- College of Horticulture, Sichuan Agricultural University, Chengdu, China
Light is one of the important environmental factors affecting the growth and development of facility vegetables. In this experiment, we investigated the effects of different light intensities on the growth, nutritional quality and flavonoid accumulation of celery under hydroponic and full LED light conditions. Four light intensities of 40, 100, 200, or 300 µmol·m-2·s-1 were set up in the experiment, and three harvest periods were set up on the basis of different light intensities, which were 15, 30, and 45 d after treatment (labeled as S1, S2, and S3, respectively). The results showed that the plant height and aboveground biomass of celery increased with the increase of light intensity, and the light intensity of 200 μmol·m-2·s-1 was beneficial to increase the contents of chlorophyll, carotenoids, total phenols, vitamin C, cellulose, total flavones and apigenin in celery. During the S1-S3 period, the activities of PAL, CHS, CHI and ANS increased gradually under 200 and 300 μmol·m-2·s-1 light intensity treatments, and the activities of FNS and CHS enzymes were the highest under 200 μmol·m-2·s-1 light intensity treatment. The expression and ANS activity of Ag3GT, a key gene for anthocyanin synthesis, reached the maximum value at 300 μmol·m-2·s-1, and the expression level and FNS activity of AgFNS, a key gene for apigenin synthesis, reached a maximum value at 200 μmol·m-2·s-1. In general, the anthocyanin content was the highest at 300 μmol·m-2·s-1, and the apigenin content was the highest at 200 μmol·m-2·s-1. In conclusion, light intensity of 200 µmol·m-2·s-1 treatment was more favorable for celery growth and nutrient synthesis.
1 Introduction
Light is an important source of energy and signaling for plants, which influences plant growth, development, and structural characteristics. The morphological structure and material accumulation of plants are closely related to light intensity, and both low and high light are detrimental to plant growth (Lv et al., 2021). When plants are exposed to low-light environments for long periods of time, they tend to develop a series of shade-tolerant mechanisms in order to adapt to such environments, such as increasing plant height to obtain more light energy, decreasing leaf area, decreasing energy consumption and transpiration, promoting plant stem elongation, decreasing root biomass, and enhancing apical dominance (Aphalo and Ballaré, 1995; Ballaré and Scopel, 1997; Collins and Wein, 2000). When the light intensity is too strong, the tissue structure of plant leaves will change, the leaves will become smaller and thicker, and the light intensity is too strong to affect the transportation and transfer of nutrients and water in the plant body, and the leaves will turn yellow or even wither (Matos et al., 2009). Studies have shown that the content of vitamin C and soluble sugars decreases when light intensity is insufficient, suggesting that low light has an inhibitory effect on the synthesis of vitamin C and soluble sugars (Fu et al., 2017; Liu et al., 2022). In addition, with the increase of light intensity, the content of ascorbic acid in lettuce showed an increasing trend, but the content of soluble protein and nitrate in lettuce showed a decreasing trend (Fu et al., 2017). In conclusion, too much or too little light can inhibit plant growth, so only at the appropriate light intensity can we better promote the quality formation of vegetables.
Flavonoids are important secondary metabolites in plants with pharmacological effects such as anticancer, antioxidant and hypoglycemic. They generally refer to a series of compounds formed by two benzene rings interconnected by a central three-carbon chain (Bast et al., 2007; Mojžiš et al., 2008). Flavonoids can be categorized into eight types according to their substituents: flavonoids, flavonols, dihydroflavonoids, chalcones, isoflavonoids, dioflavonoids, anthocyanins and flavanols (Safe et al., 2021). Anthocyanins and apigenin are important secondary metabolites in plants and belong to flavonoids. For plants, light is a crucial environmental factor affecting the synthesis of flavonoids, which plays a role in two ways: Light not only affects plant morphogenesis and growth metabolism, provides the necessary metabolic substrates for the synthesis of flavonoids, but also promotes the synthesis of flavonoids by activating enzymes that regulate flavonoid metabolism. Studies have shown that different light intensities, light qualities and photoperiods can indirectly regulate the expression of flavonoid-regulated genes and thus control the accumulation of flavonoids through the modulation of photosensitive pigments and cryptochromes (Jaakola, 2013). Benjamín Battistoni et al. demonstrated that different ratios of light quality composition favored the synthesis of spinach flavonoids (Samuolienė et al., 2012). The changes in flavonoid content in kale leaves revealed that the accumulation of quercetin glucoside analog synthesis was promoted under strong light conditions, whereas the accumulation of kaempferol glucoside analog synthesis was inhibited, but the total flavonoid content was consistently higher in strong light than in weak light (Neugart et al., 2016).
Celery (Apium graveolens L.) is a widely cultivated vegetable in the world with rich nutritional and health values. There is the efficacy of lowering blood pressure, strong antioxidant and free radical scavenging ability, cancer prevention and anti-cancer in celery. Especially, anthocyanins and apigenin are important flavonoids in celery and have the ability to improve the body’s defense against diseases. In addition to varietal differences, its components as well as content are also affected by environmental factors such as light, but there are fewer studies on the dynamics of flavonoid compounds in celery, as well as the correlation between the corresponding physiological indexes and light intensity.
In this study, Hongcheng red celery (HQ) was used as the test material to determine the effects of light intensity on the growth, basic nutrients and the content of important flavonoids in the body of celery under the conditions of plant factories. By exploring the effects of light intensity on the synthesis and accumulation of flavonoid compounds in different developmental stages of celery leaves and petioles, and then understanding the changes in the synthesis and accumulation of compounds under the treatment of different light intensities, we can provide a reference for the molecular mechanism of light regulation of the synthesis and accumulation of flavonoid compounds in celery. At the same time, it can also provide scientific basis for the promotion and cultivation management of celery in production, and provide reference value for the reasonable control of light in practice.
2 Materials and methods
2.1 Materials and seedlings cultivation
Experiments were conducted in a plant factory at Sichuan Agricultural University (Chengdu, China) in 2021. The selected material was Hongcheng red celery (Apium graveolens L.) Full and healthy celery seeds were soaked in warm broth and then germinated in a climatic chamber, where the temperatures were maintained at 18°C ± 2°C (day) and 15°C ± 2°C (night). After the seeds were dewy, they were sown in black cross seedling sponges (2.5 × 2.5 × 2.5 cm) with round holes and two seeds were sown in each hole. When the seedlings reached two leaves and one heart, they were watered with 1/2 of Hoagland’s nutrient solution. The pH of the nutrient solution was 6.5 ± 0.5 and the EC was 1.2 ± 0.5 ms·cm-1.
2.2 Experimental treatment
When the seedlings are five leaves and one heart (about 60 d after sowing), they are selected and moved into the hydroponic cycle racks of the plant factory and kept at 1 plant per hole. These seedlings were shosen in uniform growth, with free of pests and diseases. The light intensity of the light source was adjusted with a plant light analyzer. The experiment was set up with four treatments, 40 µmol·m-2·s-1, 100 µmol·m-2·s-1, 200 µmol·m-2·s-1, and 300 µmol·m-2·s-1. Each treatment had 35 seedlings and was replicated three times for a total of 105 seedlings. The environmental conditions were maintained at a temperature of 25°C/18°C (day/night), air humidity of 50%, and a photoperiod of 12/12 h (day/night) (Pre-experimental screening). After transplanting, the seedlings were watered with 1/2 Hoagland nutrient solution at the same pH as that of seedlings and an EC value of 2.0 ± 0.5 ms·cm-1. The content of flavonoids, flavonoid-related enzyme activities, and expression of related genes in celery were determined at 15, 30, and 45 d after treatment (S1, S2, and S3, respectively).
2.3 Determination of growth parameters
After 45 d of treatment with different light intensities, we harvested celery. Plant height and root length were measured with a millimeter scale. Aboveground dry and fresh weights were measured with an electronic balance, and water content was calculated using the formula: (fresh weight - dry weight)/fresh weight × 100%.
2.4 Assay for chlorophyll content
After 45 d of treatment with different light intensities, we harvested celery. For each treatment, 0.5 g of fresh leaves were weighed and the photosynthetic pigment content of celery leaves was determined with reference to the acetone-ethanol mixture immersion method (Lichtenthaler and Buschmann, 2001). The chlorophyll content was calculated as follows:
2.5 Measurement of chlorophyll fluorescence and photosynthetic parameters
After 45 d of treatment with different light intensities, five fully expanded mature leaves from each treatment were randomly selected and the maximum photosynthetic efficiency (Fv/Fm), photochemical burst (qP), non-photochemical burst (NPQ), and relative electron transfer rate (ETR) of photosystem II were determined by a portable fluorometer (Walz, PAM-2500, Effeltrich, Germany). Net photosynthetic rate (Pn), intercellular CO2 concentration (Ci) stomatal conductance (Gs), and transpiration rate (Tr) of leaves were determined with a portable photosynthesis meter (Li-6400,United State).
2.6 Measurement of essential nutrients
After 45 d of treatment with different light intensities, we divided the celery into leaves and petioles and took 0.5 g of fresh samples for the determination of the basic nutrients they contained. Nitrate content in celery leaves was measured with reference to the salicylic acid-sulfuric acid colorimetric method (Cataldo et al., 1975). Vitamin C content was determined by molybdenum blue spectrophotometry (Chen et al., 2015). The total phenolic content in methanolic extracts was determined using the Folin-Ciocalteau procedure with gallic acid as a standard (Singleton et al., 1999). Cellulose content was measured by digestion and gravimetric technique (Antia et al., 2006).
2.7 Measurement of major flavonoid compounds content
The content of major flavonoid compounds contained in celery was determined at 15, 30 and 45 d after treatment with different light intensities. The extract was carried out with methanol at a material ratio of 1:25, and the total flavonoid content was determined after ultrasonication and filtration (Ghadage et al., 2017). The anthocyanin content was determined by the method of Li (Li et al., 2012). According to: OD = (OD530 - OD620) - 0.1 × (OD650 – OD620) formula, the anthocyanin absorbance value in each sample was calculated, and the anthocyanin content in each sample was calculated according to the total anthocyanin content per 1 mg sample per 0.1 absorbance. Apigenin content was determined by liquid chromatography (Kim et al., 2008; Yan et al., 2014).
2.8 Measurement of flavonoid-related enzyme activities
The activities of flavonoid-related enzymes in celery were measured at 15, 30 and 45 days after different light intensities. The activities of phenylalanine deaminase (PAL), chalcone isomerase (CHI), chalcone synthase (CHS), flavonoid synthase (FNS) and anthocyanin synthase (ANS) during the synthesis of flavonoids were measured using ELISA kits produced by Chongqing Bonoheng Biotechnology Co.
2.9 Expression analysis of key flavonoid-related genes
Ag3GT, a key gene for anthocyanin synthesis, and AgFNS, a key gene for apigenin synthesis, were identified quantitatively (Tan, 2020). The expression levels of apigenin and anthocyanin-related genes were measured at 15, 30 and 45 days after different light intensities. Total RNA was extracted from the leaves and petioles of fresh celery by employing an RNA preparation kit (Tiangen, Beijing; 0.1 g). 1µg of total RNA was reverse transcribed to cDNA using TaKaRa reverse transcription kit, then diluted with sterile ddH2O and stored in -20°C refrigerator and used for fluorescent quantitative PCR analysis. The fluorescent dye reagent is TB GreenTM Permix Ex TapTM II (TAKARA). Use the Archimed X Series real-time PCR instruments. The amplification program was as follows: 30 s at 95°C, 40 cycles of 5 s at 95°C, 15 s at 58°C. Relative gene expression was evaluated using the 2-△△CT method. The PCR primers were designed using Primer Premier 5 software (PREMIER Biosoft, Palo Alto, CA, United States), and is listed in Table 1. Actin (AF111812) was used as an internal control.
2.11 Statistical analysis
Trials were statistically and graphically plotted using Origin 2017, and SPSS 27.0 software was used to perform analysis of significant variance (ANOVA) on the data, and Duncan’s multiple test was used to compare the means.
3 Results and analysis
3.1 Effect of light intensity on biological parameters of celery
The effects of different light intensities on the changes of various morphological indicators of celery were basically the same. With increasing light intensity, celery height, fresh weight and dry weight tended to increase and then decrease, while root length decreased (Figure 1). The above-ground fresh and dry weights of celery increased by 64.07% and 205.17%, respectively, when the light intensity was 200 µmol·m-2·s-1 compared with that of 40 µmol·m-2·s-1. This indicates that suitable light intensity can promote the growth of celery (Table 2).
The photosynthetic pigment content of celery leaves increased and then decreased with increasing light intensity, and the contents of chlorophyll a, chlorophyll b, total chlorophyll and carotenoids were highest at a light intensity of 100 µmol·m-2·s-1and lowest at a light intensity of 300 µmol·m-2·s-1 for all photosynthetic pigments except carotenoids (Table 3).
3.2 Effect of light intensity on Photosynthetic characteristics in celery
Different light intensities affected the photosynthetic capacity of celery. The values of Pn, Gs and Ci all varied in an ascending and then descending manner, reaching a maximum at a light intensity of 200 µmol·m-2·s-1(Figures 2A, B, D). The value of Tr reached a maximum at a light intensity of 300 µmol·m-2·s-1, which was higher than that at a light intensity of 40 µmol·m-2·s-1 124.43% (Figure 2C).
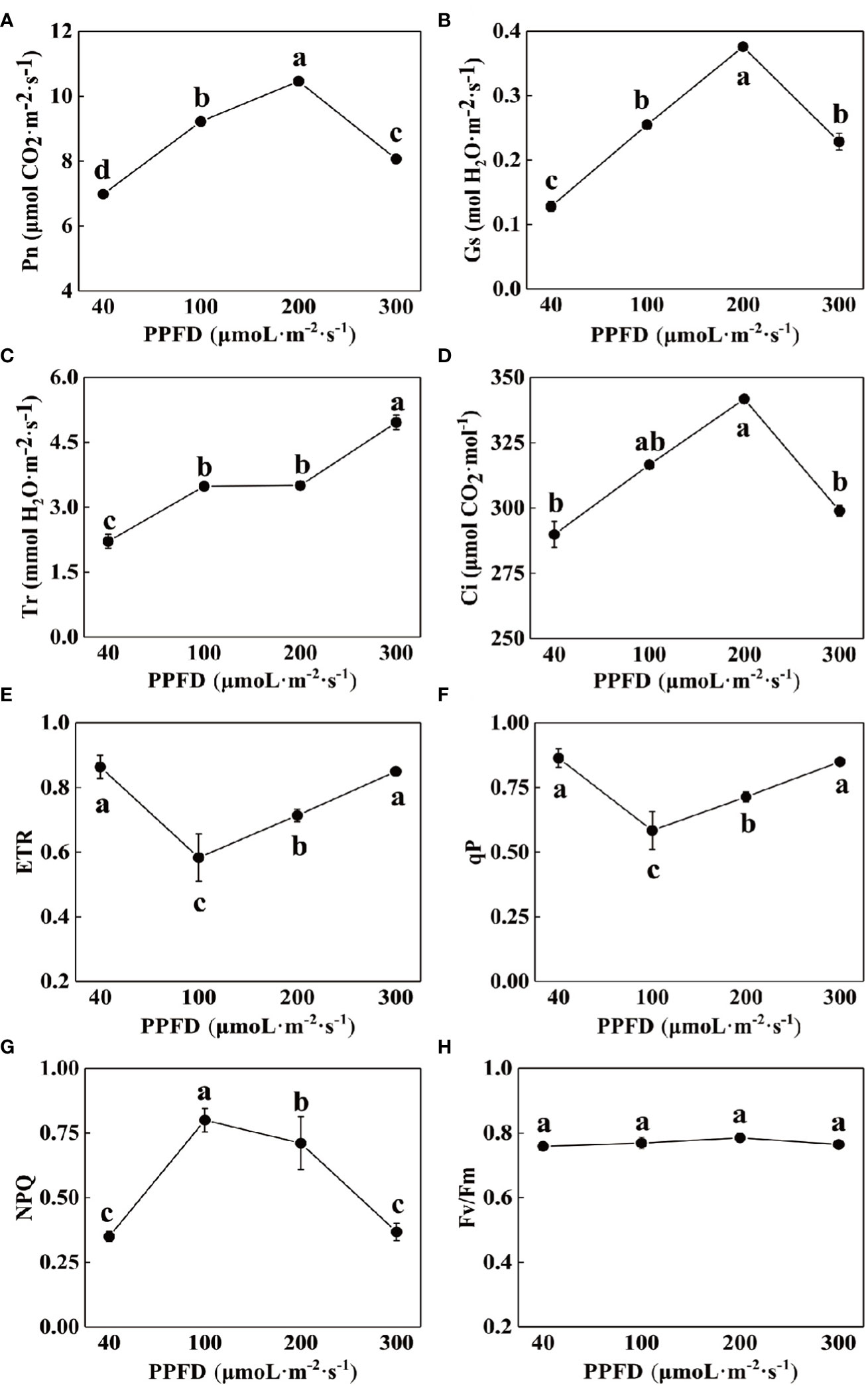
Figure 2 Effects of different light intensities on photosynthetic gas exchange parameters and chlorophyll fluorescence parameters of celery. (A) net photosynthetic rate (Pn), (B) stomatal conductance (Gs), (C) transpiration rate (Tr), (D) intercellular CO2 concentration (Ci), (E) Electron transport rate through PSII (ETR), (F) photochemical quenching coefficient (qP), (G) Non-photochemical quenching (NPQ) and (H) The maximum PSII quantum yield (Fv/Fm) of celery leaves in response to 40, 100, 200 and 300 µmol·m-2·s-1 photosynthetic photon flux density (PPFD). The mean ± SE are shown (n ≥ 3). Different letters indicate significant difference among the treatments (P< 0.05).
Chlorophyll fluorescence parameters reflect the process of absorption, transmission and dissipation of light energy by the photosystem and are often used to test the photosynthetic capacity of plants. The NPQ of celery leaves increased and then decreased with increasing light intensity, and was significantly higher than the other treatments at a light intensity of 100 µmol·m-2·s-1, 128.57%, 12.68%, and 116.22% (Figure 2G), respectively, while ETR and qP decreased and then increased (Figures 2E, F), and Fv/Fm did not change significantly (Figure 2H).
3.3 Effect of light intensity on essential nutrients in celery
Figure 3 reflects the changes in essential nutrients in celery leaves under different light intensity treatments. The vitamin C and total phenolics contents of celery leaves were higher than those of petioles (Figures 3A, B), and the cellulose and were lower than those of petioles (Figure 3D). All the indicators except nitrate showed a trend of increasing and then decreasing with increasing light intensity, reaching a maximum at a light intensity of 200 µmol·m-2·s-1. The content of nitrate decreased with increasing light intensity and was significantly lower at a light intensity of 300 µmol·m-2·s-1 (Figure 3C). Compared with a light intensity of 40 µmol·m-2·s-1, the nitrate content of leaves and petioles respectively decreased by 33.88% and 38.96%.
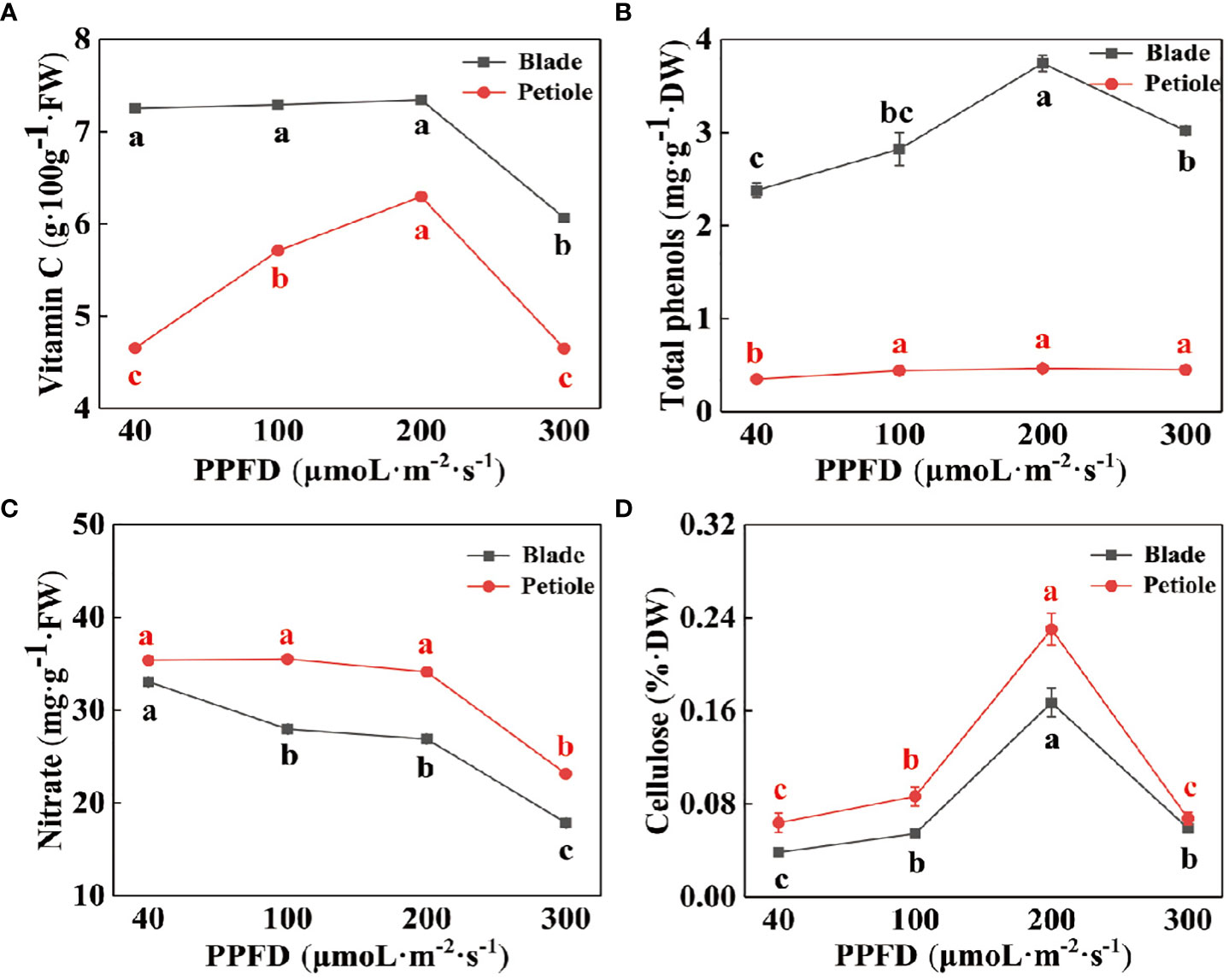
Figure 3 The contents of vitamin C (A), total phenolics (B), nitrate (C) and cellulose (D) in celery leaves and petioles under different photosynthetic photon flux density (PPFD). Different letters (a–d) on the bar plots indicate significant difference at p< 0.05 using one-way analysis of variance with Duncan’s multiple-range test.
3.4 Effect of light intensity on important flavonoid compounds
Suitable light is beneficial in promoting the synthesis of important flavonoids in celery. Celery petioles have higher levels of anthocyanins than leaves (Figure 4C), while leaves contain higher levels of apigenin than petioles (Figure 4B). During the same period, the content of total flavones and apigenin increased and then decreased with increasing light intensity, reaching a maximum at a light intensity of 200 µmol·m-2·s-1 (Figures 4A, B), while the content of anthocyanins continued to be enhanced (Figure 4C). The anthocyanin content of leaves and petioles gradually increased with increasing treatment time, but apigenin showed the opposite trend (Figure 4). In S3 period, the anthocyanin content of leaves and petioles was 344.48%, 644.44% and 47.43%, 98.13% higher at light intensity of 300 µmol·m-2·s-1 compared to S1 and S2 periods. The apigenin content was the highest at 200 μmol·m-2·s-1 in celery, and the apigenin content in leaves and petioles at S3 period decreased by 22.47 %, 37.62 %, 18.01 % and 8.23 % compared with S1 and S2 periods (Figure 4).
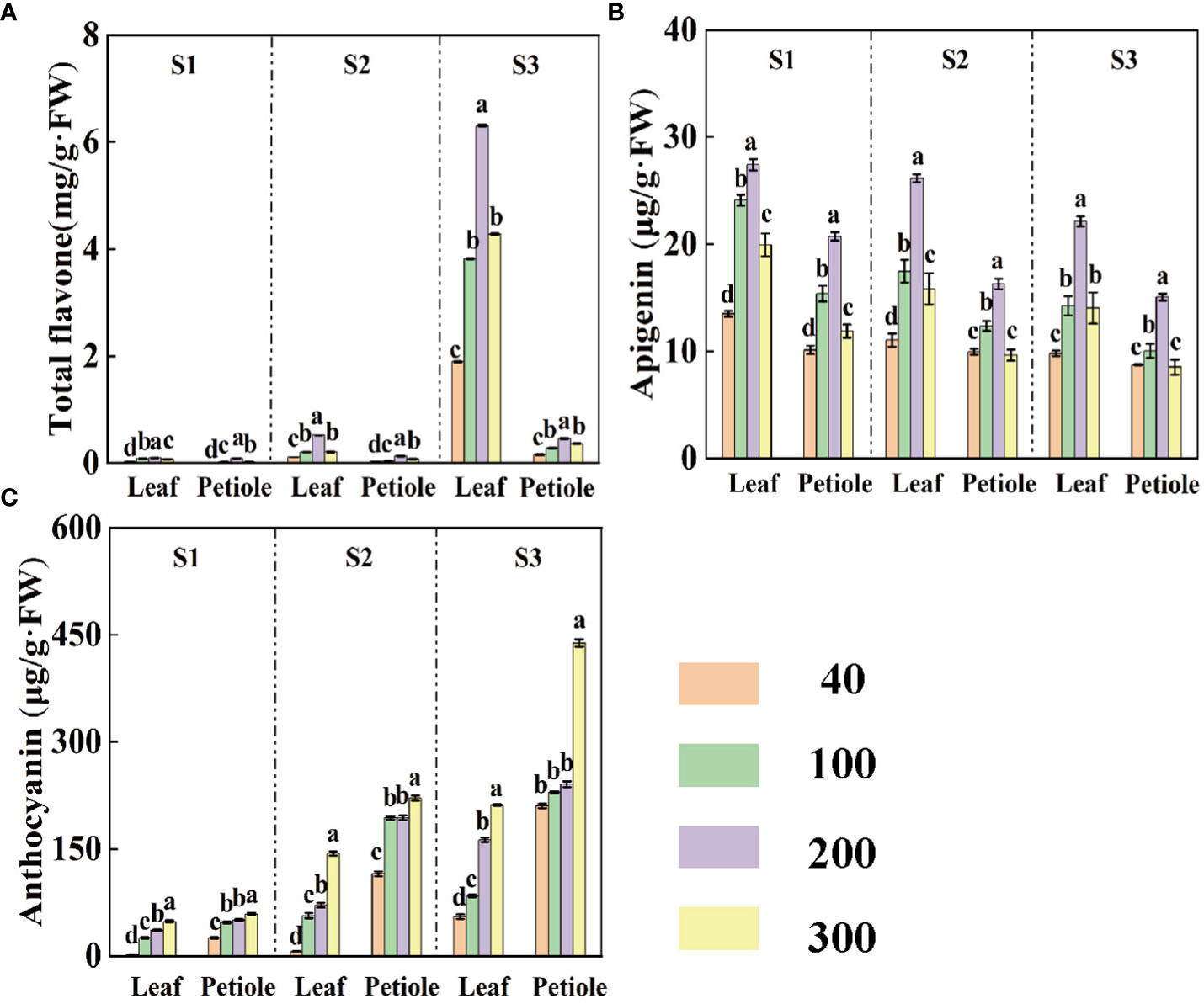
Figure 4 The contents of total flavones (A), apigenin (B) and anthocyanins (C) in celery at three different periods (S1 15d, S2 30d, S3 45d) under different light intensity treatments. Different letters (a–c) on the bar plots indicate significant difference at p< 0.05 using one-way analysis of variance with Duncan’s multiple-range test.
3.5 Effect of light intensity on PAL, CHI, CHS, FNS and ANS
Except for the ANS activity which increased with the increase of light intensity, the activities of all the enzymes showed a trend of increasing and then decreasing with the increase of light intensity, and reached the maximum at the light intensity of 200 µmol·m-2·s-1 (Figure 5). Under the same light intensity treatment, the activities of PAL, CHS, CHI and ANS were enhanced with increasing treatment time (Figures 5A–D), but the activity of FNS was gradually decreased (Figure 5E). The lowest FNS activity decreased by 56.01%, 43.01% and 14.14% and 9.59% in S3 period compared with S1 and S2, respectively (Figure 5E).
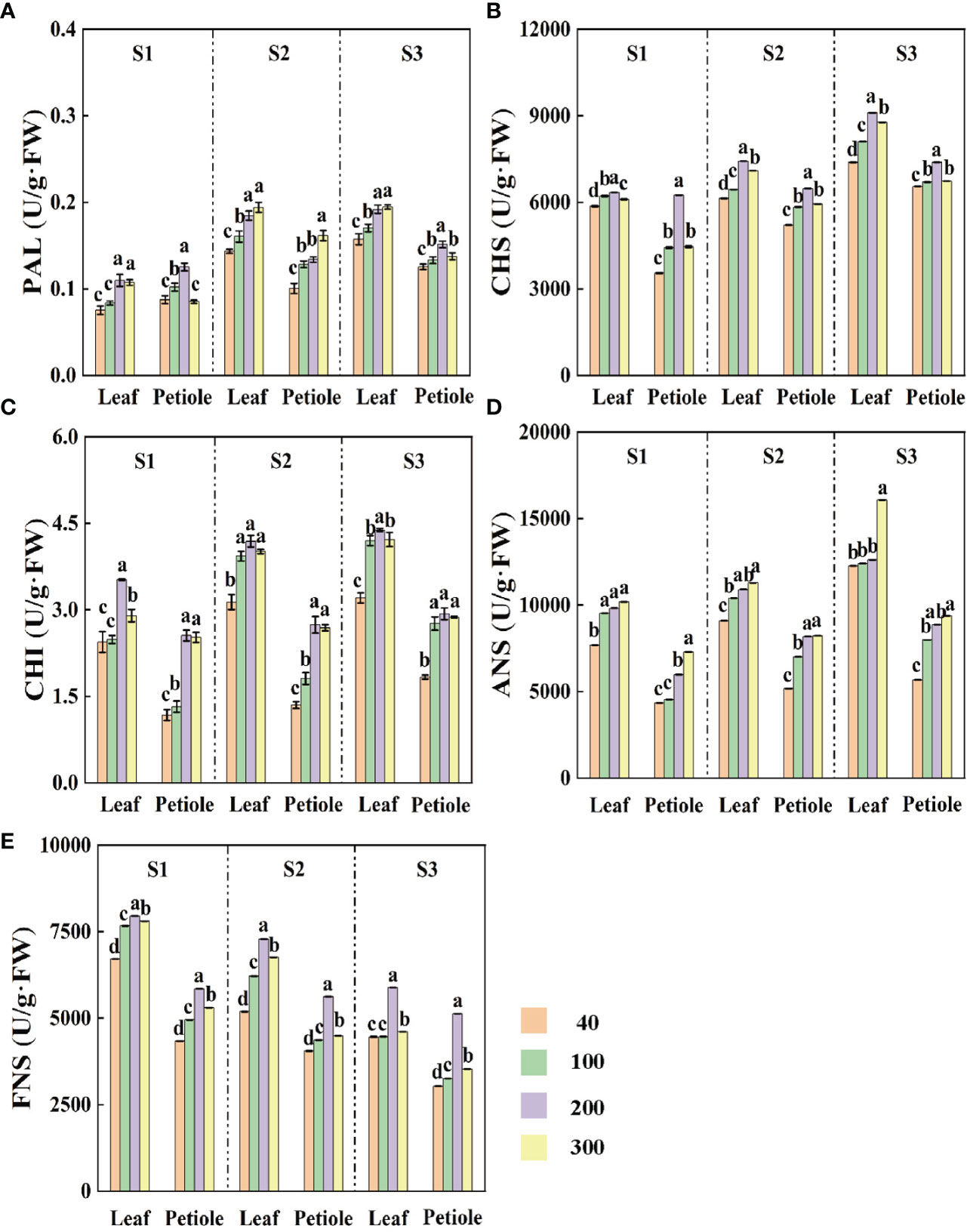
Figure 5 The effects of light intensity on (A) phenylalanine deaminase PAL, (B) chalcone synthase CHS, (C) chalcone isomerase CHI, (D) anthocyanin synthase ANS, and (E) flavonoid synthase FNS at three different periods (S1 15d, S2 30d, S3 45d). Different letters (a–e) on the bar plots indicate significant difference at p< 0.05 using one-way analysis of variance with Duncan’s multiple-range test.
3.6 Effect of light intensity on apigenin and anthocyanin gene expression
The expression of anthocyanin synthesis gene Ag3GT increased with the increase of light intensity, while the expression of apigenin synthesis gene AgFNS showed a trend of increasing and then decreasing, and reached the maximum at the light intensity of 200 µmol·m-2·s-1 (Figure 6). The expression of AgFNS showed a decreasing trend under the same light intensity treatment and the expression of AgFNS in the leaves was significantly higher than that in the petioles (Figure 6B). The expression of Ag3GT increased with the increase of treatment time under the same light intensity treatment, and the maximum expression of the gene in the leaf and petiole increased 422.86%, 760.20% and 62.59%, 102.32% in S3 period compared with S1 and S2 periods, respectively, and the maximum expression in the petiole was 2.07 times higher than that in the leaf (Figure 6A).
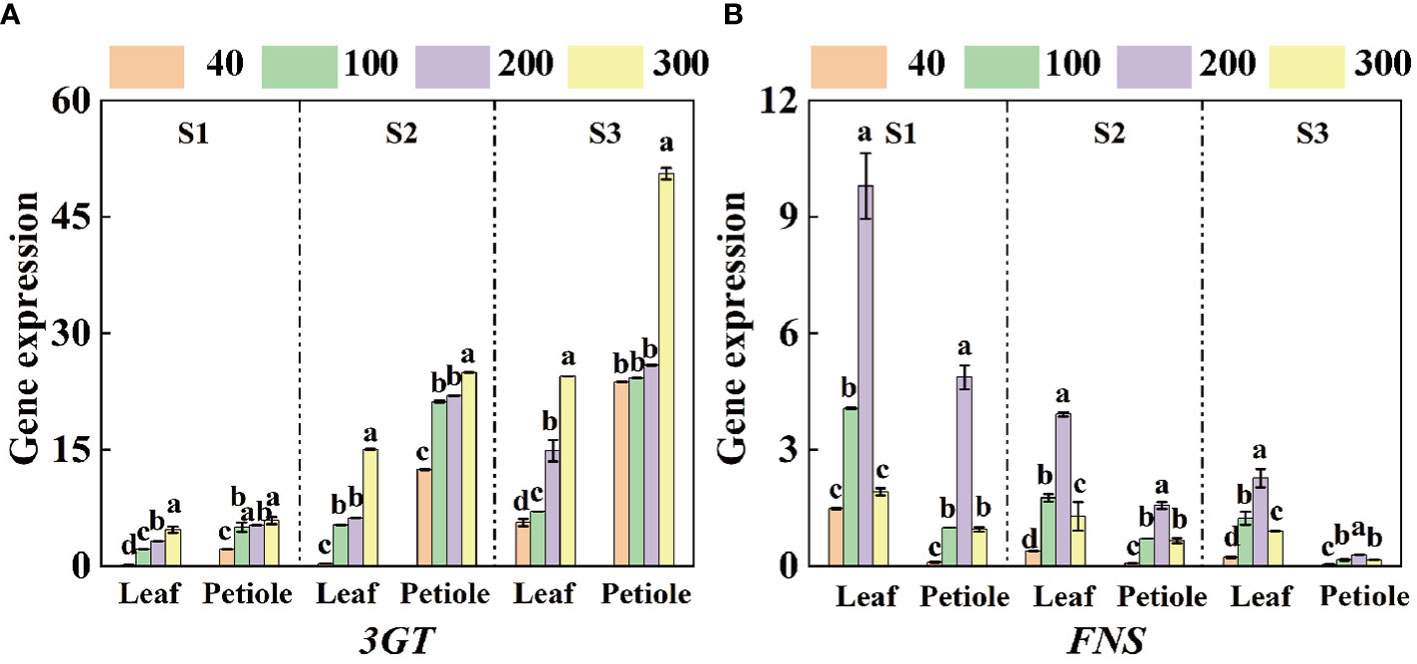
Figure 6 qRT-PCR analyzed the expression of genes related to apigenin and anthocyanin synthesis in celery blades and petioles. (A, B) The expression levels of 3GT and FNS in three different periods (S1 15d, S2 30d, S3 45d). The mean ± SE are shown (n ≥ 3). Different letters indicate significant difference among the treatments (P< 0.05).
3.7 Correlation analysis of light intensity on anthocyanin and apigenin content of celery
Figure 7 shows the correlation analysis of light intensity on apigenin and apigenin content. In celery blades (Figure 7A), the light intensity was significantly correlated with the anthocyanin content and apigenin content of celery blades. The content of apigenin in celery blades was significantly negatively correlated with the shoot water content of celery, and was positively correlated with the expression of stem thickness, aerial dry weight, Gs, Ci, Fv/Fm, blade total phenols, AgFNS, total flavonoids, PAL, CHS, FNS and AgFNS. The anthocyanin content was positively correlated with PAL activity and Ag3GT expression in blades, but negatively correlated with chlorophyll a, chlorophyll b and total chlorophyll content in blades. In celery petioles, the light intensity was significantly negatively correlated with the total chlorophyll content in celery petioles, and its ETR was significantly positively correlated.
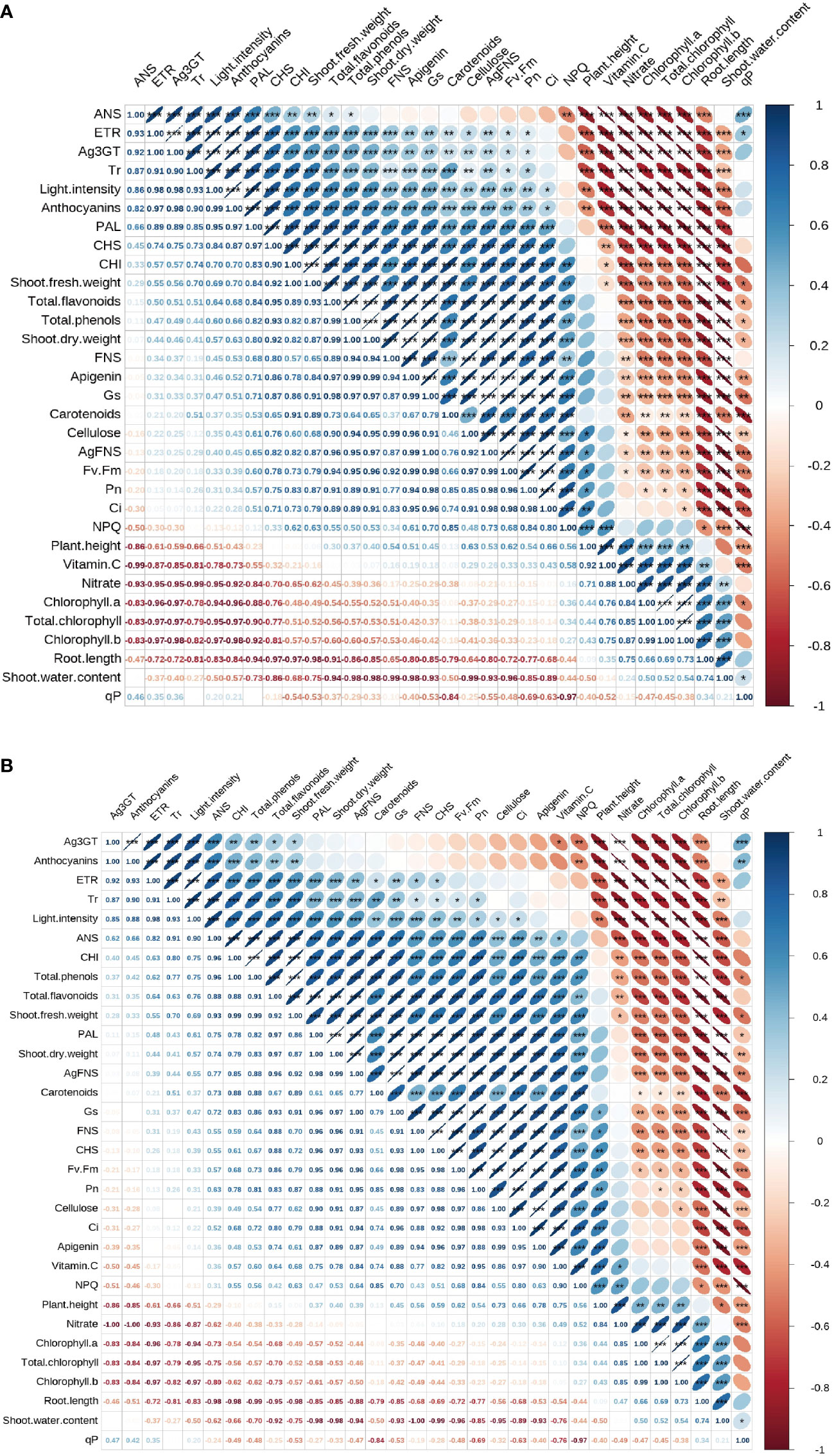
Figure 7 Correlation analysis of light intensity on anthocyanin and apigenin content. Correlation analysis of light intensity on anthocyanin and apigenin content of celery blade (A) and petioles (B).
In celery petioles (Figure 7B), light intensity was significantly negatively correlated with the total chlorophyll content in celery petioles. The content of apigenin in the petiole was positively correlated with CHS activity and cellulose content. The anthocyanin content in the petiole was significantly negatively correlated with the total chlorophyll content, and the nitrate content and Ag3GT expression in the petiole were significantly positively correlated.
4 Discussion
Light is one of the most influential environmental factors on plants, and light intensity plays a crucial role in the morphological construction and material accumulation of plants. The fresh weight of Brassica microgreens increased 34% as light intensity increased from 105 to 315 μmol·m-2·s-1 (Gerovac et al., 2016). The length and fresh weight of tartary buckwheat sprouts can be affected by light, even if the intensity is very low (50 μmol·m-2·s-1) (Tuan et al., 2013). The 50 μmol·m-2·s-1 treatment increased fresh weight and hypocotyl length of broccoli microgreens (Liu, 2021). In this study, the aboveground biomass and water content of celery were significantly reduced under low light conditions, and the height and aboveground biomass of celery plants were highest and root development was most vigorous at a light intensity of 200, while the accumulation of each biomass of celery was also significantly inhibited at a light intensity of 300 (Table 1).
Zhang found that too much or too little light treatment reduced the photosynthetic pigment content in plant leaves and inhibited photosynthesis in plants (Zhang et al., 2021). Chll a, Chll b and Chll a + b contents in celery leave were lowest at light intensity of 40, and chlorophyll content at light intensity of 300 was lower than that at light intensity of 200, indicating that chlorophyll in celery leave is less tolerant to both low light environment and high light intensity, and the decrease in chlorophyll content under strong light may be caused by damage or reduction in the number of basal grains in chloroplasts (Table 2). The appropriate light intensity can reduce the damage to the leave and the formation of related tissue structures, which in turn is conducive to promoting chlorophyll synthesis (Feng et al., 2019; Wang T. et al., 2022). The present results indicates that the transpiration rate (Tr) and net photosynthetic rate (Pn) of grass coral gradually increased to a certain level and then decreased with the increase of light intensity in a certain range of light intensity (Shi-Jian et al., 2015). In our study, we found that Pn, GS and Ci of celery all showed an increasing and then decreasing trend in the light intensity range of this experiment (Figures 2A, B, D), while Tr gradually increased with the increase of light intensity, which might be due to the differences caused by different plant species (Figure 2C), indicating that the suitable light intensity is beneficial to promote photosynthesis of celery.
Chang found that the nitrate content in leaf lettuce was reduced by increasing the light intensity appropriately, and the results of the present experiment were consistent with this (Chang et al., 2013). With increasing light intensity, nitrate content decreases and decreases significantly at a light intensity of 300 μmol·m-2·s-1 (Figure 3C). Studies have shown that the content of soluble sugar, cellulose, and VC in water spinach increases significantly with increasing light intensity (Glowacz et al., 2015). However, In this study, the contents of VC and cellulose in celery petioles and leaves increased first and then decreased with the increase of light intensity, and reached a maximum when the light intensity was 200 μmol·m-2·s-1 (Figures 3A, D). This may be due to different experimental settings or differences between plant species. Increasing light intensity is beneficial to the synthesis of soluble proteins and total phenols, but too much light intensity will play an inhibitory role (Miao et al., 2023). In this study, the total phenolic content decreased significantly when the light intensity increased to 300 µmol·m-2·s-1, which may be due to the fact that the synthesis process of phenolic compounds is regulated by phenylalanine via phenylalanine aminolysin (PAL), while high light intensity affected PAL activity (Figure 3B).
Studies have shown that the concentration of flavonoids and total phenols in lettuce is sensitive to environmental conditions (Liu et al., 2007). Under high light stress, polyphenols are often produced in plant tissues as some form of protective agent (Chalker-Scott, 1999). Anthocyanins, as one of the most abundant pigments in higher plants, increase with the increase of light intensity, which helps to improve the coloring ability and antioxidant capacity of leaves, while protecting plants from photodamage and photoinhibition in the face of excessive solar radiation (Gitelson et al., 2007; Zhang et al., 2019). In this study, the anthocyanins, total phenols and flavonoids contents of celery leaves and petioles increased with the increase of light intensity, and the activity of phenylalanine ammonia-lyase (PAL), as a key enzyme in the synthesis of phenols, increased under the induction of strong light (Figures 4A, C). Studies have shown that flavonoids contain many chemicals (Williams and Grayer, 2004). During the S3 period, there is a sudden increase in the total flavonoid content in the leaves, possibly the accumulation of other flavonoids.
The flavonoid content in Cabernet Sauvignon grapes increased significantly under high light intensity conditions (Koyama et al., 2012). PAL, CHS, CHI, FNS and ANS are the more critical enzymes for the synthesis of apigenins and anthocyanins in plants (Yan et al., 2020; Dai et al., 2022). The expression of related genes in the metabolic pathway of anthocyanin pre-synthesis is regulated by light intensity (Zhang et al., 2018). It was found that the expression of structural and regulatory genes in the anthocyanin synthesis and metabolism pathway was up-regulated in plants with increased anthocyanin content under high light conditions, while in low light or dark conditions, anthocyanin-related genes were not expressed or their expression was down-regulated leading to a significant decrease in anthocyanin (An et al., 2023). Similar to the results of the present study, PAL, CHS and CHI activities in celery increased and then decreased with increasing light intensity in a certain light intensity range under different light intensity conditions (Figure 5A–C). This indicates that the activity of these three enzymes is different in different light intensity treatments and in different parts of the plant, which is similar to the previous findings, and its activity increases with the increase of light intensity (Dong et al., 1998; Qiu, 2016).
The results showed that light intensity was the key factor affecting the synthesis of anthocyanins in tartary buckwheat, and the content of anthocyanins changed significantly (Wang D. et al., 2022). In this study, the content of anthocyanins in leaves and petioles increased with the increase of light intensity at 15, 30 and 45 days, and the activity of ANS enzyme and the expression of the key gene Ag3GT gene were also up-regulated during the synthesis of anthocyanins under strong light (Figures 5D, 6A). Besides, we found that the activity of FNS, a key enzyme for apigenin synthesis, and the expression of AgFNS, a key gene, were consistently decreased with increasing treatment time under the same light treatment, which was opposite to the expression trend of ANS, a key enzyme for anthocyanin synthesis, and Ag3GT, a key gene (Figures 5D, E, 6). This is consistent with the previous research on the competition between anthocyanins and apigenin synthesis in celery (Martens and Forkmann, 1998; Gebhardt et al., 2005).
5 Conclusion
The light intensity treatment of 200 μmol·m-2·s-1 significantly improved the yield and quality of celery, and also reduced the nitrate content. The content of nitrate and cellulose in celery leaves is lower than that of petioles, and the content of other nutrients in leaves is higher than that of petioles. The contents of apigenin and anthocyanins were higher at 200 μmol·m-2·s-1, and higher at 30 d. The light intensity of 300 μmol·m-2·s-1 inhibited the activities of CHS, CHI and FNS. The expression of AgFNS, a key gene for apigenin synthesis, was up-regulated with the increase of light intensity, and was down-regulated at 300 μmol·m-2·s-1, indicating that the expression of Ag3GT, a key gene for anthocyanin synthesis in celery, was proportional to the light intensity. Correlation analysis showed that 200 μmol·m-2·s-1 was the optimal light intensity for celery growth.
Data availability statement
The original contributions presented in the study are included in the article/supplementary material. Further inquiries can be directed to the corresponding author.
Author contributions
ZH: Conceptualization, Methodology, Project administration, Writing – review & editing. YQ: Formal analysis, Writing – original draft, Writing – review & editing. XL: Formal analysis, Writing – original draft, Writing – review & editing. CL: Writing – review & editing. SC: Writing – review & editing. QC: Writing – review & editing. SL: Writing – review & editing. DS: Investigation, Writing – review & editing. XG: Investigation, Writing – review & editing. XZ: Writing – review & editing. LS: Methodology, Writing – review & editing.
Funding
The author(s) declare financial support was received for the research, authorship, and/or publication of this article. This study was supported by the Second Tibetan Plateau Scientific Expedition and Research (2019QZKK0303) and Sichuan Luzhou expert workstation (2322339013).
Acknowledgments
The authors are thankful to the Second Tibetan Plateau Scientific Expedition and Research (2019QZKK0303) and Sichuan Luzhou expert workstation (2322339013) for financial supports.
Conflict of interest
The authors declare that the research was conducted in the absence of any commercial or financial relationships that could be construed as a potential conflict of interest.
Publisher’s note
All claims expressed in this article are solely those of the authors and do not necessarily represent those of their affiliated organizations, or those of the publisher, the editors and the reviewers. Any product that may be evaluated in this article, or claim that may be made by its manufacturer, is not guaranteed or endorsed by the publisher.
References
An, X., Tan, T., Song, Z., Guo, X., Zhang, X., Zhu, Y., et al. (2023). Physiological response of anthocyanin synthesis to different light intensities in blueberry. PloS One 18 (6), e0283284. doi: 10.1371/journal.pone.0283284
Antia, B. S., Akpan, E. J., Okon, P. A., Umoren, I. U. (2006). Nutritive and anti-nutritive evaluation of sweet potatoes (Ipomoea batatas) leaves. Pakistan J. Nutr. 5, 166–168. doi: 10.3923/PJN.2006.166.168
Aphalo, P. J., Ballaré, C. L. (1995). On the importance of information-acquiring systems in plant-plant interactions. Funct. Ecol. 9, 5–14. doi: 10.2307/2390084
Ballaré, C. L., Scopel, A. L. (1997). Phytochrome signalling in plant canopies: testing its population-level implications with photoreceptor mutants of Arabidopsis. Funct. Ecol. 11, 441–450. doi: 10.1046/J.1365-2435.1997.00108.x
Bast, A., Haenen, G., Bruynzeel, A. M., Vijgh, W. J. (2007). Protection by flavonoids against anthracycline cardiotoxicity: from chemistry to clinical trials. Cardiovasc. Toxicol. 7, 154–159. doi: 10.1007/s12012-007-0018-0
Cataldo, D. A., Haroon, M., Schrader, L. E., Youngs, V. L. (1975). Communications in soil science and plant analysis rapid colorimetric determination of nitrate in plant tissue by nitration of salicylic acid. Commun. Soil Sci. Plant Anal. 6, 71–80. doi: 10.1080/00103627509366547
Chalker-Scott, L. (1999). Environmental significance of anthocyanins in plant stress responses. Photochem. Photobiol. 70 (1), 1–9. doi: 10.1111/j.1751-1097.1999.tb01944.x
Chang, A. C., Yang, T. Y., Riskowski, G. L. (2013). Ascorbic acid, nitrate, and nitrite concentration relationship to the 24hour light/dark cycle for spinach grown in different conditions. Food Chem. 138 (1), 382–388. doi: 10.1016/j.foodchem.2012.10.036
Chen, G., Mo, L., Li, S., Zhou, W., Wang, H., Liu, J., et al. (2015). Separation and determination of reduced vitamin C in polymerized hemoglobin-based oxygen carriers of the human placenta. Artif. Cells Nanomedicine Biotechnol. 43 (3), 152–156. doi: 10.3109/21691401.2014.981269
Collins, B. S., Wein, G. R. (2000). Stem elongation response to neighbour shade in sprawling and upright polygonum species. Ann. Bot. 86, 739–744. doi: 10.1006/ANBO.2000.1233
Dai, Y., Zhang, L., Sun, X., Li, F., Zhang, S., Zhang, H., et al. (2022). Transcriptome analysis reveals anthocyanin regulation in Chinese cabbage (Brassica rapa L.) at low temperatures. Sci. Rep. 12 (1), 6308. doi: 10.1038/s41598-022-10106-1
Dong, Y. H., Beuning, L., Davies, K., Mitra, D., Morris, B., Kootstra, A. (1998). Expression of pigmentation genes and photo-regulation of anthocyanin biosynthesis in developing royal gala apple flowers. Funct. Plant Biol. 25 (2), 245–252. doi: 10.1071/pp97108
Feng, Y. H., Raza, L., Li, K., Chen, D., Khalid, B., Du, A., et al. (2019). The influence of light intensity and leaf movement on photosynthesis characteristics and carbon balance of soybean. Front Plant Sci. 9, 1952. doi: 10.3389/fpls.2018.01952
Fu, Y., Li, H., Yu, J., Liu, H., Cao, Z., Manukovsky, N., et al. (2017). Interaction effects of light intensity and nitrogen concentration on growth, photosynthetic characteristics and quality of lettuce (Lactuca sativa L. Var. youmaicai). Scientia Hortic. 214, 51–57. doi: 10.1016/j.scienta.2016.11.020
Gebhardt, Y., Witte, S., Forkmann, G., Lukacin, R., Matern, U., Martens, S. (2005). Molecular evolution of flavonoid dioxygenases in the family Apiaceae. Phytochemistry 66 (11), 1273–1284. doi: 10.1016/j.phytochem.2005.03.030
Gerovac, J. R., Craver, J. K., Boldt, J. K., Lopez, R. G. (2016). Light intensity and quality from sole-source light-emitting diodes impact growth, morphology, and nutrient content of brassica microgreens. Hortence A Publ. Am. Soc. Hortic. ence 51 (5), 497–503. doi: 10.21273/HORTSCI.51.5.497
Ghadage, D. M., Kshirsagar, P. R., Pai, S. R., Chavan, J. J. (2017). Extraction efficiency, phytochemical profiles and antioxidative properties of different parts of Saptarangi (Salacia chinensis L.) – An important underutilized plant. Biochem. Biophysics Rep. 12, 79–90. doi: 10.1016/j.bbrep.2017.08.012
Gitelson, A. A., Merzlyak, M. N., Chivkunova, O. B. (2007). Optical properties and nondestructive estimation of anthocyanin content in plant leaves. Photochem. Photobiol. 74 (1), 38–45. doi: 10.1562/0031-8655(2001)0740038opaneo2.0.co2
Glowacz, M., Mogren, L. M., Reade, J. P., Cobb, A. H., Monaghan, J. M. (2015). High- but not low-intensity light leads to oxidative stress and quality loss of cold-stored baby leaf spinach. J. Sci. Food Agric. 95 (9), 1821–1829. doi: 10.1002/jsfa.6880
Jaakola, L. (2013). New insights into the regulation of anthocyanin biosynthesis in fruits. Trends Plant Sci. 18 9, 477–483. doi: 10.1016/j.tplants.2013.06.003
Kim, J. H., Cheon, Y. M., Kim, B. G., Ahn, J. (2008). Analysis of flavonoids and characterization of theOsFNS gene involved in flavone biosynthesis in Rice. J. Plant Biol. 51, 97–101. doi: 10.1007/BF03030717
Koyama, K., Ikeda, H., Poudel, P. R., Goto-Yamamoto, N. (2012). Light quality affects flavonoid biosynthesis in young berries of Cabernet Sauvignon grape. Phytochemistry 78, 54–64. doi: 10.1016/j.phytochem.2012.02.026
Li, Y. Y., Mao, K., Zhao, C., Zhao, X. Y., Zhang, H. L., Shu, H. R., et al. (2012). MdCOP1 ubiquitin E3 ligases interact with MdMYB1 to regulate light-induced anthocyanin biosynthesis and red fruit coloration in apple. Plant Physiol. 160 (2), 1011–1022. doi: 10.1104/pp.112.199703
Lichtenthaler, H. K., Buschmann, C. (2001). Chlorophylls and carotenoids: measurement and characterization by UV-VIS spectroscopy. Curr. Protoc. Food Analytical Chem. 1 (1), F4.3.1–F4.3.8. doi: 10.1002/0471142913.faf0403s01
Liu, H. (2021). Differential effects of low light intensity on broccoli microgreens growth and phytochemicals. Agronomy 11 (3), 537. doi: 10.3390/agronomy11030537
Liu, X., Ardo, S., Bunning, M., Parry, J., Zhou, K., Stushnoff, C., et al. (2007). Total phenolic content and dpph radical scavenging activity of lettuce (lactuca sativa l.) grown in colorado. LWT- Food Sci. Technol. 40 (3), 552–557. doi: 10.1016/j.lwt.2005.09.007
Liu, K., Gao, M., Jiang, H., Ou, S., Li, X., He, R., et al. (2022). Light intensity and photoperiod affect growth and nutritional quality of brassica microgreens. Molecules (Basel Switzerland) 27 (3), 883. doi: 10.3390/molecules27030883
Lv, B., Zhu, J., Kong, X., Ding, Z. (2021). Light participates in the auxin-dependent regulation of plant growth. J. Integr. Plant Biol. 63 (5), 819–822. doi: 10.1111/jipb.13036
Martens, S., Forkmann, G. (1998). Genetic control of flavone synthase ii activity in flowers of gerbera hybrids. Phytochemistry 49 (7), 1953–1958. doi: 10.1016/S0031-9422(98)00345-8
Matos, F. S., Wolfgramm, R., Gonçalves, F. V., Cavatte, P. C., Ventrella, M. C., Damatta, F. M. (2009). Phenotypic plasticity in response to light in the coffee tree. Environ. Exp. Bot. 67 (2), 421–427. doi: 10.1016/J.ENVEXPBOT.2009.06.018
Miao, C., Yang, S., Xu, J., Wang, H., Zhang, Y., Cui, J., et al. (2023). Effects of light intensity on growth and quality of lettuce and spinach cultivars in a plant factory. Plants (Basel Switzerland) 12 (18), 3337. doi: 10.3390/plants12183337
Mojžiš, J., Varinská, L., Mojžišová, G., Kostova, I. P., & Mirossay, L. (2008). Antiangiogenic effects of flavonoids and chalcones. Pharmacol. Res. (4) 57 4, 259–265. doi: 10.1016/j.phrs.2008.02.005
Neugart, S., Krumbein, A., Zrenner, R. (2016). Influence of Light and Temperature on Gene Expression Leading to Accumulation of Specific Flavonol Glycosides and Hydroxycinnamic Acid Derivatives in Kale (Brassica oleracea var. sabellica). Front. Plant Sci. 7. doi: 10.3389/fpls.2016.00326
Qiu, S. Z. (2016). Regulative mechanism of light on anthocyanin synthesis and metabolism of Betula Royal Frost leaves (Heilongjiang: Northeast Forestry University).
Safe, S., Jayaraman, A., Chapkin, R. S., Howard, M., Mohankumar, K., Shrestha, R. (2021). Flavonoids: structure-function and mechanisms of action and opportunities for drug development. Toxicological Res. 37 (2), 147–162. doi: 10.1007/s43188-020-00080-z
Samuolienė, G., Sirtautas, R., Brazaitytė, A., Duchovskis, P. (2012). LED lighting and seasonality effects antioxidant properties of baby leaf lettuce. Food Chem. 134 (3), 1494–1499. doi: 10.1016/j.foodchem.2012.03.061
Shi-Jian, Y., Yong-Jiang, Z., Guillermo, G., Mei, S., Ren-Yi, M., Kun-Fang, C. (2015). Determinants of water circulation in a woody bamboo species: afternoon use and night-time recharge of culm water storage. Tree Physiol. 35 (9), 964–974. doi: 10.1093/treephys/tpv071
Singleton, V. L., Orthofer, R., Lamuela-Raventós, R. M. (1999). Analysis of total phenols and other oxidation substrates and antioxidants by means of Folin-Ciocalteu reagent. Methods Enzymology 299, 152–178. doi: 10.1016/S0076-6879(99)99017-1
Tan, G. F. (2020). Study on the mechanism of anthocyanins and apigenins metabolization in celery (Nan Jing: Nanjing agricultural university).
Tuan, P. A., Thwe, A. A., Kim, Y. B., Kim, J. K., Kim, S. J., Lee, S., et al. (2013). Effects of white, blue, and red light-emitting diodes on carotenoid biosynthetic gene expression levels and carotenoid accumulation in sprouts of tartary buckwheat (fagopyrum tataricum gaertn.). J. Agric. Food Chem. 61 (50), 12356–12361. doi: 10.1021/jf4039937
Wang, T., Liu, S., Tian, S., Ma, T., Wang, W. (2022). Light regulates chlorophyll biosynthesis via ELIP1 during the storage of Chinese cabbage. Sci. Rep. 12, 11098. doi: 10.1038/s41598-022-15451-9
Wang, D., Yang, T., Li, Y., Deng, F., Dong, S., Li, W., et al. (2022). Light intensity-A key factor affecting flavonoid content and expression of key enzyme genes of flavonoid synthesis in tartary buckwheat. Plants (Basel Switzerland) 11 (16), 2165. doi: 10.3390/plants11162165
Williams, C. A., Grayer, R. J. (2004). Anthocyanins and other flavonoids. Natural product Rep. 21 (4), 539–573. doi: 10.1039/b311404j
Yan, J., He, L., Xu, S., Wan, Y., Wang, H., Wang, Y., et al. (2020). Expression analysis, functional marker development and verification of AgFNSI in celery. Sci. Rep. 10 (1), 531. doi: 10.1038/s41598-019-57054-x
Yan, J., Yu, L., Xu, S., Gu, W., Zhu, W. (2014). Apigenin accumulation and expression analysis of apigenin biosynthesis relative genes in celery. Scientia. Hortic. 165, 218–224. doi: 10.1016/j.scienta.2013.11.018
Zhang, M., Whitman, C. M., Runkle, E. S. (2019). Manipulating growth, color, and taste attributes of fresh cut lettuce by greenhouse supplemental lighting. Scientia Hortic. 252, 274–282. doi: 10.1016/j.scienta.2019.03.051
Zhang, M., Wang, Y., Chen, X., Xu, F., Ding, M., Ye, W., et al. (2021). Plasma membrane H-ATPase overexpression increases rice yield via simultaneous enhancement of nutrient uptake and photosynthesis. Nat Commun. 12 (1), 735. doi: 10.1038/s41467-021-20964-4
Keywords: light intensity, celery, nutrients, quality, flavonoid
Citation: Qin Y, Liu X, Li C, Chu Q, Cheng S, Su L, Shao D, Guo X, He Z and Zhou X (2024) Effect of light intensity on celery growth and flavonoid synthesis. Front. Plant Sci. 14:1326218. doi: 10.3389/fpls.2023.1326218
Received: 24 October 2023; Accepted: 14 December 2023;
Published: 16 January 2024.
Edited by:
Heiko Lokstein, Charles University, CzechiaReviewed by:
Athanasios Koukounaras, Aristotle University of Thessaloniki, GreeceSami I. Ali, National Research Centre (Egypt), Egypt
Copyright © 2024 Qin, Liu, Li, Chu, Cheng, Su, Shao, Guo, He and Zhou. This is an open-access article distributed under the terms of the Creative Commons Attribution License (CC BY). The use, distribution or reproduction in other forums is permitted, provided the original author(s) and the copyright owner(s) are credited and that the original publication in this journal is cited, in accordance with accepted academic practice. No use, distribution or reproduction is permitted which does not comply with these terms.
*Correspondence: Zhongqun He, hzqun328@163.com
†These authors have contributed equally to this work and share first authorship