- Laboratoire de Biotechnologie Végétale, Université libre de Bruxelles, Gosselies, Belgium
Introduction: Plant A/T-rich protein and zinc-binding protein (PLATZ) are plant-specific transcription factors playing a role in plant development and stress response. To assess the role of PLATZs in vascular system development and wood formation in poplar, a functional study for PtaPLATZ18, whose expression was associated with the xylem, was carried out.
Methods: Poplar dominant repressor lines for PtaPLATZ18 were produced by overexpressing a PtaPLATZ18-SRDX fusion. The phenotype of three independent transgenic lines was evaluated at morphological, biochemical, and molecular levels and compared to the wild type.
Results: The PtaPLATZ18-SRDX lines showed increased plant height resulting from higher internode length. Besides, a higher secondary xylem thickness was also evidenced in these dominant repression lines as compared to the wild type suggesting an activation of cambial activity. A higher amount of lignin was evidenced within wood tissue as compared to the wild type, indicating an alteration in cell wall composition within xylem cell types. This latter phenotype was linked to an increased expression of genes involved in lignin biosynthesis and polymerization.
Discussion: The phenotype observed in the PtaPLATZ18-SRDX lines argues that this transcription factor targets key regulators of plant growth and vascular tissues development.
1 Introduction
PLATZ are plant-specific transcription factors (TFs) that bind A/T-rich DNA sequences. They are characterised by two conserved domains required for their zinc-dependent DNA-binding, C-X2-H-X11-C-X2-C-X(4-5)-C-X2-C-X(3-7)-H-X2-H and C-X2-C-X(10-11)-C-X3-C (Nagano et al., 2001). Some PLATZ proteins have been localized both in the nucleus, which is consistent with their function as transcriptional regulators, and in the cytoplasm, suggesting a role in cellular signaling pathways (So et al., 2015; Li et al., 2017; Kim et al., 2018; Zhou and Xue, 2020; Li et al., 2021; Han et al., 2022; Wai et al., 2022; Zhao et al., 2022). PLATZ TFs are central elements in protein accumulation by acting at two distinct regulation levels. On the one hand, they regulate the biosynthesis of transfer RNAs (tRNAs) and 5S ribosomal RNA (5S rRNA) through protein-protein interactions within the RNA polymerase III complex (Pol III) (Li et al., 2017; Wang et al., 2018). On the other hand, several PLATZ can control transcription by binding A/T-rich sequences in a Pol II-dependent process (Kim et al., 2018). PLATZ have been identified either as transcriptional activators (Kim et al., 2018; Hu et al., 2023) or repressors (Nagano et al., 2001; Liu et al., 2020; Zhao et al., 2022; Zhang et al., 2023). The size of the PLATZ family varies between species. For instance, 12 PLATZ genes have been identified in Arabidopsis (Wang et al., 2018), 17 in Zea mays (Wang et al., 2018), and 18 in Populus trichocarpa (Ma et al., 2023).
The characterization of several PLATZ from monocot and dicot plants has highlighted their role in the regulation of a large panel of stress responses and/or developmental processes. First, the expression of several PLATZ is induced under abiotic stress, and they play a role in regulating abiotic stress tolerance. For instance, in Arabidopsis, AtPLATZ1 and AtPLATZ2 have been identified as regulators of seed desiccation tolerance (González-Morales et al., 2016). AtPLATZ1/AIN1 is also involved in ABA-mediated inhibition of root elongation by modulating ROS homeostasis (Dong et al., 2021). AtPLATZ2 is involved in the regulation of stress tolerance as lines overexpressing AtPLATZ2 had an increased sensitivity to salt stress (Liu et al., 2020). AtPLATZ4 was found to promote drought tolerance by directly repressing Plasma membrane Intrinsic Protein 2;8 (PIP2;8) as well as by regulating genes involved in ABA signalling (Liu et al., 2023). In poplar, PtPLATZ1-4 and PtPLATZ8-9 are involved in cadmium tolerance as evidenced by heterologous expression in yeast and overexpression in poplar (Ma et al., 2023).
Second, PLATZ were found to regulate developmental processes including seed filling, floral development, and organ as well as plant growth. For instance, the Z. mays semi-dominant mutant floury 3 (fl3) showed a defect in endosperm development (Li et al., 2017). FL3 (also named ZmPLATZ12) interacts with proteins of the Pol III complex including RPC53, TbBRF1, NRPC2, and TFC1, and the fl3 mutant has defects in Pol III activity as shown by reduced accumulation of 5S rRNA and several tRNAs (Li et al., 2017; Zhao et al., 2020). Another maize PLATZ, ZmPLATZ2, was found to directly bind to the ZmSSI starch synthetic gene promoter and when ZmPLATZ2 was transiently expressed in maize endosperm or overexpressed in rice, an upregulation of starch synthesis genes was observed (Li et al., 2021). In O. sativa, the Osfl3 CRISPR/Cas9 knockout lines were also impacted in grain development as shown by their reduced grain weight and size (Guo et al., 2022). FL3 was positively regulated by the Triticum aestivum Positive regulator of Grain Size1 (PGS1) basic helix-loop-helix TF through binding to the E-box motif in the FL3 promoter of both wheat and rice (Guo et al., 2022). The rice GLABROUS GENE 6 (GL6)/SHORT GRAIN 6 (SG6) was found to participate in the Pol III transcription machinery by interacting with the RPC53 and TFC1 subunits to promote cell division in the spikelet hull, which positively controls grain length (Wang et al., 2019; Zhou & Xue, 2020). Finally, the ectopic expression of the soybean GmPLATZ in Arabidopsis triggers larger seeds with a 12.5-49% increase in the 1000-seed weight (Hu et al., 2023). On the opposite, these authors showed that the knockout gmpla/b soybean mutants had a ~10% lower 100-seed weight and smaller seeds with reduced cell numbers but no difference in seed nutrient composition compared to the control.
The Vitis vinifera VviPLATZ1 is involved in the control of female flower morphology as in the CRISPR/Cas9 mutants, female flowers with reflex stamens were observed instead of hermaphrodite flowers in the wild type (WT) (Iocco-Corena et al., 2021). The Arabidopsis AtPLATZ10 expression was reported to be controlled by the Agamous-mediated biotimer suggesting a role in flower development (Pelayo et al., 2023).
Phenotypic and molecular analyses of Arabidopsis activation-tagged lines and knockout mutants demonstrated that AtPLATZ3 (ORESARA 15, ORE15) controls cell proliferation during growth as well as leaf senescence and directly regulates the genes encoding the TFs GROWTH-REGULATING FACTOR (GRF) 1/4 in a Pol II-dependent process (Kim et al., 2018). Consistently, the expression of ORE15 peaks in young leaves and progressively decreases during leaf aging (Kim et al., 2018; Jun et al., 2019). In addition, ORE15 was reported to be a regulator of root growth and development as it positively controls root apical meristem size in a process involving auxin and cytokinin signalling-related pathways (Timilsina et al., 2022). Transgenic Arabidopsis overexpressing AtPLATZ4 exhibited a lower fresh weight than the WT, and it was suggested that AtPLATZ4 acts as a negative regulator of growth by suppressing the expression of EXP6 and EXP16 expansin genes thereby inhibiting the expansion of the cell wall (Liu et al., 2023).
A role of PLATZs in developmental processes linked to perennial growth, such as wood formation has not been reported yet. However, there are indications of the involvement of PLATZ in secondary cell wall (SCW) formation in several plant species. Actually, in hemp and nettle, two species harboring extraxylary fibers with a gelatinous-type SCW, several PLATZs were upregulated in thickening bast fibers, either as compared to elongating bast fibers or to the xylem core of the stem (Guerriero et al., 2017; Xu et al., 2019). These genes were among the most highly expressed TF-encoding genes in the investigated tissues, supporting a role in SCW thickening. In addition, a transcriptomic analysis made in Populus deltoides × P. euramericana along a gradient from the shoot apex to the fifth internode, where secondary growth is well developed, highlighted the preferential expression of several PLATZs in internodes with cells undergoing SCW thickening (Chao et al., 2019). As this expression pattern is comparable to those of well-described regulators of SCW biosynthesis, such as homologs of Arabidopsis NST2, MYB46, and MYB83, these authors suggested a role for some PLATZ genes in the transition from primary to secondary growth. Finally, Potri.013G078500 (PLATZ14) was detected in a shotgun analysis of the nuclear-fractionated proteome of stem-differentiating xylem tissue of P. trichocarpa (Loziuk et al., 2017).
To assess the role of PLATZs in vascular system development and wood formation in poplar (P. tremula x P. alba), a functional study for PtaPLATZ18, whose expression was associated with the xylem, was investigated. The PtaPLATZ18 dominant repression (SRDX) lines produced by expressing full-length PtaPLATZ18 in frame with the SUPERMAN repression domain X (SRDX) displayed an enhanced plant elongation. Besides, a higher secondary xylem thickness was also evidenced in the dominant repression lines of PtaPLATZ18 as compared to WT suggesting an activation of cambial activity in the transgenic lines. Finally, a significantly higher amount of lignin was evidenced within wood tissue as compared to the WT, indicating an alteration in cell wall composition within xylem cell types. This report evidences a role for PtaPLATZ18 in vascular tissues development in poplar, a woody perennial species.
2 Materials and methods
2.1 In silico expression analysis
The RNA-seq data used in this study were retrieved from Sundell et al. (2017). Hierarchical clustering and heatmapping of P. tremula PtPLATZ genes were conducted using Mev software (Howe et al., 2011) with Euclidean distance and complete linkage clustering.
2.2 Plant material
Both WT and transgenic poplars (P. tremula x P. alba clone INRA 717-1B4) were grown aseptically and then transferred in soil in the phytotron for three months, as described previously (25°C, 16h day/8h night, 55% humidity) (Baldacci-Cresp et al., 2015).
2.3 Cloning of PtaPLATZ18, gene constructs, plant transformation, and selection of homogeneous transgenic poplar lines
PtaPLATZ18 was amplified from P. tremula x P. alba 717-1B4 cDNA (primers are given in Supplementary Table 1), cloned into the pCR BLUNT II TOPO (Life Technologies, Carlsbad, CA, USA) and sequenced. For the PtaPLATZ18-SRDX fusion construct, PtaPLATZ18 was further amplified by PCR using a forward primer containing attB1 and a reverse primer containing the sequence encoding both the SRDX (LDLDLELRLGFA) peptide (Hiratsu et al., 2003) and the attB2 sequence (Supplementary Table 1), and cloned in the pK7WG2D (Karimi et al., 2002) plasmid by Gateway technology (Supplementary Figure 1). The binary vector was transformed into poplar via Agrobacterium tumefaciens (C58RifPMP90) (Leple et al., 1992). A minimum of 20 independent transgenic lines were produced and the expression level of the PLATZ18-SRDX construct was analyzed for 15 of them (Supplementary Figure 2). Subsequently, six lines were multiplied in vitro and grown for three months in soil in a phytotron (25°C, 16h day/8h night, 55% of humidity), with five biological replicates. Among them, three lines (SRDX2, SRDX19, and SRDX20), showing a significant increase in height, were selected for further characterization (Supplementary Figure 2).
2.4 Expression profile analyses by RT-qPCR
For the expression profile analysis of PLATZ genes in different organs and tissues, three biological replicates and two trees per biological replicate were sampled. Samples from the apex (first 2 cm of the stem), young stem (internodes from 2-7 cm under the apex), young leaves (numbers 10-12 under the first expanded leaf), young roots and lignified roots, as well as secondary vascular tissues from a portion of the stem taken at 15-45 cm below the apex i.e. xylem (debarked stem, scratched with a scalpel) and phloem (bark) of 3-month-old WT poplars, were collected and immediately frozen in liquid nitrogen.
For the analysis of SRDX2, SRDX19, and SRDX20 lines, young stem (internode number 1 to 4 under the first expanded leaf) and xylem (debarked stem from 30 to 60 cm from the ground, scratched with a scalpel) were sampled and immediately frozen in liquid nitrogen. Five biological replicates, made of one plant per replicate, were sampled for each SRDX line and the associated WT.
Total RNA was extracted according to Muoki et al. (2012), followed by a DNAse treatment using the TURBO DNA-Free kit (Fisher Scientific), according to the manufacturer’s instructions. For each RNA sample, reverse transcription was performed on 2 μg of total RNA, using the Protoscript II First Strand cDNA Synthesis Kit (New England Biolabs). RT-qPCR were carried out in three technical replicates in a 20 μl volume containing 25 ng of cDNA, 0.5 µl primers (10 µM), and 10 µl of Luna Universal qPCR Master Mix (NEB #M3003) using a thermocycler LightCycler 480 system (Roche). The thermal cycle was set up as follows: pre-incubation at 95°C/1 min, 40 amplification cycles of 95°C/15 s then 60°C/15 s, a melting curve step at 95°C/5 s, 65°C/1 min then heat up to 97°C at 0.11°C/s, and a cooling step to 40°C. Relative gene expression was determined by the 2-ΔΔCT method (Livak and Schmittgen, 2001), using adjusted primer efficiencies, and CDC2 as a reference gene (Baldacci-Cresp et al., 2016) for expression normalization. Monolignol biosynthesis genes were identified by Wang et al. (2018) and the genes putatively involved in monolignol polymerization were identified by Sundell et al. (2017). Genes coding for TFs associated with secondary cell wall biosynthesis in P. trichocarpa were taken from Li et al. (2012) (SND1-A2 and VND6-C1) and Payyavula et al. (2022) (MYB3 and MYB28), and primers for corresponding genes in P. tremula x P. alba were designed using PrimerQuest. Primer sequences are given in Supplementary Table 1.
2.5 Plant phenotyping
Poplar plant height was measured each week for three months. Then, mature leaves (numbers 19 to 21 under the first expanded leaf) from WT and transgenic lines were scanned and analyzed to estimate the mean leaf area, using Digimizer (https://www.digimizer.com/). The internode number was counted starting from the first expanded leaf and the internode mean length was determined by dividing plant height by internode numbers. The stem diameter was measured using a calliper at the basis of the stem. A 2 cm sample was retrieved from the internode number 6 and kept at 4°C in 70% ethanol. Stem cross sections (110 µm thick) were made using an HM 650 V vibratome and photographed by a stereomicroscope (Leica M165-FC). The pictures were analyzed using ImageJ (Schneider et al., 2012) to estimate the percentage of bark, xylem, and pith in the stem.
Stem cross sections were subjected to phloroglucinol staining as described in Speer (1987) or to Maüle staining (5 min in 4% KMnO4, three water baths, 80 sec in 3% HCl, three water baths, observation under microscope in a drop of Tris-HCl 1M) adapted from Yamashita et al. (2016), then examined and photographed under a wide-field microscope (Axio Observer Z1).
2.6 Cell wall residue preparation
The basal part (30 cm long) of the debarked stem was dried at 50°C for 48 h and then ground using a ball mill. One gram of wood powder was mixed with 10 ml of methanol:water (80:20, v/v), sonicated for 10 min, and homogenised for 4 h at room temperature. After 2 min of centrifugation at 4000 g, the pellet was washed two times with ethanol 80% and three times with pure acetone, then dried at 35°C for 24 h. The cell wall residue (CWR) quantification was performed on 300 mg of wood powder, with two technical replicates and three biological replicates per line, and the same protocol as described above. The CWR powder was used as material for both the lignin and cellulose analyses.
2.7 Lignin content
The lignin content was determined using the cysteine-assisted sulfuric acid (CASA) method (Lu et al., 2021). Briefly, 10 mg of CWR was fully dissolved with 1 mL of 10% L-cysteine (w/v) in 72% sulfuric acid after an incubation of 1 h at room temperature under agitation. The solution was diluted to 100 mL with pure water and CASA lignin content was evaluated based on the absorbance at 283 nm and an absorption coefficient of 11.23 g−1·L·cm−1. Five biological replicates and two technical replicates were performed.
2.8 Cellulose content
To determine the cellulose content, an Updegraff treatment was performed on the CWR powder. The Updegraff reagent is composed of acetic acid, nitric acid, and pure water in 8:1:2 proportions. 1.5 ml of this reagent was added to the samples and heated to 100°C for 30 min. After a centrifugation of 15 min and 4000 g, the pellet was washed three times with pure water and two times with pure acetone, then dried at 35°C for 24 h.
The cellulose content was determined on 10 mg of the obtained powder, according to the protocol described by Dampanaboina et al. (2021). The equation used here is: with [Glu] as the glucose concentration (µg/ml), A the dilution factor in anthrone solution (here: 0.5), B the sample volume taken to measure the glucose concentration (µl) (here: 0.1), and m the sample mass (mg).
3 Results
3.1 Several PLATZ are expressed within vascular tissues in poplar
As a preliminary investigation, the expression pattern of the 18 poplar PLATZ genes was studied by retrieving and examining data issued from an RNA-seq analysis performed on a series of laser-microdissected tangential stem sections from phloem to lignified xylem in a 45-year-old P. tremula (Sundell et al., 2017). As shown in Figure 1, 16 PLATZs are expressed within vascular tissues. Some of them are preferentially expressed in the cambial zone (such as PtPLATZ9) whereas the expression of others is linked to phloem, xylem, or not differential within the stem. Particularly, six of them (PtPLATZ1, PtPLATZ2, PtPLATZ7, PtPLATZ10, PtPLATZ14, and PtPLATZ18) display a high expression level in lignified xylem (secondary xylem) suggesting a role for these genes in xylem development and/or SCW formation.
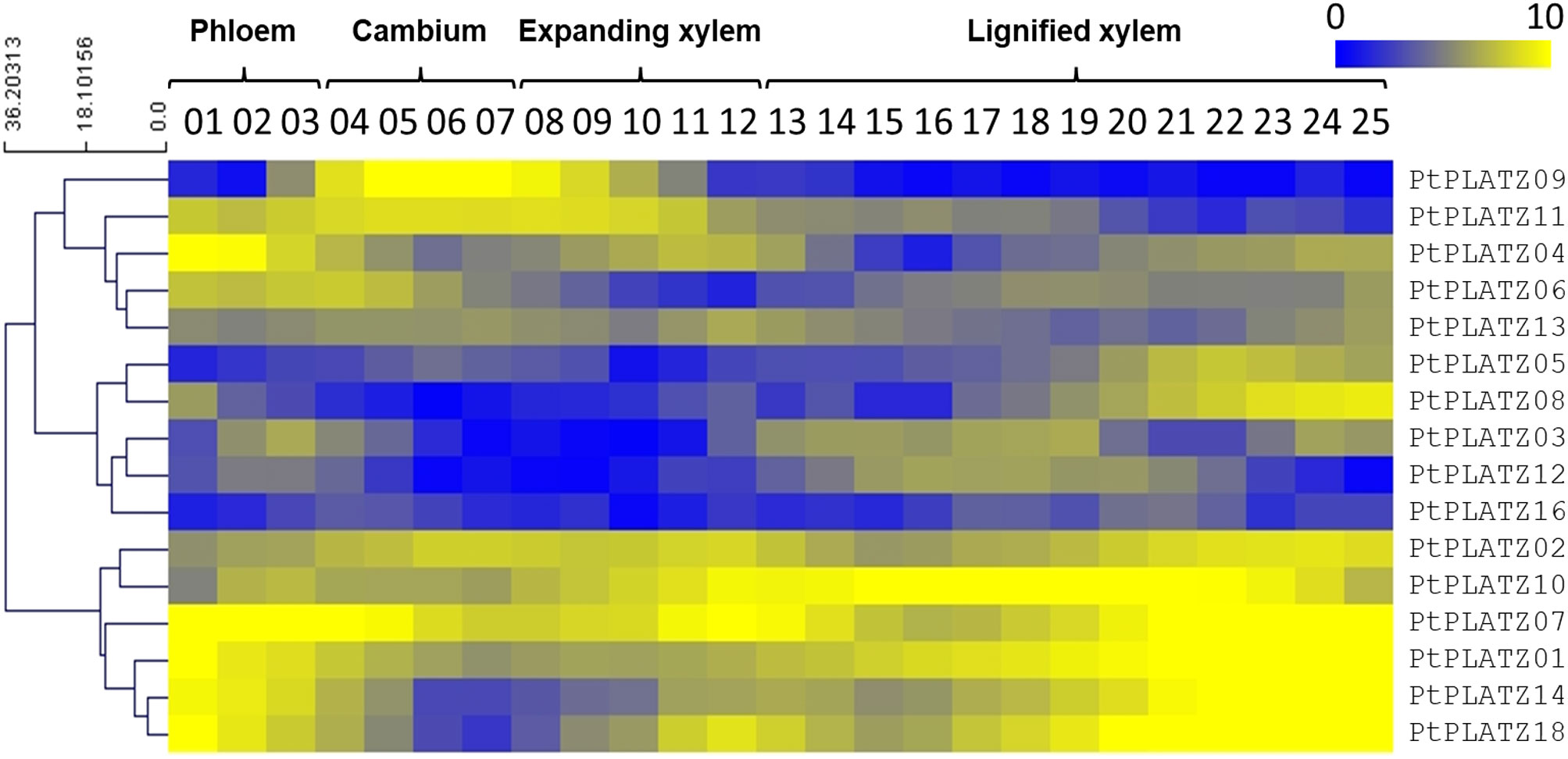
Figure 1 PLATZ expression profile as determined by RNA-seq on a series of tangential laser-microdissected sections from phloem to lignified xylem of a 45-year-old Populus tremula tree (data from Sundell et al. (2017)). There were no RNA-seq data for PtPLATZ15 and PtPLATZ17.
To assess the expression profile of these six genes in the model poplar P. tremula x P. alba grown in the phytotron for three months, a RT-qPCR was performed on secondary vascular tissues, i.e., debarked stem (made mainly of secondary xylem) and bark (including phloem). As shown in Figure 2, the six PtaPLATZ genes showed different expression profiles. Whereas PtaPLATZ2 was lowly expressed in both tissues, the five other genes, and particularly PtaPLATZ1, PtaPLATZ14, and PtaPLATZ18, had a higher expression in the xylem compared to bark. To evaluate the specificity of the expression profile of these genes, a complementary expression analysis was made on different organs i.e. apex, leaf, young stem, as well as young roots (containing no secondary vascular tissues) and old roots (containing secondary vascular tissues) (Supplementary Figure 3). PtaPLATZ1 was strongly expressed in leaves and roots compared to the apex and young stem, PtaPLATZ2 had a low but preferential expression in the apex and young roots, PtaPLATZ7 was expressed at a similar level in all samples, and PtaPLATZ10 was preferentially expressed in the young root. PtaPLATZ14 and PtaPLATZ18 were expressed in all parts but with a 2-3-fold lower relative expression level (Supplementary Figure 3) than in the xylem (Figure 2). As a case study, PtaPLATZ18 was selected as a relevant candidate gene for a functional study in the context of vascular tissue development and lignification in poplar.
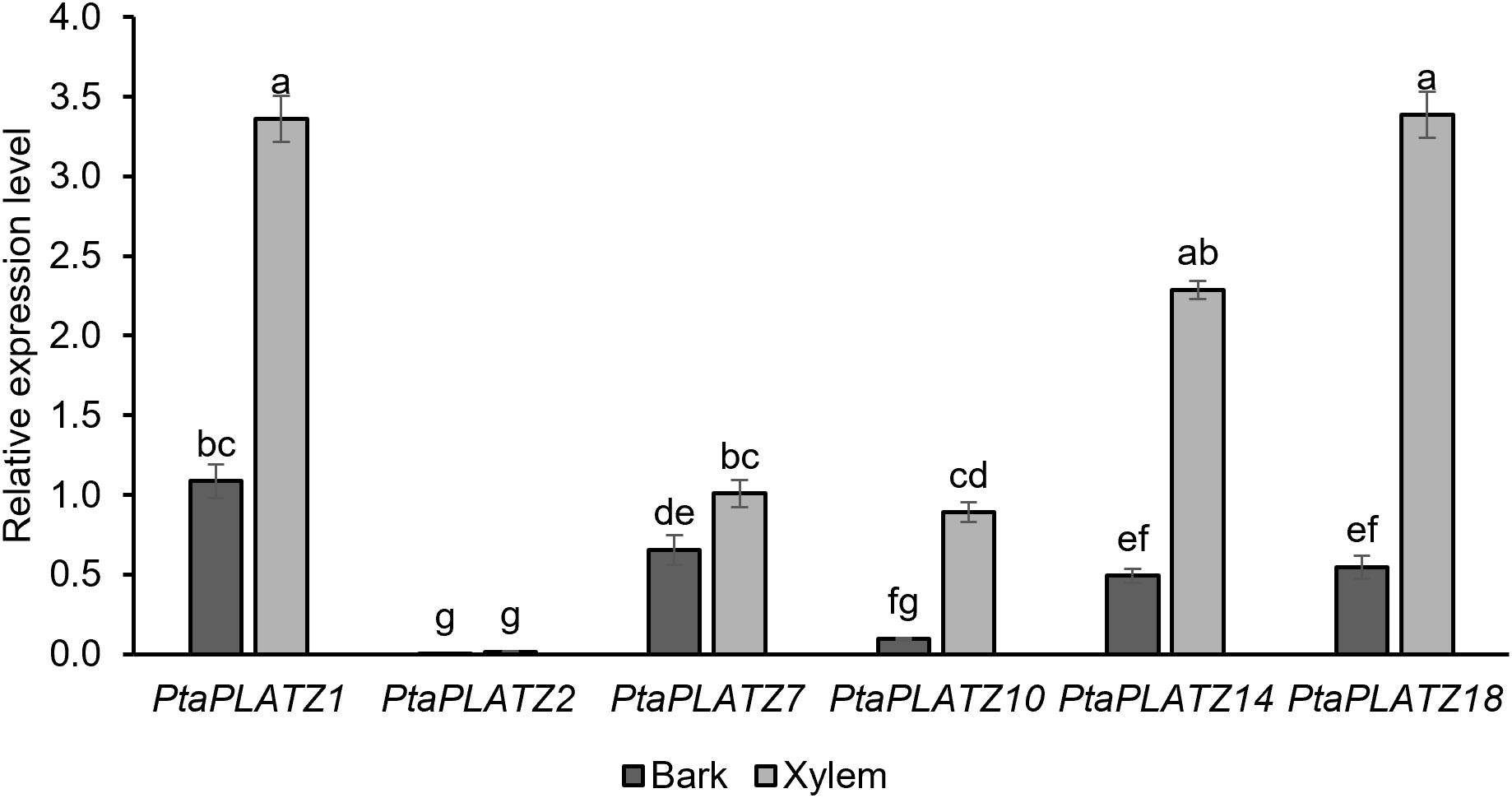
Figure 2 Expression level of six PtaPLATZ genes in bark and xylem of the stem of 3-month-old P. tremula x P. alba grown in the greenhouse, as measured by RT-qPCR. Bars represent the standard error, from five biological replicates and three technical replicates. Letters indicate the significantly different groups identified by a Kruskal-Wallis test with a Bonferroni correction (P < 0.05).
3.2 The dominant repression of PtaPLATZ18 enhances plant elongation and xylem development
To explore the function of PtaPLATZ18 in vascular tissue development and/or lignification, we produced dominant repressor lines for PtaPLATZ18 by generating poplar lines overexpressing a PtaPLATZ18-SRDX fusion. Six transgenic lines expressing PtaPLATZ18-SRDX were grown in vitro in phytotron for three months. Five of these lines were characterized by a significantly increased height (Supplementary Figure 2) and three of them (SRDX2, SRDX19, and SRDX20) were selected for further characterization. The expression level of both endogenous PtaPLATZ18 and PtaPLATZ18-SRDX was analysed in the secondary xylem by RT-qPCR. As shown in Figure 3, the endogenous PtaPLATZ18 expression level was similar for all lines whereas PtaPLATZ18-SRDX was highly expressed in the three SRDX lines and not detectable in the WT.
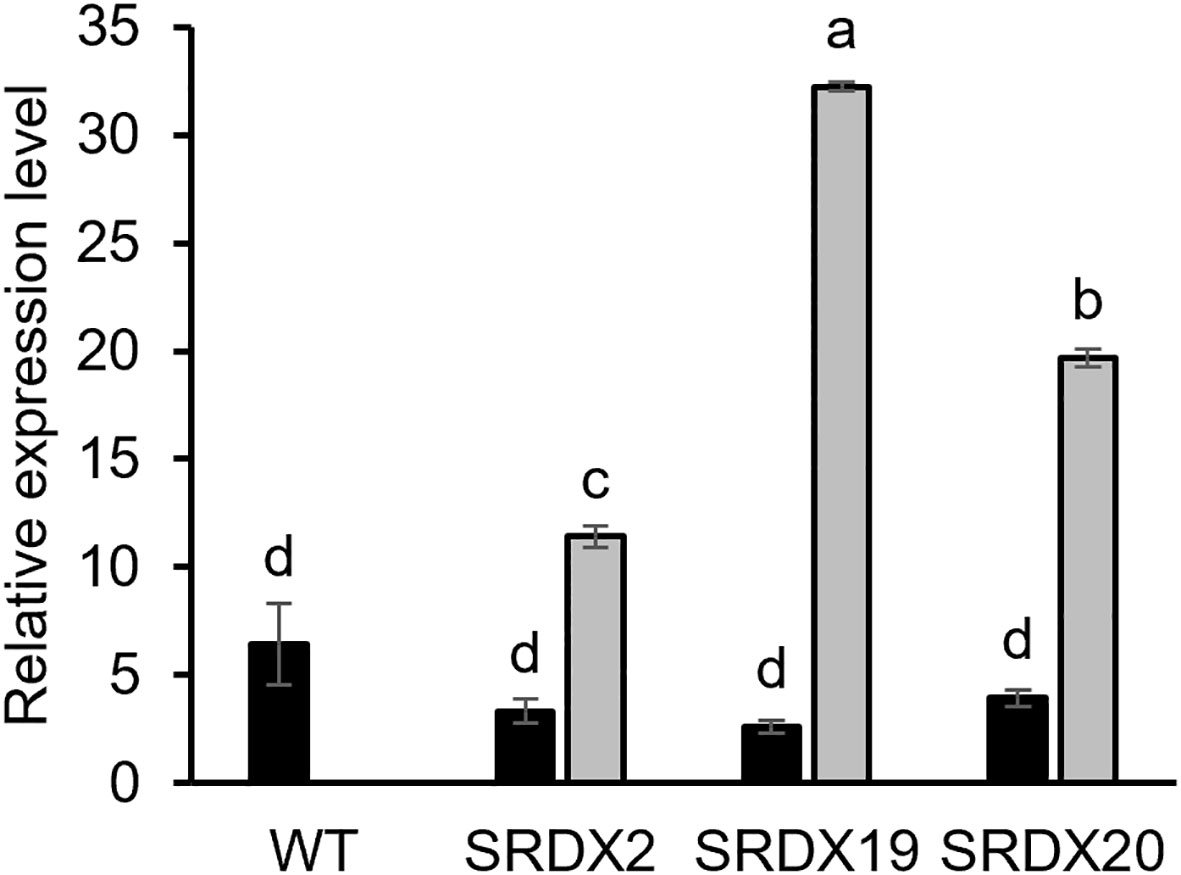
Figure 3 Relative expression level of endogenous PtaPLATZ18 (black) and PtaPLATZ18-SRDX (grey) in the xylem of 3-month-old SRDX and WT lines, as measured by RT-qPCR. Values are the mean and the standard error of five plants (biological replicates) and three technical replicates. The values have been normalized by using the CDC2 reference gene. Letters indicate the significantly different groups identified by an ANOVA and Tukey, P < 0.05.
Figure 4 shows the 3-month-old PtaPLATZ18-SRDX lines (SRDX2, SRDX19, and SRDX20) which displayed an enhanced growth compared to the WT (Figure 4A) as their height was increased by 22 to 38% (Figure 4B). This increase in height was due to an increased mean length of the internodes by 25 to 45% (Figure 4C). There was no significant difference in the diameter measured at the basis of the stem of the three transgenic lines and the WT (Figure 4D). In addition, SRDX lines exhibit smaller leaves than the WT with a leaf area decreased by 32-38% (Figure 4E).
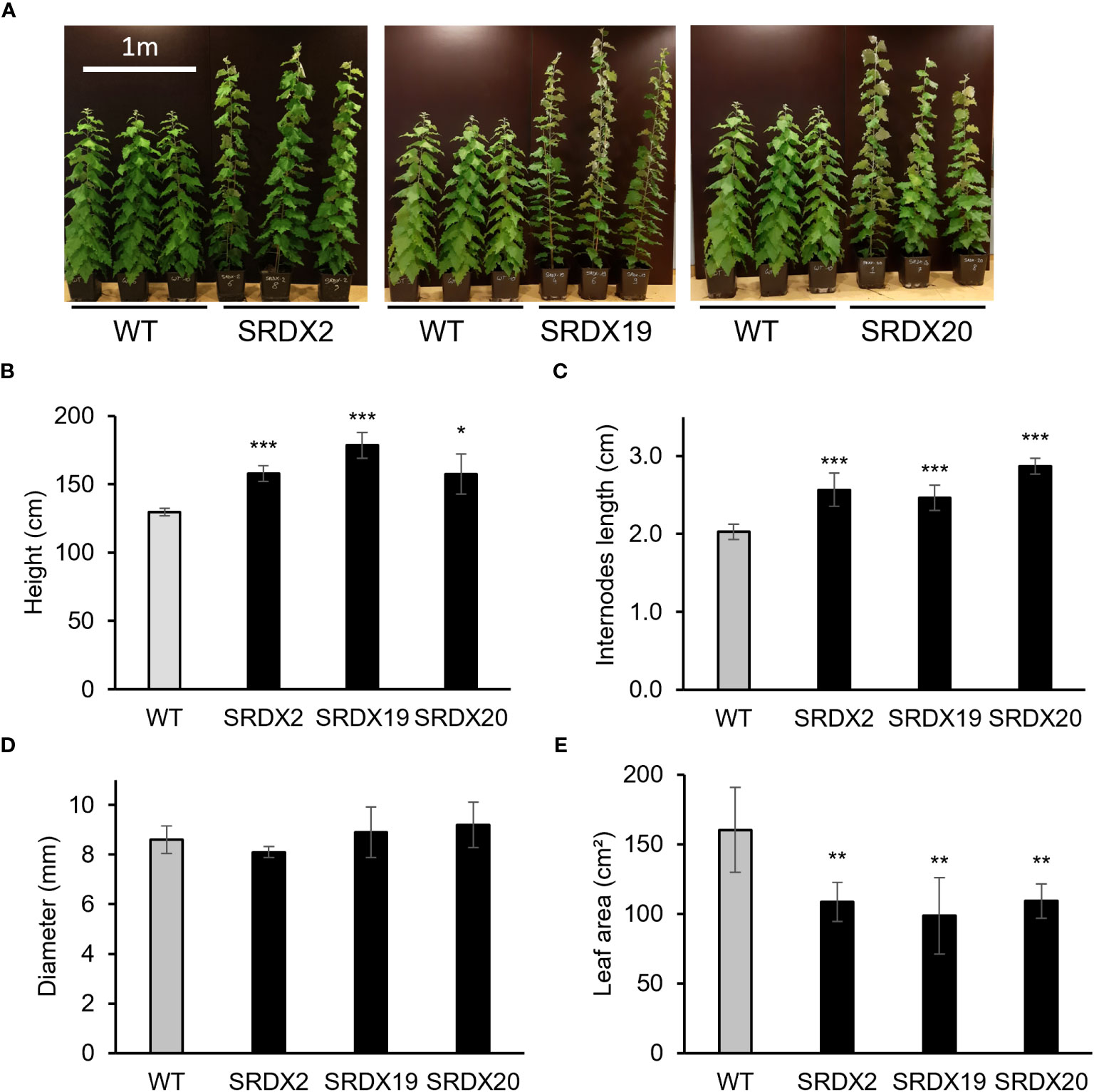
Figure 4 Phenotype of 3-month-old PtaPLATZ18-SRDX poplar lines and the WT. (A) Global phenotype. (B) Height. (C) Internodes mean length. (D) Diameter of the basal stem. (E) Mean area of leaves numbers 19 to 21. Five biological replicates. Bars = standard deviation. Student t-test was performed for each mutant line against the WT. *, P < 0.05; **, P < 0.01; ***, P < 0.001.
To characterize the SRDX lines at the anatomical level, stem cross sections were realized in the 6th internode below the first expanded leaf and stained with phloroglucinol-HCl to visualize lignified tissues. As shown in Figure 5A and Supplementary Figure 4, transgenic lines displayed a more developed secondary xylem than the WT. To quantify this observation, bark, xylem, and pith proportions were measured, providing their percentage in the stem sections (Figure 5B). A significant increase in the proportion of xylem by 38%, 21%, and 26% was observed in SRDX2, SRDX19, and SRDX20 lines respectively, compared to the WT. This increase in xylem size takes place at the expense of bark, whose proportion decreases by 13%, 16%, and 29% in the three SRDX lines, respectively, compared to the WT.
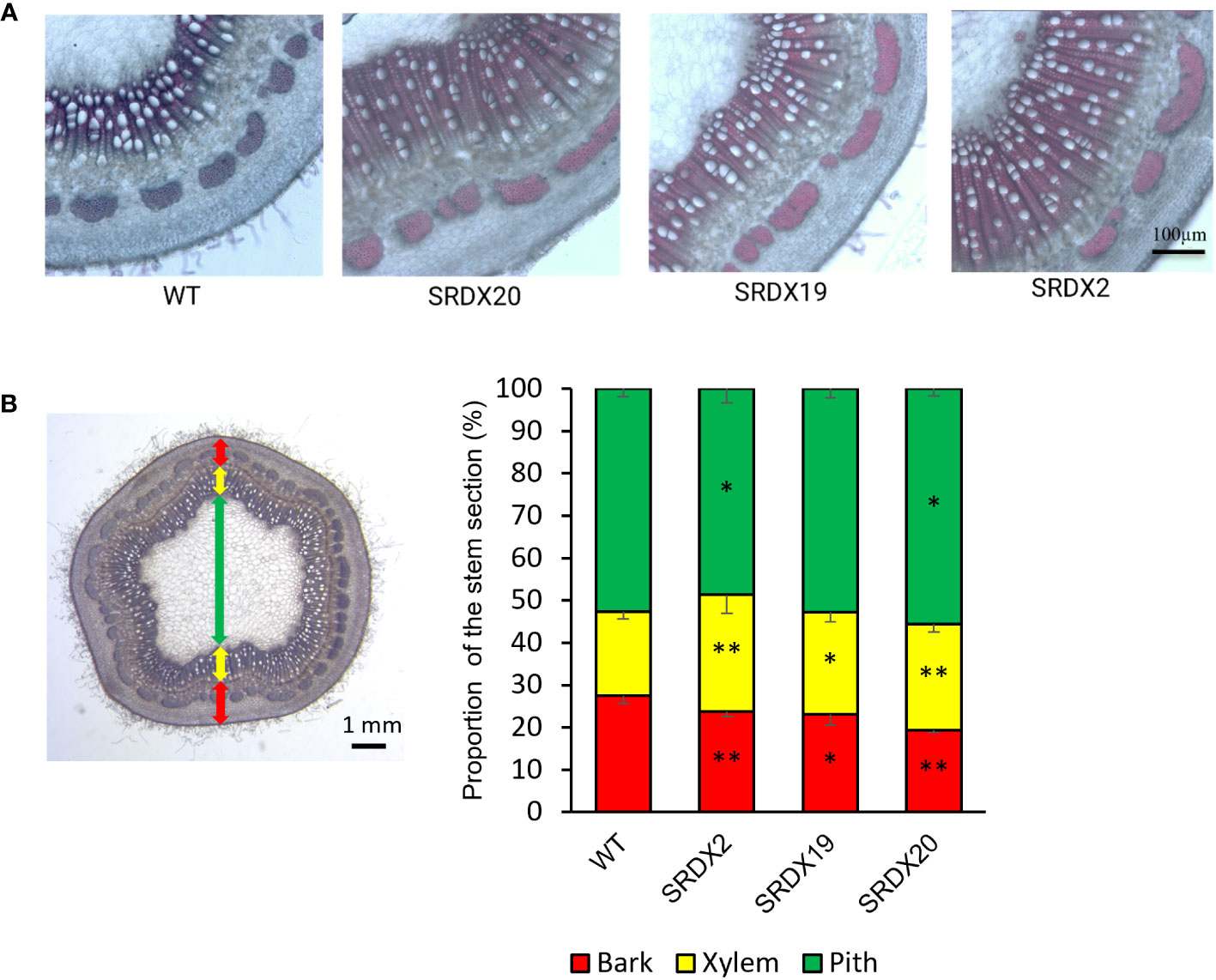
Figure 5 Enhanced xylem formation in 3-month-old PtaPLATZ18-SRDX lines compared to the WT. (A) Phloroglucinol-HCl stained stem cross sections (110 µm thickness) of the 6th internode from the apex visualized using bright field microscopy. (B) Percentage of bark, xylem, and pith stem sections. Two measures were performed on three stem sections per plant and five plants were studied per line. Student t-test was done for each mutant line against the WT. *, P < 0.05; **, P < 0.01. Bars = standard deviation.
3.3 PtaPLATZ18-SRDX lines have a higher lignin content in the stem xylem than the WT
Subsequently, the impact of the PtaPLATZ18-SRDX expression on the cell wall components accumulation was evaluated on CWR produced from wood, taken from the 30 cm basal portion of the stem. As shown in Table 1, the amount of recovered CWR was similar for the three SRDX lines and the WT. When compared to the WT, no significant modification was found regarding the cellulose content of the SRDX lines (Table 1). On the contrary, they contain a significantly higher lignin content, by 11 to 22%, depending on the lines. A Maüle staining was performed on stem cross section taken at a similar level to those for the phloroglucinol staining to evaluate possible change in lignin composition. This experiment did not reveal a clear difference in qualitative lignin composition between the WT and the SRDX lines (Supplementary Figure 5).

Table 1 Lignin, cellulose, and CWR content quantified in the WT and the three PtaPLATZ18-SRDX lines.
3.4 Higher expression of genes involved in the biosynthesis and polymerization of lignin in the xylem of PtaPLATZ18-SRDX lines
To evaluate whether the increased proportion of secondary xylem tissue and lignin accumulation in the SRDX lines were associated with a raised expression of genes involved in monolignol biosynthesis and/or polymerization, the expression level of several related genes (Supplementary Table 1) was subsequently monitored in both whole young stem (at a level comparable to stem sections of Figure 6, Supplementary Figures 4, 5) and in xylem (at a level comparable the material used for lignin quantification).
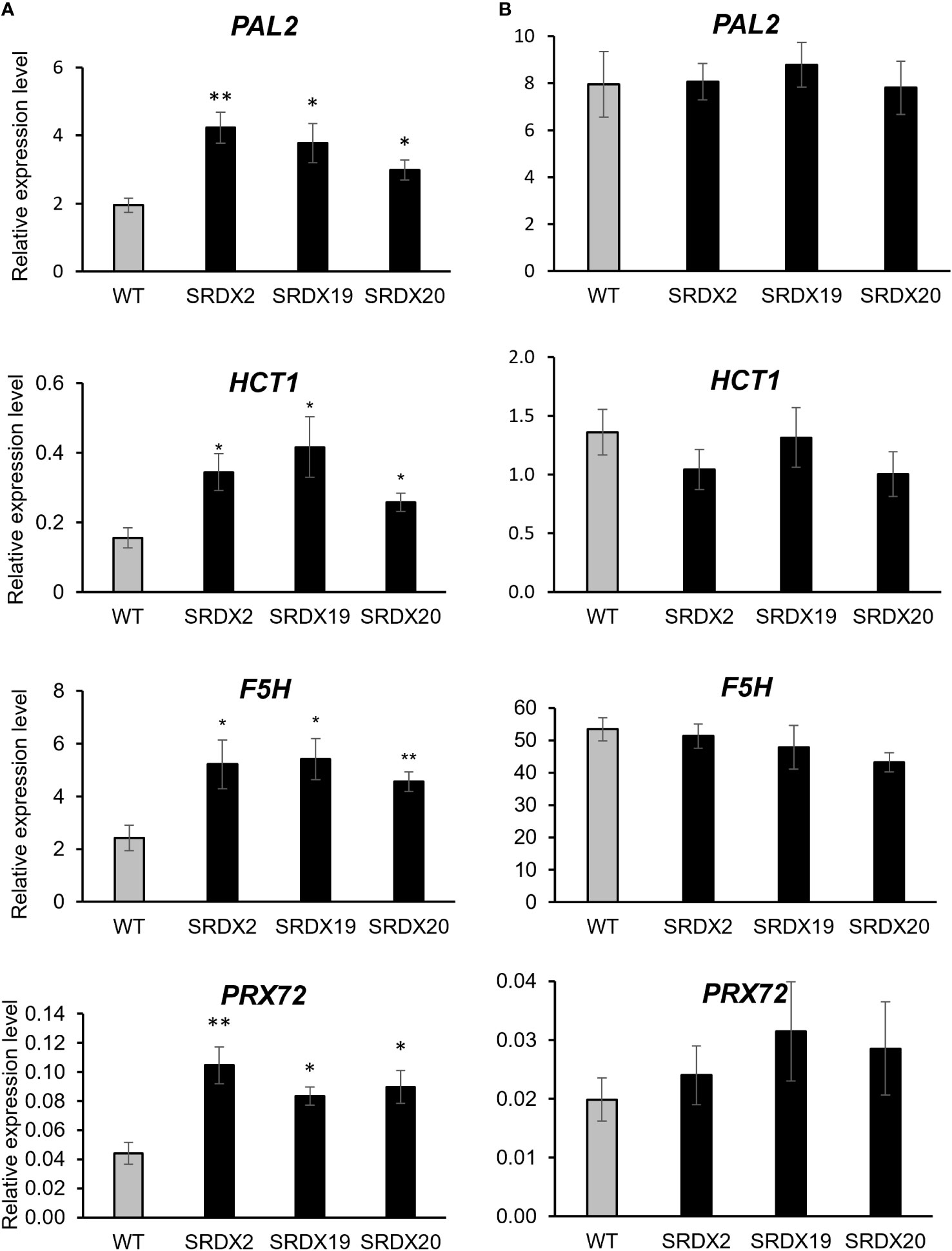
Figure 6 Relative expression level of genes involved in lignin biosynthesis and polymerization in 3-month-old WT and PtaPLATZ18-SRDX lines, as measured by RT-qPCR. (A) Young stem. (B) Xylem. Value for each line is an average of five plants (biological replicates) and three technical replicates, normalized by the CDC2 reference gene. Bars = standard error. Student t-test was performed for each mutant line against the WT. *, P < 0.05; **, P < 0.01.
In young stems of the three SRDX lines, among the 14 studied genes (Figure 6A, Supplementary Figure 6), four of them showed a significative ~2-fold increase in their expression level, compared to the WT. These genes include phenylalanine ammonia-lyase 2 (PAL2), hydroxycinnamoyl-CoA shikimate transferase 1 (HCT1), ferulate-5 hydroxylase 1 (F5H1), and the gene homologous to the A. thaliana peroxidase 72 (PRX72). The expression level of these four genes was further evaluated in xylem. As shown in Figure 6B, their expression level was higher than in the young stem and there was no difference between SRDX and WT lines. As there was no differential expression in lignin-related genes in the xylem, one can hypothesize that the higher lignin content in SRDX lines is due to the enhanced expression of lignin biosynthetic and polymerization genes within the young stem, containing mainly developing xylem.
To evaluate which regulation pathway may be altered by the expression of the PtaPLATZ18-SRDX construct, the expression level of several genes coding for TFs involved in the regulation of secondary cell wall differentiation was investigated in young stem tissues. Whereas no significant change was monitored for the expression of SND1-A2, MYB3, or MYB28, that of VND6-C1 was 1.6 to 2.6-fold higher than in the WT, depending on the line (Supplementary Figure 7).
4 Discussion
Here, we analysed the expression profile of several PLATZ genes in different tissues and organs of poplar, and selected PtaPLATZ18 as a candidate gene regulating secondary growth and/or secondary cell wall formation as it is preferentially expressed within xylem compared to other analysed plant tissues and organs (Figures 1, 2 and Supplementary Figure 3). We show that the dominant repression of PtaPLATZ18 triggers phenotypical alterations at morphological, anatomical, and biochemical levels as discussed below.
At the morphological level, dominant repression of PtaPLATZ18 results in an enhanced growth (by 22-38% depending on the line) associated with increased internode elongation (by 25-45% depending on the line) (Figure 4), suggesting that PtaPLATZ18 controls plant growth and development as already reported for other PLATZ genes. For instance, the dominant Arabidopsis ore15-1D (AtPLATZ3) activation mutant had a 28% increase in plant height when compared to the WT (Kim et al., 2018). Likewise, the overexpression of T. aestivum PLATZ1-A1 leads to an increased plant height and inversely its mutation caused a reduction of plant height compared to the WT. This gene was found to be the causative gene underlying the gibberellic acid (GA)-sensitive REDUCED HEIGHT (RHT) 25 dwarfing locus (Zhang et al., 2023). By using yeast two-hybrid and co-immunoprecipitation, these authors showed that PLATZ1-A1 interacts with RHT1, as well as with DELLA, a critical component of the GA growth-stimulating pathway. Recently, gmpla/b soybean mutants were described to have reduced leaf size and plant height, which was caused by a reduced internode elongation compared to the WT (Hu et al., 2023). GmPLATZ was found to regulate 3 A-type cyclin (GmCYCA2;2, GmCYCA2;4a and GmCYCA2;4b) and 3 D-type cyclin (GmCYCD1;1, GmCYCD4;1 and GmCYCD6;1) genes. Besides, this study reported that the expression of GmGA20OX was downregulated in the gmpla/b mutants and that GmPLATZ activates directly the promoter of GmGA20OX through the core element 5’-AATGGGCATT-3’ (Hu et al., 2023).
Stem elongation and growth are known to be regulated by GA level (Davies, 2010). For instance, in poplar, the overexpression of Arabidopsis GA20 oxidase, a key enzyme for producing bioactive GA, was reported to increase plant growth and induce xylogenesis (Eriksson et al., 2000; Cho et al., 2019). Actually, 3-month-old transgenic poplars overexpressing the Pinus densiflora PdGA20ox1 showed an increase of up to ~55% in height with no changes in stem diameter, when compared to the control (Cho et al., 2019).
Further, the dominant repression of PtaPLATZ18 results in a leaf area decreased by 32-38%, depending on the line (Figure 4E). A similar observation was reported for the ore15-2 knockout mutant (AtPLATZ3) characterized by a decreased leaf size mainly caused by an alteration of cell proliferation rather than cell expansion (Kim et al., 2018). ORE15 was found to interact directly with the promoters of GRF1 and GRF4, which are major regulators of plant growth and development including leaf development (Gonzalez et al., 2012).
Pleiotropic effects of elevated GA concentration were noticed in transgenic poplars overexpressing AtGA20ox1 such as smaller leaf area (Eriksson et al., 2000). In addition, a reduction of 38-40% in leaf area was measured in 2-month-old p35S-PdGA20ox1 poplar lines (Cho et al., 2019).
At the anatomical level, dominant repression of PtaPLATZ18 results in a significant increase in the proportion of xylem tissue (Figure 5) indicating that PtaPLATZ18 plays a role in the formation of secondary growth by controlling cambial activity. Several other genes have been found to promote cambial activity and xylem development in poplar, together with plant height. For instance, the overexpression of PagRabE1b encoding a small guanosine triphosphate (GTP)-binding protein leads to an increased xylem width by 27-50% and thicker xylem fiber cell walls (Liu et al., 2021). In another study, the overexpression of PagSAG101a induced increased plant height, as well as increased internode number and stem diameter, xylem width (by 5.1-14.7%), and secondary cell wall thickness while opposite phenotypes were observed for PagSAG10a knock-out plants (He et al., 2022). As shown by these authors, PagSAG101a gene expression is regulated by the PagC3H17 TF. The overexpression of PagC3H17 (Chai et al., 2014) gave similar results than that of PagSAG101a, indicating that the PagC3H17-PagSAG101a module plays a role in the positive gene regulatory network of secondary vascular system development (He et al., 2022). Likewise, overexpression of UNFERTILIZED EMBRYO SAC12 (UNE12) encoding a basic helix-loop-helix (bHLH) TF promoted xylem development and increased the thickness of the cell walls and the lignin content (as analyzed by FTIR and Raman microspectrometry) of secondary xylem in poplar together with a reduced plant height, shorter internodes and curled leaves, when compared to the WT (Song et al., 2023).
At the biochemical level, dominant repression of PtaPLATZ18 results in a higher lignin content as measured in the cell walls of the SRDX lines compared to the WT (Table 1). Compared to the WT, the expression of genes involved in lignin biosynthesis and polymerization of SRDX lines was higher in young stems, with early developmental steps of secondary growth (upper portion of the stem), but not in the xylem from the stem with well-established secondary growth (lower portion of the stem) (Figure 6 and Supplementary Figure 6). These genes include PAL2, the first gene of the monolignol biosynthesis pathway (Wang et al., 2014), HCT1, encoding a central enzyme for the direction of the flux towards coniferyl and sinapyl alcohols (Hoffmann et al., 2003), F5H1 encoding a key enzyme for the synthesis of sinapyl alcohol (Meyer et al., 1998) and a gene homologous to the A. thaliana peroxidase 72 (PRX72), which has been shown to play a role in monolignol polymerization (Herrero et al., 2013). Therefore, the increased lignin accumulation in older xylem tissue of the SRDX lines seems to originate from lignin metabolism activation at earlier stages of secondary growth. Besides, the gene encoding VND6-C1, belonging to the VND6 family of NAC TFs which are master regulators of xylem vessel element differentiation, was upregulated in the SRDX lines (Supplementary Figure 7), suggesting that PtaPLATZ18 acts upstream of the complex NAC-MYB network regulating xylogenesis in both Arabidopsis and poplar (Kubo et al., 2005; Ohtani et al., 2011; Li et al., 2012). A more in-depth study through RNA-seq analysis would give a comprehensive overview of the transcriptional changes induced by the alteration of PtaPLATZ18 expression.
Future research on the transcriptional activity of PtaPLATZ18, i.e. as an activator or as a repressor, on its protein interactions, and on the biological processes regulated by this TF are required to unravel its role in plant vegetative growth, secondary growth, and wood formation.
Data availability statement
The original contributions presented in the study are included in the article/Supplementary Material, further inquiries can be directed to the corresponding author. Nucleotide sequence of PtaPLATZ18 can be found in the GenBank database under the accession number OR730833.
Author contributions
CG: Conceptualization, Data curation, Formal analysis, Investigation, Methodology, Supervision, Validation, Visualization, Writing – original draft, Writing – review & editing. MBe: Conceptualization, Investigation, Methodology, Supervision, Visualization, Writing – review & editing. JS: Data curation, Formal analysis, Investigation, Validation, Visualization, Writing – original draft. AM: Investigation, Writing – review & editing. ME: Supervision, Writing – review & editing. MBa: Conceptualization, Funding acquisition, Methodology, Project administration, Supervision, Writing – original draft, Writing – review & editing.
Funding
The author(s) declare financial support was received for the research, authorship, and/or publication of this article. MBe and CG are supported by Belgian Fonds de la Recherche Scientifique (FRS-FNRS) research projects T.0068.18 and T.0010.22, respectively. MBa is a Senior Research Associate of the FRS-FNRS.
Acknowledgments
The authors acknowledge Ricardo Gameiro Da Costa, Nathanaël Sanne, and Aubry Fenestre for their technical assistance. Thanks to Louise Conrard and the CMMI team for the access to the wide-field microscope. A special thanks to Annabelle Dupas and Nathalie Ladouce from the LRSV team, for their collaboration in the wood powder preparation.
Conflict of interest
The authors declare that the research was conducted in the absence of any commercial or financial relationships that could be construed as a potential conflict of interest.
Publisher’s note
All claims expressed in this article are solely those of the authors and do not necessarily represent those of their affiliated organizations, or those of the publisher, the editors and the reviewers. Any product that may be evaluated in this article, or claim that may be made by its manufacturer, is not guaranteed or endorsed by the publisher.
Supplementary material
The Supplementary Material for this article can be found online at: https://www.frontiersin.org/articles/10.3389/fpls.2023.1302536/full#supplementary-material
References
Baldacci-Cresp, F., Moussawi, J., Leplé, J.-C., Van Acker, R., Kohler, A., Candiracci, J., et al. (2015). PtaRHE1, a Populus tremula x Populus alba RING-H2 protein of the ATL family, has a regulatory role in secondary phloem fibre development. Plant J. 82, 978–990. doi: 10.1111/tpj.12867. Article 6.
Baldacci-Cresp, F., Sacré, P.-Y., Twyffels, L., Mol, A., Vermeersch, M., Ziemons, E., et al. (2016). Poplar–root knot nematode interaction: A model for perennial woody species. Mol. Plant Microbe Interact. 29, 560–572. doi: 10.1094/MPMI-01-16-0015-R
Chai, G., Qi, G., Cao, Y., Wang, Z., Yu, L., Tang, X., et al. (2014). Poplar PdC3H17 and PdC3H18 are direct targets of PdMYB3 and PdMYB21, and positively regulate secondary wall formation in Arabidopsis and poplar. New Phytol. 203, 520–534. doi: 10.1111/nph.12825
Chao, Q., Gao, Z.-F., Zhang, D., Zhao, B.-G., Dong, F.-Q., Fu, C.-X., et al. (2019). The developmental dynamics of the Populus stem transcriptome. Plant Biotechnol. J. 17, 206–219. doi: 10.1111/pbi.12958
Cho, J.-S., Jeon, H.-W., Kim, M.-H., Vo, T. K., Kim, J., Park, E.-J., et al. (2019). Wood forming tissue-specific bicistronic expression of PdGA20ox1 and PtrMYB221 improves both the quality and quantity of woody biomass production in a hybrid poplar. Plant Biotechnol. J. 17, 1048–1057. doi: 10.1111/pbi.13036
Dampanaboina, L., Yuan, N., Mendu, V. (2021). Estimation of crystalline cellulose content of plant biomass using the Updegraff method. J. Vis. Exp. 171, 62031. doi: 10.3791/62031
Davies, P. J. (2010). Plant Hormones (Dordrecht: Springer Netherlands). doi: 10.1007/978-1-4020-2686-7
Dong, T., Yin, X., Wang, H., Lu, P., Liu, X., Gong, C., et al. (2021). ABA-INDUCED expression 1 is involved in ABA-inhibited primary root elongation via modulating ROS homeostasis in Arabidopsis. Plant Sci. 304, 110821. doi: 10.1016/j.plantsci.2021.110821
Eriksson, M. E., Israelsson, M., Olsson, O., Moritz, T. (2000). Increased gibberellin biosynthesis in transgenic trees promotes growth, biomass production and xylem fiber length. Nat. Biotech. 18, 784–788. doi: 10.1038/77355
Gonzalez, N., Vanhaeren, H., Inzé, D. (2012). Leaf size control: Complex coordination of cell division and expansion. Trends Plant Sci. 17, 332–340. doi: 10.1016/j.tplants.2012.02.003
González-Morales, S. I., Chávez-Montes, R. A., Hayano-Kanashiro, C., Alejo-Jacuinde, G., Rico-Cambron, T. Y., de Folter, S., et al. (2016). Regulatory network analysis reveals novel regulators of seed desiccation tolerance in Arabidopsis thaliana. Proc. Natl. Acad. Sci. U.S.A. 113, E5232–E5241. doi: 10.1073/pnas.1610985113
Guerriero, G., Behr, M., Hausman, J.-F., Legay, S. (2017). Textile hemp vs. Salinity: insights from a targeted gene expression analysis. Genes 8, 242. doi: 10.3390/genes8100242
Guo, X., Fu, Y., Lee, Y. J., Chern, M., Li, M., Cheng, M., et al. (2022). The PGS1 basic helix-loop-helix protein regulates Fl3 to impact seed growth and grain yield in cereals. Plant Biotechnol. J. 20, 1311–1326. doi: 10.1111/pbi.13809
Han, X., Rong, H., Tian, Y., Qu, Y., Xu, M., Xu, L. (2022). Genome-wide identification of PLATZ transcription factors in Ginkgo biloba L. and their expression characteristics during seed development. Front. Plant Sci. 13. doi: 10.3389/fpls.2022.946194
He, H., Song, X.-Q., Jiang, C., Liu, Y.-L., Wang, D., Wen, S.-S., et al. (2022). The role of senescence-associated gene101 (PagSAG101a) in the regulation of secondary xylem formation in poplar. J. Integr. Plant Biol. 64, 73–86. doi: 10.1111/jipb.13195
Herrero, J., Fernández-Pérez, F., Yebra, T., Novo-Uzal, E., Pomar, F., Pedreño, M. Á., et al. (2013). Bioinformatic and functional characterization of the basic peroxidase 72 from Arabidopsis thaliana involved in lignin biosynthesis. Planta 237, 1599–1612. doi: 10.1007/s00425-013-1865-5
Hiratsu, K., Matsui, K., Koyama, T., Ohme-Takagi, M. (2003). Dominant repression of target genes by chimeric repressors that include the EAR motif, a repression domain, in Arabidopsis. Plant J. 34, 733–739. doi: 10.1046/j.1365-313X.2003.01759.x
Hoffmann, L., Maury, S., Martz, F., Geoffroy, P., Legrand, M. (2003). Purification, cloning, and properties of an acyltransferase controlling shikimate and quinate ester intermediates in phenylpropanoid metabolism. J. Biol. Chem. 278, 95–103. doi: 10.1074/jbc.M209362200
Howe, E. A., Sinha, R., Schlauch, D., Quackenbush, J. (2011). RNA-Seq analysis in MeV. Bioinform 27, 3209–3210. doi: 10.1093/bioinformatics/btr490
Hu, Y., Liu, Y., Lu, L., Tao, J.-J., Cheng, T., Jin, M., et al. (2023). Global analysis of seed transcriptomes reveals a novel PLATZ regulator for seed size and weight control in soybean. New Phytol. 240, 2436–2454. doi: 10.1111/nph.19316
Iocco-Corena, P., Chaïb, J., Torregrosa, L., Mackenzie, D., Thomas, M. R., Smith, H. M. (2021). VviPLATZ1 is a major factor that controls female flower morphology determination in grapevine. Nat. Commun. 12, 6995. doi: 10.1038/s41467-021-27259-8
Jun, S. E., Kim, J. H., Hwang, J. Y., Huynh Le, T. T., Kim, G.-T. (2019). ORESARA15 acts synergistically with ANGUSTIFOLIA3 and separately from AINTEGUMENTA to promote cell proliferation during leaf growth. Int. J. Mol. Sci. 21, 241. doi: 10.3390/ijms21010241
Karimi, M., Inzé, D., Depicker, A. (2002). GATEWAY™ vectors for Agrobacterium-mediated plant transformation. Trends Plant Sci. 7, 193–195. doi: 10.1016/S1360-1385(02)02251-3
Kim, J. H., Kim, J., Jun, S. E., Park, S., Timilsina, R., Kwon, D. S., et al. (2018). ORESARA15, a PLATZ transcription factor, mediates leaf growth and senescence in Arabidopsis. New Phytol. 220, 609–623. doi: 10.1111/nph.15291
Kubo, M., Udagawa, M., Nishikubo, N., Horiguchi, G., Yamaguchi, M., Ito, J., et al. (2005). Transcription switches for protoxylem and metaxylem vessel formation. Genes Dev. 19, 1855–1860. doi: 10.1101/gad.1331305
Leple, J. C., Brasileiro, A. C. M., Michel, M. F., Delmotte, F., Jouanin, L. (1992). Transgenic poplars: expression of chimeric genes using four different constructs. Plant Cell Rep. 11, 137–141. doi: 10.1007/BF00232166
Li, Q., Lin, Y.-C., Sun, Y.-H., Song, J., Chen, H., Zhang, X.-H., et al. (2012). Splice variant of the SND1 transcription factor is a dominant negative of SND1 members and their regulation in Populus trichocarpa. Proc. Natl. Acad. Sci. U.S.A. 109, 14699–14704. doi: 10.1073/pnas.1212977109
Li, H., Wang, Y., Xiao, Q., Luo, L., Zhang, C., Mao, C., et al. (2021). Transcription factor ZmPLATZ2 positively regulate the starch synthesis in maize. Plant Growth Regul. 93, 291–302. doi: 10.1007/s10725-020-00687-0
Li, Q., Wang, J., Ye, J., Zheng, X., Xiang, X., Li, C., et al. (2017). The maize imprinted gene Floury3 encodes a PLATZ protein required for tRNA and 5S rRNA transcription through interaction with RNA Polymerase III. Plant Cell 29, 2661–2675. doi: 10.1105/tpc.17.00576
Liu, M., Wang, C., Ji, Z., Lu, J., Zhang, L., Li, C., et al. (2023). Regulation of drought tolerance in Arabidopsis involves the PLATZ4 -mediated transcriptional repression of plasma membrane aquaporin PIP2;8. Plant J. 115, 434–451. doi: 10.1111/tpj.16235
Liu, Y.-L., Wang, L.-J., Li, Y., Guo, Y.-H., Cao, Y., Zhao, S.-T. (2021). A small guanosine triphosphate binding protein PagRabE1b promotes xylem development in poplar. Front. Plant Sci. 12. doi: 10.3389/fpls.2021.686024
Liu, S., Yang, R., Liu, M., Zhang, S., Yan, K., Yang, G., et al. (2020). PLATZ2 negatively regulates salt tolerance in Arabidopsis seedlings by directly suppressing the expression of the CBL4/SOS3 and CBL10/SCaBP8 genes. J. Exp. Bot. 71, 5589–5602. doi: 10.1093/jxb/eraa259
Livak, K. J., Schmittgen, T. D. (2001). Analysis of relative gene expression data using real-time quantitative PCR and the 2 -ΔΔCT Method. Methods 25, 402–408. doi: 10.1006/meth.2001.1262
Loziuk, P., Hecht, E., Muddiman, D. (2017). N-linked glycosite profiling and use of Skyline as a platform for characterization and relative quantification of glycans in differentiating xylem of Populus trichocarpa. Anal. Bioanal. Chem. 409, 487–497. doi: 10.1007/s00216-016-9776-5
Lu, F., Wang, C., Chen, M., Yue, F., Ralph, J. (2021). A facile spectroscopic method for measuring lignin content in lignocellulosic biomass. Green Chem. 23, 5106–5112. doi: 10.1039/D1GC01507A. Article 14.
Ma, X., Yang, H., Bu, Y., Wu, X., Sun, N., Xiao, J., et al. (2023). Genome-wide identification of PLATZ genes related to cadmium tolerance in Populus trichocarpa and characterization of the role of PtPLATZ3 in phytoremediation of cadmium. Int. J. Biol. Macromol. 228, 732–743. doi: 10.1016/j.ijbiomac.2022.12.176
Meyer, K., Shirley, A. M., Cusumano, J. C., Bell-Lelong, D. A., Chapple, C. (1998). Lignin monomer composition is determined by the expression of a cytochrome P450-dependent monooxygenase in Arabidopsis. Proc. Natl. Acad. Sci. U.S.A. 95, 6619–6623. doi: 10.1073/pnas.95.12.6619
Muoki, R. C., Paul, A., Kumari, A., Singh, K., Kumar, S. (2012). An improved protocol for the isolation of RNA from roots of tea (Camellia sinensis (L.) O. Kuntze). Mol. Biotechnol. 52, 82–88. doi: 10.1007/s12033-011-9476-5
Nagano, Y., Furuhashi, H., Inaba, T., Sasaki, Y. (2001). A novel class of plant-specific zinc-dependent DNA-binding protein that binds to A/T-rich DNA sequences. Nucleic Acids Res. 29, 4097–4105. doi: 10.1093/nar/29.20.4097
Ohtani, M., Nishikubo, N., Xu, B., Yamaguchi, M., Mitsuda, N., Goué, N., et al. (2011). A NAC domain protein family contributing to the regulation of wood formation in poplar. Plant J. 67, 499–512. doi: 10.1111/j.1365-313X.2011.04614.x
Payyavula, R. S., Badmi, R., Jawdy, S. S., Rodriguez, M., Jr., Gunter, L., Sykes, R. W. (2022). Biomass formation and sugar release efficiency of Populus modified by altered expression of a NAC transcription factor. Plant Direct 6 (8), e419. doi: 10.1002/pld3.419
Pelayo, M. A., Morishita, F., Sawada, H., Matsushita, K., Iimura, H., He, Z., et al. (2023). AGAMOUS regulates various target genes via cell cycle–coupled H3K27me3 dilution in floral meristems and stamens. Plant Cell 35, 2821–2847. doi: 10.1093/plcell/koad123
Schneider, C. A., Rasband, W. S., Eliceiri, K. W. (2012). NIH Image to ImageJ: 25 years of image analysis. Nat. Methods 9, 671–675. doi: 10.1038/nmeth.2089. Article 7.
So, H.-A., Choi, S. J., Chung, E., Lee, J.-H. (2015). Molecular characterization of stress-inducible PLATZ gene from soybean (Glycine max L.). Plant Omics 8, 479–484. doi: 10.3316/informit.263226429190188
Song, C., Guo, Y., Shen, W., Yao, X., Xu, H., Zhao, Y., et al. (2023). PagUNE12 encodes a basic helix-loop-helix transcription factor that regulates the development of secondary vascular tissue in poplar. Plant Physiol. 192, 1046–1062. doi: 10.1093/plphys/kiad152
Speer, E. O. (1987). A method of retaining phloroglucinol proof of lignin. Stain Technol. 62, 279–280. doi: 10.3109/10520298709108008
Sundell, D., Street, N. R., Kumar, M., Mellerowicz, E. J., Kucukoglu, M., Johnsson, C., et al. (2017). AspWood: high-spatial-resolution transcriptome profiles reveal uncharacterized modularity of wood formation in Populus tremula. Plant Cell 29, 1585–1604. doi: 10.1105/tpc.17.00153
Timilsina, R., Kim, Y., Park, S., Park, H., Park, S.-J., Kim, J. H., et al. (2022). ORESARA 15, a PLATZ transcription factor, controls root meristem size through auxin and cytokinin signalling-related pathways. J. Exp. Bot. 73, 2511–2524. doi: 10.1093/jxb/erac050
Wai, A. H., Rahman, M. M., Waseem, M., Cho, L.-H., Naing, A. H., Jeon, J.-S., et al. (2022). Comprehensive genome-wide analysis and expression pattern profiling of PLATZ gene family members in Solanum Lycopersicum L. under multiple abiotic stresses. Plants 11, 3112. doi: 10.3390/plants11223112
Wang, A., Hou, Q., Si, L., Huang, X., Luo, J., Lu, D., et al. (2019). The PLATZ transcription factor GL6 affects grain length and number in rice. Plant Physiol. 180, 2077–2090. doi: 10.1104/pp.18.01574
Wang, J., Ji, C., Li, Q., Zhou, Y., Wu, Y. (2018). Genome-wide analysis of the plant-specific PLATZ proteins in maize and identification of their general role in interaction with RNA polymerase III complex. BMC Plant Biol. 18, 221. doi: 10.1186/s12870-018-1443-x
Wang, J. P., Naik, P. P., Chen, H.-C., Shi, R., Lin, C.-Y., Liu, J., et al. (2014). Complete proteomic-based enzyme reaction and inhibition kinetics reveal how monolignol biosynthetic enzyme families affect metabolic flux and lignin in Populus trichocarpa. Plant Cell 26 (3), 894–914. doi: 10.1105/tpc.113.120881
Xu, X., Backes, A., Legay, S., Berni, R., Faleri, C., Gatti, E., et al. (2019). Cell wall composition and transcriptomics in stem tissues of stinging nettle (Urtica dioica L.): Spotlight on a neglected fibre crop. Plant Direct 3 (8), e00151. doi: 10.1002/pld3.151
Yamashita, D., Kimura, S., Wada, M., Takabe, K. (2016). Improved Mäule color reaction provides more detailed information on syringyl lignin distribution in hardwood. J. Wood. Sci. 62, 131–137. doi: 10.1007/s10086-016-1536-9
Zhang, J., Li, C., Zhang, W., Zhang, X., Mo, Y., Tranquilli, G. E., et al. (2023). Wheat plant height locus RHT25 encodes a PLATZ transcription factor that interacts with DELLA (RHT1). Proc. Natl. Acad. Sci. U.S.A. 120, e2300203120. doi: 10.1073/pnas.2300203120
Zhao, H., Qin, Y., Xiao, Z., Li, Q., Yang, N., Pan, Z., et al. (2020). Loss of function of an RNA Polymerase III subunit lads to impaired maize kernel development. Plant Physiol. 184, 359–373. doi: 10.1104/pp.20.00502
Zhao, J., Zheng, L., Wei, J., Wang, Y., Chen, J., Zhou, Y., et al. (2022). The soybean PLATZ transcription factor GmPLATZ17 suppresses drought tolerance by interfering with stress-associated gene regulation of GmDREB5. Crop J. 10, 1014–1025. doi: 10.1016/j.cj.2022.03.009
Keywords: PLATZ, transcription factor, poplar, vascular tissue development, lignin, secondary growth
Citation: Guérin C, Behr M, Sait J, Mol A, El Jaziri M and Baucher M (2023) Evidence for poplar PtaPLATZ18 in the regulation of plant growth and vascular tissues development. Front. Plant Sci. 14:1302536. doi: 10.3389/fpls.2023.1302536
Received: 26 September 2023; Accepted: 29 November 2023;
Published: 21 December 2023.
Edited by:
Meng-Zhu Lu, Zhejiang Agriculture & Forestry University, ChinaReviewed by:
Igor Cesarino, University of São Paulo, BrazilYoshimi Nakano, National Institute of Advanced Industrial Science and Technology (AIST), Japan
Copyright © 2023 Guérin, Behr, Sait, Mol, El Jaziri and Baucher. This is an open-access article distributed under the terms of the Creative Commons Attribution License (CC BY). The use, distribution or reproduction in other forums is permitted, provided the original author(s) and the copyright owner(s) are credited and that the original publication in this journal is cited, in accordance with accepted academic practice. No use, distribution or reproduction is permitted which does not comply with these terms.
*Correspondence: Marie Baucher, bWFyaWUuYmF1Y2hlckB1bGIuYmU=