- 1REQUIMTE, LAQV, ICBAS, School of Medicine and Biomedical Sciences, University of Porto, Porto, Portugal
- 2REQUIMTE, LAQV, Departament of Chemistry and Biochemistry, Faculty of Sciences, University of Porto, Porto, Portugal
- 3Veterinary and Animal Research Centre (CECAV) and Associate Laboratory for Veterinary and Animal Science (AL4AnimalS), Universidade de Trás-os-Montes e Alto Douro, Quinta de Prados, Vila Real, Portugal
- 4Centre for the Research and Technology Agro-Environmental and Biological Sciences (CITAB), Universidade de Trás-os-Montes e Alto Douro, Vila Real, Portugal
Introduction: Lupins and other legumes have been considered as alternative plant-based protein sources to soybeans for both humans and livestock. Furthermore, they can contribute to more sustainable agricultural systems. The productivity and chemical composition of legumes is highly variable between species, cultivars, and with the edaphoclimatic conditions.
Methods: This work evaluated the adaptability of seven Lupinus cultivars in two different sowing locations, during two consecutive years, through the characterization of their seed, as a means of investigating their suitability to be used as a source of food and/or feed.
Results and discussion: Lupinus angustifolius cv. Tango and Lupinus luteus cv. Acos were the most stable genotypes across the environments when considering the seed and protein production, while L. luteus cv. Alburquerque and L. luteus cv. Mister showed less variation in the total alkaloid content across the environments. The edaphoclimatic conditions affected seed and protein yields, as higher rainfall resulted in high productivity. The lower temperatures observed in the first year at both locations caused a reduction in the production of alkaloids in L. luteus cv. Acos and Cardiga. Due to the high alkaloid content of some of the studied cultivars their use as food or feed can pose some safety concerns. However, these cultivars can have high levels of resistance to herbivore and insect attacks, which can be of the utmost importance for the use of these crops for recovering poor or exhausted soils.
1 Introduction
Grain legumes (Fabaceae) are valuable sources of plant-based protein for both humans and livestock, and can contribute to more sustainable and healthier low-input agricultural systems due to their ability to fix biological nitrogen through symbiotic association with soil bacteria (Ferreira et al., 2021). Despite the efforts of the European Commission to promote the production of legumes to reduce the historic dependence on imported plant protein sources (European Commission, 2018), these plants continue to be underrepresented in European cropping systems (Watson et al., 2017). However, advances achieved in recent decades regarding breeding, cultivation practices, and policy initiatives (Berger and Ludwig, 2014; MacWilliam et al., 2014; Ferreira et al., 2021) have been promoting their production and consumption. Jensen et al. (2021) have recently reviewed the European legume crop production status and concluded that an annual increase of 1% in legume yields would have a greater impact on protein production in the EU in the medium term than additional subsidies (i.e., 75€ ha−1 for soybean and leguminous crops). These figures highlight the need for a continued increase in grain legume productivity through research and development (R&D) to efficiently improve the resilience and economic sustainability of European agrifood systems.
The productivity of Fabaceae varies greatly between species and with soil and climatic conditions (French et al., 2001; Palta et al., 2004; Fraser et al., 2005). Legume crops are particularly vulnerable to extreme temperatures and drought stress during vegetative and reproductive growth (Khatun et al., 2021), which can negatively affect plant development and grain production (Savita et al., 2020). Therefore, it is of the utmost importance to identify the most appropriate legume species and cultivars for specific locations to maximize grain productivity and protein production, particularly in the Mediterranean region where the periods of severe and extreme drought and heat events have been exacerbated by climate change.
White (Lupinus albus L.), blue, or narrow-leafed (Lupinus angustifolius L.), and yellow (Lupinus luteus L.) lupins are native European grain legumes, which are well adapted to acidic and sandy soils, a trait that is of particular importance in many Mediterranean regions and that differentiates them from other grain legumes (Lema and Soengas, 2023). Lupin seeds accumulate proteins preferentially to oils or starch, being a well-balanced protein source, except for the low levels of sulfur-containing amino acids (Musco et al., 2017). Thus, lupins are an interesting source of protein for food and feed, an alternative to soybean, which is also deficient in sulfur-containing amino acids (Lucas et al., 2015; Monteiro et al., 2021). However, lupins, principally if not genetically selected, can accumulate considerable amounts of nitrogenous secondary metabolites, particularly quinolizidine and piperidine alkaloids, which are synthesized from the amino acid lysine, and to a minor extent, indole alkaloids, which are synthesized from tryptophan (Wink et al., 1995; Adhikari et al., 2012).
The alkaloids are a large group of secondary metabolites that plants produce to defend themselves against a variety of pathogenic microorganisms and predators, including insects and herbivores, and against competing plants via allelopathy (Wink and Mohamed, 2003). Alkaloid synthesis occurs predominantly in the aerial green parts of the plant, under light stimulation, and are translocated in the phloem to other parts of the plant, accumulating in seeds as they mature (Otterbach et al., 2019; Mancinotti et al., 2022). The biosynthesis of quinolizidine alkaloids begins with the decarboxylation of L-lysine to cadaverine that forms the nitrogen-containing heterocycle and then the various quinolizidine skeletons of bicyclic, tricyclic, and tetracyclic alkaloids (Frick et al., 2017; Mancinotti et al., 2022). The quinolizidine alkaloids can then be further modified by dehydrogenation, oxygenation, hydroxylation, glycosylation, or esterification to form a wide variety of structurally related quinolizidine alkaloids (reviewed in Frick et al., 2017). Cadaverine is also the precursor in the biosynthesis of piperidine alkaloids (Sato et al., 2018).
The interactions between genotype and environment are complex and alkaloids in lupins can reach considerable levels, thus rendering the plant toxic and with a bitter unpleasant taste to herbivores (Frick et al., 2017). The mechanisms of toxicity and susceptibilities to individual lupin alkaloids include anticholinergic effects and inhibition of voltage-dependent ion channels, with risk of paralysis, spasms and tremors, respiratory failure, and heart arrhythmia; the last underlying the well-established antiarrhythmic effect of sparteine (Schrenk et al., 2019). The piperidine alkaloid ammodendrine is suspected to be teratogenic and some quinolizidine alkaloids may become teratogenic in bovine animals after metabolization in the rumen (Green et al., 2012).
In this study, the adaptability and the production of grain and protein yields of seven cultivars of three Lupinus species (i.e., L. albus, L. angustifolius, and L. luteus) in two different sowing locations, during two consecutive years, were evaluated. Detailed alkaloid profiles were also determined to evaluate the impact of cultivars and environmental factors, such as soil characteristics, rainfall, and temperature, on alkaloid biosynthesis. The generated knowledge will contribute to the promotion of lupin production by identifying the best cultivar for use as a source of food and/or feed.
2 Materials and methods
2.1 Trial location, plant material, experimental design, and environment characterization
Seven cultivars of legume seeds of the genus Lupinus were acquired from local seed providers: L. albus cv. Dulce and L. luteus cv. Alburquerque were supplied by Cicytex—Centro de Investigaciones Científicas y Tecnológicas de Extremadura, Spain; L. albus cv. Estoril, L. angustifolius cv. Tango, and L. luteus cv. Mister were supplied by Fertiprado, Portugal; L. luteus cv. Acos and L. luteus cv. Cardiga were supplied by INIAV—Instituto Nacional de Investigação Agrária e Veterinária, Elvas, Portugal.
This study was carried out simultaneously over two consecutive years at two different experimental fields on the northeast of Portugal. One site was at Mirandela (MI; 41.511896, −7.161595) and the other at Vila Real (VR; 41.284747, −7.738875). In both years and locations, soils were plowed before sowing and samples (0 cm–30 cm depth) were taken for general physicochemical composition determinations (Table 1). Due to soil chemical composition, 1,600 kg of dolomitic lime and 800 kg of superphosphate were added to the soil in VR in the first year and 25 kg was added to the soil on the same site in the second year.
The legume cultivars were sown on 28 November 2016 at MI and 23 November 2016 at VR (year 1) and on 15 November 2017 at MI and 21 November 2017 at VR (year 2), using a randomized block design of plots of 10 m2 (2.5 m × 4 m). The plots were replicated four times, thus resulting in 28 plots on each location, for each year. The plots were rain fed, no supplemental irrigation was provided. The sowing densities were calculated to achieve crop plant densities of 30 plants m−2, 58 plants m−2, and 58 plants m−2, respectively, for L. albus, L. luteus, and L. angustifolius. The seeds were placed in rows 30 cm apart, and seed-to-seed distance in rows was calculated based on the targeted crop plant densities above. In addition, the seed-to-seed distance was corrected for the germination rate of each cultivar, which was determined by previous germination tests conducted at the laboratory. The germination rates and the sowing densities for both years are presented in Table S1 (Supplementary Information). The harvest occurred when all the pods in the same plot were ripe on the following dates: MI year 1, between 31 May 2017 and 22 June 2017; VR year 1, between 16 June 2017 and 6 July 2017; MI year 2, between 6 July 2018 and 17 July 2018; and VR year 2, between 12 July 2018 and 20 July 2018. The pods were collected and transported to the laboratory where seed threshing was performed by hand. The grain yield (t DM ha−1) was evaluated using a central area of 2 m2.
To better characterize each location, daily data on the average minimum and maximum temperatures (˚C) and precipitation (mm) were collected from local weather stations to calculate monthly values from September to August on both growing years (Figure S1). The temperature and precipitation data for the study of the environmental effect on productivity and alkaloid composition are provided in Table 1.
2.2 Proximate chemical composition
The grain seeds were dried in a forced-air oven at 65°C for 24 h for dry matter (DM) determination and ground to pass through a 1-mm sieve. The ground samples were analyzed according to the Association of Official Analytical Chemists (AOAC)’s (AOAC, 2000) methods for DM (934.01), ether extraction (920.39), and Kjeldahl N (954.01) content. The crude protein content was calculated as Kjeldahl N multiplied by a conversion factor (6.25). Neutral detergent fiber content was also determined according to Robertson and Soest (1981) and expressed exclusive of residual ash.
2.3 Alkaloid extraction
The alkaloids were extracted from the powdered seeds according to the procedure described by Magalhães et al. (2017). Briefly, alkaloids were extracted with 5% trichloroacetic acid for 30 min, under constant stirring. After centrifugation and alkalinization of the supernatant the crude extract was purified by liquid–liquid extraction with dichloromethane, the organic solvent was evaporated, and the alkaloid-rich residue was stored at −20°C, protected from light, until analysis. Each sample was extracted in duplicate.
2.4 GC-MS analysis
The alkaloid extracts were dissolved in dichloromethane and filtered with a 0.45-µm regenerated cellulose syringe filter before gas chromatography–mass spectrometry (GC-MS) analysis, following the conditions described by Magalhães et al. (2017) with modifications. The chromatographic analysis of the extracts was performed in a Thermo Fisher Scientific (Waltham, MA, USA) Trace 1300, ISQ single-quadrupole mass spectrometer equipped with a TraceGOLD TG-5MS column (30 m × 0.25 mm; 0.25 µm) from Thermo Fisher Scientific. The oven temperature was programmed as follows: 150°C for 1 min; followed by an increase at 5°C min−1 until the temperature reaches 235°C, it is then held for 15 min; and then the temperature is increased at 10°C min−1 until 280°C is reached, then it is held for 10 min. The injection volume was 1 µL and a split ratio of 1:5 was used. The identification of the compounds was performed by the analysis of standards or by comparison with the National Institute of Standards and Technology (NIST)’s database (2001).
2.5 Alkaloid quantification
The quantification of each alkaloid in the extracts was achieved by using calibration curves of standards prepared in dichloromethane and analyzed under the same conditions as the samples. The total peak area was plotted as a function of concentration. Gramine (99%; Sigma, St. Louis, MO, USA), lupinine (100%; Sigma), sparteine (≥ 98%; Sigma), angustifoline (> 98%; Ambinter, Orléans, France), and lupanine (> 98%; Ambinter) were quantified as themselves. The other alkaloids were quantified as equivalents of the standard from the same chemical class (indole, piperidine, bicyclic, tricyclic, or tetracyclic quinolizidine).
2.6 Statistical analysis
The statistical analyses to evaluate the effect of the environment (represented by the interaction location × year) on the genotype (cultivar) productivity and alkaloid content were performed through the analysis of variance (ANOVA) using the “stability” package (version 0.5.0) in R (software version 4.3.1; The R Foundation for Statistical Computing, Vienna, Austria). The significance was set for p-values lower than 0.05 and multiple comparisons of means was carried out using the Tukey test with the packages “multcomp” (version 1.4–25) and “multcompView” (version 0.1–9) in R. The stability of the genotypes across the environments was calculated using the coefficient of variation (CV), the ecovalence stability index (W) (Wricke, 1962; Wricke, 1964), and Shukla’s stability variance (Shukla) (Shukla, 1972). For all the indexes, the lower the value, the higher the stability of the genotype. The correlation pattern between the environmental variables and the environments was characterized via a principal component analysis (PCA) and the two PCA axes were displayed in a distance biplot performed using the packages “FactoMineR” (version 2.8) and “factoextra” (version 1.0.7) in R. The interaction effects between the genotype and the environment as a function of the environmental variables was studied by a redundancy analysis (RDA) using the package “vegan” (version 2.6–4) in R.
3 Results
3.1 Productivity and proximate chemical composition
The seed and protein yields were affected by the genotype, the environment, and the interaction genotype × environment (Table 2; p < 0.05), with the environment being the main factor responsible for the variability (73% and 74%, respectively) of the results.
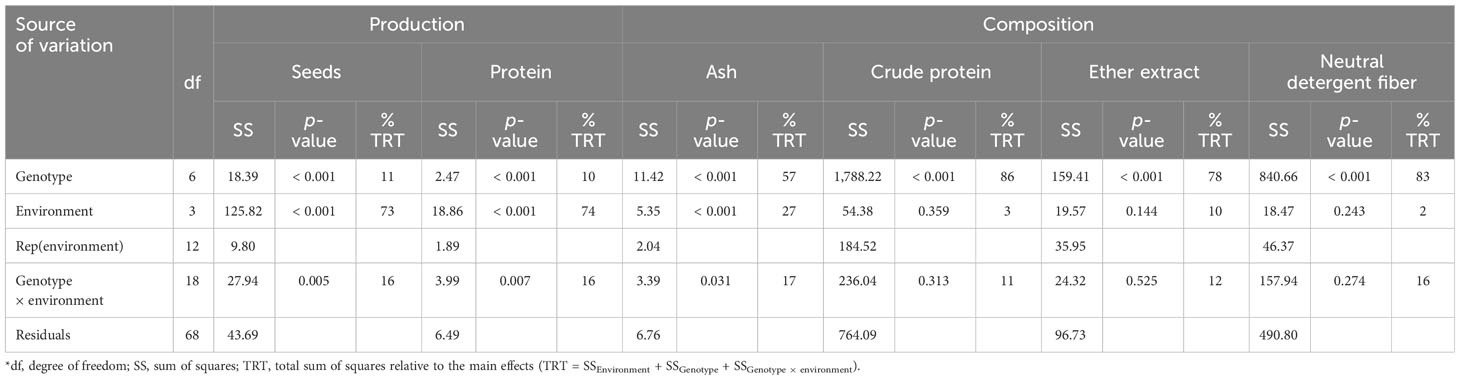
Table 2 Analysis of variance of seed and protein production (t DM ha−1), and seed chemical composition (g 100 g−1 DM) for Lupinus cultivars sown in four environments (with a combination of sowing locations and years)*.
The seed production was lower in the first year of the study, regardless of the cultivar, and seed production was the highest for L. albus cv. Estoril in VR year 2 (5.10 t DM ha−1; Figure 1A). Protein production followed the same pattern as of seed production, that is, the lowest value was found for cultivars in year 1 (0.07 t DM ha−1–0.31 t DM ha−1; Figure 1B) and the highest value was recorded in year 2 for L. albus cv. Estoril (1.88 t DM ha−1; Figure 1B).
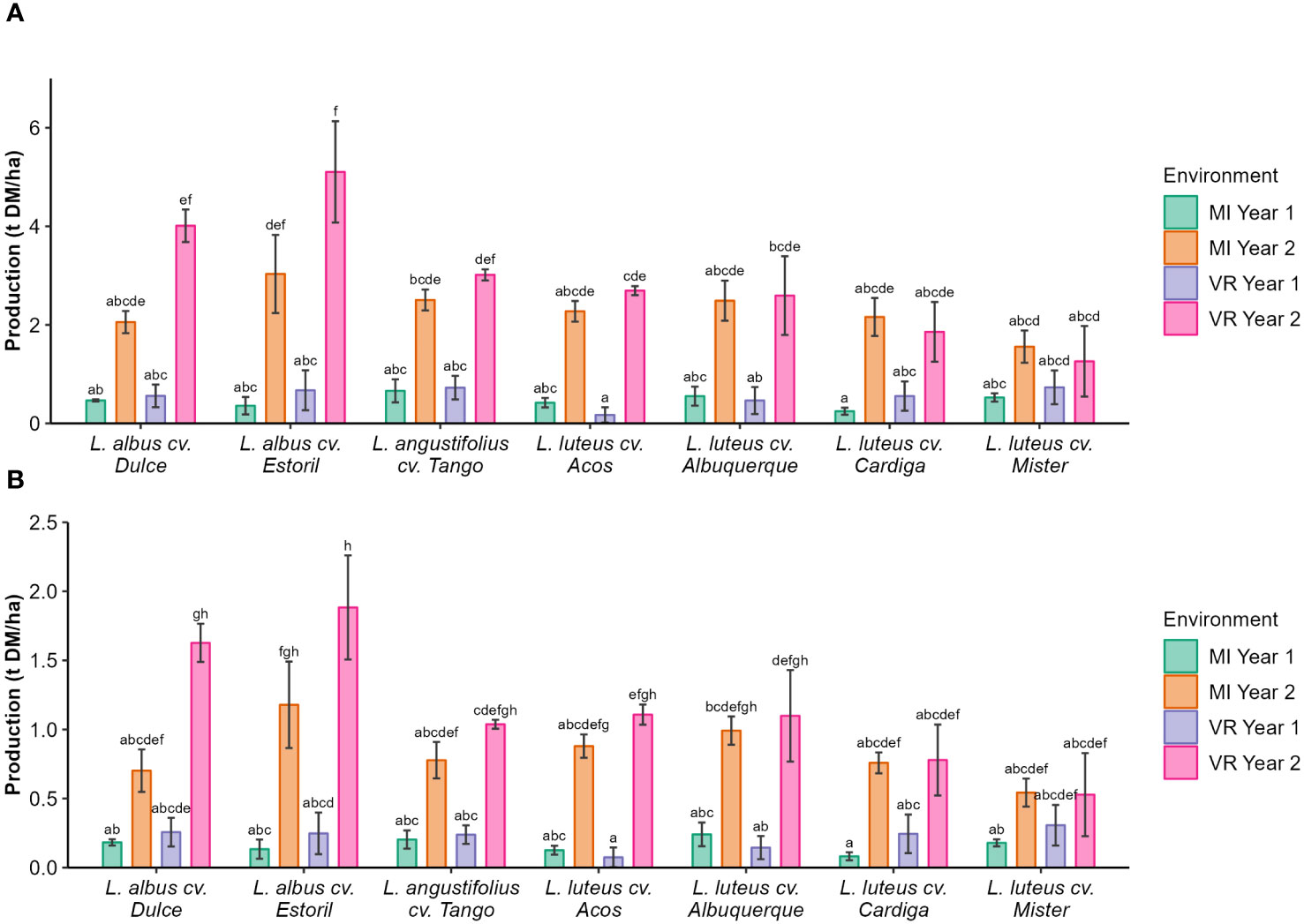
Figure 1 (A) Seed and (B) protein production (t ha−1 DM) by genotype and environment. The different letters show statistically significant differences (p < 0.05).
Concerning the chemical composition of the Lupinus seeds, ash, crude protein, ether extract, and neutral detergent fiber contents varied significantly between cultivars (p < 0.001; Table 2). Ash content was affected by the interaction genotype × environment (p = 0.031), and the ether extract content by the environment (p = 0.004; Table 2). The ash content varied between 3.60 g 100 g−1 DM and 5.28 g 100 g−1 DM (Figure S2, Supplementary Information). The crude protein content was the lowest in L. angustifolius cv. Tango (32.2 g 100 g−1 DM), followed by L. albus cv. Estoril (37.8 g 100 g−1 DM), and did not differ (p > 0.05) between the other studied cultivars (Figure S3A). Lupinus albus cultivars presented with the highest ether extract content (7.24 g 100 g−1 DM –7.28 g 100 g−1 DM; Figure S3B). The cultivars of L. angustifolius and L. luteus presented with the highest neutral detergent fiber content (25.2 g 100 g−1 DM–28.4 g 100 g−1 DM) and L. albus cultivars the lowest (20.5 g 100 g−1 DM–20.7 g 100 g−1 DM; Figure S3C).
3.2 Total alkaloid content and by chemical class
In the analyzed samples, 32 alkaloids were identified as belonging to three chemical classes (indole, piperidine, and quinolizidine). The list of the identified alkaloids in the studied Lupinus cultivars is presented in Table S2. All the classes were affected (p < 0.05) by the genotype × environment interaction (Table 3), which accounted for 25% to 42% of the variability of the results. It was also observed that the genotype was the main source of variation (48% to 74%) of the alkaloid content in Lupinus seeds (Table 3). The results of the total content of alkaloids and by chemical class are presented in Figure 2. Considering the different classes of alkaloids, the most relevant ones were the quinolizidine-based compounds, followed by the indoles and piperidines. The indole alkaloids were only present in L. luteus cultivars Acos and Cardiga, with the highest contents being found in the Cardiga cultivar in MI year 2 and VR year 2 (624 mg kg−1 DM and 581 mg kg−1 DM, respectively) and the lowest in the same cultivar in MI year 1 and VR year 1 (21.4 mg kg−1 DM and 14.5 mg kg−1 DM, respectively; Figure 2A). The piperidine alkaloids were quantified in L. albus cultivars and L. luteus cultivars Acos, Alburquerque, and Cardiga (Figure 2B). In the remaining cultivars piperidine alkaloids were either not detected or not quantified. The highest piperidine alkaloids value was found for L. luteus cv. Cardiga in MI year 1 (99.9 mg kg−1 DM), followed by the same cultivar in VR year 1 (55.4 mg kg−1 DM). The bicyclic quinolizidines were only quantified in L. luteus cultivars, with the highest being in L. luteus cv. Cardiga in MI year 2 and VR year 2 (1,531 mg kg−1 DM and 1,437 mg kg−1 DM, respectively; Figure 2C), followed by L. luteus cv. Acos in the same environments (909 mg kg−1 DM and 843 mg kg−1 DM; Figure 2C). L. albus and L. angustifolius were the species containing detectable levels of tricyclic quinolizidine alkaloids (Figure 2D), with the highest values being found for Estoril and Tango cultivars in MI year 1 and VR year 1 (90.7 mg kg−1 DM –265 mg kg−1 DM) and the lowest in L. albus cv. Estoril in MI year 2 and VR year 2 (54.9 mg kg−1 DM and 62.1 mg kg−1 DM, respectively). Tetracyclic quinolizidine alkaloid occurrence was verified in all the studied lupin cultivars (Figure 2E). L. luteus cv. Cardiga showed the highest concentration in MI year 1 (331 mg kg−1 DM), followed by the same cultivar in VR year 1 (265 mg kg−1 DM), and the lowest levels were obtained for L. luteus cv. Mister (3.23 mg kg−1 DM –14.8 mg kg−1 DM; Figure 2E). The total quinolizidine alkaloid content was the highest for L. luteus cv. Cardiga (473 mg kg−1 DM 1,746 mg kg−1 DM; Figure 2F), and L. luteus cv. Acos in MI year 2 and VR year 2 (1,097 mg kg−1 DM and 1,022 mg kg−1 DM, respectively). As the major class of alkaloids in Lupinus seeds are quinolizidines, it was verified that the same pattern was recorded for the total alkaloid content (Figure 2G).
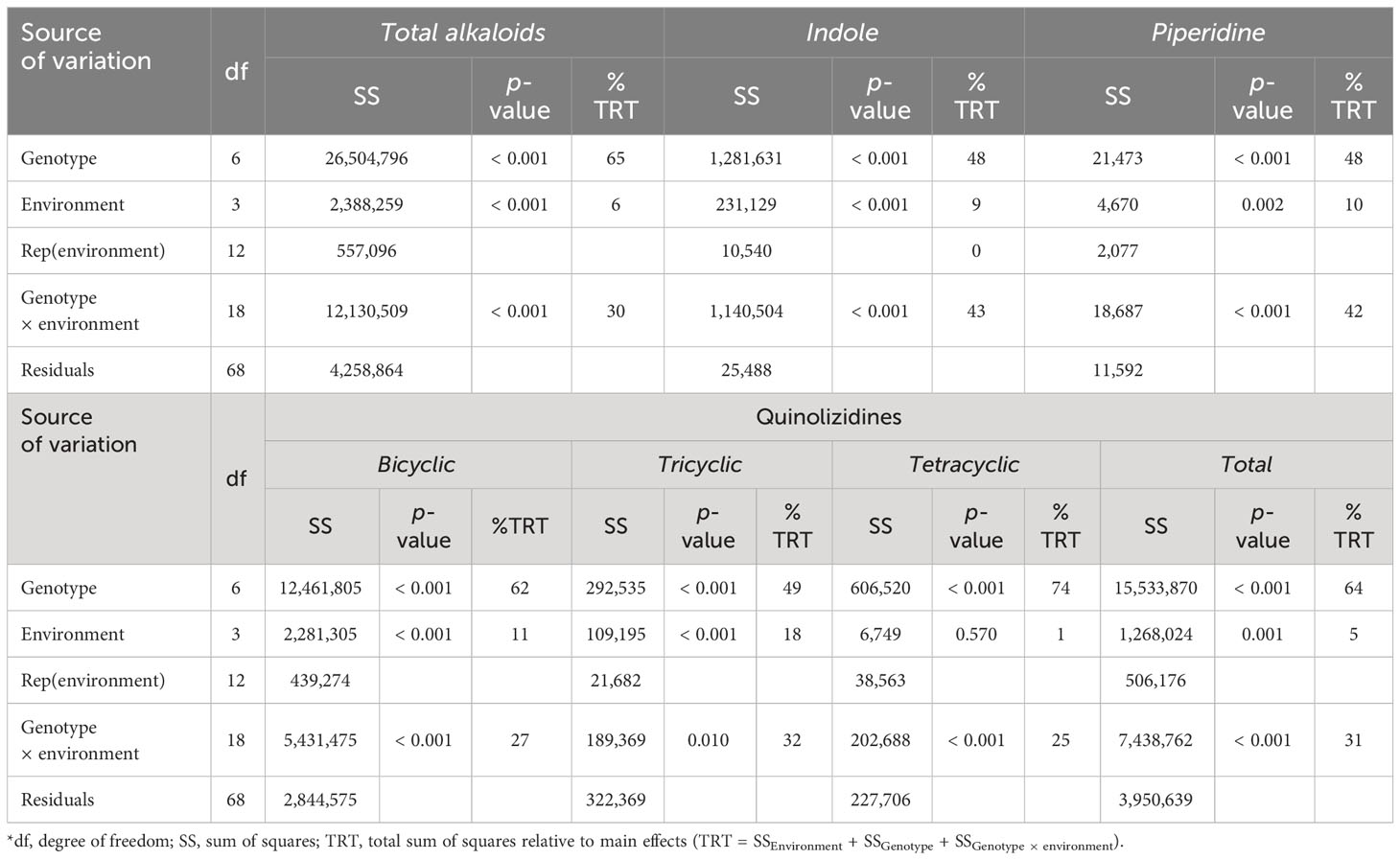
Table 3 Analysis of variance of the concentrations (mg kg−1 DM) of indole, piperidine, quinolizidine (bicyclic, tricyclic, tetracyclic, and total), and total alkaloids for Lupinus cultivars sown in four environments (with a combination of sowing locations and years)*.
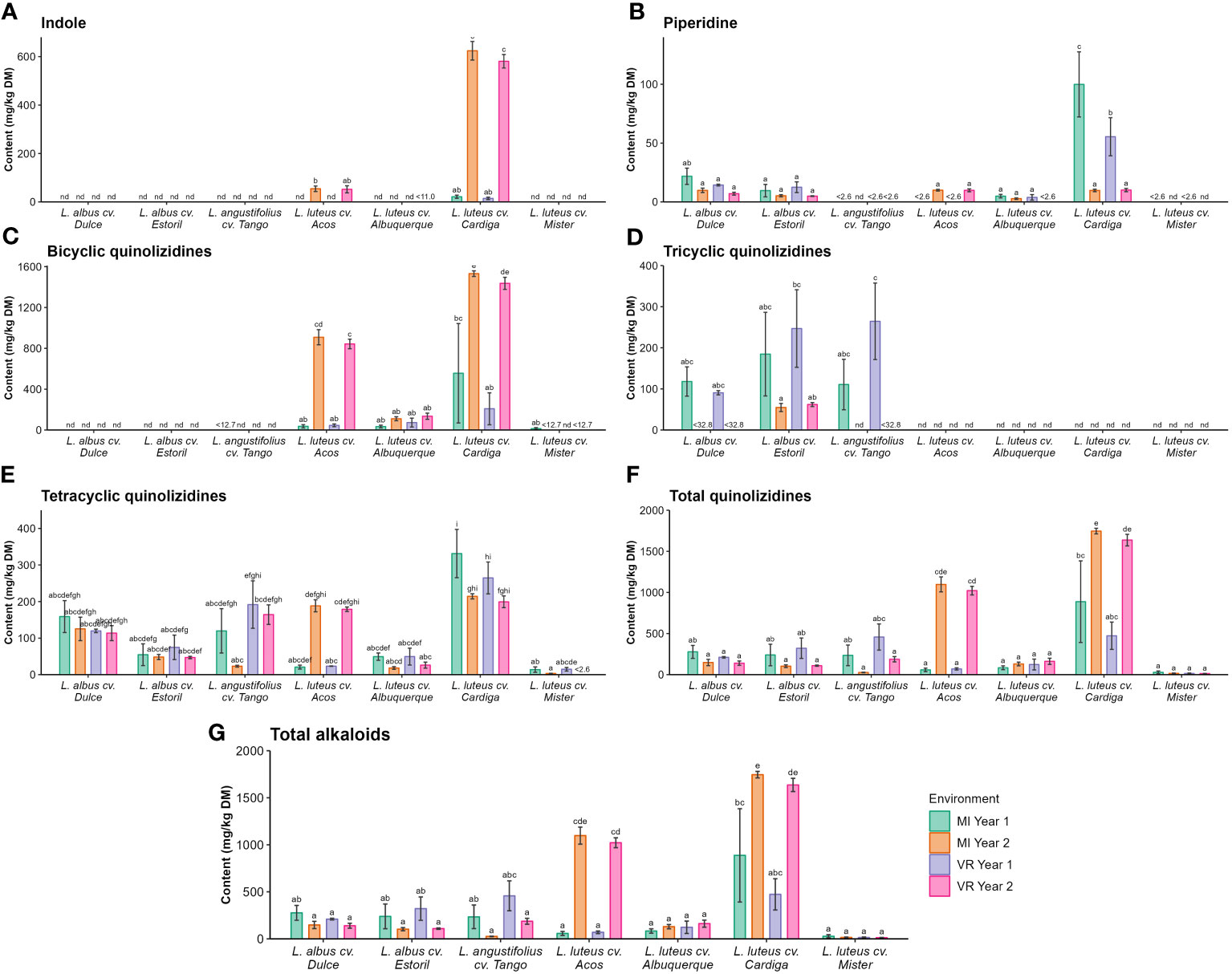
Figure 2 Concentrations (mg kg−1 DM) of (A) indole, (B) piperidine, (C) bicyclic, (D) tricyclic, and (E) tetracyclic, (F) total quinolizidine and (G) total alkaloids by Lupinus cultivar and environment. nd, not detected; <(value), below the limit of quantification. The different letters within each graph mean statistically significant differences (p < 0.05).
3.3 Content of individual alkaloids
A total of 13 individual alkaloids, identified in the analyzed Lupinus samples (Table S3), were quantified. All the individual alkaloids were affected (p <0.05) by the interaction genotype × environment (Table 4).
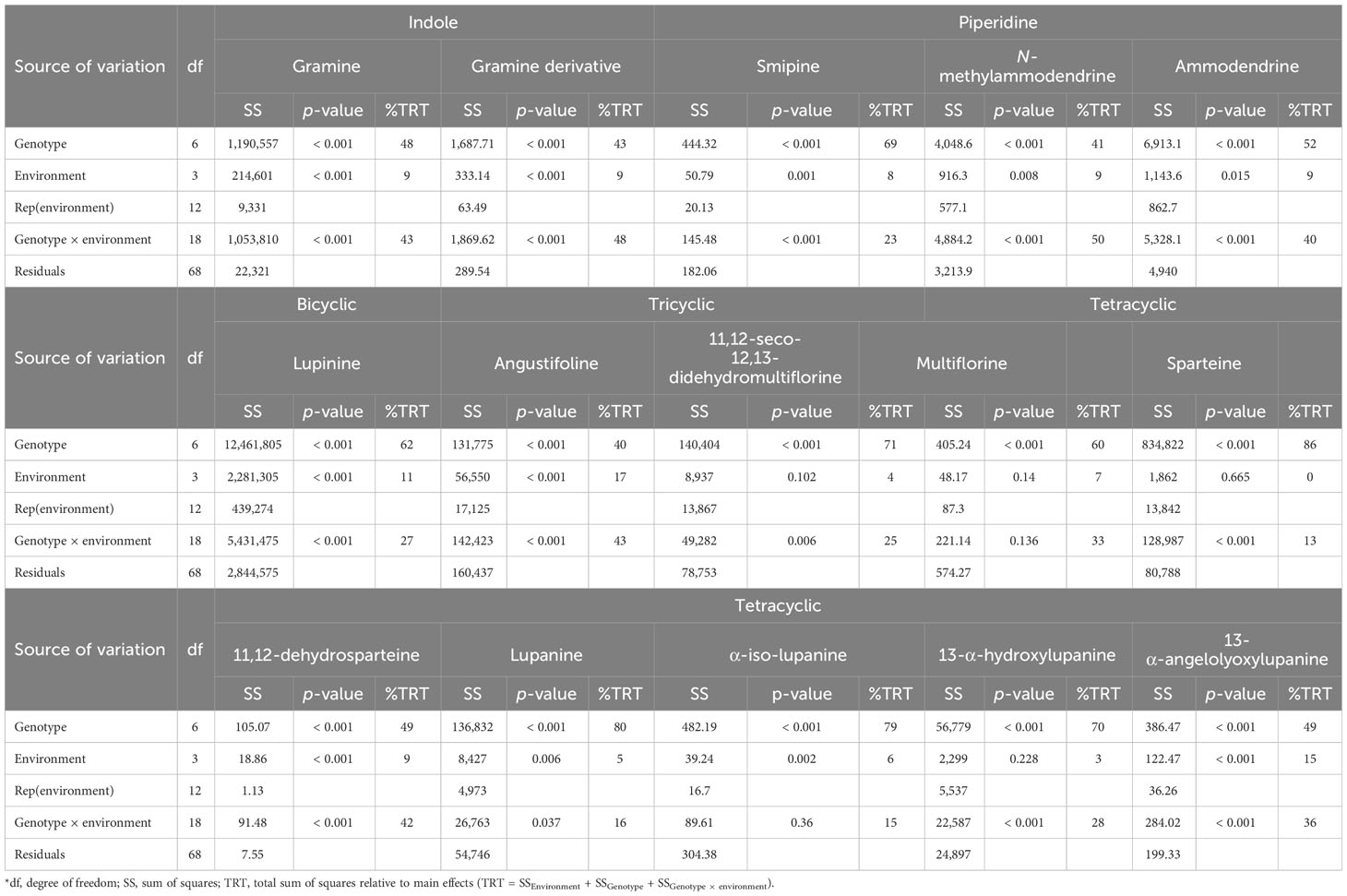
Table 4 Analysis of variance of the concentrations (mg kg−1 DM) of individual alkaloids for Lupinus cultivars sown in four environments (with a combination of sowing locations and years)*.
The complete results for the quantification of these alkaloids are presented in Table S3. Gramine was measured in L. luteus cv. Acos and L. luteus cv. Cardiga, the highest contents were found in the former for MI year 2 (596 mg kg−1 DM) and VR year 2 (564 mg kg−1 DM), as verified for the gramine derivative (27.7 mg kg−1 DM and 16.9 mg kg−1 DM, respectively, Figure 3A). Smipine characterized the L. albus cultivars, with the highest content recorded in L. albus cv. Dulce MI year 1 (11.1 mg kg−1 DM; Table S3). N-methylammodendrine was present only in L. luteus cv. Cardiga, with the highest levels recorded in MI year 1 (49.4 mg kg−1 DM). Ammodendrine (Figure 3B) was present in L. albus cultivars, and L. luteus cultivars Acos, Alburquerque, and Cardiga, with the Cardiga showing the highest content for MI year 1 (49.3 mg kg−1 DM) and VR year 1 (40.2 mg kg−1 DM). Lupinine was only detected in L. luteus cultivars (Figure 3C), while angustifoline (Figure 3D), lupanine (Figure 3E), and 13-α-hydroxylupanine (Figure 3F) were only present in the L. albus and L. angustifolius cultivars.
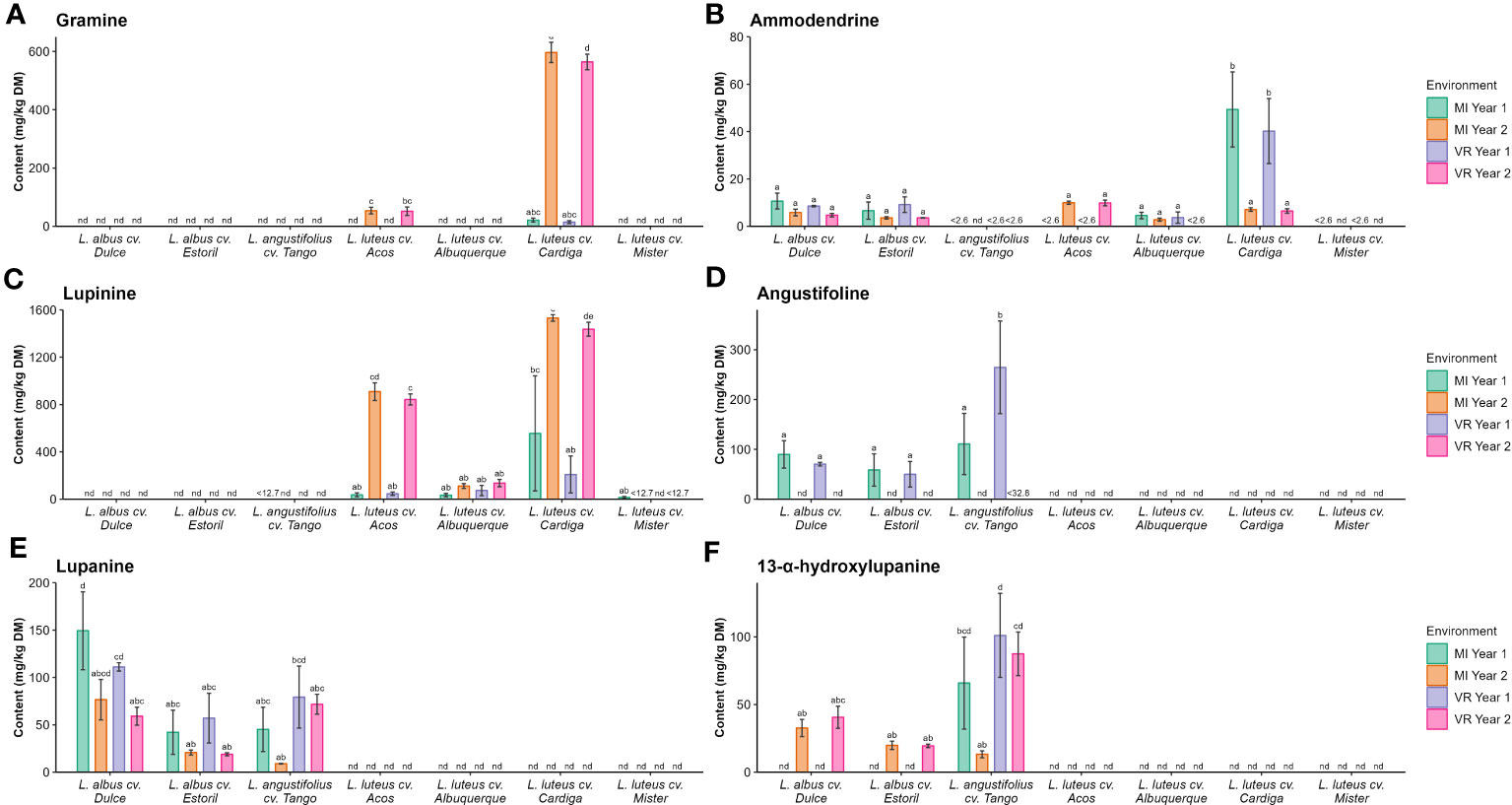
Figure 3 Concentrations (mg kg−1 DM) of (A) gramine, (B) ammodendrine, (C) lupinine, (D) angustifoline, (E) lupanine, and (F) 13-α-hydroxylupanine by Lupinus cultivar and environment. nd, not detected; <(value), below the limit of quantification. The different letters within each graph mean statistically significant differences (p < 0.05).
3.4 Effect of the environment on the productivity and alkaloid content
The stability of the cultivars across years and locations (i.e., environments) was evaluated in terms of productivity (i.e., seed and protein production) and alkaloid content. For the latter, only total alkaloid content was evaluated. The results of the ANOVA (Tables 2, 3) showed that the interaction genotype × environment was significant (p < 0.05) for seed and protein production, and for total alkaloid content. In terms of productivity indexes, the main source of variation was the environment, accounting for 73% and 74% of the total sum of squares relative to the main effects (%TRT) for seed and protein production, respectively. On the other hand, the main source of variation in alkaloid content was caused by the genotype, with %TRT values of 65%, followed by the interaction genotype × environment (30%).
The stability of the cultivars in terms of seed and protein production revealed that the most stable cultivar across environments was L. angustifolius cv. Tango (Table 5) by the three indexes for which the lower the value, the higher the stability. This cultivar was followed by L. luteus cv. Acos and L. luteus cv. Alburquerque. Although the highest productivity values were obtained for L. albus cv. Estoril (seed: 2.42 t DM ha−1; and protein: 0.909 t DM ha−1), it was considered the least stable genotype.
The content of total alkaloids was found to be more stable across environments in L. luteus cv. Alburquerque, and L. luteus cv. Mister, corresponding also to the cultivars with the lowest total alkaloid content (130 mg kg−1 DM and 19.5 mg kg−1 DM, respectively; Table 5). On the other hand, L. luteus cv. Cardiga with the highest mean value for alkaloid content (1,540 mg kg−1 DM) was considered the least stable genotype.
The correlation patterns between the edaphoclimatic variables and the differences between environments was studied by the PCA biplot, as presented in Figure 4. The first two components (PC) accounted for 89.2% of the total variability of the biplot (Figure 4), thus highlighting the differences between the environments. The variables were clustered into two main groups: (1) the climatic variables (temperature and rainfall) that distinguish the sowing years; and (2) the characteristics of soil that discriminated the locations. In each of these groups all the variables were highly correlated between them (r > 0.75), except clay. It was also possible to verify that seed and protein production was highly affected by the higher rainfall values in year 2.
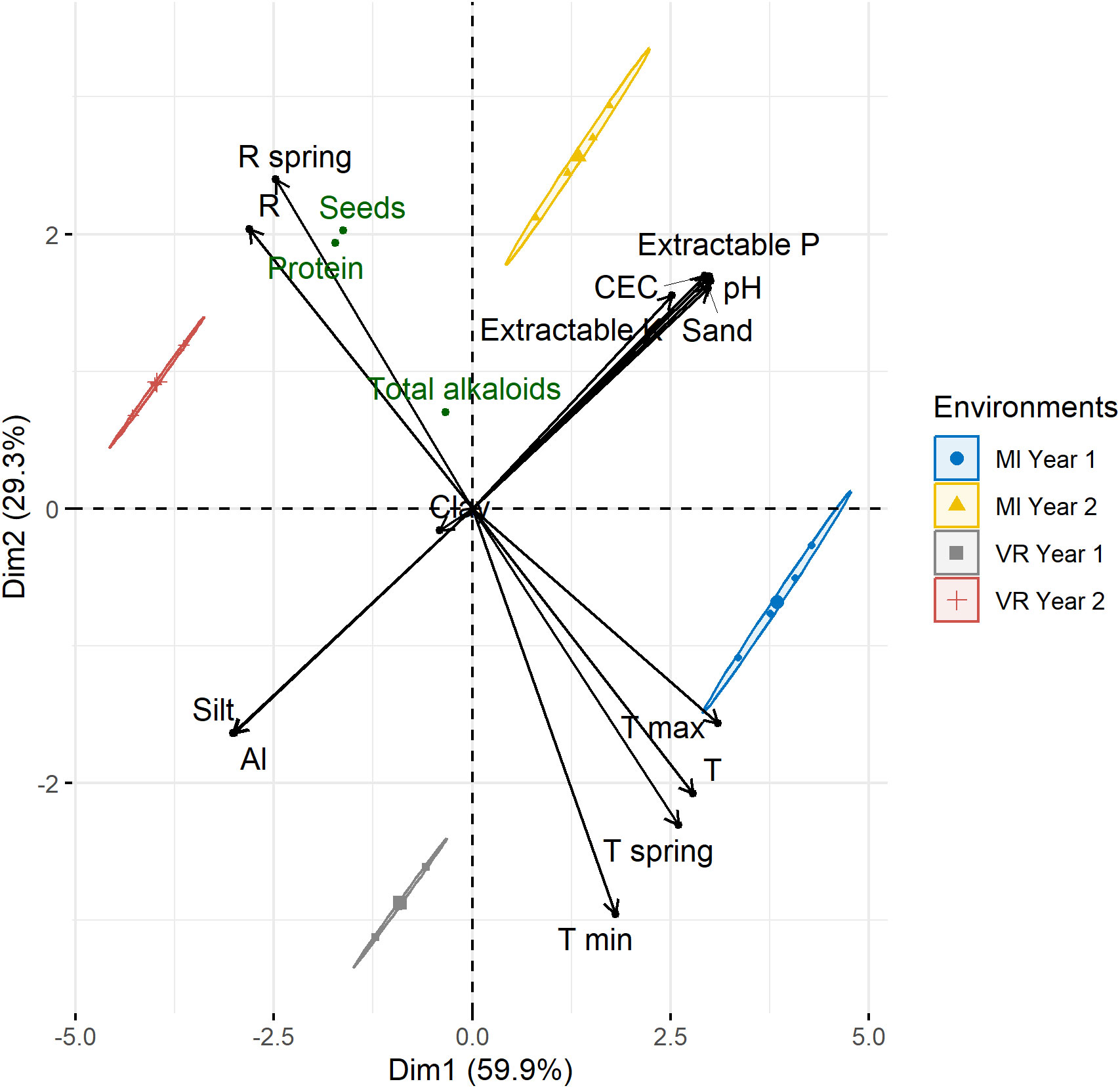
Figure 4 Principal component analysis (PCA) biplot showing the environments and the environmental variables.
The environmental variable with a significant impact on productivity, as selected for by the RDA procedure, was the total spring rainfall (R spring) for seed (Figure 5A) and protein (Figure 5B) production (p = 0.042, model R2 = 0.884 for seed and model R2 = 0.926 for protein). The biplots presented in Figures 5A, B shows the relationship between the environmental variables, the environment scores, and the genotype scores, with the first two axes accounting for 99.3% of the total genotype by environment variation. The total spring rainfall was positively correlated with year 2 at both locations, and with the increased seed and protein production in the same environments. For total alkaloid content, no environmental variable was considered to significantly (p < 0.05) affect the alkaloid production. In addition, the mean minimum temperature (T min) was shown to have a low impact on the alkaloids’ concentration (p = 0.083, model R2 = 0.775), and the results are depicted in Figure 5C. Higher T min was associated with the environments MI year 1 and VR year 1, and this had an impact on the highest content of alkaloids for L. albus cv. Dulce, L. albus cv. Estoril, L. angustifolius cv. Tango, and L. luteus cv. Mister. For total quinolizidine alkaloids, the same results were obtained (data not shown).
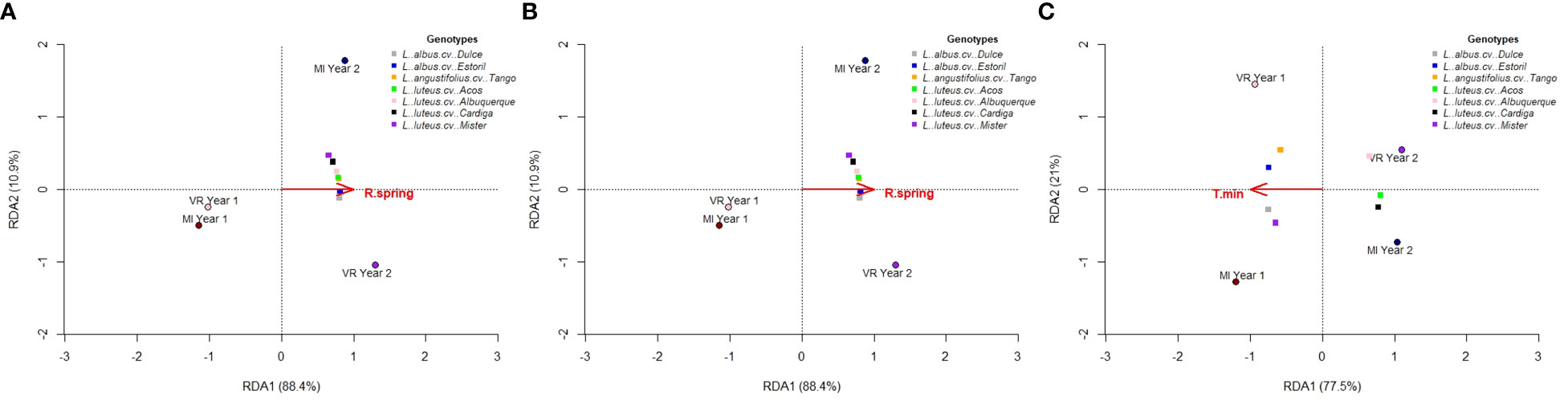
Figure 5 Reduced rank factorial regression biplots for (A) seed production, (B) protein production, and (C) total alkaloid content.
4 Discussion
4.1 Productivity and seed chemical composition
The Lupinus productivity was affected by the interaction genotype × environment, and a marked impact of the environment was verified by the ANOVA for the genotype-by-environment interaction model. The differences on the productivity were essentially observed in L. albus cultivars (Dulce and Estoril), L. angustifolius cv. Tango, and L. luteus cv. Acos, for which the productivities were higher in year 2 at both locations. In fact, L. albus cultivars were ranked as genotypes with low stability across environments, although average higher mean values of seed and protein production were obtained, as reported by other authors (Jul et al., 2003; Gresta et al., 2010). On the other hand, L. angustifolius cv. Tango and L. luteus cv. Acos were the two most stable cultivars, indicating a better adaptability to the edaphoclimatic conditions of the trial, when compared to the remaining cultivars. Although French et al. (2001) reported higher grain yields (around 51%) for L. angustifolius than L. luteus, in the present work, the production of L. angustifolius cv. Tango was similar to that of L. luteus cultivars. When studying two L. angustifolius cultivars Borweta and Bordako, Fraser et al. (2005) observed very different grain production values (3 t DM ha−1 for Borweta and 1.5 t DM ha−1 for Bordako), showing the high variability in productivity within the species. The grain productions of L. luteus cultivars were within the broad range of values reported by other authors (Chiofalo et al., 2012; Grażyna et al., 2017).
The differences in production yields are described to have been influenced by the climatic conditions (Maknickiene, 2001; Jul et al., 2003), and the soil characteristics, such as the pH (Gresta et al., 2010) and the phosphorous and aluminum concentrations (Quiñones et al., 2022). In the present study, it was observed that the higher total rainfall during spring (between March and May) observed in year 2 at both locations was responsible for the increased productivity. In Mediterranean countries, due to the low temperatures observed during the early stages of the vegetative phase (between November and February), the main growth stage of the plants occurs between March and May. The rainfall distribution during the vegetative cycle of Lupinus is recognized as having an important role on the Lupinus seed yield (Perdigão et al., 2021) as verified in the present work.
The seeds’ chemical composition was essentially affected by Lupinus cultivars, except for ash, which was significantly affected by the genotype × environment interaction, as also reported by Calabrò et al. (2015). Higher crude protein levels were observed for L. luteus cultivars (42.7 g 100 g−1 DM –44.4 g 100 g−1 DM) and L. albus cv. Dulce (42.6 g 100 g−1 DM), followed by L. albus cv. Estoril (37.8 g 100 g−1 DM), and L. angustifolius cv. Tango (32.2 g 100 g−1 DM). These values were higher than those reported by Gresta et al. (2010) for L. luteus, but within the values found by other authors (Yu et al., 2002; Beyer et al., 2015). The ether extract values were the highest and neutral detergent fiber the lowest for L. albus cultivars Dulce and Estoril, and the values were within the range reported for this species (Calabrò et al., 2015).
4.2 Effects of cultivar and edaphoclimatic conditions on the seed alkaloid content
A high variability of the total alkaloid content was observed with a significant effect of the genotype × environment, similar results were also reported by Calabrò et al. (2015) for L. albus cultivars and by Beyer et al. (2015) for L. angustifolius. The increased accumulation of alkaloids in lupins seeds has been associated with edaphoclimatic variables. Jansen et al. (2009) observed a 3.7-fold increase in the total alkaloids in L. angustifolius for an increase of about 3°C in the daily mean temperature, that is, a small increase in ambient temperature can cause a drastic increase in the production of alkaloids. Moreover, drought stress can be also responsible for the production of higher levels of alkaloids in seeds (Christiansen et al., 1997). The soil characteristics, such as pH, the elemental composition, and the type and amount of fertilizer used, can also impact alkaloid production in Lupinus plants.
In our study, total alkaloid content increased in year 2 for Cardiga and Acos L. luteus cultivars, with no differences between environments for the other cultivars, suggesting a different reaction of these cultivars to environmental conditions. L. luteus cv. Cardiga and Acos total alkaloid content has shown higher variability between environments than the other cultivars, which was confirmed by the lowest stability indexes for these genotypes across environments, thus suggesting that alkaloid accumulation in the seeds in these cultivars is dependent on the edaphoclimatic conditions. No explanation for the differences between these genotypes and the remaining in the study was found elsewhere, but it can be supposed that these cultivars show a distinct response to abiotic stress conditions caused by different profiles of temperature and rainfall during the different stages of the plant development. The environmental variables analysis by RDA showed that the mean minimum temperature in year 1 for both sowing locations had some impact on the reduced production of alkaloids in these environments. Considering the safety limit of 200 mg kg−1, which is accepted by many health authorities for the direct use of lupins in feed and food products (Pilegaard and Gry, 2009), only L. luteus cv. Alburquerque and Mister contained less than this value in both sowing years.
Total alkaloid content reflects the content of quinolizidine alkaloids, often known as “lupin alkaloids”, the most relevant ones in the Lupinus genus (Frick et al., 2017), thus deserving more attention in the context of antinutritional compounds in lupins, with most of the scientific publications and regulatory documents describing only this category of alkaloids. This alkaloid class occurs mostly in the Fabaceae family and is responsible for the protection of plants against insect pests (Philippi et al., 2015). The biosynthesis of quinolizidine alkaloids occurs via decarboxylation of L-lysine, forming the major structural compounds lupinine (bicyclic), sparteine, lupanine, and multiflorine (tetracyclic). These alkaloids can be further modified by dehydrogenation, oxygenation, hydroxylation, glycosylation, or esterification forming a wide variety of related quinolizidines (Frick et al., 2017), as those identified in the present work.
The environmental impact on the seed alkaloid content is recognized to be high, affecting the compounds’ biosynthesis and transport from the plant tissues to the seed (Frick et al., 2017). The quinolizidine biosynthesis is regulated by light and water conditions, with production being increased during the day and in conditions of low water. Although the effects of drought in Lupinus seeds are unclear and unpredictable (Christiansen et al., 1997), the increase in the temperature has been reported to have a significant impact on quinolizidine alkaloid production in seeds of narrow-leafed cultivars (Cowling and Tarr, 2004; Jansen et al., 2009; Frick et al., 2017; Frick et al., 2018), although no effect was observed in the present work. The soil characteristics also play a role on quinolizidine alkaloid content in lupin seeds, as lower soil pH and potassium deficiency increases the levels of quinolizidine alkaloids in seeds (Jansen et al., 2012), whereas phosphorous deficiency reduces them (Gremigni et al., 2003).
Indole and piperidine alkaloids are also present in Lupinus seeds along with quinolizidine alkaloids, although often disregarded. A detailed characterization of the structural diversity of alkaloids produced by lupins is pivotal to effectively assessing the full potential of Lupinus seed cultivars, as toxic effects may be expected especially relating to the indole/piperidine/quinolizidine nucleus. This information is important both for selecting the cultivars better adapted to biotic stresses during plant growth and the ones best suited for food and feed purposes. The high levels of quinolizidine alkaloids in Lupinus seeds gives them a bitter taste and this is a health concern due to their high toxicity (Schrenk et al., 2019). In this sense, most of the studied lupin cultivars should be processed for use in food purposes through specific debittering processes (between 89% and 97% of quinolizidine alkaloids present in seeds are removed by water treatment and boiling), and its inclusion level must be low when used in animal feeds (Schrenk et al., 2019). The only exceptions were the yellow lupin cultivars Alburquerque and Mister. The Lupinus species can be distinguished based on their individual alkaloid profile, agreeing with previously described alkaloid profiles for each species (Wink et al., 1995; Boschin et al., 2008; Calabrò et al., 2015; Magalhães et al., 2017; Osorio et al., 2018; Święcicki et al., 2019). Tricyclic quinolizidines were characteristic of L. albus and L. angustifolius, while angustifoline was the main alkaloid present in L. angustifolius cv. Tango, with it being detected at more than twice the content of that recorded in L. albus, with 11,12-seco-12,13-didehydromultiflorine only being detected in the L. albus species. Although 11,12-seco-12,13-didehydromultiflorine is structurally a tricyclic compound, its occurrence in plants is related to its precursor multiflorine, a tetracyclic quinolizidine, also only quantified in the L. albus samples. Wink et al. (1995) reported that these tri- and tetracyclic quinolizidine alkaloids have a restricted distribution over the Lupinus species, with L. albus being one of the species in which these alkaloids are produced. The occurrence of lupanine and related alkaloids (α-iso-lupanine, 13α-hydroxylupanine, and 13-α-angelolyoxylupanine) were essentially limited to L. albus and L. angustifolius, the profile varied between the species. Lupanine is considered to be one of the most toxic alkaloids to humans and animals (Schrenk et al., 2019), and has a large impact on aphid survival (Ridsdill-Smith et al., 2001).
The presence and formation of smipine, a piperidine alkaloid, in lupins is poorly reported. Wink et al. (1995) reported the identification of this alkaloid in the Lupinus genus; however, no results of its quantification were shown. Magalhães et al. (2017) determined low levels of this alkaloid in some L. albus cultivars (10 mg kg−1 DM–30 mg kg−1 DM), but not in L. angustifolius or L. luteus. In the present work, smipine was quantified in L. albus cv. Dulce and Estoril. Interestingly, the information about smipine occurrence in plants is very scarce; it is described to be present in Lupinus formosus (Fitch et al., 1974), the desert plant Haloxylon salicornicum (El-Shazly et al., 2005), and as one of the major alkaloids of the genus Dichilus (Van Wyk et al., 1988).
Lupinine, the most abundant alkaloid in L. luteus (Wink et al., 1995; Święcicki et al., 2019), was quantified in the range 14.1 mg kg−1 DM to 1,531 mg kg−1 DM, with the Acos and Cardiga cultivars being those with the highest contents. The strong insecticidal activity of lupinine has been reported (Campbell et al., 1933). Sparteine, which is two or three times more toxic to animals than lupanine (Schrenk et al., 2019), was produced at levels between 9% and 80% of the total alkaloid content in L. luteus cultivars (3.23 mg kg−1 DM–331 mg kg−1 DM). Sparteine toxicity to humans is largely studied due to its use in the past as an antiarrhythmic and oxytocic drug (Schrenk et al., 2019).
Gramine, rare in the Lupinus species, was quantified in L. luteus bitter cultivars (with high total alkaloid levels) Acos (51.6 mg kg−1 DM–53.8 mg kg−1 DM) and Cardiga (14.5 mg kg−1 DM–596 mg kg−1 DM). Besides, a high increase on the seeds' gramine content was observed in year 2 when compared to year 1 suggesting a high influence of the edaphoclimatic conditions on the production of this alkaloid. Similarly, gramine has been reported to occur in L. luteus, and not in L. albus or L. angustifolius (Magalhães et al., 2017; Święcicki et al., 2019). Indeed, only some L. luteus cultivars are known to produce gramine (Osorio et al., 2018), and earlier studies have shown that the introduction and cultivation of L. luteus throughout Central Europe resulted in a considerable decrease of the gramine content, as opposed to the wild forms of this species (Święcicki and Jach, 1980). From the nutritional perspective, the high levels of this alkaloid in Acos and Cardiga cultivars is not desirable, yet it can be important in plant defense mechanisms, as gramine was found to be one of the most toxic compounds to aphids, after lupanine (Ridsdill-Smith et al., 2001).
Ammodendrine was present in the three lupin species, ranging in concentration from 0.3% to 7.4% of the total alkaloid content. The high levels of ammodendrine found may preclude the direct use of the white, narrow-leafed, and yellow lupins cultivars studied for food and feed purposes, as this alkaloid is suspected to be a teratogen for both humans and livestock (Green et al., 2012).
The great discrepancy in the alkaloid content among cultivars, and also among years, either total and by chemical class, may indicate cross-pollination with spontaneous lupins increasing alkaloid levels in some cultivars (Boschin and Resta, 2013). In fact, the ancestors of L. luteus (and L. angustifolius) originated in the Iberian Peninsula and, although the origin of L. albus is not known, semi-wild cultivars of these species have been cultivated throughout the entire Mediterranean region over a long period of time (Gustafsson and Gadd, 1965). The levels of alkaloids dropped considerably during lupins’ domestication, but in the wild populations alkaloids can reach very high levels of concentration (Gustafsson and Gadd, 1965; Otterbach et al., 2019). For instance, up to 12% of the seed DM of quinolizidine alkaloids were found in wild populations of L. albus and 1.5% of total alkaloids (including gramine) were described in a semi-domesticated Spanish accession of L. luteus (Osorio et al., 2018). Also, many papers do not report piperidine alkaloids. Although most Lupinus species produce trace levels of these alkaloids (Wink et al., 1995), the present study suggests that this class may contribute for the observed differences among the cultivars.
5 Conclusions
The productivity and alkaloid content of Lupinus seeds showed to be affected by the environment, with a distinct impact in different cultivars. Lupinus angustifolius cv. Tango, L. luteus cv. Acos, and L. luteus cv. Alburquerque were the most stable cultivars across the environments in terms of seed and protein production, in opposition to L. albus cv. Estoril, the most productive cultivar but with the least stability. The productivity was mainly affected by the total rainfall during the spring months, which correspond to the vegetative phase of the plant; higher rainfall in the second year of the trial at both locations resulted in higher seed and protein production. For the total alkaloid content, L. luteus cv. Alburquerque and L. luteus cv. Mister were the genotypes with the highest stability and the lowest alkaloid content. Lupinus luteus cv. Cardiga seeds were those with the highest alkaloids levels and the lowest stability across environments. The increased accumulation of alkaloids in L. albus cv. Dulce, L. albus cv. Estoril, L. angustifolius cv. Tango, and L. luteus cv. Mister seeds in the first year of the study could be partially attributed to the higher minimum temperatures observed in those environments. Still, this finding should be carefully studied as it is postulated that the high levels of genetic variability of these cultivars can lead to different metabolic responses in the plants and, consequently, different responses to abiotic stress through alkaloid biosynthesis.
The high total alkaloid content of most of the cultivars studied limits its consumption as raw food, as a safety limit of 200 mg kg−1 in seeds for human consumption is recommended by several health authorities. In this context, only L. luteus cv. Alburquerque and Mister presented alkaloid values below this limit, implying that the other cultivars should not be consumed before alkaloid removal. Due to the high alkaloid content of some of the studied cultivars conferring resistance to pests, they can be particularly important for recovering poor or exhausted soils due to their ability to grow in highly infertile, neutral to acidic soils, thus enriching them in nitrogen and mobilizing phosphorus that can be used by non-legumes in crop rotations.
Data availability statement
The raw data supporting the conclusions of this article will be made available by the authors, without undue reservation.
Author contributions
IV, CS, MA, and MM conducted the analytical work. MA, VP, CM, and SG-S conducted the field study and sample collection. LF and CG were involved in the proximate composition analysis. AF and HT conceived and designed the experimental study. IV and CS analyzed the data. IV, MM, AC, and AF performed the statistical analysis. IV wrote the first draft of the manuscript. HT conceived and designed the field study. All authors contributed to the article and approved the submitted version.
Funding
The author(s) declare financial support was received for the research, authorship, and/or publication of this article. This work received financial support from Ministério para a Ciência e Tecnologia – FCT Fundação para a Ciência e a Tecnologia through national funds to the project UIDP/50006/2020.
Acknowledgments
The authors are grateful for the seeds made available by the Centro de Investigaciones Científicas y Tecnológicas de Extremadura (Spain), Fertiprado (Portugal), and the Instituto Nacional de Investigação Agrária e Veterinária (INIAV, Portugal). The authors would like to thank Ana Sofia Soares, Áurea Queirós, Rui Costa, Mário Sérgio and Professor Carlos Castro for their technical assistance during the field experiments, and Professor Vasco Cadavez for the assistance with the statistical analyses. The field research was supported by Project I&D INTERACT - Integrative Research in Environment, Agro-Chain and Technology though the grants of M. Almeida, C. Miranda and S. Garcia-Santos (BI/UTAD/INTERACT/ISAC/196/2016, BPD/UTAD/INTERACT/ISAC/209/2016 and BPD/UTAD/INTERACT/ISAC/198/2016, respectively), NORTE-01-0145-FEDER-000017, included at the research line entitled Innovation for Sustainable Agro-food Chains – ISAC, co-financed by the European Regional Development Fund (ERDF) through NORTE 2020 (Regional Operational Programme North 2014–2020). The research was also supported by AgriFood XXI - Development and consolidation of research in the agrifood sector in Northern Portugal I&D&I project (NORTE-01-0145-FEDER-000041) cofinanced by European Regional Development Fund (ERDF), through the NORTE 2020 (Programa Operacional Regional do Norte 2014/2020), including the contract of C. Sousa. The work was also supported by National Funds by FCT - Portuguese Foundation for Science and Technology through the projects UIDB/04033/2020, UIDB/50006/2020, UIDB/00772/2020, and the contracts of I.M. Valente and M.R.G. Maia through program DL 57/2016 – Norma transitória (Refs. SFRH/BPD/111181/2015 and SFRH/BPD/70176/2010).
Conflict of interest
The authors declare that the research was conducted in the absence of any commercial or financial relationships that could be construed as a potential conflict of interest.
Publisher’s note
All claims expressed in this article are solely those of the authors and do not necessarily represent those of their affiliated organizations, or those of the publisher, the editors and the reviewers. Any product that may be evaluated in this article, or claim that may be made by its manufacturer, is not guaranteed or endorsed by the publisher.
Supplementary material
The Supplementary Material for this article can be found online at: https://www.frontiersin.org/articles/10.3389/fpls.2023.1231777/full#supplementary-material
References
Święcicki, W., Czepiel, K., Wilczura, P., Barzyk, P., Kaczmarek, Z., Kroc, M. (2019). Chromatographic fingerprinting of the old world lupins seed alkaloids: A supplemental tool in species discrimination. Plants (Basel) 8, 548. doi: 10.3390/plants8120548
Święcicki, W., Jach, K. (1980). Variation and evolution of alkaloid complex in yellow lupine (Lupinus luteus L.) during domestication. Acta Agrobot. 33, 177–195. doi: 10.5586/aa.1980.015
Adhikari, K. N., Edwards, O. R., Wang, S., Ridsdill-Smith, T. J., Buirchell, B. (2012). The role of alkaloids in conferring aphid resistance in yellow lupin (Lupinus luteus L.). Crop Pasture Sci. 63, 444–451. doi: 10.1071/CP12189
AOAC. (2000). Official methods of analysis. 17th ed. Gaithersburg, MD, USA: Association of Official Analytical Chemists.
Berger, J. D., Ludwig, C. (2014). Contrasting adaptive strategies to terminal drought-stress gradients in Mediterranean legumes: Phenology, productivity, and water relations in wild and domesticated Lupinus luteus L. J. Exp. Bot. 65, 6219–6229. doi: 10.1093/jxb/eru006
Beyer, H., Schmalenberg, A. K., Jansen, G., Jürgens, H. U., Uptmoor, R., Broer, I., et al. (2015). Evaluation of variability, heritability and environmental stability of seed quality and yield parameters of L. angustifolius. Field Crops Res. 174, 40–47. doi: 10.1016/j.fcr.2014.12.009
Boschin, G., Annicchiarico, P., Resta, D., D'agostina, A., Arnoldi, A. (2008). Quinolizidine alkaloids in seeds of lupin genotypes of different origins. J. Agric. Food Chem. 56, 3657–3663. doi: 10.1021/jf7037218
Boschin, G., Resta, D. (2013). “Alkaloids Derived from Lysine: Quinolizidine (a Focus on Lupin Alkaloids),” in Natural Products: Phytochemistry, Botany and Metabolism of Alkaloids, Phenolics and Terpenes. Eds. Ramawat, K. G., Mérillon, J.-M. (Berlin, Heidelberg: Springer Berlin Heidelberg), 381–403.
Calabrò, S., Cutrignelli, M. I., Lo Presti, V., Tudisco, R., Chiofalo, V., Grossi, M., et al. (2015). Characterization and effect of year of harvest on the nutritional properties of three varieties of white lupine (Lupinus albus L.). J. Sci. Food Agric. 95, 3127–3136. doi: 10.1002/jsfa.7049
Campbell, F. L., Sullivan, W. N., Smith, C. R. (1933). The relative toxicity of nicotine, anabasine, methyl anabasine, and lupinine for culicine mosquito larvae. J. Econ. Entomol. 26, 500–509. doi: 10.1093/jee/26.2.500
Chiofalo, B., Presti, V. L., Chiofalo, V., Gresta, F. (2012). The productive traits, fatty acid profile and nutritional indices of three lupin (Lupinus spp.) species cultivated in a Mediterranean environment for the livestock. Anim. Feed Sci. Technol. 171, 230–239. doi: 10.1016/j.anifeedsci.2011.11.005
Christiansen, J. L., Jørnsgård, B., Buskov, S., Olsen, C. E. (1997). Effect of drought stress on content and composition of seed alkaloids in narrow-leafed lupin, Lupinus angustifolius L. Eur. J. Agron. 7, 307–314. doi: 10.1016/S1161-0301(97)00017-8
Cowling, W., Tarr, A. (2004). Effect of genotype and environment on seed quality in sweet narrow-leafed lupin(Lupinus angustifolius L.). Aust. J. Agric. Res. 55, 745–751. doi: 10.1071/AR03223
El-Shazly, A. M., Dora, G., Wink, M. (2005). Alkaloids of Haloxylon salicornicum (Moq.) Bunge ex Boiss. (Chenopodiaceae). Pharmazie 60, 949–952. doi: 10.1002/chin.200616192
European Commission (2018). Report from the commission to the council and the European Parliament on the development of plant proteins in the European Union (Brussels, Belgium: European Commission).
Ferreira, H., Pinto, E., Vasconcelos, M. W. (2021). Legumes as a cornerstone of the transition toward more sustainable agri-food systems and diets in Europe. Front. Sustain. Food Syst. 5. doi: 10.3389/fsufs.2021.694121
Fitch, W. L., Dolinger, P. M., Djerassi, C. (1974). Alkaloid studies. LXVIII. Novel piperidyl alkaloids from lupinus formosus. J. Organ. Chem. 39, 2974–2979. doi: 10.1021/jo00934a008
Fraser, M. D., Fychan, R., Jones, R. (2005). Comparative yield and chemical composition of two varieties of narrow-leafed lupin (Lupinus angustifolius) when harvested as whole-crop, moist grain and dry grain. Anim. Feed Sci. Technol. 120, 43–50. doi: 10.1016/j.anifeedsci.2004.12.014
French, R. J., French, R. J., Sweetingham, M. W., Sweetingham, M. W., Shea, G. G., Shea, G. G. (2001). A comparison of the adaptation of yellow lupin (Lupinus luteus L.) and narrow-leafed lupin (L. angustifolius L.) to acid sandplain soils in low rainfall agricultural areas of Western Australia. Aust. J. Agric. Res. 52, 945–954. doi: 10.1071/AR00084
Frick, K. M., Foley, R. C., Kamphuis, L. G., Siddique, K. H. M., Garg, G., Singh, K. B. (2018). Characterization of the genetic factors affecting quinolizidine alkaloid biosynthesis and its response to abiotic stress in narrow-leafed lupin (Lupinus angustifolius L.). Plant Cell Environ. 41, 2155–2168. doi: 10.1111/pce.13172
Frick, K. M., Kamphuis, L. G., Siddique, K. H., Singh, K. B., Foley, R. C. (2017). Quinolizidine alkaloid biosynthesis in lupins and prospects for grain quality improvement. Front. Plant Sci. 8, 87. doi: 10.3389/fpls.2017.00087
Grażyna, S., Agnieszka, F., Katarzyna, P., Jerzy, S., Wiesław, K. (2017). The productivity of two yellow lupine (Lupinus luteus L.) cultivars as an effect of different farming systems. Plant Soil Environ. 63, 552–557. doi: 10.17221/639/2017-PSE
Green, B. T., Lee, S. T., Panter, K. E., Brown, D. R. (2012). Piperidine alkaloids: human and food animal teratogens. Food Chem. Toxicol. 50, 2049–2055. doi: 10.1016/j.fct.2012.03.049
Gremigni, P., Hamblin, J., Harris, D., Cowling, W. A. (2003). The interaction of phosphorus and potassium with seed alkaloid concentrations, yield and mineral content in narrow-leafed lupin (Lupinus angustifolius L.). Plant Soil 253, 413–427. doi: 10.1023/A:1024828131581
Gresta, F., Abbate, V., Avola, G., Magazzù, G., Chiofalo, B. (2010). Lupin seed for the crop-livestock food chain. Ital. J. Agron. 5, 333–340. doi: 10.4081/ija.2010.333
Gustafsson, A. R. E., Gadd, I. (1965). Mutations and crop improvement. II. The genus Lupinus (Leguminosae). Hereditas 53, 15–39. doi: 10.1111/j.1601-5223.1965.tb01977.x
Jansen, G., Jürgens, H.-U., Ordon, F. (2009). Effects of temperature on the alkaloid content of seeds of Lupinus angustifolius cultivars. J. Agron. Crop Sci. 195, 172–177. doi: 10.1111/j.1439-037X.2008.00356.x
Jansen, G., Jürgens, H.-U., Schliephake, E., Ordon, F. (2012). Effect of the Soil pH on the Alkaloid Content of Lupinus angustifolius. Int. J. Agron. 2012, 269878. doi: 10.1155/2012/269878
Jensen, G. H., Elleby, C., Dominguez, P. I. (2021). Reducing the European Union's plant protein deficit: Options and impacts. Agric. Econ. 67, 391–398. doi: 10.17221/94/2021-AGRICECON
Jul, L. B., Flengmark, P., Gylling, M., Itenov, K. (2003). Lupin seed (Lupinus albus and Lupinus luteus) as a protein source for fermentation use. Ind. Crops Prod. 18, 199–211. doi: 10.1016/S0926-6690(02)00076-6
Khatun, M., Sarkar, S., Era, F. M., Islam, A. K. M. M., Anwar, M. P., Fahad, S., et al. (2021). Drought stress in grain legumes: Effects, tolerance mechanisms and management. Agronomy 11, 2374. doi: 10.3390/agronomy11122374
Lema, M., Soengas, P. (2023). Identification of promising accessions of wild Lupinus hispanicus Boiss. et Reut. from northwestern Spain. Genet. Resour. Crop Evol. 70, 677–690. doi: 10.1007/s10722-022-01531-2
Lucas, M. M., Stoddard, F. L., Annicchiarico, P., Frías, J., Martínez-Villaluenga, C., Sussmann, D., et al. (2015). The future of lupin as a protein crop in Europe. Front. Plant Sci. 6, 705. doi: 10.3389/fpls.2015.00705
MacWilliam, S., Wismer, M., Kulshreshtha, S. (2014). Life cycle and economic assessment of Western Canadian pulse systems: The inclusion of pulses in crop rotations. Agric. Syst. 123, 43–53. doi: 10.1016/j.agsy.2013.08.009
Magalhães, S. C. Q., Fernandes, F., Cabrita, A. R. J., Fonseca, A. J. M., Valentão, P., Andrade, P. B. (2017). Alkaloids in the valorization of European Lupinus spp. seeds crop. Ind. Crops Prod. 95, 286–295. doi: 10.1016/j.indcrop.2016.10.033
Maknickiene, Z. (2001). Effect of genotype on seed yield in lupine Lupinus luteus L., Lupinus angustifolius L. and resistance to fungal diseases (Colletotrichtum lindemuthianum Br. et Cav., Fusarium oxysporum Schl.). Biologija 3, 27–29.
Mancinotti, D., Frick, K. M., Geu-Flores, F. (2022). Biosynthesis of quinolizidine alkaloids in lupins: mechanistic considerations and prospects for pathway elucidation. Natural Prod. Rep. 39, 1423–1437. doi: 10.1039/D1NP00069A
Monteiro, A., Miranda, C., Trindade, H. (2021). Mediterranean lupines as an alternative protein source to soybean. Biol. Life. Sci. Forum 3, 38. doi: 10.3390/IECAG2021-09911
Musco, N., Cutrignelli, M. I., Calabrò, S., Tudisco, R., Infascelli, F., Grazioli, R., et al. (2017). Comparison of nutritional and antinutritional traits among different species (Lupinus albus L., Lupinus luteus L., Lupinus angustifolius L.) and varieties of lupin seeds. J. Anim. Physiol. Anim. Nutr. 101, 1227–1241. doi: 10.1111/jpn.12643
NIST. (2001) NIST Chemistry WebBook. Available at: https://webbook.nist.gov/chemistry/ [Accessed March 5, 2023].
Osorio, C. E., Amiard, V. S. E., Aravena-Calvo, J., Udall, J. A., Doyle, J. J., Maureira-Butler, I. J. (2018). Chromatographic fingerprinting of Lupinus luteus L. (Leguminosae) main secondary metabolites: a case of domestication affecting crop variability. Genet. Resour. Crop Evol. 65, 1281–1291. doi: 10.1007/s10722-018-0613-x
Otterbach, S. L., Yang, T., Kato, L., Janfelt, C., Geu-Flores, F. (2019). Quinolizidine alkaloids are transported to seeds of bitter narrow-leafed lupin. J. Exp. Bot. 70, 5799–5808. doi: 10.1093/jxb/erz334
Palta, J. A., Turner, N. C., French, R. J. (2004). The yield performance of lupin genotypes under terminal drought in a Mediterranean-type environment. Aust. J. Agric. Res. 55, 449–459. doi: 10.1071/AR03135
Perdigão, A., Pereira, J. L. S., Moreira, N., Trindade, H., Coutinho, J. (2021). A 3-year field study to assess winter cover crops as nitrogen sources for an organic maize crop in Mediterranean Portugal. Eur. J. Agron. 128, 126302. doi: 10.1016/j.eja.2021.126302
Philippi, J., Schliephake, E., Jürgens, H.-U., Jansen, G., Ordon, F. (2015). Feeding behavior of aphids on narrow-leafed lupin (Lupinus angustifolius) genotypes varying in the content of quinolizidine alkaloids. Entomol. Experiment. Applicata 156, 37–51. doi: 10.1111/eea.12313
Pilegaard, K., Gry, J. (2009). Alkaloids in edible lupin seeds : A toxicological review and recommendations (Copenhagen: Nordic Council of Ministers).
Quiñones, M. A., Lucas, M. M., Pueyo, J. J. (2022). Adaptive mechanisms make lupin a choice crop for acidic soils affected by aluminum toxicity. Front. Plant Sci. 12, 810692. doi: 10.3389/fpls.2021.810692
Ridsdill-Smith, T., Edwards, O. R., Shaofang, W., Ghisalberti, E., Reidy-Crofts, J. (2001). “Aphid response to plant defensive compounds in lupins,” in Proceedings of the Sixth International Symposium on Aphid spp (Paris: INRA), 491–498.
Robertson, J. B., Van Soest, P. J. (1981). “The detergent system of analysis,” in The analysis of dietary fiber in food. Eds James, W. P. T., Theander, O. (New York, USA: Marcel Dekker Inc.), 123–158.
Sato, H., Uchiyama, M., Saito, K., Yamazaki, M. (2018). The energetic viability of Δ1-piperideine dimerization in lysine-derived alkaloid biosynthesis. Metabolites 8, 48. doi: 10.3390/metabo8030048
Savita, Tomer, A., Singh, S. K. (2020). “Drought stress tolerance in legume crops,” in Agronomic Crops: Stress Responses and Tolerance. Ed. Hasanuzzaman, M. (Singapore: Springer Singapore), 149–155.
Schrenk, D., Bodin, L., Chipman, J. K., Del Mazo, J., Grasl-Kraupp, B., Hogstrand, C., et al. (2019). Scientific opinion on the risks for animal and human health related to the presence of quinolizidine alkaloids in feed and food, in particular in lupins and lupin-derived products. EFSA J. 17, e05860. doi: 10.2903/j.efsa.2019.5860
Shukla, G. K. (1972). Some statistical aspects of partitioning genotype-environmental components of variability. Heredity 29, 237–245. doi: 10.1038/hdy.1972.87
Van Wyk, B. E., Verdoorn, G. H., Schutte, A. L. (1988). Chemotaxonomic value of alkaloids in the genus Dichilus. Biochem. System. Ecol. 16, 471–474. doi: 10.1016/0305-1978(88)90046-4
Watson, C. A., Reckling, M., Preissel, S., Bachinger, J., Bergkvist, G., Kuhlman, T., et al. (2017). “Grain legume production and use in European agricultural systems,” in Advances in Agronomy. Ed. Sparks, D. L. (Academic Press), 235–303.
Wink, M., Meißner, C., Witte, L. (1995). Patterns of quinolizidine alkaloids in 56 species of the genus Lupinus. Phytochemistry 38, 139–153. doi: 10.1016/0031-9422(95)91890-D
Wink, M., Mohamed, G. I. A. (2003). Evolution of chemical defense traits in the Leguminosae: mapping of distribution patterns of secondary metabolites on a molecular phylogeny inferred from nucleotide sequences of the rbcL gene. Biochem. System. Ecol. 31, 897–917. doi: 10.1016/S0305-1978(03)00085-1
Wricke, G. (1962). Uber eine methode zur erfassung der okologischen streubreite in feldversuchen. Z. Pflanzenzuecht 47, 92–96.
Wricke, G. (1964). Zur berechnung der ökovalenz bei sommerweizen und hafer. Z. für Pflanzenzüchtung 52, 127–138.
Keywords: crop valorization, Fabaceae, narrow leaf lupin, yellow lupin, white lupin
Citation: Valente IM, Sousa C, Almeida M, Miranda C, Pinheiro V, Garcia-Santos S, Ferreira LMM, Guedes CM, Maia MRG, Cabrita ARJ, Fonseca AJM and Trindade H (2023) Insights from the yield, protein production, and detailed alkaloid composition of white (Lupinus albus), narrow-leafed (Lupinus angustifolius), and yellow (Lupinus luteus) lupin cultivars in the Mediterranean region. Front. Plant Sci. 14:1231777. doi: 10.3389/fpls.2023.1231777
Received: 31 May 2023; Accepted: 22 November 2023;
Published: 15 December 2023.
Edited by:
Roberto Papa, Marche Polytechnic University, ItalyReviewed by:
Karolina Susek, Polish Academy of Sciences, PolandLorenzo Raggi, University of Perugia, Italy
Copyright © 2023 Valente, Sousa, Almeida, Miranda, Pinheiro, Garcia-Santos, Ferreira, Guedes, Maia, Cabrita, Fonseca and Trindade. This is an open-access article distributed under the terms of the Creative Commons Attribution License (CC BY). The use, distribution or reproduction in other forums is permitted, provided the original author(s) and the copyright owner(s) are credited and that the original publication in this journal is cited, in accordance with accepted academic practice. No use, distribution or reproduction is permitted which does not comply with these terms.
*Correspondence: Inês M. Valente, aW5lcy52YWxlbnRlQGZjLnVwLnB0
†Present address: Carla Miranda, Associated Laboratory for Green Chemistry (LAQV-REQUIMTE), University NOVA of Lisbon, Caparica, Lisbon, Portugal
Toxicology Research Unit (TOXRUN), University Institute of Health Sciences (IUCS), CESPU, CRL, Gandra, Portugal