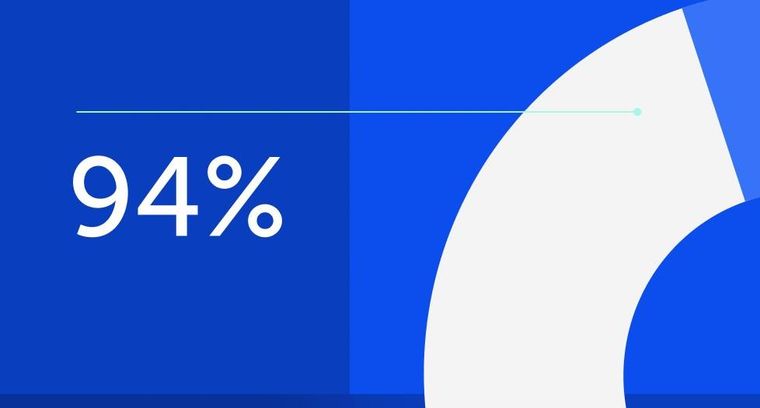
94% of researchers rate our articles as excellent or good
Learn more about the work of our research integrity team to safeguard the quality of each article we publish.
Find out more
ORIGINAL RESEARCH article
Front. Plant Sci., 05 December 2023
Sec. Functional Plant Ecology
Volume 14 - 2023 | https://doi.org/10.3389/fpls.2023.1282884
Leaf functional traits play critical roles in plant functioning. Although the functional traits of overstory trees have been extensively studied, minimal research has been conducted regarding understory species, despite the understory layer is an important component of temperate forests. Such insufficiency limit the broader understanding of processes and functions in forest ecosystems, particularly when under the increasing atmospheric nitrogen (N) deposition. Here, we investigated the responses of 18 leaf functional traits in six understory herbaceous species within young and mature stands (three species per stand) in larch (Larix principis-rupprechtii) plantations that subjected to 12 years of anthropogenic N addition. We found that N addition did not significantly impact the photosynthetic traits of understory herbaceous species in either stand; it only led to increased chlorophyll content in Geum aleppicum Jacq. Similarly, with the exception of decreases in the predawn leaf water potential of Sanguisorba officinalis L., N addition did not significantly affect leaf hydraulic traits. With the exception of changes to adaxial epidermis thickness in Potentilla chinensis Ser. (decreased) and G. aleppicum (increased), N addition had negligible effects on leaf anatomical traits and specific leaf area, however, interspecific variations in the plasticity of leaf anatomical traits were observed. Stable responses to N addition were also observed for nonstructural carbohydrates (NSC) and their components (soluble sugars and starch), with the exception of Polygonum divaricatum L., which exhibited increases in NSC. Overall, our results suggest that the functional traits of understory herbaceous species exhibit stability under conditions of long-term N enrichment in temperate plantations.
Atmospheric nitrogen (N) deposition has substantially increased in the past century because of fossil fuel combustion and the use of fertilizers in agriculture (Galloway et al., 2004). Globally, the influence of N deposition on ecosystem structure and function has been extensively studied (Bobbink et al., 2010; Lu et al., 2010a; Marklein and Houlton, 2012; Midolo et al., 2019; Liang et al., 2020). Indeed, N deposition could significantly affect many ecological properties and processes. For example, atmospheric N deposition can lead to soil acidification, imbalances between N and phosphorus (P), the loss of exchangeable base cations, and declines in biodiversity (Bobbink et al., 2010; Lu et al., 2010b; Peñuelas et al., 2013; Lu et al., 2018; Midolo et al., 2019). However, N deposition can also improve plant biomass accumulation and soil carbon sequestration in N-limited ecosystems (Thomas et al., 2010; Maaroufi et al., 2015; Zak et al., 2017; Schulte-Uebbing & de Vries, 2018).
Leaf functional traits, including photosynthetic, hydraulic, and anatomical traits, play critical roles in leaf function, which influence the regulation of plant growth and survival (Wright et al., 2010; Liu et al., 2016; Rowland et al., 2021; Sonawane et al., 2021; Xiong and Flexas, 2022). Increased N availability may affect gas exchange, as well as hydraulic and anatomical structures, with consequences for plant performance (Cai et al., 2017; Báez and Homeier, 2018; Zhang et al., 2021a). Most studies on the responses of functional traits to N addition were focused on grassland species or woody tree species (Liang et al., 2020; Zhang et al., 2021b). For example, Pivovaroff et al. (2016) observed increased predawn water potential (Ψpd), but no changes in photosynthesis, leaf hydraulic conductivity (Kleaf), or midday water potential (Ψmd), in response to long-term N addition in a Mediterranean-type ecosystem. In contrast, Zhang et al. (2021b) reported that short-term N addition increased net photosynthetic rate (An) and chlorophyll concentration (Chl), and reduced specific leaf area (SLA) and stomatal conductance (gs). Additionally, leaf anatomical traits (e.g., palisade mesophyll thickness [PMT], the diameter of the midrib vascular bundle [VBD], and leaf thickness [LT]) can influence leaf photosynthetic and hydraulic capacities; these traits are responsive to environmental changes such as N enrichment (Niinemets et al., 2007; Kröber et al., 2015; Cai et al., 2017; He et al., 2018; Sonawane et al., 2021). The stratum of understory vegetation preserves about 80% of plant biodiversity in temperate forests (Gilliam, 2007). Considering that understory vegetation is an important component of forest ecosystems, functional changes in understory vegetation can substantially affect forest structure and function, such as tree regeneration, carbon-nutrient-water cycling, and stability (Gilliam, 2007; Gilliam et al., 2016; Giuggiola et al., 2018; Landuyt et al., 2019; Blondeel et al., 2020; Xing et al., 2022). For example, in a recent review, Balandier et al. (2022) reported that understory vegetation contributed one-third of ecosystem evapotranspiration in boreal and temperate forests, and the removal of understory vegetation could reduce water competition, with subsequent consequence of increasing soil water content and stimulating sap flow and the growth of overstory tree species (e.g., mean annual radial growth increased by 4.6-fold, Giuggiola et al., 2018). Deng et al. (2016) demonstrated that, when subjected to exogenous N inputs, understory vegetation have competitive advantages in terms of resource acquisition (e.g., P) compared with the corresponding young overstory trees. However, relative to overstory tree species, understory vegetation were largely ignored during the functioning assessments of forest ecosystems, particularly under climate change (Landuyt et al., 2019).
Although suitable levels of N addition can benefit plant productivity, excessive N may weaken or constrain growth (Högberg et al., 2006; Karst et al., 2021), presumably because of nutrient imbalances and changes in functional traits induced by N addition (Högberg et al., 2006; Báez and Homeier, 2018; Li et al., 2022). A recent meta-analysis by Liang et al. (2020) revealed that N addition significantly increases photosynthetic parameters such as photosynthetic rate, gs, and transpiration rate (E). Moreover, Du et al. (2020) reported that N addition substantially reduces soluble sugars (SS) in woody plant leaves without influencing starch (ST), whereas the opposite effects were observed in herbaceous plant leaves. Notably, the responses of functional traits to climate change have been extensively concentrated on overstory tree species; in contrast, minimal research has been conducted regarding to understory vegetation, particularly with respect to anatomical and hydraulic traits, and moreover, the responses of understory and overstory species may significantly differ (Mo et al., 2020; Jacob et al., 2022). The few studies on understory vegetation showed that N enrichment had limited effects on functional traits such as SLA of forest understory community (Blondeel et al., 2020); and in most cases, long-term (~10-year) N addition exerted little impacts on leaf functional traits of understory vegetation in tropical forests (Mao et al., 2017; Mo et al., 2020). In addition, experimental duration may affect the responses of functional traits to N addition, but most studies have been conducted over relatively short temporal scales (Mao et al., 2018; Yan et al., 2018a; Tang et al., 2021; Zhang et al., 2021b); while studies of the effects of long-term N addition are rare (Blondeel et al., 2020; Liang et al., 2020). Furthermore, stand age may modulate the responses of plants to N addition (Schulte-Uebbing & de Vries, 2018; Yan et al., 2018a), presumably because of differences in environmental conditions. Therefore, analyses of changes in leaf functional traits in response to long-term N addition of understory vegetation under different aged forests would facilitate the comprehensive and predictive changes in forest ecological processes and functions under increasing atmospheric N deposition (Landuyt et al., 2019).
The total area of plantations in the temperate zone has considerably increased in recent decades, particularly in China, which contains approximately one-third of the global plantation area (Payn et al., 2015). Larch (Larix spp.) is the main timber tree species in northern China and the Northern Hemisphere (Mason and Zhu, 2014; Yan et al., 2017; Yan et al., 2018b). Here, we selected six understory herbaceous species that occurs in young and mature larch (L. principis-rupprechtii) plantations which were subjected to anthropogenic N addition for 12 consecutive years, with the aim to explore how long-term N addition affects the functional traits of understory species. We measured 18 leaf functional traits, including An, gs, E, Chl, Ψpd, Ψmd, Kleaf, adaxial and abaxial epidermis thickness (ADET and ABET), PMT, spongy mesophyll thickness (SMT), VBD, diameter of midrib (MD), LT, SLA, and nonstructural carbohydrates (NSCs) and their components (SS and ST). We hypothesized that long-term N addition would affect leaf photosynthetic, hydraulic, and anatomical traits of understory species because of the typical N-limitation of the study region (Sun et al., 2016). Moreover, we presumed that the magnitude of N-addition-induced changes in functional traits would vary according to species and stand age because of differences in environmental conditions.
The study was conducted at the Saihanba Ecological Station (42° 25’ N, 117° 15’ E, 1505 m elevation) of Peking University, located in the Saihanba National Forest Park in Hebei Province, northern China. The climate is semi-humid, with long, cold winters (November–March), and short springs and summers. The annual mean temperature is –1.0°C, and the monthly mean ranges from –21.8°C in January to 16.2°C in June. The annual mean precipitation is 460 mm. Snowfall begins in mid-October, and snow melt occurs in early April. Typically, < 30 cm of snow accumulates in winter. Ambient N deposition is 13 kg ha−1 year−1 (Deng et al., 2016).
In 2009, an 11-year-old larch (Larix principis-rupprechtii) plantation and a 45-year-old larch plantation were selected to represent young and mature stands, respectively. Both of the stands were fenced by 100 m × 100 m, and each was divided into nine 20 m × 20 m plots, with a buffer zone of approximately 10 m between adjacent plots. Nitrogen addition began in May 2010 and included three treatments: control (no N addition), low N addition (20 kg N ha−1 year−1; N20), and high N addition (50 kg N ha−1 year−1; N50). Each treatment was replicated three times. Between May 2010 and the present, liquid urea was applied each month to the N addition treatments during the growing season (May–October) using backpack sprayers to ensure homogeneity within each plot. An equal volume of water was added to the control plots. Details of the experimental design can be seen in Yan et al. (2018c).
In mid-August 2021, we sampled leaves from three understory species in each stand. Specifically, Vicia sepium L., Potentilla chinensis Ser., and Polygonum divaricatum L. were sampled from the young stand; Agrimonia pilosa Ldb., Geum aleppicum Jacq., and Sanguisorba officinalis L. were sampled from the mature stand. For trait measurements, we sampled only fully expanded, mature leaves from healthy individuals with no visible signs of pests or diseases.
We selected three individuals of each species with similar growth status in each plot, then sampled fully mature leaves from each individual. Photosynthetic parameters, including An, gs, and E, were measured in situ between 09:00 and 11:30 on sunny days with a LI-6800 portable photosynthesis system (Li-Cor Inc., Lincoln, NE, USA). The CO2 concentration in the chamber was maintained at 400 μmol mol−1, and the temperature of the leaf cuvette was maintained at 25°C. The photosynthetic photon flux density was set at 1500 μmol m−2 s−1. The relative humidity was maintained at 50–60%. After photosynthesis measurements, Chl was measured on six replicates of each species in each plot using a chlorophyll content meter (CCM-300, Opti-Sciences Inc., Hudson, NH, USA).
We also randomly selected ≥ 5 individuals of each species in each plot for leaf sampling. The leaves were cut with scissors, placed in envelopes inside a cooler, and immediately transported to the laboratory. Leaves were scanned using a digital scanner and leaf area was calculated using ImageJ software. Leaves were then oven-dried at 65°C for ≥ 48 h to obtain leaf dry mass. Specific leaf area (cm2 g−1) was obtained through the division of leaf area by leaf dry mass.
We collected healthy, intact leaves from five individuals of each species in each plot. The leaf water potential metrics Ψpd and Ψmd were measured before sunrise (06:00) and at midday (12:00–14:00), respectively, using a pressure chamber (Model 1505D, PMS Instrument Company, Albany, OR, USA). Immediately after E measurement, the leaves of three individuals were used to measure water potential. Neighboring leaves were covered with aluminum foil for 30 min prior to water potential measurements.
Kleaf (mmol m−2 s−1 MPa−1) was calculated as:
where E (mol m−2 s−1) represents the transpiration rate per unit leaf area as measured with a LI-6800 portable photosynthesis system (Li-Cor Inc.), and ΔΨ (MPa) is the difference in water potential between covered and uncovered leaves.
We assessed leaf anatomy by observing paraffin sections of fresh leaf samples under a light microscope. We sampled five healthy, fully mature leaves from five individuals of each species in each plot. We cut each leaf to extract the leaf blade tissue within 5 mm of either side of the midrib, then sliced this sample into 5 mm segments. Segments were fixed in FAA solution for ≥ 24 h; dehydrated using varying concentrations of alcohol; cleared with xylene; and embedded in paraffin blocks. The paraffin blocks were cut into 5 µm sections using a sliding microtome, stained with safranin-fast green, and sealed with gum. The prepared cross-sections were observed under a light microscope. ImageJ software was used to measure anatomical traits including adaxial epidermis thickness, abaxial epidermis thickness, palisade mesophyll thickness, spongy mesophyll thickness, diameter of midrib, diameter of the midrib vascular bundle, and leaf thickness.
NSCs were determined using the modified anthrone method. Fresh leaf samples were microwaved at 700 W for 90 s to stop enzymatic activity, then oven-dried for ≥ 48 h at 65°C to achieve a constant weight and ground with a ball mill (MM400, RETSCH GmbH, Haan, Germany). Powdered leaf samples (0.1 g) were placed into 10 ml centrifuge tubes with 2 ml of 80% ethanol. The mixture was incubated for 30 min in a water bath at 80°C, then centrifuged at 3500 rpm for 10 min. The supernatant was retained, and the residue was extracted two additional times for SS measurement. After SS extraction, the residue was subjected to ST extraction by adding 2 ml of distilled water and boiling the solution in a ~100°C water bath for 15 min, then adding 2 ml of 9.2 mol L−1 HClO4 and shaking the mixture for 15 min. Then, 4 ml of distilled water was added and the solution was centrifuged at 3500 rpm for 10 min. Subsequently, 2 ml of 4.6 mol L−1 HClO4 and 5 ml of distilled water were added to the precipitate, the solution was centrifuged at 3500 rpm for 5 min, and the supernatant was retained. Twice, we added an 5 ml of distilled water to wash the residue, then combined it into the supernatant. Concentrations of SS and ST were measured using a UV-Vis spectrophotometer with an absorbance of 620 nm. Total NSC concentrations were calculated by summing SS and ST.
Two-way analysis of variance was used to determine how functional traits were affected by N addition, species, and the interaction between those two factors. Least significant difference tests were used to assess differences among treatments. When necessary, the data were transformed to improve homogeneity of variance and/or normality. When the transformed data did not satisfy these assumptions, non-parametric Kruskal–Wallis tests were used. All analyses were conducted using SPSS 16.0 for Windows (SPSS Inc., Chicago, IL, USA), and differences were considered statistically significant at P-values< 0.05.
Long-term N addition did not significantly impact An, gs, or E in any of the six understory species in either stand (Figure 1); however, Chl was increased in G. aleppicum. We observed minimal variation in photosynthetic traits among species (Table 1).
Figure 1 Effects of N addition on An (A), gs (B), E (C), and Chl (D) of Vicia sepium (VS), Potentilla chinensis (PC), Polygonum divaricatum (PD) in the young stand, and Agrimonia pilosa (AP), Geum aleppicum (GA), and Sanguisorba officinalis (SO) in the mature stand. Asterisk represents significant differences among N addition treatments (P < 0.05). Values are means ± standard errors (n = 3).
With the exception of a reduction in Ψpd in S. officinalis, N addition did not significantly affect Ψpd, Ψmd, or Kleaf in any of the six species (Figure 2). Ψpd and Ψmd significantly varied among species in the mature and young stands, respectively, but Kleaf had no variation among species in either stand (Table 1).
Figure 2 Effects of N addition on Ψpd (A), Ψmd (B), and Kleaf (C) of Vicia sepium (VS), Potentilla chinensis (PC), Polygonum divaricatum (PD) in the young stand, and Agrimonia pilosa (AP), Geum aleppicum (GA), and Sanguisorba officinalis (SO) in the mature stand. Asterisk represents significant differences among N addition treatments (P < 0.05). Values are means ± standard errors (n = 3).
After N addition, adaxial epidermis thickness decreased in P. chinensis in the young stand, whereas it increased in G. aleppicum in the mature stand (Figure 3). Nitrogen addition had no other significant impacts on leaf anatomical traits. However, with the exception of SLA, leaf anatomical traits significantly differed among species; spongy mesophyll thickness slightly differed among species in the mature stand (P = 0.072).
Figure 3 Effects of N addition on ADET (A), ABET (B), PMT (C), SMT (D), VBD (E), MD (F), LT (G), and SLA (H) of Vicia sepium (VS), Potentilla chinensis (PC), Polygonum divaricatum (PD) in the young stand, and Agrimonia pilosa (AP), Geum aleppicum (GA), and Sanguisorba officinalis (SO) in the mature stand. Asterisk represents significant differences among N addition treatments (P < 0.05). Values are means ± standard errors (n = 3).
With the exception of significant increases in total NSC concentrations in P. divaricatum in the young stand (Figure 4), neither NSC nor its components (SS and ST) exhibited significant responses to N addition in any understory species. However, we observed significant interspecific variation in all three variables.
Figure 4 Effects of N addition on NSC (A), SS (B), and ST (C) of Vicia sepium (VS), Potentilla chinensis (PC), Polygonum divaricatum (PD) in the young stand, and Agrimonia pilosa (AP), Geum aleppicum (GA), and Sanguisorba officinalis (SO) in the mature stand. Asterisk represents significant differences among N addition treatments (P < 0.05). Values are means ± standard errors (n = 3).
Numerous studies have revealed positive correlations between leaf N concentrations and photosynthetic capacity (Evans, 1989; Reich et al., 1997; Wright et al., 2004), largely because N is an important component of photosynthetic proteins (Evans, 1989; Güsewell, 2004; Evans and Clarke, 2019). Contrary to our hypothesis, we did not observe improvements in the An of understory species after long-term N addition (Figure 1). However, N addition had significant positive effects on leaf N concentrations of understory species in the mature stand (Yan et al., 2018a). These findings indicate that N addition-induced increases in leaf N concentrations do not necessarily lead to increases in photosynthetic carbon assimilation capacity, probably due to that larger fraction of leaf N was allocated to cell walls but not photosynthetic proteins (Bauer et al., 2004; Talhelm et al., 2011; Pivovaroff et al., 2016; Onoda et al., 2017). Our results differ from the findings in other N-limited ecosystems, where higher N availability typically improves leaf physiological traits (Cooke et al., 2005; Zhang et al., 2021a,b).
The lack of changes in An in response to long-term N enrichment can be attributed to various mechanisms. Nitrogen is unlikely to be a key limiting factor for understory species in the young stand; thus, N addition may have induced few changes in leaf N and had minimal effects on the allocation of N to photosynthetic processes (Yan et al., 2018a). However, the availability of other resources, such as light, may constrain the growth of understory species (Yan et al., 2018a; De Pauw et al., 2022); in the mature stand, increased leaf N concentrations were presumably insufficient to compensate for the negative effects of increased overstory shading on photosynthesis (Palmroth et al., 2014; Yan et al., 2018a). Additionally, the excess N in leaves may have been allocated to soluble proteins and/or free amino acids, rather than chlorophyll (Mao et al., 2018). Since NSCs are the primary products of photosynthesis (Hartmann and Trumbore, 2016), and thus, NSCs did not vary (Figure 4) due to the relatively stable photosynthetic rates. The exception of the statistically significant increase in NSCs observed in P. divaricatum can presumably be attributed to corresponding improvements in leaf photosynthesis albeit not significant (Figures 1, 4).
With the exception of G. aleppicum, N addition had no impact on Chl among species in the mature stand (Figure 1) despite increased leaf N concentrations (Yan et al., 2018a). This result implies that the additional leaf N was probably incorporated into soluble proteins and/or free amino acids, rather than chlorophyll; however, further investigations into leaf N metabolism are needed to confirm this hypothesis.
Leaf water transport plays a critical role in regulating the growth and survival of plants, particularly in the context of increasing atmospheric N deposition (Sack and Holbrook, 2006; Wang et al., 2016; Jin et al., 2020). The efficiency of water transport through the leaf can be quantified using Kleaf (Sack and Holbrook, 2006). Given that water transport resistance is generally higher in leaves than in stems and shoots, Kleaf has a substantial impact on water transport at the whole-plant scale (Sack et al., 2003; Sack et al., 2005; Wang et al., 2016), particularly in understory herbaceous species. Analyses of trees have shown that N addition significantly affects Kleaf, thereby influencing whole-plant water use (Wang et al., 2016; Jin et al., 2020; Zhang et al., 2021c). However, in contrast to our hypotheses, N addition did not alter Kleaf values in understory species in either stand (Figure 2). The most probable explanation for this result arises from the lack of changes in leaf anatomical structure, such as VBD (Figure 3). Leaf anatomical traits (e.g., VBD) determine Kleaf, and plants with larger VBDs are generally characterized by higher Kleaf values (Sack and Holbrook, 2006; Sonawane et al., 2021; Xiong and Flexas, 2022). Accordingly, the lack of changes in gs and E (Figure 1) is understandable.
Leaf water potential may reflect the functional capacity of plants to respond to water availability. Among leaf water metrics, Ψpd is considered the most useful and representative one for determining whether plants suffer from drought stress (Trugman et al., 2021). With the exception of a reduction in Ψpd in S. officinalis (Figure 2), Ψpd and Ψmd remained relatively constant in both stands despite long-term N inputs. These results indicate that although S. officinalis may experience drought stress, long-term N addition had minor impacts on leaf water relations in the other understory species.
Leaf anatomical traits are associated with leaf economics (e.g., photosynthetic rate) and leaf hydraulics (e.g., hydraulic conductivity) (Niinemets et al., 2007; Liang et al., 2019; Sonawane et al., 2021; Xiong and Flexas, 2022). For example, a thicker palisade mesophyll layer may contain more chloroplasts, thereby optimizing photosynthesis; in contrast, a thinner epidermis may promote gas exchange and increase transpiration (Kröber et al., 2015; He et al., 2018). Thus, in the context of environmental change (e.g., increased N availability), we expect plants to exhibit adaptations in leaf anatomy that would maximize overall plant function (Chen et al., 2010; Cai et al., 2017; He et al., 2018). Previous studies have demonstrated that even short-term N addition affects some leaf anatomical traits, such as the diameters of the midrib and midrib vascular bundle in Arabidopsis thaliana, without affecting other traits (e.g., thicknesses of leaves, palisade and spongy mesophyll layers, and adaxial and abaxial epidermis) (Cai et al., 2017). Contrary to our hypotheses, however, few of the measured leaf anatomical traits exhibited changes despite 12 years of N addition (Figure 3). The apparent stability of leaf anatomical traits reflects the acclimation of understory species to long-term N inputs in temperate larch plantations. Our findings differ from the results of Zhang et al. (2021c), which showed that two tree species (Castanopsis chinensis and Schima superba) in a subtropical forest exhibited decreased leaf thickness after 6-year canopy N addition, and Guo et al. (2022), which reported increases in palisade mesophyll thickness, leaf thickness, and palisade-spongy mesophyll ratio for the herbaceous species of Stipa glareosa in a desert steppe after 3-year N addition. Furthermore, we observed substantial interspecific variations in leaf anatomical traits (Figure 3; Table 1), indicating that these understory species exhibited distinct adaptive strategies.
Nevertheless, we selected only 3–5 individuals per species within each plot, which may, to some extent, limit the statistical power, thereby, more replicates might be better to detect the differences. Furthermore, trait correlations and other environmental factors such as temperature, drought, and herbivore outbreaks, may have constrained the responses of leaf functional traits to N addition, which deserve further investigations.
Using a 12-year anthropogenic N addition experiment, we investigated the responses of 18 leaf functional traits in six understory herbaceous species to increased N availability in young and mature larch plantations in northern China. Contrary to our hypotheses, understory species exhibited few eco-physiological changes in response to long-term N addition. This lack of response highlights the capacity of understory species to maintain the stability of functional traits. Confirmation of the representativeness of our results requires further investigations into the plasticity of other traits and species in these larch plantations.
The raw data supporting the conclusions of this article will be made available by the authors, without undue reservation.
TY: Conceptualization, Formal Analysis, Funding acquisition, Investigation, Methodology, Project administration, Supervision, Validation, Visualization, Writing – original draft. LW: Data curation, Formal Analysis, Investigation, Methodology, Visualization. PW: Investigation, Methodology. TZ: Investigation, Methodology.
The author(s) declare financial support was received for the research, authorship, and/or publication of this article. This work was supported by the National Natural Science Foundation of China (32171762), the Natural Science Foundation of Gansu Province (22JR5RA460), and the Lanzhou University’s “Double First-Class” Guided Project Team Building-Funding-Research Startup Fee.
We thank Dr. Huanhuan Song for her helpful and critical comments and suggestions on experimental design and the earlier version of this manuscript.
The authors declare that the research was conducted in the absence of any commercial or financial relationships that could be construed as a potential conflict of interest.
All claims expressed in this article are solely those of the authors and do not necessarily represent those of their affiliated organizations, or those of the publisher, the editors and the reviewers. Any product that may be evaluated in this article, or claim that may be made by its manufacturer, is not guaranteed or endorsed by the publisher.
Báez, S., Homeier, J. (2018). Functional traits determine tree growth and ecosystem productivity of a tropical montane forest: Insights from a long-term nutrient manipulation experiment. Global Change Biol. 24, 399–409. doi: 10.1111/gcb.13905
Balandier, P., Gobin, R., Prévosto, B., Korboulewsky, N. (2022). The contribution of understorey vegetation to ecosystem evapotranspiration in boreal and temperate forests: a literature review and analysis. Eur. J. For. Res. 141, 979–997. doi: 10.1007/s10342-022-01505-0
Bauer, G. A., Bazzaz, F. A., Minocha, R., Long, S., Magill, A., Aber, J., et al. (2004). Effects of chronic N additions on tissue chemistry, photosynthetic capacity, and carbon sequestration potential of a red pine (Pinus resinosa Ait.) stand in the NE United States. For. Ecol. Manage. 196, 173–186. doi: 10.1016/j.foreco.2004.03.032
Blondeel, H., Perring, M. P., Depauw, L., De Lombaerde, E., Landuyt, D., De Frenne, P., et al. (2020). Light and warming drive forest understorey community development in different environments. Global Change Biol. 26, 1681–1696. doi: 10.1111/gcb.14955
Bobbink, R., Galloway, K. H. J., Spranger, T., Alkemade, R., Ashmore, M., Bustamante, M., et al. (2010). Global assessment of nitrogen deposition effects on terrestrial plant diversity: a synthesis. Ecol. Appl. 20, 30–59. doi: 10.1890/08-1140.1
Cai, Q., Ji, C. J., Yan, Z. B., Jiang, X. X., Fang, J. Y. (2017). Anatomical responses of leaf and stem of Arabidopsis thaliana to nitrogen and phosphorus addition. J. Plant Res. 130, 1035–1045. doi: 10.1007/s10265-017-0960-2
Chen, F. S., Zeng, D. H., Fahey, T. J., Yao, C. Y., Yu, Z. Y. (2010). Response of leaf anatomy of Chenopodium acuminatum to soil resource availability in a semi-arid grassland. Plant Ecol. 209, 375–382. doi: 10.1007/s11258-010-9778-x
Cooke, J. E. K., Martin, T. A., Davis, J. M. (2005). Short-term physiological and developmental responses to nitrogen availability in hybrid poplar. New Phytol. 167, 41–52. doi: 10.1111/j.1469-8137.2005.01435.x
Deng, M. F., Liu, L. L., Sun, Z. Z., Piao, S. L., Ma, Y. C., Chen, Y. W., et al. (2016). Increased phosphate uptake but not resorption alleviates phosphorus deficiency induced by nitrogen deposition in temperate Larix principis-rupprechtii plantations. New Phytol. 212, 1019–1029. doi: 10.1111/nph.14083
De Pauw, K., Sanczuk, P., Meeussen, C., Depauw, L., De Lombaerde, E., Govaert, S., et al. (2022). Forest understorey communities respond strongly to light in interaction with forest structure, but not to microclimate warming. New Phytol. 233, 219–235. doi: 10.1111/nph.17803
Du, Y., Lu, R. L., Xia, J. Y. (2020). Impacts of global environmental change drivers on non-structural carbohydrates in terrestrial plants. Funct. Ecol. 34, 1525–1536. doi: 10.1111/1365-2435.13577
Evans, J. R. (1989). Photosynthesis and nitrogen relationships in leaves of C3 plants. Oecologia 78, 9–19. doi: 10.1007/BF00377192
Evans, J. R., Clarke, V. C. (2019). The nitrogen cost of photosynthesis. J. Exp. Bot. 70, 7–15. doi: 10.1093/jxb/ery366
Galloway, J. N., Dentener, F. J., Capone, D. G., Boyer, E. W., Howarth, R. W., Seitzinger, S. P., et al. (2004). Nitrogen cycles: past, present, and future. Biogeochemistry 70, 153–226. doi: 10.1007/s10533-004-0370-0
Gilliam, F. S. (2007). The ecological significance of the herbaceous layer in temperate forest ecosystems. Bioscience 57, 845–858. doi: 10.1641/B571007
Gilliam, F. S., Welch, N. T., Phillips, A. H., Billmyer, J. H., Peterjohn, W. T., Fowler, Z. K., et al. (2016). Twenty-five-year response of the herbaceous layer of a temperate hardwood forest to elevated nitrogen deposition. Ecosphere 7, e01250. doi: 10.1002/ecs2.1250
Giuggiola, A., Zweifel, R., Feichtinger, L. M., Vollenweider, P., Bugmann, H., Haeni, M., et al. (2018). Competition for water in a xeric forest ecosystem-Effects of understory removal on soil micro-climate, growth and physiology of dominant Scots pine trees. For. Ecol. Manage. 409, 24–1249. doi: 10.1016/j.foreco.2017.11.002
Guo, A. X., Zuo, X. A., Hu, Y., Yue, P., Li, X. Y., Lv, P., et al. (2022). Two dominant herbaceous species have different plastic responses to N addition in a desert steppe. Front. Plant Sci. 13. doi: 10.3389/fpls.2022.801427
Güsewell, S. (2004). N:P ratios in terrestrial plants: variation and functional significance. New Phytol. 164, 243–266. doi: 10.1111/j.1469-8137.2004.01192.x
Hartmann, H., Trumbore, S. (2016). Understanding the roles of nonstructural carbohydrates in forest trees-from what we can measure to what we want to know. New Phytol. 211, 386–403. doi: 10.1111/nph.13955
He, N. P., Liu, C. C., Tian, M., Li, M. L., Yang, H., Yu, G. R., et al. (2018). Variation in leaf anatomical traits from tropical to cold-temperate forests and linkage to ecosystem functions. Funct. Ecol. 32, 10–19. doi: 10.1111/1365-2435.12934
Högberg, P., Fan, H. B., Quist, M., Binkley, D., Tamm, C. O. (2006). Tree growth and soil acidification in response to 30 years of experimental nitrogen loading on boreal forest. Global Change Biol. 12, 489–499. doi: 10.1111/j.1365-2486.2006.01102.x
Jacob, V., Choat, B., Churchill, A. C., Zhang, H. Y., Barton, C. V. M., Krishnananthaselvan, A., et al. (2022). High safety margins to drought-induced hydraulic failure found in five pasture grasses. Plant Cell Environ. 45, 1631–1646. doi: 10.1111/pce.14318
Jin, Y., Wang, C. K., Zhou, Z. H., Gu, J. C. (2020). Contrasting responses of hydraulic traits between leaf and branch to 16-year nitrogen addition in a larch plantation. For. Ecol. Manage. 475, 118461. doi: 10.1016/j.foreco.2020.118461
Karst, J., Wasyliw, J., Birch, J. D., Franklin, J., Chang, S. X., Erbilgin, N. (2021). Long-term nitrogen addition does not sustain host tree stem radial growth but doubles the abundance of high-biomass ectomycorrhizal fungi. Global Change Biol. 27, 4125–4138. doi: 10.1111/gcb.15713
Kröber, W., Heklau, H., Bruelheide, H. (2015). Leaf morphology of 40 evergreen and deciduous broadleaved subtropical tree species and relationships to functional ecophysiological traits. Plant Biol. 17, 373–383. doi: 10.1111/plb.12250
Landuyt, D., De Lombaerde, E., Perring, M. P., Hertzog, L. R., Ampoorter, E., Maes, S. L., et al. (2019). The functional role of temperate forest understorey vegetation in a changing world. Global Change Biol. 25, 3625–3641. doi: 10.1111/gcb.14756
Li, R. S., Yu, D., Zhang, Y. K., Han, J. M., Zhang, W. D., Yang, Q. P., et al. (2022). Investment of needle nitrogen to photosynthesis controls the nonlinear productivity response of young Chinese fir trees to nitrogen deposition. Sci. Total Environ. 840, 156537. doi: 10.1016/j.scitotenv.2022.156537
Liang, J., Wright, J. S., Cui, X. W., Sternberg, L., Gan, W. X., Lin, G. H. (2019). Leaf anatomical traits determine the 18O enrichment of leaf water in coastal halophytes. Plant Cell Environ. 41, 2744–2757. doi: 10.1111/pce.13398
Liang, X. Y., Zhang, T., Lu, X. K., Ellsworth, D. S., BassiriRad, H., You, C. M., et al. (2020). Global response patterns of plant photosynthesis to nitrogen addition: A meta-analysis. Global Change Biol. 26, 3585–3600. doi: 10.1111/gcb.15071
Liu, X. J., Swenson, N. G., Lin, D. M., Mi, X. C., Umaña, M. N., Schmid, B., et al. (2016). Linking individual-level functional traits to tree growth in a subtropical forest. Ecology 97, 2396–2405. doi: 10.1002/ecy.1445
Lu, X. K., Mo, J. M., Gilliam, F. S., Zhou, G. Y., Fang, Y. T. (2010b). Effects of experimental nitrogen additions on plant diversity in an old-growth tropical forest. Global Change Biol. 16, 2688–2700. doi: 10.1111/j.1365-2486.2010.02174.x
Lu, X. K., Vitousek, P. M., Mao, Q. G., Gilliam, F. S., Luo, Y. Q., Zhou, G. Y., et al. (2018). Plant acclimation to long-term high nitrogen deposition in an N-rich tropical forest. Proc. Natl. Acad. Sci. United States America 115, 5187–5192. doi: 10.1073/pnas.1720777115
Lu, M., Yang, Y. H., Luo, Y. Q., Fang, C. M., Zhou, X. H., Chen, J. K., et al. (2010a). Responses of ecosystem nitrogen cycle to nitrogen addition: a meta-analysis. New Phytol. 189, 1040–1050. doi: 10.1111/j.1469-8137.2010.03563.x
Maaroufi, N. I., Nordin, A., Hasselquist, N. J., Bach, L. H., Palmqvist, K., Gundale, M. J. (2015). Anthropogenic nitrogen deposition enhances carbon sequestration in boreal soils. Global Change Biol. 21, 3169–3180. doi: 10.1111/gcb.12904
Mao, Q. G., Lu, X. K., Mo, H., Gundersen, P., Mo, J. M. (2018). Effects of simulated N deposition on foliar nutrient status, N metabolism and photosynthetic capacity of three dominant understory plant species in a mature tropical forest. Sci. Total Environ. 610–611, 555–562. doi: 10.1016/j.scitotenv.2017.08.087
Mao, Q. G., Lu, X. K., Wang, C., Zhou, K. J., Mo, J. M. (2017). Responses of understory plant physiological traits to a decade of nitrogen addition in a tropical reforested ecosystem. For. Ecol. Manage. 401, 65–74. doi: 10.1016/j.foreco.2017.06.047
Marklein, A. R., Houlton, B. Z. (2012). Nitrogen inputs accelerate phosphorus cycling rates across a wide variety of terrestrial ecosystems. New Phytol. 193, 696–704. doi: 10.1111/j.1469-8137.2011.03967.x
Mason, W. L., Zhu, J. J. (2014). Silviculture of planted forests managed for multi-functional objectives: lessons from Chinese and British experiences (Netherlands: Springer).
Midolo, G., Alkemade, R., Schipper, A. M., Benítez-López, A., Perring, M. P., de Vries, W. (2019). Impacts of nitrogen addition on plant species richness and abundance: A global meta-analysis. Global Ecol. Biogeography 28, 398–413. doi: 10.1111/geb.12856
Mo, Q. F., Wang, W. J., Chen, Y. Q., Peng, Z. T., Zhou, Q. (2020). Response of foliar functional traits to experimental N and P addition among overstory and understory species in a tropical secondary forest. Global Ecol. Conserv. 23, e01109. doi: 10.1016/j.gecco.2020.e01109
Niinemets, Ü, Lukjanova, A., Turnbull, M. H., Sparrow, A. D. (2007). Plasticity in mesophyll volume fraction modulates light-acclimation in needle photosynthesis in two pines. Tree Physiol. 27, 1137–1151. doi: 10.1093/treephys/27.8.1137
Onoda, Y., Wright, I. J., Evans, J. R., Hikosaka, K., Kitajima, K., Niinemets, Ü, et al. (2017). Physiological and structural tradeoffs underlying the leaf economics spectrum. New Phytol. 214, 1447–1463. doi: 10.1111/nph.14496
Palmroth, S., Bach, L. H., Nordin, A., Palmqvist, K. (2014). Nitrogen-addition effects on leaf traits and photosynthetic carbon gain of boreal forest understory shrubs. Oecologia 175, 457–470. doi: 10.1007/s00442-014-2923-9
Payn, T., Carnus, J. M., Freer-Smith, P., Kimberley, M., Kollert, W., Liu, S. R., et al. (2015). Changes in planted forests and future global implications. For. Ecol. Manage. 352, 57–67. doi: 10.1016/j.foreco.2015.06.021
Peñuelas, J., Poulter, B., Sardans, J., Ciais, P., van der Velde, M., Bopp, L., et al. (2013). Human-induced nitrogen-phosphorus imbalances alter natural and managed ecosystems across the globe. Nat. Commun. 4, 2934. doi: 10.1038/ncomms3934
Pivovaroff, A. L., Santiago, L. S., Vourlitis, G. L., Grantz, D. A., Allen, M. F. (2016). Plant hydraulic responses to long-term dry season nitrogen deposition alter drought tolerance in a Mediterranean-type ecosystem. Oecologia 181, 721–731. doi: 10.1007/s00442-016-3609-2
Reich, P. B., Walters, M. B., Ellsworth, D. S. (1997). From tropics to tundra: Global convergence in plant functioning. Proc. Natl. Acad. Sci. United States America 94, 13730–13734. doi: 10.1073/pnas.94.25.13730
Rowland, L., Oliveira, R. S., Bittencourt, P. R. L., Giles, A. L., Coughlin, I., Costa, P. D., et al. (2021). Plant traits controlling growth change in response to a drier climate. New Phytol. 229, 1363–1374. doi: 10.1111/nph.16972
Sack, L., Cowan, P. D., Jaikumar, N., Holbrook, N. M. (2003). The ‘hydrology’ of leaves: co-ordination of structure and function in temperate woody species. Plant Cell Environ. 26, 1343–1356. doi: 10.1046/j.0016-8025.2003.01058.x
Sack, L., Holbrook, N. M. (2006). Leaf hydraulics. Annu. Rev. Plant Biol. 57, 361–381. doi: 10.1146/annurev.arplant.56.032604.144141
Sack, L., Tyree, M. T., Holbrook, N. M. (2005). Leaf hydraulic architecture correlates with regeneration irradiance in tropical rainforest trees. New Phytol. 167, 403–413. doi: 10.1111/j.1469-8137.2005.01432.x
Schulte-Uebbing, L., de Vries, W. (2018). Global-scale impacts of nitro-gen deposition on tree carbon sequestration in tropical, temperate, and boreal forests: A meta-analysis. Global Change Biol. 24, e416–e431. doi: 10.1111/gcb.13862
Sonawane, B. V., Koteyeva, N. K., Johnson, D. M., Cousins, A. B. (2021). Differences in leaf anatomy determines temperature response of leaf hydraulic and mesophyll CO2 conductance in phylogenetically related C4 and C3 grass species. New Phytol. 230, 1802–1814. doi: 10.1111/nph.17287
Sun, Z. Z., Liu, L. L., Peng, S. S., Peñuelas, J., Zeng, H., Piao, S. L. (2016). Age-related modulation of the nitrogen resorption efficiency response to growth requirements and soil nitrogen availability in a temperate pine plantation. Ecosystems 19, 698–709. doi: 10.1007/s10021-016-9962-5
Talhelm, A. F., Pregitzer, K. S., Burton, A. J. (2011). No evidence that chronic nitrogen additions increase photosynthesis in mature sugar maple forests. Ecol. Appl. 21, 2413–2424. doi: 10.1890/10-2076.1
Tang, S. B., Zhang, L. L., Lambers, H., Ren, W. D., Lu, X. F., Hou, E. Q., et al. (2021). Addition of nitrogen to canopy versus understorey has different effects on leaf traits of understorey plants in a subtropical evergreen broad-leaved forest. J. Ecol. 109, 692–702. doi: 10.1111/1365-2745.13496
Thomas, R. Q., Canham, C. D., Weathers, K. C., Goodale, C. L. (2010). Increased tree carbon storage in response to nitrogen deposition in the US. Nat. Geosci. 3, 13–17. doi: 10.1038/ngeo721
Trugman, A. T., Anderegg, L. D. L., Anderegg, W. R. L., Das, A. J., Stephenson, N. L. (2021). Why is tree drought mortality so hard to predict? Trends Ecol. Evol. 36, 520–532. doi: 10.1016/j.tree.2021.02.001
Wang, A. Y., Wang, M., Yang, D., Song, J., Zhang, W. W., Han, S. J., et al. (2016). Responses of hydraulics at the whole-plant level to simulated nitrogen deposition of different levels in Fraxinus mandshurica. Tree Physiol. 36, 1045–1055. doi: 10.1093/treephys/tpw048
Wright, S. J., Kitajima, K., Kraft, N. J. B., Reich, P. B., Wright, I. J., Bunker, D. E., et al. (2010). Functional traits and the growth-mortality trade-off in tropical trees. Ecology 91, 3664–3674. doi: 10.1890/09-2335.1
Wright, I. J., Reich, P. B., Westoby, M., Ackerly, D. D., Baruch, Z., Bongers, F., et al. (2004). The worldwide leaf economics spectrum. Nature 428, 821–827. doi: 10.1038/nature02403
Xing, A. J., Xu, L. C., Zhao, M. Y., Shen, H. H., Ma, S. H., Fang, J. Y. (2022). Shifts in understory plant composition induced by nitrogen addition predict soil fungal beta diversity in a boreal forest. Biol. Fertility Soils 58, 667–677. doi: 10.1007/s00374-022-01652-x
Xiong, D. L., Flexas, J. (2022). Safety-efficiency tradeoffs? Correlations of photosynthesis, leaf hydraulics, and dehydration tolerance across species. Oecologia 200, 51–64. doi: 10.1007/s00442-022-05250-4
Yan, T., Lü, X. T., Zhu, J. J., Yang, K., Yu, L. Z., Gao, T. (2018b). Changes in nitrogen and phosphorus cycling suggest a transition to phosphorus limitation with the stand development of larch plantations. Plant Soil 422, 385–396. doi: 10.1007/s11104-017-3473-9
Yan, T., Qu, T. T., Song, H. H., Ciais, P., Piao, S. L., Sun, Z. Z., et al. (2018a). Contrasting effects of N addition on the N and P status of understory vegetation in plantations of sapling and mature Larix principis-rupprechtii. J. Plant Ecol. 11, 843–852. doi: 10.1093/jpe/rty023
Yan, T., Qu, T. T., Sun, Z. Z., Dbyzinski, R., Chen, A. P., Yao, X. C., et al. (2018c). Negative effect of nitrogen addition on soil respiration dependent on stand age: Evidence from a 7-year field study of larch plantations in northern China. Agric. For. Meteorol. 262, 24–33. doi: 10.1016/j.agrformet.2018.06.029
Yan, T., Zhu, J. J., Yang, K., Yu, L. Z., Zhang, J. X. (2017). Nutrient removal under different harvesting scenarios for larch plantations in northeast China: Implications for nutrient conservation and management. For. Ecol. Manage. 400, 150–158. doi: 10.1016/j.foreco.2017.06.004
Zak, D. R., Freedman, Z. B., Upchurch, R. A., Steffens, M., Kögel-Knabner, I. (2017). Anthropogenic N deposition increases soil organic matter accumulation without altering its biochemical composition. Global Change Biol. 23, 933–944. doi: 10.1111/gcb.13480
Zhang, T., Liang, X. Y., Ye, Q., BassiriRad, H., Liu, H., He, P. C., et al. (2021c). Leaf hydraulic acclimation to nitrogen addition of two dominant tree species in a subtropical forest. Sci. Total Environ. 771. doi: 10.1016/j.scitotenv.2021.145415
Zhang, H. X., Yuan, F. H., Wu, J. B., Jin, C. J., Pivovaroff, A. L., Tian, J. Y., et al. (2021a). Responses of functional traits to seven-year nitrogen addition in two tree species: coordination of hydraulics, gas exchange and carbon reserves. Tree Physiol. 41, 190–205. doi: 10.1093/treephys/tpaa120
Zhang, Z. Z., Zhao, P., Ouyang, L., Zhao, X. H., Zhu, L. W., Ni, G. Y. (2021b). Divergent responses of dominant forest tree species to manipulated canopy and understory N additions in terms of foliage stoichiometric, photosynthetic, and hydraulic traits. J. Geophysical Res.: Biogeosci. 126, e2021JG006579. doi: 10.1029/2021JG006579
Keywords: nitrogen deposition, temperate forest, herbaceous layer, economic and hydraulic traits, stand age
Citation: Yan T, Wang L, Wang P and Zhong T (2023) Stability in the leaf functional traits of understory herbaceous species after 12-yr of nitrogen addition in temperate larch plantations. Front. Plant Sci. 14:1282884. doi: 10.3389/fpls.2023.1282884
Received: 25 August 2023; Accepted: 20 November 2023;
Published: 05 December 2023.
Edited by:
Marcin Zadworny, Poznań University of Life Sciences, PolandReviewed by:
Ning Dong, Imperial College London, United KingdomCopyright © 2023 Yan, Wang, Wang and Zhong. This is an open-access article distributed under the terms of the Creative Commons Attribution License (CC BY). The use, distribution or reproduction in other forums is permitted, provided the original author(s) and the copyright owner(s) are credited and that the original publication in this journal is cited, in accordance with accepted academic practice. No use, distribution or reproduction is permitted which does not comply with these terms.
*Correspondence: Tao Yan, eWFudEBsenUuZWR1LmNu
Disclaimer: All claims expressed in this article are solely those of the authors and do not necessarily represent those of their affiliated organizations, or those of the publisher, the editors and the reviewers. Any product that may be evaluated in this article or claim that may be made by its manufacturer is not guaranteed or endorsed by the publisher.
Research integrity at Frontiers
Learn more about the work of our research integrity team to safeguard the quality of each article we publish.