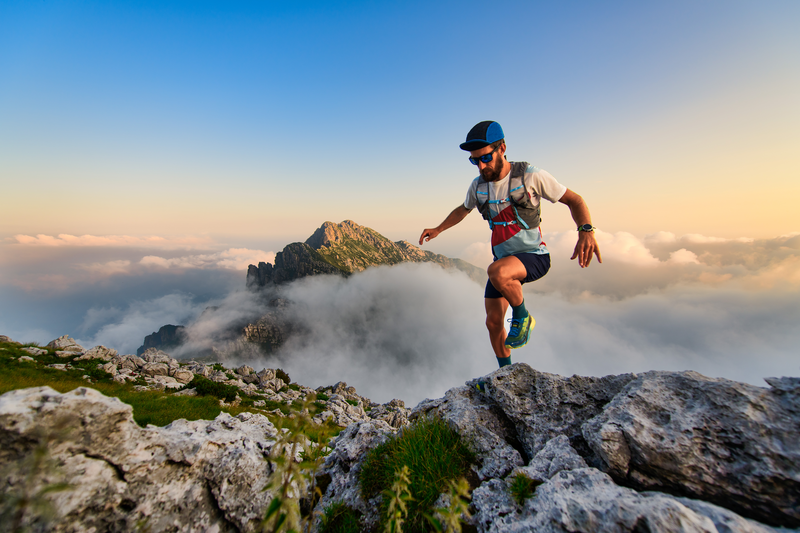
95% of researchers rate our articles as excellent or good
Learn more about the work of our research integrity team to safeguard the quality of each article we publish.
Find out more
ORIGINAL RESEARCH article
Front. Plant Sci. , 27 February 2024
Sec. Plant Development and EvoDevo
Volume 15 - 2024 | https://doi.org/10.3389/fpls.2024.1308059
Introduction: Among candidate genes underlying the control components of apomixis, APOLLO is known for its strong linkage to apomeiosis in the genus Boechera. The gene has “apo alleles,” which are characterized by a set of linked apomixis-specific polymorphisms, and “sex alleles.” All apomictic Boechera genotypes are heterozygous for the apo/sex alleles, whereas all sexual genotypes are homozygous for sex alleles.
Methods: In this study, native and synthetic APOLLO promoters were characterized by detecting the expression level of the β-glucuronidase (GUS) gene in Arabidopsis.
Results: Comparing various flower developmental stages in transgenic lines containing different constructs with 2-kb native transgenic lines revealed that changes to the APOLLO promoter causes shifts in tissue and developmental stage specificity of GUS expression. Importantly, several apomixis-specific polymorphisms in the 5′UTR change the timing and location of GUS activity from somatic to reproductive tissues.
Discussion: These synthetic data simulate a plausible evolutionary process, whereby apomixis-specific gene activity can be achieved.
In contrast to sexual reproduction and segregation of traits in his peas, Mendel’s research on Hieracium illustrated a distinct kind of inheritance in which segregation was absent and which was later identified as the parthenogenetic development of meiotically unreduced egg cells (Bicknell et al., 2016; Mittelsten Scheid, 2022; Van Dijk and Ellis, 2023). As with Hieracium, many plant species reproduce through apomixis, a natural form of asexual reproduction through which seeds are produced with embryos that are genetic clones of the mother plant (Hojsgaard and Hörandl, 2019; Underwood and Mercier, 2022).
While apomixis can arise via different mechanisms in natural plant populations (Hojsgaard and Hörandl, 2019; Underwood and Mercier, 2022), it fundamentally differs from sexual seed formation by the production of the meiotically unreduced egg cell (apomeiosis) that develops into an embryo without fertilization (parthenogenesis) in addition to endosperm development with or without fertilization of the central cell (Hojsgaard and Hörandl, 2019). Many apomicts are, furthermore, facultative (i.e., producing both sexually and apomictically derived embryos), and hence there is interplay between the reproductive pathways (Bicknell et al., 2003; Fehrer et al., 2007; Aliyu et al., 2010; Koltunow et al., 2011). Apomicts are often interspecific hybrids, which has led to the hypothesis that deregulation of genes underlying sexual seed formation underlies the genesis of apomixis (Carman, 1997). Furthermore, many apomictic plants and parthenogenetic animals are also polyploid, with implications for changes in gene expression and mutational load tolerance (among other traits) as being favorable for apomixis (Hojsgaard, 2018).
Apomixis has received much attention because of its potential ability to freeze complex hybrid genotypes (Tucker and Koltunow, 2009). It does not exist in crop plants, and the introduction of apomixis from wild apomictic relatives into crops (Underwood et al., 2022) or via de novo induction would be highly disruptive to modern agriculture (Spillane et al., 2004; Underwood et al., 2022). Recent progress in inducing apomixis properties in lettuce and rice demonstrates the importance of research in this field to establish new tools to improve global food security (Underwood et al., 2022; Vernet et al., 2022).
The genus Boechera Á. Löve & D. Löve (Boecher’s rock cress; Brassicaceae) (Dobeš et al., 2006) is an ideal model system to study the evolution of apomixis, a major reason being that sexual and apomict plants can be compared genetically at the same ploidy level (Corral et al., 2013). The genus is characterized by diploid sexuals (basic chromosome number x = 7; 2n = 2x = 14), as well as diploid (2n = 2x = 14 or 15) and triploid (2n = 3x = 21) apomicts (Kantama et al., 2007; Alexander et al., 2013; Lovell et al., 2013). A highly reticulate pattern of genetic variation on inter- and intraspecific levels is reflective of numerous diploid and triploid apomictic lineages in geographically and genetically distinct populations (Kiefer et al., 2009; Rushworth et al., 2011, 2018), whereby rare haploid apomictic pollen can spread of apomixis factors to establishment new diploid apomictic lineages (Mau et al., 2021).
The introduction of apomixis-like reproduction in plants using gene mutants (D’Erfurth et al., 2009) or through genetic constructs based on candidate loci identified in different wild apomictic species (Underwood et al., 2022) demonstrates that multiple functional pathways are available to achieve asexuality. From a functional evolutionary perspective, deregulation of genes underlying sexual seed formation likely involves multiple loci that are simultaneously co-opted to induce apomixis, with changes to tissue specificity and timing of gene expression leading to the switch from sexual to apomictic seed production in natural populations (Schmidt, 2020).
Here, we demonstrate that sex- vs. apomixis-specific polymorphisms in the 5′UTR of the apomixis candidate APOLLO gene (Corral et al., 2013) can modify timing and location of gene expression in Arabidopsis. UTR control of gene expression has been demonstrated to be significant in other plant species (Srivastava et al., 2018) as well as humans (Babu et al., 2021). For example, a novel motif in the 5′UTR of Big Root Biomass gene modulates root biomass in sesame, whereby this motif in single and duplicated copies was responsible for high and low root biomass in two different accessions, respectively (Dossa et al., 2021). Global spatial analysis of Arabidopsis natural variation revealed 5′UTR splicing of LATE ELONGATED HYPOCOTYL (LHY) in response to temperature (James et al., 2018). In addition, blue light exposure in Arabidopsis triggers variation in 5′UTR length resulting in differential transcription start site usage that, in turn, influences the post-transcriptional regulatory processes (Gregory, 2018; Kurihara et al., 2018).
In Boechera, APOLLO is part of the DEDD 3′→5′ exonuclease superfamily (Corral et al., 2013), and the expression of its ortholog in A. thaliana (AT1G74390) has been reported in flowering stages, mature plant embryos, petal differentiation and expansion stages, plant embryo bilateral stages, plant embryo cotyledonary stages, and plant embryo globular stages (https://www.arabidopsis.org). Sexual Boechera is homozygous for an APOLLO sex allele, whereas apomicts have both apo- and sex-alleles that differ in sequence composition and structure (Corral et al., 2013). In Boechera, apo-alleles of APOLLO are highly expressed in developing apomictic ovules during the megaspore mother cell stage, whereas sex-alleles are not expressed in apomictic ovules (Corral et al., 2013). No APOLLO expression occurs in the ovules of sexual plants (Corral et al., 2013). Ovule-specific expression of APOLLO in apomictic Boechera is hypothesized to be associated with differences in the 5′UTR region that differs between apo- and sex-alleles, whereby the apo-allele 5′UTR is truncated and additionally contains an internal 20-bp insertion in comparison to the sex-allele 5′UTR (Corral et al., 2013). Here, we have employed a promoter-swap experiment between apo-allele– and sex-allele–specific sequences to investigate the influence of the apomixis-specific polymorphisms on the gene expression of this exonuclease in Arabidopsis.
Arabidopsis thaliana (Columbia-0 ecotype) seeds were obtained from the Arabidopsis Biological Resource Centre (ABRC). Surface sterilized seeds were sown on MS plates containing ½ MS (Murashige & Skoog) medium (pH adjusted to 5.7 with 1 M NaOH) with 0.8% phytoagar, stratified at 4°C for 48 h and then transferred to a phytochamber at 20°C with a light cycle of 16-h light (white fluorescent bulbs, with a light intensity of 70 μmol m−2 s−1) and 8-h dark light. Two-week-old seedlings were transferred to pots containing Sunshine mix 8 and grown in the same conditions.
Native and promoter swap constructs were created using various combinations of sex- versus apo-specific polymorphisms identified in Corral et al (2013; Figure 1; Supplementary Table 1). All primers used are described in Supplementary Tables 2 and 3. All verified construct sequences have been submitted to GeneBank, as listed in Supplementary Table 4.
Figure 1 Construct pC1, pC2, pC3, pC4, pC5, pC6, and pC7. Constructs pC3 to pC7 created using SOE (Horton et al., 1989). For pC1, primer pairs of pAPOLLO AF-2 kb and pAPOLLO 1R were used to amplify 1,945 bp of DNA containing 71 bp of the 5′UTR of the Apo promoter containing the 20-bp apo-specific insertion (yellow). For pC2, primer pairs of pAPOLLO SF-2 kb and pAPOLLO 1R were used to amplify 1,970-bp DNA containing 104 bp of the 5′UTR (gray). For pC3, primer pairs used to amplify 1,880 bp of the Apo promoter are shown in green, and primer pairs used to amplify 44 bp of the 5′UTR (gray) by deleting the 20-bp apo-specific insertion (yellow) are shown in dark blue. For pC4, primer pairs used to amplify 1,917 bp of the Sex promoter are shown in green, and primer pairs used to amplify 52 bp of Sex promoter by incorporating the 20-bp apo-specific insertion (yellow) are shown in dark blue, with the 5′UTR of the sex-allele shown in gray. For pC5, primer pairs used to amplify 1,880 bp of the Apo promoter are shown in green, and primer pairs used to amplify 44 bp of the Apo promoter by incorporating a random 20-bp insertion (yellow) are shown in dark blue, with the 5′UTR of the apo-allele shown in gray. For pC6, primer pairs used to amplify 1,870 bp of the Apo-promoter are shown in green, and primer pairs used to amplify 104 bp of the Sex promoter are shown in dark blue, with the 5′UTR of the sex-allele shown in gray, with the 20-bp apo-specific insertion (yellow) deleted. For pC7, primer pairs used to amplify 1,865 bp of the Sex promoter are shown in green, and primer pairs used to amplify 71 bp 5′UTR of the Apo promoter carrying the apo-specific insertion (yellow) are shown in dark blue, with the 5′UTR of the apo-allele in shown gray color.
To build native Boechera APOLLO putative promoter constructs (C1 and C2), approximately 2 kb upstream of start codon, including the 5′UTR, was amplified from B. stricta (ES 718; sexual) and B. divaricarpa (ES 517; apomictic) genomic DNA using CloneAmp (Thermo Fischer Scientific) DNA polymerase. Specific primers were designed on the basis of BAC apo- and sex-allele sequence libraries (Corral et al., 2013). Synthetic promoter splice variants (C3 to C7) were constructed using a modified “gene Splicing by Overlap Extension” method (SOE) (Horton et al., 1989). For C3, C4, and C5, modifications to the 5′UTR indel region were created by modifying the respective primer sequences (Supplementary Figure 1). Construct pC5 was composed of the 2-kb native Apo promoter plus the 5′UTR of the apo-allele, but the apomixis-specific 20-bp insertion sequence was randomized (but ratios of base pairs remained identical to the wild-type insertion; Figure 1). Resultant PCR products were purified and cloned into a pENTR™/D-TOPO™ entry vector via directional TOPO® cloning and incorporated into the pBGWFS7 GFP : GUS reporter vector (Nakagawa et al., 2007) using Gateway™ LR Clonase™. All constructs were verified using Sanger sequencing.
Binary vectors were introduced into the Agrobacterium tumefaciens strain GV3101 by electroporation, and 8-week-old wild-type Arabidopsis Col-0 plants were transformed by floral dip (Clough and Bent, 1998). Transformed seeds were selected on the basis of the method by Harrison et al. (2006). Two-week-old glufosinate-resistant seedlings were then transferred to pots containing Sunshine mix 8, and presence of the transgene was confirmed by PCR of genomic DNA.
Tissue-specific promoter activity for all constructs was assessed by β-glucuronidase (GUS) activity; seedlings and flowers from at least 10 different independent transformation events were examined per construct. GUS staining was performed following the protocol of Jefferson (1987). Developmental staging was based on pistil and stigma maturity according to Smyth et al. (1990) and Christensen et al. (1997). We defined five developmental stages as in Rojek et al. (2021) for analyses based upon pistil length, whereby the first stage (pre-meiotic stage) had a pistil length of 0.7 mm, and, for the post-meiotic stages, the pistil lengths were 1.0 mm, 1.5 mm, 2.0 mm, and 3.0mm. Among all of these stages, the final stage is considered as post-fertilization stage (Rojek et al., 2021).
Tissues were cleared following the protocol of Malamy and Benfey (1997). Samples were mounted in 50% glycerol on glass microscope slides. Among 10 different pre-assessed transgenic lines, three were used for microscopic analysis and photography, and A. thaliana var. Columbia that was not exposed to the clearing material was used as a control. Promoter activity in the leaves and flowers of A. thaliana was measured by a GUS histochemical assay at 2 and 5 days after treatment in a minimum of three flowers per developmental stage (Smyth et al., 1990; Rojek et al., 2018). Images were obtained under a Zeiss Lumar.V12 Stereoscope and Olympus BX61 microscope and analyzed using ImageJ (https://rsb.info.nih.gov/ij/). To compare intensity of GUS-positive areas, the linear region-of-interest method was used, whereby a straight line is drawn through cell images to depict the main intensity of the color (Béziat et al., 2017). The original data were normalized by Min-Max method and analyzed using a two-way analysis of variance (two-way ANOVA). Finally, statistical differences were determined by Tukey test.
Positive GUS activity for root, shoots, and leaves of 2-week-old seedlings for transgenic lines carrying the construct pC1 (2-kb native Apo promoter) was observed in 33 lines of 2-week-old seedlings, each with four replicates (Figure 2). From this pattern (Figure 2A), three of the 33 lines were negative only for roots, shoots, or leaves, whereas five of the 33 lines were negative only for roots (Supplementary Figure 2). In contrast, no GUS activity was observed for 31 lines of 2-week-old seedlings transgenic for the construct pC2, carrying the 2-kb Sex native promoter (Figure 2B; Supplementary Figure 2).
Figure 2 Expression patterns of Boechera native promoter GUS constructs in Arabidopsis. (A) GUS distribution of 2-kb native pApo promoter (pC1) in 2-week-old plant. (B) No GUS activity observed in 2-kb native pSex promoter (pC2). (A, B) Scale bar, 0.5 mm. (C) Positive GUS activity in leaf of 6-week-old Arabidopsis transgenic for pC1. Scale bar, 2.0 mm (Zeiss Lumar.V12 Stereoscope).
There was positive GUS activity in leaves of 6-week-old Arabidopsis transgenic lines for pC1 (Figure 2C) and no GUS activity in any plants containing pC2 (Supplementary Figure 2).
No other consistent GUS activity was detected in any tissues over a 6-week growth period in three transgenic Arabidopsis lines for the 2-kb native Boechera construct pC1 (Figure 1) or for 10 transgenic lines of 6-week-old Arabidopsis containing the 2-kb native Boechera pC2 construct.
Construct pC3 (2-kb native Apo promoter plus 5′UTR with apo-specific insertion deleted; Figure 1) GUS activity was measured in eight transgenic lines, over five different developmental stages (total of 40 flower buds). No GUS activity was observed in the pre-meiotic (0.7 mm) and post-meiotic (1.0 mm) flower stages (Figures 3A, B, F, G), whereas positive GUS activity was observed for stages 3, 4, and 5 in the style and filament (including vascular tissue) with an increasing activity toward the final stages (Figures 3C–E, H–J; P ≤ 0.05, Tukey test; Supplementary Figure 3). Dispersed GUS activity in petals was excluded because they did not show a constant pattern for all samples. Thus, removal of the 20-bp apo-specific insertion from its native 2-kb allele shifts expression from somatic to both male and female reproductive tissue.
Figure 3 Expression patterns of synthetic promoter construct (pC3: GUS) in Arabidopsis flowers. Construct pC3 promoter in different developmental stages of flowers. (A) 0.7-mm flower. (B) 1.0-mm flower with GUS activity in petals. (C) 1.5-mm flower with GUS activity in style and filament. (D) 2.0-mm flower with GUS activity in style and filament. (E) 3.0-mm flower with GUS activity in style and filament. (A–E) Scale bar, 1.0 mm (Zeiss Lumar.V12 Stereoscope). (F) 0.7-mm flower. (G) 1.0-mm flower. (H) 1.5-mm flower with GUS activity in style and filament. (I) 2.0-mm flower with GUS activity in style and filament. (J) 3.0-mm flower with GUS activity in style and filament. (F–J) Scale bar, 100 μm (Olympus BX61 microscope).
Construct pC4 carried the 2-kb native pSex promoter, the 5′UTR of the sex-allele, with the 20-bp Apo-insertion added. The GUS activity for seven transgenic pC4 lines, each of which had five different developmental stages (total of 35 flower buds) was measured, and no GUS activity was detected (Supplementary Figure 4).
Construct pC5 was composed of the 2-kb native pApo promoter plus the 5′UTR of the apo-allele, but a 20-bp randomized (i.e., same nucleotide composition but different sequence) insertion was substituted for the apomixis-specific 20-bp insertion (Figure 1). GUS activity was observed in the anthers and receptacle for the pre-meiotic stage, as well as some anther-specific GUS activity for stage 2 (Figures 4A, B, F, G; Supplementary Figure 5). Style-specific GUS activity appeared in stage 3 and increased in intensity over stages 3, 4, and 5 (Figures 4C–E, H–J). GUS activity for the flower receptacle and petals was excluded for quantification because they did not show a constant pattern for all samples.
Figure 4 Expression patterns of spliced promoter construct (pC5: GUS) in different developmental stages of Arabidopsis flowers. (A) 0.7-mm flower with GUS activity in anther and receptacle. (B) 1.0-mm flower with GUS activity in anther and receptacle. (C) 1.5-mm flower with GUS activity in style and receptacle. (D) 2.0-mm flower with GUS activity in style and receptacle. (E) 3.0-mm flower with GUS activity in style and receptacle. (A–E) Scale bar, 1.0 mm (Zeiss Lumar.V12 Stereoscope). (F) 0.7-mm flower with GUS activity in anthers. (G) 1.0-mm flower with GUS activity in anthers. (H) 1.5-mm flower with GUS activity in style. (I) 2.0-mm flower with GUS activity in style. (J) 3.0-mm flower with GUS activity in style. (G–J) Scale bar, 50 µm.
Construct pC6 carried the 2-kb pApo promoter, with a swapped native 5′UTR of sex-allele (Figure 1), and was analyzed in four transgenic lines over five different developmental stages (total of 20 flower buds). Anther-specific GUS activity with varying intensity was observed for all developmental stages (Figure 5), with the strongest expression in stage 2 (Figure 5B; P ≤ 0.05; Tukey test; Supplementary Figure 6). We hypothesize that pollen activity is induced (Figures 5G–K), as is evidenced by post-dehiscence loss of activity in anthers (Figures 5D, E).
Figure 5 Expression patterns of spliced promoter construct (pC6: GUS) in different developmental stages of Arabidopsis flowers. (A) 0.7-mm flower with GUS activity in anthers. (B) 1.0-mm flower with GUS activity in anthers. (C) 1.7-mm flower with GUS activity in anthers. (D) 2.0-mm flower with GUS activity in anthers. (E) 3.0-mm flower with GUS activity in anthers. (A–E) Scale bar, 1.0 mm (Zeiss Lumar.V12 Stereoscope). (F) Arabidopsis inflorescence; scale bar, 2.0 mm. (G) 0.7-mm flower with GUS activity in anthers. (H) 1.0-mm flower with GUS activity in anthers. (I) 1.5-mm flower with GUS activity in anthers. (J) 2.0-mm flower with GUS activity in anthers. (K) 3.0-mm flower. (G–K) Scale bar, 50 µm (Olympus BX61 microscope). Pollen-specific GUS expression demonstrated by black arrows (I, J, K). .
Construct pC7 carried the 2-kb Sex promoter with a swapped apo-allele 5′UTR (Figure 1) and was analyzed in six transgenic lines over five developmental stages (total of 30 flower buds). GUS activity with a varied intensity was observed in the transmitting tract, ovules and anthers for the first two developmental stages (Figures 6A, B), whereas only ovule expression occurred in stage 3 (Figure 6C). Ovule expression began in stage one (Figure 6) and lasted for three stages (P ≤ 0.05; Tukey test; Supplementary Figure 7). In stage 4 (Figure 6D), expression in both tissues ceased. The Arabidopsis inflorescence is shown in Figure 6E, and ovule expression is visible clearly in Figure 6F.
Figure 6 Expression patterns of promoter construct (pC7: GUS) in different developmental stages of Arabidopsis flowers. (A) 0.7-mm flower with GUS activity in transmitting tract, ovules, and anthers. (B) 1.0-mm flower with GUS activity in transmitting tract, ovules, and anthers. (C) 1.5-mm flower with GUS activity in and transmitting tract and ovules. (D) 2.0-mm flower. (A–D) Scale bar, 1.0 mm. (E) The Arabidopsis inflorescence. Scale bar, 2.0 mm (Zeiss Lumar.V12 Stereoscope). (F) 1.0-mm flower showing expression in ovules (black arrows). Scale bar, 50 μm (Olympus BX61 microscope).
A comparison of gene expression in the micro-dissected ovules of apomictic versus sexual Boechera led to the identification of the APOLLO gene. The heterozygous nature and presence of apomixis-specific polymorphisms were proposed to underlay gene expression differences between pre-meiotic ovules of apomictic versus sexual Boechera (Corral et al., 2013). Here, we investigated the nature of regulatory differences between native and synthetic APOLLO promoter GUS constructs in transformed Arabidopsis to understand functional differences between conserved sex- versus apo-allele polymorphisms in the 5′UTR region of the APOLLO gene. We have made no effort here to understand sequence variation in the 2-kb promoter regions of apo- and sex-alleles from multiple accessions of Boechera, as our focus was the 5′UTR region of the APOLLO gene. A comparison of the native promoter region sequences (constructs pC1 and pC2; Supplementary Table 4), nonetheless, demonstrates that these upstream regions also differ extensively, with potential implications for specific interactions in the promoter swap constructs which have not been tested here.
These data demonstrate that evolutionarily conserved apomixis-specific polymorphisms in the 5′UTR of the apo allele in Boechera can cause changes to gene expression timing and location in A. thaliana. Importantly, coordinated expression in both male and female reproductive tissue can be achieved, thus providing a tool which can potentially be applied to reproductive genetics in Brassica plants.
Arabidopsis transgenic lines for the 2-kb native Apo promoter demonstrate expression in the vascular tissues of roots stems and leaves, and expression ceases before the development of reproductive tissues (Figure 2). In contrast, no expression was observed for the 2-kb Sex promoter in any Arabidopsis transformants.
In an analysis of the 5′UTR regions of APOLLO, Corral et al. (2013) showed that the Boechera 20-bp apomixis-specific insertion of the apo-allele shared a SORLIP1AT transcription factor binding site with the orthologous regions in A. thaliana and Brassica rapa, as well as a LIM1 transcription factor binding site with the orthologous regions in A. thaliana, whereas the sex-allele did not. Furthermore, the Boechera apo-allele transcripts are truncated at the 5′end of the 20-bp apo-specific insertion, whereas the sex-allele transcripts are approximately 35 bp longer (Corral et al., 2013). Finally, the apo-allele is characterized by exclusive expression in the ovules of apomictic Boechera (Corral et al., 2013), whereas, in transformed sexual A. thaliana, it is expressed in somatic and not reproductive tissues (Figure 2). In this case, we hypothesize that expression differences between the apo- and sex-alleles result from the different transcription factor landscape of the A. thaliana genome.
Compared to the native apo-allele promoter-5′UTR (Figures 1 and 2), removal of the 20-bp apomixis-specific insertion shifted expression from somatic to male and female reproductive tissues (Figure 3). pC3 showed GUS activity in filaments (including vascular tissue) and style in earlier and later stages of flower development respectively (Figure 3), and thus the absence of the 20-bp apo-specific insertion changes the locality and timing of expression. Deletion of the 20-bp Apo-insertion thus likely leads to a change in the recognition site for regulating interacting elements that control expression in specific floral tissues. Expression of the native Boechera apo-allele is not solely dependent on the apomixis-specific insertion, as insertion of the 20-bp apomixis-specific sequence into the native Boechera sex allele produced no GUS activity in A. thaliana transformants (Supplementary Figure 4). Alternatively, the 2-kb-upstream promoter region of the sex-allele may not be long enough to drive expression. Interestingly, the apomixis-specific insertion length is also important for expression in reproductive tissues, as randomization of the insertion sequence (with identical nucleotide composition) while maintaining length led to strong early expression in anther heads, which stopped after fertilization. Post-fertilization was then characterized by growing strong expression in the stigma (Figure 4).
Transgenic pC6 lines carrying 2 kb of the Apo promoter and the 5′UTR of the sex-allele (i.e., with no apomixis-specific 20-bp insertion) showed strong GUS staining in anthers in both pre- and post-meiotic floral buds (Figure 5A–K). Closer examination of the anthers demonstrated that strong expression occurred in the pollen grains (Figures 5I, J). This expression ceased after fertilization (Figure 5E).
Finally, transgenic pC7 lines carrying the 2-kb native Sex promoter and 5′UTR of the apo-allele with the 20-bp apo-insertion (Figure 6; Supplementary Figure 7) showed a window of GUS activity in both ovules and anthers in earlier stages of flower development, which included the fertilization stage (Figure 6). As the native sex-allele promoter (pC2) showed no activity in Arabidopsis flowers (Figure 2), these data demonstrate that the apo 5′UTR can drive both male- and female-specific expression in A. thaliana.
Considering the ortholog of the APOLLO gene in A. thaliana (AT1G74390), the NAC45/86-DEPENDENT EXONUCLEASE-DOMAIN PROTEIN 3 (or NEN3), these data suggest that polymorphisms shared between the Boechera apo 5′UTR and that of A. thaliana are functionally involved with coordinating expression of this gene (or gene family) in male and female tissues during fertilization.
Removal of the 20-bp apomixis-specific insertion from the native apo-allele promoter-5′UTR (Figure 1) shifted expression from somatic (Figure 2) to male and female reproductive tissues (Figure 3). pC3 showed GUS activity in filaments and stigma in earlier and later stages of flower development, respectively (Figure 3), and thus the absence of the 20-bp apo-specific insertion changes the locality and timing of expression. Deletion of the 20-bp apo-insertion thus likely leads to a change in the recognition site for interacting regulatory elements that control APOLLO expression in specific floral tissues.
Expression of the native Boechera apo-allele is not solely dependent on the apomixis-specific insertion, as insertion of the 20-bp apomixis-specific sequence into the native Boechera sex-allele produced no GUS activity in A. thaliana transformants (Supplementary Figure 4). Alternatively, the 2-kb-upstream promoter region of the sex-allele may not be long enough to drive expression. Interestingly, the apomixis-specific insertion length is also important for expression in reproductive tissues, as randomization of the insertion sequence while maintaining length led to strong early expression in anther heads which stopped after fertilization. Post-fertilization was then characterized by growing strong expression in the style (Figure 4).
Transgenic pC6 lines carrying 2 kb of the Apo promoter and the 5′UTR of the sex-allele (i.e., with no apomixis-specific 20-bp insertion) showed strong GUS staining in anthers in both pre- and post-meiotic floral buds (Figure 5). Closer examination of the anthers demonstrated that strong expression occurred in the pollen grains (Figures 5I, J). This expression ceased post-fertilization (Figure 5E). As the native apo-allele promoter drives expression in Arabidopsis while the native sex-allele promoter does not, the data so far demonstrate that the Apo-promoter upstream of the 5′UTR drives expression in A. thaliana, whereas the 5′UTR underlies male- or female-specific tissue expression.
The similarities between the 5′UTR sequences of the Boechera apo-allele, A. thaliana, and B. rapa suggest that the apo-allele is ancestral compared to the sex-allele and that deregulation of APOLLO in apomicts likely underlies its expression in pre-meiotic tissues (Corral et al., 2013). This is supported by the data presented here, whereby, in A. thaliana, a sexually reproducing species, removal or scrambling of the apo-specific 5′UTR insertion, and neighboring sequence induced expression in both male and female reproductive tissue. Together, these data suggest that the apo-5′UTR encodes a sequence-specific binding site for a transcriptional or translational repressor, one that may be specifically expressed in sexual meiotic cells.
The elevated heterozygosity in diploid apomictic Boechera can be explained by widespread hybridization associated with the transition from sexuality to asexuality, or it could be a byproduct of apomixis itself. In apomictic individuals, the overall heterozygosity is expected to increase over generations due to the lack of recombination and mutation accumulation (Meselson effect; Hojsgaard and Hörandl, 2015). Clear evidence for the hybrid origin of highly heterozygous apomicts has been shown (Beck et al., 2012), in addition to mutation accumulation on a genomic level in apomictic Boechera in conserved non-coding sequences (Lovell et al., 2017). Finally, rare haploid pollen produced by apomictic Boechera can transfer the complete apomictic trait in a single generation in both intra- and interspecific crosses onto emasculated female plants (Mau et al., 2021). Taken together, these data demonstrate that the conserved apo-allele of Boechera is found with different sexual alleles on a broad geographic scale, not to mention different genomic “contexts” considering the nuclear and cytoplasmic genomes in independently derived apomictic lineages (Kiefer et al., 2009; Kiefer and Koch, 2012).
Here, we show that conserved polymorphisms in the native Boechera apo-allele 5′UTR are functionally associated with driving gene expression in both male and female tissues during important reproductive stages (e.g., gametogenesis and fertilization). The interspecific “collision” of different sexual genomes may produce apomictic Boechera with structural changes such as homeologous chromosome substitutions and aneuploidy (Kantama et al., 2007). Extending divergence to regulatory genes, apomixis could result from the novel pattern of gene expressions created by the interaction of divergent transcriptional regulators (Carman, 2001) and is supported by the data reported in this study.
The datasets presented in this study can be found in online repositories. The names of the repository/repositories and accession number(s) can be found in the article/Supplementary Material.
MH: Formal analysis, Investigation, Methodology, Validation, Writing – original draft, Writing – review & editing. JA: Conceptualization, Formal analysis, Investigation, Methodology, Supervision, Validation, Writing – original draft, Writing – review & editing. TS: Conceptualization, Formal analysis, Funding acquisition, Investigation, Methodology, Project administration, Resources, Supervision, Validation, Writing – original draft, Writing – review & editing.
The author(s) declare financial support was received for the research, authorship, and/or publication of this article. This work was funded by a grant to TS from the Global Institute for Food Security and the University of Saskatchewan.
The authors declare that the research was conducted in the absence of any commercial or financial relationships that could be construed as a potential conflict of interest.
All claims expressed in this article are solely those of the authors and do not necessarily represent those of their affiliated organizations, or those of the publisher, the editors and the reviewers. Any product that may be evaluated in this article, or claim that may be made by its manufacturer, is not guaranteed or endorsed by the publisher.
The Supplementary Material for this article can be found online at: https://www.frontiersin.org/articles/10.3389/fpls.2024.1308059/full#supplementary-material
Alexander, P. J., Windham, M. D., Beck, J. B., Al-Shehbaz, I. A., Allphin, L., Bailey, C. D. (2013). Molecular phylogenetics and taxonomy of the genus Boechera and related genera (Brassicaceae: Boechereae). Systematic Bot. 38, 192–209. doi: 10.1600/036364413X661917
Aliyu, O. M., Schranz, M. E., Sharbel, T. F. (2010). Quantitative variation for apomictic reproductionin the genus Boechera (Brassicaceae). Am. J. Bot. 97, 1719–1731. doi: 10.3732/ajb.1000188
Babu, D., Vannelli, S., Fanelli, A., Mellone, S., Baffico, A. M., Corrado, L., et al. (2021). Variants in the5′UTR reduce SHOX expression and contribute to SHOX haploinsufficiency. Eur. J. Hum. Genet. 29, 110–121. doi: 10.1038/s41431-020-0676-y
Beck, J. B., Alexander, P. J., Allphin, L., Al-Shehbaz, I. A., Rushworth, C., Bailey, C. D., et al. (2012). Does hybridization drive the transition to asexuality in diploid Boechera? Evolution 66, 985–995. doi: 10.1111/j.1558-5646.2011.01507.x
Béziat, C. H., Kleine-Vehn., J., Feraru, E. (2017). Histochemical Staining of β-Glucuronidase andIts Spatial Quantification BT - Plant Hormones: Methods and Protocols. Eds. Kleine-Vehn, J., Sauer, M. (New York, NY: Springer New York), 73–80.
Bicknell, R., Catanach, A., Hand, M., Koltunow, A. (2016). Seeds of doubt: Mendel’s choice ofHieracium to study inheritance, a case of right plant, wrong trait. Theor. Appl. Genetics Springer Verlag 129, 2253–2266. doi: 10.1007/s00122-016-2788-x
Bicknell, R. A., Lambie, S. C., Butler, R. C. (2003). Quantification of progeny classes in twofacultatively apomictic accessions of Hieracium. Hereditas 138, 11–20. doi: 10.1034/j.1601-5223.2003.01624.x
Carman, J. G. (1997). Asynchronous expression of duplicate genes in angiosperms may cause apomixis,bispory, tetraspory, and polyembryony. Biol. J. Linn. Soc. 61, 51–94. doi: 10.1111/j.1095-8312.1997.tb01778.x
Carman, J. G. (2001). “The gene effect: genome collisions and apomixis,” in The Flowering of Apomixis: From Mechanisms to Genetic Engineering, Mexico, D.F.: CIMMYT, IRD, European Commission DG VI (FAIR). 95–110.
Christensen, C. A., King, E. J., Jordan, J. R., Drews, G. N. (1997). Megagametogenesis in arabidopsis wild type and the Gf mutant. Sexual Plant Reprod. 10, 49–64. doi: 10.1007/s004970050067
Clough, S. J., Bent, A. F. (1998). Floral dip: a simplified method for Agrobacterium –mediated transformation of Arabidopsis thaliana. Plant J. 16, 735–743. doi: 10.1046/j.1365-313x.1998.00343.x
Corral, J. M., Vogel, H., Aliyu, O. M., Hensel, G., Thiel, T., Kumlehn, J., et al. (2013). A conserved apomixis-specific polymorphism is correlated with exclusive exonuclease expression in premeiotic ovules of apomictic Boechera species. Plant Physiol. 163, 1660–1672. doi: 10.1104/pp.113.222430
D’Erfurth, I., Jolivet, S., Froger, N., Catrice, O., Novatchkova, M., Mercier, R. (2009). Turning meiosis into mitosis. PloS Biol. 7, e1000124. doi: 10.1371/journal.pbio.1000124
Dobeš, C. H., Marcus, A. K., Sharbel, T. F. (2006). Embryology, karyology, and modes of reproduction inthe North American genus Boechera (Brassicaceae): A compilation of seven decades of research. Annalsof Missouri Botanical Garden 93, 517–534. doi: 10.3417/0026-6493(2007)93[517:EKAMOR]2.0.CO;2
Dossa, K., Zhou, R., Li, D., Liu, A., Qin, L., Mmadi, M. A., et al. (2021). A novel motif in the 5’-UTR of an orphan gene ‘Big root biomass’ Modulates root biomass in sesame. Plant Biotechnol. J. 19, 1065–1079. doi: 10.1111/pbi.13531
Fehrer, J., Gemeinholzer, B., Chrtek, J., Bräutigam, S. (2007). Incongruent plastid and nuclear DNAphylogenies reveal ancient intergeneric hybridization in Pilosella hawkweeds (Hieracium, Cichorieae,Asteraceae). Mol. Phylogenet. Evol. 42, 347–361. doi: 10.1016/j.ympev.2006.07.004
Gregory, B. D. (2018). Shedding some blue light on alternative promoter usage in plants. PNAS 115, 7654–7656. doi: 10.1073/pnas.1809312115
Harrison, S. J., Mott, E. K., Parsley, K., Aspinall, S., Gray, J. C., Cottage, A. (2006). A rapid and robust method of identifying transformed Arabidopsis thaliana seedlings following floral dip transformation. Plant Methods 2, 19. doi: 10.1186/1746-4811-2-19
Hojsgaard, D. (2018). Transient activation of apomixis in sexual neotriploids may retain genomically altered states and enhance polyploid establishment. Front. Plant Sci. 9. doi: 10.3389/fpls.2018.00230
Hojsgaard, D., Hörandl, E. (2015). A little bit of sex matters for genome evolution in asexual plants. Front. Plant Sci. 6. doi: 10.3389/fpls.2015.00082
Hojsgaard, D., Hörandl, E. (2019). The Rise of Apomixis in natural plant populations. Front. Plant Sci. 10. doi: 10.3389/fpls.2019.00358
Horton, R. M., Henry, D., Steffan, N., Jeffry, K., Pease and Larry, R. (1989). Engineering hybrid genes without the use of restriction enzymes: gene splicing by overlap extension. Gene 77, 61–68. doi: 10.1016/0378-1119(89)90359-4.
James, A. B., Sullivan, S., Nimmo, H. G. (2018). Global spatial analysis of Arabidopsis natural variant simplicates 5′UTR splicing of LATE ELONGATED HYPOCOTYL in responses to temperature. Plant Cell Environ. 41, 1524–1538. doi: 10.1111/pce.13188
Jefferson, R. A. (1987). Assaying chimeric genes in plants: The GUS gene fusion system. Plant Mol. Biol. Report. 5, 387–405. doi: 10.1007/BF02667740
Kantama, L., Sharbel, T. F., Schranz, M. E., Mitchell-Olds, T., de Vries, S., de Jong, H. (2007). Diploid apomicts of the Boechera holboellii complex display large-scale chromosome substitutions and aberrant chromosomes. PNAS 104, 14026–14031. doi: 10.1073/pnas.0706647104
Kiefer, C. H., Dobeš, C. H., Sharbel, T. F., Koch, M. A. (2009). Phylogeographic structure of the chloroplast DNA gene pool in North American Boechera – a genus and continental-wide perspective. Mol. Phylogenet. Evol. 52, 303–311. doi: 10.1016/j.ympev.2009.03.016.
Kiefer, C. H., Koch, M. A. (2012). A continental-wide perspective: the genepool of nuclear encoded ribosomal DNA and single-copy gene sequences in North American Boechera (Brassicaceae). PloS One 7, e36491. doi: 10.1371/journal.pone.0036491
Koltunow, A. M. G., Johnson, S. D., Rodrigues, J. C. M., Okada, T., Hu, Y., Tsuchiya, T., et al. (2011). Sexual reproduction is the default mode in apomictic Hieracium subgenus Pilosella, in whichtwo dominant loci function to enable apomixis. Plant J. 66, 890–902. doi: 10.1111/j.1365-313X.2011.04556.x
Kurihara, Y., Makita, Y., Kawashima, M., Matsui, M. (2018). Transcripts from downstream alternativeTranscription start sites evade UORF-mediated inhibition of gene expression in arabidopsis. Proc. Natl. Acad. Sci. 115, 7831–7836. doi: 10.1073/pnas.1804971115
Lovell, J. T., Aliyu, O. M., Mau, M., Schranz, M. E., Koch, M., Kiefer, C., et al. (2013). On the originand evolution of apomixis in Boechera. Plant Reprod. 26, 309–315. doi: 10.1007/s00497-013-0218-7
Lovell, J. T., Williamson, R. J., Wright, S. I., McKay, J. K., Sharbel, T. F. (2017). Mutation Accumulation in an Asexual Relative of Arabidopsis. PLOS Genet. 13, e1006550.
Malamy, J. E., Benfey, P. N. (1997). Organization and cell differentiation in lateral roots of Arabidopsis thaliana. Development 124 (), 33 LP–44. Available at: http://dev.biologists.org/content/124/1/33.abstract.
Mau, M., Liiving, T., Fomenko, L., Goertzen, R., Paczesniak, D., Böttner, L., et al. (2021). The spread ofinfectious asexuality through haploid pollen. New Phytol. 230, 804–820. doi: 10.1111/nph.17174
Mittelsten Scheid, O. (2022). Mendelian and non-mendelian genetics in model plants. Plant Cell 34 (7), 2455–2461. doi: 10.1093/plcell/koac070
Nakagawa, T., Kurose, T., Hino, T., Tanaka, K., Kawamukai, M., Niwa, Y., et al. (2007). Development of series of gateway binary vectors, pGWBs, for realizing efficient construction of fusion genes for plant transformation. J. Biosci. Bioeng. 104, 34–41. doi: 10.1263/jbb.104.34
Rojek, J., Kapusta, M., Kozieradzka-Kiszkurno, M., Majcher, D., Górniak, M., Sliwinska, E., et al. (2018). Establishing the cell biology of apomictic reproduction in diploid boechera stricta(Brassicaceae). Ann. Bot. 122, 513–539. doi: 10.1093/aob/mcy114
Rojek, J., Tucker, M. R., Pinto, S. C., Rychłowski, M., Lichocka, M., Soukupova, H., et al. (2021). Rab-dependent vesicular traffic affects female gametophyte development in Arabidopsis. J. Exp. Bot. 72, 320–340. doi: 10.1093/jxb/eraa430
Rushworth, C. A., Song, B.-H., Lee, C.-R., Mitchell-Olds, T. (2011). Boechera, a model system for ecological genomics. Mol. Ecol. 20, 4843–4857. doi: 10.1111/mec.2011.20.issue-23
Rushworth, C. A., Windham, M. D., Keith, R. A., Mitchell-Olds., T. (2018). Ecological differentiation facilitates fine-scale coexistence of sexual and asexual Boechera. Am. J. Bot. 105, 2051–2064. doi: 10.1002/ajb2.1201
Schmidt, A. (2020). Controlling Apomixis: shared features and distinct characteristics of gene regulation. Genes 11, 329. doi: 10.3390/genes11030329.
Smyth, D. R., Bowman, J. L., Meyerowitz., E. M. (1990). Early flower development in Arabidopsis. Plant Cell 2, 755–767.
Spillane, C. H., Curtis, M. D., Grossniklaus, U. (2004). Apomixis technology development—Virgin Birthsin Farmers’ Fields? Nat. Biotechnol. 22, 687–691. doi: 10.1038/nbt976
Srivastava, A. K., Lu, Y., Zinta, G., Lang, Z. H., Zhu, J.-K. (2018). UTR-Dependent control of gene expression in pPlants. Trends Plant Sci. 23, 248–259. doi: 10.1016/j.tplants.2017.11.003.
Tucker, M. R., Koltunow, A. M. G. (2009). Sexual and asexual (Apomictic) seed development in flowering plants: molecular, morphological and evolutionary relationships. Funct. Plant Biol. 36, 490–504. doi: 10.1071/FP09078.
Underwood, Ch. J., Vijverberg, K., Rigola, D., Okamoto, S. H., Oplaat, C., Op den Camp, R. H. M., et al. (2022). A PARTHENOGENESIS allele from apomictic dandelion can induceEgg cell division without fertilization in lettuce. Nat. Genet. 54(1), 84–93. doi: 10.1038/s41588-021-00984-y
Underwood, Ch. J., Mercier, R., et al. (2022). Engineering apomixis: clonal seeds approaching the fields. Annu. Rev. Plant Biol. 73, 201–225.doi: 10.1146/annurev-arplant-102720-013958
Van Dijk, P. J., Ellis, T. H. N. (2023). Gregor mendel and the theory of species multiplication. Genetics 224, iyad046. doi: 10.1093/genetics/iyad046
Keywords: apomixis, APOLLO, Boechera, promoter-swap, 5’UTR, reproduction
Citation: Honari M, Ashnest JR and Sharbel TF (2024) Sex- versus apomixis-specific polymorphisms in the 5′UTR of APOLLO from Boechera shift gene expression from somatic to reproductive tissues in Arabidopsis. Front. Plant Sci. 15:1308059. doi: 10.3389/fpls.2024.1308059
Received: 05 October 2023; Accepted: 31 January 2024;
Published: 27 February 2024.
Edited by:
Concepción Gómez-Mena, Polytechnic University of Valencia, SpainReviewed by:
Steven Dreissig, Martin Luther University of Halle-Wittenberg, GermanyCopyright © 2024 Honari, Ashnest and Sharbel. This is an open-access article distributed under the terms of the Creative Commons Attribution License (CC BY). The use, distribution or reproduction in other forums is permitted, provided the original author(s) and the copyright owner(s) are credited and that the original publication in this journal is cited, in accordance with accepted academic practice. No use, distribution or reproduction is permitted which does not comply with these terms.
*Correspondence: Maryam Honari, bWhvbmFyaUBsYWtlaGVhZHUuY2E=; Timothy F. Sharbel, VGltLlNoYXJiZWxAdXNhc2suY2E=
†Present addresses: Joanne R. Ashnest, School of Agriculture, Environmental and Veterinary Sciences, Charles Sturt University, Wagga Wagga, NSW, Australia
Timothy F. Sharbel, Department of Plant Sciences, University of Saskatchewan, Saskatoon, SK, Canada
Disclaimer: All claims expressed in this article are solely those of the authors and do not necessarily represent those of their affiliated organizations, or those of the publisher, the editors and the reviewers. Any product that may be evaluated in this article or claim that may be made by its manufacturer is not guaranteed or endorsed by the publisher.
Research integrity at Frontiers
Learn more about the work of our research integrity team to safeguard the quality of each article we publish.