- 1College of Agriculture, Henan University of Science and Technology, Luoyang, Henan, China
- 2School of Agriculture and Animal Husbandry Engineering, Zhoukou Vocational and Technical College, Henan, China
- 3Henan Engineering Research Center for Rural Human Settlement, Luoyang, Henan, China
- 4Luoyang Key Laboratory of Symbiotic Microorganism and Green Development, Luoyang, Henan, China
Medicinal plants are invaluable resources for mankind and play a crucial role in combating diseases. Arbuscular mycorrhizal fungi (AMF) are widely recognized for enhancing the production of medicinal active ingredients in medicinal plants. However, there is still a lack of comprehensive understanding regarding the quantitative effects of AMF on the accumulation of medicinal active ingredients. Here we conducted a comprehensive global analysis using 233 paired observations to investigate the impact of AMF inoculation on the accumulation of medicinal active ingredients. This study revealed that AMF inoculation significantly increased the contents of medicinal active ingredients by 27%, with a particularly notable enhancement observed in flavonoids (68%) and terpenoids (53%). Furthermore, the response of medicinal active ingredients in belowground organs (32%) to AMF was more pronounced than that in aboveground organs (18%). Notably, the AMF genus Rhizophagus exhibited the strongest effect in improving the contents of medicinal active ingredients, resulting in an increase of over 50% in both aboveground and belowground organs. Additionally, the promotion of medicinal active ingredients by AMF was attributed to improvements in physiological factors, such as chlorophyll, stomatal conductance and net photosynthetic rate. Collectively, this research substantially advanced our comprehension of the pivotal role of AMF in improving the medicinal active ingredients of plants and provided valuable insights into the potential mechanisms driving these enhancements.
Introduction
The knowledge surrounding medicinal plants represents the culmination of wisdom accumulated over thousands of years (Petrovska, 2012; Zuo et al., 2020). It is recorded that humans began utilizing medicinal plants for treating diseases as early as 2,500 years ago (Wiart, 2006). The efficacy of medicinal plants has gained global recognition (Shen et al., 2022; Wang et al., 2022b). World Health Organization (WHO), (2019) reported that more than 80% of the populations in the world regularly relies on medicinal plants to combat various. It is also well known that the extraction of artemisinin from medicinal plants by Tu Youyou earned her the prestigious Nobel Prize in 2014. Artemisinin had successfully treated 22.395 million individuals by 2018, as reported by World Health Organization (WHO), (2022). Furthermore, extracts from medicinal plants such as quercetin, luteolin and acacetin have shown significant potential in enhancing the immunity of COVID-19 patients and reducing the likelihood of entering the critical stage (Luo et al., 2020; Ren et al., 2020; Runfeng et al., 2020; Khadka et al., 2021). However, in recent years, the quality of medicinal plant has experienced a sharp decline alongside the increase in cultivation yield. This decline manifests in decreased contents of medicinal active ingredients and weakened physiological activities (Zeng et al., 2013a; Wang et al., 2018). In response, various scholars have undertaken studies involving different approaches such as the use of fertilizers (Alaoui Jamali et al., 2014) and breeding techniques (Wang et al., 2020) to improve the quality of medicinal plants. Among these approaches, the use of arbuscular mycorrhizal fungi (AMF) as a green fertilizer has garnered significant attention for its potential in enhancing the quality of medicinal plants.
AMF as a ubiquitous soil microorganism plays irreplaceable roles in the management of plant quality (Davison et al., 2015; van der Heijden et al., 2015; Noceto et al., 2021). AMF enhances plant quality traits by regulating plant metabolic activity and chemical compounds, including soluble sugars, polysaccharides, flavonoids, and alkaloids (Grimoldi et al., 2006; Verlinden et al., 2018; Zhang et al., 2020a; Medina et al., 2023). Moreover, compounds such as flavonoids and alkaloids can also serve criteria for evaluating the quality of medicinal plants (Zhang et al., 2020a). It has been reported that more than 90% of medicinal plant species establish symbiotic associations with AMF (Zhang et al., 2022). Recent studies have shown that AMF can promote medicinal plant seed germination (Zhang et al., 2020b), growth (Luo et al., 2021) and secondary metabolic activity (Zeng et al., 2013b), and enhance phenols (Duc et al., 2021), flavonoids (Zhang et al., 2020a), terpenes (Xie et al., 2018a), quinones (Yang et al., 2017) and other medicinal active ingredients (Zeng et al., 2013b). AMF are also well known to help plants tolerate various environmental stressors, such as drought, salinity, or heavy metal toxicity (Porcel et al., 2015; Zhao et al., 2022). Stress mitigation indirectly supports active ingredient production by maintaining plant health. The research on improving the quality of medicinal plants by AMF inoculation has become one of the hotspots (Jeffries et al., 2003; Gosling et al., 2006; van der Heijden et al., 2015; Amani Machiani et al., 2022; Abdullahi et al., 2023).
Currently, a large number of studies on AMF and medicinal plants have been conducted to explore the effects of mycorrhizal symbiosis on medicinal active ingredients (Zeng et al., 2013b; Ostadi et al., 2020; Duc et al., 2021; Zhao et al., 2022). Most studies found that AMF inoculation increased medicinal active ingredient contents in plants (Srivastava et al., 2016; Chen et al., 2017; Xie et al., 2018a), and a few researches reported AMF had negative or no effects on medicinal active ingredients (Vieira et al., 2021). The effects of AMF inoculation on medicinal active ingredients differed from organs (aboveground and belowground organs) and medicinal components (Zubek et al., 2012; Lima et al., 2017; Xie et al., 2018b). While, the quantitative effect of AMF inoculation on medicinal active ingredients remains unclear. Filling this critical knowledge gap will help to provide a theoretical reference for enhancing the medicinal active ingredients by AMF inoculation and refine the theory of mycorrhizal symbiosis.
AMF colonization is widely perceived as one of the key ways to impact the medicinal active ingredients in plants (Zeng et al., 2013a; Zeng et al., 2013b). Previous comprehensive studies have shown the potential of AMF in enhancing medicinal active ingredients (Mandal et al., 2013; Zeng et al., 2013a; Zeng et al., 2013b). However, the responses of medicinal active ingredients in plant organs to AMF were disparate (Xie et al., 2018b; Yu et al., 2019; Merlin et al., 2020), which may due to the differences of physiological activities in different plant organs when inoculated with AMF (Chen et al., 2017; Merlin et al., 2020). Therefore, it is necessary to extensively research the effects of medicinal active ingredients in plant organs interacting with AMF, to obtain the general response model from the specific response of medicinal active ingredients in plant organs to AMF inoculation.
Previous studies have shown that AMF is universal in improving the quality of medicinal active ingredients (Vo et al., 2019; Zhang et al., 2020a; Wu et al., 2021), such as enhancing the synthesis and accumulation of terpenes and flavonoids in medicinal plants (Hristozkova et al., 2016; Wei et al., 2020; Zhang et al., 2020a). While the efficacy of AMF promoting medicinal active ingredients was diverse (Zubek et al., 2012; Hristozkova et al., 2016; Wu et al., 2021) and there is no consensus on which medicinal active ingredients are most affected by AMF or which species of AMF had the best effect on medicinal active ingredients (Hristozkova et al., 2016; Chen et al., 2017; Lima et al., 2017). The selection of the optimal AMF to enhance medicinal active ingredients has been a prominent and widely discussed topic (Lima et al., 2017; Khalediyan et al., 2021; Wu et al., 2021). Medicinal active ingredient has different responses to different AMF species (Andrade et al., 2012; Yu et al., 2019). Single or multi-AMF inoculation also has different effects on medicinal active ingredients (Zubek et al., 2012; Duc et al., 2021). Considering the inconsistent results, it is vital to synthesize the available data to reveal clear and generalizable patterns of the response of medicinal active ingredients to single or multi-AMF inoculation, and to further identify the AMF species with the most significant effects on medicinal active ingredients.
In this study, we conducted a quantitative analysis at the first time to assess the effect of AMF on medicinal active ingredients. We hypothesized that (a) the effect of AMF on medicinal active ingredients linked with plant organ and compounds of medicinal active ingredients, (b) single or multi-AMF inoculation had different effects on medicinal active ingredients. The study aims to provide a reference to increase medicinal active ingredients by AMF inoculation and theoretical foundation for the development of the pharmaceutical industry.
Materials and methods
Search strategy and eligibility criteria
This meta-analysis followed the PRISMA protocol (Moher et al., 2015). Peer-reviewed publications researching on AMF associated with medicinal plants and measured indices of medicinal active ingredients were searched using Web of Science database until December 2021 with no restriction on publication year. The search terms were ‘(arbuscular mycorrhiza) AND (active ingredients OR secondary metabolic product)’. Papers included in the analysis had to meet the following criteria: (a) the papers had to be original research and reviews were excluded; (b) experiments involved AMF treatment and a corresponding control; (c) the object of study was a medicinal plant; (d) at least one medicinal active ingredient was included in the study; (e) the number of replicates for AMF treatments and control groups can be obtained; (f) means or standard deviations (SD) or standard errors (SE) or 95% confidence interval of the AMF treatment and control groups can be obtained.
Data extraction
For the quantitative analyses, each included publication was evaluated by two reviewers (Menghan Zhang and Mingli Yuan) independently and for accuracy the searching results were cross-checked. In case of any discrepancies, a third reviewer (Zhaoyong Shi) was involved. Based on the search strategy, 28 papers were included in this systematic analysis (Figure 1, Supplementary Data 1).
According to Han et al. (2020) and Luo et al. (2006), we obtained data on medicinal active ingredient information for each study and physiological variables if reported. All original data were obtained from main text, tables, figures and Supplementary Files of literature. When the data were presented in figures, we extracted numerical data using GetData Graph Digitizer 2.26. In total, our database included 233 observations on medicinal active ingredients (detailed information were shown in Table S1).
For each observation, the components of medicinal active ingredients were classified into phenols, flavonoids, alkaloid, terpenes, organic acids, quinones and others with aboveground or belowground organs and single- or multi-AMF recorded. About single AMF species classification, we referred to the website (http://www.speciesfungorum.org/). The effect of AMF inoculation on medicinal active ingredient were analyzed from order, family and genus level. Due to data limitations, we did not study the impact of AMF at the species level and focusing on the genus level allows us to address a broad range of AMF species and their potential effects on multiple medicinal plant species. In order to investigate the physiological mechanism of AMF effecting medicinal active ingredient, we also recorded seven physiological variables including total carotenoids (TC), chlorophyl A (Chl-A), chlorophyl B(Chl-B), total carbohydrates (CHO), stomatal conductivity (Gs), net photosynthetic rate (Pn) and water utilization ratio (WUE) (detailed information were shown in Table S2).
Data analysis
The effect size of medicinal active ingredients to AMF was evaluated by the natural log response ratio (lnRR) (Hedges et al., 1999; Luo et al., 2006). The response ration was calculated as:
Where XT and XC represent the medicinal active ingredients in AMF treatment and the control treatment, respectively.
The variance (v) of every lnRR was calculated as follows:
Where ST and SC represent SD of AMF treatment and control treatment, respectively, nT and nC are the sample sizes.
The conversion equation for SD and SE is as follows:
Where n is the sample size. In several studies with no SD or SE and only 95% confidence intervals, we identified 1/10 of the mean as SD (Han et al., 2020).
This study employed the Q test and I² statistic to assess heterogeneity among the included literature. In the Q test, a larger Q value and a smaller P value indicate significant heterogeneity when P<0.01. The I² statistic quantifies the degree of heterogeneity, with values exceeding 50% considered significant heterogeneity (Higgins et al., 2003). When I²<50%, a fixed-effects model is employed; when I²>50%, it is advisable to use a random-effects model. Based on heterogeneity analysis (Tables S3, S4), a random-effects model was employed in present study (Viechtbauer, 2010; Zhang et al., 2019).
The weighted response ratio and 95% confidence interval were calculated using “metafor” package in R 3.4.1 (http://R-project.org/). If the value of the confidence interval (95%) does not coincide with 0, the effect of AMF inoculation was considered significant (Han et al., 2020).
To explore the relationship between medicinal active ingredients effect and total physiological factors (TC, ChlA&B, Gs, CHO, Pn and WUE), linear regression analysis was performed by SPSS (Version 23.0, Chicago, IL, USA).
Results
The overall effects of AMF on medicinal active ingredients
The effect size of AMF on medicinal active ingredients ranged from -2.89 to 2.44 with positive, negative and neutral effect size accounting for 55.4%, 14.2%, 30.4%, respectively (Figure 2). The overall mean effect size of AMF inoculation on medicinal active ingredients was 27% (CI [19-36%]) (Figure 3). When the plant organs were classified into aboveground and belowground organs, the result showed that the medicinal active ingredients both in aboveground and belowground organs were increased by AMF inoculation. The effect size of AM inoculation on belowground organs (18%, CI [5-32%]) was higher, but not significantly, than that on aboveground organs (34%, CI [24-45%]) (Figure 3).
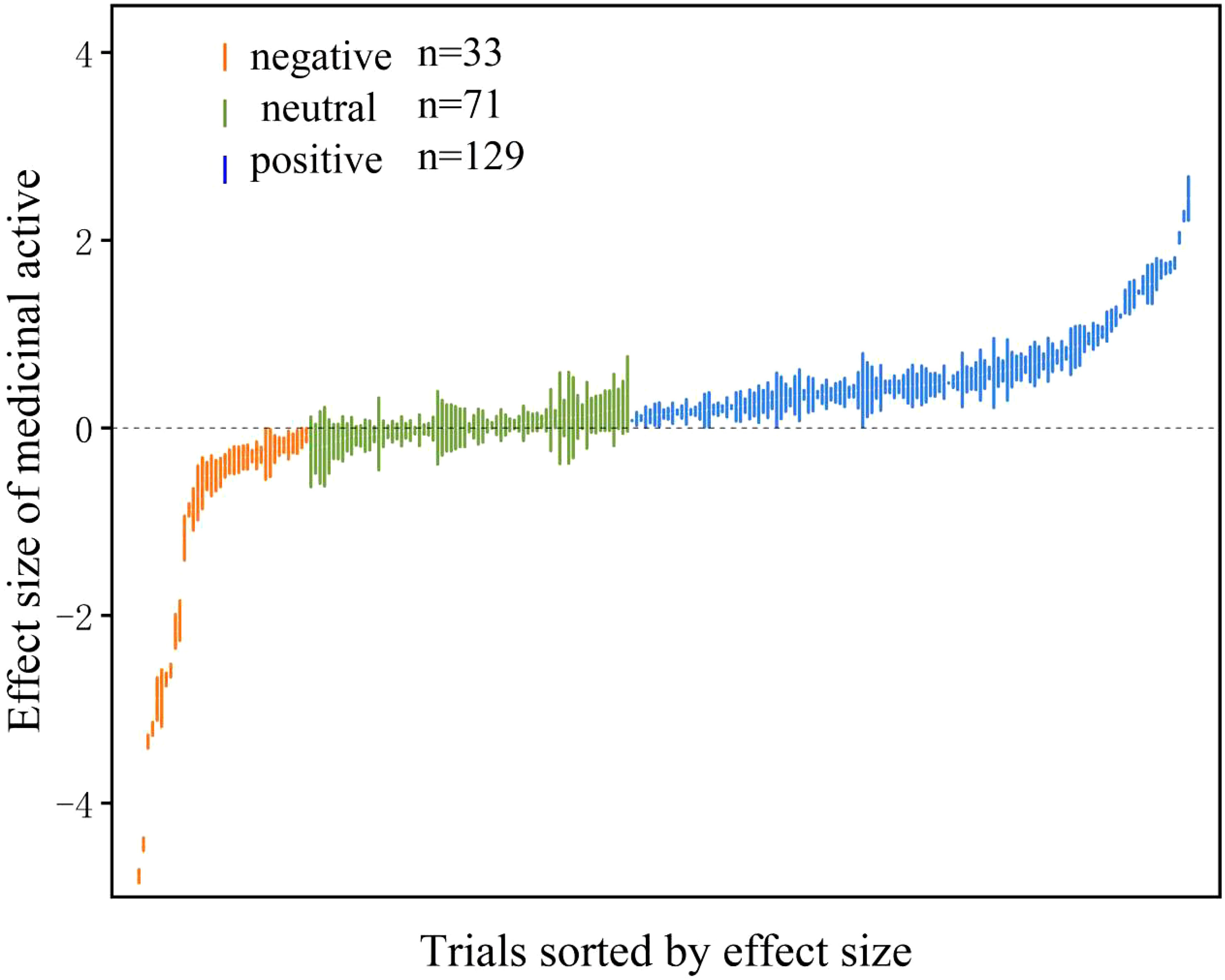
Figure 2 Effect of arbuscular mycorrhizal fungi (AMF) on medicinal active ingredient. The “n” represents trial numbers. The green, vermilion and blue data points represent the positive, neutral and negative effect, respectively.
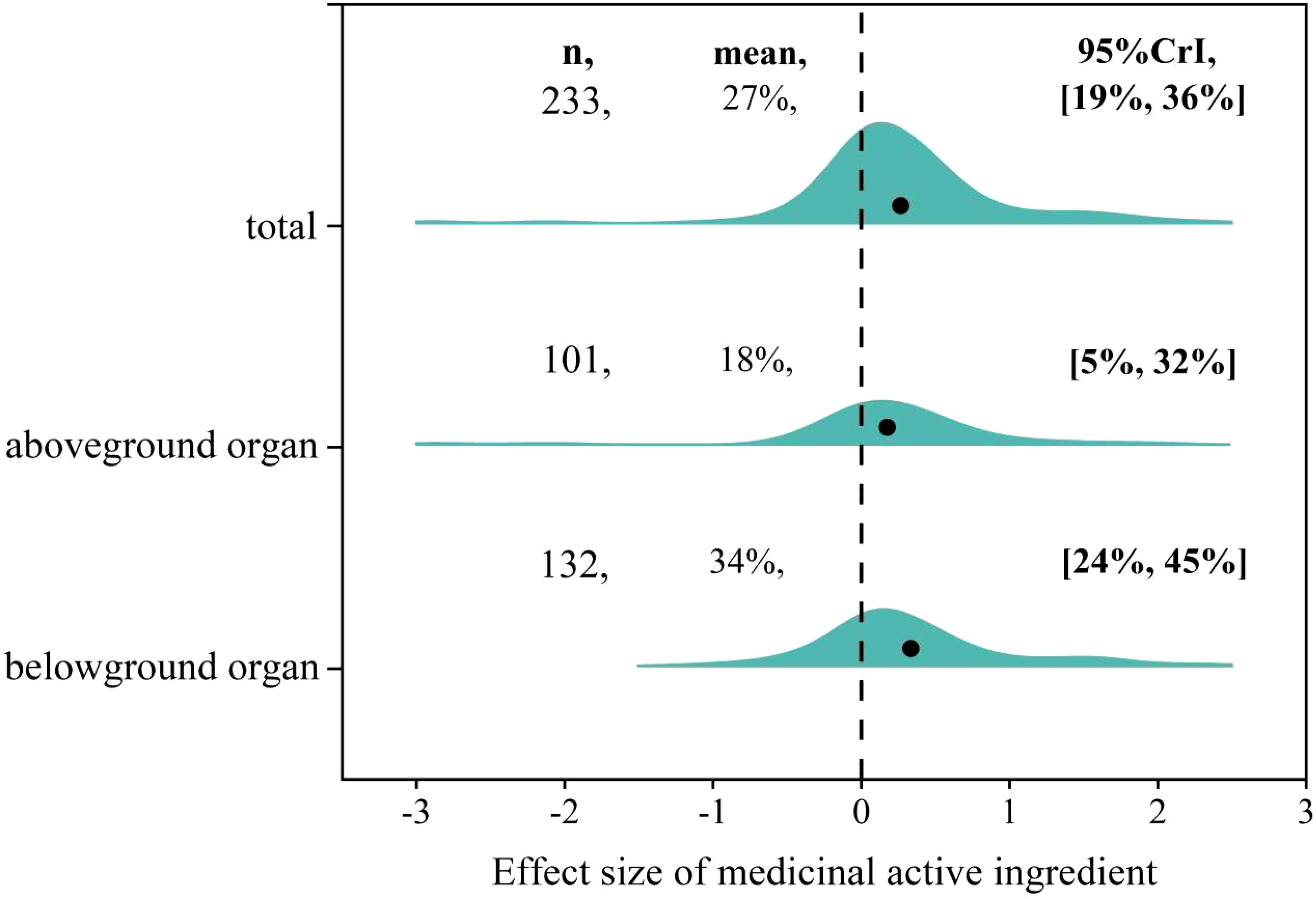
Figure 3 Overall effect of AMF on medicinal active ingredients including Total, in Aboveground and Belowground organs.
The overall effects of AMF on medicinal plants
In our current study, there are 20 different medicinal plant species across the 28 articles analyzed. From the list of the impact of AMF on these 20 medicinal plant species (Table 1), the findings revealed that AMF inoculation exerted a beneficial effect on the majority of these medicinal species. However, it should be noted that AMF inoculation appeared to have limited or ineffectual effects on a select few medical species, such as Acmella oleacea and Eclipta prostrata.
Responses of different medicinal active ingredients to AMF inoculation
We classified medicinal active ingredients into several kinds of compounds, such as phenols, flavonoids, alkaloid, terpenes, organic acids, quinones and others, to explore their responses to AMF inoculation. The results showed that AMF inoculation had prominent positive effects on flavonoids, terpenes, phenols and quinonoids (Figure 4A). The mean effect size on flavonoids was the highest (68%), followed by terpenoids (53%), and on phenols and quinones was 13% and 24%, respectively. The positive effect of AMF inoculation on organic acids was non-significant with an average effect size of 19%. When comes to alkaloids and others, the effect was negative, but not significant.
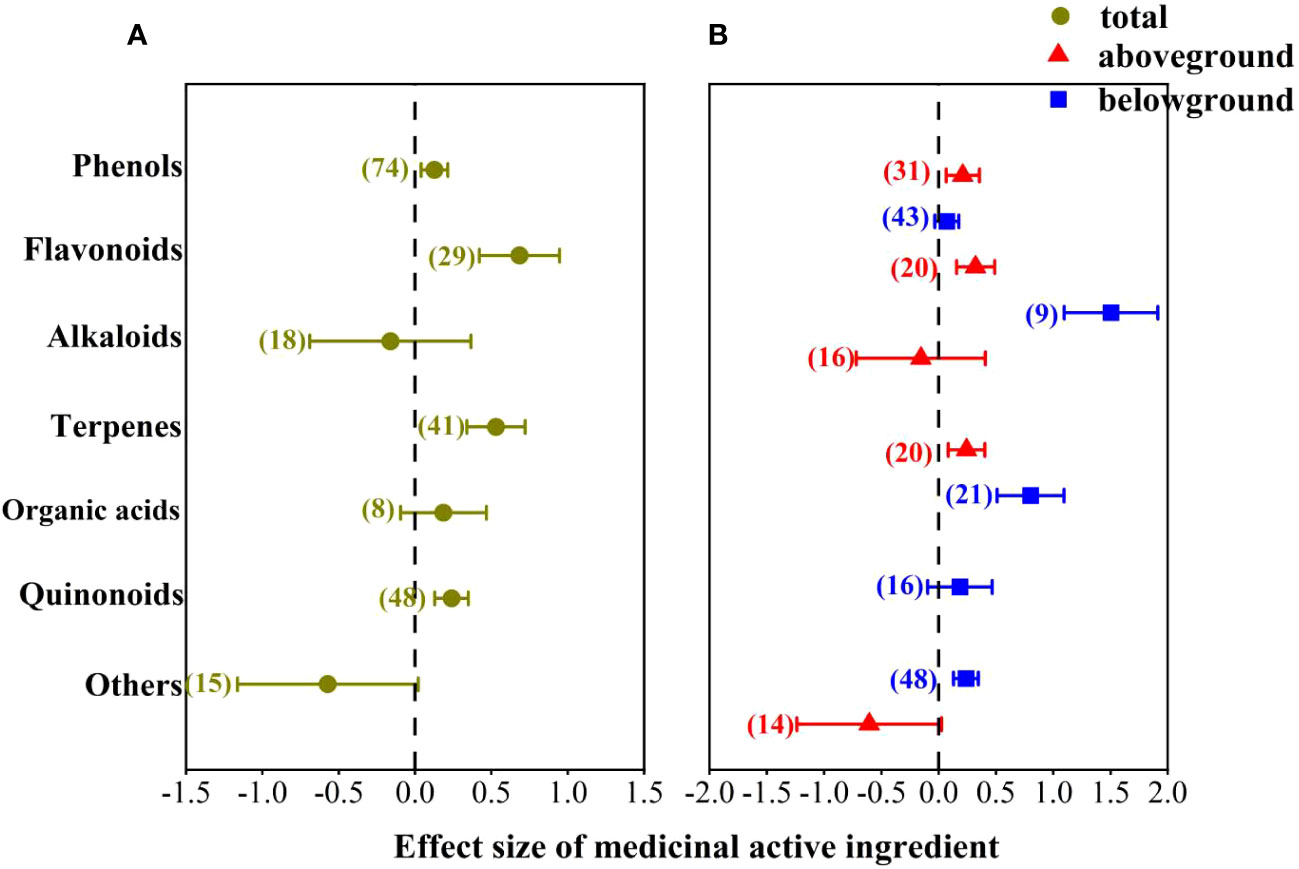
Figure 4 Effect of AMF on different compounds of medicinal active ingredients. (A) Total; (B) aboveground and underground organs.
Furthermore, we further investigated the effects of AMF inoculation on different medicinal compounds in different organs (Figure 4B), the results were similar to the overall. It’s worth noting that the effect of AMF inoculation on flavonoids and terpenoids was more effective in belowground organs, with effect size 150% and 80%, respectively. The effect of AMF inoculation on phenols was more effective in aboveground organs with effect size 21%.
The different function between single and multi-AMF inoculation
Result shows that the multi-AMF inoculation had significant effects on the medicinal active ingredients in aboveground organs with effect size of 25% (Figure 5A), while single AMF inoculation had insignificant positive effect. The single AMF inoculation significantly increased (34%) the contents of medicinal active ingredients in belowground organs. The study does not present the effect of multi-AMF inoculation on medicinal active ingredients in belowground organs, given the absence of relevant research on the subject. The effects of single AMF on the Order level were uneven (Figure 5B). Both Diversisporales and Glomerales had significant positive effects on medicinal active ingredients. And Archaeosporales had a non-significant negative effect on medicinal active ingredients. From AMF genus level, the Rhizophagus was the most effective genus that had the highest effect size on medicinal active ingredients both in aboveground (60%) and belowground organs (51%) compared with other genera (Figure 5C).
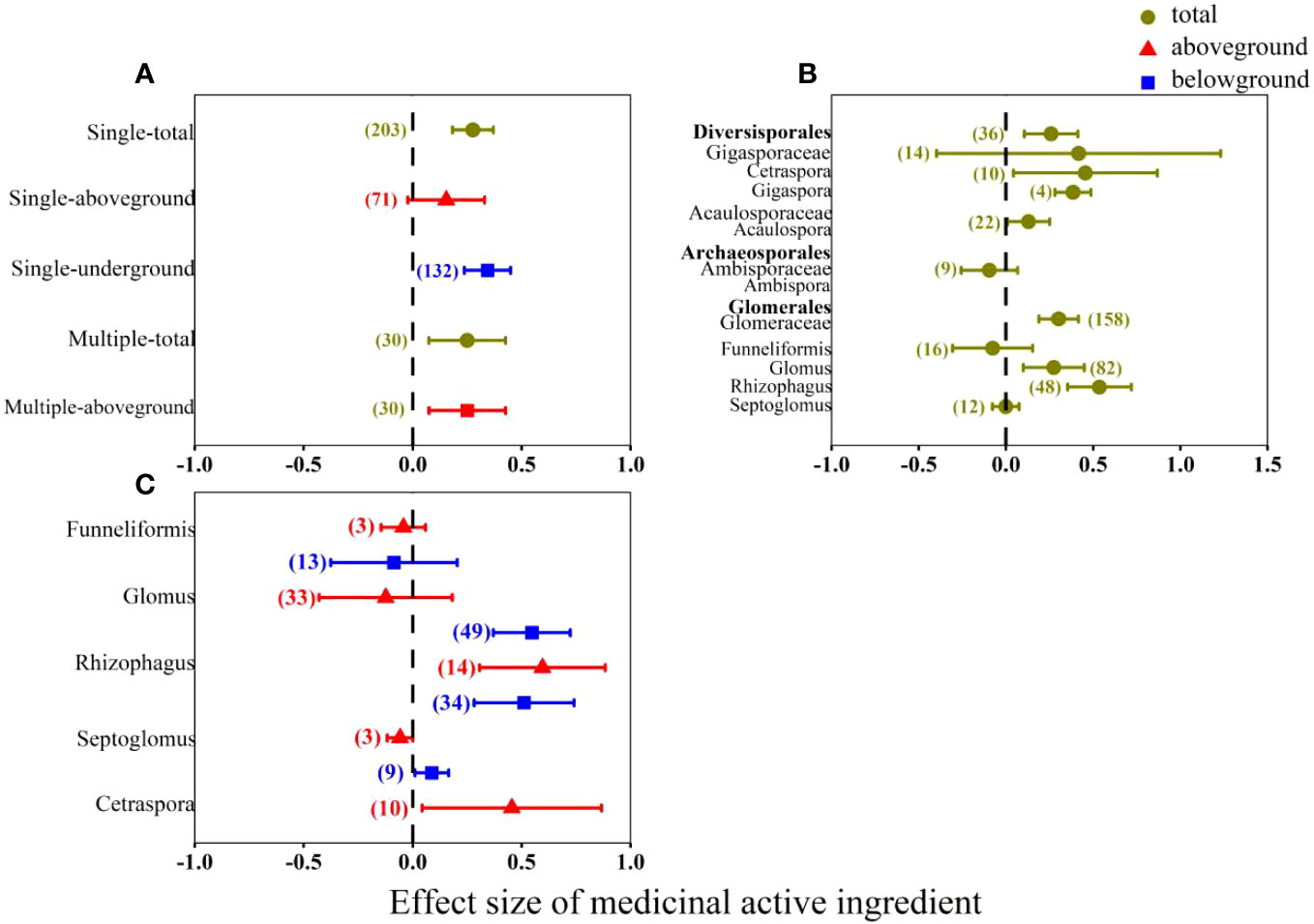
Figure 5 Effect of AMF on overall medicinal active ingredients. AMF classification: (A) signal or multi-AMF inoculation; (B) AMF inoculation on order, family and genus level; (C) AMF inoculation on genus level.
Influence of AMF inoculation on physiological factors
Physiological status of host plant was obviously improved by AMF inoculation (Figure 6). AMF inoculation significantly increased plant TC (6%), Chl A (28%), Chl B (41%), Pn (84%), Gs (94%) and WUE (96%). The results also showed that the AMF inoculation decreased CHO by 29%, but not significantly. Further, we explored the relationship between changes in plant physiological factors and changes in the content of medicinal active ingredients before and after AMF inoculation. We found that the effect size of medicinal active ingredients was positively correlated with Chl A (R2 = 0.6668, P<0.001), Chl B (R2 = 0.2424, P<0.005), Pn (R2 = 0.7755, P<0.001), Gs (R2 = 0.7674, P<0.001), but negatively correlated with TC (R2 = 0.66, P<0.001) (Figure 7).
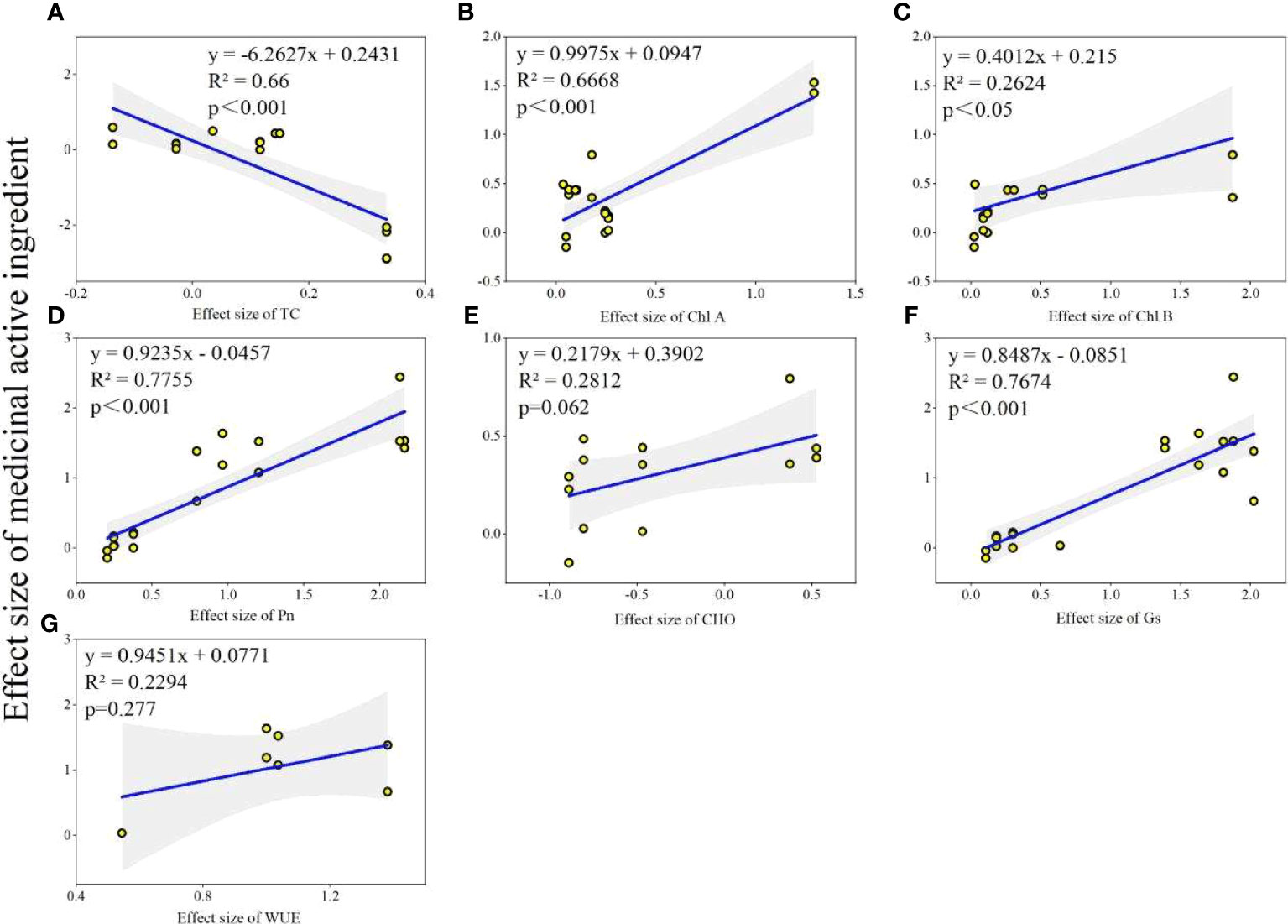
Figure 7 The relationship between effects sizes of medicinal ingredients and physiological factors. (A) TC: Total Carotenoids; (B) Chl A: Chlorophyll A; (C) Chl B: Chlorophyll B; (D) Pn: net photosynthetic rate; (E) CHO: total carbohydrates; (F) Gs: stomatal conductance; (G) WUE: water utilization ratio.
Discussion
Based on a global scale quantitative synthesis with 233 pairs of data from 28 studies, we found that AMF inoculation have a significant positive effect on majority medicinal active ingredients, especially flavonoids in plant belowground organs. The enhancement of medicinal active ingredients by AMF inoculation may be related to the positive effect of AMF inoculation on plant physiological activity. By studying single or multi-AMF inoculation from different guilds, it was found that the medicinal active ingredients had the strongest response to Rhizophagus, one genus of AMF. Collectively, these findings provide a quantitative reference for the use of AMF inoculation to increase the content of medicinal active ingredients, which are valuable for the development of pharmaceutical industry.
Nowadays, human beings have a strong demand for the high quality medicinal active ingredients to better prevent and treat diseases. AMF has gained much attention because of its positive effect on medicinal active ingredients (Zeng et al., 2013b; Lima et al., 2017; Khalediyan et al., 2021). The quantitative analysis in this study confirmed that AMF inoculation could improve the quality of medicinal plants and enhanced the accumulation of medicinal active ingredients (De la Rosa-Mera et al., 2011; Mandal et al., 2013; Ran et al., 2021). In this study, we examined a total of 20 distinct medicinal plant species across the 28 articles under analysis. Our findings indicate that AMF inoculation predominantly exerts a favorable influence on the majority of these medicinal species, which will assist in guiding more targeted and effective AMF-based cultivation strategies for specific medicinal plant species. Furthermore, when investigating the interaction between AMF and plants, the concentration of AMF inoculation is also a crucial factor that should not be disregarded. Research indicates that the inoculation concentration of AMF can affect root system architecture (RSA). RSA traits such as total length, projected area, surface area, and volume tend to increase more significantly under higher AMF inoculation concentrations (Wu et al., 2012). The alteration in RSA induced by AMF may be associated with enhanced nutrient uptake (Padilla and Encina, 2005; Schroeder and Janos, 2005) and increased salt tolerance (Wu et al., 2011). Given the limited research on contrasting the effects of different concentrations of AMF inoculation on medicinal active ingredients, our study specifically centered on assessing whether AMF inoculation had an impact on them.
The findings that AMF enhanced the content of medicinal active ingredients in aboveground and belowground organs of plants were reported in previous researches (Srivastava et al., 2016; Xie et al., 2018a; Ran et al., 2021). Chen et al. proved that AMF inoculation significantly increased medicinal active ingredient contents in different belowground organs of Glycyrrhiza uralensis (Chen et al., 2017). Furthermore, Andrade et al. (2012) confirmed that AMF colonization significantly influenced the medicinal active ingredients in aboveground organs of Catharanthus roseus, compared to the medicinal plants with non-AMF colonization. Overall, consistent with our results in the present study, AMF inoculation has positive effects on medicinal active ingredient contents and the quality of medicinal plants. Scientific evidence has confirmed that the mycorrhizal network formed by AMF and plants increases the surface area for nutrient absorption, facilitating the uptake of essential nutrients such as phosphorus, nitrogen and various micronutrients (Smith and Read, 2008). Improved nutrient acquisition is fundamental for the biosynthesis of medicinal compounds.
The contribution of AMF to the increase of medicinal active ingredient contents varied depending on medicinal organs, medicinal compounds, and single or multi-AMF. AMF had the highest average effect size on medicinal active ingredient of the belowground organs, compared with that of aboveground organs. Previous studies have suggested that AMF have more potential in improving the productivity and ecological function of the belowground organs of plants (Hiiesalu et al., 2014; Yang et al., 2021). Yu et al. also found flavonoids in belowground organs of medicinal plants was significantly increased by Rhizophagus (Yu et al., 2019). Therefore, to further optimize the production of medicinal active ingredients in medicinal plants, it is advisable to inoculate with AMF when the belowground organs are intended for use in medicinal preparations, such as Lonicera japonica, Coptis chinensis, Glycyrrhiza glabra, Angelica sinensis (Wei et al., 2016; Li et al., 2020; Sidhu et al., 2020; Tseng et al., 2022).
Single or multi-AMF inoculation had differed effects on medicinal active ingredients. Medicinal active ingredients with single AMF inoculation had the higher effect size compared to that with multi-AMF inoculation. Differences in the species and combinations of AMF were thought to be an impact factor on the medicinal active ingredient in previous studies (Zeng et al., 2013b; Lima et al., 2017; Vieira et al., 2021). Similar to our result that Rhizophagus had the best effect on increasing the concentration of medicinal active ingredients. It has been also proved to be a particularly excellent root colonizer and plant symbiotic compared to other species of AMF in the horticulture plants (Wang et al., 2022a). The genera may form particularly beneficial symbiotic relationships with medicinal plants, leading to increased production of secondary metabolites. Previous study also showed that Rhizophagus could improve quality of Commiphora leptophloeos by promoting the production of the secondary metabolites (Lima et al., 2017). Currently, little is known about the interactions between AMF and medicinal plants, but available data seem to support the idea that management of AMF rhizosphere communities may have important implications for the development of sustainable agriculture and more efficient production of medicinal plant materials (Gianinazzi et al., 2010).
The mechanism of AMF inducing changes in the concentration of medicinal active ingredients in different parts of medicinal plants can be multifaceted (Toussaint, 2007). Among them, one of the reasons is that the physiological and secondary metabolic ability of medicinal plants in the symbiotic state can be improved (Oliveira et al., 2013; Zeng et al., 2013b). Among the mentioned medicinal active ingredients in this study, flavonoids showed the strongest responses to AMF, followed by terpenoids. Similarly, previous publication reported that AMF symbiosis increased the accumulation of medicinal active ingredients, especially terpenoids and flavonoids (Xie et al., 2018b). In present study, the results showed that AMF inoculation had positive effects on most of physiological factors, such as TC, Chl, Pn, Gs and WUE. Consequently, the enhancement of medicinal active ingredients by AMF may be related to its promotion of physiological activities (Xie et al., 2018b; Yu et al., 2019; Ran et al., 2021).
Photosynthesis is one of the key critical physiological and metabolic activities in plants, and it is closely coupled with upstream and downstream physiological metabolic activities (Ferreira et al., 2004). TC and Chl are proxies of plant photosynthesis and reflect the physiological and metabolic activity of plants (Andrade et al., 2012). Abdel Latef & Chaoxing (2011) reported that the content of Chl A and Chl B were enhanced in Lycopersicon esculentum when inoculated with AMF. Ran et al. (2021) reported that the promotion of active ingredients in medicinal plants of American ginseng was closely related to Chl. It is well known that the photosynthesis of plants is affected by the conductance of Gs (Gago et al., 2016). The Gs of the plant further affects the Pn (Mathur et al., 2018). Pn is commonly used to characterize the intensity of photosynthetic physiological activity in plants (Xie et al., 2018a; Xie et al., 2018b). Xie et al. (2018b) showed that there was a certain relationship between the increase of photosynthetic intensity and the increase of medicinal active components of Glycyrrhiza uralensis. Compared with the control group, AMF inoculation increased photosynthetic physiological activity, but decreased the photosynthetic product CHO in our study. CHO is one of the most important products of plant physiological metabolism and one of the key exchanges of plant roots and AMF (Marschner and Dell, 1994). We speculate that symbiosis enhances the exchange of AMF and medicinal plant CHO, stimulates photosynthesis, and enhances physiological and metabolic activities of medicinal plants (Gupta et al., 2021). Moreover, the effect of medicinal plants on WUE was especially increased by AMF inoculation. The physiological and metabolic activities of plants are inseparable from the participation of water, and a higher WUE indicates a higher potential of physiological and metabolic activities (Suter et al., 2019). The studies indicate that an WUE in medicinal plants may serve as a mechanism through which mycorrhizas facilitate the accumulation of medicinal active ingredients. This is supported by the observation that an increased WUE in AMF-plants correlates with higher levels of medicinal active ingredients, regardless of the specific mycorrhizal species or plant species involved (Xie et al., 2018b).
Numerous studies have shown that the physiological changes, such as Chl, Pn and Gs in medicinal plants inoculated with AMF is the reason for the quality improvement of medicinal active ingredients (Xie et al., 2018a; Xie et al., 2018b; Suter et al., 2019; Ran et al., 2021). In this study, the positive relationship between the effects of AMF inoculation on medicinal active ingredients and physiological activities provided evidence that the improvement of physiological and metabolic activities contributes to the accumulation of medicinal active ingredients of plants. These effects are attributed to the complex interactions between AMF and the plant host. Chl play critical roles in photosynthesis and are essential for capturing and converting light energy into chemical energy. The improved Chl content can lead to increased photosynthetic efficiency and, subsequently, higher production of medicinal active compounds (Lichtenthaler, 1987; Blankenship, 2021). Gs is influenced by AMF through its impact on water and nutrient uptake. AMF can enhance plant’s water absorption capabilities by extending its root system through mycorrhizal hyphae (Zhao et al., 2022; Kuyper and Jansa, 2023). As a result, plants with AMF colonization tend to maintain more optimal stomatal conductance even under water-limiting conditions. AMF colonization often leads to an increased Pn, which is due to several factors, including improved nutrient availability (particularly P), enhanced water uptake, and the modulation of plant hormones by AMF (Evelin et al., 2009; Smith and Smith, 2011). The higher Pn provides more energy and carbon compounds for the biosynthesis of medicinal compounds.
In addition, the molecular-level mechanisms through which AMF regulate the synthesis of medicinal compounds are also receiving increasing attention. Research has demonstrated that secondary metabolites of plant root, serving as signal molecules, play a pivotal role in both establishing AMF symbiosis and triggering a defense response in plants, resulting in heightened secondary metabolite production (Vierheilig, 2004). Previous study found that flavonoids enhanced AMF invasion and infection rates, leading to increased secondary metabolite production in plants (Scervino et al., 2007). Strigolactone, categorized as a sesquiterpene, has been identified as a signaling molecule that fosters mycorrhizal symbiosis (Mitra et al., 2021). Besides, AMF colonization leads to changes in enzyme activities within host plants and has an impact on the expression of genes related to secondary metabolites (Shrivastava et al., 2015; Ran et al., 2022). For example, the transcription of genes responsible for isoprenoid biosynthesis was enhanced by AMF symbiosis and displayed a correlation with the quantitative levels of terpenoids (Mandal et al., 2015). In recent years, significant advancements have been made in researching the molecular-level mechanisms behind AMF-mediated enhancement of medicinal active ingredients (Mechri et al., 2015; Kapoor et al., 2017; Ran et al., 2022; Zhao et al., 2022). These developments offer fresh perspectives on enhancing the quality of medicinal plants through AMF utilization. Nevertheless, to fully unlock the potential of AMF in enhancing the quality of medicinal plants, further research in this area needs to be strengthened.
Nonetheless, there are limitations to the current study. First, our study analyzes the overall impact of AMF on medicinal plants, providing a broad perspective. It lacks specific AMF inoculation strategies tailored to individual plant species. Based on this foundation, we expect to establish more refined experiments to further screen AMF inoculation strategies that are suitable for local conditions. Additionally, it cannot be overlooked that soil quality also plays an irreplaceable role in the interaction between AMF and medical plants. Soil conditions, such as pH, temperature, available nitrogen and phosphorus etc., greatly impact the status of infection of AMF on medicinal plants (Zhao et al., 2012; Zeng et al., 2013b). AMF assist plants in enhancing mineral nutrition uptake and directly promote plant’s growth (Wei and Wang, 1991; Smith and Read, 2008; Zeng et al., 2013b; Soti et al., 2023). On the other hand, the AMF hyphae extend into the surrounding soil, potentially altering the soil structure, thereby contributing to the improvement of soil quality and fertility (Thirkell et al., 2017; Chen et al., 2018; Zhao et al., 2022). Consequently, these changes can have additional effects on medicinal plants. The interplay of soil quality, AMF, and medicinal plants involves both direct and indirect interactions, complicating the differentiation of their individual effects. In the current study, we focus solely on AMF as a variable to explore its influence on medicinal active ingredients. Therefore, the consideration of soil conditions will be encompassed in our future research endeavors.
Conclusions
This study conducted a quantitative assessment of the effect of AMF inoculation on the active ingredients of medicinal plants on a global scale. The results showed a significant increase in the medicinal active ingredients of belowground organs following AMF inoculation, with flavonoids displaying the strongest positive response to AMF. Notably, the genus Rhizophagus had the highest effect size on medicinal active ingredients. Consequently, it is recommended to inoculate medicinal plants with the genus Rhizophagus to enhance the active ingredients, particularly when targeting active ingredients from belowground organs. Furthermore, when examining the relationships between the response of medicinal active ingredients and physiological variables, we found positive correlations between that active ingredients and Chl A, Chl B, Pn and Gs. In summary, the findings of this study provide valuable insights into the utilization of AMF to enhance the active ingredients of medicinal plants. However, further research is necessary to explore the impact of physiological activities and environmental factors on the medicinal active ingredients of AMF-inoculated medicinal plants, as well as the underlying mechanisms.
Data availability statement
The original contributions presented in the study are included in the article/Supplementary Material, further inquiries can be directed to the corresponding author/s.
Author contributions
M-LY: Data curation, Formal Analysis, Writing – original draft, Conceptualization, Methodology. M-HZ: Conceptualization, Writing – original draft. Z-YS: Conceptualization, Supervision, Writing – review & editing, Funding acquisition. SY: Methodology, Software, Writing – review & editing. M-GZ: Methodology, Software, Conceptualization, Writing – review & editing. ZW: Software, Writing – review & editing. S-WW: Data curation, Methodology, Writing – review & editing. J-KG: Software, Supervision, Writing – review & editing.
Funding
The authors declare financial support was received for the research, authorship, and/or publication of this article. This research was funded by NSFC (32171620, 31670499), Scientific and technological research projects in Henan province (232102111005), University humanities and social science research project in Henan Province (2024-ZDJH-777).
Conflict of interest
The authors declare that the research was conducted in the absence of any commercial or financial relationships that could be construed as a potential conflict of interest.
Publisher’s note
All claims expressed in this article are solely those of the authors and do not necessarily represent those of their affiliated organizations, or those of the publisher, the editors and the reviewers. Any product that may be evaluated in this article, or claim that may be made by its manufacturer, is not guaranteed or endorsed by the publisher.
Supplementary material
The Supplementary Material for this article can be found online at: https://www.frontiersin.org/articles/10.3389/fpls.2023.1276918/full#supplementary-material
Abbreviations
AMF, arbuscular mycorrhizal fungi; Chl, chlorophyll; CHO, total carbohydrates; Pn, net photosynthetic rate; TC, total carotenoids; Gs stomatal conductivity; WUE, water utilization ratio.
References
Abdel Latef, A. A. H., Chaoxing, H. (2011). Effect of arbuscular mycorrhizal fungi on growth, mineral nutrition, antioxidant enzymes activity and fruit yield of tomato grown under salinity stress. Scientia Hortic. 127 (3), 228–233. doi: 10.1016/j.scienta.2010.09.020
Abdullahi, R., Kwari, J., Zubairu, A. (2023). Determination of arbuscular mycorrhizal fungi (AMF) association with some selected medicinal plants. Res. Highlights Agric. Sci. 9, 71–80. doi: 10.9734/bpi/rhas/v9/5242A
Alaoui Jamali, C., Kasrati, A., Bekkouche, K., Hassani, L., Wohlmuth, H., Leach, D., et al. (2014). Cultivation and the application of inorganic fertilizer modifies essential oil composition in two Moroccan species of Thymus. Ind. Crops Products 62, 113–118. doi: 10.1016/j.indcrop.2014.08.017
Amani Machiani, M., Javanmard, A., Habibi Machiani, R., Sadeghpour, A. (2022). Arbuscular mycorrhizal fungi and changes in primary and secondary metabolites. Plants 11 (17), 2183. doi: 10.3390/plants11172183
Andrade, S. A. L., Malik, S., Sawaya, A. C. H. F., Bottcher, A., Mazzafera, P. (2013). Association with arbuscular mycorrhizal fungi influences alkaloid synthesis and accumulation in Catharanthus roseus and Nicotiana tabacum plants. Acta Physiologiae Plantarum 35 (3), 867–880. doi: 10.1007/s11738-012-1130-8
Barbosa da Sliva, F. S., Maia, L. C. (2018). Mycorrhization and phosphorus may be an alternative for increasing the production of metabolites in Myracrodruon urundeuva. Theor. Exp. Plant Physiol. 30, 297–302. doi: 10.1007/s40626-018-0123-4
Blankenship, R. E. (2021). Molecular mechanisms of photosynthesis (Hoboken, NJ, United States: John Wiley & Sons).
Chen, M., Arato, M., Borghi, L., Nouri, E., Reinhardt, D. (2018). Beneficial services of arbuscular mycorrhizal fungi–from ecology to application. Front. Plant Sci. 9, 1270. doi: 10.3389/fpls.2018.01270
Chen, M., Yang, G., Sheng, Y., Li, P., Qiu, H., Zhou, X., et al. (2017). Glomus mosseae Inoculation Improves the Root System Architecture, Photosynthetic Efficiency and Flavonoids Accumulation of Liquorice under Nutrient Stress. Front. Plant Sci. 8. doi: 10.3389/fpls.2017.00931
Davison, J., Moora, M., Opik, M., Adholeya, A., Ainsaar, L., Ba, A., et al. (2015). Global assessment of arbuscular mycorrhizal fungus diversity reveals very low endemism. Science 349 (6251), 970–973. doi: 10.1126/science.aab1161
De la Rosa-Mera, C. J., Ferrera-Cerrato, R., Alarcón, A., de Jesús Sánchez-Colín, M., Muñoz-Muñiz, O. D. (2011). Arbuscular mycorrhizal fungi and potassium bicarbonate enhance the foliar content of the vinblastine alkaloid in Catharanthus roseus. Plant Soil 349 (1-2), 367–376. doi: 10.1007/s11104-011-0883-y
de Oliveira, P. T. F., Dos Santos, E. L., da Silva, W. A. V., Ferreira, M. R. A., Soares, L. A. L., da Silva, F. A., et al. (2019). Production of biomolecules of interest to the anxiolytic herbal medicine industry in yellow passionfruit leaves (Passiflora edulis f. flavicarpa) promoted by mycorrhizal inoculation[J]. J. Sci. Food Agric. 99 (7), 3716–3720. doi: 10.1002/jsfa.9598
Duc, N. H., Vo, A. T., Haddidi, I., Daood, H., Posta, K. (2021). Arbuscular mycorrhizal fungi improve tolerance of the medicinal plant eclipta prostrata (L.) and induce major changes in polyphenol profiles under salt stresses. Front. Plant Sci. 11. doi: 10.3389/fpls.2020.612299
Evelin, H., Kapoor, R., Giri, B. (2009). Arbuscular mycorrhizal fungi in alleviation of salt stress: A review. Ann. Bot. 104 (7), 1263–1280. doi: 10.1093/aob/mcp251
Ferreira, K. N., Iverson, T. M., Maghlaoui, K., Barber, J., Iwata, S. (2004). Architecture of the photosynthetic oxygen-evolving center. Science 303 (5665), 1831–1838. doi: 10.1126/science.1093087
Fontana, A., Reichelt, M., Hempel, S., Gershenzon, J., Unsicker, S. (2009). The Effects of Arbuscular Mycorrhizal Fungi on Direct and Indirect Defense Metabolites of Plantago lanceolata L. J. Chem. Ecol. 35, 833–843. doi: 10.1007/s10886-009-9654-0
Gago, J., Daloso, D. D., Figueroa, C. M., Flexas, J., Fernie, A. R., Nikoloski, Z. (2016). Relationships of leaf net photosynthesis, stomatal conductance, and mesophyll conductance to primary metabolism: A multispecies meta-analysis approach. Plant Physiol. 171 (1), 265–279. doi: 10.1104/pp.15.01660
Gianinazzi, S., Gollotte, A., Binet, M. N., van Tuinen, D., Redecker, D., Wipf, D. (2010). Agroecology: The key role of arbuscular mycorrhizas in ecosystem services. Mycorrhiza 20 (8), 519–530. doi: 10.1007/s00572-010-0333-3
Gosling, P., Hodge, A., Goodlass, G., Bending, G. D. (2006). Arbuscular mycorrhizal fungi and organic farming. Agriculture Ecosyst. Environ. 113 (1-4), 17–35. doi: 10.1016/j.agee.2005.09.009
Grimoldi, A. A., Kavanova, M., Lattanzi, F. A., Schaufele, R., Schnyder, H. (2006). Arbuscular mycorrhizal colonization on carbon economy in perennial ryegrass: quantification by 13CO2/12CO2 steady-state labelling and gas exchange. New Phytol. 172 (3), 544–553. doi: 10.1111/j.1469-8137.2006.01853.x
Gupta, S., Thokchom, S. D., Kapoor, R. (2021). Arbuscular mycorrhiza improves photosynthesis and restores alteration in sugar metabolism in triticum aestivum L. Grown in arsenic contaminated soil. Front. Plant Sci. 12. doi: 10.3389/fpls.2021.640379
Han, Y., Feng, J., Han, M., Zhu, B. (2020). Responses of arbuscular mycorrhizal fungi to nitrogen addition: A meta-analysis. Global Change Biol. 26 (12), 7229–7241. doi: 10.1111/gcb.15369
Hedges, L. V., Gurevitch, J., Curtis, P. S. (1999). The meta-analysis of response ratios in experimental ecology. Ecology 80 (4), 1150–1156. doi: 10.1890/0012-9658(1999)080[1150:Tmaorr]2.0.Co;2
Higgins, J. P., Thompson, S. G., Deeks, J. J., Altman, D. G. (2003). Measuring inconsistency in meta-analyses. Bmj 327 (7414), 557–560. doi: 10.1136/bmj.327.7414.557
Hiiesalu, I., Partel, M., Davison, J., Gerhold, P., Metsis, M., Moora, M., et al. (2014). Species richness of arbuscular mycorrhizal fungi: associations with grassland plant richness and biomass. New Phytol. 203 (1), 233–244. doi: 10.1111/nph.12765
Hristozkova, M., Gigova, L., Geneva, M., Stancheva, I., Velikova, V., Marinova, G. (2018). Influence of Mycorrhizal Fungi and Microalgae Dual Inoculation on Basil Plants Performance. Gesunde Pflanz. 70, 99–107. doi: 10.1007/s10343-018-0420-5
Hristozkova, M., Geneva, M., Stancheva, I., Boychinova, M., Djonova, E. (2016). Contribution of arbuscular mycorrhizal fungi in attenuation of heavy metal impact on Calendula officinalis development. Appl. Soil Ecol. 101, 57–63. doi: 10.1016/j.apsoil.2016.01.008
Jeffries, P., Gianinazzi, S., Perotto, S., Turnau, K., Barea, J.-M. (2003). The contribution of arbuscular mycorrhizal fungi in sustainable maintenance of plant health and soil fertility. Biol. Fertility Soils 37 (1), 1–16. doi: 10.1007/s00374-002-0546-5
Kapoor, R., Anand, G., Gupta, P., Mandal, S. (2017). Insight into the mechanisms of enhanced production of valuable terpenoids by arbuscular mycorrhiza. Phytochem. Rev. 16, 677–692. doi: 10.1007/s11101-016-9486-9
Khadka, D., Dhamala, M. K., Li, F. F., Aryal, P. C., Magar, P. R., Bhatta, S., et al. (2021). The use of medicinal plants to prevent COVID-19 in Nepal. J. Ethnobiology Ethnomedicine 17 (1), 1–17. doi: 10.1186/s13002-021-00449-w
Khalediyan, N., Weisany, W., Schenk, P. M. (2021). Arbuscular mycorrhizae and rhizobacteria improve growth, nutritional status and essential oil production in Ocimum basilicum and Satureja hortensis. Ind. Crops Products 160, 113163. doi: 10.1016/j.indcrop.2020.113163
Kuyper, T. W., Jansa, J. (2023). Arbuscular mycorrhiza: Advances and retreats in our understanding of the ecological functioning of the mother of all root symbioses. Plant Soil 489, 41–48. doi: 10.1007/s11104-023-06045-z
Lazzara, S., Militello, M., Carrubba, A., et al. (2017). Arbuscular mycorrhizal fungi altered the hypericin, pseudohypericin, and hyperforin content in flowers of Hypericum perforatum grown under contrasting P availability in a highly organic substrate[J]. Mycorrhiza 27 (4), 345–354. doi: 10.1007/s00572-016-0756-6
Li, Y., Li, W., Fu, C., Song, Y., Fu, Q. (2020). Lonicerae japonicae flos and Lonicerae flos: A systematic review of ethnopharmacology, phytochemistry and pharmacology. Phytochem. Rev. 19, 1–61. doi: 10.1007/s11101-019-09655-7
Lichtenthaler, H. K. (1987). [34] Chlorophylls and carotenoids: pigments of photosynthetic biomembranes (Amsterdam, Netherlands: Elsevier), 350–382.
Lima, C. S., Santos, H. R. S., Albuquerque, U. P. D., Silva, F. S. B. D. (2017). Mycorrhizal symbiosis increase the level of total foliar phenols and tannins in Commiphora leptophloeos (Mart.) J.B. Gillett seedlings. Ind. Crops Products 104, 28–32. doi: 10.1016/j.indcrop.2017.04.020
Luo, Y., Hui, D., Zhang, D. (2006). Elevated CO2 stimulates net accumulations of carbon and nitrogen in land ecosystems: A meta-analysis. Ecology 87 (1), 53–63. doi: 10.1890/04-1724
Luo, J., Li, X., Jin, Y., Traore, I., Dong, L., Yang, G., et al. (2021). Effects of arbuscular mycorrhizal fungi Glomus mosseae on the growth and medicinal components of Dysosma versipellis under copper stress. Bull. Environ. contamination Toxicol. 107, 924–930. doi: 10.1007/s00128-019-02780-1
Luo, E. D., Zhang, D. Y., Luo, H., Liu, B. W., Zhao, K. M., Zhao, Y. H., et al. (2020). Treatment efficacy analysis of traditional Chinese medicine for novel coronavirus pneumonia (COVID-19): an empirical study from Wuhan, Hubei Province, China. Chin. Med. 15 (1), 1–13. doi: 10.1186/s13020-020-00317-x
Mandal, S., Evelin, H., Girl, B., Singh, V. P., Kapoor, R. (2013). Arbuscular mycorrhiza enhances the production of stevioside and rebaudioside-A in Stevia rebaudiana via nutritional and non-nutritional mechanisms. Appl. Soil Ecol. 72, 187–194. doi: 10.1016/j.apsoil.2013.07.003
Mandal, S., Upadhyay, S., Wajid, S., Ram, M., Jain, D. C., Singh, V., et al (2014). Arbuscular mycorrhiza increase artemisinin accumulation in Artemisia annua by higher expression of key biosynthesis genes via enhanced jasmonic acid levels. Mycorrhiza 24, 345–357. doi: 10.1007/s00572-014-0614-3
Mandal, S., Upadhyay, S., Singh, V. P., Kapoor, R. (2015). Enhanced production of steviol glycosides in mycorrhizal plants: a concerted effect of arbuscular mycorrhizal symbiosis on transcription of biosynthetic genes. Plant Physiol. Biochem. 89, 100–106. doi: 10.1016/j.plaphy.2015.02.010
Marschner, H., Dell, B. (1994). Nutrient uptake in mycorrhizal symbiosis. Plant Soil 159 (1), 89–102. doi: 10.1007/bf00000098
Mathur, S., Sharma, M. P., Jajoo, A. (2018). Improved photosynthetic efficacy of maize (Zea mays) plants with arbuscular mycorrhizal fungi (AMF) under high temperature stress. J. Photochem. Photobiol. B 180, 149–154. doi: 10.1016/j.jphotobiol.2018.02.002
Mechri, B., Tekaya, M., Cheheb, H., Attia, F., Hammami, M. (2015). Accumulation of flavonoids and phenolic compounds in olive tree roots in response to mycorrhizal colonization: A possible mechanism for regulation of defense molecules. J. Plant Physiol. 185, 40–43. doi: 10.1016/j.jplph.2015.06.015
Medina, C., Mutis, A., Bardehle, L., Hormazabal, E., Borie, F., Aguilera, P., et al. (2023). Arbuscular mycorrhizal fungi enhance monoterpene production in red clover (Trifolium pratense L.): A potential tool for pest control. Natural Product Res. 37 (6), 981–984. doi: 10.1080/14786419.2022.2094375
Merlin, E., Melato, E., Lourenco, E. L. B., Jacomassi, E., Gasparotto, A. G., da Cruz, R. M. S., et al. (2020). Inoculation of arbuscular mycorrhizal fungi and phosphorus addition increase coarse mint (Plectranthus amboinicus Lour.) plant growth and essential oil content. Rhizosphere 15, 100217. doi: 10.1016/j.rhisph.2020.100217
Mitra, D., Rad, K. V., Chaudhary, P., Ruparelia, J., Sagarika, M. S., Boutaj, H., et al. (2021). Involvement of strigolactone hormone in root development, influence and interaction with mycorrhizal fungi in plant: Mini-review. Curr. Res. Microbial Sci. 2, 100026. doi: 10.1016/j.crmicr.2021.100026
Moher, D., Shamseer, L., Clarke, M., Ghersi, D., Liberati, A., Petticrew, M., et al. (2015). Preferred reporting items for systematic review and meta-analysis protocols (PRISMA-P) 2015 statement. Systematic Rev. 4 (1), 1–9. doi: 10.1186/2046-4053-4-1
Noceto, P. A., Bettenfeld, P., Boussageon, R., Heriche, M., Sportes, A., van Tuinen, D., et al. (2021). Arbuscular mycorrhizal fungi, a key symbiosis in the development of quality traits in crop production, alone or combined with plant growth-promoting bacteria. Mycorrhiza 31 (6), 655–669. doi: 10.1007/s00572-021-01054-1
Oliveira, M. D., Campos, M. A. D., de Albuquerque, U. P., da Silva, P. S. B. (2013). Arbuscular mycorrhizal fungi (AMF) affects biomolecules content in Myracrodruon urundeuva seedlings. Ind. Crops Products 50, 244–247. doi: 10.1016/j.indcrop.2013.07.041
Orujei, Y., Shabani, L., Sharifi Tehrani, M. (2013). Induction of glycyrrhizin and total phenolic compound production in licorice by using arbuscular mycorrhizal fungi. Russ. J. Plant Physiol. 60, 855–960. doi: 10.1134/S1021443713050129
Ostadi, A., Javanmard, A., Amani Machiani, M., Morshedloo, M. R., Nouraein, M., Rasouli, F., et al. (2020). Effect of different fertilizer sources and harvesting time on the growth characteristics, nutrient uptakes, essential oil productivity and composition of Mentha x piperita L. Ind. Crops Products 148, 112290. doi: 10.1016/j.indcrop.2020.112290
Padilla, I., Encina, C. (2005). Changes in root morphology accompanying mycorrhizal alleviation of phosphorus deficiency in micropropagated Annona cherimola Mill. plants. Scientia Hortic. 106 (3), 360–369. doi: 10.1016/j.scienta.2005.05.001
Pistelli, L., Ulivieri, V., Giovaneli, S., Avio, L., Giovannetti, M., Pistelli, L., et al (2017). Changes in root morphology accompanying mycorrhizal alleviation of phosphorus deficiency in micropropagated Annona cherimola Mill. plants. Plant Biol. 19 (6), 926–933. doi: 10.1111/plb.12608
Petrovska, B. B. (2012). Historical review of medicinal plants’ usage. Pharmacognosy Rev. 6 (11), 1. doi: 10.4103/0973-7847.95849
Porcel, R., Redondo-Gómez, S., Mateos-Naranjo, E., Aroca, R., Garcia, R., Ruiz-Lozano, J. M. (2015). Arbuscular mycorrhizal symbiosis ameliorates the optimum quantum yield of photosystem II and reduces non-photochemical quenching in rice plants subjected to salt stress. J. Plant Physiol. 185, 75–83. doi: 10.1016/j.jplph.2015.07.006
Ran, Z., Ding, W., Cao, S., Fang, L., Zhou, J., Zhang, Y. (2022). Arbuscular mycorrhizal fungi: Effects on secondary metabolite accumulation of traditional Chinese medicines. Plant Biol. 24 (6), 932–938. doi: 10.1111/plb.13449
Ran, Z., Yang, X., Zhang, Y., Zhou, J., Guo, L. (2021). Effects of arbuscular mycorrhizal fungi on photosynthesis and biosynthesis of ginsenoside in Panax quinquefolius L. Theor. Exp. Plant Physiol. 33 (3), 235–248. doi: 10.1007/s40626-021-00208-y
Ren, J. L., Zhang, A. H., Wang, X. J. (2020). Traditional Chinese medicine for COVID-19 treatment. Pharmacol. Res. 155, 104743. doi: 10.1016/j.phrs.2020.104743
Runfeng, L., Yunlong, H., Jicheng, H., Weiqi, P., Qinhai, M., Yongxia, S., et al. (2020). Lianhuaqingwen exerts anti-viral and anti-inflammatory activity against novel coronavirus (SARS-CoV-2). Pharmacol. Res. 156, 104761. doi: 10.1016/j.phrs.2020.104761
Scervino, J. M., Ponce, M. A., Erra-Bassells, R., Bompadre, J., Vierheilig, H., Ocampo, J. A., et al. (2007). The effect of flavones and flavonols on colonization of tomato plants by arbuscular mycorrhizal fungi of the genera Gigaspora and Glomus. Can. J. Microbiol. 53 (6), 702–709. doi: 10.1139/W07-036
Schroeder, M., Janos, D. (2005). Plant growth, phosphorus nutrition, and root morphological responses to arbuscular mycorrhizas, phosphorus fertilization, and intraspecific density. Mycorrhiza 15, 203–216. doi: 10.1007/s00572-004-0324-3
Shen, Z. J., Fu, Y. B., Hou, J. L., Lin, L. N., Wang, X. Y., Li, C. Y., et al. (2022). Integrating network pharmacology, UPLC-Q–TOF–MS and molecular docking to investigate the effect and mechanism of Chuanxiong Renshen decoction against Alzheimer's disease. Chin. Med. 17 (1), 143. doi: 10.1186/s13020-022-00698-1
Shrivastava, G., Ownley, B. H., Augé, R. M., Toler, H., Dee, M., Vu, A., et al. (2015). Colonization by arbuscular mycorrhizal and endophytic fungi enhanced terpene production in tomato plants and their defense against a herbivorous insect. Symbiosis 65, 65–74. doi: 10.1007/s13199-015-0319-1
Sidhu, P., Shankargouda, S., Rath, A., Ramamurthy, P. H., Fernandes, B., Singh, A. K. (2020). Therapeutic benefits of liquorice in dentistry. J. Ayurveda Integr. Med. 11 (1), 82–88. doi: 10.1016/j.jaim.2017.12.004
Smith, S. E., Read, D. J. (2008). Mycorrhizal Symbiosis, Third Edn (San Diego: Academic Press). doi: 10.1016/B978-012370526-6.50001-5
Smith, S. E., Smith, F. A. (2011). Roles of arbuscular mycorrhizas in plant nutrition and growth: new paradigms from cellular to ecosystem scales. Annu. Rev. Plant Biol. 62, 227–250. doi: 10.1146/annurev-arplant-042110-103846
Soti, P., Kariyat, R., Racelis, A. (2023). Effective farm management promotes native AMF and benefit organic farming systems. Agriculture Ecosyst. Environ. 342, 108240. doi: 10.1016/j.agee.2022.108240
Srivastava, S., Conlan, X. A., Cahill, D. M., Adholeya, A. (2016). Rhizophagus irregularis as an elicitor of rosmarinic acid and antioxidant production by transformed roots of Ocimum basilicum in an in vitro co-culture system. Mycorrhiza 26 (8), 919–930. doi: 10.1007/s00572-016-0721-4
Suter, B., Triolo, R., Pernet, D., Dai, Z. W., Van Leeuwen, C. (2019). Modeling stem water potential by separating the effects of soil water availability and climatic conditions on water status in grapevine (Vitis vinifera L.). Front. Plant Sci. 10, 1485. doi: 10.3389/fpls.2019.01485
Tarraf, W., Ruta, C., Tagarelli, A., De Cillis, F., De Mastro, G. (2017). Influence of arbuscular mycorrhizae on plant growth, essential oil production and phosphorus uptake of Salvia officinalis L. Ind. Crops Prod. 102, 144–153. doi: 10.1016/j.indcrop.2017.03.010
Thirkell, T. J., Charters, M. D., Elliott, A. J., Sait, S. M., Field, K. J. (2017). Are mycorrhizal fungi our sustainable saviours? Considerations for achieving food security. J. Ecol. 105 (4), 921–929. doi: 10.1111/1365-2745.12788
Toussaint, J. P. (2007). Investigating physiological changes in the aerial parts of AM plants: what do we know and where should we be heading? Mycorrhiza 17 (4), 349–353. doi: 10.1007/s00572-007-0133-6
Tseng, C.-Y., Sun, M.-F., Kao, T.-C., Li, T.-C., Lin, C.-T. (2022). Role of Coptis chinensis in antibiotic susceptibility of carbapenem-resistant Klebsiella pneumoniae. J. Microbiology Immunol. Infection 55 (5), 946–955. doi: 10.1016/j.jmii.2021.07.003
van der Heijden, M. G. A., Martin, F. M., Selosse, M. A., Sanders, I. R. (2015). Mycorrhizal ecology and evolution: the past, the present, and the future. New Phytol. 205 (4), 1406–1423. doi: 10.1111/nph.13288
Verlinden, M. S., Ven, A., Verbruggen, E., Janssens, I. A., Wallander, H., Vicca, S. (2018). Favorable effect of mycorrhizae on biomass production efficiency exceeds their carbon cost in a fertilization experiment. Ecology 99 (11), 2525–2534. doi: 10.1002/ecy.2502
Viechtbauer, W. (2010). Conducting meta-analyses in R with the metafor package. J. Stat. Software 36 (3), 1–48. doi: 10.18637/jss.v036.i03
Vieira, M. E., Freitas, M. S. M., Peçanha, D. A., Lima, T. C., Martins, M. A., Vieira, I. J. C. (2021). Arbuscular mycorrhizal fungi and phosphorus in spilanthol and phenolic compound yield in jambu plants. Horticultura Bras. 39 (2), 192–198. doi: 10.1590/s0102-0536-20210210
Vierheilig, H. (2004). Regulatory mechanisms during the plant arbuscular mycorrhizal fungus interaction. Can. J. Bot. 82 (8), 1166–1176. doi: 10.1139/b04-015
Vo, A. T., Haddidi, I., Daood, H., Mayer, Z., Posta, K. (2019). Impact of arbuscular mycorrhizal inoculation and growth substrate on biomass and content of polyphenols in eclipta prostrata. HortScience 54 (11), 1976–1983. doi: 10.21273/hortsci14227-19
Wang, C. C., Cai, H., Zhao, H., Yan, Y., Shi, J. J., Chen, S. Y., et al. (2018). Distribution patterns for metabolites in medicinal parts of wild and cultivated licorice. J. Pharm. Biomed. Anal. 161, 464–473. doi: 10.1016/j.jpba.2018.09.004
Wang, H. X., Hao, Z. P., Zhang, X., Xie, W., Chen, B. D. (2022a). Arbuscular mycorrhizal fungi induced plant resistance against fusarium wilt in jasmonate biosynthesis defective mutant and wild type of tomato. J. Fungi 8 (5), 422. doi: 10.3390/jof8050422
Wang, M., Li, L., Fan, T., Cao, L., Zhang, J., Li, S., et al. (2022b). Semisynthetic aurones A14 protects against T-cell acute lymphoblastic leukemia via suppressing proliferation and inducing cell cycle arrest with apoptosis. Chin. Med. 17 (1), 1–13. doi: 10.1186/s13020-022-00693-6
Wang, W. L., Xu, J. F., Fang, H. Y., Li, Z. J., Li, M. H. (2020). Advances and challenges in medicinal plant breeding. Plant Sci. 298, 110573. doi: 10.1016/j.plantsci.2020.110573
Wei, G., Wang, H. (1991). Effect of vesicular-arbuscular mycorrhizal fungi on growth, nutrient uptake and synthesis of volatile oil in Schizonepeta tenuifolia briq. Zhongguo Zhong Yao Za Zhi 16 (3), 139–142, 189-190.
Wei, W.-L., Zeng, R., Gu, C.-M., Qu, Y., Huang, L.-F. (2016). Angelica sinensis in China-A review of botanical profile, ethnopharmacology, phytochemistry and chemical analysis. J. Ethnopharmacology 190, 116–141. doi: 10.1016/j.jep.2016.05.023
Wei, X., Zhi-peng, H., Lan-ping, G., Xin, Z., Shu-bin, Z., You-shan, W., et al. (2020). Research advances in terpenoids synthesis and accumulation in plants as influenced by arbuscular mycorrhizal symbiosis. Biotechnol. Bull. 36 (9), 49–63. doi: 10.13560/j.cnki.biotech.bull.1985.2020-0117
World Health Organization (WHO). (2019). WHO: Neglected tropical diseases (Avenue Appia CH-1211 Geneva: World Health Organization).
World Health Organization (WHO). (2022). WHO: World malaria report (Geneva: World Health Organization).
Wu, Q.-S., He, X.-H., Zou, Y.-N., Liu, C.-Y., Xiao, J., Li, Y. (2012). Arbuscular mycorrhizas alter root system architecture of Citrus tangerine through regulating metabolism of endogenous polyamines. Plant Growth Regul. 68, 27–35. doi: 10.1007/s10725-012-9690-6
Wu, Y. H., Wang, H., Liu, M., Li, B., Chen, X., Ma, Y. T., et al. (2021). Effects of native arbuscular mycorrhizae isolated on root biomass and secondary metabolites of salvia miltiorrhiza bge. Front. Plant Sci. 12. doi: 10.3389/fpls.2021.617892
Wu, Q.-S., Zou, Y.-N., He, X.-H., Luo, P. (2011). Arbuscular mycorrhizal fungi can alter some root characters and physiological status in trifoliate orange (Poncirus trifoliata L. Raf.) seedlings. Plant Growth Regul. 65, 273–278. doi: 10.1007/s10725-011-9598-6
Xie, W., Hao, Z., Yu, M., Wu, Z., Zhao, A., Li, J., et al. (2019). Improved phosphorus nutrition by arbuscular mycorrhizal symbiosis as a key factor facilitating glycyrrhizin and liquiritin accumulation in Glycyrrhiza uralensis. Plant Soil 439 (1-2), 243–257. doi: 10.1007/s11104-018-3861-9
Xie, W., Hao, Z., Zhou, X., Jiang, X., Xu, L., Wu, S., et al. (2018). Arbuscular mycorrhiza facilitates the accumulation of glycyrrhizin and liquiritin in Glycyrrhiza uralensis under drought stress. Mycorrhiza 28 (3), 285–300. doi: 10.1007/s00572-018-0827-y
Yang, Y., Ou, X., Yang, G., Xia, Y., Chen, M., Guo, L., et al. (2017). Arbuscular mycorrhizal fungi regulate the growth and phyto-active compound of salvia miltiorrhiza seedlings. Appl. Sci. 7 (1), 68. doi: 10.3390/app7010068
Yang, S., Shi, Z., Zhang, M., Li, Y., Gao, J., Wang, X., et al. (2021). Stoichiometry of carbon, nitrogen and phosphorus in shrub organs linked closely with mycorrhizal strategy in northern China. Front. Plant Sci. 12. doi: 10.3389/fpls.2021.687347
Yu, M., Xie, W., Zhang, X., Zhang, S., Wang, Y., Hao, Z. (2019). Arbuscular mycorrhizal fungi can compensate for the loss of indigenous microbial communities to support the growth of liquorice (Glycyrrhiza uralensis fisch.). Plants. 9 (1), 7. doi: 10.3390/plants9010007
Zeng, Y., Guo, L. P., Chen, B. D., Hao, Z. P., Wang, J. Y., Huang, L. Q., et al. (2013a). Arbuscular mycorrhizal symbiosis and active ingredients of medicinal plants: current research status and prospectives. Mycorrhiza 23 (4), 253–265. doi: 10.1007/s00572-013-0484-0
Zeng, Y., Guo, L. P., Chen, B. D., Hao, Z. P., Wang, J. Y., Huang, L. Q., et al. (2013b). Arbuscular mycorrhizal symbiosis for sustainable cultivation of Chinese medicinal plants: A promising research direction. Am. J. Chin. Med. 41 (6), 1199–1221. doi: 10.1142/S0192415X1350081X
Zhang, S., Lehmann, A., Zheng, W., You, Z., Rillig, M. C. (2019). Arbuscular mycorrhizal fungi increase grain yields: A meta-analysis. New Phytol. 222 (1), 543–555. doi: 10.1111/nph.15570
Zhang, Y., Li, Y. Y., Chen, X. M., Guo, S. X., Lee, Y. I. (2020b). Effect of different mycobionts on symbiotic germination and seedling growth of Dendrobium officinale, an important medicinal orchid. Bot. Stud. 61 (1), 2. doi: 10.1186/s40529-019-0278-6
Zhang, Y., Li, Y., Guo, S. (2020a). Effects of the mycorrhizal fungus Ceratobasidium sp. AR2 on growth and flavonoid accumulation in Anoectochilus roxburghii. PeerJ 8, e8346. doi: 10.7717/peerj.8346
Zhang, M., Shi, Z., Zhang, S., Gao, J. (2022). A database on mycorrhizal traits of chinese medicinal plants. Front. Plant Sci. 13. doi: 10.3389/fpls.2022.840343
Zhao, Y., Cartabia, A., Lalaymia, I., Declerck, S. (2022). Arbuscular mycorrhizal fungi and production of secondary metabolites in medicinal plants. Mycorrhiza 32 (3-4), 221–256. doi: 10.1007/s00572-022-01079-0
Zhao, J., Cheng, X., Gu, X., Liu, B. (2012). Relationship between AM fungi of new eight medicinal plants and soil factors in Anguo of Hebei. J. Henan Agric. Sci. 41 (6), 87–91.
Zubek, S., Mielcarek, S., Turnau, K. (2012). Hypericin and pseudohypericin concentrations of a valuable medicinal plant Hypericum perforatum L. @ are enhanced by arbuscular mycorrhizal fungi. Mycorrhiza 22 (2), 149–156. doi: 10.1007/s00572-011-0391-1
Keywords: arbuscular mycorrhizal fungi, medicinal active ingredients, medicinal plant, secondary metabolites, physiological variables
Citation: Yuan M-L, Zhang M-H, Shi Z-Y, Yang S, Zhang M-G, Wang Z, Wu S-W and Gao J-K (2023) Arbuscular mycorrhizal fungi enhance active ingredients of medicinal plants: a quantitative analysis. Front. Plant Sci. 14:1276918. doi: 10.3389/fpls.2023.1276918
Received: 13 August 2023; Accepted: 22 September 2023;
Published: 20 October 2023.
Edited by:
Ankush Prasad, Palacký University Olomouc, CzechiaReviewed by:
Renuka Ramalingam Manoharan, Palacký University, CzechiaSeyyed Sasan Mousavi, Shiraz University, Iran
Wenjin Zhang, Ningxia Medical University, China
Copyright © 2023 Yuan, Zhang, Shi, Yang, Zhang, Wang, Wu and Gao. This is an open-access article distributed under the terms of the Creative Commons Attribution License (CC BY). The use, distribution or reproduction in other forums is permitted, provided the original author(s) and the copyright owner(s) are credited and that the original publication in this journal is cited, in accordance with accepted academic practice. No use, distribution or reproduction is permitted which does not comply with these terms.
*Correspondence: Zhao-Yong Shi, Shizy1116@126.com