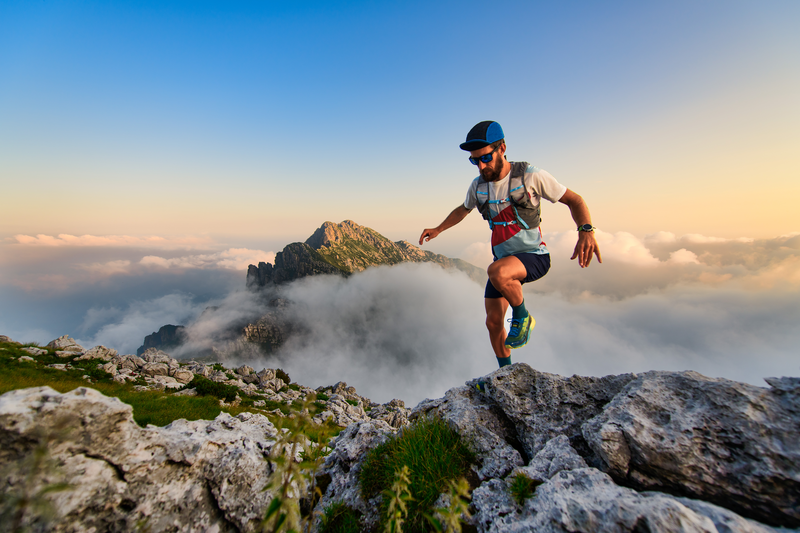
94% of researchers rate our articles as excellent or good
Learn more about the work of our research integrity team to safeguard the quality of each article we publish.
Find out more
REVIEW article
Front. Plant Sci. , 15 January 2024
Sec. Plant Breeding
Volume 14 - 2023 | https://doi.org/10.3389/fpls.2023.1273363
Black gram [Vigna mungo (L.) Hepper] is a highly nutritious grain legume crop, mainly grown in South and Southeast Asia, with the largest area in India, where the crop is challenged by several biotic and abiotic stresses leading to significant yield losses. Improving genetic gains to increase on-farm yields is the primary goal of black gram breeding programs. This could be achieved by developing varieties resistant to major diseases like mungbean yellow mosaic disease, urdbean leaf crinkle virus, Cercospora leaf spot, anthracnose, powdery mildew, and insect pests such as whitefly, cowpea aphids, thrips, stem flies, and bruchids. Along with increasing on-farm yields, incorporating market-preferred traits ensures the adoption of improved varieties. Black gram breeding programs rely upon a limited number of parental lines, leading to a narrow genetic base of the developed varieties. For accelerating genetic gain, there is an urgent need to include more diverse genetic material for improving traits for better adaptability and stress resistance in breeding populations. The present review summarizes the importance of black gram, the major biotic and abiotic stresses, available genetic and genomic resources, major traits for potential crop improvement, their inheritance, and the breeding approaches being used in black gram for the development of new varieties.
Black gram [Vigna mungo (L.) Hepper; 2n = 22] is a highly nutritious grain legume crop mainly grown in South and Southeast Asian countries including Afghanistan, Bangladesh, India, Myanmar, Pakistan, Sri Lanka, Thailand, and Vietnam (Kaewwongwal et al., 2015). The crop is also grown on a smaller scale in some African countries such as Kenya, Uganda, and Tanzania and in South American countries such as Argentina and Brazil. Black gram is also locally known as Urdbean, Urid, Mash, and Biri in India; as Mashkalai in Bangladesh; as Maas in Nepal; as Matpe in Myanmar; as Thua Khiao Piu Dam and Thua Khaek in Thailand; and as đậu muồng ăn in Vietnam. It was domesticated in India from its wild progenitor, V. mungo var. silvestris (Chandel et al., 1984) approximately 3,500 to 4,500 years ago (Fuller and Harvey, 2006). From India, black gram spread to the west coast peninsular of Thailand (Southeast Asia) approximately 2,300 years ago (Castillo, 2013). It plays an important role in vegetarian diets in South Asia due to its high nutritive value. Mature dry seeds of black gram possess approximately 24%–26% protein, 60% carbohydrates, 1.3% fats, phosphorus (345 mg/100 g), potassium, iron (8.7 mg/100 g), and calcium (185 mg/100 g) along with several essential amino acids (arginine, phenylalanine, leucine, lysine, valine, and isoleucine, etc.), vitamins such as vitamin B3 (niacin; 2 mg/100 g), vitamin A (23 IU/100 g), vitamin B1 (thiamine; 0.42 mg/100 g), and vitamin B2 (riboflavin; 0.37 mg/100 g) (USDA National Nutrient Database, 2018). It is widely consumed as dry whole grain or split grain known as daal and as unfermented and fermented flour (Khan et al., 2021). Popular Indian dishes like idli, dosa, and vada are prepared using black gram flour. It is also used as a major ingredient in several food items such as cakes, biscuits, snacks, and cookies. Its seed may be used in the food industry as functional food and nutraceutical as well as in the cosmetic and pharmaceutical industries (Pandey, 2019; Khan et al., 2021). In Thailand and Japan, black gram sprouts are preferred to mungbean sprouts because of their longer shelf life (Kaewwongwal et al., 2015).
The crop is a potential component of various cropping systems, especially in rice and wheat fallows owing to its short life cycle (70–90 days), capacity to fix atmospheric nitrogen, and relative drought tolerance. Black gram is generally intercropped with maize, sorghum, cotton, millets, and pigeonpea or rotated with cereal crops such as rice to increase soil fertility, minimize pest and disease incidence, and enhance dry matter yield of main crops (Yadav et al., 2006). India is the world’s largest producer of black gram, contributing 70% of the global production, followed by Myanmar and Pakistan. India produces approximately 2.7 million tonnes from an approximately 4.4 m ha area with an average yield of 598 kg/ha (Directorate of Economics and Statistics, 2021). Approximately 60% of the crop area is cultivated during the Kharif season; however, the rabi season cultivation is increasing due to the adoption of early maturing (75–80 days) varieties in rice fallows. Black gram contributes approximately 10% of the total pulse production in India with more than 90% of its production coming from 10 states, viz., Maharashtra, Karnataka, Madhya Pradesh, Gujarat, Uttar Pradesh, Jharkhand, Telangana, Odisha, Andhra Pradesh, and Tamil Nadu (Directorate of Economics and Statistics, 2021).
In Myanmar and Thailand, black gram has been largely cultivated as an export crop for Indian and Japanese markets since the late 1980s (Kaewwongwal et al., 2015). The crop is grown on approximately 1.1 m ha with a total production of approximately 1.58 million tonnes in lower Myanmar, especially in Bago (44%) and Ayeyarwady regions (41%) (Fujita and Okamoto, 2006; Dasgupta and Roy, 2015). In Australia and the USA, it is also grown as a fodder crop (Jansen, 2006). Due to the slow rate of production growth, India has become increasingly dependent on imports from some of the other black gram-producing countries such as Myanmar, Kenya, Mozambique, Australia, and Tanzania to satisfy domestic black gram demand (Commodity Profile for Pulses, 2019). Low and stagnant productivity (450–800 kg/ha) is one of the major stumbling blocks for vertical as well as horizontal expansion of the crop. Limited active breeding programs across major growing countries focusing on black gram improvement, lack of access to genetic and genomic resources, and poor seed systems are some of the major reasons behind low productivity levels (Kaewwongwal et al., 2015). The manuscript reviews the major production constraints, nature of inheritance of different desirable traits, and genetic and genomic resources available to enhance the genetic gains for target traits in black gram breeding.
The gap between potential and realized yield in pulses including black gram is wide and could be attributed to several biotic and abiotic stresses and the cultivation of the crop under poor crop management conditions (Rana et al., 2016). These biotic and abiotic stresses vary across the production regions depending on the cropping system and prevailing weather conditions. Understanding these production constraints is important for breeding programs to design a sound strategy to improve the yield levels of black gram.
The prevailing mono-cropping and intensive farming systems created the problem of several pests and diseases, and their intensive management through chemical measures has resulted in the development of resistance in pathogens against chemicals (Saxena et al., 2018). In India, biotic stresses including pests and diseases are reported to cause yield losses of up to 70% in black gram (Sharma et al., 2011), of which nearly 30% is due to insects (Justin et al., 2015). However, the avoidable losses due to pest incidence varied from 15.62% to 30.96% with an average of 24.03% in black gram production (Duraimurugan and Tyagi, 2014). It indicates that the loss due to the biotic stresses can be minimized through adopting resistant cultivars and integrated pest and disease management practices.
Approximately 198 insect species are reported to feed on pulse crops around the world, of which 115 are reported in India (Kooner et al., 2006). Out of these 115 species, 45 insect species have been reported on mungbean and black gram (Saxena et al., 2018), approximately 25 of them were major insect pests of black gram during the Kharif season, and 17 were reported during the spring season (Kooner et al., 2006; Duraimurugan and Tyagi, 2014). The insect species composition and their infestation vary across geography, seasons, plant phenology, and the prevailing environmental conditions such as temperature, humidity, and rainfall. The pest spectrum of black gram (Table 1) could be divided based on their feeding habit and plant parts as a) defoliators, viz., Bihar hairy caterpillar (Spilosoma obliqua Walker), red hairy caterpillar (Amsacta moorei Butler), tobacco cutworm (Spodoptera lituraFabricius), and blue butterfly (Lampides boeticus (Linnaeus)); b) sucking pests, viz., leafhopper (Empoasca kerri Pruthi), whitefly (Bemisia tabaci Gennadius), cowpea aphids (Aphis craccivora Koch), and thrips (Megalurothrip distalis Kany and Caliothrips indicus Bagnall); c) internal feeders, viz., stem fly (Ophiomyia phaseoli Tryon) and Galerucid Beetles (Madurasia obscurella Jacoby); d) pod borers/pod sucking bugs, viz., plant bugs (Riptortus pedestris (Fabricius), Nezara viridula (Linnaeus), and Clavigralla gibbosa Spinola), spotted pod borer (Maruca vitrata Fabricius), field bean pod borer (Adisura atkinsoni Moore), and bruchids (Callosobruchus chinesis Linnaeus and Callosobruchus maculatus Fabricius) (Sharma et al., 2011; Yadav et al., 2015; Saxena et al., 2018).
Sucking pests are the major group of insects that cause yield reduction in three ways: a) direct feeding/sucking on the plant parts such as leaves, flowers, young shoots, and pods could lead to poor growth and development of the plant; b) by acting as a vector for viral diseases; and c) by hampering the photosynthesis, causing poor nutrient assimilation. The flower thrips feed on the floral parts, and the flower shed before opening; thus, plants attain a bushy growth bearing few pods with shriveled seeds (Sharma et al., 2011; Srinivasan, 2014). Some of the sucking pests such as whitefly is a vector for viral diseases such as mungbean yellow mosaic disease (MYMD) and Urdbean Leaf Crinkle Virus (ULCV) and are responsible for direct as well as indirect yield losses. Dropping of young pods was reported under the severe incidence of cowpea aphid and pod bugs (Sharma et al., 2011). Cowpea aphids excrete honeydew while feeding on plants, which leads to the development of sooty molds on plants, leading to poor photosynthesis, nutrient assimilation, and yield levels (Annan et al., 1994).
The sucking pests such as whitefly and aphids, pod feeders, and pod bugs were reported to cause more damage during the rainy season, whereas thrip incidence was reported to be severe during the spring season, which could be due to higher temperatures during the spring season (Duraimurugan and Tyagi, 2014; Yadav and Patel, 2015). The stem fly is another major pest in some of the black gram growing states of India such as Punjab (Saxena et al., 2018) and Odisha and Bihar (Yadav and Patel, 2015) and is recently reported as an emerging pest in Andhra Pradesh (Manjula et al., 2019). The maggots of stem fly mine the leaves or bore into the leaf petiole and tender stem, resulting in withering, drooping, and death of the plant, leading to yield losses of 20%–60% to the complete crop failure if not managed during the seedling stage (Lal, 1985; Sharma et al., 2011; Saxena et al., 2018; Manjula et al., 2019).
The young larvae of Helicoverpa armigera damage the crop by defoliation, while the older ones prefer to feed on buds, flowers, and pods. The adults of the spotted pod borer arrive on the plants during the flowering stage to lay eggs on the flowers. Emerging larvae web the leaves and inflorescence and devour the flowers, flower buds, and pods. The larval feeding holes on pods act as entry holes for the moisture leading to the discoloration of seeds, thus reducing market quality (Lal, 1985; Sharma et al., 2011; Srinivasan, 2014; Saxena et al., 2018). Bruchids are important storage pests of black gram, which could cause 100% yield loss if not managed while storing the grains. It reduces seed weight, germination, nutritional quality, and market value, thereby rendering it unfit for human consumption, agricultural, and commercial uses (Duan et al., 2014). The study on the effect of bruchid infestation on antinutritional factors in legumes reported increases in trypsin inhibitor activity by 25%, saponin by 16%, and phytic acids by 46% (Modgil and Mehta, 1997; Baroowa and Gogoi, 2012). The seed moisture above 10% increases the susceptibility of grain to bruchids (Sharma et al., 2011). The important insect pests of black gram and respective information on the economic damage, season, and preferred plant parts for feeding are summarized in Table 1.
Among the diseases, MYMD caused by Mungbean Yellow Mosaic Virus (MYMV) and Mungbean Yellow Mosaic India Virus (MYMIV) is one of the major production constraints, responsible for more than 50% yield reductions in black gram and other Vigna species in South and Southeast Asia. In addition to black gram, MYMD is also responsible for the reduction in yields of other leguminous crops (Ramesh et al., 2017; Dikshit et al., 2020). The MYMV belongs to the Gemini group of viruses mainly transmitted by the whitefly. Initially, yellow spots appear on the leaves, and eventually, these yellow spots coalesce, turning the complete vegetation into a yellow color. The leaf yellowing reduces photosynthesis and nutrient assimilation, leading to poor flowering and pod development (Srivastava and Prajapati, 2012). Considering the devastating incidence of MYMD across production environments, it has become one of the must-have traits for breeding programs along with other economically important traits. Urdbean leaf crinkle disease (ULCD) is another viral disease considered to be very severe in black gram compared to other Vigna species (Kadian, 1980; Bashir et al., 2005). It is responsible for the reduction of seed yield from 35% to 81%, depending upon the time of infection, variety under cultivation, and growing season (Bashir et al., 1991; Reddy et al., 2005; Ashfaq et al., 2007; Gautam et al., 2016). However, some studies reported approximately 76%–100% yield reductions due to ULCD (Singh, 1980). The disease is broadly distributed across different states in India (William et al., 1968) and Pakistan (Bashir and Zubair, 1985; Ilyas et al., 1992). ULCV belongs to the Tospovirus group and is reported to be transmitted by several insects, viz., aphids (Dhingra, 1975), whiteflies, and leafhoppers (Gautam et al., 2016). The viral disease causes crinkling, curling, and puckering of leaves; the infected plants become stunted with deformed floral organs (Reddy et al., 2005). The leaf crinkle disease-susceptible plant produces smaller seeds, leading to poor yields. Disease incidence during seedling to vegetative stage caused more yield losses compared to incidence during reproductive stages (Reddy et al., 2005).
Cercospora leaf spot (CLS) caused by Cercospora canescens is another important disease that mostly affects rainy season crops owing to a reduction in yields by 25% when leaf defoliation reaches 75% (Dhar and Chaudhary, 2001; Gupta et al., 2020). This pathogen forms spots on the leaves with a brown to grayish center and reddish-brown border, which subsequently spread to petioles, pods, and stems. The disease leads to severe defoliation under favorable humid conditions (Raguchander et al., 2005; Dubey and Singh, 2010; Singh, 2010).
Powdery mildew caused by Podosphaera xanthii became another production constraint and priority for black gram breeding programs, especially in the agro-ecologies where the crop is grown in rice fallows. The symptom initiates as feeble dark spots on the leaf, which develop into small white powdery spots, coalescing to form a white powdery coating on all the aerial plant parts such as leaves, stems, and pods. Subsequently, the color of the powdery mass turns dirty white and covers the entire leaf surface, leading to reduced photosynthesis and induced early maturity and subsequent yield reduction. Unlike CLS disease, it is severe under cooler conditions (Sharma et al., 2011). In addition to these, focus also needs to be given to some of the emerging diseases such as anthracnose, Macrophomina blight, and bacterial leaf spot. Macrophomina blight is caused by the Macrophomina phaseolina responsible for dry root rot, collar rot, seedling blight, stem rot, leaf blight, and pod and stem blight (Vidhyasekaran and Arjunan, 1978; Sharma et al., 2011). The seedling rot during germination reduces the crop establishment. The infected plants form localized dark brown patches at ground level, which later encircle the stem. The sclerotial bodies appear on the outer tissue of the stem and root (Basandrai et al., 2016).
The anthracnose disease is mainly caused by Colletotrichum truncatum (Basandrai et al., 2016; Saxena et al., 2018) and seldom by Colletotrichum capsici (Mishra et al., 2011). The fungal pathogen forms characteristic circular brown sunken spots with dark centers and bright red-orange margins produced on the leaves and pods. Under severe disease situations, affected plants fall off. The pathogen is reported to be transmitted to the next generation through seeds and crop residue (Mishra et al., 2011). The summary of major diseases, their estimated yield losses, and favorable weather conditions for disease development are presented in Table 2.
Table 2 Summary of major black gram diseases, their causal agents, reported yield losses, and optimal weather conditions for disease development.
Among the abiotic stresses, drought, heat, waterlogging, and salinity stresses along with photo- and thermo-sensitivity are some of the major abiotic stresses responsible for severe yield losses in legumes across the production environment (Sultana et al., 2014; Nadeem et al., 2019). These stresses pose physiological changes to the plant and adversely affect the yield and nutritional quality of legume crops (Scheelbeek et al., 2018; Zonneveld et al., 2020). Black gram cultivation is performed year-round during rainy, post-rainy, and spring seasons across different agro-ecologies and cropping systems in India and other countries. In humid regions of North-East and coastal parts of India, black gram is grown soon after the monsoon rice crop to utilize the residual soil moisture, whereas it is grown as a rainfed crop in several parts of North India (Punjab, Haryana, Western Uttar Pradesh, and Bihar) (Singh et al., 2009). The water deficit stress is often experienced by the crop in many regions, especially during the rainfed and post-rainy season cultivation without supportive irrigation. Approximately 20%–30% of yield losses could be due to water deficit stress, which can be higher if the stress coincides with the flowering and pod development stage (Baroowa and Gogoi, 2012; Sai and Chidambaranathan, 2019; Kumar et al., 2020). It also reduces the efficiency of physiological processes such as the rate of photosynthesis and transpiration, and stomatal conductance (Durga et al., 2003; Baroowa and Gogoi, 2015). Moisture stress disturbs turgor pressure in the plant cells and affects cell enlargement, photosynthetic pigments, and membrane stability, resulting in poor plant growth. In addition to these, it also affects the stress response, defense systems of plants against pathogens, and several gene expressions and signaling pathways (Baroowa and Gogoi, 2015). The moisture deficit stress-tolerant varieties, viz., VBN4 and K1, showed a fivefold increase in production of abscisic acid (ABA) and lipid peroxidase activity compared to susceptible varieties (Sai and Chidambaranathan, 2019).
In several studies, the stress-tolerant black gram genotypes exhibited higher quantities of chlorophyll and increased leaf area index (Pratap and Sharma, 2010; Kumar et al., 2020), plant height, stress tolerance index, dry matter stress tolerance index, and assimilation rate (Baroowa and Gogoi, 2015; Yohan et al., 2018) compared to the reduction in these growth parameters in stress-susceptible genotypes (Gurumurthy et al., 2019; Shahi et al., 2019). Soil salinity reduces the chlorophyll content and affects leaf turgidity while increasing the production of proline (Ashraf, 1989).
The black gram is sensitive to low as well as higher temperature conditions. The high-temperature stress is responsible for the reduction of 38% to 82% of seed yield in black gram (Anitha et al., 2015). The pollen germination was significantly affected during higher temperatures. The plant traits such as leaf morphology (wax/pubescence), seed hardiness, pollen viability, and germination and receptivity of stigma were associated with temperature tolerance (Ganeshan et al., 2012; Choudhary et al., 2014). The higher temperature-tolerant (>38°C ± 2°C) genotype produced seed surface with shiny luster, thin and reduced cotyledon fissures, and bold and well-structured starch granules (Partheeban and Vijayaraghavan, 2020). Considering the changing climate scenario, breeding for climate-smart varieties that offer tolerance to prevailing biotic and abiotic stresses will ensure uninterrupted growth in genetic gains and supply of increasing future food demand.
Enhancement of yield under various biotic and abiotic stresses requires the analysis of trait diversity found in germplasm collections and the study of inheritance patterns of important morphological and agronomic traits. There are some studies on genetic diversity in black gram for agronomic and morphological traits (Ghafoor et al., 2001; Shafique et al., 2011; Choudhary et al., 2018; Patidar et al., 2018), seed storage protein (Ghafoor and Ahmad, 2005), grain yield (Kumar et al., 2002; Tomar et al., 2003), and resistance against biotic stresses (Vishalakshi et al., 2017). However, studies on the genetics and inheritance of qualitative and quantitative morphological and agronomic traits, the cytogenetics, and the combining ability among genotypes for different target traits are limited in black gram (Kumar et al., 2000) as compared to other Vigna species such as cowpea and mungbean. Based on the available studies, the inheritance pattern of different morphological, biotic, and abiotic stress-related traits is discussed below.
Black gram shows a spreading plant type; however, the modern cultivars are developed with erect and semi-erect plant types. The erect plant type is not completely dominant over the spreading type (Sen and Jana, 1964). It has large trifoliate leaves that are either ovate or lanceolate in shape. Ovate leaf shape is dominant over lanceolate and is mainly controlled by a single dominant gene (Verma, 1971). However, the hastate shape is reported to be dominant over the ovate and probably controlled by duplicate dominant genes (Singh and Singh, 1971) (Table 3). A fused leaf (cotton leaf type) variant was reported to be recessive to the ovate leaf shape and is controlled by a single recessive gene (Muralidharan et al., 1990). Two types of pod orientations, i.e., main stem bearing and sympodial bearing types, are found in black gram. Main stem pod bearing is controlled by a single incomplete dominant gene. Recently, the above canopy pod-bearing genotypes were also developed in black gram (Gupta et al., 2020). Three different pod colors are reported, viz., black, brown, and straw color, of which black pod color is very prominent and dominant over the straw and brown pod color (Sen and Jana, 1964; Verma, 1971; Arshad et al., 2005). The inheritance of protruded stigma and crumpled petals in naturally occurring mutants reported the involvement of a single recessive gene with a pleiotropic effect (Kumar et al., 2012). The brown seed coat color is recessive to the green seed coat color with qualitative inheritance (Sen and Jana, 1964). However, in contrast to this, brown seed coat color was reported to be dominant over green seed color by Arshad et al. (2005). Shiny seed surface is dominant over dull seed surface in black gram (Sen and Jana, 1964).
There are contrasting reports on the inheritance of resistance that include monogenic dominant (Dahiya et al., 1977; Kaushal and Singh, 1988a; Gupta et al., 2005; Gupta et al., 2013a), as well as the monogenic or digenic recessive nature of resistance to MYMD (Singh, 1981; Dwivedi and Singh, 1985; Verma and Singh, 1986; Singh et al., 1987; Pal et al., 1991; Rambabu et al., 2018) available in black gram. The duplicate and recessive epistatic gene interaction (Thamodhran et al., 2016) along with tri-genic control with inhibitory gene action (Vadivel et al., 2021) was also reported for resistance to MYMD. The chi-square goodness-of-fit test on an F2 population revealed inhibitory gene action with two genes controlling the expression of resistance to MYMD (Subramaniyan et al., 2022). This suggests that the genetics of resistance to MYMD is complex, which could be due to the presence of different strains of the causal virus and their variable pathogenicity. Genetic analysis of resistance to PM reported it to be governed by a single recessive gene (Kaushal and Singh, 1989); however, a recent report indicates that the resistance is controlled by a single dominant gene without maternal effects (Santosh, 2016). The inheritance of resistance to CLS (Kaushal and Singh, 1991) and anthracnose is controlled by a single dominant gene (Kaushal and Singh, 1988b). Two duplicate dominant genes control resistance to bruchid (C. maculatus) infestation in black gram (Dongre et al., 1996; Souframanien and Gopalakrishna, 2007). However, resistance is also reported to be controlled by the monogenic dominant gene (Tickoo et al., 2006) (Table 3).
The loss of genetic diversity during domestication and subsequent diversification of crops resulted in a decrease in the genetic diversity and useful genes/traits that were not selected during domestication. The limited information on the characterization and evaluation of germplasm accessions led to the use of fewer parents by the breeding programs, resulting in a narrow genetic base in most of the Vigna species. Screening of diverse germplasm accessions and crop wild relatives can provide valuable genetic resources to source new traits for breeding programs (Ganguly and Bhat, 2012).
As black gram originated in India, the largest number of its globally available genetic resources (3,146 accessions) are collected and conserved by the National Bureau of Plant Genetic Resources (NBPGR), New Delhi, India (Singh D et al., 2016). The ICAR-Indian Institute of Pulses Research (ICAR-IIPR), Kanpur, India, has the mandate of pulse crop improvement including black gram and also holds an active collection of approximately 829 accessions (Arora, 1988; Gupta et al., 2001). The largest black gram collection outside of India is held by the World Vegetable Center, Taiwan, comprising 884 black gram accessions that originated from different parts of the world (Jacob et al., 2015). Some other gene banks are holding black gram, viz., Plant Genetic Resources Centre (PGRC), Bangladesh Agricultural Research Institute (BARI), Bangladesh (339 accessions); Plant Genetic Resources Conservation Unit, University of Georgia, USA (304 accessions); N.I. Vavilov All-Russian Institute of Plant Genetic Resources, Russia (220 accessions); and Research Center of Genetic Resources, National Agriculture and Food Research Organization (NARO), Japan (220 accessions). Australian Grains Genebank, Australia (102 accessions) and International Center for Tropical Agriculture, Colombia (93 accessions) also hold black gram accessions (Table 4). NARO has the largest number of accessions (14) of wild black gram. However, many germplasms conserved in several gene banks are duplicates of Indian origin. There is considerable genetic diversity available among the Indian black gram accessions (Singh et al., 1991; Singh and Shukla, 1994; Nautiyal and Shukla, 1999; Kaewwongwal et al., 2015); however, its exploitation was hindered by limited access to information about the germplasm such as characterization data and classification efforts (Gupta et al., 2001).
Molecular genetic diversity analysis of 520 cultivated and 14 wild accessions from various countries using 22 simple sequence repeat (SSR) markers revealed that the diversity of germplasm from different regions was comparable, albeit the germplasm from South Asia showed the greatest gene diversity (Kaewwongwal et al., 2015). The study also revealed that the level of gene diversity in black gram is close to that in mungbean and rice bean, but lower than azuki bean.
Black gram is classified into two sub-taxa, i.e., i) V. mungo var. mungo characterized by black large seeds and early maturity and the wild type ii) V. mungo var. silvestris Lukoki, Maréchal & Otoul, with denser inflorescences, hairier and climbing nature, and small seeds with prominent raised aril (Lukoki et al., 1980; Tomooka et al., 2000). V. mungo var. silvestris is considered to be the progenitor of cultivated black gram (Lukoki et al., 1980) and was successfully used in breeding programs for the genetic improvement of several traits (Jansen, 2006). Black gram is classified into the subgenus Ceratotropis (also known as Asian Vigna). Species in this subgenus are highly diverse and distributed widely in Asia (Tomooka et al., 2000), and thus, they adapt well to various environments and can be useful gene sources for the genetic improvement of black gram. Some of the important wild species of Vigna, namely, Vigna bourneae, Vigna capensis, Vigna dalzelliana, Vigna grandis, Vigna hainiana, Vigna minima, V. mungo var. sylvestris, Vigna radiata var. sublobata (wild mungbean), and Vigna vexillata, were collected from Western and Eastern Ghats, Northwestern Plains, Central Plateau region, and Northern Himalayas in India (Dana, 1998). Several accessions of wild Vigna species, i.e., Vigna aconitifolia, V. dalzelliana, V. hainiana, Vigna khandalensis, V. mungo var. silvestris, V. radiata var. sublobata, and Vigna trilobata, were collected from diversity rich areas of Gujarat, Rajasthan, Maharashtra, Madhya Pradesh, Bihar, Odisha, and West Bengal during 1974 and 1994 by NBPGR, New Delhi (Dana, 1998). However, there is no information on the cross-compatibility between a black gram and all of these wild Vigna species, except wild black gram and wild mungbean.
The cross-compatibility study of V. mungo with other species revealed 37.5% compatibility between V. radiata (♀) and V. mungo (♂), whereas it was 11.6% between V. mungo (♀) and Vigna umbellata (♂) (Bhanu, 2018). The true interspecific F1’s among V. radiata (♀, SML 668 and SML 832) and V. mungo (♂, Mash 114 and Mash 218) reported 5.5% to 24.1% pod set, 14.29% to 30.56% germination, and 22.59% to 28.36% pollen fertility (Lekhi et al., 2018). Different kinds of pre- and post-fertilization barriers were responsible for complete sterility or low fertility in F1 hybrids between these two species (Bhanu, 2018). Rice bean (V. umbellata) genotypes RBL 1 and RBL 9 showed high crossability and percent seed set with different cultivars of black gram. Another study successfully crossed V. mungo and V. radiata, and the recovered hybrids were reciprocally crossed with Vigna angularis. The reciprocal three-way interspecific F1 hybrids were reported partially fertile (Gupta et al., 2002). The introgression of desirable traits from rice bean (RBL 1) into black gram (Mash 338 and UG 562 and 844) resulted in poor cross-compatibility and plant fertility for initial generations and improved gradually from the F2 generation onward (Singh et al., 2013). The yield evaluation of 24 uniform F7 progeny bulks exhibited −35.48% to 50.31% yield variation over the check cultivar (‘Mash 338’, a female parent) along with resistance to MYMD, CLS, and bacterial leaf spot diseases. The desirable traits such as a high number of pods per plant, seed weight, and MYMD resistance from rice bean have been successfully introgressed into the black gram.
Varieties Mash 1008 and Mash 118 released in 2004 and 2008, respectively, were developed from an interspecific cross between black gram and mungbean (Singh et al., 2016a). The variety Mash 114 was developed from an interspecific cross between black gram and rice bean and recorded a 39.45% superior yield over the check cultivar ‘Mash 338’ (female parent) across 14 multi-location trials (Singh et al., 2013). Another variety, Vamban 7, resistant to MYMD and powdery mildew was obtained from Vamban 3 × wild black gram (V. mungo var. silvestris), whereas TU-40, resistant to powdery mildew, was obtained from TU 94-2 × wild black gram (Singh et al., 2016a).
The efforts in characterization, evaluation, and screening of black gram germplasm for desirable traits identified several useful trait-specific genotypes for breeding programs. The trait-specific genotypes were identified as potential donors for some of the agronomic traits such as number of pods per plant (KL 1, UH 81-44, and IPU 99-79 with >150 pods), number of seeds per pod (IC 106088, HPU 193, HPU 2, and PLU 257 with >8 seeds per pod), early maturity (IPU96-3, IPU 31-5, PLU 710, L25-7, and STY 2848 <70 days), and MYMD resistance (IC 27026, IC 06088, UL 2, HPU 4, HPU188, STY 2848, UH 80-26, IP 99-127, PLU 62, PLU 158, and PLU227) (Gupta et al., 2001). JP219132 was reported to show extra-large seed size, leaves, and stems (Chaitieng et al., 2006; Naito et al., 2017).
Black gram germplasm identified for biotic stress resistance are compiled in Table 5. The genotype LBG-645 was reported as highly resistant to powdery mildew in glass houses and laboratory screening (Priyanka et al., 2018). The genotypes CO-5, IPU 07-3, and Mash 1-1 exhibited moderate resistance to dry root rot compared to the susceptible check VO 2135-B-BL in sick pot assay (Elmerich et al., 2022). Cultivated black gram is known to be immune to Callosobruchus chinensis infestation but highly susceptible to C. maculatus (Srinives et al., 2007). However, wild progenitor V. mungo var. silvestris is resistant to C. maculatus with the larval antibiosis mechanism of resistance (Dongre et al., 1996; Tomooka et al., 2000). The genotypes that are resistant to spotted pod borer (Krishna et al., 2006) and stem fly were also reported in black gram (Neupane et al., 2021).
Black gram germplasm identified for abiotic stress resistance is summarized in Table 5. Vamban 2 variety has been reported as tolerant to drought stress in different studies and hence could be used as a parent to develop new varieties and genetic populations to further investigate the mechanism of drought tolerance in black gram (Goyal and Khan, 2010; Solanki et al., 2011 and Singh et al., 2016b). Other genotypes such as PGRU 95016, COBG05, IPU 99209, IPU 941, and IPU 243 were also reported as drought tolerant (Gurumurthy et al., 2019). The black gram varieties ‘J.L’, ‘PDU-1’ (Dash and Shree, 2013), ‘VBG-07-001’, and ‘VBG-06-010’ (Partheeban, 2017) were found to perform well in high-temperature regimes. Gupta et al. (2021) studied a panel of 97 diverse black gram genotypes for yield under heat stress and non-stress conditions in the field and identified eight highly tolerant lines (‘UPU 85-86’, ‘IPU 94-2’, ‘IPU 98/36’, ‘NO-5731’, ‘PGRU 95014’, ‘PGRU 95016’, ‘PLU 1’, and ‘BGP 247’). The trait-specific genotypes for waterlogging tolerance (Rana et al., 2016), photo-insensitivity (Singh et al., 2016b; Dash and Shree, 2013), and thermo-insensitivity (Singh et al., 2016b; Partheeban, 2017) were reported in different studies and are listed in Table 6.
Black gram germplasms that are useful for nutritional quality improvement are shown in Table 7. The genotypes with high protein content such as LBG17, Malabiri local, Boudha local, and G. Udayagiri local with >25% protein (Kole et al., 2002) and K1 and IPU 11-02 with >24% protein content were identified in black gram (Rana et al., 2016). The genotypes UG-218 and HIM Mash were reported with better protein quality, especially high methionine and lysine content (Modgil et al., 2019). Accessions rich in iron (Fe) and zinc (Zn) were also reported in black gram, which could be used to develop biofortified varieties to achieve nutritional security by combating mineral malnutrition (Singh et al., 2017).
In India, the establishment of the All India Coordinated Pulses Improvement Project (AICPIP) in 1967 provided breeders access to improved germplasm and an opportunity to test their improved breeding lines in multi-location trials across the country. The collective efforts of State Agriculture Universities and the Indian Council of Agricultural Research-Indian Institute of Pulses Research (ICAR-IIPR) resulted in the development and release of over 85 black gram varieties. Before the establishment of AICPIP, the early efforts of varietal development resulted in the release of popular varieties such as T 9, T 27, and T 77 (Gupta et al., 2001). T 9, a selection from the material collected from Bareilly (UP) in the early 1950s, has been used extensively by different breeding programs (Goyal and Khan, 2010). The varieties T 9, ADT 1, and Co 1 had made significant contributions to black gram production in India and were extensively used in the breeding program as parents to develop new varieties (Gupta et al., 2001). In Myanmar, six varieties were developed and released to farmers including Yezin-2, Yenzin-3, Yenzin-5, Yezin 6, Yezin-7, and Pale Tun. The first four varieties were selected from accessions P-45-1 (India), P-11-30 (Myanmar), P-69-354 (Myanmar), and LBG-17 (India). Yezin-7 was developed from hybridization and selection of the cross Yezin-4 and Yezin-6. Pale Tun is a mutant of P-11-30. In Thailand, farmers, traders, and users prefer varieties with large seed sizes. U-Thong 2 and Phitsanulok 2, pure-line varieties selected from Indian varieties 68/71 and BC48 (PI 288603) and released in the late 1970s and early 1990s, respectively, have been grown widely by the farmers for nearly 25 years due to high and stable yield and large seed size, compared to local varieties. At present, Chai Nat 80, a progeny derived from hybridization between a local variety (Prajeen) and an Indian variety (NBG 5), is the most popular cultivar in Thailand. In Australia, Regur released in the mid-1970s has been grown as a single black gram variety for approximately 35 years. The list of varieties developed in India, Bangladesh, Pakistan, Thailand, Australia, and Myanmar is presented in Table 8.
In India, over 60% of black gram varieties share type 9 (T9) as one of the ancestors in their pedigree (Singh et al., 2016a). The pedigree analysis of advanced breeding lines entered for the Initial Varietal Trial in AICPIP from 1996 to 2005 revealed that T9 was used as one of the parents in over 78% of the advanced breeding lines (Katiyar et al., 2008). Another variety, D 6-7, was used as a parent in more than 12% of advanced breeding lines. The use of the limited number of parents by the breeding programs could be one of the reasons behind the narrow genetic base of available varieties. Therefore, it is an urgent need for the breeding programs to focus more on the use of diverse genetic material and recycling the newly developed advanced breeding lines into crossing nurseries.
Genetic improvement in several food legumes by either traditional or molecular approaches has been hindered due to the absence of genomic resources till the last decade (Varshney et al., 2009). The DNA marker techniques have been developed in various crops including food legumes owing to the recent developments in molecular biology. It includes the development of several different generations of molecular markers, molecular characterization of germplasm, and the development of genetic and quantitative trait locus (QTL) maps along with trait-linked diagnostic markers (Boukar et al., 2019). Genetic linkage maps and diversity within germplasm collections in black gram have been studied primarily using random amplified polymorphic DNA (RAPD) (Souframanien and Gopalakrishna, 2004), inter simple sequence repeat (ISSR) (Souframanien and Gopalakrishna, 2004), amplified fragment length polymorphism (AFLP), and SSR markers (Chaitieng et al., 2006).
Several efforts have been made to develop genomic resources and use them to assess the diversity at the genomic level in black gram. The DNA polymorphism in 18 elite genotypes using 25 RAPD and 16 ISSR markers reported 44 out of 104 scoreable fragments for RAPD and 55 out of 101 bands for ISSR as polymorphic (Souframanien and Gopalakrishna, 2004). ISSR markers have been effectively used for repeat motif analysis as well as varietal identification in black gram (Ranade and Gopalakrishna, 2001). The genetic diversity of 26 black gram landraces using AFLP markers revealed 74.5% to 93% polymorphism (Sivaprakash et al., 2004).
Since there were not many polymorphic markers in black gram, the transferability of SSR markers from other Vigna species to a black gram was tested and reported several polymorphic markers. Chaitieng et al. (2006) tested the amplification and polymorphism of 211 genomic SSR markers from azuki beans in cultivated and wild black and found that 73% and 50% of the markers were amplifiable and polymorphic, respectively. Chankaew et al. (2014) analyzed the application of 1,429 azuki bean genic SSR markers in the same black gram accessions used by Chaitieng et al. (2006) and found that 84% of the markers were amplifiable but that only 13% of them were polymorphic. Tangphatsornruang et al. (2009) detected amplification and polymorphism of 127 mungbean genomic SSR markers in cultivated and wild black gram and found that 66% and 50% of the markers were amplifiable and polymorphic, respectively. In a similar study, Somta et al. (2009) reported that as high as 92% of 85 genic SSR markers from mungbean tested in the same black gram materials reported by Tangphatsornruang et al. (2009) were amplifiable and 34% were polymorphic. Sixty-five SSR markers of cowpea were tested across different Vigna species and reported amplification of 85% (55) markers in black gram (Gupta and Gopalakrishna, 2010). The molecular diversity of six MYMD-resistant and MYMD-susceptible genotypes each from mungbean and black gram using 24 cowpea resistance gene analog (RGA) markers grouped two resistant (IPU 02-33 and IPU 6-02) and two susceptible (LBG 20 and T9) black gram genotypes into distinct clusters (Narasimhan et al., 2010). However, the limited number of SSR markers in these legumes hindered the genomic studies in the black gram.
The emergence of advanced sequencing technologies including next-generation sequencing (NGS) and third-generation sequencing (TSG) in the 2000s has revolutionized genome study in living organisms. NGS is able to sequence millions of short fragments of DNA in parallel. It provides a rapid, inexpensive, and comprehensive analysis of the genomes of individual organisms as well as complex populations. NGS is also a powerful tool in the discovery and genotyping of large numbers of single-nucleotide polymorphisms (SNPs) at a drastically reduced expense (Elshire et al., 2011) and in the construction of whole-genome sequencing, thus enhancing the genetic gain in breeding. Third-generation sequencing (TGS) (also known as single-molecule sequencing) sequences large numbers of long fragments of DNA, which is highly useful for producing high-quality genome assemblies providing scientists to explore genomes at an unprecedented resolution. The transcriptome sequencing of immature seed tissues of TU94-2 through Illumina paired-end sequencing technology generated 17.2 million paired-end reads and 48,291 transcript contigs (TCS) that could be useful for gene discovery and genic-SSR markers (Souframanien and Reddy, 2015). Another study on the transcriptome sequence of immature seed tissue in wild black gram developed a large number of genic SSR and SNP markers (Raizada and Souframanien, 2019). Genetic relatedness among 27 black gram genotypes was also evaluated using 19 genic SNPs through high-resolution melt (HRM) analysis (Raizada and Souframanien, 2021).
A whole-genome sequence (WGS) or reference genome sequence of a species is an indispensable genomic resource for gene discovery. WGSs of black grams have been developed recently. Pootakham et al. (2020) produced a high-quality, chromosome-level assembly of 499 Mb comprising 11 pseudomolecules from the black gram variety Chai Nat 80 (CN80) using NGS and TGS technologies. The genome annotation contained 32,729 predicted gene models, of which 29,411 (89.86%) were protein-coding genes. Shortly, Souframanien et al. (2021) constructed a draft genome sequence of 502 Mb of black gram variety Pant U-31 with 42,115 predicted genes. Ambreen et al. (2022) constructed a draft reference-guided genome assembly of black gram genotype ‘Uttara’ (IPU 94-1; known for its high resistance to Mungbean Yellow Mosaic Disease), with a cumulative size of 454 Mb and 28,881 predicted genes, of which 444 Mb was anchored on 11 chromosomes. Naito et al. (2022) constructed a draft genome sequence of 475 Mb of black gram variety Subsmotod with 28,227 predicted genes. The size of these black gram genome assemblies is only 79%–87% of the estimated genome size of the black gram (574 Mb; Arumuganathan and Earle, 1991). Therefore, a large part of the black gram genome still cannot be captured. In addition, the number of predicted genes in these genomes is highly different, albeit this may reflect the high genome variations among the black gram varieties. Nonetheless, these WGSs are useful genomic resources to hasten the understanding of evolutionary relationships, the development of diagnostic molecular markers, and the identification of novel genes and haplotypes in black gram.
The development of genomic resources opens opportunities for the construction of linkage maps and the identification of quantitative trait loci and diagnostic molecular markers for desirable traits (He et al., 2014). High-quality genome assembly together with information about genomic variations from germplasm enabled the use of novel breeding tools such as genomic selection (GS), marker-assisted selection (MAS), marker-assisted recurrent selection (MARS), and marker-assisted backcrossing (MABC) (Ribaut et al., 2010) and further comparative genomics and phylogenetic research into legumes (Pandey et al., 2016). Due to limited genomic resources for the black gram, especially polymorphic DNA markers, in the past, there have been few reports on gene and QTL mapping in this crop. A genetic linkage map is a basic tool for gene and QTL mapping. The first genetic linkage map of the black gram was reported in 2006 when Chaitieng and her colleagues developed linkage maps for the black gram using only 148 marker loci including 59 RFLP, 61 SSR, 27 AFLP, and one morphological marker (Chaitieng et al., 2006). Two years later, another black gram linkage map was developed using 428 marker loci (254 AFLP, 86 RAPD, 41 ISSR, and 47 SSR markers), but unfortunately, most of them were dominant (Gupta et al., 2008). Recently, a high-density linkage map of 3,675 SNP markers from specific-locus amplified fragment sequencing was developed (Somta et al., 2019). All of these maps were constructed based on a population derived from crossing between cultivated and wild black gram.
Most of the gene and QTL mapping studies in black gram involved the resistance to MYMD and bruchid (C. maculatus). By using bulked-segregant analysis (BSA), Gupta et al. (2013b) identified SSR marker CEDG180 linked to MYMD resistance. ISSR marker ISSR8111357 was identified to be linked to the MYMD resistance gene with a 6.8-cM distance, and the marker was converted into sequence characterized amplified region (SCAR) marker for validation on other MYMD resistant and susceptible genotypes (Souframanien and Gopalakrishna, 2006). Through BSA using an F2 population of a cross between T9 (MYMD resistant) and LBG-759 (MYMD susceptible), SSR marker VR9 was found to be linked to MYMD resistance (Naik et al., 2017). In another study, SSR marker CEDG185 was identified as linked with the MYMD resistance by BSA (Rambabu et al., 2018). Two major QTLs, qmymv2_60 and qmymv10_60, governing resistance to MYMD disease were mapped onto LG2 and LG10 with 20.90% and 24.90% phenotypic variation explained (PVE), respectively (Vadivel et al., 2021). Validation of these QTLs in two other mapping populations confirmed the presence of qmymv10_60. Similarly, a study on the F2 population derived from a cross between MDU 1 (susceptible) and TU 68 (resistant) identified one major QTL qMYMVD_60 on LG10 responsible for 21% PVE of MYMD variation. This QTL was delimited by SSR markers CEDG180 and CEDG116 (Subramaniyan et al., 2022). Since the QTL qmymv10_60 was confirmed and validated for its association with MYMD resistance in different studies, this QTL can be one of the potential regions for fine mapping and identification of diagnostic markers and haplotypes for MAS and haplotype-based breeding approaches.
By utilizing the genetic map developed by Gupta et al. (2008) (see above), QTLs Cmrae1.1 and Cmrae1.2 on LG3 and LG4, respectively, were detected for the percent adult emergence, and six QTLs (two (Cmrdp1.1 and Cmrdp1.2) on LG1, three (Cmrdp1.3, Cmrdp1.4, and Cmrdp1.5) on LG2, and one (Cmrdp1.6) on LG10) were identified for developmental period with PVE ranging from 8.4% to 16.4% (Souframanien et al., 2010). By using the high-density SNP-based linkage map, two QTLs, qVmunBr6.1 and qVmunBr6.2, located approximately 10 cM apart on LG6 were identified for a percentage of damaged seeds and infestation severity progress (Somta et al., 2019). Comparative genome analysis revealed qVmunBr6.1 and qVmunBr6.2 as new loci for C. maculatus resistance in Vigna species (Somta et al., 2019).
QTLs for seed weight were detected in black gram using mog (multiple-organ gigantism) mutant as the large-seeded parent. The mog mutant expressed extra-large size of seeds, leaves, and stems. QTL mapping and gene transformation studies revealed that mog gene accounted for approximately 66% of seed weight variation in the mapping population and additive gene effect of the mog increased 2.0 g of seed weight and that mog is a VmPEAPOD (VmPPD) gene (Naito et al., 2017). Loss of function caused by an 8-bp deletion on the VmPPD resulted in the mog phenotype (Naito et al., 2017). Later, major QTLs for the multiple-organ gigantism (MOG) phenotypes were reported on LG6 with the pleiotropic effect on different traits ranging from 15% for plant height to 40% for leaf size (Somta et al., 2020). The QTL for the MOG phenotype was also reported to contribute approximately 30% PVE for seed weight (Somta et al., 2020). In another study, QTLs for seed weight were identified using two F2 populations derived from crossing between cultivated and wild black grams in which 10 QTLs on seven linkage groups (LGs) were detected in total for the seed weight (Lomlek et al., 2023). The QTLs explained between 5.07% (qSd100wt7.1+) and 34.20% (qSd100wt10.2+) of the weight variation, depending on the population. Genes encoding for pentatricopeptide repeat (RPP)-containing protein, WRKY46, glutathione S-transferase U9, NAC domain-containing protein 100, calcineurin B-like protein (CBL)-interacting protein kinase, cyclin-D6-1, kinesin, histidine phosphotransfer protein, AUXIN RESPONSE FACTOR 2A, and WAVE-DAMPENED2 (WVD2)-like 4 protein are candidate genes for seed weight QTLs (Lomlek et al., 2023). Two minor QTLs for pod dehiscence, qPdt3.1− and qPdt5.1−, on LGs 3 and 5, respectively, with ≤10% PVE and a single QTL with large effect controlling approximately 20% PVE for seed dormancy were also reported in black gram (Somta et al., 2020). The QTLs detected for pod dehiscence in black gram were different than those reported in other closely related Vigna species including mungbean (Isemura et al., 2012), adzuki bean (Kaga et al., 2008), moth bean (Yundaeng et al., 2019), and cowpea (Kongjaimun et al., 2012). Recently, QTL analyses using dense SNP-based linkage maps of two mapping populations identified 12 different QTLs with PVE of 3.4% to 38.0% for flowering time in the black gram (Suamuang et al., 2023). Genes encoding zinc finger protein 10, F-box protein SKIP14, and NAC domain-containing protein 37-like are candidate genes at major QTLs for the flowering time (Suamuang et al., 2023). None of the candidates are the same as those for flowering time in other Vigna species. In addition, using the same populations and linkage maps as that reported by Suamuang et al. (2023), 10 QTLs were detected for seed weight, and three were detected for seed dormancy. Depending on populations, QTLs explained between 5.07% (qSd100wt7.1+) to 34.20% (qSd100wt10.2+) of the seed weight variation and 10.18% (qSdwa6.1−) to 43.81% (qSd100wt6.2−) of the seed dormancy variation (Lomlek et al., 2023). Several candidate genes were identified including WVD2-LIKE 4 for seed weight that appeared to be an ortholog with a candidate gene for the seed weight in mungbean.
Considering the low rate of genetic gains and on-farm yield levels, higher seed yield with early maturity (65–75 days), photo–thermo insensitivity, rapid plant growth, high biomass, high harvest index, increased seed size, synchronous maturity, resistance to MYMD, ULCD, PM, and CLS, and tolerance to drought, heat, and salinity stresses are some of the major breeding objectives for black gram breeding programs across the world. In addition to these, high protein content, higher amounts of sulfur-containing amino acids, and high Fe and Zn contents are some of the major nutritional quality traits focused on by the breeding programs. The varieties with erect semi-determinate plant types are becoming popular due to their suitability for cultivation in the sole cropping system and mechanical harvesting.
Intensive breeding programs of black gram are in India. Breeding methods such as introduction, mass selection, pure line selection, pedigree selection, backcrossing, and mutation breeding are widely used by most of the breeding programs in India and elsewhere. Initially, the varieties were developed through direct selections from local landraces and introduced germplasms. Over 50% of varieties in India from 1949 to 2000 were developed through selections from local materials (Gupta et al., 2020). T9 is one of the popular varieties developed through the introduction and mass selection, whereas ADT 1, CO 1, CO 2, CO 3, CO 5, and ADT 3 were developed through pure line selection from local populations (Gupta and Kumar, 2006; Tickoo et al., 2006). Later, the emphasis was on the use of hybridization-based techniques to create variability and to combine multiple traits in a single variety to cater to the needs of different production environments (Gupta et al., 2020). In India, KM 1 was the first variety developed through hybridization in 1977. VBN 6, 7, 8, 9, 10, and 11 are some of the varieties recently developed using hybridization followed by selection (Geetha et al., 2020). The hybridization-based approaches require prior accurate information about parental genotypes, genetics of the trait to design a sound breeding strategy, precise phenotyping procedures, and facilities for testing of segregating and advanced breeding material. These approaches have contributed to generating new recombinants with desirable traits and to the development of several popular varieties across different countries (Dikshit et al., 2020). Most of the varieties developed in black gram during the last two decades are based on recombining the traits through hybridization and phenotypic selections in early and advanced generations. The multiparent-based hybridization is now being explored by the black gram breeding programs to develop varieties with a broader genetic base. In Thailand, black gram breeding began in the mid-1970s with the major aims of improving plant type, earliness of flowering and maturity, and seed size and yield. At the early stages of breeding, germplasm from the World Vegetable Center was introduced and selected by mass selection and pure line selection leading to the release of two varieties, U-Thong 2 and Phitsanulok 2, which have been utilized for export for approximately 30 years. All the recent varieties were developed by hybridization and selection using exotic and local germplasm. At the World Vegetable Center, black gram lines (AVUB 2001 to AVUB 2030) were recently developed with improved protein quality, and resistance to bruchids and MYMD, from crosses involving VM 2164 with Mash1-1 (Boddepalli et al., 2021).
Considering the narrow genetic base of cultivated black gram and cross incompatibility with many of the wild Vigna species, mutation breeding using various mutagens has been adopted in black gram to create variability and develop improved varieties. It has played a remarkable role in genetics, genomics, and breeding research across different crops (Kharkwal and Shu, 2009). Globally, mutation breeding has contributed to the commercial release of 3,348 plant varieties across 229 different plant species in more than 75 countries (FAO/IAEA Mutant Variety Database, 2020). Approximately 18 varieties have been released through mutation breeding in black gram, and all the varieties except one have been released from India. The gamma rays were used effectively in black gram to create variability for chlorophyll content (Usharani and Kumar, 2015; Ramchander et al., 2017), methionine content (Arulbalachandran et al., 2009), pods per plant, number of seeds per plant, number of primary branches, pod length (Usharani and Kumar, 2015; Anitha and Mullainathan, 2018), seed size (Chinchest and Nakeeraks, 1991), and seed yield (Raina et al., 2018). The gamma irradiation treatment successfully created inter-population that led to the selection of two novel mutants, i.e., G7 and G13 derived from ADT 3, and G34 from TU 37-9 with improved yield and stable performance across environments (Dasarathan et al., 2021). The nutritional and battering qualities of varieties MDU 1 and VBN (Bg) 4 were increased through electron beam and gamma-ray mutants. The mutant lines, viz., ACM-014-021, ACM-015-015, ACM-15-023, ACM-015-013, ACM-015-003, ACM-015-030, ACM-014-006, and ACM-014-007, were identified to have superior albumin content, globulin content, total soluble protein, arabinose content, 100-seed weight, and seed yield per plant (Vanniarajan et al., 2021). Apart from the conventional electromagnetic radiations, like X-ray and gamma ray, the electron beam is now an alternative source of energy to induce mutations. In black gram, electron beam irradiation showed increased effectiveness and efficiency in the induction of chlorophyll and morphological mutants in comparison to gamma rays (Souframanien et al., 2016).
Ethyl methanesulfonate (EMS) was also successfully used to create variability for number of seeds per pod, number of primary branches, plant height (Usharani and Kumar, 2015; Kuralarasan et al., 2018), protein content, nitrate reductase activity (Thilagavathi and Mullainathan, 2011), methionine content (Arulbalachandran et al., 2009), and seed yield (Verma et al., 2018). Some of the other mutagens such as sodium azide (Raina et al., 2018), diethyl sulfate (Anitha and Mullainathan, 2018), colchicine, and electron beam (Thilagavathi and Mullainathan, 2011; Veni et al., 2017) were also successfully used in black gram. In India, the Bhabha Atomic Research Centre (BARC) facilitates mutation breeding in several crops including black gram. The varieties developed through mutation breeding include CO 4, Vamban 2, TU94–2, TAU-1, TAU 2, TPU-4, Ujala (OBG17), and Prasad (B3–8-8) (Reddy and Dhanasekar, 2007). The variety CO 4 has early maturity (70–80 days) along with a large seed size (6 g/100 seeds) and is resistant to powdery mildew. Three varieties, namely, Sarla, Prasad, and Vamban 2, are the mutants of a popular variety T9; Sarla and Prasad have tolerance to MYMD, whereas Vamban 2 has tolerance to drought stress. Ujala (OBG17), a mutant variety developed from Prasad (B3–8-8), offers resistance to MYMD and CLS (Gupta et al., 2020). The breeding methods based on phenotypic selections were quite successfully used for those traits where phenotypic selection is precise and easy. The phenotyping for several desirable traits (such as biotic and abiotic stresses) and nutritional quality traits is tedious and time- and resource-consuming and largely depends upon the environmental conditions and phenotyping approaches. This is one of the reasons behind low genetics gains for such desirable traits in black gram and several other crops leading to stagnant yield levels across major growing countries. In contrast to this, the trait-linked molecular markers offer a precise and accurate selection of parents and individuals of segregating populations that can supplement the phenotypic selection for target traits. MAS is one of the useful and precise methods of selection of individuals with desirable traits for genetic improvement and is now being widely used in multiple legumes such as chickpea (Thudi et al., 2014), pigeonpea (Pazhamala et al., 2015), mungbean (Schafleitner, 2020), and groundnut (Varshney et al., 2014; Deshmukh et al., 2020). Although efforts were made in the past for the identification of molecular markers linked to the desirable traits in black gram, none of them could be successfully deployed for MAS in the breeding programs. The PCR-based markers, particularly SNPsv are widely preferred by the breeding programs, as they are cost-effective for genotyping in large segregating populations (Bohar et al., 2020). A flowchart of genetic and genomic resources and their applications in black gram breeding is summarized in Figure 1. The advancement in the identification of diagnostic markers and low-cost genotyping platforms for low-, mid-, and high-density genotyping assays and high-throughput phenotyping tools are expected to help breeding programs enhance genetic gains in black gram.
Figure 1 Genetic and genomic resources and their applications in black gram breeding for accelerating the genetic gains. RIL, recombinant Inbred Lines; NIL, near-isogenic lines; NAM, nested association mapping; MAGIC, multiparent advanced generation intercross).
The desirable genes from different species or genera have been successfully transferred into high-yielding cultivars in many crop species; however, most of them are not yet commercialized due to environmental biosafety and regulatory policies across many countries (Zhang et al., 2018). In black gram, the protocols for in vitro regeneration systems and genetic transformations by Agrobacterium tumefaciens have been already developed and used by Saini et al. (2003). The transgenic black gram was developed through the transformation of shoot apices with A. tumefaciens (strain EHA105) containing a binary vector pKSB (Saini and Jaiwal, 2005). The binary vector harbored bialaphos resistance (bar) and commonbean α-amylase inhibitor-1 (αAI-1) genes leading to increased transformation efficiency of up to 6.5%. The successful transformation with 7.6% frequency was achieved using a cotyledonary node and A. tumefaciens (LBA4404) carrying the binary vector pME 524 (with nptII, bar, and uidA genes). Attempts were also made to incorporate salt tolerance by transforming black gram with glyoxalase I gene derived from Brassica juncea and the constitutive CmYLCV promoter (Bhomkar et al., 2008). A plasmid pGJ42 harboring neomycin phosphotransferase (nptII) selectable marker gene, the barley antifungal gene chitinase (AAA56786), and ribosome-inactivating protein (RIP; AAA32951) were successfully used for the transformation of black gram to develop resistance against corynespora leaf spot disease caused by Corynespora cassiicola (Chopra and Saini, 2014). The protection from diseases among transformed lines varies from 27% to 47% compared to wild-type plants. One of the Alto Keto Reductase (AKR) genes, ALDRXV4 was extracted from Xerophyta viscosa and transferred to the black gram through agrobacterium mediation. The T1 individuals were resistant to drought, salt, and H2O2-induced oxidative stresses by reducing the accumulation of toxic metabolites and upregulating the sorbitol accumulation in the plants (Singh et al., 2016b). Genome-editing tools such as CRISPR/Cas9 are becoming popular functional genomics tools among researchers, as they can create novel genetic variations with the deletion of harmful or the addition of desirable traits in plants with precision and efficiency (Chen and Gao, 2014). It is a rapidly developing technique being used for different genetic manipulation, including generating knockouts, making precise modifications, creating multiplex genome engineering, or activating and repressing genes (Arora and Narula, 2017). The use of genome editing in various crops is increasing (Chen and Gao, 2014; Zhang et al., 2018); however, there has been limited success in legume species. The CRISPR/Cas9-mediated genome editing has been successfully reported in Medicago truncatula (Michno et al., 2015; Confalonieri et al., 2021), soybean (Sun et al., 2015; Han et al., 2019; Bao et al., 2020), red clover (Dinkins et al., 2021), cowpea (Ji et al., 2019; Che et al., 2021), and chickpea (Badhan et al., 2021). In cowpea, the symbiosis receptor-like kinase target gene VuSYMRK edited gene that controls nodule symbiosis exhibited complete inhibition in nodule formation (Ji et al., 2019). This technology in association with base editors and prime editing could be used for de novo domestication of crop wild relatives (CWRs) of underutilized legumes and “reengineering of metabolism” to increase resilience and enhance nutritive value (Gasparini et al., 2021; Nasti and Voytas, 2021). The genome-editing technologies could significantly contribute to legume improvement including black gram to enhance productivity through improving biotic and abiotic stress tolerances (Jha et al., 2022; Mahto et al., 2022; Singh et al., 2023).
Legumes are versatile crops that contribute to mitigating the global food security challenges under changing climate scenarios. The development of genomic resources in legumes including black gram could help breeders to use genomic-assisted breeding tools such as diagnostic molecular markers for target traits to make precise selections in early generations. The developments in the genome sequence could enable researchers to develop low-, mid-, and high-density SNP assays that can be used for new genomic discoveries and deployment of genomic predictions for selection decisions based on breeding values. These genomic-based predictions and speed breeding approaches could enhance genetic gain in legumes (Jha et al., 2022). The novel insights into genomic variations for target traits, their evolution, domestication events, and diversification could be generated through genomic sequencing of CWRs, landraces, and improved breeding lines. Efforts are needed for the development and integration of high-throughput precision phenotyping tools to accelerate the genetic gains for complex traits. A deeper understanding of the mechanism of resistance/tolerance to various biotic and abiotic stresses will be useful in designing a sound breeding strategy. In addition to genetic gains for yields, the improvement in nutritional quality traits such as protein content and quality, and high iron and zinc content could be traits of interest to achieve nutritional security, especially in developing countries. The greater resilience of black gram to changing climatic conditions offers an opportunity for horizontal expansion of the area to enhance environmental sustainability, particularly in cereal-based cropping systems.
RN: Conceptualization, Writing – original draft, Writing – review & editing, Funding acquisition. SC: Writing – original draft, Writing – review & editing. ND: Writing – original draft. AS: Writing – original draft. AG: Writing – original draft. NB: Writing – original draft. HP: Writing – original draft. RS: Writing – original draft, Writing – review & editing. SJ: Writing – original draft, Writing – review & editing. PS: Writing – review & editing.
The author(s) declare financial support was received for the research, authorship, and/or publication of this article. The support of the long-term strategic donors of the World Vegetable Center, namely, Taiwan, United States Agency for International Development (USAID), UK Government’s Foreign, Commonwealth & Development Office (FCDO), Australian Centre for International Agricultural Research (ACIAR), Germany, Thailand, Philippines, Korea and Japan is acknowledged. NSRF via the Program Management Unit for Human Resources and Institutional Development, Research and Innovation (grant number B16F640185) of Thailand.
The authors declare that the research was conducted in the absence of any commercial or financial relationships that could be construed as a potential conflict of interest.
All claims expressed in this article are solely those of the authors and do not necessarily represent those of their affiliated organizations, or those of the publisher, the editors and the reviewers. Any product that may be evaluated in this article, or claim that may be made by its manufacturer, is not guaranteed or endorsed by the publisher.
Abhishek, K. D., Gautam, N. K., Sajad, U. N., Saurabh, K. D., Pankhuri, S., Manoj, K. Y., et al. (2020). Symptom Based Screening of Urdbean Accessions against Leaf Crinkle, Bud Deformation and Yellow Mosaic under Natural Conditions. Int. J. Curr. Microbiol. App. Sci. 9, 1583–1588. doi: 10.20546/ijcmas.2020.902.182
Ambreen, H., Oraon, P. K., Wahlang, D. R., Satyawada, R. R., Agarwal, S. K., Agarwal, M., et al. (2022). Long-read-based draft genome sequence of Indian black gram IPU-94-1 ‘Uttara’: Insights into disease resistance and seed storage protein genes. Plant Genome. 15. doi: 10.1002/tpg2.20234
Anitha, I., Mullainathan, L. (2018). Mutagenic effect of EMS and DES on black gram (Vigna mungo L. Hepper) in M1 generation. J. Phytol. 12, 6–8.
Anitha, Y., Vanaja, M., Kumar, G. V. (2015). Identification of Attributes Contributing to High Temperature Tolerance in Black gram (Vigna mungo L. Hepper) Genotypes. Int. J. Sci. Res. ISSN 5, 1021–1025.
Annan, I. B., Saxena, K. N., Schaefers, G. A., Tingey, W. M. (1994). Effects of infestation by cowpea aphid (Homoptera: Aphididae) on different growth stages of resistant and susceptible cowpea cultivars. Int. J. Trop. Insect Sci. 15, 401–410. doi: 10.1017/s1742758400015733
Arora, R. K. (1988). “The Indian gene centre - Priorities and Prospects for collection,” in Plant Genetic Resources – Indian Perspectives. Eds. Paroda, R. S., Arora, R. K., Chandel, K. P. S. (New Delhi: NBPGR), 66–5.
Arora, L., Narula, A. (2017). Gene editing and crop improvement usingCRISPR-cas9 system. Front. Plant Sci. 8. doi: 10.3389/fpls.2017.01932
Arshad, M., Ghafoor, A., Qureshi, A. S. (2005). Inheritance of qualitative traits and their linkage in black gram [Vigna mungo (L.) Hepper]. Pakistan J. Bot. 37, 41–46.
Arulbalachandran, D., Mullainathan, L., Karthigayan, S., Somasundaram, S. T., Velu, S. (2009). Evaluation of genetic variation in mutants of black gram (Vigna mungo l.) Hepper) as revealed by RAPD markers. Emirates J. Food Agric. 21, 42–50. doi: 10.9755/EJFA.V21I2.5163
Arumuganathan, K., Earle, E. D. (1991). Nuclear DNA content of some important plant species. Plant Mol. Biol. Rep. 9, 208–218. doi: 10.1007/BF02672069
Ashfaq, M., Aslam Khan, M., Mughal, S. M., Javed, N., Mukhtar, T., Bashir, M. (2007). Evaluation of urdbean germplasm for resistance against urdbean leaf crinkle virus. Pakistan J. Botany. 39 (6), 2103–2111.
Ashraf, M. (1989). The effect of NaCl on water relations, chlorophyll, and protein and proline contents of two cultivars of black gram (Vigna mungo L.). Plant Soil 1989 1192 119, 205–210. doi: 10.1007/BF02370409
Badhan, S., Ball, A. S., Mantri, N. (2021). First report of CRISPR/Cas9 mediated DNA-free editing of 4CL and RVE7 genes in chickpea protoplasts. Int. J. Mol. Sci. 22, 396. doi: 10.3390/ijms22010396
Bao, A., Zhang, C., Huang, Y., Chen, H., Zhou, X., Cao, D. (2020). Genome editing technology and application in soybean improvement. Oil Crop Science. 5, 31–40. doi: 10.1016/j.ocsci.2020.03.001
Baroowa, B., Gogoi, N. (2012). Effect of induced drought on different growth and biochemical attributes of black gram (Vigna mungo L .) and green gram (Vigna radiata L .). J. Environ. Res. Dev. 6.
Baroowa, B., Gogoi, N. (2015). Changes in plant water status, biochemical attributes and seed quality of black gram and green gram genotypes under drought. Int. Lett. Nat. Sci. 42, 1–12. doi: 10.18052/WWW.SCIPRESS.COM/ILNS.42.1
Basandrai, A. K., Sharma, V., Katoch, A., Basandrai, D., Sharma, P. N. (2016). Genetic diversity of Colletotricum truncatum infecting urdbean (Vigna mungo) in Himachal Pradesh. Indian Phytopathol. 69, 386–390.
Bashir, M., Ahmad, Z., Ghafoor, A. (2005). Sources of genetic resistance in mungbean and black gram against Urdbean Leaf Crinkle Virus (ULCV). Pakistan J. Bot. 37, 47–51.
Bashir, M., Mughal, S. M., Malik, B. A. (1991). Assesment of yield losses due to leaf crinkle virus in Urdbean, Vigna mungo (L.) Hepper. Pakistan J. Bot. 23, 140–142.
Bashir, M., Zubair, M. (1985). Survey report of Kharif pulses in Islamabad, Rawalpindi and Sialkot districts during 1985. Pulses Programme, NARC. Pakistan Agric. Res. Council Islamabad. 23.
Berani, N. K., Damor, M. P., Godhani, P. H. (2018). Impact of date of sowing on lepidopteran insect pest of black gram, Vigna mungo (L.) Hepper. J. Entomology Zoology Stud. 6, pp.24–pp.26.
Bhanu, A. N. (2018). Crossability studies of interspecific hybridization among vigna species. Biomed. J. Sci. Tech. Res. 2. doi: 10.26717/bjstr.2018.02.000818
Bhisht, I. S., Singh, M. (2013). Asian vigna. Genet. genomic Resour. grain legume improvement, 237–267. doi: 10.1016/B978-0-12-397935-3.00010-4
Bhomkar, P., Upadhyay, C. P., Saxena, M., Muthusamy, A., Shiva Prakash, N., Pooggin, M., et al. (2008). Salt stress alleviation in transgenic Vigna mungo L. Hepper by overexpression of the glyoxalase I gene using a novel Cestrum yellow leaf curling virus (CmYLCV) promoter. Mol. Breeding. 22 (2), 169–181. doi: 10.1007/s11032-008-9164-8
Boddepalli, V. N., War, A. R., Pandey, A. K., Shivanna, A., Gowda, A., Chaudhari, S., et al. (2021). “Development of black gram varieties with improved protein quality, and resistance to bruchids and mungbean yellow mosaic disease,” in Abstracts: National Web Conference on Sustaining Pulse Production for Self-sufficiency and Nutritional Security, Kanpur, U.P., India, Feb. 9-11. 97, ICAR-Indian Institute of Pulses Research.
Bohar, R., Chitkineni, A., Varshney, R. K. (2020). Genetic molecular markers to accelerate genetic gains in crops. BioTechniques 69, 159–161. doi: 10.2144/BTN-2020-0066
Boukar, O., Belko, N., Chamarthi, S., Togola, A., Batieno, J., Owusu, E., et al. (2019). Cowpea (Vigna unguiculata): Genetics, genomics, and breeding. Plant Breed. 138, 415–424. doi: 10.1111/PBR.12589
Castillo, C. (2013). The archaeobotany of Khao Sam Kaeo and Phu Khao Thong: the agriculture of late prehistoric southern Thailand (London, United Kingdom: University College London). Ph.D. Thesis.
Chaitieng, B., Kaga, A., Tomooka, N., Isemura, T., Kuroda, Y., Vaughan, D. A. (2006). Development of a black gram [Vigna mungo (L.) Hepper] linkage map and its comparison with an azuki bean [Vigna angularis (Willd.) Ohwi and Ohashi] linkage map. Theor. Appl. Genet. 2006 1137 113, 1261–1269. doi: 10.1007/S00122-006-0380-5
Chandel, K. P. S., Lester, R. N., Starling, R. J. (1984). The wild ancestors of urid and mung beans (Vigna mungo (L.) Hepper and V. radiata (L.) Wilczek). Bot. J. Linn. Soc 89, 85–96. doi: 10.1111/J.1095-8339.1984.TB01002.X
Chankaew, S., Isemura, T., Isobe, S., Kaga, A., Tomooka, N., Somta, P., et al. (2014). Detection of genome donor species of neglected tetraploid crop Vigna reflexo-pilosa (créole bean), and genetic structure of diploid species based on newly developed EST-SSR markers from azuki bean (Vigna angularis). PloS One 9, e104990. doi: 10.1371/journal.pone.0104990
Chatak, S., Banyal, D. K. (2020). Evaluation of IDM components for the management of urdbean anthracnose caused by Colletotrichum truncatum (Schwein) Andrus and Moore. Himachal Journal of Agricultural Research 46, 156–161.
Che, P., Chang, S., Simon, M. K., Zhang, Z., Shaharyar, A., Ourada, J., et al. (2021). Developing a rapid and highly efficient cowpea regeneration, transformation and genome editing system using embryonic axis explants. Plant J 106 (3), 817–830. doi: 10.1111/tpj.15202
Chen, K., Gao, C. (2014). Targeted genome modification technologies andTheir applications in crop improvements. Plant Cel. Rep. 33, 575–583. doi: 10.1007/s00299-013-1539-6
Chinchest, A., Nakeeraks, P. (1991). Mutation breeding of black gram (Vigna mungo). In Proc. mungbean meeting Department Agric. 90, 43–46.
Chopra, R., Saini, R. (2014). Transformation of black gram (Vigna mungo (L.) Hepper) by barley chitinase and ribosome-inactivating protein genes towards improving resistance to Corynespora leaf spot fungal disease. Appl. Biochem. Biotechnol. 174 (8), 2791–2800. doi: 10.1007/s12010-014-1226-2
Choudhary, A. K., Sultana, R., Chaturvedi, S. K., Sharma, R., Bhatt, B. P., Singh, S. P. (2014). “Breeding strategies to mitigate abiotic stresses in pulses,” in Emerging Challenges and Opportunities for Biotic and Abiotic Stress Management (ECOBASM). 16–21.
Choudhary, A. K., Sultana, R., Vales, M. I., Saxena, K. B., Kumar, R. R., Ratnakumar, P. (2018). Integrated physiological and molecular approaches to improvement of abiotic stress tolerance in two pulse crops of the semi-arid tropics. Crop J. 6, 99–114. doi: 10.1016/J.CJ.2017.11.002
Commodity Profile for Pulses (2019). Ministry of agriculture and farmers welfare, department of agriculture, cooperation and farmers welfare, India.
Confalonieri, M., Carelli, M., Gianoglio, S., Moglia, A., Biazzi, E., Tava, A. (2021). CRISPR/cas9-mediated targeted mutagenesis of CYP93E2 modulates the triterpene saponin biosynthesis in medicago truncatula. Front. Plant Science. 12. doi: 10.3389/fpls.2021.690231
Dahiya, B. S., Brar, J. S., Bhullar, B. S. (1977). Inheritance of protein content and its correlation with grain yield in pigeonpea (Cajanus cajan (L.) Millsp.). Qual. Plant 1977 273 27, 327–334. doi: 10.1007/BF01092325
Dasarathan, M., Geetha, S., Karthikeyan, A., Sassikumar, D., Meenakshiganesan, N. (2021). Development of Novel Black gram (Vigna mungo (L.) Hepper) Mutants and Deciphering Genotype × Environment Interaction for Yield-Related Traits of Mutants. Agron. 11, 1287. doi: 10.3390/AGRONOMY11071287
Dasgupta, Roy, I. (2015). ISBN 978-92-5-109079-4. FAO Regional Office for Asia and the Pacific, pp 222.
Dash, M., Shree, D. (2013). Temperature effect on morpho-biochemical characters in some black gram (Vigna mungo) genotypes. Int. Scholarly Res. Notices 2013. doi: 10.5402/2013/942868
Deshmukh, D. B., Marathi, B., Sudini, H. K., Variath, M. T., Chaudhari, S., Manohar, S. S., et al. (2020). Combining high oleic acid trait and resistance to late leaf spot and rust diseases in groundnut (Arachis hypogaea L.). Front. Genet. 11. doi: 10.3389/FGENE.2020.00514/BIBTEX
Dhar, V., Chaudhary, R. G. (2001). Disease resistance in Pulse CropsCurrent Status and Future Approaches (in) The Role of Resistance in Intensive Agriculture. Eds. Nagarajan, S., Singh, D. P. (New Delhi: Kalyani publishers), 144–157.
Dhingra, K. L. (1975). Transmission of urdbean leaf crinkle virus by two aphid species. Indian Phytopathology. 28 (1), 80–82.
Dikshit, H. K., Mishra, G. P., Somta, P., Shwe, T., Alam, A. K. M. M., Bains, T. S., et al. (2020). Classical genetics and traditional breeding in mungbean. in The Mungbean Genome, Compendium of Plant Genomes ed. Nair, R. M., et al (Switzerland AG: © Springer Nature), 43–54. doi: 10.1007/978-3-030-20008-4_4
Dinkins, R. D., Hancock, J., Coe, B. L., May, J. B., Goodman, J. P., Bass, W. T., et al. (2021). Isoflavone levels, nodulation and gene expression profiles of a CRISPR/Cas9 deletion mutant in the isoflavone synthase gene of red clover. Plant Cell Rep. 40 (3), pp.517–pp.528. doi: 10.1007/s00299-020-02647-4
Directorate of Economics and Statistics (2021). “Ministry of agriculture and farmers welfare department of agriculture, cooperation and farmers welfare,” in Third Advance Estimates of Production of Food grains for 2020-21 (Government of India).
Dongre, T. K., Pawar, S. E., Thakare, R. G., Harwalkar, M. R. (1996). Identification of resistant sources to cowpea weevil (Callosobruchus maculatus (F.)) in Vigna sp. and inheritance of their resistance in black gram (Vigna mungo var. mungo). J. Stored Prod. Res. 32, 201–204. doi: 10.1016/S0022-474X(96)00028-8
Duan, C. X., Zhu, Z. D., Ren, G. X., Wang, X. M., Li, D. D. (2014). Resistance of faba bean and pea germplasm to callosobruchus chinensis (Coleoptera: bruchidae) and its relationship with quality components. J. Econ. Entomol. 107, 1992–1999. doi: 10.1603/EC14113
Dubey, S. C., Singh, B. (2010). Seed treatment and foliar application of insecticides and fungicides for management of cercospora leaf spots and yellow mosaic of mungbean (Vigna radiata). Int. J. Pest Manage 56, 309–314. doi: 10.1080/09670874.2010.496875
Duraimurugan, P., Tyagi, K. (2014). Pest spectra, succession and its yield losses in mungbean and urdbean under changing climatic scenario. Legume Res. 37, 212–222. doi: 10.5958/j.0976-0571.37.2.032
Durga, K. K., Rao, M. M., Reddy, M. V., Rao, Y. K. (2003). Effect of early drought stress on morpho-physiological characters and yield of mungbean and urdbean cultivars. Indian J. Pulses Res. 16 (2), 133–135.
Dwivedi, S., Singh, D. P. (1985). Inheritance of resistance to yellow mosaic virus in a wide cross of black gram (V mungo[L] Hepper). Z Pflanzenzuchtg 95, 281–284.
Elmerich, C., Pandey, A. K., Vemula, A., Rathore, A., Nair, R. M. (2022). Black gram-macrophominaphaseolina interactions and identification of novel sources of resistance. Plant Dis 106 (11), 2911–2919. doi: 10.1094/PDIS-11-21-2588-RE
Elshire, R. J., Glaubitz, J. C., Sun, Q., Poland, J. A., Kawamoto, K., Buckler, E. S., et al. (2011). A robust, simple genotyping-by-sequencing (GBS) approach for high diversity species. PLoS ONE 6, e19379. doi: 10.1371/journal.pone.0019379
FAO/IAEA Mutant Variety Database (2020). Available at: https://mvd.iaea.org/#!Search?page=1&size=15&sortby=Name&sort=ASC.
Fernandez, G. C. J., Talekar, N. S. (1990). Genetics and breeding for bruchid resistance in asiaticSpecies. Bruchids Legume Proc. 2nd Int. Symp. Okayama 1989, 209–217. doi: 10.1007/978-94-009-2005-7_23
Fujita, K., Okamoto, I. (2006). Agricultural policies and development of Myanmar’s agricultural sector. overview Institute Developing Economies JETRO Chiba p 18.
Fuller, D. Q., Harvey, E. L. (2006). The archaeobotany of Indian pulses: identification, processing and evidence for cultivation. Environmental Archeology 11, 219–246. doi: 10.1179/174963106x123232
Ganeshan, S., Gaur, P. M., Chibbar, R. N. (2012). Pulse crops: biotechnological strategies to enhance abiotic stress tolerance. Improv. Crop Product. Sustain. Agric., 423–447. doi: 10.1002/9783527665334.CH17
Ganguly, P. R., Bhat, K. (2012). Study of the pattern of variation for microsatellite markers in black gram (Vigna mungo) germplasm. DHR International Journal Of Biomedical and LifeSciences (DHR-IJBLS) 3, 1, 1–6.
Gasparini, K., dos Reis Moreira, J., Peres, L. E., Zsögön, A. (2021). De novodomestication of wild species to create crops with increased resilience andNutritional value. Curr. Opin. Plant Biol. 60, 102006. doi: 10.1016/j.pbi.2021.102006
Gaur, P. M., Chaturvedi, S. K. (2004). Genetic options for managing biotic stresses in pulse crops. Pulses New Perspect. Proc. Natl. Symp. Crop Diversif. Nat. Resour. Manage., 91–111.
Gautam, N. K., Kumar, K., Prasad, M. (2016). Leaf crinkle disease in urdbean (Vigna mungo L. Hepper): An overview on causal agent, vector and host. Protoplasma, 729–746. doi: 10.1007/S00709-015-0933-Z
Geetha, S., Gnanamalar, R. P., Manivannan, N., Mahalingam, A., Lakshminarayanan, S., Bharathikumar, K., et al. (2020). VBN 11 - A new high-yielding and MYMV disease-resistant black gram variety. Electron. J. Plant Breed. 11, 987–992. doi: 10.37992/2020.1104.161
Ghafoor, A., Ahmad, Z. (2005). Diversity of agronomic traits and total seed protein in black gram (Vigna mungo). Acta Biol. Crac. Ser. Bot. 47, 69–75.
Ghafoor, A., Sharif, A., Ahmad, Z., Zahid, M. A., Rabbani, M. A. (2001). Genetic diversity in black gram (Vigna mungo L. Hepper). F. Crop Res. 69, 183–190. doi: 10.1016/S0378-4290(00)00141-6
Goyal, S., Khan, S. (2010). Induced mutagenesis in Urdbean (Vigna mungo L. Hepper): A review. Int. J. Bot. 6, 194–206. doi: 10.3923/IJB.2010.194.206
Guljar, D., Rashmi, D., Revanappa, S. B., Suma, M., Saabale, P. R. (2019). Identification for resistant sources against dry root rot in black gram germplasm (Vigna mungo L.). Int. J. Chem. Stud. 7, 990–992.
Gupta, S., Das, A., Pratap, A., Gupta, D. S. (2021). Urdbean. Beans Peas, 33–54. doi: 10.1016/B978-0-12-821450-3.00014-7
Gupta, S. K., Gopalakrishna, T. (2010). Development of unigene-derived SSR markers in cowpea (Vigna unguiculata) and their transferability to other Vigna species. Genome 53, 508–523. doi: 10.1139/G10-028
Gupta, S., Gupta, D. S., Anjum, T. K., Pratap, A., Kumar, J. (2013a). Inheritance and molecular tagging of MYMIV resistance gene in black gram (Vigna mungo L. Hepper). Euphytica 193, 27–37. doi: 10.1007/s10681-013-0884-4
Gupta, S., Gupta, D. S., Anjum, K. T., Pratap, A., Kumar, J. (2013b). Transferability of simple sequence repeat markers in black gram (Vigna mungo L. Hepper). Aust. J. Crop Sci. 7 (3), 345–353. doi: 10.3316/informit.261050483342258
Gupta, S., Kumar, S. (2006). “Urd bean breeding,” in Advances in mungbean and urdbean, vol. 149 . Eds. Ali, M., Kumar, S. (Kanpur: Indian Institute of Pulses Research), 168.
Gupta, D. S., Kumar, J., Parihar, A. K., Gupta, S. (2020). Breeding for high-yielding and disease-resistant urdbean cultivars. Accel. Plant Breed. 3, 173–191. doi: 10.1007/978-3-030-47306-8_6
Gupta, S., Kumar, S., Singh, R. A., Chandra, S. (2005). Identification of a single dominant gene for resistance to Mungbean Yellow Mosaic Virus in black gram (Vigna mungo (L.) Hepper). SABRAO J. Breed. Genet. 37, 85–89.
Gupta, V. P., Plaha, P., Rathore, P. K. (2002). Partly fertile interspecific hybrid between a black gram × green gram derivative and an adzuki bean. Plant Breed. 121, 182–183. doi: 10.1046/J.1439-0523.2002.00675.X
Gupta, S. R., Singh, R. A., Gupta, S., Dikshit, H. K. (2001). Variability and its characterization in Indian collections of blackgram [Vigna mungo (L.) Hepper]. Plant Genet. Resour. Newsletter. Rome Italy 1979, 20–24.
Gupta, S. K., Souframanien, J., Gopalakrishna, T. (2008). Construction of a genetic linkage map of black gram, Vigna mungo (L.) Hepper, based on molecular markers and comparative studies. Genome 51, 628–637. doi: 10.1139/G08-050
Gurumurthy, S., Sarkar, B., Vanaja, M., Lakshmi, J., Yadav, S. K., Maheswari, M. (2019). Morpho-physiological and biochemical changes in black gram (Vigna mungo L. Hepper) genotypes under drought stress at flowering stage. Acta Physiol. Plant 41, 1–14. doi: 10.1007/S11738-019-2833-X
Han, J., Guo, B., Guo, Y., Zhang, B., Wang, X., Qiu, L. J. (2019). Creation ofEarly flowering germplasm of soybean by CRISPR/cas9 technology. Front.Plant Sci. 10. doi: 10.3389/fpls.2019.01446
He, J., Zhao, X., Laroche, A., Lu, Z. X., Liu, H. K., Li, Z. (2014). Genotyping-by-sequencing (GBS), An ultimate marker-assisted selection (MAS) tool to accelerate plant breeding. Front. Plant Sci. 5. doi: 10.3389/FPLS.2014.00484/BIBTEX
Ilyas, M. B., Haq, M. A., Iftikhar, K. (1992). Studies on the responses of growth components of urdbean against leaf crinkle virus. Pakistan J. Phytopathol. 4, 51–56.
Isemura, T., Kaga, A., Tabata, S., Somta, P., Srinives, P., Shimizu, T., et al. (2012). Construction of a genetic linkage map and genetic analysis of domestication related traits in mungbean (Vigna radiata). PloS One 7, e41304. doi: 10.1371/journal.pone.0041304
Jacob, S. R., Tyagi, V., Agrawal, A., Chakrabarty, S. K., Tyagi, R. K. (2015). Indian plant germplasm on the global platter: an analysis. PloS One 10, e0126634. doi: 10.1371/JOURNAL.PONE.0126634
Jana, M. K. (1962). X-ray induced mutants of Phaseolus mungo L 2 Sterility and vital mutants. Genetica Iberica. 14, 71–104.
Jansen, P. C. M. (2006). “Vigna mungo (L.) Hepper,” in PROTA (Plant Resources of Tropical Africa / Ressources végétales de l’Afrique tropicale. Eds. Brink, M., Belay, G.(Wageningen, Netherlands). Available at: http://www.prota4u.org/search.asp. Record from PROTA4U.
Jha, U. C., Nayyar, H., PARIDA, S. K., Bakir, M., Von Wettberg, E., Siddique, K. H. (2022). Progress of genomics-driven approaches for sustaining underutilized legume crops in the post-genomic era. Front. Genet., 536. doi: 10.3389/fgene.2022.831656
Ji, J., Zhang, C., Sun, Z., Wang, L., Duanmu, D., Fan, Q. (2019). GenomeEditing in cowpea vigna unguiculata using CRISPR-cas9. Int. J. Mol. Sci. 20 (10), 2471. doi: 10.3390/ijms20102471
Justin, C. G. L., Anandhi, P., Jawahar, D. (2015). Management of major insect pests of blackgram under dryland conditions. J. Entomol. Zool. Stud. 3, 115–121.
Kadian, O. P. (1980). Studies on Leaf Crinkle Disease of Urdbean (Vigna mungo (L.) Hepper)/Mung Bean (Vigna radiata (L.) Wilczek) and its Control (College of Agriculture Chaudhary Charan Singh Haryana Agricultural University Hisar). Doctoral dissertation.
Kaewwongwal, A., Kongjaimun, A., Somta, P., Chankaew, S., Yimram, T., Srinives, P. (2015). Genetic diversity of the blackgram [Vigna mungo (L.) Hepper] gene pool as revealed by SSR markers. Breed. Sci. 65, 127–137. doi: 10.1270/JSBBS.65.127
Kaga, A., Isemura, T., Toomoka, N., Vaughan, D. A. (2008). The genetics of domestication of azuki bean (Vigna angularis). Genetics 178, 1013–1036. doi: 10.1534/genetics.107.078451
Katiyar, P. K., Dixit, G. P., Singh, B. B. (2008). Genetic base of advanced urdbean breeding lines developed in India as revealed by pedigree analysis. Indian J. Genet. Plant Breed. 68 (03), 324–326.
Kaushal, R. P., Singh, B. M. (1988a). Inheritance of disease resistance in blackgram (V. mungo) to mungbean yellow mosaic virus. Indian J. Agric. Sci. 58, 2:123–2:124.
Kaushal, R. P., Singh, B. M. (1988b). Genetics of disease resistance in urdbean (Vigna mungo (L) Hepper) to the leaf spots caused by Colletotrichum truncatum (Schw) Andrus & Moore. Euphytica. 37, 279–281. doi: 10.1007/BF00015124
Kaushal, R. P., Singh, B. M. (1989). Genetics of resistance in blackgram (Phaseolus mungo) to powdery mildew caused by Erysiphe polygoni. Indian.J. Agric. Sci. 59 (10), 684–685.
Kaushal, R. P., Singh, B. M. (1991). Heritability of resistance in urdbean to leaf spots caused by Cercospora canescens. Indian Phytopathol. 44, 1,131–1,132.
Khan, F., Nayab, M., Ansari, A. N., Zubair, M. (2021). Medicinal properties of mash (Vigna mungo (Linn.) hepper): A comprehensive review. J. Drug Deliv. Ther. 11, 121–124. doi: 10.22270/jddt.v11i3-S.4888
Kharkwal, M. C., Shu, Q. Y. (2009). “The role of induced mutations in world food security,” in Induced plant mutations in the genomics era (Rome: Food and Agriculture Organization of the United Nations), 33–38.
Kitturmath, M. S. (2008). Investigations on insecticide resistance in Spodoptera litura Fab. (Lepidoptera: Noctuidae) (Bengaluru: University Of Agricultural Sciences GKVK). Doctoral dissertation.
Kole, C., Mohanty, S. K., Pattanayak, S. K. (2002). Selection of protein rich genotypes in urdbean [Vigna mungo (L.) Hepper]. Indian J. Genet. Plant Breed. 62 (04), 345–346.
Kongjaimun, A., Kaga, A., Tomooka, N., Somta, P., Vaughan, D. A., Srinives, P. (2012). The genetics of domestication of yardlong bean, Vigna unguiculata (L.) Walp. ssp. unguiculata cv.-gr. sesquipedalis. Ann. Bot. 109, 1185–1200. doi: 10.1093/aob/mcs048
Kooner, B. S., Cheema, H. K., Kaur, R. (2006). “Insect pests and their management,” in Advances in mungbean and urdbean. Eds. Ali, M., Shivkumar (Kanpur: Indian Institute of Pulses Research), 335–401.
Krishna, Y., Rao, Y. K., Rao, V. R. S., Rajasekhar, P., Rao, V. S. (2006). Screening for resistance against spotted pod borer Maruca vitrata (Geyer). blackgram. J. Entomol. Res. 30 (1), 35–37.
Kumar, S., Gupta, S. ,. H., Datta, S., Singh, B., Singh, B. B. (2012). Inheritance of Protruded Stigma in blackgram [Vigna mungo (L.) Hepper]. Crop Sci. 52, 57–63. doi: 10.2135/CROPSCI2011.01.0033
Kumar, Y. S., Hemalatha, S., Chandrika, V., Latha, P., Karuna, S. G. (2020). Growth and yield of summer blackgram (Vigna mungo l.) as influenced by moisture stress and foliar nutrition. Andhra Pradesh J. Agril. Sci. 6, 111–114.
Kumar, R., Rathi, A. S., Singh, A., Krishna, R. (2002). Genetic divergence in relation to breeding for grain yield in urdbean (Vigna Mungo L.). Legum Res. 25 (2), 109–112.
Kumar, R., Singh, A., Rathi, A. S. (2000). Estimating genetic parameters in Urdbean. Ann. Agric. Res. 21 (3), 335–337.
Kuralarasan, V., Vanniarajan, C., Kanchana, S., Veni, K., Anandhi, L. S. (2018). Genetic divergence, heritability and genetic advance in mutant lines of urdbean [Vigna mungo (L.) hepper]. Legume Res. 41, 833–836. doi: 10.18805/LR-3794
Lal, S. S. (1985). A review of insect pests of mungbean and their control in India. Int. J. Pest Manage. 31, 105–114. doi: 10.1080/09670878509370960
Lekhi, P., Gill, R. K., Kaur, S., Bains, T. S. (2018). Generation of interspecific hybrids for introgression of mungbean yellow mosaic virus resistance in [Vigna radiata (L.) Wilczek]. Legume Res. 41, 526–531. doi: 10.18805/LR-3808
Lomlek, C., Suamuang, S., Laosatit, K., Tangphatsornruang, S., Tanadul, O., Somta, P. (2023). QTL analysis of seed weight and seed dormancy in black gram (Vigna mungo (L.) Hepper). Agr. Nat. Resour. 57 (3). doi: 10.34044/j.anres.2023.57.3.03
Lukoki, L., Marechal, R., Otoul, E. (1980). Les ancêtres sauvages des haricots cultivés: Vigna radiata (L.) Wilczek et V. mungo (L.) Hepper. Hepper. Bull. Jard. Bot. Nat. Belgique. 50, 385–391. doi: 10.2307/3667837
Mahalakshmi, M. S., Sreekanth, M., Adinarayana, M., Rao, Y. K. (2015). Efficacy of some novel insecticide molecules against incidence of whiteflies (Bemisia tabaci Genn.) and occurrence of Yellow Mosaic Virus (YMV) disease in urdbean. Int. J. Pure Appl. 3, 101–106. doi: 10.18782/2320-7051.2105
Mahto, R. K., Ambika, S. C., Chandana, B. S., Singh, R. K., Verma, S., Gahlaut, V., et al. (2022). Chickpea biofortification for cytokinin dehydrogenase via genome editing to enhance abiotic-biotic stress tolerance and food security. Front. Genet. 13. doi: 10.3389/fgene.2022.900324
Manjula, K., Prasanthi, L., John, S. M. (2019). Occurrence of Stem fly, Ophiomyia phaseoli in Major pest status in blackgram in Southern Zone of Andhra Pradesh, India. Asian J. Sci. Technol. 10 (12), 10522–10525.
Manjunath, G. N., Mallapur, C. P. (2019). Field screening of blackgram genotypes against spotted pod borer, Maruca vitrata (Geyer). J. Entomol. Zool. 7, 631–634.
Michno, J. M., Wang, X., Liu, J., Curtin, S. J., Kono, T. J., Stupar, R. M. (2015). CRISPR/Cas Mutagenesis of soybean and Medicago truncatula using a newweb-tool and a modified Cas9 enzyme. GM Crops Food. 6, 243–252. doi: 10.1080/21645698.2015.1106063
Mishra, V., Srivastava, K. C., Yadav, S. (2011). Test of plant extracts against anthracnose of urdbean caused by colletotrichum capsici. Indian Journals. Ann. Plant Prot. Sci. 19 (2), 500–501.
Modgil, R., Kaundal, S., Sandal, A. (2019). Bio-Chemical and Functional Characteristics of blackgram (Vigna mungo) Cultivars Grown in Himachal Pradesh. Int. J. Curr. Microbiol. Appl. Sci. 8, 2126–2137. doi: 10.20546/ijcmas.2019.804.250
Modgil, R., Mehta, U. (1997). Effect of Callosobruchus chinensis (Bruchid) infestation on antinutritional factors in stored legumes. Plant Foods Hum. Nutr. 1997 504 50, 317–323. doi: 10.1007/BF02436078
Muralidharan, V., Rangasamy, S. R., Babu, R. C., Murugarajendran, C., Rao., Y. K. (1990). Inheritance of fused leaflets nature in urdbean. Indian J. Pulses Res. 3 (1), 75–76.
Nadeem, M., Li, J., Yahya, M., Sher, A., Ma, C., Wang, X., et al. (2019). Research progress and perspective on drought stress in legumes: A review. Int. J. Mol. Sci. 20 (10), 2541. doi: 10.3390/IJMS20102541
Naik, B. J., Anuradha, C., Kumar, P. A., Sreedhar, V., Chary, S. (2017). Identification of simple sequence repeats (SSR) markers linked to yellow mosaic virus (YMV) resistance in blackgram [Vigna mungo (L). Hepper]. Agric. Update 12, 812–819. doi: 10.15740/has/au/12.techsear(3)2017/812-819
Naito, K., Takahashi, Y., CHaitieng, B., Hirano, K., Kaga, A., Takagi, K., et al. (2017). Multiple organ gigantism caused by mutation in VmPPD gene in blackgram (Vigna mungo). Breed Sci. 67 (2), 151–158. doi: 10.1270/jsbbs.16184
Naito, K., Wakatake, T., Shibata, T. F., Iseki, K., Shigenobu, S., Takahashi, Y., et al. (2022). Genome sequence of 12 Vigna species as a knowledge base of stress tolerance and resistance. bioRxiv 03, 28.486085. doi: 10.1101/2022.03.28.486085
Narasimhan, A., Patil, B. R., Datta, S., Kaashyap, M. (2010). Genetic diversity assessment across different genotypes of mungbean and urdbean using molecular markers. Electron J. Plant Breed. 56, 114–120.
Nasti, R. A., Voytas, D. F. (2021). Attaining the promise of plant gene editingat scale. Proc. Nat. Acad. Sci. 118 (22), e2004846117. doi: 10.1073/pnas.2004846117
Nautiyal, M. K., Shukla, A. (1999). Classification of Uridbean germplasm from diversity zones of Uttar Pradesh. Indian J. Plant Gen. Res. 12 (3), 366–370.
Negi, K. S., Muneem, K. C. (1998). Preliminary Evaluation of Black gram (Vigna mungo (L.) Hepper) Germplasm of North-West Himalaya. Indian J. Hill Farm. 11, 19–23.
Negi, H., Vishunavat, K. (2006). Urdbean leaf crinkle infection in relation to plant age, seed quality, seed transmission and yield in urdbean. Ann. Plant Sci. 14 (1), 169–172.
Neupane, S., Subedi, S., Darai, R., Sharma, T. (2021). Field assessment of blackgram (Vigna mungo L. Hepper) genotypes against major insect pests in subtropical region of Nepal. J. Agric. Nat. Resour. 4, 248–255. doi: 10.3126/janr.v4i1.33278
Pal, S. S., Dhaliwal, H. S., Bains, S. S. (1991). Inheritance of resistance to yellow mosaic virus in some vigna species. Plant Breed. 106, 168–171. doi: 10.1111/J.1439-0523.1991.TB00496.X
Pandey, S. (2019). Review on medicinal importance of Vigna genus. Plant Sci. Today 6, 450–456. doi: 10.14719/pst.2019.6.4.614
Pandey, M. K., Roorkiwal, M., Singh, V. K., Ramalingam, A., Kudapa, H., Thudi, M., et al. (2016). Emerging genomic tools for legume breeding: Current status and future prospects. Front. Plant Sci. 7. doi: 10.3389/FPLS.2016.00455/BIBTEX
Pandey, S., Sharma, M., Kumari, S., Gaur, P. M., Chen, W., Kaur, L., et al. (2009). “Integrated foliar diseasesmanagement of legumes,” in GrainLegumes: Genetic improvement,Management and Trade. Ed. Ali, M., et al (Kanpur, India: IndianSociety of Pulses Research andDevelopment, Indian Institute of PulsesResearch), 143–161.
Pandiyan, M., Geetha, S., Gnanamalar, R. P., Packiaraj, D., Mahalingam, A., Sassikumar, D., et al. (2018). A new high yielding MYMV disease resistant black gram variety VBN 8. Electron. J. Plant Breed. 9, 1272–1279. doi: 10.5958/0975-928X.2018.00159.X
Pandiyan, M., Senthil, N., Balaji, T., Veeramani, P., Savitha, B. K., Senthivel, V., et al. (2017). Studies on performance of drought tolerant genotypes under drought and normal conditions through morpho, physio and biochemical attributes of black gram (Vigna mungo. L.) and green gram (Vigna radiata L.). Int. J. Curr. Adv. Res. 5 (2), 489–496. doi: 10.21474/IJAR01/3176
Partheeban, C. (2017). Evaluation of black gram (temperature and interaction). Res. J. Recent Sci. 6 (1), 11–21.
Partheeban, C., Vijayaraghavan, H. (2020). Morphological and ultra-structural characterization of black gram [Vigna mungo (L.) hepper] genotypes grown under high temperature stress. Legume Res. 43, 757–763. doi: 10.18805/LR-4273
Pathak, G. N. (1961). Inheritance of hairy character on pods in black gram (Phaseolus mungo L). Curr. Sci. 30, 434–435.
Patidar, M., Sharma, H., Haritwal, S. (2018). Genetic variability studies in black gram (Vigna mungo L). Hepper. Int. J. Chem. Stud. 6 (2), 1501–1503.
Pawar, S. E., Manjayya, J. G., Souframanien., J., Bhatkar, S. M. (2000). “Genetic improvement of black gram using induced mutations,” in DAE-BRNS Symp. The Use of Nuclear and Molecular Techniques in Crop Improvement(Mumbai: Bhabha Atomic Research Centre), 170–174.
Pazhamala, L., Saxena, R. K., Singh, V. K., Sameerkumar, C. V., Kumar, V., Sinha, P., et al. (2015). Genomics-assisted breeding for boosting crop improvement in pigeonpea (Cajanus cajan). Front. Plant Sci. 6. doi: 10.3389/fpls.2015.00050
Ponnusamy, D., Pratap, A., Singh, S. K., Gupta, S. (2014). Evaluation of screening methods for bruchid beetle (Callosobruchus chinensis) resistance in Greengram (Vigna radiata) and Black gram (Vigna mungo) genotypes and influence of seed physical characteristics on its infestation. Vegetos 27, 60–67. doi: 10.5958/j.2229-4473.27.1.011
Pootakham, W., Nawae, W., Naktang, C., Sonthirod, Yoocha, C. T., Kongkachana, W., et al. (2020). A chromosome-scale assembly of the black gram (Vigna mungo) genome. Mol. Ecol. Resour., 1–13. doi: 10.1111/1755-0998.13243
Prasad, D., Kumar, D., Prasad, R., Kumar, B. (2005). Efficacy of Insecticides and their Combination with NSKE for the Management of Insect Pests of Black gram. Environ. Agric. 172, 171–176.
Pratap, V., Sharma, Y. K. (2010). Impact of osmotic stress on seed germination and seedling growth in black gram (Phaseolus mungo). J. Environ. Biol. 31, 721–726.
Priyanka, S., Rangaiah, S., Pavan, R. (2018). Screening black gram genotypes under artificially inoculated conditions for powdery mildew resistance. Legume Res. 41, 907–912. doi: 10.18805/LR-3796
Raguchander, T., Prabakar, K., Samiyappan, R. (2005). Held evaluation of Pseudomonas fluorescens and Bacillus subtius on the management of Cercospora leaf spot and powdery mildew in urdbean. Legume Res. - an Int. J. 28, 137–139.
Raina, A., Khursheed, S., Khan, S. (2018). Optimisation of mutagen doses for gamma rays and sodium azide in cowpea optimisation of mutagen doses for gamma rays and sodium azide in cowpea genotypes. Tren. Biosci. 11, 2386–2389.
Raizada, A., Souframanien, J. (2019). Transcriptome sequencing, de novo assembly, characterisation of wild accession of black gram (Vigna mungo var. silvestris) as a rich resource for development of molecular markers and validation of SNPs by high resolution melting (HRM) analysis. BMC Plant Biol. 19, 1–16. doi: 10.1186/s12870-019-1954-0
Raizada, A., Souframanien, J. (2021). SNP genotyping and diversity analysis based on genic-SNPs through high resolution melting (HRM) analysis in blackgram [Vigna mungo (L.) Hepper]. Genet. Resour. Crop Evol. 68, 1331–1343. doi: 10.1007/s10722-020-01064-6
Rambabu, E., Anuradha, C., Sridhar, V., Reddy, S. S. (2018). Genetics of Mungbean Yellow Mosaic Virus (MYMV) Resistance in Black gram (Vigna mungo (L.) Hepper). Int. J. Curr. Microbiol. App. Sci. 7, 3869–3872.
Ramchander, L., Shunmugavalli, N., Rajesh, S. (2017). Induced mutagenic frequency and spectrum of chlorophyll mutants in black gram (Vigna mungo). Farm Sci. J. 7 (1), 19–22.
Ramesh, S. V., Chouhan, B. S., Ramteke, R. (2017). Molecular detection of Begomovirus (family: Geminiviridae) infecting Glycine max (L.) Merr. and associated weed Vigna trilobata. J. Crop Weed. 13, 64–67.
Ramu, P. S., Swathi, K., Rao, S. G. (2018). A review on seasonal incidence and insecticidal management of spotted pod borer [Maruca vitrata (Geyer)] with special reference to urdbean (Vigna mungo L.). Indian J. Entomol. Zool. 6, 926–931.
Rana, J. C., Gautam, N. K., Gayacharan, C., Singh, M., Yadav, R., Tripathi, K., et al. (2016). Genetic resources of pulse crops in India: An overview. Indian J. Genet. Plant Breed. 76, 420–436. doi: 10.5958/0975-6906.2016.00061.4
Ranade, R., Gopalakrishna, T. (2001). Characterization of balckgram (Vigna mungo (L.) Hepper) varieties using RAPD. Plant Var. Seeds 14, 227.
Rao, Y. K. (1999). Inheritance of new plant types in black gram [Vigna mungo (L.) Hepper]. Legume Res. 22, 157–161.
Rao, Y. K. S., Ramgasamy, S. R. S., Muralidharan, V., Chandrababu, R. (1989). Inheritance of multifoliate leaf in urdbean. Int. J. Puls. Res. 2, 166–168.
Reddy, K. S., Dhanasekar, P. (2007). Induced mutations for genetic improvement of mungbean, urd bean and cowpea pulse crop in India. IANCAS Bull. 4 (4), 229–307.
Reddy, R. ,. C., Tonapi, V. A., Varanavasiappan, S., Navi, S. S., Jayarajan, R. (2005). Influence of plant age on infection and symptomatological studies on urd bean leaf crinkle virus in urd bean (Vigna mungo). Int. J. Agric. Sci. 1 (1), 1–6.
Ribaut, J. M., de Vicente, M. C., Delannay, X. (2010). Molecular breeding in developing countries: challenges and perspectives. Curr. Opin. Plant Biol. 13, 213–218. doi: 10.1016/J.PBI.2009.12.011
Sai, C. B., Chidambaranathan, P. (2019). Reproductive stage drought tolerance in black gram is associated with role of antioxidants on membrane stability. Plant Physiol. Rep. 24, 399–409. doi: 10.1007/S40502-019-00471-X
Saini, R., Jaiwal, P. K. (2005). Efficient transformation of a recalcitrant grain legume Vigna mungo L. Hepper via Agrobacterium-mediated gene transfer into shoot apical meristem cultures. Plant Cell Rep. 24, 164–171. doi: 10.1007/s00299-005-0934-z
Saini, R., Jaiwal, S., Jaiwal, P. K. (2003). Stable genetic transformation of Vigna mungo L. Hepper via Agrobacterium tumefaciens. Plant Cell Rep. 21 (9), pp.851–pp.859. doi: 10.1007/s00299-003-0574-0
Sandhu, J. S., Gill, R. K., Singh, I., Singh, S., Gupta, S. K. (2012). Mash 479, a disease resistant variety of kharif urdbean. Indian J. Genet. Plant Breed. 72, 109–109.
Santosh, C. P. (2016). Inheritance of powdery mildew resistance, grain yield and yield components in black gram (Vigna mungo L Hepper) (Rahuri, India: Mahatma Phule Krishi Vidyapeeth). MSc Thesis.
Sathya, P., Manivannan, N., Mahalingam, A., Satya, V. K., Parameswari, C. (2022). Inheritance of Urdbean Leaf Crinkle Virus Disease in Black gram [Vigna mungo (L.) Hepper]. Legume Res. 1, 4. doi: 10.18805/LR-4291
Saxena, H., Bandi, S., Devindrappa, M. (2018). Pests of pulses. Pests Their Manage., 99–136. doi: 10.1007/978-981-10-8687-8_5
Schafleitner, R. (2020). “Molecular marker resources and their application,” in The Mungbean Genome, Compendium of Plant Genomes Ed. Nair R. M. et al (Switzerland AG: © Springer Nature; ), (Springer Books), 107–124. doi: 10.1007/978-3-030-20008-4_8
Scheelbeek, P. F. D., Bird, F. A., Tuomisto, H. L., Green, R., Harris, F. B., Joy, E. J. M., et al. (2018). Effect of environmental changes on vegetable and legume yields and nutritional quality. Proc. Natl. Acad. Sci. U. S. A. 115, 6804–6809. doi: 10.1073/pnas.1800442115
Sen, N. K., Jana, M. K. (1964). Genetics of black gram (Phaseolus mungo L.). Genetica 34 (1), 46–57. doi: 10.1007/BF01664178
Shafique, S., Khan, M. R., Nisar, M., Shafiqure-Ur-Rehman (2011). Investigation of genetic diversity in black gram [Vigna mungo (L.) Hepper]. Pakistan J. Bot. 43, 1223–1232.
Shahi, S., Kumar, R., Srivastava, M. (2019). Response of black gram (Vigna mungo) to potassium under water stress condition. Ann. Plant Soil Res. 21, 93–97.
Sharma, O. P., Bambawale, O. M., Gopali, J. B., Bhagat, S., Yelshetty, S., Singh, S. K. (2011). Field Guide Mungbean and Urdbean (New Delhi: National Centre for Integrated Pest Management).
Singh, D. P. (1980). Inheritance of resistance to yellow mosaic virus in black gram (Vigna mungo (L.) Hepper). Theor. Appl. Genet. 57, 233–235. doi: 10.1007/BF00264676
Singh, D. P. (1981). Breeding for resistance to diseases in greengram and black gram. Theor. Appl. Genet. 59, 1–10. doi: 10.1007/BF00275766
Singh, A. K. (2010). Integrated management of Cercospora leaf spot of urd bean (Vigna mungo). J. Myco. Plant Pathol. 40 (4), 595.
Singh, K. K., Ali, M., Venkatesh, M. S. (2009). Pulses in cropping systems. Tech. Bulletin IIPR Kanpur 47.
Singh, S., Bisht, I. S., Dabas, B. S. (1987). Screening of urdbean germplasm for field resistance to yellow mosaic virus. Indian J. Myco. Plant Pathol. 17, 216–217.
Singh, G., Gurha, S. N., Ghosh, A. (1998). “Diseases of mungbean and urdbean and their management,” in Diseases of Field Crops and their Management. Ed. Thind, T. S. (Ludhiana, India: National Agricultural Technology Information Centre), 179–204.
Singh, C., Kumar, R., Sehgal, H., Bhati, S., Singhal, T., Gayacharan, et al. (2023). Unclasping potentials of genomics and gene editing in chickpea to fight climate change and global hunger threat. Front. Genet. Sec. Plant Genomics 14. doi: 10.3389/fgene.2023
Singh, I., Sandhu, J. S., Gupta, S. K., Singh, S. (2013). Introgression of productivity and other desirable traits from ricebean (Vigna umbellata) into black gram (Vigna mungo). Plant Breed. 132, 401–406. doi: 10.1111/PBR.12068
Singh, C., Shukla, A. (1994). Classification of germplasm in urdbean. Indian J. Pulses Res. 7, 112–115.
Singh, K. B., Singh, J. K. (1971). Inheritance of leaf shape in black gram (Phaseolus mungo L.). Sci. Cult 37, 583.
Singh, D. P., Singh, B. B., Gupta, R. P. (1991). Evaluation and classification of urdbean germplasm. Narendra Deva J. Agril. Res. 6, 138–141.
Singh, D. P., Singh, B. B., Gupta, S. (2015). Development of Pant Urd 31: a high yielding and yellow mosaic disease resistant variety of black gram. J. Food Legume 28, 1–4.
Singh, D. P., Singh, B. B., Pratap, A. (2016a). Genetic improvement of mungbean and urdbean and their role in enhancing pulse production in India. Indian J. Genet. Plant Breed. 76, 550–567. doi: 10.5958/0975-6906.2016.00072.9
Singh, P., Kumar, D., Sarin, N. B. (2016b). Multiple abiotic stress tolerance in Vigna mungo is altered by overexpression of ALDRXV4 gene via reactive carbonyl detoxification. Plant Mol. Biol. 91, 257–273. doi: 10.1007/S11103-016-0464-9
Singh, J., Kanaujia, R., Srivastava, A. K., Dixit, G. P., Singh, N. P. (2017). Genetic variability for iron and zinc as well as antinutrients affecting bioavailability in black gram (Vigna mungo (L.) Hepper). J. Food Sci. Technol 54, 1035–1042. doi: 10.1007/S13197-017-2548-1
Sirohi, A., Singh, A. (1998). Inheritance of hairy character on pods in black gram. J. Crop Improv. 25 (2), 246–246.
Sivaprakash, K. R., Prashanth, S. R., Mohanty, B. P., Parida, A. (2004). Genetic diversity of black gram (Vigna mungo) landraces as evaluated by amplified fragment length polymorphism markers. Curr. Sci. 86, 1411–1416.
Solanki, R. K., Gill, R. K., Verma, P., Singh, S. (2011). “Mutation breeding in pulses: an overview,” in Breeding of pulse crops. (Ludhiana: Kalyani Publishers), 85–103.
Somta, P., Chen, J., Yimram, T., Yundaeng, C., Yuan, X., Tomooka, N., et al. (2020). QTL Mapping for agronomic and adaptive traits confirmed pleiotropic effect of mog gene in black gram [Vigna mungo (L.) Hepper]. Front. Genet. 11. doi: 10.3389/fgene.2020.00635
Somta, P., Chen, J., Yundaeng, C., Yuan, X., Yimram, T., Tomooka, N., et al. (2019). Development of an SNP-based high-density linkage map and QTL analysis for bruchid (Callosobruchus maculatus F.) resistance in black gram (Vigna mungo (L.) Hepper). Sci. Rep. 9, 1–9. doi: 10.1038/s41598-019-40669-5
Somta, P., Seehalak, W., Srinives, P. (2009). Development, characterization and cross-species amplification of mungbean (Vigna radiata) genic microsatellite markers. Conserv. Genet. 10, 1939–1943. doi: 10.1007/s10592-009-9860-x
Souframanien, J., Gopalakrishna, T. (2004). A comparative analysis of genetic diversity in black gram genotypes using RAPD and ISSR markers. Theor. Appl. Genet. 109, 1687–1693. doi: 10.1007/S00122-004-1797-3
Souframanien, J., Gopalakrishna, T. (2006). ISSR and SCAR markers linked to the mungbean yellow mosaic virus (MYMV) resistance gene in black gram [Vigna mungo (L.) Hepper]. Plant Breed. 125, 619–622. doi: 10.1111/J.1439-0523.2006.01260.X
Souframanien, J., Gopalakrishna, T. (2007). Source for bruchid resistance and its inheritance in Trombay wild urdbean (Vigna mungo var. silvestris). J.Food. Leg 20 (1), 19.
Souframanien, J., Gupta, S. K., Gopalakrishna, T. (2010). Identification of quantitative trait loci for bruchid (Callosobruchus maculatus) resistance in black gram [Vigna mungo (L.) Hepper]. Euphytica. 176, 349–356. doi: 10.1007/S10681-010-0210-3
Souframanien, J., Gupta, S. K., Gopalakrishna, T. (2011). Breeding of black gram genotypes for resistance to bruchid beetle through molecular and conventional approaches. Indian J. Genet. Plant Breed. 71 (2), 164.
Souframanien, J., Raizada, A., Dhanasekar, P., Suprasanna, P. (2021). Draft genome sequence of the pulse crop black gram [Vigna mungo (L.) Hepper] reveals potential R-genes. Sci. Reps. 11, 11247. doi: 10.1038/s41598-021-90683-9
Souframanien, J., Reddy, K. S. (2015). De novo assembly, characterization of immature seed transcriptome and development of genic-SSR markers in black gram [Vigna mungo (L.) Hepper]. PloS One 10, 1–15. doi: 10.1371/journal.pone.0128748
Souframanien, J., Reddy, K. S., Petwal, V. C., Dwivedi, J. (2016). Comparative effectiveness and efficiency of electron beam and 60Co γ -rays in induction of mutations in black gram [Vigna mungo (L.) Hepper]. J. Food Legume 29 (1), 1–65.
Souframanien, J., Roja, G., Gopalakrishna, T. (2014). Genetic variation in raffinose family oligosaccharides and sucrose content in black gram [Vigna mungo L. (Hepper)]. J. Food Legume 27, 37–41.
Srinivasan, R. (2014). Insect and mite pests on vegetable legumes: a field guide for identification and management. Shanhua, Taiwan: AVRDC – The World Vegetable Center, AVRDC Publication No. 14-778 92 p.
Srinives, P., Somta, P., Somta, C. (2007). Genetics and breeding of resistance to bruchids (Callosobruchus spp) in Vigna crops: a review. NU Int. J. Sci. 4, 1–17.
Srivastava, P., Pandey, A., Singh, M. (2013). Identification of the RAPD Marker linked to Powdery Mildew Resistant Gene(s) in Black gram by using Bulk Segregant Analysis. Res. J. Biotechnol. 8, 9–15.
Srivastava, A. K., Prajapati, R. K. (2012). Influence of weather parameters on outbreak of mungbean yellow mosaic virus in black gram (Vigna mungo L.) of Bundelkhand Zone of Central India. J. Agric. Phys. 12 (2), 143–151.
Suamuang, S., Lomlek, C., Kongkachana, W., Tangphatsornruang, S., Laosatit, K., Tanadul, O., et al. (2023). Identification of quantitative trait loci controlling flowering time in black gram (Vigna mungo [L.] Hepper). Agric. Natural Resour. 57, 43–50. doi: 10.34044/j.anres.2023.57.1.05
Subramaniyan, R., Narayana, M., Krishnamoorthy, I., Natarajan, G., Gandhi, K. (2021). Multivariate analysis on blackgram genotypes for bruchine (Callosobruchus maculatus F.) resistance towards selection of parental lines. J. Appl. Natural Sci. 13 (4), 1206–1213. doi: 10.31018/jans.v13i4.2997
Subramaniyan, R., Narayana, M., Krishnamoorthy, I., Natarajan, G., Gandhi, K. (2022). Novel and stable QTL regions conferring resistance to MYMV disease and its inheritance in black gram (Vigna mungo (L.) Hepper). J. Genet. 101, 1–7. doi: 10.1007/S12041-022-01359-W
Sultana, R., Choudhary, A. K., Pal, A. K., Saxena, K. B., Prasad, B. D., Singh, R. (2014). Abiotic stresses in major pulses: current status and strategies. Approaches to Plant Stress their Manage., 173–190. doi: 10.1007/978-81-322-1620-9_9
Sun, X., Hu, Z., Chen, R., Jiang, Q., Song, G., Zhang, H., et al. (2015). TargetedMutagenesis in soybean using the CRISPR-cas9 system. Sci. Reps. 5, 10342. doi: 10.1038/srep10342
Tangphatsornruang, S., Somta, P., Uthaipaisanwong, P., Chanprasert, J., Sangsrakru, D., Seehalak, W., et al. (2009). Characterization of microsatellites and gene contents from genome shotgun sequences of mungbean (Vigna radiata (L.) Wilczek). BMC Plant Biol. 9, 137. doi: 10.1186/1471-2229-9-137
Thamodhran, G., Geetha, S., Ramalingam, A. (2016). Genetic study in URD bean ( Vigna Mungo (L.) Hepper) for inheritance of mungbean yellow mosaic virus resistance. Int. J. Agric. Environ. Biotechnol. 9, 33. doi: 10.5958/2230-732x.2016.00005.x
Thilagavathi, C., Mullainathan, L. (2011). Influence of physical and chemical mutagens on quantitative characters of Vigna mungo (L. Hepper). Int. Multidiscip. Res. J. 72, 111–116.
Thudi, M., Gaur, P. M., Krishnamurthy, L., Mir, R. R., Kudapa, H., Fikre, A., et al. (2014). Genomics-assisted breeding for drought tolerance in chickpea. Funct. Plant Biol. 41, 1178–1190. doi: 10.1071/FP13318
Tickoo, J. L., Lal, S. K., Chandra, N., Dikshit, H. K. (2006). “Mungbean breeding,” in Advances in mungbean and urdbean. Eds. Ali, M., Kumar, S. (Kanpur: IIPR), 110–148.
Tomar, S. S., Tiwari, S. K., Kumar, R., Singh, R. P. (2003). Cluster analysis in urdbean (Vigna mungo (L.) Hepper). Indian J. @ Agric. And Weed Sci. 2 (1), 23–25.
Tomooka, N., Kashiwaba, K., Vaughan, D. A., Ishimoto M. and Egawa, Y. (2000). The effectiveness of evaluating wild species: searching for sources of resistance to bruchids beetles in the genus Vigna subgenus Ceratotropis. Euphytica 115, 27–41. doi: 10.1023/A:1003906715119
USDA, Agricultural Research Service, National Plant Germplasm System (2021). Germplasm Resources Information Network (GRINTaxonomy) (Beltsville, Maryland: National Germplasm Resources Laboratory).
USDA National Nutrient Database (2018). Available at: https://ndb.nal.usda.gov/ndb/foods/show/4821?manu=&fgcd=&ds.
Usharani, K. S., Kumar, C. R. A. (2015). Induced polygenic variability using combination treatment of gamma rays and ethyl methane sulphonate in black gram (Vigna mungo (L.) Hepper). Afr. J. Biotechnol. 14, 1702–1709. doi: 10.5897/ajb2015.14438
Vadivel, K., Manivannan, N., Mahalingam, A., Satya, V. K., Vanniarajan, C., Ragul, S. (2021). Identification and Validation of Quantitative Trait Loci of Mungbean Yellow Mosaic Virus Disease Resistance in Black gram [Vigna mungo (L). Hepper]. Legume Res. - an Int. J., 1–7. doi: 10.18805/lr-4459
Vanniarajan, C., Chandirakala, R., Souframanien, J. (2021). Genetic and biochemical characterization of electron beam and gamma ray induced mutants for superior battering quality trait and MYMV in urdbean [Vigna mungo (L.)]. Legume Res.an Int. J., 1–7. doi: 10.18805/lr-4583
Varshney, R. K., Close, T. J., Singh, N. K., Hoisington, D. A., Cook, D. R. (2009). Orphan legume crops enter the genomics era! Curr. Opin. Plant Biol. 12, 202–210. doi: 10.1016/J.PBI.2008.12.004
Varshney, R. K., Pandey, M. K., Janila, P., Nigam, S. N., Sudini, H., Gowda, M. V. C., et al. (2014). Marker−assisted introgression of a QTL region to improve rust resistance in three elite and popular varieties of peanut (Arachis hypogaea L.). Theor. Appl. Genet. 127, 1771–1781. doi: 10.1007/s00122-014-2338-3
Veni, K., Vanniarajan, C., Souframanien, J. (2017). Probit analysis and effect of electron beam and gamma rays in black gram (Vigna mungo (L.) Hepper). Electron. J. Plant Breed. 8, 950–955. doi: 10.5958/0975-928X.2017.00112.0
Verma, S. N. P. (1971). Inheritance of photosensitivity in mungbean (Phaseolus aureus Roxb.). Mysore J. Agric. Sci. 5, 477–480.
Verma, N., Izhar, T., Chakraborty, M., Manjaya, J. G. (2018). Mutation study of gamma ray in M 3 generation of Soybean ( Glycine max . ( L .) Merrill ). J. Pharmacogn. Phytochem. 7, 3543–3547.
Verma, R. P. S., Singh, D. P. (1986). The allelic relationship of genes giving resistance to mungbean yellow mosaic virus in black gram. Theor. Appl. Genet. 72, 737–738. doi: 10.1007/BF00266537
Vidhyasekaran, P., Arjunan, G. (1978). Studies on leaf blight of urdbean caused by Macrophomina phaseoli. Indian Phytopathol. 31, 361–362.
Vishalakshi, B., Umakanth, B., Shanbhag, A. P., Ghatak, A., Sathyanarayanan, N., Madhav, M. S., et al. (2017). RAPD assisted selection of black gram (Vigna mungo L. Hepper) towards the development of multiple disease resistant germplasm. 3 Biotech. 7, 1–6. doi: 10.1007/s13205-016-0582-8
William, F. J., Grewal, J. S., Amin, K. S. (1968). Serious and new diseases of pulses crop in India in 1966. Plant Dis. Rept. 52, 300–304.
Yadav, S. K., Agnihotri, M., Bisht, R. S. (2015). Seasonal incidence of insect-pests of black gram, Vigna mungo (Linn.) and its correlation with abiotic factors. Agric. Sci. Dig. - A Res. J. 35, 146. doi: 10.5958/0976-0547.2015.00027.0
Yadav, D. S., Kushwaha, B. L., Sushant (2006). “Cropping systems,” in Advances in Mungbean and Urdbean. Eds. Masood, A., Shiv, K. (Kanpur: Indian Institute of Pulses Research), 211–229.
Yadav, S. K., Patel, S. (2015). Monitoring of insect-pest complex on black gram, Vigna mungo (Linn.) at Pantnagar. J. Entomol. Res. 39, 337–340. doi: 10.5958/0974-4576.2015.00042.0
Yohan, Y., Sudhakar, P., Umamahesh, V., Reddy, D. M., Sumathi, V. (2018). Evaluation of black gram (Vigna mungo L. Hepper) genotypes for growth parameters under imposed moisture stress condition. J. Pharmacogn. Phytochem. 7, 981–986.
Yundaeng, C., Somta, P., Amkul, K., Kongjaimun, A., Kaga, A., Tomooka, N. (2019). Construction of genetic linkage map and genome dissection of domestication-related traits of moth bean (Vigna aconitifolia), a legume crop of arid areas. Mol. Genet. Genomics 294, 621–635. doi: 10.1007/s00438-01901536-0
Zhang, Y., Massel, K., Godwin, I. D., Gao, C. (2018). Applications andPotential of genome editing in crop improvement. Genome Biol. 19, 1. doi: 10.1186/s13059-018-1586-y
Keywords: Vigna mungo, black gram, Urdbean, breeding, genetics, genomics
Citation: Nair RM, Chaudhari S, Devi N, Shivanna A, Gowda A, Boddepalli VN, Pradhan H, Schafleitner R, Jegadeesan S and Somta P (2024) Genetics, genomics, and breeding of black gram [Vigna mungo (L.) Hepper]. Front. Plant Sci. 14:1273363. doi: 10.3389/fpls.2023.1273363
Received: 06 August 2023; Accepted: 18 December 2023;
Published: 15 January 2024.
Edited by:
Sergio J. Ochatt, INRA UMR1347 Agroécologie, FranceReviewed by:
Rajendra Kumar, Indian Agricultural Research Institute (ICAR), IndiaCopyright © 2024 Nair, Chaudhari, Devi, Shivanna, Gowda, Boddepalli, Pradhan, Schafleitner, Jegadeesan and Somta. This is an open-access article distributed under the terms of the Creative Commons Attribution License (CC BY). The use, distribution or reproduction in other forums is permitted, provided the original author(s) and the copyright owner(s) are credited and that the original publication in this journal is cited, in accordance with accepted academic practice. No use, distribution or reproduction is permitted which does not comply with these terms.
*Correspondence: Ramakrishnan M. Nair, cmFtYWtyaXNobmFuLm5haXJAd29ybGR2ZWcub3Jn
Disclaimer: All claims expressed in this article are solely those of the authors and do not necessarily represent those of their affiliated organizations, or those of the publisher, the editors and the reviewers. Any product that may be evaluated in this article or claim that may be made by its manufacturer is not guaranteed or endorsed by the publisher.
Research integrity at Frontiers
Learn more about the work of our research integrity team to safeguard the quality of each article we publish.