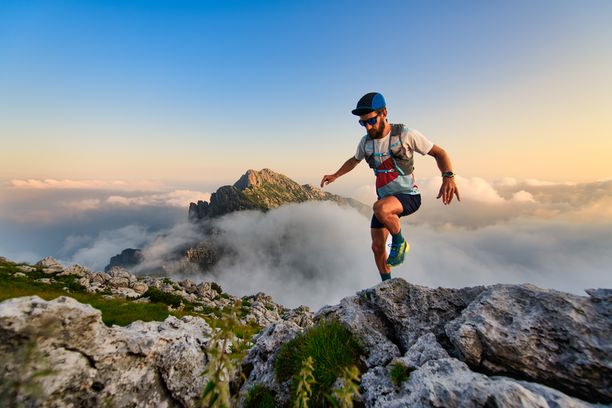
95% of researchers rate our articles as excellent or good
Learn more about the work of our research integrity team to safeguard the quality of each article we publish.
Find out more
REVIEW article
Front. Plant Sci., 12 December 2023
Sec. Plant Abiotic Stress
Volume 14 - 2023 | https://doi.org/10.3389/fpls.2023.1302046
Stem cells residing in plant apical meristems play an important role during postembryonic development. These stem cells are the wellspring from which tissues and organs of the plant emerge. The shoot apical meristem (SAM) governs the aboveground portions of a plant, while the root apical meristem (RAM) orchestrates the subterranean root system. In their sessile existence, plants are inextricably bound to their environment and must adapt to various abiotic stresses, including osmotic stress, drought, temperature fluctuations, salinity, ultraviolet radiation, and exposure to heavy metal ions. These environmental challenges exert profound effects on stem cells, potentially causing severe DNA damage and disrupting the equilibrium of reactive oxygen species (ROS) and Ca2+ signaling in these vital cells, jeopardizing their integrity and survival. In response to these challenges, plants have evolved mechanisms to ensure the preservation, restoration, and adaptation of the meristematic stem cell niche. This enduring response allows plants to thrive in their habitats over extended periods. Here, we presented a comprehensive overview of the cellular and molecular intricacies surrounding the initiation and maintenance of the meristematic stem cell niche. We also delved into the mechanisms employed by stem cells to withstand and respond to abiotic stressors.
Pluripotent stem cells, the versatile architects of multicellular organisms, orchestrate the continual regeneration of organs and tissues, mending the wear and tear of daily life. This remarkable regenerative potential, harnessed by some plants, enables them to persist for centuries or even millennia (Sang et al., 2018; McKim, 2019; Hata and Kyozuka, 2021; Umeda et al., 2021). Stem cells, nestled within their specialized microenvironments, are key to orchestrating cellular differentiation. These cells, initially undifferentiated, retain the remarkable ability for self-renewal and the generation of progeny. These progenies, in turn, may either retain stem cell properties or embark on a journey towards specialized specific functions (Aichinger et al., 2012; Wang et al., 2020; Nicolas and Laufs, 2022). However, continuous differentiation ultimately curtails the ability of cells to sustain continuous proliferation, underscoring the critical importance of precise stem cell regulation. Any deviation, whether in the form of a reduction or excessive proliferation of stem cells, can lead to pathological states, disrupting the cellular composition and impairing tissue function. Recent scientific endeavors in stem cell research have been predominantly devoted to unraveling the intricacies of initiation, maintenance, and signal transduction (Sablowski, 2011; Janocha and Lohmann, 2018; Warghat et al., 2018; Motte et al., 2019; Fujinami et al., 2020; Xue et al., 2020), often involving the participation of plant hormones (Lee et al., 2013; Xu et al., 2020; Gomes and Scortecci, 2021; Yamoune et al., 2021; Li et al., 2023).
For sessile organisms like plants, the challenge of survival hinges on their ability to adapt to a repertoire of abiotic stresses, including drought, salinity, temperature fluctuations, exposure to heavy metal ions, ultraviolet radiation, and other various physical perturbations (Sun et al., 2020; Markham and Greenham, 2021; Zhang et al., 2022; Kopecka et al., 2023). These stressors not only affect the geographical distribution of plants but also exert profound effects on their growth, development, and, in agricultural contexts, crop yields (Villalobos-Lopez et al., 2022). The molecular landscape of plant responses to abiotic stresses is intricate, including signal perception, signal transduction, transcriptional regulation, post-transcriptional processing, translation, and post-translational modifications. Given the pivotal role of stem cells in plant development, contemporary research has increasingly focused on elucidating the relationship between plant stem cells and their responses to abiotic stresses (Gong et al., 2020; Lamers et al., 2020; Li et al., 2021a; Ubogoeva et al., 2021; Shinozaki and Yamaguchi-Shinozaki, 2022; Charng et al., 2023). In this review, we summarized the knowledge surrounding the composition, initiation, and maintenance of plant stem cells, as well as the multifaceted mechanisms governing plant responses to abiotic stresses. Particular emphasis was placed on the intricacies of signal perception, with a primary focus on the relationship between plant stem cells and abiotic stresses.
In plants, the distribution of stem cells spans roots, stems, and vascular tissues, serving as the self-sustaining units for organ development. These stem cells, nestled within the apical meristem, play a pivotal role in the postembryonic development of plants, including the shoot apical meristem (SAM) responsible for aboveground growth and the root apical meristem (RAM) governing root system development. The meristems possess dual functions, orchestrating both the production of new cells and the initiation of organogenesis (Aichinger et al., 2012; Liu et al., 2018a).
The intricate mechanism of new cell generation within the shoot meristem has been illuminated through elegant cell-tracking experiments. Within the shoot meristem of Arabidopsis thaliana seedlings, cells in the SAM are distinctly organized into clonally separate layers. This arrangement, known as the tunica-corpus theory, classifies cells into the L1 and L2 layers forming the tunica and multiple layers at L3 and below constituting the corpus (Jurgens et al., 1994). This tri-layered structure characterizes Dicotyledons, while Monocotyledons possess two layers, and Gymnosperms feature just one. The vertical division of primordial cells residing in the L1 and L2 layers continuously expands the surface area of the plant shoot meristem (Wegner, 2000; Gross-Hardt and Laux, 2003). Further categorization of the SAM, according to the zoning theory, reveals the central meristem zone (CZ), rib meristem zone (RZ), and peripheral meristem zone (PZ) (Gross-Hardt and Laux, 2003). Stem cells reside at the apex of the CZ, with the cluster of cells beneath them, known as the organizer center (OC), playing a vital role in stem cell population maintenance (Kerstetter and Hake, 1997; Mayer et al., 1998; Lee and Clark, 2013; Tanaka et al., 2015; Somssich et al., 2016; Meng et al., 2017; Negin et al., 2017; Zhang et al., 2017; Su et al., 2020) (Figure 1A).
Figure 1 Structure and signaling pathways of meristem stem cells: (A) Structure of shoot meristem stem cells. CZ, central meristem zone; PZ, peripheral meristem zone; RZ, rib meristem zone; OC, organizer center. (B) Signaling pathways of shoot meristem stem cells. (C) Structure of root meristem stem cells. QC, quiescent center; CI, columella initial; ELRCI, epidermis or lateral root cap initial; CEI, cortex or endodermis initial; SI, stele initial. (D) Signaling pathways of root meristem stem cell.
The initiation of the SAM involves key regulatory factors, CLAVATA3 (CLV3) and WUSCHEL (WUS). The expression of the stem cell marker CLV3 can be detected during embryonic development in the SAM. WUS, a pivotal regulator of stem cell homeostasis, is initially expressed in a 16-cell embryonic OC, dynamically maintaining the stem cell niche size (Mayer et al., 1998; Jha et al., 2020; Hirakawa, 2021). Plant hormones, especially auxin and cytokinin, assume a crucial role in regulating stem apical meristem initiation. WOX2, a WUS homologous gene, participates in apical meristem initiation by regulating PIN1-dependent auxin transport during embryogenesis (Lee et al., 2013; Zhang et al., 2017; Xu et al., 2020; Gomes and Scortecci, 2021; Yamoune et al., 2021; Li et al., 2023). In addition, various key regulatory factors contribute to stem cell initiation, such as TOPLESS (TPL), ZWILLE (ZLL), SHOOTMERISTEMLESS (STM), and WOX2. TOPLESS (TPL) encodes a member of the Groucho/Tup1-type transcriptional corepressors. TPL functions as a co-repressor for EAR-domain-containing transcription factors (Long et al., 2006; Szemenyei et al., 2008). PLT genes are direct targets of TPL. The phenotype of tpl-1 in the shoot apex of mutant embryos is caused by ectopic expression of PLT genes (Smith and Long, 2010). Genetic interaction with tpl-1 also casts the homeodomain-leucine zipper III (HD-ZIP III) transcription factors as key regulators of shoot fates (Smith and Long, 2010). HD-ZIP III proteins function in promoting “adaxial” fates in lateral organs and “central” fates in the meristem (Engstrom et al., 2004). Domain-specific expression of HD-ZIP III and PLT transcription factors establish shoot and root fates at the poles of the globular embryo (Jeong et al., 2011). Intriguingly, the tpl-1 mutant phenotype manifests as the conversion of stems into roots, unveiling the pivotal role of the TPL protein. TPL engages in interactions with the auxin repressor IAA12/BDL, thereby mediating auxin-dependent transcriptional repression and influencing the initiation of shoot stem cells during embryogenesis (Long et al., 2006; Szemenyei et al., 2008). Another critical protagonist is the ZLL protein, whose expression is initially detected at the 2-cell and 8-cell embryonic stages. ZLL is one of the critical SAM regulators, which represses microRNA165/166 (miR165/166) for SAM maintenance. Levels of miR165/166 in the zll mutants are abnormally elevated, leading to a reduction in the quantity of HD-ZIP III gene transcripts, which is the target of miR165/166 (Liu et al., 2009). In zll mutants, elevated ARF2 transcription levels and intensified auxin responses result in the absence of the shoot meristem. Therefore, ZLL initiates stem cell formation by negatively regulating the auxin signaling pathway (Moussian et al., 1998; Lynn et al., 1999; Tucker et al., 2008; Roodbarkelari et al., 2015). The formation of shoot stem cells also hinges on the STM protein. STM is involved in a negative feedback regulatory pathway with CUP-SHAPED COTYLEDON (CUC) and plays a pivotal role in initiating shoot stem cells (Takada et al., 2001; Hibara et al., 2006). This intricate relationship involves CUC1 and CUC2 inducing STM expression (Aida et al., 1999). Concurrently, as STM protein levels rise, they indirectly repress CUC1 and CUC2 gene expressions by activating miR-164a (Spinelli et al., 2011). Furthermore, STM induces stem cell initiation in a cytokinin-dependent manner (Jasinski et al., 2005; Yanai et al., 2005). The WOX2 gene, in contrast, stimulates the up-regulation of the HD-ZIP III transcription factor, thereby regulating the expression of the cytokinin biosynthetic gene ISOPENTENYLTRANSFERASE1 (IPT1), which is pivotal in the initiation of shoot stem cells (Zhang et al., 2017). It’s worth noting that ongoing research endeavors continue to unveil new key regulatory genes involved in shoot stem cell initiation, indicating the complexity of these processes. Plant hormones, particularly auxin and cytokinin, also exert significant influence during this stage.
The maintenance of shoot stem cells is governed by the WUS/CLV negative feedback pathway. Research has shown that the WUS protein relocates from the OC to the stem cell niche, thereby activating the expression of CLV3 (Fletcher, 2018; Lopes et al., 2021), which in turn promotes stem cell production (Yadav et al., 2010; Yadav et al., 2011). Importantly, a dose-dependent relationship exists between the WUS protein levels and CLV3 activation (Perales et al., 2016). In Arabidopsis thaliana, CLV3 belongs to the CLV family, with CLV1 and CLV2 representing transmembrane protein kinases. A feedback regulatory pathway between WUS and CLV3 ensues, where an increase in WUS protein expression promotes CLV3 expression, consequently enhancing stem cell division. Conversely, escalating CLV3 protein secretion inhibits WUS expression, ensuring the maintenance of normal meristem development (Schoof et al., 2000; Song et al., 2010; Liu et al., 2013; Daum et al., 2014; Perales et al., 2016; Rodriguez et al., 2016; Su et al., 2020).
This negative feedback pathway also engages in interactions with factors in the cytokinin signaling pathway, particularly class B and class A Arabidopsis Response Regulator (ARR) transcription factors, collaboratively regulating the size of the SAM (Gordon et al., 2009; Hwang et al., 2012; Dai et al., 2017). Among these ARR proteins, class A ARRs are negative regulators of cytokinin, while class B ARRs are positive cytokinin regulators (Xie et al., 2018). The WUS protein, for instance, directly inhibits the expressions of several class A ARRs, such as ARR5, ARR6, ARR7, and ARR15 (Leibfried et al., 2005), consequently intensifying the cytokinin response in the SAM. In contrast, class B ARRs, including ARR1, ARR10, and ARR12, directly activate the WUS expression, contributing substantially to meristem homeostasis. Moreover, these ARRs directly repress the expression of YUCs, and indirectly promote WUS induction (Meng et al., 2017). This feedback regulation is critical for maintaining the SAM’s size and promoting overall stability (Chickarmane et al., 2012).
In addition to these feedback pathways, numerous other factors, frequently involving auxin or cytokinin signaling pathways, participate in the maintenance of the apical meristem. The classical auxin response model showed that intracellular auxin is transported by PIN family proteins in SAM and the auxin signal is sensed by auxin receptor TRANSPORT INHIBITOR RESPONSE1 (TIR1) (Vernoux et al., 2000; Kepinski and Leyser, 2005). Studies have illuminated that auxin can indirectly influence the WUS-CLV3 feedback loop by inhibiting the expression of ARR7/15 through MP/ARF5 (Zhao et al., 2010). The repressor ARF/ARF3, expressed in the peripheral SAM region, can directly impede the expression of the cytokinin synthesis gene ISOPENTENYLTRANSFERASE5 (AtIPT5), thereby modulating auxin and cytokinin distribution in specific SAM regions. This distribution pattern, featuring auxin predominantly in the peripheral region and cytokinin chiefly in the central region, fosters stem cell activity and ensures the stable growth of the SAM (Cheng et al., 2013). Notably, the pivotal auxin response factor, MP/ARF5, an activator in the ARF, has been identified as a negative regulator of CLV3 expression. This regulatory action operates through direct transcriptional inhibition of DORNROSCHEN/ENHANCER OF SHOOT REGENERATION 1 (DRN/ESR1) (Luo et al., 2018). In addition, the collaborative synergy between the HAIRY MERISTEM (HAM1/2), proteins with GRAS domain which function in dictating shoot stem cell initiation and proliferation, and WUS proteins assumes a crucial role in shaping the spatial expression profile of CLV3 in the stem cell region of the outer SAM layer (Zhou et al., 2018; Han et al., 2020a). In a specific context, the WUS protein initiates the activation of CLV3 exclusively within the CZ, where HAM1/2 proteins are absent. Conversely, HAM1/2 functions as gatekeepers, maintaining CLV3 expression at subdued levels within the RZ. This dual action effectively prevents the WUS-dependent activation of CLV3 and/or repressing CLV3 transcription (Zhou et al., 2018; Han et al., 2020b; Geng and Zhou, 2021). Moreover, the SPLAYED (SYD) protein, serving as an SNF2 chromatin remodeling ATPase, robustly stimulates WUS transcription. In the syd mutant, this vital transcriptional activation of WUS diminishes, resulting in reduced meristem size (Kwon et al., 2005). Collectively, these factors assume pivotal roles in the maintenance of shoot stem cell homeostasis, with many of their functions intricately entwined with the auxin signaling pathway.
STIMPY protein expression is induced by cytokinin in the Arabidopsis thaliana shoot meristem (Wu et al., 2005; Skylar et al., 2010). The deficiency of STIMPY, as evident in the stimpy mutant, leads to decreased expression of ARR5, culminating in a smaller SAM and pronounced cellular differentiation. Remarkably, this phenotypic manifestation bears a striking resemblance to that of the wus mutant phenotype, underscoring the collaborative influence of STIMPY within the cytokinin signaling pathway to maintain the activity of shoot stem cells. Additionally, pivotal genes such as KNOTTED1 in maize (Vollbrecht et al., 2000; Zinkgraf et al., 2017) and STM in Arabidopsis thaliana (Long et al., 1996) are indispensable for the steadfast development of the meristem, with their functions profoundly intertwined with the cytokinin signaling pathway (Vollbrecht et al., 2000). Furthermore, the mutant of the cytokinin synthesis gene LONELY GUY (LOG) exhibits phenotypic parallels to the wus mutant, further accentuating the profound impact of cytokinin on shoot stem cell activity (Chickarmane et al., 2012). Furthermore, recent research has unveiled the involvement of epigenetic modification in preserving stem cell fate in the SAM (Ma et al., 2019b). WUSCHEL acts via regulation of histone acetylation to control auxin signaling output in stem cells which allows cells to translate a potent and highly dynamic developmental signal into stable cell behavior (Ma et al., 2019b). Figure 1B provides a succinct visual representation of the signaling pathways governing plant shoot meristem stem cells.
Roots are indispensable for plants, as they facilitate water and nutrient absorption and promote plant development. In Arabidopsis thaliana, roots can be divided into three morphological parts: the meristematic zone (MZ), the transition zone (TZ), and the elongation zone (EZ). The meristematic zone can be subdivided into the distal meristem (DM) and the proximal meristem (PM). Within the MZ, we find the stem cell niche, comprising the quiescent center (QC) at its core, surrounded by various stem cell populations. A longitudinal section of the Arabidopsis thaliana root reveals that these surrounding stem cell populations consist of cortex or endodermis initials (CEIs), epidermis or lateral root cap initials (ELRCIs), columella initials (CIs), and stele initials (SI). These regions host complex regulatory networks to maintain QC stability and ensure normal apical meristem development (Lee et al., 2013). The formation of the apical QC and its surrounding stem cell population is essential for establishing the apical meristem (van den Berg et al., 1995; Sarkar et al., 2007) (Figure 1C).
As in the case of SAM, root apical meristem formation occurs during embryogenesis with the plant hormone auxin playing a crucial role in initiating the apical meristem (Lau et al., 2008; De Smet, 2010; Brumos et al., 2018; Gomes and Scortecci, 2021). The initiation of embryonic root stem cells is regulated by ARF5, which interacts with its auxin-labile inhibitor IAA12/BODENLOS (BDL) (Rademacher et al., 2012). Additionally, ARF5 promotes auxin transport from the embryo to the hypophysis precursor by positively regulating the expression of the auxin transporter PIN1, thus providing signals for initiating root apical stem cells (Benkova et al., 2003; Friml et al., 2004; Weijers et al., 2005; Weijers et al., 2006).
Furthermore, TMO5 and TMO7, the members of the bHLH transcription factor family, are regulated by ARF5 and function downstream of the ARF5-BDL auxin signaling pathway, controlling the initiation of root apical stem cells (Schlereth et al., 2010). The ARF5-BDL-mediated auxin signaling pathway also regulates the initiation of root stem cells through its downstream genes, such as OBERON1 (OBE1), OBE2, TITANIA1 (TTA1), TTA2, and NO transmit TRACT (NTT). Mutations in OBE1/2 and TAA1/2 lead to delayed root tip development or even root-less phenomenon, akin to the mp mutant phenotype (Saiga et al., 2008; Thomas et al., 2009; Saiga et al., 2012). Significantly, ARF5/MP can directly bind to NTT regulatory sequences, promoting its expression during root apical meristem initiation. NTT encodes a putative zinc-finger transcription factor, and its presence is essential for apical meristem formation (Crawford et al., 2015).
To maintain stem cells in the root apical meristem, ensuring the stable distribution of QC cells is essential. This stability and the normal development of roots hinge on the consistent arrangement of QC cells and surrounding stem cells in the root apical meristem. While other plant species often exhibit more complex root structures than Arabidopsis thaliana, the straightforward organization of Arabidopsis roots has made it an ideal model for plant stem cell research (Timilsina et al., 2019; Wein et al., 2020).
Laser ablation techniques provided insight into the pivotal role of QC in controlling stem cell activity (van den Berg et al., 1997). Following the ablation of QC cells, adjacent columella stem cells (CSCs) cease proliferation and differentiate into starch-containing columella cells. Simultaneously, cortex and endodermis initials (CEIs) neighboring the ablation differentiate into CEI daughter cells. Importantly, stem cell activity is maintained only when cells are in direct contact with QC. RNAi-mediated downregulation of RETINOBLASTOMARELATED (RBR) activity impedes the differentiation of stem cell progeny, resulting in several layers of undifferentiated cells adjacent to the QC. This experimental evidence highlights the pivotal role of QC-delivered stem cell-promoting signals, which are effective within a certain range of cell diameters and typically diminish in cells not in direct contact with the QC. Therefore, the QC-delivered stem cell-promoting signal represents the essential pathway for stem cell maintenance in Arabidopsis thaliana root tips (Dubrovsky and Ivanov, 2021; Matosevich and Efroni, 2021; Strotmann and Stahl, 2021; Zhai et al., 2023).
To ensure the normal growth of plants, it is imperative to uphold the unique characteristics of stem cells in the root apical meristem and SAM during the postembryonic growth phase (Yang et al., 2019; Yu et al., 2019). The WUSCHEL-RELATED HOMEOBOX5 (WOX5) gene, serving as a specific marker of the QC within the root apical meristem, plays a crucial role in regulating the equilibrium between cell division within the apical meristem and stem cell differentiation. WOX5 ensures the persistence of terminal stem cells (Sarkar et al., 2007), as evidenced by the abnormal phenotype observed in wox5 mutants, characterized by premature differentiation of columella cells. Conversely, the restoration of WOX5 expression results in delayed columella cell differentiation (Zhang et al., 2015; Zhai et al., 2020).
The maintenance of the QC necessitates the functioning of the PLT and SHR/SCR pathways. A dearth of gene expression within these two pathways can engender disruptions in QC cell distribution and the untimely cessation of root growth. The PLT gene encodes a transcription factor featuring the AP2 domain, fervently promoting cell division within the root meristem. The loss of PLT gene expression can lead to the loss of the radicle, the embryonic root. In concert, SHR and SCR, members of the GRAS transcription factor family, maintain a regulatory relationship, with SHR being the catalyst for SCR transcription. Subsequently, SCR perpetuates QC stability, independent of cell-cell interactions (Sablowski, 2007; Petricka et al., 2012). Additionally, regulating the nuclear localization of SHORTROOT (SHR) emerges as a pivotal contributor to the maintenance of the root meristem (Sabatini et al., 2003).
An intriguing observation pertains to the existence of a negative feedback regulatory pathway involving WOX5/ACR4/CLE40 in the root apical meristem. CLE40, a polypeptide akin to CLV3, inhibits the expression of WOX5 when excessively secreted by columella cells. This inhibition, in turn, mitigates stem cell proliferation and fosters cell differentiation within the root meristem, providing a critical regulatory mechanism (Stahl et al., 2009). Another significant contributor is Repressor of WUSCHEL1 (ROW1) protein. Its absence precipitates the ectopic expression of WOX5, ensuing disruption within the QC region and perturbing cell differentiation (Zhang et al., 2015). Moreover, WOX5 serves as a guardian of the root stem cell niche identity, accomplished through the repression of the differentiation factor CDF4 or the inhibition of CYCD1;1 and CYCD3;3 activity (Forzani et al., 2014; Pi et al., 2015).
Plant hormones, especially auxin and cytokinin, are intricately entwined in the preservation of stem cells in the root apical meristem (Artner and Benkova, 2019; Roychoudhry and Kepinski, 2022). Auxin regulates QC stability and meristem activity through three gene classes: PLT, ARF, and PID (Aida et al., 2004; Friml et al., 2004; Galinha et al., 2007). Among these, PLT1, PLT2, PLT3, and BBM genes are promoted by auxin concentration gradients mediated by the PIN protein. They are also positively regulated by auxin response factors, such as ARF5 and ARF7, contributing significantly to early radicle development and stem cell maintenance (Aida et al., 2004; Galinha et al., 2007). PLT gradient formation is associated with de novo organ development. The auxin–PLT network can act as a core module to regulate growth. Meanwhile, auxin-PLTs-ARRs molecular network controls the self-organized patterning of the root (Mahonen et al., 2014; Santuari et al., 2016; Salvi et al., 2020). Recent findings have revealed that auxin stimulates the expressions of tyrosylprotein sulfotransferase (TPST) and several ROOT GROWTH FACTOR (RGF) genes, thus maintaining the characteristics of root stem cells by inducing a gradient of PLT expression (Matsuzaki et al., 2010; Zhou et al., 2010). In contrast, cytokinin fosters cell differentiation, stifling root apical meristem division and expansion (Lee et al., 2013). Moreover, cytokinin has been found to mediate cell differentiation in the root meristem through the regulation of PIN5-mediated auxin intracellular transporter or via IAA-amino synthase GRETCHEN HAGEN 3.17 (GH3.17)-mediated auxin homeostasis (Di Mambro et al., 2019). At the same time, cytokinin can downregulate the expressions of PIN1 and PIN4 while positively upregulating the expression of PIN3 and PIN7, thereby orchestrating the size and activity of the root meristem (Ruzicka et al., 2009; Scacchi et al., 2010). Cytokinin activates the expressions of ARR1 and ARR2 proteins and subsequently induces the upregulation of IAA3, which, in turn, promotes cell differentiation in the root tip transition region (Dello Ioio et al., 2008; Moubayidin et al., 2010). Furthermore, cytokinin and auxin cooperate to maintain the size of the root meristem and ensure root growth through the intermediary action of the SHY2 protein, a repressor of auxin signaling which negatively regulates the PIN, that is activated by either ARR1 or ARR12 (Dello Ioio et al., 2008; Moubayidin et al., 2010). A comprehensive model elucidating the signaling pathways of plant root meristem stem cells is displayed in Figure 1D.
The preceding section provided an exhaustive examination of the architecture, initiation processes, and maintenance mechanisms of plant meristem stem cells. In this section, we delved into the kingdom of plant responses to abiotic stresses, with a particular emphasis on elucidating the intricate mechanisms governing how plants perceive and respond to these environmental challenges. Additionally, we explored the intriguing interconnections between the development of plant stem cells and their responses to various abiotic stressors (Zhu, 2016; Gong et al., 2020; Zhang et al., 2020a; Ubogoeva et al., 2021).
We emphasized the plant’s ability to perceive these stressors through specialized molecular pathways, including osmolarity, salinity, temperature, and drought. It is noteworthy that each form of abiotic stress engages unique molecular signaling pathways tailored to its specific attributes. To commence our exploration, we scrutinize the intricate process of how plants sense alterations in osmolarity. Both drought and salinity can induce high osmotic stress in plant cells. In Arabidopsis thaliana, the Reduced Hyperosmolality-Induced [Ca2+]i Increase1 (OSCA1) gene encoding the hyperosmolarity-gated calcium channel takes on the role of an osmotic pressure sensor. In osca1 mutants, intracellular Ca2+ influx is reduced, leaf transpiration is deficient, and plant root growth is inhibited under high osmotic pressure (Jojoa-Cruz et al., 2018; Liu et al., 2018b; Maity et al., 2019). Similarly, in Arabidopsis thaliana, the MSCS-LIKE 8 (MSL8) gene, encoding the membrane tension-gated ion channel, functions as a sensor of pollen membrane tension induced by low osmotic stress. Under low osmotic pressure, MSL8 expression is upregulated, facilitating ion outflow and safeguarding cells against hypoosmotic stress (Hamilton et al., 2015). These two genes assume pivotal roles in sensing osmotic pressure signals in plants.
Transitioning to the realm of salinity perception, when plants confront high-salt environments, they grapple not only with elevated osmotic stress but also with ion stress induced by Na+ ions. Typically, changes in Na+ signaling pathways coincide with Ca2+ signaling pathways. In Arabidopsis thaliana, the Monocation-Induced [Ca2+]i Increases1 (MOCA1) gene encodes a glucuronodyl transferase responsible for appending a negatively charged glucuronic acid (GlcA) group to inositol phosphorylated ceramide (IPC), resulting in the formation of glycosyl inositol phosphorylated ceramide (GIPC) sphingolipids that bind Na+ cations (Jiang et al., 2019). Research has revealed that MOCA1-dependent GIPC serves as a sensor for fluctuations in environmental Na+ levels, with this process entailing Ca2+ transporters. In Arabidopsis thaliana, two highly bona fide Ca2+ permeable transporters, ANNEXIN1 (AtANN1) and AtANN4, come into play (Laohavisit et al., 2013; Ma et al., 2019a). AtANN1 is indispensable for facilitating salt-activated Ca2+ inflow into the plasma membrane of root epidermal cells, while AtANN4, in conjunction with its interacting proteins Salt Overly Sensitive2 (SOS2) and SOS3-Like Calcium Binding Protein8 (SCaBP8) (also known as CBL10), regulates Ca2+ signaling changes induced by salt stress, facilitated through a phosphorylation-dependent negative feedback loop (Ma et al., 2019a). Salt stress not only perturbs the plasma membrane but also disrupts cell wall integrity through various mechanisms. In Arabidopsis thaliana, the LRX-RALF-FER module, including the Cell Wall Leucine-Rich Repeat Extensins3/4/5 (LRX3/4/5), Rapid Alkalinization Factor22/23 (RALF22/23), and Receptor-Like Kinase FERONIA (FER) proteins, emerges as a pivotal component in sensing salt stress and regulating salt tolerance (Feng et al., 2018; Zhao et al., 2018a).
Temperature variations, encompassing both heat and cold stress, can modulate the fluidity of the plasma membrane. Cold stress perception predominantly centers on Ca2+ channels in the plasma membrane. In rice, mutations in Cyclic Nucleotide-Gated Channel (CNGC) ion channel proteins, specifically OsCNGC9, OsCNGC14, and OsCNGC16, alter temperature stress-induced Ca2+ signaling and reduce tolerance to temperature stress (Ma et al., 2015; Cui et al., 2020; Liu et al., 2021; Wang et al., 2021). Notably, recent research has unveiled the Chilling tolerance divergence 1 (COLD1) gene as a potential low-temperature sensor. COLD1, in concert with Rice G-protein-α subunit 1 (RGA1), triggers a cold stress-induced increase in cytoplasmic Ca2+ levels (Ma et al., 2015). These findings underscore the critical role of Ca2+ signaling in temperature perception. Intriguingly, in Arabidopsis thaliana, diminished Ca2+ influx induced by cold stress is observed in atann1 mutants, resulting in reduced cold tolerance. Remarkably, AtANN1, involved in temperature perception, is also implicated in salinity perception in Arabidopsis thaliana, hinting at potential shared signaling pathways for temperature and salinity perception (Laohavisit et al., 2013). In addition, nuclear and cytoplasmic proteins, such as the histone variant H2A.Z and the photoreceptor phytochrome B (phyB), may contribute to temperature sensing (Kumar and Wigge, 2010; Jung et al., 2016; Legris et al., 2016). H2A.Z has been demonstrated to regulate gene transcription in a temperature-dependent manner (Kumar and Wigge, 2010), while phyB responds to changes in ambient temperature by altering its active state (Jung et al., 2016; Legris et al., 2016; Fujii et al., 2017). These findings suggest that photoreceptors like phyB may assume crucial roles in sensing extreme temperature fluctuations. In Arabidopsis thaliana, heat stress perception is mediated by the transcriptional suppressor Early Flowering 3 (ELF3). ELF3 is involved in regulating the expressions of numerous genes associated with growth and development. As temperatures rise, ELF3 gradually loses its inhibitory effect on downstream target genes, resulting in their activation (Jung et al., 2020). Notably, both heat and cold stress can affect various physiological and biochemical properties of plants. Among these, protein denaturation, a phenomenon unique to heat stress, triggers a specific response mediated by heat shock proteins (HSPs). These HSPs, typically inhibiting heat stress transcription factors (HSF) at normal temperatures, relinquish their hold on HSF as temperature rises, owing to the accumulation of denatured proteins. This event initiates the heat stress response (Scharf et al., 2012).
In summation, plants have exhibited a remarkable capacity to perceive abiotic stresses, such as osmotic stress, salinity stress, and temperature stress, through different signaling pathways. These perceptive processes span from ion channels at the plasma membrane, including Na+ and Ca2+ channels, to components at the cell surface, such as cell walls, and extend to intracellular compartments, encompassing the cytoplasm and nucleus. The profound hydrophilic nature of plants, manifested through their preference for habitats with high water potential, underscores their ability to sense soil moisture levels effectively. The timely activation of stress response mechanisms is essential for plant survival under drought conditions. Recent studies have indicated that Ca2+ signaling is also involved in the root hydrophilic response (Shkolnik et al., 2018). miz1 mutants, characterized by diminished hydrophilicity, exhibit weakened phloem Ca2+ signals. In contrast, mutations affecting the ER-localized type 2A Ca2+-ATPase (ECA1), which interacts with Mizu-Kussey 1 (MIZ1) and is inhibited by MIZ1, result in elevated Ca2+ levels and heightened hydrophilicity (Shkolnik et al., 2018). Therefore, MIZ1 and ECA1 emerge as key determinants influencing root hydrophilicity. However, the precise mechanisms underlying the perception of water potential and drought conditions warrant further experimental validation. As the root system senses water deficit in the soil, it can transmit this signal to aerial parts of the plant. While the exact mechanism governing this perception remains the subjects of ongoing investigation, a wealth of evidence implicates abscisic acid (ABA), H+ (pH), Ca2+, ROS, NO, lipids, small peptides, RNA molecules, and physical signals participating in this process. Of particular note, the small peptide CLAVATA3/endosperm surrounding region-related 25 (CLE25) has been observed to intensify in response to plant dehydration. Upon interaction with receptors Barely Any Meristem 1 (BAM1) and BAM3, CLE25 stimulates the expression of the Nine-Cis-Epoxycarotenoid Dioxygenase 3 (NCED3) gene, thereby promoting ABA biosynthesis and enhancing drought tolerance (Takahashi et al., 2018). While substantial progress has been made in comprehending some perception mechanisms, numerous facets of these processes merit further dedicated investigation. Figure 2 provides a concise visual representation of plant responses to abiotic stresses.
In the context of their sessile nature, plants confront environmental challenges by deploying intricate survival mechanisms. Central to these adaptive strategies are meristem cells, the linchpin of plant development governing roots, shoots, leaves, and flowers (Wang et al., 2020). However, meristem cells are acutely sensitive to abiotic stresses, including temperature fluctuations (both cold and heat), salinity, drought, ultraviolet radiation, and irradiation. These factors can inflict DNA damage and disrupt the normal division of stem cells, precipitating either stem cell mortality or aberrant plant development. Nevertheless, the plant kingdom has honed an assortment of mechanisms to ensure the persistence of stem cell activity, equipping them to survive amidst ever-changing environments. Presented below is a comprehensive elucidation of these mechanisms.
In the face of prolonged or severe stress conditions, apical stem cells manifest distinctive. Initially, the activity of root apical stem cells may dwindle or, in extreme cases, succumb to apoptosis. Concurrently, this milieu incites the division of QC cells. Etiological agents such as ultraviolet radiation, diverse forms of irradiation, and specific pharmaceutical agents, including bleomycin and zeocin, serve as instigators of DNA damage and consequent apoptotic responses in apical stem cells (Fulcher and Sablowski, 2009; Furukawa et al., 2010; Heyman et al., 2013; Canher et al., 2020). Perturbations in temperature, especially cold stress, may similarly usher in the demise of root stem cells. Nevertheless, in most cases, plants sacrifice selects columella stem cell progeny to ensure the survival of pivotal stem cells (Hong et al., 2017). Prolonged exposure to high-intensity radiation elicits QC cell division, with QC cells exhibiting greater resilience to DNA damage when juxtaposed with stem cells. QC cell demise typically materializes only under severe stress circumstances (Fulcher and Sablowski, 2009; Furukawa et al., 2010). Simultaneously, in instances of severe damage, such as the removal of the root cap (Ivanov et al., 2011; Bystrova et al., 2015), exposure to chilling stress (Hong et al., 2017), significant alterations in water osmotic potential (Mira et al., 2020), exposure to heavy metal ions (Kozhevnikova et al., 2007), and heat stress (Heyman et al., 2013; Heyman et al., 2016), the activation of QC cell division is observed, facilitating the restoration of root growth.
Importantly, the NAC family transcription factor SUPPRESSOR OF GAMMA RESPONSE1 (SOG1) assumes a central role in orchestrating plant stem cell responses to high-intensity UVB radiation, X-rays, and radiological agents (Fulcher and Sablowski, 2009; Furukawa et al., 2010; Ogita et al., 2018; Ryu et al., 2019). Root tip responses to radiation and aluminum stress also entail the cooperative engagement of two cell cycle checkpoint kinases, ATAXIA TELANGIECTASIA MUTATED (ATM) and ATM AND RAD3-RELATED (ATR) and aluminum stress (Rounds and Larsen, 2008; Sjogren et al., 2015).
Simultaneously, the transcription factor ETHYLENE RESPONSE FACTOR 115 (ERF115) assumes prominence, as it emerges as a lynchpin in facilitating plant recovery and regeneration amidst stressful conditions. Under standard conditions, ERF115 remains confined to QC cells, serving as a rate-determining regulator of QC division (Heyman et al., 2013). Upon exposure to stressors such as elevated temperatures or physical injury, ERF115 can express not only in QC cells, but also in adjoining areas of cell demise, and the inner cortex. This activation instigates a cascade effect, prompting stem cells to engage in restorative cell division (Heyman et al., 2013; Heyman et al., 2016; Zhou et al., 2019b; Canher et al., 2020). ERF109, a homologous gene of ERF115, also features prominently, as it experiences rapid induction following programmed cell death, thereby stimulating the expression of ERF115 in the proximity of dead cells and fostering the meristem regeneration (Heyman et al., 2016; Zhou et al., 2019b). Additionally, ERF115 forms heterodimers with PHYTOCHROME A SIGNAL TRANSDUCTION1 (PAT1), hereby expediting restorative cell division subsequent to root tip excision (Heyman et al., 2016).
The pivotal distribution of reactive oxygen species (ROS) profoundly influences the adaptative responses of stem cell niches to stressful conditions. ROS, metabolic by-products of aerobic metabolism, accumulate under stressful conditions, culminating in DNA damage, protein oxidation, and lipid peroxidation (Gill and Tuteja, 2010; Huang et al., 2019). Stressors, such as heat, cold, drought, heavy metals, and pathogens, rapidly perturb the redox homeostasis, resulting in pronounced ROS accumulation in plant tissues. This perturbation, in severe cases, may culminate in the senescence of plant organs (Lee et al., 2012; Kawarazaki et al., 2013; Kim and Hwang, 2014; Zhao et al., 2018b). A typical example is the flood-induced hypoxic response of apical stem cells. Under hypoxic conditions, maintaining low levels of ROS is essential for safeguarding apical meristem persistence (Sasidharan et al., 2018). In the apical meristem, both hypoxia-induced ROS and nitric oxide (NO) accumulation elicit QC cell division and meristem cell death (Mira et al., 2016). Prohibitin3 (PHB3), in this context, can restrict the spatial expression of ethylene response factor (ERF) transcription factors, ERF115, ERF114, and ERF109. These ERF factors, in turn, mediate ROS signaling in a PLT-independent manner to regulate the maintenance of stem cell niches and root growth through phytosulfokine (PSK) peptide hormones (Kong et al., 2018). Recent investigations have underscored the pivotal role of the Arabidopsis SYNTAXIN OF PLANTS81(AtSYP81) gene in root development, where it regulates peroxisome-mediated ROS production in the root tip. In atsyp81 mutants, a noticeable reduction in ROS levels is observed in the root tips, resulting in a significant decrease in apical meristem activity and the disruption of the apical stem cell niche’s identity. The supplementation of ROS donors in atsyp81 mutants effectively rectifies the impaired stem cell viability in the apical meristem. These experimental findings indicate the role of AtSYP81 in regulating root meristem activity and preserving the identity of the apical stem cell niche through the control of peroxisomes and peroxidase-mediated ROS homeostasis (Wang et al., 2023). In Arabidopsis thaliana, ROS signaling is critical for meristem development and the maintenance of stem cell identity (Ubogoeva et al., 2021). A variety of stressors can disrupt the balance of ROS in plants, leading to damage or even the senescence of plant organs. Consequently, the maintenance of a balanced ROS level assumes paramount importance for the survival of plant stem cells.
Soil salinity affects plant development, invariably diminishing crop yield. Recent scientific inquiries have shed light on the response to NaCl concentrations surpassing a specified threshold in the root tip of Arabidopsis thaliana. This incites the activation of local and systemic Ca2+ signaling pathways, which culminate in the engagement of the Salt Overly Sensitive (SOS) pathway. The SOS pathway is a conserved and important regulatory mechanism for Na+ exclusion and for alleviating long-distance transport of toxic Na+ (Shi et al., 2002; El Mahi et al., 2019). The latter comprises an ensemble of Ca2+ sensors, kinases, and Na+/H+ exchange modules that are ubiquitously distributed throughout the root. In concert with this, salt stress occasions a significant upregulation of GSO1 expression, especially in the endodermis and meristem (Chen et al., 2023). The upregulated GSO1 expression in the apical meristem, in particular, serves as a specialized conduit for intracellular Na+ detoxification via the GSO1-SOS2-SOS1 module. Activation of the SOS2-SOS1 module allows root growth by protecting the meristem to be maintained in adverse environments (Chen et al., 2023). This multifaceted receptor-like kinase GSO1-mediated mechanism is pivotal in preserving intracellular ion homeostasis and, by extension, normal root development, thereby enabling roots to sustain growth in adverse environments (Chen et al., 2023). Concurrently, it is imperative to recognize that the resilience and adaptability of plant apical meristem cells to salt stress are inextricably linked with microRNAs, the redox state, ROS, NO, and plant hormones such as auxin and cytokinin (Yang and Lee, 2023). Recent research has found that a prion-like domain (PrD) in the key shoot meristem regulator STM can stimulate it to form nuclear condensates which are required for maintaining the shoot meristem. The formation of STM condensates is enhanced upon salt stress, which allows enhanced salt tolerance and increased shoot branching (Cao et al., 2023). Salt-dependent reduction of miR165 and 166 causes a rapid increase in PHABULOSA (PHB) expression and production of the root meristem prodifferentiation hormone cytokinin (Scintu et al., 2023). A comprehensive metabolome and transcriptome analysis of three CK signal-deficient Arabidopsis ahp2,3,5 and arr1,10,12 mutants treated with salt stress showed that CK signaling induced reprogramming of gene-metabolic networks associated with Arabidopsis response to salinity (Abdelrahman et al., 2021). This intricate nexus highlights the correlation between salt stress response and signaling cascades that govern stem cell homeostasis in the plant meristem (Yang and Lee, 2023).
Temperature fluctuations exert profound control over plant development by modulating the behavior and attributes of stem cells. The programmed cell death of columella stem cell daughters (CSCDs) reestablishes the auxin maximum in the QC and preserves the functionality of the stem cell niche during chilling stress. This regulatory mechanism substantially enhances the survival prospects of plant roots when confronted with chilling stress. Furthermore, upon the restoration of normal ambient temperatures, the plant’s root system can recommence its growth phase (Hong et al., 2017).
In higher plants, the SAM perpetuates a division-centric agenda that culminates in the development of aerial plant organs. However, water assumes paramount importance in maintaining normal plant development, and drought stress can impede the proliferation of plant stem cells. In Arabidopsis thaliana, the STM gene emerges as a critical regulator in the SAM, enhancing plant tolerance to drought. In the shoot tips of Arabidopsis thaliana, the R2R3-type MYB96 transcription factor, inducible by ABA, plays a pivotal role in adapting to drought stress by regulating the transcriptional accumulation of STM. The overexpression of MYB96 substantially upregulates the expression of STM, thereby engendering enhanced drought tolerance. Conversely, myb96 mutants exhibit a marked reduction in STM expression, concomitant with diminished drought tolerance. These observations underscore the pivotal role played by the MYB96-STM axis in bolstering plant tolerance to drought stress (Lee et al., 2016).
The SAM in plants navigates a complex regulatory network that balances proliferation and differentiation processes. Cell differentiation in the developing shoot is intrinsically tied to the intracellular environment and the role of plastids. Arabidopsis thaliana mutants exhibiting chronic osmotic stress (msl2 msl3) manifest significantly enlarged and morphologically aberrant plastids in the SAM, concomitant with the formation of callus in large quantities at the shoot tips. This induction of callus formation is underpinned by a nexus of interconnected mechanisms involving cytokinin receptor AHK2, cytokinin signaling inhibitors ARR7 and ARR15, and the stem cell identity gene WUSCHEL. Furthermore, the process of callus induction precipitated by plastids under osmotic stress necessitates heightened levels of ROS and ABA biosynthesis in plastids. This intriguing interplay indicates the role of osmotic stress in activating plastid-mediated mechanisms that promote SAM development, implicating the cytokinin signaling pathway in this process (Wilson et al., 2016).
Plant hormones, especially auxin and cytokinin, wield substantial influence over the development of plant meristem cells. These phytohormones, in turn, assume varying roles in the context of stem cell responses to abiotic stresses. Auxin is essential for plants to respond to stress (Jing et al., 2023). Notably, with its maximum concentration dictating QC identity and preserving stem cell niche integrity, emerges as a critical factor in stress adaptation (Jiang and Feldman, 2005). For instance, TRYPTOPHAN AMINOTRANSFERASE OF ARABIDOPSIS 1 (TAA1) in Arabidopsis thaliana can promote auxin accumulation in the root tips under aluminum stress (Yang et al., 2014). TAA/TAR-mediated auxin biosynthesis emerges as indispensable in the root meristem’s response to ethylene stress (Brumos et al., 2018). Following root damage or removal in Arabidopsis thaliana, auxin biosynthesis in the root tips accelerates significantly, expediting the recovery of root growth (Matosevich et al., 2020). The molecular marker DR5, reflective of auxin activity in the root, exhibits decreased activity in the QC under low potassium (K+) conditions (Zhang et al., 2020b). Auxin plays an important role in a “sacrifice-for-survival” mechanism in which cold-damaged CSCs are sacrificed in order for the root growth. Low-temperature stress leads to decreased DR5 activity in the QC, which in turn precipitates CSC cleavage (Hong et al., 2017). In addition, auxin has demonstrated a protective role, safeguarding stem cells from Zeocin-induced cell death (Hong et al., 2017). The homeodomain TF gene, HB33 was identified as a positive regulator of ABA response which was repressed by auxin response factor 2 (ARF2). The arf2 mutant showed enhanced ABA sensitivity (Wang et al., 2011). ARF2-HB33 module may link the ABA and auxin signaling pathways to response the drought stress (Wang et al., 2011). Overexpression of auxin biosynthesis genes leads to increased salt tolerance (Dunlap and Binzel, 1996; Kim et al., 2013; Ke et al., 2015). The expression of auxin biosynthesis gene YUC4 is significantly increased in response to NaCl treatment in Arabidopsis (Cackett et al., 2022). The tir1/afb2/afb3 mutants, which was defective in multiple TIR/Auxin-Signaling F-box (AFB) receptors, display hypersensitivity to NaCl treatment in the RAM. The slowing of root growth caused by salt stress might be an adaptive mechanism for plants surviving (Iglesias et al., 2010; Yu et al., 2020).
In addition to auxin, the plant stem cell niche is profoundly influenced by other phytohormones, such as cytokinin, ethylene, jasmonic acid (JA), and brassinosteroid (BR). Recent investigations have revealed cytokinin’s integral role in fortifying plant responses to temperature stress, drought stress, and salt stress (Li et al., 2021b). This phytohormone’s signaling pathway encompasses CYTOKININ RESPONSE FACTOR (CRF), ARABIDOPSIS HISTIDINE CONTAINING PHOSPHOTRANSMITTER (AHP), cytokinin receptor (AHK), and ARABIDOPSIS RESPONSE REGULATOR (ARR) proteins, collectively steering plants towards tolerance and adaptability of plants under these adverse conditions (Jeon et al., 2010; Mason et al., 2010; Kang et al., 2012; Chen and Yang, 2013; Jeon and Kim, 2013; Nishiyama et al., 2013; Jeon et al., 2016; Joshi et al., 2016; Nguyen et al., 2016; Cortleven et al., 2019; Song et al., 2019; Zhou et al., 2019a; Hyoung et al., 2020; Li et al., 2021a; Li et al., 2021b). Similarly, ethylene accumulation in the root tips in response to soil compaction or flooding underscores ethylene’s pivotal role in aiding meristem adaptation to stress (Hartman et al., 2019; Pandey et al., 2021). Ethylene signaling, in this context, is integral to the scavenging of the plant globin1 (PGB1), a process essential for meristem adaptation to flood-induced hypoxia (Hartman et al., 2019). Recent studies have shown that JA plays a key role in stem cell niche regeneration (Zhou et al., 2019b). In instances where the root tip is cut off or infected by pathogens, JA and auxin synergistically activate the SCR-SHR-RBR pathway, facilitating restorative cell division and the resumption of root tip growth.
Furthermore, BR recruitment of the BRI1-EMS-SUPPRESSOR 1 (BES1) -BRASSINOSTEROIDS AT VASCULAR AND ORGANIZING CENTER (BRAVO) -ERF115 signaling module assumes a critical role in governing QC cell division and sustaining the characteristics of the apical meristem (Vilarrasa-Blasi et al., 2014). The dynamic involvement of BR signaling in plant responses to temperature, salt, and drought stresses further underscores its multifaceted influence on plant development (Planas-Riverola et al., 2019). The intricate web of responses orchestrated by plant stem cells in the face of abiotic stresses is displayed in Figure 3.
Figure 3 Model of the stem cell niche response to abiotic stress. ROS, reactive oxygen species; CK, cytokinin; ABA, abscisic acid; Eth, ethylene; JA, jasmonic acid; BR, brassinosteroid.
The initiation and maintenance of plant meristem cells represent vital processes governing plant growth and development. Advancements in technologies such as single-cell sequencing have yielded an expanding body of experimental evidence supporting the crucial role of plant stem cells, extending their implications to plant regeneration and crop yield improvement (Liu et al., 2023). These developments have contributed to a more comprehensive grasp of the initiation, maintenance, signal transduction, and other processes of plant stem cells. In the backdrop of ongoing global environmental shifts, characterized by phenomena such as drought stress, temperature fluctuations, salt stress, and exposure to heavy metal ions, plants have undergone intricate adaptations in regulatory mechanisms to safeguard their reproductive integrity. As the core components of plant development, plant stem cells play a pivotal role in enabling plants to bolster their resilience against environmental stresses. This review has furnished a comprehensive exploration of the regulatory mechanisms governing the initiation and maintenance of plant stem cells, including the root apical meristem and the shoot apical meristem. Additionally, we have summarized the response mechanisms that plants employ to counter abiotic stresses, with a particular emphasis on the processes of perception in these stresses. Furthermore, we have outlined recent insights into the mechanisms by which plant stem cells fortify tolerance and adaptability under challenging conditions. However, it is imperative to acknowledge that the study of plant responses to abiotic stresses remains an ongoing pursuit, rife with numerous outstanding questions. These inquiries span areas such as the identification of receptors that sense stress signals, the unraveling of the mechanism governing apical meristem of plant responses to stress, and the exploration of unresolved aspects of abiotic stresses that have surfaced in recent literature (Verslues et al., 2023). Solutions to these important scientific questions will undoubtedly proceed in tandem with the relentless advancement of scientific knowledge.
HKZ: Writing – original draft, Writing – review & editing. YM: Writing – review & editing. HZ: Writing – original draft, Writing – review & editing. CY: Writing – original draft, Writing – review & editing.
The author(s) declare financial support was received for the research, authorship, and/or publication of this article. The work was supported by the National Natural Science Foundation of China (32000147).
The authors declare that the research was conducted in the absence of any commercial or financial relationships that could be construed as a potential conflict of interest.
All claims expressed in this article are solely those of the authors and do not necessarily represent those of their affiliated organizations, or those of the publisher, the editors and the reviewers. Any product that may be evaluated in this article, or claim that may be made by its manufacturer, is not guaranteed or endorsed by the publisher.
Abdelrahman, M., Nishiyama, R., Tran, C. D., Kusano, M., Nakabayashi, R., Okazaki, Y., et al. (2021). Defective cytokinin signaling reprograms lipid and flavonoid gene-to-metabolite networks to mitigate high salinity in Arabidopsis. Proc. Natl. Acad. Sci. U.S.A. 118 (48). doi: 10.1073/pnas.2105021118
Aichinger, E., Kornet, N., Friedrich, T., Laux, T. (2012). Plant stem cell niches. Annu. Rev. Plant Biol. 63, 615–636. doi: 10.1146/annurev-arplant-042811-105555
Aida, M., Beis, D., Heidstra, R., Willemsen, V., Blilou, I., Galinha, C., et al. (2004). The PLETHORA genes mediate patterning of the Arabidopsis root stem cell niche. Cell 119 (1), 109–120. doi: 10.1016/j.cell.2004.09.018
Aida, M., Ishida, T., Tasaka, M. (1999). Shoot apical meristem and cotyledon formation during Arabidopsis embryogenesis: interaction among the CUP-SHAPED COTYLEDON and SHOOT MERISTEMLESS genes. Development 126 (8), 1563–1570. doi: 10.1242/dev.126.8.1563
Artner, C., Benkova, E. (2019). Ethylene and Cytokinin: Partners in root growth regulation. Mol. Plant 12 (10), 1312–1314. doi: 10.1016/j.molp.2019.09.003
Benkova, E., Michniewicz, M., Sauer, M., Teichmann, T., Seifertova, D., Jurgens, G., et al. (2003). Local, efflux-dependent auxin gradients as a common module for plant organ formation. Cell 115 (5), 591–602. doi: 10.1016/s0092-8674(03)00924-3
Brumos, J., Robles, L. M., Yun, J., Vu, T. C., Jackson, S., Alonso, J. M., et al. (2018). Local auxin biosynthesis is a key regulator of plant development. Dev. Cell 47 (3), 306–318 e305. doi: 10.1016/j.devcel.2018.09.022
Bystrova, E. I., Zhukovskaya, N. V., Rakitin, V. J., Ivanov, V. B. (2015). The role of ethylene in activation division cell quiescent center in the cut maize roots. Ontogenez 46 (2), 82–86.
Cackett, L., Cannistraci, C. V., Meier, S., Ferrandi, P., Pencik, A., Gehring, C., et al. (2022). Salt-specific gene expression reveals elevated auxin levels in Arabidopsis thaliana plants grown under saline conditions. Front. Plant Sci. 13. doi: 10.3389/fpls.2022.804716
Canher, B., Heyman, J., Savina, M., Devendran, A., Eekhout, T., Vercauteren, I., et al. (2020). Rocks in the auxin stream: Wound-induced auxin accumulation and ERF115 expression synergistically drive stem cell regeneration. Proc. Natl. Acad. Sci. U.S.A. 117 (28), 16667–16677. doi: 10.1073/pnas.2006620117
Cao, X., Du, Q., Guo, Y., Wang, Y., Jiao, Y. (2023). Condensation of STM is critical for shoot meristem maintenance and salt tolerance in Arabidopsis. Mol. Plant 16 (9), 1445–1459. doi: 10.1016/j.molp.2023.09.005
Charng, Y. Y., Mitra, S., Yu, S. J. (2023). Maintenance of abiotic stress memory in plants: Lessons learned from heat acclimation. Plant Cell 35 (1), 187–200. doi: 10.1093/plcell/koac313
Chen, B., Yang, H. (2013). 6-Benzylaminopurine alleviates chilling injury of postharvest cucumber fruit through modulating antioxidant system and energy status. J. Sci. Food Agric. 93 (8), 1915–1921. doi: 10.1002/jsfa.5990
Chen, C., He, G., Li, J., Perez-Hormaeche, J., Becker, T., Luo, M., et al. (2023). A salt stress-activated GSO1-SOS2-SOS1 module protects the Arabidopsis root stem cell niche by enhancing sodium ion extrusion. EMBO J. 42 (13), e113004. doi: 10.15252/embj.2022113004
Cheng, Z. J., Wang, L., Sun, W., Zhang, Y., Zhou, C., Su, Y. H., et al. (2013). Pattern of auxin and cytokinin responses for shoot meristem induction results from the regulation of cytokinin biosynthesis by AUXIN RESPONSE FACTOR3. Plant Physiol. 161 (1), 240–251. doi: 10.1104/pp.112.203166
Chickarmane, V. S., Gordon, S. P., Tarr, P. T., Heisler, M. G., Meyerowitz, E. M. (2012). Cytokinin signaling as a positional cue for patterning the apical-basal axis of the growing Arabidopsis shoot meristem. Proc. Natl. Acad. Sci. U.S.A. 109 (10), 4002–4007. doi: 10.1073/pnas.1200636109
Cortleven, A., Leuendorf, J. E., Frank, M., Pezzetta, D., Bolt, S., Schmulling, T. (2019). Cytokinin action in response to abiotic and biotic stresses in plants. Plant Cell Environ. 42 (3), 998–1018. doi: 10.1111/pce.13494
Crawford, B. C., Sewell, J., Golembeski, G., Roshan, C., Long, J. A., Yanofsky, M. F. (2015). Plant development. Genetic control of distal stem cell fate within root and embryonic meristems. Science 347 (6222), 655–659. doi: 10.1126/science.aaa0196
Cui, Y., Lu, S., Li, Z., Cheng, J., Hu, P., Zhu, T., et al. (2020). CYCLIC NUCLEOTIDE-GATED ION CHANNELs 14 and 16 promote tolerance to heat and chilling in rice. Plant Physiol. 183 (4), 1794–1808. doi: 10.1104/pp.20.00591
Dai, X., Liu, Z., Qiao, M., Li, J., Li, S., Xiang, F. (2017). ARR12 promotes de novo shoot regeneration in Arabidopsis thaliana via activation of WUSCHEL expression. J. Integr. Plant Biol. 59 (10), 747–758. doi: 10.1111/jipb.12567
Daum, G., Medzihradszky, A., Suzaki, T., Lohmann, J. U. (2014). A mechanistic framework for noncell autonomous stem cell induction in Arabidopsis. Proc. Natl. Acad. Sci. U.S.A. 111 (40), 14619–14624. doi: 10.1073/pnas.1406446111
Dello Ioio, R., Nakamura, K., Moubayidin, L., Perilli, S., Taniguchi, M., Morita, M. T., et al. (2008). A genetic framework for the control of cell division and differentiation in the root meristem. Science 322 (5906), 1380–1384. doi: 10.1126/science.1164147
De Smet, I. (2010). Multimodular auxin response controls lateral root development in Arabidopsis. Plant Signal. Behav. 5 (5), 580–582. doi: 10.4161/psb.11495
Di Mambro, R., Svolacchia, N., Dello Ioio, R., Pierdonati, E., Salvi, E., Pedrazzini, E., et al. (2019). The lateral root cap acts as an auxin sink that controls meristem size. Curr. Biol. 29 (7), 1199–1205 e1194. doi: 10.1016/j.cub.2019.02.022
Dubrovsky, J. G., Ivanov, V. B. (2021). The quiescent centre of the root apical meristem: conceptual developments from Clowes to modern times. J. Exp. Bot. 72 (19), 6687–6707. doi: 10.1093/jxb/erab305
Dunlap, J. R., Binzel, M. L. (1996). NaCI reduces Indole-3-Acetic Acid levels in the roots of tomato plants independent of Stress-Induced Abscisic Acid. Plant Physiol. 112 (1), 379–384. doi: 10.1104/pp.112.1.379
El Mahi, H., Perez-Hormaeche, J., De Luca, A., Villalta, I., Espartero, J., Gamez-Arjona, F., et al. (2019). A critical role of sodium flux via the plasma membrane Na+/H+ exchanger SOS1 in the salt tolerance of Rice. Plant Physiol. 180 (2), 1046–1065. doi: 10.1104/pp.19.00324
Engstrom, E. M., Izhaki, A., Bowman, J. L. (2004). Promoter bashing, microRNAs, and Knox genes. New insights, regulators, and targets-of-regulation in the establishment of lateral organ polarity in Arabidopsis. Plant Physiol. 135 (2), 685–694. doi: 10.1104/pp.104.040394
Feng, W., Kita, D., Peaucelle, A., Cartwright, H. N., Doan, V., Duan, Q., et al. (2018). The FERONIA receptor kinase maintains cell-wall integrity during salt stress through Ca2+ Signaling. Curr. Biol. 28 (5), 666–675 e665. doi: 10.1016/j.cub.2018.01.023
Fletcher, J. C. (2018). The CLV-WUS stem cell signaling pathway: A roadmap to crop yield optimization. Plants (Basel) 7 (4). doi: 10.3390/plants7040087
Forzani, C., Aichinger, E., Sornay, E., Willemsen, V., Laux, T., Dewitte, W., et al. (2014). WOX5 suppresses CYCLIN D activity to establish quiescence at the center of the root stem cell niche. Curr. Biol. 24 (16), 1939–1944. doi: 10.1016/j.cub.2014.07.019
Friml, J., Yang, X., Michniewicz, M., Weijers, D., Quint, A., Tietz, O., et al. (2004). A PINOID-dependent binary switch in apical-basal PIN polar targeting directs auxin efflux. Science 306 (5697), 862–865. doi: 10.1126/science.1100618
Fujii, Y., Tanaka, H., Konno, N., Ogasawara, Y., Hamashima, N., Tamura, S., et al. (2017). Phototropin perceives temperature based on the lifetime of its photoactivated state. Proc. Natl. Acad. Sci. U.S.A. 114 (34), 9206–9211. doi: 10.1073/pnas.1704462114
Fujinami, R., Yamada, T., Imaichi, R. (2020). Root apical meristem diversity and the origin of roots: insights from extant lycophytes. J. Plant Res. 133 (3), 291–296. doi: 10.1007/s10265-020-01167-2
Fulcher, N., Sablowski, R. (2009). Hypersensitivity to DNA damage in plant stem cell niches. Proc. Natl. Acad. Sci. U.S.A. 106 (49), 20984–20988. doi: 10.1073/pnas.0909218106
Furukawa, T., Curtis, M. J., Tominey, C. M., Duong, Y. H., Wilcox, B. W., Aggoune, D., et al. (2010). A shared DNA-damage-response pathway for induction of stem-cell death by UVB and by gamma irradiation. DNA Repair (Amst) 9 (9), 940–948. doi: 10.1016/j.dnarep.2010.06.006
Galinha, C., Hofhuis, H., Luijten, M., Willemsen, V., Blilou, I., Heidstra, R., et al. (2007). PLETHORA proteins as dose-dependent master regulators of Arabidopsis root development. Nature 449 (7165), 1053–1057. doi: 10.1038/nature06206
Geng, Y., Zhou, Y. (2021). HAM gene family and shoot meristem development. Front. Plant Sci. 12. doi: 10.3389/fpls.2021.800332
Gill, S. S., Tuteja, N. (2010). Reactive oxygen species and antioxidant machinery in abiotic stress tolerance in crop plants. Plant Physiol. Biochem. 48 (12), 909–930. doi: 10.1016/j.plaphy.2010.08.016
Gomes, G. L. B., Scortecci, K. C. (2021). Auxin and its role in plant development: structure, signalling, regulation and response mechanisms. Plant Biol. (Stuttg) 23 (6), 894–904. doi: 10.1111/plb.13303
Gong, Z., Xiong, L., Shi, H., Yang, S., Herrera-Estrella, L. R., Xu, G., et al. (2020). Plant abiotic stress response and nutrient use efficiency. Sci. China Life Sci. 63 (5), 635–674. doi: 10.1007/s11427-020-1683-x
Gordon, S. P., Chickarmane, V. S., Ohno, C., Meyerowitz, E. M. (2009). Multiple feedback loops through cytokinin signaling control stem cell number within the Arabidopsis shoot meristem. Proc. Natl. Acad. Sci. U.S.A. 106 (38), 16529–16534. doi: 10.1073/pnas.0908122106
Gross-Hardt, R., Laux, T. (2003). Stem cell regulation in the shoot meristem. J. Cell Sci. 116 (Pt 9), 1659–1666. doi: 10.1242/jcs.00406
Hamilton, E. S., Jensen, G. S., Maksaev, G., Katims, A., Sherp, A. M., Haswell, E. S. (2015). Mechanosensitive channel MSL8 regulates osmotic forces during pollen hydration and germination. Science 350 (6259), 438–441. doi: 10.1126/science.aac6014
Han, H., Geng, Y., Guo, L., Yan, A., Meyerowitz, E. M., Liu, X., et al. (2020a). The overlapping and distinct roles of HAM family genes in Arabidopsis shoot meristems. Front. Plant Sci. 11. doi: 10.3389/fpls.2020.541968
Han, H., Liu, X., Zhou, Y. (2020b). Transcriptional circuits in control of shoot stem cell homeostasis. Curr. Opin. Plant Biol. 53, 50–56. doi: 10.1016/j.pbi.2019.10.004
Hartman, S., Liu, Z., van Veen, H., Vicente, J., Reinen, E., Martopawiro, S., et al. (2019). Ethylene-mediated nitric oxide depletion pre-adapts plants to hypoxia stress. Nat. Commun. 10 (1), 4020. doi: 10.1038/s41467-019-12045-4
Hata, Y., Kyozuka, J. (2021). Fundamental mechanisms of the stem cell regulation in land plants: lesson from shoot apical cells in bryophytes. Plant Mol. Biol. 107 (4-5), 213–225. doi: 10.1007/s11103-021-01126-y
Heyman, J., Cools, T., Canher, B., Shavialenka, S., Traas, J., Vercauteren, I., et al. (2016). The heterodimeric transcription factor complex ERF115-PAT1 grants regeneration competence. Nat. Plants 2 (11), 16165. doi: 10.1038/nplants.2016.165
Heyman, J., Cools, T., Vandenbussche, F., Heyndrickx, K. S., Van Leene, J., Vercauteren, I., et al. (2013). ERF115 controls root quiescent center cell division and stem cell replenishment. Science 342 (6160), 860–863. doi: 10.1126/science.1240667
Hibara, K., Karim, M. R., Takada, S., Taoka, K., Furutani, M., Aida, M., et al. (2006). Arabidopsis CUP-SHAPED COTYLEDON3 regulates postembryonic shoot meristem and organ boundary formation. Plant Cell 18 (11), 2946–2957. doi: 10.1105/tpc.106.045716
Hirakawa, Y. (2021). CLAVATA3, a plant peptide controlling stem cell fate in the meristem. Peptides 142, 170579. doi: 10.1016/j.peptides.2021.170579
Hong, J. H., Savina, M., Du, J., Devendran, A., Kannivadi Ramakanth, K., Tian, X., et al. (2017). A sacrifice-for-survival mechanism protects root stem cell niche from chilling stress. Cell 170 (1), 102–113 e114. doi: 10.1016/j.cell.2017.06.002
Huang, H., Ullah, F., Zhou, D. X., Yi, M., Zhao, Y. (2019). Mechanisms of ROS regulation of plant development and stress responses. Front. Plant Sci. 10. doi: 10.3389/fpls.2019.00800
Hwang, I., Sheen, J., Muller, B. (2012). Cytokinin signaling networks. Annu. Rev. Plant Biol. 63, 353–380. doi: 10.1146/annurev-arplant-042811-105503
Hyoung, S., Cho, S. H., Chung, J. H., So, W. M., Cui, M. H., Shin, J. S. (2020). Cytokinin oxidase PpCKX1 plays regulatory roles in development and enhances dehydration and salt tolerance in Physcomitrella patens. Plant Cell Rep. 39 (3), 419–430. doi: 10.1007/s00299-019-02500-3
Iglesias, M. J., Terrile, M. C., Bartoli, C. G., D’Ippolito, S., Casalongue, C. A. (2010). Auxin signaling participates in the adaptative response against oxidative stress and salinity by interacting with redox metabolism in Arabidopsis. Plant Mol. Biol. 74 (3), 215–222. doi: 10.1007/s11103-010-9667-7
Ivanov, V. B., Bystrova, E. I., Mesenko, M. M., Kotova, L. M., Kotov, A. A. (2011). Activation of cell division in the quiescent center of excised maize root tip. Ontogenez 42 (5), 357–362. doi: 10.1134/S1062360411050067
Janocha, D., Lohmann, J. U. (2018). From signals to stem cells and back again. Curr. Opin. Plant Biol. 45 (Pt A), 136–142. doi: 10.1016/j.pbi.2018.06.005
Jasinski, S., Piazza, P., Craft, J., Hay, A., Woolley, L., Rieu, I., et al. (2005). KNOX action in Arabidopsis is mediated by coordinate regulation of cytokinin and gibberellin activities. Curr. Biol. 15 (17), 1560–1565. doi: 10.1016/j.cub.2005.07.023
Jeon, J., Cho, C., Lee, M. R., Van Binh, N., Kim, J. (2016). CYTOKININ RESPONSE FACTOR2 (CRF2) and CRF3 regulate lateral root development in response to cold stress in Arabidopsis. Plant Cell 28 (8), 1828–1843. doi: 10.1105/tpc.15.00909
Jeon, J., Kim, J. (2013). Arabidopsis response Regulator1 and Arabidopsis histidine phosphotransfer Protein2 (AHP2), AHP3, and AHP5 function in cold signaling. Plant Physiol. 161 (1), 408–424. doi: 10.1104/pp.112.207621
Jeon, J., Kim, N. Y., Kim, S., Kang, N. Y., Novak, O., Ku, S. J., et al. (2010). A subset of cytokinin two-component signaling system plays a role in cold temperature stress response in Arabidopsis. J. Biol. Chem. 285 (30), 23371–23386. doi: 10.1074/jbc.M109.096644
Jeong, S., Bayer, M., Lukowitz, W. (2011). Taking the very first steps: from polarity to axial domains in the early Arabidopsis embryo. J. Exp. Bot. 62 (5), 1687–1697. doi: 10.1093/jxb/erq398
Jha, P., Ochatt, S. J., Kumar, V. (2020). WUSCHEL: a master regulator in plant growth signaling. Plant Cell Rep. 39 (4), 431–444. doi: 10.1007/s00299-020-02511-5
Jiang, K., Feldman, L. J. (2005). Regulation of root apical meristem development. Annu. Rev. Cell Dev. Biol. 21, 485–509. doi: 10.1146/annurev.cellbio.21.122303.114753
Jiang, Z., Zhou, X., Tao, M., Yuan, F., Liu, L., Wu, F., et al. (2019). Plant cell-surface GIPC sphingolipids sense salt to trigger Ca2+ influx. Nature 572 (7769), 341–346. doi: 10.1038/s41586-019-1449-z
Jing, H., Wilkinson, E. G., Sageman-Furnas, K., Strader, L. C. (2023). Auxin and abiotic stress responses. J. Exp. Bot. doi: 10.1093/jxb/erad325
Jojoa-Cruz, S., Saotome, K., Murthy, S. E., Tsui, C. C. A., Sansom, M. S., Patapoutian, A., et al. (2018). Cryo-EM structure of the mechanically activated ion channel OSCA1.2. Elife 7. doi: 10.7554/eLife.41845
Joshi, R., Wani, S. H., Singh, B., Bohra, A., Dar, Z. A., Lone, A. A., et al. (2016). Transcription factors and plants response to drought stress: Current understanding and future directions. Front. Plant Sci. 7. doi: 10.3389/fpls.2016.01029
Jung, J. H., Barbosa, A. D., Hutin, S., Kumita, J. R., Gao, M., Derwort, D., et al. (2020). A prion-like domain in ELF3 functions as a thermosensor in Arabidopsis. Nature 585 (7824), 256–260. doi: 10.1038/s41586-020-2644-7
Jung, J. H., Domijan, M., Klose, C., Biswas, S., Ezer, D., Gao, M., et al. (2016). Phytochromes function as thermosensors in Arabidopsis. Science 354 (6314), 886–889. doi: 10.1126/science.aaf6005
Jurgens, G., Torres Ruiz, R. A., Berleth, T. (1994). Embryonic pattern formation in flowering plants. Annu. Rev. Genet. 28, 351–371. doi: 10.1146/annurev.ge.28.120194.002031
Kang, N. Y., Cho, C., Kim, N. Y., Kim, J. (2012). Cytokinin receptor-dependent and receptor-independent pathways in the dehydration response of Arabidopsis thaliana. J. Plant Physiol. 169 (14), 1382–1391. doi: 10.1016/j.jplph.2012.05.007
Kawarazaki, T., Kimura, S., Iizuka, A., Hanamata, S., Nibori, H., Michikawa, M., et al. (2013). A low temperature-inducible protein AtSRC2 enhances the ROS-producing activity of NADPH oxidase AtRbohF. Biochim. Biophys. Acta 1833 (12), 2775–2780. doi: 10.1016/j.bbamcr.2013.06.024
Ke, Q., Wang, Z., Ji, C. Y., Jeong, J. C., Lee, H. S., Li, H., et al. (2015). Transgenic poplar expressing Arabidopsis YUCCA6 exhibits auxin-overproduction phenotypes and increased tolerance to abiotic stress. Plant Physiol. Biochem. 94, 19–27. doi: 10.1016/j.plaphy.2015.05.003
Kepinski, S., Leyser, O. (2005). The Arabidopsis F-box protein TIR1 is an auxin receptor. Nature 435 (7041), 446–451. doi: 10.1038/nature03542
Kerstetter, R. A., Hake, S. (1997). Shoot meristem formation in vegetative development. Plant Cell 9 (7), 1001–1010. doi: 10.1105/tpc.9.7.1001
Kim, J. I., Baek, D., Park, H. C., Chun, H. J., Oh, D. H., Lee, M. K., et al. (2013). Overexpression of Arabidopsis YUCCA6 in potato results in high-auxin developmental phenotypes and enhanced resistance to water deficit. Mol. Plant 6 (2), 337–349. doi: 10.1093/mp/sss100
Kim, D. S., Hwang, B. K. (2014). An important role of the pepper phenylalanine ammonia-lyase gene (PAL1) in salicylic acid-dependent signalling of the defence response to microbial pathogens. J. Exp. Bot. 65 (9), 2295–2306. doi: 10.1093/jxb/eru109
Kong, X., Tian, H., Yu, Q., Zhang, F., Wang, R., Gao, S., et al. (2018). PHB3 maintains root stem cell niche identity through ROS-responsive AP2/ERF transcription factors in Arabidopsis. Cell Rep. 22 (5), 1350–1363. doi: 10.1016/j.celrep.2017.12.105
Kopecka, R., Kameniarova, M., Cerny, M., Brzobohaty, B., Novak, J. (2023). Abiotic stress in crop production. Int. J. Mol. Sci. 24 (7). doi: 10.3390/ijms24076603
Kozhevnikova, A. D., Seregin, I. V., Bystrova, E. I., Ivanov, V. B. (2007). Effects of heavy metals and strontium on division of root cap cells and meristem structural organization. Russian J. Plant Physiol. 54 (2), 257–266. doi: 10.1134/s1021443707020148
Kumar, S. V., Wigge, P. A. (2010). H2A.Z-containing nucleosomes mediate the thermosensory response in Arabidopsis. Cell 140 (1), 136–147. doi: 10.1016/j.cell.2009.11.006
Kwon, C. S., Chen, C., Wagner, D. (2005). WUSCHEL is a primary target for transcriptional regulation by SPLAYED in dynamic control of stem cell fate in Arabidopsis. Genes Dev. 19 (8), 992–1003. doi: 10.1101/gad.1276305
Lamers, J., van der Meer, T., Testerink, C. (2020). How plants sense and respond to stressful environments. Plant Physiol. 182 (4), 1624–1635. doi: 10.1104/pp.19.01464
Laohavisit, A., Richards, S. L., Shabala, L., Chen, C., Colaco, R. D., Swarbreck, S. M., et al. (2013). Salinity-induced calcium signaling and root adaptation in Arabidopsis require the calcium regulatory protein annexin1. Plant Physiol. 163 (1), 253–262. doi: 10.1104/pp.113.217810
Lau, S., Jurgens, G., De Smet, I. (2008). The evolving complexity of the auxin pathway. Plant Cell 20 (7), 1738–1746. doi: 10.1105/tpc.108.060418
Lee, H. G., Choi, Y. R., Seo, P. J. (2016). Increased STM expression is associated with drought tolerance in Arabidopsis. J. Plant Physiol. 201, 79–84. doi: 10.1016/j.jplph.2016.07.002
Lee, C., Clark, S. E. (2013). Core pathways controlling shoot meristem maintenance. Wiley Interdiscip Rev. Dev. Biol. 2 (5), 671–684. doi: 10.1002/wdev.110
Lee, Y., Lee, W. S., Kim, S. H. (2013). Hormonal regulation of stem cell maintenance in roots. J. Exp. Bot. 64 (5), 1153–1165. doi: 10.1093/jxb/ers331
Lee, S., Seo, P. J., Lee, H. J., Park, C. M. (2012). A NAC transcription factor NTL4 promotes reactive oxygen species production during drought-induced leaf senescence in Arabidopsis. Plant J. 70 (5), 831–844. doi: 10.1111/j.1365-313X.2012.04932.x
Legris, M., Klose, C., Burgie, E. S., Rojas, C. C., Neme, M., Hiltbrunner, A., et al. (2016). Phytochrome B integrates light and temperature signals in Arabidopsis. Science 354 (6314), 897–900. doi: 10.1126/science.aaf5656
Leibfried, A., To, J. P., Busch, W., Stehling, S., Kehle, A., Demar, M., et al. (2005). WUSCHEL controls meristem function by direct regulation of cytokinin-inducible response regulators. Nature 438 (7071), 1172–1175. doi: 10.1038/nature04270
Li, Y., Han, S., Qi, Y. (2023). Advances in structure and function of auxin response factor in plants. J. Integr. Plant Biol. 65 (3), 617–632. doi: 10.1111/jipb.13392
Li, H., Testerink, C., Zhang, Y. (2021a). How roots and shoots communicate through stressful times. Trends Plant Sci. 26 (9), 940–952. doi: 10.1016/j.tplants.2021.03.005
Li, S. M., Zheng, H. X., Zhang, X. S., Sui, N. (2021b). Cytokinins as central regulators during plant growth and stress response. Plant Cell Rep. 40 (2), 271–282. doi: 10.1007/s00299-020-02612-1
Liu, X., Bie, X. M., Lin, X., Li, M., Wang, H., Zhang, X., et al. (2023). Uncovering the transcriptional regulatory network involved in boosting wheat regeneration and transformation. Nat. Plants 9 (6), 908–925. doi: 10.1038/s41477-023-01406-z
Liu, Q., Ding, Y., Shi, Y., Ma, L., Wang, Y., Song, C., et al. (2021). The calcium transporter ANNEXIN1 mediates cold-induced calcium signaling and freezing tolerance in plants. EMBO J. 40 (2), e104559. doi: 10.15252/embj.2020104559
Liu, L., Wang, Y., Shi, Z., Zhang, M., Sun, C. (2018a). Advances in plant stem cell culture. Sheng Wu Gong Cheng Xue Bao 34 (11), 1734–1741. doi: 10.13345/j.cjb.180047
Liu, X., Wang, J., Sun, L. (2018b). Structure of the hyperosmolality-gated calcium-permeable channel OSCA1.2. Nat. Commun. 9 (1), 5060. doi: 10.1038/s41467-018-07564-5
Liu, Q., Yao, X., Pi, L., Wang, H., Cui, X., Huang, H. (2009). The ARGONAUTE10 gene modulates shoot apical meristem maintenance and establishment of leaf polarity by repressing miR165/166 in Arabidopsis. Plant J. 58 (1), 27–40. doi: 10.1111/j.1365-313X.2008.03757.x
Liu, C., Yin, H., Gao, P., Hu, X., Yang, J., Liu, Z., et al. (2013). Phosphatidylserine synthase 1 is required for inflorescence meristem and organ development in Arabidopsis. J. Integr. Plant Biol. 55 (8), 682–695. doi: 10.1111/jipb.12045
Long, J. A., Moan, E. I., Medford, J. I., Barton, M. K. (1996). A member of the KNOTTED class of homeodomain proteins encoded by the STM gene of Arabidopsis. Nature 379 (6560), 66–69. doi: 10.1038/379066a0
Long, J. A., Ohno, C., Smith, Z. R., Meyerowitz, E. M. (2006). TOPLESS regulates apical embryonic fate in Arabidopsis. Science 312 (5779), 1520–1523. doi: 10.1126/science.1123841
Lopes, F. L., Galvan-Ampudia, C., Landrein, B. (2021). WUSCHEL in the shoot apical meristem: old player, new tricks. J. Exp. Bot. 72 (5), 1527–1535. doi: 10.1093/jxb/eraa572
Luo, L., Zeng, J., Wu, H., Tian, Z., Zhao, Z. (2018). A molecular framework for auxin-controlled homeostasis of shoot stem cells in Arabidopsis. Mol. Plant 11 (7), 899–913. doi: 10.1016/j.molp.2018.04.006
Lynn, K., Fernandez, A., Aida, M., Sedbrook, J., Tasaka, M., Masson, P., et al. (1999). The PINHEAD/ZWILLE gene acts pleiotropically in Arabidopsis development and has overlapping functions with the ARGONAUTE1 gene. Development 126 (3), 469–481. doi: 10.1242/dev.126.3.469
Ma, Y., Dai, X., Xu, Y., Luo, W., Zheng, X., Zeng, D., et al. (2015). COLD1 confers chilling tolerance in rice. Cell 160 (6), 1209–1221. doi: 10.1016/j.cell.2015.01.046
Ma, Y., Miotk, A., Sutikovic, Z., Ermakova, O., Wenzl, C., Medzihradszky, A., et al. (2019b). WUSCHEL acts as an auxin response rheostat to maintain apical stem cells in Arabidopsis. Nat. Commun. 10 (1), 5093. doi: 10.1038/s41467-019-13074-9
Ma, L., Ye, J., Yang, Y., Lin, H., Yue, L., Luo, J., et al. (2019a). The SOS2-SCaBP8 complex generates and fine-tunes an AtANN4-dependent calcium signature under salt stress. Dev. Cell 48 (5), 697–709 e695. doi: 10.1016/j.devcel.2019.02.010
Mahonen, A. P., Ten Tusscher, K., Siligato, R., Smetana, O., Diaz-Trivino, S., Salojarvi, J., et al. (2014). PLETHORA gradient formation mechanism separates auxin responses. Nature 515 (7525), 125–129. doi: 10.1038/nature13663
Maity, K., Heumann, J. M., McGrath, A. P., Kopcho, N. J., Hsu, P. K., Lee, C. W., et al. (2019). Cryo-EM structure of OSCA1.2 from Oryza sativa elucidates the mechanical basis of potential membrane hyperosmolality gating. Proc. Natl. Acad. Sci. U.S.A. 116 (28), 14309–14318. doi: 10.1073/pnas.1900774116
Markham, K. K., Greenham, K. (2021). Abiotic stress through time. New Phytol. 231 (1), 40–46. doi: 10.1111/nph.17367
Mason, M. G., Jha, D., Salt, D. E., Tester, M., Hill, K., Kieber, J. J., et al. (2010). Type-B response regulators ARR1 and ARR12 regulate expression of AtHKT1;1 and accumulation of sodium in Arabidopsis shoots. Plant J. 64 (5), 753–763. doi: 10.1111/j.1365-313X.2010.04366.x
Matosevich, R., Cohen, I., Gil-Yarom, N., Modrego, A., Friedlander-Shani, L., Verna, C., et al. (2020). Local auxin biosynthesis is required for root regeneration after wounding. Nat. Plants 6 (8), 1020–1030. doi: 10.1038/s41477-020-0737-9
Matosevich, R., Efroni, I. (2021). The quiescent center and root regeneration. J. Exp. Bot. 72 (19), 6739–6745. doi: 10.1093/jxb/erab319
Matsuzaki, Y., Ogawa-Ohnishi, M., Mori, A., Matsubayashi, Y. (2010). Secreted peptide signals required for maintenance of root stem cell niche in Arabidopsis. Science 329 (5995), 1065–1067. doi: 10.1126/science.1191132
Mayer, K. F., Schoof, H., Haecker, A., Lenhard, M., Jurgens, G., Laux, T. (1998). Role of WUSCHEL in regulating stem cell fate in the Arabidopsis shoot meristem. Cell 95 (6), 805–815. doi: 10.1016/s0092-8674(00)81703-1
McKim, S. M. (2019). How plants grow up. J. Integr. Plant Biol. 61 (3), 257–277. doi: 10.1111/jipb.12786
Meng, W. J., Cheng, Z. J., Sang, Y. L., Zhang, M. M., Rong, X. F., Wang, Z. W., et al. (2017). Type-B ARABIDOPSIS RESPONSE REGULATORs specify the shoot stem cell niche by dual regulation of WUSCHEL. Plant Cell 29 (6), 1357–1372. doi: 10.1105/tpc.16.00640
Mira, M. M., El-Khateeb, E. A., Gaafar, R. M., Igamberdiev, A. U., Hill, R. D., Stasolla, C. (2020). Stem cell fate in hypoxic root apical meristems is influenced by phytoglobin expression. J. Exp. Bot. 71 (4), 1350–1362. doi: 10.1093/jxb/erz410
Mira, M. M., Hill, R. D., Stasolla, C. (2016). Phytoglobins improve hypoxic root growth by alleviating apical meristem cell death. Plant Physiol. 172 (3), 2044–2056. doi: 10.1104/pp.16.01150
Motte, H., Vanneste, S., Beeckman, T. (2019). Molecular and environmental regulation of root development. Annu. Rev. Plant Bio.l 70, 465–488. doi: 10.1146/annurev-arplant-050718-100423
Moubayidin, L., Perilli, S., Dello Ioio, R., Di Mambro, R., Costantino, P., Sabatini, S. (2010). The rate of cell differentiation controls the Arabidopsis root meristem growth phase. Curr. Biol. 20 (12), 1138–1143. doi: 10.1016/j.cub.2010.05.035
Moussian, B., Schoof, H., Haecker, A., Jurgens, G., Laux, T. (1998). Role of the ZWILLE gene in the regulation of central shoot meristem cell fate during Arabidopsis embryogenesis. EMBO J. 17 (6), 1799–1809. doi: 10.1093/emboj/17.6.1799
Negin, B., Shemer, O., Sorek, Y., Eshed Williams, L. (2017). Shoot stem cell specification in roots by the WUSCHEL transcription factor. PloS One 12 (4), e0176093. doi: 10.1371/journal.pone.0176093
Nguyen, K. H., Ha, C. V., Nishiyama, R., Watanabe, Y., Leyva-Gonzalez, M. A., Fujita, Y., et al. (2016). Arabidopsis type B cytokinin response regulators ARR1, ARR10, and ARR12 negatively regulate plant responses to drought. Proc. Natl. Acad. Sci. U.S.A. 113 (11), 3090–3095. doi: 10.1073/pnas.1600399113
Nicolas, A., Laufs, P. (2022). Meristem initiation and de novo stem cell formation. Front. Plant Sci. 13. doi: 10.3389/fpls.2022.891228
Nishiyama, R., Watanabe, Y., Leyva-Gonzalez, M. A., Ha, C. V., Fujita, Y., Tanaka, M., et al. (2013). Arabidopsis AHP2, AHP3, and AHP5 histidine phosphotransfer proteins function as redundant negative regulators of drought stress response. Proc. Natl. Acad. Sci. U.S.A. 110 (12), 4840–4845. doi: 10.1073/pnas.1302265110
Ogita, N., Okushima, Y., Tokizawa, M., Yamamoto, Y. Y., Tanaka, M., Seki, M., et al. (2018). Identifying the target genes of SUPPRESSOR OF GAMMA RESPONSE 1, a master transcription factor controlling DNA damage response in Arabidopsis. Plant J. 94 (3), 439–453. doi: 10.1111/tpj.13866
Pandey, B. K., Huang, G., Bhosale, R., Hartman, S., Sturrock, C. J., Jose, L., et al. (2021). Plant roots sense soil compaction through restricted ethylene diffusion. Science 371 (6526), 276–280. doi: 10.1126/science.abf3013
Perales, M., Rodriguez, K., Snipes, S., Yadav, R. K., Diaz-Mendoza, M., Reddy, G. V. (2016). Threshold-dependent transcriptional discrimination underlies stem cell homeostasis. Proc. Natl. Acad. Sci. U.S.A. 113 (41), E6298–E6306. doi: 10.1073/pnas.1607669113
Petricka, J. J., Winter, C. M., Benfey, P. N. (2012). Control of Arabidopsis root development. Annu. Rev. Plant Biol. 63, 563–590. doi: 10.1146/annurev-arplant-042811-105501
Pi, L., Aichinger, E., van der Graaff, E., Llavata-Peris, C. I., Weijers, D., Hennig, L., et al. (2015). Organizer-derived WOX5 signal maintains root columella stem cells through chromatin-mediated repression of CDF4 expression. Dev. Cell 33 (5), 576–588. doi: 10.1016/j.devcel.2015.04.024
Planas-Riverola, A., Gupta, A., Betegon-Putze, I., Bosch, N., Ibanes, M., Cano-Delgado, A. I. (2019). Brassinosteroid signaling in plant development and adaptation to stress. Development 146 (5). doi: 10.1242/dev.151894
Rademacher, E. H., Lokerse, A. S., Schlereth, A., Llavata-Peris, C. I., Bayer, M., Kientz, M., et al. (2012). Different auxin response machineries control distinct cell fates in the early plant embryo. Dev. Cell 22 (1), 211–222. doi: 10.1016/j.devcel.2011.10.026
Rodriguez, K., Perales, M., Snipes, S., Yadav, R. K., Diaz-Mendoza, M., Reddy, G. V. (2016). DNA-dependent homodimerization, sub-cellular partitioning, and protein destabilization control WUSCHEL levels and spatial patterning. Proc. Natl. Acad. Sci. U.S.A. 113 (41), E6307–E6315. doi: 10.1073/pnas.1607673113
Roodbarkelari, F., Du, F., Truernit, E., Laux, T. (2015). ZLL/AGO10 maintains shoot meristem stem cells during Arabidopsis embryogenesis by down-regulating ARF2-mediated auxin response. BMC Biol. 13, 74. doi: 10.1186/s12915-015-0180-y
Rounds, M. A., Larsen, P. B. (2008). Aluminum-dependent root-growth inhibition in Arabidopsis results from AtATR-regulated cell-cycle arrest. Curr. Biol. 18 (19), 1495–1500. doi: 10.1016/j.cub.2008.08.050
Roychoudhry, S., Kepinski, S. (2022). Auxin in root development. Cold Spring Harb. Perspect. Biol. 14 (4). doi: 10.1101/cshperspect.a039933
Ruzicka, K., Simaskova, M., Duclercq, J., Petrasek, J., Zazimalova, E., Simon, S., et al. (2009). Cytokinin regulates root meristem activity via modulation of the polar auxin transport. Proc. Natl. Acad. Sci. U.S.A. 106 (11), 4284–4289. doi: 10.1073/pnas.0900060106
Ryu, T. H., Go, Y. S., Choi, S. H., Kim, J. I., Chung, B. Y., Kim, J. H. (2019). SOG1-dependent NAC103 modulates the DNA damage response as a transcriptional regulator in Arabidopsis. Plant J. 98 (1), 83–96. doi: 10.1111/tpj.14201
Sabatini, S., Heidstra, R., Wildwater, M., Scheres, B. (2003). SCARECROW is involved in positioning the stem cell niche in the Arabidopsis root meristem. Genes Dev. 17 (3), 354–358. doi: 10.1101/gad.252503
Sablowski, R. (2007). The dynamic plant stem cell niches. Curr. Opin. Plant Bio.l 10 (6), 639–644. doi: 10.1016/j.pbi.2007.07.001
Sablowski, R. (2011). Plant stem cell niches: from signalling to execution. Curr. Opin. Plant Biol. 14 (1), 4–9. doi: 10.1016/j.pbi.2010.08.001
Saiga, S., Furumizu, C., Yokoyama, R., Kurata, T., Sato, S., Kato, T., et al. (2008). The Arabidopsis OBERON1 and OBERON2 genes encode plant homeodomain finger proteins and are required for apical meristem maintenance. Development 135 (10), 1751–1759. doi: 10.1242/dev.014993
Saiga, S., Moller, B., Watanabe-Taneda, A., Abe, M., Weijers, D., Komeda, Y. (2012). Control of embryonic meristem initiation in Arabidopsis by PHD-finger protein complexes. Development 139 (8), 1391–1398. doi: 10.1242/dev.074492
Salvi, E., Rutten, J. P., Di Mambro, R., Polverari, L., Licursi, V., Negri, R., et al. (2020). A self-organized PLT/Auxin/ARR-B network controls the dynamics of root zonation development in Arabidopsis thaliana. Dev. Cell 53 (4), 431–443 e423. doi: 10.1016/j.devcel.2020.04.004
Sang, Y. L., Cheng, Z. J., Zhang, X. S. (2018). iPSCs: A comparison between animals and plants. Trends Plant Sci. 23 (8), 660–666. doi: 10.1016/j.tplants.2018.05.008
Santuari, L., Sanchez-Perez, G. F., Luijten, M., Rutjens, B., Terpstra, I., Berke, L., et al. (2016). The PLETHORA gene regulatory network guides growth and cell differentiation in Arabidopsis roots. Plant Cell 28 (12), 2937–2951. doi: 10.1105/tpc.16.00656
Sarkar, A. K., Luijten, M., Miyashima, S., Lenhard, M., Hashimoto, T., Nakajima, K., et al. (2007). Conserved factors regulate signalling in Arabidopsis thaliana shoot and root stem cell organizers. Nature 446 (7137), 811–814. doi: 10.1038/nature05703
Sasidharan, R., Hartman, S., Liu, Z., Martopawiro, S., Sajeev, N., van Veen, H., et al. (2018). Signal dynamics and interactions during flooding stress. Plant Physiol. 176 (2), 1106–1117. doi: 10.1104/pp.17.01232
Scacchi, E., Salinas, P., Gujas, B., Santuari, L., Krogan, N., Ragni, L., et al. (2010). Spatio-temporal sequence of cross-regulatory events in root meristem growth. Proc. Natl. Acad. Sci. U.S.A. 107 (52), 22734–22739. doi: 10.1073/pnas.1014716108
Scharf, K. D., Berberich, T., Ebersberger, I., Nover, L. (2012). The plant heat stress transcription factor (Hsf) family: structure, function and evolution. Biochim. Biophys. Acta 1819 (2), 104–119. doi: 10.1016/j.bbagrm.2011.10.002
Schlereth, A., Moller, B., Liu, W., Kientz, M., Flipse, J., Rademacher, E. H., et al. (2010). MONOPTEROS controls embryonic root initiation by regulating a mobile transcription factor. Nature 464 (7290), 913–916. doi: 10.1038/nature08836
Schoof, H., Lenhard, M., Haecker, A., Mayer, K. F., Jurgens, G., Laux, T. (2000). The stem cell population of Arabidopsis shoot meristems in maintained by a regulatory loop between the CLAVATA and WUSCHEL genes. Cell 100 (6), 635–644. doi: 10.1016/s0092-8674(00)80700-x
Scintu, D., Scacchi, E., Cazzaniga, F., Vinciarelli, F., De Vivo, M., Shtin, M., et al. (2023). microRNA165 and 166 modulate response of the Arabidopsis root apical meristem to salt stress. Commun. Biol. 6 (1), 834. doi: 10.1038/s42003-023-05201-6
Shi, H., Quintero, F. J., Pardo, J. M., Zhu, J. K. (2002). The putative plasma membrane Na(+)/H(+) antiporter SOS1 controls long-distance Na(+) transport in plants. Plant Cell 14 (2), 465–477. doi: 10.1105/tpc.010371
Shinozaki, K., Yamaguchi-Shinozaki, K. (2022). Functional genomics in plant abiotic stress responses and tolerance: From gene discovery to complex regulatory networks and their application in breeding. Proc. Jpn. Acad. Ser. B Phys. Biol. Sci. 98 (8), 470–492. doi: 10.2183/pjab.98.024
Shkolnik, D., Nuriel, R., Bonza, M. C., Costa, A., Fromm, H. (2018). MIZ1 regulates ECA1 to generate a slow, long-distance phloem-transmitted Ca(2+) signal essential for root water tracking in Arabidopsis. Proc. Natl. Acad. Sci. U.S.A. 115 (31), 8031–8036. doi: 10.1073/pnas.1804130115
Sjogren, C. A., Bolaris, S. C., Larsen, P. B. (2015). Aluminum-dependent terminal differentiation of the Arabidopsis root tip Is mediated through an ATR-, ALT2-, and SOG1-regulated transcriptional response. Plant Cell 27 (9), 2501–2515. doi: 10.1105/tpc.15.00172
Skylar, A., Hong, F., Chory, J., Weigel, D., Wu, X. (2010). STIMPY mediates cytokinin signaling during shoot meristem establishment in Arabidopsis seedlings. Development 137 (4), 541–549. doi: 10.1242/dev.041426
Smith, Z. R., Long, J. A. (2010). Control of Arabidopsis apical-basal embryo polarity by antagonistic transcription factors. Nature 464 (7287), 423–426. doi: 10.1038/nature08843
Somssich, M., Je, B. I., Simon, R., Jackson, D. (2016). CLAVATA-WUSCHEL signaling in the shoot meristem. Development 143 (18), 3238–3248. doi: 10.1242/dev.133645
Song, X., Guo, P., Li, C., Liu, C. M. (2010). The cysteine pairs in CLV2 are not necessary for sensing the CLV3 peptide in shoot and root meristems. J. Integr. Plant Biol. 52 (9), 774–781. doi: 10.1111/j.1744-7909.2010.00978.x
Song, Y., Li, J., Liu, M., Meng, Z., Liu, K., Sui, N. (2019). Nitrogen increases drought tolerance in maize seedlings. Funct. Plant Biol. 46 (4), 350–359. doi: 10.1071/FP18186
Spinelli, S. V., Martin, A. P., Viola, I. L., Gonzalez, D. H., Palatnik, J. F. (2011). A mechanistic link between STM and CUC1 during Arabidopsis development. Plant Physiol. 156 (4), 1894–1904. doi: 10.1104/pp.111.177709
Stahl, Y., Wink, R. H., Ingram, G. C., Simon, R. (2009). A signaling module controlling the stem cell niche in Arabidopsis root meristems. Curr. Biol. 19 (11), 909–914. doi: 10.1016/j.cub.2009.03.060
Strotmann, V. I., Stahl, Y. (2021). At the root of quiescence: function and regulation of the quiescent center. J. Exp. Bot. 72 (19), 6716–6726. doi: 10.1093/jxb/erab275
Su, Y. H., Zhou, C., Li, Y. J., Yu, Y., Tang, L. P., Zhang, W. J., et al. (2020). Integration of pluripotency pathways regulates stem cell maintenance in the Arabidopsis shoot meristem. Proc. Natl. Acad. Sci. U.S.A. 117 (36), 22561–22571. doi: 10.1073/pnas.2015248117
Sun, H., Sun, X., Wang, H., Ma, X. (2020). Advances in salt tolerance molecular mechanism in tobacco plants. Hereditas 157 (1), 5. doi: 10.1186/s41065-020-00118-0
Szemenyei, H., Hannon, M., Long, J. A. (2008). TOPLESS mediates auxin-dependent transcriptional repression during Arabidopsis embryogenesis. Science 319 (5868), 1384–1386. doi: 10.1126/science.1151461
Takada, S., Hibara, K., Ishida, T., Tasaka, M. (2001). The CUP-SHAPED COTYLEDON1 gene of Arabidopsis regulates shoot apical meristem formation. Development 128 (7), 1127–1135. doi: 10.1242/dev.128.7.1127
Takahashi, F., Suzuki, T., Osakabe, Y., Betsuyaku, S., Kondo, Y., Dohmae, N., et al. (2018). A small peptide modulates stomatal control via abscisic acid in long-distance signalling. Nature 556 (7700), 235–238. doi: 10.1038/s41586-018-0009-2
Tanaka, W., Ohmori, Y., Ushijima, T., Matsusaka, H., Matsushita, T., Kumamaru, T., et al. (2015). Axillary meristem formation in rice requires the WUSCHEL ortholog TILLERS ABSENT1. Plant Cell 27 (4), 1173–1184. doi: 10.1105/tpc.15.00074
Thomas, C. L., Schmidt, D., Bayer, E. M., Dreos, R., Maule, A. J. (2009). Arabidopsis plant homeodomain finger proteins operate downstream of auxin accumulation in specifying the vasculature and primary root meristem. Plant J. 59 (3), 426–436. doi: 10.1111/j.1365-313X.2009.03874.x
Timilsina, R., Kim, J. H., Nam, H. G., Woo, H. R. (2019). Temporal changes in cell division rate and genotoxic stress tolerance in quiescent center cells of Arabidopsis primary root apical meristem. Sci. Rep. 9 (1), 3599. doi: 10.1038/s41598-019-40383-2
Tucker, M. R., Hinze, A., Tucker, E. J., Takada, S., Jurgens, G., Laux, T. (2008). Vascular signalling mediated by ZWILLE potentiates WUSCHEL function during shoot meristem stem cell development in the Arabidopsis embryo. Development 135 (17), 2839–2843. doi: 10.1242/dev.023648
Ubogoeva, E. V., Zemlyanskaya, E. V., Xu, J., Mironova, V. (2021). Mechanisms of stress response in the root stem cell niche. J. Exp. Bot. 72 (19), 6746–6754. doi: 10.1093/jxb/erab274
Umeda, M., Ikeuchi, M., Ishikawa, M., Ito, T., Nishihama, R., Kyozuka, J., et al. (2021). Plant stem cell research is uncovering the secrets of longevity and persistent growth. Plant J. 106 (2), 326–335. doi: 10.1111/tpj.15184
van den Berg, C., Willemsen, V., Hage, W., Weisbeek, P., Scheres, B. (1995). Cell fate in the Arabidopsis root meristem determined by directional signalling. Nature 378 (6552), 62–65. doi: 10.1038/378062a0
van den Berg, C., Willemsen, V., Hendriks, G., Weisbeek, P., Scheres, B. (1997). Short-range control of cell differentiation in the Arabidopsis root meristem. Nature 390 (6657), 287–289. doi: 10.1038/36856
Vernoux, T., Kronenberger, J., Grandjean, O., Laufs, P., Traas, J. (2000). PIN-FORMED 1 regulates cell fate at the periphery of the shoot apical meristem. Development 127 (23), 5157–5165. doi: 10.1242/dev.127.23.5157
Verslues, P. E., Bailey-Serres, J., Brodersen, C., Buckley, T. N., Conti, L., Christmann, A., et al. (2023). Burning questions for a warming and changing world: 15 unknowns in plant abiotic stress. Plant Cell 35 (1), 67–108. doi: 10.1093/plcell/koac263
Vilarrasa-Blasi, J., Gonzalez-Garcia, M. P., Frigola, D., Fabregas, N., Alexiou, K. G., Lopez-Bigas, N., et al. (2014). Regulation of plant stem cell quiescence by a brassinosteroid signaling module. Dev. Cell 30 (1), 36–47. doi: 10.1016/j.devcel.2014.05.020
Villalobos-Lopez, M. A., Arroyo-Becerra, A., Quintero-Jimenez, A., Iturriaga, G. (2022). Biotechnological advances to improve abiotic stress tolerance in crops. Int. J. Mol. Sci. 23 (19). doi: 10.3390/ijms231912053
Vollbrecht, E., Reiser, L., Hake, S. (2000). Shoot meristem size is dependent on inbred background and presence of the maize homeobox gene, knotted1. Development 127 (14), 3161–3172. doi: 10.1242/dev.127.14.3161
Wang, L., Hua, D., He, J., Duan, Y., Chen, Z., Hong, X., et al. (2011). Auxin Response Factor2 (ARF2) and its regulated homeodomain gene HB33 mediate abscisic acid response in Arabidopsis. PloS Genet. 7 (7), e1002172. doi: 10.1371/journal.pgen.1002172
Wang, J., Ren, Y., Liu, X., Luo, S., Zhang, X., Liu, X., et al. (2021). Transcriptional activation and phosphorylation of OsCNGC9 confer enhanced chilling tolerance in rice. Mol. Plant 14 (2), 315–329. doi: 10.1016/j.molp.2020.11.022
Wang, J., Su, Y., Kong, X., Ding, Z., Zhang, X. S. (2020). Initiation and maintenance of plant stem cells in root and shoot apical meristems. aBIOTECH 1 (3), 194–204. doi: 10.1007/s42994-020-00020-3
Wang, M., Zhang, H., Zhao, X., Zhou, J., Qin, G., Liu, Y., et al. (2023). SYNTAXIN OF PLANTS81 regulates root meristem activity and stem cell niche maintenance via ROS signaling. Plant Physiol. 191 (2), 1365–1382. doi: 10.1093/plphys/kiac530
Warghat, A. R., Thakur, K., Sood, A. (2018). Plant stem cells: what we know and what is anticipated. Mol. Biol. Rep. 45 (6), 2897–2905. doi: 10.1007/s11033-018-4344-z
Wegner, J. (2000). A theoretical approach to the genesis of cell layer arrangements in undifferentiated tissues. Plant Sci. 153 (2), 177–183. doi: 10.1016/s0168-9452(99)00264-2
Weijers, D., Sauer, M., Meurette, O., Friml, J., Ljung, K., Sandberg, G., et al. (2005). Maintenance of embryonic auxin distribution for apical-basal patterning by PIN-FORMED-dependent auxin transport in Arabidopsis. Plant Cell 17 (9), 2517–2526. doi: 10.1105/tpc.105.034637
Weijers, D., Schlereth, A., Ehrismann, J. S., Schwank, G., Kientz, M., Jurgens, G. (2006). Auxin triggers transient local signaling for cell specification in Arabidopsis embryogenesis. Dev. Cell 10 (2), 265–270. doi: 10.1016/j.devcel.2005.12.001
Wein, A., Le Gac, A. L., Laux, T. (2020). Stem cell ageing of the root apical meristem of Arabidopsis thaliana. Mech. Ageing Dev. 190, 111313. doi: 10.1016/j.mad.2020.111313
Wilson, M. E., Mixdorf, M., Berg, R. H., Haswell, E. S. (2016). Plastid osmotic stress influences cell differentiation at the plant shoot apex. Development 143 (18), 3382–3393. doi: 10.1242/dev.136234
Wu, X., Dabi, T., Weigel, D. (2005). Requirement of homeobox gene STIMPY/WOX9 for Arabidopsis meristem growth and maintenance. Curr. Biol. 15 (5), 436–440. doi: 10.1016/j.cub.2004.12.079
Xie, M., Chen, H., Huang, L., O’Neil, R. C., Shokhirev, M. N., Ecker, J. R. (2018). A B-ARR-mediated cytokinin transcriptional network directs hormone cross-regulation and shoot development. Nat. Commun. 9 (1), 1604. doi: 10.1038/s41467-018-03921-6
Xu, P., Zhao, P. X., Cai, X. T., Mao, J. L., Miao, Z. Q., Xiang, C. B. (2020). Integration of jasmonic acid and ethylene into auxin signaling in root development. Front. Plant Sci. 11. doi: 10.3389/fpls.2020.00271
Xue, Z., Liu, L., Zhang, C. (2020). Regulation of shoot apical meristem and axillary meristem development in Plants. Int. J. Mol. Sci. 21 (8). doi: 10.3390/ijms21082917
Yadav, R. K., Perales, M., Gruel, J., Girke, T., Jonsson, H., Reddy, G. V. (2011). WUSCHEL protein movement mediates stem cell homeostasis in the Arabidopsis shoot apex. Genes Dev. 25 (19), 2025–2030. doi: 10.1101/gad.17258511
Yadav, R. K., Tavakkoli, M., Reddy, G. V. (2010). WUSCHEL mediates stem cell homeostasis by regulating stem cell number and patterns of cell division and differentiation of stem cell progenitors. Development 137 (21), 3581–3589. doi: 10.1242/dev.054973
Yamoune, A., Cuyacot, A. R., Zdarska, M., Hejatko, J. (2021). Hormonal orchestration of root apical meristem formation and maintenance in Arabidopsis. J. Exp. Bot. 72 (19), 6768–6788. doi: 10.1093/jxb/erab360
Yanai, O., Shani, E., Dolezal, K., Tarkowski, P., Sablowski, R., Sandberg, G., et al. (2005). Arabidopsis KNOXI proteins activate cytokinin biosynthesis. Curr. Biol. 15 (17), 1566–1571. doi: 10.1016/j.cub.2005.07.060
Yang, Z. B., Geng, X., He, C., Zhang, F., Wang, R., Horst, W. J., et al. (2014). TAA1-regulated local auxin biosynthesis in the root-apex transition zone mediates the aluminum-induced inhibition of root growth in Arabidopsis. Plant Cell 26 (7), 2889–2904. doi: 10.1105/tpc.114.127993
Yang, S., Lee, H. (2023). Salinity-triggered responses in plant apical meristems for developmental plasticity. Int. J. Mol. Sci. 24 (7). doi: 10.3390/ijms24076647
Yang, G., Yu, Z., Gao, L., Zheng, C. (2019). SnRK2s at the crossroads of growth and stress responses. Trends Plant Sci. 24 (8), 672–676. doi: 10.1016/j.tplants.2019.05.010
Yu, Z., Duan, X., Luo, L., Dai, S., Ding, Z., Xia, G. (2020). How plant hormones mediate salt stress responses. Trends Plant Sci. 25 (11), 1117–1130. doi: 10.1016/j.tplants.2020.06.008
Yu, Z., Zhang, D., Xu, Y., Jin, S., Zhang, L., Zhang, S., et al. (2019). CEPR2 phosphorylates and accelerates the degradation of PYR/PYLs in Arabidopsis. J. Exp. Bot. 70 (19), 5457–5469. doi: 10.1093/jxb/erz302
Zhai, N., Pan, X., Zeng, M., Xu, L. (2023). Developmental trajectory of pluripotent stem cell establishment in Arabidopsis callus guided by a quiescent center-related gene network. Development 150 (5). doi: 10.1242/dev.200879
Zhai, H., Zhang, X., You, Y., Lin, L., Zhou, W., Li, C. (2020). SEUSS integrates transcriptional and epigenetic control of root stem cell organizer specification. EMBO J. 39 (20), e105047. doi: 10.15252/embj.2020105047
Zhang, M. L., Huang, P. P., Ji, Y., Wang, S., Wang, S. S., Li, Z., et al. (2020b). KUP9 maintains root meristem activity by regulating K(+) and auxin homeostasis in response to low K. EMBO Rep. 21 (6), e50164. doi: 10.15252/embr.202050164
Zhang, Y., Jiao, Y., Liu, Z., Zhu, Y. X. (2015). ROW1 maintains quiescent centre identity by confining WOX5 expression to specific cells. Nat. Commun. 6, 6003. doi: 10.1038/ncomms7003
Zhang, Z., Tucker, E., Hermann, M., Laux, T. (2017). A molecular framework for the embryonic initiation of shoot meristem stem cells. Dev. Cell 40 (3), 264–277 e264. doi: 10.1016/j.devcel.2017.01.002
Zhang, H., Zhao, Y., Zhu, J. K. (2020a). Thriving under stress: How plants balance growth and the stress response. Dev. Cell 55 (5), 529–543. doi: 10.1016/j.devcel.2020.10.012
Zhang, H., Zhu, J., Gong, Z., Zhu, J. K. (2022). Abiotic stress responses in plants. Nat. Rev. Genet. 23 (2), 104–119. doi: 10.1038/s41576-021-00413-0
Zhao, Z., Andersen, S. U., Ljung, K., Dolezal, K., Miotk, A., Schultheiss, S. J., et al. (2010). Hormonal control of the shoot stem-cell niche. Nature 465 (7301), 1089–1092. doi: 10.1038/nature09126
Zhao, C., Zayed, O., Yu, Z., Jiang, W., Zhu, P., Hsu, C. C., et al. (2018a). Leucine-rich repeat extensin proteins regulate plant salt tolerance in Arabidopsis. Proc. Nat.l Acad. Sci. U.S.A. 115 (51), 13123–13128. doi: 10.1073/pnas.1816991115
Zhao, Q., Zhou, L., Liu, J., Cao, Z., Du, X., Huang, F., et al. (2018b). Involvement of CAT in the detoxification of HT-induced ROS burst in rice anther and its relation to pollen fertility. Plant Cell Rep. 37 (5), 741–757. doi: 10.1007/s00299-018-2264-y
Zhou, M., Ghnaya, T., Dailly, H., Cui, G., Vanpee, B., Han, R., et al. (2019a). The cytokinin trans-zeatine riboside increased resistance to heavy metals in the halophyte plant species Kosteletzkya pentacarpos in the absence but not in the presence of NaCl. Chemosphere 233, 954–965. doi: 10.1016/j.chemosphere.2019.06.023
Zhou, W., Lozano-Torres, J. L., Blilou, I., Zhang, X., Zhai, Q., Smant, G., et al. (2019b). A jasmonate signaling network activates root stem cells and promotes regeneration. Cell 177 (4), 942–956 e914. doi: 10.1016/j.cell.2019.03.006
Zhou, W., Wei, L., Xu, J., Zhai, Q., Jiang, H., Chen, R., et al. (2010). Arabidopsis Tyrosylprotein sulfotransferase acts in the auxin/PLETHORA pathway in regulating postembryonic maintenance of the root stem cell niche. Plant Cell 22 (11), 3692–3709. doi: 10.1105/tpc.110.075721
Zhou, Y., Yan, A., Han, H., Li, T., Geng, Y., Liu, X., et al. (2018). HAIRY MERISTEM with WUSCHEL confines CLAVATA3 expression to the outer apical meristem layers. Science 361 (6401), 502–506. doi: 10.1126/science.aar8638
Zhu, J. K. (2016). Abiotic stress signaling and responses in plants. Cell 167 (2), 313–324. doi: 10.1016/j.cell.2016.08.029
Keywords: stem cell, meristem, DNA damage, ROS, Ca2+, abiotic stress
Citation: Zhang H, Mu Y, Zhang H and Yu C (2023) Maintenance of stem cell activity in plant development and stress responses. Front. Plant Sci. 14:1302046. doi: 10.3389/fpls.2023.1302046
Received: 26 September 2023; Accepted: 28 November 2023;
Published: 12 December 2023.
Edited by:
Omar Borsani, Universidad de la República, UruguayReviewed by:
Raffaele Dello Ioio, Sapienza University of Rome, ItalyCopyright © 2023 Zhang, Mu, Zhang and Yu. This is an open-access article distributed under the terms of the Creative Commons Attribution License (CC BY). The use, distribution or reproduction in other forums is permitted, provided the original author(s) and the copyright owner(s) are credited and that the original publication in this journal is cited, in accordance with accepted academic practice. No use, distribution or reproduction is permitted which does not comply with these terms.
*Correspondence: Caiyu Yu, eXVjYWl5dTIwMjNAb3V0bG9vay5jb20=; Hui Zhang, emhhbmdodWkyNjA3QGhlbmF1LmVkdS5jbg==
Disclaimer: All claims expressed in this article are solely those of the authors and do not necessarily represent those of their affiliated organizations, or those of the publisher, the editors and the reviewers. Any product that may be evaluated in this article or claim that may be made by its manufacturer is not guaranteed or endorsed by the publisher.
Research integrity at Frontiers
Learn more about the work of our research integrity team to safeguard the quality of each article we publish.