- 1Department of Vegetable Sciences and Floriculture, Faculty of Horticulture, Mendel University, Brno, Czechia
- 2Department of Horticulture, Faculty of Biotechnology and Horticulture, University of Agriculture, Krakow, Poland
- 3Department of Agricultural and Environmental Chemistry, Faculty of Agriculture and Economics, University of Agriculture, Krakow, Poland
- 4Department of Agricultural Sciences, University of Naples Federico II, Naples, Italy
Introduction: Smart management in crop cultivation is increasingly supported by application of arbuscular mycorrhizal fungi (AMF) and plant growth-promoting microorganisms (PGPM), which sustain soil fertility and plant performance. The aim of this study was the evaluation of the effects of consortia composed of (Claroideoglomus claroideum BEG96, Claroideoglomus etunicatum BEG92, Funneliformis geosporum BEG199, Funneliformis mosseae BEG 95, and Rhizophagus irregularis BEG140) and PGPM (Azospirillum brasilense – AZ, or Saccharothrix sp. – S) on onion cultivated in growing media with a composition corresponding to a degraded soil.
Methods: Three types of substrate formulations were used, with peat:sand ratios of 50:50, 70:30, 100:0 (v:v). The analysis of substrate parameters crucial for its fertility (pH, salinity, sorption complex capacity, and elements’ content) and characteristics reflecting onion seedlings’ performance (fresh weight, stress biomarkers, and elements’ content) was performed.
Results: AMF colonized onion roots in all treatments, showing increasing potential to form intercellular structures in the substrates rich in organic matter. Additionally, co-inoculation with PGPM microorganisms accelerated arbuscular mycorrhiza establishment. Increased antioxidant activity and glutathione peroxidase (GPOX) activity of onion roots sampled from the formulations composed of peat and sand in the ratio of 100:0, inoculated with AMF+S, and positive correlation between GPOX, fresh weight and antioxidant activity of onion roots reflected the successful induction of plant acclimatization response. Total phenols content was the highest in roots and leaves of onion grown in substrates with 70:30 peat:sand ratio, and, in the case of roots, it was correlated with AMF colonization parameters but not with antioxidant activity.
Discussion: AMF and PGPM efficiency in supporting onion growth should be linked to the increased onion root system capacity in mineral salts absorption, resulting in more efficient aboveground biomass production. AMF and PGPM consortia were effective in releasing minerals to soluble fraction in substrates rich in organic matter, making elements available for uptake by onion root system, though this phenomenon depended on the PGPM species. Microorganism consortia enhanced onion seedlings’ performance also in substrates with lower content of organic carbon through plant biofertilization and phytostimulation
1 Introduction
Microbial interactions in the rhizosphere are intensified by released plant root exudates, which are the main food source for microorganisms, increasing their population density and activity (Raaijmakers et al., 2009; Gavilanes et al., 2021). Plants benefit from root-associated microorganisms through various activities (phytohormones, nutrient supplementation, and pathogen suppression), ultimately increasing growth, health, and yield, thus decreasing the dependence on harmful chemicals and their after-effects (Raaijmakers et al., 2009; Syed and Tollamadugu, 2019). The potential of microbial symbioses is currently considered a valuable contribution to precision agriculture (Ma, 2019). Such inoculation can increase crop yield by enhancing nutrient uptake and pathogen biocontrol (Parnell et al., 2016; Nacoon et al., 2021). Significant changes in the indigenous microflora of soil by introducing single cultures of exogenous microorganisms appear to be complex, and the efforts have not always been successful (Onishchuk et al., 2017; Iturralde et al., 2019). Therefore, the physiological and ecological compatibility of beneficial and effective microorganisms is an important factor that increases the probability of shifting and controlling the “microbiological equilibrium” of the rhizosphere to encourage the growth, yield, and health of crops (Bidondo et al., 2016).
Onion (Allium cepa L.) is an economically important crop that is characterized by high environmental and cultivation demands. Onion seedlings grow relatively slowly and develop shallow weakly branched roots without root hairs, which shows a low efficiency in uptaking soil water and nutrients; thus, they are vulnerable to their deficiency since the beginning of the crop cycle (Sekara et al., 2017; Golubkina et al., 2020). Therefore, it is difficult to supply onion seedlings with sufficient amounts of soil nutrients to ensure optimum growth, especially in the early growth stages (Serra and Currah, 2002). According to Lee and Lee (2014) the optimum ranges of soil parameters should be as follows: pH 6.0–6.5; organic matter 25–35 mg kg−1; P 129–168 mg kg−1; exchangeable K, Ca, and Mg, 0.39–0.50, 5.8–6.7, and 2.1–2.7 cmolc kg−1, respectively. The rate of nutrient uptake depends on the growth stage, as the requirement for N is high during seedling production and subsequent vegetative growth (Mosse et al., 1981; Drost and Koenig, 2002). Simultaneously, high levels of N may cause leaching, denitrification, and increased susceptibility to pests and diseases (Sekara et al., 2017). Arbuscular mycorrhizal fungi (AMF) and plant growth-promoting microorganisms (PGPM) can be successful inoculants when applied at the beginning of the onion vegetation stage to balance the seedling nutritional status (Deressa and Schenk, 2008; Colo et al., 2014). However, the potential benefits justify the investigation of the mechanisms of interactions between AMF, PGPM, and onion plants (Lone et al., 2015; Rozpadek et al., 2016). The research undertaken so far covers the selection of the most effective microorganisms to establish successful symbiose/mutualism in particular environmental conditions and farming systems (Bolandnazar, 2009; Galván et al., 2009; Albrechtová et al., 2012; Caruso et al., 2018), and the implications of these ecological relationships on onion plant performance, especially with respect to bulb yield and quality (Mollavali et al., 2015; Shinde and Shinde, 2016; Fredotovic and Puizina, 2019; Petrovic et al., 2020).
Among the AMF associated with onion roots in different environments and cultivation systems, Claroideoglomus spp., Funneliformis spp., and Rhizophagus spp. are the most widespread (Charron et al., 2001; Bolandnazar et al., 2007; Bolandnazar, 2009; Galván et al., 2009; Mohamed et al., 2014; Mollavali et al., 2015). Concerning the plant growth promoting bacteria, the effects of inoculation with Azotobacter sp., Sphingobacterium sp., and Burkholderia sp. were investigated in onion (Tinna et al., 2020). Hong et al. (2019) demonstrated endophytic infection of cyst-like cells after onion inoculation with Azospirillum brasilense. Nevertheless, knowledge regarding the relationship between onions and Azospirillum spp. is limited.
Less attention has been paid to AMF and PGPM co-inoculation during the initial stages of onion ontogeny, particularly during transplant production in controlled conditions, where all types of substrates can be used, shaping distinct conditions for the establishment of ecological relationships in the rhizosphere (Joe et al., 2012; Colo et al., 2014; Mohamed et al., 2014; Ma, 2019). However, such experiments provide clear insight in soil–plant–microorganisms system and allow the identification of the most beneficial AMF and PGPM consortia for onions in the juvenile growth stage. Moreover, the established symbiosis can be continued under field conditions bringing multiple advantages during the overall growing cycle, including increased plant nutrient uptake, imparted biotic and abiotic stress tolerance, and better bulb quality characteristics (Tinna et al., 2020).
The aim of this study was to evaluate the effect of onion inoculation with AMF + A. brasilense or Saccharothrix sp. consortia on onion seedling development in relation to the substrate formulation. The influence of AMF and PGPM consortia on the growing medium was assessed with respect to the parameters crucial for fertility (pH, salinity, sorption complex capacity, and C, N, P, K, Mg, Na, and Ca concentrations) and onion seedling biochemical characteristics reflecting plant performance (fresh weight, stress biomarkers, and concentration of K, P, Mg, Na, and Ca).
2 Materials and methods
2.1 Material and experimental protocol
The onion (Allium cepa L.) cultivar ‘Stalagmit’ F1 (Moravoseed, Ltd, CZ) was used for this research. The experiment consisted of seven treatments, each with three replicates (eight plants per replicate). The experimental treatments included three non-inoculated substrate formulations (control) and four substrate formulations inoculated with consortia of arbuscular mycorrhizal fungi (AMF) and plant growth-promoting microorganisms (PGPM). The treatments and abbreviations used in this study are listed in Table 1.
Sowing peat (Klasmann, DE) and sand (local sources) were used. Calcium carbonate in remarkable amounts was used to maintain a pH of approximately 6.5. The remaining substrate parameters are shown in Supplementary Table 1. Before sowing, the substrates were autoclaved at 120°C for 60 min, and then inoculated with AMF and PGPM, namely A. brasilense (Tarrand et al., 1978) (CCM 3862) (Czech Collection of Microorganisms, Masaryk University, Brno, Czech Republic), or Saccharothrix sp. (ST2020) (AMF + S). Saccharothrix species and strain details are currently confidential because of the patent pending. AMF mix was composed of Claroideoglomus claroideum BEG96, Claroideoglomus etunicatum BEG92, Funneliformis geosporum BEG199, Funneliformis mosseae BEG 95, and Rhizophagus irregularis BEG140 (Symbiom Ltd., Lanškroun, Czech Republic). The AMF mix contained 145 spores per gram, and it was applied at a dose of 0.015 g per cm3 of substrate. Inoculation with PGPM was performed on onion seeds soaked for 30 min in A. brasilense and Saccharothrix sp. (ST2020) suspensions, respectively. A. brasilense culture were grown for one week on LuriaAgar media at 24°C (HiMedia Laboratories, Mumbai, India) and ST2020 was grown in a yeast-malt extract liquid medium (ISP2) from the International Streptomyces Project (Shirling and Gottlieb, 1966) at 28°C with agitation at 90 rpm for 10 days. Next, both cultures were homogenized using sterile ceramic beads and the concentration of both suspensions was adjusted to 108 CFU/ml in sterile physiological saline (Joe et al., 2012). The substrate parameters selected at the beginning of the experiment are listed in Supplementary Table 1.
2.2 Cultivation conditions
The seeds were sterilized for 10 min in 0.5% sodium hypochlorite, washed with sterile distilled water, and sown in Teku V9 containers (square, 9 × 9 cm; height, 8 cm; volume, 512 cm3) on 2 April 2020. Seedlings were grown in a phytochamber Fytoscope 4400 (PSI, Czech Republic) at a temperature of 20/18°C (day/night), relative air humidity 80%, light intensity 120 µmol m−2 s−1 at the germination stage; 18/16°C, 70%, 200 µmol m−2 s−1, respectively, at the beginning of the cotyledon stage, and 21/18°C, 75%, and 200 µmol m−2 s−1, respectively, after the first leaf stage, with 16 h of daylight. Seedlings were irrigated with a measured volume of tap water. Urea was used for fertilization (5 May, liquid 0.2% solution, 20 cm3 per pot in irrigation doses), later the fertilizer YaraTera Kristalon 20 + 5 + 10 + 2 (N, P, K, Mg) Azur was applied each week until 18 June as a 0.1% liquid solution at a dose of 20 cm3 per pot.
2.3 Substrate sampling and analyses
At the end of the experiment (26 June 2020), samples of homogenized substrate (150 g per treatment) were collected, air-dried, and basic parameters were determined, including pH (H2O and KCl) using the potentiometric method, salinity with the conductometric method, and the sorption complex capacity using Kappen’s method. Total N and organic C were determined via elemental analysis using a Vario Max Cube apparatus (Elementar Analysensysteme GmbH, Langenselbold, Germany). The available forms of macroelements (K, P, Mg, Na, and Ca) after extraction with acetic acid were determined by inductively coupled plasma atomic emission spectrometry using a Perkin Elmer Optima 7600 spectrometer (PerkinElmer, US).
2.4 Plant material sampling
Onion plants were collected on 26 June 2020, all leaves were cut with scissors, and roots were completely extracted from the substrate and washed with distilled H2O. Samples were stored immediately after harvest in a deep freezer (TSE240VGP, Thermo Fisher Scientific, USA) at temperature −80°C until analysis.
2.5 Fresh weight
Total leaf and root fresh weights (FWs) per plant were measured using a Sartorius A120S balance (Sartorius AG, Germany).
2.6 Staining and microscopy
For colonization analysis, four randomly selected 10 mm long root section per replicate were sampled, fixed in a formaldehyde:ethanol:acetic acid 10%:50%:5% v/v solution (FAA) and stored in the dark at 4°C before staining for microscopy (Schmidt et al., 2008). The roots were then rinsed in distilled H2O, cleared in 2% KOH for 1 h at 50°C, and washed in distilled H2O (4 × 3 min). Roots were stained in a tube with a mixture consisting of WGA AF 594 conjugate (Invitrogen, USA) (50 μg ml−1), concanavalin A AF 647 (Invitrogen, USA) (50 μg ml−1), and acid fuchsine (3%) at a ratio of 1:1:1 for 4–5 h at room temperature, rinsed in 1×PBS (4 × 3 min), and incubated for 12 h in 1×PBS to remove all excess stain. Before mounting on the slide, a few drops of Hoechst stain were added to the slides with the roots (Vierheilig et al., 2005). Mycorrhizal colonization was quantification by evaluating four root segments per replication for mycelia, vesicles, arbuscules, and spore presence. Ten observations were made at each root segment, and the calculation of colonization was based on the ratio of segments with AMF structures to roots without fungal structures (Alarcón and Cuenca, 2005).
Confocal microscopy was completed using the LSM 800 (Carl Zeiss, Germany) microscope at 590/617 nm excitation/emission for WGA AF 594, 650/668 nm for concanavalin A AF 647, and 350/461 nm for Hoechst stain. The lens used was a ×20/0.8 NA. Images were processed using the Zen Blue software (Carl Zeiss, Germany).
2.7 Analyses of stress biomarkers
The antioxidant activity against 2,2-diphenyl-1-picrylhydrazyl (DPPH radical) was measured in onion root and leaf samples. For the extraction, 2.5 g of homogenized onion roots or leaves per repetition was weighed, ground with 10 ml 80% methanol, and centrifuged (3,492×g, 10 min, 4°C). The mixture containing 0.1 cm3 supernatant and 4.9 cm3 0.1 mM DPPH in 80% methanol was incubated in darkness at 20–22°C after 15 min, the absorbance was measured at λ = 517 nm using a UV–VIS Helios Beta spectrophotometer (Thermo Fisher Scientific, Inc., US). Antioxidant activity was calculated using the following formula: DPPH (%) = ((A0 − A1)/A0) × 100, where A0 is the absorbance of the reference solution and A1 is the absorbance of the test solution (Molyneux, 2004).
Total phenol content was determined using the modified Folin–Ciocalteu colorimetric method (Djeridane et al., 2006). For the extraction, a 2.5 g sample of plant material was ground, mixed with 10 cm3 of 80% methanol, and centrifuged (3,492×g, 15 min, 4°C). The glass tubes were filled with 0.1 cm3 of the supernatant and 2 cm3 of sodium carbonate, left for 5 min, and then 0.1 cm3 of Folin–Ciocalteu’s reagent mixed with deionized water (1:1 v/v) was added. After 45 min, phenols were determined by the colorimetric method at 750 nm using a UV–VIS spectrophotometer against a reference solution. The total phenol value was expressed as gallic acid equivalents (mg GAE) per gram of FW.
Glutathione peroxidase activity (GPOX) was measured according to Lück (1962). Plant samples (2.5 g) were ground in an ice bath with 20 cm3 of a 0.05 M potassium phosphate buffer and centrifuged (3,492×g, 15 min, 4°C). The reaction mixture contained diluted supernatant, 0.05 M potassium phosphate buffer, p-phenylenediamine, and hydrogen peroxide. The absorbance at 485 nm was recorded at 60 s intervals for 2 min using a UV–VIS spectrophotometer. GPOX activity is expressed as units (U) per g FW per min.
2.8 Element concentration in plant tissues
To determine the element concentrations in onion roots and shoots, 0.5 g dry weight (DW) of plant material samples were mineralized in a mixture of HNO3 and H2O2 at 1:3 (v:v), then 2 cm3 HNO3 per 100 cm3 distilled water was added. The samples were concentrated 5-fold, and then the concentrations of elements (K, P, Mg, Na, and Ca) were determined by atomic emission spectroscopy (ICP) with an Optima 7600 spectrophotometer (Perkin Elmer, US) using the method described by Pasławski and Migaszewski (2006).
2.9 Statistical analysis
The substrate and plant samples were analyzed for every treatment in three technical replicates, each consisting of eight plants. The data were tested for normality of distribution according to the Shapiro–Wilk method and homogeneity of variances using the Levene test. The ANOVA was applied to test significance levels at p ≤0.05 (*), p ≤0.01 (**), or p ≤0.001 (***) and non-significant (ns), followed by Tukey’s honest significant difference (HSD) test to separate means into homogenous groups. The results were also examined using Pearson’s correlation coefficient (r) between the analyzed parameters. Principal component analysis (PCA) and cluster analysis (CA) were performed to precisely demonstrate and analyze the data and their relationships. Correlations and PCA were used as supplementary statistical methods that enabled and expanded the analysis of the presented data and made additional relationships visible between the experimental treatments and variables. The results using raw data are presented for PCA because no substantial differences appeared between the raw and standardized data. Modeling was performed with “general regression models (GRM)”, a stepwise regression procedure with backward elimination was performed to remove the least significant variables. Multiple regression screening, based on the investigated variables, was performed to determine the most accurate compatibility. Coefficients of determination (R2) and standard errors of estimation (SEe) were calculated to assess the accuracy of the models. All analyses were performed using Statistica 13.3 (Dell, Inc., USA).
We used multiple linear regression analysis to develop models that describe how the y-variable (FW of leaves, roots, and leaves + roots of onion seedlings) is related to several explanatory variables xn (substrate and plant parameters). After stepwise regression analysis with backward elimination of all investigated parameters variables, did not allow to formulate clear model, so variables affecting simultaneously the FW were screened and two models were developed. The first model for onion FW focused on whole-seedling FW and had the following form: FW of roots + leaves = 1.120 ∗ Mg in substrate − 0.004 ∗ Na in the substrate. The input data were the mean content of elements in the substrate and mycorrhizal parameters. The coefficient of determination is high (R2 = 0.968). This means that the regression line approximates the real data points quite well and that the model can explain approximately 97% of the onion seedling DW variation with p ≤0.05.
The second model was revealed for onion root FW, with input data including only the colonization rate, arbuscule abundance, and vesicle abundance:
The coefficient of determination is high (R2 = 0.944). with p ≤0.05. According to multiple regression models, the total onion seedling FW can be predicted based on the Mg and Na content in the substrate, whereas root FW can be predicted based on the analyzed AMF parameters.
3 Results
3.1 Fresh weight of onion as affected by AMF and PGPM application to investigated growing media
The fresh weight (FW) of onion leaves and roots at harvest was 10.94 g and 8.54 g on average, respectively, and exhibited significant dependence on the experimental treatments and plant tissues (Figure 1; Supplementary Table 2). The lowest root FW value was recorded in the AMF + AZ 50 treatment (0.72 g) and the decrease in root FW was 43.3% compared to the control C 50 treatment (1.26 g). The roots of onions sampled from all treatments inoculated with PGPM (AMF + AZ 70, AMF + AZ 100, and AMF + S 100) developed similar root FW as the non-inoculated control with corresponding peat:sand formulations (C 70 and C 100). The FW of onion seedling leaves grown on all substrates inoculated with AMF and PGPM (AMF + AZ 50, AMF + AZ 70, AMF + AZ 100, and AMF + S 100) were significantly higher than in the corresponding controls, where similar peat:sand ratios were used (C 50, C 70, and C 100, respectively). The differences in FW accounted for 18.0% for the AMF + AZ 50 treatment, 22.0% for AMF + AZ 70, 19.7% for AMF + Z 100, and 20.6% for AMF + S 100. Analysis of the total onion seedling FW (leaf + root) revealed that the highest values were recorded for AMF + AZ 100 and AMF + S 100 treatments, 3.40 and 3.57 g, respectively). Onion root FW was negatively correlated with Ca and Mg content in the leaves (Supplementary Table 3). Leaf FW was positively correlated with Ca and Mg content in roots, but negatively correlated with Na content in roots. Moreover, leaf FW was positively affected by Ca, Mg, Na, K, and P content in the substrate, AMF colonization rate, arbuscule, and vesicle abundance in the roots.
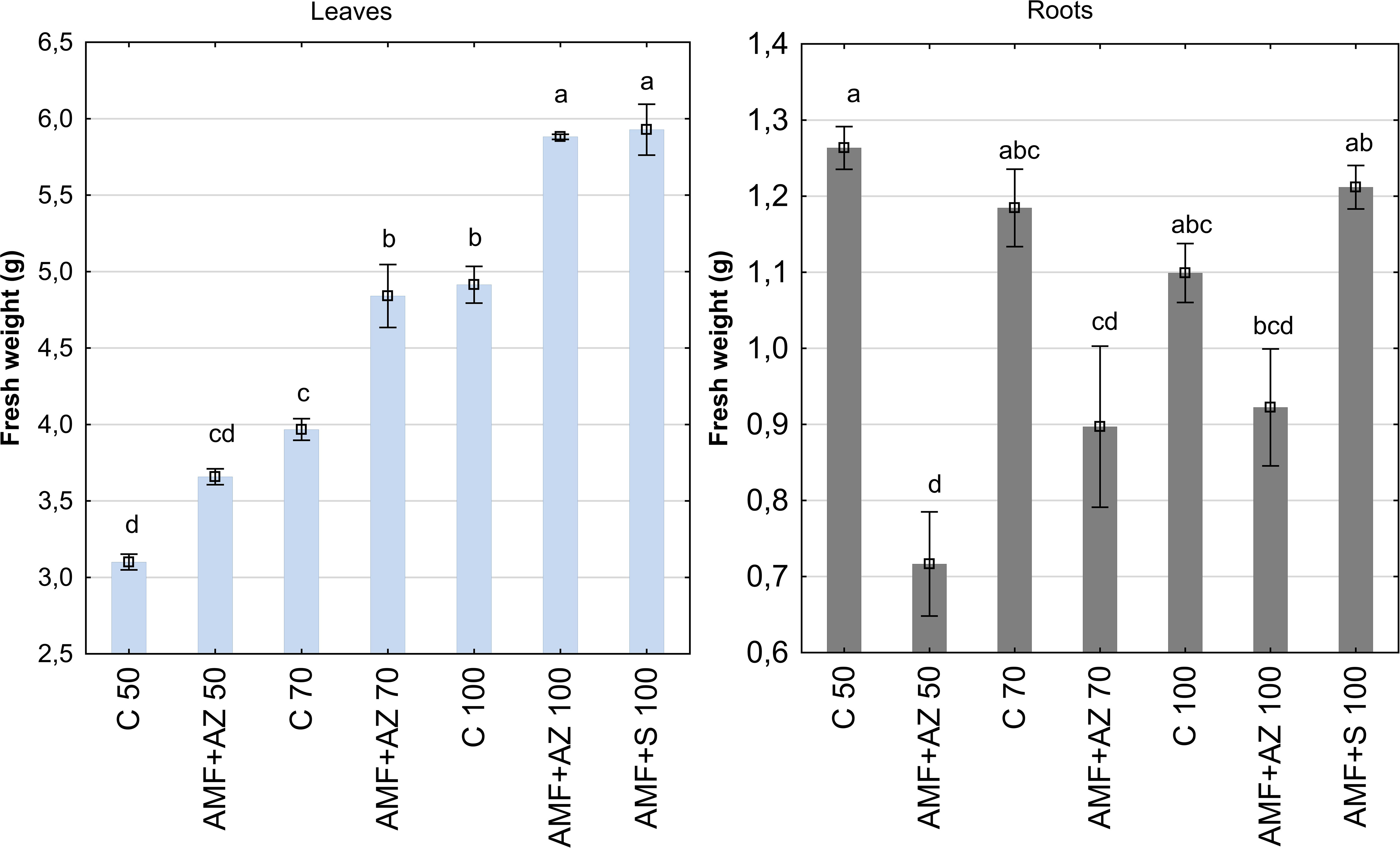
Figure 1 Mean values (± SE) of the fresh weight of onion leaves (left) and roots (right). Bars marked with different letters represent different values at p ≤0.05, according to Tukey’s test. C 50, peat:sand ratio 50:50 (v:v) without inoculation; AMF + AZ 50, peat:sand ratio 50:50 (v:v) inoculated with arbuscular mycorrhizal fungi (AMF) and Azospirillum brasilense (AZ); C 70, peat:sand ratio 70:30 (v:v) without inoculation; AMF + AZ 70, peat:sand ratio 70:30 (v:v) inoculated with AMF and AZ; C 100, peat:sand ratio 100:0 (v:v) without inoculation; AMF + AZ 100, peat:sand ratio 100:0 (v:v) inoculated with AMF and AZ; AMF + S 100 peat:sand ratio 100:0 (v:v) inoculated with AMF and ST2020 (S).
3.2 Root colonization of onion as affected by AMF and PGPM application to investigated growing media
The data in Figure 2 show a high level of root colonization rate in the AMF + AZ 100 and AMF + S 100 treatments (88.3 and 90.0%, respectively). Results of observations performed with confocal microscopy showed successful root colonization of AMF in all inoculated treatments (Figure 3). No colonization was observed in the control treatment.
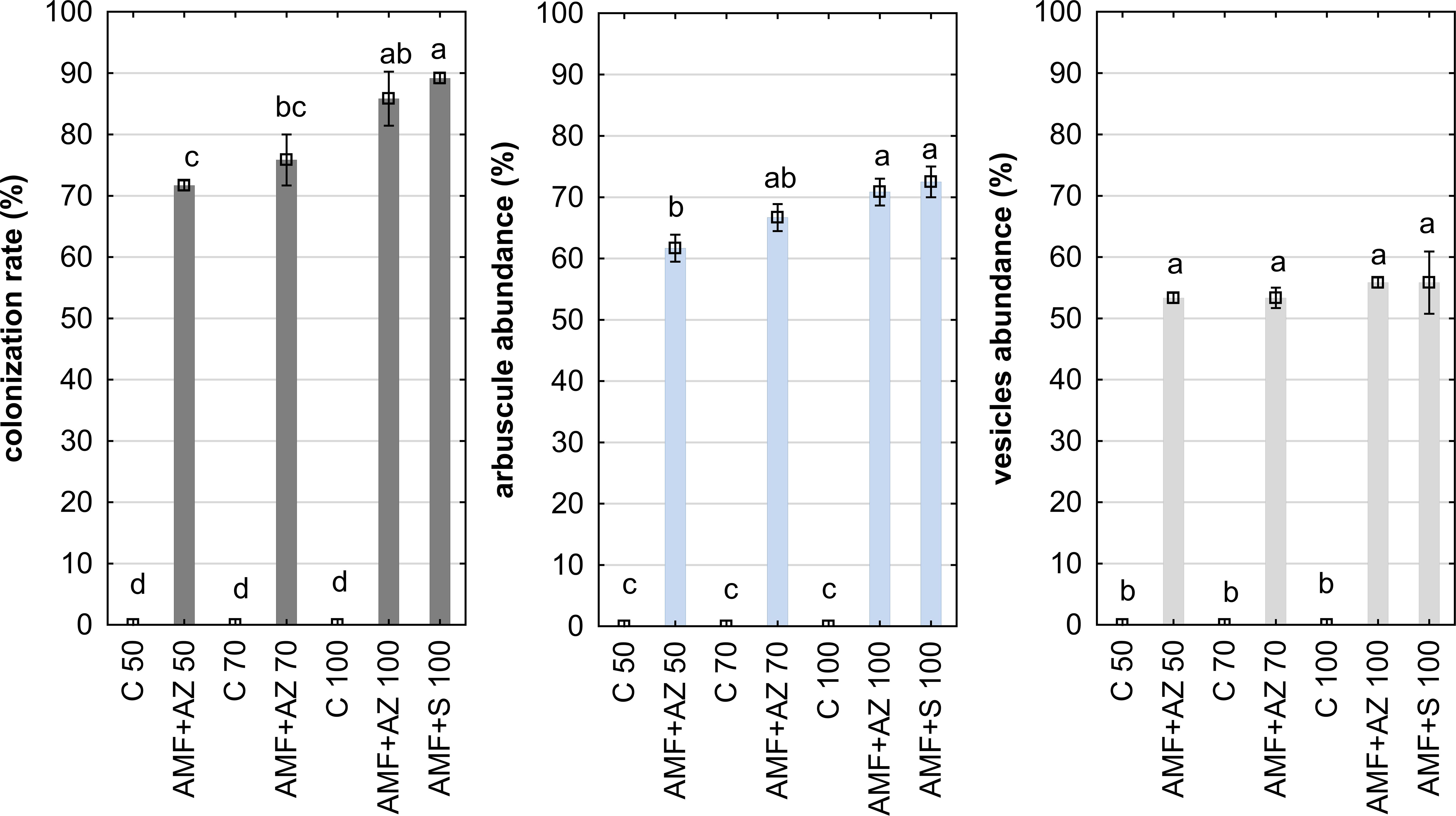
Figure 2 Mean values ( ± SE) of the colonization rate (left), arbuscule abundance (center), and vesicle abundance (right). Bars marked with different letters represent different values at p ≤0.05, according to Tukey’s test. Abbreviations are explained in Figure 1 and Table 1.
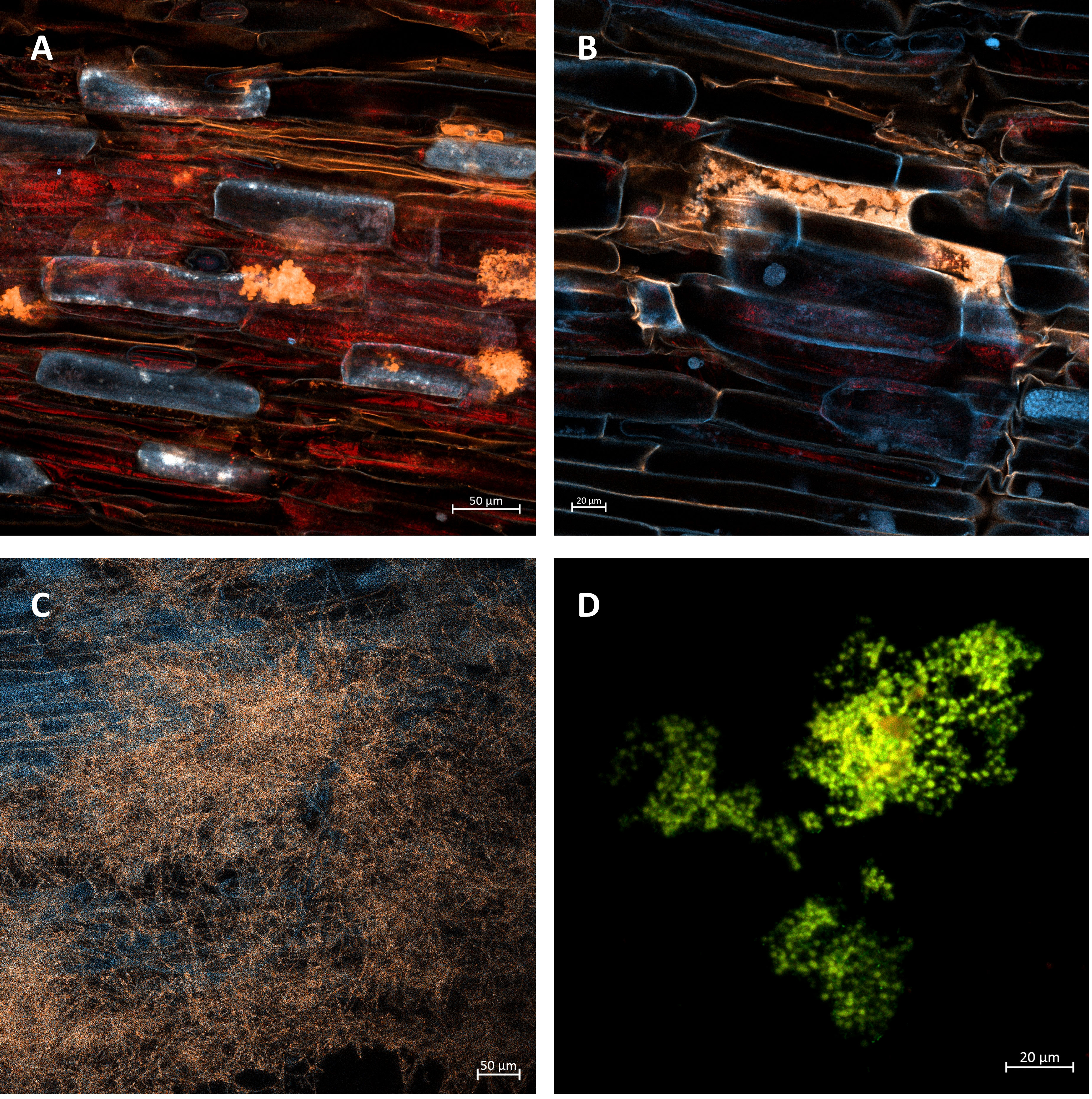
Figure 3 (A) Arbuscules (in orange color) developed within the tissue of onion root. Treatment 50 AMF + AZ. Bar = 50 µm. (B) Details of arbusculum in particular root cells (orange structure), treated with 100 AMF + AZ. Bar = 20 µm. (C) Grid of mycorrhizal mycelia surrounding the onion roots treated with 50 AMF + AZ. Bar = 50 µm. (D) Colonies of Azospirillum brasilense in the root-hair area. Treatment with 100 AMF + AZ. Scale bar = 20 µm.
The arbuscules were present in all treatments inoculated with AMF, with the highest value observed in AMF + AZ 100 and AMF + S 100 treatment (73%) (Figure 2). Vesicles were observed in onion roots sampled from all inoculated substrate formulations in similar amounts 53%–60% (Figure 2). Described parameters indicated the intensive process of mutual symbiosis resulting in the parallel development of AMF, namely colonization rate, arbuscule abundance, and vesicles abundance. This process was additionally confirmed by PCA analysis, showing a close distance between the eigenvectors relevant to colonization rate, arbuscule, and vesicle abundance. Mycorrhization parameters of onion roots (colonization rate, arbuscule abundance, and vesicle abundance) were positively correlated with K content in the substrate. Mycorrhization parameters were positively correlated with antioxidant activity and total phenols in root tissues but negatively correlated with root FW (Supplementary Table 3). Concerning leaf tissues, mycorrhization parameters were positively correlated with leaf FW and Ca, Mg, Na, and P.
3.3 Analyses of stress biomarkers of onion as affected by AMF and PGPM application to investigated growing media
In general, the experimental treatments significantly affected the antioxidant activity of onion seedlings, although no general tendencies were observed. The antioxidant activity, measured as DPPH scavenging activity, was higher in onion seedling roots sampled from the treatments inoculated with AMF and PGPM, namely AMF + AZ 50 (11.2%), AMF + AZ 100 (18.0%), and AMF + S 100 (19.8%), compared to the corresponding controls (C 50 and C 100) at a significance level of 0.05. For onion leaves, the antioxidant activity of plant samples collected from the AMF + AZ 70, AMF + AZ 100 treatments was lower (6.6% and 17.5%, respectively, at a significance level of 0.05) than that of the corresponding controls (C 70 and C 100) (Figure 4A; Supplementary Table 2). The antioxidant activity of onion root extracts was positively correlated with K content in the substrate, AMF colonization rate, arbuscule and vesicle abundance, and root K, Ca, Mg, and GPOX activity (Supplementary Table 3). The antioxidant activity of onion leaf extracts was not affected by soil characteristics or AMF symbiosis, but it was positively correlated with K, Mg, and P content in roots, as well as with GPOX activity in root tissues.
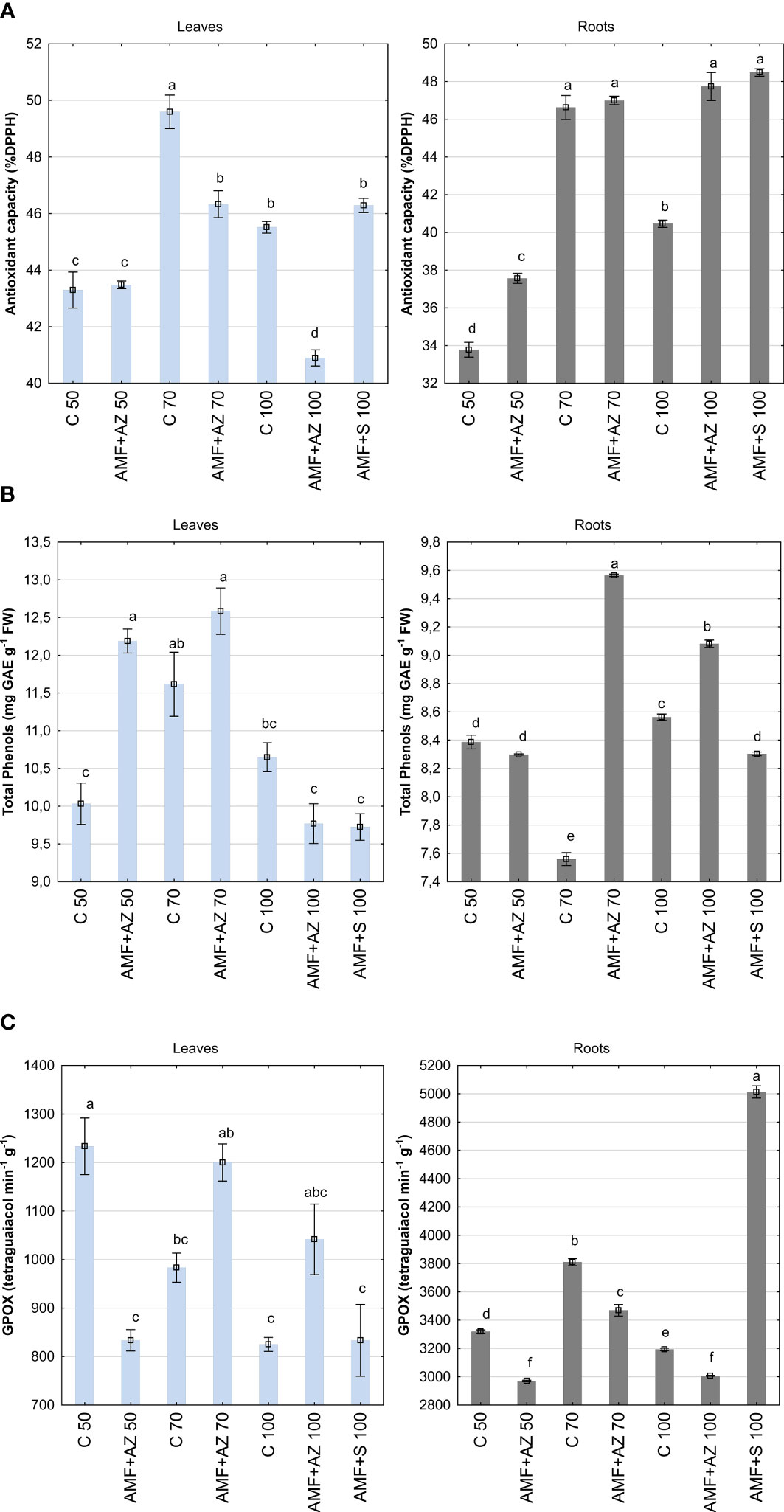
Figure 4 Mean values ( ± SE) of antioxidant activity (A), total phenols (B), and glutathione peroxidase (GPOX) activity (C) in the leaves (left) and roots (right) of onion seedlings. Bars marked with different letters are significantly different at p ≤0.05, according to Tukey’s test. Abbreviations are explained in Figure 1 and Table 1.
The total phenol content was 35.7% higher in onion seedling leaves than in roots based on the main effect analysis (Figure 4B; Supplementary Table 2). Onion roots sampled from plants inoculated with AMF + AZ 70 accumulated the highest number of phenolic compounds (9.56 g), 26.5% higher than the corresponding uninoculated control (C 70). In onion leaves, the highest number of total phenols was determined in AMF + AZ 50 and AMF + AZ 70 treatments (12.19 and 12.58 r, respectively), but only in the case of AMF + AZ 50, the mentioned compounds were accumulated at 21.5% higher extent than in plant leaves of corresponding control (C 50). Total phenols of onion root extracts were positively correlated with Ca content in roots, as well as K and Na contents in leaves (Supplementary Table 3). A negative correlation was noted between the total phenol content and all elements analyzed in the substrate.
The onion seedling roots showed three-fold higher glutathione peroxidase (GPOX) activity than the leaves, concerning the main effects (Figure 4C; Supplementary Table 2). The highest GPOX activity was noted in root samples from the AMF + S 100 treatment (3,470 µmol tetraguaiacol min−1 g−1). The GPOX activity in roots sampled from inoculated AMF + AZ 50 and AMF + AZ 70 treatments was lower (by 5% and 8%, respectively) than that in samples from the corresponding non-inoculated controls (C 50 and C 70). The lowest GPOX activity was noted in onion leaf tissues sampled from treatments with addition of inoculants, namely AMF + AZ 50 and AMF + S 100 (833.3 and 833.8 µmol tetraguaiacol min−1 g−1, respectively) as well as from non-inoculated C 100 treatment (825.0 µmol tetraguaiacol min−1 g−1). The GPOX activity in onion leaves of AMF + AZ 70 and AMF + AZ 100 treatments was higher (by 22.0% and 26.3%, respectively) than that recorded in plant samples from the corresponding controls (C 70 and C 100). The GPOX activity of onion root extracts was positively correlated with Mg and negatively correlated with Na content in the roots (Supplementary Table 3). No positive correlations were observed between GPOX activity in onion leaf tissues and other soil or plant characteristics. The correlations between the investigated stress biomarkers and the other onion seedling root and leaf characteristics are illustrated in Figures 5C, D by the magnitude of the angles between the relevant eigenvectors.
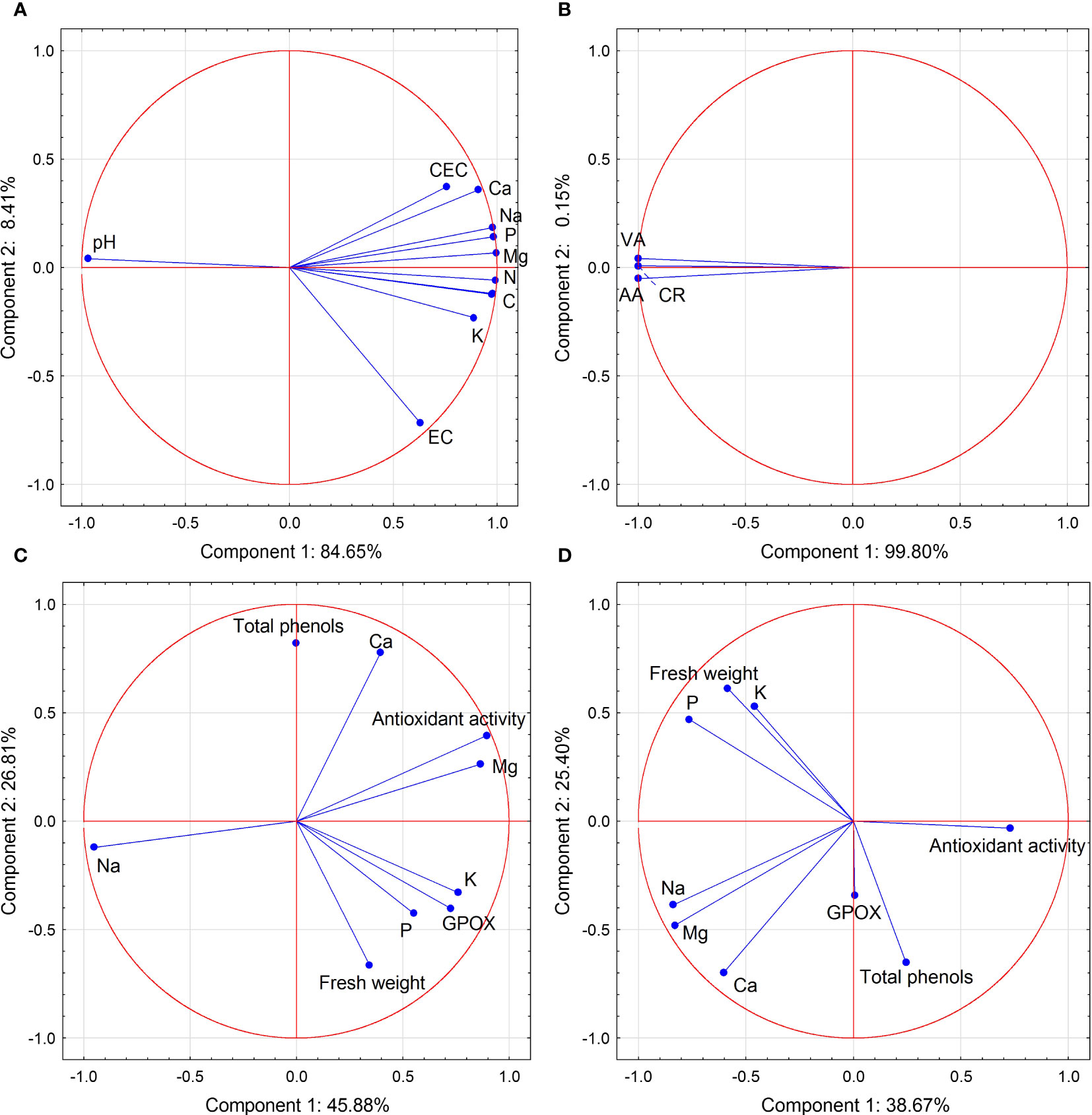
Figure 5 Biplot of PCA of the feature space built using data on the biochemical characteristics of the substrate after cultivation (A), root colonization (B), onion roots (C), and leaves (D). Circles represent observations in the principal component space, whereas vectors indicate the contributions of each feature to the first (x axis) and second (y axis) PCs. EC, cation exchange capacity; EC, electrical conductivity; VA, vesicle abundance; AA, arbuscule abundance; CR, colonization rate; GPOX, glutathione peroxidase activity.
3.4 Element’s concentration in onion tissues as affected by AMF and PGPM application to investigated growing media
The mineral content of onion seedlings was significantly affected by substrate composition and microbial inoculation. K and Ca accumulated in higher amounts in onion seedling leaves than in roots. In contrast, Mg, Na, and P contents were higher in the onion seedling roots than in the leaves (Table 2).
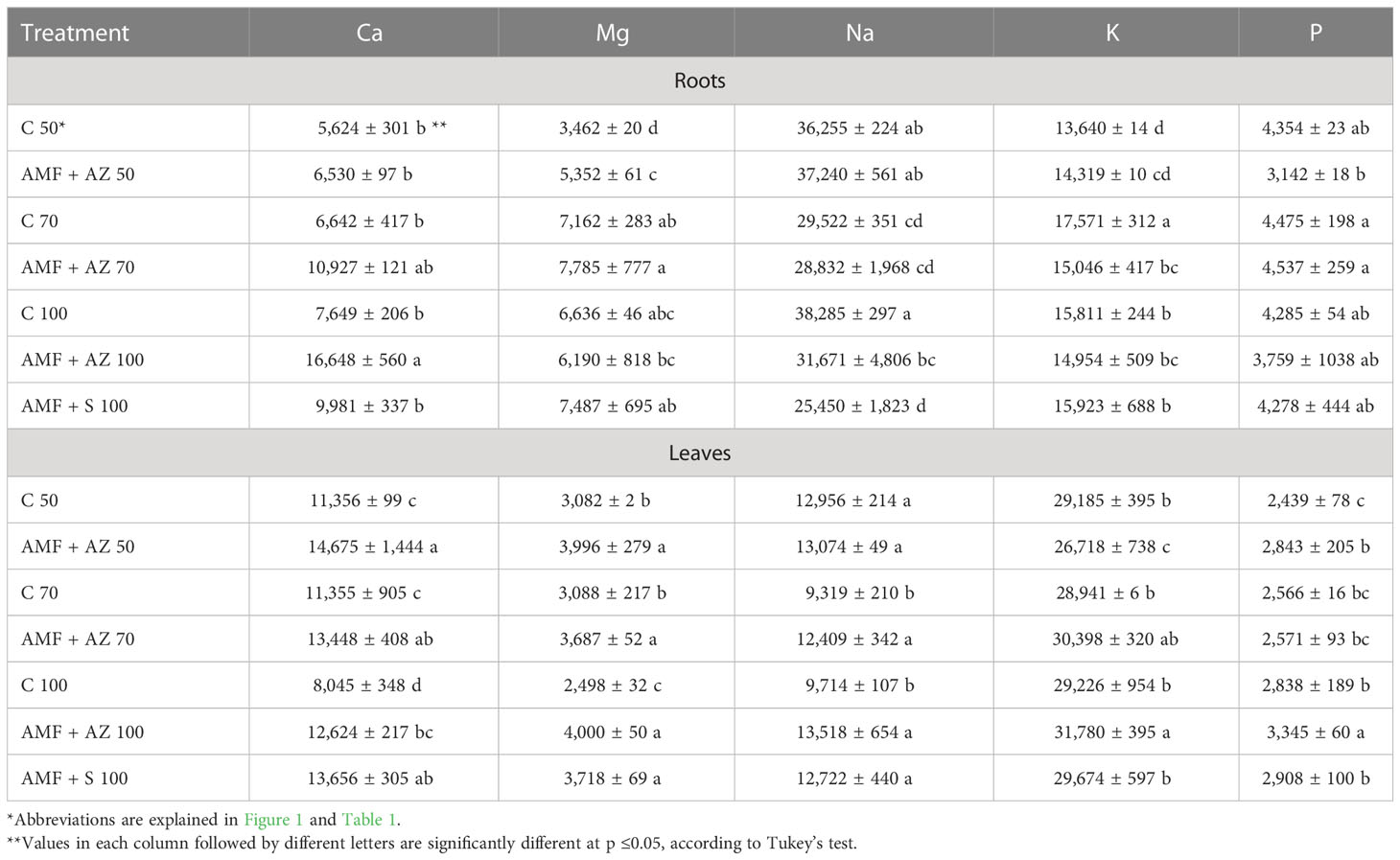
Table 2 Effects of soil microbial inoculants on mineral content in onion seedling roots and leaves (mg kg−1 DW) (mean values ± SD).
The calcium content in the onion leaves and roots ranged from 5,624 to 16,648 mg kg−1 DW (Table 2; Supplementary Table 4). Under the conditions of the peat content in the substrate at the level of 50%, the Ca content in the roots was 5,624 mg kg−1 DW, while when cultivated on pure peat, this value increased to 7,649 mg kg−1 DW. In onion roots cultivated in substrates with inoculation (AMF + AZ 70 and AMF + AZ 100), a significant increase in Ca content was observed in comparison to the corresponding controls (C 70 and C 100). Concerning the Ca content in onion leaves, a significant increase was found in plant material collected from the AMF-S 100 treatment compared to the C 100 treatment.
The Mg content in the tested plants ranged from 2,498 to 7,785 mg kg−1 DW (Table 2; Supplementary Table 4). A significant increase in the concentration of Mg in the onion root tissues by 25%, and in the leaf tissues by 15% was observed as a result of inoculation. The roots collected from the inoculated AMF + AZ 70 and AMF + S 100 treatments contained the highest amount of Mg, but these values were not significantly different from those of the corresponding controls (C 70 and C 100). In the case of onion leaves, inoculation significantly increased Mg content in the AMF + AZ 100 and AMF + S 100 treatments compared to that in the corresponding control (C 100).
In general, approximately 30% more sodium was observed in plants inoculated with substrates than in the corresponding control without mycorrhiza. The level of Na determined in onion roots of the inoculated AMF + AZ 100 and AMF + S 100 treatments was significantly lower than that in the plant material from the corresponding control (C 100). In the case of Na content in onion seedling leaves, no significant differences were noted for the treatments.
There was no difference in the K content of the biomass of plants grown on substrates with and without mycorrhiza. There was also no effect of increasing the proportion of peat in the substrate on the shaping of K content, which ranged from 13,626 to 32,175 mg kg−1 DW. Onion roots sampled from the AMF + AZ 70 treatment contained lower amounts of K than the corresponding control (C 70). Similarly, onion leaves of AMF + AZ 70 accumulated less K than those of the corresponding control (C 70); however, in the case of AMF + AZ 100, the dependence was reversed. In the case of K content in onion seedling leaves, no significant differences were noted between the treatments.
The phosphorus content in plant roots ranged from 2,721 to 4,796 mg kg−1 DW, whereas that in leaves ranged from 2,360 to 3,405 mg kg−1 DW. It was found that the P content was approximately 10% higher inplants collected from substrates subjected to inoculation. P accumulation in onion roots sampled from the inoculated AMF + AZ 50 treatment was lower than that in the samples from the corresponding control (C 50). No significant differences were recorded for the treatments concerning P content in the onion seedling leaves.
The analysis of correlation coefficients indicated a positive relationship between Ca content in the growing medium and P content in the leaves (Supplementary Table 3). Ca content in onion roots was positively correlated with Mg, Na, K, and P in the growing medium and with Mg, K, and P content in leaves.
3.5 Substrate properties after finishing of the growing cycle
The sum of alkaline and acid cations in the sorption complex resulted from the share of the organic fraction in the substrate formulations, and was the highest in the C 100, AMF + AZ 100, and AMF + S 100 treatments, with an organic carbon content of approximately 50%. Total nitrogen was determined in the range of 4.5%–5.0%, and electrical conductivity (EC) 1.38–2.15 mS (Table 3). Organic C was positively correlated with the sum of alkaline and acid cations in the sorption complex and with each of the investigated cations (N, Ca, Mg, Na, K, and P) (Supplementary Table 5).
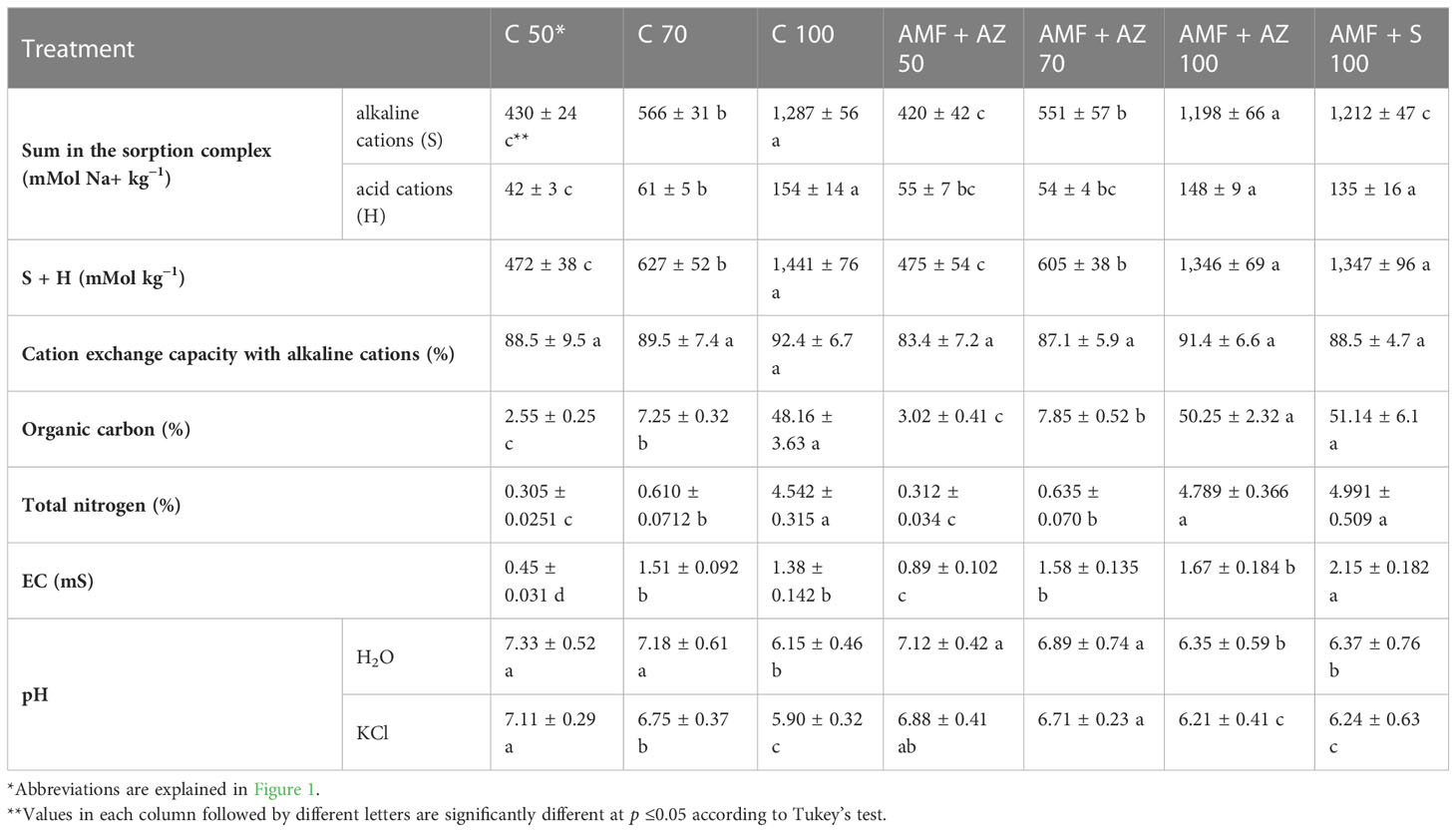
Table 3 Effects of soil microbial inoculants on substrate physical and chemical characteristics after onion seedling cultivation (mean ± SD).
The contents of Ca, Mg, Na, K, and P forms available to plants in the growing medium analyzed after cultivation were the highest in the formulations composed of peat:sand at a ratio of 100:0 (v:v), namely C 100, AMF + AZ 100, and AMF + S 100 (Table 3). Potassium content in the substrates after plant cultivation ranged from 21 to 147 mg kg−1 DW. Moreover, substrates composed of peat:sand in the ratio of 100:0 (v:v), with the addition of beneficial microorganisms (AMF + AZ 100 and AMF + S 100), contained the highest level of K after cultivation. In the case of treatments composed of peat:sand at a ratio of 70:30 (v:v), higher contents of Ca (9.6%), Mg (30.4%), Na (15.1%), and K (53.2%) were determined in growing media inoculated with PGPM (AMF + AZ 70), compared to the non-inoculated control (C 70), whereas P content was similar in the corresponding inoculated and non-inoculated treatments.
A statistically significant correlation was observed between the contents of all examined elements in their available forms in the media. The most common was a negative correlation between the pH measured in KCl and the sum of cations in the sorption complex, as well as the content of Ca, Mg, Na, K, and P forms available to plants (Table 4). These dependencies are illustrated in Figure 5A by the wide angles between the eigenvectors relevant to pHKCl and other soil characteristics.
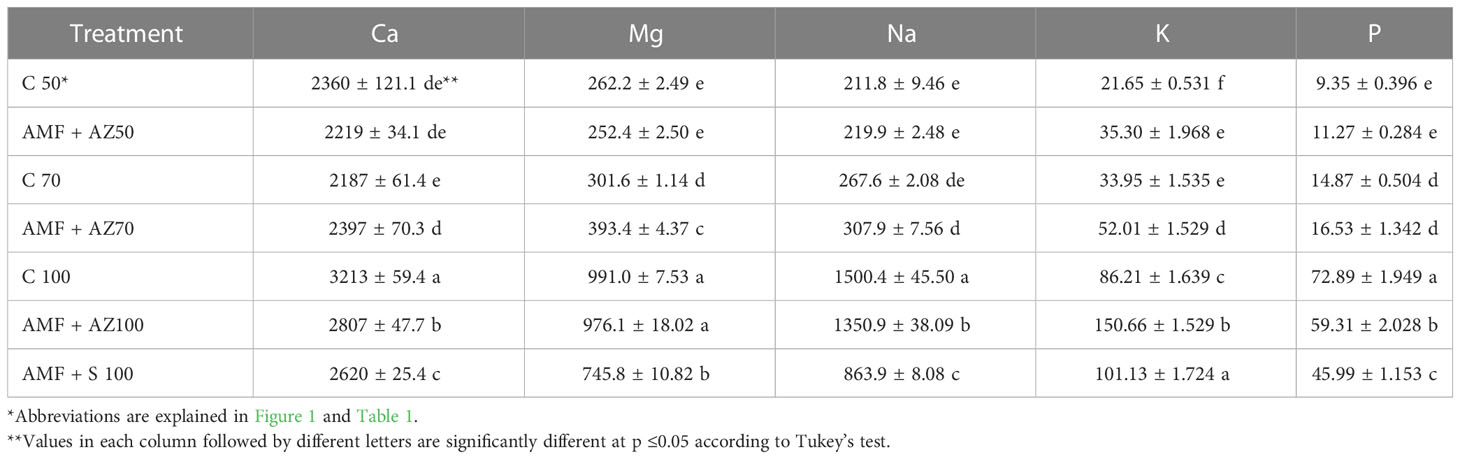
Table 4 Effects of soil microbial inoculants on soluble minerals (mg kg−1 DW) in the substrate after onion seedling cultivation (mean ± SD).
3.6 PCA and cluster analyses
The PCA biplot (Figure 5) shows the contributions of each determined parameter to the first and second PCs separately for soil characteristics, AMF colonization, and onion seedling root and leaf parameters. The data revealed that PC 1 and PC 2 for substrate characteristics accounted for 93.06% of the total variance within the data set, and pHKCl spread below 0 for PC 1, while the remaining factors contributed positively to this component (Figure 5A). All AMF colonization parameters contributed negatively to PC 1 (99.80% of total variance) (Figure 5B). PCA analysis of onion root characteristics showed that all variables, except Na and total phenols, had positive input to PC 1 (84.65% of total variance), while total phenols, antioxidant activity, Ca, and Mg content had positive output to PC 2 (26.81% of total variance) (Figure 5C). In the case of onion leaf characteristics, antioxidant activity and total phenols had a positive input to PC 1, although its share of total variance was only 38.54%. FW, P, and K contents brought positive load to PC 2 (Figure 5D).
In the dendrogram presented in Figure 6, the y-axis shows the distances between the treatments whereas the x-axis represents the analyzed treatments. Based on the cluster analysis (CA) method applied, two main clusters were distinguished based on the substrate characteristics (Figure 6A): the first cluster was formed by substrates with a peat:sand ratio of 50:50 and 70:30 (v:v), and the second was formed with a substrate composed of a peat:sand ratio of 100 (v:v). The CA was obtained from the experimental design. CA analysis of root colonization parameters also distinguished two main clusters: the first was composed of non-inoculated treatments (C 50, C 70, and C 100), and the second was inoculated substrate formulations, with the closest distance between the AMF + AZ 70 and AMF + S 100 treatments (Figure 6B). The root characteristics of the CA also form two main clusters. The first included the C 50, C 100, and AMF + AZ 50 formulations, and the second included the remaining treatments. CA covering leaf characteristics resulted in grouping of the investigated substrate formulations into clusters separating C 50 and C 70 from the other treatments, while the closest relationship was recorded for AMF + AZ 70 and AMF + S 100 treatments. In general, the fact that certain objects belong to clusters demonstrates their considerable similarity within the group in question.
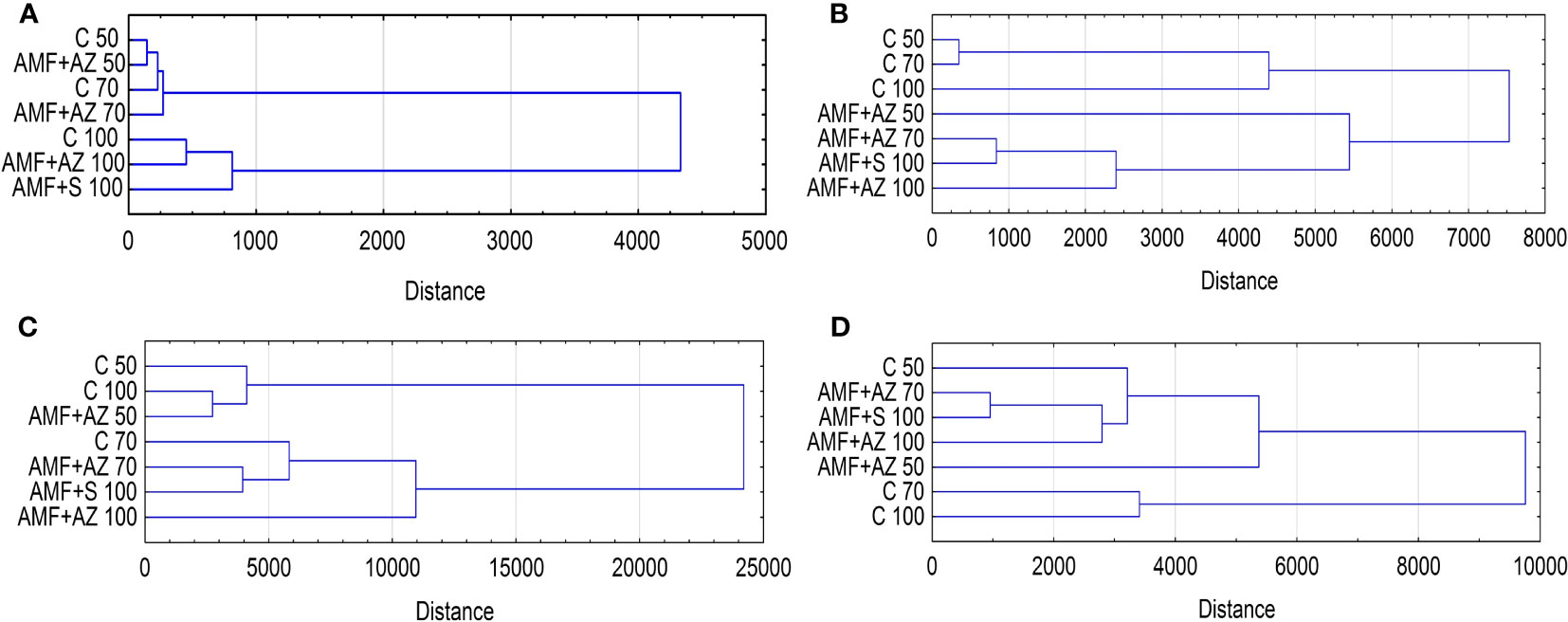
Figure 6 Dendrogram (Euclidean distance, Ward’s method) showing the extent of distance between experimental treatments based on biochemical characteristics of soil after cultivation (A), root colonization (B), onion root (C), and leaf characteristics (D). Abbreviations are explained in Figure 1 and Table 1.
4 Discussion
4.1 Fresh weight of onion as affected by AMF and PGPM application to investigated growing media
In the present experiment, the roots of onions from all treatments inoculated with AMF and PGPM had FW similar to that of the uninoculated control treatments in every peat:sand combination. However, the leaf FW of onion seedlings grown on all inoculated substrates was significantly higher than that in the corresponding controls. Albrechtová et al. (2012) confirmed the synergism between AMF and saprotrophic fungi, resulting in a two-fold increase in the onion yield in the presence of organic matter. The mechanism behind the positive effect of inoculation on onion FW can be the synergistic action of AMF, which induces an accumulation of secondary metabolites, vitamins, and minerals (Baslam et al., 2013), and bacteria, such as N-fixing A. brasilense, which significantly enhances the length of root hairs and seedling weight by increasing the availability of minerals (Ribaudo et al., 2006). Crops inoculated with Azospirillum spp. experience changes in root morphology via siderophore production which regulates plant growth as well as vitamins such as thiamine and riboflavin (Sahoo et al., 2014a; Sahoo et al., 2014b). The application of PGPM in vegetables caused a yield increase of above ground crops by 8%–21%, and underground plant parts by approximately 25%–50%, as well as improved nutrient use efficiency by 12%–36% of N, 18%–29% of P, and 9%–15% of K (Rai et al., 2016). The present study confirmed that leaf FW was positively affected by substrate mineral composition, AMF colonization rate, and arbuscule abundance in onion roots. Onion seedling FW was linked to better efficiency but not development of the root system, resulting in higher aboveground biomass production after AMF + PGPM inoculation. Moreover, total seedling biomass can be predicted with the proposed regression equations, including Mg and Na content in the substrate, and root fresh weight can be predicted using regression equations, including AMF parameters (colonization rate, arbuscule abundance, and vesicle abundance).
4.2 Root colonization of onion as affected by AMF and PGPM application to investigated growing media
The results of observations performed with confocal microscopy showed successful root colonization of AMF in onion seedlings in all treatments. However, the colonization rate, arbuscule abundance, and vesicle abundance were the highest in substrate formulations based on the peat medium. Paranavithana et al. (2020) confirmed that AMF colonization of rice roots is more effective in soils rich in organic carbon. The described parameters indicated the intensive process of mutual symbiosis resulting in the parallel development by AMF mentioned morphological structures, i.e., arbuscules, that the primary sites of nutrient interchange between roots and fungi (Akiyama and Hayashi, 2006). Additionally, the bacterial strains used in the inocula formulation could accelerate mycorrhiza establishment and development because of their ability to decompose chitin and chitosan, the two main constituents of AMF spore walls, thus supporting spore germination in the onion rhizosphere (Battini et al., 2016). Although Saccharothrix spp. produce dithiolopyrolone derivatives with antifungal activity (Merrouche et al., 2017), including PGPM in the formulation used in the present study, the presence of antagonism with AMF was not confirmed. In contrast, such consortium application resulted in improved activity of the enzyme, which is related to lignin formation. Cluster analysis revealed a close distance between AMF + AZ 70 and AMF + S 100 treatments with respect to onion root and leaf characteristics; therefore, AMF and Saccharothrix sp. The consortium was efficient in substrates rich in organic C, while AMF and Azotobacter sp. could be applied to substrates with lower organic C content. According to a study by Zhou et al. (2020), AMF increase plant carbon sequestration. The combination of AMF and Saccharothrix sp. was more effective in organic carbon sequestration and substrate colonization, although other substrate characteristics discussed in the previous subchapter should also be considered. For example, the highest soluble K content in the substrate of AMF + S 100 treatments was analyzed after the experiment. Moreover, the very high antioxidant activity and GPOX activity in onion roots sampled from the AMF + S 100 treatment indicated the successful induction of plant acclimatization, probably linked to Saccharothrix sp. and AMF interaction. In a previous study, Merrouche et al. (2017) reported the antagonism of Saccharothrix against fungi (e.g., Fusarium spp.) and bacteria. Based on present results, it can be concluded that Saccharothrix versus AMF interactions can evolve from parasitism under less optimal conditions to synergism under optimal conditions, in which competition for mineral resources is low or absent.
4.3 Stress biomarkers of onion as affected by AMF and PGPM application to investigated growing media
Onion seedlings grown in substrates reflecting degraded soil are under abiotic stress, including nutrient imbalances, low water-holding capacity, high concentrations of toxic heavy metals, such as Mn, Fe, and Al, high salinity, and pH fluctuations (Enkhtuya et al., 2000; Lehman et al., 2015). These factors modify the growth, function, and mineral absorption of the root system. AMF and PGPM can improve soil parameters and even compensate for chemical fertilizers, especially in onions, which are described as a highly mycorrhizal-dependent crop (Bidondo et al., 2016). The present study showed a new aspect of this relationship because onion seedling roots grown in substrates inoculated with AMF + AZ 50, AMF + AZ 100, and AMF + S 100 showed higher antioxidant activity than the corresponding controls. The latter indicates the acceleration of anti-stress processes leading to the synthesis of reactive oxygen species scavengers in onion roots. However, in onion leaves of the AMF + AZ 100, and AMF + S 100 treatments, antioxidant activity was significantly lower in leaves than in roots. Antioxidant potency is a measure of the ability of the plants to reverse the toxicity induced by stress factors (Veskoukis et al., 2019). In light of the present results, reinforcing the antioxidant defense of tissues was sufficiently efficient in onion roots in the AMF + AZ 100, and AMF + S 100 treatments, which did not need to be initiated to a similar degree in the onion leaves. AMF and PGPM seem to be moderators of these processes, acting in the rhizosphere by providing mineral nutrients and expressing a variety of enzymes that contribute to the control of cellular ROS levels, as reported by Pozo and Azcón-Aguilar (2007). AMF enhances the concentration of phenolic compounds in the roots (Lokhandwala et al., 2014). In the present study, onion roots sampled from the AMF + AZ 70 treatment accumulated the highest amount of phenol compounds. In onion leaves, the highest number of total phenols was found in AMF + AZ 50 and AMF + AZ 70. Phenolic compounds can scavenge reactive oxygen, which is why the phenolic profile is commonly correlated with the antioxidant activity of plant tissues (Veskoukis et al., 2019). In the present study, total phenols of onion root extracts were positively correlated with AMF (colonization rate, arbuscule, and vesicle abundance), but negatively correlated with antioxidant activity. This phenomenon can be explained by the activity of AMF and PGPM to transform phenols into simple compounds, which are ecologically active in soils through stabilization of free enzymes, modification of the transport and bioavailability of nutrients, and enhancement of element mineralization and humus formation (Raaijmakers et al., 2009).
Additionally, the antioxidant activity of onions is linked to sulfur derivates other than polyphenols (Fredotovic and Puizina, 2019). Moreover, phenols accelerate nutrient uptake by roots by chelating metallic ions and enhancing active absorption sites and soil porosity (Seneviratne and Jayasinghearachchi, 2003). Indeed, the present data revealed a positive correlation between total phenols in onion roots and Ca content in roots, as between K and Na content in leaves. This phenomenon can be explained using polyphenol compounds by AMF as substrates to modify biochemical conditions in the rhizosphere environment (Stewart and Stewart, 2008; Raaijmakers et al., 2009; Fredotovic and Puizina, 2019).
GPOX catalyzes lignin formation and establishes a structural barrier by producing reactive oxygen and nitrogen species (Marjamaa et al., 2009). In the present study, the highest GPOX activity was recorded in root samples from the AMF + S 100 treatment, and the elevated GPOX activity under AMF colonization indicates alleviated oxidative stress and therefore a higher resistance, as was reported by Domokos et al. (2018) for Artemisia annua inoculated with R. irregularis. Islam et al. (2015) stated that PGPM activated plant antioxidant defense by regulating the activity of GPOX, as a crucial antioxidant enzyme. The next issue is that the GPOX activity in roots sampled from inoculated treatment AMF + AZ 70 was higher as compared to values determined in samples from corresponding controls. AMF and Saccharothrix sp. consortium were more effective in organic carbon sequestration of peat media and, in turn, colonization. Moreover, the high antioxidant activity and GPOX activity in onion roots sampled from the AMF + S 100 treatment indicated the successful induction of plant response to stress factors, probably linked to Saccharothrix sp. and AMF interaction. In addition, the co-inoculation of onion seedlings with AMF and A. brasilense could cause increased GPOX activity in treatments with a lower amount of organic carbon, reflecting abiotic stress. These results are consistent with those previously reported in other plant species, inoculated separately with AMF (Domokos et al., 2018), A. brasilense (Checchio et al., 2021), or Saccharothrix spp. (Enebe and Babalola, 2019), but no similar effect has been demonstrated for inoculation with AMF and PGPM consortia.
4.4 Element’s concentration in onion Tissues as affected by AMF and PGPM application to investigated growing media
Elemental content in plant products is one of the most important quality parameters (Szeląg-Sikora et al., 2016). The chemical composition of plants determines their nutritional value as well as their suitability for processing or long-term storage (Niemiec et al., 2015). Under the conditions of intensive horticulture, a deficiency of macroelements is very common, which results in a reduction in the yield of plants and deterioration in the usable quality of the crop. Deficiency of macroelements in plants occurs even under conditions of high substrate content (Sikora et al., 2020). The level of bioaccumulation of elements in plants depends not only on their amount in the biotope, but also on their properties (Niemiec et al., 2020a). Increasing the degree of absorption of micronutrients is a strategic aspect of the development of agricultural sciences and production practices (Niemiec et al., 2020b; Seymen et al., 2021). The observed macronutrient contents in the onions of all objects were at the optimal level (Wang et al., 2020; Romo-Péreza et al., 2021); however, samples were taken for analysis in the early stages of development. In the case of macronutrients such as calcium or phosphorus, deficiencies are often visible in the later stages of vegetative development or even in the stage of generative development. An increase in the accumulation of macronutrients in young plants reduces the risk of deficiency at harvest maturity (Bana et al., 2022). Research has demonstrated that co-inoculants of AMF and PGPM enhance the nutrient-use efficiency of fertilizers and reduce chemical fertilizer application rates (Bhardwaj et al., 2014). In general, AMF and PGPM directly affect mineral absorption by the host plant by improving plant growth through nutrient acquisition by the fungus, or indirectly by modifying nutrient mobilization from organic substrates, by enhancing fertilizer use efficiency, or by beneficial association with other microorganisms (Vishwakarma et al., 2017). The analysis of available forms of nutrients in the substrate, their uptake by onion roots, and their translocation to shoots was performed in the framework of the present experiment. The onions collected from the substrate with a peat:sand ratio of 100:0, co-inoculated with AMF + AZ, and AMF + S contained the highest levels of Ca and Mg (but lower Na) in leaves. Azospirillum-inoculated plants have been reported to cause acidification of the root surroundings, which increases macronutrient and micronutrient uptake (Dobbelaere and Okon, 2007). El-Batanomy (2009) revealed that a mixture of microbial cultures showed the highest nitrogenase activity and mycorrhizal infection in onion roots. The total essential nutrient content in onion dry shoots increased in mixed inocula of Azospirillum lipoferum, A. chrococcoum, Bacillus circulans, B. polymyxa, Rhizobium sp., and AMF compared to that in the fertilized control. Moreover, Adesemoye et al. (2009) determined another possible mechanism of PRPM and AMF cooperation; namely, the former can act as a vehicle to spread non-mycorrhizal microorganisms throughout the rhizosphere. Bettoni et al. (2014) demonstrated the increase of P level on onion seedlings inoculated with Glomus intraradices and organic matter amendment. In the present study, Ca content in onion roots was positively correlated with other elements in the substrate, as well as AMF colonization parameters, antioxidant activity, total phenols in roots, FW of leaves, and K, Mg, and P content in leaves. Moreover, Ca, Mg, K, P, and Na contents in onion leaves were positively correlated with AMF colonization parameters. Surprisingly, relatively few increases in P uptake into the seedlings were observed, which was one of the strongest effects of AMF inoculation. Growing medium composition had a more significant effect on P availability and uptake than AMF-related accumulation of this element by onion seedlings. Mentioned interdependence was reflected in highest soluble P content after cultivation cycle in substrates based on peat (100%) with and without AMF inoculation. This effect is due to competition between the decomposition products of organic matter and P for soil sorption sites, resulting in increased soil solution P concentrations (Guppy et al., 2005). Additionally, the differences between AMF-inoculated and uninoculated plants can be considered the costs paid by plants for AMF-root associations. According to Al-Karakis (2002), AMF inoculation of garlic plants was highest when soil P was lowest, and decreased with increasing P application. Moreover, AMF selectively uptake or make available essential cations to plants, which act as osmotic equivalents for toxic Na ions (Hammer et al., 2011). Moreover, Na ions are compartmentalized in cell vacuoles and mycorrhizal fungal hyphae to avoid translocation to the shoots (Estrada et al., 2013). The interactions between AMF and PGPM concerning mineral salt uptake and synergism can be managed, but one must be aware that synergetic interactions between these microbes could also be manifested.
4.5 Substrate characteristics
Onions are among the most sensitive crops to soil conditions, especially salinity, which affects plant growth particularly at the seedling stage (Onishchuk et al., 2017). The physiological and chemical properties of the substrate, including its biomass and chemical composition influence plant growth in a multidirectional manner (Niemiec et al., 2020a). In the present study, the available forms of Ca, Mg, Na, K, and P in the substrate analyzed after cultivation were highest in the formulations composed of peat:sand at a ratio of 100:0 (v:v). Uzinger et al. (2020) demonstrated that the combination of compost and plant growth promoting bacteria resulted in higher P and K availability. In the present study, AMF + PGPM was effective in releasing minerals into the soluble fraction in substrates rich in organic matter, although this capacity was dependent on the consortium of microorganisms used. AMF + AZ 100 and AMF + S 100 application resulted in the highest level of K after cultivation, whereas AMF + AZ 70 inoculants increased the soluble forms of Ca, Mg, Na, and K compared to the non-inoculated control. The P content was similar among the treatments mentioned above. A significant and positive correlation between the soluble elements in substrates and organic carbon, while a negative correlation with pHKCl, confirmed the crucial significance of organic matter and reaction for element availability to plants. This phenomenon has been noted by numerous researchers (Guaya et al., 2020; Niemiec et al., 2020b; Lei et al., 2021). Additionally, in the present study, the mineral soluble forms were not reduced in formulations with higher EC values, particularly in the inoculated treatments. Balliu et al. (2015) stated that uptake of elements was enhanced in inoculated tomato seedlings even under moderate salt stress. Despite the large differences in the organic fraction content of the substrates and the capacity of the sorption complex, slight differences in substrate pH occurred after the experiment. In general, a wide spectrum of soil properties converge to create synergistic effects, leading to the reshaping of microbiome/plant interactions.
5 Conclusions
Alliaceae crops encounter various challenges, and limiting conditions can result in serious economic losses at every growth stage. Research on plant growth-promoting microbes (PGPM) as a potential component of mainstream agriculture is important and essential. Inoculation with AMF and PGPM consortia resulted in significant improvement in onion seedling performance, i.e., higher aboveground biomass production and better stress adaptation in cultivation in substrates with lower organic carbon content. Our results demonstrated that AMF and Saccharothrix sp. were effective in substrates containing 70%–100% peat, while AMF and Azotobacter sp. can be recommended to inoculate substrates with 50% organic C to enhance onion seedling performance. Differences between AMF- and PGPM-inoculated plants concerning fresh weight, stress biomarkers, and element concentration were considered the costs paid by plants due to AMF–root associations linked with growing medium-related availability of nutrients. The present findings have revealed a significant aspect of soil/plant management, i.e., that organic/beneficial microbe synergy might be the future key for effective nutrient management and sustainable production. Although we demonstrated the benefits of AMF and PGPM inoculation on onion seedlings, the maintenance of symbiosis after transplanting can be the goal of subsequent investigations, as field conditions create new challenges to plant–microorganism interactions.
Data availability statement
The raw data supporting the conclusions of this article will be made available by the authors, without undue reservation.
Author contributions
Conceptualization, RP, AS, AK, and LR. Methodology, RP and LR. Validation, AS and RP. Formal analysis, MN, LR, MJ, and MK. Resources, AS. Data curation, RP and AS. Writing—original draft preparation, RP and AS. Writing—review and editing, LR, MK, MG, MJ, MN, and GC. Supervision, RP. Project administration, AS. Funding acquisition, RP and AS. All authors listed have made a substantial, direct, and intellectual contribution to the work and approved it for publication.
Funding
This study was supported by the project of the Internal Grant Agency of the Faculty of Horticulture, Mendel University in Brno IGA-ZF/2021-SI1004. Microscopic analyses were performed on infrastructure supported by the project OP VVV CZ.02.1.01/0.0/0.0/16_017/0002334 Research infrastructure for young scientists was financed from structural funds of the EU and Ministry of Education of the Czech Republic. The study was supported by the Polish-Czech Joint Research Project PPN/BCZ/2019/1/00014; Microorganism biodiversity impact on soil conservation in agricultural crop system.
Conflict of interest
The authors declare that the research was conducted in the absence of any commercial or financial relationships that could be construed as a potential conflict of interest.
Publisher’s note
All claims expressed in this article are solely those of the authors and do not necessarily represent those of their affiliated organizations, or those of the publisher, the editors and the reviewers. Any product that may be evaluated in this article, or claim that may be made by its manufacturer, is not guaranteed or endorsed by the publisher.
Supplementary material
The Supplementary Material for this article can be found online at: https://www.frontiersin.org/articles/10.3389/fpls.2023.1222557/full#supplementary-material
References
Adesemoye, A. O., Torbert, H. A., Kloepper, J. W. (2009). Plant growth promoting rhizobacteria allow reduced application rates of chemical fertilizers. Microb. Ecol. 58, 921–929. doi: 10.1007/s00248-009-9531-y
Akiyama, K., Hayashi, H. (2006). Strigolactones: chemical signals for fungal symbionts and parasitic weeds in plant roots. Ann. Bot. Lond. 97, 925–931. doi: 10.1093/aob/mcl063
Alarcón, C., Cuenca, G. (2005). Arbuscular mycorrhizas in coastal sand dunes of the paraguanä peninsula, Venezuela. Mycorrhiza 16 (1), 1–9. doi: 10.1007/s00572-005-0005-x
Albrechtová, L. S. J., Latr, A., Nedorost, Ľ., Pokluda, R., Posta, K., Vosátka, M. (2012). Dual inoculation with mycorrhizal and saprotrophic fungi applicable in sustainable cultivation improves the yield and nutritive value of onion. Sci. World J. 2012, 1–8. doi: 10.1100/2012/374091
Al-Karakis, G. N. (2002). Benefit, cost, and phosphorus use efficiency of mycorrhizal field–grown garlic at different soil phosphorus levels. J. Plant Nutri. 25 (6), 1175–1184. doi: 10.1081/PLN-120004381
Balliu, A., Sallaku, G., Rewald, B. (2015). AMF inoculation enhances growth and improves the nutrient uptake rates of transplanted, salt–stressed tomato seedlings. Sustainability 7 (12), 15967–15981. doi: 10.3390/su71215799
Bana, R., Grover, M., Kumar, V., Jat, G., Kuri, B., Singh, D., et al. (2022). Multi–micronutrient foliar fertilization in eggplant under diverse fertility scenarios: effects on productivity, nutrient biofortification and soil microbial activity. Sci. Hortic. 294, 110781. doi: 10.1016/j.scienta.2021.110781
Baslam, M., Garmendia, I., Goicoechea, N. (2013). Enhanced accumulation of vitamins, nutraceuticals and minerals in lettuces associated with arbuscular mycorrhizal fungi (AMF): a question of interest for both vegetables and humans. Agriculture 3 (1), 188–209. doi: 10.3390/agriculture3010188
Battini, F., Cristani, C., Giovannetti, M., Agnolucci, M. (2016). Multifunctionality and diversity of culturable bacterial communities strictly associated with spores of the plant beneficial symbiont Rhizophagus intraradices. Microbiol. Res. 183, 68–79. doi: 10.1016/j.micres.2015.11.012
Bettoni, M. M., Mogor, Á.F., Pauletti, V., Goicoechea, N. (2014). Growth and metabolism of onion seedlings as affected by the application of humic substances, mycorrhizal inoculation and elevated CO2. Sci. Hortic. 180, 227–235. doi: 10.1016/j.scienta.2014.10.037
Bhardwaj, D., Ansari, M. A., Sahoo, R. K., Tuteja, N. (2014). Biofertilizers function as key player in sustainable agriculture by improving soil fertility, plant tolerance and crop productivity: a review. Microb. Cell Factories 13, 66–74. doi: 10.1186/1475-2859-13-66
Bidondo, L. F., Colombo, R., Bompadre, J., Benavides, M., Scorza, V., Silvani, V., et al. (2016). Cultivable bacteria associated with infective propagules of arbuscular mycorrhizal fungi. implications for mycorrhizal activity. Appl. Soil Ecol. 105, 86–90. doi: 10.1016/j.apsoil.2016.04.013
Bolandnazar, S. (2009). The effect of mycorrhizal fungi on onion (Allium cepa l.) growth and yield under three irrigation intervals at field condition. J. Food Agric. Environ. 7, 360–362.
Bolandnazar, S., Aliasgarzad, N., Neishabury, M., Chaparzadeh, N. (2007). Mycorrhizal colonization improves onion (Allium cepa l.) yield and water use efficiency under water deficit condition. Sci. Hortic. 114, 11–15. doi: 10.1016/j.scienta.2007.05.012
Caruso, G., Golubkina, N., Seredin, T., Sellitto, B. (2018). Utilization of AMF in production of Allium species. Veg Crop Russ. 3, 85–90. doi: 10.18619/2072-9146-2018-3-93-98
Charron, G., Furlan, V., Bernier-Cardou, M., Doyon, G. (2001). Response of onion plants to arbuscular mycorrhizae. effects of inoculation method and phosphorus fertilization on biomass and bulb firmness. Mycorrhiza 11, 187–197. doi: 10.1007/s005720100122
Checchio, M. V., de Cássia Alves, R., de Oliveira, K. R., Moro, G. V., Santos, D. M. M. D., Gratão, P. L. (2021). Enhancement of salt tolerance in corn using Azospirillum brasilense: an approach on antioxidant systems. J. Plant Res. 134 (6), 1279–1289. doi: 10.1007/s10265-021-01332-1
Colo, J., Hajnal–Jafari, T. I., Duric, S., Stamenov, D., Hamidovic, S. (2014). Plant growth promotion rhizobacteria in onion production polish J. Microbiol 63 (1), 83–88. doi: 10.33073/PJM-2014-012
Deressa, T. G., Schenk, M. K. (2008). Contribution of roots and hyphae to phosphorus uptake of mycorrhizal onion (Allium cepa l.)-a mechanistic modeling approach. J. Plant Nutr. Soil Sci. 171, 810–820. doi: 10.1002/jpln.200700209
Djeridane, A., Yousfi, M., Nadjemi, B., Boutassouna, D., Stocker, P., Vidal, N. (2006). Antioxidant activity of some Algerian medicinal plants extracts containing phenolic compound. Food Chem. 97, 654–660. doi: 10.1016/j.foodchem.2005.04.028
Dobbelaere, S., Okon, Y. (2007). “The plant growth promoting effects and plant responses,” in Nitrogen fixation: origins, applications and research progress, associative and endophytic nitrogen–fixing bacteria and cyanobacterial associations, vol. V . Eds. Elmerich, C., Newton, W. E. (Heidelberg: Springer).
Domokos, E., Jakab-Farkas, L., Darkó, B., Bíró-Janka, B., Mara, G., Albert, C., et al. (2018). Increase in artemisia annua plant biomass artemisinin content and guaiacol peroxidase activity using the arbuscular mycorrhizal fungus rhizophagus irregularis. Front. Plant Sci. 9, 478. doi: 10.3389/fpls.2018.00478
Drost, D., Koenig, R. (2002). : nitrogen use efficiency and onion yield increased with a polymer–coated nitrogen source. HortScience 37, 338–342. doi: 10.21273/HORTSCI.37.2.338
El-Batanomy, N. (2009). Synergistic effect of plant-growth promoting rhizobacteria and arbuscular mycorrhiza fungi on onion (Allium cepa) growth and its bulbs quality after storage. New Egypt J. Microbiol. 23, 163–182.
Enebe, M. C., Babalola, O. O. (2019). The impact of microbes in the orchestration of plants’ resistance to biotic stress: a disease management approach. Appl. Microbiol. Biotechnol. 103 (1), 9–25. doi: 10.1007/s00253-018-9433-3
Enkhtuya, B., Rydlová, J., Vosátka, M. (2000). Effectiveness of indigenous and non-indigenous isolates of arbuscular mycorrhizal fungi in soils from degraded ecosystems and man–made habitats. Appl. Soil Ecol. 14 (3), 201–211. doi: 10.1016/S0929-1393(00)00057-3
Estrada, B., Arroca, R., Maathuis, F. J. M. (2013). Arbuscular mycorrhizal fungi native from a Mediterranean saline area enhance maize tolerance to salinity through improved ionhomeostasis. Plant Cell Environ. 36 (10), 1771–1782. doi: 10.1111/pce.12082
Fredotovic, Ž., Puizina, J. (2019). Edible Allium species: chemical composition, biological activity and health effects. Ital J. Food Sci. 31, 19–39. doi: 10.14674/IJFS-1221
Galván, G. A., Parádi, I., Burger, K., Baar, J., Kuyper, T. W., Scholten, O. E., et al. (2009). Molecular diversity of arbuscular mycorrhizal fungi in onion roots from organic and conventional farming systems in the Netherlands. Mycorrhiza 19, 317–328. doi: 10.1007/s00572-009-0237-2
Gavilanes, F., Amaral, H., García, M., Araujo-Junior, C., Zanão Júnior, L., Nomura, R., et al. (2021). “Interactions between edaphoclimatic conditions and plant-microbial inoculants and their impacts on plant growth, nutrient uptake, and yields,” in Advances in the domain of environmental biotechnology. Environmental and microbial biotechnology. Edc. N. R. Maddela, , L. C. García Cruzatty and S. Chakraborty (Singapore: Springer), 591–633. doi: 10.1007/978-981-15-8999-7_22
Golubkina, N., Krivenkov, L., Sekara, A., Vasileva, V., Tallarita, A., Caruso, G. (2020). Prospects of arbuscular mycorrhizal fungi utilization in production of Allium. Plants 9 (2), 279. doi: 10.3390/plants9020279
Guaya, D., Mendoza, A., Valderrama, C., Farran, A., Sauras-Yera, T., Cortina, J. (2020). Use of nutrient–enriched zeolite (NEZ) from urban wastewaters in amended soils: evaluation of plant availability of mineral elements. Sci. Total Environ. 727, 138646. doi: 10.1016/j.scitotenv.2020.138646
Guppy, C., Menzies, N., Moody, P., Blamey, F. (2005). Competitive sorption reactions between phosphorus and organic matter in soil: a review. Soil Res. 43 (2), 189. doi: 10.1071/SR04049
Hammer, E., Nasr, H., Pallon, J., Olsson, P., Wallander, H. (2011). Elemental composition of arbuscular mycorrhizal fungi at high salinity. Mycorrhiza 21, 117–129. doi: 10.1007/s00572-010-0316-4
Hong, L., Orikasa, Y., Sakamoto, H., Ohwada, T. (2019). Plant tissue localization and morphological conversion of Azospirillum brasilense upon initial interaction with Allium cepa l. Microorganisms 7, 275. doi: 10.3390/microorganisms7090275
Islam, F., Yasmeen, T., Arif, M. S., Ali, S., Ali, B., Hameed, S., et al. (2015). Plant growth promoting bacteria confer salt tolerance in Vigna radiata by up-regulating antioxidant defense and biological soil fertility. Plant Growth Regul. 80, 23–36. doi: 10.1007/s10725-015-0142-y
Iturralde, E. T., Covelli, J. M., Alvarez, F., Pérez-Giménez, J., Arrese–Igor, C., Lodeiro, A. R. (2019). Soybean–nodulating strains with low intrinsic competitiveness for nodulation, good symbiotic performance, and stress–tolerance isolated from soybean-cropped soils in Argentina. Front. Microbiol. 10, 1061. doi: 10.3389/fmicb.2019.01061
Joe, M., Karthikeyan, B., Chauhan, P., Shagol, C., Islam, M., Deiveekasundaram, M., et al. (2012). Survival of Azospirillum brasilense flocculated cells in alginate and its inoculation effect on growth and yield of maize under water deficit conditions. Eur. J. Soil Biol. 50, 198–206. doi: 10.1016/j.ejsobi.2012.03.002
Lee, J., Lee, S. (2014). Correlations between soil physico-chemical properties and plant nutrient concentrations in bulb onion grown in paddy soil. Sci. Hortic. 179, 158–162. doi: 10.1016/j.scienta.2014.09.019
Lehman, R. M., Cambardella, C. A., Stott, D. E., Acosta-Martinez, V., Manter, D. K., Buyer, J. S., et al. (2015). Understanding and enhancing soil biological health: the solution for reversing soil degradation. Sustainability 7 (1), 988–1027. doi: 10.3390/su7010988
Lei, M., Wang, Y., Guo, G., Zhang, D., Zhao, X. (2021). The bio-availability and accumulation of the trace elements in rock-soil-fruit system in carbonatite regions of different stratums: critical soil factors and transfer models. Sci. Total Environ. 760, 143328. doi: 10.1016/j.scitotenv.2020.143328
Lokhandwala, S., Kareliya, N., Patel, T., Rana, M. (2014). Phenol production and antimicrobial activity of fulleniformis mosseae inoculated allium opea roots. Int. J. Rec. Biotechnol. 2 (1), 35–37.
Lone, R., Shuab, R., Wani, K., Ganaie, M. A., Tiwari, A., Koul, K. (2015). Mycorrhizal influence on metabolites, indigestible oligosaccharides, mineral nutrition and phytochemical constituents in onion (Allium cepa l.) plant. Sci. Hortic. 193, 55–61. doi: 10.1016/j.scienta.2015.06.032
Lück, H. (1962). “Peroxydase,” in Methoden der enzymatischen analyse (Weinheim: Verlag Chemie GmbH).
Ma, Y. (2019). Seed coating with beneficial microorganisms for precision agriculture. Biotechnol. Adv. 37 (7), 107423. doi: 10.1016/j.biotechadv.2019.107423
Marjamaa, K., Kukkola, E. M., Fagerstedt, K. V. (2009). The role of xylem class III peroxidases in lignification. J. Exp. Bot. 60, 367–376. doi: 10.1093/jxb/ern278
Merrouche, R., Yekkour, A., Lamari, L., Zitouni, A., Mathieu, F., Sabaou, N. (2017). Efficiency of Saccharothrix algeriensis NRRL b–24137 and its produced antifungal dithiolopyrrolones compounds to suppress Fusarium oxysporum–induced wilt disease occurring in some cultivated crops. Arab. J. Sci. Engin. 42 (6), 2321–2327. doi: 10.1007/s13369-017-2504-4
Mohamed, A. A., Eweda, W. E., Heggo, A., Hassan, E. A. (2014). Effect of dual inoculation with arbuscular mycorrhizal fungi and sulphur–oxidising bacteria on onion (Allium cepa l.) and maize (Zea mays l.) grown in sandy soil under green house conditions. Ann. Agric. Sci. 59, 109–118. doi: 10.1016/j.aoas.2014.06.015
Mollavali, M., Bolandnazar, S., Nazemieh, H., Aliasgharzad, N. (2015). The effect of mycorrhizal fungi on antioxidant activity of various cultivars of onion (Allium cepa l.). Int. J. Biosci. 6, 66–79. doi: 10.12692/ijb/6.1.66-79
Molyneux, P. (2004). The use of the stable free radical diphenylpicrylhydrazyl (DPPH) for estimating antioxidant activity. Songklanakarin J. Sci. Technol. 26, 211–219.
Mosse, B., Stribley, D., LeTacon, F. (1981). Ecology of mycorrhizae and mycorrhizal fungi. Adv. Microb. Ecol. 5, 137–210. doi: 10.1007/978-1-4615-8306-6_4
Nacoon, S., Jogloy, S., Riddech, N., Mongkolthanaruk, W., Ekprasert, J., Cooper, J., et al. (2021). Combination of arbuscular mycorrhizal fungi and phosphate solubilizing bacteria on growth and production of Helianthus tuberosus under field condition. Sci. Rep. 11 (1), 6501. doi: 10.1038/s41598-021-86042-3
Niemiec, M., Chowaniak, M., Zuzek, D., Komorowska, M., Mamurovich, G. S., Kodirov Gafurovich, K., et al. (2020a). Evaluation of the chemical composition of soil as well as vine leaves and berries from the selected commercial farms in the republic of Tajikistan. J. Elementol. 25 (2), 675–686. doi: 10.5601/jelem.2019.24.4.1810
Niemiec, M., Komorowska, M., Mudryk, K., Jewiarz, M., Sikora, J., Szeląg-Sikora, A., et al. (2020b). “Evaluation of the fertilizing potential of products based on torrefied biomass and valorized with mineral additives,” in Springer proceedings in energy (Cham: Springer), 267–275.
Niemiec, M., Szeląg-Sikora, A., Cupiał, M. (2015). “Evaluation of the efficiency of celeriac fertilization with the use of slow-acting fertilizers,” in Farm machinery and processes management in sustainable agriculture, 7th international scientific symposium. Eds. Huyghebaert, B., Lorencowicz, E., Uziak, J. (Amsterdam: Agriculture and Agricultural Science Procedia Elsevier), 177–183.
Onishchuk, O. P., Vorobyov, N. I., Provorov, N. A. (2017). Nodulation competitiveness of nodule bacteria: genetic control and adaptive significance. Appl. Biochem. Microbiol. 53, 131–139. doi: 10.1134/S0003683817020132
Paranavithana, T. M., Marasinghe, S., Perera, G. A. D., Ratnayake, R. R. (2020). Effects of crop rotation on enhanced occurrence of arbuscular mycorrhizal fungi and soil carbon stocks of lowland paddy fields in seasonally dry tropics. Paddy Water Environ. 19 (1), 217–226. 10.1007/s10333-020-00833-4
Parnell, J., Berka, R., Young, H., Sturino, J., Kang, Y., Barnhart, D., et al. (2016). From the Lab to the farm: an industrial perspective of plant beneficial microorganisms. Front. Plant Sci. 7. doi: 10.3389/fpls.2016.01110
Pasławski, P., Migaszewski, Z. M. (2006). The quality of element determinations in plant materials by instrumental methods. Pol. J. Environ. Stud. 15 (2a), 154–164.
Petrovic, B., Sękara, A., Pokluda, R. (2020). Biofertilizers enhance quality of onion. Agronomy 10 (12), 1937. doi: 10.3390/agronomy10121937
Pozo, M., Azcón-Aguilar, C. (2007). Unraveling mycorrhiza-induced resistance. Curr. Opin. Plant Biol. 10 (4), 393–398. doi: 10.1016/j.pbi.2007.05.004
Raaijmakers, J. M., Paulitz, T. C., Steinberg, C., Alabouvette, C., Moënne-Loccoz, Y. (2009). The rhizosphere: playground and battlefield for soilborne pathogens and beneficial microorganisms. Plant Soil 321 (1–2), 341–361. doi: 10.1007/s11104-008-9568-6
Rai, S., Rani, P., Kumar, M., Rai, A., Shahi, S. K. (2016). Effect of integrated nutrient management on nutrients uptake and productivity of onion. Nat. Environ. Poll. Technol. 15 (2), 573.
Ribaudo, C. M., Krumpholz, E. M., Cassán, F. D., Bottini, R., Cantore, M. L., Cura, J. A. (2006). Azospirillum sp. promotes root hair development in tomato plants through a mechanism that involves ethylene. J. Plant Growth Regul. 25, 175–185. doi: 10.1007/s00344-005-0128-5
Romo-Péreza, M. L., Weinert, C. H., Egert, B., Franzisky, B. L., Kulling, S. E., Zörb, C. (2021). Sodium accumulation has minimal effect on metabolite profile of onion bulbs. Plant Physiol. Biochem. 168, 423–431. doi: 10.1016/j.plaphy.2021.10.031
Rozpadek, P., Rapala-Kozik, M., Wezowicz, K., Grandin, A., Karlsson, S., Wazny, R., et al. (2016). Arbuscular mycorrhiza improves yield and nutritional properties of onion (Allium cepa). Plant Physiol. Biochem. 107, 264–272. doi: 10.1016/j.plaphy.2016.06.006
Sahoo, R. K., Ansari, M. W., Dangar, T. K., Mohanty, S., Tuteja, N. (2014a). Phenotypic and molecular characterization of efficient nitrogen fixing Azotobacter strains of the rice fields. Protoplasma 251 (3), 511–523. doi: 10.1007/s00709-013-0547-2
Sahoo, R. K., Ansari, M. W., Pradhan, M., Dangar, T. K., Mohanty, S., Tuteja, N. (2014b). Phenotypic and molecular characterization of efficient native Azospirillum strains from rice fields for crop improvement. Protoplasma 251 (4), 943–953. doi: 10.1007/s00709-013-0607-7
Schmidt, S. K., Sobieniak-Wiseman, L. C., Kageyama, S. A., Halloy, S. R. P., Schadt, C. W. (2008). Mycorrhizal and dark–septate fungi in plant roots above 4270 meters elevation in the Andes and rocky mountains. Arctic Antarctic Alpine Res. 40 (3), 576–583. doi: 10.1657/1523-0430(07-068)[SCHMIDT]2.0.CO;2
Sekara, A., Pokluda, R., Del Vacchio, L., Somma, S., Caruso, G. (2017). Interactions among genotype, environment and agronomic practices on production and quality of storage onion (Allium cepa l.)-a review. Hortic. Sci. (Prague) 201, 44, 21–42. doi: 10.17221/92/2015-HORTSCI
Seneviratne, G., Jayasinghearachchi, H. S. (2003). Mycelial colonization by bradyrhizobia and azorhizobia. J. Biosci. 28, 243–247. doi: 10.1007/BF02706224
Serra, A. D. B., Currah, L. (2002). “Agronomy of onions,” in Allium crop science: recent advances (Wallingford, UK: CABI), 187–232.
Seymen, M., Erdinç, Ç., SaitKurtar, E. S., Kal, Ü., Şensoy, S., Türkmen, Ö. (2021). “Chapter 12-potential effect of microbial biostimulants in sustainable vegetable production,” in Microbiome stimulants for crops, mechanisms and applications, (Amsterdam, Netherlands: Woodhead Publishing (Elsevier)) 193–237.
Shinde, S. K., Shinde, B. P. (2016). Consequence of arbuscular mycorrhiza on enhancement, growth and yield of onion (Allium cepa L.). Int. J. Life–Sci. Sci. Res. 2, 206–211.
Shirling, E. T., Gottlieb, D. (1966). Methods for characterization of streptomyces species. Int. J. Sys. Bacteriol. 16 (3), 313–340. doi: 10.1099/00207713-16-3-313
Sikora, J., Niemiec, M., Tabak, M., Grodek-Szostak, Z., Szeląg–Sikora, A., Kuboń, M., et al. (2020). Assessment of the efficiency of nitrogen slow–release fertilizers in integrated production of carrot depending on fertilization strategy. Sustainability 12 (5), 1982. doi: 10.3390/su12051982
Stewart, A. J., Stewart, R. F. (2008). “Phenols,” in Encyclopedia of ecology. Eds. Jørgensen, S. E., Fath, B. D. (Amsterdam: Academic Press). doi: 10.1016/B978–008045405–4.00417–1
Syed, S., Tollamadugu, N. P. (2019). “Role of plant growth–promoting microorganisms as a tool for environmental sustainability,” in Recent developments in applied microbiology and biochemistry. Ed. Viswanath, B. (New York: Academic Press), 209–222.
Szeląg-Sikora, A., Niemiec, M., Jakub, S. (2016). Assessment of the content of magnesium, potassium, phosphorus and calcium in water and algae from the black Sea in selected bays near Sevastopol. J. Elem. 21 (3), 915–926. doi: 10.5601/jelem.2015.20.4.969
Tarrand, J. J., Krieg, N. R., Dobereiner, J. (1978). A taxonomic study of the Spirillum lipoferum group, with descriptions of a new genus, Azospirillum gen. nov. and two species, Azospirillum lipoferum (Beijerinck) comb. nov. and Azospirillum brasilense sp. nov. Can. J. Microbiol. 24, 967–980. doi: 10.1139/m78-160
Tinna, D., Garg, N., Sharma, S., Pandove, G., Chawla, N. (2020). Utilization of plant growth promoting rhizobacteria as root dipping of seedlings for improving bulb yield and curtailing mineral fertilizer use in onion under field conditions. Sci. Hortic. 270, 109432. doi: 10.1016/j.scienta.2020.109432
Uzinger, N., Takács, T., Szili-Kovács, T., Radimszky, L., Füzy, A., Draskovits, E., et al. (2020). Fertility impact of separate and combined treatments with biochar, sewage sludge compost and bacterial inocula on acidic sandy soil. Agronomy 10 (10), 1612. doi: 10.3390/agronomy10101612
Veskoukis, A., Kerasioti, E., Priftis, A., Kouka, P., Spanidis, Y., Makri, S., et al. (2019). A battery of translational biomarkers for the assessment of the in vitro and in vivo antioxidant action of plant polyphenolic compounds: the biomarker issue. Curr. Opi. Toxicol. 13, 99–109. doi: 10.1016/j.cotox.2018.10.001
Vierheilig, H., Schweiger, P., Brundrett, M. (2005). An overview of methods for the detection and observation of arbuscular mycorrhizal fungi in roots. Physiol. Plant 125, 393–404. doi: 10.1111/j.1399-3054.2005.00564.x
Vishwakarma, K., Sharma, S., Kumar, V., Upadhyay, N., Kumar, N., Mishra, R., et al. (2017). “Current scenario of root exudate–mediated plant-microbe interaction and promotion of plant growth,” in Probiotics in agroecosystem (Singapore: Springer).
Wang, Y., Deng, C., Cota-Ruiza, K., Peralta-Videa, J. R., Sun, Y., Rawat, S., et al. (2020). Improvement of nutrient elements and allicin content in green onion (Allium fistulosum) plants exposed to CuO nanoparticles. Sci. Total Environ. 725, 138387. doi: 10.1016/j.scitotenv.2020.138387
Keywords: Allium cepa L., mycorrhiza, Azospirillum brasilense, degraded soil, stress biomarkers
Citation: Pokluda R, Ragasová LN, Jurica M, Kalisz A, Komorowska M, Niemiec M, Caruso G, Gąstoł M and Sekara A (2023) The shaping of onion seedlings performance through substrate formulation and co-inoculation with beneficial microorganism consortia. Front. Plant Sci. 14:1222557. doi: 10.3389/fpls.2023.1222557
Received: 14 May 2023; Accepted: 26 June 2023;
Published: 12 July 2023.
Edited by:
Sunil Mundra, United Arab Emirates University, United Arab EmiratesReviewed by:
Tünde Takács, Hungarian Academy of Sciences (MTA), HungaryUgo De Corato, Energy and Sustainable Economic Development (ENEA), Italy
Copyright © 2023 Pokluda, Ragasová, Jurica, Kalisz, Komorowska, Niemiec, Caruso, Gąstoł and Sekara. This is an open-access article distributed under the terms of the Creative Commons Attribution License (CC BY). The use, distribution or reproduction in other forums is permitted, provided the original author(s) and the copyright owner(s) are credited and that the original publication in this journal is cited, in accordance with accepted academic practice. No use, distribution or reproduction is permitted which does not comply with these terms.
*Correspondence: Lucia Nedorost Ragasová, bHVjaWEucmFnYXNvdmFAbWVuZGVsdS5jeg==