- 1Laboratory of Advanced Studies in Vertical Agriculture, Goiano Federal Institute of Education, Science and Technology, Rio Verde, Brazil
- 2Department of Biomolecules, Goiano Federal Institute of Education, Science and Technology, Iporá, Brazil
- 3Laboratory of Plant Physiology, Goiano Federal Institute of Education, Science and Technology, Rio Verde, Brazil
- 4PostGraduate Program in Plant Production, University of Rio Verde, Rio Verde, Brazil
- 5Biodiversity Metabolism and Genetics Laboratory, Goiano Federal Institute of Education, Science and Technology, Rio Verde, Brazil
- 6Research Center for Exact and Technological Sciences, Franca University, Franca, Brazil
Urban vertical agriculture with lighting system can be an alternative green infrastructure to increase local food production irrespective of environmental and soil conditions. In this system, light quality control can improve the plant physiological performance, well as induce metabolic pathways that contribute to producing phenolic compounds important to human health. Therefore, this study aimed to evaluate the influence of RBW (red, blue and white) and monochromatic (red and blue; R and B, respectively) light associated or not with UV-B on photosynthetic performance and phenolic compound production in microtomato fruits cultivated via vertical agriculture. The experimental design adopted was completely randomized, with six replicates illuminated with 300 µmol·m−2·s−1 light intensities (RBW, RBW + UV, B, B + UV, R, and R + UV), 12 h photoperiod, and 3.7 W·m−2 UV-B irradiation for 1 h daily for the physiological evaluations. Twenty-six days after the installation, gas exchange, chlorophyll a fluorescence and nocturnal breathing were evaluated. Fruits in different ripening stages (green, orange, and red) were collected from microtomato plants grown under with different light qualities, to evaluate the physiological performance. The identification and quantification of the phenolic compound rutin was also performed to investigate their metabolic response. This study identified that plants grown under B + UV had high photosynthetic rates (A=11.57 µmol·m−2·s−1) and the fruits at all maturation stages from plants grown under B and B + UV had high rutin content. Meanwhile, the activation of suppressive mechanisms was necessary in plants grown under R because of the high nocturnal respiration and unregulated quantum yield of the non-photochemical dissipation of the photosystem II. These results highlight the importance of selecting light wavelength for vegetable cultivation to produce fruits with a high content of specialized metabolites that influence color, flavor, and health promotion, which is of special interest to farmers using sustainable cropping systems.
Introduction
Specialized metabolites, traditionally referred to as secondary metabolites, are compounds present in some natural sources that benefit both survival and reproduction in different environments. These molecules are derived from the primary metabolism, and plants synthesize approximately 200,000 to 1 million such compounds (Garagounis et al., 2021). Phenolic compounds, particularly phenolic acids and flavonoids are a class of compounds of interest due to their high antioxidant capacity that modulates and/or regulates oxidative stress (Ribeiro et al., 2019; Shih and Morgan, 2020). Rutin, a glycoside flavonoid widely distributed in the plant kingdom, is a natural molecule of great interest due to its pharmacological activities and used for treating varicose veins, internal bleeding, and hemorrhoids. It also has antioxidant, anti-inflammatory, antidiabetic, and cardioprotective activities (Semwal et al., 2021).
Elicitors have been a promising strategy to increase bioactive compound production. Light is one of the most studied factors; thus, information is available for several plant species grown under different wavelengths (Liu et al., 2019). Besides being a key factor in regulating growth and development, light also acts as an environmental signal that triggers the production of specific molecules in response to the environment and influences plant development stage (Silva et al., 2020). Wavelength differences are perceived due to the action of several photoreceptors, such as phytochromes (that absorb red and far-red light), cryptochromes, and phototropins (that absorb blue and UV-A light), and UVR8 (that absorbs UV-B light) (Wang et al., 2020). Light also strongly affects the biosynthesis and accumulation of several secondary metabolites in plants, which is related to both increased photosynthesis that generates more carbon skeletons for plant metabolism, including secondary metabolism, and the action of photoreceptors that can perceive photons and trigger signaling pathways, which in turn change gene expression levels. Thus, light condition modulation can stimulate both growth and production of specific compounds of interest (Silva et al., 2020; Thoma et al., 2020). Several studies confirm that photosynthesis can be modulated, and secondary metabolite synthesis is enhanced in species grown under light-emitting diodes (LEDs), particularly when monochromatic blue or red lights are used instead of white light. The individual effects of each wavelength vary depending on the species studied and their growing conditions (Wu et al., 2007; Li and Kubota, 2009; Johkan et al., 2010; Bian et al., 2015; Lobiuc et al., 2017).
Ultraviolet radiation also induces bioactive compound synthesis, since it induces the synthesis of flavonoids and phenolic compounds, which work both as antioxidants and defense molecules for plants (Neugart and Schreiner, 2018). UV-B doses can be manipulated to control secondary metabolite production and plant performance; for example, plants can initially be grown under optimal conditions until they reach a certain degree of development, and then be subjected to UV-B treatment to produce the compounds of interest, thus reducing the morphological impacts of UV-B (Mosadegh et al., 2018). Suzuki et al. (2005) observed a 122% increase in rutin content in UV-B irradiated buckwheat. Moreover, the rutin content also increased in lettuce grown under continuous blue light (Naoya Fukuda et al., 2022). However, high levels of UV-B can be deleterious to plants and are able to influence growth and development, as well as alter genetic, physiological and biochemical aspects, leading to damage to photosynthetic machinery and compromising the photosynthetic rate, triggering photoinhibition and photoprotection responses (Nocchi et al., 2020).
Controlled growing environments mainly circumvent the light-related issues since multiple factors can be controlled. Thus, LEDs can be used because they efficiently convert electrical energy into light energy in addition to having a narrow and specific emission band (Zou et al., 2020). Their use in plant cultivation and the number of studies on the most suitable wavelengths have increased (Thwe et al., 2014; Kim et al., 2015; Ko et al., 2015; Liu et al., 2016; Kim et al., 2018; Liu et al., 2018; Taulavuori et al., 2016; Nam et al., 2018; Taulavuori et al., 2018). Additionally, this protected cultivation environment also circumvents the effects of current climate change scenarios that are expected to worsen in the coming decades, considering that the frequency of natural disasters such as droughts and storms has increased (Fang et al., 2020). It is already well established that blue (425-490 nm) and red (610-700 nm) light are the best spectral qualities for promoting the photosynthetic process of plants (Shafiq et al., 2021). The light environment includes the intensity, quality, and direction of light, with light intensity being the key factor for plant growth (Pan et al., 2020). High light intensities can lead to increased thickness and reduction in leaf area to avoid damage caused by excess light (Matos et al., 2009). Yasar & Uzal (2023) investigated the effect of increased light intensity on tomato plants grown under LED lights with a spectrum similar to the sun and concluded that high intensities can be beneficial, but only if they are within the tolerable limits of the species, since thus the antioxidant system is able to reduce or eliminate the harmful effects of reactive oxygen species (ROS) generated under stress conditions, like that of excess light. For the microtomato, Ke et al. (2022) and Ke et al. (2023) found that 300 μmol.m-2.s-1 is the appropriate intensity for both the vegetative and reproductive stages, favoring plant growth and increase in fruit biomass. High luminous intensity (700 μmol.m-2.s-1) led to reduced chlorophyll content and reduced photosynthetic capacity.
Tomato (Solanum lycopersicum L.), one of the most consumed vegetables worldwide (>180 million ton being produced yearly), is one of the many crops that can be favored by a controlled environment using LEDs. Its cultivation has become highly effective due to the development of new varieties from breeding programs initiated in the 20th century and the introduction of advanced cultivation technologies (Mata-Nicolás et al., 2020). The main agricultural challenge in the last 20 years has been to produce sufficient nutritious food for a growing population and, at the same time, meet the requirements of sustainable cultivation, thereby reducing environmental impacts (Ronga et al., 2020).
Although rutin is found in many species, few plants are known as major rutin sources (Kianersi et al., 2020). Its concentration increases in UVB-irradiated plants, which reinforces its protective role against UV-B (Chua, 2013). Thus, investigating strategies to increase the production of compounds of interest, such as rutin, is essential to produce nutraceutical foods that will contribute to human health.
In this scenario, we hypothesize that different light wavelengths promote contrasting responses in the production of secondary metabolites such as rutin, the associated use of UV-B light may enhance the secondary metabolite production by increasing plant stress, and using different light wavelengths and UV-B promotes different physiological responses in plants. Thus, this study aimed to evaluate the influence of RBW and monochromatic wavelengths associated with UV-B on the photosynthetic performance and phenolic compound production in microtomato fruits grown in vertical farming.
Materials and methods
Plant material and growing conditions
The experiment was conducted at the Plant Biotechnology Laboratory Complex of the Goiano Federal Institute/Rio Verde campus (17°48′10.9″S, 50°54′21.8″W, 755 m altitude). Initially, Micro-Tom (MT) wild type microtomato (MTwt) cultivar seeds were sown in trays containing Bioplant Plus® substrate (BioPlant Agrícola Ltd., Nova Ponte, Brazil) and germinated for 15 days. The seedlings were transplanted to 350 cm³ pots containing the same substrate, supplemented with 0.5 g 4:14:8 NPK, and grown until 39 days in a greenhouse under controlled irradiance, temperature (approximately 25°C), and relative humidity (approximately 60%).
Then, the plants were transferred to an indoor vertical farming system at 50 ± 5% relative humidity and 25 ± 2°C (the room was cooled with air conditioning, to ensure constant temperature and humidity. In addition, the environments with the microtomato plants presented a thermohygrometer for constant monitoring of these variables) in insulated 1.00×0.75 m² vertical boxes with adjustable height and illuminated with LEDs (SG4 – SpectraGrow). Each booth was separated by an expanded polystyrene board (Styrofoam®). The plants were exposed to 300 µmol·m−2·s−1 broad spectrum control LEDs [RBW light (RBW) and RBW + UV-B (RBWUV)], 480 nm blue light (B), blue light + UV-B (BUV), 660 nm red light (R), and red light + UV-B (RUV) for 40 days at constant light intensity, creating approximately 12.96 mol·m−2·d−1 daily light integral (DLI) and 12 h photoperiod (7:00 AM to 7:00 PM). UV-B light (UV-B Broadband lamp – Philips) was administered daily from 11:00 AM to 12:00 PM (mean intensity: 3.7 W·m−2).
The experiment was conducted in an entirely randomized design, with six light qualities and six repetitions. The physiological parameters were evaluated using the leaves 26 days after exposure, considering that most fruits were at their full size. The fruits were collected for rutin content analysis at each growing condition, according to the ripeness level as green, orange, and red.
Gas exchange and chlorophyll a fluorescence
Gas exchanges were evaluated to record the photosynthesis rate (A, µmol·m−2·s−1), transpiration rate (E, mmol·m−2·s−1), stomatal conductance (gs, mol H2O·m−2·s−1), internal CO2 concentration (Ci), and nocturnal respiration rate (Rn, µmol CO2·m−2·s−1). These data were used to calculate the water use efficiency (WUE = A/E). Measurements were made using a LI-6800 XT portable gas exchange meter (IRGA, Li-Cor Inc., Nebraska, USA) between 08:00 AM and 11:00 AM on a fully expanded leaf at 25°C, 1000 µmol·m−2·s−1 artificial photosynthetically active radiation (PAR), approximately 400 μmol·mol−1 atmospheric CO2 concentration (Ca), and approximately 65% relative humidity.
Chlorophyll a fluorescence was evaluated on the same leaf in which gas exchange was measured using an IRGA. The fluorescence obtained was the effective quantum yield of photochemical energy conversion in photosystem II (FSII) (YII = (Fm′−F)/Fm′). The quantum yields of regulated energy dissipation (YNPQ = (F/Fm′)−(F/Fm)), and unregulated energy dissipation (YNO = F/Fm), were calculated according to the protocols reported by Genty et al. (1989) and Hendrickson et al. (2004), respectively. The ΔF/Fm′ was further used to estimate the apparent electron transport rate (ETR = ΔF/Fm′ PAR.LeafABS.0.5) (Bilger et al., 1995), where PAR is the photon flux density (µmol·m−2·s−1) incident on the leaf; LeafABS corresponds to the incident light fraction absorbed by the leaves; and 0.5 is the excitation energy fraction distributed to the FSII (Laisk and Loreto, 1996). The apparent electron transport rate to CO2 assimilation (ETR/A) rate was calculated to estimate photosynthesis at risk of oxidative stress (Geissler et al., 2015).
Chloroplast pigment index
Chlorophyll a, b, and total indices were obtained using a CLOROFILOG chlorophyll meter (CFL; Falker 1030, Porto Alegre, Brazil) on a fully expanded leaf, in triplicate. Rutin content analysis
Chromatographic conditions
Chromatographic analyses were performed on a Shimadzu® high efficiency liquid chromatograph with SPD-M20A photodiode detector and Zorbax Eclipse Plus C18 analytical column (250×4.6 mm², 5 µm; Agilent®) coupled to Zorbax Eclipse Plus C18 pre-column (12.5×4.6 mm², 5 µm; Agilent®). The chromatographic conditions were: 10 µL injection volume, 30°C oven temperature, 200–400 nm scanning range, and monitored readings at 254 nm. The mobile phase was eluted at 1.0 mL·min−1 flow rate, with phase A comprising 0.1% acetic acid UV/HPLC/spectroscopic grade (Proquimios®) and mobile phase B comprising HPLC-grade methanol (J.T. Baker®). The elution gradient of mobile phase B increased from 10 to 66% over 32 min, decreased from 66 to 10% in 3 min, and remained isocratic at 10% for 5 min; thus, the total run time was 40 min. The mobile phase with acidified water was filtered using a vacuum mobile filtration system (Restek) with a cellulose filter membrane (47 mm × 0.45 µm, Whatman®). The HPLC-grade methanol was filtered through a polytetrafluoroethylene (PTFE) membrane (47 mm × 0.45 µm, Whatman®).
Analytical curves and sample preparation
The rutin standard (standard bank of the Natural Products Group of the Franca University) was serially diluted to 100, 50, 25, 12.5, 6.25, and 3.125 µg·mL−1 using HPLC-grade methanol to construct calibration curves. The resulting solutions were injected in triplicate into the chromatograph. Calibration curves were constructed by plotting the standard chromatographic peak areas versus concentrations in the Microsoft® Excel® software for Microsoft 365 version 2010 for Windows®. Linearity was verified by the coefficient of determination (R2) and the correlation coefficient (r), which should be above 0.99 according to RDC No. 166/2017 (BRASIL, 2017), with relative standard deviation (RSD) lower than 5%. The resulting equations for each curve were estimated by the least squares method (LSM).
Methanolic extracts were prepared by suspending approximately 0.1 g freeze-dried microtomato in 2 mL HPLC-grade methanol in an ultrasonic bath (Saners Medical, Sonic Clean 2PS) for 30 min. The supernatant was transferred to a 3.0 mL syringe and directly filtered using a syringe containing a Nylon filter (13 mm diameter; 0.45 µm pore diameter; Analítica®). Then, 10 μL sample extracts were injected in duplicate in the high-performance liquid chromatograph. The compounds were detected by comparing with the standard retention peak periods and the resulting spectra and quantified through the peak areas and equations obtained from respective analytical curves.
Statistical analyses
The data obtained were analyzed by the Shapiro–Wilk and Komolgorov–Smirnov tests for normality, and then the homogeneity of variance was verified by the Levene test. These were the necessary assumptions for analysis of variance. These tests showed that the assumptions were not met. Thus, the non-parametric Kruskal–Wallis test was followed by the Dunn (1964) test for multiple comparisons, with methods to control a false discovery rate (FDR) (Benjamini & Hochberg, 1995). The test considered a significance level α equal to 5%. The R statistical software version 4.3.0 (R Core Team, 2023) was used for statistical analyses using the “FSA” (Ogle et al., 2020), “PMCMRplus” (Pohlert, 2018), and “ggplot2” (Wickham, 2011) packages.
Results
The MT life cycle is accelerated compared to that of conventional tomatoes, since the fruits begin to develop at 39 days and many fruits begin to ripen at 65 days. MT plants were sensitive to R, which was intensified under RUV; thus, evaluating their physiological characteristics were difficult.
Different wavelength lights with and without UV-B modify the chloroplast pigment index
Microtomato plants grown under RBW had higher chlorophyll a, b, and total chlorophyll index (Figures 1A–C), compared to plants grown under B.
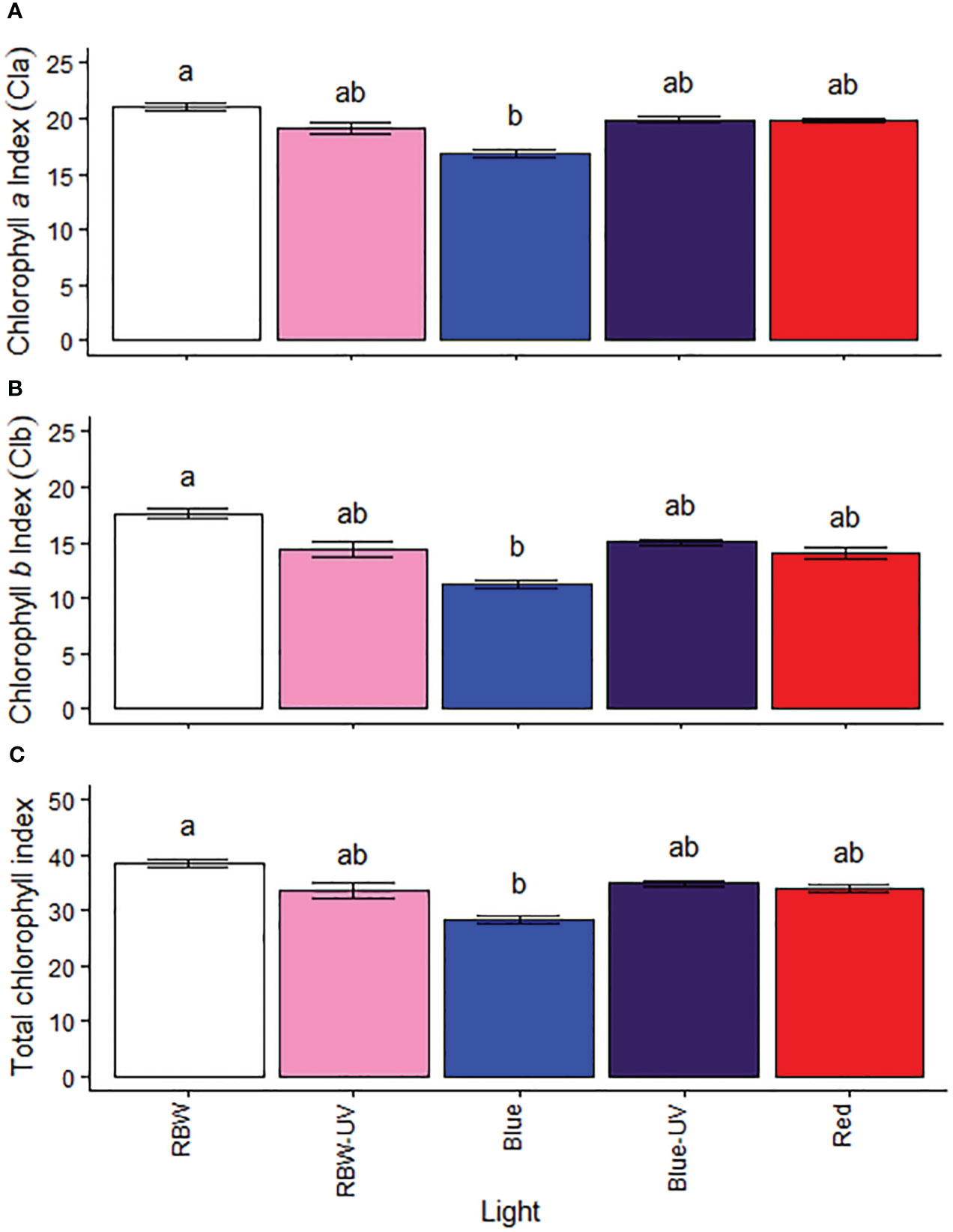
Figure 1 (A) Chlorophyll a, (B) chlorophyll b, and (C) total chlorophyll indexes of microtomato plants after cultivating for 26 days under different light sources with or without UV-B radiation. Means followed by the same letter do not differ by the Dunn’s test at 5% probability.
Different wavelength lights with and without UV-B change the light energy used for photosynthesis
Chlorophyll a fluorescence was measured to estimate the proportion of light used in photosynthetic processes and its regulatory mechanisms. The maximum FSII quantum yield (Fv/Fm) was low in plants grown under R (Figure 2A), indicating sensitivity to light source. However, the plants exposed to BUV and R had lower YII (Figure 2B), photochemical quenching (qP, Figure 2C), and ETR (Figure 2D) than those grown under RBWUV. No difference was observed in quantum yield of regulated energy dissipation (Figure 2E). In response to a low YII, qP, and ETR, plants mainly grown under R showed non-regulated energy dissipation (Figure 2F), while the lowest values were evident under B.
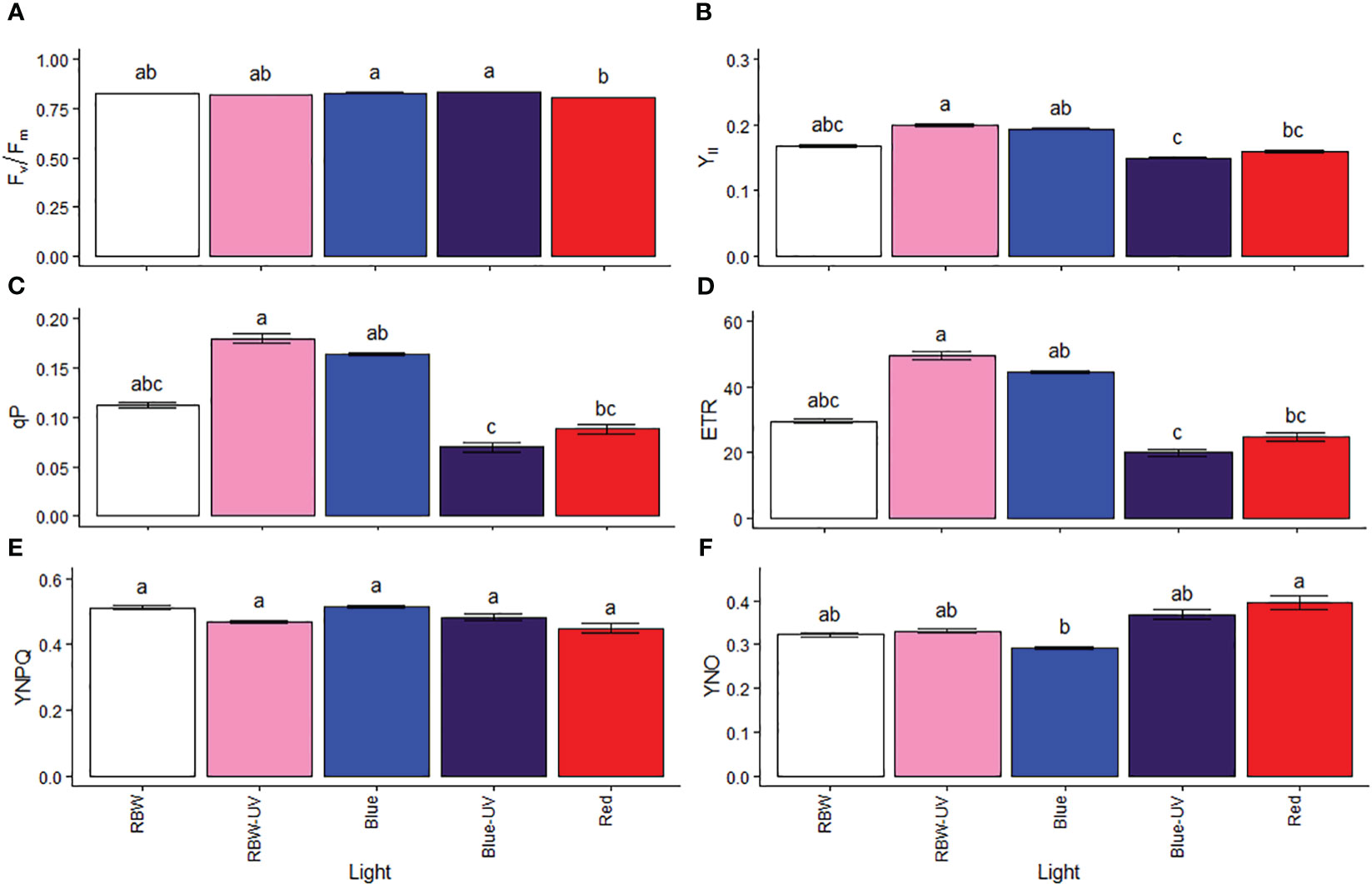
Figure 2 (A) Potential quantum yield of photosystem II (Fv/Fm), (B) effective quantum yield of photosystem II (YII), (C) photochemical quenching (qP), electron transport rate (ETR), quantum yield of regulated energy dissipation (YNPQ), and quantum yield of non-regulated energy dissipation (YNO) in microtomato plants after cultivating for 26 days under different light sources with or without UV-B radiation. Means followed by the same letter do not differ by Dunn’s test at 5% probability.
Different wavelength lights with and without UV-B affect gas exchange
Gas exchange variables showed that plants grown under BUV maintained gs (Figure 3A), resulting in high A (Figure 3B) and E (Figure 3C). Plants grown under RBWUV showed lower Ci than plants grown under BUV (Figure 3D). In turn, the increased ETR/A (Figure 3E) in plants grown under RBWUV may indicate oxidative stress risk.
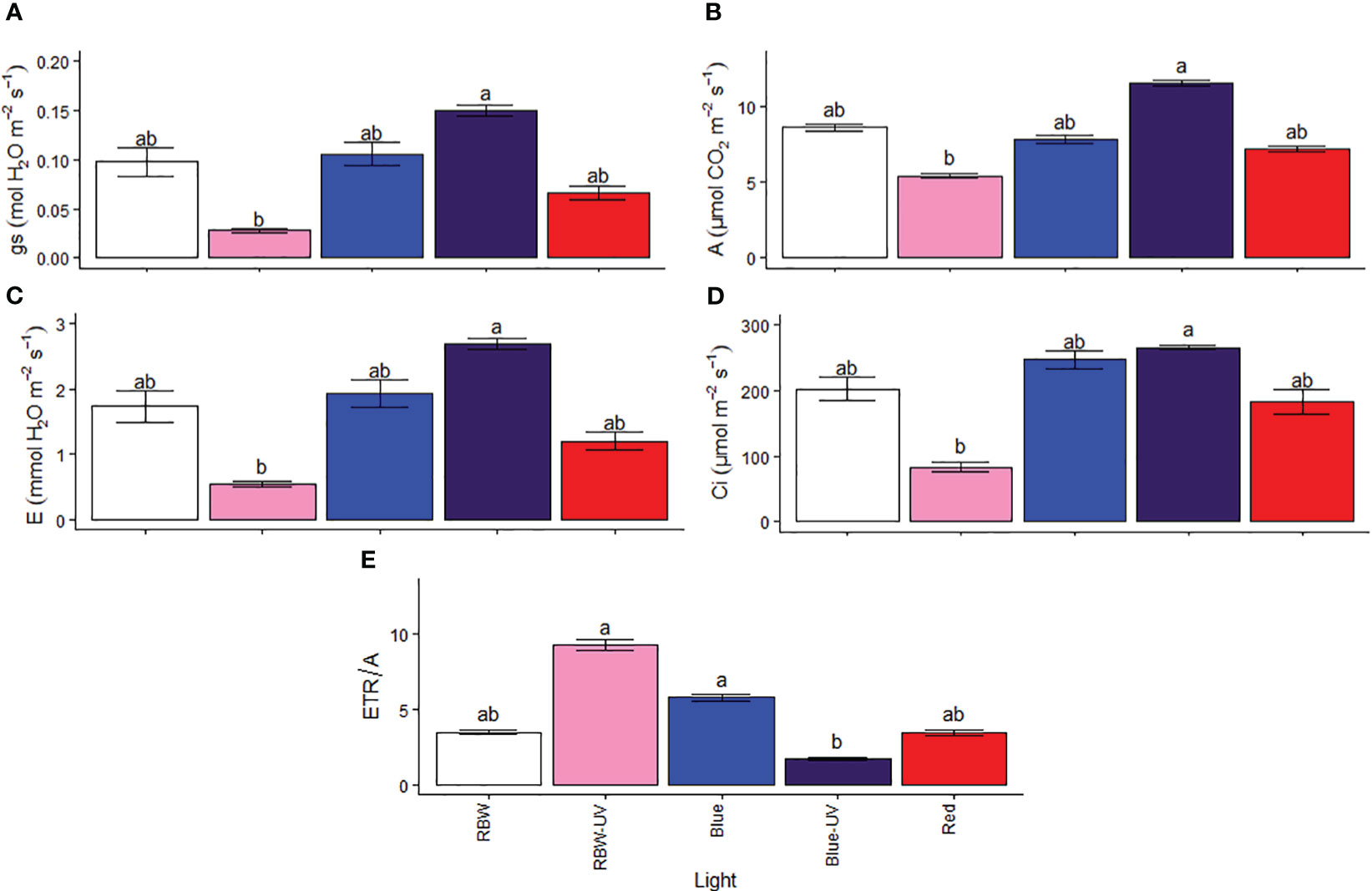
Figure 3 (A) Stomatal conductance (gs), (B) photosynthetic rate (A), (C) transpiration rate (E), (D) internal CO2 concentration (Ci), and (E) electron transport to photosynthetic ratio (ETR/A) for microtomato plants after cultivating for 26 days under different light sources with or without UV-B radiation. Means followed by the same letter do not differ by the Dunn’s test at 5% probability.
Nocturnal respiration rate changes under monochromatic red light
Rn and Rn/A were higher in the plants grown under R, while they were the lowest in the plants grown under BUV (Figures 4A, B).
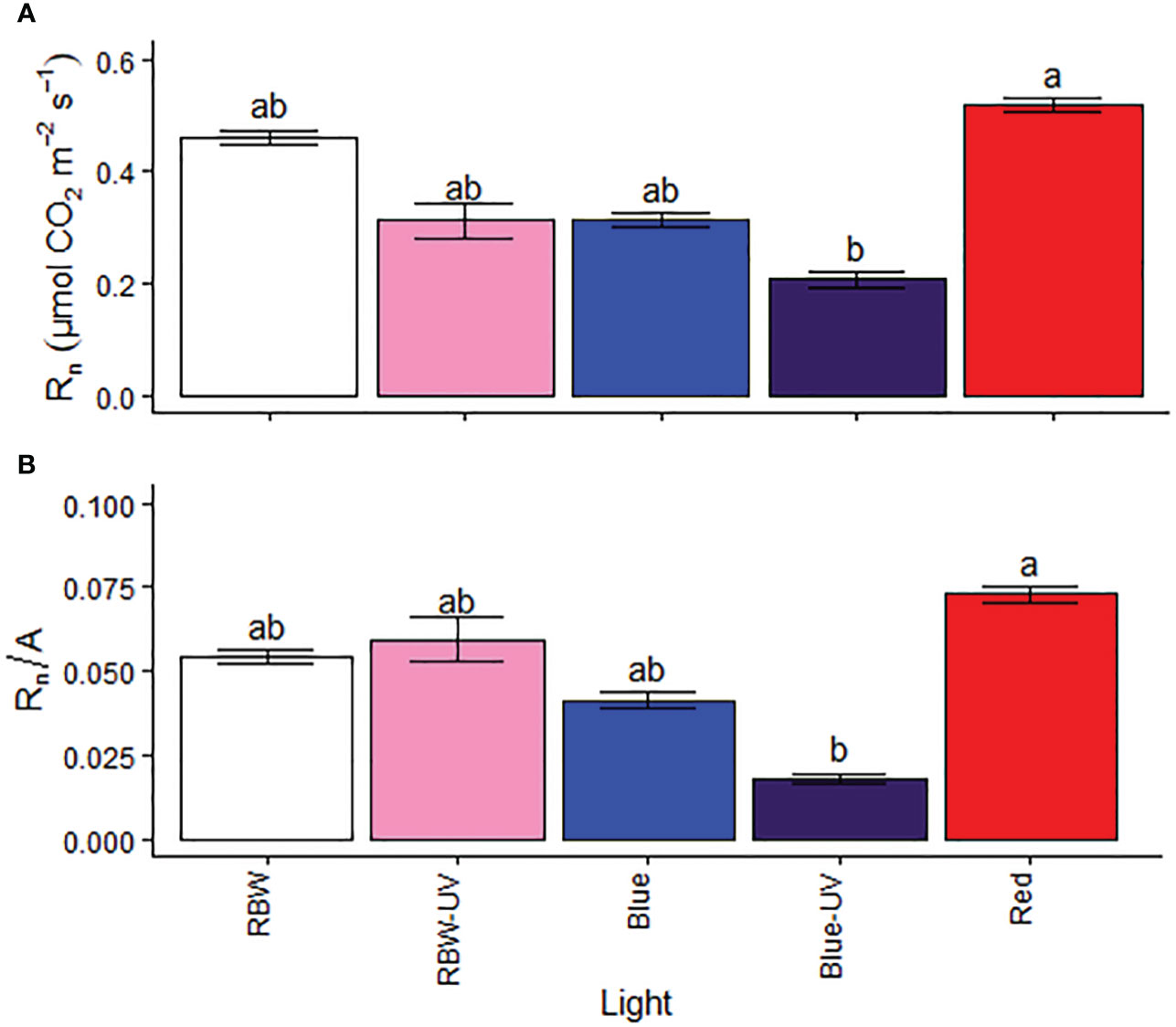
Figure 4 (A) Respiration rate (Rn) and (B) respiration to photosynthetic ratio (Rn/A) of microtomato plants after cultivating for 26 days under different light sources with or without UV-B radiation. Means followed by the same letter do not differ by the Dunn’s test at 5% probability.
Principal component analysis of the physiological variables evaluated
The first two dimensions of the principal components (DIM1 and DIM2) together explained 80.3% (DIM1 47.9% and DIM2 32.4%) data variation (Figures 5A, B). DIM1 variation was mainly explained by the high influence of BUV on the variables A, gs, and E. W influenced chloroplast pigments (Chla, Chlb, and total chlorophyll). B had the greatest influence on the variables F0, ETR, qP, and YII with DIM2. R directly influenced unregulated energy dissipation and negatively influenced Rn (Figure 4A). No variables were influenced by RBWUV. The representation quality of the variable performance (cos², Figure 5B) show that A, gs, E, YII, ETR, qP, Ci, F0, and Chla were well-represented in DIM1 and DIM2. B and BUV were the most dominant contributors (Figure 5C). A high percentage of contribution (Figure 5D) was observed, while Chla, total chlorophyll, YNO, Chlb, YNPQ, and Rn had a low representation (Figure 5B).
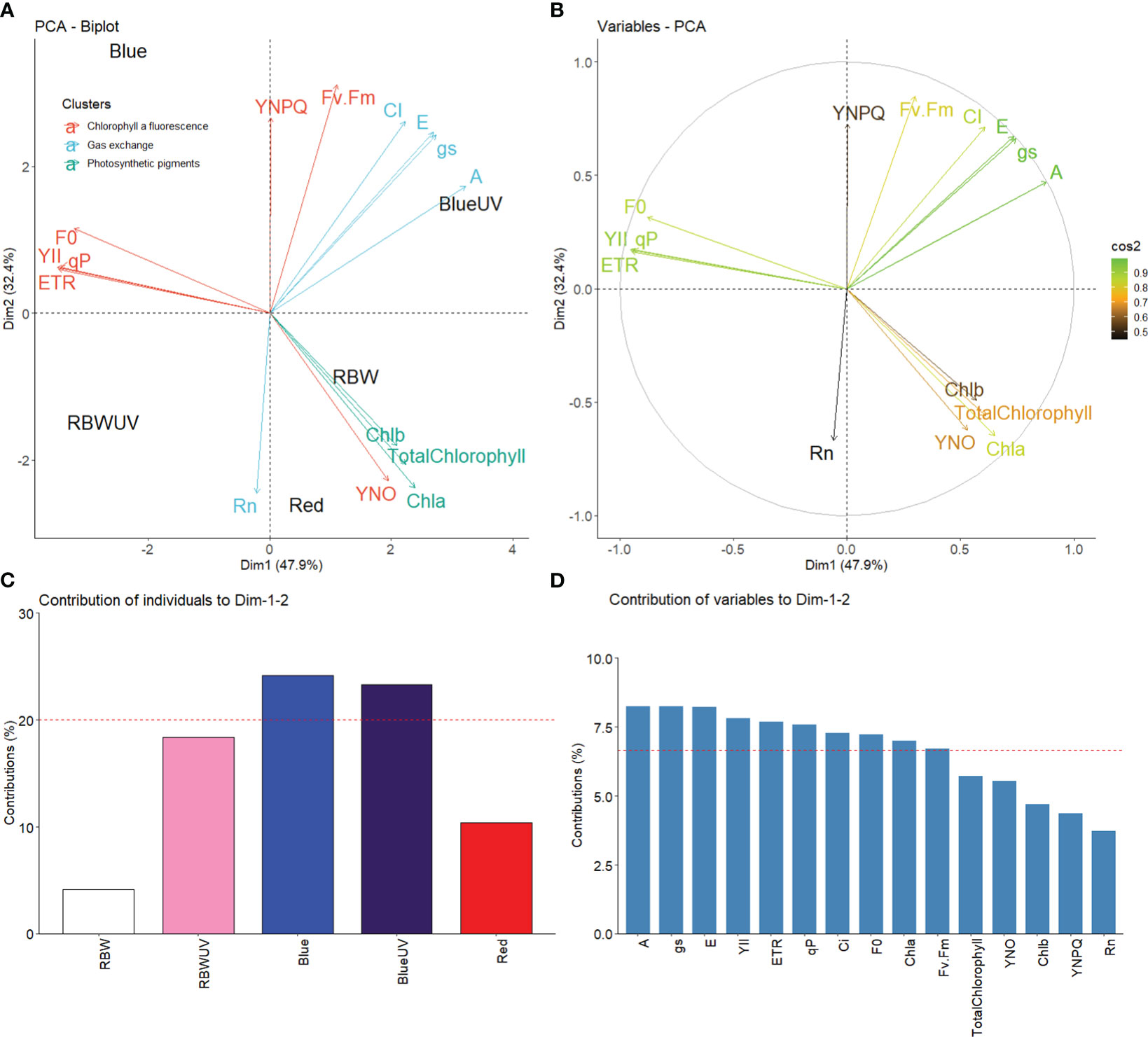
Figure 5 (A) Principal component analysis of microtomato chloroplast pigments, chlorophyll a fluorescence, and gas exchange under different light sources with or without UV-B radiation; (B) representation quality (cos²) of the variables DIM1 and DM2. The color gradient indicates the quality of variable representations; (C) plot of contribution of spectral qualities of light with or without UV-B radiation; (D) plot of the contribution of the variables DIM1 and DIM2. The dashed line indicates the expected measured contribution.
Monochromatic blue light and UV-B radiation improve rutin levels in MT fruit
Rutin content was evaluated in green, orange, and red (ripe) MT fruits, except those grown under RUV, due to plant death. In the chromatographic analyzes for the quantification of rutin (RT = 26.9 min e λMax = 255 e 355 nm), the response of the UV detector at 255 nm was linear from 3,125-100 µg,mL-1. The obtained regression equation was y = 21183x – 9983.1 with a correlation coefficient (R2) of 0.9999 (Supplementary Figures S1–S3). Higher content of rutin was found in fruits at all maturation stages, both in plants under B and BUV lights, highlighting the green fruits grown under B and orange fruits grown under BUV had the highest rutin concentrations (853.141 ± 37.5 and 882.059 ± 62.2 mg.kg-1, respectively) (Figure 6).
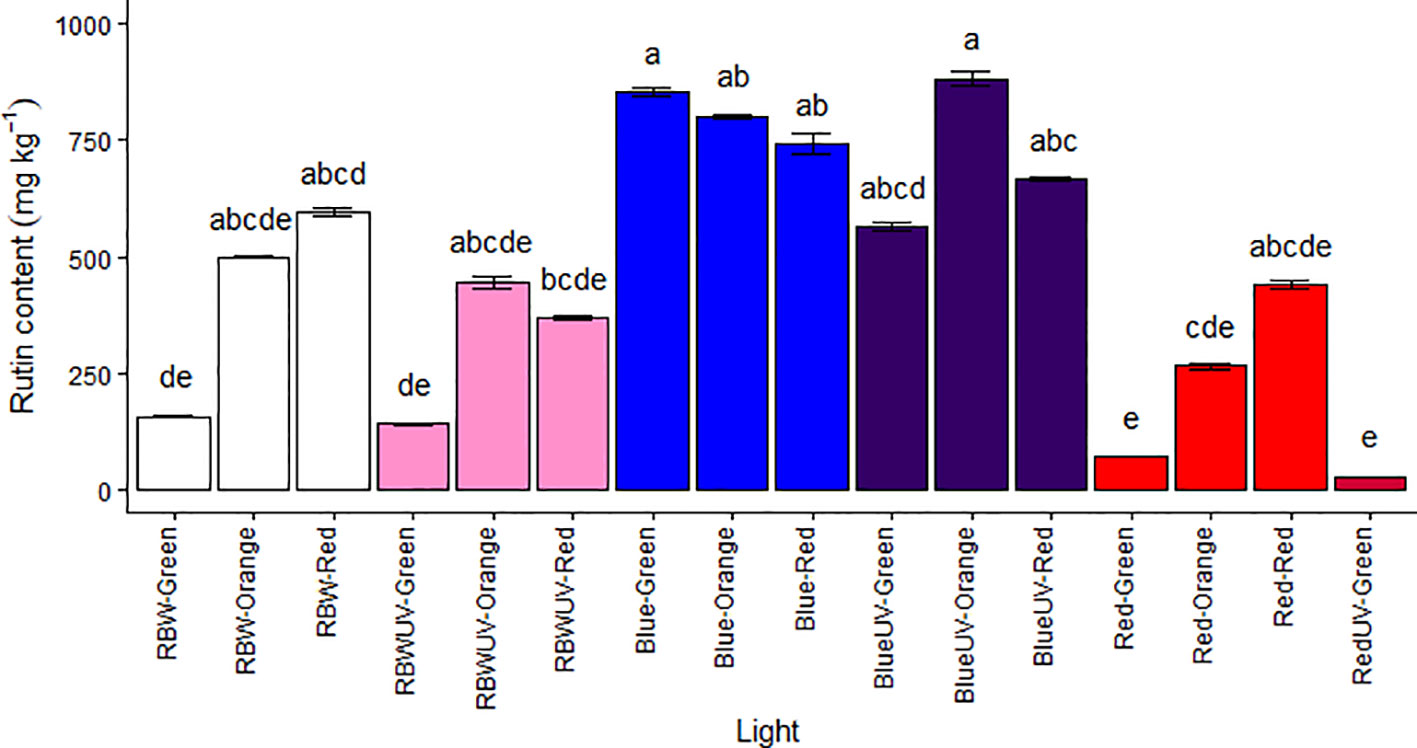
Figure 6 Rutin content (mg·kg−1) in the fruits of microtomato plants subjected to six light treatments [RBW light (RBW), RBW + ultraviolet light (RBWUV), blue light (B), blue light + ultraviolet light (BUV), red light (R), and red light + ultraviolet light (RUV)] at different harvesting stages: green tomato (G), orange tomato (O), and red tomato (R). Means followed by the same letter do not differ by the Dunn’s test at 5% probability.
The response surface graphs for rutin content (mg.kg-1) as function of collection stages and light treatments combined or not with UV show that blue light (represented in the graph by the number 2) was the quality of light that most contributed to production of rutin in the three stages of maturation (Figures S4, S5). Therefore, they corroborate the results shown in figure 6. Moreover, the green fruits grown under R and RUV had the lowest rutin concentrations (68.234 ± 0.352 and 27.357 ± 1.43 mg.kg-1, respectively). Furthermore, the green fruits grown under RBW and RBWUV also had low rutin concentrations (Figure 6 and Supplementary Figures S4, S5).
Discussion
Various studies have focused on the effects of light spectral quality on the physiological and biochemical performance of plants. Improving the production of bioactive compounds, such as rutin, in fruits is a key step toward healthy food production. This study indicated that despite the positive effects of light wavelength and UV-B radiation on rutin concentration in microtomato fruits, the blue light alone and/or associated with UV-B considerably increased its content. This is probably because monochromatic lights affect photosynthetic parameters, morphology, and stomatal opening rates (Liu et al., 2011; XiaoYing et al., 2011; Wang et al., 2016). Lanoue et al. (2017) have reported high E and Ci in tomatoes grown under monochromatic lights, corroborating the results of this study, in which plants grown under BUV had the highest E (Figure 3). Photoreceptors play a fundamental role in plant responses to the environment. Cryptochromes are primarily responsible for mediating blue light-induced changes in gene expression, phenolic compound accumulation, and flowering regulation. Phototropins mediate chloroplast movement and phototropism, both responsible for mediating stoma opening and inhibiting hypocotyl growth. In addition, UVR8 mediates UV-B-induced responses, also changing gene expression and favoring the biosynthesis and accumulation of flavonoids such as rutin (Rai et al., 2021). Blue light-stimulated stomatal opening increases the efficiency of photosynthesis, which are associated with the high absorption capacity of this wavelength by photosynthetic pigments (chlorophylls and carotenoids), leading to decreased chlorophyll content for many species and reduced FSII efficiency (Landi et al., 2020), without affecting CO2 fixation efficiency, as observed in this study. The results indicate that pigment and FSII efficiency reductions were adjustments made by the plants to maintain their high photosynthetic efficiency due to high stoma regulation. In contrast, the plants grown under RBWUV light had low gs and A and high FSII efficiency, ETR, and ETR/A, demonstrating that many electrons are transported for energy and NADPH production, not being used for CO2 fixation. Shadow avoidance syndrome is often observed in plants grown under R and the negative effects attributed to high phytochrome stimulation are observed in plants whose development have been affected by the absence of far-red light (Izzo et al., 2020).
Hoffmann et al. (2015) have reported that high blue light intensity improved the photosynthetic performance of pepper. MT plants grown under BUV showed similar behavior, with higher gs and, consequently, higher A and E than those grown under other wavelengths (Figure 3). This behavior may be related to low photosynthetic pigment degradation and increased production of ultraviolet light-absorbing compounds, which are usually expressed in greater amounts in the presence of both blue light and UV-B light (Nascimento et al., 2013; Ouzounis et al., 2014; Hoffmann et al., 2015). In this study, BUV favored both photosynthetic process and rutin accumulation.
Although the photosynthetic pigments observed in this study (chlorophyll a, b, and total chlorophyll; Figure 4) were not the highest in the plants grown under B, they probably induced the production of other pigments, such as carotenoids, which are accessory pigments that invariably increase in response to UV-B because they are associated with light absorption in this spectrum (Kurinjimalar et al., 2019). Palma et al. (2021) have reported that the chlorophyll content variation in peppers treated with associated UV-B and white light is similar to those reported in this study, as the plants grown under RBW had the highest chlorophyll content (Figure 4). Although contrary results have been reported in several previous studies, they focus on the use of different UV-B dosages, and the different light to UV-B ratio can lead to different results.
Yang et al. (2018) reported that YII decreased and YNO increased in tomato plants grown under blue and purple light with increasing light intensity compared to those grown under white light. Moreover, the authors reported increased non-photochemically dissipated YNPQ in plants grown under blue and purple light, which indicates photoprotection induction. The results obtained in this study showed similar behavior in microtomato plants grown under BUV with reduced YII and increased YNO, suggesting that the photoprotective capacity of plants grown under BUV improved their development and the accumulation of rutin, a photoprotective metabolite.
According to Igamberdiev et al. (2014), respiration and photorespiration are regulated by gene expression, oxidation state changes, metabolites produced by the photosynthetic process, and photorespiration. Phytochromes mediate the expression of these genes, proving that light perception plays a crucial role in these steps. The high respiration rates in plants grown under R reported in this study indicate high energy demand to handle the stress imposed by this wavelength.
Phenolic compounds are influenced by genetic factors, being associated with the ripening process and phenolic compound metabolism (Scalzo et al., 2005; Atkinson et al., 2006; Paula et al., 2015). Several factors, such as pest attacks, weather stress, ultraviolet radiation, cultivation site, agricultural practices, harvest and storage, processing and preparation conditions and methods, analytical variability, can cause the quantitative variation of a specific flavonoid in a plant; moreover, location and cultivar accounts for 25% and 33% variability, respectively (Haytowitz et al., 2013; Verma et al., 2019). This study confirmed and quantified high rutin contents, particularly in plants grown under B and BUV. Several studies reported similar results, with rutin being the main phenolic compound quantified (Blasa et al., 2010; Vallverdú-Queralt et al., 2012; Flores et al., 2021). Durán et al. (2018) reported higher phenolic compound accumulation in tomato peel than those in seeds and pulp. The whole fruit was analyzed in this study.
Rutin is a compound found in asparagus, buckwheat, Fava d’anta fruit, blackberry, rue, apple, passion fruit flower, grape, and citrus fruits such as orange, lime, lemon, grapefruit. Buckwheat is considered the main rutin source, which despite being present in most plants, is rarely found in the edible parts (Patel & Patel, 2019). Polyphenolic compounds are poorly absorbed or metabolized before reaching their sites of action, due to their low water solubility. Its bioavailability is low in the gastrointestinal tract; thus, approximately 500 mg rutin should be consumed daily, distributed twice a day, to provide its beneficial effects (Remanan & Zhu, 2021). The extraction of bioactive compounds such as rutin requires care, and a series of steps, such as efficiency, cost-effectiveness, and molecule integrity, must be considered for its preservation in the plant (Gullón et al., 2017). Thus, producing fruits with high rutin content is an excellent advantage since it provides health benefits without the need to be extracted. Naoya Fukuda et al. (2022) observed increased expression of genes regulated by blue light. Cryptochromes can induce reactive oxygen species (ROS) synthesis to adapt to stressful environments. Under stress conditions, they can produce phenolic compounds through flavonoid activation and phenylpropanoid biosynthesis. This defense mechanism corroborates the results observed in this study, because the plants grown under B and BUV had high rutin content.
The results of this study show that MT cultivation under blue light with or without UV increases the photosynthetic rate and bioactive compound production. Derived from primary metabolism, compounds that are part of a specialized metabolism use the carbon skeletons generated mainly by the photosynthetic process to be produced. Furthermore, light is an effective elicitor for the increased production of these molecules, as demonstrated in this study and corroborated by many others. The results shown here are fundamental to understanding the influence of different light wavelengths on different crops. In addition, producing bioactive compound-rich foods is important to improve the quality of life of the population that will consume these foods. These results provide a theoretical basis to further elucidate the mechanism behind rutin synthesis regulation by blue light and associated UV-B in tomatoes and establish a new generation of technological innovations for enhancing specialized metabolite production in crops. This work opens the opportunity to improve the nutraceutical quality of fruits, working with the manipulation of phytochromes and with plants more responsive to UVR8 receptors. Furthermore, in future studies it would be possible to verify the effects of increased light intensity, as well as to vary the time of exposure to UV-B to obtain new responses, in addition to those observed in this study. Thus, the tolerable limit for the microtomato plant could be identified, without observing deleterious effects on plants.
Data availability statement
The original contributions presented in the study are included in the article/Supplementary Material. Further inquiries can be directed to the corresponding authors.
Author contributions
IL: Formal Analysis, Investigation, Methodology, Writing – original draft. AA: Investigation, Methodology, Writing – original draft. ER: Investigation, Methodology, Writing – review & editing. FBS: Investigation, Writing – review & editing. FF: Investigation, Writing – review & editing, Formal Analysis. LS: Investigation, Resources, Writing – original draft. MRo: Writing – original draft, Conceptualization, Methodology. MRe: Writing – original draft, Data curation, Formal Analysis. LB: Data curation, Formal Analysis, Writing – original draft. TO: Resources, Writing – original draft, Investigation. AJ: Conceptualization, Supervision, Visualization, Writing – review & editing. FGS: Conceptualization, Supervision, Writing – review & editing, Funding acquisition.
Funding
The author(s) declare financial support was received for the research, authorship, and/or publication of this article. The project was funded by the Coordination for the Improvement of Higher Education Personnel (CAPES, Finance Code 001), The National Council for Scientific and Technological Development (CNPq, Grant # 408285/2021-4), and by the Financier of Studies and Projects/ the Research Support Foundation of the State of Goiás (FINEP/FAPEG Tecnova II Grant # 202010267000346).
Acknowledgments
The authors thank the Goiano Federal Institute of Education, Science and Technology - Rio Verde CAMPUS, the Coordination for the Improvement of Higher Education Personnel (CAPES), The National Council for Scientific and Technological Development (CNPq), and the Financier of Studies and Projects (FINEP), the Research Support Foundation of the State of Goiás (FAPEG) for the funding, and SpectraGrow Inc. represented by Audax Electronics for the partnership in the supply of LEDs lights.
Conflict of interest
The authors declare that the research was conducted in the absence of any commercial or financial relationships that could be construed as a potential conflict of interest.
Publisher’s note
All claims expressed in this article are solely those of the authors and do not necessarily represent those of their affiliated organizations, or those of the publisher, the editors and the reviewers. Any product that may be evaluated in this article, or claim that may be made by its manufacturer, is not guaranteed or endorsed by the publisher.
Supplementary material
The Supplementary Material for this article can be found online at: https://www.frontiersin.org/articles/10.3389/fpls.2023.1261174/full#supplementary-material
References
Atkinson, C. J., Dodds, P. A., Ford, Y. Y., Le Mière, J., Taylor, J. M., Blake, P. S., et al. (2006). Effects of cultivar, fruit number and reflected photosynthetically active radiation on Fragaria x ananassa productivity and fruit ellagic acid and ascorbic acid concentrations. Ann. Bot. 97, 429–441. doi: 10.1093/aob/mcj046
Benjamini, Y., Hochberg, Y. (1995). Controlling the false discovery rate: a practical and powerful approach to multiple testing. J. R. Stat. Soc Ser. B Stat. Methodol. 57, 289–300. doi: 10.2307/2346101
Bian, Z. H., Yang, Q. C., Liu, W. K. (2015). Effects of light quality on the accumulation of phytochemicals in vegetables produced in controlled environments: A review. J. Sci. Food Agric. 95, 869–877. doi: 10.1002/jsfa.6789
Bilger, W., Schreiber, U., Bock, M. (1995). Determina,on of the quantum efficiency of photosystem II and of non-photochemical quenching of chlorophyll fluorescence in the field. Oecologia 102, 425–432. doi: 10.1007/BF00341354
Blasa, M., Gennari, L., Angelino, D., Ninfali, P. (2010). “Fruit and vegetable antioxidants in health,” in Bioactive foods in promoting health. Eds. Watson, R. R., Preedy, V. R. (Amsterdam: Academic Press), 37–58. doi: 10.1016/B978-0-12-374628-3.00003-7
Chua, L. S. (2013). A review on plant-based rutin extraction methods and its pharmacological activities. J. Ethnopharmacol. 150, 805–817. doi: 10.1016/j.jep.2013.10.036
Dunn, O. J. (1964). Multiple comparisons using rank sums. Technometrics. 6, 241–252. doi: 10.2307/1266041
Durán, D. I. R., Cárdenas, S. A., Martinez, P. R., Ochoa, F. G., del Socorro López Cortez, M. (2018). Antioxidant content in skin and seeds of three tomato varieties grown in two regions of Mexico. Int. J. Food Nutr. Sci. 7, 1–14.
Fang, M.-H., De Guzman, G. N. A., Bao, Z., Majewska, N., Mahlik, S., Grinberg, M., et al. (2020). Ultra-high-efficiency near-infrared Ga2O3:Cr3+phosphor and controlling of phytochrome. J. Mater. Chem. C. 8, 11013–11017. doi: 10.1039/d0tc02705g
Flores, I. R., Vásquez-Murrieta, M. S., Franco-Hernández, M. O., Márquez-Herrera, C. E., Ponce-Mendoza, A., del Socorro López-Cortéz, M. (2021). Bioactive compounds in tomato (Solanum lycopersicum) variety saladette and their relationship with soil mineral content. Food Chem. 344, 128608. doi: 10.1016/j.foodchem.2020.128608
Garagounis, C., Delkis, N., Papadopoulou, K. K. (2021). Unraveling the roles of plant specialized metabolites: using synthetic biology to design molecular biosensors. New Phytol. 231 (4), 1338–1352. doi: 10.1111/nph.17470
Geissler, N., Hussin, S., El-Far, M. M. M., Koyro, H. W. (2015). Elevated atmospheric CO2 concentra,on leads to different salt resistance mechanisms in a C3 (Chenopodium quinoa) and a C4 (Atriplex nummularia) halophyte. Environ Exp Bot 118, 67–77. doi: 10.1016/j.envexpbot.2015.06.003
Genty, B., Briantais, J. M., Baker, N. R. (1989). The relationship between the quantum yield of photosynthetic electron transport and quenching of chlorophyll fluorescence. Biochim Biophys Acta Gen Subj 990, 87–92. doi: 10.1016/S0304-4165(89)80016-9
Gullón, B., Lú-Chau, T. A., Moreira, M. T., Lema, J. M., Eibes, G. (2017). Rutin: A review on extraction, identification and purification methods, biological activities and approaches to enhance its bioavailability. Trends Food Sci. Technol. 67, 220–235. doi: 10.1016/j.tifs.2017.07.008
Haytowitz, D. B., Bhagwat, S., Holden, J. M. (2013). Sources of variability in the flavonoid content of foods. Proc. Food Sci. 2, 46–51. doi: 10.1016/j.profoo.2013.04.008
Hendrickson, L., Furbank, R. T., Chow, W. S. (2004). A simple alternative approach to assessing the fate of absorbed light energy using chlorophyll fluorescencee. Photosynth Res 82, 73–81. doi: 10.1023/B:PRES.0000040446.87305.f4
Hoffmann, A. M., Noga, G., Hunsche, M. (2015). High blue light improves acclimation and photosynthetic recovery of pepper plants exposed to UV stress. Environ. Exp. Bot. 109, 254–263. doi: 10.1016/j.envexpbot.2014.06.017
Igamberdiev, A. U., Eprintsev, A. T., Fedorin, D. N., Popov, V. N. (2014). Phytochrome-mediated regulation of plant respiration and photorespiration. Plant Cell Environ. 37, 290–299. doi: 10.1111/pce.12155
Izzo, L. G., Mele, B. H., Vitale, L., Vitale, E., Arena, C. (2020). The role of monochromatic red and blue light in tomato early photomorphogenesis and photosynthetic traits. Environ. Exp. Bot. 179, 104195. doi: 10.1016/j.envexpbot.2020.104195
Johkan, M., Shoji, K., Goto, F., Hashida, S.-N., Yoshihara, T. (2010). Blue light-emitting diode light irradiation of seedlings improves seedling quality and growth after transplanting in red leaf lettuce. HortScience 45, 1809–1814. doi: 10.21273/hortsci.45.12.1809
Ke, X., Yoshida, H., Hikosaka, S., Goto, E. (2022). Optimization of photosynthetic photon flux density and light quality for increasing radiation-use efficiency in dwarf tomato under led light at the vegetative growth stage. Plants 11, 121. doi: 10.3390/plants11010121
Ke, X., Yoshida, H., Hikosaka, S., Goto, E. (2023). Photosynthetic photon flux density affects fruit biomass radiation-use efficiency of dwarf tomatoes under LED light at the reproductive growth stage. Front. Plant Sci. 14. doi: 10.3389/fpls.2023.1076423
Kianersi, F., Abdollahi, M. R., Mirzaie-Asl, A., Dastan, D., Rasheed, F. (2020). Identification and tissue-specific expression of rutin biosynthetic pathway genes in Capparis spinosa elicited with salicylic acid and methyl jasmonate. Sci. Rep. 10, 1–15. doi: 10.1038/s41598-020-65815-2
Kim, Y. J., Kim, H. M., Kim, H. M., Jeong, B. R., Lee, H.-J., Kim, H.-J., et al. (2018). Ice plant growth and phytochemical concentrations are affected by light quality and intensity of monochromatic light-emitting diodes. Hortic. Environ. Biotechnol. 59, 529–536. doi: 10.1007/s13580-018-0058-3
Kim, Y. J., Kim, Y. B., Li, X., Choi, S. R., Park, S., Park, J. S., et al. (2015). Accumulation of phenylpropanoids by white, blue, and red light irradiation and their organ-specific distribution in Chinese cabbage (Brassica rapa ssp. pekinensis). J. Agric. Food Chem. 63, 6772–6778. doi: 10.1021/acs.jafc.5b02086
Ko, E. Y., Nile, S. H., Sharma, K., Li, G. H., Parka, S. W. (2015). Effect of different exposed lights on quercetin and quercetin glucoside content in onion (Allium cepa L.). Saudi J. Biol. Sci. 22, 398–403. doi: 10.1016/j.sjbs.2014.11.012
Kurinjimalar, C., Ganapathy, K., Rangaraja, T., Govindaswamy, K., Ramasamy, R. (2019). Impact of ultraviolet-B radiation on growth and biochemical composition of Botryococcus braunii Kutz. Curr. Sci. 116, 89–95. doi: 10.18520/cs/v116/i1/89-95
Laisk, A., Loreto, F. (1996). Determining photosynthetic parameters from leaf CO2 exchange and chlorophyll fluorescence (Ribulose-1,5-Bisphosphate Carboxylase/Oxygenase Specificity Factor, Dark Respiration in the Light, Excitation Distribution between Photosystems, Alternative Electron Transport Rate, and Mesophyll Diffusion Resistance). Plant Physiol. 110, 903–912. doi: 10.1104/pp.110.3.903
Landi, M., Zivcak, M., Sytar, O., Brestic, M., Allakhverdiev, S. I. (2020). Plasticity of photosynthetic processes and the accumulation of secondary metabolites in plants in response to monochromatic light environments: A review. Biochimica et Biophysica Acta - BioenergeBcs. 1861, 148131. doi: 10.1016/j.bbabio.2019.148131
Lanoue, J., Leonardos, E. D., Ma, X., Grodzinski, B. (2017). The effect of spectral quality on daily patterns of gas exchange, biomass gain, and water-use-efficiency in tomatoes and lisianthus: An assessment of whole plant measurements. Front. Plant Sci. 8. doi: 10.3389/fpls.2017.01076
Li, Q., Kubota, C. (2009). Effects of supplemental light quality on growth and phytochemicals of baby leaf lettuce. Environ. Exp. Bot. 67, 59–64. doi: 10.1016/j.envexpbot.2009.06.011
Liu, X. Y., Chang, T. T., Guo, S. R., Xu, Z. G., Li, J. (2011). Effect of different light quality of LED on growth and photosynthetic character in cherry tomato seedling. Acta Hortic. 907, 325–330. doi: 10.17660/ActaHortic.2011.907.53
Liu, H. K., Chen, Y. Y., Hu, T. T., Zhang, S. J., Zhang, Y. H., Zhao, T. Y., et al. (2016). The influence of light-emitting diodes on the phenolic compounds and antioxidant activities in pea sprouts. J. Funct. Foods. 25, 459–465. doi: 10.1016/j.jff.2016.06.028
Liu, Y., Fang, S., Yang, W., Shang, X., Fu, X. (2018). Light quality affects flavonoid production and related gene expression in Cyclocarya paliurus. J. Photochem. Photobiol. B. 179, 66–73. doi: 10.1016/j.jphotobiol.2018.01.002
Liu, H. K., Kang, Y. F., Zhao, X. Y., Liu, Y. P., Zhang, X. W., Zhang, S. (2019). Effects of elicitation on bioactive compounds and biological activities of sprouts. J. Funct. Foods. 53, 136–145. doi: 10.1016/j.jff.2018.12.019
Lobiuc, A., Vasilache, V., Pintilie, O., Stoleru, T., Burducea, M., Oroian, M., et al. (2017). Blue and red LED illumination improves growth and bioactive compounds contents in acyanic and cyanic Ocimum basilicum L. Microgreens. Molecules 22, 2111. doi: 10.3390/molecules22122111
Mata-Nicolás, E., Montero-Pau, J., Gimeno-Paez, E., Garcia-Carpintero, V., Ziarsolo, P., Menda, N., et al. (2020). Exploiting the diversity of tomato: the development of a phenotypically and genetically detailed germplasm collection. Hortic. Res. 7, 66. doi: 10.1038/s41438-020-0291-7
Matos, F. S., Wolfgramm, R., Gonçalves, F. V., Cavatte, P. C., Ventrella, M. C., DaMatta, F. M. (2009). Phenotypic plasticity in response to light in the coffee tree. Environ. Exp. Bot. 67, 421–427. doi: 10.1016/j.envexpbot.2009.06.018
Mosadegh, H., Trivellini, A., Ferrante, A., Lucchesini, M., Vernieri, P., Mensuali, A. (2018). Applications of UV-B lighting to enhance phenolic accumulation of sweet basil. Sci. Hortic. 229, 107–116. doi: 10.1016/j.scienta.2017.10.043
Nam, T. G., Kim, D. O., Eom, S. H. (2018). Effects of light sources on major flavonoids and antioxidant activity in common buckwheat sprouts. Food Sci. Biotechnol. 27, 169–176. doi: 10.1007/s10068-017-0204-1
Naoya Fukuda, M. E., Yoshida, H., Kusano, M. (2022). Effects of light quality, photoperiod, CO2 concentration, and air temperature on chlorogenic acid and rutin accumulation in young lettuce plants. Plant Physiol. Biochem. 186, 290–298. doi: 10.1016/j.plaphy.2022.07.010
Nascimento, L. B. S., Leal-Costa, M. V., Coutinho, M. A., Moreira, N., Lage, C. L., dosS., N., et al. (2013). Increased antioxidant activity and changes in phenolic profile of Kalanchoe pinnata (Lamarck) Persoon (Crassulaceae) specimens grown under supplemental blue light. Photochem. Photobiol. 89, 391–399. doi: 10.1111/php.12006
Neugart, S., Schreiner, M. (2018). UVB and UVA as eustressors in horticultural and agricultural crops. Sci. Hortic. 234, 370–381. doi: 10.1016/j.scienta.2018.02.021
Nocchi, N., Duarte, H. M., Pereira, R. C., Konno, T. U. P., Soares, A. R. (2020). Effects of UV-B radiation on secondary metabolite production, antioxidant activity, photosynthesis and herbivory interactions in Nymphoides humboldtiana (Menyanthaceae). J. Photochem. Photobiol. B 212, 112021. doi: 10.1016/J.JPHOTOBIOL.2020.112021
Ogle, D., Wheeler, P., Dinno, A. (2020) FSA: Simple fisheries stock assessment methods. Available at: https://cran.r-project.org/web/packages/FSA/index.html (Accessed February 2, 2023).
Ouzounis, T., Fretté, X., Rosenqvist, E., Ottosen, C. O. (2014). Spectral effects of supplementary lighting on the secondary metabolites in roses, chrysanthemums, and campanulas. J. Plant Physiol. 171, 1491–1499. doi: 10.1016/j.jplph.2014.06.012
Palma, C. F. F., Castro-Alves, V., Morales, L. O., Rosenqvist, E., Ottosen, C. O., Strid, Å. (2021). Spectral composition of light affects sensitivity to UV-B and photoinhibition in cucumber. Front. Plant Sci. 11. doi: 10.3389/fpls.2020.610011
Pan, T., Wang, Y., Wang, L., Ding, J., Cao, Y., Qin, G., et al. (2020). Increased CO2 and light intensity regulate growth and leaf gas exchange in tomato. Physiologia Plantarum 168 (3), 694–708. doi: 10.1111/ppl.13015
Patel, K., Patel, D. K. (2019). “The beneficial role of rutin, a naturally occurring flavonoid in health promotion and disease prevention: A systematic review and update,” in Bioactive food as dietary interventions for arthritis and related inflammatory diseases. Eds. Watson, R. R., Preedy, V. R. (Amsterdam: Elsevier Inc), 457–479. doi: 10.1016/B978-0-12-813820-5.00026-X
Paula, J. T., Resende, J. T. V., Faria, M. V., Figueiredo, A. S. T., Schwarz, K., Neumann, E. R. (2015). Características físico-químicas e compostos bioativos em frutos de tomateiro colhidos em diferentes estádios de maturação (Physicochemical characteristics and bioactive compounds in tomato fruits harvested at different ripeness stages). Hortic. Bras. 33, 434–440. doi: 10.1590/s0102-053620150000400005
Pohlert, T. (2018) PMCMRplus: calculate pairwise multiple comparisons of mean rank sums extended. Available at: https://CRAN.Rproject.org/package=PMCMRplus (Accessed February 2, 2023).
R Core Team (2023) The R project for statistical computing. Available at: https://www.R-project.org/ (Accessed February 2, 2023).
Rai, N., Morales, L. O., Aphalo, P. J. (2021). Perception of solar UV radiation by plants: photoreceptors and mechanisms. Plant Physiol. 186, 1382–1396. doi: 10.1093/PLPHYS/KIAB162
Remanan, M. K., Zhu, F. (2021). Encapsulation of rutin using quinoa and maize starch nanoparticles. Food Chem. 353, 128534. doi: 10.1016/j.foodchem.2020.128534
Ribeiro, V. R., Mari, I. P., Stafussa, A. P., Rossetto, R., Maciel, G. M. (2019). Bringing together Saccharomyces cerevisiae and bioactive compounds from plants: A new function for a well-known biosorbent. J. Funct. Foods. 60, 103433. doi: 10.1016/j.jff.2019.103433
Ronga, D., Caradonia, F., Parisi, M., Bezzi, G., Parisi, B., Allesina, G., et al. (2020). Using digestate and biochar as fertilizers to improve processing tomato production sustainability. Agronomy 10, 138. doi: 10.3390/agronomy10010138
Scalzo, J., Politi, A., Pellegrini, N., Mezzetti, B., Battino, M. (2005). Plant genotype affects total antioxidant capacity and phenolic contents in fruit. Nutrition. 21, 207–213. doi: 10.1016/j.nut.2004.03.025
Semwal, R., Joshi, S. K., Semwal, R. B., Semwal, D. K. (2021). Health benefits and limitations of rutin - A natural flavonoid with high nutraceutical value. Phytochem. Lett. 46, 119–128. doi: 10.1016/j.phytol.2021.10.006
Shafiq, I., Hussain, S., Raza, M. A., Iqbal, N., Asghar, M. A., Raza, A., et al. (2021). ‘Crop photosynthetic response to light quality and light intensity’. J. Integr. Agric. 20 (1), 4–23. doi: 10.1016/S2095-3119(20)63227-0
Shih, M. L., Morgan, J. A. (2020). Metabolic flux analysis of secondary metabolism in plants. Metab. Eng. Commun. 10, e00123. doi: 10.1016/j.mec.2020.e00123
Silva, T. D., Batista, D. S., Fortini, E. A., Castro, K. M., Felipe, S. H. S., Fernandes, A. M., et al. (2020). Blue and red light affects morphogenesis and 20-hydroxyecdisone content of in vitro Pfaffia glomerata accessions. J. Photochem. Photobiol. B. 203, 111761. doi: 10.1016/j.jphotobiol.2019.111761
Suzuki, T., Honda, Y., Mukasa, Y. (2005). Effects of UV-B radiation, cold and desiccation stress on rutin concentration and rutin glucosidase activity in tartary buckwheat (Fagopyrum tataricum) leaves. Plant Sci. 168, 1303–1307. doi: 10.1016/j.plantsci.2005.01.007
Taulavuori, K., Hyöky, V., Oksanen, J., Taulavuori, E., Julkunen-Tiitto, R. (2016). Species-specific differences in synthesis of flavonoids and phenolic acids under increasing periods of enhanced blue light. Environ. Exp. Bot. 121, 145–150. doi: 10.1016/j.envexpbot.2015.04.002
Taulavuori, K., Pyysalo, A., Taulavuori, E., Julkunen-Tiitto, R. (2018). Responses of phenolic acid and flavonoid synthesis to blue and blue-violet light depend on plant species. Environ. Exp. Bot. 150, 183–187. doi: 10.1016/j.envexpbot.2018.03.016
Thoma, F., Somborn-Schulz, A., Schlehuber, D., Keuter, V., Deerberg, G. (2020). Effects of light on secondary metabolites in selected leafy greens: A review. Front. Plant Sci. 11. doi: 10.3389/fpls.2020.00497
Thwe, A. A., Kim, Y. B., Li, X., Seo, J. M., Kim, S. J., Suzuki, T., et al. (2014). Effects of light-emitting diodes on expression of phenylpropanoid biosynthetic genes and accumulation of phenylpropanoids in Fagopyrum tataricum sprouts. J. Agric. Food Chem. 62, 4839–4845. doi: 10.1021/jf501335q
Vallverdú-Queralt, A., Jáuregui, O., Medina-Remón, A., Lamuela-Raventós, R. M. (2012). Evaluation of a method to characterize the phenolic profile of organic and conventional tomatoes. J. Agric. Food Chem. 60, 3373–3380. doi: 10.1021/jf204702f
Verma, M. L., Sharma, S., Saini, R., Rani, V., Kushwaha, R. (2019). “Bioflavonoids: Synthesis, functions and biotechnological applications,” in Biotechnological production of bioactive compounds. Eds. Verma, M. L., Chandel, A. K. (Amsterdam: Elsevier), 69–105. doi: 10.1016/B978-0-444-64323-0.00003-5
Wang, P., Chen, S., Gu, M., Chen, X., Chen, X., Yang, J., et al. (2020). Exploration of the effects of different blue led light intensities on flavonoid and lipid metabolism in tea plants via transcriptomics and metabolomics. Int. J. Mol. Sci. 21, 1–18. doi: 10.3390/ijms21134606
Wang, J., Lu, W., Tong, Y., Yang, Q. (2016). Leaf morphology, photosynthetic performance, chlorophyll fluorescence, stomatal development of lettuce (Lactuca sativa L.) exposed to different ratios of red light to blue light. Front. Plant Sci. 7. doi: 10.3389/fpls.2016.00250
Wickham, H. (2011). ggplot2. Wiley interdiscip. Rev. Comput. Stat. 3, 180–185. doi: 10.1002/wics.147
Wu, M.-C., Hou, C.-Y., Jiang, C.-M., Wang, Y.-T., Wang, C.-Y., Chen, H.-H., et al. (2007). A novel approach of LED light radiation improves the antioxidant activity of pea seedlings. Food Chem. 101, 1753–1758. doi: 10.1016/j.foodchem.2006.02.010
XiaoYing, L., ShiRong, G., ZhiGang, X., XueLei, J., Tezuka, T. (2011). Regulation of chloroplast ultrastructure, cross-section anatomy of leaves, and morphology of stomata of cherry tomato by different light irradiations of light-emitting diodes. HortScience 46, 217–221. doi: 10.21273/hortsci.46.2.217
Yang, X., Hui, X. H., Shao, L., Li, T., Wang, Y., Wang, R. (2018). Response of photosynthetic capacity of tomato leaves to different LED light wavelength. Environ. Exp. Bot. 150, 161–171. doi: 10.1016/j.envexpbot.2018.03.013
Yasar, F., Uzal, O. (2023). Oxidative Stress and Antioxidant Enzyme Activities in Tomato (Solanum lycopersicum) Plants Grown at Two Different Light Intensities. Gesunde Pflanzen 75 (3), 479–485. doi: 10.1007/s10343-022-00716-0
Keywords: flavonoids, LEDs, tomato, rutin, vertical agriculture, Solanum lycopersicum
Citation: Lima IHA, Rodrigues AA, Resende EC, da Silva FB, Farnese FdS, Silva LdJ, Rosa M, Reis MNO, Bessa LA, de Oliveira TC, Januário AH and Silva FG (2023) Light means power: harnessing light spectrum and UV-B to enhance photosynthesis and rutin levels in microtomato plants. Front. Plant Sci. 14:1261174. doi: 10.3389/fpls.2023.1261174
Received: 19 July 2023; Accepted: 14 August 2023;
Published: 04 September 2023.
Edited by:
Jose R Peralta-Videa, The University of Texas at El Paso, United StatesReviewed by:
Yuqing Ye, Rice University, United StatesNubia Zuverza, Connecticut Agricultural Experiment Station, United States
Copyright © 2023 Lima, Rodrigues, Resende, da Silva, Farnese, Silva, Rosa, Reis, Bessa, de Oliveira, Januário and Silva. This is an open-access article distributed under the terms of the Creative Commons Attribution License (CC BY). The use, distribution or reproduction in other forums is permitted, provided the original author(s) and the copyright owner(s) are credited and that the original publication in this journal is cited, in accordance with accepted academic practice. No use, distribution or reproduction is permitted which does not comply with these terms.
*Correspondence: Fabiano Guimarães Silva, ZmFiaWFuby5zaWx2YUBpZmdvaWFuby5lZHUuYnI=; Ana Helena Januário, YW5hLmphbnVhcmlvQHVuaWZyYW4uZWR1LmJy