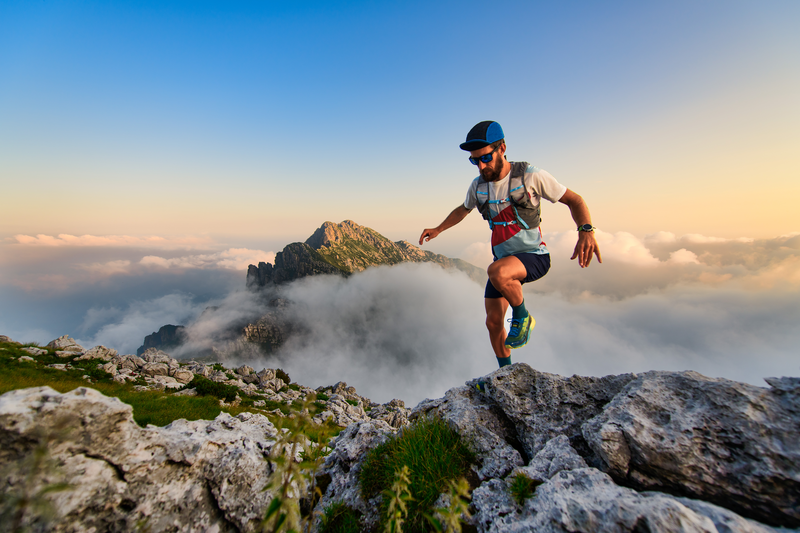
94% of researchers rate our articles as excellent or good
Learn more about the work of our research integrity team to safeguard the quality of each article we publish.
Find out more
ORIGINAL RESEARCH article
Front. Plant Sci. , 29 January 2024
Sec. Functional Plant Ecology
Volume 15 - 2024 | https://doi.org/10.3389/fpls.2024.1335524
Introduction: Canopy species need to shift their ecological adaptation to improve light and water resources utilization, and the study of intraspecific variations in plant leaf functional traits based at individual scale is of great significance for evaluating plant adaptability to climate change.
Methods: In this study, we evaluate how leaf functional traits of giant trees relate to spatial niche specialization along a vertical gradient. We sampled the tropical flagship species of Parashorea chinensis around 60 meters tall and divided their crowns into three vertical layers. Fourteen key leaf functional traits including leaf morphology, photosynthetic, hydraulic and chemical physiology were measured at each canopy layer to investigate the intraspecific variation of leaf traits and the interrelationships between different functional traits. Additionally, due to the potential impact of different measurement methods (in-situ and ex-situ branch) on photosynthetic physiological parameters, we also compared the effects of these two gas exchange measurements.
Results and discussion: In-situ measurements revealed that most leaf functional traits of individual-to-individual P. chinensis varied significantly at different canopy heights. Leaf hydraulic traits such as midday leaf water potential (MWP) and leaf osmotic potential (OP) were insignificantly correlated with leaf photosynthetic physiological traits such as maximal net assimilation rate per mass (Amass). In addition, great discrepancies were found between in-situ and ex-situ measurements of photosynthetic parameters. The ex-situ measurements caused a decrease by 53.63%, 27.86%, and 38.05% in Amass, and a decrease of 50.00%, 19.21%, and 27.90% in light saturation point compared to the in-situ measurements. These findings provided insights into our understanding of the response mechanisms of P. chinensis to micro-habitat in Xishuangbanna tropical seasonal rainforests and the fine scale adaption of different resultant of decoupled traits, which have implications for understanding ecological adaption strategies of P. chinensis under environmental changes.
Forests are highly diversified ecosystems that play a key role in the carbon and hydrologic cycle (Needham et al., 2022). Some far anthropogenic influences on global climate change have led to longer and more frequent climate extremes, which may adversely affect the structure and function of forest communities. In response, plants adjust physiologically and morphologically to changes in their surrounding climate, thereby forming specific functional traits, reflecting adaptation strategies to different environment stresses. Consequently, trait-based ecological research has potential to account for plant species distribution and function, perfect global vegetation patterns, and to predict ecosystem processes (Wright et al., 2004; Díaz et al., 2016; Shi et al., 2020; Hagan et al., 2023). However, prior studies mainly consider the trait characteristics by hypothesizing that the individuals of plant species are the same (Anderegg et al., 2018). This approach overlooks the fact that intraspecific variations in plant functional traits across individuals can contribute significantly to the total trait variation of a plant population even a forest community (Huang et al., 2023). Intraspecific variations represent plant genetic diversity and the different phenotypes produced by the same genotype under variable ambient conditions, which is well known as phenotypic plasticity (Sobral and Sampedro, 2022). The overlook in the intraspecific variation may lead to inaccurate estimation on trait diversity (Lasky et al., 2014; Siefert et al., 2015). In comparison with the patterns observed at the species level, the diversity of individual traits may provide more compelling evidence for ecological adaptation and indicate the different axes of niche division (Koch et al., 2004; Lasky et al., 2014; Pfautsch et al., 2018).
Prior studies focusing on functional trait variations in leaves have greatly advanced our comprehension of plant performance and ecological adaptation. Among various leaf-level traits, those related to CO2 and water exchange and light capture have probably received more attention (Li et al., 2015; Xu et al., 2021). Leaf economics and hydraulic traits directly affect plant nutrient absorption and carbon assimilation rate, and control water transport to resist atmospheric drought (Wright et al., 2004). This in turn sets limitations on key aspects of plant architecture such as maximum height, allometry and canopy physiology (Koch et al., 2004; de Bittencourt et al., 2022). Forest microenvironment is an important driver of the intraspecific variation of leaf functional traits (Kenzo et al., 2015; Zuleta et al., 2022). Forest ecosystems experience notable environmental heterogeneity along vertical gradients, with increasing light radiation and vapor pressure difference (VPD) and decreasing atmospheric humidity (Duan et al., 2022). Within the same forest community, changes in local environmental gradients, which often follow the same patterns as those found at large spatial scales, can strongly influence ecological adaptation strategies (Kenzo et al., 2015; Lu et al., 2015; Chen et al., 2019). Moreover, leaf also play a pivotal role in niche differentiation (Ishii et al., 2013; Hess et al., 2022). In tropical and subtropical regions, ecological adaptation along a fine-scale vertical gradient can be explained by habitat filtering based on leaf traits (Kenzo et al., 2015; Feng et al., 2022). Leaves in these regions are highly specialised to the microenvironment conditions, exhibiting contrasting physiological characteristics found under strong selective pressures (Koch et al., 2004). Thus, traits related to how efficiently plants carbon assimilation and resist drought stress are highlighted as critical factors on the mechanism of species coexistence in a community (Li et al., 2015).
Given that leaf economics and hydraulic traits are both related to the process of water-CO2 exchange, the relationship of these two sets of traits has garnered widespread attention (Li et al., 2015). From the perspective of a single economics spectrum, there is a strong coordination between leaf economics and hydraulic traits across comprehensive species, implying that leaves with higher photosynthetic rates tend to have more effective hydraulic systems (Wright et al., 2004; Reich, 2014). Nevertheless, leaf functional traits may not always change in this coordinated way. Increasing evidence supports multiple dimensions of plant functional traits (Sack et al., 2003; Sack and Scoffoni, 2013). Recent studies in tropical-subtropical forest systems suggested that there was a decoupling between leaf hydraulic and economics traits among different species (Li et al., 2015). Blackman et al. (2016) further found that leaf functional trait coordination at intraspecific scale was also decoupled in response to environmental change. This decoupling helps for understanding the ecological adaptation strategies of species under climate change, because these independent trait dimensions may be selected by different environmental filters (Li et al., 2015). Despite this, whether this phenomenon also exists at the individual scale remains to be discussed, as there is little research on the relationship between different types of functional traits in specific species (Wright et al., 2004). In complex forest ecosystems where resource space is multidimensional and highly heterogeneous, to improve the performance of whole tree performance, different combinations of leaf functional traits at the individual species level is likely to be necessary to adapt to various changes in fine-scale habitats (Martinez and Fridley, 2018; Pritzkow et al., 2020).
Parashorea chinensis H. Wang, belonging to the family Dipterocarpaceae, is the flagship species in the Asian tropics (Tang et al., 2008; de Bittencourt et al., 2022). P. chinensis is distributed in the tropical seasonal rainforest of China, especially in Xishuangbanna, Yunnan Province. Adult individuals of P. chinensis in the canopy may reach heights above 60 m (Deng et al., 2020), which is also the tallest tree of tropical seasonal rainforest in China and means a high proportion of aboveground carbon storage (Tang et al., 2008). Meanwhile, P. chinensis also plays an essential role in maintaining biodiversity and the stability of ecological networks in community (Hu et al., 2022; Shen et al., 2022). Data reveal that large trees are more susceptible to the impact of drought due to changes in precipitation time and amplitude, as well as increasing temperatures (Bennett et al., 2015; Liu et al., 2021). Given that the structure of these forests is highly variable across the vertical gradient (Deng et al., 2020; Shen et al., 2022), if the adult P. chinensis fails to respond adaptively to keep up with the increasing stresses imposed by fine spatial scales, the trees may be rapidly at risk from the microenvironment apart from climate change. However, to this day, there are only limited studies about how different leaf functional traits within adult-individual-level P. chinensis shift with microenvironmental gradients. The handful of functional traits studies of the P. chinensis from vertical gradients in China which measure water-use efficiency, leaf morphological structures and nutrient allocation strategies do suggest that there is a strong fierce pressure on plant function across vertical gradients (Deng et al., 2020). However, these studies usually focused on diverse size classes of trees with comparatively few leaf photosynthetic traits, which limit our capacity to understand intraspecific variations and niche differentiation in leaf functional traits. Therefore, there is a need to note that the investigation on the intraspecific variation and correlation between leaf functional traits will enable better understanding of the trade-off between adaptive characteristics and functional traits of P. chinensis for different vertical gradient.
Benefiting by using the canopy crane, we can access canopy leaves to measure leaf hydraulic and economics traits of sample trees of P. chinensis in the forest at different vertical heights directly (in-situ measurement). In the past, it was hard to measure photosynthesis-related traits in-situ, and gas exchange observations performed on branches that have been excised from the tree are widespread (ex-situ measurement) (Santiago and Mulkey, 2003; Missik et al., 2020), consequently. The method of cutting branches assumes that cutting branches underwater can minimize damage to the branches hydraulic system (Hanson et al., 2013; Sperry et al., 2016). However, some researchers suggest that compared with intact branches, leaf photosynthetic traits such as stomatal conductance and net rate of photosynthetic CO2 assimilation on detached branches are significantly distinct (Santiago and Mulkey, 2003; Missik et al., 2020). Related excision response could vary due to factors such as the length of the excised branches and the canopy position (Woodruff et al., 2004; Buckley, 2005; Missik et al., 2020). Regardless of the mechanisms, there is still less agreement about which species may be affected minimally this measurement. Thereby, it is reasonable to expect that large trees like the P. chinensis under greater risk of hydraulic failure may exhibits remarkably significant divergent results by using different measurement methods.
In this study, we assessed key leaf economics and hydraulic traits (Table 1) of adult trees of P. chinensis at three heights (Table S1) and addressed whether variation in leaf photosynthetic traits is determined by tree height, different measurements and their interaction. To achieve the above goals, we examined (i) the variations in leaf functional traits along vertical gradients at the individual species level; (ii) the interrelationships between different functional traits at the individual level; (iii) the effect of different measurement methods on photosynthetic physiological parameters of P. chinensis.
We conducted this study in the Xishuangbanna National Natural Reserve (101°34’N, 21°36’E) in Yunnan Province, China, which is a virgin tropical seasonal rainforest and P. chinensis is the dominant canopy tree species therein (Tang et al., 2008; Deng et al., 2020). The Xishuangbanna National Natural Reserve experiences a tropical seasonal climate with an obvious dry season (November-April) and a rainy season (May-October) which provides 80% of annual precipitation; the mean annual temperature is 21°C, and the mean annual rainfall is 1532 mm (Deng et al., 2020).
The canopy crane is located in the same tropical seasonal rainforest (TCT7015-10E, Zoomlion Heavy Industry, Changsha, China), which maximum operational height is 80 m and the job length is 60 m. A dynamics plot of 1.44 ha was established in 2014, centered around the crane base, for plant species investigation. Among all these 6928 individuals of 217 woody tree species with a diameter at breast height (DBH) ≥ 1 cm in this plot, P. chinensis had the highest relative importance values and the largest basal area (Tang et al., 2008).
In July 2022, which is the main growing season for most local plants. We first selected 5 adult-individual P. chinensis from the plot and divided their canopy into three equal parts from top to bottom. Afterwards, we sampled and measured southfacing branches and sun-exposed leaves of the canopy of these 5 trees across three vertical gradients. Before measuring leaves when sunny days, we operated the crane and measured every selected tree height with a tapeline.
After reaching the different canopy heights (Table S1) using the forest tower crane (TCT7015-10E, Zoomlion Heavy Industry, Changsha, China), we used both uncut and detached branches to inspect the influence of any height-related and method-related effect on the gas exchange parameters. The light-response curve was measured with a portable photosynthesis-fluorescence meter (LI-6800, LI-COR, Inc, Lincoln, NE, USA) and we maintained a stable CO2 concentration in the fluorescence leaf chamber (400-440ppm) with a CO2 buffer bottle. The relative humidity was 75%–90% (Deng et al., 2020; Shen et al., 2022), the air flow was 500 µmol·s−1, the leaf temperature was approximately 30°C and the VPD was 1.3 ± 0.2 kPa (Deng et al., 2020). After light adaptation under 1000 µmol·m-2s-1, the PPFD gradient was set as follows: 2300, 1800, 1300, 800, 600, 400, 200, 100, 50, 30, and 0 µmol·m-2s-1. Before measurement, the instrument needed to be matched. At each PPFD gradient, the leaves were allowed to balance for at least 3 minutes in view of the steadiness of stomatal conductance (Gs). We analyze the light response curves by using the model proposed by Ye (2007), as denoted as Ye model hereinafter, following measurement to calculate the maximal net assimilation rate (Amax), the light saturation point (LSP), the light compensation point (LCP) and the respiration rate (Rd).
The maximal net assimilation rate per mass is calculated as follows:
The Gs was recorded when measuring the photosynthetic physiological traits of leaves. We took measurements of the leaf thickness (LT) using a digital micrometer (SWSIWI, China) and avoided the main leaf vein. Leaf lamina area (LA) was measured using a leaf area meter (LI-3000A, Li-Cor, USA). We also measured leaf relative water content by weighing fresh leaves, soaking them in ultra-pure water for 2 hours, weighing the saturated fresh weight, then drying at them 65°C for 72 hours and weighing the dry mass. From these measurements, we calculated leaf dry mass per area (LMA), leaf density (LD), and leaf relative water content (LWC).
The calculation equations are as follows:
Nitrogen content (Nmass) in the dried and ground leaves was analyzed using an elemental analyzer (Vario ISOTOPE Cube, Elementar Analysensysteme GmbH, Langenselbold, Germany).
We measured midday leaf water potential (MWP) immediately after collecting leaves by using a pressure chamber (Model 1505, PMS, Albany, USA) between 12:00-1:30 PM. We also measured leaf osmotic potential (OP) by using a vapor pressure osmometer (Vapro 5600, Wescor, USA). For different canopy layers of each sampled tree, we measured three to five sun-exposed leaves. All measurements were taken on the same sunny day.
Leaf trait data were tested for the normality of distribution using the Shapiro–Wilk tests. Initially multiple comparisons (ANOVA) of leaf traits at different heights were carried out using Tukey’s test with 95% confidence intervals. Then we conducted a principal component analysis (PCA) to examine how leaf traits varied among the different elevations. A permutational multivariate analysis of variance (PERMANOVA) was used to test the difference in leaf traits at different heights. To examine the relationships between different types of leaf functional traits at the individual level, we conducted Pearson correlation analysis. To investigate the impact of different measurement methods on the parameters of leaf photosynthetic-related traits, we employed a linear mixed model (Zuur et al., 2009), treating the individual as a random factor and canopy height and measurement method as fixed effects (Shi et al., 2021). We first constructed a model including all factors and their interactions, and then compared this with the original model after sequentially removing two-factor interactions and single factors, to test the significance of factors or interactions (Lu et al., 2020). Furthermore, we performed the same statistical analysis on the functional trait parameters obtained from detached measurements as from in-situ measurements, to test the differences in statistical results under different measurement methods. All data analysis and visualizations were carried out using R v.4.2.1 (R Core Team, 2022).
In-situ measurements revealed that most leaf functional traits of P. chinensis at the individual level varied significantly across different canopy heights. For instance, within the leaf morphological and structural traits, the LA, LD, and Gs increased 1.16-, 1.29-, and 1.73-fold, respectively (Figures 1A–F), from the low to high canopy, but the LT and LWC of high canopy were 1.45 and 1.13 times those at the lower canopy, oppositely. Leaf photosynthetic physiological traits, such as Amass, LCP, and Rdmass, showed significant difference between low and high canopy (Figures 1G–J), whereas no obvious discrepancies were observed in most of those parameters between the low and middle height (Figures 1G–J). Contrary to the trends for LWC, leaf hydraulic traits, including OP and MWP, declined with the increasing height (Figures 1K, L). Leaf chemical traits, such as Nmass, was less affected by the vertical gradient.
Figure 1 Differences in leaf functional traits of adult-individual level of P. chinensis at 3 vertical heights as measured in-situ. Differences for each trait were determined using the Tukey’s test, with a confidence level of 95%. High, high canopy; Middle, middle canopy; Low, lower canopy. LA, Leaf area (A); LMA, leaf mass per area (B); LT, leaf thickness (C); LD, leaf density (D); Gs, stomatal conductance (E); LWC, leaf water content (F); Amass, maximum net photosynthetic rate per unit mass (G); LSP, light saturation point (H); LCP, light compensation point (I); Rdmass, dark respiration rate per unit mass (J); OP, leaf osmotic potential (K); MWP, midday leaf water potential (L); Nmass, nitrogen content per unit mass (M); PNUE, photosynthetic nitrogen use efficiency (N). Error bars represent standard errors. Significant differences between each height (ANOVA, P< 0.05) are indicated by different letters.
Our PCA revealed the individual-level variation of 14 leaf traits across multiple scales and divided them into two nearly decoupled dimensions (Figure 2). The first leading dimension of leaf adaptation to height, which accounted for 49.6% of the variation, was mainly dominated by leaf carbon assimilation and hydraulic safety (loaded traits such as Gs, PNUE, Amass, LD, MWP, OP, LSP, Rdmass, LCP, LMA, and LWC). The second principal component of resource acquisition, explaining 17.3% of the variation, was mainly represented by LA, LSP and Nmass. The PCA revealed the existence of two leading independent dimensions of the variation in leaf traits. Along the first axis, the high height was separated from the other two heights, with significant differences among all three heights supported by PERMANOVA (R2 = 0.22, P = 0.036, Table S2).
Figure 2 PCA analysis results of 14 leaf functional traits at different vertical heights measured in-situ. Blue, gray, and orange represent the high, middle, and lower vertical heights, respectively. The ellipses indicate a 95% confidence interval. High, high canopy; Middle, middle canopy; Low, lower canopy. LA, Leaf area; LMA, leaf mass per area; LT, leaf thickness; LD, leaf density; Amass, maximum net photosynthetic rate per unit mass; LSP, light saturation point; LCP, light compensation point; Rdmass, dark respiration rate per unit mass; Gs, stomatal conductance; LWC, leaf water content; MWP, midday leaf water potential; OP, leaf osmotic potential; Nmass, nitrogen content per unit mass; PNUE, photosynthetic nitrogen use efficiency.
Among all leaf hydraulic traits, MWP had no correlation with OP (Figures 2, 3). Among all leaf economic traits, LT was significantly negatively related to LD and Gs, whereas a positive relationship was found between Amass and PNUE. Contrary to these significant correlations, neither MWP nor OP was uncorrelated with Amass.
Figure 3 Results of the correlation tests between different leaf functional traits of P. chinensis measured in-situ. LA, Leaf area; LMA, leaf dry mass per area; LT, leaf thickness; LD, leaf density; Amass, maximum net photosynthetic rate per unit mass; LSP, light saturation point; LCP, light compensation point; Rdmass, dark respiration rate per unit mass; Gs, stomatal conductance; LWC, leaf water content; MWP, midday leaf water potential; OP, leaf osmotic potential; Nmass, nitrogen content per unit mass; PNUE, photosynthetic nitrogen use efficiency. Significance is denoted by asterisks::*P< 0.05; **P< 0.01; and ***P< 0.001.
There were significant differences in most parameters of the leaf photosynthetic physiological traits of P. chinensis between different measurement methods, particularly Amass and LSP (Figure 4, Table S4). As shown in Table S4, from the lower to the upper canopy, the ex-situ measurements resulted in a decrease by 53.63%, 27.86%, and 38.05% in Amass, and a decrease by 50.00%, 19.21%, and 27.90% in LSP compared to the in-situ measurements, separately. We found that the multivariate permutation test of the differences in leaf functional traits at different canopy heights significantly changed when using detached branches (R2 = 0.15, P=0.12, Figure S1, Table S2), although different methods did not substantially alter the patterns of functional coordination between Amass and leaf hydraulic traits (Figure S2).
Figure 4 Parameters of light response curves of leaves at different heights under different measurement methods. Amass, maximum net photosynthetic rate per unit mass (A); LSP, light saturation point (B); LCP, light compensation point (C); Rdmass, dark respiration rate per unit mass (D); in-situ, in-situ measurement; ex-situ, ex-situ measurement; High: high canopy; Middle, middle canopy; Low, lower canopy. The P-value represents the impact of measurement methods on leaf functional traits of the overall tree canopy. The significance of different canopy layers are denoted by asterisks: *P< 0.05; **P< 0.01; ***P< 0.001; and ns, not significant.
Leaf photosynthetic physiological traits such as LCP and Rdmass are key indicators reflecting plant adaptation to light (Givnish, 1988). As canopy height increases, light availability also promoted. The transmittance of diffuse light varied from 0.3% to 100%, and the light intensity varied from 2.3 W·m-2 to 208W·m-2 (Deng et al., 2020; Shen et al., 2022). The leaves of P. chinensis exhibit low LCP and Rdmass in the low canopy layer, which help to conserve carbon better than leaves in the high canopy and to gain carbon better at lower light levels (Koch et al., 2004). The trade-off between low LCP, respiration rates and high maximum photosynthetic rates of the leaves of P. chinensis in habitat shift from low canopy to high canopy reflected its change in ecological adaptation, which are similar to the pattern of the strategy of different sizes of trees from understory to canopy (Deng et al., 2020). The leaf morphological and structural trait is also likely to express the responses to the resource gradients (Wright et al., 2004; Reich, 2014), P. chinensis increased the thickness of the palisade tissue to enhance CO2 diffusion pathways and dissolution area (Zou et al., 2022), promoting leaf light capture ability and the concentration of photosynthesis-related enzymes (Jiao et al., 2022), leading to LT increased and LD declined (Poorter et al., 2009). In general, plants will invest more nitrogen for photosynthesis on strong light conditions to increase photosynthetic efficiency. However, in comparison with the high canopy, although the LSP and Nmass did not change with vertical gradients, Amass and PNUE significantly decreased. These results are perhaps because the light conditions have reached at the status of saturation at the canopy layer, and the plants may allocate more nitrogen for non-metabolic functions such as light protection mechanisms (Roig-Oliver et al., 2021).
In addition to light condition, water resource along vertical gradient could shape the ecological adaption strategies (Koch et al., 2004; Pfautsch et al., 2018). Shen et al. (2022) have reported that the relative humidity above ground was obviously decreased as a result of increasing tree height in this plot, which ranged from 53.6% at 62m to 99.9% at 2 m. Hydraulic safety, as measured by leaf water potential like MWP and OP, is a trait linked to the degree of endurance to drought stress (Giles et al., 2021). We found that the leaves of P. chinensis in the low canopy adjust to being higher by decreasing leaf water potentials, resulting in lower MWP and OP in the high canopy (Figure 1). The unimodal pattern for leaf hydraulic traits is also consistent with the result from Sequoia sempervirens (Koch et al., 2004) and Eucalyptus grandis (Pfautsch et al., 2018). This indicates that large trees compensate for increasing water stress with height by adjusting their structures (Koch et al., 2004; Stovall et al., 2019). Moreover, leaves had a decrease in LA with tree height, but the LT still increased with tree height, suggesting that intraspecific variations, in addition to adaptations in light resources, might play an important role in adapting to maintain hydraulic safety function with increasing vertical gradients. Decreases in LMA with height were not found among different vertical layers, which suggests the decreases in MWP and OP with height were driven by adjustments to LT, LD and LA. This capacity may allow P. chinensis to have more flexibility in maintaining a trade-off between carbon balance and longevity, all of which change with LMA.
Leaf economics and hydraulic traits are important in plant growth and ecological adaptation. It has been proposed that they covary along a single axis of trait variation (Reich, 2014), which was based on the theory that hydraulic traits are the foundation of a key aspect of leaf economics spectrum (Wright et al., 2004). Although traits within each kind were closely associated, in this study, we found that leaf photosynthetic trait (Amass) and leaf hydraulic traits (MWP, OP) were statistically decoupled. This indicated that key leaf functional traits could not align themselves along a single axis of variation of P. chinensis, as recently proposed for functional trait variation across species (Reich, 2014). Instead, it suggests that different kinds of leaf functional trait can vary independently from each other even within an adult-individual level, which are consistent with studies that have observed independence among leaf functional traits across diverse sets of species and genotypes (Sack et al., 2003; Li et al., 2015; Blackman et al., 2016).
We found that leaf hydraulic traits such as OP were unrelated to net photosynthesis and stomatal conductance among P. chinensis individuals. These results support emerging reports of weak coordination between leaf hydraulic traits and leaf gas exchange across some sets of angiosperms (Gleason et al., 2016), which suggest that the driving factors linking hydraulic and gas exchange have not yet been fully understood. Yin et al. (2018) argued that adequate water availability may be a key factor leading to decoupling between functional traits by comparing and analyzing the relationship between the leaf economic and hydraulic traits in tropical-subtropical regions and the Loess Plateau. In moist area, plants tend to have more diverse and freer trait combinations to acclimate finely divided environmental gradients. For example, the Amass may vary strongly with light availability (Feng et al., 2022), while the MWP changes with drought stress (Giles et al., 2021). We also found that Amass was related to neither LMA nor Nmass, indicating that photosynthetic capacity can vary among vertical gradients without covariation in key facets of total leaf investment. These results contrast previous research showed that positive relationships between leaf economics traits among species with structurally similar leaves (Wyka et al., 2012). At last, stomatal conductance was intimately related to LT and LD, implying that the leaf morphology and structure has a potential impact on the CO2-water exchange process (Zwieniecki and Boyce, 2014).
Among leaf functional traits comparisons across species, decoupling may be caused by the result of physical separation of leaf structures. Li et al. (2015) proposed that leaves typically contain two functional modules and represent two functional subsystems. The two may be influenced by evolutionary divergence. The upper palisade tissue is associated with leaf photosynthetic physiological traits, while the lower spongy tissue is associated with leaf hydraulic traits. Both of these may be influenced by evolutionary differences and the processes within each sublayer may not necessarily change simultaneously or at the same rate in different growth conditions. Such explanations are relatively limited within individual species, yet. Within species, independent functional trait dimensions may be caused by differential expression of genotypes, which is very attractive for understanding how multiple trait combinations are generated. Blackman et al. (2016) utilized this perspective to explain why the lack of significant correlation between vein density and maximum stomatal conductance across genotypes even if the functions on both sides of the leaf are the same. However, our results provide insight that the change in ecological adaption caused by microenvironment discrepancy on vertical gradients at individual scale may also alter the relationship between leaf structure, hydraulics, and gas exchange characteristics (Brodribb et al., 2013), which allows trees to adjust functions more freely to adapt to the particular set of fine-scale environment and thus maintain overall canopy performance and enhances ecological adaption in turn. It is apparent that these results are potentially extended to different large trees species, which have implications for understanding biogeochemical processes in global models.
Performing on detached branches is a common practice for gas-exchange measurements, especially for extremely tall trees like P. chinensis. These data allow us to further study the environmental adaptability and plasticity of plants (Missik et al., 2020), and provide a basis for local or even global terrestrial ecological models (Walker et al., 2014). However, the data indicate that the conclusions drawn from this method tend to cause significant biases (Figure 4, Table S3), which were also reported previously (Missik et al., 2020).
In Table S3, the data suggest that canopy position may also be the possible driver affecting measurement parameters. Species with high photosynthetic capacity also demonstrate high water-CO2 exchange capacity to avoid photosynthetic constraints (Xu et al., 2021). Large trees must transport water to higher heights to resist the effects of gravity and path length related resistance (Liu et al., 2019), thus more prone to hydraulic challenges such as xylem cavitation and embolism (Bennett et al., 2015; Liu et al., 2021). The leaves of the upper canopy of the P. chinensis have lower OP and MWP (Figure 1), indicating greater cavitation vulnerability. This to some extent explains why there is a greater deviation in the results ex-situ measured at the upper crown layer compared to the lower layer of the tree crown (Figure 3). Even though branches are immediately placed in water and recut after pruning from the tree, it may still cause xylem embolism and hydraulic conductivity (Santiago and Mulkey, 2003), which are closely related to Gs (Hernandez-Santana et al., 2016). Given that the Gs measured in ex-situ branches are greatly affected (Table S4), which in turn affects photosynthesis, it is not possible to accurately reflect the differences in leaf ecological adaptability of different canopy heights. Besides, a decrease in water transport capacity also affects the transport of hormones and nutrients (Santiago and Mulkey, 2003), thereby probably inhibiting photosynthesis (Nam et al., 2021).
Our results suggest that in trait-based ecological research, especially when it comes to leaf photosynthetic physiological traits, in-situ measurement should be used as much as possible. When on-site conditions are limited, we should cut branches that exceed the length of the vessel (Santiago and Mulkey, 2003). Theoretically longer detached branches could decrease biases, which is partly because that if the length of the excised branch is much longer than xylem elements, the leaves on the cut longer branches may be farther away from the xylem elements at the distal end of the branches, which may be less affected by embolism (Santiago and Mulkey, 2003; Missik et al., 2020). Unfortunately, cutting long branches is constrained by the current protection and management policies of P. chinensis. In addition, in light of the varying degrees of resection bias between species caused by differences in xylem (Hacke et al., 2001, Santiago and Mulkey, 2003; Missik et al., 2020), comparisons between species that rely on ex-situ branch data may be unreliable. For future research, we suggest conducting comparative tests on branches that can be used for in-situ measurement. Previous work has also shown that compared to observation results collected in the field shortly after branch cutting (Niinemets et al., 2005), pre-treatment of cut branches under low light and constant temperature for 2-3 days may reduce bias related to cutting, which is not allowed to observe the gas exchange rate at the in-situ stress level.
Our study showed that Parashorea chinensis leaf functional traits varied substantially with different canopy heights in the same community. The individual variation could determine the photosynthetic capacity for P. chinensis to survive in environments with differential light and water availability and contributes to the evidence of ecological adaptation observed along those fine-scale environmental gradients. We also provided evidence that key leaf functional traits can vary independently even at the individual level, which may allow different resultant of adaptive traits in response to changing environments. In addition, the data also showed that different measurement results can significantly influence the results of the data analysis associated with measurement parameters, so differences in measurement methods should be weighed when determining the functional traits of P. chinensis. In summary, understanding individual variations in P. chinensis can contribute considerably to deeply understood ecological adaptation strategies of canopy species under the background of climate change.
The raw data supporting the conclusions of this article will be made available by the authors, without undue reservation.
NJ: Conceptualization, Data curation, Formal analysis, Validation, Visualization, Writing – original draft, Writing – review & editing. XY: Investigation, Writing – original draft. JD: Investigation, Writing – original draft. MD: Conceptualization, Data curation, Investigation, Supervision, Visualization, Writing – original draft. YM: Writing – review & editing. LF: Writing – original draft. RB: Writing – original draft. JZ: Writing – original draft. JS: Writing – original draft. GD: Writing – original draft. HL:Conceptualization, Funding acquisition, Methodology, Supervision, Writing – original draft, Writing – review & editing.
The author(s) declare financial support was received for the research, authorship, and/or publication of this article. This study was supported by the Yunnan Revitalization Talent Support Program (HL), the National Natural Science Foundation of China (No.31872685), the 14th Five- Year Plan of the Xishuangbanna Tropical Botanical Garden ChineseAcademy of Sciences (E3ZKFF7B, E3ZKFF2B, E3ZKFF9B), the CAS-UCLA Scholarship (HL), and the Yunnan Natural Science Foundation (2019FB037).
The authors declare that the research was conducted in the absence of any commercial or financial relationships that could be construed as a potential conflict of interest.
All claims expressed in this article are solely those of the authors and do not necessarily represent those of their affiliated organizations, or those of the publisher, the editors and the reviewers. Any product that may be evaluated in this article, or claim that may be made by its manufacturer, is not guaranteed or endorsed by the publisher.
The Supplementary Material for this article can be found online at: https://www.frontiersin.org/articles/10.3389/fpls.2024.1335524/full#supplementary-material
Anderegg, L. D. L., Berner, L. T., Badgley, G., Sethi, M. L., Law, B. E., HilleRisLambers, J. (2018). Within-species patterns challenge our understanding of the leaf economics spectrum. Ecol. Lett. 21, 734–744. doi: 10.1111/ele.12945
Bennett, A. C., McDowell, N. G., Allen, C. D., Anderson-Teixeira, K. J. (2015). Larger trees suffer most during drought in forests worldwide. Nat. Plants. 1, 15139. doi: 10.1038/nplants.2015.139
Bittencourt, P. R. D., Bartholomew, D. C., Banin, L. F., Bin Suis, M. F. A., Nilus, R., Burslem, D. F. R. P., et al. (2022). Divergence of hydraulic traits among tropical forest trees across topographic and vertical environment gradients in Borneo. New Phytol. 235, 2183–2198. doi: 10.1111/nph.18280
Blackman, C. J., Aspinwall, M. J., de Dios, V. R., Smith, R. A., Tissue, D. T. (2016). Leaf photosynthetic, economics and hydraulic traits are decoupled among genotypes of a widespread species of eucalypt grown under ambient and elevated CO2. Funct. Ecol. 30, 1491–1500. doi: 10.1111/1365-2435.12661
Brodribb, T. J., Holbrook, N. M. (2003). Stomatal closure during leaf dehydration, correlation with other leaf physiological traits. Plant Physiol. 132, 2166–2173. doi: 10.1104/pp.103.023879
Brodribb, T. J., Jordan, G. J., Carpenter, R. J. (2013). Unified changes in cell size permit coordinated leaf evolution. New Phytol. 199, 559–570. doi: 10.1111/nph.12300
Buckley, T. N. (2005). The control of stomata by water balance. New Phytol. 168, 275–292. doi: 10.1111/j.1469-8137.2005.01543.x
Chen, Q., Sun, J. Q., Song, L., Liu, W. Y., Yu, F. H., Li, S., et al. (2019). Trait acclimation of the clonal fern Selliguea griffithiana to forest epiphytic and terrestrial habitats. Ecol Res. 34, 406–414. doi: 10.1111/1440-1703.12002
Deng, Y., Deng, X. B., Dong, J. L., Zhang, W. F., Hu, T., Nakamura, A., et al. (2020). Detecting growth phase shifts based on leaf trait variation of a canopy dipterocarp tree species (Parashorea chinensis). Forests 11, 1145. doi: 10.3390/f11111145
Díaz, S., Kattge, J., Cornelissen, J. H. C., Wright, I. J., Lavorel, S., Dray, S., et al. (2016). The global spectrum of plant form and function. Nature 529, 167–171. doi: 10.1038/nature16489
Duan, M. C., Li, L., Ding, G. G., Ma, Z. Q. (2022). Leading nutrient foraging strategies shaping by root system characteristics along the elevations in rubber (Hevea brasiliensis) plantations. Tree Physiol. 42, 2468–2479. doi: 10.1093/treephys/tpac081
Feng, J. Y., Lian, J. Y., Mei, Q. M., Cao, H. L., Ye, W. H. (2022). Vertical variation in leaf traits and crown structure promote the coexistence of forest tree species. Forests 13, 1548. doi: 10.3390/f13101548
Giles, A. L., Rowland, L., Bittencourt, P. R. L., Bartholomew, D. C., Coughlin, I., Costa, P. B., et al. (2021). Small understorey trees have greater capacity than canopy trees to adjust hydraulic traits following prolonged experimental drought in a tropical forest. Tree Physiol. 42, 537–556. doi: 10.1093/treephys/tpab121
Givnish, T. J. (1988). Adaptation to sun and shade: A whole-plant perspective. Aust. J. Plant Physiol. 15, 63–92. doi: 10.1071/PP9880063
Gleason, S. M., Blackman, C. J., Chang, Y., Cook, A. M., Laws, C. A., Westoby, M. (2016). Weak coordination among petiole, leaf, vein, and gas-exchange traits across Australian angiosperm species and its possible implications. Ecol. Evol. 6, 267–278. doi: 10.1002/ece3.1860
Hacke, U. G., Sperry, J. S. (2001). Functional and ecological xylem anatomy. Perspect. Plant Ecol. Evol. Syst. 4, 97–115. doi: 10.1078/1433-8319-00017
Hagan, J. G., Henn, J. J., Osterman, W. H. A. (2023). Plant traits alone are good predictors of ecosystem properties when used carefully. Nat. Ecol. Evol. 7, 332–334. doi: 10.1038/s41559-022-01920-x
Hanson, T. D., Green, E. L., Pockman, W. T. (2013). Spatio-temporal decoupling of stomatal and mesophyll conductance induced by vein cutting in leaves of Helianthus annuus. Front. Plant Sci. 4. doi: 10.3389/fpls.2013.00365
Hernandez-Santana, V., Rodriguez-Dominguez, C. M., Fernández, J. E., Diaz-Espejo, A. (2016). Role of leaf hydraulic conductance in the regulation of stomatal conductance in almond and olive in response to water stress. Tree Physiol. 36, 725–735. doi: 10.1093/treephys/tpv146
Hess, C., Levine, J. M., Turcotte, M. M., Hart, S. P. (2022). Phenotypic plasticity promotes species coexistence. Nat. Ecol. Evol. 6, 1256–1261. doi: 10.1038/s41559-022-01826-8
Hu, H. X., Mo, Y. X., Shen, T., Wu, Y., Shi, X. M., Ai, Y. Y., et al. (2022). Simulated high-intensity phorophyte removal mitigates the robustness of epiphyte community and destroys commensal network structure. For Ecol. Manage. 526, 120586. doi: 10.1016/j.foreco.2022.120586
Huang, L. C., Ratkowsky, D. A., Hui, C., Gielis, J., Lian, M., Yao, W. H., et al. (2023). Inequality measure of leaf area distribution for a drought-tolerant landscape plant. Plants 12, 3143. doi: 10.3390/plants12173143
Ishii, H., Azuma, W., Nabeshima, E. (2013). The need for a canopy perspective to understand the importance of phenotypic plasticity for promoting species coexistence and light-use complementarity in forest ecosystems. Ecol. Res. 28, 191–198. doi: 10.1007/s11284-012-1025-6
Jiao, X. C., Yu, X. M., Yuan, Y. J., Li, J. M. (2022). Effects of vapor pressure deficit combined with different N levels on tomato seedling anatomy, photosynthetic performance, and N uptake. Plant Sci. 324, 111448. doi: 10.1016/j.plantsci.2022.111448
Kenzo, T., Inoue, Y., Yoshimura, M., Yamashita, M., Tanaka-Oda, A., Ichie, T. (2015). Height-related changes in leaf photosynthetic traits in diverse Bornean tropical rain forest trees. Oecologia 177, 191–202. doi: 10.1007/s00442-014-3126-0
Koch, G. W., Sillett, S. C., Jennings, G. M., Davis, S. D. (2004). The limits to tree height. Nature 428, 851–854. doi: 10.1038/nature02417
Lasky, J. R., Yang, J., Zhang, G. C., Cao, M., Tang, Y., Keitt, T. H. (2014). The role of functional traits and individual variation in the co-occurrence of Ficus species. Ecology 95, 978–990. doi: 10.1890/13-0437.1
Li, L., McCormack, M. L., Ma, C. G., Kong, D. L., Zhang, Q., Chen, X. Y., et al. (2015). Leaf economics and hydraulic traits are decoupled in five species-rich tropical-subtropical forests. Ecol. Lett. 18, 899–906. doi: 10.1111/ele.12466
Liu, L. Y., Chen, X. Z., Ciais, P., Yuan, W. P., Maignan, F., Wu, J., et al. (2021). Tropical tall forests are more sensitive and vulnerable to drought than short forests. Glob Chang Biol. 28, 1583–1595. doi: 10.1111/gcb.16017
Liu, H., Gleason, S. M., Hao, G. Y., Hua, L., He, P. C., Goldstein, G., et al. (2019). Hydraulic traits are coordinated with maximum plant height at the global scale. Sci. Adv. 5, eaav1332. doi: 10.1126/sciadv.aav1332
Lu, H. Z., Brooker, R., Song, L., Liu, W. Y., Sack, L., Zhang, J. L., et al. (2020). When facilitation meets clonal integration in forest canopies. New Phytol. 225, 135–142. doi: 10.1111/nph.16228
Lu, H. Z., Liu, W. Y., Yu, F. H., Song, L., Xu, X. L., Wu, C. S., et al. (2015). Higher clonal integration in the facultative epiphytic fern Selliguea griffithiana growing in the forest canopy compared with the forest understorey. Ann Bot. 116, 113–122. doi: 10.1093/aob/mcv059
Martinez, K. A., Fridley, J. D. (2018). Acclimation of leaf traits in seasonal light environments: Are non-native species more plastic? J. Ecol. 106, 2019–2030. doi: 10.1111/1365-2745.12952
Missik, J. E. C., Oishi, A. C., Benson, M. C., Meretsky, V. J., Phillips, R. P., Novick, K. A. (2020). Performing gas-exchange measurements on excised branches - evaluation and recommendations. Photosynthetica 59, 61–73. doi: 10.32615/ps.2020.079
Nam, H., Shahzad, Z., Dorone, Y., Clowez, S., Zhao, K. M., Bouain, N., et al. (2021). Interdependent iron and phosphorus availability controls photosynthesis through retrograde signaling. Nat. Commun. 12, 7211. doi: 10.1038/s41467-021-27548-2
Needham, J. F., Arellano, G., Davies, S. J., Fisher, R. A., Hammer, V., Knox, R., et al. (2022). Tree crown damage and its effects on forest carbon cycling in a tropical forest. Glob Chang Biol. 28, 5560–5574. doi: 10.1111/gcb.16318
Niinemets, Ü., Cescatti, A., Rodeghiero, M., Tosens, T. (2005). Leaf internal diffusion conductance limits photosynthesis more strongly in older leaves of Mediterranean evergreen broad-leaved species. Plant Cell Environ. 28, 1552–1566. doi: 10.1111/j.1365-3040.2005.01392.x
Pfautsch, S., Aspinwall, M. J., Drake, J. E., Chacon-Doria, L., Langelaan, R. J. A., Tissue, D. T., et al. (2018). Traits and trade-offs in whole-tree hydraulic architecture along the vertical axis of Eucalyptus grandis. Ann. Bot. 121, 129–141. doi: 10.1093/aob/mcx137
Poorter, H., Niinemets, Ü., Poorter, L., Wright, I. J., Villar, R. (2009). Causes and consequences of variation in leaf mass per area (LMA): a meta-analysis. New Phytol. 182, 565–588. doi: 10.1111/j.1469-8137.2009.02830.x
Pritzkow, C., Williamson, V., Szota, C., Trouvé, R., Arndt, S. K. (2020). Phenotypic plasticity and genetic adaptation of functional traits influences intra-specific variation in hydraulic efficiency and safety. Tree Physiol. 40, 215–229. doi: 10.1093/treephys/tpz121
R Core Team (2022). R: A language and environment for statistical computing (Vienna, Austria: R Foundation for Statistical Computing). Available at: https://www.R-project.org/.
Reich, B. P. (2014). The world-wide ‘fast–slow’ plant economics spectrum: a traits manifesto. J. Ecol. 102, 275–301. doi: 10.1111/1365-2745.12211
Ripullone, F., Grassi, G., Lauteri, M., Borghetti, M. (2003). Photosynthesis-nitrogen relationships: interpretation of different patterns between Pseudotsuga menziesii and Populus x euroamericana in a mini-stand experiment. Tree Physiol. 23, 137–144. doi: 10.1093/treephys/23.2.137
Roig-Oliver, M., Fullana-Pericàs, M., Bota, J., Flexas, J. (2021). Adjustments in photosynthesis and leaf water relations are related to changes in cell wall composition in Hordeum vulgare and Triticum aestivum subjected to water deficit stress. Plant Sci. 311, 111015. doi: 10.1016/j.plantsci.2021.111015
Sack, L., Cowan, P. D., Jaikumar, N., Holbrook, N. M. (2003). The ‘hydrology’ of leaves: co-ordination of structure and function in temperate woody species. Plant Cell Environ. 26, 1343–1356. doi: 10.1046/j.0016-8025.2003.01058.x
Sack, L., Holbrook, N. M. (2006). Leaf hydraulics. Annu. Rev. Plant Biol. 57, 361–381. doi: 10.1146/annurev.arplant.56.032604.144141
Sack, L., Scoffoni, C. (2013). Leaf venation: structure, function, development, evolution, ecology and applications in the past, present and future. New Phytol. 198, 983–1000. doi: 10.1111/nph.12253
Santiago, L. S., Mulkey, S. S. (2003). A test of gas exchange measurements on excised canopy branches of ten tropical tree species. Photosynthetica 41, 343–347. doi: 10.1023/B:PHOT.0000015457.92479.eb
Shen, T., Corlett, R. T., Collart, F., Kasprzyk, T., Guo, X. L., Patiño, J., et al. (2022). Microclimatic variation in tropical canopies: A glimpse into the processes of community assembly in epiphytic bryophyte communities. J. Ecol. 110, 3023–3038. doi: 10.1111/1365-2745.14011
Shi, P. J., Jiao, Y. B., Diggle, P. J., Turner, R., Wang, R., Niinemets, Ü. (2021). Spatial distribution characteristics of stomata at the areole level in Michelia cavaleriei var. platypetala (Magnoliaceae). Ann. Bot. 128, 875–885. doi: 10.1093/aob/mcab106
Shi, P. J., Preisler, H. K., Quinn, B. K., Zhao, J., Huang, W. W., Röll, A., et al. (2020). Precipitation is the most crucial factor determining the distribution of moso bamboo in Mainland China. Glob Ecol. Conserv. 22, e00924. doi: 10.1016/j.gecco.2020.e00924
Siefert, A., Violle, C., Chalmandrier, L., Albert, C. H., Taudiere, A., Fajardo, A., et al. (2015). A global meta-analysis of the relative extent of intraspecific trait variation in plant communities. Ecol. Lett. 18, 1406–1416. doi: 10.1111/ele.12508
Sobral, M., Sampedro, L. (2022). Phenotypic, epigenetic, and fitness diversity within plant genotypes. Trends Plant Sci. 27, 843–846. doi: 10.1016/j.tplants.2022.06.008
Sperry, J. S., Wang, Y. J., Wolfe, B. T., Mackay, D. S., Anderegg, W. R. L., McDowell, N. G., et al. (2016). Pragmatic hydraulic theory predicts stomatal responses to climatic water deficits. New Phytol. 212, 577–589. doi: 10.1111/nph.14059
Stovall, A. E. L., Shugart, H., Yang, X. (2019). Tree height explains mortality risk during an intense drought. Nat. Commun. 10, 4385. doi: 10.1038/s41467-019-12380-6
Tang, J. W., Shi, J. P., Zhang, G. M., Bai, K. J. (2008). Density, structure and biomass of Papashorea chinensis population in different patches in Xishuangbanna, SW. J. Plant Ecol. 32, 40–54. doi: 10.3773/j.issn.1005-264x.2008.01.005
Walker, A. P., Beckerman, A. P., Gu, L. H., Kattge, J., Cernusak, L. A., Domingues, T. F., et al. (2014). The relationship of leaf photosynthetic traits - V cmax and J max - to leaf nitrogen, leaf phosphorus, and specific leaf area: a meta-analysis and modeling study. Ecol. Evol. 4, 3218–3235. doi: 10.1002/ece3.1173
Wang, Z. Q., Huang, H., Wang, H., Penuelas, J., Sardans, J., Niinemets, Ü., et al. (2022). Leaf water content contributes to global leaf trait relationships. Nat. Commun. 13, 5525. doi: 10.1038/s41467-022-32784-1
Wilson, P. J., Thompson, K., Hodgson, J. G. (1999). Specific leaf area and leaf dry matter content as alternative predictors of plant strategies. New Phytol. 149, 155–162. doi: 10.1046/j.1469-8137.1999.00427.x
Woodruff, D. R., Bond, B. J., Meinzer, F. C. (2004). Does turgor limit growth in tall trees? Plant Cell Environ. 27, 229–236. doi: 10.1111/j.1365-3040.2003.01141.x
Wright, I. J., Reich, P. B., Westoby, M., Ackerly, D. D., Baruch, Z., Bongers, F., et al. (2004). The worldwide leaf economics spectrum. Nature 428, 821–827. doi: 10.1038/nature02403
Wyka, T. P., Oleksyn, J., Zytkowiak, R., Karolewski, P., Jagodziński, A. M., Reich, P. B. (2012). Responses of leaf structure and photosynthetic properties to intra-canopy light gradients: a common garden test with four broadleaf deciduous angiosperm and seven evergreen conifer tree species. Oecologia 170, 11–24. doi: 10.1007/s00442-012-2279-y
Xu, H. Y., Wang, H., Prentice, I. C., Harrison, S. P., Wright, I. J. (2021). Coordination of plant hydraulic and photosynthetic traits: confronting optimality theory with field measurements. New Phytol. 232, 1286–1296. doi: 10.1111/nph.17656
Ye, Z. P. (2007). A new model for relationship between irradiance and the rate of photosynthesis in Oryza sativa. Photosynthetica 45, 637–640. doi: 10.1007/s11099-007-0110-5
Yin, Q. L., Wang, L., Lei, M. L., Dang, H., Quan, J. X., Tian, T. T., et al. (2018). The relationships between leaf economics and hydraulic traits of woody plants depend on water availability. Sci. Total Environ. 621, 245–252. doi: 10.1016/j.scitotenv.2017.11.171
Zou, Q. Q., Liu, D. H., Sang, M., Jiang, C. D. (2022). Sunflower leaf structure affects chlorophyll a fluorescence induction kinetics in vivo. Int. J. Mol. Sci. 23, 14996. doi: 10.3390/ijms232314996
Zuleta, D., MullerLandau, H. C., Duque, A., Caro, N., Cardenas, D., Castaño, N., et al. (2022). Interspecific and intraspecific variation of tree branch, leaf and stomatal traits in relation to topography in an aseasonal Amazon forest. Funct. Ecol. 36, 2955–2968. doi: 10.1111/1365-2435.14199
Zuur, A. F., Ieno, E. N., Walker, N., Saveliev, A. A., Smith, G. M. (2009). Mixed effects models and extensions in ecology with R (New York: Springer).
Keywords: canopy physiology, intraspecific variation, leaf hydraulic traits, leaf photosynthetic physiological traits, vertical distribution, ecological adaptation
Citation: Jin N, Yu X, Dong J, Duan M, Mo Y, Feng L, Bai R, Zhao J, Song J, Dossa GGO and Lu H (2024) Vertical variation in leaf functional traits of Parashorea chinensis with different canopy layers. Front. Plant Sci. 15:1335524. doi: 10.3389/fpls.2024.1335524
Received: 09 November 2023; Accepted: 08 January 2024;
Published: 29 January 2024.
Edited by:
Xin-Sheng Chen, Anhui University, ChinaCopyright © 2024 Jin, Yu, Dong, Duan, Mo, Feng, Bai, Zhao, Song, Dossa and Lu. This is an open-access article distributed under the terms of the Creative Commons Attribution License (CC BY). The use, distribution or reproduction in other forums is permitted, provided the original author(s) and the copyright owner(s) are credited and that the original publication in this journal is cited, in accordance with accepted academic practice. No use, distribution or reproduction is permitted which does not comply with these terms.
*Correspondence: Huazheng Lu, bHVodWF6aGVuZ0B4dGJnLmFjLmNu
Disclaimer: All claims expressed in this article are solely those of the authors and do not necessarily represent those of their affiliated organizations, or those of the publisher, the editors and the reviewers. Any product that may be evaluated in this article or claim that may be made by its manufacturer is not guaranteed or endorsed by the publisher.
Research integrity at Frontiers
Learn more about the work of our research integrity team to safeguard the quality of each article we publish.