- 1School of Agriculture, Sun Yat-sen University, Shenzhen, China
- 2Laboratory of Plant Breeding and Genetics, Graduate School of Agricultural and Life Sciences, University of Tokyo, Tokyo, Japan
- 3Laboratory of Breeding and Genetics, Graduate School of Agriculture, Osaka Metropolitan University, Sakai, Osaka, Japan
- 4Education and Research Field, School of Agriculture, Osaka Metropolitan University, Sakai, Osaka, Japan
Hybrid lethality is a type of reproductive isolation barrier observed in two developmental stages, hybrid embryos (hybrid seeds) and hybrid seedlings. Hybrid lethality has been reported in many plant species and limits distant hybridization breeding including interspecific and intergeneric hybridization, which increases genetic diversity and contributes to produce new germplasm for agricultural purposes. Recent studies have provided molecular and genetic evidence suggesting that underlying causes of hybrid lethality involve epistatic interaction of one or more loci, as hypothesized by the Bateson–Dobzhansky–Muller model, and effective ploidy or endosperm balance number. In this review, we focus on the similarities and differences between hybrid seed lethality and hybrid seedling lethality, as well as methods of recovering seed/seedling activity to circumvent hybrid lethality. Current knowledge summarized in our article will provides new insights into the mechanisms of hybrid lethality and effective methods for circumventing hybrid lethality.
1 Introduction
Distant or wide hybridization including interspecific and intergeneric hybridization increases genetic diversity and contributes to produce new germplasm by transferring resistance to disease or novel and useful phenotypes for agricultural purposes. However, the formation and evolution of reproductive isolation has prevented gene flow between species through distant hybridization (Lafon-Placette et al., 2017; Kulmuni et al., 2020). Despite its evolutionary importance, reproductive isolation is also an obstacle to distant hybridization breeding in plants. Reproductive isolation involves various pre-mating, post-mating prezygotic, and postzygotic isolating barriers in plants (Coyne and Orr, 2004; Savolainen et al., 2006; Rieseberg and Willis, 2007; Rieseberg and Blackman, 2010). Ecogeographic isolation and pollinator isolation (pollinator fidelity in a natural mixed population) are typical examples of pre-mating isolation barriers (Ramsey et al., 2003; Coyne and Orr, 2004; Savolainen et al., 2006; Streisfeld and Kohn, 2007; Zhang et al., 2022a), whereas interspecific pollen-pistil incompatibility, conspecific pollen precedence, gametic incompatibility, and pistil-length mismatch are examples of post-mating prezygotic isolating barriers (Rieseberg and Willis, 2007; Lee et al., 2008; Rieseberg and Blackman, 2010; Huang et al., 2023). Postzygotic isolation barriers include hybrid seed lethality (Bikard et al., 2009; Dziasek et al., 2021), immature fruit abscission (Gupta et al., 1996; He et al., 2019; Kawaguchi et al., 2021), hybrid seedling lethality or inviability (Tezuka et al., 2010; Tezuka, 2013; Xiao et al., 2017; Deng et al., 2019; Mino et al., 2022), hybrid weakness (Ichitani et al., 2011; Shiragaki et al., 2020b), hybrid sterility (Yamagata et al., 2010; Koide et al., 2018; Li et al., 2020), and hybrid breakdown (Li et al., 1997; Kubo and Yoshimura, 2005; Zhang et al., 2021).
Hybrid lethality has been widely studied in various plant species, including genera Nicotiana (Tezuka et al., 2010; He et al., 2019), Arabidopsis (Bomblies et al., 2007; Dilkes et al., 2008), Oryza (Nadir et al., 2018), Triticum (Hermsen, 1963b; Tikhenko et al., 2005), and Capsella (Dziasek et al., 2021). Hybrid lethality can be observed in hybrid embryos during seed development and in hybrid seedlings or plants during plant development without infection. Hybrid lethality in embryo results in seed abortion and is often called hybrid seed lethality. Regarding hybrid lethality in seedlings, different terms, such as hybrid weakness, hybrid necrosis, or others, are used depending on the severity of the symptom and plant species. Because of the different growth and developmental stages during which hybrid lethality is observed, the similarities and differences between hybrid seed lethality and hybrid seedling lethality are topics of interest for future research.
Recent evidence has shown that hybrid lethality is often caused by epistatic gene interaction of two or more loci, as explained by the Bateson–Dobzhansky–Muller (BDM) model (Figure 1A) (Johnson and Porter, 2000; Johnson, 2002; Bomblies and Weigel, 2007; Sweigart and Willis, 2012). In this model, hybrid lethality is caused by genes that were present in an ancestral population but evolved differences in isolated descendent populations. Hybrid lethality in seeds and seedlings may be caused by developmental defects due to genes encoding disease resistance proteins (Bomblies et al., 2007; Chen et al., 2014; Deng et al., 2019), such as duplicate copies of critical genes for development, which were formed during divergent evolution, including photosynthetic gene (Zuellig and Sweigart, 2018) and histidinol-phosphate amino-transferase gene (HPA) (Bikard et al., 2009).
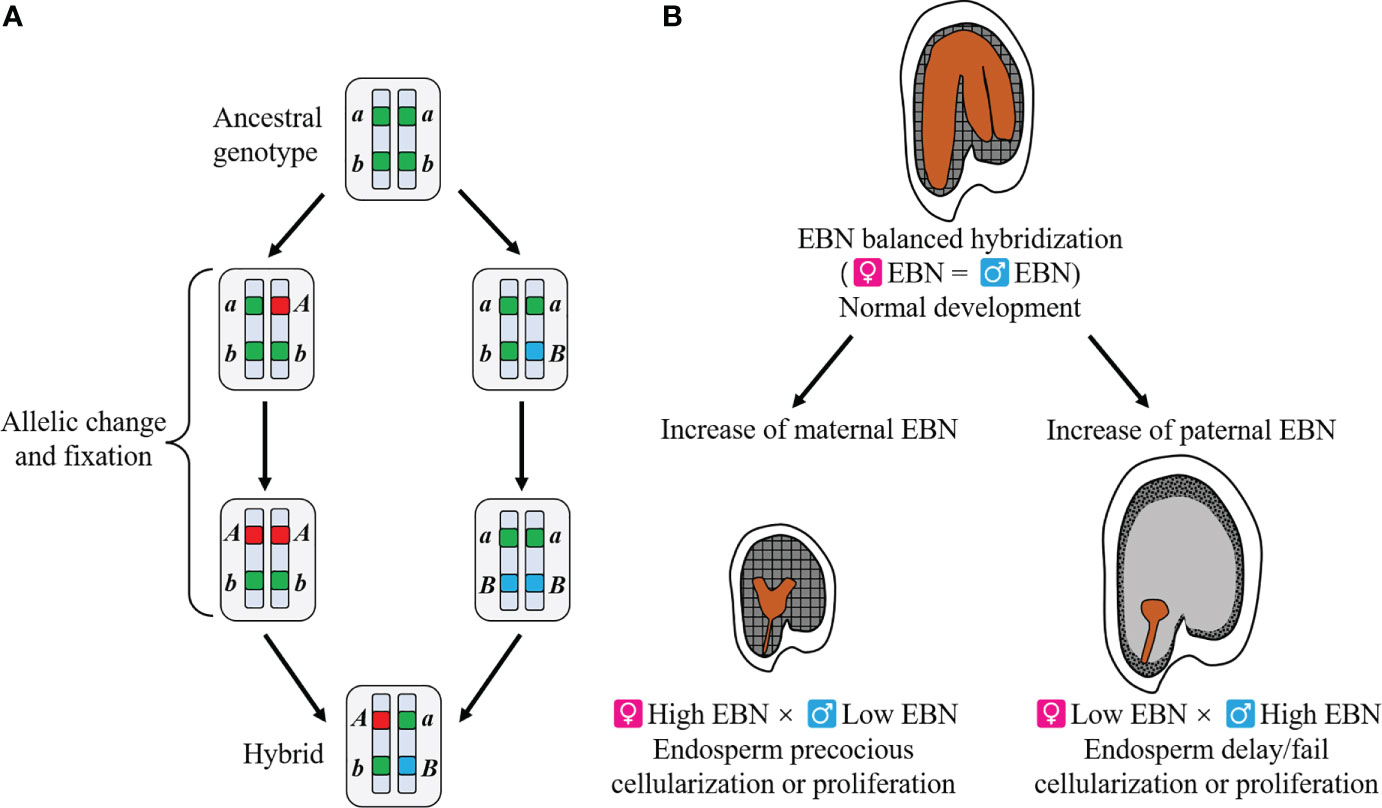
Figure 1 Overview of two theories explaining genetic incompatibilities. (A) Bateson–Dobzhansky–Muller model. An ancestral population showed the genotype of aabb. Each lineage evolved to independent mutations (a to A and b to B). When hybridization occurs between the newly formed two species, deleterious interaction of the two alleles, A and B, causes genetic incompatibilities. In this model, allelic interaction of a single locus as well as two or more loci might be responsible for genetic incompatibilities. (B) Endosperm balance number (EBN) hypothesis. Balanced effective ploidy or EBN between parents yields normal endosperm, leading to normal embryo development and thus normally germinable seeds. When EBN of parents is unbalanced, the timing of endosperm cellularization or proliferation is disturbed, resulting in embryo arrest and hybrid seed lethality. This hypothesis is applicable to both interspecific and interploidy hybridizations.
In this review, we focused on and summarized the current knowledge on hybrid lethality in seeds and seedlings, particularly but not exclusively in Nicotiana (tobacco) species. We also introduce recent molecular advances for finding similarities and/or differences in mechanisms between both types of hybrid lethality. Finally, we discuss the methods to overcome or circumvent hybrid lethality and the active application of hybrid lethality to prevent gene flow.
2 Hybrid seed lethality
2.1 Two categories of hybrid seed lethality
Hybrid seed lethality during seed development is observed after successful fertilization in both interspecies and interploidy hybridizations and has been well studied in Nicotiana (Kostoff, 1930; McCray, 1933; He et al., 2020), Arabidopsis (Bikard et al., 2009; Lafon-Placette et al., 2018), Oryza (Ishikawa et al., 2011), Triticum (Tikhenko et al., 2008; Tikhenko et al., 2017a), and Mimulus (Coughlan et al., 2020). Hybrid seed lethality is characterized by embryo arrest and/or endosperm defects. Based on underlying causes, hybrid seed lethality is classified into two main categories: (1) hybrid seed lethality with underlying causes in embryo itself (impaired or arrested embryo development, but normal endosperm development are observed), and (2) hybrid seed lethality caused by defective endosperm development, leading to embryo arrest (Table 1).
2.1.1 Hybrid seed lethality with underlying causes in the embryo itself
Several studies have reported that early embryo abnormal development is the main cause of hybrid seed lethality in Arabidopsis (Bikard et al., 2009) and tribe Triticeae (Tikhenko et al., 2008). In intergeneric hybridization between wheat (Triticum durum) and rye (Secale cereale), both belonging to tribe Triticeae, despite normal endosperm development, the development of shoot apical meristem (SAM) in hybrid embryo is arrested, leading to incompletely differentiated embryos and non-germinating seeds (Tikhenko et al., 2005; Tikhenko et al., 2008). Hybrid embryo lethality in this cross is caused by the interaction between two alleles from two loci, i.e., wheat embryo lethality (Eml)-A1 and rye Eml-R1, which are likely to be orthologous genes (Tikhenko et al., 2010; Tikhenko et al., 2011). Gene mapping results implied that Eml-A1 is involved in SAM maintenance, and thus, the underlying cause of hybrid seed lethality is the embryo itself (Tikhenko et al., 2017a).
Similarly, in hybridization between Arabidopsis thaliana accessions Columbia (Col) and Cape Verde Islands (Cvi), embryo arrest, resulting in hybrid seed lethality, is caused by duplicate genes LD1.1 and LD 1.5, which encode histidinol-phosphate amino-transferase that catalyzes an important step in the biosynthetic pathway leading to an essential amino acid histidine (Bikard et al., 2009). Homozygous states for both silenced Col allele at the LD1.1 locus and silenced Cvi allele at the LD1.5 locus causes embryo lethality (Bikard et al., 2009). Hence, hybrid seed lethality with underlying causes in embryo agrees with the BDM model and, at least in some cases known so far, is caused by gene functional alteration.
2.1.2 Hybrid seed lethality with underlying causes in endosperm
Polyploidy is widely acknowledged in plant evolution. Although several mechanisms are considered to be related to polyploidization, the most likely mechanism is the participation of unreduced 2n gametes. However, production of polyploids through combination of n and 2n gametes is often prevented. Even if a few polyploids survive, the polyploids have difficulty producing viable offspring in hybridization with diploid progenitors. This reproductive barrier, which is called the triploid block, often involves incompatibility in the endosperm or hybrid seed lethality and is considered to act as an instant reproductive barrier (Köhler et al., 2010; Schatlowski and Köhler, 2012).
Research to date has implicated that in many cases, hybrid seed lethality is caused by abnormal endosperm development (Scott et al., 1998; Bushell et al., 2003; Ishikawa et al., 2011; Rebernig et al., 2015; Lafon-Placette et al., 2017). In angiosperms, endosperm is essentially a triploid tissue developed after the fusion of two polar nuclei with a sperm nucleus and is mainly responsible for providing nutrition for embryo growth and germination. Based on differences in its developmental patterns, endosperm is classified into three types: free nuclear, ab initio cellular, and helobial (Vijayaraghavan and Prabhakar, 1984; Floyd and Friedman, 2000). Hybrid seed lethality has been studied in plants with free nuclear or ab initio cellular endosperm. In free nuclear endosperm, fertilized triploid endosperm nucleus divides without formation of cell wall and later become cellularized, whereas the cell wall is coordinately formed with every endosperm nucleus division in ab initio cellular endosperm. In plants with free nuclear endosperm, hybrid seed lethality is characterized by a disturbance in the timing of endosperm cellularization, which is an important developmental transition stage for embryo development in this type of endosperm (Ishikawa et al., 2011; Sekine et al., 2013; Lafon-Placette et al., 2017; İltaş et al., 2021). In the ab initio cellular endosperm, hybrid seed lethality shows impaired endosperm cell proliferation (Oneal et al., 2016; Roth et al., 2018).
2.1.2.1 Endosperm balance number is involved in hybrid seed lethality
Hybrid seed lethality caused by defective endosperm development is well explained by effective ploidy or endosperm balance number (EBN) hypothesis proposed by Johnston et al. (1980) (Figure 1B). EBN is an arbitrary number allocated to each species and the normal development of endosperm requires a relative maternal (m):paternal (p) EBN ratio of 2:1 (Johnston et al., 1980; Städler et al., 2021). In intraspecies-interploidy hybridization, m:p genome ratio deviates from 2:1; thus, EBN ratio also deviates from 2:1, resulting in endosperm developmental failure. Maternal EBN excess generally results in precocious developmental transition in the endosperm and the production of smaller seeds compared to self-pollinated parental seeds, whereas paternal EBN excess generally results in delayed or failed endosperm development and the production of bigger seeds. Such examples that can be explained by the EBN hypothesis have been reported in many plant species including Arabidopsis (Scott et al., 1998), Oryza (Sekine et al., 2013), and Mimulus (Coughlan et al., 2020). Similarly, the EBN hypothesis fits well with hybrid seed lethality in interspecies hybridizations, even if the parental species have the same ploidy level (Scott et al., 1998; Rebernig et al., 2015; Garner et al., 2016). Therefore, differences in parental EBN rather than differences in parental ploidy levels are assumed to be responsible for hybrid seed lethality.
Nicotiana species have ab initio cellular endosperm (Sehgal and Gifford, 1979). In this genus, hybrid seed lethality is widely observed in interspecies hybridizations (McCray, 1933; Reed and Collins, 1978; Subhashini et al., 1985; Iwai et al., 1986; Subhashini et al., 1986; He et al., 2020). Recently, two types of hybrid seed lethality, types I and II hybrid seed lethality, which show different severity of symptoms dependent on the maternal accessions, were reported in hybridizations between octoploid Nicotiana suaveolens and allotetraploid N. tabacum (He et al., 2020). Type I seed lethality occurs in hybridization between N. suaveolens PI 555561 (8x) and N. tabacum (4x) and is characterized by precocious developmental transition and subsequent developmental arrest of the endosperm and abnormal hypertrophy of the embryo remaining in globular state. In contrast, type II seed lethality occurs in another hybridization between N. suaveolens accession PI 555565 (8x) and N. tabacum (4x) and is characterized by symptoms more severe than those of type I seed lethality, such as precocious developmental transition of the endosperm and subsequent narrowing of the endosperm region as if pressed by surrounding cells, and embryo growth arrest in the early globular stage. Both type I and type II seed lethality are related to maternal ploidy level as witnessed by the experiments using ploidy manipulated lines. Even in the case of hybridization producing normal seeds, phased increase of ploidy levels in maternal plants subsequently causes type I and type II seed lethality in both interspecies and interploidy hybridizations. The two types of hybrid seed lethality are reversed by an increase in ploidy levels of paternal parents. Thus, hybrid seed lethality in Nicotiana is well explained by EBN hypothesis and higher ploidy levels in maternal parents than in paternal parents cause type I or type II seed lethality (He et al., 2020; He et al., 2022).
Previous studies have demonstrated that parent-of-origin specific hybrid endosperm defect is observed in hybrid seed lethality in reciprocal hybridizations and have implied that genomic imprinting is involved in the endosperm defect (Bushell et al., 2003; Josefsson et al., 2006; Lafon-Placette et al., 2017). Genomic imprinting is the epigenetic phenomenon modifying the expression of genes in a parent-of-origin manner. Genomic imprinting mainly includes DNA methylation, histone modification, and non-coding RNA regulation, and disturbed balance of these modification is expected to cause hybrid seed lethality (Lauria et al., 2004; Erdmann et al., 2017; Jiang et al., 2017; Martinez et al., 2018; Dziasek et al., 2021). Current epigenetic analysis in plants suggested that the regulation of imprinting in plants is likely to be explained through a combination of several different epigenetic mechanisms, and these mainly include DNA methylation and trimethylation of lysine 27 of histone H3 (H3K27me3) (reviewed by Batista and Köhler (2020)).
EBN might be related to parental conflict hypothesis, where maternal and paternal genomes have opposite effects on offspring development: the maternal parent is equally related to all of their progeny and thus should allocate equally, whereas the paternal parents are only related to their own progeny, but not to the competing half-siblings, and thus should somehow direct the maternal parent to allocate differentially (Haig and Westoby, 1989; Haig and Westoby, 1991). In plants, the parental conflict hypothesis predicts that the maternal genome excess or maternally expressed genes (MEGs) lead to reduced nutrient flow to the embryo, reduced seed size, and potentially reduced seed set under unfavorable conditions. Conversely, the paternal genome excess or paternally expressed genes (PEGs) promotes nutrient flow to the embryo and increases seed size and seed set (Schatlowski and Köhler, 2012; Bai and Settles, 2015). This is consistent with the endosperm and embryo observation in interploidy hybridizations using parental plants with stepwise changes in EBN (Scott et al., 1998; He et al., 2022).
Several key genes for EBN-based hybrid seed lethality have been identified, suggesting that BDM-type mechanism might be related to endosperm of incompatible species (Köhler et al., 2010). Hybrid seed lethality in paternal excess hybridization can be circumvented by using PEG mutants, such as PICKLE RELATED 2 (PKR2 which encodes a CHD3 chromatin remodeler), PHERES1 (PHE1, the type I MADS-box transcription factor), and ADMETOS (ADM which encodes a protein belonging to the diverse family of molecular chaperones called J-domain proteins) (Kradolfer et al., 2013b; Huang et al., 2017; Batista et al., 2019). In contrast, MEG mutants, such as MEDEA (MEA, the Polycomb group gene), are effective for circumventing hybrid seed lethality in maternal excess hybridization (Kradolfer et al., 2013a). Additionally, the mutant for TRANSPARENT TESTA GLABRA2 (TTG2; which encodes a WRKY transcription factor controlling epidermal cell fate with pleiotropic effects on seed development and trichome production) has also been reported to partially rescue hybrid seeds from lethality through reduced integument cell elongation and precocious endosperm cellularization in paternal excess hybridization (Garcia et al., 2005; Dilkes et al., 2008). Similarly, the mutant for TRANSPARENT TESTA 4 (TT4; which encodes the enzyme chalcone synthase) has also been reported to rescue hybrid seeds in paternal excess hybridization (Scott et al., 2013; Doughty et al., 2014). The ttg2 and tt4 mutants are characterized by the lack of flavonoid seed pigmentation in the seed coat because they do not accumulate proanthocyanidins, suggesting the involvement of flavonoid biosynthesis pathway in hybrid seed lethality (Scott et al., 2013). A recent study demonstrated that mutants of other genes in the flavonoid biosynthesis pathway also rescued hybrid seeds from lethality; in particular, tt8 mutants completely rescued hybrid seeds unlike mutants of other genes, suggesting that auxin flux and signaling, rather than flavonoid biosynthesis pathway, might be involved in hybrid seed lethality because TT8 interacts with genes expressed in seed coat including the repressor IAA27/PAP2, a canonical Aux/IAA (Zumajo-Cardona et al., 2023). However, despite these extensive studies, underlying molecular mechanisms of EBN differences are largely unknown. Hence, elucidating the detailed mechanism of hybrid seed lethality caused by endosperm defect in interspecific and interploidy hybridizations remains challenging.
2.1.2.2 Immature fruit abscissions caused by hybrid seed lethality
Plants have evolved sophisticated organ abscission to respond to seed/fruit dispersal, pathogen attack, and environment stress. Abscission is a universal and physiological process in plant development that occurs through loosening of adjacent cell walls within the abscission zone (AZ) and subsequent cell separation (Addicott, 1982; Taylor and Whitelaw, 2001). It takes place under various developmental signals, such as fertilization, senescence, and ripening, and environmental signals, such as light, pathogen, and temperature (reviewed by Sawicki et al. (2015)). Abscission is also observed in developing ovaries (immature fruits) in interspecies and interploidy hybridizations in Nicotiana, which exhibit type II hybrid seed lethality (He et al., 2020; He et al., 2022). The post-pollination developing fruit or pod abscission has also been reported in other interspecific crosses of the genera Cicer (Mallikarjuna, 1999), Lupinus (Gupta et al., 1996), Phaseolus (Mok et al., 1978), and Vigna (Barone et al., 1992), all of which belong to the Fabaceae family. Studies in Nicotiana have clearly demonstrated that manipulation of parental ploidy levels not only affects hybrid seed lethality (type II, not type I, in the Nicotiana case) but also affects immature fruit abscission in interspecies and interploidy hybridizations (He et al., 2020; He et al., 2022). This implies that the parental conflict hypothesis could also be applied to fruit maturation and abscission, which are maternal processes. However, the detailed process by which hybrid seed lethality causes immature fruit abscission is unclear.
Failure of embryo development (seed abortion) may cause ovary or fruit abscission during plant reproduction (Sawicki et al., 2015). In general, plant hormones, such as auxin, ethylene, gibberellins (GAs), and abscisic acid (ABA), play important roles in organ abscission. Ethylene and ABA act as abscission-accelerating hormones (Vernieri et al., 1992; Zacarias et al., 1995; Taylor and Whitelaw, 2001; Zhu et al., 2010), whereas auxin and GAs are well-known inhibitors of abscission (Mahouachi et al., 2009; Basu et al., 2013; Liang et al., 2020). In particular, the interaction between auxin and ethylene plays important roles in regulation of abscission (Taylor and Whitelaw, 2001). A basipetal auxin flux through AZ is considered to prevent abscission by rendering the AZ insensitive to ethylene. When seed abortion occurs, the auxin flux is suppressed, leading to the enhancement of the AZ sensitivity to ethylene and activation of AZ (Sawicki et al., 2015). Similarly, it has been demonstrated that auxin is a trigger of seed development in many plant species; auxin biosynthesis genes are activated after fertilization, then auxin is accumulated and auxin signaling is activated in seed tissues (reviewed by Figueiredo and Kohler (2018)). Fruit abscission occurs when only small number of seeds are contained in the fruit owing to the insufficient supply of auxin from seeds or ovary (Din et al., 2019). Therefore, immature fruit abscission in interspecies and interploidy hybridizations may also be controlled by auxin and ethylene. In the case of Nicotiana, when the ovary was occupied by seeds exhibiting type I hybrid seed lethality, a sufficient amount of auxin to prevent immature fruit abscission might be somehow transported to the AZ. On the other hand, when the ovary was occupied by seeds exhibiting type II hybrid seed lethality, the supply of auxin from seeds or ovary might be insufficient, leading to immature fruit abscission. Although involvement of auxin in immature fruit abscission has been suggested because immature fruit abscission, but not type II hybrid seed lethality, was suppressed by exogenous auxin treatments (He et al., 2022), further studies are needed to validate this hypothesis.
2.2 Methods of recovering seed activity to circumvent hybrid seed lethality
Circumventing hybrid seed lethality is valuable in achieving distant hybridization to transfer desirable genes to crop germplasm. For example, genes of rye (S. cereale) have been introduced into wheat (T. durum) germlines to improve resistance to pathogens and pests (reviewed by Crespo-Herrera et al. (2017)). Hence, to circumvent hybrid seed lethality, several methods have been developed, including (1) hybrid embryo rescue technique, and (2) restoration of endosperm development to indirectly rescue the hybrid embryo (Figure 2, Table 1).
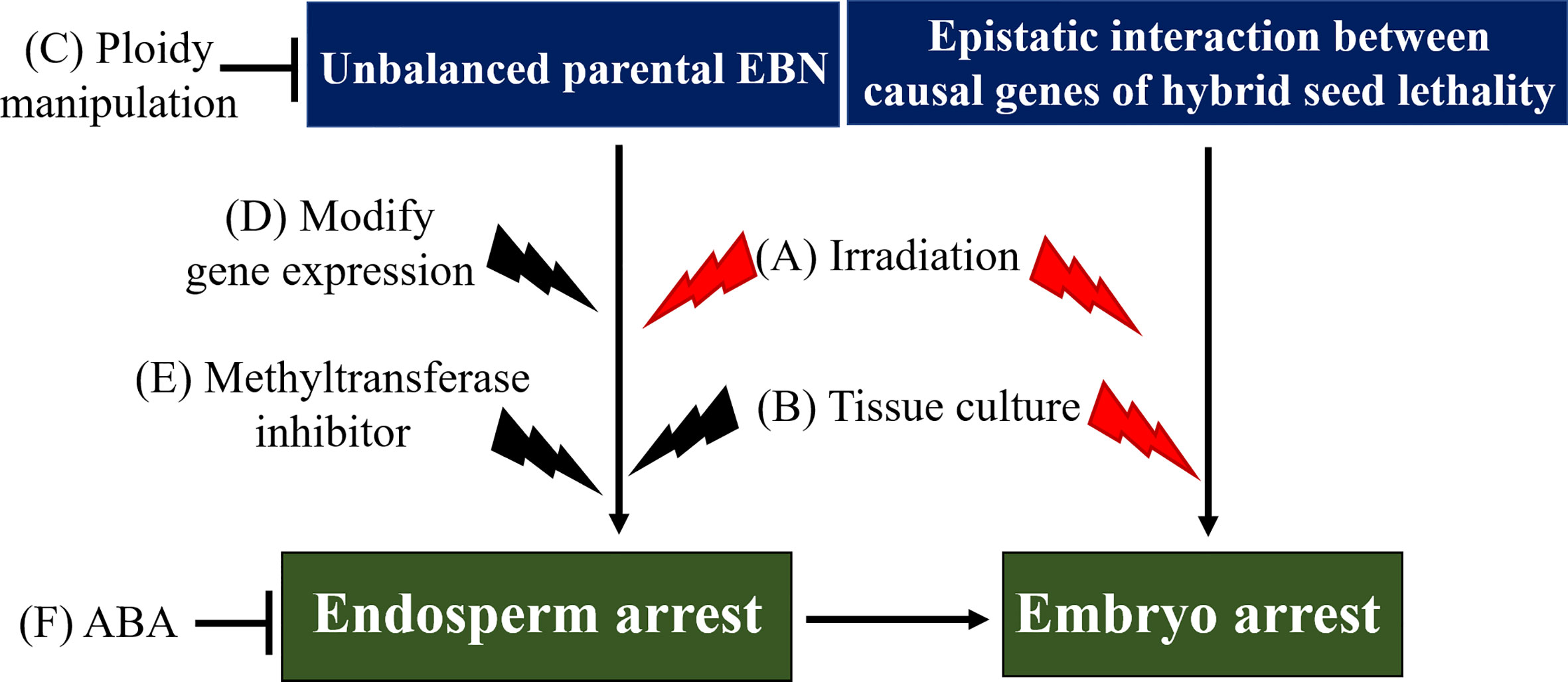
Figure 2 Model of hybrid seed lethality and methods to overcome or circumvent hybrid seed lethality. Two pathways are involved in the induction of hybrid seed lethality: (1) epistatic interaction between causal genes of hybrid seed lethality directly affecting hybrid embryos, and (2) unbalanced parental EBN, which causes endosperm arrest, leading to hybrid embryo arrest. Irradiation (A) and tissue culture (embryo rescue technique, (B) may be effective to overcome hybrid seed lethality caused by epistatic interaction between causal genes. Tissue culture and ploidy manipulation (C) are widely used to overcome or circumvent EBN-based hybrid seed lethality. Additionally, modification of gene expression (D), increase in endogenous ABA levels (F), application of methyltransferase inhibitor (E) and exogenous ABA, and possibly irradiation may be effective in some hybridization combinations at least. Black thunderbolt icons represent induction of mutations. Possible methods to overcome hybrid seed lethality are represented by red thunderbolt icons. EBN, endosperm balance number; ABA, abscisic acid.
2.2.1 Hybrid seed rescue through the embryo rescue technique
Before hybrid embryos become defective, the immature embryos are isolated and cultured on the medium for their recovery and growth. This embryo rescue technique is effective to rescue embryos from the endosperm defective hybrid seeds and has been widely used in various plant species, including wheat (Polgári et al., 2014) and melon (Nuñez-Palenius et al., 2006). When embryos are too small to isolate, ovule or ovary culture is available for hybrid embryo rescue (Figure 2B). For example, in several interspecific hybridizations in Nicotiana, ovule culture was successful to obtain hybrid seedlings by circumvent hybrid seed lethality (Reed and Collins, 1978; Shizukuda and Nakajima, 1982; Subhashini et al., 1985; Iwai et al., 1986; Subhashini et al., 1986; Chung et al., 1988). In interspecific hybridization of Lilium, embryo rescue by ovary culture was effective to obtain hybrid seedlings (Fernandez et al., 1998). Effectiveness of embryo rescue appears to depend on the severity of hybrid seed lethality because ovule culture was successful in type I hybrid seed lethality but not in type II hybrid seed lethality in Nicotiana interspecific-interploidy hybridizations (He et al., 2020). Based on the nutritional requirements of developing embryo, embryonic development is divided into two phases, a heterotrophic phase and autotrophic phase. The embryo is still heterotrophic at the globular stage, and only in the late heart-shaped stage, along with the beginning of cotyledonary development and the consequent internal differentiation, the embryo becomes autotrophic (Raghavan, 1966). Embryo rescue is usually conducted in the heterotrophic phase, where the young embryo still depends at the expense of the endosperm, and can be accomplished in vitro by providing, through culture medium, the complex nutritional factors, including amino acids, carbohydrates, vitamins, and other growth factors that would normally be supplied by endosperm. Hence, embryo rescue technique is an effective way to circumvent hybrid seed lethality due to endosperm defective.
In the case where the causal genes are directly involved in embryo development, embryo rescue might not be effective. When embryo rescue was applied to circumvent hybrid seed lethality caused by Eml-A1 and Eml-R1 in hybridization between wheat (T. aestivum) and rye (S. cereale), no positive effect was observed on embryo development (Tikhenko et al., 2008). This further implies that embryo rescue technique is only effective when used to complement the role of the endosperm in supporting the developing embryo.
2.2.2 Hybrid seed rescue through endosperm restoration
Restoration of endosperm development to recover the embryo viability is widely applied in the interploidy and interspecific hybridization. Endosperm developmental defect can be bypassed by altering the EBN of parental species by ploidy manipulation (Figure 2C) (Johnston and Hanneman, 1982; Bushell et al., 2003; Lafon-Placette et al., 2017). In interspecific-interploidy hybridization between N. suaveolens (8x) and N. tabacum (4x) (ab initio cellular endosperm), type I and type II seed lethality could be overcome through restoring endosperm and embryo development by increasing paternal N. tabacum ploidy from 4x to 8x (He et al., 2020). Another study provides evidence that decreasing maternal EBN can also restore endosperm development and hybrid seed lethality in Nicotiana (He et al., 2022).
As described above, endosperm abnormal development responsible for hybrid seed lethality in nuclear endosperm is well studied in Arabidopsis and Oryza. In this endosperm developmental mode, a disturbance in the timing of endosperm cellularization is the primary cause observed histologically (Ishikawa et al., 2011; Sekine et al., 2013; Lafon-Placette et al., 2017; İltaş et al., 2021). Hence, recovering the endosperm development by manipulation of parental ploidy levels is effective to support nutrition for embryo growth and germination. In interspecific hybridization between diploid species O. sativa and O. longistaminata, the manipulation to increase the O. sativa ploidy levels restored the endosperm cellularization and hybrid seed viability (Ishikawa et al., 2011; Tonosaki et al., 2018). Additionally, several mutants producing unreduced gametes have been identified (Köhler et al., 2010; Schatlowski and Köhler, 2012). Such a mutants might also be effective to circumvent hybrid seed lethality by balancing EBN ratio in endosperm.
In polyploidization, another mechanism for bypassing triploid block may be polyspermy, a fertilization of one egg with two sperm. In A. thaliana, triploid block can be circumvented by polyspermy because polyspermy selectively polyploidizes the egg cell while rendering the genome size of endosperm unaffected (Mao et al., 2020).
Besides ploidy manipulation, chemical treatment can also restore endosperm development. Recent study showed that epimutagenesis chemically induced by treatment with DNA methyltransferase inhibitor 5-azacytidine was successful in circumventing hybrid seed lethality in Arabidopsis interploidy hybridization and Capsella interspecific hybridization (Figure 2E) (Huc et al., 2022). In Arabidopsis interploidy hybridization, endosperm cellularization could be restored by increasing endogenous ABA levels using mutants for ABA hydroxylase-encoding gene CYP707A2 or by exogenous application of ABA, leading to suppression of the triploid block and hybrid embryo arrest (Figure 2F) (Xu et al., 2023). Hence, endosperm defects can be restored by ploidy alteration or specific reagent treatment, leading to recovery of embryo viability and circumventing hybrid seed lethality.
Additionally, temperature might influence hybrid seed lethality. In interspecies hybridizations in Arabidopsis, hybridizations using parental plants growing at low temperatures resulted in suppression of endosperm-based hybrid seed lethality (Bjerkan et al., 2020). This low temperature effect may be attributed to the increased levels of ABA (Xu et al., 2023).
3 Hybrid seedling lethality
3.1 Mechanisms of hybrid seedling lethality
Hybrid lethality in seedlings has been reported for over 100 years in many plant species, such as Triticum-Aegilops complex (Sax, 1921), Nicotiana (Malloch and Malloch, 1924; Christoff, 1928; Kostoff, 1930), Crepis (Hollingshead, 1930), Gossypium (Gerstel, 1954), Oryza (Oka, 1957), Solanum (Day, 1958; Sawant, 1958), Cucurbita (Whitaker and Bemis, 1964), Capsicum (Hirose et al., 1960; Pickersgill, 1971), Papaver (Mcnaughton and Harper, 1960), Hordeum (Takahashi et al., 1970), Phaseolus (Shii et al., 1980; Gepts and Bliss, 1985), and Camellia (Nadamitsu et al., 1986). Several terms representing hybrid lethality or developmental defects of hybrid seedlings are used by researchers. Although these terms are used differently according to plant species, they seem to be mainly used according to the severity of phenotypic symptoms. The general symptoms of hybrid lethality are apparent death of seedlings and are typically observed in interspecific hybridizations in Nicotiana (Yamada et al., 1999; Tezuka, 2012; Tezuka, 2013). Hybrid weakness is characterized by the weak growth of seedlings compared to both parents and is typically observed in Oryza (Chu and Oka, 1972; Chen et al., 2013; Nadir et al., 2019; Shiragaki et al., 2019), Capsicum (Shiragaki et al., 2020b; Shiragaki et al., 2021; Shiragaki et al., 2022), and Phaseolus (Koinange and Gepts, 1992; Reiber and Neuman, 1999a). Hybrid necrosis may refer to both types and is mainly used for the Triticum-Aegilops complex (Tsunewaki and Kihara, 1962; Toxopeus and Hermsen, 1964; Mizuno et al., 2010; Zhang et al., 2022b) and Arabidopsis (Bomblies et al., 2007; Świadek et al., 2017). Such hybrid seedling lethality and other similar phenomena are observed in F1 hybrid seedlings. When F1 hybrids are normal but their F2 and later progeny contain individuals showing seedling lethality, this phenomenon is called hybrid breakdown (hybrid breakdown is also used to refer sterility in such a generation) (Fukuoka et al., 1998; Plötner et al., 2017; Matsubara, 2020). The molecular mechanism of hybrid seedling lethality has been unknown for a long time and has only recently become somewhat clear. Recent research has revealed that developmental defects of seedlings, which has referred to using several terms, could also be a phenomenon with a similar mechanism.
3.1.1 Epistatic interaction causing hybrid seedling lethality
Hybrid seedling lethality is generally genetically simple. In many cases, hybrid seedling lethality is caused by deleterious epistatic interaction of two dominant alleles of different loci as proposed by the BDM model (Hollingshead, 1930; Sawant, 1956; Oka, 1957; Tsunewaki, 1960; Hermsen, 1963a; Takahashi et al., 1970; Chu and Oka, 1972; Shii et al., 1980; Lee, 1981; Hu et al., 2016). Several reports have stated that epistatic interaction of alleles at a single locus causes hybrid seedling lethality as hypothesized by the BDM model (Smith et al., 2011; Chae et al., 2014; Todesco et al., 2014). Similarly, seedling lethality in hybrid breakdown are also caused by epistatic interaction of recessive alleles (Oka, 1957; Matsubara, 2020). Additionally, nuclear-cytoplasmic interaction has been reported to cause hybrid seedling lethality (Inai et al., 1993), but no further data are available. Studies to date have isolated several genes and allowed us to understand hybrid seedling lethality from their functions.
3.1.2 Hybrid seedling lethality related to autoimmune response
Hybrid seedling lethality is observed in many interspecific hybridizations in Nicotiana (Tezuka, 2012; Tezuka, 2013). In this genus, five types of hybrid lethality, which exhibit different phenotypic abnormalities, have been recognized (Yamada et al., 1999; Tezuka and Marubashi, 2012). Among the lethality types, type II hybrid seedling lethality has been extensively studied using interspecies hybridizations between cultivated tobacco species N. tabacum and its wild relatives. The type II hybrid seedling lethality is characterized by browning of hypocotyl and roots in hybrid seedlings during early growth stages, and the hybrid seedlings eventually die. A series of studies have demonstrated that the type II hybrid seedling lethality is accompanied by vacuole-mediated programmed cell death with features of apoptotic cell death and autophagy (Yamada et al., 2000; Tezuka and Marubashi, 2004; Mino et al., 2005; Tezuka and Marubashi, 2006a; Mino et al., 2007b; Ueno et al., 2016) and is related to ethylene (Yamada and Marubashi, 2003), reactive oxygen species (Mino et al., 2002; Mino et al., 2004), and nitrogen oxide (Yamamoto et al., 2017). Taken together, type II hybrid seedling lethality resembles hypersensitive response or cell death, a type of plant defense response with a rapid localized cell death that occurs at the position of pathogen infection (Balint-Kurti, 2019; Salguero-Linares and Coll, 2019). A mitogen-activated protein kinase (MAPK) cascade that functions in plant immunity is reported to be involved in the type II hybrid seedling lethality (Mino et al., 2007a). Furthermore, it has been revealed that several genes related to disease resistance are involved in the type II hybrid seedling lethality (Mino et al., 2002; Masuda et al., 2007; Shiragaki et al., 2020a).
Identification of the causal gene provides further evidence of the involvement of resistance and immune responses in the type II hybrid seedling lethality in Nicotiana. N. tabacum, an allotetraploid species with S and T genomes, has an allele at the Hybrid Lethality 1 (NtHL1) locus on chromosome H (Ma et al., 2020) of the T genome, or more likely, on chromosome Q of the S genome (based on the analysis using simple sequence repeat (SSR) markers in the N. tabacum linkage map) (Marubashi and Onosato, 2002; Tezuka and Marubashi, 2006b; Tezuka et al., 2007; Tezuka et al., 2010; Tezuka et al., 2012), whereas its wild relatives have Hla1-1 or other alleles at the Hybrid Lethality A1 (HLA1) locus (Iizuka et al., 2012). Epistatic interaction of the two alleles at the two loci causes the type II hybrid seedling lethality. Recently, NtHL1 was isolated by transposon tagging; this gene codes a coiled-coil nucleotide-binding site-leucine-rich repeat (CC-NBS-LRR) protein, which is probably involved in disease resistance (Ma et al., 2020). Its counterpart, HLA1, has been mapped by the linkage analysis (Tezuka et al., 2021). Identification of the HLA1 will enable a deeper understanding of the interaction between NtHL1 and HLA1.
Another interesting aspect of the involvement of immune response in type II hybrid seedling lethality has been reported by Katsuyama et al. (2021). The molecular chaperone, heat shock protein 90 (HSP90), and two interacting co-chaperons, required for Mla12 resistance (RAR1) and suppressor of G2 allele of skp1 (SGT1), form a complex that interacts with many NBS-LRRs, also known as NLRs (Kadota et al., 2010; Kadota and Shirasu, 2012; Balint-Kurti, 2019). Agrobacterium-mediated transient expression of RAR1 as well as SGT1 derived from N. gossei (a wild relative of N. tabacum) induced cell death in N. tabacum, whereas the transient expression of those genes derived from N. tabacum did not (Katsuyama et al., 2021). Additionally, a specific inhibitor of HSP90, geldanamycin, suppressed cell death in type II hybrid seedling lethality. These findings suggest the involvement of HSP90-SGT1-RAR1 complex in type II hybrid seedling lethality. Because each species has established an appropriate set of chaperons, the chaperons may be incompatible with those from other species (Katsuyama et al., 2021; Mino et al., 2022).
Similar to Nicotiana, hybrid seedling lethality in other genera is often associated with autoimmune response (Table 2). In hybrid seedling lethality under a two-locus model, at least one causal gene encodes NLR or leucine-rich repeat receptor-like kinase (LRR-RLK) involved in plant immunity (Bomblies et al., 2007; Chen et al., 2014; Sicard et al., 2015; Deng et al., 2019; Si et al., 2021). In hybrid seedling lethality under a one-locus model, the causal gene also encodes NLR or RLK (Smith et al., 2011; Chae et al., 2014). Physiological and molecular studies have also indicated the involvement of plant defense response in hybrid seedling lethality (Mizuno et al., 2010; Shiragaki et al., 2019; Shiragaki et al., 2020b; Shiragaki et al., 2021; Xiao et al., 2021). Therefore, it is apparent that plant defense systems play an important role in the establishment of hybrid seedling lethality systems, which act as reproductive isolation barriers.
3.1.3 Hybrid seedling lethality not resulting from autoimmune response
Although studies on hybrid seedling lethality other than that involving autoimmune response are less advanced, some studies have been reported. As mentioned above, embryo lethality (hybrid breakdown) is caused by duplicate genes LD1.1 and LD 1.5 in hybridization between Arabidopsis thaliana accessions (Bikard et al., 2009). Epistatic interaction of these genes also causes weak growth of seedlings in case of the homozygous for Col allele at the LD1.1 locus and heterozygous (Col and Cvi alleles) at the LD1.5 locus. This is due to the reduced histidine quantity in the seedlings because they have only a single functional copy of histidinol-phosphate amino-transferase gene, and the weak phenotype is restored by supplementation of the histidine (Bikard et al., 2009).
In interspecies hybridization in Mimulus, F2 hybrid seedlings show lethality (hybrid breakdown) owing to a complete lack of chlorophyll production. The lethality is caused by two duplicate genes of PLASTID TRANSCRIPTIONALLY ACTIVE CHROMOSOME 14 (pTAC14), a gene critical for chloroplast development, and F2 hybrid seedlings die when they lack a functional copy of pTAC14 (Zuellig and Sweigart, 2018). In rice intersubspecific hybridizations, weak growth by hybrid breakdown is caused by duplicate recessive genes hwe1 and hwe2. HWE1 and HWE2 encode the Esa1-associated factor 6 (EAF6) protein, which is a component of histone acetyltransferase complexes and possibly plays a pivotal role in the transcriptional regulation of essential genes for plant development. hwe1 and hwe2 alleles lack the function and cause hybrid breakdown not resulting from autoimmune response (Kubo et al., 2022).
Relationships between plant growth and hybrid seedling lethality has also been reported in another hybridization. Chrysanthemum morifolium and Leucanthemum paludosum have different flowering seasons. Intergeneric hybrids of the two species flowered at the two seasons by compound expression of FLOWERING LOCUS T (FT)-like genes derived from both parents, which leads to short vegetative growth. However, the continuous flowering appeared to cause hybrid weakness (Li et al., 2023). Collectively, lack of key genes or a complex combination of genes for plant growth and development could cause hybrid seedling lethality; however, accumulation of more data is necessary to systematize other mechanisms of hybrid seedling lethality than the autoimmune response mechanism.
3.2 Methods to overcome or circumvent hybrid seedling lethality
It seems that when hybrid seedling lethality is caused by lack of important elements for plant growth, supplementing the elements, if possible, could be effective to overcome or circumvent hybrid seedling lethality. However, other methods would be needed to overcome or circumvent hybrid seedling lethality related to autoimmune response. Although several methods have been reported to date, the mechanisms underlying these methods have not been fully elucidated. Here, we introduce the methods to overcome or circumvent hybrid seedling lethality and discuss why these methods are effective.
In some methods, hybrid seedling lethality may be suppressed during the crosstalk between defense and growth responses in plants (Figure 3, Table 2). Elevated temperatures compared with normal growth temperatures suppress hybrid seedling lethality in many cases (Phillips, 1977; Dhaliwal et al., 1986; Tezuka and Marubashi, 2004; Bomblies et al., 2007; Saito et al., 2007; Jeuken et al., 2009; Deng et al., 2019; Shiragaki et al., 2021; Yan et al., 2021); however, in a certain case, low temperature suppressed hybrid seedling lethality in rice (Chen et al., 2014). Several disease resistance responses are temperature-sensitive and suppressed at high temperatures (Figure 3D) (Samuel, 1931; Erickson et al., 1999; Sawada et al., 2004). Phytochrome B acting as a temperature sensor is biologically active by high temperature and can activate the expression of PHYTOCHROME INTERACTING FACTOR 4, which promotes growth and suppresses defense response (Gangappa et al., 2017). Therefore, it is possible that elevated temperatures may suppress excessive defense response in hybrid seedling lethality through such a mechanism.
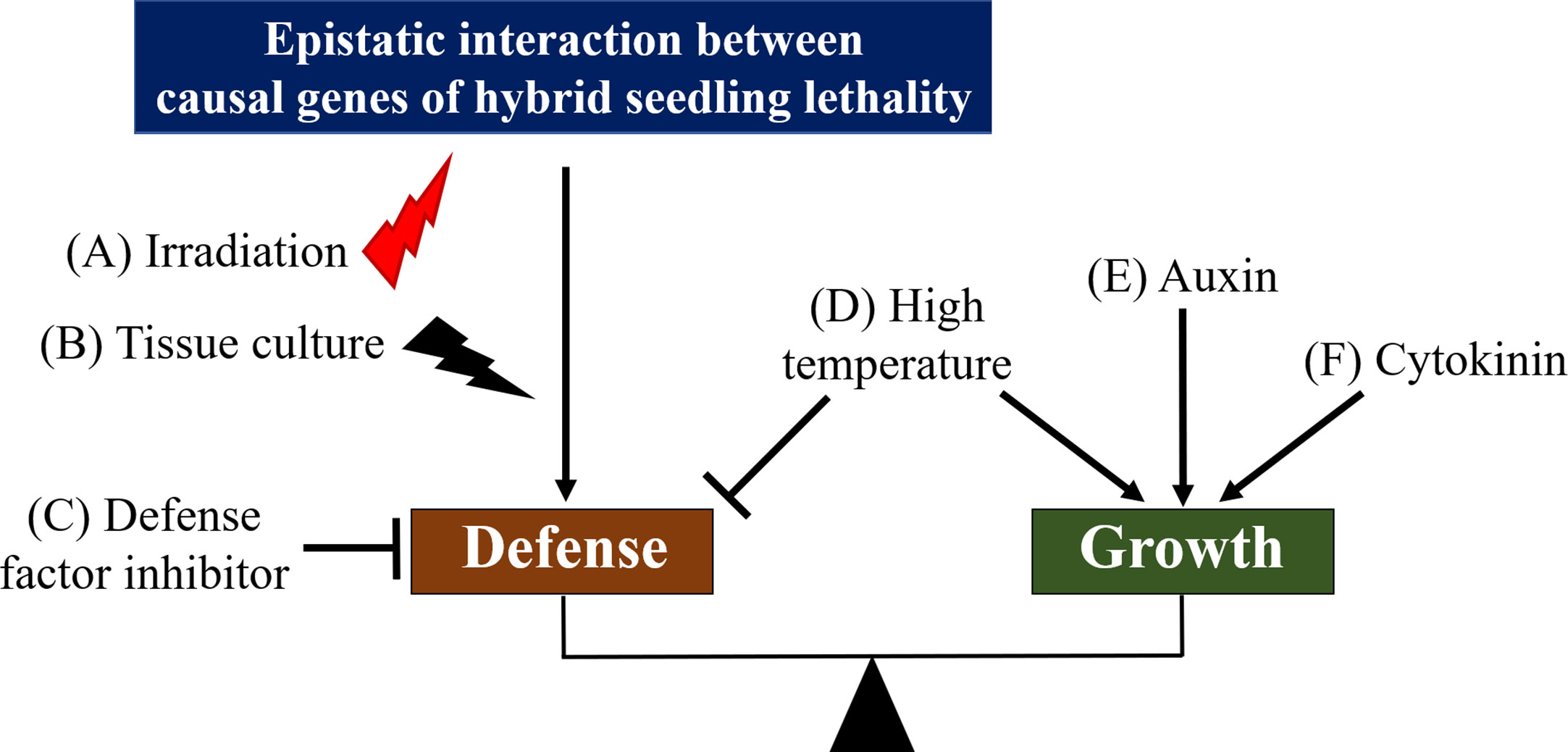
Figure 3 Hypothetical model for the crosstalk between defense and growth pathway in hybrid seedling lethality related to autoimmune response. Known factors or methods to overcome hybrid seedling lethality are shown on the crosstalk diagram. Irradiation (A) and tissue culture (B) can induce mutations or deletions of causal genes for hybrid seedling lethality or important genes in defense response during hybrid seedling lethality. Application of defense factor inhibitors (C), such as those for phenylalanine ammonia-lyase and ethylene biosynthesis, may be effective to suppress hybrid seedling lethality because these suppress defense response. On the other hand, high temperature (D) may suppress defense response and promote seedling growth, and auxin (E) and cytokinin (F) may activate the growth pathway of seedlings. Effective methods to overcome hybrid seed lethality by irradiation and tissue culture are represented by red (potential induction of mutations) and black (induction of mutations) thunderbolt icons, respectively.
The permanent application of auxin (Figure 3E) (Zhou et al., 1991) or temporary application of cytokinin (Figure 3F) (Inoue et al., 1994; Inoue et al., 1997; Kobori et al., 2007) suppresses or overcomes hybrid seedling lethality in Nicotiana, respectively; however, their suppression mechanisms remain unclear. Treatment of hybrid seedlings with an inhibitor of phenylalanine ammonia-lyase, L-2-aminooxy-3-phenylpropionic acid, suppressed hybrid lethality in Nicotiana, most likely via the inactivation of the salicylic acid pathway involved in defense response (Figure 3C) (Shiragaki et al., 2020a). Similarly, treatment of Nicotiana hybrid seedlings with inhibitors of ethylene biosynthesis, amino-oxyacetic acid, and amino-ethoxy vinyl glycine suppressed hybrid seedling lethality via the inactivation of the ethylene pathway involved in defense response (Yamada and Marubashi, 2003). Considering the tight involvement of defense response in hybrid seedling lethality, we predict that auxin and cytokinin activate the growth pathway (Vega et al., 2019), and defense responses in hybrid seedling lethality are suppressed by the crosstalk between growth and defense responses (Figure 3). In hybridizations between Phaseolus vulgaris cultivars, hybrid seedling lethality involving defense responses can be suppressed by application of cytokinin using hydroponic culture as well as by grafting of hybrid seedlings onto the parents as rootstock (Hannah et al., 2007). This may be effective because cytokinins transported from roots of the rootstocks to shoots influence growth of hybrid seedlings (Shii et al., 1981; Reiber and Neuman, 1999b). Additionally, Inoue et al. (1997) suggested other possibilities: cytokinin induces mutations in causal genes for hybrid seedling lethality or enables the screening of variant cells that carry a spontaneous mutation in the causal genes.
Irradiation with γ-rays and ion beams has been used to overcome hybrid seedling lethality (Figure 3A). Viable hybrid seedlings were obtained using irradiated pollen or egg cells of parents in Nicotiana (Shintaku et al., 1988; Shintaku et al., 1989; Kitamura et al., 2003); by irradiation of hybrid seeds in Triticum (Sharma, 1969) and by irradiation of hybrid shoots from the hybridization between Japanese pear and apple (Gonai et al., 2006). The tissue culture method is also effective to overcome hybrid seedling lethality (Figure 3B). Viable hybrid seedlings were obtained via calli after culture of germinated seeds, cotyledons, or leaves in Nicotiana (Ternovskii et al., 1972; Lloyd, 1975; Ternovskii et al., 1976; Iwai et al., 1985; Deverna et al., 1987). In Triticum, hybrid seedling lethality was overcome through regeneration from calli obtained by culture of immature hybrid embryos (Chen et al., 1989). Although mechanisms of overcoming hybrid seedling lethality by irradiation and tissue culture methods are not well understood, these methods may give rise to mutations or deletions of causal genes for hybrid seedling lethality or important genes in defense response during hybrid seedling lethality (Figure 3).
In some hybridization combinations, viable hybrids have been obtained at very low frequency without overcome and circumvent methods (Burk et al., 1979; Tezuka and Marubashi, 2006a; Tezuka et al., 2010; He et al., 2019). In some cases, this can be attributed to the deletion of the entire chromosome or certain regions of the chromosome where causal genes for hybrid lethality are located (Tezuka et al., 2010; Tezuka et al., 2012; Hancock et al., 2015). In hybridization between N. suaveolens and N. tabacum, deletion of the chromosomal region containing a hybrid seedling lethality gene could be caused by reciprocal translocations between homoeologous chromosomes of N. tabacum in viable hybrid seedlings obtained spontaneously very rarely as well as those obtained by tissue-culture (Nakata et al., 2021). Nevertheless, other mechanisms may also be involved in the spontaneous generation of very rare viable hybrid seedlings.
4 Comparison of hybrid lethality in seeds and seedlings
It would be interesting to see whether hybrid lethality in seeds and seedlings can be induced by similar mechanisms. Although much remains unknown about the genetic basis of hybrid lethality, one case shows obvious link between hybrid seed lethality and hybrid seedling lethality, i.e., hybrid lethality associated with duplicate LD1.1 and LD1.5 loci, leading to lack of histidine, as summarized above (Bikard et al., 2009). This indicates that both types of hybrid lethality are induced when the causal genes are involved in both seed and seedling stages.
However, it seems that there are no similarities in hybrid lethality between seeds and seedlings for other mechanisms. Current studies have demonstrated that in many cases, autoimmune responses are involved in hybrid seedling lethality. Nevertheless, such a mechanism has not yet been reported in hybrid seed lethality, although some cases may involve epistatic interaction as explained by BDM model. This might be attributed to the behavior of the autoimmune responses, which usually function in seedlings. The situation is similar for EBN-based hybrid seed lethality; there have been no reports stating that EBN is involved in hybrid seedling lethality. In hybridizations between N. suaveolens and N. tabacum, the use of N. suaveolens accessions with different EBNs did not change the severity of hybrid seedling lethality (He et al., 2019). Parental EBNs directly and indirectly affect endosperm and embryo development, respectively, in hybrid seeds but do not affect hybrid seedling development.
5 Possible methods to overcome or circumvent hybrid seed lethality and hybrid seedling lethality
Although several methods have been developed to overcome or circumvent EBN-based hybrid lethality caused by endosperm defects, fewer effective methods have been reported for overcoming lethality caused by defects in embryo itself. To overcome this situation, it may be possible to apply methods developed for hybrid seedling lethality (Figure 2). Considering that hybrid seedling lethality can be overcome by regeneration from calli formed on explants, it may be possible to overcome hybrid seed lethality through regeneration from calli induced by culture of immature hybrid embryos. In fact, hybrid seed lethality due to epistatic interaction of Eml-A1 and Eml-R1 can be overcome by such a method (Tikhenko et al., 2017b); however, whether this method is effective for other hybridizations remains to be investigated. Additionally, irradiation which causes DNA damage led to mutations may be effective for hybrid seed lethality as in the case of hybrid seedling lethality (Figure 2A). These two methods, which possibly induce new mutations, may also be effective to overcome EBN-based hybrid seed lethality because several reports have indicated that modification of the MEG or PEG expression can partially or completely rescue hybrid seeds from lethality as mentioned above. Fleming and Burrows (2023) discussed the relationships between epigenetics and mutagenesis and indicated that epigenetic modifications require DNA repair pathways for erasure, and oxidative DNA damage can alter and affect gene expression.
Several methods have been developed to overcome or circumvent hybrid seedling lethality related to autoimmune response, some of which can be effective possibly by crosstalk between defense and growth responses (Figure 3). Meanwhile, fewer effective methods have been reported for overcoming lethality caused by mechanisms other than autoimmune response. Hybrid seedling lethality can occur when important genes for life, such as metabolism-related genes, are redundantly copied, leading to generation of loss-of-function alleles at each locus, thereby resulting in hybrid seedlings with the set of loss-of-function alleles. This lethality can be avoided or overcome if additional substances are added to compensate for the loss of function (Bikard et al., 2009). Moreover, if it is difficult to compensate for the loss of function, the alleles at the causal loci can be changed to functional alleles using mutagens, genome editing, or genetic modification. Alternatively, cross breeding can be performed by selecting breeding lines to be free of the loss-of-function alleles.
6 Active application of hybrid lethality to prevent gene flow
In nature, gene flow occurs among cultivars and/or wild and weedy relatives. If hybridization occurs between cultivars or between cultivar and its relatives, genetic purity and characteristic traits of the cultivar is diminished. Furthermore, gene flow from non-transgenic herbicide resistant sorghum (Sorghum bicolor) and sunflower (Helianthus annuus) to weedy relatives is a concrete problem, although two types of strategy, physical containment (such as mesh on greenhouses and isolation distance in the field) and biological containment (such as chloroplast transformation and male sterility), have been proposed to prevent gene or transgene flow (Bozic et al., 2015; Gressel, 2015). The use of hybrid seedling lethality genes has been proposed to prevent genetic contamination (Sato and Inarura, 1989; Yonezawa et al., 1990). To achieve this, the cultivar possessing an epistatic gene which causes hybrid seedling lethality with its counterpart is developed. When the cultivar is pollinated by pollen from other cultivars or its relatives which possess the counterpart gene, hybrid seedlings show hybrid lethality and the inflow of alien genes to the cultivar can be suppressed. In this system, a set of epistatic genes causing F1 hybrid seedling lethality is available, but those causing hybrid breakdown are not useful. Such a system would also be effective to suppress outflow of foreign genes in transgenic plants to cultivars and wild and weedy relatives in the field.
For practical application of this system, sufficient severity of hybrid seedling lethality is required to prevent progeny from surviving (Sato and Inarura, 1989; Yonezawa et al., 1990). Additionally, high stability of hybrid seedling lethality would be required because viable hybrids might emerge at very low frequency as mentioned above in interspecies crosses in Nicotiana. Therefore, use of additional set of epistatic genes causing hybrid seedling lethality might be effective. In Nicotiana, very rare survival hybrids seem unlikely to emerge when two sets of epistatic genes are possibly involved in hybrid seedling lethality (Tezuka and Marubashi, 2012). Additionally, use of other isolation mechanisms may also be effective, although there may also be loopholes in these mechanisms. For example, germinable seeds may be rarely produced even though the hybridization combination almost always produces hybrid seeds showing EBN-based hybrid seed lethality (He et al., 2019). However, combination of hybrid seed lethality and hybrid seedling lethality is expected to suppress gene flow more robustly than each lethality alone.
7 Conclusions and perspectives
Hybrid lethality has been considered to occur in embryos and seedlings after hybridizations between different lineages. However, similarities and differences between hybrid seed lethality and hybrid seedling lethality remain poorly understood. Recent molecular evidences indicated that evolution of genes derived from a common ancestor can lead to deleterious epistatic interaction leading to both hybrid seed lethality and hybrid seedling lethality. Additionally, hybrid seed lethality involves a unique mechanism related to endosperm, whereas hybrid seedling lethality involves a unique mechanism related to autoimmune response. For plant breeding, hybrid lethality is an obstacle which needs to be overcome or circumvented, as well as a valuable resource to prevent gene flow. In this context, it is necessary to identify other genes involved in hybrid lethality and investigate whether other mechanisms are involved in hybrid lethality.
Author contributions
TT proposed the concept. All authors conceived the manuscript. HH and TT wrote the manuscript. KS wrote part of the manuscript regarding methods to overcome hybrid seedling lethality. HH and KS prepared the figures. All authors reviewed and edited the manuscript. All authors have read and agreed to the published version of the manuscript.
Funding
This review was partly supported by JSPS KAKENHI Grant Number JP20K05988 from the Japan Society for the Promotion of Science.
Acknowledgments
We thank Dr. Masanobu Mino (Osaka Metropolitan University) for careful review of the manuscript.
Conflict of interest
The authors declare that the research was conducted in the absence of any commercial or financial relationships that could be construed as a potential conflict of interest.
Publisher’s note
All claims expressed in this article are solely those of the authors and do not necessarily represent those of their affiliated organizations, or those of the publisher, the editors and the reviewers. Any product that may be evaluated in this article, or claim that may be made by its manufacturer, is not guaranteed or endorsed by the publisher.
References
Świadek, M., Proost, S., Sieh, D., Yu, J., Todesco, M., Jorzig, C., et al. (2017). Novel allelic variants in ACD6 cause hybrid necrosis in local collection of Arabidopsis thaliana. New Phytol. 213, 900–915. doi: 10.1111/nph.14155
Bai, F., Settles, A. M. (2015). Imprinting in plants as a mechanism to generate seed phenotypic diversity. Front. Plant Sci. 5. doi: 10.3389/fpls.2014.00780
Balint-Kurti, P. (2019). The plant hypersensitive response: concepts, control and consequences. Mol. Plant Pathol. 20, 1163–1178. doi: 10.1111/mpp.12821
Barone, A., Del Giudice, A., Ng, N. Q. (1992). Barriers to interspecific hybridization between Vigna unguiculata and Vigna vexillata. Sex Plant Reprod. 5, 195–200. doi: 10.1007/BF00189811
Basu, M. M., González-Carranza, Z. H., Azam-Ali, S., Tang, S., Shahid, A. A., Roberts, J. A. (2013). The manipulation of auxin in the abscission zone cells of arabidopsis flowers reveals that indoleacetic acid signaling is a prerequisite for organ shedding. Plant Physiol. 162, 96–106. doi: 10.1104/pp.113.216234
Batista, R. A., Köhler, C. (2020). Genomic imprinting in plants–revisiting existing models. Genes Dev. 34, 24–36. doi: 10.1101/gad.332924.119
Batista, R. A., Moreno-Romero, J., Qiu, Y., Van Boven, J., Santos-González, J., Figueiredo, D. D., et al. (2019). The MADS-box transcription factor PHERES1 controls imprinting in the endosperm by binding to domesticated transposons. eLife 8, e50541. doi: 10.7554/eLife.50541
Bikard, D., Patel, D., Le, M. C., Giorgi, V., Camilleri, C., Bennett, M. J., et al. (2009). Divergent evolution of duplicate genes leads to genetic incompatibilities within A. thaliana. Science 323, 623–626. doi: 10.1126/science.1165917
Bjerkan, K. N., Hornslien, K. S., Johannessen, I. M., Krabberød, A. K., Van Ekelenburg, Y. S., Kalantarian, M., et al. (2020). Genetic variation and temperature affects hybrid barriers during interspecific hybridization. Plant J. 101, 122–140. doi: 10.1111/tpj.14523
Bomblies, K., Lempe, J., Epple, P., Warthmann, N., Lanz, C., Dangl, J. L., et al. (2007). Autoimmune response as a mechanism for a dobzhansky-muller-type incompatibility syndrome in plants. PloS Biol. 5, e236. doi: 10.1371/journal.pbio.0050236
Bomblies, K., Weigel, D. (2007). Hybrid necrosis: autoimmunity as a potential gene-flow barrier in plant species. Nat. Rev. Genet. 8, 382–393. doi: 10.1038/nrg2082
Bozic, D., Pavlovic, D., Bregola, V., Di Loreto, A., Bosi, S., Vrbnicanin, S. (2015). Gene flow from herbicide-resistant sunflower hybrids to weedy sunflower. J. Plant Dis. Prot 122, 183–188. doi: 10.1007/BF03356548
Burk, L. G., Gerstel, D. U., Wernsman, E. A. (1979). Maternal haploids of Nicotiana tabacum l. from seed. Science 206, 585. doi: 10.1126/science.206.4418.585
Bushell, C., Spielman, M., Scott, R. J. (2003). The basis of natural and artificial postzygotic hybridization barriers in Arabidopsis species. Plant Cell 15, 1430–1442. doi: 10.1105/tpc.010496
Chae, E., Bomblies, K., Kim, S. T., Karelina, D., Zaidem, M., Ossowski, S., et al. (2014). Species-wide genetic incompatibility analysis identifies immune genes as hot spots of deleterious epistasis. Cell 159, 1341–1351. doi: 10.1016/j.cell.2014.10.049
Chen, C., Chen, H., Lin, Y. S., Shen, J. B., Shan, J. X., Qi, P., et al. (2014). A two-locus interaction causes interspecific hybrid weakness in rice. Nat. Commun. 5, 3357. doi: 10.1038/ncomms4357
Chen, C., Chen, H., Shan, J. X., Zhu, M. Z., Shi, M., Gao, J. P., et al. (2013). Genetic and physiological analysis of a novel type of interspecific hybrid weakness in rice. Mol. Plant 6, 716–728. doi: 10.1093/mp/sss146
Chen, Z., Evans, D. A., Vasconcelos, A. (1989). Use of tissue culture to bypass wheat hybrid necrosis. Theor. Appl. Genet. 78, 57–60. doi: 10.1007/BF00299753
Christoff, M. (1928). Cytological studies in the genus Nicotiana. Genetics 13, 233–277. doi: 10.1093/genetics/13.3.233
Chu, Y. E., Oka, H. I. (1972). The distribution and effects of genes causing F1 weakness in Oryza breviligulata and O. glaberrima. Genetics 70, 163–173. doi: 10.1093/genetics/70.1.163
Chung, C. S., Nakajima, T., Takeda, G. (1988). Interspecific hybridization between Nicotiana trigonophylla dun. and N. tabacum l. through ovule culture. Jpn J. Breed 38, 319–326. doi: 10.1270/jsbbs1951.38.319
Coughlan, J. M., Wilson Brown, M., Willis, J. H. (2020). Patterns of hybrid seed inviability in the Mimulus guttatus sp. complex reveal a potential role of parental conflict in reproductive isolation. Curr. Biol. 30, 83–93.e85. doi: 10.1016/j.cub.2019.11.023
Crespo-Herrera, L. A., Garkava-Gustavsson, L., Åhman, I. (2017). A systematic review of rye (Secale cereale l.) as a source of resistance to pathogens and pests in wheat (Triticum aestivum l.). Hereditas 154, 14. doi: 10.1186/s41065-017-0033-5
Day, P. R. (1958). Autogenous necrosis in the tomato. Plant Pathol. 7, 57–58. doi: 10.1111/j.1365-3059.1958.tb00826.x
Deng, J., Fang, L., Zhu, X., Zhou, B., Zhang, T. (2019). A CC-NBS-LRR gene induces hybrid lethality in cotton. J. Exp. Bot. 70, 5145–5156. doi: 10.1093/jxb/erz312
Deverna, J. W., Myers, J. R., Collins, G. B. (1987). Bypassing prefertilization barriers to hybridization in Nicotiana using in vitro pollination and fertilization. Theor. Appl. Genet. 73, 665–671. doi: 10.1007/BF00260773
Dhaliwal, H. S., Sharma, S. K., Randhawa, A. S. (1986). How to overcome hybrid necrosis in wheat? Wheat Inf. Service 61-62, 27–28.
Dilkes, B. P., Spielman, M., Weizbauer, R., Watson, B., Burkart-Waco, D., Scott, R. J., et al. (2008). The maternally expressed WRKY transcription factor TTG2 controls lethality in interploidy crosses of Arabidopsis. PloS Biol. 6, 2707–2720. doi: 10.1371/journal.pbio.0060308
Din, S., Wani, R. A., Nisar, F., Farwah, S., Rizvi, S., Wani, T. F., et al. (2019). Fruit set and development: pre-requisites and enhancement in temperate fruit crops. J. Pharmacogn Phytochem. 8, 1203–1216.
Doughty, J., Aljabri, M., Scott, R. J. (2014). Flavonoids and the regulation of seed size in Arabidopsis. Biochem. Soc. Trans. 42, 364–369. doi: 10.1042/bst20140040
Dziasek, K., Simon, L., Lafon-Placette, C., Laenen, B., Wärdig, C., Santos-González, J., et al. (2021). Hybrid seed incompatibility in Capsella is connected to chromatin condensation defects in the endosperm. PloS Genet. 17, e1009370. doi: 10.1371/journal.pgen.1009370
Erdmann, R. M., Satyaki, P. R. V., Klosinska, M., Gehring, M. (2017). A small RNA pathway mediates allelic dosage in endosperm. Cell Rep. 21, 3364–3372. doi: 10.1016/j.celrep.2017.11.078
Erickson, F. L., Holzberg, S., Calderon-Urrea, A., Handley, V., Axtell, M., Corr, C., et al. (1999). The helicase domain of the TMV replicase proteins induces the N-mediated defence response in tobacco. Plant J. 18, 67–75. doi: 10.1046/j.1365-313x.1999.00426.x
Fernandez, A.-M. A., Tanisaka, T., Nakazaki, T., Lkehashi, H. (1998). Efficient hybridization between Lilium × elegance and L. longlflorum through in vitro ovary slice culture. Breed Sci. 48, 71–75. doi: 10.1270/jsbbs1951.48.71
Figueiredo, D. D., Kohler, C. (2018). Auxin: a molecular trigger of seed development. Genes Dev. 32, 479–490. doi: 10.1101/gad.312546.118
Fleming, A. M., Burrows, C. J. (2023). DNA Modifications walk a fine line between epigenetics and mutagenesis. Nat. Rev. Mol. Cell Biol. 24, 449–450. doi: 10.1038/s41580-023-00590-2
Floyd, S. K., Friedman, W. E. (2000). Evolution of endosperm developmental patterns among basal flowering plants. Int. J. Plant Sci. 161, S57–S81. doi: 10.1086/317579
Fukuoka, S., Namai, H., Okuno, K. (1998). Geographical variation of the genes controlling hybrid breakdown and genetic differentiation of the chromosomal regions harboring these genes in Asian cultivated rice, Oryza sativa l. Genes Genet. Syst. 73, 211–217. doi: 10.1266/ggs.73.211
Gangappa, S. N., Berriri, S., Kumar, S. V. (2017). PIF4 coordinates thermosensory growth and immunity in Arabidopsis. Curr. Biol. 27, 243–249. doi: 10.1016/j.cub.2016.11.012
Garcia, D., Fitz Gerald, J. N., Berger, F. (2005). Maternal control of integument cell elongation and zygotic control of endosperm growth are coordinated to determine seed size in arabidopsis. Plant Cell 17, 52–60. doi: 10.1105/tpc.104.027136
Garner, A. G., Kenney, A. M., Fishman, L., Sweigart, A. L. (2016). Genetic loci with parent-of-origin effects cause hybrid seed lethality in crosses between Mimulus species. New Phytol. 211, 319–331. doi: 10.1111/nph.13897
Gepts, P., Bliss, F. A. (1985). F1 hybrid weakness in the common bean: differential geographic origin suggets two gene pools in cultivated bean germplasm. J. Hered 76, 447–450. doi: 10.1093/oxfordjournals.jhered.a110142
Gerstel, D. U. (1954). A new lethal combination in interspecific cotton hybrids. Genetics 39, 628–639. doi: 10.1093/genetics/39.5.628
Gonai, T., Manabe, T., Inoue, E., Hayashi, M., Yamamoto, T., Hayashi, T., et al. (2006). Overcoming hybrid lethality in a cross between Japanese pear and apple using gamma irradiation and confirmation of hybrid status using flow cytometry and SSR markers. Sci. Hortic. 109, 43–47. doi: 10.1016/j.scienta.2006.02.026
Gressel, J. (2015). Dealing with transgene flow of crop protection traits from crops to their relatives. Pest Manag Sci. 71, 658–667. doi: 10.1002/ps.3850
Gupta, S., Buirchell, B., Cowling, W. (1996). Interspecific reproductive barriers and genomic similarity among the rough-seeded Lupinus species. Plant Breed 115, 123–127. doi: 10.1111/j.1439-0523.1996.tb00886.x
Haig, D., Westoby, M. (1989). Parent-specific gene expression and the triploid endosperm. Am. Nat. 134, 147–155. doi: 10.1086/284971
Haig, D., Westoby, M. (1991). Genomic imprinting in endosperm: its effect on seed development in crosses between species, and between different ploidies of the same species, and its implications for the evolution of apomixis. Philos. Trans. R Soc. Lond B 333, 1–13. doi: 10.1098/rstb.1991.0057
Hancock, W. G., Kuraparthy, V., Kernodle, S. P., Lewis, R. S. (2015). Identification of maternal haploids of Nicotiana tabacum aided by transgenic expression of green fluorescent protein: evidence for chromosome elimination in the N. tabacum × N. africana interspecific cross. Mol. Breed 35, 179. doi: 10.1007/s11032-015-0372-8
Hannah, M. A., Krämer, K. M., Geffroy, V., Kopka, J., Blair, M. W., Erban, A., et al. (2007). Hybrid weakness controlled by the dosage-dependent lethal (DL) gene system in common bean (Phaseolus vulgaris) is caused by a shoot-derived inhibitory signal leading to salicylic acid-associated root death. New Phytol. 176, 537–549. doi: 10.1111/j.1469-8137.2007.02215.x
He, H., Iizuka, T., Maekawa, M., Sadahisa, K., Morikawa, T., Yanase, M., et al. (2019). Nicotiana suaveolens accessions with different ploidy levels exhibit different reproductive isolation mechanisms in interspecific crosses with Nicotiana tabacum. J. Plant Res. 132, 461–471. doi: 10.1007/s10265-019-01114-w
He, H., Sadahisa, K., Yokoi, S., Tezuka, T. (2022). Parental genome imbalance causes hybrid seed lethality as well as ovary abscission in interspecific and interploidy crosses in Nicotiana. Front. Plant Sci. 13. doi: 10.3389/fpls.2022.899206
He, H., Yokoi, S., Tezuka, T. (2020). A high maternal genome excess causes severe seed abortion leading to ovary abscission in Nicotiana interploidy-interspecific crosses. Plant Direct 4, e00257. doi: 10.1002/pld3.257
Hermsen, J. (1963a). The genetic basis of hybrid necrosis in wheat. Genetica 33, 245–287. doi: 10.1007/BF01725765
Hermsen, J. (1963b). Hybrid necrosis as a problem for the wheat breeder. Euphytica 12, 1–16. doi: 10.1007/BF00033587
Hirose, T., Nishi, S., Takashima, S. (1960). Studies on the interspecies crossing in cultivated Capsicum. i. crossability. Sci. Rep. Kyoto Prefectural University. Agric. 12, 40–46. (in Japanese with English summary).
Hollingshead, L. (1930). A lethal factor in Crepis effective only in an interspecific hybrid. Genetics 15, 114–140. doi: 10.1093/genetics/15.2.114
Hu, Y., Xue, Y., Liu, J., Fang, Z., Yang, L., Zhang, Y., et al. (2016). Hybrid lethality caused by two complementary dominant genes in cabbage (Brassica oleracea l.). Mol. Breed 36, 73. doi: 10.1007/s11032-016-0498-3
Huang, J., Yang, L., Yang, L., Wu, X., Cui, X., Zhang, L., et al. (2023). Stigma receptors control intraspecies and interspecies barriers in brassicaceae. Nature 614, 303–308. doi: 10.1038/s41586-022-05640-x
Huang, F., Zhu, Q. H., Zhu, A., Wu, X., Xie, L., Wu, X., et al. (2017). Mutants in the imprinted PICKLE RELATED 2 gene suppress seed abortion of fertilization independent seed class mutants and paternal excess interploidy crosses in arabidopsis. Plant J. 90, 383–395. doi: 10.1111/tpj.13500
Huc, J., Dziasek, K., Pachamuthu, K., Woh, T., Kohler, C., Borges, F. (2022). Bypassing reproductive barriers in hybrid seeds using chemically induced epimutagenesis. Plant Cell 34, 989–1001. doi: 10.1093/plcell/koab284
Ichitani, K., Taura, S., Tezuka, T., Okiyama, Y., Kuboyama, T. (2011). Chromosomal location of HWA1 and HWA2, complementary hybrid weakness genes in rice. Rice 4, 29–38. doi: 10.1007/s12284-011-9062-2
Iizuka, T., Kuboyama, T., Marubashi, W., Oda, M., Tezuka, T. (2012). Nicotiana debneyi has a single dominant gene causing hybrid lethality in crosses with N. tabacum. Euphytica 186, 321–328. doi: 10.1007/s10681-011-0570-3
İltaş, Ö., Svitok, M., Cornille, A., Schmickl, R., Lafon Placette, C. (2021). Early evolution of reproductive isolation: a case of weak inbreeder/strong outbreeder leads to an intraspecific hybridization barrier in Arabidopsis lyrata. Evolution 75, 1466–1476. doi: 10.1111/evo.14240
Inai, S., Ishikawa, K., Nunomura, O., Ikehashi, H. (1993). Genetic analysis of stunted growth by nuclear-cytoplasmic interaction in interspecific hybrids of Capsicum by using RAPD markers. Theor. Appl. Genet. 87, 416–422. doi: 10.1007/BF00215086
Inoue, E., Marubashi, W., Niwa, M. (1994). Simple method for overcoming the lethality observed in the hybrid between Nicotiana suaveolens and N. tabacum. Breed Sci. 44, 333–336. doi: 10.1270/jsbbs1951.44.333
Inoue, E., Marubashi, W., Niwa, M. (1997). Improvement of the method for overcoming the hybrid lethality between Nicotiana suaveolens and N. tabacum by culture of F1 seeds in liquid media containing cytokinins. Breed Sci. 47, 211–216. doi: 10.1270/jsbbs1951.47.211
Ishikawa, R., Ohnishi, T., Kinoshita, Y., Eiguchi, M., Kurata, N., Kinoshita, T. (2011). Rice interspecies hybrids show precocious or delayed developmental transitions in the endosperm without change to the rate of syncytial nuclear division. Plant J. 65, 798–806. doi: 10.1111/j.1365-313X.2010.04466.x
Iwai, S., Kishi, C., Nakata, K., Kawashima, N. (1986). Production of Nicotiana tabacum × Nicotiana acuminata hybrid by ovule culture. Plant Cell Rep. 5, 403–404. doi: 10.1007/BF00268613
Iwai, S., Kishi, C., Nakata, K., Kubo, S. (1985). Production of a hybrid of Nicotiana repanda willd. × N. tabacum l. by ovule culture. Plant Sci. 41, 175–178. doi: 10.1016/0168-9452(85)90085-8
Jeuken, M. J. W., Zhang, N. W., Mchale, L. K., Pelgrom, K., Den, B. E., Lindhout, P., et al. (2009). Rin4 causes hybrid necrosis and race-specific resistance in an interspecific lettuce hybrid. Plant Cell 21, 3368–3378. doi: 10.1105/tpc.109.070334
Jiang, H., Moreno-Romero, J., Santos-González, J., De Jaeger, G., Gevaert, K., Van De Slijke, E., et al. (2017). Ectopic application of the repressive histone modification H3K9me2 establishes post-zygotic reproductive isolation in Arabidopsis thaliana. Genes Dev. 31, 1272–1287. doi: 10.1101/gad.299347.117
Johnson, N. A. (2002). Sixty years after “Isolating mechanisms, evolution and temperature”: muller’s legacy. Genetics 161, 939–944. doi: 10.1093/genetics/161.3.939
Johnson, N. A., Porter, A. H. (2000). Rapid speciation via parallel, directional selection on regulatory genetic pathways. J. Theor. Biol. 205, 527–542. doi: 10.1006/jtbi.2000.2070
Johnston, S. A., Den Nijs, T. P., Peloquin, S. J., Hanneman, R. E., Jr. (1980). The significance of genic balance to endosperm development in interspecific crosses. Theor. Appl. Genet. 57, 5–9. doi: 10.1007/bf00276002
Johnston, S. A., Hanneman, R. E., Jr. (1982). Manipulations of endosperm balance number overcome crossing barriers between diploid solanum species. Science 217, 446–448. doi: 10.1126/science.217.4558.446
Josefsson, C., Dilkes, B., Comai, L. (2006). Parent-dependent loss of gene silencing during interspecies hybridization. Curr. Biol. 16, 1322–1328. doi: 10.1016/j.cub.2006.05.045
Kadota, Y., Shirasu, K. (2012). The HSP90 complex of plants. Biochim. Biophys. Acta 1823, 689–697. doi: 10.1016/j.bbamcr.2011.09.016
Kadota, Y., Shirasu, K., Guerois, R. (2010). NLR sensors meet at the SGT1-HSP90 crossroad. Trends Biochem. Sci. 35, 199–207. doi: 10.1016/j.tibs.2009.12.005
Katsuyama, Y., Doi, M., Shioya, S., Hane, S., Yoshioka, M., Date, S., et al. (2021). The role of chaperone complex HSP90-SGT1-RAR1 as the associated machinery for hybrid inviability between Nicotiana gossei domin and N. tabacum l. Gene 776, 145443. doi: 10.1016/j.gene.2021.145443
Kawaguchi, K., Ohya, Y., Maekawa, M., Iizuka, T., Hasegawa, A., Shiragaki, K., et al. (2021). Two Nicotiana occidentalis accessions enable gene identification for type II hybrid lethality by the cross to N. sylvestris. Sci. Rep. 11, 17093. doi: 10.1038/s41598-021-96482-6
Kitamura, S., Inoue, M., Ohmido, N., Fukui, K., Tanaka, A. (2003). Chromosomal rearrangements in interspecific hybrids between Nicotiana gossei domin and N. tabacum l., obtained by crossing with pollen exposed to helium ion beams or gamma-rays. Nucl. Instrum Methods Phys. Res. B 206, 548–552. doi: 10.1016/S0168-583X(03)00818-8
Kobori, S., Masuda, Y., Horii, M., Marubashi, W. (2007). High levels of the cytokinin BAP suppress programmed cell death in hybrid tobacco cells (Nicotiana suaveolens × n. tabacum) expressing hybrid lethality. Plant Biotech. 24, 375–381. doi: 10.5511/plantbiotechnology.24.375
Köhler, C., Mittelsten Scheid, O., Erilova, A. (2010). The impact of the triploid block on the origin and evolution of polyploid plants. Trends Genet. 26, 142–148. doi: 10.1016/j.tig.2009.12.006
Koide, Y., Ogino, A., Yoshikawa, T., Kitashima, Y., Saito, N., Kanaoka, Y., et al. (2018). Lineage-specific gene acquisition or loss is involved in interspecific hybrid sterility in rice. Proc. Natl. Acad. Sci. U.S.A. 115, E1955–E1962. doi: 10.1073/pnas.1711656115
Koinange, E. M. K., Gepts, P. (1992). Hybrid weakness in wild Phaseolus vulgaris l. J. Hered 83, 135–139. doi: 10.1093/oxfordjournals.jhered.a111173
Kostoff, D. (1930). Ontogeny, genetics, and cytology of Nicotiana hybrids. Genetica 12, 33–139. doi: 10.1007/BF01508072
Kradolfer, D., Hennig, L., Köhler, C. (2013a). Increased maternal genome dosage bypasses the requirement of the FIS polycomb repressive complex 2 in arabidopsis seed development. PloS Genet. 9, e1003163. doi: 10.1371/journal.pgen.1003163
Kradolfer, D., Wolff, P., Jiang, H., Siretskiy, A., Köhler, C. (2013b). An imprinted gene underlies postzygotic reproductive isolation in Arabidopsis thaliana. Dev. Cell 26, 525–535. doi: 10.1016/j.devcel.2013.08.006
Kubo, T., Yoshimura, A. (2005). Epistasis underlying female sterility detected in hybrid breakdown in a japonica-indica cross of rice (Oryza sativa l.). Theor. Appl. Genet. 110, 346–355. doi: 10.1007/s00122-004-1846-y
Kubo, T., Yoshimura, A., Kurata, N. (2022). Loss of OsEAF6, a subunit of the histone acetyltransferase complex, causes hybrid breakdown in intersubspecific rice crosses. Front. Plant Sci. 13. doi: 10.3389/fpls.2022.866404
Kulmuni, J., Butlin, R. K., Lucek, K., Savolainen, V., Westram, A. M. (2020). Towards the completion of speciation: the evolution of reproductive isolation beyond the first barriers. Philos. Trans. R Soc. Lond B Biol. Sci. 375, 20190528. doi: 10.1098/rstb.2019.0528
Lafon-Placette, C., Hatorangan, M. R., Steige, K. A., Cornille, A., Lascoux, M., Slotte, T., et al. (2018). Paternally expressed imprinted genes associate with hybridization barriers in Capsella. Nat. Plants 4, 352–357. doi: 10.1038/s41477-018-0161-6
Lafon-Placette, C., Johannessen, I. M., Hornslien, K. S., Ali, M. F., Bjerkan, K. N., Bramsiepe, J., et al. (2017). Endosperm-based hybridization barriers explain the pattern of gene flow between Arabidopsis lyrata and Arabidopsis arenosa in central Europe. Proc. Natl. Acad. Sci. U.S.A. 114, E1027–E1035. doi: 10.1073/pnas.1615123114
Lauria, M., Rupe, M., Guo, M., Kranz, E., Pirona, R., Viotti, A., et al. (2004). Extensive maternal DNA hypomethylation in the endosperm of Zea mays. Plant Cell 16, 510–522. doi: 10.1105/tpc.017780
Lee, J. A. (1981). Genetics of D3 complementary lethality in Gossypium hirsutum and G. barbadense. J. Hered 72, 299–300. doi: 10.1093/oxfordjournals.jhered.a109510
Lee, C. B., Page, L. E., Mcclure, B. A., Holtsford, T. P. (2008). Post-pollination hybridization barriers in Nicotiana section Alatae. Sex Plant Reprod. 21, 183–195. doi: 10.1007/s00497-008-0077-9
Li, Z., Mao, C., Wu, X., Zhou, H., Zhao, K., Jiang, J., et al. (2023). Hybrid weakness and continuous flowering caused by compound expression of FTLs in Chrysanthemum morifolium × Leucanthemum paludosum intergeneric hybridization. Front. Plant Sci. 14. doi: 10.3389/fpls.2023.1120820
Li, Z., Pinson, S. R., Paterson, A. H., Park, W. D., Stansel, J. W. (1997). Genetics of hybrid sterility and hybrid breakdown in an intersubspecific rice (Oryza sativa l.) population. Genetics 145, 1139–1148. doi: 10.1093/genetics/145.4.1139
Li, J., Zhou, J., Zhang, Y., Yang, Y., Pu, Q., Tao, D. (2020). New insights into the nature of interspecific hybrid sterility in rice. Front. Plant Sci. 11. doi: 10.3389/fpls.2020.555572
Liang, Y., Jiang, C., Liu, Y., Gao, Y., Lu, J., Aiwaili, P., et al. (2020). Auxin regulates sucrose transport to repress petal abscission in rose (Rosa hybrida). Plant Cell 32, 3485–3499. doi: 10.1105/tpc.19.00695
Lloyd, R. (1975). Tissue culture as a means of circumventing lethality in an interspecific Nicotiana hybrid. Tob Sci. 19, 4–6.
Ma, J., Hancock, W. G., Nifong, J. M., Kernodle, S. P., Lewis, R. S. (2020). Identification and editing of a hybrid lethality gene expands the range of interspecific hybridization potential in Nicotiana. Theor. Appl. Genet. 133, 2915–2925. doi: 10.1007/s00122-020-03641-w
Mahouachi, J., Iglesias, D. J., Agustí, M., Talon, M. (2009). Delay of early fruitlet abscission by branch girdling in citrus coincides with previous increases in carbohydrate and gibberellin concentrations. Plant Growth Regul. 58, 15–23. doi: 10.1007/s10725-008-9348-6
Mallikarjuna, N. (1999). Ovule and embryo culture to obtain hybrids from interspecific incompatible pollinations in chickpea. Euphytica 110, 1–6. doi: 10.1023/A:1003621908663
Malloch, W. S., Malloch, F. W. (1924). Species crosses in Nicotiana, with particular reference to N. longiflora × N. tabacum, N. longiflora × N. sanderae, N. tabacum × N. glauca. Genetics 9, 261–291. doi: 10.1093/genetics/9.3.261
Mao, Y., Gabel, A., Nakel, T., Viehöver, P., Baum, T., Tekleyohans, D. G., et al. (2020). Selective egg cell polyspermy bypasses the triploid block. Elife 9, e52976. doi: 10.7554/eLife.52976
Martinez, G., Wolff, P., Wang, Z., Moreno-Romero, J., Santos-González, J., Conze, L. L., et al. (2018). Paternal easiRNAs regulate parental genome dosage in Arabidopsis. Nat. Genet. 50, 193–198. doi: 10.1038/s41588-017-0033-4
Marubashi, W., Onosato, K. (2002). Q chromosome controls the lethality of interspecific hybrids between Nicotiana tabacum and N. suaveolens. Breed Sci. 52, 137–142. doi: 10.1270/jsbbs.52.137
Masuda, Y., Yamada, T., Kuboyama, T., Marubashi, W. (2007). Identification and characterization of genes involved in hybrid lethality in hybrid tobacco cells (Nicotiana suaveolens × N. tabacum) using suppression subtractive hybridization. Plant Cell Rep. 26, 1595–1604. doi: 10.1007/s00299-007-0352-5
Matsubara, K. (2020). How hybrid breakdown can be handled in rice crossbreeding? Front. Plant Sci. 11. doi: 10.3389/fpls.2020.575412
McCray, F. A. (1933). Embryo development in Nicotiana species hybrids. Genetics 18, 95–110. doi: 10.1093/genetics/18.2.95
Mcnaughton, I. H., Harper, J. L. (1960). The comparative biology of closely related species living in the same area. II. aberrant morphology and a virus-like syndrome in hybrids between Papaver rhoeas l. and P. dubium l. New Phytol. 59, 27–41. doi: 10.1111/j.1469-8137.1960.tb06198.x
Mino, M., Abe, S., Suzuki, T., Yokoyama, H., Kaminaka, H., Ogawa, K., et al. (2004). Hydrogen peroxide functions as a cell death signal for hybrid lethality in the F1 of Nicotiana gossei × N. tabacum. Plant Sci. 167, 267–274. doi: 10.1016/j.plantsci.2004.03.028
Mino, M., Kubota, M., Nogi, T., Zhang, S., Inoue, M. (2007a). Hybrid lethality in interspecific F1 hybrid Nicotiana gossei × N. tabacum involves a MAP-kinases signalling cascade. Plant Biol. 9, 366–373. doi: 10.1055/s-2006-924725
Mino, M., Maekawa, K., Ogawa, K., Yamagishi, H., Inoue, M. (2002). Cell death process during expression of hybrid lethality in interspecific F1 hybrid between Nicotiana gossei domin and Nicotiana tabacum. Plant Physiol. 130, 1776–1787. doi: 10.1104/pp.006023
Mino, M., Misaka, Y., Ueda, J., Ogawa, K., Inoue, M. (2005). Hybrid lethality of cultured cells of an interspecific F1 hybrid of Nicotiana gossei domin and N. tabacum l. Plant Cell Rep. 24, 179–188. doi: 10.1007/s00299-005-0923-2
Mino, M., Murata, N., Date, S., Inoue, M. (2007b). Cell death in seedlings of the interspecific hybrid of Nicotiana gossei and N. tabacum; possible role of knob-like bodies formed on tonoplast in vacuolar-collapse-mediated cell death. Plant Cell Rep. 26, 407–419. doi: 10.1007/s00299-006-0261-z
Mino, M., Tezuka, T., Shomura, S. (2022). The hybrid lethality of interspecific F1 hybrids of Nicotiana: a clue to understanding hybrid inviability–a major obstacle to wide hybridization and introgression breeding of plants. Mol. Breed 42, 10. doi: 10.1007/s11032-022-01279-8
Mizuno, N., Hosogi, N., Park, P., Takumi, S. (2010). Hypersensitive response-like reaction is associated with hybrid necrosis in interspecific crosses between tetraploid wheat and Aegilops tauschii coss. PloS One 5, e11326. doi: 10.1371/journal.pone.0011326
Mok, D. W., Mok, M. C., Rabakoarihanta, A. (1978). Interspecific hybridization of Phaseolus vulgaris with P. lunatus and P. acutifolius. Theor. Appl. Genet. 52, 209–215. doi: 10.1007/bf00273891
Nadamitsu, S., Andoh, Y., Kond, K., Segawa, M. (1986). Interspecific hybrids between Camellia vietnamensis and C. chrysantha by cotyledon culture. Jpn J. Breed 36, 309–313. doi: 10.1270/jsbbs1951.36.309
Nadir, S., Khan, S., Zhu, Q., Henry, D., Wei, L., Lee, D. S., et al. (2018). An overview on reproductive isolation in Oryza sativa complex. AoB Plants 10, ply060. doi: 10.1093/aobpla/ply060
Nadir, S., Li, W., Zhu, Q., Khan, S., Zhang, X. L., Zhang, H., et al. (2019). A novel discovery of a long terminal repeat retrotransposon-induced hybrid weakness in rice. J. Exp. Bot. 70, 1197–1207. doi: 10.1093/jxb/ery442
Nakata, K., Nagashima, H., Inaba, N., Yamashita, H., Shinozaki, Y., Kanekatsu, M., et al. (2021). Analysis of the possible cytogenetic mechanism for overcoming hybrid lethality in an interspecific cross between Nicotiana suaveolens and Nicotiana tabacum. Sci. Rep. 11, 7812. doi: 10.1038/s41598-021-87242-7
Nuñez-Palenius, H. G., Klee, H. J., Cantliffe, D. J. (2006). Embryo-rescue culture of the ‘Galia’ muskmelon (Cucumis melo l. var. reticulatus ser.) male parental line. Plant Cell Tiss Org Cult 85, 345–352. doi: 10.1007/s11240-006-9086-2
Oka, H. I. (1957). Phylogenetic differentiation of cultivated rice. XV. complementary lethal genes in rice. Jpn J. Genet. 32, 83–87. doi: 10.1266/jjg.32.83
Oneal, E., Willis, J. H., Franks, R. G. (2016). Disruption of endosperm development is a major cause of hybrid seed inviability between Mimulus guttatus and Mimulus nudatus. New Phytol. 210, 1107–1120. doi: 10.1111/nph.13842
Phillips, L. L. (1977). Interspecific incompatibility in Gossypium. IV. temperature-conditional lethality in hybrids of G. klotzschianum. Am. J. Bot. 64, 914–915. doi: 10.1002/j.1537-2197.1977.tb11935.x
Pickersgill, B. (1971). Relationships between weedy and cultivated forms in some species of chili peppers (genus Capsicum). Evolution 25, 683–691. doi: 10.1111/j.1558-5646.1971.tb01926.x
Plötner, B., Nurmi, M., Fischer, A., Watanabe, M., Schneeberger, K., Holm, S., et al. (2017). Chlorosis caused by two recessively interacting genes reveals a role of RNA helicase in hybrid breakdown in Arabidopsis thaliana. Plant J. 91, 251–262. doi: 10.1111/tpj.13560
Polgári, D., Cseh, A., Szakács, É., Jäger, K., Molnár-Láng, M., Sági, L. (2014). High-frequency generation and characterization of intergeneric hybrids and haploids from new wheat-barley crosses. Plant Cell Rep. 33, 1323–1331. doi: 10.1007/s00299-014-1618-3
Raghavan, V. (1966). Nutrition, growth and morphogenesis of plant embryos. Biol. Rev. 41, 1–54. doi: 10.1111/j.1469-185X.1966.tb01536.x
Ramsey, J., Bradshaw, H. D., Jr., Schemske, D. W. (2003). Components of reproductive isolation between the monkeyflowers Mimulus lewisii and M. cardinalis (Phrymaceae). Evolution 57, 1520–1534. doi: 10.1111/j.0014-3820.2003.tb00360.x
Rebernig, C. A., Lafon-Placette, C., Hatorangan, M. R., Slotte, T., Köhler, C. (2015). Non-reciprocal interspecies hybridization barriers in the Capsella genus are established in the endosperm. PloS Genet. 11, e1005295. doi: 10.1371/journal.pgen.1005295
Reed, S. M., Collins, G. B. (1978). Interspecific hybrids in Nicotiana through in vitro culture of fertilized ovules: N. stocktonii × N. tabacum; N. nesophila × N. tabacum; N. repanda × N. tabacum. J. Hered 69, 311–315. doi: 10.1093/oxfordjournals.jhered.a108956
Reiber, J. M., Neuman, D. S. (1999a). Hybrid weakness in Phaseolus vulgaris l. i. disruption of development and hormonal allocation. J. Plant Growth Regul. 18, 101–106. doi: 10.1007/PL00007056
Reiber, J. M., Neuman, D. S. (1999b). Hybrid weakness in Phaseolus vulgaris. II. disruption of root-shoot integration. J. Plant Growth Regul. 18, 107–112. doi: 10.1007/PL00007057
Rieseberg, L. H., Blackman, B. K. (2010). Speciation genes in plants. Ann. Bot. 106, 439–455. doi: 10.1093/aob/mcq126
Rieseberg, L. H., Willis, J. H. (2007). Plant speciation. Science 317, 910–914. doi: 10.1126/science.1137729
Roth, M., Florez-Rueda, A. M., Griesser, S., Paris, M., Städler, T. (2018). Incidence and developmental timing of endosperm failure in post-zygotic isolation between wild tomato lineages. Ann. Bot. 121, 107–118. doi: 10.1093/aob/mcx133
Saito, T., Ichitani, K., Suzuki, T., Marubashi, W., Kuboyama, T. (2007). Developmental observation and high temperature rescue from hybrid weakness in a cross between Japanese rice cultivars and Peruvian rice cultivar ‘Jamaica’. Breed Sci. 57, 281–288. doi: 10.1270/jsbbs.57.281
Salguero-Linares, J., Coll, N. S. (2019). Plant proteases in the control of the hypersensitive response. J. Exp. Bot. 70, 2087–2095. doi: 10.1093/jxb/erz030
Samuel, G. (1931). Some experiments on inoculating methods with plant viruses, and on local lesions. Ann. Appl. Biol. 18, 494–507. doi: 10.1111/j.1744-7348.1931.tb02320.x
Sato, Y., Inarura, T. (1989). An attempt for prevention of spreading gene pollution by natural hybridization in rice. Jpn J. Breed 39, 389–394. doi: 10.1270/jsbbs1951.39.389. in Japanese with English summary.
Savolainen, V., Anstett, M. C., Lexer, C., Hutton, I., Clarkson, J. J., Norup, M. V., et al. (2006). Sympatric speciation in palms on an oceanic island. Nature 441, 210–213. doi: 10.1038/nature04566
Sawada, H., Takeuchi, S., Hamada, H., Kiba, A., Matsumoto, M., Hikichi, Y. (2004). A new tobamovirus-resistance gene, L1a, of sweet pepper (Capsicum annuum l.). J. Jpn Soci Hortic. Sci. 73, 552–557. doi: 10.2503/jjshs.73.552
Sawant, A. C. (1956). Semilethal complementary factors in a tomato species hybrid. Evolution 10, 93–96. doi: 10.2307/2406100
Sawant, A. C. (1958). Cytogenetics of interspecific hybrids, Lycopersicon esculentum mill. × L. hirsutum humb. and bonpl. Genetics 43, 502–514. doi: 10.1093/genetics/43.3.502
Sawicki, M., Aït Barka, E., Clément, C., Vaillant-Gaveau, N., Jacquard, C. (2015). Cross-talk between environmental stresses and plant metabolism during reproductive organ abscission. J. Exp. Bot. 66, 1707–1719. doi: 10.1093/jxb/eru533
Sax, K. (1921). Sterility in wheat hybrids. i. sterility relationships and endosperm development. Genetics 6, 399–416. doi: 10.1093/genetics/6.4.399
Schatlowski, N., Köhler, C. (2012). Tearing down barriers: understanding the molecular mechanisms of interploidy hybridizations. J. Exp. Bot. 63, 6059–6067. doi: 10.1093/jxb/ers288
Scott, R. J., Spielman, M., Bailey, J., Dickinson, H. G. (1998). Parent-of-origin effects on seed development in Arabidopsis thaliana. Development 125, 3329–3341. doi: 10.1242/dev.125.17.3329
Scott, R. J., Tratt, J. L., Bolbol, A. (2013). “Seed development in interploidy hybrids,” in Polyploid and hybrid genomics. Eds. Chen, Z. J., Birchler, J. A. (Oxford, UK: John Wiley & Sons, Inc), 271–290.
Sehgal, C., Gifford, J. E. (1979). Developmental and histochemical studies of the ovules of Nicotiana rustica l. Bot. Gaz 140, 180–188. doi: 10.1086/337074
Sekine, D., Ohnishi, T., Furuumi, H., Ono, A., Yamada, T., Kurata, N., et al. (2013). Dissection of two major components of the post-zygotic hybridization barrier in rice endosperm. Plant J. 76, 792–799. doi: 10.1111/tpj.12333
Sharma, D. (1969). Use of radiations for breaking hybrid necrosis in wheat. Euphytica 18, 66–70. doi: 10.1007/BF00021983
Shii, C. T., Mok, M. C., Mok, D. W. S. (1981). Developmental controls of morphological mutants of Phaseolus vulgaris l.: differential expression of mutant loci in plant organs. Dev. Genet. 2, 279–290. doi: 10.1002/dvg.1020020306
Shii, C. T., Mok, M. C., Temple, S. R., Mok, D. W. S. (1980). Expression of developmental abnormalities in hybrids of Phaseolus vulgaris l. interaction between temperature and allelic dosage. J. Hered 71, 218–222. doi: 10.1093/oxfordjournals.jhered.a109353
Shintaku, Y., Yamamoto, K., Nakajima, T. (1988). Interspecific hybridization between Nicotiana repanda willd. and N. tabacum l. through the pollen irradiation technique and the egg cell irradiation technique. Theor. Appl. Genet. 76, 293–298. doi: 10.1007/BF00257858
Shintaku, Y., Yamamoto, K., Takeda, G. (1989). Chromosomal variation in hybrids between Nicotiana repanda willd. and N. tabacum l. through pollen and egg-cell irradiation techniques. Genome 32, 251–256. doi: 10.1139/g89-436
Shiragaki, K., Furukawa, H., Yokoi, S., Tezuka, T. (2021). Temperature-dependent sugar accumulation in interspecific Capsicum F1 plants showing hybrid weakness. J. Plant Res. 134, 1199–1211. doi: 10.1007/s10265-021-01340-1
Shiragaki, K., Iizuka, T., Ichitani, K., Kuboyama, T., Morikawa, T., Oda, M., et al. (2019). HWA1-and HWA2-mediated hybrid weakness in rice involves cell death, reactive oxygen species accumulation, and disease resistance-related gene upregulation. Plants 8, 450. doi: 10.3390/plants8110450
Shiragaki, K., Nakamura, R., Nomura, S., He, H., Yamada, T., Marubashi, W., et al. (2020a). Phenylalanine ammonia-lyase and phenolic compounds are related to hybrid lethality in the cross Nicotiana suaveolens × N. tabacum. Plant Biotech. 37, 327–333. doi: 10.5511/plantbiotechnology.20.0606a
Shiragaki, K., Seko, S., Yokoi, S., Tezuka, T. (2022). Capsicum annuum with causal allele of hybrid weakness is prevalent in Asia. PloS One 17, e0271091. doi: 10.1371/journal.pone.0271091
Shiragaki, K., Yokoi, S., Tezuka, T. (2020b). A hypersensitive response-like reaction is involved in hybrid weakness in F1 plants of the cross Capsicum annuum × Capsicum chinense. Breed Sci. 70, 430–437. doi: 10.1270/jsbbs.19137
Shizukuda, N., Nakajima, T. (1982). Productioin of interspecific hybrids between Nicotiana rustica l. and N. tabacum l. through ovule culture. Jpn J. Breed 32, 371–377. doi: 10.1270/jsbbs1951.32.371. in Japanese with English summary.
Si, Y., Zheng, S., Niu, J., Tian, S., Gu, M., Lu, Q., et al. (2021). Ne2, a typical CC-NBS-LRR-type gene, is responsible for hybrid necrosis in wheat. New Phytol. 232, 279–289. doi: 10.1111/nph.17575
Sicard, A., Kappel, C., Josephs, E. B., Lee, Y. W., Marona, C., Stinchcombe, J. R., et al. (2015). Divergent sorting of a balanced ancestral polymorphism underlies the establishment of gene-flow barriers in capsella. Nat. Commun. 6, 7960. doi: 10.1038/ncomms8960
Smith, L. M., Bomblies, K., Weigel, D. (2011). Complex evolutionary events at a tandem cluster of Arabidopsis thaliana genes resulting in a single-locus genetic incompatibility. PloS Genet. 7, e1002164. doi: 10.1371/journal.pgen.1002164
Städler, T., Florez-Rueda, A. M., Roth, M. (2021). A revival of effective ploidy: the asymmetry of parental roles in endosperm-based hybridization barriers. Curr. Opin. Plant Biol. 61, 102015. doi: 10.1016/j.pbi.2021.102015
Streisfeld, M. A., Kohn, J. R. (2007). Environment and pollinator-mediated selection on parapatric floral races of Mimulus aurantiacus. J. Evol. Biol. 20, 122–132. doi: 10.1111/j.1420-9101.2006.01216.x
Subhashini, U., Venkateswarlu, T., Anjani, K. (1986). Embryo rescue in Nicotiana hybrids by in-vitro culture. Plant Sci. 43, 219–222. doi: 10.1016/0168-9452(86)90021-X
Subhashini, U., Venkateswarlu, T., Anjani, K., Prasad, G. S. R. (1985). In vitro hybridization in an incompatible cross Nicotiana glutinosa × Nicotiana megalosiphon. Theor. Appl. Genet. 71, 545–549. doi: 10.1007/BF00251203
Sweigart, A. L., Willis, J. H. (2012). Molecular evolution and genetics of postzygotic reproductive isolation in plants. F1000 Biol. Rep. 4, 23. doi: 10.3410/b4-23
Takahashi, R., Hayashi, J., Moriya, I. (1970). Studies on lethal seedlings of barley by complementary genes. i. mode of inheritance and the geographical distribution of the lethal genes. Nogaku Kenkyu 53, 197–204. in Japanese.
Taylor, J. E., Whitelaw, C. A. (2001). Signals in abscission. New Phytol. 151, 323–340. doi: 10.1046/j.0028-646x.2001.00194.x
Ternovskii, M. F., Butenko, R. G., Moiseeva, M. E. (1972). The use of tissue culture to overcome the barrier of incompatibility between species and sterility of interspecies hybrids. Sov Genet. 8, 27–33. Translated from Genetika.
Ternovskii, M. F., Shinkareva, I. K., Lar’kina, N. I. (1976). Production of interspecific tobacco hybrids by the pollination of ovules in vitro. Sov Genet. 12, 1209–1213. Translated from Genetika.
Tezuka, T. (2012). “Hybrid lethality in the genus nicotiana,” in Botany. Ed. Mworia, J. K. (Rijeka, Croatia: InTech), 191–210.
Tezuka, T. (2013). “Hybrid lethality in nicotiana: a review with special attention to interspecific crosses between species in sect. suaveolentes and n. tabacum,” in Herbaceous plants: cultivation methods, grazing and environmental impacts. Ed. Wallner, F. (New York: Nova Science Publishers), 69–94.
Tezuka, T., Kitamura, N., Imagawa, S., Hasegawa, A., Shiragaki, K., He, H., et al. (2021). Genetic mapping of the HLA1 locus causing hybrid lethality in Nicotiana interspecific hybrids. Plants 10, 2062. doi: 10.3390/plants10102062
Tezuka, T., Kuboyama, T., Matsuda, T., Marubashi, W. (2007). Possible involvement of genes on the q chromosome of Nicotiana tabacum in expression of hybrid lethality and programmed cell death during interspecific hybridization to Nicotiana debneyi. Planta 226, 753–764. doi: 10.1007/s00425-007-0522-2
Tezuka, T., Kuboyama, T., Matsuda, T., Marubashi, W. (2010). Seven of eight species in Nicotiana section Suaveolentes have common factors leading to hybrid lethality in crosses with Nicotiana tabacum. Ann. Bot. 106, 267–276. doi: 10.1093/aob/mcq114
Tezuka, T., Marubashi, W. (2004). Apoptotic cell death observed during the expression of hybrid lethality in interspecific hybrids between Nicotiana tabacum and N. suaveolens. Breed Sci. 54, 59–66. doi: 10.1270/jsbbs.54.59
Tezuka, T., Marubashi, W. (2006a). Genomic factors lead to programmed cell death during hybrid lethality in interspecific hybrids between Nicotiana tabacum and N. debneyi. SABRAO J. Breed Genet. 38, 69–81.
Tezuka, T., Marubashi, W. (2006b). Hybrid lethality in interspecific hybrids between Nicotiana tabacum and N. suaveolens: evidence that the q chromosome causes hybrid lethality based on q-chromosome-specific DNA markers. Theor. Appl. Genet. 112, 1172–1178. doi: 10.1007/s00122-006-0219-0
Tezuka, T., Marubashi, W. (2012). Genes in s and T subgenomes are responsible for hybrid lethality in interspecific hybrids between Nicotiana tabacum and Nicotiana occidentalis. PloS One 7, e36204. doi: 10.1371/journal.pone.0036204
Tezuka, T., Matsuo, C., Iizuka, T., Oda, M., Marubashi, W. (2012). Identification of Nicotiana tabacum linkage group corresponding to the q chromosome gene(s) involved in hybrid lethality. PloS One 7, e37822. doi: 10.1371/journal.pone.0037822
Tikhenko, N., Poursarebani, N., Rutten, T., Schnurbusch, T., Börner, A. (2017a). Embryo lethality in wheat-rye hybrids: dosage effect and deletion bin mapping of the responsible wheat locus. Biol. Plant 61, 342–348. doi: 10.1007/s10535-016-0691-6
Tikhenko, N., Rutten, T., Senula, A., Rubtsova, M., Keller, E. R. J., Börner, A. (2017b). The changes in the reproductive barrier between hexaploid wheat (Triticum aestivum l.) and rye (Secale cereale l.): different states lead to different fates. Planta 246, 377–388. doi: 10.1007/s00425-017-2694-8
Tikhenko, N., Rutten, T., Voylokov, A., Houben, A. (2008). Analysis of hybrid lethality in F1 wheat-rye hybrid embryos. Euphytica 159, 367–375. doi: 10.1007/s10681-007-9528-x
Tikhenko, N., Tsvetkova, N., Priyatkina, S., Voylokov, A., Börner, A. (2011). Gene mutations in rye causing embryo lethality in hybrids with wheat: allelism and chromosomal localisation. Biol. Plant 55, 448–452. doi: 10.1007/s10535-011-0109-4
Tikhenko, N. D., Tsvetkova, N. V., Voylokov, A. V. (2005). Genetic control of embryo lethality in crosses between common wheat and rye. Russ J. Genet. 41, 877–884. doi: 10.1007/s11177-005-0175-9
Tikhenko, N., Tsvetkova, N., Voylokov, A., Dobrovolskaya, O., Zaynali Nezhad, K., Röder, M. S., et al. (2010). Embryo lethality in wheat × rye hybrids–mode of inheritance and the identification of a complementary gene in wheat. Euphytica 176, 191–198. doi: 10.1007/s10681-010-0202-3
Todesco, M., Kim, S. T., Chae, E., Bomblies, K., Zaidem, M., Smith, L. M., et al. (2014). Activation of the Arabidopsis thaliana immune system by combinations of common ACD6 alleles. PloS Genet. 10, e1004459. doi: 10.1371/journal.pgen.1004459
Tonosaki, K., Sekine, D., Ohnishi, T., Ono, A., Furuumi, H., Kurata, N., et al. (2018). Overcoming the species hybridization barrier by ploidy manipulation in the genus Oryza. Plant J. 93, 534–544. doi: 10.1111/tpj.13803
Toxopeus, H., Hermsen, J. G. T. (1964). Chloroplast degeneration as a consequence of “hybrid necrosis” in wheat. Euphytica 13, 29–32. doi: 10.1007/BF00037515
Tsunewaki, K. (1960). Monosomic and conventional analyses in common wheat. III. lethality. Jpn J. Genet. 35, 71–75. doi: 10.1266/jjg.35.71
Tsunewaki, K., Kihara, H. (1962). Comparative gene analysis of common wheat and its ancestral species, i. necrosis. Jpn J. Genet. 37, 474–484. doi: 10.1266/jjg.37.474
Ueno, N., Nihei, S., Miyakawa, N., Hirasawa, T., Kanekatsu, M., Marubashi, W., et al. (2016). Time course of programmed cell death, which included autophagic features, in hybrid tobacco cells expressing hybrid lethality. Plant Cell Rep. 35, 2475–2488. doi: 10.1007/s00299-016-2048-1
Vega, A., O’brien, J. A., Gutiérrez, R. A. (2019). Nitrate and hormonal signaling crosstalk for plant growth and development. Curr. Opin. Plant Biol. 52, 155–163. doi: 10.1016/j.pbi.2019.10.001
Vernieri, P., Tagliasacchi, A. M., Forino, L., Lanfranchi, A., Lorenzi, R., Avanzi, S. (1992). Abscisic acid levels and cell structure in single seed tissues of shedding affected fruits of Malus domestica borkh. J. Plant Physiol. 140, 699–706. doi: 10.1016/S0176-1617(11)81026-6
Vijayaraghavan, M. R., Prabhakar, K. (1984). “The endosperm,” in Embryology of angiosperms. Ed. Johri, B. M. (Berlin, Heidelberg: Springer), 319–376.
Whitaker, T. W., Bemis, W. (1964). Virus-like syndromes of Cucurbita species hybrids. Heredity 19, 229–236. doi: 10.1038/hdy.1964.27
Xiao, Z., Hu, Y., Zhang, X., Xue, Y., Fang, Z., Yang, L., et al. (2017). Fine mapping and transcriptome analysis reveal candidate genes associated with hybrid lethality in cabbage (Brassica oleracea). Genes 8, 147. doi: 10.3390/genes8060147
Xiao, Z., Liu, X., Fang, Z., Yang, L., Zhang, Y., Wang, Y., et al. (2021). Transcriptome and plant hormone analyses provide new insight into the molecular regulatory networks underlying hybrid lethality in cabbage (Brassica oleracea). Planta 253, 96. doi: 10.1007/s00425-021-03608-1
Xu, W., Sato, H., Bente, H., Santos-González, J., Köhler, C. (2023). Endosperm cellularization failure induces a dehydration stress response leading to embryo arrest. Plant Cell. 35, 874–888. doi: 10.1093/plcell/koac337
Yamada, T., Marubashi, W. (2003). Overproduced ethylene causes programmed cell death leading to temperature-sensitive lethality in hybrid seedlings from the cross Nicotiana suaveolens × N. tabacum. Planta 217, 690–698. doi: 10.1007/s00425-003-1035-2
Yamada, T., Marubashi, W., Niwa, M. (1999). Detection of four lethality types in interspecific crosses among Nicotiana species through the use of three rescue methods for lethality. Breed Sci. 49, 203–210. doi: 10.1270/jsbbs.49.203
Yamada, T., Marubashi, W., Niwa, M. (2000). Apoptotic cell death induces temperature-sensitive lethality in hybrid seedlings and calli derived from the cross of Nicotiana suaveolens × N. tabacum. Planta 211, 614–622. doi: 10.1007/s004250000329
Yamagata, Y., Yamamoto, E., Aya, K., Win, K. T., Doi, K., Sobrizal, et al. (2010). Mitochondrial gene in the nuclear genome induces reproductive barrier in rice. Proc. Natl. Acad. Sci. U.S.A. 107, 1494–1499. doi: 10.1073/pnas.0908283107
Yamamoto, T., Shomura, S., Mino, M. (2017). Cell physiology of mortality and immortality in a Nicotiana interspecific F1 hybrid complies with the quantitative balance between reactive oxygen and nitric oxide. J. Plant Physiol. 210, 72–83. doi: 10.1016/j.jplph.2017.01.001
Yan, X., Li, M., Zhang, P., Yin, G., Zhang, H., Gebrewahid, T. W., et al. (2021). High-temperature wheat leaf rust resistance gene Lr13 exhibits pleiotropic effects on hybrid necrosis. Mol. Plant 14, 1029–1032. doi: 10.1016/j.molp.2021.05.009
Yonezawa, K., Sato, Y. L., Nomura, T., Morishima, H. (1990). Computer-simulated evaluation of the hybrid weakness gene system as a means of preventing genetic contamination of rice cultivars. Plant Breed 104, 241–247. doi: 10.1111/j.1439-0523.1990.tb00429.x
Zacarias, L., Talon, M., Ben-Cheikh, W., Lafuente, M. T., Primo-Millo, E. (1995). Abscisic acid increases in non-growing and paclobutrazol-treated fruits of seedless mandarins. Physiol. Plant 95, 613–619. doi: 10.1111/j.1399-3054.1995.tb05530.x
Zhang, M., Lv, S., Wang, Y., Wang, S., Chen, C., Wang, C., et al. (2022b). Fine mapping and distribution analysis of hybrid necrosis genes Ne1 and Ne2 in wheat in China. Theor. Appl. Genet. 135, 1177–1189. doi: 10.1007/s00122-021-04023-6
Zhang, H. P., Tao, Z. B., Trunschke, J., Shrestha, M., Scaccabarozzi, D., Wang, H., et al. (2022a). Reproductive isolation among three nocturnal moth-pollinated sympatric Habenaria species (Orchidaceae). Front. Plant Sci. 13. doi: 10.3389/fpls.2022.908852
Zhang, M., Wei, H., Liu, J., Bian, Y., Ma, Q., Mao, G., et al. (2021). Non-functional GoFLA19s are responsible for the male sterility caused by hybrid breakdown in cotton (Gossypium spp.). Plant J. 107, 1198–1212. doi: 10.1111/tpj.15378
Zhou, W. M., Yoshida, K., Shintaku, Y., Takeda, G. (1991). The use of IAA to overcome interspecific hybrid inviability in reciprocal crosses between Nicotiana tabacum l. and N. repanda willd. Theor. Appl. Genet. 82, 657–661. doi: 10.1007/BF00226805
Zhu, H., Yuan, R., Greene, D. W., Beers, E. P. (2010). Effects of 1-methylcyclopropene and naphthaleneacetic acid on fruit set and expression of genes related to ethylene biosynthesis and perception and cell wall degradation in apple. J. Am. Soc. Hort Sci. 135, 402–409. doi: 10.21273/jashs.135.5.402
Zuellig, M. P., Sweigart, A. L. (2018). Gene duplicates cause hybrid lethality between sympatric species of Mimulus. PloS Genet. 14, e1007130. doi: 10.1371/journal.pgen.1007130
Keywords: Bateson-Dobzhansky-Muller model, endosperm balance number, hybrid seed lethality, hybrid seedling lethality, reproductive isolation
Citation: He H, Shiragaki K and Tezuka T (2023) Understanding and overcoming hybrid lethality in seed and seedling stages as barriers to hybridization and gene flow. Front. Plant Sci. 14:1219417. doi: 10.3389/fpls.2023.1219417
Received: 09 May 2023; Accepted: 20 June 2023;
Published: 05 July 2023.
Edited by:
Tzung-Fu Hsieh, North Carolina State University, United StatesReviewed by:
Elen Oneal, Duke University, United StatesYu-Hung Hung, Donald Danforth Plant Science Center, United States
Miguel Angel Flores-Vergara, North Carolina State University, United States
Copyright © 2023 He, Shiragaki and Tezuka. This is an open-access article distributed under the terms of the Creative Commons Attribution License (CC BY). The use, distribution or reproduction in other forums is permitted, provided the original author(s) and the copyright owner(s) are credited and that the original publication in this journal is cited, in accordance with accepted academic practice. No use, distribution or reproduction is permitted which does not comply with these terms.
*Correspondence: Takahiro Tezuka, tezuka@omu.ac.jp