- 1Department of Botany, Faculty of Science, Mansoura University, Mansoura, Egypt
- 2Department of Biology, College of Science, Princess Nourah bint Abdulrahman University, Riyadh, Saudi Arabia
- 3Department of Biology, College of Science, Qassim University, Buraydah, Saudi Arabia
- 4Biology Department, College of Science, Jouf University, Sakaka, Saudi Arabia
- 5Department of Biology, Faculty of Science, Al-Baha University, Al-Baha, Saudi Arabia
- 6Department of Botany and Microbiology, Faculty of Science, Assiut University, Assiut, Egypt
- 7Botany and Microbiology Department, Faculty of Science, Helwan University, Cairo, Egypt
- 8School of Ecology and Environment, Northwestern Polytechnical University, Xi’an, China
- 9Botany and Microbiology Department, Faculty of Science, Damietta University, Damietta, Egypt
One of the most crucial steps in the practical conservation of endangered endemic mountain plants is to address their population size status and habitat requirements concurrently with understanding their response to future global warming. Three endangered Silene species—Silene leucophylla Boiss., S. schimperiana Boiss., and S. oreosinaica Chowdhuri—in Egypt were the focus of the current study. These species were examined for population status change, habitat quality variables (topography, soil features, and threats), and predictive current and future distributions. To find population size changes, recent field surveys and historical records were compared. Using Random Forest (RF) and Canonical Correspondence Analysis (CCA), habitat preferences were assessed. To forecast present-day distribution and climate change response, an ensemble model was used. The results reported a continuous decline in the population size of the three species. Both RF and CCA addressed that elevation, soil texture (silt, sand, and clay fractions), soil moisture, habitat-type, chlorides, electric conductivity, and slope were among the important variables associated with habitat quality. The central northern sector of the Saint Catherine area is the hotspot location for the predictive current distribution of three species with suitable areas of 291.40, 293.10, and 58.29 km2 for S. leucophylla, S. schimperiana, and S. oreosinaica, respectively. Precipitation-related variables and elevation were the key predictors for the current distribution of three Silene species. In response to climate change scenarios, the three Silene species exhibited a gradual contraction in the predictive suitable areas with upward shifts by 2050 and 2070. The protection of these species and reintroduction to the predicted current and future climatically suitable areas are urgent priorities. Ex-situ conservation and raised surveillance, as well as fenced enclosures may catapult as promising and effective approaches to conserving such threatened species.
1 Introduction
Climate change, land use, and over-exploitation all contribute to global biodiversity loss (Dawson et al., 2011; Cahill et al., 2013; Dagnino et al., 2020). Recognizing the influence of environmental factors on the regional distribution of plants is one of the main goals of conservation biology. The climate is among the environmental factors that affect plants’ habitat, distribution, ecology, and phenology (Hu et al., 2015; Liu et al., 2021). Climate change will continue to have an apparent impact on living organisms and ecosystems at all levels of ecological organization: shifts in geographic range, population, and life-history changes, changes in the structure and functioning of ecosystems, and changes in the species composition of communities (McCarty, 2001). Therefore, one of the most important challenges in conserving biodiversity is to anticipate how to face the expected climate change and search for precautionary measures to reduce its effect on species and communities (Walther et al., 2002; Root et al., 2003; Thuiller, 2004).
As a result of climate change, some plants may expand or contract their geographic range, while others may shift their range. In particular, endemic plant species have narrow ecological niches and poor dispersal abilities within well-defined habitats. Therefore, at global, national, or even local scales, endemic species are included in the prioritization of conservation efforts (Orsenigo et al., 2018). Endemic montane plants may not be qualified to adapt and maintain pace with new climate conditions and consequently, might migrate/shift to more favorable habitats or become more susceptible to habitat loss (Engler et al., 2009; Dullinger et al., 2012; Cuena-Lombraña et al., 2018; Hoorn et al., 2018; Abdelaal et al., 2019). Moreover, endemic species with isolated populations at mountain barriers and seed dispersal limitations are more vulnerable to extinction risk (Trew and Maclean, 2021). Hence, identifying and understanding the potential effects of upcoming climate change scenarios on species distribution ranges, particularly, endemic plant species, are critical steps in conserving and restoring these species (Behroozian et al., 2020; Dhyani et al., 2021).
The Saint Catherine Protectorate (St. Catherine) is located within the Sinai Peninsula in the Sinaico-Arabian biogeographic sector and Sinaic subsector of Egypt (Abdelaal et al., 2020b). It is considered Egypt’s most floristically rich territory, hosting 472 vascular plant species, including 14 exclusive endemics (Fayed et al., 2004; Mosallam, 2007; Abdelaal et al., 2018). Such phytodiversity is driven by the presence of different microhabitats such as wadi beds, terraces, rocky cliffs, slopes, gorges, caves, and basins (Shaltout et al., 2015) with an elevation gradient up to ca. 2641 m above sea level (a.s.l.). For Egypt (including St. Catherine), during a few decades (1971–2000), the average annual temperature rose by 0.62°C, which exceeded the typical rise worldwide of 0.17°C (Domroes and El-Tantawi, 2005; IPCC, 2014). Therefore, Egypt is an arid country vulnerable to the effects of climate change in particular.
The genus Silene L. (Family Caryophyllaceae) consists of ca. 850–900 species distributed in the Mediterranean region and Asia (Oxelman et al., 2000; Jafari et al., 2020; Martín-Gómez et al., 2020). In the flora of Egypt, Silene is represented by 29 species, three of which (Silene leucophylla Boiss., S. schimperiana Boiss., and S. oreosinaica Chowdhuri) are exclusive endemics to the St. Catherine Protectorate (Boulos, 2009). These three endemics are the focus of the current study.
Silene leucophylla is a perennial hemicryptophyte, up to 40 cm tall, with obovate entire rosette leaves (Boulos, 2009). It is restricted to rocky cliffs within mountains. Silene leucophylla is used as a grazing plant and in traditional medicine to treat diarrhea, leprosy, and inflammation (Mosallam, 2007). It is categorized as an endangered plant (Saker et al., 2011). Silene schimperiana is a perennial hemicryptophyte with stems 50–80 cm long and narrow linear-spathulate rosette leaves, confined to rocky crevices of mountains (Boulos, 2009). It is restricted to granite rocky soils within mountains. It is also assessed as an endangered plant (Mosallam, 2007). Silene oreosinaica is a perennial caespitose chamaephyte, 20–35 cm tall, with oblong-lanceolate rosette leaves. It is restricted to St. Catherine and assessed as a critically endangered plant (Radford et al., 2011).
Species Distribution Models (SDMs) have been established as one of the most commonly used tools in conservation ecology (Guisan and Thuiller, 2005). By correlating species occurrence data with environmental factors, SDMs can predict the potential distribution ranges of species under current and future conditions (Guisan and Zimmermann, 2000), help to identify the most contributing environmental factors and assist in recognizing suitable sites for restoration or translocation of endemic and rare species (Mccain and Colwell, 2011; Fois et al., 2015, Fois et al., 2018; Abdelaal et al., 2019; Wang et al., 2019; Abdelaal et al., 2020a). Occasionally, ecological data are complex, unbalanced, and contain missing values. Therefore, as being SDMs limitations, modeling techniques might originate non-significant predictions, leaving high amounts of unexplained variation leading to underfit models, having insufficient suppleness to describe observed occurrence–environment relationships, and originating a misinterpretation of the factors shaping species distributions (Dutra Silva et al., 2017).
To the best of our knowledge, no earlier research has addressed the population status, habitat preferences, and potential predictive current and future distributions of the three target Silene species. In this context, the objectives of this study were to: a) detect the changes in population size of three Silene species as compared with previous historical data, b) determine the habitat preferences for study Silene species; c) model the potential current distribution and key environmental variables; and d) identify the potential geographic changes in response to climate change.
2 Materials and methods
2.1 Study site, occurrence records and population status of three Silene species
The present study was conducted in the Saint Catherine Protectorate in the Sinaic biogeographic subsector of Egypt (Figure 1). St. Catherine is an igneous massif with an approximate surface area of 5196 km2 and an elevation of up to 2641 m a.s.l. Due to its location, high mountains, and arid to hyper-arid climates, St. Catherine is characterized by unique habitats, biodiversity, endemism, landforms, and geologic structure (Moustafa and Klopatek, 1995; Moustafa et al., 2001). The mean annual rainfall (1970–2020) reaches 50.20 mm during the winter. However, during the years 2014 (Moustafa et al., 2017) and 2020 (personal observation), unpredictable one-day floods with snowfall on the mountain tops have been recorded. The average annual temperature fluctuated from 8.6°C in January to 25.5°C in August. The foremost threats in St. Catherine include drought, overgrazing, overcollection, and tourist activities.
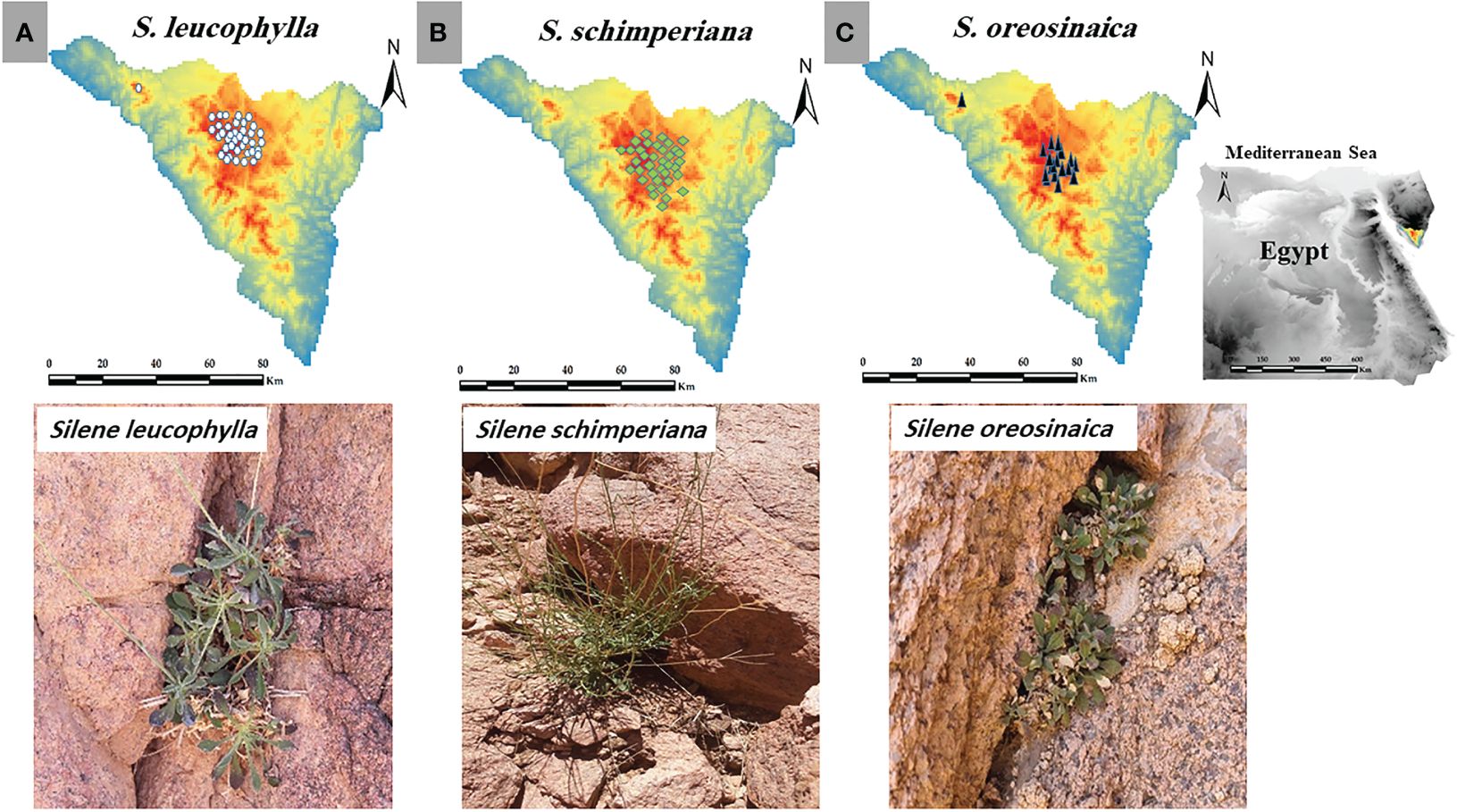
Figure 1 Study area, current occurrence records and morphology of (A) Silene leucophylla, (B) S. schimperiana, and (C) S. oreosinaica.
After consultation with literature, websites, and local and international herbaria, the georeferenced occurrence records of all populations of S. leucophylla, S. schimperiana, and S. oreosinaica were collected from direct field surveys (Figure 1). Silene leucophylla, S. schimperiana, and S. oreosinaica were recorded in 8, 6, and 3 sites, respectively. For each Silene species, the biannual historic (2016–2020) population data (total number of individuals) were obtained from previous databases and reports of the last five years (unpublished data), while the current (2021–2022) population size was directly obtained from field observations. In addition, the importance value index (IVI, out of 200) was evaluated for each species by sum of both relative density and relative cover within several permanent plots of 25 m2 each (Kent, 2011).
2.2 Habitat preferences for three Silene species
In each site, elevation, slope, and aspect were recorded or calculated. For soil analysis, bulk soil samples were collected, sieved, and air-dried. Soil physical (texture and moisture content) and chemical features (pH, electric conductivity, organic matter, calcium carbonates, chlorides, sulphates, potassium, calcium, and magnesium) were estimated. pH and electric conductivity(EC) were measured in 1:5 w/v soil solutions by pH meter (Lutron pH 206) and conductivity meter (Corning, NY, USA), respectively. Organic matter (OM) was estimated by ignition in a muffle furnace at 450°C for 4 h. Soil analyses were carried out according to Burt (2004). Microhabitat type was recorded and ranked as follows: (1): slopes, (2): gorges, and (3): terraces. Field observations on threat type sign was ranked as follow: (1): drought, (2): over-grazing, and (3): over-collection, and distance to the nearest track/road were also documented for each Silene species in all localities. All the commonly associated species in the surveyed plots with the three Silene species were recorded. All variables were tested against normality and transformed when necessary. To find the most important variable for the habitat preference of three Silene species, the “randomForest” (RF) package (Breiman, 2001) in R software was used. In addition, Canonical Correspondence Analysis (CCA) in the “vegan” package was used to address the relationship between measured environmental variables and the abundance of three Silene species. One–way ANOVA was applied to test the significance of habitat quality variables.
2.3 Predictive current and future distributions using ensemble modelling
To avoid overfitting bias, the occurrence records of each Silene species were filtered by selecting a random point within a single raster cell of 1 km × 1 km in ArcGIS 10.7.1 (Fois et al., 2018; Smeraldo et al., 2018; Abdelaal et al., 2019). To create predictive models, 90, 75, and 40 occurrence records for S. leucophylla, S. schimperiana, and S. oreosinaica, respectively, were used. For the current period (1970–2000), 19 bioclimatic variables (BIO1–BIO19) were downloaded from the WorldClim v.2.1 portal at a resolution of ~1 km2 (http://www.worldclim.org) (Fick and Hijmans, 2017) (Supplementary Table S1). In addition, topographical factors (elevation, aspect, and slope) were retrieved through the Shuttle Radar Topographic Mission (SRTM) from EarthEnv at 1 km2 resolution (https://www.earthenv.org/topography) (Amatulli et al., 2018).
On the other hand, to avoid highly correlated variables, a Variance Inflation Factor (VIF) with a threshold of VIF< 5 was applied using the ‘usdm’ package (Naimi, 2017) in the R-environment (R Core Team, 2022). In the end, 12 variables: temperature seasonality (BIO4); the maximum temperature of the warmest month (BIO5), mean temperature of the wettest quarter (BIO8), mean temperature of the warmest quarter (BIO10), mean temperature of the coldest quarter (BIO11), precipitation of the driest month (BIO14), precipitation of the wettest month, precipitation seasonality (BIO15), precipitation of the driest quarter (BIO17), precipitation of the warmest quarter (BIO18), precipitation of the coldest quarter (BIO19), elevation and aspect were kept for the modelling process (Table 1).
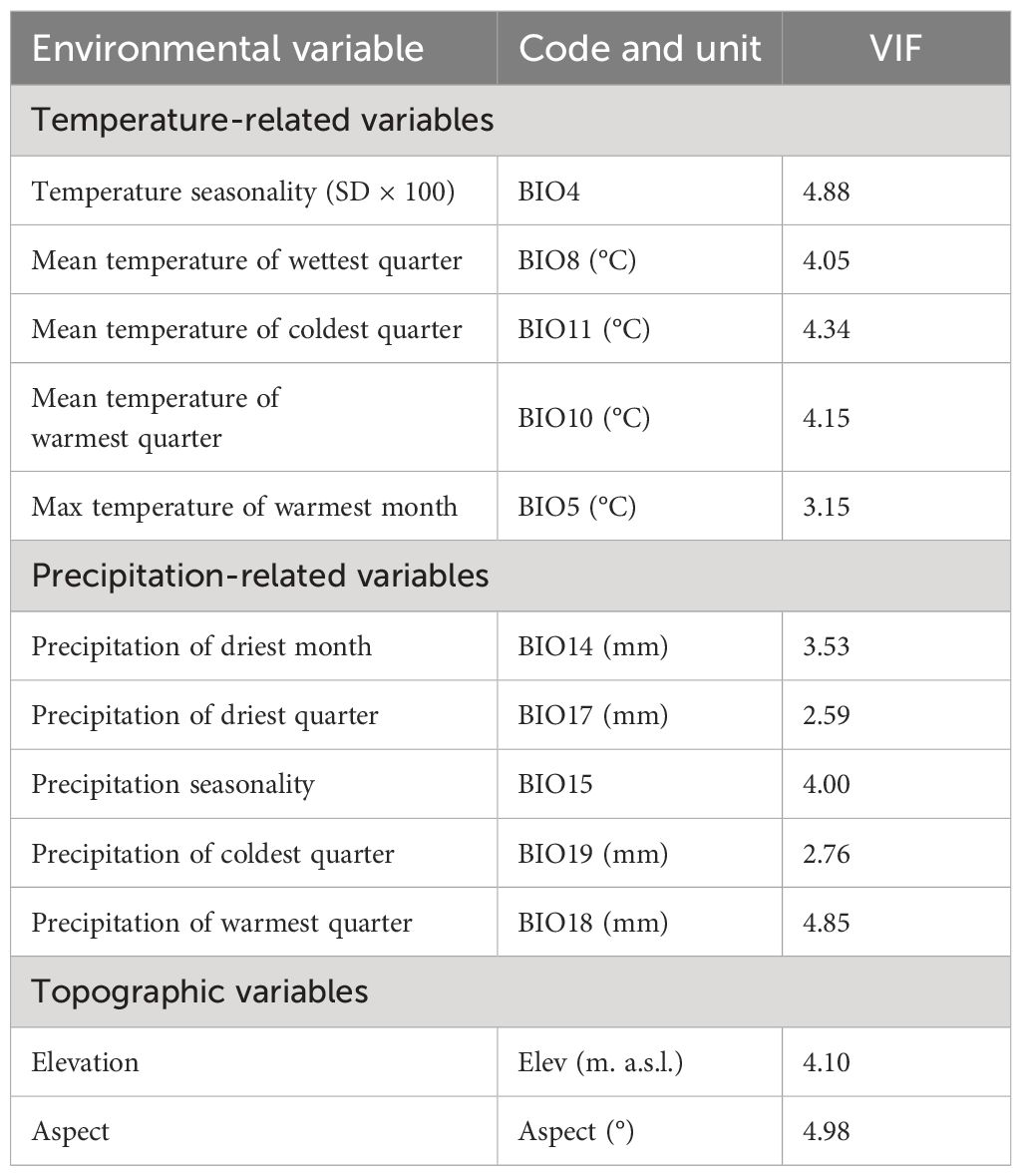
Table 1 List of 12 environmental predictors used to model the potentially suitable areas for the studied Silene species with their Variance Inflation Factors (VIF< 5).
For future climate projections, the ensemble average of the outputs of CCSM4 and HadGEM2-CC global climate models (GCMs) were used to represent the future potential distributions of the three Silene species in Egypt. In addition, two Representative Concentration Pathways (RCPs): optimistic RCP2.6 and pessimistic RCP8.5, for two periods: 2050 and 2070 were downloaded from WorldClim v.2 (http://www.worldclim.org) at ~1 km2 resolution. The RCP2.6 scenario represents a low forcing level of 3 W m-2 maximum, whereas the RCP8.5 scenario depicts a rising radiative forcing level of 8.5 W m-2 by 2100 (van Vuuren et al., 2011).
To reduce bias and uncertainties in model prediction, an ensemble model of three algorithms was constructed (Thuiller et al., 2019) to predict the current and future distributions of Silene species. The three algorithms included GLM (Generalized Linear Model), RF (Random Forest), and BRT (Boosting Regression Trees). The GLM is a regression model, while RF and BRT are machine learning methods. The three selected models (GLM, RF and BRT) have excellent stability and transferability than other models (El-Barougy et al., 2023). Using the R sdm package (Naimi and Araújo, 2016), 70% training data, and 30% testing data, the species distribution models were conducted. The maximum training sensitivity plus specificity (MTSS) threshold was applied, which is an excellent selection method to avoid over-prediction and under-prediction errors (Liu et al., 2016; Guisan et al., 2017). To assess the models’ performance, two metrics were considered: Area Under the Curve (AUC) and True Skill Statistics (TSS) (Allouche et al., 2006; Guisan et al., 2017). The TSS and AUC values are not affected by the prevalence of the species occurrence points or the size of the study region. Higher values of both metrics (close to 1) imply high models’ accuracy and a strong correlation between model prediction and species’ real distributions. Depending on the MTSS values and by using the reclassify tool in ArcGIS, the probability maps under current and future conditions for each Silene species were categorized into four suitability classes as follows: unsuitable, low, moderate, and high. To evaluate the spatial range changes in the predicted distributions of the studied species under current and future climate scenarios (RCP2.6 and RCP8.5), two suitability classes were distinguished after applying the MTSS threshold: unsuitable and suitable areas. The predictive models were compared, and changes in suitable areas were calculated.
3 Results
3.1 Population status change of three Silene species
As compared with the last five years, there is a gradual loss in the population size of three Silene species (Figure 2). For S. leucophylla, the current population size is 1000 individuals with loss percentages of 28.6, 9.1, and 5.2% when compared with the years 2016, 2018, and 2020, respectively. The current population sizes for S. schimperiana and S. oreosinaica are 900 and 60 individuals, respectively.
According to recent field observations (2021–2022), the population and microhabitat features for three Silene species were recorded (Supplementary Table S2). The population features varied among Silene species. For S. leucophylla, the total number of individuals is 1000 individuals with 69 mature individuals, and distributed in eight sites in St. Catherine. Silene leucophylla survives at an elevation extent between 1700 and 2200 m a.s.l. and grows in terraces, slopes, and gorges microhabitats in the rocky crevices of mountains in all aspect directions except south and flat. On the other hand, the current population size of S. schimperiana is 900 individuals with 51 mature individuals, spread across six sites in St. Catherine. It grows in rocky crevices at an elevation range of 1300–2300 m inside terraces, gorges, and slopes microhabitats. Silene oreosinaica had the smallest population size of 60 individuals, including 28 matures, and was found only in three sites on slopes microhabitats with a north-facing aspect and a narrow elevation range of 2000 to 2300 m.
Additionally, from our field observations, the populations of all target Silene species were sporadically distributed and severely fragmented by mountain barriers, with a continuous decline in the quality of their habitats and population size. The main threats to the three species were overgrazing by feral donkeys and domestic animals, drought, and overcollection for scientific research. Except for S. schimperiana, there are no fenced enclosures established for long-term monitoring of S. leucophylla and S. oreosinaica. The other microhabitat features and commonly associated species are displayed in Supplementary Table S2.
3.2 Habitat preferences for Silene species
For three Silene species, Random Forest (RF) predicted the most important habitat variables (Figure 3A). Elevation, clay fraction, silt fraction, moisture content, sand fraction, and slope are the most important variables with high values of Mean Decrease Gini values (>1.0). In addition, all other variables highly contribute to the habitat quality of Silene species with Mean Decrease Gini importance values ≥0.33.
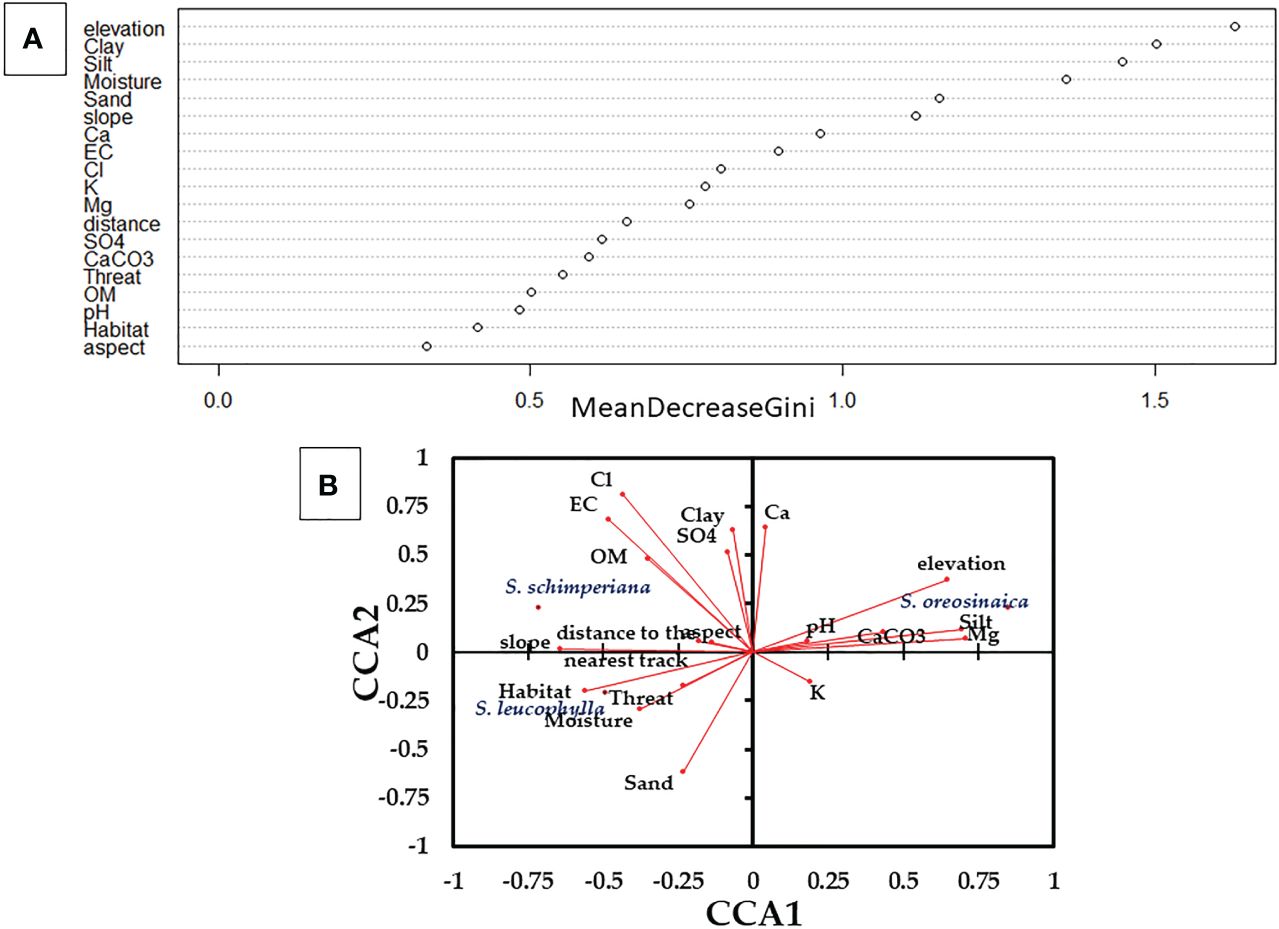
Figure 3 Variables importance that affect the occurrences and abundance of three Silene species derived from (A) Random Forest (RF); and (B) Canonical Correspondence Analysis (CCA). Eigenvalues for CCA1 and CCA2 are 31.46% and 56.48%, respectively. OM, organic matter; and EC, electric conductivity.
In addition, the Canonical Correspondence Analysis (CCA) elucidated the correlation between the abundance of Silene species and measured variables (Figure 3B). S. leucophylla demonstrated a strong correlation with habitat-type, sand fraction, and moisture content, whereas S. schimperiana showed positive correlations with chlorides, electric conductivity, slope, organic matter and clay fraction. On the other hand, S. oreosinaica exhibited positive correlations with elevation, silt fraction, and magnesium, and negative correlations with slope and habitat type.
On the other hand, the habitat quality variables of three Silene species are displayed in Supplementary Table S3. Except for sand fraction, pH, electric conductivity, SO4, Ca2+, aspect, slope, and distance to the nearest track, the other measured variables were significantly diverse at p≤ 0.05. The habitat of S. leucophylla had the highest values of moisture content (20.07%) and slope (30.71%), but the lowest values of CaCO3 (8.31%), Mg+2 (4.96 mEq/L) and elevation (1826 m). S. schimperiana habitat recorded the highest mean values of clay fraction (14.67%), organic matter (4.62%), Cl- (14.27 mEq/L), habitat-type (2.0), and threat-type (1.70). Additionally, the habitat of S. oreosinaica attained the highest mean values of silt fraction (5.32%), CaCO3 (18.47%), K+ (6.87 mEq/L), Mg+2 (9.16 mEq/L) and elevation (2184 m).
3.3 Predictive current distribution, model performance, and key environmental predictors
The potential current distributions and suitability classes for three Silene species in St. Catherine, Egypt are shown in Figure 4. The middle northern sector of St. Catherine is identified as the hotspot location for the distribution of three Silene species with high potential suitable areas. According to the potentially suitable areas, S. schimperiana had the largest areas (293.10 km2, 5.64% of St. Catherine’s total area), followed by S. leucophylla (291.40 km2), while S. oreosinaica attained the lowest suitable areas (56.63 km2).
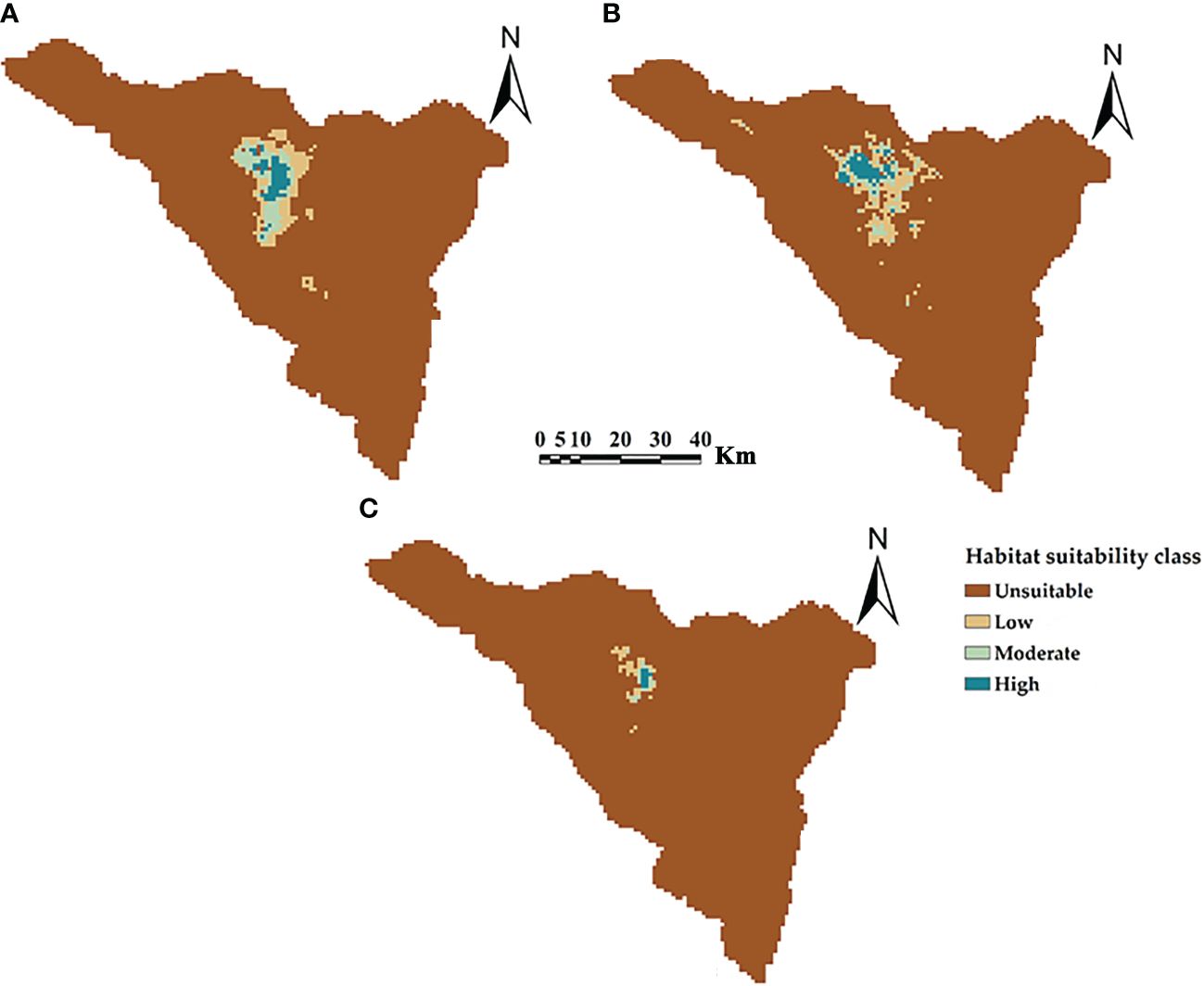
Figure 4 The predictive maps for the current potential distribution of three Silene species: (A) S. leucophylla; (B) S. schimperiana; and (C) S. oreosinaica in St. Catherine Protectorate, Egypt. Maximum training sensitivity plus specificity (MTSS) threshold values are 0.20, 0.16 and 0.30 for S. leucophylla, S. schimperiana, and S. oreosinaica, respectively.
The average AUC and TSS values of single models and ensemble models for three Silene species were calculated (Supplementary Table S4). As compared with the three single models (GLM, RF and BRT), the ensemble models for three Silene species achieved higher values of AUC and TSS, indicating the high accuracy of ensemble models. The ensemble models’ AUC for S. leucophylla, S. schimperiana, and S. oreosinaica were 0.985, 0.983, and 0.997, respectively (Figure 5). These values revealed excellent model performance for three Silene species. A similar pattern of TSS values (0.890 for S. leucophylla, 0.885 for S. schimperiana, and 0.898 for S. oreosinaica) was obtained, confirming the reliability of the predictive models.
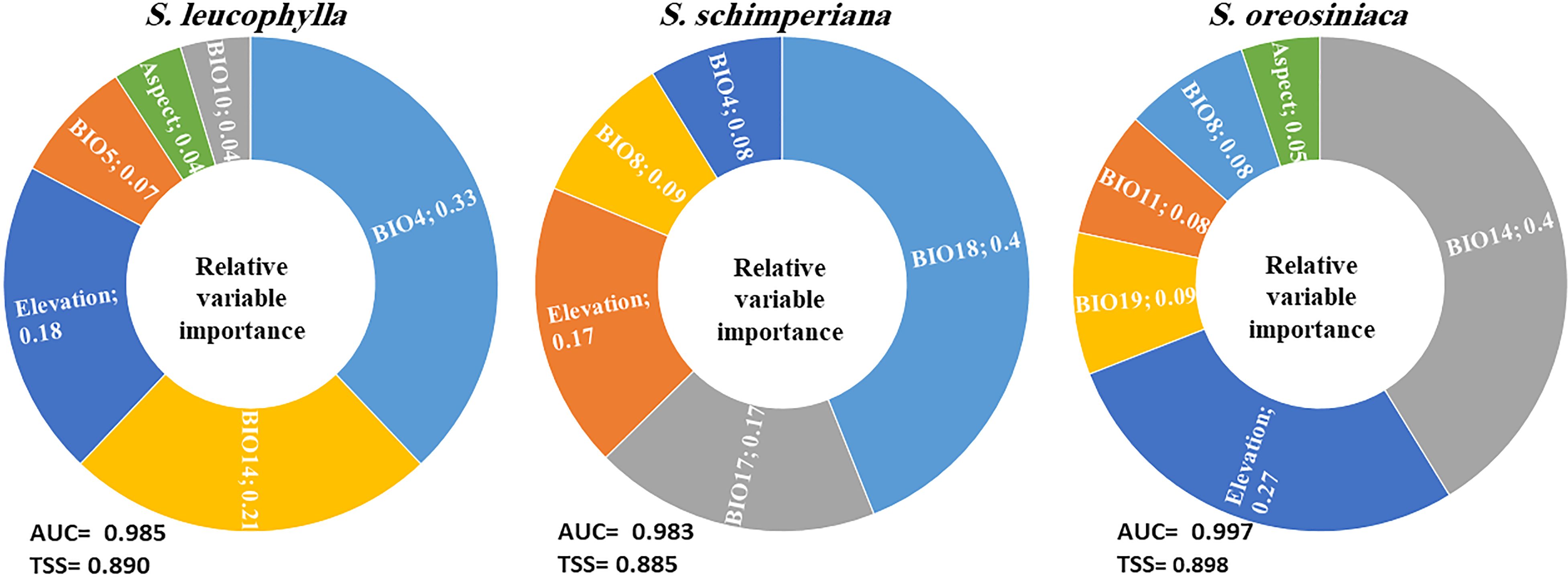
Figure 5 The relative importance of the environmental predictors explaining the potential distribution of three Silene species. The averages of the AUC and TSS indicate the accuracy of the ensemble models. BIO4, temperature seasonality; BIO14, precipitation of the driest month; BIO5, maximum temperature of the warmest month; BIO18, precipitation of the warmest quarter; BIO17, precipitation of the driest quarter; BIO8, mean temperature of the wettest quarter; BIO19, precipitation of the coldest quarter; and BIO11, mean temperature of the coldest quarter.
Concerning relative variables importance, the most important environmental variables for S. leucophylla were temperature seasonality (BIO4), precipitation of the driest month (BIO14), and elevation (Figure 5). For S. schimperiana, precipitation of the warmest quarter (BIO18), precipitation of the driest quarter (BIO17), and elevation were the main variables with a total importance of 74%. Among the selected variables, precipitation of the driest month (BIO14), elevation, and precipitation of the coldest quarter (BIO19) were the most imperative variables affecting the potential distribution of S. oreosinaica (Figure 5).
3.4 Predictive distribution range change under climate change
By subtracting the predicted current distribution (Figure 4) from the predicted future distribution (Figure 6) yields the change in the distribution range of three Silene species (Table 2). The predicted future distribution of three Silene species in all climate scenarios (RCP2.6 and RCP8.5) over time (2050 and 2070) showed a progressive loss in suitable areas than the predicted current distribution.
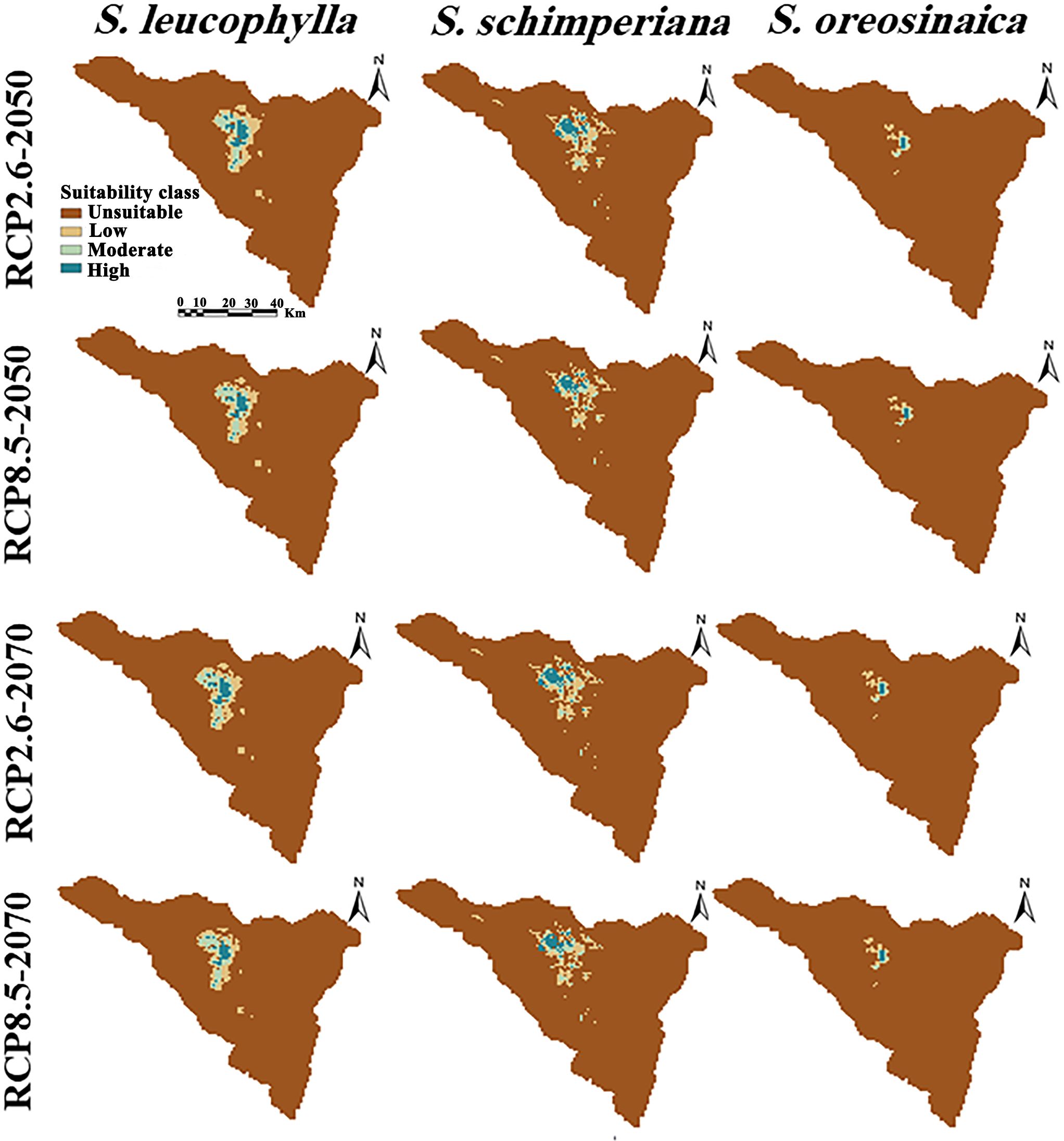
Figure 6 Potential future distributions of three Silene species under the optimistic low (RCP2.6) and pessimistic high (RCP8.5) emission scenarios. Columns from left to right show the projections of S. leucophylla, S. schimperiana, and S. oreosinaica, respectively.
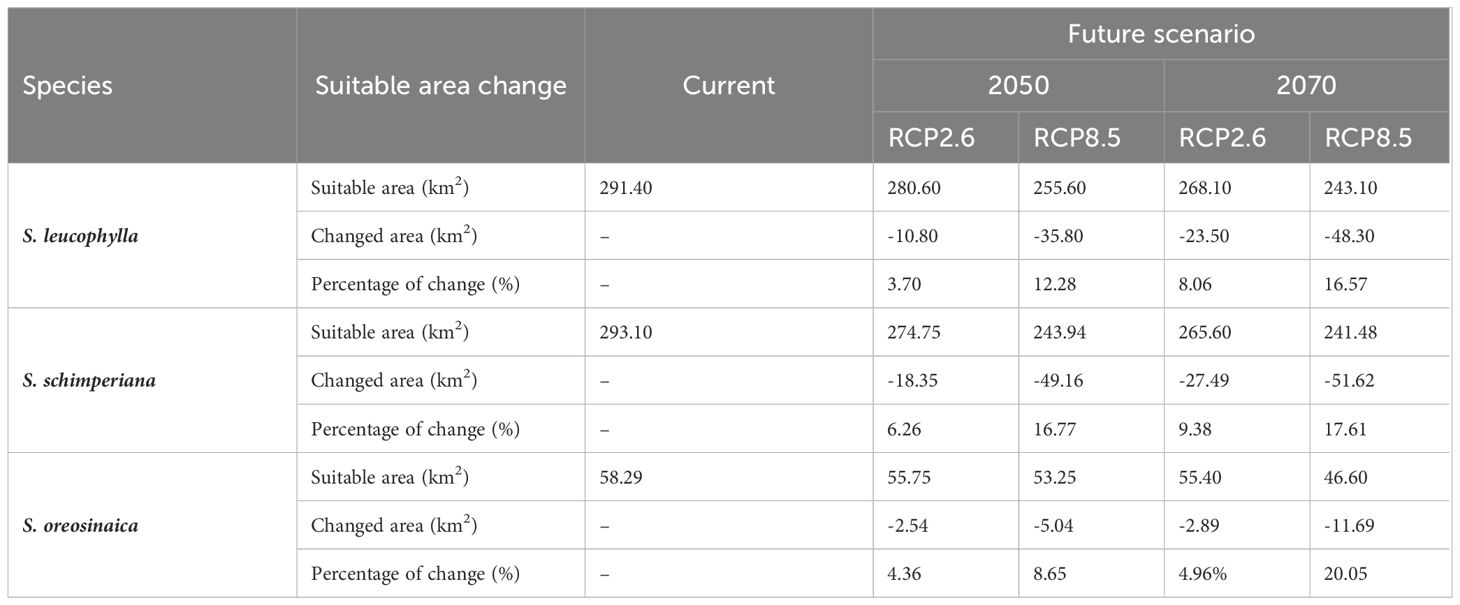
Table 2 The estimation of fluctuations in the potential distribution of three Silene species under two future climate change scenarios.
Under RCP2.6–2050, RCP2.6–2070, RCP8.5–2050, and RCP8.5–2070 scenarios, the current suitable area of S. leucophylla was projected to decrease by 3.70%, 8.06%, 12.28%, and 16.57%, respectively. By 2050 and 2070, under the two RCP2.6 and RCP8.5 scenarios, the potential current suitable areas for S. schimperiana would decrease by 6.26%, 16.77%, 9.38%, and 17.61%, respectively. A comparable configuration was also established for S. oreosinaica, where the suitable areas would decrease by 4.36%, 4.96%, 8.65%, and 20.05%, respectively, under RCP2.6–2050, RCP2.6–2070, RCP8.5–2050, and RCP8.5–2070 scenarios (Table 2).
For three Silene species, no range expansion or gain in suitable areas was observed in the future under two climate change scenarios (RCP2.6 and RCP8.5) of 2050 and 2070. However, there is a gradually shrinking range in the north-central parts of St. Catherine with an upward shift to future climatically high-elevation areas. According to extinction risk, the studied Silene species can be ranked S. oreosinaica, then S. schimperiana, and finally S. leucophylla.
4 Discussion
By comparing current field observations with previous historical data, there is a continuous decline in the total number of individuals, with few mature individuals among the three studied Silene species. Moreover, based on current distribution modelling, the distribution ranges of three Silene species are highly fragmented due to mountain barriers, which are common in the study area (Zahran and Willis, 2009). Owing to their feeding properties as grazing plants, and the ruthless long-term drought, the populations of three Silene species are declining. Due to overgrazing and overcollection, the flowering and fruiting organs are eradicated, so the plants lose the ability to regenerate and hamper their life cycle. Moreover, the narrow distribution ranges at high elevations in rocky crevices with small and highly fragmented populations may also impede the expansion of their range and dispersal. Therefore, due to range restrictions, continuous human threats, and predicted climate change, the studied Silene species are vulnerable to extinction risk.
Both RF and CCA displayed the importance of elevation, soil texture (silt, sand and clay fractions), soil moisture, habitat-type, chlorides, electric conductivity and slope as among the main predictors affecting the habitat preferences and distribution of three Silene species in St. Catherine, Egypt. The three Silene species preferred rocky slope soil with a sandy texture, alkaline, and slightly saline soil. The significant variation in some soil features (silt fraction, clay, moisture, organic matter, calcium carbonates, and chlorides) among the three Silene species may be attributed to topography and plant composition (Shaltout et al., 2015). Many ecological factors and microhabitats support the existence of endemic plants within St. Catherine (Khedr, 2021). For example, rocky ground such as granite, cliffs, eroded soil, and a higher altitude range of mountains, as well as a unique geological structure, made St. Catherine an important area of endemism in Egypt (Moustafa and Klopatek, 1995; Danin, 2008; Hosni et al., 2013; Abdelaal et al., 2018).
In general, the St. Catherine mountain species are susceptible to temperature, and rainfall changes (Moustafa and Klopatek, 1995; Grainger and Gilbert, 2008; Abdelaal et al., 2020a). Moreover, some studies have emphasized the significance of microhabitat specificity for endemic species of the South Sinai (Ayyad et al., 2000; Khedr, 2007; Shaltout et al., 2015, Shaltout et al., 2016). These microhabitats are constructed by elevation, slope degree, and aspect direction. Our field observation showed that S. leucophylla and S. schimperiana share the same microhabitats, where they were recorded in slopes, gorges, and terraces in all aspects except flat and south-facing sites, while S. oreosinaica was only recorded in a slope microhabitat in the north direction. This finding was also confirmed by previous studies (Fakhry et al., 2019; Rabei et al., 2021). Thus, these species might receive little solar radiation, low temperatures, and high moisture as compared with steep south-facing sites where hotter and drier conditions prevail (Khedr, 2007; Abdelaal et al., 2020a).
For current climate conditions, the three Silene species inhabit the higher-elevation areas within the central northern sector of St. Catherine. These findings were consistent with our field observations and historical distributions reported in the literature (Moustafa et al., 2001; Zahran et al., 2015; Abdelaal et al., 2018; Fakhry et al., 2019; Rabei et al., 2021). Both S. leucophylla and S. schimperiana have wide distribution ranges as compared with S. oreosinaica.
On the other hand, the relative importance of the investigated bioclimatic and topographic predictors varied among Silene species. In addition to elevation, the predicted current distribution of S. leucophylla was more sensitive to temperature seasonality, and precipitation of the driest month, however, precipitation-related variables (BIO14, BIO17, and BIO18) were among the important variables controlling the current distribution of both S. schimperiana and S. oreosinaica. This indicates that the three Silene species could be more vulnerable to global warming (i.e. an increase in temperature and a decrease in precipitation) than other common mountain species in the study area. However, evidence is required to support whether the studied Silene species are physiologically able to resist some degree of hot and dry conditions. These findings are in accordance with other studies that applied SDM to address the potential geographical distributions for rare, endemic, and medicinal plant species in similar and related environments such as Daphne mucronata in Central Iran (Abolmaali et al., 2018), Nepeta binaloudensis in Khorassan–Kopet Dagh (Erfanian et al., 2021), Pomatosace filicula in the Qinghai–Tibet Plateau (Chen et al., 2022) and Allium species in Iran (Nasab et al., 2022), where they reported the vital role of climatic factors and elevation in shaping the plants’ distribution. A similar finding was also reported for endemic plants in the Kashmir Himalaya (Mir et al., 2020), which underlined the importance of temperature seasonality (BIO4), precipitation of the driest month (BIO14), and elevation. Furthermore, in Egypt, the spatial distributions of medicinal plants (Kaky and Gilbert, 2016, Kaky and Gilbert, 2017; Kaky et al., 2020) and endemic plants (e.g. Rosa arabica and Primula boveana) (Abdelaal et al., 2019; Abdelaal et al., 2020a) were closely controlled by precipitation and temperature-related variables and elevation.
The predicted current suitable distribution areas for three Silene species were characterized by moist conditions, low temperatures, and high elevation ranges (≥1600 m for S. leucophylla, ≥1550 for S. schimperiana, and ≥2000 m for S. oreosinaica) (Figure 4). These findings are in line with several studies (Moustafa and Klopatek, 1995; Moustafa et al., 2001; Zahran et al., 2015; Abdelaal et al., 2020a) that documented that, most endemic species of St. Catherine prefer moist microhabitats in rock crevices at high elevations with cold temperatures and moderate rainfall. Moreover, the models’ results for the current potential distribution of three Silene species exhibited some climatically topographically suitable sites within St. Catherine, despite our field surveys and literature that did not indicate the occurrence of these species in this mountain range. This may be due to three hypotheses: firstly, the possible extinction or disappearance of these species in those suitable sites (Rabei et al., 2021). Second, due to poor dispersal, the endemic species did not occupy all the available space and have not been recorded before in these suitable sites and conditions, or finally, a lack of adequate field surveys, especially for high-elevation rugged mountain areas. A similar concern has been addressed for Rosa arabica and Primula boveana in the same study area (Abdelaal et al., 2019; Abdelaal et al., 2020a) and Nepeta binaloudensis in Iran (Erfanian et al., 2021). However, additional floristic surveys are encouraged at these sites to look for new Silene populations or to find out what circumstances have made it difficult for these species to recruit or colonize all suitable areas.
In response to climate change, some species might benefit through range expansion and acquiring additional suitable areas for their survival and persistence, while others are negatively impacted by range contraction or habitat loss (Thuiller et al., 2005; Sintayehu, 2018). The three Silene species showed range-shift by moving upward to high-elevation areas in response to the increasing severity of climate change scenarios from RCP2.6 up to RCP8.5. However, this upward range shift was accompanied by a gradual contraction in their geographical range. Montane plant species that populate high-elevation areas are more susceptible to range shifts or extinction threats owing to climate warming (Lenoir et al., 2008; Dullinger et al., 2012; Dakhil et al., 2021). A similar pattern was also reported for the two endemic species, Rosa arabica and Primula boveana (Abdelaal et al., 2019; Abdelaal et al., 2020a). Range contraction was also predicted for several species in mountain areas and related arid environments, for example, Nepeta binaloudensis in Iran (Erfanian et al., 2021), Tilia species in Turkey (Canturk and Kulaç, 2021), and Horsfieldia tetratepala in China (Cai et al., 2022). Range-restricted and mountain-top species respond differently to climate change, either through range contraction or shifting their range to higher elevations. A study investigating climate change influences on the flora of the South Sinai documented a considerable upslope shift in its elevation-limits (Coals et al., 2018). As a result, it is predicted that, global warming could have a negative effect on narrow-range and endemic plants in St. Catherine, where mesic microhabitats and high altitudes exist.
Despite the St. Catherine is home to 14 exclusive endemic vascular plants and is accounted as the most floristically rich region in Egypt, coupled with the availability of laws that forbid the illegal collection, exploitation, and overgrazing of these species, their strict implementation is negligible. Threats such as narrow-range distribution, habitat specificity, drought, and overgrazing, all contribute to the ongoing population decline of three Silene species. Therefore, the studied Silene species are threatened and subsequently endangered according to the International Union for Conservation of Nature (IUCN, 2023). Thus, a sustainable conservation agenda is required to prevent the further loss of these species.
In conclusion, this study reported the continuous decline in the population size of S. leucophylla, S. schimperiana, and S. oreosinaica. Furthermore, the potential geographic distribution of three Silene species might undergo upward range shifts in response to climate change. Therefore, as high-altitude sensitive plants, the three Silene species may not be able to withstand future global warming. In order to reduce the risk of extinction, a thorough survey, ex-situ conservation, and translocation actions with regular monitoring of species are needed. The potential suitable areas under both current and future climates could be considered a decision-support tool for conservation planning and restoration strategies for the three Silene species. Besides, fenced enclosures and tissue culture propagation, followed by regular monitoring, are required after reintroduction into the proposed refugia. These actions should be supported by increased public awareness and policy initiatives to mitigate the effects of human activity. Finally, our methods could be replicated for other endemic and endangered species all over the world and can be done by conservationists, ecologists or relevant researchers. Regarding the conservation interest, our study highlighted the most important environmental variables and predicted suitable sites that should be considered during the reintroduction, or in-situ conservation of three Silene species in response to climate change, as well as giving an alarm to the rate of population decline of the three Silene species.
Data availability statement
The original contributions presented in the study are included in the article/Supplementary Material. Further inquiries can be directed to the corresponding author.
Author contributions
MA: Conceptualization, Data curation, Formal analysis, Investigation, Methodology, Software, Writing – original draft, Writing – review & editing. AA-H: Formal analysis, Funding acquisition, Investigation, Methodology, Project administration, Writing – review & editing, Conceptualization, Writing – original draft. SA: Investigation, Validation, Visualization, Writing – review & editing, Supervision. HA-H: Investigation, Validation, Visualization, Writing – review & editing. SA-R: Investigation, Validation, Visualization, Writing – review & editing. AA: Data curation, Investigation, Validation, Visualization, Writing – review & editing. MD: Data curation, Validation, Writing – review & editing, Formal analysis. RE-B: Data curation, Validation, Writing – review & editing, Formal analysis. AY: Data curation, Validation, Visualization, Writing – review & editing, Formal analysis.
Funding
The author(s) declare financial support was received for the research, authorship, and/or publication of this article. This research was funded by Princess Nourah bint Abdulrahman University Researchers Supporting Project number (PNURSP2024R93), Princess Nourah bint Abdulrahman University, Riyadh, Saudi Arabia. The authors extend also their appreciation to the Deputyship for Research & Innovation, Ministry of Education in Saudi Arabia for funding this research work through project number 223202.
Acknowledgments
The authors express their gratitude to Princess Nourah bint Abdulrahman University Researchers Supporting Project number (PNURSP2024R93), Princess Nourah bint Abdulrahman University, Riyadh, Saudi Arabia. The authors would like to thank St. Catherine researchers and rangers for their help during field trips.
Conflict of interest
The authors declare that the research was conducted in the absence of any commercial or financial relationships that could be construed as a potential conflict of interest.
Publisher’s note
All claims expressed in this article are solely those of the authors and do not necessarily represent those of their affiliated organizations, or those of the publisher, the editors and the reviewers. Any product that may be evaluated in this article, or claim that may be made by its manufacturer, is not guaranteed or endorsed by the publisher.
Supplementary material
The Supplementary Material for this article can be found online at: https://www.frontiersin.org/articles/10.3389/fpls.2024.1336911/full#supplementary-material
References
Abdelaal, M., Fois, M., Dakhil, M. A., Bacchetta, G., El-Sherbeny, G. A. (2020a). Predicting the potential current and future distribution of the endangered endemic vascular plant Primula boveana Decne. ex Duby in Egypt. Plants 9, 957. doi: 10.3390/plants9080957
Abdelaal, M., Fois, M., Fenu, G., Bacchetta, G. (2018). Critical checklist of the endemic vascular plants of Egypt. Phytotaxa 360, 19–34. doi: 10.11646/phytotaxa.360.1.2
Abdelaal, M., Fois, M., Fenu, G., Bacchetta, G. (2019). Using MaxEnt modeling to predict the potential distribution of the endemic plant Rosa arabica Crép. Egypt. Ecol. Inform. 50, 68–75. doi: 10.1016/j.ecoinf.2019.01.003
Abdelaal, M., Fois, M., Fenu, G., Bacchetta, G. (2020b). Biogeographical characterisation of Egypt based on environmental features and endemic vascular plants distribution. Appl. Geogr. 119, 102208. doi: 10.1016/j.apgeog.2020.102208
Abolmaali, S. M. R., Tarkesh, M., Bashari, H. (2018). MaxEnt modeling for predicting suitable habitats and identifying the effects of climate change on a threatened species, Daphne mucronata, in central Iran. Ecol. Inform. 43, 116–123. doi: 10.1016/j.ecoinf.2017.10.002
Allouche, O., Tsoar, A., Kadmon, R. (2006). Assessing the accuracy of species distribution models: Prevalence, kappa and the true skill statistic (TSS). J. Appl. Ecol. 43, 1223–1232. doi: 10.1111/j.1365-2664.2006.01214.x
Amatulli, G., Domisch, S., Tuanmu, M. N., Parmentier, B., Ranipeta, A., Malczyk, J., et al. (2018). Data Descriptor: A suite of global, cross-scale topographic variables for environmental and biodiversity modeling. Sci. Data 5, 1–15. doi: 10.1038/sdata.2018.40
Ayyad, M. A., Fakhry, A. M., Moustafa, A.-R. A. (2000). Plant biodiversity in the Saint Catherine area of the Sinai Peninsula, Egypt. Biodivers. Conserv. 9, 265–281. doi: 10.1023/A:1008973906522
Behroozian, M., Ejtehadi, H., Townsend Peterson, A., Memariani, F., Mesdaghi, M. (2020). Climate change influences on the potential distribution of Dianthus polylepis Bien. ex Boiss. (Caryophyllaceae), an endemic species in the Irano-Turanian region. PLoS One 15, e0237527. doi: 10.1371/JOURNAL.PONE.0237527
Boulos, L. (2009). Flora of Egypt Checklist, revised annotated edition (Cairo, Egypt: Al Hadara Publishing).
Burt, R. (2004). Soil survey laboratory methods manual. Soil survey investigations report 42, version 4.0. United States Department of Agriculture (USDA). Nat. Resour. Conserv. Serv. Natl. Soil Surv. Cent., 1–736.
Cahill, A. E., Aiello-Lammens, M. E., Caitlin Fisher-Reid, M., Hua, X., Karanewsky, C. J., Ryu, H. Y., et al. (2013). How does climate change cause extinction? Proc. R. Soc B Biol. Sci. 280, 20121890. doi: 10.1098/RSPB.2012.1890
Cai, C., Zhang, X., Zha, J., Li, J., Li, J. (2022). Predicting climate change impacts on the rare and endangered Horsfieldia tetratepala in China. Forests 13, 1051. doi: 10.3390/f13071051
Canturk, U., Kulaç, Ş. (2021). The effects of climate change scenarios on Tilia ssp. in Turkey. Environ. Monit. Assess. 193, 1–15. doi: 10.1007/s10661-021-09546-5
Chen, K., Wang, B., Chen, C., Zhou, G. (2022). MaxEnt modeling to predict the current and future distribution of Pomatosace filicula under climate change scenarios on the Qinghai–Tibet Plateau. Plants 11, 670. doi: 10.3390/plants11050670
Coals, P., Shmida, A., Vasl, A., Duguny, N. M., Gilbert, F. (2018). Elevation patterns of plant diversity and recent altitudinal range shifts in Sinai’s high-mountain flora. J. Veg. Sci. 29, 255–264. doi: 10.1111/jvs.12618
Cuena-Lombraña, A., Fois, M., Fenu, G., Cogoni, D., Bacchetta, G. (2018). The impact of climatic variations on the reproductive success of Gentiana lutea L. in a Mediterranean mountain area. Int. J. Biometeorol. 62, 1283–1295. doi: 10.1007/s00484-018-1533-3
Dagnino, D., Guerrina, M., Minuto, L., Mariotti, M. G., Médail, F., Casazza, G. (2020). Climate change and the future of endemic flora in the South Western Alps: relationships between niche properties and extinction risk. Reg. Environ. Change 20, 1–12. doi: 10.1007/s10113-020-01708-4
Dakhil, M. A., Halmy, M. W. A., Hassan, W. A., El-Keblawy, A., Pan, K., Abdelaal, M. (2021). Endemic Juniperus montane species facing extinction risk under climate change in southwest China: Integrative approach for conservation assessment and prioritization. Biol. (Basel). 10, 63. doi: 10.3390/biology10010063
Danin, A. (2008). Desert rocks–a habitat which supports many species that were new to science in the last 40 years. Turk. J. Bot. 32, 459–464.
Dawson, T. P., Jackson, S. T., House, J. I., Prentice, I. C., Mace, G. M. (2011). Beyond predictions: biodiversity conservation in a changing climate. Sci. (80-.). 332, 53–58. doi: 10.1126/science.1200303
Dhyani, A., Kadaverugu, R., Nautiyal, B. P., Nautiyal, M. C. (2021). Predicting the potential distribution of a critically endangered medicinal plant Lilium polyphyllum in Indian Western Himalayan Region. Reg. Environ. Change 21, 1–11. doi: 10.1007/s10113-021-01763-5
Domroes, M., El-Tantawi, A. (2005). Recent temporal and spatial temperature changes in Egypt. Int. J. Climatol. 25, 51–63. doi: 10.1002/joc.1114
Dullinger, S., Gattringer, A., Thuiller, W., Moser, D., Zimmermann, N. E., Guisan, A., et al. (2012). Extinction debt of high-mountain plants under twenty-first-century climate change. Nat. Clim. Change 28, 619–622. doi: 10.1038/nclimate1514
Dutra Silva, L., Brito de Azevedo, E., Bento Elias, R., Silva, L. (2017). Species distribution modeling: Comparison of fixed and mixed effects models using INLA. ISPRS Int. J. geo-Information 6, 391. doi: 10.3390/ijgi6120391
El-Barougy, R. F., Dakhil, M. A., Halmy, M. W. A., Cadotte, M., Dias, S., Farahat, E. A., et al. (2023). Potential extinction risk of Juniperus phoenicea under global climate change: Towards conservation planning. Glob. Ecol. Conserv. 46, e02541. doi: 10.1016/j.gecco.2023.e02541
Engler, R., Randin, C. F., Vittoz, P., Czáka, T., Beniston, M., Zimmermann, N. E., et al. (2009). Predicting future distributions of mountain plants under climate change: Does dispersal capacity matter? Ecography (Cop.). 32, 34–45. doi: 10.1111/j.1600-0587.2009.05789.x
Erfanian, M. B., Sagharyan, M., Memariani, F., Ejtehadi, H. (2021). Predicting range shifts of three endangered endemic plants of the Khorassan-Kopet Dagh floristic province under global change. Sci. Rep. 11, 1–13. doi: 10.1038/s41598-021-88577-x
Fakhry, A. M., El-Keblawy, A., Shabana, H. A., El Gamal, I., Shalouf, A. (2019). Microhabitats affect population size and plant vigor of three critically endangered endemic plants in Southern Sinai mountains, Egypt. Land 8, 86. doi: 10.3390/land8060086
Fayed, A. A., El-Garf, I. A., Abdel-Khalik, K. N., Osman, A. K. (2004). Floristic survey of the mountainous region of South Sinai, St Katherine’s Protectorate. Medicinal Plants Conservation Project. Egypt, Report, 146.
Fick, S. E., Hijmans, R. J. (2017). WorldClim 2: new 1-km spatial resolution climate surfaces for global land areas. Int. J. Climatol. 37, 4302–4315. doi: 10.1002/joc.5086
Fois, M., Cuena-Lombraña, A., Fenu, G., Bacchetta, G. (2018). Using species distribution models at local scale to guide the search of poorly known species: Review, methodological issues and future directions. Ecol. Modell. 385, 124–132. doi: 10.1016/j.ecolmodel.2018.07.018
Fois, M., Fenu, G., Cuena Lombraña, A., Cogoni, D., Bacchetta, G. (2015). A practical method to speed up the discovery of unknown populations using Species Distribution Models. J. Nat. Conserv. 24, 42–48. doi: 10.1016/j.jnc.2015.02.001
Grainger, J., Gilbert, F. (2008). “Around the sacred mountain: The St. Katherine Protectorate in South Sinai, Egypt,” in Protected Landscapes and Cultural and Spiritual Values. Volume 2 in the series Values of Protected Landscapes and Seascapes. IUCN, GTZ and Obra Social de Caixa Catalunya. Ed. J.-M. Mallarach (Kasparek Verlag, Heidelberg), 21–37.
Guisan, A., Thuiller, W. (2005). Predicting species distribution: Offering more than simple habitat models. Ecol. Lett. 8, 993–1009. doi: 10.1111/j.1461-0248.2005.00792.x
Guisan, A., Thuiller, W., Zimmermann, N. E. (2017). Habitat Suitability and Distribution Models: With Applications in R (Cambridge, UK: Cambridge University Press). doi: 10.1017/9781139028271
Guisan, A., Zimmermann, N. E. (2000). Predictive habitat distribution models in ecology. Ecol. Modell. 135, 147–186. doi: 10.1016/S0304-3800(00)00354-9
Hoorn, C., Perrigo, A., Antonelli, A. (2018). Mountains, Climate and Biodiversity (Hoboken, USA: John Wiley & Sons).
Hosni, H., Hosny, A., Shamso, E., Hamdy, R. (2013). Endemic and near-endemic taxa in the flora of Egypt. Egypt J. Bot. 53, 1–21.
Hu, X.-G., Jin, Y., Wang, X.-R., Mao, J.-F., Li, Y. (2015). Predicting impacts of future climate change on the distribution of the widespread conifer Platycladus orientalis. PLoS One 10, e0132326. doi: 10.1371/journal.pone.0132326
IUCN (2023) The IUCN Red List of Threatened Species. Version 2023-1. Available online at: https://www.iucnredlist.org (Accessed April 9, 2023).
Jafari, F., Zarre, S., Gholipour, A., Eggens, F., Rabeler, R. K., Oxelman, B. (2020). A new taxonomic backbone for the infrageneric classification of the species-rich genus Silene (Caryophyllaceae). Taxon 69, 337–368. doi: 10.1002/tax.12230
Kaky, E., Gilbert, F. (2016). Using species distribution models to assess the importance of Egypt’s protected areas for the conservation of medicinal plants. J. Arid Environ. 135, 140–146. doi: 10.1016/j.jaridenv.2016.09.001
Kaky, E., Gilbert, F. (2017). Predicting the distributions of Egypt’s medicinal plants and their potential shifts under future climate change. PLoS One 12, e0187714. doi: 10.1371/journal.pone.0187714
Kaky, E., Nolan, V., Alatawi, A., Gilbert, F. (2020). A comparison between Ensemble and MaxEnt species distribution modelling approaches for conservation: A case study with Egyptian medicinal plants. Ecol. Inform. 60, 101150. doi: 10.1016/j.ecoinf.2020.101150
Kent, M. (2011). Vegetation Description and Data Analysis: A Practical Approach (Hoboken, USA: John Wiley & Sons).
Khedr, A. (2007). “Assessment, classification, and analysis of microhabitats supporting globally significant plant species,” in Conserv. Sustain. Use Med. Plants Arid Semi-arid Eco-systems Proj. Saint Katherine Prot. Egypt, Final Rep. (EEAA, GEF UNDP, Cairo, Egypt).
Khedr, A. (2021). “Microhabitats supporting endemic plants in Sinai, Egypt,” in Management and Development of Agricultural and Natural Resources in Egypt’s Desert (Netherlands: Springer), 369–381.
Lenoir, J., Gégout, J. C., Marquet, P. A., De Ruffray, P., Brisse, H. (2008). A significant upward shift in plant species optimum elevation during the 20th century. Sci. 320, 1768–1771. doi: 10.1126/science.1156831
Liu, C., Newell, G., White, M. (2016). On the selection of thresholds for predicting species occurrence with presence-only data. Ecol. Evol. 6, 337–348. doi: 10.1002/ece3.1878
Liu, L., Guan, L., Zhao, H., Huang, Y., Mou, Q., Liu, K., et al. (2021). Modeling habitat suitability of Houttuynia cordata Thunb (Ceercao) using MaxEnt under climate change in China. Ecol. Inform. 63, 101324. doi: 10.1016/j.ecoinf.2021.101324
Martín-Gómez, J. J., Rewicz, A., Rodríguez-Lorenzo, J. L., Janoušek, B., Cervantes, E. (2020). Seed morphology in Silene based on geometric models. Plants 9, 1787. doi: 10.3390/plants9121787
Mccain, C. M., Colwell, R. K. (2011). Assessing the threat to montane biodiversity from discordant shifts in temperature and precipitation in a changing climate. Ecol. Lett. 14, 1236–1245. doi: 10.1111/ele.2011.14.issue-12
McCarty, J. P. (2001). Ecological consequences of recent climate change. Conserv. Biol. 15, 320–331. doi: 10.1046/j.1523-1739.2001.015002320.x
Mir, A. H., Tyub, S., Kamili, A. N. (2020). Ecology, distribution mapping and conservation implications of four critically endangered endemic plants of Kashmir Himalaya. Saudi J. Biol. Sci. 27, 2380–2389. doi: 10.1016/j.sjbs.2020.05.006
Mosallam, H. A. M. (2007). Assessment of target species in Saint Katherine protectorate, Sinai, Egypt. J. Appl. Sci. Res. 3, 456–469.
Moustafa, A., Klopatek, J. M. (1995). Vegetation and landforms of the Saint Catherine area, southern Sinai, Egypt. J. Arid Environ. 30, 385–395. doi: 10.1006/jare.1995.0033
Moustafa, A., Zaghloul, M. S., E. Wahab, R. H. A., Shaker, M. (2001). Evaluation of plant diversity and endemism in Saint Catherine Protectorate, South Sinai, Egypt. Egypt. J. Bot. 2002 41, 121–139.
Moustafa, A., Zaghloul, M., Mansour, S., Alsharkawy, D., Alotaibi, M. (2017). Long term monitoring of Rosa arabica populations as a threatened species in South Sinai, Egypt. J. Biodivers. Endanger. Species 05, 1–8. doi: 10.4172/2332-2543.1000197
Naimi, B. (2017). Package ‘usdm’. Uncertainty Analysis for Species Distribution Models (Wien). Available at: https://cran.r-project.org/web/packages/usdm/index.html.
Naimi, B., Araújo, M. B. (2016). Sdm: A reproducible and extensible R platform for species distribution modelling. Ecography (Cop.). 39, 368–375. doi: 10.1111/ecog.01881
Nasab, F., Mehrabian, A., Mostafavi, H., Neemati, A. (2022). The influence of climate change on the suitable habitats of Allium species endemic to Iran. Environ. Monit. Assess. 194, 1–20. doi: 10.1007/s10661-022-09793-0
Orsenigo, S., Montagnani, C., Fenu, G., Gargano, D., Peruzzi, L., Abeli, T., et al. (2018). Red listing plants under full national responsibility: Extinction risk and threats in the vascular flora endemic to Italy. Biol. Conserv. 224, 213–222. doi: 10.1016/j.biocon.2018.05.030
Oxelman, B., Lidén, M., Rabeler, R. K., Popp, M. (2000). A revised generic classification of the tribe Sileneae (Caryophyllaceae). Nord. J. Bot. 20, 743–748. doi: 10.1111/j.1756-1051.2000.tb00760.x
Rabei, S. H., El Gamal, I. A., Nada, R. M. (2021). Effect of genetic diversity on the distribution of endemic species of the genus Silene (Caryophyllaceae) in Saint Katherine Protectorate, Sinai, Egypt. Plant Mol. Biol. Rep. 39, 364–375. doi: 10.1007/s11105-020-01253-8
Radford, E. A., Catullo, G., Montmollin, B. (2011). Important plant areas of the south and east Mediterranean region: priority sites for conservation (IUCN Gland (Suiza) WWF, Gland (Suiza).
R Core Team (2022). R: A language and environment for statistical computing (Vienna, Austria: R Foundation for Statistical Computing). Available at: http://www.r-project.org.
Root, T. L., Price, J. T., Hall, K. R., Schneider, S. H., Rosenzweig, C., Pounds, J. A. (2003). Fingerprints of global warming on wild animals and plants. Nature 421, 57–60. doi: 10.1038/nature01333
Saker, M., El-Demerdash, M., Allam, M. A. (2011). In vitro propagation and genetic characterization as effective tools for conservation of Silene leucophylla, grown in St. Katherine Protected Area, Sinai, Egypt. J. Genet. Eng. Biotechnol. 9, 21–27. doi: 10.1016/j.jgeb.2011.05.011
Shaltout, K. H., Ahmed, D. A., Shabana, H. A. (2015). Population structure and dynamics of the endemic species Phlomis aurea Decne in different habitats in southern Sinai Peninsula, Egypt. Glob. Ecol. Conserv. 4, 505–515. doi: 10.1016/j.gecco.2015.10.002
Shaltout, K. H., Ahmed, D. A., Shabana, H. A. (2016). Distribution of the species associated with Phlomis aurea Decne along an elevation gradient in southern Sinai, Egypt. Ecol. Mediterr. 42, 65–77. doi: 10.3406/ecmed.2016.1233
Sintayehu, D. W. (2018). Impact of climate change on biodiversity and associated key ecosystem services in Africa: A systematic review. Ecosyst. Heal. Sustain. 4, 225–239. doi: 10.1080/20964129.2018.1530054
Smeraldo, S., Di Febbraro, M., Bosso, L., Flaquer, C., Guixé, D., Lisón, F., et al. (2018). Ignoring seasonal changes in the ecological niche of non-migratory species may lead to biases in potential distribution models: Lessons from bats. Biodivers. Conserv. 27, 2425–2441. doi: 10.1007/s10531-018-1545-7
Thuiller, W. (2004). Patterns and uncertainties of species’ range shifts under climate change. Glob. Change Biol. 10, 2020–2027. doi: 10.1111/j.1365-2486.2004.00859.x
Thuiller, W., Guéguen, M., Renaud, J., Karger, D. N., Zimmermann, N. E. (2019). Uncertainty in ensembles of global biodiversity scenarios. Nat. Commun. 10, 1446. doi: 10.1038/s41467-019-09519-w
Thuiller, W., Lavorel, S., Araújo, M. B., Sykes, M. T., Prentice, I. C. (2005). Climate change threats to plant diversity in Europe. Proc. Natl. Acad. Sci. 102, 8245–8250. doi: 10.1073/pnas.0409902102
Trew, B. T., Maclean, I. M. D. (2021). Vulnerability of global biodiversity hotspots to climate change. Glob. Ecol. Biogeogr. 30, 768–783. doi: 10.1111/geb.13272
van Vuuren, D. P., Edmonds, J., Kainuma, M., Riahi, K., Thomson, A., Hibbard, K., et al. (2011). The representative concentration pathways: An overview. Clim. Change 109, 5–31. doi: 10.1007/s10584-011-0148-z
Walther, G.-R., Post, E., Convey, P., Menzel, A., Parmesan, C., Beebee, T. J. C., et al. (2002). Ecological responses to recent climate change. Nature 416, 389–395. doi: 10.1038/416389a
Wang, H. H., Wonkka, C. L., Treglia, M. L., Grant, W. E., Smeins, F. E., Rogers, W. E. (2019). Incorporating local-scale variables into distribution models enhances predictability for rare plant species with biological dependencies. Biodivers. Conserv. 28, 171–182. doi: 10.1007/s10531-018-1645-4
Zahran, M. A., Wafaa, A. M., Samy, A. A., Omran, G. N. (2015). Endemic species in Sinai peninsula, Egypt, with particular reference to Saint Katherine protectorate: I-ecological features. J. Environ. Sci. Mansoura Univ. 44, 589–609.
Keywords: arid region, conservation, Egypt, ensemble model, global warming
Citation: Abdelaal M, AL-Huqail AA, Alghanem SMS, Alhaithloul HAS, Al-Robai SA, Abeed AHA, Dakhil MA, El-Barougy RF and Yahia AA (2024) Population status, habitat preferences and predictive current and future distributions of three endangered Silene species under changing climate. Front. Plant Sci. 15:1336911. doi: 10.3389/fpls.2024.1336911
Received: 12 November 2023; Accepted: 05 June 2024;
Published: 20 June 2024.
Edited by:
Dun Wang, Northwest A&F University, ChinaReviewed by:
Gabriele Casazza, University of Genoa, ItalyRui Bento Elias, University of the Azores, Portugal
Copyright © 2024 Abdelaal, AL-Huqail, Alghanem, Alhaithloul, Al-Robai, Abeed, Dakhil, El-Barougy and Yahia. This is an open-access article distributed under the terms of the Creative Commons Attribution License (CC BY). The use, distribution or reproduction in other forums is permitted, provided the original author(s) and the copyright owner(s) are credited and that the original publication in this journal is cited, in accordance with accepted academic practice. No use, distribution or reproduction is permitted which does not comply with these terms.
*Correspondence: Arwa Abdulkreem AL-Huqail, arwaalhuqail049@gmail.com; aaalhuqail@pnu.edu.sa
†These authors have contributed equally to this work