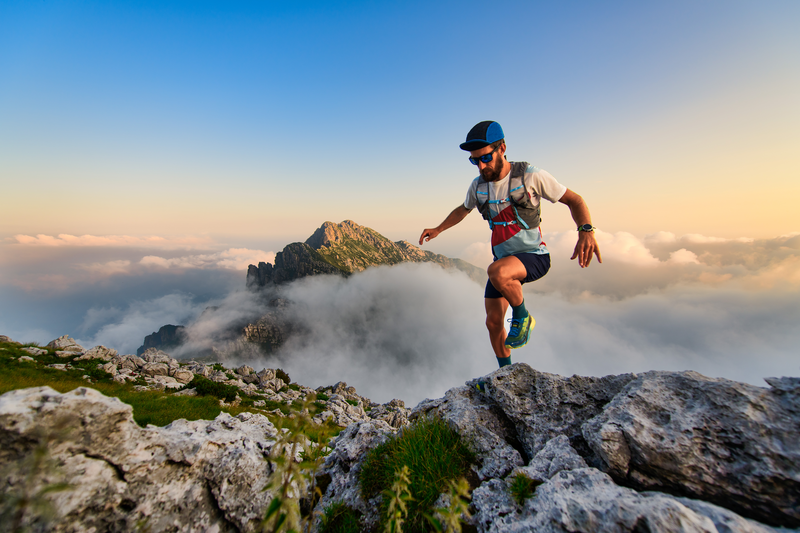
95% of researchers rate our articles as excellent or good
Learn more about the work of our research integrity team to safeguard the quality of each article we publish.
Find out more
ORIGINAL RESEARCH article
Front. Plant Sci. , 15 September 2023
Sec. Plant Breeding
Volume 14 - 2023 | https://doi.org/10.3389/fpls.2023.1228844
Induced polyploidy usually results in larger vegetative and reproductive plant organs. In order to study the effect of chromosome doubling on Thymus vulgaris, three levels of colchicine concentration including 0.1, 0.3 and 0.5% (w/v) were applied for 6, 12 and 24 hours on apical meristem of 2- and 4-leaf seedlings. Ploidy level was evaluated by flow cytometry and microscopic chromosome counting. Chemical composition of essential oils extracted by hydro-distillation was analyzed by gas Chromatography/mass spectrometry (GC/MS) and gas Chromatography (GC). The application of 0.3% colchicine at 4-leaf seedling for 6 hours resulted in the highest survival rate and the highest number of tetraploid plants. Cytogenetic and flow cytometry analyses confirmed the increase of chromosome number from 2n=2x=30 in diploids to 2n=4x=60 in induced tetraploids. Tetraploid plants had larger leaves, taller and thicker stems, dense branching, longer trichome, larger stomata, larger guard cells, and decreased number of stomata. The number of chloroplasts and mitochondria increased significantly in tetraploid plants by 1.66 and 1.63 times, respectively. The expression of CYP71D178, CYP71D180 and CYP71D181 increased in tetraploids by 3.27, 7.39 and 2.15 times, respectively, probably resulting in higher essential oil compounds, as tetraploids outyielded the diploid plants by 64.7% in essential oil, 40.9% in thymol and 18.6% in carvacrol content.
Garden thyme (Thymus vulgaris L.) is one of the most important medicinal and aromatic plants. Besides common culinary and seasoning use, the antibacterial, anti-spasmolytic, antitumor and antiviral properties have made it very useful for pharmaceuticals especially for cough remedy (Satyal et al., 2016; Salma et al., 2017; Salehi et al., 2018; Mondak et al., 2020; Nieto, 2020; Galovičová et al., 2021). Very few efforts, however, have been made towards genetic improvement of this plant.
Increasing the number of chromosomes which is called ploploidy is one of the tools for plants improvement. Polyploidy has played an important role in plant breeding and also plant evolution (Sleper and Poehlman, 2006; Iannicelli et al., 2020; Madani et al., 2021). Winkler (1916) coined the term “polyploidy” in a study of vegetative grafts in Solanum, when he discovered tetraploid plants regenerated from callus tissue on cut stems of diploid S. nigrum. Winge (1917) proposed that spontaneous chromosome doubling, resulting from accidental somatic doubling in mitosis or non-reduction in meiosis, could convert interspecific sterile hybrids into fertile types. Grant (1981) postulated that 47% of all flowering plants were of polyploidy origin and proposed that 58% of monocots and 43% of dicots were polyploid. As polyploidization has made a great contribution to the evolution of many species, plant breeders try to take advantage of induced polyploidy in their programs. The hypothesis is that polyploidy may lead to useful characteristics like larger vegetative and reproductive organs (Adaniya and Shirai, 2001; Liqin et al., 2019; Madani et al., 2021). In addition, more than 90% of the reviewed experimental data point toward a positive impact of ploidy level on the ability of plants to cope with different kinds of biotic and abiotic stresses (Tossi et al., 2022).
Polyploidization can be induced by quite a few antimitotic agents. The most frequently used antimitotic chemicals are colchicine, trifluralin, and oryzalin. The whole method of induced chromosome doubling consists of a series of steps, including an induction phase, regrowth phase, and a confirmation technique to evaluate the rate of achievement (Salma et al., 2017). Blakeslee and Avery (1937) were among pioneers discovered the induction of polyploidy by colchicine. Induced polyploidy has been tried in several crop plants. Chen et al. (1945) generated autotetraploid barley plants by the use of colchicine and observed various irregularities in chromosome configuration as well as regular bivalents in a few lines. They also reported that the fertility of the 4n plants as a whole was lower than that of the diploids.
Compared with the original diploid, autotetraploid rice showed superior agronomic characters, such as larger panicle, larger seed and stronger stem, while seed-setting rate and fertility was lower than that in its diploid varieties (Song and Zhang, 1992; Chen et al., 2021).
Guo and Birchler (1994) studied dosage effects on gene expression in monoploid, diploid, triploid, and tetraploid maize plants and showed that for most tested genes, the transcript level per cell was directly proportional to structural gene dosage. Study of phenotypic and proteome changes in 2X, 4X and 6X ploidy series of maize revealed that plants of higher ploidy exhibited increased cell size but slower growth rate, later flowering, fewer tassel branches as well as reduced stature and fertility while the expression of most proteins increased linearly with ploidy (Yao et al., 2011).
Javadian et al. (2017) reported that induced tetraploidy increased podophyllotoxin content in the leaves and stems of Linum album by upregulating the expression level and enzyme activity of genes involved in the pathway.
Sabzehzari et al. (2019) demonstrated that induced tetraploid plants of Ispaghul (Plantago psyllium) were larger than their intact diploids in terms of height, leaf thickness, seed size, pollen grain, seeds per spike, number of guard cells and stomata size as well as chlorophyll and carotenoid content.
Besides experimental studies, induced polyploidy has long been used by plant breeders in commercial genetic improvement of sugar beet, seedless watermelon, rye, red clovers and ryegrass (Sattler et al., 2016). Polyploidy induction has also been widely applied to ornamental crops breeding, resulting in the extension of flower longevity, larger flower size and deep flower colors. Tulips and azalea are two examples with large number of induced polyploid commercial cultivars (Jones et al., 2007; Marasek-Ciolakowska et al., 2012)
Due to increased number of gene copies, artificially induced polyploidy may increase enzymatic content and activity that contribute to enhanced secondary metabolites of medicinal plants (Dhawan and Lavania, 1996; Iannicelli et al., 2020). The tetraploid forms of Chamomile (Matricaria chamomilla L) outperform their diploid relatives in both biochemical and morphological traits including essential oils, bisaboloids, chamazulene, apigenin, favonoids, height, capitulum size and weight and dry seed weight. Currently almost one quarter of the chamomile varieties cultivated worldwide are tetraploids induced by colchicine, highlighting the success of polyploidy breeding establishment in this medicinal crop (Das, 2014). The cultivated tetraploid chamomile varieties, Lutea and Goral, for instance, have been shown to produce 20% higher chamazulene (one of the valuable medicinal components of chamomile essential oil) than diploid cultivars (Gosztola et al., 2006). Tavan et al. (2015) used colchicine for ploidy induction in Thymus persicus nodal shoot tip explants and observed a higher amount of pentacyclic triterpenoids in induced tetraploids. Braynen et al. (2021) showed that the key genes promoting floral formation were down-regulated, whereas floral transition genes and a flowering locus were up-regulated in autotetraploid Brassica rapa. Iannicelli et al. (2020) reviewed the application of polyploidy in breeding of aromatic and medicinal species. Dhawan and Lavania (1996); Salma et al. (2017) and Madani et al. (2021) also reviewed the influence of artificial polyploidization on secondary metabolites content in medicinal plants.
Alsahlany et al. (2019) and Kramer and Bamberg (2019) studied guard cell size and number of chloroplasts per guard cell in potato trying to find a reliable simple tool to identify ploidy level. There has been very few attempts in the literature to induce polyploidy in Thymus vulgaris. Shmeit et al. (2020) treated nodal segments of thyme with 80μM centration of oryzalin for 24 hours and regenerated tetraploid plants on MS culture medium. They reported increased plant size and secondary metabolites in tetraploids. Navrátilová et al. (2021) tried in vitro polyploidization of diploid thyme explants by 0.346 and 1.73 mg L−1 oryzalin and developed tetraploid plants containing higher proportions of thymol and carvacrol. As both researches were carried out under resource- and facility-demanding in vitro condition, we aimed at this study to develop a more efficient colchicine-based protocol for in vivo polyploidization of Thymus vulgaris and to evaluate the its effect on physiological, anatomical, and phytochemical features.
Thymus vulgaris seeds were planted in small pots filled with cocopeat and kept in a normal glass house with 16 hours day length, 26/18°C (day/night). Plants at 2- and 4-leaf stages were selected for colchicine treatment. Stock colchicine (Sigma®) solutions were prepared in sterilized distilled water. Two drops of 2% (v/v) dimethyl sulfoxide (DMSO) and Tween20 were added to increase cell permeability and colchicine absorption (Głowacka et al., 2009). 5 mL colchicine solution was dropped on apical meristem of seedlings and secured by a piece of cotton covered with aluminum foil to avoid evaporation. Three levels of colchicine concentration including 0.1, 0.3 and 0.5% (w/v) were applied for 6, 12 and 24 hours. Each treatment was run in 5 replications. Seedlings were then rinsed smoothly with water spray. Two weeks after treatment, plants were moved to bigger pots filled with soil, sand and cocopeat (3:2:1).
Three months after seedlings treatment, plants showing atypical morphology (i.e. leaf thickness, shape, color, and size; shorter internodes) were selected as putative tetraploids. Ploidy level of selected plants were analyzed according to the method described by Loureiro et al. (2007) and Javadian et al. (2017). Soybean (Glycine max) variety Polanka (2C DNA = 2.50 pg) was used as standard reference plant (Dolezel et al., 1994). 25 mg leaf samples from putative plants as well as 25 mg from standard soybean were submerged in one mL Woody Buffer Plant (Loureiro et al., 2007) and chopped with a sharp razor blade. The suspension of isolated nuclei was filtered through a Partec 30 μm nylon mesh filters (Partec, Munster, Germany) to remove cell debris. The nuclear suspension of each sample was treated with 50 μg mL-1 RNase (Sigma-Aldrich Corporation, MO, USA) to prevent staining of double-stranded RNA and stained with μg mL-1 propidium iodide (PI, Fluka). Samples were then incubated on ice and analyzed by BDFACanto ™ flow cytometer (BD Biosciences, Bedford, MA, USA), using the BD FACSDiva™ Software. More than 5000 stained nuclei were analyzed per sample. Data were first transferred to Flowing Software version 2.5.0, followed by Partec FloMax Software version 2.4e (Partec, Munster, Germany). Instable plants with both diploid and tetraploid cells (mixoploids) were removed.
To confirm tetraploidy, chromosomes of the plants demonstrating DNA doubling in flow cytometry as well as control diploid plants were counted. 5-6 cm cuttings were made from each plant and regenerated to root. 1 cm long root tips were sampled and pretreated with 1% α-monobromonaphthalene for 3h at 4°C temperature followed by rinsing with distilled water for 10 min. Chromosome fixation was carried out using Carnoy’s solution consisting of 1part glacial acetic acid and 3 parts ethanol (95 to 100%) for 20 min at 4°C followed by 10 min rinsing with distilled water. Roots, then, hydrolyzed in 1 N HCl for 15 min at 60°C followed by 30 min rinsing. Specimens were stained with aceto orcein (2%) solution for 4 h. The stained root tips, 2 mm long, were squashed on a glass slide. The number of chromosomes was counted under Olympus DP70 light microscope equipped with a camera. Survival rate and tetraploid induction rate was calculated by the number of achieved tetraploid plants from each treatment divided by all treated plants.
All diploid and tetraploid plants were carefully monitored for important morphological traits after colchicine treatment. Plant height, leaf length, width and thickness, main stem diameter and internode distance were measured four months after treatment when the plants has become stable.
Six diploid and six tetraploid plants (derived from 0.3% colchicine treatment for 6 hours at 4-leaf seedling) were selected for further cellular, physiological and biochemical measurements. Stomata length, width and density, trichome length and intensity as well as guard cells length and number (in a microscopic view scope) were measured using epidermis layer of three expanded leaves by colorless varnish (Smith et al., 1989). To make the epidermis layer prepared, the expanded leaves were dried, and the epidermis was separated using a piece of banderol. Separated epidermis were fixed on glass slides. The number of chloroplasts and mitochondria were counted in 150-200 guard cells in diploid and tetraploid plants’ leaves (Figure 1). 1% Janus Green powder (Merck™) solution was used to stain mitochondria.
Thyme samples were dried at room condition (20–25° C) under shade for 72 hours. To assess the essential oil content, the shade dried samples were subjected to the hydro-distillation method for 3 hours with 250 mL water, using a Clevenger-type apparatus according to the British Pharmacopoeia (HMSO, 1988). Essential oil content was calculated by dividing mass essential oil to mass dry matter. The isolated oils were dried over anhydrous sodium sulfate and stored in tightly closed dark vials at 4°C for further analyses.
Gas chromatography (GC) analysis was performed using a Thermoquest gas chromatograph equipped with fused silica capillary DB-5 column (25 m×0.25 mm i.d.; film thickness 0.25 µm). The injector and detector temperatures were kept at 250 and 280°C, respectively. Nitrogen was used as the carrier gas at a flow rate of 1.1 mL/min; oven temperature program was 60–250°C at the rate of 4°C/min and finally held isothermally for 10 min; split ratio was 1:50.
Gas chromatography-mass spectrometer (GC-MS) analysis was carried out by use of Thermoquest-Finnigan gas chromatograph equipped with fused silica capillary DB-5 column (60 m×0.25 mm i.d.; film thickness 0.25 µm) coupled with a TRACE mass (Manchester, UK). Helium was used as carrier gas with ionization voltage of 70 eV. Ion source and interface temperatures were 200 and 250°C, respectively. Mass range was from 35 to 456 amu. Oven temperature program was the same as mentioned above for the GC. The constituents of the essential oils were calculated according to Hadian et al. (2014).
Total Chlorophyll (a+b) content was evaluated in tetraploid and diploid plants by the method described by Lichtenthaler (1987) using spectrophotometer (UV/Vis 2100 model). The absorbance was read by spectrophotometer (UV/Vis 2100 model) at 645 and 663 nm wavelengths. The pigments content was calculated based on the equations elucidated in Lichtenthaler and Wellburn (1983) and expressed as mg/g fresh weight.
Total RNA was isolated and purified from thyme leaves using RNeasy plant mini kit (Qiagen, Valencia, CA, USA). The quality of the RNA samples was assessed in an agarose gel 2%, and its concentration was determined with a nanodrop. To remove contaminating DNA, RNAs were treated with DNaseI, RNase-free (Thermo. Fisher Scientific, Fermentas). DNase-treated RNA samples (1 μg) were reverse-transcribed using RevertAid™ First Strand cDNA Synthesis kit (Fermentas, K1622) to finally obtain a 20 μg cDNA. Specific primers for three cytochromes involved in thymol and carvacrol Biosynthesis including CYP71D178, CYP71D180 and CYP71D181 were designed based on Crocoll et al. (2010) and were checked by Primer3 Software with the following parameters: melting temperature of 60 °C, PCR product length range 120–200 bp pairs and Guanine-Cytosine contents from 50 to 60%. Real-time PCR (RT-PCR) was performed using the fluorescent SYBRGreen dye with BIOPRAD System. Relative quantification of gene expression was calculated by the 2-ΔΔCT method (Livak and Schmittgen, 2001) considering Brassica napus actin gene as the internal standard
The impact of different concentration and duration of colchicine treatments at 2- and 4-leaf growth stages on survival rate and the number of tetraploid plants were statistically analyzed on the basis of completely randomized design ANOVA. The mean values were compared using the Duncan’s test by SAS 9 software (SAS Institute Inc. 2013).
The higher concentration of colchicine resulted in less plant survival at both 2-leaf and 4-leaf stages (Figure 2). Out of 18 treatments including three concentrations of colchicine (0.1, 0.3 and 0.5%), three times of application (6, 12 and 24 hours), and two stages (2- and 4-leaf), six treatments failed to raise any polyploid plants. Only application for 6 hours of 0.3% colchicine at 2- and 4-leaf stage and 0.1% at 4-leaf stage appeared to be successful for inducing polyploidization, the very first being the most efficient one (Figure 3).
Figure 2 The impact of treatment time: 6 h (black bars), 12 h (dotted bars) and 24 h (hatched bars) and colchicine concentration on plants survival. The treatments with the same letter on top have no significant difference based on Duncan’s test in 0.01 significant level.
Figure 3 Percentage of tetraploid plants obtained from different treatments: 0.3% colchicine for 6 hours at 2-leaf stage (dotted bars), 0.1% colchicine for 6 hours at 4-leaf stage (hatched bars), and 0.3% colchicine for 6 hours at 4-leaf stage (black bars). The treatments with different letter on top are significantly different based on Duncan’s test by 0.01 significant level.
Plants showing morphological changes e.g., darker leaf color, growth stunt, and low internode distance were chosen as candidate tetraploid plants and their ploidy level checked by flow cytometry and chromosome counting. The mean 2C DNA content of diploid thyme was found to be 1.88 picograms (pg) being very close to 1.54 pg reported by Marie and Brown (1993). The observations demonstrated that genome size in tetraploid plants had been successfully doubled to 3.74 pg confirming the effectiveness of colchicine treatment (Figure 4).
Figure 4 Flow cytometry analysis of thyme cell nuclei, (A) peak1 and 2: diploid and standard. (B) peak1 and 2: standard and tetraploid plant) (C) peak1-3: diploid, standard and tetraploid plants, respectively in a mixoploid. Soybean Polanka variety (2C DNA = 2.50 pg) was used as standard. Peaks C.V. kept less than 5%.
To ensure the ploidy level of plants, the number of chromosomes was counted in root tip cells of candidate tetraploid plants confirmed by flow cytometry. The number of chromosomes in diploid thyme was 2n = 2x = 30. Although some plants had 57 or, 58 chromosomes, plants with exactly 60 chromosomes were chosen as autotetraploid (Figure 5).
There was a growth stunt in tetraploid plants up to two months after treatment. Tetraploid plants exhibited taller and thicker stems, dense branching, and larger leaves (Table 1).
The results showed that tetraploid plants had lower number but larger stomata than diploid plants. Trichome size was also significantly increased (Table 2). The number of chloroplasts and mitochondria in tetraploid plants increased by 1.66 and 1.63 times, respectively (Table 2) while there was no significant difference in their size compared to control diploid ones.
Real-time PCR of Cytochrome genes involved in thymol and carvacrol biosynthesis indicated that the expression of CYP71D178, CYP71D180 and CYP71D181 genes increased by 3.27-fold, 7.39-fold, and 2.15-fold in tetraploid plants compared to diploid controls, respectively (Figure 6).
Figure 6 Expression level of three cytochrome genes; (A) CYP71D178, (B) CYP71D180 and (C) CYP71D81 in thyme; diploid (hatched bars) and tetraploid (black bars).
Content of main compounds in tetraploid and diploid plants are shown in Table 3. Essential oil content, thymol, carvacrol, γ-terpinene, and linalool increased in tetraploid plants, while p-Cymene, Camphor and chlorophyll content were decreased.
Induced polyploidy has been used by scientists in several field, medicinal and ornamental crops in order to enlarge the plant organs or to enhance secondary metabolites production (Chen et al., 1945; Dhawan and Lavania, 1996; Zeng et al., 2006; Sattler et al., 2016; Salma et al., 2017; Madani et al., 2021). The probable decline in seed setting of thyme induced polyploids would not make any barrier in its cultivation, as the plant can be asexually propagated through cuttings.
In this research, we made an attempt to double the number of chromosomes in Thymus vulgaris in an in vivo condition. The results indicated that applying 0.3% colchicine on apical meristem of four-leaf stage seedlings for 6 hours was optimum treatment for polyploidy induction leading to maximum survival and the number of tetraploid plants (Figures 2, 3). Tavan et al. (2015) also found 0.3% colchicine (although for 12 h) as the most efficient condition for inducing polyploidy in Thymus persicus nodal shoot tip explants. Flow cytometry output (Figure 4) demonstrated doubled genome size in tetraploid plants (3.74 pg) and cytogenetic analysis confirmed the increase of chromosome number from 2n=2x=30 in diploids to 2n=4x=60 in induced tetraploids (Figure 5).
Previous studies on T. vulgaris have reported tetraploid induction and its effect on essential oil production, but those procedures involved nodal segments and oryzalin, instead of colchicine (Shmeit et al., 2020; Navrátilová et al., 2021).
One of the concerns of in vitro ploidy induction is the low number of polyploid plants finally obtained, as too many plantlets are lost during tissue culture procedure from explants until mature plants. For example, polyploidy rate has been reported 7.5%, 2.3-10% and 7.8% in Shmeit et al. (2020); Navrátilová et al. (2021), and Tavan et al. (2015) experiments, respectively, while it was 24.8% in our study (Figure 3). In vivo polyploidization does not need any well-equipped tissue culture lab and this might be another privilege.
There was a growth stunt in tetraploid plants up to two months after treatment probably due to toxic damage and degradation caused by colchicine (Swanson, 1957). Chen and Gao (2007) also found a growth retardation in Astragalus membranaceus in early weeks and later normal growth following ploidy induction.
Tetraploid plants exhibited taller and thicker stems, dense branching, and larger leaves (Table 1), likely as a result of increased cell size (Comai, 2005). This result is in close agreement with previous studies. Shmeit et al. (2020) reported increased plant weight, height, leaf length, breadth and thickness and Navrátilová et al. (2021) demonstrated shorter internodes, darker and rounded leaves, and more compact appearance in induced tetraploid plants of thyme.
A correlation between ploidy level and morphological characteristics has been reported in numerous plant species such as Phlox subulata (Zhang et al., 2008), Tanacetum parthenium (Majdi et al., 2010), Gerbera jamesonii (Gantait et al., 2011), Lychnis senno (Nonaka et al., 2011), Ullucus tuberosus (Viehmannova et al., 2011), and Ocimum basilicum and Dracocephalum moldavica (Omidbaigi et al., 2010; Omidbaigi et al., 2012). Larger leaves in induced tetraploids has been reported in Platanus acerifolia (Liu et al., 2007), Tanacetum parthenium (Majdi et al., 2010), Dracocephalum moldavica (Omidbaigi et al., 2010). Lower height, shorter roots, thicker stems and darker leaves have been observed in Thymus persicus induced tetraploids by Tavan et al. (2015). Sabzehzari et al. (2019) also concluded that induced tetraploid plants of Ispaghul (Plantago psyllium) were larger than their intact diploids in terms of height, leaf thickness and seed size.
It has been observed by several researches that tetraploid induction may significantly alter the length, width and number of stomata (Cohen and Yao, 1996; Beck et al., 2003; Yang et al., 2006; Liu et al., 2007). Tetraploid plants exhibited a reduced number of stomata compared to diploid plants, but the individual stomata were larger in size (Table 2). Longer and wider stomata and reduced stomatal density were reported by Tavan et al. (2015) in induced tetraploids of Thymus persicus. Javadian et al. (2017) detected increased size of leaves and stomatal cells, while decreased stomata density in Linum album tetraploids. Studies indicate that in line with the increase in ploidy level, the cell incorporates more chromosomes into the nucleus, leading to an increase in cell size (Byrne et al., 1981; Kondorosi et al., 2000; Comai, 2005; Ng et al., 2011).
Our findings revealed that the number of chloroplasts and mitochondria increased by 1.66 and 1.63 times in tetraploid plants, respectively (Table 2) without any alteration in their size compared to control diploid ones. Increased chloroplast number in polyploid plants have been reported in tomato (Koornneef et al., 1989), Triticum monococum (Hawke and Leech, 1990), watermelon (Sari et al., 1999), Medicago spp (Ghanavati et al., 2004), and Onobrychis spp (Ghanavati and Eskandari (2011). A positive correlation between the mean number of chloroplasts and the number of chromosomes was discovered by Frandsen (1968) in potato. Ordoez et al. (2017); Alsahlany et al. (2019) and Kramer and Bamberg (2019) recently recommend chloroplast counting as an efficient and inexpensive method for breeders to easily differentiate ploidy between diploid and tetraploid potato. Increased number of chloroplasts (and mitochondria) in tetraploids could be arisen form increase in the copy number of genes controlling their biogenesis in the nucleus (Leech et al., 1981; Ghanavati et al., 2004).
Evidence suggest that polyploidy activates some mechanisms in cell effecting DNA template and also transcription and translation leading to decrease, increase or even silence the gene expression so that it can influence physiological and morphological traits of the plant (Adams and Wendel, 2005). In line with our findings, an enhance in transcription level of benzylisoquinoline biosynthesis genes in Papaver bracteatum tetraploid plants has been observed by Madani et al. (2019). Braynen et al. (2021) reported the up-regulation of floral transition genes in autotetraploid Brassica rapa, too.
The expression of CYP71D178, CYP71D180 and CYP71D181 genes appeared to be much higher in tetraploid plants compared to diploid controls in our study (Figure 6). This result justifies the higher amount of thymol and carvacrol in tetraploid plants (Table 3). CYP71D178, CYP71D179 and CYP71D82 are proposed to be thymol synthases while CYP71D180 and CYP71D181 being carvacrol synthases Crocoll et al. (2011).
Cytochrome genes are known to express enzymes catalyzing a number of oxidations of terpene metabolism and are likely to be involved in the reactions from γ-terpinene to thymol and carvacrol. Crocoll et al. (2010) isolated eleven cytochrome P450 gene sequences from oregano, thyme and marjoram and suggested a pathway for thymol and carvacrol starting with the formation of γ-terpinene by a monoterpene synthase. The second step appeared to be catalyzed by cytochrome P450s in a two-step oxidation. Krause et al. (2021) elucidated biosynthetic pathway of thyme main compounds suggesting that the aromatic backbone of thymol and carvacrol is formed by P450 mono-oxygenases in combination with a dehydrogenase via an unstable intermediate. Two additional P450s of the CYP76S and CYP736A subfamilies catalyze the hydroxylation of thymol and carvacrol to thymohydroquinone.
Essential oil content in tetraploid plants was 64.7% higher than that in diploid plants as the content increased from 0.85 to 1.4 (Table 3). These results are almost the same as findings of Shmeit et al. (2020) who reported 0.81% and 1.19% essential oil in diploid and tetraploid thyme plants, respectively. Thymol, carvacrol and γ-terpinene, as main medicinal compounds of thyme (Galovičová et al., 2021), were found to increase by 40.9%, 18.6% and 29.3%, respectively, in tetraploid plants (Table 3). The content of thymol and carvacrol increased by 18.01% and 0.49%, respectively in tetraploid thyme plants developed by Shmeit et al. (2020). Navrátilová et al. (2021), however, observed no difference in total essential oil between diploids and induced tetraploids, although single analyte concentrations e.g., thymol and carvacrol were increased. Tohidi et al. (2021) found that carvacrol in natural tetraploid populations of thyme was higher than diploid ones.
Evidence suggests that γ-terpinene is primary substrate for to thymol and carvacrol synthesis (Crocoll et al., 2010; Lima et al., 2013). So, its rise in tetraploids seems to have increased thymol and carvacrol content as shown in Table 3. An increase of 35.6% and 24% in essential oil have been reported in Carum carvi (Dijkstra and Speckmann, 1980) and Dracocephalum moldavica (Omidbaigi et al., 2010) in induced tetraploids, respectively. A 50% rise in artemisinin content in Artemisa annua (Lin et al., 2011), 5.8-times higher thebaine in Centella asiatica (Madani et al., 2019), and 52–152% increase in alkaloid production in Datura stramonium (Berkov and Philipov, 2002) have been documented in the literature, too.
Chlorophyll content was decreased from 2.01 to 1.6 mgg-1 fresh weight (20.4%). Decline in chlorophyll content justifies the darker color in tetraploid plants leave as reported in Urgenia indica (Phulari, 2011), lemon (Afshar Mohammadian et al., 2012), Thymus persicus (Tavan et al., 2015) and Thymus vulgaris (Navrátilová et al., 2021).
In this research the number of chromosomes of Thymus vulgaris was doubled from 30 to 60 by an in vivo protocol and its impact on morphology, anatomy, phytochemistry and gene expression was studied. Application of 0.3% colchicine for 6 hours at 4-leaf seedling appeared to be optimum treatment for polyploid induction leading to maximum survival and the number of tetraploid plants Number of chloroplasts and mitochondria, the number of stomata cells and guard cells length increased. Tetraploid plants outperformed the diploid plants by 64.7% in essential oil, 40.9% in thymol and 18.6% in carvacrol content. The findings of this research suggest that induced polyploidy has the potential to enhance the production of secondary metabolites in thyme, thus providing opportunities for its genetic improvement.
The raw data supporting the conclusions of this article will be made available by the authors, without undue reservation.
VM has proposed the idea and was project leader and the main author. ST, MA, and HM conducted the experiment under the supervision of VM. All authors contributed to the article and approved the submitted version.
The authors declare that the research was conducted in the absence of any commercial or financial relationships that could be construed as a potential conflict of interest.
All claims expressed in this article are solely those of the authors and do not necessarily represent those of their affiliated organizations, or those of the publisher, the editors and the reviewers. Any product that may be evaluated in this article, or claim that may be made by its manufacturer, is not guaranteed or endorsed by the publisher.
Adams, K. L., Wendel, J. F. (2005). Novel patterns of gene expression in polyploid plants. Trends Genet. 21 (10), 539–543. doi: 10.1016/j.tig.2005.07.009
Adaniya, S., Shirai, D. (2001). In vitro induction of tetraploid ginger (Zingiber officinalis) and its pollen fertility and germinability. Sci. Hortic. 88, 277–287. doi: 10.1016/S0304-4238(00)00212-0
Afshar Mohammadian, M., Pourakbari Kasmaei, R., Omidi, Z., Ghanati, F., Tarang, A. (2012). Morphologic and physiologic effects on polyploidy induction in Citrus ourantifolia. Plant Biol. J. 12, 13–24.
Alsahlany, M., Zarka, D., Coombs, J., Douches, D. (2019). Comparison of methods to distinguish diploid and tetraploid potato for applied diploid breeding. Am. J. Potato Res. 96, 419–426. doi: 10.1007/s12230-018-09710-7
Beck, S., Dunlop, R., Fossey, A. (2003). Stomatal length and frequency as a measure of ploidy level in black wattle, Acacia mearnsii (de Wild). Botanical J. Linn. Soc. 141, 177–181. doi: 10.1046/j.1095-8339.2003.00132.x
Berkov, S., Philipov, S. (2002). Alkaloid production in diploid and autotetraploid plants of Datura stramonium. Pharm. Biol. 40, 617–621. doi: 10.1076/phbi.40.8.617.14650
Blakeslee, A. F., Avery, A. G. (1937). Methods of inducing doubling of chromosomes in plants: By treatment with colchicine. J. Hered. 28, 393–411. doi: 10.1093/oxfordjournals.jhered.a104294
Braynen, J., Yang, Y., Yuan, J. (2021). Comparative transcriptome analysis revealed differential gene expression in multiple signaling pathways at flowering in polyploid Brassica rapa. Cell Biosci. 11, 1–13. doi: 10.1186/s13578-021-00528-1
Byrne, M. C., Nelson, C. J., Randall, D. (1981). Ploidy effects on anatomy and gas exchange of tall fescue leaves. Plant Physiol. 68, 891–893. doi: 10.1104/pp.68.4.891
Chen, R., Feng, Z., Zhang, X., Song, Z., Cai, D. (2021). A new way of rice breeding: polyploid rice breeding. Plants 10 (3), 422. doi: 10.3390/plants10030422
Chen, L., Gao, S. (2007). In vitro tetraploid induction and generation of tetraploids from mixoploids in Astragalus membranaceus. Scientia Hortic. 112, 339–344. doi: 10.1016/J.SCIENTA.2006.12.045
Chen, S., Shen, S., Tang, P. S. (1945). Studies on colchicine-induced autotetraploid barley. I and II. Cytological and morphological observations. Am. J. Bot. 32 (3), 103–106. doi: 10.2307/2437527
Cohen, D., Yao, J. L. (1996). In vitro chromosome doubling of nine Zantedeschia cultivars. Plant Cell Tiss Organ Cult. 47, 43–49. doi: 10.1007/BF02318964
Comai, L. (2005). The advantages and disadvantages of being polyploid. Nat. Rev. Genet. 6, 836–846. doi: 10.1038/nrg1711
Crocoll, C., Asbach, J., Novak, J., Gershenzon, J., Degenhardt, J. (2010). Terpene synthases of oregano (Origanum vulgare L.) and their roles in the pathway and regulation of terpene biosynthesis. Plant Mol. Biol. 73 (6), 587–603. doi: 10.1007/s11103-010-9636-1
Das, M. (2014). Chamomile: medicinal, biochemical, and agricultural aspects (New york: CRC Press), 295.
Dhawan, O., Lavania, U. (1996). Enhancing the productivity of secondary metabolites via induced polyploidy: a review. Euphytica 87, 81–89. doi: 10.1007/BF00021879
Dijkstra, H., Speckmann, G. I. (1980). Autotetrapliody in caraway (Carum carvi) for the increase of the aetheric oil content of the seed. Euphytica 29, 89–96. doi: 10.1007/BF00037252
Dolezel, J., Dolezelova, M., Novak, F. J. (1994). Flow cytometric estimation of nuclear DNA amount in diploid bananas. Biol. Plant 36, 351–357. doi: 10.1007/BF02920930
Frandsen, N. O. (1968). Die Plastidenzahl als Merkmal bei der Kartoffel. Theor. Appl. Genet. 38, 153–167. doi: 10.1007/BF00933811
Galovičová, L., Borotová, P., Valková, V., Vukovic, N., Vukic, M., Štefániková, J., et al. (2021). Thymus vulgaris essential oil and its biological activity. Plants 10 (9), 1959. doi: 10.3390/plants10091959
Gantait, S., Mandal, N., Bhattacharyya, S., Das, P. K. (2011). Induction and identification of tetraploids using in vitro colchicine treatment of Gerbera jamesonii Bolus cv. Sciella. Plant Cell Tiss Organ Cult. 106, 485–493. doi: 10.1007/s11240-011-9947-1
Ghanavati, F., Eskandari, H. (2011). Relationship between the chloroplast number in stomatal guard cells, flow cytometry and ploidy level in Onobrychis spp. Seed Plant J. 27, 427–439. doi: 10.22092/spij.2017.111073
Ghanavati, F., Mozafari, J., Masoumi, A. (2004). Relationship between ploidy level and the chloroplast number in stomatal guard cells of Medicago spp. Seed Plant J. 20, 117–127. doi: 10.22092/spij.2017.110611
Głowacka, K., Jezowski, S., Kaczmarek, Z. (2009). Polyploidization of Miscanthus sinensis and Miscanthus giganteus by plant colchicine treatment. Ind. Crops Prod. 30, 444–446. doi: 10.1016/j.indcrop.2009.07.011
Gosztola, B., Nemeth, E., Sarosi, S. Z., Szabo, K., Kozak, A. (2006). Comparative evaluation of chamomile (Matricaria recutita L.) populations from different origin. Int. J. Hortic. Sci. 12, 91–95. doi: 10.31421/IJHS/12/1/629
Guo, M., Birchler, J. A. (1994). Trans-Acting dosage effects on the expression of model gene systems in maize aneuploids. Science 266, 1999–2002. doi: 10.1126/science.266.5193.1999
Hadian, J., Bigdeloo, M., Nazeri, V., Khadivi-Khub, A. (2014). Assessment of genetic and chemical variability in Thymus caramanicus. Mol. Biol. Rep. 41 (5), 3201–3210. doi: 10.1007/s11033-014-3180-z
Hawke, J. C., Leech, R. M. (1990). Acetyl coenzyme A carboxylase in species of Triticum of different ploidy. Planta 181, 543–546. doi: 10.1007/BF00193008
Iannicelli, J., Guariniello, J., Tossi, V. E., Regalado, J. J., Di Ciaccio, L., van Baren, C. M., et al. (2020). The polyploid effect in the breeding of aromatic and medicinal species. Sci. Hortic. 260, 108854. doi: 10.1016/j.scienta.2019.108854
Javadian, N., Karimzadeh, G., Sharifi, M., Moeini, A., Behmanesh, M. (2017). In vitro polyploidy induction: changes in morphology, podophyllotoxin biosynthesis, and expression of the related genes in Linum album. Planta 245 (6), 1165–1178. doi: 10.1007/s00425-017-2671-2
Jones, J. R., Ranney, T. G., Lynch, N. P., Krebs, S. L. (2007). Ploidy levels and relative genome sizes of diverse species, hybrids, and cultivars of Rhododendron. J. Am. Rhododendron Soc. 61, 220–227.
Kondorosi, E., Roudier, F., Gendreau, E. (2000). Plant cell-size: growing by ploidy? Curr. Opin. Plant Biol. 3, 488–492. doi: 10.1016/S1369-5266(00)00118-7
Koornneef, M., van Diepen, J. A. M., Hanhart, C. J., Kieboom-de Waart, A. C., Martinelli, L., Schoenmakers, H. C. H., et al. (1989). Chromosomal instability in cell- and tissue cultures of tomato haploids and diploids. Euphytica 43, 179–186. doi: 10.1007/bf00037911
Kramer, L. J., Bamberg, J. (2019). Comparing methods of ploidy estimation in potato species. Am. J. Potato Res. 96, 419–426. doi: 10.1007/s12230-019-09729-4
Krause, S. T., Liao, P., Crocoll, C., Boachon, B., Förster, C., Leidecker, F., et al. (2021). The biosynthesis of thymol, carvacrol, and thymohydroquinone in Lamiaceae proceeds via cytochrome P450s and a short-chain dehydrogenase. Proc. Natl. Acad. Sci. 28, 118. doi: 10.1073/pnas.2110092118
Leech, R. M., Thomson, W., Platt-Aloia, K. (1981). Observations on the mechanism of chloroplast division in higher plants. New Phytol. 87, 1–9. doi: 10.1111/j.1469-8137.1981.tb01686.x
Lichtenthaler, H. (1987). Chlorophylls and carotenoids: Pigments of photosynthetic biomembranes. Methods Enzymol. 148, 350–382. doi: 10.1016/0076-6879(87)48036-1
Lichtenthaler, H., Wellburn, A. R. (1983). Determinations of total carotenoids and chlorophylls a and b of leaf extracts in different solvents. Biochem. Soc. 11 (5), 591–592. doi: 10.1042/bst0110591
Lima, A. S., Schimmel, J., Lukas, B., Novak, J., Barroso, J. G., Figueiredo, A. C., et al. (2013). Genomic characterization, molecular cloning and expression analysis of two terpene synthases from Thymus caespititius. Planta 238 (1), 191–204. doi: 10.1007/s00425-013-1884-2
Lin, X., Zhou, Y., Zhang, J., Lu, X., Zhang, F., Shen, Q., et al. (2011). Enhancement of artemisinin content in tetraploid Artemisia annua plants by modulating the expression of genes in artemisinin biosynthetic pathway. Biotechnol. Appl. Biochem. 58, 50–57. doi: 10.1002/bab.13
Liqin, G., Jianguo, Z., Xiaoxia, L. (2019). Polyploidy-related differential gene expression between diploid and synthesized allotriploid and allotetraploid hybrids of Populus. Mol. Breed. 39, 69. doi: 10.1007/s11032-019-0975-6
Liu, G., Li, Z., Bao, M. (2007). Colchicine-induced chromosome doubling in Platanus acerifolia and its evect on plant morphology. Euphytica 157, 145–154. doi: 10.1007/s10681-007-9406-6
Livak, K. J., Schmittgen, T. D. (2001). Analysis of relative gene expression data using Real-Time Quantitative PCR and the 2–ΔΔCT Method. Methods 25 (4), 402–408. doi: 10.1006/meth.2001.1262
Loureiro, J., Rodriguez, E., Dolezel, J., Santos, C. (2007). Two new nuclear isolation buffers for plant DNA flow cytometry: a test with 37 species. Ann. Bot. 100, 875–888. doi: 10.1093/aob/mcm152
Madani, H., Escrich, A., Hosseini, B., Sanchez-Muñoz, R., Khojasteh, A., Palazon, J. (2021). Effect of polyploidy induction on natural metabolite production in medicinal plants. Biomolecules 11 (6), 899. doi: 10.3390/biom11060899
Madani, H., Hosseini, B., Karimzadeh, G., Rahimi, A. (2019). Enhanced thebaine and noscapine production and modulated gene expression of tyrosine/dopa decarboxylase and salutaridinol 7-O-acetyltransferase genes in induced autotetraploid seedlings of Papaver bracteatum. Acta Physiol. Plant 41, 1–12. doi: 10.1007/s11738-019-2984-9
Majdi, M., Karimzadeh, G., Malboobi, M. A., Omidbaigi, R., Mirzaghaderi, G. (2010). Induction of tetraploidy to feverfew (Tanacetum parthenium schulz-bip.): morphological, physiological, cytological, and phytochemical changes. Hort Sci. 45 (1), 16–21. doi: 10.21273/HORTSCI.45.1.16
Marasek-Ciolakowska, A. R., Ramanna, M. S., Arens, P., Tuyl, J. V. (2012). Breeding and cytogenetics in the genus Tulipa. Floricult Ornamental Biotech. 6, 90–97.
Marie, D., Brown, S. C. (1993). A cytometric exercise in plant DNA histograms, with 2C values for 70 species. Biol. Cell 78 (1–2), 41–51. doi: 10.1016/0248-4900(93)90113-S
Mondak, B., Mohammadi, V., Hadian, J., Zeinali, H. (2020). The impact of temperature on metabolites and the expression of genes involved in thymol and carvacrol biosynthesis pathway in Thymus vulgaris. Iranian J. Field Crop Sci. 51 (1), 195–205. doi: 10.22059/ijfcs.2019.267935.654536
Navrátilová, B., Švécarová, M., Bednář, J., Ondřej, V. (2021). In Vitro plyploidization of Thymus vulgaris L. and its effect on composition of essential oils. Agronomy 11 (3), 596. doi: 10.3390/agronomy11030596
Ng, D. W., Zhang, C., Miller, M., Shen, Z., Briggs, S., Chen, Z. J. (2011). Proteomic divergence in Arabidopsis autopolyploids and allopolyploids and their progenitors. Heredity108 4), 419–430. doi: 10.1038/hdy.2011.92
Nieto, G. (2020). A review on applications and uses of thymus in the food industry. Plants (Basel) 9, 961. doi: 10.3390/plants9080961
Nonaka, T., Oka, E., Asano, M., Kuwayama, S., Tasaki, H., Han, D., et al. (2011). Chromosome doubling of Lychnis spp. by in vitro spindle toxin treatment of nodal segments. Sci. Hortic. 129, 832–839. doi: 10.1016/j.scienta.2011.05.004
Omidbaigi, R., Mirzaee, M., Hassani, M. E., SedghiMoghadam, M. (2012). Induction and identification of polyploidy in basil (Ocimum basilicum L.) by colchicine treatment. Int. J. Plant Production 4 (2), 87–98. doi: 10.22069/ijpp.2012.686
Omidbaigi, R., Yavari, S., Hassani, M., Yavari, S. (2010). Induction of autotetraploidy in dragonhead (Dracocephalum moldavica L.) by colchicine treatment. J. Fruit Ornamental Plant Res. 18 (1), 23–35.
Ordoez, B., Orrillo, M., Bonierbale., M. (2017). Manual on potato reproductive and cytological biology (Lima (Peru: International Potato Center (CIP), 65.
Phulari, S. S. (2011). Polyploidy breeding in Urgenia indica: to study the effect of colchicine treatment on morphological character. Botany 1, 207–210.
Sabzehzari, M., Hoveidamanesh, S., Modarresi, M., Mohammadi, V. (2019). Morphological, anatomical, physiological, and cytological studies in diploid and tetraploid plants of Plantago psyllium. Plant Cell Tissue Organ Culture 139 (1), 131–137. doi: 10.1007/s11240-019-01670-y
Salehi, B., Mishra, A., Shukla, I., Sharifi-Rad, M., Contreras, M., Segura-Carretero, A., et al. (2018). Thymol, thyme, and other plant sources: Health and potential uses. Phytother. Res. 32, 1688–1706. doi: 10.1002/ptr.6109
Salma, U., Kundu, S., Mandal, N. (2017). Artificial polyploidy in medicinal plants: Advancement in the last two decades and impending prospects. J. Crop Sci. Biotechnol. 20, 9–19. doi: 10.1007/s12892-016-0080-1
Sari, N., Abak, K., Pitrat, M. (1999). Comparison of ploidy level screening methods in watermelon. J. Scientia Horticult. 82, 265–277. doi: 10.1016/S0304-4238(99)00077-1
Sattler, M., Carvalho, C., Clarindo, W. (2016). The polyploidy and its key role in plant breeding. Planta 243, 281–296. doi: 10.1007/s00425-015-2450
Satyal, P., Murray, B. L., McFeeters, R. L., Setzer, W. N. (2016). Essential oil characterization of Thymus vulgaris from various geographical locations. Foods 5, 70. doi: 10.3390/foods5040070
Shmeit, Y. H., Fernandez, E., Novy, P., Kloucek, P., Orosz, M., Kokoska, L. (2020). Autopolyploidy effect on morphological variation and essential oil content in Thymus vulgaris L. Scientia Hortic. 263, 109095. doi: 10.1016/j.scienta.2019.109095
Sleper, D. A., Poehlman, J. M. (2006). Breeding field crops. 5th Edition (USA: Wiley-Blackwell Inc).
Smith, S., Weyers, J., Berry, W. G. (1989). Variation in stomatal characteristics over the lower surface of Commelina communis leaves. J. Plant Cell Environ. 12, 653–654. doi: 10.1111/j.1365-3040.1989.tb01234.x
Song, W. C., Zhang, Y. H. (1992). Rice tetraploidy and its effect on agronomic traits and nutritional constituents. Acta Agronom Sin. (in Chinese) 18, 137–144.
Swanson, C. P. (1957). Cytology and Cytogenetics (Englewood Cliffs, NJ: Prentice-Hall, Inc.), 526–532.
Tavan, M., Mirjalili, M. H., Karimzadeh, G. (2015). In vitro polyploidy induction: changes in morphological, anatomical and phytochemical characteristics of Thymus persicus (Lamiaceae). Plant Cell Tiss Organ Cult. 122, 573–583. doi: 10.1007/s11240-015-0789-0
Tohidi, B., Rahimmalek, M., Arzani, A. (2021). Variation in phytochemical, morphological, and ploidy levels of Iranian Thymus species. Chem. Biodiver. 18, e2000911. doi: 10.1002/cbdv.202000911
Tossi, V., Martínez Tosar, L., Laino, L., Iannicelli, J., Regalado, J., Escandón, A., et al. (2022). Impact of polyploidy on plant tolerance to abiotic and biotic stresses. Front. Plant Sci. 13. doi: 10.3389/fpls.2022.869423
Viehmannova, I., Travnickova, M., Špatenkova, E., Monika, C., Travnícek, P. (2011). Induced polyploidization and its influence on yield, morphological, and qualitative characteristics of microtubers in Ullucus tuberosus. Plant Cell Tissue Organ Culture 109, 83–90. doi: 10.1007/s11240-011-0076-7
Winge, Ö. (1917). The chromosomes: Their number and general importance. Compt. Rend. Trav. Lab. Carlsberg 13, 131–275.
Winkler, H. (1916). Über die experimentelle Erzeugung von Pflanzen mit abweichenden Chromosomenzahlen. Z. Bot. 8, 417–531.
Yang, X. M., Cao, Z. Y., An, L. Z., Wang, Y. M., Fang, X. W. (2006). In vitro tetraploid induction via colchicine treatment from diploid somatic embryos in grapevine (Vitis vinifera L.). Euphytica 152, 217–224. doi: 10.1007/s10681-006-9203-7
Yao, H., Kato, A., Mooney, B., Birchler, J. A. (2011). Phenotypic and gene expression analyses of a ploidy series of maize inbred Oh43. Plant Mol. Biol. 75, 237–251. doi: 10.1007/s11103-010-9722-4
Zeng, S., Chen, C. W., Hong, L., Liu, J. H., Deng, X. (2006). In vitro induction, regeneration and analysis of autotetraploids derived from protoplasts and callus treated with colchicine in Citrus. Plant Cell Tissue Organ Cult. 87, 85. doi: 10.1007/s11240-006-9142-y
Keywords: Thymus vulgaris, induced polyploidy, gene expression, thymol, carvacrol
Citation: Mohammadi V, Talebi S, Ahmadnasab M and Mollahassanzadeh H (2023) The effect of induced polyploidy on phytochemistry, cellular organelles and the expression of genes involved in thymol and carvacrol biosynthetic pathway in thyme (Thymus vulgaris). Front. Plant Sci. 14:1228844. doi: 10.3389/fpls.2023.1228844
Received: 25 May 2023; Accepted: 23 August 2023;
Published: 15 September 2023.
Edited by:
Sergio J. Ochatt, INRA UMR1347 Agroécologie, FranceReviewed by:
Helena Trindade, University of Lisbon, PortugalCopyright © 2023 Mohammadi, Talebi, Ahmadnasab and Mollahassanzadeh. This is an open-access article distributed under the terms of the Creative Commons Attribution License (CC BY). The use, distribution or reproduction in other forums is permitted, provided the original author(s) and the copyright owner(s) are credited and that the original publication in this journal is cited, in accordance with accepted academic practice. No use, distribution or reproduction is permitted which does not comply with these terms.
*Correspondence: Valiollah Mohammadi, dm1vaGFtbWFkaUB1dC5hYy5pcg==
Disclaimer: All claims expressed in this article are solely those of the authors and do not necessarily represent those of their affiliated organizations, or those of the publisher, the editors and the reviewers. Any product that may be evaluated in this article or claim that may be made by its manufacturer is not guaranteed or endorsed by the publisher.
Research integrity at Frontiers
Learn more about the work of our research integrity team to safeguard the quality of each article we publish.