- Jiangsu Key Laboratory of Crop Cultivation and Physiology/Co-Innovation Center for Modern Production Technology of Grain Crops/Innovation Center of Rice Cultivation Technology in Yangtze Valley, Research Institute of Rice Industrial Engineering Technology, Yangzhou University, Yangzhou, China
Zinc oxide nanoparticles (ZnO NPs) have been widely used in agriculture as a new type of Zn fertilizer, and many studies were conducted to evaluate the effect of ZnO NPs on plant growth. However, there are relatively few studies on the effects of application methods and appropriate dosages of ZnO NPs on rice yield, quality, grain Zn content, and distribution. Therefore, in the 2019 and 2020, field trials were conducted with six ZnO NPs basal application dosages of no ZnO NPs, 3.75 kg hm−2, 7.5 kg hm−2, 15 kg hm−2, 30 kg hm−2, and 60 kg hm−2, and the effects of ZnO NPs application on rice yield, quality, grain Zn content, and distribution were investigated. The results demonstrated that applying ZnO NPs in Zn-deficient soils (available Zn < 1.0 mg kg−1) increased rice grain yield by 3.24%–4.86% and 3.51%–5.12% in 2019 and 2020, respectively. In addition, ZnO NPs improved the quality of rice by increasing the head milling rate, reducing chalky grain percentage, and increasing the taste value and breakdown of rice. In terms of Zn accumulation in rice, ZnO NPs application significantly increased the Zn content in both milled rice and brown rice, compared with no Zn treatment, in 2019 and 2020, Zn content in milled rice significantly increased by 20.46%–41.09% and 18.11%–38.84%, respectively, and in brown rice significantly increased by 25.78%–48.30% and 20.86%–42.00%, respectively. However, the Zn fertilizer utilization gradually decreased with increasing ZnO NPs application dosage. From the perspective of yield, rice quality, Zn fertilizer utilization, and Zn accumulation, basal application of 7.5 kg–30 kg hm−2 ZnO NPs is beneficial for rice yield and quality improvement and rice Zn accumulation. This study effectively demonstrated that ZnO NPs could be a potential high‐performed fertilizer for enhancing rice yield, quality, and zinc content of edible grain fraction synergistically.
1 Introduction
Zinc (Zn) is an essential micronutrient for human health, and its deficiency can lead to various adverse effects (Prasad, 2013). Food crops, such as rice, are the main source of dietary Zn (Hall and King, 2022). However, the Zn content in rice is insufficient to meet the body’s requirements (Yang et al., 2021). Zn is also crucial for the growth and development of rice, affecting yield and quality (Naik and Das, 2008; Bana et al., 2021; Tuiwong et al., 2021). Low soil Zn levels (DTPA-Zn < 0.5 mg kg−1) contribute to crop Zn deficiency, resulting in negative effects on rice, including poor tillering, reduced root development, low seed-setting rate, and insufficient Zn content in plants (Kumar et al., 2022). Approximately half of the world’s soils are estimated to be deficient or potentially deficient in Zn, posing a significant challenge to public health and the nutritional value of rice (Rehman et al., 2012; Tulchinsky, 2017).
Increasing the Zn content in crops can be achieved through agronomic practices and breeding techniques (Palmgren et al., 2008; Bouis et al., 2011). Adequate application of Zn fertilizer can improve rice yield (Guo et al., 2016), reduce chalkiness, increase protein and amylose content (Ghasal et al., 2016), and enhance Zn uptake and translocation to grains (Ahsin et al., 2020). However, the Zn content in rice grains often falls below the desired levels for human nutrition (Singh et al., 2020). The effectiveness of different Zn fertilizer application methods varies (Elmer and White, 2016), with traditional ionic Zn fertilizers (ZnSO4, ZnCl2, etc.) being less efficient due to poor migration and absorption by rice roots (Patel et al., 2022). Foliar application shows promise but is affected by environmental factors (Zhang et al., 2017; Soltani et al., 2022). Hence, it is crucial to acquire expertise in the efficient application of Zn fertilizer to enhance rice yield, quality, and Zn content. This knowledge is vital for addressing Zn deficiency in the human body and ensuring food security.
Nanomaterials are widely used in industry (in plant growth), and regulation of its physiological responses is an attempt of recent years (Upadhyaya et al., 2017). Nanomaterials can enter plants through roots, leaves, and other organs, which can improve plant resistance, reduce the degree of plant diseases, and increase the photosynthetic rate of plants. The fertilizer effect of nanomaterials is also reflected in the promotion of plant uptake and utilization, reducing environmental risks (Wang et al., 2018; Bala et al., 2019; Zhang et al., 2022). As a new type of Zn fertilizer, Zn oxide nanoparticles (ZnO NPs) are less affected by soil texture, organic matter, and colloidal material than common Zn fertilizers due to their high-specific surface area (SSA) and surface activity and are easily absorbed and utilized by the rice root system (Zhang et al., 2021). It was found that ZnO NPs application promoted rice yield as well as rice grain Zn content and Zn accumulation better than ionic Zn at the same Zn application rate (Yang et al., 2021). ZnO NPs also improve the salt tolerance of rice pre-growth and further reduces the effect of salt on rice seedling growth vigor, nutritional growth, and flowering and fruit set (Singh et al., 2022). In addition, ZnO NPs are also effective in mitigating the uptake and accumulation of Cd and As in rice seeds (Ma et al., 2020).
Upon application or release into the environment, ZnO NPs undergo rapid dissolution and conversion. Some NPs attach to the crop root surface, while others are taken up by plants as Zn phosphate. Notably, ZnO NPs do not exhibit specific toxicity compared with ZnSO4 (Du et al., 2019). Analysis of Zn distribution in the soil reveals that ZnO NPs convert to Zn ions within a short time, penetrating the plant’s tissues and reaching the aboveground parts through the xylem (Lv et al., 2021). The dissolution rate of ZnO NPs is influenced by soil properties, particularly pH and ZnO NPs concentration (Wang et al., 2013). ZnO NPs demonstrate greater efficacy in acidic soils compared with alkaline soils (Sun et al., 2020). They can benefit crop growth and yield at low concentrations but may exhibit toxicity at high concentrations, albeit to a lesser extent than ZnSO4. ZnO NPs increase Zn content in crop grains without posing a nano-risk (Du et al., 2019).
Current research on nanomaterials in rice production primarily focuses on their role in promoting rice growth and development, as well as their toxic mechanisms and negative impacts on rice. However, there is limited research on the application methods, appropriate dosages, and their effects on rice yield, quality, and Zn content in the edible portion of the grain. Therefore, this study aimed to investigate the effects of basal application of ZnO NPs on rice yield, quality, and edible grain Zn content at different application rates through field experiments. The objective was to determine the optimal application rate that enhances rice yield, improves quality, and fortifies the edible grain with Zn. The findings of this study will provide a scientific and effective theoretical foundation for the application of ZnO NPs in rice production.
2 Materials and methods
2.1 Materials
The ZnO NPs used in this study were purchased by the researchers from shanghai Chaowei Nanotechnology Co. (ZnO NPs purity ≥ 99.95%). The ZnO NPs were used as received without any additional purification steps. Under scanning electron microscopy (SEM), the ZnO NPs were spherical with a particle size of 20 nm–50 nm and an SSA of 133.6 m2 g−1. Conventional urea (46% N), calcium superphosphate (12.5% P2O5), and potassium chloride (60% K2O) were purchased from local fertilizer outlet.
2.2 Experimental location and design process
2.2.1 Experimental location
Field trials were conducted in the 2019 and 2020 rice growing seasons at the experimental farm (32°23.4’ N, 119°25.2’ E) of Yangzhou University, Jiangsu Province, China. The soil type was sandy loam with pH 6.76, 1.30 g kg−1 total nitrogen, 24.4 g kg−1 organic matter, 104.2 mg kg−1 alkaline hydrolysable nitrogen, 35.4 mg kg−1 Olsen-P, 72.5 mg kg−1 exchangeable K, and 0.87 mg kg−1 available Zn. The area has a subtropical monsoon climate with a mild climate and abundant rainfall, with an average annual temperature of about 15.2°C and an annual precipitation of about 1020 mm. Meteorological data for the growing season of this crop are shown in Table 1.
2.2.2 Design process
The rice variety used in this study was Nanjing 9108, a late-maturing medium japonica rice. It was selected by the Institute of Grain Crops, Jiangsu Academy of Agricultural Sciences in Jiangsu, China. Nanjing 9108 is characterized as a multi-spike japonica variety. Rice was planted at a density of 12.5 cm × 25 cm with four seedlings per hole. There were six treatments in the field trial: with no ZnO NPs as control (CK); application ZnO NPs 3.75 kg hm−2 as T1; application ZnO NPs 7.5 kg hm−2 as T2; application ZnO NPs 15 kg hm−2 as T3; application ZnO NPs 30 kg hm−2 as T4; and application ZnO NPs 60 kg hm−2 as T5. The 2019 trial was sown on May 22 and transplanted on June 22, and the 2020 trial was sown on May 19 and transplanted on June 20. The rice was harvested on 18 October 2019 and 16 October 2020, respectively. According to what was observed on the field, the grain‐filling period of rice was 46 days. The N fertilizer in the experiment was 270 kg hm−2 and was applied with basal, tillering, and panicle fertilizer at a ratio of 3:3:4 at the relevant growth stage. The potash fertilizer was applied at 270 kg hm−2, while the phosphate fertilizer application level was 135 kg hm−2. Moreover, the potash fertilizer was applied as 50% basal fertilizer and 50% panicle fertilizer. The phosphate fertilizer was applied entirely as basal fertilizer. During the specific application procedure, the ZnO NPs were uniformly applied by mixing them with quartz sand sieved through a 0.5-mm mesh, according to the predetermined dosage of ZnO NPs. The amount of fertilizer applied and the method of application remained the same for 2 years for each treatment. Treatments were performed on plots of 25 m2 (5 m × 5 m), and each treatment was replicated three times. Each plot was separated by a soil monopoly (35 cm wide and 20 cm high) and covered with plastic film. The test plots were flooded after transplanting and the flooding continued until 7 days before maturity. Pests, pathogens, and weeds were controlled with commonly used chemical treatments.
2.3 Sampling and data collection
2.3.1 Yield and yield components
At maturity, each plot was harvested manually for 4 m2 and weighing the actual yield (14.5% moisture content). Each plot was collected for 1 m2 (excluding marginal plants) to examine the number of panicles that was investigated. Thirty representative plants were selected consecutively in each plot to investigate the spikelets per panicle, seed-setting rate and 1,000-grain weight.
2.3.2 Dry matter accumulation
Five consecutive plant samples were collected from each plot at the jointing, heading, and maturity stages, and all samples were separated into leaf, stem (internode plus sheath), and spike tissue. Each sample of rice plants was bagged separately and dried at 105°C for 30 min, then at 80°C to a constant weight.
2.3.3 Rice quality
The rice grains were naturally air-dried, stored at room temperature for 90 days, and then air-selected with a winnowing machine to determine the rice grain quality. The rice quality traits were determined according to China’s National Standard (GB/T17891-2017). A 100-g sample of rice grains was passed through a de-husker for polishing and then separated into broken and unbroken grains. The brown rice rate, milled rice rate, and head rice rate were expressed as percentages of the total (100 g) rice grains. The values for the appearance quality (chalky grain percentage and chalkiness degree) were calculated using a rice appearance quality detector (Hangzhou Wanshen Detection Technology Co., Ltd., Hangzhou, China). The protein and amylose content of milling rice were determined using a 1241 NIR rapid quality analyzer (Infrared 1241 grain analyzer) manufactured by FOSS TECATOR, Sweden. The rice taste meter (Satake Corporation, Higashi-Hiroshima, Japan) was used to automatically measure the taste value of rice. The pasting properties of the rice flour were determined using a rapid viscosity analyzer (RVA, Super3, Newport Scientific, Warriewood, Australia).
2.3.4 Determination of Zn content
Concentration of Zn in stalks, leaves, seeds, hulls, brown rice, paste layer, and milled rice: 0.5 g of the sample was weighed, added to 5 mL of good quality pure nitric acid and subjected to high-temperature digestion in a microwave digester (CEM-MARS 5, USA). The concentration of Zn in the filtrate was determined by plasma emission spectroscopy-atomic absorption (iCAP 6300, USA) after dilution of the digestion solution.
2.4 Data analysis
One‐way analysis of variance (ANOVA) was applied using SPSS 20.0 to analyze the data. The LSD test was applied at a 0.05 probability level to establish the significance of discrepancy among each mean.
3 Results
3.1 Rice yield and its components
The data in Table 2 depict the influence of ZnO NPs on grain yield and yield component of rice. All 2 years of ZnO NPs increased rice grain yield, with a trend of increasing then decreasing with increasing ZnO NPs. In the 2019, T3 and T4 treatments significantly increased by 4.46%–4.86%, and in the 2020, T1–T5 significantly increased by 3.51%–5.12%, all with the highest yield of rice under T4 treatment.
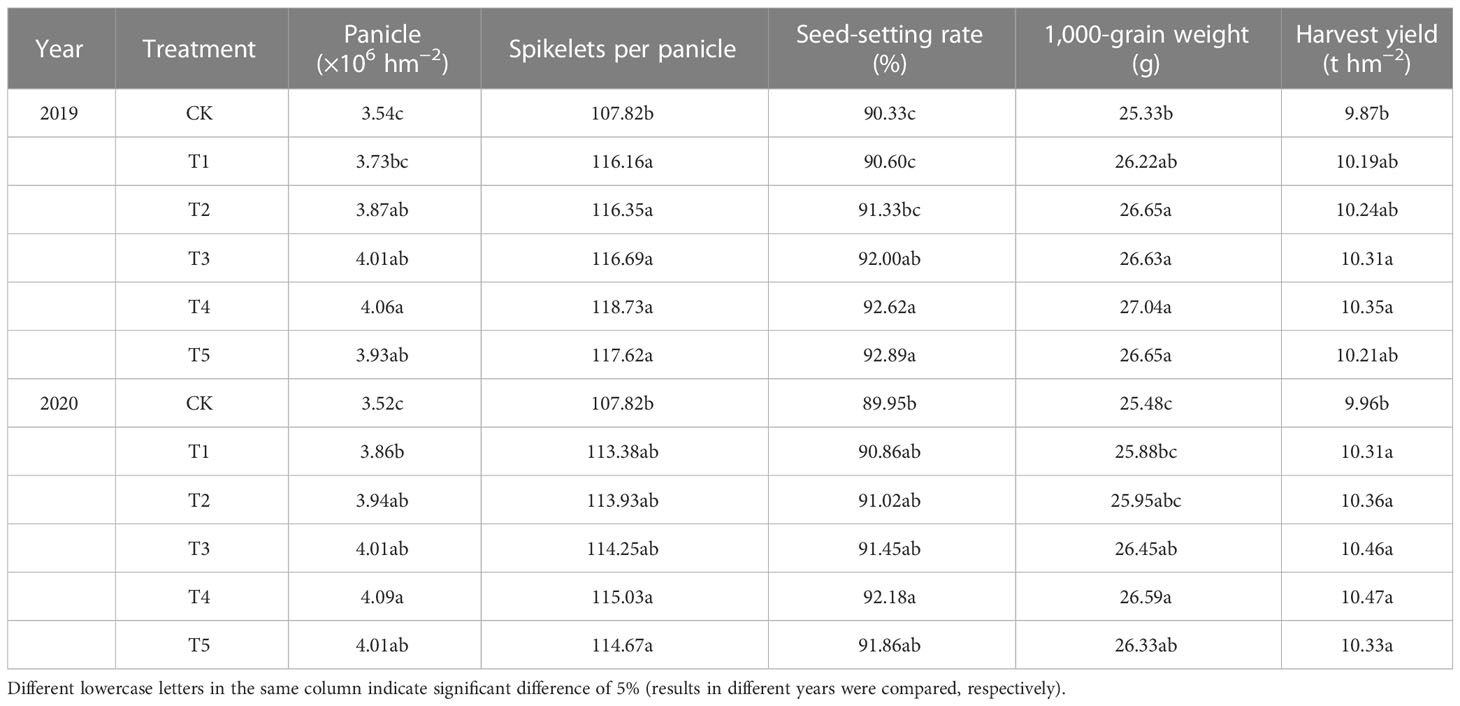
Table 2 Effects of ZnO NPs different application dosage on yield and yield components of japonica rice.
In terms of yield components, the number of panicles increased by 5.37%–14.69% and 9.66%–16.19%, the number of spikelets per panicle increased by 7.74%–10.12% and 5.16%–6.69%, the seed-setting rate increased by 0.3%–2.83% and 1.01%–2.48%, and the 1,000-grain weight increased by 3.51%–6.75% and 1.57%–4.36%, respectively. It is worth noting that, in the 2-year experiment, the number of panicle and the number of spikelets per panicle tended to increase and then decrease with increasing ZnO NPs application in both years, and the best results were obtained under the T4 treatment in both years. In the 2019, the seed-setting rate increased with increasing ZnO NPs application, while in the 2020, the seed-setting rate tended to increase and then decrease with increasing ZnO NPs application, with a significant increase of 2.48% in the T4 treatment; the 1,000-grain weight of the ZnO NPs treatment did not show a regular pattern in the 2019, with a significant increase of 2.13%–6.75% in the T2–T4 treatment compared with the CK, while in the 2020, with increasing ZnO NPs application, the 1,000-grain weight tended to increase and then decrease with increasing ZnO NPs application in the 2020, with the best effect under the T4 treatment with a significant increase of 4.36%.
3.2 Dry matter accumulation
Dry matter accumulation in rice is the key to rice yield formation. As shown in Table 3, application of ZnO NPs increased dry matter accumulation at the jointing, heading, and maturity, by 10.28%–16.45% and 4.21%–9.41% at the jointing, 11.05%–23.60% and 2.63%–6.32% at the heading, and 9.36%–12.12% and 3.44%–7.00% at the maturity in 2 years, respectively, compared with CK. Different ZnO NPs treatments had no significant effect on the dry matter accumulation of rice at each growth stage. The higher dry matter growth rate at heading stage indicated that ZnO NPs played an important role in rice yield formation during this period. The dry matter accumulation and proportion of rice at different growth stages in Table 3 also confirmed this hypothesis. The dry matter accumulation and proportion of rice were the highest at heading-maturity stage. The weight of dry matter in a certain period reflected the growth status of the ZnO NPs treatments during that period; the dry matter accumulation and proportion of rice in this period reflect the growth characteristics of rice. Therefore, higher dry matter accumulation at the jointing–heading stage will increase the final yield, which is consistent with the grain yield results. In addition, the dry matter accumulation of rice at the jointing and maturity stages was also higher than that of CK, indicating that ZnO NPs also improved dry matter accumulation in the early and late growth stages of rice.
3.3 Processing and appearance quality
The brown rice rate, the milling rice rate and the head milling rate are important criteria for judging the quality of rice processing. In the 2-year trail, the application of ZnO NPs increased the brown rice rate by 0.65%–1.14% and 0.07%–0.77% and the head milling rate by 2.82%–3.54% and 1.91%–2.99%, respectively. Except for T5 treatment in 2019, other ZnO NPs treatments in the 2-year experiment increased the milling rice rate and were highest in the T1 treatment, while in the 2020 trial, the milling rice rate gradually increased with the increase in the amount of ZnO NPs applied, with the T4–T5 treatments achieving significant levels of improvement compared with CK (as shown in Table 4).
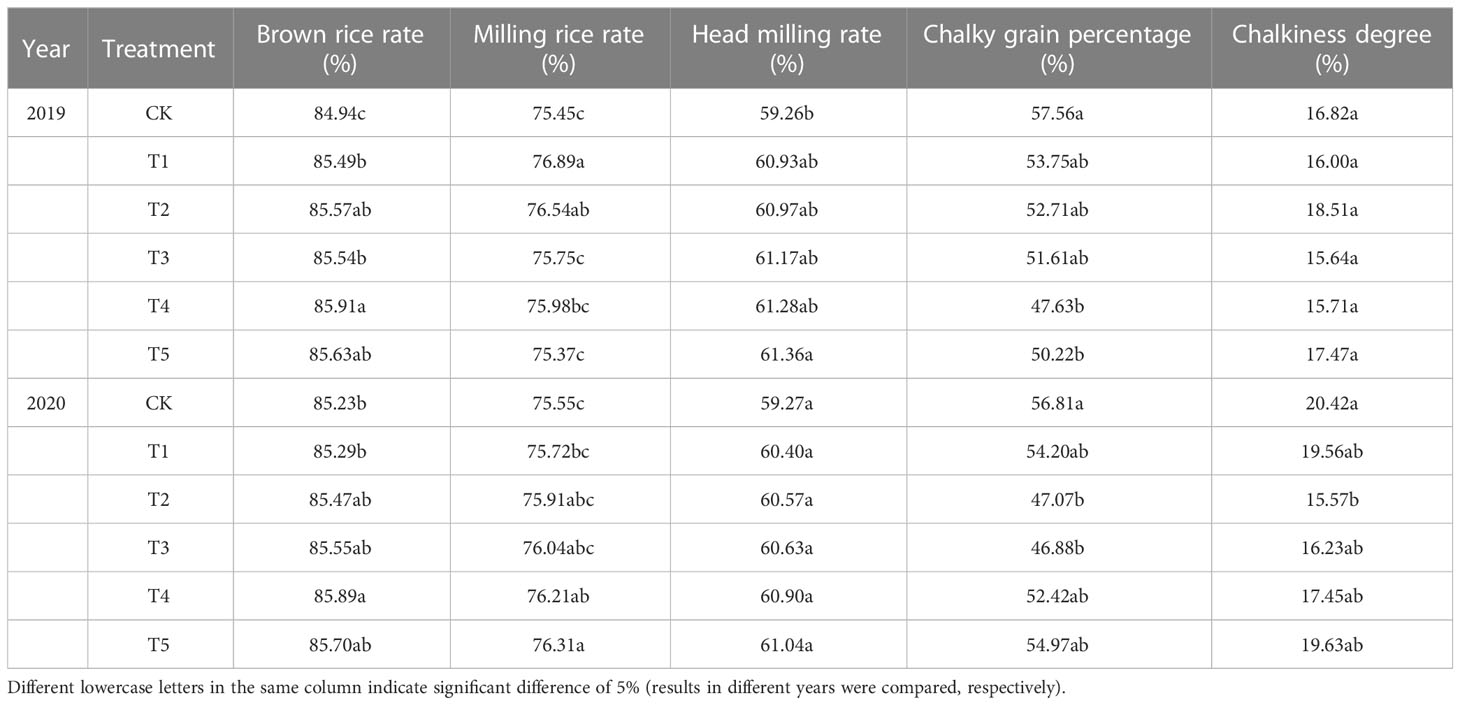
Table 4 Effects of ZnO NPs different application dosage on processing and appearance qualities of japonica rice.
Further comparison of the effects of ZnO NPs on rice appearance quality revealed that ZnO NPs application reduced chalky grain percentage and showed a decreasing and then increasing trend with increasing ZnO NPs application, with chalky grain percentage reaching the lowest in treatment T4 in the 2019 trial and the lowest in treatment T3 in the 2020 trial. There was no significant change in chalky grain percentage with increasing ZnO NPs application in the 2019 trial, while in the 2020 trial, chalky grain percentage tended to decrease and then increase with increasing ZnO NPs application, with the lowest chalky grain percentage being achieved in the T2 treatment.
3.4 Nutritional and taste quality
In the 2019 trail, compared with CK, the application of ZnO NPs increased the protein content of rice, and the protein content of rice in treatments T3–T5 increased significantly (4.95%–12.79%). In the 2020 trial, rice protein content showed a trend of increasing and then decreasing with increasing ZnO NPs application. In the 2019, the amylose content showed a trend of increasing and then decreasing with the increase in ZnO NPs application, with a significant increase of 6.87%–10.56% compared with CK. In the 2020, the overall trend of ZnO NPs application treatments showed a decrease compared with CK, and none of them reached a significant difference level, with the T5 treatment having the lowest amylose content, with a decrease of 4.24% compared with CK.
Compared with CK, the application of ZnO NPs improved the taste value of rice by 7.30%–10.82% in 2019, with the T2 treatment having the highest taste value; in the 2020, the T2–T4 treatment significantly increased the taste value of rice by 6.80%–8.60% compared with CK, with the T4 treatment having the highest taste value (as shown in Table 5). Further analysis of the rice taste value indicators revealed that the increase in taste value was mainly achieved by improving the appearance, stickiness and balance of the rice and reducing the hardness of the rice.
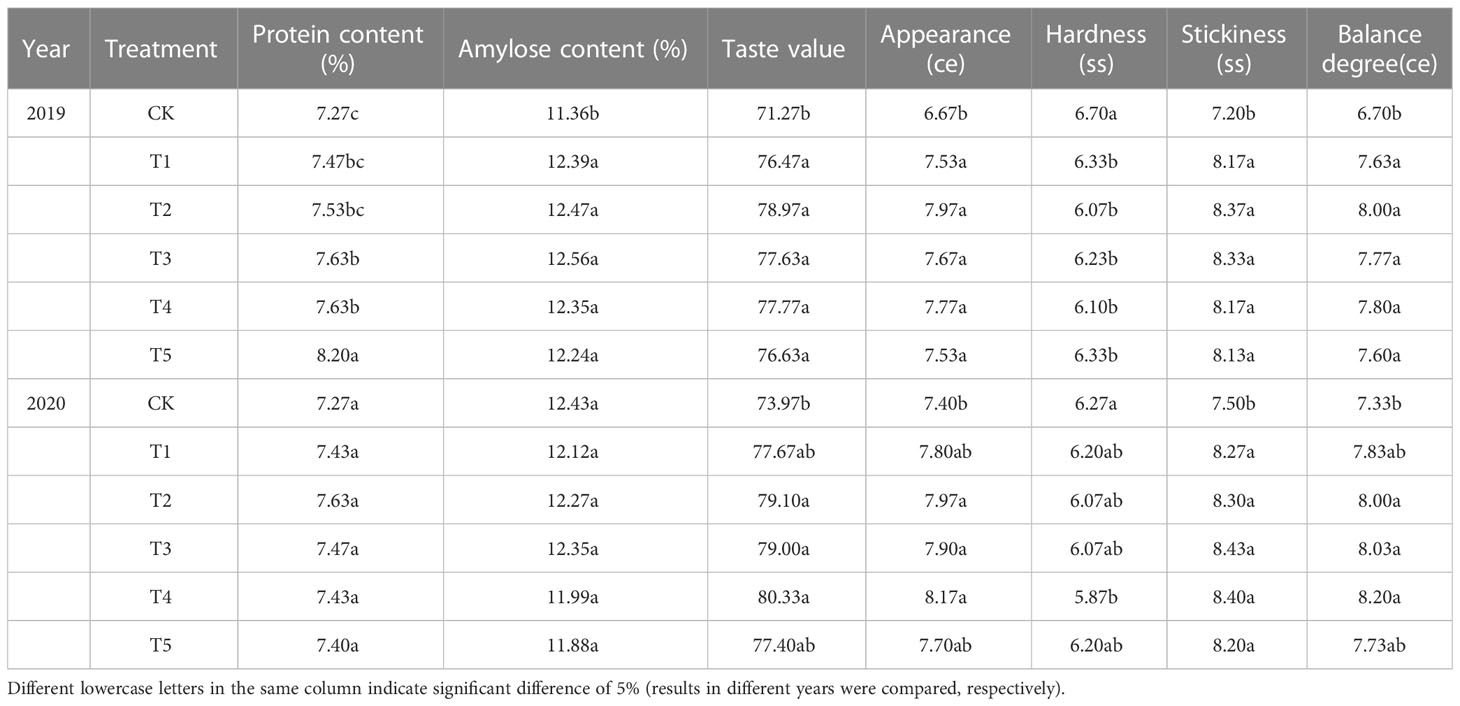
Table 5 Effects of ZnO NPs different application dosage on nutrition and eating qualities of japonica rice.
3.5 RVA parameters
The RVA profile characteristics are an important indicator of the quality of rice cooking. Compared with CK, the application of ZnO NPs could improve the breakdown, which increased by 17.63%–50.59% in 2019 compared with CK; in the 2020, the breakdown significantly increased by 24.52%–31.83% compared with CK. There was a difference in the pattern of change in peak viscosity among the treatments with ZnO NPs, with ZnO NPs increasing peak viscosity in 2019 compared with CK (2.41%–3.39%); in the 2020, compared with the CK, peak viscosity decreased in all the ZnO NPs treatments, except for T4 treatment, with a significant decrease in peak viscosity in T1 3.13%. ZnO NPs application reduced through viscosity by 2.49%–11.86% and 10.62%–14.49% in both years, compared with CK, there is no significant difference in 2019 and a significant difference in 2020. Application of ZnO NPs reduced final viscosity by 0.46%–6.98% and 2.87%–12.96% in both years, respectively. All treatments of ZnO NPs application reduced the setback except for T2 treatment in 2019, and T5 treatment reached a significant difference level compared with CK in 2020. Compared with CK, ZnO NPs application increased the pasting temperature by 0.37%–2.23% and 0.07%–1.96% in both years respectively (as shown in Table 6).
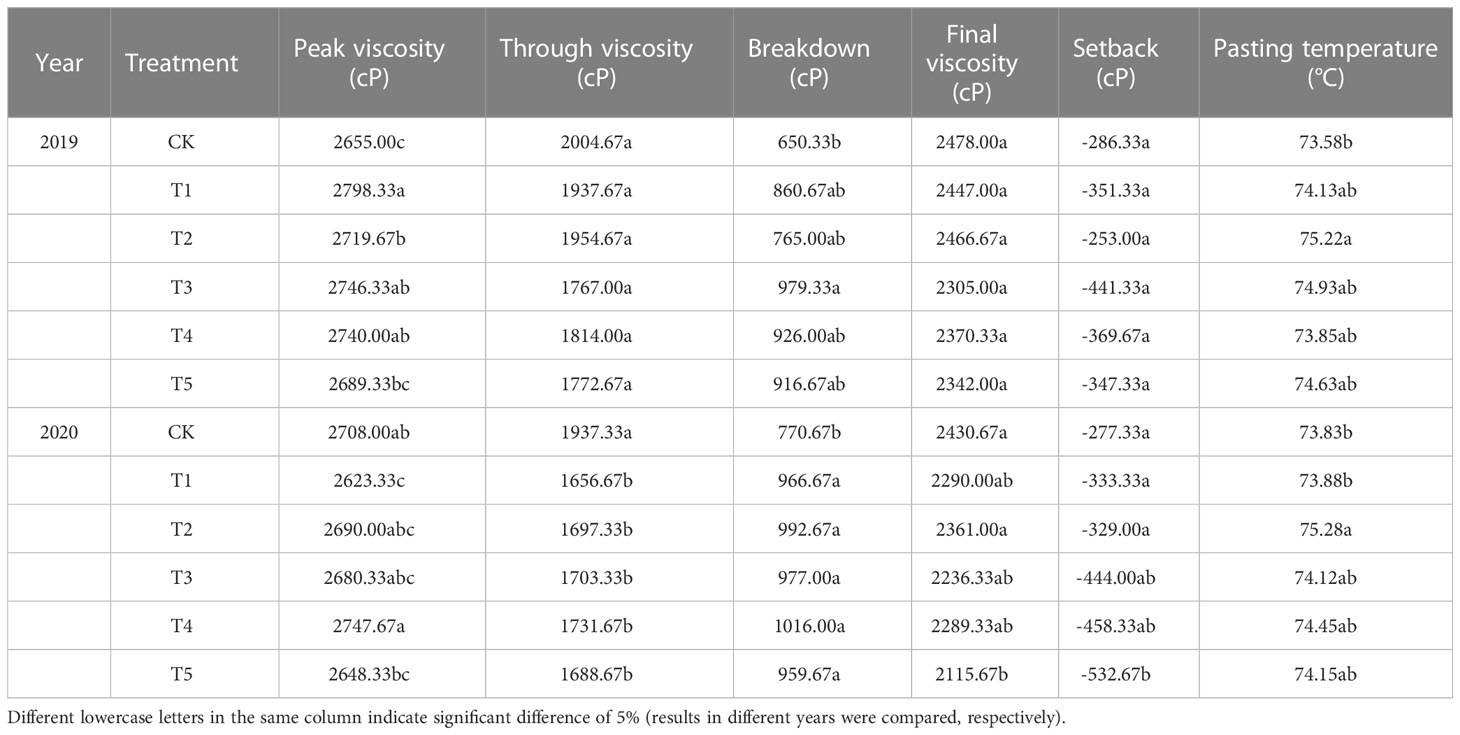
Table 6 Effects of ZnO NPs different application dosage on RVA profile characteristics of japonica rice.
3.6 Aboveground Zn accumulation in rice
As presented in Table 7, the Zn content of aboveground rice plants treated with ZnO NPs increased with the increase of ZnO NPs application. In the 2019, Zn content in rice stalks and leaves of T3–T5 treatments were significantly increased by 64.95%–92.48% and 50.20%–116.61%, respectively, compared with CK; in the 2020, the Zn content in rice stems treated with T4 and T5 was significantly increased by 90.28%–128.02% compared with CK, and the Zn content in rice leaves treated with T4 and T5 was significantly increased by 112.86%–156.79%. The Zn content in rice grains increased with increasing ZnO NPs application, with significant increases of 16.67%–35.35% and 12.49%–30.27% compared with CK in both years, respectively. Zn accumulation in aboveground rice plants also increased with the increase of ZnO NPs application, compared with CK, Zn accumulation in above ground plants was significantly increased by 39.26%–124.00% and 33.80%–118.38% in both years by the application of ZnO NPs, respectively.
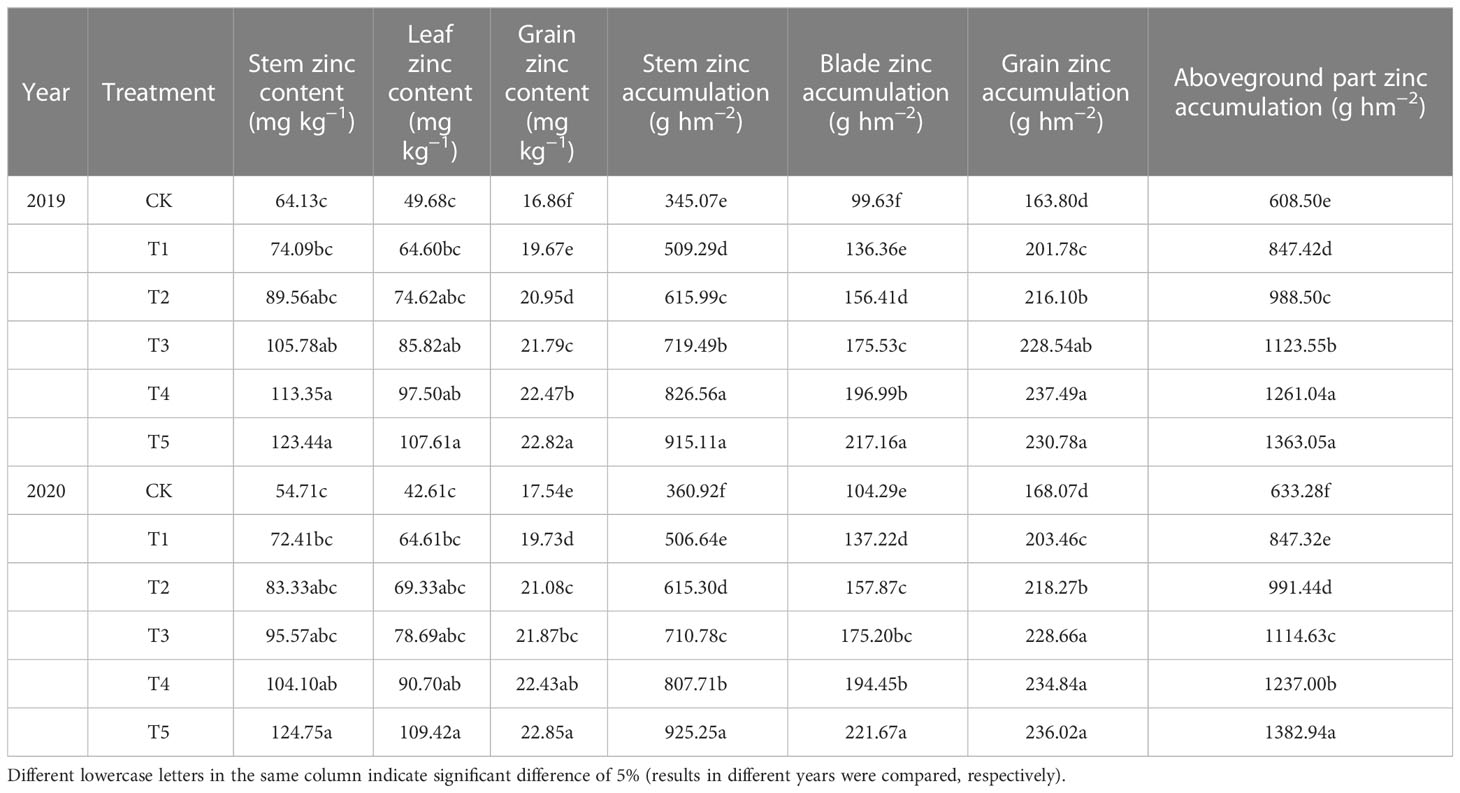
Table 7 Effect of ZnO NPs different application dosage on Zn accumulation in aboveground plants of japonica rice.
3.7 Zn content and proportion of Zn content of each part of the grain
The application of ZnO NPs all significantly increased the Zn content in the milled rice, brown rice, grain coat, and aleurone layer. Compared with CK, the application of ZnO NPs significantly increased the Zn content of milled rice by 20.46%–41.09% and 18.11%–38.84%, respectively. In the 2019 trail, Zn content in milled rice showed an increasing and then decreasing trend with increasing ZnO NPs application, with the highest Zn content in T4 treatment, while 2020 did not show a clear pattern with increasing ZnO NPs application. Compared with CK, the Zn content of brown rice increased by 25.78%–48.30% and 20.86%–42.00% in both years, respectively, and the Zn content of brown rice and grain coat and aleurone layer showed an increasing and then decreasing trend with the increase of ZnO NPs application, and reached the maximum in T4 treatment. The Zn content increasing of brown rice was remarkably lower than the vegetative organs, indicating that more Zn was sediment in the vegetative organs and not efficiently positioned in rice grains. The Zn content of rice hull did not show a significant pattern of change, and compared with CK, all the treatments with ZnO NPs application increased the Zn content of rice hull, except for T1 and T4 treatments in 2020 (as shown in Table 8).
In the 2-year trial, except for the T2 treatment, the application of ZnO NPs was able to reduce the proportion of Zn in the rice hull and all reached a significant level of difference. Except for the T2 treatment in 2020, all applied ZnO NPs treatments increased the proportion of grain and aleurone layer to grain Zn to varying degrees. Except for the T2 treatment in 2019, all treatments with ZnO NPs application significantly increased the proportion of milled rice to grain Zn (as shown in Figure 1). This result may be due to the fact that ZnO NPS facilitated the transfer of Zn to the milled rice, which is conducive to promoting greater human intake of Zn from rice.
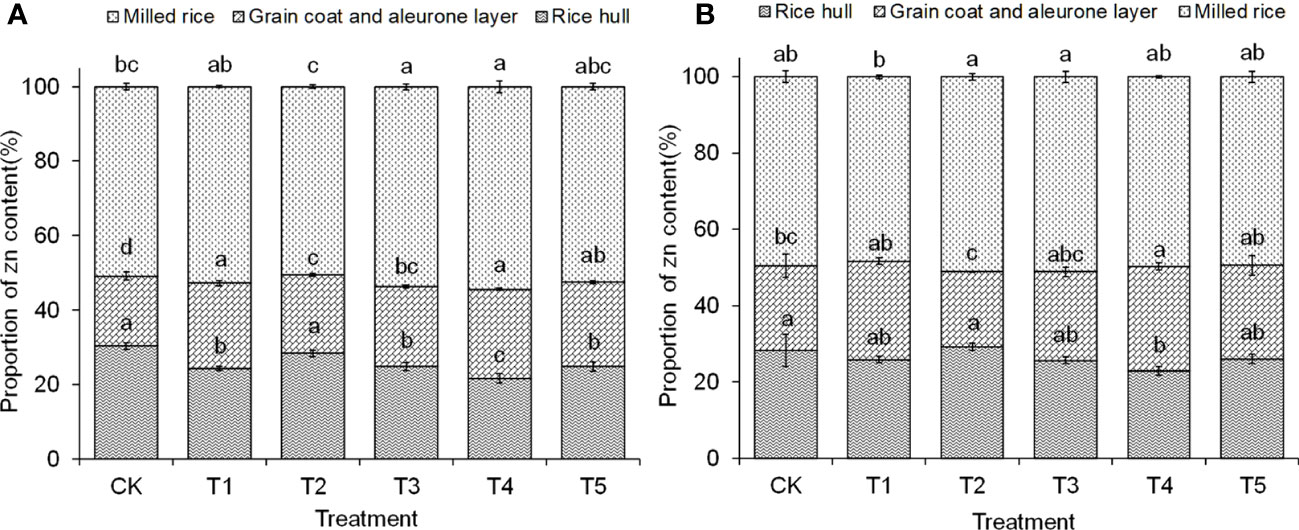
Figure 1 Effects of ZnO NPs dosages on the ratio of zinc content in different parts of japonica rice grain. (A), 2019; (B), 2020. Different lowercase letters in the same column indicate significant difference of 5% (results in different years were compared, respectively).
3.8 Zn fertilizer utilization
In the 2-year trial, Zn fertilizer utilization decreased with increasing ZnO NPs application, with all treatments showing a significant decrease in the 2019 except for the T5 treatment, while in the 2020, the T3 treatment showed a significant decrease and the remaining treatments did not show a significant decrease and showed a slow decrease (as shown in Figure 2).
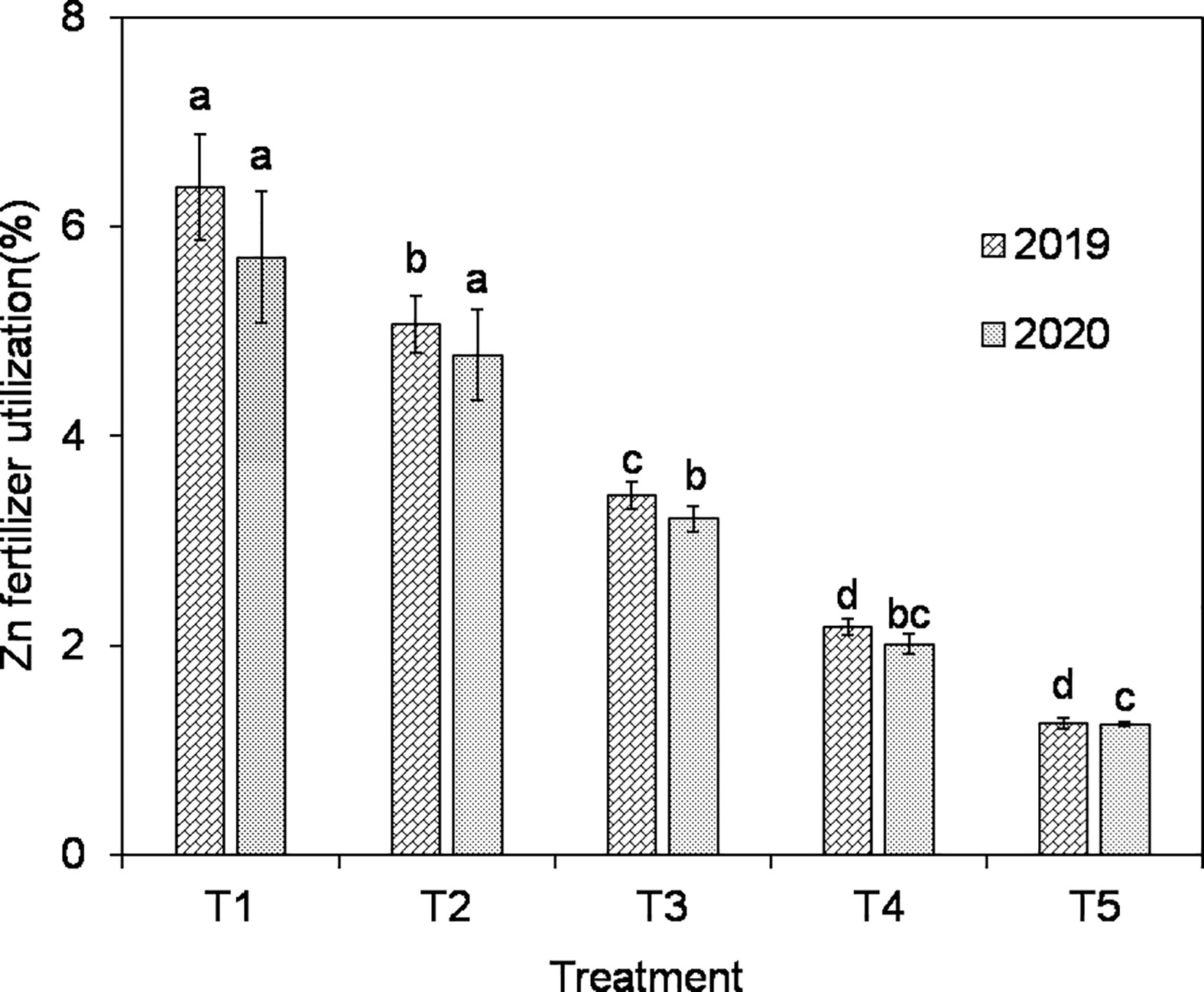
Figure 2 Effects of ZnO NPs dosages on utilization rate of Zn fertilizer in mature stage of japonica rice. Different lowercase letters in the same column indicate significant difference of 5% (results in different years were compared, respectively).
4 Discussion
4.1 Grain yield
In the present study, the application of ZnO NPs resulted in increases in rice grain yield of 3.24%–4.86% and 3.51%–5.12% in the 2-year trials compared with the control (CK). The tested soil belonged to the effective area of Zn fertilizer according to the Zn fertilizer application classification in China (Liu, 1994). Supplementing ZnO NPs fertilizer on Zn-deficient soils can enhance crop yields. Previous research by Guo et al. (2016) also demonstrated that Zn fertilizer application significantly improves rice yield components. In our study, ZnO NPs application on potentially Zn-deficient soils increased rice yield by promoting panicle and spikelet development, leading to a larger population of glumes in rice. Higher dry matter accumulation is associated with higher rice yields, as the formation of rice yield is primarily a process of dry matter production and distribution (Cheng et al., 2022). Our results indicated that ZnO NPs applications at the jointing, heading, and maturity stages enhanced dry matter accumulation, suggesting the positive impact of ZnO NPs on final yield. Zn plays a crucial role in various enzymatic processes within plants, including chlorophyll synthesis and carbohydrate metabolism. By increasing the application of Zn fertilizer, photosynthesis and the efficiency of photosynthetic assimilate synthesis in rice are enhanced. This, in turn, promotes the accumulation of dry matter in rice, which is essential for achieving high yields. There was a significant positive correlation between rice yield and Zn application within a certain range, but excessive Zn application can have toxic effects on rice and may not be beneficial for yield increase (Bana et al., 2021; Tuiwong et al., 2021). In our experiment, rice yield increased and then decreased with increasing ZnO NPs application. The highest rice yield was achieved at an application rate of 30 kg hm−2 of ZnO NPs and further increases in ZnO NPs led to a decrease in yield, with the lowest yield observed at an application rate of 60 kg hm−2. However, even at the highest application rate of ZnO NPs, the yield remained higher than when 3.75 kg hm−2 of ZnO NPs was applied. This could be attributed to the active adsorption capacity of ZnO NPs, which promotes the uptake of elemental phosphorus by the plant through its interaction with phosphate ions, while also reducing the toxic effects of ZnO NPs on rice (Akram et al., 2020).
The price of ZnO NPs on the market is ¥120 kg−1, compared with the application of ZnO NPs 3.75 kg hm−2, when applying 60 kg hm−2, the increase in income in the 2-year trial is only ¥56 (the market price of rice is calculated as ¥2.8 kg−1), but the application of ZnO NPs fertilizer costs ¥6,750 more, in terms of economic efficiency, 60 kg hm−2 of ZnO NPs is not the most appropriate amount. As a new type of fertilizer, ZnO NPs can greatly improve the ecological benefits of the soil, enhance the water and fertilizer retention capacity of the soil, and promote sustainable crop production. In terms of price, ordinary ionic Zn costs ¥10–30 kg−1 and ZnO NPs is four to 12 times more expensive; ZnO NPs does not have an advantage. However, by reducing the purity of ZnO NPs in agricultural production, finding suitable application rates for ZnO NPs, or achieving large-scale production, ZnO NPs can be used to achieve higher yield and ecological benefits at a lower capital investment.
4.2 Grain quality
The quality of rice encompasses various aspects such as processing quality, appearance quality, nutritional quality, taste quality, and cooking quality. Previous research has demonstrated that the application of Zn fertilizer can enhance the processing quality of rice by increasing the percentage of brown rice and milled rice (Zhen et al., 2012). In line with these findings, the results of our study indicate that the application of ZnO NPs leads to improvements in the brown rice rate and milling rice rate, with some impact on the head milling rate as well. This improvement can be attributed to the promotion of grain filling and a reduction in grain breakage during the milling process, thus enhancing the overall processing quality of the rice. Moreover, the application of ZnO NPs enhances the assimilation of photosynthesis products in rice, facilitating their transfer to the kernels (Asif et al., 2005). This leads to a reduction in kernel breakage during the milling process, thereby improving the processing quality of rice. Additionally, the increased degree of grain assimilation contributes to improved appearance quality of the rice.
Chalkiness in rice grains, caused by uneven assimilate distribution, affect appearance quality (Wang et al., 2021). In our study, ZnO NPs application in 2019 did not show a significant trend in chalkiness. However, in 2020, increasing ZnO NPs application led to a decrease followed by an increase in chalkiness. The best appearance quality was observed at a ZnO NPs application rate of 15 kg hm−2, while higher rates resulted in a decline. Zn ion concentration affects assimilate transport efficiency, with moderate Zn fertilization enhancing photosynthesis and assimilate synthesis (Tondey et al., 2022). Conventional Zn fertilizers may not be effective in Zn-deficient soils due to rapid immobilization (Baddar and Unrine, 2021). In contrast, ZnO NPs slowly dissolve, acting as a sustained Zn supply, promoting plant growth and grain filling (Zhang et al., 2018). Zn enrichment improves grain assimilate filling, but excessive Zn can be toxic, reducing protein content and disrupting photosynthesis (Li et al., 2022a). Zn fertilizer application within a certain range increases protein and starch accumulation in grains (Singh et al., 2021). In 2019, ZnO NPs increased rice protein content, while in 2020, it showed an increasing-then-decreasing trend. Amylose content followed a similar pattern in 2019, with an overall decreasing trend in 2020. Nitrogen-Zn interactions and rainfall may influence nitrogen metabolism and affect protein and amylose content (Liang et al., 2015; Kuroha and Ashikari, 2020). Zn treatment may also modulate genes and enzymes involved in amylose synthesis (Zhao et al., 2022). Further investigation is needed to determine the exact causes.
ZnO NPs application had positive effects on the cooking and eating quality of rice, resulting in several improvements. The treated rice exhibited enhanced taste, appearance, stickiness, and balance, while its hardness was reduced (Ge et al., 2022). These quality enhancements can be attributed to the role of Zn as a cofactor for flavor enzymes and an activator of these enzymes. At specific temperatures, Zn and other trace element ions activate flavor enzymes, leading to the breakdown of sugars, fats, and proteins in rice. This breakdown process increases the presence of fatty acids, amino acids, aldehydes, ketones, vinegars, and other flavor compounds in the rice, ultimately improving its taste (Suganya et al., 2020). In comparison with traditional ionic Zn, ZnO NPs more effectively increase the Zn concentration in the grains, thereby promoting the activation of flavor enzymes and enhancing the overall taste value of rice.
The characteristics of the RVA spectrum, such as breakdown and setback, are closely associated with the taste quality of steamed rice (Xuan et al., 2020; Xu et al., 2021). Generally, rice with better taste quality tends to exhibit higher peak viscosities, lower breakdown, and lower setback values. Studies have shown that a decrease in amylose content promotes water absorption and swelling of rice, resulting in enhanced stickiness and increased breakdown, which ultimately improves the palatability of rice (Derycke et al., 2005). In this study, the application of ZnO NPs in 2019 led to enhanced peak rice viscosity, increased breakdown, and higher pasting temperature. All treatments, except for the application of Zn at a rate of 7.5 kg hm−2, reduced rice setback. In 2020, the application of ZnO NPs increased rice breakdown and pasting temperature while reducing rice setback. However, there was no significant pattern of change observed in peak viscosity. These variations in results between the two years may be attributed to environmental factors. Heavy rainfall in 2020 reduced the plants’ photosynthetic capacity, resulting in a limited availability of sugar sources. This decrease in sugar sources could have inhibited starch expansion, leading to a reduction in amylose content. Consequently, the lack of sugar sources and limited starch expansion may have contributed to the absence of significant changes in peak rice viscosity (Ai et al., 2023).
4.3 Grain Zn content
The additional application of Zn fertilizer has been observed to increase the Zn content and accumulation in plants, indicating its influence on Zn translocation and distribution within the plant (Yang et al., 2021; Zhang et al., 2021). Studies have shown that the Zn content and accumulation in aboveground rice plants increased with the application of ZnO NPs (Li et al., 2022b). Even when the external Zn levels reached toxic levels for the plants, rice plants continued to uptake and accumulate exogenous Zn uncontrollably. It has been established that externally added Zn fertilizer serves as the main source of Zn in Zn-deficient or potentially Zn-deficient soils (Montalvo et al., 2016). When applied as a novel Zn fertilizer, ZnO NPs not only have the potential to be directly absorbed by rice roots but also gradually release Zn ions, thereby supporting the growth and development of rice (Du et al., 2019).
Zn content and accumulation in different parts of the rice plant increased with Zn application, with stalks showing higher levels compared with leaves and grains (Paramesh et al., 2020; Zhang et al., 2021). In our study, Zn content followed the order: stalk > leaf > grain, and Zn accumulation followed the order: stalk > grain > leaf, with increasing ZnO NPs application. The enrichment of Zn in stalks can be attributed to their role as the primary pathway for Zn transport to the grains. ZnO NPs application may affect the translocation process, as they can interact with organic acids in the roots and become fixed in the roots (Palmgren et al., 2008). This interaction influences the availability of Zn from ZnO NPs as a fertilizer. These findings highlight the potential impact of ZnO NPs on Zn distribution within the rice plant, particularly in the stalks. Understanding the mechanisms involved is essential for optimizing the use of ZnO NPs as a fertilizer in rice cultivation.
As the amount of ZnO NPs applied increased, there was a gradual decrease in the utilization of Zn fertilizer. This decline can be attributed to several factors. First, the application of higher levels of ZnO NPs inhibited plant biomass accumulation, and there was a correlation between Zn accumulation and biomass presence (Repkina et al., 2023). This indicates that, as the concentration of ZnO NPs increased, the ability of the plants to effectively utilize the Zn fertilizer decreased. Additionally, it was observed that the ZnO NPs applied to the soil rapidly converted into a form that was not readily available to the plant root system, leading to the loss of Zn fertilizer and making it difficult for rice to absorb and utilize the Zn to its maximum extent (Zhang et al., 2018). Moreover, the neutral pH of the test soil (pH = 6.76) in this study could have reduced the effectiveness of ZnO NPs. The 2-year trial consistently showed an increase in soil pH with increasing ZnO NPs application (as shown in Figure 3). This increase in soil pH can be attributed to the dissolution of Zn ions from ZnO NPs, which raises the pH and subsequently hampers Zn fertilizer utilization (Sheteiwy et al., 2021). Furthermore, the transport process of Zn in the soil involves complex interactions between the soil, plants, and seeds, influenced by factors such as root secretions and organic acids produced by the plants (Palmgren et al., 2008). Despite the increased uptake of Zn by the plants, the overall utilization of Zn fertilizer showed a gradual decrease compared with the increase in ZnO NPs application.
Increasing the Zn content in milling rice is important for consumption. The application of ZnO NPs in this study significantly increased the Zn content in milling rice, showing a range of 20.46%–41.09% and 18.11%–38.84% higher compared with the control group in the 2-year trial. The most effective increase in Zn content in milling rice was observed at 30 and 60 kg hm-2 of ZnO NPs. After being applied to the soil, ZnO NPs were partially absorbed directly by the rice plant roots. During root uptake and transportation within the plant, the NPs were broken down by organic acids present in the plant, and the released Zn was utilized in the form of soluble Zn2+ or organic Zn (Dimkpa et al., 2013). Another portion of ZnO NPs dissolved in the soil due to acidic substances and combined with phosphate ions, resulting in Zn phosphate that was then used by rice plants. Amino acids, as organic molecules, can enhance plant response and absorption of Zn after reacting with it, thereby increasing the Zn content in milled rice (Xiao et al., 2022). Previous studies (Dimkpa et al., 2013; Wang et al., 2013) have shown that when ZnO NPs are applied to the soil, plants take up Zn in the form of soluble Zn2+ rather than as ZnO NPs. This indicates that the application of ZnO NPs increases the concentration of Zn in rice grains without posing risks to human consumption or health.
Phytic acid, such as inositol hexakisphosphate, can limit the bioavailability of Zn and hinder its uptake by humans (Jaksomsak et al., 2018). In rice grains, Zn is unevenly distributed, with higher levels found in the embryo and dextrin layer, and lower levels in the endosperm, leading to Zn enrichment in non-edible parts such as the rice hull and bran (Zhang et al., 2012; Castro-Alba et al., 2019). In this study, the application of ZnO NPs significantly increased the Zn content in all parts of the grain, particularly in brown rice and milled rice, while reducing the proportion of Zn in the rice hull. However, a substantial portion of Zn still remained in non-edible parts such as the grain coat and aleurone layer, accounting for over 45% of the total Zn content. Despite this, the ZnO NPs treatment improved rice processing quality, increasing the proportion of brown rice and milled rice, which may contribute to improved Zn delivery and human health benefits.
5 Conclusion
In this study, field experiments were conducted to evaluate the effects of basal application of ZnO NPs at different rates on rice yield, quality, and edible grain Zn content to determine the optimal application rate with potential for application. The findings of this study provide strong evidence for the positive effects of ZnO NPs application on rice production, quality, and Zn content. Applying ZnO NPs at rates between 7.5 kg and 30 kg hm−2 resulted in increased rice yield, improved grain quality, and enhanced Zn accumulation in the grains. The application of ZnO NPs led to increase the number of panicles, spikelets per panicle, seed-setting rate and 1,000-grain weight, all of which contributed to higher rice yields. Additionally, ZnO NPs application promoted dry matter accumulation throughout the growth period of rice, further supporting increased yields. The use of ZnO NPs also had noticeable benefits on rice processing, appearance, nutrition, taste, and steaming quality. The increased Zn content in the grain, brown rice, and milled rice indicated the effectiveness of ZnO NPs in enhancing the nutritional value of rice. However, it is essential to consider the soil environment when applying ZnO NPs in the field, as high concentrations of ZnO NPs in the rhizosphere may have adverse effects on rice roots. Further research is required to determine the optimal application methods to ensure the safe and effective use of ZnO NPs for different rice varieties and soil conditions. Overall, the results highlight the potential of ZnO NPs as a promising trace element fertilizer for achieving high-yielding, high-quality, and Zn-enriched rice production.
Data availability statement
The original contributions presented in the study are included in the article/supplementary material. Further inquiries can be directed to the corresponding author.
Author contributions
KM and XY: experimental design, data analysis and edited and improved the manuscript. QW, CD, and RW: participate in data collection, validation and investigation. SY and YY: writing the original draft and data curation. HPZ and HCZ: validation, project management, funding acquisition and supervision. All authors contributed to the article and approved the submitted version.
Funding
This work received financial support from the National Natural Science Foundation of China (31901447), The Key Research and Development Program of Jiangsu Province (BE2022338), Innovation and Entrepreneurship Program of Jiangsu Province (JSSCBS20211062), Earmarked Fund for Jiangsu Agricultural Industry Technology System (JATS[2022]485), and Project Funded by the Priority Academic Program Development of Jiangsu Higher Education Institutions.
Conflict of interest
The authors declare that the research was conducted in the absence of any commercial or financial relationships that could be construed as a potential conflict of interest.
Publisher’s note
All claims expressed in this article are solely those of the authors and do not necessarily represent those of their affiliated organizations, or those of the publisher, the editors and the reviewers. Any product that may be evaluated in this article, or claim that may be made by its manufacturer, is not guaranteed or endorsed by the publisher.
References
Ahsin, M., Hussain, S., Rengel, Z., Amir, M. (2020). Zinc status and its requirement by rural adults consuming wheat from control or zinc-treated fields. Environ. Geochem. Hlth. 42, 1877–1892. doi: 10.1007/s10653-019-00463-8
Ai, X. F., Xiong, R. Y., Tan, X. M., Wang, H. X., Zeng, Y. J., Huang, S., et al. (2023). Low temperature and light combined stress after heading on starch fine structure and physicochemical properties of late-season indica rice with different grain quality in southern China. Food Res. Int. 164, 112320. doi: 10.1016/j.foodres.2022.112320
Akram, M., Xu, X., Gao, B. Y., Yue, Q. Y., Shang, Y. N., Khan, R., et al. (2020). Adsorptive removal of phosphate by the bimetallic hydroxide nanocomposites embedded in pomegranate peel. J. Environ. Sci. 91, 189–198. doi: 10.1016/j.jes.2020.02.005
Asif, M., Yilmaz, O., Ozturk, L. (2017). Potassium deficiency impedes elevated carbon dioxide-induced biomass enhancement in well watered or drought-stressed bread wheat. J. Plant Nutr. Soil Sci. 180, 474–481. doi: 10.1002/jpln.201600616
Baddar, Z. E., Unrine, J. M. (2021). Effects of Soil pH and Coatings on the Efficacy of Polymer coated ZnO Nanoparticulate fertilizers in Wheat (Triticum aestivum). Environ. Sci. Technol. 55, 13532–13540. doi: 10.1021/acs.est.1c00443
Bala, R., Kalia, A., Dhaliwal, S. S. (2019). Evaluation of efficacy of znO nanoparticles as remedial zinc nanofertilizer for rice. J. Soil Sci. Plant Nutt. 19, 379–389. doi: 10.1007/s42729-019-00040-z
Bana, R. C., Gupta, A. K., Bana, R. S., Shivay, Y. S., Bamboriya, S. D., Thakur, N. P., et al. (2021). Zinc-coated urea for enhanced zinc biofortification, nitrogen use efficiency and yield of basmati rice under typic fluvents. Sustainability 14, 104. doi: 10.3390/su14010104
Bouis, H. E., Hotz, C., McClafferty, B., Meenakshi, J. V., Pfeiffer, W. H., Eozenou, P., et al. (2011). Biofortification: A new tool to reduce micronutrient malnutrition. Food Nutr. Bull. 32, S31–S40. doi: 10.1177/15648265110321S105
Castro-Alba, V., Lazarte, C. E., Bergenstahl, B., Granfeldt, Y. (2019). Phytate, iron, zinc, and calcium content of common Bolivian foods and their estimated mineral bioavailability. Food Sci. Nutr. 7, 2854–2865. doi: 10.1002/fsn3.1127
Cheng, F. W., Bin, S. Y., Iqbal, A., He, L. J., Wei, S. Q., Zheng, H., et al. (2022). High sink capacity improves rice grain yield by promoting nitrogen and dry matter accumulation. Agronomy 12, 1688. doi: 10.3390/agronomy12071688
Derycke, V., Veraverbeke, W. S., Vandeputte, G. E., De Man, W., Hoseney, R. C., Delcour, J. A. (2005). Impact of proteins on pasting and cooking properties of nonparboiled and parboiled rice. Cereal Chem. 82, 468–474. doi: 10.1094/CC-82-0468
Dimkpa, C. O., Latta, D. E., McLean, J. E., Britt, D. W., Boyanov, M. I., Anderson, A. J. (2013). Fate of cuO and znO nano- and microparticles in the plant environment. Environ. Sci. Technol. 47, 4734–4742. doi: 10.1021/es304736y
Du, W., Yang, J. Y., Peng, Q. Q., Liang, X. P., Mao, H. (2019). Comparison study of zinc nanoparticles and zinc sulphate on wheat growth: From toxicity and zinc biofortification. CHEMOSPHERE 227, 109–116. doi: 10.1016/j.chemosphere.2019.03.168
Elmer, W. H., White, J. C. (2016). The use of metallic oxide nanoparticles to enhance growth of tomatoes and eggplants in disease infested soil or soilless medium. Environ. Sci-Nano. 3, 1072–1079. doi: 10.1039/c6en00146g
Ge, J. L., Zhang, X. B., Wei, H. H., Dai, Q. G. (2022). A Decreased Nitrogen Rate with Increased Planting Density Facilitated Better Palatability of Conventional japonica Rice at High Yield Levels. Agriculture 12, 1292. doi: 10.3390/agriculture12091292
Ghasal, P. C., Shivay, Y. S., Pooniya, V., Kumar, P., Verma, R. K. (2016). Zinc fertilization enhances growth and quality parameters of aromatic rice (Oryza sativa L.) varieties. Indian J. Plant Physiol. 1, 323–332. doi: 10.1007/s40502-016-0243-2
Guo, J. X., Feng, X. M., Hu, X. Y., Tian, G. L., Ling, N., Wang, J. H., et al. (2016). Effects of soil zinc availability, nitrogen fertilizer rate and zinc fertilizer application method on zinc biofortification of rice. J. Agr. Sci. 154, 584–597. doi: 10.1017/S0021859615000441
Hall, A. G., King, J. C. (2022). Zinc fortification: current trends and strategies. Nutrients 14, 3895. doi: 10.3390/nu14193895
Jaksomsak, P., Tuiwong, P., Rerkasem, B., Guild, G., Palmer, L., Stangoulis, J., et al. (2018). The impact of foliar applied zinc fertilizer on zinc and phytate accumulation in dorsal and ventral grain sections of four thai rice varieties with different grain zinc. J. Cereal Sci. 79, 6–12. doi: 10.1016/j.jcs.2017.09.004
Kumar, S., Verma, S. K., Yadav, A., Taria, S., Alam, B., Banjara, T. R. (2022). Tillage based crop establishment methods and zinc application enhances productivity, grain quality, profitability and energetics of direct-seeded rice in potentially zinc-deficient soil in the subtropical conditions of India. Commun. Soil Sci. Plan. 53, 1085–1099. doi: 10.1080/00103624.2022.2043340
Kuroha, T., Ashikari, M. (2020). Molecular mechanisms and future improvement of submergence tolerance in rice. Mol. Breed. 40, 41. doi: 10.1007/s11032-020-01122-y
Li, H. H., Li, Z., Xie, S. C., Huang, Y. X., Chen, M. F., Xie, T. H., et al. (2022b). Accumulation and distribution of zinc in rice plants (Oryza sativa L.) growing in zinc contaminated paddy soils with biochar. J. Environ. Chem. Eng. 10, 106811. doi: 10.1016/j.jece.2021.106811
Li, X. Y., Zhang, L., Ren, H. Y., Wang, X. Y., Mi, F. G. (2022a). Zinc toxicity response in Ceratoides arborescens and identification of CaMTP, a novel zinc transporter. Front. Plant Sci. 13. doi: 10.3389/fpls.2022.976311
Liang, C. G., Liu, J., Wang, Y., Xiong, D., Ding, C. B., Li, T. (2015). Low Light During Grain Filling Stage Deteriorates Rice Cooking Quality, but not Nutritional Value. Rice Sci. 22, 197–206. doi: 10.1016/j.rsci.2015.04.003
Liu, Z. (1994). Regularities of content and distribution of zinc in soils of China. Sci. Agric. Sin. 27, 30–37. doi: CNKI:SUN:ZNYK.0.1994-01-005
Lv, Z. Y., Sun, H. D., Du, W., Li, R. Y., Mao, H., Kopittke, P. M. (2021). Interaction of different-sized ZnO nanoparticles with maize (Zea mays) Accumulation, biotransformation and phytotoxicity. Sci. Total Environ. 796, 148927. doi: 10.1016/j.scitotenv.2021.148927
Ma, X. M., Sharifan, H., Dou, F. G., Sun, W. J. (2020). Simultaneous reduction of arsenic (As) and cadmium (Cd) accumulation in rice by zinc oxide nanoparticles. Chem. Eng. J. 384, 123802. doi: 10.1016/j.cej.2019.123802
Montalvo, D., Degryse, F., da Silva, R. C., Baird, R., McLaughlin, M. J. (2016). Agronomic effectiveness of zinc sources as micronutrient fertilizer. Adv. Agron. 139, 215–267. doi: 10.1016/bs.agron.2016.05.004
Naik, Sk., Das, D. K. (2008). Relative performance of chelated zinc and zinc sulphate for lowland rice (Oryza sativa L.). Nutr. Cycl. Agroecosys. 81, 219–227. doi: 10.1007/s10705-007-9158-7
Palmgren, M. G., Clemens, S., Williams, L. E., Kraemer, U., Borg, S., Schjorring, J. K., et al. (2008). Zinc biofortification of cereals: problems and solutions. Trends Plant Sci. 13, 464–473. doi: 10.1016/j.tplants.2008.06.005
Paramesh, V., Dhar, S., Dass, A., Kumar, B., Kumar, A., El-Ansary, D. O., et al. (2020). Role of integrated nutrient management and agronomic fortification of zinc on yield, nutrient uptake and quality of wheat. Sustainability 12, 3513. doi: 10.3390/su12093513
Patel, P. S., Singh, S. K., Patra, A., Jatav, S. S. (2022). Root dipping, foliar and soil application of zinc increase growth, yields, and grain zinc in rice (Oryza sativa L.) grown in moderate zinc soil of inceptisol order. Commun. Soil Sci. Plan. 53, 1917–1929. doi: 10.1080/00103624.2022.2069800
Prasad, A. S. (2013). Discovery of human zinc deficiency: its impact on human health and disease. Adv. Nutr. 4, 176–190. doi: 10.3945/an.112.003210
Rehman, H. U., Aziz, T., Farooq, M., Wakeel, A., Rengel, Z. (2012). Zinc nutrition in rice production systems: a review. Plant Soil. 361, 203–226. doi: 10.1007/s11104-012-1346-9
Repkina, N., Nilova, I., Kaznina, N. (2023). Effect of zinc excess in substrate on physiological responses of sinapis alba L. Plants-Basel 12, 211. doi: 10.3390/plants12010211
Sheteiwy, M. S., Shaghaleh, H., Hamoud, Y. A., Holford, P., Shao, H. B., Qi, W. C., et al. (2021). Zinc oxide nanoparticles: potential effects on soil properties, crop production, food processing, and food quality. Environ. Sci. pollut. Res. 28, 36942–36966. doi: 10.1007/s11356-021-14542-w
Singh, A., Sengar, R. S., Rajput, V. D., Minkina, T., Singh, R. K. (2022). Zinc oxide nanoparticles improve salt tolerance in rice seedlings by improving physiological and biochemical indices. Agriculture 12, 1014. doi: 10.3390/agriculture12071014
Singh, A., Shivay, Y. S., Prasanna, R., Kumar, A. (2021). Basmati rice quality enhancement by zinc fertilization and green manuring on a sub-tropical inceptisol in indo-gangetic plains of India. J. Agr. Sci. 13, 125. doi: 10.5539/JAS.V13N5P125
Singh, K., Verma, G., Manchanda, J. S. (2020). Soil and foliar zinc application for enhancing grain zinc content of aromatic rice genotypes grown on zinc deficient and sufficient soil. J. Soil Water. Conserv. 19, 223–227. doi: 10.5958/2455-7145.2020.00030.2
Soltani, S. M., Molki, M. T. K. A., Allahgholipour, M., Kavoosi, M., Komuleh, A. S. (2022). The effects of foliar application of zinc and phosphorous on the morphology and yield of the dominant Iranian rice variety (Hashemi). J. Plant Nutr. 45, 3145–3158. doi: 10.1080/01904167.2022.2058536
Suganya, A., Saravanan, A., Manivannan, N. (2020). Role of zinc nutrition for increasing zinc availability, uptake, yield, and quality of maize (Zea mays L.) grains: an overview. Commun. Soil Sci. Plan. 51, 2001–2021. doi: 10.1080/00103624.2020.1820030
Sun, H. D., Du, W., Peng, Q. Q., Lv, Z. Y., Mao, H., Kopittke, P. M. (2020). Development of znO nanoparticles as an efficient zn fertilizer: using synchrotron-based techniques and laser ablation to examine elemental distribution in wheat grain. J. Agric. Food. Chem. 68, 5068–5075. doi: 10.1021/acs.jafc.0c00084
Tondey, M., Kalia, A., Singh, A., Abd-Elsalam, K., Hassan, M. M., Dheri, G. S. (2022). A comparative evaluation of the effects of seed invigoration treatments with precursor zinc salt and nano-sized zinc oxide (ZnO) particles on vegetative growth, grain yield, and quality characteristics of Zea mays. J. Anal. Sci. Technol. 13, 40. doi: 10.1186/s40543-022-00346-1
Tuiwong, P., Lordkaew, S., Prom-u-thai, C. (2021). Improving grain zinc concentration in wetland and upland rice varieties grown under waterlogged and well-drained soils by applying zinc fertilizer. Agronomy 11, 554. doi: 10.3390/agronomy11030554
Tulchinsky, T. H. (2017). Correction to: micronutrient deficiency conditions: Global Health issues. Public Health Rev. 38, 25. doi: 10.1186/s40985-017-0071-6
Upadhyaya, H., Roy, H., Shome, S., Tewari, S., Bhattacharya, M. K., Panda, S. K. (2017). Physiological impact of Zinc nanoparticle on germination of rice (Oryza sativa L.) seed. J. Plant Sci. 1, 062–070. doi: 10.29328/journal.jpsp.1001008
Wang, P., Menzies, N. W., Lombi, E., McKenna, B. A., Johannessen, B., Glover, C. J., et al. (2013). Fate of znO nanoparticles in soils and cowpea (Vigna unguiculata). Environ. Sci. Technol. 47, 13822–13830. doi: 10.1021/es403466p
Wang, X. X., Sun, W. J., Zhang, S., Sharifan, H., Ma, X. M. (2018). Elucidating the effects of cerium oxide nanoparticles and zinc oxide nanoparticles on arsenic uptake and speciation in rice (Oryza sativa) in a hydroponic system. Environ. Sci. Technol. 52, 10040–10047. doi: 10.1021/acs.est.8b01664
Wang, X. Q., Wang, K. L., Yin, T. Y., Zhao, Y. F., Liu, W. Z., Shen, Y. Y., et al. (2021). Nitrogen fertilizer regulated grain storage protein synthesis and reduced chalkiness of rice under actual field warming. Front. Plant Sci. 12. doi: 10.3389/fpls.2021.715436
Xiao, Y. S., Zhou, B., Han, Z. Z., Liu, S. Z., Ding, C., Jia, F. F., et al. (2022). Microbial mechanism of zinc fertilizer input on rice grain yield and zinc content of polished rice. Front. Plant Sci. 13. doi: 10.3389/fpls.2022.962246
Xu, H., Xu, N., Wu, L., Yu, Z. W., Wang, X. D., Lu, J. H., et al. (2021). Genome sequencing of rice subspecies and genetic analysis of recombinant lines reveals regional rapid visco analyser (RVA) associated loci. Plant Growth Regul. 95, 1–11. doi: 10.1007/s10725-021-00738-0
Xuan, Y., Yi, Y., Liang, H., Wei, S. Q., Chen, N. P., Jiang, L. G., et al. (2020). Amylose content and RVA profile characteristics of noodle rice under different conditions. Agron. J. 112, 117–129. doi: 10.1002/agj2.20079
Yang, G. Y., Yuan, H. Y., Ji, Ht., Liu, H. J., Zhang, Y. F., Wang, G. D., et al. (2021). Effect of ZnO nanoparticles on the productivity, Zn biofortification, and nutritional quality of rice in a life cycle study. Plant Physiol. Bioch. 163, 87–94. doi: 10.1016/j.plaphy.2021.03.053
Zhang, X., Jiang, B., Ma, Y. B. (2017). Aging of zinc added to soils with a wide range of different properties: Factors and modeling. Environ. Toxicol. Chem. 36, 2925–2933. doi: 10.1002/etc.3896
Zhang, T., Sun, H. D., Lv, Z. Y., Cui, L. L., Mao, H., Kopittke, P. M. (2018). Using synchrotron-based approaches to examine the foliar application of znSO4 and znO nanoparticles for field-grown winter wheat. J. Agric. Food Chem. 66, 2572–2579. doi: 10.1021/acs.jafc.7b04153
Zhang, H. P., Wang, R., Chen, Z. Q., Cui, P. Y., Lu, H., Yang, Y. J., et al. (2021). The effect of zinc oxide nanoparticles for enhancing rice (Oryza sativa L.) yield and quality. Agriculture 11, 1247. doi: 10.3390/agriculture11121247
Zhang, H. P., Wang, R., Chen, Z. Q., Pu, J. L., Wang, J. J., Zhang, H. C., et al. (2022). Nanoscale molybdenum oxide improves plant growth and increases nitrate utilisation in rice (Oryza sativa L.). Food Energy Secur. 11, e383. doi: 10.1002/fes3.383
Zhang, Y., Xu, Y. H., Yi, H. Y., Gong, J. M. (2012). Vacuolar membrane transporters OsVIT1 and OsVIT2 modulate iron translocation between flag leaves and seeds in rice. Plant J. 72, 400–410. doi: 10.1111/j.1365-313X.2012.05088.x
Zhao, G. C., Xie, S. F., Zong, S. P., Wang, T., Mao, C. J., Shi, J. X., et al. (2022). Mutation of TL1, encoding a novel C2H2 zinc finger protein, improves grains eating and cooking quality in rice. Theor. Appl. Genet. 135, 3531–3543. doi: 10.1007/s00122-022-04198-6
Keywords: zinc oxide nanoparticles, soil application, rice quality, zinc distribution, zinc bioavailability
Citation: Mi K, Yuan X, Wang Q, Dun C, Wang R, Yang S, Yang Y, Zhang H and Zhang H (2023) Zinc oxide nanoparticles enhanced rice yield, quality, and zinc content of edible grain fraction synergistically. Front. Plant Sci. 14:1196201. doi: 10.3389/fpls.2023.1196201
Received: 29 March 2023; Accepted: 31 July 2023;
Published: 18 August 2023.
Edited by:
Min Huang, Hunan Agricultural University, ChinaReviewed by:
Zhaowen Mo, South China Agricultural University, ChinaMin Xi, Anhui Academy of Agricultural Sciences (CAAS), China
Copyright © 2023 Mi, Yuan, Wang, Dun, Wang, Yang, Yang, Zhang and Zhang. This is an open-access article distributed under the terms of the Creative Commons Attribution License (CC BY). The use, distribution or reproduction in other forums is permitted, provided the original author(s) and the copyright owner(s) are credited and that the original publication in this journal is cited, in accordance with accepted academic practice. No use, distribution or reproduction is permitted which does not comply with these terms.
*Correspondence: Haipeng Zhang, aHB6aGFuZ0B5enUuZWR1LmNu