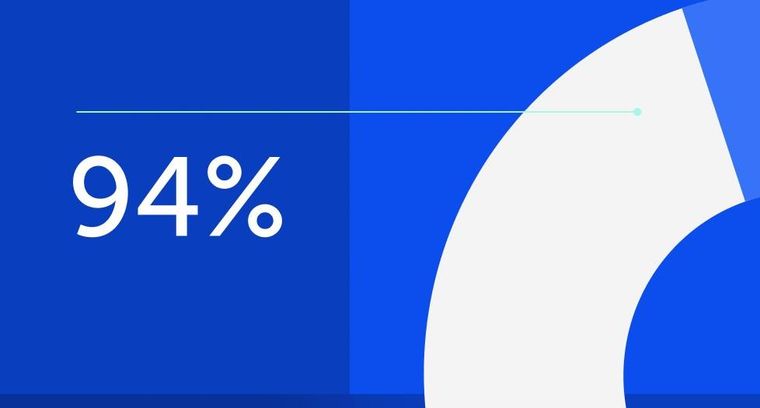
94% of researchers rate our articles as excellent or good
Learn more about the work of our research integrity team to safeguard the quality of each article we publish.
Find out more
ORIGINAL RESEARCH article
Front. Plant Sci., 14 February 2024
Sec. Plant Systematics and Evolution
Volume 15 - 2024 | https://doi.org/10.3389/fpls.2024.1303175
The genus Tetradesmus (Scenedesmaceae; Sphaeropleales) comprises one of the most abundant green algae in freshwater environments. It includes morphologically diverse species that exhibit bundle-like, plane-arranged coenobia, and unicells, because several different Scenedesmus-like groups were integrated into this genus based on phylogenetic analysis. Nevertheless, there is no clear information regarding the phylogenetic relationship of Tetradesmus species, determined using several marker genes, because of low phylogenetic support and insufficient molecular data. Currently, genome information is available from diverse taxa, which could provide high-resolution evolutionary relationships. In particular, phylogenetic studies using chloroplast genomes demonstrated the potential to establish high-resolution phylogenetic relationships. However, only three chloroplast genomes are available from the genus Tetradesmus. In this study, we newly generated 9 chloroplast genomes from Tetradesmus and constructed a high-resolution phylogeny using a concatenated alignment of 69 chloroplast protein sequences. We also report one novel species (T. lancea), one novel variety (T. obliquus var. spiraformis), and two novel formae (T. dissociatus f. oviformis, T. obliquus f. rectilineare) within the genus Tetradesmus based on morphological characteristics (e.g., cellular arrangements and coenobial types) and genomic features (e.g., different exon–intron structures in chloroplast genomes). Moreover, we taxonomically reinvestigated the genus Tetradesmus based on these results. Altogether, our study can provide a comprehensive understanding of the taxonomic approaches for investigating this genus.
The genus Tetradesmus G.M.Smith (Scenedesmaceae; Sphaeropleales) comprises a ubiquitous group of unicellular green algae found in aquatic and terrestrial environments, including rocks, sand, and soil (Tsarenko and John, 2011). This genus was characterized by spindle-shaped cells that generally form a bundle-like coenobium (Smith, 1913). Phylogenetic analyses have led to the integration of several Scenedesmus-like species, including Tetradesmus wisconsinensis G.M.Smith as the type species, into the genus Acutodesmus (Hegewald) Tsarenko. Formerly a subgenus within the genus Scenedesmus Myen, Acutodesmus now encompasses not only coenobia with a bundle-like structure but also those arranged on a plane, along with unicells, showing diverse morphological characteristics (Tsarenko and Petlevanny, 2001; Hegewald et al., 2013). However, ten Acutodesmus taxa have once again been relocated to the genus Tetradesmus because the genus Acutodesmus established in 2001, incorporating the type species A. wisconsinensis, but this species was initially described as the type species for the earlier genus Tetradesmus by G.M.Smith in 1913 (Wynne and Hallan, 2015; Wynne and Guiry, 2016; Lewis and Flechtner, 2019). Because of the complicated taxonomic history of the genus Tetradesmus, synonyms of Tetradesmus species are still used, especially in biotechnological research [e.g., Scenedesmus dimorphus (Turpin) Kützing and Acutodesmus obliquus (Turpin) Hegewald & Hanagata; Talarek-Karwel et al., 2020; Hu et al., 2021].
The phylogenetic relationships of Tetradesmus species have been extensively investigated using 18S ribosomal RNA or ITS2 sequences (Hegewald and Hanagata, 2000; Eliaš et al., 2010; Hegewald et al., 2010; Hegewald et al., 2013) and chloroplast genes (e.g., rbcL and tufA; Sciuto et al., 2015; Terlova and Lewis, 2019; Shetty et al., 2021). However, the phylogenetic relationships still remain unclear because of insufficient molecular data to construct a high-resolution phylogeny of the genus Tetradesmus. Furthermore, several molecular data (e.g., marker genes) in public databases were generated from morphologically unverified species (Mai et al., 2023). Such unverified sequences could cause confusions in interpreting the phylogenetic relationships of Tetradesmus species. Currently, genome sequencing information from diverse taxa is available because of the reduction of costs for genome sequencing approaches. In particular, the phylogenetic analysis using chloroplast genomes was useful to construct high-resolution evolutionary relationships (Lemieux et al., 2007; Lee et al., 2016; Song et al., 2016). Nonetheless, only three chloroplast genomes (NC_008101, CM007919, and MK514088) are available from the genus Tetradesmus (de Cambiaire et al., 2006; Starkenburg et al., 2017).
In this study, we generated complete chloroplast genomes from 9 Tetradesmus strains and analyzed the morphologies and phylogenetic relationships of Tetradesmus species. Based on our data, we provide a clear phylogenetic relationship and reestablish a complicated taxonomic history of the genus Tetradesmus, including diverse (e.g., unicellular, plane-arranged, and bundle-like) cellular types. We also newly report T. obliquus f. rectilineare H.S.Cho & J.M.Lee, f. nov., T. obliquus var. spiraformis H.S.Cho & J.M.Lee, var. nov., T. dissociatus f. oviformis H.S.Cho & J.M.Lee, f. nov., and T. lancea H.S.Cho & J.M.Lee, sp. nov. as novel formae, variety, and species in the genus Tetradesmus. Our results can provide a comprehensive understanding of taxonomic approaches for exploring the genus Tetradesmus.
The algal strains for genome sequencing were obtained from Freshwater Bioresources Culture Collection (FBCC) at the Nakdonggang National Institute of Biological Resources (Republic of Korea), Culture Collection of Algae and Protozoa (CCAP, UK), Culture Collection of Algae at the University of Texas (UTEX, USA), and Culture Collection of Algae at the University of Göttingen (SAG, Germany). All algal strains were cultured in 3 N Bold’s Basal Medium (Nichols and Bold, 1965) under 12:12 light/dark photocycle with a light intensity of 100 µmol·m−2·s−1 in a 20°C incubation chamber.
The morphological observation of Tetradesmus cells was performed under a light microscope (LM; ECLIPSE Ni-U, Nikon, Tokyo, Japan) and a scanning electron microscope (SEM; SU8220, Hitachi Ltd., Tokyo, Japan). To prepare samples for SEM observation, cells were treated with 2% glutaraldehyde solution for fixation and dehydrated through a series of ethanol concentrations (20%, 30%, 40%, 50%, 60%, 70%, 80%, 90%, and 100% ethanol) followed by critical-point drying (CPD; HCP-2, Hitachi Ltd., Tokyo, Japan). Then, the samples were coated with platinum. To measure the cell sizes (length and width) of Tetradesmus species, 100 cells were observed under the light microscope.
The illustrations of Tetradesmus species were drawn based on original descriptions and related literature (Supplementary Table 1; Bohlin, 1897; Kützing, 1834; Lagerheim, 1882; Lemmermann, 1898; Meyen, 1829; Turpin, 1828; Chodat, 1902; Reinhard, 1904; Collins, 1909; Chodat, 1913; Smith, 1913; Wołoszyńska, 1914; Printz, 1915; West, 1915; Smith, 1916; Chodat, 1926; Khristjuk, 1926; Huber-Pestalozzi, 1936; Hortobágyi, 1941; Prescott, 1944; Korshikov, 1953; Shen, 1956; Verses and Trainor, 1966; Fott and Komárek, 1974; Wang, 1990; Hu, 1992; Holtmann, 1994; Ettl and Gärtner, 1995; Hegewald and Hanagata, 2000; Wasser and Tsarenko, 2000; Tsarenko and Petlevanny, 2001; Lewis and Flechtner, 2004; Tsarenko et al., 2005; Hegewald et al., 2013; Wynne and Hallan, 2015; Wynne and Guiry, 2016; Lewis and Flechtner, 2019; Mikhailyuk et al., 2019; Taşkın, 2019; Terlova and Lewis, 2019).
Genomic DNA was extracted using the DNeasy Plant Mini Kit (Qiagen, Hilden, Germany) according to the manufacturer’s instructions. Genome sequencing data were generated using the NovaSeq6000 sequencing platform (Illumina, San Diego) with a 151–bp paired-end sequencing library. The sequencing raw reads were assembled using SPAdes Assembler (v3.14.2; Bankevich et al., 2012) and NOVOplasty (v4.3.1; Dierckxsens et al., 2017). Chloroplast genome contigs of Tetradesmus species were sorted by local-BLASTn search (e-value cutoff=1.e-05) with the chloroplast genome of T. obliquus (NC_008101). From the sorted contigs, complete chloroplast genome sequences were reassembled using Geneious Prime (v2022.2). The chloroplast genome of SAG 38.81 was reconstructed from the genome assembly (GCA_902809745.2; Astafyeva et al., 2020). The prediction of protein-coding genes in the chloroplast genomes was performed by BLASTx search (e-value cutoff=1.e-05; NCBI codon table 11) and annotated using Geneious Prime (v2022.2). Ribosomal RNA (rRNA) sequences (5S, 16S, and 23S) in the chloroplast genomes were predicted by local-BLASTn search (e-value cutoff=1.e-05) with rRNA sequences in the chloroplast genome of T. obliquus (NC_008101). Transfer RNA (tRNA) sequences were predicted using the ARAGORN program (Laslett and Canback, 2004).
To construct a concatenated alignment of chloroplast genes, we used conserved 69 chloroplast protein sequences from 13 Tetradesmus and three outgroup species, and each homologous gene set was aligned using MAFFT (v7.450) under default options (Katoh and Standley, 2013). The maximum likelihood (ML) tree was constructed using 69 concatenated amino acid sequences (partition information with -q option) using the IQ-tree program (v1.6.12; Nguyen et al., 2015). The phylogenetic model was determined using the model test option (-m TEST), and ultrafast bootstrap search was conducted with 1,000 replications (-bb 1,000).
We successfully constructed the circular chloroplast genomes of our target Tetradesmus strains (Table 1), which exhibited conserved genome structures and gene contents (CDS, rRNA, and tRNA) compared with the chloroplast genome (NC_008101) of T. obliquus f. rectilineare f. nov. UTEX 393 (detailed descriptions of novel forma are given in the next section). The chloroplast genomes of Tetradesmus species varied in size from 148,816 to 196,309 bp (Table 1). Interestingly, the psaA gene showed several different types of exon-intron boundaries and exon orders (Figure 1A). The exon orders of psaA in the chloroplast genomes of Tetradesmus species are roughly divided into two types as follows: the first exon is located in the large single copy (LSC) region or the small single copy (SSC) region (Figure 1A). In T. obliquus (Turpin) M.J.Wynne, T. obliquus f. rectilineare f. nov., T. obliquus var. spiraformis var. nov., and T. distendus (T.Holtmann) M.J.Wynne, the first exon of psaA is located in the downstream region (i.e., the last position) of its following exons on the LSC region (Figure 1A). The dipartite exon structure of psaA has been reported in the genus Tetradesmus (de Cambiaire et al., 2006). In another type from Tetradesmus species, the first exon is located in the SSC region, and the second exon is located in the downstream region of its following exons on the LSC region (Figure 1A). The discontinuous and (independently transcribed) tripartite exon structures of psaA have been reported in the CS clade (Chlamydomonadales and Sphaeropleales clade) of the class Chlorophyceae (Kück et al., 1987; Brouard et al., 2010; Fučíková et al., 2016; Watanabe et al., 2016). Although several types of exon order exist in psaA of Tetradesmus species, the amino acid sequences are highly conserved compared with those of single-exon psaA genes in green algal species from the non-CS clade (Figure 1B; NP_045850, YP_001382199, QUO99137, YP_003795480, and YP_009774535). All 13 Tetradesmus strains in this study contained the rpl32 gene, including two exons, with the first exon located in the SSC region and the second exon located in the LSC region (Figure 1A). However, the direction of the second exon in rpl32 is reversed in five Tetradesmus strains [T. obliquus, T. obliquus f. rectilineare, T. obliquus var. spiraformis, T. major f. lunatus (Korshikov) Fott & Komárek, and T. reginae (T.Holtmann) M.J.Wynne; asterisk in Figure 1A]. The amino acid sequences of rpl32 are highly conserved in the Tetradesmus species (Figure 1B). The discontinuous structure of rpl32 has been previously reported in Jenufa minuta Nemcová, M.Eliás, Skaloud & Neustupa (Chlorophyceae, incertae sedis; Turmel et al., 2020). We postulate that diverse exon structures of psaA and rpl32 in the genus Tetradesmus could be derived from species-specific (or infraspecific) genetic variations. Moreover, T. obliquus UTEX 3031 and T. obliquus f. rectilineare UTEX 393 exhibit different exon-intron structures in psbA and psaB in the chloroplast genomes (Supplementary Figure 1). Therefore, the chloroplast genomes can provide information regarding genetic and structural variations for taxonomic identification at variety- or forma-level in the genus Tetradesmus.
Figure 1 Exon-intron structures in the chloroplast genomes of Tetradesmus species. (A) Discontinuous exon structures of psaA and rpl32. The opposite direction of the second exon in rpl32 is indicated by asterisk. (B) Multiple sequence alignments of psaA and rpl32 from Tetradesmus species with the single-exon genes (psaA: NP_045850, QUO99137, YP_001382199, YP_003795480, and YP_009774535; rpl32: NP_045889, QUO99139, YP_001382159, YP_003795477, and YP_009774556).
To explore the phylogenetic relationship of Tetradesmus species, we conducted a multigene phylogeny using 69 conserved protein sequences found in the chloroplast genomes (Figure 2). The phylogenetic relationships of the 13 Tetradesmus strains are clearly addressed, and all nodes are fully supported (100% bootstrap supporting values; BS; Figure 2). In the multigene phylogeny, the early diverged clade is composed of T. dimorphus (Turpin) M.J.Wynne and T. lanceae, and the sister clade is divided into two monophyletic groups as follows: (group 1) T. arenicola Mikhailyuk & P.M.Tsarenko, T. bajacalifornicus L.A.Lewis & Flechtner, T. lagerheimii M.J.Wynne & Guiry, T. dissociatus f. oviformis, and T. deserticola L.A.Lewis & Flechtner; (group 2) T. obliquus, T. obliquus f. rectilineare, T. obliquus var. spiraformis, T. distendus, T. major f. lunatus, and T. reginae (Figure 2). In group 1, T. arenicola and T. bajacalifornicus show a monophyletic clade, and T. lagerheimii is their sister branch. T. dissociatus f. oviformis and T. deserticola are monophyly as a sister clade of the other taxa in group 1. In group 2, T. obliquus species exhibit a monophyletic group, and T. obliquus var. spiraformis SAG 22.81 is indicated as an early diverged taxon. The T. obliquus clade and T. distendus are monophyly, and their sister clade includes T. major f. lunatus and T. reginae (Figure 2). To investigate the phylogenetic relationship of Tetradesmus species with broad taxon samples, we constructed an ML tree (IQ-tree v1.6.12; Nguyen et al., 2015) using the nucleotide sequences of the ITS (ITS1-5.8S-ITS2) region and two chloroplast genes (rbcL and tufA; Table 2, Figure 3). Although supporting values in several branches were relatively low, the phylogenetic tree constructed using the marker genes (Figure 3) could yield the same phylogenetic relationship of Tetradesmus species as that of the multigene phylogeny using the 69 chloroplast protein-coding genes (Figure 2).
Figure 2 Maximum likelihood tree of Tetradesmus species constructed using a concatenated alignment of 69 chloroplast protein sequences (1,000 bootstrap replications).
Figure 3 Phylogenetic analysis and microscopic observation of Tetradesmus species. The maximum likelihood tree was constructed using the ITS (ITS1-5.8S-ITS2) region, rbcL, and tufA sequences (1,000 bootstrap replications; only >70% bootstrap supporting values are shown). Scale bars in the images of light microscopy indicate 10 µm (image no. 1–12, 15–16, and 21–24) and 5 µm (image no. 13–14 and 17–20).
Although UTEX 393 and SAG 22.81 were previously labeled as T. obliquus, we suggest that these strains are a forma and a variety of T. obliquus, respectively, based on different exon-intron structures in the chloroplast genomes (i.e., psaA, psaB, and psbA; Figure 1, Supplementary Figure 1) and their distinct morphological characteristics (Figure 3). T. obliquus (basionym: Achnanthes obliqua) was characterized by spindle-shaped cells with acute apices, and their cells are typically arranged on a flat plane in two rows as four- or eight-celled coenobia (Table 3, Figure 3; Turpin, 1828; Smith, 1916). Especially, an eight-celled coenobium of T. obliquus (UTEX 3031) displays an oblique arrangement between each four-celled unit (Figure 3, Supplementary Figure 2), which was illustrated in their original description (Turpin, 1828). However, the morphological characteristics of UTEX 393 in the eight-celled coenobia exhibit rectilinear cellular arrangements in two rows (Table 3, Figure 3, Supplementary Figure 2). These strains also present distinct exon-intron structures (psbA and psaB; Supplementary Figure 1). Therefore, we propose that the distinct morphological characteristics and exon-intron structures between UTEX 3031 and UTEX 393 are regarded as irreversible infraspecific variations, specifically in exon-intron structures. Despite these distinctive features, several representative marker genes between UTEX 3031 and UTEX 393 remain conserved, with a few nucleotide differences (identical in 18S rRNA, 1 bp in ITS2, 5 bp in rbcL, and 3 bp in tufA). Particularly, the most representative marker gene, 18S rRNA (commonly used for species identification), is identical in these strains, thus UTEX 393 should be considered as a novel forma of T. obliquus, specifically T. obliquus f. rectilineare. Furthermore, the cells of SAG 22.81 exhibit bundle-like and twisted coenobia (Table 3, Figure 3, Supplementary Figure 2). Previously, Hegewald and Hanagata (2000) and Sciuto et al. (2015) suggested SAG 22.81 as T. reginae and T. obliquus, respectively, but we propose that SAG 22.81 is T. obliquus var. spiraformis as a novel variety of T. obliquus. This proposal is based on genetic variations in marker genes (4 bp in 18S rRNA, 2 bp in ITS2, 10 bp in rbcL, and 8 bp in tufA), exon-intron boundaries with exon orders (psaA; Figure 1A) in their chloroplast genomes, and distinct morphological differences (e.g., bundle-like coenobia) observed in SAG 22.81 compared to T. obliquus UTEX 3031.
The detailed descriptions of T. obliquus f. rectilineare and T. obliquus var. spiraformis are given at the end of this section. The sister clade of T. obliquus strains is composed of T. distendus strains (FBCC-A1020 and SAG 2003), which possess similar morphological characteristics (e.g., cell shape and coenobial arrangement) as those of T. obliquus f. rectilineare; however, T. distendus displays more curved marginal cells and acute apices (Table 3, Figure 3, Supplementary Figure 2). The morphological characteristics of FBCC-A1035 correspond to the original description of T. major f. lunatus (Fott and Komárek, 1974), which typically exhibits arcuate and crescent-shaped cells (Table 3, Figure 3, Supplementary Figure 2). T. major f. lunatus (FBCC-A1035) has more curved cells with acute apices and a slightly larger cell size than that of T. reginae (CCAP 276/66; Hegewald et al., 2013), although these two species commonly display bundle-like coenobia (Table 3, Figure 3, Supplementary Figure 2).
The SAG 3.99 strain was previously labeled as T. wisconsinensis in the culture collection (Culture Collection of Algae at the University of Göttingen, SAG, Germany), which exhibits lemon-shaped unicells with an oval outline and pointed ends (Figure 3); however, T. wisconsinensis typically displays crescent-shaped cells and bundle-like coenobia (Smith, 1913). The morphological characteristics of SAG 3.99 correspond to the reference strain of T. bajacalifornicus LG2-VF16, which exhibits crescent- to lemon-shaped unicells with pointed ends and apical thickening (Table 3; Lewis and Flechtner, 2004; Lewis and Flechtner, 2019). Moreover, SAG 3.99 is closely clustered with T. bajacalifornicus strains (ZA1-2, ZA1-5, ZA1-7, and BCP-LG2-VF16) in the phylogenetic analysis (Figure 3). Hence, we suggest that SAG 3.99 can be considered T. bajacalifornicus. A sister branch of the T. bajacalifornicus clade is T. arenicola (SAG 2564 as the reference strain), which could exhibit lemon-shaped, crescent-shaped, or fusiform cells with pointed ends (Figure 3, Supplementary Figure 2). Furthermore, T. arenicola frequently forms both unicells and bundle-like coenobia with variation of cellular arrangements (Mikhailyuk et al., 2019). The sister branches of the clade, which includes T. bajacalifornicus and T. arenicola, are composed of Tetradesmus sp. CCAP 276/35 (BS 99%), T. adustus Terlova & L.A.Lewis (JT2-VF29; BS 72%), and T. lagerheimii SAG 38.81 (BS 85%; Figure 3). SAG 38.81 was labeled as Scenedesmus acuminatus (a synonym of T. lagerheimii) in the culture collection. SAG 38.81 displays crescent-shaped cells with acute apices and forms four-celled coenobia on a strongly curved plane (Figure 3, Supplementary Figure 2), which completely correspond to the typical morphological characteristics of T. lagerheimii (Lagerheim, 1882; Chodat, 1902). Nevertheless, the cells of SAG 38.81 are mostly solitary, and their cell size is considerably smaller than previous reports (Table 3). Therefore, we suggest that SAG 38.81 can be provisionally considered T. cf. lagerheimii, and hence further study is required to explore whether this strain is T. lagerheimii with cell size variation or a different Tetradesmus species. The sister clade of these taxa includes T. deserticola BCP-SNI-2 and T. dissociatus f. oviformis SAG 5.95 (Figure 3). SAG 5.95 was identified as T. dissociatus based on the comparison of ITS2 sequences with those of the reference strain, UTEX 1537 (Sciuto et al., 2015). However, the morphological characteristics of SAG 5.95 show ellipsoidal cells without apical thickening and pointed ends (Table 3, Figure 3). These characteristics are considerably different from the morphology of T. dissociatus UTEX 1537, particularly in the presence of long bridges at the pointed ends (Supplementary Figures 3A, B; Verses and Trainor, 1966). Moreover, the rRNA region of T. dissociatus UTEX 1537 contains intron sequences (784 bp; OR600236), which is distinct from SAG 5.95 (Supplementary Figure 3C), but 18S rRNA region is identical in these strains. Hence, we propose that SAG 5.95 is T. dissociatus f. oviformis as a novel forma of T. dissociatus. This proposal is based on genetic variations in 18S rRNA region (i.e., intron insertion) and morphological differences observed between SAG 5.95 and UTEXT1537.
The cells of FBCC-A330 are arranged on a slightly curved plane as four- and eight-celled coenobia, and the apices of marginal cells are curved (i.e., a crescent shape), in contrast to straight inner cells (Figure 3, Supplementary Figure 2). The cells of four-celled coenobia are arranged in one row, whereas the cells of eight-celled coenobia are arranged in two rows (Figure 3). The morphological characteristics of FBCC-A330 correspond to the original description of T. dimorphus, although there was no description of the plane arrangement (Turpin, 1828; Tsarenko and John, 2011). Smith (1916) described that the cells of T. dimorphus are arranged on a flat plane. However, we postulate that the illustrations indicate a slightly curved plane because their two marginal cells in the four-celled coenobia are arranged slightly ahead (or behind) of the inner cells (Smith, 1916), similar to that in FBCC-A330 (Figure 3, Supplementary Figure 2). Therefore, we identified FBCC-A330 as T. dimorphus. Nevertheless, several different types of sequences, which are labeled as T. dimorphus, were reported on the NCBI database, and most of them are unpublished data without morphological descriptions (i.e., morphologically unverified species). To compare our result and T. dimorphus-related sequences, we constructed an ML tree using the rRNA sequences (18S-ITS1-5.8S-ITS2 region) of T. dimorphus FBCC-A330 and their homologous sequences (BLASTn search e-value cutoff=1.e-10; Supplementary Figure 4; IQ-tree v1.6.12; Nguyen et al., 2015). Although no monophyletic taxon with T. dimorphus FBCC-A330 was observed in the ML tree, the cells of the four-celled coenobia of all previously reported T. dimorphus, which were morphologically described (green color in Supplementary Figure 4), are arranged in two rows (Ahamefule et al., 2018; Alam et al., 2019; Sehgal et al., 2019; Khaw et al., 2020; Chanu et al., 2021; Huang et al., 2022). The one-row arrangement in the four-celled coenobia is the distinguishing morphological characteristics of T. dimorphus (Turpin, 1828; Smith, 1916; Tsarenko and John, 2011). Thus, we suggest that, to date, only FBCC-A330 is morphologically verified T. dimorphus, corresponding to their morphological descriptions (Turpin, 1828; Smith, 1916; Tsarenko and John, 2011). The morphological characteristics of FBCC-A708 are similar to those of T. dimorphus FBCC-A330, but FBCC-A708 has longer cells and slightly less curved marginal cells (Table 3, Figure 3). Furthermore, both the four- and eight-celled coenobia in FBCC-A708 are arranged on a slightly curved plane in slightly two rows (Figure 3). Hence, we propose that FBCC-A708 is T. lancea as a novel species in the genus Tetradesmus.
Tetradesmus obliquus f. rectilineare H.S.Cho & J.M.Lee f. nov.
Description: Cells are spindle-shaped with acute apices. The cells of four- and eight-celled coenobia are arranged on a flat plane in two rows. The rectilinear cellular arrangement in two rows in the eight-celled coenobium is a significant morphological difference compared with that in T. obliquus. The length of cells is 6.8–17.9 μm (average 13.8 μm), and their width is 2.3–5.1 μm (average 3.8 μm). The cells contain one pyrenoid each (Figure 3, Supplementary Figure 2).
Holotype: The permanent slide (NNIBRCL23411) of strain UTEX 393 is deposited in the Freshwater Bioresources Culture Collection (FBCC; https://fbp.nnibr.re.kr/fbcc/) at Nakdonggang National Institute of Biological Resources, Sangju, Gyeongsangbuk-do, Republic of Korea.
Reference strain: UTEX 393 from the Culture Collection of Algae at the University of Texas (UTEX, USA).
Etymology: The specific epithet “rectilineare” is derived from the Latin word “rectilineare” (rectilinear), which indicates the morphological characteristics of the cellular arrangement in the eight-celled coenobium.
Type locality and habitat: Freshwater (the locality is not available).
Representative DNA sequence: rRNA sequences (18S rRNA partial, ITS1, 5.8S rRNA, ITS2, and 28S rRNA partial; KP645233) and chloroplast genome (NC_008101).
Tetradesmus obliquus var. spiraformis H.S.Cho & J.M.Lee var. nov.
Description: Cells are spindle-shaped with acute apices. Four- or eight-celled and twisted bundle-like coenobia are typical, which indicates a significant morphological difference compared with that in T. obliquus. The length of the cells is 10.9–16.9 μm (average 13.2 μm), and their width is 3.1–6.4 μm (average 4.1 μm). The cells contain one pyrenoid each (Figure 3, Supplementary Figure 2).
Holotype: The permanent slide (NNIBRCL23412) of strain SAG 22.81 is deposited in the Freshwater Bioresources Culture Collection (FBCC; https://fbp.nnibr.re.kr/fbcc/) at Nakdonggang National Institute of Biological Resources, Sangju, Gyeongsangbuk-do, Republic of Korea.
Reference strain: SAG 22.81 from the Culture Collection of Algae at the Georg-August-University Göttingen (Göttingen, Germany).
Etymology: The specific epithet “spiraformis” is derived from a compound word of the Latin words “spiralis” (spiral) and “-formis” (-form), which indicate the morphological characteristics of the coenobia.
Type locality and habitat: Freshwater, Laguna Pataccocha, Apurimac, Peru (13°44’06.74”S, 73°06’43.97”W).
Representative DNA sequence: rRNA sequences (18S rRNA, ITS1, 5.8S rRNA, ITS2, and 28S rRNA; OR530173) and chloroplast genome (OR502672).
Tetradesmus dissociatus f. oviformis H.S.Cho & J.M.Lee f. nov.
Description: Cells are oval or ellipsoidal in shape with pointed ends. The length of cells is 6.8–12.4 μm (average 8.5 μm), and their width is 3.6–8.3 μm (average 5.5 μm). The cells contain one pyrenoid each (Figure 3, Supplementary Figure 2).
Holotype: The permanent slide (NNIBRCL23413) of strain SAG 5.95 is deposited in the Freshwater Bioresources Culture Collection (FBCC; https://fbp.nnibr.re.kr/fbcc/) at Nakdonggang National Institute of Biological Resources, Sangju, Gyeongsangbuk-do, Republic of Korea.
Reference strain: SAG 5.95 from the Culture Collection of Algae at the Georg-August-University Göttingen (Göttingen, Germany).
Etymology: The specific epithet “oviformis” is derived from a compound word of the Latin words “ovi” (egg) and “-formis” (-form), which indicate the morphological characteristics of the cell outline.
Type locality and habitat: Freshwater, Bordeaux, France (the detailed locality is not available).
Representative DNA sequence: rRNA sequences (18S rRNA, ITS1, 5.8S rRNA, ITS2, and 28S rRNA; OR530172) and chloroplast genome (OR502667).
Tetradesmus lancea H.S.Cho & J.M.Lee sp. nov.
Description: Spindle-shaped inner and slightly curved marginal cells with typical acuminate apices. The cells of four-celled coenobia are arranged on a slightly curved plane in one row, and those of eight-celled coenobia are arranged in slightly two rows. The marginal cells of coenobia are generally longer than the inner cells. The length of cells is 21.7–38.2 μm (average 29.7 μm), and their width is 3.0–8.0 μm (average 4.8 μm). The cells contain one pyrenoid each (Figure 3, Supplementary Figure 2).
Holotype: The permanent slide (NNIBRCL19693) of strain FBCC-A708 is deposited in the Freshwater Bioresources Culture Collection (FBCC; https://fbp.nnibr.re.kr/fbcc/) at Nakdonggang National Institute of Biological Resources, Sangju, Gyeongsangbuk-do, Republic of Korea.
Reference strain: FBCC-A708 from FBCC at the Nakdonggang National Institute of Biological Resources (Republic of Korea).
Etymology: The specific epithet “lancea” is derived from the Latin word “lancea” (a light spear or lance), which indicates the morphological characteristics of the cell outline.
Type locality and habitat: Riverside, Gapyeong-gun, Gyeonggi-do, Republic of Korea (37°48′54.7′′N, 127°31′17.3′′E); freshwater.
Representative DNA sequence: rRNA sequences (18S rRNA, ITS1, 5.8S rRNA, ITS2, and 28S rRNA; OR530171) and chloroplast genome (OR502671).
The genus Tetradesmus was initially established based on the bundle-like coenobia of spindle-shaped cells (Smith, 1913). However, T. wisconsinensis (the type species of Tetradesmus) and several Scenedesmus-like species were integrated into the genus Acutodesmus (Tsarenko and Petlevanny, 2001; Hegewald et al., 2013); hence, this genus was composed of species with different types of cellular arrangements such as unicells, bundle-like, and plane-type (Supplementary Figure 5). Nonetheless, most Acutodesmus taxa, including A. wisconsinensis, were transferred to the genus Tetradesmus again because the genus Acutodesmus established in 2001, which occurred after the initial report of the type species T. wisconsinensis in 1913 (Supplementary Figure 5; Wynne and Hallan, 2015; Wynne and Guiry, 2016; Lewis and Flechtner, 2019). Here, we revisited the morphological characteristics of cellular arrangements in the genus Tetradesmus and clarified three types (i.e., bundle-type, plane-type, and unicell-type) of coenobial/cellular arrangements (Figure 4).
The representative coenobial type of the genus Tetradesmus is bundle-type (Figure 4), which corresponds to the coenobial formation of the type species T. wisconsinensis (Smith, 1913). The bundle-type coenobia are also present in T. acuminatus, T. arenicola, T. cumbricus G.S.West, T. formosanus Shen, T, major (Fischer) Fott & Komárek, T. obliquus var. spiraformis, T. reginae, and T. smithii Prescott (West, 1915; Prescott, 1944; Shen, 1956; Fott and Komárek, 1974; Hu, 1992; An et al., 1999; Mikhailyuk et al., 2019). The plane-type coenobia are divided into three categories (i.e., strongly curved, slightly curved, and flat plane) based on the degree of curve in the four-celled coenobial arrangements (Figure 4). For instance, the coenobial cells of T. lagerheimii are arranged on a strongly curved plane, and those of T. dimorphus and T. lancea are arranged on a slightly curved plane. Coenobial cells arranged on a flat plane are present in T. bernardii (G.M.Smith) M.J.Wynne, T. distendus, T. nygaardii (Huber-Pestalozzi) M.J.Wynne, T. obliquus, and T. obliquus f. rectilineare (Turpin, 1828; Smith, 1916; Huber-Pestalozzi, 1936; Holtmann, 1994). The two-row or slightly two-row arrangement (i.e., almost one line as in T. lancea) of the plane-type cells is recognized by arrangements of the inner cells (Figure 4). Moreover, the coenobial cells in two rows could exhibit oblique (e.g., T. obliquus) and rectilinear (e.g., T. dimorphus and T. obliquus f. rectilineare) arrangements (Figure 4). The unicell-type cells are typically present in T. adustus, T. bajacalifornicus, T. deserticola, T. dissociatus, and T. dissociatus f. oviformis (Verses and Trainor, 1966; Lewis and Flechtner, 2004; Lewis and Flechtner, 2019; Terlova and Lewis, 2019).
Although diverse coenobial types are present in the genus Tetradesmus, this trait is not phylogenetically conserved; hence, coenobial formations independently diverged irrespective of their evolutionary relationships (Figures 2, 3). For instance, bundle-like coenobia are present in T. obliquus var. spiraformis, T. major f. lunatus, T. reginae, and T. arenicola, but most of their closely related taxa show unicell-type (e.g., T. bajacalifornicus) or plane-type (e.g., T. obliquus and T. distendus) cellular arrangements except T. major f. lunatus and T. reginae (Figures 2, 3). Therefore, we postulate that the morphological characteristics of the coenobial types are generally species/variety/forma-specific features, which is suitable for taxonomic identifiers in the genus Tetradesmus, rather than for evolutionary interpretations such as morphological differentiations based on phylogenetic relationships.
Although we reinvestigated the taxonomy of Tetradesmus species based on morphological characteristics and chloroplast genomes, we recognized that potential taxonomic issues in several reports remain, as described in the following cases. For instance, T. almeriensis Turiel, Garrido-Cardenas, Gómez-Serrano, Acién, Carretero-Paulet & S.Blanco (the reference strain CCAP 276/24), which was reported as a new species in the genus Tetradesmus, displays oval or ellipsoidal cells without acuminate cell poles rather than spindle cells (Turiel et al., 2021). The oval or ellipsoidal cells are typical traits of the genus Scenedesmus (Tsarenko and John, 2011). Furthermore, the phylogenetic analysis using rbcL and ITS sequences revealed that T. almeriensis shows a monophyletic relationship with Scenedesmus species (Turiel et al., 2021). We confirmed the same phylogenetic relationship using ITS1-5.8S-ITS2, rbcL, and tufA genes (Supplementary Figure 6); hence, we suggest transferring T. almeriensis CCAP 276/24 to the genus Scenedesmus as follows.
Scenedesmus almeriensis (Turiel, Garrido-Cardenas, Gómez-Serrano, Acién, Carretero-Paulet & S.Blanco) H.S.Cho & J.M.Lee comb. nov.
Basionym: Tetradesmus almeriensis Turiel, Garrido-Cardenas, Gómez-Serrano, Acién, Carretero-Paulet & S.Blanco, Processes 9(11): 2006. 2021.
Several Tetradesmus strains were previously labeled (e.g., UTEX 393 and SAG 22.81) as T. obliquus (or its synonym Scenedesmus obliquus) without accurate taxonomic and molecular investigations. For example, Lürling (2003) suggested that T. obliquus show a high degree of phenotypic plasticity among T. obliquus strains, including UTEX 393, but this Tetradesmus strain is newly identified as a different forma T. obliquus f. rectilineare in our study based on their distinct morphological characteristics. Specifically, we found significant genetic variations in exon-intron structures and exon order in the chloroplast genome of T. obliquus f. rectilineare UTEX393 compared to T. obliquus UTEX 3031, but we defined these differences as infraspecific variation, given the identical sequences of their 18S rRNA regions. The phenotypic plasticity in various T. obliquus strains requires further study to determine whether it is reversible or irreversible, both within a strain and between strains, in terms of morphological variations. In addition, it is essential to address how many genetic variations accumulate and their significance (e.g., nucleotide level, intron insertion, exon-intron structure, and exon order). Moreover, the investigation should explore how many similar or identical genetic variations can be identified among infraspecific taxa. We suggest that a more diverse analysis of genetic variations in chloroplast genomes among infraspecific taxa could address these points (or establish a fundamental background for further steps). Furthermore, this approach is more efficient than the analysis of nuclear genomes. As another aspect of morphological variations, several types of morphological changes (e.g., the number of colonial cells) are generally possible in diverse algal culture strains, including Tetradesmus species, depending on their cell division progression and culture conditions. We recommend that the identification of Tetradesmus species, when the target shows coenobial formation, is carried out based on four- or eight-celled coenobia derived from culture conditions, which should not typically exceed 2 weeks after subculture, with sufficient nutrients. To comprehensively verify the phenotypic plasticity within the genus Tetradesmus, further studies are required, including detailed morphological observations and culture experiments across diverse Tetradesmus strains that are correctly identified by both morphological and molecular evidence.
Several taxonomically accepted Tetradesmus species were described based on only morphological characteristics with no molecular data as described below. To review the morphological characteristics of Tetradesmus species, we redrew the illustrations of Tetradesmus species based on original descriptions and related literature (Supplementary Figure 7; references used for the illustrations are described in Supplementary Table 1 and the Methods section). Most Tetradesmus cells are spindle-shaped with diverse cellular types (unicell, plane-type, and bundle-type), but the cells of T. smithii and T. obliquus var. flexuosus (Lemmermann) Taşkin & Alp show exceptional morphological features in the genus Tetradesmus. For instance, T. smithii exhibits a bundle-like coenobium, but its cells have rounded apices (Prescott, 1944). T. obliquus var. flexuosus exhibits oval cells as a chain-like formation (Lemmermann, 1898; Collins, 1909; Taşkın, 2019), which is completely different from the typical traits of the genus Tetradesmus (Supplementary Table 1, Supplementary Figure 7). Nevertheless, we cannot clearly determine whether these taxa have exceptional morphologies within the genus Tetradesmus or belong to other taxonomic groups because there is no available strain or sequence data from these taxa. Therefore, in further studies, molecular evidence (e.g., marker genes and chloroplast genomes) will be useful to identify and compare Tetradesmus species/varieties/formae, including the diverse morphological characteristics that could cause confusions with other taxonomic groups.
We provide a clear phylogenetic relationship of Tetradesmus species using chloroplast genomes and report a novel species, a novel variety, and two novel formae from this genus based on morphological characteristics such as cellular arrangements and exon-intron structures of the chloroplast genes. Our results and approaches will provide a comprehensive taxonomic understanding of the genus Tetradesmus and help identify cryptic species or variety from this genus. As interest in a wide range of applications using Tetradesmus species (e.g., T. obliquus) increases, our study will also help clarify species identification for biotechnological studies (Escorsim et al., 2018; Talarek-Karwel et al., 2020; Gouveia et al., 2021; Oliveira et al., 2021).
The data presented in the study are deposited in the NCBI database, accession numbers OR502664 - OR502672.
HC: Data curation, Formal analysis, Investigation, Methodology, Software, Validation, Visualization, Writing – original draft, Writing – review & editing. JL: Conceptualization, Funding acquisition, Project administration, Resources, Supervision, Validation, Writing – original draft, Writing – review & editing.
The author(s) declare financial support was received for the research, authorship, and/or publication of this article. This work was supported by Korea Environment Industry & Technology Institute (KEITI) through the Project to make multi-ministerial national biological research resources more advanced, funded by Korea Ministry of Environment (MOE) (2021003420004; JL), and the National Research Foundation of Korea (NRF) grant (RS-2023-00209930; JL) funded by the Korean government (MSIT).
We thank for technical supports to YeongJun Jang (Marine Ecological Genomics Lab., Department of Oceanography, Kyungpook National University, Daegu, South Korea).
The authors declare that the research was conducted in the absence of any commercial or financial relationships that could be construed as a potential conflict of interest.
All claims expressed in this article are solely those of the authors and do not necessarily represent those of their affiliated organizations, or those of the publisher, the editors and the reviewers. Any product that may be evaluated in this article, or claim that may be made by its manufacturer, is not guaranteed or endorsed by the publisher.
The Supplementary Material for this article can be found online at: https://www.frontiersin.org/articles/10.3389/fpls.2024.1303175/full#supplementary-material
Ahamefule, S. C., Ogbonna, J. C., Moneke, A. N., Ossai, N. I. (2018). Application of photosynthetic microalgae as efficient pH bio-stabilizers and bio-purifiers in sustainable aquaculture of Clarias gariepinus (African catfish) fry. Niger. J. Biotechnol. 35, 139–150. doi: 10.4314/njb.v35i2.17
Alam, M. M., Mumtaz, A. S., Hallenbeck, P. C., Shah, S. A., Abidin, S. Z. U., Inam, F. (2019). Morphological characterization, growth appraisal, and probing biofuels potential of newly isolated Scenedesmus sp. from desert Cholistan. Microsc. Res. Tech. 82, 2079–2088. doi: 10.1002/jemt.23380
An, S. S., Friedl, T., Hegewald, E. (1999). Phylogenetic relationships of Scenedesmus and Scenedesmus-like coccoid green algae as inferred from ITS-2 rDNA sequence comparisons. Plant Biol. 1, 418–428. doi: 10.1111/j.1438-8677.1999.tb00724.x
Astafyeva, Y., Alawi, M., Indenbirken, D., Danso, D., Grundhoff, A., Hanelt, D., et al. (2020). Draft genome sequence of the green alga Scenedesmus acuminatus SAG 38.81. Microbiol. Resour. Announc. 9, e01278–e01219. doi: 10.1128/mra.01278-19
Bankevich, A., Nurk, S., Antipov, D., Gurevich, A. A., Dvorkin, M., Kulikov, A. S., et al. (2012). SPAdes: a new genome assembly algorithm and its applications to single-cell sequencing. J. Comput. Biol. 19, 455–477. doi: 10.1089/cmb.2012.0021
Bohlin, K. (1897). “Die Algen der Ersten Regnell’schen Expedition. I. Protococcoideen,” in Bih. Kongl. Sven. Vetensk., (Stockholm, Sweden) vol. 23. , 1–47. Akad. Handl.
Brouard, J. S., Otis, C., Lemieux, C., Turmel, M. (2010). The exceptionally large chloroplast genome of the green alga Floydiella terrestris illuminates the evolutionary history of the Chlorophyceae. Genome Biol. Evol. 2, 240–256. doi: 10.1093/gbe/evq014
Chanu, N. K., Mandal, M. K., Chaurasia, N. (2021). Correlating the influence of biochemical parameters in environment with pesticide tolerance of non-target algae. Biologia 76, 307–319. doi: 10.2478/s11756-020-00568-x
Chodat, R. (1902). Algues vertes de la Suisse. Pleurococcoïdes - Chroolépoïdes Beiträge Kryptogamenflora der Schweiz. Ed. Wyss, K. J. (Bern, Switzerland: Berne).
Chodat, R. (1913). Monographies d’algues en culture pure. Materiaux pour la flore cryptogamique Suisse (Vol 5). Ed. Wyss, K. J. (Bern, Switzerland: Berne).
Chodat, R. (1926). Scenedesmus. Étude de génétique de systématique expérimentale et d’hydrobiologie. Rev. Hydrobiol. 3, 71–258.
de Cambiaire, J. C., Otis, C., Lemieux, C., Turmel, M. (2006). The complete chloroplast genome sequence of the chlorophycean green alga Scenedesmus obliquus reveals a compact gene organization and a biased distribution of genes on the two DNA strands. BMC Evol. Biol. 6, 1–15. doi: 10.1186/1471-2148-6-37
Dierckxsens, N., Mardulyn, P., Smits, G. (2017). NOVOPlasty: de novo assembly of organelle genomes from whole genome data. Nucleic Acids Res. 45, e18. doi: 10.1093/nar/gkt371
Eliaš, M., Němcová, Y., Škaloud, P., Neustupa, J., Kaufnerova, V., Šejnohová, L. (2010). Hylodesmus Singaporensis gen. et sp. nov., a new autosporic subaerial green alga (Scenedesmaceae, Chlorophyta) from Singapore. Int. J. Syst. Evol. Microbiol 60, 1224–1235. doi: 10.1099/ijs.0.012963-0
Escorsim, A. M., da Rocha, G., Vargas, J. V., Mariano, A. B., Ramos, L. P., Corazza, M. L., et al. (2018). Extraction of Acutodesmus obliquus lipids using a mixture of ethanol and hexane as solvent. Biomass Bioenergy 108, 470–478. doi: 10.1016/j.biombioe.2017.10.035
Ettl, H., Gärtner, G. (1995). Syllabus der Boden-, Luft- und Flechtenalgen. Ed. Fischer, G. (Stuttgart, New York, USA).
Fott, B., Komárek, J. (1974). Die Gattungen Lauterborniella Schmidle und Tetradesmus GM Smith und ihre Stellung in der Familie der Scenedesmaceae (Chlorococcales). Preslia 46, 198–209.
Fučíková, K., Lewis, L. A., Lewis, P. O. (2016). Comparative analyses of chloroplast genome data representing nine green algae in Sphaeropleales (Chlorophyceae, Chlorophyta). Data Br. 7, 558–570. doi: 10.1016/j.dib.2016.03.014
Gouveia, L., Jazić, J. M., Ferreira, A., Maletić, S., Cvetković, D., Vidović, S., et al. (2021). Green approach for the valorization of microalgae Tetradesmus obliquus. Sustain. Chem. Pharm. 24, 100556. doi: 10.1016/j.scp.2021.100556
Hegewald, E., Bock, C., Krienitz, L. (2013). A phylogenetic study on Scenedesmaceae with the description of a new species of Pectinodesmus and the new genera Verrucodesmus and Chodatodesmus (Chlorophyta, Chlorophyceae). Fottea 13, 149–164. doi: 10.5507/fot.2013.013
Hegewald, E., Hanagata, N. (2000). Phylogenetic studies on scenedesmaceae (Chlorophyta). Algol. Stud. 100, 29–49. doi: 10.1127/algol_stud/100/2000/29
Hegewald, E., Wolf, M., Keller, A., Friedl, T., Krienitz, L. (2010). ITS2 sequence-structure phylogeny in the Scenedesmaceae with special reference to Coelastrum (Chlorophyta, Chlorophyceae), including the new genera Comasiella and Pectinodesmus. Phycologia 49, 325–335. doi: 10.2216/09-61.1
Holtmann, T. (1994). Revision des subgenus Acutodesmus, Gattung Scenedesmus (Grünalgen, Chlorophyceae). [Doctoral thesis] (Essen, Germany: University of Essen).
Hortobágyi, T. (1941). Neuere Beiträge zur qualitativen Untersuchungen des Phytoplanktons im toten Theiss-Arms “Nagyfa”. Bot. Kzl. 38, 151–170.
Hu, Z.-Y. (1992). Two new species of Tetradesmus (Chlorococcales) from China. Acta Hydrobiol. Sin. 16, 377–378.
Hu, D., Zhang, J., Chu, R., Yin, Z., Hu, J., Nugroho, Y. K., et al. (2021). Microalgae Chlorella vulgaris and Scenedesmus dimorphus co-cultivation with landfill leachate for pollutant removal and lipid production. Bioresour. Technol. 342, 126003. doi: 10.1016/j.biortech.2021.126003
Huang, M. M., Xin, X., Shi, H. L., Lin, Y. M., Ling, H. Z., Ge, J. (2022). Identification of six novel microalgal strains and characterization of its potential for development of high-value compounds. S. Afr. J. Bot. 147, 1–7. doi: 10.1016/j.sajb.2021.12.007
Huber-Pestalozzi, G. (1936). Phytoplankton aus Seen und Sümpfen Javas, gesammelt von Prof. C. Schröter-Zürich. Ber. Schweiz. Bot. Gesellsch. 46, 131–168.
Katoh, K., Standley, D. M. (2013). MAFFT multiple sequence alignment software version 7: improvements in performance and usability. Mol. Biol. Evol. 30, 772–780. doi: 10.1093/molbev/mst010
Khaw, Y. S., Khong, N. M. H., Shaharuddin, N. A., Yusoff, F. M. (2020). A simple 18S rDNA approach for the identification of cultured eukaryotic microalgae with an emphasis on primers. J. Microbiol. Methods 172, 105890. doi: 10.1016/j.mimet.2020.105890
Khristjuk, P. M. (1926). Novye formy roda Scenedesmus Meyen iz reki Dona. Izvestija Severo-Kavkazskoj Gosudarstvennogo Universiteta 11, 1–49.
Korshikov, A. A. (1953). Identification Manual of Freshwater Algae of Ukrainian SSR (Kiev: Vydannja Akademii Nauk URSR).
Kück, U., Choquet, Y., Schneider, M., Dron, M., Bennoun, P. (1987). Structural and transcription analysis of two homologous genes for the P700 chlorophyll a-apoproteins in Chlamydomonas reinhardii: evidence for in vivo trans-splicing. EMBO J. 6, 2185–2195. doi: 10.1002/j.1460-2075.1987.tb02489.x
Kützing, F. T. (1834). Synopsis Diatomearum oder, Versuch einer systematischen Zusammenstellung der Diatomeen. Linnaea 8, 529–620.
Lürling, M. (2003). Phenotypic plasticity in the green algae Desmodesmus and Scenedesmus with special reference to the induction of defensive morphology. Ann. Limnol. - Int. J. Lim. 39, 85–101. doi: 10.1051/limn/2003014
Lagerheim, N. G. (1882). Bidrag till kännedomen om stockholmstraktens Pediastreer, Protococcaceer och Palmellaceer. Öfversigt af. Kongl. Vetenskaps-Akademiens Förhandlingar 39, 47–81.
Laslett, D., Canback, B. (2004). ARAGORN, a program to detect tRNA genes and tmRNA genes in nucleotide sequences. Nucleic Acids Res. 32, 11–16. doi: 10.1093/nar/gkh152
Lee, J. M., Cho, C. H., Park, S. I., Choi, J. W., Song, H. S., West, J. A., et al. (2016). Parallel evolution of highly conserved plastid genome architecture in red seaweeds and seed plants. BMC Biol. 14, 1–16. doi: 10.1186/s12915-016-0299-5
Lemieux, C., Otis, C., Turmel, M. (2007). A clade uniting the green algae Mesostigma viride and Chlorokybus atmophyticus represents the deepest branch of the Streptophyta in chloroplast genome-based phylogenies. BMC Biol. 5, 1–17. doi: 10.1186/1741-7007-5-2
Lemmermann, E. (1898). Der grosse Waterneverstorfer Binnensee. Eine biologische Studie. Forschungsberichte aus der Biologischen Station zu Plön 6, 166–205.
Lewis, L. A., Flechtner, V. R. (2004). Cryptic species of Scenedesmus (Chlorophyta) from desert soil communities of western north America. J. Phycol. 40, 1127–1137. doi: 10.1111/j.1529-8817.2004.03235.x
Lewis, L. A., Flechtner, V. R. (2019). Tetradesmus bajacalifornicus LA Lewis and Flechtner, sp. nov. and Tetradesmus deserticola LA Lewis and Flechtner, sp. nov. (Scenedesmaceae, Chlorophyta). Not. Algarum 88, 1–2.
Mai, X. C., Shen, C. R., Liu, C. L., Trinh, D. M., Nguyen, M. L. (2023). “DNA Signaturing” database construction for Tetradesmus species identification and phylogenetic relationships of Scenedesmus-like green microalgae (Scenedesmaceae, Chlorophyta). J. Phycol. 59, 775–784. doi: 10.1111/jpy.13354
Meyen, F. J. F. (1829). Beobachtungen über einige niedere Algenformen. Nova Acta Phys.-Med. Acad. Caes. Leop.-Carol. Nat. Cur. 14, 768–778.
Mikhailyuk, T., Glaser, K., Tsarenko, P., Demchenko, E., Karsten, U. (2019). Composition of biological soil crusts from sand dunes of the Baltic Sea coast in the context of an integrative approach to the taxonomy of microalgae and cyanobacteria. Eur. J. Phycol. 54, 263–290. doi: 10.1080/09670262.2018.1557257
Nguyen, L. T., Schmidt, H. A., Von Haeseler, A., Minh, B. Q. (2015). IQ-TREE: a fast and effective stochastic algorithm for estimating maximum-likelihood phylogenies. Mol. Biol. Evol. 32, 268–274. doi: 10.1093/molbev/msu300
Nichols, H. W., Bold, H. C. (1965). Trichosarcina polymorpha gen. et sp. nov. J. Phycol. 1, 34–38. doi: 10.1111/j.1529-8817.1965.tb04552.x
Oliveira, C. Y. B., Oliveira, C. D. L., Prasad, R., Ong, H. C., Araujo, E. S., Shabnam, N., et al. (2021). A multidisciplinary review of Tetradesmus obliquus: a microalga suitable for large-scale biomass production and emerging environmental applictions. Rev. Aquac. 13, 1594–1618. doi: 10.1111/raq.12536
Prescott, G. W. (1944). New species and varieties of Wisconsin algae. Farlowia 1, 347–385. doi: 10.5962/p.315984
Printz, H. (1915). Beiträge zur Kenntnis der Chlorophyceen und ihrer Verbreitung in Norwegen. Kongel. Norske Vidensk. Selsk. Skr. 1915, 1–76.
Reinhard, L. V. (1904). Pervyja Svěděnija o Fytoplanktoně r. S. Donca: Zur Kenntnis des Phytoplankton von Donjec. Trudy Obščestva Ispytatelej Prirody pri Imperatorskom Char’kovskom Universitete 39, 1–28.
Sciuto, K., Lewis, L. A., Verleyen, E., Moro, I., La Rocca, N. (2015). Chodatodesmus australis sp. nov. (Scenedesmaceae, Chlorophyta) from Antarctica, with the emended description of the genus Chodatodesmus, and circumscription of Flechtneria rotunda gen. et sp. nov. J. Phycol. 51, 1172–1188. doi: 10.1111/jpy.12355
Sehgal, A., Goswami, K., Pal, M., Chikkaputtaiah, C., Chetia, P., Boruah, H. P. D. (2019). Morpho-taxonomic, genetic, and biochemical characterization of freshwater microalgae as potential biodiesel feedstock. 3 Biotech. 9, 137. doi: 10.1007/s13205-019-1664-1
Shen, E. Y. F. (1956). Studies on the freshwater algae of Taiwan Scenedesmaceae from Tainan. Rep. Institute Fishery Biol. Taiwan Taipei 1, 47–61.
Shetty, P., Farkas, A., Pap, B., Hupp, B., Ördög, V., Bíró, T., et al. (2021). Comparative and phylogenomic analysis of nuclear and organelle genes in cryptic Coelastrella vacuolata MACC-549 green algae. Algal Res. 58, 102380. doi: 10.1016/j.algal.2021.102380
Smith, G. M. (1913). Tetradesmus, a new four-celled coenobic alga. Bull. Torrey Bot. Club 40, 75–87. doi: 10.2307/2479812
Smith, G. M. (1916). A monograph of the algal genus Scenedesmus based upon pure culture studies. Trans. Wis. Acad. Sci. Arts Lett. 18, 422–530.
Song, H. J., Lee, J. M., Graf, L., Rho, M., Qiu, H., Bhattacharya, D., et al. (2016). A novice’s guide to analyzing NGS-derived organelle and metagenome data. Algae 31, 137–154. doi: 10.4490/algae.2016.31.6.5
Starkenburg, S. R., Polle, J. E. W., Hovde, B., Daligault, H. E., Davenport, K. W., Huang, A., et al. (2017). Draft nuclear genome, complete chloroplast genome, and complete mitochondrial genome for the biofuel/bioproduct feedstock species Scenedesmus obliquus strain DOE0152z. Genome Announc. 5, e00617–e00617. doi: 10.1128/genomea.00617-17
Talarek-Karwel, M., Bajguz, A., Piotrowska-Niczyporuk, A. (2020). 24-Epibrassinolide modulates primary metabolites, antioxidants, and phytochelatins in Acutodesmus obliquus exposed to lead stress. J. Appl. Phycol. 32, 263–276. doi: 10.1007/s10811-019-01966-8
Terlova, E. F., Lewis, L. A. (2019). A new species of Tetradesmus (Chlorophyceae, Chlorophyta) isolated from desert soil crust habitats in southwestern North America. Plant Fungal Syst. 64, 25–32. doi: 10.2478/pfs-2019-0004
Tsarenko, P. M., Hegewald, E., Braband, A. (2005). Scenedesmus-like algae of Ukraine. 1. Diversithy of taxa from water bodies in Volyn Polissia. Algol. Stud. 118, 1–45. doi: 10.1127/1864-1318/2006/0118-0001
Tsarenko, P. M., John, D. M. (2011). “Phylum Chlorophyta. Order Sphaeropleales sensu lato,” in The freshwater algal flora of the British Isles. An identification guide to freshwater and terrestrial algae, 2nd ed. Ed. Whitton, B. A. (New York, USA: Cambridge University Press), 419–475.
Tsarenko, P. M., Petlevanny, O. A. (2001). Addition to the diversity of algae of Ukraine. Algologia, Supplement. (Kyiv, Ukrine) 1–130.
Turiel, S., Garrido-Cardenas, J. A., Gómez-Serrano, C., Acién, F. G., Carretero-Paulet, L., Blanco, S. (2021). A polyphasic characterisation of Tetradesmus almeriensis sp. nov. (Chlorophyta: Scenedesmaceae). Processes 9, 2006.
Turmel, M., Vincent, A. T., Otis, C., Lemieux, C. (2020). The complete plastome of the coccoid green alga Jenufa minuta (Chlorophyceae, incertae sedis) unveils a noncanonical genetic code and a previously unrecognized trans-spliced group II intron in the rpl32 gene. Mitochondrial. DNA Part B 5, 1728–1730. doi: 10.1080/23802359.2020.17491652
Turpin, P. J. F. (1828). de la description de plusieurs genres et espèces nouvelles très remarquables, découverte parmi les productions végétales et microscopiques. Mém. Mus. Natl. Hist. Nat. 16, 295–312.
Verses, P. A., Trainor, F. R. (1966). Dactylococcus dissociatus, a new species from a Connecticut cornfield soil. Phycologia 6, 79–82. doi: 10.2216/i0031-8884-6-1-79.1
Watanabe, S., Fučíková, K., Lewis, L. A., Lewis, P. O. (2016). Hiding in plain sight: Koshicola spirodelophila gen. et sp. nov. (Chaetopeltidales, Chlorophyceae), a novel green alga associated with the aquatic angiosperm Spirodela polyrhiza. Am. J. Bot. 103, 865–875. doi: 10.3732/ajb.1500481
Wołoszyńska, J. (1914). Studien über das Phytoplankton des Viktoriasees. Schröders Zellpflanzen Ostafrikas. Hedwigia 55, 184–223.
Wynne, M. J., Guiry, M. D. (2016). Tetradesmus lagerheimii M.J.Wynne and Guiry, nomen novum, a replacement name for Tetradesmus acuminatus (Lagerheim) M.J.Wynne, nom. illeg. (Sphaeropleales, Chlorophyta). Not. Algarum 12, 1.
Keywords: Tetradesmus, cellular arrangement, coenobial type, chloroplast genome, phylogeny
Citation: Cho HS and Lee J (2024) Taxonomic reinvestigation of the genus Tetradesmus (Scenedesmaceae; Sphaeropleales) based on morphological characteristics and chloroplast genomes. Front. Plant Sci. 15:1303175. doi: 10.3389/fpls.2024.1303175
Received: 28 September 2023; Accepted: 19 January 2024;
Published: 14 February 2024.
Edited by:
Frederik Leliaert, Botanic Garden Meise, BelgiumReviewed by:
Louise A. Lewis, University of Connecticut, United StatesCopyright © 2024 Cho and Lee. This is an open-access article distributed under the terms of the Creative Commons Attribution License (CC BY). The use, distribution or reproduction in other forums is permitted, provided the original author(s) and the copyright owner(s) are credited and that the original publication in this journal is cited, in accordance with accepted academic practice. No use, distribution or reproduction is permitted which does not comply with these terms.
*Correspondence: JunMo Lee, anVubW9sZWVAa251LmFjLmty
Disclaimer: All claims expressed in this article are solely those of the authors and do not necessarily represent those of their affiliated organizations, or those of the publisher, the editors and the reviewers. Any product that may be evaluated in this article or claim that may be made by its manufacturer is not guaranteed or endorsed by the publisher.
Research integrity at Frontiers
Learn more about the work of our research integrity team to safeguard the quality of each article we publish.