- 1Key Laboratory of Crop Genetics and Physiology of Jiangsu Province, Key Laboratory of Crop Cultivation and Physiology of Jiangsu Province, College of Agriculture, Yangzhou University, Yangzhou, China
- 2Key Laboratory of Crop Water Use and Regulation, Farmland Irrigation Research Institute, Chinese Academy of Agricultural Sciences, Ministry of Agriculture and Rural Affairs, Xinxiang, Henan, China
- 3Department of Botany, University of Lakki Marwat, Lakki Marwat, Pakistan
- 4Department of Land and Water Management, Faculty of Agricultural Engineering, Sindh Agricultural University, Tandojam, Pakistan
Introduction: Agricultural activities in the North China Plain are often challenged by inadequate irrigation and nutrient supply. Inadequate and improper resource utilization may impose negative impacts on agricultural sustainability. To counteract the negative impacts, a deeper understanding of the different resource management strategies is an essential prerequisite to assess the resource saving potential of crops.
Methods: We explored plausible adaptation strategies including drip irrigation lateral spacings of 40 and 80 cm (hereafter referred to as LS40 and LS80, respectively), irrigating winter wheat after soil water consumption of 20 and 35 mm (hereafter represented as IS20 and IS35, respectively), and nitrogen fertilization scheme of a) applying 50% nitrogen as a basal dose and 50% as a top-dressing dose (NS50:50), b) 25% nitrogen as a basal dose and 75% as a topdressing dose (NS25:75), and c) no nitrogen application as a basal dose and 100% application as a top-dressing dose (NS0:100).
Results and discussion: The consecutive 2 years (2017–2018 and 2018–2019) of field study results show that growing winter wheat under LS40 enhanced the water use efficiency (WUE), grain yield, 1,000-grain weight, and number of grains per spike by 15.04%, 6.95%, 5.67%, and 21.59% during the 2017–2018 season, respectively. Additionally, the corresponding values during the 2018–2019 season were 12.70%, 7.17%, 2.66%, and 19.25%, respectively. Irrigation scheduling of IS35 treatment improved all the growth-related and yield parameters of winter wheat. Further, treating 25% nitrogen as a basal dose and application of 75% as a top-dressing dose positively influenced the winter wheat yield. While NS0:100 increased the plant height, leaf area index (LAI), and aboveground biomass as compared to the other application strategies, but high nitrogen was observed in deeper soil layers. Regarding soil environment, the lowest soil moisture and nitrate nitrogen contents were observed in LS80 during both growing seasons. Overall, coupling the IS35 with NS25:75 under 40-cm lateral spacing is a suitable choice for sustainable winter wheat production in theNorth China Plain. The results of our study may be helpful in advancing the knowledge of the farmer community for winter wheat production. The findings can also aid in advancing new insights among scientists working on soil water and nitrogen distribution in drip irrigation for better productivity.
1 Introduction
The burgeoning population demand for more supply of staple food, energy, and water is a serious challenge. This challenge is more chronic in regions where water resources are less and food demand is high. Wheat (Triticum aestivum L.) is a chief food source having a share of 20% in global calorific consumption by the world’s population (Liu et al., 2020a; Liu et al., 2020b). According to The Food and Agriculture Organization of the United Nations (FAO) (2020), China is a leading wheat producer in the world with 134.2 million tons of production on an area of 23.3 million hectares in 2020. Additionally, the North China Plain (NCP) produces approximately 50%–61% of China’s total wheat production (Wang et al., 2012). Sustainable water management is a big challenge in NCP, especially where 70% of freshwater is being exploited for agricultural activities and the groundwater table is dropping at a rate of approximately 1 m/year (Du et al., 2015; Yang et al., 2017). However, water scarcity due to low precipitation and drought especially during the winter wheat growth period is a major reason to limit the wheat yield in NCP (Si et al., 2020). Water deficiency negatively affects crop productivity, as drought condition leads to many physiological and biochemical reactions in plants (Jiang et al., 2020; Sidhu et al., 2021). Therefore, water-saving practices that give sustainable crop productivity and improve water use efficiency (WUE) should be developed.
Drip irrigation systems that have high performance are encouraged to tackle water scarcity challenges and for high water productivity as opposed to conventional irrigation methods. Previously, drip irrigation has been applied to low-density crops like cotton and corn and in fruit production. Currently, the application of drip irrigation in cereals was gradually adopted to resolve the water shortage issues in arid and semi-arid climates (Dar et al., 2017; Mostafa et al., 2018). Many scientists have reported drip irrigation application in wheat crops and found that drip irrigation saves more water compared to conventional irrigation methods. For example, Li et al. (2018) compared the traditional flood irrigation method with drip irrigation and found that wheat plants under drip irrigation have high root density, which improves the water and nutrient absorption in deep soil layers. Increasing irrigation frequency under drip irrigation sustains high moisture, especially in upper soil layers, and enhances crop productivity (Fang et al., 2018). Drip irrigation is conducive to saving irrigation water and in the improvement of dry matter accumulation, crop photosynthesis, and ultimately crop productivity (Cao et al., 2021; Li et al., 2021; Yan et al., 2022). In a comparison of drip irrigation with border irrigation by Yang et al. (2020), it was noticed that drip irrigation decreased soil evaporation, transpiration, and wheat evapotranspiration by 18.5, 22 mm, and 3.5 per year, respectively. Drip irrigation improves the winter wheat yield by 5%–13% and significantly saves irrigation water of approximately 45.9–114.8 mm, which ultimately improves the WUE as compared to the level-basin irrigation method (Wang et al., 2013). Wang et al. (2013) further added that more water productivity can be achieved through drip irrigation by maintaining the soil moisture up to 50%–60% field capacity.
Uniform distribution of water and nutrients around the drip tubes must be followed in order to gain high crop productivity and resource use efficiency. The consistent distribution of moisture under a drip irrigation system is remarkably dependent on the strip width or lateral spacings between the drip irrigation pipes and also on other irrigation strategies such as intervals between irrigation and irrigation levels (Payero et al., 2008; Wang et al., 2013). To date, there are few studies on drip irrigation systems in winter wheat. Thus, more research is required to assess the performance of drip irrigation systems for sustainable agricultural development in NCP.
Fertilizer application, especially nitrogen supply, also directly or indirectly exerts an impact on normal crop growth and productivity (Jia et al., 2014; Wang et al., 2021). Usually, farmers apply nitrogen fertilizer according to their own know-how without worrying about environmental penalties. Lassaletta et al. (2014) stated that immoderate nitrogen application leads to a loss of nitrogen by >50%, which causes environmental contamination. Ju et al. (2009) also stated that denitrification, nitrate nitrogen leaching, and ammonia volatilization cause 15%–45% of the nutrient loss in NCP, which might lead to non-point source groundwater contamination. Inappropriate nitrogen fertilization not only disturbs the environmental compartments but also becomes a factor of high economic inputs. The strategic application of nitrogen to wheat crops may extend the grain-filling stage and enhance the photosynthetic capacity, which results in better productivity (Zhang et al., 2020). Blandino et al. (2015) reported that top dressing of nitrogen can improve wheat productivity as compared to the basal application, while they further added that basal nitrogen application results in nitrogen loss via volatilization. Further, Chen et al. (2018) found that the application of nitrogen in 4:4:2 ratios at the sowing, jointing, and anthesis stages remarkably improved the yield as compared to the 4:6 and 6:4 application ratios. However, there is no clear information about nitrogen application for winter wheat specifically in NCP.
Many studies reported that water and nitrogen management approaches show interactive effects on crop growth and productivity, but these studies were conducted under conventional irrigation systems (Fang et al., 2013; Hamzei, 2018). Fertigation is an advanced technology that saves resources and provides more yield by delivering water and nutrients directly to the root zone (Wolff et al., 2017) and reducing leaching losses (Gärdenäs et al., 2005). Further, nitrogen distribution in the soil varies in drip irrigation as opposed to conventional flooding methods, and nitrogen distribution essentially depends on water movement and application methods (Gasser et al., 2002). Therefore, it is crucial to find out the best irrigation scheduling practice in integration with the nitrogen application scheme on wheat productivity in NCP.
Although extensive work on irrigation scheduling has been performed in this region for better winter wheat production (Jha et al., 2019; Mehmood et al., 2019; Si et al., 2020), to date, efforts have not been made to determine the combined effects of nitrogen application schemes and irrigation scheduling on wheat production under different drip irrigation lateral spacings. Thus, the current field experiment aimed to i) investigate the suitable nitrogen application strategy and irrigation scheduling for better winter wheat productivity, ii) quantify the distribution of soil moisture and nitrogen under different lateral spacings at various irrigation and nitrogen treatments, and iii) reveal the consequences of different irrigation scheduling and nitrogen treatments on growth and yield of wheat and water use efficiency under various lateral spacings.
2 Materials and methods
2.1 Experimental site description
The experiment was conducted in 2017–2018 and 2018–2019 growing seasons of winter wheat at the Experimental Station of Farmland Irrigation Research Institute, Chinese Academy of Agricultural Sciences (CAAS). The experimental site is located in Qiliying (35°08′N, 113°45′E; altitude 81 m) in Xinxiang City of Henan province, PR China. The physicochemical properties of the experimental site are given in Table 1. Soil texture is sandy loam and alkaline (8.5 pH) with 257.6 μs/cm electrical conductivity and is low in organic matter (1.10%). The available nitrogen, phosphorous, and potassium contents in the soil profile (average of 1-m soil depth) are 40.20, 11.90, and 100.51 mg/kg, respectively.
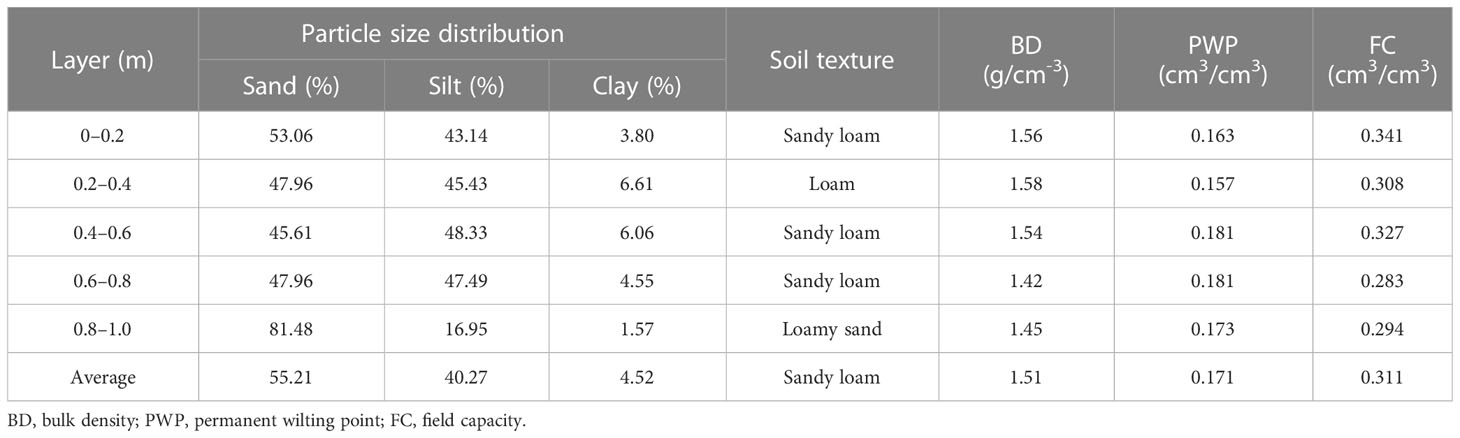
Table 1 Particle size distribution, bulk density, permanent wilting point, and field capacity of the experimental site at 1-m depth.
The weather data including air temperature (both maximum and minimum) and precipitation were collected on a daily basis from a meteorological station located at the experimental site. Generally, the rainfall at the experimental site during the winter wheat growing season varies from 60 to 200 mm, while the average seasonal temperature varies from 10°C to 12°C. However, precipitation during the 2017–2018 and 2018–2019 growing seasons was 238 and 117 mm, respectively, while average air temperature during 2017–2018 was 9.81°C and during 2018–2019 was 9.30°C. The seasonal variations in daily maximum and minimum temperature and precipitation of both growing seasons are given in Figure 1.
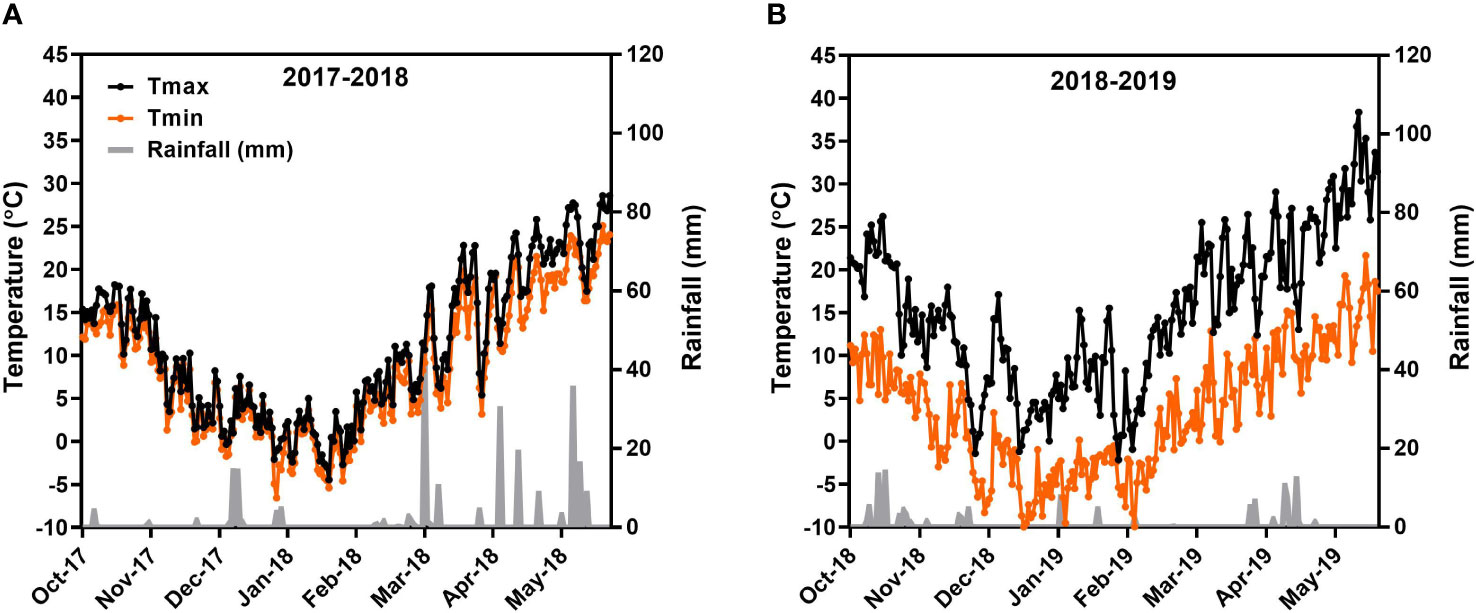
Figure 1 Weather situations during the course of field experiments. Part (A) represents 2017–2018 growing season, and part (B) represents 2018–2019 growing season.
2.2 Experimental set-up and crop management
The experiment had a split–split plot design for 12 treatments (three replications in each treatment) that combined two lateral spacings, irrigation scheduling, and nitrogen application practices. The lateral spacing treatments were included in main plots, while irrigation and nitrogen application were in sub-plots and sub-sub plots, respectively. Two lateral spacings were LS40 and LS80 with lateral tube spacing of 40 and 80 cm, respectively. There were two irrigation scheduling treatments including IS20 and IS35 (i.e., irrigating the crop when soil water consumption reached 20 and 35 mm, respectively). The treatments related to the nitrogen application scheme were NS50:50 (50% of the total as basal dose and the remaining 50% as top-dressing dose), NS25:75 (25% of total nitrogen as basal dose and the remaining 75% as top-dressing dose), and NS0:100 (all of total nitrogen as a top-dressing dose). The basal nitrogen application was broadcasted at bed preparation, while the top-dressing dose was applied by fertigation in three splits at returning green stage, jointing stage, and grain filling stage.
The Zhoumai 22 cultivar of winter wheat was sown on 15 October at 180 kg/ha seed rate and 20-cm row-to-row spacing during both growing seasons. Wang et al. (2017) conducted a literature survey on nitrogen application rate and winter wheat yield in the North China Plain. They found that the application of nitrogen at 247.6 kg/ha would be the best rate for optimum wheat yield. However, keeping in view the soil characteristics, the tested amount of nitrogen fertilizer was 270 kg/ha, which was applied in the field as per experimental treatments. The top dressing of nitrogen fertilizer was performed by fertigation as dissolving urea (46.7% N) in water up to its complete dissolution, and then dissolved urea was added to the fertilizer tank. The phosphorous application rate was 105 kg/ha as calcium superphosphate (14% P2O5), and the potassium application rate was 105 kg/ha as potassium sulfate (50% K2O) at basal. Experimental plots were harvested on 31 May during the 2017–2018 season and on 3 June in the 2018–2019 season.
The irrigation in the field was made through groundwater drawn by underground pipelines. The pipelines were linked to pressure-regulated pumps with pressure controllers to sustain the groundwater pressure level. Further, pipelines were interlinked to polyethylene drip irrigation tubes having a diameter of 16 mm and working operating pressure of 0.1–0.15 MPa. The emitter on drip irrigation tubes was at a 20-cm distance with a discharge rate of 2.2 L/h. In each plot, the water flow meter with valve and pressure gauge was installed to monitor the accurate supply of irrigation water.
Generally, winter wheat in NCP is irrigated on soil moisture status at returning green stage (Gao et al., 2014). By considering the local irrigation practice, the first irrigation in our experiment was carried out at the same time in all experimental treatments. However, further irrigation was performed as per the experimental design. The irrigation amount applied for IS20 and IS35 was 60 and 80 mm, respectively, during the 2017–2018 season, while the corresponding values during 2018–2019 were 100 and 140 mm, respectively. The details of the nitrogen application scheme for both seasons are given in Table 2.
2.3 Data collection
2.3.1 Wheat growth and yield parameters
After returning to the green stage of winter wheat, leaf area index (LAI) and plant height were recorded at 10–15 days of intervals. At each sampling, 10 plants were randomly collected from each plot for winter wheat growth measurement. The leaf area index was recorded as measured by Jha et al. (2019). Ten plots were harvested each time, and a ruler was used to determine the length (L) and width (W) of each leaf. The leaf area per plant (LA) was recorded as per the following equation:
Here, n represents the number of plants to measure leaf area (n = 10), m represents the number of leaves in an ith plant, Ai represents the leaf area of an ith plant, and Lj and Wj depict the length and width (both calculated in cm) of a jth leaf in an ith plant, respectively.
Further, leaf area index was measured as the ratio of leaf area to total land area.
where N is the total number of plants in a row of 1 m and S represents the row spacing fixed to 20 cm in our experiment.
Plant height was observed from 10 plants, and here, an average of their heights was provided. During the initial growth stages, plant height was taken from the ground surface to the top, while further at heading stages, plant height was considered from the ground surface to spikelet top (without awns). Further, to determine the yield components at harvest like the number of grains per spike and spike length, 10 plants were selected in each plot. The 1-m2 area was harvested from each plot to assess 1,000-grain weight (g), number of spikes per unit area, number of grains per spike, aboveground biomass (t/ha), and grain yield (t/ha). The grain yield was determined at 12% of moisture content after natural sun drying.
Wheat plants having uniform growth were collected randomly to measure the plant nitrogen content. Plant parts were separated and bagged in an oven at 105°C for 0.5 h and then dried till constant weight at 80°C. Afterward, the dry weight was measured, and the total nitrogen content was determined by micro-Kjeldahl digestion, distillation, and titration methods (Huang et al., 2008).
2.3.2 Measurement of soil nitrogen, crop evapotranspiration, and water use efficiency
Soil samples were taken to 1-m soil depth after 5 days of irrigation to determine soil moisture and soil nitrate nitrogen (NO3−) contents. Soil samples were excavated horizontally at 0, 10, and 20 cm to the emitter in LS40 while at 0, 20, and 40 cm to the emitter in LS80. Two equal sub-samples were derived from each main sample to determine the soil moisture and nitrate nitrogen contents. The weight of the sub-sample was measured by electronic weight balance and was subjected to an oven for drying at 105°C for 24 h. The NO3− contents were measured by keeping the soil sample with 2 M of KCl (1:10) for 1 h. Then, the NO3− concentration was assessed via AAR Flow Analyzer (AA3-HR; Seal Analytical Inc., Mequon, WI, USA).
Crop evapotranspiration (ETc) was calculated by soil water balance equation as followed by Chen et al. (2015):
Here, P and I represent precipitation and applied irrigation, respectively; U is the capillary rise of groundwater upward; R represents surface runoff; D is the downward flow; ΔS represents the change in soil water storage up to 100-cm soil depth. In our study, surface runoff and downward drainage were neglected owing to low irrigation amount and no heavy rainfall. Further, the upward capillary rise was also considered zero, as the water-storing capacity of the experimental site was more than what restricts upward flow.
Soil water consumption was measured by subtracting the currently measured soil moisture contents from previous moisture content as given in Equation 4.
WUE was calculated according to El-Rahman (2009) by dividing the grain yield by evapotranspiration.
Here, Y represents the grain yield.
2.4 Statistical analysis
The collected data were organized and analyzed in Statistics 8.1 under a split–split plot design. The drip lateral spacings data were kept in the main plot, while the irrigation scheduling treatments and nitrogen application scheme were placed in the sub-plot and sub-sub plot, respectively. To assess the impacts of irrigation scheduling, nitrogen treatments, and lateral spacings on the growth, yield, and WUE of winter wheat, an analysis of variance (ANOVA) was executed. Further, treatment means were compared by using the least significant difference (LSD) at a significance level (p = 0.05) to measure any significant differences.
3 Results
3.1 Effects of LS, IS, and NS on soil environment
3.1.1 Soil moisture
The seasonal variations in soil moisture content during the 2017–2018 and 2018–2019 periods are illustrated in Figures 2 and 3, respectively. Changes in soil moisture content were notably affected due to various treatments. It was found that soil moisture contents were lower at harvest than during that the re-greening stage, as moisture contents start declining, especially after the stem-elongation stage. Due to high rainfall in the 2017–2018 growing season, soil moisture was high during the first growing season as opposed to the 2018–2019 growing season. Overall, the soil moisture was more in LS40 as compared to the LS80 during the whole growing season. Variations in soil moisture content were more prominent in upper (0–20 cm) and deeper (80–100 cm) soil depths. We observed minimum soil moisture between 20 and 60 cm and high soil moisture at the upper and deeper layers. Further, LS40 distributed more moisture contents in deeper soil layers than LS80.
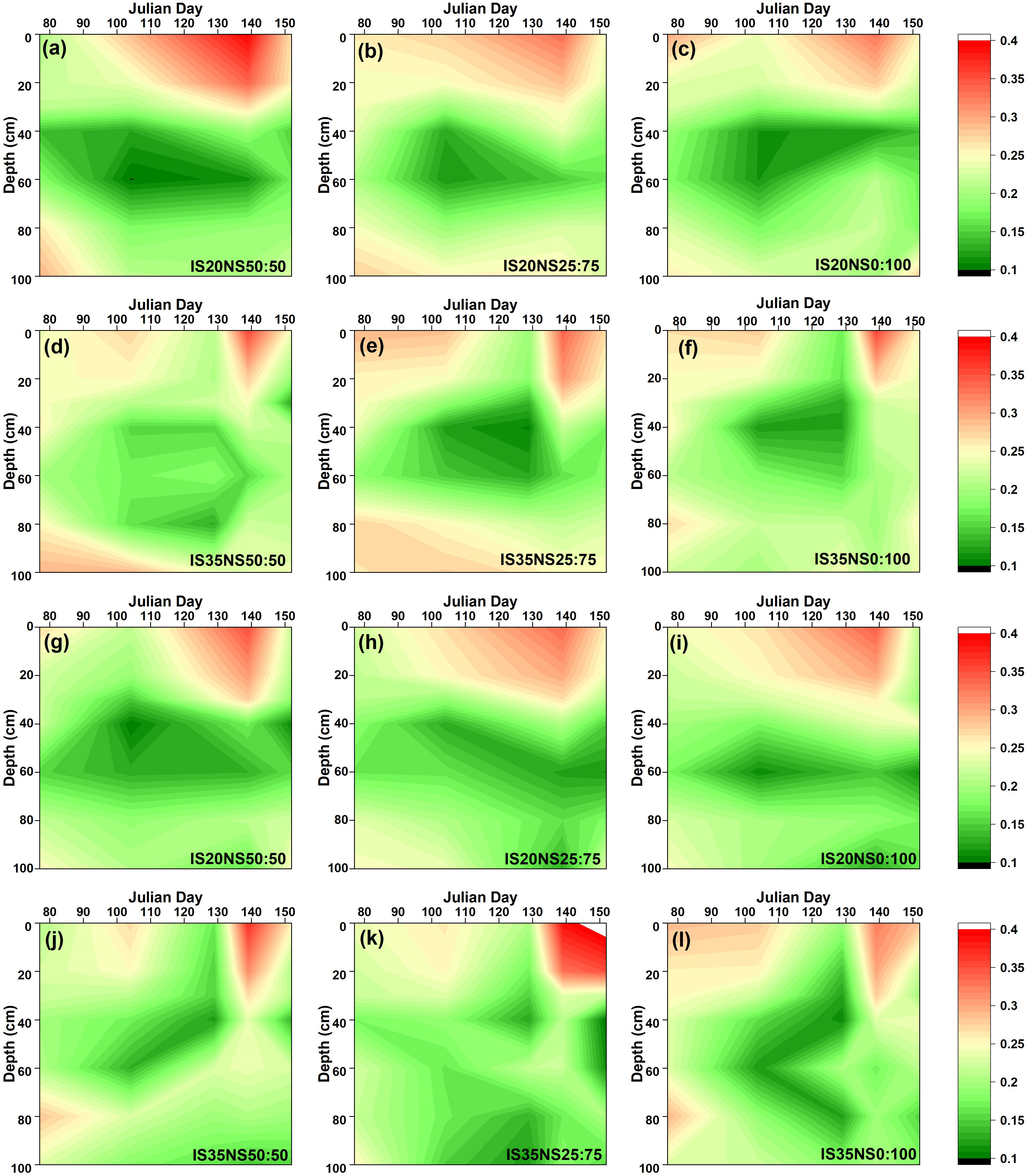
Figure 2 Changes in soil moisture (m3/m3) under different irrigation scheduling and nitrogen application schemes in LS40 (A–F) and LS80 (G–L) during 2017–2018 growing season.
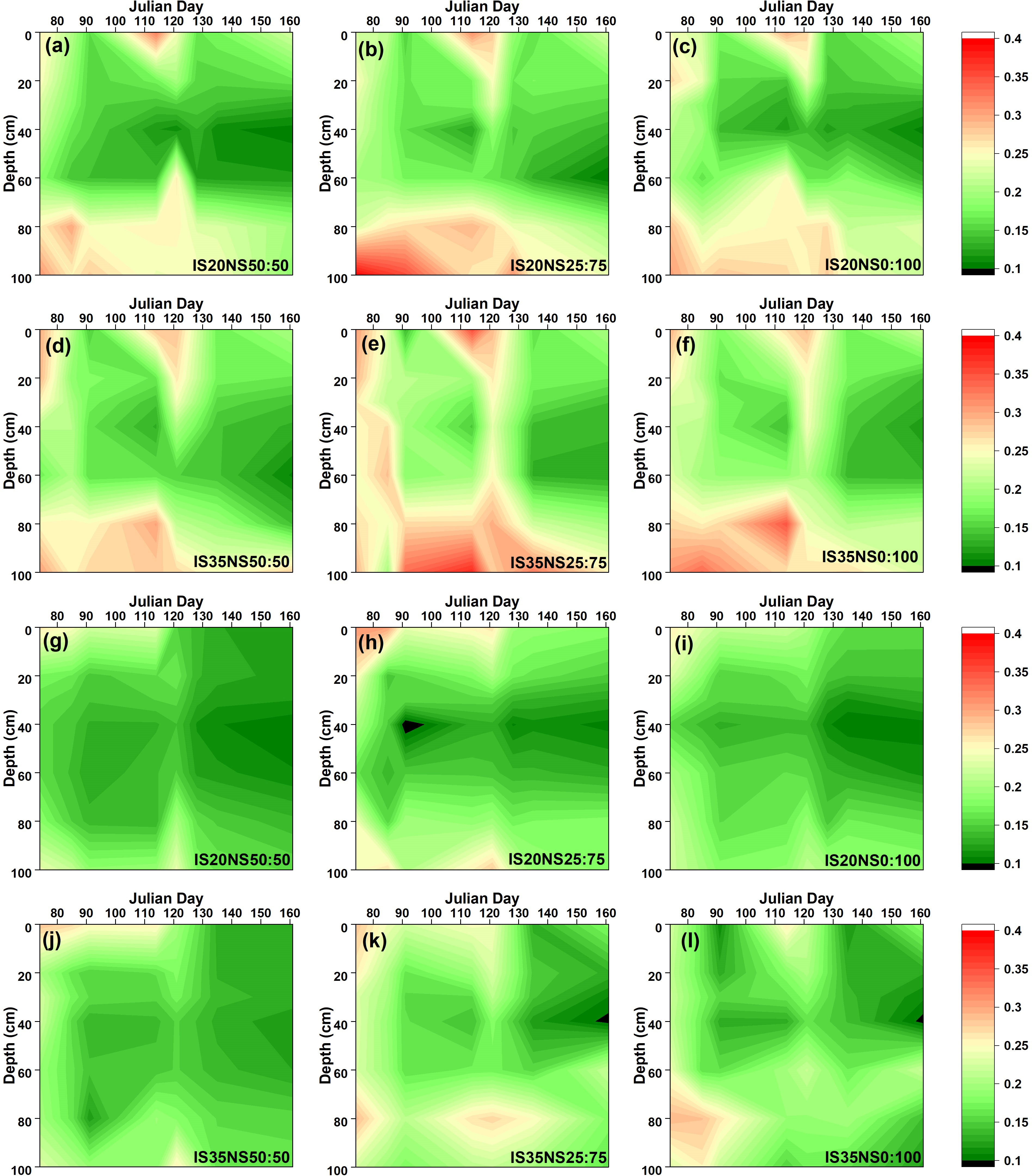
Figure 3 Changes in soil moisture (m3/m3) under different irrigation scheduling and nitrogen application schemes in LS40 (A–F) and LS80 (G–L) during 2018–2019 growing season.
3.1.2 Soil nitrogen
The seasonal changes in nitrate nitrogen (NO3−–N) contents because of different irrigation scheduling, nitrogen application scheme, and drip irrigation lateral spacing are provided in Figures 4 and 5, respectively. Data presented in Figures 4, 5 show significant differences among nitrogen application regimes. Generally, nitrate nitrogen contents increased by changing the nitrogen application from NS50:50 to NS25:75 and from NS25:75 to NS0:100. Among different lateral spacing, LS40 has high soil nitrogen contents during both growing seasons as compared to the LS80. The nitrogen application regime with more basal nitrogen application has the highest soil nitrogen at the winter wheat re-greening stage and has the lowest nitrate nitrogen at other growth stages. Overall, NO3 contents in IS35 and NS0:100 under 40-cm lateral spacing were the highest than that of other irrigation and nitrogen treatments. As per the experimental design, after the application of nitrogen at jointing and grain filling, soil nitrogen contents were also significantly increased. Under both irrigation treatments, soil nitrate nitrogen was mainly distributed in 0–30- and 60–100-cm soil layers. However, in IS35, nitrogen contents were high at a depth of 80–100 cm. Further, soil nitrogen contents observed in 40–60-cm depths were lower than those recorded in other soil depths. For most of the treatments, nitrogen contents initially showed a trend of decreasing with an increase in soil depth and then increasing in deep layers.
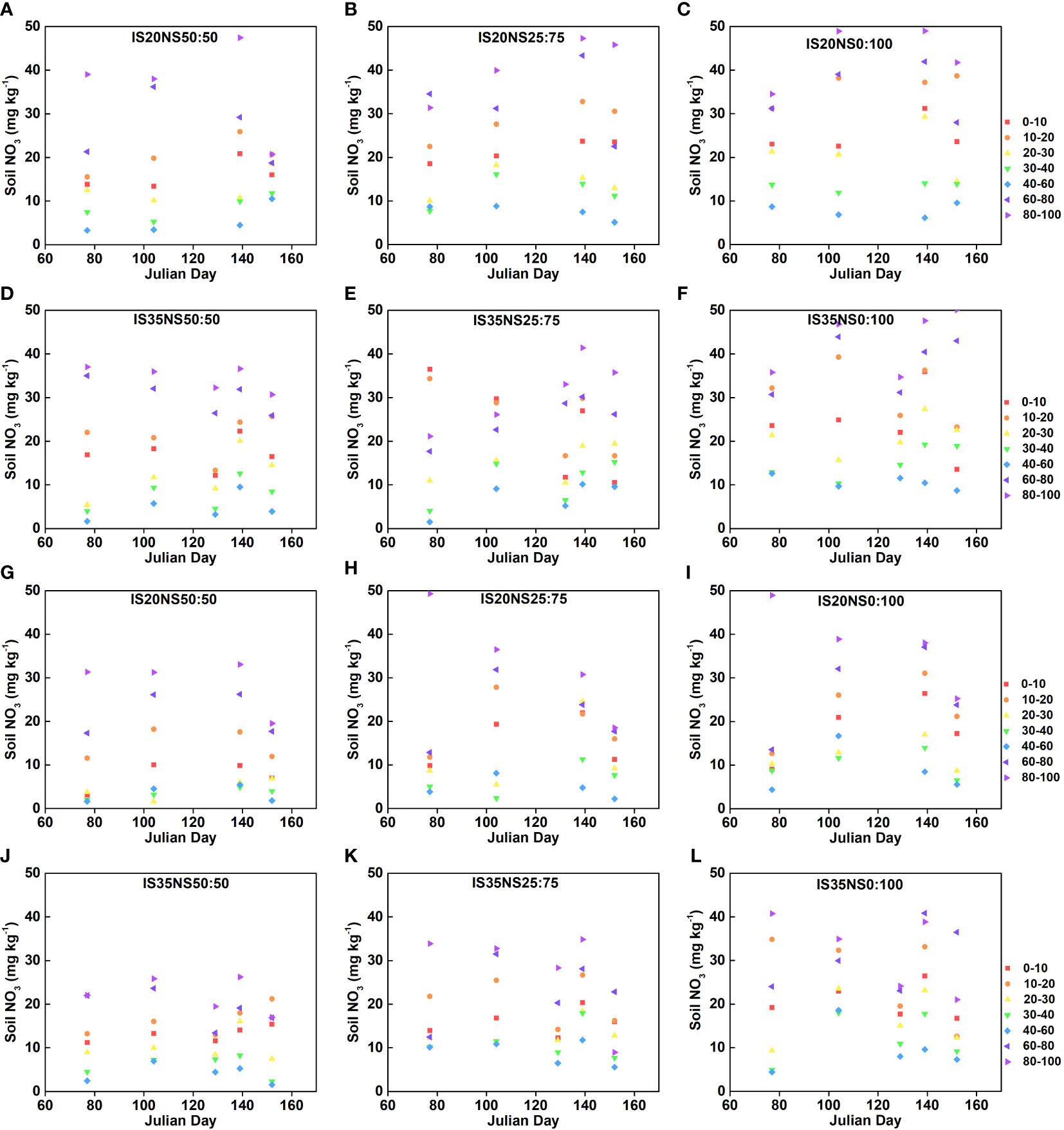
Figure 4 Soil nitrogen contents (mg/kg) at 100-cm depth under different irrigation scheduling and nitrogen application schemes in LS40 (A–F) and LS80 (G–L) during 2017–2018 growing season.
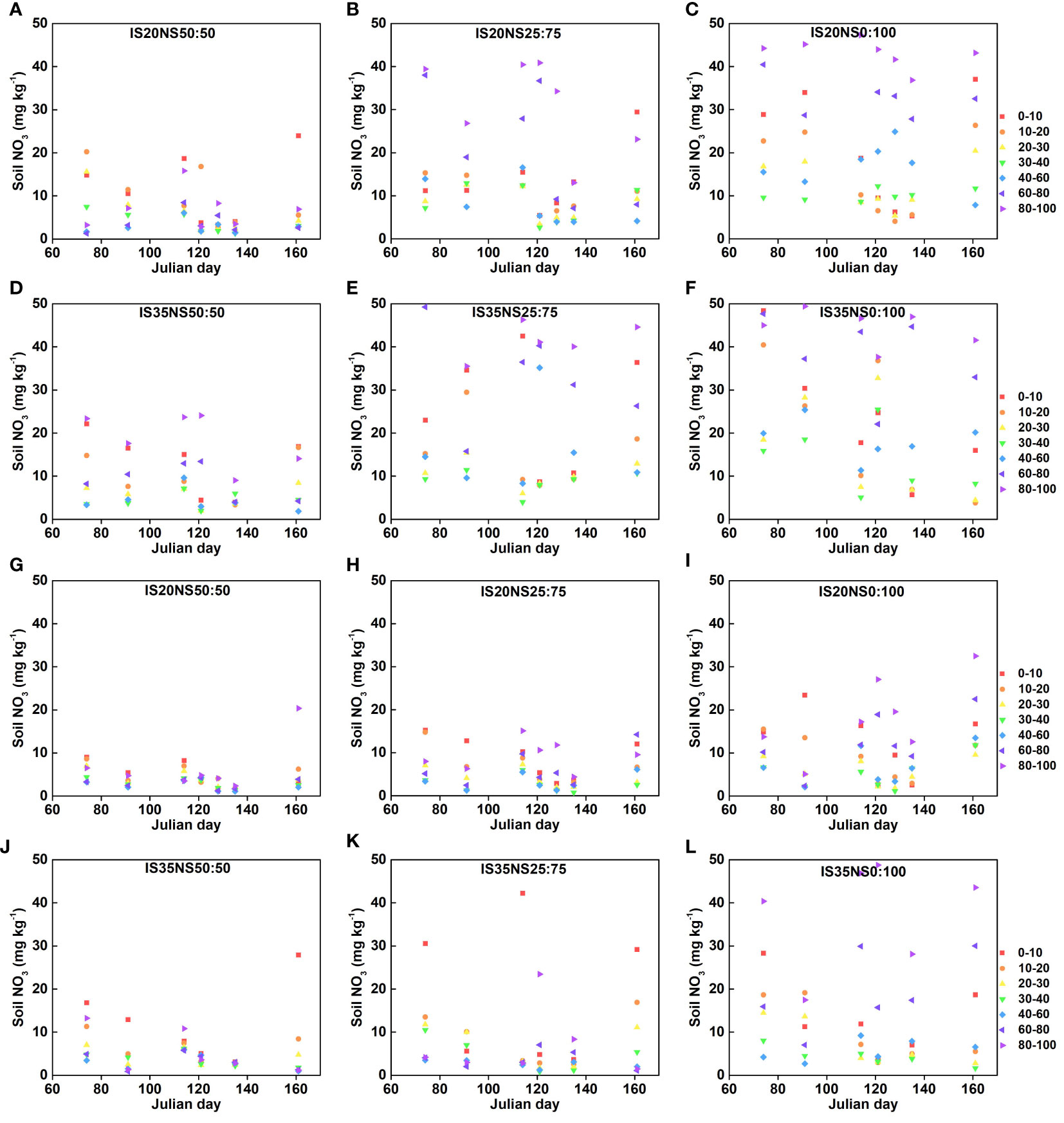
Figure 5 Soil nitrogen contents (mg/kg) at 100-cm depth under different irrigation scheduling and nitrogen application schemes in LS40 (A–F) and LS80 (G–L) during 2018–2019 growing season.
3.2 Response of crop growth indicators to different treatments
Data presented in Figures 6, 7 represent the seasonal changes in winter wheat growth characteristics (leaf area index and plant height) during the 2017–2018 and 2018–2019 growing seasons under various irrigation scheduling, nitrogen application, and lateral spacing regimes. Among different treatments, leaf area index and plant height under NS50:50 were evidently high at the winter wheat re-greening stage. However, growth under NS50:50 starts decreasing and was the lowest at wheat maturity during both study seasons. The highest leaf area index and plant height were recorded in NS0:100 as compared to other nitrogen application scenarios. In the case of irrigation scheduling, IS35 increased crop growth as compared to IS20. Further, LS40 has a high plant height and leaf area index as opposed to LS80 during both study periods. Leaf area index values were the highest at the booting stage under all treatments. Afterward, it gradually decreased till harvesting during both growing seasons. A similar trend was noticed for growth characteristics during both study periods.
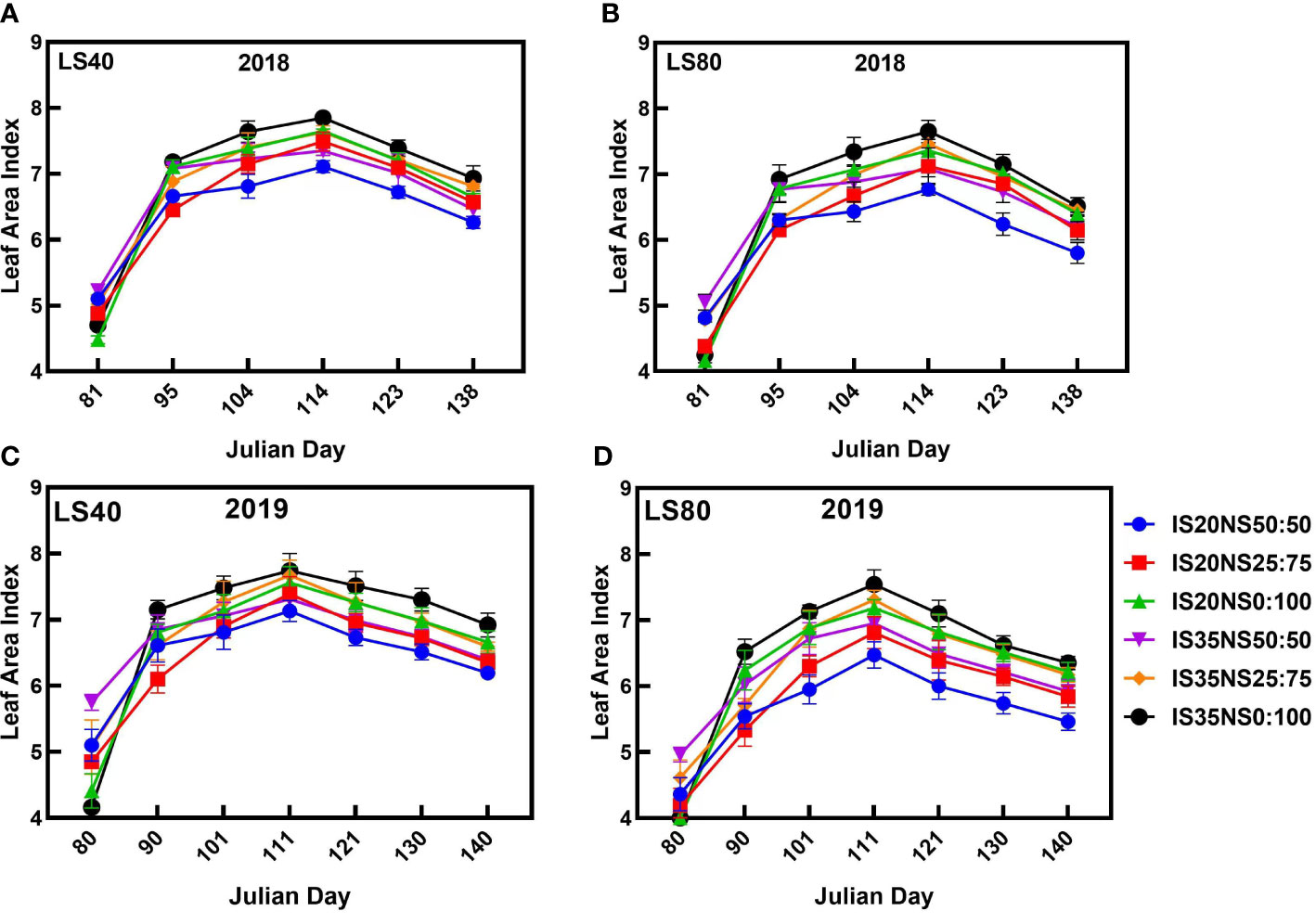
Figure 6 Leaf area index of winter wheat under different lateral spacings, irrigation scheduling, and nitrogen application schemes during 2018 (A, B) and 2019 (C, D) growing seasons.
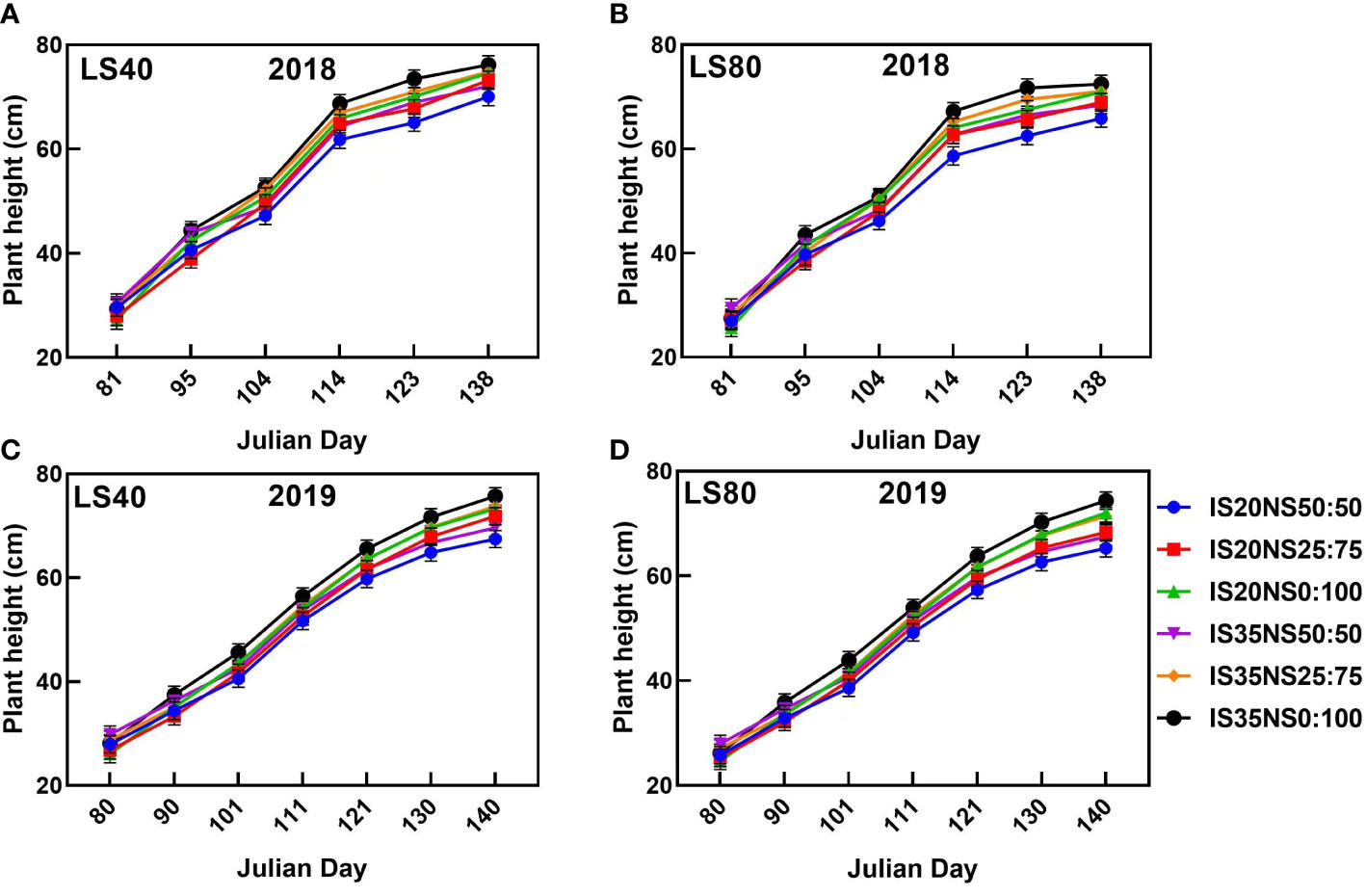
Figure 7 Plant height (cm) of winter wheat under different lateral spacings, irrigation scheduling, and nitrogen application schemes during 2018 (A, B) and 2019 (C, D) growing seasons.
The impacts of different irrigation, nitrogen application scheme, and drip irrigation lateral spacing on the aboveground biomass of winter wheat are given in Table 3. Data presented in Table 3 reveal that aboveground biomass was markedly affected due to lateral spacings (p< 0.01 and p< 0.01), irrigation scheduling (p< 0.01 and p< 0.01), and nitrogen application scheme (p< 0.001 and p< 0.001) in both growing seasons. The interaction effect of all three factors was also significant at p< 0.001 and p< 0.01 during both study seasons. We observed that increasing the irrigation amount and applying all of the total nitrogen at the re-greening, jointing, and grain-filling stages significantly enhanced winter wheat biomass in both lateral spacings. However, increasing the lateral spacing from LS40 to LS80 decreased the winter wheat aboveground biomass by 20.69% and 20.39% in both seasons, respectively. The highest wheat biomass (18.88 and 19.05 t/ha) was recorded in IS35NS0:100 under LS40 during the first and second growing seasons, respectively. However, the lowest aboveground biomass (8.95 and 9.26 t/ha) was noticed in IS20NS50:50 under LS80 during the 2017–2018 and 2018–2019 seasons, respectively.
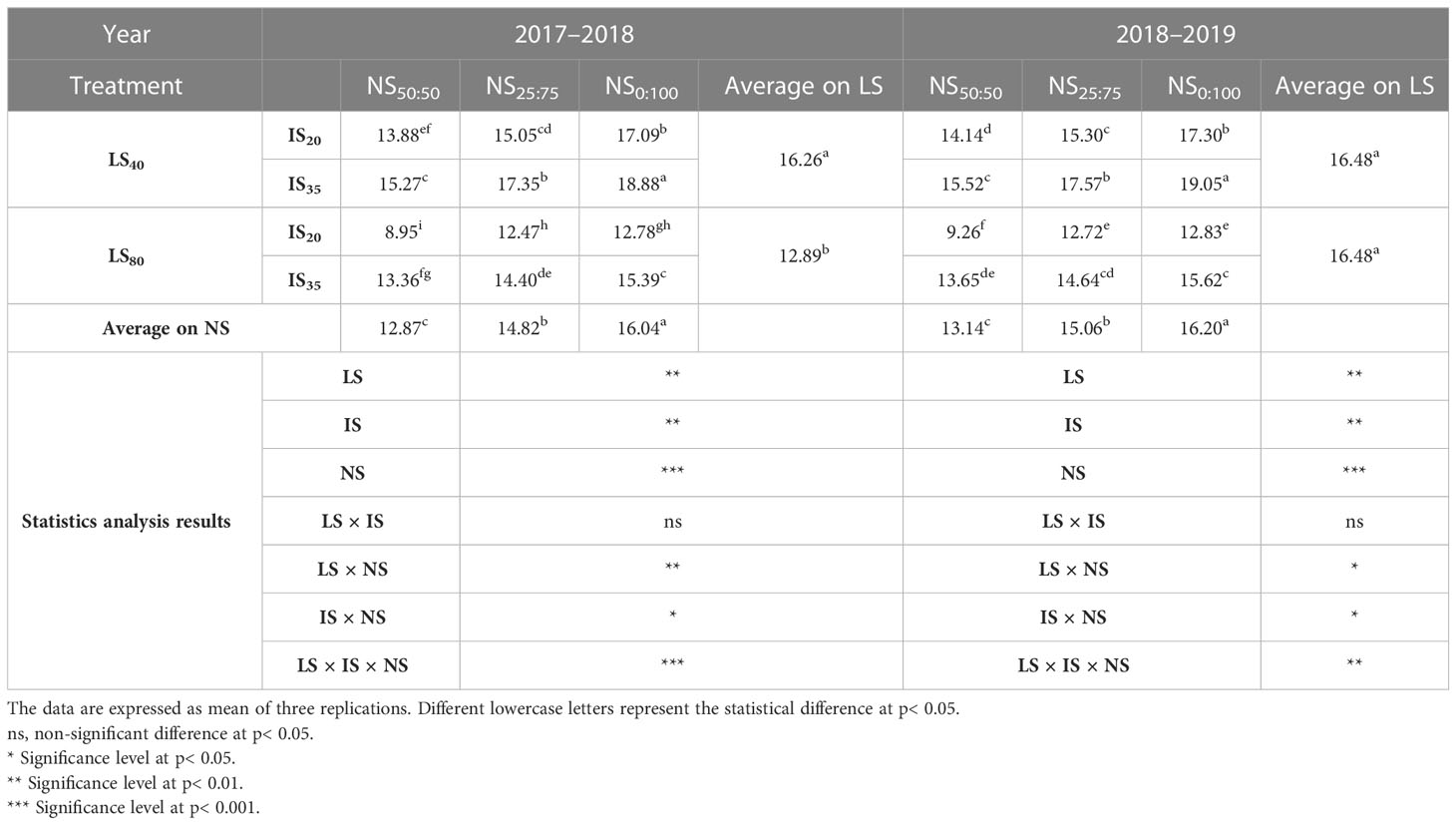
Table 3 Aboveground biomass (t/ha) variability in wheat under lateral spacings, irrigation, and nitrogen application strategies.
3.3 Grain yield and its components
Different nitrogen application scenarios at (p< 0.001 and p< 0.001), irrigation scheduling treatments at (p< 0.001 and p< 0.001), and lateral spacings at (p< 0.05 and p< 0.01) inserted a significant effect on wheat grain yield during both years (Table 4). The combined interactive effect of all factors was also significant at p< 0.001 and p< 0.05 during both study periods. The grain yield values ranged from 6.89 to 8.86 t/ha during 2017–2018 and from 7.42 to 9.61 t/ha during the second study year. We found that the change in lateral spacing from LS80 to LS40 cm notably increased the grain yield by 6.95% and 7.17% in two seasons, respectively. In the case of irrigation scheduling treatments, irrigating the winter wheat at IS35 improved the grain yield by 15.19% and 14.18% compared with that of IS20 treatment during both study periods. Further, applying 25% of total nitrogen as a basal dose and the remaining 75% as a top-dressing dose evidently improved the grain yield more than other nitrogen application schemes. Overall, the highest grain yield (8.86 and 9.61 t/ha) was observed at IS35NS25:75 under 40-cm lateral spacing during both study seasons. Further, minimum winter wheat grain yield (6.89 and 7.42 t/ha) was noticed in IS20LS50:50 under LS80 treatment.
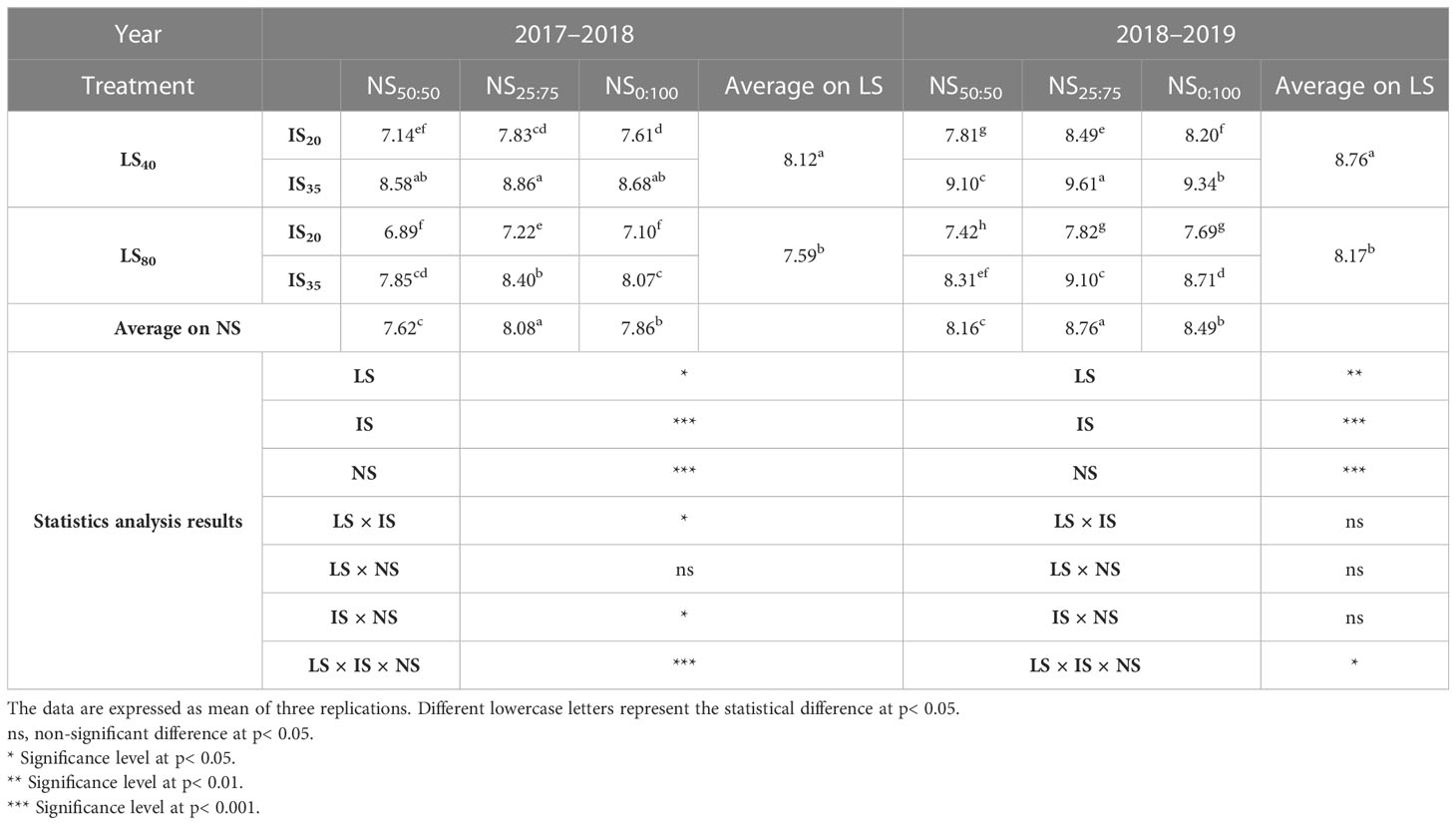
Table 4 Grain yield (t/ha) variability in wheat under lateral spacings, irrigation scheduling, and nitrogen application strategies.
A mean comparison of different yield-related components including spike length, number of grains per spike, 1,000-grain weight, and spikes in a unit area under various regimes is presented in Table 5. Data showed that all of the yield-related indices were almost significantly changed (p< 0.01) due to lateral spacings and by p< 0.001 due to irrigation scheduling and nitrogen application scheme during both growing seasons. The combined interactive effect for spikes per unit area was non-significant in both seasons. However, the combined interactive effect for grains per spike and 1,000-grain weight was not significant during the first and second study periods, respectively. We found that a change in lateral spacings from LS40 cm to LS80 results in decreased yield components. Further, escalating the top-dressing amount of total nitrogen from 50% to 75% enhanced all yield-related parameters, but the application of all concentrations of nitrogen as a top-dressing dose reduced productivity. Application of IS35NS25:75 treatments under LS40 resulted in the highest spike length (8.68 cm), grains per spike (41), spikes per unit area (561 * 104 ha−1), and 1,000-grain weight (52.94 g) during the 2017–2018 growing season; however, the corresponding values during the 2018–2019 growing season were 9.20 cm, 46.8, 711.67 * 104 ha−1, and 53.70 g, respectively. In contrast, the lowest values for all aforementioned yield components were observed in IS20NS50:50 under 80-cm lateral spacing during both growing seasons.
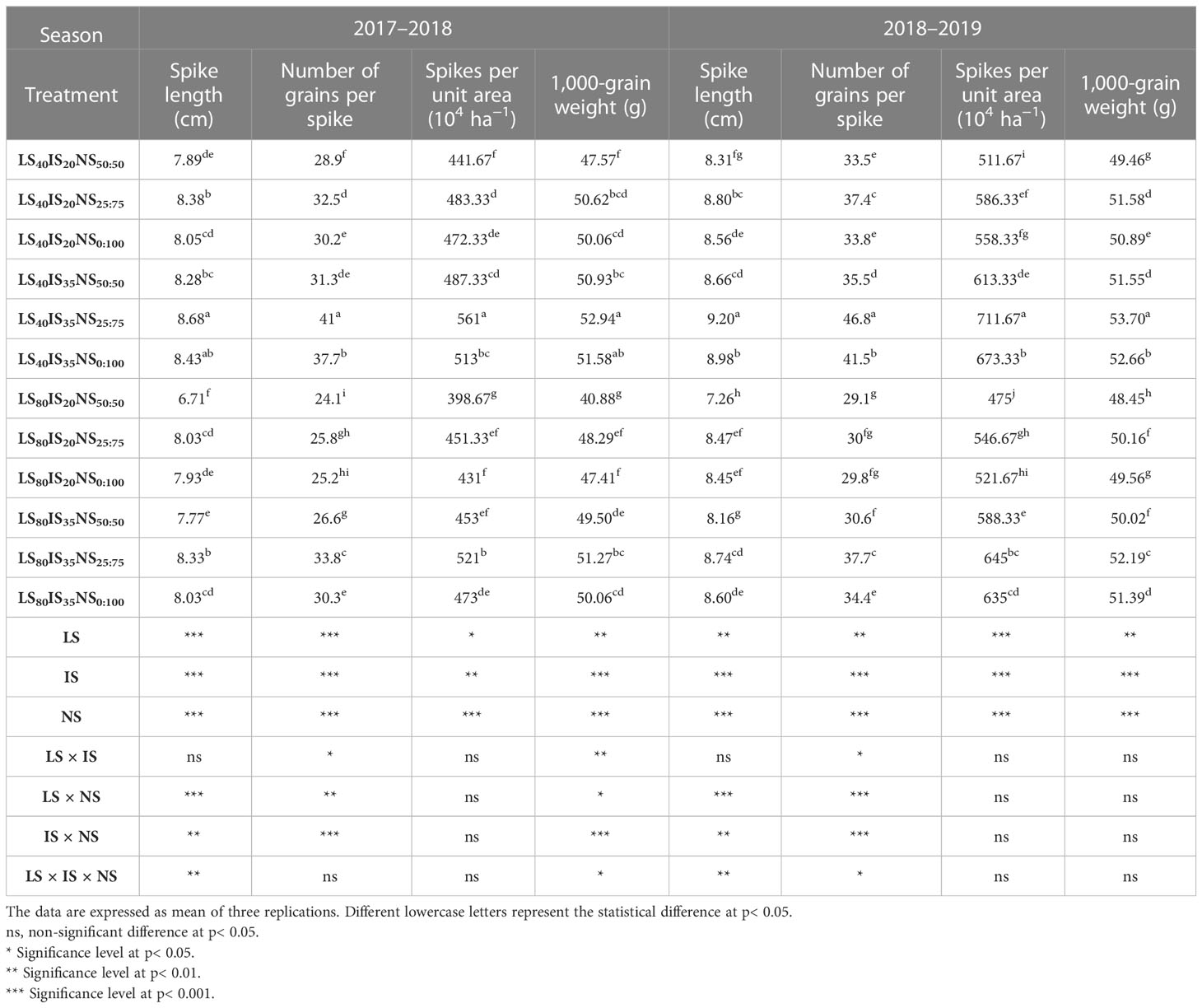
Table 5 Interactive effects of lateral spacings, irrigation scheduling, and nitrogen application strategies on grain yield-related attributes of wheat.
3.4 Changes in plant nitrogen
The results regarding plant total nitrogen of both growing seasons are presented in Figure 8. Data revealed that irrigation scheduling, nitrogen application scheme, and lateral spacing have significantly affected the plant nitrogen content. However, their interactive effect was insignificant during both study years. In the case of drip irrigation lateral spacing, growing winter wheat at 40-cm lateral spacing improved the plant nitrogen content by 7% and 11% compared with those of LS80 during the first and second study periods, respectively. Between different irrigation scheduling treatments, IS35 improved the plant nitrogen content by 5% and 7% as opposed to IS20 during both study years. We found that 100% nitrogen application as a top-dressing dose enhanced the total plant nitrogen contents by 5%–10% and 6%–13% as compared to NS25:75 and NS50:50 during both seasons, respectively.
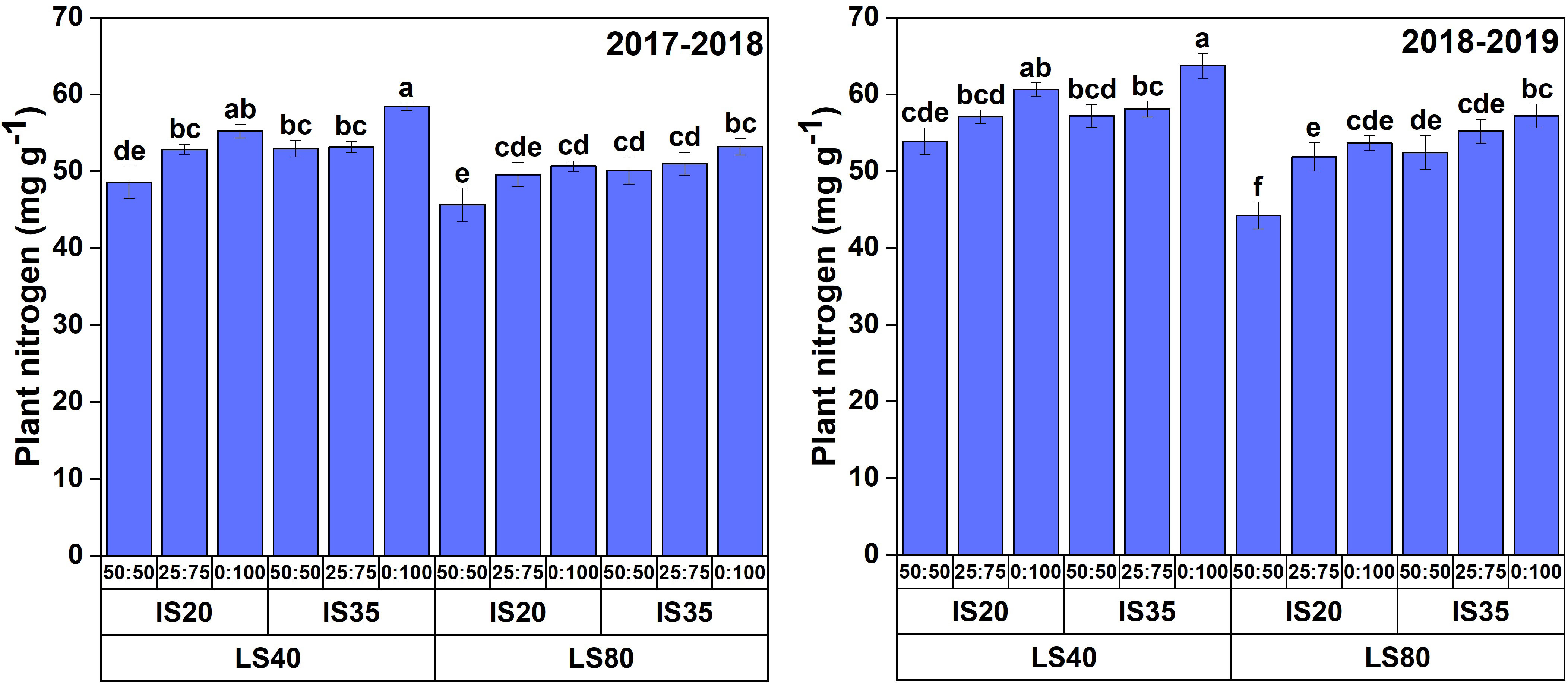
Figure 8 Plant nitrogen contents (mg/g) in winter wheat under various lateral spacings, irrigation scheduling, and nitrogen application regimes in 2017–2018 and 2018–2019 growing seasons. Different lowercase letters represent the statistical difference at p< 0.05.
3.5 Effects of LS, IS, and NS on evapotranspiration and water use efficiency
The variance analysis of ETc showed that crop evapotranspiration was significantly affected due to lateral spacings (p< 0.01 and p< 0.001), irrigation scheduling (p< 0.001 in both seasons), and nitrogen application scheme (p< 0.001 in both seasons) (Table 6). However, their interactive effect was not significant during both study years. The average ETc values in LS40 and LS80 were 439 and 472 mm, respectively, during the first growing season, while the corresponding values in the second study period were 427 and 449 mm, respectively. In the case of irrigation scheduling treatments, irrigating the crop at IS35 enhanced the ETc by 11% more than that of IS20 in 2017–2018 and 2018–2019. The average values for ETc under IS20 and IS35 were 430 and 480 mm, respectively, during the first study period, while the corresponding values during the second study period were 414 and 462 mm, respectively. Further, increasing the top-dressing ratio also increased the ETc, as NS0:100 enhanced the ETc by 2% and 4% as compared to the NS50:50 during the first and second study years, respectively.
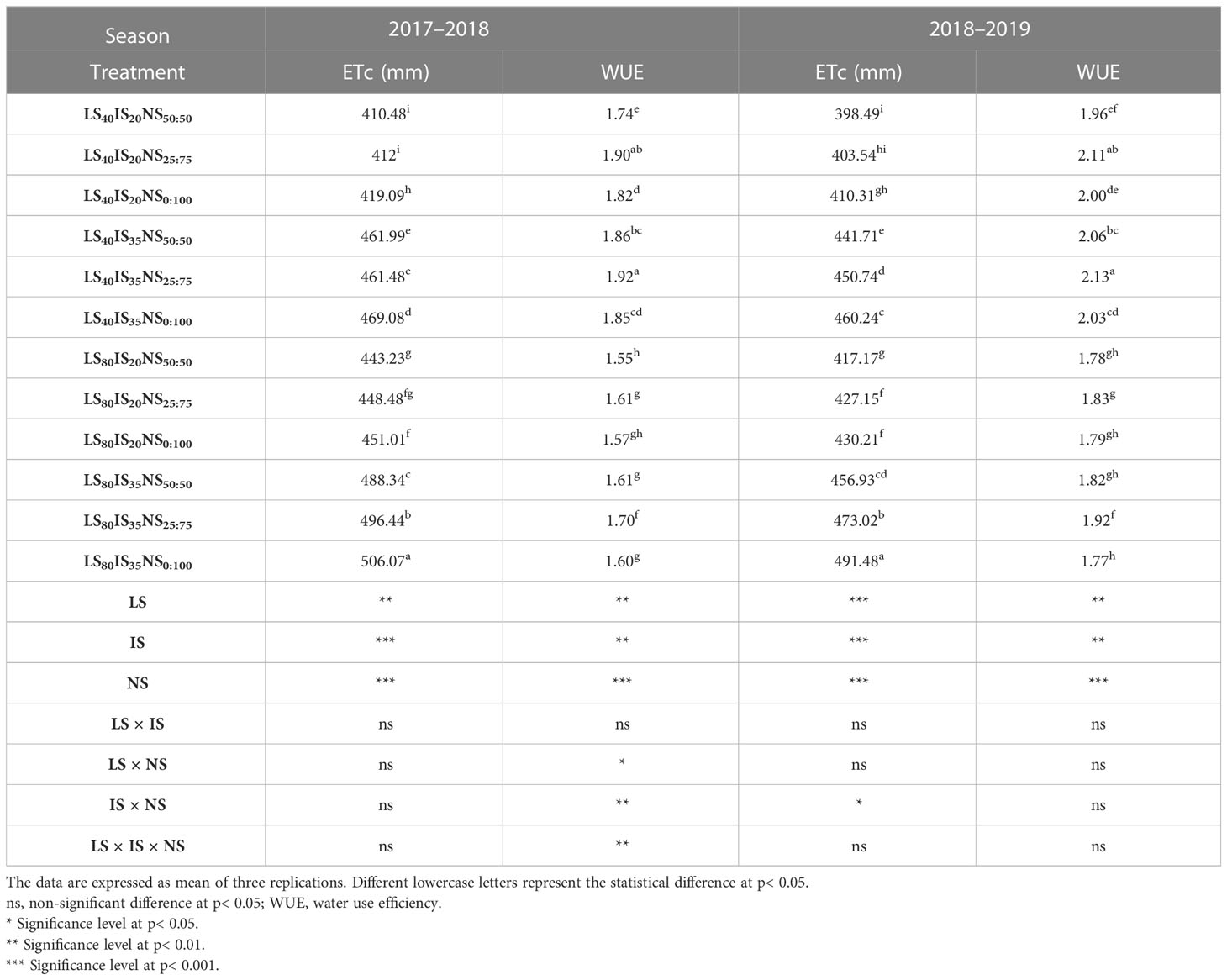
Table 6 Interactive effects of lateral spacings, irrigation scheduling, and nitrogen application strategies on ETc and WUE.
Data presented in Table 6 revealed that water use efficiency was evidently influenced by applying different irrigation scheduling (p< 0.01), nitrogen application scheme (p< 0.001), and lateral spacing (p< 0.01) treatments to winter wheat during both seasons. However, the interactive effect of all three factors was significant (p< 0.01) in the 2017–2018 growing season. An increase in WUE was observed under closer lateral spacings, as LS40 gave higher WUE than LS80. We found that LS40 improved the WUE by 15% and 12% as opposed to LS80 during both growing seasons. On average across the LS and NS, IS35 substantially improved the WUE by 3% and 2% as compared to IS20 during the 2017–2018 and 2018–2019 study periods, respectively. Concerning the NS, escalating top-dressing nitrogen from NS50:50 to NS25:75 notably improved the WUE by 5% in the first and second study seasons, respectively.
4 Discussion
4.1 Soil environment
We found that soil moisture distribution was larger in LS40 as compared to LS80. The dense lateral pipes might allow uniform moisture distribution, which results in more soil moisture content in LS40. These findings corroborate the findings of Chouhan et al. (2015). Different crop behaviors under various lateral spacing have also led to varied soil moisture distribution. Interestingly, we noticed that soil water contents in LS80 depleted slightly earlier than LS40, which indicates a short stress to winter wheat plants during the whole growing season.
Nitrogen applied to the agricultural system has mainly three destinations: uptake by plants, residual soil nitrogen, and loss by the soil system. Garnier et al. (2010) and Van Der Laan et al. (2010) stated that loss of nitrogen from the soil system might lead to a marked increase in groundwater nitrogen accumulation and can be a threat to aquatic life. However, the main form of nitrogen for plant uptake is NO3−–N, which can affect plant water and nutrient uptake (Nie et al., 2012). We found high nitrogen contents in deep soil layers under NS50:50 at the re-greening stage. This indicates that during the early development stages, wheat plants were very small and did not require much nitrogen. Thus, the application of 50% nitrogen as basal in NCP might be a loss of nitrogen resources. Zhao and Si (2015) found that nitrogen is lost through immobilization, ammonia volatilization, and denitrification during 5 months’ time span between the sowing and re-greening stages of winter wheat. The low soil nitrogen in NS25:75 treatment could be caused by better nitrogen utilization by wheat crops. Ma et al. (2021) stated that an adequate supply of nitrogen fertilizer guaranteed reduced nitrogen losses, rational soil distribution during crop growth, and notably improved wheat biomass in later growth stages. Further, NS0:100 treatment also had environmental pollution risk mainly because of surplus nitrogen in deeper soil layers at maturity. These results are similar to the findings of Shi et al. (2012) and Zain et al. (2021). As the nitrogen contents were more in deeper soil layers, we could not conclude about no leaching beyond the wheat root zone. Further, loss of nitrogen through gas emission should be recommended to address the sustainable utilization of nitrogen in winter wheat production.
4.2 Crop growth
Aboveground biomass is an important crop growth indicator, as it represents the inclusive contribution of plant height and leaf area index. The growth indicators of wheat including leaf area index, plant height, and aboveground biomass are presented in Figures 6, 7 and Table 3. We found that closer lateral spacings, IS35, and application of all the total nitrogen as the top-dressing dose guaranteed higher winter wheat growth as compared to the other treatments. Consistent with our findings, Chen et al. (2015) and Lv et al. (2019) also reported high LAI under closer drip irrigation lateral spacings. This might be probably because of water deficit conditions due to more evaporation under LS80 that results in reduced crop growth. We found the highest crop growth indicators under IS35 and NS0:100, implying that sufficient irrigation and nitrogen were present in the field for winter wheat growth. Abrar et al. (2020) and Si et al. (2020) found that nitrogen availability positively affects crop growth, while water deficiency negatively influenced crop growth. Deficient irrigation and fertilizer lead to a reduction in different cell processes, like cell division and cell elongation (Farooq et al., 2009), thereby reducing the LAI, plant height, and aboveground biomass under IS20 and NS50:50 treatments. Xu et al. (2021) also reported improved crop growth with a top-dressing dose of nitrogen fertilizer under a wheat–maize intercropping system. High leaf area index values and plant height under NS50:50 at the re-greening stage and then lower corresponding values at the next growth stages indicate that applying all of the total nitrogen as top-dressing dose could be just beneficial for an increase in winter wheat growth indicators.
4.3 Plant N uptake
An adequate nitrogen supply is critical for the normal growth and development of crops. Srivastava et al. (2018) reported that the fertilizer amount available for plant uptake directly influences crop growth and development, thereby affecting the final crop yield. We found that increasing the top-dressing ratio led to higher nitrogen accumulation in wheat plants. Previously, Rasmussen et al. (2015); Liu et al. (2017), and Yin et al. (2020) reported that an increase in nitrogen application results in high nitrogen uptake. Zhang et al. (2020) also stated that plant nitrogen concentration was significantly increased by top-dressing nitrogen under various irrigation treatments. In the current study, IS35 and NS0:100 significantly enhanced the nitrogen uptake by wheat plants. These results are similar to those of Guo et al. (2015), who reported higher nitrogen uptake in maize plants with an increase in irrigation and nitrogen supply. To the best of our knowledge, this is the first study in which nitrogen uptake by wheat plants was analyzed under different drip irrigation lateral spacings. We found higher nitrogen uptake by plants under LS40, which might be caused by the optimum availability of water content as compared to the water availability in LS80. Conclusively, considering the wheat yield and WUE, applying 25% of total nitrogen as basal and the remaining 75% as top-dressing dose can be a suitable scheme for sustainable winter wheat productivity.
4.4 Grain yield and its components
Elucidating the most efficient lateral spacing, irrigation scheduling practice, and nitrogen application scheme was the key concern of this study. The ultimate objective of the study was to improve the grain yield for better sustainable agriculture. We found that grain yield was highly variable across different experimental treatments (Table 4). The rise in grain yield under LS40 might be attributed to high values of grain yield components under closer lateral spacings. Xu et al. (2018) stated that grain yield is closely associated with spikelets per spike and the number of grains per spike. In our study, yield components, especially the number of grains per spike and 1,000-grain weight, showed poor performance under LS80. These results are similar to the findings of Lv et al. (2019), who found 9.96% and 8.52% reduction in grain yield of widened lateral spacings compared with closer lateral tubes. The reduced number of grains per spike and 1,000-grain weight were the major drivers for this decrease in yield as presented in Table 5. Irrigation scheduling has also an important role in winter wheat productivity, as the highest yield was obtained under IS35-related treatments. Mostafa et al. (2018) found that continuous availability of moisture in wheat root zone led to highest grain yield and yield-related parameters while applying double lateral lines than that of single drip lateral line.
The presence of enough nitrogen is also very important to achieve better winter wheat production. The continuous decreasing trend in NS50:50 was noticed in the current study, as the leaf area index, plant height, aboveground biomass, and grain yield components were all lower in NS50:50 as compared to the other nitrogen application scheme. We found the best winter wheat grain yield by applying 75% nitrogen as top-dressing dose rather than 50% at top-dressing dose. These results are in line with the findings of Liu et al. (2019), who stated that decreasing basal application and increasing top-dressing dose remarkably enhance the yield components and finally the yield of winter wheat. Moreover, growth indicators were high in NS0:100, but the grain yield and yield-related attributes were not better in this application scheme. More application of nitrogen as top-dressing dose might delay the wheat maturity and enhance the growth characteristics rather than yield parameters. Thus, the top-dressing dose of all nitrogen is not a good application strategy for winter wheat. Liang et al. (2017) and Zhang et al. (2017) stated that the application of more nitrogen at the booting stage might influence the grain filling and ultimately the grain yield by delaying maturity.
4.5 ETc and WUE
The ETc values were high in the 2017–2018 growing season as compared to the 2018–2019 season. This could be mainly because of more precipitation (approximately 238 mm) in the first study season than 117-mm rainfall in the second growing season. A significant difference in ETc between LS40 and LS80 was observed during both growing seasons. The higher ETc values in LS80 might be due to the more evaporation losses, as the distance among drip laterals was so large to not facilitate the proper moisture content distribution for wheat growth and productivity, while less ETc values under 40-cm drip lateral spacing may relate to proper moisture availability for wheat plants. In the case of the nitrogen application scheme, the application of all nitrogen as top-dressing dose notably enhanced the ETc during both growing seasons. This is likely a result of high plant growth under this treatment, as high plant growth requires much water from the soil and also results in more transpiration. The highest plant growth under NS0:100 could be the reason for more ETc in this treatment (Qi et al., 2009). Further, our results are in line with the findings of Rathore et al. (2017), who found 8% enhanced ETc in plants treated with more nitrogen as compared to plants treated with low nitrogen.
In terms of low grain yield and high ETc under LS80, WUE was also low under this drip irrigation lateral spacing. However, the higher WUE under LS40 could be mainly because of more grain yield and less ETc. Mostafa et al. (2018) found that growing the plants in double drip lateral lines notably enhanced the WUE more than that of single lateral lines. Among different nitrogen application treatments, increasing the top-dressing ratio from 50% to 75% significantly improved the WUE, but a further increase in nitrogen application from 75% to 100% as top-dressing dose decreased the WUE. This is likely caused by more grain yield under NS25:75 and less ETc than NS0:100 treatment. Further, these findings are in parallel with the results of El-Hendawy et al. (2008) and Zhang et al. (2017). We suggest that proper splitting of total nitrogen fertilizer is advantageous to improve the WUE rather than increase in application rate.
5 Conclusion
Soil water and nitrogen resources are suffering from overuse; alarmingly, this situation is projected to worsen in the near future. Therefore, management of these resources by doing field studies is necessary for sustainable agricultural productivity. In our study, we highlighted that different drip lateral spacings, irrigation scheduling, and nitrogen fertilization scheme applied over two consecutive growing seasons have a clear influence on winter wheat growth and productivity. The irrigation scheduling whenever soil water consumption reached 35 mm plus 25% nitrogen as the basal dose and 75% as the top-dressing dose under drip lateral spacing of 40 cm evidently facilitated the winter wheat yield, yield-related parameters, and water use efficiency. In contrast, growing winter wheat under 80-cm lateral spacing with other irrigation scheduling and nitrogen fertilization treatments was not a suitable choice for winter wheat productivity. Further, in order to prevent nitrogen loss in winter wheat, the treatment with NS0:100 is not recommended, as this treatment just improved the growth characteristics of winter wheat and did not produce suitable grain yield. Further, studies on the physiological and biochemical processes of winter wheat under different irrigation scheduling and nitrogen fertilization management strategies should be considered for a better understanding of winter wheat growth and yield pattern under varied soil and environmental conditions. We also suggest that nitrogen loss through greenhouse gas emission should also be quantified by conducting experiments under various split nitrogen application regimes. Finally, genetic expression must be taken into consideration to better understand the underlying mechanisms under different treatments.
Data availability statement
The original contributions presented in the study are included in the article/supplementary material. Further inquiries can be directed to the corresponding authors.
Author contributions
Investigation: MZ. Methodology: MZ and FM. Data curation: MZ, CM, and AK. Formal analysis: MZ, ZS, HM, FM, and CS. Writing—original draft: MZ and ZS. Visualization: ZS. Software: HM, CM, and AK. Supervision: DA and CS. Writing—review and editing: DA and CS. All authors contributed to the article and approved the submitted version.
Funding
The research was funded by the National Natural Science Foundation of China (51879267, 32172110, 32001465, and 31872852), Key Research and Development Program (Modern Agriculture) of Jiangsu Province (BE2020319), China Agricultural Research System (CARS-03), and Basic Scientific Research Project of the Chinese Academy of Agricultural Sciences for funding (FIRI2016-05 and FIRI2017-09).
Acknowledgments
The authors are thankful to the following for providing the funds: National Natural Science Foundation of China (51879267, 32172110, 32001465, and 31872852), Key Research and Development Program (Modern Agriculture) of Jiangsu Province (BE2020319), China Agricultural Research System (CARS-03), and Basic Scientific Research Project of the Chinese Academy of Agricultural Sciences (FIRI2016-05 and FIRI2017-09).
Conflict of interest
The authors declare that the research was conducted in the absence of any commercial or financial relationships that could be construed as a potential conflict of interest.
Publisher’s note
All claims expressed in this article are solely those of the authors and do not necessarily represent those of their affiliated organizations, or those of the publisher, the editors and the reviewers. Any product that may be evaluated in this article, or claim that may be made by its manufacturer, is not guaranteed or endorsed by the publisher.
References
Abrar, M. M., Saqib, M., Abbas, G., Atiq-Ur-Rahman, M., Mustafa, A., Shah, S., et al. (2020). Evaluating the contribution of growth, physiological, and ionic components towards salinity and drought stress tolerance in Jatropha curcas. Plants 9, 1574. doi: 10.3390/plants9111574
Blandino, M., Vaccino, P., Reyneri, A. (2015). Late-season nitrogen increases improver common and durum wheat quality. Agron. J. 107, 680–690. doi: 10.2134/agronj14.0405
Cao, Y., Cai, H., Sun, S. (2021). Effects of growth-stage-based limited irrigation management on the growth, yields, and radiation utilization efficiency of winter wheat in northwest China. J. Sci. Food Agric. 101, 5819–5826. doi: 10.1002/jsfa.11233
Chen, R., Cheng, W., Cui, J., Liao, J., Fan, H., Zheng, Z., et al. (2015). Lateral spacing in drip-irrigated wheat: The effects on soil moisture, yield, and water use efficiency. Field Crops Res. 179, 52–62. doi: 10.1016/j.fcr.2015.03.021
Chen, X., Wang, J., Wang, Z., Li, W., Wang, C., Yan, S., et al. (2018). Optimized nitrogen fertilizer application mode increased culms lignin accumulation and lodging resistance in culms of winter wheat. Field Crops Res. 228, 31–38. doi: 10.1016/j.fcr.2018.08.019
Chouhan, S. S., Awasthi, M. K., Nema, R. K. (2015). Studies on water productivity and yields responses of wheat based on drip irrigation systems in clay loam soil. Indian J. Sci. Tech. 8, 650. doi: 10.17485/ijst/2015/v8i7/64495
Dar, E. A., Brar, A. S., Mishra, S. K., Singh, K. B. (2017). Simulating response of wheat to timing and depth of irrigation water in drip irrigation system using CERES-Wheat model. Field Crops Res. 214, 149–163. doi: 10.1016/j.fcr.2017.09.010
Du, T., Kang, S., Zhang, J., Davies, W. J. (2015). Deficit irrigation and sustainable water-resource strategies in agriculture for China’s food security. J. Exp. Bot. 66, 2253–2269. doi: 10.1093/jxb/erv034
El-Hendawy, S. E., Hokam, E. M., Schmidhalter, U. (2008). Drip irrigation frequency: The effects and their interaction with nitrogen fertilization on sandy soil water distribution, maize yield and water use efficiency under Egyptian conditions. J. Agron. Crop Sci. 194, 180–192. doi: 10.1111/j.1439-037X.2008.00304.x
El-Rahman, G. A. (2009). Water use efficiency of wheat under drip irrigation systems at Al-Maghara area, North Sinai, Egypt. American-Eurasian J.Sci. Res. 5, 664–670. doi: 10.21608/jssae.2009.90464
Fang, Q., Ma, L., Yu, Q., Hu, C., Li, X., Malone, R., et al. (2013). Quantifying climate and management effects on regional crop yield and nitrogen leaching in the North China Plain. J.Environ. Qual. 42, 1466–1479. doi: 10.2134/jeq2013.03.0086
Fang, Q., Zhang, X., Shao, L., Chen, S., Sun, H. (2018). Assessing the performance of different irrigation systems on winter wheat under limited water supply. Agric. Water Manage. 196, 133–143. doi: 10.1016/j.agwat.2017.11.005
FAO (Food and Agriculture Organization) (2020) FAOSTAT. Available at: http://www.fao.org/faostat/.
Farooq, M., Wahid, A., Kobayashi, N., Fujita, D., Basra, S. (2009). Plant drought stress: effects, mechanisms and management. Agron. Sustain. Dev. 29, 185–212 (2009). doi: 10.1051/agro:2008021
Gao, Y., Yang, L., Shen, X., Li, X., Sun, J., Duan, A., et al. (2014). Winter wheat with subsurface drip irrigation (SDI): Crop coefficients, water-use estimates, and effects of SDI on grain yield and water use efficiency. Agric. Water Manage. 146, 1–10. doi: 10.1016/j.agwat.2014.07.010
Gärdenäs, A., Hopmans, J., Hanson, B., Šimůnek, J. (2005). Two-dimensional modeling of nitrate leaching for various fertigation scenarios under micro-irrigation. Agric. Water Manage. 74, 219–242. doi: 10.1016/j.agwat.2004.11.011
Garnier, M., Recanatesi, F., Ripa, M. N., Leone, A. (2010). Agricultural nitrate monitoring in a lake basin in Central Italy: a further step ahead towards an integrated nutrient management aimed at controlling water pollution. Environ. Monit. Assess. 170, 273–286. doi: 10.1007/s10661-009-1231-z
Gasser, M., Caron, J., Laverdiere, M., Lagace, R. (2002). Solute transport modeling under cultivated sandy soils and transient water regime. J. Environ. Qual. 31, 1722–1730. doi: 10.2134/jeq2002.1722
Guo, B., Gao, H., Tang, C., Liu, T., Chu, G. (2015). Response of water coupling with N supply on maize nitrogen uptake, water and N use efficiency, and yield in drip irrigation condition. Ying Yong Sheng tai xue bao= J.Appl. Ecol. 26, 3679–3686.
Hamzei, J. (2018). Nitrogen rate applied affects dry matter translocation and performance attributes of wheat under deficit irrigation. J. Plant Nutt. 41, 807–817. doi: 10.1080/01904167.2017.1382533
Huang, J., He, F., Cui, K., Buresh, R. J., Xu, B., Gong, W., et al. (2008). Determination of optimal nitrogen rate for rice varieties using a chlorophyll meter. Field Crops Res. 105, 70–80. doi: 10.1016/j.fcr.2007.07.006
Jha, S. K., Ramatshaba, T. S., Wang, G., Liang, Y., Liu, H., Gao, Y., et al. (2019). Response of growth, yield and water use efficiency of winter wheat to different irrigation methods and scheduling in North China Plain. Agric. Water Manage. 217, 292–302. doi: 10.1016/j.agwat.2019.03.011
Jia, X., Shao, L., Liu, P., Zhao, B., Gu, L., Dong, S., et al. (2014). Effect of different nitrogen and irrigation treatments on yield and nitrate leaching of summer maize (Zea mays L.) under lysimeter conditions. Agric. Water Manage. 137, 92–103. doi: 10.1016/j.agwat.2014.02.010
Jiang, T., Liu, J., Gao, Y., Sun, Z., Chen, S., Yao, N., et al. (2020). Simulation of plant height of winter wheat under soil Water stress using modified growth functions. Agric. Water Manage. 232, 106066. doi: 10.1016/j.agwat.2020.106066
Ju, X. T., Xing, G. X., Chen, X. P., Zhang, S. L., Zhang, L. J., Liu, X. J., et al. (2009). Reducing environmental risk by improving N management in intensive Chinese agricultural systems. Proceed. Nat. Acad. Sci. 106, 3041–3046. doi: 10.1073/pnas.0813417106
Lassaletta, L., Billen, G., Grizzetti, B., Anglade, J., Garnier, J. (2014). 50 year trends in nitrogen use efficiency of world cropping systems: the relationship between yield and nitrogen input to cropland. Environ. Res. Let. 9, 105011. doi: 10.1088/1748-9326/9/10/105011
Li, J., Wang, Z., Yao, C., Zhang, Z., Liu, Y., Zhang, Y. (2021). Micro-sprinkling irrigation simultaneously improves grain yield and protein concentration of winter wheat in the North China Plain. Crop J. 9, 1397–1407. doi: 10.1016/j.cj.2020.12.009
Li, J., Xu, X., Lin, G., Wang, Y., Liu, Y., Zhang, M., et al. (2018). Micro-irrigation improves grain yield and resource use efficiency by co-locating the roots and N-fertilizer distribution of winter wheat in the North China Plain. Sci. Total Environ. 643, 367–377. doi: 10.1016/j.scitotenv.2018.06.157
Liang, W., Zhang, Z., Wen, X., Liao, Y., Liu, Y. (2017). Effect of non-structural carbohydrate accumulation in the stem pre-anthesis on grain filling of wheat inferior grain. Field Crops Res. 211, 66–76. doi: 10.1016/j.fcr.2017.06.016
Liu, Z., Chen, Z., Ma, P., Meng, Y., Zhou, J. (2017). Effects of tillage, mulching and N management on yield, water productivity, N uptake and residual soil nitrate in a long-term wheat-summer maize cropping system. Field Crops Res. 213, 154–164. doi: 10.1016/j.fcr.2017.08.006
Liu, Z., Gao, F., Liu, Y., Yang, J., Zhen, X., Li, X., et al. (2019). Timing and splitting of nitrogen fertilizer supply to increase crop yield and efficiency of nitrogen utilization in a wheat–peanut relay intercropping system in China. Crop J. 7, 101–112. doi: 10.1016/j.cj.2018.08.006
Liu, X., Wang, Y., Yan, X., Hou, H., Liu, P., Cai, T., et al. (2020b). Appropriate ridge-furrow ratio can enhance crop production and resource use efficiency by improving soil moisture and thermal condition in a semi-arid region. Agric. Water Manage. 240, 106289. doi: 10.1016/j.agwat.2020.106289
Liu, M., Wu, X., Li, C., Li, M., Xiong, T., Tang, Y. (2020a). Dry matter and nitrogen accumulation, partitioning, and translocation in synthetic-derived wheat cultivars under nitrogen deficiency at the post-jointing stage. Field Crops Res. 248, 107720. doi: 10.1016/j.fcr.2020.107720
Lv, Z., Diao, M., Li, W., Cai, J., Zhou, Q., Wang, X., et al. (2019). Impacts of lateral spacing on the spatial variations in water use and grain yield of spring wheat plants within different rows in the drip irrigation system. Agric. Water Manage. 212, 252–261. doi: 10.1016/j.agwat.2018.09.015
Ma, Q., Wang, M., Zheng, G., Yao, Y., Tao, R., Zhu, M., et al. (2021). Twice-split application of controlled-release nitrogen fertilizer met the nitrogen demand of winter wheat. Field Crops Res. 267, 108163. doi: 10.1016/j.fcr.2021.108163
Mehmood, F., Wang, G., Gao, Y., Liang, Y., Chen, J., Si, Z., et al. (2019). Nitrous oxide emission from winter wheat field as responded to irrigation scheduling and irrigation methods in the North China Plain. Agric. Water Manage. 222, 367–374. doi: 10.1016/j.agwat.2019.06.015
Mostafa, H., El-Nady, R., Awad, M., El-Ansary, M. (2018). Drip irrigation management for wheat under clay soil in arid conditions. Ecolog. Eng. 121, 35–43. doi: 10.1016/j.ecoleng.2017.09.003
Nie, S.-W., Eneji, A. E., Chen, Y.-Q., Peng, S., Huang, J.-X., Huang, S.-M. (2012). Nitrate leaching from maize intercropping systems with N fertilizer over-dose. J. Integ. Agric. 11, 1555–1565. doi: 10.1016/S2095-3119(12)60156-7
Payero, J. O., Tarkalson, D. D., Irmak, S., Davison, D., Petersen, J. L. (2008). Effect of irrigation amounts applied with subsurface drip irrigation on corn evapotranspiration, yield, water use efficiency, and dry matter production in a semiarid climate. Agric. Water Manage. 95, 895–908. doi: 10.1016/j.agwat.2008.02.015
Qi, L., Dang, T., Chen, L. (2009). The water use characteristics of winter wheat and response to fertilization on dryland of Loess Plateau. Res. Soil Water Conserv. 16, 105.
Rasmussen, I. S., Dresbøll, D. B., Thorup-Kristensen, K. (2015). Winter wheat cultivars and nitrogen (N) fertilization—Effects on root growth, N uptake efficiency and N use efficiency. Europ. J. Agron. 68, 38–49. doi: 10.1016/j.eja.2015.04.003
Rathore, V. S., Nathawat, N. S., Bhardwaj, S., Sasidharan, R. P., Yadav, B. M., Kumar, M., et al. (2017). Yield, water and nitrogen use efficiencies of sprinkler irrigated wheat grown under different irrigation and nitrogen levels in an arid region. Agric. Water Manage. 187, 232–245. doi: 10.1016/j.agwat.2017.03.031
Shi, Z., Jing, Q., Cai, J., Jiang, D., Cao, W., Dai, T. (2012). The fates of 15N fertilizer in relation to root distributions of winter wheat under different N splits. Europ. J. Agron. 40, 86–93. doi: 10.1016/j.eja.2012.01.006
Si, Z., Zain, M., Mehmood, F., Wang, G., Gao, Y., Duan, A. (2020). Effects of nitrogen application rate and irrigation regime on growth, yield, and water-nitrogen use efficiency of drip-irrigated winter wheat in the North China Plain. Agric. Water Manage. 231, 106002. doi: 10.1016/j.agwat.2020.106002
Sidhu, R. K., Kumar, R., Rana, P. S., Jat, M. (2021). Automation in drip irrigation for enhancing water use efficiency in cereal systems of South Asia: Status and prospects. Adv. Agron. 167, 247–300. doi: 10.1016/bs.agron.2021.01.002
Srivastava, R., Panda, R., Chakraborty, A., Halder, D. (2018). Enhancing grain yield, biomass and nitrogen use efficiency of maize by varying sowing dates and nitrogen rate under rainfed and irrigated conditions. Field Crops Res. 221, 339–349. doi: 10.1016/j.fcr.2017.06.019
Van Der Laan, M., Stirzaker, R., Annandale, J., Bristow, K., Du Preez, C. (2010). Monitoring and modelling draining and resident soil water nitrate concentrations to estimate leaching losses. Agric. Water Manage. 97, 1779–1786. doi: 10.1016/j.agwat.2010.06.012
Wang, J., Gong, S., Xu, D., Yu, Y., Zhao, Y. (2013). Impact of drip and level-basin irrigation on growth and yield of winter wheat in the North China Plain. Irri. Sci. 31, 1025–1037. doi: 10.1007/s00271-012-0384-7
Wang, J., Wang, E., Yang, X., Zhang, F., Yin, H. (2012). Increased yield potential of wheat-maize cropping system in the North China Plain by climate change adaptation. Clim. Change 113, 825–840. doi: 10.1007/s10584-011-0385-1
Wang, H., Wu, L., Wang, X., Zhang, S., Cheng, M., Feng, H., et al. (2021). Optimization of water and fertilizer management improves yield, water, nitrogen, phosphorus and potassium uptake and use efficiency of cotton under drip fertigation. Agric. Water Manage. 245, 106662. doi: 10.1016/j.agwat.2020.106662
Wang, H., Zhang, Y., Chen, A., Liu, H., Zhai, L., Lei, B., et al. (2017). An optimal regional nitrogen application threshold for wheat in the North China Plain considering yield and environmental effects. Field Crops Res. 207, 52–61. doi: 10.1016/j.fcr.2017.03.002
Wolff, M. W., Hopmans, J. W., Stockert, C. M., Burger, M., Sanden, B. L., Smart, D. R. (2017). Effects of drip fertigation frequency and N-source on soil N2O production in almonds. Agric. Ecosys. Environ. 238, 67–77. doi: 10.1016/j.agee.2016.08.001
Xu, K., Chai, Q., Hu, F., Fan, Z., Yin, W. (2021). N-fertilizer postponing application improves dry matter translocation and increases system productivity of wheat/maize intercropping. Sci. Rep. 11, 22825. doi: 10.1038/s41598-021-02345-5
Xu, X., Zhang, M., Li, J., Liu, Z., Zhao, Z., Zhang, Y., et al. (2018). Improving water use efficiency and grain yield of winter wheat by optimizing irrigations in the North China Plain. Field Crops Res. 221, 219–227. doi: 10.1016/j.fcr.2018.02.011
Yan, S., Wu, Y., Fan, J., Zhang, F., Guo, J., Zheng, J., et al. (2022). Quantifying grain yield, protein, nutrient uptake and utilization of winter wheat under various drip fertigation regimes. Agric. Water Manage. 261, 107380. doi: 10.1016/j.agwat.2021.107380
Yang, X.-L., Chen, Y.-Q., Steenhuis, T. S., Pacenka, S., Gao, W.-S., Ma, L., et al. (2017). Mitigating groundwater depletion in North China plain with cropping system that alternate deep and shallow rooted crops. Front. Plant Sci. 8, 980. doi: 10.3389/fpls.2017.00980
Yang, D., Li, S., Kang, S., Du, T., Guo, P., Mao, X., et al. (2020). Effect of drip irrigation on wheat evapotranspiration, soil evaporation and transpiration in Northwest China. Agric. Water Manage. 232, 106001. doi: 10.1016/j.agwat.2020.106001
Yin, X., Kersebaum, K.-C., Beaudoin, N., Constantin, J., Chen, F., Louarn, G., et al. (2020). Uncertainties in simulating N uptake, net N mineralization, soil mineral N and N leaching in European crop rotations using process-based models. Field Crops Res. 255, 107863. doi: 10.1016/j.fcr.2020.107863
Zain, M., Si, Z., Li, S., Gao, Y., Mehmood, F., Rahman, S.-U., et al (2021). The coupled effects of irrigation scheduling and nitrogen fertilization mode on growth, yield and water use efficiency in drip-irrigated winter wheat Sustainability 13, 2742. doi: 10.3390/su13052742
Zhang, Y., Wang, J., Gong, S., Xu, D., Sui, J. (2017). Nitrogen fertigation effect on photosynthesis, grain yield and water use efficiency of winter wheat. Agric. Water Manage. 179, 277–287. doi: 10.1016/j.agwat.2016.08.007
Zhang, Z., Zhang, Y., Shi, Y., Yu, Z. (2020). Optimized split nitrogen fertilizer increase photosynthesis, grain yield, nitrogen use efficiency and water use efficiency under water-saving irrigation. Sci. Rep. 10, 1–14. doi: 10.1038/s41598-020-75388-9
Keywords: drip irrigation, irrigation scheduling, lateral spacing, nitrogen fertilization scheme, winter wheat
Citation: Zain M, Si Z, Ma H, Cheng M, Khan A, Mehmood F, Duan A and Sun C (2023) Developing a tactical irrigation and nitrogen fertilizer management strategy for winter wheat through drip irrigation. Front. Plant Sci. 14:1231294. doi: 10.3389/fpls.2023.1231294
Received: 30 May 2023; Accepted: 21 July 2023;
Published: 10 August 2023.
Edited by:
Xiukang Wang, Yan’an University, ChinaReviewed by:
Peng Zhang, Northwest A&F University, ChinaElsayed Mansour, Zagazig University, Egypt
Muhammad Rizwan, Qatar University, Qatar
Copyright © 2023 Zain, Si, Ma, Cheng, Khan, Mehmood, Duan and Sun. This is an open-access article distributed under the terms of the Creative Commons Attribution License (CC BY). The use, distribution or reproduction in other forums is permitted, provided the original author(s) and the copyright owner(s) are credited and that the original publication in this journal is cited, in accordance with accepted academic practice. No use, distribution or reproduction is permitted which does not comply with these terms.
*Correspondence: Aiwang Duan, duanaiwang@aliyun.com; Chengming Sun, cmsun@yzu.edu.cn
†These authors have contributed equally to this work