Corrigendum: Sensor-based precision nutrient and irrigation management enhances the physiological performance, water productivity, and yield of soybean under system of crop intensification
- 1ICAR–Indian Agricultural Research Institute, New Delhi, India
- 2ICAR-Directorate of Groundnut Research, Regional Station, Ananatpur, Andhra Pradesh, India
- 3ICAR-Indian Institute of Seed Science, Regional Station, Bengaluru, India
- 4ICAR-Central Potato Research Institute, Shimla, India
- 5ICAR-National Dairy Research Institute, Karnal, India
- 6ICAR-National Rice Research Institute, Cuttack, India
- 7Department of Agricultural Research, Regional Research Centre, Aung Ban, Myanmar
Sensor-based decision tools provide a quick assessment of nutritional and physiological health status of crop, thereby enhancing the crop productivity. Therefore, a 2-year field study was undertaken with precision nutrient and irrigation management under system of crop intensification (SCI) to understand the applicability of sensor-based decision tools in improving the physiological performance, water productivity, and seed yield of soybean crop. The experiment consisted of three irrigation regimes [I1: standard flood irrigation at 50% depletion of available soil moisture (DASM) (FI), I2: sprinkler irrigation at 80% ETC (crop evapo-transpiration) (Spr 80% ETC), and I3: sprinkler irrigation at 60% ETC (Spr 60% ETC)] assigned in main plots, with five precision nutrient management (PNM) practices{PNM1-[SCI protocol], PNM2-[RDF, recommended dose of fertilizer: basal dose incorporated (50% N, full dose of P and K)], PNM3-[RDF: basal dose point placement (BDP) (50% N, full dose of P and K)], PNM4-[75% RDF: BDP (50% N, full dose of P and K)] and PNM5-[50% RDF: BDP (50% N, full P and K)]} assigned in sub-plots using a split-plot design with three replications. The remaining 50% N was top-dressed through SPAD assistance for all the PNM practices. Results showed that the adoption of Spr 80% ETC resulted in an increment of 25.6%, 17.6%, 35.4%, and 17.5% in net-photosynthetic rate (Pn), transpiration rate (Tr), stomatal conductance (Gs), and intercellular CO2 concentration (Ci), respectively, over FI. Among PNM plots, adoption of PNM3 resulted in a significant (p=0.05) improvement in photosynthetic characters like Pn (15.69 µ mol CO2 m−2 s−1), Tr (7.03 m mol H2O m−2 s−1), Gs (0.175 µmol CO2 mol−1 year−1), and Ci (271.7 mol H2O m2 s−1). Enhancement in SPAD (27% and 30%) and normalized difference vegetation index (NDVI) (42% and 52%) values were observed with nitrogen (N) top dressing through SPAD-guided nutrient management, helped enhance crop growth indices, coupled with better dry matter partitioning and interception of sunlight. Canopy temperature depression (CTD) in soybean reduced by 3.09–4.66°C due to adoption of sprinkler irrigation. Likewise, Spr 60% ETc recorded highest irrigation water productivity (1.08 kg ha−1 m−3). However, economic water productivity (27.5 INR ha−1 m−3) and water-use efficiency (7.6 kg ha−1 mm−1 day−1) of soybean got enhanced under Spr 80% ETc over conventional cultivation. Multiple correlation and PCA showed a positive correlation between physiological, growth, and yield parameters of soybean. Concurrently, the adoption of Spr 80% ETC with PNM3 recorded significantly higher grain yield (2.63 t ha−1) and biological yield (8.37 t ha−1) over other combinations. Thus, the performance of SCI protocols under sprinkler irrigation was found to be superior over conventional practices. Hence, integrating SCI with sensor-based precision nutrient and irrigation management could be a viable option for enhancing the crop productivity and enhance the resource-use efficiency in soybean under similar agro-ecological regions.
Introduction
Soybean (Glycine max (L.) Merill), an introduced crop to India, fits well in all agro-ecological regions of the country (Agarwal et al., 2013; Kumar et al., 2013). In India, it is cultivated in an area of 12.81 million hectares with the annual production of 12.9 million tons (DAFW GOI, 2021). The versatility of soybean to be used in a variety of food sectors, dietary supplements, medicines, and bio-materials will result in a significant increase in demand in the years to come (Rezaei et al., 2002; Raghuvanshi and Bisht, 2010). Yet, India’s average soybean yield is just approximately 1 t ha−1, while the global average is 2–3 t ha−1. As a result, there is a paucity of soybean grain availability (Bianchi and Szpak, 2017). Input resource conservation has always been the current generation’s top priority if we have to feed the projected 9–10 billion people by 2050 on the same amount of land, water, and other resources (Martens, 2001; Misra, 2014). The advent of new varieties and hybrids with higher harvest index and shorter life cycles has enhanced the agricultural production; concomitantly, they have also increased the ecological footprint by accelerating the depletion of natural resources (Triboi and Triboi-Blondel, 2002; Fess et al., 2011; Singh et al., 2019). With the roots in the system of rice intensification (SRI), the system of crop intensification (SCI) has gained popularity in recent years as a win–win strategy that optimizes crop yields while minimizing ecological footprints and achieving sustainable yields that have fewer inputs by resource requirements (Table 1).
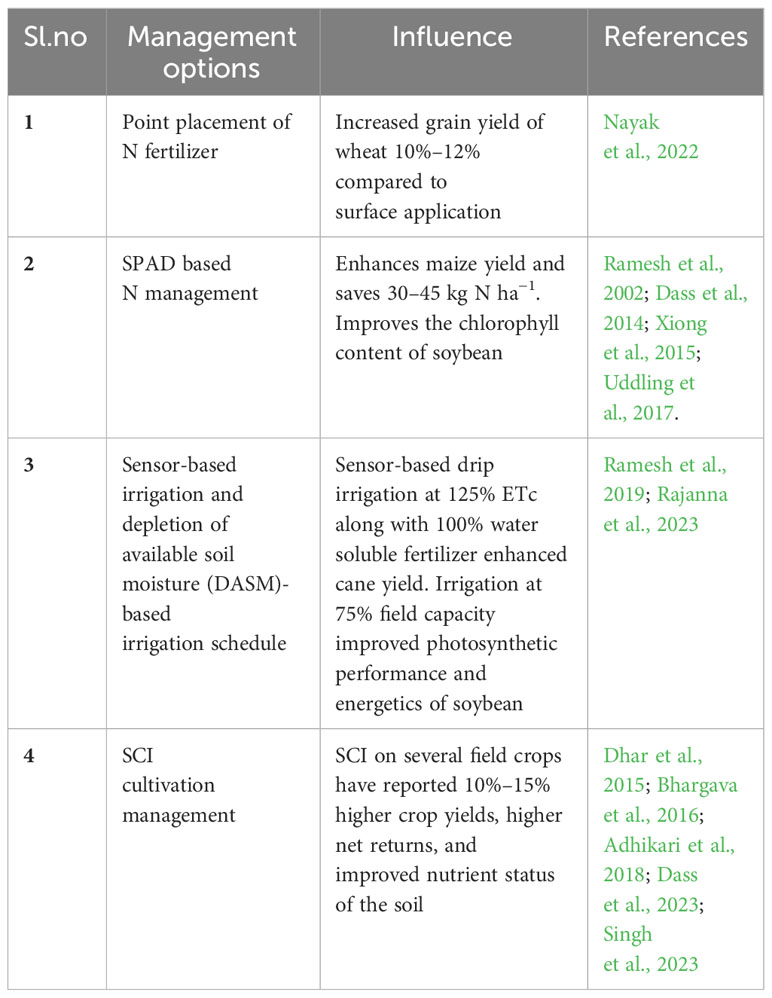
Table 1 Influence of precise nutrient, irrigation management, and SCI practices on crop performance, available nutrient status, and resource use efficiency.
Many physiological functions influence crop productivity, such as photosynthesis, transpiration, and stomatal conductance, and have an impact on crop output (Dass and Bhattacharyya, 2017; Pohare et al., 2018). Good agronomic practices can change the physiological performance of the crop by introducing sensible nutrient and irrigation management practices (Singh et al., 2002; Ramesh et al., 2017; Bangre and Singh, 2020; Devaraj, 2020). Furthermore, the application of high rates of fertilizers and irrigation water contributes to greenhouse gas emission, soil salinity, and alkalinity problems, and makes fertile land to be barren that will contribute to climate change (Bennett, 2000; Wang et al., 2002; Ramesh et al., 2017; Jat et al., 2018; Larbi and Green, 2018; Cheng et al., 2021). Therefore, to combat the ill effects of climate change, the use of modern precision tools for nutrient management, such as SPAD meter, GreenSeeker, and leaf color chart (LCC), which correlate the spectral characters of the crop for analyzing the sufficiency or deficiency of particular nutrients in standing crops, could enhance and conserve the available resources (Table 1). Precision irrigation, viz., automated irrigation, sensor-based and simulation modeling irrigation, is gaining popularity among the farmers in developing countries in view of its ease of operation and water saving under scarce condition. India has more potential for precession irrigation in the future for effective irrigation water management because of the country’s large area under micro-irrigation (13.78 m ha), which includes drip and sprinkler systems (PIB, GOI, 2022). Henceforth, sensor-based tools for irrigation, such as infrared thermometer and soil moisture meter for instant quick profile soil moisture determination, can lead the way for successful in-season precision nutrient and irrigation management with optimum resource efficiency (Bryant et al., 2017; Hou et al., 2018; Wood et al., 2020). However, coming to the adaption of sensor-based irrigation depends on resource base of the farmer, institutional policies, and support from the local government organization through provision of incentives. Proper extension education and spread of knowledge through proper information will help in extending the area under sensor-based irrigation. Furthermore, the precise application of fertilizers through point placement reduces fertilizer requirements and enhances FUE and energetics by maximising crop nutrient uptake (Shafagh et al., 2008; Nayak et al., 2019; Gebre and Earl, 2021). Studies have reported that sprinkler irrigation will enhance the WUE of the crops by 20%–30% and saves water by 30%–45% (Zhao et al., 2009; Li et al., 2019; Solgi et al., 2022). Nevertheless, most of the studies focus on yield and soil fertility status wherein there is not much focus on basic physiological relationships, which finally defines the yield of crop. Hence, the present study throws much insights on growth and physiology of soybean crop with the objectives: to study the applicability of SPAD meter, green seeker, infrared thermometer, and soil moisture meter as an alternative tool to conventional practices for enhancing nutrient and water-use efficiencies and to study the impact of sensor-based tools on net photosynthesis, transpiration, dry-matter accumulations, and other growth indices and their influences on the crop yield.
Materials and methods
Site and weather conditions
A 2-year field experiment was conducted in the experimental farm of ICAR-IARI New Delhi, India (latitude of 28°.38′ N and longitude of 77°.09′ E) during the rainy months of the years 2020 and 2021. The experimental site is situated in the semi-arid region of the Indo-Gangetic plains with dry-hot summers and cold winters. The soil of the experimental site was sandy loam in texture and belonged to Typic Ustochrepts. The top 0–15-cm soil layer physicochemical characteristics were estimated using standard analytical techniques (Rana et al., 2014) and presented in Table 2. The weekly minimum temperature during the cropping period ranged between 10°C and 28°C, while the maximum temperature ranged from 28°C to 37°C. The cumulative of 587.3 mm was received during kharif 2020 (June to October) and 1,379.4 mm rainfall was received during kharif 2021 (June to October). The cumulative rainfall received during the second season was higher compared to the normal rainfall (Figure 1). The daily pan evaporation ranged from 2.3 to 7.4 mm day−1. The maximum and minimum relative humidity ranged from 76% to 93% and 32% to 80%, respectively, during the cropping period (Supplementary Tables S1, S2).
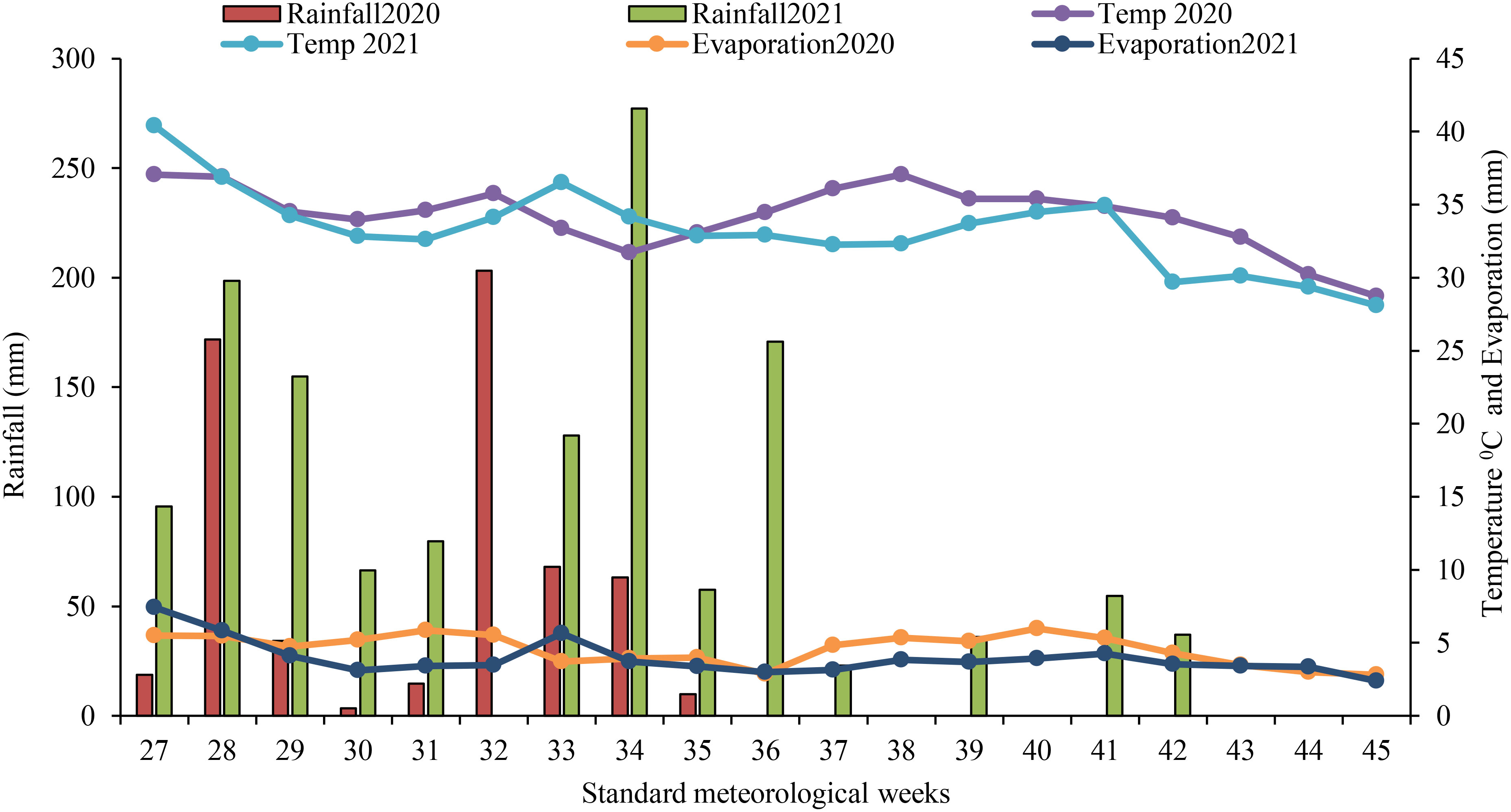
Figure 1 Weekly rainfall, temperature, and evaporation during the kharif 2020 and 2021 (Agro meteorological Observatory, Division of Agricultural Physics, ICAR-IARI, New Delhi).
Study details
The present study was carried out in a split-plot design, comprising three main plots (315 m2 each) and five sub-plots (21 m2 each). The main plots were assigned to irrigation (I): I1, 50% depletion of available soil moisture (DASM) (FI); I2, sprinkler irrigation at 80% ETC (crop evapotranspiration) (Spr 80% ETc), and iii), sprinkler irrigation at 60% ETC (Spr 60% ETc). The total gross plot area with three replications were 945 m2.The sub-plots were allotted with precision nutrient management (PNM1–5) as given in Table 3. Portable sprinklers (Spr 80% ETc and Spr 60% ETc) were used for irrigating the crops. In addition, there was an absolute control plot (conventional management practices both for irrigation and nutrient management). The soybean–wheat cropping system has been followed in the present study under the system of crop intensification (SCI) practices.
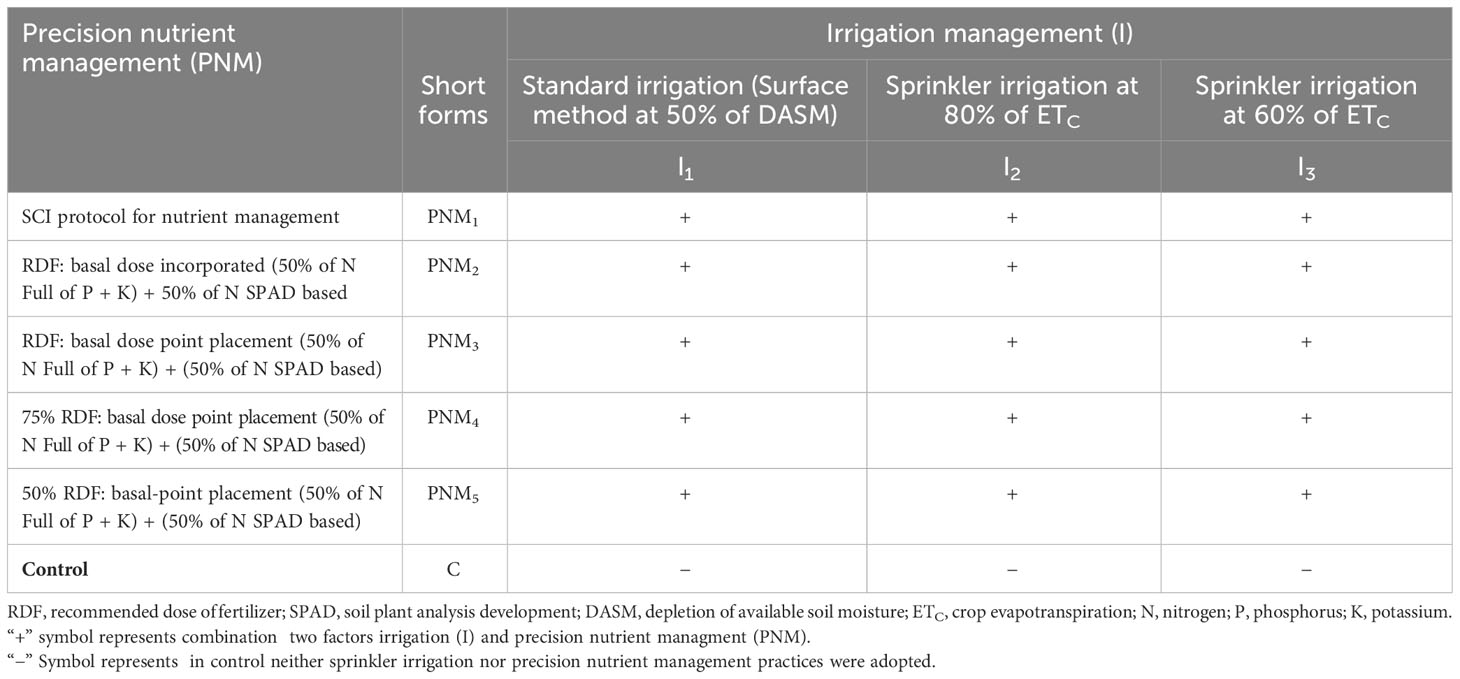
Table 3 Details of treatment combination of precision nutrient and irrigation management adopted in soybean under system of crop intensification (SCI).
The standard package of practices for system of crop intensification (SCI) cultivation of soybean was adopted as earlier used by Singh et al. (2023). Under SCI, seeds were treated with the solution made from the combination of vermicompost (2.5 kg), 5 L of cow urine, and 2.5 kg of jaggery in 20–25 L of hot water (60°C). The vermicompost and water solution were prepared separately, and after cooling, both solutions were mixed, and the soybean seeds were soaked in the mixed solution for 2 h. Furthermore, the seeds were separated from the solution by filtering. Then, the seeds were treated with Rhizobium and phosphorus-solubilizing bacteria (PSB) and allowed them to shade dry overnight; then, the pre-germinated seeds were used for sowing. Two pre-germinated seeds were dibbled at 30×30 cm per hill by hand sowing. The nutrients were applied as per the treatment given in Table 4. After 7 days of sowing, gap filling (pre-germinated seeds) was done to maintain the ideal plant population. The seed treatment was uniformly followed in all the treatments. In SCI nutrient management (PNM1), the recommended amount of nutrients was administered during field preparation using vermicompost at 2.5 t ha−1 treated with Trichoderma (2.5 kg t−1), and the remaining amount of phosphorus was supplied through single super phosphate at 250 kg ha−1. The recommended dose of fertilizer (RDF) for soybean under New Delhi region based on soil-test crop response (STCR)-based recommendation is 52:72:40 (N:P2O5:K2O kg ha−1), respectively. For PNM2–5, STCR-based RDF was practiced. The source of N and P2O5 fertilizer was di-ammonium phosphate (DAP), and K2O fertilizer was muriate of potash (MOP). Based on treatment combinations, N dose was split into two parts, one part was applied basally at the time of sowing along with full amount of P and K, for PNM2–5 treatments. The remaining 50% of N was top dressed based on the SPAD readings; SPAD value ≤ 30 was taken as standard reference reading for top dressing of N in soybean (Dass et al., 2022; Rajanna et al., 2022a), The fertilizers were placed into soil in two ways: (i) broadcasting and ii) point placement (fertilizers placed manually around root zone of plant in circular manner). Under the control treatment, the crop was grown with standard package of practices. The total amount of nutrients supplied for each PNM is presented in Table 3. Hand hoeing and manual weeding were done twice at 20 DAS and 40 DAS; standard management practices were followed for other pest and disease management.
Water use and soil moisture measurement
A soil moisture meter (Delta-T Devices Ltd., Burwell, Cambridge, CB5 0EJ, UK) was used to measure the volumetric soil water content (v/v) to monitor depletion of available soil moisture. Each plot’s volumetric changes in soil moisture were tracked using a soil moisture sensor at intervals of 0–10 cm, 10–20 cm, 20–30 cm, and 30–40 cm soil depth. Soil moisture sensor (access) tubes were inserted into soil up to 1 m depth between the crop rows using a post-hole auger, containing one tube in each main plot. The Delta-T (DL-6) PR 2 soil moisture probe (Delta-T Devices Ltd., Burwell, Cambridge, CB5 0EJ, UK) was used to measure the volumetric moisture content of the soil at various crop growth stages. In FI, when soil moisture approaches 50% depletion of available soil moisture, irrigation was commissioned. In each irrigation, 5 cm depth equivalent amount of water was applied. For scheduling Spr 80% ETc and Spr 60% ETC, sprinkler irrigation ETC was computed by using ET0 (reference evapotranspiration) and Kc (Crop coefficient). ET0 was worked out by using the Penman -Monteith method to determine the KC. The stages of crop development are identified as early stage (Kc ini), development stage, mid-season stage (Kc mid), and late season stage (Kc end), along with their lengths and appropriate Kc coefficients. The chosen Kc coefficients are then adjusted for the frequency of wetting or climatic conditions for each stage of the soybean. Finally, the Kc curve was constructed against different stages of crop growth for any time during the growing season (Figure 2). ETc was calculated as the product of ET0 and Kc (Allen et al., 1998).
A total of four irrigations were given to the crops in both the cropping cycles. Sprinkler irrigation was scheduled twice a week (especially during dry spells) as determined by the ETc of the crop accordingly 80% ETc and 60% ETc.
SPAD reading (chlorophyll content) and normalized difference vegetation index
Five healthy tagged plants were used to measure the chlorophyll content or leaves greenness by placing the SPAD meter (Konica-Minolta SPAD-502, Osaka, Japan) on the middle part of the fully expanded trifoliate leaf, avoiding the main vein. Five readings from different leaves within the plant were taken, and the average SPAD reading was calculated for each plant. The SPAD readings were taken regularly at weekly intervals between 30–45 DAS and 60–90 DAS and used for analysis.
The NDVI was calculated using a handheld optical crop sensor Trimble GreenSeeker® (Trimble, Sunnyvale, CA, USA). To achieve the average NDVI values, the GreenSeeker® device was moved along the rows of crops while being held at the same height above the crop canopy (50 cm).The GreenSeeker® emits near-infrared (NIR) and red light toward the vegetation canopy. Different wavelengths of light were absorbed based on the chlorophyll content in the plant leaves and other plant pigments. The amount and spectral properties of the reflected light depend on the health and physiological status of the plants, including factors such as leaf chlorophyll content, and canopy structure. The NDVI readings taken regularly at weekly intervals from 30 to 45 DAS and 60 to 90 DAS were used for analysis.
Photosynthetic characters and net assimilation rate
The fully opened index leaves of the soybean plant were put in the sensor chamber of the instrument i.e., infrared gas analyzer (IRGA) [LI 6400 XT], a portable photosynthetic system. The photosynthetic characteristics of the crop were measured during the flowering stage (R2) of the crop on a bright sunny day between 900 hours and 1100 hours. The CO2 concentration was maintained at ambient, the air flowrate through the chamber was 500 mol s−1, and the relative humidity was between 70% and 75%. Photosynthetic characters recorded include the following: i) net photosynthetic rate (Pn)—amount of CO2 consumed by leaves per unit area per unit time (µ mol CO2 m−2 s−1); ii) transpiration rate (Tr)—the amount of water consumed by leaves per unit area per unit time (m mol H2O m−2 s−1); iii) stomatal conductance (Gs)—gas exchange of stomata per unit area per unit time (mol H2O m−2 s−1); and iv) inter-cellular CO2 concentration (iC)—concentration of CO2 inside leaf (mol H2O m2 s−1).
Canopy temperature depression
The relative temperature of the CTD to the surrounding air was determined using a handheld infrared thermometer; five measurements of canopy temperature were taken from each experimental plot between12:00 and 14:00 on sunny days only. The temperature of the surrounding air was also measured simultaneously. The following formula was used to compute the canopy temperature depression.
Photosynthetically active radiation
Photosynthetically active radiation (PAR) denotes the fraction of solar radiation, i.e., the number of moles of photons in the radiant energy in a specific spectral range (400–700 nm). It was measured using the canopy analyzer LP-80 Accu PAR Line Quantum Sensor of 1 m length connected with a data logger and expressed in μmol (photons) m−2 s−1. PAR was measured by placing the Line Quantum Sensor in between the plant rows to record transmitted solar radiation (at bottom of the crop canopy), while incident solar radiation was measured at top of the crop canopy. Five readings per plot were recorded at 30–45 DAS and 60–90 DAS between 12:00 and 13:00 on a clear sunny day.
Crop growth indices
Dry matter accumulation was calculated by taking dry matter at 60 DAS from the net plot area and finally expressed in g m−2. Five representative plants from plot were randomly sampled at 30 and 60 DAS and oven-dried at 60–70°C. Based on plant dry weight, crop growth indices, such as leaf area index, RGR, CGR, and NAR, were worked out using Equations 3–5 as given by Watson (1952);
where W1 and W2 are dry weights of plant, LA1 and LA2 are the leaf area, T1 and T2 are the time intervals in days, and S is the land area occupied by the plants.
The leaf area was measured using an LI-3100C leaf area meter (Li-COR, Lincoln, NE, USA) as cm2 plant−1, and the leaf area index (LAI) was calculated as per Rana et al. (2014).
Yield estimation
The crop was harvested from the net-plot area (18.3 m2) leaving behind the two border rows and dried in the field for 7–10 days, and the whole biomass weight was recorded for each treatment and designated as biological yield. The dried samples were threshed plotwise by using Pullman thresher.
Water productivity
For the purpose of estimating water productivity, the irrigation water provided to each plot was measured, and different calculations were made using the rainfall data gathered from the ICAR-Indian Agricultural Research Institute’s meteorological observatory in New Delhi.
(a) Irrigation water productivity (IWP) (kg ha−1 m−3).
The computation of irrigation water productivity for soybean crop was done utilizing the formula used by Dass et al. (2013).
(b) Economic water productivity (INR ha−1 m−3).
The calculation of economic water productivity (EWP) for soybean was carried out following the methodology described by Igbadun et al. (2006).
(c) Water-use efficiency (WUE) (kg ha–1 mm–1 day−1).
Water-use efficiency was worked out as the ratio of yield to evapotranspiration of crop (Dass et al., 2013).
Statistical analysis
The difference between the treatments was statistically analyzed through ANOVA by using JMP® software from SAS. The significant difference between the two treatment mean values was determined by using least significance difference (LSD) by performing Tukey’s honestly significant difference (HSD) test (p<0.005) and indicated by different letters. A comparison was made between control versus SCI and control versus the rest of the treatment combinations to compare the performance of crop under SCI versus conventional cultivation. For better understanding of the relationship between different growth, physiological, and yield parameters, multiple variate analysis (MVA) and principle component analysis (PCA) were performed using JMP®software.
Results
Irrigation effects
The adoption of ETc-based sprinkler irrigation significantly enhanced photosynthetic characters of soybean during 2020 and 2021. Net photosynthetic rate (Pn) at flowering stage under sprinkler irrigation (Spr 80% Etc) showed 25.6% and 42.5% higher Pn value compared to FI (standard flood irrigation at 50% DASM) during 2020 and 2021, respectively (Table 5). Likewise, the adoption of Spr 80% Etc irrigation schedule enhanced transpiration rate (Tr) from 5.59 to 6.42 m mol H2O m2 s−1 during both study years. Similarly, the practice of Spr 80% ETc exhibited significantly (p>0.05) higher Tr (6.66 and 7.33 m mol H2O m2 s−1), stomatal conductance (Gs) (0.17 and 0.21 mol CO2 mol−1 year−1) and intercellular CO2 concentration (Ci) (246.6 and 319.4 mol H2O m2 s−1) than flood irrigation during both crop seasons, respectively. However, FI and Spr 60% ETc did not show any significant difference between them for Gs and Ci in both crop seasons. In both years, there was a notable increase of 14.9% and 16.3% in NAR in soybean under Spr 80% ETc compared to FI.
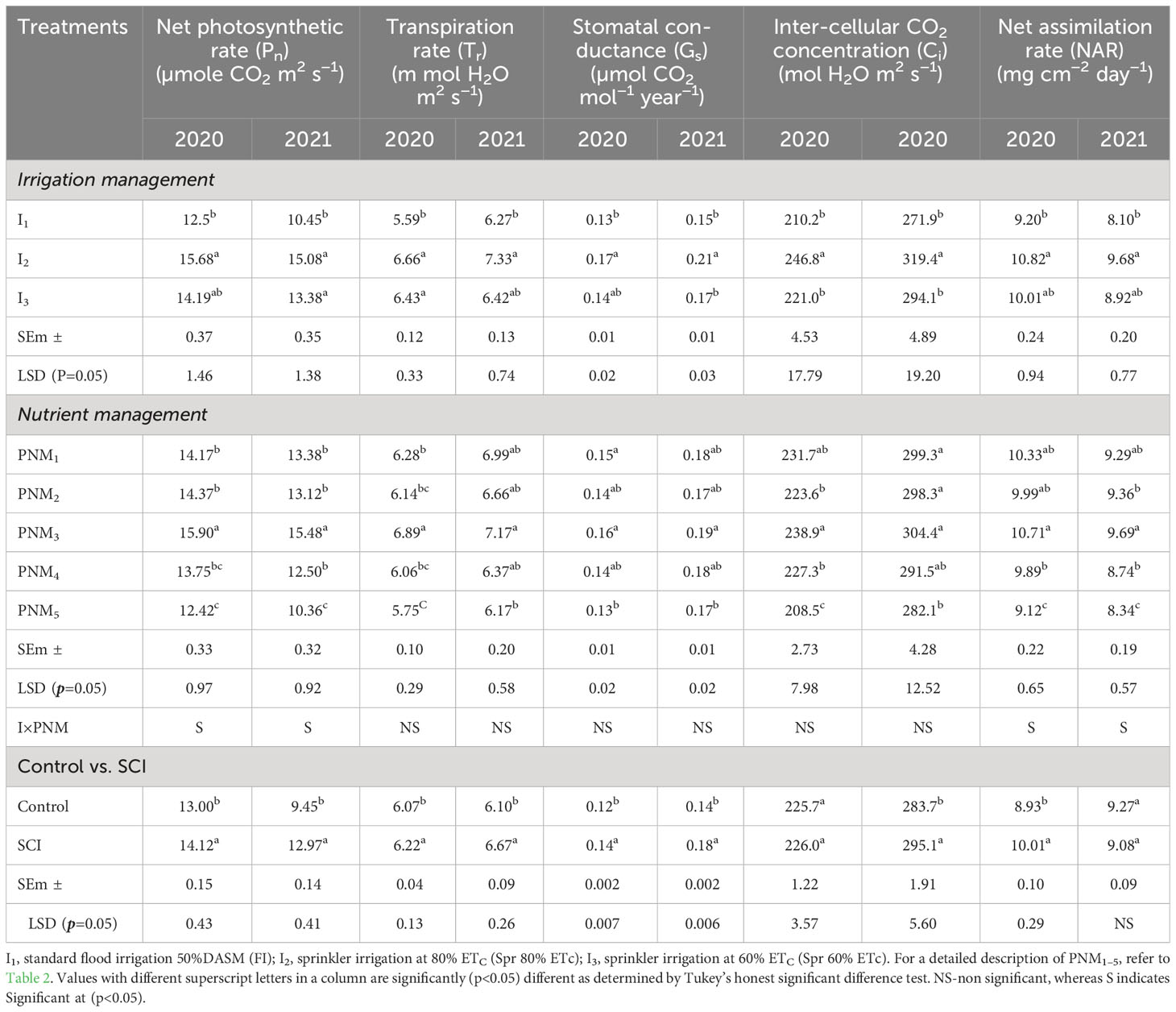
Table 5 Effect of precision nutrient and irrigation management on photosynthetic character, viz., net photosynthetic rate Pn, transpiration rate Tr, stomatal conductance Gs, inter-cellular CO2 concentration Ci, and net assimilation rate at flowering stage of soybean under SCI.
The SPAD values varied from 27.6 to 31.7 at 30–45 DAS among irrigation regimes across study years. The treatment Spr 80% ETc recorded significantly (p=0.05) higher SPAD values over FI (Table 6). In general, the chlorophyll content (SPAD) followed the order of Spr 80% ETc > Spr 60% ETc >FI. Using sprinkler irrigation at Spr 80% ETc increased NDVI values by 23.6%–27.7% and 12.7%–16.1% at 30–45 DAS and 60–90 DAS, respectively, over FI treatment. There was a reduction in canopy temperature of soybean ranging from 3.12°C to 4.44°C over the entire crop growth duration (Table 6). The CTD with Spr 80% ETc was significantly (p=0.05) lower 24.4%–25.9% and 33.9%–42.3% at 30–45 DAS and 60–90 DAS, respectively over standard flood irrigation plots. Similarly, photo synthetically active radiation (PAR) and transmitted and intercepted light were recorded at 30–45 DAS and 60–90 DAS period (Figures 3A, B). At 30–45 DAS, FI exhibited significantly greater (p=0.05) light transmittance vis-à-vis less light interception by the crop canopy than others. With the advancement of the crop age (60–90 DAS), greater crop canopy resulted more light interception vis-à-vis less transmission of the incident light through the canopy. PAR interception in Spr 80% ETc showed an increment of 14.4%–27.0% and 13.2%–21.8% at 30–45 DAS and 60–90 DAS over FI.
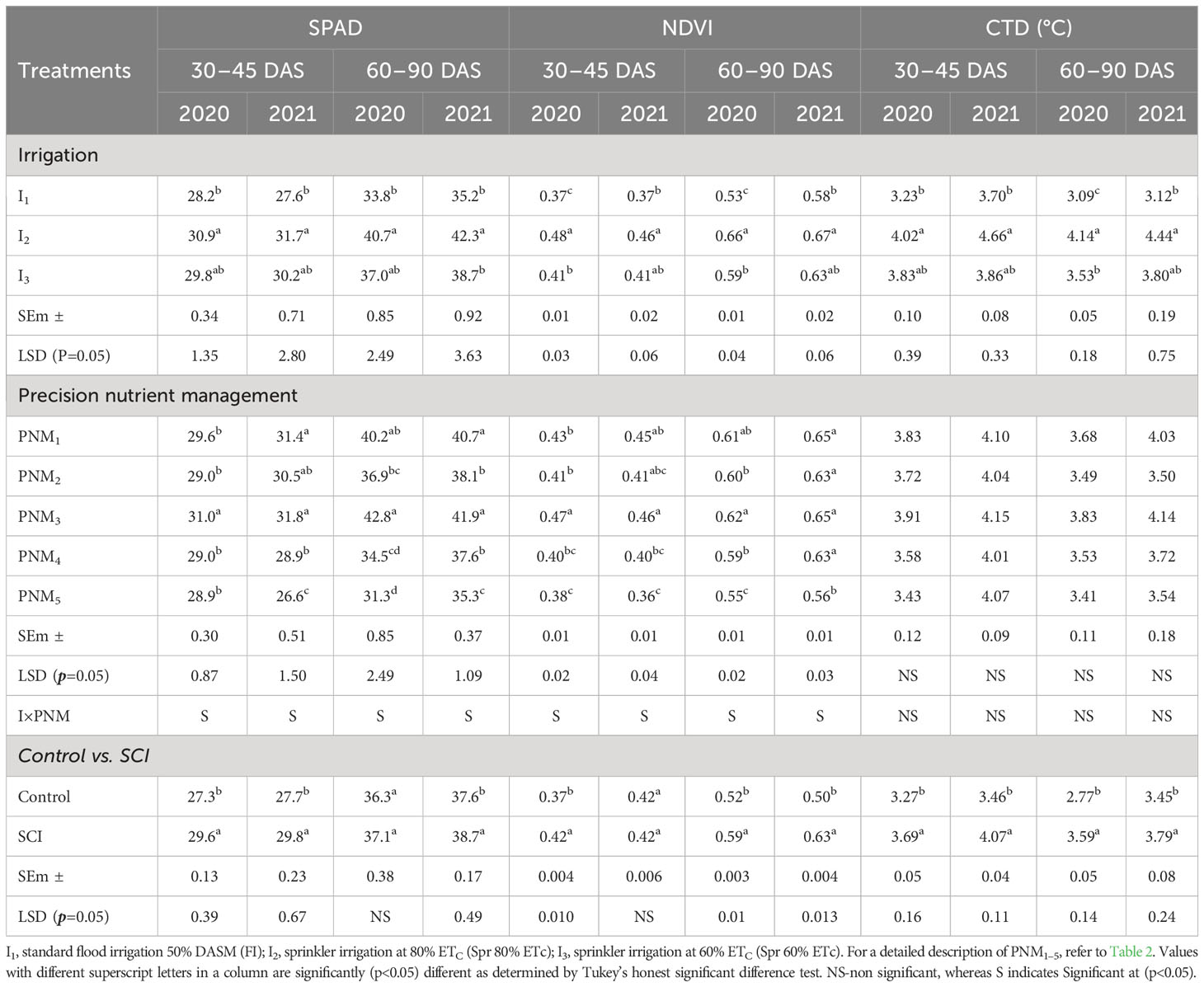
Table 6 Effect of precision nutrient and irrigation management on SPAD, normalized difference vegetation index (NDVI), and canopy temperature depression (CTD) at 30–45 DAS and 60–90 DAS of soybean crop.
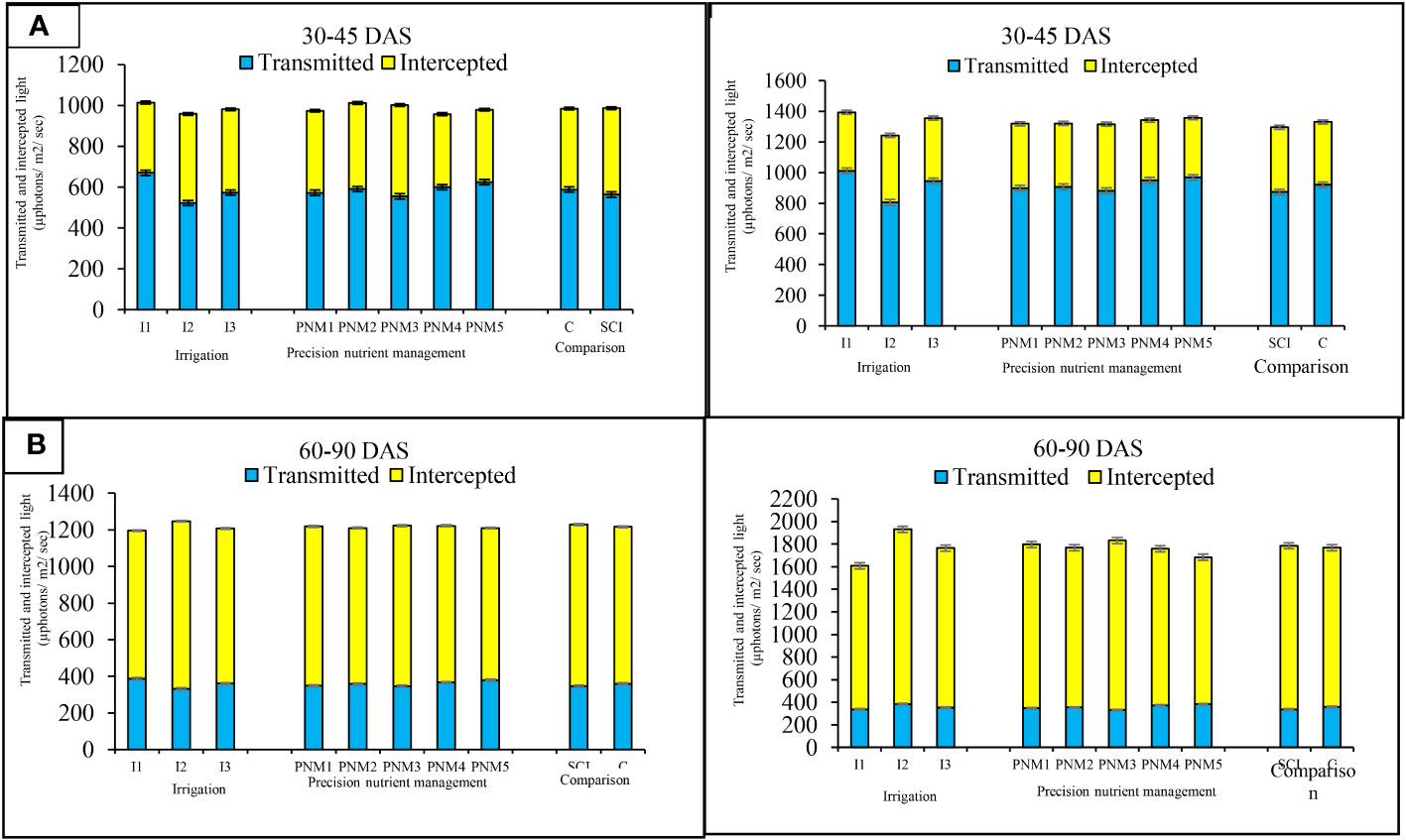
Figure 3 Effect of precision nutrient and irrigation management on transmission and interception photosynthetically active radiation (PAR) by soybean crop canopy at 30–45 DAS (A) and 60–90 DAS (B) during 2020 and 2021. For a detailed description of I (irrigation) and PNM (precision nutrient management), refer to Table 2. Any treatment difference that exceeds the range of the bar within a given year is significantly different, as indicated by the LSD 0.05 bar above each column.
The higher crop growth indices (CGR, RGR, and LAI), dry matter accumulation (DMA), and dry matter partitioning (DMP) of soybean were recorded in Spr 80% ETc, which was followed by Spr 60% ETc and FI during study period (Table 7). Spr 80% ETc exhibited significantly (p=0.05) higher CGR (8.33 and 8.42 g m−2 day) and RGR (77.28 and 66.70 mg g−1 day−1) over FI during 2020 and 2021. Likewise, plots with Spr 80% Etc irrigation recorded significantly higher LAI at 60 DAS with increments ranging from 26.2% to 28.3% and 29.4% and 43.1% during 2020 and 2021, respectively. The DMA at harvest of soybean also followed a similar trend in both years. The percent increase in stem dry weight (SDW) for Spr 80% ETc was 19.5%–20.7% and 6.1%–6.7% (mean of 2 years) as compared to FI and Spr 60% ETc, respectively (Figure 4). Pod dry weight (PDW) increased by 18.7%–19.7% and 11.5%–5.3% than FI and Spr 60% ETc in 2020 and 2021, respectively. No significant difference was observed in LDW (leaf dry weight) in both years.
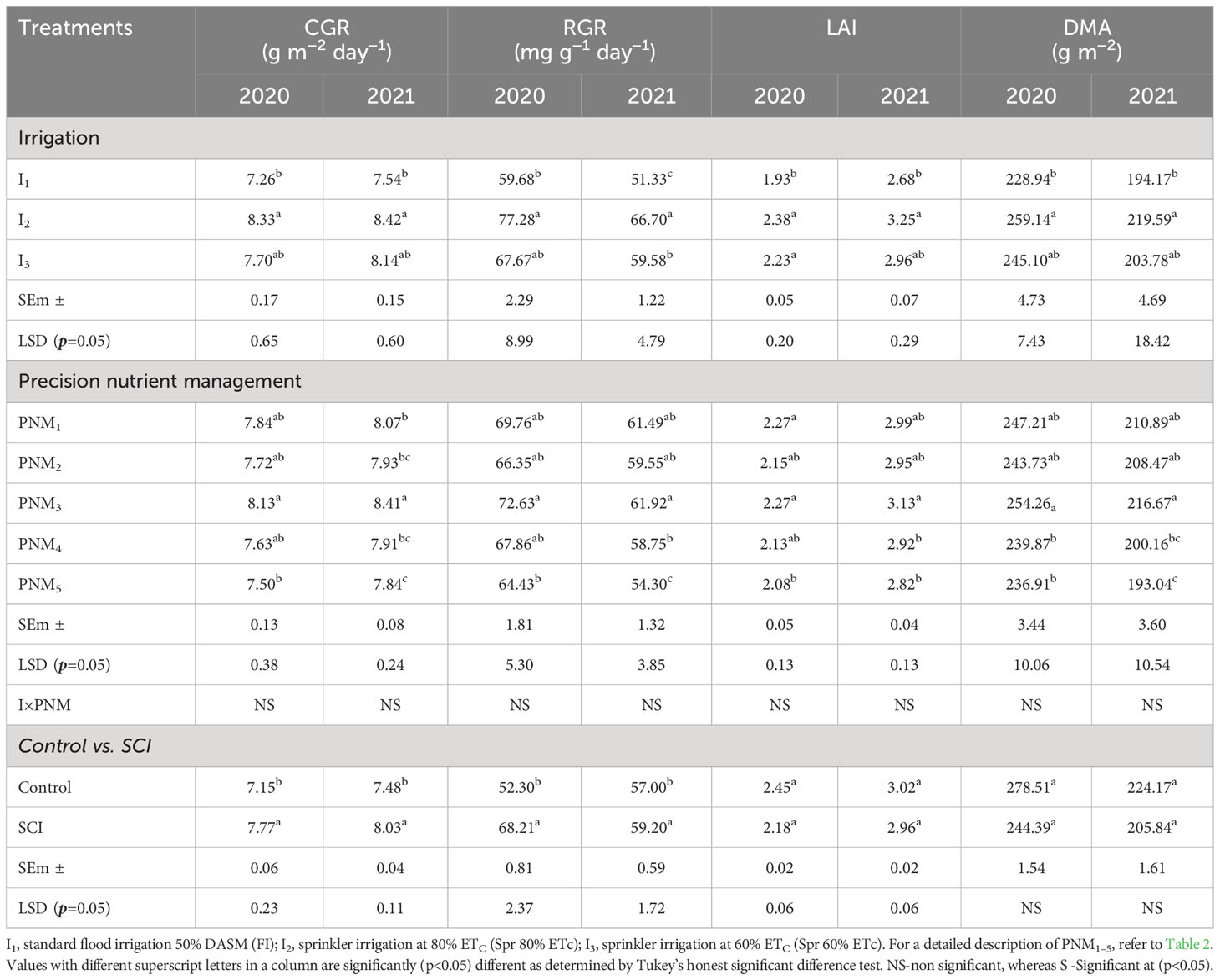
Table 7 Effect of precision nutrient and irrigation management on crop growth rate (CGR), relative growth rate (RGR), leaf area index (LAI), and dry matter accumulation (DMA) in soybean.
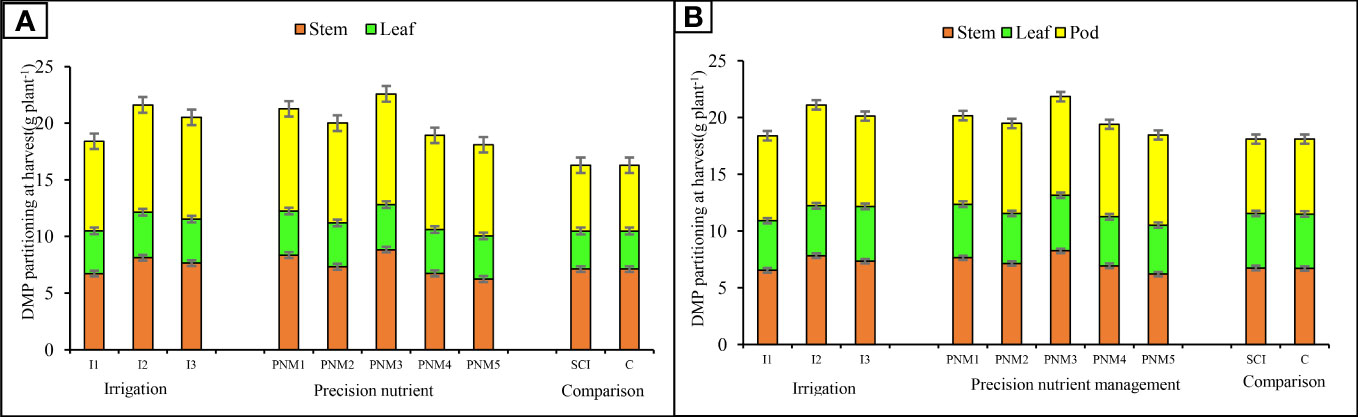
Figure 4 Effect of precision nutrient and irrigation management on dry matter partitioning of soybean (stem, leaves and pods) at harvest 2020 (A) and 2021 (B). For a detailed description of I (irrigation) and PNM (precision nutrient management), refer to Table 2. Any treatment difference that exceeds the range of the bar within a given year is significantly different, as indicated by the LSD 0.05 bar above each column.
Significantly higher grain yields of 2.45 t ha–1 and 2.56 t ha–1 were recorded with the application of Spr 80% ETc than FI, and it was at par with Spr 60% ETc (2.15 t ha–1 and 2.30 t ha–1). The percent increase in grain yield ranged from 23.1% to 29.1% under Spr 80% ETc over FI plots. A significantly lower grain yield was recorded in FI (1.97 t ha–1 and 1.99 t ha–1) in both years (Supplementary Table S9). The highest soybean biological yield was recorded in the Spr 80% ETc (7.61 t ha–1 and 7.72 t ha–1), which was 13.7%–18.5% higher than FI and 16.0%–20.0% higher than Spr 60% ETc. The FI recorded the least soybean biological yield among the irrigation management practices (6.69 t ha–1 and 6.51 t ha–1) in both years (Supplementary Table S9). The grain and biological yield under various irrigation treatments followed the Spr 80% ETc > Spr 60% ETc> FI. Similarly, IWP followed the trend as Spr 60% ETc > Spr 80% ETc > FI across years. Soybean, crop under Spr 60% ETc resulted in highest IWP (0.95; 1.21 kg ha−1 m−3), whereas Spr 80% ETc recorded the highest EWP (24.8; 18.1 INR ha−1 m−3) and WUE (7.1; 8.1 kg ha–1 mm–1 day−1), respectively, during 2020 and 2021 (Table 8). Likewise, the Spr 80 ETc showed an increment IWP, EWP, and WUE to the extent of 32.6%, 31.08%, and 49.06% over FI (Table 8).
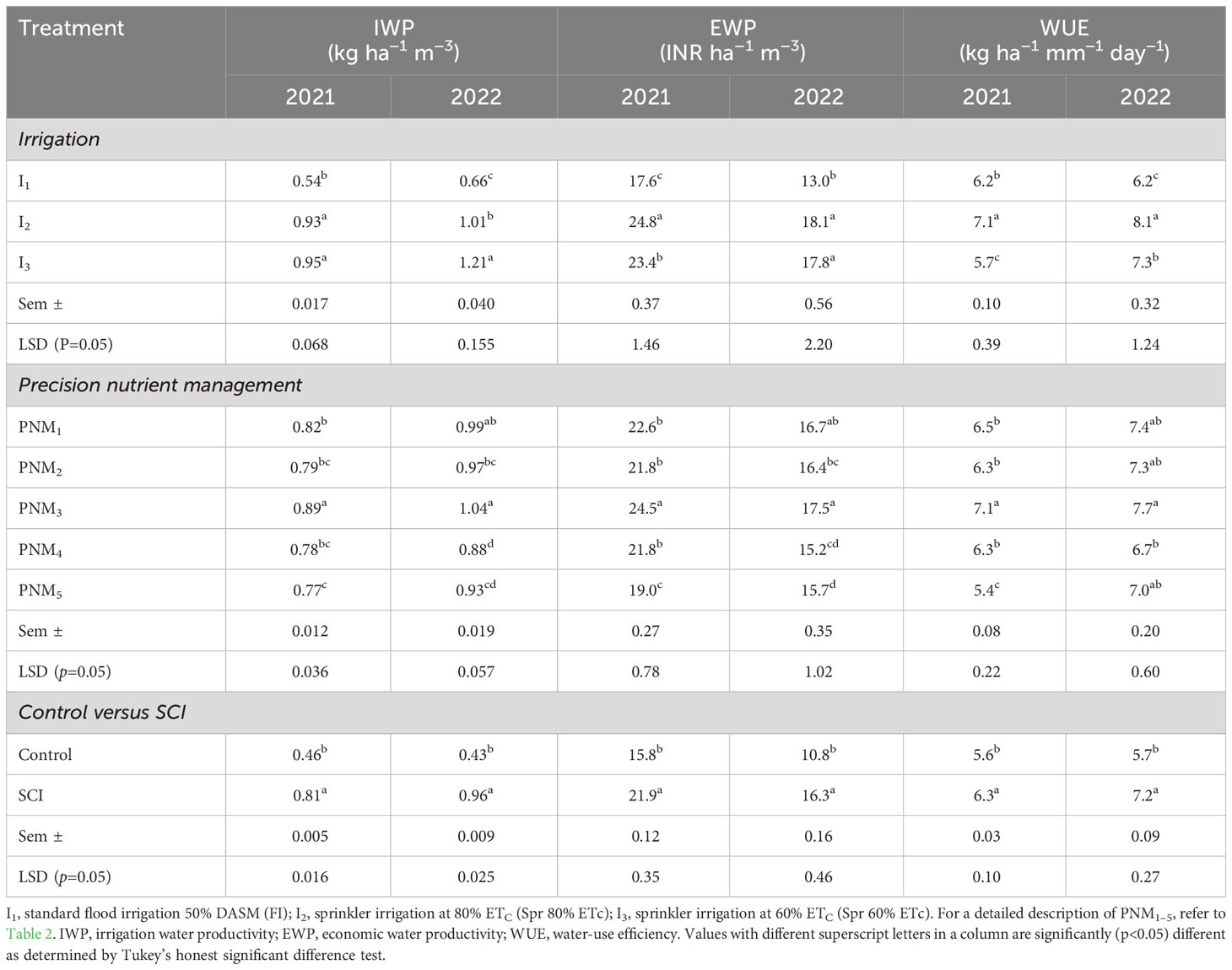
Table 8 Effect of precision nutrient and irrigation management on total water productivity, irrigation water productivity, and economic water productivity in soybean under SCI.
Precision nutrient management effects
Precision nutrient management (PNM) practices exhibited significant (p=0.05) improvement in photosynthetic characteristics like Pn, Tr, Gs, Ci, and NAR during 2020 and 2021. The adoption of PNM practices enhanced Pn in soybean from 10.36 to 15.90 µmol CO2 m2 s−1 (Table 5). Likewise, the adoption of PNM3 [point placement of fertilizers and 50% SPAD based N top dressing] exhibited significantly higher Pn compared to PNM5 with the percent increment ranging from 28.2% and 49% during both study years, respectively. Likewise, soybean leaves under PNM treatments transpired at the rate of 6.22–6.67 m mol H2O m2 s−1 during both years. However, PNM3 exhibited 18.9% and 16.1% greater Tr rate than PNM5. Similarly, the adoption of PNM3 practice enhanced GS by 23.1% and 11.8%, Ci by 14.5% and 7.9%, and NAR by 17.4% and 16.2% over PNM5 in both seasons (Table 5). However, PNM2 and PNM4 were statistically comparable across both seasons for most of the photosynthetic parameters. Likewise, PNM practices also enhanced SPAD and NDVI values in soybean. The chlorophyll content and NDVI values in PNM practices followed the order of PNM3> PNM1>PNM2> PNM4> PNM5 at 30–45 DAS and 60–90 DAS in both seasons (Table 6). The SPAD and NDVI values of soybean peaked at 60–90 DAS resulting from SPAD-meter-assisted N top dressing. The SPAD values under PNM ranged from 29 to 38 and 35.3 to 42.8 at 30–45 DAS and 60–90 DAS, respectively, during the study period. NDVI values were significantly higher under PNM3 at 30–45 DAS (0.47) and 60–90 DAS (0.64). There was no significant difference in CTD under PNM treatments during any of the study years (Table 6), whereas PNM3 practice enhanced percent interception of PAR than its PNM5 at all the measurement intervals in both years.
The crop growth indices (CGR, RGR, and LAI), DMA, and DMP of soybean were significantly (p=0.05) higher under PNM practices during 2020 and 2021 (Table 7). The adoption of PNM3 recorded significantly higher CGR (7.3% and 3.8% higher), RGR (11.8% and 2.3% higher), and DMA (8.8% and 2.7% higher) over PNM5 and PNM1 in both the years (Table 9). Likewise, superior LAI under PNM3 (2.27 and 2.13) was observed than under other PNM treatments. Dry matter fractions of soybean at harvest in both years showed no significant difference in LDW among the PNM treatments (Figure 4). However, PNM3 showed an increment of 6.5%, 15.3%, and 27.1% over PNM1, PNM2, and PNM5 in SDW. Likewise, significantly (p=0.05) higher PDW was recorded in PNM3 over PNM5.
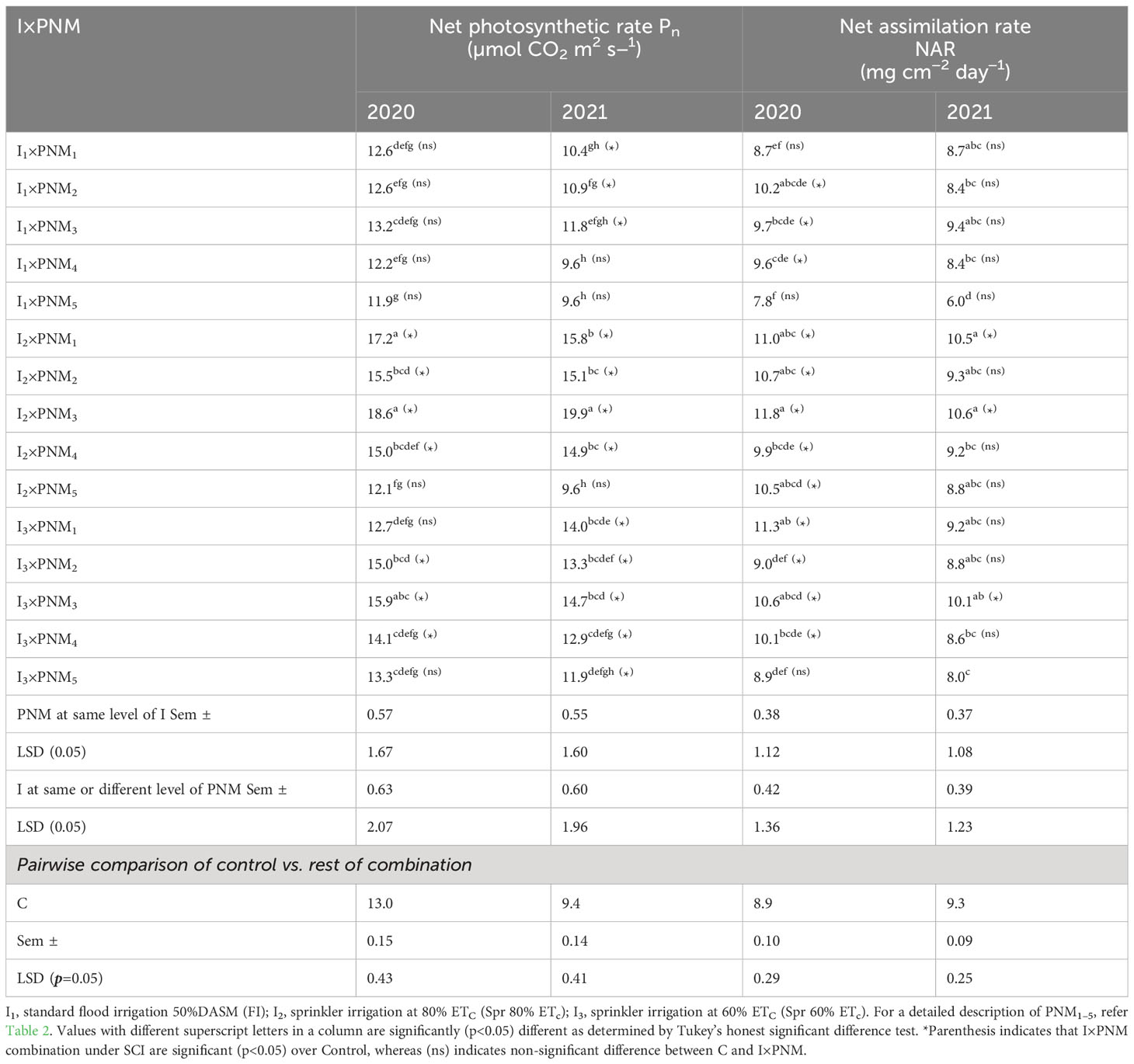
Table 9 Interaction effects precision nutrient (PNM) and irrigation management (I) on net photosynthetic rate and net assimilation rate of soybean under SCI.
The grain yield was significantly (p=0.05) higher in PNM3 (2.45 t ha–1 and 2.43 t ha–1) followed by PNM1 (2.43 t ha–1 and 2.12 t ha–1). Likewise, soybean crop under PNM3 recorded 7.67 t ha–1 and 7.65 t ha–1 of biological yield, which was 26.8% and 20.3% higher than soybean biological yield recorded under PNM5 in 2020 and 2021, respectively (Supplementary Table S9). The enhancement in soybean yields under PNM treatments followed the trend PNM3>PNM1>PNM2>PNM4>PNM5 during the study period. A similar trend with respect to water productivity was observed under PNM practices, where adoption of PNM3 recorded significantly higher IWP (0.89 and 1.04 kg ha−1 m−3), EWP (24.5 and 17.5 INR ha−1 m−3), and WUE (7.1 and 7.7 kg ha–1 mm–1 day−1) during 2020 and 2021, respectively. The IWP, EWP, and WUE of soybean under PNM3 were 15.6%–11.8%, 11.5%–28.9%, and 10.0%–30.1% higher than PNM5 during both study years.
Interaction effects
The photosynthetic characters like net photosynthetic rate (Pn) and net assimilation rate (NAR) at flowering stage were significantly (p=0.05) affected by the interaction effect of irrigation management and PNM practices (Table 9). A combination of irrigations and PNM practice enhanced Pn values from 18.61 to 19.97 mol CO2 m2 s−1and NAR values from 6 to 11.8 mg cm−2 day−1during 2020 and 2021. In both years, the Pn (17.2; 15.8 µmol CO2 m2 s−1) and NAR (11.8; 10.6 mg cm−2 day−1) of soybean plants under the I2×PNM3 treatment were higher than other combinations (Table 10), whereas the lowest Pn and NAR were recorded with the combination of I1×PNM5. Likewise, SPAD and NDVI values exhibited significant interaction effect irrigation and PNM practices at 30–45 DAS and 60–90 DAS (Table 10). The increase in SPAD due to I2×PNM3 over I1×PNM5 varied from 20.97% to 54.7% and 54.3% to 53.2% at 30–45 DAS and 60–90 DAS. Likewise, I2×PNM3 registered 57%–74% and 52.1%–53.1% increase in NDVI at 30–45 DAS and 60–90 DAS over I1×PNM5. Pair-wise comparison of individual combination with conventional cultivation showed that Pn, NAR, SPAD, and NDVI were significantly (p=0.05) improved by most of the irrigation × PNM combinations over control. Significantly higher grain yield was recorded with the combination of I2×PNM3 (2.59 and 2.67 t ha–1) than other combinations during both study years. The lowest grain yield of 1.7 and 2.01 t ha–1 was recorded with I1×PNM5. Likewise, the highest biological yield was recorded under I2×PNM3 (8.3 and 8.42 t ha–1). The interaction effect of irrigation and PNM practices showed that I3×PNM3 recorded the highest IWP (0.65 and 0.89 kg ha−1 m−3), whereas I2×PNM3 showed highest EWP (29.2 and 33.9 INR ha–1 m3) and WUE (5.74 and 6.43 kg ha–1 mm–1 day−1) during the study period (Supplementary Table S10).
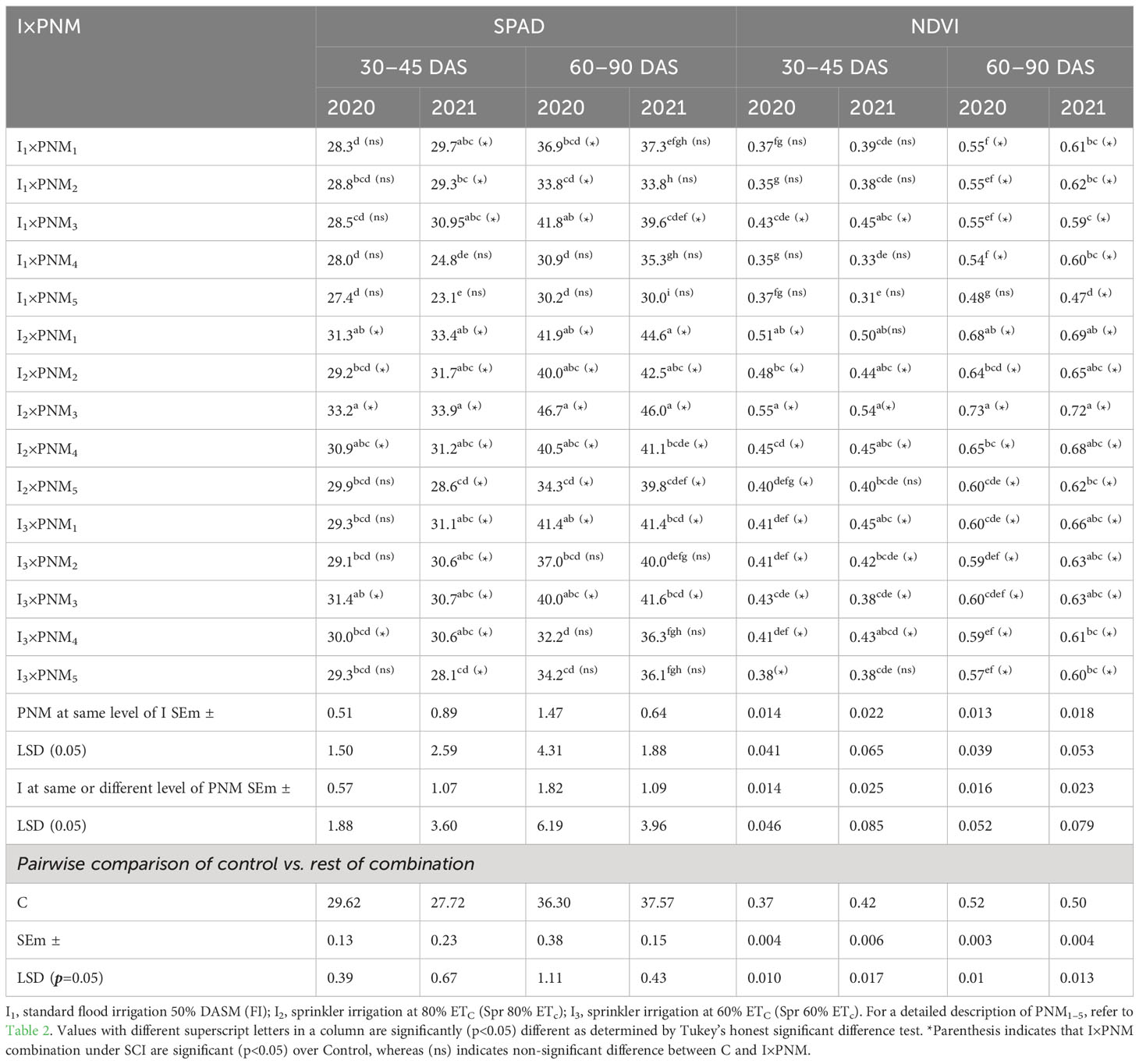
Table 10 Irrigation management (I) × precision nutrient (PNM) interaction effects on SPAD and NDVI values of soybean under SCI.
Comparison of SCI versus control
A comparative study of SCI and conventional cultivation of soybean showed that all the photosynthetic characters (Pn, Tr, Gs, and Ci) under study showed significant (p=0.05) improvement under SCI over control during both years of study. Likewise, crop growth indices, viz., CGR, RGR, and DMP, also exhibited significant (p=0.05) edge over control, whereas growth indices like LAI, DMA, and PAR interception were found to be similar in SCI and conventional practices. A comparison of SCI versus control evidenced significantly higher grain yield (2.20 and 2.28 t ha–1) with the percent increase of 13% and 27% in both the cropping season (Supplementary Table S9). During 2020, there was no significant difference between SCI and conventional for biological, while in the second year, SCI cultivation (6.96 t ha–1) produced significantly higher biological yield than conventional cultivation. Water productivity improved significantly (p=0.05) under SCI over control.
Multivariate analysis and principal component analysis
The association of the relationship between different physiological parameters, crop growth indices, and productivity was studied through multivariate analysis (MVA) (Figure 5). Predominantly, most of the physiological and growth indices variables were positively correlated with grain yield (GY) and biological yield (BY) (Figure 5). Nevertheless, a strong positive correlation was observed in Pn (r = 0.77 and 0.88), Tr (r=0.80 and 0.74), CTD (r=0.81 and 0.63), and CGR (0.79 and 0.71) for GY and BY, respectively. Furthermore, chlorophyll index (SPAD) and NDVI exhibited a strong association with GY (r = 0.80 and 0.70); however, SPAD showed intense association with BY (r= 0.82), while the NDVI showed stronger association with GY (r=0.80). Dimension reduction in data set and the most important variables contributing yield was obtained by principal component analysis (PCA) (Figure 6). PCA showed the superiority of sprinkler irrigation (Spr 80% ETc) and precision nutrient management (PNM) over FI ×PNM combinations. GY is closely related with Tr, Pn, NDVI, CTD, CGR, RGR, and RGR except BY and SPAD, which are found to be less associated.
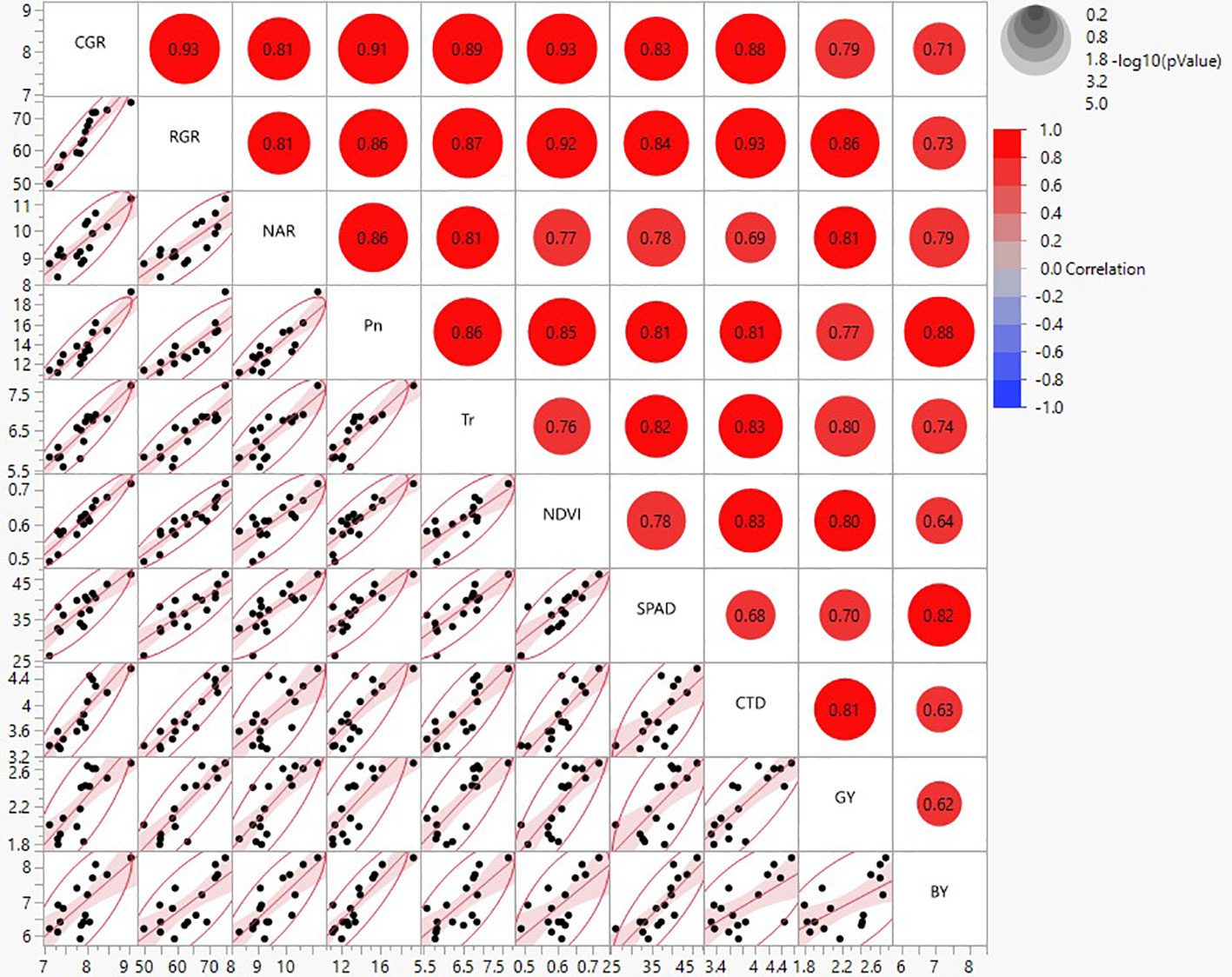
Figure 5 Multivariate analysis showing correlation between various crop growth indices (CGR, RGR, and NAR at 60 DAS), physiological, sensor parameters (Pn, Tr, NDVI, SPAD, and CTD at flowering stage), grain (GY), and biological yield (BY) during 2020 and 2021. The upper triangle displays the significant circles with correlation coefficient (p=0.05) (2-year pooled data), while the lower triangle displays the scatter plot matrix with line fit.
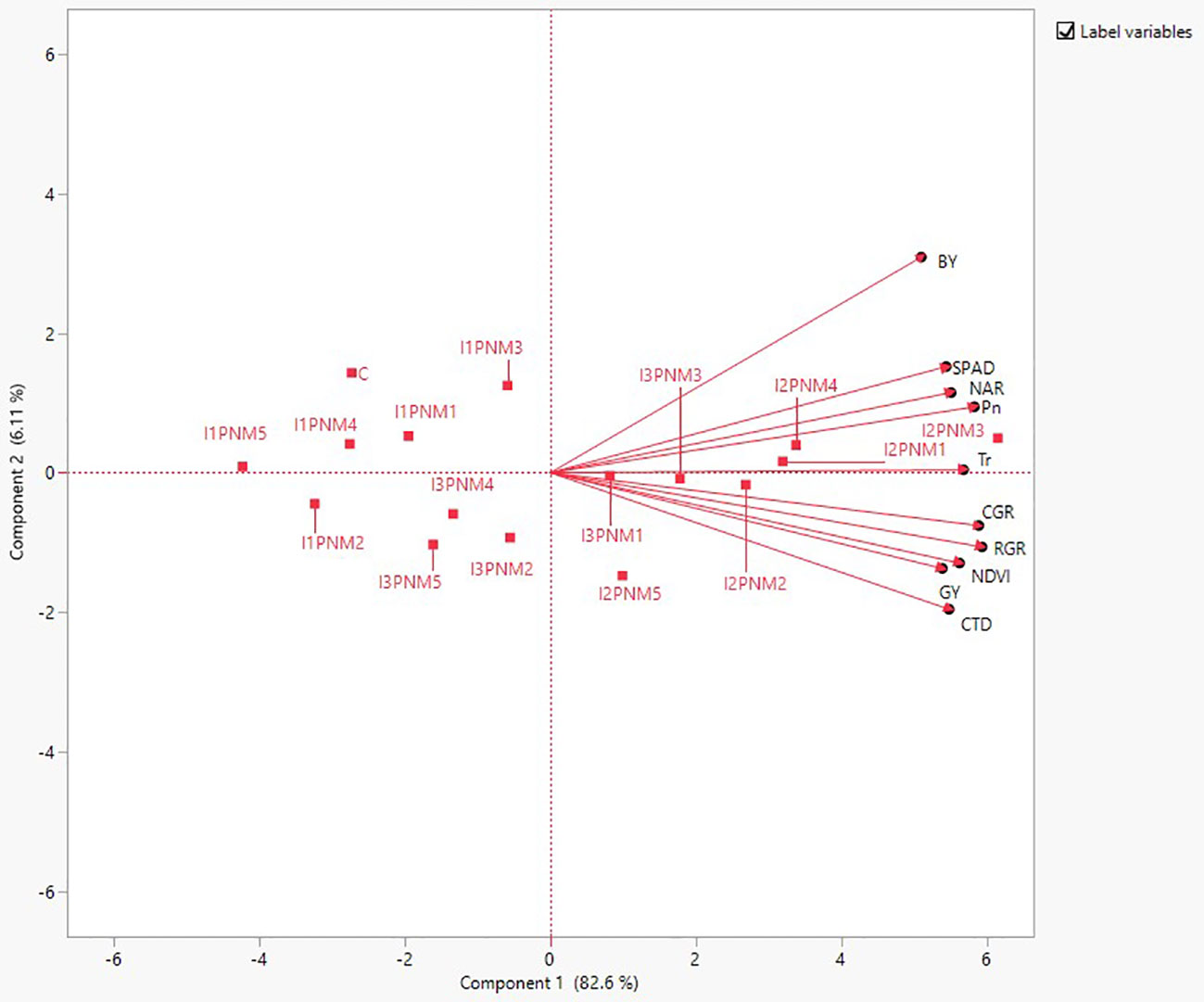
Figure 6 PCA biplots on the effect of precision nutrient and irrigation management on physiological and sensor parameters (Pn, Tr, NAR, SPAD, NDVI, and CTD) on grain yield (GY) and biological yield (BY) of soybean from the pooled data of 2020 and 2021. Squares in red color indicate treatment combinations of I×PNM, and C indicates control.
Discussion
Irrigation effect
Among the irrigation management practices, the adoption of sprinkler irrigation at 80% ETc (Spr 80% ETc) resulted in an increment of 25.6%, 17.6%, 35.4%, and 17.5% in Pn, Tr, Gs, and Ci compared to standard flood irrigation at 50% DASM (FI). Sprinkler irrigation alters the crop’ s microclimate by lowering canopy temperature (Table 7) and maintaining plant water status (Li et al., 2013; Bana et al., 2020; Liu et al., 2021; Tang et al., 2022) and eventually leads to better net-photosynthetic rate in soybean (Cotrim et al., 2021; Rajanna et al., 2022b; Wang et al., 2023). Additionally, Pinnamaneni et al. (2022) observed that soybean cultivated in a rainfed environment or without irrigation exhibited a decrease in stomatal conductance and transpiration, which in turn decreased the crop’s ability to perform photosynthetically. The enhancement in SPAD and NDVI values under sprinkler irrigation could be attributed to sufficient availability of moisture, and the solubility of nutrients helped the crops to grow luxuriantly, which was reflected in higher SPAD and NDVI values at various growth stages (Supplementary Tables S3–S6).
The improved photosynthetic characters and chlorophyll content of the plant helps in better assimilation of nutrients from the soil, thereby improving the crop growth indices (Table 7). The improved crop growth indices and DMA in Spr 80% Etc and Spr 60% ETc irrigation applied plots could be attributed to optimum soil profile moisture and good aeration throughout the effective root zone of the crop (Bhattarai et al., 2004; Daisy et al., 2013; He et al., 2017; Montoya et al., 2017). Earlier studies show that under deficit irrigation or moisture stress condition crop growth get hampered (Xu et al., 2016; Ahmed et al., 2019; Birthal et al., 2021). The adoption of ETc-based irrigation application in soybean has a direct relationship with transpiration, where the greater the ETc, the more the transpiration, which, in turn, enhances the photosynthesis through better exchange of gases and greater chlorophyll content. Therefore, sprinkler irrigation based on ETc (Spr 80% ETc and Spr 60% ETC) resulted in improved crop growth and development (Sincik et al., 2008; Irmak et al., 2013; Anda et al., 2020; Da Silva et al., 2022; Sandhu and Irmak, 2022; Tavares et al., 2022). Moreover, it reduces the amount of nutrients that are lost through leaching because irrigation water was applied depending on the ETc of the crop through sprinklers, avoiding an excessive application as opposed to normal flood irrigation (Table 4). Yang et al. (2020) showed that sprinkler irrigation reduced the nitrate leaching loss (44%) below the root zone, which is attributed to the fact that the wetting front is shallow under sprinkler irrigation compared to furrow irrigation. Application of excess water more than the crop needs resulted in deep percolation in FI leading to leaching loss of applied nutrients along with water (Katoh et al., 2004; Alva et al., 2006; Zhou et al., 2006). Irrigation of crop with Spr 80% ETc and Spr 60% ETc practice resulted in better photosynthates partitioning toward sink (pod dry weight) over FI (Figure 4). The positive correlation between CGR, NAR, and DMA with grain yield showed positive impact of sprinkler irrigation on yield of soybean (Figure 5). The lowest grain yield was recorded in FI (1.97 t ha−1 and 1.99 t ha−1); even though the crop under FI received higher amount of irrigation water, the greater part of it might not be effectively utilized by the crop for its metabolic process because of deeper percolation loss of applied irrigation water beyond the effective root zone under the influence of gravity (Abbasi et al., 2003). The higher water requirements coupled with greater evapo-transpiration under FI resulted in less water productivity (Table 8). Ghazanfar et al. (2010) explained that the significant increase in applied water under FI could be due to the higher evaporation during the growing period, while the sprinkler irrigation minimizes evaporation losses from the soil and from crop canopy surface and also applying irrigation based on crop water needs (ETc) improved WUE of soybean under Spr 60% ETc (Kang et al., 2014; Gajic et al., 2018; Uddin and Murphy, 2020; Djaman et al., 2022). The irrigation management practices had greatly influenced the yield of the soybean crop in our current study through enhanced physiological process (net photosynthesis and transpiration) and resource-use efficiency (water productivity and water-use efficiency).
PNM effect
Basal point placement of fertilizer coupled with top dressing of remaining 50% N fertilizer with SPAD-based management (PNM3) exhibited significantly higher Pn, Tr, Gs, and Ci over PNM5 (Table 5). Therefore, the point placement of fertilizer around the root zone of the crop and need-based application of N fertilizer through SPAD-guided N top dressing helps in maximum acquisition of applied nutrients by the crop. This resulted in quick canopy development by interception of maximum photosynthetically active radiation (PAR) (Hergert et al., 2012; Nkebiwe et al., 2016; Bechtaoui et al., 2021). Higher PAR interception (Figures 3A, B) corroborates longer photosynthetic duration resulting in improved photosynthetic characters like Pn, Tr, Gs, and Ci (Richards, 2000; Thakur et al., 2010; Zhu et al., 2010; Bhagat et al., 2017; Elicin et al., 2021). Likewise, an increment of 29.2% in chlorophyll content (SPAD) at 60–90 DAS was recorded under PNM treatments after SPAD-assisted top dressing of N (Table 6). The increase in chlorophyll content is attributed to the advancement in crop age, as there is more chlorophyll biosynthesis due to greater leaf size of the plant (Buttery and Buzzell, 1977; Costa et al., 2001; Pramanik and Bera, 2013; Islam et al., 2014; Kandel, 2020). Therefore, nitrogen application by using a SPAD meter would help save nitrogen fertilizer and enhance the growth and yield of different crops (Behera et al., 2007; Islam et al., 2014; Ali, 2020; Dass et al., 2022; Rajanna et al., 2022a). Thus, enhancement in NDVI values at 60–90 DAS, which has a positive correlation (r=0.78) with SPAD (Figure 5, Table 7), shows that sensor-based PNM helps in improving chlorophyll content of the plant.
PNM practices also help in enhancing crop growth indices (CGR, RGR, NAR, and LAI) through better acquisition of nutrients (Table 7). Siyal et al. (2012) found that the placement of fertilizer in the root zone of crop reduces nitrogen leaching from 0% to 33% over surface application. Likewise, point placement of fertilizer resulted in improved nutrient-use efficiency, since higher concentration of nutrients around the root zone results in better uptake of nutrients and minimizes losses (Schmitt et al., 2001; Fengqin et al., 2018; Dass et al., 2019; Nayak et al., 2022; Paramesh et al., 2023). Similarly, in our current study, point placement of fertilizer (PNM3) performed significantly (p=0.05) superior in nutrient concentration as against PNM2, PNM4, and PNM5, since the nutrient forage area of roots in PNM2,4,5 is reduced, resulting in suboptimal crop growth (CGR, RGR, and NAR) and DMA. Furthermore, frequent hoeing and PNM1 (SCI nutrient management), which combined organic and inorganic nutrient management practices, led to better root growth, which might have increased nutrient uptake when fertilizer is applied in specific locations (Singh et al., 2018; Dass et al., 2019; Parihar et al., 2020). Point placement of fertilizer coupled with SPAD-assisted N management resulted in higher resource-use efficiency with minimum wastage of resource, which corroborated the improvement in yield of soybean (Dass et al., 2014; Dass et al., 2019; Rajanna et al., 2022a). Optimum resource-use efficiency augmented higher water productivity that, in turn, enhanced crop yields with less water requirement (Burt et al., 2005; Liu et al., 2011; Jha et al., 2019). Precise application of nutrients around the root zone of crop resulted in optimum crop growth and development and enabled the plant roots to extract moisture from deeper layers, which ultimately enhanced crop water productivity, viz., IWP, EWP, and WUE (Mishra and Salokhe, 2011; Parihar et al., 2017; Jat et al., 2018; Sadhukhan et al., 2023). The precise nutrient management practices had greatly influenced the yield of the soybean crop in our current study through enhanced physiological process (net photosynthesis and transpiration) and resource-use efficiency, viz., water productivity and nutrient-use efficiency through site-specific application.
Irrigation and PNM Interaction effect
The significant interaction effect of Pn, NAR, SPAD, and NDVI reiterates the positive effect of I ×PNM on crop growth performances (Table 9, Table 10). Addendum of all the above sensor based precision nutrient and irrigation management helps in better performance of crop under SCI. Prior studies also showed similar results regarding SPAD, NDVI, and chlorophyll content in improving the performance of crop under sensor-based management system (Raun et al., 2005; Bandyopadhyay et al., 2010; Mishra and Salokhe, 2011; Baral et al., 2021; Rajanna et al., 2022a; Farias et al., 2023). The interaction effect on grain and biological yield corroborates that the integration of I and PNM practice along with SCI significantly helped in improving the grain and biological yield of soybean (Figure 7). The application of nutrients and irrigation water as per the needs of the crop through sensor-guided tools, viz., SPAD, NDVI, infrared thermometer, and moisture meter helps in significantly higher yield of crop with minimum wastage of resources (Ma et al., 1995; Sapkota et al., 2014; Shah et al., 2021; Varinderpal et al., 2021).
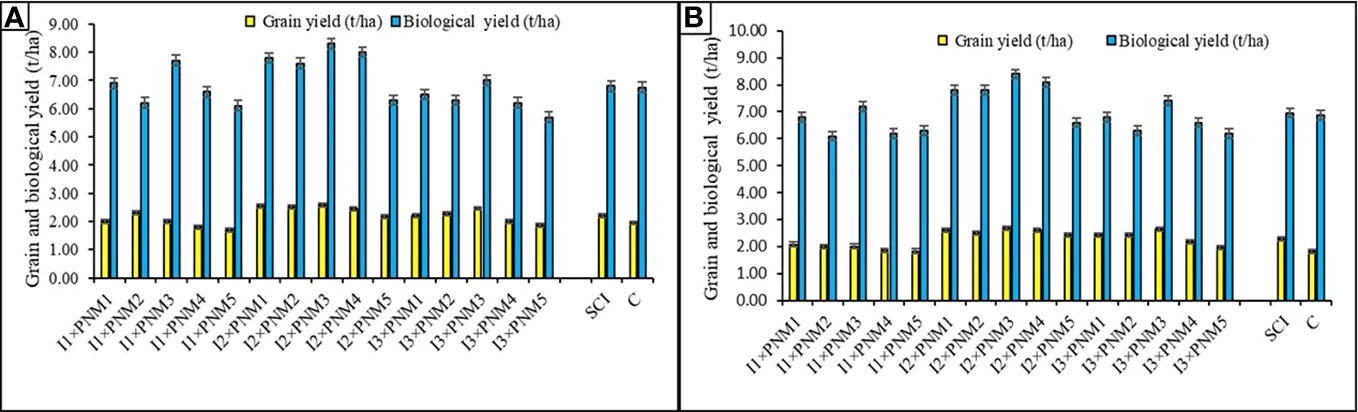
Figure 7 Irrigation management (I) × precision nutrient (PNM) interaction effects on grain yield and biological yield of soybean under SCI 2020 (A) and 2021 (B). For a detailed description of I (irrigation) and PNM (precision nutrient management), refer to Table 2. Any treatment difference that exceeds the range of the bar within a given year is significantly different, as indicated by the LSD 0.05 bar above each column.
SCI versus conventional cultivation
The cultivation of soybean under SCI improved photosynthetic characters in both years. Similarly, in rice under SRI system, wider spacing resulted in better root development, which resulted in higher transportation and accumulation of N and cytokinin from root to shoot, thereby increasing the Pn of the rice plant (Stoop et al., 2002; Dass and Chandra, 2013). Similarly, in our current study, soybean under SCI showed improved photosynthetic characters over control. SCI exhibited increment of 7.4%, 13.5%, 4.3%, and 13.7% in CGR, RGR, NAR, and LAI, respectively (Table 7), which could be attributed to reduced plant to plant competition for nutrient, moisture, and better interception of PAR (Bullock et al., 1988; Stoop et al., 2002; Singh et al., 2023). However, DMA and PAR interception (Figures 3A, B) were higher in conventional cultivation (control) plots compared to SCI cultivation, since plant population per unit area is higher under control, which significantly (p=0.05) contributed to DMA (Table 7), whereas under SCI, wider spacing (30×30 cm) resulted in lower DMA (Abraham et al., 2014). A reduction in interplant competition under SCI cultivation resulting from optimum crop geometry contributes significantly higher photosynthates partitioning toward sink (Kumar et al., 2011; Divya et al., 2015; Nayaka et al., 2021). SCI practices have positive impact on growth and yield of crops by providing optimum space for individual plant to exploit the native available resources (Dobermann, 2004; Kuttamani and Velayudham, 2016; Dass et al., 2023) coupled with better interception of light and improved acquisition of moisture and nutrients (Singh et al., 2018). Thus, sensor-based precision nutrient and irrigation management under SCI favored the physiology, growth, and development of soybean with improved utilization of resources that ultimately enhances crop productivity. Crop management practices also affect the irrigation water requirement through water consumption by changing soil permeability, and evaporation water consumption of plants; likewise, repeated inter-cultivation under SCI resulted in conserving soil moisture by acting as soil mulch (Singh et al., 2022).
Limitation of the present study
The limitation of the current study offers the scope for the development of machine that will carry out both sowing and fertilizer placement simultaneously, which in turn reduces the sowing and fertilizer placement cost of labor. The lack of proper regulation over the sprinkler system, since it is portable, and the measurement of photosynthetic parameters at regular intervals of crop phenology will help in better understanding and give better insights how sensor tools help in providing favorable microclimate for crop growth.
Conclusion
The current work provides valuable insights into the mechanisms by which sensor-guided instruments enable precise water and nutrition utilization in soybean crop intensification (SCI). Utilizing SCI enhances the crop productivity through improved physiological and growth parameters. Similarly, the adoption of sprinkler irrigation at 80% ETC (Spr 80% ETc) showed superiority for all the physiological, growth, and yield parameters over sprinkler irrigation at 60% (Spr 60% ETC) ETC and FI (standard flood irrigation at 50% DASM).Thus, the application of irrigation at Spr 80% ETc resulted in an increment of 25.6%, 17.6% 35.4%, and 17.5% in photosynthetic rate, transpiration rate, stomatal conductance, and intercellular CO2 concentration over flood irrigation plots. Among the PNM, PNM3 showed significant improvement in photosynthetic characters, SPAD, NDVI, including grain and stover yield. A comparison of SCI versus control showed that crop productivity was enhanced significantly (p = 0.05) under SCI cultivation due to improved photosynthetic characters, growth, and development of crop. The enhancement in the grain yield under SCI was to the tune of 19.7% over control. The trend of performance for irrigation (I) and precision nutrient management (PNM) for photosynthetic characters, SPAD (greenness), NDVI, crop growth indices, and yield was Spr 80% ETc >Spr 60% ETC>FI and P3>P1> P2> P4>P5. However, for water productivity under irrigation, the trend was Spr 60% ETc> Spr 80% ETc>FI. Therefore, using sensor-based precision nutrient and irrigation management technique appears to be a successful strategy for improving crop production and maximizing resource utilization in the cultivation of soybeans across a wide range of geographical regions.
Data availability statement
The original contributions presented in the study are included in the article/Supplementary Material. Further inquiries can be directed to the corresponding author.
Author contributions
SKS: Conceptualization, Investigation, Methodology, Writing – review & editing, Resources, Writing – original draft. AD: Conceptualization, Investigation, Methodology, Project administration, Supervision, Writing – review & editing. SD: Conceptualization, Methodology, Project administration, Writing – review & editing. RG: Methodology, Writing – review & editing. TS: Writing – original draft. SS: Writing – original draft. MS: Formal analysis, Writing – review & editing. AC: Formal analysis, Writing – review & editing. HK: Data curation, Writing – original draft. PBR: Writing – review & editing. SP: Formal analysis, Writing – review & editing. VKS: Formal analysis, Writing – review & editing. VP: Formal analysis, Writing – review & editing. PK: Data curation, Writing – original draft. MK: Writing – review & editing. RS: Data curation, Writing – original draft. VT: Writing – review & editing. KK: Writing – review & editing. KNK: Data curation, Writing – original draft. A-AS: Data curation, Writing – original draft. ADD: Writing – review & editing.
Funding
The author(s) declare that no financial support was received for the research, authorship, and/or publication of this article.
Acknowledgments
The authors are thankful to ICAR–Indian Agricultural Research Institute, New Delhi for arranging necessary experimental field and laboratory facilities. The first author is thankful to the IARI, New Delhi for providing Senior Research Fellowship during the course of study.
Conflict of interest
The authors declare that the research was conducted in the absence of any commercial or financial relationships that could be construed as a potential conflict of interest.
Publisher’s note
All claims expressed in this article are solely those of the authors and do not necessarily represent those of their affiliated organizations, or those of the publisher, the editors and the reviewers. Any product that may be evaluated in this article, or claim that may be made by its manufacturer, is not guaranteed or endorsed by the publisher.
Supplementary material
The Supplementary Material for this article can be found online at: https://www.frontiersin.org/articles/10.3389/fpls.2023.1282217/full#supplementary-material
Glossary
References
Abbasi, F., Adamsen, F. J., Hunsaker, D. J., Feyen, J., Shouse, P., Van Genuchten, M. T. (2003). Effects of flow depth on water flow and solute transport in furrow irrigation: Field data analysis. J. Irrig. Drain. Eng. 129 (4), 237–246. doi: 10.1061/(ASCE)0733-9437(2003)129:4(237
Abraham, B., Araya, H., Berhe, T., Edwards, S., Gujja, B., Khadka, R. B., et al. (2014). The system of crop intensification: reports from the field on improving agricultural production, food security, and resilience to climate change for multiple crops. Agric. Food. Secur. 3 (1), 1–12. doi: 10.1186/2048-7010-3-4
Adhikari, P., Araya, H., Aruna, G., Balamatti, A., Banerjee, S., Baskaran, P., et al. (2018). System of crop intensification for more productive, resource-conserving, climate-resilient, and sustainable agriculture: Experience with diverse crops in varying agroecologies. Int. J. Agric. Sustain. 16 (1), 1–28. doi: 10.1080/14735903.2017.1402504
Agarwal, D. K., Billore, S. D., Sharma, A. N., Dupare, B. U., Srivastava, S. K. (2013). Soybean: introduction, improvement, and utilization in India—problems and prospects. Agric. Res. 2 (4), 293–300. doi: 10.1007/s40003-013-0088-0
Ahmed, N., Zhang, Y., Li, K., Zhou, Y., Zhang, M., Li, Z. (2019). Exogenous application of glycine betaine improved water use efficiency in winter wheat (Triticum aestivum L.) via modulating photosynthetic efficiency and antioxidative capacity under conventional and limited irrigation conditions. Crop J. 7 (5), 635–650. doi: 10.1016/j.cj.2019.03.004
Ali, A. M. (2020). Using hand-held chlorophyll meters and canopy reflectance sensors for fertilizer nitrogen management in cereals in small farms in developing countries. J. Sens. 20 (4), 1127. doi: 10.3390/s20041127
Allen, R. G., Pereira, L. S., Raes, D., Smith, M. (1998). Crop evapotranspiration-Guidelines for computing crop water requirements-FAO Irrigation and drainage paper 56 Vol. 300 (Rome: FAO).
Alva, A. K., Paramasivam, S., Fares, A., Delgado, J. A., Mattos, D., Jr., Sajwan, K. (2006). Nitrogen and irrigation management practices to improve nitrogen uptake efficiency and minimize leaching losses. J. Crop Improv. 15 (2), 369–420. doi: 10.1300/J411v15n02_11
Anda, A., Simon, B., Soos, G., Teixeira da Silva, J. A., Farkas, Z., Menyhart, L. (2020). Assessment of soybean evapotranspiration and controlled water stress using traditional and converted evapotranspirometers. J. Atmos. 11 (8), 830. doi: 10.3390/atmos11080830
Bana, R. C., Yadav, S. S., Shivran, A. C., Singh, P., Kudi, V. K. (2020). Site-specific nutrient management for enhancing crop productivity. Int. Res. J. Pure Appl. Chem. 21, 17–25. doi: 10.9734/IRJPAC/2020/v21i1530249
Bandyopadhyay, K. K., Misra, A. K., Ghosh, P. K., Hati, K. M., Mandal, K. G., Moahnty, M. (2010). Effect of irrigation and nitrogen application methods on input use efficiency of wheat under limited water supply in a Vertisol of Central India. Irrig. Sci. 28, 285–299. doi: 10.1007/s00271-009-0190-z
Bangre, J., Singh, B. (2020). Performance of Soybean [Glycine max (L.) Merrill] under organic and inorganic nutrient management in semi-arid tropical conditions in central India. J. Exp. Agric. Int. 42 (9), 235–242.
Baral, B. R., Pande, K. R., Gaihre, Y. K., Baral, K. R., Sah, S. K., Thapa, Y. B., et al. (2021). Real-time nitrogen management using decision support-tools increases nitrogen use efficiency of rice. Nutr. Cycl. Agroecosyst. 119, 355–368. doi: 10.1007/s10705-021-10129-6
Bechtaoui, N., Rabiu, M. K., Raklami, A., Oufdou, K., Hafidi, M., Jemo, M. (2021). Phosphate-dependent regulation of growth and stresses management in plants. Front. Plant Sci. 12. doi: 10.3389/fpls.2021.679916
Behera, U. K., Sharma, A. R., Pandey, H. N. (2007). Sustaining productivity of wheat–soybean cropping system through integrated nutrient management practices on the Vertisols of central India. Int. J. Plant Soil Sci. 297, 185–199. doi: 10.1007/s11104-007-9332-3
Bennett, A. J. (2000). Environmental consequences of increasing production: some current perspectives. Agric. Ecosyst. Environ. 82, 89–95. doi: 10.1016/S0167-8809(00)00218-8
Bhagat, K. P., Bal, S. K., Singh, Y., Potekar, S., Saha, S., Ratnakumar, P., et al. (2017). Effect of reduced PAR on growth and photosynthetic efficiency of soybean genotypes. J. Agrometeorol. 19 (1), 1–9. doi: 10.54386/jam.v19i1.734
Bhargava, C., Deshmukh, G., Sawarkar, S. D., Alawa, S. L., Ahirwar, J. (2016). The system of wheat intensification in comparison with convention method of wheat line sowing to increase wheat yield with low input cost. Plant Arch. 16 (2), 801–804.
Bhattarai, S. P., Huber, S., Midmore, D. J. (2004). Aerated subsurface irrigation water gives growth and yield benefits to zucchini, vegetable soybean and cotton in heavy clay soils. Ann. Appl. Biol. 144 (3), 285–298. doi: 10.1111/j.1744-7348.2004.tb00344.x
Bianchi, E., Szpak, C. (2017). Soybean prices, economic growth and poverty in Argentina and Brazil (Winnipeg, Manitoba, Canada: FAO), 49. Available at: http://www.fao.org/3/I8316EN/i8316en.pdf.
Birthal, P. S., Hazrana, J., Negi, D. S., Pandey, G. (2021). Benefits of irrigation against heat stress in agriculture: evidence from wheat crop in India. Agric. Water Manage. 255, 106950. doi: 10.1016/j.agwat.2021.106950
Bryant, C. J., Krutz, L. J., Falconer, L., Irby, J. T., Henry, C. G., Pringle, III, H. C., et al. (2017). Irrigation water management practices that reduce water requirements for Mid-South furrow-irrigated soybean. Crop Forage Turfgrass Manage. 3 (1), 1–7. doi: 10.2134/cftm2017.04.0025
Bullock, D. G., Nielsen, R. L., Nyquist, W. E. (1988). A growth analysis comparison of corn grown in conventional and equidistant plant spacing. Crop Sci. 28 (2), 254. doi: 10.2135/cropsci1988.0011183X002800020015x
Burt, C. M., Mutziger, A. J., Allen, R. G., Howell, T. A. (2005). Evaporation research: Review and interpretation. J. Irrig. Drain. Eng. 131 (1), 37–58. doi: 10.1061/(ASCE)0733-9437(2005)131:1(37
Buttery, B. R., Buzzell, R. I. (1977). The relationship between chlorophyll content and rate of photosynthesis in soybeans. Can. J. Plant Sci. 57 (1), 1–5. doi: 10.4141/cjps77-001
Cheng, M., Wang, H., Fan, J., Zhang, S., Liao, Z., Zhang, F., et al. (2021). A global meta-analysis of yield and water use efficiency of crops, vegetables and fruits under full, deficit and alternate partial root-zone irrigation. Agric. Water Manag .248, 106771. doi: 10.1016/j.agwat.2021.106771
Costa, C., Dwyer, L. M., Dutilleul, P., Stewart, D. W., Ma, B. L., Smith, D. L. (2001). Inter-relationships of applied nitrogen, SPAD, and yield of leafy and non-leafy maize genotypes. J. Plant Nutr. 24 (8), 1173–1194. doi: 10.1081/PLN-100106974
Cotrim, M. F., Gava, R., Campos, C. N. S., de David, C. H. O., Reis, I. D. A., Teodoro, L. P. R., et al. (2021). Physiological performance of soybean genotypes grown under irrigated and rainfed conditions. J. Agron. Crop Sci. 207 (1), 34–43. doi: 10.1111/jac.12448
DAFW GOI. (2021). Department of agriculture and farmers welfare Government of India. First advanced estimate of food grain production 2020-21. Available at: https://agricoop.nic.in/en (Accessed June 10, 2023).
Daisy, M., Thavaprakaash, N., Velayudham, K., Divya, V. (2013). Effect of System of Crop Intensification (SCI) practices on growth, yield attributes and yield of castor hybrid YRCH 1. Int. J. Adv. Life. Sci. 6 (4), 366–374.
Da Silva, J. A., de Carvalho, L. G., Ribeiro Andrade, F. (2022). Gas exchanges and growth of soybean as affected by irrigation depths and soil compaction. Chil. J. Agric. Res. 82 (3), 502–512. doi: 10.4067/S0718-58392022000300502
Dass, A., Bhattacharyya, R. (2017). Wheat residue mulch and anti-transpirants improve productivity and quality of rainfed soybean in semi-arid north-Indian plains. Field Crops Res. 210, 9–19. doi: 10.1017/S0014479713000252
Dass, A., Chandra, S. (2013). Irrigation, spacing and cultivar effects on net photosynthetic rate, dry matter partitioning and productivity of rice under system of rice intensification in mollisols of northern India. Exp. Agric. 49 (04), 504–523. doi: 10.1017/S0014479713000252
Dass, A., Dey, D., Lal, S., Rajanna, G. (2019). Tank-mix insecticide and herbicide application effects on weeds, insect-pest menace and soybean productivity in semi-arid northern plains of India. Legume Res. 42 (3), 385–391. doi: 10.18805/LR-3855
Dass, A., Kaur, R., Bana, R. S., Rana, K. S., Choudhary, A. K., Pooniya, V., et al. (2013). “Irrigation Water Management – A practical Manual,” in Division of Agronomy (New Delhi-110 012, India: ICAR–Indian Agricultural Research Institute), 104.
Dass, A., Kushwaha, H. L., Sahoo, P. K., Dhar, S., Choudhary., A. K., Khura, T. K., et al. (2023). Comparative analysis of machine-planted and manual-planted wheat on crop and water productivity, and profitability under system of wheat intensification management. Front. Sustain. Food Syst. 7. doi: 10.3389/fsufs.2023.1187647
Dass, A., Rajanna, G. A., Babu, S., Lal, S. K., Choudhary, A. K., Singh, R., et al. (2022). Foliar application of macro-and micronutrients improves the productivity, economic returns, and resource-use efficiency of soybean in a semiarid climate. Sustainability 14 (10), 5825. doi: 10.3390/su14105825
Dass, A., Suri, V. K., Choudhary, A. K. (2014). Site specific nutrient management approaches for enhanced nutrient-use efficiency in agricultural crops. RRJoCST 3 (3), 1–6.
Devaraj, A. (2020). Influence of spacing and nutrient levels on yield, physiological parameters and nutrient uptake in short duration varieties of red gram (Cajanus cajan L.). J. pharmacogn. phytochem. 9 (5), 1463–1467.
Dhar, S., Barah, B. C., Vyas, A. K., Uphoff, N. (2015). Comparing System of Wheat Intensification (SWI) with standard recommended practices in the northwestern plain zone of India. Arch. Agron. Soil Sci. 62 (7), 994–1006. doi: 10.1080/03650340.2015.1101518
Divya, V., Velayudham, K., Thavaprakaash, N., Daisy, M. (2015). Growth, yield attributes, yield and economics of sesame (Sesamum indicum l.) As influenced by system of crop intensification (SCI) practices. APJAS 1 (2), 62–66.
Djaman, K., Koudahe, K., Saibou, A., Darapuneni, M., Higgins, C., Irmak, S. (2022). Soil water dynamics, effective rooting zone, and evapotranspiration of sprinkler irrigated potato in a sandy loam soil. J. Agron. 12 (4), 864. doi: 10.3390/agronomy12040864
Dobermann, A. (2004). A critical assessment of the system of rice intensification (SRI). Agric. Syst. 79 (3), 261–281. doi: 10.5958/0976-4666.2015.00033.9
Elicin, A. K., Ozturk, F., Kizilgeçi, F., Koca, Y. K. (2021). Soybean (Glycine max.(L.) Merrill) vegetative growth performance under chemical and organic manures nutrient management system. Fresenius Environ. Bull. 30 (11), 12684–12690.
Farias, G. D., Bremm., C., Bredemeier, C., de Lima Menezes, J., Aquino Alves, L., Tiecher, T., et al. (2023). Normalized Difference Vegetation Index (NDVI) for soybean biomass and nutrient uptake estimation in response to production systems and fertilization strategies. Front. Sustain. Food Syst. 6. doi: 10.3389/fsufs.2022.959681
Fengqin, H. U., Huoyan, W. A., Pu, M. O., Jianmin, Z. H. (2018). Nutrient composition and distance from point placement to the plant affect rice growth. Pedosphere 28 (1), 124–134. doi: 10.1016/S1002-0160(17)60393-X
Fess, T. L., Kotcon, J. B., Benedito, V. A. (2011). Crop breeding for low input agriculture: a sustainable response to feed a growing world population. Sustainability 3 (10), 1742–1772. doi: 10.3390/su3101742
Gajic, B., Kresovic, B., Tapanarova, A., Zivotic, L., Todorovic, M. (2018). Effect of irrigation regime on yield, harvest index and water productivity of soybean grown under different precipitation conditions in a temperate environment. Agric. Water Manage. 210, 224–231. doi: 10.1016/j.agwat.2018.08.002
Gebre, M. G., Earl, H. J. (2021). Soil water deficit and fertilizer placement effects on root biomass distribution, soil water extraction, water use, yield, and yield components of soybean [Glycine max (L.) Merr.] grown in 1-m rooting columns. Front. Plant Sci. 12. doi: 10.3389/fpls.2021.581127
Ghazanfar, M., Asoodar, M. A., Alami Saeed, K. H. (2010). The effect of conservation tillage, planting and irrigation methods on water use efficiency and wheat grain yield in north of Ahwaz. J. Eng. Appl. Sci. 5 (2), 101–105. doi: 10.3923/jeasci.2010.101.105
He, J., Du, Y. L., Wang, T., Turner, N. C., Yang, R. P., Jin, Y., et al. (2017). Conserved water use improves the yield performance of soybean (Glycine max (L.) Merr.) under drought. Agric. Water Manage. 179, 236–245. doi: 10.1016/j.agwat.2016.07.008
Hergert, G. W., Wortmann, C. S., Ferguson, R. B., Shapiro, C. A., Shaver, T. M. (2012). Using starter fertilizers for corn, grain sorghum, and soybeans. Neb Guide G361 (Lincoln: University of Nebraska). Available at: https://extension.unl.edu/publications.
Hou, M., Tian, F., Zhang, L., Li, S., Du, T., Huang, M., et al. (2018). Estimating crop transpiration of soybean under different irrigation treatments using thermal infrared remote sensing imagery. Agronomy 9 (1), 8. doi: 10.3390/agronomy9010008
Igbadun, H. E., Mahoo, H. F., Tarimo, A. K. P. R., Salim, B. A. (2006). Crop water productivity of an irrigated maize crop in Mkoji sub–catchment of the Great Ruaha River Basin Tanzania. Agric. Water Manage. 85, 141–150. doi: 10.1016/j.agwat.2006.04.003
Irmak, S., Odhiambo, L. O., Specht, J. E., Djaman, K. (2013). Hourly and daily single and basal evapotranspiration crop coefficients as a function of growing degree days, days after emergence, leaf area index, fractional green canopy cover, and plant phenology for soybean. Trans. ASABE 56 (5), 1785–1803. doi: 10.13031/trans.56.10219
Islam, M. R., Haque, K. S., Akter, N., Karim, M. A. (2014). Leaf chlorophyll dynamics in wheat based on SPAD meter reading and its relationship with grain yield. Sci. Agric. 8 (1), 13–18. doi: 10.15192/PSCP.SA.2014.4.1.1318
Jat, R. D., Jat, H. S., Nanwal, R. K., Yadav, A. K., Bana, A., Choudhary, K. M., et al. (2018). Conservation agriculture and precision nutrient management practices in maize-wheat system: Effects on crop and water productivity and economic profitability. Field Crops Res. 222, 111–120. doi: 10.1016/j.fcr.2018.03.025
Jha, S. K., Ramatshaba, T. S., Wang, G., Liang, Y., Liu, H., Gao, Y., et al. (2019). Response of growth, yield and water use efficiency of winter wheat to different irrigation methods and scheduling in North China Plain. Agric. Water Manage. 217, 292–302. doi: 10.1016/j.agwat.2019.03.011
Kandel, B. P. (2020). “SPAD value varies with age and leaf of maize plant and its relationship with grain yield,” in BMC Research Notes, vol. 13. (Springer Nature, New York, USA: Springer publication), 1–4. doi: 10.1186/s13104-020-05324-7
Kang, L. Y., Yue, S. C., Li, S. Q. (2014). Effects of phosphorus application in different soil layers on root growth, yield, and water–use efficiency of winter wheat grown under semi–arid conditions. J. Integr. Agric. 13 (9), 2028–2039. doi: 10.1016/S2095-3119(14)60751-6
Katoh, M., Murase, J., Hayashi, M., Matsuya, K., Kimura, M. (2004). Nutrient leaching from the plow layer by water percolation and accumulation in the subsoil in an irrigated paddy field. J. Soil Sci. Plant Nutr. 50 (5), 721–729. doi: 10.1080/00380768.2004.10408528
Kumar, S., Patel, N. R., Sarkar, A., Dadhwal, V. K. (2013). Geospatial approach in assessing agro-climatic suitability of soybean in rainfed agro-ecosystem. J. Indian Soc Remote. Sens. 41, 609–618. doi: 10.1007/s12524-012-0249-9
Kumar, A., Raj, R., Dhar, S., Pandey, U. C. (2011). Performance of system of wheat intensification (SWI) and conventional wheat sowing under north eastern plain zone of India. Ann. Agric. Res. 36 (3), 258–262.
Kuttimani, R., Velayudham, K. (2016). System of crop intensification (SCI) techniques on yield and economics of greengram. Indian J. Agric. Vet. Sci. 9 (7), 01–04. doi: 10.9790/2380-0907020104
Larbi, P. A., Green, S. (2018). Time series analysis of soybean response to varying atmospheric conditions for precision agriculture. Precis. Agric. 19, 1113–1126. doi: 10.1007/s11119-018-9577-2
Li, D., Liu, H., Qiao, Y., Wang, Y., Dong, B., Cai, Z., et al. (2013). Physiological regulation of soybean (Glycine max L. Merr.) growth in response to drought under elevated CO2. J. Food Agric. Environ. 11, 649–654.
Li, H., Issaka, Z., Jiang, Y., Tang, P., Chen, C. (2019). Overview of emerging technologies in sprinkler irrigation to optimize crop production. Int. J. Agric. Biol. 12 (3), 1–9. doi: 10.25165/j.ijabe.20191203.4310
Liu, H., Yu, L., Luo, Y., Wang, X., Huang, G. (2011). Responses of winter wheat (Triticum aestivum L.) evapotranspiration and yield to sprinkler irrigation regimes. Agric. Water Manage. 98 (4), 483–492. doi: 10.1016/j.agwat.2020.106498
Liu, Z., Jiao, X., Zhu, C., Katul, G. G., Ma, J., Guo, W. (2021). Micro-climatic and crop responses to micro-sprinkler irrigation. Agric. Water Manage. 243, 106498. doi: 10.1016/j.agwat.2020.106498
Ma, B. L., Morrison, M. J., Voldeng, H. D. (1995). Leaf greenness and photosynthetic rates in soybean. Crop Sci. 35 (5), 1411–1414. doi: 10.2135/cropsci1995.0011183X003500050025x
Martens, D. A. (2001). Nitrogen cycling under different soil management systems. Adv. Agron. 70 (1), 143–192. doi: 10.1016/S0065-2113(01)70005-3
Mishra, A., Salokhe, V. M. (2011). Rice root growth and physiological responses to SRI water management and implications for crop productivity. Paddy Water Environ. 9, 41–52. doi: 10.1007/s10333-010-0240-4
Misra, A. K. (2014). Climate change and challenges of water and food security. Int. J. Sustain. Environ. 3 (1), 153–165. doi: 10.1016/j.ijsbe.2014.04.006
Montoya, F., García, C., Pintos, F., Otero, A. (2017). Effects of irrigation regime on the growth and yield of irrigated soybean in temperate humid climatic conditions. Agric. Water Manage. 193, 30–45. doi: 10.1016/j.agwat.2017.08.001
Nayak, H. S., Parihar, C. M., Jat, S. L., Lata, B. N., Mandal, Singh, V. K., et al. (2019). Effect of nitrogen point placement on energetic and soil enzymatic activities on long-term conservation agriculture based maize (Zea mays) - wheat (Triticum aestivum) system of western Indo-Gangetic plains. Indian J. Agric. Sci. 89 (12), 2102–2106. doi: 10.56093/ijas.v89i12.96282
Nayak, H. S., Parihar, C. M., Mandal, B. N., Patra, K., Jat, S. L., Singh, R., et al. (2022). Point placement of late vegetative stage nitrogen splits increase the productivity, N-use efficiency and profitability of tropical maize under decade long conservation agriculture. Eur. J. Agron. 133, 126417. doi: 10.1016/j.eja.2021.126417
Nayaka, G. V., Reddy, G. P., Kumar, R. M. (2021). Dry matter production and partitioning in different plant parts of rice cultivars under irrigation regimes and systems of cultivation. Indian J. Agric. Sci. 55 (3), 347–352. doi: 10.18805/IJARe.A-5316
Nkebiwe, P. M., Weinmann, M., Bar-Tal, A., Muller, T. (2016). Fertilizer placement to improve crop nutrient acquisition and yield: A review and meta-analysis. Field Crops Res. 196, 389–401. doi: 10.1016/j.fcr.2016.07.018
Paramesh, V., Kumar, M. R., Rajanna, G. A., Gowda, S., Nath, A. J., Madival, Y., et al. (2023). Integrated nutrient management for improving crop yields, soil properties, and reducing greenhouse gas emissions. Front. Sustain. Food Syst. 7. doi: 10.3389/fsufs.2023.1173258
Parihar, C. M., Jat, H. S., Jat, S. L., Kakraliya, S. K., Nayak, H. S. (2020). Precision nutrient management for higher nutrient use efficiency and farm profitability in irrigated cereal-based cropping systems. Indian J. Fertilisers 16 (10), 1000–1014.
Parihar, C. M., Jat, S. L., Singh, A. K., Majumdar, K., Jat, M. L., Saharawat, Y. S., et al. (2017). Bio–energy, water–use efficiency and economics of maize–wheat–mungbean system under precision–conservation agriculture in semi–arid agro–ecosystem. Energy 119, 245–256. doi: 10.1016/j.energy.2016.12.068
PIB, GOI. (2022). Press Information Bureau, Government of India. Coverage under Drip and Micro Irrigation. Available at: https://pib.gov.in/PressReleaseIframePage.aspx?PRID=1795499 (Accessed November 13, 2023).
Pinnamaneni, S. R., Anapalli, S. S., Reddy, K. N. (2022). Photosynthetic response of soybean and cotton to different irrigation regimes and planting geometries. Front. Plant Sci. 13. doi: 10.3389/fpls.2022.894706
Pohare, V. B., Thawal, D. W., Kamble, A. B. (2018). Physiological response of soybean (Glycine max L.) as influenced by integrated nutrient management practices. J. Agrometeorol. 20 (3), 202–205. doi: 10.54386/jam.v20i3.544
Pramanik, K., Bera, A. K. (2013). Effect of seedling age and nitrogen fertilizer on growth, chlorophyll content, yield and economics of hybrid rice (Oryza sativa L.). Int. J. Agron. Plant Prod. 4 (5), 3489–3499.
Raghuvanshi, R. S., Bisht, K. B. (2010). “Uses of soybean: products and preparation,” in The soybean: botany, production and uses (Wallingford UK: Cabi), 404–426.
Rajanna, G. A., Dass, A., Singh, V. K., Choudhary, A. K., Paramesh, V., Babu, S., et al. (2023). Energy and carbon budgeting in a soybean–wheat system in different tillage, irrigation and fertilizer management practices in South-Asian semi-arid agroecology. Eur. J. Agron. 148 (2023), 126877. doi: 10.1016/j.eja.2023.126877
Rajanna, G. A., Dass, A., Suman, A., Babu, S., Venkatesh, P., Singh, V. K., et al. (2022a). Co-implementation of tillage, irrigation, and fertilizers in soybean: Impact on crop productivity, soil moisture, and soil microbial dynamics. Field Crops Res. 288, 108672. doi: 10.1016/j.fcr.2022.108672
Rajanna, G. A., Manna, S., Singh, A., Babu, S., Singh, V. K., Dass, A., et al. (2022b). Biopolymeric superabsorbent hydrogels enhance crop and water productivity of soybean–wheat system in Indo-Gangetic plains of India. Sci. Rep. 12, 11955. doi: 10.1038/s41598-022-16049-x
Ramesh, K., Ashok, K. P., Ashis, K. (2017). Best management practices for soybean under soybean–wheat system to minimize the impact of climate change. Indian J. Fertilisers 13 (2), 42–55.
Ramesh, N., Baradhan, G., Suresh Kumar, S. M. (2019). Soil moisture sensor based drip irrigation on sugarcane at different fertilizer levels. Plant Arch. 19 (1), 808–812.
Ramesh, K., Chandrasekaran, B., Balasubramanian, T. N., Bangarusamy, U., Sivasamy, R., Sankaran, N. (2002). Chlorophyll dynamics in rice (Oryza sativa) before and after flowering based on SPAD (chlorophyll) meter monitoring and its relation with grain yield. J. Agron. Crop Sci. 188 (2), 102–105. doi: 10.1046/j.1439-037X.2002.00532.x
Rana, K. S., Choudhary, A. K., Sepat, S., Bana, R. S., Dass, A. (2014). Methodological and analytical agronomy. Post Graduate School Indian Agric. Res. Institute New Delhi., 228.
Raun, W. R., Solie, J. B., Stone, M. L., Martin, K. L., Freeman, K. W., Mullen, R. W., et al. (2005). Optical sensor-based algorithm for crop nitrogen fertilization. Commun. Soil Sci. Plant Anal. . 36 (19-20), 2759–2781. doi: 10.1080/00103620500303988
Rezaei, K., Wang, T., Johnson, L. A. (2002). Combustion characteristics of candles made from hydrogenated soybean oil. J. American. Oil. Chem. Soc 79, 803–808. doi: 10.1007/s11746-002-0562
Richards, R. A. (2000). Selectable traits to increase crop photosynthesis and yield of grain crops. J. Exp. Bot. 51 (1), 447–458. doi: 10.1093/jexbot/51.suppl_1.447
Sadhukhan, R., Kumar, D., Sen, S., Sepat, S., Ghosh, A., Shivay, Y. S., et al. (2023). Precision nutrient management in zero–till direct–seeded rice influences the productivity, profitability, nutrient, and water use efficiency as well as the environmental footprint in the indo gangetic plain of India. Agriculture 13 (4), 784. doi: 10.3390/agriculture13040784
Sandhu, R., Irmak, S. (2022). Effects of subsurface drip-irrigated soybean seeding rates on grain yield, evapotranspiration and water productivity under limited and full irrigation and rainfed conditions. Agric. Water Manage. 267, 107614. doi: 10.1016/j.agwat.2022.107614
Sapkota, T. B., Majumdar, K., Jat, M. L., Kumar, A., Bishnoi, D. K., McDonald, A. J., et al. (2014). Precision nutrient management in conservation agriculture based wheat production of Northwest India: Profitability, nutrient use efficiency and environmental footprint. Field Crops Res. 155, 233–244. doi: 10.1016/j.fcr.2013.09.001
Schmitt, M. A., Lamb, J. A., Randall, G. W., Orf, J. H., Rehm, G. W. (2001). In-season fertilizer nitrogen applications for soybean in Minnesota. J. Agron. 93 (5), 983–988. doi: 10.2134/agronj2001.935983x
Shafagh, K. J., Zehtab, S. S., Javanshir, A., Moghaddam, M., Nasab, A. D. M. (2008). Effects of nitrogen and duration of weed interference on grain yield and SPAD (chlorophyll) value of soybean (Glycine max (L.) Merrill.). J. Food Agric. Environ. 6 (3-4), 368–373.
Shah, J., Wang, X., Khan, S. U., Khan, S., Gurmani, Z. A., Fiaz, S., et al. (2021). Optical-sensor-based nitrogen management in oat for yield enhancement. Sustainability 13 (12), 6955. doi: 10.3390/su13126955
Sincik, M., Candogan, B. N., Demirtas, C., Buyukcangaz, H., Yazgan, S., Goksoy, A. T. (2008). Deficit irrigation of soya bean [Glycine max (L.) Merr.] in a sub-humid climate. J. Agron. Crop Sci. 194 (3), 200–205. doi: 10.1111/j.1439-037X.2008.00307.x
Singh, R. K., Dhar, S., Dass, A., Sharma, V. K., Kumar, A., Gupta, G., et al. (2018). Productivity and profitability of soybean (Glycine max) and wheat (Triticum aestivum) genotypes grown in sequence under system of crop intensification. Indian J. Agric. Sci. 88 (9), 1407–1412. doi: 10.56093/ijas.v88i9.83500
Singh, R. K., Dhar, S., Upadhyay, P. K., GA, D. R., Singh, V. K., Kumar, R., et al. (2022). Soybean crop intensification for sustainable above–ground–under–ground plant–soil interactions. Front. Sustain. Food Syst. 7. doi: 10.3389/fsufs.2023.1194867
Singh, S. R. K., Mishra, A., Suryawanshi, A., Shrivastava, V. (2019). Effect of varietal intervention on the yield of soybean crop across agro-climatic zones in Madhya Pradesh: A cluster approach. J. pharmacogn. Phytochem. 8 (2), 408–410.
Singh, B., Singh, Y., Ladha, J. K., Bronson, K. F., Balasubramanian, V., Singh, J., et al. (2002). Chlorophyll meter– and leaf colour chart– based nitrogen management for rice and wheat in north–western India. J. Agron. 94, 821–829. doi: 10.2134/agronj2002.8210
Singh, R. K., Upadhyay, P. K., Dhar, S., Rajanna, G. A., Singh, V. K., Kumar, R., et al. (2023). Soybean crop intensification for sustainable aboveground-underground plant–soil interactions. Front. Sustain. Food Syst. 7. doi: 10.3389/fsufs.2023.1194867
Siyal, A. A., Bristow, K. L., Simunek, J. (2012). Minimizing nitrogen leaching from furrow irrigation through novel fertilizer placement and soil surface management strategies. Agric. Water Manage. 115, 242–251. doi: 10.1016/j.agwat.2012.09.008
Solgi, S., Ahmadi, S. H., Sepaskhah, A. R., Edalat, M. (2022). Wheat yield modeling under water-saving irrigation and climatic scenarios in transition from surface to sprinkler irrigation systems. J. Hydrol. 612, 128053. doi: 10.1016/j.jhydrol.2022.128053
Stoop, W. A., Uphoff, N., Kassam, A. (2002). A review of agricultural research issues raised by the system of rice intensification (SRI) from Madagascar: opportunities for improving farming systems for resource-poor farmers. Agric. Syst. 71 (3), 249–274. doi: 10.1016/S0308-521X(01)00070-1
Tang, X., Liu, H., Yang, L., Li, L., Chang, J. (2022). Energy balance, microclimate, and crop evapotranspiration of winter wheat (Triticum aestivum L.) under sprinkler irrigation. Agriculture 12 (7), 1–23. doi: 10.3390/agriculture12070953
Tavares, C. J., Ribeiro Junior, W. Q., Ramos, M. L., Pereira, L. F., Casari, R. A., Pereira, A. F., et al. (2022). Water stress alters morph physiological, grain quality and vegetation indices of soybean cultivars. Plants 11 (4), 559. doi: 10.3390/plants11040559
Thakur, A. K., Uphoff, N., Antony, E. (2010). An assessment of physiological effects of system of rice intensification (SRI) practices compared with recommended rice cultivation practices in India. Exp. Agric. 46 (1), 77–98. doi: 10.1017/S0014479709990548
Triboi, E., Triboi-Blondel, A. M. (2002). Productivity and grain or seed composition: a new approach to an old problem. Eur. J. Agron. 16 (3), 163–186. doi: 10.1016/S1161-0301(01)00146-0
Uddin, M. J., Murphy, S. R. (2020). Evaporation losses and evapotranspiration dynamics in overhead sprinkler irrigation. J. Irrig. Drain. Eng. 146 (8), 04020023. doi: 10.1061/(ASCE)IR.1943-4774.0001469
Uddling, J., Gelang-Alfredsson, J., Piikki, K., Pleijel, H. (2007). Evaluating the relationship between leaf chlorophyll concentration and SPAD-502 chlorophyll meter readings. Photosynthesis Res. 91, 37–46. doi: 10.1007/s11120-006-9077-5
Varinderpal, S., Kunal, Gosal, S. K., Choudhary, R., Singh, R., Adholeya, A. (2021). Improving nitrogen use efficiency using precision nitrogen management in wheat (Triticum aestivum L.). J. Plant Nutr. Soil Sci. 184 (3), 371–377. doi: 10.1002/jpln.202000371
Wang, L., Wang, R., Luo, C., Dai, H., Xiong, C., Wang, X., et al. (2023). Effects of different soil water and heat regulation patterns on the physiological growth and water use in an apple–soybean intercropping system. Agronomy 13 (2), 511. doi: 10.3390/agronomy13020511
Wang, D., Wilson, C., Shannon, M. C. (2002). Interpretation of salinity and irrigation effects on soybean canopy reflectance in visible and near-infrared spectrum domain. Int. J. Remote Sens. 23 (5), 811–824. doi: 10.1080/01431160110070717
Watson, D. J. (1952). The physiological basis of variation in yield. Adv. Agron. 4, 101–145. doi: 10.1016/S0065-2113(08)60307-7
Wood, C. W., Krutz, L. J., Henry, W. B., Irby, T., Orlowski, J. M., Bryant, C. J., et al. (2020). Developing sensor-based irrigation scheduling that maximizes soybean grain yield, irrigation water use efficiency, and returns above irrigation costs. Crop Forage Turfgrass Manage. 6 (1), 20029. doi: 10.1002/cft2.20029
Xiong, D., Chen, J., Yu, T., Gao, W., Ling, X., Li, Y., et al. (2015). SPAD-based leaf nitrogen estimation is impacted by environmental factors and crop leaf characteristics. Sci. Rep. 5 (1), 13389. doi: 10.1038/srep13389
Xu, C., Tao, H., Tian, B., Gao, Y., Ren, J., Wang, P. (2016). Limited-irrigation improves water use efficiency and soil reservoir capacity through regulating root and canopy growth of winter wheat. Field Crops Res. 196, 268–275. doi: 10.1016/j.fcr.2016.07.009
Yang, W., Jiao, Y., Yang, M., Wen, H., Gu, P., Yang, J., et al. (2020). Minimizing soil nitrogen leaching by changing furrow irrigation into sprinkler fertigation in potato fields in the Northwestern China Plain. Water 12 (8), 2229. doi: 10.3390/w12082229
Zhao, L., Wu, L., Li, Y. S., Lu, X. H., Zhu, D. F., Uphoff, N. (2009). Influence of the system of rice intensification on rice yield and nitrogen and water use efficiency with different application rates. Exp. Agric. 45, 275–286. doi: 10.1017/S0014479709007583
Zhou, J.-B., Xi, J.-G., Chen, Z.-J., Li, S.-X. (2006). Leaching and transformation of nitrogen fertilizers in soil after application of N with irrigation: A soil column method. Pedosphere 16 (2), 245–252. doi: 10.1016/S1002-0160(06)60050-7
Keywords: precision nutrient management, sprinkler irrigation, SPAD, photosynthetic rate, PAR interception, SCI, water productivity, soybean yield
Citation: Sachin KS, Dass A, Dhar S, Rajanna GA, Singh T, Sudhishri S, Sannagoudar MS, Choudhary AK, Kushwaha HL, Praveen BR, Prasad S, Sharma VK, Pooniya V, Krishnan P, Khanna M, Singh R, Varatharajan T, Kumari K, Nithinkumar K, San A-A and Devi AD (2023) Sensor-based precision nutrient and irrigation management enhances the physiological performance, water productivity, and yield of soybean under system of crop intensification. Front. Plant Sci. 14:1282217. doi: 10.3389/fpls.2023.1282217
Received: 23 August 2023; Accepted: 27 November 2023;
Published: 18 December 2023.
Edited by:
Min Huang, Hunan Agricultural University, ChinaReviewed by:
Wei Li, Jiangsu University, ChinaMuhammad Ishaq Asif Rehmani, Ghazi University, Pakistan
Copyright © 2023 Sachin, Dass, Dhar, Rajanna, Singh, Sudhishri, Sannagoudar, Choudhary, Kushwaha, Praveen, Prasad, Sharma, Pooniya, Krishnan, Khanna, Singh, Varatharajan, Kumari, Nithinkumar, San and Devi. This is an open-access article distributed under the terms of the Creative Commons Attribution License (CC BY). The use, distribution or reproduction in other forums is permitted, provided the original author(s) and the copyright owner(s) are credited and that the original publication in this journal is cited, in accordance with accepted academic practice. No use, distribution or reproduction is permitted which does not comply with these terms.
*Correspondence: Anchal Dass, YW5jaGFsX2RAcmVkaWZmbWFpbC5jb20=