- 1College of Forestry, Central South University of Forestry and Technology, Changsha, China
- 2Hunan Monitoring Center of Forest Resources and Ecological Environment, Hunan Prospecting Designing and Research General Institute for Agriculture Forestry and Industry, Changsha, China
- 3Guangxi Key Laboratory of Plant Conservation and Restoration Ecology in Karst Terrain, Guangxi Institute of Botany, Guangxi Zhuang Autonomous Region and Chinese Academy of Sciences, Guilin, China
- 4Royal Botanic Gardens, Kew, Richmond, United Kingdom
Boehmeria is a taxonomically challenging group within the nettle family (Urticaceae). The polyphyly of the genus has been proposed by previous studies with respect to five genera (Debregeasia, Cypholophus, Sarcochlamys, Archiboehmeria, and Astrothalamus). Extensive homoplasy of morphological characters has made generic delimitation problematic. Previous studies in other plant groups suggest that plastome structural variations have the potential to provide characters useful in reconstructing evolutionary relationships. We aimed to test this across Boehmeria and its allied genera by mapping plastome structural variations onto a resolved strongly supported phylogeny. In doing so, we expanded the sampling of the plastome to include Cypholophus, Sarcochlamys, Archiboehmeria, and Astrothalamus for the first time. The results of our phylogenomic analyses provide strong support for Sarcochlamys as being more closely related to Leucosyke puya than to Boehmeria and for the clustering of Boehmeria s.l. into four subclades. The sizes of the plastomes in Boehmeria s.l. ranged from 142,627 bp to 170,958 bp. The plastomes recovered a typical quadripartite structure comprising 127~146 genes. We observe several obvious structural variations across the taxa such as gene loss and multiple gene duplication, inverted repeat (IR) contraction and wide expansions, and inversions. Moreover, we recover a trend for these variations that the early clades were relatively conserved in evolution, whereas the later diverging clades were variable. We propose that the structural variations documented may be linked to the adaptation of Boehmeria s.l. to a wide range of habitats, from moist broadleaf forests in Asia to xeric shrublands and deserts in Africa. This study confirms that variation in plastome gene loss/duplication, IR contraction/expansion, and inversions can provide evidence useful for the reconstruction of evolutionary relationships.
1 Introduction
Boehmeria Jacq., the largest genus in the tribe Boehmerieae (Urticaceae) with 51 accepted species (POWO, 2024), is native to Asia, Africa, and the Americas. It encompasses a wide range of life forms including perennial herbs, shrubs, and trees. Boehmeria occurs in diverse biomes, tropical and subtropical moist broadleaf forests, temperate broadleaf forests, tropical dry broadleaf forests, and subtropical dry broadleaf forests (Kravtsova et al., 2000; Wilmot-Dear and Friis, 2013). Boehmeria species have been utilized for a multitude of purposes such as fiber production, forage, restoration, and consumption as a green vegetable and beverage, as well as in traditional medicine (Rehman et al., 2019; Arsul et al., 2021; Lee et al., 2022; Liu et al., 2022; Zhu et al., 2022). Notably, two species, Boehmeria oblongifolia and B. leiophylla, are classified as second-class protected plants in China (https://www.gov.cn/zhengce/zhengceku/2021–09/09/content_5636409.htm).
Boehmeria is considered a taxonomically challenging group, with uncertainty over the rank of taxonomic entities and a non-phylogenetic infrageneric classification, and as such warrants further research (Kravtsova et al., 2000; Wilmot-Dear et al., 2010; Wilmot-Dear and Friis, 2013). Recent phylogenetic studies based on several loci have revealed the poly- or paraphyletic nature of the genus in relation to five other genera (Debregeasia, Cypholophus, Sarcochlamys, Archiboehmeria, and Astrothalamus) (Wu et al., 2013, 2015, 2018b). In these studies, Boehmeria and allied genera are consistently recovered in three strongly supported clades (see Figures 1A, B: 1A1 to 1A3) in which clade 1A1 was inferred as a sister group to a group comprising clades 1A2 and 1A3. Wu et al. (2015) evaluated the informativeness of 19 morphological characters, all of which were recovered as homoplastic. Cypholophus (approximately 30 species) and Debregeasia (nine species) are the only genera with a higher species diversity, while the remaining three genera are monotypic (POWO, 2024). Of the genera allied to Boehmeria, all are shrubs or trees, primarily distributed in the tropical and subtropical moist broadleaf forests of Indomalaya and the Palearctic, and only Debregeasia extends into dry biomes (dry broadleaf forests, xeric shrublands, and deserts in the Afrotropics) (POWO, 2024).
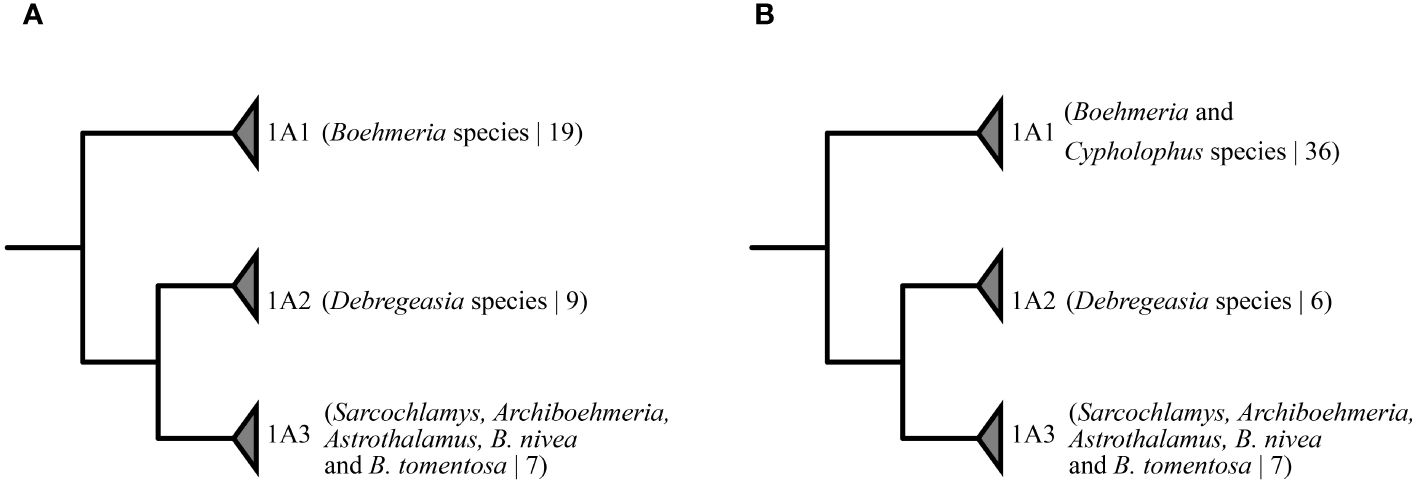
Figure 1 Phylogenetic relationships of the three clades formed by Boehmeria and its related genera from previous analyses based on combined data of four chloroplast, two nuclear, and one mitochondrial loci: (A) Wu et al. (2013), (2015); (B) Wu et al. (2018b). Numeric values in brackets correspond to the total number of accessions sampled in the clade.
Plastomes have emerged as valuable tools in resolving phylogenetic relationships in plants. Their moderate nucleotide substitution rates, smaller genome sizes, and high copy number make them ideal for phylogenetic study (Twyford and Ness, 2017; Guo et al., 2022). While plastome structural variations have been identified as phylogenetically informative characters that can aid in understanding evolutionary relationships (Jansen and Palmer, 1987; Wu et al., 2024), they have been rarely used to do so. Plastome structural variations include, among others, contraction or expansion of inverted repeats (IRs), gene loss or duplication, and inversions (Villarreal et al., 2013; Dugas et al., 2015; Choi and Choi, 2017; Sinn et al., 2018; Wu et al., 2024). Plastome evolution has been linked to speciation events, suggesting its role in diversification within plant lineages (Bock et al., 2014; Scobeyeva et al., 2021). Although a few Boehmeria plastomes have been assembled, analyses have focused on single species and/or inferred phylogenetic relationships based on very limited taxon sampling (Wu et al., 2018a; He et al., 2021). Whole plastome data have been used to elucidate phylogenetic relationships within the Urticaceae, specifically the Urticeae tribe and three specific genera (Oreocnide, Debregeasia, and Pilea) (Wang et al., 2020; Li et al., 2021; Ogoma et al., 2022; Wu et al., 2022). Little attention, however, has been given to the plastome’s structural variations in these studies, with only IR expansion/contraction, a few gene losses, and inversions being mentioned. In summary, while plastome data have been utilized for phylogenetic inference within the Urticaceae family, including Boehmeria, there remains an untested opportunity for the application of plastome structural information.
For the above reasons, we sampled species of Boehmeria and the related genera (Debregeasia, Cypholophus, Archiboehmeria, Astrothalamus, and Sarcochlamys), aiming to evaluate the power of the plastome dataset in elucidating evolutionary relationships. Our main tasks were as follows: 1) reconstruct robust phylogenetic relationships with different data matrixes and analytical methods and 2) investigate plastome structural variations.
2 Materials and methods
2.1 Sampling and sequencing
Previous studies have recovered a polyphyletic Boehmeria related to five other genera. We re-evaluated these relationships using whole plastome data. A plastome phylogenomic study about one of these genera (Debregeasia) has been conducted previously (Wang et al., 2020). Thus, we sampled representatives from the remaining genera, and a total of 11 newly sequenced species were included (six from Boehmeria and the remaining five from Debregeasia, Cypholophus, Archiboehmeria, Astrothalamus, and Sarcochlamys, respectively). Detailed sampling information is provided in Supplementary Table 1. Total genomic DNA (gDNA) was extracted from silica-gel-dried leaves using the modified CTAB method (Arseneau et al., 2017). The quality and quantity of DNA were measured on 1% Tris–acetate–ethylenediamine tetraacetic acid (TAE) agarose gels using the Qubit fluorometric quantification (Invitrogen, Carlsbad, CA, United States). The gDNA was fragmented and library size was selected for 350-bp inserts. Sequencing with 2 × 150-bp paired-end (PE) reads was performed on the Illumina HiSeq 2500/X-Ten at the Beijing Genomics Institute (BGI) in Shenzhen, China. The raw data underwent preprocessing to eliminate adapter sequences and low-quality bases and reads, yielding approximately 2 Gb of clean data per sample.
2.2 Assembly and annotation
Clean reads were used to conduct de-novo assembly in GetOrganelle v1.7.6.1 with a kmer length of 65–121 bp (Jin et al., 2020). Next, the well-assembled circular sequence was annotated using both PGA and CPGAVAS2 with Boehmeria spicata (NC_036989), B. umbrosa (NC_036990), and Debregeasia saeneb (NC_062311) as reference genomes (Qu et al., 2019; Shi et al., 2019). Subsequently, we examined the accuracy and did manual corrections, where necessary, in CPGView and Geneious v9.0.2 (Kearse et al., 2012; Liu et al., 2023). The annotated plastomes were submitted to NCBI to acquire accession numbers (Supplementary Table 2).
2.3 Phylogenetic inference based on plastome data
2.3.1 Other published plastome data sampling and reannotation
To clarify and confirm the relationships of Boehmeria and other genera, 22 representatives from other genera and tribes in Urticaceae as well as eight and six published Boehmeria and Debregeasia species, respectively, were included as ingroups, and three species from Moraceae were used as outgroups. Accession numbers can be found in Supplementary Table 2.
Although plastomes are relatively conservative, structural variations (like inversion and IR contraction/expansion) are very common in some taxa, which will lead to aligning difficulty and errors when applying whole plastomes to infer phylogenetic relationships. Considering this, we usually separately extract and align protein-coding sequences (CDS), genes, and intergenetic regions (IGS) according to the annotation information of plastomes. However, annotation errors frequently occur in published plastomes, which is not conducive to downstream analysis. Therefore, we checked and re-annotated these downloaded plastomes in Geneious following the steps in Qu et al. (2023).
2.3.2 Phylogeny reconstruction
Previous studies have shown changed substitution rates for genes transferred to the IR or SC region due to the boundary shift (Guo et al., 2021), indicating that these genes in different taxa or lineage may suffer from heterotachy (Wang et al., 2019; Kapli et al., 2020; Steenwyk et al., 2023). Applying such genes can impact the phylogenetic reconstruction (Lockhart et al., 2006; Zhong et al., 2010; Wu et al., 2011). Therefore, we prepared two matrixes to test whether boundary genes can twist phylogenetic relationships in our studied taxa—matrix1: 78-CDS (common CDS) and matrix2: 59-CDS (removing CDS from 19 genes located at IR/SC boundaries). To fully utilize phylogenetic information from the whole plastome, another two matrixes were also prepared—matrix3: genes+IGS and matrix4: genes+IGS-SNP.
Empirical studies on the structural and functional attributes of plastomes indicate that the plastome may not evolve as a single locus and may be subject to divergent evolutionary pressures (Sloan et al., 2012; Dugas et al., 2015; Weng et al., 2016; Gonçalves et al., 2019). Additionally, plastid gene tree discordance has been found in several studies (Lockhart et al., 2006; Wu et al., 2011; Zhang et al., 2020). Therefore, we employed both concatenation and coalescent-based methods. Sequence alignment was carried out in MAFFT v7.407 (Katoh and Standley, 2013), and poorly aligned regions were trimmed with default settings in trimAL v1.4.1 (Capella-Gutiérrez et al., 2009). For concatenation-based methods, Geneious Prime v9.0.2 was used to concatenate data into one matrix (Kearse et al., 2012). The maximum likelihood (ML) tree was constructed in IQ-TREE v1.6.12 (Nguyen et al., 2015) with 5,000 bootstrap replicates and automatically selecting the best model. The BI tree was obtained in MrBayes v3.2.7a (Ronquist et al., 2012) by running 100 million generations and sampling every 1,000 generations. The best substitution model was determined based on the Bayesian information criterion (BIC) (Aho et al., 2014) in jModelTest2 v. 2.1.7 (Darriba et al., 2012). All best-fit models can be found in Table 1. Two independent runs were performed, each consisting of four Markov chain Monte Carlo (MCMC) chains. The beginning 25% of trees were discarded as burn-in, while the remaining trees were used for generating a consensus tree. The convergence of the MCMC chains of each run was determined when the average standard deviation of split frequencies (ASDSF) achieved ≤0.01. For coalescent-based methods, single gene trees were inferred in IQ-TREE with 1,000 bootstrap replicates and automatically selecting the best models. Nodes with less than 20% support were collapsed using Newick Utilities v1.6 (Junier and Zdobnov, 2010) as this can help improve gene tree accuracy. Species tree inference was conducted in ASTRAL III v5.7.8 (Zhang et al., 2018), node support was assessed by local posterior probability (LPP; Sayyari and Mirarab, 2016), and normalized quartet score (NQS) and quartet frequencies were used to reflect the level of gene tree discordance.
2.4 Plastome structural variation analyses
According to the delimitation results of plastome-based phylogenetic inference, a total of 23 plastomes were used to perform structural variation analyses. Basic structural features were summarized in Geneious, including plastome size, GC content (of the whole, SC, and IR), gene numbers, and gene duplication and loss. The four junctions between IR and SC of those 23 plastomes were compared and visualized in CPJSDRAW (Li et al., 2023). Whole genome alignments for the 23 plastomes and the reference Hemistylus odontophylla (MN189963) were performed using progressiveMauve v. 2.3.1 (Darling et al., 2004).
3 Results
3.1 Assembly and annotation condition
Four genera (Cypholophus and three monotypic genera: Archiboehmeria, Astrothalamus, and Sarcochlamys) are sequenced at the genomic level for the first time. All samples were successfully assembled except Astrothalamus reticulatus, with only a few scaffolds assembled. These successfully assembled plastomes have a typical quadripartite structure ranging from 142,627 bp to 170,051 bp, containing 82–98 protein-coding genes (PCGs), 37 tRNAs, and 8 rRNAs (Table 2). Although A. reticulatus is not completely assembled, 48 PCGs are retained from scaffolds, which still can be used in the downstream analysis.
3.2 Phylogenetic relationships
Both matrix1 (Figures 2A, B) and matrix2 (Supplementary Figures 1A, B) recovered congruent tree topologies in concatenated and coalescent methods, respectively, with most nodes receiving high support values (MLBS = 100; PP = 1; LPP = 1). An exception was the relationship between Archiboehmeria atrata and B. nivea: in the concatenated ML tree of matrix2, the two were separated with no support value while they clustered into sisters in all other trees. The overall support value of matrix2 was moderately lower than matrix1.
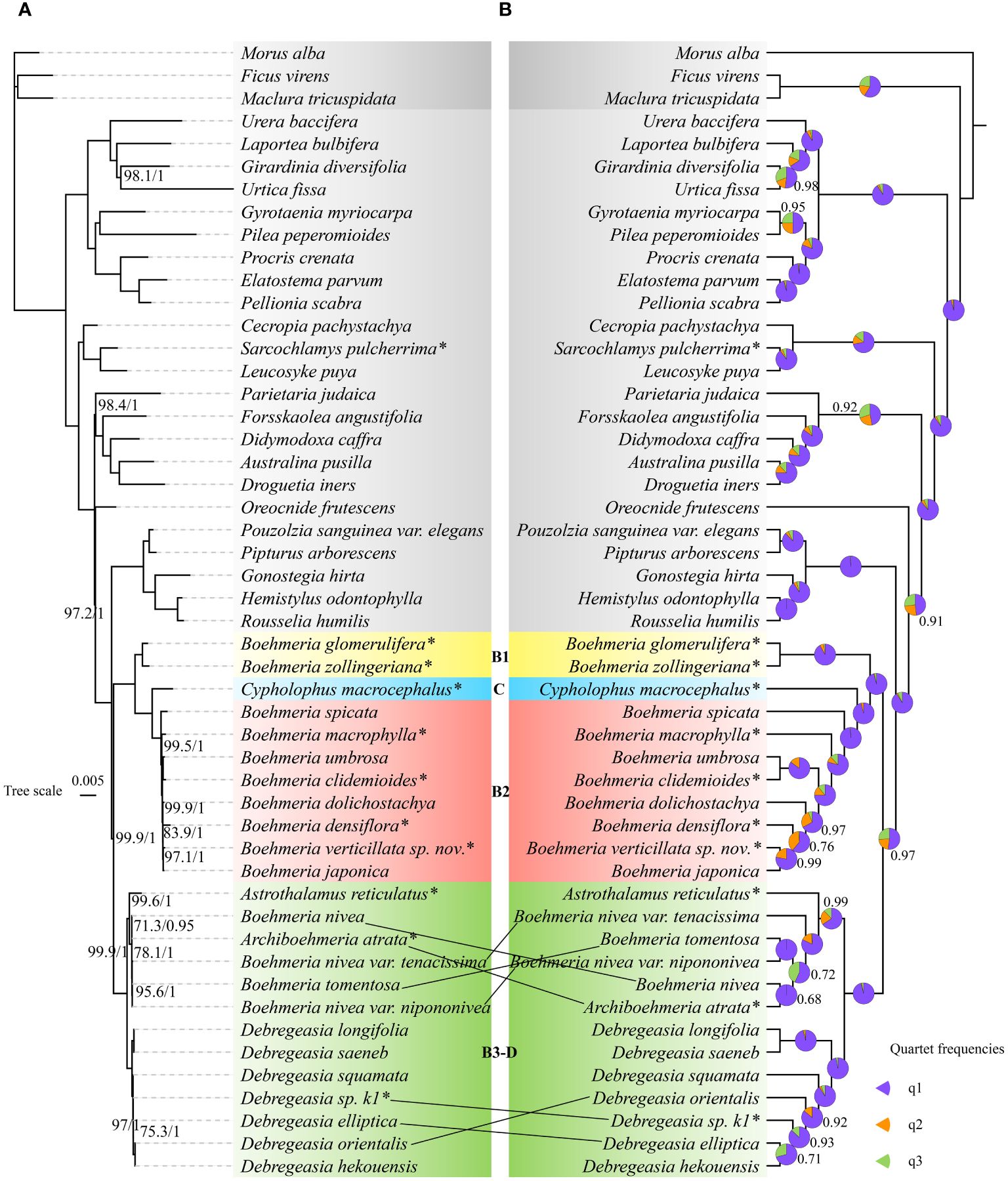
Figure 2 Phylogenetic relationships of Boehmeria and its related genera inferred by matrix1 (78-CDS): (A) concatenated tree produced by maximum likelihood (ML) analysis—numbers associated with branches (ML_BS/BI_PP) are assessed by maximum likelihood bootstrap (ML_BS) and Bayesian posterior probabilities (BI_PP); (B) coalescent tree—numbers associated with branches denote local posterior probability (LPP) support values, and pie charts show the relative frequencies of the three quartet topologies around the branch (purple = congruent with the species tree, orange = first alternative topology, green = second alternative topology). Branches with no support values are maximally supported. *Asterisks indicate the newly sequenced species.
Matrix3 (Supplementary Figures 2A, B) and matrix4 (Supplementary Figures 3A, B) present the same topologies as matrix1 in concatenated methods with only a few moderate differences in support values. In the coalescent method, these three matrixes also show congruent topologies. All NQSs of the coalescent method are >0.92 (Table 1). The topologies of concatenated and coalescent methods are also congruent.
All trees recovered Boehmeria as polyphyletic with respect to Astrothalamus, Archiboehmeria, Cypholophus, and Debregeasia, while Sarcochlamys pulcherrima was inferred as sister to Leucosyke puya (non-Boehmeriaea species) with full support (MLBS = 100; PP = 1; LPP = 1). Boehmeria and its related genera (Boehmeria sensu lato) formed two large clades. To better aid subsequent analyses, we further divided them into four subclades, namely, B1, C, B2, and B3-D (Figure 2). Clade B1 consisted of two Boehmeria species, clade C contained Cypholophus species, and clade B2 consisted of eight Boehmeria species; clade B1 and clade C were successive sisters to clade B2. Clade B3-D formed two subclades (B3 and D), one containing Archiboehmeria, Astrothalamus, Boehmeria tomentosa, and B. nivea and the other containing exclusively Debregeasia species. Clade B3-D was a sister group to the clade consisting of clades B1, C, and B2.
3.3 Plastome structural variations
3.3.1 General plastome characteristics of Boehmeria s.l.
Great variations in the plastome size were found in Boehmeria s.l. (Table 2). The smallest plastome occurred in clade C (Cypholophus macrocephalus, 142,627 bp), and the largest occurred in clade B2 (B. spicata, 170,958 bp), which showed larger plastome size than the other three clades. The smallest IR occurred also in clade C (C. macrocephalus, 16,948 bp), and the largest in clade B2 (B. umbrosa, 41,807 bp), which showed a larger IR size than the other three clades (Table 2, Figure 3). The LSC and SSC regions showed relatively little variation, ranging from 68,844 bp to 88,506 bp and 17,822 bp to 20,049 bp, respectively. The GC content of plastomes also varied among these clades, with clade B2 having a lower GC content in the whole plastome, LSC, SSC, and IR regions compared to the other clades (Table 2).
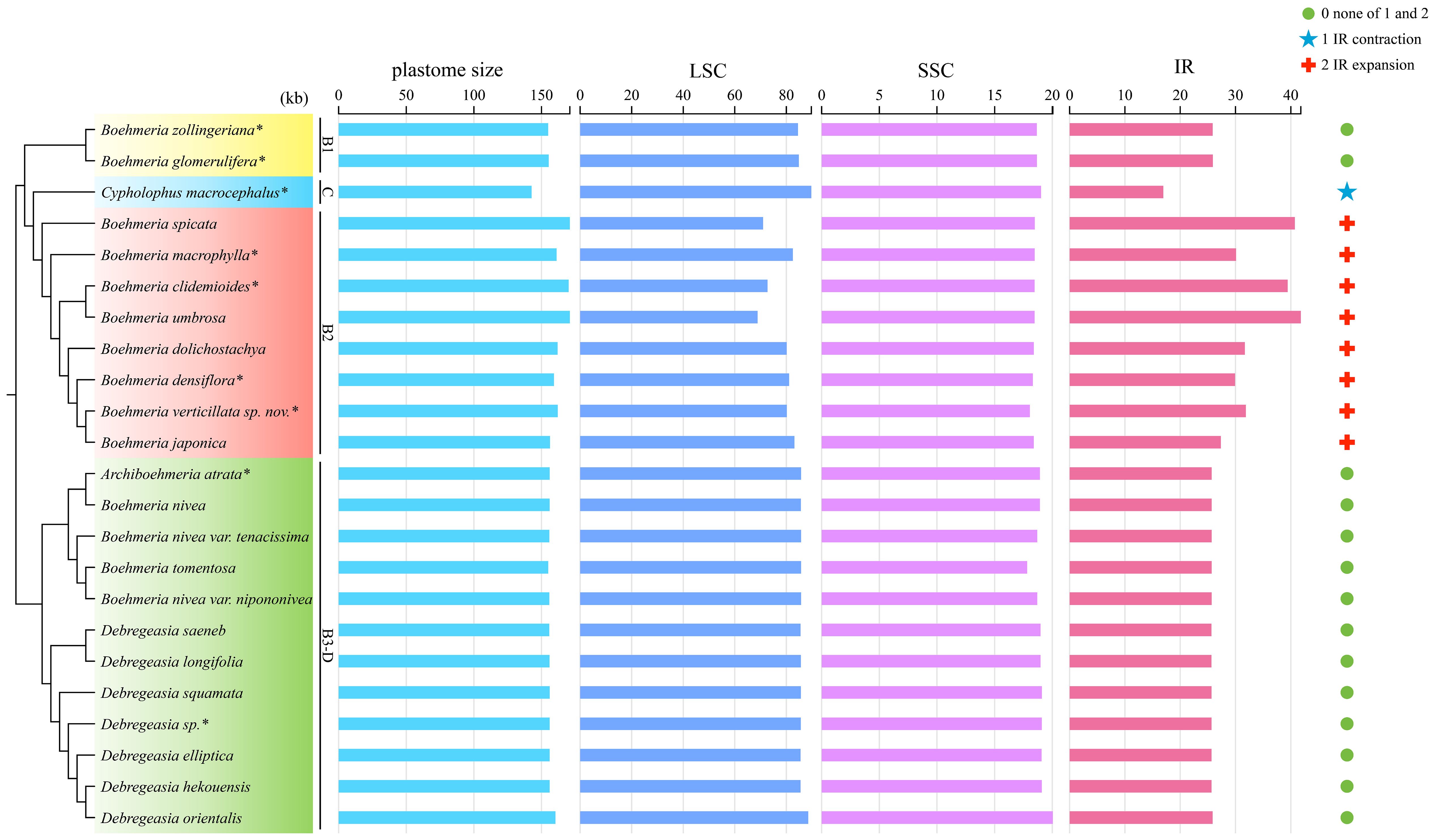
Figure 3 Plastome size variation and IR contraction and expansion in the four clades. The cladogram (left) was converted from the concatenated method phylogram (Figure 2A); the histogram (medium) shows the comparison of the whole size and three different regions of plastomes; the symbol matrix (right) shows the conditions of IR contraction and expansion. kb, kilobases. *Asterisks indicate the newly sequenced species.
3.3.2 Multiple gene duplications and several gene losses
Comparison of the 23 plastomes of Boehmeria s.l. showed that they encoded a set of 127 to 146 genes, including 84 to 101 PCGs, 36 to 38 tRNAs, and 8 rRNAs (Table 2). Clades B1 and B3-D had the same conserved 84 PCGs, while clades C and B2 experienced PCG loss and duplication (Table 2, Figure 4). Clade C experienced both gene loss and duplication, including loss of one copy of ycf2, rpl2, rpl23, and trnI-CAU and duplication of psbA and trnH-GUG. Clade B2 experienced multiple gene duplications, including a total of 17 PCGs and 1 tRNA (Figure 4). These gene losses and duplications were all caused by IR boundary shifts. The LSC/IRb (JLB) border of C. macrocephalus (clade C) moved toward IRb leading to four genes (ycf2, rpl23, rpl2, and trnI-CAU), originally located in IRb, being relocated in the LSC region; therefore, each lost one copy in the IRa. The IRa/LSC (JLA) border moved toward LSC leading to two genes (psbA, trnH-GUG), originally located in the LSC, being relocated in IRa; thus, each had the second copy in the IRb. The multiple gene duplications of all members in clade B2 were only due to the JLB border moving toward LSC resulting in multiple genes originally located in the LSC being relocated in IRb, and thus, each had an extra copy in IRa. Additionally, B. tomentosa of clade B3-D lost trnL-UAG, which was the only observed loss in clade B3-D.
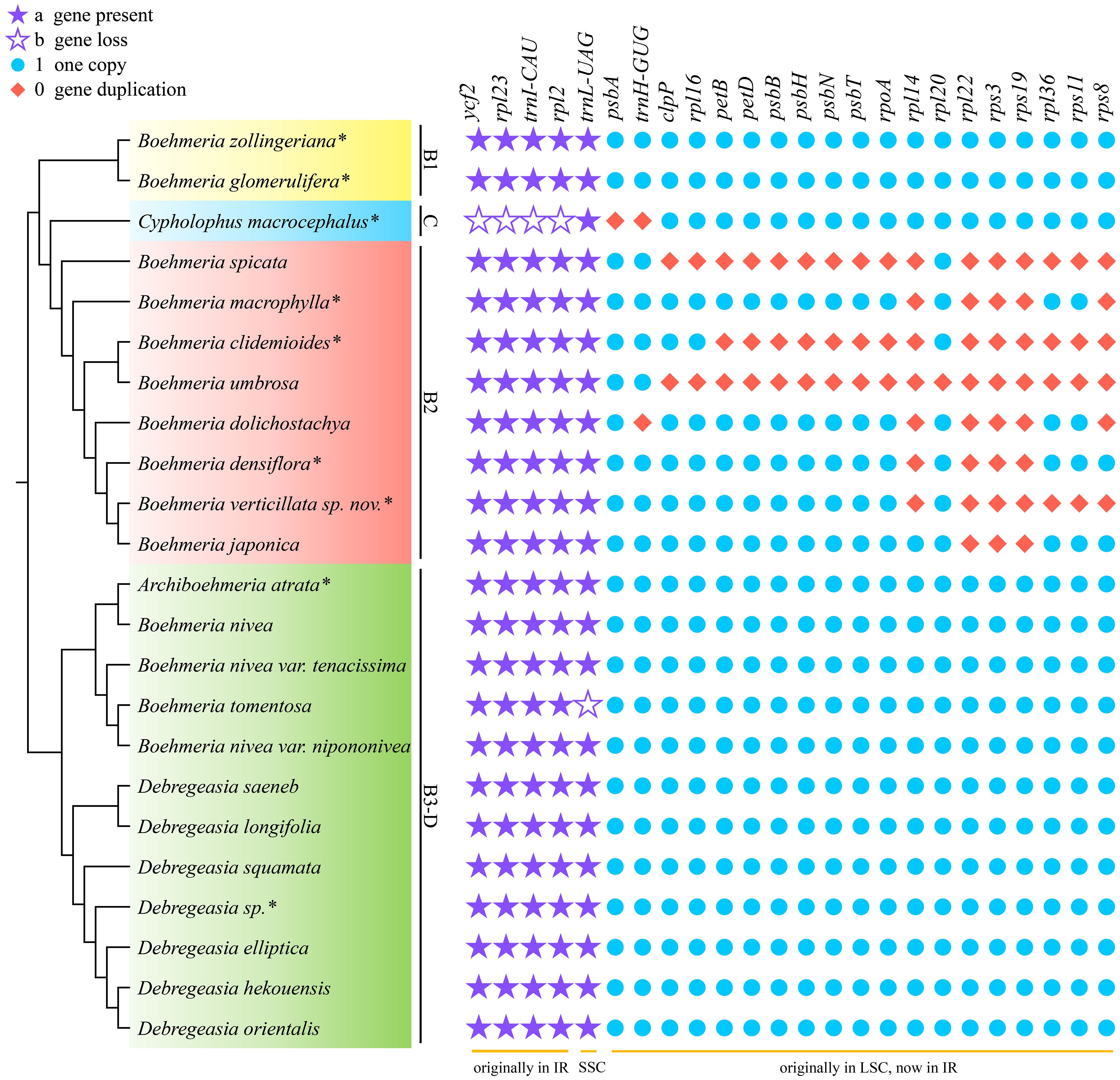
Figure 4 Gene loss and duplication in the four clades. The cladogram (left) was converted from the concatenated method phylogram (Figure 2A); the symbol matrix (right) shows the conditions of gene loss and duplication. *Asterisks indicate the newly sequenced species.
3.3.3 IR expansion/contraction and different boundary types
Both the expansion and contraction of IR were recovered in our studied taxa. Clades B1 and B3-D exhibited relatively conserved IR sizes with averages of 25,891 bp and 25,692 bp, respectively. The IR of C. macrocephalus in clade C contracted to 16,948 bp, while its sister clade (B2) showed a marked expansion with an average IR size of 34,113 bp (Table 2, Figure 3).
The SSC/IRa (JSA) boundary was conservative in all four clades with ycf1 located here (Figure 5). IRb/SSC (JSB) was also conservative with ndhF across the border, except for C. macrocephalus of clade C with this gene completely located in the SSC region; the direction of it was forward (boundary type 2) (Figure 5). JLA was conservative in clades B1 and B3-D with rpl2 and trnH-GUG near this border, but it was diverse in clades C and B2. JLB was also conservative in clades B1 and B3-D, with rpl22 near and rps19 across the boundary (boundary type 1) and rps19 across and rpl2 near the boundary (boundary type 3), but JLB was also diverse in clades C and B2.
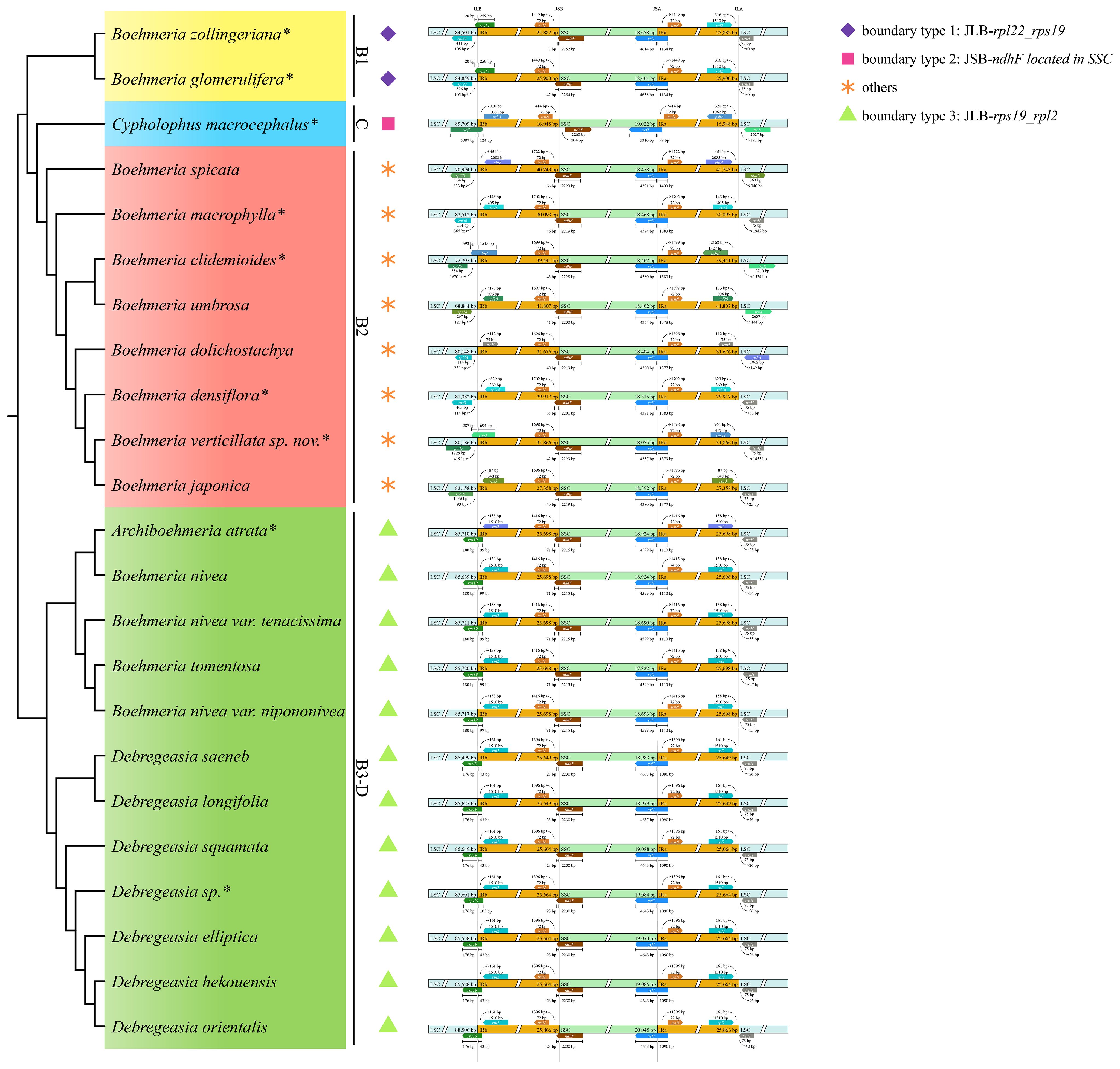
Figure 5 IR/SC boundary comparison in the four clades. The cladogram (left) was converted from the concatenated method phylogram (Figure 2A); the symbol matrix (medium) shows the different IR/SC boundary types; the image (right) shows the four junctions of plastomes; JLB, JSB, JSA, and JLA represent the junction sites of LSC/IRb, IRb/SSC, SSC/IRa, and IRa/LSC, respectively. *Asterisks indicate the newly sequenced species.
3.3.4 Single gene inversions and large-scale inversions
Clades B1 and B3-D were conservative with no inversion, while clade C and all members of clade B2 showed inversions (Figure 6). A total of four inversion events were detected, including single gene inversions (ndhF and trnC-GCA) and large-scale inversions (Figure 6; Supplementary Figure 4). The ndhF inversion solely occurred in C. macrocephalus; the trnC-GCA inversion was shared by clades C and B2. Boehmeria spicata of clade B2 possessed two inversions (trnC-GCA inversion; the large-scale inversion trnH-GUG_ndhC, which overlapped with the former inversion), causing the direction of trnC-GCA being finally different from other members in this clade. The large-scale inversion of trnH-GUG_ndhC (~51 kb) was recovered in B. spicata, while the remaining large-scale inversion of trnH-GUG_trnK-UUU (~5 kb) was shared by B. clidemioides and B. umbrosa. All these inversions occurred in the LSC region.
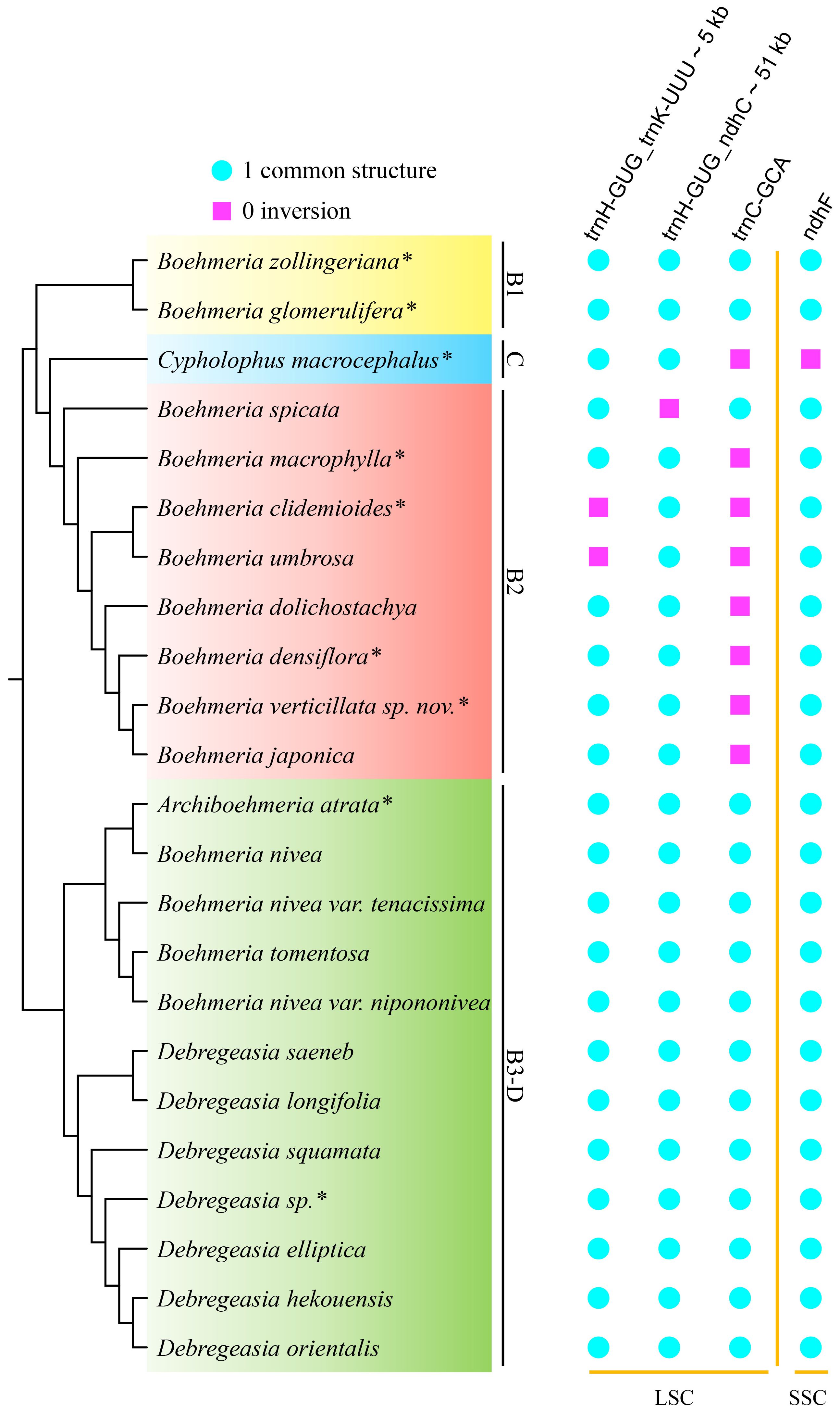
Figure 6 Inversions in the four clades. The cladogram (left) was converted from the concatenated method phylogram (Figure 2A); the symbol matrix (right) shows the conditions of inversions. *Asterisks indicate the newly sequenced species.
4 Discussion
4.1 Plastome phylogenomics inferred the polyphyly of Boehmeria
Our results showed congruent topologies between matrix1 and matrix2 both in the concatenated and coalescent methods, but the support values become lower after removing boundary genes. This probably means that the boundary genes may not suffer from heterotachy or the effect of heterotachy was too little to twist phylogenetic reconstruction in our studied taxa. Moreover, the NQSs are all >0.92, indicating only slight gene incongruence. Still, the use of such genes needs to be done cautiously, since they can lead to incongruent and misleading tree topologies (Lockhart et al., 2006; Wu et al., 2011).
Our four data matrixes derived from the plastome recovered consistent tree topologies in both concatenated and coalescent methods with strong support at most nodes, revealing Boehmeria as polyphyletic with respect to the other four genera (Cypholophus, Astrothalamus, Archiboehmeria, and Debregeasia). Species of Boehmeria and the four genera formed two large clades: one is B1-C-B2, which is dominated by Boehmeria species and corresponds to clade 1A1 in Wu et al. (2013), (2015), (2018b); the other formed B3 and D, which corresponds to 1A3 and 1A2 of Wu et al. (2013), (2015), (2018b). Cypholophus (C) was nested within Boehmeria, which was consistent with Wu et al. (2018b). Archiboehmeria was recovered within a clade that also includes B. nivea and Astrothalamus, and this clade (B3) was recovered as a sister to Debregeasia (D). As above, this was consistent with Wu et al. (2013), (2015), (2018b). The position of Sarcochlamys, sister to Leucosyke within a clade sister to the Cecropieae, differed from Wu et al. (2013), (2015), (2018b) but is congruent with an analysis of 353 nuclear markers (Monro et al., in preparation). That incongruence with Wu et al. (2013), (2015), (2018b) may be attributed to the insufficient phylogenetic information derived from limited gene loci used in their studies (Guo et al., 2022; Steenwyk et al., 2023). Short branches were consistently recovered for species within Boehmeria s.l., suggesting rapid diversification, which corresponds to and may be associated with the relatively diverse range of biomes that they occupy, from the moist broadleaf forests of Asia to African xeric biomes.
Our results demonstrated that plastome data can be a reliable tool for phylogenetic reconstruction, given that the resulting phylograms were highly resolved. A similar conclusion can be reached from previous Urticaceae phylogenies based on plastome data (Wang et al., 2020; Li et al., 2021; Ogoma et al., 2022; Wu et al., 2022).
4.2 Plastome structural variation provides further support for phylogenetic relationship
4.2.1 Diverse plastome structural variation in Boehmeria s.l.
Considerable plastome size variation was detected for this group, which ranged from 142,627 bp to 170,958 bp, representing both the smallest and the largest ones recorded for the Urticaceae to date (NCBI, 2024). This is greater than the range of 145,419–161,930 bp of 57 plastomes in the Urticeae tribe (Ogoma et al., 2022) and is indicative of distinct structural differences (Ruhlman and Jansen, 2018). Changes in IR size are considered one of the main causes of plastome size variation (Jansen and Ruhlman, 2012), and in our study, they contribute to the large plastomes documented for clade B2. It has been suggested that large IR increases the stability of plastomes through homologous recombination-induced repair mechanisms (Maréchal and Brisson, 2010; Wicke et al., 2011; Zhu et al., 2016). However, we observed a lower GC content in this clade (B2). Lower GC content in larger plastomes has also been observed in Pelargonium, Plantago, and Silene (Magee et al., 2010; Zhu et al., 2016), where it has been ascribed to natural selection responding to the higher biochemical costs for GC base synthesis (Šmarda et al., 2014).
Gene loss and duplication was a distinct variation of clade C-B2, while clades B1 and B3-D were conserved in gene content. The duplications observed in clade B2 were mainly concentrated in the rpl, rps, and psb genes (Figure 4). These genes are involved in self-replication and photosynthesis, respectively (Zhang et al., 2020). The duplication of these functional genes may strengthen their adaptive ability to a wide range of habitats, from moist broadleaf forests to xeric deserts, by improving their stress response and light utilization capacity (Saha et al., 2017; Xiong et al., 2021). These gene losses and duplications were all caused by IR boundary shifts, which are common in plants (Dugas et al., 2015; Sinn et al., 2018; Guo et al., 2021; Ogoma et al., 2022; Wu et al., 2024). The loss of trnL-UAG in B. tomentosa was the only observed gene loss in clade B3-D. It occurred independent of any IR boundary shift, and since it is restricted to a single species, it has no phylogenetic implication. Compared with the divergent clade C-B2, clades B1 and B3-D had no obvious IR boundary shifts and had conserved IR size, boundary type, and gene content. The shift of IR/SC boundaries may, therefore, be linked to the diversification of clade C-B2. Considering the distinct features of gene loss and duplication and IR contraction and expansion among these clades, they could be used as effective phylogenetic informative characters.
Clade C-B2 also differed from its sister clades in the presence of a shared trnC-GCA inversion that may be associated with a key evolutionary event (Jansen and Palmer, 1987), which led to the divergence of clade C-B2 from B1. Our results recovered very rare large-scale inversions in Urticaceae occurring in the plastomes of B. spicata, B. clidemioides, and B. umbrosa (clade B2), which are however very common in other families such as Campanulaceae (Knox, 2014), Geraniaceae (Röschenbleck et al., 2017; Ruhlman and Jansen, 2018), Fabaceae (Charboneau et al., 2021; Lee et al., 2021), Passifloraceae (Rabah et al., 2019), and Juncaceae (Wu et al., 2024). Inversions have been demonstrated to represent homoplasies (Pinaceae, Wu et al., 2011; Fabaceae, Charboneau et al., 2021; Passifloraceae, Rabah et al., 2019) and homologies (Asteraceae, Jansen and Palmer, 1987; Geraniaceae, Röschenbleck et al., 2017; Poaceae, Wu et al., 2024; Campanulaceae, Xu et al., 2022). In this study, the trnC-GCA inversion was homologous for clade C-B2, and the large-scale inversion trnH-GUG_trnK-UUU was homologous for the clade comprising B. clidemioides and B. umbrosa. In contrast, the large-scale trnH-GUG_ndhC inversion and single ndhF inversion occurred independently in B. spicata and C. macrocephalus, respectively, which need deeper investigation with more species diversity.
4.2.2 Trends of variations in the plastome evolution of Boehmeria s.l.
Plastomes of Boehmeria s.l. exhibit extensive variation in genome size, from gene content to GC content. Moreover, our results showed a general trend of size variation where the early clades (B1 and B3-D) were relatively conserved in evolution, whereas the later diverging groups (clades C and B2) showed a high degree of variation. This pattern was also observed for gene content, IR contraction and expansion, and IR boundary types and inversions. The plastome structural variation that we documented may be associated with its diversification and evolution that enabled members of this group to shift biome to more xeric environments and to exhibit diverse morphology and life forms (from herbs to trees). Diverse plastome structural variations were also detected in Poales, and these variations exhibit a trend of small–large–moderate pattern (Wu et al., 2024). Since the plastome bears the primary photosynthetic function, which is fundamental to the survival of green plants, knowing plastome evolution would be highly useful for us to better understand the evolutionary process of these plants.
Data availability statement
The datasets presented in this study can be accessed at NCBI GenBank; the list of SRA accession numbers can be found in Supplementary Table 1 and plastome accession numbers can be found in Supplementary Table 2.
Author contributions
MZ: Formal analysis, Visualization, Writing – original draft, Writing – review & editing. LX: Data curation, Formal analysis, Writing – review & editing. J-JZ: Investigation, Resources, Writing – review & editing. QZ: Resources, Writing – review & editing. X-MQ: Methodology, Writing – review & editing. X-WL: Investigation, Writing – review & editing. LW: Conceptualization, Funding acquisition, Project administration, Resources, Supervision, Writing – review & editing. AM: Conceptualization, Writing – review & editing. L-FF: Conceptualization, Writing – review & editing.
Funding
The author(s) declare financial support was received for the research, authorship, and/or publication of this article. This study was supported by the Light of West China Program of the Chinese Academy of Sciences ([2020]82), the Guangxi Natural Science Foundation Program (2022GXNSFAA035440), the Fund of Guangxi Key Laboratory of Plant Conservation and Restoration Ecology in Karst Terrain (22–035-26), the Natural Science Foundation of Hunan (2021JJ31152), and the Basic Research Fund of Guangxi Academy of Sciences (CQZ-C-1901).
Acknowledgments
We are grateful to Herbaria K for providing leaf materials of some key taxa. We would like to thank Xue-Jun Jiang (Natural Resources Bureau of Dongan County, Hunan, China) and Wei-Dong Zhang (Natural Resources Bureau of Dongan County, Hunan, China) for helping in the field investigation.
Conflict of interest
The authors declare that the research was conducted in the absence of any commercial or financial relationships that could be construed as a potential conflict of interest.
Publisher’s note
All claims expressed in this article are solely those of the authors and do not necessarily represent those of their affiliated organizations, or those of the publisher, the editors and the reviewers. Any product that may be evaluated in this article, or claim that may be made by its manufacturer, is not guaranteed or endorsed by the publisher.
Supplementary material
The Supplementary Material for this article can be found online at: https://www.frontiersin.org/articles/10.3389/fpls.2024.1297499/full#supplementary-material
Supplementary Figure 1 | Phylogenetic relationships of Boehmeria and its related genera inferred by matrix2 (59-CDS): (A) concatenated tree produced by Maximum Likelihood (ML) analysis, numbers associated with branches (ML_BS/BI_PP) are assessed by Maximum Likelihood Bootstrap (ML_BS) and Bayesian posterior probabilities (BI_PP); (B) coalescent tree, numbers associated with branches denote local posterior probability (LPP) support values, pie charts show relative frequencies of the three quartet topologies around the branch (purple = congruent with the species tree, orange = first alternative topology, green = second alternative topology). Branches with no support values are maximally supported.
Supplementary Figure 2 | Phylogenetic relationships of Boehmeria and its related genera inferred by matrix3 (gene+IGS): (A) concatenated tree produced by Maximum Likelihood (ML) analysis, numbers associated with branches (ML_BS/BI_PP) are assessed by Maximum Likelihood Bootstrap (ML_BS) and Bayesian posterior probabilities (BI_PP); (B) coalescent tree, numbers associated with branches denote local posterior probability (LPP) support values, pie charts show relative frequencies of the three quartet topologies around the branch (purple = congruent with the species tree, orange = first alternative topology, green = second alternative topology). Branches with no support values are maximally supported.
Supplementary Figure 3 | Phylogenetic relationships of Boehmeria and its related genera inferred by matrix4 (gene+IGS-SNP): (A) concatenated tree produced by Maximum Likelihood (ML) analysis, numbers associated with branches (ML_BS/BI_PP) are assessed by Maximum Likelihood Bootstrap (ML_BS) and Bayesian posterior probabilities (BI_PP); (B) coalescent tree, numbers associated with branches denote local posterior probability (LPP) support values, pie charts show relative frequencies of the three quartet topologies around the branch (purple = congruent with the species tree, orange = first alternative topology, green = second alternative topology). Branches with no support values are maximally supported.
Supplementary Figure 4 | Mauve alignment showing gene arrangements within the four clades with Hemistylus odontophylla (MN189963) used as reference (length indicated above). Large colored boxes represent the gene blocks and the colored lines indicate the linear position of different genes in the plastome.
References
Aho, K., Derryberry, D., Peterson, T. (2014). Model selection for ecologists: the worldviews of AIC and BIC. Ecology 95, 631–636. doi: 10.1890/13-1452.1
Arseneau, J. R., Steeves, R., Laflamme, M. (2017). Modified low-salt CTAB extraction of high-quality DNA from contaminant-rich tissues. Mol. Ecol. Resour 17, 686–693. doi: 10.1111/1755-0998.12616
Arsul, M. I., Insanu, M., Fidrianny, I. (2021). Phytochemistry and pharmacological activities of Boehmeria genus: an update review. Pharmacognosy J. 13, 1533–1541. doi: 10.5530/pj
Bock, D. G., Andrew, R. L., Rieseberg, L. H. (2014). On the adaptive value of cytoplasmic genomes in plants. Mol. Ecol. 23, 4899–4911. doi: 10.1111/mec.12920
Capella-Gutiérrez, S., Silla-Martínez, J. M., Gabaldón, T. (2009). trimAl: a tool for automated alignment trimming in large-scale phylogenetic analyses. Bioinformatics 25, 1972–1973. doi: 10.1093/bioinformatics/btp348
Charboneau, J. L. M., Cronn, R. C., Liston, A., Wojciechowski, M. F., Sanderson, M. J. (2021). Plastome structural evolution and homoplastic inversions in neo-astragalus (Fabaceae). Genome Biol. Evol. 13, evab215. doi: 10.1093/gbe/evab215
Choi, I. S., Choi, B. H. (2017). The distinct plastid genome structure of Maackia fauriei (Fabaceae: Papilionoideae) and its systematic implications for genistoids and tribe Sophoreae. PloS One 12, e0173766. doi: 10.1371/journal.pone.0173766
Darling, A. C. E., Mau, B., Blattner, F. R., Perna, N. T. (2004). Mauve: multiple alignment of conserved genomic sequence with rearrangements. Genome Res. 14, 1394–1403. doi: 10.1101/gr.2289704
Darriba, D., Taboada, G. L., Doallo, R., Posada, D. (2012). JModelTest 2: more models, new heuristics and parallel computing. Nat. Methods 9, 772. doi: 10.1038/nmeth.2109
Dugas, D., Hernandez, D., Koenen, E. J. M., Schwarz, E., Straub, S., Hughes, C. E., et al. (2015). Mimosoid legume plastome evolution: IR expansion, tandem repeat expansions, and accelerated rate of evolution in clpP. Sci. Rep. 5, 16958. doi: 10.1038/srep16958
Gonçalves, D. J. P., Simpson, B. B., Ortiz, E. M., Shimizu, G. H., Jansen, R. K. (2019). Incongruence between gene trees and species trees and phylogenetic signal variation in plastid genes. Mol. Phylogenet Evol. 138, 219–232. doi: 10.1016/j.ympev.2019.05.022
Guo, C., Luo, Y., Gao, L. M., Yi, T. S., Li, H. T., Yang, J. B., et al. (2022). Phylogenomics and the flowering plant tree of life. J. Integr. Plant Biol. 65, 299–323. doi: 10.1111/jipb.13415
Guo, Y. Y., Yang, J. X., Bai, M. Z., Zhang, G. Q., Liu, Z. J. (2021). The chloroplast genome evolution of Venus slipper (Paphiopedilum): IR expansion, SSC contraction, and highly rearranged SSC regions. BMC Plant Biol. 21, 248. doi: 10.1186/s12870-021-03053-y
He, X., Zheng, Z., Wang, Q., Zhou, M., Liao, G., Ge, Y., et al. (2021). Complete chloroplast genome sequence of the medicinal plant ramie (Boehmeria nivea L. gaud) and its phylogenetic relationship to other Urticaceae species. Mitochondrial DNA B Resour 6, 1136–1137. doi: 10.1080/23802359.2021.1878959
Jansen, R. K., Palmer, J. D. (1987). A chloroplast DNA inversion marks an ancient evolutionary split in the sunflower family (Asteraceae). Proc. NatI Acad. Sci. U.S.A. 84, 5818–5822. doi: 10.1073/pnas.84.16.5818
Jansen, R. K., Ruhlman, T. A. (2012). Plastid genomes of seed plants. Genomics chloroplasts mitochondria 35, 103–126. doi: 10.1007/978-94-007-2920-9_5
Jin, J. J., Yu, W., Yang, J. B., Song, Y., Depamphilis, C. W., Yi, T. S., et al. (2020). GetOrganelle: a fast and versatile toolkit for accurate de novo assembly of organelle genomes. Genome Biol. 21, 241. doi: 10.1186/s13059-020-02154-5
Junier, T., Zdobnov, E. M. (2010). The Newick utilities: high-throughput phylogenetic tree processing in the UNIX shell. Bioinformatics 26, 1669–1670. doi: 10.1093/bioinformatics/btq243
Kapli, P., Yang, Z., Telford, M. J. (2020). Phylogenetic tree building in the genomic age. Nat. Rev. Genet. 21, 428–444. doi: 10.1038/s41576-020-0233-0
Katoh, K., Standley, D. M. (2013). MAFFT multiple sequence alignment software version 7: improvements in performance and usability. Mol. Biol. Evol. 30, 772–780. doi: 10.1093/molbev/mst010
Kearse, M., Moir, R., Wilson, A., Stones-Havas, S., Cheung, M., Sturrock, S., et al. (2012). Geneious Basic: An integrated and extendable desktop software platform for the organization and analysis of sequence data. Bioinformatics 28, 1647–1649. doi: 10.1093/bioinformatics/bts199
Knox, E. B. (2014). The dynamic history of plastid genomes in the Campanulaceae sensu lato is unique among angiosperms. Proc. Natl. Acad. Sci. U.S.A. 111, 11097–11102. doi: 10.1073/pnas.1403363111
Kravtsova, T. I., Friis, I., Wilmot, C. M. (2000). Morphology and anatomy of fruits in new world Boehmeria in relation to taxonomy. Kew Bull. 55, 43–62. doi: 10.2307/4117760
Lee, C., Choi, I. S., Cardoso, D., de Lima, H. C., de Queiroz, L. P., Wojciechowski, M. F., et al. (2021). The chicken or the egg? Plastome evolution and an independent loss of the inverted repeat in papilionoid legumes. Plant J. 107, 861–875. doi: 10.1111/tpj.15351
Lee, S. K., Lee, Z. X., Lim, Y. Y., Liew, K. B., Akowuah, G. A., Chew, Y. L. (2022). Ramie (Boehmeria nivea): a functional food and herbal medicine for inflammatory bowel disease (IBD) and the promotion of optimal health. Nat. Prod J. 12, 53–61. doi: 10.2174/2210315512666220302160241
Li, H., Guo, Q., Xu, L., Gao, H., Liu, L., Zhou, X. (2023). CPJSdraw: analysis and visualization of junction sites of chloroplast genomes. PeerJ 11, e15326. doi: 10.7717/peerj.15326
Li, J., Tang, J., Zeng, S., Han, F., Yuan, J., Yu, J. (2021). Comparative plastid genomics of four Pilea (Urticaceae) species: insight into interspecific plastid genome diversity in Pilea. BMC Plant Biol. 21, 25. doi: 10.1186/s12870-020-02793-7
Liu, S., Ni, Y., Li, J., Zhang, X., Yang, H., Chen, H., et al. (2023). CPGView: a package for visualizing detailed chloroplast genome structures. Mol. Ecol. Resour. 23, 694–704. doi: 10.1111/1755-0998.13729
Liu, Z., Zeng, Z., Yang, X., Zhu, S., Liu, T., Wang, Y. (2022). Genetic insights into the crude protein and fiber content of ramie leaves. Front. Plant Sci. 13. doi: 10.3389/fpls.2022.969820
Lockhart, P., Novis, P., Milligan, B. G., Riden, J., Rambaut, A., Larkum, T. (2006). Heterotachy and tree building: A case study with plastids and eubacteria. Mol. Biol. Evol. 23, 40–45. doi: 10.1093/molbev/msj005
Magee, A. M., Aspinall, S., Rice, D. W., Cusack, B. P., Sémon, M., Perry, A. S., et al. (2010). Localized hypermutation and associated gene losses in legume chloroplast genomes. Genome Res. 20, 1700–1710. doi: 10.1101/gr.111955.110
Maréchal, A., Brisson, N. (2010). Recombination and the maintenance of plant organelle genome stability. New Phytol. 186, 299–317. doi: 10.1111/j.1469-8137.2010.03195.x
NCBI. (1988). (Bethesda (MD) National Library of Medicine (US), National Center for Biotechnology Information). Available online at: https://www.ncbi.nlm.nih.gov/.
Nguyen, L. T., Schmidt, H. A., Von Haeseler, A., Minh, B. Q. (2015). IQ-TREE: A fast and effective stochastic algorithm for estimating maximum-likelihood phylogenies. Mol. Biol. Evol. 32, 268–274. doi: 10.1093/molbev/msu300
Ogoma, C. A., Liu, J., Stull, G. W., Wambulwa, M. C., Oyebanji, O., Milne, R. I., et al. (2022). Deep insights into the plastome evolution and phylogenetic relationships of the tribe urticeae (Family urticaceae). Front. Plant Sci. 13. doi: 10.3389/fpls.2022.870949
POWO. (2024). Plants of the world online (London, United Kingdom: Royal Botanic Gardens, Kew, Plants of the World Online). Available at: http://www.plantsoftheworldonline.org/.
Qu, X. J., Moore, M. J., Li, D. Z., Yi, T. S. (2019). PGA: a software package for rapid, accurate, and flexible batch annotation of plastomes. Plant Methods 15, 50. doi: 10.1186/s13007-019-0435-7
Qu, X. J., Zou, D., Zhang, R. Y., Stull, G. W., Yi, T. S. (2023). Progress, challenge and prospect of plant plastome annotation. Front. Plant Sci. 14. doi: 10.3389/fpls.2023.1166140
Rabah, S. O., Shrestha, B., Hajrah, N. H., Sabir, M. J., Alharby, H. F., Sabir, M. J., et al. (2019). Passiflora plastome sequencing reveals widespread genomic rearrangements. J. Syst. Evol. 57, 1–14. doi: 10.1111/jse.12425
Rehman, M., Gang, D., Liu, Q., Chen, Y., Wang, B., Peng, D., et al. (2019). Ramie, a multipurpose crop: potential applications, constraints and improvement strategies. Ind. Crops Prod 137, 300–307. doi: 10.1016/j.indcrop.2019.05.029
Ronquist, F., Teslenko, M., van der Mark, P., Ayres, D. L., Darling, A., Höhna, S., et al. (2012). Mrbayes 3.2: Efficient bayesian phylogenetic inference and model choice across a large model space. Syst. Biol. 61, 539–542. doi: 10.1093/sysbio/sys029
Röschenbleck, J., Wicke, S., Weinl, S., Kudla, J., Müller, K. F. (2017). Genus-wide screening reveals four distinct types of structural plastid genome organization in pelargonium (Geraniaceae). Genome Biol. Evol. 9, 64–76. doi: 10.1093/GBE/EVW271
Ruhlman, T. A., Jansen, R. K. (2018). Aberration or analogy? The atypical plastomes of geraniaceae. Adv. Bot. Res. 85, 223–262. doi: 10.1016/bs.abr.2017.11.017
Saha, A., Das, S., Moin, M., Dutta, M., Bakshi, A., Madhav, M. S., et al. (2017). Genome-wide identification and comprehensive expression profiling of ribosomal protein small subunit (RPS) genes and their comparative analysis with the large subunit (RPL) genes in rice. Front. Plant Sci. 8. doi: 10.3389/fpls.2017.01553
Sayyari, E., Mirarab, S. (2016). Fast coalescent-based computation of local branch support from quartet frequencies. Mol. Biol. Evol. 33, 1654–1668. doi: 10.1093/molbev/msw079
Scobeyeva, V. A., Artyushin, I., Krinitsina, A. A., Nikitin, P. A., Antipin, M. I., Kuptsov, S. V., et al. (2021). Gene loss, pseudogenization in plastomes of genus allium (Amaryllidaceae), and putative selection for adaptation to environmental conditions. Front. Genet. 12. doi: 10.3389/fgene.2021.674783
Shi, L., Chen, H., Jiang, M., Wang, L., Wu, X., Huang, L., et al. (2019). CPGAVAS2, an integrated plastome sequence annotator and analyzer. Nucleic Acids Res. 47, W65–W73. doi: 10.1093/nar/gkz345
Sinn, B. T., Sedmak, D. D., Kelly, L. M., Freudenstein, J. V. (2018). Total duplication of the small single copy region in the angiosperm plastome: Rearrangement and inverted repeat instability in Asarum. Am. J. Bot. 105, 71–84. doi: 10.1002/ajb2.1001
Sloan, D. B., Alverson, A. J., Wu, M., Palmer, J. D., Taylor, D. R. (2012). Recent acceleration of plastid sequence and structural evolution coincides with extreme mitochondrial divergence in the angiosperm genus Silene. Genome Biol. Evol. 4, 294–306. doi: 10.1093/gbe/evs006
Šmarda, P., Bureš, P., Horová, L., Leitch, I. J., Mucina, L., Pacini, E., et al. (2014). Ecological and evolutionary significance of genomic GC content diversity in monocots. Proc. Natl. Acad. Sci. U.S.A. 111, E4096–E4102. doi: 10.1073/pnas.1321152111
Steenwyk, J. L., Li, Y., Zhou, X., Shen, X. X., Rokas, A. (2023). Incongruence in the phylogenomics era. Nat. Rev. Genet. 24, 834–850. doi: 10.1038/s41576-023-00620-x
Twyford, A. D., Ness, R. W. (2017). Strategies for complete plastid genome sequencing. Mol. Ecol. Resour 17, 858–868. doi: 10.1111/1755-0998.12626
Villarreal, J. C., Forrest, L. L., Wickett, N., Goffinet, B. (2013). The plastid genome of the hornwort Nothoceros aenigmaticus (Dendrocerotaceae): Phylogenetic signal in inverted repeat expansion, pseudogenization, and intron gain. Am. J. Bot. 100, 467–477. doi: 10.3732/ajb.1200429
Wang, R. N., Milne, R. I., Du, X. Y., Liu, J., Wu, Z. Y. (2020). Characteristics and mutational hotspots of plastomes in debregeasia (Urticaceae). Front. Genet. 11. doi: 10.3389/fgene.2020.00729
Wang, H. C., Susko, E., Roger, A. J. (2019). The relative importance of modeling site pattern heterogeneity versus partition-wise heterotachy in phylogenomic inference. Syst. Biol. 68, 1003–1019. doi: 10.1093/sysbio/syz021
Weng, M. L., Ruhlman, T. A., Jansen, R. K. (2016). Plastid-nuclear interaction and accelerated coevolution in plastid ribosomal genes in Geraniaceae. Genome Biol. Evol. 8, 1824–1838. doi: 10.1093/gbe/evw115
Wicke, S., Schneeweiss, G. M., dePamphilis, C. W., Müller, K. F., Quandt, D. (2011). The evolution of the plastid chromosome in land plants: Gene content, gene order, gene function. Plant Mol. Biol. 76, 273–297. doi: 10.1007/s11103-011-9762-4
Wilmot-Dear, C. M., Friis, I. (2013). The Old World species of Boehmeria (Urticaceae, tribus Boehmerieae). A taxonomic revision. J. Plant Taxonomy Plant Geogr. 58, 85–216. doi: 10.3767/000651913X674116
Wilmot-Dear, C. M., Friis, I., Thomas, Z. (2010). New species in old world Boehmeria (Urticaceae). Edinb J. Bot. 67, 431–450. doi: 10.1017/S0960428610000144
Wu, Z. Y., Du, X. Y., Milne, R. I., Liu, J., Li, D. Z. (2018a). Complete chloroplast genome sequences of two Boehmeria species (Urticaceae). Mitochondrial DNA B Resour 3, 939–940. doi: 10.1080/23802359.2018.1502635
Wu, H., Li, D. Z., Ma, P. F. (2024). Unprecedented variation pattern of plastid genomes and the potential role in adaptive evolution in Poales. BMC Biol. 22, 97. doi: 10.1186/s12915-024-01890-5
Wu, Z. Y., Liu, J., Provan, J., Wang, H., Chen, C. J., Cadotte, M. W., et al. (2018b). Testing Darwin’s transoceanic dispersal hypothesis for the inland nettle family (Urticaceae). Ecol. Lett. 21, 1515–1529. doi: 10.1111/ele.13132
Wu, Z. Y., Milne, R. I., Chen, C. J., Liu, J., Wang, H., Li, D. Z. (2015). Ancestral state reconstruction reveals rampant homoplasy of diagnostic morphological characters in Urticaceae, conflicting with current classification schemes. PloS One 10, e0141821. doi: 10.1371/journal.pone.0141821
Wu, Z. Y., Milne, R. I., Liu, J., Slik, F., Yu, Y., Luo, Y. H., et al. (2022). Phylogenomics and evolutionary history of Oreocnide (Urticaceae) shed light on recent geological and climatic events in SE Asia. Mol. Phylogenet Evol. 175, 107555. doi: 10.1016/j.ympev.2022.107555
Wu, Z. Y., Monro, A. K., Milne, R. I., Wang, H., Yi, T. S., Liu, J., et al. (2013). Molecular phylogeny of the nettle family (Urticaceae) inferred from multiple loci of three genomes and extensive generic sampling. Mol. Phylogenet Evol. 69, 814–827. doi: 10.1016/j.ympev.2013.06.022
Wu, C. S., Wang, Y. N., Hsu, C. Y., Lin, C. P., Chaw, S. M. (2011). Loss of different inverted repeat copies from the chloroplast genomes of pinaceae and cupressophytes and influence of heterotachy on the evaluation of gymnosperm phylogeny. Genome Biol. Evol. 3, 1284–1295. doi: 10.1093/gbe/evr095
Xiong, W., Lan, T., Mo, B. (2021). Extraribosomal functions of cytosolic ribosomal proteins in plants. Front. Plant Sci. 12. doi: 10.3389/fpls.2021.607157
Xu, X., Li, X., Wang, D. (2022). New insights into the backbone phylogeny and character evolution of corydalis (Papaveraceae) based on plastome data. Front. Plant Sci. 13. doi: 10.3389/fpls.2022.926574
Zhang, C., Rabiee, M., Sayyari, E., Mirarab, S. (2018). ASTRAL-III: Polynomial time species tree reconstruction from partially resolved gene trees. BMC Bioinf. 19, 153. doi: 10.1186/s12859-018-2129-y
Zhang, X., Sun, Y. X., Landis, J. B., Lv, Z., Shen, J., Zhang, H., et al. (2020). Plastome phylogenomic study of Gentianeae (Gentianaceae): widespread gene tree discordance and its association with evolutionary rate heterogeneity of plastid genes. BMC Plant Biology 20, 1–15. doi: 10.1186/s12870-020-02518-w
Zhong, B., Yonezawa, T., Zhong, Y., Hasegawa, M. (2010). The position of gnetales among seed plants: Overcoming pitfalls of chloroplast phylogenomics. Mol. Biol. Evol. 27, 2855–2863. doi: 10.1093/molbev/msq170
Zhu, A., Guo, W., Gupta, S., Fan, W., Mower, J. P. (2016). Evolutionary dynamics of the plastid inverted repeat: The effects of expansion, contraction, and loss on substitution rates. New Phytol. 209, 1747–1756. doi: 10.1111/nph.13743
Keywords: Boehmeria, polyphyly, plastome, phylogenetic relationship, structural variations, Urticaceae
Citation: Zhan M, Xue L, Zhou J-J, Zhang Q, Qin X-M, Liao X-W, Wu L, Monro AK and Fu L-F (2024) Polyphyly of Boehmeria (Urticaceae) congruent with plastome structural variation. Front. Plant Sci. 15:1297499. doi: 10.3389/fpls.2024.1297499
Received: 20 September 2023; Accepted: 08 July 2024;
Published: 30 July 2024.
Edited by:
Gerald Matthias Schneeweiss, University of Vienna, AustriaReviewed by:
Qiang Fan, Sun Yat-sen University, ChinaIbrar Ahmed, Alpha Genomics Private Limited, Pakistan
Copyright © 2024 Zhan, Xue, Zhou, Zhang, Qin, Liao, Wu, Monro and Fu. This is an open-access article distributed under the terms of the Creative Commons Attribution License (CC BY). The use, distribution or reproduction in other forums is permitted, provided the original author(s) and the copyright owner(s) are credited and that the original publication in this journal is cited, in accordance with accepted academic practice. No use, distribution or reproduction is permitted which does not comply with these terms.
*Correspondence: Lei Wu, d3VsZWlpYmtAMTYzLmNvbQ==; Long-Fei Fu, bG9uZ2ZlaWZ1QDEyNi5jb20=; Alexander K. Monro, YS5tb25yb0BrZXcub3Jn