- 1Department of Biotechnology, Institute of Natural and Applied Sciences, Çukurova University, Adana, Türkiye
- 2Laboratory of Biotechnology, Environment, Agri-Food and Health, Faculty of Sciences Dhar El Mehraz, Sidi Mohammed Ben Abdellah University, Fez, Morocco
- 3Laboratory of Natural Substances, Pharmacology, Environment, Modeling, Health & Quality of Life, Faculty of Sciences Dhar El Mehraz, Sidi Mohamed Ben Abdallah University, Fez, Morocco
- 4Mycology unit Biological Control Research Institute, Adana, Türkiye
- 5Laboratory of Biotechnology, Conservation and Valorisation of Natural Resources, Faculty of Sciences Dhar El Mehraz, Sidi Mohamed Ben Abdallah University, Fez, Morocco
- 6Department of Botany and Microbiology College of Science, King Saud University, Riyadh, Saudi Arabia
- 7Department of Food Science, Faculty of Agricultural and Food Sciences, Laval University, Quebec City, QC, Canada
- 8Laboratory of Chemisty and Biochemistry, Faculty of Medicine and Pharmacy, Ibn Zohr University, Laayoune, Morocco
- 9Department of Food Engineering, Faculty of Agriculture, Çukurova University, Adana, Türkiye
Perennial ryegrass (Lolium perenne L.) is a valuable forage and soil stabilisation crop. Perennial crops have long been associated with good environmental performance and ecosystem stability. Vascular wilt diseases caused by Fusarium species are the most damaging plant diseases affecting both woody perennials and annual crops. Therefore, the aim of the present study was the assessment of the preventive and growth-promoting effects of carvacrol against Fusarium oxysporum, F. solani, and F. nivale (phylogenetically analyzed on the basis of internal transcribed spacer (ITS) regions) causing vascular wilt of ryegrass in vitro and under greenhouse conditions. To accomplish this aim, various parameters were monitored including coleoptile development, rhizogenesis, the incidence of coleoptile lesions, disease index, the visual appearance of ryegrass health, ryegrass organic matter and soil fungal load. The results obtained showed that F. nivale was highly harmful to ryegrass seedlings compared to other Fusarium species. Furthermore, carvacrol with 0.1 and 0.2 mg/mL protected significantly the seedlings against Fusarium wilt diseases both in vitro and in the greenhouse. Simultaneously, carvacrol also functioned as a seedling growth promoter, as is reflected in all monitored parameters, such as the recovery of seedling height and root length, and the development of new leaf buds and secondary roots. Carvacrol proved to be effective plant growth promoter and a bio-fungicide against Fusarium vascular diseases.
Introduction
Perennial ryegrass (Lolium perenne L.) is a cool-season grass grown throughout temperate regions of the world (Wilkins & Humphreys, 2003; Wiering et al., 2021). Perennial plants have long been associated with good environmental performance and improved ecosystem health, perennials protect and stabilizer the soil against wind and water erosion, while increasing soil quality and organic matter thanks to biomass accumulation (Blanco-canqui, 2010). An increased proportion of perennials increase biodiversity by providing habitat for animals and insects. Additionally, perennial crops can increase the quantity and diversity of mineral nutrients available in the rhizosphere by establishing complex and often long-term relationships with the microbiome (Davis et al., 2010; Nehls et al., 2010). Perennial grasses with their large and active root system, play a crucial role in scavenging available nutrients and preventing them from leaching away where they may become pollutants (Heaton et al., 2010). Further, though a popular species in the turf industry, perennial ryegrass has desirable grazing characteristics and high nutritive value (Sampoux et al., 2011). Therefore, the main role of this species is to provide forage for ruminant animals. Consequently, perennial ryegrass forage breeders have focused on improving biomass, nutritive value, persistence, disease resistance, and winter-hardiness (Sampoux et al., 2011).
Vascular wilt diseases caused by fungal pathogens are the most damaging plant diseases that affect both woody perennials and annual crops (Boutaj et al., 2022). The Fusarium genus is one of the utmost complex and adaptive genus in the Eumycota (Gordon, 2017). Fusarium species which cause destructive vascular wilts, rots, and damping-off diseases, are ubiquitous soil-borne pathogens of a wide range of horticultural and food crops (Bodah, 2017; Saghrouchni et al., 2021). In addition to the damages caused in the pre-harvest period, many Fusarium species are capable of producing mycotoxins in agricultural and food commodities, as well as in perennial forage (Juraschek et al., 2022). These phytopathogens attack the vascular system made up of xylem arteries, tracheary elements that transport water and minerals from the roots to the photosynthetic organs, and phloem elements, which transport organic photosynthesis products (Agrios, 2005). High number of harmful mycotoxins produced by Fusarium can be commonly detected in ruminant diets, such as deoxynivalenol and fumonisins, which can interfere with ruminant animals and human health (Escrivá et al., 2015; Gallo et al., 2021).
Fusarium spp., Microdochium spp., and Neocosmospora spp. can cause destructive vascular wilt disease. Fusarium nivale (Fr.) Samuels and I.C. Hallet, Fusarium oxysporum described by Schltdl., and Fusarium solani (Mart.) L. Lombard and Crous, three species owning to the “Fusarium complex” have devastating effects on species belonging to wild gramineae, Lolium perenne L. (ryegrass) (Saghrouchni et al., 2021). These species usually limit the production of economically important crops since these strains attack several types of plants especially gramineae family including rice, maize, wheat, barley, oats, and rye, and therefore induce significant losses especially when the environmental conditions are favourable (Servin et al., 2015).
To control Fusarium wilt, several strategies were used such as soil solarisation and fumigation with various pesticides. However, the presence of chemical residues is harmful to the public health and can affect the quality of soil and groundwater (Boutaj et al., 2022). These products can be used before planting to treat soil and/or during cultivation, e.g., chloropicrin and benomyl (Dorugade et al., 2021; Karki et al., 2022). Due to excessive use, some fungicides can no longer be effective towards resistant strains among fungal species (Chaves et al., 2022). Therefore, it is necessary to identify alternative weapons to control Fusarium wilt disease.
Pathogen control and disease management can only be achieved through an integrative approach in which biological control can play a major role. Nowadays, biological agents including botanical agents (Plant extracts, essential oils and their major compounds) are used as alternative solutions (Lecomte et al., 2016; Agour et al., 2020; Jawhari et al., 2021; Amrati et al., 2021). Carvacrol is a natural low-molecular weight product, this secondary metabolite derived from plants (Thymus vulgaris and Origanum vulgare described by Linnaeus). Carvacrol has been the subject of many investigations that place a higher priority on natural products with biological activities to control such diseases. This monoterpene has been shown to possess a wide range of biological effects, such as antibacterial, antifungal, insecticidal, antioxidant, antimutagenic, antigenotoxic, and antitumor (Aprotosoaie et al., 2019). Previous investigations demonstrated that carvacrol is one of the potent monoterpenes that can be used to control fungal species (Saghrouchni et al., 2021; Gonçalves et al., 2021).
Based on the previously discussed reasons, the main aim of the current paper was to evaluate the preventive and stimulatory effects of carvacrol on Fusarium wilt disease of ryegrass in vitro and under greenhouse conditions. For this purpose, an infestation of three fungal strains was carried out on ryegrass pre-treated with carvacrol, and several parameters were monitored including coleoptile development and rhizogenesis, incidence of lesions on coleoptiles, disease index, visual aspect of the health status, the weight of organic matter and the Fusarium load of the soil.
Material and methods
Chemicals
The agar medium used in this study was 3% malt extract agar (MEA) amended with 0.5% mycological peptone, and potato dextrose agar (PDA) (Biokar, France). The monoterpene carvacrol (99%) used in this study was obtained from Flagresso, Austria.
Fungal strains
Fusarium oxysporum, F. solani, and F. nivale strains used in the present study were isolated from diseased leaves, stems, and roots of ryegrass and from soil samples before being identified. For morphological characterisation, macroscopic traits such as the colony appearance, colour, pigmentation and growth rate were observed on potato dextrose agar (PDA) according to Leslie & Summerell (2006). Morphological identification was also performed based on the morphological characteristics observed at optical microscope as described by Booth (1971) and Leslie & Summerell (2006).
Fungal DNA extraction
The morphological identification was further confirmed by DNA sequencing. Single hyphal tip isolates were grown on cellophane overlain on PDA for 10 days. The mycelium was lyophilized and 10 mg of lyophilized mycelium, grinded with 5 mm iron beads. Then, fungal DNA was extracted using a DNeasy Plant Mini Kit (Qiagen, Hilden, Germany) according to the manufacturer’s protocol (Fallahi et al., 2019). The quality and quantity of the DNA obtained were evaluated by measuring the concentration (ng/μL) in a NanoDrop ND-1000 spectrophotometer (Thermo Fisher Scientific). The quality of the DNA yielded by each method was determined by gel electrophoresis in a 1% agarose gel at 90 V for 15-20 min, stained with Ethidium bromide
Molecular identification
To confirm the identity of the fungus, ITS-5 (5`-GGAAGTAAAAGTCGTAACAAGG-3`-) and ITS-4 (5`-CCTCCGCTTATTGATATGC-3’) were used to amplify complete internal transcribed spacer (ITS) as described by White et al. (1990). The ITS region was amplified in a 25 μL reaction using 25 ng DNA, 1 x Dream Taq buffer (1.5 mM MgCl2), 0.3 μM pimer, 200 μM dNTP, 0.2 U Taq DNA Polymerase. The PCR conditions were as follows: 95°C for 1 min, 35 cycles of 95°C for 15 s, 55°C for 30 s, and 72°C for 90 s, and a final cycle at 72°C for 5 min. PCR products were electrophoresed on a 1% (w/v) agarose gel in 1x THE (20 mM Tris-HEPES, pH 8.06) at 90 V for 1 hour. The product was purified and then sequenced. The nitrogen base sequence was analyzed using an automated DNA sequencer. Sequencing data were trimmed and assembled using ChromasPro program version 1.5. The program Molecular Evolutionary Genetic Analysis software, ver. 5.0 (MEGA4.0; http://www.megasoftware.net) was performed in order to edit and align the sequence files, which were manually adjusted. In order to assess the relationships between the major taxa, ambiguous parts of the ITS regions were removed from further analysis and more conserved and alignable parts of the region and all genes were used to generate phylogenetic trees containing representative taxa from major groups. The assembled data were BLASTED with genomic data that has been registered in NCBI/National Center for Biotechnology Information (http://www.ncbi.nlm.nih.gov/BLAST/). The aligned sequences were BLAST in GenBank database, to identify all the strains. In this study, phylogenetic tree was generated using maximum parsimony (MP) in MEGA5.0 (Tamura et al., 2011). Bootstrap values for the maximum parsimony tree (MPT) were calculated for 1,000 replicates (Felsenstein, 1985). The edited ITS sequences were compared with other available Fusarium species sequences in the GenBank. Furthermore, the sequences of some known species were downloaded from GenBank and used to reconstruct a combined ITS region and phylogenetic trees.
Preparation of fungal inoculants
Sporulation was obtained by culturing the fungal strains in a MEA medium after 7 days of incubation at 28°C (Pujol et al., 1997). Afterwards, the spores were collected by flooding the plate with 1 mL water, including 0.05% Tween 20, using a sterile spread rod. Then, the number of spores was counted manually with a haemocytometer (Merck, Germany) with light microscopy (Optika, Italy) before being diluted to an inoculum of about 106 spores/mL (Noman et al., 2016).
Plant material
Ryegrass seeds of the English Ryegrass variety were kindly offered by the technical service of the Golf Royal Dar Es-salaam, Rabat, Morocco.
Culturing substrate
The substrate used for the cultivation of the ryegrass consists of 100% vegetable soil. Topsoil sieved through a 2-mm sieve was autoclaved twice at 121°C/0.1MPa for 30 minutes.
Evaluation in vitro of the growth-promoting and preventive effects of carvacrol on ryegrass
Thirty seeds were disinfected with 0.5% sodium hypochlorite solution for 20 min and then rinsed twice with sterile distilled water for 10 min and dried before being transferred to Petri plates containing sterile layers of filter paper (Kordali et al., 2007; Kordali et al., 2008). Afterward, each group received 1 mL of sterile water daily. After seeds germination, 1 mL of 99% carvacrol diluted in agar 0.2% (1:10) was dispensed into the plates. Afterwards, the infestation of the groups was carried out by adding 1 mL of spores’ suspension (106 spores/mL) of the F. oxysporum, F. solani, and F. nivale and with a consortium (1:1:1) of all the spores’ strains. The experiment was carried out for 4 weeks under ambient temperature (28 ± 2°C). The experimental protocol has been presented in the Table 1. Plant height (PH), root length (RL), percentage of second leaf appearance (SL) and percentage of second root appearance (SR) were measured weekly. Moreover, the lesions developed on the coleoptile were also noted and the incidence of the disease (I) was estimated by the following equation (Mironenko, 1960):
Where n1 and n2, respectively, represent the total number of seeds per Petri plate and the number of seeds with unaltered coleoptile of seedlings.
Evaluation of the preventive effect of carvacrol on ryegrass grown in soil under greenhouse conditions
One gram of ryegrass’ seeds (approximately 200 seeds) was seeded into pots containing 150 g of topsoil. Each group received a constant volume of 15 mL (in order to wet the soil completely) of carvacrol (diluted in agar 0.2% (1:10)) twice a week. After that, infection was carried out with 15 mL of the spores’ suspension (106 spores/mL) of F. oxysporum, F. solani, and F. nivale and with a mixture of all strain’s spores. The experimental protocol has been presented in the Table 2. The evolution of the fungal charge of Fusarium was followed once a week using the serial dilution technique. One gram from each sample was transferred to test tube containing 9 mL of 0.9% NaCl. From this solution, a series of dilutions (10-2 to 10-6) were made (El Barnossi et al., 2019). From each dilution 0.1 mL was spread on Petri plates containing MEA medium. The plates were incubated at 27°C for 4 days. Colonies of Fusarium species were then counted by the enumeration technique. Consequently, plates containing 15 to 150 colonies were selected for counting and the result was expressed in log (CFU/g) of three replicates (Ribeiro et al., 2020). As regards the virulence of each fungal strain, it was estimated after 7 weeks based on the disease index (DI) technique using the 0-4 scale established by Hernandez et al. (1998) which was based on visible symptoms on each seedling, where code 4: dead turf, code 3: 67 to 99% affected plant, code 2: 34 to 66% affected plant, code 1: 1 to 33% affected plant and code 0: no visible symptoms. The experiment was carried out under greenhouse conditions using both normal topsoil and disinfected topsoil.
Measurements of fresh and dry weights
To determine the fresh shoots and roots weight, the shoots and roots were carefully washed under running tap water. Prior to weight determination, excess moisture was blotted using paper towels, and the weight was determined. While for the dry weight determination, the samples were dried in an oven at 70°C for 72 h till constant weight was reached after the dry weight was determined (Huang et al., 2017).
Statistical analysis
Quantitative results were expressed as means, of triplicate experiments ± SD (standard deviation). To determine statistical significance, Two way-ANOVA and Tukey’s multiple range tests at p< 0.05 were performed using GraphPad Prism 8.0.1 (Graph Pad Software Inc., San Diego, USA).
Results
Molecular identification
Molecular identification was confirmed by sequencing the ITS. BLAST analysis using ITS sequences revealed a threshold of 100% similarity between the sequences from the isolates in this study and sequences from strains which were previously deposited in Genbank. The ITS sequences were deposited in GenBank as: F. solani (accession no. OP810954), F. oxysporum (accession no. OP824842), and F. nivale (accession no. OP810953).
All strains isolated from soils and ryegrass represented two different lineages (Figure 1). The isolates of F. solani and F. oxysporum were divided into subgroups. F. nivale was included in other branches. Phylogenetic tree demonstrated that all strains are closely related with a strong bootstrap support (100%). All isolates described as F. solani, F. oxysporum and F. nivale, based on morphological characteristics, were identified as F. solani, F. oxysporum and F. nivale through molecular analyses.
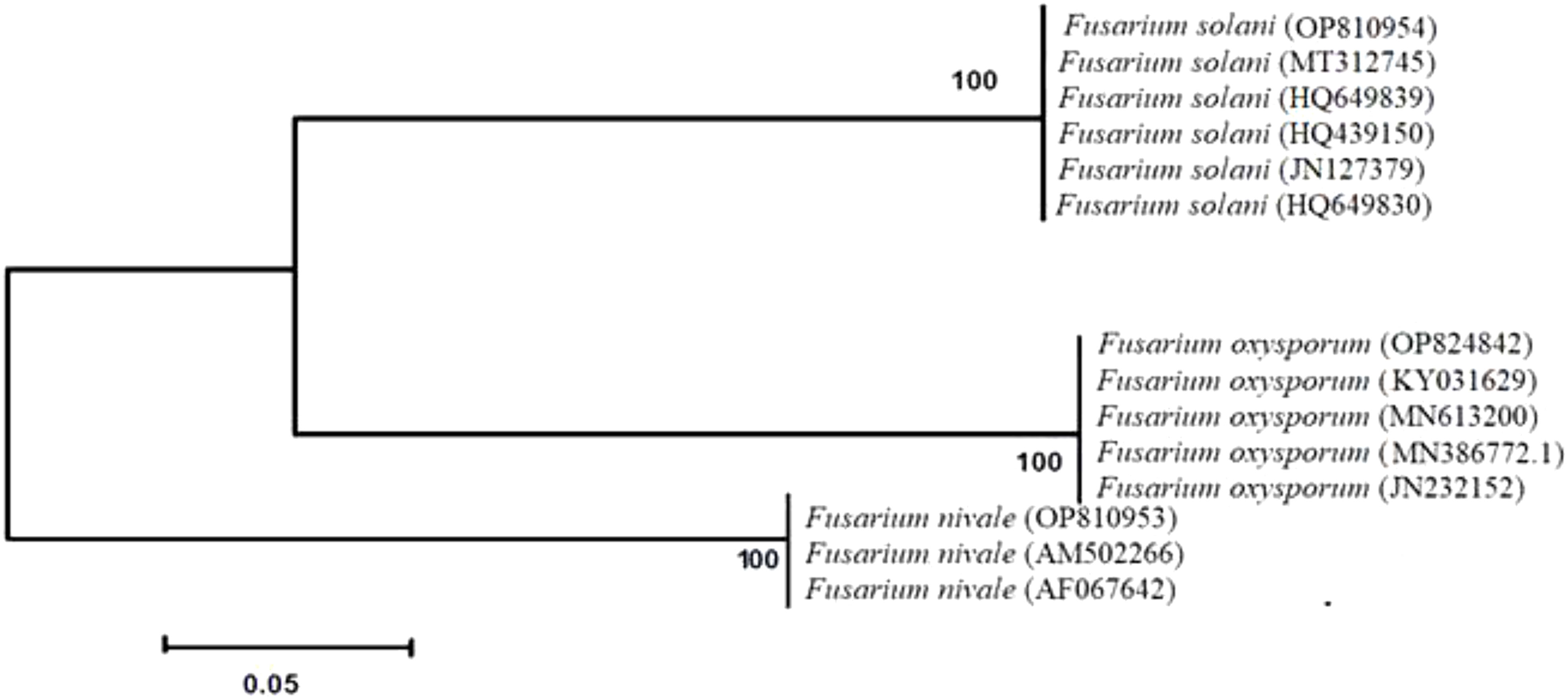
Figure 1 Phylogenetic analysis obtained by sequence analysis of Fusarium isolates 18S rDNA-ITS-region. Bootstrap tests were performed with 1,000 replications.
Evaluation in vitro of the growth-promoting and preventive effects of carvacrol on ryegrass
Table 3 shows the results of the effect of carvacrol treatment on the coleoptile growth and on rhizogenesis of ryegrass in-vitro. After infestation of the seedlings with fungal spores, Fusarium significantly reduced plant height and root length for all tested strains compared to the negative control, especially F. nivale which was the most virulent on ryegrass seedlings. In contrast, after treatment, plant height and root length recovered well with all tested concentration of carvacrol. As a consequence, the measurement values of the treated plants were almost equal to those of the negative control and sometimes better.
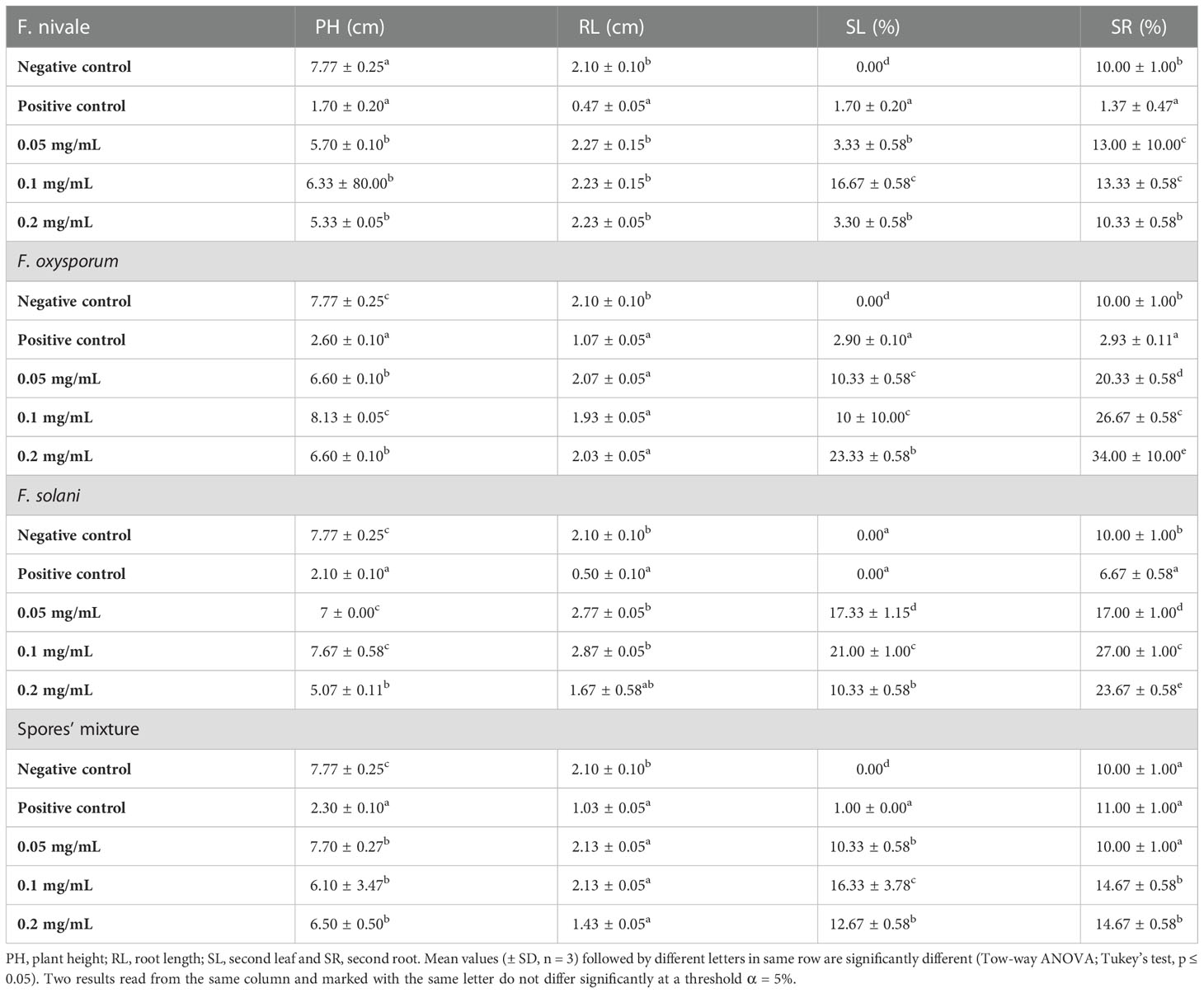
Table 3 The effect of carvacrol treatment on the coleoptile growth and on rhizogenesis of the ryegrass in vitro.
On the other hand, Table 3 shows also the results of the carvacrol effect on plant development. According to the result portrayed by the table plants in both the positive and negative control did not develop a second leaf. However, in the treated groups between 10 and 23.33% of the plants have developed a second leaf during the experiment time. In addition, as regards the carvacrol effect on the appearance of the second root, 10% of the plants in the negative control developed the second root. After infestation with F. nivale, the fungus inhibited the development of the second root, and consequently the percentage was decreased to 1.37%. Treatment with 0.05, 0.1, and 0.2 mg/mL carvacrol significantly allowed the emergence of the second root in all plants with a percentage reaching 20.33, 26.67 and 34% respectively.
Regarding the incidence of lesions on coleoptiles of ryegrass seedlings, the Figure 2 shows that the incidence was high with F. nivale with a percentage of 50%. Fusarium solani, F. oxysporum and the spores mixtures did not induce severe lesions on coleoptiles, where the incidence percentage ranged between 23 an 33%.
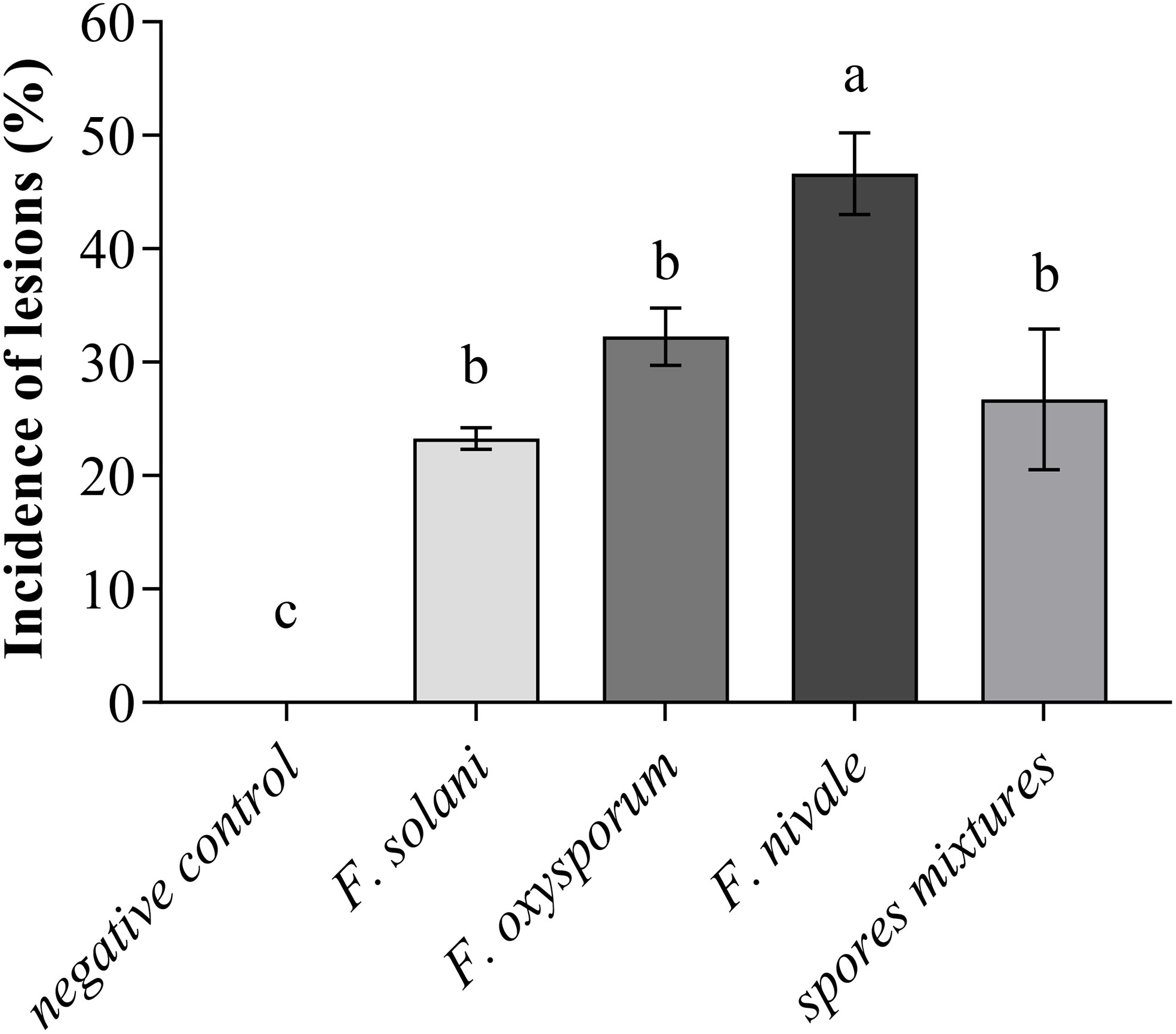
Figure 2 Incidence of lesions on coleoptiles of ryegrass seedlings. Means (± SD, n = 3) denoted by the same letter indicate no significant difference according to Tukey’s multiple range tests at p< 0.05.
According to the results, carvacrol showed protective effect on ryegrass against Fusarium wilt disease. Furthermore, carvacrol showed growth-promoting effect on both the leaves and the rhizogenesis.
Evaluation of the preventive effect of carvacrol on ryegrass grown in soil under greenhouse conditions
To evaluate the preventive effect of carvacrol and the virulence of the Fusarium species, the disease index (DI) was used. This index is an indication of the health status of the plant, the more it tends towards 4 the more affected the plant is. The results presented in Figure 3 demonstrate that before infestation, the DI was equal to 2, whereas, after the infestation the DI was increased to 3 and 4. In contrast, after the treatment with carvacrol, the DI decreased to 1 and 0. In the disinfected soil, F. nivale - with a DI tending towards 4 - was found to be the most virulent, however in the normal soil this virulence was reduced which was reflected by the elevation of DI to 2 as the negative control. The aggresivity of F. nivale is manifested by the total death of almost all plants of the infested and untreated lot in the disinfected soil (Figure 4). Regarding the most effective concentrations of carvacrol, 0.1 and 0.2 mg/mL were shown to have a better protective effect with a DI ranging between 1 and 0 against all tested strains and in both normal and disinfected soil.
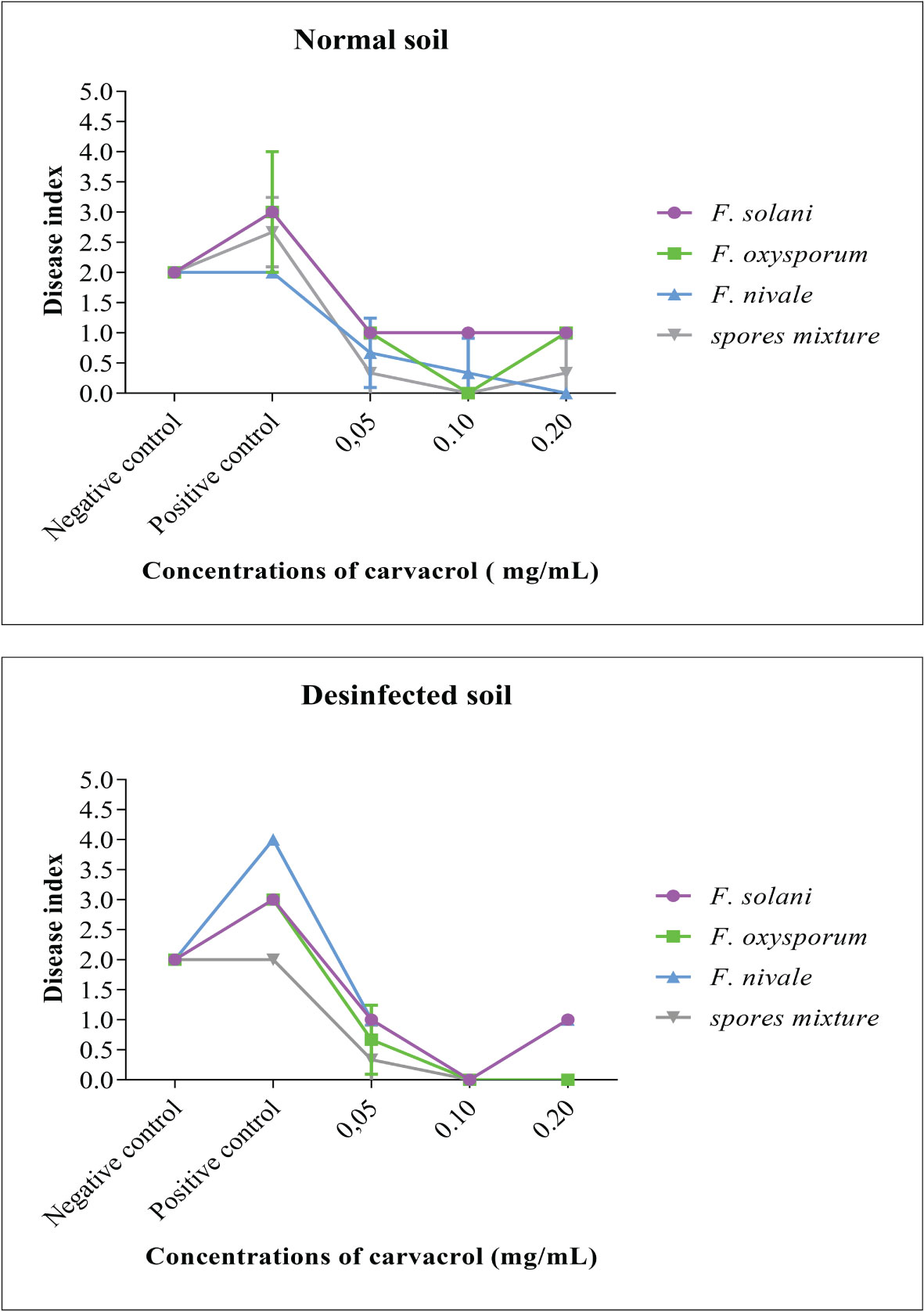
Figure 3 The disease index and the evolution of the average symptom severity on infested ryegrass after 7 weeks of preventive treatment with carvacrol. The results differ significantly at a threshold α = 5% (Two-way ANOVA, Turkey test). Error bars show standard deviations.
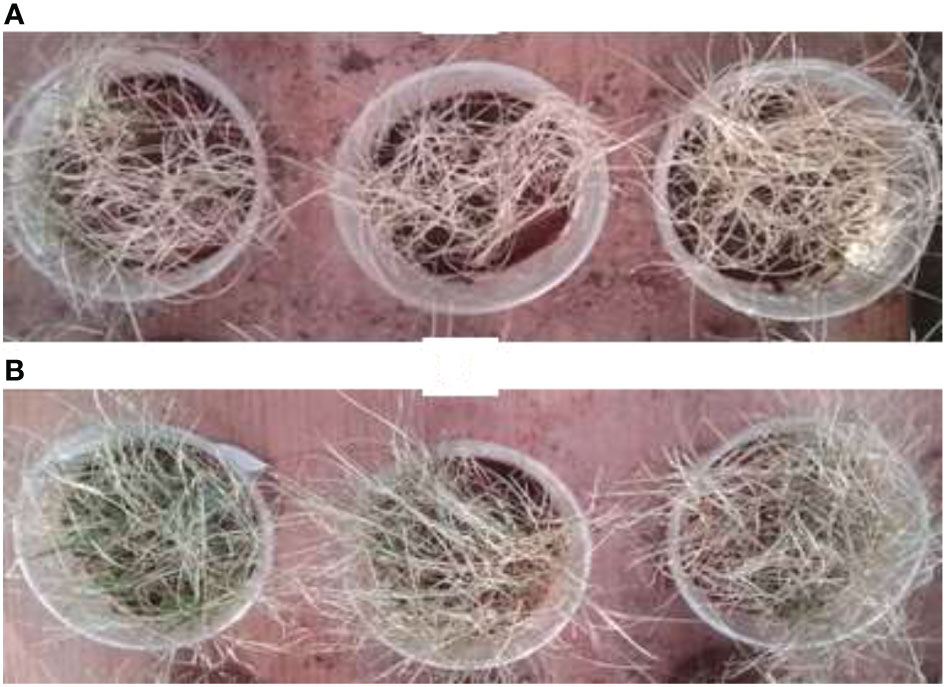
Figure 4 Visual appearance of the health status of ryegrass in disinfected soil. (A): dead plants due to fusarium wilt disease induced by (F) nivale and (B): negative control.
Figures 5, 6 show the appearance of the health status of all pots in the greenhouse in disinfected and normal soil. The pictures show that the plants in the infested and treated lots with carvacrol were healthier than those in the infested and untreated lots.
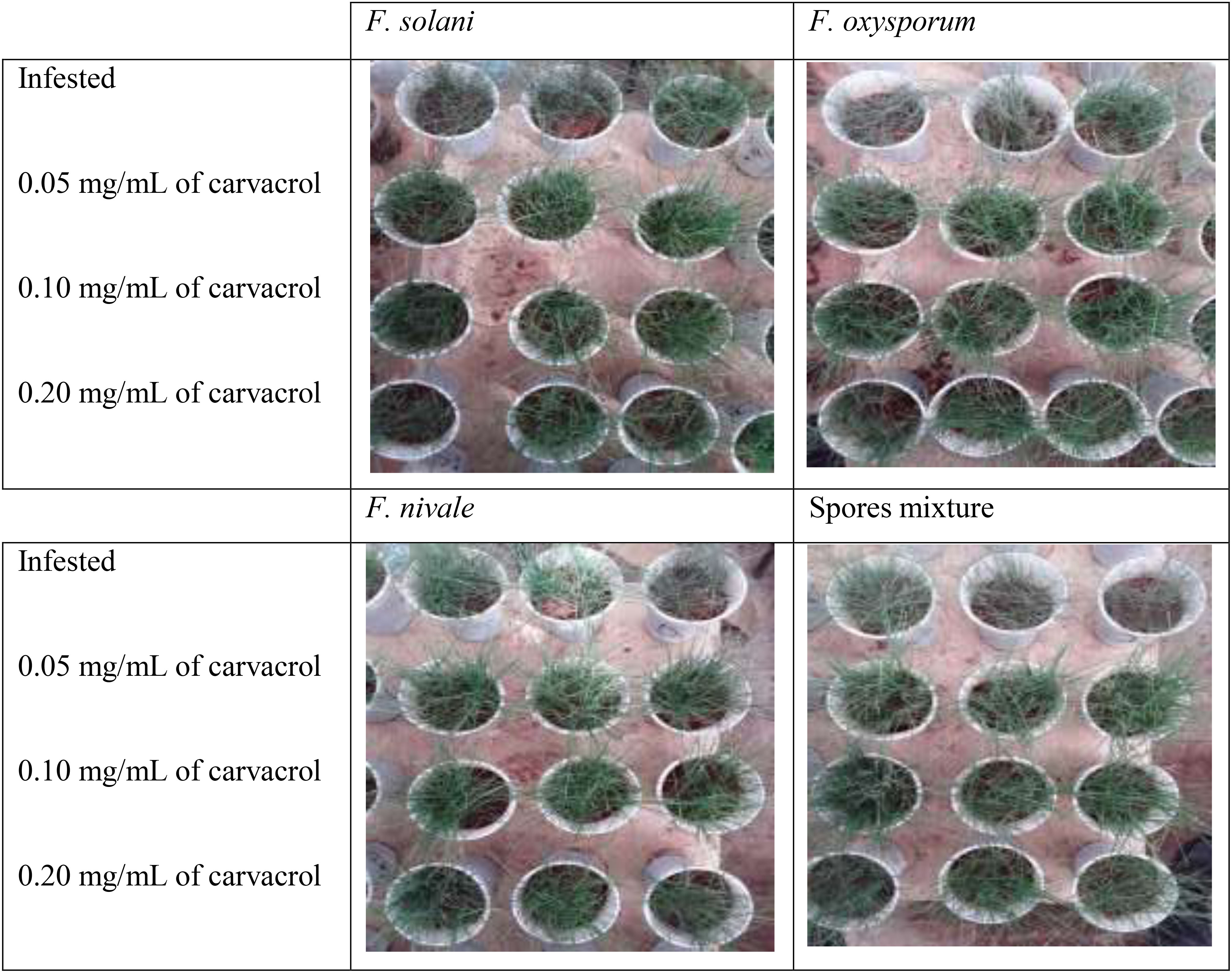
Figure 5 The visual appearance of the health status of ryegrass in normal soil after 4 weeks of preventive treatment with carvacrol under greenhouse conditions.
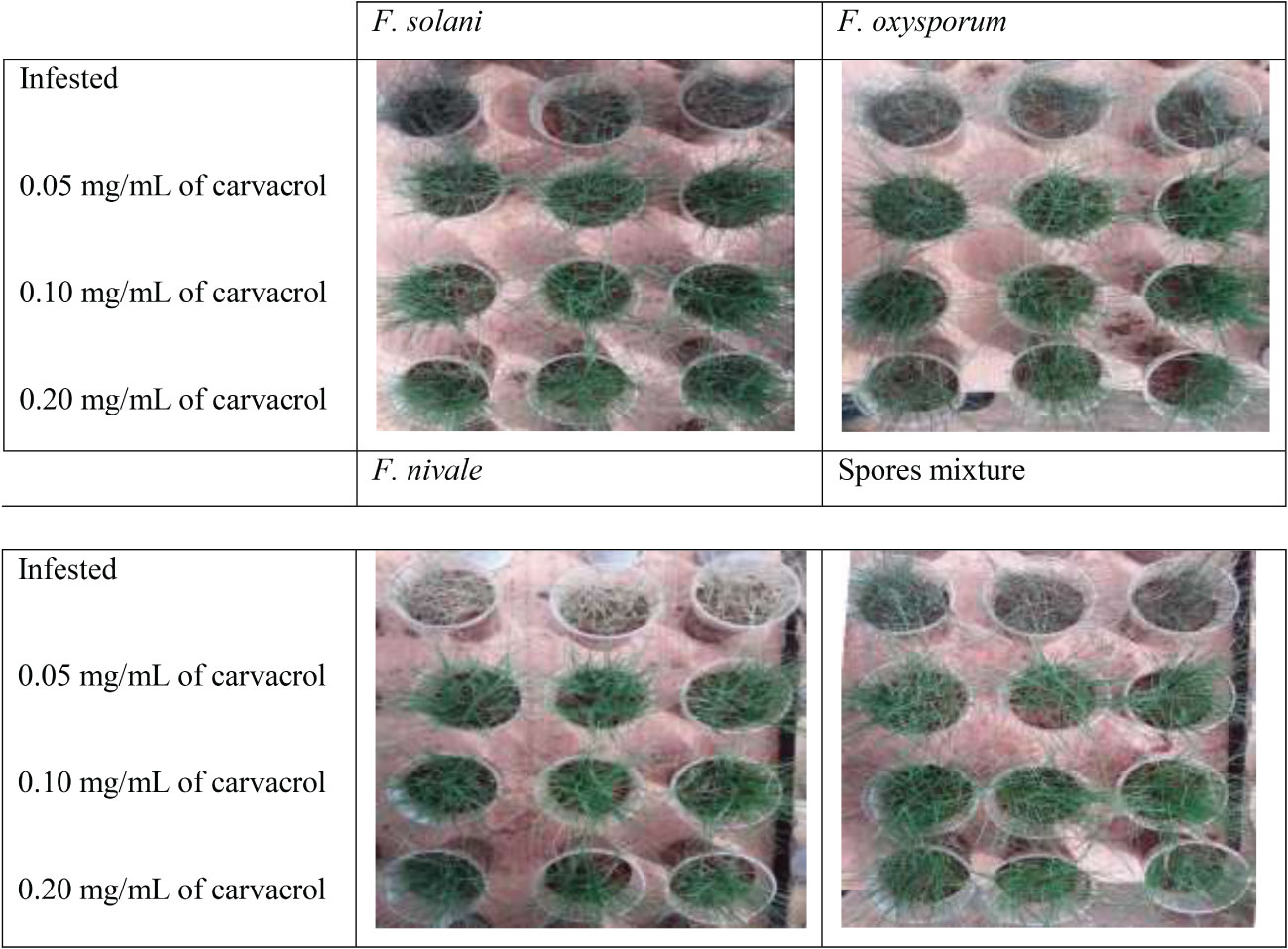
Figure 6 The visual appearance of the health status of ryegrass in disinfected soil after 4 weeks of preventive treatment with carvacrol under greenhouse conditions.
The fungal load of Fusarium was measured during the whole period of ryegrass cultivation. Figures 7, 8 show the results of the follow-up of this load in both disinfected and normal soil. According to the figures, after the infestation, the Fusarium load was much higher in the disinfected soil than in the normal soil compared with negative control. In the normal soil, after treatment, the load of F. solani became null from the third week of treatment with 0.2 mg/mL and at the end of the fourth week the load was nulled with 0.1 mg/mL of carvacrol. In the disinfected soil, F. solani was eliminated with 0.2 mg/mL of carvacrol from the second week. Furthermore, with this concentration, the fungal load of all the spores’ mixture was well eliminated after three weeks in both soil types. We noticed also that the disinfected soil remained without any apparition of fungal charge until the last week, we found a little of Fusarium charge (Figure 8).
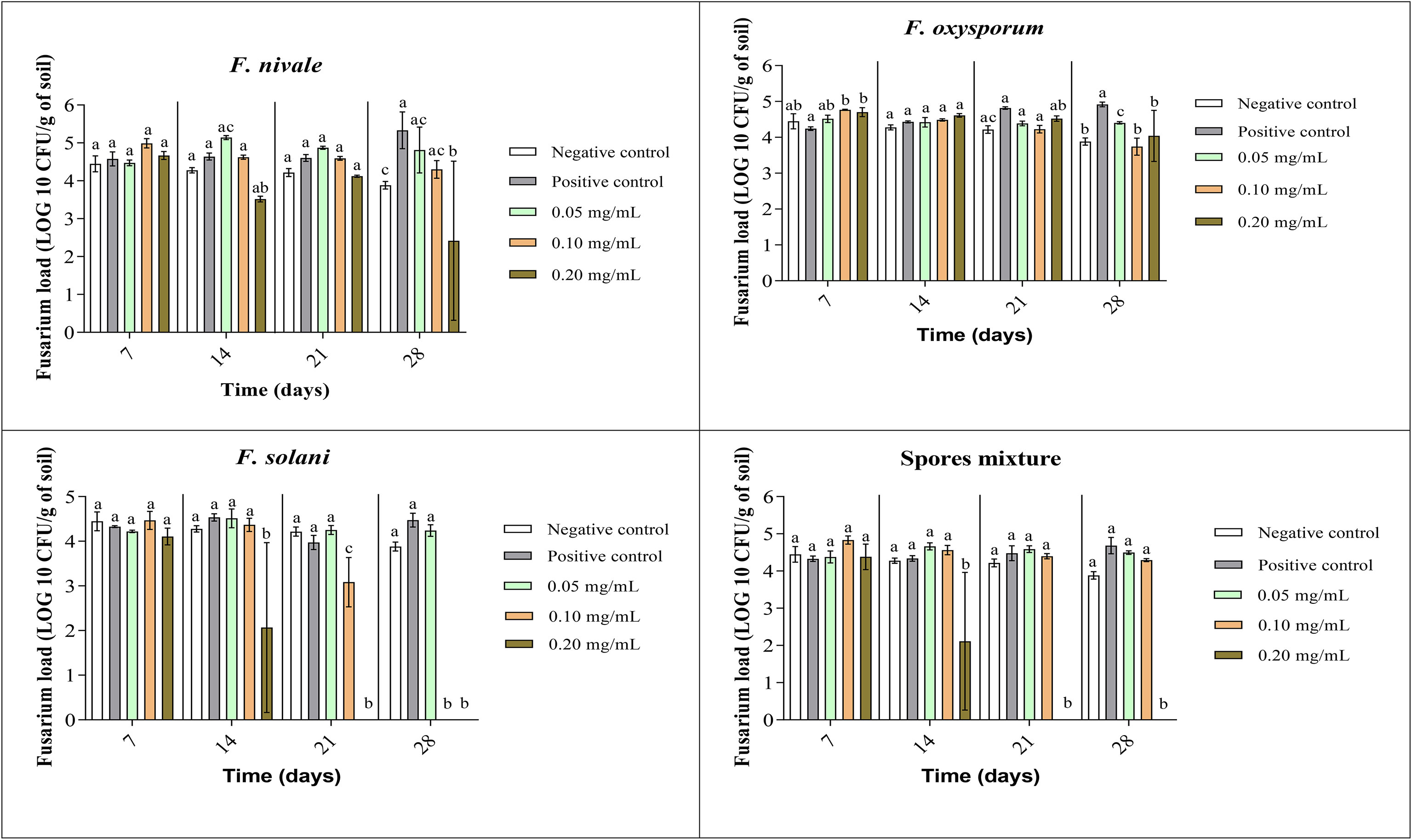
Figure 7 Fusarium load during treatment of ryegrass pots with carvacrol for four weeks in normal soil under greenhouse. Means (± SD, n = 3) denoted by the same letter indicate no significant difference according to Tukey’s multiple range tests at p< 0.05.
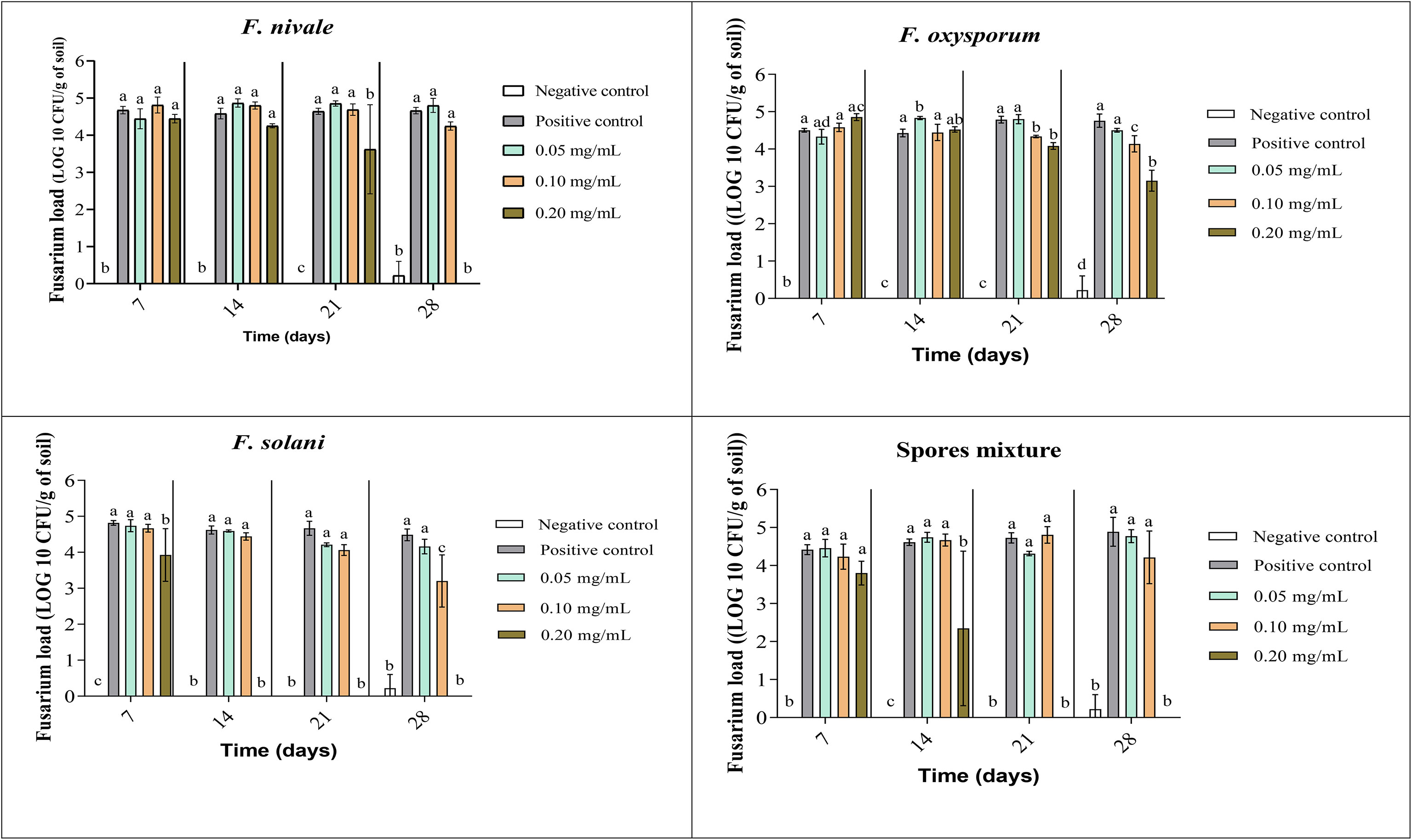
Figure 8 Fusarium load during treatment of ryegrass pots with carvacrol for four weeks in disinfested soil under greenhouse. Means (± SD, n = 3) denoted by the same letter indicate no significant difference according to Tukey’s multiple range tests at p< 0.05.
Measurements of fresh and dry weights
Figures 9, 10 show the results of the quantitative evaluation of the vegetative growth variables such as fresh and dry weight of ryegrass shoots and roots after 4 weeks of treatment with carvacrol in normal and disinfected soil under greenhouse. In Figure 9, where we used normal soil, after infestation with Fusarium spores, the amount of organic matter has reduced significantly for all the groups and also for all growth parameters. After treatment, the amount of organic matter recovered and sometimes even increased especially with 0.1 and 0.2 mg/mL of carvacrol, compared to the negative control. On the other hand, in disinfected soil (Figure 10), F. nivale showed more damage on ryegrass. As a result, the amount of organic matter decreased from 7.4 to 4.9 g, from 2.17 to 0.26 g and from 1.87 to 0.17 g for the fresh shoots weight, dry shoots weight and dry root weight respectively.
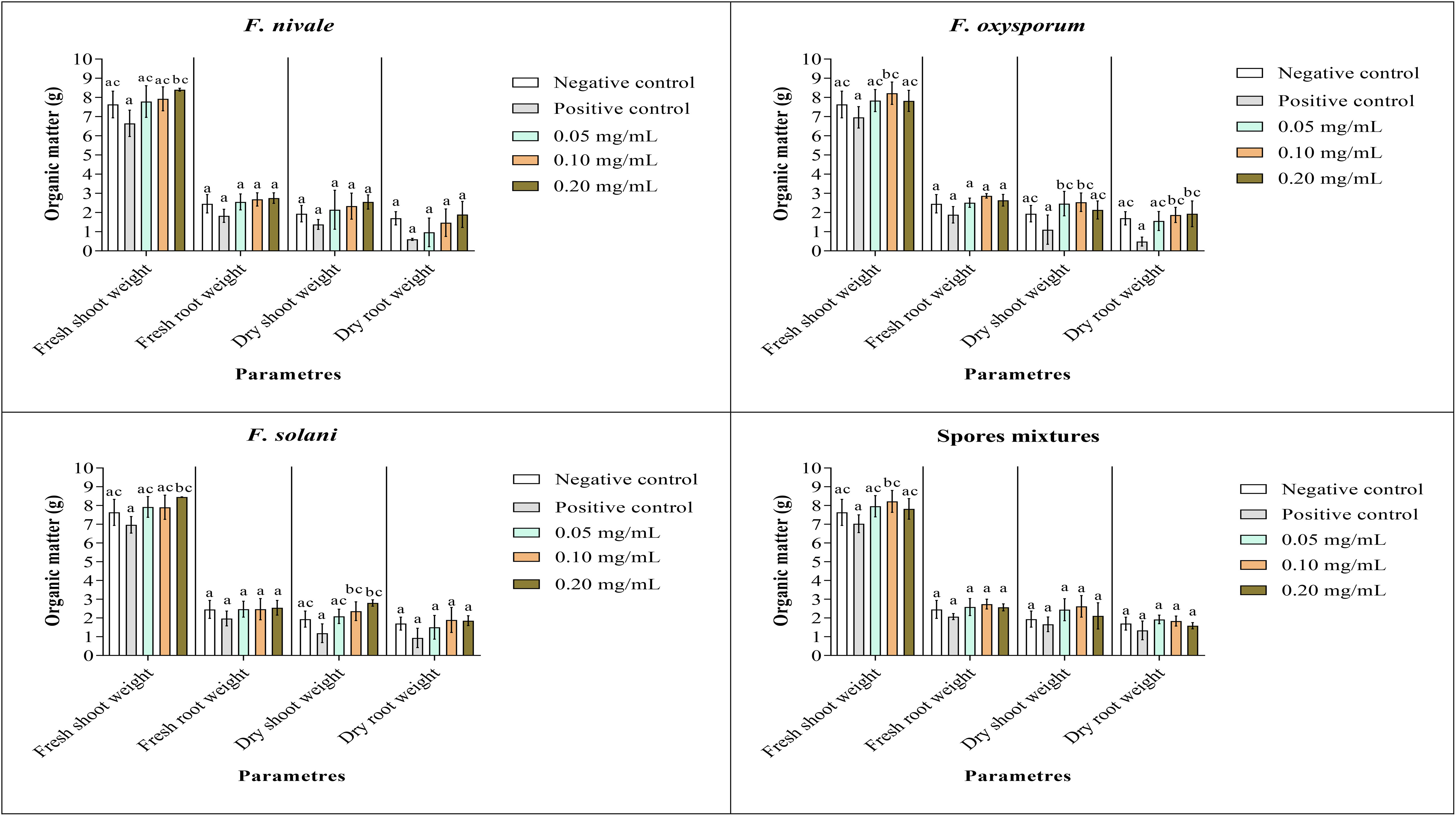
Figure 9 Organic matter of ryegrass after four weeks of treatment with carvacrol in normal soil under greenhouse. Means (± SD, n = 3) denoted by the same letter indicate no significant difference according to Tukey’s multiple range tests at p< 0.05.
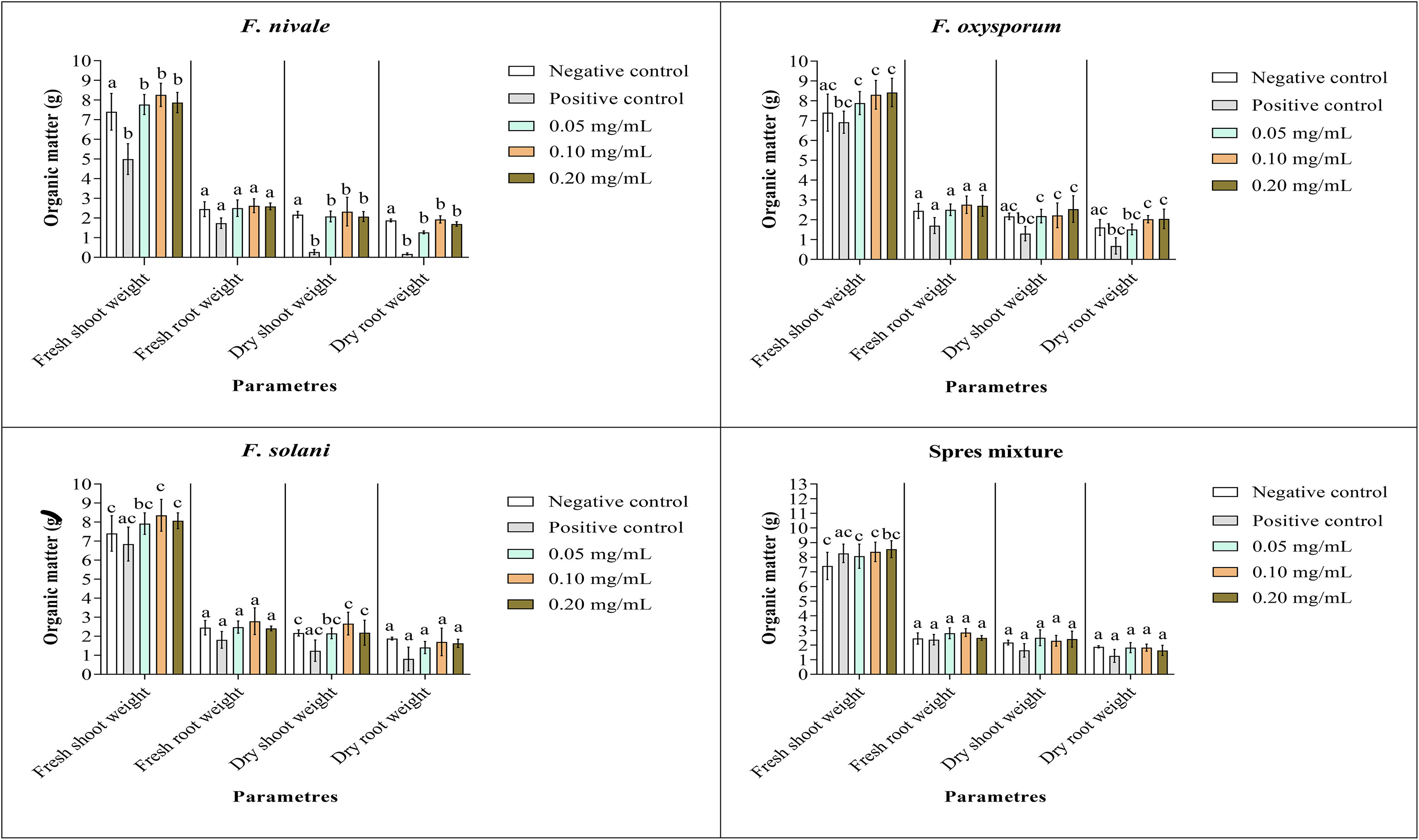
Figure 10 Organic matter of ryegrass after four weeks of treatment with carvacrol in disinfected soil under greenhouse. Means (± SD, n = 3) denoted by the same letter indicate no significant difference according to Tukey’s multiple range tests at p< 0.05.
Discussion
From the results obtained, we found that all three strains tested negatively influenced the development and growth of coleoptiles and seedling roots. Furthermore, the incidence of lesions on coleoptiles was very high for F. nivale. After in vitro treatment with carvacrol, the height of the seedlings was restored and also the length of the roots. During the treatment we noticed that the carvacrol treated seedlings fared better than the negative control plants due to the effect of carvacrol on the development of new leaf buds and also on the emergence of secondary roots.
The results obtained were in agreement with what Boudoudou et al. (2009) reported, infestation with F. graminearum, F. nivale, F. solani and F. semitectum influenced coleoptile and root development of seedlings of five rice varieties. The length of coleoptiles decreased from 4.0-6.1 to 2.6-5.3 cm. The number of roots decreases from 6.0-8.4 to 4.0-7.5 and the length of roots decreases from 5.5-7.0 to 3.2-6.9 cm. This decrease was high with F. graminearum and F. nivale in contrast to F. solani. In the same study, the researchers evaluated the lesion incidence on coleoptiles, therefore F. solani did not induce lesions on coleoptiles as opposed to the other three species. The incidence was high with F. semitectum for two rice varieties (28.5 and 25.1%) and with F. nivale for one variety (29.9%). All varieties were affected by F. graminearum (6.5 to 21.46%). The fact that Fusarium penetrates through the roots of the plant and damages the whole plant through the vessels of the plant, is why it is called vascular disease (Shaban et al., 2018). Therefore, we thought to apply a systematic (in soil) preventive treatment in order to avoid the penetration of Fusarium into the roots and thus the establishment of the disease.
The results obtained on the disease index showed that infected plants treated with 0.1 and 0.2 mg/mL carvacrol were well protected. It should also be noted that the isolates expressed more virulence in the disinfected soil than in the normal soil. This would be in favour of a competition between the Fusarium and the soil flora which would exert an inhibition on Fusarium. And this was reflected by the visual appearance of the ryegrass seedlings.
The improvement of the disease index following the carvacrol treatment can be explained by the results obtained on the Fusarium load of the soil. Indeed, the fungal load of the soil was decreased in the function of the dose and time. These results are in accordance with other study findings, the treatment of disinfected soil with carvacrol resulted in a reduction in dose-dependence of F. oxysporum load. 0.8 mg/mL of carvacrol was capable of reducing the load to 0 after 3 weeks. In normal soil, the total disinfection has taken 4 weeks of treatment with the same concentration (Saghrouchni et al., 2020). In another study undertaken on soil disinfection using thymol, another major compound of T. vulgaris and O. vulgare. the treated lot with 0.5 mg/mL of thymol has shown a decrease after 21 days from 5.26 Log10 to 3.56 Log10. For the treated lot with 2 mg/mL of thymol, a total disappearance of F. oxysporum occurred after 14 days (Oukhouia et al., 2017).
After providing an idea of the visual and qualitative aspects of ryegrass, we assessed vegetative growth variables such as fresh and dry shoots and root weights of the ryegrass. The results of the quantitative evaluation of the vegetative growth variables showed that the amount of organic matter was recovered after being decreased after infestation and sometimes even increased compared to negative control especially with 0.1 and 0.2 mg/mL of carvacrol. These findings evidently showed that carvacrol is an effective plant growth promoter. Therefore, this monoterpene can be used as biostimulator for plant development. Nowadays, there is increasing interest in the use of naturally occurring ‘biostimulators’ for enhancing the growth of agricultural and horticultural crops (Kurepin et al., 2014). The activity of biostimulators to promote plant growth is often attributed to their ability to directly or indirectly provide mineral nutrients (mostly N, but also P, S and other macro- and micro-nutrients) to plants. Although optimal growth of plants depends on the availability of adequate mineral nutrients, that growth (and also development, including reproduction) is also regulated by plant hormones (phytohormones), including gibberellins, auxins, cytokinins, abscisic acid, ethylene and other phytohormones (Bassanezi et al., 2021; Wu et al., 2021; Liu et al., 2022). For example, auxin is involved in the stimulation of cell elongation in shoots and root initiation. Gibberellins, can also increase stem elongation. Cytokinins, which are usually found in high concentrations in meristematic regions and other areas of active growth, such as actively expanding portions of roots and stem, young leaves, developing fruits and seeds, are involved in the stimulation of cell division, shoot growth, root development, leaf expansion and the inhibition or slowing of leaf or organ senescence.
Carvacrol and other plant products can be useful in crop production due to their antimicrobial and growth-promoting potential. On the other hand, in livestock production, the use of medicinal plants is gaining more recognition as a result of numerous efforts (Oluwafemi et al., 2020). The worldwide interest on botanical products has grown significantly as described by (Oluwafemi et al., 2020), cattle, goat, sheep, horses and pigs represents about 70% of the animals treated with herbal remedies followed by poultry (9.1%), dogs (5.3%) and rabbits (4.3%). Medicinal plants are mostly used especially by rural and small-scale farmers because they are inexpensive, readily available and effective. Recently, researchers have suggested the use plant extracts and essential oils as alternative growth promoters in poultry feed because they have antimicrobial, anti-parasitic, antioxidant and anti-helminthic properties due to the presence of phytochemical or bioactive chemicals in plants (Oluwafemi et al., 2020).
Fusarium appears to be phytopathogenic, It alters the early stages of development of the ryegrass seedlings tested (Saghrouchni et al., 2021). This genus is capable of invading other host plants with intense virulence, including other grasses and cereals. Treating seedlings with carvacrol has effectively protected them against Fusarium wilt disease. In addition to the anti-Fusarium action, carvacrol is thought to exert a growth-promoting effect on the seedlings. When left untreated, the fungus contained in the seeds becomes fully established. Thus, damaged seeds may harbour masses of conidia, when the seeds were able to germinate, diseased seedlings emerge (Saghrouchni et al., 2021). To control Fusarium, seeds treatment has been recommended (Mironenko, 1960). The alterations observed in seedlings from inoculated seeds show that these species can be troublesome in the early stages of seedling development. Therefore, it is important to monitor their development especially when using susceptible varieties.
Conclusion
Carvacrol and other plant products may be utilized in controlling diseases like Fusarium wilt, as well as improving plant yield. Further, to understand how carvacrol act as a growth-promotor and biostimulator for enhancing coleoptile growth and rhizogenesis, future studies are recommended. Carvacrol may interact with phytohormones such as auxins, cytokinins, gibberellins, etc. It remains a molecule of plant origin!
Data availability statement
The raw data supporting the conclusions of this article will be made available by the authors, without undue reservation.
Author contributions
HS, Writing—original draft preparation; HS, IL, and TA, formal analysis; HS, MB, AB, IM, AA, AH, IV, and HN, writing—review and editing. All authors contributed to the article and approved the submitted version.
Acknowledgments
The authors extend their appreciation to the Researchers supporting Project number (RSP-2023/98) King Saud University, Riyadh, Saudi Arabia for financial support. The authors also gratefully thank Biological Control Research Institute Adana, Turkey for their kind collaboration during the conduction of the study.
Conflict of interest
The authors declare that the research was conducted in the absence of any commercial or financial relationships that could be construed as a potential conflict of interest.
Publisher’s note
All claims expressed in this article are solely those of the authors and do not necessarily represent those of their affiliated organizations, or those of the publisher, the editors and the reviewers. Any product that may be evaluated in this article, or claim that may be made by its manufacturer, is not guaranteed or endorsed by the publisher.
References
Agour, A., Mssillou, I., Saghrouchni, H., Bari, A., Lyoussi, B., Derwich, E. (2020). Chemical composition, antioxidant potential and antimicrobial properties of the essential oils of haplophyllum tuberculatum (Forsskal) a. juss from Morocco. Trop. J. Natural Product Res. Available 4 (12), 1108–1115. doi: 10.26538/tjnpr/v4i12.13
Amrati, F. E.-Z., Bourhia, M., Saghrouchni, H., Slighoua, M., Grafov, A., Ullah, R., et al. (2021). Caralluma europaea (Guss.) N.E.Br.: Anti Inflammatory, Antifungal, and Antibacterial Activities against Nosocomial Antibiotic‐Resistant Microbes of Chemically Characterized Fractions. Molecules (26), 636. doi: 10.3390/molecules26030636
Aprotosoaie, A. C., Vlad, S., Adriana, T., Anca, M. (2019). “Antigenotoxic potential of some dietary non-phenolic phytochemicals,” in Studies in natural products chemistry (Elsevier: USA), 223–297.
Bassanezi, R. B., Primiano, I. V., Vescove, H. V. (2021). Effect of enhanced nutritional programs and exogenous auxin spraying on huanglongbing severity , fruit drop , yield and economic profitability of orange orchards. Crop Prot. 145, 105609. doi: 10.1016/j.cropro.2021.105609
Blanco-canqui, H. (2010). Energy crops and their implications on soil and environment. Agron. J. 102 (2), 403–419. doi: 10.2134/agronj2009.0333
Bodah, T. E. (2017). Root rot diseases in plants: A review of common causal agents and management strategies. Agric. Res. Technol. 5 (3), 1–9. doi: 10.19080/artoaj.2017.05.555661
Boudoudou, H., Hassikou, R., Ouazzani Touhami, A., Badoc, A., Douira, A. (2009). First demonstrations of fusariose on rice germination and plantlets. Bull. Soc Pharm. Bordeaux 148, 45–54.
Boutaj, H., Meddich, A., Roche, J., Mouzeyar, S., El, C. (2022). The effects of mycorrhizal fungi on vascular wilt diseases. Crop Prot. 155, 105938. doi: 10.1016/j.cropro.2022.105938
Chaves, M. A., Reginatto, P., Souza, B., Itiki, R. (2022). Fungicide resistance in fusarium graminearum species complex. Curr. Microbiol. 79 (2), 1–9. doi: 10.1007/s00284-021-02759-4
Davis, S. C., Parton, W. J., Dohleman, F. G., Candice, M., Grosso, S., Del, Kent, A. D., et al. (2010). Comparative biogeochemical cycles of bioenergy crops reveal nitrogen-fixation and low greenhouse gas emissions in a miscanthus 3 giganteus. Ecosystems 13, 144–156. doi: 10.1007/s10021-009-9306-9
Dorugade, S., Sardesai, V., Kamble, S. (2021). Survival ability of benoyl resistant isolate of fusarium solani causing dry rot of elephant foot yam. Bioinfolet 18, 197–198.
El Barnossi, A., Saghrouchni, H., Moussaid, F., Chahmi, N., Housseini, A. I. (2019). Microbiological study of effects of solid organic waste (chicken droppings and sheep manure) decomposed in the soil used for pisum sativum cultivation. Int. J. Environ. Stud. 77 (5), 830–842. doi: 10.1080/00207233.2019.1704116
Escrivá, L., Font, G., Manyes, L. (2015). In vivo toxicity studies of fusarium mycotoxins in the last decade : A review. Food Chem. Toxicol. 78, 185–206. doi: 10.1016/j.fct.2015.02.005
Fallahi, M., Saremi, H., Javan-Nikkhah, M., Somma, S., Haidukowski, M., Logrieco, A. F., et al. (2019). Isolation, molecular identification and mycotoxin profile of fusarium species isolated from maize kernels in Iran. Toxins 11 (5), 297. doi: 10.3390/toxins11050297
Felsenstein, J. (1985). Confidence limits on phylogenies: an approach using the bootstrap. Evolution 39, 783–791.
Gallo, A., Ghilardelli, F., Doupovec, B., Faas, J., Schatzmayr, D., Masoero, F. (2021). Kinetics of gas production in the presence of fusarium mycotoxins in rumen fluid of lactating dairy cows kinetics of gas production in the presence of fusarium mycotoxins in rumen fluid of lactating dairy cows. JDS Commun. 2, 243–247. doi: 10.3168/jdsc.2021-0100
Gonçalves, D. C., Tebaldi de Queiroz, V., Costa, A. V., Lima, W. P., Belan, L. L., Moraes, W. B., et al. (2021). Reduction of fusarium wilt symptoms in tomato seedlings following seed treatment with origanum vulgare l. essential oil and carvacrol. Crop Prot. 141 (3), 105487. doi: 10.1016/j.cropro.2020.105487
Gordon, T. R. (2017). Fusarium oxysporum and the fusarium wilt syndrome. Annu. Rev. Phytopathol. 55, 23–39. doi: 10.1146/annurev-phyto-080615-095919
Heaton, E. A., Dohleman, F. G., Miguez, A. F., Juvik, J. A., Lozovaya, V., Widholm, J., et al. (2010). “Miscanthus : A promising biomass crop,” in A role for biomass crops, vol. Vol. 56. , 76–136). Advances in Botanical Research. St. Louis, MO, USA. doi: 10.1016/B978-0-12-381518-7.00003-0
Hernandez, M. E. S., D´avila, A. R., Algaba, A. P., de L´opez, M. A. B., Casas, A. T. (1998). Occurrence and etiology of death of young olive trees in southern Spain. Eur. J. Plant Pathol. 104, 347–357.
Huang, P., de-Bashan, L., Crocker, T., Kloepper, J. W., Bashan, Y. (2017). Evidence that fresh weight measurement is imprecise for reporting the effect of plant growth-promoting (rhizo)bacteria on growth promotion of crop plants. Biol. Fertility Soils 53 (2), 199–208. doi: 10.1007/s00374-016-1160-2
Jawhari, F. Z., Moussaoui, A. E. L., Bourhia, M., Imtara, H., Saghrouchni, H., Ammor, K., et al. (2021). Anacyclus pyrethrum var. pyrethrum (l.) and anacyclus pyrethrum var. depressus (ball) maire: Correlation between total phenolic and flavonoid contents with antioxidant and antimicrobial activities of chemically characterized extracts. Plants 10 (149). doi: 10.3390/plants10010149
Juraschek, L. M., Kappenberg, A., Amelung, W. (2022). Mycotoxins in soil and environment. Sci. Total Environ. 814, 152425. doi: 10.1016/j.scitotenv.2021.152425
Karki, K., Grant, J., Luiz, A., Ribeiro, B., Petkar, A., Hajihassani, A., et al. (2022). Evaluation of pic-clor 60 [ choloropicrin pre-mixed with 1 , 3 dicholoropropene ] and soil-applied fungicides for the fusarium wilt management in watermelon. Crop Prot. 154, 105894. doi: 10.1016/j.cropro.2021.105894
Kordali, S., Cakir, A., Ozer, H., Cakmakci, R., Kesdek, M., Mete, E. (2008). Antifungal, phytotoxic and insecticidal properties of essential oil isolated from Turkish origanum acutidens and its three components, carvacrol, thymol and p-cymene. Bioresource Technol. 99 (18), 8788–8795. doi: 10.1016/j.biortech.2008.04.048
Kordali, S., Cakir, A., Sutay, S. (2007). Inhibitory effects of monoterpenes on seed germination and seedling growth. Z. Fur Naturforschung - Section C J. Biosci. 62 (3–4), 207–214. doi: 10.1515/znc-2007-3-409
Kurepin, L. V., Zaman, O., Pharis, R. P. (2014). Phytohormonal basis for the plant growth promoting action of naturally occurring biostimulators. Soc. Chem. Industry 94, 1715–1722. doi: 10.1002/jsfa.6545
Lecomte, C., Alabouvette, C., Edel-hermann, V., Robert, F., Steinberg, C. (2016). Biological control of ornamental plant diseases caused by fusarium oxysporum : A review. Biol. Control 101, 17–30. doi: 10.1016/j.biocontrol.2016.06.004
Liu, S., Adibi, M., Huijser, P., Smith, R. S., Tsiantis, M., Adibi, M., et al. (2022). Cytokinin promotes growth cessation in the arabidopsis root. Curr. Biol. 32, 1–12. doi: 10.1016/j.cub.2022.03.019
Mironenko, P. V. (1960). Fusarium wilt of rice in the caspian area. Trud. Vses. Inst. Zashch. Rast. 14, 123–128.
Nehls, U., Gohringer, F., Wittulsky, S., Dietz, S. (2010). Fungal carbohydrate support in the ectomycorrhizal symbiosis : a review. Plant Biol. 12, 292–301. doi: 10.1111/j.1438-8677.2009.00312.x
Noman, E. A., Rahman, N. N. N. A., Shahadat, M., Nagao, H., Al-Karkhi, A. F. M., Al-Gheethi, A., et al. (2016). Supercritical fluid CO2 technique for destruction of pathogenic fungal spores in solid clinical wastes. Clean - Soil Air Water 44 (12), 1700–1708. doi: 10.1002/clen.201500538
Oluwafemi, R. A., Olawale, A. I., Alagbe, J. O. (2020). Recent trends in the utilization of medicinal plants as growth promoters in poultry nutrition – a review. J. Anim. Physiol. Anim. Nutr. 104 (1), 5–11.
Oukhouia, M., Hamdani, H., Remmal, A., Sennouni, C., Jabeur, I. (2017). In-vitro study of anti-fusarium effect of thymol, carvacrol, eugenol and menthol. J. Plant Pathol. Microbiol. 8 (423), 1–7. doi: 10.4172/2157-7471.1000423
Pujol, I., Guarro, J., Sala, J., Riba, M. D. (1997). Effects of incubation temperature, inoculum size, and time of reading on broth microdilution susceptibility test results for amphotericin b against fusarium. Antimicrobial Agents Chemotherapy 41 (4), 808–811. doi: 10.1128/aac.41.4.808
Ribeiro, M. S. S., Freitas-Silva, O., Castro, I. M., Teixeira, A., Marques-da-Silva, S. H., Sales-Moraes, A. C. S., et al. (2020). Efficacy of sodium hypochlorite and peracetic acid against aspergillus nomius in Brazil nuts. Food Microbiol. 90, 103449. doi: 10.1016/j.fm.2020.103449
Saghrouchni, H., El Barnossi, A., Chefchaou, H., Mzabi, A., Tanghort, M., Remmal, A., et al. (2020). Study the effect of carvacrol, eugenol and thymol on fusariums sp responsible for lolium perenne fusariosis. EM Int. 26 (3), 2020–1059.
Saghrouchni, H., El Barnossi, A., Salamatullah, A. M., Bourhia, M., Alzahrani, A., Alkaltham, M. S., et al. (2021). Carvacrol : A promising environmentally friendly agent to fight seeds damping-off diseases induced by fungal species. Agronomy 11, 985. doi: 10.3390/agronomy11050985
Sampoux, J. P., Baudouin, P., Bayle, B., Béguier, V., Bourdon, P., Chosson, J. F., et al. (2011). Breeding perennial grasses for forage usage: An experimental assessment of trait changes in diploid perennial ryegrass (Lolium perenne l.) cultivars released in the last four decades. Field Crops Res. 123, 117–129. doi: 10.1016/j.fcr.2011.05.007
Servin, A., Elmer, W., Mukherjee, A., de la Torre-Roche, R., Hamdi, H., White, J. C., et al. (2015). A review of the use of engineered nanomaterials to suppress plant disease and enhance crop yield. J. Nanoparticle Res. 17, 1–21. doi: 10.1007/s11051-015-2907-7
Shaban, M., Miao, Y., Ullah, A., Khan, A. Q., Menghwar, H., Khan, A. H., et al. (2018). Physiological and molecular mechanism of defense in cotton against verticillium dahliae. Plant Physiol. Biochem. 125, 193–204. doi: 10.1016/j.plaphy.2018.02.011
Tamura, K., Peterson, D., Peterson, N., Stecher, G., Nei, M., Kumar, S. (2011). MEGA5: Molecular evolutionary genetics analysis using maximum likelihood, evolutionary distance, and maximum parsimony methods. Mol. Biol. Evol. 28 (10), 2731–2739. doi: 10.1093/molbev/msr121
White, T. J., Bruns, T., Lee, S., Taylor, J. W. (1990). “Amplification and direct sequencing of fungal ribosomal RNA genes for phylogenetics,” in PCR proto- cols: A guide to methods and applications, vol. 64 . Eds. Innis, M., Gelfand, D., Sninsky, J., White, T. (New York: Academic Press, Inc.), 315–322.
Wiering, N. P., Sheaffer, C. C., Ehlke, N. J., Catalano, D., Martinson, K. (2021). Forage potential of winter-hardy perennial ryegrass populations in monoculture and binary alfalfa mixture. Agron. J. 113, 5183–5195. doi: 10.1002/agj2.20837
Wilkins, P. W., Humphreys, M. O. (2003). Progress in breeding perennial forage grasses for temperate agriculture. J. Agric. Sci. 140, 129–150. doi: 10.1017/S0021859603003058
Keywords: Lolium perenne, perennial ryegrass, fusarium wilt, carvacrol, bio-fungicide, plant growth promotor
Citation: Saghrouchni H, Barnossi AE, Mssillou I, Lavkor I, Ay T, Kara M, Alarfaj AA, Hirad AH, Nafidi H-A, Bourhia M and Var I (2023) Potential of carvacrol as plant growth-promotor and green fungicide against fusarium wilt disease of perennial ryegrass. Front. Plant Sci. 14:973207. doi: 10.3389/fpls.2023.973207
Received: 21 June 2022; Accepted: 12 January 2023;
Published: 10 February 2023.
Edited by:
Rachid Lahlali, Ecole Nationale d’Agriculture de Meknès, MoroccoReviewed by:
Göksel Özer, Abant Izzet Baysal University, TürkiyeSaid Ibrahim Behiry, Alexandria University, Egypt
Copyright © 2023 Saghrouchni, Barnossi, Mssillou, Lavkor, Ay, Kara, Alarfaj, Hirad, Nafidi, Bourhia and Var. This is an open-access article distributed under the terms of the Creative Commons Attribution License (CC BY). The use, distribution or reproduction in other forums is permitted, provided the original author(s) and the copyright owner(s) are credited and that the original publication in this journal is cited, in accordance with accepted academic practice. No use, distribution or reproduction is permitted which does not comply with these terms.
*Correspondence: Hamza Saghrouchni, hsaghrouchni@student.cu.edu.tr; Mohammed Bourhia, bouriamohammed@gmail.com