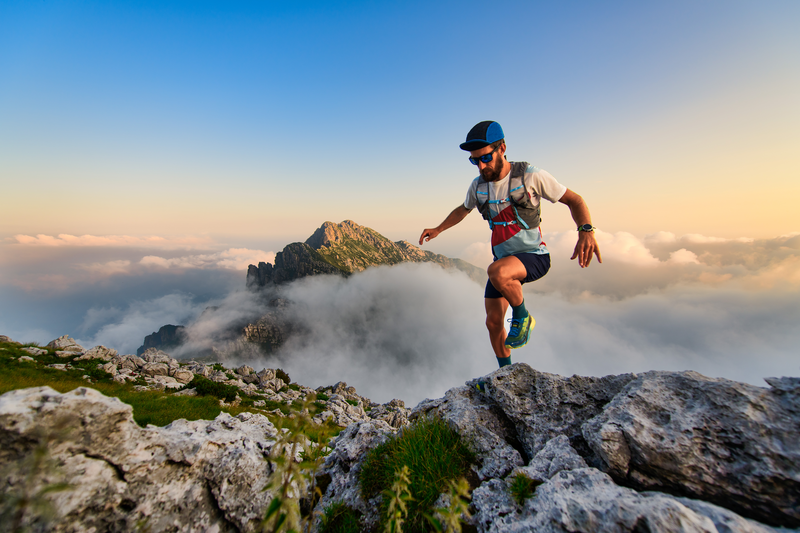
94% of researchers rate our articles as excellent or good
Learn more about the work of our research integrity team to safeguard the quality of each article we publish.
Find out more
ORIGINAL RESEARCH article
Front. Plant Sci. , 05 January 2024
Sec. Plant Symbiotic Interactions
Volume 14 - 2023 | https://doi.org/10.3389/fpls.2023.1326766
This article is part of the Research Topic Plant-Rhizobia Symbiosis and Nitrogen Fixation in Legumes View all 12 articles
The Lotus japonicus population carrying new Lotus retrotransposon 1 (LORE1) insertions represents a valuable biological resource for genetic research. New insertions were generated by activation of the endogenous retroelement LORE1a in the germline of the G329-3 plant line and arranged in a 2-D system for reverse genetics. LORE1 mutants identified in this collection contributes substantially to characterize candidate genes involved in symbiotic association of L. japonicus with its cognate symbiont, the nitrogen-fixing bacteria Mesorhizobium loti that infects root nodules intracellularly. In this study we aimed to identify novel players in the poorly explored intercellular infection induced by Agrobacterium pusense IRBG74 sp. For this purpose, a forward screen of > 200,000 LORE1 seedlings, obtained from bulk propagation of G329-3 plants, inoculated with IRBG74 was performed. Plants with perturbed nodulation were scored and the offspring were further tested on plates to confirm the symbiotic phenotype. A total of 110 Lotus mutants with impaired nodulation after inoculation with IRBG74 were obtained. A comparative analysis of nodulation kinetics in a subset of 20 mutants showed that most of the lines were predominantly affected in nodulation by IRBG74. Interestingly, additional defects in the main root growth were observed in some mutant lines. Sequencing of LORE1 flanking regions in 47 mutants revealed that 92 Lotus genes were disrupted by novel LORE1 insertions in these lines. In the IM-S34 mutant, one of the insertions was located in the 5´UTR of the LotjaGi5g1v0179800 gene, which encodes the AUTOPHAGY9 protein. Additional mutant alleles, named atg9-2 and atg9-3, were obtained in the reverse genetic collection. Nodule formation was significantly reduced in these mutant alleles after M. loti and IRBG74 inoculation, confirming the effectiveness of the mutant screening. This study describes an effective forward genetic approach to obtain novel mutants in Lotus with a phenotype of interest and to identify the causative gene(s).
Plant mutant collections have become a key resource to perform functional genomics and characterization of genes-of-interest in various biological processes. Mutagenized populations can be obtained with physical, chemical or biological mutagens (Chaudhary et al., 2019). The availability of mutant collections in the model legumes Medicago truncatula and Lotus japonicus (Lotus) have contributed importantly to understand the genetic networks that govern legume-rhizobia symbiosis (Roy et al., 2020). In the M. truncatula R108 line, a large mutant population was generated with the tobacco retrotransposon element Tnt1 (Tadege et al., 2008; Pislariu et al., 2012). In the Lotus accession Gifu, genotyping of plants regenerated from tissue culture (Madsen et al., 2005), identified new insertions of an endogenous LORE1 retrotransposon and further investigation showed epigenetic activation of the LORE1a element in the germline (Fukai et al., 2008; Fukai et al., 2010). Subsequent studies exploited this discovery, generating a reverse genetic mutant collection of 134,682 individual plants from the G329-3 line carrying an active LORE1a. In this collection, information of the insertion sites was obtained by sequencing, facilitating identification and characterization of mutants disrupted in genes-of-interest (Fukai et al., 2012; Urbanski et al., 2012; Malolepszy et al., 2016).
Lotus establishes a mutualistic association with its cognate symbiont Mesorhizobium loti. The symbiotic association occurs in the rhizosphere, where both organisms exchange chemical signals for the specific recognition. This compatibility activates a symbiotic signaling pathway that allows intracellular rhizobial infection via root-hair infection threads (ITs) and initiation of the nodule organogenesis program. Bacteria migrate through the ITs towards the dividing cortical cells in the nodule primordia, that give rise to a nitrogen-fixing nodule. In the nodule cells, rhizobia are encapsuled into membranous structures called symbiosomes, where they convert atmospheric nitrogen into ammonia (Downie, 2014). Lotus is able to establish symbiotic associations with a broad spectrum of rhizobial species (Gossmann et al., 2012; Sandal et al., 2012; Zarrabian et al., 2022), and it was recently shown that is effectively nodulated by IRBG74, an Agrobacterium pusense strain isolated from Sesbania cannabina nodules (Cummings et al., 2009). IRBG74 induces massive root hair curling in Lotus, followed by intercellular infection of the epidermal cells (Montiel et al., 2021). Intercellular colonization is an entry mode observed in approximately 25% of the legume species investigated and although this group includes major legume crops, genetic control remains largely unexplored (Sprent, 2007). However, recent discoveries in intercellularly infected legumes such as Arachys hypogaea, Aeschynomene evenia and Lotus, have attracted attention to this entry mode and provided valuable information about the process (Chaintreuil et al., 2016; Peng et al., 2017; Bertioli et al., 2019; Chen et al., 2019; Karmakar et al., 2019; Montiel et al., 2021; Quilbe et al., 2021; Raul et al., 2022; Montiel et al., 2023). While intra- and intercellular rhizobial invasion share some genetic components and transcriptional responses, relevant differences were found among these processes (Quilbe et al., 2022).
Lotus is an optimal organism with abundant resources to analyze different plant-microbe interactions, such as germline activation of endogenous LORE1a retrotransposon, where new insertion sites can be tracked in the genome with a relatively simple and quick sequence-based method (Urbanski et al., 2012). Here we assess the potential use of a LORE1a activated population in a bulk forward screening. We present a collection of novel mutants impaired in intercellular symbiotic colonization by IRBG74 and compare the nodulation phenotype with that obtained by intracellular M. loti colonization. The genome mapping of the LORE1 insertion sites allowed us to identify ATG9 as a novel regulator in Lotus-rhizobia symbioses.
Seeds from the line G329-3 were collected during several harvesting periods to obtain a mixed and balanced population. Batches with thousands of LORE1 seeds were scarified with hydrochloric acid for 20 min, followed by several washes with distilled sterile water. The seeds were placed in square Petri dishes with moistened paper and two days later, transferred to autoclaved square boxes (40x40x40 cm) filled with Leca. The swollen seeds were inoculated with IRBG74 (O.D. 0.05) and the boxes were maintained in a growth room with controlled temperature, photoperiod (21° C; 16:8 h), intensity light (100 µmol/m2/s). The plants were watered once per week with B & D solution (Broughton and Dilworth, 1971). This procedure was repeated for several months until approximately 200,000 plants were screened. We selected mutants with a visible nodulation phenotype such as: Nod-, hypernodulation or Fix-. This latter manifested by white nodules, reduced nodulation, short plant size and yellow leaves. The selected lines were kept at the greenhouse for seed production. Ten offspring plants from each mutant were subsequently tested by a nodulation assay in plate with IRBG74 (O. D. 0.05).
To track the LORE1 elements in the mutant collection, we followed the protocol previously described by Urbanski et al. (2012). First, total DNA was isolated from 3-5 plant of each mutant with hexadecyltrimethylammonium bromide (CTAB) (Rogers and Bendich, 1985). The DNA was quantified and shared with a Covaris S-series instrument to obtain 600-800 bp fragments. The DNA was blunted, end-repaired and adenylated with a T4 DNA polymerase, T4 DNA polynucleotide kinase and Taq polymerase, respectively, following the manufacturer´s instructions. A ligation was performed to incorporate a splinkerette intermediate adaptor (IA) to the DNA sequences (Mikkers et al., 2002), using a T4 DNA ligase. The flanking LORE1 fragments were obtained by sequential PCR reactions. The first PCR products were amplified with the Splink1 and P2 oligonucleotides, obtaining amplicons of 500-600 bp, that were excised from the agarose gels and used as template for a nested PCR using the Splink2 and P3 primers (Supplementary Table S2). For each DNA sample, a unique P3 oligonucleotide harboring a molecular barcode was used. With this procedure, PCR products of 200-400 bp were amplified and pooled at equimolar concentrations for Illumina sequencing (Supplementary Table S2). The sequencing data was processed to detect the LORE1, adaptor and barcode sequences. These regions were trimmed and only the genomic region was mapped to the L. japonicus Gifu genome using bowtie2 with the following parameters: –end-to-end -X 500 -N 1 -L 28 -D 20 (Kamal et al., 2020). Only insertion with ≥ 4 reads were considered for the analysis.
The L. japonicus accession Gifu (Handberg and Stougaard, 1992) and LORE1 lines were germinated as described above. For nodulation tests, seedlings of 3-5 days post-germination (dpg) were transferred to 12x12 cm square Petri dishes (10 seedlings per plate) containing 1.4% (w/v) agar slant with ¼ B & D medium (Broughton and Dilworth, 1971) and inoculated with 1 ml per plate of a bacterial suspension (M. loti R7A or IRBG74; OD600 = 0.05). The plants were kept in a growth room at 21° C with photoperiod (16/8 h). The nodule numbers were recorded weekly using a stereomicroscope. For the root growth dynamics, instead of B & D solution, the agar was supplemented with Gamborg’s B-5 basal medium (Sigma-Aldrich, G5893) and the progression of the apical main root was monitored weekly.
PCR reactions were performed to genotype the Nod- lines IM-A39, IM-N10 and IM-D22 using specific oligonucleotides for symbiotic genes (Supplementary Table S2), with the Phire Plant Direct PCR Mix (Thermofisher), following the manufacturer’s instructions. Nested PCRs were done for NFR5 in the IM-A39 mutant to flank the LORE2 insertion and the PCR product was sequenced. The LORE1 lines, 30164676 (atg9-2) and 30077209 (atg9-3), affected in the LotjaGi5g1v0179800 gene (LjATG9) were genotyped according to the Lotus base guidelines (Mun et al., 2016).
Progeny of the G329-3 line was successfully used to generate the LORE1 reverse genetics mutant collection in Lotus, composed of 134,682 individual plants (Urbanski et al., 2012; Malolepszy et al., 2016). To explore the potential of LORE1 for forward genetics, cuttings of the G329-3 line were cultivated and harvested in bulk. In this study, several batches of these seeds were used in a large-scale forward screen, searching for mutant plants perturbed in the symbiotic association with IRBG74, a Rhizobium sp. that induce nitrogen-fixing nodules in Lotus through intercellular infection (Montiel et al., 2021). More than 200,000 LORE1 seedlings were inoculated in boxes with IRBG74 and plants with aberrant nodulation phenotypes at 6-8 weeks post-inoculation (wpi) were screened out. Focusing especially on Fix- mutants and delayed nodulation likely to carry mutations in genes required for intercellular infection, 1,200 putative mutants were selected and transferred to the greenhouse for seed production. Large-scale mutant screenings tend to have a variable proportion of false positive selection, therefore, to confirm the symbiotic phenotype of the selected lines, ten offspring plants from each mutant were inoculated with IRBG74 in plates (Figure 1). Since this is an ongoing project, the nodulation phenotype of the progeny of 629 mutants, selected in the first screening still needs to be validated. However, in the remaining 571 lines, the plate test confirmed the nodulation phenotype in 110 mutants (Figure 1, Supplementary Figure S1). In this mutant population, 105 lines showed a Fix- phenotype manifested in white nodule appearance, however, we also identified 4 Nod- mutants without visible nodules and 1 hypernodulating mutant (Supplementary Figure S1).
Figure 1 Scheme of the LORE1 mutant screening to identify lines affected in the intercellular infection by IRBG74. Approximately 200,000 LORE1 plants were inoculated with IRBG74, selecting 1,200 mutants with a Nod- or Fix- phenotype at 6-8 wpi. The offspring seeds of 571 selected lines were inoculated on plates (2nd screening), confirming the nodulation phenotype in 110 lines. For this study, we conducted the FST poolit method to identify the LORE1 insertions sites in the genome of 47 selected mutants.
In this work, we identified 110 LORE1 mutants affected in the nodulation performance with IRBG74. Since handling this number of mutant lines represents a technical challenge, we focused our attention on a subset of 20 randomly chosen mutants. First, we explored the specificity of the symbiotic phenotype, by analyzing the nodulation kinetics on plates at 2-6 weeks post-inoculation (wpi) with M. loti and IRBG74 (Supplementary Figures S2A-E), which colonize Lotus roots intra- and intercellularly, respectively (Montiel et al., 2021). In 12 lines, the number of pink nodules was significantly reduced at any timepoint with both rhizobial strains compared to Gifu (Figures 2A, B). However, nodule formation by M. loti was only affected in IM-53, IM-A19 and IM-D25 mutants. By contrast, the nodulation induced by IRBG74 was exclusively altered in IM-D08, IM-D11, IM-D26 and IM-N12 lines (Figures 2A, B, Supplementary Figures S2A-E). Since perturbances in the nodulation kinetics, nodule organogenesis and nitrogen fixation can negatively impact the plant growth, we measured the shoot length in the LORE1 mutants at 6 wpi with both rhizobial strains. All the mutants tested showed a significantly shorter shoot length compared to Gifu at 6 wpi with IRBG74 (Figure 2A). On contrary, the aerial part was significantly affected in 11 lines inoculated with M. loti respect to Gifu (Figure 2A). These results suggest that all the mutants selected were affected in the Lotus-IRBG74, manifested by a delayed/reduced nodule formation or inefficient plant-growth promotion. Additionally, we observed significant differences in the root growth of several mutants at 6 wpi with IRBG74. The length was reduced in 7 lines and increased in 5 mutants (Supplementary Figure S3).
Figure 2 Significant reductions of nodule number and shoot length in LORE1 mutants. (A) Graphical summary represented by shades of green of the significant differences observed by Mann–Whitney U-test for the parameters recorded in Supplementary Figures S2A-F. (B) Venn diagrams of the LORE1 lines perturbed in the nodule formation induced by M. loti (green) and IRBG74 (pink).
Our study revealed that most of the selected LORE1 mutants were perturbed in the symbiotic program triggered by IRBG74 compared to M. loti. Nonetheless, during our analysis we noticed that certain mutants might be also affected in root development (Supplementary Figure S3). We explored whether any of these mutants have additional defects in their root growth under non-symbiotic conditions, by monitoring the main root length at 2-5 weeks post-germination (wpg) on plates supplemented with 12 mM KNO3. In 14 lines, the root length was significantly shorter compared to Gifu plants of similar age at different timepoints. However, the length of the main root remained shorter throughout the analysis in the mutants IM-58, IM-D09, IM-N4, IM-O7, IM-O33 and IM-S30 (Figure 3). These results prompted us to measure the length of the aerial part in the plants grown in the above referred conditions. Although the shoot length was significantly different in 10 LORE1 mutants compared to Gifu at 5 wpg, only in IM-58, IM-O33 and IM-S30 lines, the size was shorter (Supplementary Figure S4). Our data indicates that shoot and root growth is affected in several LORE1 lines.
Figure 3 Differential root growth dynamics of LORE1 mutants. The progression of main root growth on nitrogen-repleted medium was recorded in Gifu and LORE1 lines at 2-5 weeks post-germination. In boxplots, the center line represents means values of 3 independent experiments; box limits, upper and lower quartiles; whiskers, 1.5× interquartile range; points represent individual data points. The number of plants tested is shown below the violin graphs. The asterisk indicates statistical significance between the LORE1 mutants and Gifu according to Mann–Whitney U-test (*P < 0.05; **P < 0.01; ***P < 0.001).
Mutants analyzed in this study, showed significant alterations in the root growth and plant-microbe associations. To get further insight into the genes associated to these phenotypes, the retrotransposon insertions were tracked in a subset of 20 mutants that were analyzed in more detail, along with 27 additional lines, randomly selected from the screening with IRBG74 (Figure 1, Supplementary Figure S1). For this purpose, we followed the FST poolit protocol that was previously developed to determine the insertion sites in the LORE1 mutant collection (Urbanski et al., 2012; Malolepszy et al., 2016). The endogenous LORE1 sequences and their respective position in the Lotus genome were successfully identified in all samples tested, confirming the effectiveness of the protocol (Supplementary Table S1). In 14 lines only the original LORE1 elements were detected, however, in the remaining 33 mutants, 226 novel retrotransposon insertions were identified, 128 of them unique (Supplementary Table S1). These retrotransposons were distributed in introns, UTR, promoter and intergenic regions of the six chromosomes (Figures 4A, B). A total of 92 Lotus genes were disrupted in these 47 mutants and, each mutant line had in average 4.8 novel LORE1 insertions (Figure 4C). Interestingly, an inspection of the expression profiles in the sequences disrupted by LORE1 insertion in the Lotus database, revealed that certain genes were transcriptionally induced during symbiotic associations with rhizobia or pathogens. Therefore, these genes, interfered by LORE1 elements, are potentially important for symbiosis (Supplementary Figure S5).
Figure 4 Distribution profile of retrotransposon elements in sequenced LORE1 lines. (A) Number of different LORE1 insertions detected in the genome of each mutant, including novel and endogenous elements. Venn diagrams with the number of unique LORE1 elements found in exon, intron, UTR+Promoter, intergenic regions (B) and chromosomes (C).
The nodulation kinetics showed that the IM-A39 mutant was unable to develop any nodules after M. loti and IRBG74 inoculation (Figure 2). Similarly, we noticed that IM-D22 and IM-N10 lines were Nod-. The LORE1 mapping in these plants indicates that IM-D22 contained 13 novel LORE1 insertions, one located in the first exon of CYCLOPS, a crucial transcription factor for the nodulation process (Yano et al., 2008). However, the IM-A39 mutant only had endogenous LORE1 elements and the IM-N10 line contained one intergenic and one exonic insertion, that was not located in a known early symbiotic gene (Supplementary Table S1). These findings suggest that non-LORE1-mediated mutations occurred in IM-A39 and caused the Nod- phenotype. Nod- mutants have been previously documented by different research groups, and this condition is generally caused by the loss-of-function in the early symbiotic genes NSP1, NSP2, NFR5, SYMRK, CCAMK, NIN and NFR1 (Schauser et al., 1999; Radutoiu et al., 2003; Kalo et al., 2005; Heckmann et al., 2006; Yano et al., 2008; Capoen et al., 2009; Singh et al., 2014). Specific primers were designed to amplify by PCR each gene from the start to the stop predicted codon, using as template genomic DNA. The expected amplicons were obtained for all the tested genes in the different genotypes, except for NFR5 in IM-A39. In Gifu, IM-D22 and IM-N10 mutants, a PCR product of 1.7 Kb was amplified, while in IM-A39 the amplicon size was approximately 7 Kb (Figure 5). Sequencing of nested PCRs products for the NFR5 gene in the IM-A39 line revealed a LORE2 insertion within the gene. This finding indicates that additional genomic modifications occurred in some mutants.
Figure 5 Genotyping of early symbiotic genes in LORE1 mutants with a Nod- phenotype. Gel electrophoresis of PCR products amplified with specific oligonucleotides for NSP1, NPS2, NFR5, SYMRK, CCAMK, NIN and NFR1, using as template genomic DNA isolated from leaves of Gifu, IM-A39 (A), IM-D22 (D) and IM-N10 (N) plants. The amplicons encompass from the first to the last predicted codons for each gene. A larger fragment was obtained for the NFR5 in the IM-A39 mutant, revealing a potential insertion within the gene.
Identification of insertion sites in 47 selected LORE1 mutants provided valuable information to discover genes associated with phenotype observed. This list was compared with a collection of homozygous mutants that we have developed in our group in recent years, by genotyping LORE1 lines previously published (Malolepszy et al., 2016). We noticed that we had two additional mutant alleles for one of the insertions found in the IM-S34 line. This mutant contained a LORE1 insertion in the 5´UTR of the predicted AUTOPHAGY9 gene (ATG9), while the additional mutant alleles were affected by a retrotransposon element in the 5´UTR (atg9-2) and the second exon (atg9-3) (Figure 6A). This prompted us to evaluate if the symbiotic phenotype in the IM-S34 mutant (atg9-1) was caused by the disruption of ATG9. Importantly, the IM-S34 line had a low seed production and germination rate that impeded us in performing robust nodulation kinetics with M. loti and IRBG74. This phenotype was probably caused by additional LORE1 elements detected in genes highly expressed in pod and seed (Supplementary Figure S6A). On contrary, ATG9 was mainly expressed in nodules at 21 dpi and induced in different root tissues at 10 dpi with M. loti (Supplementary Figure S6B; Frank et al., 2023).
Figure 6 Nodulation, shoot and root phenotype of atg9-2 and atg9-3 mutants after inoculation with M. loti and IRBG74. (A) Scheme of the LjATG9 gene structure. Pink rectangles, UTRs; Green rectangles, exons; Black lines, introns. Total number of nodules in Gifu (n=20), atg9-2 (n=20) and atg9-3 (n=10) at 2-6 wpi with IRBG74 (B) and M. loti (C). Representative images of nodules formed in Gifu, atg9-2 and atg9-3 at 5 wpi with IRBG74 (D) and M. loti (E). Scale, 1 mm. Root and shoot length measurements with representative images of Gifu, atg9-2 and atg9-3 at 6 wpi with IRBG74 (F–H) and M. loti (I–K). Scale, 1 cm. In boxplots, the center line represents means values of 3 independent experiments; box limits, upper and lower quartiles; whiskers, 1.5× interquartile range; points represent individual data points. The asterisk indicates statistical significance between the LORE1 mutants and Gifu according to Mann–Whitney U-test (*P < 0.05; **P < 0.01; ***P < 0.001).
The nodules numbers in the atg9-2 and atg9-3 mutants were recorded at 2-5 wpi with M. loti and IRBG74. In both mutants nodule development was delayed after IRBG74 inoculation, and the nodule numbers were significantly lower at 3-5 wpi with both rhizobial strains respect to Gifu (Figures 6B, C). Additionally, the nodules formed in these mutants were smaller and pale pink compared to Gifu (Figures 6D, E). The deficient nodulation in these mutants apparently had a negative impact on the growth of the plants harvested at 6 wpi with both inocula, since the shoot length was significantly shorter in atg9-2 and atg9-3 compared to Gifu (Figures 6F, H, I, K). Interestingly, the root length was affected in the mutants inoculated with IRBG74 but not with M. loti (Figures 6G, H, J, K). These results indicate that LjATG9 directly or indirectly affects symbiotic program induced by M. loti and IRBG74.
The screening of legume mutants disturbed in the symbiotic association with rhizobia have provided relevant information on the complex genetic network required for rhizobial infection and nodule organogenesis in model legumes (Schauser et al., 1998; Catoira et al., 2001; Kawaguchi et al., 2002; Lombardo et al., 2006; Starker et al., 2006; Pislariu et al., 2012; Domonkos et al., 2013). In this study, we conducted a large forward mutant screen, with 200,000 LORE1 lines, scoring plants affected in the nodulation program by IRBG74. A great advantage of this approach is the relatively simple and fast insertion site sequencing methodology for identification of causative LORE1 insertions (Urbanski et al., 2012). Compared to map-based cloning, TILLING and whole genome resequencing (Hayashi et al., 2001; Sandal et al., 2002; Sandal et al., 2006; Uchida et al., 2011; Sandal et al., 2012), the FST poolit methods is cheap, robust and fast. Different reports indicate that LORE1 elements have a significant higher preference for genic regions, representing 55-76% of the total novel insertions (Fukai et al., 2012; Urbanski et al., 2012; Malolepszy et al., 2016). Similarly, we observed in the 47 sequenced lines, that 71% of the novel LORE1 copies were inserted in genic regions, distributed in the different chromosomes. We detected an average of 4.8 novel insertions per plant in our mutant collection, which is comparable to the 2.7-4.7 media described in previous studies (Fukai et al., 2012; Urbanski et al., 2012; Malolepszy et al., 2016). These results reflect the efficacy of the method employed and the persistence of the retrotransposon activity in the germline of the G329-3 line.
In our selected mutant collection, 92 Lotus genes with LORE1 insertions were identified. The genes compromised belong to various molecular processes, where 63 of them showed the highest expression levels under symbiotic conditions. Interestingly, we detected in this gene list two members of the cellulose synthase family, LotjaGi6g1v0183200 and LotjaGi4g1v0062500. It was recently shown that mutants affected in a CELLULOSE SYNTHASE-LIKE D1 (CSLD1) gene, develop abnormal root hairs and root nodule symbiosis (Karas et al., 2021). Among the genes affected by LORE1 elements we also found LotjaGi3g1v0273500, which encodes a predicted RhoGEF protein that shares homology with LjSPK1. In Lotus, SPK1 interacts with ROP6 to coordinate the polarized growth of ITs (Liu et al., 2020). Additionally, we also identified known symbiotic players. The Nod- phenotype of the IM-D22 mutant, correlates with the LORE1 element detected in the coding region of CYCLOPS, a key transcription factor required for successful rhizobial infection and nodule organogenesis (Yano et al., 2008). However, we found that in 29% of the sequenced lines only the endogenous LORE1 copies were detected and no additional LORE1 insertions. This number is slightly higher to the 10-20% proportion observed in previous studies (Urbanski et al., 2012; Malolepszy et al., 2016). Therefore, the symbiotic phenotype in this set of mutants is likely mediated by non-LORE1 mutations. This hypothesis is supported by the evidence obtained in the Nod- mutant IM-A39, where a LORE2 element was detected in the coding region of NFR5, the NF receptor (Radutoiu et al., 2003). This finding is not surprising since transposition of LORE2 elements has been documented in regenerated Gifu plants (Fukai et al., 2008). In the Nod- mutant IM-N10, large insertions/deletions were not detected in the early symbiotic genes tested, however, we cannot exclude mutations in the flanking regions of the sequences or other mutations that might impact the ORF of the genes.
In Lotus, the colonization by M. loti occurs via root-hair ITs, an intracellular infection process that has been extensively studied. However, a recent working model in Lotus was developed to study the intercellular infection in legumes, a largely unknown process that exists in approximately 25% of all the legume genera (Sprent, 2007). Although, the intra- and intercellular colonization in Lotus share some genetic components, remarkable differences have been observed among both processes (Quilbe et al., 2022). For instance, the rinrk1 and ern1 mutants show a more severe symbiotic phenotype with M. loti than with IRBG74 and by contrast, several cytoskeleton and cytokinin-related mutants are more affected in the intercellular infection (Copeland, 2021; Montiel et al., 2021). In this work, the large-scale mutant screening in boxes followed by a more stringent evaluation on plate led to the identification of 110 mutants potentially affected in the nodulation program induced by IRBG74, which colonizes Lotus intercellularly. A more detailed analysis in a subset of 20 mutants confirmed one of the selection criteria used in this study, since all the lines showed a significant shorter shoot length respect to Gifu after IRBG74 inoculation. Similarly, the nodule numbers were significantly reduced in 16 lines inoculated with IRBG74 in comparison to Gifu. Although the nodulation kinetics with IRBG74 was not affected in IM-53, IM-A19, IM-D25 and IM-O33 mutants, these lines showed symptoms of nitrogen starvation such as leaf chlorosis and shorter aerial part. Additionally, the nodule formation with M. loti was negatively impacted at different timepoints in IM-53, IM-A19 and IM-D25 lines. Only the nodulation performance of IM-O33 mutant was not affected with any rhizobial inoculum, and the compromised growth of the aerial part in this line is apparently not related to defects in mutualistic interactions with rhizobia, since the short size was also observed in nitrogen-replete conditions. The combined evidence indicates that our screening approaches showed a 95% success (19/20) to select novel mutants affected in the Lotus-rhizobia symbiosis, most of them perturbed in the symbiotic association of Lotus with IRBG74.
Autophagy is a complex coordinated process occurring in eukaryotic organisms, to degrade and recycle cytoplasmic material, which can be induced under adverse conditions, developmental processes, or pathogenic interactions (Wang et al., 2021). We found that in the IM-S34 mutant, a LORE1 element was inserted in the 5´UTR of the LjATG9 gene, a putative orthologue of Arabidopsis thaliana ATG9, which is a component of the autophagosome (Zhuang et al., 2017). The analysis performed in additional mutant alleles, atg9-2 and atg9-3, confirmed the relevance of ATG9 in the symbiotic association of Lotus with M. loti and IRBG74. The role of autophagy has been marginally analyzed in the legume-rhizobia symbiosis, however, it was shown in Phaseolus vulgaris that silencing of the autophagy-related genes PI3K and BECLIN1/ATG6, results in aborted rhizobial infection in root hairs, and reduced nodule formation (Estrada-Navarrete et al., 2016). The recycling of cellular components during rhizobial infection and nodule organogenesis is likely to occur in this complex symbiotic process, however, further research is needed to unveil the precise mechanism of autophagy during legume-rhizobia symbiosis, including a deeper characterization of LjATG9.
L. japonicus is an excellent model legume with several characteristics that have facilitated the research of the legume-rhizobia symbiosis, including abundant expression data, genetic amenability and the Lotus Base, a portal that integrates transcriptomic and genomic data (Mun et al., 2016). These valuable features have been boosted with the generation of the LORE1 mutant collection (Urbanski et al., 2012; Malolepszy et al., 2016). This resource has been widely employed by the scientific community to characterise genes-of-interest, since the retrotransposon elements can be tracked in the genome. In this study, we successfully exploited the germline activity of LORE1a and information in the Lotus base to identify novel mutants disturbed in the intercellular colonization of IRBG74 in Lotus roots. Importantly, the mapping of LORE1 flanking sites revealed that uncharacterized genes transcriptionally upregulated during nodulation are likely associated to the phenotypes observed. These results reinforce the notion that certain molecular components are recruited by the plant host, depending on the infection mechanism, intracellular or intercellular. We also discovered that additional traits of interest can be tested with LORE1 lines, such as plant development.
The original contributions presented in the study are included in the article/Supplementary Material. Further inquiries can be directed to the corresponding authors.
IG-S: Conceptualization, Investigation, Methodology, Writing – original draft. SA: Conceptualization, Resources, Software, Supervision, Writing – review & editing. EM-M: Methodology, Writing – review & editing. MR-G: Methodology, Writing – review & editing. JG: Methodology, Writing – review & editing. NA-B: Methodology, Writing – review & editing. MS: Funding acquisition, Resources, Writing – review & editing. JS: Conceptualization, Funding acquisition, Project administration, Resources, Supervision, Visualization, Writing – original draft, Writing – review & editing. JM: Conceptualization, Funding acquisition, Investigation, Methodology, Resources, Writing – original draft.
The author(s) declare financial support was received for the research, authorship, and/or publication of this article. This work was supported by the grant Engineering the Nitrogen Symbiosis for Africa made to the University of Cambridge by the Bill & Melinda Gates Foundation (ENSA; OPP11772165), the European Research Council (ERC) under the European Union’s Horizon 2020 research and innovation programme (grant agreement no. 834221), the Dirección General de Asuntos del Personal Académico (DGAPA)-Universidad Nacional Autónoma de México (UNAM) – Programa de Apoyo a Proyectos de Investigación e Innovación Tecnológica (PAPIIT, grant IA200723). IG-S was granted with a PhD scholarship of CONACyT.
We thank Finn Pedersen and Nanna Walther with the assistance of LORE1 mutant propagation at the greenhouse, and Noor de Jong, with the setup and screening of LORE1 mutants.
The authors declare that the research was conducted in the absence of any commercial or financial relationships that could be construed as a potential conflict of interest.
The author(s) declared that they were an editorial board member of Frontiers, at the time of submission. This had no impact on the peer review process and the final decision.
All claims expressed in this article are solely those of the authors and do not necessarily represent those of their affiliated organizations, or those of the publisher, the editors and the reviewers. Any product that may be evaluated in this article, or claim that may be made by its manufacturer, is not guaranteed or endorsed by the publisher.
The Supplementary Material for this article can be found online at: https://www.frontiersin.org/articles/10.3389/fpls.2023.1326766/full#supplementary-material
Supplementary Table 1 | Mapping of LORE1 elements in the mutant collection.
Supplementary Table 2 | List of primers used for LORE1 genome mapping and genotyping.
Bertioli, D. J., Jenkins, J., Clevenger, J., Dudchenko, O., Gao, D., Seijo, G., et al. (2019). The genome sequence of segmental allotetraploid peanut Arachis hypogaea. Nat. Genet. 51, 877–884. doi: 10.1038/s41588-019-0405-z
Broughton, W. J., Dilworth, M. J. (1971). Control of leghemoglobin synthesis in snake beans. Biochem. J. 125, 1075–1080. doi: 10.1042/bj1251075
Capoen, W., Den Herder, J., Sun, J., Verplancke, C., De Keyser, A., De Rycke, R., et al. (2009). Calcium spiking patterns and the role of the calcium/calmodulin-dependent kinase CCaMK in lateral root base nodulation of Sesbania rostrata. Plant Cell 21, 1526–1540. doi: 10.1105/tpc.109.066233
Catoira, R., Timmers, A. C., Maillet, F., Galera, C., Penmetsa, R. V., Cook, D., et al. (2001). The HCL gene of Medicago truncatula controls Rhizobium-induced root hair curling. Development 128, 1507–1518. doi: 10.1242/dev.128.9.1507
Chaintreuil, C., Rivallan, R., Bertioli, D. J., Klopp, C., Gouzy, J., Courtois, B., et al. (2016). A gene-based map of the Nod factor-independent Aeschynomene evenia sheds new light on the evolution of nodulation and legume genomes. DNA Res. 23, 365–376. doi: 10.1093/dnares/dsw020
Chaudhary, J., Deshmukh, R., Sonah, H. (2019). Mutagenesis approaches and their role in crop improvement. Plants (Basel) 8. doi: 10.3390/plants8110467
Chen, X., Lu, Q., Liu, H., Zhang, J., Hong, Y., Lan, H., et al. (2019). Sequencing of Cultivated Peanut, Arachis hypogaea, Yields Insights into Genome Evolution and Oil Improvement. Mol. Plant 12, 920–934. doi: 10.1016/j.molp.2019.03.005
Copeland, C. (2021). Same but different: examining the molecular mechanisms of intercellular rhizobial infection. Plant Physiol. 185, 754–756. doi: 10.1093/plphys/kiaa097
Cummings, S. P., Gyaneshwar, P., Vinuesa, P., Farruggia, F. T., Andrews, M., Humphry, D., et al. (2009). Nodulation of Sesbania species by Rhizobium (Agrobacterium) strain IRBG74 and other rhizobia. Environ. Microbiol. 11, 2510–2525. doi: 10.1111/j.1462-2920.2009.01975.x
Domonkos, A., Horvath, B., Marsh, J. F., Halasz, G., Ayaydin, F., Oldroyd, G. E., et al. (2013). The identification of novel loci required for appropriate nodule development in Medicago truncatula. BMC Plant Biol. 13, 157. doi: 10.1186/1471-2229-13-157
Estrada-Navarrete, G., Cruz-Mireles, N., Lascano, R., Alvarado-Affantranger, X., Hernandez-Barrera, A., Barraza, A., et al. (2016). An autophagy-related kinase is essential for the symbiotic relationship between phaseolus vulgaris and both rhizobia and arbuscular mycorrhizal fungi. Plant Cell 28, 2326–2341. doi: 10.1105/tpc.15.01012
Frank, M., Fechete, L. I., Tedeschi, F., Nadzieja, M., Norgaard, M. M. M., Montiel, J., et al. (2023). Single-cell analysis identifies genes facilitating rhizobium infection in Lotus japonicus. Nat. Commun. 14, 7171. doi: 10.1038/s41467-023-42911-1
Fukai, E., Dobrowolska, A. D., Madsen, L. H., Madsen, E. B., Umehara, Y., Kouchi, H., et al. (2008). Transposition of a 600 thousand-year-old LTR retrotransposon in the model legume Lotus japonicus. Plant Mol. Biol. 68, 653–663. doi: 10.1007/s11103-008-9397-2
Fukai, E., Soyano, T., Umehara, Y., Nakayama, S., Hirakawa, H., Tabata, S., et al. (2012). Establishment of a Lotus japonicus gene tagging population using the exon-targeting endogenous retrotransposon LORE1. Plant J. 69, 720–730. doi: 10.1111/j.1365-313X.2011.04826.x
Fukai, E., Umehara, Y., Sato, S., Endo, M., Kouchi, H., Hayashi, M., et al. (2010). Derepression of the plant Chromovirus LORE1 induces germline transposition in regenerated plants. PloS Genet. 6, e1000868. doi: 10.1371/journal.pgen.1000868
Gossmann, J. A., Markmann, K., Brachmann, A., Rose, L. E., Parniske, M. (2012). Polymorphic infection and organogenesis patterns induced by a Rhizobium leguminosarum isolate from Lotus root nodules are determined by the host genotype. New Phytol. 196, 561–573. doi: 10.1111/j.1469-8137.2012.04281.x
Handberg, K., Stougaard, J. (1992). Lotus japonicus, an autogamous, diploid legume species for classical and molecular genetics. Plant J. 2, 487–496. doi: 10.1111/j.1365-313X.1992.00487.x
Hayashi, M., Miyahara, A., Sato, S., Kato, T., Yoshikawa, M., Taketa, M., et al. (2001). Construction of a genetic linkage map of the model legume Lotus japonicus using an intraspecific F2 population. DNA Res. 8, 301–310. doi: 10.1093/dnares/8.6.301
Heckmann, A. B., Lombardo, F., Miwa, H., Perry, J. A., Bunnewell, S., Parniske, M., et al. (2006). Lotus japonicus nodulation requires two GRAS domain regulators, one of which is functionally conserved in a non-legume. Plant Physiol. 142, 1739–1750. doi: 10.1104/pp.106.089508
Kalo, P., Gleason, C., Edwards, A., Marsh, J., Mitra, R. M., Hirsch, S., et al. (2005). Nodulation signaling in legumes requires NSP2, a member of the GRAS family of transcriptional regulators. Science 308, 1786–1789. doi: 10.1126/science.1110951
Kamal, N., Mun, T., Reid, D., Lin, J. S., Akyol, T. Y., Sandal, N., et al. (2020). Insights into the evolution of symbiosis gene copy number and distribution from a chromosome-scale Lotus japonicus Gifu genome sequence. DNA Res. 27. doi: 10.1093/dnares/dsaa015
Karas, B. J., Ross, L., Novero, M., Amyot, L., Shrestha, A., Inada, S., et al. (2021). Intragenic complementation at the Lotus japonicus CELLULOSE SYNTHASE-LIKE D1 locus rescues root hair defects. Plant Physiol. 186, 2037–2050. doi: 10.1093/plphys/kiab204
Karmakar, K., Kundu, A., Rizvi, A. Z., Dubois, E., Severac, D., Czernic, P., et al. (2019). Transcriptomic analysis with the progress of symbiosis in 'Crack-entry' Legume arachis hypogaea highlights its contrast with 'Infection thread' Adapted legumes. Mol. Plant Microbe Interact. 32, 271–285. doi: 10.1094/MPMI-06-18-0174-R
Kawaguchi, M., Imaizumi-Anraku, H., Koiwa, H., Niwa, S., Ikuta, A., Syono, K., et al. (2002). Root, root hair, and symbiotic mutants of the model legume Lotus japonicus. Mol. Plant Microbe Interact. 15, 17–26. doi: 10.1094/MPMI.2002.15.1.17
Liu, J., Liu, M. X., Qiu, L. P., Xie, F. (2020). SPIKE1 activates the GTPase ROP6 to guide the polarized growth of infection threads in lotus japonicus. Plant Cell 32, 3774–3791. doi: 10.1105/tpc.20.00109
Lombardo, F., Heckmann, A. B., Miwa, H., Perry, J. A., Yano, K., Hayashi, M., et al. (2006). Identification of symbiotically defective mutants of Lotus japonicus affected in infection thread growth. Mol. Plant Microbe Interact. 19, 1444–1450. doi: 10.1094/MPMI-19-1444
Madsen, L. H., Fukai, E., Radutoiu, S., Yost, C. K., Sandal, N., Schauser, L., et al. (2005). LORE1, an active low-copy-number TY3-gypsy retrotransposon family in the model legume Lotus japonicus. Plant J. 44, 372–381. doi: 10.1111/j.1365-313X.2005.02534.x
Malolepszy, A., Mun, T., Sandal, N., Gupta, V., Dubin, M., Urbanski, D., et al. (2016). The LORE1 insertion mutant resource. Plant J. 88, 306–317. doi: 10.1111/tpj.13243
Mikkers, H., Allen, J., Knipscheer, P., Romeijn, L., Hart, A., Vink, E., et al. (2002). High-throughput retroviral tagging to identify components of specific signaling pathways in cancer. Nat. Genet. 32, 153–159. doi: 10.1038/ng950
Montiel, J., Garcia-Soto, I., James, E. K., Reid, D., Cardenas, L., Napsucialy-Mendivil, S., et al. (2023). Aromatic amino acid biosynthesis impacts root hair development and symbiotic associations in Lotus japonicus. Plant Physiol. 193, 1508–1526. doi: 10.1093/plphys/kiad398
Montiel, J., Reid, D., Gronbaek, T. H., Benfeldt, C. M., James, E. K., Ott, T., et al. (2021). Distinct signaling routes mediate intercellular and intracellular rhizobial infection in Lotus japonicus. Plant Physiol. 185, 1131–1147. doi: 10.1093/plphys/kiaa049
Mun, T., Bachmann, A., Gupta, V., Stougaard, J., Andersen, S. U. (2016). Lotus Base: An integrated information portal for the model legume Lotus japonicus. Sci. Rep. 6, 39447. doi: 10.1038/srep39447
Peng, Z., Liu, F., Wang, L., Zhou, H., Paudel, D., Tan, L., et al. (2017). Transcriptome profiles reveal gene regulation of peanut (Arachis hypogaea L.) nodulation. Sci. Rep. 7, 40066. doi: 10.1038/srep40066
Pislariu, C. I., Murray, J. D., Wen, J., Cosson, V., Muni, R. R., Wang, M., et al. (2012). A Medicago truncatula tobacco retrotransposon insertion mutant collection with defects in nodule development and symbiotic nitrogen fixation. Plant Physiol. 159, 1686–1699. doi: 10.1104/pp.112.197061
Quilbe, J., Lamy, L., Brottier, L., Leleux, P., Fardoux, J., Rivallan, R., et al. (2021). Genetics of nodulation in Aeschynomene evenia uncovers mechanisms of the rhizobium-legume symbiosis. Nat. Commun. 12, 829. doi: 10.1038/s41467-021-21094-7
Quilbe, J., Montiel, J., Arrighi, J. F., Stougaard, J. (2022). Molecular mechanisms of intercellular rhizobial infection: novel findings of an ancient process. Front. Plant Sci. 13, 922982. doi: 10.3389/fpls.2022.922982
Radutoiu, S., Madsen, L. H., Madsen, E. B., Felle, H. H., Umehara, Y., Gronlund, M., et al. (2003). Plant recognition of symbiotic bacteria requires two LysM receptor-like kinases. Nature 425, 585–592. doi: 10.1038/nature02039
Raul, B., Bhattacharjee, O., Ghosh, A., Upadhyay, P., Tembhare, K., Singh, A., et al. (2022). Microscopic and transcriptomic analyses of dalbergoid legume peanut reveal a divergent evolution leading to nod-factor-dependent epidermal crack-entry and terminal bacteroid differentiation. Mol. Plant Microbe Interact. 35, 131–145. doi: 10.1094/MPMI-05-21-0122-R
Rogers, S. O., Bendich, A. J. (1985). Extraction of DNA from milligram amounts of fresh, herbarium and mummified plant tissues. Plant Mol. Biol. 5, 69–76. doi: 10.1007/BF00020088
Roy, S., Liu, W., Nandety, R. S., Crook, A., Mysore, K. S., Pislariu, C. I., et al. (2020). Celebrating 20 years of genetic discoveries in legume nodulation and symbiotic nitrogen fixation. Plant Cell 32, 15–41. doi: 10.1105/tpc.19.00279
Sandal, N., Jin, H., Rodriguez-Navarro, D. N., Temprano, F., Cvitanich, C., Brachmann, A., et al. (2012). A set of Lotus japonicus Gifu x Lotus burttii recombinant inbred lines facilitates map-based cloning and QTL mapping. DNA Res. 19, 317–323. doi: 10.1093/dnares/dss014
Sandal, N., Krusell, L., Radutoiu, S., Olbryt, M., Pedrosa, A., Stracke, S., et al. (2002). A genetic linkage map of the model legume Lotus japonicus and strategies for fast mapping of new loci. Genetics 161, 1673–1683. doi: 10.1093/genetics/161.4.1673
Sandal, N., Petersen, T. R., Murray, J., Umehara, Y., Karas, B., Yano, K., et al. (2006). Genetics of symbiosis in Lotus japonicus: recombinant inbred lines, comparative genetic maps, and map position of 35 symbiotic loci. Mol. Plant Microbe Interact. 19, 80–91. doi: 10.1094/MPMI-19-0080
Schauser, L., Handberg, K., Sandal, N., Stiller, J., Thykjaer, T., Pajuelo, E., et al. (1998). Symbiotic mutants deficient in nodule establishment identified after T-DNA transformation of Lotus japonicus. Mol. Gen. Genet. 259, 414–423. doi: 10.1007/s004380050831
Schauser, L., Roussis, A., Stiller, J., Stougaard, J. (1999). A plant regulator controlling development of symbiotic root nodules. Nature 402, 191–195. doi: 10.1038/46058
Singh, S., Katzer, K., Lambert, J., Cerri, M., Parniske, M. (2014). CYCLOPS, a DNA-binding transcriptional activator, orchestrates symbiotic root nodule development. Cell Host Microbe 15, 139–152. doi: 10.1016/j.chom.2014.01.011
Sprent, J. I. (2007). Evolving ideas of legume evolution and diversity: a taxonomic perspective on the occurrence of nodulation. New Phytol. 174, 11–25. doi: 10.1111/j.1469-8137.2007.02015.x
Starker, C. G., Parra-Colmenares, A. L., Smith, L., Mitra, R. M., Long, S. R. (2006). Nitrogen fixation mutants of Medicago truncatula fail to support plant and bacterial symbiotic gene expression. Plant Physiol. 140, 671–680. doi: 10.1104/pp.105.072132
Tadege, M., Wen, J., He, J., Tu, H., Kwak, Y., Eschstruth, A., et al. (2008). Large-scale insertional mutagenesis using the Tnt1 retrotransposon in the model legume Medicago truncatula. Plant J. 54, 335–347. doi: 10.1111/j.1365-313X.2008.03418.x
Uchida, N., Sakamoto, T., Kurata, T., Tasaka, M. (2011). Identification of EMS-induced causal mutations in a non-reference Arabidopsis thaliana accession by whole genome sequencing. Plant Cell Physiol. 52, 716–722. doi: 10.1093/pcp/pcr029
Urbanski, D. F., Malolepszy, A., Stougaard, J., Andersen, S. U. (2012). Genome-wide LORE1 retrotransposon mutagenesis and high-throughput insertion detection in Lotus japonicus. Plant J. 69, 731–741. doi: 10.1111/j.1365-313X.2011.04827.x
Wang, P., Wang, T., Han, J., Li, M., Zhao, Y., Su, T., et al. (2021). Plant autophagy: an intricate process controlled by various signaling pathways. Front. Plant Sci. 12, 754982. doi: 10.3389/fpls.2021.754982
Yano, K., Yoshida, S., Muller, J., Singh, S., Banba, M., Vickers, K., et al. (2008). CYCLOPS, a mediator of symbiotic intracellular accommodation. Proc. Natl. Acad. Sci. U.S.A. 105, 20540–20545. doi: 10.1073/pnas.0806858105
Zarrabian, M., Montiel, J., Sandal, N., Ferguson, S., Jin, H., Lin, Y. Y., et al. (2022). A promiscuity locus confers Lotus burttii nodulation with rhizobia from five different genera. Mol. Plant Microbe Interact 11, 1006–1017. doi: 10.1094/MPMI-06-22-0124-R
Keywords: symbiosis, legume (nodules), mutant screening, autophagy, intercellular infection, Lotus japonicus
Citation: García-Soto I, Andersen SU, Monroy-Morales E, Robledo-Gamboa M, Guadarrama J, Aviles-Baltazar NY, Serrano M, Stougaard J and Montiel J (2024) A collection of novel Lotus japonicus LORE1 mutants perturbed in the nodulation program induced by the Agrobacterium pusense strain IRBG74. Front. Plant Sci. 14:1326766. doi: 10.3389/fpls.2023.1326766
Received: 23 October 2023; Accepted: 12 December 2023;
Published: 05 January 2024.
Edited by:
Maria Jose Soto, Spanish National Research Council (CSIC), SpainReviewed by:
María Isabel Rubia, Public University of Navarre, SpainCopyright © 2024 García-Soto, Andersen, Monroy-Morales, Robledo-Gamboa, Guadarrama, Aviles-Baltazar, Serrano, Stougaard and Montiel. This is an open-access article distributed under the terms of the Creative Commons Attribution License (CC BY). The use, distribution or reproduction in other forums is permitted, provided the original author(s) and the copyright owner(s) are credited and that the original publication in this journal is cited, in accordance with accepted academic practice. No use, distribution or reproduction is permitted which does not comply with these terms.
*Correspondence: Jesús Montiel, am1vbnRpZWxAY2NnLnVuYW0ubXg=; Jens Stougaard, c3RvdWdhYXJkQG1iZy5hdS5kaw==
Disclaimer: All claims expressed in this article are solely those of the authors and do not necessarily represent those of their affiliated organizations, or those of the publisher, the editors and the reviewers. Any product that may be evaluated in this article or claim that may be made by its manufacturer is not guaranteed or endorsed by the publisher.
Research integrity at Frontiers
Learn more about the work of our research integrity team to safeguard the quality of each article we publish.