- 1Tree Fruit Research and Extension Center, Washington State University, Wenatchee, WA, United States
- 2Department of Horticulture, Washington State University, Pullman, WA, United States
Rootstocks are used in modern apple production to increase productivity, abiotic and biotic stress tolerance, and fruit quality. While dwarfing for apple rootstocks has been well characterized, the physiological mechanisms controlling dwarfing have not. Previous research has reported rootstock effects on scion water relations. Root architecture and variability in soil moisture across rooting depths can also contribute to these differences among rootstocks in the field. To exclude these effects on rootstock behavior, scions were grafted onto four different rootstocks with varying effects on scion vigor (B.9, M.9, G.41 and G.890). Non-grafted rootstocks were also grown to examine whether the effects of rootstock occurred independently from scion grafting. Plants were grown in a greenhouse under near steady-state hydroponic conditions. Carbon (δ13C), oxygen (δ18O) and nitrogen (δ15N) isotope composition were evaluated and relationships with carbon assimilation, water relations, and shoot growth were tested. Rootstocks affected scion shoot growth, aligning with known levels of vigor for these four rootstocks, and were consistent between the two scion cultivars. Furthermore, changes in water relations influenced by rootstock genotype significantly affected leaf, stem, and root δ13C, δ18O, and δ15N. Lower δ13C and δ18O were inconsistently associated with rootstock genotypes with higher vigor in leaves, stems, and roots. G.41 had lower δ15N in roots, stems, and leaves in both grafted and ungrafted trees. The effects of rootstock on aboveground water relations were also similar for leaves of ungrafted rootstocks. This study provides further evidence that dwarfing for apple rootstocks is linked with physiological limitations to water delivery to the developing scion.
Introduction
The use of composite plants consisting of a rootstock and scion is necessary for modern tree fruit production. Rootstocks impart beneficial traits such as resistance or tolerance to abiotic or biotic stresses (Garner, 2013), yield efficiency, and fruit quality (Palmer and Wertheim, 1980; Sansavini et al., 1980; Mika et al., 2000; Reig et al., 2018; Reig et al., 2020). Dwarfing rootstocks can allocate more energy to fruit production increasing both fruit quality and yield (Melnyk and Meyerowitz, 2015). Dwarfing rootstocks limit scion vegetative growth (Cohen and Naor, 2002; Tworkoski and Miller, 2007; Hayat et al., 2020) and this effect has been extensively described (Reviewed in Marini and Fazio, 2018). Still, the mechanisms controlling rootstock effects on vigor are poorly understood and may be multifaceted (Beakbane and Thompson, 1940; Webster, 2002; Gregory et al., 2013). Rootstocks may influence hormone transport limiting scion growth (Tworkoski and Fazio, 2016; Foster et al., 2017; Lordan et al., 2017; Adams et al., 2018). In some cases, the graft union itself may also limit xylem connectivity that may impede water and mineral movement to the scion (Atkinson et al., 2003; Tworkoski and Fazio, 2008; Bauerle et al., 2011). In other examples, root architecture and distribution can also affect nutrient and water uptake which could also affect scion growth and development (Hayat et al., 2020). Lower hydraulic conductivity has been reported for dwarfing rootstocks compared to more vigorous rootstocks (Atkinson and Else, 2001; Atkinson et al., 2003). These effects have also been reported in olives (Nardini et al., 2006), grapes (Shtein et al., 2017), and citrus (Martínez-Alcántara et al., 2013). The common effects among these proposed mechanisms are limitations to water and solute movement through the soil-plant-atmosphere continuum (Atkinson and Else, 2001; Xu and Ediger, 2021; Xu et al., 2021).
Water relations are regulated with direct transport of water from the soil to the leaves through the xylem (Olien and Lakso, 1986). Resistance has been reported to increase as the dwarfing effect increases (Atkinson et al., 2003; Tworkoski and Fazio, 2015). Furthermore, Olien and Lakso (1984) suggested that lower water potential for dwarfing rootstocks is not caused by resistance to water transport over the whole tree but exclusively by the rootstock (Olien and Lakso, 1984). Dwarfing rootstocks generally have less leaf area compared with vigorous rootstocks. Moreover, dwarfing effect is associated with scion precosity and, thus, earlier partitioning of photosynthates to fruit production occurs (Tworkoski and Fazio, 2015). However, dwarfing traits can also be observed in non-fruiting trees. The effect of dwarfing rootstocks is hypothesized to be associated with water relations, but the location of these limitations remains unclear.
Stable isotope composition can be useful for evaluating the effect of rootstock on scion growth and is an integrated measure of water-use efficiency and water relations. During carbon assimilation, 12C is discriminated against over the heavier isotope 13C at both the diffusion step into the mesophyll and during fixation by Rubisco, so δ13C of fixed carbon becomes depleted relative to atmospheric carbon dioxide (Farquhar et al., 1989; Wallace et al., 2013). Discrimination during carbon fixation is largely regulated by stomatal conductance. More conservative plants limit the inflow of CO2 into the leaf and thus, reduce the plant discrimination rates against 13C. Stomatal conductance is directly affected by plant water status, thus δ¹³C serves as a proxy method for water relations studies in plants (Farquhar et al., 1989; Wallace et al., 2013). Oxygen isotope composition can be used to separate physiological processes like leaf stomatal conductance and transpiration rate, especially when integrated with δ13C (Barbour, 2007). Since δ13C provides an integrative record of supply and demand for CO2, combining δ13C and δ18O could enable the separation of stomatal and photosynthetic effects from the rootstock (Barbour, 2007) and as consequence, acquire a stronger understanding of water use efficiency within the plant (Cernusak, 2020). Nitrogen isotope composition can be an indicator of N assimilation processes (Evans, 2001; Kalcsits et al., 2014) and the linkage between these three isotope measurements has been established for agricultural crops (Yousfi et al., 2013). These isotopes are often integrators of environmental conditions in the soil. However, soil-based heterogeneity can often mask rootstock-driven differences in isotope composition which may help shed light on the mechanisms driving tolerance to abiotic stress and environmental plasticity.
Being the interface between soil water supplies and photosynthetic tissues above-ground, rootstocks clearly have a significant role in improving tolerance to abiotic stresses such as water limitations under a changing climate. The objective for this study was to assess how rootstocks affect plant water relations and stable isotope composition when grown in a near steady-state hydroponic conditions. These approaches will help discern between architectural and soil-based interactions that can occur in the field from rootstocks and physiological limitations occurring in the root or graft union. We hypothesized that dwarfing rootstocks limit water uptake to aboveground tissue thus reducing transpiration rate, stomatal conductance, and photosynthesis and that rootstock effects are present when a scion is grafted to the rootstock or are left ungrafted.
Materials and methods
Greenhouse hydroponic system and growth conditions
The hydroponic system was comprised of six custom-built 455 L holding tanks placed in temperature-controlled greenhouse at Washington State University – Tree Fruit Research and Extension Center (WSU-TFREC), Wenatchee, WA (47.438127, -120.346656) with temperatures maintained between 20-23°C (Table 1). The wooden containers were lined with black rubber pond liners (Total Pond, West Palm Beach, Florida) and covered by a 75 x 75 cm tray (Botanicare-Agron, Aurora, CO) containing 24.5 L pots filled with perlite (PVP Industries, Orwell, OH). The hydroponics solution was a modified 1/10th Hoagland’s nutrient solution with concentrations of the following mineral salts: 60 mg L-1 of KNO3, 50 mg L-1 of MgSO4*7H2O, 95 mg L-1 of CaNO3*4H2O, 15 mg L-1 of (NH4)3PO4. For micronutrients, a stock solution was prepared at 1000x the target concentrations and then 1 mL L-1 of stock solution was added to each container with final concentrations of: 500 μg L-1 of KCl, 250 μg L-1 of H3Bo3, 50 μg L-1 of MnCl2, 20 μg L-1 of ZnSO4*7H2O, 5 μg L-1 of CuSO4*5H2O, 1 μg L-1 of H2MoO4 (85%), 500 g L-1 of FeSO4*7H2O and 300 μg L-1 of NaEDTA. pH was maintained between 6.5-7.0. The solution was circulated from the holding tank to the hydroponic tray using two submersible water pumps (3500L H-1, 60W) (Vivosun, Ontario, CA) per container through three 8-Port NPT Irrigation Manifold (Orbit, North Salt Lake, UT) placed on the front and sides of each tray (Figure 1). An air pump (EcoPlus 13500 LPH, Sunlight Supply Inc., Vancouver, WA) was connected to four air stone (Active Aqua ASCM Medium Air Stones, Hydrofarm Inc, Petaluma, Ca) which provided aeration at all times. Solution constantly circulated from the holding tanks to each pot during the experiment. Weather conditions such as air temperature and relative humidity (RH) were collected using an ATMOS 14 temperature & humidity sensor (Decagon Devices, Pullman, WA).
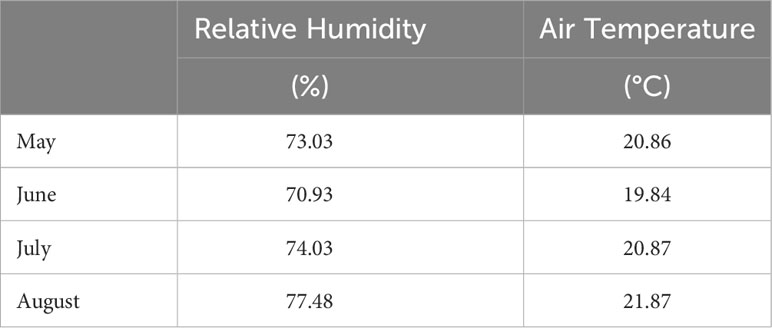
Table 1 Average greenhouse relative humidity and air temperature during May to August of 2019 during the experiment.
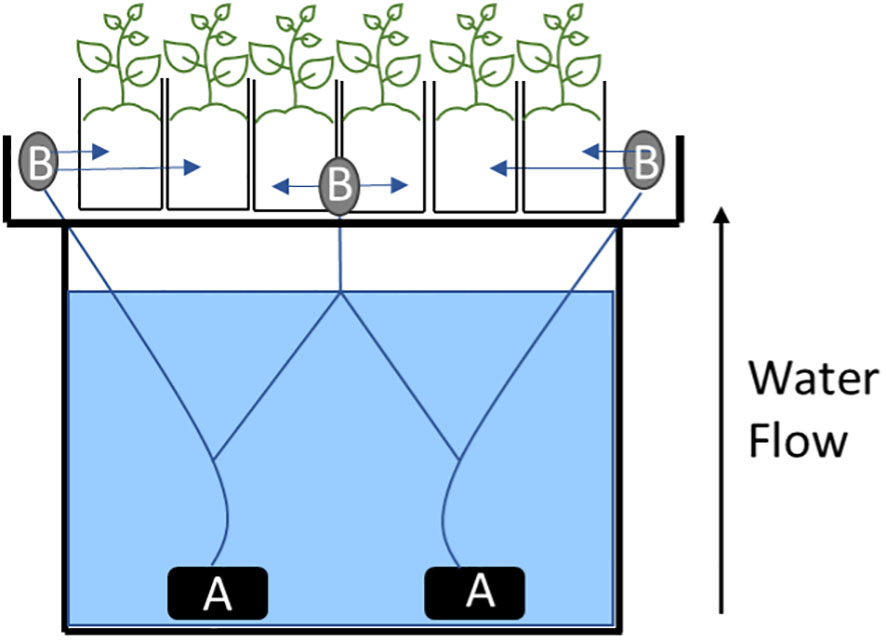
Figure 1 Schematic for the hydroponic system. (A) two water pumps used to propel water up to the plants. (B) three 8-Port NPT Irrigation Manifold used to distribute the water equally into each one of the 24 pots.
Plant material and experimental design
Budagovsky 9 (B.9), Geneva 41 (G.41), Geneva 890 (G.890) and Malling M.9-T337 (M.9) rootstocks were selected to provide a range of known vigor levels among rootstocks. The accepted order in vigor from highest to lowest is; G.890>G.41>M.9-T337>B.9. Prior to grafting, dormant rootstock cuttings were stored for two months at 2°C in dark room. Budwood from two contrasting scion cultivars, ‘Honeycrisp’® and `WA 38`®, was collected from Sunrise orchard (WSU-TFREC Sunrise Orchard, Rock Island, WA (47.311574, -120.067855) in February and placed in dark room until the time of the grafting. The two cultivars were grafted onto the rootstocks using a cleft graft. Nine composite plants were created for each combination of cultivar and rootstock. Eighteen other rootstock clones for each rootstock remained ungrafted. Three weeks after grafting, 72 ungrafted rootstocks (Experiment 1) and 72 grafted plants (experiment 2), were transferred to the greenhouse (Figure 2). The trees were placed into containers in a randomized complete block design (RCBD) with three containers as separate experimental blocks. Measurements were made after trees were grown for 60 days under hydroponic conditions.
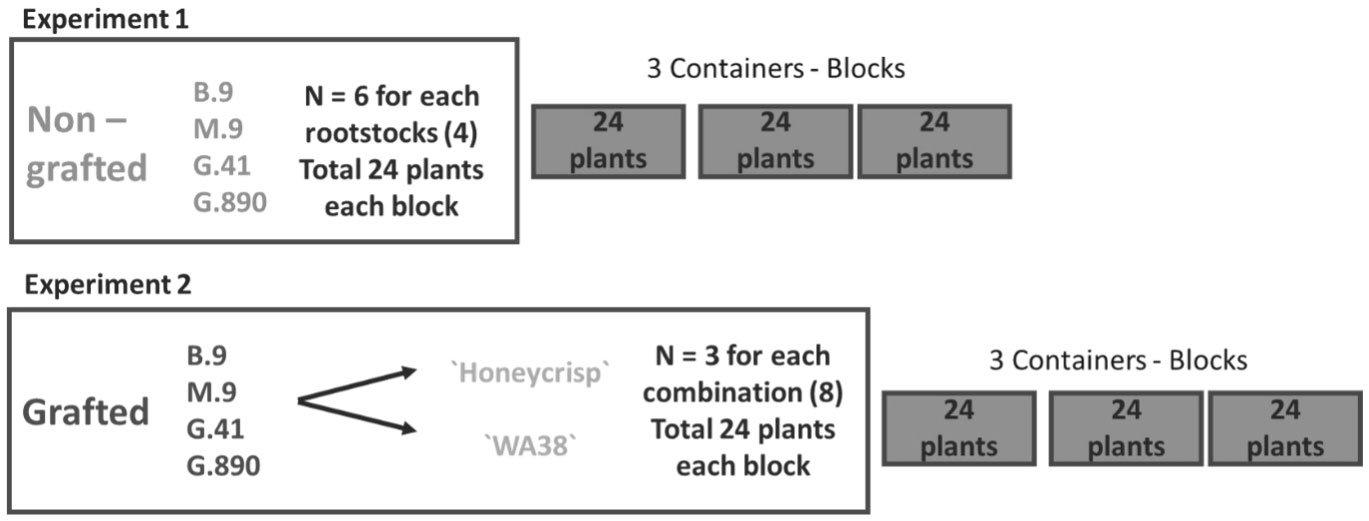
Figure 2 Experimental design for two greenhouse experiments. Experiment 1: Non-grafted plants experiment with six replicates for each rootstock placed individually in 24 pots for each experimental block. Experiment 2: grafted plants experiment with three replicates of each rootstock-scion combination placed individually in 24 pots for each the three experimental blocks.
Tree measurements
Gas exchange was measured on one mature, fully expanded leaf from each of the trees to estimate CO2 assimilation, stomatal conductance, and intrinsic water use efficiency (iWUE as µmol CO2 mol H2O-1) (Equation 1), estimated as the ratio of net photosynthetic CO2 assimilation (A) to stomatal conductance (gs) using a Licor-6400XT (Li-Cor, Inc., Lincoln, NE, USA). Air flow was constant at 400 µmol s−1, reference CO2 concentration was set at 400 ppm, leaf temperature at 25°C, and photosynthetic photon flux density inside the chamber was set to 1,500 μmol m−2 s−1. After placing the leaf in the gas exchange chamber, the leaf was allowed to equilibrate until reference and sample values stabilized. Measurements were made every two weeks between 10:00 and 12:00 for two months on cloud-free days. Means were calculated for all sampling times during the season.
Equation 1
Stem water potential was measured on one fully mature expanded leaf selected from the bottom of each tree by placing individual leaves in a silver reflective bag for at least 90 minutes. At solar noon, stem water potential was measured using a Scholander System Pressure Chamber Instrument (PMS Instrument Co., Albany, OR, USA). Shoot length was measured from the bud scar of the only shoot allowed to develop to the apical meristem for each plant.
Isotope analysis
Two mature leaves, vegetative stems (10 cm), and 1/3 of each root system were sampled for isotope composition. Samples were placed in paper bags and brought to the laboratory and dried. Dry leaves, stems and roots were well-mixed, and 2 g subsamples were ground to a fine powder using a VWR Homogenizer (VWR, Radnor, PA).
Oxygen isotope composition (δ18O) in biomass
0.6-0.8 mg samples for leaves and 1 mg samples for stems and roots was weighed using a high-precision analytical balance (XSE105 DualRange, Metler Toledo, Greifensee, Switzerland) into into 4x6 mm silver capsules (Costech Analystycal Technologies, Inc., Valencia, CA, USA). Prepared capsules were shipped for analysis to the Stable Isotope Core Laboratory at Washington State University. The samples were analyzed with continuous-flow pyrolysis using TC/EA interfaced with an IRMS (Delta Plus, ThermoFinnigan, Bremen, Germany) through a continuous flow device (Conflo-III, ThermoFinnigan,Bremen, Germany). Oxygen isotope ratios of each sample were then determined, and the values reported in “delta” notation as δ values in parts per thousand (‰):
Equation 2
δ18O was calculated as the ratio of the heavy isotope (18O) over the light oxygen isotope (16O) in the samples divided by the heavy isotope (18O) over the light oxygen isotope (16O) of the standard. The δ18O was compared with the standard Vienna-Standard Mean Ocean Water (VSMOW), the international standard commonly used for oxygen isotope analysis.
Carbon(δ13C) and nitrogen (δ15N) isotope composition in biomass
3 mg samples for leaves and 4 mg samples for stems and roots were weighed using a high-precision analytical balance (XSE105 DualRange, Metler Toledo, Greifensee, Switzerland) into 5mm x 9mm tin capsules (Costech Analystycal Technologies, Inc., Valencia, CA, USA). Prepared capsules were shipped for analysis to the Stable Isotope Core Laboratory at Washington State University. Samples were analyzed using an elemental analyzer (ECS 4010, Costech Analytical, Valencia, CA, USA) coupled with a continuous flow isotope ratio mass spectrometer (Delta PlusXP Thermofinnigan, Bremen). Carbon and nitrogen isotope ratios were determined using the standard Vienna PeeDee belemnite (VPDB) and the values reported in “delta” notations as δ values in permil (‰) as described in Equation 3 and 4 respectively:
Equation 3
Equation 4
Statistical analysis
The experiment with rootstocks and scions was a randomized complete block design (RCBD) containing rootstocks (N=4) and scion (N=2) as factors with three blocks and three replicates of each rootstock-scion combination for each block. The experiment with non-grafted plants were also arranged as a RCBD with only rootstock (N=4) as a factor with three blocks and six replicates for each rootstock. Grafted and non-grafted plants were analyzed as separate experiments. Data were analyzed for effects of rootstocks and scions using two-way analysis of variance (ANOVA) on SAS 9.4 PROC GLM software (SAS Campus Drive Cary, NC, USA) for shoot length, net carbon assimilation, stomatal conductance, transpiration rate, midday stem water potential and δ13C, δ18O and δ15N. All treatment means were separated using Tukey`s means separation test with a confidence limit of 95%. Although cultivar was a factor in this statistical model, there were no interactions among cultivar and rootstock and, therefore, to focus on rootstock relations, scion cultivar effects were pooled and presented together. Linear regression was used to explore the relationships between variables and figures were prepared using OriginPro 2021 Data Analysis and Graphing Software (OriginLab Corporation, MA, USA).
Results
Water relations, gas exchange, and N content
Midday stem water potential (Ψm) significantly varied among rootstocks even under hydroponic conditions. For grafted plants, Ψm ranged from -0.68 MPa for G.890 to -0.84 MPa for B.9 (P< 0.05) (Figure 3; Table 2). M.9, G.41 and G.890 were not significantly different from one another. Similar patterns were also observed for stomatal conductance between G.890 and B.9. G.890 had higher stomatal conductance compared to B.9 (P<0.001) (Figure 3). For non-grafted trees, differences among rootstocks in stem water potential and stomatal conductance were smaller than for grafted trees. For ungrafted trees, M.9 had lower Ψm compared to B.9 (P = 0.05) (Figure 3). Moreover, M.9 had higher stomatal conductance than G.41 (P< 0.05) (Figure 3). Nitrogen content was highest for G.890 and lowest for B.9 with M.9 and G.41 with moderate N content when ungrafted (Figure 4). However, grafting dampened the effect of rootstock on leaf N content. There were no differences among rootstocks when they had a scion grafted onto them (P>0.05).
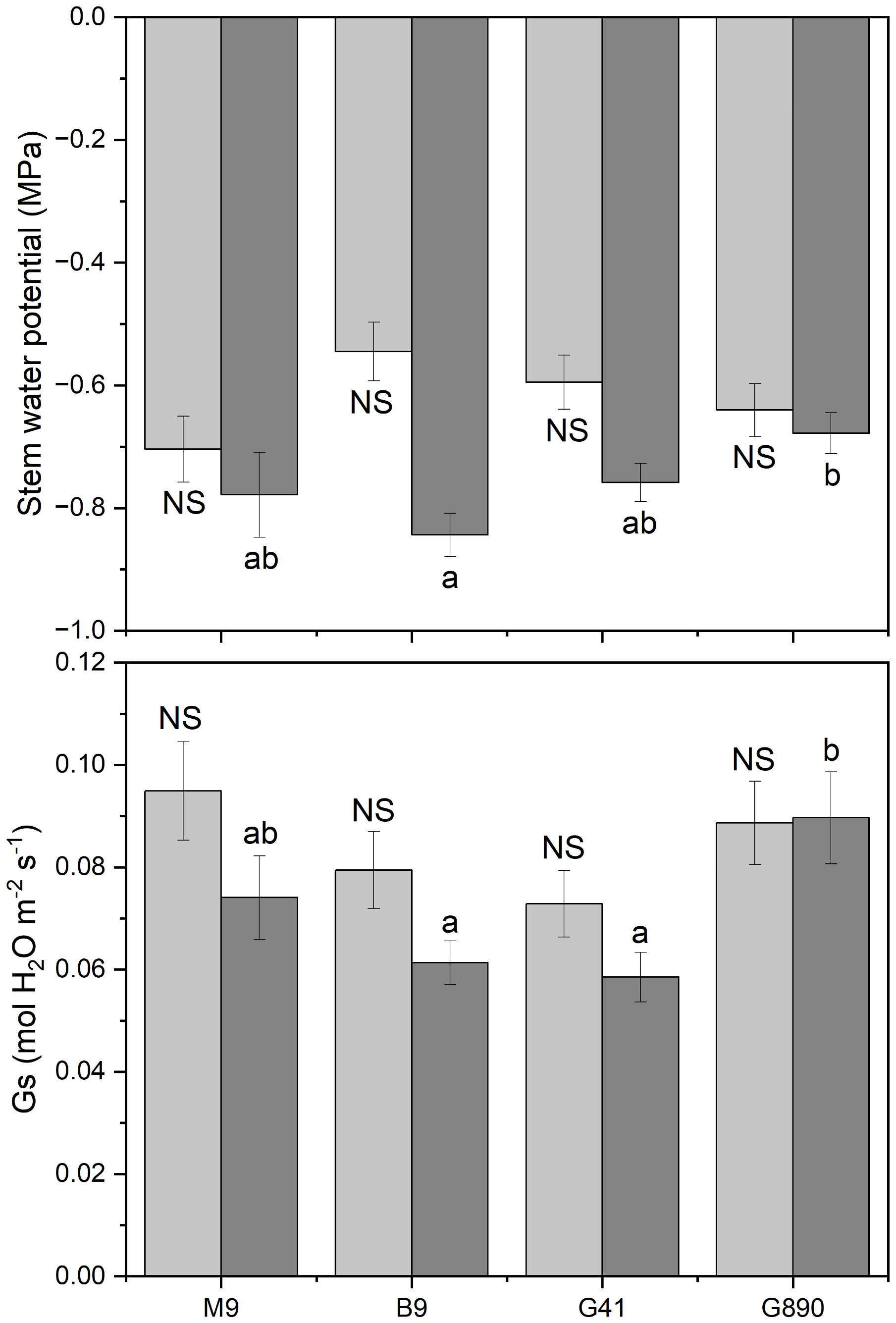
Figure 3 Stem water potential and stomatal conductance for non-grafted trees (light grey) and grafted trees (dark grey) for B.9, M.9, G.41 and G.890 rootstocks measured. Error bars denote standard error (N=3). Large and small letters indicate significance differences among means for ungrafted and grafted rootstocks, respectively, determined using a Tukey`s HSD mean separation test (α=0.05). ns indicates that the means are not significantly different.
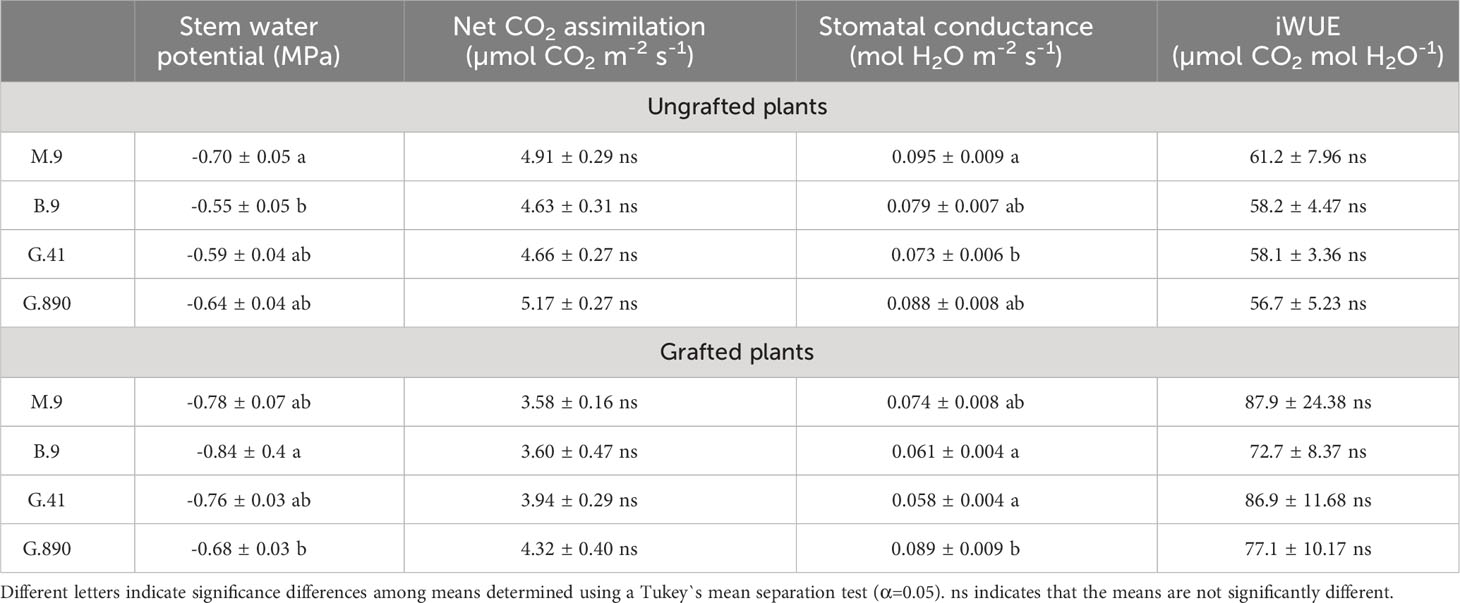
Table 2 Mean stem water potential (MPa), CO2 assimilation (µmol CO2 m-2 s-1), Stomatal conductance (mol H2O m-2 s-1) and Intrinsic Water use efficiency (iWUE; µmol CO2 mol H2O-1) ( ± SEM; N=6) for apple trees on M.9, B.9, G.41 and G.890 rootstocks.
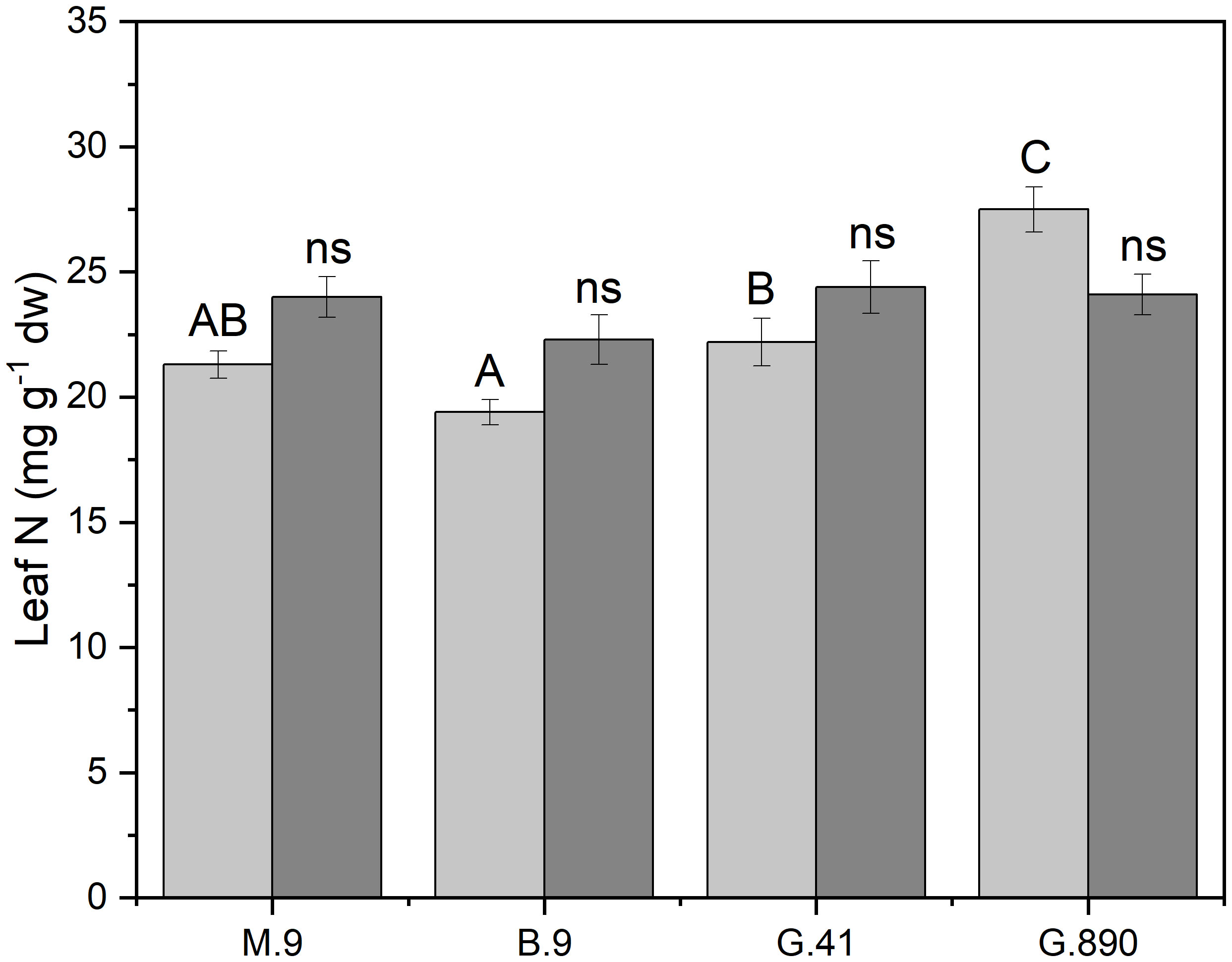
Figure 4 Leaf nitrogen content (mg g-1 dw) non-grafted trees (light grey) and grafted trees (dark grey) for B.9, M.9, G.41 and G.890 rootstocks measured. Error bars denote standard error (N=3). Large and small letters indicate significance differences among means for ungrafted and grafted rootstocks, respectively, determined using a Tukey`s HSD mean separation test (α=0.05). ns indicates that the means are not significantly different.
Tree growth and leaf, stem and root δ13C, δ18O, and δ15N
Whether a scion was grafted or left ungrafted, rootstocks strongly mediated shoot length (Figure 5). M.9 had the shortest shoot length among all rootstocks with an average shoot length of 25.8 cm for ungrafted trees (P< 0.001). Mean shoot length for ungrafted G.890, G.41 and B.9 were not significantly different. The same rankings for shoot length among rootstocks were also observed in grafted trees. Shoot length of M.9 was the shortest among all rootstocks with an average of 21.9 cm (P<0.01) and G.890, G.41 and B.9 did not differ statistically. However, for both grafted and ungrafted trees, G.890 had more shoot growth compared to the other rootstocks (Figure 5).
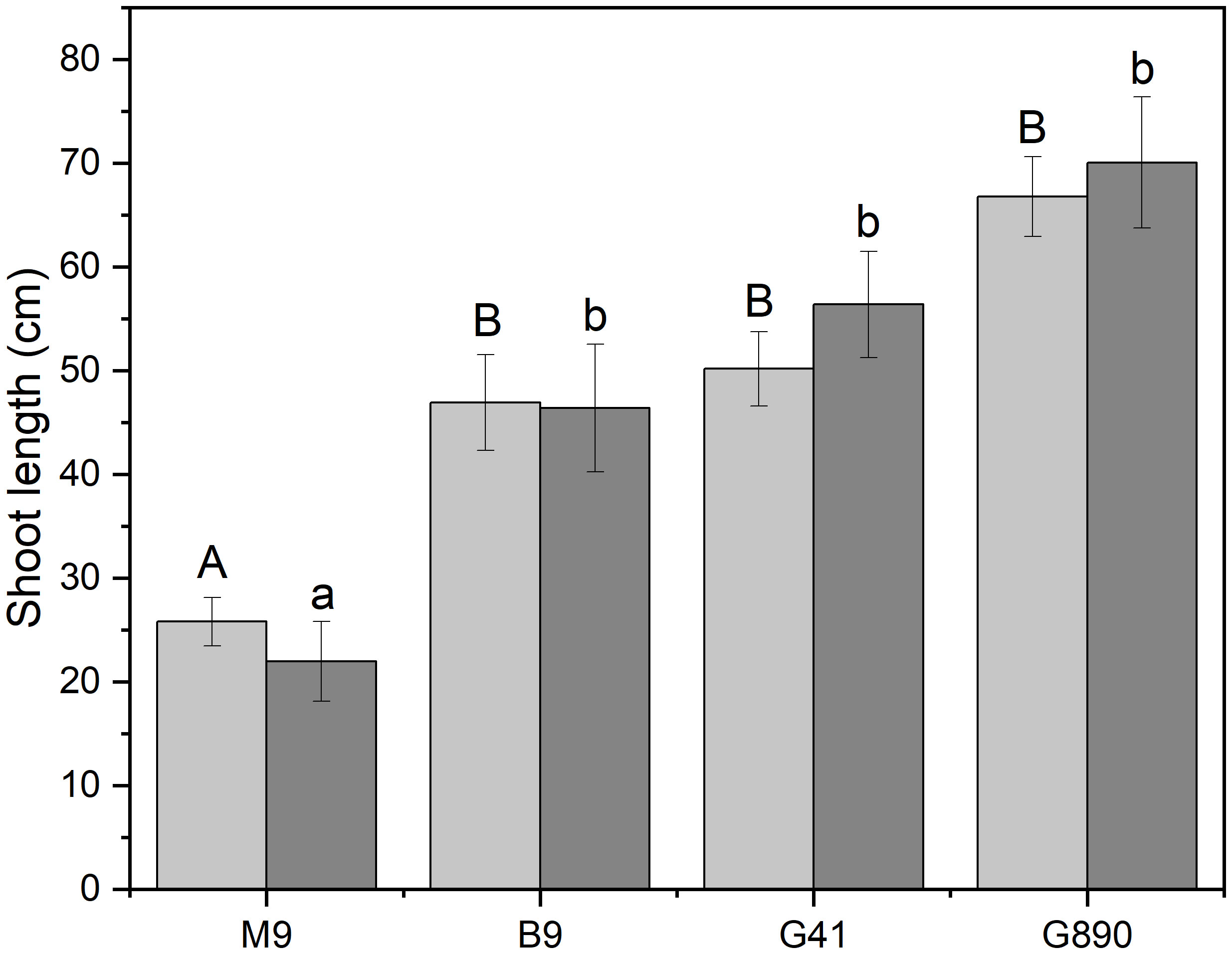
Figure 5 Shoot length for non-grafted trees (light grey) and grafted trees (dark grey) for B.9, M.9, G.41 and G.890 rootstocks. Error bars denote standard error (N=3). Large and small letters indicate significance differences among means for ungrafted and grafted rootstocks, respectively, determined using a Tukey`s HSD mean separation test (α=0.05).
Leaf δ13C was not different among rootstocks when trees were grafted (Figure 6). However, when trees were left ungrafted, mean leaf δ13C ranged from -30 to -32 ‰. Leaf δ13C was more enriched for M.9 and B.9 and was most depleted for G.890. The same patterns were observed for stems and roots. Stem δ13C ranged from -27 to -30 ‰ with G.41 and G.890 more depleted compared to M.9 and B.9. Similarly, root δ13C averaged between -28 to -30 ‰ and G.890 and G.41 were more depleted compared to M.9 and B.9. Interestingly, root δ13C for grafted trees was not statistically different among rootstocks. However, stem δ13C of grafted trees, which belonged to the scion, was like those of ungrafted trees with M.9 and B.9 being more depleted compared to G.890. Moreover, stem δ13C was more enriched than leaf δ13C for both grafted and ungrafted trees and was strongly affected by rootstocks (Table 3). Similarly, the same effect can be observed in the positive correlation between leaf and stem δ13C for ungrafted and grafted plants (P<0.001; r = 0.605; P< 0.05, r = 0.353) respectively (Figure 7). Overall, grafting appeared to dampen the effects of rootstock on both leaf and stem isotope composition, but not roots. The differences between the rootstocks with the most and least enriched leaf and stem δ13C were greater when rootstocks remained ungrafted. Roots δ13C was also correlated positively with leaf δ13C (P<0.001; r = 0.596; P< 0.05, r = 0.383; Figure 7) and stems δ13C (P<0.001; r = 0.793; P< 0.05, r = 0.416; Figure 8).
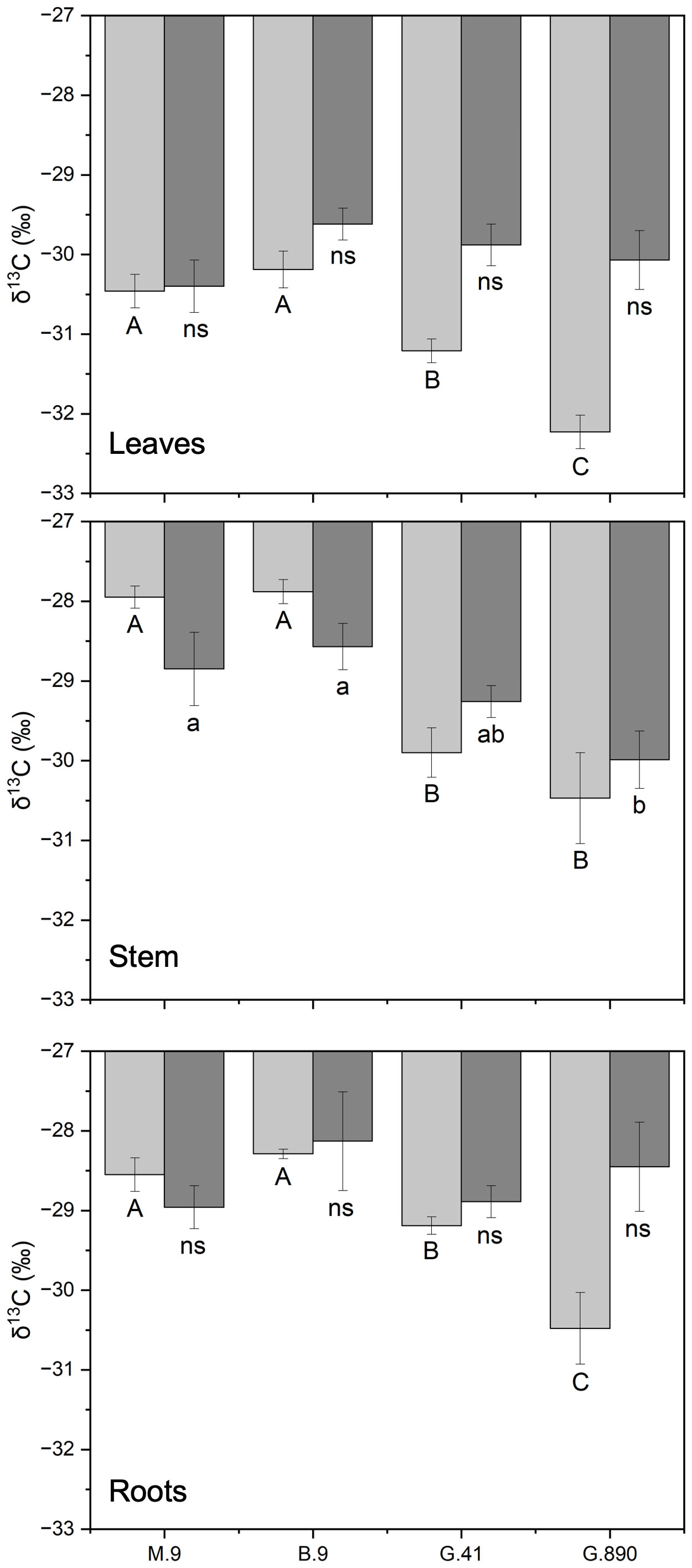
Figure 6 δ13C of non-grafted (light grey bars) and grafted trees (dark grey bars) for leaves, stems and roots for B.9, M.9, G.41 and G.890 rootstocks. Error bars denote standard error (N=3). Large and small letters indicate significance differences among means for ungrafted and grafted rootstocks, respectively, determined using a Tukey`s HSD mean separation test (α=0.05). ns indicates that the means are not significantly different.
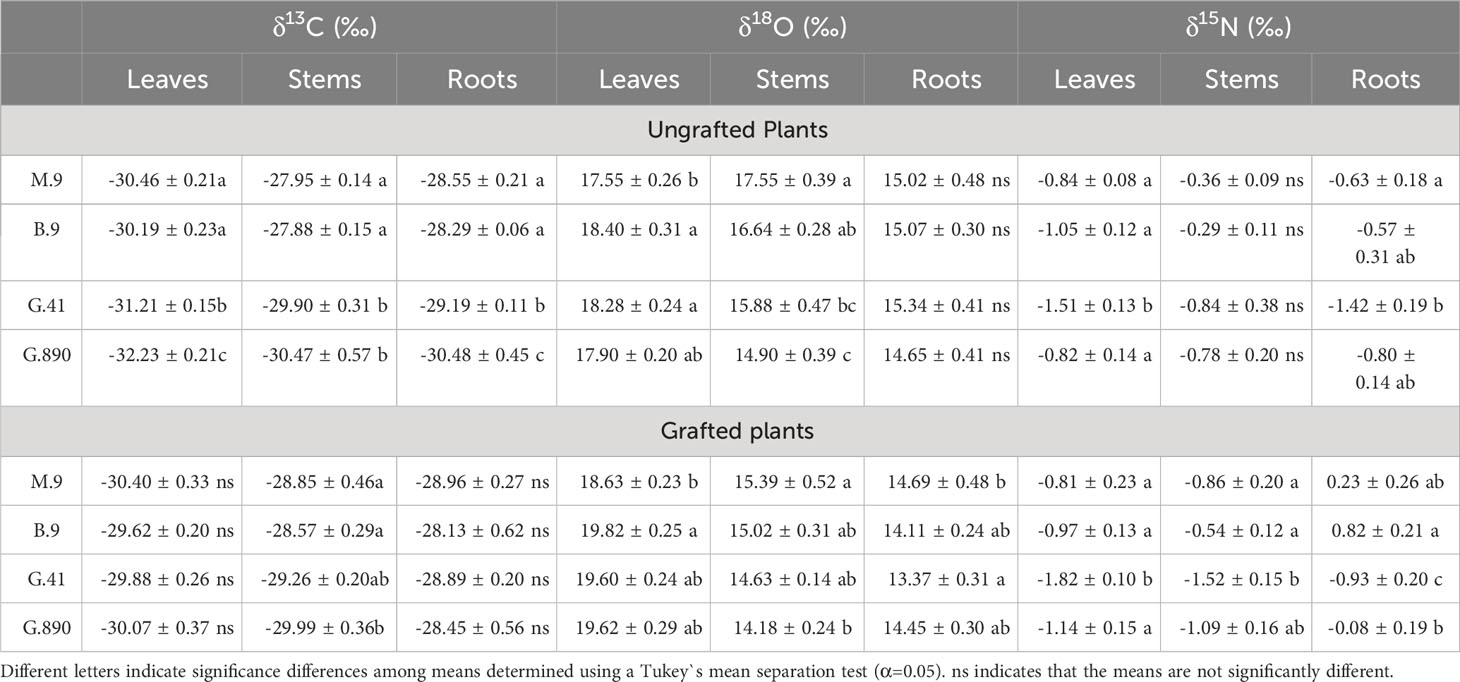
Table 3 Mean carbon (δ13C), oxygen (δ18O), and nitrogen (δ15N) isotope composition ( ± SEM, N=6) for apple trees on B.9, M.9, G.41 and G.890 rootstocks.
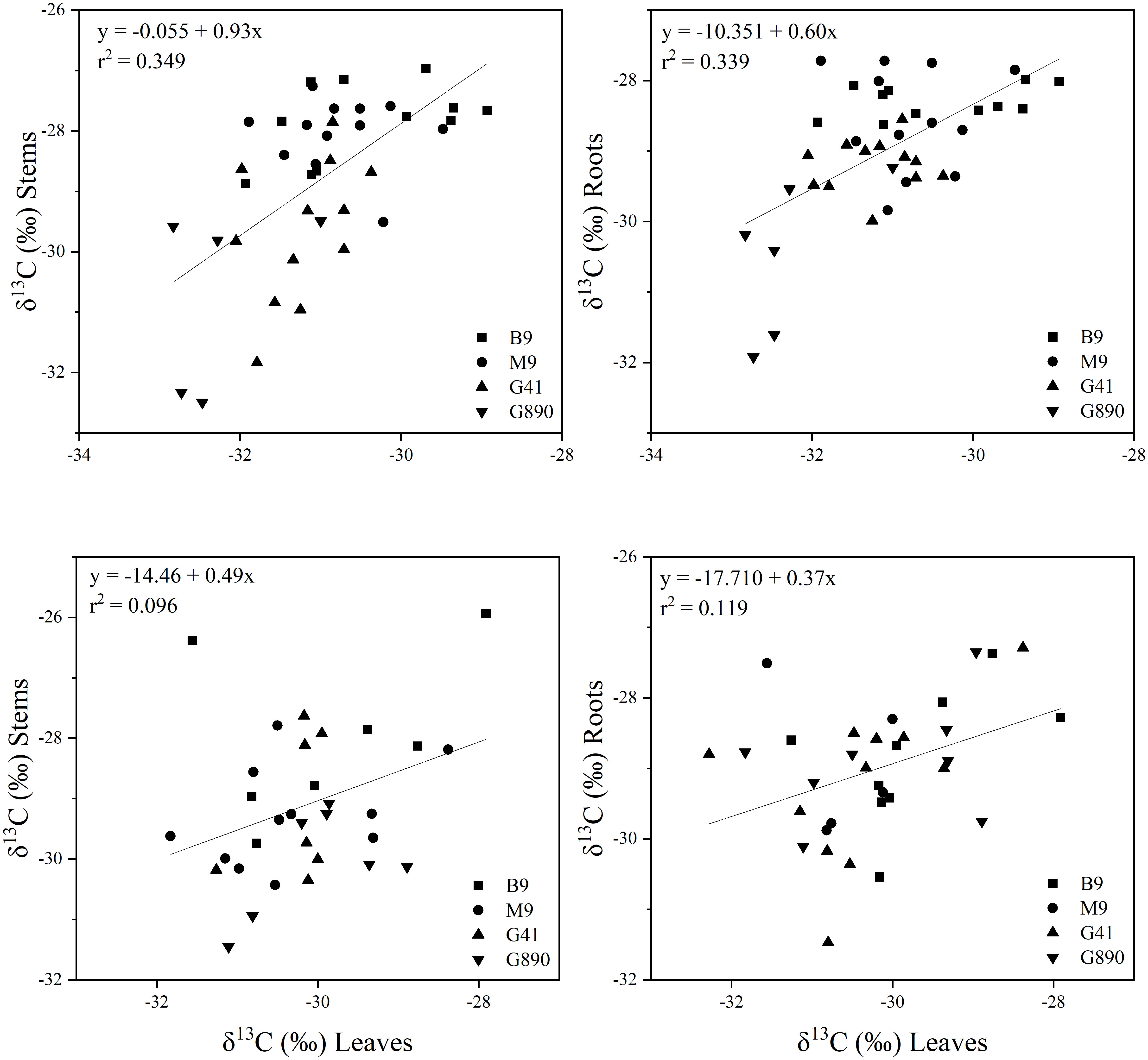
Figure 7 Top graphs: the relationship between δ13C leaves and stems (r= 0.605; P<0.001) (Top left) and δ13C leaves and roots (r = 0.596; P<0.001) (Top right) for non-grafted trees and between δ13C leaves and stems (r = 0.596; P<0.001) (Bottom left) and δ13C leaves and roots (r = 0.383; P<0.05) (Bottom right) for grafted trees for B.9, M.9, G.41 and G.890 rootstocks. The lines indicate the best-fit linear relationship for the combined data points.
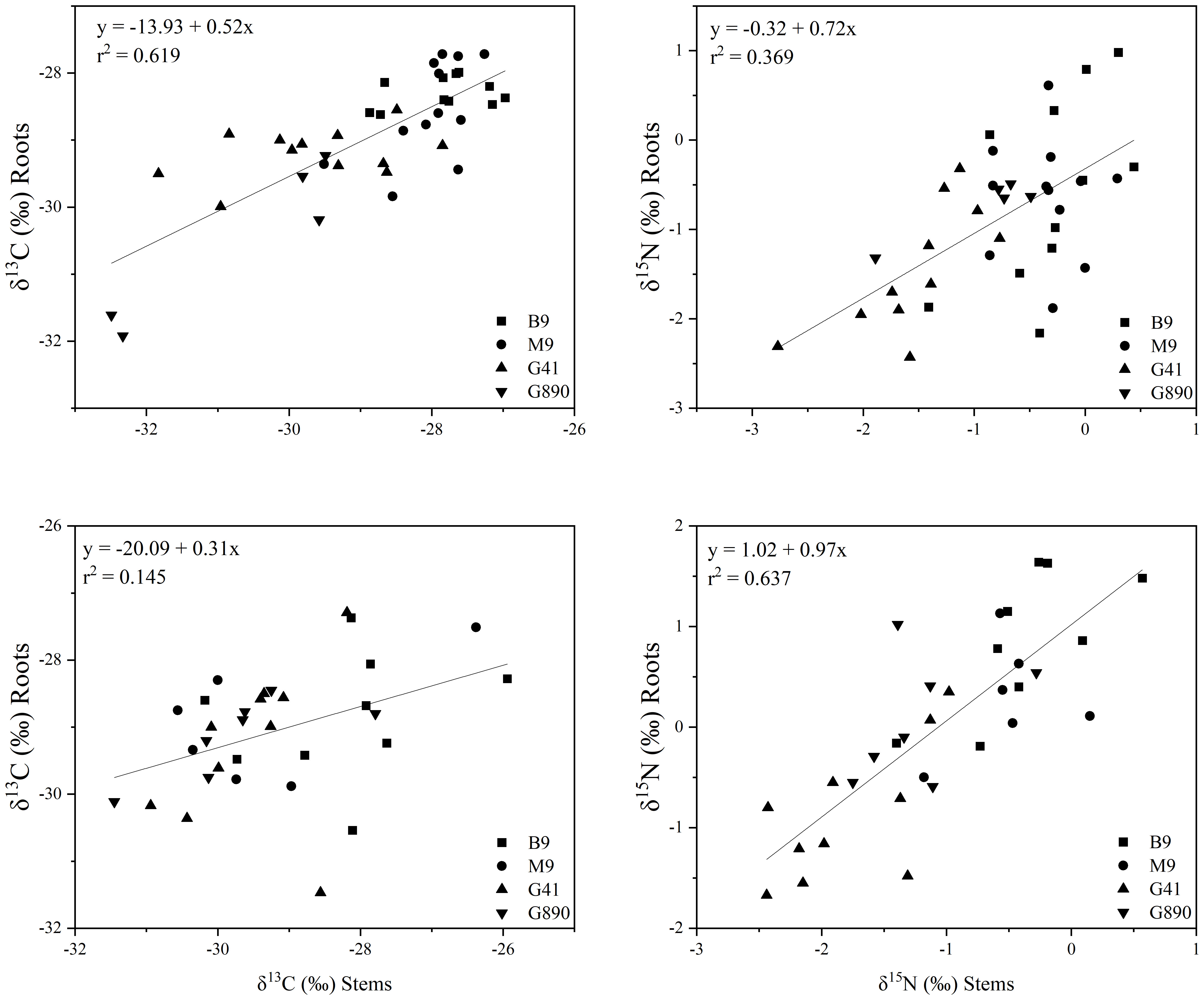
Figure 8 The relationship between δ13C stems and roots (r = 0.793; P<0.001) (Top left) and δ15N stems and roots (r = 0.613; P<0.001) (Top right) for non-grafted trees. Bottom graphs: relationship between δ13C stems and roots (r = 0.416; P<0.05) (Bottom left) and δ15N stems and roots (r = 0.416; P<0.05) (Bottom right) for grafted trees for B.9, M.9, G.41 and G.890 rootstocks. The lines indicate the best-fit linear relationship for the combined data points.
Leaf biomass δ18O was also significantly affected by rootstock. These differences were consistent whether the rootstock was grafted or not. For non-grafted trees, leaf δ18O was the lowest for M.9. (Figure 9). The same trend was observed for grafted trees with M.9 lower than B.9, G.41, and G.890 (P< 0.05). Differences in stem δ18O were also observed among rootstocks. δ18O for G.890 was lower compared to M.9, B.9 and G.41 for ungrafted trees (P< 0.001). Similar differences were also observed for grafted trees where G.890 was more depleted compared to B.9 (P = 0.01) (Figure 9). Root δ18O was not different among rootstocks when trees were grafted (Figure 9). However, when trees were left ungrafted, mean root δ18O ranged from 13 to 15 ‰ and was highest for M.9 and G.890 (Figure 9). For δ18O for leaves and stems, leaves were more enriched than stems for both ungrafted and grafted plants, contrary to what was observed for δ13C.
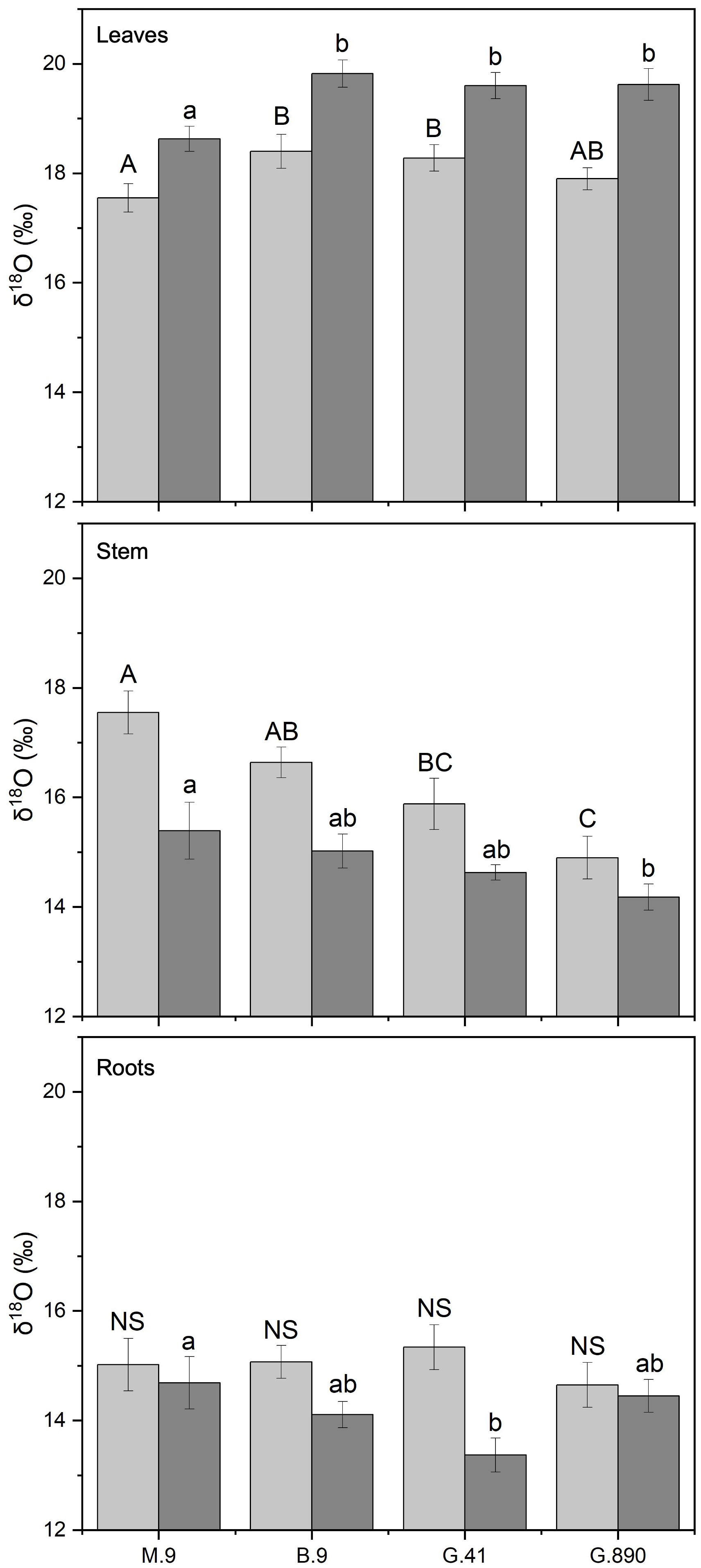
Figure 9 δ18O of non-grafted (light grey bars) and grafted trees (dark grey bars) for leaves, stems and roots for B.9, M.9, G.41 and G.890 rootstocks. Error bars denote standard error (N=3). Large and small letters indicate significance differences among means for ungrafted and grafted rootstocks, respectively, determined using a Tukey`s HSD mean separation test (α=0.05). ns indicates that the means are not significantly different.
Differences in δ15N were consistently different among rootstocks for both grafted and ungrafted trees. Leaf δ15N for G.41 was significantly more depleted compared to G.890, M.9 and B.9 for both grafted (P< 0.001) and ungrafted trees (P<0.001) (Figure 10). Leaf δ15N for G.41 was approximately 0.8‰ less than other rootstocks. G.41 also showed remarkably negative values compared to M.9, B.9 and G.890, especially for grafted trees (Figure 10).
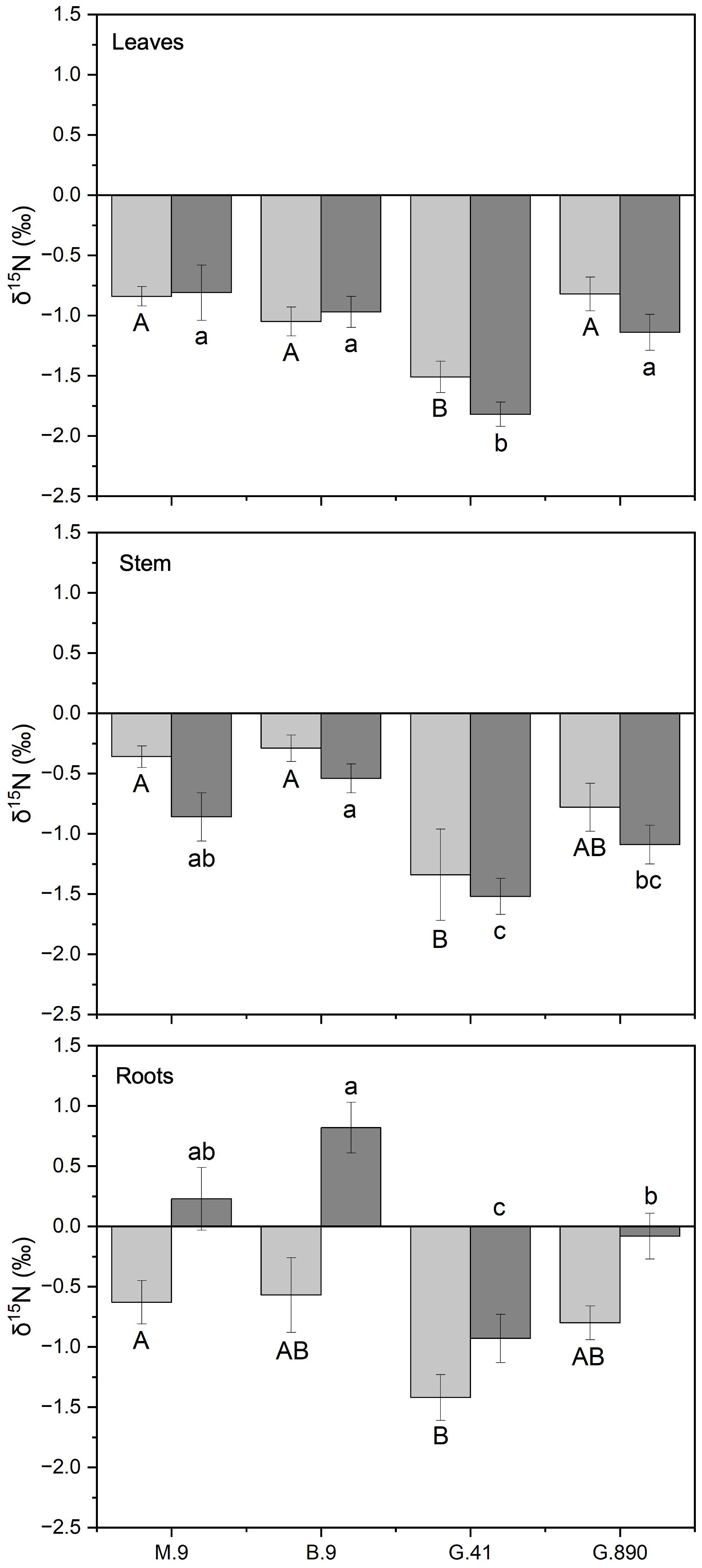
Figure 10 δ15N of non-grafted (light grey bars) and grafted trees (dark grey bars) for leaves, stems and roots for B.9, M.9, G.41 and G.890 rootstocks. Error bars denote standard error (N=3). Large and small letters indicate significance differences among means for ungrafted and grafted rootstocks, respectively, determined using a Tukey`s HSD mean separation test (α=0.05). ns indicates that the means are not significantly different.
Leaf δ15N correlated positively with stem δ15N for ungrafted and grafted plants (P<0.001; r = 0.552; P< 0.001, r = 0.743; Figure 11) and with root δ15N (P<0.001; r = 0.713; P< 0.001, r = 0.707). There were no patterns of enrichment of stem δ15N compared to leaf δ15N like there was for δ13C. Stem δ15N also correlated positively with root δ15N for ungrafted and grafted plants (P<0.01; r = 0.442; P< 0.05, r = 0.416) respectively (Figure 11).
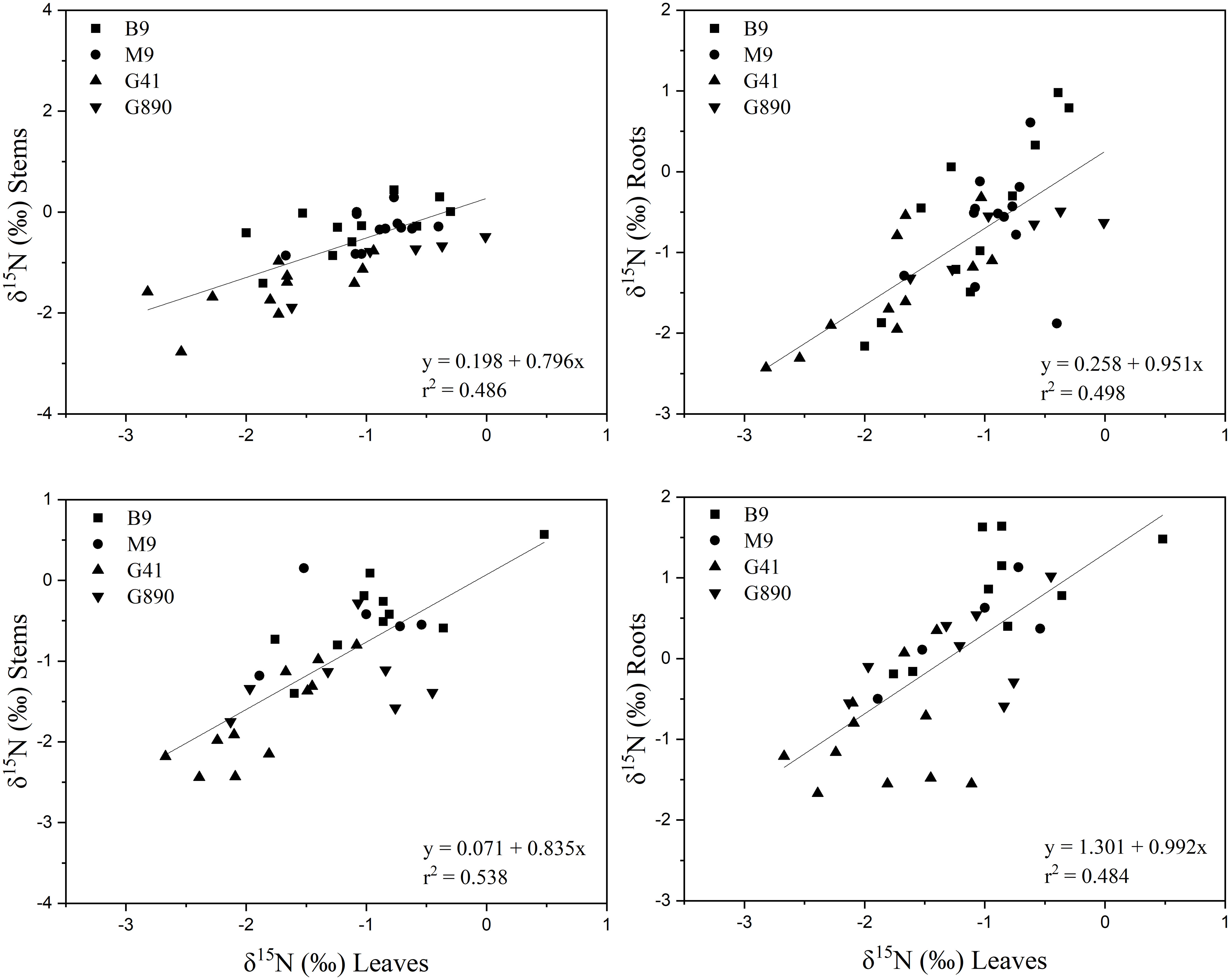
Figure 11 Top graphs: the relationship between δ15N leaves and stems (r = 0.552; P<0.001)(left) and δ15N leaves and roots (r = 0.713; P<0.001)(right) for non-grafted trees. Bottom graphs: the relationship between δ13C leaves and stems (r = 0.743; P<0.001)(left) and δ13C leaves and roots (r = 0.707; P<0.001) (right) for grafted trees for B.9, M.9, G.41 and G.890 rootstocks. The lines indicate the best-fit linear relationship for the combined data points.
Discussion
Here, we report a clear effect of rootstocks on above ground apple growth and physiology under controlled greenhouse conditions. These differences were consistent whether a scion was grafted to the rootstock or the rootstock was left ungrafted. There were strong differences in not only δ13C, but also δ18O and δ15N. Rootstock differences in functional physiology and δ13C and δ18O corresponded to known differences in vigor among the rootstocks used in these experiments.
G.890 was the most vigorous of the four rootstocks used in this study which is consistent with previous rootstock research (Robinson et al., 2012; Kviklys et al., 2014; Valverdi and Kalcsits, 2021). B.9 was expected to have the lowest vigor (Czynczyk et al., 2000; Xu and Ediger, 2021; Casagrande Biasuz and Kalcsits, 2022). However, M.9 was significantly smaller than the other rootstocks. These results aligned with other studies that reported similar patterns when grafted with ‘Goldspur Delicious’, ‘Redspur Delicious’ progeny (Tworkoski and Miller, 2007), ‘Golden Delicious’, ‘Honeycrisp’, and ‘Fuji’ cultivars (Dallabetta et al, 2021). Slow growth has been reported for M.9 under greenhouse conditions (Valverdi et al., 2019). In this study, root health also appeared to be affected compared to other rootstocks (data not shown). That may have an impact on growth that was inconsistent with dwarfing characteristics observed under field conditions.
Like what was observed previously (Biasuz and Kalcsits, 2022), δ13C was related to shoot vigor. Carbon isotope composition can indicate differences in intrinsic water-use efficiency (Gonçalves et al, 2007; Seibt et al, 2008; Martínez-Alcántara et al, 2013; Foster et al, 2017; Xu and Ediger, 2021). Depleted δ13C can indiacate elevated stomatal conductance (Farquhar et al, 1989; Arndt and Wanek, 2002; Liu et al, 2012; Cernusak et al, 2013). However, according to the dual isotope model (Scheidegger et al., 2000), uncoupling of δ13C and δ18O can indicate that stomatal conductance is not responsible for variations in δ13C. Poor agreement between δ13C and δ18O in leaves suggests that stomatal conductance may not be the key driver of variation in δ13C and that another trait imparted by the rootstock itself may have contributed to observed differences in δ13C. In support, we observed depleted δ13C non-grafted plants for G.890 and enriched values for M.9 and B.9. However, these differences in δ13C were not observed for grafted plants with the same scion.
Stem δ13C more closely reflected differences among rootstocks in shoot vigor than leaf δ13C. Moreover, stems were enriched compared to leaves for δ13C. Cernusak et al. (2009) suggested that post-photosynthetic carbon fractionation may be caused by seasonal separation of growth (Cernusak et al., 2009). These patterns of enrichment were also observed for apple under field conditions (Kalcsits et al., 2022). Under field conditions, development and growth of leaves occur in spring with plentiful soil water content. The development and growth of stem tissue occurs later when warmer temperatures and elevated VPD reduce stomatal conductance at leaf level resulting to a less carbon isotope discrimination (Cernusak et al., 2009). Moreover, isotope fractionation can occur in leaves and may change during leaf development. Mature leaves can be the source for carbon used to synthesize heterotrophic tissues like stems which become enriched relative to newly developed leaves on the terminal end of the stem (Cernusak et al., 2009; Werner and Gessler, 2011).
Stem δ18O closely followed the same differences observed for δ13C. For both grafted and non-grafted plants, we observed depleted values for vigorous G.890 and enriched values for M.9 and B.9 indicating differences in water and carbon assimilation in leaves. Consequently, stem δ13C was enriched and δ18O was depleted compared to leaves. However, these differences were only observed for higher vigor rootstocks like G.41 and G.890 and were not observed for M.9 and B.9. Not only these effects were consistent for both grafted and ungrafted trees, G.890 had depleted stem δ13C and enriched δ18O values for both grafted and ungrafted trees, which demonstrates that the impact of rootstock on whole plant water relations can be independent of the scion or the presence of a graft union.
G.41 had significantly more depleted δ15N compared to the other two rootstocks and these results were consistent between grafted and ungrafted trees. Evans (2001) and Kalcsits et al. (2014) have described the physiological mechanisms underlying variation in nitrogen isotope discrimination. The δ15N (‰) of each source nitrogen used here was -0.73‰, 2.51‰, and 0.03‰ for calcium nitrate, ammonium phosphate, and potassium nitrate, respectively. This experiment was close to an open-source experiment like Kalcsits and Guy (2016) but with smaller nitrogen supply. Therefore, according to proposed nitrogen isotope discrimination models, more depleted leaf and stem δ15N could indicate higher efflux from the roots. However, more research will be required to delineate the underlying reasons for different δ15N for G.41 compared to the other three rootstocks in this study.
Water limitations imposed by dwarfing rootstocks have been previously reported in woody plants (Bongi et al., 1994; Padgett-Johnson et al., 2000; Gibberd et al., 2001; Cohen and Naor, 2002; Ma et al., 2010; Liu et al., 2012; Galbignani et al., 2016; Peccoux et al., 2018; Villalobos-González et al., 2019; Frioni et al., 2020; Reig et al., 2022). However, close associations between stem water potential and gas exchange were not always observed. Under greenhouse conditions in this study, there were no consistent trends in the association between rootstock-induced vigor and stomatal conductance or stem water potential. The controlled conditions of this study may have dulled previously reported rootstock responses (Casagrande Biasuz and Kalcsits, 2022). Other studies had compared water potential among dwarfing and vigorous rootstocks under different soil conditions. More dwarfing rootstocks generally show more negative stem water potential than the more vigorous rootstocks (Olien and Lakso, 1986). Differences on stem water potential of dwarfing and vigorous rootstocks occur during the day between pre-dawn and midday (Basile et al., 2003). ‘Fuji’ showed lower water potential on M.9 than on MM.111 at pre-dawn, however, under drought conditions both ‘Gala’ and ‘Fuji’ had less negative water potential on M.9 than on MM.111 (Tworkoski and Fazio, 2016). Trees under well-watered conditions showed higher leaf water potential for dwarfing rootstocks and more negative for vigorous rootstocks and at the same time, stomatal conductance was greater for more vigorous rootstocks (Atkinson et al., 2000). Basile et al. (2003) and Giulivo et al. (1984) observed higher water potentials in trees grafted on more dwarfing rootstocks compared to more vigorous rootstocks. Nevertheless, the latter showed higher stomatal conductance associated with higher use of water on trees grafted on vigorous while those on dwarfing were more conservative. Stomatal insensitivity to decreasing leaf water potential provides a means by which growth on vigorous rootstocks is maximized until water supplies are depleted (Atkinson et al., 2000).
Rootstocks can modulate water relations through an impact on the soil-plant-atmosphere continuum. Here, we observed similarities in differences in leaf gas exchange and isotope composition among rootstocks for both grafted and ungrafted plants. Even though graft union may affect the growth and development of plants in some cases, the consistent differences in above ground growth between grafted and non-grafted plants in this study highlighted that roots are a significant contributor to tree related vigor, gas exchange and isotope composition that may be independent of the graft union. Even though Atkinson and Else (2001) observed higher resistance to water flow through the graft union on some dwarfing rootstocks like M.27 compared to the vigorous rootstock MM.106 and M.9 being an intermediate between these two rootstocks, this may not be the case for other studies. Carlson and Oh (1975) reported dwarfing for M.8 rootstocks used as an interstem compared to when M.8 was not used. These reported observations would indicate that physiological limitation in the rootstock itself or the graft union rather than root-based traits contribute to limitations in tree vigor. Cohen et al. (2007) and Adams et al. (2018) both observed a minor resistance to water flow at the graft union. Fresh grafted trees may be more resistant immediately after grafting due to wound formation, but after two months of maturation, trees did not show any sustained hydraulic resistance at the graft union (Gascó et al., 2007). In general, studies on mature trees conclude that the graft union does not play a major role in the dwarfing effect (Nardini et al., 2006; Else et al., 2018).
Conclusions
Here, both grafted and ungrafted apple trees highlighted the effect of rootstock on above ground growth and gas exchange and its consequential effects on carbon, oxygen, and nitrogen isotope discrimination under controlled hydroponic conditions. This work separated out confounding effects that can sometimes be observed in the field. Although the graft union may affect scion vigor in some cases, rootstock differences in isotope composition were also observed in ungrafted trees indicating that root-based traits are contributing to changes in plant water status and leaf functional physiology. Even under controlled conditions, these effects were associated with rootstock induced shoot vigor and were consistent between grafted and ungrafted trees. These results have implications in understanding the contributions of potential dwarfing mechanisms for apple rootstocks and their effect on gas exchange and water relations with longer term implications for selecting rootstocks that are more tolerant of abiotic stresses.
Data availability statement
The raw data supporting the conclusions of this article will be made available by the authors, without undue reservation.
Author contributions
EC: Conceptualization, Data curation, Formal analysis, Investigation, Methodology, Software, Visualization, Writing – original draft, Writing – review & editing. LK: Conceptualization, Data curation, Funding acquisition, Methodology, Project administration, Resources, Supervision, Validation, Visualization, Writing – original draft, Writing – review & editing.
Funding
The author(s) declare financial support was received for the research, authorship, and/or publication of this article. This research was funded by the U.S. Department of Agriculture (USDA), National Institute of Food and Agriculture-Specialty Crop Research Initiative project “AppleRoot2Fruit: Accelerating the development, evaluation, and adoption of new apple rootstocks” (2016-51181-25406). LK was also supported by the USDA National Institute of Food and Agriculture, Hatch/State project 1014919 and Hatch/Multistate project 1011062.
Acknowledgments
The authors would like to extend appreciation to all members of the Kalcsits lab for help and support during these experiments.
Conflict of interest
The authors declare that the research was conducted in the absence of any commercial or financial relationships that could be construed as a potential conflict of interest.
Publisher’s note
All claims expressed in this article are solely those of the authors and do not necessarily represent those of their affiliated organizations, or those of the publisher, the editors and the reviewers. Any product that may be evaluated in this article, or claim that may be made by its manufacturer, is not guaranteed or endorsed by the publisher.
References
Adams, S., Lordan, J., Fazio, G., Bugbee, B., Francescatto, P., Robinson, T. L., et al. (2018). Effect of scion and graft type on transpiration, hydraulic resistance and xylem hormone profile of apples grafted on Geneva® 41 and M. 9-NIC™ 29 rootstocks. Scientia Hortic. 227, 213–222. doi: 10.1016/j.scienta.2017.09.052
Arndt, S. K., Wanek, W. (2002). Use of decreasing foliar carbon isotope discrimination during water limitation as a carbon tracer to study whole plant carbon allocation. Plant Cell Environ. 25 (5), 609–616. doi: 10.1046/j.1365-3040.2002.00838.x
Atkinson, C., Else, M. (2001). Understanding how rootstocks dwarf fruit trees. Compact Fruit Tree 34 (2), 46–49.
Atkinson, C. J., Else, M. A., Taylor, L., Dover, C. J. (2003). Root and stem hydraulic conductivity as determinants of growth potential in grafted trees of apple (Malus pumila Mill.). J. Exp. Bot. 54 (385), 1221–1229. doi: 10.1093/jxb/erg132
Atkinson, C. J., Policarpo, M., Webster, A. D., Kingswell, G. (2000). Drought tolerance of clonal Malus determined from measurements of stomatal conductance and leaf water potential. Tree Physiol. 20 (8), 557–563. doi: 10.1093/treephys/20.8.557
Barbour, M. M. (2007). Stable oxygen isotope composition of plant tissue: a review. Funct. Plant Biol. 34 (2), 83–94. doi: 10.1071/FP06228
Basile, B., Marsal, J., DeJong, T. M. (2003). Daily shoot extension growth of peach trees growing on rootstocks that reduce scion growth is related to daily dynamics of stem water potential. Tree Physiol. 23 (10), 695–704. doi: 10.1093/treephys/23.10.695
Bauerle, T. L., Centinari, M., Bauerle, W. L. (2011). Shifts in xylem vessel diameter and embolisms in grafted apple trees of differing rootstock growth potential in response to drought. Planta 234 (5), 1045–1054. doi: 10.1007/s00425-011-1460-6
Beakbane, A. B., Thompson, E. C. (1940). Anatomical studies of stems and roots of hardy fruit trees II. The internal structure of the roots of some vigorous and some dwarfing apple rootstocks, and the correlation of structure with vigour. J. Pom Hort Sci. 17 (2), 141–149. doi: 10.1080/03683621.1947.11513669
Bongi, G., Palliotti, A., Rocchi, P., Roselli, G. (1994). Evaluation of WUE in peach grafted on different interspecific hybrid rootstocks. Plant Physiol. Biochem. 32, 149–157.
Carlson, R. F., Oh, S. D. (1975). Influence of interstem lengths of M.8 clone Malus sylvestris Mill, on growth, precocity, yield, and spacing of 2 apple cultivars. J. Amer. Soc Hortic. Sci. 100 (5), 450–452. doi: 10.21273/JASHS.100.5.450
Casagrande Biasuz, E., Kalcsits, L. A. (2022). Apple rootstocks affect functional leaf traits with consequential effects on carbon isotope composition and vegetative vigor. AoB Plants 14 (4). doi: 10.1093/aobpla/plac020
Cernusak, L. A. (2020). Gas exchange and water-use efficiency in plant canopies. Plant Biol. 22, 52–67.). doi: 10.1111/plb.12939
Cernusak, L. A., Tcherkez, G., Keitel, C., Cornwell, W. K., Santiago, L. S., Knohl, A., et al. (2009). Why are non-photosynthetic tissues generally 13C enriched compared with leaves in C3 plants? Review and synthesis of current hypotheses. Funct. Plant Biol. 36 (3), 199–213. doi: 10.1071/FP08216
Cernusak, L. A., Ubierna, N., Winter, K., Holtum, J. A., Marshall, J. D., Farquhar, G. D. (2013). Environmental and physiological determinants of carbon isotope discrimination in terrestrial plants. New Phytol. 200 (4), 950–965. doi: 10.1111/nph.12423
Cohen, S., Naor, A. (2002). The effect of three rootstocks on water use, canopy conductance and hydraulic parameters of apple trees and predicting canopy from hydraulic conductance. Plant Cell Environ. 25, 17–28. doi: 10.1046/j.1365-3040.2002.00795.x
Cohen, S., Naor, A., Bennink, J., Grava, A., Tyree, M. T. (2007). Hydraulic resistance components of mature apple trees on rootstocks of different vigors. J. Exp. Bot. 58, 4213–4224. doi: 10.1093/jxb/erm281
Czynczyk, A., Bielicki, P., Bartosiewicz, B. (2000). Testing new dwarfing apple rootstocks from Polish and foreign breeding programmes. Acta Hortic. 557, 83–90. doi: 10.17660/ActaHortic.2001.557.9
Dallabetta, N., Guerra, A., Pasqualini, J., Fazio, G. (2021). Performance of semi-dwarf apple rootstocks in two-dimensional training systems. HortScience 56 (2), 234–241. doi: 10.21273/HORTSCI15492-20
Else, M. A., Taylor, J. M., Young, S., Atkinson, C. J. (2018). The effect of the graft union on hormonal and ionic signalling between rootstocks and scions of grafted apple (Malus pumila L. Mill.). Environ. Exp. Bot. 156, 325–336. doi: 10.1016/j.envexpbot.2018.07.013
Evans, R. D. (2001). Physiological mechanisms influencing plant nitrogen isotope composition. Trends Plant Sci. 6 (3), 121–126. doi: 10.1016/S1360-1385(01)01889-1
Farquhar, G. D., Ehleringer, J. R., Hubick, K. T. (1989). Carbon isotope discrimination and photosynthesis. Ann. Rev. Plant Biol. 40 (1), 503–537. doi: 10.1146/annurev.pp.40.060189.002443
Foster, T. M., McAtee, P. A., Waite, C. N., Boldingh, H. L., McGhie, T. K. (2017). Apple dwarfing rootstocks exhibit an imbalance in carbohydrate allocation and reduced cell growth and metabolism. Hort Res. 4 (1), 1–13. doi: 10.1038/hortres.2017.9
Frioni, T., Biagioni, A., Squeri, C., Tombesi, S., Gatti, M. S., Poni, S. (2020). Grafting cv. Grechetto gentile vines to new M4 rootstock improves leaf gas exchange and water status as compared to commercial 1103P rootstock. Agronomy 10 (708), 1–14. doi: 10.3390/agronomy10050708
Galbignani, M., Merli, M., Magnanini, E., Bernizzoni, F., Talaverano, I., Gatti, M., et al. (2016). Gas exchange and water—Use efficiency of cv. Sangiovese grafted to rootstocks of varying water—Deficit tolerance. Irr Sci. 34, 105–116. doi: 10.1007/s00271-016-0490-z
Gascó, A., Nardini, A., Raimondo, F., Gortan, E., Motisi, A., Gullo, M. L., et al. (2007). Hydraulic kinetics of the graft union in different Olea europaea L. scion/rootstock combinations. Environ. Exp. Bot. 60 (2), 245–250. doi: 10.1016/j.envexpbot.2006.10.011
Gibberd, M. R., Walker, R. R., Blackmore, D. H., Condon, A. G. (2001). Transpiration efficiency and carbon-isotope discrimination of grapevines grown under well-watered conditions in either glasshouse or vineyard. Aust. J. Grape Wine R 7, 110–117. doi: 10.1111/j.1755-0238.2001.tb00197.x
Giulivo, C., Ponchia, G., Gianola, A., Pitacco, A. (1984). Effect of rootstock on water balance of Golden Delicious apple trees. Acta Hortic. 171, 399–404. doi: 10.17660/ActaHortic.1985.171.37
Gonçalves, B., Correia, C. M., Silva, A. P., Bacelar, E. A., Santos, A., Ferreira, H., et al. (2007). Variation in xylem structure and function in roots and stems of scion–rootstock combinations of sweet cherry tree (Prunus avium L.). Trees 21 (2), 121–130. doi: 10.1007/s00468-006-0102-2
Gregory, P. J., Atkinson, C. J., Bengough, A. G., Else, M. A., Fernández-Fernández, F., Harrison, R. J., et al. (2013). Contributions of roots and rootstocks to sustainable, intensified crop production. J. Exp. Bot. 64 (5), 1209–1222. doi: 10.1093/jxb/ers385
Hayat, F., Asghar, S., Yanmin, Z., Xue, T., Nawaz, M. A., Xu, X., et al. (2020). Rootstock induced vigour is associated with physiological, biochemical and molecular changes in ‘Red Fuji’ apple. Int. J. Agric. Biol. 24, 1823–1834. doi: 10.17957/IJAB/15.1627
Kalcsits, L. A., Buschhaus, H. A., Guy, R. D. (2014). Nitrogen isotope discrimination as an integrated measure of nitrogen fluxes, assimilation and allocation in plants. Physiol. Plant 151 (3), 293–304. doi: 10.1111/ppl.12167
Kalcsits, L. A., Guy, R. D. (2016). Variation in fluxes estimated from nitrogen isotope discrimination corresponds with independent measures of nitrogen flux in Populus balsamifera L. Plant Cell Environ. 39 (2), 310–319. doi: 10.1111/pce.12614
Kalcsits, L., Valverdi, N., Reid, M. (2022). Timing of water limitations affect source to sink differences in δ13C composition in apple. Acta Hortic. 1335, 437–444. doi: 10.17660/ActaHortic.2022.1335.54
Kviklys, D., Robinson, T. L., Fazio, G. (2014). Apple rootstock evaluation for apple replant disease. Act Hortic. 1130, 425–430. doi: 10.17221/71/2014-PSE
Liu, B. H., Cheng, L., Ma, F. W., Liang, D., Zou, Y. J. (2012). Influence of rootstock on drought response in young ‘Gale Gala’ apple (Malus domestica Borkh.) trees. J. Sci. Food Agric. 92, 2421–2427. doi: 10.1002/jsfa.5647
Lordan, J., Fazio, G., Francescatto, P., Robinson, T. L. (2017). Effects of apple (Malus × domestica) rootstocks on scion performance and hormone concentration. Sci. Hortic. 225, 96–105. doi: 10.1016/j.scienta.2017.06.050
Ma, X., Ma, F., Li, C., Mi, Y., Bai, T., Shu, H. (2010). Biomass accumulation, allocation, and water-use efficiency in 10 malus rootstocks under two watering regimes. Agroforestry Syst. 80 (2), 283–294. doi: 10.1007/s10457-010-9306-y
Marini, R. P., Fazio, G. (2018). Apple rootstocks: history, physiology, management, and breeding. Hortic. Rev. 45, 197–312. doi: 10.1002/9781119431077.ch6
Martínez-Alcántara, B., Rodriguez-Gamir, J., Martínez-Cuenca, M. R., Iglesias, D. J., Primo-Millo, E., Forner-Giner, M. A. (2013). Relationship between hydraulic conductance and citrus dwarfing by the Flying Dragon rootstock (Poncirus trifoliata L. Raft var. monstruosa). Trees 27 (3), 629–638. doi: 10.1016/j.scienta.2014.07.032
Melnyk, C. W., Meyerowitz, E. M. (2015). Plant grafting. Curr. Biol. 25 (5), 183–188. doi: 10.1016/j.cub.2015.01.029
Mika, A., Buler, Z., Chlebowska, D. (2000). Effects of within row spacing and training systems of plum trees grafted on vigorous and semidwarf rootstocks. Acta Hortic. 557, 275–280. doi: 10.17660/ActaHortic.2001.557.35
Nardini, A., Gascó, A., Raimondo, F., Gortan, E., Lo Gullo, M. A., Caruso, T., et al. (2006). Is rootstock-induced dwarfing in olive an effect of reduced plant hydraulic efficiency? Tree Physiol. 26 (9), 1137–1144. doi: 10.1093/treephys/26.9.1137
Olien, W. C., Lakso, A. N. (1984). A comparison of the dwarfing character and water relations of five apple rootstocks. Acta Hortic. 146, 151–158. doi: 10.17660/ActaHortic.1984.146.16
Olien, W. C., Lakso, A. N. (1986). Effect of rootstock on apple (Malus domestica) tree water relations. Physiol. Plant 67 (3), 421–430. doi: 10.1111/j.1399-3054.1986.tb05757.x
Padgett-Johnson, M., Williams, L. E., Walker, M. A. (2000). The influence of Vitis riparia rootstock on water relations and gas exchange of Vitis vinifera cv. Carignane scion under non-irrigated conditions. Am. J. Enol Vit 51, 137–143. doi: 10.5344/ajev.2000.51.2.137
Palmer, J. W., Wertheim, S. J. (1980). Effects of tree density on fruit quality. Acta Hortic. 114, 139–142.
Peccoux, A., Loveys, B., Zhu, J., Gambetta, G. A., Delrot, S., Vivin, P., et al. (2018). Dissecting the rootstock control of scion transpiration using model-assisted analyses in grapevine. Tree Physiol. 38, 1026–1040. doi: 10.1093/treephys/tpx153
Reig, G., Iglesias, I., Zazurca, L., Torguet, L., Martinez, G., Miarnau, X. (2022). Physiological and agronomical responses of ‘Vairo’almond and ‘Big Top’nectarine cultivars grafted onto different prunus rootstocks and grown under semiarid Mediterranean conditions. Agronomy 12 (4), 821. doi: 10.3390/agronomy12040821
Reig, G., Lordan, J., Fazio, G., Grusak, M. A., Hoying, S., Cheng, L., et al. (2018). Horticultural performance and elemental nutrient concentrations on ‘Fuji’grafted on apple rootstocks under New York State climatic conditions. Scientia Hortic. 227, 22–37. doi: 10.1016/j.scienta.2017.07.002
Reig, G., Lordan, J., Hoying, S., Fargione, M., Donahue, D. J., Francescatto, P., et al. (2020). Long-term performance of ‘Delicious’ apple trees grafted on Geneva® rootstocks and trained to four high-density systems under New York State climatic conditions. HortScience 55 (10), 1538–1550. doi: 10.21273/HORTSCI14904-20
Robinson, T. L., Fazio, G., Aldwinckle, H. S. (2012). Characteristics and performance of four new apple rootstocks from the Cornell-USDA apple rootstock breeding program. Acta Hortic. 1058, 651–656. doi: 10.17660/ActaHortic.2014.1058.85
Sansavini, S., Bassi, D., Giunchi, L. (1980). Tree efficiency and fruit quality in high-density apple orchards. Acta Hortic. 114, 114–136. doi: 10.17660/ActaHortic.1981.114.13
Scheidegger, Y., Saurer, M., Bahn, M., Siegwolf, R. (2000). Linking stable oxygen and carbon isotopes with stomatal conductance and photosynthetic capacity: a conceptual model. Oecologia 125, 350–357. doi: 10.1007/s004420000466
Seibt, U., Rajabi, A., Griffiths, H., Berry, J. A. (2008). Carbon isotopes and water use efficiency: sense and sensitivity. Oecologia 155 (3), 441–454. doi: 10.1007/s00442-007-0932-7
Shtein, I., Hayat, Y., Munitz, S., Harcavi, E., Akerman, M., Drori, E., et al. (2017). From structural constraints to hydraulic function in three Vitis rootstocks. Trees 31 (3), 851–861. doi: 10.1007/s00468-016-1510-6
Tworkoski, T., Fazio, G. (2008). Physiological and morphological effects of size-controlling rootstocks on 'Fuji' apple scions. Acta Hortic. 903, 865–872. doi: 10.17660/ActaHortic.2011.903.120
Tworkoski, T., Fazio, G. (2015). Effects of size-controlling apple rootstocks on growth, abscisic acid, and hydraulic conductivity of scion of different vigor. Int. J. Fruit Sci. 15 (4), 369–381. doi: 10.1080/15538362.2015.1009973
Tworkoski, T., Fazio, G. (2016). Hormone and growth interactions of scions and size-controlling rootstocks of young apple trees. Plant Growth Reg. 78 (1), 105–119. doi: 10.1007/s10725-015-0078-2
Tworkoski, T., Miller, S. (2007). Rootstock effect on growth of apple scions with different growth habits. Scientia Hortic. 111, 335–343. doi: 10.1016/j.scienta.2006.10.034
Valverdi, N. A., Cheng, L., Kalcsits, L. (2019). Apple scion and rootstock contribute to nutrient uptake and partitioning under different belowground environments. Agronomy 9 (8), 415. doi: 10.3390/agronomy9080415
Valverdi, N. A., Kalcsits, L. (2021). Apple rootstock genotype affects scion responses to water limitations under field conditions. Acta Physiol. Plant 43 (7), 1–14. doi: 10.1007/s11738-021-03266-6
Villalobos-González, L., Muñoz-Araya, M., Franck, N., Pastenes, C. (2019). Controversies in midday water potential regulation and stomatal behavior might result from the environment, genotype, and/or rootstock: evidence from Carménère and Syrah grapevine varieties. Front. Plant Sci. 10, 15–22. doi: 10.3389/fpls.2019.01522
Wallace, M., Jones, G., Charles, M., Fraser, R., Halstead, P., Heaton, T. H., et al. (2013). Stable carbon isotope analysis as a direct means of inferring crop water status and water management practices. World Archaeol 45 (3), 388–409. doi: 10.1080/00438243.2013.821671
Webster, A. D. (2002). Vigour mechanisms in dwarfing rootstocks for temperate fruit trees. In I International Symposium on Rootstocks for Deciduous Fruit Tree Species. 658, pp. 29–41.
Werner, C., Gessler, A. (2011). Diel variations in the carbon isotope composition of respired CO2 and associated carbon sources: a review of dynamics and mechanisms. Biogeosciences 8, 2437–2459. doi: 10.5194/bg-8-2437-2011
Xu, H., Ediger, D. (2021). Rootstocks with different vigor influenced scion–water relationships and stress responses in AmbrosiaTM apple trees (Malus domestica var. Ambrosia). Plants 10 (4), 614. doi: 10.3390/plants10040614
Xu, H., Ediger, D., Singh, A., Pagliocchini, C. (2021). Rootstock–scion hydraulic balance influenced scion vigor and yield efficiency of Malus domestica cv. Honeycrisp on Eight Rootstocks. Horticulturae 7 (5), 99. doi: 10.3390/horticulturae7050099
Keywords: Malus domestica, carbon isotope composition, oxygen isotope composition, nitrogen isotope composition, leaf gas exchange, scion-rootstock interactions
Citation: Biasuz EC and Kalcsits L (2023) Rootstock effects on leaf function and isotope composition in apple occurred on both scion grafted and ungrafted rootstocks under hydroponic conditions. Front. Plant Sci. 14:1274195. doi: 10.3389/fpls.2023.1274195
Received: 07 August 2023; Accepted: 27 November 2023;
Published: 13 December 2023.
Edited by:
María Angeles Moreno, Spanish National Research Council (CSIC), SpainReviewed by:
Gemma Reig, Institute of Agrifood Research and Technology (IRTA), SpainMi Xun, Shandong Agricultural University, China
Juan Pedro Ferrio, Fundacion Agencia Aragonesa para la Investigacion y el Desarrollo, Spain
Copyright © 2023 Biasuz and Kalcsits. This is an open-access article distributed under the terms of the Creative Commons Attribution License (CC BY). The use, distribution or reproduction in other forums is permitted, provided the original author(s) and the copyright owner(s) are credited and that the original publication in this journal is cited, in accordance with accepted academic practice. No use, distribution or reproduction is permitted which does not comply with these terms.
*Correspondence: Lee Kalcsits, lee.kalcsits@wsu.edu