- 1Herbario JBB, Jardín Botánico de Bogotá, Bogotá, Colombia
- 2Royal Botanic Gardens, Kew, Richmond, United Kingdom
- 3Herbario Icesi, Departamento de Biología, Universidad Icesi, Cali, Colombia
- 4Laboratorio de Genética de la Conservación, Instituto de Investigaciones de Recursos Biológicos Alexander von Humboldt, Bogotá, Colombia
- 5Gothenburg Global Biodiversity Centre, Department of Biological and Environmental Sciences, University of Gothenburg, Gothenburg, Sweden
- 6Department of Biology, University of Oxford, Oxford, United Kingdom
Seasonally tropical dry forests (SDTFs) in the American tropics are a highly diverse yet poorly understood and endangered ecosystem scattered from Northern Mexico to Southern Argentina. One floristic element of the STDFs is the genus Magoniella (Polygonaceae), which includes two liana species, M. laurifolia and M. obidensis, which have winged fruits and are distributed from Costa Rica to Southern Brazil. In a field expedition to the SDTFs of the Colombian Caribbean in 2015, morphologically distinctive individuals of Magoniella were found. In this study, we investigated the species boundaries within Magoniella and determined the phylogenetic position of these morphologically distinctive individuals in the tribe Triplaridae. We compiled morphological trait data across 19 specimens of both species and produced newly sequenced nuclear–plastid DNA data for M. obidensis. Morphometric analyses revealed significant differences in fruit length and perianth size among individuals from the Colombian Caribbean compared to M. obidensis and bract length when compared to M. laurifolia. Maximum likelihood analysis of non-conflicting nuclear and plastid datasets placed the Colombian Caribbean individuals as sister to M. obidensis with maximum statistical support. Additionally, pairwise sequence comparisons of the nuclear ribosomal ITS and the lfy2i loci consistently showed 15-point mutations (10 transitions, five transversions) and six 2 bp-long substitutions that differ between M. obidensis and the Colombian Caribbean individuals. Our morphological and molecular evidence thus suggests that the Colombian Caribbean individuals of Magoniella represent a divergent population from M. laurifolia and M. obidensis, which we describe and illustrate as a new species, M. chersina. Additionally, we provide nomenclatural updates for M. laurifolia and M. obidensis. This study highlights the power of combining morphological and molecular evidence in documenting and naming plant diversity.
Introduction
In the American tropics (the Neotropics), seasonally tropical dry forests (SDTFs) constitute a diverse yet disjunct biome, found in fragmented areas from Mexico in the North to Argentina in the South (Pennington et al., 2009; Linares-Palomino et al., 2011). This biome is characterised by a mean annual temperature above 17°C (Holdridge, 1947) and annual precipitation below ~1800 mm, with a dry season lasting 3–6 months, during which the monthly precipitation is less than 100 mm (Banda-R et al., 2016). The vegetation in SDTFs is deciduous or semi-deciduous during the dry season. Many trees and shrubs shed their leaves, and herbs usually survive as seeds or underground. This biome can be distinguished from more open, grass-rich savannas by its closed canopy during the wet season, as well as the presence of cacti and other succulents. These are not well adapted to recurrent fires characteristic of savannas, and there are fewer grasses (Pennington et al., 2010; Banda-R et al., 2016).
Compared to the two other major lowland neotropical biomes—rainforests and savannas—SDTFs exhibit distinctive phylogenetic and biogeographic patterns (Pennington and Lavin, 2016). Species found in STDF fragments usually have a longer evolutionary persistence, resulting in narrower distribution ranges due to limited dispersal or eco-physiological barriers, preventing their range expansions. These fragmented SDTF areas are often referred to as “enclaves” or “nuclei” (Pennington et al., 2009). Based on environmental and floristic characteristics, the Caribbean coast of Colombia and Venezuela’s STDF nuclei are treated as a single area of dry forest, distinct from the adjacent northern inter-Andean valleys of Colombia and Venezuela (Pennington et al., 2004; Linares-Palomino et al., 2011; Banda-R et al., 2016; Dinerstein et al., 2017; González-M et al., 2018; Pérez-Escobar et al., 2022). Banda-R et al. (2016) categorized both nuclei in the “higher level northern group,” yet their somewhat disjunct distribution suggests that the Amazonian–Chocoan rainforests have served as a barrier for biotic exchange between these SDTF nuclei (Pérez-Escobar et al., 2019).
Among the numerous Neotropical plants with extensive distributions that are characteristic of certain biomes, the genus Magoniella Adr.Sanchez (Polygonaceae) stands out. It was erected to encompass species previously classified under the genus Ruprechtia C.A.Mey., based on morphological and phylogenetic evidence presented in a study focussed on the tribe Triplarideae (Sanchez and Kron, 2011). This tribe currently comprises three additional genera: Triplaris Loefl., Ruprechtia and Salta Adr.Sanchez. Collectively, these four genera include 53 species (Govaerts et al., 2021; POWO, 2023) of dioecious trees, shrubs and lianas, all characterised by stipules (ochreas) protecting the stem and leaf buds, three-winged fruits, flowers with six tepals and six stamens, and three-lobed or simple seeds.
Each of these genera displays distinct preferences within lowland neotropical biomes. For instance, Salta triflora (Griseb.) Adr.Sanchez, the sole species in its genus, occurs mainly in forests and shrublands in the Chaco savannas, occasionally appearing in seasonally flooded forests (Pendry, 2004). In contrast, most Triplaris species are found in seasonally flooded forests in Amazonia, along rivers and open areas, while those outside of Amazonia are located in patches of dry forests in the Andean region (Brandbyge, 1986; Sanchez and Kron, 2011). Similarly, Ruprechtia species are distributed in various SDTF regions, with some restricted to particular dry forest enclaves, while others have a wide distribution in the humid rainforest of Central and South America (Pendry, 2004). Magoniella obidensis (Huber) Adr.Sanchez and M. lauriflora (Cham. & Schltdl.) Adr.Sanchez, the only two recognised species in the genus (Pendry, 2004; Sanchez and Kron, 2011), are found in the tropical rainforests of the Amazon basin and the Atlantic Forest, respectively. The genus differs from its sister genera in the Triplarideae by its liana habit, hollow stems, and large green fruits with red sepals (Sanchez and Kron, 2011).
During floristic exploratory expeditions conducted in the Colombian Caribbean region between 2015 and 2016, a specimen of an unusual Polygonaceae plant was collected (Aguilar-Cano et al., 2016). Its partial infructescence featuring two fruits, subtended by a single tubular bracteole, suggested a morphological similarity with Ruprechtia. However, its strict liana habit and large green fruits with red sepals corresponded to diagnostic traits of Magoniella. Two additional specimens found in the K and MO herbaria (Thiers, 2021, continuously updated) had also been collected from two other localities in the tropical dry forest of the Caribbean coast of Colombia and Venezuela. These specimens had been overlooked as a distinct species by taxonomists for nearly 100 years but displayed morphological affinities with the Magoniella specimen collected by Aguilar-Cano (Aguilar-Cano et al., 2016).
In this study, we compiled morphological and molecular phylogenetic evidence to propose a new species of Magoniella to science and to ascertain its phylogenetic position within the tribe Triplaridae. To achieve this, we produced nuclear and plastid DNA sequences and collected data on morphological traits to investigate species boundaries. We employed an integrative taxonomic approach, using operational criteria to assess lineage separation, integrate multiple lines of evidence, and document biodiversity (De Queiroz, 2007; Freudenstein et al., 2016; Grace et al., 2021; Wells et al., 2021). Specifically, we sampled two populations of the putative new species, included 198 sequences from 33 species (representing the entire generic diversity of Triplarideae), and assessed their morphological variation and distribution ranges. As a result, we unequivocally placed the potential new species in the genus Magoniella and confirmed that these collections from the Colombian and Venezuelan Caribbean dry forests indeed represent a distinct species, Magoniella chersina.
Materials and methods
DNA extraction, amplification, and sequencing
DNA extraction from leaf tissue samples was carried out using the CTAB protocol from Ivanova et al. (2008). Two plastid markers, the ndhF coding region and the rps16-trnK noncoding spacers, and two nuclear regions, the internal transcribed spacer (ITS) and the second intron of the low-copy nuclear region Leafy (lfy2i), were amplified and sequenced using the primer combinations reported in Supplementary Table S1. A total of 20 new sequences were generated in this study (voucher information provided in Supplementary Table S2). Amplification of selected regions was performed in a final volume of 15 μL containing the following: 2 μL of template DNA (~10–50 ng), 1X Taq buffer ((NH4) 2SO4), 200 μM of each deoxynucleoside triphosphate, 2 mM MgCl2, 0.2 μM of each primer, 0.4 μg/μL bovine serum albumin, and 1 U of Taq DNA polymerase. The PCR cycling conditions consisted of a first cycle of denaturation at 94°C for 3 min; followed by 35 cycles of 94°C for 30 s, TA (50°C rps16-trnk, ndhF, ITS; 56°C lfy2i) for 40 s, and 72°C for 2 min; and a final extension cycle at 72°C for 5 min. The sequences obtained were first assembled and edited using Geneious software version 10.26 (http://www.geneious.com, Kearse et al., 2012).
Phylogenetic analysis
Our newly generated sequences were combined with the DNA dataset of Sanchez and Kron (2011), which targeted four plastid (matK, ndhF, trnV-ndhC, and rps16-trnK) and two nuclear (ITS, lfy2i) DNA regions and represented 62% of known Triplarideae species. The combined DNA dataset sampled 33 putative species: nine from Triplaris (of the 19 known to science [POWO, 2023]), 17 from Ruprechtia (of the 31 [POWO, 2023]), one from the monotypic genus Salta, and two from Magoniella, as well as four outgroup species in family Polygonaceae used by Sanchez and Kron (2011): Antigonon leptopus Hook. & Arn., Coccoloba swartzii Meisn., Eriogonum alatum Torr., and Gymnopodium floribundum Rolfe. Species names, voucher specimens (for new sequences generated by this study), and all GenBank accession numbers of sequences included in phylogenetic analyses are listed in Supplementary Table S2.
Multiple sequence DNA alignments were conducted with the software MAFFT v. 7.453 (Katoh et al., 2002), using a maximum of 1,000 refinement iterations (flag—maxiter), and the option globalpair (i.e., G-INS-s high accuracy strategy). Maximum likelihood (ML) analyses were performed using the software RAXML-HPC v.8.2.4 (Stamatakis, 2014), using the general time-reversible nucleotide substitution model, a gamma distribution model (20 categories) and 500 non-parametric bootstraps (MLBS) as implemented in the CIPRES Science Gateway computing facility (Miller et al., 2015) (https://www.phylo.org/). Three ML phylogenetic analyses were carried out from three datasets: (i) combined plastid dataset, (ii) combined nuclear dataset, and (iii) total non-conflicting combined nuclear and plastid dataset following an analysis on topological discordance between both datasets [i.e., Pérez-Escobar et al. (2016, 2017)], using the software PACo (Balbuena et al., 2013) (http://www.uv.es/cophylpaco/) as implemented in R.
Morphological characters and geographical distribution
Species delimitation in Magoniella has predominantly relied on the morphological variation observed in fruits, because variability in vegetative and male reproductive characters is minimal (Pendry, 2004). Specimens of Magoniella previously deposited at the herbaria FMB and K (Thiers, 2021) were studied, including digitised type and reference specimens available at JSTOR Global Plants (http://plants.jstor.org/), Reflora (http://reflora.jbrj.gov.br/reflora/herbarioVirtual), MO (https://www.tropicos.org/), NYBG (http://sweetgum.nybg.org/science/vh/), and biovirtual (http://www.biovirtual.unal.edu.co/es/colecciones/search/plants/). These specimens included typological material of all accepted species in Magoniella (Supplementary Table S3).
We investigated six characters of ripe fruits, including ripe fruit length, perianth tube length, perianth tube width, fruit sepal length, fruit sepal width, and bracteole length. These measurements were obtained from digital images using the ImageJ v. 2.8.0 software (Schneider et al., 2012). A total of 19 Magoniella specimens were measured, with three different fruits studied for each specimen (n = 74 fruits). To assess biologically significant differences, we employed the approximate Two-Sample Fisher-Pitman Permutation Test (Fisher, 1936). Given that our comparative DNA and morphometric analyses clearly indicated that the studied specimens diverge from individuals of closely related species (see Results below), we followed Pendry (2004) terminology to construct a morphological description of the new species. The putative new species was diagnosed based on a unique combination of identified features (Donoghue, 1985). We derived the geographical distribution ranges of Magoniella from the examined specimens in this study and botanical monographs (Supplementary Table S4, Pendry, 2004).
Preliminary conservation status
We estimated the conservation category of the new taxon by calculating its extent of occurrence (EOO) and area of occupancy using the GeoCAT tool (Bachman et al., 2011) and following the IUCN Red List Categories criteria (IUCN Standards and Petitions Subcommittee, 2013).
Results
Phylogenetic relationships in Triplaridae
The combined nuclear alignment contained 2,076 base pairs, with 1,204 variable and 451 parsimony informative sites. The combined plastid alignment contained 3,363 base pairs, of which 171 were parsimony informative. ML analyses independently inferred from the concatenated nuclear (Figure 1a) and plastid (Figure 1b) datasets recovered the monophyly of Triplarideae [maximum likelihood bootstrap support (MLBS): 100%–99%, respectively]. Within the Triplarideae, three strongly supported clades were recovered, supporting the monophyly of Triplaris and Ruprechtia s.s. (Figure 1).
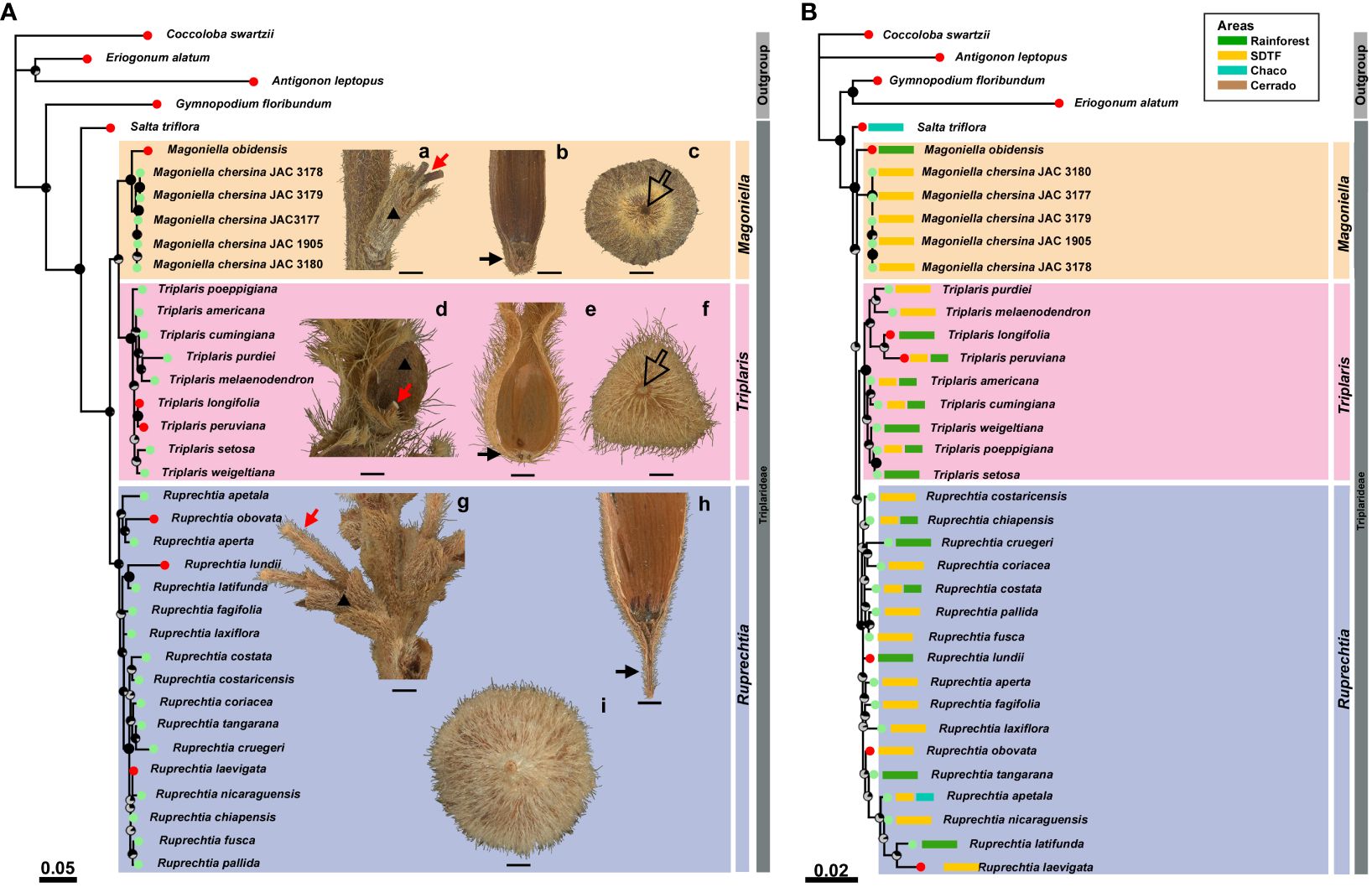
Figure 1 Maximum likelihood phylograms inferred from combined (A) ITS + lfy2i nuclear loci and (B) combined matK + ndhF + rps16-trnK + ndhC-trnV plastid loci. Fully black pie charts at nodes indicate nodes attaining maximum statistical support. Circles preceding species names indicate potential outlier (red circle) and congruent (green circle) terminals. Pictures in front of each clade show morphological characters of the partial infructescence and ripe fruits of the new species plus representative species of Ruprechtia and Triplaris. Magoniella chersina (FMB-124471): (a) Partial infructescence with pedicels (red arrow) longer than tubular bracteole (black triangle). (b) Longitudinal view of the perianth tube of ripe fruits with the base abruptly taper to scars, showing a narrow, somewhat sharpen base of up to 2.5 mm long (black arrow). (c) Inferior view of the basal part of the fruiting perianth tube (open arrow indicates scar from abscised pedicel). Triplaris weigeltiana (FMB-24212): (d) partial infructescence with bracteole fissured abaxially (black triangle) and a single pedicel (red arrow). (e) Longitudinal view of ripe fruits showing the base of the perianth tube truncate (black arrow). (f) Inferior view of the basal part of the fruiting perianth tube (open arrow indicates scar from abscised pedicel). Ruprechtia ramiflora (FMB-124421): (g) Partial infructescence with two pedicels (red arrow) subtended by a single tubular bracteole (black triangle). (h) Longitudinal view of ripe fruits showing a perianth tube base extending into a stalk (black arrow). (i) Inferior view of the basal part of the fruiting perianth tube (open arrow indicates scar from abscised pedicel). Black bars represent 1 mm. Geographical distribution in Neotropical biomes of sampled species is shown in front of tree terminals with coloured boxes preceding species names (see Supplementary Figure S2).
A visual comparison between the nuclear and plastid phylogenies revealed multiple topological incongruences. To quantify the level of topological discordance, we conducted an analysis in PACo, which revealed that 15 terminals were potentially conflicting. Twelve of these terminals belonged to Ruprechtia s.s. and three to Triplaris (two outliers were misclassified by PACo as conflicting: Ruprechtia pallida and Ruprechtia fusca). In particular, the nuclear phylogeny placed Salta triflora as sister to the remainder of the tribe, where Magoniella and Triplaris clustered in a poorly supported clade (MLBS: 56%) sister to Ruprechtia (Figure 1a). In contrast, the plastid phylogeny recovered Magoniella as a polyphyletic group, placing M. obidensis as sister to Ruprechtia, Magoniella and Triplaris, and with the relationship of Triplaris + Ruprechtia recovered as poorly supported (MLBS: 42%; Figure 1b). Because the incongruent terminals were exclusively placed in poorly supported branches (Figure 1), no data were excluded from the nuclear–plastid supermatrix used for ML phylogenetic inference.
The ML analysis from the nuclear–plastid matrix yielded an overall strongly supported phylogeny (Figure 2). The results presented below are therefore based on this phylogeny. Here, the tribe Triplarideae was recovered as monophyletic (MLBS: 100) and segregated into three main lineages corresponding to Magoniella, Triplaris and Ruprechtia. Salta triflora was placed as sister to these three maximally supported clades. Within Triplaridaea, Magoniella clustered in a maximally supported clade (MLBS: 100); Ruprechtia s.s. was recovered as sister to Triplaris in a poorly supported clade (MLBS: 45; Figure 2).
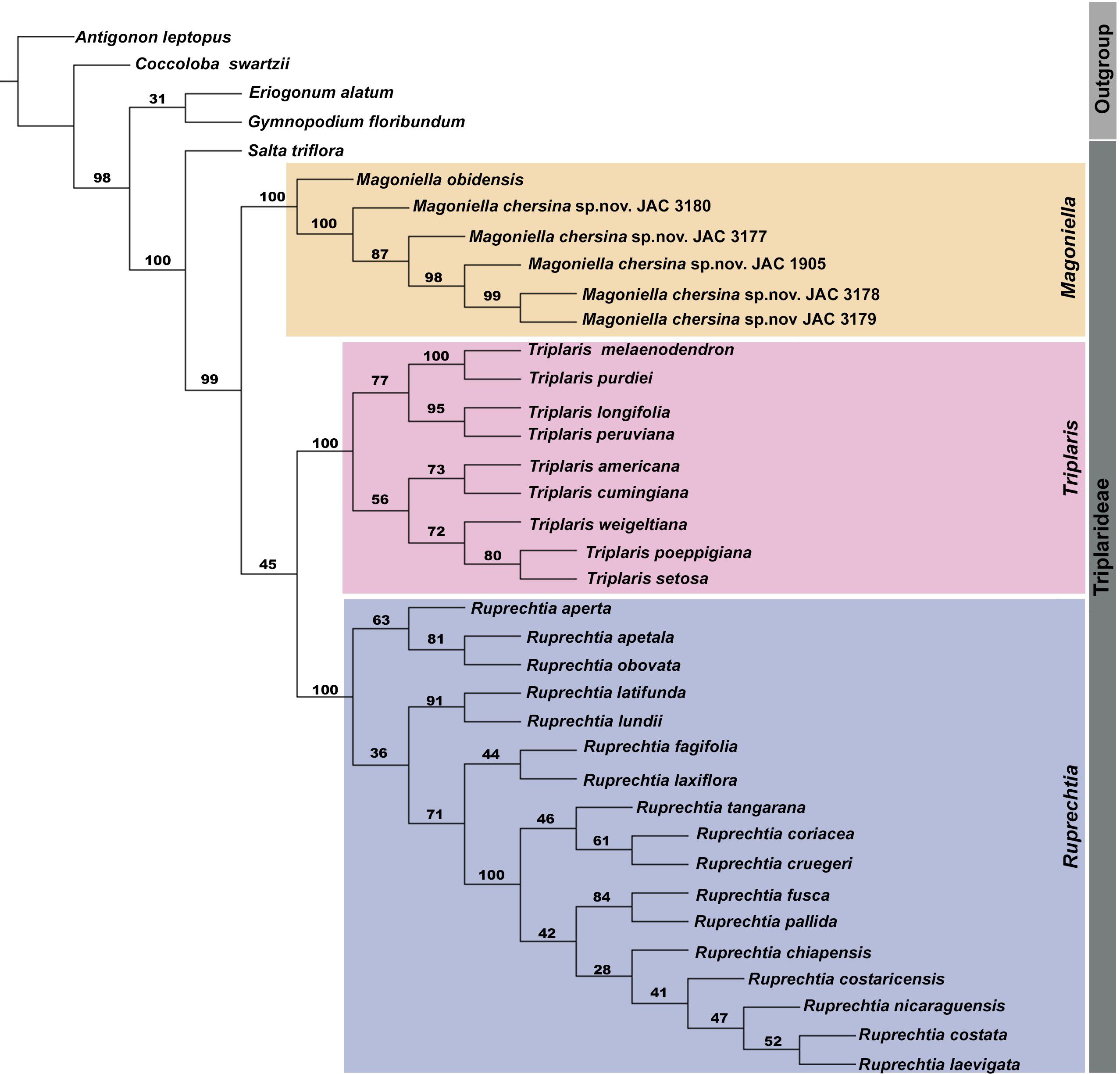
Figure 2 Maximum likelihood tree showing the phylogenetic relationships of Triplaridae inferred from a nuclear-plastid supermatrix (ITS + lfy2i + matK + ndhF + rps16-trnK + ndhC-trnV). The values above branches indicate bootstrap support percentages.
Geographical distribution and morphological differentiation
We found distinct geographical ranges among the three Magoniella species. Our geographical distribution records confirmed that M. obidensis and M. lauriflora are exclusively found in the tropical rainforests of the Brazilian and Bolivian Amazon basin, and the Atlantic Forest in southeastern Brazil, respectively. In contrast, the specimens of the newly described species, M. chersina, are limited to the STDF of the Colombian and Venezuelan Caribbean (Figures 1b, 3, 4). This new species stands out for its widely disjunct northern distribution, which is notable both for the significant geographical distance from the other two species and its presence in an eco-physiologically distinct biome.
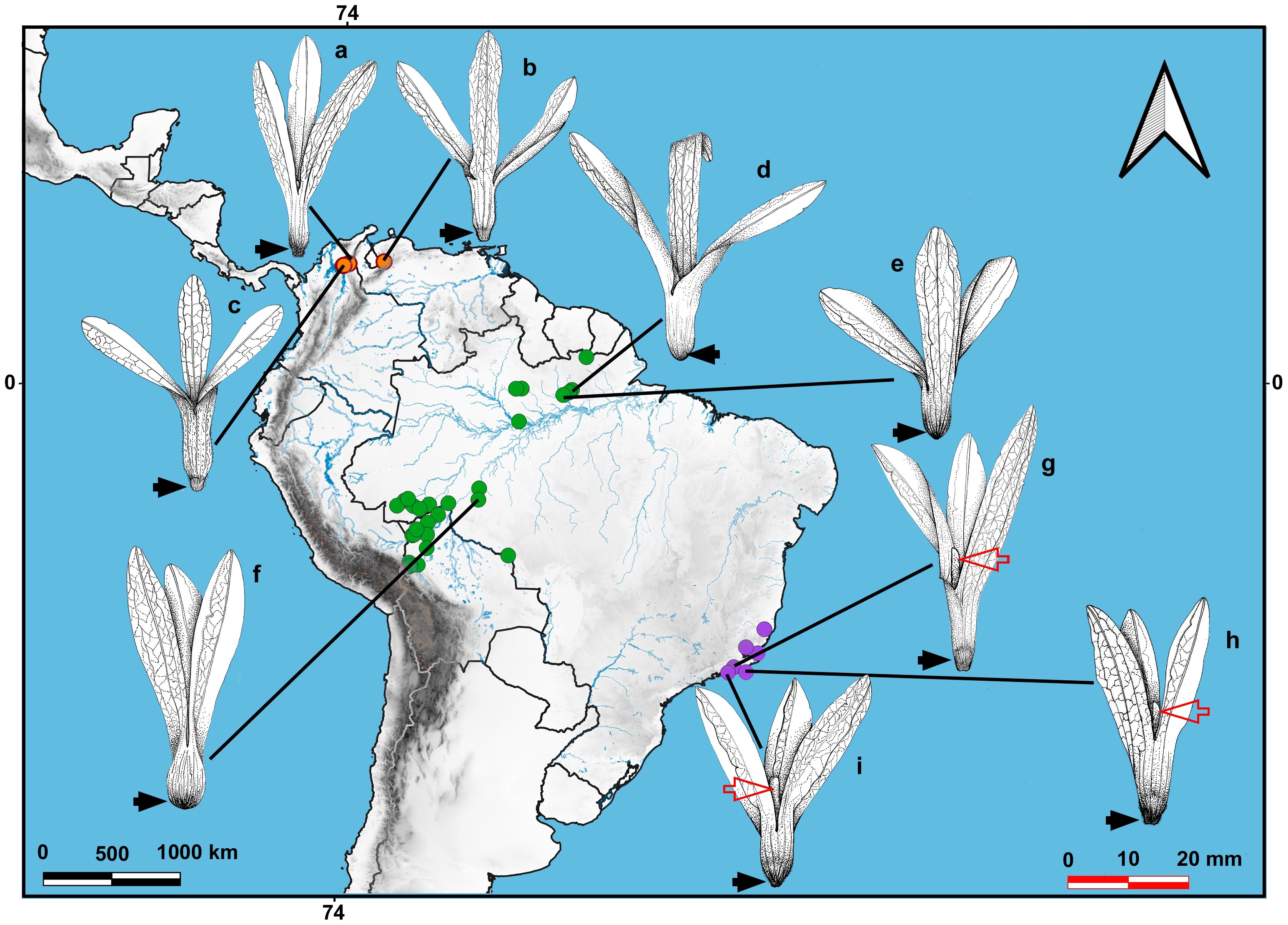
Figure 3 Geographical distribution and ripe fruit shape variation of Magoniella species. Fruit illustrations are based on herbarium specimens, as follows: Magoniella chersina: (a) Allen 929 (K-H3651/6019). (b) Aguilar-Cano 1905 (FMB-124471). (c) Pittier 13302 (MO-983676). Magoniella obidensis: (d) Ducke 2899 (F-602321). (e) Ducke 19542 (K). (f) Prance 6991 (K). Magoniella laurifolia: (g) Petroa 6703 (K). (h) Sucre 3512 (K). (j) Maquete 634 (K). Illustrations drawn by M. Paula Mendivil to a uniform scale (red–white bar). Key features, including the base of the perianth tube (black arrow) in the fruit abruptly or gradually tapering to the abscission zone, resulting in a narrow, somewhat sharp or broad obtuse base, and the sepaloid fruiting petals (red arrow), distinguishes M. chersina (orange dots) from M. laurifolia (purple dots) and M. obidensis (green dots).
The new species shares several characteristics with the other two species (Magoniella obidensis and M. laurifolia), including a strict lianaceous habit, leaf shape, hollow stems, and large green fruits with chartaceous reddish sepals (Figures 4A–D) (Pendry, 2004; Sanchez & Kron, 2011). However, it possesses a unique combination of fruit traits not found in any Magoniella species (Figures 3a–c, 4, 5; see species diagnosis and key to the species in the Taxonomic treatment section). In our comparative analysis, we conducted the Fisher-Pit permutation test implemented on measurements of six morphological characters for all Magoniella species. The results of the our comparative analysis revealed that bracteole length differed significantly between M. chersina and M. laurifolia, M. chersina and M. obidensis, and M. laurifolia and M. obidensis (Figure 6, Supplementary Table S5; p ≤ 0.05) and that M. obidensis has significantly larger fruits than those found in the new species and in M. laurifolia (Figure 6, Supplementary Table S5; p ≤ 0.05). The remaining five characters of M. chersina were significantly different from M. obidensis (Figures 6, Supplementary Table S5; p ≤ 0.05), but not from M. laurifolia (Figure 6, Supplementary Table S5; p ≤ 0.05). In summary, based on the morphological differences, disjunct geographical range, and phylogenetic divergence of the individuals found in the SDTFs of Colombia and Venezuela compared to their sister species, we formally describe these populations as a new species to science (see Taxonomic treatment).
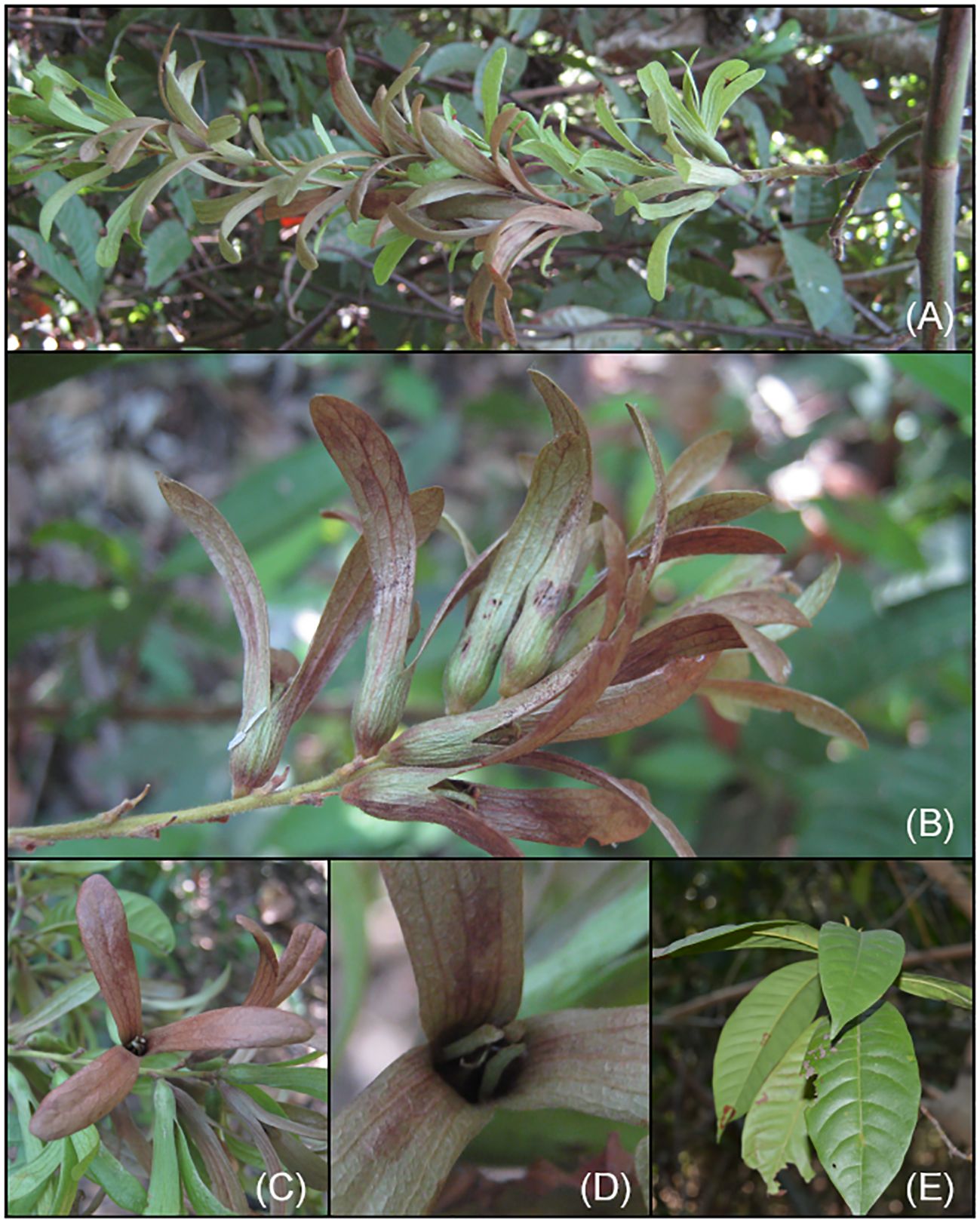
Figure 4 Magoniella chersina Aguilar-Cano & O.Pérez. (A) Habit and fruiting branch. (B) Infructescence. (C) Fruits seen from above showing sepals. (D) Fruit detail seen from above showing petals. (E) Leaves on the abaxial side of a juvenile individual.
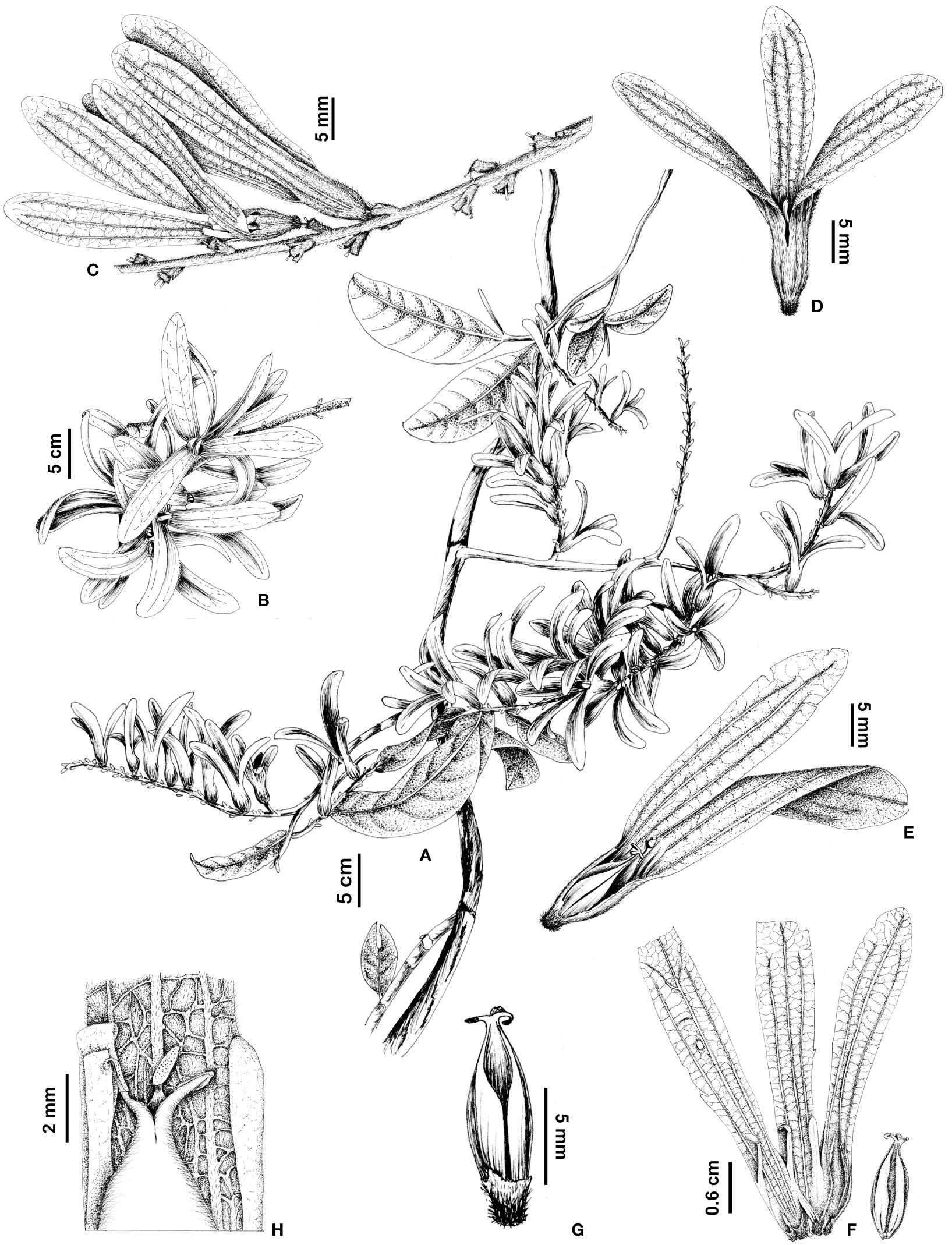
Figure 5 Magoniella chersina Aguilar-Cano & O.Pérez. (A) Habit and fruiting branch. (B) Infructescence. (C) Partial infructescence. (D) Fruit. (E) Longitudinal section of fruit. (F) Fruit with the sepals opened and seed. (G) Seed with basal extension of the calyx remnant. (H) Zoom-in on a seeds showing the upper portion. Illustration by Camila Pizano from the holotype specimen.
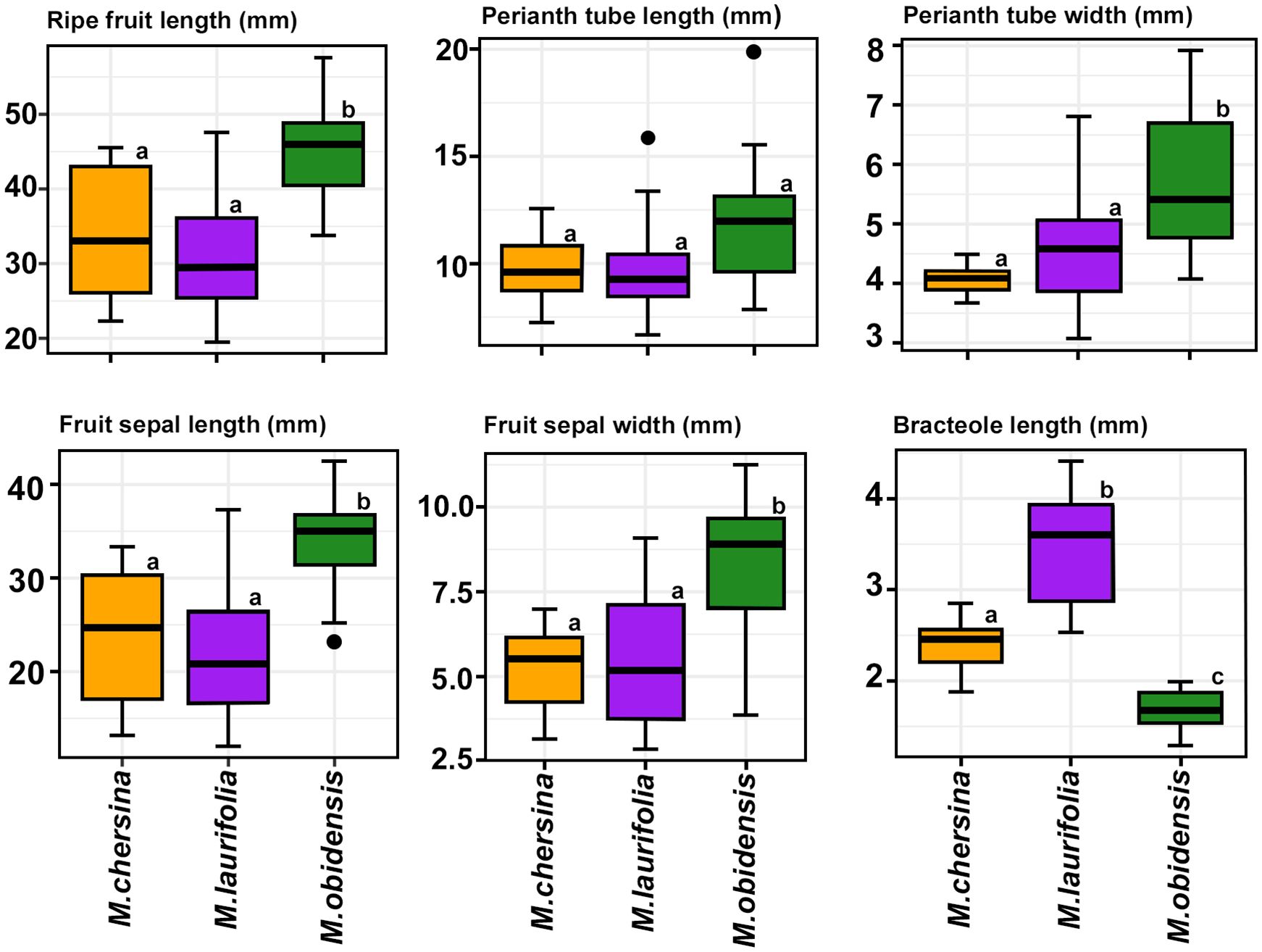
Figure 6 Differences in six morphological characters of ripe fruits among species of Magoniella (M. chersina, M. laurifolia, M. obidensis). The lowercase letters (a-c) above the boxes indicate statistically significant differences (there were no significant differences between species that are marked with the same letter). Note that bracteole length was the discriminatory trait for the three species.
Discussion
Our phylogenetic analyses revealed that Magoniella chersina and M. obidensis form a strongly supported clade, corroborating their exclusion from Ruprechtia, as previously reported by Sanchez and Kron (2011). Despite having a limited sample of M. obidensis in our analyses, these results further establish the placement of the new species within the monophyletic genus Magoniella (Figure 2). Moreover, while we were unable to include sequences from M. laurifolia in our phylogenetic analysis due to their absence in the NCBI database and our inability to collect specimens in the field or sequence DNA from historical specimens, the new species demonstrates a clear relationship with M. laurifolia. This is supported by shared morphological features (dense indumentum on the principal axis of the inflorescences, bracts, and base of the perianth tube). However, M. chersina can be distinguished by a set of fruit morphological characters, whereby its pedicels are longer than the bracteoles, clearly visible after the ripe fruits have fallen (Figure 5C) (vs. shorter than the bracteoles, not noticeable after the ripe fruits have fallen in M. laurifolia) and fruits with shorter linear petals, 10–11 mm (Figures 5D, F) (vs. petals sepaloid, variable, 17–30 mm in M. laurifolia).
The presence of the strict liana habit, not observed in any other species of Triplarideae, stands as a synapomorphy of Magoniella as previously proposed by Sanchez and Kron (2011) based on the species known at that time. The five accessions of M. chersina coalesced into a highly supported clade within Magoniella (Figure 2). Similar clustering patterns have been recovered in various unrelated lineages characterised by high levels of endemism across distinct regions of Neotropical SDTFs (Pennington et al., 2004). Our study contributed further evidence of the distinct phylogeographic structure often associated with STDF lineages, characterised by having long internal branches and terminal with short branch lengths (Pennington et al., 2004). These findings reinforce the notion that STDF species exhibit marked isolation and dispersal limitations, leading to in-situ speciation and strong niche phylogenetic conservatism (Pennington et al., 2004; Pennington and Lavin, 2016; Särkinen et al., 2011b).
The discordance in nuclear-plastid tree topologies observed in our datasets aligns with findings in numerous angiosperm lineages (Pérez-Escobar et al., 2017, 2021; Stull et al., 2023; Zuntini et al., 2024) (Figure 1). Here, the nuclear phylogeny strongly reflects relationship patterns supported by morphological evidence, such as partial infructescence, fruiting perianth tube and basal part of the fruiting perianth across the three genera. The Magoniella and Triplaris clades includes species with the base of perianth tube not extending into a narrow stalk terminated by scar from the abscised pedicel (Figures 1A, b, e). In contrast, the Ruprechtia s.s clade includes species with the base of the perianth tube extended into a pedicel-like structure, a stalk (Figures 1a–h). Notably, the Magoniella clade differs from other two major Triplarideae clades in having the basal part of the fruiting perianth tube either abruptly or gradually tapering to the abscission zone, resulting in a narrow or broad obtuse base (Figures 3a-i). This base never extends into a stalk, as in the Ruprechtia clade, or terminates abruptly into a truncate base, as in the Triplaris clade (Figures 3a-i).
Interestingly, the plastid tree appears to match well with the geographical distributions of the two lineages within Magoniella. M. obidensis occurs in humid forest of the Amazon basin, whilst M. chersina is exclusively distributed in disjunct locations within the SDTFs of the Colombian and Venezuelan Caribbean (Figure 1B). Plastid trees reflecting geographical clustering patterns at shallow phylogenetic levels as compared to nuclear trees is a phenomenon that has been reported for several plant lineages (e.g., Araceae: Nauheimer et al., 2012; Asteraceae: Fehrer et al., 2007; Orchidaceae: Pérez-Escobar et al., 2017; Polemoniaceae: Rose et al., 2020), including legumes (Gu et al., 2024). Here, the often-localised female gamete dispersal mode in angiosperms compared with the longer travelling male gametes could explain why uniparentally inherited organellar genomes better track geographical distribution patterns than phylogenies produced from biparentally inherited genomes (i.e., nuclear). Although the nuclear results show that M. chersina is distinct from sister M. obidensis (Figure 1B), when considered alone, the plastid phylogenomic evidence further strongly supports the hypothesis that M. chersina is indeed a divergent lineage from M. obidensis. Magoniella chersina shows no geographical overlap with the other two species in the genus (Figure 1B).
Colombian seasonally dry forests are estimated to have originated around 20,000 years ago, likely during the Last Glacial Maximum (Werneck et al., 2010), and have served as a cradle of rapidly speciating orchid lineages (Pérez-Escobar et al., 2024). However, this chronology contradicts phylogenetic evidence for dry-adapted Andean legumes, which suggests that legume lineages have been geographically isolated for several million years, spanning from 4.0 to 18.8 million years ago (da Cruz et al., 2018; Särkinen et al., 2011a). Furthermore, Pennington et al. (2004) suggested that Ruprechtia species from the Caribbean coast of Colombia and Venezuela comprise a lineage of post-Pleistocene diversification. This discrepancy merits further investigation, including a molecular dating analysis of tribe Triplaridae. This analysis will enable an evaluation of whether Magoniella chersina could have evolved in pockets of dry vegetation older than the Last Glacial Maximum, or initially thrived in humid forests and gradually adapted to the dry conditions of Colombian seasonally dry forests. As Antonelli et al. (2018) have demonstrated, transitions from forest to open habitats have been commonplace throughout the history of Neotropical plant evolution, which could provide an explanation for the unique case of Magoniella chersina.
Taxonomic treatment
1. Magoniella chersina Aguilar-Cano & O.Pérez sp. nov.
Type
COLOMBIA, Cesar, municipio de Chimichagua, vereda Santo Domingo Hacienda Rincón Grande. Fragmento de bosque inundable en las márgenes de la Ciénaga de Zapatosa, vegetación secundaria semiabierta dominada por Attalea butyracea y Cecropia peltata, 9°14’5.74”N 73°50’10.14”W, 29 m, 3 March 2015, José Aguilar Cano, Sandra Medina & Luis Morelo 1905 (holotype FMB-124471!, isotypes COL-615779, K) (Figures 4, 5).
Diagnosis
The new species differs from the other Magoniella species by the basal part of the fruiting perianth tube abruptly tapering to abscission zone, resulting in a narrow, somewhat sharp base of up to 2.5 mm long, completely covered with densely sericeous-pubescence of erect, longer trichomes and pedicels longer than the tubular bracteole, clearly visible after the ripe fruits have fallen. The nuclear ribosomal ITS region differs from that of M. obidensis by a) the GT → TA (176–178 bp), TC → CT (217–219 bp), TA → AT (274–276 bp), CA → AC (277–279 bp) substitutions; b) 5-point transitions (T → C: 445 bp, 492 bp, 592 bp; C → T: 225 bp,; A → G: 574 bp); and c) 1 point transversion (G → T: 670 bp). Additionally, the lfy2 gene of M. chersina differs from that of M. obidensis by containing 5-point transitions (A → G: 250 bp; G → A: 350 bp; C → T: 488 bp, 954 bp; T → C: 1,173 bp) and 4 transversion (G → T: 426 bp, 584 bp; T → G: 820 bp; A → T: 926 bp).
Description
Liana, young individuals erect to 50 cm tall; tap root; twigs terete, hollow, green when very young and pale griseous with age and inhabited by ants in dilated nodes, slightly lenticellate, young branches slightly pubescent, trichomes appressed, golden-brown, glabrescent to completely glabrous with age.
Stipules persistent ochreas, entire, 5–6 (–7) mm long, encircling the twigs, caducous with age, slightly pubescent especially towards the margin, long trichomes appressed, glabrescent with age. Leaves alternate, membranaceous to sub-chartaceous, elliptic, leaf blade 18–27.5 × 7–10.5 cm in erect young individuals and 6.5–18.5 × 2.2–7 cm in lianescentes adult individuals, base cuneate, sometimes short decurrent at petiole, apex acuminate, margins moderately undulate, adaxial leaf surface glabrous, abaxially glabrescent, sometimes with minute dark glands, pubescent especially along the midrib and secondary veins, short trichomes appressed; venation brochidodromus, midrib elevated adaxially, secondary veins prominent abaxially, 12–23, veins per side, slightly sunken adaxially with the lamina bullate, tertiary venation faint and dense scalariform-reticulate; petioles 0.5–1.5 cm long, glabrous adaxially, pubescent abaxially, trichomes appressed. Male inflorescences axillary, usually simple, rarely branched at the base, 13–26 cm long; flowers arranged in partial inflorescences along the principal axis, internodes to 3–5 mm long, densely pubescent, erect or wavy short trichomes; each partial inflorescences 3–5 flowered, bearing by a minute bract, 1–1.5 mm long, acute, densely pubescent, appressed trichomes. Male flowers notably pedicellate, 2.7–3.0 mm long, remaining after the flowers have been fallen, glabrescent; subtended by tubular bracteoles, 2 mm long, glabrescent; sepals 3, ovate, up 1.0 mm long, pubescent; petals 3, ovate, 0.8–1.3 mm long, sparsely pubescent; stamens 9 in two whorls (6 outer and 3 inner), filaments 0.5–0.9 mm long; anthers 0.35–0.40 mm long. Female flowers not seen. Infructescence axillary simple, when terminal branched, up to 20 cm long; lax, fruits arranged in partial infructescence along the principal axis, internodes 2–10 mm long, densely pubescent, erect or wavy short trichomes; partial infructescence bearing by a bract, to 2 mm long, acute, densely pubescent, appressed trichomes, each partial infructescence with 2 fruits, subtended by single tubular bracteole, 1.9–2.8 mm long, densely pubescent, appressed trichomes; pedicels longer than the bracteoles, 2.4–2.9 mm long, densely sericeous at the base, glabrous towards the apex, remaining after fruits have been fallen. Fruits winged achenes, 37–41 mm long, completely green to green with variegate reddish immature, completely red when ripe; perianth tube of ripe fruits narrowly elongate, campanulate, 6–8 mm long, sparsely pubescent, appressed trichomes, the basal part of the fruiting perianth tube shortly tapering to abscission zone, resulting in a narrow, somewhat sharp base up to 2.5 mm long, completely covered with densely sericeous-pubescence with erect, longer trichomes; sepals of ripe fruits diverging from a slightly constricted zone above of seed, 25–35 × 6–9.5 mm long, obovate, chartaceous, abaxially glabrescent, adaxially glabrous, main and secondary venation evident, tertiary venation, densely reticulate; fruiting petals persist, linear, 10–11 mm long, oblong, adnate to the inside of the perianth tube, the free part 5.5–6.5 × 0.7–0.8 mm long, abaxially glabrescent, adaxially glabrous; staminodes ca. 0.5 mm long in fruit; disk glabrous; seeds three-lobed, 11–12 mm long, smooth, glabrous, stigmas persist in fruit, linear to ellipsoid 0.8–1.0 mm long.
Additional specimens examined (paratypes)
COLOMBIA, Cesar, municipio de Chimichagua, vereda Santo Domingo, Vereda Santo Domingo. Hacienda Rincón Grande. Fragmento de bosque inundable en las márgenes de la Ciénaga de Zapatosa, vegetación secundaria semiabierta dominada por Attalea butyracea y Cecropia peltata.9°14’5.74”N 73°50’10.14”W, 29 m, 15 de abril 2016, José Aguilar-Cano, Sandra Medina, Felipe Villegas & Juan Carlos Roble 3177 (FMB-124472; COL); 9°14’5.74”N 73°50’10.14”W, 29 m, 15 de abril 2016, José Aguilar-Cano, Sandra Medina, Felipe Villegas & Juan Carlos Roble 3180 (FMB-124474); vereda Ojo de agua. Predios de Asopeagro, vegetación secundaria abierta asociada a las márgenes de la Ciénaga de Zapatosa, compuestas por densos entramados de bejucos y arbustos, el único elemento emergente corresponde a la palma Attalea butyracea”. 9°15’32.98”N 73°46’38.39”W, 27 m, 15 abril 2016, José Aguilar Cano, Sandra Medina, Felipe Villegas & Juan Carlos Roble 3178 (FMB-124473); 9°15’32.98”N 73°46’38.39”W, 27 m, 15 abril 2016, José Aguilar-Cano, Sandra Medina & Felipe Villegas 3179 (FMB-124475); municipio de Chiriguaná, Poponte, [9°23’38.90”N 73°22’49.32”W, 93 m], 1 de febrero 1925, C. Allen 929 (K). VENEZUELA, Trujillo, between Quebrada Seca bridge and Motatán, [9°32’22.56”N 70°36’37.76”W, 400 m], 1 de febrero 1929, R., Pittier 13302; 13299 (F, GH, M, MO, NY, VEN).
Distribution and ecology
Magoniella chersina is restricted to the Neotropical dry forest (SDTF) nuclei of the Caribbean coast of Colombia and Venezuela. In Colombia, it is known from three localities in Cesar; two populations ca 7 km apart (including the type locality) exist in Chimichagua municipality, situated at the north of the Zapatosa floodplain lake complex. These regions are 15–29 m in elevation, and represent one of the largest freshwater marshes in Colombia. Notably, this area has been designated as a Ramsar wetland of conservation priority. An additional population is located in the Chiriguana municipality, at the lower part of the western foothills from the Perijá massif, northernmost of the Cordillera Oriental, at ca 46 m of elevation.
The populations from the holotype and paratype were collected in seasonally inundated flooded areas near the wetland edges in quite disturbed forest openings, with sites mostly dominated by the palm Attalea butyracea and the pioneer tree Cecropia peltata, on which the new species climbs (Figures 7A, B). Burning for agriculture seemed to be frequent in the type locality (Figure 7B), potentially posing a threat to the new species and its native ecosystem. The record of the Perijá massif was collected almost a century ago by C. Allen in 1925 and deposited in the K herbarium. Several researchers observed the material of the previously undescribed species but did not formally describe it, incorrectly identifying it as Ruprechtia laurifolia, R. obidensis, and Triplaris scandens. Similarly, Henri Pittier collected two specimens in 1929, which were initially identified as M. obidensisis, and these were found in the lower part of the western foothills of the northern areas in the Venezuelan Cordillera of Mérida, near to the Maracaibo Lake (Pendry, 2004). As a result, all known populations of the new species are disjunct in their distribution compared to populations of M. obidensis and M. laurifolia, which are primarily restricted to the Amazon basin and the Atlantic Forest, respectively (Figure 3). The geographical range of Magoniella chersina falls completely within the distinctive dry forest vegetation (Figure 1b), and does not share its habitat with any close relatives in the Caribbean coast of Colombia and Venezuela (Figure 3). Moreover, none of the species of Ruprechtia s.s. and Triplaris that occur in the neotropical SDTF nuclei could be confused with M. chersina. Therefore, the new species is considered endemic to the Caribbean coast of Colombia and Venezuela, representing the first record of the genus Magoniella in the flora of those two countries.
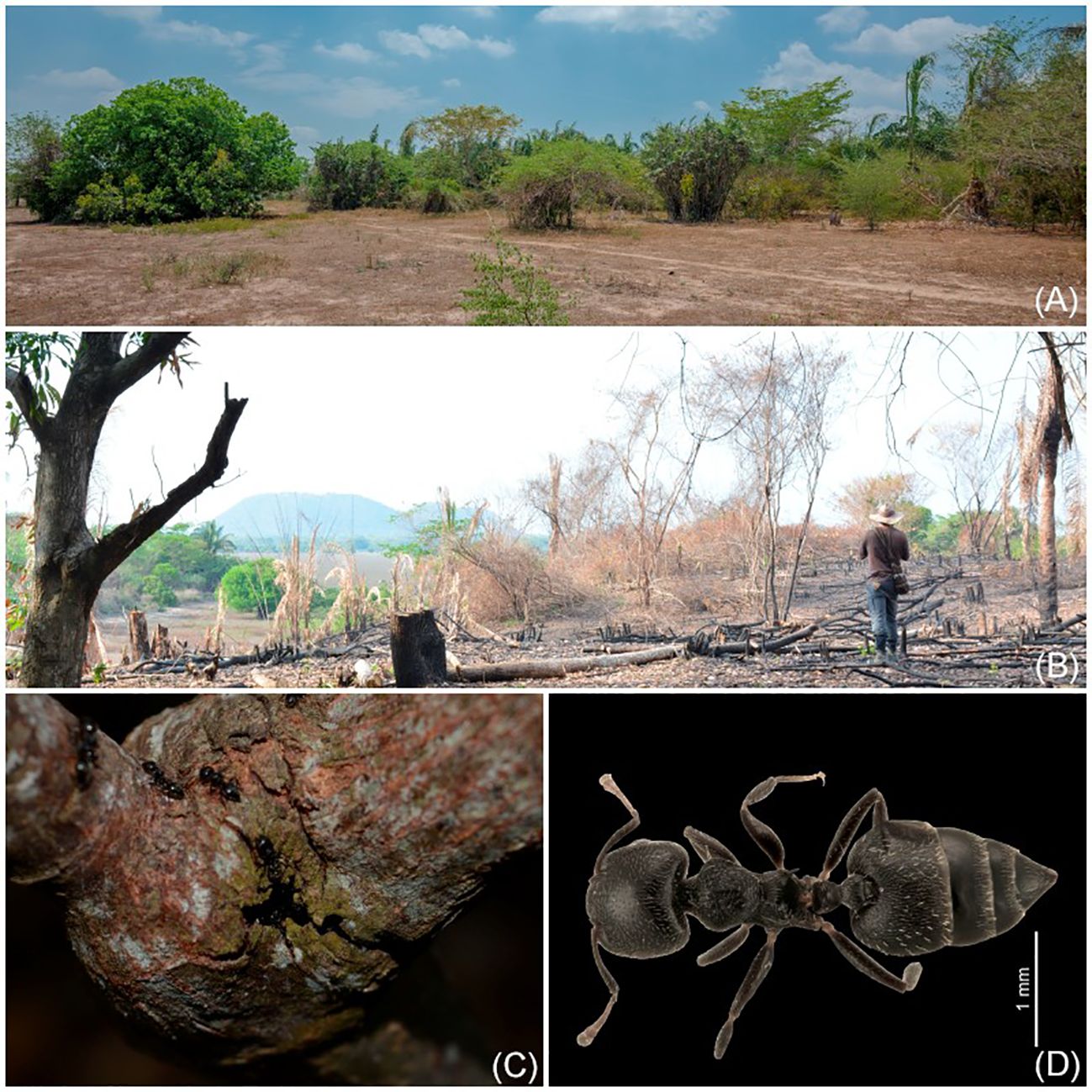
Figure 7 (A) Habitat of Magoniella chersina in its the type locality. (B) Habitat of M. chersina at the north of the Zapatosa floodplain lake complex in Cesar (Colombia), affected by slash-and-burn agriculture. (C) Stems with hollow nodes inhabited by ants. (D) Crematogaster erecta Mayr, 1866, [IAvH-E-261079; IAvH-E-261080], dorsal. Photos: Felipe Villegas (A, B), José Aguilar-Cano (C), Jhon Neita (D).
Hollow internodes have been found in seven species of Ruprechtia, but only R. tangarana, a western Amazonian species, has been reported to be inhabited by Pseudomyrmex ants (Brandbyge and Øllgaard, 1984; Brandbyge, 1989). Additionally, R. cruegeri, R. laurifolia, R. lundii, R. maracensis, and R. latifunda appear to host ants, but the species found belong to the genus Crematogaster (Formicidae, Myrmicinae) (Pendry, 2004; Sanchez and Kron, 2011). In Magoniella, only M. obidensis has been reported to harbor ants (Pendry, 2004; Sanchez and Kron, 2011). During our study, we observed the presence of Crematogaster distans (Mayr 1870) (Figure 7D) associated with ten vines of the new species. These ants were found in hollow internodes and in the unvascularised areas near the nodes, where the stem widens and develops cracks, allowing ants to enter and exit the host plant (Figure 7C).
While opportunistic ants might be found in any plant with hollow cavities (Mayer et al., 2014), in the case of Triplaris, a close relative of Magoniella, there appears to be a tendency for related species of Pseudomyrmex ants to prefer the same plant species (Ward, 1999; Torres and Sanchez, 2017). Most ants show a broader host range, indicating that there are also varying degrees of ant-host specificity (Sanchez, 2015). Although it is uncertain whether these ants offer any protection from herbivory in Magoniella chersina or are obligate mutualists, we found them always nesting inside the plants. Therefore, the association between Magoniella chersina and Crematogaster distans cannot be definitively classified as obligate or specific mutualism, and further collections are warranted to clarify the nature of the interaction and its mode of evolution between these two organisms.
Phenology
The fruits of the new species were collected in March and historical specimens were collected in fruit in February. Flowering does not seem to be annual, given that in 2015 the plant was fruiting, but the following year (April 2016) it was found in a sterile state and with abundant regeneration from the previous year’s flowering and fruiting. In contrast, Magoniella obidensis and M. laurifolia typically undergo flowering and fruiting in June and October. These differences in phenological seasonality between M. chersina and its sister species are noteworthy.
Dispersal
The winged achenes of the Triplarideae species develop from the perianth, and their wind dispersal is associated with different levels of flight performance, dependent on the relationship between symmetry, wing angle, and fruit weight. Generally, mature fruits are dispersed a few meters from the mother plant (Pendry, 2004; Finalé, 2016). The winged achenes in Magoniella (Figure 3) also appear adapted to wind-dispersal, yet their dry wings and low density also allow them to float on the water surface and to be transported by water currents. Therefore, we propose that the fruits of M. chersina (Figures 3, 5) are mainly dispersed by water, the primary dispersal method for species in riverine forests that lack or have limited long-distance wind dispersal. While most dry forest lianaceous species rely on wind for dispersal (Gentry, 1995), water dispersal is more commonly associated with rheophytic plants in swamps, watercourses, and shorelines, playing a limited role in the dispersal of seeds and fruits of terrestrial plants (van der Pijl, 1982; Weberling, 1989). In its wetland edge habitat, the new species likely relies on water for its dispersal, with wind potentially important only for short-distance dispersal.
Etymology
The epithet is derived from the Greek root chersin (=dry). This choice reflects current knowledge of the species’ habitat preference for dry areas, unlike the other two species of the genus that inhabit humid rainforests.
Conservation status
This species is endemic to the STDF of the Colombian and Venezuelan Caribbean. We observed that two of the four currently known populations of the new species occur in the Zapatosa floodplain lake complex, a Ramsar wetland conservation area corresponding to the type localities. These two populations have a combined total of ca 15 adult individuals, with a continuous recruitment of new individuals from seed. Young individuals are densely aggregated in populations of between 25 and 75 young individuals, clustered around parent plants, showing that populations of the new species seem to have high seedling recruitment. However, seasonally inundated flooded areas near the wetland edges are highly fragmented and the few remnants that persist are surrounded by roads, croplands, and cattle pastures (Rivera et al., 2013) and exposed to continuous threats, such as habitat loss because of non-sustainable activities, logging, fire, and illegal settlements (Figures 7A, B).
Two additional herbarium specimens were collected in the 1920s in areas that now appear on satellite imagery to have little forest cover left, with land use changes, including agriculture, mining, or urban settlements. New surveys at the known localities for this species are recommended to confirm the species’ distribution and establish the current population size and trends.
Almost two-thirds of dry forest in Colombia and Venezuela have been lost, primarily due to agriculture and cattle grazing, and less than 5% is under protection (Fajardo et al., 2005; Rodríguez et al., 2008; García et al., 2014; González-M et al., 2018). This means that modern threats in this area are common and frequent, and the habitat of this species is in continuous decline. The EOO of this species has been estimated at 2,265 km2, below the threshold for the Endangered category, and its habitat has been continually declining, with less than five current locations. However, information on populations is incomplete, and the species could not be assessed based on its population size and decline. We suspect that the population has been declining, based on the species’ habitat preference and habitat loss in its region of occurrence. We suggest that M. chersina should be considered as Endangered [EN B1ab (iii)], due to its restricted range and considering the lowest number of locations combined with an inferred continuing decline in its habitat. We recommend that a formal IUCN Red List assessment is carried out swiftly.
2. Magoniella obidensis (Huber) Adr. Sanchez
Basionym: Ruprechtia obidensis Huber in Bol. Mus. Paraense Nat. Hist. 5: 344. 1909. TYPE: BRAZIL. Para: Obidos, capueira, 31 Jul 1902, A. Ducke 2899 (lectotype, here designated: MG 002899!; isolectotypes: BM 571400!, F 602321 (photo & fragment)!, G 00437689!. Ruprechtia apetala var. sprucei Meisn. in C.F.P.von Martius & auct. suc. (eds.), Fl. Bras. 5(1): 57. 1855. —TYPE: Brazil. Para: circa Santarém, Jun 1850, Spruce s.n. (holotype: G 00437688!, Field Museum negative no. 7414: F, GH 00036827! GOET 006066! NY 00260344!. Ruprechtia macrocalyx Huber, Bol. Mus. Goeldi Hist. Nat. Ethnogr. 5: 345. 1909. —TYPE: Brazil. Para: Faro, capoeira, 27 Aug 1907, Ducke 8540 (lectotype, here designated: MG 008539!; isolectotypes: BM 000571404!, F 651468 (photo & fragment)!, G 00437692!. Ruprechtia scandens Rusby, Mem. New York Bot. Gard. 7: 237. 1927. —TYPE: Bolivia. El Beni: Huachi, head of the Beni River, 1800 ft, 18 Aug 1921, Rusby & White 972 (lectotype designated by Pendry, 2004: NY 00260320! (two sheets); isolectotypes: B 100250422, BKL 00004118, GH 00036834!, GH 00036833, MICH 1111770!, NY 00260321!, NY 00260319!, K 000585097, K 000585098!, 00260319! US)!. Coccoloba zernyi Standl. in Publ. Field Mus. Nat. Hist., Bot. Ser. 22: 18 (1940). Ruprechtia zernyi (Standley) R. A. Howard, J. Arnold Arbor. 61: 390. 1960. —TYPE: Brazil. Amazonas: Taperinha, near Santarém, 13 Aug 1927, Zerny 890 (holotype: F934804!; isotype: GH 00055152 (photo & fragment)!.
Nomenclatural notes
The combination Magoniella obidensis (Huber) Adr.Sanchez, was published using Ruprechtia obidensis Huber, its older basionym (Sanchez and Kron, 2011). However, this author incorrectly cited an exsiccatae collected by Henry H. Rusby as the type specimen, the latter corresponded to a heterotypic synonym of R. scandendes Rusby from Bolivia (Mem. New York Bot. Gard. 7: 237. 1927). Therefore, the Rusby specimen does not correspond to the specimens cited by Huber in the protologue of R. obidensis (A. Ducke 2899, 2901). Whilst an erroneously cited specimen does not invalidate a combination (Turland et al., 2018, Art. 41.6), we here provide an exsiccatae correction through a basionym citation to avoid further ambiguity.
Huber (1909) described Ruprechtia obidensis using two specimens, Ducke 2899 ♀ and Ducke 2901 ♂; both were cited in the protologue. They did not designate either of the two as the holotype, so both specimens constitute syntypes according to Article 40, Note 1 of the International Code of Nomenclature for algae, fungi, and plants (ICN) (Turland et al., 2018). Pendry (2004) refers to the collection deposited in MG as the holotype specimen, with an isotype located in the BM herbarium. However, this cannot be considered an inadvertent lectotypification because the publication does not state that a lectotype is being designated and was published after 1 January 2001 (see Turland et al., 2018). Therefore, a lectotype still needs to be designated.
Subsequently, Pendry (2004) cited specimens collected by Ducke (8539 ♂ and 8540 ♀) stored at the herbarium BM, with isotypes in F, G, GH, MO, NY, as the holotype of Ruprechtia macrocalyx. However, when Huber (1909) described this species, they did not designate in the protologue any of the two specimens as the holotype. Again, according to Article 40, Note 1 of the ICN (Turland et al., 2018), both specimens are thus considered syntypes, and the designation of a lectotype is required. Because Pendry’s publication predates 1 January 2001, it also cannot be considered an inadvertent lectotypification. Therefore, the specimen Ducke 2899, ♀ (deposited in MG, accession 002899) and Ducke 8540, ♀ (deposited in MG, accession 008539) are selected as the lectotype of R. obidensis and R. macrocalyx respectively. These lectotypifications are done following Article 8.1 of the ICN (Turland et al., 2018), giving priority to the specimen that comes from the female plant since in this group the species diagnosis falls mostly on female reproductive characters. Additionally, we can check duplicates for both species at BM, F, and G.
3. Magoniella scandens (Vell.) Aguilar-Cano comb. nov
Basionym: Magonia scandens Vell. in Fl. Flum.: 165. 1825. Triplaris scandens (Vell.) Cocucci, Revista Fac. Ci. Exact. 19: 361. 1957. Magoniella laurifolia (Cham. & Schltdl.) Adr. Sanchez, Syst. Bot. 36 (3): 708. 2011, comb. inval. nom. illeg.— TYPE: Vellozo’s illustration, Flora Fluminensis icones 4: t. 60 (lectotype designated by Pendry, 2004. Triplaris laurifolia Cham. & Schltdl, Linnaea 3: 55. 1828. Ruprechtia laurifolia (Cham. & Schltdl.) C. A. Meyer, Mém. Acad. Imp. Sei. Saint-Pétersbourg, Sér. 6, Sei. Math., Seconde Pt. Sei. Nat. 6: 150. 1840, syn nov. —TYPE: BRAZIL. “Brasilia aequinoctialis” Sello s.n. (lectotype designated by Pendry, 2004: B 101001716!; isolectotypes: B 101001717!, BR 5288069!, HAL 0098197)!.
Nomenclatural notes
Magoniella laurifolia (Cham. & Schltdl) Adr. Sanchez, is a combination proposed by Sanchez and Kron (2011) based on Magonia scandens Vell. (“Fl. Flum.: 165. 1825. — TYPE: Vellozo’s illustration, Flora Fluminensis icones 4: t. 60 [lectotype designated by Pendry, 2004]”) as the intended basionym. However, Sanchez proposed this combination based on Triplaris laurifolia Cham. & Schldl (in Sanchez & Kron, 2011; IPNI, 2022), therefore M. laurifolia is a name that is not validly published (comb. inval.), because Sanchez did not correctly cite the author of this basionym. The nomenclatural action of Sanchez represents an invalid combination due a mistake in the author’s name (T. laurifolia Cham. & Schldl., see: Turland et al., 2018, Art. 41.5). Additionally, according to Article 11.4 of the ICN, the epithet “scandens” is the earliest legitimate name available for a transfer to Magoniella even though it was published under Magonia Vell., a later homonym of Magonia A. St.-Hil. (Sapindaceae), Kuntze (1898) (Turland et al., 2018, Art. 55.1). Therefore, the new combination provided here under Article 6.10 (Turland et al., 2018), must replace Magoniella laurifolia (Cham. & Schltdl.) Adr. Sanchez. This name is nomenclaturally superfluous sensu the current taxonomy of Magoniella (Turland et al., 2018, Art. 52) because the name M. laurifolia included the type of a heterotypic synonym, whose epithet was available and not already pre-empted in the genus Magoniella. Hence, we here propose the new combination Magoniella scandens (Vell.) Aguilar-Cano based on Magonia scandens Vell.
Key to the species of Magoniella
1. Pedicels shorter than the bracteoles, not noticeable after the ripe fruits have fallen……………………………………………………………………………………….3. M. scandens.
1’. Pedicels longer than the tubular bracteole, clearly visible after the ripe fruits have fallen……………………………………………………………………………………………2.
2. Base of the perianth tube in the fruit abruptly taper to scars, resulting in a narrow, somewhat sharp base, completely covered with a dense indumentum; bracts 1.9–2.8 mm long……………………………………………………………………………………………….1. M. chersina.
2’. Base of the perianth tube in the fruit no tapering to scar, resulting in a broad obtuse base, glabrescent to completely glabrous; bracts 1.3–2.8 mm long……….2. M. obidensis.
Data availability statement
The datasets presented in this study can be found in online repositories. The names of the repository/repositories and accession number(s) can be found in the article/Supplementary Material.
Author contributions
JA-C and OAP-E conceived the study. JA-C produced the morphological diagnosis of the new species. OAP-E and JA-C produced the molecular diagnosis of the new species. JA-C developed the taxonomic treatment and conducted morphometrical analyses. JA-C and ET conducted wet-lab work. JA-C and OAP-E conducted molecular analyses. CP provided the pen and ink illustrations of this new species. JA-C prepared figures. JA-C and OAP-E wrote the manuscript, with contributions from CP, AA, and ET. All authors contributed to the article and approved the submitted version.
Funding
The author(s) declare financial support was received for the research, authorship, and/or publication of this article. The first author is grateful to the Jardín Botánico de Bogotá (JBB) for financial support for the preparation of this manuscript; Juan José Aguilar-Pachón for the support in the edition of the figures and maps; OAP-E is supported by the Orchid Sainsbury Fellowship at the Royal Botanic Gardens, Kew, and the Swiss Orchid Foundation.
Acknowledgments
To Julio Betancur for their help accessing the herbarium COL and Gerardo Aymard for providing advice on taxonomic nomenclature. John Neita identified the ant specimens found in the hollow stems of M. chersina.
Conflict of interest
The authors declare that the research was conducted in the absence of any commercial or financial relationships that could be construed as a potential conflict of interest.
Publisher’s note
All claims expressed in this article are solely those of the authors and do not necessarily represent those of their affiliated organizations, or those of the publisher, the editors and the reviewers. Any product that may be evaluated in this article, or claim that may be made by its manufacturer, is not guaranteed or endorsed by the publisher.
Supplementary material
The Supplementary Material for this article can be found online at: https://www.frontiersin.org/articles/10.3389/fpls.2024.1253260/full#supplementary-material
SUPPLEMENTARY FIGURE S1 | Maximum likelihood phylograms inferred from combined (A) ITS + lfy2i nuclear loci and (B) combined matK + ndhF + rps16-trnK + ndhC-trnV plastid loci. Fully black pie charts at nodes indicate nodes attaining maximum statistical support. Circles preceding species names indicate potential outlier (red circle) and congruent (blue circle) terminals.
SUPPLEMENTARY FIGURE S2 | Map Neotropical biomes of sampled species shown in front of tree terminals with coloured boxes preceding species names in Figure 1a. Based and adapted from terrestrial biomes proposed by Dinerstein et al. (2017).
References
Aguilar-Cano, J., Diaz-Pulido, A., Mesa-S, L., Fernanda González, M. (2016). “Biodiversidad local,” in Catálogo de biodiversidad de la región caribe. Serie Planeación ambiental para la conservación de la biodiversidad en áreas operativas de Ecopetrol. Proyecto Planeación ambiental para la conservación de la biodiversidad en las áreas operativas de Ecopetrol, vol. 3, eds. Mesa-S., L., Santamaría, M., García-Martínez, H., Aguilar-Cano, J. (Instituto de Investigación de Recursos Biológicos Alexander von Humboldt, Bogotá), 225–259.
Antonelli, A., Zizka, A., Carvalho, F. A., Scharn, R., Bacon, C. D., Silvestro, D., et al. (2018). Amazonia is the primary source of Neotropical biodiversity. Proc. Natl. Acad. Sci. U. S. A. 115, 6034–6039. doi: 10.1073/pnas.1713819115
Bachman, S., Moat, J., Hill, A., de la Torre, J., Scott, B. (2011). Supporting Red List threat assessments with GeoCAT: geospatial conservation assessment tool. ZK 150, 117–126. doi: 10.3897/zookeys.150.2109
Balbuena, J. A., Míguez-Lozano, R., Blasco-Costa, I. (2013). PACo: A novel procrustes application to cophylogenetic analysis. PloS One 8, e61048. doi: 10.1371/journal.pone.0061048
Banda-R, K., Delgado-Salinas, A., Dexter, K. G., Linares-Palomino, R., Oliveira-Filho, A., Prado, D., et al. (2016). Plant diversity patterns in neotropical dry forests and their conservation implications. Science. 353, 1383–1387. doi: 10.1126/science.aaf5080
Brandbyge, J. (1989). Notes on the genus Ruprechtia (Polygonaceae). Nordic. J. Bot. 9, 57–61. doi: 10.1111/j.1756–1051.1989.tb00985.x
Brandbyge, J. (1986). A revision of the genus Triplaris (Polygonaceae). Nordic. J. Bot. 6, 545–570. doi: 10.1111/j.1756–1051.1986.tb00454.x
Brandbyge, J., Øllgaard, B. (1984). Inflorescence structure and generic delimitation of Triplaris and Ruprechtia (Polygonaceae). Nordic. J. Bot. 4, 765–769. doi: 10.1111/j.1756–1051.1984.tb02007.x
da Cruz, D. T., Idárraga, Á., Banda-R, K., Cogollo, Á., van den Berg, C., de Queiroz, L. P., et al. (2018). Ancient speciation of the papilionoid legume Luetzelburgia jacana, a newly discovered species in an inter-Andean seasonally dry valley of Colombia. Taxon. 67, 931–943. doi: 10.12705/675.6
De Queiroz, K. (2007). Species concepts and species delimitation. Syst. Biol. 56, 879–886. doi: 10.1080/10635150701701083
Dinerstein, E., Olson, D., Joshi, A., Vynne, C., Burgess, N. D., Wikramanayake, E., et al. (2017). An ecoregion-based approach to protecting half the terrestrial realm. BioScience. 67, 534–545. doi: 10.1093/biosci/bix014
Donoghue, M. J. (1985). A critique of the biological species concept and recommendations for a phylogenetic alternative. Bryol. 88, 172. doi: 10.2307/3243026
Fajardo, L., Gonzalez, V., Nassar, J. M., Lacabana, P., Portillo Q., C. A., Carrasquel, F., et al. (2005). Tropical dry forests of Venezuela: characterization and current conservation status. Biotropica. 37, 531–546. doi: 10.1111/j.1744–7429.2005.00071.x
Fehrer, J., Gemeinholzer, B., Chrtek, J. C. J. R., Bräutigam, S. (2007). Incongruent plastid and nuclear DNA phylogenies reveal ancient intergeneric hybridization in Pilosella hawkweeds (Hieracium, Cichorieae, Asteraceae). Mol. Phylogenet. Evol. 42, 347–361. doi: 10.1016/j.ympev.2006.07.004
Finalé, G. L. (2016). Desempeño de vuelo de los frutos de Triplaris americana (Polygonacea) según su morfología / Flight performance of Triplaris americana (Polygonacea) fruits according to their morphology. Rev. Del. Jardín. Botánico. Nacional. 37, 47–51.
Fisher, R. A. (1936). The use of multiple measurements in taxonomic problems. Ann. Eugenics. 7, 179–188. doi: 10.1111/j.1469–1809.1936.tb02137.x
Freudenstein, J. V., Broe, M. B., Folk, R. A., Sinn, B. T. (2016). Biodiversity and the species concept—Lineages are not enough. Syst. Biol. 66, 644–656. doi: 10.1093/sysbio/syw098
García, H., Corzo, G., Isaacs-Cubides, P. J., Etter, A. (2014). “Distribución y estado actual de los remanentes del bioma de Bosque Seco Tropical en Colombia: insumos para su gestión,” in El Bosque Seco Tropical en Colombia, eds. Pizano, C., García, H. (Instituto de Investigación de Recursos Biológicos Alexander von Humboldt, Bogotá), 229–251.
Gentry, A. (1995). “Diversity and floristic composition of neotropical dry forest,” in Seasonally Dry Tropical Forests, eds. Bullock, S. H., Mooney, H. A., Medina, E. (Cambridge University Press, Cambridge), 146–194.
González-M, R., García, H., Isaacs, P., Cuadros, H., López-Camacho, R., Rodríguez, N., et al. (2018). Disentangling the environmental heterogeneity, floristic distinctiveness and current threats of tropical dry forests in Colombia. Environ. Res. Lett. 13, 045007. doi: 10.1088/1748–9326/aaad74
Govaerts, R., Nic Lughadha, E., Black, N., Turner, R., Paton, A. (2021). The World Checklist of Vascular Plants, a continuously updated resource for exploring global plant diversity. Sci. Data. 8, 215. doi: 10.1038/s41597–021-00997–6
Grace, O. M., Pérez-Escobar, O. A., Lucas, E. J., Vorontsova, M. S., Lewis, G. P., Walker, B. E., et al. (2021). Botanical Monography in the Anthropocene. Trends Plant Sci. 26, 433–441. doi: 10.1016/j.tplants.2020.12.018
Gu, S.-R., Zeng, Q.-B., Clark, R., Jiang, K.-W., Pérez-Escobar, O. A., Li, S.-J., et al. (2024). Phylogeny and re-circumscription of Cheniella (Leguminosae: Cercidoideae) based on plastomes data and morphology, with description of three new species. Taxon. 73, 475–502. doi: 10.1002/tax.13177
Holdridge, L. R. (1947). Determination of World Plant Formations From Simple Climatic Data. Science. 105, 367–368. doi: 10.1126/science.105.2727.367
Huber, J. (1909). Materiaes para a Flora amazonica VII. Plantae Duckeanae austro-guyanenses. Enumeração das plantas siphonogmas colleccionadas de 1902 a 1907 na Guyana brasiieira pelo Sr. Adolpho Ducke e determinados pelo Dr J. Huber. Bol. Mus. Goeldi Hist. Nat. Ethnogr. 5, 294–436.
IPNI. (2022). International Plant Names Index. Available online at: http://www.ipni.org (Accessed June 5, 2022).
IUCN Standards and Petitions Subcommittee. (2013). Guidelines for using the IUCN Red List Categories and Criteria. Version 10.1. Available online at: http://www.iucnredlist.org/documents/RedListGuidelines.pdf (Accessed November 25, 2021).
Ivanova, N. V., Fazekas, A. J., Hebert, P. D. N. (2008). Semi-automated, membrane-based protocol for DNA isolation from plants. Plant Mol. Biol. Rep. 26, 186–198. doi: 10.1007/s11105–008-0029–4
Katoh, K., Misawa, K., Kuma, K., Miyata, T. (2002). MAFFT: a novel method for rapid multiple sequence alignment based on fast Fourier transform. Nucleic Acids Res. 15, 3059–3066. doi: 10.1093/nar/gkf436
Kearse, M., Moir, R., Wilson, A., Stones-Havas, S., Cheung, M., Sturrock, S., et al. (2012). Geneious Basic: An integrated and extendable desktop software platform for the organization and analysis of sequence data. Bioinformatics 28, 1647–1649. doi: 10.1093/bioinformatics/bts199
Linares-Palomino, R., Oliveira-Filho, A. T., Pennington, R. T. (2011). “Neotropical seasonally dry forests: diversity, endemism, and biogeography of woody plants.” in Seasonally. Dry. Trop. Forests., ed. Dirzo, R., Youmg, H. S., Mooney, H. S., Ceballos, G. (Washington: Island Press). doi: 10.5822/978–1-61091–021-7_1
Mayer, V. E., Frederickson, M. E., McKey, D., Blatrix, R. (2014). Current issues in the evolutionary ecology of ant–plant symbioses. New Phytol. 202, 749–764. doi: 10.1111/nph.12690
Miller, M. A., Schwartz, T., Pickett, B. E., He, S., Klem, E. B., Scheuermann, R. H., et al. (2015). A restful API for access to phylogenetic tools via the CIPRES science gateway. Evol. Bioinform. 11, 43–48. doi: 10.4137/ebo.s21501
Nauheimer, L., Boyce, P. C., Renner, S. S. (2012). Giant taro and its relatives: A phylogeny of the large genus Alocasia (Araceae) sheds light on Miocene floristic exchange in the Malesian region. Mol. Phylogenet. Evol. 63, 43–51. doi: 10.1016/j.ympev.2011.12.011
Pendry, C. A. (2004). Monograph of Ruprechtia (Polygonaceae). Syst. Bot. Monogr. 67, 1. doi: 10.2307/25027911
Pennington, R. T., Lavin, M., Oliveira-Filho, A. (2009). Woody plant diversity, evolution, and ecology in the tropics: perspectives from seasonally dry tropical forests. Annu. Rev. Ecol. Evol. Syst. 40, 437–457. doi: 10.1146/annurev.ecolsys.110308.120327
Pennington, R. T., Lavin, M., Prado, D. E., Pendry, C. A., Pell, S. K., Butterworth, C. A. (2004). Historical climate change and speciation: neotropical seasonally dry forest plants show patterns of both Tertiary and Quaternary diversification. Phil. Trans. R. Soc Lond. B. 359, 515–538. doi: 10.1098/rstb.2003.1435
Pennington, R. T., Lavin, M., Särkinen, T., Lewis, G. P., Klitgaard, B. B., Hughes, C. E. (2010). Contrasting plant diversification histories within the Andean biodiversity hotspot. Proc. Natl. Acad. Sci. U.S.A. 107, 13783–13787. doi: 10.1073/pnas.1001317107
Pennington, R., Lavin, M. (2016). The contrasting nature of woody plant species in different neotropical forest biomes reflects differences in ecological stability. New Phytol 210, 25–37.
Pérez-Escobar, O. A., Balbuena, J. A., Gottschling, M. (2016). Rumbling orchids: how to assess divergent evolution between chloroplast endosymbionts and the nuclear host. Syst. Biol. 65, 51–65. doi: 10.1093/sysbio/syv070
Pérez-Escobar, O. A., Bogarín, D., Przelomska, N. A. S., Ackerman, J. D., Balbuena, J. A., Bellot, S., et al. (2024). The origin and speciation of orchids. New Phytol. 242, 700–716. doi: 10.1111/nph.19580
Pérez-Escobar, O. A., Dodsworth, S., Bogarín, D., Bellot, S., Balbuena, J. A., Schley, R. J., et al. (2021). Hundreds of nuclear and plastid loci yield novel insights into orchid relationships. Am. J. Bot. 108, 1166–1180. doi: 10.1002/ajb2.1702
Pérez-Escobar, O. A., Gottschling, M., Chomicki, G., Condamine, F. L., Klitgård, B. B., Pansarin, E., et al. (2017). Andean mountain building did not preclude dispersal of lowland epiphytic orchids in the neotropics. Sci. Rep. 7, 4919. doi: 10.1038/s41598–017-04261-z
Pérez-Escobar, O. A., Lucas, E., Jaramillo, C., Monro, A., Morris, S. K., Bogarín, D., et al. (2019). The origin and diversification of the hyperdiverse flora in the Chocó Biogeographic region. Front. Plant Sci. 10. doi: 10.3389/fpls.2019.01328
Pérez-Escobar, O. A., Zizka, A., Bermúdez, M. A., Meseguer, A. S., Condamine, F. L., Hooen, C., et al. (2022). The Andes through time: evolution and distribution of Andean floras. Tr. Pl. Sci. 27, 364. doi: 10.1016/j.tplants.2021.09.010
POWO. (2023). Plants of the World Online (Kew: Royal Botanic Gardems). Available online at: http://www.plantsoftheworldonline.org/ (Accessed March 27, 2023).
Rivera, D. O., Rangel, J., Avella-Muñoz, A., García, J., Castro, S. (2013). “Las plantas con flores del complejo cenagoso Zapatosa -Incluye localidades de Mata de Palma y La Pachita,” in Colombia Diversidad Biótica XIII. Complejo cenagoso Zapatosa y ciénagas del Sur del Cesar. Biodiversidad, conservación y manejo, ed. Rangel, J. (Bogota: Instituto de Ciencias Naturales, Universidad Nacional de Colombia), 203–243.
Rodríguez, J. P., Nassar, J. M., Rodríguez-Clark, K. M., Zager, I., Portillo-Quintero, C. A., Carrasquel, F., et al. (2008). Tropical dry forests in Venezuela: assessing status, threats and future prospects. Envir. Conserv. 35, 311. doi: 10.1017/s0376892908005237
Rose, J. P., Toledo, C. A. P., Lemmon, E. M., Lemmon, A. R., Sytsma, K. J. (2020). Out of sight, out of mind: widespread nuclear and plastid-nuclear discordance in the flowering plant genus Polemonium (Polemoniaceae) suggests widespread historical gene flow despite limited nuclear signal. Syst. Biol. 70, 162–180. doi: 10.1093/sysbio/syaa049
Sanchez, A. (2015). Fidelity and promiscuity in an ant-plant mutualism: A case study of Triplaris and Pseudomyrmex. PloS One 10, e0143535. doi: 10.1371/journal.pone.0143535
Sanchez, A., Kron, K. A. (2011). Phylogenetic relationships of Triplaris and Ruprechtia: re-delimitation of the recognized genera and two new genera for tribe triplarideae (Polygonaceae). Syst. Bot. 36, 702–710. doi: 10.1600/036364411x583664
Särkinen, T. E., Marcelo-Peña, J. L., Yomona, A. D., Simon, M. F., Pennington, T. P., Hughes, C. E. (2011b). Underestimated endemic species diversity in the dry inter-Andean valley of the Río Marañón, northern Peru: An example from Mimosa (Leguminosae, Mimosoideae). Taxon. 60, 139–150. doi: 10.5167/UZH-51346
Särkinen, T., Pennington, R. T., Lavin, M., Simon, M. F., Hughes, C. E. (2011a). Evolutionary islands in the Andes: persistence and isolation explain high endemism in Andean dry tropical forests. J. Biogeography 39, 884–900. doi: 10.1111/j.1365–2699.2011.02644.x
Schneider, C. A., Rasband, W. S., Eliceiri, K. W. (2012). NIH Image to ImageJ: 25 years of image analysis. Nat. Methods 9, 671–675. doi: 10.1038/nmeth.2089
Stamatakis, A. (2014). RAxML version 8: a tool for phylogenetic analysis and post-analysis of large phylogenies. Bioinformatics 30, 1312–1313. doi: 10.1093/bioinformatics/btu033
Stull, G. W., Pham, K. K., Soltis, P. S., Soltis, D. E. (2023). Deep reticulation: the long legacy of hybridization in vascular plant evolution. Plant J. 114, 743–766. doi: 10.1111/tpj.16142
Thiers, B. M. (2021). Index Herbariorum: A global directory of public herbaria and associated staff (New York botanical garden's virtual herbarium). Available online at: http://sweetgum.nybg.org/science/ih/ (Accessed December 12, 2021).
Torres, M. F., Sanchez, A. (2017). Neotropical ant-plant Triplaris americana attracts Pseudomyrmex mordax ant queens during seedling stages. Insect. Soc 64, 255–261. doi: 10.1007/s00040–017-0542–2
Turland, N., Wiersema, J., Barrie, F., Greuter, W., Hawksworth, D., Herendeen, P., et al. (2018). International Code of Nomenclature for algae, fungi, and plants (Glashütten: Koeltz Botanical Books). doi: 10.12705/code.2018
van der Pijl, L. (1982). Principles of dispersal in Higher Plants (Berlin Heidelberg: Springer). doi: 10.1007/978–3-642–87925-8
Ward, P. S. (1999). Systematics, biogeography and host plant associations of the Pseudomyrmex viduus group (Hymenoptera: Formicidae), Triplaris- and Tachigali-inhabiting ants. Zool. J. Linn. Soc. 126, 451–540. doi: 10.1111/j.1096–3642.1999.tb00157.x
Weberling, F. (1989). Morphology of flowers and inflorescences. Ed. Pankhurst, R. J. (Cambridge: Cambridge University Press).
Wells, T., Carruthers, T., Muñoz-Rodríguez, P., Sumadijaya, A., Wood, J. R. I., Scotland, R. W. (2021). Species as a heuristic: reconciling theory and practice. Syst. Biol. 71, 1233–1243. doi: 10.1093/sysbio/syab087
Werneck, F. P., Costa, G. C., Colli, G. R., Prado, D. E., Sites, J. W., Jr (2010). Revisiting the historical distribution of Seasonally Dry Tropical Forests: new insights based on palaeodistribution modelling and palynological evidencegeb. Global Ecol. Biogeography. 20, 272–288. doi: 10.1111/j.1466–8238.2010.00596.x
Keywords: dry tropical forests, systematics, molecular diagnosis, phylogenetics, Triplarideae, taxonomy, deforestation, extinction risk
Citation: Aguilar-Cano J, Pérez-Escobar OA, Pizano C, Tovar E and Antonelli A (2024) Isolated, neglected, and likely threatened: a new species of Magoniella (Polygonaceae) from the seasonally dry tropical forests of Northern Colombia and Venezuela revealed from nuclear, plastid, and morphological data. Front. Plant Sci. 15:1253260. doi: 10.3389/fpls.2024.1253260
Received: 05 July 2023; Accepted: 13 May 2024;
Published: 23 July 2024.
Edited by:
Maximilian Weigend, University of Bonn, GermanyReviewed by:
Adriana Sanchez, Rosario University, ColombiaHengchang Wang, Chinese Academy of Sciences (CAS), China
Copyright © 2024 Aguilar-Cano, Pérez-Escobar, Pizano, Tovar and Antonelli. This is an open-access article distributed under the terms of the Creative Commons Attribution License (CC BY). The use, distribution or reproduction in other forums is permitted, provided the original author(s) and the copyright owner(s) are credited and that the original publication in this journal is cited, in accordance with accepted academic practice. No use, distribution or reproduction is permitted which does not comply with these terms.
*Correspondence: José Aguilar-Cano, jose.aguilarcano@gmail.com; Oscar Alejandro Pérez-Escobar, o.perez-escobar@kew.org
†Present address: Camila Pizano, Department of Biology, Lake Forest College, Lake Forest, IL, United States
‡These authors have contributed equally to this work and share senior authorship