- 1Department of Grassland Science, College of Animal Science, Guizhou University, Guiyang, China
- 2Institute for Forest Resources and Environment of Guizhou, Guizhou University, Guiyang, China
- 3College of Agriculture, Guizhou University, Guiyang, China
- 4Key Lab Forest Ecology Tibet Plateau, Ministry Education, Tibet Agriculture & Animal Husbandry University, Nyingchi, China
Sophora moorcroftiana is a leguminous plant endemic to the Qinghai-Tibet Plateau. It has excellent abiotic stress tolerance and is considered an ideal species for local ecological restoration. However, the lack of genetic diversity in the seed traits of S. moorcroftiana hinders its conservation and utilization on the plateau. Therefore, in this study, genotypic variation and phenotypic correlations were estimated for nine seed traits among 15 accessions of S. moorcroftiana over two years, 2014 and 2019, respectively from 15 sample points. All traits evaluated showed significant (P< 0.05) genotypic variation. In 2014, accession mean repeatability was high for seed perimeter, length, width, and thickness, and 100-seed weight. In 2019, mean repeatability for seed perimeter and thickness, and 100-seed weight were high. The estimates of mean repeatability for seed traits across the two years ranged from 0.382 for seed length to 0.781 for seed thickness. Pattern analysis showed that 100-seed weight was significantly positively correlated with traits such as seed perimeter, length, width, and thickness, and identified populations with breeding pool potential. In the biplot, principal components 1 and 2 explained 55.22% and 26.72% of the total variation in seed traits, respectively. These accessions could produce breeding populations for recurrent selection to develop S. moorcroftiana varieties suitable for restoring the fragile ecological environment of the Qinghai-Tibet Plateau.
1 Introduction
The Qinghai-Tibet Plateau, with an average altitude of more than 4,000 m above sea level (m a.s.l.), is called the “Roof of the World” or the “Third-Pole on Earth” (Chen et al., 2020). The area is approximately 2.5 million km2, accounting for a fourth of China’s total territorial land (Dong et al., 2020; Shi et al., 2021). Owing to its characteristics of high terrain and low oxygen, the unique biological resources of the Qinghai-Tibet Plateau play a vital role in global biodiversity (Tao et al., 2020). At the same time, although different terrain and topography create a large number of diverse habitats for plants, the ecosystem in this region is fragile, and the vegetation is extremely sensitive to global climate change (Chen et al., 2020; Deng et al., 2020).
Sophora moorcroftiana (Benth.) Baker, is a perennial deciduous dwarf shrub of the legume family endemic to the Qinghai-Tibet Plateau. It has strong ecological adaptability, such as drought resistance, barren tolerance, and wind and sand resistance. It is mainly distributed in the valleys and hillsides of the Yarlung Zangbo River Basin at an altitude of 2,800–4,500 m above sea level (m a.s.l.) and is a dominant pioneer plant among drought-tolerant shrubs (Liu et al., 2020) and the preferred tree species for ecological restoration in the plateau. Further, low-polarity compounds contained in the seeds, such as matrine and sophocarpine, can be used to treat Echinococcus granulosus infections (Luo et al., 2018).
Seeds provide plants with an evolutionary advantage that allows them to survive and develop in drier places/times, store energy and nutrients to support initial development and growth, increase offspring fitness, and allow colonization and survival in adverse environments (Niklas et al., 2008; Lamont and Groom, 2013; Saatkamp et al., 2019). A range of seed morphological characteristics (e.g., seed size and epidermal characteristics) and physiological traits can coordinate the timing of seed germination under conditions suitable for seedling establishment (Saatkamp et al., 2019). In addition, both seed shape and size traits are useful for analyzing plant biodiversity and can be used to characterize intra- and inter-species variation as well as genotypic discrimination, and their correlation information is important for breeding, targeting seed yield and quality (Cervantes et al., 2016; Saatkamp et al., 2019; Khamassi et al., 2021). For example, seed mass has been identified as a key plant fitness-related trait, with larger seeds conferring advantages to plants in properties such as drought tolerance during seedling establishment, compared to small-seeded plants (Cochrane et al., 2015). This trait may reflect a trade-off for plants to develop short-term reductions in reproductive success (e.g., reduced seed production) with reduced long-term risk (Venable, 2007).
Compared to other plant organs such as flowers and leaves, using seed traits to characterize the genetic diversity of species has certain advantages because seeds are relatively easier to collect and store (Grillo et al., 2010). Pinna et al. (2014) used seed morphology parameters to analyze the interspecific, specific, and intraspecific levels of 10 Juniperus populations collected from the Mediterranean. Khamassi et al. (2021) characterized and evaluated the seed morphology of 24 local faba bean (Vicia faba) accessions and found that accessions with a white hilum were associated with lower mature grain content. Bacchetta et al. (2008) measured seed morphological characteristics for 220 accessions in the Sardinian Germplasm Bank using digital image analysis techniques and concluded that the method could be used to identify very similar taxa in these species with an accuracy of 83.7%–100%. At present, the precise quantification of seed morphological characteristics is facilitated by the development and use of digital techniques, quantification, and modeling methods (Cervantes et al., 2016).
Therefore, in this study, the variation in seed morphological characteristics of 15 S. moorcroftiana populations collected from different locations on the Qinghai-Tibet Plateau was studied using digital image analysis techniques. Our research focused on estimating the genetic variation within and among populations. In addition, a combination of potentially beneficial seed traits has been evaluated in breeding programs. This study aimed to provide data support for the genetic diversity and taxonomy of S. moorcroftiana, and to provide valuable parameters and information for the selection and breeding of strong adaptability S. moorcroftiana varieties.
2 Materials and methods
2.1 Germplasm
The seed resources of 15 accessions were evaluated in this study. S. moorcroftiana seeds were collected at 15 sampling points during October 1–7, 2014, and October 1–7, 2019 (Figure 1). The collected seeds were dried to a moisture content of 6%–8% and stored at 4°C and 30%–50% relative humidity. The climate data of the sampling points are provided by the meteorological data center of the China meteorological administration.
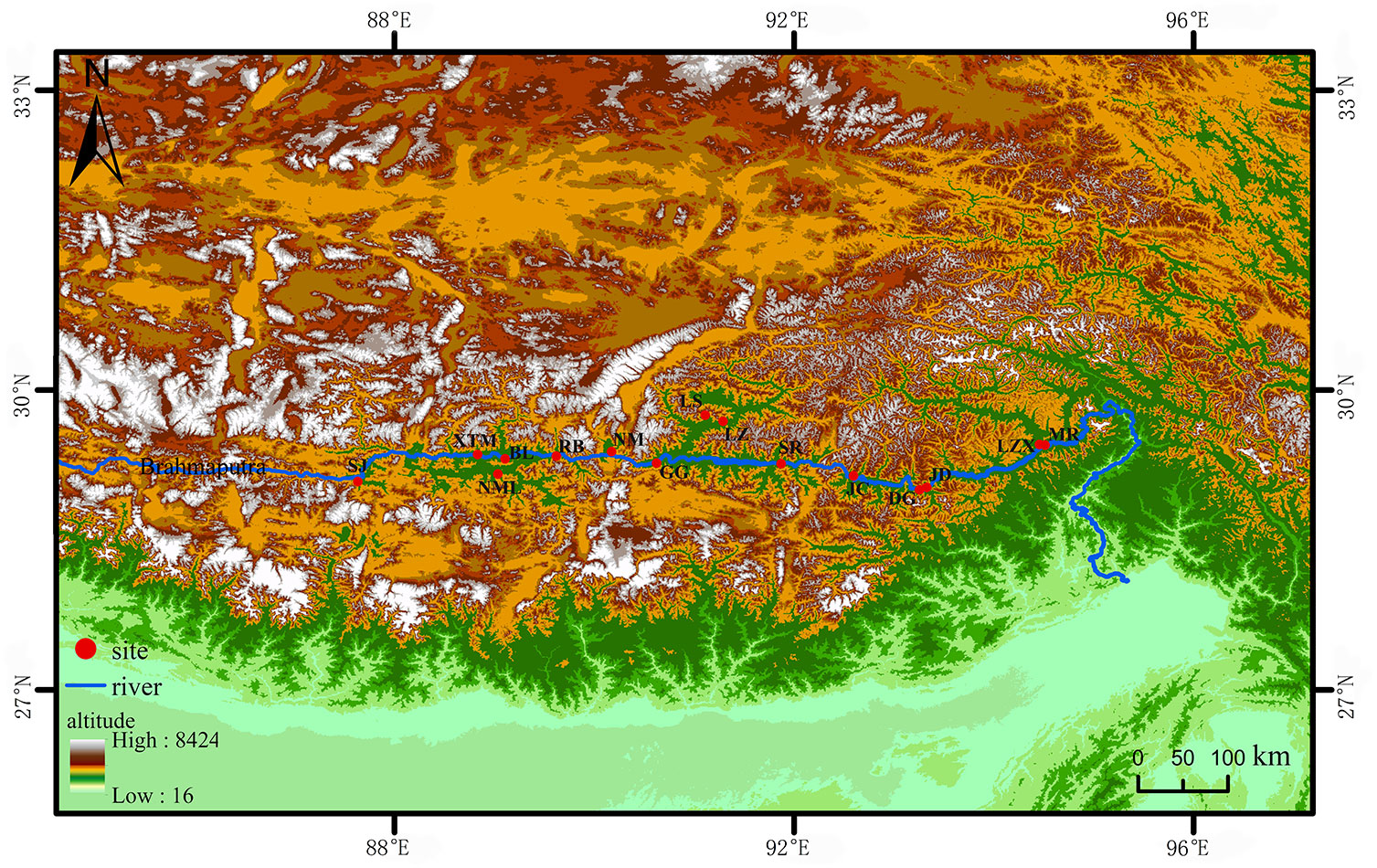
Figure 1 The distribution of 15 S. moorcroftiana accessions used in this study. The red dots represent the sampling points. LZX, Lin zhi; NML, Nan mu lin; GG, Gong ga; RB, Ren bu; JC, Jia cha; LS, La sa; JD, Jin dong; BL, Bai lang; XTM, Xie tong men; DG, Dong ga; SJ, Sa jia; NM, Ni mu; SR, Sang ri; MR, Mi rui; LZ, Lin zhou.
2.2 Trait measurements
Nine seed traits were measured: SL, seed length (mm); SW, seed width (mm); W/L, seed width to seed length ratio; HL, hilum length (mm); HW, hilum width (mm); HW/HL, hilum width to hilum length ratio; Pe, perimeter (mm); ST, seed thickness (mm) and SY, 100-seed weight (g). 100 seeds were manually counted. Use an electronic balance (Sartorius, BSA224S-CW, China) for weighing. Before trait measurements, a flatbed scanner (EPSON GT-15000) was used to obtain digital images of the seed samples. During the scan, the seeds were allowed to equilibrate before measurement (room temperature was maintained at 20 ± 3°C and 40 ± 5% relative humidity) (Grillo et al., 2010). The scanned image resolution was 200 dpi, and the number of pixels was 1024 × 1024.
Three replicates were scanned for each population and each replicate included 100 seeds. Seed samples were prepared and scanned according to methods described by Venora et al. (2007); Bacchetta et al. (2008), and Dong et al. (2016). A WinSEEDLE 2011 image analysis system was used to process the acquired images (Dong et al., 2016).
2.3 Data analysis
Data analysis was based on (1) variance component analysis to assess the magnitude and significance of genotypic variation between populations and (2) pattern analysis, including a combination of clustering and principal component analysis (PCA) (Dong et al., 2016; Dong et al., 2019) to provide a graphical summary of the multi-trait data matrices.
Data on seed traits from 15 S. moorcroftiana population accessions were analyzed within and over two years (2014 and 2019). The analyses were conducted using the variance component analysis procedure, residual maximum likelihood (REML), in GenStat 7.1 (2003) (Dong et al., 2019). Analysis of data over the years was performed using a mixed linear model (Dong et al., 2019).
All seed trait means were derived from the best linear unbiased predictor (BLUP) analysis (White and Hodge, 1989; Dong et al., 2019). These BLUP values were used to build a population × trait mean matrix adjusted for population × year interaction effects.
Referring to Fehr (1987) method, the estimated genotypic (), genotype × year interaction (), experimental error (), nl (number of years), and nr (number of replications) obtained from REML analysis were used to estimate the population accession mean repeatability (R).
Accession mean repeatability within a single year:
Accession mean repeatability across years:
Phenotypic correlation (rp) analysis was performed using GenStat 7.1 (2003), and multivariate analysis of variance (MANOVA) was used to assess accessions for 15 populations over two years, resulting in the sum of the estimated cross-products of the multi-trait data matrix.
Pattern analysis is a combination of cluster analysis and principal component analysis (PCA): a) based on the variance components over the two years 2014 and 2019 to obtain an adjusted mean matrix of genotype × trait BLUP and finally obtain a graphical summary of accession traits for eight populations; and b) to analyze the type of association (positive or negative) among the nine seed traits in 2014 and 2019.
3 Results
3.1 Genotypic variance components and the mean repeatability of nine seed traits of S. moorcroftiana
The genotypic variance components of the nine seed traits in 2014 and 2019 showed significant differences (P< 0.05) for all the traits evaluated in the 15 S. moorcroftiana accessions (Tables 1, 2).
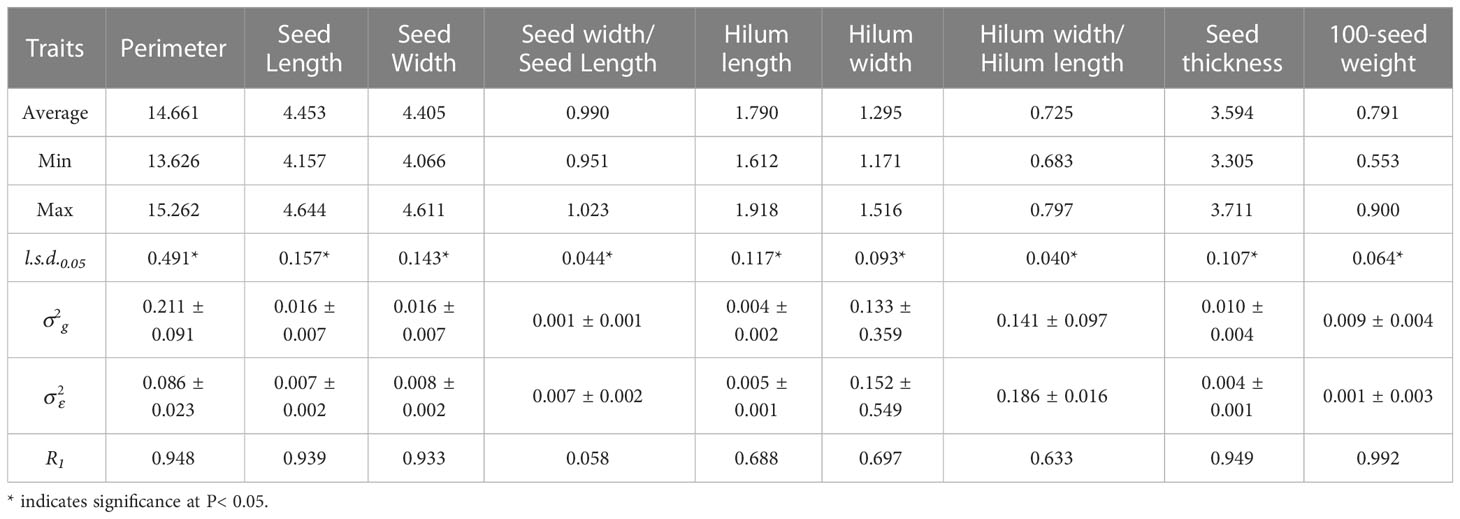
Table 1 Average, maximum, minimum, least significant differences (l.s.d.0.05), estimated genotypic () and experimental error () variance components and associated standard errors ( ± SE), and mean repeatability (R1) estimated from the 15 S. moorcroftiana accessions, evaluated in 2014.
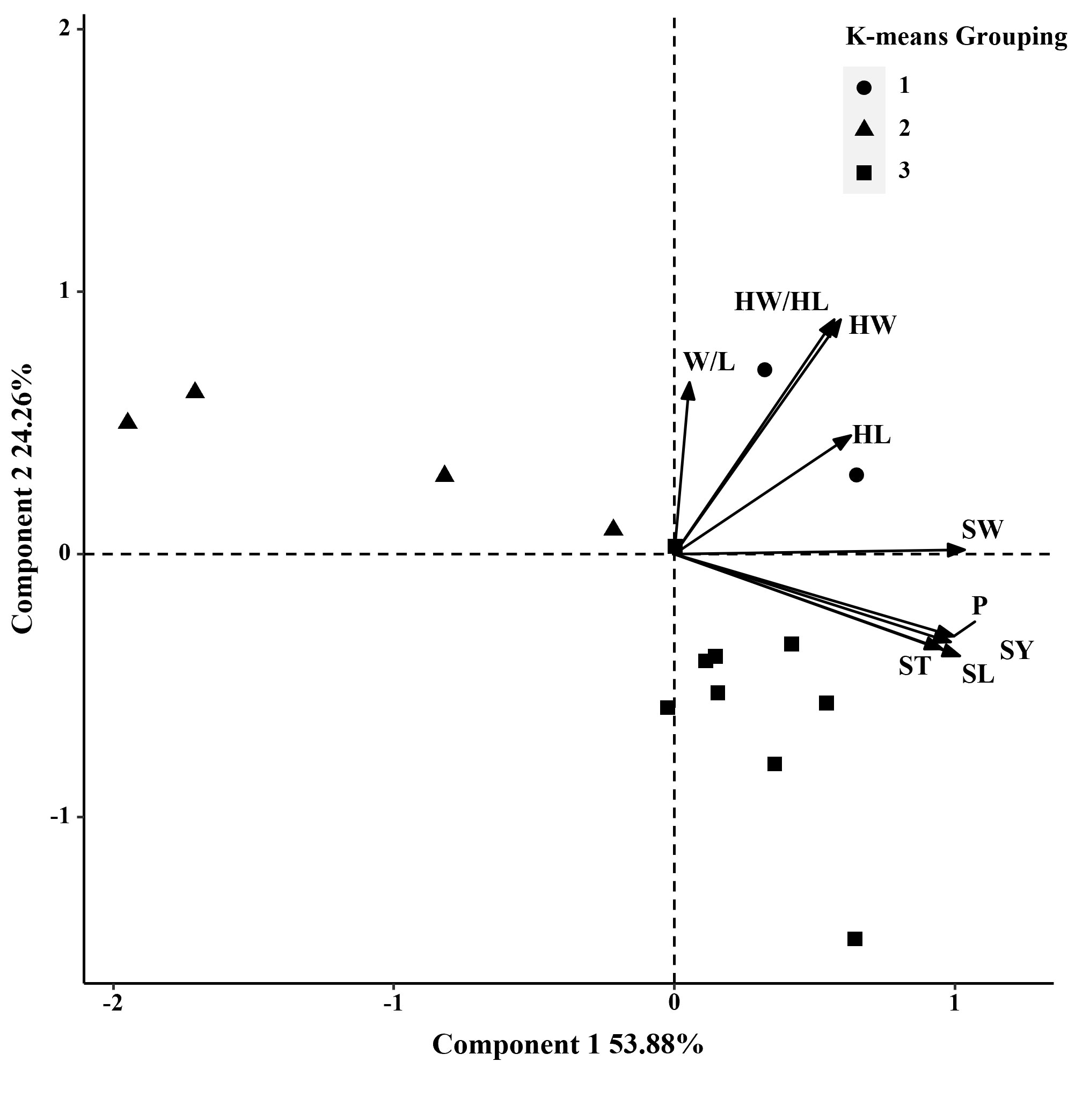
Figure 2 Biplot generated using standardized Best Linear Unbiased Predictor values for nine seed traits measured from 15 S. moorcroftiana accessions, evaluated in 2014. Components I and II account for 53.88% and 24.26% of total variation, respectively. The different symbols indicate progeny Groups 1 to 3 generated from cluster analysis.
In 2014, accession mean repeatability (R1) was high for seed perimeter, length, width, and thickness, and 100-seed weight, ranging from 0.933 to 0.992 (Table 1; Supplementary Figure 1A). The R1 values for the hilum length, width, and hilum length/width ratio were intermediate, ranging from 0.633 to 0.697. R1 for seed width/seed length was the lowest at 0.058. In 2019, R1 for seed perimeter and thickness, and 100-seed weight were high, ranging from 0.846 to 0.991 (Table 2; Supplementary Figure 1B). R1 for seed length, hilum length and width, and hilum length/width ratio were intermediate, ranging from 0.604 to 0.767. R1 values for seed width and seed width/length ratio were lower than those of the other traits (0.489 and 0.054, respectively).
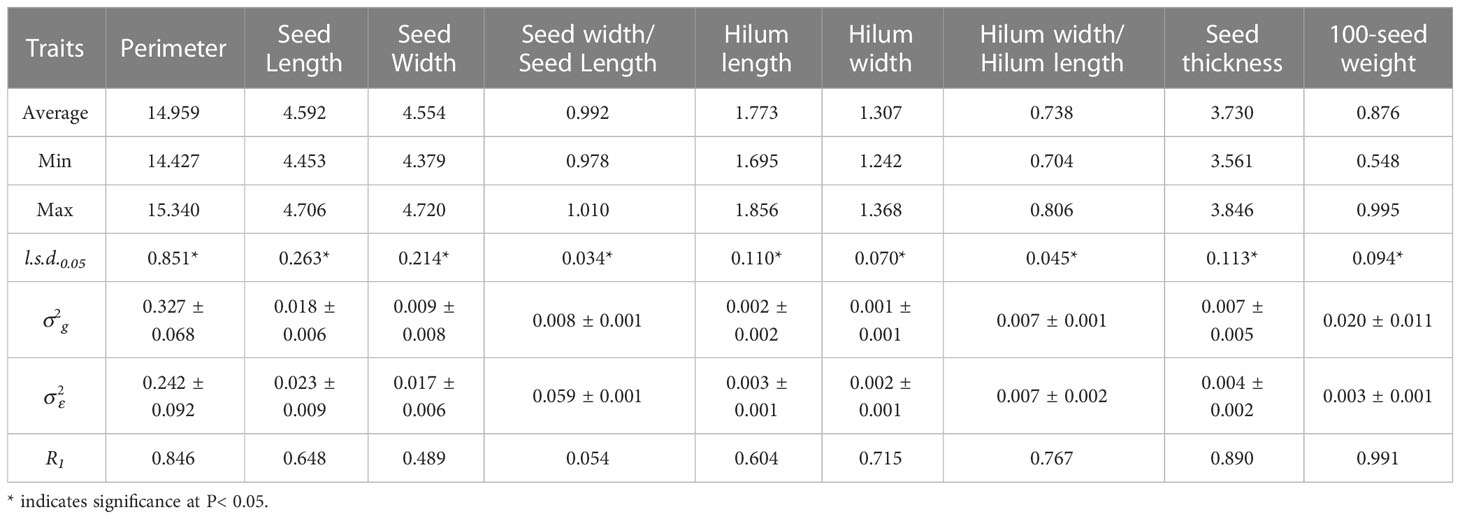
Table 2 Average, maximum, minimum, least significant differences (l.s.d.0.05), estimated genotypic () and experimental error () variance components and associated standard errors ( ± SE), and mean repeatability (R1) estimated from the 15 S. moorcroftiana accessions, evaluated in 2019.
Analysis of variance for over two years, 2014 and 2019, showed significant genotypic variation (P< 0.05) among the 15 S. moorcroftiana accessions for nine seed traits (Table 3). The mean repeatability (R2) of hilum length/width and seed thickness was higher than that of the other traits (0.757 and 0.781, respectively). The R2 values for the hilum length and width, and 100-seed weight were intermediate at 0.597, 0.643, and 0.626, respectively. R2 for seed perimeter, length, width, and width/length ratio were lower than those for the other traits, ranging from 0.097 to 0.493.
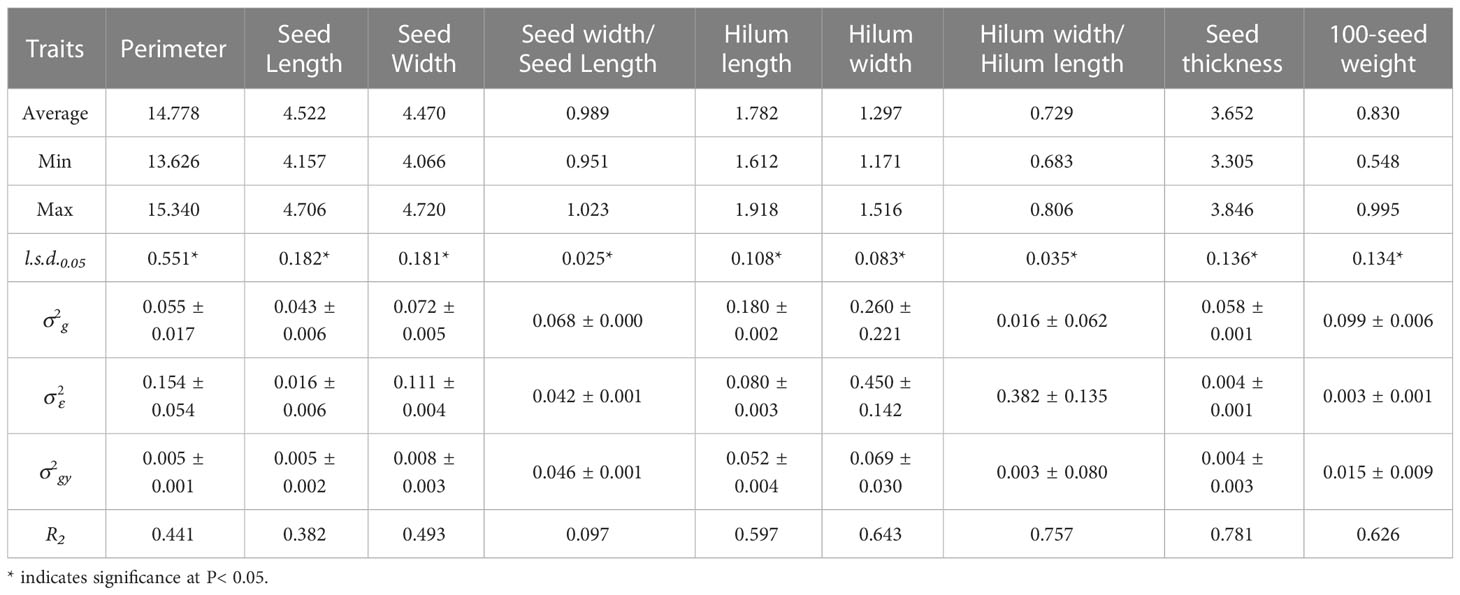
Table 3 Average, maximum, minimum, least significant differences (l.s.d.0.05), estimated genotypic (), genotype × year interaction () and experimental error () variance components and associated standard errors ( ± SE), and mean repeatability (R2) estimated from the 15 S. moorcroftiana accessions, evaluated across two years, 2014 and 2019.
3.2 Pattern analysis and phenotypic correlation of S. moorcroftiana
In 2014, based on phenotypic correlation analysis, there was a positive correlation between 100-seed weight and seed perimeter, length, width, and thickness (Table 4). Seed perimeter, length, and width also exhibited strong positive correlations at the phenotypic level. In the biplot, principal components 1 and 2 explained 53.88% and 24.26% of the total variation in seed traits, respectively (Figure 2). The BLUP mean matrix of nine seed traits was used for the cluster analysis grouping of 15 S. moorcroftiana accessions in 2014, truncated at the group three level. According to the trait means for each group, Group 1 had the highest mean seed perimeter, length, width, and width/length ratio, hilum length, width, and length/width ratio, and 100-seed weight and included two accessions (Supplementary Table 1).
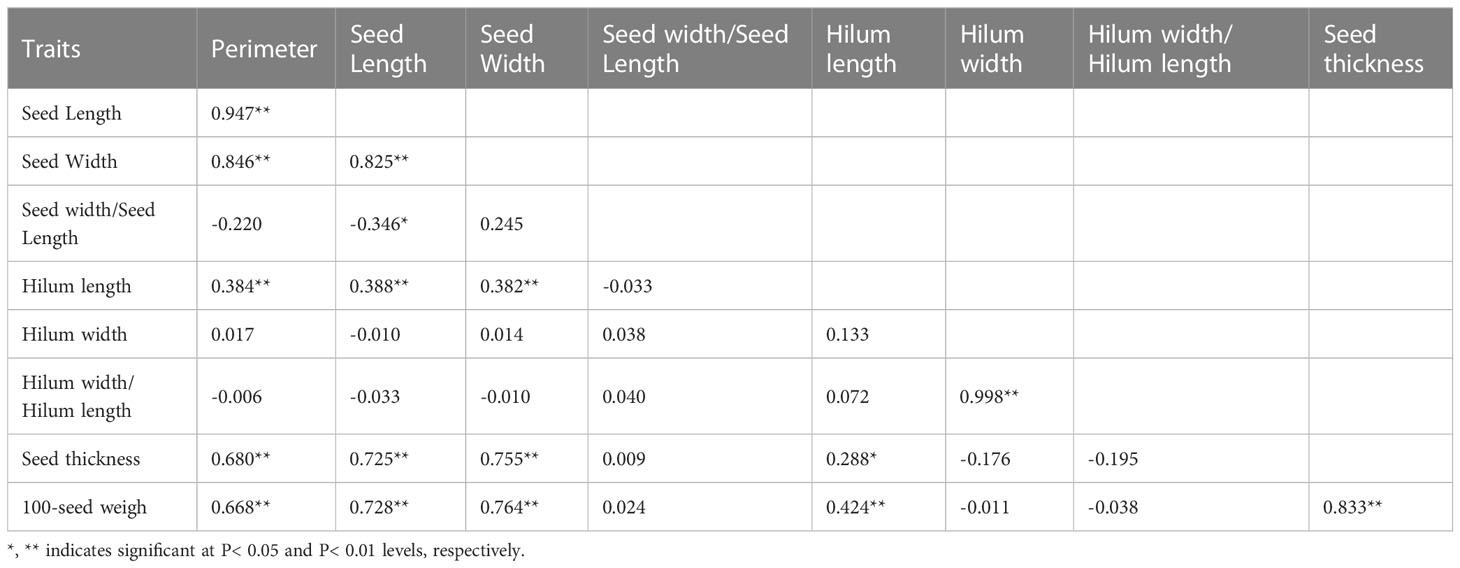
Table 4 Phenotypic (rp) correlation coefficients, between traits based on the 15 S. moorcroftiana accessions, evaluated in 2014.
In 2019, the phenotypic correlation analysis showed that 100-seed weight, seed perimeter, length, width, and thickness showed strong positive correlations at the phenotypic level (Table 5). In the biplot, principal component 1 explained 70.74% of the total seed trait variation and principal component two explained 15.39% (Figure 3). The 15 S. moorcroftiana accession groups generated from the cluster analysis were truncated at the two-group level. The results showed that the second group had a higher seed perimeter, length, width, thickness and width/length ratio, hilum length, and 100-seed weight (Supplementary Table 2).
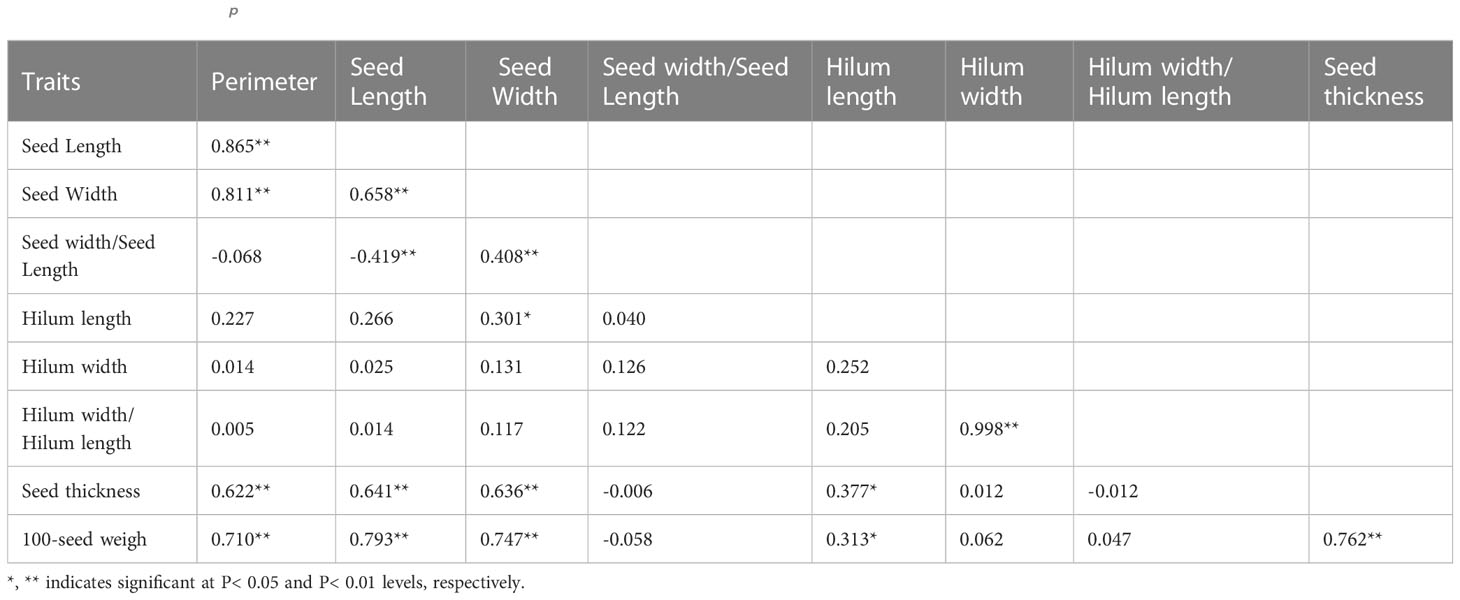
Table 5 Phenotypic (rp) correlation coefficients, between traits based on the 15 S. moorcroftiana accessions, evaluated in 2019.
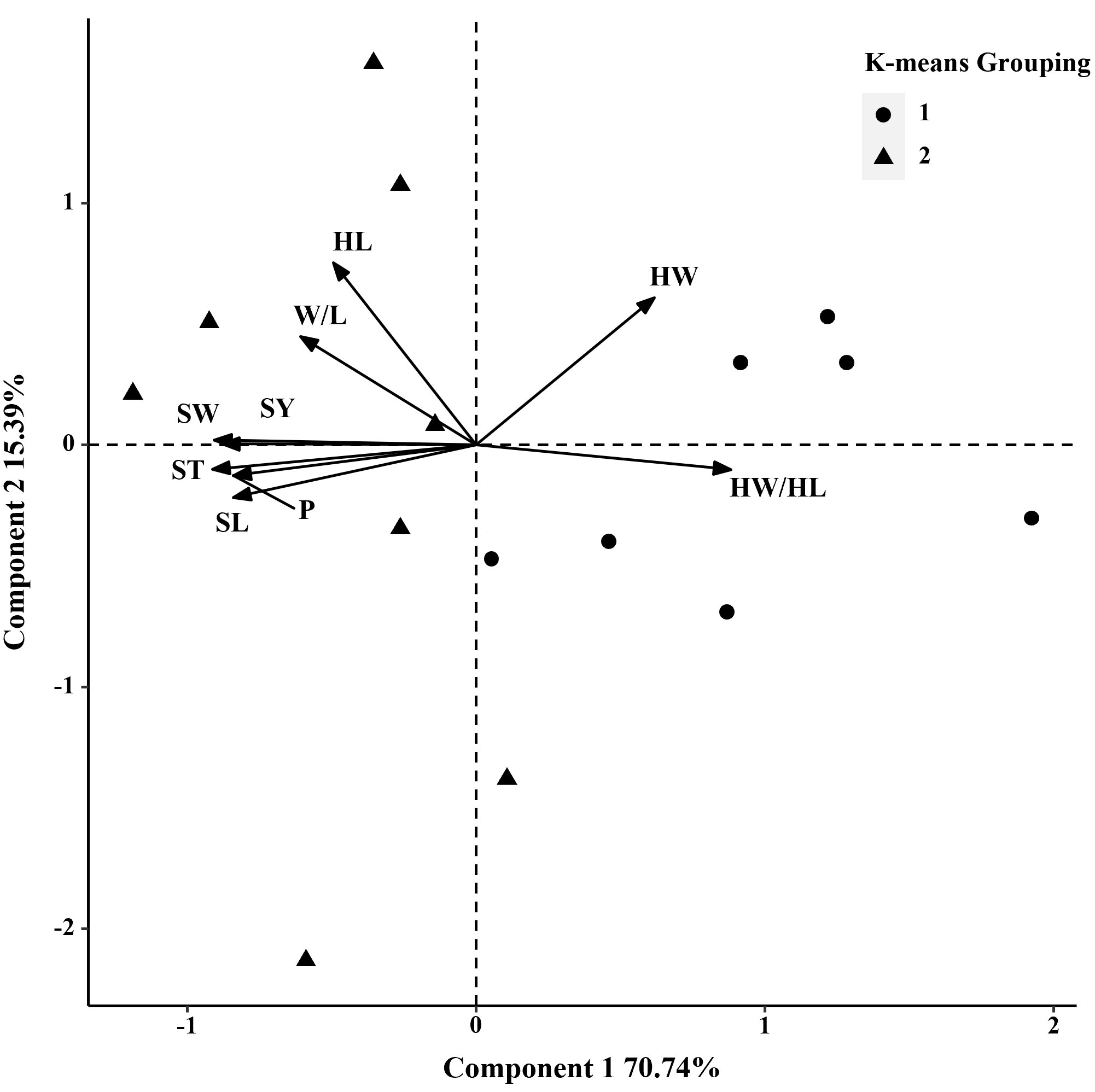
Figure 3 Biplot generated using standardized Best Linear Unbiased Predictor values for nine seed traits measured from 15 S. moorcroftiana accessions, evaluated in 2019. Components I and II account for 70.74% and 15.39% of total variation, respectively. The different symbols indicate progeny Groups 1 and 2 generated from cluster analysis.
In 2014 and 2019, based on the phenotypic correlation analysis, 100-seed weight showed a strong positive correlation with seed perimeter, length, width, and thickness at the phenotypic level and a strong negative correlation with hilum length/width ratio (Table 6). In the biplot, principal components 1 and 2 explained 55.22% and 26.72% of the total variation in seed traits, respectively (Figure 4). The 15 S. moorcroftiana accession groups generated from the cluster analysis were truncated at the three-group level. The third group of accessions had higher seed perimeter, seed length, width, width/length ratio, thickness, and 100-seed weight, including five accessions (Supplementary Table 3). Furthermore, in 2014 and 2019, the seed traits perimeter, hilum length/width ratio, seed thickness, and 100-seed weight were all significantly correlated with altitude, and hilum length/width ratio, seed thickness, and 100-seed were significantly correlated with the monthly average maximum temperature, monthly average minimum temperature, and monthly average temperature during the growing season (Supplementary Figure 2).
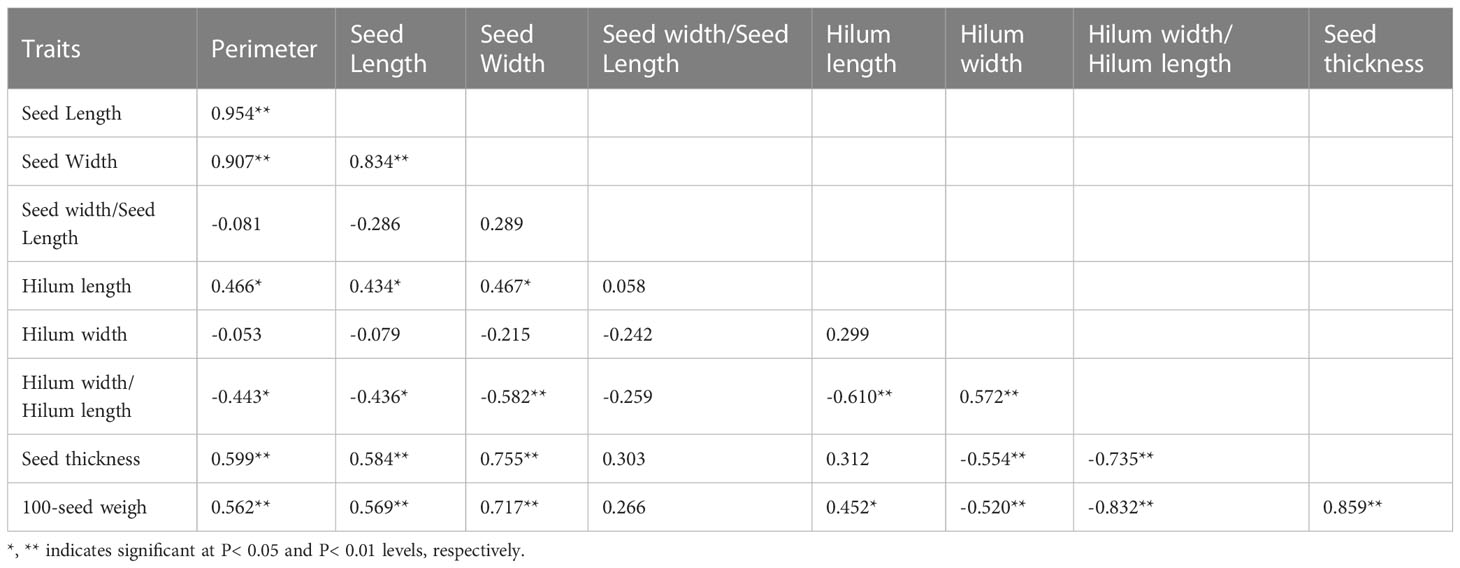
Table 6 Phenotypic (rp) correlation coefficients, between traits based on the 15 S. moorcroftiana accessions, evaluated across two years, 2014 and 2019.
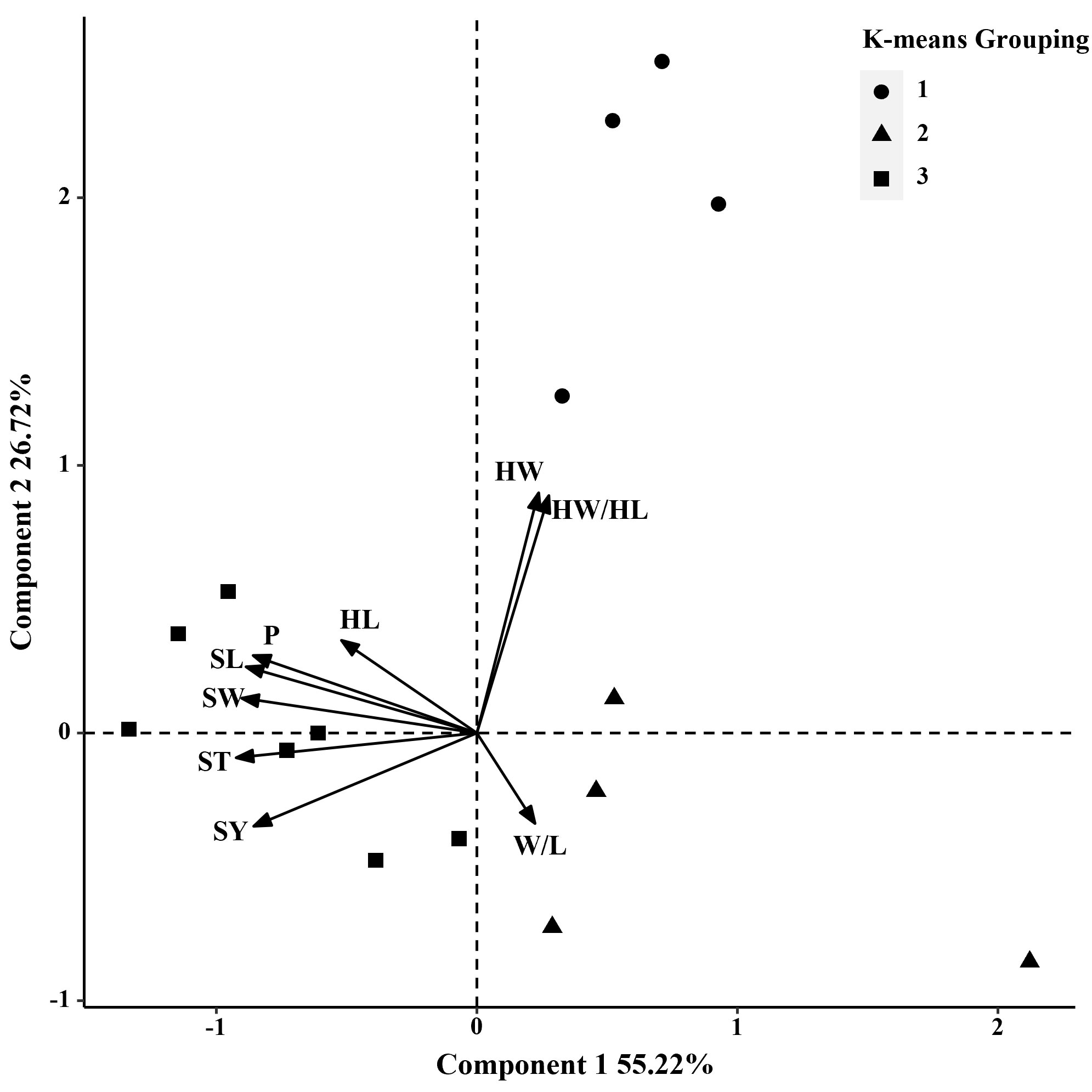
Figure 4 Biplot generated using standardized Best Linear Unbiased Predictor values for nine seed traits measured from 15 S. moorcroftiana accessions, evaluated across two years, 2014 and 2019. Components I and II account for 55.22% and 26.72% of total variation, respectively. The different symbols indicate progeny Groups 1 to 3 generated from cluster analysis.
4 Discussion
Numerous studies have shown that structural diversity in seed traits helps characterize both intra- and inter-species variation. Therefore, the study of macro- and micro-seed traits is important in quantifying genetic diversity and plant taxonomy (Barthlott, 1981; Grillo et al., 2010).
Previous studies on S. moorcroftiana have mainly focused on its population distribution (Liu et al., 2020; Xin et al., 2021; Yang et al., 2021), soil seed banks (Zhao et al., 2007), medicinal functions (Wang et al., 2014; Luo et al., 2018), analysis of transcriptome (Li et al., 2015) and verification of gene functions (Li et al., 2017). In this study, we report, for the first time, the phenotypic and genotypic variations in nine seed traits and the mean repeatability of 15 accessions of S. moorcroftiana.
In nature, plants growing in various environments have evolved adaptive traits related to seed morphology and physiology to cope with adverse environments, such as variability in seed size, seed dormancy characteristics, and a special structure that maintains the reproduction and spread of the population (Venable and Brown, 1988; Luzuriaga et al., 2006). These seed traits are mainly determined by the seed genotype and parental environment (Schmitt et al., 1992). At the same time, parental effects also include the result of the interaction of genotype and maternal environment. The influence of parents on offspring is partly determined by genes; therefore, they are evolvable (Lacey, 1998). In the present study, seed perimeter, seed thickness, and 100-seed weight all had high R1 values in a single year, whereas hilum length/hilum width and seed thickness had high R2 values across years. The relatively high genotypic variation in these traits indicated potential genetic variation among the 15 S. moorcroftiana accessions that could be used for selection and breeding (Dong et al., 2019). Furthermore, these seed trait variation reflects the result of genetic variation and phenotypic plasticity in response to environmental variation (Wang et al., 2023). This information helps to understand the response mechanism and variation rules of plants to the environment, which is important for the collection, preservation and evaluation of germplasm resources.
The size and weight of seeds produced by different plant species vary widely. A previous study found that seed size showed different characteristics during the growth and development of plant offspring (Moles et al., 2005). Small-seeded plant species can produce more seeds than large-seeded plant species for a given amount of energy. However, seedlings of large-seeded plant species are more resilient to biotic and abiotic stresses during their establishment. Small-seeded plant species adopt another strategy for winning by quantity: producing as many offspring as possible to ensure their survival. This suggests that traits such as seed size and weight of different species grown in a specific environment can have a major impact on seedling establishment and survival (Dong et al., 2016). Therefore, information on the genetic variation in key seed-size traits in breeding materials will facilitate the execution of various developmental programs (Odiaka, 2005). In this study, the ranges of seed perimeter, seed thickness, and 100-seed weight reflecting seed size and quality were 13.626–15.262 mm, 3.305–3.711 mm, and 0.553–0.900 g in 2014 and 14.427–15.340 mm, 3.561–3.846 mm, and 0.548–0.995 g in 2019, respectively; the R1 of these traits was higher than 0.8, and the R2 of seed thickness and 100-seed weight were higher than 0.6. This suggests that these traits are mainly affected by the genotype and can provide valuable information for S. moorcroftiana breeding. In addition, seed thickness, length, width, and perimeter, and 100-seed weight showed extremely significant positive correlations between the two years, indicating that changes in any trait may significantly affect seed weight. This result indicated that these traits were mainly determined by seed genotype and that changes in either trait could significantly affect seed weight. This positive correlation has important commercial and practical implications for breeding programs (Amiri et al., 2010; Dong et al., 2019). At the same time, variability in seed size affects seed dispersal in a variety of ways. Because smaller seeds are usually dispersed further by abiotic factors such as water and wind. This is closely related to the external environment such as the altitude, slope, temperature and rainfall of the population (Liao et al., 2020; Yang et al., 2021). This was also supported by the correlations between seed traits and altitude and temperature in this study. This phenomenon has important implications for individual reproductive success, community structure, and biodiversity patterns of plants (Snell et al., 2019).
In previous studies, pattern analysis has been successfully used to analyze nine environmental and nine genotype trait data matrices (Jahufer et al., 1997; Zhang et al., 2006; Luo et al., 2016). Jahufer et al. (1997) used pattern analysis to analyze 439 white clover germplasm resources and screened out germplasm populations characterized by large leaves, tall plants, and thick stolons, which could be used to develop varieties that can tolerate summer drought stress environments. Dong et al. (2019) analyzed the genotypic and phenotypic variation of 18 traits of 418 common vetch germplasms based on pattern analysis and obtained germplasm populations with low shattering rates, high seed yields, and high plant dry weights, which can be used for common vetch breeding programs with high seed yield and high dry plant weight. Similarly, in this study, we obtained germplasm populations with higher seed sizes and 100-seed weights using pattern analysis. These accessions could be used in S. moorcroftiana breeding programs with high seedling establishment success rates to adapt to the harsh natural conditions of the Qinghai-Tibet Plateau.
Seed size, shape, and epidermal surface characteristics of plants play important roles in plant morphological diversity, and these seed morphological characteristics can provide data for taxa at different taxonomic levels (Ocampo et al., 2014). In addition, the seed characteristics of plants are different from their floral features, which are generally considered to be more conserved and thus can provide valuable information on the evolutionary history of flowering plants (Barthlott, 1981). Becquer et al. (2014) studied seed shape and size, raphe shape and size, and seed coat surface morphology data of 47 Compositae species from the Antilles, providing information for phylogenetic reconstruction and trait evolution analysis. In this study, the genotypic variation in different seed traits of each accession was significantly different (P < 0.05), which may help to investigate their taxonomic relationships. Analysis of the seed morphological characteristics of S. moorcroftiana showed that the JC, JD, SR, and BL accessions were significantly different (P < 0.05) from the GG, RB, NML, XTM, NM, DG, and SJ accessions, which could be divided into two groups. Our study shows that these heterogeneous seed traits can provide valuable information on the evolutionary relationship of S. moorcroftiana, and the seed morphology database has the potential for taxonomic screening (Dell'Aquila, 2007).
Nondestructive studies based on the plant seed characterization have proven to be an informative, noninvasive, and suitable tool for differentiating germplasm resources (Sinkovič et al., 2019). The results obtained in this study are serving as the useful information on genetic diversity, plant classification and breeding of S. moorcroftiana accessions, which could be used for future research on the evolution, classification and population restoration of S. moorcroftiana.
5 Conclusion
This study estimated the phenotypic correlation, genotypic variation, and mean repeatability of nine seed traits in 15 S. moorcroftiana accessions. Seed perimeter, seed thickness, and 100-seed weight showed high mean repeatability over two years (2014 and 2019), indicating their potential for genetic improvement. Pattern analysis showed that the 100-seed weight was significantly and positively correlated with seed perimeter, length, width, and thickness. The significant correlation between these traits provides key information for S. moorcroftiana breeding programs that focus on developing varieties with high seedling establishment success rates. This study not only deepens our understanding of the genetic diversity of S. moorcroftiana seed morphological traits but also provides important information for the development of breeding banks.
Data availability statement
The original contributions presented in the study are included in the article/Supplementary Material. Further inquiries can be directed to the corresponding author.
Author contributions
RD and HL conceived the experiment. QG and JL collected the seeds. WZ and CL carried out the experiment. RD and QG analyzed the data. RD and HL wrote the paper. All the authors contributed to the manuscript and approved the submitted version. All authors contributed to the article and approved the submitted version.
Funding
This research was supported by the National Natural Science Foundation of China (31960320 and 32060392) and the Guizhou Province Science and Technology Projects (Qian Ke He Zhi Cheng [2020]1Y074), Qian Ke He Cheng Guo ([2022] Zhong Dian 005), and the GZMARS-Forage Industry Technology System of Guizhou Province.
Acknowledgments
We thank Baocheng Jin and Jinping Zhang (Guizhou University, China) for their valuable help and advice on the GS figures.
Conflict of interest
The authors declare that the research was conducted in the absence of any commercial or financial relationships that could be construed as a potential conflict of interest.
Publisher’s note
All claims expressed in this article are solely those of the authors and do not necessarily represent those of their affiliated organizations, or those of the publisher, the editors and the reviewers. Any product that may be evaluated in this article, or claim that may be made by its manufacturer, is not guaranteed or endorsed by the publisher.
Supplementary material
The Supplementary Material for this article can be found online at: https://www.frontiersin.org/articles/10.3389/fpls.2023.1185393/full#supplementary-material
References
Amiri, R., Vahdati, K., Mohsenipoor, S., Mozaffari, M. R., Leslie, C. (2010). Correlations between some horticultural traits in walnut. HortScience 45, 1690–1694. doi: 10.21273/HORTSCI.45.11.1690
Bacchetta, G., Grillo, O., Mattana, E., Venora, G. (2008). Morpho-colorimetric characterisation by image analysis to identify diaspores of wild plant species. Flora. 203, 669–682. doi: 10.1016/j.flora.2007.11.00
Barthlott, W. (1981). Epidermal and seed surface characters of plants: systematic applicability and some evolutionary aspects. Nord. J. Bot. 1, 345–355. doi: 10.1111/j.1756-1051.1981.tb00704.x
Becquer, E. R., Michelangeli, F. A., Borsch, T. (2014). Comparative seed morphology of the antillean genus Calycogonium (Melastomataceae: miconieae) as a source of characters to untangle its complex taxonomy. Phytotaxa 166, 241–258. doi: 10.11646/phytotaxa.166.4.1
Cervantes, E., Martín, J. J., Saadaoui, E. (2016). Updated methods for seed shape analysis. Scientifica 2016, 5691825. doi: 10.1155/2016/5691825
Chen, F., Zhang, J., Liu, J., Cao, X., Hou, J., Zhu, L., et al. (2020). Climate change, vegetation history, and landscape responses on the Tibetan plateau during the Holocene: a comprehensive review. Quat. Sci. Rev. 243, 106444. doi: 10.1016/j.quascirev.2020.106444
Cochrane, A., Yates, C. J., Hoyle, G. L., Nicotra, A. B. (2015). Will among-population variation in seed traits improve the chance of species persistence under climate change? Glob. Ecol. Biogeogr. 24, 12–24. doi: 10.1111/geb.12234
Dell'Aquila, A. (2007). Towards new computer imaging techniques applied to seed quality testing and sorting. Seed Sci. Technol. 35, 519–538. doi: 10.15258/sst.2007.35.3.01
Deng, T., Wu, F., Zhou, Z., Su, T. (2020). Tibetan Plateau: an evolutionary junction for the history of modern biodiversity. Sci. China Earth Sci. 63, 172–187. doi: 10.1007/s11430-019-9507-5
Dong, R., Jahufer, M. Z. Z., Dong, D. K., Wang, Y. R., Liu, Z. P. (2016). Characterisation of the morphological variation for seed traits among 537 germplasm accessions of common vetch (Vicia sativa l.) using digital image analysis. New Z. J. Agric. Res. 59, 422–435. doi: 10.1080/00288233.2016.1229682
Dong, S., Shang, Z., Gao, J., Boone, R. B. (2020). Enhancing sustainability of grassland ecosystems through ecological restoration and grazing management in an era of climate change on qinghai-Tibetan plateau. Agric. Ecosyst. Environ. 287, 106684. doi: 10.1016/j.agee.2019.106684
Dong, R., Shen, S. H., Jahufer, M. Z., Dong, D. K., Luo, D., Zhou, Q., et al. (2019). Effect of genotype and environment on agronomical characters of common vetch (Vicia sativa l.). Genet. Resour. Crop Evol. 66, 1587–1599. doi: 10.1007/s10722-019-00789-3
Grillo, O., Mattana, E., Venora, G., Bacchetta, G. (2010). Statistical seed classifiers of 10 plant families representative of the Mediterranean vascular flora. Seed Sci. Technol. 38, 455–476. doi: 10.15258/sst.2010.38.2.19
Jahufer, M. Z. Z., Cooper, M., Harch, B. D. (1997). Pattern analysis of the diversity of morphological plant attributes and herbage yield in a world collection of white clover (Trifolium repens l.) germplasm characterised in a summer moisture stress environment of Australia. Genet. Resour. Crop Evol. 44, 289–300. doi: 10.1023/A:1008692629734
Khamassi, K., Babay, E., Rouissi, M., Dakhlaoui, A., Ben Ayed, R., Hanana, M. (2021). Genetic variability of Tunisian faba beans (Vicia faba l.) based on seeds’ morphophysical properties as assessed by statistical analysis. J. Food Qual. 2021, 1–10. doi: 10.1155/2021/9493607
Lacey, E. P. (1998). “What is an adaptive environmentally induced parental effect?,” in Maternal effects as adaptations. Eds. Mousseau, T. A., Fox, C. W. (New York: Oxford Univ. Press), 54–66.
Lamont, B., Groom, P. (2013). Seeds as a source of carbon, nitrogen, and phosphorus for seedling establishment in temperate regions: a synthesis. AJPS. 4, 30–40. doi: 10.4236/ajps.2013.45A005
Li, H., Yao, W., Fu, Y., Li, S., Guo, Q. (2015). De novo assembly and discovery of genes that are involved in drought tolerance in Tibetan sophora moorcroftiana. PloS One 10, e111054. doi: 10.1371/journal.pone.0111054
Li, H., Zhang, Y., Guo, Q., Yao, W. (2017). Molecular characterisation of a DREB gene from sophora moorcroftiana, an endemic species of plateau. Protoplasma 254, 1735–1741. doi: 10.1007/s00709-016-1065-9
Liao, C., Li, H., Lv, G., Tian, M., Tian, J., Shi, H., et al (2020) Stability and micro-topography effects of sophora moorcroftiana community for fixation of sandy land under natural restoration, southern Tibetan plateau. Authorea. doi: 10.22541/au.158679907.75173603
Liu, Y., Yi, F., Yang, G., Wang, Y., Pubu, C., He, R., et al. (2020). Geographic population genetic structure and diversity of sophora moorcroftiana based on genotyping-by-sequencing (GBS). PeerJ 8, e9609. doi: 10.7717/peerj.9609
Luo, K., Jahufer, M. Z. Z., Wu, F., Di, H. Y., Zhang, D. Y., Meng, X. C., et al. (2016). Genotypic variation in a breeding population of yellow sweet clover (Melilotus officinalis). Front. Plant Sci. 7. doi: 10.3389/fpls.2016.00972
Luo, Y., Zhang, G., Liu, X., Yuan, M., Gao, Q., Gao, H., et al. (2018). Therapeutic and immunoregulatory effects of water-soluble alkaloids E2-a from sophora moorcroftiana seeds as a novel potential agent against echinococcosis in experimentally protoscolex-infected mice. Vet. Res. 49, 1–13. doi: 10.1186/s13567-018-0596-9
Luzuriaga, A. L., Escudero, A., Pérez-García, F. (2006). Environmental maternal effects on seed morphology and germination in sinapis arvensis (Cruciferae). Weed Res. 46, 163–174. doi: 10.1111/j.1365-3180.2006.00496.x
Moles, A. T., Ackerly, D. D., Webb, C. O., Tweddle, J. C., Dickie, J. B., Westoby, M. (2005). A brief history of seed size. Sci. 307, 576–580. doi: 10.2307/3840005
Niklas, K. J., Leck, M. A., Parker, V. T., Simpson, R. L. (2008). Embryo morphology and seedling evolution. seedling ecology and evolution. Eds. Leck, M. A., Parker, V. T., Simpson, R. L. (Cambridge: Cambridge University Press), 103–129.
Ocampo, G., Michelangeli, F. A., Almeda, F. (2014). Seed diversity in the tribe miconieae (Melastomataceae): taxonomic, systematic, and evolutionary implications. PloS One 9, e100561. doi: 10.1371/journal.pone.0100561
Odiaka, N. (2005). Morphological diversity among local germplazm of fluted pumpkin collected in makurdi, Nigeria. J. Food Agr Environ. 3, 199–204.
Pinna, M. S., Grillo, O., Mattana, E., Cañadas, E. M., Bacchetta, G. (2014). Inter-and intraspecific morphometric variability in Juniperus l. seeds (Cupressaceae). System. Biodivers. 12, 211–223. doi: 10.1080/14772000.2014.904827
Saatkamp, A., Cochrane, A., Commander, L., Guja, L. K., Jimenez-Alfaro, B., Larson, J., et al. (2019). A research agenda for seed-trait functional ecology. New Phytol. 221, 1764–1775. doi: 10.1111/nph.15502
Schmitt, J., Niles, J., Wulff, R. D. (1992). Norms of reaction of seed traits to maternal environments in plantago lanceolata. Am. Nat. 139, 451–466. doi: 10.1086/285338
Shi, W., Qiao, F., Zhou, L. (2021). Identification of ecological risk zoning on qinghai-tibet plateau from the perspective of ecosystem service supply and demand. Sustainability 13, 5366–466. doi: 10.3390/su13105366
Sinkovič, L., Pipan, B., Sinkovič, E., Meglič, V. (2019). Morphological seed characterization of common (Phaseolus vulgaris l.) and runner (Phaseolus coccineus l.) bean germplasm: a Slovenian gene bank example. BioMed. Res. Int. 1-13, 6376948. doi: 10.1155/2019/6376948
Snell, R. S., Beckman, N. G., Fricke, E., Loiselle, B. A., Carvalho, C. S., Jones, L. R., et al. (2019). Consequences of intraspecific variation in seed dispersal for plant demography, communities, evolution and global change. AoB Plants 11, plz016. doi: 10.1093/aobpla/plz016
Tao, R., Xu, C., Wang, Y., Sun, X., Li, C., Ma, J., et al (2020). Spatiotemporal differentiation of alpine butterfly Parnassius glacialis (Papilionidae: Parnassiinae) in China: Evidence from mitochondrial DNA and nuclear single nucleotide polymorphisms. Genes 11, 188. doi: 10.3390/genes11020188
Venable, D. (2007). Bet hedging in a guild of desert annuals. Ecology 88, 1086–1090. doi: 10.1890/06-1495
Venable, D. L., Brown, J. S. (1988). The selective interactions of dispersal, dormancy, and seed size as adaptations for reducing risk in variable environments. Am. Nat. 131, 360–383. doi: 10.1086/284795
Venora, G., Grillo, O., Shahin, M. A., Symons, S. J. (2007). Identification of Sicilian landraces and Canadian cultivars of lentil using an image analysis system. Food Res. Int. 40, 161–166. doi: 10.1016/j.foodres.2006.09.001
Wang, C., Gong, H., Feng, M., Tian, C. (2023). Phenotypic variation in leaf, fruit and seed traits in natural populations of Eucommia ulmoides, a relict chinese endemic tree. Forests 14, 462. doi: 10.3390/f14030462
Wang, S. Y., Sun, Z. L., Liu, T., Gibbons, S., Zhang, W. J., Qing, M. (2014). Flavonoids from sophora moorcroftiana and their synergistic antibacterial effects on MRSA. Phytother. Res. 28, 1071–1076. doi: 10.1002/ptr.5098
White, T. L., Hodge, G. R. (1989). Predicting breeding values with applications in forest tree improvement (Boston: Springer science business media).
Xin, F., Liu, J., Chang, C., Wang, Y., Jia, L. (2021). Evaluating the influence of climate change on sophora moorcroftiana (Benth.) baker habitat distribution on the Tibetan plateau using maximum entropy model. Forests 12, 1230. doi: 10.3390/f12091230
Yang, L., Li, H., Li, Q., Guo, Q., Li, J. (2021). Genetic diversity analysis and potential distribution prediction of Sophora moorcroftiana endemic to qinghai–Tibet plateau, China. Forests 12, 1106. doi: 10.3390/f12081106
Zhang, Y., He, Z., Zhang, A., van Ginkel, M., Peña, R. J., Ye, G. (2006). Pattern analysis on protein properties of Chinese and CIMMYT spring wheat cultivars sown in China and CIMMYT. Aust. J. Agr. Res. 57, 811–822. doi: 10.1071/AR05372
Keywords: Sophora moorcroftiana, seed traits, genotypic variation, image analysis, digital technologies
Citation: Dong R, Guo Q, Li H, Li J, Zuo W and Long C (2023) Estimation of morphological variation in seed traits of Sophora moorcroftiana using digital image analysis. Front. Plant Sci. 14:1185393. doi: 10.3389/fpls.2023.1185393
Received: 13 March 2023; Accepted: 02 May 2023;
Published: 29 May 2023.
Edited by:
Philipp Von Gillhaussen, Interantional Plant Phenotyping Network (IPPN), GermanyReviewed by:
Fiona R. Hay, Aarhus University, DenmarkRupesh Tayade, Kyungpook National University, Republic of Korea
Copyright © 2023 Dong, Guo, Li, Li, Zuo and Long. This is an open-access article distributed under the terms of the Creative Commons Attribution License (CC BY). The use, distribution or reproduction in other forums is permitted, provided the original author(s) and the copyright owner(s) are credited and that the original publication in this journal is cited, in accordance with accepted academic practice. No use, distribution or reproduction is permitted which does not comply with these terms.
*Correspondence: Huie Li, bGlodWllc2hAMTI2LmNvbQ==