- 1Lushan Botanical Garden, Chinese Academy of Sciences, Jiujiang, China
- 2College of Life Science, Nanchang University, Nanchang, China
- 3China-USA Citrus Huanglongbing Joint Laboratory (A Joint Laboratory of The University of Florida’s Institute of Food and Agricultural Sciences and Gannan Normal University), National Navel Orange Engineering Research Center, Gannan Normal University, Ganzhou, Jiangxi, China
1 Introduction
Citrus production faces numerous environmental challenges including the devastating huanglongbing (HLB) disease. HLB, also known as citrus greening, affects health, growth and fruit quality of Citrus plants (Wang, 2019). Citrus crops have a long history of cultivation as grafted trees on selected rootstock cultivars that can improve tree performance and provide some degree of resistance to HLB (Shokrollah et al., 2011; Bowman and Albrecht, 2020; Bowman et al., 2021). Recently, several transgenic approaches have made significant progress in combating HLB. However, public acceptance of genetically modified (GM) crops is very low, and many consumers prefer to eat GM-free food (Lucht, 2015). In this article, we explore the potential of different approaches to combat HLB by grafting non-transgenic scions on transgenic and non-transgenic rootstocks.
2 HLB, a pathogen-triggered immune disease
HLB is caused by the infection with the phloem-colonizing bacterium Candidatus Liberibacter (CL) resulting in phloem cell death that is the key for most HLB symptoms. Early symptoms of the HLB disease include a yellow blotchy pattern in leaves and yellow shoots. In later stages, infected trees bear small-sized, deformed, and poorly colored (greening) fruits. A recent study demonstrates that HLB is an immune-mediated plant disease triggered by CL infection that stimulates systemic and chronic immune response in phloem tissues, including reactive oxygen species (ROS) production, callose deposition and induction of immune-related genes (Ma W. et al., 2022). HLB pathogens are transmitted by Asian citrus psyllid (Diaphorina citri), an insects of the Psyllidae family (Nadarasah and Stavrinides, 2011). The life cycle of the Psyllids depends on the Citrus plants, whose young flushes are the food and nursery of the Psyllid nymphs, while the adult insects suck sap from the phloem. As primary endosymbionts of the Psyllidae, CL bacteria are contained within the bacteriome, an interspecies organ comprising a close association of insect and bacterial cells (Ramsey et al., 2015). The Psyllids transmit the CL bacteria by sucking the phloem sap of Citrus plants. The propagation of the CL bacteria depends entirely on the Psyllid and the Citrus hosts. All attempts to culture CL failed until recently, making research and combat of HLB very challenging (Ha et al., 2019).
3 Limited effects of non-transgenic Citrus rootstock on HLB symptoms in scions
While some Citrus rootstocks, such as C. trifoliata and some of its hybrids, are tolerant to CL infection as seedlings, this tolerance to infection generally does not result in a large improvement in the HLB tolerance of trees, when the rootstock is grafted with a CL-sensitive scion, such as sweet orange (Bowman and Joubert, 2020). Therefore, using HLB-tolerant rootstocks only partially increases the overall tree tolerance to CL in the field. However, this approach can significantly enhance yield, fruit quality, and tree size (Bowman et al., 2016). Rootstocks can also improve the quality of fruit juice in HLB-affected Citrus by enhancing metabolites such as flavonoids and terpenoids (Huang et al., 2020). Ground application of manganese to rootstocks enhances root density and deteriorates CL populations, promoting healthier trees and improves fruit yield (Zambon et al., 2019). Hence, the choice of HLB-tolerant rootstocks and enhanced nutrition can significantly contribute to a better overall tree tolerance. Still, achieving full resistance to HLB requires additional strategies.
4 Recent advances in combating HLB by transgenic approaches involving salicylic acid-dependent defense pathways
Root-originated RNAs, proteins, and secondary metabolites can be transported via the vascular system to the shoot. This opens the doors for approaches using transgenic rootstocks to combat HLB disease in non-transgenic scions (Figure 1A). Recent studies have demonstrated that the exogenous application of the phytohormone salicylic acid (SA) to Citrus rootstocks and overexpression of components of the SA signal transduction pathway can improve the resistance to HLB and other Citrus pathogens. In general, SA signaling is the core of the plant defense mechanisms against pathogens, which can activate locally hypersensitive response (HR) and systemic acquired resistance (SAR) (Gao et al., 2015). Phloem-specific and constitutive expression of Arabidopsis NONEXPRESSOR OF PR1 (NPR1), which encodes the primary SA receptor in plants (Wu et al., 2012), significantly decrease the severity of HLB infections. Some transgenic plants have remained disease-free for more than three years (Dutt et al., 2015). Overexpression of NPR1 activates SA-induced defense genes in Citrus, while the interaction of NPR1 protein with CsNPR3 and CsTGA5 in the nucleus demonstrates that the SA network orchestrating the defense mechanism against pathogens is evolutionary conserved in Citrus (Qiu et al., 2020). Since NPR1 can induce SAR, increasing pathogen resistance in the whole plant, the expression of NPR1 in transgenic rootstocks might be sufficient to combat CL in non-transgenic scions. However, a transport system may be required to deliver NPR1 to the HLB infection sites in the scions to unleash the full potential of NPR1 as a SA receptor and key regulator of SA-triggered defense.
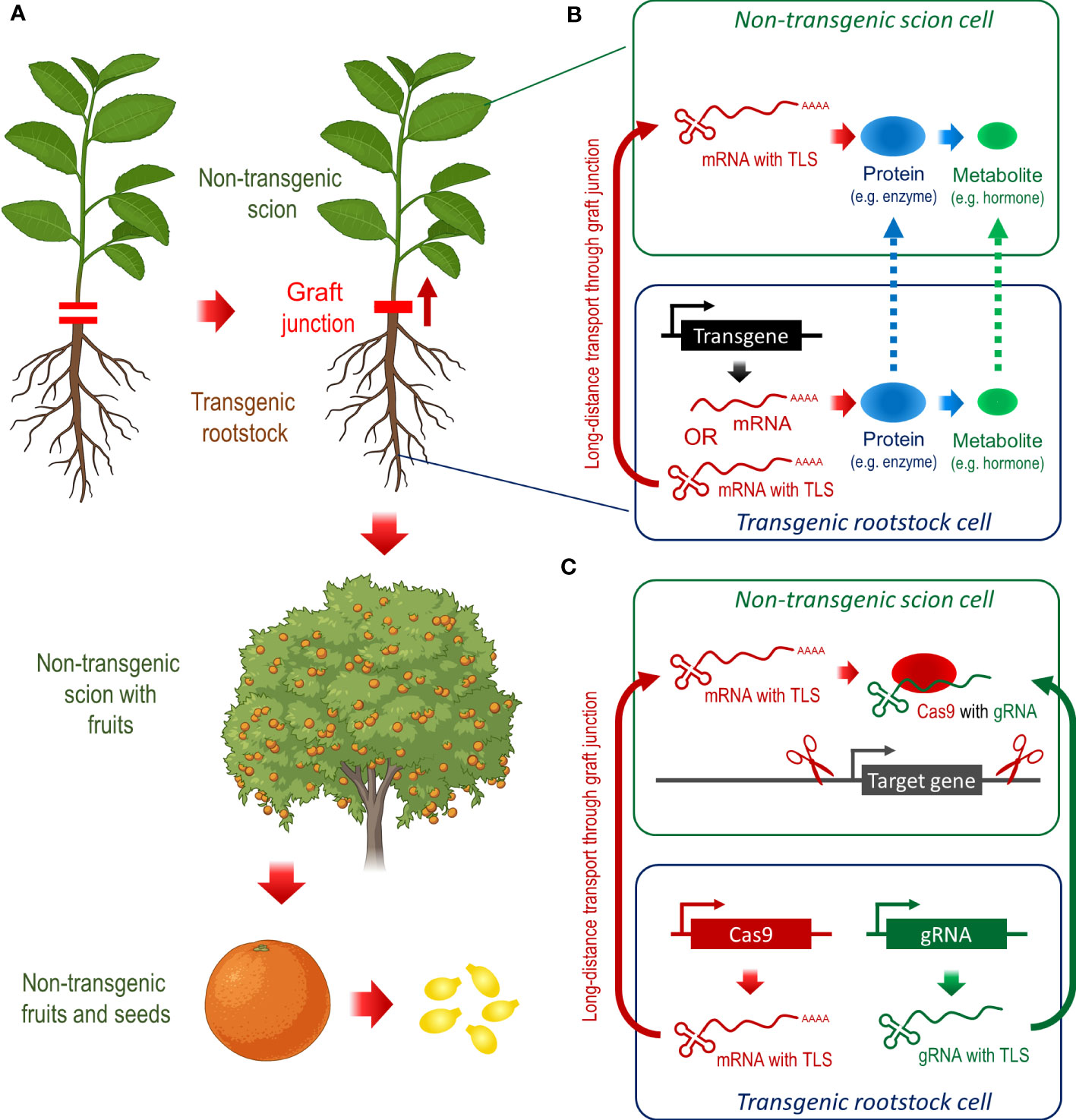
Figure 1 Transgenic rootstocks can provide desirable traits in non-transgenic scions of fruit trees, which is a powerful tool to combat HLB in Citrus. (A) Grafting of non-transgenic scion to transgenic rootstocks can improve traits of scion, fruits, and other products, which remain transgene-free. (B) Theoretically, mRNAs, proteins, and/or secondary metabolites can transmit the transgene function from the rootstock to the scion, but the fusion of RNAs to TLS sequences seems to be the most promising approach for this task. (C) Heritable transgene-free genome editing by grafting and TLS-dependent mobility of components of the CRISPR/Cas9 genome editing system.
Maintaining SA homeostasis plays a critical role in normal growth, development, and various physiological processes in plants (Rivas-San Vicente and Plasencia, 2011; Vlot et al., 2021). SA carboxyl methyltransferases (SAMTs) convert SA into methyl salicylate (MeSA) that serves as an important long-distance signal in SAR response (Chen et al., 2003; Park et al., 2007). Overexpression of CsSAMT1 leads to increased concentrations of both SA and MeSA, upregulating the transcription of disease resistance genes during stress conditions. This results in reduced HLB symptoms, smaller CL populations, and less hyperplasia of phloem cells (Zou et al., 2021). Since MeSA is a mobile SAR signal, overexpression of SAMTs in transgenic rootstocks might be sufficient to trigger similar SAR responses throughout the entire Citrus tree, including the non-transgenic scion. These findings highlight the potential of transgenic approaches to combat HLB through the constitutive expression of SA- and SAR-related genes, which are also good candidates for rootstock-specific expression in future approaches.
5 Root-to-shoot transport of RNAs from transgenic donor rootstocks can fight HLB in non-transgenic scions
Plants use long-distance signaling to coordinate responses to external biotic and abiotic stress (Takahashi and Shinozaki, 2019). This long-distance communication may include metabolites, hormones, proteins, peptides, electrical signals, and small RNAs, while messenger RNAs (mRNAs) might be the most common signal vehicle (Heeney and Frank, 2023). tRNA-like structures (TLSs) are sufficient to mediate mRNA transport and are essential for the mobility of a large number of transcripts that can move through the graft junction between rootstock and scion. Transgenic mRNAs, which harbor a characteristic TLS, can move from roots into wild-type scions, where these mRNAs can be translated into proteins (Zhang et al., 2016). Hence, engineering TLS-mRNA hybrids in Citrus can ensure the transport of rootstock-generated transgenic transcripts to the scion, and ultimately, the translation to proteins such as NPR1 or SAMT1, while the scion remains transgene-free (Figure 1B).
The concept of ‘using transgenic rootstocks to produce products of non-transgenic scions in fruit trees’ was established in cherry using a RNA interference (RNAi) vector: The rootstock-to-scion transfer of the hairpin RNA (hpRNA)-derived small interfering RNAs (siRNAs) was sufficient to enhance virus resistance in the non-transgenic scion (Zhao and Song, 2014). In Citrus, expression of hpRNAs in the interstock strengthens the tolerance to Citrus psorosis virus in the whole tree (Francesco et al., 2020). This finding demonstrates that expression of RNAi vectors in Citrus rootstock or interstocks is sufficient to knock-down target genes in non-transgenic scions. In contrast to NPR1, NPR3 and NPR4 function as transcriptional co-repressors of SA-induced genes (Ding et al., 2018). Targeting NPR3/4 by rootstock-derived RNAi could boost the SA-dependent defense response against HLB in the non-transgenic scion.
6 Heritable transgene-free genome editing by grafting can generate HBL-resistant Citrus
Genome editing via CRISPR/Cas9 is a powerful biotechnology, widely applied to improve traits in crop plants, including fruit trees. Currently, the prevalent method for delivering CRISPR/Cas9 components involves integration of the bacterial T−DNA into the host plant genome (Dalla Costa et al., 2020). In Citrus, CRISPR/Cas9 has been used to modify development and disease resistance genes. For instance, it was employed to reduce the expression of the LOB1 by removing promoter enhancer regions in sweet orange and grapefruit, decreasing their susceptibility to Citrus canker disease (Peng et al., 2017; Jia et al., 2022). Similarly, the knock-out of CsWRKY22 enhances resistance to Citrus canker (Wang et al., 2019). Although these examples demonstrate the potential of CRISPR/Cas9 genome editing in Citrus, they all employed Agrobacterium-mediated transformation. To obtain transgene-free Citrus mutants, removing the CRISPR/Cas9 transgene cassettes by outcrossing would be extremely time-consuming.
A more direct approach for producing transgene-free genome-edited plants is to deliver CRISPR/Cas9 components in the form of mRNA (Hu and Gao, 2023). The CRISPR/Cas9 system basically comprises two components: (i) the CRISPR-associated protein 9 (Cas9) nuclease that can generate DNA double-strand breaks at specific genomic sequences by employing (ii) guide RNAs (gRNAs), resulting in edited DNA sequences (Mali et al., 2013). Combining Cas9 mRNA and gRNAs with TLS motifs provides the capability for RNA movement from transgenic rootstocks to distal parts in wild-type scions through graft junctions (Figure 1C). Here, Cas9 mRNA is translated, and Cas9 protein with the gRNAs can produce heritable mutations at target loci (Zaman et al., 2023). This concept has recently been proved by grafting wild-type shoots of Arabidopsis thaliana and Brassica rapa onto transgenic rootstocks of Arabidopsis thaliana containing the TLS-modified CRISPR/Cas9 transgene cassettes. Ultimately, the flowers successfully produced transgene-free seeds with heritable mutations (Yang et al., 2023). For the combat of HLB in Citrus, we propose transgene-free CRISPR/Cas9 approaches targeting negative regulators of SA signaling and SAR response, such as NPR3 and NPR4. Using a new transformation technique, which directly transforms regenerating roots (Ma H. et al., 2022), can significantly accelerate the generation of CRISPR/Cas9 donor rootstocks. After successful genome editing, transgene-free mutant scions can be grafted to transgene-free rootstocks.
7 Conclusion and outlook
The choice of rootstocks can provide limited resilience to HLB in Citrus scions. It remains unclear whether this partial HLB tolerance results only from significantly healthier root system indirectly promoting an overall better tree health or is provided by rootstock-originated RNAs, proteins, and/or secondary metabolites such as the stress hormone SA (Melnyk and Meyerowitz, 2015; Bowman and Joubert, 2020). Recent studies demonstrate that transgenic approaches could achieve full HLB resistance in commercial Citrus trees. Still, public and regulatory concerns about GM plants prevent their widespread commercial deployment. Using transgenic rootstocks to produce products from non-transgenic scions in Citrus and other fruit trees seems to be a reasonable alternative. Recent emerging technologies like TLS-RNA fusions could facilitate approaches with transgenic rootstocks (Zhang et al., 2016). The TLS technology can either be used to deliver the product from the transgenic rootstock to the non-transgenic scion tissue or combined with CRISPR/Cas9 genome editing to generate transgene-free mutants (Yang et al., 2023). Both strategies can be powerful tools to fight against HLB in Citrus and can even be combined, while the Citrus fruits and other products of the scion shoot will remain transgene-free. However, the TLS technology was never used in Citrus and implementation might be challenging. Several obstacles have to be overcome before TLS-based approaches can be considered for combating HLB in commercial Citrus production. First, it would be required to test, whether TLS sequences of Citrus or other plant species can promote RNA movement upwards through the graft junction. If successful, the transportation of TLS-RNAs and the translation in the scion phloem may face limitation in efficiency in comparison to the direct overexpression of the transgene in the scion. Furthermore, the choice of the transgene will influence the outcome of the TLS-based approaches. Based on recent studies, SA- and SAR-related genes seem to be good candidates to stop HLB infections at the beginning. Nevertheless, there is the risk that SA- and SAR-related approaches could aggravate the HLB symptoms, since HLB seems mostly a pathogen-triggered immune disease (Ma W. et al., 2022). Therefore, SA/SAR-independent approaches could be an alternative such as targeting the Psyllidae by TLS-delivered RNAi approaches (Shaffer, 2020; Wang, 2019). Despite of all these obstacles, we believe that the TLS technology have the potential to be a powerful tool for combating HLB and solving other Citrus threads, and this perspective should motivate us to work in this field.
Author contributions
RG: Visualization, Writing – original draft, Writing – review & editing. XL: Writing – review & editing. SD: Writing – review & editing. QX: Writing – review & editing. RM: Conceptualization, Visualization, Writing – original draft, Writing – review & editing.
Funding
The author(s) declare financial support was received for the research, authorship, and/or publication of this article. This work was kindly funded by Jiangxi Province Recruitment Program of Foreign Experts (project no jxsq2023104003), the National Natural Science Foundation of China (project nos 31640054 and 31771602), and Starting Grants of the Lushan Botanical Garden, Chinese Academy of Sciences, Jiujiang (project nos 2021ZWZX24 and 2021ZWZX25) and Jiangxi Province Lushan Botanical Garden, CAS, National Demonstration Platform for Attracting Talents and Intelligence (project no YZJD2023020), and Jiangxi Province Natural Science Foundation (project no S2023ZRYCL0074).
Conflict of interest
The authors declare that the research was conducted in the absence of any commercial or financial relationships that could be construed as a potential conflict of interest.
Publisher’s note
All claims expressed in this article are solely those of the authors and do not necessarily represent those of their affiliated organizations, or those of the publisher, the editors and the reviewers. Any product that may be evaluated in this article, or claim that may be made by its manufacturer, is not guaranteed or endorsed by the publisher.
References
Bowman, K. D., Albrecht, U. (2020). Rootstock influences on health and growth following candidatus liberibacter asiaticus infection in young sweet orange trees. Agronomy 10, 1907. doi: 10.3390/agronomy10121907
Bowman, K. D., Joubert, J. (2020). “Citrus rootstocks,” in The Genus Citrus (Elsevier: United Kingdom). doi: 10.1016/b978-0-12-812163-4.00006-1
Bowman, K. D., McCollum, G., Albrecht, U. (2016). Performance of ‘Valencia’ orange (Citrus sinensis [L.] Osbeck) on 17 rootstocks in a trial severely affected by Huanglongbing. Scientia Hortic. 201, 355–361. doi: 10.1016/j.scienta.2016.01.019
Bowman, K. D., McCollum, G., Albrecht, U. (2021). SuperSour: A new strategy for breeding superior citrus rootstocks. Front. Plant Sci. 12. doi: 10.3389/fpls.2021.741009
Chen, F., D'Auria, J. C., Tholl, D., Ross, J. R., Gershenzon, J., Noel, J. P., et al. (2003). An Arabidopsis thaliana gene for methylsalicylate biosynthesis, identified by a biochemical genomics approach, has a role in defense. Plant J. 36, 577–588. doi: 10.1046/j.1365-313X.2003.01902.x
Dalla Costa, L., Piazza, S., Pompili, V., Salvagnin, U., Cestaro, A., Moffa, L., et al. (2020). Strategies to produce T-DNA free CRISPRed fruit trees via Agrobacterium tumefaciens stable gene transfer. Sci. Rep. 10, 1607. doi: 10.1038/s41598-020-77110-1
Ding, Y., Sun, T., Ao, K., Peng, Y., Zhang, Y., Li, X., et al. (2018). Opposite roles of salicylic acid receptors NPR1 and NPR3/NPR4 in transcriptional regulation of plant immunity. Cell 173, 1454–1467.e15. doi: 10.1016/j.cell.2018.03.044
Dutt, M., Barthe, G., Irey, M., Grosser, J., Ezura, H. (2015). Transgenic citrus expressing an arabidopsis NPR1 gene exhibit enhanced resistance against huanglongbing (HLB; Citrus greening). PLoS One 10, e0137134. doi: 10.1371/journal.pone.0137134
Francesco, A., Simeone, M., Gómez, C., Costa, N., García, M. L. (2020). Transgenic Sweet Orange expressing hairpin CP-mRNA in the interstock confers tolerance to citrus psorosis virus in the non-transgenic scion. Transgenic Res. 29, 215–228. doi: 10.1007/s11248-020-00191-1
Gao, Q.-M., Zhu, S., Kachroo, P., Kachroo, A. (2015). Signal regulators of systemic acquired resistance. Front. Plant Sci. 06. doi: 10.3389/fpls.2015.00228
Ha, P. T., He, R., Killiny, N., Brown, J. K., Omsland, A., Gang, D. R., et al. (2019). Host-free biofilm culture of “Candidatus Liberibacter asiaticus,” the bacterium associated with Huanglongbing. Biofilm 1, 100005. doi: 10.1016/j.bioflm.2019.100005
Heeney, M., Frank, M. H. (2023). The mRNA mobileome: Challenges and opportunities for deciphering signals from the noise. Plant Cell 35, 1817–1833. doi: 10.1093/plcell/koad063
Hu, J., Gao, C. (2023). CRISPR-edited plants by grafting. Nat. Biotechnol. 41, 909–910. doi: 10.1038/s41587-022-01516-7
Huang, L., Grosser, J., Gmitter, F. G., Sims, C. A., Wang, Y. (2020). Effects of scion/rootstock combination on flavor quality of orange juice from Huanglongbing (HLB)-affected trees: A two-year study of the targeted metabolomics. J. Agric. Food Chem. 68, 3286–3296. doi: 10.1021/acs.jafc.9b07934
Jia, H., Omar, A. A., Orbović, V., Wang, N. (2022). Biallelic editing of the LOB1 promoter via CRISPR/cas9 creates canker-resistant ‘Duncan’ Grapefruit. Phytopathology® 112, 308–314. doi: 10.1094/PHYTO-04-21-0144-R
Lucht, J. (2015). Public acceptance of plant biotechnology and GM crops. Viruses 7, 4254–4281. doi: 10.3390/v7082819
Ma, H., Meng, X., Xu, K., Li, M., Gmitter, F. G., Liu, N., et al. (2022). Highly efficient hairy root genetic transformation and applications in citrus. Front. Plant Sci. 13. doi: 10.3389/fpls.2022.1039094
Ma, W., Pang, Z., Huang, X., Xu, J., Pandey, S. S., Li, J., et al. (2022). Citrus Huanglongbing is a pathogen-triggered immune disease that can be mitigated with antioxidants and gibberellin. Nat. Commun. 13, 109. doi: 10.1038/s41467-022-28189-9
Mali, P., Esvelt, K. M., Church, G. M. (2013). Cas9 as a versatile tool for engineering biology. Nat. Methods 10, 957–963. doi: 10.1038/nmeth.2649
Melnyk, C. W., Meyerowitz, E. M. (2015). Plant grafting. Curr. Biol. 25, R183–R188. doi: 10.1016/j.cub.2015.01.029
Nadarasah, G., Stavrinides, J. (2011). Insects as alternative hosts for phytopathogenic bacteria. FEMS Microbiol. Rev. 35, 555–575. doi: 10.1111/j.1574-6976.2011.00264.x
Park, S.-W., Kaimoyo, E., Kumar, D., Mosher, S., Klessig, D. F. (2007). Methyl salicylate is a critical mobile signal for plant systemic acquired resistance. Science 318, 113–116. doi: 10.1126/science.1147113
Peng, A., Chen, S., Lei, T., Xu, L., He, Y., Wu, L., et al. (2017). Engineering canker-resistant plants through CRISPR /Cas9-targeted editing of the susceptibility gene Cs LOB 1 promoter in citrus. Plant Biotechnol. J. 15, 1509–1519. doi: 10.1111/pbi.12733
Qiu, W., Soares, J., Pang, Z., Huang, Y., Sun, Z., Wang, N., et al. (2020). Potential mechanisms of atNPR1 mediated resistance against Huanglongbing (HLB) in citrus. IJMS 21, 2009. doi: 10.3390/ijms21062009
Ramsey, J. S., Johnson, R. S., Hoki, J. S., Kruse, A., Mahoney, J., Hilf, M. E., et al. (2015). Metabolic interplay between the Asian citrus psyllid and its Profftella symbiont: an Achilles’ Heel of the citrus greening insect vector. PloS One 10, e0140826. doi: 10.1371/journal.pone.0140826
Rivas-San Vicente, M., Plasencia, J. (2011). Salicylic acid beyond defence: Its role in plant growth and development. J. Exp. Bot. 62, 3321–3338. doi: 10.1093/jxb/err031
Shaffer, L. (2020). RNA-based pesticides aim to get around resistance problems. Proc. Natl. Acad. Sci. U.S.A. 117, 32823–32826. doi: 10.1073/pnas.2024033117
Shokrollah, H., Lee Abdullah, T., Sijam, K., Akmar Abdullah, S. N. (2011). Potential use of selected citrus rootstocks and interstocks against HLB disease in Malaysia. Crop Prot. 30, 521–525. doi: 10.1016/j.cropro.2010.09.005
Takahashi, F., Shinozaki, K. (2019). Long-distance signaling in plant stress response. Curr. Opin. Plant Biol. 47, 106–111. doi: 10.1016/j.pbi.2018.10.006
Vlot, A. C., Sales, J. H., Lenk, M., Bauer, K., Brambilla, A., Sommer, A., et al. (2021). Systemic propagation of immunity in plants. New Phytol. 229, 1234–1250. doi: 10.1111/nph.16953
Wang, L., Chen, S., Peng, A., Xie, Z., He, Y., Zou, X. (2019). CRISPR/Cas9-mediated editing of CsWRKY22 reduces susceptibility to Xanthomonas citri subsp. citri in Wanjincheng orange (Citrus sinensis (L.) Osbeck). Plant Biotechnol. Rep. 13, 501–510. doi: 10.1007/s11816-019-00556-x
Wang, N. (2019). The citrus Huanglongbing crisis and potential solutions. Mol. Plant 12, 607–609. doi: 10.1016/j.molp.2019.03.008
Wu, Y., Zhang, Di, Chu, J. Y., Boyle, P., Wang, Y., Brindle, I. D., et al. (2012). The Arabidopsis NPR1 protein is a receptor for the plant defense hormone salicylic acid. Cell Rep. 1, 639–647. doi: 10.1016/j.celrep.2012.05.008
Yang, L., Machin, F., Wang, S., Saplaoura, E., Kragler, F. (2023). Heritable transgene-free genome editing in plants by grafting of wild-type shoots to transgenic donor rootstocks. Nat. Biotechnol. 346, 1258096. doi: 10.1038/s41587-022-01585-8
Zaman, Q. U., Raza, A., Gill, R. A., Hussain, M. A., Wang, H. F., Varshney, R. K. (2023). New possibilities for trait improvement via mobile CRISPR-RNA. Trends Biotechnol. 41, 1335–1338. doi: 10.1016/j.tibtech.2023.05.001
Zambon, F. T., Kadyampakeni, D. M., Grosser, J. W. (2019). Ground application of overdoses of manganese have a therapeutic effect on sweet orange trees infected with Candidatus liberibacter asiaticus. horts 54, 1077–1086. doi: 10.21273/HORTSCI13635-18
Zhang, W., Thieme, C. J., Kollwig, G., Apelt, F., Yang, L., Winter, N., et al. (2016). TRNA-related sequences trigger systemic mRNA transport in plants. Plant Cell 28, 1237–1249. doi: 10.1105/tpc.15.01056
Zhao, D., Song, G.-q. (2014). Rootstock-to-scion transfer of transgene-derived small interfering RNA s and their effect on virus resistance in nontransgenic sweet cherry. Plant Biotechnol. J. 12, 1319–1328. doi: 10.1111/pbi.12243
Keywords: Citrus fruit trees, Candidatus Liberibacter (CL), Citrus greening, grafting, root-to-shoot transport, tRNA-like structures (TLSs), CRISPR/Cas9, heritable transgene-free genome editing
Citation: Gill RA, Li X, Duan S, Xing Q and Müller-Xing R (2024) Citrus threat huanglongbing (HLB) - Could the rootstock provide the cure? Front. Plant Sci. 15:1330846. doi: 10.3389/fpls.2024.1330846
Received: 31 October 2023; Accepted: 23 January 2024;
Published: 09 February 2024.
Edited by:
Elena Palomo Ríos, Universidad de Málaga, SpainReviewed by:
Laurent G. Deluc, Oregon State University, United StatesCopyright © 2024 Gill, Li, Duan, Xing and Müller-Xing. This is an open-access article distributed under the terms of the Creative Commons Attribution License (CC BY). The use, distribution or reproduction in other forums is permitted, provided the original author(s) and the copyright owner(s) are credited and that the original publication in this journal is cited, in accordance with accepted academic practice. No use, distribution or reproduction is permitted which does not comply with these terms.
*Correspondence: Qian Xing, UVhpbmdAbHNiZy5jbg==; Ralf Müller-Xing, UmFsZi5NdWVsbGVyQGhodS5kZQ==