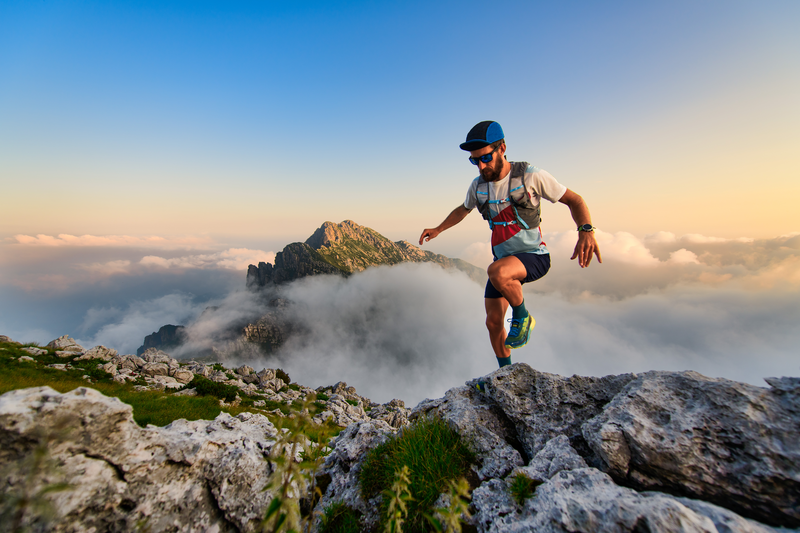
95% of researchers rate our articles as excellent or good
Learn more about the work of our research integrity team to safeguard the quality of each article we publish.
Find out more
ORIGINAL RESEARCH article
Front. Plant Sci. , 04 October 2023
Sec. Plant Pathogen Interactions
Volume 14 - 2023 | https://doi.org/10.3389/fpls.2023.1274770
This article is part of the Research Topic The Use of Microbial Volatile Compounds for Controlling Plant Pathogens View all 8 articles
Gray mold and brown rot, caused respectively by Botrytis cinerea and Monilinia spp., are fungal diseases responsible for significant losses during the storage of fruit and vegetables. Nowadays, the control of postharvest diseases is shifting towards more sustainable strategies, including the use of plant secondary metabolites. In this study, the antifungal activity of Origanum vulgare, Thymus vulgaris, Thymus serpyllum, Melaleuca alternifolia, Lavandula officinalis, Lavandula hybrida, Citrus bergamia, Rosmarinus officinalis, Cinnamomum zeylanicum essential oils (EOs) in vapor phase was tested in vitro against B. cinerea, Monilinia fructicola, Monilinia fructigena, and Monilinia laxa. For the experiments, a protocol using a volatile organic compounds (VOC) chamber was designed. Results indicate a dose-dependent inhibitory activity of all the tested EOs, with O. vulgare, T. vulgaris, and T. serpyllum being the most active ones, with minimum inhibitory concentrations (MIC) of 22.73, 45.45, and 22.73 µl/L, respectively, against B. cinerea and a range between 5.64 and 22.73 µl/L against the three Monilinia spp. Overall, B. cinerea presented lower sensitivity to vapor-phase EOs than any of the Monilinia strains, except for the C. zeylanicum EO, which consistently showed higher inhibition against B. cinerea. Among the three Monilinia spp., M. fructicola was the least sensitive, while M. fructigena was the most sensitive. The use of VOC chambers proved to be a reliable protocol for the assessment of antimicrobial activities of EOs. These results suggest that the VOC emitted by the tested EOs are effective towards important decay-causing fungi, and that they could be used for the control of gray mold and brown rot in in vivo trials.
Essential oils (EOs) are complex and diverse mixtures of chemical compounds, many of them of volatile nature, which can be obtained from plant organs and tissues through different extraction methods (El Khetabi et al., 2022). They present relevant biological activities, such as attraction or repellency, antioxidant, insecticidal, and antimicrobial effects. EOs have been proven to produce significant antimicrobial activity against many phytopathogenic fungi, both in direct liquid contact and in vapor phase (Reyes-Jurado et al., 2020; El Khetabi et al., 2021; Ma et al., 2022). This action depends on the specific sensitivity of each microbial strain and on several characteristics of the EOs, including their composition, the proportion of each individual compound, and their ability to diffuse and reach the microbial target (Pinto et al., 2020; El Khetabi et al., 2022).
EO composition vary not only according to the plant species, but also to the variety, cultivar, plant part, physiological state, and extraction method (Kloucek et al., 2012; El Khetabi et al., 2022; Reyes-Jurado et al., 2020). This overwhelming heterogeneity leads to an intricate overlapping of modes of action that can impair microbial growth. EOs affect different target structures in microorganisms, damaging cell membranes, the cell wall, genetic material, or membrane proteins (Ayala-Zavala et al., 2008; Usall et al., 2016), thus disrupting important physiological and metabolic processes leading to the inactivation of essential enzymes, leakage of cell content, depletion of proton motive active sites, spore disruption, or the induction of apoptotic pathways, among others (Usall et al., 2016; Spadaro et al., 2021). As a result, EOs and their individual compounds usually present additive or synergistic antimicrobial activity (Derbassi et al., 2022). On the contrary, a few studies have reported fungal growth promotion or an increase in disease incidence induced by some EOs in certain conditions (Santoro et al., 2018). Therefore, dosage needs to be carefully evaluated and controlled to avoid unwanted adverse effects in eventual commercial applications.
EO complexity leads to challenges in both the study and subsequent application of EO VOCs for microbial control. EO hydrophobicity and volatility compromise their effectivity in direct contact, affecting the availability and diffusion of the individual active compounds, and imposing the use of solvents or emulsifiers that may affect the resulting outcome (Kloucek et al., 2012). The testing of EO VOCs against postharvest pathogens relies mostly on in vitro methodologies derived from the protocol proposed by Maruzzella and Sicurella (1960), using an upside-down Petri dish, or the introduction of plates inside bigger containers (Schmidt et al., 2017; Song et al., 2019). In general, the study of volatile interactions traditionally faces methodological difficulties such as the leakage of active compounds, limitation of oxygen availability and gas exchange, determination of the real concentrations reached inside the experimental units, and a general lack of standardized materials and protocols (Kai and Piechulla, 2009; Kloucek et al., 2012).
Nevertheless, biological interactions mediated by volatile organic compounds (VOCs) from plants, fungi, yeasts and bacteria have been explored for decades (Dennis and Webster, 1971; Avalos et al., 2018; Tilocca et al., 2020). Although studies which have focused on the direct contact of EOs with the fungal strains via growth media, liquid mixtures, or coatings are still prevalent (Kapetanakou et al., 2019; Gonçalves et al., 2021), a great interest is arising towards the fumigant antifungal activity of EO volatiles, and for their potential use in postharvest treatment of fruits and vegetables (Cindi et al., 2015; Taghavi et al., 2018; Reyes-Jurado et al., 2020; Ma et al., 2022).
This control of postharvest spoilage is of the utmost importance, as it is estimated that nearly half of all fruits and vegetables produced on the planet are lost or not consumed (FAO, 2021). Plant diseases are responsible for significant losses, which annually cost the global economy over $220 billion. Gray mold and brown rot, caused respectively by Botrytis cinerea Pers. and Monilinia spp. can be counted among some of the most destructive and widespread postharvest fungal diseases (Santoro et al., 2018; Roca-Couso et al., 2021; De Miccolis Angelini et al., 2022).
Botrytis cinerea is a ubiquitous phytopathogen that affects a vast range of agricultural plants as well as causing postharvest decay of fruits and vegetables. This pathogen can occur in the field and remain in a latent state up until later phases of postharvest management, where it emerges as a pathogen (Powelson, 1960). At this stage, the infection can quickly spread to neighboring fruits in a process known as nesting, with the subsequent increase in product loss. Moreover, this fungus can grow at low temperatures, further compromising the storage and marketing stages (Roca-Couso et al., 2021). Monilinia fructicola (G. Winter) Honey, Monilinia fructigena (Pers.) Honey, and Monilinia laxa (Aderh. and Ruhland) Honey are among the most common postharvest fungal pathogens affecting stone and pome fruits, and are the causal agents of brown rot and blossom blight on fruit trees, resulting in important yield losses, postharvest fruit waste, and reduced shelf life (Santoro et al., 2018; Casals et al., 2022; De Miccolis Angelini et al., 2022).
Nowadays, the focus in the control of postharvest diseases is shifting towards more sustainable strategies, including the use of biological control agents and natural compounds such as plant and microbial extracts and secondary metabolites (Romanazzi et al., 2022). Among them, plant essential oils (EOs) have been proven to mediate diverse biological interactions, standing out their significant antimicrobial activity against many phytopathogenic fungi, both in direct liquid contact and in vapor phase (Reyes-Jurado et al., 2020; El Khetabi et al., 2021; Moumni et al., 2021). Numerous plant EOs in volatile phase present antifungal effects against postharvest pathogens, including B. cinerea (Servili et al., 2017; Pinto et al., 2020; Di Francesco et al., 2022) and Monilinia spp. (Spadaro et al., 2021; Santoro et al., 2018; Khumalo et al., 2017).The strong antifungal effect of vapor-phase EOs from T. vulgaris, C. zeylanicum, O. vulgare and other Origanum species on B. cinerea has been extensively reported (Munhuweyi et al., 2017; Zhao et al., 2021; Elsayed et al., 2022; Kaharamanoglu et al., 2022; Kara et al., 2022). Inhibitory effects of some EOs volatiles against M. fructicola have been also reported, such as T. vulgaris (Sellamuthu et al., 2013; Spadaro et al., 2021) and M. alternifolia (Xu et al., 2022). O. vulgare (Carović-Stanco et al., 2013) and T. vulgaris (Khumalo et al., 2017; Pinto et al., 2020) EO VOCs also inhibited M. laxa growth. Nevertheless, there are many plant EOs that have not been tested yet in volatile phase against these major phytopathogenic fungi. This is especially true for M. fructicola, M. laxa, and M. fructigena, for which very few EO volatiles have been assayed to date. Therefore, there is a need to further explore the antifungal activity of EOs in volatile phase against these postharvest pathogens in order to develop alternative strategies for their control.
The main objectives of the present work were: (i) to develop an effective protocol for the evaluation of these interactions using VOC chambers; and (ii) to evaluate and compare the in vitro antifungal activity of the VOCs released by twelve EOs and a commercial mixture against the postharvest pathogens B. cinerea, M. fructicola, M. fructigena, and M. laxa.
Four postharvest pathogenic fungi were evaluated in the present work for their susceptibility to EOs in vapor phase: B. cinerea (strain B05.10; Netherlands), and M. fructicola, M. fructigena, and M. laxa isolated from infected nectarines in Italy (collection of Plant Pathology Unit, Marche Polytechnic University, Italy). These strains had been previously identified in our laboratory by morphological and molecular methods using multiplex PCR (Makau et al., 2023) following the method proposed by Côté et al. (2004). They were stored at 4 °C and refreshed by growing them on potato dextrose agar (PDA; 40 g/L; Scharlab S.L., Sentmenat, Spain) at 22 °C ± 2 °C prior to their use in the experiments.
Twelve commercial essential oils were tested individually to assess their vapor-phase antifungal activity against the referred fungal strains: Origanum vulgare, Thymus vulgaris, Thymus serpyllum, Melaleuca alternifolia, Lavandula officinalis, Lavandula hybrida, Citrus bergamia, Rosmarinus officinalis, and Cinnamomum zeylanicum. EOs from two different providers were tested in the case of O. vulgare, T. vulgaris, and M. alternifolia. A commercial mixture (MIX) composed of 25% M. alternifolia, 25% O. vulgare, 25% C. zeylanicum, and 25% T. vulgaris was also assayed. The EOs, including the mixture, were kindly provided by Flora srl (Pisa, Italy) and GreenVet (Forli, Italy). The extraction method, plant material, and purity grade of the tested EOs (100% pure) were certified by the suppliers (Table 1).
Table 1 Essential oils information regarding origin, year and method of extraction, and main components.
Fungi were exposed to the volatiles of the listed EOs (Table 1) using non-vented VOC chambers (Álvarez-García et al., 2021) (JD. Catalán S.L., Madrid; Spain. Produced specifically by request for these experiments). An adequate dose of pure EO was placed on top of a microscopy glass slide (Thermo Scientific, Gerhard Menzel GmbH, Braunschweig, Germany) inside a Petri dish. This plate formed the lower base of the VOC Chamber, while its lid was substituted by a VOC chamber central piece (Figures 1, 2). On the other side, an 8 mm diameter plug of the corresponding fungal strain was inoculated onto the center of another Petri dish containing 20 mL of PDA. These Petri dishes were flipped over and placed upside down on top of the central piece, forming the upper plate of the fully assembled VOC chamber (Figures 1, 2). In this way, the lower plate containing the EO and the upper plate with the fungi are held together facing each other and connected by the hole in the central piece, allowing the free flow of VOCs from one compartment to the other (Figures 1, 2). The chambers were sealed with two layers of Parafilm (Amcor-Bemis, USA) and put into a thermostatic cabinet (Lovibond TC255S, Tintometer GmbH, Germany) at 22 °C ± 2 °C.
Figure 1 Graphical representation of the new protocol using non-vented VOC chambers (Álvarez-García et al., 2021) for the exposure of Botrytis cinerea, Monilinia fructicola, Monilinia fructigena, and Monilinia laxa to the VOCs released by Origanum vulgare, Thymus vulgaris, Thymus serpyllum, Melaleuca alternifolia, Lavandula officinalis, Lavandula hybrida, Citrus bergamia, Rosmarinus officinalis, and Cinnamomum zeylanicum EOs; and a mixture (MIX) of 25% M. alternifolia, 25% O. vulgare, 25% C. zeylanicum, and 25% T. vulgaris. Dpi, days post inoculation.
Figure 2 (A) Closed VOC Chamber with EO spread on a glass slide in the lower plate, upper plate containing the fungal plug cultured on 20 mL PDA medium, and a central piece holding both plates together and sealed with parafilm. (B) Open VOC Chamber showing the glass slide with EO in the lower plate and a fungal colony growing in the upper one. The hole that allows the flow of VOCs between plates can be clearly seen in the central piece.
From three up to eight doses were assessed depending on the specific EO, having been selected considering the bibliography and the sensitivity of each fungal strain in accordance with preliminary assays. Concentrations are expressed as microliters of EO per liter of air inside the VOC chamber (µL/L) by calculating the air volume of the headspace (volume of the two 90mm Petri dishes forming the chamber minus 20 ml corresponding to the PDA, giving a final volume of 0.11 L of air per VOC chamber). Lowest concentrations were set up at 2.82 µL/L for all EOs, while the highest ones were determined by assessing the inhibitory activity of each individual treatment. The EO volumes were measured using a micropipette (DLAB Scientific Co., ltd., China). The specific concentrations tested for each EO and fungal strain can be found in the results section alongside their corresponding percentages of inhibition (PIs, %). Inoculated plates without any EO were used as controls. Three replicates were performed per treatment in a single assay. PIs were determined using the following equation: PI = [(C - T)/C] x 100 (Gotor-Vila et al., 2017). Where C is the diameter of the control colony and T that of the treatment, both after subtracting 8 mm corresponding to the diameter of the inoculation plug, as proposed by Mutawila et al. (2016).
Growth was assessed by measuring two perpendicular diameters of each replicate, considering the edge of the colony. Data were collected 3 days post inoculation (dpi) for B. cinerea; and 5 dpi for M. fructicola, M. fructigena, and M. laxa, when mycelial growth of one of the treatments reached the edge of the plate, and PIs were subsequently calculated in comparison to the growth of the untreated control. The Minimum Inhibitory Concentration (MIC) for each EO and fungal strain was determined as the lowest tested concentration that showed no mycelial growth inhibition at the last day of the experiment (Fontana et al., 2021). A rank analysis (Conover and Iman, 1981) was performed considering the MIC for each fungus and treatment.
The inoculation plugs from those treatments presenting complete inhibition were re-inoculated on fresh PDA to evaluate whether the activity of the volatile EOs was fungicidal or fungistatic (Moumni et al., 2021), and eventual growth was recorded 5 dpi.
After testing the data normality and equality of variances with Kolmogorov-Smirnov´s test and Levene´s test, statistical analyses were carried out using one-way analysis of variance (ANOVA), and means were separated with Tukey’s post hoc test (p ≤ 0.05). A non-parametric Kruskal–Wallis H-test was used, and means were separated with the Mann-Whitney U-test (p ≤ 0.05) when data were not normally distributed or homoscedastic. All statistical analyses were performed using IBM SPSS Statistics 26 (Armonk, NY, United States).
Results indicate a dose-dependent inhibitory activity of VOCs from all tested EOs against the fungal strains. Overall, they exerted lower antifungal effects against B. cinerea in comparison to all three Monilinia spp. (Table 2). The rank analysis showed the following order of sensitivity: B. cinerea < M. fructicola < M. laxa < M. fructigena. O. vulgare, T. serpyllum and T. vulgaris showed lower MICs, ranging from 22.73 to 45.45 μL/L for B. cinerea and from 5.54 to 22.73 μL/L against the different Monilinia spp. strains. C. zeylanicum rendered moderate results, with MICs of 45.45 μL/L against B. cinerea and M. fructigena, and of 90.91 μL/L for M. fructicola and M. laxa. This was the only treatment in which some of the Monilinia spp., specifically M. fructicola and M. laxa, presented higher MIC than B. cinerea. M. alternifolia, L. officinalis, and L. hybrida EOs presented higher MICs, being 181.82 to 363.64 μL/L against B. cinerea and 90.91 to 181.82 μL/L for Monilinia spp., while those of C. bergamia and R. officinalis presented an MIC of 363.64 μL/L against B. cinerea, M. fructicola, and M. fructigena, and of 181.82 μL/L for M. laxa. The commercial mixture (MIX) exerted a considerable inhibition, mostly in the range of the two tested Thymus spp., with MICs: 45.45 μL/L against B. cinerea, 22.73 μL/L for M. fructicola and M. laxa, and 11.76 μL/L against M. fructigena. Some MIC differences were also observed between EOs derived from the same plant but from a different provider (O. vulgare, T. vulgaris, M. alternifolia). MICs of all the tested EOs against the four fungal strains are presented in Table 2.
Table 2 Minimum Inhibitory Concentration (MIC) of VOCs from twelve individual essential oils and a commercial mixture against Botrytis cinerea (3 dpi), Monilinia fructicola, Monilinia fructigena, and Monilinia laxa (5 dpi).
The antifungal effects of all tested EOs were demonstrated to be dose dependent, with percentage of inhibition (PI) rising as concentrations increased (Tables 3–6).
Table 3 Percentage of Inhibition (PI, %) produced by the tested EOs in vapor phase against Botrytis cinerea.
Table 4 Percentage of Inhibition (PI, %) produced by the tested EOs in vapor phase against Monilinia fructicola.
Table 5 Percentage of Inhibition (PI, %) produced by the tested EOs in vapor phase against Monilinia fructigena.
Table 6 Percentage of Inhibition (PI, %) produced by the tested EOs in vapor phase against Monilinia laxa.
B. cinerea (Table 3) was usually less affected by EO volatiles than all three Monilinia spp. (Tables 4–6), with differences up to more than 20% regarding the same concentration and treatment. For example, 5.64 μL/L of O. vulgare 1 EO showed inhibitions of 82.9% against B. cinerea and 100%, 96.3%, and 96.6% against M. fructicola, M. fructigena and M. laxa, respectively; or 22.73 μL/L of M. alternifolia 1 EO produced PIs of 23.6% against B. cinerea and 57.3% for M. fructicola, 87.6% for M. fructigena, and 44.6% for M. laxa. M. fructicola and M. laxa had lower PIs than B. cinerea when exposed to some concentrations of certain EOs, such as M. alternifolia 2, L. hybrida, C. bergamia, or R. officinalis, especially in their lower doses. C. zeylanicum EO was the only treatment that consistently showed higher inhibitory activity towards B. cinerea than to the tested Monilinia spp. For example, using the lowest concentration of 2.82 μL/L, the results were 0.0%, 3.0%, 1.0%, and 10.1% for M. fructicola, M. fructigena, and M. laxa, respectively, and 37.9% for B. cinerea. These differences were even higher in subsequent concentrations, with 11.36 μL/L of C. zeylanicum EO producing PIs of 20.6%, 12.6%, and 31.2% against M. fructicola, M. fructigena, and M. laxa, and of 69.9% against B. cinerea. In general, M. fructigena (Table 5) was the most inhibited among the three Monilinia spp. strains, although it presented a higher MIC value for T. vulgaris EO and higher than M. laxa for C. bergamia and R. officinalis (Table 2).
No fungicidal activity was observed for any of the EOs at the tested concentrations, since all fungal plugs from treatments with complete inhibition were able to grow after re-inoculation on fresh PDA, demonstrating the fungistatic effect of the EO VOCs.
This study indicates that EO VOCs show antimicrobial activity toward B. cinerea, M. fructicola, M. fructigena, and M. laxa, and a dose-dependent inhibitory activity. B. cinerea usually showed a lower sensitivity to the tested EOs in comparison to the three tested Monilinia spp. This result confirms previous studies, in which M. fructicola proved to be more affected by several EO VOCs than B. cinerea (Santoro et al., 2018). These authors reported an increased incidence of gray mold in both nectarines and peaches treated with thyme and savory EOs, which they related to the reduction in brown rot derived from the treatment. T. vulgaris EO MIC on B. cinerea (26.7 μL/L) was reported to be double that on M. laxa (13.3 μL/L) (Pinto et al., 2020), which are very much in line with our results (22.73-45.45 μL/L for B. cinerea and 11.36 μL/L for M. laxa). These differences were even higher when testing the effects of its individual components, namely thymol and p-cymene. Conversely, in the present study, C. zeylanicum EO VOCs were the only ones that produced a consistently higher antifungal activity on B. cinerea than against any of the tested Monilinia spp. strains. These results could point to a different mode of action, thus further research would be of interest to understand the specific traits of this EO.
O. vulgare, T. vulgaris, and T. serpyllum presented the highest antifungal activity of all the tested EOs. This is again in accordance with previous research that showed their strong inhibitory effects (Paris et al., 2020; Pinto et al., 2020: Yan et al., 2021; Di Francesco et al., 2022; Elsayed et al., 2022; Kara et al., 2022; Zanotto et al., 2023). The commercial mixture of M. alternifolia, O. vulgare, C. zeylanicum and T. vulgaris presented quite high inhibitory activity, although this was lower than that of the most active of its components (O. vulgare), similar to that of T. vulgaris, but stronger than that of M. alternifolia and C. zeylanicum. These results do not indicate a synergistic activity of the listed EOs. Previous studies have reported both synergistic and non-synergistic results concerning microbial inhibition from EO mixtures (Goñi et al., 2009).
Among the four tested fungi, B. cinerea is the most studied one concerning the antifungal activity of vapor phase EOs, both in vitro and in vivo (Di Francesco et al., 2022; Elsayed et al., 2022). Out of the twelve applied EOs, T. serpyllum and C. bergamia seem to be the only ones that had not yet been tested against this pathogen. The strong antifungal effect of vapor-phase EOs from O. vulgare and other Origanum species on B. cinerea has been extensively reported (Munhuweyi et al., 2017; Zhao et al., 2021; Elsayed et al., 2022; Kaharamanoglu et al., 2022; Kara et al., 2022). Zhao et al. (2021) found significant inhibitory activity of O. vulgare EO VOCs on B. cinerea and proved that this activity was significantly higher in volatile rather than in direct contact, although the MIC they reported was considerably higher than the obtained in the present study (around 250 μg/L in comparison to 22.73 μL/L), while they reported a MIC of around 15.63 μg/L for carvacrol and thymol. We could hypothesized that these differences derive from the lower level of carvacrol in the O. vulgare EO used by these authors (15% in comparison to 69% and 79% in the present study). Several studies have also demonstrated the strong antifungal effect of T. vulgaris and C. zeylanicum EOs against B. cinerea (Paris et al., 2020; Pinto et al., 2020; Di Francesco et al., 2022; Elsayed et al., 2022), which is confirmed in our investigations. Elsayed et al. (2022) found significant disease reduction on grapes treated with these EOs, both applied with spray or fumigation. Yan et al. (2021) reported in vitro PIs close to 100% at 50 μL/L for T. vulgaris and Origanum heracleoticum EOs. In our case, this inhibition was reached at lower doses (22.73 to 45.45 μL/L). These same authors reported that EOs from Melaleuca spp., Lavandula spp., and R. officinalis were less effective against B. cinerea, as also found in our trials. For example, they reported a PI of 72.3%, very similar to the 78.4% we observed at 181.82 μL/L for R. officinalis EO. Other studies further support these findings, with L. hybrida showing a half maximal inhibitory concentration (IC50) around one order of magnitude higher that of T. vulgaris (Maietti et al., 2013), and a similar outcome was reported for R. officinalis and Lavandula angustifolia (Di Francesco et al., 2022). Yu et al. (2015) reported that the treatment with M. alternifolia EO constituents led to pronounced alterations in cellular ultrastructure, mycelial morphology, and membrane permeability, linked to a reduction in ergosterol levels.
Concerning M. fructicola, previous studies indicate that T. vulgaris EO inhibits its growth both in vitro and in vivo, being able to reduce brown rot incidence in nectarines and peaches, while promoting gray mold (Santoro et al., 2018). This was an effect that the authors related to the higher resistance of B. cinerea to the volatiles, which would also benefit from the niche left by the Monilinia sp. This same study reported that thyme and savory EOs effectively inhibit spore germination and germ tube elongation of M. fructicola. The inhibitory activity of T. vulgaris EO against M. fructicola has been further reported by other studies (Sellamuthu et al., 2013; Spadaro et al., 2021). M. alternifolia EO has been tested in vapor phase against M. fructicola (Xu et al., 2022). These authors used an EO-liposome formulation, obtaining PIs at least one order of magnitude lower than those from our investigation. They ascribed this low inhibitory activity to the slow release of volatiles from the liposomes (Xu et al., 2022). Xiong et al. (2021) reported a relevant inhibitory activity of lavender EO on M. fructicola, both in direct contact and with fumigation. These authors associated the antifungal effects of Lavandula sp. EO to cytoplasm leakage, hyphal and spore distortion, and cell membrane damage. They also reported an increased expression of apoptosis related genes in the exposed colonies. As far as we know, no previous vapor phase studies have been conducted with O. vulgare, T. serpyllum, C. bergamia, R. officinalis, or C. zeylanicum EO VOCs on M. fructicola, although some of them have been tested in direct contact (Lazar-Baker et al., 2011; Grulová et al., 2020; Xu et al., 2021). In this regard, Xu et al. (2021) reported that EOs such as T. vulgaris and M. alternifolia affect the structure of M. fructicola cell membrane, leading to changes in mycelial morphology, membrane permeability, and levels of intracellular reactive oxygen species.
The reported general higher PIs for M. fructigena in comparison to the other fungi suggest a higher sensitivity to most vapor-phase EOs than that shown by B. cinerea and the other tested Monilinia spp. These results cannot be compared with previous work, since we have found no published research assessing the effect of EOs in the vapor phase against M. fructigena. However, there are some studies concerning the antifungal activity of some EOs on this pathogen using the agar dilution method. El Khetabi et al. (2021) found small differences between M. fructigena and M. laxa, reporting similar growth inhibition for both fungi exposed to several EOs, including T. vulgaris, R. officinalis, and other species from the genus Origanum, Lavandula, and Citrus. Elshafie et al. (2015a; 2015b) also reported the important inhibitory activity of O. vulgare and T. vulgaris EOs and some of their constituents, such as carvacrol and thymol, against M. fructicola, M. fructigena, and M. laxa both in vitro and in vivo, although they did not highlight relevant differences among these fungal species.
With regard to M. laxa, as far as we know, out of the twelve individual EOs tested in our investigation, only T. vulgaris and C. zeylanicum had already been assayed in vapor phase against this pathogen. Pinto et al. (2020) observed a strong in vitro inhibitory activity of T. vulgaris EO VOCs and its individual components thymol, p-cymene, and ɣ-terpinene on M. laxa. These authors reported an MIC of 13.3 μL/L, which is similar to the one we obtained (11.36 μL/L). In vivo assays indicate that T. vulgaris and C. zeylanicum EO VOCs can also protect peaches from brown rot caused by M. laxa via the induction of physiological and defensive responses in the fruit (Cindi et al., 2016; Khumalo et al., 2017). EO VOCs from different Ocimum basilicum varieties also demonstrated in vitro antifungal activity against this pathogen (Carović-Stanco et al., 2013).
When considering their major components, our results suggest that EOs with significant levels of carvacrol and thymol (namely O. vulgare, T. vulgaris, and T. serpyllum) show higher antifungal activities than the others. This is supported by previous reports, in which these individual compounds demonstrated strong antifungal activity (Elshafie et al., 2015b; Pinto et al., 2020; Buonsenso et al., 2023), even presenting in some cases a MIC one order of magnitude lower than the whole essential oil, for example thymol MIC against M. laxa was 1.6 μL/L, while thyme EO MIC was 13.3 μL/L (Pinto et al., 2020). Kara et al. (2022) have also highlighted the inhibitory effects of EOs rich in carvacrol and thymol.
As far as we know, this is the first report in which VOC chambers have been used to evaluate the antimicrobial effects of volatile compounds from plant EOs. They have proven to be an effective alternative methodology for the study of these interactions. This protocol could be especially useful for a second phase of in vitro experiments after using a simpler screening method (Kloucek et al., 2012; Cernava et al., 2015), or as a transition for in vivo or ex vivo trials (Álvarez-García et al., 2022a).
VOC chambers proved to be an effective method to evaluate the antimicrobial activity of volatile EOs. The tested EOs presented significant concentration-dependent antifungal activity against B. cinerea, M. fructicola, M. fructigena, and M. laxa. Overall, B. cinerea was less inhibited than the three Monilinia spp., except for C. zeylanicum EO, which consistently showed higher inhibition against B. cinerea, with a MIC of 45.45 μL/L in comparison to 90.91 μL/L against M. laxa and M. fructicola. Among the Monilinia strains, M. fructigena was the most sensitive, followed by M. laxa, and being M. fructicola the most resistant one.
O. vulgare, T. vulgaris, and T. serpyllum EOs volatiles presented the highest inhibitory activity, with a MIC of 22.73, 45.45, and 22.73 µL/L, respectively, against B. cinerea and a range between 5.64 and 22.73 μL/L against the three Monilinia spp. M. alternifolia, L. officinalis and L. hybrida EOs showed intermediate antifungal activity, with MICs ranging from 181.82 to 363.64 μL/L against B. cinerea and from 90.91 to 181.82 in the case of Monilinia spp. C. bergamia and R. officinalis EOs were the least effective ones, with a MIC of 363.64 μL/L against B. cinerea, M. fructicola, and M. fructigena; and 181.82 μL/L against M. laxa. Further in vivo assays should be conducted to elucidate whether some of these EOs could be of use for the postharvest control of gray mold and brown rot infections in fruits and vegetables.
The raw data supporting the conclusions of this article will be made available by the authors, without undue reservation.
VOC Chambers will be made available to researchers upon reasonable request, unless commercial agreements reached with third parties regarding the patent exploitation prohibit it (in which case the VOC Chambers should be available in the market).
SÁ-G: Conceptualization, Validation, Data curation, Formal Analysis, Investigation, Methodology, Software, Visualization, Writing – original draft. MM: Formal Analysis, Investigation, Software, Validation, Writing – review & editing. GR: Validation, Writing – review & editing, Conceptualization, Funding acquisition, Project administration, Resources, Supervision.
The authors declare financial support was received for the research, authorship, and/or publication of this article. This work was conducted within PRIMA StopMedWaste “Innovative Sustainable technologies TO extend the shelf-life of Perishable MEDiterranean fresh fruit, vegetables and aromatic plants and to reduce WASTE”, which was funded by the Partnership for Research and Innovation in the Mediterranean Area (PRIMA), Project ID: 1556, a program supported by the European Union, and Euphresco BasicS “Basic substances as an environmentally friendly alternative to synthetic pesticides for plant protection” projects, and by Italy-China bilateral project “Biosynthesis regulation of metabolic markers and correlation with quality safety during fruit decay (BioQuaSa)”, funded by Italian Ministry of Foreign Affairs and International Cooperation. We acknowledge the Margarita Salas postdoctoral grant awarded to SÁ-G (Ministerio de Universidades; Plan de Recuperación, Transformación y Resiliencia; Universidad de León; Spain) financed by the EU-NextGenerationEU.
SÁ -G possesses a 55% share as main inventor.
The remaining authors declare that the research was conducted in the absence of any commercial or financial relationships that could be construed as a potential conflict of interest.
The authors declare the existence of potential financial competing interests derived from the patent held by the University of León regarding the VOC Chamber. The funders had no role in the design of the study; in the collection; analyses; or interpretation of data; in the writing of the manuscript; or in the decision to publish the results.
The author(s) declared that they were an editorial board member of Frontiers, at the time of submission. This had no impact on the peer review process and the final decision.
All claims expressed in this article are solely those of the authors and do not necessarily represent those of their affiliated organizations, or those of the publisher, the editors and the reviewers. Any product that may be evaluated in this article, or claim that may be made by its manufacturer, is not guaranteed or endorsed by the publisher.
Álvarez-García, S., Manga-Robles, A., Encina, A., Gutiérrez, S., Casquero, P. A. (2022a). Novel culture chamber to evaluate in vitro plant-microbe volatile interactions: Effects of Trichoderma harzianum volatiles on wheat plantlets. Plant Sci. 320. doi: 10.1016/j.plantsci.2022.111286
Álvarez-García, S., Mayo-Prieto, S., Carro-Huerga, G., Rodríguez-González, Á., González-López, Ó., Gutiérrez, S., et al. (2021). Volatile organic compound chamber: a novel technology for microbiological volatile interaction assays. J. Fungi 7 (4), 248. doi: 10.3390/jof7040248
Avalos, M., van Wezel, G. P., Raaijmakers, J. M., Garbeva, P. (2018). Healthy scents: microbial volatiles as new frontier in antibiotic research? Curr. Opin. Microbiol. 45, 84–91. doi: 10.1016/j.mib.2018.02.011
Ayala-Zavala, J. F., Oms-Oliu, G., Odriozola-Serrano, I., Gonza-lez-Aguilar, G. A., Álvarez-Parrilla, E., Martın-Belloso, et al. (2008). Bio-preservation of fresh-cut tomatoes using natural antimicrobials. Eur. Food Res. Technol. 226 (5), 1047–1055. doi: 10.1007/s00217-007-0630-z
Buonsenso, F., Schiavon, G., Spadaro, D. (2023). Efficacy and mechanisms of action of essential oils’ vapours against blue mould on apples caused by Penicillium expansum. Int. J. Mol. Sci. 24 (3), 2900. doi: 10.3390/ijms24032900
Carović-Stanco, K., Fruk, G., Satovic, Z., Ivic, D., Politeo, O., Sever, Z., et al. (2013). Effects of Ocimum spp. essential oil on Monilinia laxa in vitro. J. Essential Oil Res. 25 (2), 143–148. doi: 10.1080/10412905.2012.751057
Casals, C., Torres, R., Teixidó, N., De Cal, A., Segarra, J., Usall, J. (2022). Brown rot on stone fruit: From epidemiology studies to the development of effective control strategies. Scientia Hortic. 301. doi: 10.1016/j.scienta.2022.111096
Cernava, T., Aschenbrenner, I. A., Grube, M., Liebminger, S., Berg, G. (2015). A novel assay for the detection of bioactive volatiles evaluated by screening of lichen-associated bacteria. Front. Microbiol. 6. doi: 10.3389/fmicb.2015.00398
Cindi, M. D., Shittu, T., Sivakumar, D., Bautista-Baños, S. (2015). Chitosan boehmite-alumina nanocomposite films and thyme oil vapour control brown rot in peaches (Prunus persica L.) during postharvest storage. Crop Prot. 72, 127–131. doi: 10.1016/j.cropro.2015.03.011
Cindi, M. D., Soundy, P., Romanazzi, G., Sivakumar, D. (2016). Different defense responses and brown rot control in two Prunus persica cultivars to essential oil vapours after storage. Postharvest Biol. Technol. 119, 9–17. doi: 10.1016/j.postharvbio.2016.04.007
Conover, W. J., Iman, L. R. (1981). Rank transformation as a bridge between parametric and nonparametric statistics. Am. Statistician 35, 124–129. doi: 10.2307/2683975
Côté, M., Tardif, F., Meldrum, A. (2004). Identification of Monilinia fructigena, M. fructicola, M. laxa, and Monilia polystroma on inoculated and naturally infected fruit using multiplex PCR. Plant Dis. 88, 1219–1225. doi: 10.1094/PDIS.2004.88.11.1219
De Miccolis Angelini, R. M., Landi, L., Raguseo, C., Pollastro, S., Faretra, F., Romanazzi, G. (2022). Tracking of diversity and evolution in the brown rot fungi Monilinia fructicola, Monilinia fructigena, and Monilinia laxa. Front. Microbiol. 13. doi: 10.3389/fmicb.2022.854852
Dennis, C., Webster, J. (1971). Antagonistic properties of species-groups of Trichoderma. II Production of volatile antibiotics. Trans. Br. Mycological Soc. 57 (1), 41–48. doi: 10.1016/S0007-1536(71)80078-5
Derbassi, N. B., Pedrosa, M. B., Heleno, S., Carocho, M., Ferreira, I. C. F. R., Barros, L. (2022). Plant volatiles: Using Scented molecules as food additives. Trends Food Sci. Technol. 122, 97–103. doi: 10.1016/j.tifs.2022.02.002
Di Francesco, A., Aprea, E., Gasperi, F., Parenti, A., Placì, N., Rigosi, F., et al. (2022). Apple pathogens: Organic essential oils as an alternative solution. Scientia Hortic. 300, 111075. doi: 10.1016/j.scienta.2022.111075
El Khetabi, A., Ezrari, S., El Ghadraoui, L., Tahiri, A., Haddou, L. A., Belabess, Z., et al. (2021). In vitro and in vivo antifungal activities of nine commercial essential oils against brown rot in apples. Horticulturae 7, 1–18. doi: 10.3390/horticulturae7120545
El Khetabi, A., Lahlali, R., Ezrari, S., Radouane, N., Lyousfi, N., Banani, H., et al. (2022). Role of plant extracts and essential oils in fighting against postharvest fruit pathogens and extending fruit shelf life: A review. Trends Food Sci. Technol. 120, 402–417. doi: 10.1016/j.tifs.2022.01.009
Elsayed, M. I., Al-Qurashi, A. D., Almasaudi, N. M., Abo-Elyousr, K. A. M. (2022). Efficacy of essential oils against gray mold and effect on fruit quality during cold storage in table grapes. South Afr. J. Bot. 146, 481–490. doi: 10.1016/j.sajb.2021.11.046
Elshafie, H. S., Mancini, E., Camele, I., De Martino, L., De Feo, V. (2015a). In vivo antifungal activity of two essential oils from Mediterranean plants against postharvest brown rot disease of peach fruit. Ind. Crops Products 66, 11–15. doi: 10.1016/j.indcrop.2014.12.031
Elshafie, H. S., Mancini, E., Sakr, S., De Martino, L., Mattia, C. A., De Feo, V., et al. (2015b). Antifungal activity of some constituents of Origanum vulgare L. essential oil against postharvest disease of peach fruit. J. Medicinal Food 18 (8), 929–934. doi: 10.1089/jmf.2014.0167
FAO (2021). Fruit and vegetables – your dietary essentials. The International Year of Fruits and Vegetables 2021, background paper (Rome: FAO, Food and Agriculture Organiz).
Fontana, D. C., Dourado Neto, D., Milani Pretto, M., Mariotto, A. B., Caron, B. O., Kulczynski, S. M., et al. (2021). Using essential oils to control diseases in strawberries and peaches. Int. J. Food Microbiol. 338, 108980. doi: 10.1016/j.ijfoodmicro.2020.108980
Gonçalves, D. C., Ribeiro, W. R., Gonçalves, D. C., Menini, L., Costa, H. (2021). Recent advances and future perspective of essential oils in control Colletotrichum spp.: A sustainable alternative in postharvest treatment of fruits. Food Res. Int. 150, 110758. doi: 10.1016/j.foodres.2021.110758
Goñi, P., López, P., Sánchez, C., Gómez-Lus, R., Becerril, R., Nerín, C. (2009). Antimicrobial activity in the vapour phase of a combination of cinnamon and clove essential oils. Food Chem. 116 (4), 982–989. doi: 10.1016/j.foodchem.2009.03.058
Gotor-Vila, A., Teixidó, N., Di Francesco, A., Usall, J., Ugolini, L., Torres, R., et al. (2017). Antifungal effect of volatile organic compounds produced by Bacillus amyloliquefaciens CPA-8 against fruit pathogen decays of cherry. Food Microbiol. 64, 219–225. doi: 10.1016/j.fm.2017.01.006
Grulová, D., Caputo, L., Elshafie, H. S., Baranová, B., De Martino, L., Sedlák, V., et al. (2020). Thymol chemotype Origanum vulgare L. essential oil as a potential selective bio-based herbicide on monocot plant species. Molecules 25 (3), 595. doi: 10.3390/molecules25030595
Kaharamanoglu, I., Panfilova, O., Kesimci, T. G., Bozhüyük, A. U., Gürbüz, R., Alptekin, H. (2022). Control of postharvest gray mold at strawberry fruits caused by Botrytis cinerea and improving fruit Storability through Origanum onites L. and Ziziphora clinopodioides L. Volatile Essential Oils. Agronomy 12 (2), 389. doi: 10.3390/agronomy12020389
Kai, M., Piechulla, B. (2009). Plant growth promotion due to rhizobacterial volatiles - An effect of CO2? FEBS. Letters 583 (21), 3473–3477. doi: 10.1016/j.febslet.2009.09.053
Kapetanakou, A. E., Nestora, S., Evageliou, V., Skandamis, P. N. (2019). Sodium alginate–cinnamon essential oil coated apples and pears: Variability of Aspergillus carbonarius growth and ochratoxin A production. Food Res. International 119, 876–885. doi: 10.1016/j.foodres.2018.10.072
Kara, M., Türkmen, M., Soylu, S. (2022). Chemical compositions and in vitro antifungal activities of essential oils obtained from different Origanum species against postharvest gray mold rot of persimmon fruit. Acta Hortic. 1338, 283–290. doi: 10.17660/ActaHortic.2022.1338.41
Khumalo, K. N., Tinyane, P., Soundy, P., Romanazzi, G., Glowacz, M., Sivakumar, D. (2017). Effect of thyme oil vapour exposure on the brown rot infection, phenylalanine ammonia-lyase (PAL) activity, phenolic content and antioxidant activity in red and yellow skin peach cultivars. Scientia Hortic. 214, 195–199. doi: 10.1016/j.scienta.2016.11.044
Kloucek, P., Smid, J., Frankova, A., Kokoska, L., Valterova, I., Pavela, R. (2012). Fast screening method for assessment of antimicrobial activity of essential oils in vapor phase. Food Res. Int. 47 (2), 161–165. doi: 10.1016/j.foodres.2011.04.044
Lazar-Baker, E. E., Hetherington, S. D., Ku, V. V., Newman, S. M. (2011). Evaluation of commercial essential oil samples on the growth of postharvest pathogen Monilinia fructicola (G. Winter) Honey. Lett. Appl. Microbiol. 52 (3), 227–232. doi: 10.1111/j.1472-765X.2010.02996.x
Ma, Q., Xu, Y., Xiao, H., Mariga, A. M., Chen, Y., Zhang, X., et al. (2022). Rethinking of botanical volatile organic compounds applied in food preservation: Challenges in acquisition, application, microbial inhibition and stimulation. Trends Food Sci. Technol. 125, 166–184. doi: 10.1016/j.tifs.2022.05.007
Maietti, S., Rossi, D., Guerrini, A., Useli, C., Romagnoli, C., Poli, F., et al. (2013). A multivariate analysis approach to the study of chemical and functional properties of chemo-diverse plant derivatives: Lavender essential oils. Flavour Fragrance J. 28 (3), 144–154. doi: 10.1002/ffj.3145
Makau, S. M., D’Ortenzio, A. L., Mancini, V., Murolo, S., Landi, L., Romanazzi, G. (2023). Molecular identification of Monilinia laxa, Monilinia fructigena and Monilinia fructicola from stone fruits in the Marche Region, Central-Eastern Italy. Acta Hortic. 1363, 45–50. doi: 10.17660/ActaHortic.2023.1363.7
Maruzzella, J. C., Sicurella, N. A. (1960). Antibacterial activity of essential oil vapors. J. Am. Pharmacists Assoc. 49 (11), 692–694. doi: 10.1002/jps.3030491103
Moumni, M., Romanazzi, G., Najar, B., Pistelli, L., Ben Amara, H., Mezrioui, K., et al. (2021). Antifungal activity and chemical composition of seven essential oils to control the main seedborne fungi of cucurbits. Antibiotics 10, 1–16. doi: 10.3390/antibiotics10020104
Munhuweyi, K., Caleb, O. J., Lennox, C. L., van Reenen, A. J., Opara, U. L. (2017). In vitro and in vivo antifungal activity of chitosan-essential oils against pomegranate fruit pathogens. Postharvest Biol. Technol. 129, 9–22. doi: 10.1016/j.postharvbio.2017.03.002
Mutawila, C., Vinale, F., Halleen, F., Lorito, M., Mostert, L. (2016). Isolation, production and in vitro effects of the major secondary metabolite produced by Trichoderma species used for the control of grapevine trunk diseases. Plant Pathol. 65, 104–113. doi: 10.1111/ppa.12385
Paris, M. J., Ramírez-Corona, N., Palou, E., López-Malo, A. (2020). Modelling release mechanisms of cinnamon (Cinnamomum zeylanicum) essential oil encapsulated in alginate beads during vapor-phase application. J. Food Eng. 282. doi: 10.1016/j.jfoodeng.2020.110024
Pinto, L., Bonifacio, M. A., De Giglio, E., Cometa, S., Logrieco, A. F., Baruzzi, F. (2020). Unravelling the antifungal effect of red thyme oil (Thymus vulgaris L.) compounds in vapor phase. Molecules 25, 1–16. doi: 10.3390/molecules25204761
Powelson, R. L. (1960). Initiation of strawberry fruit rot caused by Botrytis cinerea. Phytopathology 50 (7), 491–494.
Reyes-Jurado, F., Navarro-Cruz, A. R., Ochoa-Velasco, C. E., Palou, E., López-Malo, A., Ávila-Sosa, R. (2020). Essential oils in vapor phase as alternative antimicrobials: A review. Food Sci. Nutr. 60 (10), 1641–1650. doi: 10.1080/10408398.2019.1586641
Roca-Couso, R., Flores-Félix, J. D., Rivas, R. (2021). Mechanisms of action of microbial biocontrol agents against Botrytis cinerea. J. Fungi 7 (12). doi: 10.3390/jof7121045
Romanazzi, G., Orçonneau, Y., Moumni, M., Davillerd, Y., Marchand, P. A. (2022). Basic substances, a sustainable tool to complement and eventually replace synthetic pesticides in the management of pre and postharvest diseases: reviewed instructions for users. Molecules 27 (11), 3484. doi: 10.3390/molecules27113484
Santoro, K., Maghenzani, M., Chiabrando, V., Bosio, P., Gullino, M. L., Spadaro, D., et al. (2018). Thyme and savory essential oil vapor treatments control brown rot and improve the storage quality of peaches and nectarines, but could favor gray mold. Foods 7, 1–17. doi: 10.3390/foods7010007
Schmidt, R., De Jager, V., Zühlke, D., Wolff, C., Bernhardt, J., Cankar, K., et al. (2017). Fungal volatile compounds induce production of the secondary metabolite Sodorifen in Serratia plymuthica PRI-2C. Sci. Rep. 7 (862), 1–14. doi: 10.1038/s41598-017-00893-3
Sellamuthu, P. S., Sivakumar, D., Soundy, P. (2013). Antifungal activity and chemical composition of thyme, peppermint, and citronella oils in vapor phase against avocado and peach postharvest pathogens. J. Food Saf. 33 (1), 86–93. doi: 10.1111/jfs.12026
Servili, A., Feliziani, E., Romanazzi, G. (2017). Exposure to volatiles of essential oils alone or under hypobaric treatment to control postharvest gray mold of table grapes. Postharvest Biol. Technol. 133, 36–40. doi: 10.1016/j.postharvbio.2017.06.007
Song, G. C., Riu, M., Ryu, C. M. (2019). Beyond the two compartments Petri dish: optimising growth promotion and induced resistance in cucumber exposed to gaseous bacterial volatiles in a miniature greenhouse system. Plant Methods 15, 9. doi: 10.1186/s13007-019-0395-y
Spadaro, D., Banani, H., Santoro, K., Garibaldi, A., Gullino, M. L. (2021). Essential oils to control postharvest diseases of apples and peaches: Elucidation of the mechanism of action. Acta Hortic. 1323, 35–41. doi: 10.17660/ActaHortic.2021.1323.7
Taghavi, T., Kim, C., Rahemi, A. (2018). Role of natural volatiles and essential oils in extending shelf life and controlling postharvest microorganisms of small fruits. Microorganisms 6 (4). doi: 10.3390/microorganisms6040104
Tilocca, B., Cao, A., Migheli, Q. (2020). Scent of a Killer: Microbial volatilome and Its role in the biological control of plant pathogens. Front. Microbiol. 11. doi: 10.3389/fmicb.2020.00041
Usall, J., Ippolito, A., Sisquella, M., Neri, F. (2016). Physical treatments to control postharvest diseases of fresh fruits and vegetables. Postharvest Biol. Technol. 122, 30–40. doi: 10.1016/j.postharvbio.2016.05.002
Xiong, X., Zhang, L., Li, X., Zeng, Q., Deng, R., Ren, X., et al. (2021). Antifungal mechanisms of lavender essential oil in the inhibition of rot disease caused by Monilinia fructicola in postharvest flat peaches. Can. J. Microbiol. 67, 724–736. doi: 10.1139/cjm-2020-0484
Xu, Y., Wei, Y., Jiang, S., Xu, F., Wang, H., Shao, X. (2022). Preparation and characterization of tea tree oil solid liposomes to control brown rot and improve quality in peach fruit. LWT - Food Sci. Technol. 162, 113442. doi: 10.1016/j.lwt.2022.113442
Xu, Y., Wei, J., Wei, Y., Han, P., Dai, K., Zou, X., et al. (2021). Tea tree oil controls brown rot in peaches by damaging the cell membrane of Monilinia fructicola. Postharvest Biol. Technol. 175, 111474. doi: 10.1016/j.postharvbio.2021.111474
Yan, J., Wu, H., Chen, K., Feng, J., Zhang, Y. (2021). Antifungal activities and mode of action of Cymbopogon citratus, Thymus vulgraris, and Origanum heracleoticum essential oil vapors against Botrytis cinerea and their potential application to control postharvest strawberry gray mold. Foods 10 (10). doi: 10.3390/foods10102451
Yu, D., Wang, J., Shao, X., Xu, F., Wang, H. (2015). Antifungal modes of action of tea tree oil and its two characteristic components against Botrytis cinerea. J. Appl. Microbiol. 119 (5), 1253–1262. doi: 10.1111/jam.12939Zanotto
Zanotto, A. W., Kanemaru, M. Y. S., de Souza, F. G., Duarte, M. C. T., de Andrade, C. J., Pastore, G. M. (2023). Enhanced antimicrobial and antioxidant capacity of Thymus vulgaris, Lippia sidoides, and Cymbopogon citratus emulsions when combined with mannosylerythritol a lipid biosurfactant. Food Res. Int. 163. doi: 10.1016/j.foodres.2022.112213
Keywords: cinnamon, fumigation, lavender, oregano, rosemary, tea tree, thyme, VOC chamber
Citation: Álvarez-García S, Moumni M and Romanazzi G (2023) Antifungal activity of volatile organic compounds from essential oils against the postharvest pathogens Botrytis cinerea, Monilinia fructicola, Monilinia fructigena, and Monilinia laxa. Front. Plant Sci. 14:1274770. doi: 10.3389/fpls.2023.1274770
Received: 08 August 2023; Accepted: 13 September 2023;
Published: 04 October 2023.
Edited by:
Alessandra Di Francesco, University of Udine, ItalyReviewed by:
Neringa Rasiukeviciute, Lithuanian Research Centre for Agriculture and Forestry, LithuaniaCopyright © 2023 Álvarez-García, Moumni and Romanazzi. This is an open-access article distributed under the terms of the Creative Commons Attribution License (CC BY). The use, distribution or reproduction in other forums is permitted, provided the original author(s) and the copyright owner(s) are credited and that the original publication in this journal is cited, in accordance with accepted academic practice. No use, distribution or reproduction is permitted which does not comply with these terms.
*Correspondence: Gianfranco Romanazzi, Zy5yb21hbmF6emlAdW5pdnBtLml0
Disclaimer: All claims expressed in this article are solely those of the authors and do not necessarily represent those of their affiliated organizations, or those of the publisher, the editors and the reviewers. Any product that may be evaluated in this article or claim that may be made by its manufacturer is not guaranteed or endorsed by the publisher.
Research integrity at Frontiers
Learn more about the work of our research integrity team to safeguard the quality of each article we publish.