- 1Shandong Institute of Pomology, Taian, China
- 2College of Horticulture, Shenyang Agricultural University, Shenyang, China
Photosynthetic products in most fleshy fruits are unloaded via the apoplasmic pathway. Sugar transporters play an important role in the apoplasmic unloading pathway and are involved in sugar transport for fruit development. The MdSWEET23, cloned from ‘‘Hanfu’’ apple (Malus × domestica Borkh.) fruits, belongs to Clade III of the SWEET family. Subcellular localization revealed that MdSWEET23 is localized on the plasma membrane. β-glucuronidase activity assays showed that MdSWEET23 was primarily expressed in the sepal and carpel vascular bundle of apple fruits. Heterologous expression assays in yeast showed that MdSWEET23 functions in sucrose transport. The overexpression of MdSWEET23 in the ‘‘Orin” calli increased the soluble sugar content. The silencing of MdSWEET23 significantly reduced the contents of sucrose and sorbitol in apple fruits. Ectopic overexpression of MdSWEET23 in tomato altered sugar metabolism and distribution in leaves and fruits, causing a reduction in photosynthetic rates and plant height, enhanced cold stress tolerance, and increased the content of sucrose, fructose, and glucose in breaking color fruits, but did not increase sugar sink potency of tomato fruits.
Introduction
In higher plants, the synthesis, transport, and distribution of photosynthetic products are important physiological processes. For economically valuable fruits, phloem unloading and post-phloem transport play important roles in fruit quality improvement and yield formation (Patrick, 1997; Zhang et al., 2004; Ma et al., 2019). Zhang et al. (2018) pointed out that the transport of carbohydrates is more important than their synthesis during sugar accumulation in fruits. Therefore, enhancing the unloading capacity of sink organs is more beneficial for improving crop yield and quality than increasing the photosynthetic efficiency of source organs. For fruits that accumulate high levels of sugar, especially in later fruit development, the apoplasmic pathway facilitates continuous sugar accumulation and prevents sugar reflux from fruits (Zhang et al., 2004). For example, in apple (Zhang et al., 2004) and pear (Zhang et al., 2014), phloem unloading is the apoplasmic pathway throughout fruit development. Although phloem unloading is the symplasmic pathway in early fruit development in tomato (Ruan and Patrick, 1995) and grapes (Zhang et al., 2006), the apoplasmic unloading pathway is utilized in late fruit development. In Jujube fruits, a transient symplasmic unloading pathway exists in the middle of development, and the pathway in the early and late developmental stages is the apoplasmic pathway (Nie et al., 2010).
Sugar transporters have vital roles in the transmembrane transport of sugars. There are three types of sugar transporters in plants: monosaccharide transporters (MSTs), sucrose transporters (SUTs), and Sugars Will Eventually be Exported Transporters (SWEETs). Unlike the MSTs and SUTs, SWEET proteins are considered to be bidirectional uniporters by which sugar can be transported across the cell membrane along a concentration gradient without depending on pH and adenosine triphosphate (Chen, 2014; Chen H. Y. et al., 2015). SWEETs are involved in numerous plant biological processes, including growth, nectar secretion, seed nutrient filling, fruit development, and response to biotic and abiotic stresses (Chen, 2014; Yang et al., 2018; Wang S. et al., 2019; Zhang W. et al., 2019; Breia et al., 2021; Li et al., 2022). SWEETs mediate the transport of sugar from SE/CC complexes to sink tissues in apoplasmic phloem unloading. In Arabidopsis, AtSWEET9 has a distinct role in nectar secretion (Breia et al., 2021); AtSWEET11, AtSWEET12, and AtSWEET15 participate in seed development by mediating sucrose transport from the seed coat to the embryo (Breia et al., 2021). In tomato, glucose transporter SlSWEET1a is highly expressed in the veins of young leaves (sink) and plays a key role in glucose transport from the apoplast to the parenchyma cells (Ho et al., 2019). In cucumber, a hexose transporter CsSWEET7a that was localized to the phloem region of the flowers participates in the apoplasmic phloem unloading during flower anthesis. CsSWEET7a was also involved in sugar phloem unloading in cucumber fruit (Li et al., 2022). Hu et al. (2022) reported that CsSWEET2 plays an important role in improving plant cold tolerance. In tea plant, the expression levels of CsSWEET16, CsSWEET1a, and CsSWEET17 are altered under cold stress (Wang et al., 2018; Yao et al., 2020). In rice, OsSWEET13 and OsSWEET15 are involved in the response to drought and salt stress by interacting with the ABA-responsive transcription factor OsbZIP72 (Mathan et al., 2021).
In our previous study, 27 SWEETs were identified from the apple genome, and MdSWEET1, 6, 8, 9, 10, 18, 20, 23, 26, and 27 may play roles at different fruit developmental stages (Nie et al., 2022). Zhen et al. (2018) reported that MdSWEET9b and MdSWEET15a may be involved in regulating sugar accumulation in apple fruits. MdSWEET9b, a sucrose transporter, influences fruit sugar accumulation by binding their promoters to MdWRKY9, which interacted with MdbZIP23/46 at the protein and DNA levels (Zhang et al., 2023). Our previous findings suggested that MdSWEET23 is involved in sugar unloading in apple fruit, as the expression of it was positively correlated with the fruit sucrose, glucose, fructose, and soluble sugar contents. (Nie et al., 2022). In this study, we characterized the MdSWEET23 gene in apple and found that ectopic overexpression of MdSWEET23 in tomato improved the cold resistance in the seedling stage and increased sugar content in breaking color (BC) fruits.
Materials and methods
Plant materials and growth conditions
The apple fruit samples utilized in this study were collected from 10-year-old ‘‘Hanfu’’ apple trees (M. domestica) cultivated at the Shenyang Agricultural University experimental station (123.88°E, 41.88°N). The ‘Orin’ calli in this study were grown on MS medium with 0.8 mg·L−1 6-BA and 1.5 mg·L−1 2,4-D, in darkness at 25°C. Nicotiana benthamiana plants used for subcellular localization were cultivated with a 16 h/8 h (light/dark) photoperiod at 25 ± 2°C and a relative humidity of 50%–70%. In this study, tomato (Solanum lycopersicum L. ‘Micro-Tom’) seeds were used for genetic transformation. Both wild-type (WT) plants and transgenic tomato lines were grown in an artificial climate chamber under a 16 h/8 h (day/night) photoperiod with a temperature of 25 ± 2°C/18 ± 2°C (day/night) and a relative humidity of 50%–70%. All collected samples were immediately frozen in liquid nitrogen and stored at -80°C until use.
RNA extraction, cDNA synthesis, and quantitative real-time PCR
RNA extraction, cDNA synthesis, and qRT-PCR were performed using our previously described method (Nie et al., 2022). All primers used are presented in Table S1. Gene expression levels were analyzed using the 2-ΔΔCT method (Livak and Schmittgen, 2001). The experiment was repeated three times.
Cloning of MdSWEET23, sequence alignment, and phylogenetic analysis
The sequence of MdSWEET23 was acquired from the Genome Database for Rosaceae. The cDNA of MdSWEET23 was amplified from ‘‘Hanfu’’ apple fruits. The pRI101-MdSWEET23-GFP recombinant plasmid was constructed for subcellular localization and genetic transformation. The sequence of MdSWEET23 was submitted to the online websites MOTIF search (https://www.genome.jp/tools/motif/), DeepTMHMM (https://dtu.biolib.com/DeepTMHMM), and ProtParam tool (http://web.expasy.org/protaram/) for analysis of conserved motifs, transmembrane domains, molecular weight, theoretical isoelectric point (PI), and grand average of hydropathicity. Separate multiple sequence alignments were performed for MdSWEET23 and 17 Arabidopsis SWEET sequences (AtSWEET1-17) using ClustalW in MEGA X software. Subsequently, a phylogenetic tree was constructed using the Neighbor-joining method (Kumar et al., 2018).
Subcellular localization of MdSWEET23
The pRI101-MdSWEET23-GFP fusion protein was transiently expressed in Nicotiana benthamiana and onion epidermal cells as previously described (Zhang et al., 2021). Empty vectors expressing non-targeted GFP were used as controls. Fluorescent signals were observed and photographed using a fluorescent microscope system (NikonNi-E, Nikon, Japan).
Promoter cloning, sequence analysis, and GUS histochemical staining
An 1863 bp sequence upstream of the start codon (ATG) of the MdSWEET23 gene was amplified from the apple genome DNA. The primers used for this extraction are listed in Table S1. The sequences of the cloned promoter were submitted to the PlantCARE website for online analysis of cis-acting elements. The PCR product was cloned into the pBI121 vector upstream of the β-glucuronidase (GUS) gene to construct the pBI121-pMdSWEET23-GUS recombinant plasmid. The cDNA of MdSWEET23 was cloned into the plasmid pBI121-pMdSWEET23-GUS downstream pMdSWEET23 to construct the pBI121-pMdSWEET23-MdSWEET23-GUS plasmid. The recombinant plasmids were transformed into ‘‘Hanfu’’ apple fruits using the Agrobacterium tumefaciens LBA4404 and the method described by Spolaore et al. (2001). After 3 days of storage in the dark, the injected fruits were sampled for GUS histochemical staining. Subsequently, the tissues expressing GUS were examined and photographed.
Yeast complementation assay
Yeast functional complementation assays were conducted following the methods described in a previous study (Loqué et al., 2007). Briefly, the recombinant plasmid pDR196-MdSWEET23 was constructed, with the primers used in the construction process presented in Table S1. Subsequently, the recombinant plasmid pDR196-MdSWEET23 or the empty pDR196 vector (as a control) was individually introduced into the yeast mutant strains, SUSY7/ura and EBY.VW4000. SUSY7/ura and EBY.VW4000 yeast cells carrying pDR196-MdSWEET23 were inoculated on a solid SD/Ura medium supplemented with different carbon sources. Transformants were incubated at 30°C for 2–4 days and then observed and imaged.
VIGS test
A 210 bp fragment of MdSWEET23 cDNA fragment corresponding to bases 675 bp–885 bp of MdSWEET23 was PCR amplified from ‘‘Hanfu’’ apple fruit cDNA. The PCR products obtained were successfully ligated into the pTRV2 vector. Agrobacterium strain GV3101 was separately transformed with pTRV1, pTRV2, and pTRV2-MdSWEET23. Subsequently, TRV1- and TRV2-MdSWEET23 Agrobacterium strains were co-inoculated into 140 days after bloom (DAB) ‘‘Hanfu’’ apple fruit by infiltrated using a needleless 1 mL syringe at the maximum diameter of apple fruit, with each fruit being injected three times at evenly distributed locations. The fruits injected with pTRV1 and pTRV2 Agrobacterium strains were used as controls. For each treatment, 10 fruits were injected and bagged. After 5 days, RNA was extracted from the samples for qRT-PCR analysis to detect the MdSWEET23 silencing efficiency. Three fruits with high gene silencing efficiency were selected to determine sorbitol, fructose, glucose, and sucrose contents and MdSDH5, MdSDH6, and MdSOT1 expression levels. The experiment was repeated three times.
‘‘Orin’’ calli transformation
The pRI101-MdSWEET23-GFP was transformed into ‘‘Orin’’ calli using the Agrobacterium strain LBA4404, and then the transformed calli was cultured on MS medium containing 50 mg·L-1 kanamycin for transgenic selection. The transgenic lines were confirmed using genomic PCR analysis with specific primers shown in Table S1. Transcript levels of MdSWEET23 in these lines were examined using qRT-PCR. Samples were collected to determine the sorbitol, fructose, glucose, and sucrose contents. The experiment was repeated three times.
Ectopic overexpression of MdSWEET23 in tomato
Micro-tomato transformation was conducted using the methods described by Sun et al. (2006). Two transgenic lines of the T3 generation were selected and used for the phenotypic observation. Plant height was determined 60 days after seeding. Net photosynthetic rate (Pn) was determined using the portable photosynthesis system (CIRAS-2, PP systems, USA) at photosynthetically active radiation of 800 μmol·m-2·s-1. The leaf chlorophyll concentration was measured with a SPAD-502 meter (Konica Minolta Sensing, Japan) and expressed as SPAD values. Fruits at the mature green (MG), BC, and red ripe (RR) stages (Figure 1A) were used for analysis of sugar contents, analysis of sugar-metabolizing enzyme activities, and the expression levels of sugar transporter genes. The experiment was repeated three times.
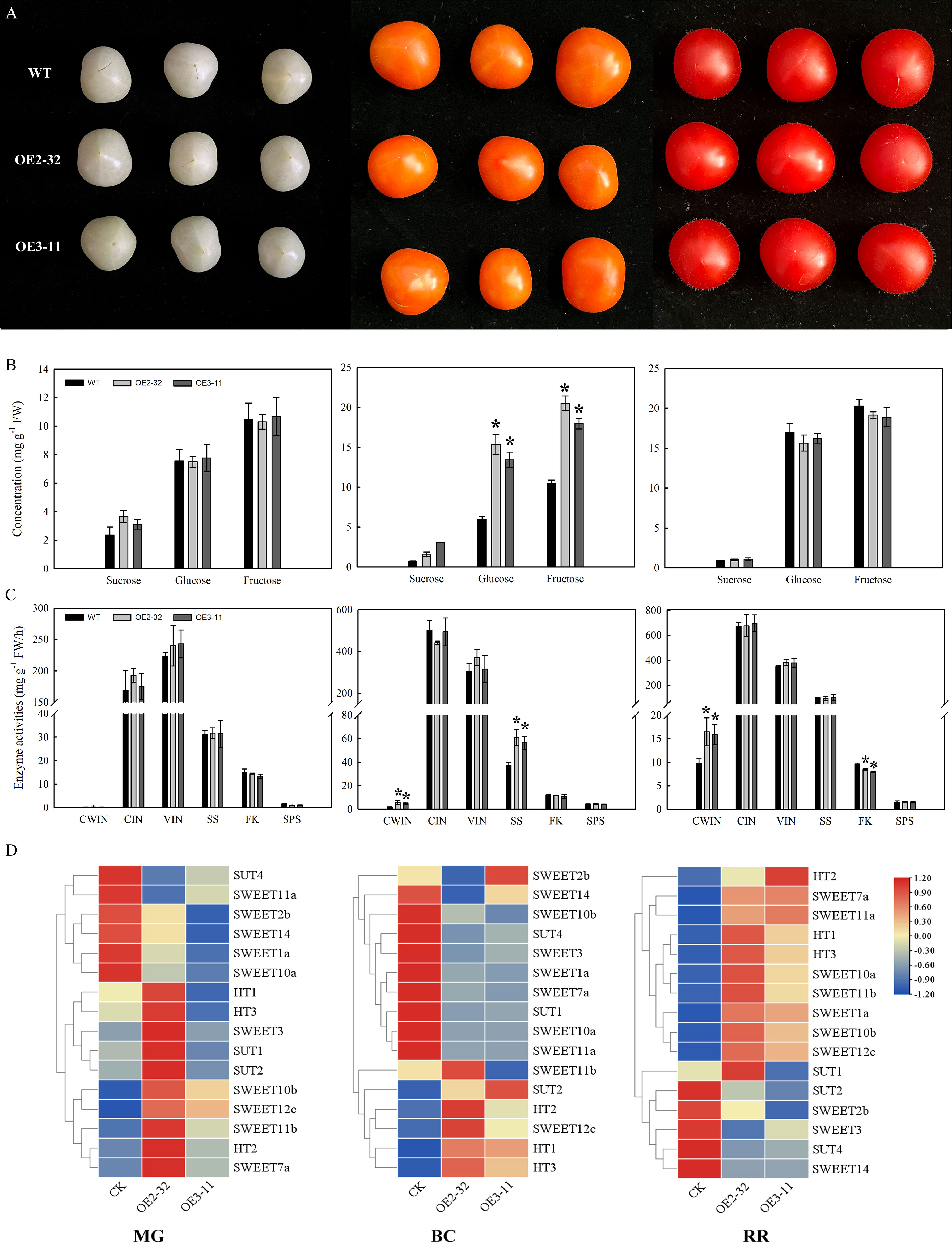
Figure 1 Sugar concentration, enzyme activities, and expression levels of sugar transporters in fruits of MdSWEET23-OE lines and WT. (A) The phenotype of MG, BC, and RR fruits of MdSWEET23-OE lines and WT. (B) Contents of sucrose, glucose, and fructose in MG, BC, and RR fruits of MdSWEET23-OE lines and WT. (C) Enzyme activities in MG, BC, and RR fruits of MdSWEET23-OE lines and WT. (D) Heat map illustrating the expression levels of sugar transporters in MG, BC, and RR fruits of MdSWEET23-OE lines and WT. The significance compared to WT was determined using Student’s t-test at *P<0.05.
Cold treatments
Seedlings of the WT and two MdSWEET23-overexpressing (OE) transgenic plants were grown under normal conditions for 60 days, followed by cold treatment (3.5°C) for 3.5–4 h. Leaves were collected to measure the levels of relative electrolyte leakage (REL), soluble sugar, and starch. The REL was determined by the method of Hu et al. (2022). The experiment was repeated three times.
Enzyme assays and determination of sugars and starch
The enzymatic activities of cell wall invertase (CWIN), sucrose synthase (SS), cytosolic invertase (CIN), fructokinase (FK), vacuolar invertase (VI), and sucrose phosphate synthase (SPS) were measured following the kit instructions of Suzhou Grace Biotechnology Co. The soluble sugar and starch content were determined by the methods of Zhang et al. (2021). The sorbitol, fructose, glucose, and sucrose contents were determined using high-performance liquid chromatography (HPLC) (1260 series, Agilent Technologies), a method referenced by Li et al. (2020).
Statistical analysis
All experiments were conducted with three independent biological replicates. Error bars represent the standard deviation (SD) of the three replicates, and values are presented as the mean ± SD. All statistical analyses were performed using IBM SPSS Statistics 23. Additionally, a t-test for independent samples was used for significant differences between the two groups of data, and significant differences were expressed as * P<0.05. One-way analysis of variance was performed on the data using Duncan`s multiple range test, and the results are indicated by letters. Histograms were plotted using Sigma Plot 10.0.
Results
MdSWEET23 encodes a Clade III SWEET protein
The MdSWEET23 cDNA was cloned from the total RNA of “Hanfu” apple fruits, and it consists of an 897 bp open reading frame that encodes a polypeptide of 298 amino acids (Table S2). The polypeptide has a molecular weight, an isoelectric point, and a grand average hydropathicity of 33.48 kDa, 8.82, and 0.497, respectively (Table S3). MOTIF search and TMHMM analysis showed that MdSWEET23 contained seven α-helical transmembrane domains with two conserved MtN3/saliva motifs (Figures 2A, B), which are unique to the typical plant SWEET proteins. Phylogenetic analysis revealed that MdSWEET23 has the highest similarity to AtSWEET13 with a similarity of approximately 50.50% (Table S4), and MdSWEET23 is classified as a member of the Clade III in the SWEET family (Figure 2C). Han et al. (2017) reported the 2.8-Å resolution crystal structure of AtSWEET13 in the inward-facing conformation with a substrate analog, 2′-deoxycytidine 5′-monophosphate, bound in the central cavity. Four residues (Val23TM1, Ser54TM2, Val145TM5, and Ser176TM6) inside the binding pocket in AtSWEET13 lead to a larger cavity than monosaccharide transporters, which have Leu, Asn, Met, and Asn in the corresponding sites, and these residues are most likely involved in substrate selectivity. MdSWEET23 has the same residues as AtSWEET13 in the corresponding sites by amino acid sequence alignment (Figure 2D). Thus, it is assumed that the transport activity of MdSWEET23 toward sucrose may be higher than that toward glucose.
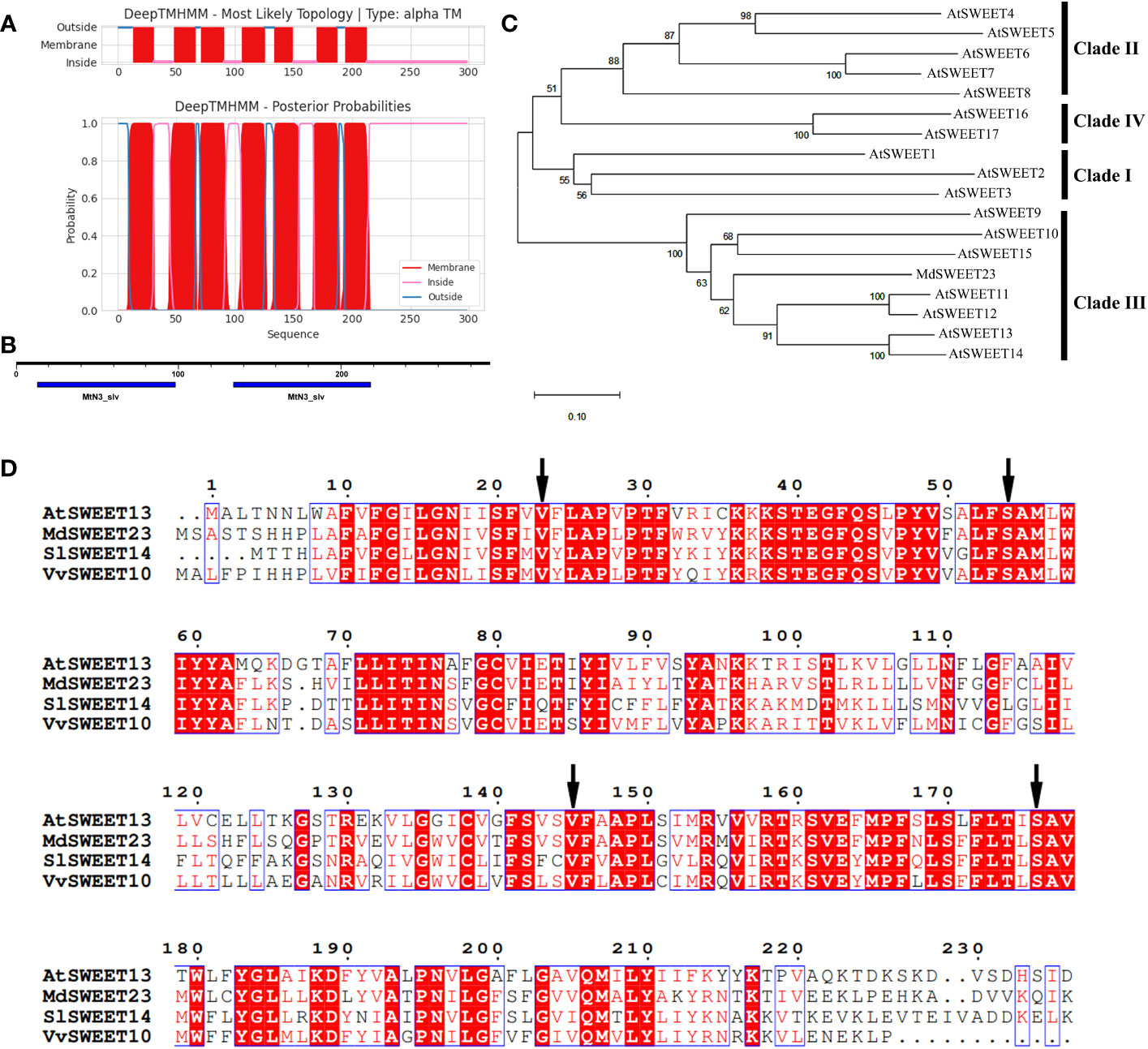
Figure 2 Sequence analysis of MdSWEET23. (A) TMs of the MdSWEET23 protein. (B) Conserved motifs of MdSWEET23. (C) Phylogenetic analysis of SWEET proteins between MdSWEET23 and AtSWEETs (AtSWEET1-17). (D) Multiple sequences alignment of SWEET proteins from Malus domestica (MdSWEET23), Arabidopsis thaliana (AtSWEET13), Solanum lycopersicum (SlSWEET14), and Vitis vinifera (VvSWEET10). The arrow indicates the position of Leu, Asn, Met, and Asn.
Identification of promoter and cis-regulatory elements of MdSWEET23
The promoter regions (1863 bp) of MdSWEET23 were cloned (Table S5), and its cis-acting elements were analyzed using the PlantCARE program. Hormone-, stress-, and light-responsive and organ-specific cis-acting elements were identified in the MdSWEET23 promoter. Thus, it is speculated that the expression of MdSWEET23 may be in response to light, hormones (such as MeJA, auxin, and ABA), and abiotic stresses (such as low-temperature and drought). Phloem tissue-specific cis-acting elements (as-1) were also identified in the MdSWEET23 promoter, which implied that it might be a phloem-specific promoter (Table 1).
Spatial expression of MdSWEET23
To confirm the spatial expression patterns of MdSWEET23, we fused its promoter sequence with the GUS gene to generate two constructs, pMdSWEET23-GUS and pMdSWEET23:MdSWEET23-GUS. The GUS activity in transgenic apple fruits was predominantly detected within the vascular bundle region, particularly in the sepal and carpel vascular bundles (Figure 3).
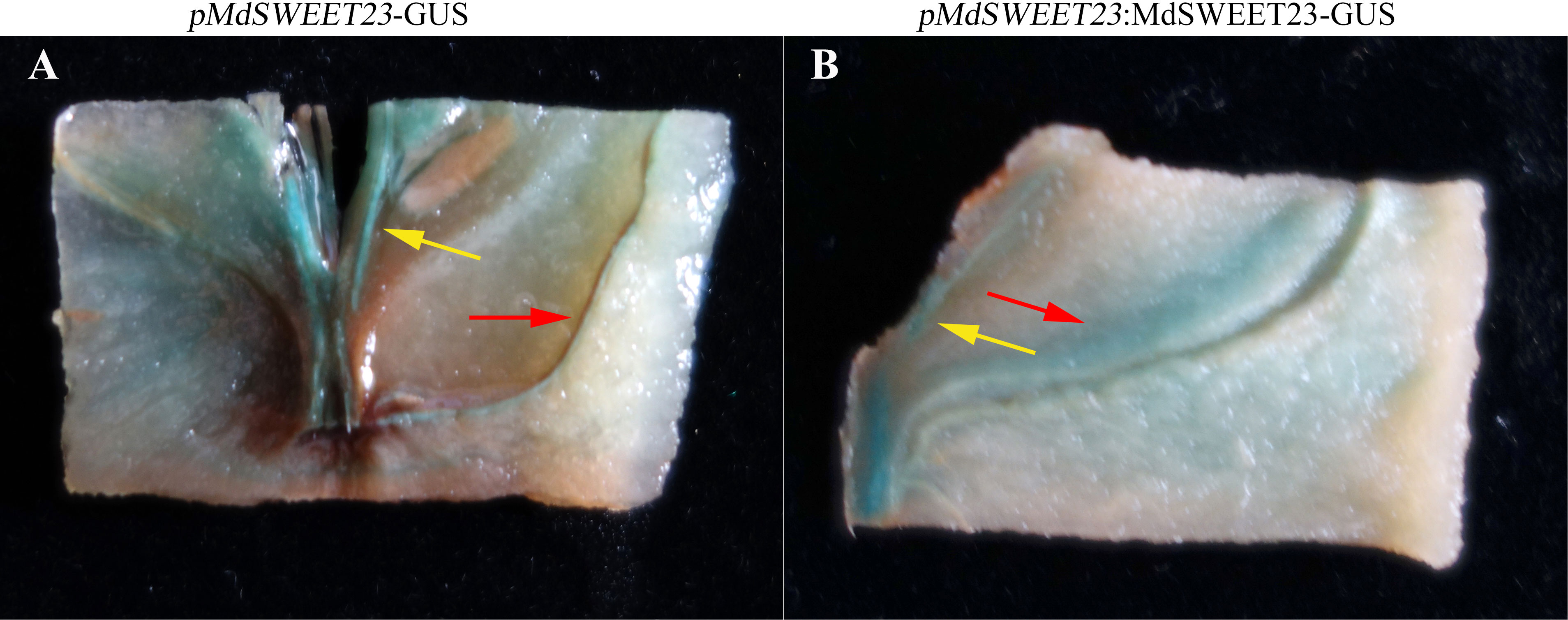
Figure 3 Spatial expression of MdSWEET23. (A) pMdSWEET23-GUS staining pattern in ‘‘Hanfu’’ apple fruit. (B) pMdSWEET23:MdSWEET23-GUS staining pattern in ‘‘Hanfu’’ apple fruit. The red arrows indicate the sepal vascular bundle and the yellow arrows indicate the carpel vascular bundle.
MdSWEET23 is localized on the plasma membrane
To determine the subcellular localization of MdSWEET23, a MdSWEET23-GFP fusion protein was transiently expressed in both Nicotiana benthamiana and onion epidermal cells. Fluorescence signals from the MdSWEET23-GFP fusion protein were only detected on the plasma membrane in Nicotiana benthamiana and onion epidermal cells (Figure 4B, D), indicating that MdSWEET23 is likely localized on the plasma membrane.
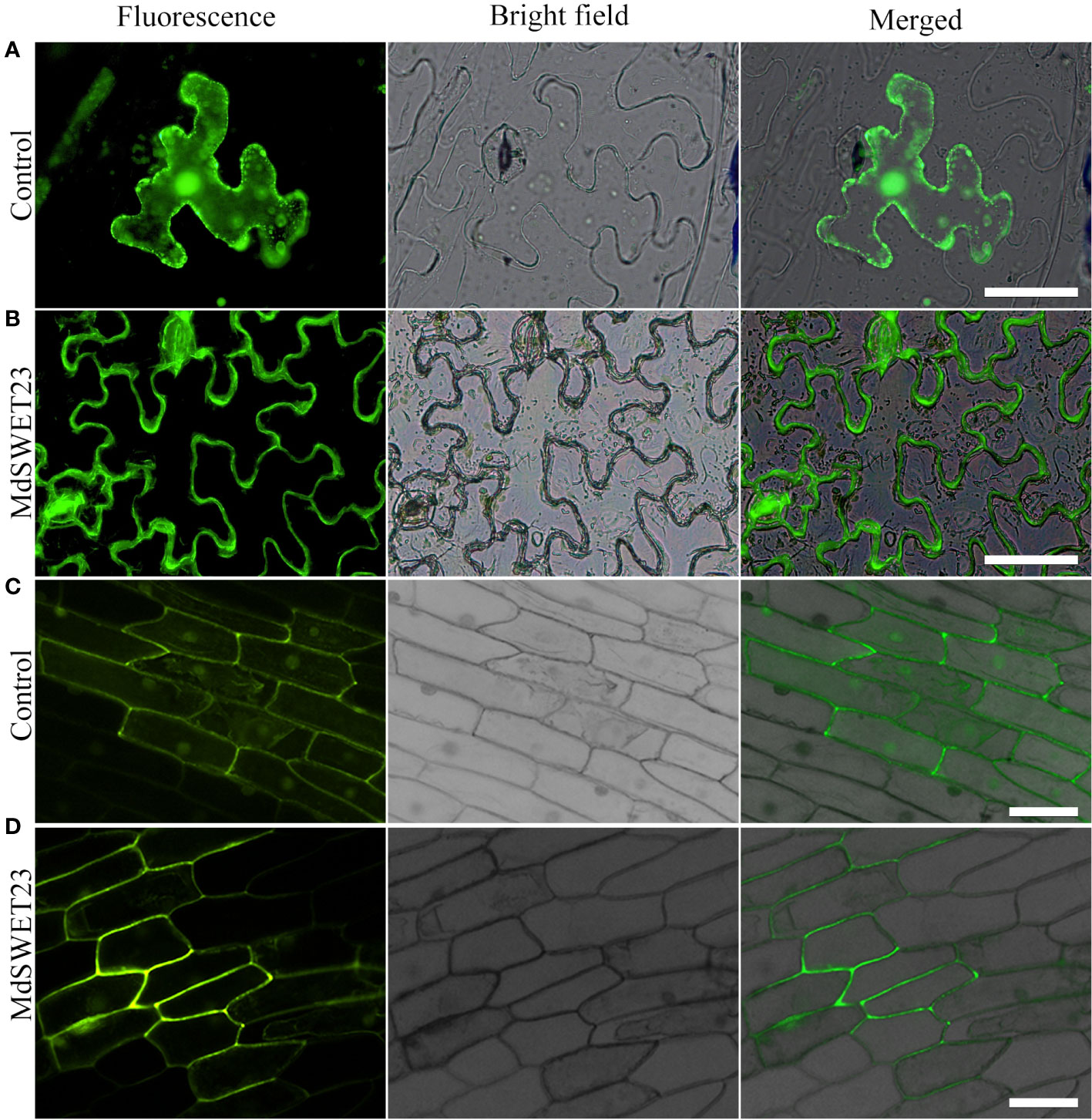
Figure 4 Subcellular localization of MdSWEET23. (A, B) MdSWEET23-GFP fusion protein was transiently expressed in tobacco leaves. (C, D) MdSWEET23-GFP fusion protein was transiently expressed in onion epidermal cells. Transient expression of GFP alone (35S: GFP) was used as a control. The scale bar in the figure is 100 μm.
MdSWEET23 transports sucrose in yeast
The functionality of MdSWEET23 in sugar transport was examined by transforming yeast strains, including EBY.VW4000 and SUSY7/ura3 with the pDR196-MdSWEET23 fusion vector. The yeast mutant strain, EBY.VW4000 displayed the inability to grow on monosaccharides but exhibited growth on maltose (Wieczorke et al., 1999). In contrast, the mutant strain SUSY7/ura3 could not use external sucrose as the sole carbon source (Riesmeier et al., 1992). A spotting assay showed that EBY.VW4000 cells transformed with pDR196-MdSWEET23 and the empty pDR196 vector (as negative control) exhibited comparable growth rates on the culture medium containing 2% (w/v) maltose, galactose, glucose, and fructose (Figure 5A). The SUSY7/ura3 cells transformed either with pDR196-MdSWEET23 or the empty vector pDR196 could grow well on 2% (w/v) glucose, whereas pDR196-MdSWEET23 transformants grew better than the negative control when given 2% (w/v) sucrose (Figure 5B). These results indicate that MdSWEET23 is involved in sucrose transport.
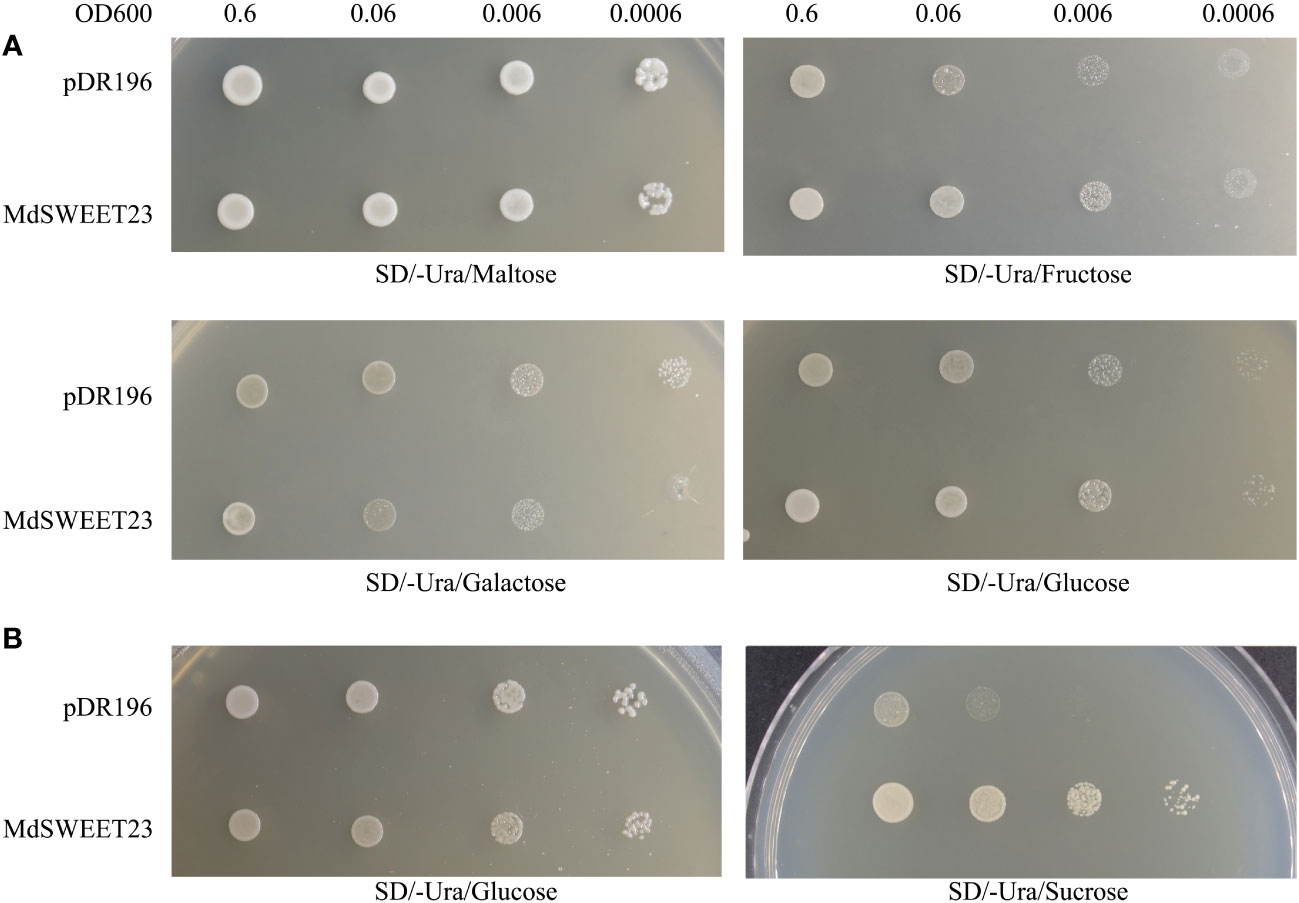
Figure 5 Heterologous expression of MdSWEET23 in yeast strain EBY.VW4000 and SUSY7/ura. (A) Yeast cells EBY.VW4000 with pDR196-MdSWEET23 or pDR196 vector (as a negative control) were grown on (SD)/-uracil (Ura) solid medium containing 2% (V/W)maltose, 2% (V/W)fructose, 2% (V/W)galactose, or 2% (V/W)glucose as sole carbon source. (B) Yeast cells SUSY7/ura with pDR196-MdSWEET23 or pDR196 vector (as a negative control) were grown on SD/-Ura solid medium containing 2% (V/W) glucose, or 2% (V/W) sucrose as sole carbon source.
Silencing MdSWEET23 influences the sugar accumulation in apple fruits
The expression of MdSWEET23 in VIGS-treated fruits was examined using qRT-PCR. The results showed that VIGS treatment resulted in a significant reduction in the transcript level of the MdSWEET23, which was only 25.28% of the control level (Figure 6A). Silencing of MdSWEET23 significantly reduced sucrose and sorbitol contents in fruits; however, it had no significant effect on the accumulation of fructose and glucose (Figure 6B). MdSWEET23 silencing resulted in a significant increase in the expression of MdSOT1, which was approximately 4 times higher than that of the control, while the expression of MdSDH6 was significantly decreased (Figure 6C). MdSOT1 functions in the unloading of sorbitol in apple fruits (Wei et al., 2014). While, MdSDH6 encodes a sorbitol dehydrogenase (SDH) that converts sorbitol to fructose (Wang et al., 2009). Therefore, the silencing of MdSWEET23 not only reduced the accumulation of sucrose in ‘‘Hanfu’’ apple fruits but also affected the transport and metabolism of sorbitol.
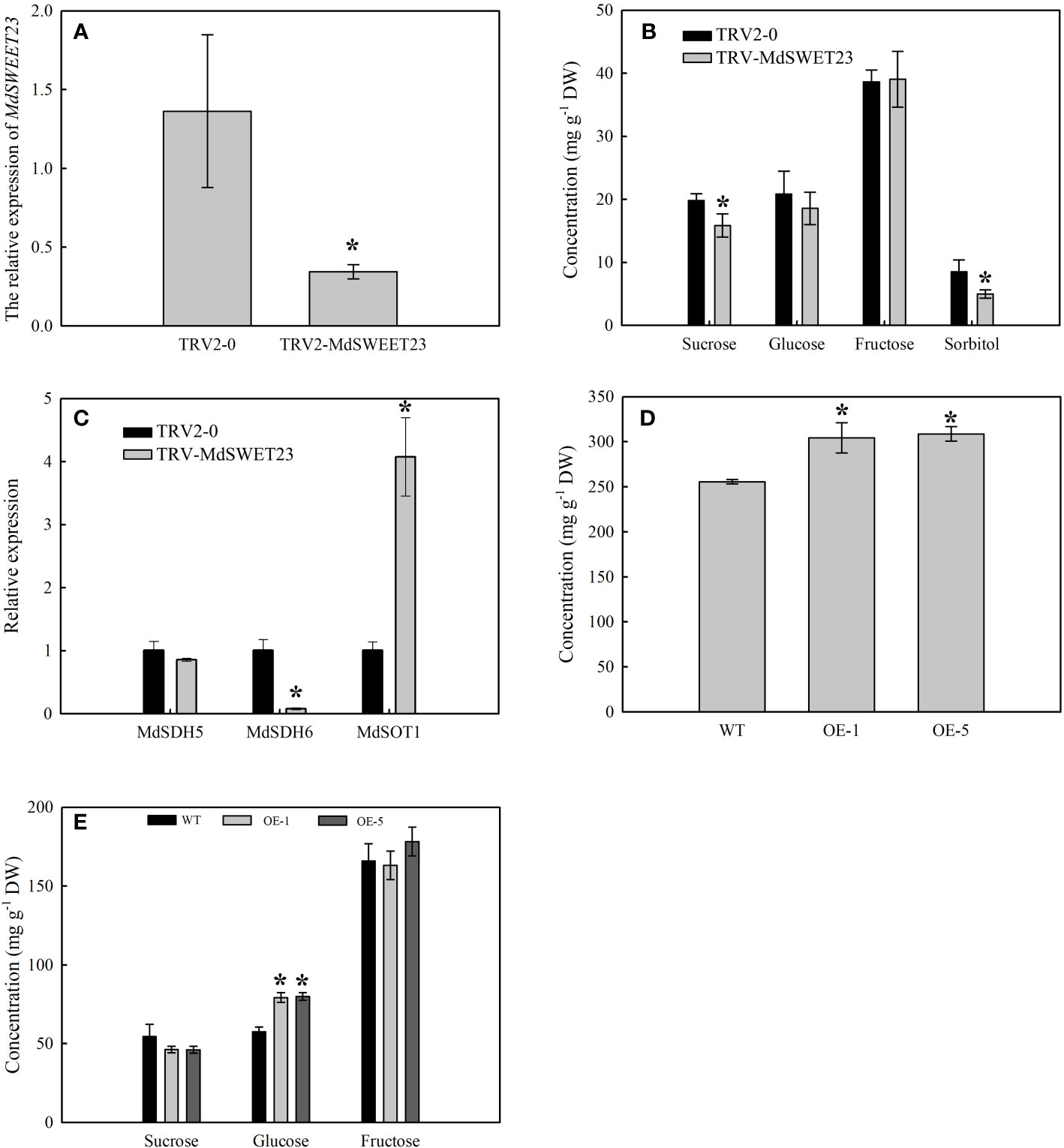
Figure 6 Functional analysis of MdSWEET23. (A–C) The expression levels of MdSWEET23 (A), MdSDH5, MdSDH6, and MdSOT1 (C), and the sugar content (B) in MdSWEET23 silencing apple fruits. (D, E) The content of total soluble sugar (D) and the contents of sucrose, glucose, and fructose (E) in MdSWEET23-OE ‘‘Orin’’ calli. The significance compared to WT was determined using Student's t-test at *P<0.05.
Overexpression of MdSWEET23 increased the soluble sugar content in the ‘‘Orin” calli
A pRI101-MdSWEET23 recombinant plasmid was constructed and transformed into ‘‘Orin” calli to verify the function of MdSWEET23. After the PCR identification, four overexpression lines were obtained, and OE-1 and OE-5 were selected for further experiments (Supplementary Figure 1A). The growth of ‘‘Orin” calli lines of OE-1 and OE-5 is shown in Supplementary Figure 1B. The contents of soluble sugars and glucose in both OE-1 and OE-5 lines were significantly higher than those in the WT plants (Figures 6D, E). Since only sucrose was added as a carbon source in the medium, the accumulation of glucose in the calli might be due to sucrose hydrolysis. The overexpression of MdSWEET23 in the “Orin” calli promoted the accumulation of soluble sugars.
MdSWEET23 overexpression reduced plant height and photosynthetic rates in tomato
To determine the impact of MdSWEET23 expression on sugar accumulation in plants, transgenic tomato lines overexpressing MdSWEET23 driven by the CaMV35S promoter were generated. After PCR identification, eight MdSWEET23-overexpression (MdSWEET23-OE) lines were obtained (Supplementary Figure 2), and OE2-32 and OE3-11 in T3 generation were used for further experiments. Compared with WT plants, the plant height in lines OE2-32 and OE3-11 decreased by 24.42% and 36.88%, respectively (Figures 7A, B), and Pn of mature leaves decreased by 45.43% and 53.84%, respectively (Figure 7E). The contents of sucrose, glucose, fructose, and starch in mature leaves of lines OE2-32 and OE3-11 were significantly higher than those in WT plants. Particularly, the starch concentration in these lines was 2.51 and 2.19 times higher than that in WT plants, respectively (Figure 7C).
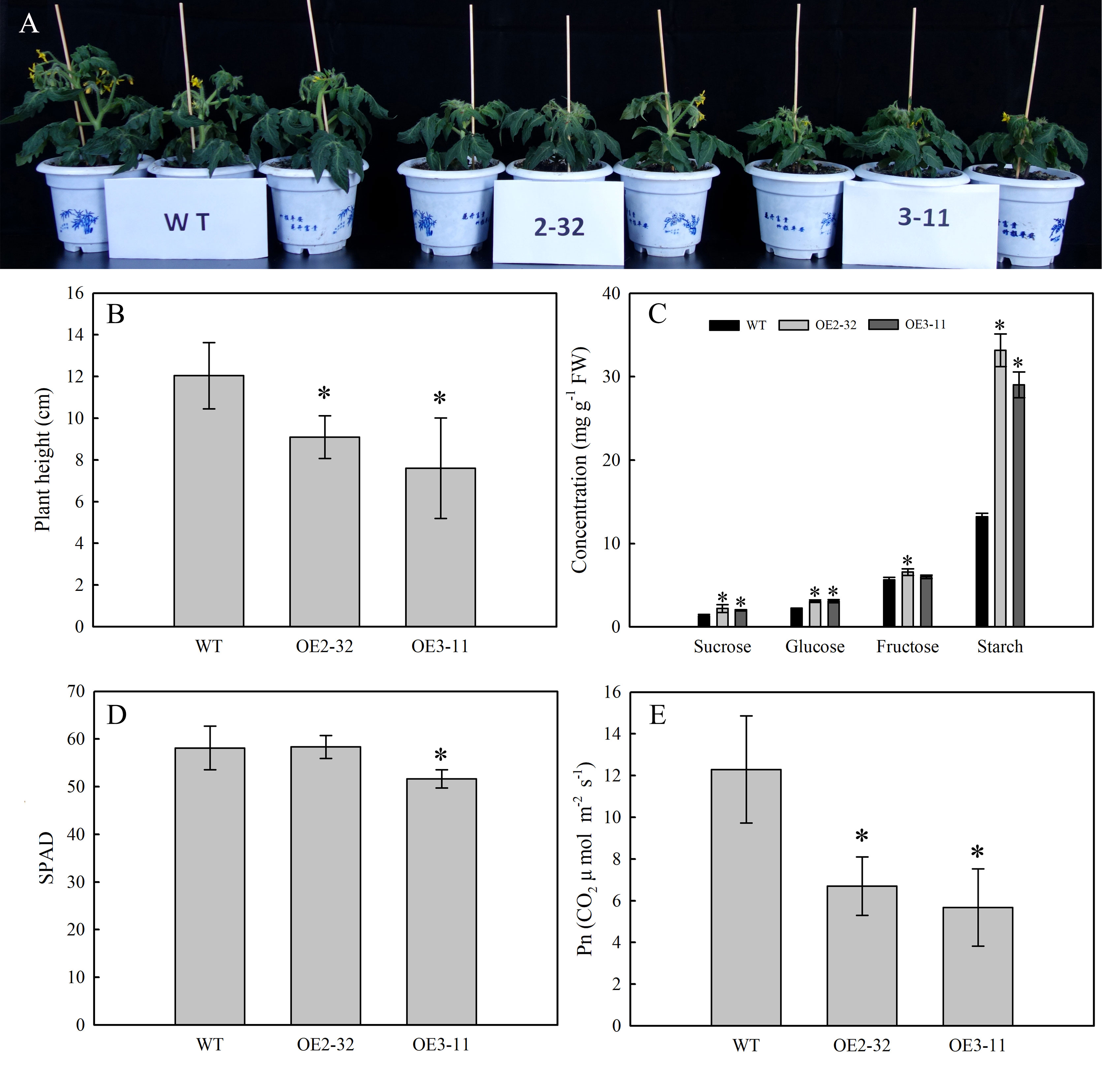
Figure 7 Phenotype and physiological characterization of MdSWEET23-OE tomato lines. (A) Phenotypic characteristics of MdSWEET23-OE lines and WT. (B) Plant height of MdSWEET23-OE lines and WT. (C) Contents of sugar and starch in leaves of MdSWEET23-OE lines and WT. (D) Leaf SPAD values of MdSWEET23-OE lines and WT. (E) Pn values of MdSWEET23-OE lines and WT. The significance compared to WT was determined using Student's t-test at *P<0.05.
MdSWEET23 overexpression in tomato results in sugar accumulation increased in BC fruits
We examined the sugar content in MG, BC, and RR fruits (Figure 1A) of MdSWEET23-OE lines and WT. The sucrose content in MG fruits of OE2-32 and OE3-11 was significantly higher than that of WT, but no significant differences were observed in glucose and fructose contents between them (Figure 1B). At the BC stage, MdSWEET23-OE lines exhibited significantly higher levels of sucrose, glucose, and fructose than the WT (Figure 1B). In contrast, the sucrose, glucose, and fructose contents of RR fruits showed no significant difference between MdSWEET23-OE lines and WT (Figure 1B).
To investigate the reasons for the sugar level alteration in MdSWEET23-OE lines, we further examined the activity of sugar metabolism-related enzymes and the expression levels of genes related to sugar transport. In MG fruits, no significant difference was found in enzyme activities between MdSWEET23-OE lines and WT (Figure 1C). In BC fruits, the CWIN and SS activities of MdSWEET23-OE lines were significantly higher than those of the WT, with 3.84 and 1.56 times increases, respectively. In RR fruits, the CWIN activity of MdSWEET23-OE lines was significantly higher than that of the WT, with a 1.66-fold increase, while the FK activity was significantly lower than that of the WT, at approximately 86% of the WT.
Compared with WT, the HT1 and HT2 expression levels in MdSWEET23-OE lines were notably higher in BC fruits, while no significant changes were observed in their expression in RR and MG fruits. In BC fruits of MdSWEET23-OE lines, the expression of SUT2 was upregulated, while the expression of SUT1 was downregulated; and the transcript levels of SUT4 were notably downregulated in MG, BC, and RR fruits of the MdSWEET23-OE lines. The overexpression of MdSWEET23 also affected the expression of several SWEETs (Figure 1D).
MdSWEET23 overexpression in tomato improves tolerance to cold stress at the seedling stage
Under cold stress (3.5°C for 4 h), no significant change was found in the leaves of OE2-32 and OE3-11 lines; however, the leaves of WT showed wilting symptoms (Figure 8A). The release of electrolytes can characterize the damage to plant cells (Hu et al., 2022). Before cold stress treatment, there was no significant difference in REL levels between the two transgenic lines and WT (Figure 8B). After cold treatment, the REL levels of OE2-32 and OE3-11 lines were lower than those of WT (Figure 8B). We also compared the changes in sugar and starch contents of leaves between MdSWEET23-OE lines and WT before and after cold treatment. The results showed that the soluble sugar content in leaves of MdSWEET23-OE lines was only slightly higher than that of WT under cold treatment (Figure 8C). The starch content in leaves of MdSWEET23-OE lines and WT all decreased when exposed to cold treatment; however, the starch content in leaves of OE2-32 and OE3-11 decreased from 58.27 to 28.61 mg·g-1 FW (by 50.89%) and from 53.81 to 29.16 mg·g-1 FW (by 45.81%), respectively, whereas that of WT only decreased from 20.27 to 13.23 mg·g-1 FW (by 34.73%) (Figure 8D).
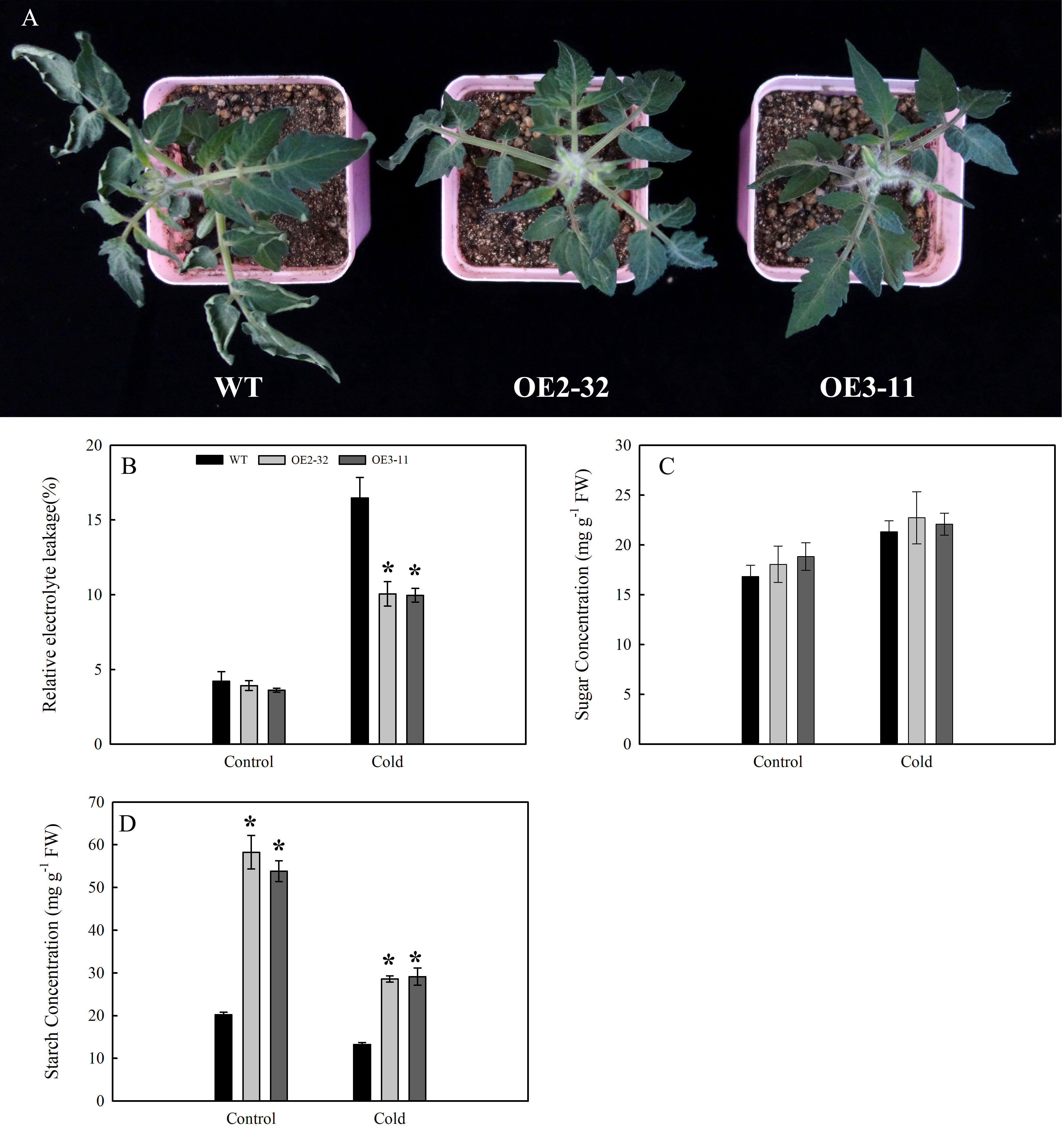
Figure 8 Changes in growth and physiological characteristics of MdSWEET23-OE lines and WT under cold treatment. (A) Phenotypic changes of MdSWEET23-OE lines and WT plants under cold treatment. (B) REL levels of MdSWEET23-OE lines and WT plants under cold treatment. (C) Content of total soluble sugars in leaves of MdSWEET23-OE lines and WT plants under cold treatment. (D) Content of starch in leaves of MdSWEET23-OE lines and WT plants under cold treatment. The significance compared to WT was determined using Student's t-test at *P<0.05.
Discussion
MdSWEET23 is localized on the plasma membrane in vascular bundle tissues and involved in sucrose transport in apple
Fruit growth is a high-priority sink in assimilate partitioning. An apoplasmic phloem unloading strategy is employed in the fleshy fruits of apples, which requires sugar transporters to export sugar into the apoplasm. Many SWEETs function in flesh fruits, such as SlSWEET7a and SlSWEET14 in tomato (Zhang et al., 2021), VvSWEET10 in grape (Zhang Z. et al., 2019), and MdSWEET9b in apple (Zhang et al., 2023). CsSWEET7a localized on the plasma membrane in companion cells (CCs) of the phloem has recently been reported to be involved in sugar phloem unloading in cucumber fruit by exporting hexoses from CCs to the apoplasmic space (Li et al., 2021). In our previous study, MdSWEET23 could play an important role during the progress of apple fruits maturation with starch breaks down and soluble sugars accumulate massively (Nie et al., 2022). Here, MdSWEET23, isolated from a cDNA library of apple fruits, belongs to clade III and is phylogenetically most closely related to AtSWEET13 (Figure 2). AtSWEET13, which also belongs to clade III, is plasma membrane-localized and transports both sucrose and GA (Kanno et al., 2016). But a recent study found that sucrose rather than GA transported by AtSWEET13 and AtSWEET14 directly affects anther development and seed yield (Wang et al., 2022). Our heterologous expression assay in yeast showed that MdSWEET23 functioned in sucrose transport rather than glucose and fructose transport and could restore SUSY7/ura3 growth on media supplemented with sucrose (Figure 5). Moreover, the overexpression of MdSWEET23 in ‘‘Orin” calli promoted sugars accumulation in calli, which grown on MS medium with sucrose as the carbon source (Figure 6). These results indicate that MdSWEET23 is a functional sucrose transporter. In previous studies, it has been found that most SWEETs in clade III are capable of transporting sucrose. Such as AtSWEET11, 12, and 13 in Arabidopsis (Chen et al., 2012; Han et al., 2017), SbSWEET13a, 13b, and 13c in sorghum, and StSWEET11 in potato (Abelenda et al., 2019), but their functions are different.
The functions of SWEETs also largely depend on their subcellular and tissue localization. SWEET proteins are mainly localized on the plasma and the tonoplast, while, a few members are also localized on the endoplasmic reticulum and the Golgi membranes (Chen L. Q. et al., 2015; Jeena et al., 2019; Breia et al., 2021). AtSWEET13 (Kanno et al., 2016) in Arabidopsis, PbSWEET4 (Ni et al., 2020) and PuSWEET15 (Li et al., 2020) in pear, BsSWEET15 and BsSWEET16 (Lu et al., 2022) in Bletilla striata, and SlSWEET14 (Zhang et al., 2021) in tomato, are also localized on the plasma membrane. The localization of sugar transporter ClVST1 shifted from tonoplast in wild-type watermelon to plasma membrane in sweet watermelon, which increased sugar sink potency (Ren et al., 2020). MdSWEET23 was localized on the plasma membrane (Figure 4) and mainly expressed in the vascular bundle, especially in the sepal and carpel vascular bundles of apple fruit (Figure 3). The carpel vascular bundle is the major channel for nutrients transporting toward the seeds, and the sepal vascular bundle is the main channel for nutrient and water transport for fruit expansion (Wang et al., 2015). In Arabidopsis, AtSWEET11, 12, and 15 are all highly expressed in the seed coat and play key roles in seed development (Le Hir et al., 2015). Therefore, it is speculated that MdSWEET23 may participate in apple seed development. The expression of MdSWEET23 in the sepal vascular bundle region indicates that it may be involved in the unloading of sucrose to the fleshy tissue of apple fruits. In apple, MdSWEET9b functions on the SE/CC, and its surrounding parenchyma cells is involved in fruit sugar accumulation (Zhang et al., 2023). In cucumber, CsSWEET7a, localized to the plasma membrane in CCs of the phloem, is involved in sugar phloem unloading in cucumber fruit by removing hexoses from CCs to the apoplasmic space (Li et al., 2021).
The silencing of MdSWEET23 in “Hanfu” apple fruits significantly reduced sucrose and sorbitol content, which further confirmed the involvement of MdSWEET23 in sucrose transport and accumulation in apple fruits. Further, whether the decrease in sorbitol content (Figure 6B) was due to MdSWEET23 silencing and whether MdSWEET23 was involved in sorbitol transport and accumulation in apple fruits? The expression of MdSOT1 significantly increased, whereas that of MdSDH6 substantially decreased in the MdSWEET23-silenced fruits. These results indicate that MdSWEET23 silencing affects sorbitol metabolism and transport in apple fruits. However, more direct evidence is needed to prove whether MdSWEET23 can transport sorbitol. In apple, the carbohydrates used for long-distance transportation are sucrose and sorbitol. Sucrose and sorbitol arriving in the SE/CC complex are pumped into the apoplasmic space by SUT/SOT (Fan et al., 2009; Chen et al., 2012) after that sucrose is broken down to hexose by CWIN, which was transported to the phloem parenchyma cells by the hexose transporters (Zhang et al., 2004). We speculate that MdSWEET23 may play a role in sucrose or sorbitol transport along the sugar concentration gradient from the apoplasmic space to the phloem parenchyma cells.
Ectopic overexpression of MdSWEET23 in tomatoes caused altered sugar metabolism and distribution
Sugar transport can influence the distribution of carbohydrates throughout the plant, which is important for plant growth, and the disorganization of sugar distribution will lead to abnormal plant growth. The overexpression of ZjSWEET2.2 in jujube led to a significant increase in leaf photosynthetic assimilate (Geng et al., 2020), whereas the overexpression of PbSWEET4 in strawberry reduced sugar content in leaves (Ni et al., 2020). Klemens et al. (2013) found that the overexpression of AtSWEET16 in Arabidopsis resulted in significantly increased growth compared to the WT. While, overexpression of OsSWEET14 and OsSWEET5 in rice significantly reduced plant height (Zhou et al., 2014; Kim et al., 2021). Silencing of SlSWEET7a and SlSWEET14 in tomato resulted in a 35%–48% increase in plant height compared to the WT (Zhang et al., 2021). The ectopic overexpression of MdSWEET23 in tomato led to an over-accumulation of carbohydrates in leaves, with a decrease in photosynthetic rate and plant height (Figure 7). In apple, MdSWEET23 is expressed mainly in the sepal and carpel vascular bundles of fruits (sink organs) (Figure 3). While, in transgenic tomato lines, the expression of the MdSWEET23 was driven by the CaMV35S promoter, a constitutive promoter, and thus MdSWEET23 expression in tomato lacks temporal and spatial regulation. In source organs, SWEETs are mainly responsible for transporting sucrose from the synthesis site to the loading site (Chen et al., 2012). The expression of MdSWEET23 in tomato functional leaves (source organ) may have affected the loading process of photosynthetic assimilates, too much sucrose was transported to the loading site and caused the abnormal accumulation of starch and soluble sugars in mature leaves. The increases in soluble sugar and starch content in leaves negatively regulate the photosynthetic rate, causing a decrease in the net photosynthetic rate of the leaves (Sonnewald, 2001). In maize, CST1 belongs to the Clade I of the SWEET protein family, is specifically expressed in subsidiary cells, and positively regulates stomatal opening and source capacity at the grain-filling stage (Wang H. et al., 2019). At the seedling stage, phenotypes of the MdSWEET23-OE plants might partially or wholly be ascribed to the disordered sugar distribution.
Previous genome-wide studies in different plants have found that SWEETs exhibit variable expression patterns under cold treatments, which suggests that SWEETs are involved in cold-induced sugar-signaling responses (Zhang W. et al., 2019; Jiang et al., 2021). Overexpression of AtSWEET16 (Klemens et al., 2013) and AtSWEET17 (Chardon et al., 2013) in Arabidopsis elevated sugar content and increased cold tolerance. Hu et al. (2022) found that cold-tolerant cucumber had a higher expression level of CsSWEET2 than cold-sensitive cucumber, and the overexpression of CsSWEET2 in Arabidopsis enhanced the cold resistance. The MdSWEET23-OE lines showed higher resistance to cold stress. Ectopic overexpression of MdSWEET23 accelerated starch degradation in leaves under cold stress, but leaf soluble sugar content in OE lines showed no significant difference with WT. We speculated that the overexpression of MdSWEET23 in tomato may have resulted in facilitating the process of sugar loading during cold treatment, which in turn allowed more sugar to be transported out of the source organ, and could potentially influence starch hydrolysis through the feedback regulation mechanism. Overexpression of MdSWEET23 in tomato may alter sucrose transport and distribution in OE lines under cold stress, thereby maintaining sugar homeostasis in response to cold stress. The possible mechanisms of increased cold tolerance of MdSWEET23-OE tomato lines need to be further investigated.
Based on the characteristics of MdSWEET23 in substrate transport, subcellular and tissue localization (Figures 3–5), we speculated that MdSWEET23 could be involved in the uptake of sucrose through the plasma membrane in sepal and carpel vascular bundles of apple fruits. Ectopic overexpression of MdSWEET23 in tomato significantly increased the sugar content of the BC fruits but had no significant effect on the sugar content of the RR fruits (Figure 1). In pear, overexpression of PuSWEET15 led to a significant increase in sucrose content in fruits (Li et al., 2020). Similarly, overexpression of VvSWEET10 resulted in a significant enhancement of sugar content in grape calli and tomato fruits (Zhang Z. et al., 2019). Silencing of SlSWEET7a and SlSWEET14 in tomato increased the sugar content of the fruits(Zhang et al., 2021). Sugars can act as signaling molecules that control distinct aspects of plant development. Several recent studies have reported that downstream sugar transporters could affect the sugar metabolism, by influencing upstream sugar metabolic enzyme activities and expression of sugar transporters through the feedback regulation mechanisms. For example, in cucumber, the overexpression of CsSWEET7a could shift the enzymatic reactions to produce more hexoses due to its overexpression leading to more hexose being unloaded from CCs, and less hexose retained in the cytosol of CCs (Li et al., 2021). MdERDL6-1, a tonoplast H+/glucose symporter, was highly expressed in apple fruits, and the overexpressing of it up-regulated expression of the H+/sugar antiporter genes, TST1 and TST2, by exporting glucose from vacuole to cytosol, which in turn led to increased glucose, fructose and sucrose in transgenic apple and tomato leaves and fruits (Zhu et al., 2021). In this study, we observed that overexpression of MdSWEET23 in tomato could affect upstream sugar metabolic enzymes and the expression of sugar transporters. During the hexose accumulation phase in tomato, sugar unloading is the apoplastic pathway (Ruan and Patrick, 1995). Sucrose arrived in the SE/CC complex unloaded into the apoplasm by SUTs or catabolized to hexose by CWIN, and then hexose was transported to parenchyma cells by HTs (Zhang et al., 2021). In BC fruits of the MdSWEET23-OE tomato lines, more sucrose may unload into the apoplasm by MdSWEET23, leading to high CWIN activity to hydrolyze sucrose into hexoses(Figure 1C), which might be the cause of more accumulation of hexose in fruits of transgenic lines. Meanwhile, the expressions of hexoses transporter genes HT1, HT2, and HT3 were also stimulated (Figure 1D)., although CWIN activity remained significantly higher as compared to WT. Ectopic overexpression of MdSWEET23 may generate an unusual situation affecting the expression of sucrose transporter genes SUT1, SUT2, and SUT4 (Figure 1D). Down-regulated SUT1, SUT2, and SUT4 in RR fruits may negatively affect sucrose unloading, thereby influencing the enrichment of sucrose against the concentration gradient in fruits. Ectopic overexpression of MdSWEET23 in tomato caused altered sugar metabolism and distribution but did not increase sugar sink potency in tomato fruits.
Conclusions
This study demonstrates that MdSWEET23, derived from apples, functions as a sucrose transporter and is localized on the plasma membrane in vascular bundle tissues. It appears to participate in the unloading of sucrose in the phloem. Overexpression of this gene in apple calli significantly increased the sugar content. Conversely, when the gene was silenced during the phase of rapid sugar accumulation in the fruit, it negatively influenced sugar accumulation therein. The findings reveal that MdSWEET23 possesses significant potential for enhancing the sugar content in fruit.
Data availability statement
The original contributions presented in the study are included in the article/Supplementary Files, further inquiries can be directed to the corresponding authors.
Author contributions
PN: Conceptualization, Data curation, Formal Analysis, Methodology, Project administration, Writing – original draft. LW: Data curation, Software, Formal Analysis, Validation, Writing – original draft. ML: Investigation, Methodology, Writing – review & editing, Data curation. DL: Investigation, Supervision, Writing – review & editing. SQ: Methodology, Project administration, Resources, Writing – review & editing. XX: Conceptualization, Funding acquisition, Writing – review & editing.
Funding
The authors declare financial support was received for the research, authorship, and/or publication of this article. This research was supported by the Shandong Province Key R&D Program (2022TZXD008), the China Agriculture Research System of MOF and MARA (CARS-27), and the Shandong Academy of Agricultural Sciences Agricultural Science and Technology Innovation Project: (CXGC2023B02).
Acknowledgments
We would like to express our special thanks to Professor Xiaolei Sui from China Agricultural University for providing the yeast strains SUSY7/ura3 and EBY.VW4000.
Conflict of interest
The authors declare that the research was conducted in the absence of any commercial or financial relationships that could be construed as a potential conflict of interest.
The reviewer X-FW declared a past co-authorship with the author XX to the handling editor.
Publisher’s note
All claims expressed in this article are solely those of the authors and do not necessarily represent those of their affiliated organizations, or those of the publisher, the editors and the reviewers. Any product that may be evaluated in this article, or claim that may be made by its manufacturer, is not guaranteed or endorsed by the publisher.
Supplementary material
The Supplementary Material for this article can be found online at: https://www.frontiersin.org/articles/10.3389/fpls.2023.1266194/full#supplementary-material
References
Abelenda, J. A., Bergonzi, S., Oortwijn, M., Sonnewald, S., Du, M., Visser, R. G. F., et al. (2019). Source-sink regulation is mediated by interaction of an FT Homolog with a SWEET protein in potato. Curr. Biol. 29, 1178–1186.e6. doi: 10.1016/j.cub.2019.02.018
Breia, R., Conde, A., Badim, H., Fortes, A. M., Gerós, H., Granell, A. (2021). Plant SWEETs: from sugar transport to plant–pathogen interaction and more unexpected physiological roles. Plant Physiol. 186, 836–852. doi: 10.1093/plphys/kiab127
Chardon, F., Bedu, M., Calenge, F., Klemens, P. A., Spinner, L., Clement, G., et al. (2013). Leaf fructose content is controlled by the vacuolar transporter SWEET17 in Arabidopsis. Curr. Biol. 23, 697–702. doi: 10.1016/j.cub.2013.03.021
Chen, L. Q. (2014). SWEET sugar transporters for phloem transport and pathogen nutrition. New Phytol. 201, 1150–1155. doi: 10.1111/nph.12445
Chen, H. Y., Huh, J. H., Yu, Y. C., Ho, L. H., Chen, L. Q., Tholl, D., et al. (2015). The Arabidopsis vacuolar sugar transporter SWEET2 limits carbon sequestration from roots and restricts Pythium infection. Plant J. 83, 1046–1058. doi: 10.1111/tpj.12948
Chen, L. Q., Lin, I. W., Qu, X. Q., Sosso, D., McFarlane, H. E., Londoño, A., et al. (2015). A cascade of sequentially expressed sucrose transporters in the seed coat and endosperm provides nutrition for the Arabidopsis embryo. Plant Cell 27, 607–619. doi: 10.1105/tpc.114.134585
Chen, L. Q., Qu, X. Q., Hou, B. H., Sosso, D., Osorio, S., Fernie, A. R., et al. (2012). Sucrose efflux mediated by SWEET proteins as a key step for phloem transport. Science 335, 207–211. doi: 10.1126/science.1213351
Fan, R. C., Peng, C. C., Xu, Y. H., Wang, X. F., Li, Y., Shang, Y., et al. (2009). Apple sucrose transporter SUT1 and sorbitol transporter SOT6 interact with cytochrome b5 to regulate their affinity for substrate sugars. Plant Physiol. 150, 1880–1901. doi: 10.1104/pp.109.141374
Geng, Y. Q., Wu, M. J., Zhang, C. M. (2020). Sugar transporter ZjSWEET2.2 mediates sugar loading in leaves of Ziziphus jujuba Mill. Front. Plant Sci. 11. doi: 10.3389/fpls.2020.01081
Ho, L. H., Klemens, P. A. W., Neuhaus, H. E., Ko, H. Y., Hsieh, S. Y., Guo, W. J.. (2019). SlSWEET1a is involved in glucose import to young leaves in tomato plants. J. Exp. Bot. 70, 3241–3254.
Han, L., Zhu, Y. P., Liu, M., Zhou, Y., Lu, G. Y., Lan, L., et al. (2017). Molecular mechanism of substrate recognition and transport by the AtSWEET13 sugar transporter. Proc. Natl. Acad. Sci. U. S. A. 114, 10089–10094. doi: 10.1073/pnas.1709241114
Hu, L. P., Zhang, F., Song, S. H., Yu, X. L., Ren, Y., Zhao, X. Z., et al. (2022). CsSWEET2, a hexose transporter from cucumber (Cucumis sativus L.), affects sugar metabolism and improves cold tolerance in Arabidopsis. Int. J. Mol. Sci. 23, 3886. doi: 10.3390/ijms23073886
Jeena, G. S., Kumar, S., Shukla, R. K. (2019). Structure, evolution and diverse physiological roles of SWEET sugar transporters in plants. Plant Mol. Biol. 100, 351–365. doi: 10.1007/s11103-019-00872-4
Jiang, L., Song, C., Zhu, X., Yang, J. (2021). SWEET transporters and the potential functions of these sequences in tea (Camellia sinensis). Front. Genet. 12. doi: 10.3389/fgene.2021.655843
Kanno, Y., Oikawa, T., Chiba, Y., Ishimaru, Y., Shimizu, T., Sano, N., et al. (2016). AtSWEET13 and AtSWEET14 regulate gibberellin-mediated physiological processes. Nat. Commun. 7, 13245. doi: 10.1038/ncomms13245
Kim, P., Xue, C. Y., Song, H. D., Gao, Y., Feng, L., Li, Y., et al. (2021). Tissue-specific activation of DOF11 promotes rice resistance to sheath blight disease and increases grain weight via activation of SWEET14. Plant Biotechnol. J. 19, 409–411. doi: 10.1111/pbi.13489
Klemens, P. A. W., Patzke, K., Deitmer, J., Spinner, L., Le Hir, R. L., Bellini, C., et al. (2013). Overexpression of the vacuolar sugar carrier AtSWEET16 modifies germination, growth, and stress tolerance in Arabidopsis. Plant Physiol. 163, 1338–1352. doi: 10.1104/pp.113.224972
Kumar, S., Stecher, G., Li, M., Knyaz, C., Tamura, K. (2018). MEGA X: Molecular evolutionary genetics analysis across computing platforms. Mol. Biol. Evol. 35, 1547–1549. doi: 10.1093/molbev/msy096
Le Hir, R., Spinner, L., Klemens, P. A. W., Chakraborti, D., Marco, F., Vilaine, F., et al. (2015). Disruption of the sugar transporters AtSWEET11 and AtSWEET12 affects vascular development and freezing tolerance in Arabidopsis. Mol. Plant 8, 1687–1690. doi: 10.1016/j.molp.2015.08.007
Li, X. Y., Guo, W., Li, J. C., Yue, P. T., Bu, H. D., Jiang, J., et al. (2020). Histone acetylation at the promoter for the transcription factor PuWRKY31 affects sucrose accumulation in pear fruit. Plant Physiol. 182, 2035–2046. doi: 10.1104/pp.20.00002
Li, Y., Liu, H., Yao, X., Sun, L., Sui, X. (2022). The role of sugar transporter CsSWEET7a in apoplasmic phloem unloading in receptacle and nectary during cucumber anthesis. Front. Plant Sci. 12. doi: 10.3389/fpls.2021.758526
Li, Y., Liu, H., Yao, X., Wang, J., Feng, S., Sun, L., et al. (2021). Hexose transporter CsSWEET7a in cucumber mediates phloem unloading in companion cells for fruit development. Plant Physiol. 186, 640–654. doi: 10.1093/plphys/kiab046
Livak, K. J., Schmittgen, T. D. (2001). Analysis of relative gene expression data using real-time quantitative PCR and the 2(-Delta Delta C(T)) Method. Methods 25, 402–408. doi: 10.1006/meth.2001.1262
Loqué, D., Lalonde, S., Looger, L. L., von Wirén, N., Frommer, W. B. (2007). A cytosolic trans-activation domain essential for ammonium uptake. Nature 446, 195–198. doi: 10.1038/nature05579
Lu, C., Ye, J., Chang, Y., Mi, Z., Liu, S., Wang, D., et al. (2022). Genome-wide identification and expression patterns of the SWEET gene family in Bletilla striata and its responses to low temperature and oxidative stress. Int. J. Mol. Sci. 23, 10057. doi: 10.3390/ijms231710057
Ma, S., Li, Y. X., Li, X., Sui, X. L., Zhang, Z. X. (2019). Phloem unloading strategies and mechanisms in crop fruits. J. Plant Growth Regul. 38, 494–500. doi: 10.1007/s00344-018-9864-1
Mathan, J., Singh, A., Ranjan, A. (2021). Sucrose transport in response to drought and salt stress involves ABA-mediated induction of OsSWEET13 and OsSWEET15 in rice. Physiol. Plant 171, 620–637. doi: 10.1111/ppl.13210
Ni, J. P., Li, J. M., Zhu, R. X., Zhang, M. Y., Qi, K. J., Zhang, S. L., et al. (2020). Overexpression of sugar transporter gene PbSWEET4 of pear causes sugar reduce and early senescence in leaves. Gene 743, 144582. doi: 10.1016/j.gene.2020.144582
Nie, P. X., Wang, X. Y., Hu, L. P., Zhang, H. Y., Zhang, J. X., Zhang, Z. X., et al. (2010). The predominance of the apoplasmic phloem-unloading pathway is interrupted by a symplasmic pathway during Chinese jujube fruit development. Plant Cell Physiol. 51, 1007–1018. doi: 10.1093/pcp/pcq054
Nie, P. X., Xu, G. X., Yu, B., Lyu, D. G., Xue, X. M., Qin, S. J. (2022). Genome-wide identification and expression profiling reveal the potential functions of the SWEET gene family during the sink organ development period in apple (Malus × domestica Borkh.). Agronomy 12, 1747. doi: 10.3390/agronomy12081747
Patrick, J. W. (1997). Phloem unloading: sieve element unloading and post-sieve element transport. Annu. Rev. Plant Physiol. Plant Mol. Biol. 48, 191–222. doi: 10.1146/annurev.arplant.48.1.191
Ren, Y., Sun, H., Zong, M., Guo, S., Ren, Z., Zhao, J., et al. (2020). Localization shift of a sugar transporter contributes to phloem unloading in sweet watermelons. New Phytol. 227, 1858–1871. doi: 10.1111/nph.16659
Riesmeier, J. W., Willmitzer, L., Frommer, W. B. (1992). Isolation and characterization of a sucrose carrier cDNA from spinach by functional expression in yeast. EMBO J. 11, 4705–4713. doi: 10.1002/j.1460-2075.1992.tb05575.x
Ruan, Y. L., Patrick, J. W. (1995). The cellular pathway of postphloem sugar transport in developing tomato fruit. Planta 196, 434–444. doi: 10.1007/BF00203641
Sonnewald, U. (2001). Sugar sensing and regulation of photosynthetic carbon metabolism (The Netherlands: Kluwer Academic Publishers). doi: 10.1007/0-306-48148-0_6
Spolaore, S., Trainotti, L., Casadoro, G. (2001). A simple protocol for transient gene expression in ripe fleshy fruit mediated by Agrobacterium. J. Exp. Bot. 52, 845–850. doi: 10.1093/jexbot/52.357.845
Sun, H. J., Uchii, S., Watanabe, S., Ezura, H. (2006). A highly efficient transformation protocol for Micro-Tom, a model cultivar for tomato functional genomics. Plant Cell Physiol. 47, 426–431. doi: 10.1093/pcp/pci251
Wang, X. L., Xu, Y. H., Peng, C. C., Fan, R. C., Gao, X. Q. (2009). Ubiquitous distribution and different subcellular localization of sorbitol dehydrogenase in fruit and leaf of apple. J. Exp. Bot. 60, 1025–1034. doi: 10.1093/jxb/ern347
Wang, J., Xue, X. Y., Zeng, H. Q., Li, J. K., Chen, L. Q. (2022). Sucrose rather than GA transported by AtSWEET13 and AtSWEET14 supports pollen fitness at late anther development stages. New Phytol. 236, 525–537. doi: 10.1111/nph.18368
Wang, H., Yan, S., Xin, H., Huang, W., Zhang, H., Teng, S., et al. (2019). A subsidiary cell-localized glucose transporter promotes stomatal conductance and photosynthesis. Plant Cell 31, 1328–1343. doi: 10.1105/tpc.18.00736
Wang, L., Yao, L., Hao, X., Li, N., Qian, W., Yue, C., et al. (2018). Tea plant SWEET transporters: Expression profiling, sugar transport, and the involvement of CsSWEET16 in modifying cold tolerance in Arabidopsis. Plant Mol. Biol. 96, 577–592. doi: 10.1007/s11103-018-0716-y
Wang, Y. F., Ye, Z., Liu, H., Liu, Q. L., Zhang, B., Hao, Y. Y. (2015). Changes of vascular bundles structure and water transport of apple fruit in different development period. Plant Physiol. J. 51, 1414–1418. doi: 10.13592/j.cnki.ppj.2015.0367
Wang, S., Yokosho, K., Guo, R., Whelan, J., Ruan, Y. L., Ma, J. F., et al. (2019). The soybean sugar transporter GMSWEET15 mediates sucrose export from endosperm to early embryo. Plant Physiol. 180, 2133–2141. doi: 10.1104/pp.19.00641
Wei, X. Y., Liu, F. L., Chen, C., Ma, F. W., Li, M. J. (2014). The Malus domestica sugar transporter gene family: identifications based on genome and expression profiling related to the accumulation of fruit sugars. Front. Plant Sci. 5. doi: 10.3389/fpls.2014.00569
Wieczorke, R., Krampe, S., Weierstall, T., Freidel, K., Hollenberg, C. P., Boles, E. (1999). Concurrent knock-out of at least 20 transporter genes is required to block uptake of hexoses in Saccharomyces cerevisiae. FEBS Lett. 464, 123–128. doi: 10.1016/S0014-5793(99)01698-1
Yang, J., Luo, D. P., Yang, B., Frommer, W. B., Eom, J. S.. (2018). SWEET11 and 15 as key players in seed filling in rice. New Phytol. 218, 604–615.
Yao, L., Ding, C., Hao, X., Zeng, J., Yang, Y., Wang, X., et al. (2020). CsSWEET1a and CsSWEET17 mediate growth and freezing tolerance by promoting sugar transport across the plasma membrane. Plant Cell Physiol. 61, 1669–1682. doi: 10.1093/pcp/pcaa091
Zhang, C. M., Bian, Y., Hou, S. H., Li, X. G. (2018). Sugar transport played a more important role than sugar biosynthesis in fruit sugar accumulation during Chinese jujube domestication. Planta 248, 1187–1199. doi: 10.1007/s00425-018-2971-1
Zhang, X. S., Feng, C. Y., Wang, M. N., Li, T. L., Liu, X., Jiang, J. (2021). Plasma membrane-localized SlSWEET7a and SlSWEET14 regulate sugar transport and storage in tomato fruits. Hortic. Res. 8, 186. doi: 10.1038/s41438-021-00624-w
Zhang, L. Y., Peng, Y. B., Pelleschi-Travier, S., Fan, Y., Lu, Y. F., Lu, Y. M., et al. (2004). Evidence for apoplasmic phloem unloading in developing apple fruit. Plant Physiol. 135, 574–586. doi: 10.1104/pp.103.036632
Zhang, X. Y., Wang, X. L., Wang, X. F., Xia, G. H., Pan, Q. H., Fan, R. C., et al. (2006). A shift of Phloem unloading from symplasmic to apoplasmic pathway is involved in developmental onset of ripening in grape berry. Plant Physiol. 142, 220–232. doi: 10.1104/pp.106.081430
Zhang, S., Wang, H., Wang, T., Zhang, J., Liu, W., Fang, H., et al. (2023). Abscisic acid and regulation of the sugar transporter gene MdSWEET9b promote apple sugar accumulation. Plant Physiol. 192, 2081–2101. doi: 10.1093/plphys/kiad119
Zhang, W., Wang, S., Yu, F., Tang, J., Shan, X., Bao, K., et al. (2019). Genome-wide characterization and expression profiling of SWEET genes in cabbage (Brassica oleracea var. capitata L.) reveal their roles in chilling and clubroot disease responses. BMC Genomics 20, 93. doi: 10.1186/s12864-019-5454-2
Zhang, H. P., Wu, J. Y., Tao, S. T., Wu, T., Qi, K.-j., Zhang, S. J., et al. (2014). Evidence for apoplasmic phloem unloading in pear fruit. Plant Mol. Biol. Rep. Biol. Rep. 32, 931–939. doi: 10.1007/s11105-013-0696-7
Zhang, Z., Zou, L. M., Ren, C., Ren, F. R., Wang, Y., Fan, P., et al. (2019). VvSWEET10 mediates sugar accumulation in Grapes. Genes (Basel). 10, 255. doi: 10.3390/genes10040255
Zhen, Q. L., Fang, T., Peng, Q., Liao, L., Zhao, L., Owiti, A., et al. (2018). Developing gene-tagged molecular markers for evaluation of genetic association of apple SWEET genes with fruit sugar accumulation. Hortic. Res. 5, 14. doi: 10.1038/s41438-018-0024-3
Zhou, Y., Liu, L., Huang, W. F., Yuan, M., Zhou, F., Li, X. H., et al. (2014). Overexpression of OsSWEET5 in rice causes growth retardation and precocious senescence. PloS One 9, e94210. doi: 10.1371/journal.pone.0094210
Zhu, L. C., Li, B. Y., Wu, L. M., Li, H. X., Wang, Z. Y., Wei, X. Y., et al. (2021). MdERDL6-mediated glucose efflux to the cytosol promotes sugar accumulation in the vacuole through up-regulating TSTs in apple and tomato. Proc. Natl. Acad. Sci. U. S. A. 118, e2022788118. doi: 10.1073/pnas.2022788118
Keywords: MdSWEET23, apple fruit, sucrose transporter, plasma membrane, cold stress
Citation: Nie P, Wang L, Li M, Lyu D, Qin S and Xue X (2023) MdSWEET23, a sucrose transporter from apple (Malus × domestica Borkh.), influences sugar metabolism and enhances cold tolerance in tomato. Front. Plant Sci. 14:1266194. doi: 10.3389/fpls.2023.1266194
Received: 24 July 2023; Accepted: 15 September 2023;
Published: 03 October 2023.
Edited by:
Fei Shen, Beijing Academy of Agricultural and Forestry Sciences, ChinaReviewed by:
Meng Li, Henan Agricultural University, ChinaXin Li, China Agricultural University, China
Xiao-Fei Wang, Shandong Agricultural University, China
Copyright © 2023 Nie, Wang, Li, Lyu, Qin and Xue. This is an open-access article distributed under the terms of the Creative Commons Attribution License (CC BY). The use, distribution or reproduction in other forums is permitted, provided the original author(s) and the copyright owner(s) are credited and that the original publication in this journal is cited, in accordance with accepted academic practice. No use, distribution or reproduction is permitted which does not comply with these terms.
*Correspondence: Sijun Qin, qsj1975@syau.edu.cn; Xiaomin Xue, xuexiaomin79@126.com