- 1Faculty of Agricultural, Environmental and Food Sciences, Free University of Bolzano - Bozen, Bolzano, Italy
- 2Swiss Federal Institute for Forest, Snow and Landscape Research WSL, Birmensdorf, Switzerland
- 3Oeschger Centre for Climate Change Research, University of Bern, Bern, Switzerland
- 4Istituto di Ricerca sugli Ecosistemi Terrestri (IRET), Consiglio Nazionale Ricerche, Sesto Fiorentino, Italy
- 5National Biodiversity Future Center (NBFC), Palermo, Italy
- 6Department of Forest and Wood Science, Faculty of AgriSciences, Stellenbosch University, Stellenbosch, South Africa
- 7Department of Land Environment Agriculture and Forestry, University of Padova, Legnaro, Italy
- 8Chair of Forest Growth and Woody Biomass Production, Technische Universität Dresden, Tharandt, Germany
- 9Department of Silviculture, Institute of Forest Sciences, Warsaw University of Life Sciences-SGGW, Warsaw, Poland
- 10School of Life Sciences, Chair for Forest Growth and Yield Science, Technical University of Munich (TUM), Freising, Germany
- 11Bavarian State Institute of Forestry (LWF), Freising, Germany
- 12Instituto Universitario de Investigación en Gestión Forestal Sostenible (iuFOR). Escuela Técnica Superior de Ingenierías Agrarias de Palencia, Universidad de Valladolid, Palencia, Spain
Introduction: Forests are threatened by increasingly severe and more frequent drought events worldwide. Mono-specific forests, developed as a consequence of widespread management practices established early last century, seem particularly susceptible to global warming and drought compared with mixed-species forests. Although, in several contexts, mixed-species forests display higher species diversity, higher productivity, and higher resilience, previous studies highlighted contrasting findings, with not only many positive but also neutral or negative effects on tree performance that could be related to tree species diversity. Processes underlying this relationship need to be investigated. Wood anatomical traits are informative proxies of tree functioning, and they can potentially provide novel long-term insights in this regard. However, wood anatomical traits are critically understudied in such a context. Here, we assess the role of tree admixture on Pinus sylvestris L. xylem traits such as mean hydraulic diameter, cell wall thickness, and anatomical wood density, and we test the variability of these traits in response to climatic parameters such as temperature, precipitation, and drought event frequency and intensity.
Methods: Three monocultural plots of P. sylvestris and three mixed-stand plots of P. sylvestris and Quercus sp. were identified in Poland and Spain, representing Continental and Mediterranean climate types, respectively. In each plot, we analyzed xylem traits from three P. sylvestris trees, for a total of nine trees in monocultures and nine in mixed stands per study location.
Results: The results highlighted that anatomical wood density was one of the most sensitive traits to detect tree responses to climatic conditions and drought under different climate and forest types. Inter-specific facilitation mechanisms were detected in the admixture between P. sylvestris and Quercus sp., especially during the early growing season and during stressful events such as spring droughts, although they had negligible effects in the late growing season.
Discussion: Our findings suggest that the admixture between P. sylvestris and Quercus sp. increases the resilience of P. sylvestris to extreme droughts. In a global warming scenario, this admixture could represent a useful adaptive management option.
1 Introduction
Forest vulnerability and dieback induced by global warming and drought events are increasing worldwide (Allen et al., 2010; Allen et al., 2015). Drought severity and frequency are expected to increase in the next decades (Dai et al., 2018), and Central and Southern Europe are predicted to be strongly affected (Giorgi, 2006; Somorowska, 2022).
An increasing number of studies have consistently documented higher productivity (Del Río and Sterba, 2009; Bielak et al., 2014) and greater resilience to global climate changes in mixed forests versus monocultures (e.g., Pretzsch et al., 2013b; Pardos et al., 2021). The functional benefits derived from tree species diversity rely on the niche complementarity hypothesis, which states that diversity promotes inter-specific facilitation by enhancing access to resources via niche differentiation (Tilman, 1999; Zhang et al., 2012; Pretzsch et al., 2013b). It could be expected that individuals belonging to different species and having dissimilar resource needs show lower competition in comparison with the intra-specific competition among individuals within monocultures. In addition, in mixed forests, niche complementarity could improve growth and increase overall ecosystem resilience. It is known that benefits of increased biodiversity on tree performance vary in space, time, and with different species (Forrester, 2014). Tree species-specific vulnerabilities against various kinds of stressors (i.e., pests, windstorms and drought) are less severe in mixed-forest ecosystems, making them more resilient to perturbations. Studies on species interactions in mixed forests showed contrasting results in terms of growth/productivity and resilience to drought events. As an example, in the works of Bielak et al. (2014) and Pretzsch et al. (2015), the admixture of species increased tree productivity compared with pure stands. In the work of Zeller et al. (2017), the increase in productivity occurred only for one species (P. sylvestris), whereas the opposite was shown for the other (Fagus sylvatica). Non-significant differences in productivity between mixed and pure forests were reported by Giberti et al. (2022). Pretzsch et al. (2013b) showed that, in mixed- forest tree, resilience to drought is affected by species-specific interactions and that different reactions to drought can be observed depending on the species considered. Similar results were presented by Bello et al. (2019) and Thurm et al. (2016), who found beneficial effects of tree admixture during drought events for Q. petraea and Pseudotsuga menziesii, but not for P. sylvestris and F. sylvatica. Instead, Merlin et al. (2015) did not find significant effects of tree admixture on tree resistance and resilience to drought when considering mixed P. sylvestris –Q. petraea stands. According to Callaway and Walker (1958), benefits of increased biodiversity could be more apparent under harsher conditions such as under resource limitation. This hypothesis was supported by Paquette and Messier (2011), who showed the beneficial effects of complementarity to be more important in stressful environments (boreal forest), rather than in more stable environments (temperate forest), and by Pretzsch et al. (2013b), who showed that facilitation effects related to mixed-forest composition emerged during episodic stressful events such as drought. These documented experiences demonstrate that tree performance in mixed forests can vary greatly depending on specific conditions and further studies are required to assess the influence of different species under variable soil and climate conditions.
Common parameters adopted to understand whether mixed forests can be more productive and/or resilient than pure forests include direct measures of tree growth, i.e., ring width, basal area increment, and derived measures such as volume increment per hectare (Del Río and Sterba, 2009; Michelot et al., 2012; Pretzsch et al., 2013a; Pretzsch et al., 2013b; Merlin et al., 2015; Steckel et al., 2019). These proxies are only related to tree growth and do not allow inferences on possible trade-offs occurring among functional tree ring properties. The assessment of xylem traits can provide a higher-resolution insight into the processes that relate to tree resilience. Physiological parameters such as isotopic signature, predawn leaf water potential, and wood density were scarcely explored in the context of investigating tree resilience under climatic alterations in mixed forests (Toïgo et al., 2015; Zalloni et al., 2018; Bello et al., 2019; Giberti et al., 2022). The influence of tree admixture on xylem traits has not been explored yet, although xylem trait variability provides information about physiological processes during acclimation, in response to changing abiotic and biotic conditions (Fonti et al., 2010; Cuny et al., 2014; Pellizzari et al., 2016; Pacheco et al., 2018a; Castagneri et al., 2020; Guérin et al., 2020).
Pinus sylvestris L. (Scots pine) is a pioneer, light-demanding evergreen species that is of great ecological and economic importance. It is one of the most widely distributed Eurasian pine species, occurring under a variety of different environmental conditions, showing high genetic variability, high ecological plasticity (Cheddadi et al., 2006), and local acclimation to climate change (Martínez-Vilalta et al., 2009; Martín et al., 2010; Matisons et al., 2019). In recent times, mortality and decline of P. sylvestris induced by drought were reported across its southern distribution (Martínez-Vilalta and Piñol, 2002; Eilmann et al., 2009; Allen et al., 2010; Hereş et al., 2014; Pellizzari et al., 2016; Petrucco et al., 2017). On the basis of current climate projections, a further significant decline in Central Europe is being forecasted (Buras and Menzel, 2019). The admixture between P. sylvestris and Quercus sp. has been investigated focusing on complementarity in water uptake (Bello et al., 2019), in growth and yield (Del Río and Sterba, 2009; Merlin et al., 2015; Pretzsch et al., 2020), and in wood density (Toïgo et al., 2015; Giberti et al., 2022). Inter-specific facilitation mechanisms occur due to the complementary realized by differences in leaf phenology, wood anatomy (Michelot et al., 2012), and root stratification and morphology (Merlin et al., 2015; Bello et al., 2019), with P. sylvestris having a shallow root system (0 cm to 40 cm) and a strong first taproot (Merlin et al., 2015) and Quercus sp. having a generally deeper root system. The two species show different seasonal growth patterns and different strategies for coping with drought (Eilmann et al., 2009; Merlin et al., 2015; Bello et al., 2019). These characteristics could provide an overall reduction in competition during drought events. Because the inter-specific interactions can change depending on the growing season, a careful assessment of the extent and the timing of drought is fundamental to forecasting the response of mixed forests to extreme droughts that are likely to occur more frequently in the next decades (Merlin et al., 2015).
In this study, we analyze the effect of tree admixture on P. sylvestris xylem traits under two different climatic conditions: 1) Continental (Poland), which has optimum climatic conditions for P. sylvestris and lies in the core of the native range of this species (Caudullo et al., 2017); and 2) Mediterranean (Spain), at the southern edge of P. sylvestris distribution range (Caudullo et al., 2017). At both sites, we identified pure P. sylvestris forests of local provenance as well as mixed forests of P. sylvestris and Quercus petraea L. (Poland) and Quercus pyrenaica WILLD (Spain). Quercus petraea is partially shade- tolerant, well adapted to occasional droughts. Quercus pyrenaica is drought-tolerant; under transitional climatic zones, between sub-humid temperate and semi-arid Mediterranean, it is adapted to shade (Caudullo et al., 2017).
The following xylem traits were selected for this study as they are likely candidates to elucidate physico-mechanical xylem response mechanisms to climatic factors such as drought events:
i) Mean hydraulic diameter (DH), for the assessment of tree hydraulics, because according to Hagen-Poiseuille law, the conductivity of the hydraulic conduit is directly proportional to its radius raised to the fourth power (Zimmermann, 2013). The wider the conduits, the more efficient the hydraulic system, but the more prone it putatively becomes to cavitation, which could eventually lead to drought-induced dieback (Eilmann et al., 2009; Desoto et al., 2011; Bryukhanova and Fonti, 2013).
ii) Cell wall thickness (CWT; averaged over all walls) related to mechanical strength preventing cell collapse (Hacke et al., 2001) followed by cavitation and to the amount of carbon allocated to the xylem (Cuny et al., 2015).
iii) Anatomical wood density {AD; i.e., cell wall area/[cell wall area + lumen area (LA)]} as proxy for absolute wood density (Björklund et al., 2017; Björklund et al., 2021).
Trees in mixed forests could have greater access to environmental resources, which can buffer and help trees to endure stressful events such as drought (Magruder et al., 2013; Pretzsch et al., 2013b; Thurm et al., 2016). Hence, we expect P. sylvestris in mixed forests to show reduced climate sensitivity (enhanced climatic resistance/resilience), thereby presenting a wood architecture with no or only minor xylem trait adjustments induced by drought stress. On the contrary, P. sylvestris growing in pure stands could show higher climate sensitivity, thus presenting specific xylem trait adjustments induced by drought stress. Trees growing in semi-arid conditions are characterized by an inconstant growth rate due to regularly occurring drought stress (Biondi and Qeadan, 2008). Hence, we also expect to find generally higher xylem trait variability in P. sylvestris trees in the Spanish site compared with that in the Polish site. In addition, trees under drought stress tend to show high xylem trait variability (Levanič et al., 2011; Hereş et al., 2014; Guérin et al., 2020; Buras et al., 2023), and we expect that pines growing in pure stands could present this feature more strongly.
More specifically we hypothesize the following.
1. At both sites, xylem traits of P. sylvestris in pure forests will show higher sensitivity to climate (i.e., temperature, precipitation, and aridity index) and also higher variability compared with P. sylvestris growing in mixed forests. As response to drought, pure P. sylvestris xylem morphology will show reduced ring width, reduced DH, and increased CWT and AD. In P. sylvestris trees growing in mixed stands, these traits will present reduced or none xylem trait adjustments to drought events.
2. Under Mediterranean climate, summer drought occurs naturally, and we expect P. sylvestris to show higher xylem traits variability compared with P. sylvestris grown under continental climate.
2 Materials and methods
2.1 Study areas, forest, and species characteristics
The first study site was in Poland, at the Rogów Forest Experimental Station (51°48′42.30 ″ N, 19°49′55.55 ″ E, 200 meter above the sea level (m a.s.l.)), situated 100 km southwest of Warsaw, managed by the Warsaw University of Life Sciences-SGGW (Figure 1). Soils are silty and sandy loams developed over sandstone bedrock and are classified as Luvisols (Anjos et al., 2014). Following Köppen’s classification, the climate is Dfb, i.e., “warm-summer humid continental” (Beck et al., 2018). The site includes a pure P. sylvestris forest and a mixed one with P. sylvestris and Q. petraea. Pine trees were sowed in 1936 and in 1944 after clearcutting an oak stand, whereas oak trees regenerated naturally. The site did not undergo significant disturbance by wind storms or pests. The second site was in Spain, at Palacio de Valdellorma (42°48′54.50″ N, 5° 6′45.64″ W, 916 m a.s.l.), in León province, in an experimental mixed forest managed by the Sustainable Forest Management Research Institute, Universidad de Valladolid (Figure 1). Soils are Humic loamy Cambisols, with an impermeable layer below 150 cm (Zádorová et al., 2021). Following Köppen’s classification, the climate is Csb, i.e., “warm-summer Mediterranean” (Beck et al., 2018). The site hosted a pure P. sylvestris and a mixed P. sylvestris and Q. pyrenaica forest. Pine trees were sown in 1971–1972 following a partial clear-cut of pre-existing oak trees.
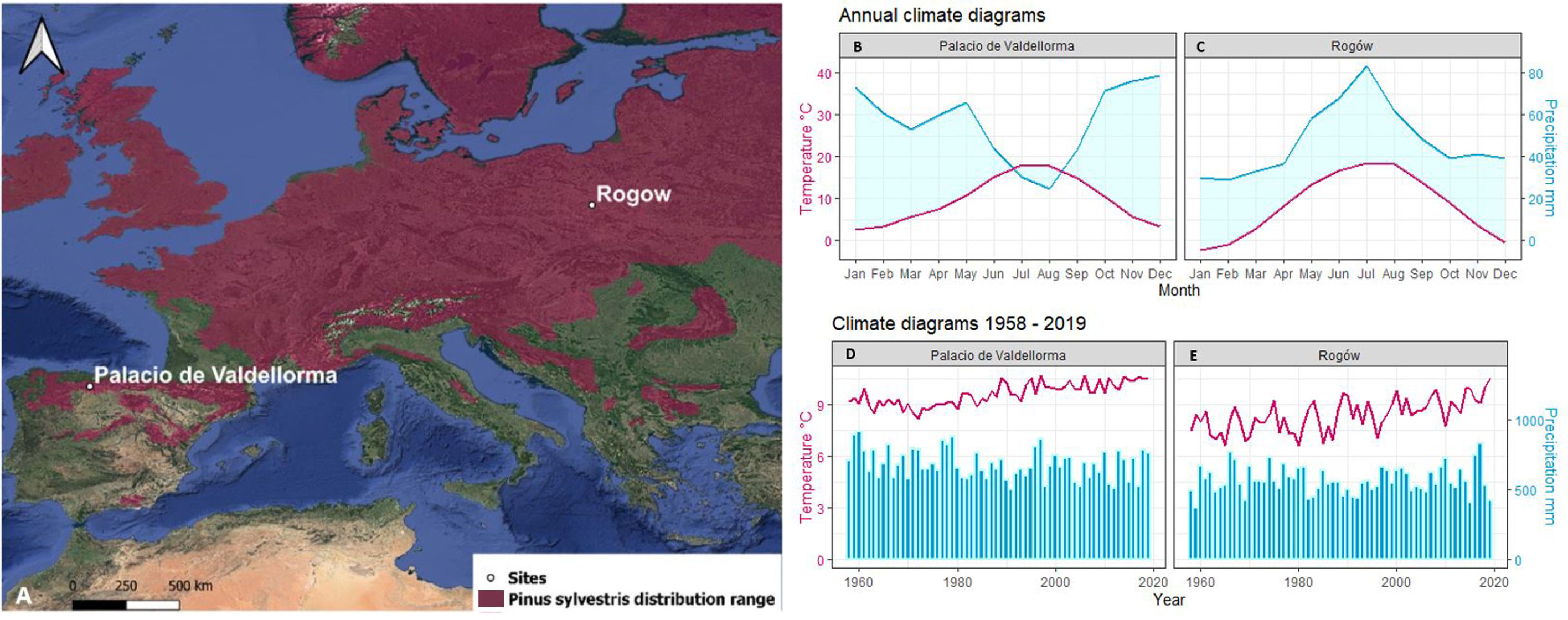
Figure 1 (A) Site location: Rogòw, 51°48′42.30″N 19°49′55.55″E, 200 m a.s.l., Palacio de Valdellorma 42°48′54.50″ N, 5° 6′45.64″ W, 916 m a.s.l. on distribution range of Pinus sylvestris (Caudullo et al., 2017). Relative annual climatogram: (B) Palacio de Valdellorma and (C) Rogów; climatogram for the time span 1958–2019: (D) Palacio de Valdellorma and (E) Rogów. The climatic records were obtained from the local weather station for Rogów and from the Terraclimate dataset for Palacio de Valdellorma.
2.1.1 Pinus sylvestris
The Central European P. sylvestris is a fast growing high-performance species reaching considerable height at maturity (Wachowiak et al., 2018). Being evergreen, able to photosynthesize during warm winters (Michelot et al., 2012), it benefited from recent-winter and late winter temperature increases. Indeed, late- winter and early- spring temperatures are considered among the main drivers supporting P. sylvestris development across Central Europe (Koprowski, 2012; Misi and Náfrádi, 2016; Misi et al., 2019; Giberti et al., 2022), where it showed vulnerability to water deficit during the early growing season (Michelot et al., 2012). At this latitude, budburst usually occurs by mid-May (Perot et al., 2021). At lower latitudes, P. sylvestris tend to slow growth, to reduce height, and to establish earlier budset and budburst compared with central European populations (Wachowiak et al., 2018). Under Mediterranean climate, an extended growing season was reported, with xylem cell production from the mid of April until late October (Camarero et al., 2011). Under these climatic conditions, spring rains always positively influenced pine growth, whereas drought reduced it (Bogino et al., 2009; Camarero et al., 2011).
2.1.2 Quercus petraea and Quercus pyrenaica
Being ring-porous, the growth of these oak species is not regular during the growing season: in springtime, they grow fast and form wide earlywood (EW) cells (González-González et al., 2013) but tend to be highly sensitive to spring drought (Merlin et al., 2015). Quercus petraea is a mesophilic oak, well adapted to drought events (Merlin et al., 2015). In Central Europe, it shows xylem cell production before budburst (Michelot et al., 2012), which usually occurs around early to mid-April (Perot et al., 2021; Gafenco et al., 2022), whereas complete leaf unfolding occurs in late April (Gafenco et al., 2022). Quercus pyrenaica is more drought-tolerant than the former species. At similar latitude and conditions to the Spanish site, cell production in Q. pyrenaica starts in mid-March, prior to budburst, which occurs a few weeks later (Pérez-de-Lis et al., 2016), and leaf expansion is generally completed in May but can eventually last until late June. The last EW cell forms by the end of June, and latewood (LW) production stops in mid-September, even if cell lignification continued for one more month (González-González et al., 2013). Drought- tolerant oaks (anisohydric) tend to show a contrasting water-use strategy in comparison with drought- avoiding (isohydric) pine; this different intra-annual drought sensitivity may produce contrasting drought response.
2.2 Climatic data
Polish climatic data were obtained from two weather stations located in the proximity: Łódź, at 38 km west, and Skierniewice, at 20 km northeast of the study site. The data were assembled by applying an inversely weighted mean by distance to monthly data derived from the two weather stations covering the period 1952–2019. No weather stations were in close proximity to the Spanish site; monthly climatic data were extracted from the online dataset Terraclimate (Abatzoglou et al., 2018), a 4- km-gridded, high-resolution dataset designed to calculate water balance, available for the time span 1958 –2019. For consistency, the correlation between Polish weather station climatic records and the ones of the corresponding 4-km grid cell (centered in 51.8125 N, 19.8542 E) available for the Polish site in Terraclimate were assessed by monthly correlation analysis for the period 1958– 2019. The majority of the climatic variables showed high correlations (R > 0.90).
Rogów is characterized by a mean annual temperature of 8.3°C and an annual precipitation of 565 mm. The study site climatogram covering the time span 1958 –2019 (Figure 1) showed a consistent linear increase in mean annual temperature over time (p< 0.001), observed especially during spring and summer months (Chojnacka-Ożga and Ożga, 2018). Precipitation did not show clear trends over time, but it tended to increase during spring. The climate in Palacio de Valdellorma is characterized by a mean annual temperature of 9.2°C and annual precipitation of 679 mm. For the time span 1958 –2019, mean annual temperature showed a linear increase over time (p< 0.001), and annual precipitation a linear decrease (p< 0.03).
The following climatic variables were considered: monthly precipitation and temperature and the Standardized Precipitation Evapotranspiration Index (SPEI), a multi-scalar index for estimating drought magnitude with respect to normal conditions. SPEI was calculated with R package “SPEI” (Beguería et al., 2017), using a 3- and a 6-month window because they show the highest correlations based on an initial screening. Years characterized by severe drought were identified using SPEI at 3-month resolution<− 1.5 (Potop et al., 2014, Figure 2).
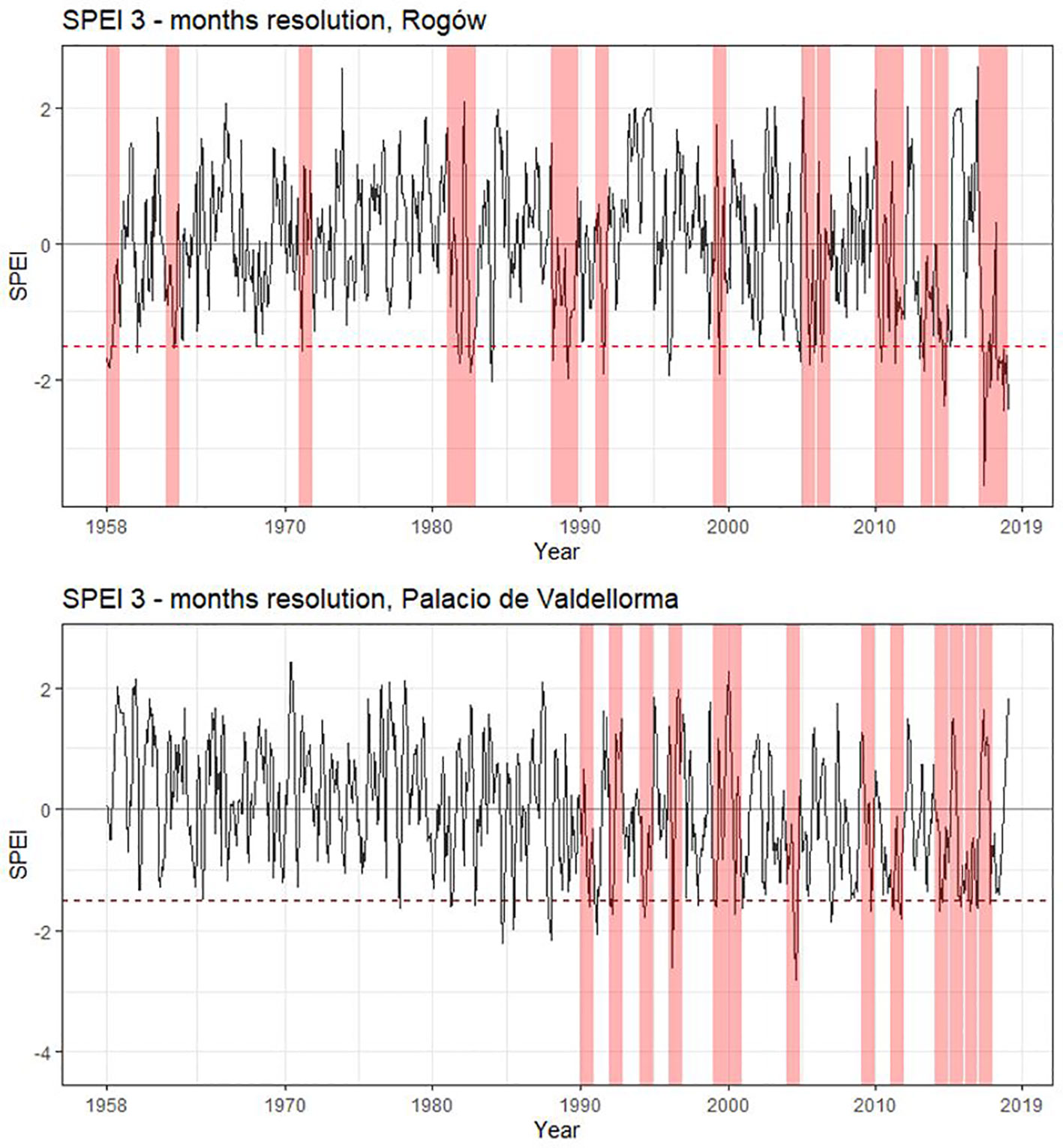
Figure 2 Standardized Precipitation Evapotranspiration Index for the two study sites. SPEI calculated with a 3- month window starting from March to October. Drought years (SPEI< −1.5) are highlighted in pink. Rogów: 1959, 1963, 1972, 1982, 1983, 1989, 1990, 1992, 2000, 2006, 2007, 2011, 2012, 2014, 2015, and 2018. Palacio de Valdellorma: 1991, 1993, 1995, 1997, 2000, 2001, 2005, 2010, 2012, 2015, 2016, 2017, and 2018.
2.3 Sampling design and lab work
At both sites, we selected six pre-existing plots (three in pure forest and three in mixed forest) close to each other. The plots were flat, homogeneous in terms of altitude, exposition, climate, and soil characteristics. From every plot, we selected six dominant and healthy P. sylvestris trees, i.e., the tallest trees with large diameter at breast height, thus having comparable social class and excluding other sources of variation except for climate and tree competition given by tree density and different tree species. Overall, we cored 36 trees per site in pure and the same amount in mixed stands. In mixed forest, we selected trees surrounded only by Q. petraea and Q. pyrenaica, in Poland and Spain, respectively. The characteristics of the plots are summarized in Table 1. The Stand Density Index (calculated with Reineke formula, Reineke, 1933), was employed to determine stand density. This index showed a similar competition level in both forest types in the Polish site (741 in pure forest and 742 in mixed forest) but a different competition level for the two forest types in the Spanish site (935 in pure forest and 1,413 in mixed forest). We collected one core of 1 cm diameter at 1 m height from the northern side of every study tree using an increment borer.
The cores were analyzed at the Technical University of Munich Chair for Forest Growth and Yield Science, with LIGNOSTATION™, a high- frequency densitometry assessment instrument, which allows for non-destructive and quick measure of ring width and ring density (Schinker et al., 2003; Zeller et al., 2017). All ring series were cross-dated with TSAP-Win software (Rinntech®, 2010).
We selected a subsample of three trees per plot, whose cores showed no signs of damage, for a total of 18 cores per site (nine in pure and nine in mixed forest). The characteristics of the selected trees and the corresponding cores are summarized in Table 2. The cores were divided into 4 cm-long pieces soaked in hot water to soften the wood and to remove resin before producing 15 μm -thick transverse sections with a rotary microtome (HistoCore MULTICUT, Leica Biosystems, Nussloch, Germany). The sections were stained with a solution of safranine and astrablue (1% and 0.5% in distilled water, respectively), rinsed with distilled water and then with increasing ethanol concentrations: 50% –96%, and mounted permanently on glass microscope slides with Eukitt (BiOptica, Milan, Italy) following von Arx et al. (2016). High-quality images were obtained with a slide scanner at 100× magnification and 1.99 pixels μ–1 resolution (D-sight 2.0 System, Menarini Diagnostics, Florence, Italy). The images were processed with ROXAS v. 3.0.454, a software that allows for quantitative investigation of wood anatomical traits (von Arx and Carrer, 2014; Prendin et al., 2017, Figure 3). In total, 3.1 million tracheids (2.0 × 106 for Poland, 1.1 × 106 for Spain) were measured. To avoid redundancy and to select more informative xylem traits, cross-correlation analysis was performed on the following traits: LA, DH, average CWT and AD. Given the high correlation between LA and DH, radial cell wall thickness, tangential cell wall thickness, and the different cell wall measurements (R2 > 0.97), we retained only DH, CWT, and AD. Wood anatomical trait chronology was visually compared with the chronology previously obtained with LIGNOSTATION™ for a careful assessment of the calendar years.
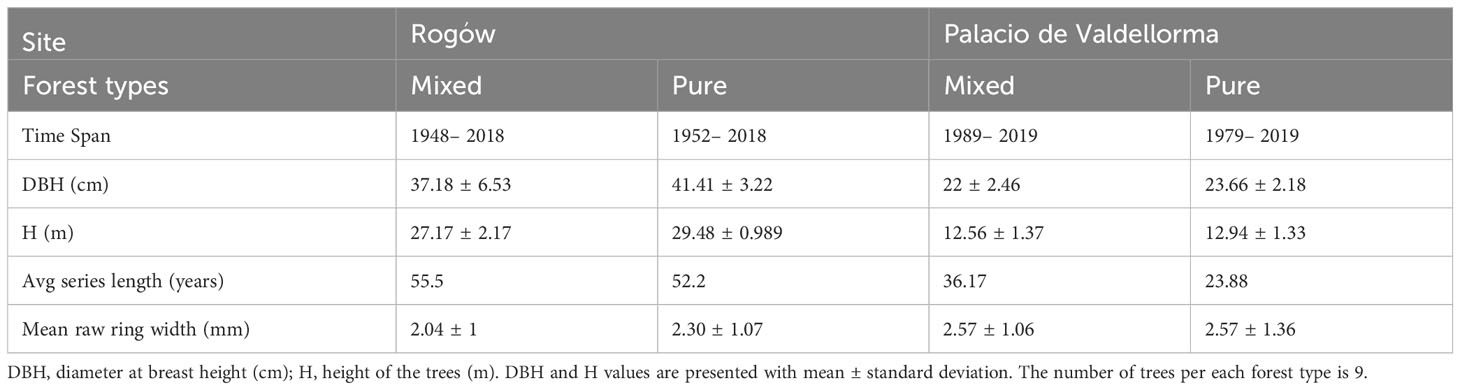
Table 2 Characteristics of the trees and the cores selected for wood anatomical analyses summarized per forest type at the two sites, in Rogów and Palacio de Valdellorma.
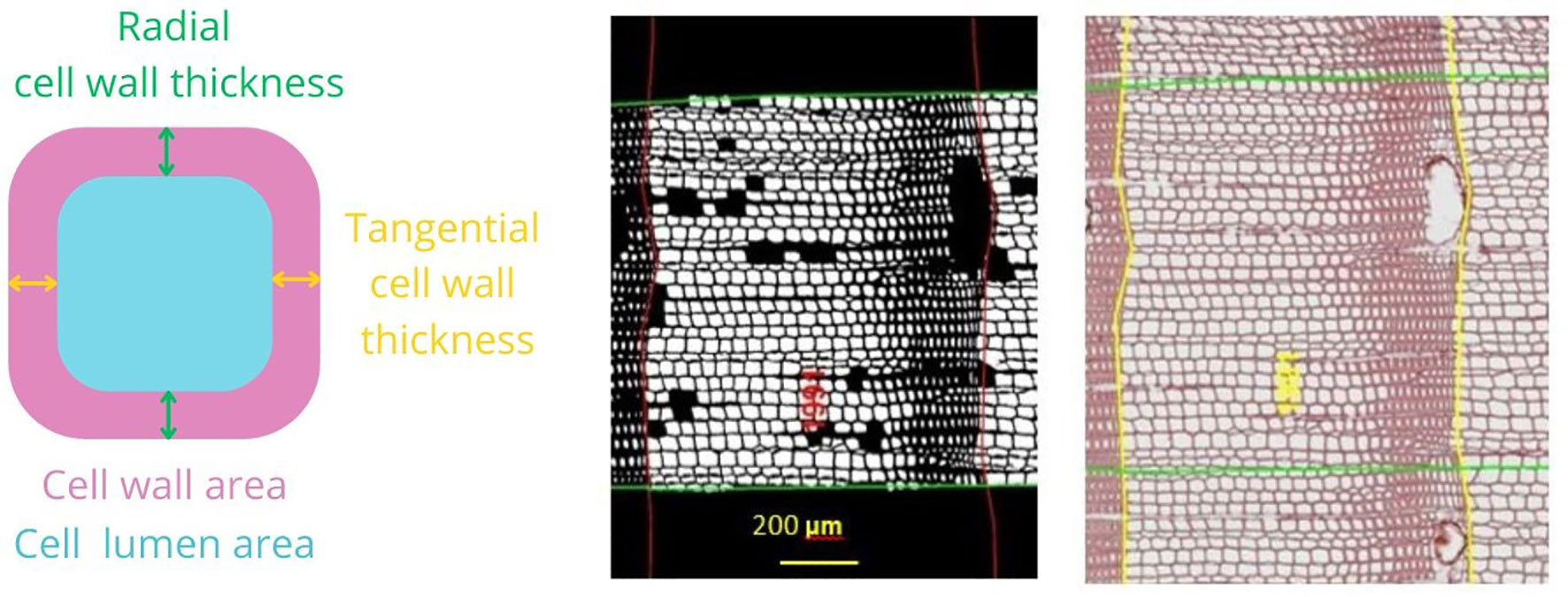
Figure 3 Illustration of anatomical measurements. At the right an image of a stained section where ring borders are drawn (yellow) and calendar years are assigned using ROXAS. The green area represents the area of interest where wood anatomical traits were analyzed. At the middle, there is a black-and-white image where only the cells used in the analysis are highlighted. Scale bar, 200 μm. At the left, there is a schematic representation of the wood anatomical parameters selected for the analysis. Mean hydraulic diameter (DH) = sum (cell hydraulic diameter 5)/sum (cell hydraulic diameter 4); cell wall thickness (CWT) = (radial cell wall thickness + tangential cell wall thickness)/2; anatomical wood density (AD) = cell wall area/(cell wall area + lumen area).
2.4 Ring width and wood anatomical trait analyses
The analyses were performed with R v. 4.0.2 (R: The R Project for Statistical Computing, 2020) using the packages dplyr (Wickham et al., 2023) and dplR (Bunn et al., 2023). Each ring was divided into 100 equal-width tangential sectors, based on the relative position of each cell in the ring (Pacheco et al., 2018b). The intra-annual profile of the wood anatomical traits was carefully inspected; sometimes, EW cells were present in LW and vice versa, due to some bad quality images. On the basis of conduit size, we identified and removed from the dataset the outliers related to those cells. Further outliers were identified in EW and LW sectors, respectively, when the data resulted greater than the Upper Quartile (75th percentile of data distribution) multiplied by 1.5 or lower than the Lower Quartile (25th percentile of data distribution) multiplied by 1.5.
2.4.1 EW-LW anatomical chronologies and relationship with climate
To explore the relationship between wood anatomical traits and climate, each sector was assigned to EW (mainly related to water transport functions) or LW (mainly related to mechanical support functions) based on Mork’s Index (Denne, 1989). Median EW and LW values were calculated for each individual tree. The median was preferred to the mean to reduce the impact of skewed parameter distributions. The raw chronologies are presented in Figure 4. The age-related trend that characterizes the EW and LW anatomical series was removed using a detrending procedure. This technique accounts also for the axial widening of wood anatomical traits, thus allowing for a comparison between traits collected at different distances from the apex (Petit et al., 2022), as in our case (Table 2). Each series was fitted with a cubic smoothing spline with 50% frequency –response cutoff of 30 years. Detrended series were calculated as a ratio between raw and fitted values for each year (Fritts, 1976; Cook and Kairiūkštis, 1990). Finally, the mean chronologies per forest type were obtained by first removing the autocorrelation (“prewhitening” in dplR) and then applying a bi-weight robust mean (Cook and Kairiūkštis, 1990). To assess the correlation between climate and detrended wood anatomical traits at annual EW and LW resolution, the function “dcc” from the R package treeclim (Zang and Biondi, 2015) was used. Pearson’s correlation was applied to the interval 1958 –2019 for the Polish site and for the period 1990 –2018 for the Spanish one, according to the age of the trees and to the availability of climatic records. Confidence intervals and significance (α< 0.05) of the correlation were calculated by means of bootstrapping (Biondi and Waikul, 2004). The climatic variables considered for the analyses were mean monthly temperature, monthly precipitation, and SPEI, calculated at a 6-monthly mean window, because the best correlations were found at this resolution. For this analysis, the climatic variables were detrended identically to the tree-ring and wood anatomical series to remove the same amount of frequency from the series and avoid distortion in correlation coefficients (Ols et al., 2023).
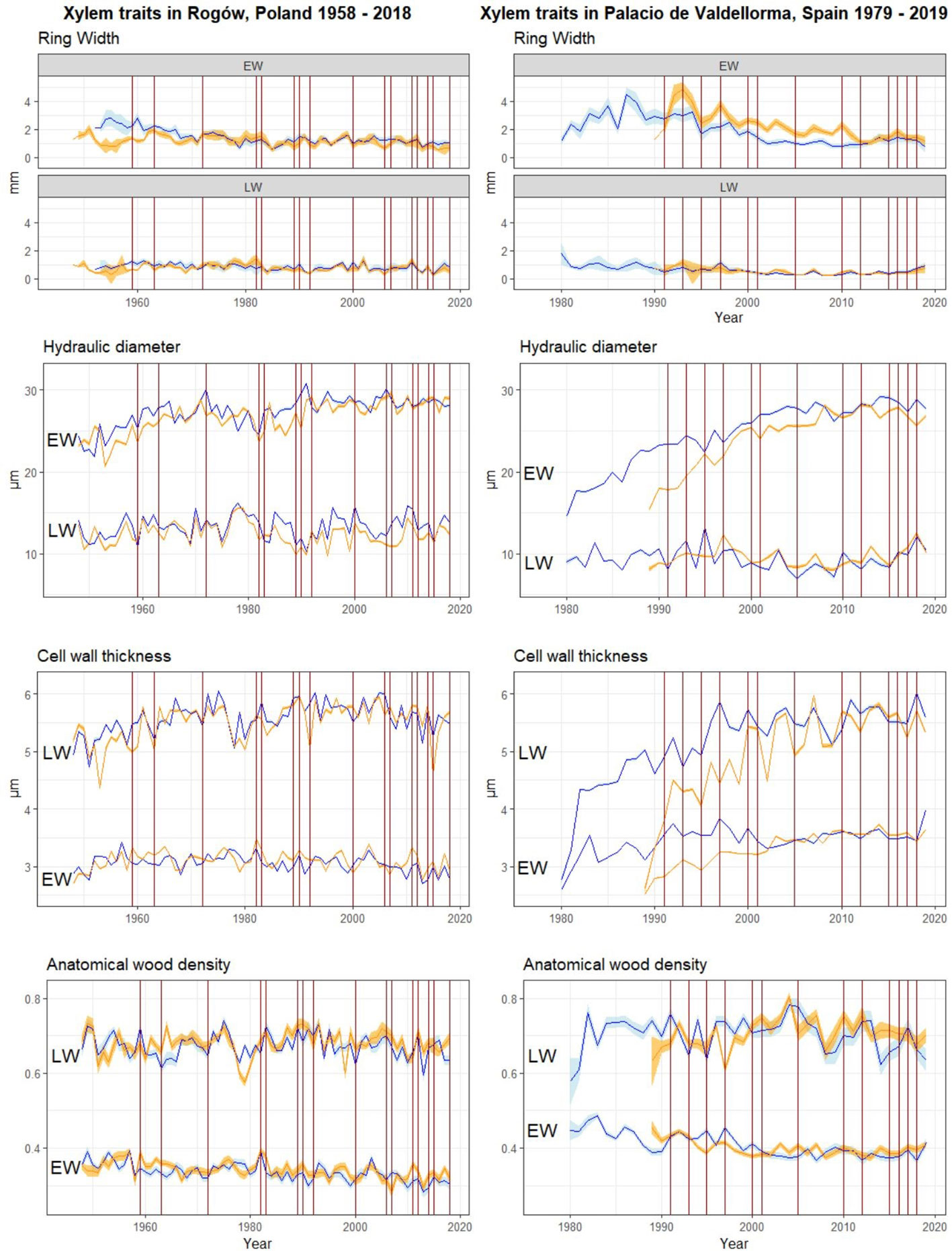
Figure 4 Raw chronologies of EW and LW traits. Orange lines, P. sylvestris in mixed forest; blue lines, P. sylvestris in pure forest. From top to bottom: EW and LW width, mean hydraulic diameter, cell wall thickness, and anatomical wood density. The vertical red lines indicate the years when drought occurred (cf. Figure 2). On the left panel, P. sylvestris in Rogów, Poland. On the right panel, P. sylvestris in Palacio de Valdellorma, Spain.
2.4.2 Intra-annual resolution analyses to detect drought effects between forest types and sites
To assess possible differences in intra-annual profiles of xylem traits between the two forest types, median values for each sector per tree were normalized by means of the minimum–maximum technique to account for individual tree variability and for the axial widening of xylem traits. Therefore, the minimum value of the wood anatomical trait profile of a given ring (x) gets converted to 0, whereas the maximum value gets converted to 1. Every remaining value gets converted into a decimal between 0 and 1 by means of the following formula:
An intra-annual profile per forest type, spanning the whole chronology, was obtained (Castagneri et al., 2020) by averaging the normalized values of each tree. Each intra-annual profile was smoothed with a loess function to reduce high-frequency variability and plotted with its confidence interval set at 95%. The non-parametric Komolgorov–Smirnov test was used to detect differences in the distribution of intra-annual xylem traits during drought between pines growing in pure and mixed forests in both sites. Drought years were identified by SPEI, calculated at 3-month resolution, starting from March to October, when the index started showing values below −1.5. In addition, intra-annual profiles of wood anatomical traits during drought and during non-drought were obtained by averaging the intra-annual drought year profiles and the non-drought ones. The Gini coefficient was computed to assess the degree of inequality of intra-annual xylem trait profiles during drought and non-drought periods. The Gini coefficient measures the inequality of a distribution varying between 0 to 1, where 0 represents perfect congruence and 1 represents the perfect incongruence (Biondi and Qeadan, 2008). Confidence intervals were calculated using the percentile, bias-corrected, and accelerated bootstrapping procedures.
Finally, to detect possible legacy effects on xylem traits related to drought events, intra-annual xylem trait profiles per forest type were obtained before, during, and after the year affected by drought. These profiles were obtained by averaging the intra-annual wood anatomical trait profiles 1 year before drought occurrence, the drought year, and the year after the drought event. Loess fit intra-annual profiles of wood anatomical traits were plotted 1 year before, during, and 1 year after the drought. Possible significant differences between xylem trait profiles of pines growing in pure and mixed forests at both sites were evaluated by applying the Kolmogorov–Smirnov test.
3 Results
3.1 Sensitivity of EW-LW anatomical traits to climate
The descriptive statistics of non-detrended wood anatomical traits in pure and mixed P. sylvestris stands are summarized in Table 3. In the correlation analyses, AD showed the highest responsiveness and was the best discriminant between forest types and sites. In the Polish site, temperature and SPEI strongly influenced EW traits, whereas LW traits also showed correlations with precipitation. In the Spanish site, all climatic variables were correlated with xylem traits (Figures 5A, B; for more statistical results, see Table S1 in Supplementary Materials).
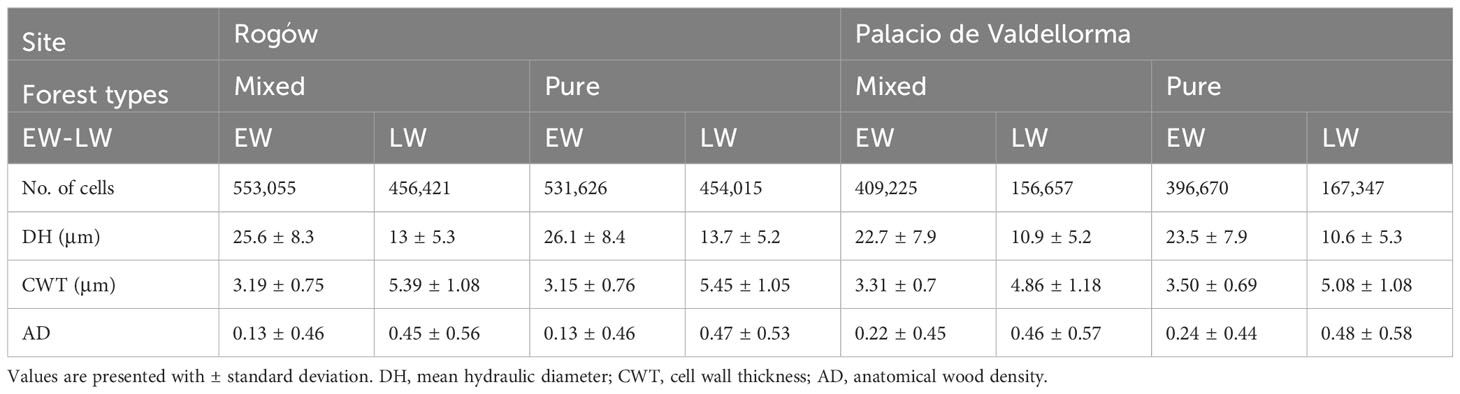
Table 3 Descriptive statistics of raw wood anatomical traits grouped by site, forest type, and earlywood/latewood.
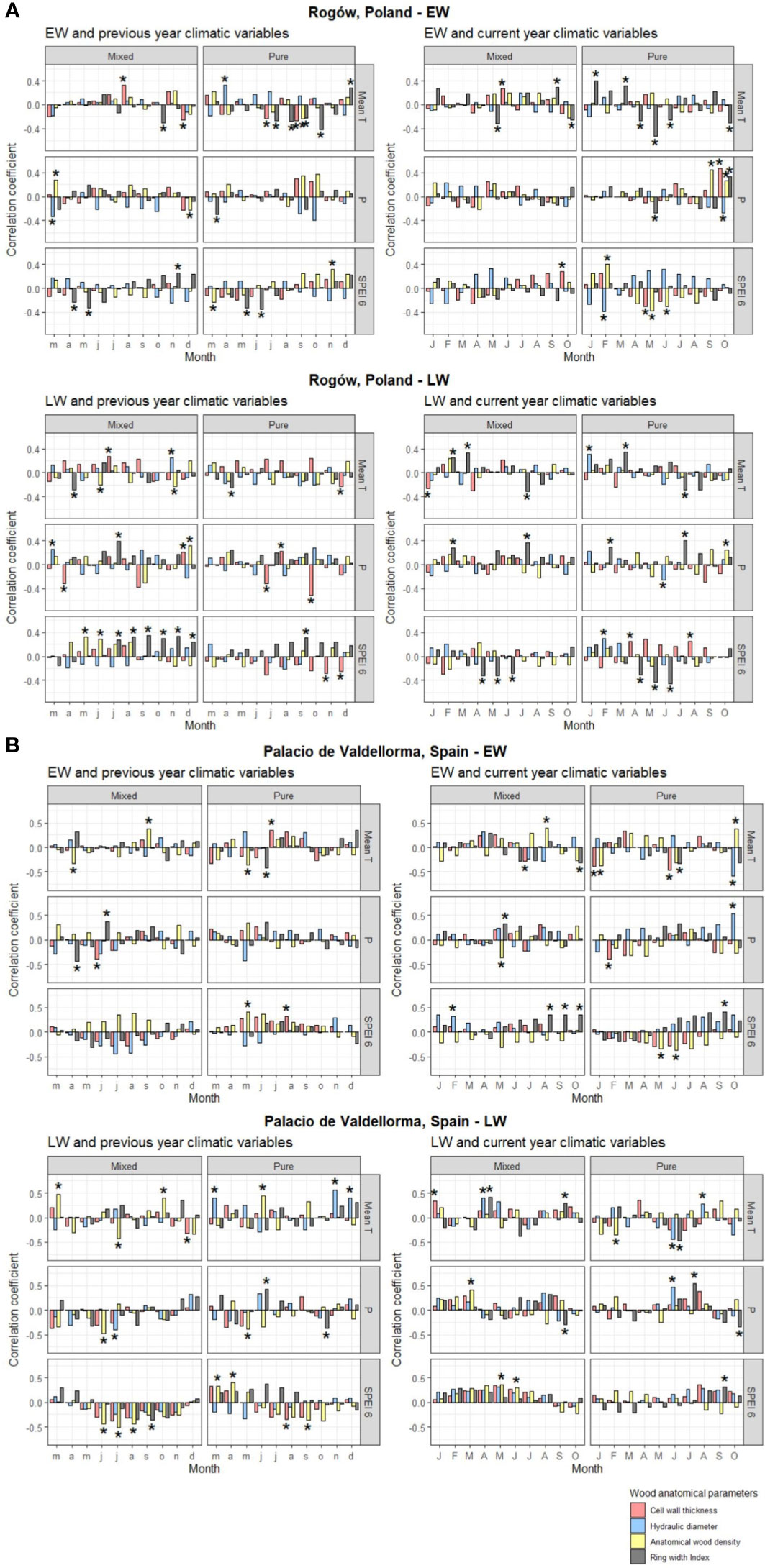
Figure 5 Pearson’s correlation for pines growing in Rogòw, Poland (A), and Palacio de Valdellorma, Spain (B), between monthly mean temperature, monthly precipitation, and Standardized Precipitation Evapotranspiration Index calculated at 6-month resolution (SPEI averaged within the previous 6 months starting from the month considered) of the previous year March to December (left) and current year January to October (right), and indexed chronologies of ring width, mean hydraulic diameter, cell wall thickness, and anatomical wood density of P. sylvestris in pure and mixed forest. The top panel shows correlations with EW traits and bottom panel with LW traits. Correlations were performed with the dcc function from “treeclim” R package, where the coefficients are univariate estimates of Pearson’s product moment correlation. The height of the bars represents the correlation coefficient, the bootstrap method applied provides confidence intervals, and the significance of the correlation is indicated by asterisks when p< 0.05. Time span considered for the analysis for the pines at the Polish site: 1958 –2018; for pines at the Spanish site: 1990– 2018.
3.1.1 Inter-annual responses of EW to climate
Pines in pure forest stands presented similar responses to a set of climatic variables in both sites indicating that, when droughts occurred in May to June, trees produced EW with higher AD (and increased carbon investment into CWT in Poland). EW traits of such pines showed stronger correlations with previous and current year’s temperatures (and current SPEI in the case of Poland) compared with pines growing in mixed stands (Figures 5A, B). In pure pine stands, previous May to July temperatures decreased EW width in both sites. They also reduced CWT and AD in trees growing in Polish (July) and Spanish (May) sites, respectively (Figures 5A, B).
3.1.2 Inter-annual responses of LW to climate
Tree admixture minimally influenced LW traits, which showed similar responses between sites and forest types. As an example, CWT and AD negatively correlated with previous April and July precipitation (mixed and pure forest, respectively) in the Polish site and with previous June and May precipitation (mixed and pure forest, respectively) in the Spanish site (Figures 5A, B). LW traits also showed different responses in each site, independent of tree admixture. In Poland, at both forest types, current February/July precipitation and temperature increased LW width. Conversely, it was reduced by the previous April/current July temperature and by previous summer droughts (Figure 5A). In the Spanish site, in both forest types, AD in LW increased following previous summer droughts (in pure forest also CWT; Figure 5B).
3.1.3 Site-dependent EW and LW responses to climate
The following different results in climate correlation analyses between forest types highlighted the role of tree admixture in pine response to local climate. In the Polish site, only in the pure pine stands, EW width increased with temperature in January and March, whereas higher April to May and June temperature reduced it (Figure 5A).
In the Spanish site, xylem trait–climate associations were less dependent on admixture and stand density. The EW CWT and AD negatively correlated with precipitation for pines growing in pure (January) and in mixed forest (May), respectively. In the latter, EW width increased with May precipitation. In both forest types, a rise in previous April/May temperature reduced EW AD, whereas higher temperature in July and September increased carbon investment into CWT (pure forest) and coincided with an increase in AD (mixed forest; Figure 5B). Previous rise in November/December temperature favored larger DH in LW in the pure pine forests and was related to a decrease in CWT in LW in pines in mixed forest. Previous summer droughts increased AD in LW in both forest types (also CWT for pure forest pine). AD was reduced in LW for pines in pure forest when drought occurred during previous March/April and similarly for pines in mixed forest, when drought occurred during current May/June (Figure 5B).
3.2 Analyses of intra-annual wood anatomical profiles
3.2.1 Intra-annual responses of wood anatomical traits to drought
At the Polish site, severe drought events occurred in the following years: 1959, 1963, 1972, 1982, 1983, 1989, 1990, 1992, 2000, 2006, 2007, 2011, 2012, 2014, 2015, and 2018; at the Spanish site: 1991, 1993, 1995, 1997, 2000, 2001, 2005, 2010, 2012, 2015, 2016, 2017, and 2018 (cf. Figure 2). The intra-annual profiles of wood anatomical traits in pure and mixed forests partly overlapped for most of the years in both sites, showing no evident pattern linked to tree admixture (Polish and Spanish sites, Figures S1A–C; Poland and Spain, Figures S2A–C; Supplementary Materials). Trees grown at the Spanish site showed age differences; therefore, we plotted intra-annual profiles following their ontogenesis (Figures S3A–C). Pine profiles growing in the two forest types showed no difference. A Kolmogorov–Smirnov test applied to the years characterized by drought highlighted significant differences between pines growing in the two forest types but no consistent pattern among forest types and sites (Figures S1A–C, S2A–C).
Table 4 summarizes the Gini coefficients per intra-annual profiles aggregated by sites, forest types, and drought events. At the Polish site, the Gini coefficient did not reveal significant differences in variability between the profiles. At the Spanish site, the Gini coefficient did not highlight differences in wood anatomical traits except for ring width index (RWI). During the absence of drought, pines in mixed forests showed lower Gini coefficients compared with pines in pure forests. On the contrary, during drought, pines in pure forests showed lower Gini coefficients compared with pines in mixed forests (Figure 6).
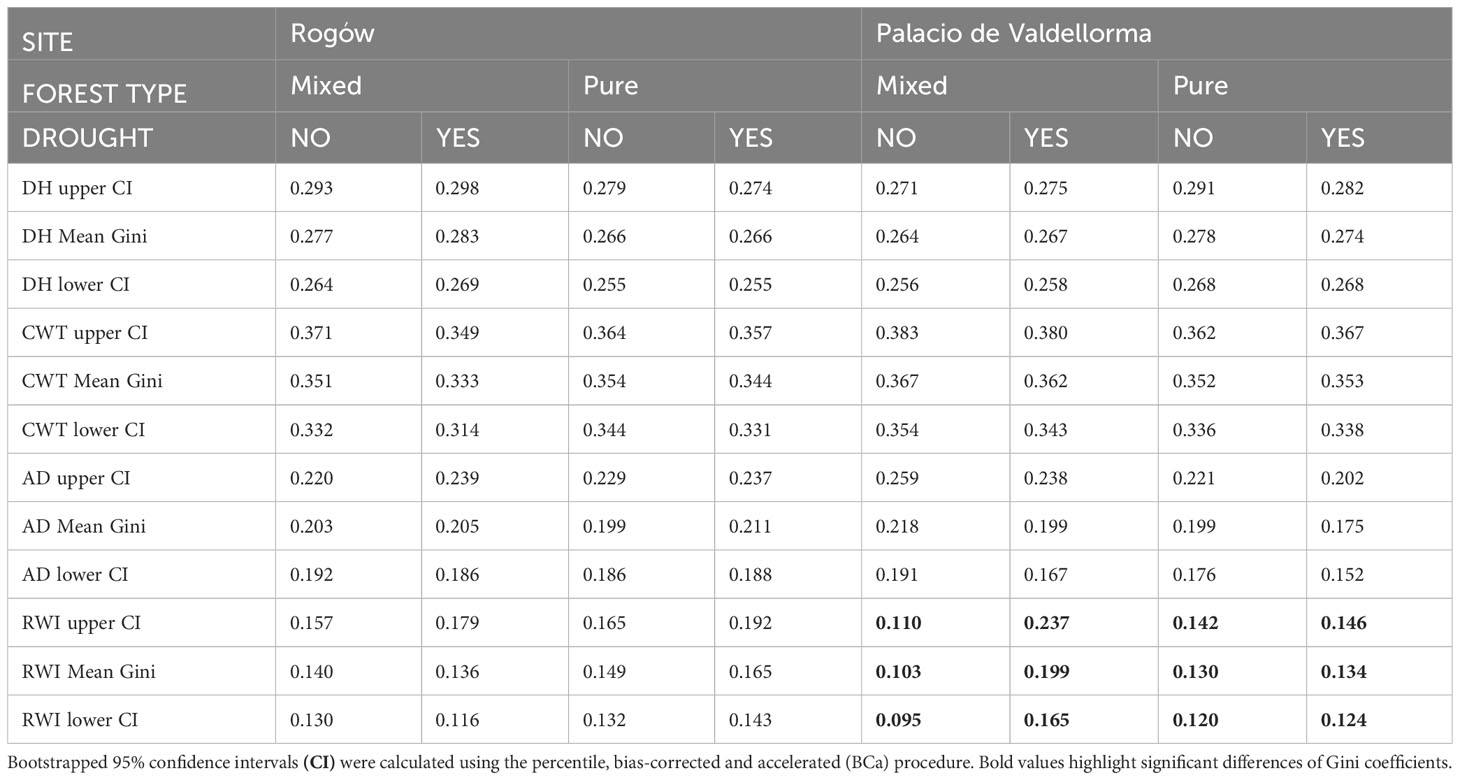
Table 4 Gini coefficient calculated for mean hydraulic diameter (DH), cell wall thickness (CWT), anatomical wood density (AD), and ring width index (RWI) in mixed and pure forests during years with absence of drought (NO) and drought conditions (YES).
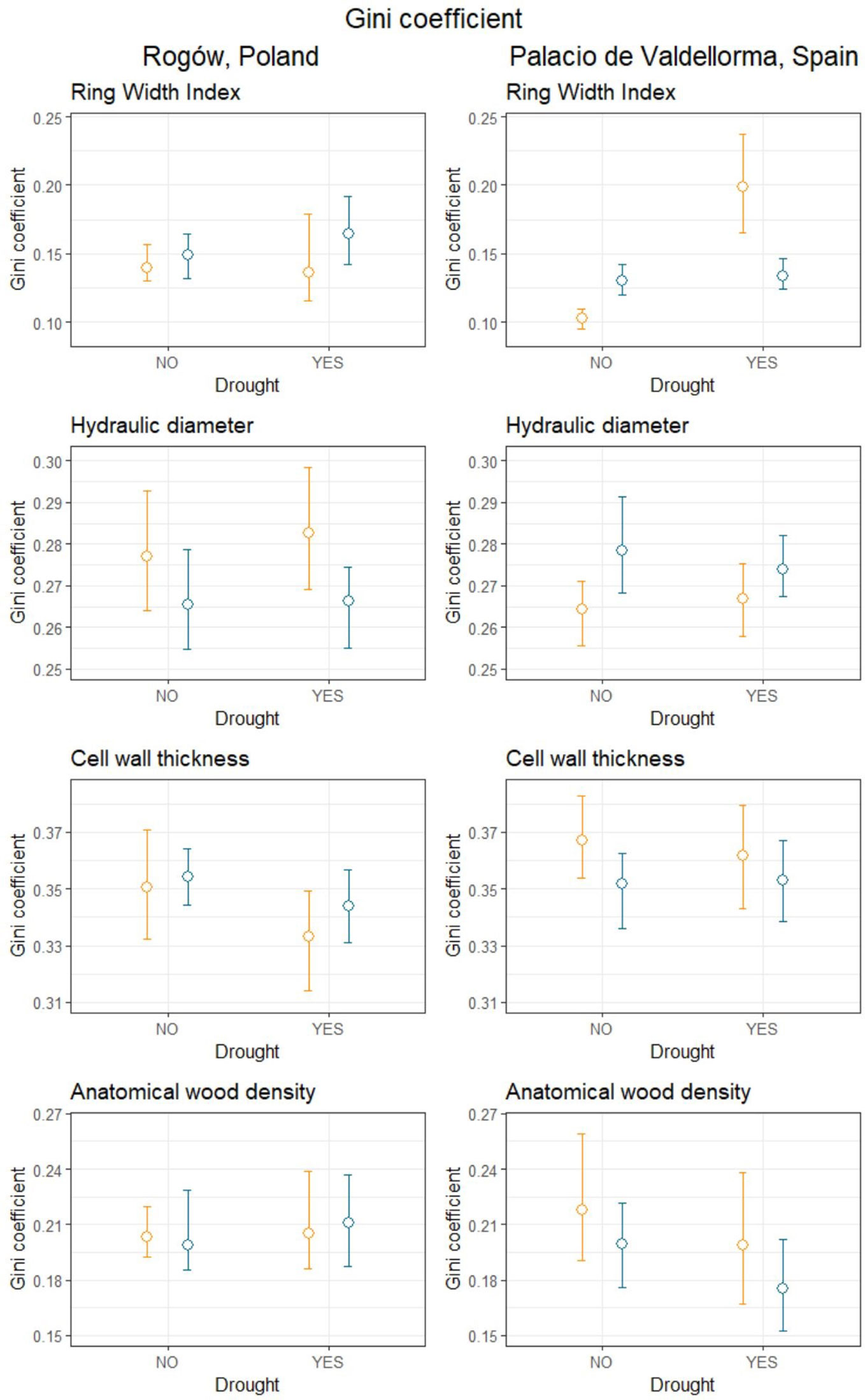
Figure 6 Gini coefficient calculated for mean hydraulic diameter, cell wall thickness, anatomical wood density and ring width index during years when drought occurred (YES) and during years characterized by absence of drought (NO), divided into mixed (orange) and pure (blue) forests, in Poland (left panel) and in Spain (right panel). Empty circles represent the mean Gini coefficient, and the upper and lower bound represent the bootstrapped 95% confidence intervals calculated using the percentile, bias-corrected and accelerated (BCa) method.
3.2.2 Drought legacy effects on intra-annual wood anatomical traits
The intra-annual analyses of xylem trait profiles conducted 1 year before, during, and 1 year after drought events revealed AD to be a fundamental trait showing contrasting legacy effects related to drought events for the two forest types (Figure 7; Table S2 in Supplementary Materials).
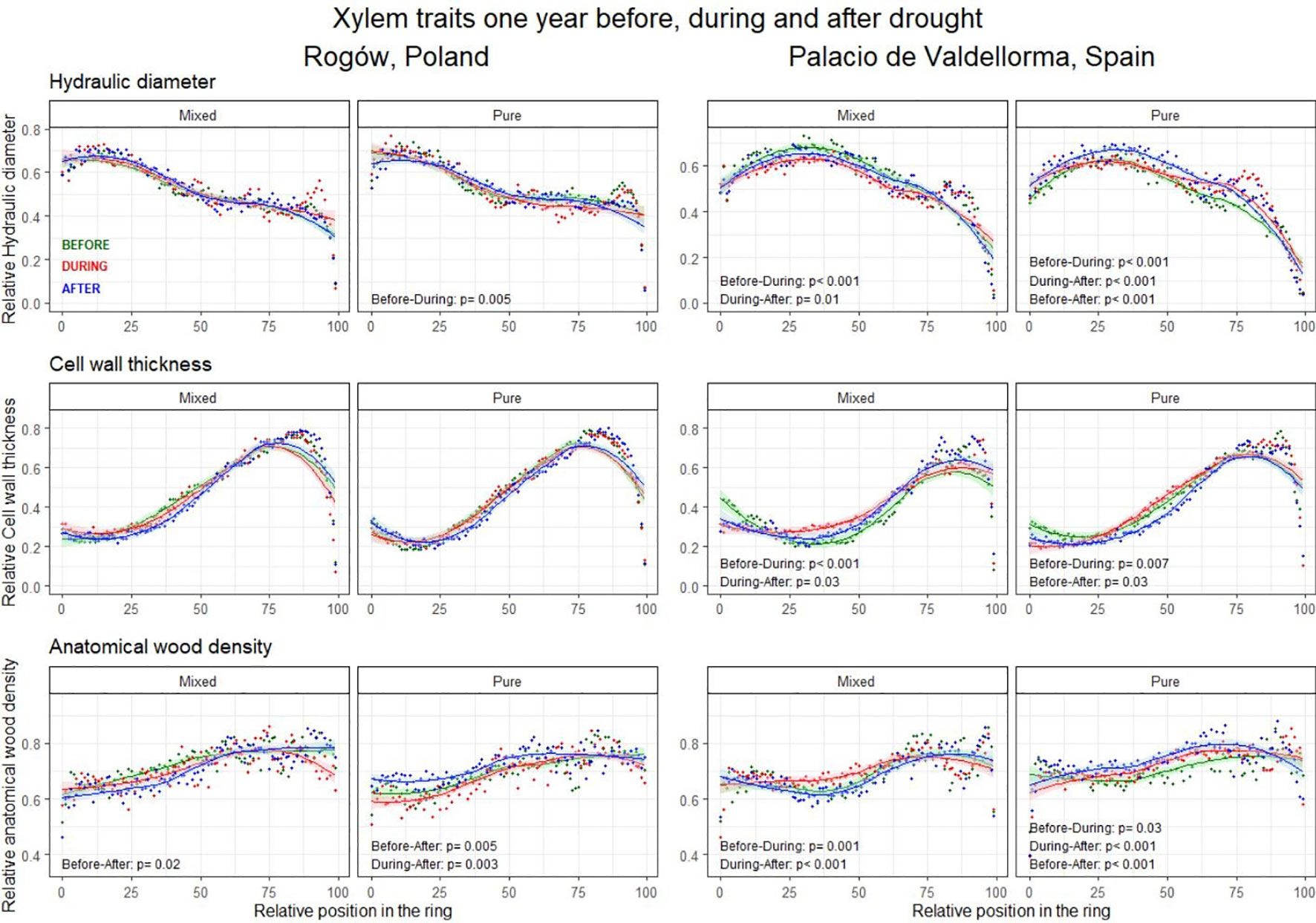
Figure 7 Intra-annual profiles of mean hydraulic diameter (top), cell wall thickness (middle), and anatomical wood density (bottom) aggregated per drought occurrences. The green lines represent the intra-annual profile 1 year before the drought, the red lines during the drought, and the blue lines 1 year after the drought. Possible differences between wood anatomical trait profiles are highlighted with the Kolmogorov–Smirnov test and presented when significant (p< 0.05).
In pure pine stands, on both sites, AD increased significantly 1 year after the drought compared with the pre-drought profile (Polish site, p = 0.005; Spanish site, p< 0.001) and to the during-drought profile (Polish site, p = 0.003; Spanish site, p< 0.001). Conversely, in mixed pine stands, AD decreased significantly 1 year after the drought compared with the pre-drought profile and to the year when drought occurred (p = 0.02 and p< 0.001, Polish and Spanish sites, respectively).
At the Polish pure pine stand, the DH profile decreased significantly during drought compared with the pre-drought profile (p = 0.005). CWT profiles did not present significant differences.
At the Spanish site, the DH profiles in the two forest types differed. In the mixed pine forest, they decreased significantly during drought compared to the previous year (p< 0.001). In the pure pine stand, they showed the opposite trend, although the increase was only significant in the last sectors of the ring. In pure pine stands, DH showed a further increase 1 year after the drought compared with drought and pre-drought conditions (p< 0.001). In both forest types, CWT profiles increased during drought compared with the previous year (p< 0.01). CWT decreased significantly 1 year after the drought compared with drought and pre-drought conditions (p = 0.03; in mixed and pure forests, respectively). In addition, AD increased during drought compared with the pre-drought profile in both forest types (pure forest, p = 0.001; mixed forest, p = 0.03; Figure 7).
4 Discussion
We aimed to understand whether tree admixture influences P. sylvestris responses to climatic variability and drought events, under contrasting climatic conditions, namely, Continental versus Mediterranean. Considering previous studies, ring width usually decreased during drought events, because the carbon sequestration is considerably reduced during drought (Bréda et al., 2006; Brunner et al., 2015), leading to the formation of narrow rings. On the basis of our results, ring width was not directly affected by the observed droughts, and it did not show a consistent decrease in response to these events (Figure 4). Under such conditions, xylem traits provide higher-sensitivity and higher-resolution information on ecophysiological responses to climatic variability and drought, a feature often lacking in the most common studies based on ring-width proxies (Carrer et al., 2014).
At both sites, mixed and pure forest stands were very close to each other. Consequently, elevation and exposition, soil composition, and texture are homogeneous factors that do not represent sources of variability influencing tree responses to climate. At the Polish site, tree age and degree of tree competitiveness (measured with the Stand Density index) were comparable between forest types, and we discarded them as sources of variability (Tables 1, 2). At the Spanish site, pine trees in pure stands were older than in mixed stands (36 and 23 years old, respectively; Table 2) and were in the juvenile growth phase, which usually feature significantly different growth dynamics and climate responses than older trees (Carrer and Urbinati, 2004). Concerning xylem traits, they are known to be “size-dependent” rather than “age-dependent” (Anfodillo et al., 2013). In our study, tree height varies (Table 2), and standardization techniques that we applied are prerequisites to accurately interpret and compare xylem traits (Carrer et al., 2014). Regarding the Spanish site, the Stand Density Index was significantly higher in pure stands than in mixed stands (Table 1). In this case, a different level of competition between forest types did not produce a univocal correlation of the results to the tree admixture.
4.1 Similar wood anatomical trait responses to climate and drought at both sites
Our findings showed that, at both sites, EW traits correlated strongly with previous-year climatic conditions, especially temperature, in accordance with previous studies (Martin-Benito et al., 2013; Pellizzari et al., 2016; Björklund et al., 2017), likely due to the role of photoassimilates accumulated during the previous vegetative season (Kagawa et al., 2006; von Arx et al., 2017).
Overall, pines growing in pure forest showed a stronger correlation with temperature compared with pines in mixed stands, which, in turn, showed lower sensitivity to temperature variability (Figures 5A, B). This reduced sensitivity could suggest increased resistance of mixed-forest pines to climate variability (Chmura et al., 2011; Magruder et al., 2013; Thurm et al., 2016), which could be related to higher structural heterogeneity, increased canopy plasticity, and extended canopy occupation compared to monospecific stands (Pretzsch et al., 2015). Canopy cover is a key factor influencing forest microclimate by buffering low and high temperature extremes, and its effects are more pronounced during the vegetative season (Greiser et al., 2018). Only few studies considered the effects of different canopy cover on forest microclimate. Different temperatures were observed in pure pine versus pine-broadleaved stands (Porté et al., 2004; Perot et al., 2021). In the latter study, substantial differences were observed regarding tree phenology due to a different temperature perception experienced by trees in mixed forest.
Our results suggested that, in mixed forest, more stable microclimatic conditions occurred, and climatic resistance of trees improved, confirming our first hypothesis stating that P. sylvestris in mixed forest shows lower climate sensitivity. This indicates that mixed P. sylvestris forests are desirable in the face of climate change to buffer against the climatic extremes that are projected for the near future.
Despite the limited plasticity of EW traits in response to current climatic conditions, they are known to strongly depend on water availability, especially tracheid size (Bryukhanova and Fonti, 2013; Cabon et al., 2020). When drought occurs during spring, cavitation can impair EW functionality, which is the main tissue responsible for water transport (Martín et al., 2010). The sensitivity of P. sylvestris to spring drought was reported under both climatic conditions –Continental and Mediterranean– considered in this study (Camarero et al., 2011; Michelot et al., 2012). At both our sites, late-spring drought increased the current AD (in Polish site, also CWT) in EW in pure forest, whereas pines in mixed forest did not respond in these parameters (Figures 5A, B). Intra-annual wood anatomical trait analyses conducted to assess drought legacy effects on pines consistently revealed that pines growing in pure forest produced denser wood 1 year after the drought (Figure 7). These adjustments, performed by pines growing in pure forest, generated a safer wood with reduced cell implosion and thus cavitation risk (Hacke et al., 2001). They also indicated higher sensitivity to drought and legacy effects, which can remain visible for several years in wood anatomical traits (Pacheco et al., 2018a; Castagneri et al., 2020). Different competitive ability between trees growing in pure and mixed forests emerged during stressful events such as during spring drought (Paquette and Messier, 2011; Pretzsch et al., 2013b). This led to a harsher level of competition for pines growing in pure forest but ameliorated conditions for pines growing in mixed forest. Indeed, the latter presented a reduction in AD 1 year after the drought, showing no legacy effects (Figure 7). During drought periods, in mixed forests, contrasting water-use strategies displayed by the two tree species could have been improving the efficiency of water use. Complementarity in root plasticity and distribution could increase nutrient availability and uptake (Pretzsch et al., 2013b; Bello et al., 2019). Canopy interaction and complementarity are thought to produce lower competition for light (Pretzsch et al., 2020) and alter microclimatic conditions by shading and by reducing evapotranspiration (van Loon et al., 2014; Hatfield and Dold, 2019); these processes could be improving conditions for pines in mixed stands. In those pines, carbon resources could be allocated in other tree compartments, i.e., the canopies (Merlin et al., 2015), promoting needle elongation and primary growth, likely increasing tree competitiveness (Matisons et al., 2019). Such processes occur during late spring and could be impeded or slowed down by spring drought (Richardson, 2000).
At the Spanish site, the pure forest stands were denser than the mixed forest stands. Higher density could imply that pure pine stands could experience a higher level of competition exacerbated by drought stress. Indeed, our results showed higher sensitivity to drought of pines growing in pure stands compared with mixed stands. This makes it difficult to interpret the results univocally, and, although they would be in line with our expectation, the higher sensitivity to drought seen in pines growing in pure stands at the Spanish site cannot be attributed only to tree admixture as a single cause but also to stand density. Hence, our first hypothesis stating that P. sylvestris growing in pure stands will present higher xylem modifications in response to drought events compared with P. sylvestris growing in admixtures with Quercus sp. can only be partially confirmed.
LW traits showed similar correlations with climatic variables at both sites and in both forest types. Pine responded to previous spring and summer precipitation without allocating carbon resources to reinforce the hydraulic system, showing a decrease in CWT and in AD. This is in line with previous studies (Björklund et al., 2017; Știrbu et al., 2022), and it indicates the presence of a time lag in the influence that previous-year climatic conditions have on LW traits, despite their high responsiveness to current climatic conditions (Eilmann et al., 2006; Seo et al., 2008; Martin-Benito et al., 2013; Cuny and Rathgeber, 2016). These results also showed that P. sylvestris LW traits respond in similar ways to spring and to summer precipitation, thereby presenting precise regulatory mechanisms, which likely integrate local genetic adaptation and phenotypic plasticity into a coordinated response to climate; despite the different provenances of the trees, the local climate, and the different stand density at the Spanish site.
Correlation with climatic variables, coupled with the trend of the intra-annual wood anatomical profiles, where most changes occurred in the first two/third sectors of the ring, indicated a negligible effect of tree admixture when droughts occurred during the late growing season. This indicates that the benefits of inter-specific facilitation mechanisms become relevant during the first part of the growing season and are mainly visible in EW traits and during stressful events, such as spring drought. This inference is coherent with the stress gradient hypothesis stating that facilitation mechanisms could be more evident under harsher conditions such as under resource limitation (Callaway and Walker, 1958; Paquette and Messier, 2011; Pretzsch et al., 2013b). Our findings suggested that inter-specific facilitation mechanisms are likely influenced by the phenology of the species considered (Merlin et al., 2015), a factor that was neglected in the most studies that investigated the effect of tree admixture on tree performance (Del Río and Sterba, 2009; Pretzsch et al., 2013a; Bielak et al., 2014; Pretzsch et al., 2015; Thurm et al., 2016; Zeller et al., 2017; Pretzsch et al., 2020).
4.2 Site-dependent wood anatomical trait response to climate variability and drought
Cell enlargement tends to vary significantly with species and with site conditions and is highly sensitive to water stress (Cabon et al., 2020). At the Polish site, small variations of DH were reported (Figures 5A, 7). This suggested that the site was not water-limited and that P. sylvestris wood anatomical traits were mainly under the influence of temperature, as assessed by several studies on conifer species in temperate climate (Seo et al., 2008; Liang et al., 2013; Björklund et al., 2017). Temperature influences many physiological processes, from photosynthate availability to wall thickening (Cuny and Rathgeber, 2016) for which it is considered a fundamental driver (Körner, 2003; Cuny et al., 2015). In Central Poland, high winter/late-winter temperatures positively influence P. sylvestris growth, lengthening the growing season (Koprowski, 2012; Misi and Náfrádi, 2016; Misi et al., 2019; Giberti et al., 2022). In our study, we found interesting local differences driven by the influence of tree admixture on xylem traits. Only pure-stand P. sylvestris EW width correlated positively with temperatures in January and March. On the contrary, pines growing in mixed stands did not show such correlations (Figure 5A). In mixed forests, during winter and late winter, we expect slightly higher air temperature: given the absence of oak leaves, the sunlight penetrates more efficiently in comparison with closed canopies of the pure P. sylvestris forests. Increase in winter temperatures presumably provided P. sylvestris in the pure stands with an amount of energy sufficient to anticipate and lengthen the growing season. Conversely, LW width presented similar correlations in both forest types. It was positively correlated with precipitation in spring and summer, and negatively correlated with current temperature and drought, in line with previous studies (Liang et al., 2013; Martin-Benito et al., 2013; Matisons et al., 2019), re-confirming the negligible effect of tree admixture on LW traits.
At the Spanish site, xylem traits responded similarly to the climatic variables for both forest types, despite the different tree admixture and the different stand density (Figure 5B). Under a Mediterranean climate, water availability in summer is a primary tree growth limiting factor (Bogino et al., 2009; Martin-Benito et al., 2013; Cuny et al., 2015), and higher sensitivity is expected toward increased summer temperatures that can eventually become associated to seasonal drought. This is also what we observed in our case, where the previous summer temperature increased carbon investment into CWT (pure forest), and increased AD (mixed forest) in EW. Carbon investment into CWT was reduced following precipitation, showing adjustments that reduced hydraulic safety (Hacke and Sperry, 2001; Hacke et al., 2001; Figure 5B). Analyses of wood anatomical trait profiles of pines for both forest types showed that AD and CWT increased during drought, as observed in previous studies (Hacke and Sperry, 2001; Hacke et al., 2001; Desoto et al., 2011; Hereş et al., 2014; Pellizzari et al., 2016, Figure 7), probably improving the safety of the hydraulic system against cavitation risks. Understandably, pines in mixed forests also showed a smaller DH during drought events, whereas pure pine forests showed an increase in DH during drought. The maximization of hydraulic efficiency during drought, at the risk of cavitation, was also observed in pines in previous studies (Eilmann et al., 2009; Eilmann et al., 2011; Martin-Benito et al., 2013; Martin-Benito et al., 2017; Petrucco et al., 2017; Candel-Pérez et al., 2018; Guérin et al., 2020; Buras et al., 2023). However, a closer inspection of the intra-annual DH profile revealed that this trait increased only in the last portion of the ring (Figure 7). Pinus sylvestris growing under Mediterranean and Sub-Mediterranean climates shows secondary growth extended until October (Camarero et al., 2011; Prislan et al., 2016). In case of summer drought, a larger lumen and prolonged cambial activity could supply P. sylvestris hydraulic efficiency at the end of summer or early autumn, when better conditions in terms of temperature and water availability occur.
4.3 Intra-annual wood anatomical trait responses to drought at both sites
According to our second hypothesis, we expected harsher conditions in terms of drought at the Spanish site, which is located under a Mediterranean climate at the southern margin of P. sylvestris’ range. Consequently, we expected higher xylem trait variability for P. sylvestris trees, given the recurring drought that they are subject to. We could not confirm this hypothesis because we did not find evidence of higher xylem trait variability in pines in Spain (Table 4; Figure 6). In addition, we did not find consistent patterns at both sites suggesting that drought induces higher variability in xylem traits. Significant changes were reported at the Spanish site, where trees growing in mixed forests showed less constant growth rates (RWI) during drought than pines in pure forest, whereas the opposite occurred in the absence of drought; no differences were reported regarding other traits. Higher xylem trait variability was found in previous studies in dying or dead trees, in comparison with surviving ones after drought stress (Levanič et al., 2011; Hereş et al., 2014). During our study, we selected healthy and dominant trees to reduce the variability related to abiotic and biotic conditions; we chose sites with no evident limiting factors trying to isolate the sole effects of climate and tree admixture on pines.
Similar responses to climate were observed in pure pine xylem traits to both our sites. The influence of tree admixture was prominent in pines growing at the Polish rather than the Spanish site. The sampling strategy conducted at the Polish site allowed us to attribute xylem trait change between forest types to tree admixture as the sole factor, whereas, for the Spanish site, stand density could also play a role. Despite these sources of variability, pines growing at the Spanish site showed similarities in climate and drought responses, which could eventually indicate limited phenotypic plasticity. In the work of Richter et al. (2012), the Continental and Mediterranean populations of P. sylvestris were subject to water deficit in a common garden experiment. The authors reported reduced phenotypic plasticity in the southern P. sylvestris populations and hypothesized that southern provenance showed limited plasticity as a strategy to cope with the stressful environment where water deficit is recurrent. In our case, xylem trait adjustment was of modest magnitude at both sites, suggesting moderate or non-stressed conditions perceived by the trees (Martin-Benito et al., 2013).
Signs of tree mortality can be visible in xylem traits far in advance of the actual death (Pellizzari et al., 2016). Long-term risk of dieback following multiple single drought events may occur with the increase in severity and frequency of droughts (Petrucco et al., 2017; Dai et al., 2018). In this context, our findings provide interesting insights on xylem trait adjustments toward climatic variability and drought and the role that tree admixture plays in this context. For future studies, we recommend including suppressed and dying trees after drought stress along different stand density levels, to fully understand the role of tree admixture in modulating xylem traits and to investigate possible changes in inter-specific facilitation effects.
5 Conclusion
This study was carried out to understand whether tree admixture can influence wood anatomical trait responses to climatic variability and drought events. Among the wood anatomical traits considered, AD, being the synthesis of the cell wall and the lumen area, was one of the most sensitive traits to detect climate variability and drought responses under different climatic conditions and forest types. This corroborates the relevance of this trait as a proxy in the context of dendro-anatomical studies (Castagneri et al., 2020).
We showed that tree admixture significantly influenced xylem responses to climatic variables, and exerted legacy effects detectable during the year following drought events. Our findings highlighted that inter-specific facilitation mechanisms that occurred in the admixture between P. sylvestris and Quercus sp. become relevant in the first part of the growing season, during stressful events such as spring droughts and detectable in EW traits. This was evident considering the Polish site, although further investigations are needed for the Spanish site to disentangle the role of tree admixture from the one of stand density. In contrast, LW traits showed similarities in both forest types and sites, and this suggested negligible effects of tree admixture during the late growing season. On the basis of our findings, we suggest that inter-specific facilitation mechanisms are likely modulated by the phenology that characterizes the species considered.
A closer inspection of the intra-annual variability of xylem traits showed the potential role that tree admixture has in modulating xylem traits response in the face of climatic variability and drought events. This gains importance when considering that signs of tree mortality in xylem traits can occur far in advance of the actual death of trees (Pellizzari et al., 2016). Future climatic projections foresee a northward migration of P. sylvestris, and its decline in Central Europe by the end of the century (Buras and Menzel, 2019). Our results suggested that P. sylvestris could be subject to harsher competition in monospecific stands than that in mixed stands with Quercus sp., and this is in agreement with the findings of previous studies. Under current climatic conditions, this admixture could be adopted as a solution for an adaptive management strategy to increase the resilience of P. sylvestris to drought events.
Data availability statement
The original contributions presented in the study are included in the article/Supplementary Material. Further inquiries can be directed to the corresponding authors.
Author contributions
GT and CW initiated the collaboration project. CW developed the research idea for this study. GT, CW, KB, and FB planned the sampling design and the research activity. KB and FB were responsible for the dendrometric measurements of the experimental sites in Poland and Spain. GSG, GT, CW, KB, and BdT performed sample campaigns. EU was responsible for the first dendrochronological analyses of the cores with LIGNOSTATION™. GSG produced the wood anatomical samples. GSG, MC, and LU worked on the anatomical image acquisition from samples. The quantitative wood anatomical analyses were performed by GSG supervised by GA, AG, CW, and BT. GSG wrote the first draft of the manuscript and elaborated tables and figures with the help of AG, CW, KB, BT, and GA. CW supervised the study. All authors contributed to the revision of the manuscript and approved the final version.
Funding
The CARE4C project has received funding from the European Union’s Horizon 2020 research and innovation program under the Marie Skłodowska-Curie grant agreement No 778322. This research was carried out under the PhD scholarship (Mountain Environment and Agriculture, XXXIV° cycle) funded by the Free University of Bozen-Bolzano. K B was additionally supported by the Polish Government MniSW 2018–2021 Matching Fund No. 117/H2020/2018. FB contribution has been partly funded by the Junta de Castilla y León (Spain) through the projects “CLU-2019-01 and CL-EI-2021-05 iuFOR Institute Unit of Excellence of the University of Valladolid” and co-financed by the European Regional Development Fund and by the Spanish Ministry of Science and Innovation through the project IMFLEX (PID2021-126275OB-C22) and co-financed by the European Union. This work was supported by the Open Access Publishing Fund of the Free University of Bozen-Bolzano.
Acknowledgments
We are grateful to all Carbon smart forestry under climate change (CARE4C) partners who allowed this work to be conducted; Haile Shebeshe Assefa and Francesco Giammarchi, Faculty of Agricultural, Environmental and Food Sciences, Free University of Bolzano – Bozen; Monika Bradatsch and William Dario Aguirre Ráquira, Chair of Forest Growth and Yield Science, Technical University of Munich; Marcin Czacharowski and Wojciech Ożga, Department of Silviculture, Warsaw University of Life Sciences-SGGW; Cristobal Ordóñez Alonso and Ana Martín Ariza, Sustainable Forest Research Institute, University of Valladolid and Nic Pacini, Department of Environmental Engineering, University of Calabria.
Conflict of interest
The authors declare that the research was conducted in the absence of any commercial or financial relationships that could be construed as a potential conflict of interest.
Publisher’s note
All claims expressed in this article are solely those of the authors and do not necessarily represent those of their affiliated organizations, or those of the publisher, the editors and the reviewers. Any product that may be evaluated in this article, or claim that may be made by its manufacturer, is not guaranteed or endorsed by the publisher.
Supplementary material
The Supplementary Material for this article can be found online at: https://www.frontiersin.org/articles/10.3389/fpls.2023.1213814/full#supplementary-material
References
Abatzoglou, J. T., Dobrowski, S. Z., Parks, S. A., Hegewisch, K. C. (2018). TerraClimate, a high-resolution global dataset of monthly climate and climatic water balance from 1958-2015. Sci. Data 5, 170191. doi: 10.1038/sdata.2017.191
Allen, C. D., Breshears, D. D., McDowell, N. G. (2015). On underestimation of global vulnerability to tree mortality and forest die-off from hotter drought in the Anthropocene. Ecosphere 6 (8), 1–55. doi: 10.1890/ES15-00203.1
Allen, C. D., Macalady, A. K., Chenchouni, H., Bachelet, D., McDowell, N., Vennetier, M., et al. (2010). A global overview of drought and heat-induced tree mortality reveals emerging climate change risks for forests. For. Ecol. Manage. 259 (4), 660–684. doi: 10.1016/j.foreco.2009.09.001
Anfodillo, T., Petit, G., Crivellaro, A. (2013). Axial conduit widening in woody species: a still neglected anatomical pattern. IAWA J. 34 (4), 352–364. doi: 10.1163/22941932-00000030
Anjos, L., Gaistardo, C. M., Deckers, J., Dondeyne, S., Eberhardt, E., Gerasimova, M., et al. (2014). World reference base for soil resources 2014 International soil classification system for naming soils and creating legends for soil maps. (Rome (Italy): FAO), JRC91947. Available at: https://publications.jrc.ec.europa.eu/repository/handle/JRC91947.
Beck, H. E., Zimmermann, N. E., McVicar, T. R., Vergopolan, N., Berg, A., Wood, E. F. (2018). Present and future köppen-geiger climate classification maps at 1-km resolution. Sci. Data 5, 180214. doi: 10.1038/sdata.2018.214
Beguería, S., Vicente-Serrano, S. M., Beguería, M. S. (2017). Package ‘spei.’ Calculation of the Standardised Precipitation-Evapotranspiration Index.
Bello, J., Hasselquist, N. J., Vallet, P., Kahmen, A., Perot, T., Korboulewsky, N. (2019). Complementary water uptake depth of Quercus petraea and Pinus sylvestris in mixed stands during an extreme drought. Plant Soil 437 (1–2), 93–115. doi: 10.1007/s11104-019-03951-z
Bielak, K., Dudzinska, M., Pretzsch, H. (2014). Mixed stands of scots pine (Pinus Sylvestris L.) and Europe spruce [Picea Abies (L.) karst] can be more productive than monocultures. Evidence from over 100 years of observation of long-term experiments. For. Syst. 23 (3), 573–589. doi: 10.5424/fs/2014233-06195
Biondi, F., Qeadan, F. (2008). Inequality in paleorecords. Ecology 89, 1056–1067. doi: 10.1890/07-0783.1
Biondi, F., Waikul, K. (2004). DENDROCLIM2002: A C++ program for statistical calibration of climate signals in tree-ring chronologies. Comput. Geosciences 30 (3), 303–311. doi: 10.1016/j.cageo.2003.11.004
Björklund, J., Seftigen, K., Schweingruber, F., Fonti, P., von Arx, G., Bryukhanova, M., et al. (2017). Cell size and wall dimensions drive distinct variability of earlywood and latewood density in Northern Hemisphere conifers. New Phytol. 216 (3), 728–740. doi: 10.1111/nph.14639
Björklund, J., Fonti, M. V., Fonti, P., Van den Bulcke, J., von Arx, G. (2021). Cell wall dimensions reign supreme: cell wall composition is irrelevant for the temperature signal of latewood density/blue intensity in Scots pine. Dendrochronologia 65, 125785. doi: 10.1016/j.dendro.2020.125785
Bogino, S., Fernández Nieto, M. J., Bravo, F. (2009). Climate effect on radial growth of Pinus sylvestris at its southern and western distribution limits. Silva Fennica 43 (4), 609–623. doi: 10.14214/sf.183
Bréda, N., Huc, R., Granier, A., Dreyer, E. (2006). Temperate forest trees and stands under severe drought: A review of ecophysiological responses, adaptation processes and long-term consequences. Ann. For. Sci. 63 (6), 625–644). doi: 10.1051/forest:2006042
Brunner, I., Herzog, C., Dawes, M. A., Arend, M., Sperisen, C. (2015). How tree roots respond to drought. Front. Plant Sci. 6. doi: 10.3389/fpls.2015.00547
Bryukhanova, M., Fonti, P. (2013). Xylem plasticity allows rapid hydraulic adjustment to annual climatic variability. Trees – Structure Funct. 27 (3), 485–496. doi: 10.1007/s00468-012-0802-8
Bunn, A., Korpela, M., Biondi, F., Campelo, F., Mérian, P., Qeadan, F., et al. (2023). dplR: Dendrochronology Program Library in R. R package version 1.7.5. Available at: https://CRAN.R-project.org/package=dplR.
Buras, A., Menzel, A. (2019). Projecting tree species composition changes of Eropean forests for 2061–2090 under RCP 4.5 and RCP 8.5 scenarios. Front. Plant Sci. 9. doi: 10.3389/fpls.2018.01986
Buras, A., Rehschuh, R., Fonti, M., Lange, J., Fonti, P., Menzel, A., et al. (2023). Enhanced drought vulnerability of forest-edge Scots pine trees indicated by quantitative wood anatomy and stable carbon isotopes. Front. For. Glob. Change. 6. doi: 10.3389/ffgc.2023.1233052
Cabon, A., Fernández-de-Uña, L., Gea-Izquierdo, G., Meinzer, F. C., Woodruff, D. R., Martínez-Vilalta, J., et al. (2020). Water potential control of turgor-driven tracheid enlargement in Scots pine at its xeric distribution edge. New Phytol. 225 (1), 209–221. doi: 10.1111/nph.16146
Callaway, R. M., Walker, L. R. (1958). Competition and facilitation: a synthetic approach to interactions in plant communities. Special Feature Ecol. 78 (7), 1958–1965. doi: 10.1890/0012-9658(1997)078[1958:CAFASA]2.0.CO;2
Camarero, J. J., Bigler, C., Linares, J. C., Gil-Pelegrín, E. (2011). Synergistic effects of past historical logging and drought on the decline of Pyrenean silver fir forests. For. Ecol. Manage. 262 (5), 759–769. doi: 10.1016/j.foreco.2011.05.009
Candel-Pérez, D., Lo, Y. H., Blanco, J. A., Chiu, C. M., Camarero, J. J., de Andrés, E. G., et al. (2018). Drought-induced changes in wood density are not prevented by thinning in scots pine stands. Forests 9 (1). doi: 10.3390/f9010004
Carrer, M., Urbinati, C. (2004). Age-dependent tree-ring growth responses to climate in Larix decidua and Pinus cembra. Ecology 85, 730–740. doi: 10.1890/02-0478
Carrer, M., von Arx, G., Castagneri, D., Petit, G. (2014). Distilling allometric and environmental information from time series of conduit size: The standardization issue and its relationship to tree hydraulic architecture. Tree Physiol. 35 (1), 27–33). doi: 10.1093/treephys/tpu108
Castagneri, D., Prendin, A. L., Peters, R. L., Carrer, M., von Arx, G., Fonti, P. (2020). Long-term impacts of defoliator outbreaks on larch xylem structure and tree-ring biomass. Front. Plant Sci. 11. doi: 10.3389/fpls.2020.01078
Caudullo, G., Welk, E., San-Miguel-Ayanz, J. (2017). Chorological maps for the main European woody species. Data Brief 12, 662–666. doi: 10.1016/j.dib.2017.05.007
Cheddadi, R., Vendramin, G. G., Litt, T., François, L., Kageyama, M., Lorentz, S., et al. (2006). Imprints of glacial refugia in the modern genetic diversity of Pinus sylvestris. Global Ecol. Biogeography 15 (3), 271–282. doi: 10.1111/j.1466-822X.2006.00226.x
Chmura, D. J., Anderson, P. D., Howe, G. T., Harrington, C. A., Halofsky, J. E., Peterson, D. L., et al. (2011). Forest responses to climate change in the northwestern United States: Ecophysiological foundations for adaptive management. For. Ecol. Manage. 261 (7), 1121–1142). doi: 10.1016/j.foreco.2010.12.040
Chojnacka-Ożga, L., Ożga, W. (2018). Air temperature anomalies in experimental forests in Rogów in 1924–2015. For Res. Pap 79, 37–44. doi: 10.2478/frp-2018-0005
Cuny, H. E., Rathgeber, C. B. K. (2016). Xylogenesis: Coniferous trees of temperate forests are listening to the climate tale during the growing season but only remember the last words! Plant Physiol. 171 (1), 306–317. doi: 10.1104/pp.16.00037
Cuny, H. E., Rathgeber, C. B. K., Frank, D., Fonti, P., Fournier, M. (2014). Kinetics of tracheid development explain conifer tree-ring structure. New Phytol. 203 (4), 1231–1241. doi: 10.1111/nph.12871
Cuny, H. E., Rathgeber, C. B. K., Frank, D., Fonti, P., Makinen, H., Prislan, P., et al. (2015). Woody biomass production lags stem-girth increase by over one month in coniferous forests. Nat. Plants 1, 1–6. doi: 10.1038/nplants.2015.160
Dai, A., Zhao, T., Chen, J. (2018). Climate change and drought: a precipitation and evaporation perspective. Curr. Climate Change Rep. 4 (3), 301–312). doi: 10.1007/s40641-018-0101-6
Del Río, M., Sterba, H. (2009). Comparing volume growth in pure and mixed stands of Pinus sylvestris and Quercus pyrenaica. Ann. For. Sci. 66 (5), 502–502. doi: 10.1051/forest/2009035
Denne, M. P. (1989). Definition of latewood according to Mork (1928). IAWA J. 10, 59–62. doi: 10.1163/22941932-90001112
Desoto, L., de la Cruz, M., Fonti, P. (2011). Intra-annual patterns of tracheid size in the mediterranean tree Juniperus thurifera as an indicator of seasonal water stress. Can. J. For. Res. 41 (6), 1280–1294. doi: 10.1139/x11-045
Eilmann, B., Weber, P., Rigling, A., Eckstein, D. (2006). Growth reactions of Pinus sylvestris L. and Quercus pubescens Willd. to drought years at a xeric site in Valais, Switzerland. Dendrochronologia 23 (3), 121–132. doi: 10.1016/j.dendro.2005.10.002
Eilmann, B., Zweifel, R., Buchmann, N., Fonti, P., Rigling, A. (2009). Drought-induced adaptation of the xylem in Scots pine and pubescent oak. Tree Physiol. 29 (8), 1011–1020. doi: 10.1093/treephys/tpp035
Eilmann, B., Zweifel, R., Buchmann, N., Graf Pannatier, E., Rigling, A. (2011). Drought alters timing, quantity, and quality of wood formation in Scots pine. J. Exp. Bot. 62 (8), 2763–2771. doi: 10.1093/jxb/erq443
Fonti, P., von Arx, G., García-González, I., Eilmann, B., Sass-Klaassen, U., Gärtner, H., et al. (2010). Studying global change through investigation of the plastic responses of xylem anatomy in tree rings. New Phytol. 185 (1), 42–53. doi: 10.1111/j.1469-8137.2009.03030.x
Forrester, D. I. (2014). The spatial and temporal dynamics of species interactions in mixed-species forests: From pattern to process. For. Ecol. Manage. 312, 282–292). doi: 10.1016/j.foreco.2013.10.003
Fritts, H. C. (1976). "The statistics of ring-width and climatic data," in Tree rings and climate, (London: Academic Press), 246–311. doi: 10.1016/B978-0-12-268450-0.50011-2
Gafenco, I. M., Pleșca, B. I., Apostol, E. N., Șofletea, N. (2022). Spring and autumn phenology in sessile oak (Quercus petraea) near the eastern limit of its distribution range. Forests 13 (7), 1125. doi: 10.3390/f13071125
Giberti, G. S., Wellstein, C., Giovannelli, A., Bielak, K., Uhl, E., Aguirre-Ráquira, W., et al. (2022). Annual carbon sequestration patterns in trees: A case study from scots pine monospecific stands and mixed stands with sessile oak in central Poland. Forests 13 (4), 582. doi: 10.3390/f13040582
Giorgi, F. (2006). Climate change hot-spots. Geophysical Res. Lett. 33 (8). doi: 10.1029/2006GL025734
González-González, B. D., García-González, I., Vázquez-Ruiz, R. A. (2013). Comparative cambial dynamics and phenology of Quercus robur L. and Q. pyrenaica Willd. in an Atlantic forest of the northwestern Iberian Peninsula. Trees 27, 1571–1585. doi: 10.1007/s00468-013-0905-x
Greiser, C., Meineri, E., Luoto, M., Ehrlén, J., Hylander, K. (2018). Monthly microclimate models in a managed boreal forest landscape. Agric. For. Meteorology 250–251, 147–158. doi: 10.1016/J.AGRFORMET.2017.12.252
Guérin, M., von Arx, G., Martin-Benito, D., Andreu-Hayles, L., Griffin, K. L., McDowell, N. G., et al. (2020). Distinct xylem responses to acute vs prolonged drought in pine trees. Tree Physiol. 40 (5), 605–620. doi: 10.1093/treephys/tpz144
Hacke, U. G., Sperry, J. S. (2001). Functional and ecological xylem anatomy. Perspect. Plant Ecology Evol. Systematics 4 (2), 97–115. doi: 10.1078/1433-8319-00017
Hacke, U. G., Sperry, J. S., Pockman, W. T., Davis, S. D., McCulloh, K. A. (2001). Trends in wood density and structure are linked to prevention of xylem implosion by negative pressure. Oecologia 126 (4), 457–461. doi: 10.1007/s004420100628
Hatfield, J. L., Dold, C. (2019). Water-use efficiency: Advances and challenges in a changing climate. Front. Plant Sci. 10. doi: 10.3389/fpls.2019.00103
Hereş, A. M., Camarero, J. J., López, B. C., Martínez-Vilalta, J. (2014). Declining hydraulic performances and low carbon investments in tree rings predate Scots pine drought-induced mortality. Trees - Structure Funct. 28 (6), 1737–1750. doi: 10.1007/s00468-014-1081-3
Kagawa, A., Sugimoto, A., Maximov, T. C. (2006). 13CO2 pulse-labelling of photoassimilates reveals carbon allocation within and between tree rings. Plant Cell Environ. 29 (8), 1571–1584. doi: 10.1111/j.1365-3040.2006.01533.x
Koprowski, M. (2012). Long-term increase of march temperature has no negative impact on tree rings of european larch (Larix decidua) in lowland Poland. Trees 26, 1895–1903. doi: 10.1007/s00468-012-0758-8
Körner, C. (2003). Carbon limitation in trees. J. Ecol. 91 (1), 4–17). doi: 10.1046/j.1365-2745.2003.00742.x
Levanič, T., Čater, M., McDowell, N. G. (2011). Associations between growth, wood anatomy, carbon isotope discrimination and mortality in a Quercus robur forest. Tree Physiol. 31 (3), 298–308. doi: 10.1093/treephys/tpq111
Liang, W., Heinrich, I., Simard, S., Helle, G., Liñán, I. D., Heinken, T. (2013). Climate signals derived from cell anatomy of scots pine in NE Germany. Tree Physiol. 33 (8), 833–844. doi: 10.1093/treephys/tpt059
Magruder, M., Chhin, S., Palik, B., Bradford, J. B. (2013). Thinning increases climatic resilience of red pine. Can. J. For. Res. 43 (9), 878–889. doi: 10.1139/cjfr-2013-0088
Martín, J. A., Esteban, L. G., de Palacios, P., Fernández, F. G. (2010). Variation in wood anatomical traits of Pinus sylvestris L. between Spanish regions of provenance. Trees - Structure Funct. 24 (6), 1017–1028. doi: 10.1007/s00468-010-0471-4
Martin-Benito, D., Anchukaitis, K. J., Evans, M. N., del Río, M., Beeckman, H., Cañellas, I. (2017). Effects of drought on xylem anatomy and water-use efficiency of two co-occurring pine species. Forests 8 (9). doi: 10.3390/f8090332
Martin-Benito, D., Beeckman, H., Cañellas, I. (2013). Influence of drought on tree rings and tracheid features of Pinus nigra and Pinus sylvestris in a mesic Mediterranean forest. Eur. J. For. Res. 132 (1), 33–45. doi: 10.1007/s10342-012-0652-3
Martínez-Vilalta, J., Cochard, H., Mencuccini, M., Sterck, F., Herrero, A., Korhonen, J. F. J., et al. (2009). Hydraulic adjustment of Scots pine across Europe. New Phytol. 184 (2), 353–364. doi: 10.1111/j.1469-8137.2009.02954.x
Martínez-Vilalta, J., Piñol, J. (2002). Drought-induced mortality and hydraulic architecture in pine populations of the NE Iberian Peninsula. For. Ecol. Manage. 161, 247–256. doi: 10.1016/S0378-1127(01)00495-9
Matisons, R., Krišāns, O., Kārkliņa, A., Adamovičs, A., Jansons, Ā., Gärtner, H. (2019). Plasticity and climatic sensitivity of wood anatomy contribute to performance of eastern Baltic provenances of Scots pine. For. Ecol. Manage. 452, 117568. doi: 10.1016/j.foreco.2019.117568
Merlin, M., Perot, T., Perret, S., Korboulewsky, N., Vallet, P. (2015). Effects of stand composition and tree size on resistance and resilience to drought in sessile oak and Scots pine. For. Ecol. Manage. 339, 22–33. doi: 10.1016/j.foreco.2014.11.032
Michelot, A., Bréda, N., Damesin, C., Dufrêne, E. (2012). Differing growth responses to climatic variations and soil water deficits of Fagus sylvatica, Quercus petraea and Pinus sylvestris in a temperate forest. For. Ecol. Manage. 265, 161–171. doi: 10.1016/j.foreco.2011.10.024
Misi, D., Náfrádi, K. (2016). Possibility of identification of negative extreme climatic events using pinus sylvestris tree-rings in transdanubia, Hungary. Dendrobiology 75, 45–54. doi: 10.12657/denbio.075.005
Misi, D., Puchałka, R., Pearson, C., Robertson, I., Koprowski, M. (2019). Differences in the climate-growth relationship of scots pine: A case study from Poland and Hungary. Forests 10 (3), 243. doi: 10.3390/f10030243
Ols, C., Klesse, S., Girardin, M. P., Evans, M. E., DeRose, R. J., Trouet, V. (2023). Detrending climate data prior to climate–growth analyses in dendroecology: A common best practice? Dendrochronologia 79, 126094. doi: 10.1016/j.dendro.2023.126094
Pacheco, A., Camarero, J. J., Carrer, M. (2018a). Shifts of irrigation in Aleppo pine under semi-arid conditions reveal uncoupled growth and carbon storage and legacy effects on wood anatomy. Agric. For. Meteorology 253–254, 225–232. doi: 10.1016/j.agrformet.2018.02.018
Pacheco, A., Camarero, J. J., Ribas, M., Gazol, A., Gutierrez, E., Carrer, M. (2018b). Disentangling the climate-driven bimodal growth pattern in coastal and continental Mediterranean pine stands. Sci. Total Environ. 615, 1518–1526. doi: 10.1016/j.scitotenv.2017.09.133
Paquette, A., Messier, C. (2011). The effect of biodiversity on tree productivity: From temperate to boreal forests. Global Ecol. Biogeography 20 (1), 170–180. doi: 10.1111/j.1466-8238.2010.00592.x
Pardos, M., del Río, M., Pretzsch, H., Jactel, H., Bielak, K., Bravo, F., et al. (2021). The greater resilience of mixed forests to drought mainly depends on their composition: Analysis along a climate gradient across Europe. For. Ecol. Manage. 481, 118687. doi: 10.1016/j.foreco.2020.118687
Pellizzari, E., Camarero, J. J., Gazol, A., Sangüesa-Barreda, G., Carrer, M. (2016). Wood anatomy and carbon-isotope discrimination support long-term hydraulic deterioration as a major cause of drought-induced dieback. Global Change Biol. 22 (6), 2125–2137. doi: 10.1111/gcb.13227
Pérez-de-Lis, G., Rossi, S., Vázquez-Ruiz, R. A., Rozas, V., García-González, I. (2016). Do changes in spring phenology affect earlywood vessels? Perspective from the xylogenesis monitoring of two sympatric ring-porous oaks. New Phytol. 209 (2), 521–530. doi: 10.1111/nph.13610
Perot, T., Balandier, P., Couteau, C., Delpierre, N., Jean, F., Perret, S., et al. (2021). Budburst date of Quercus petraea is delayed in mixed stands with Pinus sylvestris. Agric. For. Meteorology 300, 108326. doi: 10.1016/j.agrformet.2021.108326
Petit, G., Zambonini, D., Hesse, B. D., Häberle, K. (2022). No xylem phenotypic plasticity in mature Picea abies and Fagus sylvatica trees after 5 years of throughfall precipitation exclusion. Global Change Biol. 28 (15), 4668–4683. doi: 10.1111/gcb.16232
Petrucco, L., Nardini, A., von Arx, G., Saurer, M., Cherubini, P. (2017). Isotope signals and anatomical features in tree rings suggest a role for hydraulic strategies in diffuse drought-induced die-back of Pinus nigra. Tree Physiol. 37 (4), 523–535. doi: 10.1093/treephys/tpx031
Porté, A., Huard, F., Dreyfus, P. (2004). Microclimate beneath pine plantation, semi-mature pine plantation and mixed broadleaved-pine forest. Agric. For. Meteorology 126 (1–2), 175–182. doi: 10.1016/j.agrformet.2004.06.001
Potop, V., Boroneanţ, C., Možný, M., Štěpánek, P., Skalák, P. (2014). Observed spatiotemporal characteristics of drought on various time scales over the Czech Republic. Theor. Appl. Climatology 115 (3–4), 563–581. doi: 10.1007/s00704-013-0908-y
Prendin, A. L., Petit, G., Carrer, M., Fonti, P., Björklund, J., von Arx, G. (2017). New research perspectives from a novel approach to quantify tracheid wall thickness. Tree Physiol. 37 (7), 976–983. doi: 10.1093/treephys/tpx037
Pretzsch, H., Bielak, K., Block, J., Bruchwald, A., Dieler, J., Ehrhart, H. P., et al. (2013a). Productivity of mixed versus pure stands of oak (Quercus petraea (Matt.) Liebl. and Quercus robur L.) and European beech (Fagus sylvatica L.) along an ecological gradient. Eur. J. For. Res. 132 (2), 263–280. doi: 10.1007/s10342-012-0673-y
Pretzsch, H., del Río, M., Ammer, C., Avdagic, A., Barbeito, I., Bielak, K., et al. (2015). Growth and yield of mixed versus pure stands of Scots pine (Pinus sylvestris L.) and European beech (Fagus sylvatica L.) analysed along a productivity gradient through Europe. Eur. J. For. Res. 134 (5), 927–947. doi: 10.1007/s10342-015-0900-4
Pretzsch, H., Schütze, G., Uhl, E. (2013b). Resistance of European tree species to drought stress in mixed versus pure forests: Evidence of stress release by inter-specific facilitation. Plant Biol. 15 (3), 483–495. doi: 10.1111/j.1438-8677.2012.00670.x
Pretzsch, H., Steckel, M., Heym, M., Biber, P., Ammer, C., Ehbrecht, M., et al. (2020). Stand growth and structure of mixed-species and monospecific stands of Scots pine (Pinus sylvestris L.) and oak (Q. robur L., Quercus petraea (Matt.) Liebl.) analysed along a productivity gradient through Europe. Eur. J. For. Res. 139 (3), 349–367. doi: 10.1007/s10342-019-01233-y
Prislan, P., Gričar, J., de Luis, M., Novak, K., del Castillo, E. M., Schmitt, U., et al. (2016). Annual cambial rhythm in Pinus halepensis and Pinus sylvestris as indicator for climate adaptation. Front. Plant Sci. 7 (DECEMBER2016). doi: 10.3389/fpls.2016.01923
R: The R Project for Statistical Computing. (2020). Available at: https://www.r-project.org/.
Reineke, L. H. (1933). Perfecting a stand-density index for evenaged forests. J. Agric. Res. 46, 627–638.
Richter, S., Kipfer, T., Wohlgemuth, T., Guerrero, C. C., Ghazoul, J., Moser, B. (2012). Phenotypic plasticity facilitates resistance to climate change in a highly variable environment. Oecologia 169, 269–279. doi: 10.1007/s00442-011-2191-x
Rinntech® (2010). TSAP-WIN™: Time series analysis and presentation for dendrochronology and related applications (Heidelberg, Germany). Available at: http://www.rinntech.com.
Schinker, M. G., Hansen, N., Spiecker, H. (2003). High-Frequency densitometry-a new method for the rapid evaluation of wood density variations. IAWA J. 24 (3), 231–239. doi: 10.1163/22941932-90001592
Seo, J. W., Eckstein, D., Jalkanen, R., Rickebusch, S., Schmitt, U. (2008). Estimating the onset of cambial activity in Scots pine in northern Finland by means of the heat-sum approach. Tree Physiol. 28 (1), 105–112. doi: 10.1093/treephys/28.1.105
Somorowska, U. (2022). Amplified signals of soil moisture and evaporative stresses across Poland in the twenty-first century. Sci. Total Environ. 812, 151465. doi: 10.1016/j.scitotenv.2021.151465
Steckel, M., Heym, M., Wolff, B., Reventlow, D. O. J., Pretzsch, H. (2019). Transgressive overyielding in mixed compared with monospecific Scots pine (Pinus sylvestris L.) and oak (Quercus robur L., Quercus petraea (Matt.) Liebl.) stands – Productivity gains increase with annual water supply. For. Ecol. Manage. 439, 81–96. doi: 10.1016/j.foreco.2019.02.038
Știrbu, M. I., Roibu, C. C., Carrer, M., Mursa, A., Unterholzner, L., Prendin, A. L. (2022). Contrasting climate sensitivity of pinus cembra tree-ring traits in the carpathians. Front. Plant Sci. 13. doi: 10.3389/fpls.2022.855003
Thurm, E. A., Uhl, E., Pretzsch, H. (2016). Mixture reduces climate sensitivity of Douglas-fir stem growth. For. Ecol. Manage. 376, 205–220. doi: 10.1016/j.foreco.2016.06.020
Tilman, D. (1999). The ecological consequences of changes in biodiversity: a search for general principles 1. Ecology 80 (5), 1455–1474. doi: 10.2307/176540
Toïgo, M., Vallet, P., Tuilleras, V., Lebourgeois, F., Rozenberg, P., Perret, S., et al. (2015). Species mixture increases the effect of drought on tree ring density, but not on ring width, in Quercus petraea-Pinus sylvestris stands. For. Ecol. Manage. 345, 73–82. doi: 10.1016/j.foreco.2015.02.019
van Loon, M. P., Schieving, F., Rietkerk, M., Dekker, S. C., Sterck, F., Anten, N. P. R. (2014). How light competition between plants affects their response to climate change. New Phytol. 203 (4), 1253–1265. doi: 10.1111/nph.12865
von Arx, G., Arzac, A., Fonti, P., Frank, D., Zweifel, R., Rigling, A., et al. (2017). Responses of sapwood ray parenchyma and non-structural carbohydrates (NSC) of Pinus sylvestris to drought and long-term irrigation. Funct. Ecol. 31, 1371–1382. doi: 10.1111/1365-2435.12860
von Arx, G., Carrer, M. (2014). ROXAS – A new tool to build centuries-long tracheid-lumen chronologies in conifers. Dendrochronologia 32 (3), 290–293. doi: 10.1016/J.DENDRO.2013.12.001
von Arx, G., Crivellaro, A., Prendin, A. L., Čufar, K., Carrer, M. (2016). Quantitative wood anatomy—practical guidelines. Front. Plant Sci. 7. doi: 10.3389/fpls.2016.00781
Wachowiak, W., Perry, A., Donnelly, K., Cavers, S. (2018). Early phenology and growth trait variation in closely related European pine species. Ecol. Evol. 8 (1), 655–666. doi: 10.1002/ece3.3690
Wickham, H., François, R., Henry, L., Müller, K., Vaughan, D. (2023). dplyr: A Grammar of Data Manipulation. R package version 1.1.0. Available at: https://CRAN.R-project.org/package=dplyr.
Zádorová, T., Skála, J., Žížala, D., Vaněk, A., Penížek, V. (2021). Harmonization of a large-scale national soil database with the World Reference Base for Soil Resources 2014. Geoderma 384, 114819. doi: 10.1016/J.GEODERMA.2020.114819
Zalloni, E., Battipaglia, G., Cherubini, P., Saurer, M., de Micco, V. (2018). Contrasting physiological responses to Mediterranean climate variability are revealed by intra-annual density fluctuations in tree rings of Quercus ilex L. and Pinus pinea L. Tree Physiol. 38 (8), 1213–1224. doi: 10.1093/treephys/tpy061
Zang, C., Biondi, F. (2015). Treeclim: An R package for the numerical calibration of proxy-climate relationships. Ecography 38 (4), 431–436. doi: 10.1111/ecog.01335
Zeller, L., Ammer, C., Annighöfer, P., Biber, P., Marshall, J., Schütze, G., et al. (2017). Tree ring wood density of Scots pine and European beech lower in mixed-species stands compared with monocultures. For. Ecol. Manage. 400, 363–374. doi: 10.1016/j.foreco.2017.06.018
Zimmermann, M. H. (2013). Xylem structure and the ascent of sap. (Springer Science & Business Media).
Keywords: climate change, drought, inter-specific facilitation, mixed forest, Pinus sylvestris, quantitative wood anatomy
Citation: Giberti GS, von Arx G, Giovannelli A, du Toit B, Unterholzner L, Bielak K, Carrer M, Uhl E, Bravo F, Tonon G and Wellstein C (2023) The admixture of Quercus sp. in Pinus sylvestris stands influences wood anatomical trait responses to climatic variability and drought events. Front. Plant Sci. 14:1213814. doi: 10.3389/fpls.2023.1213814
Received: 28 April 2023; Accepted: 24 October 2023;
Published: 16 November 2023.
Edited by:
Huanjiong Wang, Institute of Geographic Sciences and Natural Resources Research (CAS), ChinaReviewed by:
Raquel Lobo-do-Vale, University of Lisbon, PortugalAnna Lintunen, University of Helsinki, Finland
Copyright © 2023 Giberti, von Arx, Giovannelli, du Toit, Unterholzner, Bielak, Carrer, Uhl, Bravo, Tonon and Wellstein. This is an open-access article distributed under the terms of the Creative Commons Attribution License (CC BY). The use, distribution or reproduction in other forums is permitted, provided the original author(s) and the copyright owner(s) are credited and that the original publication in this journal is cited, in accordance with accepted academic practice. No use, distribution or reproduction is permitted which does not comply with these terms.
*Correspondence: Giulia Silvia Giberti, Z2l1bGlhc2lsdmlhLmdpYmVydGlAdW5pYnouaXQ=; Camilla Wellstein, Y2FtaWxsYS53ZWxsc3RlaW5AdW5pYnouaXQ=
†Deceased