- 1Institute of Botany, Heinrich Heine University, Düsseldorf, Germany
- 2Heinrich Heine University, Center of Excellence on Plant Sciences (CEPLAS), Düsseldorf, Germany
Cellular homeostasis of the micronutrient iron is highly regulated in plants and responsive to nutrition, stress, and developmental signals. Genes for iron management encode metal and other transporters, enzymes synthesizing chelators and reducing substances, transcription factors, and several types of regulators. In transcriptome or proteome datasets, such iron homeostasis-related genes are frequently found to be differentially regulated. A common method to detect whether a specific cellular pathway is affected in the transcriptome data set is to perform Gene Ontology (GO) enrichment analysis. Hence, the GO database is a widely used resource for annotating genes and identifying enriched biological pathways in Arabidopsis thaliana. However, iron homeostasis-related GO terms do not consistently reflect gene associations and levels of evidence in iron homeostasis. Some genes in the existing iron homeostasis GO terms lack direct evidence of involvement in iron homeostasis. In other aspects, the existing GO terms for iron homeostasis are incomplete and do not reflect the known biological functions associated with iron homeostasis. This can lead to potential errors in the automatic annotation and interpretation of GO term enrichment analyses. We suggest that applicable evidence codes be used to add missing genes and their respective ortholog/paralog groups to make the iron homeostasis-related GO terms more complete and reliable. There is a high likelihood of finding new iron homeostasis-relevant members in gene groups and families like the ZIP, ZIF, ZIFL, MTP, OPT, MATE, ABCG, PDR, HMA, and HMP. Hence, we compiled comprehensive lists of genes involved in iron homeostasis that can be used for custom enrichment analysis in transcriptomic or proteomic studies, including genes with direct experimental evidence, those regulated by central transcription factors, and missing members of small gene families or ortholog/paralog groups. As we provide gene annotation and literature alongside, the gene lists can serve multiple computational approaches. In summary, these gene lists provide a valuable resource for researchers studying iron homeostasis in A. thaliana, while they also emphasize the importance of improving the accuracy and comprehensiveness of the Gene Ontology.
Introduction
Gene Ontology (GO) is a collection of attributes (terms) with which genes are associated based on several levels of evidence (Ashburner et al., 2000). Gene Ontology is divided into the three main categories “Molecular Function,” “Cellular Component,” and “Biological Process.” While Cellular Component GO terms exclusively indicate the location of gene products from the tissue down to the subcellular location, Molecular Function GO terms describe the specific molecular activity of gene products without referencing the location, biological background, or conditions under which these activities occur. Therefore, depending on the research question, the main category Biological Process may be more relevant, in which the GO terms describe overarching processes that are usually accomplished by the interplay and/or interaction of several distinct gene products. GO terms are hierarchically organized in an upside-down tree-like structure where the upper “parent” terms are more generic and the lower “child” terms are the more specific ones.
Different GO terms are linked if a relationship exists between them. In the QuickGo database, possible relationships between GO terms include “is a,” “is part of,” “regulates,” “positively regulates,” “negatively regulates,” “occurs in,” “capable of,” and “capable of part of” (Binns et al., 2009). However, there may be variations in these relationships depending on the specific database. GO terms may also be viewed as linked containers, which include all the genes that are associated with this term. Therefore, genes associated with a GO term are often designated as “in” this term. Entries in the Gene Ontology are based on evidence codes such as “Inferred from Sequence or structural Similarity” (ISS), “Inferred from Sequence Orthology” (ISO), “Inferred from Electronic Annotation” (IEA), “Inferred from Direct Assay” (IDA), “Inferred from Mutant Phenotype” (IMP), “Inferred from Expression Pattern” (IEP), and many more (Gene Ontology, C., 2023a). We focus on a Gene Ontology’s Biological Process category. Possible relationship types of genes within this category, are “involved in,” “acts upstream of or within,” “acts upstream of or within, positive effect,” “acts upstream of or within, negative effect,” “acts upstream of,” “acts upstream of, positive effect,” or “acts upstream of, negative effect” (Gene Ontology, C., 2023b). Since GO terms have been set up to be consistent across several organisms, including plants, and curated for a plethora of different species, the GO annotations for single species are not always comprehensive. Therefore, depending on the currently available evidence for that species and the time that passes between periodic updates, GO terms may contain one or more genes or they can still be empty.
GO has been widely used for enrichment analysis of transcriptomic or proteomic data. This is possible due to the fact that the number of genes in a GO term can be used in conjunction with the total number of known genes and the respective number of regulated genes or proteins for statistical evaluation and to obtain p-values that allow us to infer whether or not a GO term is enriched in a set of regulated genes or proteins. This is usually accomplished by using tests for independence, with which measured frequencies or distributions are compared with expected frequencies or distributions, such as the hypergeometric test (Hahne et al., 2008), the binomial test (Aitken and Gonin, 1936), the χ2 test (Pearson, 1900), Fisher’s exact test (Fisher, 1935), and others (Huang da et al., 2009). Due to the relative simplicity and reliability of these tests, the hypergeometric test and Fisher’s exact test are among the most commonly used statistical tests in publicly available tools for GO term or, more generally, gene set enrichment analysis (Rivals et al., 2007; Huang da et al., 2009).
Among the most widely studied model plants, Arabidopsis thaliana is one of the best-annotated species in terms of gene annotations and GO terms. The Arabidopsis Information Resource (TAIR) database offers various tools for browsing or downloading ontologies and performing GO term enrichment analysis, among others (Lamesch et al., 2012). Although there are other excellent tools and databases, such as MapMan (Usadel et al., 2009) and the Kyoto Encyclopedia of Genes and Genomes (KEGG) database (Kanehisa and Goto, 2000), which are very suitable for molecular function and biochemical pathway analysis, the Gene Ontology remains the most commonly used option when analyzing biological processes. The A. thaliana-specific Gene Ontology database is accessible through the TAIR website (https://www.arabidopsis.org > Browse > Ontologies/Keywords) (Lamesch et al., 2012).
In the past, GO term enrichment analyses have proven to be an invaluable tool in the iron field, unearthing new insights and shedding light on previously unknown connections such as the crucial role of ribosomal proteins in the context of iron deficiency (Wang et al., 2013), the identification of overlapping gene modules in the intricate interplay between phosphate and iron homeostasis (Li and Lan, 2015), and the discovery of iron-responsive genes that also respond to synthetic community of bacterial commensals (SynCom) and coumarins (Harbort et al., 2020). Moreover, it is worth noting that stress responses often result in a significant shift in iron management (Kanwar et al., 2021), further underscoring the importance of iron in many fundamental processes in plants, such as nutrition, stress response, and development. Given these results, it is clear that further investigations into iron deficiency as well as iron excess stress using GO term enrichment analysis is imperative. They also show that it is important to have a reliable and accurate resource of iron homeostasis genes for enrichment analysis of omics datasets. We used the TAIR ontology browser to investigate the GO category Biological Process for the model plant A. thaliana with respect to iron homeostasis and found striking gaps and shortcomings in the listed genes that we addressed to build a new resource.
Organization of iron homeostasis in the A. thaliana Gene Ontology category biological process
We utilized the Gene Ontology browser provided by the TAIR database (Lamesch et al., 2012) and the visualization tool QuickGO (Binns et al., 2009) to obtain tree images. We analyzed the minimal tree structures of iron-specific GO terms for A. thaliana and discovered that GO terms related to iron homeostasis were distributed among five distinct paths (Figure 1). The uppermost iron-specific parent GO terms of these paths were “response to iron ion” (GO:0010039) (Figure 1A), “iron transport” (GO:0006826) (Figure 1B), “multicellular organismal-level iron ion homeostasis” (GO:0060586) (Figure 1C), “intracellular iron ion homeostasis” (GO:0006879) (Figure 1D), and “response to iron ion starvation” (GO:1990641) (Figure 1E). In their respective paths, multicellular organismal-level iron ion homeostasis (GO:0060586) (Figure 1C) and response to iron ion starvation (GO:1990641) (Figure 1E) both contained 29 and 17 genes, respectively, with no additional genes present in any of the children terms. Thus, these were the only iron-specific GO terms in their respective paths that contained genes.
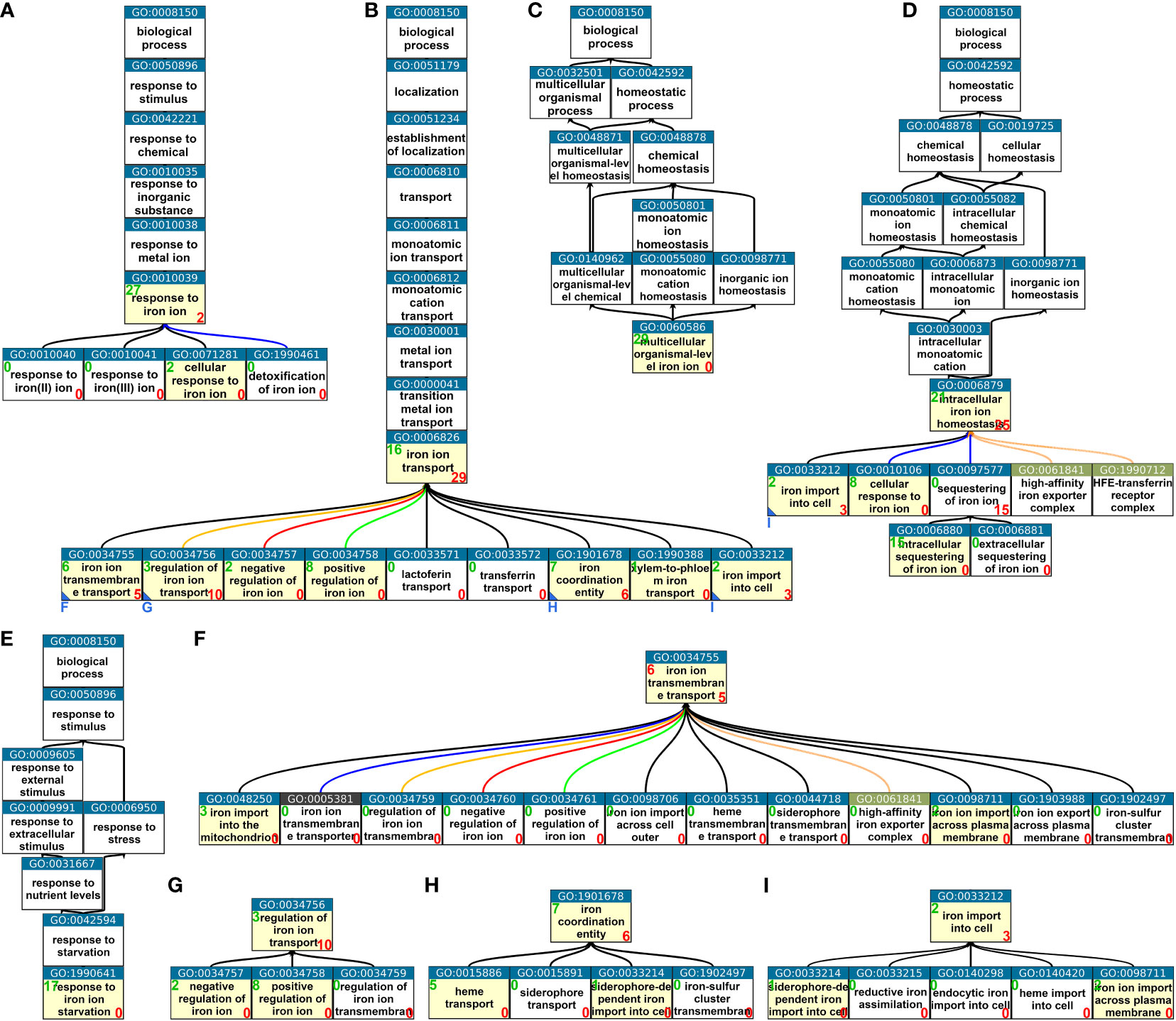
Figure 1 Iron homeostasis-related GO terms from the category “Biological Process.” (A–E) Minimal trees of Biological Process GO terms leading to the lowest possible hierarchy level with nonempty iron homeostasis-related GO terms. (F–I) Extensions of the minimal trees (A–E) where further children terms could not be displayed due to space restrictions. Small blue letters and blue triangles at the bottom left corner of a box of the minimal trees (B, D) indicate in which subfigure (F–I) the respective tree is completed starting with the indicated GO term. Possible children terms are not displayed if they were all empty. Yellow boxes indicate that the GO term has direct member genes, whereas white boxes indicate GO terms with no direct member genes. This does not apply to more unspecific parent terms. Green numbers at the top left corner of a box indicate the number of direct member genes. Red numbers at the bottom right corner of a box indicate the number of genes in direct children terms. Overlaps between direct members of a GO term and members of children terms may occur but do not necessarily have to occur. GO terms relating to iron but not iron homeostasis such as iron–sulfur cluster-related or very unspecific GO terms such as “iron binding” are not displayed. The images were created using the publicly available tool QuickGO (https://www.ebi.ac.uk/QuickGO), and the most recent Gene Ontology annotations were assessed from the TAIR database (https://www.arabidopsis.org → Browse → Ontologies/Keywords).
The GO term response to iron ion (GO:0010039) contained 27 genes, with two genes present in its direct child term cellular response to iron ion (GO:0071281) and no other genes in any of the other children terms (Figure 1A). The path leading to the GO term intracellular iron ion homeostasis (GO:0006879) was further subdivided and contained genes downstream in two more levels of children terms (Figures 1D, I). The largest branch was the one leading over “iron ion transport (GO:0006826). Seven direct children GO terms and eight downstream GO terms contained genes (Figures 1B, F–I). To summarize, the iron-specific Biological Response GO terms were organized into five distinct aspects of iron homeostasis: the general aspect of the response, the more specific aspects of the multicellular and intracellular response, the even more specific aspect of the response to iron starvation, and the most specific aspect of iron transport.
Distribution of genes in iron homeostasis-related GO terms of the category biological process
By searching the TAIR gene ontology browser, we obtained the gene models in each iron homeostasis-specific GO term of the Biological Process category (Figure 1) and compiled them in the Supplementary Tables S1-S19. Although the number of splicing variants associated with iron-specific GO terms added up to a greater number, the total number of actual gene loci represented was only 113 (Supplementary Table S1). This resulted from multiple gene associations with different GO terms and multiple gene models for the same gene locus listed in a single GO term. For example, FIT, the gene of the central and essential regulator of the iron uptake machinery in A. thaliana (Colangelo and Guerinot, 2004; Jakoby et al., 2004; Bauer et al., 2007), was present in both response to iron ion (GO:0010039) and regulation of iron ion transport (GO:0034756) (Supplementary Tables S5, S11) while PYE, another regulator of iron uptake (Long et al., 2010), had multiple gene models in multicellular organismal-level iron ion homeostasis (GO:0060586) (Supplementary Table S15). In the following text, we repeatedly state that genes are represented in at least one of the iron homeostasis-related GO terms. These statements are meant to refer to Supplementary Table S1, although no reference is attached to respective statements.
Upon closer inspection of individual pairs of parent and child GO terms, we found that some genes were present in both parent and child terms, but often, genes present in the parent term were not present in the direct child term, and vice versa. For instance, a member of the YELLOW STRIPE LIKE (YSL) gene family, YSL2, was present in the parent term iron ion transport (GO:0006826) but not in its child term “iron ion transmembrane transport” (GO:0034755), whereas YSL4 and YSL6 were in the child term iron ion transmembrane transport (GO:0034755) but not in its parent term iron ion transport (GO:0006826) (Supplementary Tables S2, S10). However, YSL2 encodes a transmembrane protein that transports iron–nicotianamine complexes (DiDonato et al., 2004), and we would thus expect it to be present in both iron ion transport (GO:0006826) and iron ion transmembrane transport (GO:0034755). Conversely, we would expect YSL4 and YSL6 to be found not only in the child term iron ion transmembrane transport (GO:0034755) but also in its parent term iron ion transport (GO:0006826), as they encode transmembrane proteins that export iron from chloroplasts (DiDonato et al., 2004; Divol et al., 2013). Despite this evidence having existed for many years, the information has not yet been transferred to the Gene Ontology.
One observation is that some genes may be present in iron-specific GO terms despite lacking direct evidence of involvement in iron homeostasis. For instance, the gene of a Mitochondrial substrate carrier family protein, AT1G07025, which has no symbol as of now, is listed in the “iron import into the mitochondrion” (GO:0034755) term with the evidence code “Inferred from Biological aspects of Ancestor.” While there is no direct evidence, other family members like MIT1 and MIT2 have been shown to transport iron into mitochondria (Jain et al., 2019). Another example is the gene ABCB26 (AT1G70610), which is included in the iron ion transport GO term with the evidence code Inferred from Biological aspects of Ancestor and is mentioned alongside the MIT1 gene. However, a BLAST alignment between the protein sequences of ABCB26 and MIT1 did not reveal significant similarity (data not shown), casting doubt on the involvement of ABCB26 in iron homeostasis. Although the close similarity of a gene with others that have been demonstrated to participate in iron homeostasis may indicate a putative role in iron homeostasis or in the response to iron, the unattended automatic annotation may be prone to error. As a result, erroneous additions of genes to a GO term could affect the reliability and results of GO term enrichment analyses, particularly in GO terms with a limited number of genes.
Representation of important regulators of iron homeostasis
We compiled a comprehensive list of all gene loci represented in iron homeostasis-related GO terms, excluding terms related to iron utilization, such as “iron-sulfur cluster assembly” (GO:0016226). The resulting list contained 113 loci (Supplementary Table S1) and provided lists of genes in each iron homeostasis-related GO term (Supplementary Tables S2-S19). We compared this list to the literature and found that many important genes with known functions in iron homeostasis were missing (Table 1). Specifically, we looked at known regulators of iron homeostasis and found that certain transcription factor genes from the bHLH IVc family, including BHLH034 (IDT1) (Li et al., 2016), BHLH104 (Zhang et al., 2015; Li et al., 2016; Wang et al., 2017), and BHLH105 (ILR3) (Tissot et al., 2019; Gao et al., 2020), were not represented in iron-specific GO terms. Additionally, the gene BHLH110, which binds heme (Shimizu et al., 2020), was absent from the list. We also observed that repressors of iron uptake, MYB28 and MYB29 (Coleto et al., 2021), were not present in iron-related GO terms. Lastly, although it has been demonstrated that MYC1 interacts with FIT and negatively regulates the iron uptake machinery (Song et al., 2023), the MYC1 gene was not present in the list.
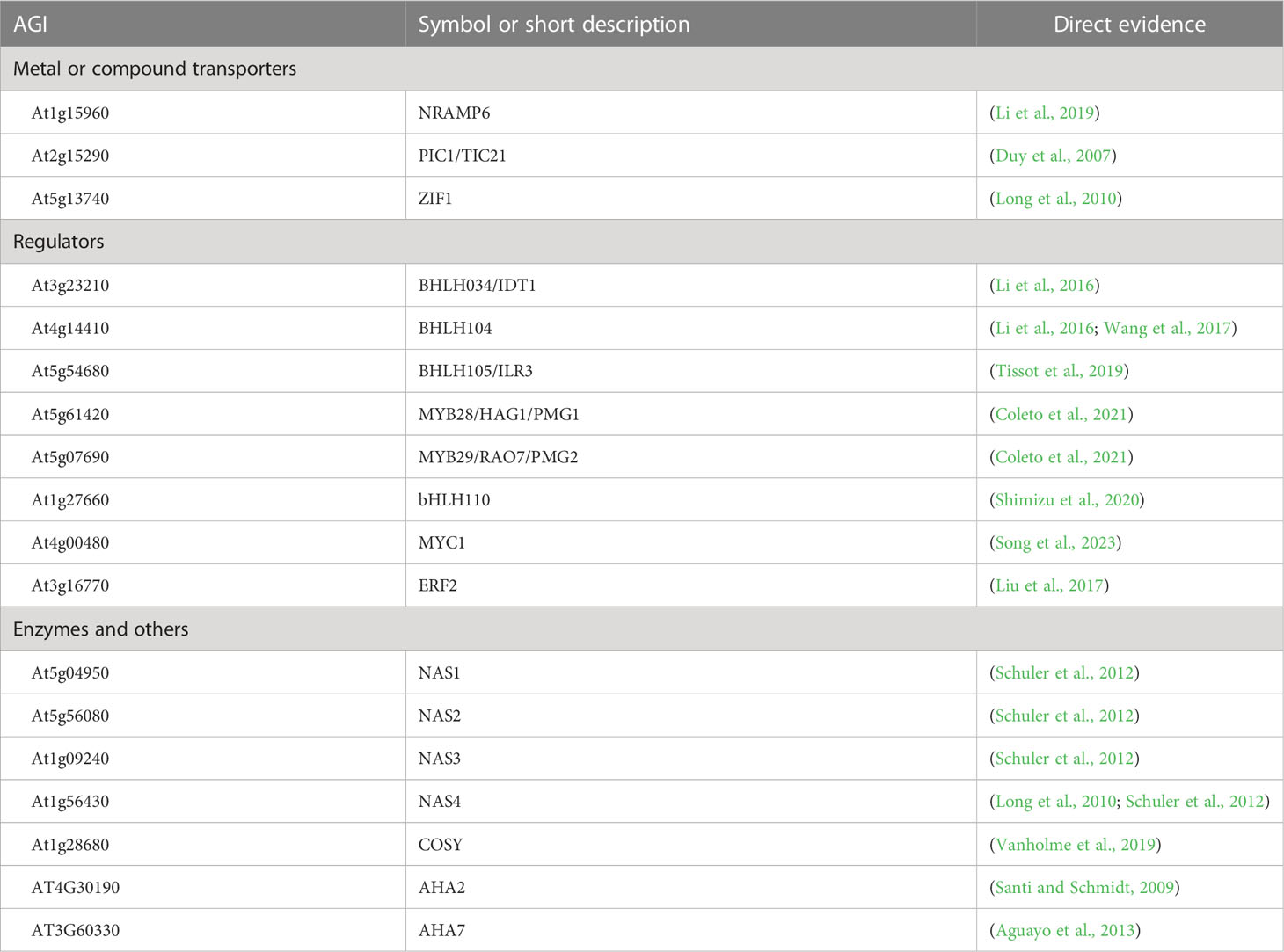
Table 1 Genes with direct evidence of involvement in iron homeostasis in A. thaliana that were not represented in the Gene Ontology in any of the iron homeostasis-related GO terms but should be represented in at least one iron homeostasis-related GO term with the evidence code “Inferred from Direct Assay” (IDA) or “Inferred from Mutant Phenotype” (IMP).
Representation of important transporters involved in iron homeostasis
In Arabidopsis, transporter genes are crucial for iron homeostasis as they control the amount of iron taken up by the plant and how it is distributed or sequestered. The spatial and temporal expression of these genes are decisive in this process. Among the NATURAL RESISTANCE-ASSOCIATED MACROPHAGE PROTEIN (NRAMP) transporter genes, NRAMP1, NRAMP3, and NRAMP4 (Curie et al., 2000; Lanquar et al., 2005; Cailliatte et al., 2010) were associated with iron homeostasis-related GO terms, while NRAMP6 (Li et al., 2019) was not. Similarly, YSL transporter genes YSL1, YSL2, YSL3, YSL4, and YSL6 (Le Jean et al., 2005; Schaaf et al., 2005; Waters et al., 2006; Conte et al., 2013; Divol et al., 2013) were included in the list, but there is a high chance that NRAMP2 and NRAMP5 as well as YSL5, YSL7, and YSL8 might also be involved in iron homeostasis, given their membership in their respective paralog groups. These genes should be included in the list with the Inferred from Biological aspects of Ancestor evidence code. ZIF1 is a direct PYE target and plays a role in nicotianamine sequestration in iron homeostasis (Long et al., 2010; Haydon et al., 2012). However, it was not included in the iron homeostasis-associated GO terms. On the other hand, genes coding for transporters of the IREG paralogs, IREG1, IREG2, and IREG3 (Wintz et al., 2003; Schaaf et al., 2006; Kim et al., 2021), were all associated with iron-specific GO terms.
From the VIT transporter family genes, VIT1 (Kim et al., 2006; Roschzttardtz et al., 2009), VTL1–5 (Gollhofer et al., 2011; Gollhofer et al., 2014), and genes of the ER-body-localized VIT family members MEB1, MEB2, and AT4G27870 (Yamada et al., 2013) were in the list. However, there is no direct evidence for VTL4 and VTL5 and for AT4G27870. According to the TAIR ontology browser, only VTL1, VTL2, and VTL5 were entered with the reference to direct evidence (Gollhofer et al., 2014), while the others were annotated with the evidence level Inferred from Biological aspects of Ancestor. The Zrt- and Irt-related protein (ZIP) family members and metal transporter genes IRT1–3 (Eide et al., 1996; Lin et al., 2009; Vert et al., 2009) were present in the list. Additionally, members of the MATE transporter family are involved in iron homeostasis, and the three known genes of the citrate efflux transporter gene MATE43/FRD3 (Roschzttardtz et al., 2011), the GOLGI-located iron transporter gene MATE48 (Seo et al., 2012), and the gene MATE52 (Wang et al., 2016) were included in iron homeostasis-related GO terms. OPT3 is essential for the phloem loading of iron (Zhai et al., 2014), and the gene is also represented in the Gene Ontology. Finally, the gene for the coumarin efflux transporter PDR9 (Rodríguez-Celma et al., 2013; Fourcroy et al., 2014) was included in the list. Among mitochondrial iron transporter genes, NAP14 (Voith von Voithenberg et al., 2019) was present, but PIC1 (Duy et al., 2007) was absent. MTP8, which mediates iron redistribution during seed development and germination (Chu et al., 2017; Eroglu et al., 2017), was also represented in GO.
Representation of enzymes and other genes with important functions in iron homeostasis
Several genes involved in iron homeostasis encode enzymes in biosynthetic pathways of compounds required for the solubilization or transport of iron, while others function in the reduction of Fe and/or other heavy metals. However, some of the well-known genes involved in iron homeostasis, such as NAS1–NAS4 (Schuler et al., 2012), were not represented in the list. Among the coumarin biosynthesis genes, F6’H1 (Schmid et al., 2013; Schmid et al., 2014), S8H (Rajniak et al., 2018; Siwinska et al., 2018; Tsai et al., 2018), and CYP82C4 (Rajniak et al., 2018) were present, but COSY (Vanholme et al., 2019) was not. FRO2, a well-known member of the iron uptake machinery in Arabidopsis (Robinson et al., 1999), was present in iron-specific GO terms, but other ferric chelate reductases were absent. However, other FRO genes are potentially involved in iron homeostasis, as FRO3 was found to be induced under iron deficiency in Arabidopsis (Mukherjee et al., 2006), and FRO4 and FRO5 were demonstrated to be regulated by FIT in conjunction with the bHLH Ib transcription factors bHLH38/39/100/101 (Cai et al., 2021). Additionally, FRO3 is a direct target of PYE (Long et al., 2010). Therefore, we suggest that the FRO family should be entirely represented in at least one of the iron homeostasis-related GO terms.
Although the phytochelatin synthase genes PCS1 and PCS2 have been annotated in TAIR in conjunction with Fe(III), there is no evidence that they play a role in iron homeostasis, and they have not been listed among the iron homeostasis-related genes in GO. The ubiquitin E3 ligases that interact with and target other regulators of the BHLH transcription factor family for degradation, such as BTS (Long et al., 2010; Selote et al., 2015), BTSL1, and BTSL2 (Hindt et al., 2017; Rodríguez-Celma et al., 2019; Lichtblau et al., 2022), were also represented in the list of genes in iron homeostasis-related GO terms. In iron uptake, one of the concerted steps is the solubilization of iron by acidification of the rhizosphere by a plasma membrane H+-ATPase. In Arabidopsis, this is accomplished by AHA2, which is induced by iron deficiency (Santi and Schmidt, 2009). Iron deficiency-induced upregulation of another plasma membrane H+-ATPase gene family member, AHA7, implies that it might also participate in iron homeostasis but is not responsible for rhizosphere acidification (Santi and Schmidt, 2009). However, none of them was represented in iron homeostasis-related GO terms.
Representation of FIT-regulated genes
Next, we investigated the genes that are regulated by FIT (Colangelo and Guerinot, 2004; Mai et al., 2016a) and found that some of them were and others were not represented in iron homeostasis-related GO terms (Table 2). Among the FIT-regulated genes according to Colangelo and Guerinot (2004), ATAVT6B (putative amino acid transporter), ATL35, GRF11, CYP71B5, GSTL1, FOLB1, PME41, AT3G61930 (hypothetical protein), MWL-2, SAUR18, AT1G14190 (glucose-methanol-choline (GMC) oxidoreductase family protein), AT1G73120 (F-box/RNI superfamily protein), UGT72E1, AT3G07720 (kelch-repeat protein), HMA3, COPT2, and MTP3/MTPa2 were not represented in iron-specific GO terms.
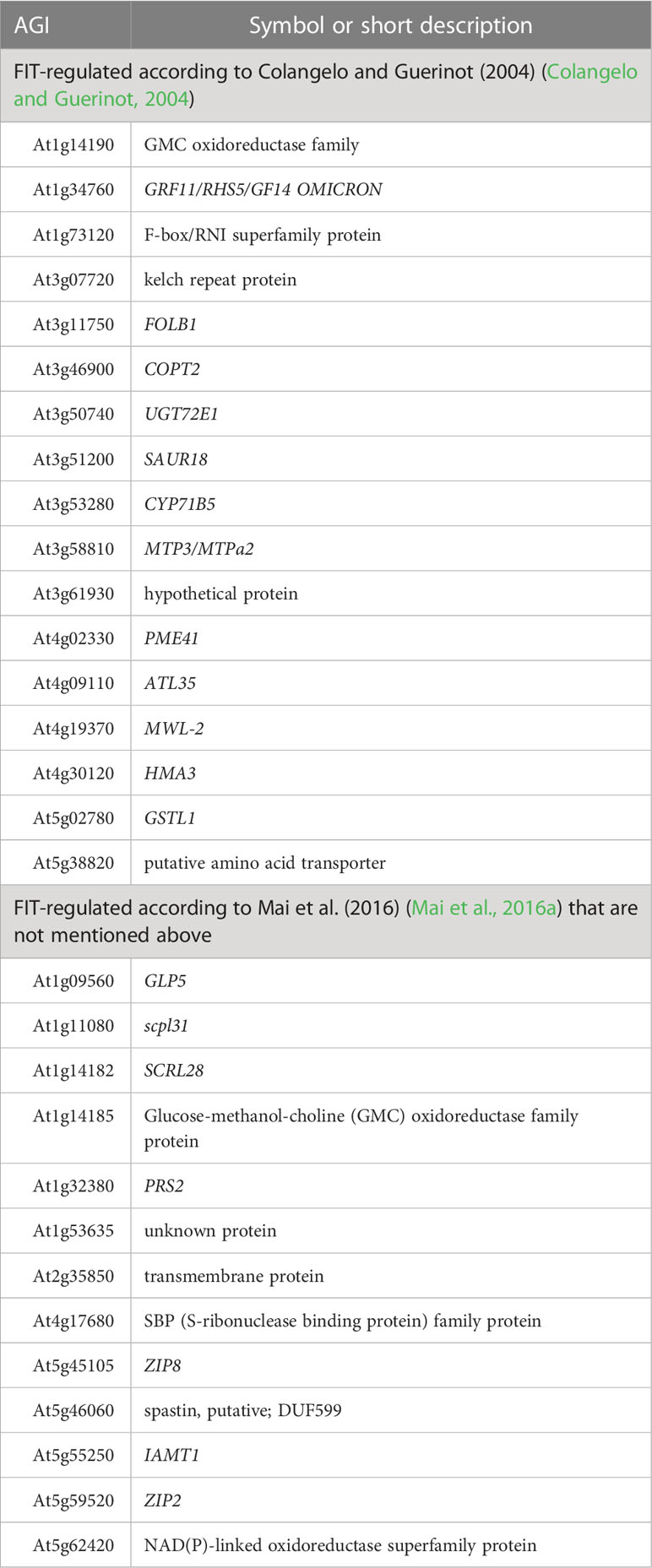
Table 2 FIT-regulated and iron deficiency-induced genes that were not represented in the Gene Ontology in at least one iron homeostasis-related GO term but should be represented in at least one iron homeostasis-specific GO term with the evidence code “Inferred from Expression Pattern” (IEP).
From the genes that were additionally found to be regulated by FIT according to Mai et al. (2016a), the positively FIT-regulated genes GLP5, SCRL28, AT1G14185 (GMC oxidoreductase family protein), PRS2, AT1G53635 (hypothetical protein), AT2G35850 (transmembrane protein), AT4G17680 (S-ribonuclease-binding protein), ZIP8, AT5G46060 (spastin, putative), IAMT1, and AT5G62420 (NAD(P)-linked oxidoreductase superfamily protein) were not found in any of the iron homeostasis-related GO terms. The robustly FIT-repressed genes ZIP2 and SCPL31 were also not present. From the most robustly iron deficiency-induced genes (Ivanov et al., 2012; Mai et al., 2016b) that have not been mentioned above, AT3G06890 (transmembrane protein) was not represented in any of the iron homeostasis-specific GO terms.
Representation of FIT-regulated metal transporter genes
Remarkably, the above-mentioned FIT-regulated genes that were not represented in any of the iron homeostasis-related GO terms (Table 2) include metal transporters known to transport other metals or putatively transport heavy metals, such as MTP3/MTPa2. Due to the low specificity of IRT1, which transports zinc and cobalt besides iron (Korshunova et al., 1999), MTP3 mediates the exclusion of zinc and cobalt from the shoot under Fe deficiency (Arrivault et al., 2006). This clearly shows that transporters not transporting iron but other metals may play important roles in the context of iron homeostasis in Arabidopsis and probably contribute to an increase in the chance of survival under iron deficiency. Such genes should be included at least in the GO term “response to iron starvation.”
The fact that MTP3 is regulated by FIT underlines its importance. Such crosstalk between Fe homeostasis and other metals is also exemplified by the finding that with COPT2 and the FERRIC REDUCTASE OXIDASE (FRO) gene family members FRO4 and FRO5, copper uptake is induced by FIT in conjunction with the Ib transcription factors BHLH038, 39, 100, and 101 due to increased demand for copper under iron deficiency (Cai et al., 2021). It has been hypothesized that CuSOD can functionally replace FeSOD under low iron conditions (Waters et al., 2012). Likewise, the potential roles of ZIP transporters under iron deficiency have been demonstrated (Yang et al., 2010). Among the ZIP transporter genes, ZIP2 is negatively and ZIP8 is positively regulated by FIT (Mai et al., 2016a). HMA3 is a member of the HEAVY METAL ATPASE (HMA) family of genes. HMA3 is also regulated by FIT (Colangelo and Guerinot, 2004), and it was demonstrated to contribute to cobalt, cadmium, zinc, and lead tolerance by sequestration of the metals into the vacuole (Morel et al., 2009).
The lower evidence codes if direct experimental evidence is lacking
Many genes in the TAIR-hosted Gene Ontology database were found to have been entered with the evidence code “Inferred from Biological aspects of Ancestor” (IBA). Moreover, there are more evidence codes that can be applied if direct evidence is lacking, such as ISO, ISS, or IEP. Automatic unattended entries are often useful and increase the speed by which new entries are made in Gene Ontology. However, some of the entries may be erroneous, as exemplified further above, and some prominent gene families from which several members are involved in iron homeostasis in A. thaliana as demonstrated in the literature, were not represented in any of the iron homeostasis-specific GO terms. Besides the NAS ortholog group, which was missing entirely, members of the YSL gene family (YSL5, YSL7, and YSL8) were absent in iron homeostasis GO terms. Additionally, we could not find all members of the NRAMP transporter family (NRAMP2, NRAMP5, and NRAMP6).
Furthermore, members of the FRO gene family (FRO1 and FRO3–8) were not represented, although there is evidence for some of them that they play important roles, at least in the context of iron starvation (Waters et al., 2012; Cai et al., 2021). Besides COPT2, one of the FIT-regulated genes, there is evidence that the two COPPER TRANSPORTER (COPT) genes, COPT1 and COPT3, influence iron homeostasis (Perea-Garcia et al., 2020). However, none of the applicable evidence codes have been used to enter these genes into any of the iron homeostasis-specific GO terms. We strongly suggest that one of these codes are applied to the above-mentioned genes, if applicable, to make the iron homeostasis-related GO terms more complete, and reliable. We suggest to not only add the missing genes but also their respective ortholog/paralog groups to the Gene Ontology with the evidence code Inferred from Biological aspects of Ancestor, Inferred from Sequence Orthology, or Inferred from Sequence or structural Similarity (Table 3).
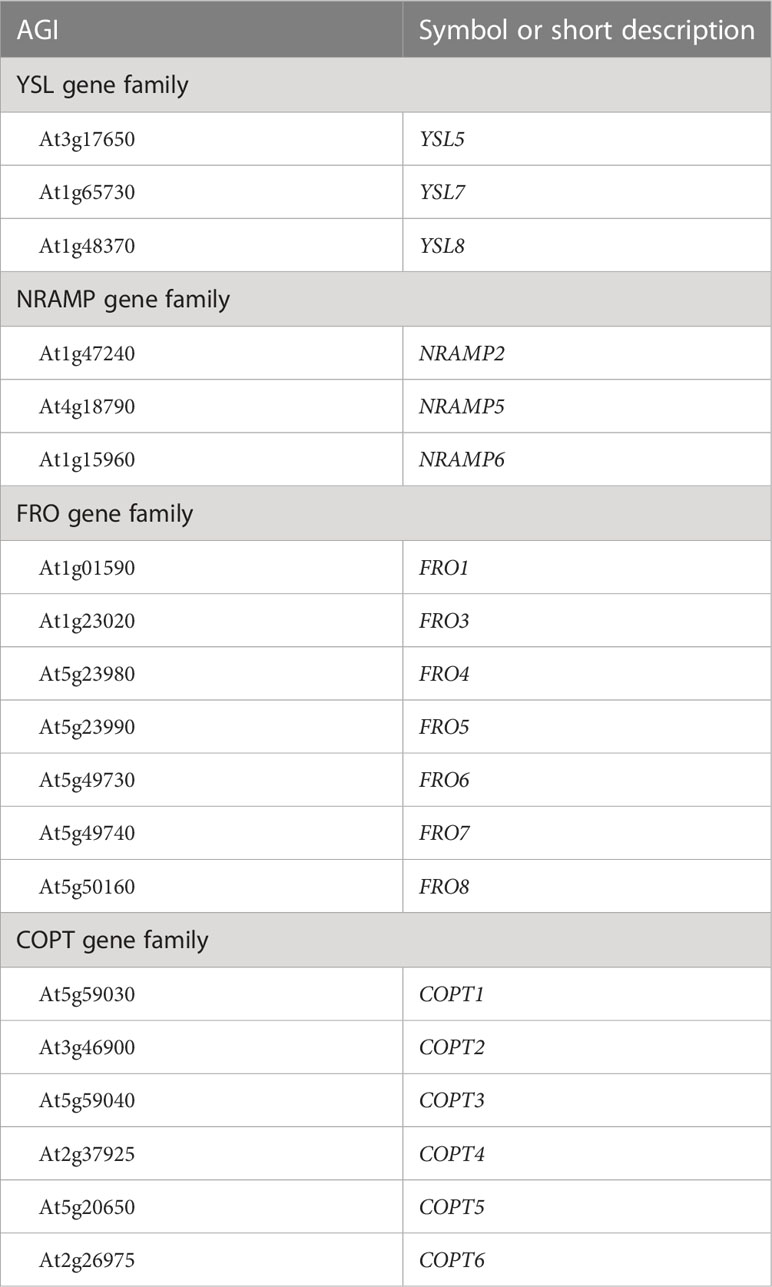
Table 3 Genes from gene families that were not represented in iron homeostasis-related GO terms but should be represented in at least one such term with the evidence code “Inferred from Biological aspects of Ancestor” (IBA), “Inferred from Sequence Orthology” (ISO), “Inferred from Sequence or structural Similarity” (ISS), or “Inferred from Expression Pattern” (IEP), where applicable.
Gene groups and families with a high likelihood of finding new iron homeostasis-relevant members
Iron homeostasis in A. thaliana has been the subject of extensive research. Notable progress has been made in the sub-field of iron homeostasis regulation, particularly in the roots (Liang, 2022; Li et al., 2023). However, the mechanisms of iron homeostasis in the shoot are not yet well understood. This includes the translocation, partitioning, and redistribution of iron from source to sink organs at different developmental stages, the role of transporters and enzymes, and the regulatory mechanisms involved. As there are gene families and ortholog/paralog groups whose members have been demonstrated to participate in iron homeostasis, or that have been shown to participate in homeostasis of other transition metals, we want to point out some of the ones that are interesting to consider in further studies.
We propose the family of ZIP transporter genes as a potential candidate for further investigation in iron homeostasis. In particular, ZIP2 and ZIP8 have been reported to be negatively and positively regulated by FIT (Mai et al., 2016a), respectively, and ZIP9 was induced under iron-deficient conditions and combined iron and zinc deficiency in roots (Yang et al., 2010). Although none of these genes were represented in any of the iron homeostasis-related GO terms, they may still play a crucial role in shoot iron homeostasis. Another group of interest is the small ZINC-INDUCED FACILITATOR (ZIF) and zinc-induced facilitator-like (ZIFL) transporter family, with ZIF1 being a direct PYE target that affects the intracellular distribution of iron (Long et al., 2010; Haydon et al., 2012). However, ZIF1 was not represented in any iron homeostasis-related GO terms.
The METAL TOLERANCE PROTEIN (MTP) transporter family includes MTP8/MTPc3, which participates in intracellular iron and manganese distribution in roots and shoots, is induced under iron deficiency, and its expression is FIT-dependent (Colangelo and Guerinot, 2004; Yang et al., 2010; Chu et al., 2017; Eroglu et al., 2017). MTP3/MTPa2, a vacuolar zinc transporter gene, is also regulated by FIT and belongs to the most robustly iron deficiency-induced genes (Colangelo and Guerinot, 2004; Mai et al., 2016a). While MTP8/MTPc3 was represented in iron homeostasis-related GO terms, MTP3/MTPa2 was not.
The oligopeptide transporter (OPT) family includes OPT3, which loads iron into the phloem and is required for shoot-to-root iron signaling (Zhai et al., 2014; Khan et al., 2018). From the Multidrug and Toxic Compound Extrusion (MATE) transporter family, MATE43/FRD3, MATE48/BCD1, and MATE52/ESL1 play roles in iron homeostasis in A. thaliana (Roschzttardtz et al., 2011; Seo et al., 2012; Wang et al., 2016), and they were represented in iron homeostasis GO terms. The ATP-BINDING CASSETTE subfamily G (ABCG) transporter gene family, including the subfamily of PLEIOTROPIC DRUG RESISTANCE (PDR) transporter genes, is another interesting gene family. In particular, ABCG37/PDR9 was demonstrated to be essential for exporting coumarins into the rhizosphere, where they enhance the mobilization of iron from the soil, especially at high pH (Rodríguez-Celma et al., 2013; Fourcroy et al., 2014; Schmid et al., 2014; Rajniak et al., 2018).
Finally, the diverse group of HEAVY METAL-ASSOCIATED (HMA) H+-ATPase transporter proteins, partly members of the Heavy metal transport/detoxification superfamily protein (HMP) superfamily, includes HMA3, which is regulated by FIT and induced under iron deficiency (Colangelo and Guerinot, 2004). Although the direct involvement of HMA3 in iron homeostasis has not yet been demonstrated, its FIT-dependent induction under iron deficiency implies its potential importance in this context.
We estimate that there is a high chance of finding the abovementioned or other members of the ZIP, ZIF, ZIFL, MTP, OPT, MATE, ABCG, PDR, HMA, and HMP gene families/orthologs/paralogs to be crucial in the context of iron homeostasis in general or specifically in response to iron starvation, or in the response to excess iron (for which there is no GO term as of now), even if they might act on other metals or in the transport of other chemical compounds.
Entries not present in their most appropriate GO terms
Some genes related to iron homeostasis were not represented in the most appropriate GO terms. For instance, BTSL1 and BTSL2 were categorized under iron ion transport (GO:0006826) and “regulation of iron ion transport” (GO:0034756) with the evidence code “inferred from mutant phenotype” and the relationship type “acts upstream of or within.” However, they were not categorized under “negative regulation of iron ion transport” (GO:0034757), which is what they have been reported to do (Rodríguez-Celma et al., 2019). Similarly, FIT was not classified under iron ion transport (GO:0006826), where it acts as a regulator. Instead, it was placed under regulation of iron ion transport (GO:0034756), which fits the function of FIT (Colangelo and Guerinot, 2004; Jakoby et al., 2004), but not in its most appropriate GO term, “positive regulation of iron ion transport” (GO:0034758).
IMA/FEP peptide genes were categorized under their most appropriate GO term, positive regulation of iron ion transport (GO:0034758), but they were not present in iron ion transport (GO:0006826), where they act as upstream regulators (Grillet et al., 2018; Gautam et al., 2021). Interestingly, BTSL1 and 2 were the only genes categorized under “iron import across plasma membrane” (GO:0098711), but genes like IRT1 or FRO2, which are directly involved in iron uptake, were not present. The relationship type acts upstream of or within is also equally valid for all the genes encoding transcription factors involved in the regulation of iron transmembrane transporters, but they were not categorized as such.
The genes mentioned above illustrate that the exact location of genes in the tree of connected GO terms and the completeness of their individual entries are crucial. As of now, the way iron homeostasis-related GO terms were populated with genes heavily impacts the detection of enrichment of the biological process of iron homeostasis as relevant GO terms are underpopulated or empty. This decreases the chances of detecting terms and increases the chances of falsely detecting terms if they have only one or very few members.
Custom sets of genes for enrichment analysis of iron homeostasis
As almost all of the genes currently represented in any of the iron homeostasis-related Biological Process GO terms somehow participate in the response to iron ion, they should at least be listed in that particular GO term (GO:0010039). However, since this only applies to 48 of the 113 genes represented, we suggest manually performing enrichment analysis with a custom list of genes that includes all 113 previously represented genes (Supplementary Table S20). To make the gene set more complete, we also suggest extending the list with missing genes for which there is direct evidence of iron homeostasis participation (Table 1), resulting in a more complete set (Supplementary Table S21, “extended gene list 1”).
Although crosstalk between iron and other metals is important and some genes have been shown to be regulated by FIT with potential functions in the context of iron homeostasis, none of these genes were represented in iron homeostasis-related GO terms. Therefore, we would expect them to be included at least in the generic terms “response to iron ion” (GO:0010039) and “response to iron ion starvation” (GO:1990641), at least with the evidence code IEP or others if applicable. To account for this, we created an even further extended gene set (Supplementary Table S22, “extended gene list 2”), containing the genes from the previous set (Supplementary Table S21, “extended gene list 1”) and all FIT-regulated genes that were not previously represented in one of the iron homeostasis-related GO terms (Table 2).
We also suggest representing the smaller paralog/ortholog groups/families in iron homeostasis-related GO terms (Table 3), and we have created another further extended list that contains these genes (Supplementary Table S23, “extended gene list 3”) in addition to the ones in the previous list (Supplementary Table S22, “extended gene list 2”). Finally, we suggest adding the larger gene families that may have further yet unknown participants in iron homeostasis in the broadest sense (Table 4) and have assembled an experimental list that includes all of the previously mentioned gene lists (Supplementary Table S23, extended gene list 3) and the gene families from (Table 4).
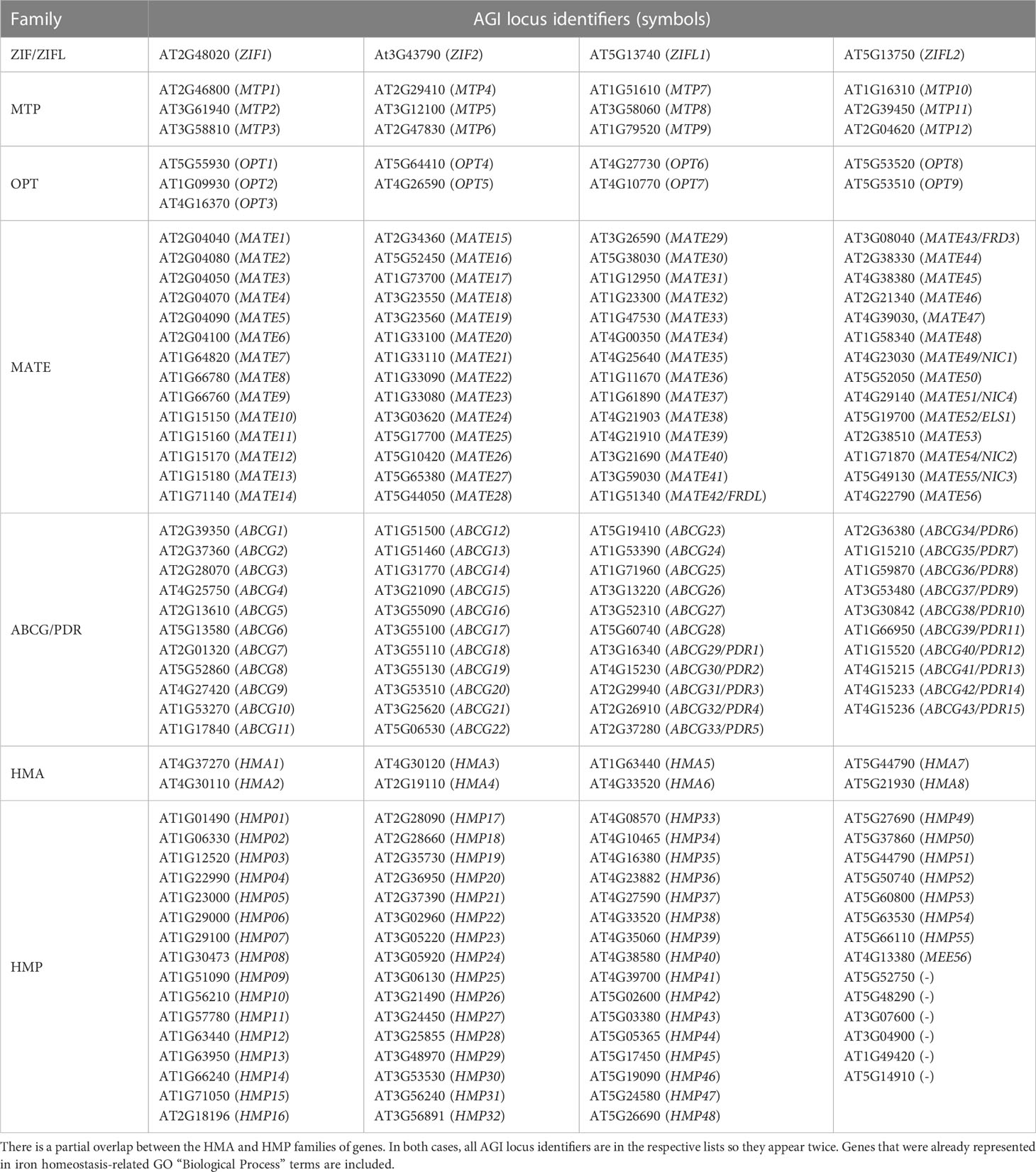
Table 4 Gene families from which we estimate more members might be detected to play roles in the context of iron homeostasis in the future based on the fact that they transport transition metals or that known members transport other chemical compounds that are in the context of iron homeostasis.
These lists can be used to perform enrichment analysis of iron homeostasis using simple 2 × 2 contingency table tests for independence, such as Fisher’s exact test or the χ2 test, which are easily performed and available in the most commonly used software and frameworks for statistical analyses. We performed such enrichment analyses using Fisher’s exact test. We performed the analysis with the original set of 113 genes (Supplementary Table S1) and with our extended list 2 (Supplementary Table S22). This was applied to genes induced by iron deficiency vs. sufficient iron supply in the roots of 6-week-old Arabidopsis plants (Mai et al., 2016b). We showed that our list performed better than the original list since more genes are detected. Furthermore, enrichment was revealed with a lower p-value and a higher odds ratio (Supplementary Table S24).
Conclusions and prospects
We investigated the iron homeostasis-specific GO terms from the Biological Process category in the Arabidopsis thaliana Gene Ontology database hosted by TAIR, excluding the iron–sulfur cluster and similar GO terms. We observed that a total of 113 loci were represented in 17 such GO terms that were organized into five distinct paths starting from the root of the Biological Process category and covering different aspects of iron homeostasis. Comparison of the genes represented in the relevant GO terms with the current literature revealed that important players were not represented, although evidence for their roles in iron homeostasis has been published for years. This included iron transporters NRAMP6, PIC1/TIC21, and ZIF1, transcription factors such as BHLH034/IDT1, BHLH104, BHLH105/ILR3, MYB28/HAG1/PMG1, MYB29/RAO7/PMG2, BHLH110, MYC1, and ERF2, and important enzymes such as the whole NAS ortholog group (NAS1-4), COSY, AHA2, and AHA7 (Table 1). Furthermore, many of the FIT target genes were also not present in the iron homeostasis-related GO terms (Table 2). However, it can be assumed that their functions may be important in the context of iron homeostasis, as they are regulated by the central and essential transcription factor of the iron uptake machinery in roots.
It is not surprising that new findings take time to be transferred from the literature to databases like the Gene Ontology, particularly if they require human assistance. However, there were entries with evidence from the literature as recent as 2022 (Supplementary Table S1), while the four NAS genes, which have been known to participate in iron homeostasis for over a decade (Schuler and Bauer, 2011), were not included. This inconsistency highlights one of the main challenges of the Gene Ontology: limited coverage. We also found that some genes were represented in iron homeostasis-related GO terms but not in their most appropriate terms, such as IRT1 and FRO2, which reveals another challenge: some terms in the Gene Ontology can be vague or ambiguous, making it difficult to accurately annotate genes. Additionally, we noticed that while there is a GO term for “response to iron ion starvation” (GO:1990641), there is currently no GO term covering the response to excess iron. This further highlights the shortcomings of the Gene Ontology, specifically inconsistencies in GO terms and an incomplete representation of biological processes. Finally, the Gene Ontology can be biased towards well-studied genes and processes. Additionally, there may be large gaps in knowledge transfer, possibly due to different sources of data and annotation practices, which is particularly evident in the field of iron homeostasis in A. thaliana. Taken together, these limitations can lead to underrepresentation or overrepresentation of certain genes and terms in the Gene Ontology and can ultimately lead to erroneous results, such as false positives and false negatives, in GO term enrichment analyses.
In order to address the limitations of the current Gene Ontology in representing the complex biological process of iron homeostasis in A. thaliana, we have compiled a series of comprehensive lists of genes that can be used for enrichment analysis in transcriptomic or proteomic studies. Our lists include genes with varying levels of evidence, ranging from those with direct experimental evidence of participation in iron homeostasis to those with potential roles in this process that are yet to be fully characterized.
Our most extensively validated list (Supplementary Table S20) includes all genes represented in iron homeostasis-specific terms in the Gene Ontology (Supplementary Table S1) as well as all those with direct evidence of participation in iron homeostasis in A. thaliana that were not previously represented (Table 1). Our more inclusive lists additionally incorporate genes that are known to be regulated by FIT or are robustly induced by iron deficiency (Table 2), which were surprisingly absent from the Gene Ontology (Supplementary Table S21), and missing members of small gene families or ortholog/paralog groups (Table 3), which could have easily been added to the Gene Ontology with the appropriate evidence codes (Supplementary Table S22). These lists provide a more comprehensive view of the genes involved in iron homeostasis in A. thaliana and highlight the gaps in the current Gene Ontology. Finally, we have further included a list of gene families of which we anticipate that members may have important roles in iron homeostasis but are currently underrepresented in the Gene Ontology (Table 4). This “experimental” gene list (Supplementary Table S23) provides an interesting resource for future studies in this field.
Overall, our lists can be used in transcriptomic and proteomic studies to perform custom enrichment analyses of iron homeostasis, which are easily performed with the most commonly used software for statistical analyses (example: Supplementary Table S24). Additionally, they can be used to simply look up all the hitherto known iron homeostasis-related genes in a set of genes without having to go through them manually. They provide a valuable tool for researchers studying iron homeostasis in A. thaliana and highlight the need for continued efforts to improve the completeness and accuracy of the Gene Ontology.
Author contributions
H-JM and DB wrote the manuscript, H-JM and DB collected and assembled data, PB reviewed the manuscript and provided funding.
Funding
This work was funded by the Deutsche Forschungsgemeinschaft (DFG, German Research Foundation) – Project-ID 456082119 – TRR 341/1, and by Deutsche Forschungsgemeinschaft grants International Research Training group 2466, F0205120 (NextPlant, project ID 391465903/GRK 2466) (DB is a member of the Next Plant graduate school). Support was also provided by Germany´s Excellence Strategy – EXC-2048/1 – project ID 390686111.
Conflict of interest
The authors declare that the research was conducted in the absence of any commercial or financial relationships that could be construed as a potential conflict of interest.
Publisher’s note
All claims expressed in this article are solely those of the authors and do not necessarily represent those of their affiliated organizations, or those of the publisher, the editors and the reviewers. Any product that may be evaluated in this article, or claim that may be made by its manufacturer, is not guaranteed or endorsed by the publisher.
Supplementary material
The Supplementary Material for this article can be found online at: https://www.frontiersin.org/articles/10.3389/fpls.2023.1204723/full#supplementary-material
References
Aguayo, M. F., Ampuero, D., Mandujano, P., Parada, R., Munoz, R., Gallart, M., et al. (2013). Sorbitol dehydrogenase is a cytosolic protein required for sorbitol metabolism in Arabidopsis thaliana. Plant Sci. 205-206, 63–75. doi: 10.1016/j.plantsci.2013.01.012
Aitken, A., Gonin, H. (1936). XI.—On Fourfold Sampling with and without replacement. Proc. R. Soc. Edinburgh 55, 114–125. doi: 10.1017/S0370164600014413
Arrivault, S., Senger, T., Kramer, U. (2006). The Arabidopsis metal tolerance protein AtMTP3 maintains metal homeostasis by mediating Zn exclusion from the shoot under Fe deficiency and Zn oversupply. Plant J. 46 (5), 861–879. doi: 10.1111/j.1365-313X.2006.02746.x
Ashburner, M., Ball, C. A., Blake, J. A., Botstein, D., Butler, H., Cherry, J. M., et al. (2000). Gene ontology: tool for the unification of biology. The Gene Ontology Consortium. Nat. Genet. 25 (1), 25–29. doi: 10.1038/75556
Bauer, P., Ling, H. Q., Guerinot, M. L. (2007). FIT, the FER-LIKE IRON DEFICIENCY INDUCED TRANSCRIPTION FACTOR in arabidopsis. Plant Physiol. Biochem. 45 (5), 260–261. doi: 10.1016/j.plaphy.2007.03.006
Binns, D., Dimmer, E., Huntley, R., Barrell, D., O'Donovan, C., Apweiler, R. (2009). QuickGO: a web-based tool for Gene Ontology searching. Bioinformatics 25 (22), 3045–3046. doi: 10.1093/bioinformatics/btp536
Cai, Y., Li, Y., Liang, G. (2021). FIT and bHLH Ib transcription factors modulate iron and copper crosstalk in Arabidopsis. Plant Cell Environ. 44 (5), 1679–1691. doi: 10.1111/pce.14000
Cailliatte, R., Schikora, A., Briat, J. -F., Mari, S., Curie, C. (2010). High-affinity manganese uptake by the metal transporter NRAMP1 is essential for Arabidopsis growth in low manganese conditions. Plant Cell 22 (3), 904–917. doi: 10.1105/tpc.109.073023
Chu, H. H., Car, S., Socha, A. L., Hindt, M. N., Punshon, T., Guerino, M. L. (2017). The Arabidopsis MTP8 transporter determines the localization of manganese and iron in seeds. Sci. Rep. 7 (1), 11024. doi: 10.1038/s41598-017-11250-9
Colangelo, E. P., Guerinot, M. L. (2004). The essential basic helix-loop-helix protein FIT1 is required for the iron deficiency response. Plant Cell 16 (12), 3400–3412. doi: 10.1105/tpc.104.024315
Coleto, I., Bejarano, I., Marin-Pena, A. J., Medina, J., Rioja, C., Burow, M., et al. (2021). Arabidopsis thaliana transcription factors MYB28 and MYB29 shape ammonium stress responses by regulating Fe homeostasis. New Phytol. 229 (2), 1021–1035. doi: 10.1111/nph.16918
Conte, S. S., Chu, H. H., Rodriguez, D. C., Punshon, T., Vasques, K. A., Salt, D. E., et al. (2013). Arabidopsis thaliana Yellow Stripe1-Like4 and Yellow Stripe1-Like6 localize to internal cellular membranes and are involved in metal ion homeostasis. Front. Plant Sci. 4, 283. doi: 10.3389/fpls.2013.00283
Curie, C., Alonso, J. M., JEAN, M. L., Ecker, J. R., Briat, J. -F. (2000). Involvement of NRAMP1 from Arabidopsis thaliana in iron transport. Biochem. J. 347 (3), 749–755. doi: 10.1042/bj3470749
DiDonato, R. J. Jr., Roberts, L. A., Sanderson, T., Eisley, R. B., Walker, E. L. (2004). Arabidopsis Yellow Stripe-Like2 (YSL2): a metal-regulated gene encoding a plasma membrane transporter of nicotianamine-metal complexes. Plant J. 39 (3), 403–414. doi: 10.1111/j.1365-313X.2004.02128.x
Divol, F., Couch, D., Conejero, G., Roschzttardtz, H., Mari, S., Curie, C. (2013). The Arabidopsis YELLOW STRIPE LIKE4 and 6 transporters control iron release from the chloroplast. Plant Cell 25 (3), 1040–1055. doi: 10.1105/tpc.112.107672
Duy, D., Wanner, G., Meda, A. R., von Wiren, N., Soll, J., Philippar, K. (2007). PIC1, an ancient permease in Arabidopsis chloroplasts, mediates iron transport. Plant Cell 19 (3), 986–1006. doi: 10.1105/tpc.106.047407
Eide, D., Broderius, M., Fett, J., Guerinot, M. L. (1996). A novel iron-regulated metal transporter from plants identified by functional expression in yeast. Proc. Natl. Acad. Sci. U.S.A. 93 (11), 5624–5628. doi: 10.1073/pnas.93.11.5624
Eroglu, S., Giehl, R. F. H., Meier, B., Takahashi, M., Terada, Y., Ignatyev, K., et al. (2017). Metal tolerance protein 8 mediates manganese homeostasis and iron reallocation during seed development and germination. Plant Physiol. 174 (3), 1633–1647. doi: 10.1104/pp.16.01646
Fisher, R. A. (1935). Statistical methods for research workers Vol. 98 (Edinburgh: Royal Statistical Society), S. 39–S. 54.
Fourcroy, P., Siso-Terraza, P., Sudre, D., Saviron, M., Reyt, G., Gaymard, F., et al. (2014). Involvement of the ABCG37 transporter in secretion of scopoletin and derivatives by Arabidopsis roots in response to iron deficiency. New Phytol. 201 (1), 155–167. doi: 10.1111/nph.12471
Gao, F., Robe, K., Bettembourg, M., Navarro, N., Rofidal, V., Santoni, V., et al. (2020). The Transcription Factor bHLH121 Interacts with bHLH105 (ILR3) and Its Closest Homologs to Regulate Iron Homeostasis in Arabidopsis. Plant Cell 32 (2), 508–524. doi: 10.1105/tpc.19.00541
Gautam, C. K., Tsai, H. H., Schmidt, W. (2021). IRONMAN tunes responses to iron deficiency in concert with environmental pH. Plant Physiol. 187 (3), 1728–1745. doi: 10.1093/plphys/kiab329
Gene Ontology, C. (2023a) Guide to GO evidence codes. Available at: http://geneontology.org/docs/guide-go-evidence-codes/.
Gene Ontology, C (2023b) Annotation Relations - GO Wiki. Available at: https://wiki.geneontology.org/Annotation_Relations.
Gollhofer, J., Schlawicke, C., Jungnick, N., Schmidt, W., Buckhout, T. J. (2011). Members of a small family of nodulin-like genes are regulated under iron deficiency in roots of Arabidopsis thaliana. Plant Physiol. Biochem. 49 (5), 557–564. doi: 10.1016/j.plaphy.2011.02.011
Gollhofer, J., Timofeev, R., Lan, P., Schmidt, W., Buckhout, T. J. (2014). Vacuolar-Iron-Transporter1-Like proteins mediate iron homeostasis in Arabidopsis. PloS One 9 (10), e110468. doi: 10.1371/journal.pone.0110468
Grillet, L., Lan, P., Li, W., Mokkapati, G., Schmidt, W. (2018). IRON MAN is a ubiquitous family of peptides that control iron transport in plants. Nat. Plants 4 (11), 953–963. doi: 10.1038/s41477-018-0266-y
Hahne, F., Huber, W., Gentleman, R., Falcon, S., Falcon, S., Gentleman, R. (2008). Hypergeometric testing used for gene set enrichment analysis. Bioconductor Case Stud., 207–220. doi: 10.1007/978-0-387-77240-0-14
Harbort, C. J., Hashimoto, M., Inoue, H., Niu, Y., Guan, R., Rombolà, A. D., et al. (2020). Root-secreted coumarins and the microbiota interact to improve iron nutrition in Arabidopsis. Cell Host Microbe 28 (6), 825–837.e6. doi: 10.1016/j.chom.2020.09.006
Haydon, M. J., Kawachi, M., Wirtz, M., Hillmer, S., Hell, R., Krämer, U. (2012). Vacuolar nicotianamine has critical and distinct roles under iron deficiency and for zinc sequestration in Arabidopsis. Plant Cell 24 (2), 724–737. doi: 10.1105/tpc.111.095042
Hindt, M. N., Akmakjian, G. Z., Pivarski, K. L., Punshon, T., Hell, I., Baxter, D. E., et al. (2017). BRUTUS and its paralogs, BTS LIKE1 and BTS LIKE2, encode important negative regulators of the iron deficiency response in Arabidopsis thaliana. Metallomics 9 (7), 876–890. doi: 10.1039/C7MT00152E
Huang da, W., Sherman, B. T., Lempicki, R. A. (2009). Bioinformatics enrichment tools: paths toward the comprehensive functional analysis of large gene lists. Nucleic Acids Res. 37 (1), 1–13. doi: 10.1093/nar/gkn923
Ivanov, R., Brumbarova, T., Bauer, P. (2012). Fitting into the harsh reality: regulation of iron-deficiency responses in dicotyledonous plants. Mol. Plant 5 (1), 27–42. doi: 10.1093/mp/ssr065
Jain, A., Dashner, Z. S., Connolly, E. L. (2019). Mitochondrial iron transporters (MIT1 and MIT2) are essential for iron homeostasis and embryogenesis in Arabidopsis thaliana. Front. Plant Sci. 10, 1449. doi: 10.3389/fpls.2019.01449
Jakoby, M., Wang, H. Y., Reidt, W., Weisshaar, B., Bauer, P. (2004). FRU (BHLH029) is required for induction of iron mobilization genes in Arabidopsis thaliana. FEBS Lett. 577 (3), 528–534. doi: 10.1016/j.febslet.2004.10.062
Kanehisa, M., Goto, S. (2000). KEGG: kyoto encyclopedia of genes and genomes. Nucleic Acids Res. 28 (1), 27–30. doi: 10.1093/nar/28.1.27
Kanwar, P., Baby, D., Bauer, P. (2021). Interconnection of iron and osmotic stress signalling in plants: is FIT a regulatory hub to cross-connect abscisic acid responses? Plant Biol. 23, 31–38. doi: 10.1111/plb.13261
Khan, M. A., Castro-Guerrero, N. A., McInturf, S. A., Nguyen, N. T., Dame, A. N., Wang, J., et al. (2018). Changes in iron availability in Arabidopsis are rapidly sensed in the leaf vasculature and impaired sensing leads to opposite transcriptional programs in leaves and roots. Plant Cell Environ. 41 (10), 2263–2276. doi: 10.1111/pce.13192
Kim, S. A., Punshon, T., Lanzirotti, A., Li, L., Alonso, J. M., Ecker, J. R., et al. (2006). Localization of iron in Arabidopsis seed requires the vacuolar membrane transporter VIT1. Science 314 (5803), 1295–1298. doi: 10.1126/science.1132563
Kim, L. J., Tsuyuki, K. M., Hu, F., Park, E. Y., Zhang, J., Iraheta, J. G., et al. (2021). Ferroportin 3 is a dual-targeted mitochondrial/chloroplast iron exporter necessary for iron homeostasis in Arabidopsis. Plant J. 107 (1), 215–236. doi: 10.1111/tpj.15286
Korshunova, Y. O., Eide, D., Clark, W. G., Guerinot, M. L., Pakrasi, H. B. (1999). The IRT1 protein from Arabidopsis thaliana is a metal transporter with a broad substrate range. Plant Mol. Biol. 40 (1), 37–44. doi: 10.1023/A:1026438615520
Lamesch, P., Berardini, T. Z., Li, D., Swarbreck, D., Wilks, C., Sasidharan, R., et al. (2012). The Arabidopsis Information Resource (TAIR): improved gene annotation and new tools. Nucleic Acids Res. 40 (Database issue), D1202–D1210. doi: 10.1093/nar/gkr1090
Lanquar, V., Lelièvre, F., Bolte, S., Hamès, C., Alcon, C., Neumann, D., et al. (2005). Mobilization of vacuolar iron by AtNRAMP3 and AtNRAMP4 is essential for seed germination on low iron. EMBO J. 24 (23), 4041–4051. doi: 10.1038/sj.emboj.7600864
Le Jean, M., Schikora, A., Mari, S., Briat, J. F., Curie, C. (2005). A loss-of-function mutation in AtYSL1 reveals its role in iron and nicotianamine seed loading. Plant J. 44 (5), 769–782. doi: 10.1111/j.1365-313X.2005.02569.x
Li, X., Zhang, H., Ai, Q., Liang, G., Yu, D. (2016). Two bHLH Transcription Factors, bHLH34 and bHLH104, Regulate Iron Homeostasis in Arabidopsis thaliana. Plant Physiol. 170 (4), 2478–2493. doi: 10.1104/pp.15.01827
Li, J., Wang, Y., Zheng, L., Li, Y., Zhou, X., Li, J., et al. (2019). The intracellular transporter AtNRAMP6 is involved in Fe homeostasis in Arabidopsis. Front. Plant Sci. 10, 1124. doi: 10.3389/fpls.2019.01124
Li, M., Watanabe, S., Gao, F., Dubos, C. (2023). Iron nutrition in plants: towards a new paradigm? Plants 12 (2), 384. doi: 10.3390/plants12020384
Li, W., Lan, P. (2015). Genome-wide analysis of overlapping genes regulated by iron deficiency and phosphate starvation reveals new interactions in Arabidopsis roots. BMC Res. Notes 8 (1), 1–16. doi: 10.1186/s13104-015-1524-y
Liang, G. (2022). Iron uptake, signaling, and sensing in plants. Plant Commun. 3 (5), 100349. doi: 10.1016/j.xplc.2022.100349
Lichtblau, D. M., Schwarz, B., Baby, D., Endres, C., Sieberg, C., Bauer, P. (2022). The iron deficiency-regulated small protein effector FEP3/IRON MAN1 modulates interaction of BRUTUS-LIKE1 with bHLH subgroup IVc and POPEYE transcription factors. Front. Plant Sci. 13. doi: 10.3389/fpls.2022.930049
Lin, Y. F., Liang, H. M., Yang, S. Y., Boch, A., Clemens, S., Chen, C. C., et al. (2009). Arabidopsis IRT3 is a zinc-regulated and plasma membrane localized zinc/iron transporter. New Phytol. 182 (2), 392–404. doi: 10.1111/j.1469-8137.2009.02766.x
Liu, W., Li, Q., Wang, Y., Wu, T., Yang, Y., Zhang, X., et al. (2017). Ethylene response factor AtERF72 negatively regulates Arabidopsis thaliana response to iron deficiency. Biochem. Biophys. Res. Commun. 491 (3), 862–868. doi: 10.1016/j.bbrc.2017.04.014
Long, T. A., Tsukagoshi, H., Busch, W., Lahner, B., Salt, D. E., Benfey, P. N. (2010). The bHLH transcription factor POPEYE regulates response to iron deficiency in Arabidopsis roots. Plant Cell 22 (7), 2219–2236. doi: 10.1105/tpc.110.074096
Mai, H. J., Pateyron, S., Bauer, P. (2016a). Iron homeostasis in Arabidopsis thaliana: transcriptomic analyses reveal novel FIT-regulated genes, iron deficiency marker genes and functional gene networks. BMC Plant Biol. 16, 1–22. doi: 10.1186/s12870-016-0899-9
Mai, H. J., Pateyron, S., Bauer, P. (2016b). Iron homeostasis in Arabidopsis thaliana: transcriptomic analyses reveal novel FIT-regulated genes, iron deficiency marker genes and functional gene networks. BMC Plant Biol. 16 (1), 211. doi: 10.1186/s12870-016-0899-9
Morel, M., Crouzet, J., Gravot, A., Auroy, P., Leonhardt, N., Vavasseur, A., et al. (2009). AtHMA3, a P1B-ATPase allowing Cd/Zn/Co/Pb vacuolar storage in Arabidopsis. Plant Physiol. 149 (2), 894–904. doi: 10.1104/pp.108.130294
Mukherjee, I., Campbell, N. H., Ash, J. S., Connolly, E. L. (2006). Expression profiling of the Arabidopsis ferric chelate reductase (FRO) gene family reveals differential regulation by iron and copper. Planta 223 (6), 1178–1190. doi: 10.1007/s00425-005-0165-0
Pearson, K. (1900). X. On the criterion that a given system of deviations from the probable in the case of a correlated system of variables is such that it can be reasonably supposed to have arisen from random sampling. London Edinburgh Dublin Philos. Magazine J. Sci. 50 (302), 157–175. doi: 10.1080/14786440009463897
Perea-Garcia, A., Andres-Borderia, A., Vera-Sirera, F., Perez-Amador, M. A., Puig, S., Penarrubia, L. (2020). Deregulated high affinity copper transport alters iron homeostasis in arabidopsis. Front. Plant Sci. 11, 1106. doi: 10.3389/fpls.2020.01106
Rajniak, J., Giehl, R. F. H., Chang, E., Murgia, I., von Wiren, N., Sattely, E. S.. (2018). Biosynthesis of redox-active metabolites in response to iron deficiency in plants. Nat. Chem. Biol. 14 (5), 442–450. doi: 10.1038/s41589-018-0019-2
Rivals, I., Personnaz, L., Taing, L., Potier, M. -C. (2007). Enrichment or depletion of a GO category within a class of genes: which test? Bioinformatics 23 (4), 401–407. doi: 10.1093/bioinformatics/btl633
Robinson, N. J., Procter, C. M., Connolly, E. L., Guerinot, M. L. (1999). A ferric-chelate reductase for iron uptake from soils. Nature 397 (6721), 694–697. doi: 10.1038/17800
Rodríguez-Celma, J., Lin, W. -D., Fu, G. -M., Abadia, J., López-Millán, A. -F., Schmidt, W. (2013). Mutually exclusive alterations in secondary metabolism are critical for the uptake of insoluble iron compounds by Arabidopsis and Medicago truncatula. Plant Physiol. 162 (3), 1473–1485. doi: 10.1104/pp.113.220426
Rodríguez-Celma, J., Connorton, J. M., Kruse, I., Green, R. T., Franceschetti, M., Chen, Y. -T. (2019). Arabidopsis BRUTUS-LIKE E3 ligases negatively regulate iron uptake by targeting transcription factor FIT for recycling. Proc. Natl. Acad. Sci. 116 (35), 17584–17591. doi: 10.1073/pnas.1907971116
Rodríguez-Celma, H., Conejero, G., Curie, C., Mari, S. (2009). Identification of the endodermal vacuole as the iron storage compartment in the Arabidopsis embryo. Plant Physiol. 151 (3), 1329–1338. doi: 10.1104/pp.109.144444
Roschzttardtz, H., Séguéla-Arnaud, M., Briat, J. -F., Vert, G., Curie, C. (2011). The FRD3 citrate effluxer promotes iron nutrition between symplastically disconnected tissues throughout Arabidopsis development. Plant Cell 23 (7), 2725–2737. doi: 10.1105/tpc.111.088088
Santi, S., Schmidt, W. (2009). Dissecting iron deficiency-induced proton extrusion in Arabidopsis roots. New Phytol. 183 (4), 1072–1084. doi: 10.1111/j.1469-8137.2009.02908.x
Schaaf, G., Schikora, A., Haberle, J., Vert, G., Ludewig, U., Briat, J. F., et al. (2005). A putative function for the arabidopsis Fe-Phytosiderophore transporter homolog AtYSL2 in Fe and Zn homeostasis. Plant Cell Physiol. 46 (5), 762–774. doi: 10.1093/pcp/pci081
Schaaf, G., Honsbein, A., Meda, A. R., Kirchner, S., Wipf, D., von Wiren, N. (2006). AtIREG2 encodes a tonoplast transport protein involved in iron-dependent nickel detoxification in Arabidopsis thaliana roots. J. Biol. Chem. 281 (35), 25532–25540. doi: 10.1074/jbc.M601062200
Schmid, N. B., Giehl, R. F., Doll, S., Mock, H. P., Strehmel, N., Scheel, D., et al. (2013). F6'H1-dependent coumarins mediate iron acquisition from alkaline substrates in Arabidopsis thaliana. Plant Physiol. 164 (1), 160–172. doi: 10.1104/pp.113.228544
Schmid, N. B., Giehl, R. F., Doll, S., Mock, H. P., Strehmel, N., Scheel, D., et al. (2014). Feruloyl-CoA 6′-Hydroxylase1-dependent coumarins mediate iron acquisition from alkaline substrates in Arabidopsis. Plant Physiol. 164 (1), 160–172. doi: 10.1104/pp.113.228544
Schuler, M., Bauer, P. (2011). Heavy metals need assistance: the contribution of nicotianamine to metal circulation throughout the plant and the arabidopsis NAS gene family. Front. Plant Sci. 2, 69. doi: 10.3389/fpls.2011.00069
Schuler, M., Rellan-Alvarez, R., Fink-Straube, C., Abadia, J., Bauer, P. (2012). Nicotianamine functions in the Phloem-based transport of iron to sink organs, in pollen development and pollen tube growth in Arabidopsis. Plant Cell 24 (6), 2380–2400. doi: 10.1105/tpc.112.099077
Selote, D., Samira, R., Matthiadis, A., Gillikin, J. W., Long, T. A. (2015). Iron-binding E3 ligase mediates iron response in plants by targeting basic helix-loop-helix transcription factors. Plant Physiol. 167 (1), 273–286. doi: 10.1104/pp.114.250837
Seo, P. J., Park, J., Park, M. J., Kim, Y. S., Kim, S. G., Jung, J. H., et al. (2012). A Golgi-localized MATE transporter mediates iron homoeostasis under osmotic stress in Arabidopsis. Biochem. J. 442 (3), 551–561. doi: 10.1042/BJ20111311
Shimizu, T., Yasuda, R., Mukai, Y., Tanoue, R., Shimada, T., Imamura, S., et al. (2020). Proteomic analysis of haem-binding protein from Arabidopsis thaliana and Cyanidioschyzon merolae. Philos. Trans. R Soc. Lond B Biol. Sci. 375 (1801), 20190488. doi: 10.1098/rstb.2019.0488
Siwinska, J., Siatkowska, K., Olry, A., Grosjean, J., Hehn, A., Bourgaud, F., et al. (2018). Scopoletin 8-hydroxylase: a novel enzyme involved in coumarin biosynthesis and iron-deficiency responses in Arabidopsis. J. Exp. Bot. 69 (7), 1735–1748. doi: 10.1093/jxb/ery005
Song, H., Geng, Q., Wu, X., Hu, M., Ye, M., Xu, X., et al. (2023). The transcription factor MYC1 interacts with FIT to negatively regulate iron homeostasis in Arabidopsis thaliana. Plant J. 114 (1), 193–208. doi: 10.1111/tpj.16130
Tissot, N., Robe, K., Gao, F., Grant-Grant, S., Boucherez, J., Bellegarde, F., et al. (2019). Transcriptional integration of the responses to iron availability in Arabidopsis by the bHLH factor ILR3. New Phytol. 223 (3), 1433–1446. doi: 10.1111/nph.15753
Tsai, H. -H., Rodríguez-Celma, J., Lan, P., Wu, Y. -C., Vélez-Bermúdez, I. C., Schmidt, W. (2018). Scopoletin 8-hydroxylase-mediated fraxetin production is crucial for iron mobilization. Plant Physiol. 177 (1), 194–207. doi: 10.1104/pp.18.00178
Usadel, B., Poree, F., Nagel, A., Lohse, M., Czedik-Eysenberg, A., Stitt, M. (2009). A guide to using MapMan to visualize and compare Omics data in plants: a case study in the crop species, Maize. Plant Cell Environ. 32 (9), 1211–1229. doi: 10.1111/j.1365-3040.2009.01978.x
Vanholme, R., Sundin, L., Seetso, K. C., Kim, H., Liu, X., Li, J., et al. (2019). COSY catalyses trans-cis isomerization and lactonization in the biosynthesis of coumarins. Nat. Plants 5 (10), 1066–1075. doi: 10.1038/s41477-019-0510-0
Vert, G., Barberon, M., Zelazny, E., Seguela, M., Briat, J. F., Curie, C. (2009). Arabidopsis IRT2 cooperates with the high-affinity iron uptake system to maintain iron homeostasis in root epidermal cells. Planta 229 (6), 1171–1179. doi: 10.1007/s00425-009-0904-8
Voith von Voithenberg, L., Park, J., Stube, R., Lux, C., Lee, Y., Philippar, K. (2019). A novel prokaryote-type ECF/ABC transporter module in chloroplast metal homeostasis. Front. Plant Sci. 10, 1264. doi: 10.3389/fpls.2019.01264
Wang, J., Lan, P., Gao, H., Zheng, L., Li, W., Schmidt, W. (2013). Expression changes of ribosomal proteins in phosphate-and iron-deficient Arabidopsis roots predict stress-specific alterations in ribosome composition. BMC Genomics 14 (1), 1–14. doi: 10.1186/1471-2164-14-783
Wang, Z., Qian, C., Guo, X., Liu, E., Mao, K., Mu, C., et al. (2016). ELS1, a novel MATE transporter related to leaf senescence and iron homeostasis in Arabidopsis thaliana. Biochem. Biophys. Res. Commun. 476 (4), 319–325. doi: 10.1016/j.bbrc.2016.05.121
Wang, C., et al. (2017). Fe-deficiency-induced expression of bHLH104 enhances Fe-deficiency tolerance of Arabidopsis thaliana. Planta 246 (3), 421–431. doi: 10.1007/s00425-017-2703-y
Waters, B. M., Chu, H. -H., DiDonato, R. J., Roberts, L. A., Eisley, R. B., Lahner, B., et al. (2006). Mutations in Arabidopsis yellow stripe-like1 and yellow stripe-like3 reveal their roles in metal ion homeostasis and loading of metal ions in seeds. Plant Physiol. 141 (4), 1446–1458. doi: 10.1104/pp.106.082586
Waters, B. M., McInturf, S. A., Stein, R. J. (2012). Rosette iron deficiency transcript and microRNA profiling reveals links between copper and iron homeostasis in Arabidopsis thaliana. J. Exp. Bot. 63 (16), 5903–5918. doi: 10.1093/jxb/ers239
Wintz, H., Fox, T., Wu, Y. Y., Feng, V., Chen, W., Chang, H. S., et al. (2003). Expression profiles of Arabidopsis thaliana in mineral deficiencies reveal novel transporters involved in metal homeostasis. J. Biol. Chem. 278 (48), 47644–47653. doi: 10.1074/jbc.M309338200
Yamada, K., Nagano, A. J., Nishina, M., Hara-Nishimura, I., Nishimura, M. (2013). Identification of two novel endoplasmic reticulum body-specific integral membrane proteins. Plant Physiol. 161 (1), 108–120. doi: 10.1104/pp.112.207654
Yang, T. J., Lin, W. D., Schmidt, W. (2010). Transcriptional profiling of the Arabidopsis iron deficiency response reveals conserved transition metal homeostasis networks. Plant Physiol. 152 (4), 2130–2141. doi: 10.1104/pp.109.152728
Zhai, Z., Gayomba, S. R., Jung, H. -i., Vimalakumari, N. K., Piñeros, M., Craft, E., et al. (2014). OPT3 is a phloem-specific iron transporter that is essential for systemic iron signaling and redistribution of iron and cadmium in Arabidopsis. Plant Cell 26 (5), 2249–2264. doi: 10.1105/tpc.114.123737
Keywords: gene ontology, GO term, biological process, Arabidopsis thaliana, iron homeostasis, enrichment analysis, gene set
Citation: Mai H-J, Baby D and Bauer P (2023) Black sheep, dark horses, and colorful dogs: a review on the current state of the Gene Ontology with respect to iron homeostasis in Arabidopsis thaliana. Front. Plant Sci. 14:1204723. doi: 10.3389/fpls.2023.1204723
Received: 12 April 2023; Accepted: 04 July 2023;
Published: 24 July 2023.
Edited by:
Felipe Klein Ricachenevsky, Federal University of Rio Grande do Sul, BrazilReviewed by:
Maria Bernal, Ruhr University Bochum, GermanyJeeyon Jeong, Amherst College, United States
Copyright © 2023 Mai, Baby and Bauer. This is an open-access article distributed under the terms of the Creative Commons Attribution License (CC BY). The use, distribution or reproduction in other forums is permitted, provided the original author(s) and the copyright owner(s) are credited and that the original publication in this journal is cited, in accordance with accepted academic practice. No use, distribution or reproduction is permitted which does not comply with these terms.
*Correspondence: Hans-Jörg Mai, aGFucy1qb2VyZy5tYWlAaGh1LmRl