- National Agrobiodiversity Center, National Institute of Agricultural Sciences, Rural Development Administration, Jeonju-si, Jeollabuk-do, Republic of Korea
The past decade has seen an observable loss of plant biodiversity which can be attributed to changing climate conditions, destroying ecosystems to create farmlands and continuous selective breeding for limited traits. This loss of biodiversity poses a significant bottleneck to plant biologists across the globe working on sustainable solutions to address the current barriers of agricultural productivity. Plant genetic resources centers or genebanks that conserve plant germplasm can majorly contribute towards addressing this problem. Second only to soybean, Brassica remains the largest oil-seed crop and is cultivated across 124 countries, and FAO estimates for a combined gross production values of broccoli, cabbages, cauliflower, mustard and rape seeds stands at a staggering 67.5 billion US dollars during the year 2020. With such a global status, wide variety of uses and more recently, growing importance in the health food sector, the conservation of diverse genetic resources of Brassica appeals for higher priority. Here we review the current status of Brassica conservation across plant genebanks. At present, at least 81,752 accessions of Brassica are recorded to be conserved in 148 holding institutes spread across only 81 countries. Several aspects that need to be addressed to improve proper conservation of the Brassica diversity was well as dissemination of germplasm are discussed. Primarily, the number of accessions conserved across countries and the diversity of Brassica taxa most countries has been highly limited which may lead to biodiversity loss in the longer run. Moreover, several practical challenges in Brassica germplasm conservation especially with respect to taxonomic authorities have been discussed. The current review identifies and highlights areas for progress in Brassica conservation, which include but are not limited to, distribution of conserved Brassica biodiversity, challenges faced by conservation biologists, conservation methods, technical hurdles and future avenues for research in diverse Brassica species.
1 Introduction
Brassica is one of the highly diverse and largest genera of plants, cultivated and consumed all over the world in its different forms (Fahey, 2016; El-Esawi, 2017). Members of the genus Brassica include, but are not limited to morphologically diverse crops including bok choy, broccoli, Brussel sprouts, cabbage, cauliflower, canola, Chinese cabbage, kale, kohlrabi, mustard, rapeseed, and turnips. These plants, given their enormity in diversity and distribution, have equally substantial uses to mankind both directly as health foods, in cuisines, source of oil, source of therapeutics and indirectly as energy crops, in pest control as well as biofumigants (Dixon, 2006; Cornblatt et al., 2007; Szczygłowska et al., 2011; Fahey, 2016; Hagos et al., 2020; Wijaya et al., 2020). Therefore, it is important to study the diverse Brassica species to tap their genetic potential for making the best use of them in the future. To accelerate worldwide research on Brassica, conservation and dissemination of Brassica genetic resources becomes essential with the responsibility falling on plant genetic resource centers or plant genebanks to ensure adequate supply of germplasm. Also, given the narrowing gene pool in Brassica crops as a result of continuous breeding for limited selective traits and overall loss of biodiversity due to changing climate conditions, genebanks offer a great choice for conservation of its plant genetic resources including cultivars, crop wild relatives (CWR) and landraces. The International Treaty on Plant Genetic Resources for Food and Agriculture (Plant Treaty) was framed in 2001 for sustainable use of the plant genetic resources for food and agriculture (PGRFA) across the world (Panis et al., 2020; Pathirana and Carimi, 2022). Currently, the Consortium of International Agricultural Research Centers (CGIAR) maintains a network among 15 research centers and with a presence in 89 countries conserves more than 770,000 accessions of plants (CGIAR, 2022). On the other hand, the WIEWS (World Information and Early Warning System) of FAO connects 22,708 member institutes spread across 114 countries that conserve 5.7 million accessions of plant species (WIEWS, 2022). Both of these institutions strive conservation and adequate supply of the plant genetic resources (PGRs) to the global community.
With regards to conservation of PGRs, continuous research is being carried out to 1. Optimize the storage conditions for different crop germplasm as several factors influence the quality of conserved germplasm during long-term storage; 2. Improve the data availability and networking by optimizing the current gene bank information management systems (Kameswara Rao et al., 2017; Weise et al., 2020). This has led to adequate resources being published for proper documentation as well as conservation of PGRs in genebanks (Reed et al., 2004; Food and Agriculture Organization of the United Nations, 2013; Kameswara Rao et al., 2017; Langridge and Waugh, 2019; Volk et al., 2019). In the present agricultural scenario, the responsibility of conserving the genetic diversity of specific plant species and distribution to breeders as well as plant researchers fall majorly on the genebanks. In this present review, we try to summarize the current status of Brassica conservation at genebanks in terms of availability and diversity, the challenges faced by the genebanks in Brassica germplasm, hurdles in field collections, and future need for conservation of Brassica biodiversity.
2 Current status of Brassica conservation
2.1 Data collection
To collect updated information on Brassica genetic resources, the following webpages were accessed: FAO-WIEWS, ITIS (Integrated Taxonomic Information System), POWO (Plants of the world online), Genesys, EURISCO, GRIN (Germplasm Resources Information Network), GBIS-IPK (Genebank Information System of the IPK - Institut Für Pflanzengenetik Und Kulturpflanzenforschung Gatersleben), UKVGB (United Kingdom Vegetable Gene bank), RDA (Rural Development Administration, S. Korea), NARO (National Agriculture and Food Research Organization), VIR (N.I. Vavilov All-Russian Scientific Research Institute of Plant Industry), WVC (World Vegetable Center), and CGN (Centre for Genetic Resources, the Netherlands) were accessed. For literature review the keywords used were “Brassica germplasm”, “Brassica conservation”, “Plant genetic resources”, “Brassica biodiversity” at Google scholar website (www.scholar.google.com). Only publications that discussed Brassica physiology or germplasm conservation strategies and/or policies were chosen. Preference was given to publications on policies and germplasms status updates published after the year 2017.
2.2 Plant genetic resource centers and Brassica germplasm collections
While WIEWS remains to be the global platform for information on conserved plant germplasm, two online data repositories, Genesys, managed by the Crop Trust and the European Search Catalogue for Plant Genetic Resources (EURISCO) also cater as major online directories for obtaining ordering information on specific accessions. Genesys database provides information on about 4.1 million accessions conserved in 450 genebanks all over the world of which approximately less than 1% (0.96%, 41,487 accessions as on 1st December, 2022) belong to Brassica (Genesys, 2017) (Figure 1). EURISCO stores data of about 2 million accessions of plants and their wild relatives, preserved in 400 institutes located in 43 member countries of the European Cooperative Programme for Plant Genetic Resources (ECPGR) (Weise et al., 2017). EURISCO stores only 26,321 accessions of Brassica (as on 1st December, 2022) which constitute to only 1.3% of the total accessions in the database (Figure 1). Brassica also does not appear among the list of top ten crops with at least 100,000 accessions conserved worldwide (Börner and Khlestkina, 2019). However, European nations are major contributors to the overall production of Broccoli, cauliflower and rapeseed/canola in the world with a combined output of over 7.6 million tons of agricultural crop production in 2021 (Figure 1) (FAOSTAT, 1997). There is an evident underrepresentation of Brassica accessions among the conserved germplasm despite their nature of being such a diverse and anthropologically important genus of crop plants (Kellingray et al., 2017; Wijaya et al., 2020; Salehi et al., 2021).
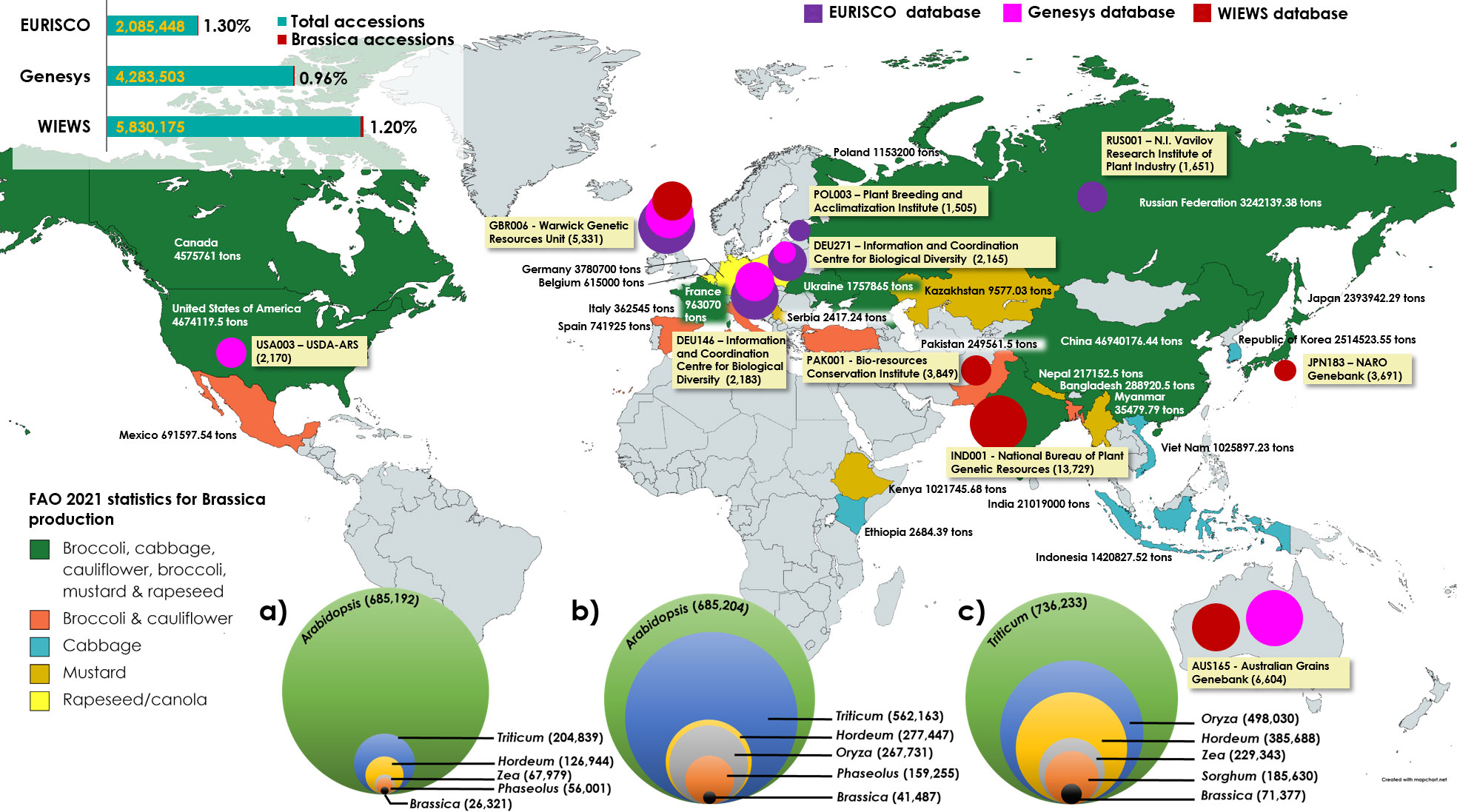
Figure 1 Brassica production statistics (FAOSTAT) during the year 2021 and top five Brassica plant genetic resource holding centers according to FAO-WIEWS, Genesys and EURISCO databases. Labels contain the institutional code followed by the name of the organization and number of Brassica accessions held (in parentheses). Highest plant germplasms conserved according to FAO-WIEWS, Genesys and EURISCO databases (a, b & c) respectively. The world map in the background was created at www.mapchart.net under Creative Commons Attribution-ShareAlike 4.0 International License which permits it to be free to copy and redistribute.
A comparison of overall Brassica production in 2021 and major plant genetic resources centers involved in conserving Brassica biodiversity across the world indicate that Asia, the United States and Europe remain to be major producers of Brassica crops (Figure 1). The WIEWS official data from the SDG 2.5.1.a indicates that a total of 71,378 accessions of Brassica are currently maintained ex situ all over the world (Table S1). Primary observation may indicate that the highest number of accessions being conserved in genebanks in Europe and Asia, but in terms of species diversity, after Europe, North America (USDA-ARS) conserves diverse species of Brassica germplasm (Table S1). Further analysis indicate that less than 10 accessions are conserved in genebanks from Africa (Niger, Nigeria, Eswatini, South Africa, Libya), Middle East (Cyprus, Tajikistan, Jordan, Lebanon), Eastern Europe (Georgia, Montenegro, Armenia) and South America (Argentina, Mexico) (WIEWS, 2022). However, these parts of the world also contribute significantly to the world Brassica production and fall among the top 5 producers of crops such as Broccoli, cauliflower, cabbage and mustard seeds (Figure 1). Improving the available Brassica species biodiversity and dissemination could considerably advance further breeding and research in these parts of the world.
Currently, for ordering Brassica germplasm, the Germplasm Resources Information Network (GRIN) and Gene bank Information System (GBIS) from the US (maintained by Unites States Department of Agriculture - Agricultural Research Service (USDA-ARS) and Leibniz Institute of Plant Genetics and Crop Plant Research serve as channels where one can directly request Brassica germplasm for research purposes (Postman et al., 2010; Oppermann et al., 2015). In addition to these widely used genebanks, several other facilities are also available to source Brassica germplasm locally and internationally albeit the diversity being very limited (Liu et al., 2020). The current status of Brassica germplasm in major genebanks are given in the table below (Table 1). A Principal Component Analysis (PCA) of the available Brassica germplasm information indicated that country such as India conserves very high number of accessions (accession) whereas Korea, Germany, Great Britain conserves high diversity of Brassica species (unique). At the same time, high number of accessions conserved in Korea and Japan possess data on their biological status (status) (Figure 2A). Comparison of species indicates that B. juncea, remains the highly conserved Brassica taxon followed by B. napus, B. rapa and unsegregated B. spp contribute to the highest number of accessions stored in genebanks (Figure 2B). A majority of these Brassica germplasm are maintained as ex situ collections by germplasm collections and materials are preserved in short, medium and long term storage and available as seeds, plant material, and/or DNA (Walters et al., 2004; Acker et al., 2017; Lusty et al., 2021). A search on Genesys and EURISCO webpages indicate that a majority of Brassica germplasm are stored as long term storage, followed by seed collections and mid-term storage (Figure 2C). The major plant material conserved in all these three strategies are of seed type and seeds are the easiest way for exchange or dissemination of Brassica germplasm.
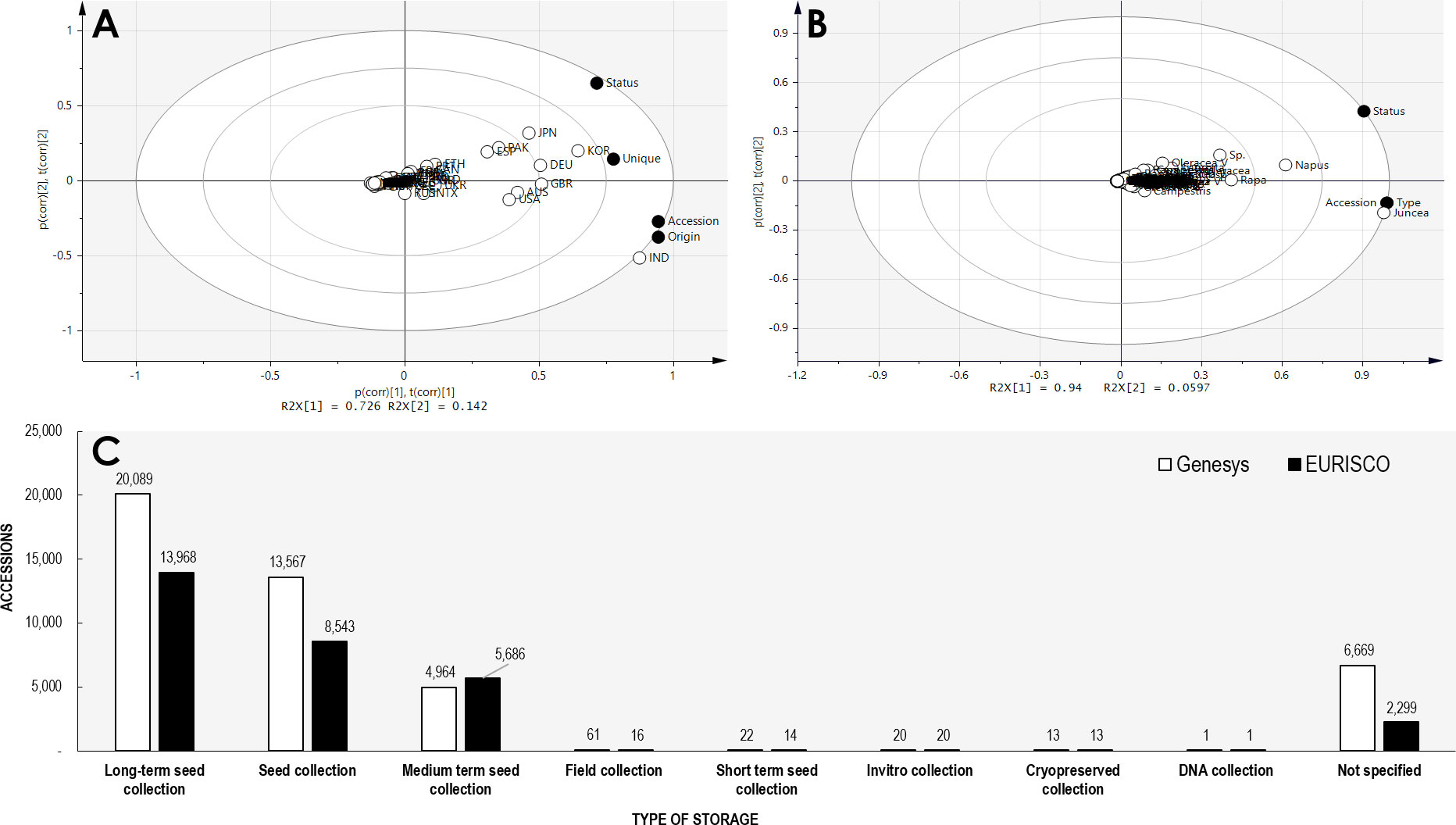
Figure 2 Principal component analysis of Brassica germplasm accessions. (A) Comparison of conserved Brassica accession by countries (accession – total accessions; unique – unique taxon of Brassica; origin – accessions with origin data available; status – accession with their biological status available). (B) Comparison of conserved Brassica accessions by taxon (accession – total accessions; status – accession with their biological status available; type – type of storage- long or short term data available). (C) Type of germplasm storage of Brassica germplasm in plant genebanks associated with online directories GENESYS and EURISCO (as accessed on 1st December, 2022).
2.3 Worldwide assessment of conserved Brassica germplasm
Currently, comparison of the three online directories yields a total of 81,752 Brassica accessions stored in 81 countries across the world in addition to 3 regional and 3 international research centers (data summarized from WIEWS, Genesys & EURISCO). Additionally, few genebanks such as The National Crop Genebank of China (NCGC) and National Agrobiodiversity Center-RDA, South Korea also conserve Brassica germplasm but are limited for sharing of resources due to governmental policies. Historically, the six most common species of Brassica explained by the U triangle are well documented and are widely conserved all across the world (U, 1935) (Figure S1). It can be observed that genebanks that conserve high number of accessions of species such as Brassica napus, B. oleracea and B. carinata are located in vicinity to their geographical origins (Figure S2). Across Asia, green leafy vegetable crops of B. rapa (subsp. chinensis and pekinensis), oil producing rape B. napus and mustards of B. juncea with local origins are highly conserved in genebanks of India, Japan, Pakistan, Taiwan and South Korea (Figure S2). Co-ordination and dissemination of Brassica germplasm have resulted in countries such as Australia, Canada and the United States of America (USA) which are geographically far from the natural areas of origin of Brassica species to accumulate in both number and diversity (Table 1; Figure 3).
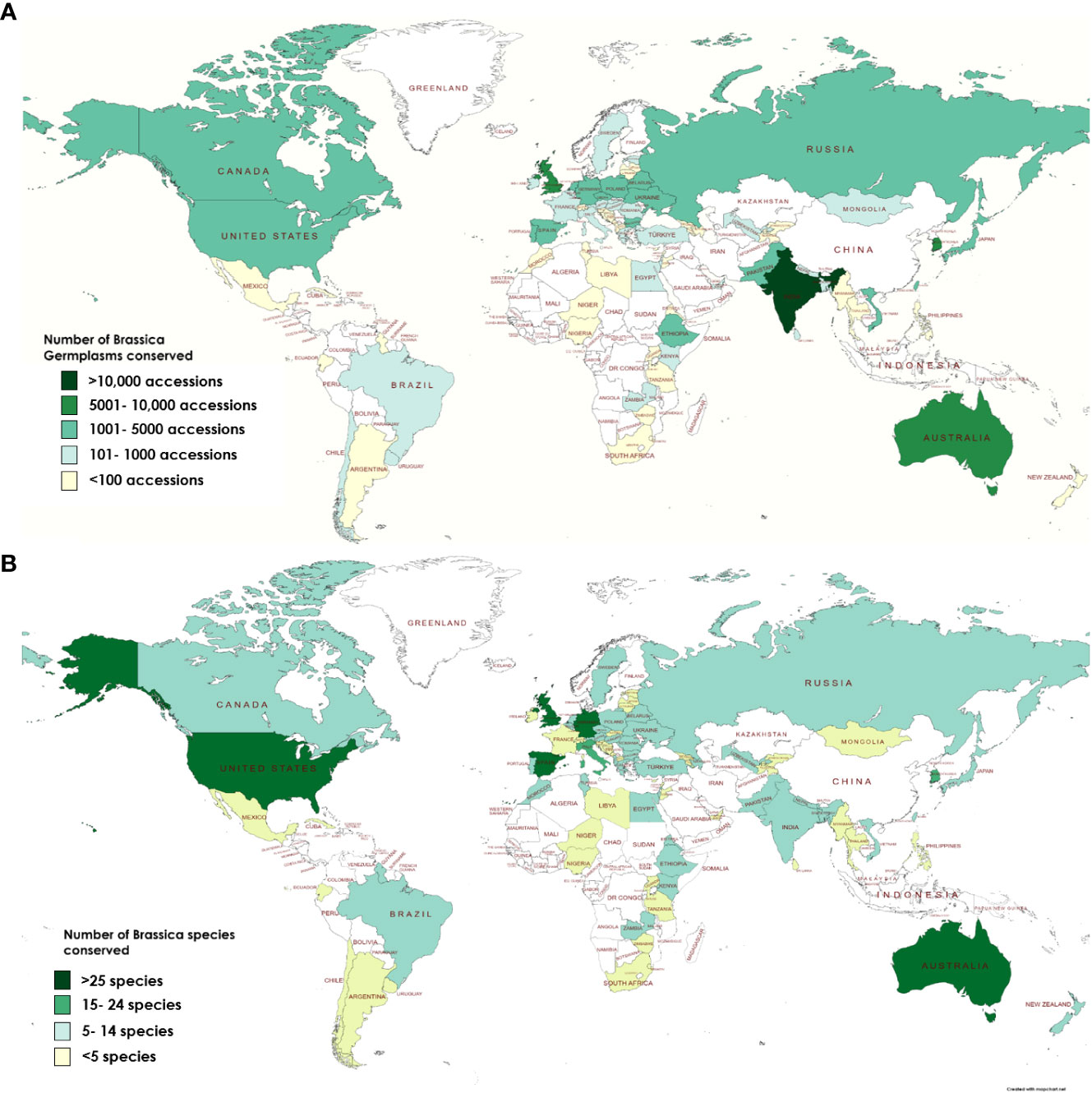
Figure 3 Conservation of Brassica biodiversity in terms of (A) Number of accessions stored (B) Species diversity across plant genebanks around the world.
A country wise comparison of the WIEWS and Genesys databases for conserved Brassica accessions indicate that Australia, India, Germany, United Kingdom (UK), USA and Taiwan conserve the largest number of Brassica germplasm (Figure 3A). In terms of species diversity, the most common Brassica crop seeds conserved are B. oleracea (WIEWS: 17,389 accessions; Genesys 14,798 accessions); B. rapa (WIEWS: 15,132 accessions; Genesys 8,991 accessions) and B. juncea (WIEWS: 14,810 accessions; Genesys 4,698 accessions). However other Brassica species such as B. tyrrhena, B. taurica, B. bivoniana, B. atlantica, B. nivalis, and B. aucheri etc. are not highly conserved (<10 accessions). Moreover, there also exists disproportion in the biodiversity conserved at the plant genebanks. Among 146 plant genebanks recorded to conserve Brassica >38% geenbanks (56) have a Brassica biodiversity of less than 10 taxons (including subspecies and varieties) and largely conserve B. oleracea, B. rapa and B. juncea genetic resources. Data from FAO-WIEWS indicate that Australia, Germany, Spain, UK and USA conserve more than 25 representative species of Brassica including wild types (Figure 3B). Although the FAO promotes sharing of plant genetic resources for food and agriculture (PGRFA) through its sustainable developmental goals (SDGs), much work has to be done to ensure that the currently available biodiversity of Brassica species is not lost. Crop wild types are excellent sources for breeding for development of disease tolerant and abiotic stress tolerant plants that would be suitable for fresh challenges posed by the changing climate conditions.
3 Conservation methods for Brassica germplasm
The Seed Information Database (SID) maintained by Millennium Seed Bank of the Royal Botanic Gardens, Kew, UK designate all the 48 taxa of Brassica (species and subspecies) stored by them to be orthodox seeds. The orthodox nature of seeds from Brassica has in fact been well established (Genesys, 2017; Solberg et al., 2020). Therefore, long-term storage at -18 to -20°C for 40-60 year range is possible (Solberg et al., 2020). This explains the nature of storage of Brassica seeds, where a majority of germplasms are currently held in long term storage (Figure 2C) (Weise et al., 2017). However, other types of storages such as in vitro collection, DNA collection, cryopreservation are also employed to preserve Brassica germplasm, but scarcely used. Further studies or research are warranted on conserved germplasms to not only understand the characteristics and traits of stored seeds, but also to improve the currently followed storage practices (Acker et al., 2017; Panis et al., 2020). Several studies have been conducted in the past on monitoring dormancy, re-generation and suitability/effects of different temperatures as well as periods of storage for varied plants (Ellis et al., 2018; van Treuren et al., 2018; Everingham et al., 2021). Germplasms of Brassica conserved in gene banks have also been subjected to studies to optimize their storage conditions, regulation of seed dormancy and elucidation of useful characteristics such as metabolite profiling.
Seeds from members of family Brassicaceae, have been reported to commonly have a half-life (P50) of 54 years and some plants such as cabbage (Brassica oleracea L.) can even be maintained at +20°C and 50% RH up to 5 years in paper bags (Nagel and Börner, 2010; Solberg et al., 2020). Seeds of B. villosa ssp. drepanensis exhibited a high germination rate (92%) when stored at -20°C with a moisture content of 3% to 6% for 16 years. However, room temperature stored seeds succumbed to rapid deterioration after 3 to 4 years (Scialabba et al., 2016). Study of long term (36 years) stored Brassicaceae members showed that desiccation to reduce seed water content to less than 2.5% of fresh weight significantly improved germination at the end of storage. This effect was enhanced by enrichment of the storage environment with carbon di oxide (CO2) (Gonzalez-Benito et al., 2011). Hermitic storage of 37 Brassicaceae members including B. napus, B. fruticulosa and B. fruticulosa glaberrima at −5°C to −10°C with seed moisture content of 0.3 to 3%, 20% RH, indicated sustenance of seed viability over a period of 38-40 years (Pérez-García et al., 2007). Similar studies on seeds of Brassica fruticulosa and B. repanda stored at −5°C to −10°C with seed moisture content of <3%, 20% RH, over a period of 40 years from 1966 to 2006 also indicated sustained viability, displaying a higher germination rate of over 70% without use of dormancy-breaking agents such as gibberellic acid (Pérez-García et al., 2009). A study comparing Brassica rapa L. ssp. pekinensis and Capsicum annuum L. long term (10 years) seed storage using different packaging material indicated that B. rapa L. ssp. pekinensis was the most affected by storage at ambient temperatures even when packaged in vacuum-sealed aluminum pouches, but could be successfully stored for up to 10 years at 5°C/RH 30, using vacuum-sealed aluminum pouches (Soh et al., 2014). From these studies it can be understood that while some Brassica plants such as Cabbage (Brassica oleracea L.) can be stored under ambient temperatures (+20°C) it may not be suitable for others plants such as B. rapa L. ssp. pekinensis and B. villosa ssp. drepanensis which are to be stored at much lower temperatures of -5°C to -20°C. Other optimal conditions reported for Brassica members include a relative humidity of less than 50%, moisture content of <6%, use of CO2 if stored in sealed containers or use of vacuum sealed aluminum pouches. Also, extreme desiccation of seeds during storage have been seen to have a detrimental effects on seed longevity on plants such as B. repanda (Mira et al., 2015).
Other studies on Brassica species conserved in gene banks are found to be on the biochemical changes that occur in seeds during long term storage and screening of collections for their metabolites. Studies on seed lipid peroxidation and membrane permeability in Brassicaceae members during long term storage and their effect on seed germination, vigor indicated that increased membrane permeability to occur in Brassica repanda seeds which led to reduced germination % as well as vigor loss (Mira et al., 2011). In another study, a seed lipid thermal fingerprinting using differential scanning calorimetry (DSC) was proposed, which used several biophysical markers to predict performance of oily seeds of Brassicaceae during long-term storage (8-44 years) (Mira et al., 2019). A study on 168 accessions of Brassica rapa on understanding relationships between genetic markers and metabolites developed a set of genetic markers could be used for rapid selection of specific metabolite producing B. rapa genotypes (Pino Del Carpio et al., 2011).
4 Challenges in Brassica germplasm conservation
4.1 Taxonomic predicament of Brassica germplasm in genebanks
A major concern with worldwide Brassica germplasm collections often is the uncertainty associated with the naming of plant genetic resources. Descriptions of the origins, phenotypic and genotypic diversity of several Brassica members have been published (Dixon, 2006; Bonnema et al., 2011). However, naming of the Brassica members hitherto remains complex and often ambiguous due to differences in the naming conventions. Proper phylogenetic organization of the members of Brassica has been arduous due to extensive crossing and hybridization over centuries which occurred across geographically isolated microenvironments causing difficulties in tracing and comparison (Bonnema et al., 2011; Fahey, 2016). There is also an overlap of plant forms and morphological traits across species which further complicates phenotypical phylogenetic assignment and organization (OECD, 2016). The origins of modern Brassica vegetables and their taxonomy continues to be unsettled and regarded to be in a state of constant flux (Fahey, 2016).
At present, only 13 subordinate taxa in the rank of species are recognized by the Integrated Taxonomic Information System (ITIS) in the genus Brassica (ITIS, 2022) (as on 05th October, 2022) (Table S2). The ITIS is a taxonomic database maintained by the United States government which partners with other governmental agencies of Canada and Mexico as well as organizations dedicated to biodiversity such as GBIF (Global Biodiversity Information Facility) (GBIF, 2020). However, other well-recognized online catalogues as well as germplasm collections do not necessarily follow these naming conventions. For example, as on 05th October, 2022, the Plants of the World Online-Royal Botanical Gardens, Kew in collaboration with World Flora Online project, provides taxonomic recognition of 41 species of Brassica (Borsch et al., 2020; POWO, 2022) (Table S2). These names are accepted and added to its ‘World Checklist of Seed Plants’ (Govaerts et al., 2022). Another repository BrassiBase, dedicated towards developing taxonomic, evolutionary, systematics and germplasm knowledge systems specifically for Brassicaceae, reports 45 species of Brassica (Kiefer et al., 2013) (Table S2). The GRIN-taxonomy resource which provides taxonomic information on plants conserved by the United States National Plant Germplasm System (USNPGS) remains as the only provider of taxonomic information along with conserving germplasm. The GRIN-taxonomy also currently recognizes 41 species of Brassica. But they also are not completely in agreement with the accepted list of Brassica species given the World Checklist of Seed plants. On a comparison of the Brassica taxa recognized by the plant taxonomy authorities, online PGR directories and plant genebanks we recognized issues related with the naming of Brassica taxa at species level (Figure 4). There are 18 Brassica species that are present only among the plant taxonomy lists (ITIS, POWO, BrassiBase & GRIN-Taxonomy) which are not conserved under the designated name at any germplasm conservation center or present in the list of Brassica species in the online repositories (Genesys & EURISCO) (Figure 4A). Similarly, it can also be observed that there are 15 Brassica species which are listed only in the online PGR directories (Genesys & EURISCO). This could be solved as they all of them are subspecies of Brassica, particularly Brassica oleracea and B. rapa which have been misnamed or updated to a new taxon (Figure 4B). Same is the case of Brassica sylvestris which was formerly designated as Brassica rapa subsp. sylvestris which is now recognized only in plant genebanks but not by plant taxonomy authorities or online PGR directories. The NCBI taxonomy database under Brassica (Taxonomy ID:3705) indicates 129 distinct taxa which after removing species crosses and taxa marked ambiguous names such as groups provide 87 unique taxa (Figure 5). These 87 unique taxa fail to include all the Brassica species conserved in plant genebanks.
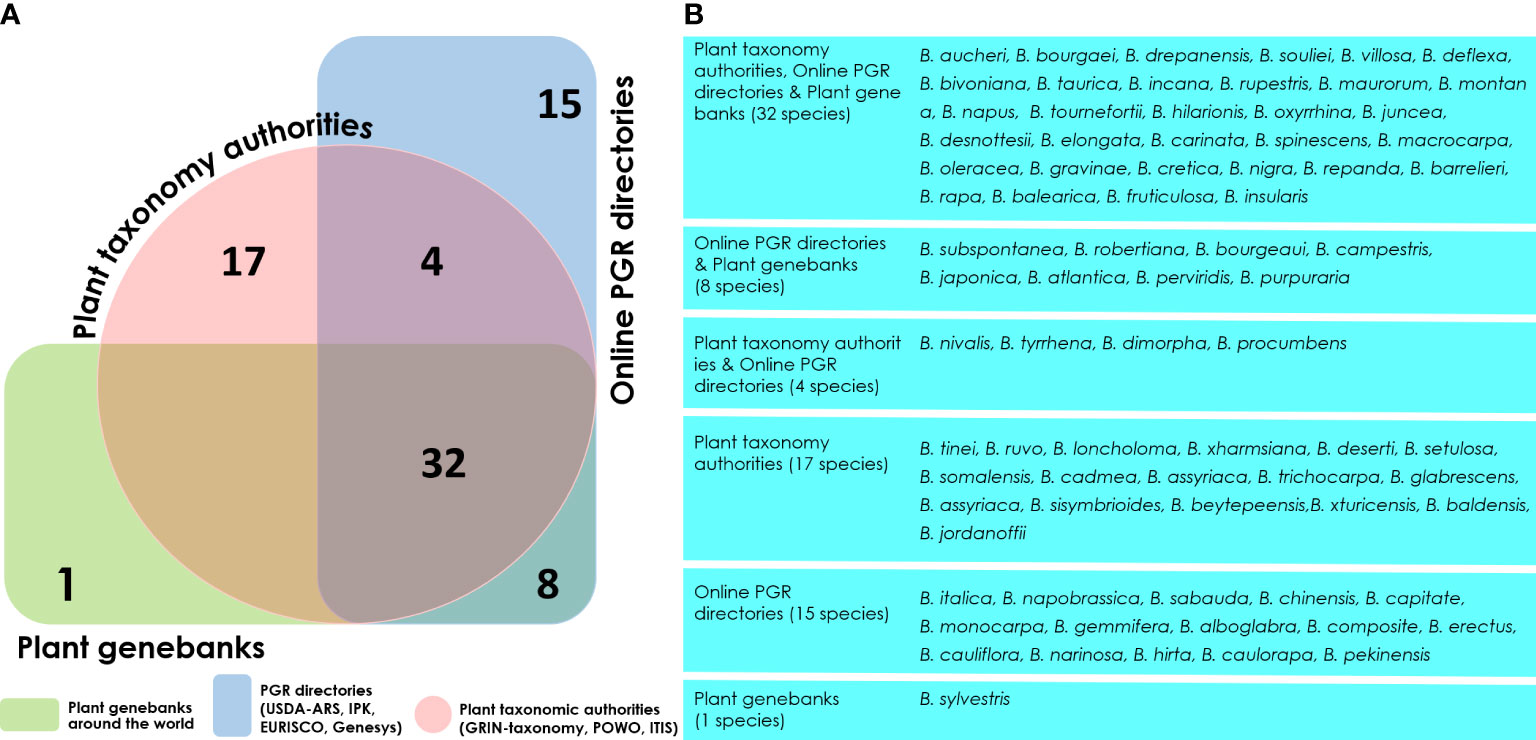
Figure 4 Venn diagram illustrating the distribution of Brassica species. (A) number of Brassica species available across Plant taxonomy authorities (Integrated Taxonomic Information System-ITIS, Plants of the world online-POWO, GRIN taxonomy), online plant genetic resource directories (WIEWS database, Genesys and EURISCO) and plant genebanks. (B) List of Brassica taxa in groups specified in the Venn diagram.
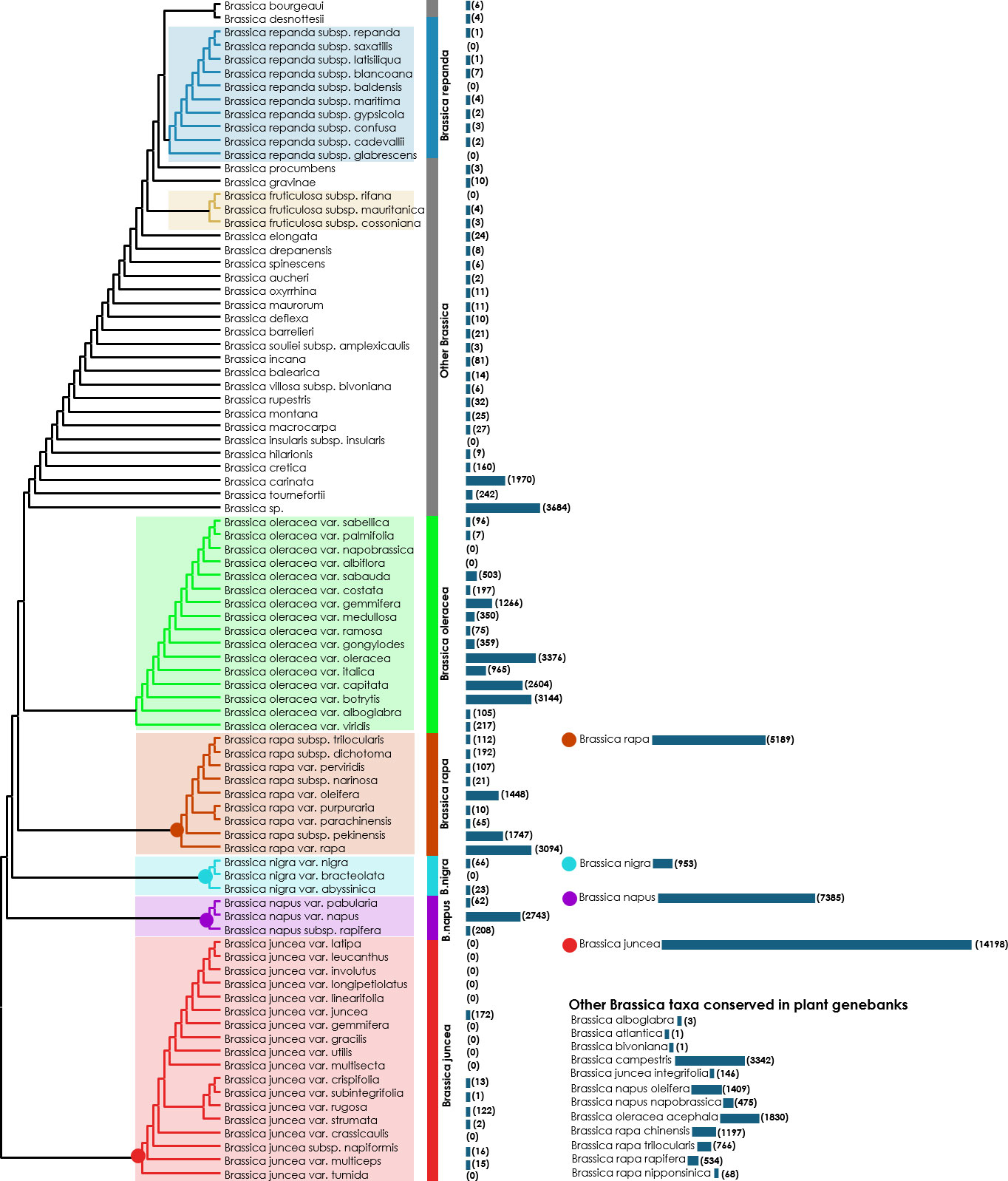
Figure 5 A common tree drawn using the NCBI taxonomy database (Sayers et al., 2019; Schoch et al., 2020). The base Newick’s tree was downloaded from the NCBI taxonomy database and redrawn using MEGA v. 11. Bars along with numbers in the parentheses indicate the number of accessions available according to the WIEWS database.
The ambiguity of Brassica naming also continues to exist at the ground level. To begin with, the plant genetic resources storage centers around the world which are responsible for conservation and distribution of germplasm do not reflect the diverse species richness in Brassica germplasm conserved by them. The Gene bank Information System of the Leibniz Institute of Plant Genetics and Crop Plant Research (GBIS-IPK) indicates storing of accessions belonging to 31 species of Brassica whereas the United States Department of Agriculture- Germplasm Resource Information Network (USDA-GRIN) stores 24 different species of Brassica (excluding Brassica sp.) (Brassica-dataset, 2015; Oppermann et al., 2015) (Table S2). It is understandable that plant genebanks concentrate towards conserving local resources and rely on crop nomenclature. However, as most of them follow the ex situ model of conservation, they can also be used as an effective tool in protecting and documenting the Brassica biodiversity. For example, as several of the accepted taxa of Brassica such as Brassica assyriaca, B. baldensis, B. beytepeensis etc. have been officially added as verified and accepted species (Govaerts et al., 2022), plant genetic resource centers around the world can attempt to work towards taxonomic reclassification and updating of information on the bioresources which they conserve.
The problem of taxonomic uncertainties becomes much more complex across genebanks all around the world when describing the status at much lower taxonomic levels such as subspecies, cultivars and varieties which are often interchanged depending on the author/gene bank accessed. Such issues at times can complicate the taxonomic assignment after breeding or during research. Introduction of universal code for naming at this juncture, would be a tedious as well as an incredible task. A polymerase chain reaction (PCR) based approach for identification of Brassica species has been proposed albeit its efficacy is limited within the species from the U triangle (Koh et al., 2017). There is a possibility that this problem could be solved by help of genetic sequencing approaches. Since the advancement of sequencing technologies, genomic component based typing followed by distinctive universal taxonomic assignments have helped to overcome such barriers. This would also shorten the time required by traditional identification using phenotyping. The current requirement on Brassica taxonomy would therefore involve a polyphasic approach for phylogenetic organization which would combine more than one of the following: phenotyping or studying morphological traits; use of cytological markers, karyotyping; phytochemical, storage protein profiling; studying isozyme markers; microsatellite markers such as SSRs (short tandem repeats), SNPs (single nucleotide polymorphisms); and sequencing methods such as specific-locus amplified fragment sequencing (SLAF-seq) and using large scale genomic analyses and fingerprinting to categorize Brassica species (Pino Del Carpio et al., 2011; Liu et al., 2013; El-Esawi, 2017; Fotev et al., 2018; Yang et al., 2018; Bhandari et al., 2020; Chao et al., 2020; He et al., 2021; Rakshita et al., 2021; Hong et al., 2022; Li et al., 2022)
4.2 Diverse morphotypes and cytogenetics of Brassica
The richness of both species and morphotypes in Brassica can be understood to have arisen after the whole genome triplication (WGT) event that has been accepted (Arias et al., 2014; Cheng et al., 2014). Among the speciation concepts of Brassica, the U triangle is the most famous and accepted concept of cytogenetic relationships between the species belonging to the genus Brassica (Figure S1). The genome types of the ancestral diploid and tetraploid as explained in the triangle of U has been well discussed (Branca and Cartea, 2011). In addition to the six species of Brassica explained by the U triangle, other species of Brassica have also been continually described (Govaerts et al., 2022) (Figure 6). These are morphologically distinct compared to the six species and not much information is available about them for scientific research. There is a marked morphological diversity among various species of Brassica where some form heads or curd or stem and lead modifications. This variation among the species can be observed in their leaves – size, shape, margins, presence of anthocyanin; stems; habit; flower color and roots. To give few examples, the Brassica species B. spinescens and B. balearica form slight succulent leaves which is unique among various Brassica and B. maurorum produces minute flowers which look like an inflorescence (Figure 6). Some plants grow more than 2 meters in height (B. rapa) where are some (B. elongata, B. balearica and B. maurorum) do not grow more than 30 cm in height. Despite these plants having a long history of documentation and exhibiting significant difference in their morphology, habit and growth periods, they have not been studied in detail or conserved in large number in plant genebanks. In the recent decades, adequate emphasis has been given in literature to the importance of conservation of wild type species. These other Brassica species are vital as they can help to deal with the bottleneck problems associated with Brassica breeding which warrants the need for conservation of these wild type species of Brassica.
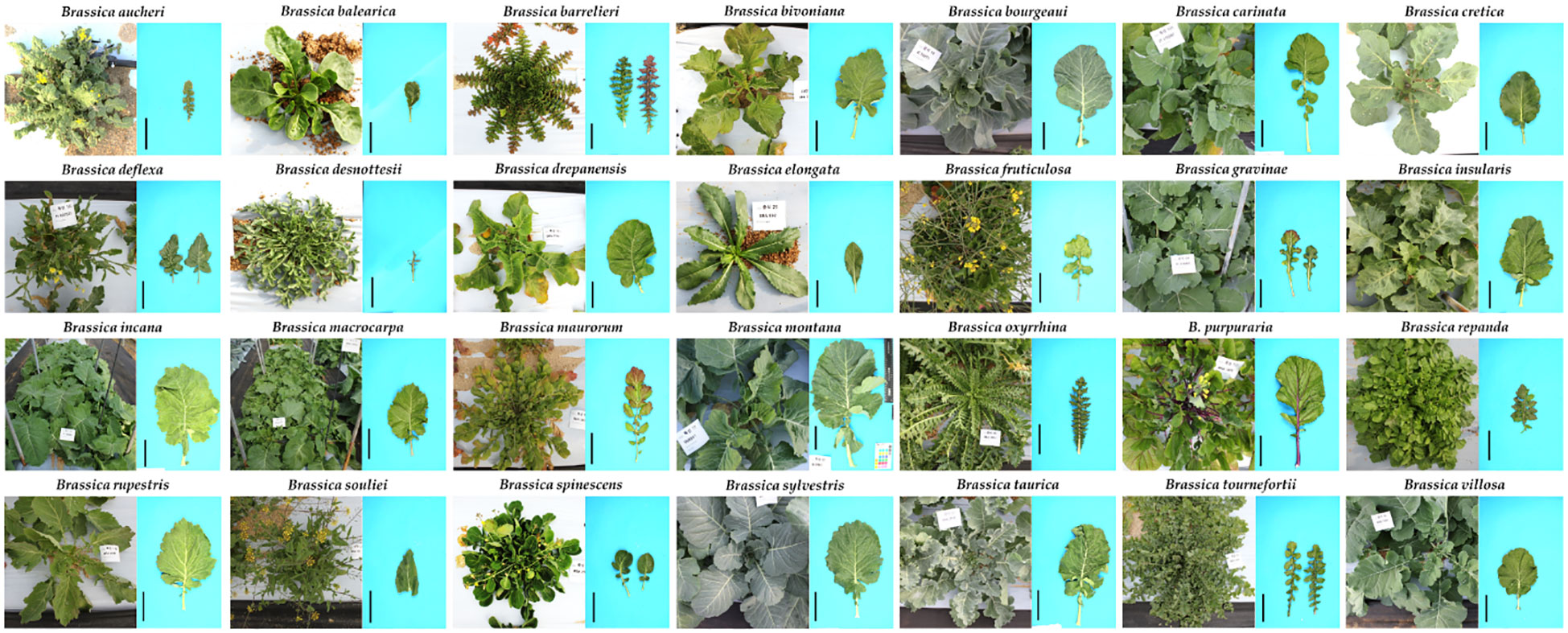
Figure 6 Morphologically varied wild type species of Brassica. The scale bars (in black) adjacent to the individual leaves represent a length of 10 cm and were included using ImageJ software (Unpublished data).
4.3 Technical hurdles in cultivation of Brassica species
Members of the family Brassicaceae family especially the vegetable crops, prefer to grow under cooler temperate conditions (15–20°C), with high humidity, but some species are also found to grow also under subtropical and even tropical zones (Fahey, 2016; Lin et al., 2018; Razzaq et al., 2021). For example Chinese cabbage plants grow well at a temperatures range of 13–20°C but prefer warmer growing conditions and often bolt at lower temperatures (Salehi et al., 2021). Brassica juncea or mustard plants widely grown on northern parts of India also prefer warmer temperatures as high as 27°C and can tolerate temperatures as low as 6°C (Shekhawat et al., 2012). Among other species, Brussels sprouts and kale are the most resistant to low temperatures, as they can tolerate temperatures as low as −12°C. In addition to preference of longer photoperiods for optimized growth, Brassica vegetables such as kohlrabi, Chinese cabbage, cabbage have a weaker root system and require humus rich or loamy soil with pH near neutral (6.2-7.0) and high water retention properties (Dhaliwal, 2017; Razzaq et al., 2021). Therefore, propagation of diverse Brassica species requires environment controllable facilities and not all of them can be multiplied in outdoor fields in all countries.
It has been reported that some Brassica species are self-fertile but most of them are self-incompatible and may require cross pollination to obtain seed (Stewart, 2002). This pollination can occur through wind, animals or insects or through human intervention. Though the human intervention aspects are related to breeding, cross-pollination by wind or insects such as bees are undesirable and can contaminate varieties. In case of wind pollination, isolation of plants is very helpful to avoid cross-pollination and incase of B. napus outcross could be reduced to less than 4% on separating plants by 120 cm; in oil seed rape almost 10% of pollen could be detected at a distance of even 360 m from the edge of the field; in radish also, the minimal distance for 0% crossing was at least 50 m (Stewart, 2002). The plant B. napus is highly prone to cross-pollination and spontaneous inter-specific hybridization of B. napus is possible with sexually compatible species (Meglic and Pipan, 2018). To produce pure seeds, Brassica plants need to be grown in isolation and cross-pollination risk reduces with the distance or introducing physical barriers in the flowering plants. However, as explained earlier, cross pollination is not a common phenomenon and rates are very low among Brassica spp. (Stewart, 2002). Avoidance of cross pollination can be carried by using a number of strategies including creating topographical barriers such as edges that would restrict or limit wind and insects, shifting of bee hives, establishment of border zones at the edges of the where seeds will not be collected from the plants in the zones and planting fields in such a way that the flowering time and period do not overlap (Stewart, 2002).
4.4 Diseases and pests
Almost all the Brassica vegetables are relatively susceptible to the same or similar diseases as well as insect pests (Fahey, 2016). Overall, pests are a serious threat to agricultural productivity and lately, with the exponential growth of Brassica cultivation, the distribution of Brassica vegetable pests have also increased (Witzel et al., 2021). The common microbial diseases occurring in Brassica species are tabulated (Table S3). The most common microbial infections in Brassica species are most caused by fungi especially Leptosphaeria (Blackleg disease), Sclerotinia (rot) and Verticillium (wilt). However, clubroot disease caused by Plasmodiophora is the highly reported disease among Brassica plants. Among bacterial pathogens Pesudomonas and Pectobacterium are the most common and Turnip yellow virus is the most common viral pathogen. In addition to diseases, Brassica crops are also attacked by a variety of pests that affect the growth as well as yield of Brassica members (Table S4). These causes a variety of issues ranging from feeding on the leaf tissues to killing the whole plants by feeding on plant roots. The most common insect pests of Brassica include aphids, worms, mites and weevils. However, there are also several beneficial microorganisms and insects that aid growth of Brassica species. These help the plants grow and prevent predators from feeding on the plants. Microorganisms including bacteria and fungi have been reported to help Brassica species by stimulating plant growth through mechanisms such as nitrogen fixation, siderophore production, alleviate chilling and heavy metal stress, and more importantly act as biocontrol agents against several bacterial and fungal diseases (Card et al., 2015). In addition to microbial interactions leading to enhanced growth of Brassica plants, some of the insects have also been reported to aid Brassica plants by acting as major predators of pests and feed on eggs of pests, aphids, small caterpillars, thrips and mites that attack the plants (Table S5).
5 Importance of conserving Brassica biodiversity
The culinary and therapeutic use of species in the U triangle has been well established and needs no emphasis. However, potential uses of other species are also currently gaining importance. For example, wild type accessions of Brassica montana and B. balearica were reported to exhibit resistance against the black rot caused by Xanthomonas campestris which particularly affects B. oleracea crops (Sheng et al., 2020). Similarly, B. fruticulosa, B. hilarionis, B. macrocarpa, B. montana, B. spinescens and B. villosa have been reported to exhibit antibiosis against the cabbage root fly (Delia radicum) which is a major threat to cabbage production in parts of Western Europe, the United States and Canada (Shuhang et al., 2016). Moderate resistance against other plant pathogen Sclerotinia sclerotiorum have reported in other Brassica species including B. atlantica, B. bourgeai, B. incana, B. macrocarpa, and B. vilosa (Taylor et al., 2018). Presence and applications of glucosinolates from Brassica has been well established in B. oleracea, B. juncea and B. rapa. The species B. bourgeaui has also been studied for their glucosinoates and have been reported to contain significantly high total glucosinotes than several B. oleracea crops in particular gluconapin, glucoraphanin and neoglucobrassicin (Tortosa et al., 2017). Glucosinolates have also been studied in B. desnottesii, B. drepanensis, B. elongata, and B. gravinae (Montaut et al., 2017; Malfa et al., 2022). All of the above studies have been made on germplasm obtained from plant genebanks and therefore, further research on the prospects of wild type Brassica can be significantly improved if these accessions are locally available for researchers.
6 Conclusions
The genus Brassica has given rise to a number of agriculturally important vegetable crops since ancient times. Moreover, it also provides the second major oilseed crop next only to soybean (Gupta, 2016). Conservation of Brassica germplasm and its biodiversity has still room for progress at the current scenario. Brassica vegetables has consistently been gaining popularity especially in the health food sector and are currently consumed across the Americas, Asia, and several European countries. Several parts of the plants are economically important depending on the species and those include leaves, seeds, oils. Thus their economic importance has multiplied and are now grown along with cereal crops to meet global demand (Salehi et al., 2021). With respect to medicine, members of genus Brassica are rich in biologically active metabolites which have been reported to possess potential health-promoting properties (Lee et al., 2013; Solov’yeva et al., 2013; Lee et al., 2014; Klopsch et al., 2017; Bhandari et al., 2020). Bioactive metabolites other than glucosinolates have also been identified and reported in Brassica which include alkaloids, anthocyanins, carotenoids, flavonoids, folates, other phenols, phytoalexins, phytosteroids and tocopherols (Pino Del Carpio et al., 2011; Branca et al., 2018; Lotti et al., 2018; Ramirez et al., 2020; Salehi et al., 2021). In addition to the health benefits of Brassica plants, in recent times, their commercial importance has grown in the food industry in which their morphological appeal to the consumers remains an important factor. This includes the appetizing appearance which is a combination of properties such as color, size, shape of the edible parts of the plant. Gene bank germplasm can therefore be screened for accessions with such attractive morphological traits. Earlier screening has been carried out for form/appearance significant Brassica vegetables including Kale and cauliflower (Thorwarth et al., 2018; Witzel et al., 2021). Further studies have also been made which included morphological properties of Chinese broccoli (B. oleracea alboglabra), and B. rapa (Fotev et al., 2018; Witzel et al., 2021). As crop wild relatives can serve as potential source of both biomedical compounds as well to improve commercial important phenotypic characteristics, conservation of the available biodiversity of Brassica becomes a prerequisite. In addition, they can also serve as a source of several useful traits which can be exploited to adapt crops to changing climate and improve biotic as well as abiotic resistances in Brassica crop species (Quezada-Martinez et al., 2021). Current status indicates conserved Brassica to be highly concentrated on few species and unevenly distributed across countries. Plant genebanks can provide an excellent solution to preserve the Brassica biodiversity for research, breeding, and commercial purposes.
Author contributions
B-SH was involved in the conceptualization of the manuscript. PS and B-SH were involved in writing of the manuscript. S-HK was involved with data analysis, preparation of figures and tables. All authors contributed to the article and submitted and approved the submitted section.
Funding
The study was supported by the grant (PJ01672201) funded by the National Agrobiodiversity Center, Rural Development Administration, Republic of Korea. This study was also supported by the 2023 Postdoctoral Fellowship Program (P.S.) of the National Institute of Agricultural Sciences, RDA, Republic of Korea.
Conflict of interest
The authors declare that the research was conducted in the absence of any commercial or financial relationships that could be construed as a potential conflict of interest.
Publisher’s note
All claims expressed in this article are solely those of the authors and do not necessarily represent those of their affiliated organizations, or those of the publisher, the editors and the reviewers. Any product that may be evaluated in this article, or claim that may be made by its manufacturer, is not guaranteed or endorsed by the publisher.
Supplementary material
The Supplementary Material for this article can be found online at: https://www.frontiersin.org/articles/10.3389/fpls.2023.1220134/full#supplementary-material
References
Acker, J. P., Adkins, S., Alves, A. A. C., Horna, D., Toll, J. (2017). Feasibility study for a Safety back-up Cryopreservation facility. Independent expert report: July 2017. Rome (Italy): Bioversity International. 100p.
Arias, T., Beilstein, M. A., Tang, M., McKain, M. R., Pires, J. C. (2014). Diversification times among Brassica (Brassicaceae) crops suggest hybrid formation after 20 million years of divergence. Am. J. Bot. 101 (1), 86–91. doi: 10.3732/ajb.1300312
Bhandari, S. R., Rhee, J., Choi, C. S., Jo, J. S., Shin, Y. K., Lee, J. G. (2020). Profiling of Individual Desulfo-Glucosinolate Content in Cabbage Head (Brassica Oleracea var. capitata) Germplasm. Molecules 25 (8) 1860. doi: 10.3390/Fmolecules25081860
Bonnema, A., Del Carpio, D., Zhao, J. (2011). Diversity analysis and molecular taxonomy of Brassica vegetable crops, in Genetics, Genomics and Breeding of Vegetable Brassicas. New York: CRC Press. pp. 81– 124.
Börner, A., Khlestkina, E. K. (2019). Ex-situ genebanks—Seed treasure chambers for the future. Russian J. Genet. 55 (11), 1299–1305. doi: 10.1134/S1022795419110036
Borsch, T., Berendsohn, W., Dalcin, E., Delmas, M., Demissew, S., Elliott, A., et al. (2020). World Flora Online: Placing taxonomists at the heart of a definitive and comprehensive global resource on the world's plants. TAXON 69 (6), 1311–1341. doi: 10.1002/tax.12373
Branca, F., Cartea, E. (2011). “Brassica,” in Wild Crop Relatives: Genomic and Breeding Resources: Oilseeds. Ed. Kole, C. (Berlin, Heidelberg: Springer Berlin Heidelberg), 17–36.
Branca, F., Chiarenza, G. L., Cavallaro, C., Gu, H., Zhao, Z., Tribulato, A. (2018). Diversity of Sicilian broccoli (Brassica oleracea var. italica) and cauliflower (Brassica oleracea var. botrytis) landraces and their distinctive bio-morphological, antioxidant, and genetic traits. Genet. Resour. Crop Evol. 65 (2), 485–502. doi: 10.1007/s10722-017-0547-8
Brassica-dataset (2015). USDA Agricultural Research Service, (2015) (Germplasm Resources Information Network (GRIN). ". USDA Agricultural Research Service).
Card, S. D., Hume, D. E., Roodi, D., McGill, C. R., Millner, J. P., Johnson, R. D. (2015). Beneficial endophytic microorganisms of Brassica – A review. Biol. Control 90, 102–112. doi: 10.1016/j.biocontrol.2015.06.001
CGIAR (2022) CGIAR: Results dashboard - Genebanks. Available at: https://www.cgiar.org/food-security-impact/results-dashboard/.
Chao, H., Li, T., Luo, C., Huang, H., Ruan, Y., Li, X., et al. (2020). BrassicaEDB: A gene expression database for Brassica crops. Int. J. Mol. Sci. 21 (16), 5831. doi: 10.3390/Fijms21165831
Cheng, F., Wu, J., Wang, X. (2014). Genome triplication drove the diversification of Brassica plants. Horticult. Res. 1 (1), 14024. doi: 10.1038/hortres.2014.24
Cornblatt, B. S., Ye, L., Dinkova-Kostova, A. T., Erb, M., Fahey, J. W., Singh, N. K., et al. (2007). Preclinical and clinical evaluation of sulforaphane for chemoprevention in the breast. Carcinogenesis 28 (7), 1485–1490. doi: 10.1093/carcin/bgm049
El-Esawi, M. A. (2017). Genetic diversity and evolution of Brassica genetic resources: from morphology to novel genomic technologies – a review. Plant Genet. Resour. 15 (5), 388–399. doi: 10.1017/S1479262116000058
Ellis, R. H., Nasehzadeh, M., Hanson, J., Woldemariam, Y. (2018). Medium-term seed storage of 50 genera of forage legumes and evidence-based genebank monitoring intervals. Genet. Resour. Crop Evol. 65 (2), 607–623. doi: 10.1007/s10722-017-0558-5
Everingham, S. E., Offord, C. A., Sabot, M. E. B., Moles, A. T. (2021). Time-traveling seeds reveal that plant regeneration and growth traits are responding to climate change. Ecology 102 (3), e03272. doi: 10.1002/ecy.3272
Fahey, J. W. (2016). “Brassica: characteristics and properties,” in Encyclopedia of Food and Health. Eds. Caballero, B., Finglas, P. M., Toldrá, F. (Oxford: Academic Press), 469–477.
Food and Agriculture Organization of the United Nations (2013). Genebank standards for plant genetic resources for food and agriculture (Rome: Food and Agriculture Organization of the United Nations).
Fotev, Y. V., Artemyeva, A., Fateev, D., Bugrovskaya, G., Belousova, V., Kukushkina, T. (2018). Results of SSR analysis, properties of plant morphology and biochemical composition of Chinese broccoli–a new vegetable crop for Russia. Veg. Crops Russia 1, 12–19. doi: 10.18619/2072-9146-2018-1-12-19
Genesys (2017). Genesys Global Portal on Plant Genetic Resources. Crop Trust. https://www.genesys-pgr.org/.
Gonzalez-Benito, M. E., Pérez-García, F., Tejeda, G., Gomez-Campo, C. (2011). Effect of the gaseous environment and water content on seed viability of four Brassicaceae species after 36 years storage. Seed Sci. Technol. 39, 443–451. doi: 10.15258/sst.2011.39.2.16
Govaerts, R., Dransfield, J., Zona, S., Hodel, D. R., Henderson, A. (2022). World Checklist of Selected Plant Families (Royal Botanic Gardens, Kew).
Gupta, S. K. (2016). “Chapter 3 - Brassicas,” in Breeding Oilseed Crops for Sustainable Production. Ed. Gupta, S. K. (San Diego: Academic Press), 33–53.
Hagos, R., Shaibu, A. S., Zhang, L., Cai, X., Liang, J., Wu, J., et al. (2020). Ethiopian mustard (Brassica carinata A. Braun) as an alternative energy source and sustainable crop. Sustainability 12 (18), 7492. doi: 10.3390/su12187492
He, Z., Ji, R., Havlickova, L., Wang, L., Li, Y., Lee, H. T., et al. (2021). Genome structural evolution in Brassica crops. Nat. Plants 7 (6), 757–765. doi: 10.1038/s41477-021-00928-8
Hong, S., Choi, S. R., Kim, J., Jeong, Y.-M., Kim, J.-S., Ahn, C.-H., et al. (2022). Identification of accession-specific variants and development of KASP markers for assessing the genetic makeup of Brassica rapa seeds. BMC Genomics 23 (1), 326. doi: 10.1186/s12864-022-08567-9
ITIS (2022). Intergrated Taxonomic Information System. National Museum of Natural History, Smithsonian Institution. https://www.itis.gov/.
Kameswara Rao, N., Dulloo, M. E., Engels, J. M. M. (2017). A review of factors that influence the production of quality seed for long-term conservation in genebanks. Genet. Resour. Crop Evol. 64 (5), 1061–1074. doi: 10.1007/s10722-016-0425-9
Kellingray, L., Tapp, H. S., Saha, S., Doleman, J. F., Narbad, A., Mithen, R. F. (2017). Consumption of a diet rich in Brassica vegetables is associated with a reduced abundance of sulphate-reducing bacteria: A randomised crossover study. Mol. Nutr. Food Res. 61 (9). doi: 10.1002/mnfr.201600992
Kiefer, M., Schmickl, R., German, D. A., Mandáková, T., Lysak, M. A., Al-Shehbaz, I. A., et al. (2013). BrassiBase: introduction to a novel knowledge database on Brassicaceae evolution. Plant Cell Physiol. 55 (1), e3–e3. doi: 10.1093/pcp/pct158
Klopsch, R., Witzel, K., Börner, A., Schreiner, M., Hanschen, F. S. (2017). Metabolic profiling of glucosinolates and their hydrolysis products in a germplasm collection of Brassica rapa turnips. Food Res. Int. 100, 392–403. doi: 10.1016/j.foodres.2017.04.016
Koh, J. C. O., Barbulescu, D. M., Norton, S., Redden, B., Salisbury, P. A., Kaur, S., et al. (2017). A multiplex PCR for rapid identification of Brassica species in the triangle of U. Plant Methods 13 (1), 49. doi: 10.1186/s13007-017-0200-8
Langridge, P., Waugh, R. (2019). Harnessing the potential of germplasm collections. Nat. Genet. 51 (2), 200–201. doi: 10.1038/s41588-018-0340-4
Lee, J. G., Bonnema, G., Zhang, N., Kwak, J. H., de Vos, R. C. H., Beekwilder, J. (2013). Evaluation of glucosinolate variation in a collection of turnip (Brassica rapa) germplasm by the analysis of intact and desulfo glucosinolates. J. Agric. Food Chem. 61 (16), 3984–3993. doi: 10.1021/jf400890p
Lee, M.-K., Chun, J.-H., Byeon, D. H., Chung, S.-O., Park, S. U., Park, S., et al. (2014). Variation of glucosinolates in 62 varieties of Chinese cabbage (Brassica rapa L. ssp. pekinensis) and their antioxidant activity. LWT - Food Sci. Technol. 58 (1), 93–101. doi: 10.1016/j.lwt.2014.03.001
Li, G., Yue, L., Cai, X., Li, F., Zhang, H., Zhang, S., et al. (2022). Fingerprint construction through genotyping by sequencing for applied breeding in Brassica rapa. Genome 65 (2), 105–113. doi: 10.1139/gen-2021-0021%M34648727
Lin, Y.-r., Lee, J.-y., Tseng, M.-c., Lee, C.-y., Shen, C.-h., Wang, C.-s., et al. (2018). Subtropical adaptation of a temperate plant (Brassica oleracea var. italica) utilizes non-vernalization-responsive QTLs. Sci. Rep. 8 (1), 13609. doi: 10.1038/s41598-018-31987-1
Liu, U., Cossu, T. A., Davies, R. M., Forest, F., Dickie, J. B., Breman, E. (2020). Conserving orthodox seeds of globally threatened plants ex situ in the Millennium Seed Bank, Royal Botanic Gardens, Kew, UK: the status of seed collections. Biodiver. Conserv. 29 (9), 2901–2949. doi: 10.1007/s10531-020-02005-6
Liu, B., Wang, Y., Zhai, W., Deng, J., Wang, H., Cui, Y., et al. (2013). Development of InDel markers for Brassica rapa based on whole-genome re-sequencing. Theor. Appl. Genet. 126 (1), 231–239. doi: 10.1007/s00122-012-1976-6
Lotti, C., Iovieno, P., Centomani, I., Marcotrigiano, A. R., Fanelli, V., Mimiola, G., et al. (2018). Genetic, bio-agronomic, and nutritional characterization of kale (Brassica oleracea L. var. Acephala) diversity in Apulia, Southern Italy. Diversity 10 (2), 25. doi: 10.3390/d10020025
Lusty, C., van Beem, J., Hay, F. R. (2021). A performance management system for long-term germplasm conservation in CGIAR genebanks: aiming for quality, efficiency and improvement. Plants 10 (12), 2627. doi: 10.3390/plants10122627
Malfa, G. A., De Leo, M., Tundis, R., Braca, A., Loizzo, M. R., Di Giacomo, C., et al. (2022). Biological Investigation and Chemical Study of Brassica villosa subsp. drepanensis (Brassicaeae) Leaves. Molecules 27 (23), 8447. doi: 10.3390/molecules27238447
Meglic, V., Pipan, B. (2018). Spatial and Temporal Assessment of Brassica napus L. Maintaining Genetic Diversity and Gene Flow Potential: An Empirical Evaluation. Intechopen Publishers. https://www.intechopen.com/chapters/59678.
Mira, S., Estrelles, E., González-Benito, M. E. (2015). Effect of water content and temperature on seed longevity of seven Brassicaceae species after 5 years of storage. Plant Biol. 17 (1), 153–162. doi: 10.1111/plb.12183
Mira, S., Estrelles, E., González-Benito, M. E., Corbineau, F. (2011). Biochemical changes induced in seeds of Brassicaceae wild species during ageing. Acta Physiologiae Plantarum 33 (5), 1803–1809. doi: 10.1007/s11738-011-0719-7
Mira, S., Nadarajan, J., Liu, U., González-Benito, M. E., Pritchard, H. W. (2019). Lipid thermal fingerprints of long-term stored seeds of Brassicaceae. Plants 8 (10), 414. doi: 10.3390/Fplants8100414
Montaut, S., Blažević, I., Ruščić, M., Rollin, P. (2017). LC–MS profiling of glucosinolates in the seeds of Brassica elongata Ehrh., and of the two stenoendemic B. botteri Vis and B. cazzae Ginzb. & Teyber. Natural Product Res. 31 (1), 58–62. doi: 10.1080/14786419.2016.1212032
Nagel, M., Börner, A. (2010). The longevity of crop seeds stored under ambient conditions. Seed Sci. Res. 20 (1), 1–12. doi: 10.1017/S0960258509990213
OECD (2016). Safety Assessment of Transgenic Organisms in the Environment. Organisation for Economic Co-operation and Development, Vol. 5. https://www.oecd.org/chemicalsafety/safety-assessment-of-transgenic-organisms-in-the-environment-volume-5-9789264253018-en.htm.
Oppermann, M., Weise, S., Dittmann, C., Knüpffer, H. (2015). GBIS: the information system of the German Genebank. Database 2015. doi: 10.1093/database/bav021
Panis, B., Nagel, M., Van den houwe, I. (2020). Challenges and prospects for the conservation of crop genetic resources in field genebanks, in in vitro collections and/or in liquid nitrogen. Plants 9 (12), 1634. doi: 10.3390/plants9121634
Pathirana, R., Carimi, F. (2022). Management and utilization of plant genetic resources for a sustainable agriculture. Plants 11 (15), 2038. doi: 10.3390/plants11152038
Pérez-García, F., Gómez-Campo, C., Ellis, R. (2009). Successful long-term ultra dry storage of seed of 15 species of Brassicaceae in a genebank: variation in ability to germinate over 40 years and dormancy. Seed Sci. Technol. 37 (3), 640–649. doi: 10.15258/sst.2009.37.3.12
Pérez-García, F., Gonzalez-Benito, M. E., Gómez-Campo, C. (2007). High viability recorded in ultra-dry seeds of 37 species of Brassicaceae after almost 40 years of storage. Seed Sci. Technol. 35, 143–153. doi: 10.15258/sst.2007.35.1.13
Pino Del Carpio, D., Basnet, R. K., De Vos, R. C. H., Maliepaard, C., Paulo, M. J., Bonnema, G. (2011). Comparative methods for association studies: A case study on metabolite variation in a Brassica rapa core collection. PloS One 6 (5), e19624. doi: 10.1371/journal.pone.0019624
Postman, J., Hummer, K., Ayala-Silva, T., Bretting, P., Franko, T., Kinard, G., et al. (2010). GRIN-Global: An international project to develop a global plant genebank information management system. Acta Hortic. 859, 49–55. doi: 10.17660/ActaHortic.2010.859.4
POWO (2022). Plants of the World Online. Royal Botanic Gardens, Kew. https://powo.science.kew.org/.
Quezada-Martinez, D., Addo Nyarko, C. P., Schiessl, S. V., Mason, A. S. (2021). Using wild relatives and related species to build climate resilience in Brassica crops. Theor. Appl. Genet. 134 (6), 1711–1728. doi: 10.1007/s00122-021-03793-3
Rakshita, K. N., Singh, S., Verma, V. K., Sharma, B. B., Saini, N., Iquebal, M. A., et al. (2021). Agro-morphological and molecular diversity in different maturity groups of Indian cauliflower (Brassica oleracea var. botrytis L.). PloS One 16 (12), e0260246. doi: 10.1371/journal.pone.0260246
Ramirez, D., Abellán-Victorio, A., Beretta, V., Camargo, A., Moreno, D. A. (2020). Functional ingredients from Brassicaceae species: overview and perspectives. Int. J. Mol. Sci. 21 (6), 1998. doi: 10.3390/ijms21061998
Razzaq, H., Armstrong, S. J., Saleem, H. (2021). “Chapter 10 - Brassicas: A complete guide to the potential of their wild relatives,” in Wild Germplasm for Genetic Improvement in Crop Plants. Eds. Azhar, M. T., Wani, S. H. (Academic Press), 187–199.
Reed, B. M., Engelmann, F., Dulloo, E., Engels, J. (2004). Technical guidelines for the management of field and in vitro germplasm collections. Handbooks for Genebanks. n.7, 106 p. International Plant Genetic Resources Institute, 95. https://hdl.handle.net/10568/105045.
Salehi, B., Quispe, C., Butnariu, M., Sarac, I., Marmouzi, I., Kamle, M., et al. (2021). Phytotherapy and food applications from Brassica genus. Phytother. Res. 35 (7), 3590–3609. doi: 10.1002/ptr.7048
Sayers, E. W., Cavanaugh, M., Clark, K., Ostell, J., Pruitt, K. D., Karsch-Mizrachi, I. (2019). GenBank. Nucleic Acids Res. 47 (D1), D94–D99. doi: 10.1093/nar/gky989
Schoch, C. L., Ciufo, S., Domrachev, M., Hotton, C. L., Kannan, S., Khovanskaya, R., et al (2020). NCBI Taxonomy: a comprehensive update on curation, resources and tools. Database (Oxford) 2020. doi: 10.1093/database/baaa062
Scialabba, A., Giorgetti, L., Bellani, L. M. (2016). Stress integrated tests and cytological analyses reveal Brassica villosa subsp. drepanensis seed quality decrease upon long-term storage. Plant Biosyst. - Int. J. Dealing all Aspects Plant Biol. 150 (4), 757–766. doi: 10.1080/11263504.2014.991359
Shekhawat, K., Rathore, S. S., Premi, O. P., Kandpal, B. K., Chauhan, J. S. (2012). Advances in agronomic management of indian mustard (Brassica juncea (L.) czernj. Cosson): an overview. Int. J. Agron. 2012, 408284. doi: 10.1155/2012/408284
Sheng, X.-G., Branca, F., Zhao, Z.-Q., Wang, J.-S., Yu, H.-F., Shen, Y.-S., et al. (2020). Identification of black rot resistance in a wild Brassica species and its potential transferability to cauliflower. Agronomy 10 (9), 1400. doi: 10.3390/agronomy10091400
Shuhang, W., Voorrips, R. E., Steenhuis-Broers, G., Vosman, B., van Loon, J. J. A. (2016). Antibiosis resistance against larval cabbage root fly, Delia radicum, in wild Brassica-species. Euphytica 211 (2), 139–155. doi: 10.1007/s10681-016-1724-0
Soh, E. H., Lee, W. M., Park, K.w., Choi, K.-J., Yoon, M. K. (2014). Change of germination rate for chili pepper and chinese cabbage seed in relation to packaging materials and storage conditions over 10 years. Hortic. Sci. Technol. 32 (6), 864–871. doi: 10.7235/hort.2014.14091
Solberg, S.Ø., Yndgaard, F., Andreasen, C., von Bothmer, R., Loskutov, I. G., Asdal, Å. (2020). Long-term storage and longevity of orthodox seeds: A systematic review. Front. Plant Sci. 11. doi: 10.3389/fpls.2020.01007
Solov’yeva, A. E., Artem’yeva, A. M., Schuetze, W. (2013). Peculiar properties of glucosinolate accumulation in the Brassicaceae family. Russian Agric. Sci. 39 (5), 419–422. doi: 10.3103/S1068367413050169
Stewart, A. (2002). A review of Brassica species, cross-pollination and implications for pure seed production in New Zealand. Agronomy New Zealand 32/33, 63–82.
Szczygłowska, M., Piekarska, A., Konieczka, P., Namieśnik, J. (2011). Use of Brassica plants in the phytoremediation and biofumigation processes. Int. J. Mol. Sci. 12 (11), 7760–7771. doi: 10.3390/ijms12117760
Taylor, A., Rana, K., Handy, C., Clarkson, J. P. (2018). Resistance to Sclerotinia sclerotiorum in wild Brassica species and the importance of Sclerotinia subarctica as a Brassica pathogen. Plant Pathol. 67 (2), 433–444. doi: 10.1111/ppa.12745
Thorwarth, P., Yousef, E. A., Schmid, K. J. (2018). Genomic prediction and association mapping of curd-related traits in gene bank accessions of cauliflower. G3: Genes Genomes Genet. 8 (2), 707–718. doi: 10.1534/g3.117.300199
Tortosa, M., Velasco, P., Afonso, D., Padilla, G., Ríos, D., Soengas, P. (2017). Characterization of a Spanish Brassica oleracea collection by using molecular and biochemical markers. Scientia Hortic. 219, 344–350. doi: 10.1016/j.scienta.2017.03.021
U, N. (1935). Genome analysis in Brassica with special reference to the experimental formation of B. napus and peculiar mode of fertilization. Japanese J. Bot. 7, 389–452.
van Treuren, R., Bas, N., Kodde, J., Groot, S. P. C., Kik, C. (2018). Rapid loss of seed viability in ex situ conserved wheat and barley at 4°C as compared to –20°C storage. Conserv. Physiol. 6 (1). doi: 10.1093/conphys/coy033
Volk, G. M., Namuth-Covert, D., Byrne, P. F. (2019). Training in plant genetic resources management: A way forward. Crop Sci. 59 (3), 853–857. doi: 10.2135/cropsci2018.11.0689
Walters, C., Wheeler, L., Stanwood, P. C. (2004). Longevity of cryogenically stored seeds. Cryobiology 48 (3), 229–244. doi: 10.1016/j.cryobiol.2004.01.007
Weise, S., Lohwasser, U., Oppermann, M. (2020). Document or lose it—On the importance of information management for genetic resources conservation in genebanks. Plants 9 (8), 1050. doi: 10.3390/plants9081050
Weise, S., Oppermann, M., Maggioni, L., van Hintum, T., Knüpffer, H. (2017). EURISCO: The European search catalogue for plant genetic resources. Nucleic Acids Res. 45 (D1), D1003–D1008. doi: 10.1093/nar/gkw755
WIEWS (2022). WIEWS - World Information and Early Warning System on Plant Genetic Resources for Food and Agriculture (Food and Agriculture Organisation of the United Nations).
Wijaya, H., Rouw, R., Kadir, A. (2020). Brassica box food products as a healthy local food innovation in The Covid-19 pandemic period. IOP Conf. Series: Earth Environ. Sci. 575, 12011. doi: 10.1088/1755-1315/575/1/012011
Witzel, K., Kurina, A. B., Artemyeva, A. M. (2021). Opening the Treasure Chest: The Current Status of Research on Brassica oleracea and B. rapa Vegetables From ex situ Germplasm Collections. Front. Plant Sci. 12. doi: 10.3389/fpls.2021.643047
Keywords: plant genetic resources, genebank, Brassica biodiversity, taxonomy, Brassica conservation, Brassica species
Citation: Subramanian P, Kim S-H and Hahn B-S (2023) Brassica biodiversity conservation: prevailing constraints and future avenues for sustainable distribution of plant genetic resources. Front. Plant Sci. 14:1220134. doi: 10.3389/fpls.2023.1220134
Received: 10 May 2023; Accepted: 06 July 2023;
Published: 27 July 2023.
Edited by:
Svein Øivind Solberg, Evenstad Campus, NorwayReviewed by:
Ana María Fita, Universitat Politècnica de València, SpainSurinder Banga, Punjab Agricultural University, India
Copyright © 2023 Subramanian, Kim and Hahn. This is an open-access article distributed under the terms of the Creative Commons Attribution License (CC BY). The use, distribution or reproduction in other forums is permitted, provided the original author(s) and the copyright owner(s) are credited and that the original publication in this journal is cited, in accordance with accepted academic practice. No use, distribution or reproduction is permitted which does not comply with these terms.
*Correspondence: Bum-Soo Hahn, bshahn@korea.kr
†These authors have contributed equally to this work