- 1Key Laboratory of Agro-Forestry Environmental Processes and Ecological Regulation of Hainan Province, Center for Eco-Environment Restoration Engineering of Hainan Province, School of Ecological and Environmental Sciences, Hainan University, Haikou, China
- 2School of Life Sciences, Hainan University, Haikou, China
- 3School of Plant Protection, Hainan University, Haikou, China
At present, establishing planted forests, typically composed of not more than two tree species, to avoid forest losses has received increasing attention. In addition, investigating the impact of environmental stress such as waterlogging on different planting patterns is essential for improving wetland ecosystem resilience. Knowledge about the impact of waterlogging on planted forests is crucial for developing strategies to mitigate its adverse effects. Here, we conducted experimentally a simulated pure and mixed planting system composed of two contrasting WL-tolerant species (Cleistocalyx operculatus and Syzygium cumini) to determine their ecophysiological responses based on the type of interaction. Results showed that the aboveground growth performance of S. cumini was better than that of C. operculatus under well-watered conditions regardless of the planting model, which is contrary to the belowground accumulation that was significantly improved in C. operculatus. Intra- and interspecific interactions in different planting models facilitated the growth performance of C. operculatus while provoking a significant competition in S. cumini under waterlogging. Such phenomenon was explained through the remarkable ability of C. operculatus to naturally increase its root network under stress on non-stress conditions compared with S. cumini. In this study, two main factors are proposed to play key roles in the remarkable performance of C. operculatus compared with S. cumini following the planting model under waterlogging. The high level of nitrogen and phosphor absorption through C. operculatus primary roots and the significant starch biosynthesis constituted the key element that characterized the facilitation or competition within the intra- or interspecific interactions shown in C. operculatus compared with S. cumini. Furthermore, the intraspecific competition is more pronounced in S. cumini than in C. operculatus when grown in a pure planting pattern, particularly when subjected to waterlogging. However, when the two species are planted together, this competition is alleviated, resulting in enhanced waterlogging tolerance.
Introduction
Plant–plant interactions play a crucial role in regulating plant growth, species coexistence, and community composition (Wang and Li, 2016). Numerous studies have focused on competition as the most crucial interaction among neighboring plants (Zhang et al., 2013; Li et al., 2023b). In general, competition intensifies when significant similarities are found in resource needs (Levine and HilleRisLambers, 2009) or when plants exhibit kin recognition (Mahall and Callaway, 1992; Murphy and Dudley, 2009). Plant neighboring could trigger an increased investment into competitive organs (Poorter et al., 2012). For example, in the presence of Suaeda salsa, Phragmites australis allocated more biomass into the roots for soil water absorption (Guo et al., 2022). However, positive plant–plant interactions (facilitation) have been widely explored in the past two decades (Bertness and Callaway, 1994). In addition, positive interactions among plants under harsh conditions can create a beneficial environment that supports the growth of certain species, leading to the potential expansion of their geographic range (Bulleri et al., 2016; Filazzola et al., 2018). Plant–plant interactions can be altered possibly because of abiotic factors (e.g., water, nutrients, light, or space) and neighbor or target plant characteristics (Pennings et al., 2003; Li et al., 2015). Hence, obtaining a comprehensive understanding of the changes occurring in plant interactions is crucial when exposed to external abiotic factors such as waterlogging and in the presence of neighboring plants.
Waterlogging impedes gas exchange between the soil and atmosphere, leading to energy deficiency in plant roots and accumulation of toxic metabolites and reactive oxygen species (ROS) (Subbaiah and Sachs, 2003). In addition, waterlogging interferes with plant growth (Parad et al., 2013), reducing root activity (Li et al., 2022) and affecting the photosynthesis (Yordanova et al., 2005; Yordanova and Popova, 2007), uptake, and transport of mineral elements (Patrick et al., 1985; Pezeshki, 2001); nutrient distribution patterns (Smethurst et al., 2005; Pierce et al., 2010); and other physiological processes, thereby leading to plant death. Nevertheless, plants do not passively accept the damages caused by waterlogging stress. Plants exhibit various morphological and physiological responses mainly through “quiescence” and “escape” strategies (Garssen et al., 2015). This phenomenon has been observed in Crataeva tapia L. (Capparaceae) seedlings, hypertrophied lenticels, and adventitious roots (ARs) during waterlogging (Parolin, 2001a; Parolin, 2001b). Moreover, these species undergo leaf shedding and produce new leaves as a response to waterlogging (Parolin, 2002), indicating their resilience to prolonged waterlogging. In tropical areas, summer waterlogging is a factor influencing plant–plant interactions, which often represent an important bottleneck for species survival. The frequency and intensity of future extreme rainfall events will continue to increase as global climate change intensifies (Kreuzwieser and Rennenberg, 2014). Consequently, such an increase may have further impacts on plant–plant interactions. A previous study has shown that the interspecific interactions between Phragmites australis and Spartina alterniflora varied under environmental conditions, and the intensity of competition was affected by the level of the tidal zones (Yuan et al., 2013). Yang et al. (2022) reported that waterlogging induced the competitive relationship under well-watered conditions into a mutualistic relationship between Cleistocalyx operculatus and Syzygium jambos; both species showed improved tolerance to waterlogging stress. By contrast, Yue et al. (2019) discovered that waterlogging on dry lands can significantly improve the competitiveness of invasive Bidens pilosa L. over native Bidens biternate (Lour.) Merr. These disparate findings imply an incomplete comprehension of the mechanisms governing plant–plant interactions in the presence of waterlogging.
Syzygium cumini and C. operculatus are medicinal tropical terrestrial tree species belonging to the family Myrtaceae, which are primarily distributed in South China and other tropical areas (Li et al., 2022). Our previous studies confirmed that both species are waterlogged tolerant, and C. operculatus showed more tolerance to waterlogging than S. cumini (Li et al., 2022; Li et al., 2023a; Li et al., 2023b; Li et al., 2023c). However, the effects of waterlogging stress on the neighboring relationships between C. operculatus and S. cumini remain unexplored. Cleistocalyx operculatus and S. cumini are forest species that might be suitable in different wetland and riparian forest areas. They can be used in different planted forest systems (mixed or pure planted forest). Planted forests have emerged as a viable solution to combat deforestation and promote sustainable land use. By carefully selecting and planting specific tree species, these forests can restore and preserve crucial ecosystem functions. Nevertheless, the successful establishment and long-term survival of planted forests rely heavily on the intricate relationship among different selected tree species. Despite the growing emphasis on establishing planted forests, typically composed of not more than two tree species, to avoid forest losses, the characterization and understanding of the intra- or interspecific interactions among plant species under abiotic stresses such as waterlogging are still lacking. The key concern in planted forests in wetland ecosystems is the limited knowledge regarding the mechanism by which tree species interact with one another to establish their adaptation and resilience against submergence conditions. Here, we provided a rare study that deciphers the ecophysiological responses of tree species against waterlogging and the type of planting pattern based on a comprehensive understanding of how different tree species interact with one another with regard to resource competition and facilitation. By unraveling the intricacies of these interactions, researchers and forest managers can make informed decisions regarding tree species selection, planting patterns, and forest management practices, thereby enhancing the resilience and productivity of planted forests. Furthermore, characterizing the interactions within planted forests can shed light on potential synergies and trade-offs among different tree species. Thus, several hypotheses have been raised: Does the combination of C. operculatus and S. cumini species exhibit cooperative relationships, where they mutually benefit from each other’s presence, leading to enhanced growth and survival? Is it possible that the combination of C. operculatus and S. cumini may result in competition for resources or negative interactions, which could hinder their productivity?
Materials and methods
Plant materials and experimental designs
Two-year-old saplings of C. operculatus and S. cumini were collected from a local commercial tree nursery in February 2022. We cut each sapling at 5 cm above the soil surface to ensure uniform growth after re-tillering. The roots were washed carefully with tap water. In this study, well-watered and waterlogging treatments were used, and the three planting patterns were monocultures, pure planting, and mixed planting. For each species, the well-watered and waterlogging treatments were marked as CK-S and WL-S, respectively, in the single planting pattern; CK-P and WL-P, respectively, in the pure planting pattern; and CK-M and WL-M, respectively, in the mixed planting pattern. Two saplings were planted 8 cm apart in each pot (10 L, upper bore 258 mm, lower bore 230 mm × 270 mm high), and each pot was filled with 8 kg of soil (red soil:sand = 2:1, v/v). The single plant was planted in a 5-L pot, which was filled with 4 kg of soil (upper bore 225 mm, lower bore 205 mm × 150 mm high). All the treatments were placed in the experimental greenhouse at Hainan University (20°03′33.2″N, 110°20′16.9″E). The area has a typical tropical monsoon climate, and healthy saplings with almost uniform growth were selected for the waterlogging experiment after 3 months.
Waterlogging treatment started on 17 May 2022, and the water level of the mixture and monoculture plants was maintained at 5 cm above the soil surface. A total of 15 saplings (five biological replications, each with at least three saplings) were used for each treatment in each species, and the waterlogging treatment ended up on 27 August 2022. The treatments lasted for 130 days.
Analysis of biomass accumulation
At the end of the waterlogging experiment, we collected and recorded the stem height increment (SHI), adventitious root fresh weight (ARFW), primary root fresh weight (PRFW), stem fresh weight (SFW), leaf fresh weight (LFW), total biomass (TB), and the ratio of aboveground to belowground fresh weight (A/B). The total leaf area (TLA) in each plant was determined using the LI-3000 C Area Meter (LI-COR Inc., USA).
Determination of gas exchange and chlorophyll content
On 15–16 August 2022, the net photosynthetic rate (A), stomatal conductance (gs), and transpiration rate (E) were measured on the youngest, fully expanded leaves of each sapling with an open gas exchange system (LI-6400XT, LI-COR Inc.) between 08:30 a.m. to 11:30 a.m. In ensuring the scientific accuracy of the measurement, the leaf temperature was set at 28°C, the optical quantum flux density was set at 1,500 μmol m−2 s−1, and the relative humidity was controlled at approximately 65%–70%. The chlorophyll contents were extracted using 80% (v/v) chilled acetone. The absorbance of chlorophyll a (Chla), chlorophyll b (Chlb), and carotenoids (Caro) was recorded at 663, 646, and 470 nm, respectively. The total chlorophyll (TChl) was calculated as the sum of Chla and Chlb (Li et al., 2023a).
Determination of the emergence time, activity, lignin content of AR, and porosity of primary roots
During the experiment, the emergence time of ARs as the point at which root primordia on the stems of waterlogged plants measured ≥5 mm was recorded. For each plant, we meticulously recorded the emergence time of AR. The root porosity (% of the volume of gas per unit of tissue volume) was determined at the end of the experiment in accordance with the method of Munir et al. (2019). The weight biomass recorded above and the formula proposed by Jensen et al. (1969) were used to calculate root porosity:
where Mb is the mass of the water-filled specific gravity bottle, Ma is the ARFW, Ma+b is the mass of ARs placed into the water-filled specific gravity bottle, and Mh is the mass of the specific gravity bottle filled with the homogenate of ARs.
The lignin contents of ARs were measured using the acetyl bromide method (Li et al., 2022). The absorbance was determined at 280 nm using a spectrophotometer, and the unit of lignin content was expressed as A280·g−1·FW. The root activity of ARs and primary roots was measured using the method described by Li et al. (2022). The absorbance was recorded at 485 nm and compared with that of the calibration curve, and the root activity was expressed as mg·g−1·h−1·FW.
Calculation of competitive relationships
The relative interaction intensity (RII), relative competition intensity (RCI), and aggressivity between C. operculatus and S. cumini in different planting patterns were calculated separately to determine the competitive relationship. The calculations were based on the following formula:
where PW and PS are the performance of plants with and without neighbors, respectively. The formulas used were in accordance with the method of Zhang et al. (2020).
where a and b represent S. cumini and C. operculatus, respectively; Yab and Yba represent the mixed planting biomass of S. cumini or C. operculatus; and Yaa and Ybb represent the same species planting biomass. Zab and Zba are the proportion of S. cumini and C. operculatus in the mixed planting system, and A indicates aggressivity. In addition, the RCI was analyzed in accordance with the method of Grace (1995), and aggressivity was calculated in accordance with the method of McGilchrist and Trenbath (1971).
Determination of soluble protein, proline, peroxidase, ascorbate peroxidase, and superoxide dismutase
All operations were performed at 4°C. Cells (0.2 g) of plant leaves were homogenized in a mortar with 5 mL of 50 mM phosphate buffer at pH 7. The filtrate was centrifuged at 15,000×g for 15 min at 4°C. Soluble protein, free proline, peroxidase (POD), and superoxide dismutase (SOD) were measured as described by our previous reports (Yang et al., 2022). Ascorbate peroxidase was measured as described by Lin and Pu (2010).
Determination of superoxide radical, malondialdehyde, soluble sugar, starch, and midday leaf water potential
Superoxide radical (O2·−), malondialdehyde (MDA), soluble sugar, and starch were measured as described by our previous reports (Li et al., 2022). Midday leaf water potential (Ψ md) was measured in the leaf used for the determination of gas exchange and chlorophyll fluorescence using a potentiometer (WP4C; Decagon Devices, Inc., Pullman, WA, USA) in accordance with a previously described protocol (Liao et al., 2019).
Determination of soil and root mineral element content
At the end of the experiment, the soil and roots from CK and waterlogging treatments were collected and then oven-dried at 80°C for 72 h to a constant weight. The samples were ground in a mortar and passed through a 100-mesh sieve. Approximately 0.5 g of powdered soil and 0.1 g of root sample were digested with 5 mL H2SO4 for the determination of total nitrogen and total phosphorus contents by using the semi-micro-Kjeldahl method and then determined by using a fully automated flow analyzer (PROXIMA 1022/1/1, ALLIANCE Instruments, France).
Statistical analysis
SPSS 25.0 (SPSS, Chicago, IL, USA) was used to perform statistical analyses. Data were checked for normality and homogeneity of variances before the analysis and Ln-transformed if these assumptions were not satisfied. One-way ANOVAs were used to determine differences between the two treatments, and Duncan’s multiple range test was employed to detect possible differences among means. An independent-sample t-test was used to compare the differences between the two species. Differences and correlations were considered to be significant at P<0.05.
Structural equation modeling (SEM) analysis was performed using IBM SPSS AMOS Ver. 26. The chi-square test (χ2) was used to test the overall fit of the SEM. If the model fits the χ2/df index between 0.00 and 2.00 and the P-value is greater than 0.05, then the model was considered acceptable. Based on the Bonferroni correction, the correlation of the probability level is at P< 0.01 = 0.05/5, which was considered to be significant by SEM analysis. The root mean square error of approximation, the comparative fit index, Tucker–Lewis’s index, and the optimal range of values are as described by Fan et al. (2016).
Results
Comparative analysis of the morphological traits and biomass accumulation between Syzygium cumini and Cleistocalyx operculatus among the treatments
Significant interspecific differences between the levels of TB and A/B were found in all treatments (Table 1). Compared with the CK-S treatment, the CK-P treatment significantly decreased the SHI, PRFW, SFW, TLA, LFW, and TB in S. cumini, as well as the TLA, LFW, and A/B in C. operculatus, but significantly increased the A/B in S. cumini and the PRFW in C. operculatus. The CK-M treatment significantly decreased the SHI, PRFW, SFW, TLA, LFW, and TB in S. cumini, but not in C. operculatus. In addition, compared with the CK-P treatment, the CK-M treatment significantly increased the PRFW, TLA, LFW, and TB in both species and the SFW in C. operculatus. The A/B significantly decreased in both species. Furthermore, compared with the WL-S treatment, the WL-P treatment significantly decreased the SHI in both species, as well as the ARFW, PRFW, SFW, TLA, LFW, and TB in S. cumini, but increased the A/B in S. cumini, as well as the PRFW, LFW, and TB in C. operculatus. The WL-M treatment significantly decreased the SHI, ARFW, SFW, TLA, LFW, and TB in S. cumini, as well as the A/B in C. operculatus, and increased the ARFW, PRFW, TLA, LFW, and TB in C. operculatus. Compared with the WL-P treatment, the PRFW and TLA in both species, the LFW in S. cumini, and the ARFW and TB in C. operculatus increased significantly; the A/B in both species significantly decreased.
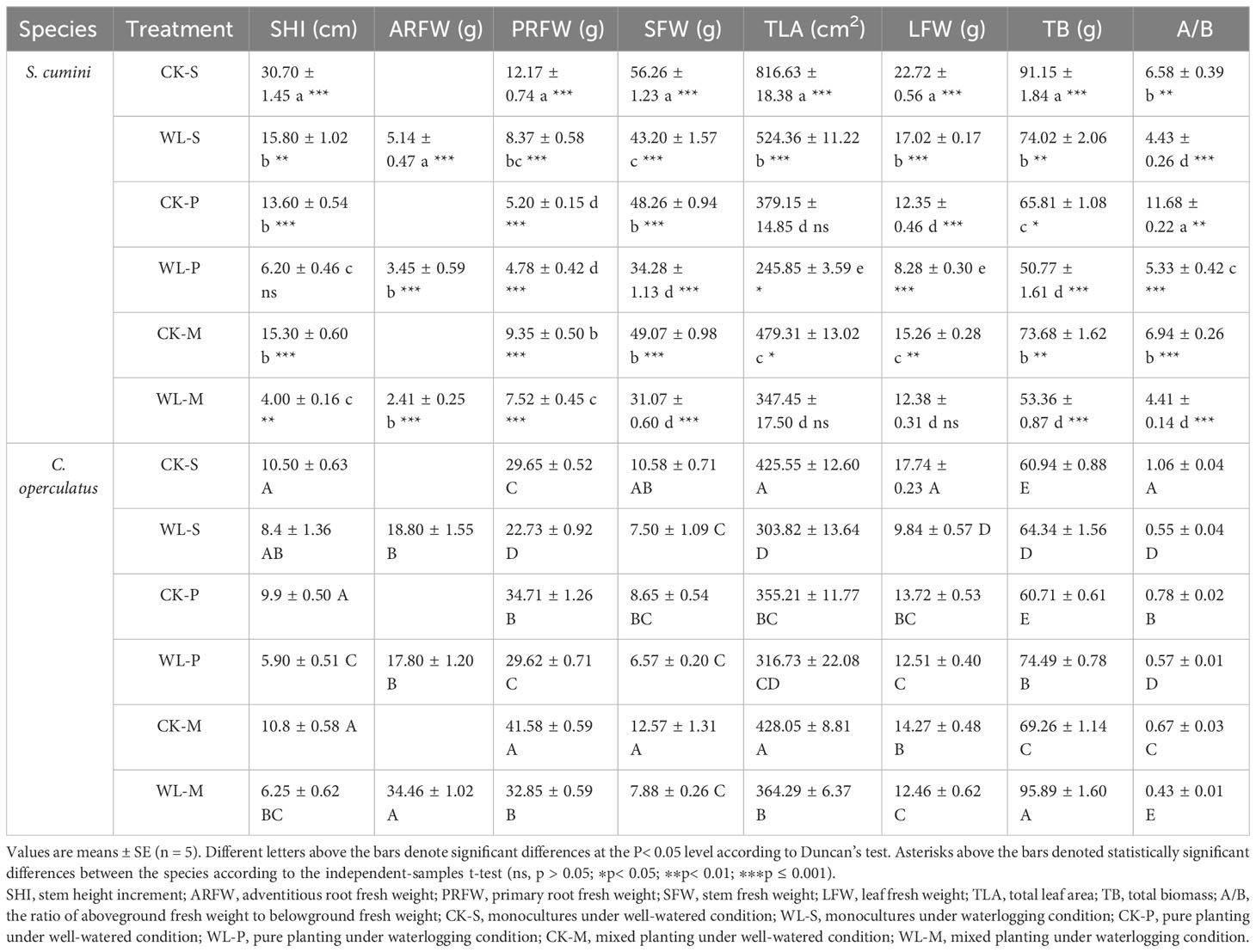
Table 1 Comparative analysis of biomass allocation between Syzygium cumini and Cleistocalyx operculatus among the treatments.
Comparative analyses on the emergence time of AR, AR activity, AR lignin, primary root activity, and primary root porosity between Syzygium cumini and Cleistocalyx operculatus among the treatments
As shown in Figure 1, the emergence time of ARs under waterlogging of C. operculatus saplings was significantly shorter than that of S. cumini, and insignificant differences in the emergence time of ARs were detected among the WL-S, WL-P, and WL-M treatments in C. operculatus. Compared with the WL-S treatment, the WL-P treatment shortened the emergence time of ARs in S. cumini, significantly increased the lignin content of ARs, and significantly decreased the AR activity in both species. In addition, compared with the WL-P treatment, the WL-M treatment significantly increased the emergence time of ARs in S. cumini, as well as the AR activity, but significantly decreased the lignin content in both species.
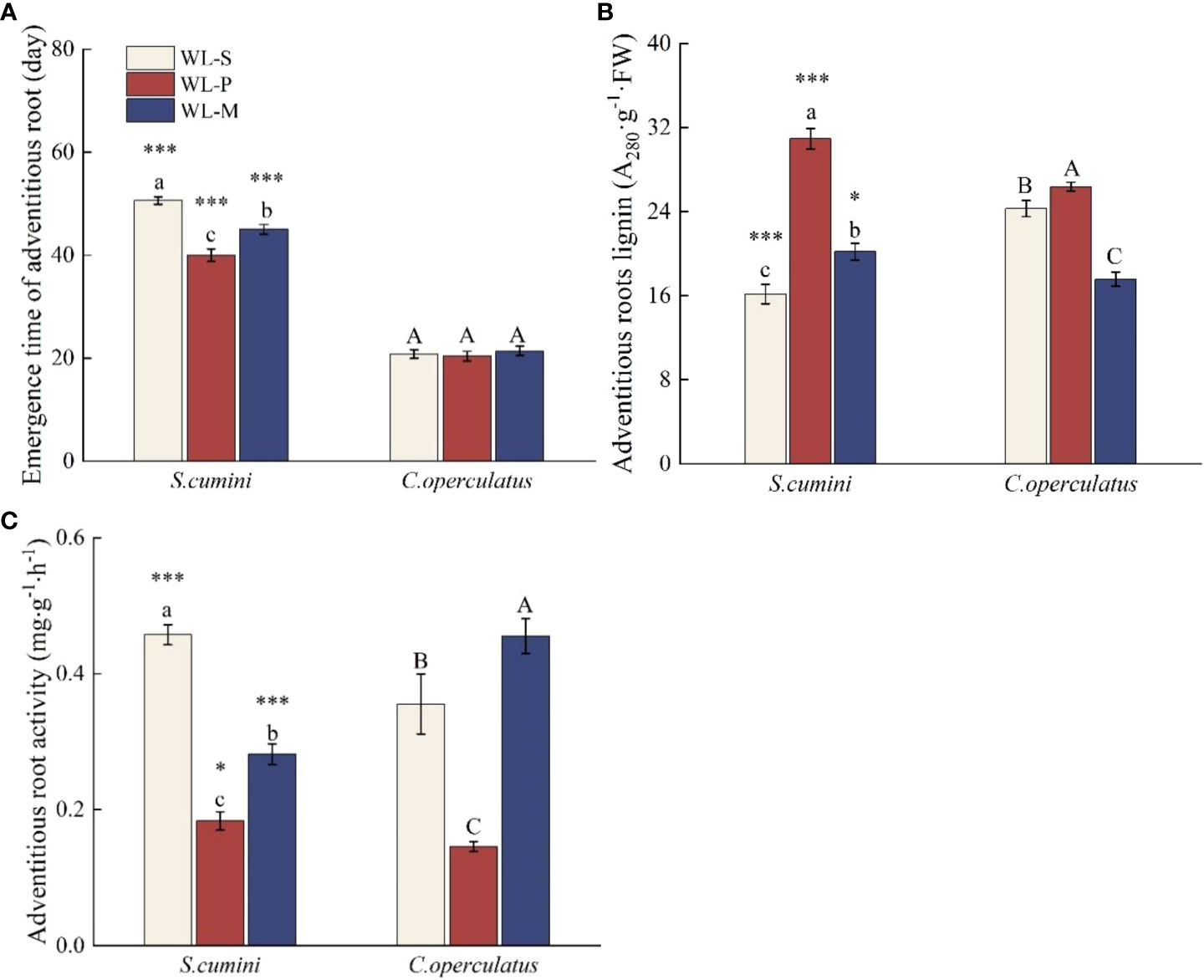
Figure 1 Emergence time of adventitious root (A), adventitious root activity (B), and adventitious root lignin (C), between Syzygium cumini and Cleistocalyx operculatus among the treatments. CK-S, monocultures under well-watered condition; WL-S, monocultures under waterlogging condition; CK-P, pure planting under well-watered condition; WL-P, pure planting under waterlogging condition; CK-M, mixed planting under well-watered condition; WL-M, mixed planting under waterlogging condition. Values are expressed as means ± SE (n = 5). Bars with a different letter within the same species group indicate a significant difference among the treatments at P< 0.05, according to ANOVA, followed by Duncan’s test. Asterisks above the bars denote statistically significant differences between the species at P< 0.05 according to independent-samples t-test (ns, P > 0.05; ∗P< 0.05; ∗∗∗P ≤ 0.001).
Comparative analyses of primary root activity and primary root porosity between Syzygium cumini and Cleistocalyx operculatus among the treatments
For the primary roots (Figure 2), compared with the CK-S treatment, the CK-P treatment significantly decreased the primary root activity and significantly increased the primary root porosity in both species. Meanwhile, the CK-M treatment significantly increased the primary root activity in C. operculatus and increased the root porosity in S. cumini. Compared with the CK-P treatment, the CK-M treatment significantly increased the primary root activity of C. operculatus and decreased the primary root porosity. Furthermore, compared with the WL-S treatment, the WL-P and WL-M treatments significantly increased the primary root porosity in both species. Compared with the WL-P treatment, the WL-M treatment significantly increased the primary root porosity in both species.
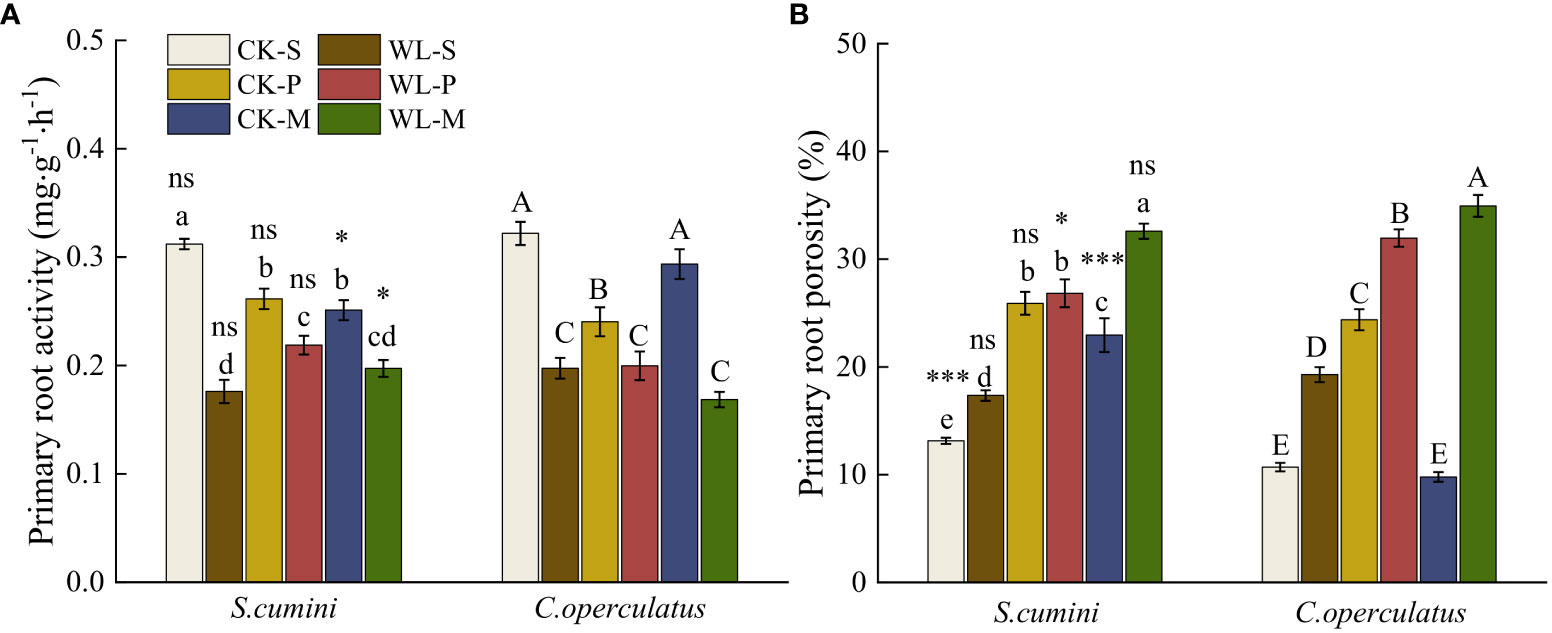
Figure 2 Primary root activity (A) and primary root porosity (B) between Syzygium cumini and Cleistocalyx operculatus among the treatments. For abbreviations explanation of treatments are the same as shown in Figure 1. Values are expressed as means ± SE (n = 5). Bars with a different letter within the same species group indicate a significant difference among the treatments at P< 0.05, according to ANOVA, followed by Duncan’s test. Asterisks above the bars denote statistically significant differences between the species at P< 0.05 according to independent-samples t-test (ns, P > 0.05; *P < 0.05; ***P ≤ 0.001).
Comparative analyses of photosynthetic pigments between Syzygium cumini and Cleistocalyx operculatus among the treatments
Significant differences in the content of chlorophyll a (Chla), chlorophyll b (Chlb), carotenoids (Caro), and the total of chlorophyll (TChl) were found in all treatments between S. cumini and C. operculatus (except for TChl under the CK-M treatment, Figure 3). Compared with the CK-S treatment, the CK-P treatment significantly decreased the content of Chla and TChl in both species and the content of Chlb in S. cumini but significantly increased the content of Chlb in C. operculatus; the CK-M treatment significantly decreased the content of Chla, Chlb, and TChl in S. cumini but significantly increased Caro content in C. operculatus. Compared with the CK-P treatment, the CK-M treatment significantly increased the content of Chla, Caro, and TChl in C. operculatus. Furthermore, compared with the WL-S treatment, the content of Chla and TChl in both species and Chlb in S. cumini significantly decreased under the WL-P treatment, whereas the WL-M treatment significantly increased Chla and TChl contents. Compared with the WL-P treatment, WL-M significantly increased Chla and TChl contents in both species, as well as Chlb in S. cumini.
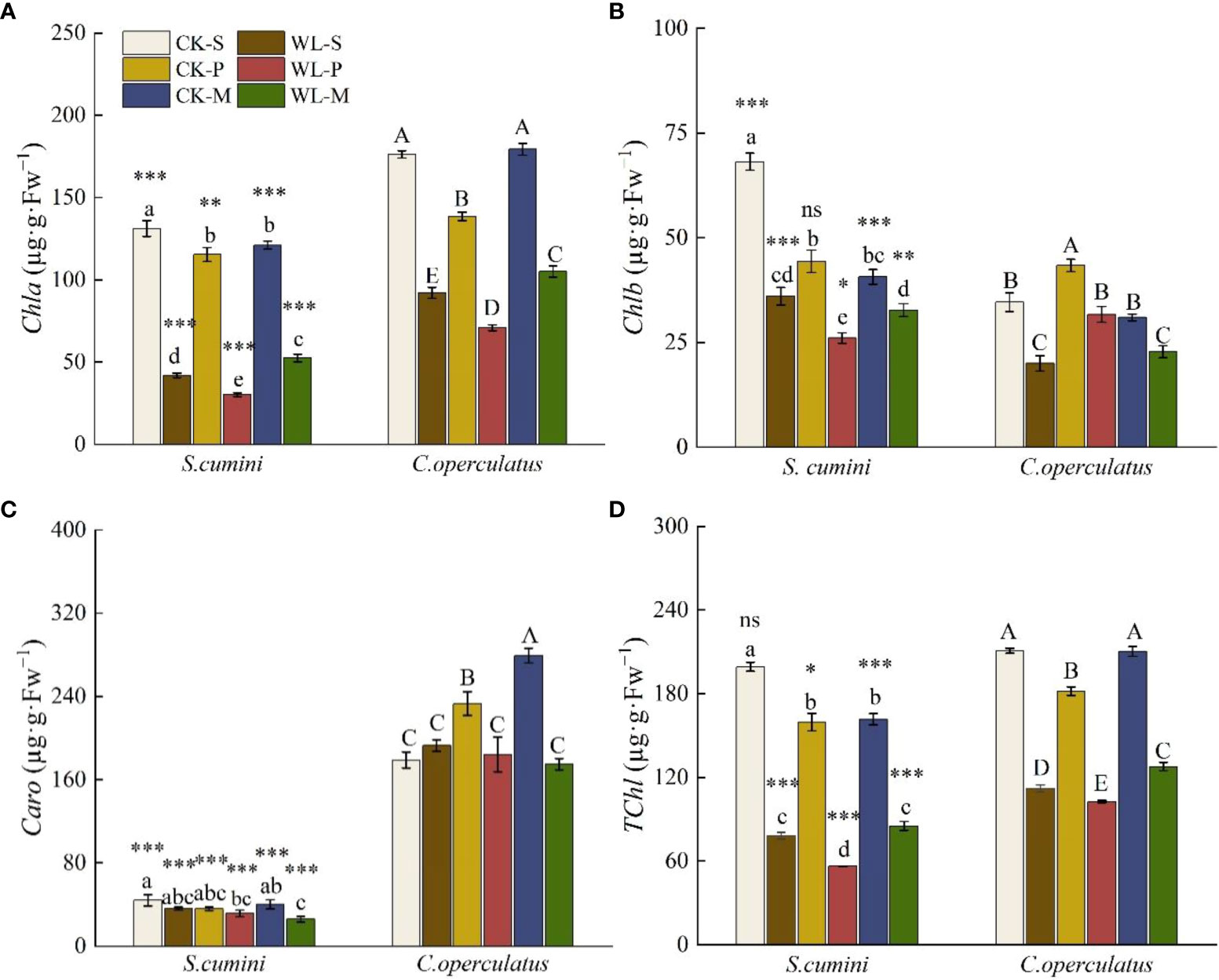
Figure 3 Variations in chlorophyll a (Chla) (A), chlorophyll b (Chlb) (B), total of chlorophyll (TChl) (C), and carotenoids (Caro) (D) contents between Syzygium cumini and Cleistocalyx operculatus among the treatments. For abbreviations explanation of treatments are the same as shown in Figure 1. Values are expressed as means ± SE (n = 5). Bars with a different letter within the same species group indicate a significant difference among the treatments at P< 0.05, according to ANOVA, followed by Duncan’s test. Asterisks above the bars denote statistically significant differences between the species at P< 0.05 according to independent-samples t-test (ns, P > 0.05; *P < 0.05; **P < 0.05; ***P ≤ 0.001).
Comparative analyses on photosynthetic traits and leaf water potential between Syzygium cumini and Cleistocalyx operculatus among the treatments
Significant differences in the net photosynthetic rate (A), transpiration rate €, and stomatal conductance (gs) were found in all treatments (except for E under the CK-M treatment and gs under the WL-S treatment, Figure 4). CK-S treatment showed the highest values of A, E, and gs and the lowest leaf water potential in both species. Compared with CK-S, the CK-P treatment significantly decreased A, E, gs, and leaf water potential in both species. Similarly, CK-M significantly decreased A, gs, and leaf water potential in S. cumini, as well as E in C. operculatus. Compared with the CK-P treatment, CK-M significantly increased A and leaf water potential, as well as A and E in S. cumini, but significantly decreased E in C. operculatus and gs in S. cumini. Furthermore, compared with the WL-S treatment, the WL-P treatment significantly decreased A and gs in S. cumini as well as E and leaf water potential in both species. Compared with the WL-P treatment, significant increases in A, E, gs, and leaf water potential were observed in both species in the WL-M treatment.
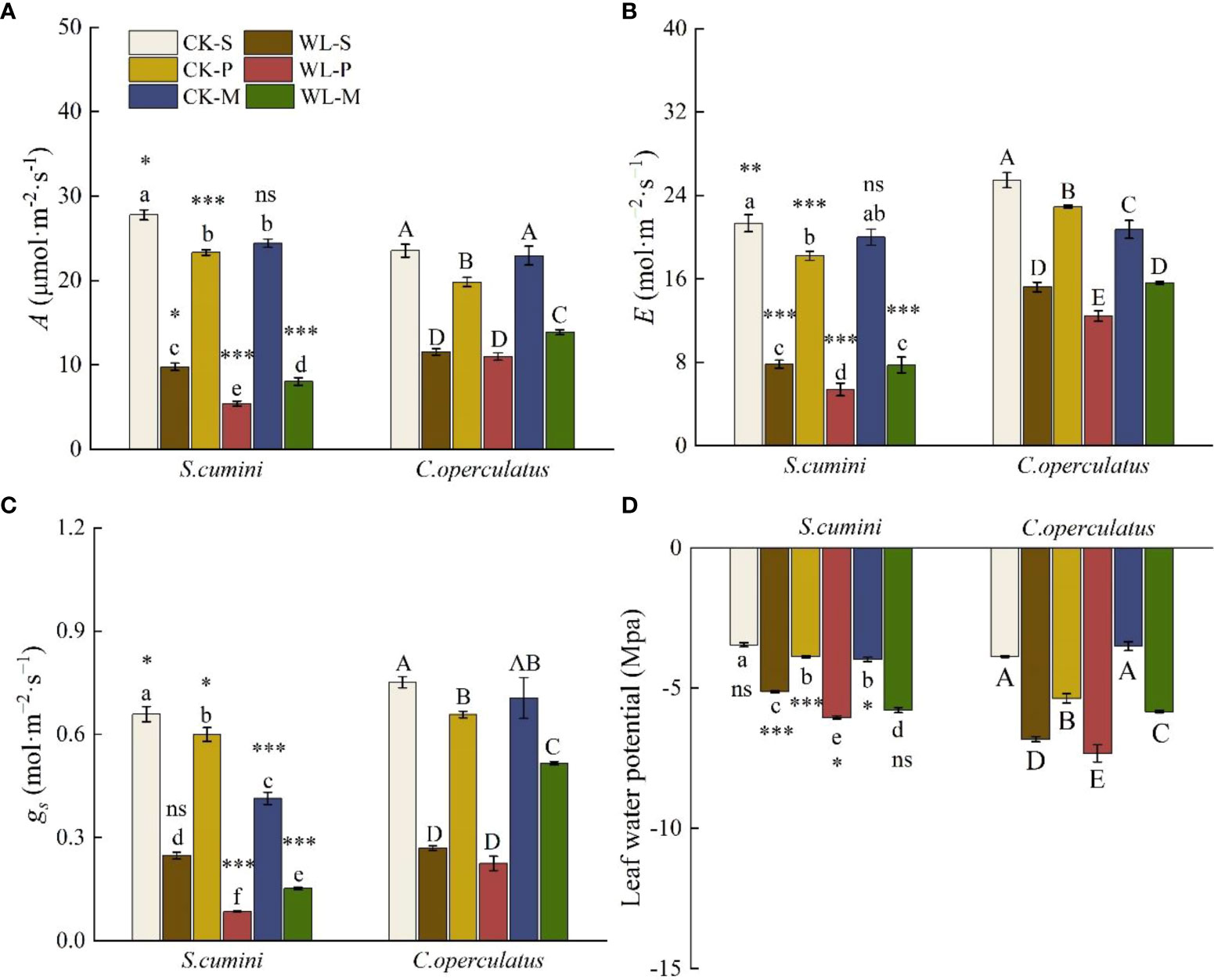
Figure 4 Variations in the leaves’ net photosynthetic rate (A) (A), transpiration rate (E) (B), stomatal conductance (gs) (C), and leaf water potential (D) between Syzygium cumini and Cleistocalyx operculatus among the treatments. Values are expressed as means ± SE (n = 5). Bars with a different letter within the same species group indicate a significant difference among the treatments at P< 0.05, according to ANOVA, followed by Duncan's test. Asterisks above the bars denote statistically significant differences between the species at P< 0.05 according to independent-samples t-test (ns, P > 0.05; *P < 0.05; **P < 0.05; ***P ≤ 0.001). For abbreviations explanation of treatments are the same as shown in Figure 1.
Comparative analyses on the antioxidant enzymatic activities between Syzygium cumini and Cleistocalyx operculatus among the treatments
As shown in Figure 5, significant interspecific differences in the activities of ascorbate peroxidase (APX), POD, and SOD were found between the two species in all treatments (except for WL-M in SOD and WL-S in POD). Compared with the CK-S treatment, the CK-P treatment significantly decreased the APX activity of S. cumini but significantly increased that of C. operculatus. In addition, the SOD activity of S. cumini was significantly increased; the CK-M treatment significantly decreased the APX activity of S. cumini and the SOD activity of C. operculatus; the POD activity in both species was significantly increased. Compared with the CK-P treatment, the CK-M treatment significantly decreased the SOD activity in both species and the POD activity of S. cumini. Furthermore, compared with the WL-S treatment, WL-P and WL-M significantly decreased the SOD and POD activities in WL-P of S. cumini; however, WL-P and WL-M significantly increased the APX and POD activities of C. operculatus. Moreover, compared with the WL-P treatment, WL-M significantly decreased the SOD activity of S. cumini and increased the SOD activity of C. operculatus, as well as the POD activity in both species.
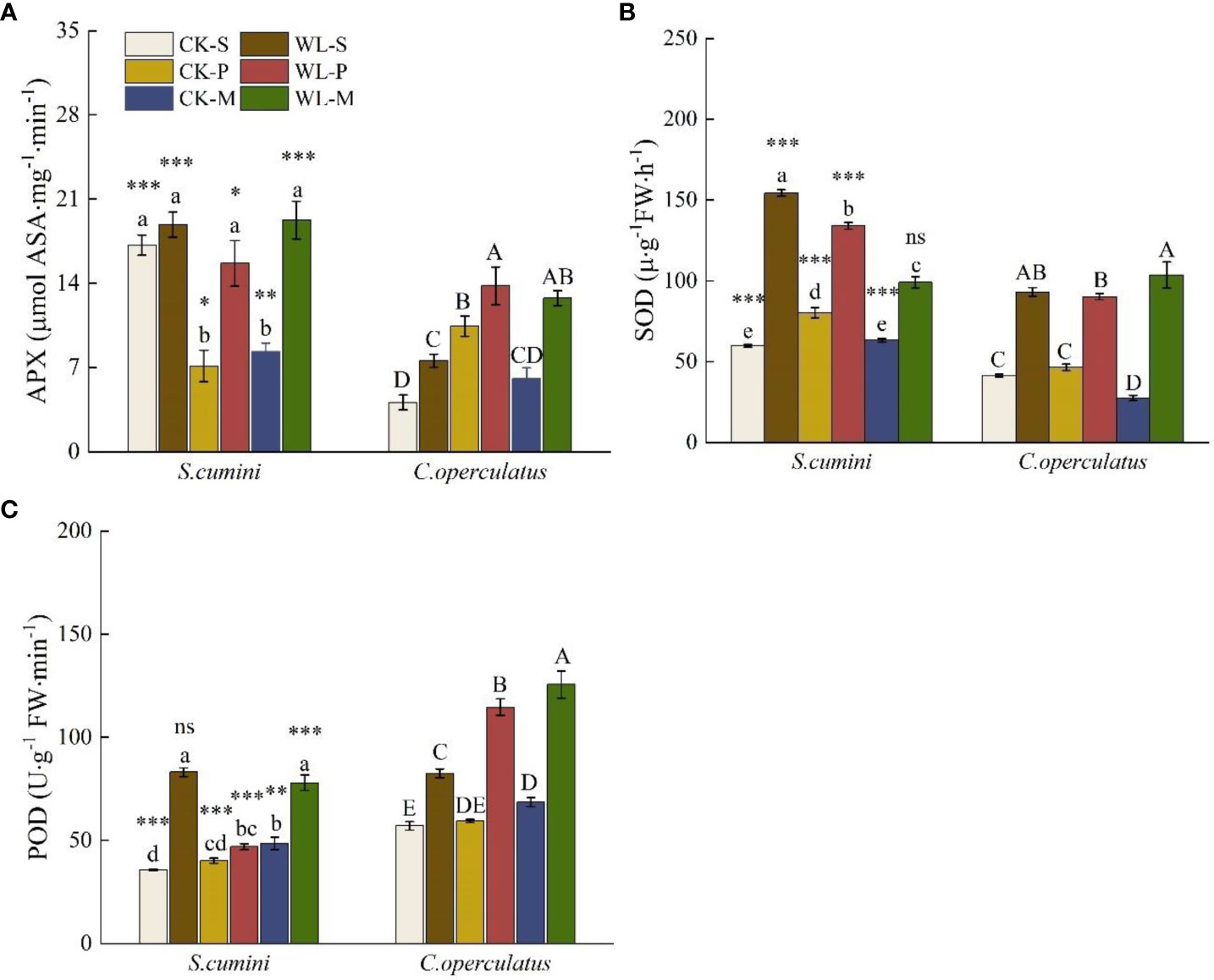
Figure 5 Variations in ascorbate peroxidase (APX) (A), superoxide dismutase (SOD) (B), and peroxidase (POD) (C) activities between Syzygium cumini and Cleistocalyx operculatus among the treatments. Values are expressed as means ± SE (n = 5). Bars with a different letter within the same species group indicate a significant difference among the treatments at P< 0.05, according to ANOVA, followed by Duncan’s test. Asterisks above the bars denote statistically significant differences between the species at P< 0.05 according to independent-samples t-test (ns, P > 0.05; *P < 0.05; **P < 0.05; ***P ≤ 0.001). For abbreviations explanation of treatments are the same as shown in Figure 1.
Comparative analyses on MDA, O2·−, soluble protein, and proline contents between Syzygium cumini and Cleistocalyx operculatus among the treatments
As shown in Figure 6, compared with the CK-S treatment, the CK-P treatment significantly increased O2·− of the two species and soluble protein in C. operculatus; the CK-M treatment significantly increased the content of MDA in S. cumini and soluble protein in C. operculatus but significantly decreased the soluble protein content in S. cumini. Moreover, compared with the CK-P treatment, the CK-M treatment significantly increased the MDA content in S. cumini and significantly decreased the O2·− content in C. operculatus and the proline content in S. cumini. In addition, compared with the WL-S treatment, the WL-P treatment significantly increased the MDA content in S. cumini, the O2·− content in C. operculatus, and soluble protein and proline contents in both species; WL-M significantly increased the MDA content in S. cumini but significantly decreased the O2·− content in S. cumini. Compared with the WL-P treatment, WL-M significantly decreased the O2·− content of the two species, as well as the soluble protein and proline contents in S. cumini.
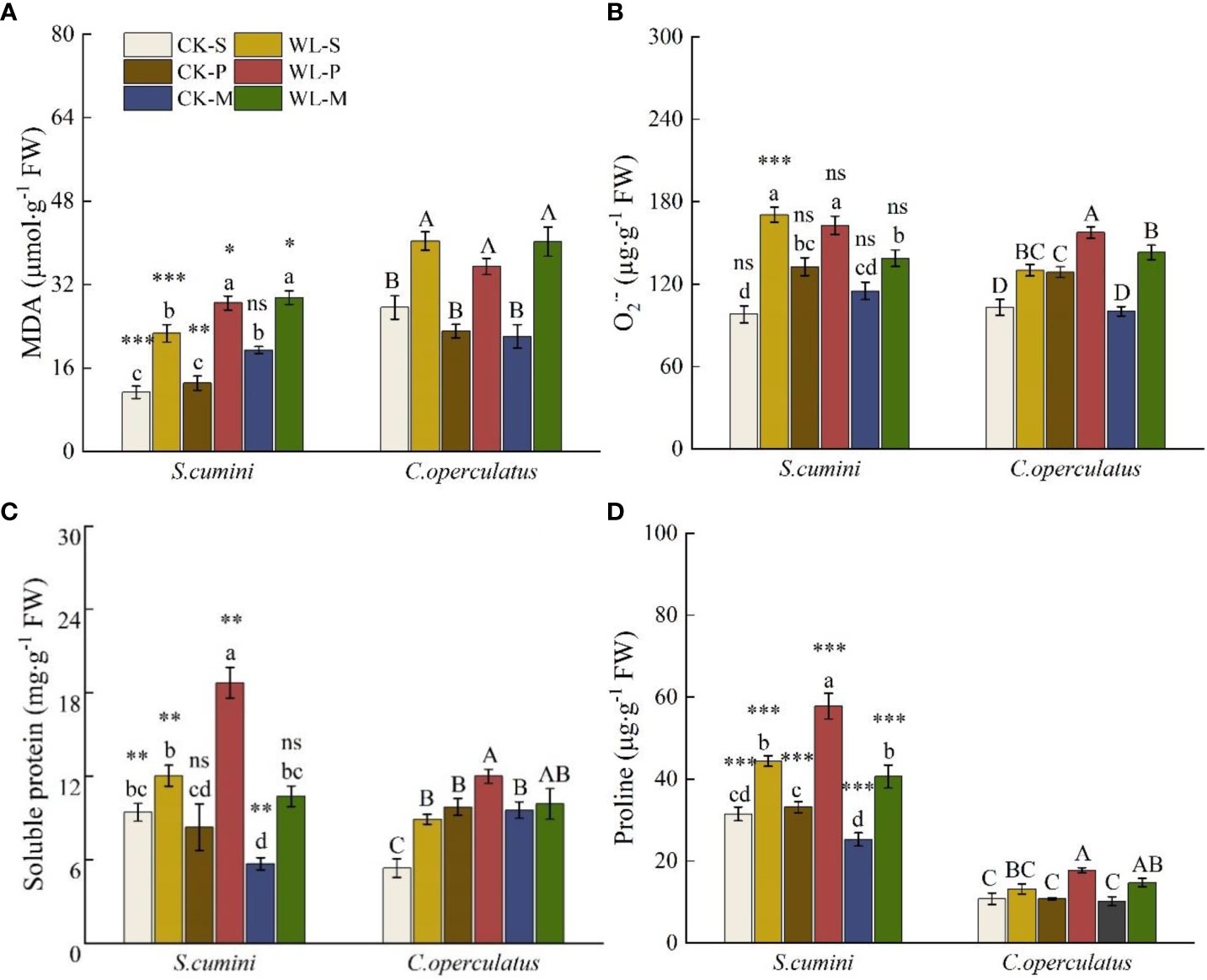
Figure 6 Variations in malondialdehyde (MDA) (A), superoxide radical (O2·−) (B), soluble protein (C), and proline (D) contents between Syzygium cumini and Cleistocalyx operculatus among the treatments. Values are expressed as means ± SE (n = 5). Bars with a different letter within the same species group indicate a significant difference among the treatments at P< 0.05, according to ANOVA, followed by Duncan’s test. Asterisks above the bars denote statistically significant differences between the species at P< 0.05 according to independent-samples t-test (ns, P > 0.05; *P < 0.05; **P < 0.05; ***P ≤ 0.001). For abbreviations explanation of treatments are the same as shown in Figure 1.
Comparative analysis on non-structural carbohydrate content in the primary roots between Syzygium cumini and Cleistocalyx operculatus among the treatments
Significant interspecific differences in the levels of soluble sugar, starch, and non-structural carbohydrate contents were found in all treatments (except for soluble sugar in the CK-P treatment, starch in the WL-S and WL-P treatments, and non-structural carbohydrate in the CK-S treatment; Table 2). Compared with the CK-S treatment, the CK-P treatment significantly increased the soluble sugar and non-structural carbohydrate contents in C. operculatus; CK-M significantly increased the soluble sugar content in S. cumini, as well as starch and non-structural carbohydrate contents in C. operculatus. Compared with the CK-P treatment, soluble sugar and non-structural carbohydrate contents significantly increased in S. cumini, and the CK-M treatment significantly increased the starch and non-structural carbohydrate contents in C. operculatus. In addition, WL-P significantly increased the soluble sugar and non-structural carbohydrate contents in S. cumini, and the WL-S treatment significantly increased the starch content; the WL-M treatment significantly decreased the soluble sugar, starch, and non-structural carbohydrate contents in S. cumini, but significantly increased the starch and non-structural carbohydrate contents in C. operculatus. Compared with the WL-P treatment, the WL-M treatment significantly decreased the soluble sugar, starch, and non-structural carbohydrate contents in S. cumini but significantly increased the starch and non-structural carbohydrate contents in C. operculatus.
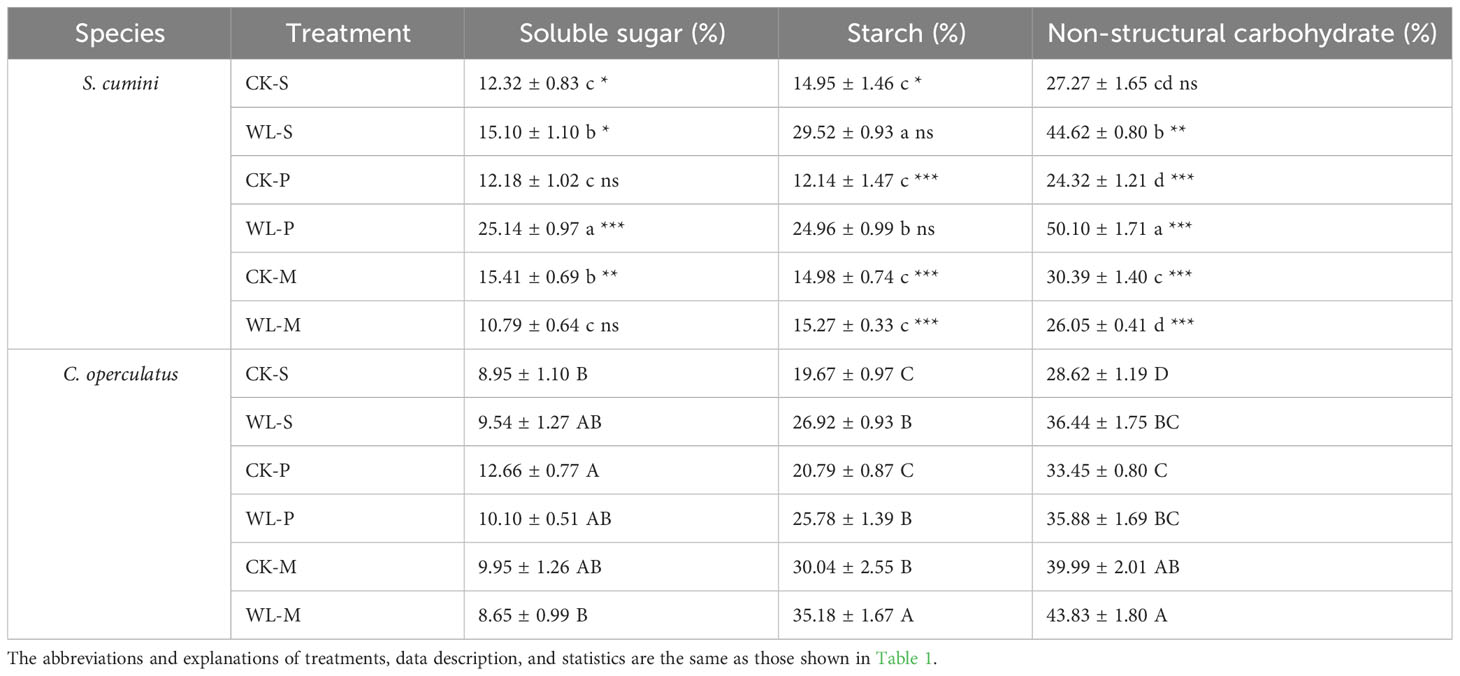
Table 2 Variations in soluble sugar, starch, and non-structural carbohydrate contents in the primary root between Syzygium cumini and Cleistocalyx operculatus among the treatments.
Comparative analyses on total N and total P in the primary roots and ARs between Syzygium cumini and Cleistocalyx operculatus among the treatments
The ARs of S. cumini have more total N and P contents than the primary roots (Table 3). Compared with the CK-S treatment, total N under the CK-P treatment and total P under the CK-M treatment significantly decreased in S. cumini. Cleistocalyx operculatus significantly increased the total N content in the CK-P and CK-M treatments; compared with the CK-P treatment, CK-M significantly increased the total N content and significantly decreased the total P content in S. cumini. In addition, compared with the WL-S treatment, the WL-P treatment significantly increased the total N content of the primary roots of S. cumini, as well as the total P under the WL-M treatment. Meanwhile, WL-M significantly reduced the total N content of the primary roots of C. operculatus. Compared with the WL-P treatment, the WL-M treatment significantly increased the total N content in S. cumini but significantly decreased the total N content of the primary roots in C. operculatus and the total P content in S. cumini. As for ARs, the WL-M treatment had the lowest total N and total P contents in S. cumini, whereas the WL-S treatment had the lowest total N and total P contents in C. operculatus.
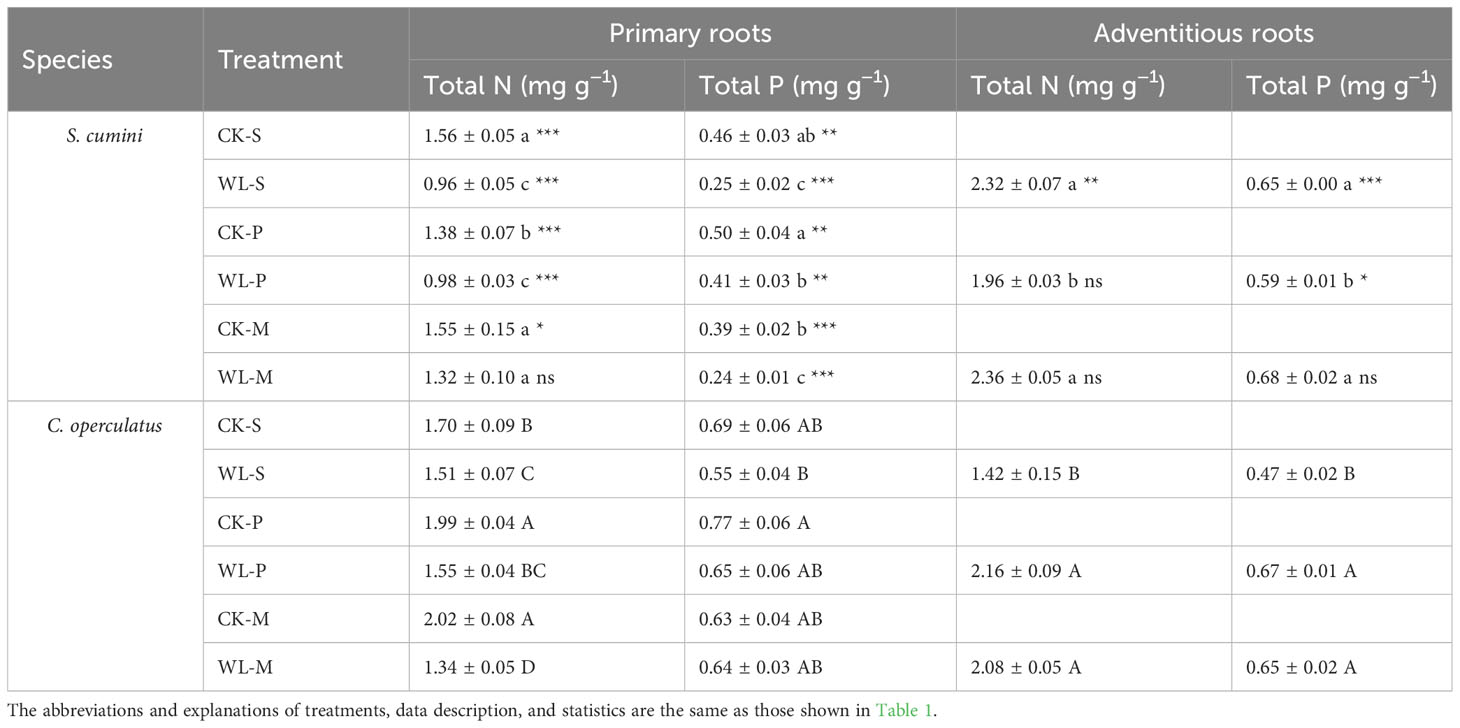
Table 3 Variations in total N and total P contents in the primary and adventitious root between Syzygium cumini and Cleistocalyx operculatus among the treatments.
Comparative analyses on the relative competitive intensity, aggressivity, and RII between Syzygium cumini and Cleistocalyx operculatus among the treatments
Under the CK-M and WL-M treatments, the RCI of both species was less than zero; the aggressivity in S. cumini was less than zero, whereas that in C. operculatus was higher than zero (Figure 7). Compared with the CK-M treatment, WL-M significantly increased the RCI in S. cumini and aggressivity in C. operculatus but significantly decreased the RCI in C. operculatus and aggressivity in S. cumini. Moreover, as shown in Figure 8, significant differences in interaction strength were observed between S. cumini and C. operculatus in all treatments; C. operculatus was always higher than zero, and S. cumini was consistently less than zero in all treatments. Compared with the CK-P treatment, CK-M significantly increased the interaction strength in both species. In addition, compared with the WL-P treatment, the WL-M treatment significantly increased the interaction strength of C. operculatus, and an increasing trend was observed in S. cumini.
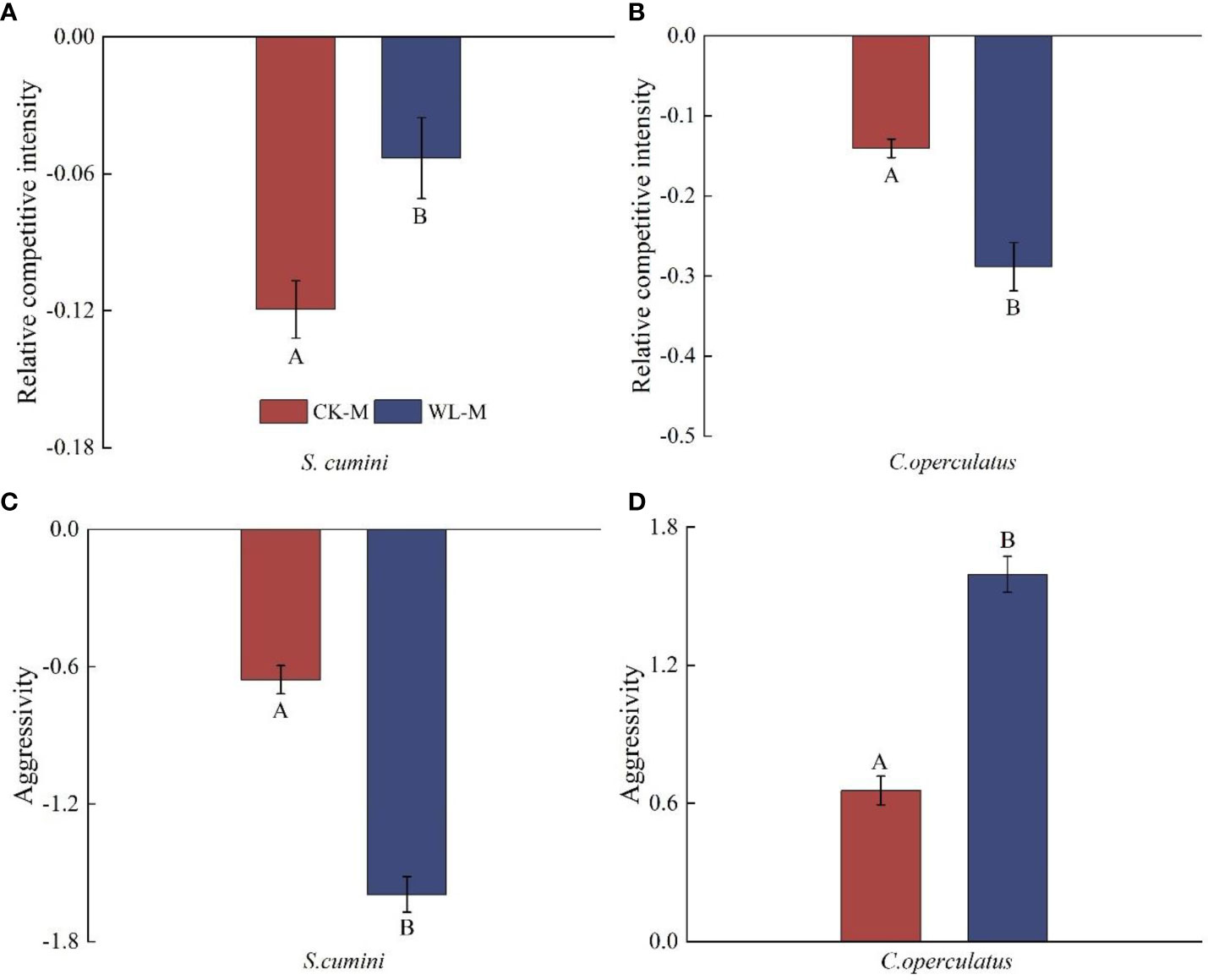
Figure 7 Relative competitive intensity (A, B) and aggressivity (C, D) between Syzygium cumini and Cleistocalyx operculatus among the treatments. The abbreviations and explanations of treatments, data description, and statistics are the same as those shown in Figure 1.
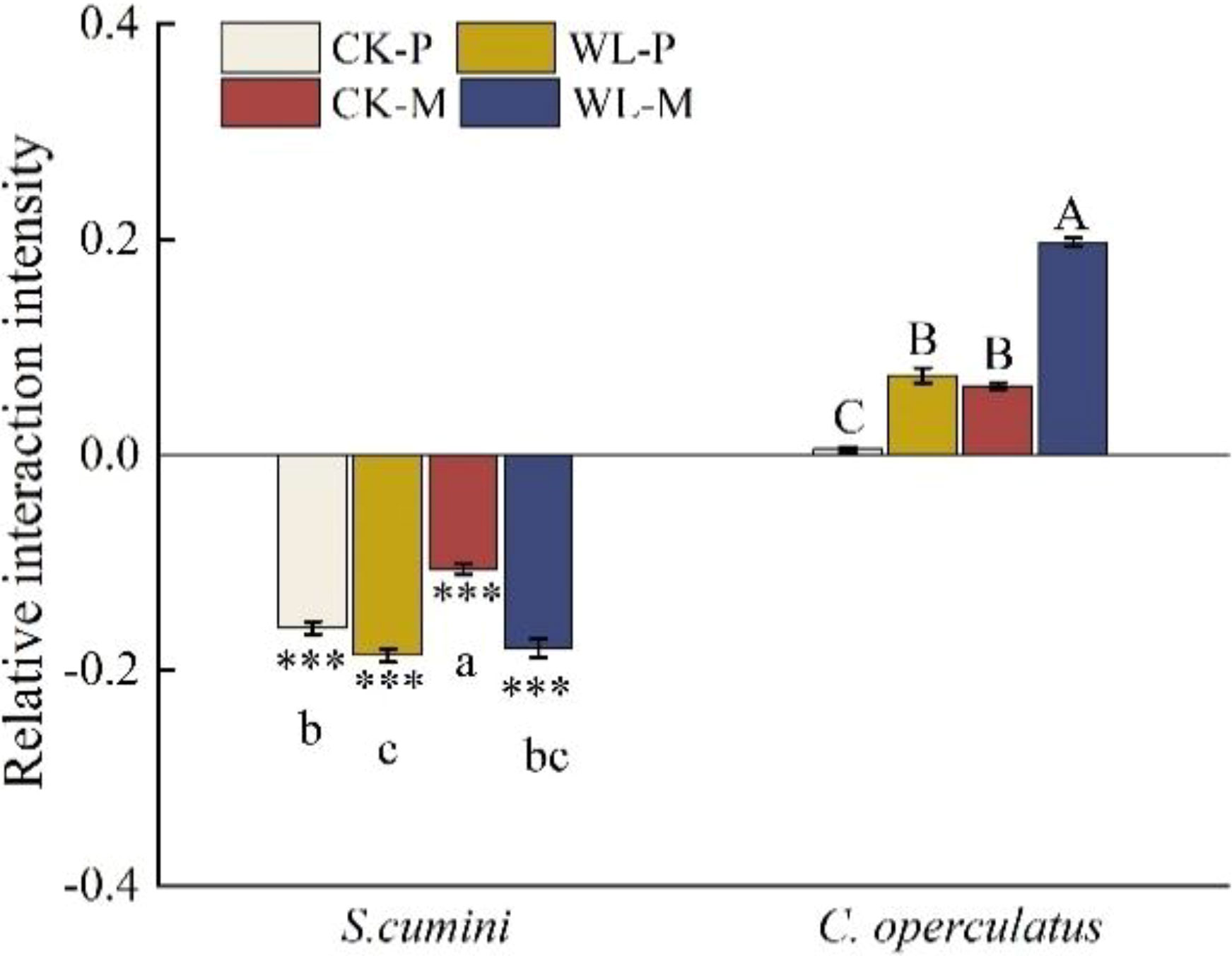
Figure 8 Relative interaction intensity between Syzygium cumini and C. operculatus among the treatments. For abbreviations explanation of treatments are the same as shown in Figure 1. Values are expressed as means ± SE (n = 5). Bars with a different letter within the same species group indicate a significant difference among the treatments at P< 0.05, according to ANOVA, followed by Duncan’s test. Asterisks above the bars denote statistically significant differences between the species at P< 0.05 according to independentsamples t-test (***P ≤ 0.001).
The SEM of ARs and a comparative comprehensive evaluation between Syzygium cumini and Cleistocalyx operculatus among different treatments following the planting pattern
The SEM shows the relationship among ARs’ nitrogen, phosphorus, and lignin contents and root activity (Figure 9). We used TB as the plant’s response to competition and stress. As shown by the SEM, ARs’ lignin content was significantly and negatively correlated with nitrogen and phosphorus contents in both species. Similarly, ARs’ activity was also negatively correlated with nitrogen and phosphorus contents (more significantly in C. operculatus) but significantly and positively correlated with TB. Morphological and physiological traits were reduced to four principal components that explained 87.44% of the variance (Table 4). Syzygium cumini had higher CE values than C. operculatus in the CK-S and WL-S treatments. Compared with the CK-S, CK-P, and CK-M treatments, the CE values of S. cumini under the WL-S, WL-P, and WL-M treatments showed a greater tendency to decrease than C. operculatus. Moreover, C. operculatus always had higher CE values than S. cumini under the WL-P and WL-M conditions.
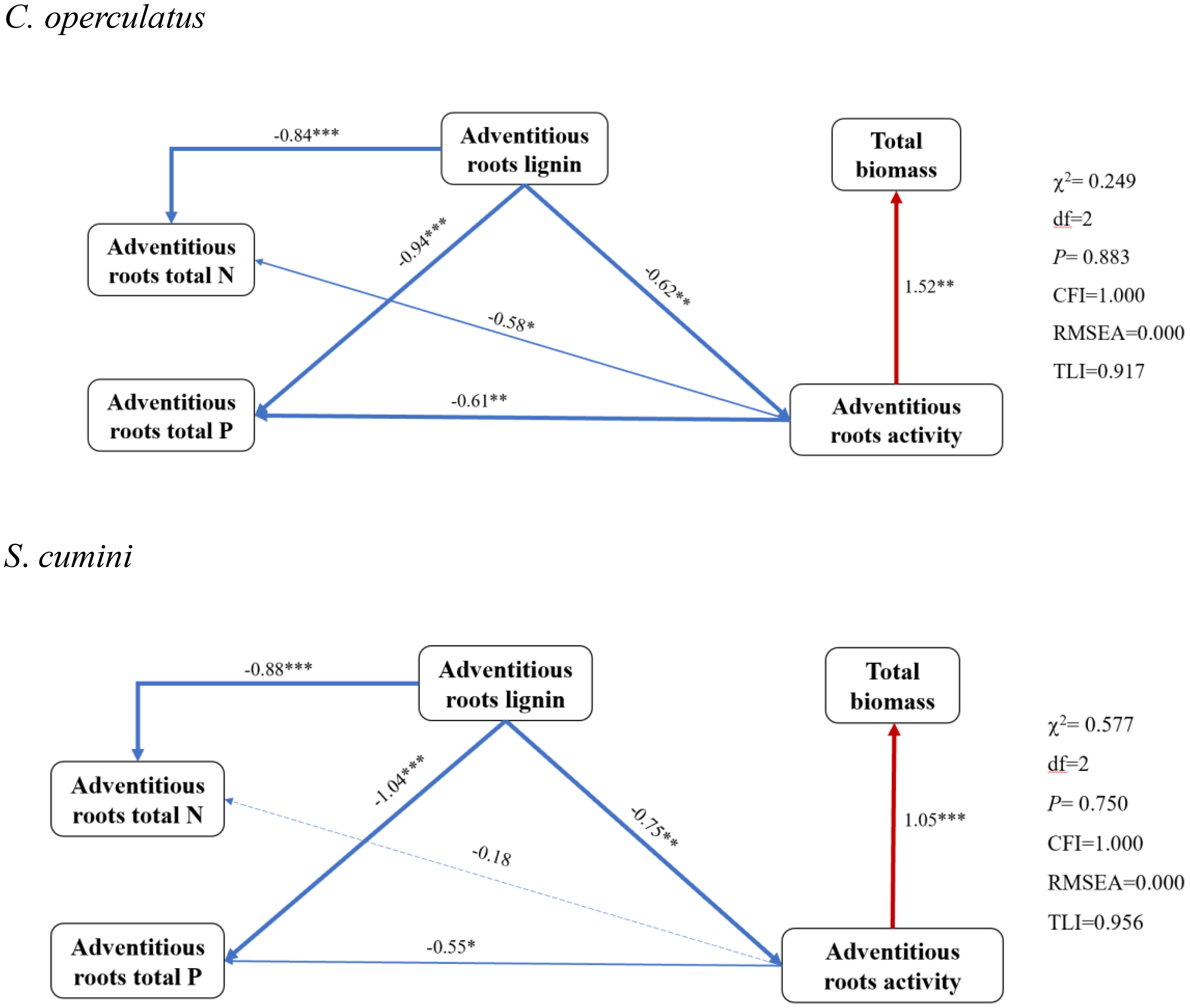
Figure 9 The SEM of adventitious roots of the two species. The solid blue line indicates a negative correlation, the solid red line indicates a positive correlation, and the dashed line indicates no significant correlation. The thickness of the line indicates the degree of correlation.
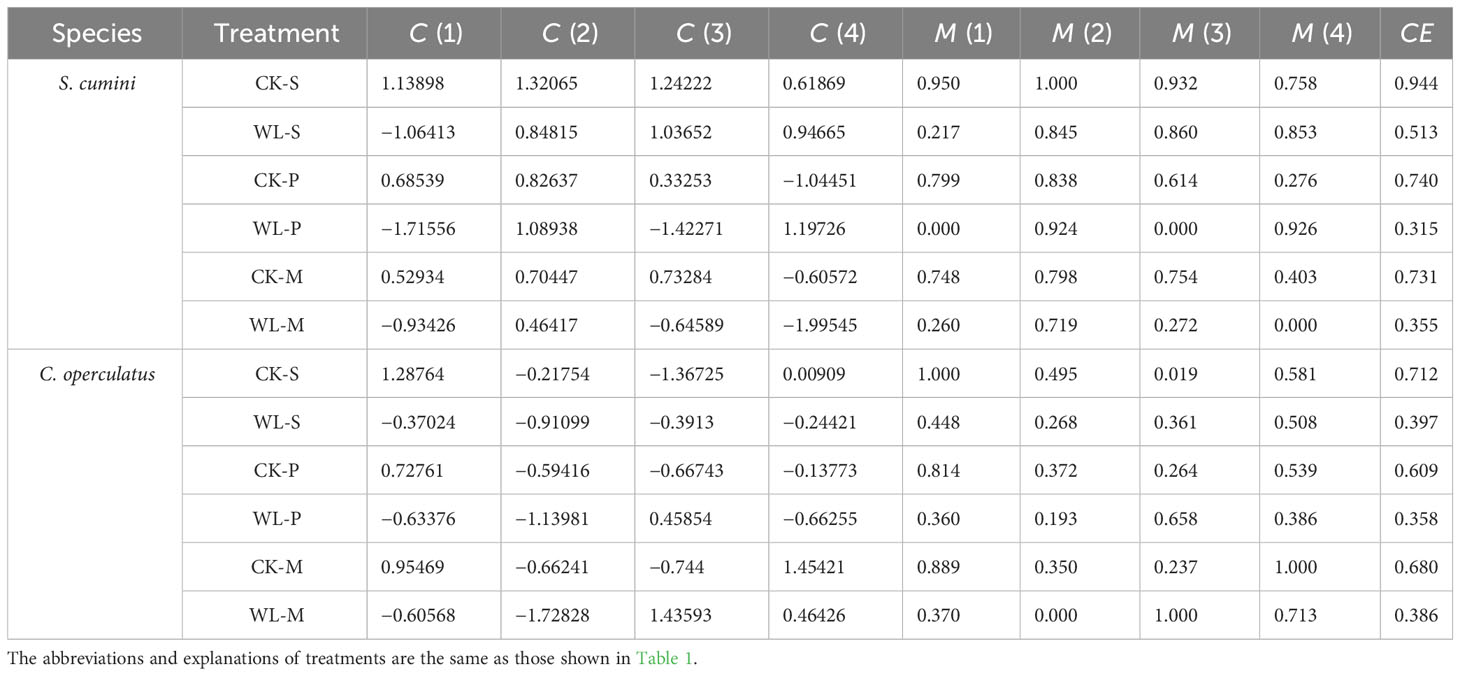
Table 4 Comparative analyses of the value of the principal component [C (m)], membership function value [M (m)], and comprehensive evaluation value (CE) of S. cumini and C. operculatus among all treatments.
Discussion
Although ecologists have primarily emphasized competition as a pivotal process in forest communities, the significance of facilitation cannot be undermined, particularly in certain ecological niches exposed to drastic environmental changes. The magnitude and nature of interactions among different species may be influenced by environmental factors and species tolerance to abiotic stresses. This knowledge is essential for ensuring the long-term success and sustainability of planted forests in a changing climate, thereby promoting forest conservation and ecosystem restoration.
Under well-watered conditions, the intraspecific interaction of Syzygium cumini in the pure planting pattern was negative compared with that of Cleistocalyx operculatus
Different plants have different ways of coping with intraspecific competition. We found that well-watered pure planting treatment affects the growth and physiology of both species. Closely related individuals growing in close proximity may engage in more intense competition because of their similar genotypes and patterns of resource utilization (Young, 1981). In addition, for the root system, according to Mahall and Callaway (1991), individuals of this species strongly reduced their root growth (Table 1) when these roots encountered the roots of another species individual (Mahall and Callaway, 1992). However, C. operculatus increased the fresh weight of the primary roots under well-watered pure planting conditions, but it is not a so-called “tragedy of the commons” in C. operculatus, which has a better trade-off strategy under intraspecific competition, as we found that in two-thirds of the treatments the size of the roots of two C. operculatus in the same pot produced a difference in biomass, thereby avoiding competition for limited resources (Table 1). Moreover, C. operculatus exhibited elevated nitrogen levels (Table 3). An optimal water–nitrogen (N) supply can enhance plant biomass accumulation through the improvement of root growth (Chen et al., 2018). Therefore, our results demonstrate that C. operculatus exhibits superior adaptation to intraspecific competition compared with S. cumini.
Interspecific interactions are beneficial for both species under well-watered conditions
Montesinos-Navarro et al. (2019) have reported that reducing the competition among distantly related plants is a prevalent mechanism enabling plant coexistence. Syzygium cumini possesses a larger leaf area and LFW (Table 1), providing a larger photosynthetic area for the accumulation of resources and energy necessary for growth. In addition, C. operculatus exhibits heightened primary root activity (Figure 2) and leaf water potential (Figure 4), which aids water absorption from the soil (Samui and Kar, 1981), and improves photosynthesis (Figure 4) to promote its growth. In the presence of non-self and non-kin neighbors, certain plant species exhibit an enhanced root allocation at the cost of reproductive investment, suggesting their ability to recognize neighbor identity at the root level (Chen et al., 2012). Dudley and File (2007) suggested that plant roots play a role in the “kin recognition” of neighboring species through the exchange of recognition substances such as root exudates and potentially common mycorrhizal networks (Anten and Chen, 2021). Although C. operculatus increased the fresh weight of the primary roots, no significant change in N uptake capacity was observed, a phenomenon that has been explained as a foraging strategy of the plant (Zhang et al., 2016). Although S. cumini and C. operculatus belong to the Myrtaceae family, their genetic relatedness is not as close as that within the same species. Mixed planting separates their respective relatives, thereby reducing resource use similarity. Furthermore, mixed planting with diverse neighbors alleviates the competitive pressure on both species (Figures 7, 8).
Mixed planting alleviates the negative effects of waterlogging on both species, compared with the pure planting pattern
Waterlogging primarily affects the root system of plants at the onset. ARs are important morphologically adaptable characteristics of plant response to waterlogging, which contribute to O2 diffusion, as well as nutrient and water uptake, and reduce the accumulation of toxic substances (Zhang et al., 2015). In this study, we observed the formation of ARs in S. cumini and C. operculatus under waterlogged conditions. In addition, C. operculatus exhibited a greater number of ARs that emerged at a shorter time compared with S. cumini (Figure 1). SEM analysis revealed a more significant correlation between the activity of ARs and their nitrogen and phosphorus contents in C. operculatus than in S. cumini (Figure 9), indicating that ARs of C. operculatus could play a greater role. Moreover, the significant increase in APX and POD activities and Caro content in C. operculatus (Figures 3, 5) suggested that this species could enable the efficient removal of a substantial amount of H2O2 and protect the photosynthetic apparatus against ROS (Liao et al., 2019; Hasanuzzaman et al., 2020). These phenomena may be related to the increase of lignin and the reduction of root activity that were more pronounced in the ARs of S. cumini compared with C. operculatus (Figure 1), thereby facilitating the increase of oxygen exchange between the root system and water (Ayi et al., 2016). Consequently, waterlogging intensifies intraspecific competition in S. cumini, while C. operculatus demonstrates superior adaptation to waterlogging stress.
The growth of both species was clearly promoted under waterlogging conditions in the mixed planting pattern (Table 4). It significantly mitigated the negative effects of flooding on photosynthesis in both species. In addition, both species exhibited a notable increase in AR activity (Figure 1). This enhanced activity facilitated the transportation of oxygen and nutrients, effectively reducing peroxide accumulation (Figure 6). Moreover, starch in the plant roots serves as a reserve material, providing sugars for the anoxic metabolism of the root system when the plant is subjected to waterlogging (Irfan et al., 2010). Prolonged waterlogging inhibits plant respiration caused by anoxia, thereby reducing ATP production. In response to this condition, water-tolerant plant roots gradually decrease their consumption of soluble sugars and convert them into complex sugars, particularly starch, for storage. This adaptation aids in maintaining appropriate energy metabolism in the roots. The increase in carbohydrate content has been attributed to the high waterlogging tolerance (Angelov et al., 1996). Cleistocalyx operculatus, being a more WL-tolerant species, exhibits increased root starch content under the mixed planting pattern (Table 2). Conversely, pure planting of S. cumini hinders its photosynthesis and leads to the accumulation of a high carbohydrate content in the roots (Table 2), possibly because of a more severe inhibition of aerobic respiration in the primary root system, which hampers carbohydrate consumption (Wu et al., 2022). Moreover, the accumulation of high levels of carbohydrates under stress could be induced by reduced root growth (Araki et al., 2012). The mixed planting pattern promotes the consumption of carbohydrates by S. cumini to meet its growth requirements. Therefore, the mixed planting pattern under waterlogging conditions is beneficial for the growth of both species.
Conclusion
In the pure planting pattern, intraspecific competition occurs within S. cumini, which is further intensified by waterlogging. Conversely, the competition between C. operculatus species in the pure planting pattern was less pronounced. The ecological niche differentiation of the two species enables them to utilize different resources and occupy distinct niches because of species affinities, which enhances their growth. The mixed planting system between the two species relieved the pressures of intraspecific competition under waterlogging, thereby improving their tolerance to waterlogging.
Data availability statement
The raw data supporting the conclusions of this article will be made available by the authors, without undue reservation.
Author contributions
MT: Data curation, Formal Analysis, Investigation, Methodology, Writing – original draft. DL: Investigation, Writing – review & editing. E-HC: Data curation, Formal Analysis, Writing – review & editing. LM: Writing – review & editing, Investigation, Methodology. WY: Investigation, Writing – review & editing. JZ: Investigation, Writing – review & editing. BC: Investigation, Writing – review & editing. LL: Investigation, Writing – review & editing. HT: Investigation, Writing – review & editing. BY: Investigation, Writing – review & editing. FY: Writing – review & editing, Funding acquisition, Supervision.
Funding
The author(s) declare financial support was received for the research, authorship, and/or publication of this article. The experiments were financially supported by the National Science Foundation of China (Nos. 32060240 and 31660165) and the Hainan Provincial Natural Science Foundation of China (Nos. 421RC1033 and 320RC507).
Conflict of interest
The authors declare that the research was conducted in the absence of any commercial or financial relationships that could be construed as a potential conflict of interest.
Publisher’s note
All claims expressed in this article are solely those of the authors and do not necessarily represent those of their affiliated organizations, or those of the publisher, the editors and the reviewers. Any product that may be evaluated in this article, or claim that may be made by its manufacturer, is not guaranteed or endorsed by the publisher.
References
Angelov, M. N., Sung, S. J. S., Doong, R. L., Harms, W. R., Kormanik, P. P., Black, C. C., Jr. (1996). Long-and short-term flooding effects on survival and sink–source relationships of swamp-adapted tree species. Tree Physiol. 16 (5), 477–484. doi: 10.1093/treephys/16.5.477
Anten, N. P., Chen, B. J. (2021). Detect thy family: mechanisms, ecology and agricultural aspects of kin recognition in plants. Plant Cell Environ. 44 (4), 1059–1071. doi: 10.1111/pce.14011
Araki, H., Hossain, M. A., Takahashi, T. (2012). Waterlogging and hypoxia have permanent effects on wheat root growth and respiration. J. Agron. Crop Sci. 198 (4), 264–275. doi: 10.1111/j.1439-037X.2012.00510.x
Ayi, Q., Zeng, B., Liu, J., Li, S., van Bodegom, P. M., Cornelissen, J. H. (2016). Oxygen absorption by adventitious roots promotes the survival of completely submerged terrestrial plants. Ann. Bot. 118 (4), 675–683. doi: 10.1093/aob/mcw051
Bertness, M. D., Callaway, R. (1994). Positive interactions in communities. Trends Ecol. Evol. 9 (5), 191–193. doi: 10.1016/0169-5347(94)90088-4
Bulleri, F., Bruno, J. F., Silliman, B. R., Stachowicz, J. J. (2016). Facilitation and the niche: implications for coexistence, range shifts and ecosystem functioning. Funct. Ecol. 30 (1), 70–78. doi: 10.1111/1365-2435.12528
Chen, B. J., During, H. J., Anten, N. P., Plant Science (2012). Detect thy neighbor: identity recognition at the root level in plants. Plant Sci. 195, 157–167. doi: 10.1016/j.plantsci.2012.07.006
Chen, Z., Tao, X., Khan, A., Tan, D. K., Luo, H. (2018). Biomass accumulation, photosynthetic traits and root development of cotton as affected by irrigation and nitrogen-fertilization. Front. Plant Sci. 9. doi: 10.3389/fpls.2018.00173
Dudley, S. A., File, A. L. (2007). Yes, kin recognition in plants! Biol. Lett. 4 (1), 69–70. doi: 10.1098/rsbl.2007.0232
Fan, Y., Chen, J., Shirkey, G., John, R., Wu, S. R., Park, H., et al. (2016). Applications of structural equation modeling (SEM) in ecological studies: an updated review. Ecol. Processes 5, 1–12. doi: 10.1186/s13717-016-0063-3
Filazzola, A., Sotomayor, D. A., Lortie, C. J. (2018). Modelling the niche space of desert annuals needs to include positive interactions. Oikos 127 (2), 264–273. doi: 10.1111/oik.04688
Garssen, A. G., Baattrup-Pedersen, A., Voesenek, L. A., Verhoeven, J. T., Soons, M. B. (2015). Riparian plant community responses to increased flooding: A meta-analysis. Global Change Biol. 21 (8), 2881–2890. doi: 10.1111/gcb.12921
Grace, J. B. (1995). On the measurement of plant competition intensity. Ecology 76 (1), 305–308. doi: 10.2307/1940651
Guo, H., Gao, F., Pang, J., Wang, H., Wang, H., Wang, Y., et al. (2022). Plant-plant interactions of Phragmites australis and Suaeda salsa as mediated by combined influences of salinity and tidal level changes. Plant Soil 474 (1-2), 141–161. doi: 10.1007/s11104-022-05321-8
Hasanuzzaman, M., Bhuyan, M. B., Zulfiqar, F., Raza, A., Mohsin, S. M., Mahmud, J. A., et al. (2020). Reactive oxygen species and antioxidant defense in plants under abiotic stress: Revisiting the crucial role of a universal defense regulator. Antioxidants 9 (8), 681. doi: 10.3390/antiox9080681
Irfan, M., Hayat, S., Hayat, Q., Afroz, S., Ahmad, A. (2010). Physiological and biochemical changes in plants under waterlogging. Protoplasma 241, 3–17. doi: 10.1007/s00709-009-0098-8
Jensen, C. R., Luxmoore, R. J., Vangundy, S. D., Stolzy, L. H. (1969). Root air space measurements by a pycnometer method 1. Agron. J. 61 (3), 474–475. doi: 10.2134/agronj1969.00021962006100030045x
Kreuzwieser, J., Rennenberg, H. (2014). Molecular and physiological responses of trees to waterlogging stress. Plant Cell Environ. 37 (10), 2245–2259. doi: 10.1111/pce.12310
Levine, J. M., HilleRisLambers, J. (2009). The importance of niches for the maintenance of species diversity. Nature 461 (7261), 254–257. doi: 10.1038/nature08251
Li, D., Cisse, E. H. M., Guo, L., Zhang, J., Miao, L., Yang, F. (2022). Comparable and adaptable strategies to waterlogging stress regulated by adventitious roots between two contrasting species. Tree Physiol. 42 (5), 971–988. doi: 10.1093/treephys/tpab165
Li, D., Guo, L., Tian, M., Miao, L., Xiang, L., Yang, F. (2023c). Effects of Waterlogging Stress on the Neighboring Relationships between Cleistocalyx operculatus (Roxb.) Merr. and Dalbergia odorifera T. Chen Saplings. Forests 14 (2), 377. doi: 10.3390/f14020377
Li, D., Miao, L., Cisse, E. H. M., Li, L., Chen, B., Yang, F. (2023b). Dissecting the below-and aboveground specific responses of two waterlogging-tolerant arbor species to nutrient supply under waterlogging conditions. Tree Physiol. 43 (3), 390–403. doi: 10.1093/treephys/tpac127
Li, D., Miao, L., Tian, M., Zhou, J., Yang, W., Yang, F. (2023a). Differences in eco-physiological responses to the removal of adventitious roots between Syzygium nervosum A. Cunn. ex DC. and Syzygium cumini (L.) Skeels saplings under waterlogging. Ann. For. Sci. 80 (1), 1–14. doi: 10.1186/s13595-023-01180-0
Li, F., Yang, G., Xie, Y., Chen, X., Deng, Z., Hu, J. (2015). Competition between two wetland macrophytes under different levels of sediment saturation. J. Limnology 74 (3), 623–630. doi: 10.4081/jlimnol.2015.1023
Liao, J., Song, H., Tang, D., Zhang, S. (2019). Sexually differential tolerance to water deficiency of Salix paraplesia—A female-biased alpine willow. Ecol. Evol. 9 (15), 8450–8464. doi: 10.1002/ece3.5175
Lin, K. H., Pu, S. F. (2010). Tissue-and genotype-specific ascorbate peroxidase expression in sweet potato in response to salt stress. Biol. Plantarum 54, 664–670. doi: 10.1007/s10535-010-0118-8
Mahall, B. E., Callaway, R. M. (1991). Root communication among desert shrubs. Proc. Natl. Acad. Sci. 88 (3), 874–876. doi: 10.1073/pnas.88.3.874
Mahall, B. E., Callaway, R. M. (1992). Root communication mechanisms and intracommunity distributions of two Mojave Desert shrubs. Ecology 73 (6), 2145–2151. doi: 10.2307/1941462
McGilchrist, C. A., Trenbath, B. R. (1971). A revised analysis of plant competition experiments. Biometrics 27, 659–671. doi: 10.2307/2528603
Montesinos-Navarro, A., Valiente-Banuet, A., Verdú, M. (2019). Plant facilitation through mycorrhizal symbiosis is stronger between distantly related plant species. New Phytol. 224 (2), 928–935. doi: 10.1111/nph.16051
Munir, R., Konnerup, D., Khan, H. A., Siddique, K. H., Colmer, T. D. (2019). Sensitivity of chickpea and faba bean to root-zone hypoxia, elevated ethylene, and carbon dioxide. Plant Cell Environ. 42 (1), 85–97. doi: 10.1111/pce.13173
Murphy, G. P., Dudley, S. A. (2009). Kin recognition: competition and cooperation in Impatiens (Balsaminaceae). Am. J. Bot. 96 (11), 1990–1996. doi: 10.3732/ajb.0900006
Parad, G. A., Zarafshar, M., Striker, G. G., Sattarian, A. (2013). Some physiological and morphological responses of Pyrus boissieriana to flooding. Trees 27, 1387–1393. doi: 10.1007/s00468-013-0886-9
Parolin, P. (2001a). Seed germination and early establishment of 12 tree species from nutrient-rich and nutrient-poor Central Amazonian floodplains. Aquat. Bot. 70 (2), 89–103. doi: 10.1016/S0304-3770(01)00150-4
Parolin, P. (2001b). Morphological and physiological adjustments to waterlogging and drought in seedlings of Amazonian floodplain trees. Oecologia 128, 326–335. doi: 10.1007/s004420100660
Parolin, P. (2002). Submergence tolerance vs. escape from submergence: two strategies of seedling establishment in Amazonian floodplains. Environ. Exp. Bot. 48 (2), 177–186. doi: 10.1016/S0098-8472(02)00036-9
Patrick, W. H., Jr., Mikkelsen, D. S., Wells, B. R. (1985). Plant nutrient behavior in flooded soil. Fertilizer Technol. Use, 197–228. doi: 10.2136/1985.fertilizertechnology.c6
Pennings, S. C., Selig, E. R., Houser, L. T., Bertness, M. D. (2003). Geographic variation in positive and negative interactions among salt marsh plants. Ecology 84 (6), 1527–1538. doi: 10.1890/0012-9658(2003)084[1527:GVIPAN]2.0.CO;2
Pezeshki, S. R. (2001). Wetland plant responses to soil flooding. Environ. Exp. Bot. 46 (3), 299–312. doi: 10.1016/S0098-8472(01)00107-1
Pierce, S. C., Moore, M. T., Larsen, D., Pezeshki, S. R. (2010). Macronutrient (N, P, K) and redoximorphic metal (Fe, Mn) allocation in Leersia oryzoides (Rice Cutgrass) grown under different flood regimes. Water Air Soil pollut. 207, 73–84. doi: 10.1007/s11270-009-0120-y
Poorter, H., Niklas, K. J., Reich, P. B., Oleksyn, J., Poot, P., Mommer, L. (2012). Biomass allocation to leaves, stems and roots: meta-analyses of interspecific variation and environmental control. New Phytol. 193 (1), 30–50. doi: 10.1111/j.1469-8137.2011.03952.x
Samui, R. P., Kar, S. (1981). Soil and plant resistance effects on transpirational and leaf water responses by groundnut to soil water potential. Soil Res. 19 (1), 51–60. doi: 10.1071/SR9810051
Smethurst, C. F., Garnett, T., Shabala, S. (2005). Nutritional and chlorophyll fluorescence responses of lucerne (Medicago sativa) to waterlogging and subsequent recovery. Plant Soil 270, 31–45. doi: 10.1007/s11104-004-1082-x
Subbaiah, C. C., Sachs, M. M. (2003). Molecular and cellular adaptations of maize to flooding stress. Ann. Bot. 91 (2), 119–127. doi: 10.1093/aob/mcf210
Wang, C. H., Li, B. (2016). Salinity and disturbance mediate direct and indirect plant–plant interactions in an assembled marsh community. Oecologia 182, 139–152. doi: 10.1007/s00442-016-3650-1
Wu, J., Wang, J., Hui, W., Zhao, F., Wang, P., Su, C., et al. (2022). Physiology of plant responses to water stress and related genes: A review. Forests 13 (2), 324. doi: 10.3390/f13020324
Yang, F., Zhang, J., Cisse, E. H. M., Li, D. D., Guo, L. Y., Xiang, L. S., et al. (2022). Competitive relationship between Cleistocalyx operculatus and Syzygium jambos under well-watered conditions transforms into a mutualistic relationship under waterlogging stress. Front. Plant Sci. 13. doi: 10.3389/fpls.2022.869418
Yordanova, R. Y., Popova, L. P. (2007). Flooding-induced changes in photosynthesis and oxidative status in maize plants. Acta Physiologiae Plantarum 29, 535–541. doi: 10.1007/s11738-007-0064-z
Yordanova, R. Y., Uzunova, A. N., Popova, L. P. (2005). Effects of short-term soil flooding on stomata behaviour and leaf gas exchange in barley plants. Biol. Plantarum 49, 317–319. doi: 10.1007/s10535-005-7319-6
Young, J. P. W. (1981). Sib competition can favour sex in two ways. J. Theor. Biol. 88 (4), 755–756. doi: 10.1016/0022-5193(81)90249-6
Yuan, Y., Wang, K., Li, D., Pan, Y., Lv, Y., Zhao, M., et al. (2013). Interspecific interactions between Phragmites australis and Spartina alterniflora along a tidal gradient in the Dongtan Wetland, Eastern China. PloS One 8 (1), e53843. doi: 10.1371/journal.pone.0053843
Yue, M., Shen, H., Li, W., Chen, J., Ye, W., Tian, X., et al. (2019). Waterlogging tolerance of Bidens pilosa translates to increased competitiveness compared to native Bidens biternata. Plant Soil 437, 301–311. doi: 10.1007/s11104-019-03967-5
Zhang, W. P., Jia, X., Damgaard, C., Morris, E. C., Bai, Y. Y., Pan, S., et al. (2013). The interplay between above-and below-ground plant–plant interactions along an environmental gradient: insights from two-layer zone-of-influence models. Oikos 122 (8), 1147–1156. doi: 10.1111/j.1600-0706.2012.20877.x
Zhang, L., Liu, Q., Tian, Y., Xu, X., Ouyang, H. (2016). Kin selection or resource partitioning for growing with siblings: implications from measurements of nitrogen uptake. Plant Soil 398, 79–86. doi: 10.1007/s11104-015-2641-z
Zhang, D., Lyu, Y., Li, H., Tang, X., Hu, R., Rengel, Z., et al. (2020). Neighbouring plants modify maize root foraging for phosphorus: coupling nutrients and neighbours for improved nutrient-use efficiency. New Phytol. 226 (1), 244–253. doi: 10.1111/nph.16206
Keywords: competition, facilitation, forest species, inter-specific, intra-specific, neighboring relationship, waterlogging
Citation: Tian M, Li D, Cisse E-HM, Miao L, Zhou J, Yang W, Chen B, Li L, Tian H, Ye B and Yang F (2023) Intra- and interspecific ecophysiological responses to waterlogging stress in two contrasting waterlogging-tolerant arbor species. Front. Plant Sci. 14:1257730. doi: 10.3389/fpls.2023.1257730
Received: 12 July 2023; Accepted: 12 October 2023;
Published: 13 November 2023.
Edited by:
Yu-Long Feng, Shenyang Agricultural University, ChinaReviewed by:
Honglang Duan, Guizhou University, ChinaXiao Xu, China West Normal University, Nanchong, China
Copyright © 2023 Tian, Li, Cisse, Miao, Zhou, Yang, Chen, Li, Tian, Ye and Yang. This is an open-access article distributed under the terms of the Creative Commons Attribution License (CC BY). The use, distribution or reproduction in other forums is permitted, provided the original author(s) and the copyright owner(s) are credited and that the original publication in this journal is cited, in accordance with accepted academic practice. No use, distribution or reproduction is permitted which does not comply with these terms.
*Correspondence: Fan Yang, ZmFueWFuZ21sZjYzMDNAMTYzLmNvbQ==