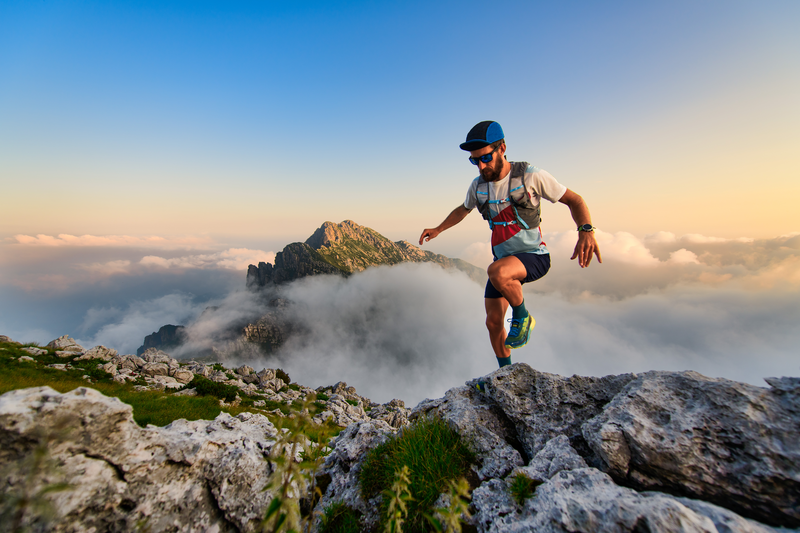
95% of researchers rate our articles as excellent or good
Learn more about the work of our research integrity team to safeguard the quality of each article we publish.
Find out more
EDITORIAL article
Front. Plant Sci. , 20 July 2023
Sec. Plant Abiotic Stress
Volume 14 - 2023 | https://doi.org/10.3389/fpls.2023.1246964
This article is part of the Research Topic Identification and Functional Analysis of Differentially Expressed Genes in Plant Response to Abiotic Stresses View all 24 articles
Editorial on the Research Topic
Identification and functional analysis of differentially expressed genes in plant response to abiotic stresses
As the impact of climate change intensifies, plants face increasing challenges in adapting to a rapidly changing environment. Abiotic stresses, such as drought, salinity, extreme temperatures, and nutrient deficiency, pose significant threats to crop productivity and global food security. It has been reported that more than 8% of the world’s agricultural land was salinized, and the area of salt-affected land is predicted to double by 2050 (Rengasamy, 2006; Guan et al., 2014). In response to these stresses, plants have evolved intricate molecular mechanisms to sense and respond to environmental cues, thereby enhancing their survival and productivity. The identification and functional analysis of differentially expressed genes (DEGs) in plant responses to abiotic stresses has become an essential area of research, aiming to unravel the underlying genetic basis of stress tolerance and facilitate the development of resilient crop varieties (Zhang et al., 2022).
Our Research Topic titled “Identification and Functional Analysis of Differentially Expressed Genes in Plant Response to Abiotic Stresses” (40012) was published on 22 July 22, 2022, and completed on 12 February, 2023. A total of 62 authors confirmed the e-mails, and finally 35 manuscripts were successfully submitted, among which 23 manuscripts were accepted for publication. In this topic, numerous differentially expressed genes (DEGS) were identified and functionally analyzed in many types of plants, such as maize, sunflower, cotton, soybean, rice, Solanum lycopersicum L., Salix matsudana, Passiflora edulis Sims, wheat, sweet potatoes, Panax ginseng, Santalum album, sorghum, and Populus, providing more valuable information for molecular mechanism research of plants in responding to abiotic stresses.
In recent years, advances in high-throughput sequencing technologies, such as RNA sequencing (RNA-seq), have revolutionized our ability to comprehensively characterize the plant transcriptome. RNA-seq enables the simultaneous quantification of gene expression levels across the entire genome, providing an unbiased and precise snapshot of gene activity under specific stress conditions. Through comparative transcriptomic analyses between stressed and control plants, researchers have successfully identified numerous DEGs involved in stress response pathways. These findings have offered crucial insights into the molecular networks governing plant adaptation to abiotic stresses.
Identifying DEGs is just the first step towards understanding their functional roles in the plant stress response. Functional analysis, including gene ontology (GO) enrichment, pathway analysis, and gene regulatory network reconstruction, plays a pivotal role in deciphering the intricate mechanisms underlying stress tolerance. GO enrichment analysis reveals the biological processes, molecular functions, and cellular components associated with the identified DEGs, highlighting the key biological pathways involved in stress adaptation. Pathway analysis provides a holistic view of the interconnected metabolic and signaling pathways orchestrating stress responses, aiding in the identification of critical genes and regulatory hubs. Additionally, gene regulatory network reconstruction elucidates the intricate interactions and hierarchical relationships among stress-responsive genes, offering a systems-level understanding of stress adaptation.
The complexity of abiotic stress responses necessitates the integration of multiple omics approaches to achieve a comprehensive understanding. Transcriptomics, proteomics, metabolomics, and epi-genomics collectively provide a multi-dimensional view of the stress response, unraveling the interplay between genes, proteins, metabolites, and epigenetic modifications. Integration of these omics datasets allows researchers to identify key regulatory nodes, discover novel stress-responsive molecules, and gain insights into the cross-talk between different layers of regulation. By adopting a systems biology perspective, we can uncover emergent properties and regulatory modules that would remain elusive when studying individual components in isolation.
Corn is the oil and feed crop with the largest sown area in China, and it plays an important role in agricultural production (Mousavi et al., 2022). High seed vigor and germination rates are crucial for corn production for its potential in high quality and yield of crops. Jin et al. performed whole-genome-wide identification of miRNAs and their targets associated with maize seed vigor, finally a total of 791 mature miRNAs were gained, among of which 286 miRNAs were newly identified to be closely related with seed vigor. Ding et al. identified 129 maize NAC protein-coding genes, of which 15 and 20 NAC genes were differentially expressed between the two genotypes H082183 and Lv28 based on transcriptome analysis. Jasmonic acid (JA), one of the Phytohormones, is always involved in modulating physiological and molecular responses to abiotic stresses (Wolters and Jurgens, 2009; Garrido-Bigotes et al., 2019). Song et al. conducted the phylogenetic analysis and expression profiles of 139 putative JAZ genes, and the results indicated that JAZ genes in sunflower were conserved in sequence but varied in their expression among duplicated HaJAZ genes, suggesting that these JAZ genes may confer neo-functionalization in the responses to abiotic stresses. Environment factors, such as temperature, drought, salt, sunshine duration, significantly influenced oil and FA compositions in soybean, thus Zuo et al. identified QTN-by-environment (QEIs) interactions and their candidate genes for soybean seed oil-related traits using 3VmrMLM method, providing important information for genetic basis, molecular mechanisms, and soybean breeding. In rice, two density of direct seeding with high and normal density were selected by Cui et al. to identify the differentially expressed genes (DEGS) involved in shade-avoidance syndrome. The results indicated that simulation of shade environment could cause rapid decrease in the expression of phytochrome genes, expression changes of multiple miR156 or miR172 genes and photoperiod-related genes. Normal growth and development of tomatoes (Solanum lycopersicum L.) as well as fruit quality and yield are limited by salt stress, so Wang et al. revealed the transcriptional regulatory network of hormones and genes under salt stress in tomato plants.
Phenotypes of Salix matsudana females and males are obviously different under salinity stress, and the molecular mechanisms were decoded by Liu et al. based on the root transcriptome of males and females. Yang et al. conducted genome-wide characterization and identification of Trihelix transcription factors and expression profiling in response to abiotic stresses in Chinese Willow (Salix matsudana Koidz) to reveal the molecular mechanism when responding to various abiotic stresses. Wheat (Triticum aestivum L.) is one of the world’s most widely cultivated crops (Alghabari et al., 2015). At present, no documents about chromosome condensation 1 (RCC1) domain proteins have been found. An et al. performed genome-wide identification and expression analysis of the regulator of RCC1 gene family in wheat. MYB TFs, common regulators of gene transcription, form a large gene family involved in a variety of biological processes in plants (Dai et al., 2023). Identification of the passion fruit (Passiflora edulis Sims) MYB family in fruit development and abiotic stress was performed by Zhang et al., laying the foundation for further analysis of the biological functions of PeMYBs involved in stress resistance in passion fruit. Tang et al. identified 144 R2R3-MYB genes and investigated their characteristics and expression patterns in Santalum album. Sweet potato, regarded as the seventh most important food crop, is a popular food source in many countries, i.e., China, India, and Kenya. Liu et al. revealed the molecular mechanism of two sweet potatoes with different drought tolerances using transcriptome analysis. Tong et al. also used transcriptome analysis to obtain 672 upregulated genes and 526 downregulated genes in the roots of rusty ginseng when compared with the healthy ginseng roots. Jiao et al. revealed the regulation mechanism of the flavonoid biosynthesis pathway in responding to cadmium stress by integration analysis of transcriptome and metabolome in sorghum. Guo et al. identified a total of 30 heat shock transcription factor (HSF) members in poplar, and overexpression of PsnHSF21 conferred salt tolerance in Populus simonii × P. nigra by functional analysis.
Cotton is the main raw material of textile industry in China and as well as the world in general, and it plays a pivotal role in our national economy (Lu et al., 2019). In this topic, a total of eight articles related to cotton tolerance were published. Yan et al. reported that GhCDPK60 positively regulated drought stress tolerance in both transgenic Arabidopsis and cotton by regulating proline content and ROS level. The GhSRS21 gene was proved to negatively control cotton salt tolerance by regulating the balance between ROS production and scavenging by Sun et al. Documents demonstrated that subtilisin-like proteases (SBTs) are a large family of serine peptidases that are unique to plants and are closely associated with environmental responses (Page and Di Cera, 2008; Jin et al., 2021). Dai et al. identified 120 and 112 SBTs in the tetraploid cotton species G. hirsutum and G. barbadense, while 67 and 69 SBTs were identified in the diploid species G. arboreum and G. raimondii, respectively. In several plant species, NITRATE TRANSPORTER 1/PEPTIDE TRANSPORTER (NPF) genes are reported to play crucial roles in plant growth, development and resistance to various stresses, involving in the transport of nitrate (NO3-) and peptides (O’brien et al., 2016; Fan et al., 2017). Liu et al. identified 201 genes encoding NPF proteins with a peptide transporter (PTR) domain in three different Gossypium species, and investigated expression profiles of the NPF genes. Cui et al. identified a total of 260 Terpene synthases (TPS) in four cotton species and conducted their expression profiles for their crucial characteristics in response to flooding stress. Trihelix transcription factors (TTFs) play important roles in abiotic stress responses in many plant species. Li et al. reported that the overexpression of the cotton trihelix transcription factor GhGT23 in Arabidopsis could mediate salt and drought stress tolerance by binding to GT and MYB promoter elements in stress-related genes. In addition, GhSS9 and GhLDAP2 were reported to enhance drought tolerance of cotton by Dai et al. and Zhao et al., respectively.
In summary, the work published in this Research Topic showed many differentially expressed genes (DEGs) and transcription factors were identified and functionally analyzed in responding salinity, drought, temperatures, and waterlogging stress. As we all know, plant tolerance is a complex quantitative trait, involving a series of DEGs and networks. Although several important research progresses were published in topic, it is far from enough to elucidate how plant tolerances are formed. Based on this Research Topic, it is very necessary to carry out further research into the following issues: 1) manuscripts related to proteomics, miRNAs, DNA methylation, and gene editing should be encouraged; 2) regulation networks of multiple genes should be constructed; 3) interaction mechanism of stress-related genes; 4) the influence of environmental factors on subcellular structures in plants.
XL conceived and wrote the paper. LL, YC, TZ and W.M contributed to the article arrangement and revision. All authors listed have made a substantial, direct, and intellectual contribution to the work and approved it for publication.
This work was supported by the National Natural Science Foundation of China (32001460), and National Engineering Research Center of Cotton Biology Breeding and Industrial Technology / Institute of Cotton Research of CAAS (NERC010103).
The authors declare that the research was conducted in the absence of any commercial or financial relationships that could be construed as a potential conflict of interest.
All claims expressed in this article are solely those of the authors and do not necessarily represent those of their affiliated organizations, or those of the publisher, the editors and the reviewers. Any product that may be evaluated in this article, or claim that may be made by its manufacturer, is not guaranteed or endorsed by the publisher.
Alghabari, F., Ihsan, M. Z., Hussain, S., Aishia, G., Daur, I. (2015). Effect of Rht alleles on wheat grain yield and quality under high temperature and drought stress during booting and anthesis. Environ. Sci. pollut. Res. Int. 22, 15506–15515. doi: 10.1007/s11356-015-4724-z
Dai, Y., Liu, S., Zuo, D., Wang, Q., Lv, L., Zhang, Y., et al. (2023). Identification of MYB gene family and functional analysis of GhMYB4 in cotton (Gossypium spp.). Mol. Genet. Genomics 298, 755–766. doi: 10.1007/s00438-023-02005-5
Fan, X., Naz, M., Fan, X., Xuan, W., Miller, A. J., Xu, G. (2017). Plant nitrate transporters: from gene function to application. J. Exp. Bot. 68, 2463–2475. doi: 10.1093/jxb/erx011
Garrido-Bigotes, A., Valenzuela-Riffo, F., Figueroa, C. R. (2019). Evolutionary analysis of JAZ proteins in plants: an approach in search of the ancestral sequence. Int. J. Mol. Sci. 20(20), 5060. doi: 10.3390/ijms20205060
Guan, R. X., Qu, Y., Guo, Y., Yu, L. L., Liu, Y., Jiang, J. H., et al. (2014). Salinity tolerance in soybean is modulated by natural variation in GmSALT3. Plant J. 80, 937–950. doi: 10.1111/tpj.12695
Jin, X., Liu, Y., Hou, Z., Zhang, Y., Fang, Y., Huang, Y., et al. (2021). Genome-wide investigation of SBT family genes in pineapple and functional analysis of AcoSBT1.12 in floral transition. Front. Genet. 12. doi: 10.3389/fgene.2021.730821
Lu, X., Fu, X., Wang, D., Wang, J., Chen, X., Hao, M., et al. (2019). Resequencing of cv CRI-12 family reveals haplotype block inheritance and recombination of agronomically important genes in artificial selection. Plant Biotechnol. J. 17, 945–955. doi: 10.1111/pbi.13030
Mousavi, S. M. N., Illes, A., Bojtor, C., Demeter, C., Zsuzsanna, B., Vad, A., et al. (2022). Quantitative and qualitative yield in sweet maize hybrids. Braz. J. Biol. 84, e265735. doi: 10.1590/1519-6984.265735
O’brien, J. A., Vega, A., Bouguyon, E., Krouk, G., Gojon, A., Coruzzi, G., et al. (2016). Nitrate transport, sensing, and responses in plants. Mol. Plant 9, 837–856. doi: 10.1016/j.molp.2016.05.004
Page, M. J., Di Cera, E. (2008). Serine peptidases: classification, structure and function. Cell Mol. Life Sci. 65, 1220–1236. doi: 10.1007/s00018-008-7565-9
Rengasamy, P. (2006). World salinization with emphasis on Australia. J. Exp. Bot. 57, 1017–1023. doi: 10.1093/jxb/erj108
Wolters, H., Jurgens, G. (2009). Survival of the flexible: hormonal growth control and adaptation in plant development. Nat. Rev. Genet. 10, 305–317. doi: 10.1038/nrg2558
Keywords: abiotic stress, differentially expressed genes, response, mechanism, function analysis
Citation: Lu X, Li L, Cui Y, Zhao T and Malik WA (2023) Editorial: Identification and functional analysis of differentially expressed genes in plant response to abiotic stresses. Front. Plant Sci. 14:1246964. doi: 10.3389/fpls.2023.1246964
Received: 25 June 2023; Accepted: 13 July 2023;
Published: 20 July 2023.
Edited and Reviewed by:
Binying Fu, Chinese Academy of Agricultural Sciences, ChinaCopyright © 2023 Lu, Li, Cui, Zhao and Malik. This is an open-access article distributed under the terms of the Creative Commons Attribution License (CC BY). The use, distribution or reproduction in other forums is permitted, provided the original author(s) and the copyright owner(s) are credited and that the original publication in this journal is cited, in accordance with accepted academic practice. No use, distribution or reproduction is permitted which does not comply with these terms.
*Correspondence: Xuke Lu, bHV4dWtlMTk4NzYxN0AxNjMuY29t
Disclaimer: All claims expressed in this article are solely those of the authors and do not necessarily represent those of their affiliated organizations, or those of the publisher, the editors and the reviewers. Any product that may be evaluated in this article or claim that may be made by its manufacturer is not guaranteed or endorsed by the publisher.
Research integrity at Frontiers
Learn more about the work of our research integrity team to safeguard the quality of each article we publish.