- 1State Key Laboratory of Resource Insects, Institute of Sericulture and Systems Biology, Southwest University, Chongqing, China
- 2Resource Institute for Chinese & Ethnic Materia Medica, Guizhou University of Traditional Chinese Medicine, Guiyang, China
- 3The National Engineering Laboratory of Crop Resistance Breeding, School of Life Sciences, Anhui Agricultural University, Hefei, China
The phytohormone abscisic acid (ABA) is vital in regulating root elongation, seed germination, and abiotic stress responses in plants. Conversely, the mechanisms of ABA in mulberry root growth, seed germination, and abiotic stress responses are poorly understood. Here, we reported that exogenous ABA and drought treatment inhibited the growth of mulberry seedlings but significantly increased the ratio of root/stem. Inhibition of ABA synthesis by fluridone and sodium tungstate resulted in the decrease of root/stem ratio. We also showed that the expression of MaNCED1 in the root was strongly induced by drought and salt stress. Increasing the expression of MaNCED1 in tobacco using overexpression leads to increased root elongation and reduced seed germination. Compared with the wild type, the accumulation of H2O2 and MDA was reduced, while the POD activity and proline content was increased in the transgenic plants after drought and salt treatment. Further studies revealed increased resistance to drought and salt stress in MaNCED1 overexpressed tobaccos. Meanwhile, the auxin and ethylene signal pathway-related gene expression levels increased in MaNCED1 overexpressed tobaccos. This study demonstrated the roles of mulberry MaNCED1 in regulating plant development and abiotic stress responses. It gave further insights into the coordinated regulation of ABA, auxin, and ethylene in seed growth and germination.
1 Introduction
Abiotic stress was one of the most critical factors to affect plant development and growth. Every year many crops growing worldwide suffers disasters caused by drought and soil salinity (Golldack et al., 2014; Guo et al., 2014; Zandalinas et al., 2018; Molnár et al., 2021). Currently, the lands under drought and salinity stresses increased continuously. Abiotic stress affects plants’ physiological and biochemical processes (Araújo et al., 2011; Gonzalez-Villagra et al., 2022). Consequently, crop yields were reduced dramatically (Perez-Alfocea et al., 1993; Venkatappa et al., 2021). The plant had developed an effective mechanism to survive in an adverse environment with long-term evolution (Zhu, 2002; Krasensky and Jonak, 2012). Abscisic acid (ABA), as a plant hormone, plays an essential role in regulating plant growth, development, seed germination (Son et al., 2016), fruit ripening (Gan et al., 2020), and stress resistance (Liu et al., 2021; De Oliveira et al., 2022). In stylosanthes guianensis, the enzyme activity of SOD and APX were increased after ABA treatment (Zhou and Guo, 2005). However, the improvement activity of SOD and APX were suppressed after being treated with ABA biosynthesis inhibitor-sodium tungstate. Meanwhile, the contents of endogenous ABA were decreased (Zhou and Guo, 2005). In Zea mays and Oryza sativa, ABA treatment could improve the resistance to cold stress (Anderson et al., 1994; Huang et al., 2021). In addition, studies have found that ABA content is associated with seed dormancy (Wang et al., 2020).
ABA can not only inhibit plant growth but also can restrain seed dormancy. Previous research has shown that mulberry seedlings treated with exogenous ABA can shorten seedling root lengths, and the inhibition was increased along with the ABA concentration (Liu et al., 2014). In Solanum lycopersicum, exogenous ABA treatment inhibits plant growth and reduces lateral root growth, improving the root-to-shoot ratio (Hooker and Thorpe, 1998). The expression levels of some lateral root initiation genes were reduced in seedlings after being treated with the ABA. These genes were increased after auxin induction, indicating that ABA and auxin play antagonism roles in lateral root development (Zhang et al., 2009).
The biosynthesis pathway of ABA is well understood in higher plants. Two possible routes for ABA biosynthesis have been suggested, including direct and indirect ones (Seo and Koshiba, 2002). Studies have revealed that the biosynthesis of ABA in higher plants primarily via an indirect pathway, the synthesis of ABA begins with the production of violaxanthin catalyzed by zeaxanthin epoxidase from C40 carotenoids. Violaxanthin was cleavaged by 9-cis-epoxycarotenoid dioxygenase (NCED) to generate 9-cis-neoxanthin. Finally, 9-cis-neoxanthin was catalyzed by aldehyde oxidase and formed ABA (Seo and Koshiba, 2002; Xiong and Zhu, 2003). NCED is the critical enzyme in ABA synthesis. Treating with an NCED specificity inhibitor, nordihydroguaiaretic acid (NDGA) decreased endogenous ABA contents (Zhang et al., 2009).
Since the first NCED gene was isolated from the maize vp14 mutant (Tan et al., 1997), it has been cloned in various plant species, such as avocado, Arabidopsis thaliana, and Solanum lycopersicum (Burbidge et al., 1999; Chernys and Zeevaart, 2000; Iuchi et al., 2001; Estrada-Melo et al., 2015). In abiotic stress conditions such as salt, drought, and heat, the same up-regulate pattern was found in NCEDs (Xia et al., 2014; Hwang et al., 2018). In cucumber, the expression of CsNCED1 and CsNCED2 was up-regulated on the third day after treatment with exogenous ABA, and the expression of CsNCED1 and CsNCED2 was up-regulated after water stress treatment (Wang et al., 2013). A similar increased expression was found in sweet cherry and Malus domestica after drought treatment (Ren et al., 2010; Kondo et al., 2012). In A. thaliana, drought stress induced the expression of the AtNCED3 gene, which plays a vital role in ABA biosynthesis. Overexpression of the AtNCED3 gene increased ABA content in A. thaliana, promoted the expression of drought- and ABA-induced genes, reduced the transpiration rate, and increased resistance to drought (Iuchi et al., 2001). The plants showed high sensitivity to drought in AtNCED3-silenced transgenic A. thaliana (Iuchi et al., 2001).
Mulberry is an important economic tree in China. The leaves are the main feed of silkworms, and the fruit has high edible and medicinal value for its abundant nutrients, active substances, and good mouthfeel. Additionally, mulberry was also used for ecological control due to its strong ability to resist stresses. ABA is central to regulating plant development and stress tolerance (Xiong and Zhu, 2003). However, the function of MaNCED1 in mulberry development and stress response processes has not been reported to date. In this study, we studied the effects of different treatments on the growth of mulberry seedlings and analyzed the expression of the MaNCED1 gene under various stresses. In addition, the drought and salt stress resistance ability was evaluated in the ectopic expression of MaNCED1 transgenic tobacco.
2 Materials and methods
2.1 Plant material and treatments
Mulberry (Morus atropurpurea Roxb.) variety (Guisangyou 62) was selected as the experimental material. Seeds were soaked in sterile water at 4°C for 24 h, sown in a sterile petri dish lined with moist filter paper, and cultured at 25°C/22°C and 16 h light/8 h dark photoperiod incubator. After 7 days, the seedlings of Guisangyou 62 with uniform growth states were selected and treated with 60 μM fluridone, 100 mg/L ABA, 200 mM mannitol, 1 mM sodium tungstate, and 100 mM NaCl, respectively (Hooker and Thorpe, 1998; Zhang et al., 2009). Materials were collected 5 days after treatment.
To analyze the expression of MaNCED1 under salt and drought conditions, the seedlings germinating for 7 days were selected and treated with 20% (w/v) PEG 6000 and 100 mM NaCl. Then, the seedlings were collected at 0 h, 1 h, 6 h, 12 h, 24 h, and 48 h after treatment. The roots and shoots were immediately separated with a scalpel, frozen in liquid nitrogen, and stored at -80°C for further use.
2.2 Determination of root and stem length of mulberry seedlings after treatment
To observe the effects of ABA, fluridone, sodium tungstate, NaCl, and mannitol treatments on mulberry seedling growth, the root and stem lengths of the seedlings treated for 5 days were measured by a ruler.
2.3 Cloning and bioinformatics analysis of MaNCED1 gene
According to the manufacturer’s procedures (Invitrogen, Carlsbad, CA, USA), total RNA was extracted from mulberry seedlings and tobacco. The total RNA was used as a template to synthesize the first strand of cDNA using PrimeScript™ RT Reagent Kit (Takara Bio., Shiga, Japan). The primers were designed according to the sequence obtained from the M. notabilis genome database ((http://morus.swu.edu.cn/morusdb/). The complete MaNCED1 gene coding sequence was obtained from Guisangyou 62 cDNA. Using the deduced amino acid sequences of MaNCED1 as queries to search in National Center for Biotechnology Information and obtained homolog amino acid sequences from other plant species (http://blast.ncbi.nlm.nih.gov/Blast.cgi). Multiple sequence alignment was performed using ClustalX software. A MEGA 4.0 software was used to construct the phylogenetic tree with a neighbour-joining method.
2.4 Plasmid construction and plant transformation
The full-length coding sequence of MaNCED1 from mulberry was cloned into the pLGNL expression vector by KpnI and SpeI restriction enzyme. Then, the recombinant plasmid was transformed into Agrobacterium tumefaciens strain LBA4404. The positive A. tumefaciens harbouring the MaNCED1 plasmid was transformed into tobacco (K326) plants using a leaf disk co-cultivation method (Li, 2011). Positive transgenic tobacco was confirmed by target gene and kanamycin resistance gene PCR assay and β-D-glucosidase (GUS) staining. Additionally, the expression levels of MaNCED1 in transgenic tobacco plants were detected by real-time quantitative reverse transcription PCR (qRT-PCR) analysis. The endogenous ABA content was determined as described by Zhu et al. (2017).
2.5 Determination of germination rate and growth analysis of transgenic tobacco
The collected WT and T2 generation transgenic seeds were germinated on a petri dish covered with absorbent cotton and filter paper. The germination rates were statistics at 3 days, 4 days, and 5 days, respectively. To observe the effects of overexpressed MaNCED1 on tobacco growth, the seedlings were incubated in a petri dish for 10 days, and the root length, stem length, and ratio of root/stem were counted.
2.6 Stress treatment of transgenic tobacco
The five-week-old WT and transgenic seedlings were chosen and independently irrigated with 30% PEG and 200 mM NaCl for 14 d. Each treatment was replicated three times. After the stress treatment, 1 g of treated tobacco leaves were collected. According to the manufacturer’s instructions, the malonaldehyde (MDA), H2O2, proline contents, and peroxidase (POD) activity were measured using test kits (Jiancheng Bioengineering Institute, Nanjing, China). Additionally, the expression levels of NtSOD and NtCAT in PEG and NaCl-treated plants were analyzed by qRT-PCR.
2.7 Quantitative real-time PCR analysis
The specific primers were designed using NCBI Primer-BLAST (https://www.ncbi.nlm.nih.gov/tools/primer-blast/index.cgi) (Supplementary Table 1). The treated mulberry and tobacco cDNA served as the qRT-PCR template. qRT-PCR analysis was performed according to the instructions of the SYBR Green Reagent Kit (Takara Bio.). The test was performed on a StepOnePlus real-time PCR machine (ABI Company). MaACTIN3 (HQ163776) and NtActin (U60489) were used as the internal control for mulberry and tobacco. The relative expression was calculated using the 2-ΔΔCt method.
2.8 Statistical analyses
All the experiments in this study were repeated at least three times. The results were collected and calculated by Excel 2013 (Microsoft, Redmond, WA, USA). The final results are shown as means ± standard deviations (SD). Statistical analysis was performed by SPSS Statistics 17.0 software (SPSS Inc., Chicago, IL, USA). The graphs were created using GraphPad Prism 5 software (GraphPad Software Inc., La Jolla, CA, USA).
3 Results
3.1 Effects of ABA, fluridone, sodium tungstate, mannitol, and NaCl on the growth of mulberry seedlings
The growth of the seedlings treated with ABA, fluridone, sodium tungstate, mannitol, and NaCl was inhibited in varying degrees, mainly manifested in the reduction of the average length of roots and stems compared with the control (Figure 1). However, the ratio of root/stem was significantly increased under mannitol and exogenous ABA treatments (Figure 1D). In contrast, the ratio of root/stem was decreased considerably after sodium tungstate, fluridone, and NaCl treatment (Figures 1D, I). We further examined the changes in gene expression of MaNCED1 under different treatments. As shown in Figures 1E, J, the expression of MaNCED1 increased significantly after mannitol and NaCl treatment and was only slightly induced after ABA treatment. Notably, treated with the ABA synthesis inhibitors, fluridone and sodium tungstate significantly reduced MaNCED1 expression (Figure 1J).
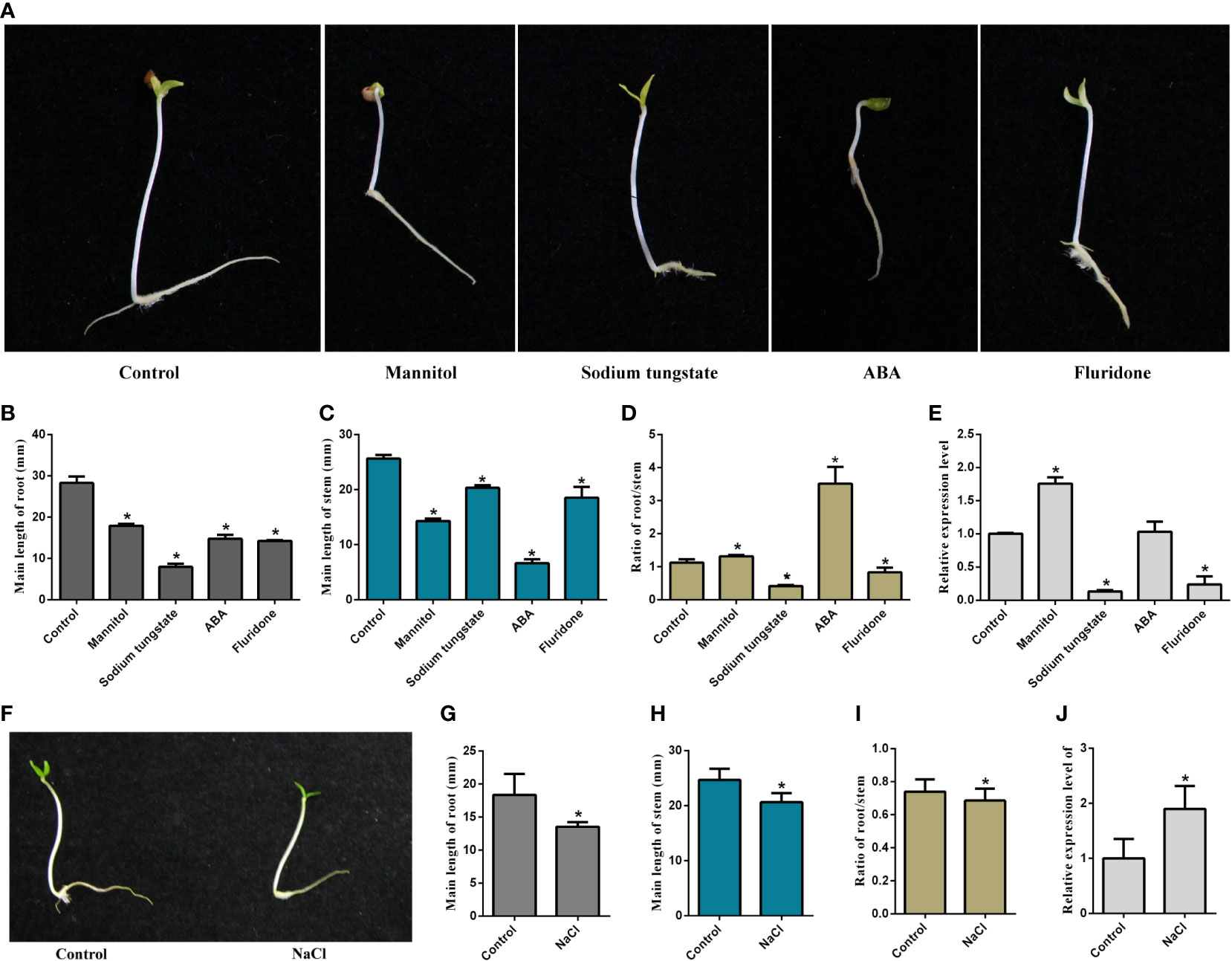
Figure 1 Effects of various reagents on mulberry seedlings growth. (A, F) Phenotypes change of seeding after being treated with mannitol, sodium tungstate, ABA, fluridone, ddH2O, and NaCl for 5 days; (B, G) Root length of seedlings after treatments; (C, H) Stem length of seedlings after treatments; (D, I) Ratio of root/stem of seedlings after treatments; (E, J) Expression analysis of MaNCED1 gene in root after treatments. The data were indicated as mean ± SD from three replications (n=30). *p < 0.05.
In order to understand whether the MaNCED1 gene is involved in abiotic stress response, we detected the expression of MaNCED1 after PEG and NaCl treatment. The expression level of MaNCED1 decreased at 1 h in shoot after PEG and NaCl treatment and then up-regulation at 12 h and 24 h (Figures 2A, B). In the root, the transcript of MaNCED1 was up-regulated by PEG and NaCl treatment and peaked at 24 h (Figures 2C, D). These results indicated that MaNCED1 responded to drought and salt stress, and its expression was strongly induced in the root.
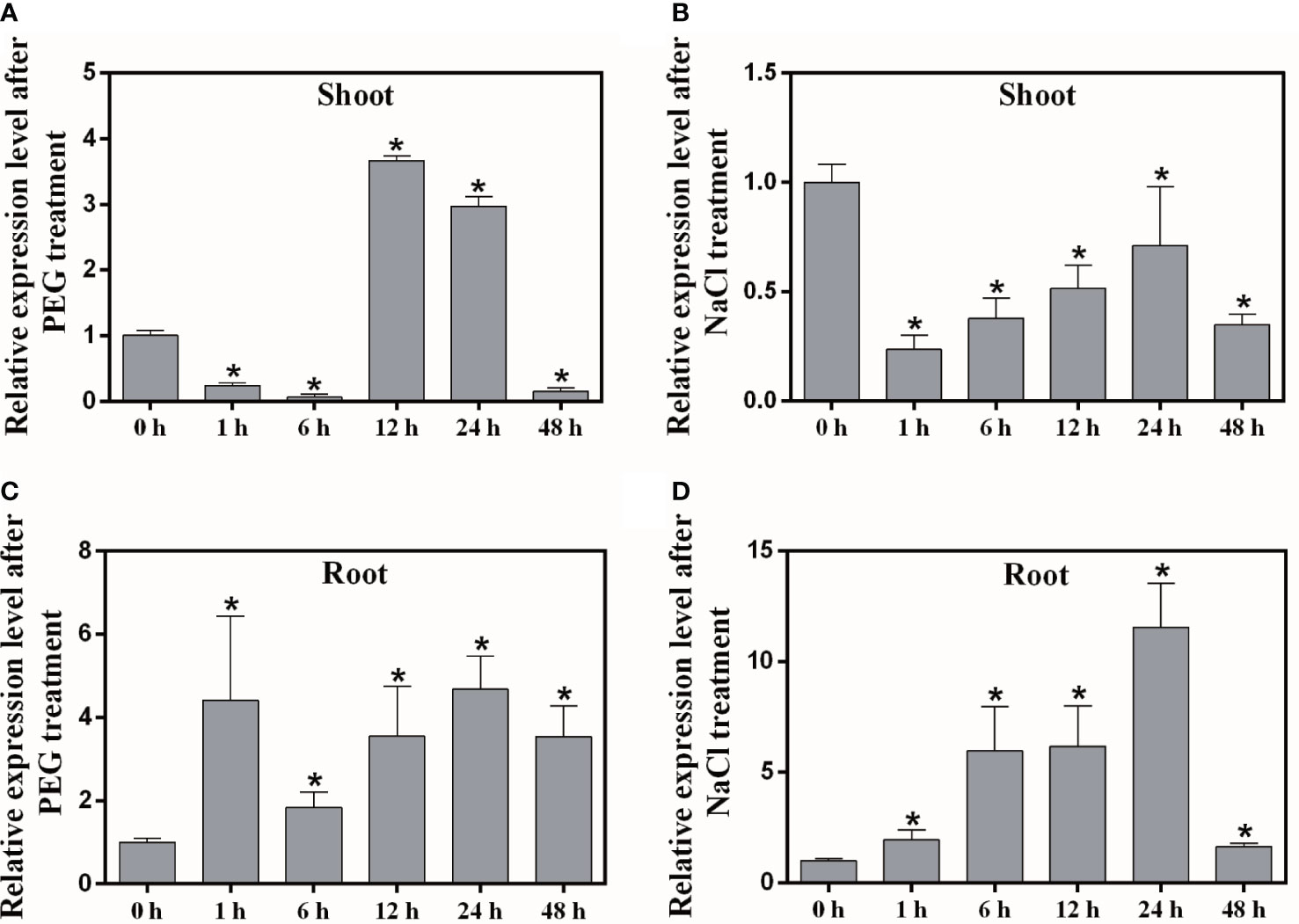
Figure 2 Expression analysis of MaNCED1 gene in mulberry shoots and roots after PEG and NaCl treatments. (A, B) Transcription levels of MaNCED1 gene in shoot after PEG and NaCl treatment for 0 h, 1 h, 6 h, 12 h, 24 h, and 48 h, respectively. (C, D) Transcription levels of MaNCED1 gene in root after PEG and NaCl treatment for 0 h, 1 h, 6 h, 12 h, 24 h, and 48 h, respectively. The data were indicated as mean ± SD from three replications. Significant differences are marked with an asterisk (p < 0.05).
3.2 Phylogenetic and sequence alignment analysis of MaNCED1
The full-length sequence of the MaNCED1 (GenBank accession number: KX181538.1) gene was obtained by amplification in the cDNA of Guisangyou 62. To examine the evolutionary relationship between MaNCED1 and other plant NCED proteins, we constructed a phylogenetic tree using MEGA 4.0 software. The results showed that these plant NCED proteins were classified into two main groups. The MaNCED1 is closely related to the NCEDs from Malus domestican, Rosa chinensis, and Solanum lycopersicum and belongs to dicotyledonous plants. The monocotyledonous plants such as Z. mays, O. sativa, Sorghum bicolor, Setaria italic, and Triticum aestirum were classified into the second group (Supplementary Figure 1). We further compared the amino acid sequences of MaNCED1 with other NCEDs. The amino acid sequence of MaNCED1 shared 76.92% identity with Citrus sinensis, 71.09% identity with Solanum lycopersicum, and 73.95% identity with Malus domestica. These four putative NCED proteins showed high similarity to each other and the same conserved structural domain (Supplementary Figure 2).
3.3 Determination of transgenic tobacco plants
To evaluate the roles of the MaNCED1 gene in plant tolerance to drought and salt stress, the full-length sequence was cloned into the pLGNL expression vector and transformed into wild-type (WT) tobacco plants. Eventually, three independent transgenic plants were obtained using GUS staining and qRT-PCR analyses (Figures 3A-D). The expression level of MaNCED1 increased significantly in transgenic plants and was 475.5 folds, 31.9 folds, and 158.3 folds higher than that of WT, respectively (Figure 3D). Meanwhile, the content of ABA in MaNCED1 overexpressing plants was significantly higher than in WT, indicating that MaNCED1 was overexpressed in tobacco successfully (Figure 3C).
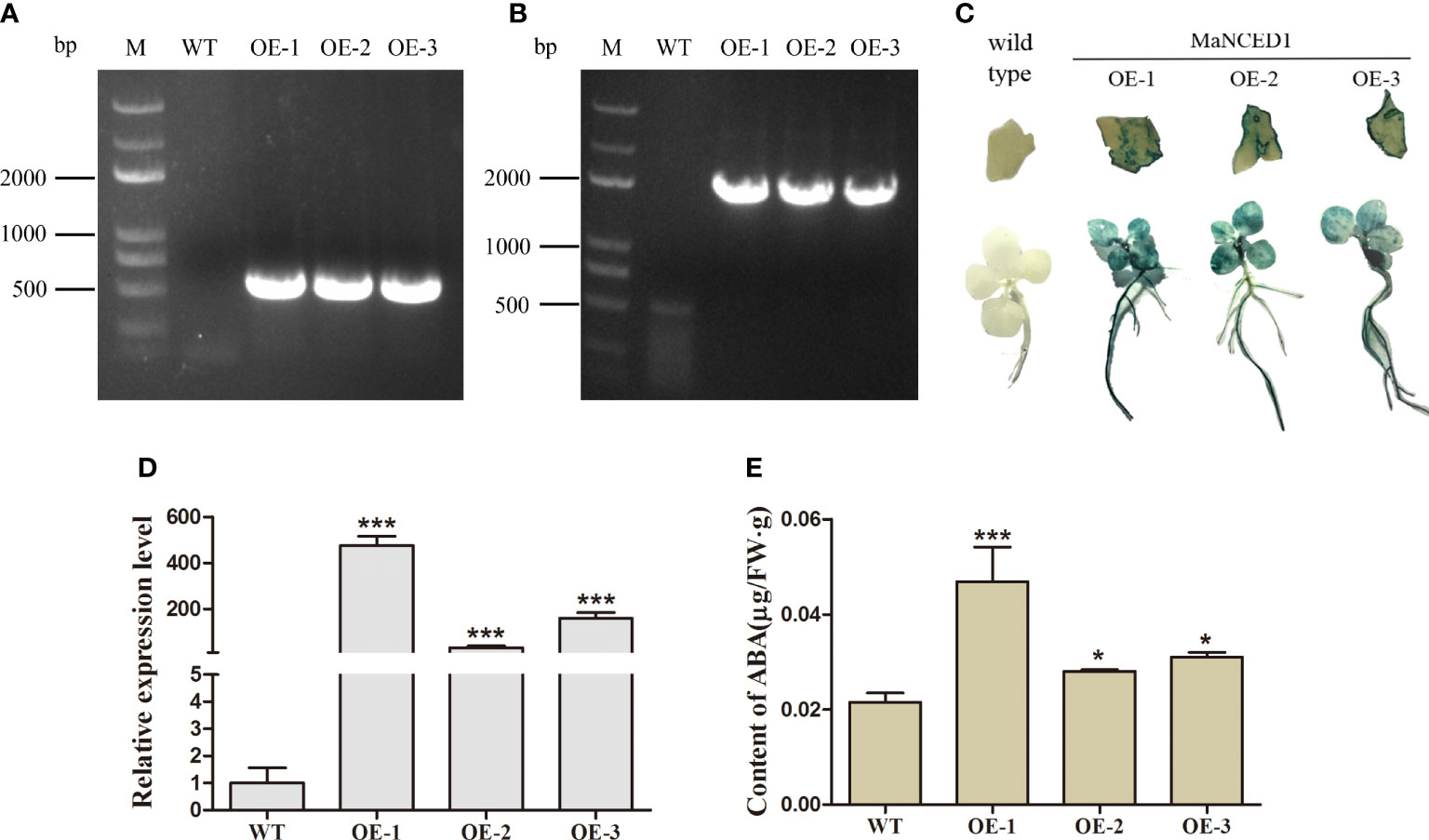
Figure 3 Confirmation of transgenic tobacco plants. (A) Amplification of the kanamycin resistance gene and (B) MaNCED1 from the genome of transgenic plants. (C) Histochemical GUS staining of transgenic lines. (D) Quantitative real-time PCR analysis of MaNCED1. (E) ABA contents in WT and MaNCED1 overexpressing tobaccos. Data represent the means ± SD (n = 3), *p < 0.05, ***p < 0.001.
3.4 Overexpression of MaNCED1 reduces tobacco germination rate and promotes root growth
To determine whether MaNCED1 is involved in regulating seed germination, the germination rates of wild-type and transgenic lines were counted. The germination rates of transgenic tobacco were significantly lower than that of wild-type (Figure 4). Furthermore, we calculated the root and stem lengths of the tobacco seedlings. Compared with the wild type, the root length of transgenic tobacco increased significantly (Figures 5A, B). However, the stem length of transgenic plants showed no apparent changes compared with WT (Figure 5C). It is worth noting that the ratio of root and stem was significantly increased compared with WT (Figure 5D).
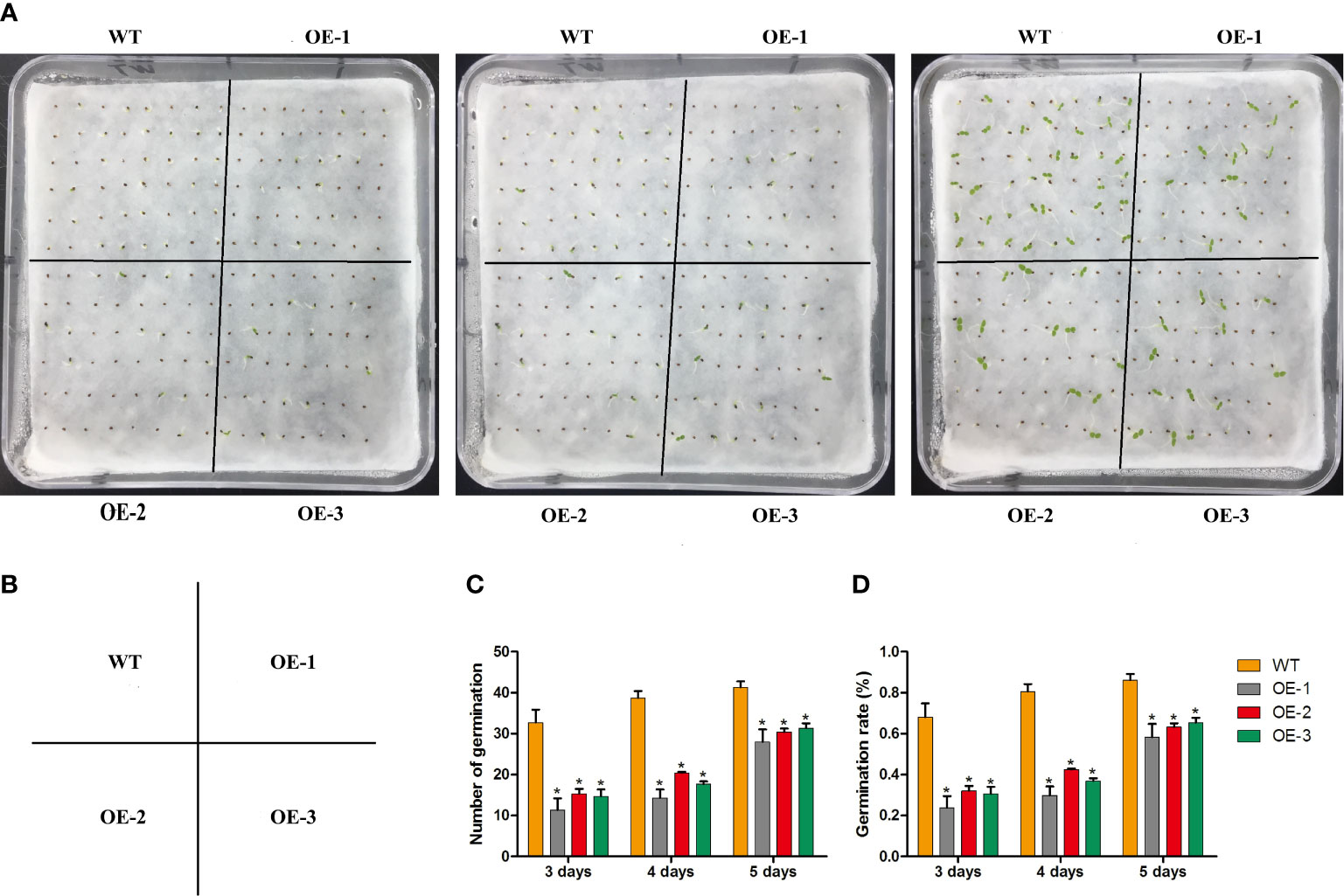
Figure 4 Statistical analysis of seed germination rates of WT and MaNCED1 overexpression plants. (A) The germination phenotype of WT and MaNCED1 overexpression plants. (B) The distribution diagram of WT and MaNCED1 overexpression plants. (C) The number of germinated seeds and (D) seed germination rates of WT and MaNCED1 overexpression plants. Seeds were germinated on filter paper soaked with sterile water, and the numbers of germinated seeds were counted at 3 days, 4 days, and 5 days after sowing. Data represent the means ± SD of three biological repetitions (n=40), *p < 0.05.
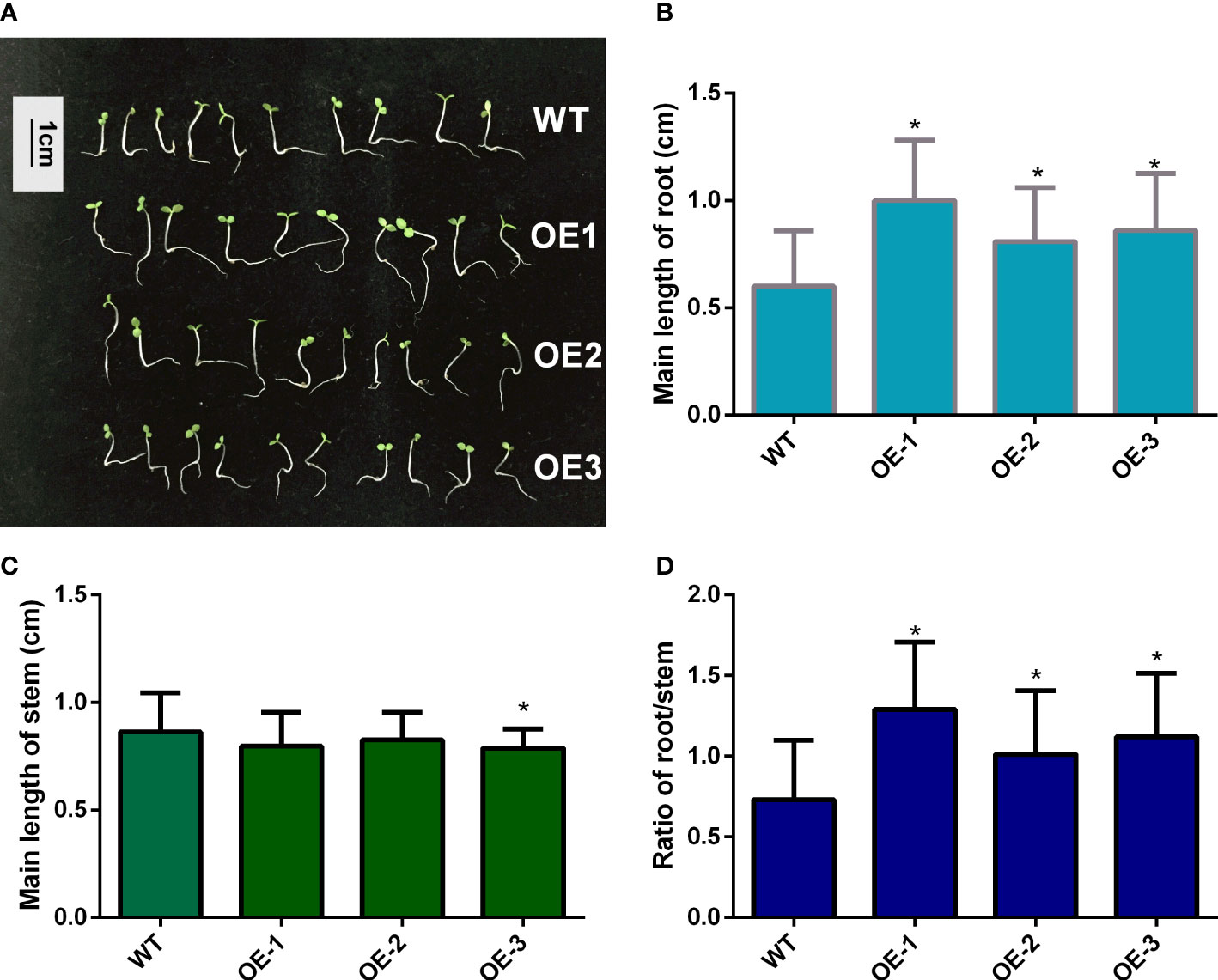
Figure 5 Root and stem length statistics of transgenic tobacco and WT seedlings. (A) The phenotype of WT and MaNCED1 overexpression plants; (B) Root and (C) stem length of WT and MaNCED1 overexpression seedlings; (D) Ratio of root/stem of WT and MaNCED1 overexpression seedlings. Seeds germinated on filter paper soaked with sterile water, and the root and stem length were counted 10 days after sowing. Data represent the means ± SD of three biological repetitions (n=10), *p < 0.05.
3.5 The overexpression of MaNCED1 increases plant tolerance to drought and salt stress
To visualize the relationship between MaNCED1 genes and plant stress resistance, five-week-old WT and two transgenic lines (highest and lowerest expressed of MaNCED1) were treated with 200 mM NaCl and 30% polyethene glycol (PEG) for 14 d. As shown in Figure 6A, the growth of MaNCED1 overexpression plants is significantly better than that of the wild type (Figure 6A). These results suggested that overexpression of MaNCED1 improved tobacco tolerance to drought and salt stresses. H2O2, proline, superoxide dismutase (SOD), POD, catalase (CAT), ascorbate peroxidase (APX), and MDA are the key enzymes involved in the scavenging of reactive oxygen species and improving plant resistance under stress conditions (Conde et al., 2011; Hai et al., 2022). The accumulation of H2O2 and MDA was reduced in MaNCED1 overexpression plants compared with WT. At the same time, the POD activity and proline content was increased in the transgenic plants after drought and salt treatment (Figures 6B-G). Additionally, the expression levels of the superoxide dismutase encoding gene (NtSOD) and catalase encoding gene (NtCAT) in MaNCED1 overexpressing tobacco were significantly increased after NaCl or PEG treatment (Figure 7).
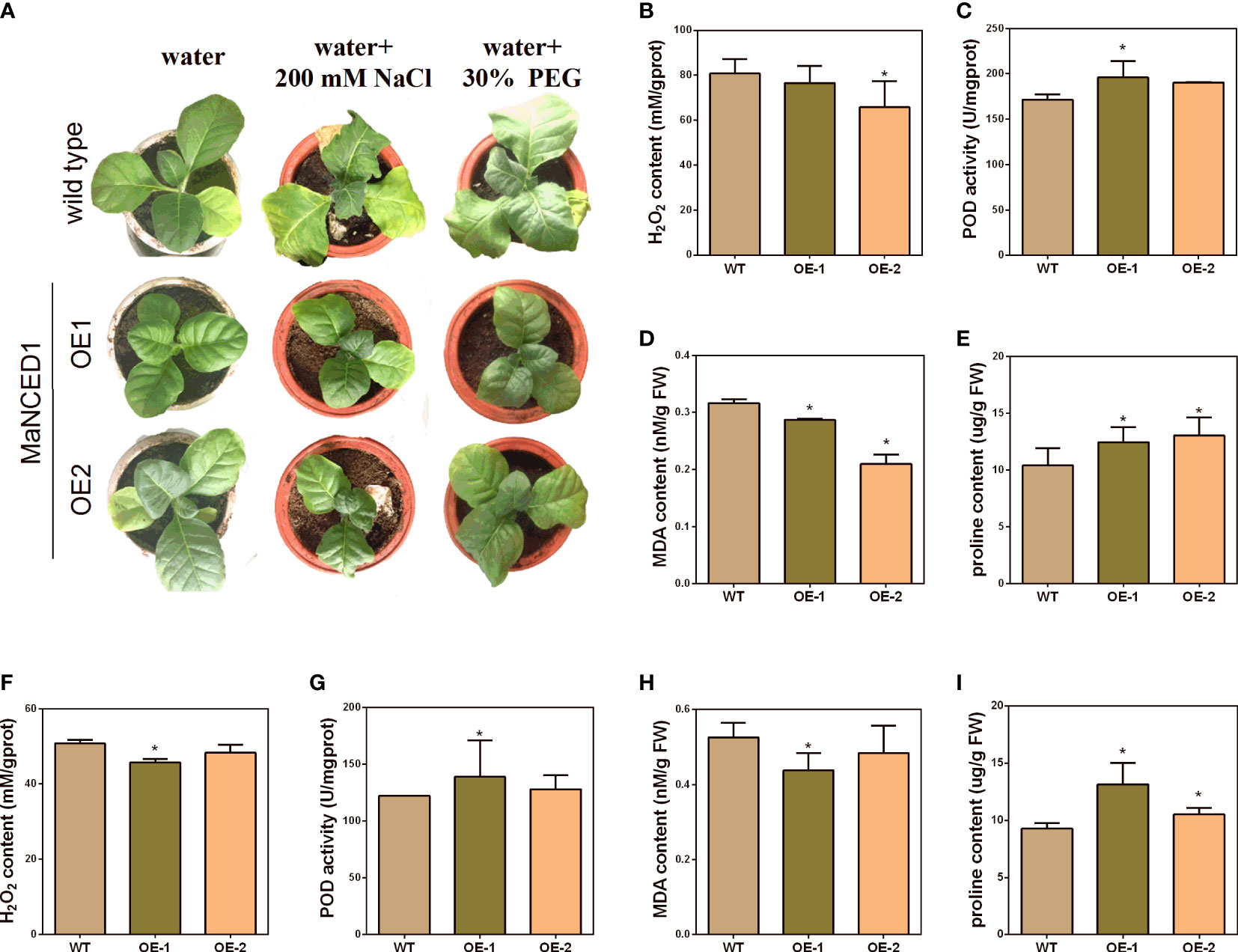
Figure 6 Stress tolerance analyses of MaNCED1 overexpressed tobacco plants. (A) The growth of transgenic tobacco and WT plants under stress conditions. (B) The H2O2 content, (C) POD activity, (D) MDA content, and (E) proline content in MaNCED1 overexpressed and WT plants under salt stress. (F) The H2O2 content, (G) POD activity, (H) MDA content, and (I) proline content in MaNCED1 overexpressed and WT plants under drought stress. Data represent the means ± SD of three biological repetitions, *p < 0.05.
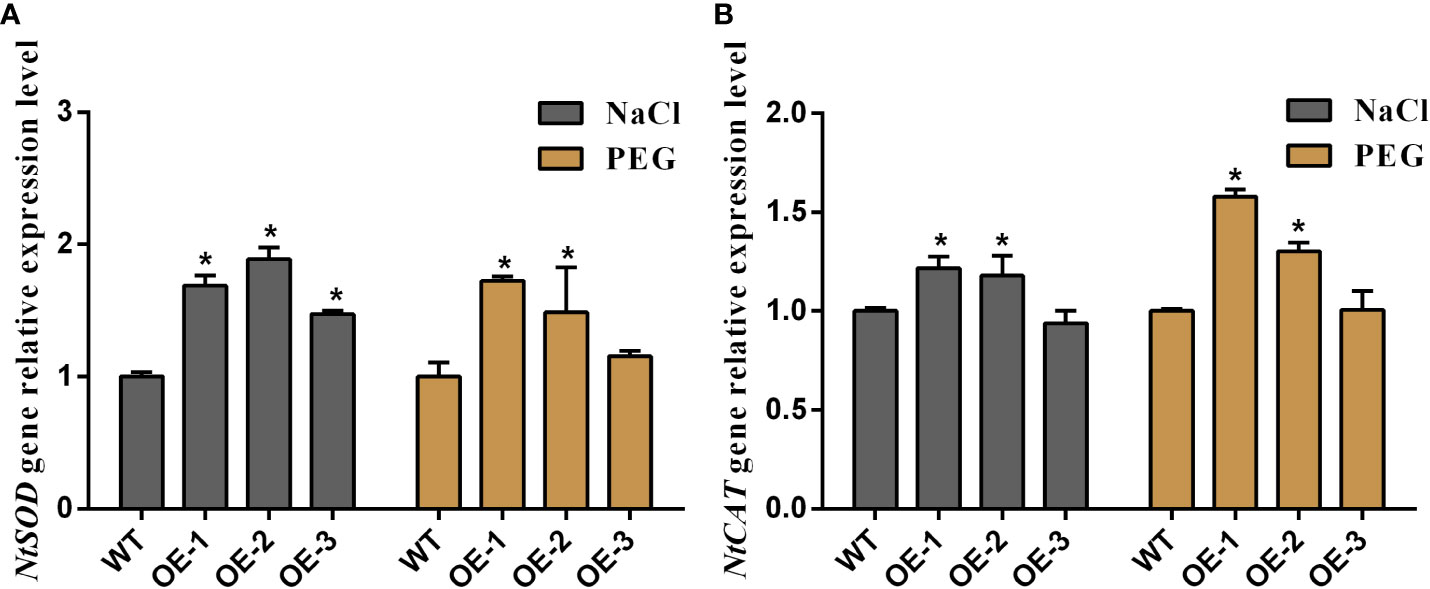
Figure 7 Relative expression levels of (A) NtSOD and (B) NtCAT genes in transgenic tobacco and WT plants after NaCl and PEG treatment, respectively. Data represent the means ± SD of three biological repetitions, *p < 0.05.
3.6 Overexpression of MaNCED1 alters the expression of genes involved in seed germination and root growth in tobacco
As shown in Figure 8, four auxin transporter-like protein genes (NtLAX1, NtLAX2, NtLAX3, and NtLAX4), one plastidal glycolate/glycerate translocator gene (NtPLGG1), and one ethylene insensitive 2 (NtEIN2) gene which involved in the regulation of seed germination and root growth were selected for analysis. Compared with WT, the expression levels of NtAUX1, NtAUX2, NtAUX3, NtAUX4, and NtEIN2 genes in transgenic tobaccos were increased, while the expression of NtPLGG1 was slightly decreased (Figure 8). Thus, MaNCED1 might regulate the growth and seed germination by affecting the expression of auxin and ethylene signalling related genes in mulberry.
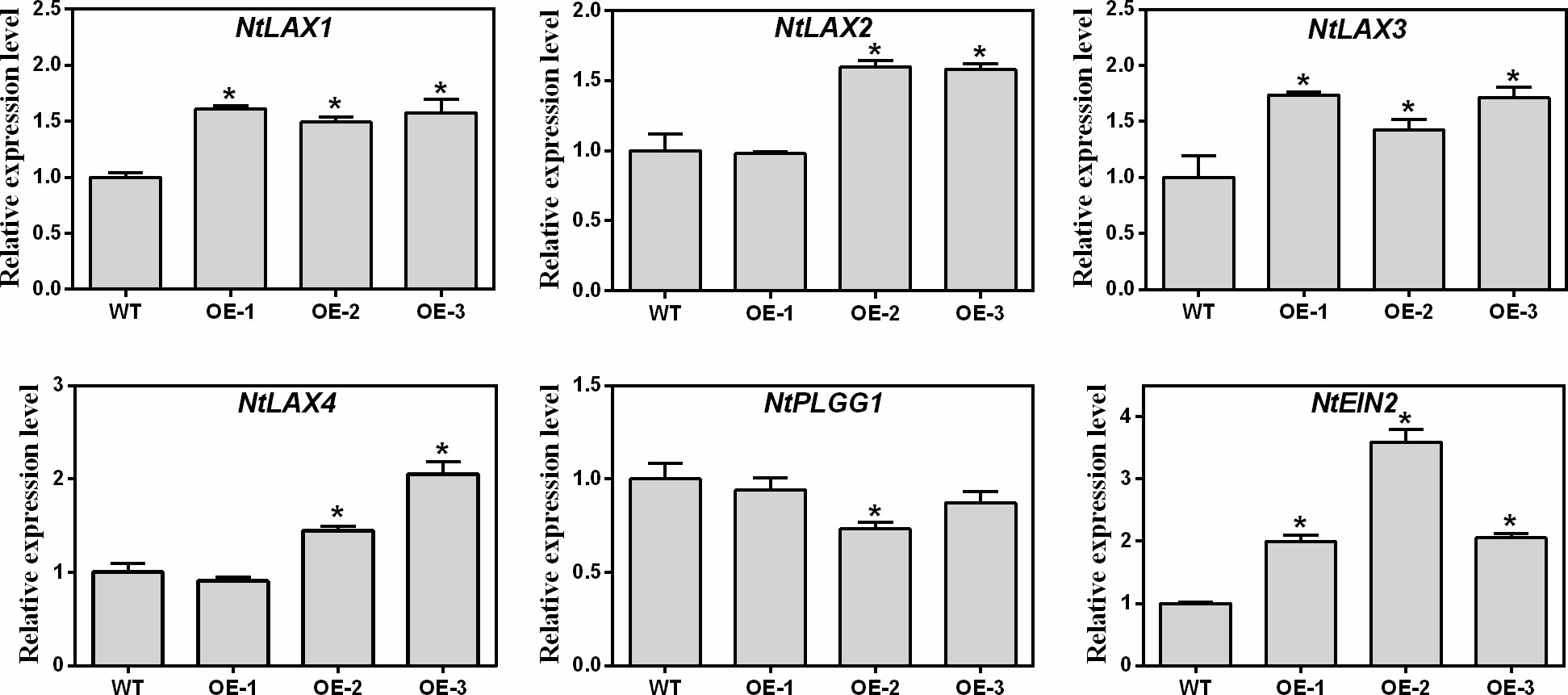
Figure 8 Relative expression levels of genes related to seed germination and root growth in transgenic and WT tobacco. Data represent the means ± SD of three biological repetitions, *p < 0.05.
4 Discussion
Salinity and drought are the major abiotic stress influencing the productivity and quality of crops worldwide (Perez-Alfocea et al., 1993; Araújo et al., 2011; Venkatappa et al., 2021; Gonzalez-Villagra et al., 2022). So, it is urgent to characterize salt- and drought-tolerant mechanisms that can be used to develop salt and drought-tolerant crops. As an important plant hormone, ABA regulates plant growth and development, seed germination, and stress resistance (Zhu, 2002; Son et al., 2016). In Arabidopsis, the primary root growth was suppressed by ABA treatment (Kim et al., 2016). However, foliage-derived ABA was found to promote root growth and inhibit the development of lateral roots (McAdam et al., 2016). The birch cultured on a medium supplement with ABA increased root length and lateral root number without adversely affecting shoot growth or adventitious root formation (Vaičiukynė et al., 2019). In this study, mulberry seedlings treated with ABA and mannitol significantly increased the ratio of root length to stem length. At the same time, ABA synthesis inhibitors (fluridone and sodium tungstate) and NaCl treatment reduced the root/shoot ratio. However, root and stem growth was inhibited in all these treatments. Longer root length is vital for a plant’s survival from drought stress, allowing the plant to absorb more water. In previous studies, exogenous ABA treatment caused stomatal closure and improved plant tolerance to drought (Li et al., 2020). Overexpression of GmCAMTA12 promoted root growth under drought conditions and enhanced drought tolerance in Arabidopsis and Soybeans (Noman et al., 2019). This result suggests that ABA regulates mulberry root growth and enhances drought resistance by increasing the root/shoot ratio.
NCED is the key rate-limiting enzyme in the ABA biosynthetic pathway (Huang et al., 2018). Silencing of NCED inhibited ABA biosynthesis, reducing ABA accumulation in plants (Gan et al., 2020). In this study, drought and salinity treatment strongly induced the expression of MaNCED1 in the root. Overexpression of MaNCED1 in tobacco significantly increased ABA content and improved drought and salt stress tolerance. Similar results have been found in rice and Arabidopsis (Iuchi et al., 2001; Estrada-Melo et al., 2015; Hwang et al., 2018). Reactive oxygen species (ROS), indispensable for plant growth and development, are also active in plant resistance to biotic or abiotic stress within limiting and normal concentrations (Choudhury et al., 2017). On the contrary, excessive ROS concentrations can lead to plant cell damage or cell death once the concentrations exceed the scavenging capacity of the plant’s antioxidant system. Various enzymes are involved in scavenging reactive oxygen species and improving plant resistance under stress conditions, such as H2O2, proline, SOD, POD, CAT, APX, and MDA. They are usually used as indicative parameters for evaluating the oxidative damage of plants under drought and salt stresses (Conde et al., 2011). In this study, overexpressed MaNCED1 reduced the accumulation of H2O2 and MDA under drought and salt stress compared with WT. At the same time, the POD activity and proline content was increased in the transgenic plants, suggesting the more comprehensive protection of the transgenic lines from oxidative stress and cell damage. Furthermore, the expression levels of NtSOD and NtCAT were significantly increased compared with WT, indicating that overexpressing of MaNCED1 enhanced the tolerance of tobacco to abiotic stresses. Similar results were found in Arabidopsis, Malus, and rice (Iuchi et al., 2001; Huang et al., 2018; Hwang et al., 2018).
Dormancy is a crucial process allowing plants to adapt to changing conditions and enables plants to survive under adverse environmental conditions and sustain the species (Wang et al., 2011; Shu et al., 2013). ABA is an important inhibitor during seed germination (Shu et al., 2013). Overexpression of NCED promotes ABA accumulation and delays seed germination (Wang et al., 2011), which is consistent with the results of this study. Previous reports indicate that ABA controls root elongation by regulating auxin biosynthesis, distribution and transport (Chen et al., 2016; Fei et al., 2023). High concentrations of exogenous auxin inhibit seed germination in A. thaliana, while low concentrations promote seed germination (He et al., 2012). Auxin is required for ABA-mediated inhibition of seed germination, and its deficient mutants show increased resistance to ABA (Thole et al., 2014). Additionally, auxin negatively regulates seed germination and positively regulates seed dormancy (Liu et al., 2013). In this study, MaNCED1 overexpression leads to increased expression of auxin transporter-like protein genes (NtLAX1-4) in tobacco. These results suggested that auxin may coordinate with ABA to inhibit the germination of mulberry seedlings. In addition, evidence indicates that ABA affects root growth and germination and stress response by activating ethylene biosynthesis (Luo et al., 2014). Overexpressing MaNCED1 in tobacco increases the expression level of NtEIN2, implying the crosstalk between ABA and ethylene in regulating plant growth and stress tolerance. PLGG1 encodes a chloroplast protein involved in ABA-inhibited seed germination and drought tolerance (Dong et al., 2018). However, only a slight reduction of NtPLGG1 was found in transgenic plants. All these results indicate that NCED perhaps affects plant growth and stress response by regulating ethylene and auxin signals.
In conclusion, our results suggest that ABA play an important role in mulberry seedling’s growth. Exogenous ABA treatment inhibited seedling growth but significantly increased the root/stem ratio. Overexpressing MaNCED1 in tobacco promoted root elongation, inhibited seedings germination and improved salt and drought stress tolerance. ABA might interact with ethylene and auxin to regulate the seed’s germination and abiotic stress tolerance. The present study will provide insights into the functions of NCED from mulberry and other plants in root development and abiotic stress tolerances.
Data availability statement
The datasets presented in this study can be found in online repositories. The names of the repository/repositories and accession number(s) can be found in the article/Supplementary material.
Author contributions
PZ, CW, and AZ conceived and designed the experiments, PZ, RL, WF, and ZX performed the experiments, PZ, RL, JL, CW, and AZ writing and editing the article. All authors contributed to the article and approved the submitted version.
Funding
This work was supported by the Hainan Province Science and Technology Special Fund (grant number ZDYF2022SHFZ319), the State Key Laboratory of Silkworm Genome Biology (sklsgb-2019KF09) and the Earmarked Fund for CARS (CARS-18-ZJ0201).
Conflict of interest
The authors declare that the research was conducted in the absence of any commercial or financial relationships that could be construed as a potential conflict of interest.
Publisher’s note
All claims expressed in this article are solely those of the authors and do not necessarily represent those of their affiliated organizations, or those of the publisher, the editors and the reviewers. Any product that may be evaluated in this article, or claim that may be made by its manufacturer, is not guaranteed or endorsed by the publisher.
Supplementary material
The Supplementary Material for this article can be found online at: https://www.frontiersin.org/articles/10.3389/fpls.2023.1228902/full#supplementary-material
References
Anderson, M. D., Prasad, T. K., Martin, B. A., Stewart, C. R. (1994). Differential gene expression in chilling-acclimated maize seedlings and evidence for the involvement of abscisic acid in chilling tolerance. Plant Physiol. 105, 331–339. doi: 10.1104/pp.105.1.331
Araújo, W. L., Tohge, T., Ishizaki, K., Leaver, C. J., Fernie, A. R. (2011). Protein degradation - an alternative respiratory substrate for stressed plants. Trends Plant Sci. 16, 489–498. doi: 10.1016/j.tplants.2011.05.008
Burbidge, A., Grieve, T. M., Jackson, A., Thompson, A., McCarty, D. R., Taylor, I. B. (1999). Characterization of the ABA-deficient tomato mutant notabilis and its relationship with maize Vp14. Plant J. 17, 427–431. doi: 10.1046/j.1365-313x.1999.00386.x
Chen, B., Ma, J., Xu, Z., Wang, X. (2016). Abscisic acid and ethephon regulation of cellulase in the endosperm cap and radicle during lettuce seed germination. J. Integr. Plant Biol. 58, 859–869. doi: 10.1111/jipb.12479
Chernys, J. T., Zeevaart, J. A. (2000). Characterization of the 9-cis-epoxycarotenoid dioxygenase gene family and the regulation of abscisic acid biosynthesis in avocado. Plant Physiol. 124, 343–353. doi: 10.1104/pp.124.1.343
Choudhury, F. K., Rivero, R. M., Blumwald, E., Mittler, R. (2017). Reactive oxygen species, abiotic stress and stress combination. Plant J. 90, 856–867. doi: 10.1111/tpj.13299
Conde, A., Chaves, M. M., Geros, H. (2011). Membrane transport, sensing and signaling in plant adaptation to environmental stress. Plant Cell Physiol. 52, 1583–1602. doi: 10.1093/pcp/pcr107
De Oliveira, F. K., Da-Silva, C. J., Garcia, N., Agualongo, D., De Oliveira, A. C. B., Kanamori, N., et al. (2022). The overexpression of NCED results in waterlogging sensitivity in soybean. Plant Stress 3, 100047. doi: 10.1016/j.stress.2021.100047
Dong, H., Bai, L., Chang, J., Song, C. P. (2018). Chloroplast protein PLGG1 is involved in abscisic acid-regulated lateral root development and stomatal movement in Arabidopsis. Biochem. Biophys. Res. Commun. 495, 280–285. doi: 10.1016/j.bbrc.2017.10.113
Estrada-Melo, A. C., Ma, C., Reid, M. S., Jiang, C. Z. (2015). Overexpression of an ABA biosynthesis gene using a stress-inducible promoter enhances drought resistance in petunia. Hortic. Res. 2, 15013. doi: 10.1038/hortres.2015.13
Fei, R., Guan, S., Duan, S., Ge, J., Sun, T., Sun, X. (2023). Elucidating biological functions of 9-cis-Epoxycarotenoid Dioxygenase genes involved in seed dormancy in Paeonia lactiflora. Plants (Basel) 12, 710. doi: 10.3390/plants12040710
Gan, Z., Shan, N., Fei, L., Wan, C., Chen, J. (2020). Isolation of the 9-cis-epoxycarotenoid dioxygenase (NCED) gene from kiwifruit and its effects on postharvest softening and ripening. Scientia Hortic. 261, 109020. doi: 10.1016/j.scienta.2019.109020
Golldack, D., Li, C., Mohan, H., Probst, N. (2014). Tolerance to drought and salt stress in plants: Unraveling the signaling networks. Front. Plant Sci. 5. doi: 10.3389/fpls.2014.00151
González-Villagra, J., Omena-Garcia, R. P., Rodrigues-Salvador, A., Nunes-Nesi, A., Cohen, J. D., Reyes-Díaz, M. M. (2022). Differential physiological and metabolic responses in young and fully expanded leaves of Aristotelia chilensis plants subjected to drought stress. Environ. Exp. Bot. 196, 104814. doi: 10.1016/j.envexpbot.2022.104814
Guo, J., Ling, H., Wu, Q., Xu, L., Que, Y. (2014). The choice of reference genes for assessing gene expression in sugarcane under salinity and drought stresses. Sci. Rep. 4, 7042. doi: 10.1038/srep07042
Hai, X., Mi, J., Zhao, B., Zhang, B., Zhao, Z., Liu, J. (2022). Foliar application of spermidine reduced the negative effects of salt stress on oat seedlings. Front. Plant Sci. 13. doi: 10.3389/fpls.2022.846280
He, J., Duan, Y., Hua, D., Fan, G., Wang, L., Liu, Y., et al. (2012). DEXH box RNA helicase-mediated mitochondrial reactive oxygen species production in Arabidopsis mediates crosstalk between abscisic acid and auxin signaling. Plant Cell 24, 1815–1833. doi: 10.1105/tpc.112.098707
Hooker, T. S., Thorpe, T. A. (1998). Effects of fluridone and abscisic acid on lateral root initiation and root elongation of excised tomato roots culturedin vitro. Plant Cell Tissue Organ Culture 52, 199–203. doi: 10.1023/A:1006033430898
Huang, Y., Guo, Y., Liu, Y., Zhang, F., Wang, Z., Wang, H., et al. (2018). 9-cis-epoxycarotenoid dioxygenase 3 regulates plant growth and enhances multi-abiotic stress tolerance in rice. Front. Plant Sci. 9. doi: 10.3389/fpls.2018.00162
Huang, S., Hu, L., Zhang, S., Zhang, M., Jiang, W., Wu, T., et al. (2021). Rice OsWRKY50 mediates ABA-dependent seed germination and seedling growth, and ABA-independent salt stress tolerance. Int. J. Mol. Sci. 22, 8625. doi: 10.3390/ijms22168625
Hwang, S. G., Lee, C. Y., Tseng, C. S. (2018). Heterologous expression of rice 9-cis-epoxycarotenoid dioxygenase 4 (OsNCED4) in Arabidopsis confers sugar oversensitivity and drought tolerance. Bot. Stud. 59, 2. doi: 10.1186/s40529-018-0219-9
Iuchi, S., Kobayashi, M., Taji, T., Naramoto, M., Seki, M., Kato, T., et al. (2001). Regulation of drought tolerance by gene manipulation of 9-cis-epoxycarotenoid dioxygenase, a key enzyme in abscisic acid biosynthesis in Arabidopsis. Plant J. 27, 325–333. doi: 10.1046/j.1365-313x.2001.01096.x
Kim, D., Ntui, V. O., Xiong, L. (2016). Arabidopsis YAK1 regulates abscisic acid response and drought resistance. FEBS Lett. 590, 2201–2209. doi: 10.1002/1873-3468.12234
Kondo, S., Sugaya, S., Sugawa, S., Ninomiya, M., Kittikorn, M., Okawa, K., et al. (2012). Dehydration tolerance in apple seedlings is affected by an inhibitor of ABA 8'-hydroxylase CYP707A. J. Plant Physiol. 169, 234–241. doi: 10.1016/j.jplph.2011.09.007
Krasensky, J., Jonak, C. (2012). Drought, salt, and temperature stress-induced metabolic rearrangements and regulatory networks. J. Exp. Bot. 63, 1593–1608. doi: 10.1093/jxb/err460
Li, X. (2011). Infiltration of Nicotiana benthamiana protocol for transient expression via Agrobacterium. Bio-protocol 1 (e95). doi: 10.21769/BioProtoc.95
Li, S., Li, X., Wei, Z., Liu, F. (2020). ABA-mediated modulation of elevated CO2 on stomatal response to drought. Curr. Opin. Plant Biol. 56, 174–180. doi: 10.1016/j.pbi.2019.12.002
Liu, C., Hu, J., Fan, W., Zhu, P., Cao, B., Zheng, S., et al. (2021). Heterotrimeric G-protein γ subunits regulate ABA signaling in response to drought through interacting with PP2Cs and SnRK2s in mulberry (Morus alba L.). Plant Physiol. Biochem. 161, 210–221. doi: 10.1016/j.plaphy.2021.02.017
Liu, C., Lyu, R., Zhu, P., Fan, W., Li, J., Wang, X., et al. (2014). Isolation and expression of mulberry (Morus alba L.) EIN2 gene. Acta Agronomica Sinica. 40, 1205–1212. doi: 10.3724/SP.J.1006.2014.01205
Liu, X., Zhang, H., Zhao, Y., Feng, Z., Li, Q., Yang, H. Q., et al. (2013). Auxin controls seed dormancy through stimulation of abscisic acid signaling by inducing ARF-mediated ABI3 activation in Arabidopsis. Proc. Natl. Acad. Sci. U.S.A. 110, 15485–15490. doi: 10.1073/pnas.1304651110
Luo, X., Chen, Z., Gao, J., Gong, Z. (2014). Abscisic acid inhibits root growth in Arabidopsis through ethylene biosynthesis. Plant J. 79, 44–55. doi: 10.1111/tpj.12534
McAdam, S. A., Brodribb, T. J., Ross, J. J. (2016). Shoot-derived abscisic acid promotes root growth. Plant Cell Environ. 39, 652–659. doi: 10.1111/pce.12669
Molnár, I., Cozma, L., Dénes, T.É., Vass, I., Vass, I. Z., Rakosy-Tican, E. (2021). Drought and saline stress tolerance induced in somatic hybrids of Solanum chacoense and Potato Cultivars by using mismatch repair deficiency. Agriculture 11, 696. doi: 10.3390/agriculture11080696
Noman, M., Jameel, A., Qiang, W. D., Ahmad, N., Liu, W. C., Wang, F. W., et al. (2019). Overexpression of GmCAMTA12 enhanced drought tolerance in Arabidopsis and Soybean. Int. J. Mol. Sci. 20, 4849. doi: 10.3390/ijms20194849
Perez-Alfocea, F., Estan, M. T., Santa Cruz, A., Bolarin, M. (1993). Effects of salinity on nitrate, total nitrogen, soluble protein and free amino acid levels in tomato plants. Int. J. Hortic. Sci. 60, 1021–1027. doi: 10.1080/00221589.1993.11516443
Ren, J., Sun, L., Wu, J., Zhao, S., Wang, C., Wang, Y., et al. (2010). Cloning and expression analysis of cDNAs for ABA 8'-hydroxylase during sweet cherry fruit maturation and under stress conditions. J. Plant Physiol. 167, 1486–1493. doi: 10.1016/j.jplph.2010.05.027
Seo, M., Koshiba, T. (2002). Complex regulation of ABA biosynthesis in plants. Trends Plant Sci. 7, 41–48. doi: 10.1016/s1360-1385(01)02187-2
Shu, K., Zhang, H., Wang, S., Chen, M., Wu, Y., Tang, S., et al. (2013). ABI4 regulates primary seed dormancy by regulating the biogenesis of abscisic acid and gibberellins in Arabidopsis. PloS Genet. 9, e1003577. doi: 10.1371/journal.pgen.1003577
Son, S., Chitnis, V. R., Liu, A., Gao, F., Nguyen, T. N., Ayele, B. T. (2016). Abscisic acid metabolic genes of wheat (Triticum aestivum L.): identification and insights into their functionality in seed dormancy and dehydration tolerance. Planta 244, 429–447. doi: 10.1007/s00425-016-2518-2
Tan, B. C., Schwartz, S. H., Zeevaart, J. A., McCarty, D. R. (1997). Genetic control of abscisic acid biosynthesis in maize. Proc. Natl. Acad. Sci. U S A. 94, 12235–12240. doi: 10.1073/pnas.94.22.12235
Thole, J. M., Beisner, E. R., Liu, J., Venkova, S. V., Strader, L. C. (2014). Abscisic acid regulates root elongation through the activities of auxin and ethylene in Arabidopsis thaliana. G3 (Bethesda) 4, 1259–1274. doi: 10.1534/g3.114.011080
Vaičiukynė, M., Žiauka, J., Žūkienė, R., Vertelkaitė, L., Kuusienė, S. (2019). Abscisic acid promotes root system development in birch tissue culture: a comparison to aspen culture and conventional rooting-related growth regulators. Physiol. Plant 165, 114–122. doi: 10.1111/ppl.12860
Venkatappa, M., Sasaki, N., Han, P., Abe, I. (2021). Impacts of droughts and floods on croplands and crop production in Southeast Asia - An application of google earth engine. Sci. Total Environ. 795, 148829. doi: 10.1016/j.scitotenv.2021.148829
Wang, Y., Hou, Y., Qiu, J., Wang, H., Wang, S., Tang, L., et al. (2020). Abscisic acid promotes jasmonic acid biosynthesis via a 'SAPK10-bZIP72-AOC' pathway to synergistically inhibit seed germination in rice (Oryza sativa). New Phytol. 228, 1336–1353. doi: 10.1111/nph.16774
Wang, L., Hua, D., He, J., Duan, Y., Chen, Z., Hong, X., et al. (2011). Auxin Response Factor 2 (ARF2) and its regulated homeodomain gene HB33 mediate abscisic acid response in Arabidopsis. PloS Genet. 7, e1002172. doi: 10.1371/journal.pgen.1002172
Wang, Y., Wang, Y., Ji, K., Dai, S., Hu, Y., Sun, L., et al. (2013). The role of abscisic acid in regulating cucumber fruit development and ripening and its transcriptional regulation. Plant Physiol. Biochem. 64, 70–79. doi: 10.1016/j.plaphy.2012.12.015
Xia, H., Wu, S., Ma, F. (2014). Cloning and expression of two 9-cis-epoxycarotenoid dioxygenase genes during fruit development and under stress conditions from Malus. Mol. Biol. Rep. 41, 6795–6802. doi: 10.1007/s11033-014-3565-z
Xiong, L. M., Zhu, J. K. (2003). Regulation of abscisic acid biosynthesis. Plant Physiol. 133, 29–36. doi: 10.1104/pp.103.025395
Zandalinas, S. I., Mittler, R., Balfagón, D., Arbona, V., Gómez-Cadenas, A. (2018). Plant adaptations to the combination of drought and high temperatures. Physiol. Plant 162, 2–12. doi: 10.1111/ppl.12540
Zhang, M., Leng, P., Zhang, G., Li, X. (2009). Cloning and functional analysis of 9-cis-epoxycarotenoid dioxygenase (NCED) genes encoding a key enzyme during abscisic acid biosynthesis from peach and grape fruits. J. Plant Physiol. 166, 1241–1252. doi: 10.1016/j.jplph.2009.01.013
Zhou, B., Guo, Z. (2005). Effect of ABA and its biosynthesis inhibitor on chilling resistance and antioxidant enzymes activity. Acta Prataculturae Sin. 14, 94–99. doi: 10.3321/j.issn:1004-5759.2005.06.015
Zhu, J. K. (2002). Salt and drought stress signal transduction in plants. Annu. Rev. Plant Biol. 53, 247–273. doi: 10.1146/annurev.arplant.53.091401.143329
Keywords: mulberry NCED, plant hormones, abiotic stress, seed germination, root elongation
Citation: Zhu P, Li R, Fan W, Xia Z, Li J, Wang C and Zhao A (2023) A mulberry 9-cis-epoxycarotenoid dioxygenase gene MaNCED1 is involved in plant growth regulation and confers salt and drought tolerance in transgenic tobacco. Front. Plant Sci. 14:1228902. doi: 10.3389/fpls.2023.1228902
Received: 25 May 2023; Accepted: 13 July 2023;
Published: 28 July 2023.
Edited by:
Sushil Satish Chhapekar, University of Missouri, United StatesReviewed by:
Zhi Gang Meng, Biotechnology Research institute of CAAS, ChinaRu Li, Guangxi University, China
Copyright © 2023 Zhu, Li, Fan, Xia, Li, Wang and Zhao. This is an open-access article distributed under the terms of the Creative Commons Attribution License (CC BY). The use, distribution or reproduction in other forums is permitted, provided the original author(s) and the copyright owner(s) are credited and that the original publication in this journal is cited, in accordance with accepted academic practice. No use, distribution or reproduction is permitted which does not comply with these terms.
*Correspondence: Aichun Zhao, emhhb2FpY2h1bkBob3RtYWlsLmNvbQ==;; emhhb2FpY2h1bkBzd3UuZWR1LmNu; Chuanhong Wang, NTc4NzQ4NjQ1QHFxLmNvbQ==
†These authors have contributed equally to this work