- 1Universidade Católica Portuguesa, CBQF - Centro de Biotecnologia e Química Fina – Laboratório Associado, Escola Superior de Biotecnologia, Porto, Portugal
- 2Ecological Sciences, James Hutton Institute, Dundee, United Kingdom
Legumes are essential to healthy agroecosystems, with a rich phytochemical content that impacts overall human and animal well-being and environmental sustainability. While these phytochemicals can have both positive and negative effects, legumes have traditionally been bred to produce genotypes with lower levels of certain plant phytochemicals, specifically those commonly termed as ‘antifeedants’ including phenolic compounds, saponins, alkaloids, tannins, and raffinose family oligosaccharides (RFOs). However, when incorporated into a balanced diet, such legume phytochemicals can offer health benefits for both humans and animals. They can positively influence the human gut microbiome by promoting the growth of beneficial bacteria, contributing to gut health, and demonstrating anti-inflammatory and antioxidant properties. Beyond their nutritional value, legume phytochemicals also play a vital role in soil health. The phytochemical containing residues from their shoots and roots usually remain in-field to positively affect soil nutrient status and microbiome diversity, so enhancing soil functions and benefiting performance and yield of following crops. This review explores the role of legume phytochemicals from a ‘one health’ perspective, examining their on soil- and gut-microbial ecology, bridging the gap between human nutrition and agroecological science.
1 Introduction
Legumes are a diverse group of plants grown worldwide and consumed as an essential source of food and feed for thousands of years, playing a vital role in developing agriculture and human civilization (Ferreira et al., 2021). Belonging to the Fabaceae family, they comprise over 19,500 species and 750 genera and account for the third largest family of flowering plants (Bruneau et al., 2013). Pulses are a subgroup of grain legumes harvested for dried grains, such as pea (Pisum sativum L.), lentil (Lens culinaris L.), chickpea (Cicer arietinum L.) and faba bean (Vicia faba L.). Cultivated by early civilizations, legumes spread worldwide providing a reliable source of balanced nutritional provision, as well as serving as key component of many traditional cuisines (Ferreira et al., 2021).
Nowadays, the importance of legumes for food security has not wavered, with the United Nations General Assembly declaring 2016 as the International Year of the Pulses and declaring World Pulses Day every February 10th thereafter (Calles et al., 2019). Legumes are valuable sources of protein, fiber, carbohydrates and fatty acids, regularly associated as key components of a healthy and balanced diet (Geraldo et al., 2022). Also, they contain several bioactive ‘non-nutrients’ often considered as toxins or ‘antifeedants’, that lately have been associated with beneficial bioactive properties for gut microbiome function and human health (Rodríguez-Daza et al., 2021; Geraldo et al., 2022). Research suggests that flavonoids, plant sterols, oligosaccharides and others phytochemicals can act as prebiotics, promoting the growth and activity of beneficial gut bacteria (Pei et al., 2020). Scientific evidence regarding the potential of pulses phytochemicals to improve digestive health, strengthening the immune system, and potentially lowering the risk of gastrointestinal disorders through increased consumption of grain legumes is expanding rapidly (Rodríguez-Daza et al., 2021).
In contrast to pulses, forage legumes are primarily cultivated to serve as animal feed, with the entire aboveground part of the plant used as a source of nutrition for livestock. This type of legumes is also gaining popularity as a pivotal crop to realise regenerative agriculture practices, where they are recognized for their potential to enhance soil functions and qualities, serving as integrated pest management tools thus reducing the need for pesticides. Forage legumes like alfalfa (Medicago sativa L.) and clover (Trifolium L. spp.) are typically harvested at an earlier stage before they reach full flowering and seeding. This stage results in these legumes having a higher fiber content (Capstaff and Miller, 2018) and various bioactive phytochemicals, including phenolic compounds (PC), polysaccharides, and phytoestrogens (Tedeschi et al., 2021; Horvat et al., 2022). In line with their impact on human health, these compounds have been reported to have positive effects on the health of ruminants’ digestive systems and also contribute to improved soil functions (Clemensen et al., 2022; Mei et al., 2022).
Grain and forage legumes alike play a key role in providing ecosystem services. Through symbiosis with specific types of soil bacteria contained within legume root nodules, often referred collectively as rhizobia (Calles et al., 2019), inert atmospheric di-nitrogen (N) gas is ‘fixed’ into biologically useful forms in a process called biological N fixation (BNF); the primary natural source of N-cycling to support ecological systems (Iannetta et al., 2016). Compared to long-term cereal-based cropping systems, which are critical contributors for greenhouse gases (GHGs) (Dutta et al., 2022), legumes have been shown to lower emissions (Rahman et al., 2022). Additionally, incorporating the wild range of legume phytochemicals via intercropping strategies can help enhance agroecosystem resilience through improved soil fertility, biodiversity and nutrient cycling (Chamkhi et al., 2022). Consequently, legumes have been identified as pivotal tools to enhance the intended transition towards ‘healthy soil’ by 2030 (European Soil Mission) (Fendrich et al., 2023). Despite this, the production of legumes in Europe remains limited and below the desired thresholds for optimal ecological function, with grain legumes accounting for less than 3% of arable land across the EU, and are mainly used for animal feed (Notz et al., 2023).
Moreover, to cater to the demand for intensive meat production, where highly digestible diets are desired (Squire et al., 2019), commercially significant legume crops are still targeted/bred to reduce their levels of specific non-nutritional phytochemicals (commonly termed ‘antifeedants’), despite the previously mentioned potential of such chemical compounds in crop and health-protection (Geraldo et al., 2022). Breeders seek to reduce non-nutritional factors in crops for several reasons. These compounds can contribute to bitterness and astringency (Robinson et al., 2019), affect colour stability (Huang et al., 2022), hinder food processing (Poljsak et al., 2021), and, in some cases, have allergenic or toxic effects (Kumar et al., 2013). Additionally, high phenolic levels in forage crops may impact livestock health and feed palatability (Lamy et al., 2011). By minimizing specific phytochemicals, breeders were aiming to enhance the overall quality, taste, and marketability of crops while addressing potential health concerns. Notwithstanding, this approach may inadvertently undermine the overall nutritional quality of legumes and soil health-promoting effects, as discussed in the present review. Furthermore, along with the removal of such phytochemicals, other desirable traits such as yield and seed size, may be compromised (Duraiswamy et al., 2023).
Legumes therefore epitomize the causal and functional processes which exist between human-, animal-, and environmental-health within the ‘One Health’ framework – and since they also play a natural and pivotal role mediating connected soil- and climate-based chemical- and water-cycles (Lerner and Berg, 2015). As this holistic approach acknowledges that the health of these domains is intricately linked; the ‘One Health’ concept is continually to gain traction while it emphasizes the need for more-collaborative efforts across disciplines to address complex health challenges (Gibbs, 2014). By recognizing the shared health vulnerabilities across different species, ecosystems and processes, One Health advocates for integrated solutions that account for such interconnectedness and with a view to ‘global health’ - aiming to achieve optimal health outcomes for all living beings while safeguarding aspects of biodiversity (i.e., including biochemical diversity), to help promote and enhance environmental sustainability and resilience (Mumford et al., 2023). Whether through enhanced gut microbiome function, or their potential to serve as favourable factors for soil microbiome diversity and soil processes to better soil-plant performance, legume phytochemicals act as crucial determining agents of ‘One Health’ in praxis.
In this review we aim to describe the qualitative profile of the highly diverse legume phytochemicals considering their biochemistry, as well as their biological and ecological significance. Scrutinising both soil and gut microbial ecology, this review critically evaluates the integration of legumes and their phytochemicals within agricultural (soil-based) systems and emphasizing their dual role as a staple in human diets and essential animal feed. Understanding legumes influence in these capacities becomes pivotal in advancing the realization of the overarching ‘One Health’ concept, underlining the interconnectedness of human, animal, and environmental health.
2 Legume phytochemicals, their biochemistry, and significance
Phytochemicals are biologically active compounds naturally produced in all plants. Notwithstanding the inference of such chemicals as ‘non-essential’, there has been an increasing interest in legumes due to their characterisation as a particularly rich source of such compounds (Tor-Roca et al., 2020). These specialised metabolites, alongside their impact on gut, soil ecology and considerable pharmaceutical importance (Eljounaidi and Lichman, 2020), can play various roles in the plants themselves. For instance, they support plant defence (Basu et al., 2022), might suppress the growth of competing plants, providing legumes with a competitive advantage (Al-Deliamy and Abdul-Ameer, 2023) and regulate certain stages of legume growth and development (Mohammed et al., 2019; Misra et al., 2023). Legumes present a wide variety of such phytochemicals and there are several ways in which these may be characterised and classified, whether by function, chemical structure, or functional groups. Main classes of such phytochemicals (Figure 1) include: oligosaccharides, phenolic compounds, carotenoids, alkaloids, terpenoids, saponins, phytosterols and others (Huang et al., 2016).
2.1 Phenolic compounds
One of the most notable and studied class of phytochemicals are phenolic compounds (PC), which from a chemical perspective are characterised by one or more hydroxyl groups (-OH) attached to an aromatic hydrocarbon group. Phenol (C6H6O) stands as the fundamental chemical structure on which this group (ranging from simple phenolics to complex and highly polymerized compounds) is based (Vermerris and Nicholson, 2006). Through LC-MS and GC-MS identification, PC were by far the most frequently described phytochemicals in legume varieties with phenolic acids, flavonoids and condensed tannins the primary sub-classes found in seeds (Tor-Roca et al., 2020).
Phenolic acids are relatively small molecules when compared to other PC such as flavonoids or tannins, and they are characterised by a phenol with a carboxylic acid functional group (Vermerris and Nicholson, 2006). They include hydroxybenzoic acid derivatives, gallic, vanillic and syringic acids and hydroxycinnamic acid derivatives such as caffeic, ferulic, p-coumaric and sinapic acids (Singh et al., 2017). Flavonoids, in turn, stand out as one of the most prevalent group of PC which is particularly abundant in various types of legumes (Tor-Roca et al., 2020). With two benzene rings linked together by a group of three carbons they are divided into anthocyanin- (coloured), and anthoxanthin- (colourless) compounds. Anthoxanthins are divided further into flavones, flavans, flavonols, and isoflavones, the latter almost exclusively found in leguminous plants (Mutha et al., 2021). Daidzein and genistein, major isoflavones present in soybean (Glycine max), have been linked to reduced risk of certain types of cancer, namely prostate (Adjakly et al., 2013), and breast cancer (Sohel et al., 2022).
Tannins are a class of compounds that have a wide structural diversity and share the ability to conjugate with polysaccharides and proteins. They are usually divided into two groups: hydrolysable- or condensed-tannins, with the former being able to undergo hydrolysis, and produce carbohydrates and phenolic acids. In contrast, condensed tannins are more stable and obtained through the condensation of flavan-3-ols (Hill, 2003). Like almost all PC, tannins have shown antioxidant properties and bioactive potential. Nevertheless, the complexes formed between tannin-protein can reduce protein digestibility and inactivate digestive enzymes, thus the perception of these compounds as (solely) ‘anti-nutritional’ factors (Samtiya et al., 2020). Regardless, it is important to assess their health benefits alongside possible undesired effects (Geraldo et al., 2022).
Lignans are part of a group of diphenolic compounds and have been extensively studied for their health benefits due to their steroid-analogous chemical structure (Senizza et al., 2020). They share some chemical and structural characteristics with lignin which is a phenolic polymer found in the cell walls of legumes and other plants, and it is the third most abundant biopolymer on Earth (Pérez et al., 2002; Vermerris and Nicholson, 2006). As a complex polymer possessing a cross-linked structure, lignin is relatively resistant to mineralisation or decomposition, and can be found in high concentrations in woody tissues, contributing to both plant- and soil-health (Frei, 2013).
The biological activities of PC are frequently centred on extractable phenolic compounds, meaning compounds that can be easily extracted from the matrix using water or organic solvents, or a mixture of these. Yet, it is now recognized that this approach overlooks the non-extractable PCs or bound phenolics - a considerable proportion of dietary polyphenolics, which are not extracted using traditional methods (Silva et al., 2018). Several legume crop species have been shown to possess high amounts of both PC and dietary fibre (polysaccharides) in the food and in-field residue components, which in turn leads to bound phenolics in their matrices. Legume PCs interact with each other and other compounds, such as carbohydrates, proteins, or lipids, through reversible noncovalent and mostly irreversible covalent interactions, impairing the bioavailability of these bound phenolics (Rocchetti et al., 2022).
2.2 Alkaloids
Alkaloids are a diverse group of chemicals that possess alkali-like properties and consist of at least one N atom within a heterocyclic ring structure. While not all legumes contain alkaloids, some species are known to produce these compounds, with significant contribution to plant growth and defence. For instance, faba beans and peas have residual alkaloids concentrations but, quinolizidine alkaloids are common and well-documented among commercially important lupin genotypes. They have garnered significant attention from farmers and breeders due to their potent bitter taste and potential toxicity at high doses (Pereira et al., 2022), which is why it is recommend they remain below the industry threshold of 0.02% (Boschin et al., 2008). Nevertheless, recent studies show that lupanine, one of the prevalent alkaloids of the genus Lupinus in both their foliage and their seed (Hill, 2003), has been shown to have anti-inflammatory and anti-cancer properties (Guerra-Ávila et al., 2023). Sparteine, another lupin alkaloid, has been associated with antiarrhythmic and anticonvulsant properties (Frick et al., 2017), showcasing the dual nature of alkaloids in legumes as both anti-nutritional factors and potential pharmaceutical agents.
2.3 Saponins
Saponins (a group of terpenoids) in legumes are present in the form of triterpenoid glycosides or steroids that have a variety of biological activities (Mustafa et al., 2022). Due to their surfactant character, they have the ability to produce foam, and are often responsible for the soapy taste and bitter flavour of some legume species (Shi et al., 2004). Some of the most studied saponins in legumes include soyasaponins, which are found in soybeans and other members of the legume family, such as lentil and pea (Tor-Roca et al., 2020). These compounds have been shown to have a range of biological activities, including cholesterol-lowering effects, anti-cancer properties, and anti-inflammatory effects (Guang et al., 2014). However, in addition to their bitter taste, saponins can be toxic when present in high concentrations and may exert adverse effects on nutrient absorption. This is primarily due to their capacity to inhibit metabolic and digestive enzymes, as well as their tendency to bind to essential nutrients like zinc (Samtiya et al., 2020), emphasising the trade-off that we have with most of these phytochemicals between positive and disadvantageous traits.
2.4 Phytosterols
Legumes are also a high dietary source of phytosterols (steroid compounds derived from plants), and these comprise the bulk of unsaponifiable substances found in plant lipids (Salehi et al., 2020). Structurally similar to cholesterol, they are thought to have cholesterol-lowering effects (Gupta et al., 2011), hence they are regarded as important dietary elements for upholding good cardiovascular health. Lentil hulls have been found to contain elevated concentrations of phytosterols, namely β-Sitosterol (Mustafa et al., 2022), which has potential as a notable drug of the future due to their action as a neuroprotective and chemopreventive agent (i.e., preventing, reversing of blocking invasive cancers) (Babu and Jayaraman, 2020).
2.5 Phytic acid
Phytic acid, also known as phytate, myo-inositol hexakisposphate or IP6, is present in several legumes but its concentration can vary greatly among pulses, with considerable discrepancies between high-phytic acid species as common bean or pea and low-phytic acid species, like faba bean and lentils (Sinkovič et al., 2023). Phytic acid can also complex both micro- and macro-elements, potentially leading to a reduction in the bioavailability of minerals, protein function, and ion cofactors to ensure proper enzymatic function (Shi et al., 2018). When consumed within the recommended daily doses, nonetheless, phytates have important health benefits, either chelating toxic metals or through anticarcinogenic properties and antioxidant activity (Geraldo et al., 2022). The majority of the phosphorus contained within seeds exists in the form of phytic acid (Gupta et al., 2015).
2.6 Raffinose family of oligosaccharides
Oligosaccharides from the raffinose family, also known as α-galactosides, have bonds between galactose residues ranging from one to six. In humans, the enzyme needed to break down these compounds is not found in the small intestine. As a result, consuming grains like fava beans and peas (Carbas et al., 2021), which contain these oligosaccharides, often leads to excessive quantities of intestinal gas, and stomach discomfort (Pereira et al., 2022). Despite these unwanted side effects, legume-derived raffinose family oligosaccharides are increasingly being included in pro- and pre-biotics. They also show potential in combating obesity and diabetes and preventing non-alcoholic fatty liver disease (Elango et al., 2022).
3 Soil- and gut microbes and functional diversity concept
Soil and gut microbiomes are complex and diverse ecosystems that play vital roles in various biogeochemical processes, while contributing significantly to both animal and human health. Soils represent the largest repository of organic matter and biological diversity on Earth, home to a vast array of microbial communities characterised by high functional diversity that play crucial roles in soil fertility, nutrient cycling, and plant health (Mishra et al., 2023). However, soil is a non-renewable natural bio-resource, that had to carry the relentless burden of intensified agricultural production to meet the rapid increase of the human population and rising consumption levels. With food demands estimated to increase up to 70% by 2050 (Giller et al., 2021), a shift from conventional or intensive high agrochemical input agriculture (which severely contributes to climate change through GHG production, eutrophication through N- and phosphorous-losses, including via soil loss, and soil function) to regenerative agriculture is of increased interest (Newton et al., 2020). Common regenerative agriculture practices include reduced synthetic (nitrogen) fertiliser use, adoption of intercropping systems (Brooker et al., 2015) and reduced tillage, well-known practices that are increasingly implemented. Nevertheless, soil microbiome manipulation in regenerative agriculture should be given due consideration as an underlying tool for providing various essential benefits to soil (Hermans et al., 2023).
On the other hand, gut microbiome refers to the diverse and active microbial communities inhabiting the gastrointestinal tract of humans and animals. Although the ratio is likely to vary among individuals, the gut microbiome constitutes a significant niche of overall human microbiota (Santhiravel et al., 2022), typically maintaining a 1:1 ratio with the number of human cells (Walker and Hoyles, 2023). Known for its high functional diversity, which is attributed to the multiplicity of microorganisms inhabiting, gut microbiome is key to human health, with a critical role in digestion, modulation of the immune system, barrier function against pathogens and metabolism (Sudheer et al., 2022).
The concept of ‘functional diversity’ within a microbiome encompasses the vast range of purposes that a community of microorganisms can perform, beyond simply the types of microbes that are present, since both soil and gut microbes’ function is crucial for maintaining the sustainability of natural ecosystems and the health of individuals, respectively (Blum et al., 2019), with certain aspects of this functional diversity of soil and gut microbes being impacted by legume phytochemicals in various ecological and physiological processes.
It should also be noted that the influence these distinct phytochemicals have on microbial ecology could be substantially different; and, even within the same class of compounds, mechanisms of action vary. Taking flavonoids as an example, an influential group of phytochemicals (Mutha et al., 2021), their influence on the gut microbiome appears to involve distinct mechanisms that differ from their effects on the soil microbiome. While in the soil, flavonoids promote rhizobia movement and enhance nodule organogenesis (Wang et al., 2022), their actions within the gut exhibit diverse pathways, fostering symbiotic relationships, modulating the growth of beneficial bacteria in the gut and potentially contributing to the overall health of the host organism (Pei et al., 2020). Such nuanced disparity underscores the multifunctionality of flavonoids, showcasing their differential impact on distinct microbial ecosystems. In the next section, we discuss the specific impacts of different fractions of phenolic compounds and other legume phytochemicals on soil and gut microbial communities.
3.1 Legume phytochemicals impact on the functional diversity of soil microbes
With agroecological outcomes ranging from local (impacts on soil health and fertility) to global scales (emission of greenhouse gases) (Berihu et al., 2023), the soil microbiome and its interaction with plants and their phytochemicals is of upmost importance, due to their potential to support sustainable and regenerative agriculture, and downstream food- and feed-systems.
The symbiotic relationship between legumes and soil bacteria is a well-established and vital component of sustainable agroecosystems. To study the rhizosphere, the soil zone influenced by plant roots, researchers use techniques such as DNA-based assays or transcriptomics to identify changes in microbial communities and gene expression. In addition, rhizobia are one of the most studied soil microbe types considering their role in initiating nodule formation in the roots (or stems) of legumes, where the aforementioned BNF occurs. This process involves a series of biochemically directed steps, which includes colonization and nodule organogenesis, where various phytochemicals come into play, with flavonoids being one example. When released by legume roots as component of their exudate, flavonoids can act as chemo-attractants, facilitating the movement of compatible rhizobia (Figure 2) towards the roots and enhancing the colonization and infection process, root nodule formation and so the efficiency of BNF (Cooper, 2004; Abdel-Lateif et al., 2012). Conversely, legume flavonoids may exhibit antimicrobial activities that influence the overall recruitment and functioning of the root microbiome. This negative effect on specific taxa recruitment, for example on parasitic nematodes, fungus, and pathogenic bacterial species (e.g. Pseudomonas mars-prunorum and P. phaseolicola) (Shah and Smith, 2020) highlights the complex interplay of these different mechanisms of action, underscoring the intricate relationship between legume phytochemicals and microbial populations in the soil. Additionally, flavonoids can even act as regulators of nodulation (nod genes) in rhizobia, which are essential for producing species-specific Nod factors. These factors, in turn, trigger specific changes in plant tissue, such as root cortical cell division and root hair curling, which are critical modifications for nodule organogenesis (Hassan and Mathesius, 2012). The ability to modulate certain microbial communities through flavonoid metabolism is an area of increasing interest. This research has potential applications, including extending nodulation to plants beyond legumes (Abdel-Lateif et al., 2012) or engineering plant phytochemicals to influence beneficial microbiomes (Wang et al., 2022).
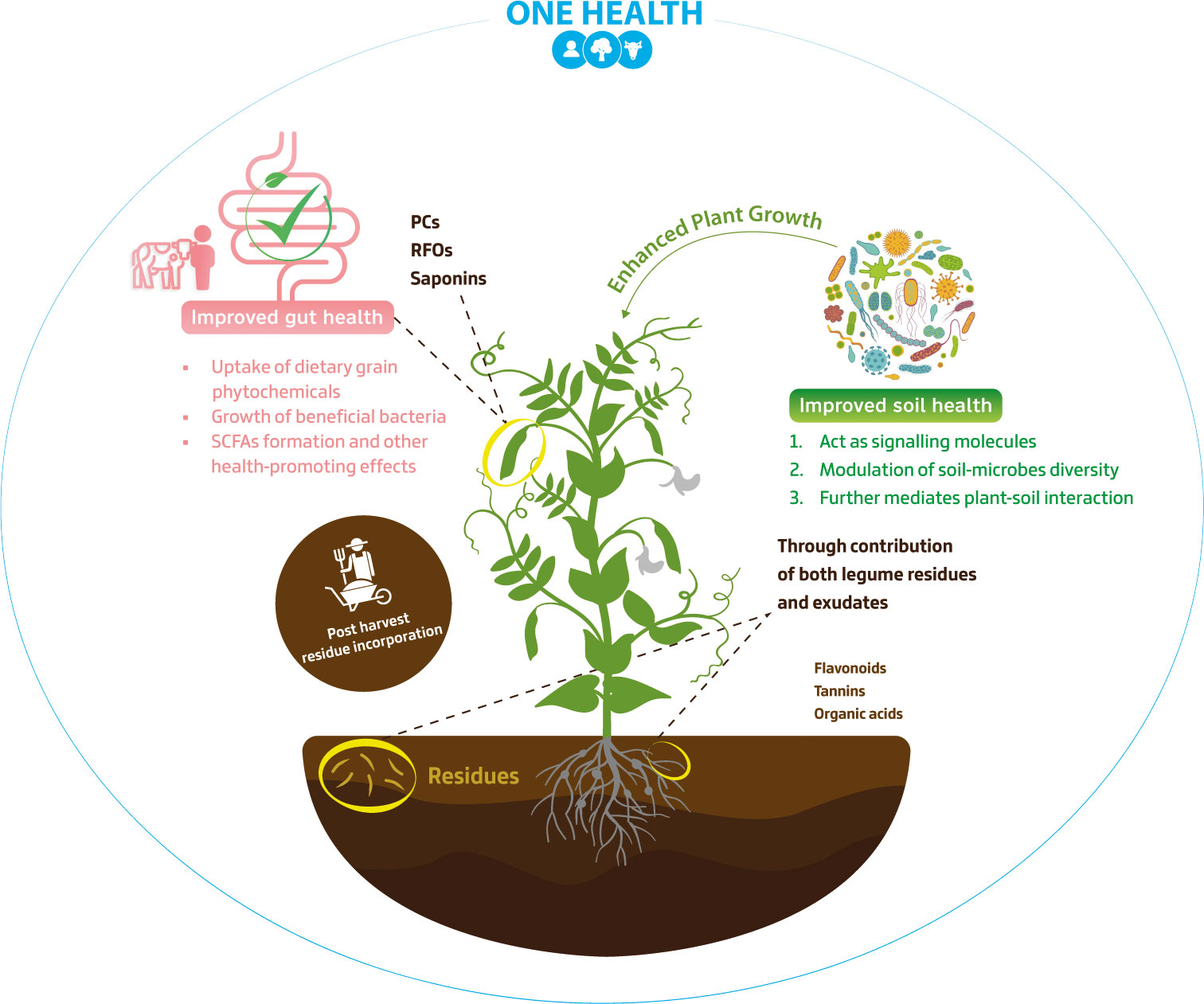
Figure 2 Soil and gut microbiomes as impacted by legume phytochemicals- One Health Approach. This figure explores the interplay between soil and gut microbiomes influenced by legume phytochemicals. The diverse impact of phytochemicals, e.g., phenolic compounds (PCs) and raffinose family oligosaccharides (RFOs), is depicted, encompassing factors such as short-chain fatty acids (SCFAs) production and exudate molecules influence on soil microbial diversity. Notably, ‘Post-harvest residue incorporation’ section emphasizes the significance of integrating legume residues into the soil after harvest, fostering microbial activity and nutrient recycling.
Phenolic acids are also commonly presented by legume roots as exudate, and may accumulate in soil, to be used as a source of carbon by several rhizobia species, influencing legume-microbe symbiosis (Seneviratne and Jayasinghearachchi, 2003). In some instances, specific taxa as Rhizobia and Pseudomonas, have been identified as capable of breaking down flavonoids compounds into simpler structures, namely phenolic acids (Wang et al., 2022). For example, protocatechuic acid was found to be responsible for the indirect allelopathic effects of catechin, a flavonoid (Wang et al., 2014). Research suggests that the accumulation of phenolic acids led to an increased relative abundance of Bacteroidetes and Firmicutes, which utilize these compounds as growth substrates (Bai et al., 2019), and have been correlated with detrimental impacts on soil micro-ecological environment. An increase in Pseudomonas population, on the other hand, was shown to promote plant growth while degrading phenolic acids present in the rhizosphere (Wang Y. et al., 2021). These findings collectively highlight that the accumulation of phenolic acids alters microbial community structure, even as the specific mechanism of influence and their consequential effects on soil ecology and plant growth remains unclear.
Although most research has focused on the regulation by flavonoid signalling rather than by phenolic acids, there is growing evince for phenolics as determinants of nodulation. A recent study based on ultra-high-performance liquid chromatography-tandem-quadrupole mass spectrometry has reported an increased plant growth and number of nodules in L. japonicus, due to the action of three phenolic acids (p-coumaric, caffeic, and ferulic) enhancing nod genes expression, thus being considered a novel type of nod inducers (Shimamura et al., 2022). The wide range of phenolic compound classes reflects their multifaceted impact on the soil microbial community, which is important to prevent or mitigate dysbiosis in soil microbiomes that would then affect plant yield and overall nutritional quality (Giovannetti et al., 2023). Given the close link between plant nutrition and human health (Hoffmann et al., 2022), this foreshadows the importance of legume phytochemicals with microbe-modulating properties to support the One Health approach (Figure 2).
Besides phenolics, organic acids are key determinants on soil microbiome structure and function. It was reported in a metagenomic approach that organic acid exudation by legume roots, when compared to wheat, explained 15% of the observed variance in rhizobacterial abundance in a microcosm (Schaedel et al., 2021). Small molecules like malic or oxalic acid, impact not only microbial activity, and are also capable of improving soil fertility too such as via mobilizing less-labile forms of P (Yu et al., 2021), promoting nutrient cycling, soil structure, and overall ecosystem resilience. Legume symbiosis with other soil microbes, such as arbuscular mycorrhizal fungi (AMF), is also highly influenced by specific molecules in root exudates. AMF are natural root symbionts, forming symbiotic relationships with a vast number of plants and helping to increase inorganic phosphorus acquisition (Wang et al., 2022). Such enhancement of root performance is in-turn helpful to nodules as they have high demand for energy (ATP-consuming), and so inorganic phosphate too. Some types of flavonoids are capable to stimulate AMF colonization, such is the case of quercetin (Tian et al., 2021) and isoflavones (daidzein and genistein) (Sugiyama, 2019), both present in root exudates, leading to an overall increase of plant biomass. Phenolic acids (Mandal et al., 2010) and strigolactones (group of sesquiterpenes) can also stimulate germination of spores and hyphal growth (Steinkellner et al., 2007), being another example of chemical-induced shifts in plant-microbial interactions leading to functional changes in the ecosystems.
Recent discoveries regarding soyasaponins, a type of saponin found in the root exudates of legume species, have highlighted their functional potential too. These compounds can disperse over greater distances in the rhizosphere, compared to isoflavones. They can influence bacterial communities by increasing the populations of bacteria that promote plant growth, such as Novosphingobium which induces the breakdown of organic matter in soil leading to the production of nutrients that plants can uptake via their roots (Fujimatsu et al., 2020). Phytochemicals, acting as antioxidants and metal chelators, also have the capacity to regulate microbial activity and soil properties, including the formation of soil organic matter and nutrient cycles (Vukicevich et al., 2016). For example, certain phytochemical-rich legume species, impact the availability of soil minerals by chelating and oxidizing/reducing metals. Isoflavonoids found in alfalfa can dissolve ferric phosphate, making both phosphate and iron accessible to the plant (Hassan and Mathesius, 2012). Tannins, on the other hand, have been shown to counteract the toxic effects of some metals through chelation, thereby positively influencing soil function and plant health (Halvorson et al., 2012). Conversely, alkaloids especially present in lupin crops can potentially have a detrimental environmental impact on plant-associated microbiota due to their mobility in soil environments. They have been recently identified as aquatic micropollutants (Günthardt et al., 2018; Hama et al., 2022).
Overall, the impact of legume phytochemicals on the soil microbiome structure and function can be complex and depend on the specific compounds produced by the legume, as well as the microbial populations present in the soil. Further research should prioritise the study of legume phytochemicals to better comprehend their influence on soil microbial relationships. Consequently, this understanding can enable the selection of select specific phytochemical-rich legumes that selectively promote the growth of beneficial microbial populations and/or soil functions of agroecosystems.
3.1.1 Legume phytochemicals impact as part of a residue management strategy
Currently, there is a growing emphasis on production systems that prioritise the sustainable management of natural resources to meet the needs of both present and future generations. This objective aligns the increased cultivation of cover crops, that is, species mixtures which usually include a high-density of legumes, sown before or after cash-crops, to cover and protect the soil between main harvest seasons, and serve as a plant-based manure (Kocira et al., 2020). By utilising cover crops effectively, European farmers can contribute to achieving the EU mission’s goals and fostering more sustainable and resilient agricultural systems (Fendrich et al., 2023). When applied efficiently legumes, such as clover, vetch, and alfalfa, are popular choices for cover cropping (Scavo et al., 2022), considering their lower C:N ratio and high levels of biomass produced. Nevertheless, legumes cover crops are more than merely good sources of bio-accumulated N for the following crop, as they present several phytochemicals that can impact the decomposition or mineralisation rates of their residues - and which overall improve serve as a ‘nature-based solution’ (Cohen-Shacham et al., 2016), to enhance soil health, crop-protection and increase productivity (Figure 2). For instance, tannins which are commonly found in forage legumes, are a group of polyphenolic compounds that can inhibit microbial decomposition, leading to a longer-lasting residue cover on the soil surface. This can offer protection against erosion, reduce weed growth, and enhance moisture retention (Halvorson et al., 2012; Erktan et al., 2017). Although not exclusive to the Fabaceae family, legumes show a greater lignin content than grasses, which also contributes to the persistence of residues in the soil and the aforementioned beneficial traits (Döring et al., 2013). Recently, cover crops residues (often called green manure) were also found to possess various antimicrobial secondary metabolites such as quercetin (a flavonoid) that help reduce the population of Escherichia coli in contaminated agricultural soils (Zhao et al., 2023) and although not applied on the soil saponins extract from soybean residues have shown a eco-friendly potential for washing pesticide residues off fresh-cut vegetables and fruits industry (Hsu et al., 2022).
Soil is a very complex system and the interplay between biotic and abiotic stresses has an enormous influence on how the regulation and signalling of these phytochemicals occurs. For instance, it has been established, that flavonoids present in root exudates and cover crops residues are critically involved in several different pathways that mediate plant-soil interaction. However, in soils where an excessive amount of organic matter accumulates, this can result in a dampening of flavonoid signalling, which in turn, can reduce important ecological processes like nodulation and overall plant growth (Del Valle et al., 2020).
In conclusion, the intricate dynamics within the complex soil system highlight the continued significance of legume phytochemicals study on microbiome modulation (Table 1). Moreover, these shifts on soil microbial diversity may result in a transmission of soil-endemic microbes, leading to a more diverse gut microbial structure and distinct bacterial composition in people closely associated with gardening activities, a primary source of soil contact, in contrast with a non-gardener group (Brown et al., 2022). As gut and the soil microbiome share similar bacteria phyla (Bacillota, Bacteroidota, Pseudomonadota, and Actinomycetota) and they can act interlinked on human health, it is important to avoid poor soil maintenance/practices, as healthy soils often lead to healthy guts (Blum et al., 2019).
3.2 Legume phytochemicals impact on the functional diversity of gut microbes
When viewed through the lens of the One Health approach (Figure 2), it is clear how legumes are key drivers of human, animal, and environmental health, emphasising that the health of one component directly impacts the others (Mackenzie and Jeggo, 2019). Nevertheless, for such a complex system to thrive, it has to be resilient enough to adapt long-term human interference (Rendon et al., 2020). Considering that, ‘functional redundancy’ has often been regarded as one of the promoters of ecological resilience, either through communities with redundant species (those that perform similar functions) (Biggs et al., 2020) or even metabolic networks that have evolved to have redundant genes for key metabolic pathways (Sambamoorthy and Raman, 2018). In this context, we propose that legume phytochemicals also exhibit a degree of redundancy, as it was already discussed that various classes of compounds fulfil analogous roles. This redundancy is crucial to the capacity of legumes and their phytochemicals to serve as a pivotal bridge to enable better choices to both environmental and human dietary concerns.
Acting within the intestine, legume phytochemicals modulate gut microbiota structure and function through several cellular signalling pathways, with absorption and metabolism by the gut microbiome being a complex topic and an area of active research (Sudheer et al., 2022). Taking PC or RFOs as examples, some are absorbed in the small intestine and enter the bloodstream, but many others (due to their complex chemical structure) pass to the colon where gut bacteria metabolize them. There, a variety of enzymes can break down the parent compounds into smaller molecules and intermediates that are more easily absorbed into the blood stream (Figure 2). These metabolites can have different biological activities compared to the original compounds, and some may have even stronger health-promoting effects (Santhiravel et al., 2022). In vitro models of the human gastrointestinal tract are key tools to simulate normal conditions, and study how these phytochemicals interact with gut microbes (Qi et al., 2023). Additionally, the utility of such models can be extended when allied with techniques such as high-throughput sequencing to discern the genetic basis of underpinning mechanisms of different phytochemicals. For instance, gut antioxidant function can be associated with both PC and carotenoids (Singh et al., 2017), while lignans and/or flavonoids can interact with hormonal receptors due to their hormone-like and anti-hormone effects (Liu et al., 2022). It is also important to mention that some phytochemicals, like phenolics (Manco et al., 2023), are more present in the hulls (i.e. seed coats of legume grains) compared to kernels (i.e. seeds without coats), which are isolated after dehulling (or decortication). The hulls are a coproduct which is often processed for use as a significant component of ruminant and pet-food diets (Halmemies-Beauchet-Filleau et al., 2018), but could contribute to a better health, considering the biological properties of their rich phytochemical profile.
3.2.1 Prebiotic effect
More than two decades ago, a certain group of non-digestible compounds (although selectively hydrolysed by gut microbes) were acknowledged for their capacity to enhance the microbiota and health of the host organism and became known as ‘prebiotics’ (Davani-Davari et al., 2019; Voss et al., 2022). Most prebiotics are also phytochemicals and the first recognised as such were oligosaccharides, as RFOs (Gibson et al., 2017). Raffinose family oligosaccharides, as well as sucrose, are the primary soluble carbohydrates present in legumes (Carbas et al., 2021; Elango et al., 2022). Their influence can be observed through various distinct effects and thanks to metabolomic analysis in specific, researchers have been able to identify and quantify such small molecules produced during the interaction of prebiotics with gut microbes (Rodríguez-Daza et al., 2021). Ciceritol, a common oligosaccharide of chickpea, was reported to enhance the growth of beneficial bacteria, such as Lactobacillus, Enterococcus and Bifidobacterium spp., while boosting the production of short chain fatty acids (SCFAs) (Zhang et al., 2017), key components of a healthy gut (Gomes et al., 2022). Similarly, lupin kernel RFOs such as stachyose and verbascose, have the potential to increase the Bifidobacterium population in the colon (Martínez-Villaluenga et al., 2005; Pereira et al., 2022). Note too, that there is often overlap between different phytochemical categories, and many compounds may have multiple and similar properties and functions in the body. Legumes intra-specific phytochemical diversity, home cooking, and industrial processing treatments also affect significantly in vitro digestibility of food- and feed-stuffs. So, it is important to consider these parameters when assessing gut microbiota structural shifts, and SCFAs production (Chen et al., 2020). Recently added to the prebiotic concept, PC also have the capacity to stimulate selective beneficial bacteria (Rodríguez-Daza et al., 2021), e.g. Eubacterium spp., Lactobacillus spp., Akkermansia spp., Bifidobacterium spp., Clostridium spp. and Bacteroidetes spp (Queiroz-Monici et al., 2005; Moreno-Indias et al., 2016; Lordan et al., 2020; Calderón-Pérez et al., 2021; Dong et al., 2022; Rocchetti et al., 2022; Rodríguez-Daza and de Vos, 2022).
3.2.2 Effect of dietary phytochemicals on disease
Good gut health relies on the optimal structure and functioning of its microbial community. The diverse range of microorganisms residing in the gut is acknowledged as a key factor in determining the level of risk from health conditions such as cancer (Hanahan, 2022), obesity (Liu et al., 2021), diabetes (Zhou et al., 2022), and inflammatory bowel diseases (Wang L. et al., 2021). As mentioned in the earlier section, phytochemicals have shown significant effectiveness in altering the composition of the intestinal microbiota, which in-turn affects an individual’s susceptibility to diseases and their response to them through various pathways. After changes in gut microbiome diversity (in terms of the balance and richness of different species), there’s also an impact on its function.
Compounds such as flavonoids, for instance, have been shown to increase the population density of Akkermansia muciniphila in the gut. This increase has been associated with protective effects against obesity development, and the improvement of the gut-blood barrier’s integrity (Juárez-Fernández et al., 2021). Similarly, the gut microbiota also plays a role in the production of equol, a metabolite of legume isoflavones, which is generated by bacteria such as Adlercreutzia equolifaciens and Bifidobacterium bifidum. Individuals who produce equol have been linked to better lipid metabolism and a lower prevalence of dyslipidaemias (high cholesterol) (Zheng et al., 2019), as well as protection against autoimmune encephalomyelitis (inflammation of the brain and spinal cord) (Jensen et al., 2021) and cardiovascular diseases (Pilšáková et al., 2010). Another isoflavone, genistein, is metabolised by gut bacteria and has been found to improve insulin signalling and reduce fat accumulation in the liver, a known risk factor for obesity and type 2 diabetes (Arunkumar et al., 2013). On the other hand, it has been reported that soy isoflavone-rich extracts do not significantly reduce lipid accumulation in cell cultures, but do seem to have anti-inflammatory potential by decreasing TNF-α (tumour necrosis factor alpha) and increasing IL-10 (an anti-inflammatory cytokine) (Angelotti et al., 2020).
Phytochemicals from various legumes, when processed by intestinal microbiota, can produce similar effects while generating different intermediate compounds. This is evident in the case of saponins. In lentil extracts, saponins are transformed into sapogenins, which have the ability to influence the growth of specific intestinal bacteria (Navarro del Hierro et al., 2020). On the other hand, soyasaponins, which are commonly found in soybean, have been observed to reduce inflammation caused by allergies by positively impacting the composition of the intestinal flora (Nagano et al., 2017). Additionally, other bioactive compounds, like β-sitosterol, a phytosterol commonly present in legume ingredients found in Mediterranean diets (Jiménez-Escrig et al., 2006), have demonstrated the capability to inhibit certain bacterial genera that possess the genes responsible for a major pathway leading to atherosclerosis (the build-up of lipids in arteries) (Wu et al., 2022). Plant sterols have also been associated with an increase in the abundance of Eubacterium hallii (Cuevas-Tena et al., 2018), an anaerobic bacterium currently classified as Anaerobutyricum hallii, known for producing butyrate, which has positive implications for insulin sensitivity treatments in obese and diabetic mice (Udayappan et al., 2016).
While lignans are typically classified as polyphenols, they have a chemical structure resembling steroids, akin to phytosterols. Consequently, they are referred to as natural phytoestrogens. The inherent bioactive potential of the parent compound is limited, but when certain bacteria capable of hydrolysing lignans, such as Lactobacillus spp. and Bifidobacterium spp., act on them, they are transformed into enterodiol and enterolactone. Enterolignans share structural similarities with estrogen steroid hormones and function as natural ligands for estrogen receptors. They have the potential to reduce LDL (low density lipoprotein) cholesterol levels and lower the risk of cardiovascular diseases (Creus-Cuadros et al., 2017; Senizza et al., 2020).
Phytochemicals may also have potential anticancer effects. Bacterial transformation of lignan was reported to alleviate tumour burden in a rat model of breast cancer (Mabrok et al., 2012) and inhibited the proliferation of prostate tumour cells (Sepporta et al., 2013), with underlying mechanisms requiring further investigation. Also, highlighting the protective impact of a diet rich in lignans, urinary enterolactone levels were inversely associated with ‘all-cause mortality’ (death due to any disease, complication, or hazardous exposure), in a prospective study conducted on over 6000 adults (Liu et al., 2020). However, impact on the functional diversity of gut microbiota lacks long-term human studies and follow-ups on short-term dietary intervention.
Overall, gut microbiome shifts related with the metabolism of phytochemicals have important implications for human health, since communication between different organs and the gut is paramount. Moreover legumes are a vast family of plants with an even wider range of phytochemicals and although there is some research in this area, a full evaluation of the genetic and phytochemical diversity offered by legume crops remains to be fully elaborated, with potentially phytochemical-rich underutilised crops or legume processing by-products also requiring more detailed investigation (Ebert, 2014). In fact, when we explore the extensive effects of legume dietary compounds on human health, it naturally prompts us to consider broader implications, including how these legume phytochemicals might have similar effects on the microbiomes of different animal species (Sherasia et al., 2018).
3.2.3 Animal gut microbiome
Livestock accounts for approximately 40% of the total value of agricultural production worldwide (Kulkarni et al., 2018) and despite new influx of public policies for the inclusion of legume grains as protein sources, the European Union still heavily relies on high protein grain imports to meet their livestock (and aquaculture) protein requirements (Jouan et al., 2020). For the last few decades, legume incorporation in animal feed forages was often discouraged due to their impact on animal gastrointestinal function via factors like tannins. Tannins, specially condensed tannins, are common molecules found in forage legumes and as previously discussed, play a significant role on astringency and bitter taste. Although, oral sensitivity in ruminant species is not well documented, this process can lead to a decrease in preference and eventually a reduction of forage consumption by some livestock species (Lamy et al., 2011). Moreover, the two most cultivated forage legumes, which happen to be low in tannins, white clover and lucerne, have been related with bloat1, a common digestive disorder in ruminants that causes damage to gut function (Caradus et al., 2022). On the positive side, legume species presenting high levels of tannin can inhibit the activity of rumen microorganisms and biofilm development, and slowing initial rates of digestion which is a distinguishing bloat-causing feature (Wang et al., 2012). Furthermore, tannins have the ability to reduce the negative impacts of gut parasites, such as for example gastrointestinal nematodes, by inhibiting the activity of key parasite enzymes (Mueller-Harvey et al., 2019). Legume tannins can also reduce methane emissions in agricultural systems directly or indirectly, by either inhibiting gut methanogens (methane producers) or/and protozoal (generates intermediates used by methanogens) populations (Ku-Vera et al., 2020; Caradus et al., 2022). Consequently, the ‘anti-nutritional’ view of phytochemicals (as is the case for tannins) is shifting to the point where they are now often considered beneficial for ecological and physiological wellbeing - reducing GHGs emissions and the impacts of climate change, and protecting ruminant health, when consumed in adequate amounts (Singh and Bhat, 2001).
Legume saponins have also been described as toxic to gut protozoa (Ramos-Morales et al., 2017) therefore lowering methane outputs. Additionally, flavonoids, well-known by now for their anti-inflammatory and antimicrobial properties, are believed to improve overall productivity and growth of ruminants (Ku-Vera et al., 2020). Most beneficial in periods of stress, flavonoids can also select against Gram-positive microorganisms leading to an increase in the production of propionate (compared to acetate), increasing the efficiency of ruminant digestion and so performance (Olagaray and Bradford, 2019).
These examples illustrate how legume phytochemicals are not only significant to human health but can also highly influence animal gut microbiome-ecology and -function (Table 2). However, more studies are needed to better understand the impact of other secondary metabolites, such as terpenes and alkaloids (Tedeschi et al., 2021).
4 Conclusions and future perspectives
In this comprehensive exploration of legume phytochemicals and their significant role in shaping the functional diversity of soil and gut microbes, we have elaborated on intricate functional interplay between plants, environment, plus human and farmed-animal health. These concepts are crucial not only for the One Health approach but also for achieving the goals of the European ‘Soil Mission’ (EuropeanComission, 2023). Legume phytochemicals, including saponins, flavonoids, other polyphenols, and RFOs, have a multifaceted impact. Notably, the impact of legume phytochemicals extends beyond the edible portion of the plant. Flavonoids released by legume roots function as signalling molecules, initiating a molecular dialogue that results in the formation of nodules and BNF. Furthermore, phytochemicals can shape the competitive landscape of soil microbiome, favouring certain microbial groups while inhibiting others. Some phytochemicals, like organic acids involved in nutrient cycling and tannins with their metal-chelating properties, enhance overall soil health and fertility. However, there are also examples of phytochemicals, such as alkaloids, that have the potential to negatively impact plant-associated microbiota.
From a dietary standpoint, legume phytochemicals can support beneficial gut bacteria like Bifidobacterium and Lactobacillus. This, in turn, leads to the production of SCFAs, such as acetate, propionate and butyrate, which contribute to maintaining gut health. In addition to their prebiotic potential, these compounds have anti-inflammatory properties and health-promoting effects that, when consumed as part of a balanced diet, can reduce the risk of various diseases, including gastrointestinal disorders.
While our understanding of the impact of legume phytochemicals on soil and gut microbes has greatly expanded, numerous challenges and avenues for future research remains. The complex dynamics of microbial communities in response to these compounds are not fully understood, and there is a need for investigations into the specific mechanisms through which legume phytochemicals influence human physiology. Additionally, there is a wealth of underutilised legume crops that have not been thoroughly explored, and which may possess richer phytochemical profiles which are crucial for tailoring agricultural and dietary strategies to influence microbial communities.
In conclusion, legume phytochemicals play a significant role in bridging the gap between agriculture, human and animal health. Moving forward, interdisciplinary collaboration among researchers, farmers, ecologists, and public health advocates will be essential to harness the full potential of these remarkable plant compounds for a sustainable and healthier future.
Author contributions
RD: Writing – original draft, Writing – review & editing. PI: Writing – review & editing. AG: Writing – review & editing. MV: Writing – original draft, Writing – review & editing.
Funding
The author(s) declare financial support was received for the research, authorship, and/or publication of this article. This research was supported by the European Union’s Horizon 2020 Research and Innovation Action (RIA) projects; ‘Realising Dynamic Value Chains for Underutilised Crops’ (RADIANT; www.radiantproject.eu), and ‘Valorising and balancing the ecosystem service benefits offered by legumes, and legume-based cropped systems’ (www.legumES-project.eu, Grant Agreement numbers 101000622 and 101135512, respectively. Also, by the Fundação para a Ciência e Tecnologia (FCT, Portugal) through PhD scholarship 2021.06831.BD. The James Hutton Institute (Scotland, UK) is supported by the Rural and Environment Science and Analytical Services (RESAS), a division of the Scottish Government. PPMI is also supported by: the EU Horizon Europe RIA, ‘ECOlogically sustainable NUTRIent management in agriculture aiming to prevent, mitigate and eliminate pollution in soils, water and air’ (www.econutriproject.eu, Grant Agreement number 101081858; and, ‘Nitrogen efficient plants for Climate Smart arable cropping systems’ (NCS) Project (http://bofin.org.uk/the-ncs-project/, Grant Agreement number 10043778. funded by DEFRA (UK Government’s Department for Environment, Food, and Rural Affairs, UK) under the InnovateUK Competition ‘Farming Futures R&D Fund, Climate Smart Farming’. We would also like to thank the scientific collaboration under the FCT project UIDB/50016/2020.
Conflict of interest
The authors declare that the research was conducted in the absence of any commercial or financial relationships that could be construed as a potential conflict of interest.
The author(s) declared that they were an editorial board member of Frontiers, at the time of submission. This had no impact on the peer review process and the final decision.
Publisher’s note
All claims expressed in this article are solely those of the authors and do not necessarily represent those of their affiliated organizations, or those of the publisher, the editors and the reviewers. Any product that may be evaluated in this article, or claim that may be made by its manufacturer, is not guaranteed or endorsed by the publisher.
Footnotes
- ^ Bloat is a condition which results from the complex interactions of feed qualities, rumen microbial diversity, and physiological (and genetic) aspects of the host. Factors leading to bloat include (high) soluble protein levels (in feed), digestion rates, microbial slime production in the rumen, and the passage rate of digested material (Wang, Y., Majak, W., & McAllister, T. A. (2012). Frothy bloat in ruminants: cause, occurrence, and mitigation strategies. Animal feed science and technology, 172(1-2), 103-114.
References
Abdel-Lateif, K., Bogusz, D., Hocher, V. (2012). The role of flavonoids in the establishment of plant roots endosymbioses with arbuscular mycorrhiza fungi, rhizobia and Frankia bacteria. Plant Signal Behav. 7, 636–641. doi: 10.4161/psb.20039
Adjakly, M., Ngollo, M., Boiteux, J. P., Bignon, Y. J., Guy, L., Bernard-Gallon, D. (2013). Genistein and daidzein: different molecular effects on prostate cancer. Anticancer Res. 33, 39–44.
Al-Deliamy, A. O. A., Abdul-Ameer, M. A. (2023). “The allelopathic effect of root exudates of mungbean and maize plants affected by metabiolic, fertilizer and mechanical stress factors on some indicators of germination of cowpea seeds,” in IOP Conference Series: Earth and Environmental Science (Mosul, Iraq: IOP Publishing Ltd). 1213, 012026. doi: 10.1088/1755-1315/1213/1/012026
Angelotti, J. A. F., Dias, F. F. G., Sato, H. H., Fernandes, P., Nakajima, V. M., Macedo, J. (2020). Improvement of aglycone content in soy isoflavones extract by free and immobilized B-glucosidase and their effects in lipid accumulation. Appl. Biochem. Biotechnol. 192, 734–750. doi: 10.1007/s12010-020-03351-5
Arunkumar, E., Karthik, D., Anuradha, C. V. (2013). Genistein sensitizes hepatic insulin signaling and modulates lipid regulatory genes through p70 ribosomal S6 kinase-1 inhibition in high-fat–high-fructose diet-fed mice. Pharm. Biol. 51, 815–824. doi: 10.3109/13880209.2013.766896
Babu, S., Jayaraman, S. (2020). An update on β-sitosterol: A potential herbal nutraceutical for diabetic management. Biomed. Pharmacother. 131, 110702. doi: 10.1016/j.biopha.2020.110702
Bai, Y., Wang, G., Cheng, Y., Shi, P., Yang, C., Yang, H., et al. (2019). Soil acidification in continuously cropped tobacco alters bacterial community structure and diversity via the accumulation of phenolic acids. Sci. Rep. 9, 12499. doi: 10.1038/s41598-019-48611-5
Basu, S., Lee, B. W., Clark, R. E., Bera, S., Casteel, C. L., Crowder, D. W. (2022). Legume plant defenses and nutrients mediate indirect interactions between soil rhizobia and chewing herbivores. Basic Appl. Ecol. 64, 57–67. doi: 10.1016/j.baae.2022.08.005
Berihu, M., Somera, T. S., Malik, A., Medina, S., Piombo, E., Tal, O., et al. (2023). A framework for the targeted recruitment of crop-beneficial soil taxa based on network analysis of metagenomics data. Microbiome 11, 8. doi: 10.1186/s40168-022-01438-1
Biggs, C. R., Yeager, L. A., Bolser, D. G., Bonsell, C., Dichiera, A. M., Hou, Z., et al. (2020). Does functional redundancy affect ecological stability and resilience? A review and meta-analysis. Ecosphere 11, e03184. doi: 10.1002/ecs2.3184
Blum, W. E. H., Zechmeister-Boltenstern, S., Keiblinger, K. M. (2019). Does soil contribute to the human gut microbiome? Microorganisms 7. doi: 10.3390/microorganisms7090287
Bonanomi, G., De Filippis, F., Cesarano, G., La Storia, A., Zotti, M., Mazzoleni, S., et al. (2019). Linking bacterial and eukaryotic microbiota to litter chemistry: Combining next generation sequencing with 13C CPMAS NMR spectroscopy. Soil Biol. Biochem. 129, 110–121. doi: 10.1016/j.soilbio.2018.11.013
Boschin, G., Annicchiarico, P., Resta, D., D’Agostina, A., Arnoldi, A. (2008). Quinolizidine alkaloids in seeds of lupin genotypes of different origins. J. Agric. Food Chem. 56, 3657–3663. doi: 10.1021/jf7037218
Brooker, R. W., Bennett, A. E., Cong, W.-F., Daniell, T. J., George, T. S., Hallett, P. D., et al. (2015). Improving intercropping: a synthesis of research in agronomy, plant physiology and ecology. New Phytol. 206, 107–117. doi: 10.1111/nph.13132
Brown, M. D., Shinn, L. M., Reeser, G., Browning, M., Schwingel, A., Khan, N. A., et al. (2022). Fecal and soil microbiota composition of gardening and non-gardening families. Sci. Rep. 12, 1595. doi: 10.1038/s41598-022-05387-5
Bruneau, A., Doyle, J. J., Herendeen, P., Hughes, C., Kenicer, G., Lewis, G., et al. (2013). Legume phylogeny and classification in the 21st century: Progress, prospects and lessons for other species–rich clades. TAXON 62, 217–248. doi: 10.12705/622.8
Calderón-Pérez, L., Llauradó, E., Companys, J., Pla-Pagà, L., Pedret, A., Rubió, L., et al. (2021). Interplay between dietary phenolic compound intake and the human gut microbiome in hypertension: A cross-sectional study. Food Chem. 344, 128567. doi: 10.1016/j.foodchem.2020.128567
Calles, T., Xipsiti, M., del Castello, R. (2019). Legacy of the international year of pulses. Environ. Earth Sci. 78, 124. doi: 10.1007/s12665-019-8106-6
Capstaff, N. M., Miller, A. J. (2018). Improving the yield and nutritional quality of forage crops. Front. Plant Sci. 9. doi: 10.3389/fpls.2018.00535
Caradus, J. R., Voisey, C. R., Cousin, G. R., Kaur, R., Woodfield, D. R., Blanc, A., et al. (2022). The hunt for the “holy grail”: Condensed tannins in the perennial forage legume white clover (Trifolium repens L.). Grass Forage Sci 77, 111–123. doi: 10.1111/gfs.12567
Carbas, B., MaChado, N., Pathania, S., Brites, C., Rosa, E. A. S., Barros, A. I. (2021). Potential of legumes: nutritional value, bioactive properties, innovative food products, and application of eco-friendly tools for their assessment. Food Rev. Int. 1-29, 1–12. doi: 10.1080/87559129.2021.1901292
Chamkhi, I., Cheto, S., Geistlinger, J., Zeroual, Y., Kouisni, L., Bargaz, A., et al. (2022). Legume-based intercropping systems promote beneficial rhizobacterial community and crop yield under stressing conditions. Ind. Crops Prod. 183, 114958. doi: 10.1016/j.indcrop.2022.114958
Chen, Y., Chang, S. K. C., Zhang, Y., Hsu, C.-Y., Nannapaneni, R. (2020). Gut microbiota and short chain fatty acid composition as affected by legume type and processing methods as assessed by simulated in vitro digestion assays. Food Chem. 312, 126040. doi: 10.1016/j.foodchem.2019.126040
Clemensen, A., Villalba, J. J., Lee, S., Provenza, F., Duke, S., Reeve, J. (2022). How do tanniferous forages influence soil processes in forage cropping systems? Crop Forage Turfgrass Manage. 8, e20166. doi: 10.1002/cft2.20166
Cohen-Shacham, E., Walters, G., Maginnis, S., Janzen, C. (2016). Nature-based Solutions to address global societal challenges (Switzerland: International Union for Conservation of Nature (IUCN)).
Cooper, J. E. (2004). “Multiple responses of rhizobia to flavonoids during legume root infection,” in Advances in Botanical Research (Wuhan, China: Academic Press), 1–62.
Creus-Cuadros, A., Tresserra-Rimbau, A., Quifer-Rada, P., Martínez-González, M. A., Corella, D., Salas-Salvadó, J., et al. (2017). Associations between both lignan and yogurt consumption and cardiovascular risk parameters in an elderly population: observations from a cross-sectional approach in the PREDIMED study. J. Acad. Nutr. Diet 117, 609–622.e601. doi: 10.1016/j.jand.2016.11.003
Cuevas-Tena, M., Gómez del Pulgar, E. M., Benítez-Páez, A., Sanz, Y., Alegría, A., Lagarda, M. J. (2018). Plant sterols and human gut microbiota relationship: An in vitro colonic fermentation study. J. Funct. Foods 44, 322–329. doi: 10.1016/j.jff.2018.03.023
Davani-Davari, D., Negahdaripour, M., Karimzadeh, I., Seifan, M., Mohkam, M., Masoumi, S. J., et al. (2019). Prebiotics: definition, types, sources, mechanisms, and clinical applications. Foods 8. doi: 10.3390/foods8030092
Del Valle, I., Webster, T. M., Cheng, H. Y., Thies, J. E., Kessler, A., Miller, M. K., et al. (2020). Soil organic matter attenuates the efficacy of flavonoid-based plant-microbe communication. Sci. Adv. 6, eaax8254. doi: 10.1126/sciadv.aax8254
Dong, L., Qin, C., Li, Y., Wu, Z., Liu, L. (2022). Oat phenolic compounds regulate metabolic syndrome in high fat diet-fed mice via gut microbiota. Food Biosci. 50, 101946. doi: 10.1016/j.fbio.2022.101946
Döring, T., Baddeley, J., Brown, R., Collins, R., Crowley, O., Cuttle, S., et al. (2013). Using legume-based mixtures to enhance the nitrogen use efficiency and economic viability of cropping systems (Edinburgh: Higher Education Press).
Duraiswamy, A., Sneha A., N. M., Jebakani K., S., Selvaraj, S., Pramitha J., L., Selvaraj, R., et al. (2023). Genetic manipulation of anti-nutritional factors in major crops for a sustainable diet in future. Front. Plant Sci. 13. doi: 10.3389/fpls.2022.1070398
Dutta, A., Trivedi, A., Nath, C. P., Gupta, D. S., Hazra, K. K. (2022). A comprehensive review on grain legumes as climate-smart crops: Challenges and prospects. Environ. Challenges 7, 100479. doi: 10.1016/j.envc.2022.100479
Ebert, A. W. (2014). Potential of underutilized traditional vegetables and legume crops to contribute to food and nutritional security, income and more sustainable production systems. Sustainability 6. doi: 10.3390/su6010319
Elango, D., Rajendran, K., van der Laan, L., Sebastiar, S., Raigne, J., Thaiparambil, N. A., et al. (2022). Raffinose family oligosaccharides: friend or foe for human and plant health? Front. Plant Sci. 13. doi: 10.3389/fpls.2022.829118
Eljounaidi, K., Lichman, B. R. (2020). Nature's chemists: the discovery and engineering of phytochemical biosynthesis. Front. Chem. 8. doi: 10.3389/fchem.2020.596479
Erktan, A., Balmot, J., Merino-Martín, L., Monnier, Y., Pailler, F., Coq, S., et al. (2017). Immediate and long-term effect of tannins on the stabilization of soil aggregates. Soil Biol. Biochem. 105, 197–205. doi: 10.1016/j.soilbio.2016.11.017
Fendrich, A. N., Matthews, F., Van Eynde, E., Carozzi, M., Li, Z., d'Andrimont, R., et al. (2023). From regional to parcel scale: A high-resolution map of cover crops across Europe combining satellite data with statistical surveys. Sci. Total Environ. 873, 162300. doi: 10.1016/j.scitotenv.2023.162300
Ferreira, H., Pinto, E., Vasconcelos, M. (2021). Legumes as a cornerstone of the transition toward more sustainable agri-food systems and diets in Europe. Front. Sustain. Food Syst. 5. doi: 10.3389/fsufs.2021.694121
Frei, M. (2013). Lignin: characterization of a multifaceted crop component. ScientificWorldJournal 2013, 436517. doi: 10.1155/2013/436517
Frick, K. M., Kamphuis, L. G., Siddique, K. H. M., Singh, K. B., Foley, R. C. (2017). Quinolizidine alkaloid biosynthesis in lupins and prospects for grain quality improvement. Front. Plant Sci. 8. doi: 10.3389/fpls.2017.00087
Fujimatsu, T., Endo, K., Yazaki, K., Sugiyama, A. (2020). Secretion dynamics of soyasaponins in soybean roots and effects to modify the bacterial composition. Plant Direct 4, e00259. doi: 10.1002/pld3.259
Geraldo, R., Santos, C. S., Pinto, E., Vasconcelos, M. W. (2022). Widening the perspectives for legume consumption: the case of bioactive non-nutrients. Front. Plant Sci. 13. doi: 10.3389/fpls.2022.772054
Gibbs, E. P. J. (2014). The evolution of One Health: a decade of progress and challenges for the future. Vet. Rec. 174, 85–91. doi: 10.1136/vr.g143
Gibson, G. R., Hutkins, R., Sanders, M. E., Prescott, S. L., Reimer, R. A., Salminen, S. J., et al. (2017). Expert consensus document: The International Scientific Association for Probiotics and Prebiotics (ISAPP) consensus statement on the definition and scope of prebiotics. Nat. Rev. Gastroenterol. Hepatol. 14, 491–502. doi: 10.1038/nrgastro.2017.75
Giller, K. E., Delaune, T., Silva, J. V., Descheemaeker, K., van de Ven, G., Schut, A. G. T., et al. (2021). The future of farming: Who will produce our food? Food Secur. 13, 1073–1099. doi: 10.1007/s12571-021-01184-6
Giovannetti, M., Salvioli di Fossalunga, A., Stringlis, I. A., Proietti, S., Fiorilli, V. (2023). Unearthing soil-plant-microbiota crosstalk: Looking back to move forward. Front. Plant Sci. 13. doi: 10.3389/fpls.2022.1082752
Gomes, S., Baltazar, F., Silva, E., Preto, A. (2022). Microbiota-derived short-chain fatty acids: new road in colorectal cancer therapy. Pharmaceutics 14. doi: 10.3390/pharmaceutics14112359
Guang, C., Chen, J., Sang, S., Cheng, S. (2014). Biological functionality of soyasaponins and soyasapogenols. J. Agric. Food Chem. 62, 8247–8255. doi: 10.1021/jf503047a
Guerra-Ávila, P. L., Guzmán, T. J., Domínguez-Rosales, J. A., García-López, P. M., Cervantes-Garduño, A. B., Wink, M., et al. (2023). Combined gamma conglutin and lupanine treatment exhibits in vivo an enhanced antidiabetic effect by modulating the liver gene expression profile. Pharmaceuticals 16, 117. doi: 10.3390/ph16010117
Günthardt, B. F., Hollender, J., Hungerbühler, K., Scheringer, M., Bucheli, T. D. (2018). Comprehensive toxic plants–phytotoxins database and its application in assessing aquatic micropollution potential. J. Agric. Food Chem. 66, 7577–7588. doi: 10.1021/acs.jafc.8b01639
Gupta, R. K., Gangoliya, S. S., Singh, N. K. (2015). Reduction of phytic acid and enhancement of bioavailable micronutrients in food grains. J. Food Sci. Technol. 52, 676–684. doi: 10.1007/s13197-013-0978-y
Gupta, A. K., Savopoulos, C. G., Ahuja, J., Hatzitolios, A. I. (2011). Role of phytosterols in lipid-lowering: current perspectives. QJM: Int. J. Med. 104, 301–308. doi: 10.1093/qjmed/hcr007
Halmemies-Beauchet-Filleau, A., Rinne, M., Lamminen, M., Mapato, C., Ampapon, T., Wanapat, M., et al. (2018). Review: Alternative and novel feeds for ruminants: nutritive value, product quality and environmental aspects. Animal 12, s295–s309. doi: 10.1017/S1751731118002252
Halvorson, J. J., Gollany, H. T., Kennedy, A. C., Hagerman, A. E., Gonzalez, J. M., Wuest, S. B. (2012). Sorption of tannin and related phenolic compounds and effects on extraction of soluble-N in soil amended with several carbon sources. Agriculture 2. doi: 10.3390/agriculture2010052
Hama, J. R., Jorgensen, D. B. G., Diamantopoulos, E., Bucheli, T. D., Hansen, H. C. B., Strobel, B. W. (2022). Indole and quinolizidine alkaloids from blue lupin leach to agricultural drainage water. Sci. Total Environ. 834, 155283. doi: 10.1016/j.scitotenv.2022.155283
Hanahan, D. (2022). Hallmarks of cancer: new dimensions. Cancer Discov. 12, 31–46. doi: 10.1158/2159-8290.CD-21-1059
Hassan, S., Mathesius, U. (2012). The role of flavonoids in root–rhizosphere signalling: opportunities and challenges for improving plant–microbe interactions. J. Exp. Bot. 63, 3429–3444. doi: 10.1093/jxb/err430
Hermans, S. M., Lear, G., Case, B. S., Buckley, H. L. (2023). The soil microbiome: An essential, but neglected, component of regenerative agroecosystems. iScience 26, 106028. doi: 10.1016/j.isci.2023.106028
Hill, G. D. (2003). “Plant antinutritional factors | Characteristics,” in Encyclopedia of Food Sciences and Nutrition. Ed. Caballero, B. (New Zealand: Oxford: Academic Press), 4578–4587.
Hoffmann, V., Paul, B., Falade, T., Moodley, A., Ramankutty, N., Olawoye, J., et al. (2022). A one health approach to plant health. CABI Agric. Biosci. 3, 62. doi: 10.1186/s43170-022-00118-2
Horvat, D., Viljevac Vuletić, M., Andrić, L., Baličević, R., Kovačević Babić, M., Tucak, M. (2022). Characterization of forage quality, phenolic profiles, and antioxidant activity in alfalfa (Medicago sativa L.). Plants 11, 2735. doi: 10.3390/plants11202735
Hsu, W.-H., Chen, S.-Y., Lin, J.-H., Yen, G.-C. (2022). Application of saponins extract from food byproducts for the removal of pesticide residues in fruits and vegetables. Food Control 136, 108877. doi: 10.1016/j.foodcont.2022.108877
Huang, P.-H., Cheng, Y.-T., Chan, Y.-J., Lu, W.-C., Li, P.-H. (2022). Effect of heat treatment on nutritional and chromatic properties of mung bean (Vigna radiata L.). Agronomy 12. doi: 10.3390/agronomy12061365
Huang, Y., Xiao, D., Burton-Freeman, B. M., Edirisinghe, I. (2016). “Chemical changes of bioactive phytochemicals during thermal processing,” in Reference Module in Food Science (Amsterdam: Elsevier).
Hwang, H. J., Lee, S. R., Yoon, J. G., Moon, H. R., Zhang, J., Park, E., et al. (2022). Ferulic acid as a protective antioxidant of human intestinal epithelial cells. Antioxid. (Basel) 11. doi: 10.3390/antiox11081448
Iannetta, P. P. M., Young, M., Bachinger, J., Bergkvist, G., Doltra, J., Lopez-Bellido, R. J., et al. (2016). A comparative nitrogen balance and productivity analysis of legume and non-legume supported cropping systems: the potential role of biological nitrogen fixation. Front. Plant Sci. 7. doi: 10.3389/fpls.2016.01700
Jensen, S. N., Cady, N. M., Shahi, S. K., Peterson, S. R., Gupta, A., Gibson-Corley, K. N., et al. (2021). Isoflavone diet ameliorates experimental autoimmune encephalomyelitis through modulation of gut bacteria depleted in patients with multiple sclerosis. Sci. Adv. 7, eabd4595. doi: 10.1126/sciadv.abd4595
Jiménez-Escrig, A., Santos-Hidalgo, A. B., Saura-Calixto, F. (2006). Common sources and estimated intake of plant sterols in the Spanish diet. J. Agric. Food Chem. 54, 3462–3471. doi: 10.1021/jf053188k
Jouan, J., Ridier, A., Carof, M. (2020). Legume production and use in feed: Analysis of levers to improve protein self-sufficiency from foresight scenarios. J. Clean. Prod. 274, 123085. doi: 10.1016/j.jclepro.2020.123085
Juárez-Fernández, M., Porras, D., Petrov, P., Román-Sagüillo, S., García-Mediavilla, M. V., Soluyanova, P., et al. (2021). The Synbiotic Combination of Akkermansia muciniphila and Quercetin Ameliorates Early Obesity and NAFLD through Gut Microbiota Reshaping and Bile Acid Metabolism Modulation. Antioxid. (Basel) 10. doi: 10.3390/antiox10122001
Kholif, A. E. (2023). A review of effect of saponins on ruminal fermentation, health and performance of ruminants. Vet. Sci. 10. doi: 10.3390/vetsci10070450
Kocira, A., Staniak, M., Tomaszewska, M., Kornas, R., Cymerman, J., Panasiewicz, K., et al. (2020). Legume cover crops as one of the elements of strategic weed management and soil quality improvement. A review. Agriculture 10. doi: 10.3390/agriculture10090394
Kulkarni, K. P., Tayade, R., Asekova, S., Song, J. T., Shannon, J. G., Lee, J.-D. (2018). Harnessing the potential of forage legumes, alfalfa, soybean, and cowpea for sustainable agriculture and global food security. Front. Plant Sci. 9. doi: 10.3389/fpls.2018.01314
Kumar, S., Verma, A. K., Das, M., Jain, S. K., Dwivedi, P. D. (2013). Clinical complications of kidney bean (Phaseolus vulgaris L.) consumption. Nutrition 29, 821–827. doi: 10.1016/j.nut.2012.11.010
Ku-Vera, J. C., Jiménez-Ocampo, R., Valencia-Salazar, S. S., Montoya-Flores, M. D., Molina-Botero, I. C., Arango, J., et al. (2020). Role of secondary plant metabolites on enteric methane mitigation in ruminants. Front. Vet. Sci. 7. doi: 10.3389/fvets.2020.00584
Lamy, E., Rawel, H., Schweigert, F. J., Capela, E. S. F., Ferreira, A., Costa, A. R., et al. (2011). The effect of tannins on Mediterranean ruminant ingestive behavior: the role of the oral cavity. Molecules 16, 2766–2784. doi: 10.3390/molecules16042766
Lerner, H., Berg, C. (2015). The concept of health in One Health and some practical implications for research and education: what is One Health? Infect. Ecol. Epidemiol. 5, 25300. doi: 10.3402/iee.v5.25300
Liu, B. N., Liu, X. T., Liang, Z. H., Wang, J. H. (2021). Gut microbiota in obesity. World J. Gastroenterol. 27, 3837–3850. doi: 10.3748/wjg.v27.i25.3837
Liu, F., Peng, Y., Qiao, Y., Huang, Y., Song, F., Zhang, M., et al. (2022). Consumption of flavonoids and risk of hormone-related cancers: a systematic review and meta-analysis of observational studies. Nutr. J. 21, 27. doi: 10.1186/s12937-022-00778-w
Liu, B., Rong, S., Sun, Y., Wallace, R., Snetselaar, L., Bao, W. (2020). Gut microbiota metabolites of dietary lignans and risk of all-cause and cause-specific mortality in adults. Curr. Developments Nutr. 4, nzaa061_067. doi: 10.1093/cdn/nzaa061_067
Lordan, C., Thapa, D., Ross, R. P., Cotter, P. D. (2020). Potential for enriching next-generation health-promoting gut bacteria through prebiotics and other dietary components. Gut Microbes 11, 1–20. doi: 10.1080/19490976.2019.1613124
Mabrok, H. B., Klopfleisch, R., Ghanem, K. Z., Clavel, T., Blaut, M., Loh, G. (2012). Lignan transformation by gut bacteria lowers tumor burden in a gnotobiotic rat model of breast cancer. Carcinogenesis 33, 203–208. doi: 10.1093/carcin/bgr256
Mackenzie, J. S., Jeggo, M. (2019). The one health approach-why is it so important? Trop. Med. Infect. Dis. 4. doi: 10.3390/tropicalmed4020088
Manco, A., Gerardi, C., Romano, G., D'Amico, L., Blanco, A., Milano, F., et al. (2023). Phenolic profile of whole seeds and seed fractions of lentils and its impact on antioxidant activity. Food Biosci. 54, 102887. doi: 10.1016/j.fbio.2023.102887
Mandal, S. M., Chakraborty, D., Dey, S. (2010). Phenolic acids act as signaling molecules in plant-microbe symbioses. Plant Signal Behav. 5, 359–368. doi: 10.4161/psb.5.4.10871
Martínez-Villaluenga, C., Frías, J., Vidal-Valverde, C., Gómez, R. (2005). Raffinose family of oligosaccharides from lupin seeds as prebiotics: application in dairy products. J. Food Prot 68, 1246–1252. doi: 10.4315/0362-028x-68.6.1246
Mei, L., Zhang, N., Wei, Q., Cao, Y., Li, D., Cui, G. (2022). Alfalfa modified the effects of degraded black soil cultivated land on the soil microbial community. Front. Plant Sci. 13. doi: 10.3389/fpls.2022.938187
Mishra, A., Singh, L., Singh, D. (2023). Unboxing the black box—one step forward to understand the soil microbiome: A systematic review. Microb. Ecol. 85, 669–683. doi: 10.1007/s00248-022-01962-5
Misra, D., Dutta, W., Jha, G., Ray, P. (2023). Interactions and regulatory functions of phenolics in soil-plant-climate nexus. Agronomy 13. doi: 10.3390/agronomy13020280
Mohammed, M., Jaiswal, S. K., Dakora, F. D. (2019). Insights into the Phylogeny, Nodule Function, and Biogeographic Distribution of Microsymbionts Nodulating the Orphan Kersting's Groundnut [Macrotyloma geocarpum (Harms) Marechal & Baudet] in African Soils. Appl. Environ. Microbiol. 85. doi: 10.1128/aem.00342-19
Moreno-Indias, I., Sánchez-Alcoholado, L., Pérez-Martínez, P., Andrés-Lacueva, C., Cardona, F., Tinahones, F., et al. (2016). Red wine polyphenols modulate fecal microbiota and reduce markers of the metabolic syndrome in obese patients. Food Funct. 7, 1775–1787. doi: 10.1039/c5fo00886g
Mueller-Harvey, I., Bee, G., Dohme-Meier, F., Hoste, H., Karonen, M., Kölliker, R., et al. (2019). Benefits of condensed tannins in forage legumes fed to ruminants: importance of structure, concentration, and diet composition. Crop Sci. 59, 861–885. doi: 10.2135/cropsci2017.06.0369
Mumford, E. L., Martinez, D. J., Tyance-Hassell, K., Cook, A., Hansen, G. R., Labonté, R., et al. (2023). Evolution and expansion of the One Health approach to promote sustainable and resilient health and well-being: A call to action. Front. Public Health 10. doi: 10.3389/fpubh.2022.1056459
Mustafa, A. M., Abouelenein, D., Acquaticci, L., Alessandroni, L., Angeloni, S., Borsetta, G., et al. (2022). Polyphenols, saponins and phytosterols in lentils and their health benefits: an overview. Pharm. (Basel) 15. doi: 10.3390/ph15101225
Mutha, R. E., Tatiya, A. U., Surana, S. J. (2021). Flavonoids as natural phenolic compounds and their role in therapeutics: an overview. Futur. J. Pharm. Sci. 7, 25. doi: 10.1186/s43094-020-00161-8
Nagano, T., Katase, M., Tsumura, K., Saito, M., Matsuda, T. (2017). Inhibitory effects of dietary soyasaponin on 2,4-dinitrofluorobenzene-induced contact hypersensitivity in mice. Exp. Dermatol. 26, 249–254. doi: 10.1111/exd.13205
Navarro del Hierro, J., Cueva, C., Tamargo, A., Núñez-Gómez, E., Moreno-Arribas, M. V., Reglero, G., et al. (2020). In vitro colonic fermentation of saponin-rich extracts from quinoa, lentil, and fenugreek. Effect on sapogenins yield and human gut microbiota. J. Agric. Food Chem. 68, 106–116. doi: 10.1021/acs.jafc.9b05659
Newton, P., Civita, N., Frankel-Goldwater, L., Bartel, K., Johns, C. (2020). What is regenerative agriculture? A review of scholar and practitioner definitions based on processes and outcomes. Front. Sustain. Food Syst. 4. doi: 10.3389/fsufs.2020.577723
Notz, I., Topp, C. F. E., Schuler, J., Alves, S., Gallardo, L. A., Dauber, J., et al. (2023). Transition to legume-supported farming in Europe through redesigning cropping systems. Agron. Sustain. Dev. 43, 12. doi: 10.1007/s13593-022-00861-w
Olagaray, K. E., Bradford, B. J. (2019). Plant flavonoids to improve productivity of ruminants – A review. Anim. Feed Sci. Technol. 251, 21–36. doi: 10.1016/j.anifeedsci.2019.02.004
Pei, R., Liu, X., Bolling, B. (2020). Flavonoids and gut health. Curr. Opin. Biotechnol. 61, 153–159. doi: 10.1016/j.copbio.2019.12.018
Pereira, A., Ramos, F., Sanches Silva, A. (2022). Lupin (Lupinus albus L.) seeds: balancing the good and the bad and addressing future challenges. Molecules 27. doi: 10.3390/molecules27238557
Pérez, J., Muñoz-Dorado, J., de la Rubia, T., Martínez, J. (2002). Biodegradation and biological treatments of cellulose, hemicellulose and lignin: an overview. Int. Microbiol. 5, 53–63. doi: 10.1007/s10123-002-0062-3
Pilšáková, L., Riečanský, I., Jagla, F. (2010). The physiological actions of isoflavone phytoestrogens. Physiol. Res. 59, 651–664. doi: 10.33549/physiolres.931902
Poljsak, B., Kovač, V., Milisav, I. (2021). Antioxidants, food processing and health. Antioxid. (Basel) 10. doi: 10.3390/antiox10030433
Qi, Y., Yu, L., Tian, F., Zhao, J., Zhai, Q. (2023). In vitro models to study human gut-microbiota interactions: Applications, advances, and limitations. Microb. Res. 270, 127336. doi: 10.1016/j.micres.2023.127336
Queiroz-Monici, K., Costa, G. E. A., da Silva, N., Reis, S. M. P. M., de Oliveira, A. C. (2005). Bifidogenic effect of dietary fiber and resistant starch from leguminous on the intestinal microbiota of rats. Nutrition 21, 602–608. doi: 10.1016/j.nut.2004.09.019
Rahman, M. M., Alam, M. S., Islam, M. M., Kamal, M. Z. U., Rahman, G. K. M. M., Haque, M. M., et al. (2022). “Chapter 20 - Potential of legume-based cropping systems for climate change adaptation and mitigation,” in Advances in Legumes for Sustainable Intensification. Eds. Meena, R. S., Kumar, S. (Cambridge, Massachusetts: Academic Press), 381–402.
Ramos-Morales, E., de la Fuente, G., Duval, S., Wehrli, C., Bouillon, M., Lahmann, M., et al. (2017). Antiprotozoal effect of saponins in the rumen can be enhanced by chemical modifications in their structure. Front. Microbiol. 8. doi: 10.3389/fmicb.2017.00399
Rendon, P., Steinhoff-Knopp, B., Saggau, P., Burkhard, B. (2020). Assessment of the relationships between agroecosystem condition and the ecosystem service soil erosion regulation in Northern Germany. PloS One 15, e0234288. doi: 10.1371/journal.pone.0234288
Robinson, G. H. J., Balk, J., Domoney, C. (2019). Improving pulse crops as a source of protein, starch and micronutrients. Nutr. Bull. 44, 202–215. doi: 10.1111/nbu.12399
Rocchetti, G., Gregorio, R. P., Lorenzo, J. M., Barba, F. J., Oliveira, P. G., Prieto, M. A., et al. (2022). Functional implications of bound phenolic compounds and phenolics–food interaction: A review. Compr. Rev. Food Sci. Food Saf. 21, 811–842. doi: 10.1111/1541-4337.12921
Rodríguez-Daza, M. C., de Vos, W. M. (2022). Polyphenols as drivers of a homeostatic gut microecology and immuno-metabolic traits of Akkermansia muciniphila: from mouse to man. Int. J. Mol. Sci. 24. doi: 10.3390/ijms24010045
Rodríguez-Daza, M. C., Pulido-Mateos, E. C., Lupien-Meilleur, J., Guyonnet, D., Desjardins, Y., Roy, D. (2021). Polyphenol-mediated gut microbiota modulation: toward prebiotics and further. Front. Nutr. 8. doi: 10.3389/fnut.2021.689456
Salehi, B., Quispe, C., Sharifi-Rad, J., Cruz-Martins, N., Nigam, M., Mishra, A. P., et al. (2020). Phytosterols: from preclinical evidence to potential clinical applications. Front. Pharmacol. 11. doi: 10.3389/fphar.2020.599959
Sambamoorthy, G., Raman, K. (2018). Understanding the evolution of functional redundancy in metabolic networks. Bioinformatics 34, i981–i987. doi: 10.1093/bioinformatics/bty604
Samtiya, M., Aluko, R. E., Dhewa, T. (2020). Plant food anti-nutritional factors and their reduction strategies: an overview. Food Prod. Process. Nutr. 2, 6. doi: 10.1186/s43014-020-0020-5
Santhiravel, S., Bekhit, A. E. A., Mendis, E., Jacobs, J. L., Dunshea, F. R., Rajapakse, N., et al. (2022). The impact of plant phytochemicals on the gut microbiota of humans for a balanced life. Int. J. Mol. Sci. 23. doi: 10.3390/ijms23158124
Scavo, A., Fontanazza, S., Restuccia, A., Pesce, G. R., Abbate, C., Mauromicale, G. (2022). The role of cover crops in improving soil fertility and plant nutritional status in temperate climates. A review. Agron. Sustain. Dev. 42, 93. doi: 10.1007/s13593-022-00825-0
Schaedel, M., Hidrobo, G., Grossman, J. (2021). From microns to meters: exploring advances in legume microbiome diversity for agroecosystem benefits. Front. Sustain. Food Syst. 5. doi: 10.3389/fsufs.2021.668195
Seneviratne, G., Jayasinghearachchi, H. S. (2003). Phenolic acids: Possible agents of modifying N2-fixing symbiosis through rhizobial alteration? Plant Soil 252, 385–395. doi: 10.1023/A:1024725511783
Senizza, A., Rocchetti, G., Mosele, J. I., Patrone, V., Callegari, M. L., Morelli, L., et al. (2020). Lignans and gut microbiota: an interplay revealing potential health implications. Molecules 25. doi: 10.3390/molecules25235709
Sepporta, M. V., Mazza, T., Morozzi, G., Fabiani, R. (2013). Pinoresinol inhibits proliferation and induces differentiation on human HL60 leukemia cells. Nutr. Cancer 65, 1208–1218. doi: 10.1080/01635581.2013.828089
Shah, A., Smith, D. L. (2020). Flavonoids in agriculture: chemistry and roles in, biotic and abiotic stress responses, and microbial associations. Agronomy 10. doi: 10.3390/agronomy10081209
Sherasia, P. L., Garg, M. R., Bhanderi, B. M. (2018). Pulses and their By-Products as Animal Feed (United Nations: FAO).
Shi, L., Arntfield, S. D., Nickerson, M. (2018). Changes in levels of phytic acid, lectins and oxalates during soaking and cooking of Canadian pulses. Food Res. Int. 107, 660–668. doi: 10.1016/j.foodres.2018.02.056
Shi, J., Arunasalam, K., Yeung, D., Kakuda, Y., Mittal, G., Jiang, Y. (2004). Saponins from edible legumes: chemistry, processing, and health benefits. J. Med. Food 7, 67–78. doi: 10.1089/109662004322984734
Shimamura, M., Kumaki, T., Hashimoto, S., Saeki, K., Ayabe, S. I., Higashitani, A., et al. (2022). Phenolic acids induce nod factor production in Lotus japonicus-mesorhizobium symbiosis. Microbes Environ. 37. doi: 10.1264/jsme2.ME21094
Silva, S., Oliveira, A. L. S., Ribeiro, T., Madureira, A. R., Pintado, M. M. (2018). “Non-extractable phenolic compounds as food ingredients,” in Non-extractable Polyphenols and Carotenoids: Importance in Human Nutrition and Health. Eds. Saura-Calixto, F., Pérez-Jiménez, J. (London: The Royal Society of Chemistry).
Singh, B., Bhat, T. (2001). Tannins revisited-changing perceptions of their effects on animal system. Anim. Nutr. Feed Technol. 1, 3–18.
Singh, B., Singh, J. P., Kaur, A., Singh, N. (2017). Phenolic composition and antioxidant potential of grain legume seeds: A review. Food Res. Int. 101, 1–16. doi: 10.1016/j.foodres.2017.09.026
Sinkovič, L., Pipan, B., Šibul, F., Nemeš, I., Tepić Horecki, A., Meglič, V. (2023). Nutrients, phytic acid and bioactive compounds in marketable pulses. Plants 12. doi: 10.3390/plants12010170
Sohel, M., Biswas, P., Al Amin, M., Hossain, M. A., Sultana, H., Dey, D., et al. (2022). Genistein, a Potential Phytochemical against Breast Cancer Treatment-Insight into the Molecular Mechanisms. Processes 10, 415. doi: 10.3390/pr10020415
Squire, G. R., Quesada, N., Begg, G. S., Iannetta, P. P. M. (2019). Transitions to greater legume inclusion in cropland: Defining opportunities and estimating benefits for the nitrogen economy. Food Energy Secur. 8, e00175. doi: 10.1002/fes3.175
Steinkellner, S., Lendzemo, V., Langer, I., Schweiger, P., Khaosaad, T., Toussaint, J.-P., et al. (2007). Flavonoids and strigolactones in root exudates as signals in symbiotic and pathogenic plant-fungus interactions. Mol. (Basel Switzerland) 12, 1290–1306. doi: 10.3390/12071290
Sudheer, S., Gangwar, P., Usmani, Z., Sharma, M., Sharma, V. K., Sana, S. S., et al. (2022). Shaping the gut microbiota by bioactive phytochemicals: An emerging approach for the prevention and treatment of human diseases. Biochimie 193, 38–63. doi: 10.1016/j.biochi.2021.10.010
Sugiyama, A. (2019). The soybean rhizosphere: Metabolites, microbes, and beyond—A review. J. Adv. Res. 19, 67–73. doi: 10.1016/j.jare.2019.03.005
Tedeschi, L. O., Muir, J. P., Naumann, H. D., Norris, A. B., Ramírez-Restrepo, C. A., Mertens-Talcott, S. U. (2021). Nutritional aspects of ecologically relevant phytochemicals in ruminant production. Front. Vet. Sci. 8. doi: 10.3389/fvets.2021.628445
Tian, B., Pei, Y., Huang, W., Ding, J., Siemann, E. (2021). Increasing flavonoid concentrations in root exudates enhance associations between arbuscular mycorrhizal fungi and an invasive plant. ISME J. 15, 1919–1930. doi: 10.1038/s41396-021-00894-1
Tor-Roca, A., Garcia-Aloy, M., Mattivi, F., Llorach, R., Andres-Lacueva, C., Urpi-Sarda, M. (2020). Phytochemicals in legumes: A qualitative reviewed analysis. J. Agric. Food Chem. 68, 13486–13496. doi: 10.1021/acs.jafc.0c04387
Udayappan, S., Manneras-Holm, L., Chaplin-Scott, A., Belzer, C., Herrema, H., Dallinga-Thie, G. M., et al. (2016). Oral treatment with Eubacterium hallii improves insulin sensitivity in db/db mice. NPJ Biofilms Microb. 2, 16009. doi: 10.1038/npjbiofilms.2016.9
Vermerris, W., Nicholson, R. (2006). “Families of phenolic compounds and means of classification,” in Phenolic Compound Biochemistry. Eds. Vermerris, W., Nicholson, R. (New York City: Springer), 1–34.
Voss, G. B., MaChado, D., Barbosa, J. C., Campos, D. A., Gomes, A. M., Pintado, M. (2022). “Chapter9 - Interplay between probiotics and prebiotics for human nutrition and health,” in Probiotics for Human Nutrition in Health and Disease. Eds. Leite de Souza, E., de Brito Alves, J. L., Fusco, V. (Amsterdam: Academic Press), 231–254.
Vukicevich, E., Lowery, T., Bowen, P., Úrbez-Torres, J. R., Hart, M. (2016). Cover crops to increase soil microbial diversity and mitigate decline in perennial agriculture. A review. Agron. Sustain. Dev. 36, 48. doi: 10.1007/s13593-016-0385-7
Walker, A. W., Hoyles, L. (2023). Human microbiome myths and misconceptions. Nat. Microbiol. 8, 1392–1396. doi: 10.1038/s41564-023-01426-7
Wang, L., Chen, M., Lam, P.-Y., Dini-Andreote, F., Dai, L., Wei, Z. (2022). Multifaceted roles of flavonoids mediating plant-microbe interactions. Microbiome 10, 233. doi: 10.1186/s40168-022-01420-x
Wang, L., Gao, M., Kang, G., Huang, H. (2021). The potential role of phytonutrients flavonoids influencing gut microbiota in the prophylaxis and treatment of inflammatory bowel disease. Front. Nutr. 8. doi: 10.3389/fnut.2021.798038
Wang, C.-M., Li, T.-C., Jhan, Y.-L., Weng, J.-H., Chou, C.-H. (2014). The impact of microbial biotransformation of catechin in enhancing the allelopathic effects of Rhododendron formosanum. PloS One 8, e85162. doi: 10.1371/journal.pone.0085162
Wang, Y., Majak, W., McAllister, T. A. (2012). Frothy bloat in ruminants: Cause, occurrence, and mitigation strategies. Anim. Feed Sci. Technol. 172, 103–114. doi: 10.1016/j.anifeedsci.2011.12.012
Wang, Y., Zhang, W., Zhang, Z., Wang, W., Xu, S., He, X. (2021). Isolation, identification and characterization of phenolic acid-degrading bacteria from soil. J. Appl. Microbiol. 131, 208–220. doi: 10.1111/jam.14956
Wu, W., Liu, W., Wang, H., Wang, W., Chu, W., Jin, J. (2022). β-sitosterol inhibits trimethylamine production by regulating the gut microbiota and attenuates atherosclerosis in ApoE–/– mice. Front. Cardiovasc. Med. 9. doi: 10.3389/fcvm.2022.986905
Yu, H., Wang, F., Shao, M., Huang, L., Xie, Y., Xu, Y., et al. (2021). Effects of rotations with legume on soil functional microbial communities involved in phosphorus transformation. Front. Microbiol. 12. doi: 10.3389/fmicb.2021.661100
Zhang, Y., Su, D., He, J., Dai, Z., Asad, R., Ou, S., et al. (2017). Effects of ciceritol from chickpeas on human colonic microflora and the production of short chain fatty acids by in vitro fermentation. LWT Food Sci. Technol. 79, 294–299. doi: 10.1016/j.lwt.2017.01.040
Zhao, Y., Haley, O. C., Xu, X., Jaberi-Douraki, M., Rivard, C., Pliakoni, E. D., et al. (2023). The potential for cover crops to reduce the load of Escherichia coli in contaminated agricultural soil. J. Food Prot. 86, 100103. doi: 10.1016/j.jfp.2023.100103
Zheng, W., Ma, Y., Zhao, A., He, T., Lyu, N., Pan, Z., et al. (2019). Compositional and functional differences in human gut microbiome with respect to equol production and its association with blood lipid level: a cross-sectional study. Gut Pathog. 11, 20. doi: 10.1186/s13099-019-0297-6
Keywords: legumes, phytochemicals, soil microbiome, gut microbiome, One Health, functional diversity
Citation: Duarte RDC, Iannetta PPM, Gomes AM and Vasconcelos MW (2024) More than a meat- or synthetic nitrogen fertiliser-substitute: a review of legume phytochemicals as drivers of ‘One Health’ via their influence on the functional diversity of soil- and gut-microbes. Front. Plant Sci. 15:1337653. doi: 10.3389/fpls.2024.1337653
Received: 13 November 2023; Accepted: 30 January 2024;
Published: 21 February 2024.
Edited by:
Karl H. Mühling, University of Kiel, GermanyReviewed by:
Michael A. Grusak, Agricultural Research Service (USDA), United StatesPraveen Guleria, DAV University, India
Silvio Waschina, University of Kiel, Germany
Copyright © 2024 Duarte, Iannetta, Gomes and Vasconcelos. This is an open-access article distributed under the terms of the Creative Commons Attribution License (CC BY). The use, distribution or reproduction in other forums is permitted, provided the original author(s) and the copyright owner(s) are credited and that the original publication in this journal is cited, in accordance with accepted academic practice. No use, distribution or reproduction is permitted which does not comply with these terms.
*Correspondence: Marta W. Vasconcelos, bXZhc2NvbmNlbG9zQHVjcC5wdA==