- 1Jiangsu Province Key Laboratory of Agricultural Meteorology, Nanjing, China
- 2Collaborative Innovation Center of Meteorological Disaster Forecasting and Assessment, Nanjing University of Information Science and Technology, Nanjing, China
- 3School of Applied Meteorology, Nanjing University of Information Science and Technology, Nanjing, China
- 4Meteorological Bureau of Heze City, Heze, China
High relative humidity (RH) and high temperature are expected more frequently due to climate change, and can severely affect the growth of chrysanthemums. In order to analyze the interactive effects of RH and high temperature on the photosynthetic performance of chrysanthemum, a completely randomized block experiment was conducted with three factors, namely temperature (Day/night temperature, 35°C/18°C, 38°C/18°C, 41°C/18°C), RH (Whole day RH, 50%, 70%, 90%), and treatment duration (3d, 6d, 9d). The control (CK) temperature was 28°C/18°C and RH was 50%. The results showed that with the increase of temperature, the apparent quantum efficiency (AQE), maximum net photosynthetic rate (Pn-max), net photosynthetic rate (Pn), transpiration rate (Tr), water use efficiency (WUE), maximal recorded fluorescence intensity (Fm), PSII maximal photochemical efficiency (Fv/Fm), absorption flux per cross section (ABS/CSm), trapped energy flux per cross section (TRo/CSm), electron transport flux per cross section (ETo/CSm) and photosynthetic pigment content of leaves significantly decreased, the minimal recorded fluorescence intensity (Fo), fluorescence intensity at point J of the OJIP curve (Fj) and non-photochemical quenching per cross section (DIo/CSm) significantly increased, the fluorescence difference kinetics of the OJ phase of chrysanthemum leaves showed K-bands. Pn, AQE, Fm, Fv, Fv/Fm, ABS/CSm, TRo/CSm, ETo/CSm and photosynthetic pigment content were higher at 70% RH than the other two RH conditions. The dominant factor causing the decrease of Pn in leaves was stomatal limitation at 35°C,38°C, three RH conditions, 3d and 6d, but non-stomatal limitation at 41°C and 9d. There was an interaction between temperature and RH, with a significant impact on Pn. The temperature had the greatest impact on Pn, followed by RH. This study confirms that heat stress severely affects the photosynthesis of chrysanthemum leaves, and when the temperature reaches or exceeds 35°C, adjusting the RH to 70% can effectively reduce the impact of heat stress on chrysanthemum photosynthesis.
1 Introduction
Chrysanthemum (Chrysanthemum morifolium Ramat.) is one of the four main cut flowers in the world (Fanourakis et al., 2022). It has been cultivated for more than 3,000 years, and over 3,000 varieties have been developed (Su et al., 2019). In China, it has a long history of use as a medicinal and edible plant (Ning et al., 2023). In Europe, the cultivation of chrysanthemum for cut flower production is a highly profitable industry (Castello et al., 2022). Chrysanthemum is one of the main flowers exported from China, with as many as 1.911 million chrysanthemums exported in 2019 alone (Dong, 2020). Hence, chrysanthemum is an economically important plant.
The optimal temperature for the growth of chrysanthemum is 25°C (Lee et al., 2013). Chrysanthemum growth is inhibited above 25°C and basically stops growing at 40°C (Li et al., 2021). Chrysanthemums ‘Shenma’ are typically short-day plants, and if the light duration is longer than 14.5 hours, its flowering will be delayed (Nakano et al., 2013). In order to meet the market supply demand and prompt chrysanthemums to bloom in the long sunshine season, black shading materials are often used for photoperiodic treatment, but the high temperature environment caused by shading in summer often causes chrysanthemums to wilt. Janka et al. (Janka et al., 2015) found that excessive irradiation and high temperatures above 28°C produced photoinhibition of chrysanthemum. The right relative humidity (RH) is equally important for the growth and development of chrysanthemum, and excessive RH can increase the incidence of white rust (Yoo and Roh, 2014). Influenced by the East Asian Monsoon, high temperature and high RH are main characteristics in greenhouse environment in southern China, which severely affect crops growth in glasshouses (Zhang et al., 2022). With global warming, extreme surface temperatures and duration in East Asia will increase more frequently (Chevuturi et al., 2018), and thus, the frequency of high temperature and high RH environments in greenhouses will be more frequent (Zheng et al., 2023). March to June is the main period for the growth of chrysanthemum seedlings in greenhouse in China and the impact of high temperature and high RH environment on the growth of greenhouse-grown chrysanthemum seedlings should be noticed. In previous studies, yield increase through moisture control has been demonstrated in strawberries (Zucchi et al., 2016) and roses (Mortensen and Gislerød, 2000), but not in chrysanthemum cultivation.
High temperature and RH affect various metabolic and physiological processes in plants. Photosynthetic parameters derived from the light response curve are important indicators of the light energy utilization capacity of plants in the study of adversity stress (Xu et al., 2022). Weng et al. (Weng et al., 2021) found that net photosynthetic rate (Pn) of melon seedlings was inhibited at 42°C and 90% RH, compared to 30°C and 60% RH. In the study of stress, photosynthetic pigment content is an important index for assessing the extent of damage to photosynthetic organs, and chlorophylls are considered important components of stress biology in higher plants (Agathokleous et al., 2020). Yang et al. (Yang et al., 2022) found that lettuce chlorophyll a and chlorophyll b were reduced at 35°C and growth was inhibited, compared to 22°C. Chlorophyll fluorescence is inextricably linked to plant photosynthesis and can respond to changes in the photosynthetic system of a plant under adversity stress (Baker, 2008; Hazrati et al., 2016). Sun et al. (Sun et al., 2008) found that high temperatures reduced the PSII potential activity (Fv/Fo) and maximum photochemical efficiency (Fv/Fm) of chrysanthemum leaves, chrysanthemum protected reaction centers from damage by reducing the capture of light energy with the efficiency of electron transfer through PSII.
The two main reasons for limiting photosynthetic rate are stomatal and non-stomatal limitation. The exploration of stomatal and non-stomatal limitation under different adverse environments has been the focus of research, especially under combined stressors. Zubaidi et al. (Zubaidi et al., 2021) found that compared to 25°C, reduction in Pn in wheat at 32°C was not only due to lower stomatal conductance, but also non-stomatal effects as mesophyll conductance and quantum yield were lower. In addition, Barker et al. (Barker, 1990) found that increasing the RH of greenhouse significantly increased the stomatal conductance (Gs), which improved the heat tolerance of tomato. While high temperatures have many detrimental impacts on plants, damage can be mitigated by regulating RH (Suzuki et al., 2015; Shamshiri et al., 2018). Zheng et al. (Zheng et al., 2020) found that increasing the RH to 70% at 35°C reduced gibberellin concentration (GA3) and increased abscisic concentration (ABA) in tomato shoots, which was favorable to the growth of tomato plants, compared to 28°C and 50% RH. Similarly, Xu et al. (Xu et al., 2020) found that high RH was effective in alleviating the limitation of tomato growth by high temperature and improving the root to crown ratio, compared to 50% RH. In rice, increasing RH by mist spray under heat stress increased chlorophyll content, Pn and yield (Jiang et al., 2020). When the effect of low, medium and high humidity on flowering and fruiting of tomato plants was studied (Peet et al., 2003), 50% RH was the optimum humidity at 35°C.
To our knowledge, there have been many studies on chrysanthemums under various factors of stress, but almost all of them are single-factor. The study of multifactorial stresses is more useful for chrysanthemum cultivation due to the complex environment in greenhouses. We hypothesized that high temperature could inhibit the photosynthesis of chrysanthemums, and changing RH could regulate it. The objective of this study is to analyze the interaction between RH and high temperature on the photosynthetic performance of chrysanthemums, analyze the dominant factors affecting chrysanthemum photosynthesis, and select the optimal RH for chrysanthemum photosynthesis in high temperature environments.
2 Materials and methods
2.1 Experimental design
The experiment was carried out in March 2022 at the Agricultural Meteorological Experiment Station of Nanjing University of Information Science and Technology (118°42′E, 32°12′N). Seedlings of C. morifolium cv. ‘Shenma’ were first raised in a greenhouse seedbed with substrate soil, vermiculite: peat mixture of 1:1 (v: v) in a Venlo-type glass greenhouse. When the seedlings had four true leaves, they were planted into pots of 17.5 cm (height) × 15.0 cm (diameter). The contents of organic carbon, available phosphorus, available potassium and nitrogen of the soil substrate were 10,450, 32.8, 89.4 and 1540 mg·kg-1. Soil texture was medium loam. Soil pH was 6.7. One plant was planted in each pot. Two weeks after planting, the plants were moved into artificial climate chambers (TGP-1260, Australia) in a laboratory.
The experiment was conducted to simulate a summer greenhouse by setting the environmental parameters of artificial climate chambers. There were three temperature (day/night temperature) conditions set as 35°C/18°C, 38°C/18°C and 41°C/18°C, and three RH conditions, namely 50%, 70% and 90%, in combination with each temperature condition. Moreover, the temperature treatment was conducted for 3, 6 and 9 d to study the time dependency of response to temperature and RH. The experiment was a completely randomized group design with a total of 27 treatment combinations (Table 1). The control (CK) temperature and RH were 28°C/18°C and 50% respectively, which are the optimum temperature and normal RH for chrysanthemums cultivation in Nanjing. The environmental conditions and management measures in the artificial climate chambers remained the same during the experiment, except for different settings of temperature and relative air humidity. The photoperiod (day/night time) was set to 7:00 a.m. - 17:00 p.m./18:00 p.m. - 6:00 a.m.The light intensity in the artificial climate chamber was 800 μmol·m-2·s-1 during daytime and 0 μmol·m-2·s-1 during nighttime.
2.2 Measurement
The light response curve, gas exchange parameters, photosynthetic pigment content and chlorophyll fluorescence of chrysanthemum leaves were measured at 4, 7 and 10 d after the start of the artificial control experiment.
2.2.1 Light response curves
With three LI-6400 portable gas exchange analyzers (LI-COR Biosciences Inc, USA), the light response curves of leaves were recorded from 9:00 a.m.-11:30 a.m. on each observation day. Inside the leaf chamber, the CO2 level was maintained at 400 μmol·mol-1. The levels of photosynthetically active radiation (PAR) (μmol·m-2·s-1) inside the leaf chamber were 1200, 1000, 800, 600, 400, 300, 200, 100, and 0. The maximum wait time after each change of light intensity was set to 180s and the minimum wait time was 120s. Photosynthetic parameters like apparent quantum efficiency (AQE), light saturation point (LSP), maximum net photosynthetic rate (Pn-max), light compensation point (LCP) and dark respiration rate (Rd) were estimated using photosynthetic model simulations of Ye (Ye, 2010). The third leaf that was fully expanded before the experiment began was measured by the light response curve. One leaf per plant and three plants per treatment were measured.
Where, Pn (μmol·m-2·s-1) means net photosynthetic rate. θ means curvature of the curve. I (μmol·m-2·s-1) means light intensity. Rd (μmol·m-2·s-1) means dark respiration rate. α means initial quantum efficiency. Pn-max (μmol·m-2·s-1) means maximum net photosynthetic rate.
2.2.2 Gas exchange parameters
Gas exchange parameters of chrysanthemum leaves were measured using the transparent leaf chamber that comes with the LI-6400 portable gas exchange analyzer (LI-COR Biosciences Inc, USA). Measurement time, leaf position was the same as described in section 2.2.1. The net photosynthetic rate (Pn), stomatal conductance (Gs), intercellular CO2 concentration (Ci), atmospheric CO2 concentration (Ca) and transpiration rate (Tr) were measured. Vapor pressure deficit (VPD) was calculated as formula 2 (Howell and Dusek, 1995; Kadam et al., 2015).The stomatal restriction values (Ls) were calculated as formula 3 (Berry, 1982). The water use efficiency (WUE) was calculated as formula 4 (R A Fischer and Turner, 1978).
2.2.3 Photosynthetic pigment content
The photosynthetic pigment content of the leaves was measured according to the method of Li (Li, 2000). A randomly derived amount of 0.2g of leaf tissue was placed into 25ml of 95% ethanol left in dark at room temperature for 48h until the photosynthetic pigments in the leaves were completely leached out. The absorbance was measured colorimetrically at 665, 649 and 470 nm using a UV-1800 instrument (SHIMADZU, Japan). The photosynthetic pigment concentration (mg·g-1) was calculated using formulas 5-9.
Where, Chla (mg·g-1), Chlb (mg·g-1), Car (mg·g-1) and Chl (mg·g-1) mean the concentrations of chlorophyll a, chlorophyll b, carotenoids and chlorophyll (a+b), respectively. D665, D649, and D470 mean the absorbance of the extracts at 665, 649, and 470 nm, respectively.
2.2.4 Chlorophyll fluorescence parameters
Chlorophyll fluorescence induction curves can provide insight into know the response of the plant to changes in environmental factors. Therefore, here we measured the chlorophyll fluorescence of each treatment. Chlorophyll fluorescence parameters were measured by a plant efficiency analyzer (Pocket PEA, Hansatech, UK). Measurement time and blade position were the same as the photosynthetic parameters. Leaves were dark-adapted with leaf clips for 30 minutes before measurement. Following a 5000 μmol·m-2·s-1 light pulse, the fast chlorophyll fluorescence-induced kinetic curve (OJIP) and its related fluorescence parameters of chrysanthemum leaves were measured with a duration of 1s. The minimal recorded fluorescence intensity (Fo), fluorescence intensity at point J of the OJIP curve (Fj), maximal recorded fluorescence intensity (Fm), PSII maximal photochemical efficiency (Fv/Fm) were measured. The JIP-test indices and terminology mentioned in this paper are presented in Table 2 (Strasser et al., 2010). The variable fluorescence intensities were O-J normalized according to formula 10-11 (Yusuf et al., 2010).
Where, Woj means fluorescence difference kinetics. Ft means instantaneous fluorescence at any moment. Fo means minimal recorded fluorescence intensity. Fj means fluorescence intensity at point J of the OJIP curve.
2.3 Data processing
All values were the means of three replicates per treatment. In order to make comparisons across treatments with different temperature, RH and treatment duration, this study used the calculation of mean values of relevant treatment indicators for comparison. The values of T35°C, T38°C and T41°C were the means of treatments which day/night temperature was set as 35°C/18°C, 38°C/18°C and 41°C/18°C, respectively. The values of RH50%, RH70% and RH90% were the means of treatments which RH condition was set as 50%, 70% and 90%, respectively. The values of L3d, L6d and L9d were the means of treatments which duration was set as 3d, 6d and 9d, respectively. Duncan’s multiple range test at the 0.05 level of significance was used to detect differences between all treatments using SPSS 26.0 software (SPSS Inc., Chicago, IL). Figures were drawn using Origin Pro 2023b (Origin Lab Corporation, Northampton, MA, USA).
19 (10), 2149-21543 Results
3.1 Interactive effects of relative humidity and high temperature on light response curves
Table 3 shows the parameters of light response curves of chrysanthemum leaves under different treatments. As to temperature, the values of LSP, AQE and Pn-max in CK treatment were significantly higher than that of all temperature treatments. AQE and Pn-max values decreased with increasing temperature. The values of LSP, AQE, and Pn-max in T41°C were the lowest among all temperature treatments, which were 36.67%, 47.79% and 57.61% lower than that of CK treatment. The values of LCP and Rd in three temperature treatments were higher than that of CK treatment.
Among RH treatments, the values of AQE in RH70% were highest and significantly larger than that of other RH treatments. Although statistically non-significant, RH70% had the highest values of LSP and Pn-max, followed by the RH50% and RH90%. The value of Rd in RH70% was lower than that of RH90%, indicating that the respiratory consumption of leaves was low and photosynthetic activity was high at 70% RH, while the respiratory consumption of leaves was high at 90% RH.
Regarding the treatment duration, although statistically non-significant, the values of LSP, AQE, and Pn-max decreased over time. The values of LSP, AQE, and Pn-max in L9d were the lowest among L3d, L6d and L9d, which were 34.82%, 39.82% and 49.11% lower than that of CK treatment.
The results of ANOVA are also shown in Table 3. Temperature and RH had a significant (P< 0.01) impact on light response curve parameters. Treatment duration had a significant (P< 0.01) impact on LCP, LSP and Rd. The interaction between temperature and RH was significant for Pn-max (P< 0.01) and LSP (P< 0.05). Besides, the interaction between temperature and treatment duration had a significant impact on Pn-max (P< 0.01), LSP and AQE (P< 0.05). Moreover, the interaction between RH and treatment duration had a significant impact on AQE and Pn-max (P< 0.01) and LCP (P< 0.05). Further, the interaction of all three factors (temperature, RH and treatment duration) was significant for AQE and Pn-max (P< 0.05). Contributions of temperature, RH and treatment duration are shown in Figure 1. Temperature had the most impact, since it contributed 57.14%, 36.70%, 45.89%, 63.01% and 46. 42% of the variation in LSP, AQE, Pn-max, LCP and Rd, followed by RH, and treatment duration was the least.
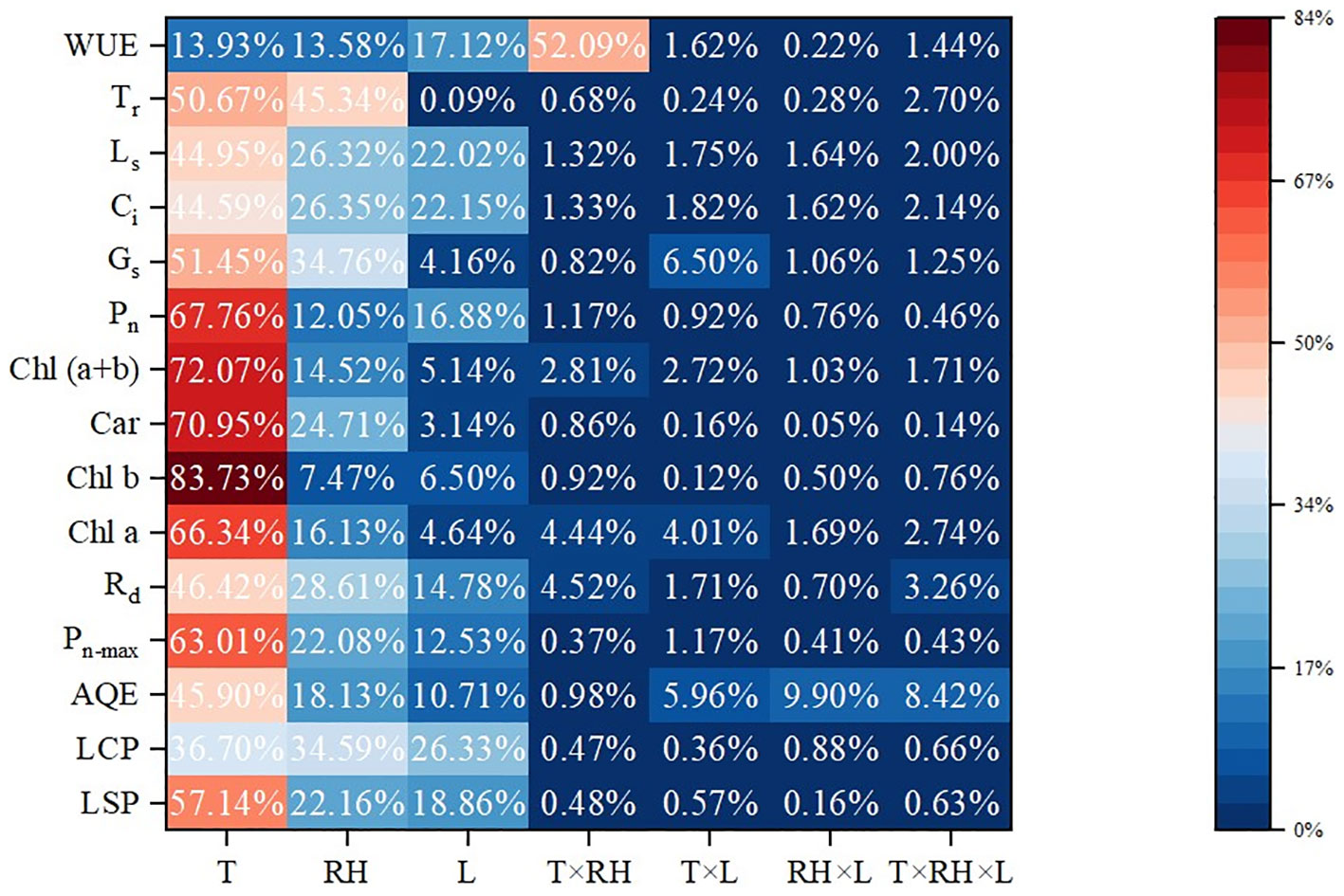
Figure 1 Contributions of temperature, RH and treatment duration. Contribution rate (%) =SSF×100/(SST-SSE-SSB). SSF means sum of squares away from the mean difference. SST, SSE and SSB mean sum of squares for total, error and block, respectively. T, RH and L represent temperature, relative humidity and treatment duration, respectively.
The interaction between temperature and RH was significant for Pn-max (P< 0.01). Interactions affecting Pn-max from looking at temperature effects at a given RH and RH effects at a given temperature were shown in Figure 2. At a given temperature, the values of Pn-max at 70% RH were highest, followed by 50% RH and 90% RH, which indicated that 70% RH mitigated the negative effect of high temperature on Pn-max, while high RH aggravated. At a given RH, the values of Pn-max decreased with increasing temperature, which indicated that increasing temperature aggravated the effect of RH on Pn-max. In addition, the values of Pn-max decreased with increasing treatment duration. The value of Pn-max was lowest at 41°C and 90% RH.
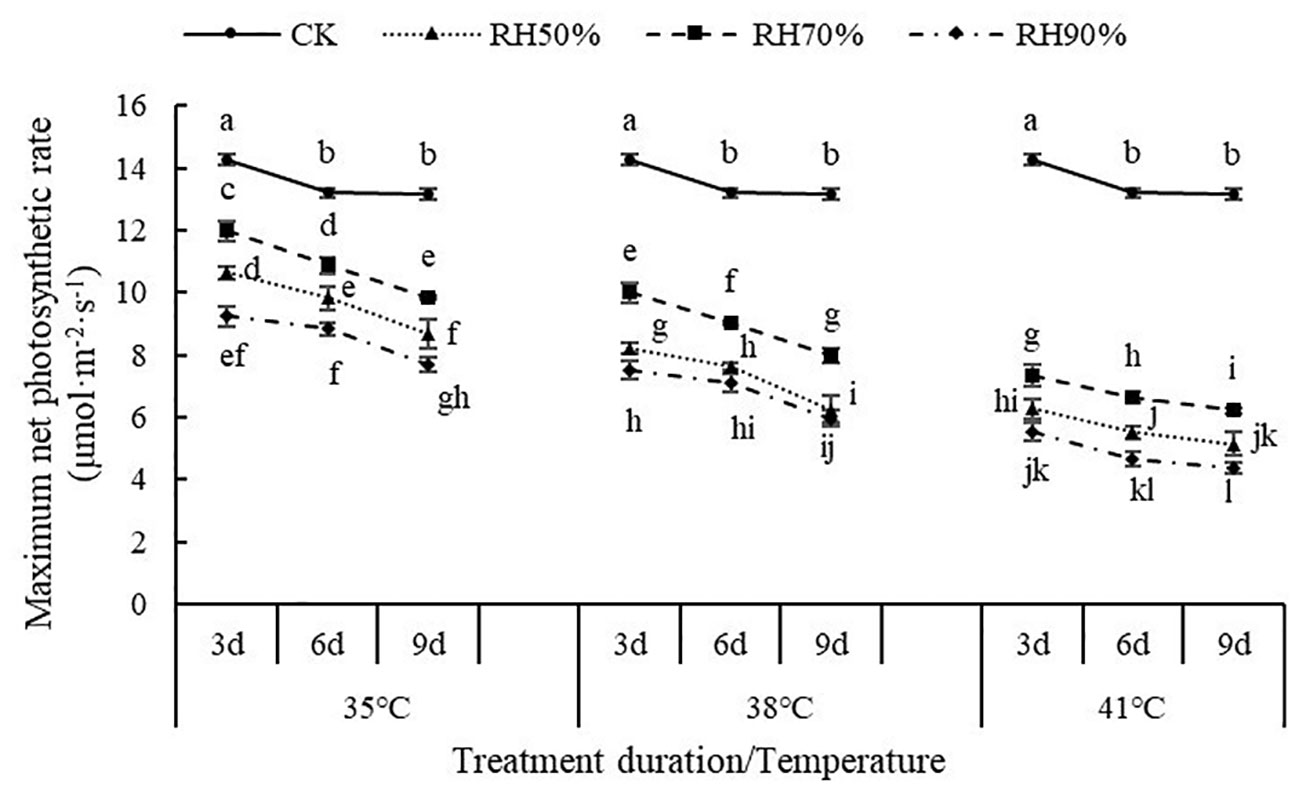
Figure 2 Effect of interaction between temperature and RH on maximum net photosynthetic rate of chrysanthemum leaves.
3.2 Interactive effects of relative humidity and high temperature on photosynthetic pigment content
Figure 3 shows the photosynthetic pigment contents of chrysanthemum leaves under different treatments. The values of chlorophyll a, chlorophyll b, carotenoids, and chlorophyll (a+b) generally decreased with increasing temperature. The values of chlorophyll a, chlorophyll b, carotenoids, and chlorophyll (a+b) in T41°C were the lowest among three temperature treatments, which were 44.86%, 40.63%, 47.62% and 43.78% lower than that of CK treatment.
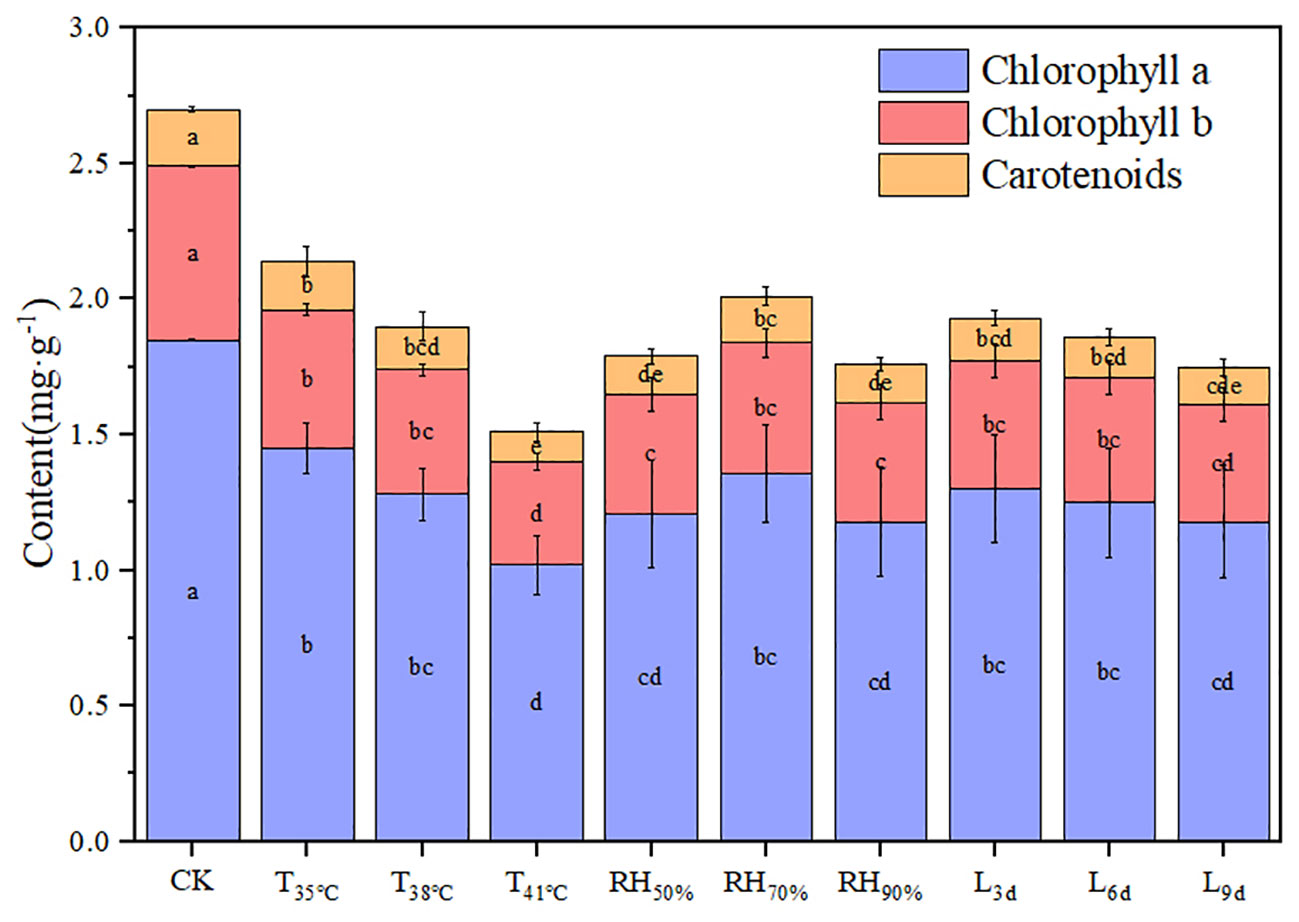
Figure 3 Effect of different temperature, RH and treatment duration on photosynthetic pigment content of chrysanthemum leaves.
The value of carotenoids in RH70% was highest and significantly higher than that of other RH treatments. Although without statistically significant, the values of chlorophyll a, chlorophyll b, and chlorophyll (a+b) decreased in RH50% and RH90%, compared to RH70%. There was no significant effect of treatment duration on photosynthetic pigment content.
Temperature had the most contribution to the variation in the values of chlorophyll a, chlorophyll b, carotenoids and chlorophyll (a+b), which was 66.34%, 83.731%, 70.95% and 72.07%, followed by RH, and treatment duration was the least.
The interaction between temperature and RH was significant for carotenoids (P< 0.01). Interactions affecting carotenoids from looking at temperature effects at a given RH and RH effects at a given temperature were shown in Figure 4. At a given temperature, the values of carotenoids at 70% RH were highest, which indicated that 70% RH mitigated the negative effect of high temperature on carotenoids. The values of carotenoids at 50% RH and 90% RH were significantly lower than that of CK, which indicated that 50% RH and 90% RH aggravated the negative effect of high temperature on carotenoids. At a given RH, the values of carotenoids decreased with increasing temperature, which indicated that increasing temperature aggravated the effect of RH on carotenoids. In addition, the values of carotenoids decreased with increasing treatment duration.
3.3 Interactive effects of relative humidity and high temperature on gas exchange parameters
Table 4 shows the gas exchange parameters of chrysanthemum leaves under different treatments. The value of Pn in CK treatment was significantly higher than that of all treatments. With increasing temperature, the value of Pn significantly decreased and to reach the lowest in T41°C, which was 57.61% lower than that of CK. Among RH treatments, although statistically non-significant, the value of Pn was highest in RH70%, followed by RH50%, and RH90% was the lowest. There was no significant effect of treatment duration on Pn.
Compared to CK treatment, the values of Gs and Ci in all treatments significantly decreased. The lowest values of Gs and Ci were recorded in T38°C, which were 52.00% and 42.40% lower than that of CK treatment. Among RH treatments, the value of Gs was highest in RH70%, followed by RH50%, and RH90% was the lowest. The value of Ci in RH70% was higher than that of RH90%. The values of Gs and Ci in L6d were the lowest among L3d, L6d and L9d, which were 48.00% and 38.66% lower than that of CK treatment.
The value of Ls in CK treatment was significantly lower than that of all treatments. Among temperature treatments, the value of Ls was highest in T38°C, which was 79.17% higher than that of CK treatment, followed by T41°C, and T35°C was the lowest. The value of Ls in RH70% was lower than that of RH50% and RH90%. There was no significant effect of treatment duration on Ls.
In order to determine whether the decrease in Pn is caused by obstruction of CO2 diffusion or decrease in enzyme activity, we conducted stomatal and non-stomatal limitation analyses of photosynthesis. The evaluation of stomatal and non-stomatal limitation mainly depends on the change direction of Ci and Ls (Xu, 1997).The increase of Ls and the decrease of Ci indicate that the main reason for Pn decrease is stomatal limitation, while the decrease of Ls and the increase of Ci indicate the reason is non-stomatal. Compared to CK, Ls increased and Ci decreased in T35°C, indicating that stomatal limitation was the reason for Pn decrease. In addition, the reason for T38°C was also stomatal limitation because Ls increased and Ci decreased, compared to T35°C. In T41°C, Ls decreased while Ci increased, compared to T38°C, indicating that the non-stomatal limitation was the reason for Pn decrease. Under three RH conditions, compared to CK, Ls increased and Ci decreased, indicating that RH affects Pn through stomatal factors. Ls increased and Ci decreased under L3d and L6d treatments, indicating that the major factor for Pn decrease from 0d to 6d was stomatal limitation. Compared to L6d, Ls decreased and Ci increased in L9d treatment, indicating that the decrease in Pn from 6d to 9d was non-stomatal limitation.
For the water regimes of chrysanthemums, the values of Tr and WUE in CK treatment were significantly higher than that of all treatments. Among temperature treatments, the value of Tr was highest in T35°C, followed by T41°C, and T38°C was the lowest, which was 12.07% lower than that of CK treatment. With increasing temperature, the value of WUE significantly decreased and reached the lowest in T41°C, which was 54.98% lower than that of CK treatment. The value of Tr in RH50% and RH70% was significantly higher than that of RH90%. Although statistically non-significant, the value of WUE was highest in RH70%, followed by RH50%, and RH90% was the lowest. The value of Tr was highest in L3d, followed by L9d, and L6d was the lowest, which was 12.07% lower than that of CK treatment. There was no significant effect of treatment duration on WUE.
The results of ANOVA are also shown in Table 4. Temperature and RH had significant impacts (P<0.01) on Pn, Gs, Ci and Ls. Temperature and treatment duration had significant impacts (P<0.01) on Tr. The interaction of temperature and RH was significant for Pn and WUE (P< 0.01). Furthermore, the interaction of temperature and treatment duration was significant for Gs and Pn (P< 0.05). Temperature had the greatest impacts on Pn, Gs, Ci, Ls and Tr with contribution rates of 67.76%, 51.45%, 44.59%, 44.95% and 50.67%, followed by RH. The interaction of temperature and RH had the greatest impact on WUE with contribution rates of 52.09%.
The interaction between temperature and RH was significant for Pn (P< 0.01). Interactions affecting Pn from looking at temperature effects at a given RH and RH effects at a given temperature were shown in Figure 5. At a given RH, the values of Pn decreased with increasing temperature, which indicated that increasing temperature aggravated the effect of RH on Pn. At a given temperature, the values of Pn at 70% RH were highest, followed by 50% RH and 90% RH, which indicated that 70% RH mitigated the negative effect of high temperature on Pn, while high RH aggravated. In addition, the values of Pn decreased with increasing treatment duration. The value of Pn was lowest at 41°C and 90% RH.
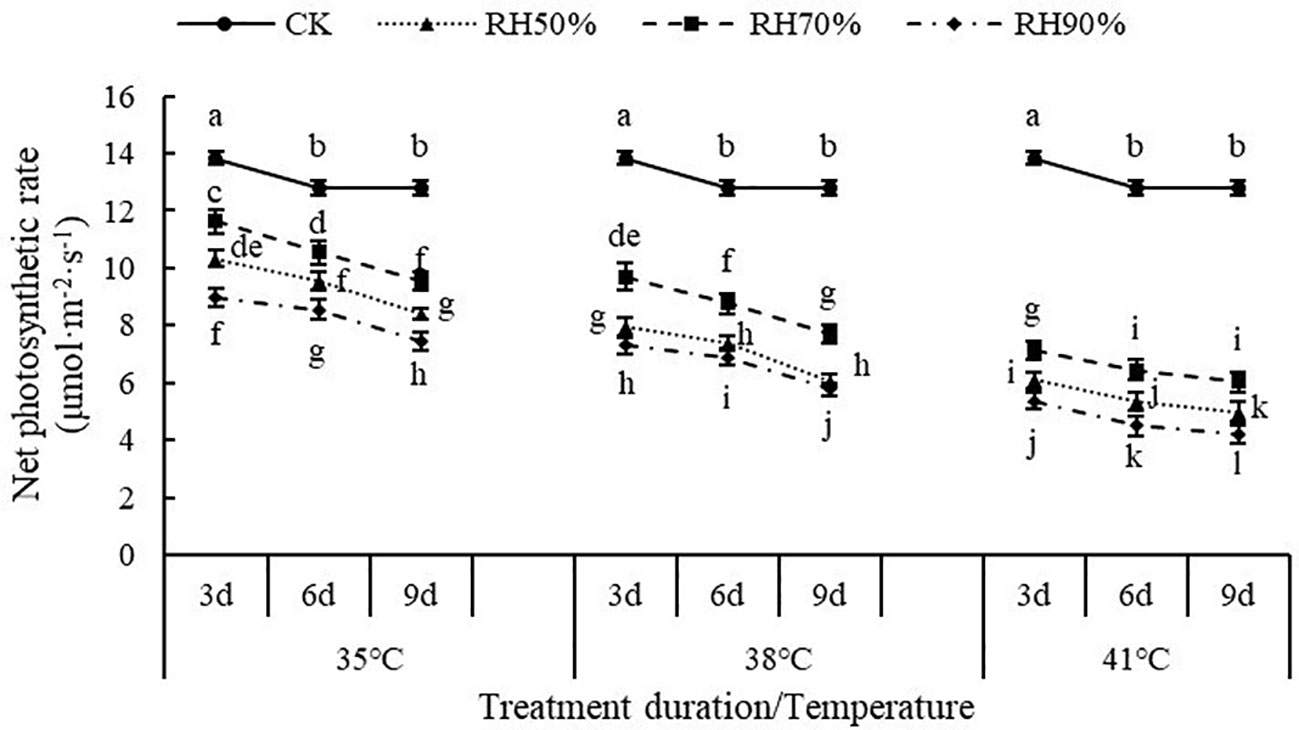
Figure 5 Effect of interaction between temperature and RH on net photosynthetic rate of chrysanthemum leaves.
3.4 Interactive effects of relative humidity and high temperature on chlorophyll fluorescence
Chlorophyll fluorescence kinetic curves respond to the photosynthetic efficiency and potential of chrysanthemum leaves. As seen in Figure 6A, the fluorescence values of curve O-P in CK treatment, T35°C, T38°C and T41°C were 318~1595, 336~1547, 367~1470 and 393~1264, indicating that the fluorescence values of the leaves decreased with increasing temperature. As seen in Figure 6B, the fluorescence values of curve O-P in RH50%, RH70% and RH90% were 356~1475, 322~1604 and 363~1441. It showed that the fluorescence value of curve O-P in RH70% was highest, followed by RH50%, and RH90% was the lowest. As seen in Figure 6C, the fluorescence values of curve O-P in L3d, L6d and L9d were 332~1570, 355~1385 and 389~1282, indicating that the fluorescence values of chrysanthemum leaves decreased with increasing treatment duration.
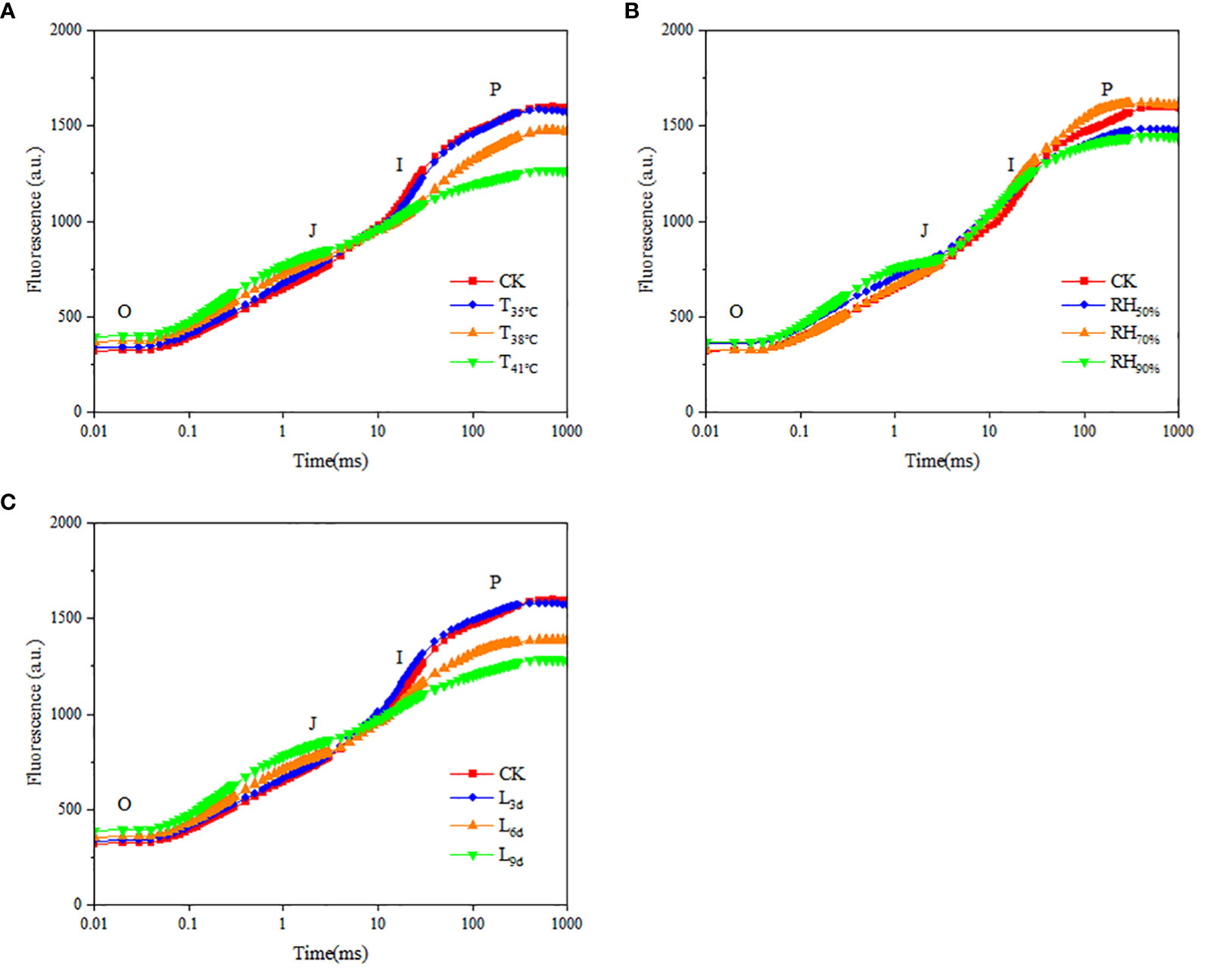
Figure 6 Effect of different temperature (A), RH (B) and treatment duration (C) on rapid fluorescence kinetic curve of chrysanthemum leaves. T, RH and L represent temperature, relative humidity and treatment duration, respectively.
As seen in Table 5, Fo and Fj were lower in CK treatment than that of all temperature treatments. With temperature increasing, Fo and Fj increased and reached the highest in T41°C, which were 23.53% and 13.93% higher than that of CK treatment. Fo and Fj in RH70% were close to that of CK treatment, while lower than that of other RH treatments. With treatment duration increasing, Fo and Fj increased and reached the highest in L9d, which were 22.29% and 15.59% higher than that of CK treatment. Fm and Fv/Fm were higher in CK than that of all temperature treatments. With temperature increasing, Fm and Fv/Fm decreased and reached the lowest in T41°C, which were 20.69% and 13.75% lower than that of CK treatment. Fm and Fv/Fm in RH70% were close to that of CK treatment, while higher than that of other RH treatments. With treatment duration increasing, Fm and Fv/Fm decreased and reached the lowest in L9d, which were 19.56% and 13.75% lower than that of CK treatment.
As seen in Figure 7A, ABS/CSm, TRo/CSm and ETo/CSm were higher in CK treatment than that of all treatments, while DIo/CSm was lowest. With temperature increasing, ABS/CSm, TRo/CSm and ETo/CSm decreased and reached the lowest in T41°C, which were 20.69%, 31.87% and 49.37% lower than that of CK treatment. While DIo/CSm increased with temperature increasing, the highest DIo/CSm was observed in T41°C, which was 23.53% higher than that of CK treatment. As seen in Figure 7B, ABS/CSm, TRo/CSm, ETo/CSm, and DIo/CSm in RH70% were close to that of CK treatment. ABS/CSm, TRo/CSm and ETo/CSm in RH50% and RH50% treatments were significantly lower than that of CK treatment, while DIo/CSm was higher. As seen in Figure 7C, With treatment duration increasing, ABS/CSm, TRo/CSm and ETo/CSm decreased and reached the lowest in L9d, which were 19.56%, 30.15% and 48.69% lower than that of CK treatment. While DIo/CSm increased with treatment duration increasing, the highest DIo/CSm was observed in L9d, which was 22.29% higher than that of CK treatment.
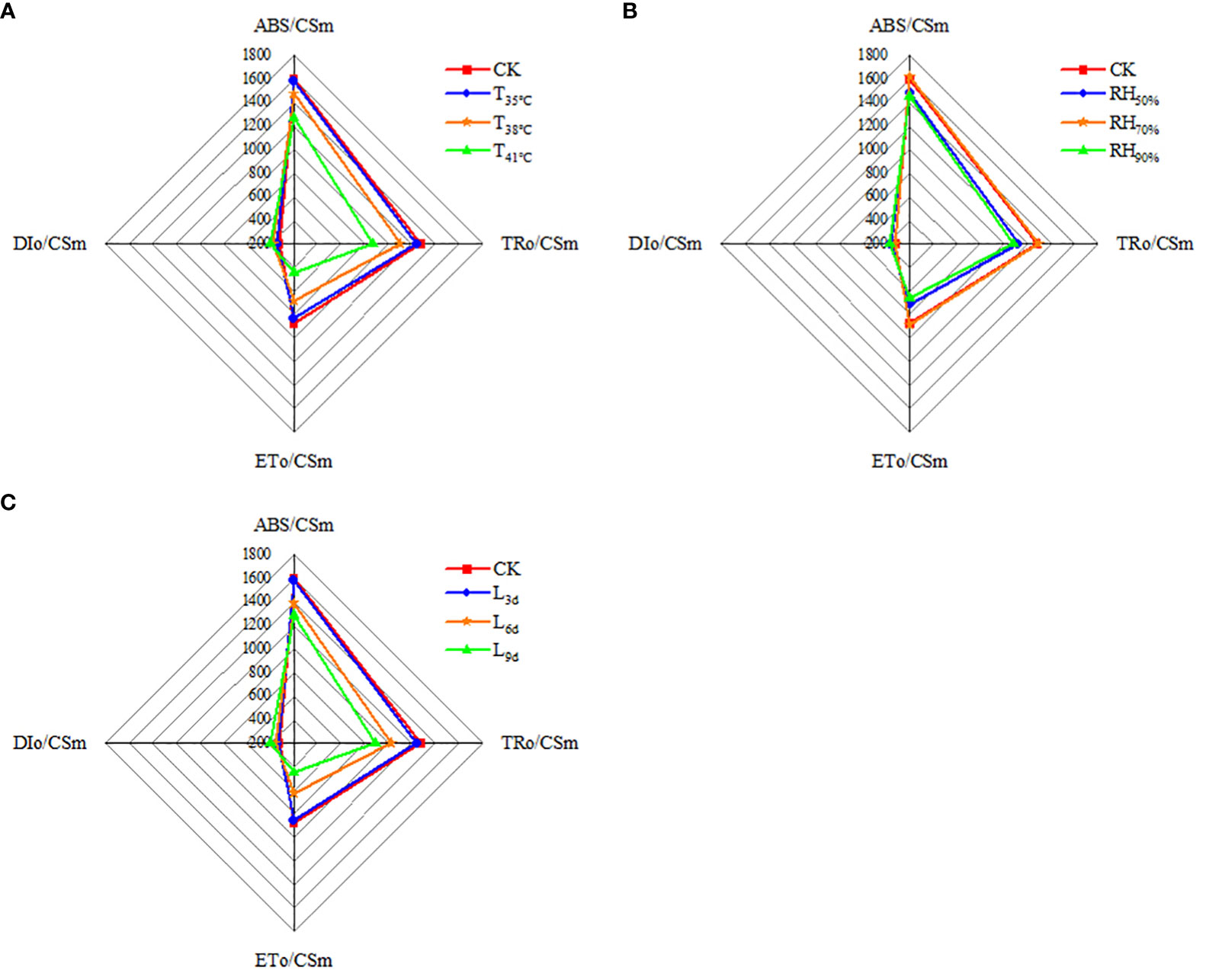
Figure 7 Effect of different temperature (A), RH (B) and treatment duration (C) on light energy absorption, capture, and transferenergy of chrysanthemum leaves. ABS/CSm means absorption flux per cross section. TRo/CSm means trapped energy flux per cross section. ETo/CSm means electron transport flux per cross section. DIo/CSm means non-photochemical quenching per cross section. T, RH and L represent temperature, relative humidity and treatment duration, respectively.
The fluorescence difference kinetics ΔWOJ of the different treated OJ phases reveal their respective K-bands. As seen in Figure 8A, the ΔWOJ curves in T38°C and T41°C followed the same trend and both showed a K-band, while ΔWOJ curves in T35°C did not show a clear K-band. As seen in Figure 8B, the ΔWOJ curves in RH50% and RH90% followed the same trend and both showed a K-band, while ΔWOJ curves in RH70% did not show a clear K-band. As seen in Figure 8C, the ΔWOJ curves in L6d and L9d followed the same trend and both showed a K-band, while ΔWOJ curves in L3d did not show a clear K-band.
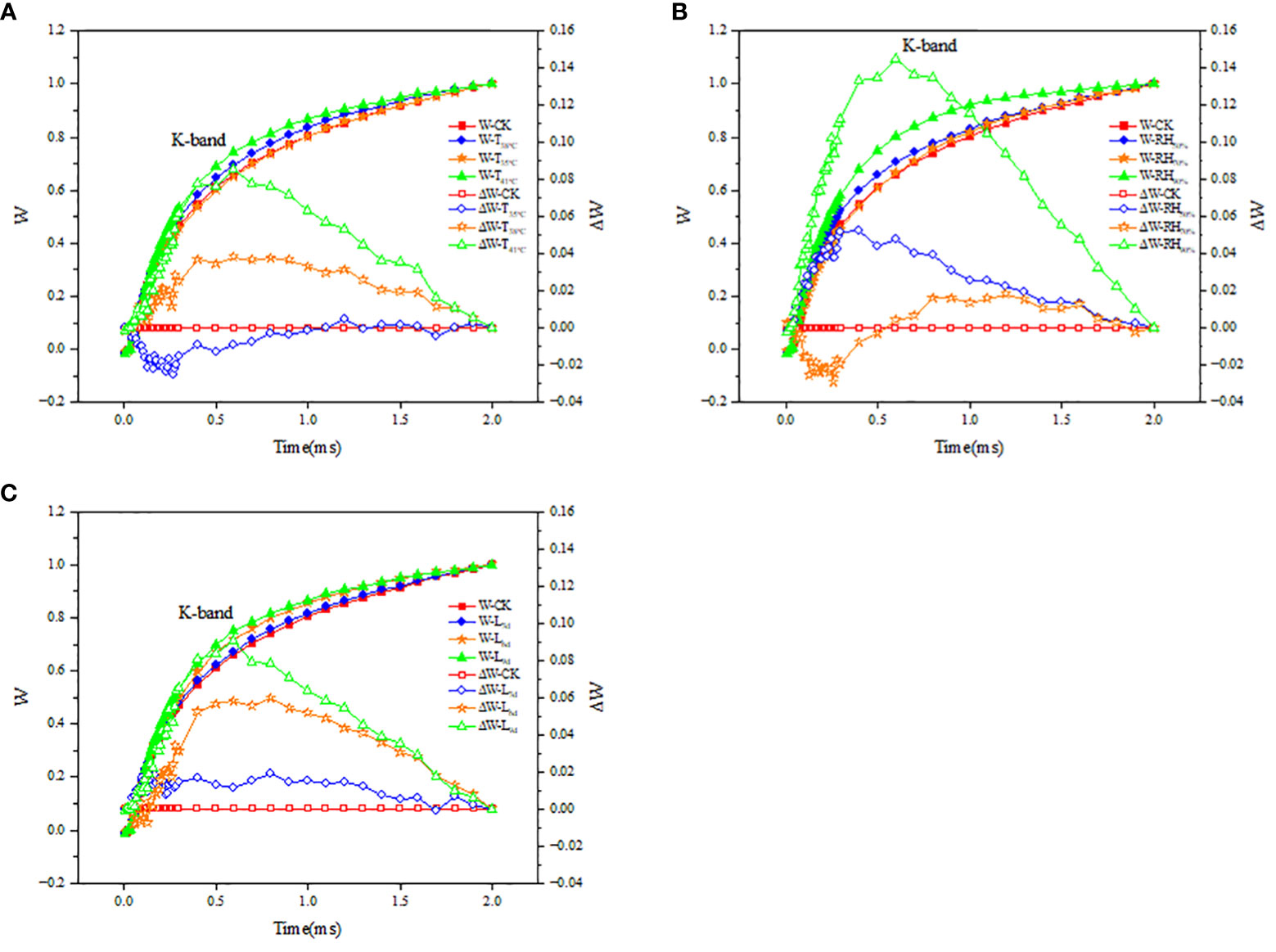
Figure 8 Effect of different temperature (A), RH (B) and treatment duration (C) on the fluorescence differential kinetics of OJ phase of chrysanthemum leaves. T, RH and L represent temperature, relative humidity and treatment duration, respectively.
4 Discussion
The light response curves parameters are important indicators that reflect the light energy utilization capacity and efficiency of plants under different environmental conditions. High temperatures affect the photosynthetic properties of plants (Li and Li, 2009). This study showed that values of AQE and Pn-max decreased significantly with increasing temperature, while the value of Rd increased. This indicated that the respiratory consumption of chrysanthemum leaves was high and photosynthetic activity was low under high temperature environments, which is in accordance with the study of Su et al. (Su and Liu, 2005) RH also affects plant photosynthesis. For example, 60% RH is the optimal level for cabbage to grow under high temperature environments, while at 90% RH under high temperature environments, cabbage was damaged and Pn was reduced (Han et al., 2019). In this study, AQE at 70% RH were higher than that at 50% and 90% RH. This suggested that adjusting the RH to 70% could alleviate the inhibition of high temperature stress on photosynthesis. The values of LSP, AQE, and Pn-max were lowest at 90% RH, suggesting that the inhibition of photosynthesis was greater under high RH environments. Similar findings were reported in different studies too (Shin et al., 2007). The prolongation of the stress time increases the damage to plant photosynthesis (Han et al., 2018). The values of LSP, AQE, and Pn-max were decreased with increasing treatment duration. This indicated that the degree of photosynthetic inhibition increased with prolonged stress.
Chlorophyll content is an important indicator to assess stress in photosynthetic organs and usually reflects chloroplast development and photosynthetic performance (Lu et al., 2019). Chlorophyll synthesis is a series of enzymatic reactions, and high temperature stress causes protein denaturation and lipid peroxidation of cell membranes, reducing the rate of chlorophyll synthesis (Liao et al., 2004). This study showed that the values of chlorophyll a, chlorophyll b, and chlorophyll (a+b) decreased with increasing temperature. It suggested that chloroplasts were damaged or the rate of chlorophyll synthesis was reduced under high temperature environments, contributing to the decrease in Pn, which is in line with the study of Yang et al. (Yang et al., 2019).
High temperature leads to the decrease in chlorophyll content of plant leaves, firstly, because high temperature reduces the rate of chlorophyll synthesis (Li et al., 2020), and secondly, because the accumulation of reactive oxygen species at high temperature accelerates the degradation of chlorophyll (Yuan et al., 2017). Carotenoids are both photosynthetic pigments and endocytic source of antioxidants, which can absorb excess energy in chloroplast, quench reactive oxygen species and prevent membrane lipid peroxidation (Zhou et al., 2016). This study showed that the content of carotenoid decreased with increasing temperature, indicating that carotenoids were damaged by high temperature stress and their functions were damaged, which can lead to reactive oxygen accumulation. This is consistent with the findings of Lokesha (Lokesha et al., 2019). This also indicated that under high temperature environments, the presence of non-stomatal limiting factors for photosynthesis inhibition. Moreover, this study found that the value of carotenoids at 70% RH was highest under high temperatures. It indicated that 70% RH was able to maintain the carotenoid content of chrysanthemum leaves under high temperatures, thus absorbing excess energy from chloroplasts, quenching reactive oxygen species, and increasing Pn.In this study, it was found that the values of chlorophyll a, chlorophyll b, and chlorophyll (a+b) decreased at 50% and 90% RH, compared to 70% RH. Hence, 70% RH could alleviate the damage of chloroplasts in chrysanthemum leaves by high temperature. The difference of photosynthetic pigment content between RH50% and RH90% was statistically non-significant. A recent study pointed out that at 46°C, lower RH amplifies the inhibition of the photosystem by high temperature (Lysenko et al., 2023). For high or low RH, further experiments are needed as to which has a greater effect on chlorophyll content.
Stomata are channels for the exchange of carbon and water between chloroplasts and the atmosphere and have an impact on plant physiology (Du et al., 2018). This study showed that the dominant factor in the decrease of Pn at 35°C and 38°C was stomatal limitation, which is concordance with the work of Fan et al. (Fan et al., 2010). In this environment, high temperature led to a large number of stomatal closures, reduced Gs and blocked CO2 diffusion, which contributed to the reduction of Pn. Wu et al. (Wu et al., 2001) found that stomatal limitation caused inhibition of photosynthesis when plants were under mild heat stress, but inhibition of photosynthesis under extreme heat stress was caused by non-stomatal limitation. In this study, when the temperature increased to 41°C, the dominant factor was non-stomatal limitation. As can be seen from the discussion below, this dominant factor was high temperature led to disruption of the internal structure of PSII and inhibition of its activity. Stomatal morphological characteristics are related to their function and also influenced by VPD (Sinclair et al., 2007). Alineaeifard et al. (Aliniaeifard and van Meeteren, 2016) found that chrysanthemums exposed to low VPD (0.23 kPa) had larger stomatal sizes, wider pore diameters, and greater stomatal densities, resulting in higher Gs compared to chrysanthemums grown in a 1.05 kPa VPD environment. This study showed that the value of Gs was higher in a 2.00 kPa VPD environment (RH70%) than that in a 3.34kPa VPD environment (RH50%). Among three RH conditions, the value of Pn at 70% RH was the maximum, while Rd was the minimum, suggesting that 70% RH mitigated the inhibitory effect of high temperature on Pn. High and low RH in high temperature environments can exacerbate the effects (Bunce, 2002). The study showed that the value of Pn decreased at 90% and 50% RH, indicating that high and low RH inhibit photosynthesis in high temperature environments. The dominant factor causing the decrease in Pn from 0d to 6d was stomatal limitation, but non-stomatal limitation from 6 d to 9 d. As can be seen from the discussion below, the dominant factor for the decrease in Pn from 6d to 9d was that prolonged stress led to disruption of the internal structure of PSII and inhibition of its activity.
WUE is the amount of CO2 assimilated per unit mass of water lost by leaf transpiration (Hatfield and Dold, 2019). Given that stomata control water balance, stomatal behavior has a significant effect on WUE (Li and Liu, 2022). This study showed that the value of Tr decreased at 35°C and 38°C. This was due to that stomatal limitation at 35°C and 38°C was a major factor in reducing Pn, and the massive closure of stomata led to the decrease of Tr. While the value of Tr increased at 41°C, because that non-stomatal limitation at 41°C was a major factor in reducing Pn, and the increase of Gs led to the increase of Tr. The value of WUE decreased with increasing temperature. This may be related to chrysanthemum leaf senescence under high temperature environments. Bunce et al. (Bunce, 2002) found that under excessively high RH conditions, increased VPD was caused by higher Tr, which lead to the increase of Gs. This study showed that VPD and the value of Tr were lower at 90% RH than that of other RH conditions. This mean that 90% RH was not favorable for transpiration of chrysanthemum leaves. Numerically, the value of WUE was higher at 70% RH than 50% RH, suggesting that 70% RH may be better for water utilization by chrysanthemum leaves than 50% RH. The value of Tr decreased at 3d and 6d, because that stomatal limitation at 3d and 6d was a major factor in reducing Pn, and the massive closure of stomata led to the decrease of Tr. While the value of Tr increased at 9d, because that non-stomatal limitation at 9d was a major factor in reducing Pn, and the increase of Gs led to the increase of Tr.
By analyzing chlorophyll fluorescence, it is possible to know the response of the plant to changes in environmental factors (Maxwell and Johnson, 2000). PS II is one of the most sensitive parts of the photosynthetic system to temperature stress and is closely linked to chlorophyll fluorescence (Murata et al., 2007). This study found that with increasing temperature, Fo and Fj increased. Photoinactivation usually leads to oxidative damage and inactivation of PSII reaction centers, which further leads to an increase in Fo (Liu et al., 2022). It indicated that high temperature resulted in inactivation of PSII reaction centers. Changes in cystoid membrane structure and organization may result in changes in Fm during many stress treatments (Baker, 2008). This study found that with increasing temperature, Fm decreased, suggesting that high temperature resulted in the changes in cystoid membrane structure and organization. Fv/Fm is used to measure the maximum efficiency of PSII (Liu et al., 2020). A decrease in Fv/Fm is often observed when plants are in a stress state, which represents impaired PSII function (Guidi et al., 2019). This study found that with increasing temperature, Fv/Fm decreased, suggesting that high temperature resulted in impaired PSII function. In addition, this study found that ABS/CSm, TRo/CSm and ETo/CSm decreased, and DIo/CSm increased in chrysanthemum leaves under high temperature environments. This suggested that high temperatures may affect the structure of the PSII functional antenna, which reduces the ability to capture light (ABS/CSm), leading to a decrease in the excitation energy of the reduced QA (TRo/CSm) and its ability to be used for electron transfer (ETo/CSm), and an increase in heat dissipation (DIo/CSm). To further analyze the changes of PSII in chrysanthemum leaves, experiments were conducted to investigate the kinetics of fluorescence differences in the OJ phases of different treatments. ΔWOJ can reflect the PSII functional antenna size and the activity of the manganese complex-dominated exocytosis complex. It was shown that K-bands appeared and ΔWOJ > 0 at 38 °C and 41°C, indicating that the oxygen release complex of PSII in chrysanthemum leaves was inactivated under high temperature environments (Wakjera et al., 2013), the efficiency of plastoquinone QA in transferring electrons was decreased (Hermans et al., 2005), and PSII functional antenna size changed (Li et al., 2009). It also suggested that the main factor for the decrease in Pn at 41°C was that high temperature led to disruption of the internal structure of PSII and inhibition of its activity. For the different RH conditions, Fo, Fj, Fm and Fv/Fm were close to those of CK at 70% RH, indicating that 70% RH mitigated the effect of high temperature on PSII activity. While Fo, Fj, and DIo/CSm increased, Fm, Fv/Fm, ABS/CSm, TRo/CSm and ETo/CSm decreased at 50% and 90% RH. It was also shown that K-bands appeared and ΔWOJ > 0 at 50% and 90% RH, indicating that both 50% and 90% RH inactivated the oxygen release complex of PSII, reduced the electron transfer efficiency of QA and PSII functional antenna size changed. With treatment duration increasing, Fo, Fj, and DIo/CSm increased, while Fm, Fv/Fm, ABS/CSm, TRo/CSm and ETo/CSm decreased. It was also shown that K-bands appeared and ΔWOJ > 0 at 6d and 9d. K-band at 9d was higher than that at 6d, suggesting that the inhibition of PSII activity was exacerbated with prolonged stress. It also suggested that the main factor for the decrease in Pn from 6d to 9d was that prolonged stress led to disruption of the internal structure of PSII and inhibition of its activity.
Under high temperature environment, photosynthesis is often suppressed before other cellular functions are compromised. And RH can influence photosynthesis differently according to environmental changes (Rodrigues et al., 2016). The results of this study showed that high temperature had a greater effect on photosynthesis in chrysanthemum seedlings than RH. This indicates that high temperature dominates the effect of photosynthesis under high temperature and high RH. Meanwhile, the study also showed that there was an interactive effect of high temperature and RH on photosynthesis of chrysanthemum leaves, and the interaction of the two had a significant effect on Pn (P<0.01). This indicates that changes in RH under high temperature conditions can significantly affect the photosynthetic rate. Excessive RH aggravated the inhibitory effect of high temperature on photosynthetic rate. This was mainly due to the fact that high RH led to low VPD, the closure of stomata, the reduction of Tr, and the reduction of CO2 exchange between inside and outside the leaves (Carvalho et al., 2015). In addition, high RH produces leaf thermal overload. Elevated leaf temperatures exacerbate damage to leaf photosynthetic functions. Lieten et al. (Lieten, 2002) found that strawberry leaves under high RH conditions showed leaf tip burn. In this study, we found that the inhibition of photosynthesis rate at 90% RH was also significantly higher at 50% RH than at 70% RH under high temperature conditions. This was because stomatal closure affected leaf CO2 exchange and heat dissipation under low RH conditions (Ferrante and Mariani, 2018). At 70% RH, Gs was significantly greater than that at 50% and 90% RH, which was favorable to alleviate the inhibition of photosynthesis by high temperature.
5 Conclusion
This experiment investigated the effects of high temperature, RH and treatment duration on photosynthesis of “Shenma” chrysanthemum leaves. The results showed that heat stress above 35°C affects chlorophyll fluorescence parameters of chrysanthemum leaves, significantly reduced the content of photosynthetic pigments, and severely inhibited photosynthesis. Under high temperature environment, the decrease of Pn and photosynthetic pigment content at 70% RH was lower than the other two RH conditions and it reduced the damage of high temperatures to photosynthesis system. The dominant factor causing the decrease of Pn in leaves was stomatal limitation at 35°C,38°C, three RH conditions, 3d and 6d, but non-stomatal limitation at 41°C and 9d. There was an interaction between temperature and RH, with a significant impact on Pn (P<0.01). Under high temperature and RH environments, temperature is the main factor affecting photosynthesis, followed by RH. When the temperature reached or exceeded 35°C, adjusting the RH to 70% could effectively reduce the damage of high temperature stress on chrysanthemum leaves.
Data availability statement
The original contributions presented in the study are included in the article/supplementary material. Further inquiries can be directed to the corresponding author.
Author contributions
JZ: Conceptualization, Formal Analysis, Investigation, Writing – original draft, Writing – review & editing, Data curation. XJ: Conceptualization, Funding acquisition, Investigation, Methodology, Supervision, Writing – original draft, Writing – review & editing, Data curation. EA: Writing – review & editing. XL: Writing – review & editing. YZ: Funding acquisition, Methodology, Writing – review & editing. RL: Funding acquisition, Writing – review & editing.
Funding
The author(s) declare financial support was received for the research, authorship, and/or publication of this article. This research was funded by the National Key Research and Development Plan of China (2019YFD1002202), Jiangsu Key Laboratory of Agricultural Meteorology Foundation (JKLAM2003).
Acknowledgments
We thank the reviewers for their constructive comments and suggestions on revising the manuscript.
Conflict of interest
The authors declare that the research was conducted in the absence of any commercial or financial relationships that could be construed as a potential conflict of interest.
Publisher’s note
All claims expressed in this article are solely those of the authors and do not necessarily represent those of their affiliated organizations, or those of the publisher, the editors and the reviewers. Any product that may be evaluated in this article, or claim that may be made by its manufacturer, is not guaranteed or endorsed by the publisher.
References
Agathokleous, E., Feng, Z., Peñuelas, J. (2020). Chlorophyll hormesis: Are chlorophylls major components of stress biology in higher plants? Sci. Total Environ. 726, 138637. doi: 10.1016/j.scitotenv.2020.138637
Aliniaeifard, S., van Meeteren, U. (2016). Stomatal characteristics and desiccation response of leaves of cut chrysanthemum (Chrysanthemum morifolium) flowers grown at high air humidity. Scientia Hortic. 205, 84–89. doi: 10.1016/j.scienta.2016.04.025
Baker, N. R. (2008). Chlorophyll fluorescence: a probe of photosynthesis in vivo. Annu. Rev. Plant Biol. 59, 89–113. doi: 10.1146/annurev.arplant.59.032607.092759
Barker, J. C. (1990). Effects of day and night humidity on yield and fruit quality of glasshouse tomatoes (Lycopersicon esculentum Mill.). J. Hortic. Sci. 65 (3), 323–331. doi: 10.1080/00221589.1990.11516061
Berry, J. A. (1982). Environmental regulation of photosynthesis. Photosynthesis 2, 263–343. doi: 10.1016/B978-0-12-294302-7.50017-3
Bunce, J. (2002). Effects of humidity on short-term response of stomatal conductance to an increase in carbon dioxide concentration. Plant Cell Environ. 21, 115–120. doi: 10.1046/j.1365-3040.1998.00253.x
Carvalho, D. R. A., Torre, S., Kraniotis, D., Almeida, D. P. F., Heuvelink, E., Carvalho, S. M. P. (2015). Elevated air movement enhances stomatal sensitivity to abscisic acid in leaves developed at high relative air humidity. Front. Plant Sci. 6. doi: 10.3389/fpls.2015.00383
Castello, I., D’Emilio, A., Baglieri, A., Polizzi, G., Vitale, A. (2022). Management of chrysanthemum verticillium wilt through vif soil mulching combined with fumigation at label and reduced rates. Agriculture 12 (2), 141. doi: 10.3390/agriculture12020141
Chevuturi, A., Klingaman, N. P., Turner, A. G., Hannah, S. (2018). Projected changes in the Asian-Australian monsoon region in 1.5°c and 2.0°c global-warming scenarios. Earth's Future 6 (3), 339–358. doi: 10.1002/2017EF000734
Dong, Y. (2020). Statistical analysis of chinese flower import and export data in 2019. China Flowers Hortic. 11), 24–25.
Du, Q., Xing, G., Jiao, X., Song, X., Li, J. (2018). Stomatal responses to long-term high vapor pressure deficits mediated most limitation of photosynthesis in tomatoes. Acta Physiologiae Planta. 40 (8), 1–12. doi: 10.1007/s11738-018-2723-7
Fan, P., Li, L., Duan, W., Li, W., Li, S. (2010). Photosynthesis of young apple trees in response to low sink demand under different air temperatures. Tree Physiol. 30, 313–325. doi: 10.1093/treephys/tpp114
Fanourakis, D., Papadakis, V. M., Psyllakis, E., Tzanakakis, V. A., Nektarios, P. A. (2022). The role of water relations and oxidative stress in the vase life response to prolonged storage: a case study in chrysanthemum. Agriculture 12 (2), 185. doi: 10.3390/agriculture12020185
Ferrante, A., Mariani, L. (2018). Agronomic management for enhancing plant tolerance to abiotic stresses: high and low values of temperature, light intensity, and relative humidity. Horticulturae 4 (3), 21. doi: 10.3390/horticulturae4030021
Fischer, R. A., Turner, N. C. (1978). Plant productivity in the arid and semiarid zones. Annu. Rev. Plant Physiol. 29 (1), 277–317. doi: 10.1146/annurev.pp.29.060178.001425
Guidi, L., Lo Piccolo, E., Landi, M. (2019). Chlorophyll fluorescence, photoinhibition and abiotic stress: does it make any difference the fact to be a C3 or C4 species? Front. Plant Sci. 10. doi: 10.3389/fpls.2019.00174
Han, W., Sun, C. X., Zhao, H. L., Hu, Q., Zheng, Q. Z., Song, X. L. (2018). Compensatory ability and defense mechanism of Chinese cabbage under high temperature stress. Chin. J. Agrometeorol. 39, 119–128. doi: 10.3969/j.issn.1000-6362.2018.02.006
Han, W., Yang, Z., Huang, L., Sun, C., Yu, X., Zhao, M. (2019). Fuzzy comprehensive evaluation of the effects of relative air humidity on the morpho-physiological traits of Pakchoi (Brassica chinensis L.) under high temperature. Scientia Hortic. 246, 971–978. doi: 10.1016/j.scienta.2018.11.079
Hatfield, J. L., Dold, C. (2019). Water-use efficiency: advances and challenges in a changing climate. Front. Plant Sci. 10. doi: 10.3389/fpls.2019.00103
Hazrati, S., Tahmasebi-Sarvestani, Z., Modarres-Sanavy, S. A., Mokhtassi-Bidgoli, A., Nicola, S. (2016). Effects of water stress and light intensity on chlorophyll fluorescence parameters and pigments of Aloe vera L. Plant Physiol. Biochem. 106, 141–148. doi: 10.1016/j.plaphy.2016.04.046
Hermans, C., Johnson, G., Strasser, R., Verbruggen, N. (2005). Physiological characterisation of magnesium deficiency in sugar beet: Acclimation to low magnesium differentially affects photosystems I and II. Planta 220, 344–355. doi: 10.1007/s00425-004-1340-4
Howell, T. A., Dusek, D. A. (1995). Comparison of vapor-pressure-deficit calculation methods—southern high plains. J. Irrigation Drainage Eng. 121 (2), 191–198. doi: 10.1061/(ASCE)0733-9437(1995)121:2(191
Janka, E., Körner, O., Rosenqvist, E., Ottosen, C. O. (2015). Using the quantum yields of photosystem II and the rate of net photosynthesis to monitor high irradiance and temperature stress in chrysanthemum (Dendranthema grandiflora). Plant Physiol. Biochem. 90, 14–22. doi: 10.1016/j.plaphy.2015.02.019
Jiang, X., Hua, M., Yang, X., Hu, N., Qiu, R., Yang, S. (2020). Impacts of mist spray on rice field micrometeorology and rice yield under heat stress condition. Sci. Rep. 10 (1), 1579. doi: 10.1038/s41598-020-58578-3
Kadam, N. N., Yin, X., Bindraban, P. S., Struik, P. C., Jagadish, K. S. V. (2015). Does morphological and anatomical plasticity during the vegetative stage make wheat more tolerant of water deficit stress than rice? Plant Physiol. 167 (4), 1389–1401. doi: 10.1104/pp.114.253328
Lee, S., Choi, C., Lee, J. G., Jang, Y., Lee, H., Lee, H. J., et al. (2013). Influence of air temperature on yield and phytochemical content of red chicory and garland chrysanthemum grown in plant factory. Horticulture 54, 399–404. doi: 10.1007/s13580-013-0095-x
Li, H. (2000). Principles and Techniques of Plant Physiology and Biochemistry Experiments (China: Higher Education Press).
Li, P., Cheng, L., Gao, H., Jiang, C., Peng, T. (2009). Heterogeneous behavior of PSII in soybean (Glycine max) leaves with identical PSII photochemistry efficiency under different high temperature treatments. J. Plant Physiol. 166 (15), 1607–1615. doi: 10.1016/j.jplph.2009.04.013
Li, S., Liu, F. (2022). Vapour pressure deficit and endogenous ABA level modulate stomatal responses of tomato plants to soil water deficit. Environ. Exp. Bot. 199, 104889. doi: 10.1016/j.envexpbot.2022.104889
Li, T., Li, M. (2009). Effect of short-term daytime sub-high temperature stress on photosynthesis of tomato leaves. Nongye Gongcheng Xuebao/Transactions Chin. Soc. Agric. Eng. 25, 220–225. doi: 10.3969/j.issn.1002-6819.2009.09.039
Li, X., Liao, F., Ma, Q., Jiang, B., Pan, Y., Luo, C., et al. (2021). Proteomic analysis of high temperature stress-responsive proteins in chrysanthemum leaves. Phyton 90, 1415–1423. doi: 10.32604/phyton.2021.016143
Li, Y.-T., Xu, W.-W., Ren, B.-Z., Zhao, B., Zhang, J., Liu, P., et al. (2020). High temperature reduces photosynthesis in maize leaves by damaging chloroplast ultrastructure and photosystem II. J. Agron. Crop Sci. 206 (5), 548–564. doi: 10.1111/jac.12401
Liao, F. Y., Li, H., He, P. (2004). Effect of high irradiance and high temperature on chloroplast composition and structure of Dioscorea zingiberensis. Photosynthetica 42, 487–492. doi: 10.1007/S11099-005-0002-5
Lieten, P. (2002). The effect of humidity on the performance of greenhouse grown strawberry. Acta Hortic. 567, 479–482. doi: 10.17660/ActaHortic.2002.567.101
Liu, Y. Y., Li, J., Liu, S. C., Yu, Q., Tong, X. J., Zhu, T., et al. (2020). Sugarcane leaf photosynthetic light responses and their difference between varieties under high temperature stress. Photosynthetica 58, 1009–1018. doi: 10.32615/ps.2020.038
Liu, X., Zhang, J., Wang, Q., Chang, T., Shaghaleh, H., Hamoud, Y. A. (2022). Improvement of photosynthesis by biochar and vermicompost to enhance tomato (Solanum Lycopersicum L.) Yield under greenhouse conditions. Plants 11 (23), 3214. doi: 10.3390/plants11233214
Lokesha, A. N., Shivashankara, K. S., Laxman, R. H., Geetha and Shankar, G. A. (2019). Effect of high temperature on fruit quality parameters of contrasting tomato genotypes. Int. J. Curr. Microbiol. Appl. Sci. 8, 1019–1029. doi: 10.20546/ijcmas.2019.803.124
Lu, T., Yu, H., Li, Q., Chai, L., Jiang, W. (2019). Improving plant growth and alleviating photosynthetic inhibition and oxidative stress from low-light stress with exogenous gr24 in tomato (Solanum lycopersicum L.) seedlings. Front. Plant Sci. 10. doi: 10.3389/fpls.2019.00490
Lysenko, E. A., Kozuleva, M. A., Klaus, A. A., Pshybytko, N. L., Kusnetsov, V. V. (2023). Lower air humidity reduced both the plant growth and activities of photosystems I and II under prolonged heat stress. Plant Physiol. Biochem. 194, 246–262. doi: 10.1016/j.plaphy.2022.11.016
Maxwell, K., Johnson, G. (2000). Chlorophyll fluorescence - a practical guide. J. Exp. Bot. 51, 659–668. doi: 10.1093/jexbot/51.345.659
Mortensen, L., Gislerød, H. (2000). Effect of air humidity on growth, keeping quality, water relations, and nutrient content of cut roses. Gartenbauwissenschaft 65, 40–44. doi: 10.1023/A:1008744114648
Murata, N., Takahashi, S., Nishiyama, Y., Allakhverdiev, S. I. (2007). Photoinhibition of photosystem II under environmental stress. Biochim. Biophys. Acta (BBA) - Bioenergetics 1767 (6), 414–421. doi: 10.1016/j.bbabio.2006.11.019
Nakano, Y., Higuchi, Y., Sumitomo, K., Hisamatsu, T. (2013). Flowering retardation by high temperature in chrysanthemums: involvement of FLOWERING LOCUS T-like 3 gene repression. J. Exp. Bot. 64 (4), 909–920. doi: 10.1093/jxb/ers370
Ning, X., Su, J., Zhang, X., Wang, H., Guan, Z., Fang, W., et al. (2023). Evaluation of volatile compounds in tea chrysanthemum cultivars and elite hybrids. Scientia Hortic. 320, 112218. doi: 10.1016/j.scienta.2023.112218
Peet, M., Sato, S., Clément, C., Pressman, E. (2003). Heat stress increases sensitivity of pollen, fruit and seed production in tomatoes (Lycopersicon Esculentum Mill.) to non-optimal vapor pressure deficits. Acta Hortic. 618, 209–215. doi: 10.17660/ActaHortic.2003.618.23
Rodrigues, C. R. F., Silveira, J. A. G., Viégas, R. A., Moura, R. M., Aragão, R. M., Silva, E. N. (2016). Combined effects of high relative humidity and K+ supply mitigates damage caused by salt stress on growth, photosynthesis and ion homeostasis in J. curcas plants. Agric. Water Manage. 163, 255–262. doi: 10.1016/j.agwat.2015.09.027
Shamshiri, R., Jones, J., Thorp, K., Ahmad, D., Che Man, H., Taheri, S. (2018). Review of optimum temperature, humidity, and vapour pressure deficit for microclimate evaluation and control in greenhouse cultivation of tomato: A review. Int. Agrophysics 32, 287–302. doi: 10.1515/intag-2017-0005
Shin, Y., Liu, R. H., Nock, J. F., Holliday, D., Watkins, C. B. (2007). Temperature and relative humidity effects on quality, total ascorbic acid, phenolics and flavonoid concentrations, and antioxidant activity of strawberry. Postharvest Biol. Technol. 45 (3), 349–357. doi: 10.1016/j.postharvbio.2007.03.007
Sinclair, T., Fiscus, E., Wherley, B., Durham, M., Rufty, T. (2007). Atmospheric vapor pressure deficit is critical in predicting growth response of "cool-season" grass Festuca arundinacea to temperature change. Planta 227 (1), 273–276. doi: 10.1007/s00425-007-0645-5
Strasser, R. J., Tsimilli-Michael, M., Qiang, S., Goltsev, V. (2010). Simultaneous in vivo recording of prompt and delayed fluorescence and 820-nm reflection changes during drying and after rehydration of the resurrection plant Haberlea rhodopensis. Biochim. Biophys. Acta 1797 (6-7), 1313–1326. doi: 10.1016/j.bbabio.2010.03.008
Su, J., Jiang, J., Zhang, F., Liu, Y., Ding, L., Chen, S., et al. (2019). Current achievements and future prospects in the genetic breeding of chrysanthemum: a review. Hortic. Res. 6 (1), 109. doi: 10.1038/s41438-019-0193-8
Su, P., Liu, X. (2005). Photosynthetic characteristics of linze jujube in conditions of high temperature and irradiation. Scientia Hortic. 104 (3), 339–350. doi: 10.1016/j.scienta.2004.08.012
Sun, X., Zheng, C., Wang, X. (2008). Effects of high temperature stress on photosynthesis and chlorophyll fluorescence of cut flower chrysanthemum (Dendranthema grandiflora 'Jinba'). Chin. J. Appl. Ecol. 19 (10), 2149–2154.
Suzuki, M., Umeda, H., Matsuo, S., Kawasaki, Y., Ahn, D., Hamamoto, H., et al. (2015). Effects of relative humidity and nutrient supply on growth and nutrient uptake in greenhouse tomato production. Scientia Hortic. 187, 44–49. doi: 10.1016/j.scienta.2015.02.035
Wakjera, E., Körner, O., Rosenqvist, E., Ottosen, C.-O. (2013). High temperature stress monitoring and detection using chlorophyll a fluorescence and infrared thermography in chrysanthemum (Dendranthema grandiflora). Plant Physiol. Biochem. PPB / Societe francaise physiologie vegetale 67C, 87–94. doi: 10.1016/j.plaphy.2013.02.025
Weng, J., Li, P., Rehman, A., Wang, L., Gao, X., Niu, Q. (2021). Physiological response and evaluation of melon (Cucumis melo L.) germplasm resources under high temperature and humidity stress at seedling stage. Scientia Hortic. 288, 110317. doi: 10.1016/j.scienta.2021.110317
Wu, H., Shou, S., Zhu, Z., Yang, X. (2001). Effects of high temperature stress on photosynthesis and chlorophyll fluorescence in sweet pepper. Acta Hortic. Sin. 28 (6), 517–521. doi: 10.3321/j.issn:0513-353X.2001.06.006
Xu, D. (1997). Some problems in stomatal limitation analysis of photosynthesis. Plant Physiol. J. 33 (4), 241–244. doi: 10.13592/j.cnki.ppj.1997.04.001
Xu, M., Wang, Q., Yang, F., Zhang, T., Zhu, X., Cheng, C., et al. (2022). The responses of photosynthetic light response parameters to temperature among different seasons in a coniferous plantation of subtropical China. Ecol. Indic. 145, 109595. doi: 10.1016/j.ecolind.2022.109595
Xu, C., Yang, Z. Q., Yang, S. Q., Wang, L., Wang, M. T. (2020). High humidity alleviates photosynthetic inhibition and oxidative damage of tomato seedlings under heat stress. Photosynthetica 58, 146–155. doi: 10.32615/ps.2019.168
Yang, X., Han, Y., Hao, J., Qin, X., Liu, C., Fan, S. (2022). Exogenous spermidine enhances the photosynthesis and ultrastructure of lettuce seedlings under high-temperature stress. Scientia Hortic. 291, 110570. doi: 10.1016/j.scienta.2021.110570
Yang, Z. Q., Xu, C., Wang, M. T., Zhao, H. L., Zheng, Y. J., Huang, H. J., et al. (2019). Enhancing the thermotolerance of tomato seedlings by heat shock treatment. Photosynthetica 57, 1184–1192. doi: 10.32615/ps.2019.127
Ye, Z. (2010). A review on modeling of responses of photosynthesis to light and CO2. Chin. J. Plant Ecol. 34, 6, 727–740. doi: 10.3773/j.issn.1005-264x.2010.06.012
Yoo, Y., Roh, Y. (2014). Occurrence of white rust and growth of chrysanthemum 'Baekma' under various relative humidity and temperature conditions in the greenhouse. Korean J. Hortic. Sci. Technol. 32, 803–811. doi: 10.7235/hort.2014.14138
Yuan, L., Yuan, Y., Liu, S., Wang, J., Zhu, S., Chen, G.-H., et al. (2017). Influence of high temperature on photosynthesis, antioxidative capacity of chloroplast, and carbon assimilation among heat-tolerant and heat-susceptible genotypes of nonheading chinese cabbage. HortScience 52, 1464–1470. doi: 10.21273/HORTSCI12259-17
Yusuf, M., Kumar, D., Rajwanshi, R., Strasser, R., Tsimilli-Michael, M., Govindjee, G., et al. (2010). Overexpression of γ-tocopherol methyl transferase gene in transgenic Brassica juncea plants alleviates abiotic stress: Physiological and chlorophyll a fluorescence measurements. Biochim. Biophys. Acta (BBA) - Bioenergetics 1797, 1428–1438. doi: 10.1016/j.bbabio.2010.02.002
Zhang, Q., Zhang, X. Y., Yang, Z. Q., Huang, Q. Q., Qiu, R. J. (2022). Characteristics of plastic greenhouse high-temperature and high-humidity events and their impacts on facility tomatoes growth. Front. Earth Sci. 10. doi: 10.3389/feart.2022.848924
Zheng, Y., Yang, Z., Luo, J., Zhang, Y., Jiang, N., Khattak, W. A. (2023). Transcriptome analysis of sugar and acid metabolism in young tomato fruits under high temperature and nitrogen fertilizer influence. Front. Plant Sci. 14. doi: 10.3389/fpls.2023.1197553
Zheng, Y., Yang, Z., Xu, C., Wang, L., Huang, H., Yang, S. (2020). The interactive effects of daytime high temperature and humidity on growth and endogenous hormone concentration of tomato seedlings. Hortscience 55 (10), 1575–1583. doi: 10.21273/hortsci15145-20
Zhou, H., Guo, S., An, Y., Shan, X., Wang, Y., Shu, S., et al. (2016). Exogenous spermidine delays chlorophyll metabolism in cucumber leaves (Cucumis sativus L.) under high temperature stress. Acta Physiologiae Planta. 38, 224. doi: 10.1007/s11738-016-2243-2
Zubaidi, A., Anugrahwati, D., McDonald, G., Gill, G. (2021). “Yield and photosynthetic rate of wheat under continuously high temperature,” in IOP Conference Series: Earth and Environmental Science, Vol. 648. (UK: IOPScience), 012126. doi: 10.1088/1755-1315/648/1/012126
Keywords: heat stress, humidity stress, chrysanthemum, photosynthesis, stomatal limitation, chlorophyll fluorescence
Citation: Zhou J, Jiang X, Agathokleous E, Lu X, Yang Z and Li R (2023) High temperature inhibits photosynthesis of chrysanthemum (Chrysanthemum morifolium Ramat.) seedlings more than relative humidity. Front. Plant Sci. 14:1272013. doi: 10.3389/fpls.2023.1272013
Received: 03 August 2023; Accepted: 15 November 2023;
Published: 05 December 2023.
Edited by:
Rong Zhou, Nanjing Agricultural University, ChinaReviewed by:
José Manuel Moutinho-Pereira, University of Trás-os-Montes and Alto Douro, PortugalSigita Jurkonienė, Nature Research Centre, Lithuania
Martijn Slot, Smithsonian Tropical Research Institute, Panama
Copyright © 2023 Zhou, Jiang, Agathokleous, Lu, Yang and Li. This is an open-access article distributed under the terms of the Creative Commons Attribution License (CC BY). The use, distribution or reproduction in other forums is permitted, provided the original author(s) and the copyright owner(s) are credited and that the original publication in this journal is cited, in accordance with accepted academic practice. No use, distribution or reproduction is permitted which does not comply with these terms.
*Correspondence: Xiaodong Jiang, jiangxd@nuist.edu.cn
†These authors share first authorship