- 1Genebank Department, Leibniz Institute of Plant Genetics and Crop Plant Research (IPK) OT Gatersleben, Seeland, Germany
- 2Centre for Genetic Resources, the Netherlands (CGN), Wageningen University & Research, Wageningen, Netherlands
Rapeseed is one of the most important agricultural crops and is used in many ways. Due to the advancing climate crisis, the yield potential of rapeseed is increasingly impaired. In addition to changing environmental conditions, the expansion of cultivated areas also favours the infestation of rapeseed with various pests and pathogens. This results in the need for continuous further development of rapeseed varieties. To this end, the potential of the rapeseed gene pool should be exploited, as the various species included in it contain promising resistance alleles against pests and pathogens. In general, the biodiversity of crops and their wild relatives is increasingly endangered. In order to conserve them and to provide impulses for breeding activities as well, strategies for the conservation of plant genetic resources are necessary. In this study, we investigated to what extent the different species of the rapeseed gene pool are conserved in European genebanks and what gaps exist. In addition, a niche modelling approach was used to investigate how the natural distribution ranges of these species are expected to change by the end of the century, assuming different climate change scenarios. It was found that most species of the rapeseed gene pool are significantly underrepresented in European genebanks, especially regarding representation of the natural distribution areas. The situation is exacerbated by the fact that the natural distributions are expected to change, in some cases significantly, as a result of ongoing climate change. It is therefore necessary to further develop strategies to prevent the loss of wild relatives of rapeseed. Based on the results of the study, as a first step we have proposed a priority list of species that should be targeted for collecting in order to conserve the biodiversity of the rapeseed gene pool in the long term.
1 Introduction
Crop plants are major components of human and animal nutrition (Grusak and DellaPenna, 1999), and play an important role as renewable resources or as basic ingredients for chemical or pharmaceutical industry (Metzger and Bornscheuer, 2006; Tilman et al., 2006). Rapeseed or canola (Brassica napus L.) is rated amongst the most important agricultural crops. It is used as high-quality edible oil, as high-protein feed for livestock breeding, as biofuel or as raw material for chemical industry like surfactants, softening agents or biodegradable varnishes (Bell, 1982; Piazza and Foglia, 2001; Link, 2008).
Amongst oil plants, rapeseed holds the second largest global market share after soybean (USDA, 2023). In addition, it is also one of the most important sources of protein for animal feed (Hu et al., 2021). Rapeseed provides an average of 40–50% of oil on a dry basis and a protein content of 17–26%. This was achieved by means of breeding programmes starting in the 1970s, which resulted in a significant reduction of harmful glucosinolates and erucic acid (Link, 2008; Jahreis and Schäfer, 2011; Barthet, 2016; Dhillon et al., 2016), in double-low (also called double-zero) cultivars. Rapeseed is the major crop for oilseed production in Europe (Carré and Pouzet, 2014).
Due to the progressing climate crisis, the yield potential of rapeseed is increasingly affected. In particular, rising temperatures, shifting rainfall patterns and increasing incidence of extreme weather events lead to unfavourable effects, such as decreasing yield (Qian et al., 2018). Changed environmental conditions and the extension of acreage foster the infestation of rapeseed with different pests and pathogens (Link, 2008; Williams, 2010). Amongst the most important pathogens are the beet western yellows virus, the Phoma lingam and Verticillium species, respectively (Gilligan et al., 1980; Heale and Karapapa, 1999; Fitt et al., 2006; Hwang et al., 2016).
In order to cope with the increasing demand for rapeseed and with climatic changes, it is necessary to improve rapeseed varieties continuously. Therefore, it is promising to exploit the potential of its gene pool for breeding through crossing with related species from the primary, secondary and tertiary gene pool, respectively (Chen and Heneen, 1990; Girke et al., 2012). The high potential of crop wild relative (CWR) species was first recognised in the 1920s by the Russian geneticist Nikolay Ivanovich Vavilov (Vavilov, 1926). CWRs usually have a broader genetic variability than crop plants domesticated over hundreds of years (Singh, 2001; McCouch, 2004; Vollbrecht and Sigmon, 2005). The importance of CWRs is continuously growing through scientific progress, e.g. by biotechnological methods enabling gene transfer between distantly related species (Hajjar and Hodgkin, 2007; Ford-Lloyd et al., 2011; Maxted et al., 2012).
In order to simplify CWR classification, Harlan and de Wet proposed to categorise the total available gene pool of a crop and its related species into three groups depending on their degree of relationship with the crop of interest (Harlan and de Wet, 1971). The primary gene pool contains the cultivated species and taxa with which it is completely inter-fertile, thus allowing easy inter-crossing. The secondary gene pool comprises taxa from different species, which are nonetheless closely related. These species can be used for crossing with at least some fertile hybrids. Gene transfer is difficult and may require the use of biotechnological techniques. The tertiary gene pool consists of more distantly related species. Crossing is only possible through the use of biotechnological techniques, such as embryo rescue or bridge crossing, requiring considerable effort.
The gene pool of rapeseed contains species with a large variety of promising resistance alleles against pests and pathogens. Related species, such as Brassica elongata Ehrh., Brassica nigra (L.) W. D. J. Koch, Brassica juncea (L.) Czern., Sinapis alba L. or Sinapis arvensis L., are known to harbour resistance genes against P. lingam whereas Brassica rapa L. has been found to show resistances against Verticillium wilt (Gerdemann-Knörck et al., 1994; Diederichsen and Sacristan, 1996; Snowdon et al., 2000; Chen et al., 2007; Rygulla et al., 2007; Wei et al., 2010). However, the biological diversity of crop wild relatives is increasingly threatened, not only by the changing climate but also by population expansion, urbanisation and environmental pollution, respectively (Bakarr et al., 2007; Jarvis et al., 2008; van Treuren et al., 2012).
In order to prevent the extinction of species on the one hand and to provide new impulses to breeding programmes on the other hand, it is indispensable to further develop strategies for preserving plant genetic resources for food and agriculture (PGRFA) (Maxted et al., 2012; Parra-Quijano et al., 2012). Important contributions to the preservation of PGRFA are the collection, the maintenance and the characterisation of crop plants and crop wild relatives. In particular, genebanks play an important role for the long-term conservation of PGRFA (Hoisington et al., 1999). There are about 1,800 collections conserving PGRFA around the world. Thereof, about 625 collections are maintained in Europe comprising more than 2 million accessions (Engels and Maggioni, 2012).
A widely accepted goal for the conservation of plant genetic resources is to conserve 95% of all alleles of a random locus that occur in a target population at a frequency of more than 5% (Nagel et al., 2022). However, for decades there has been controversy about what this means in terms of the minimum number of genebank accessions required. When collecting species, there is a tension between the goal of representing the greatest possible genetic diversity of the individual species and the simultaneous practical requirement of having to limit the size of the samples to a manageable level (Allard, 1970). Marshall and Brown (1975) have suggested sampling 50 populations within an ecogeographical region, collecting 50 individual plants per population. In contrast, Crossa et al. (1993) consider that a sample size of 160-210 plants is sufficient. Lawrence et al. (1995), in turn, conclude that 172 randomly sampled plants from a population of a species are sufficient to maintain genetic diversity. When collecting several populations, it is sufficient to take no more than 172 plants per population divided by the number of populations. Irrespective of this discussion, even in the case of a very intensive collection of individual species, the question arises to what extent genetic diversity is covered by a large number of accessions alone (Maxted et al., 2008). For this purpose, other evaluation criteria must also be taken into account, for example the taxonomic composition of the gene pool, the threat status or ecogeographical aspects. It is assumed that sampling populations from distant sites and different habitats will give a more representative coverage of the genetic diversity of a taxon (Maxted et al., 1995). However, most of the existing collections of a target crop hardly contain a representation of the entire known population of the target crop.
In that regard, gap analysis is an important aspect of genetic resources management. In general, gap analysis is a technique to identify shortcomings in biodiversity conservation actions, e.g. missing biodiversity in plant genetic resources collections or in protected areas (Jennings, 2000; Margules and Pressey, 2000; Maxted et al., 2008). It comprises various steps (Burley, 1988): (1) to identify the biodiversity within a region; (2) to examine existing conservation approaches, e.g. protected areas; (3) to determine, which elements of the biodiversity are underrepresented by the existing conservation approaches; (4) to define additional conservation actions.
In principle, gap analysis is applicable to both ex situ and in situ conservation. The present paper focusses on the ex situ conservation of PGRFA in genebank collections. In this context, gap analysis helps to identify the geographical distribution of species of interest and allows comparing with existing genebank holdings. Detected gaps can then be closed, e.g. by organising collecting expeditions.
Comprehensive information about the composition of European germplasm collections is available from the European Search Catalogue for Plant Genetic Resources (EURISCO) (Weise et al., 2017; Kotni et al., 2023). EURISCO is an information system, which documents more than two million accessions maintained ex situ in more than 400 collections. It is maintained on behalf of the European Cooperative Programme for Plant Genetic Resources (ECPGR) and is based on a network of National Inventories of 43 member countries from Europe and beyond.
To prioritise species for the improvement of genebank collections, the expected effects of climate change on the distribution of species in their natural environment should be taken into account. In addition, other threat assessments should also be taken into consideration, such as the IUCN Red List of Threatened Species (IUCN, 2023).
Since the expected climate changes depend on a large number of factors, various scenarios have been developed that are referred to as Representative Concentration Pathways (RCPs). Four scenarios have become established (RCP 2.6, RCP 4.5, RCP 6.0 and RCP 8.5), which predict possible changes in greenhouse gas emissions up to the year 2100 in relation to the pre-industrial age around the year 1750 (van Vuuren et al., 2011). This paper uses only the two most contrasting scenarios RCP 2.6 and RCP 8.5 to assess the impacts of climate change. RCP 2.6 is an optimistic scenario assuming that greenhouse gas emissions will decline after 2020. In this case, a global temperature increase of 0.3 to 1.7°C is expected between 2081 and 2100. In contrast, in the pessimistic scenario RCP 8.5, greenhouse gas emissions continue to rise resulting in a temperature increase of 2.6 to 4.8°C over the same period (Stocker et al., 2013). There’s nowadays little doubt that climate change will have significant effects on the distribution of species in their natural habitats, as the current environmental conditions will very likely be affected by climate change. Such effects can be estimated using Species Distribution Models (SDMs). In these models, the presence of a particular species at geographical locations is related to the local environmental conditions, such as temperature and precipitation parameters, after which these relationships can be used to predict species occurrence at other locations. When climate change scenarios are included in the modelling, predictions can be made of the future distribution of a species (Aguirre-Gutiérrez et al., 2017).
Here, we aim to analyse the representation of rapeseed and its wild relatives in European genebank collections and to identify gaps. In addition, ecological niche modelling was used to predict the effects of climate change on future species distributions in order to prioritise relevant species for conservation.
2 Materials and methods
2.1 Species data
To determine the composition of the primary, secondary and tertiary gene pool of rapeseed, the Crop Wild Relative Inventory (Vincent et al., 2013; CWR, 2023) of the Global Crop Diversity Trust was used. According to the Crop Wild Relative Inventory, the gene pool of rapeseed comprises various taxa from 16 genera. For improving the accuracy of further comparisons with data on germplasm holdings, these taxa were checked for synonym names based on The World Flora Online (WFO, 2023), which resulted in about 900 additional names (including subtaxa). Based on the identified composition of the rapeseed gene pool, germplasm collections across Europe were examined for gaps, i.e. countries in the distribution range of a species for which no accession are available in PGR collections. The emphasis was on European collections, because rapeseed originated from natural crossings of B. rapa and Brassica oleracea L (Neuffer, 2001). both occurring in the Mediterranean area. Also, the other species of the rapeseed gene pool mainly occur in European temperate areas. Therefore, the natural occurrence ranges of the species of the rapeseed gene pool were determined using the Euro+Med PlantBase (Euro+Med, 2023) and GRIN Taxonomy (GRIN, 2023). Information about the origin countries of genebank accessions, the countries maintaining the accessions as well as the numbers of available accessions was extracted from EURISCO (EURISCO, 2023). This data was then compared with the natural ranges of the species. In addition, the species of the rapeseed genepool were checked against the IUCN Red List of Threatened Species (IUCN, 2023).
2.2 Species distribution modelling
Species distribution modelling (or ecological niche modelling) was used to predict the effects of climate change on the future distribution of the wild relatives of B. napus in Europe and countries bordering the Mediterranean Sea. Modelling procedures followed the methods described by Aguirre-Gutiérrez et al. (2017) and van Treuren et al. (van Treuren et al., 2017; van Treuren et al., 2020). Table 1 lists 51 taxa related to rapeseed (B. napus). For the modelling, geographic occurrence data of these species were downloaded from the Global Biodiversity Information Facility (GBIF) (GBIF, 2023), with the exception of the five cultivated species (Brassica carinata A. Braun, B. juncea, B. napus, B. oleracea and Raphanus sativus L.) where it is impossible to distinguish natural occurrences from escapes from cultivation. Five taxa (Crambe hispanica subsp. abyssinica (Hochst. ex R.E.Fr.) Prina, Erucastrum abyssinicum R. E. Fr., Orychophragmus violaceus (L.) O.E. Schulz, Physaria fendleri (A. Gray) OKane & Al-Shehbaz and Rorippa indica (L.) Hiern) had no occurrence data within the studied region. From five taxa (Brassica dimorpha Coss. & Durieu, Brassica deserti Danin & Hedge, Brassica desnottesii Emb. & Maire, Brassica souliei Batt. subsp. souliei Batt. and Brassica hilarionis Post) the number of georeferenced locality data was insufficient for distribution modelling. Brassica souliei Batt. (exluding subsp. amplexicaulis) was used instead of Brassica souliei Batt. subsp. souliei Batt. Occurrence data of Hirschfeldia incana (L.) Lagr.-Foss. were downloaded using its synonym Sinapis incana L. Records from outside the studied region as well as records with missing or incorrect geographic information were removed. For nine taxa having low numbers of occurrences some additional records could be georeferenced using the locality descriptions and Google Earth. A spatial resolution, corresponding to a grid size of 2.5 min of a degree of longitude and latitude in the WorldClim dataset (Hijmans et al., 2005), was used to process the occurrence data. Multiple occurrence data per grid cell were reduced to one observation. To avoid spatial autocorrelation, only records separated by at least one grid cell were used for the distribution modelling, using seven bioclimatic variables (related to temperature and precipitation) and two soil variables (van Treuren et al., 2020). In the supplementary data, the downloads from GBIF and the number of grid cells used are given for each taxon1. The manually georeferenced records have been made available as well. The R programming language (R_Core_Team, 2019) was used for distribution modelling with the Biomod2 package (Thuiller et al., 2009). Details of the modelling procedures are provided by Aguirre-Gutiérrez et al. (2017) and van Treuren et al. (2017). Predicted occurrences are solely based on the expected suitability of geographic locations as a result of the examined bioclimatic and soil variables. Other factors that may influence species occurrence, such as dispersal ability or geographic barriers, are not taken into account.
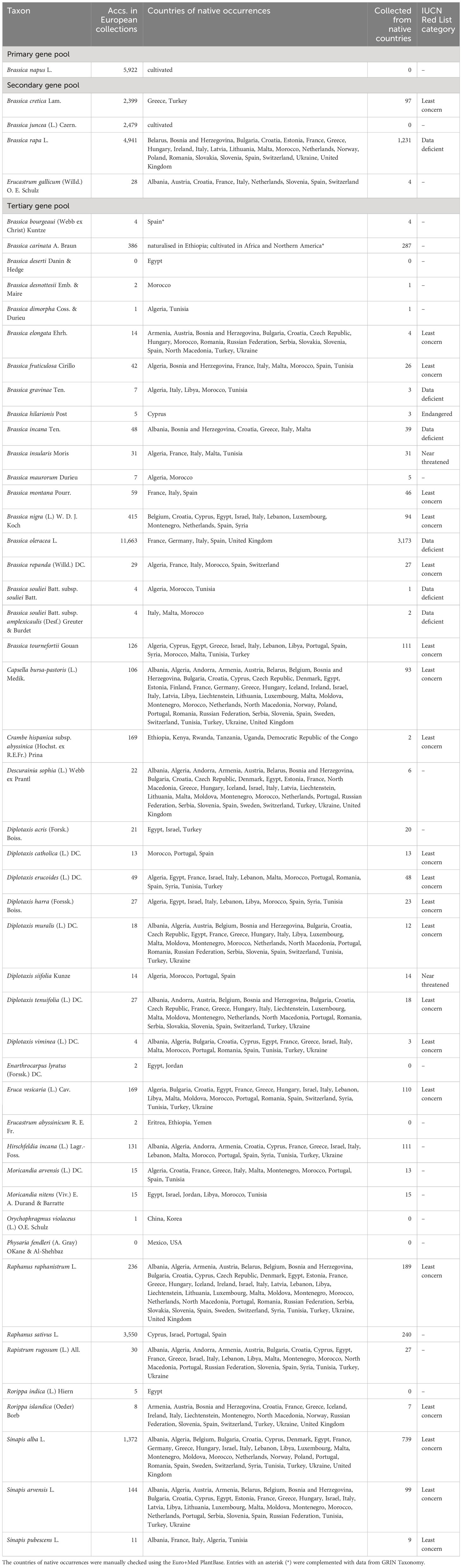
Table 1 Genepool of Brassica napus L. and its representation in European genebanks based on EURISCO data.
3 Results and discussion
Europe and especially the Mediterranean area provide a great richness of species. Coincidently, the most critical collection gaps are related to this area (Castañeda-Álvarez et al., 2016). The Cruciferous (Brassicaceae) family currently comprises 338 genera and 3,709 species (Al-Shehbaz et al., 2006). Thereof, 39 accepted species names belong to the genus Brassica (Warwick and Francis, 2006). This number considerably increases when taking into account the large number of existing synonyms. The origins of the Brassica species are the area of the Mediterranean and southwest Asia (Al-Shehbaz et al., 2006). Many of the species of the genus Brassica are economically important, especially B. rapa, B. nigra, B. oleracea, B. juncea, B. napus and B. carinata (Cheng et al., 2014). This is also reflected by the number of holdings in European germplasm collections. The majority of the Brassica species are wild relatives which are not economically significant and therefore collected to a much lesser extent. However, they are of great importance for resistance to abiotic and biotic stresses and have the potential to improve resilience in modern cultivars (Quezada-Martinez et al., 2021). In addition, some of the wild Brassicas could even serve as a source of a new crop (Razzaq et al., 2021).
3.1 Data basis used
The present study is based on freely accessible data. The main basis is passport data from the EURISCO system, which provides comprehensive data on the majority of European genebank collections. EURISCO is an aggregator database that is unique in terms of the quantity and quality of data available and the underlying network. Nevertheless, there are limitations that need to be considered. Collections of plant genetic resources are sometimes very old and documented to varying degrees. Therefore, it cannot be assumed that there is complete information about the countries of origin and the sites where the genebank samples were found. For this reason, only those accessions could be considered for which collecting information is available. Despite all limitations, this still represents the best possible data available.
Furthermore, data on natural occurrence countries of the different Brassica species were used. These data are not necessarily complete either, as they depend heavily on the available literature sources. The fact that a country, in contrast to its immediate neighbours, is not listed as a natural area of origin does not necessarily mean that the corresponding material does not exist there, but only that it has not been described there so far. As mentioned above, an attempt was made to complement data from several sources.
3.2 Gene pool inventory and representation in genebanks for rapeseed
Results of the inventory are shown in Table 1. The rapeseed gene pool comprises 51 species, of which a total of 34,777 accessions are included in EURISCO. Subtaxa were not considered, as corresponding information is only available for a part of the accessions. No accessions of B. deserti and P. fendleri were found in European genebank collections. If the inventory is further restricted to accessions originating from the native occurrence areas and marked as collected material, the total number of accessions is 7,001. In this context, only areas known for native occurrences (represented by countries) were considered. Areas, in which those species were introduced or are being cultivated, were ignored.
The primary gene pool of rapeseed only consists of the cultivated species B. napus, which is represented by 5,922 accessions in European genebanks. These numbers include not only oil types but also 564 swede accessions (B. napus var. napobrassica (L.) Rchb.) as well as 65 Siberian kale accessions (B. napus var. pabularia (DC.) Rchb.).
The secondary gene pool comprises four species, including 9,847 accessions in total. B. juncea is a cultivated species, for which 2,479 accessions are preserved. With 2,399 accessions, Brassica cretica Lam. is represented with high numbers. However, only 97 of them were collected in native occurrence countries, corresponding to 4% of the total number of accessions of this species. For Erucastrum gallicum (Willd.) O. E. Schulz, only 28 accessions are preserved in European genebanks with four of them collected from native occurrence countries. In the case of B. rapa, which is partially cultivated, 1,231 (25%) out of 4,941 accessions were collected from native countries.
The tertiary gene pool comprises 46 species, which are represented by 19,008 accessions. Despite some highly represented species, such as the economically important B. oleracea (11,663 accessions), B. nigra (415 acc.), B. carinata (386 acc.) as well as R. sativus (3,550 acc.) and S. alba (1,372 acc.), the majority of species are only maintained in relatively low numbers in European genebanks (Table 1). 35% of the species of the tertiary gene pool are represented by less than 10 accessions and 50% by less than 20 accessions (Figure 1).
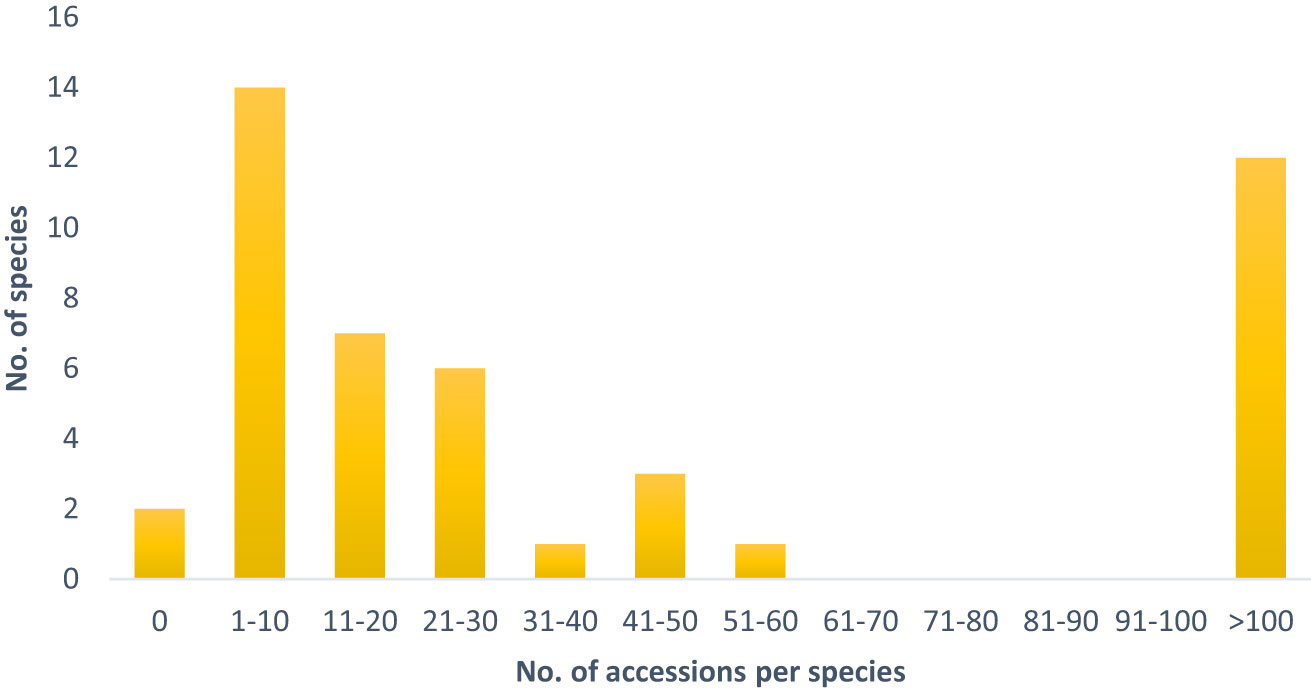
Figure 1 Number of accessions per species of the tertiary gene pool, which are maintained in European germplasm collections.
Even if the natural regions of origin are not considered, it is obvious that the majority of species of the Brassica gene pool are underrepresented in European genebanks although collecting missions were carried out in the 1970s (Razzaq et al., 2021). To solve this problem, a global strategy for the conservation of Brassica genetic resources was developed by the Global Crop Diversity Trust (Allender and Giovannini, 2023), confirming the importance of CWRs. Countries such as Italy have recognised the importance of Brassica CWRs and have established action plans (Ciancaleoni et al., 2021; Perrino and Wagensommer, 2022). Further collecting strategies for the whole of Europe need to be developed to improve ex situ conservation of at least some underrepresented species like E. gallicum from the secondary genepool which has only four accessions from native countries but nine countries where the species naturally occurs. The CWRs are also important for other Brassica genepools beside rapeseed because of the close evolutionary relationship. Rapeseed originated from an interspecific hybridisation of B. rapa and B. oleracea (Quezada-Martinez et al., 2021).
3.3 Conservation status
The Sampled Red List Index shows that approximately 22% of the plant species are threatened with extinction (Brummitt et al., 2015). Out of 1,350 European plant species, more than half are expected to be vulnerable or threatened by 2080 (Thuiller et al., 2005).
The species of the rapeseed gene pool were investigated for their conservation status. According to the IUCN Red List of Threatened Species, B. hilarionis is classified as endangered, while Brassica insularis Moris and Diplotaxis siifolia Kunze are classified as near threatened. With 5, 31 and 14 accessions, respectively, these species are maintained in low numbers in European collections. This refers only to the total accession numbers (34,777, see above); the natural occurrence countries are not taken into account here either. If these are additionally included in the considerations, the underrepresentation is further aggravated.
In general, the numbers presented in Table 1 indicate that rapeseed gene pool accessions collected at native occurrence countries of the respective species are underrepresented in European genebanks. This situation is even worse when looking at the representation of individual countries belonging to the natural distribution area (Supplementary Table 1). For example, the near threatened species, B. insularis natively occurs in five different countries, but was only collected in three of them. The near threatened species D. siifolia was collected in three out of four countries only, which is a cause for concern given the low number of accessions. B. hilarionis (endangered) is endemic to Cyprus and occurs nowhere else. Underrepresentation was also evident for species that currently are of least concern. For B. elongata, 17 countries were identified for native occurrences, but only in three of them accessions were collected. For Descurainia sophia (L.) Webb ex Prantl, 38 countries were identified, but accessions were only collected in six.
It should be noted here that the data situation regarding the endangerment status only allows limited statements to be made. Most species of the Brassica genepool are not endangered or near threatened. 21 out of the 51 species are assigned to the IUCN Red List category of least concern. For six other species, data is indicated as deficient, while 21 species are not listed in the Red List at all. Only three species are endangered or near threatened, respectively. Nevertheless, it is important to improve the future conservation, as they play a major role in crop improvement (Raggi et al., 2022). In this context, of course, conservation under in situ conditions should not be ignored, especially for wild species. However, it must be taken into account that survival under in situ conditions is by no means guaranteed (e.g. due to climate change). In addition, access to in situ material is also difficult for users. A strong focus on ex situ conservation is therefore indispensable. Appropriate strategies need to be developed for this. Therefore, as a first step, we propose a priority list for the targeted collecting (see 3.5.)
3.4 Effects of climate change on species distribution
Figure 2 shows the results of the niche modelling for the wild species of the secondary gene pool. B. juncea. was not considered because it is a cultivated species. B. rapa is not shown in Figure 2 since it is partly cultivated. In the case of B. cretica the simulation results show that the range remains almost constant at RCP 2.6 (expansion of 0.03%), but shrinks by 29.4% at RCP 8.5, assuming full migration potential. If there is no migration, however, the range will decrease by 33.9% (RCP 2.6) or 63.7% (RCP 8.5). Even more dramatic are the changes in E. gallicum (reduction of 29.5% and 71.5% respectively with migration; reduction of 40.6% and 89.4% respectively without migration) and B. rapa (reduction of 9.6% and 26.3% respectively with migration; reduction of 26.2% and 42.6% respectively without migration) (Table 2).
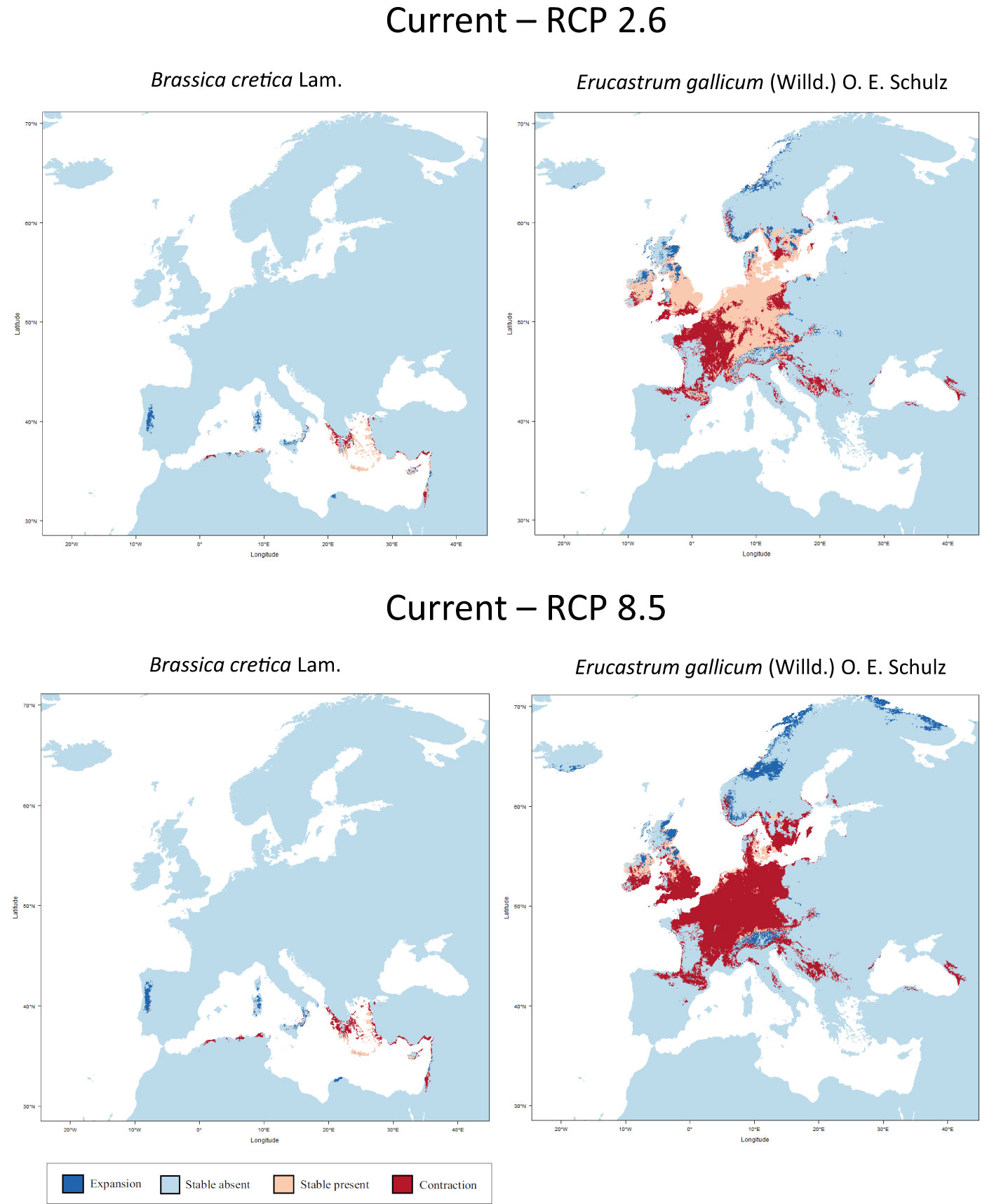
Figure 2 Predicted areas of distribution for the species of the secondary gene pool B. cretica and E. gallicum under the climate change scenarios RCP 2.6 (optimistic) and RCP 8.5 (pessimistic) for the year 2070 as compared to current conditions.
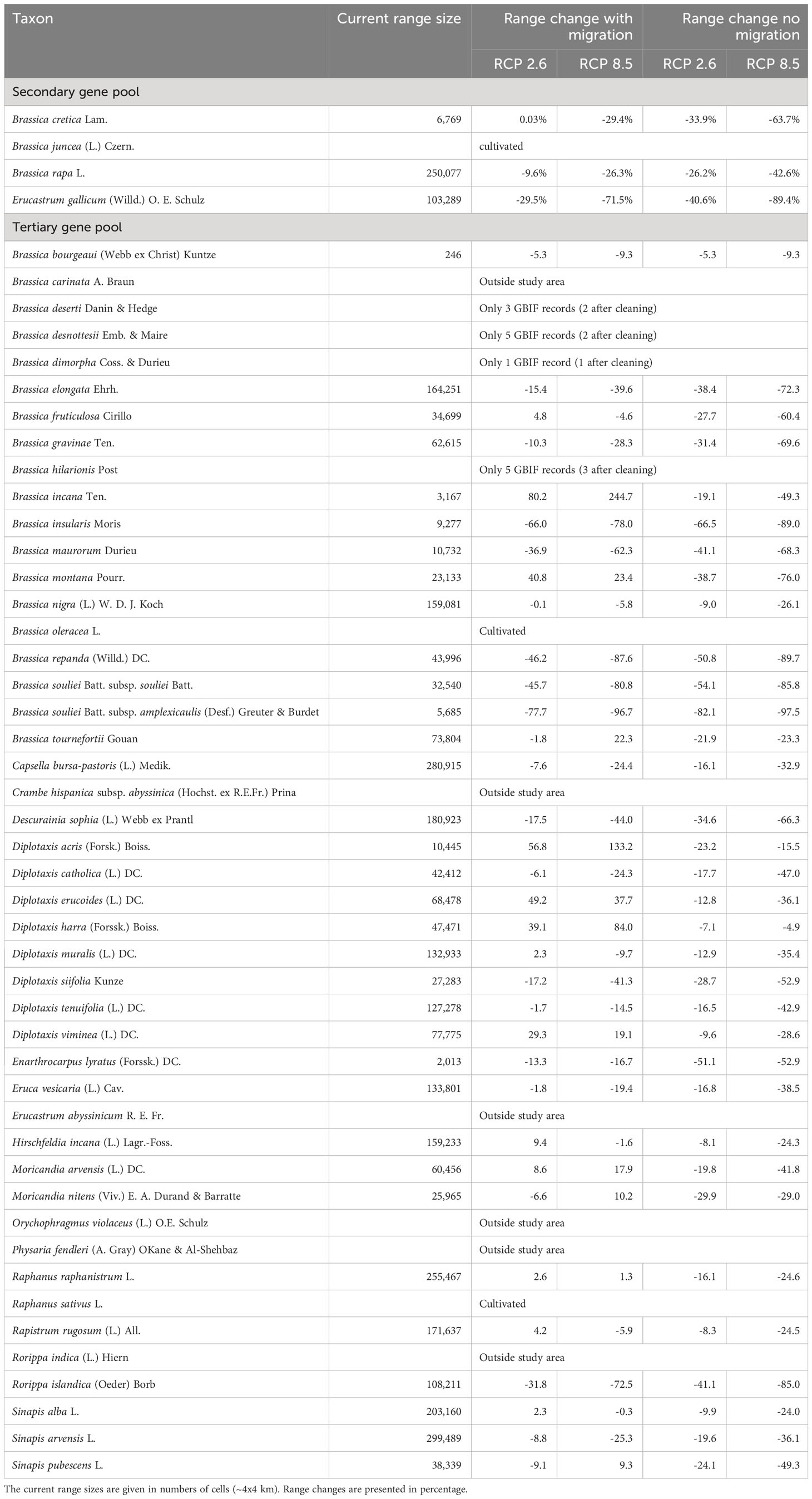
Table 2 Predicted changes of the distribution area of the species of the secondary and tertiary gene pool in 2070 under RCP 2.6 (optimistic scenario) and RCP 8.5 (pessimistic scenario), both with and without migration.
At this point, the three species listed in the IUCN Red List of Threatened Species as endangered or near threatened deserve closer examination. All three belong to the tertiary gene pool. For B. hilarionis, no niche modelling could be performed because the available occurrence data was insufficient. For B. insularis, the calculations indicate that the distribution area will decrease by 66.0% (RCP 2.6) or 78.0% (RCP 8.5) assuming full migration potential. Without migration, the decline will even be 66.5% (RCP 2.6) and 89.0% (RCP 8.5), respectively. In the case of D. siifolia, a significant reduction of the distribution area is also to be expected (reduction of 17.2% and 41.3% respectively with migration; reduction of 28.7% and 52.9% respectively without migration) (Figure 3). This is particularly dramatic against the background of the rather low number of germplasm accessions of these species in European collections. Modelling results of all species of the tertiary gene pool are shown in Table 2. In addition to the figures given there, the Supplementary Data also shows the predicted changes in the distribution areas for each species on maps.
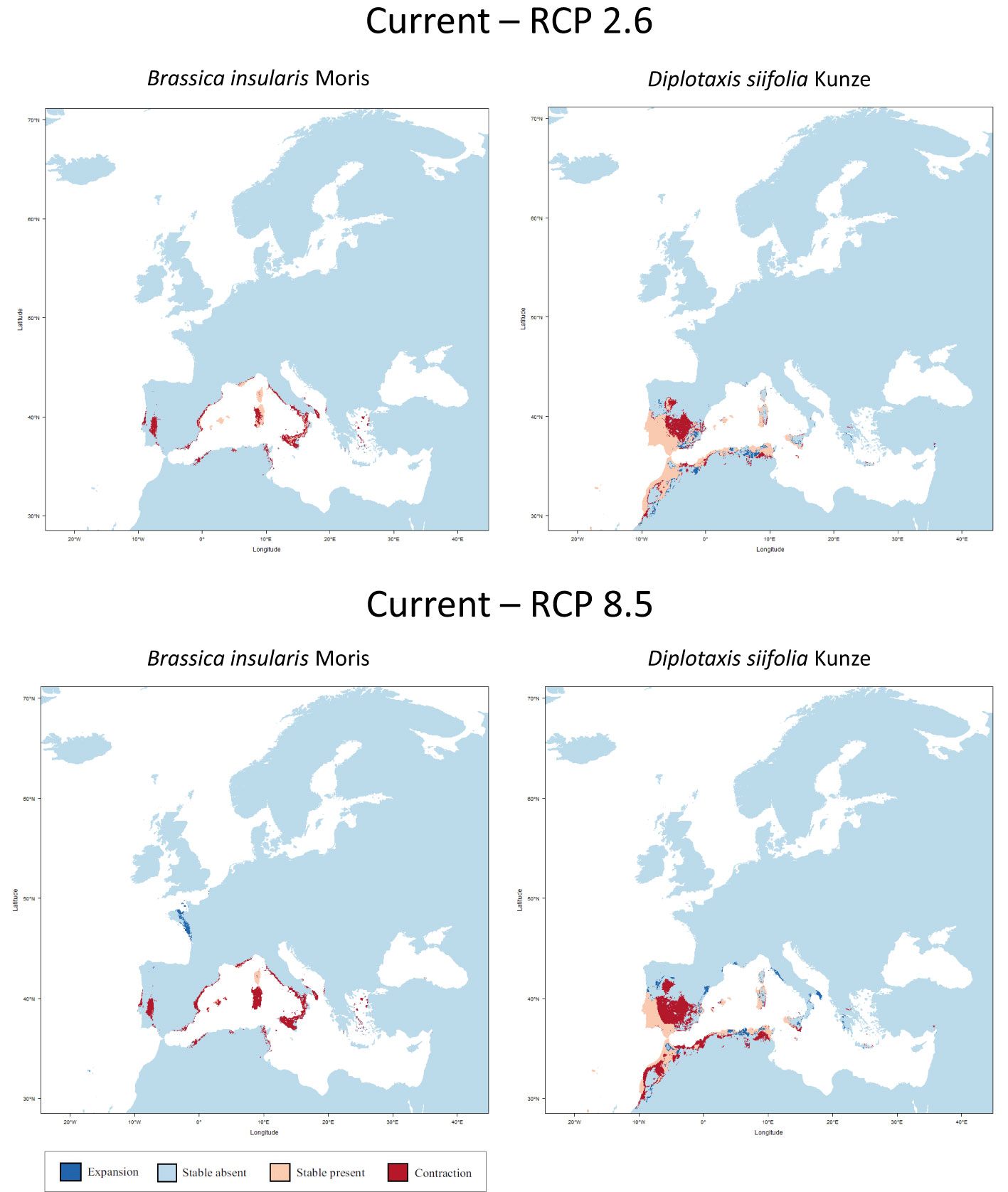
Figure 3 Predicted areas of distribution for Red List species of the tertiary gene pool under the two climate change scenarios RCP 2.6 (optimistic) and RCP 8.5 (pessimistic) for the year 2070 as compared to current conditions. B. hilarionis was omitted from the calculations due to insufficient occurrence data.
Niche modelling shows the expected loss of distribution, but also the potential for new areas of occurrence. However, the dispersal ability of species or geographic barriers that restrict migration are not considered. For the studied region, it is not known whether the species are able to reach the areas where favourable climatic conditions prevail.
However, against the background of climate change and its likely effects in the whole of Europe, there is an urgent need for action, which was made evident by the niche modelling carried out. In this context, it should be noted that the modelling results of the optimistic scenario (RCP 2.6) are probably less likely compared to those of the pessimistic scenario (RCP 8.5). The long-term effects of global warming, which is taking place in the 21st century, will also have an impact on the following centuries (Schwalm et al., 2020; Lyon et al., 2022). The niche modelling used here offers the opportunity to react in advance to upcoming changes and to adjust the collection development of the European genebanks accordingly. Based on the results of niche modelling, the development of a collecting strategy for the endangered as well as underrepresented species is therefore urgently needed.
3.5 Implications for conservation
As described in the previous sections, the majority of species of the rapeseed gene pool are underrepresented in European genebanks. This concerns in particular CWRs from the secondary and tertiary gene pool, which are also important for other Brassica species besides rapeseed due to the close evolutionary relationship between B. napus, B. rapa and B. oleracea. In addition, the CWRs have great potential for future crop improvement.
Based on the composition of the rapeseed gene pool, the information on the IUCN Red List status, the representation of the species in European genebanks (including consideration of the natural countries of origin) and the predicted effects of climate change on the future distribution ranges, we would like to propose a priority list for targeted collection. For this purpose, the species were assigned to three priority groups (high, medium, low). Cultivated species were excluded from this consideration. B. rapa is partly cultivated and was therefore also excluded from the priority list. All species listed as endangered or near threatened on the IUCN Red List were assigned to the highest priority.
For all other species, the considerations included how many accessions from the natural occurrence countries are maintained in European genebanks. As already noted in the introduction, a major difficulty in this context is that for decades there has been controversy about the minimum number of accessions required to maintain the natural diversity of a species. Maxted et al. (2020) summarises these discussions very well. In addition to the existing literature, many years of experience from practical genebank work were therefore also taken into account when drawing up the priority list.
Furthermore, the predicted future reduction of the natural distribution ranges was included in the considerations. Based on the fact that human-induced global warming has increased at an unprecedented rate within the last ten years (Forster et al., 2023), we used the niche modelling results of the RCP 8.5 scenario and also assumed that no species migration takes place.
The procedure is described in detail in the Supplementary File “Priority list creation.pdf”, the creation of the priority list in Supplementary Table 2. The results are summarised in Table 3, which lists species from the rapeseed gene pool that should be collected with priority in their natural occurrence countries.
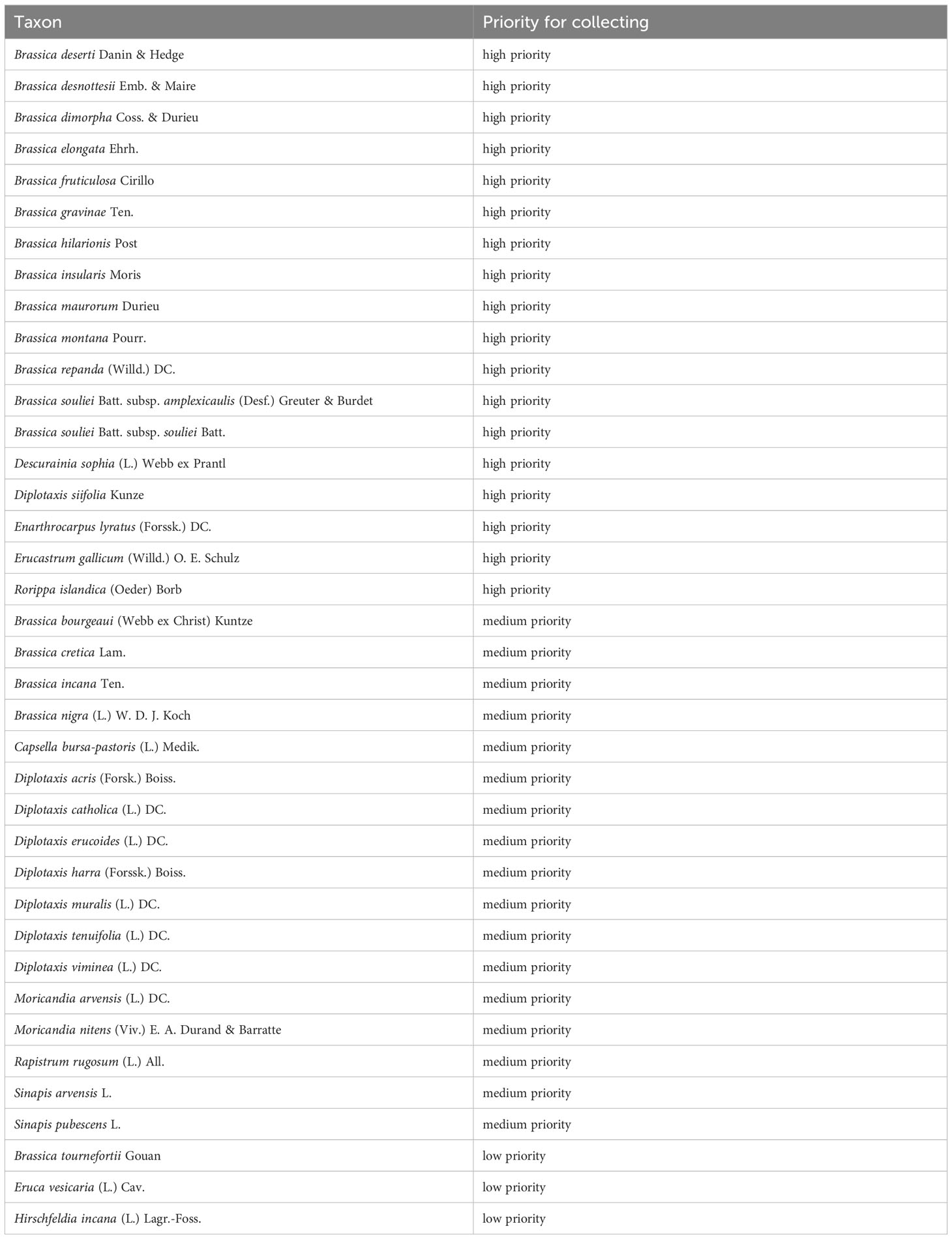
Table 3 Species from the rapeseed gene pool that should be collected with priority in their natural occurrence areas.
As a result, 18 species of the rapeseed gene pool were assigned to the highest category of the priority list. Here, it is reasonable to target the natural areas of origin and to collect additional material to be maintained ex situ in genebanks.
Therefore, further conservation and collecting strategies need to be developed for Europe at large. It should be taken into account that, especially for wild species, in situ conservation can play an important additional role, but reliable ex situ conservation is essential in any case. Data on the endangerment status can be used as a supplement in this context, but is not sufficient for a variety of species. An important role in the development of collecting strategies is taken by the expected effects of climate change on the natural distribution areas of the rapeseed gene pool species, as predicted by niche modelling. This makes it possible to react to future changes and to adapt the collection development of the genebanks accordingly.
4 Conclusion
In this study, we analysed the rapeseed gene pool and investigated to what extent the different species are conserved in European genebanks and which gaps exist. This also included the natural distribution ranges and it was found that most species of the rapeseed gene pool are significantly underrepresented in European genebanks. In addition, a niche modelling approach was used to investigate how the natural ranges of these species are likely to change by the end of the century under the assumption of various climate change scenarios. In some cases, considerable changes in the natural distribution areas were predicted. In order to close the existing gaps, a priority list was proposed. In addition to collecting trips, which are of course indispensable, sustainable conservation of CWRs requires a combination of ex situ and in situ efforts.
In general, various actions need to be taken to preserve CWRs, strategies are necessary to avoid loss of wild relatives of rapeseed: (1) Safeguarding the maintenance of all available CWR accessions in the genebanks in order to avoid further loss of material including regular regeneration and storage of a safety duplicate in Svalbard Global Seed Vault (2) Planning of collecting missions to increase the number of CWRs in genebanks for ex situ conservation. This should follow the priority list starting with the high priority species. (3) Undertaking also in situ conservation to increase the number of individuals in wild populations. (4) Protecting of natural habitats to prevent extinction. (5) Based on the niche modelling monitoring of the natural habitats. In case of loss due to climate change programmes for recolonisation or creation of new habitats.
Data availability statement
The datasets used for the niche modelling as well as the detailed results were published in an online repository. They are accessible by the DOI: 10.5281/zenodo.8081795.
Author contributions
SW coordinated the draft; SW, RH, KK, MO, RT and UL conceived and wrote the manuscript. All authors contributed to the article and approved the submitted version.
Funding
Costs for open access publishing were partially funded by the Deutsche Forschungsgemeinschaft (DFG, German Research Foundation, grant 491250510).
Acknowledgments
We would like to thank Stefanie Kreide and Manuela Nagel for fruitful discussions.
Conflict of interest
The authors declare that the research was conducted in the absence of any commercial or financial relationships that could be construed as a potential conflict of interest.
Publisher’s note
All claims expressed in this article are solely those of the authors and do not necessarily represent those of their affiliated organizations, or those of the publisher, the editors and the reviewers. Any product that may be evaluated in this article, or claim that may be made by its manufacturer, is not guaranteed or endorsed by the publisher.
Supplementary material
The Supplementary Material for this article can be found online at: https://www.frontiersin.org/articles/10.3389/fpls.2023.1244467/full#supplementary-material
Footnotes
- ^ The supplementary file “Niche modelling data.zip” is available from Zenodo by the DOI 10.5281/zenodo.8081795.
References
Aguirre-Gutiérrez, J., van Treuren, R., Hoekstra, R., van Hintum, T. J. L. (2017). Crop wild relatives range shifts and conservation in Europe under climate change. Diversity Distribut. 23 (7), 739–750. doi: 10.1111/ddi.12573
Allard, R. W. (1970). “Population structure and sampling methods, in Genetic Resources in Plants,” in Their exploration and conservation. Eds. Frankel, O. H., Bennet, E. (Blackwell: Oxford and Edinburgh), 97–107.
Allender, C., Giovannini, P. (2023). Global strategy for the conservation of brassica genetic resources (Bonn, Germany: Global Crop Diversity Trust).
Al-Shehbaz, I. A., Beilstein, M. A., Kellogg, E. A. (2006). Systematics and phylogeny of the Brassicaceae (Cruciferae): an overview. Plant Syst. Evol. 259 (2-4), 89–120. doi: 10.1007/s00606-006-0415-z
Bakarr, M. I., Bennun, L. A., Brooks, T. M., Clay, R. P., Darwall, W. R.T., De Silva, N., et al. (2007). Identification and gap analysis of key biodiversity areas: targets for comprehensive protected area systems. Best practice protected area guidelines series. Ed. Valentine, P. (Gland, Switzerland: IUCN), 116.
Barthet, V. J. (2016). Canola: overview, in encyclopedia of food grains. 2nd ed. Ed. Wrigley, C., et al (Oxford: Academic Press), 237–241.
Bell, J. M. (1982). From rapeseed to canola: A brief history of research for superior meal and edible oil. Poultry Sci. 61 (4), 613–622. doi: 10.3382/ps.0610613
Brummitt, N., Bachman, S. P., Aletrari, E., Chadburn, H., Griffiths-Lee, J., Lutz, M., et al. (2015). The Sampled Red List Index for Plants, phase II: ground-truthing specimen-based conservation assessments. Philos. Trans. R. Soc. B: Biol. Sci. 370 (1662), 20140015. doi: 10.1098/rstb.2014.0015
Burley, F. W. (1988). “Monitoring biological diversity for setting priorities in conservation,” in Biodiversity. Eds. Wilson, E. O., Peter, F. M. (Washington, DC: The National Academies Press), 227–230.
Carré, P., Pouzet, A. (2014). Rapeseed market, worldwide and in Europe. OCL 21 (1), D102. doi: 10.1051/ocl/2013054
Castañeda-Álvarez, N. P., Khoury, C. K., Achicanoy, H. A., Bernau, V., Dempewolf, H., Eastwood, R. J., et al. (2016). Global conservation priorities for crop wild relatives. Nat. Plants 2, 16022. doi: 10.1038/nplants.2016.22
Chen, B.-Y., Heneen, W. K. (1990). Resynthesized Brassica napus L.: A review of its potential in breeding and genetic analysis. Hereditas 111 (3), 255–263. doi: 10.1111/j.1601-5223.1990.tb00404.x
Chen, H.-F., Wang, H., Li, Z.-Y. (2007). Production and genetic analysis of partial hybrids in intertribal crosses between Brassica species (B. rapa, B. napus) and Capsella bursa-pastoris. Plant Cell Rep. 26 (10), 1791–1800. doi: 10.1007/s00299-007-0392-x
Cheng, F., Wu, J., Wang, X. (2014). Genome triplication drove the diversification of Brassica plants. Horticul. Res. 1, 14024. doi: 10.1038/hortres.2014.24
Ciancaleoni, S., Raggi, L., Barone, G., Donnini, D., Gigante, D., Domina, G., et al. (2021). A new list and prioritization of wild plants of socioeconomic interest in Italy: toward a conservation strategy. Agroecol. Sustain. Food Syst. 45 (9), 1300–1326. doi: 10.1080/21683565.2021.1917469
Crossa, J., Hernandez, C. M., Bretting, P., Eberhart, S. A., Taba, S. (1993). Statistical genetic considerations for maintaining germ plasm collections. Theor. Appl. Genet. 86 (6), 673–678. doi: 10.1007/BF00222655
CWR (2023). Crop Wild Relative Inventory. Available at: https://www.cwrdiversity.org/checklist/ (Accessed Jan 2023).
Dhillon, G. S., Kaur, S., Oberoi, H. S., Spier, M. R., Brar, S. K. (2016). “Agricultural-based protein by-products: characterization and applications,” in Protein byproducts. Ed. Dhillon, G.S. (Amsterdam; Boston; Heidelberg; London; New York; Oxford; Paris; San Diego; San Francisco; Singapore; Sydney; Tokyo: Academic Press), 21–36. doi: 10.1016/B978-0-12-802391-4.00002-1
Diederichsen, E., Sacristan, M. D. (1996). Disease response of resynthesized Brassica napus L. lines carrying different combinations of resistance to Plasmodiophora brassicae Wor. Plant Breed. 115 (1), 5–10. doi: 10.1111/j.1439-0523.1996.tb00862.x
Engels, J. M. M., Maggioni, L. (2012). “AEGIS: a regionally based approach to PGR conservation,” in Agrobiodiversity conservation: securing the diversity of crop wild relatives and landraces. Ed. Maxted, N., et al (Wallingford, UK; CABI), 321–326.
EURISCO (2023). European Search Catalogue for Plant Genetic Resources. Available at: http://eurisco.ecpgr.org/ (Accessed Jan 2023).
Euro+Med (2023) Euro+Med PlantBase - the information resource for Euro-Mediterranean plant diversity. Available at: https://www.europlusmed.org/ (Accessed Jan 2023).
Fitt, B. D. L., Brun, H., Barbetti, M. J., Rimmer, S. R. (2006). World-Wide Importance of Phoma Stem Canker (Leptosphaeria maculans and L. biglobosa) on Oilseed Rape (Brassica napus). Eur. J. Plant Pathol. 114 (1), 3–15. doi: 10.1007/s10658-005-2233-5
Ford-Lloyd, B. V., Dulloo, E., Toledo, Ã. (2011). Crop wild relatives - undervalued, underutilized and under threat? BioScience 61 (7), 559–565. doi: 10.1525/bio.2011.61.7.10
Forster, P. M., Smith, C. J., Walsh, T., Lamb, W. F., Lamboll, R., Hauser, M., et al. (2023). Indicators of Global Climate Change 2022: annual update of large-scale indicators of the state of the climate system and human influence. Earth Syst. Sci. Data 15 (6), 2295–2327. doi: 10.5194/essd-15-2295-2023
GBIF (2023) Global Biodiversity Information Facility. Available at: https://www.gbif.org/ (Accessed Jan 2023).
Gerdemann-Knörck, M., Sacristan, M. D., Braatz, C., Schieder, O. (1994). Utilization of Asymmetric Somatic Hybridization for the Transfer of Disease Resistance from Brassica nigra to Brassica napus. Plant Breed. 113 (2), 106–113. doi: 10.1111/j.1439-0523.1994.tb00712.x
Gilligan, C. A., Pechan, P. M., Day, R., Hill, S. A. (1980). Beet western yellows virus on oilseed rape. Plant Pathol. 29 (1), 53. doi: 10.1111/j.1365-3059.1980.tb01138.x
Girke, A., Schierholt, A., Becker, H. C. (2012). Extending the rapeseed genepool with resynthesized Brassica napus L. I: Genetic diversity. Genet. Resour. Crop Evol. 59 (7), 1441–1447. doi: 10.1007/s10722-011-9772-8
GRIN (2023) Taxonomic information on cultivated plants in GRIN-Global. Available at: https://npgsweb.ars-grin.gov/gringlobal/taxon/taxonomysearch/ (Accessed Jan 2023).
Grusak, M. A., DellaPenna, D. (1999). Improving the nutrient composition of plants to enhace human nutrition and health. Annu. Rev. Plant Physiol. Plant Mol. Biol. 50 (1), 133–161. doi: 10.1146/annurev.arplant.50.1.133
Hajjar, R., Hodgkin, T. (2007). The use of wild relatives in crop improvement: a survey of developments over the last 20 years. Euphytica 156 (1-2), 1–13. doi: 10.1007/s10681-007-9363-0
Harlan, J. R., de Wet, J. M. J. (1971). Toward a rational classification of cultivated plants. Taxon 20 (4), 509–517. doi: 10.2307/1218252
Heale, J. B., Karapapa, V. K. (1999). The verticillium threat to Canada’s major oilseed crop: canola. Can. J. Plant Pathol. 21 (1), 1–7. doi: 10.1080/07060661.1999.10600114
Hijmans, R. J., Cameron, S. E., Parra, J. L., Jones, P. G., Jarvis, A. (2005). Very high resolution interpolated climate surfaces for global land areas. Int. J. Climatol. 25 (15), 1965–1978. doi: 10.1002/joc.1276
Hoisington, D., Khairallah, M., Reeves, T., Ribaut, J.-M., Skovmand, B., Taba, S., et al. (1999). Plant genetic resources: What can they contribute toward increased crop productivity? Proc. Natl. Acad. Sci. 96 (11), 5937–5943. doi: 10.1073/pnas.96.11.5937
Hu, D., Jing, J., Snowdon, R. J., Mason, A. S., Shen, J., Meng, J., et al. (2021). Exploring the gene pool of Brassica napus by genomics-based approaches. Plant Biotechnol. J. 19 (9), 1693–1712. doi: 10.1111/pbi.13636
Hwang, S.-F., Strelkov, S. E., Peng, G., Ahmed, H., Zhou, Q., Turnbull, G., et al. (2016). Blackleg (Leptosphaeria maculans) severity and yield loss in canola in alberta, Canada. Plants 5 (3), 31. doi: 10.3390/plants5030031
IUCN (2023) The IUCN Red List of threatened species. Available at: https://www.iucnredlist.org/ (Accessed Jan 2023).
Jahreis, G., Schäfer, U. (2011). “Rapeseed (Brassica napus) Oil and its Benefits for Human Health,” in Nuts and seeds in health and disease prevention. Eds. Preedy, V. R., Watson, R. R., Patel, V. B. (San Diego: Academic Press), 967–974.
Jarvis, A., Lane, A., Hijmans, R. J. (2008). The effect of climate change on crop wild relatives. Agricul. Ecosyst. Environ. 126 (1-2), 13–23. doi: 10.1016/j.agee.2008.01.013
Jennings, M. D. (2000). Gap analysis: concepts, methods, and recent results. Landscape Ecol. 15 (1), 5–20. doi: 10.1023/A:1008184408300
Kotni, P., van Hintum, T., Maggioni, L., Oppermann, M., Weise, S. (2023). EURISCO update 2023: the European Search Catalogue for Plant Genetic Resources, a pillar for documentation of genebank material. Nucleic Acids Res. 51 (D1), D1465–D1469. doi: 10.1093/nar/gkac852
Lawrence, M. J., Marshall, D. F., Davies, P. (1995). Genetics of genetic conservation. I. Sample size when collecting germplasm. Euphytica 84 (2), 89–99. doi: 10.1007/BF01677945
Link, K. (2008). “Nutzung genetischer Diversität in Raps (Brassica napus) für Assoziationsstudien zur Resistenz gegen die Wurzelhals- und Stängelfäule (Leptosphaeria maculans),” in Institut für Pflanzenbau und Pflanzenzüchtung I. (Germany: Giessen University).
Lyon, C., Saupe, E. E., Smith, C. J., Hill, D. J., Beckerman, A. P., Stringer, L. C., et al. (2022). Climate change research and action must look beyond 2100. Global Change Biol. 28 (2), 349–361. doi: 10.1111/gcb.15871
Margules, C. R., Pressey, R. L. (2000). Systematic conservation planning. Nature 405, 243–253. doi: 10.1038/35012251
Marshall, D. R., Brown, H. D. (1975). “Optimum sampling strategies in genetic conservation,” in Crop genetic resources for today and tomorrow. Eds. Frankel, O. H., Hawkes, J. G. (Cambridge, UK: Cambridge University Press), 53–80.
Maxted, N., Dulloo, E., Ford-Lloyd, B. V., Iriondo, J. M., Jarvis, A. (2008). Gap analysis: a tool for complementary genetic conservation assessment. Diversity Distribut. 14 (6), 1018–1030. doi: 10.1111/j.1472-4642.2008.00512.x
Maxted, N., Kell, S., Ford-Lloyd, B., Dulloo, E., Toledo, Ã. (2012). Toward the systematic conservation of global crop wild relative diversity. Crop Sci. 52 (2), 774–785. doi: 10.2135/cropsci2011.08.0415
Maxted, N., Hunter, D., Ortiz Ríos, R. (2020). Plant genetic conservation (Cambridge: Cambridge University Press).
Maxted, N., van Slageren, M. W., Rihan, J. (1995). Ecogeographic surveys, in Collecting plant genetic diversity: technical guidelines. Eds. Guarino, L., Rao, V.R., Reid, R. (Wallingford: CAB International), 255–286.
McCouch, S. (2004). Diversifying selection in plant breeding. PloS Biol. 2 (10), e347. doi: 10.1371/journal.pbio.0020347
Metzger, J. O., Bornscheuer, U. (2006). Lipids as renewable resources: current state of chemical and biotechnological conversion and diversification. Appl. Microbiol. Biotechnol. 71 (1), 13–22. doi: 10.1007/s00253-006-0335-4
Nagel, M., Dulloo, M. E., Bissessur, P., Gavrilenko, T., Bamberg, J., Ellis, D., et al. (2022). Global strategy for the conservation of potato (Bonn, Germany: Global Crop Diversity Trust).
Neuffer, B. (2001). Vom Wildkohl zum Kohlrabi: Nutzpflanzen der Kreuzblütler in der Antike und heute. Schriftenreihe des Botanischen Gartens Osnabrück (Osnabrueck, Germany: Botanischer Garten der Universität Osnabrück).
Parra-Quijano, M., Iriondo, J. M., Torres, E. (2012). Improving representativeness of genebank collections through species distribution models, gap analysis and ecogeographical maps. Biodiversity Conserv. 21 (1), 79–96. doi: 10.1007/s10531-011-0167-0
Perrino, E. V., Wagensommer, R. P. (2022). Crop wild relatives (CWRs) threatened and endemic to Italy: urgent actions for protection and use. Biology 11 (2), 193. doi: 10.3390/biology11020193
Piazza, G. J., Foglia, T. A. (2001). Rapeseed oil for oleochemical usage. Eur. J. Lipid Sci. Technol. 103 (7), 450–454. doi: 10.1002/1438-9312(200107)103:7<450::AID-EJLT450>3.0.CO;2-D
Qian, B., Jing, Q., Bélanger, G., Shang, J., Huffman, T., Liu, J., et al. (2018). Simulated canola yield responses to climate change and adaptation in Canada. Agron. J. 110 (1), 133–146. doi: 10.2134/agronj2017.02.0076
Quezada-Martinez, D., Addo Nyarko, C. P., Schiessl, S. V., Mason, A. S. (2021). Using wild relatives and related species to build climate resilience in Brassica crops. Theor. Appl. Genet. 134 (6), 1711–1728. doi: 10.1007/s00122-021-03793-3
R_Core_Team (2019). R: A language and environment for statistical computing (Vienna, Austria: R Foundation for Statistical Computing).
Raggi, L., Zucchini, C., Gigante, D., Negri, V. (2022). In situ occurrence and protection of crop wild relatives in Italian sites of natura 2000 network: Insights from a data-driven approach. Front. Plant Sci. 13, 1080615. doi: 10.3389/fpls.2022.1080615
Razzaq, H., Armstrong, S. J., Saleem, H. (2021). “Brassicas: A complete guide to the potential of their wild relatives,” in Wild germplasm for genetic improvement in crop plants. Eds. Azhar, M. T., Wani, S. H. (Elsevier, Academic Press), 187–199. doi: 10.1016/B978-0-12-822137-2.00010-2
Rygulla, W., Snowdon, R. J., Eynck, C., Koopmann, B., von Tiedemann, A., Lühs, W., et al. (2007). Broadening the Genetic Basis of Verticillium longisporum Resistance in Brassica napus by Interspecific Hybridization. Phytopathology 97 (11), 1391–1396. doi: 10.1094/PHYTO-97-11-1391
Schwalm, C. R., Glendon, S., Duffy, P. B. (2020). RCP8.5 tracks cumulative CO2 emissions. Proc. Natl. Acad. Sci. 117 (33), 19656–19657. doi: 10.1073/pnas.2007117117
Singh, S. P. (2001). Broadening the genetic base of common bean cultivars: A review. Crop Sci. 41 (6), 1659–1675. doi: 10.2135/cropsci2001.1659
Snowdon, R. J., Winter, H., Diestel, A., Sacristán, M. D. (2000). Development and characterisation of Brassica napus-Sinapis arvensis addition lines exhibiting resistance to Leptosphaeria maculans. Theor. Appl. Genet. 101 (7), 1008–1014. doi: 10.1007/s001220051574
Stocker, T. F., Qin, D., Plattner, G.-K., Tignor, M. M. B., Allen, S. K., Boschung, J., et al. (2013). Climate change 2013: the physical science basis. Contribution of working group I to the fifth assessment report of the intergovernmental panel on climate change (United Kingdom and New York, NY, USA: Cambridge University Press).
Thuiller, W., Lavorel, S., Araújo, M. B., Sykes, M. T., Prentice, I. C. (2005). Climate change threats to plant diversity in Europe. Proc. Natl. Acad. Sci. U.S.A. 102 (23), 8245–8250. doi: 10.1073/pnas.0409902102
Thuiller, W., Lafourcade, B., Engler, R., Araújo, M. B. (2009). BIOMOD – a platform for ensemble forecasting of species distributions. Ecography 32 (3), 369–373. doi: 10.1111/j.1600-0587.2008.05742.x
Tilman, D., Hill, J., Lehman, C. (2006). Carbon-negative biofuels from low-input high-diversity grassland biomass. Science 314 (5805), 1598. doi: 10.1126/science.1133306
USDA (2023). Oilseeds: world markets and trade (Washington DC: United States Department of Agriculture, Foreign Agricultural Service).
van Treuren, R., Coquin, P., Lohwasser, U. (2012). Genetic resources collections of leafy vegetables (lettuce, spinach, chicory, artichoke, asparagus, lamb’s lettuce, rhubarb and rocket salad): composition and gaps. Genet. Resour. Crop Evol. 59 (6), 981–997. doi: 10.1007/s10722-011-9738-x
van Treuren, R., Hoekstra, R., Wehrens, R., van Hintum, T. (2020). Effects of climate change on the distribution of crop wild relatives in the Netherlands in relation to conservation status and ecotope variation. Global Ecol. Conserv. 23, e01054. doi: 10.1016/j.gecco.2020.e01054
van Treuren, R., Hoekstra, R., van Hintum, T. J. L. (2017). Inventory and prioritization for the conservation of crop wild relatives in The Netherlands under climate change. Biol. Conserv. 216, 123–139. doi: 10.1016/j.biocon.2017.10.003
van Vuuren, D. P., Edmonds, J., Kainuma, M., Riahi, K., Thomson, A., Hibbard, K. (2011). The representative concentration pathways: an overview. Climatic Change 109 (1), 5. doi: 10.1007/s10584-011-0148-z
Vavilov, N. I. (1926). Studies in the origin of cultivated plants (Leningrad: Institute of Applied Botany and Plant Breeding), 248.
Vincent, H., Wiersema, J., Kell, S., Fielder, H., Dobbie, S., Castañeda-Álvarez, N. P., et al. (2013). A prioritized crop wild relative inventory to help underpin global food security. Biol. Conserv. 167, 265–275. doi: 10.1016/j.biocon.2013.08.011
Vollbrecht, E., Sigmon, B. (2005). Amazing grass: developmental genetics of maize domestication. Biochem. Soc. Trans. 33 (6), 1502–1506. doi: 10.1042/BST0331502
Warwick, S. I., Francis, A. (2006). I.A. Al-Shehbaz, Brassicaceae: Species checklist and database on CD-Rom. Plant Syst. Evol. 259 (2-4), 249–258. doi: 10.1007/s00606-006-0422-0
Wei, W., Li, Y., Wang, L., Liu, S., Yan, X., Mei, D., et al. (2010). Development of a novel Sinapis arvensis disomic addition line in Brassica napus containing the restorer gene for Nsa CMS and improved resistance to Sclerotinia sclerotiorum and pod shattering. Theor. Appl. Genet. 120 (6), 1089–1097. doi: 10.1007/s00122-009-1236-6
Weise, S., Oppermann, M., Maggioni, L., van Hintum, T., Knüpffer, H. (2017). EURISCO: The European search catalogue for plant genetic resources. Nucleic Acids Res. 45 (D1), D1003–D1008. doi: 10.1093/nar/gkw755
WFO (2023). World Flora Online. Available at: http://www.worldfloraonline.org/ (Accessed Jan 2023).
Keywords: rapeseed, plant genetic resources, gap analysis, niche modelling, Brassica
Citation: Weise S, Hoekstra R, Kutschan KJ, Oppermann M, van Treuren R and Lohwasser U (2023) Analysis of gaps in rapeseed (Brassica napus L.) collections in European genebanks. Front. Plant Sci. 14:1244467. doi: 10.3389/fpls.2023.1244467
Received: 23 June 2023; Accepted: 06 September 2023;
Published: 09 October 2023.
Edited by:
Manjusha Verma, National Bureau of Plant Genetic Resources (ICAR), IndiaReviewed by:
Dagmar Janovská, Crop Research Institute (CRI), CzechiaMohammad Ehsan Dulloo, Alliance Bioversity International and CIAT, France
Copyright © 2023 Weise, Hoekstra, Kutschan, Oppermann, van Treuren and Lohwasser. This is an open-access article distributed under the terms of the Creative Commons Attribution License (CC BY). The use, distribution or reproduction in other forums is permitted, provided the original author(s) and the copyright owner(s) are credited and that the original publication in this journal is cited, in accordance with accepted academic practice. No use, distribution or reproduction is permitted which does not comply with these terms.
*Correspondence: Stephan Weise, d2Vpc2VAaXBrLWdhdGVyc2xlYmVuLmRl
†Present address: Kim Jana Kutschan, IDT Biologika GmbH, Dessau, Germany