Introduction
As a central epigenetic modification, histone acetylation affects the expression of genes with a wide range of functions across all life forms (Shen et al., 2015). Histone acetylation is mediated by histone acetylases and, generally, promotes DNA-templated transcriptional activity. Histone deacetylases (HDAs) reverse this process, leading to an inactive chromatin state and decreased transcriptional activity. Acetylation and deacetylation are central regulatory switches that govern responses to various environmental cues, orchestrating chromatin dynamics and gene activity to modulate the phenotypic readout.
The Arabidopsis genome harbours 18 HDAs, which are organized in three superfamilies comprised of the HDA classes 1-4 (Seto and Yoshida, 2014). HISTONE DEACETYLASE 19 (HDA19) belongs to the class 1 of the Reduced Potassium Dependence3/Histone Deacetylase-1 (RPD3/HDA1) superfamily and is possibly the most intensively studied HDA. HDA19 has reported roles across a broad landscape of processes and was shown to be a crucial player in seed development (Long et al., 2006; Zhou et al., 2020; Chen X. et al., 2023), pathogen response (Zhou et al., 2005), phosphate deficiency (Chen et al., 2015), light signalling (Jing et al., 2020), the regulation of flowering time (Ning et al., 2019), hormone responses (Mehdi et al., 2016; Kim et al., 2019), and floral organ identity genes (Krogan et al., 2012; Bollier et al., 2018; Ning et al., 2019). Similar to other RPD3-type HDACs, HDA19 forms various complexes that play pivotal roles in various stress responses (Feng et al., 2021; Vélez-Bermúdez and Schmidt, 2021), some of which possess yet unexplored stress-specific compositions.
In this Data Report, we provide an immunoprecipitation (IP)-based analysis of the HDA19 interactome in etiolated Arabidopsis seedlings. HDA19 is directly involved in hypocotyl elongation during photomorphogenesis and, based on our findings, also in hypocotyl elongation during skotomorphogenesis (Vélez-Bermúdez and Schmidt, 2021). The exact role of HDA19 in the latter process is still under investigation. For this reason, we conducted an immunoprecipitation experiment that provides new insights regarding the role of HDA19 in skotomorphogenesis and allows for comparisons of HDA19 targets in hypocotyl elongation during skoto- and photomorphogenesis. We believe that the catalogue of HDA19-interacting proteins presented here provides a valuable resource for follow-up research on novel interacting partners of this central protein. The material used for this experiment was derived from hypocotyls of 6-day-old etiolated Arabidopsis seedlings. The dataset contains a total of 6 files, 3 independent biological replicates of each Col-0 (control plants) and 35S::HDA19-GFP plants.
Materials and methods
Plant materials and growth conditions
Arabidopsis thaliana Col-0 and the transgenic line 35S::HDA19-GFP were used in this study. 35S::HDA19-GFP lines have been described previously (Zhou et al., 2005). Seeds were soaked in 35% bleach for 5 min, washed five times for 5 min with sterile water, and resuspended in 1 mL of sterile water for further use. Seeds were subsequently placed on a growth medium (Estelle and Somerville, 1987; ES medium) containing 5 mM KNO3, 2 mM MgSO4, 2 mM Ca (NO3)2, 2.5 mM KH2PO4, 70 μM H3BO3, 14 μM MnCl2, 1 μM ZnSO4, 0.5 μM CuSO4, 0.01 μM CoCl2, 0.2 μM Na2MoO4, and 40 μM Fe-EDTA, solidified with 0.4% Gelrite Pure. MES (1 g/L) and 1.5% (w/v) sucrose were added, and the pH was adjusted to 5.5 with KOH. Seeds were stratified on plates for 2 days at 4°C in the dark and grown at 22°C in vertical position in the dark with 70% relative humidity.
Immunoprecipitation
Experiments were carried out with the μMACS Epitope GFP tag protein isolation kit (MACSmolecular) following the manufacturer’s instructions with minor modifications. Hypocotyls were collected by dissection in dark conditions within ca. 5 minutes per plate (~25 seedlings). Samples from each plate were frozen immediately in liquid nitrogen. A total of 25 plates were used to obtain 0.5 gram of tissue. Hypocotyls were ground with liquid nitrogen and resuspended in 500 μL of extraction buffer (50 mM Tris/HCl, pH 7.5, 150 mM NaCl, 1% Triton X-100, 2X complete protease inhibitor cocktail EDTA-free (ROCHE), 1 mM PMSF, and 50 μM MG132). The samples were incubated on ice for 30 minutes with occasional mixing and centrifuged for 20 minutes at 10,000 x g at 4°C. The supernatants were individually collected in fresh tubes, and 400 μL of each input was added to 50 μl of anti-GFP microbeads and incubated for 1 hour and 30 minutes in a mixer set to 60 rpm at 4°C, while 100 μL of each input was kept to be used for Western blots using anti-GFP as a control. The samples were eluted in 50 μL denaturing elution buffer supplied with the kit.
S-Trap sample digestion and protein identification
The protocol was conducted as described previously (Chen C. W. et al., 2023). Briefly, 50 μL eluted IP sample was resuspended in 30 µL of lysis buffer (5% SDS (w/v) in 50 mM triethylammonium bicarbonate (TEAB), pH 8.5), transferred to a 1.7 mL tube, sonicated 10 times for 10 sec each, centrifuged at 16,000 g at 4°C for 20 min, and the supernatant was collected. The IP sample protein amount was determined by using a bicinchoninic acid assay (Thermo Fisher Scientific, Waltham, MA). The IP protein digestion was performed in the S-Trap micro column following the manufacturer’s protocol with some modifications. Shortly, 10 μg of protein in lysis buffer was reduced and alkylated using 1.6 μL of 200 mM tris(2-carboxyethyl)phosphine hydrochloride (TCEP) and 1.6 μL of 800 mM 2-chloroacetamide (CAA) at 45°C for 15 min. After alkylation, 3.3 µL of 55.5% (v/v) phosphoric acid (PA) was added, and the pH (~1) was controlled by means of pH paper. After acidification, the sample was mixed with 198 μL of binding buffer (100 mM TEAB in 90% (v/v), MeOH). After gentle vortexing, the sample was loaded onto an S-trap micro column and centrifuged at 4,000 g for 2 min to trap the proteins. The sample was then washed three times in the column with 150 µL of binding buffer and centrifuged at 4,000 g for 2 min each time. An additional centrifugation step (4,000 g for 2 min) was added to fully remove residual binding buffer. The S-trap column was transferred to a fresh 1.7 mL sample tube for the digestion, and 20 µL of protease solution (Lys-C + trypsin, 50 mM TEAB) was added into individual S-traps containing the samples. The cap of each S-trap was loosely closed to limit evaporative loss, and the samples were incubated for 2.5 h at 47°C. The column was removed from the incubator, and 40 µL of of three buffers were added consecutively to the column: 50 mM TEAB, elution buffer 2 (0.2% formic acid in H2O) and elution buffer 3 (50% acetonitrile (ACN) in ultrapure water). The column was centrifuged at 4,000 g for 2 min and the elution solution was collected in a new tube, dried by speed vacuum, resuspended in 100 µL of 0.1% formic acid, desalted, and loaded into a C18 Ziptip pipette tip. The elution was dried down under vacuum, the pellet was re-dissolved in 10 µL of 0.1% (v/v) formic acid (FA) with 3% (v/v) acetonitrile (ACN), and the liquid chromatography was performed by injecting 4 µL of sample in the LC-nESI-Q Exactive mass spectrometer model (Thermo Fisher Scientific) coupled with an on-line nanoUHPLC (Dionex UltiMate 3000 Binary RSLCnano). The Acclaim PepMap 100 C18 trap column (75 µm x 2.0 cm, 3 µm, 100 Å, Thermo Scientific) and the Acclaim PepMap RSLC C18 nano LC column (75 µm x 25 cm, 2 µm, 100 Å) were used to deliver solvent and separate tryptic peptides with a linear gradient from 5% to 35% of acetonitrile in 0.1% (v/v) formic acid for 60 min at a flow rate of 300 nl/min. The acquisition cycle for MS data was performed in the data-dependent mode with a full survey MS scan followed by 10 MS/MS scans of the top 10 precursor ions from the scan. The mass spectrometer was operated in full scan mode (m/z 350-1,600) in the Orbitrap analyser at a resolution of 70,000. Data-dependent MS/MS acquisitions were performed with a 2 m/z isolation window, 27% NCE (normalized collision energy), and 17,500 resolving power.
Data analysis and identification of putative interactors
Raw data were analysed with the Proteome Discoverer™ Software 2.2 (Thermo Fisher) using the Sequest search algorism. The Arabidopsis protein database (Araport11) was used to conduct the searches; only high confidence proteins were selected for the analysis. All peptide spectrum matches were filtered with a q-value threshold of 0.05 (5% FDR), and the proteins were filtered with high confidence threshold (0.05 q-value, 5% FDR). Nuclear proteins identified in more of two biological replicates were considered as putative interacting partners of HDA19. Localization of the proteins was gathered from published experimental evidence or–in cases where such information was unavailable–prediction inferred from the Subcellular Location of Proteins in Arabidopsis Database (SUBA).
Gene ontology
Gene Ontology (GO) analysis was conducted using the AgriGO v2.0 toolkit web-server (Tian et al., 2017). Significantly enriched GO categories were visualised using REVIGO (Supek et al., 2011) as previously described (Vélez-Bermúdez and Schmidt, 2021).
Protein-protein interaction network
The PPI network was constructed using STRING (https://string-db.org). Only nucleus-located partners of HDA19 (as listed in Table 1) were considered.
Dataset description
The identification of HDA19 protein partners via IP-LC-MS/MS relies on the capacity to distinguish true interactors from non-specific binders. To produce the current IP dataset, we used a powerful system to reduce the background in the IP samples. Samples from Arabidopsis thaliana Col-0 wild-type and 35S::HDA19-GFP plants were immunoprecipitated using the MultiMACS GFP isolation kit system with µMACS MicroBeads conjugated to an anti-GFP monoclonal antibody for faster and effective magnetic labelling of GFP-tagged fusion proteins. The complete procedure is depicted in Figure 1A. The IP samples were digested, subjected to LC-MS/MS analysis, and HDA19-binding proteins were identified using the Proteome Discoverer software. The dataset provided a total of 371 putative interactors that were identified with high confidence (Supplementary Data File 1). Please note that the proteins identified in the IP using Col-0 (present in the deposited data set) were removed as background. Considering only candidate proteins that are preferentially or exclusively located to the nucleus according to experimental results or predictions, a subset of 52 putatively interacting proteins was identified in at least two biological replicates (Table 1). A GO enrichment analysis of this subset revealed that besides predicted processes such as ‘histone modification’, ‘chromatin organization’, and ‘negative regulation of transcription’, proteins in the categories ‘multicellular organism development’, ‘cell cycle’, ‘protein modification’ and ‘reproduction’ were overrepresented (Figure 1B).
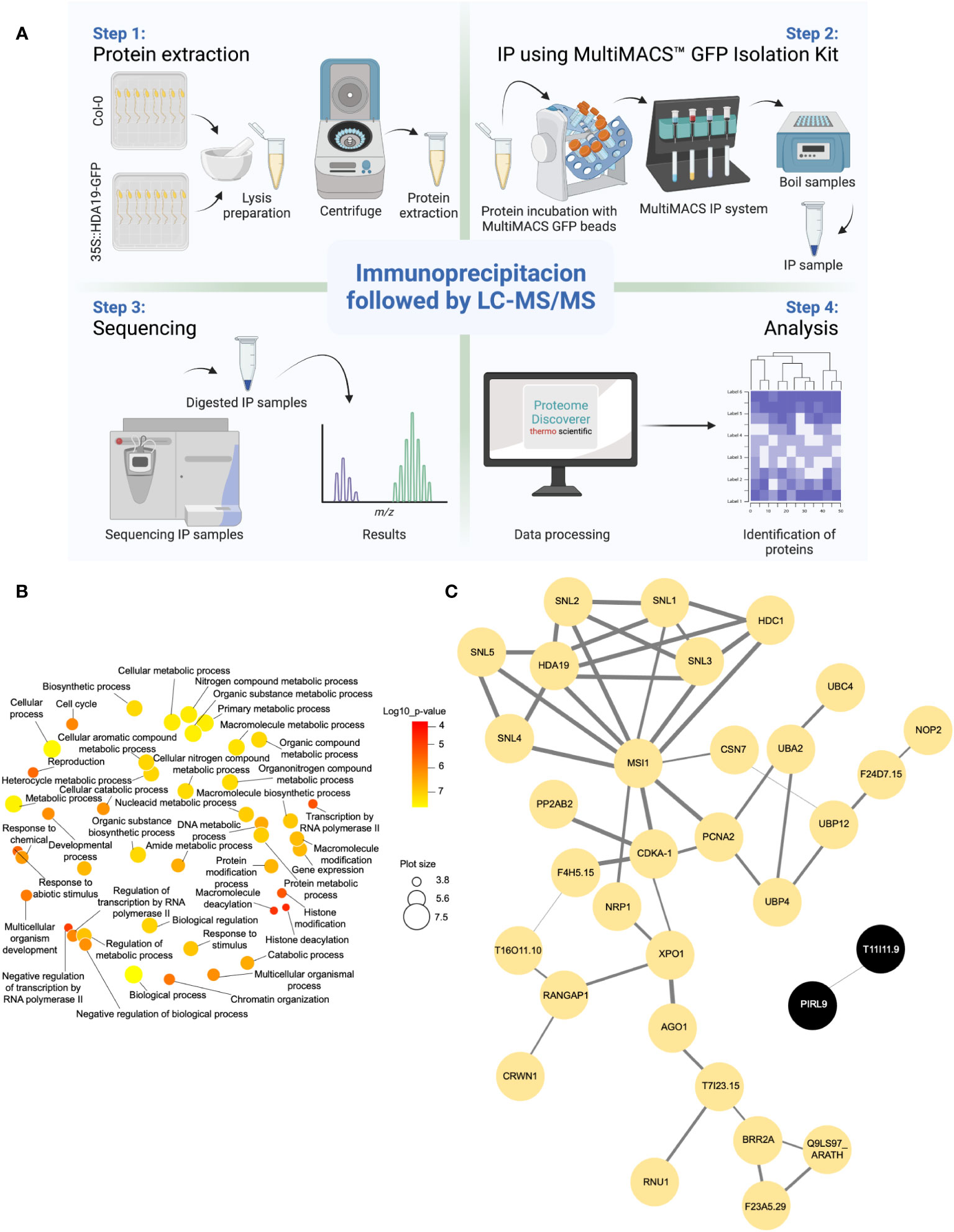
Figure 1 Identification of HDA19-interacting proteins. (A) Experimental flow of the immunoprecipitation analysis. (B) Overrepresented GO categories of putative nuclear-localised HDA19 interactors. Plot color indicates the log10 P value of enrichment, the size indicates the frequency of the GO term in the underlying GO annotation database (plots of more general terms are larger). (C) PPI network of putative nuclear-localised HDA19 interactors.
A protein-protein interaction (PPI) network constructed from this subset of proteins shows a suite of well-known partners of HDA19, including five members of the SIN-LIKE (SNL) family. SNL proteins were shown to be involved in the repression of AP2 family transcription factors that repress FLOWERING LOCUS T (FT) expression through histone deacetylation (Figure 1C) (Huang et al., 2019; Jing et al., 2021). We also identified HDC1, a component of histone deacetylase complexes that interacts with HDA6 and HDA19 (Perrella et al., 2016). A bimolecular fluorescence complementation approach revealed that HDC1 binds to the linker histone H1 (Perrella et al., 2016), which was identified as a putative interactor of HDA19 in the current dataset. The WD-40 repeat containing protein MULTICOPY SUPRESSOR OF IRA1 (MSI1), a conserved subunit of Polycomb Repressive Complex 2 (Xu et al., 2022), and PROLIFERATING CELL NUCLEAR ANTIGEN 2 (PCNA2), involved in DNA replication and damage repair (Xue et al., 2015) were identified as central nodes of the PPI network (Figure 1C).
The current dataset identified a large suite of putative novel interacting partners of a key regulator of plant development and stress responses, HDA19. The identification of SNL members, HDC1, and histone H1 can be considered as validation of the current IP assay. A surprisingly large subset of (predicted) non-nuclear proteins was identified with high confidence, suggesting that some of these proteins may transiently associate with chromatin. Besides expected binding partners such as HCD1, H1, and SNLs, we found that HDA19 interacts with proteins involved in chromatin remodelling, nuclear protein export/import, protein ubiquitination associated with DNA damage repair, and chloroplast-nucleus signalling, suggesting a wide range of largely unexplored functions of HDA19 in etiolated Arabidopsis seedlings.
Data availability statement
The mass spectrometry proteomics data have been deposited to the ProteomeXchange Consortium via the PRIDE (Perez-Riverol et al., 2016; Perez-Riverol et al., 2022; Deutsch et al., 2023) partner repository with the dataset identifier PXD045454 and can be accessed through the following link: http://www.ebi.ac.uk/pride/archive/projects/PXD045454.
Author contributions
WS: Conceptualization, Funding acquisition, Supervision, Writing – original draft, Writing – review & editing. IV: Conceptualization, Data curation, Formal Analysis, Methodology, Visualization, Writing – review & editing.
Funding
The author(s) declare financial support was received for the research, authorship, and/or publication of this article. Work in the Schmidt lab is supported by Academia Sinica and the National Science and Technology Council.
Acknowledgments
We thank Chin-Wen Chen and Chuan-Chih Hsu from the IPMB Proteomics Core Laboratory for their help with on-bead trypsin digestion and protein identification. Part A of Figure 1 was created with BioRender.com. Seeds of 35S::HDA19-GFP were a kind gift of Prof. Keqiang Wu, National Taiwan University, Taipei.
Conflict of interest
The authors declare that the research was conducted in the absence of any commercial or financial relationships that could be construed as a potential conflict of interest.
The author(s) declared that they were an editorial board member of Frontiers, at the time of submission. This had no impact on the peer review process and the final decision.
Publisher’s note
All claims expressed in this article are solely those of the authors and do not necessarily represent those of their affiliated organizations, or those of the publisher, the editors and the reviewers. Any product that may be evaluated in this article, or claim that may be made by its manufacturer, is not guaranteed or endorsed by the publisher.
Supplementary material
The Supplementary Material for this article can be found online at: https://www.frontiersin.org/articles/10.3389/fpls.2023.1296767/full#supplementary-material
Supplementary Data Sheet 1 | Putative identified interaction partners of HDA19.
References
Bollier, N., Sicard, A., Leblond, J., Latrasse, D., Gonzalez, N., Gévaudant, F., et al. (2018). At-MINI ZINC FINGER2 and Sl-INHIBITOR OF MERISTEM ACTIVITY, a conserved missing link in the regulation of floral meristem termination in Arabidopsis and Tomato. Plant Cell 30, 83–100. doi: 10.1105/tpc.17.00653
Chen, C.-W., Tsai, C.-F., Lin, M.-H., Lin, S.-Y., Hsu, C.-C. (2023). Suspension trapping-based sample preparation workflow for in-depth plant phosphoproteomics. Anal. Chem. 95, 12232–12339. doi: 10.1021/acs.analchem.3c00786
Chen, C. Y., Wu, K., Schmidt, W. (2015). The histone deacetylase HDA19 controls root cell elongation and modulates a subset of phosphate starvation responses in Arabidopsis. Sci. Rep. 5, 15708. doi: 10.1038/srep15708
Chen, X., MacGregor, D. R., Stefanato, F. L., Zhang, N., Barros-Galvão, T., Penfield, S. (2023). A VEL3 histone deacetylase complex establishes a maternal epigenetic state controlling progeny seed dormancy. Nat. Commun. 14, 2220. doi: 10.1038/s41467-023-37805-1
Deutsch, E. W., Bandeira, N., Perez-Riverol, Y., Sharma, V., Carver, J., Mendoza, L., et al. (2023). The ProteomeXchange Consortium at 10 years: 2023 update. Nucleic Acids Res. 51 (D1), D1539–D1548. doi: 10.1093/nar/gkac1040
Estelle, M. A., Somerville, C. (1987). Auxin-resistant mutants of Arabidopsis thaliana with an altered morphology. Mol. Genet. Genom. 206, 200–206. doi: 10.1007/BF00333575
Feng, C., Cai, X.-W., Su, Y.-N., Li, L., Chen, S., He, X.-J. (2021). Arabidopsis RPD3-like histone deacetylases form multiple complexes involved in stress response. J. Genet. Genomics 48, 369–383. doi: 10.1016/j.jgg.2021.04.004
Huang, F., Yuan, W., Tian, S., Zheng, Q., He, Y. (2019). SIN3 LIKE genes mediate long-day induction of flowering but inhibit the floral transition in short days through histone deacetylation in Arabidopsis. Plant J. 100, 101–113. doi: 10.1111/tpj.14430
Jing, Y., Guo, Q., Lin, R. (2021). The SNL-HDA19 histone deacetylase complex antagonizes HY5 activity to repress photomorphogenesis in Arabidopsis. New Phytol. 229, 3221–3236. doi: 10.1111/nph.17114
Kim, H., Shim, D., Moon, S., Lee, J., Bae, W., Choi, H., et al. (2019). Transcriptional network regulation of the brassinosteroid signaling pathway by the BES1–TPL–HDA19 co-repressor complex. Planta 250, 1371–1377. doi: 10.1007/s00425-019-03233-z
Krogan, N. T., Hogan, K., Jeff, A. (2012). APETALA2 negatively regulates multiple floral organ identity genes in Arabidopsis by recruiting the co-repressor TOPLESS and the histone deacetylase HDA19. Development 139, 4180–4190. doi: 10.1242/dev.085407
Long, J. A., Ohno, C., Smith, Z. R., Meyerowitz, E. M. (2006). TOPLESS regulates apical embryonic fate in Arabidopsis. Science 312, 1520–1523. doi: 10.1126/science.1123841
Mehdi, S., Derkacheva, M., Ramström, M., Kralemann, L., Bergquist, J., Hennig, L. (2016). The WD40 domain protein MSI1 functions in a histone deacetylase complex to fine-tune abscisic acid signaling. Plant Cell 28, 42–54. doi: 10.1105/tpc.15.00763
Ning, Y. Q., Chen, Q., Lin, R. N., Li, Y. Q., Li, L., Chen, S., et al. (2019). The HDA19 histone deacetylase complex is involved in the regulation of flowering time in a photoperiod-dependent manner. Plant J. 98, 448–464. doi: 10.1111/tpj.14229
Perez-Riverol, Y., Bai, J., Bandla, C., Hewapathirana, S., García-Seisdedos, D., KamatChinathan, S., et al. (2022). The PRIDE database resources in 2022: A Hub for mass spectrometry-based proteomics evidences. Nucleic Acids Res. 50 (D1), D543–D552. doi: 10.1093/nar/gkab1038
Perez-Riverol, Y., Xu, Q. W., Wang, R., Uszkoreit, J., Griss, J., Sanchez, A., et al. (2016). PRIDE Inspector Toolsuite: moving towards a universal visualization tool for proteomics data standard formats and quality assessment of ProteomeXchange datasets. Mol. Cell. Proteomics 15, 305–317. doi: 10.1074/mcp.O115.050229
Perrella, G., Carr, C., Asensi-Fabado, M. A., Donald, N. A., Páldi, K., Hannah, M. A., et al. (2016). The histone deacetylase complex 1 protein of Arabidopsis has the capacity to interact with multiple proteins including histone 3-Binding proteins and histone 1 variants. Plant Physiol. 171, 62–70. doi: 10.1104/pp.15.01760
Seto, E., Yoshida, M. (2014). Erasers of histone acetylation: the histone deacetylase enzymes. Cold Spring Harb. Perspect. Biol. 6, a018713. doi: 10.1101/cshperspect.a018713
Shen, Y., Wei, W., Zhou, D. X. (2015). Histone acetylation enzymes coordinate metabolism and gene expression. Trends Plant Sci. 20, 614–621. doi: 10.1016/j.tplants.2015.07.005
Supek, F., Bošnjak, M., Škunca, N., Šmuc, T. (2011). REVIGO summarizes and visualizes long lists of gene ontology terms. PloS One 6, e21800. doi: 10.1371/journal.pone.0021800
Tian, T., Liu, Y., Yan, H., You, Q., Yi, X., Du, Z., et al. (2017). agriGO v2.0: a GO analysis toolkit for the agricultural community 2017 update. Nucleic Acids Res. 45, W122–W129. doi: 10.1093/nar/gkx382
Vélez-Bermúdez, I. C., Schmidt, W. (2021). Chromatin enrichment for proteomics in plants (ChEP-P) implicates the histone reader ALFIN-LIKE 6 in jasmonate signalling. BMC Genom. 22, 845. doi: 10.1186/s12864-021-08160-6
Xu, Y., Li, Q., Yuan, L., Huang, Y., Hung, F. Y., Wu, K., et al. (2022). MSI1 and HDA6 function interdependently to control flowering time via chromatin modifications. Plant J. 109, 831–843. doi: 10.1111/tpj.15596
Xue, C., Liang, K., Liu, Z., Wen, R., Xiao, W. (2015). Similarities and differences between Arabidopsis PCNA1 and PCNA2 in complementing the yeast DNA damage tolerance defect. DNA Repair 28, 28–36. doi: 10.1016/j.dnarep.2015.02.003
Zhou, Y., Yang, P., Zhang, F., Luo, X., Xie, J. (2020). Histone deacetylase HDA19 interacts with histone methyltransferase SUVH5 to regulate seed dormancy in Arabidopsis. Plant Biol. J. 22, 1062–1071. doi: 10.1111/plb.13158
Keywords: immunoprecipitation, proteomics, histone acetylation, etiolated hypocotyl, skotomorphogenesis
Citation: Vélez-Bermúdez IC and Schmidt W (2023) The interactome of histone deacetylase HDA19 in dark-grown Arabidopsis seedlings. Front. Plant Sci. 14:1296767. doi: 10.3389/fpls.2023.1296767
Received: 19 September 2023; Accepted: 03 November 2023;
Published: 23 November 2023.
Edited by:
Sara Farrona, University of Galway, IrelandReviewed by:
Vicente Rubio, Spanish National Research Council (CSIC), SpainJordi Moreno-Romero, Autonomous University of Barcelona, Spain
Giorgio Perrella, University of Milan, Italy
Copyright © 2023 Vélez-Bermúdez and Schmidt. This is an open-access article distributed under the terms of the Creative Commons Attribution License (CC BY). The use, distribution or reproduction in other forums is permitted, provided the original author(s) and the copyright owner(s) are credited and that the original publication in this journal is cited, in accordance with accepted academic practice. No use, distribution or reproduction is permitted which does not comply with these terms.
*Correspondence: Isabel Cristina Vélez-Bermúdez, icvb@gate.sinica.edu.tw