- 1The New Zealand Institute for Plant & Food Research Ltd (PFR), Auckland, New Zealand
- 2The New Zealand Institute for Plant & Food Research Ltd (PFR), Motueka, New Zealand
- 3School of Biological Sciences, The University of Auckland, Auckland, New Zealand
There have been a considerable number of studies that have successfully sped up the flowering cycle in woody perennial horticultural species. One particularly successful study in apple (Malus domestica) accelerated flowering using a silver birch (Betula pendula) APETALA1/FRUITFULL MADS-box gene BpMADS4, which yielded a good balance of vegetative growth to support subsequent flower and fruit development. In this study, BpMADS4 was constitutively expressed in European pear (Pyrus communis) to establish whether this could be used as a tool in a rapid pear breeding program. Transformed pear lines flowered within 6–18 months after grafting onto a quince (Cydonia oblonga) rootstock. Unlike the spindly habit of early flowering apples, the early flowering pear lines displayed a normal tree-like habit. Like apple, the flower appearance was normal, and the flowers were fertile, producing fruit and seed upon pollination. Seed from these transformed lines were germinated and 50% of the progeny flowered within 3 months of sowing, demonstrating a use for these in a fast breeding program.
1 Introduction
Woody perennials undergo a long juvenile stage before they acquire reproductive competence (Bergonzi and Albani, 2011; van Nocker and Gardiner, 2014). Apple (Malus domestica) can take from 5 to 12 years before first flowering (Fischer, 1994). Similarly, European pear (Pyrus communis) has a long juvenile phase and can take over 10 years to first flowering (Visser, 1964; Freiman et al., 2011). The average juvenile period for European pear seedlings grown on their own roots is eight years (Brewer et al., 2008), but many pears require as much as 14 years for first flowering, or they fail to flower in research glasshouse conditions. Orchard management and agronomic practices can help reduce juvenility, with crops like apple being grafted onto a dwarfing rootstock such as ‘Malling 9’ (‘M9’) to promote faster flowering (Beakbane and Thompson, 1940). However, there are currently no pear rootstocks conferring substantial vigor control and precocity to the grafted scion cultivar (Watson et al., 2012; Knäbel et al., 2015).
Transgenic overexpression of flowering genes can promote floral induction in trees (Putterill and Varkonyi-Gasic, 2016). The molecular regulation of flowering time in plants has been very well established and is mostly evolutionary conserved, with FLOWERING LOCUS T (FT) peptides strongly promoting flowering, and highly similar but strong competitive antagonists TERMINAL FLOWER (TFL) and CENTRORADIALIS (CEN) inhibiting flowering (Wickland and Hanzawa, 2015). Overexpression or viral delivery of FT homologs accelerated flowering in woody perennials, including apple, poplar, citrus, plum, pear, kiwifruit, and Eucalyptus (Endo et al., 2005; Böhlenius et al., 2006; Hsu et al., 2006; Zhang et al., 2010; Srinivasan et al., 2012; Song et al., 2013; Wenzel et al., 2013; Yamagishi et al., 2014; Klocko et al., 2016; Voogd et al., 2017; Moss et al., 2018; Herath et al., 2023). These methods significantly reduced juvenility and induced flowering, but they have often proven to be impractical, giving rise to plants that were flowering in the tissue culture stage and commonly developing aberrant flowers. A similar effect was achieved using silencing of the TERMINAL FLOWER1 (TFL1) genes in apple and pear (Kotoda et al., 2006; Freiman et al., 2011; Flachowsky et al., 2012; Yamagishi et al., 2016). Recently, gene editing of CEN genes gave rise to early flowering kiwifruit species (Varkonyi-Gasic et al., 2019; Herath et al., 2023), whilst mutagenesis of CEN1 and overexpression of FT1 or FT2 resulted in precocious flowering in poplar (Sheng et al., 2023). Editing of TFL1 for early flowering was also performed in apple and pear (Charrier et al., 2019); however, this approach has problems including too early (in vitro) flowering, complex gene editing profiles and mosaicism, low transformation efficiency for pear, and the recessive nature of the mutation.
Ectopic expression of MADS-box genes associated with early floral development was also successfully used to promote maturity in trees, while providing a good balance of vegetative growth before floral production. For example, Arabidopsis APETALA1 (AP1) drastically reduced the length of the juvenile phase in a Citrus rootstock, which flowered within 12–18 months instead of 6–7 years (Peña et al., 2001). The transgenic adult trees displayed normal development as they retained the ability to respond to environmental cues that induce flowering every year, and flowering corresponded with the spring season. The flowers were regular and fertile, and the fast-maturity trait was inherited as dominant. Overexpression of another MADS box gene, the silver birch (Betula pendula) AP1/FRUITFUL (AP1/FUL)-like BpMADS4 was able to induce early flowering in heterologous species (Elo et al., 2007) and is used for accelerated breeding in apple (Flachowsky et al., 2007; Flachowsky et al., 2011).
Long juvenility is a major constraint for pear breeding (Fischer, 2009). This is despite opportunities arising from the sequencing of pear genomes (Wu et al., 2013; Chagné et al., 2014; Gao et al., 2021) and targeted genome editing opportunities (Charrier et al., 2019). To establish whether we could get precocious flowering in pear, we first cloned the BpMADS4 gene in a new transformation vector and tested the ability to promote flowering in the apple ‘Royal Gala’ cultivar. This construct was then used to generate pear lines to test whether similar precocious flowering could be achieved in pears to vastly accelerate pear breeding.
2 Materials and methods
2.1 Plant material and growth conditions
Plant material from M. domestica Borkh. ‘Royal Gala’ (apple) and P. communis L. ‘Conference’ (European pear) was used for transformation. Tissue culture plants were grown in 290 mL clear, wide-mouth plastic vessels with snap-on lids containing approximately 50 mL of media. Tissue culture plant material was maintained at 25°C and a photoperiod of 16 h light (40 μmol m−2 s−1, as provided by cool-white, fluorescent light) and 8 h dark periods. Tissue culture-grown apple and pear shoots were grafted onto ‘M9’ apple rootstock and Cydonia oblonga ‘Quince C’ rootstock, respectively. Grafting was performed as shown (https://youtu.be/bphgFprxtXA). Glasshouse conditions had supplementary light and heat during the winter periods and were maintained at temperature min. 18°C/max. 30°C, 14 h/10 h light/dark. Seeds were harvested from the fruit and allowed to dry for 7 days. Imbibed seeds were placed in damp vermiculite and placed at 4°C for 12 weeks until they germinated. Seedlings were then transferred to potting mix and grown in the glasshouse.
2.2 Construct design
The BpMADS4 full-length coding sequence (Elo et al., 2001) (Genbank accession X99654, nucleotides 83-865) with added BamHI and XbaI restriction enzyme sites was synthesized (GenScript, https://www.genscript.com/) and cloned into pSAK778, positioning it between the CaMV 35S promoter and OCS terminator. The construct identity was confirmed by sequencing, then it was transformed into Agrobacterium tumefaciens strains LBA4404 by electroporation. Single colonies were selected and checked for the correct plasmid by sequencing. The Agrobacterium culture was grown overnight (250 rpm, 28°C) in YEB liquid medium (Vervliet et al., 1975), pelleted, resuspended and adjusted to an optical density at 600 nm (OD600) of 0.6 with sterile water.
2.3 Plant transformation
Apple leaf sections were transformed with pSAK778 35S:BpMADS4 using A. tumefaciens-mediated transformation and selected on media containing kanamycin according to the previously described protocol (Yao et al., 1995). Regenerated apple shoots were grown on selection medium before grafting onto ‘M9’ apple rootstock. Wild-type (WT) stock plants grown on media without kanamycin selection and grafted onto ‘M9’ rootstock were used as control.
To establish pear shoot cultures, shoots with single nodes were collected from glasshouse-grown plants, surface‐sterilized using sodium hypochlorite (1.5%) for 12 minutes, washed in sterile water and grown in a micropropagation medium composed of Murashige and Skoog (MS) (Murashige and Skoog, 1962) macro- and micronutrients, supplemented with additional macronutrients: MgSO4·7H2O (0.185 g l−1), CaCl2·2H2O (0.44 g l−1) and KH2PO4 (0.17 g l−1); B5 vitamins as described by Gamborg et al. (1968); hormones N6-benzyladenine (BA) (1.0 mg l−1) and indole-3-butyric acid (IBA) (0.1 mg l−1); sucrose (3%), Difco agar (3 g l-1) and Phytagel (1.7 g l-1), with pH adjusted to 5.8. Fully opened young expanding leaves excised from 4- to 5-week-old shoot cultures were used as explants for genetic transformation.
For pear transformation, a protocol developed by Zhu (2000) and Zhu and Welander (2004) was used, with modifications. Regeneration media containing Quoirin & Lepoivre (QL) macronutrients modified according to Leblay et al. (1991), supplemented with Fe-Na-EDTA (40 mg l-1), vitamins modified from Brisset et al. (1988): myo-inositol (100 mg l-1), thiamine (0.8 mg l-1), nicotinic acid (1 mg l-1), pyridoxine HCI (1 mg l-1), glycine (4 mg l-1); variable MS micronutrient (Murashige and Skoog, 1962) and hormone thidiazuron (TDZ) and 1-naphthaleneacetic acid (NAA) composition (Table 1) were evaluated. All media for transformation contained sorbitol (40 mg l-1), Phytagel (2.5 g l-1), and were adjusted to pH 5.8 prior to autoclaving at 121°C for 20 minutes, followed by addition of filter-sterilized antibiotic solutions (where required). Components for tissue culture media were purchased from Merck (Rahway, NJ, USA) and Sigma-Aldrich (St. Louis, MO, USA).
For transformation, fully opened young expanding leaves were cut transversely into three fragments in a sterile Petri dish (90 × 20 mm) containing sterile water. The water was removed, and the leaf sections were inoculated with Agrobacterium cultures for 15 to 20 min at room temperature with gentle shaking. The inoculated leaf sections were blotted using a sterile filter paper, placed with abaxial side on the surface of the regeneration medium without antibiotics and maintained at 25°C for 5 to 7 days. After co-cultivation, the explants were washed with sterile water, blot-dried and transferred to regeneration media supplemented with kanamycin 50 mg l-1 and cefotaxime 350 mg l-1. After 4 weeks, the explants were transferred to fresh regeneration media, and subsequently subcultured every 4 weeks to fresh media with the same selection (kanamycin 50 mg l-1 and cefotaxime 350 mg l-1) until regenerated adventitious shoots were formed. The regenerated shoots were transferred to micropropagation media (as described above) supplemented with kanamycin 100 mg l-1 and cefotaxime 350 mg l-1 for micropropagation and further selection to eliminate any remaining Agrobacterium. After 4–6 months, the surviving adventitious shoots were grafted onto ‘Quince C’ rootstock. WT explants propagated on media with no antibiotic selection were grafted and used as controls.
2.4 Isolation of nucleic acids and PCR analysis
Genomic DNA was extracted from young leaves of glasshouse-grown plants using the DNeasy Plant Mini Kit (Qiagen, Hilden, Germany) following the manufacturer’s instructions. To confirm the presence of the transgene, PCR amplification was performed using the PlatinumTaq DNA Polymerase (ThermoFisher Scientific, Waltham, MA, USA) and BpMADS4 gene‐specific oligonucleotide primers (Flachowsky et al., 2007), with an initial denaturing step at 95°C for 5 min, then 35 cycles of 95°C for 15 s, 56°C for 15 s, and 72°C for 1 min. Amplification products were separated by gel electrophoresis. Total RNA was extracted from young leaves using the Spectrum Plant Total RNA kit (Sigma-Aldrich, St. Louis, MO, USA). For cDNA synthesis, 1 µg of total RNA was reverse-transcribed in a total volume of 20 µL using the QuantiTect Reverse Transcription Kit (Qiagen, Hilden, Germany) and real-time PCR was performed using the FastStart DNA MasterPLUS SYBR Green I reaction mix on a LightCycler® 1.5 instrument (Roche Diagnostics, Basel, Switzerland), with a non-template control included in each run. Amplification was carried out with an initial denaturing step at 95°C for 5 min, then 40 cycles of 95°C for 5 s, 60°C for 5 s, and 72°C for 10 s. Relative expression was calculated using the LightCycler Software version 4 (Roche Diagnostics), normalized to ACTIN (forward primer 5′-GAACTATGAGCTTCCCGATGGC-3′, reverse primer 5′-CCAGCAGCTTCCATTCCAATGAG-3′) and presented as means ± standard error of three biological replicates.
3 Results
3.1 Efficacy of the 35S:BpMADS4 construct in apple
The pSAK778 35S:BpMADS4 construct (35S:BpMADS4) was used to transform ‘Royal Gala’ apple leaves. Fifty independent lines were generated and a range of precocious flowering was seen in the lines. Flowering was observed in tissue culture (Figure 1A) or withing weeks after grafting the shoots onto ‘M9’ rootstocks (Figure 1B). The presence of the BpMADS4 transgene was confirmed in the early-flowering lines by DNA amplification compared with the WT controls (Figure 1C). Despite severe precocity and a spindly appearance (Figure 1B), most 35S:BpMADS4 lines produced flowers with normal morphology (Figure 1D); however, the floral clusters often contained more flowers than the typical five flower cluster observed in apple cultivars. Pollinated flowers produced apple fruit smaller than untransformed apples but typically had a similar shape to untransformed apples (Figure 1E). Approximately 50% of the seeds from these lines gave rise to early flowering seedlings, confirming that the BpMADS4 transformants conferred a flowering phenotype that could be passed to the progeny.
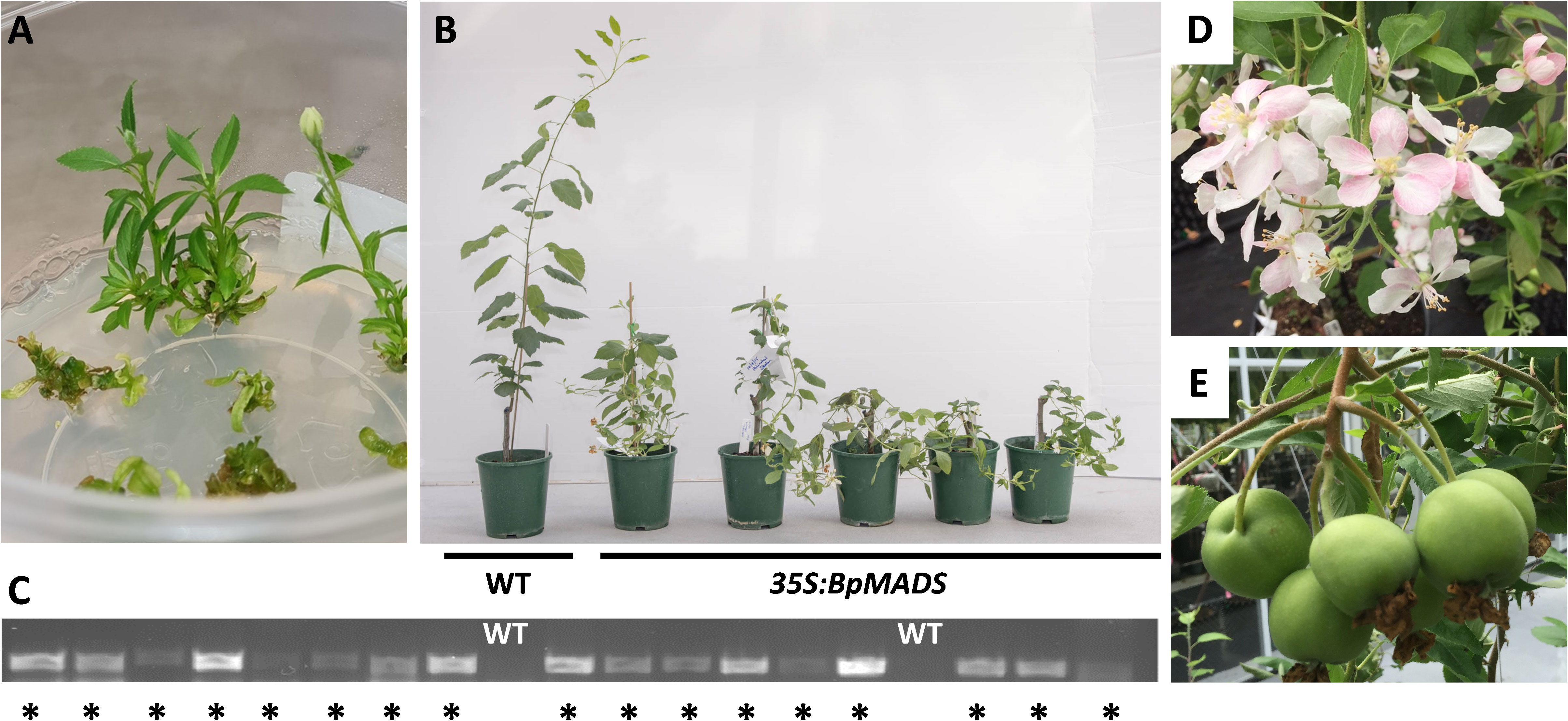
Figure 1 Early flowering apple. (A) Flower development on shoots grown in vitro. (B) Early flowering and spindly stems in 35S:BpMADS4 lines grown in the glasshouse. WT indicates the wild-type control. (C) Gel electrophoresis of amplified BpMADS4 DNA fragments. Asterisks denote lines that flowered within 6 months after establishment in the glasshouse. (D) Normal flower development. (E) Fruit set after pollination.
3.2 Early flowering 35S:BpMADS4 pear
Pear has typically been more recalcitrant to transformation than apple. Several protocols using various Pyrus species and cultivars, with different transformation efficiencies have been reported (Mourgues et al., 1996; Zhu, 2000; Murayama et al., 2003; Zhu and Welander, 2004; Yancheva et al., 2006; Nakajima et al., 2013; Chevreau et al., 2019). To test whether a more efficient protocol could be developed, leaf fragments from ‘Conference’ pears were inoculated with Agrobacterium containing 35S:BpMADS4 and placed on four different selection media (RE1, RE2, RE3 and RE4, Table 1), starting with no selection for 5–7 days, followed by selection on kanamycin 50 mg l-1 and cefotaxime 350 mg l-1. All media gave rise to kanamycin-resistant shoots (Figure 2). Roughly half of explants produced shoots on RE1, RE2 and RE3, with slightly lower regeneration efficiency noted with RE4 (Figure 2). However, less hyperhydricity was visually observed on tissue propagated on media RE2 and RE4, containing lower concentration of TDZ and NAA.
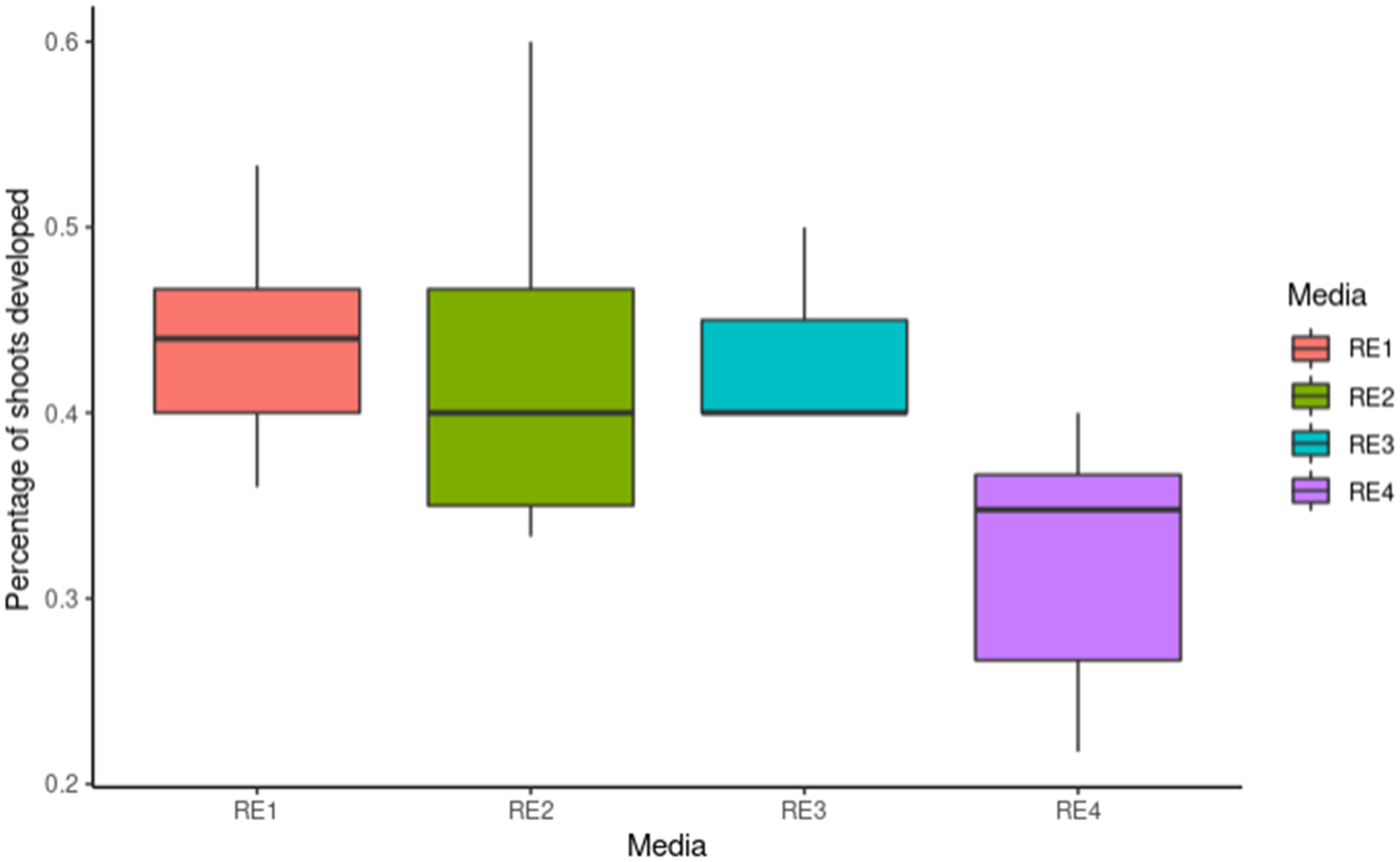
Figure 2 Transformation of ‘Conference’ pear. Y-axis represents frequencies of shoots regenerating from explants (n=30) propagated on different media containing kanamycin 50 mg l-1 for 10 weeks. The median is represented by bold horizontal line, the box indicates the interquartile range, and the minimum and maximum are shown as vertical lines.
The observed phenotypes in pear shoots grown in vitro and in the glasshouse are shown in Figure 3. Occasional flowering was observed on some of the shoots developing in vitro, with flower and leaf morphology ranging from aberrant to mostly normal (Figure 3A). None of the lines demonstrating in vitro flowering survived. Sixteen independent lines that did not flower in tissue culture and seven controls were grafted onto quince rootstock and grown in the glasshouse. The presence of the BpMADS4 transgene was confirmed by DNA amplification compared to the WT controls (Figure 3B). Of the 16 transgenic lines, seven started flowering within 6–18 months, while none of the controls flowered within this time (Figures 3B–F). All the transgenic lines appeared normal, with upright stems and branches and were comparable to WT control (Figures 3C, E). The early flowering lines had a normal flower morphology and were producing an expected cluster of five flowers per inflorescence (Figure 4A). The flowers were pollinated and produced medium-sized pears (150–230 g) with expected elongated shape (Figure 4B).
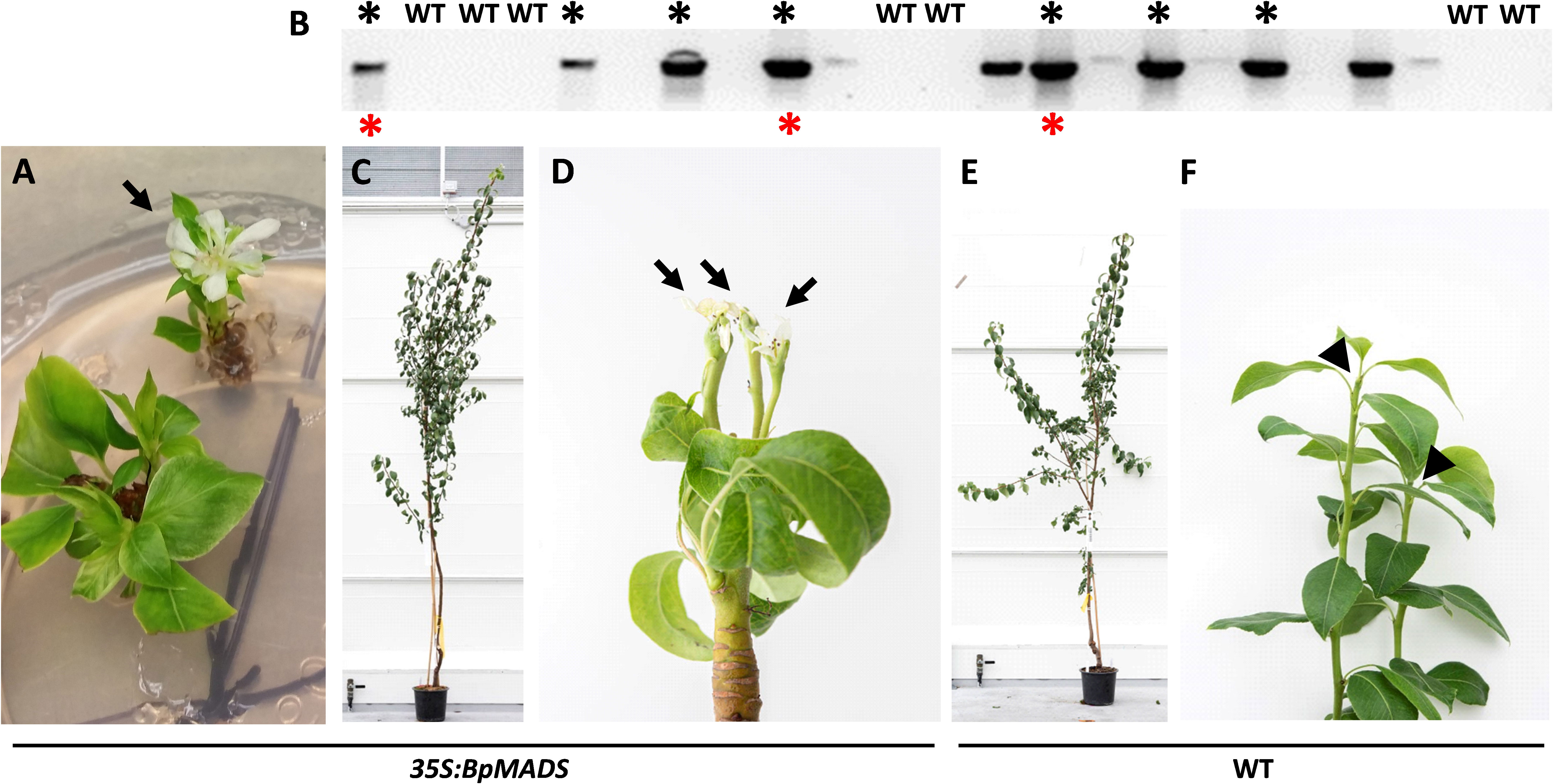
Figure 3 Early flowering pear. (A) Flower (arrow) developing in vitro. (B) Gel electrophoresis of amplified BpMADS4 DNA fragments in kanamycin-resistant and wild-type (WT) control plants. Black asterisks denote lines that flowered 6–18 months after establishment in the glasshouse. Red asterisks indicate lines confirmed to give rise to early flowering progeny. C, (D) Appearance of an early flowering 35S:BpMADS4 plant (C) with terminal flowers (D, arrows). E, (F) Appearance of a WT control (E) with vegetative shoot tips (F, arrowheads).
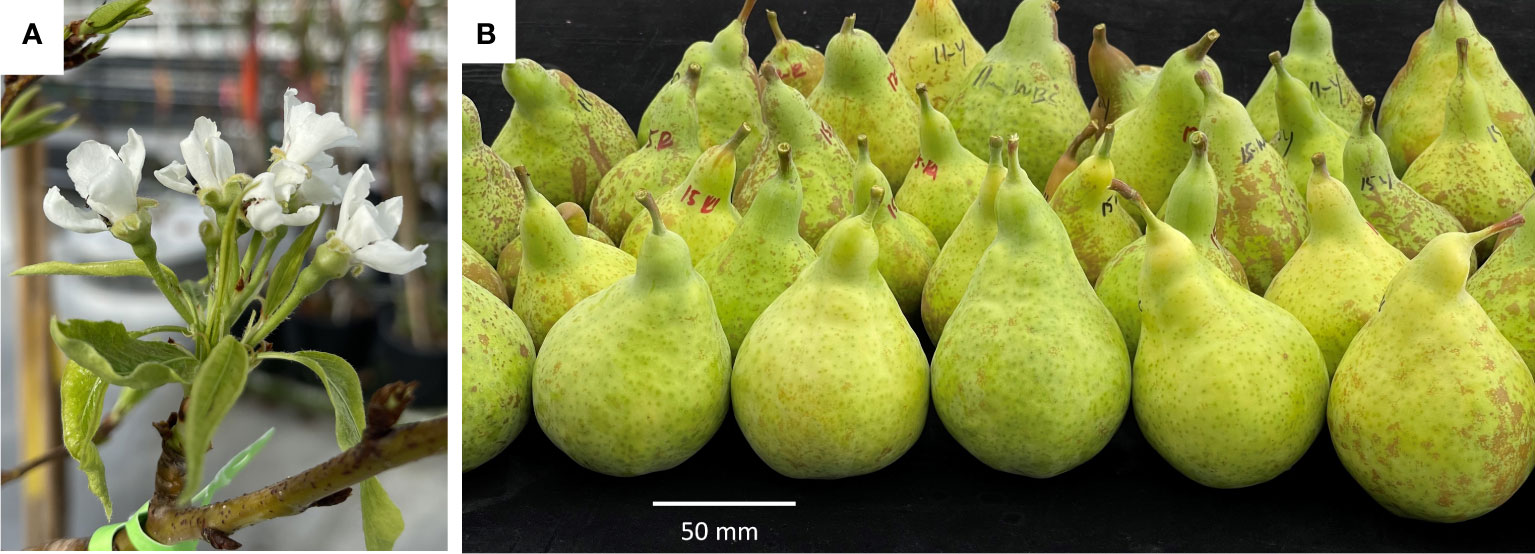
Figure 4 Reproductive development in the early flowering pear. (A) Normal flower morphology on a representative early flowering pear line. (B) Appearance of fruit collected from early flowering lines.
To test whether the transgene conferred early flowering in the first-generation hybrids, a total of 48 seeds were collected from three lines (Figure 3B) and placed into moist conditions to germinate. A total of 40 seedlings were germinated and 15 of those seedlings flowered early, producing a terminal flower cluster 6–8 weeks after transfer to soil (Figure 5A). Expression of the transgene was detected in early flowering progeny (Figure 5B; Supplementary Figure 1).
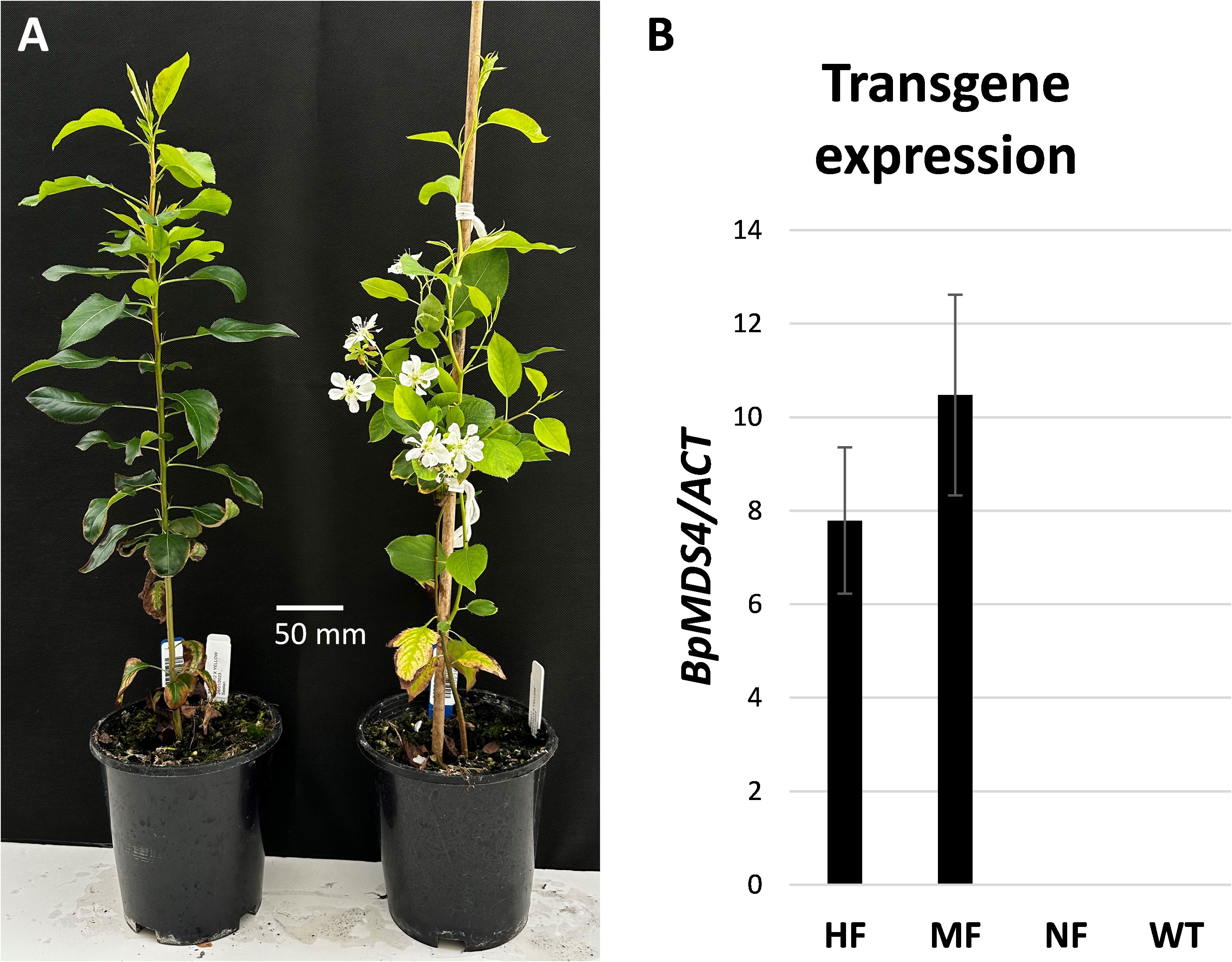
Figure 5 Appearance of first-generation hybrids. (A) Non-early flowering (left) and early flowering (right) seedlings 4 months after germination. (B) Relative BpMADS4 expression normalized to ACTIN (ACT) and presented as mean ± SE of three biological replicates in early flowering highly floral (HF), moderately floral (MF) and non-flowering (NF) pear seedlings. WT, wild type.
4 Discussion
The pear industry is facing increasing obstacles such as global warming, changes in water availability, pathogens and diseases. To overcome these obstacles, traditional breeding techniques are being used to develop new and improved pear varieties. However, the slow maturation of pear seedlings is a major hurdle to conventional breeding. To produce new varieties that are resistant to pests, it is often necessary to cross to less domesticated species, resulting in multigenerational crosses needed to re-establish quality eating pears. Due to this and the long breeding cycles, conventional breeding can take several decades to produce a new pear variety. In this study, we have shown that the silver birch MADS-box gene BpMADS4 can be used to reduce the breeding cycles to less than 2 years, greatly speeding up pear breeding. This new tool will allow wider crosses to be made, and faster introgression of desirable traits into elite lines.
Pear transformation is more challenging than other fruit crops such as apple, because of factors such as low regeneration capacity, low transformation efficiency, and genotype-dependent responses. In the majority of protocols, pear transformation is carried out using A. tumefaciens strains EHA101 or EHA105 and several different pear genotypes have been transformed, with relatively high transformation efficiencies reported for ‘Conference’ (Mourgues et al., 1996; Yancheva et al., 2006). An increase in transformation efficiency was reported for Agroinfiltration (Agrobacterium-mediated vacuum infiltration) of the explants (Chevreau et al., 2019). Here, we performed transformation using A. tumefaciens strain LBA4404 and evaluated four growth media for the pear explants. We found that decreased cytokinin and auxin may reduce the occurrence of hyperhydric (glassy) shoots, whilst gradually increasing kanamycin was able to improve transgenic shoot survival. Further optimization and testing of protocols may boost this efficiency further. However, propagation on all tested media resulted in ‘Conference’ transformation efficiencies comparable to those reported previously (Mourgues et al., 1996; Yancheva et al., 2006).
The early flowering observed in pear was generally later than that of the ‘Royal Gala’ transformants, with most apple lines flowering within 6 months of grafting and pear ranging from 6–18 months from grafting. Given that the pears showed some flowering in tissue culture similar to apple, we assume that the ‘M9’ rootstock used in apple assists the rapid flowering, compared with the quince rootstock. This is further supported by the seedlings from the rapid flowering pears flowering at an earlier developmental period than the pear grafted transgenics. Interestingly, the pear growth habit was less affected by the BpMADS4 transgene than that of apple, with primary transgenic pear showing an upright, tree-like habit. The early flowering pear seedlings were also more robust than the apples, suggesting that the spindly vine morphology reported in apple containing BpMADS4 had a different interaction with plant development processes. Expression of the transgene was detected in the early flowering progeny, but no clear correlation could be established between transgene expression and flower numbers or plant stature, demonstrating that the presence of BpMADS4 transcript rather than small differences in its level of transcription promote the development of flowers. This was consistent with previous findings in apple (Flachowsky et al., 2007).Overall, the development of a transgenic pear with a heritable early flowering trait has the potential to significantly reduce the duration of the breeding cycle and accelerate the development of new pear varieties (Figure 6). Furthermore, the early flowering lines may be developed as a model pear for fruit research, opening avenues for the application of new breeding techniques such as CRISPR/Cas-mediated gene editing for further improvement of traits of interest (Figure 6). Finally, they can be utilized for introgression of the early flowering trait into other Pyrus spp. such as Asian pear (Pyrus pyrifolia), which is difficult to transform.
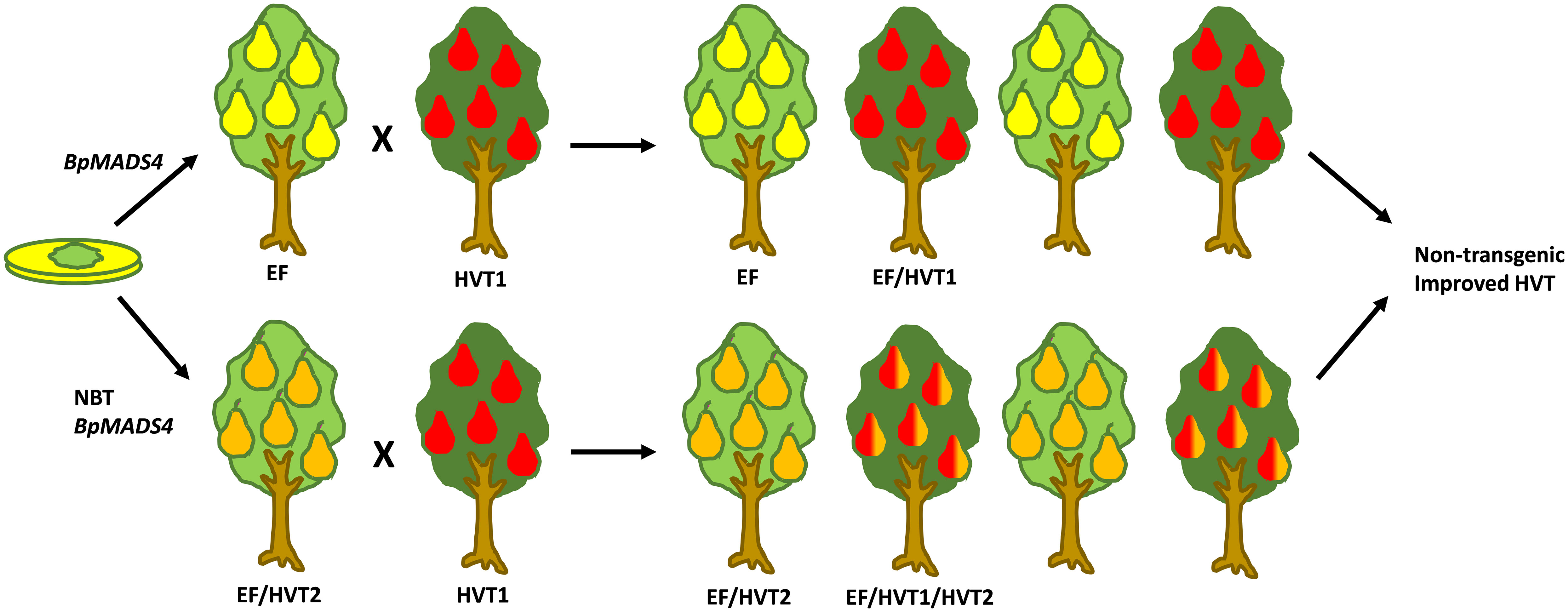
Figure 6 A schematic diagram representing the strategies for fast breeding and improvement of pear using early flowering BpMADS4 lines (EF). Top, Fast breeding cycles to introduce a high value trait (HVT). Half of the progeny is transgenic (EF), with half of those inheriting the HVT (EF/HVT). Alternatively, HVTs are introduced by new breeding technologies (NBT), e.g. gene editing (bottom). After a desired number of breeding cycles, non-transgenic (not EF) improved HVT lines are selected. Different fruit colors indicate HVTs.
Data availability statement
The raw data supporting the conclusions of this article will be made available by the authors, without undue reservation.
Author contributions
EV-G and RS conceived the study, led the research and wrote the manuscript. Y-YW and EV-G designed the constructs and Y-YW performed the cloning. ST developed the tissue culture protocols, generated transgenic plants and contributed to writing of the manuscript. KG performed the genotyping, pollinated the transgenic plants and maintained the seedlings. MD performed the grafting. LG performed the statistical analysis. All authors contributed to the article and approved the submitted version.
Funding
This work was funded by the New Zealand Ministry of Business, Innovation and Employment grant C11X1602 and internal funding from The New Zealand Institute for Plant and Food Research Limited.
Acknowledgments
We would like to thank Geeta Chiba for media preparation, Ben Lawrence, Donna Gibson and Tracey Phelps for filming and editing the Supplementary Video, and Janine Johnson and Vincent Bus for critically reading the manuscript. We thank Henryk Flachowsky and Andreas Peil for their help and advice with the BpMADS4 construct.
Conflict of interest
The authors declare that the research was conducted in the absence of any commercial or financial relationships that could be construed as a potential conflict of interest.
Publisher’s note
All claims expressed in this article are solely those of the authors and do not necessarily represent those of their affiliated organizations, or those of the publisher, the editors and the reviewers. Any product that may be evaluated in this article, or claim that may be made by its manufacturer, is not guaranteed or endorsed by the publisher.
Supplementary material
The Supplementary Material for this article can be found online at: https://www.frontiersin.org/articles/10.3389/fpls.2023.1235963/full#supplementary-material
Supplementary Video | Showing pipfruit micrografting can be accessed using the following link: https://youtu.be/bphgFprxtXA. Grafting was performed by Monica Dragulescu. The video was prepared by Ben Lawrence, Donna Gibson and Tracey Phelps at The New Zealand Institute for Plant and Food Research Limited, Auckland, New Zealand.
References
Beakbane, A. B., Thompson, E. C. (1940). Anatomical studies of stems and roots of hardy fruit trees II. The internal structure of the roots of some vigorous and some dwarfing apple rootstocks, and the correlation of structure with vigour. J. Pomol. Hortic. Sci. 17, 141–149. doi: 10.1080/03683621.1940.11513535
Bergonzi, S., Albani, M. C. (2011). Reproductive competence from an annual and a perennial perspective. J. Exp. Bot. 62, 4415–4422. doi: 10.1093/jxb/err192
Böhlenius, H., Huang, T., Charbonnel-Campaa, L., Brunner, A. M., Jansson, S., Strauss, S. H., et al. (2006). CO/FT regulatory module controls timing of flowering and seasonalgrowth cessation in trees. Science 312, 1040–1043. doi: 10.1126/science.1126038
Brewer, L. R., Morgan, C., Alspach, P. A., Volz, R. K., White, A. G. (2008). Interspecific pear breeding for flavour and texture. Acta Hortic. 800, 461–468. doi: 10.17660/ActaHortic.2008.800.58
Brisset, M.-N., Paulin, J.-P., Duron, M. (1988). Feasibility of rating fire blight susceptibility of pear cultivars (Pyrus communis) on in vitro microcuttings. Agronomie 8, 707–710. doi: 10.1051/agro:19880806
Chagné, D., Crowhurst, R. N., Pindo, M., Thrimawithana, A., Deng, C., Ireland, H., et al. (2014). The draft genome sequence of European pear (Pyrus communis L. ‘Bartlett’). PloS One 9, e92644. doi: 10.1371/journal.pone.0092644
Charrier, A., Vergne, E., Dousset, N., Richer, A., Petiteau, A., Chevreau, E. (2019). Efficient targeted mutagenesis in apple and first time edition of pear using the CRISPR-cas9 system. Front. Plant Sci. 10. doi: 10.3389/fpls.2019.00040
Chevreau, E., Dousset, N., Joffrion, C., Richer, A., Charrier, A., Vergne, E. (2019). Agroinfiltration is a key factor to improve the efficiency of apple and pear transformation. Sci. Hortic. 251, 150–154. doi: 10.1016/j.scienta.2019.03.003
Elo, A., Lemmetyinen, J., Novak, A., Keinonen, K., Porali, I., Hassinen, M., et al. (2007). BpMADS4 has a central role in inflorescence initiation in silver birch (Betula pendula). Physiol. Plantarum 131, 149–158. doi: 10.1111/j.1399-3054.2007.00947.x
Elo, A., Lemmetyinen, J., Turunen, M.-L., Tikka, L., Sopanen, T. (2001). Three MADS-box genes similar to APETALA1 and FRUITFULL from silver birch (Betula pendula). Physiol. Plantarum 112, 95–103. doi: 10.1034/j.1399-3054.2001.1120113.x
Endo, T., Shimada, T., Fujii, H., Kobayashi, Y., Araki, T., Omura, M. (2005). Ectopic expression of an FT homolog from citrus confers an early flowering phenotype on trifoliate orange (Poncirus trifoliata L. Raf.). Transgenic Res. 14, 703–712. doi: 10.1007/s11248-005-6632-3
Fischer, C. (1994). “Shortening of the juvenile period in apple breeding,” in Progress in Temperate Fruit Breeding: Proceedings of the Eucarpia Fruit Breeding Section Meeting held at Wädenswil/Einsiedeln, Switzerland from August 30 to September 3, 1993. Eds. Schmidt, H., Kellerhals, M. (Dordrecht: Springer Netherlands), 161–164.
Fischer, M. (2009). “Pear breeding,” in Breeding Plantation Tree Crops: Temperate Species (New York, NY: Springer New York), 135–160.
Flachowsky, H., Le Roux, P.-M., Peil, A., Patocchi, A., Richter, K., Hanke, M.-V. (2011). Application of a high-speed breeding technology to apple (Malus × domestica) based on transgenic early flowering plants and marker-assisted selection. New Phytol. 192, 364–377. doi: 10.1111/j.1469-8137.2011.03813.x
Flachowsky, H., Peil, A., Sopanen, T., Elo, A., Hanke, V. (2007). Overexpression of BpMADS4 from silver birch (Betula pendula Roth.) induces early-flowering in apple (Malus × domestica Borkh.). Plant Breed. 126, 137–145. doi: 10.1111/j.1439-0523.2007.01344.x
Flachowsky, H., Szankowski, I., Waidmann, S., Peil, A., Tränkner, C., Hanke, M. V. (2012). The MdTFL1 gene of apple (Malus × domestica Borkh.) reduces vegetative growth and generation time. Tree Physiol. 32, 1288–1301. doi: 10.1093/treephys/tps080
Freiman, A., Shlizerman, L., Golobovitch, S., Yablovitz, Z., Korchinsky, R., Cohen, Y., et al. (2011). Development of a transgenic early flowering pear (Pyrus communis L.) genotype by RNAi silencing of PcTFL1-1 and PcTFL1-2. Planta 235, 1239–1251. doi: 10.1007/s00425-011-1571-0
Gamborg, O. L., Miller, R. A., Ojima, K. (1968). Nutrient requirements of suspension cultures of soybean root cells. Exp. Cell Res. 50, 151–158. doi: 10.1016/0014-4827(68)90403-5
Gao, Y., Yang, Q., Yan, X., Wu, X., Yang, F., Li, J., et al. (2021). High-quality genome assembly of ‘Cuiguan’ pear (Pyrus pyrifolia) as a reference genome for identifying regulatory genes and epigenetic modifications responsible for bud dormancy. Horticult. Res. 8, 197. doi: 10.1038/s41438-021-00632-w
Herath, D., Wang, T., Voogd, C., Peng, Y., Douglas, M., Putterill, J., et al. (2023). Strategies for fast breeding and improvement of Actinidia species. Horticult. Res. 10 (3), uhad016. doi: 10.1093/hr/uhad016
Hsu, C. Y., Liu, Y., Luthe, D. S., Yuceer, C. (2006). Poplar FT2 shortens the juvenile phase and promotes seasonal flowering. Plant Cell 18, 1846–1861. doi: 10.1105/tpc.106.041038
Klocko, A. L., Ma, C., Robertson, S., Esfandiari, E., Nilsson, O., Strauss, S. H. (2016). FT overexpression induces precocious flowering and normal reproductive development in Eucalyptus. Plant Biotechnol. J. 14, 808–819. doi: 10.1111/pbi.12431
Knäbel, M., Friend, A. P., Palmer, J. W., Diack, R., Wiedow, C., Alspach, P., et al. (2015). Genetic control of pear rootstock-induced dwarfing and precocity is linked to a chromosomal region syntenic to the apple Dw1 loci. BMC Plant Biol. 15, 230. doi: 10.1186/s12870-015-0620-4
Kotoda, N., Iwanami, H., Takahashi, S., Abe, K. (2006). Antisense expression of MdTFL1, a TFL1-like gene, reduces the juvenile phase in apple. J. Am. Soc Hortic. Sci. 131, 74–81. doi: 10.21273/JASHS.131.1.74
Leblay, C., Chevreau, E., Raboin, L. M. (1991). Adventitious shoot regeneration from in vitro leaves of several pear cultivars (Pyrus communis L.). Plant Cell Tissue Organ Culture 25, 99–105. doi: 10.1007/BF00042180
Moss, S. M. A., Wang, T., Voogd, C., Brian, L. A., Wu, R., Hellens, R. P., et al. (2018). AcFT promotes kiwifruit in vitro flowering when overexpressed and Arabidopsis flowering when expressed in the vasculature under its own promoter. Plant Direct 2, e00068. doi: 10.1002/pld3.68
Mourgues, F., Chevreau, E., Lambert, C., De Bondt, A. (1996). Efficient Agrobacterium-mediated transformation and recovery of transgenic plants from pear (Pyrus communis L.). Plant Cell Rep. 16, 245–249. doi: 10.1007/s002990050216
Murashige, T., Skoog, F. (1962). A revised medium for rapid growth and bio assays with tobacco tissue cultures. Physiol. Plantarum 15, 473–497. doi: 10.1111/j.1399-3054.1962.tb08052.x
Murayama, H., Toyomasu, T., Mitsuhashi, W., Dandekar, A. M., Gao, M., Matsuta, N., et al. (2003). transformation of pear (Pyrus communis cv. ‘La France’) with genes involved in ethylene biosynthesis. Acta Horticulurae 625, 387–393. doi: 10.17660/ActaHortic.2003.625.46
Nakajima, I., Sato, Y., Saito, T., Moriguchi, T., Yamamoto, T. (2013). Agrobacterium-mediated genetic transformation using cotyledons in Japanese pear (Pyrus pyrifolia). Breed Sci. 63, 275–283. doi: 10.1270/jsbbs.63.275
Peña, L., Martín-Trillo, M., Juárez, J., Pina, J. A., Navarro, L., Martínez-Zapater, J. M. (2001). Constitutive expression of Arabidopsis LEAFY or APETALA1 genes in citrus reduces their generation time. Nat. Biotechnol. 19, 263–267. doi: 10.1038/85719
Putterill, J., Varkonyi-Gasic, E. (2016). FT and florigen long-distance flowering control in plants. Curr. Opin. Plant Biol. 33, 77–82. doi: 10.1016/j.pbi.2016.06.008
Sheng, X., Mahendra, R. A., Wang, C.-T., Brunner, A. M. (2023). CRISPR/Cas9 mutants delineate roles of Populus FT and TFL1/CEN/BFT family members in growth, dormancy release and flowering. Tree Physiol. 43 (6), 1042–1054. doi: 10.1093/treephys/tpad027
Song, G.-Q., Walworth, A., Zhao, D., Jiang, N., Hancock, J. F. (2013). The Vaccinium corymbosum FLOWERING LOCUS T-like gene (VcFT): a flowering activator reverses photoperiodic and chilling requirements in blueberry. Plant Cell Rep. 32, 1759–1769. doi: 10.1007/s00299-013-1489-z
Srinivasan, C., Dardick, C., Callahan, A., Scorza, R. (2012). Plum (Prunus domestica) trees transformed with poplar FT1 result in altered architecture, dormancy requirement, and continuous flowering. PloS One 7, e40715. doi: 10.1371/journal.pone.0040715
van Nocker, S., Gardiner, S. E. (2014). Breeding better cultivars, faster: applications of new technologies for the rapid deployment of superior horticultural tree crops. Horticult. Res. 1, 14022. doi: 10.1038/hortres.2014.22
Varkonyi-Gasic, E., Wang, T., Voogd, C., Jeon, S., Drummond, R. S. M., Gleave, A. P., et al. (2019). Mutagenesis of kiwifruit CENTRORADIALIS-like genes transforms a climbing woody perennial with long juvenility and axillary flowering into a compact plant with rapid terminal flowering. Plant Biotechnol. J. 17, 869–880. doi: 10.1111/pbi.13021
Vervliet, G., Holsters, M., Teuchy, H., Van Montagu, M., Schell, J. (1975). Characterization of different plaque-forming and defective temperate phages in agrobacterium strains. J. Gen. Virol. 26, 33–48. doi: 10.1099/0022-1317-26-1-33
Visser, T. (1964). Juvenile phase and growth of apple and pear seedlings. Euphytica 13, 119–129. doi: 10.1007/bf00033299
Voogd, C., Brian, L. A., Wang, T., Allan, A. C., Varkonyi-Gasic, E. (2017). Three FT and multiple CEN and BFT genes regulate maturity, flowering, and vegetative phenology in kiwifruit. J. Exp. Bot. 68, 1539–1553. doi: 10.1093/jxb/erx044
Watson, A. E., Seleznyova, A. N., Dayatilake, G. A., Tustin, D. S. (2012). Rootstocks affect pear (Pyrus communis) tree growth through extent of node neoformation and flowering with key differences to apple. Funct. Plant Biol. 39, 493–502. doi: 10.1071/FP12031
Wenzel, S., Flachowsky, H., Hanke, M.-V. (2013). The Fast-track breeding approach can be improved by heat-induced expression of the FLOWERING LOCUS T genes from poplar (Populus trichocarpa) in apple (Malus × domestica Borkh.). Plant Cell Tissue Organ Culture (PCTOC) 115, 127–137. doi: 10.1007/s11240-013-0346-7
Wickland, D. p., Hanzawa, Y. (2015). The FLOWERING LOCUS T/TERMINAL FLOWER gene family: functional evolution and molecular mechanisms. Mol. Plant 8, 983–997. doi: 10.1016/j.molp.2015.01.007
Wu, J., Wang, Z., Shi, Z., Zhang, S., Ming, R., Zhu, S., et al. (2013). The genome of the pear (Pyrus bretschneideri Rehd.). Genome Res. 23, 396–408. doi: 10.1101/gr.144311.112
Yamagishi, N., Kishigami, R., Yoshikawa, N. (2014). Reduced generation time of apple seedlings to within a year by means of a plant virus vector: a new plant-breeding technique with no transmission of genetic modification to the next generation. Plant Biotechnol. J. 12, 60–68. doi: 10.1111/pbi.12116
Yamagishi, N., Li, C., Yoshikawa, N. (2016). Promotion of flowering by Apple latent spherical virus vector and virus elimination at high temperature allow accelerated breeding of apple and pear. Front. Plant Sci. 7. doi: 10.3389/fpls.2016.00171
Yancheva, S. D., Shlizerman, L. A., Golubowicz, S., Yabloviz, Z., Perl, A., Hanania, U., et al. (2006). The use of green fluorescent protein (GFP) improves Agrobacterium-mediated transformation of ‘Spadona’ pear (Pyrus communis L.). Plant Cell Rep. 25, 183–189. doi: 10.1007/s00299-005-0025-1
Yao, J.-L., Cohen, D., Atkinson, R., Richardson, K., Morris, B. (1995). Regeneration of transgenic plants from the commercial apple cultivar Royal Gala. Plant Cell Rep. 14, 407–412. doi: 10.1007/BF00234044
Zhang, H., Harry, D. E., Ma, C., Yuceer, C., Hsu, C.-Y., Vikram, V., et al. (2010). Precocious flowering in trees: the FLOWERING LOCUS T gene as a research and breeding tool in Populus. J. Exp. Bot. 61, 2549–2560. doi: 10.1093/jxb/erq092
Zhu, L. H. (2000). Adventitious shoot regeneration of two dwarfing pear rootstocks and the development of a transformation protocol. J. Hortic. Sci. Biotechnol. 75, 745–752. doi: 10.1080/14620316.2000.11511317
Keywords: pear, flowering, MADS-box gene, BpMADS4, fast breeding
Citation: Tomes S, Gunaseelan K, Dragulescu M, Wang Y-Y, Guo L, Schaffer RJ and Varkonyi-Gasic E (2023) A MADS-box gene-induced early flowering pear (Pyrus communis L.) for accelerated pear breeding. Front. Plant Sci. 14:1235963. doi: 10.3389/fpls.2023.1235963
Received: 07 June 2023; Accepted: 28 August 2023;
Published: 25 September 2023.
Edited by:
Elena Palomo Ríos, University of Malaga, SpainReviewed by:
Lorenzo Burgos, Spanish National Research Council (CSIC), SpainLeandro Peña, Polytechnic University of Valencia, Spain
Copyright © 2023 Tomes, Gunaseelan, Dragulescu, Wang, Guo, Schaffer and Varkonyi-Gasic. This is an open-access article distributed under the terms of the Creative Commons Attribution License (CC BY). The use, distribution or reproduction in other forums is permitted, provided the original author(s) and the copyright owner(s) are credited and that the original publication in this journal is cited, in accordance with accepted academic practice. No use, distribution or reproduction is permitted which does not comply with these terms.
*Correspondence: Erika Varkonyi-Gasic, erika.varkonyi-gasic@plantandfood.co.nz