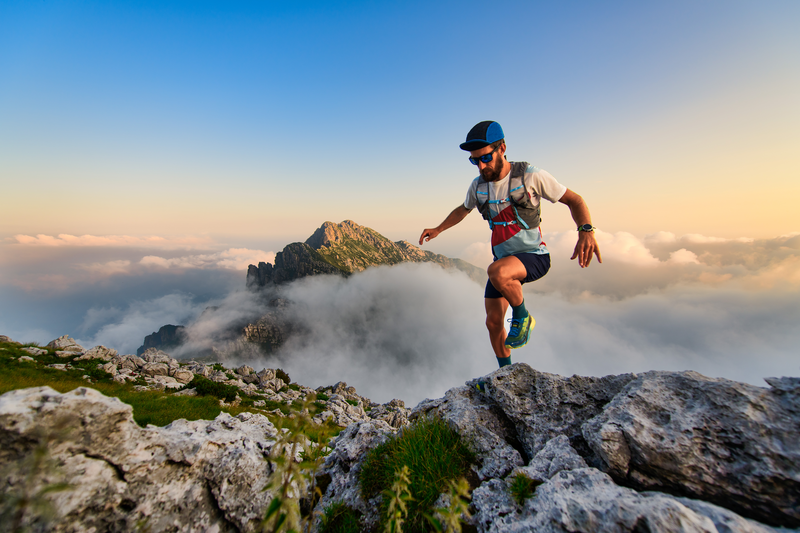
95% of researchers rate our articles as excellent or good
Learn more about the work of our research integrity team to safeguard the quality of each article we publish.
Find out more
REVIEW article
Front. Plant Sci. , 25 October 2023
Sec. Plant Breeding
Volume 14 - 2023 | https://doi.org/10.3389/fpls.2023.1277922
This article is part of the Research Topic Trends and Perspectives for the Use of Crop Wild Relatives in Crop Breeding View all 13 articles
Brassica juncea (L.) Czern. & Coss. (Indian mustard) is an economically important edible oil crop. Over the years, plant breeders have developed many elite varieties of B. juncea with better yield traits, but research work on the introgression of stress resilience traits has largely been lagging due to scarcity of resistant donors. Crop wild relatives (CWRs) are the weedy relatives of domesticated plant species which are left unutilized in their natural habitat due to the presence of certain undesirable alleles which hamper their yield potential, and thus, their further domestication. CWRs of B. juncea namely include Sinapis alba L. (White mustard), B. tournefortii Gouan. (African mustard), B. fruticulosa Cirillo (Twiggy turnip), Camelina sativa L. (Gold-of-pleasure), Diplotaxis tenuisiliqua Delile (Wall rocket), D. erucoides L. (White wall rocket), D. muralis L. (Annual wall rocket), Crambe abyssinica R.E.Fr. (Abyssinian mustard), Erucastrum gallicum Willd. (Common dogmustard), E. cardaminoides Webb ex Christ (Dogmustard), Capsella bursa-pastoris L. (Shepherds purse), Lepidium sativum L. (Garden Cress) etc. These CWRs have withstood several regimes of biotic and abiotic stresses over the past thousands of years which led them to accumulate many useful alleles contributing in resistance against various environmental stresses. Thus, CWRs could serve as resourceful gene pools for introgression of stress resilience traits into Indian mustard. This review summarizes research work on the introgression of resistance against Sclerotinia stem rot (caused by Sclerotinia sclerotiorum), Alternaria blight (caused by Alternaria brassicae), white rust (caused by Albugo candida), aphid attack, drought and high temperature from CWRs into B. juncea. However, various pre- and post-fertilization barriers due to different ploidy levels are major stumbling blocks in the success of such programmes, therefore, we also insightfully discuss how the advances made in -omics technology could be helpful in assisting various breeding programmes aiming at improvisation of stress resilience traits in B. juncea.
Indian mustard [Brassica juncea (L.) Czern & Coss.] is an economically important, edible oilseed crop of Brassicaceae family belonging to the rapeseed-mustard (RM) group. B. juncea is cultivated in India, China, Bangladesh, Pakistan, Australia, Canada and some European countries (Choudhury et al., 2023). It is predominantly cultivated as an oilseed crop in India over an estimated area of 6.2 million hectare, contributing to >85% of RM acreage (Singh et al., 2022). Indian mustard oil is widely consumed as an edible oil. Mustard oil has a unique ratio of omega-3 and omega-6 fatty acids with many benefits related to cardiac health (Manchanda and Passi, 2016). Mustard oil is also rich in alpha-tocopherol and antioxidants offering many other additional health benefits. Besides edible purposes, mustard oil is also used in body massages for increasing muscle strength and treating skin problems, and in manufacturing processes of paints and varnishes (Thakur et al., 2020). After oil extraction, the left-out seed meal is used as a protein-rich feed for farm animals, especially poultry. The vegetative part of mustard is consumed as a very popular delicious cuisine known as ‘Sarson Ka Saag’ in the northern part of India (Ananthanarayan et al., 2019).
The actual yield potential of B. juncea can be realized upto 3500-4000 kg/ha, but despite continuous breeding efforts over a period of last four decades, the national average productivity of Indian mustard still hovers around 1400 Kg/ha (Sinha et al., 2020). The overall production and productivity of this oilseed crop is hampered by several biotic (Alternaria blight caused by Sclerotinia sclerotiorum, stem rot caused by Alternaria brassicae, white rust caused by Albugo candida, powdery mildew caused by Erysiphe cruciferarum, aphids) and abiotic factors (drought, high temperature stress - both at seedling stage and terminal stage, salinity and frost) (Thakur et al., 2020). While, due to taste preferences for mustard oil, an escalating per-capita consumption, and thus market demand is being constantly observed. India is importing large quantities of edible oil from different countries to meet the huge edible oil demands of its population. This is amounting to washing out a large exchequer of money (Sharma et al., 2022). It has been estimated that by 2025, the demand of edible oil in India will increase upto 34 million tonnes, and out of this, around 14 million tonnes had to be met by Indian mustard alone (Singh et al., 2022). Throughout the globe, various Brassica research groups are making concerted efforts for developing elite high-yielding varieties of B. juncea having resistance against biotic and abiotic stresses (Chauhan et al., 2011). Among different biotic stressors, except for some Brassica germplasm showing resistance against white rust, no other crossable germplasm sources have been identified for developing B. juncea varieties which could be resistant to Alternaria blight, stem rot and powdery mildew. Among abiotic stresses, several salinity tolerant B. juncea varieties including CS 52, CS 54, CS 56 and CS 58 have been released in India (Tripathi et al., 2012). However, no robust donor line has been identified to develop resistance against drought, frost and high temperature stress in B. juncea.
Crop wild relatives (CWRs) are the weedy, wild relatives of domesticated plants, which usually occur and are maintained mostly in their centers of origin (Kashyap et al., 2022; Jain et al., 2023). CWRs have been left unexploited in nature because of the presence of some undesirable genes/alleles contributing as yield barriers. CWRs of B. juncea majorly include Sinapis alba L. (White mustard), B. tournefortii Gouan. (African mustard), B. fruticulosa Cirillo (Twiggy turnip), Camelina sativa L. (Gold-of-pleasure), Diplotaxis tenuisiliqua Delile (Wall rocket), D. erucoides L. (White wall rocket), D. muralis L. (Annual wall rocket), Crambe abyssinica R.E.Fr. (Abyssinian mustard), Erucastrum gallicum Willd. (Common dogmustard), E. cardaminoides Webb ex Christ (Dog mustard), Capsella bursa-pastoris L. (Shepherds purse), Lepidium sativum L. (Garden Cress) etc. (Singh et al., 2021a). Over the years, these CWRs have withstood the selection pressure of various biotic and abiotic stress factors, developed the resistance mechanisms gradually and inherited it over the generations. A list of CWRs of B. juncea, their common name, ploidy level, genome size, chromosome number and economic importance is given in Table 1. The rigorous use of these CWRs as potential allele donors in conventional breeding programmes is hampered due to their different ploidy levels, or certain pre- and post-fertilization barriers. Further, the introduction of undesirable alleles from the CWRs into B. juncea due to linkage drag might result in heavy yield penalties (Bohra et al., 2022). In the past few decades, certain technological interventions have been devised for the introgression of resistance traits from CWRs into the cultivated varieties of B. juncea. These techniques include protoplast fusion, embryo rescue, repeated back-cross breeding with the recurrent parent followed by open pollination and new omics techniques. This review summarizes the efforts of various research groups in utilizing CWRs for combating the adverse effects of biotic and abiotic stresses on Indian mustard yield.
Table 1 List of CWRs of Brassica juncea, their common name, ploidy level, genome size, chromosome number and economic importance.
Various biotic stresses adversely affect the yield of Indian mustard. In India, more than thirty diseases are known to affect mustard growth and yields (Saharan et al., 2005). Among them, few inflict serious damages upon mustard production depending upon their prevalence over vast geographical locations. Namely, stem rot, Alternaria leaf spot and white rust are major diseases caused by fungal pathogens which may amount to more than 90% yield losses in this oilseed crop (Saharan and Mehta, 2008; Kumar et al., 2012; Kamoun et al., 2015; Jyoti et al., 2021; Singh et al., 2021a). Besides these, parasitic feeding by aphid pest Lipaphis erysimi Kalt. leads to stunted growth, very less seed formation, and decreased oil content in Indian mustard. In the following section we discuss the efforts made by various research groups for introducing resistance traits from CWRs into Indian mustard.
White rot or stem rot caused by S. sclerotiorum is a serious fungal disease of Indian mustard. Due to changes in climatic conditions, Sclerotinia stem rot (SSR) has become a very serious disease of mustard. This necrotrophic fungus causes yield losses ranging from 5-100% in mustard production (Saharan and Mehta, 2008; Uloth et al., 2016; Sharma et al., 2018; Singh et al., 2021a). It also impacts mustard oil quality and reduces oil content (Inturrisi et al., 2021). Due to prolonged survival and broad infection ability, the management of SSR utilizing cultural and chemical practices could be less rewarding (Kamal et al., 2016; Singh et al., 2020). S. sclerotiorum is a broad host range phytopathogen which overpowers the plant defense mechanism through their interacting virulence factors (Rodriguez-Moreno et al., 2018). Recently, Gupta et al. (2022) reported the draft genome sequence of S. sclerotiorum “ESR-01”, an Indian isolate, and its secretory effector repertoire. It highlights the secretome, effector, carbohydrate active enzymes and PHI-base repertoire associated with the S. sclerotiorum genome. This knowledge has been quite helpful in refining the understanding of S. sclerotiorum-Brassica interaction.
Inadequate variation is found in Brassica germplasm for complete genetic resistance against S. sclerotiorum infection (Rana et al., 2017; Atri et al., 2019). The wild allies of Brassicaceae, viz., B. fruticulosa, C. bursa-pastoris, D. tenuisiliqua, E. gallicum, E. cardaminoides carry high levels of resistance against stem rot pathogen infection (Chen et al., 2007; Garg et al., 2010; Mei et al., 2011). Some of them are being utilized in different research programmes for transfer of SSR into B. juncea. In a study by Kumari et al. (2020a), two fertile and stable allohexaploid were generated by protoplast fusion between B. juncea and S. alba. These symmetric hybrids (2n = 60) remained stable throughout consecutive generations and displayed significant resistance against SSR (Kumari et al., 2020a). In another attempt, the SSR resistance from B. fruticulosa has been introgressed into Indian mustard (Rana et al., 2017). The developed introgressed lines (ILs) of B. juncea-B. fruticulosa had been assessed for their resistance against Sclerotinia. The cytogenetic characterization of 28 ILs showed substitution of predominantly terminal B. fruticulosa segments located on B-genome chromosomes (Rana et al., 2017). Resistance responses to S. sclerotiorum were repeat-evaluated and different ILs were genotyped for 202 transferable and 60 candidate gene simple sequence repeats. A total of 10 highly significant marker trait associations (MTAs) were achieved by association mapping. Selected ILs showed high levels of resistance against SSR (Rana et al., 2017). It has now become possible to exploit historical recombination events by utilizing genome-wide association studies (GWAS) to achieve improved mapping resolution (Tibbs Cortes et al., 2021). Genotyping by sequencing (GBS) of 88 ILs of B. juncea-B. fruticulosa helped in studying marker trait associations (MTA’s) and reported 49 significant SNPs corresponding to different loci on different chromosomes (Atri et al., 2019). This also helped in prediction of the candidate disease genes belonging to various protein families and developed an understanding of the immune responses against S. sclerotiorum in mustard. The generated marker datasets could be utilized for assisted transfer of introgressed resistant loci associated with SSR resistance into superior Brassica cultivars (Atri et al., 2019).
E. cardaminoides, another wild relative of B. juncea, is a likely source of resistance to many diseases including SSR (Gomez-Campo et al., 1999; Chandra et al., 2004). A set of B. juncea-E. cardaminoides ILs with genomic regions associated with SSR resistance was developed by Rana et al. (2019). The ILs had been evaluated for resistance responses against SSR over three crop seasons. Different SNPs associated with leucine rich repeat-receptor like kinases (LRR-RLK) genes, genetic factors associated with pathogen-associated molecular patterns (PAMPs) and effector-triggered immunity (ETI) belonging to three R-genes encoding toll-interleukin receptor- nucleotide-binding site- leucine-rich repeat (TIR-NBS-LRR) proteins have been identified in this study (Rana et al., 2019). These could be major contributing factors in SSR resistance responses. However, it is likely that some of these marker-trait associations may involve small SSR resistance responses from gene pool already present in Indian mustard. Significantly higher resistance in developed B. juncea-E. cardaminoides ILs indicated that majority of this response is due to introgression of genes from wild E. cardaminoides (Rana et al., 2019). Accelerated transcriptomic and genomics research on the developed B. juncea-CWR ILs would clarify the mechanisms underlying pathogenesis of S. sclerotiorum in B. juncea. Rana et al. (2019) have proposed taking up transcriptome-based research on S. sclerotiorum-B. juncea interaction. It would help the researchers in understanding how S. sclerotiorum infection interferes with different hormone signaling pathways to hijack B. juncea defense system. Further, spatial, and temporal changes in gene expression would be helpful in gaining advanced insights into events that lead to disease development and colonization of tissues by this pathogen. As proposed, use of E. cardaminoides specific oligo-probes for cytogenetical mapping of all the introgression sites in developed ILs would be helpful in understanding the CWR-specific contribution to S. sclerotiorum resistance in developed ILs.
The seed borne fungal pathogens of Alternaria spp. are causal agents of Alternaria leaf blight disease of B. juncea. Both, Alternaria brassicicola and A. brassicae cause Alternaria leaf blight (Sharma et al., 2022). Among the two, A. brassicae is reported to be more virulent, while, A. brassicicola co-inhabits the infected plant tissue (Sharma et al., 2002). An estimated crop-damage of upto 10-70% in B. juncea is inflicted by Alternaria leaf blight alone (Kumar and Kolte, 2012; Gupta et al., 2020), making it a serious disease of oilseed mustard. Disease infected plants are characterized by concentric ring spots which merge to develop big necrotic patches (Bohra et al., 2022). Susceptibility to Alternaria leaf blight is shown by almost all cultivated varieties of Indian mustard (Jyoti et al., 2021). Resistance to Alternaria blight is a polygenic character, therefore, introgression of traits through horizontal breeding could be a more appropriate way to transfer these traits into cultivated Brassicas. However, there is a lack of resistant crossable germplasm in cultivated B. juncea. Many cruciferous wild relatives are known to exhibit significant levels of resistance against Alternaria leaf blight. These include B. desnottesii, Camelina sativa, Diplotaxis berthautii, D. catholica, D. cretacea, D. erucoides, and E. gallicum (Sharma et al., 2002). Yet, various compatibility barriers (both pre- and post-fertilization barriers) impede the research programs aiming introgression of Alternaria blight resistance traits from CWRs into cultivated B. juncea (Vasupalli et al., 2017).
Embryo rescue techniques have been proposed as a strategy for introgression of resistant traits from CWRs into cultivated Indian mustard (Kumar et al., 2001; Bhat et al., 2006; Vasupalli et al., 2017). S. alba, a wild ally of crop Brassicas, carries significant resistance against Alternaria black spot. In the past, attempts have been made for the development of symmetric somatic hybrids with Indian mustard. However, such attempts mostly resulted in production of male sterile hybrids, hybrids with variable pollen fertility and seed set, overall unstable hybrids (Gaikwad et al., 1996). D. erucoides, a CWR of Brassicas, shows high levels of resistance against the Alternaria blight pathogen, A. brassicae. Bhat et al. (2006) attempted to introgress Alternaria blight resistance from D. erucoides to B. juncea using B. rapa as bridging species. After two successive backcrossing of the progenies with the recurrent parent i.e., B. juncea, the BC2 interspecific hybrids were advanced to BC2F7 generation and simultaneously screened for Alternaria blight resistance, which resulted in some promising resistant Indian mustard lines (Bhat et al., 2006). Kumari et al. (2020b) generated B. juncea-S. alba hybrids through protoplast fusion. Two generated hybrids were reported to be symmetric, while, third had significant resemblance to B. juncea and thus was found asymmetric. The hybrids exhibited complete male and female fertile characteristics and normal back crossing progenies. High resistance to A. brassicae infection was observed in both (Kumari et al., 2020b).
An assessment of transcriptional activation of glucosinolate biosynthetic genes and glucosinolate accumulation in Alternaria resistant D. erucoides and susceptible B. juncea species has highlighted few potential candidate genes which augment resistance to Alternaria blight (Choudhury et al., 2022). More jasmonate defense signaling-mediated transcriptional activation of candidate secondary metabolites and glucosinolate (GSL) biosynthesis genes is being observed in D. erucoides than B. juncea. The higher accumulation of defense-related GSL compounds was also reported in D. erucoides in comparison to B. juncea when infected with A. brassicae (Choudhury et al., 2022). The study is important in highlighting a few possible candidate genes for engineering defense responses of susceptible mustard cultivars against Alternaria blight (Choudhury et al., 2022). It becomes a herculean task to screen the successive progenies for the introgressed target trait in the crosses involving B. juncea and CWRs. This is because the cross-combination of different ploidy levels of the parent species results in a large amount of heterogeneity of the progenies. Thus, more area, time and labor inputs are required for screening of the progenies. The screening process can be facilitated by development of molecular markers which are closely associated with the target trait. Recently, Choudhury et al. (2023) developed sequence-tagged sites (STS) markers closely associated with the introgressed target trait i.e. Alternaria blight resistance by resequencing of a resistant and a susceptible line of BC2F7 generation of a cross between B. juncea and D. erucoides.
It is evident from the above cited literature that robust screening and scoring of CWRs-A. brassicae pathogen-host interactions, for the identification of reliable R-gene sources in the Brassica wild allies is needed. Further studies on generation of symmetric and stable somatic hybrids between B. juncea and wild allies through successful bridging of ploidy gaps would be helpful in successful introgression of Alterneria blight resistance into cultivated B. juncea varieties. Improving knowledge about elicitors and effectors for A. brassicae would also be important for devising successful disease management strategies for Alternaria blight disease in Indian mustard.
White rust is another major disease of Indian mustard. It is caused by biotrophic oomycete fungal pathogen Albugo candida (Kamoun et al., 2015). White rust is characterized by appearance of white to pale-colored pustules containing zoospores on the abaxial surface of leaves, stems and inflorescence. Therefore, the disease is sometimes also termed as white blister rust (Holub et al., 1995). Chlorosis is also observed over adaxial leaf surfaces. Systemic infection may cause stagehead leading to loss of seed formation (Verma et al., 1975; Chand et al., 2022). In B. juncea, 23-89% yield losses were reported by white rust infection (Lakra and Saharan, 1989; Chand et al., 2022).
Due to the persisting racial variation of A. candida, the known genes which offer resistance against this pathogen are often ineffective (Mehta et al., 2023). It is being suggested that among the wild relatives, B. tournefortii, could be utilized in breeding programmes for introgression of tolerance/resistance to white rust disease (Kumar, 2015). Vasupalli et al. (2017) developed B. juncea introgression lines using resistant D. erucoides as donor and susceptible B. juncea as the recurrent parent. Firstly, to bridge the ploidy gap between donor and the recurrent parent, B. rapa was used as bridging species. D. erucoides × B. rapa hybrid was developed by embryo rescue, and amphiploids were developed by colchicine doubling. This amphidiploid ‘eru-rapa’ was crossed reciprocally with B. juncea. After backcrossing with B. juncea, the BC1F1 progenies obtained were further backcrossed. The BC2F1 progeny was selfed and further progenies progressed through selfing (Bhat et al., 2006; Vasupalli et al., 2017). Mehta et al. (2023) utilized these ILs for studying their resistance characteristics against A. candida. Among these ILs, ERJ 39, ERJ 12, and ERJ 15, have shown resistance when inoculated with multiple isolates of the pathogen, A. candida at cotyledonary leaf, true leaf, and adult plant stages. While, ERJ 108, ERJ 157, ERJ 159, ERJ 13, and ERJ 32 exhibited resistance against single isolates of A. candida (Mehta et al., 2023). Thus, these ILs could be useful sources of genetic resistance against A. candida.
In India, consistent B. juncea germplasm exploration and pre-breeding efforts, followed by rigorous screening of germplasm and breeding lines at multi locations, both at the uniform disease nurseries (UDN) and national disease nurseries (NDN) levels under the All India Crop Improvement Programme on Rapeseed-Mustard (AICRP-RM) has led to the identification of more than twenty five white rust resistance (WRR) donors among B. juncea germplasm which are being registered at ICAR-NBPGR, New Delhi (Kumar et al., 2019). These white rust resistant donors are being utilized in different WRR breeding programmes of B. juncea in India. Currently, CWRs could be a less sought after solution for resistance against white rust due to availability of WRR germplasm of B. juncea.
Among the various insect species which infest Brassica species, mustard aphid, Lipaphis erysimi Kalt. is reported to cause major losses to Brassica yield (Patel et al., 2004; Patel et al., 2019). Fast multiplication of these aphids along with phloem sap-feeding mechanism affects plant growth, and leads to poor seed formation with low oil content (Rohilla et al., 2004; Rana, 2005). Across different agro-climatic conditions, and severity of infection and crop growth stage, L. erysimi is known to cause damage ranging from 35.4-91.3% to mustard crop (Ahuja et al., 2010; Kular and Kumar, 2011). Use of synthetic insecticides and other chemical control measures for checking the spread of this pest causes serious environmental pollution which imposes health hazards (Abbaszadeh et al., 2011). This caters the need for robust screening of plant genomic sources for successful introgression of traits for aphid resistance into Indian mustard.
During initial attempts, embryo rescue had been deployed for developing inter-specific hybrids between B. juncea and B. tournefortii. One of the partially fertile hybrids obtained out of these crosses exhibited tolerance to aphid attack (Kumar et al., 2001). A study pertaining to screening of weedy and wild allies for resistance to L. erysimi, identified B. fruticulosa and B. montana as most promising CWRs for this trait (Kumar et al., 2011). B. fruticulosa was crossed with B. juncea as a donor parent to synthesize the amphiploid AD-4. Resistance to L. erysimi, was exhibited by both B. juncea introgression and amphiploid lines. High accumulation of lectins was proposed to be the resistance mechanism in B. fruticulosa and amphiploid AD-4 (Kumar et al., 2011). In further studies, Atri et al. (2012) attempted introgression of aphid resistance from B. fruticulosa into B. juncea. They deployed the artificially synthesized amphiploid, AD-4 (B. fruticulosa × B. rapa var. brown sarson), as a bridge to transfer resistant traits from B. fruticulosa to B. juncea (Atri et al., 2012). Normal meiosis and pollen grain fertility was exhibited by many introgression lines which carried the euploid chromosome number (2n = 36). Intriguing transcriptomic studies on B. juncea infestation with a natural host (L. erysimi) and non-natural host cowpea aphid (A. craccivora) has highlighted the mechanistic differences of defense response activation in B. juncea by both species (Duhlian et al., 2020). This has shed light on transcriptional reprogramming of the host with respect to the genes regulating oxidative homeostasis, defense hormones and secondary metabolite pathways in B. juncea during aphid invasion (Duhlian et al., 2020).
Due to scarcity of high yielding aphid-resistant cultivars, the menace of aphid attack in Indian mustard is mainly managed by spray of harmful insecticides (Sachan and Purwar, 2007). This further necessitates the need for a genetic solution for resistance against aphid attack. For reducing the economic losses posed by aphids, CWRs could prove to be a sought-after genetic solution. Therefore, primarily, it is important to mine the genetic resistance resources among CWRs of Indian mustard. We have tabulated recent research on the utilization of crop wild relatives for the introgression of biotic and abiotic stress resistance into B. juncea (Table 2).
Table 2 Recent research on the utilization of crop wild relatives for the introgression of biotic and abiotic stress resistance into B. juncea.
Environmental factors, like high temperatures, water scarcity and soil salinity cause significant losses to crop yields (Yadav et al., 2020). Constant efforts are being made to explore abiotic stress regulation responses in B. juncea, which mostly are multi-genetically regulated. Due to changes in global environmental conditions, there is an urgent need to develop water use efficient and heat stress tolerant Indian mustard genotypes (Singh and Choudhary, 2003; Gill et al., 2012; Singh et al., 2021b). Genetic diversity available among CWRs could be a useful resource for introgression of these abiotic stress resilience traits into high yielding cultivars of Indian mustard (Kapazoglou et al., 2023; Kashyap et al., 2023).
According to Shekhawat et al. (2012), B. juncea is cultivated in nearly 85% of total harvested area, out of which, 37% is mainly rain-fed. Thus, the crop produces and quality of Indian mustard is intensively affected by drought stress. Impacts of drought stress are more severe in the eastern and western parts of India which are more prone to drought (Chauhan et al., 2007). Water stress during stem elongation and pod development stages affects pod setting, thus affecting overall yield of mustard. Drought stress causes yield losses ranging from 17-94% in Indian mustard (Akanksha et al., 2020). Introgression of drought tolerance traits into Indian mustard might include transfer of different morpho-physio and biochemical traits which control drought-tolerance characteristics (Singh and Choudhary, 2003). There is an urgent need for the development of water-use-efficient Indian mustard varieties, and CWRs might serve as useful resources for developing ones. Research studies have highlighted the differential regulation of certain genes and transcription factors under drought stress in Indian mustard (Bhardwaj et al., 2015; Wei et al., 2023). This has shed light on the coding transcripts which could be associated with drought tolerance traits in B. juncea.
Previous studies report S. alba, a wild relative of Indian mustard, to be drought tolerant (Warwick, 1993). Recently, a study involving wild species and U-triangle species of Brassica for their potential tolerance to drought during germination and early seedling stage reported B. fruticulosa as drought tolerant (Kashyap et al., 2023). Under PEG-induced drought stress conditions, B. fruticulosa exhibited increased proline content than the control plants (Kashyap et al., 2023). In another study, the genome-wide transcriptional profiling of S. alba leaves under drought and rewatering conditions revealed numerous gene expression changes under such conditions (Dong et al., 2012). Overall, down-regulation of 309 genes and up-regulation of 248 genes was reported under these conditions. Identified differentially expressed genes were shown to be involved in cell division, catalytic and metabolic process functions (Dong et al., 2012). Broad classification highlighted two categories of gene functions in this study, one encoding protective proteins, like, oxidoreductase, and another encoding regulatory proteins, like, transcription factors (Dong et al., 2012). Further, studies might be helpful in detailed revelation of B. fruticulosa and S. alba genetic factors which control the drought tolerance characteristics in these CWRs.
The potential of somatic hybridization and embryo rescue has not yet been fully utilized for introgression of drought tolerance traits from CWRs into the cultivated high yielding varieties of B. juncea because of post-fertilization barriers leading to embryo abortion. In this regard, the potential of S. alba and B. fruticulosa reported to exhibit drought resistance traits could be explored in future research programmes.
Mostly grown in rabi season, Indian mustard grows well in tropical and sub-tropical regions as winter oilseed crop (Thakur et al., 2020). Temperature growth conditions for optimal germination and seed set ranges between 25°C to 33°C (Wilson et al., 2014). Heat stress affects B. juncea growth both at early (germination and seedling stage) and late (flowering and seed ripening stage) stages, resulting in economic losses to mustard production (Sandhu et al., 2019). Crop wild relatives (CWRs) could serve as useful resources for transfer of heat resilience traits into B. juncea. Along with some other stress resistant traits, S. alba also possesses heat stress tolerance traits. Through protoplast fusion, Kumari et al. (2018) developed hybrids between B. juncea and S. alba for transferring of genes responsible for A. Brassicae and heat stress tolerance. They were successful in generating somatic hybrids showing resistance against A. brassicae, which were able to set seeds at temperatures greater than 38°C, thus, also exhibiting characteristics related to heat tolerance. A recent study conducted by Kashyap et al. (2023) highlights heat stress tolerance characteristics of another wild ally of Indian mustard, B. tournefortii (Rawa). When exposed to heat stress, B. tournefortii (Rawa) showed maximum percent increase in germination (38.46%). This study highlights heat tolerance capacities of B. tournefortii (Rawa) during early stages of growth, i.e. germination (Kashyap et al., 2023).
Studies pertaining to utilizing wild allies for introgression of heat stress resilience into Indian mustard are still in infancy. The above surveyed literature suggests that at this stage, it would be beneficial to take up studies concerning evaluation of different B. juncea CWRs for their heat tolerance traits. The advances made in transcriptomics and genomics could be utilized for identification of specific genes responsible for regulation of heat stress tolerance in CWRs. This would largely facilitate further research work on introgression of heat stress tolerance traits from CWRs into B. juncea. Figure 1 illustrates the utilization of specific CWRs for the introgression of biotic/abiotic stress resistance traits into B. juncea.
Figure 1 Utilization of specific CWRs for the introgression of biotic/abiotic stress resistance traits into B. juncea and modern -omics approaches which could be further helpful in assisting successful introgression.
Several wild plant species exhibit sexual incompatibility with crop species, thereby rendering the genes found in wild forms inaccessible (Branca and Cartea, 2010). Reproductive compatibility in Brassica species is complex, with intricate partial barriers between many of them (Han et al., 2021). This is difficult due to the pre- and post-fertilization barriers and abortion of hybrid embryos obtained after inter-specific crosses. Due to the advancements made in in vitro culture and embryo rescue, some success has been obtained in development of interspecific hybrids (Pen et al., 2018; Akmal, 2021). The embryo culture technique has proved to be a powerful tool for overcoming post-fertilization barriers between distantly related species, enabling successful hybridization (Pen et al., 2018; Akmal, 2021).
By continuous refinement of organ culture protocols, gradual progress is being achieved in obtaining stable inter-generic hybrids utilizing in vitro embryo culture methods (Akmal, 2021). Interspecific hybrid production through embryo rescue is often deployed in Brassica to overcome post-fertilization barriers. The success of this technique relies on the developmental stage of the rescued embryos (Katche et al., 2019). It is being suggested that embryo rescue can be deployed as early as 10 to 30 days after pollination in Brassica (Quazi, 1988; Inomata, 1993; Yuping and Wojciechowski, 2000). Different groups reported use of embryo culture and ovary culture for production of interspecific hybrids between B. juncea and B. campestris, and between B. juncea and B. napus (Mohapatra and Bajaj, 1988; Zhang et al., 2001; Zhang et al., 2003). Literature discussed in the above sections of this review reports successful utilization of embryo rescue for the transfer of useful traits related to climate resilience from CWRs into B. juncea (Kumar et al., 2001; Bhat et al., 2006; Vasupalli et al., 2017). However, till yet, the efforts to produce interspecific hybrids between CWRs and cultivated Brassica are still in infancy. The success of embryo rescue is largely dependent upon the stage of embryos, composition of the medium, and on the genotype to some extent (Katche et al., 2019; Ripa et al., 2020). Therefore, further standardization of protocols pertaining to these parameters would be largely helpful in obtaining successful inter-specific hybrids between B. juncea and CWRs. Figure 2 depicts the stress-resistance traits associated with S. alba and protocol for generation of inter-generic hybrids of B. juncea and S. alba using ovary culture and embryo rescue technique.
Figure 2 Abiotic and biotic stress tolerance traits associated with S. alba and protocol for generation of inter-generic hybrids of B. juncea and S. alba using ovary culture and embryo rescue technique.
Strong hybrid vigor is shown by Brassicaceae crops which have long been subject to F1 hybrid breeding. Cytoplasmic male sterility (CMS) system is often relied upon for successful F1 seed production in Brassicaceae (Yamagishi and Bhat, 2014). When cytoplasm of an alien species is present in the genetic background of another species, this confers CMS (Yamagishi and Bhat, 2014). During earlier attempts, B. juncea var. ‘Pusa Bold’, with the cytoplasmic background of Diplotaxis siifolia was developed through wide hybridization (Rao et al., 1994). The cytoplasmic substitution was achieved by repeated backcross of synthetic alloploid (D. siifolia x B. juncea) with B. juncea (Rao et al., 1994). Later, another CMS system was developed in B. juncea following repeated backcrossing of the somatic hybrid Moricandia arvensis (2n = 28, MM) + B. juncea (2n = 36, AABB), carrying mitochondria and chloroplasts from M. arvensis, to B. juncea (Prakash et al., 1998). Subsequently, Malik et al. (1999) developed two CMS lines using the bridgecross hybrids (D. erucoides × B. campestris) × B. juncea and (D. berthautii × B. campestris) × B. juncea. These were backcrossed with B. juncea (Malik et al., 1999). Further, an improved cytoplasmic male sterile (CMS) system of B. juncea having cytoplasm of the wild species D. berthautii was developed by Bhat et al. (2008). The fertility restorers of M. arvensis and D. catholica-based alloplasmic CMS systems of B. juncea were deployed for restoring the male fertility in these developed CMS lines (Bhat et al., 2008).
Among the various CMS systems adopted in breeding programmes of Brassica crops (Ogura, 1968; Yamagishi and Bhat, 2014; Katche et al., 2019), Ogura CMS system has been the most widely utilized. In this system, alien cytoplasm has been obtained by crossing B. napus to Japanese radish (Raphanus sativus) (Ogura, 1968). Complete pollen abortion, ease of transfer and high progeny sterility rates, reaching 100%, are the various advantages associated with Ogura CMS system (Ren et al., 2022). This system has been extensively deployed in B. napus, B. juncea and B. oleracea (Yamagishi and Bhat, 2014). Wild relatives are often utilized for the development of male sterile lines to help hybrid production (Katche et al., 2019). Among the various CMS systems developed in B. juncea, one CMS system is developed by incorporation of cytoplasm from wild relative B. fruticulosa (Atri et al., 2016). This has been achieved by the backcross substitution of B. juncea (2n = 36; AABB) nucleus into the cytoplasm of B. fruticulosa. Complete and stable male sterility was observed in the B. juncea genotypes which developed rudimentary anthers with sterile pollen grains and no observed changes in other vegetative parts (Atri et al., 2016). Successful B. fruticulosa introgression was documented in at least three chromosomes of B. juncea. Further, F1, F2 and test cross progenies were developed by hybridization between cytoplasmic male sterile and fertility restoring genotypes (Atri et al., 2016). The CMS-fertility-restorer system developed utilizing wild relatives of B. juncea holds significant potential for hybrid seed production in Indian mustard. Detailed studies on evolutionary relationships of CMS and fertility restorer genes would be helpful in establishing efficient F1 hybrid breeding systems in Brassicaceae crops.
In the context of global climate change, CWRs could be promising genetic resources of abiotic and biotic stress resistance (Kapazoglou et al., 2023). In the recent past, successful breeding efforts have certainly helped in introgression of stress-resistant traits from CWRs into B. juncea. However, genetic bottlenecks have always been an issue (Kumar et al., 2011; Atri et al., 2012; Kapazoglou et al., 2023). The primary step for the introduction of any novel gene into a crop involves successful identification of reliable genetic resources associated with these traits. Future research on transcriptomic profiling (RNA-seq) of CWRs alone, and in comparison with B. juncea cultivars would unravel the molecular mechanisms specifically associated with stress tolerance in CWRs. This would be helpful in the generation of a comparative transcriptomic-profile of the genomic regions, specific stress-responsive genes and biochemical pathways associated with stress tolerance in CWRs and cultivated counterparts of B. juncea. Whole genome sequencing and super-pangenomic research would help in gaining an insight into the total gene pool and the available genetic diversity of CWRs of B. juncea (Khan et al., 2020). It would also facilitate development of molecular markers which could be utilized for targeted B. juncea breeding programs. Additionally, it would be helpful in enhancing our existing knowledge on the complex genetic relationships and genomic introgression events which have occurred between CWRs and B. juncea.
In the recent past, cutting-edge genomics research has led to accurate functional characterization of various genes. It has also helped in elucidating the molecular regulators which may underlie biotic/abiotic stress tolerance (Tirnaz et al., 2022; Kapazoglou et al., 2023). Modern genomics research could be helpful in elucidation of novel gene functions in wild relatives of B. juncea. In this regard, CWR mutant and over-expression lines could be deployed for assessment of accurate gene functions associated with oil and fatty acid composition and quality. Till today, any research on this aspect is largely lacking. The identified novel-genes could be used for genetic transformation of B. juncea with the aim of bio-fortification of oil quality. Bohra et al. (2022) have reviewed in detail how the genetic potential of CWRs can be reaped for producing future crops.
Due to reduced stringency for the selection of yield-related traits, CWRs of B. juncea are valuable sources of resistance against various biotic and abiotic stresses. As reviewed, different crop wild relatives viz. B. fruticulosa, B. tournefortii, B. montana, D. erucoides, S. alba and E. cardaminoides have been used as donors for introgression of stress resistance traits into cultivated B. juncea. However, researchers need to design a pragmatic approach, case-by-case basis for resolving the pre- and post-fertilization barriers for successful transfer of traits of interest. Taking into consideration the ploidy levels of the donor and recipient species, some researchers have opted for utilization of bridge species like B. rapa (diploid species). While, others deployed protoplast fusion and embryo rescue for development of inter-generic hybrids of B. juncea and CWRs with improved stress resistance. For the stabilization of newly introduced traits many generations of recurrent parent back-crossing and open-pollination is further required in these hybrids. Therefore, in every generation, it becomes a herculean task to screen the desired hybrid plants expressing the trait of interest by crop genotyping and phenotyping.
In the past few years, the advances made in omics technologies have discovered novel information on the molecular regulators of biotic and abiotic stress tolerance in different crop plants. These -omics advances have yet not been fully utilized for mining of genes and molecular regulators of stress tolerance in B. juncea CWRs. While, use of transcriptomics would enhance our knowledge on differential regulation of various genes under stress, further genomics studies would be helpful in functional characterization of these genes, followed by their targeted transfer into elite high yielding varieties of B. juncea using biotechnological tools. Further, whole genome sequencing of CWRs and pangenomics would increase our understanding of how genotypic and phenotypic diversity was shaped during domestication/selection processes from CWRs to B. juncea. This would also be helpful in solving the challenges related to breeding and conservation of genetic resources. The whole genome sequencing of CWRs would pave the way for identification of the genes governing resistance to a particular trait and thus suitable molecular markers/candidate-gene specific markers may be deployed for selection of the desired plants, ensuring the trait introgression.
SV: Conceptualization, Resources, Writing – review & editing, Writing – original draft. ND: Writing – original draft, Writing – review & editing. KS: Writing – review & editing. NP: Writing – review & editing. LS: Writing – review & editing. DS: Writing – review & editing. DR: Writing – review & editing. KT: Writing – review & editing. DV: Writing – review & editing. AT: Writing – review & editing, Conceptualization, Resources, Writing – original draft.
The author(s) declare financial support was received for the research, authorship, and/or publication of this article. The work was funded by ICAR-Extra-mural Research Scheme on Pre-Breeding to KS.
Authors are thankful to the Director, ICAR-Directorate of Rapeseed-Mustard Research, Bharatpur, Rajasthan and ICAR Headquarter, New Delhi for providing necessary support.
The authors declare that the research was conducted in the absence of any commercial or financial relationships that could be construed as a potential conflict of interest.
All claims expressed in this article are solely those of the authors and do not necessarily represent those of their affiliated organizations, or those of the publisher, the editors and the reviewers. Any product that may be evaluated in this article, or claim that may be made by its manufacturer, is not guaranteed or endorsed by the publisher.
Abbaszadeh, G., Ameri, A., Torabizadeh, M. (2011). Evaluation of different groups of insecticides on Asian Citrus Psylla, Diaphorina citri K. (Homptera: Psyllidae). Pestic Res. J. 23, 52–54.
Ahuja, I., Rohloff, J., Bones, A. M. (2010). Defence mechanisms of Brassicaceae: implications for plant-insect interactions and potential for integrated pest management: A review. Agron. Sustain Dev. 2, 623–670. doi: 10.1051/agro/2009025
Akanksha, K., Srivastava, K., Srivastava, A., Sinha, B. (2020). Analysis of drought susceptibility index in Indian mustard [Brassica juncea (L.) czern and coss]. Indian J. Agric. 55, 446–451. doi: 10.18805/IJARe.A-5526
Akmal, M. (2021). “Embryo culture and embryo rescue in brassica,” in Brassica Breeding and Biotechnology (London, UK: IntechOpen). doi: 10.5772/intechopen.96058
Ananthanarayan, L., Dubey, K. K., Muley, A. B., Singhal, R. S. (2019). “Indian Traditional Foods: Preparation, Processing and Nutrition,” in Traditional Foods. Eds. Al-Khusaibi, M., Al-Habsi, N., Shafiur Rahman, M. (Cham: Food Engineering Series, Springer), 127–199. doi: 10.1007/978-3-030-24620-4_6
Atri, C., Akhatar, J., Gupta, M., Gupta, N., Goyal, A., Rana, K., et al. (2019). Molecular and genetic analysis of defensive responses of Brassica juncea - B. fruticulosa introgression lines to Sclerotinia infection. Sci. Rep. 19, 17089. doi: 10.1038/s41598-019-53444-3
Atri, C., Kaur, B., Sharma, S., Gandhi, N., Verma, H., Goyal, A., et al. (2016). Substituting nuclear genome of Brassica juncea (L.) Czern & Coss. in cytoplasmic background of Brassica fruticulosa results in cytoplasmic male sterility. Euphytica 209, 31–40. doi: 10.1007/s10681-015-1628-4
Atri, C., Kumar, B., Kumar, H., Kumar, S., Sharma, S., Banga, S. S. (2012). Development and characterization of Brassica juncea–fruticulosa introgression lines exhibiting resistance to mustard aphid (Lipaphis erysimi Kalt). BMC Genet. 13, 1–9. doi: 10.1186/1471-2156-13-104
Bhardwaj, A. R., Joshi, G., Kukreja, B., Malik, V., Arora, P., Pandey, R., et al. (2015). Global insights into high temperature and drought stress regulated genes by RNA-Seq in economically important oilseed crop Brassica juncea. BMC Plant Biol. 15, 1–15. doi: 10.1186/s12870-014-0405-1
Bhat, S. R., Kumar, P., Prakash, S. (2008). An improved cytoplasmic male sterile (Diplotaxis berthautii) Brassica juncea: identification of restorer and molecular characterization. Euphytica 159, 145–152. doi: 10.1007/s10681-007-9467-6
Bhat, S. R., Vijayan, P., Dwivedi, K. K., Prakash, S. (2006). Diplotaxis erucoides-induced cytoplasmic male sterility in Brassica juncea is rescued by the Moricandia arvensis restorer: genetic and molecular analyses. Plant Breed. 125, 150–155. doi: 10.1111/j.1439-0523.2006.01184.x
Bohra, A., Kilian, B., Sivasankar, S., Caccamo, M., Mba, C., McCouch, S. R., et al. (2022). Reap the crop wild relatives for breeding future crops. Trends Biotechnol. 40, 412–431. doi: 10.1016/j.tibtech.2021.08.009
Branca, F., Cartea, E. (2010). “Brassica,” in Wild Crop Relatives: Genomic and Breeding Resources: Oilseeds. Ed. Kole, C. (Berlin: Springer), 17–36.
Chand, S., Singh, N., Prasad, L., Nanjundan, J., Meena, V. K., Chaudhary, R., et al. (2022). Inheritance and allelic relationship among gene (s) for white rust resistance in Indian mustard [Brassica juncea (L.) Czern & Coss]. Sustainability 14, 11620. doi: 10.3390/su141811620
Chandra, A., Gupta, M. L., Ahuja, I., Kaur, G., Banga, S. S. (2004). Intergeneric hybridization between Erucastrum cardaminoides and two diploid crop Brassica species. Theor. Appl. Genet. 108, 1620–1626. doi: 10.1007/s00122-004-1592-1
Chauhan, J. S., Singh, K. H., Singh, V. V., Kumar, S. (2011). Hundred years of rapeseed-mustard breeding in India: accomplishments and future strategies. Indian J. Agric. Sci. 81, 1093–1109.
Chauhan, J. S., Tyagi, M. K., Kumar, A., Nashaat, N. I., Singh, M., Singh, N. B., et al. (2007). Drought effects on yield and its components in Indian mustard (Brassica juncea L.). Plant Breed. 126, 399–402. doi: 10.1111/j.1439-0523.2007.01394.x
Chen, H. F., Wang, H., Li, Z. Y. (2007). Production and genetic analysis of partial hybrids in intertribal crosses between Brassica species (B. rapa, B. napus) and Capsella bursa-pastoris. Plant Cell Rep. 26, 1791–1800. doi: 10.1007/s00299-007-0392-x
Choudhury, S., Asrani, P., Kashyap, A., Rao, M., Prasad, K., Pant, U., et al. (2023). Whole genome resequencing of advanced introgression lines of Brassica juncea L. Czern. for characterizing alien introgression from Diplotaxis erucoides L. DC. Indian J. Genet. Plant Breed. 83, 217–223. doi: 10.31742/ISGPB.83.2.7
Choudhury, S., Rao, M., Kashyap, A., Ahmaed, S., Prasad, L., Singh, N., et al. (2022). Jasmonate mediated inducible accumulation of indole glucosinolates confers resistance against Alternaria blight disease in cruciferous wild species Diplotaxis erucoides. Physiol. Mol. Plant Pathol. 122, 101904. doi: 10.1016/j.pmpp.2022.101904
Dong, C. H., Li, C., Yan, X. H., Huang, S. M., Huang, J. Y., Wang, L. J., et al. (2012). Gene expression profiling of Sinapis alba leaves under drought stress and rewatering growth conditions with Illumina deep sequencing. Mol. Biol. Rep. 39, 5851–5857. doi: 10.1007/s11033-011-1395-9
Duhlian, L., Koramutla, M. K., Subramanian, S., Chamola, R., Bhattacharya, R. (2020). Comparative transcriptomics revealed differential regulation of defense related genes in Brassica juncea leading to successful and unsuccessful infestation by aphid species. Sci. Rep. 10, 10583. doi: 10.1038/s41598-020-66217-0
Gaikwad, K., Kirti, P. B., Prakash, S., Chopra, V. L. (1996). Cytological and molecular investigations on somatic hybrids of Sinapis alba and Brassica juncea and their backcross progeny. Plant Breed. 115, 480–483. doi: 10.1111/j.1439-0523.1996.tb00961.x
Garg, H., Atri, C., Sandhu, P. S., Kaur, B., Renton, M., Banga, S. K. (2010). High level of resistance to Sclerotinia sclerotiorum in introgression lines derived from hybridization between wild crucifers and the crop Brassica species B. napus and B. juncea. Field Crops Res. 117, 51–58. doi: 10.1016/j.fcr.2010.01.013
Gill, S. S., Gill, R., Kumar, G., Pareek, A., Sharma, P. C., Anjum, N. A., et al. (2012). Mustard: approaches for crop improvement and abiotic stress tolerance. Improving Crop Resistance to Abiotic Stress 1, 2:1351–2:1368. doi: 10.1002/9783527632930.ch52
Gomez-Campo, C., Tortosa, M. E., Tewari, I., Tewari, J. P. (1999). Epicuticular wax columns in cultivated Brassica species and in their close wild relatives. Ann. Bot. 83, 515–519. doi: 10.1006/anbo.1999.0849
Gupta, S., Didwania, N., Singh, D. (2020). Biological control of mustard blight caused by Alternaria brassicae using plant growth promoting bacteria. Curr. Plant Biol. 23. doi: 10.1016/j.cpb.2020.100166
Gupta, N. C., Yadav, S., Arora, S., Mishra, D. C., Budhlakoti, N., Gaikwad, K., et al. (2022). Draft genome sequencing and secretome profiling of Sclerotinia sclerotiorum revealed effector repertoire diversity and allied broad-host range necrotrophy. Sci. Rep. 12, 21855. doi: 10.1038/s41598-022-22028-z
Han, F., Zhang, X., Yang, L., Zhuang, M., Zhang, Y., Liu, Y., et al. (2021). Genome-wide characterization and analysis of the anthocyanin biosynthetic genes in Brassica oleracea. Planta 254, 1–14. doi: 10.1007/s00425-021-03746-6
Holub, E. B., Brose, E., Tör, M., Clay, C., Crute, I. R., Beynon, J. L. (1995). Phenotypic and genotypic variation in the interaction between Arabidopsis thaliana and Albugo candida. Mol. Plant Microbe Interact. 8, 916–928. doi: 10.1094/mpmi-8-0916
Inomata, N. (1993). Embryo rescue techniques for wide hybridization. In Breeding Oilseed Brassicas Vol. 19 (Berlin, Heidelberg: Springer), 94–107. doi: 10.1007/978-3-662-06166-4_7
Inturrisi, F. C., Barbetti, M. J., Tirnaz, S., Patel, D. A., Edwards, D., Batley, J. (2021). Molecular characterization of disease resistance in Brassica juncea–The current status and the way forward. Plant Pathol. 70, 13–34. doi: 10.1111/ppa.13277
Jain, S. K., Wettberg, E., Punia, S. S., Parihar, A. K., Lamichaney, A., Kumar, J., et al. (2023). Genomic-mediated breeding strategies for global warming in chickpeas (Cicer arietinum L.). Agriculture 13, 1721. doi: 10.3390/agriculture13091721
Jyoti, S. D., Sultana, N., Hassan, L., Robin, A. H. K. (2021). “Epidemiology, genetics and resistance of Alternaria blight in oilseed Brassica,” in Brassica Breeding and Biotechnology. (IntechOpen), 1–174. doi: 10.5772/intechopen.96454
Kamal, M. M., Savocchia, S., Lindbeck, K. D., Ash, G. J. (2016). Biology and biocontrol of Sclerotinia sclerotiorum (Lib.) de Bary in oilseed Brassicas. Australas. Plant Pathol. 45, 1–14. doi: 10.1007/s13313-015-0391-2
Kamoun, S., Furzer, O., Jones, J. D., Judelson, H. S., Ali, G. S., Dalio, R. J., et al. (2015). The top 10 oomycete pathogens in molecular plant pathology. Mol. Plant Pathol. 16, 413–434. doi: 10.1111/mpp.12190
Kapazoglou, A., Gerakari, M., Lazaridi, E., Kleftogianni, K., Sarri, E., Tani, E., et al. (2023). Crop wild relatives: A valuable source of tolerance to various abiotic stresses. Plants 12, 328. doi: 10.3390/plants12020328
Kashyap, A., Garg, P., Tanwar, K., Sharma, J., Gupta, N. C., Ha, P. T. T., et al. (2022). Strategies for utilization of crop wild relatives in plant breeding programs. Theor. Appl. Genet. 135, 4151–4167. doi: 10.1007/s00122-022-04220-x
Kashyap, A., Kumari, S., Garg, P., Kushwaha, R., Tripathi, S., Sharma, J., et al. (2023). Indexing resilience to heat and drought stress in the wild relatives of rapeseed-mustard. Life 13, 738. doi: 10.3390/life13030738
Katche, E., Quezada-Martinez, D., Katche, E. I., Vasquez-Teuber, P., Mason, A. S. (2019). Interspecific hybridization for Brassica crop improvement. Crop Breed. Genet. Genom. 1, e190007. doi: 10.20900/cbgg20190007
Khan, A. W., Garg, V., Roorkiwal, M., Golicz, A. A., Edwards, D., Varshney, R. K. (2020). Super-pangenome by integrating the wild side of a species for accelerated crop improvement. Trends Plant Sci. 25, 148–158. doi: 10.1016/j.tplants.2019.10.012
Kular, J. S., Kumar, S. (2011). Quantification of avoidable yield losses in oilseed Brassica caused by insect pests. J. Plant Prot. Res. 51, 38–43. doi: 10.2478/v10045-011-0007-y
Kumar, A. (2015). Wide hybridization for resistance against disease in Brassicas. In ICAR Sponsored Winter School on New Paradigms in Diseases Management: Conventional and Molecular Approaches for Rapeseed Mustard Production organized by ICAR-Directorate of Rapeseed-Mustard Research, Bharatpur, pp 7186.
Kumar, S., Atri, C., Sangha, M. K., Banga, S. S. (2011). Screening of wild crucifers for resistance to mustard aphid, Lipaphis erysimi (Kaltenbach) and attempt at introgression of resistance gene (s) from Brassica fruticulosa to Brassica juncea. Euphytica 179, 461–470. doi: 10.1007/s10681-011-0351-z
Kumar, R., Chowdhury, J. B., Jain, R. K. (2001). Interspecific hybridization in Brassica juncea and Brassica tournefortii through embryo rescue and their evaluation for biotic and abiotic stress tolerance. Indian J. Exp. Biol. 39, 911–915.
Kumar, B., Kolte, S. J. (2012). Progression of Alternaria blight of mustard in relation to components of resistance. Indian Phytopathol. 54, 329–331.
Kumar, V., Nanjundan, J., Thakur, A. K., Singh, K. H. (2019) Rapeseed-mustard germplasm information system. Available at: http://www.drmr.res.in/rmpgis/index.php.
Kumar, P., Naresh, V., Srinivasan, R., Bhat, S. R. (2012). An evolutionarily conserved mitochondrial orf108 is associated with cytoplasmic male sterility in different alloplasmic lines of Brassica juncea and induces male sterility in transgenic Arabidopsis thaliana. J. Exp. Bot. 63, 2921–2932. doi: 10.1093/jxb/err459
Kumari, P., Bisht, D. S., Bhat, S. R. (2018). Stable, fertile somatic hybrids between Sinapis alba and Brassica juncea show resistance to Alternaria brassicae and heat stress. Plant Cell Tiss. Organ Cult. 133, 77–86. doi: 10.1007/s11240-017-1362-9
Kumari, P., Singh, K. P., Bisht, D., Kumar, S. (2020b). Somatic hybrids of Sinapis alba and Brassica juncea: Study of backcross progenies for morphological variation, chromosome constitution and reaction to Alternaria brassicae. Euphytica 216, 93. doi: 10.1007/s10681-020-02629-3
Kumari, P., Singh, K. P., Kumar, S., Yadava, D. K. (2020a). Development of a yellow-seeded stable allohexaploid brassica through inter-generic somatic hybridization with a high degree of fertility and resistance to Sclerotinia sclerotiorum. Front. Plant Sci. 11. doi: 10.3389/fpls.2020.575591
Lakra, B. S., Saharan, G. S. (1989). Correlation of leaf and staghead infection intensities of white rust with yield and yield components of mustard. Indian J. Mycol. Plant Pathol. 19, 279–281.
Malik, M., Vyas, P., Rangaswamy, N. S., Shivanna, K. R. (1999). Development of two new cytoplasmic male-sterile lines in Brassica juncea through wide hybridization. Plant Breed. 118, 75–78. doi: 10.1046/j.1439-0523.1999.118001075.x
Manchanda, S. C., Passi, S. J. (2016). Selecting healthy edible oil in the Indian context. Indian Heart J. 68, 447. doi: 10.1016/j.ihj.2016.05.004
Mehta, S., Dhawi, F., Garg, P., Rao, M., Bhattacharya, R. C., Akthar, J., et al. (2023). Potential source of resistance in introgressed, mutant and synthetic Brassica juncea L. lines against diverse isolates of white rust pathogen, Albugo candida. Agronomy 13, 1215. doi: 10.3390/agronomy13051215
Mei, J., Qian, L., Disi, J. O., Yang, X., Li, Q., Li, J., et al. (2011). Identification of resistant sources against Sclerotinia sclerotiorum in Brassica species with emphasis on B. oleracea. Euphytica 177, 393–399. doi: 10.1007/s00122-012-2000-x
Mohapatra, D., Bajaj, Y. P. S. (1988). Hybridization in Brassica juncea× Brassica campestris through ovary culture. Euphytica 37, 83–88. doi: 10.1007/bf00037228
Ogura, H. (1968). Studies on the new male sterility in Japanese radish with special reference to the utilization of this sterility towards the practical raising of hybrid seeds. Mem. Fac. Agric. Kagoshima Univ. 6, 40–75.
Patel, S. R., Awasthi, A. K., Tomar, R. K. S. (2004). Assessment of yield losses in mustard (Brassica juncea L.) due to mustard aphid (Lipaphis erysimi Kalt.) under different thermal environments in Eastern Central India. Appl. Ecol. Environ. Res. 2, 1–15. doi: 10.15666/aeer/02001015
Patel, S., Singh, C. P., Yadav, S. K. (2019). Monitoring of insect-pest complex on rapeseed-mustard at Pantnagar. J. Ent. Res. 43, 73–76. doi: 10.5958/0974-4576.2019.00014.8
Pen, S., Nath, U. K., Song, S., Goswami, G., Lee, J. H., Jung, H. J., et al. (2018). Developmental stage and shape of embryo determine the efficacy of embryo rescue in introgressing orange/yellow color and anthocyanin genes of Brassica species. Plants 7, 99. doi: 10.3390/plants7040099
Prakash, S., Kirti, P. B., Bhat, S. R., Gaikwad, K., Kumar, V. D., Chopra, V. L. (1998). A Moricandia arvensis–based cytoplasmic male sterility and fertility restoration system in Brassica juncea. Theor. Appl. Genet. 97, 488–492. doi: 10.1007/s001220050921
Purnamasari, M. I., Erskine, W., Croser, J. S., You, M. P., Barbetti, M. J. (2019). Comparative reaction of Camelina sativa to Sclerotinia sclerotiorum and Laptosphaeria maculans. Plant Dis. 103, 2884–2892. doi: 10.1094/PDIS-03-19-0664-RE
Quazi, M. H. (1988). Interspecific hybrids between Brassica napus L. and B. oleracea L. developed by embryo culture. Theor. Appl. Genet. 75, 309–318. doi: 10.1007/BF00303970
Rana, J. S. (2005). Performance of Lipaphis erysimi (Homoptera: Aphididae) on different Brassica species in a tropical environment. J. Pest Sci. 78, 155–160. doi: 10.1007/s10340-005-0088-3
Rana, K., Atri, C., Akhatar, J., Kaur, R., Goyal, A., Singh, M. P., et al. (2019). Detection of first marker trait associations for resistance against Sclerotinia sclerotiorum in Brassica juncea–Erucastrum cardaminoides introgression lines. Front. Plant Sci. 10, 1015. doi: 10.3389/fpls.2019.01015
Rana, K., Atri, C., Gupta, M., Akhatar, J., Sandhu, P. S., Kumar, N., et al. (2017). Mapping resistance responses to Sclerotinia infestation in introgression lines of Brassica juncea carrying genomic segments from wild Brassicaceae B. fruticulose. Sci. Rep. 7, 5904. doi: 10.1038/s41598-017-05992-9
Rao, G. U., Batra-Sarup, V., Prakash, S., Shivanna, K. R. (1994). Development of a new cytoplasmic male-sterility system in Brassica juncea through wide hybridization. Plant Breed. 112, 171–174. doi: 10.1111/j.1439-0523.1994.tb00666.x
Ren, W., Si, J., Chen, L., Fang, Z., Zhuang, M., Lv, H., et al. (2022). Mechanism and utilization of ogura cytoplasmic male sterility in cruciferae crops. Int. J. Mol. Sci. 23, 9099. doi: 10.3390/ijms23169099
Ripa, R. S., Arif, M. R., Islam, M. T., Robin, A. H. K. (2020). Embryo rescue response and genetic analyses in interspecific crosses of oilseed Brassica species. In Vitro Cell. Dev. Biol. - Plant 56, 682–693. doi: 10.1007/s11627-020-10116-6
Rodriguez-Moreno, L., Ebert, M. K., Bolton, M. D., Thomma, B. P. (2018). Tools of the crook-infection strategies of fungal plant pathogens. Plant J. 93, 664–674. doi: 10.1111/tpj.13810
Rohilla, H. R., Bhatnagar, P., Yadav, P. R. (2004). Chemical control of mustard aphid with newer and conventional insecticides. Indian J. Entomol. 66, 30.
Sachan, G. C., Purwar, J. P. (2007). “Integrated insect pest management in rapeseed and mustard,” in Entomology: novel approaches, vol. 7. (New Delhi: New India Publishing Agency), 399–423.
Saharan, G. S., Mehta, N. (2008). Sclerotinia diseases of crop plants: biology, ecology and disease management (Springer, Dordrecht: Springer Science & Business Media). doi: 10.1007/978-1-4020-8408-9
Saharan, G. S., Mehta, N., Sangwan, M. S. (2005). Diseases of oilseed crops (New Delhi: Indus Publication Co.).
Samarappuli, D., Zanetti, F., Berzuini, S., Berti, M. T. (2020). Crambe (Crambe abyssinica Hochst): A non-food oilseed crop with great potential: a review. Agronomy 10, 1380. doi: 10.3390/agronomy10091380
Sandhu, S. K., Pal, L., Kaur, J., Bhatia, D. (2019). Genome wide association studies for yield and its component traits under terminal heat stress in Indian mustard (Brassica juncea L.). Euphytica 215, 1–15. doi: 10.1007/s10681-019-2489-z
Sharma, G., Dinesh Kumar, V., Haque, A., Bhat, S. R., Prakash, S., Chopra, V. L. (2002). Brassica coenospecies: a rich reservoir for genetic resistance to leaf spot caused by Alternaria brassicae. Euphytica 125, 411–417. doi: 10.1023/A:1016050631673
Sharma, D., Nanjundan, J., Singh, L., Parmar, N., Singh, K. H., Verma, K. S., et al. (2022). Genetic diversity and population structure analysis in Indian Mustard germplasm using phenotypic traits and SSR markers. Plant Mol. Biol. Rep. 40, 579–594. doi: 10.1007/s11105-022-01339-5
Sharma, P., Samkumar, A., Rao, M., Singh, V. V., Prasad, L., Mishra, D. C., et al. (2018). Genetic diversity studies based on morphological variability, pathogenicity and molecular phylogeny of the Sclerotinia sclerotiorum population from Indian mustard (Brassica juncea). Front. Microbiol. 9. doi: 10.3389/fmicb.2018.01169
Shekhawat, K., Rathore, S. S., Premi, O. P., Kandpal, B. K., Chauhan, J. S. (2012). Advances in agronomic management of Indian mustard (Brassica juncea (L.) Czernj. Cosson): an overview. Int. J. Agron. 2012. doi: 10.1155/2012/408284
Singh, M., Avtar, R., Pal, A., Punia, R., Singh, V. K., Bishnoi, M. (2020). Genotype-specific antioxidant responses and assessment of resistance against Sclerotinia sclerotiorum causing Sclerotinia rot in Indian mustard. Pathogens 9, 892. doi: 10.3390/pathogens9110892
Singh, S. P., Choudhary, A. K. (2003). Selection criteria for drought tolerance in Indian mustard [Brassica juncea (L.) Czern and Coss]. Indian J. Genet. Plant Breed. 63, 263–264.
Singh, K. P., Kumari, P., Rai, P. K. (2021a). Current status of the disease-resistant gene(s)/QTLs, and strategies for improvement in Brassica juncea. Front. Plant Sci. 12. doi: 10.3389/fpls.2021.617405
Singh, B., Sran, A. S., Sohi, G. S. (2021b). “Innovative strategies to develop abiotic and biotic stress tolerance in Mustard (Brassicaceae),” in Brassica Breeding and Biotechnology. (London, UK: IntechOpen), 41–48.. doi: 10.5772/intechopen.95973
Singh, K. H., Singh, L., Parmar, N., Kumar, S., Nanjundan, J., Singh, G., et al. (2022). Molecular characterization and genetic diversity analysis in Indian mustard (Brassica juncea L. Czern & Coss.) varieties using SSR markers. PloS One 17, e0272914. doi: 10.1371/journal.pone.0272914
Sinha, S., Kumar, A., Sohane, R. K., Kumar, R. (2020). Breeding of oilseeds: a challenge for selfsufficiency. In Proceedings-cum-Abstract Book on Breeding of oilseeds: A challenge for self-sufficiency organized by Bihar Agricultural University, Sabour, Bhagalpur, Bihar, India. Available at: https://bausabour.ac.in/album/File105_04_202112_29_10PIMG1.pdf.
Thakur, A. K., Parmar, N., Singh, K. H., Nanjundan, J. (2020). Current achievements and future prospects of genetic engineering in Indian mustard (Brassica juncea L. Czern & Coss.). Planta 252, 1–20. doi: 10.1007/s00425-020-03461-8
Tibbs Cortes, L., Zhang, Z., Yu, J. (2021). Status and prospects of genome-wide association studies in plants. Plant Genome 14, e20077. doi: 10.1002/tpg2.20077
Tirnaz, S., Miyaji, N., Takuno, S., Bayer, P. E., Shimizu, M., Akter, M., et al. (2022). Whole-genome DNA methylation analysis in Brassica rapa subsp. perviridis in response to Albugo candida infection. Front. Plant Sci. 13. doi: 10.3389/fpls.2022.849358
Tripathi, R. S., Gautam, R. K., Kulshreshtha, N., Sharma, P. C., Qadar, A. (2012). Impact of salt tolerant crop varieties on food grains production under salty environment of India. J. Soil Salin. Water Qual. 8, 194–201.
Uloth, M. B., Clode, P. L., You, M. P., Barbetti, M. J. (2016). Attack modes and defence reactions in pathosystems involving Sclerotinia sclerotiorum, Brassica carinata, B. juncea and B. napus. Ann. Bot. 117, 79–95. doi: 10.1093/aob/mcv150
Vasupalli, N., Rao, M., Chamola, R., Pant, U., Bhattacharya, R., Bhat, S. R. (2017). Development and validation of donor-specific STS markers for tracking alienintrogressions into Brassica juncea (L.) Czern. Mol. Breed. 37, 1–10. doi: 10.1007/s11032-017-0714-9
Verma, P. R., Harding, H., Petrie, G. A., Williams, P. H. (1975). Infection and temporal development of mycelium of Albugo candida in cotyledons of four Brassica species. Canad. J. Bot. 53, 1016–1020. doi: 10.1139/b75-119
Warwick, S. I. (1993). Wild species in the tribe Brassicaceae (Cruciferae) as sources of agronomic traits, in guide to the wild germplasm of brassica and allied crops Vol. 17 (Ottawa Canada: Center for Land and Biological Resources Research Branch, Agriculture Canada), 1–19.
Wei, J., Xu, L., Shi, Y., Cheng, T., Tan, W., Zhao, Y., et al. (2023). Transcriptome profile analysis of Indian mustard (Brassica juncea L.) during seed germination reveals the drought stress-induced genes associated with energy, hormone, and phenylpropanoid pathways. Plant Physiol. Biochem. 200, 107750. doi: 10.1016/j.plaphy.2023.107750
Wilson, R. A., Sangha, M. K., Banga, S. S., Atwal, A. K., Gupta, S. (2014). Heat stress tolerance in relation to oxidative stress and antioxidants in Brassica juncea. J. Environ. Biol. 35, 383.
Yadav, S., Modi, P., Dave, A., Vijapura, A., Patel, D., Patel, M. (2020). Effect of abiotic stress on crops. Sustain. Crop Prod. 3. doi: 10.5772/intechopen.88434
Yadav, R., Nanjundan, J., Gupta, A. K., Rao, M., Akhtar, J., Rana, J. C., et al. (2019). Novel source of biotic stress resistance identified from Brassica species and its wild relatives. Proceedings 36, 195. doi: 10.3390/proceedings2019036195
Yamagishi, H., Bhat, S. R. (2014). Cytoplasmic male sterility in Brassicaceae crops. Breed Sci. 64, 38–47. doi: 10.1270/jsbbs.64.38
Yuping, C., Wojciechowski, A. (2000). Obtained interspecific hybrid of B. napus and B. oleracea var. italica by embryo and ovule in vitro culture. J. Huazhong (Cent. China) Agric. Univ. 19, 274–278.
Zhang, G. Q., Zhou, W. J., Gu, H. H., Song, W. J., Momoh, E. J. J. (2003). Plant regeneration from the hybridization of Brassica juncea and B. napus through embryo culture. J. Agron. Crop Sci. 189, 347–350. doi: 10.1046/j.1439-037X.2003.00059.x
Keywords: Brassica juncea, crop wild relatives (CWRs), biotic stress, abiotic stress, protoplast fusion, embryo rescue, cytoplasmic male sterility
Citation: Verma S, Dubey N, Singh KH, Parmar N, Singh L, Sharma D, Rana D, Thakur K, Vaidya D and Thakur AK (2023) Utilization of crop wild relatives for biotic and abiotic stress management in Indian mustard [Brassica juncea (L.) Czern. & Coss.]. Front. Plant Sci. 14:1277922. doi: 10.3389/fpls.2023.1277922
Received: 15 August 2023; Accepted: 11 October 2023;
Published: 25 October 2023.
Edited by:
Mahesh Rao, Indian Council of Agricultural Research, IndiaReviewed by:
Cengiz Toker, Akdeniz University, TürkiyeCopyright © 2023 Verma, Dubey, Singh, Parmar, Singh, Sharma, Rana, Thakur, Vaidya and Thakur. This is an open-access article distributed under the terms of the Creative Commons Attribution License (CC BY). The use, distribution or reproduction in other forums is permitted, provided the original author(s) and the copyright owner(s) are credited and that the original publication in this journal is cited, in accordance with accepted academic practice. No use, distribution or reproduction is permitted which does not comply with these terms.
*Correspondence: Ajay Kumar Thakur, dGhha3VyYWsyMDEwQGdtYWlsLmNvbQ==
†These authors have contributed equally to this work
Disclaimer: All claims expressed in this article are solely those of the authors and do not necessarily represent those of their affiliated organizations, or those of the publisher, the editors and the reviewers. Any product that may be evaluated in this article or claim that may be made by its manufacturer is not guaranteed or endorsed by the publisher.
Research integrity at Frontiers
Learn more about the work of our research integrity team to safeguard the quality of each article we publish.