the “rioja” package in R (Juggins, 2022).
For further analysis, we selected the IVs of woody taxa from the quadrat investigation and the percentage of woody taxa from the pollen identification. Both datasets underwent square-root transformation to stabilize variances and optimize the “signal” to “noise” ratio. To test the dissimilarity originating from different vegetation types, we employed the Multi-response Permutation Procedure (MRPP) utilizing the Bray-Curtis coefficient as a dissimilarity measure (Warton et al., 2012). To assess the consistency of taxa between plant IVs and pollen signals, we conducted ordination-based Procrustes rotation analysis. Initially, all taxa present in both the vegetation and pollen datasets were included in the ordination analysis to compare site consistency. Subsequently, only taxa appearing in both datasets were considered in the data subsets to evaluate the differing contributions of shared taxa.
Detrended Correspondence Analysis was initially applied, with the first axis lengths for the IVs and pollen datasets measuring 3.43 and 1.42, respectively. Consequently, Principal Component Analysis (PCA) was performed. The first two PCA axes were explored for both vegetation and pollen data. To measure the similarity between vegetation and pollen, we performed Procrustes rotation analysis using a standard method of multidimensional scaling on the first four PCA axes (Peres-Neto and Jackson, 2001). The significance of the similarity between the two datasets was assessed using the protest procedure. All analyses were performed using R software (version 4.3.1; R Core Team, 2023) with the “vegan” package (Oksanen et al., 2022).
3 Results
3.1 Vegetation composition
3.1.1 Coniferous forest (JH1 – JH9)
The Pin. taiwanensis forest (JH1 – JH3), situated at elevations above 1000 m, exhibited distinct community stratification (Figure 2). The tree layer is predominantly composed of Pin. taiwanensis, with a canopy density ranging from 65% to 80%. The shrub layer boasts a coverage of 50–60% and is mainly characterized by species such as Rhododendron, Eurya japonica Thunb., Lindera erythrocarpa Makino, Euscaphis japonica Thunberg, and Lindera reflexa Hemsl. Meanwhile, the herbaceous layer exhibits coverage varying from 35% to 90%, with prominent species including Rubus buergeri Miquel, Ru. pacificus Hance, Justicia quadrifaria (Nees) T. Anderson, Achyranthes bidentata Blume, and fern taxa belonging to Dryopteris Adanson and Athyrium Roth.
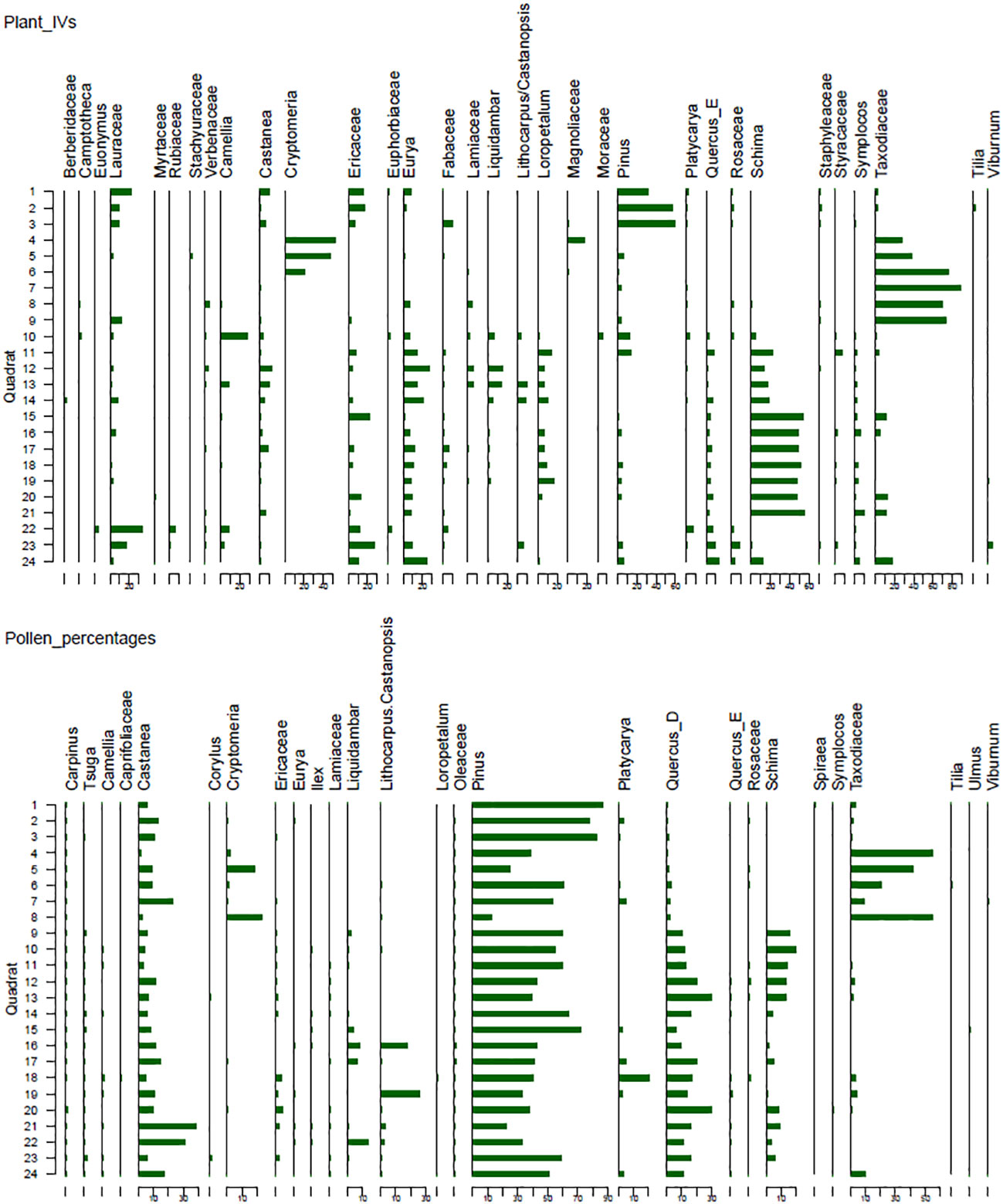
Figure 2 Stratigraphic diagram showing plant IVs and pollen percentages of selected taxa. Taxa with max IVs above 2 and max pollen percentage above 0.5% in at least one quadrat were included.
In the Cryptomeria japonica (Thunb. ex L. f.) D. Don forest (JH4 – JH5), which is distributed between 900 and 1000 m in elevation, the dominant plants in the tree layer consist of Cr. japonica and Cu. lanceolata. The shrub layer, characterized by a coverage ranging from 5% to 40%, is primarily dominated by Cr. japonica, whereas Cu. lanceolata, Lin. erythrocarpa, E. japonica, and Magnolia officinalis Rehder & E. H. Wilson are dominant. The herbaceous layer is primarily comprised of Ach. bidentata, Rehmannia glutinosa Gaertner, Reynoutria japonica Houtt., Dryopteris and Athyrium.
The Cu. lanceolata forest (JH6-JH9) is situated at elevations ranging from 711 to 1100 m. The tree layer is predominantly characterized by Cu. lanceolata, with a canopy density of 85–90%. Shrub coverage varies from 5% to 65% and primarily comprises Lindera glauca Siebold and Zuccarini, Lin. reflexa, Rhododendron, Hydrangea strigosa Rehder, Camellia oleifera Abel, and Castanea seguinii. The herbaceous layer, covering 60–90% of the area, is primarily composed of Rungia densiflora H. S. Lo, J. quadrifaria, Ach. bidentata, Viola arcuata Blume, Athyrium, and Diplazium donianum (Mett.) Tardieu.
3.1.2 Mixed forest (JH10 – JH14)
The evergreen deciduous coniferous broad-leaved mixed forest (JH10) is characterized by a community of Pin. massoniana and Liq. formosana. The canopy density of the tree layer is 80%. In addition to Pin. massoniana and Liq. formosana, taxa such as Platycarya strobilacea Siebold & Zucc., Castanea henryi, and Camptotheca acuminata Decne. are also abundant. The coverage of shrub layer is 70%, dominated by Came. oleifera, E. muricata, Maclura tricuspidata Carrière and Lin. glauca. Vine plants, such as Trachelospermum jasminoides (Lindl.) Lem., Wisteria sinensis (Sims) Sweet, Gynostemma pentaphyllum (Thunb.) Makino, and Rosa cymosa Tratt. can also be observed. The herbaceous layer mainly comprises Liriope spicata (Thunb.) Lour., and Athyrium filix-femina (L.) Roth, with a coverage of 35%.
The evergreen coniferous broad-leaved mixed forest (JH11), dominated by Sch. superba and Pin. massoniana, primarily occurs at an altitude of approximately 567 m. The tree layer boasts a canopy density of 90%, primarily consisting of Sch. superba, Pin. massoniana, Quercus pubescens Willd., Loropetalum chinense (R. Br.) Oliv., and Styrax confuses Hemsl. The shrub layer, with a coverage of 65%, comprising Eur. muricata, Sch. superba, Lor. chinense, Rh. simsii, Symplocos sumuntia Buch.-Ham. ex D. Don, and Lyonia ovalifolia (Wall.) Drude. The herbaceous layer, with modest coverage of 7%, occasionally features bamboo and ferns.
The evergreen deciduous broad-leaved mixed forest (JH12 – JH14) spans an altitude range of 550-658 m on Jinhua Mountain. The dominant communities are the Sch. superba–Liq. formosana–Castanea henryi forest and the Sch. superba–Quercus serrata Thunb.–Liq. formosana forest. The tree layer comprises mainly the Sch. superba, Liq. formosana, Castanea henryi, Q. serrata, with canopy density of 85 - 90%. The shrub layer, with coverage ranging from 50% to 80%, is mainly composed of Eur. muricata, Sch. superba, Lor. chinense, Callicarpa bodinieri H. Lév., Rhododendron ovatum (Lindl.) Planch. ex Maxim., and Clerodendrum cyrtophyllum Turcz. Vine plants consist of Gy. pentaphyllum and Rosa cymosa. The herbaceous layer, with coverage varying from 7% to 35%, primarily comprises Lir. spicata, Carex L., Pteris L., and Dryopteris.
3.1.3 Evergreen broad-leaved forest (JH15 – JH21)
The predominant community within the evergreen broad-leaved forest of Jinhua Mountain is Sch. superba, primarily distributed within an altitude range of 520–644 m. The tree layer exhibited a canopy density ranging from 85% to 90%, prominently featuring Sch. superba as the dominant and indicator species. It was complemented by Cu. lanceolata, Q. serrata, Castanea henryi, and Dal. hupeana. The shrub layer, with coverage varying from 45% to 60%, is mainly composed of Sch. superba, Lor. chinense, Eur. muricata, Rh. ovatum, Albizia kalkora (Roxb.) Prain, Symplocos anomala Brand, Sy. sumuntia, and Quercus glauca Thunb. The herbaceous layer exhibits coverage ranging from 7% to 30% and is mainly characterized by Polygonatum odoratum (Mill.) Druce, Carex, Pteris, and Dryopteris.
3.1.4 Shrubland (JH22 – JH24)
The shrubland primarily consists of Lin. glauca deciduous shrub (JH21), Rh. ovatum–Lin. glauca–Rh. simsii shrub (JH23), Q. serrata, Eurya rubiginosa Hung T. Chang shrub (JH24). The canopy density in these shrublands varied from 45% to 90%. The dominant taxa include Lin. glauca, Rh. ovatum, Rh. simsii, Q. serrata, Eur. rubiginosa, Serissa serissoides (DC.) Druce, and Euonymus carnosus Hemsl. Vine plants, such as W. sinensis, Actinidia chinensis Planch., and Celastrus gemmatus Loes. can be found in these shrublands. The coverages of the herbaceous layer range from 2% to 30%.
3.2 Pollen composition
A total of 109 pollen taxa have been identified in the soil samples, with the majority belonging to the arboreal taxa (Figure 2). The tree taxa primarily include Pinus L., Taxodiaceae Warming, Cryptomeria D. Don, Tsuga (Endl.) Carrière, Schima Reinw. ex Blume, Castanea Mill., Liquidambar L., deciduous Quercus (Quercus_D), Platycarya, evergreen Quercus (Quercus_E), Lithocarpus Blume/Castanopsis Spach, Betula L., Corylus L., and Euphorbiaceae Juss. There are also 23 shrub taxa, mainly composed of Ericaceae Juss., Caprifoliaceae Juss., Viburnum L., Rosaceae Juss., Ilex L., Camellia L., Eurya Thunb., Moraceae Gaudich., and Lamiaceae Martinov. The herbaceous taxa include Asteraceae Bercht. & J. Presl, Artemisia L., Polygonaceae Juss., Brassicaceae Burnett, Poaceae Barnhart, Acanthaceae Juss., and Saxifragaceae Juss. Fern taxa are abundant, with frequent occurrences of Polypodiaceae J. Presl & C. Presl, Dryopteris, Hicriopteris Presl/Dicranopteris Bernh., Pteris and Lygodium Sw.
3.2.1 Coniferous forest (JH1 – JH9)
The Pin. taiwanensis forest (JH1 – JH3) is characterized by a substantial content of coniferous pollen taxa, notably Pinus (82.1%) and Taxodiaceae (2.3%). Broad-leaved taxa are mainly composedprimarily consisted of Castanea (10%), Platycarya (1.6%), Quercus_D (1%), and Eurya (0.5%). The shrub taxa can be found of Rhododendron and Ericaceae. The content of herbaceous taxa is low, mainly including Artemisa, Asteraceae, and Saxifragaceae. Fern taxa comprise mainly Hicriopteris/Dicranopteris and Polypodiaceae.
In the Cr. japonica forest (JH4 – JH5), there are elevated contents of Taxodiaceae (48.6%), Pinus (31.6%), and Cryptomeria (10.2%). Deciduous arboreal taxa mainly include Castanea (5.4%), Quercus_D (0.9%), Corylus, and Platycarya. The content of evergreen broad-leaved taxa is limited, mainly comprising Eurya and Quercus_E. The shrub taxa consist of Rosaceae (0.6%), Caprifoliaceae, and Salix. Herbaceous taxa are composed mainly of Artemisa (1.9%) and Aster (0.5%). Hicriopteris/Dicranopteris (2.9%) and Polypodiaceae (2.7%) are abundant.
Within the Cu. lanceolata forest (JH6 -JH9), there are notable contents of Pinus (46.8%), Taxodiaceae (21.2%), and Cryptomeria (6.1%). Broad-leaved taxa mainly comprise Castanea (10.1%), Quercus_D (4.5%), Schima (4.1%), Platycarya (1.6%), Liquidambar (0.6), Lithocarpus/Castanopsis, Betula, and Corylus. Herbaceous taxa mainly include Artemisia (3.5%), Asteraceae (1.1%), and Aster L. (0.5%). The fern taxa are mainly composed of Hicriopteris/Dicranopteris (9.6%), Polypodiaceae (9.2%), and Athyrium (1.6%).
3.2.3 Mixed forest (JH10 – JH14)
In the evergreen deciduous coniferous broad-leaved mixed forest (J10), there is a notable presence of coniferous pollen taxa including Pinus (54.6%), Tsuga (0.7%), and Taxodiaceae (0.6%). Broad-leaved trees and shrub taxa mainly comprise Schima (20.1%), Quercus_D (12%), Castanea (4.4%), Ericaceae (1.2%), Liquidambar (0.9%), Camellia (0.8%), and Corylus, Platycarya, Ulmus, and Eurya. The herbaceous taxa mainly comprise Artemisia (1.1%). Fern taxa includes Polypodiaceae (27.2%), Hicriopteris/Dicranopteris (13.3%), and Dryopteris (5.9%).
In the evergreen coniferous broad-leaved mixed forest (JH11), high contents of Pinus (60.1%), Schima (14.5%), and Quercus_D (13.2%) are prevalent. Taxa, such as Castanea (3.1%), Liquidambar (1.1%), Rhododendron (1.1%), Taxodiaceae (0.9%), Camellia (0.8%), Tsuga (0.8%), Rosaceae (0.7%), Corylus (0.6%), and Labiatae (0.5%) are also abundant. Herbaceous taxa mainly comprise Artemisia (1.5%), whereas fern taxa is mainly composed of Hicriopteris/Dicranopteris (8.3%).
Within the evergreen deciduous broad-leaved mixed forest (JH12 – JH14), the contents of arboreal pollen are high, including Pinus (48.8%), Quercus_D (22.1%), Schima (10.4%), Castanea (8.3%), Taxodiaceae (1.9%), Ericaceae (1.5%), Lamiaceae (0.9%), Tsuga (0.9%), Rosaceae (0.9%), Quercus_E (0.7%), Corylus (0.6%) and Liquidambar (0.5%). Herbaceous taxa are low, mainly composed of Artemisia (1.6%) and Asteraceae. The content of fern taxa is high, with Polypodiaceae of 16.3% and Hicriopteris/Dicranopteris of 16.2%.
3.2.4 Evergreen broad-leaved forest (JH15 – JH21)
Pollen composition mainly comprises Pinus (41.5%), Quercus_D (16.1%) and Castanea (14.2%). The evergreen components, including Lithocarpus/Castanopsis (6.9%), Platycarya (4.5%), and Schima (3.9%), are substantial. Additionally, there were abundant taxa, such as Liquidambar (2.9%), Ericaceae (2%), Taxodiaceae (1.6%), Tsuga (0.8%), Camellia (0.6%), Quercus_E (0.6%), Eurya (0.5%), and Oleaceae (0.5%). Herbaceous taxa mainly include Artemisa (1.5%), whereas fern taxa comprise mainly Hicriopteris/Dicranopteris (17.8%).
3.2.5 Shrubland (JH22 – JH24)
The pollen assemblage is characterized by high contents of Pinus (47.8%), Castanea (18%), Quercus_D (12.8%), Liquidambar (4.8%), Taxodiaceae (3.7%), Schima (3.3%), Platycarya (1.3%), Ericaceae (1.2%), Lithocarpus/Castanopsis (1%), Corylus (0.7%), Lamiaceae (0.6%), Quercus_E (0.6%), Camellia (0.5%), and Eurya (0.5%). Artemisa (2.7%) and Asteraceae (0.9%) are abundant. The fern taxa mainly comprise Hicriopteris/Dicranopteris (20.5%) and Polypodiaceae (3%).
3.3 Correlation between vegetation and pollen
MRPP analysis revealed significant differences in both plant IVs and pollen signals among the various vegetation types (p < 0.001). In general, the compositional differences among plants within the same community are smaller than those observed among different vegetation types (Figure 3). This trend is also reflected in the differences in pollen composition. However, it is noteworthy that the compositional distance in the pollen analysis is notably smaller than that observed in the plant composition. For example, the compositional distance between coniferous forests and other communities typically exceeds 0.6 in quadrat data, contrasting with a distance of 0.3 in pollen analysis. Similarly, the compositional difference between broad-leaved forest and broad-leaved and coniferous mixed forest is low in both plant and pollen results, with mean distances of 0.3 and 0.3-0.6, respectively.
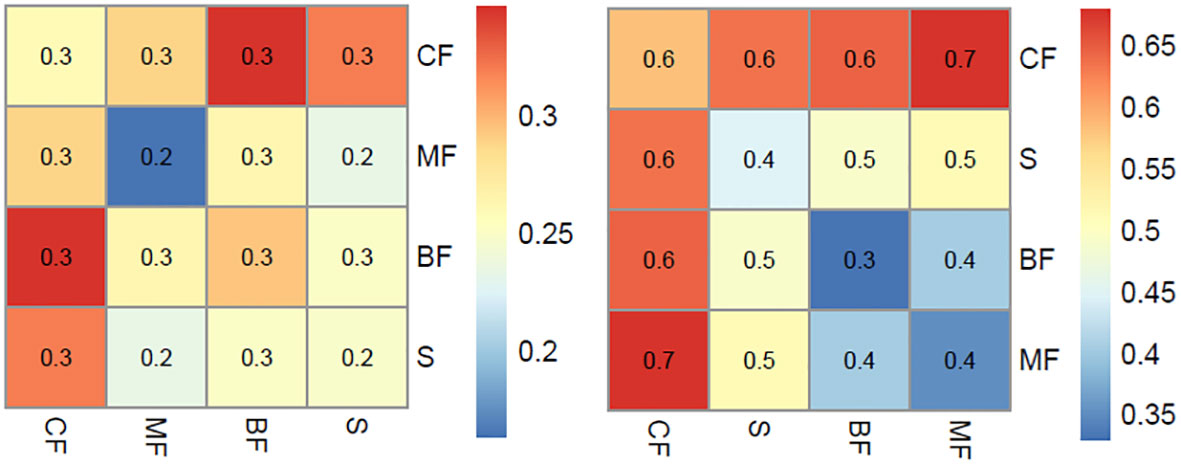
Figure 3 Mean distance within same and among different vegetation types for pollen (left) and vegetation (right).
Ordination analysis effectively segregated quadrats with different vegetation types. Both plant IVs and pollen signals can be differentiated based on their compositional differences, with the first axes explaining approximately 44% (IVs) and 37% (pollen) of the variations, respectively. Coniferous forests are generally characterized by high contents of Pinus, Taxodiaceae, and Cryptomeria, which are distinctly different from those in broad-leaved forests (Figure 4).
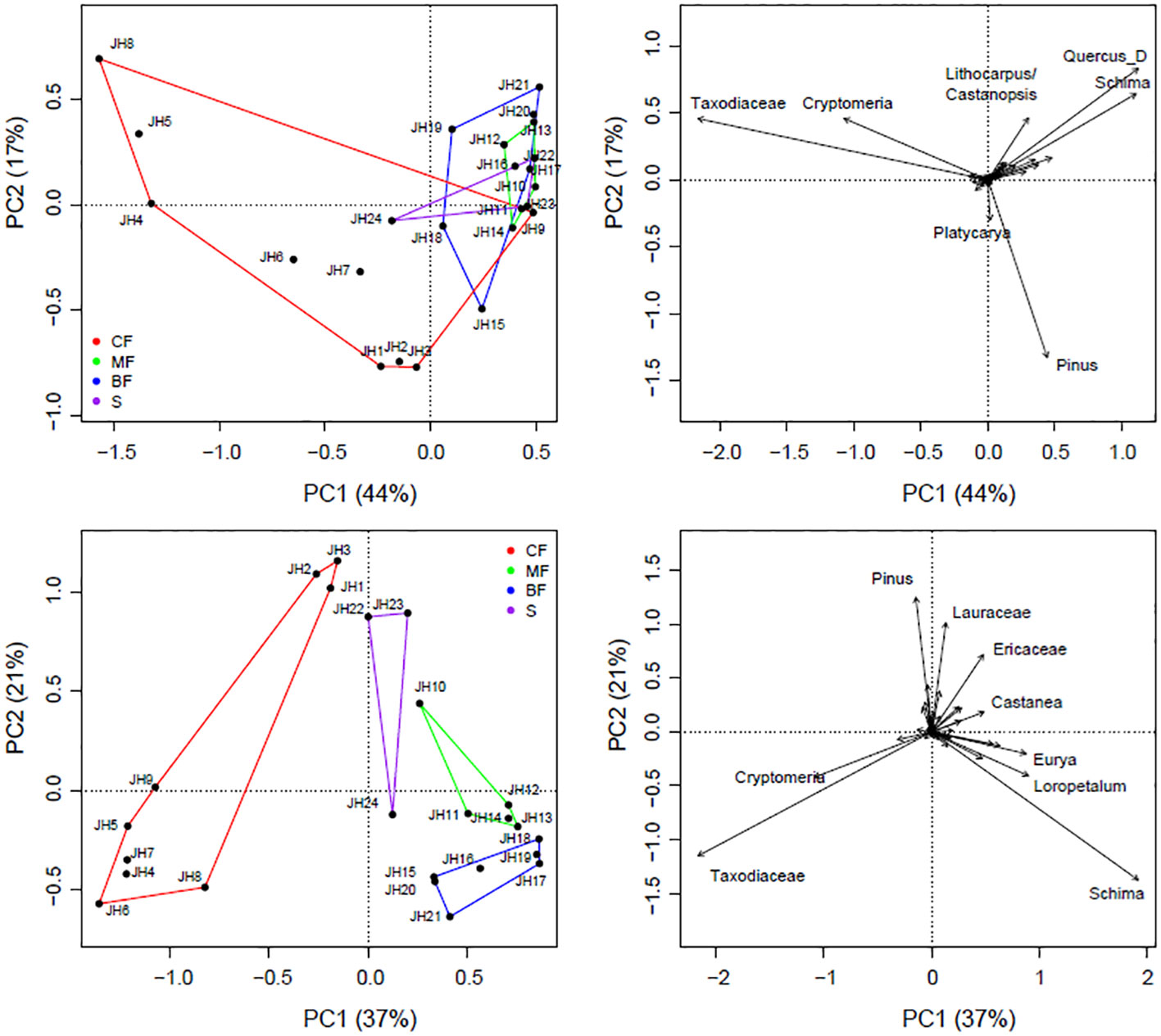
Figure 4 PCA results of pollen (upper) and vegetation (lower) composition from 24 quadrats of Jinhua Mountain (JH1-24). Only selected taxa labeled.
The procrustes and protest results indicate a significant similarity between plant IVs and pollen percentages (Figure 5). Correlations in symmetric Procrustes rotation are 0.67 at the site level, considering all pollen and plant taxa. When the analysis is conducted with the 44 shared taxa between plants and pollen, the correlations in symmetric Procrustes rotation increase to 0.69 at the site level and 0.85 at the taxa level, respectively. Quadrats of JH6, JH7, JH9, JH15, and JH22 are with high residuals, as well as taxa of Schima, Loropetalum, Quercus_E, Eurya, and Ericaceae.
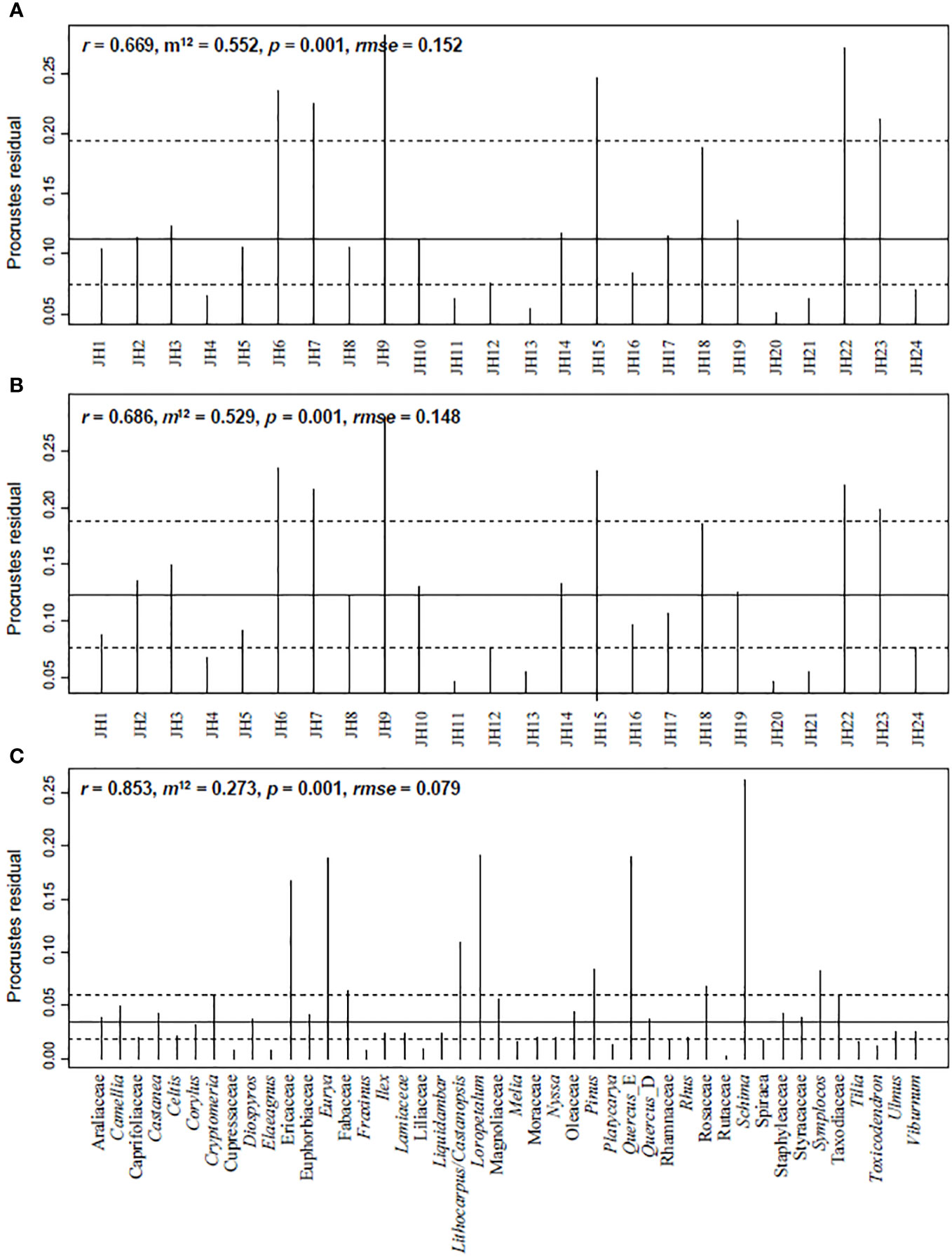
Figure 5 Procrustes residual results illustrating the similarity between plant IVs and pollen signals from quadrat data in Jinhua Mountain. (A) Based on all the plant and pollen taxa; (B, C) Based on 44 shared taxa between plant and pollen. r, correlation in a symmetric Procrustes rotation; m12, Procrustes sum of squares; rmse, Procrustes root mean squared error.
4 Discussion
Pollen analysis is a significant botanical and ecological tool for studying the dynamics of biota and biotic systems (Prentice et al., 1998; Seddon et al., 2014; Kong et al., 2018; Birks, 2019). By comparing pollen analysis with quadrat investigations, our results unequivocally demonstrated that pollen signals can accurately reflect the major plant community composition and emphasize the significance of specific plant taxa. Consequently, pollen analysis in subtropical forests holds ecological significance as it bridges the gap between current and paleoecology to some extent, bolstering our confidence in studying long-term plant community and vegetation composition changes using fossil pollen in subtropical forests.
In agreement with previous pollen analyses in subtropical China (Liu et al., 2001; Yu et al., 2002; Zheng et al., 2002; Zheng et al., 2007; Zheng et al., 2008; Ding et al., 2011; Fang et al., 2015; Wan et al., 2020), pollen composition in surface soil from Jinhua Mountain closely aligns with modern vegetation, indicating that pollen signals vary according to different plant communities. The landscape of subtropical China is characterized by moderate mountainous and hilly terrain, where mountain vegetation exhibits distinct variations along altitude gradients. On Jinhua Mountain, both vegetation investigations and pollen analyses reveal vertical variations in plant composition signals. In summary, a higher prevalence of evergreen broad-leaved taxa has been observed in the lower regions, whereas cold-tolerant coniferous taxa tend to be dominated at higher elevations (Figure 2). This phenomenon corresponds to pollen analyses conducted on Tianmu Mountain in Zhejiang Province (Guo, 2021), Yushan Mountain in Taiwan (Yu et al., 2002), Xishan Mountain in Jiangxi Province (Li et al., 2016), and Shennongjia Mountain in Hubei Province (Liu et al., 2001). Pollen composition in surface soil effectively reflects the different vegetation communities, such as pine forests and Schima forests, and their geographic distribution closely mirrors that of actual vegetation. The prevalence of Pinus pollen, particularly in pine forests, is evident in nearly all the samples. Considering that Pin. taiwanness forest is situated at higher altitudes and that Pin. massoniana can also be found sporadically in other plant communities on Jinhua Mountain (Fan et al., 2019), the high presence of Pinus pollen in our study region is justified. This finding is consistent with previous researches indicating that a high proportion of Pinus pollen is indicative of pine forests or isolated occurrence within the study region (Li and Yao, 1990; Xu et al., 2007; Xiao et al., 2011). In contrast to Pinus pollen, a high content of Taxodiaceae pollen is primarily associated with the cedar forests (Figure 2). This may be attributed to both low pollen productivity and the limited dispersal ability of Cu. lanceolata within modern vegetation (Xiao et al., 2011; Li et al., 2018).
Furthermore, our results illustrate that pollen percentage data aligns with the IVs derived from quadrat-based surveys of different plant communities. Pollen percentages provide quantitative information about pollen composition, enabling complex numerical analyses to reveal potential dynamics and driving forces. Therefore, this method is the most convenient and widely applied approach for pollen analysis. In synecology, IV serves as a comprehensive quantitative indicator of a given taxon’s status and role in the community (Song, 2016), synthesizing relative density, dominance, and frequency (Curtis and Mclntosh, 1951). Owing to its simplicity and clarity, IV has been a common tool in community ecology for many years (Song, 2016). According to the protest results, there is a significant correlation between the pollen percentages and IVs (Figure 5). This correlation is reasonable because dominant and abundant taxa are more likely to produce a greater number of pollen grains than rare taxa within the community (Andersen, 1970). For example, Cr. japonica, a dominant and edificator species in JH4-JH5, corresponds well with the high Cryptomeria pollen percentage in the soil samples. Similarly, high IVs for Cu. lanceolata, Castanea, and Liquidambar are consistent with the elevated pollen percentages of Taxodiaceae, Castanea, and Liquidambar in the same quadrats. This suggests that the commonly used pollen percentage inherently preserves the quantitative characteristics of the source plants and can effectively reflect the importance of dominant taxa in the plant community.
It is essential to note the discrepancies between pollen composition and plant signals in our study, including overall compositional levels and individual numeric features (Figures 2, 5, 6). Our results revealed that plant taxa do not precisely align with pollen composition. This discrepancy is particularly evident for certain rare taxa such as Alangium Lam., Rubiaceae Juss., and Verbenaceae J. St.-Hil. Conversely, some pollen taxa, including Alnus, Salix, and Pterocarya, were absent in the plant investigation. We propose that these mismatches stem from the limitations of the quadrat area and dispersal ability of pollen (Andersen, 1970; Jackson and Kearsley, 1998). In subtropical China, the RSAP is estimated to range from approximately 290-500 m (Chen, 2019; Jiang et al., 2020; Guo, 2021). Consequently, a forest quadrat area of 30 × 30 m may not be sufficient to capture all existing taxa (Fan et al., 2019). Concerning individual numeric features, pollen percentages do not always align with the IVs for specific taxa. The taxa absent in the pollen data exhibited an average IV of 8, contrasting with an average percentage of 1.8% for unique pollen taxa not recorded in the plant investigation (Figure 6). Strikingly, Lauraceae Juss. possesses an average IV of 5.8 in 24 quadrats, yet its pollen is absent in the quadrat soils (Figure 6). Lauraceae is common in tropical and subtropical forests (Tan et al., 2023), and many plants in this family are insect-pollinated. In our dataset, the Lauraceae plants mainly comprise Lin. glauca, Lin. erythrocarpa, and Lin. Reflexa, all of which are insect-pollinated characterized by small, fragrant flowers with floral glands. Similarly, Sch. superba is a dominant species and edificator in evergreen broad-leaved forests on Jinhua Mountain, but its pollen percentage does not align with IVs (Figure 5). Like Lauraceae, Sch. superba is a xenogamous plant that is highly depended on insect pollinators for seed production (Bai et al., 2022). In contrast to Lauraceae and Schima pollen, Pinus is the dominant species in pine forests, yet its pollen can be found high percentages in nearly all samples. These observations underscore another critical issue: the different pollen dispersal strategies of plants might affect the relationship between pollen percentages and plant IVs (Ackerman, 2000). In subtropical and tropical forests, the high frequency of insect-pollinated taxa (Wang et al., 2023) can introduce substantial uncertainties in the relationship between pollen and vegetation, leading to the underestimation of insect-pollinated taxa, such as Lauraceae, in pollen analysis.
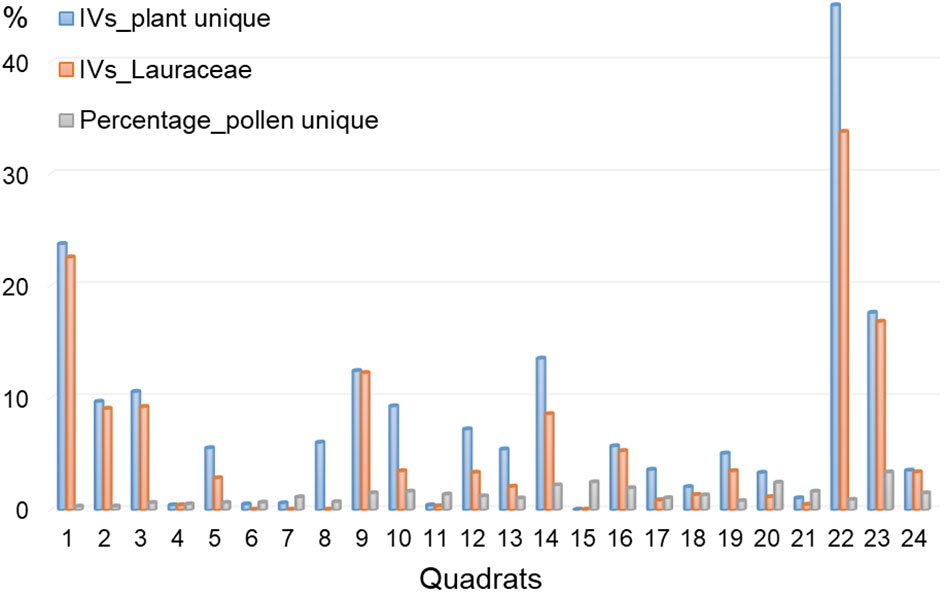
Figure 6 Unique plant IVs and pollen percentages in different quadrats revealing overall taxa mismatch between two data sets.
The application of recently published RPP and R values to pollen percentage data yielded limited improvements in our results. Fossil pollen grains preserved in soil and other archives are subject to varying pollen productivity, transportation processes, and preservation conditions, resulting in nonlinear relationships between pollen and vegetation, and uncertainties in pollen percentages when representing the importance of selected taxa (Sugita, 1994; Bunting et al., 2004). RPP estimations for selected taxa have been reported (Li et al., 2018; Wieczorek and Herzschuh, 2020), as have regional variations in pollen R values in China (Xia and Ni, 2024). We checked the RPP results from tropical and subtropical forests (Chen, 2019; Jiang et al., 2020; Guo, 2021). We found that applying these RPPs to Pinus, Castanea, Castanopsis/Lithocarpus, and Moraceae pollen percentage data led to changes and introduced additional biases. Given that pollen-plant abundance data were collected from regions with different forest compositions, this calibration should be avoided (Sugita, 1994). A similar situation arises with R values in China, which exhibit significant regional variability (Xia and Ni, 2024). As a result, we refrained from this calibration and focused on the raw pollen percentages. Nevertheless, our results indicated a significant consistency between pollen percentages and plant IVs (Figure 5), which may hold significance for pollen analysis across different vegetation types within a small region.
Despite the inherent uncertainties, the consistency between pollen percentages and plant IVs in subtropical forests from Jinhua Mountain is superior to that observed in open landscapes from the Northeast China Transect studied by Tan (2020). We propose that the main reason for this disparity lies in the different RPP and RSAP values of the two regions. For instance, Artemisia and Amaranthaceae Juss. (Chenopodiaceae) are indicative pollen taxa for typical grasslands in northern China, characterized by high RPPs and RSAPs that can extend over 1000 km (Xu et al., 2016; Cao et al., 2017). The influx of long-distance pollen into local pollen rain undoubtedly introduces bias into the relationship between pollen percentages and plant IVs Tan (2020). In the current study, the plant investigation data did not include herbaceous and fern species because of their limited coverage and biomass. The average percentages of herbaceous pollen and ferns in the pollen data are 0.1% and 1.6%, respectively. Therefore, we propose that our data exhibits regional representativeness for subtropical forests. Consequently, pollen analysis based on lake or mountain peat in subtropical China, which often maintains a high percentage of herbaceous pollen, may have underscored the development of local grasslands/wetlands while underestimating forest coverage.
In summary, our results demonstrated a significant correlation between pollen percentages and plant IVs, reinforcing the utility of pollen analysis in reflecting the ecological importance of specific taxa within a plant community. Discrepancies between pollen composition and plant signals exist, which probably stem from the limitations of the quadrat area and pollen dispersal ability. Despite inherent uncertainties, the consistency between pollen percentages and plant IVs in subtropical forests from Jinhua Mountain is superior to that observed in open landscapes. These relationships may also hold promise in paleoecology, where pollen analysis can be combined with geochemical and molecular information from natural archives to reconstruct ecological and evolutionary systems deep into the past.
Data availability statement
The original contributions presented in the study are included in the article/Supplementary Material. Further inquiries can be directed to the corresponding author.
Author contributions
KL: Formal analysis, Funding acquisition, Methodology, Writing – original draft, Writing – review & editing. BT: Investigation, Methodology, Writing – review & editing. ML: Writing – review & editing, Methodology. JN: Conceptualization, Funding acquisition, Supervision, Writing – review & editing.
Funding
The author(s) declare that financial support was received for the research, authorship, and/or publication of this article. National Natural Science Foundation of China (grant number. 31870462), key project of Zhejiang Normal University (grant number 2017PT009) and State Key Laboratory of Lake Science and Environment.
Acknowledgments
We appreciate the help of Zhaochen Kong and Yun Zhang from the Institute of Botany, Chinese Academy of Sciences, for their guidance on pollen identification.
Conflict of interest
The authors declare that the research was conducted in the absence of any commercial or financial relationships that could be construed as a potential conflict of interest.
Publisher’s note
All claims expressed in this article are solely those of the authors and do not necessarily represent those of their affiliated organizations, or those of the publisher, the editors and the reviewers. Any product that may be evaluated in this article, or claim that may be made by its manufacturer, is not guaranteed or endorsed by the publisher.
Supplementary material
The Supplementary Material for this article can be found online at: https://www.frontiersin.org/articles/10.3389/fpls.2024.1348182/full#supplementary-material
References
Ackerman, J. D. (2000). Abiotic pollen and pollination: ecological, functional and evolutionary perspectives. Plant Syst. Evol. 222, 167–185. doi: 10.1007/BF00984101
Andersen, S. T. (1970). The relative pollen productivity and pollen representation of north European trees, and correction factors for tree pollen spectra. Determined by surface pollen analyses from forests. Dan Geol Unders II Rskke. 96, 1–99. doi: 10.34194/raekke2.v96.6887
Bai, Q. S., He, B. X., Cai, Y. L., Lian, H. M., Zhang, Q., Lian, H. M., et al. (2022). Genetic diversity and population structure of Schima superba from southern China. Front. Ecol. Evol. 10. doi: 10.3389/fevo.2022.879512
Birks, H. J. B. (2019). Contributions of Quaternary botany to modern ecology and biogeography. Plant Eco Divers. 12, 189–385. doi: 10.1080/17550874.2019.1646831
Bunting, M. J., Gaillard, M. J., Sugita, S., Middleton, R., Broström, A. (2004). Vegetation structure and pollen source area. Holocene 14, 651–660. doi: 10.1191/0959683604hl744rp
Cao, X. Y., Tian, F., Telford, R. J., Ni, J., Xu, Q. H., Chen, F. H., et al. (2017). Impacts of the spatial extent of pollen-climate calibration-set on the absolute values, range and trends of reconstructed Holocene precipitation. Qua Sci. Rev. 178, 37–53. doi: 10.1016/j.quascirev.2017.10.030
Chen, H. (2019). Relevant source area of pollen and relative pollen productivities in Dabie Mountains. [master's thesis]. Hefei (AH): Anhui University. doi: 10.17521/cjpe.2021.0024
Chen, H. Y., Xu, D. Y., Liao, M. N., Li, K., Ni, J., Cao, X. Y., et al. (2021). A modern pollen dataset of China. Chin. J. Plant Ecology. 45, 799–808. doi: 10.17521/cjpe.2021.0024
Chevalier, M., Davis, B. A. S., Heiri, O., Seppä, H., Chase, B. M., Gajewski, K., et al. (2020). Pollen-based climate reconstruction techniques for late Quaternary studies. Earth-Sci Rev. 210, 103384. doi: 10.1016/j.earscirev.2020.103384
Cui, Q. Y., Zhao, Y., Qin, F., Liang, C., Li, Q., Geng, R. W. (2020). Characteristics of the modern pollen assemblages from different vegetation zones in Northeast China: Implications for pollen-based climate reconstruction. Sci. China: Earth Sci. 62, 1564–1577. doi: 10.1007/s11430-018-9386-9
Curtis, J. H., Mclntosh, R. P. (1951). An upland forest continuum in the prairie-forest border region of Wisconsin. Ecology 32, 476–496. doi: 10.2307/1931725
Davis, M. (1963). On the theory of pollen analysis. Am. J. Sci. 261, 897–912. doi: 10.2475/ajs.261.10.897
Ding, W., Pang, R. M., Xu, Q. H., Li, Y. C., Cao, X. Y. (2011). Surface pollen assemblages as indicators of human impact in the warm temperate hilly areas of eastern China. Chin. Sci. Bull. 56, 996–1004. doi: 10.1007/s11434-011-4350-1
Fan, H. D. (2020). Vegetation mapping and quantitative analysis of dominant plant communities on the south slope of Jinhua Mountain. [master’s thesis]. Jinhua (ZJ): Zhejiang Normal University
Fan, H. D., Chen, H. Y., Wu, Y. N., Liu, J. F., Xu, D. Y., Cao, J. Y., et al. (2019). Community characteristics of main vegetation types on the southern slope of Beishan Mountain in Jinhua, Zhejiang, China. Chin. J. Plant Ecology. 43, 921–928. doi: 10.17521/cjpe.2019.0114
Fang, Y. M., Ma, C. M., Sun, W. F. (2015). Differences of modern pollen spectra between the surface soils and the pollen traps in the hilly areas of Nanjing, Jiangsu Province. Acta Micropalaeontol. Sin. 32, 147–160. doi: 10.16087/j.cnki.1000-0674.2015.02.004
Guo, Y. L. (2021). Relevant source area of pollen and relative pollen productivity estimates of main plant in Tianmu Mountains. [master’s thesis.] Hefei (AH): Anhui University.
Hornick, T., Richter, A., Harpole, W. S., Bastl, M., Bohlmann, S., Bonn, A., et al. (2021). An integrative environmental pollen diversity assessment and its importance for the Sustainable Development Goals. Plants People Planet. 4, 110–121. doi: 10.1002/ppp3.10234
Jackson, S. T., Kearsley, J. B. (1998). Quantitative representation of local forest composition in forest-floor pollen assemblages. J. Ecol. 86, 474–490. doi: 10.1046/j.1365-2745.1998.00277.x
Jiang, F. Y., Xu, Q. H., Zhang, S. R., Li, F., Zhang, K., Wang, M., et al. (2020). Relative pollen productivities of the major plant taxa of subtropical evergreen–deciduous mixed woodland in China. J. Quaternary Science. 35, 526–538. doi: 10.1002/jqs.3197
Juggins, S. (2022) Package "rioja". Available online at: https://github.com/nsj3/rioja.
Kong, Z. C., Zhang, Y., Wang, L., Duan, X. H., Li, Y. M. (2018). The past, present and future of palynology in China: concentrate on Quaternary palynology. Chin. Sci. Bull. 63, 164–171. doi: 10.1360/N972017-00812
Li, F. R., Gaillard, M. J., Sugita, S., Mazier, F., Xu, Q. H., Zhou, Z. Z., et al. (2017). Relative pollen productivity estimates for major plant taxa of cultural landscapes in central eastern China. Veget Hist Archaeobot. 26, 587–605. doi: 10.1007/s00334-017-0636-9
Li, F. R., Gaillard, M. J., Xu, Q. H., Bunting, M. J., Li, Y. C., Li, J., et al. (2018). A review of relative pollen productivity estimates from temperate China for pollen-based quantitative reconstruction of past plant cover. Front. Plant Sci. 9. doi: 10.3389/fpls.2018.01214
Li, Y. Y., Ma, C. M., Fang, Y. M., Cui, A. N. (2016). Surface soil pollen in Xishan, Nanchang, Jiangxi Province, East China and its relationship with the vegetation pattern. Acta Micropalaeontol. Sin. 33, 138–151. doi: 10.16087/j.cnki.1000-0674.2016.02.003
Li, W. Y., Yao, Z. J. (1990). A study on the quantitive relationship between Pinus pollen in surface sample and Pinus vegetation. Acta Bot. Sin. 32, 943–950.
Liu, H. P., Tang, X. C., Pan, A. D., Sun, D. W., Wang, K. F. (2001). A study on the soil polynofloras of the south slope of Shennongjia, central China. Acta Sedimentol. Sin. 19, 107–112.
Oksanen, J., Simpson, G. L., Blanchet, F. G., Kindt, R. L., Legendre, P., Minchin, P. R., et al. (2022) Package "vegan" v2.6-4. Available online at: https://github.com/vegandevs/vegan.
Parsons, R. W., Prentice, I. C. (1981). Statistical approaches to R-values and the pollen— vegetation relationship. Rev. Palaeobot. Palynol. 32, 127–152. doi: 10.1016/0034-6667(81)90001-4
Peres-Neto, P. R., Jackson, D. A. (2001). How well do multivariate data sets match? The advantages of a Procrustean superimposition approach over the Mantel test. Oecologia 129, 169–178. doi: 10.1007/s004420100720
Prentice, I. C. (1985). Pollen representation, source area, and basin size: Toward a unified theory of pollen analysis. Quat. Res. 23, 76–86. doi: 10.1016/0033-5894(85)90073-0
Prentice, I. C., Harrison, S. P., Jolly, D., Guiot, J. (1998). The climate and biomes of Europe at 6000yr BP: comparison of model simulations and pollen-based reconstructions. Quat. Sci. Rev. 17, 659–668. doi: 10.1016/S0277-3791(98)00016-X
Prentice, I. C., Parsons, R. W. (1983). Maximum likelihood linear calibration of pollen spectra in terms of forest composition. Biometrics 39, 1051–1057. doi: 10.2307/2531338
Prentice, I. C., Webb, T., III (1986). Pollen percentages, tree abundances and the Fagerlind effect. J. Quat. Sci. 1, 35–43. doi: 10.1002/jqs.3390010105
R Core Team (2023). R: A language and environment for statistical computing (Vienna, Austria: R Foundation for Statistical Computing). Available at: http://www.r-project.org.
Seddon, A. W. R., Mackay, A. W., Baker, A. G., Birks, H. J. B., Breman, E., Buck, C. E., et al. (2014). Looking forward through the past: identification of 50 priority research questions in palaeoecology. J. Ecol. 102, 256–267. doi: 10.1111/1365-2745.12195
Sugita, S. (1994). Pollen representation of vegetation in Quaternary sediments: theory and method in patchy vegetation. J. Ecol. 82, 881–897. doi: 10.2307/2261452
Sugita, S. (2007a). Theory of quantitative reconstruction of vegetation I: pollen from large sites REVEALS regional vegetation composition. Holocene 17, 229–241. doi: 10.1177/0959683607075837
Sugita, S. (2007b). Theory of quantitative reconstruction of vegetation II: all you need is LOVE. Holocene 17, 243–257. doi: 10.1177/0959683607075838
Tan, B. (2020). Relationships between surface pollen and modern vegetation at quadrat scale: case studies in northeast China Transect and Beishan Mountain of Jinhua. [master’s thesis]. Jinhua (ZJ): Zhejiang Normal University.
Tan, C., Ferguson, D. K., Tang, Z., Yang, Y. (2023). Distribution and conservation of the lauraceae in China. Global Ecol. Conserv. 46, e02566. doi: 10.1016/j.gecco.2023.e02566
Wan, Q. C., Zhang, Y. Z., Huang, K. Y., Sun, Q. W., Zang, X., Gaillard, M., et al. (2020). Evaluating quantitative pollen representation of vegetation in the tropics: a case study on the Hainan Island, tropical China. Ecol. Indic. 114, 106297. doi: 10.1016/j.ecolind.2020.106297
Wang, Y. Y., Li, Y. C., Luo, A., Lyu, T., Freckleton, R. P., Dimitrov, D., et al. (2023). Evolutionary history and climate co-determine the geographical variation in pollination modes of angiosperms in China. Global Ecol. Biogeogr. 32, 2189–2198. doi: 10.1111/geb.13760
Warton, D. I., Wright, S. T., Wang, Y. (2012). Distance-based multivariate analyses confound location and dispersion effects. Methods Ecol. Evol. 3, 89–101. doi: 10.1111/j.2041-210X.2011.00127.x
Wieczorek, M., Herzschuh, U. (2020). Compilation of relative pollen productivity (RPP) estimates and taxonomically harmonised RPP datasets for single continents and Northern Hemisphere extratropics. Earth Syst. Sci. Data. 12, 3515–3528. doi: 10.5194/essd-12-3515-2020
Xia, J., Ni, J. (2024). Regional features of pollen R-values in China. Sci. China: Earth Sci. 54, 309–322. doi: 10.1007/s11430-022-1191-8
Xiao, X. Y., Shen, J., Wang, S. M. (2011). Spatial variation of modern pollen from surface lake sediments in Yunnan and southwestern Sichuan Province, China. Rev. Palaeobot. Palynol. 165, 224–234. doi: 10.1016/j.revpalbo.2011.04.001
Xu, Q. H., Li, Y. C., Yang, X. L., Zheng, Z. H. (2007). Quantitative relationship between pollen and vegetation in northern China. Sci. China Ser. D: Earth Sci. 50, 582–599. doi: 10.1007/s11430-007-2044-y
Xu, Q. H., Zhang, S. R., Gaillard, M. J., Li, M. Y., Cao, X. Y., Tian, T., et al. (2016). Studies of modern pollen assemblages for pollen dispersal- deposition- preservation process understanding and for pollen-based reconstructions of past vegetation, climate, and human impact: A review based on case studies in China. Quat. Sci. Rev. 149, 151–166. doi: 10.1016/j.quascirev.2016.07.017
Yu, G., Tang, L. Y., Yang, X. D., Ke, X. K., Harrison, S. P. (2001). Modern pollen samples from alpine vegetation on the Tibetan Plateau. Global Ecol. Biogeogr. 10, 503–519. doi: 10.1046/j.1466-822X.2001.00258.x
Yu, G., Xue, B., Liu, P. M., Li, Y. Z. (2002). Surface soil pollen and vegetation reconstruction in the vertical zone of mountainous vegetation in central and northern Taiwan. Chin. Sci. Bull. 47, 1663–1666. doi: 10.1360/csb2002-47-21-1663
Zhao, Y., Herzschuh, U., Li, Q. (2015). Complex vegetation responses to climate change on the Tibetan Plateau: a paleoecological perspective. Nat. Sci. Rev. 2, 400–402. doi: 10.1093/nsr/nwv057
Zheng, Z., Cour, P., Zou, H. P., Tan, Z. F. (2002). Modern pollen rain in Hainan Island, southern China: altitudinal pollen distribution in the tropical rain forest. Acta Palaeontol. Sin. 41, 487–496. doi: 10.3969/j.issn.0001-6616.2002.04.003
Zheng, Z., Huang, K. Y., Deng, W., Cour, P., Huang, C. X., Duzer, D., et al. (2007). Relationship between dust pollen dispersal patterns and current vegetation in continental-scale north-south sample zones in eastern China. Sci. China: Earth Sci. 37, 534–543. doi: 10.1360/zd2007-37-4-534
Zheng, Z., Huang, K. Y., Xu, Q. H., Lu, H. Y., Rachid, C., Luo, Y. L., et al. (2008). Climate indications of the geographic distribution of topsoil pollen versus established plants in China. Sci. China: Earth Sci. 38, 701–714. doi: 10.1360/zd2008-38-6-701
Zheng, Z., Ma, T., Roberts, P., Li, Z., Yue, Y. F., Peng, H. H., et al. (2021). Anthropogenic impacts on Late Holocene land-cover change and floristic biodiversity loss in tropical southeastern Asia. Proc. Natl. Acad. Sci. 118, e2022210118. doi: 10.1073/pnas.2022210118
Keywords: plant community, plant important value, pollen analysis, quadrat investigation, subtropical China
Citation: Li K, Tan B, Liao M and Ni J (2024) Quadrat soil pollen signal reflects plant important values in forests and shrublands from subtropical China. Front. Plant Sci. 15:1348182. doi: 10.3389/fpls.2024.1348182
Received: 02 December 2023; Accepted: 11 March 2024;
Published: 20 March 2024.
Edited by:
Lucian Copolovici, Aurel Vlaicu University of Arad, RomaniaReviewed by:
Ujjwal Layek, Rampurhat College, IndiaPrakash Karmakar, Vidyasagar University, India
Stoyan Vergiev, Technical University of Varna, Bulgaria
Copyright © 2024 Li, Tan, Liao and Ni. This is an open-access article distributed under the terms of the Creative Commons Attribution License (CC BY). The use, distribution or reproduction in other forums is permitted, provided the original author(s) and the copyright owner(s) are credited and that the original publication in this journal is cited, in accordance with accepted academic practice. No use, distribution or reproduction is permitted which does not comply with these terms.
*Correspondence: Jian Ni, bmlqaWFuQHpqbnUuZWR1LmNu