- 1School of Pharmacy, Anhui University of Chinese Medicine, Hefei, China
- 2State Key Laboratory for Quality Ensurance and Sustainable Use of Dao-di Herbs, National Resource Center for Chinese Materia Medica, China Academy of Chinese Medical Sciences, Beijing, China
- 3Key Scientific Research Base of Traditional Chinese Medicine Heritage (Institute of Chinese Materia Medica, China Academy of Chinese Medical Sciences), State Administration of Cultural Heritage, Beijing, China
- 4Department of Traditional Chinese Medicine, Anhui Province Key Laboratory of Research and Development of Chinese Medicine, Hefei, China
- 5National Resource Center for Chinese Materia Medica, China Academy of Chinese Medical Sciences, Beijing, China
The genus Peucedanum L. (Apiaceae) is a large group comprising more than 120 species distributed worldwide. Many plants of the genus Peucedanum have been studied and used in traditional Chinese medicine. In 2020, a new species, Peucedanum huangshanense Lu Q. Huang, H. S. Peng & S. S. Chu, was found in the Huangshan Mountains of Anhui Province, China. However, little is known about its medicinal properties. Thus, the objective of this study is to explore the potential medicinal value of P. huangshanense and its relationship with other Peucedanum species. Through textual research on illustrations of Qianhu in Bencao literature, it can be inferred that at least five species of genus Peucedanum have been used in Chinese medicine. Therefore, we chose these five species of Peucedanum and P. huangshanense together for subsequent research. We conducted morphological, chloroplast genome, and chemical analyses of six Peucedanum species, including the newly discovered P. huangshanense. The chloroplast genomes of Peucedanum showed a typical tetrad structure, and the gene structure and content were similar and conservative. There were significant differences in genome size and the expansion of the inverted repeat boundary. Through nucleotide polymorphism analysis, we screened 14 hotspot mutation regions that have the potential to be used as specific molecular markers for the taxonomy of Peucedanum. Our results showed an inversion of the trnD-trnY-trnE gene in the P. huangshanense chloroplast genome, which can be developed as a specific molecular marker for species identification. Phylogenetic analysis showed that the phylogenetic trees had high support and resolution, which strongly supports the view that Peucedanum is not a monophyletic group. P. huangshanense had the closest genetic relationship to P. ampliatum K. T. Fu, followed by P. harry-smithii Fedde ex Wolff. Furthermore, the main coumarins of P. huangshanense were most similar to those of P. japonicum Thunb. and P. harry-smithii. In summary, our research lays a foundation for the systematic classification of Peucedanum and sheds light on the medicinal value of P. huangshanense.
1 Introduction
The genus Peucedanum L. (Apiaceae) has more than 120 species and grows in Europe, Asia, and Africa. Most species of the genus Peucedanum have important medicinal value attributed to coumarin, flavonoids, phenolic compounds, and essential fatty acids (Sarkhail, 2014). For instance, the leaf and rhizome of P. ostruthium (L.) W. D. J. Koch were developed to treat Alzheimer’s disease and dermatosis in central and southern Europe (Palmioli et al., 2019; Danna et al., 2022). In addition, P. chenur Have is an endemic species of Kurdistan in western Iran, aerial parts of P. chenur has inhibitory effect on human colon cancer cells (Yadegari et al., 2020). Moreover, the volatile oils in the fruits of P. dhana A. Ham have anti-inflammatory and antioxidant activities as well as cytotoxic effects on cancer cells; they are used to treat colon adenocarcinoma in Thailand (Khruengsai et al., 2021).
Peucedani Radix (Qianhu in Chinese) is a famous Chinese medicine and has a long history of medicinal use in China. Qianhu was first recorded in Ming Yi Bie Lu over 1500 years ago. Qianhu has the effect of down bearing Qi, resolving phlegm, dispersing wind, and clearing heat. It is commonly used to treat phlegm-heat cough, asthma, and wind-heat cough with sputum in China (Committee for the Pharmacopoeia of PR China, 2020). Qianhu also has medicinal records in Korea and Japan (Kanazawa et al., 2022). Qianhu is derived from the roots of P. praeruptorum Dunn and generally using the root of vegetative growth stage (not the bolting stage) (The State Pharmacopoeia Committee of China, 2020). Many species of Peucedanum are mixed with P. praeruptorum because of their similar characteristics. For example, the roots of P. turgeniifolium Wolff, P. medicum Dunn, P. cavaleriei H. Wolff (Ligusticopsis brachyloba), and P. harry-smithii var. subglabrum Shan et Sheh are still regarded as medicinal Qianhu in the Sichuan, Yunnan, Gansu Provinces. Therefore, it is particularly important to identify the characteristics of reproductive organs such as flowers and fruits. P. praeruptorum is rich in active ingredients, such as Praeruptorin A and Praeruptorin B, which are characteristic components of genuine Qianhu (Lee et al., 2015). These chemical components differ from those found in other species of the genus Peucedanum. It is a controversial issue to use the roots of other species of Peucedanum as substitutes for Qianhu. Therefore, it is important to accurately identify and differentiate common adulterants derived from the Peucedanum genus to ensure the safety of clinical medication.
Since the Fourth Chinese Materia Medica Resources Survey was conducted in China, more than 163 new species have been discovered. The chemical characteristics and potential medicinal value of these new species are of concern. A study of 79 new species found in the census in the “Discovery and efficacy study of new resources” showed that 60% of the new species had potential medicinal value. In 2020, P. huangshanense Lu Q. Huang, H. S. Peng & S. S. Chu, a new species of the genus Peucedanum, was discovered in Huangshan, Anhui Province, China, where the species grows on the forest margins and cliffs (elev.ca. 1600 m). Detailed morphological comparisons have shown that it is similar to P. praeruptorum but has larger compound umbels (5–14 cm across), rays up to 25, and long-ovoid mericarps with lateral, narrowly winged ribs (Chu et al., 2020). To explore the phylogenetic position of this species, the nuclear ribosomal DNA internal transcribed spacer (ITS) region was sequenced for P. huangshanense. Morphological and molecular evidence supports the hypothesis that P. huangshanense is a new distinct species (Chu et al., 2020). Additionally, the root of P. huangshanense is similar to that of P. praeruptorum, whether it has the similar effect is worth studying.
Since ancient times, many herbs have been regarded as the original plants of Qianhu and have been studied for their medicinal properties (Song et al., 2015). Additionally, the roots of some congeneric species of P. praeruptorum are still regarded as substitutes for Qianhu in clinical practice because of their similar chemical composition. Thus, further study of the similarities between P. huangshanense and these alternative species is warranted for the development of P. huangshanense. Forty species belong to the genus Peucedanum in China. The genus is extremely heterogeneous and exhibits great diversity in life-forms, leaf and fruit structures, and chemical constituents (Wen et al., 2021). A previous study first attempted to comprehensively investigate the plastome features and infer phylogeny using plastome data from the Peucedanum genus (Liu et al., 2022). The results of Liu’s research conclusively demonstrate the efficacy and power of plastome data in enhancing support and resolution within the phylogeny of Peucedanum. In the present study, we investigated the herbal drawings of Qianhu recorded in ancient and modern herbal books and records. Subsequently, we collected six medicinal species used as Qianhu for complete chloroplast genome and internal transcribed spacer (ITS) analysis. In addition, the contents of ten coumarins from these Peucedanum species were determined. Combining genetic characteristics with chemical information could speculate on the medicinal value of P. huangshanense. The study will promote the discovery of new medicinal resources, and is of great significance to the sustainable development of traditional Chinese medicine resources.
2 Materials and methods
2.1 Plant materials
We collected 33 accessions of genus Peucedanum from Anhui, Fujian, Hunan and Shanxi provinces, China, including P. huangshanense, P. praeruptorum, P. japonicum Thunb, P. medicum, P. wawrae (Wolff) Su and P. harry-smithii Fedde ex Wolff. Voucher information of the samples are shown in Supplementary Table 1. The morphology of six species is shown in Supplementary Figure 1. The fresh and healthy leaves were collected for DNA extraction, and the new chloroplast genomes generated in this paper were deposited in GenBank under accession numbers: OQ473752–OQ473757. The underground parts of samples were dried and ground into powders (50 mesh). Then powders were stored under dry conditions at room temperature before analysis.
2.2 DNA extraction
Total DNA was extracted from silica-dried leaves material. Genomic DNA was extracted using the Plant Genomic DNA Kit (Tiangen Biotech, Beijing, China) according to the manufacturer’s protocol. Then, 1% (w/v) agarose gel electrophoresis was used to test DNA integrity, and concentrations were determined using a NanoPhotometer® spectrophotometer (Implen, München, Germany). The extracted DNA was stored in the refrigerator at -20°C.
2.3 ITS amplification, sequencing and data analysis
The ITS sequence was chosen in this study, since it is one of the universal DNA barcode markers for land plants. PCR amplifications of ITS were performed using universal primer 5F (5’-GGAAGTAAAAGTCGTAACAAGG-3’) and 4R (5’-TCCTCCGCTTATTGATATGC-3’) (Zhou et al., 2014). The reaction mixture was as follows: total volume 20μL, including 2X M5 HiPer plus Taq HiFi PCR mix (with blue dye) (Mei5bio, Beijing, China) 10μL, Nuclease-free ddH2O 8μL, DNA template 1μL, forward primer 0.5μL and reverse primer 0.5μL. PCR program: pre-denatured at 95°C, denatured at 94°C, denatured at 10s, annealed at 55°C, extended at 72°C for 20 s, cycle 35 times, extended at 72°C for 5 min. The amplified PCR products were checked by 1% agarose gel electrophoresis to detect whether the bands were bright and single and whether the fragment size was correct. The successfully amplified PCR with bright target band were purified and sequenced at General Biology (Chuzhou, China).
ITS sequences were assembled using SeqMan v7 (Swindell and Plastere, 1997), and then aligned and manually refined in BioEdit v7.2 (Elkins, 2013). The genetic distance was conducted using the Kimura 2-parameter model (Kimura, 1980). This analysis involved 17 nucleotide sequences of Peucedanum. Codon positions included were 1st+2nd+3rd+Noncoding. The number of base substitutions per site from between sequences are shown. ModelFinder (Kalyaanamoorthy et al., 2017) was used to select the best-fit model using BIC criterion. Maximum likelihood (ML) phylogenetic inference was performed using IQ-TREE v1.6.8 (Nguyen et al., 2015) with 1000 bootstrap replicates based on the TIM2e+G4 nucleotide substitution model to assess branch support. Bayesian inference (BI) phylogenies were inferred using MrBayes v3.2.6 (Ronquist et al., 2012) under GTR+I+G+F model (2 parallel runs, 2,000,000 generations), in which the initial 25% of sampled data were discarded as burn-in.
2.4 Chloroplast genomes sequencing, assembly and annotation
Genomic DNA was fragmented into 350 bp to construct the pair-end library, and then sequenced using the Illumina NovaSeq platform at Novogene (Tianjin, China). Raw data was filtered using fastP v0.15.0 (-n 10 and -q 15) to obtain high quality reads (Chen et al., 2018). Then clean data was used to assemble the whole chloroplast genome with GetOrganelle (Jin et al, 2020) with the K-values parameter of 21, 55, 85, and 115. The chloroplast genome of P. praeruptorum (NC_060841.1) downloaded from the National Center for Biotechnology Information (NCBI) was used as reference. The assembled genome was annotated using CPGAVAS2 (http://47.96.249.172:16019/analyzer/home ) (Shi et al, 2019), and drew a circular physical map using OGDRAW (https://chlorobox.mpimp-golm.mpg.de/OGDraw.html) (Greiner et al., 2019).
2.5 Comparison of the chloroplast genomes and phylogenetic analysis
We compared the boundaries of the LSC, SSC and IR regions among the chloroplast genomes of genus Peucedanum in IRscope (Amiryousefi et al., 2018). We used MIcroSAtellite identification tool (MISA) (Mudunuri and Nagarajaram, 2007) to visualize Simple Sequence Repeat (SSRs). The parameter of MISA was set as follows: ten repeat units for mononucleotide repeat SSRs, five repeat units for dinucleotide repeat SSRs, four repeat units for trinucleotide repeat SSRs, and three repeat units for tetra-, penta-, and hexanucleotide repeat SSRs. The max length of sequence between two SSRs, registered as compound SSR, was 100 bp. The DNA rearrangements among chloroplast genomes were detected using Mauve v2.4.0 (Darling et al., 2004). Sequence divergence were investigated using the mVISTA (Frazer et al., 2004) with P. ampliatum (OK336475.1) as reference. The nucleotide diversity was calculated based on the sliding window using the DnaSP v5.10 (Rozas et al, 2017).
Forty-eight chloroplast genomes were used for phylogenetic analysis in this study, including 13 samples of 21 species in genus Peucedanum and other 27 species from Apiaceae. Cicutavirosa L. (KX352466) and Cryptotaenia japonica Hassk. (MK629764) were used as outgroup. All chloroplast genomes were aligned using MAFFT v7.313 (Katoh and Standley, 2013). The ambiguous alignment regions were trimmed using trimAl tool of PhyloSuite (Zhang et al., 2020). The phylogenetic analyses were performed using Maximum likelihoods (ML) and Bayesian inference (BI) methods based on whole chloroplast genomes. The optimal model GTR+F+I+G4 was calculated by Modelfinder. ML analyses were performed by IQ-tree v1.6.8, and the sampling was repeated 1000 times. BI analyses were performed by MrBayes v3.2.8. The result was visualized with FigTree v1.4.4 (http://tree.bio.ed.ac.uk/software/figtree/).
2.6 High-performance liquid chromatography analysis
The underground part of samples was washed, dried, ground into powders (50 mesh) and stored under dry conditions at room temperature until analysis. Standard compounds (purity≥98%) of nodakenin, peucedanol, umbelliferone, psoralen, xanthotoxin, bergapten, imperatorin, praeruptorin A, praeruptorin B, praeruptorin E were purchased from Chengdu Lemeitian Pharmaceutical Technology Co., Ltd. (Chengdu, China). Ultrapure water was prepared using a Direct-Pure Water System (RephiLe, Shanghai, China). HPLC-grade acetonitrile was supplied by Oceanpak (Gothenburg, Sweden). Other reagents were analytical grade.
The standard compounds were weighed and dissolved in pure methanol, and make the concentration of each standard solution approximately 1.0 mg/mL. Then, in order to construct the calibration curves, each standard solution was diluted to gradient concentrations with methanol. The powdered sample (0.2 g) was mixed with 10mL methanol, subjected to ultrasonic (40 kHz, 200 W) treatment for 30 min, and allowed to cool, the methanol was added to compensate for the lost weight. The mixture was filtered and the filtrate was collected as the solution to be tested. Then, all solutions were filtered through a 0.45 μm millipore filter and stored at 4°C until analysis (Chu et al., 2020).
An Agilent 1260 Infinity II Diode Array Detector WR (Agilent Technologies Inc., Santa Clara, United States) equipped with an Agilent 5 HC-C18 column (4.6 mm × 250 mm, 5 µm) was used for High-performance liquid chromatography (HPLC) analysis. Chromatographic separation was conducted in a flow rate of 1.0 mL/min at 28°C with a mixture of acetonitrile (A) and water (B) as the mobile phase. The gradient elution condition was optimized as follows: 0–10 min, 20%–40% A; 10–12 min, 40%–45% A; 12–17 min, 45%–65% A; 17–40 min, 65% A; 40–43 min, 65%–20% A; 43–50 min, 20% A. The injection volume was 20 μL. The detection wavelength was 297 nm for 1–23 min, and 321 nm for 23–50 min (Chen et al., 2019; Chu et al., 2020). TBtools software (Chen et al., 2020) was used to generate a heatmap based on a hierarchical clustering analysis.
3 Results
3.1 Textual research on illustrations of Qianhu in the Materia Medica
Qianhu was first recorded by Ming Yi Bie Lu (approximately 500 A.D.), thus having a medicinal history of more than 1500 years. We reviewed the records of Qianhu in the Materia Medica, focusing on its medicinal plants. There are many narrative descriptions of Qianhu, but only a few illustrations of Qianhu are present. The method of combining pictures and text provides more accurate evidence for exploring the origin of Qianhu.
Ben Cao Tu Jing (1061) of the Song Dynasty described the morphology of the original Qianhu plant, documenting five illustrations from different locations (Supplementary Figure 2). The roots of Zizhou Qianhu are conical and branched, with a multistrip erect stem, alternate and bipinnate compound leaves, and small leaves. During the Song Dynasty, the jurisdiction of Zizhou included Zichuan, Zouping, Gaoqing County, and Zibo City in Shandong Province, where three species of Peucedanum are found. The original Zizhou Qianhu plants were similar to P. wawrae. Jianghzou Qianhu has a stout conical root with residual petiole fibers, solitary stem branches, alternate leaves, bipinnate compound leaves, and compound umbellate inflorescence. The areas under Jiangzhou’s jurisdiction during the Song Dynasty included Xinjiang, Quwo, Xiangfen, Yicheng, Jishan, Jiangxian, and Yuanqu counties in Shanxi Province, where P. harry-smithii is distributed and has morphological characteristics consistent with that of “Jiangzhou Qianhu.” Jiangzhou Qianhu has conical and branched roots, a blade that is oblong-ovate to broadly triangular-ovate, 2–3-ternate-pinnate leaves, and compound umbellate inflorescences. This plant is not a Peucedanum but should belong to the Apiaceae family, specifically Ostericum citriodorum (Hance) Yuan et Shan. Qianhu in Chengzhou has a thick main root, an erect stem, a stem base with residual petiole fibers, alternate and broad leaves, three compound leaves, and a compound umbellate inflorescence terminal. Undoubtedly, it belongs to Apiaceae, but it is not a plant of the genus Peucedanum, according to its leaf shape. The root of Qianhu in the Jiangning Prefecture is conical, with a striped single leaf without a petiole, and a stem base covered with petiole residual fibers. The morphology is similar to that of Chaihu in Jiangning Prefecture, so the picture should be of the Bupleurum L. plant.
The root of Qianhu recorded by Jiu Huang Ben Cao (1406) of the Ming Dynasty is multibranched, with a single erect stem, alternate leaves, bipinnate compound leaves, parted leaves, and a compound umbellate inflorescence. Based on these morphological characteristics, it is speculated that the original plant may be P. praeruptorum. The picture of “Zizhou Qianhu” by Ben Cao Pin Hui Jing Yao (1505) is similar to the picture of “Zizhou Qianhu” by Ben Cao Tu Jing. Thus, we speculated that this species may be P. wawrae based on its morphological characteristics.
Ben Cao Yuan Shi (1612) drew a diagram of the medicinal herbs of P. praeruptorum. The root is conical with branches, and the end gradually tapers. It is marked with the words “the root is black outside, yellow or white inside,” which is similar to the description of Qianhu by Jiu Huang Ben Cao, so it is speculated that the plant is P. praeruptorum. In the diagram of “Qianhu” painted by Zhi Wu Ming Shi Tu Kao (1848) of the Qing Dynasty, the root is thick and branching, and the end tapers gradually. It has several erect stems, small leaves parted deeply, and a compound umbellate inflorescence, and there is the word “Qianhu” in the painting; thus, it is speculated to be P. praeruptorum. The original plant of “Fangkui” by Qing Dao Zhong Cao Yao Shou Ce is P. japonicum (Figure 1). Therefore, it can be inferred from the literature that at least four species of Peucedanum have been recorded for medicinal applications.
3.2 Phylogenetic analysis based on the ITS sequence
In the present study, we obtained ITS fragments from 18 accessions of the genus Peucedanum. The genetic distance among 17 species of Peucedanum ranged from 0–0.2578 (Supplementary Table 2). The genetic distance between P. huangshanense and other species was large, ranging from 0.0714–0.2578. The genetic distance between P. huangshanense and P. insolens was the greatest, with a value of 0.2578. The genetic distance between P. huangshanense and P. elegans was 0.2302. In addition, the genetic distances between P. huangshanense and P. longshengense, as well as P. huangshanense and P. formosanum Hayata, were the closest, with a value of 0.0714. A total of 44 species from Apiaceae were used for phylogenetic analysis, including Cicutavirosa L. (OL473017) and Cryptotaenia japonica Hassk. (MH711328), which were used as the outgroup. The alignment matrix contained 732 positions and ranged in length from 698 bp to 725 bp. Phylogenetic analysis (Supplementary Figure 3) revealed that the genus Peucedanum was not a monophyletic group because its accessions were mixed with other species. However, the samples of P. huangshanense were clustered into a monophyletic group by approximately 100% bootstrap values, and they had the closest relationship with P. longshengense and P. formosanum.
3.3 Assembly, annotation, and feature analysis of chloroplast genomes
In this study, we assembled six chloroplast genomes from P. huangshanense, P. praeruptorum, P. japonicum, P. medicum, P. wawrae, and P. harry-smithii. Seven other chloroplast genomes of the genus Peucedanum were downloaded from NCBI, including P. longshengense (OK336479), P. harry-smithii var. grande (K. T. Fu) Shan et Sheh (OK336476), P. delavayi Franch. (MT843765), P. angelicoides Wolff ex Kretschm. (OK336474), P. ampliatum K. T. Fu (OK336475), P. mashanense Shan et al. (OK336478), and P. terebinthaceum (MT671397).
These 13chloroplast genomes (142,494–155,552 bp, 37.4–37.7% GC) have a typical quadripartite structure consisting of a large single-copy region (LSC, 85,276–99,934 bp), a small single-copy region (SSC, 17,372–17,658 bp), and a pair of inverted repeat regions (IRa and IRb; 12,594–25,394 bp) (Figure 2). They contained 114 genes, including 80 protein-coding genes, four rRNA genes, and 30 tRNA genes (Table 1; Supplementary Table 3). However, rps19 was missing from P. delavayi, and trnT-GGU was missing from P. praeruptorum and P. harry-smithii.
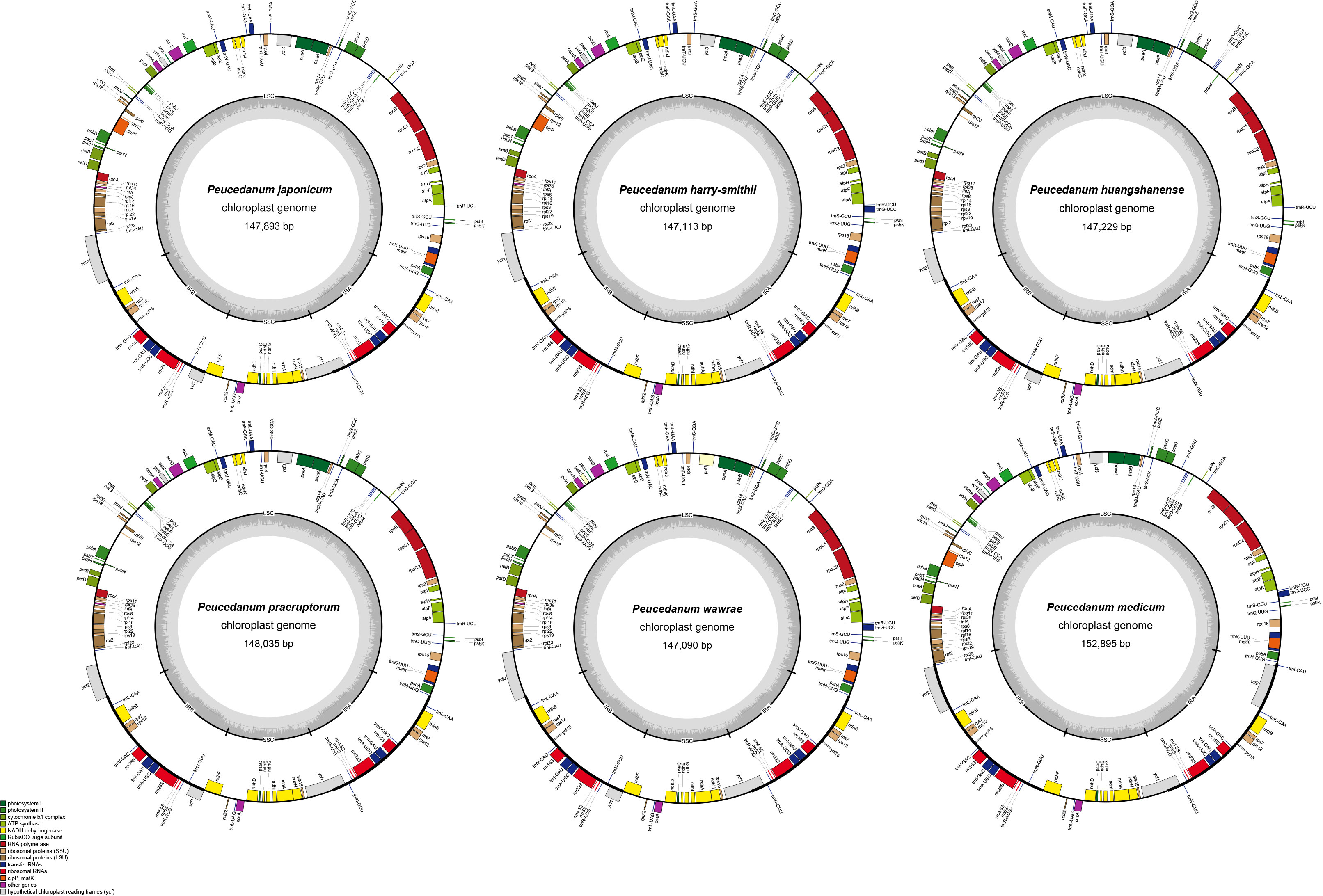
Figure 2 Chloroplast genome maps of Peucedanum. Genes shown outside of the outer layer circle are transcribed clockwise, while those insides are transcribed counterclockwise. The genes belonging to different functional groups are color-coded. The dark gray area of the inner circle denotes the GC content of chloroplast.
3.4 Chloroplast genome comparison analysis
The borders of IRa/SSC, IRb/SSC, and IRb/LSC among the 13 chloroplast genomes were slightly conserved, and the boundaries of IRb/SSC fell within the ycf1 gene. The IRa/SSC junctions of most samples were located between the ycf1 gene and the ndhF gene but expanded into the ndhF gene in P. delavayi, P. angelicoides, P. medicum, and P. harry-smithii. The IRa/LSC borders of most samples were located between genes trnL and trnH, but they expanded into psbA in P. angelicoides. However, the junctions of IRb/LSC in chloroplasts within the genus Peucedanum were divergent and could be classified into four types. The junctions of IRb/LSC in most remaining plants of Peucedanum fell into the ycf2 gene but contracted to the intergenic regions of ycf2-trnL in P. terebinthaceum, belonging to type I. The IRb/LSC borders fell within the rps19 gene in P. delavayi, which belongs to type II. IRb/LSC borders contracted to the intergenic region of trnV-trnH in P. angelicoides (type III) and moved to the intergenic regions of rpl23 in P. mashanense and P. medicum (type IV) (Figure 3).
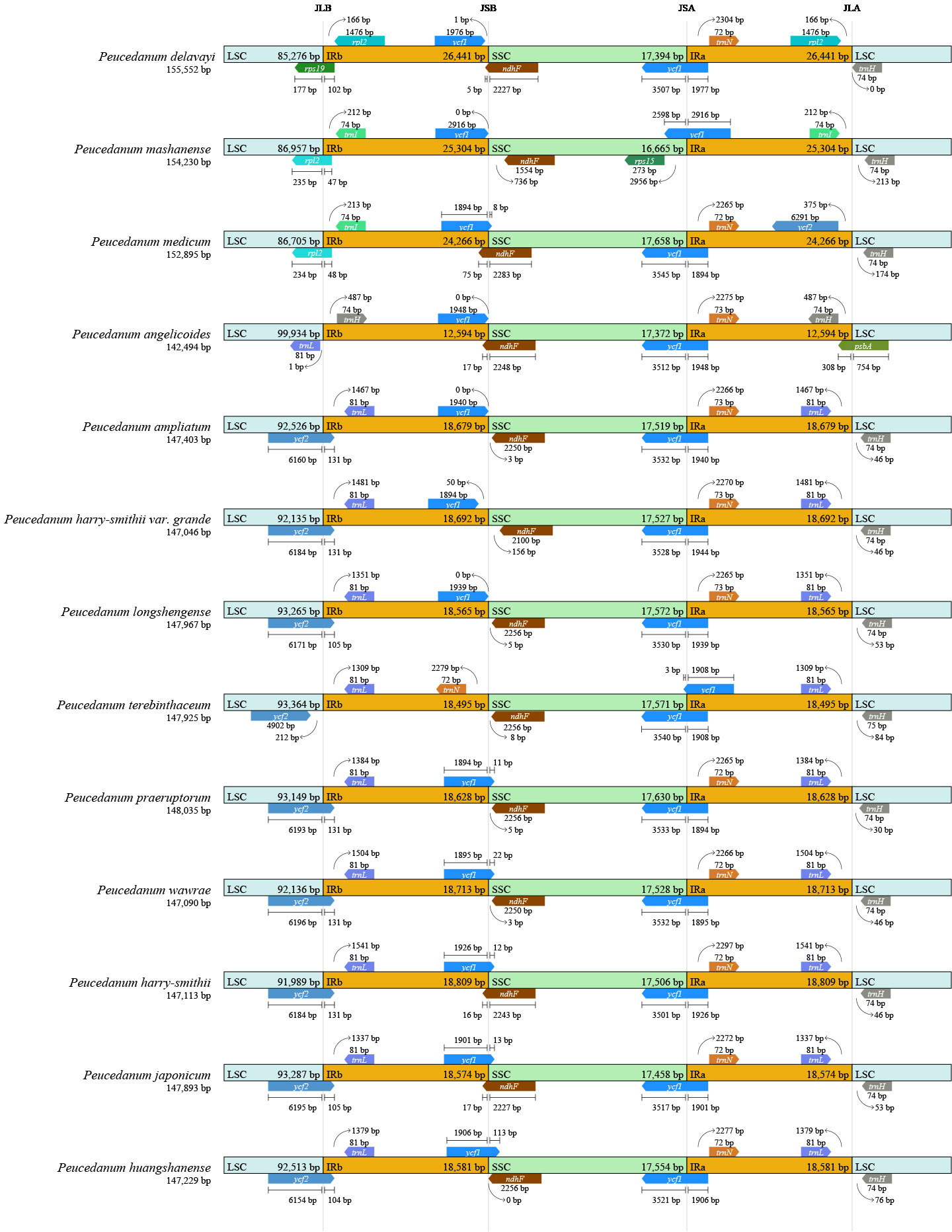
Figure 3 Comparison of the borders of the LSC, SSC, and IR regions among thirteen Peucedanum chloroplast genomes.
The total number of Simple Sequences Repeat (SSR) ranged from 57 to 89 in 13 Peucedanum chloroplast genomes. Most of the SSRs were distributed in the LSC region for all chloroplasts (Supplementary Figure 4A). Among these SSRs, mononucleotide repeats were the most abundant (28–54), followed by dinucleotides (14–20) (Supplementary Figure 4B). In addition, bases A and T were the dominant elements for all SSRs identified in the 13 chloroplast genomes, based on the MIAS analysis results.
According to the results of the collinearity analysis, the gene arrangement of the 13 Peucedanum chloroplast genomes was relatively conserved (Supplementary Figure 5). The sequence identities of the six chloroplast genomes assembled in this study were compared using mVISTA, with P. angelicoides (OK336474) as a reference. The results indicated that the chloroplast genomes of all Peucedanum species showed a high degree of conservation (Figure 4). According to the sequence divergences, the 14 mutation hotspot regions were selected as candidate DNA barcodes, including nine protein-coding genes (matK, rps16, petL, psbH, rps8, rpl22, ycf2, ycf1, and rpl32; all with Pi > 0.00800) (Supplementary Figure 6A) and five internal gene space genes (psbA-trnK, trnH-psbA, ycf2-trnL, psbK-psbI, and ycf4-cemA; all with Pi > 0.03000) (Supplementary Figure 6B).
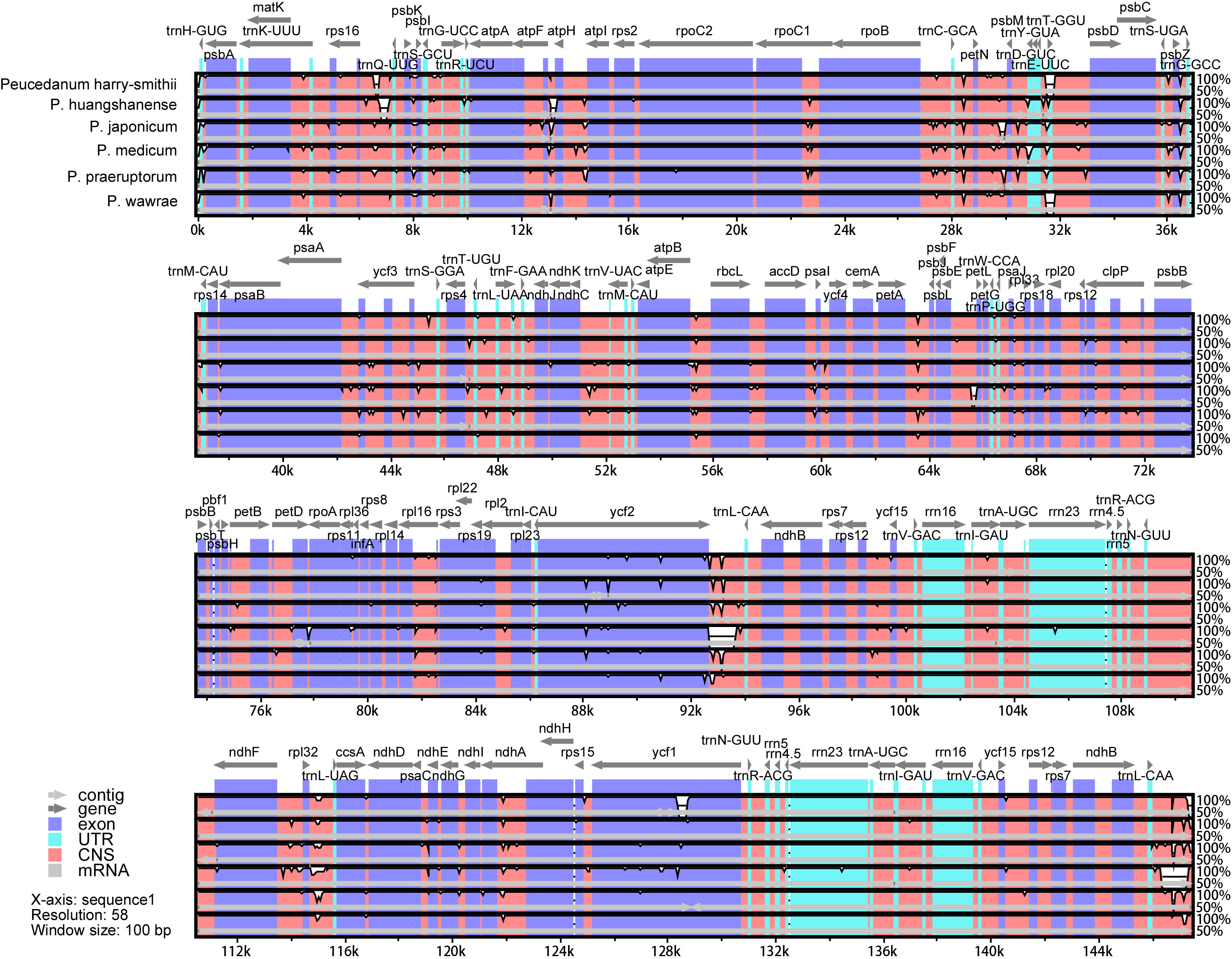
Figure 4 Sequence alignment of the whole chloroplast genomes of six taxa of Peucedanum using the LAGAN alignment algorithm in mVISTA, with P. ampliatum as the reference. Grey arrows and thick black lines above the alignment indicate gene orientation; The Y-axis represents percent identity in the 50—100% range; Purple bars represent exons, blue bars represent UTRs, and pink bars represent non-coding sequences.
3.5 Phylogenetic analysis based on chloroplast genomes
In this study, based on complete chloroplast genomes, 48 Apiaceae species were used for phylogenetic analysis. These topologies of the ML and BI trees were consistent (Figure 5). Both analyses robustly supported that the accessions of the Peucedanum genus were not clustered as monophyletic but fell into four clades. Most of the species of Peucedanum belonged to the tribe Selineae, while the samples of Selineae were also not clustered into a clade. P. franchetii and P. pubescens clustered into a clade with Ligusticum L., which was distant from all other Peucedanum members. P. chujaense, P. hakuunense, P. elegans, and P. stepposum clustered into a clade. P. mashanense was clustered with P. medicum, while P. wawrae, P. harry-smithii var. grande, P. formosanum (IUCN Red List of Threatened Species, NT), P. harry-smithii, P. ampliatum (IUCN, CR), P. huangshanense, P. praeruptorum, P. terebinthaceum, P. japonicum, and P. longshengense also formed a clade. First, the sister groups of P. praeruptorum and P. terebinthaceum, P. longshengense and P. japonicum, diverged from the others. Then, P. huangshanense, P. ampliatum, P. harry-smithii, P. formosanum, P. harry-smithii var. grande, and P. wawrae were separated. According to the results of the phylogenetic analysis based on the complete chloroplast genome, P. huangshanense had the closest genetic relationship to P. ampliatum, followed by P. harry-smithii. In addition, P. insolens belonged to the Arcuatopterus clade. P. delavayi was found to be a sister group of Pterygopleurum neurophyllum (Maxim.) Kitag., which belonged to the Acronema clade. P. angelicoides was a sister group of Semenovia transiliensis Regel & Herder belonging to the Tordyliinae clade.
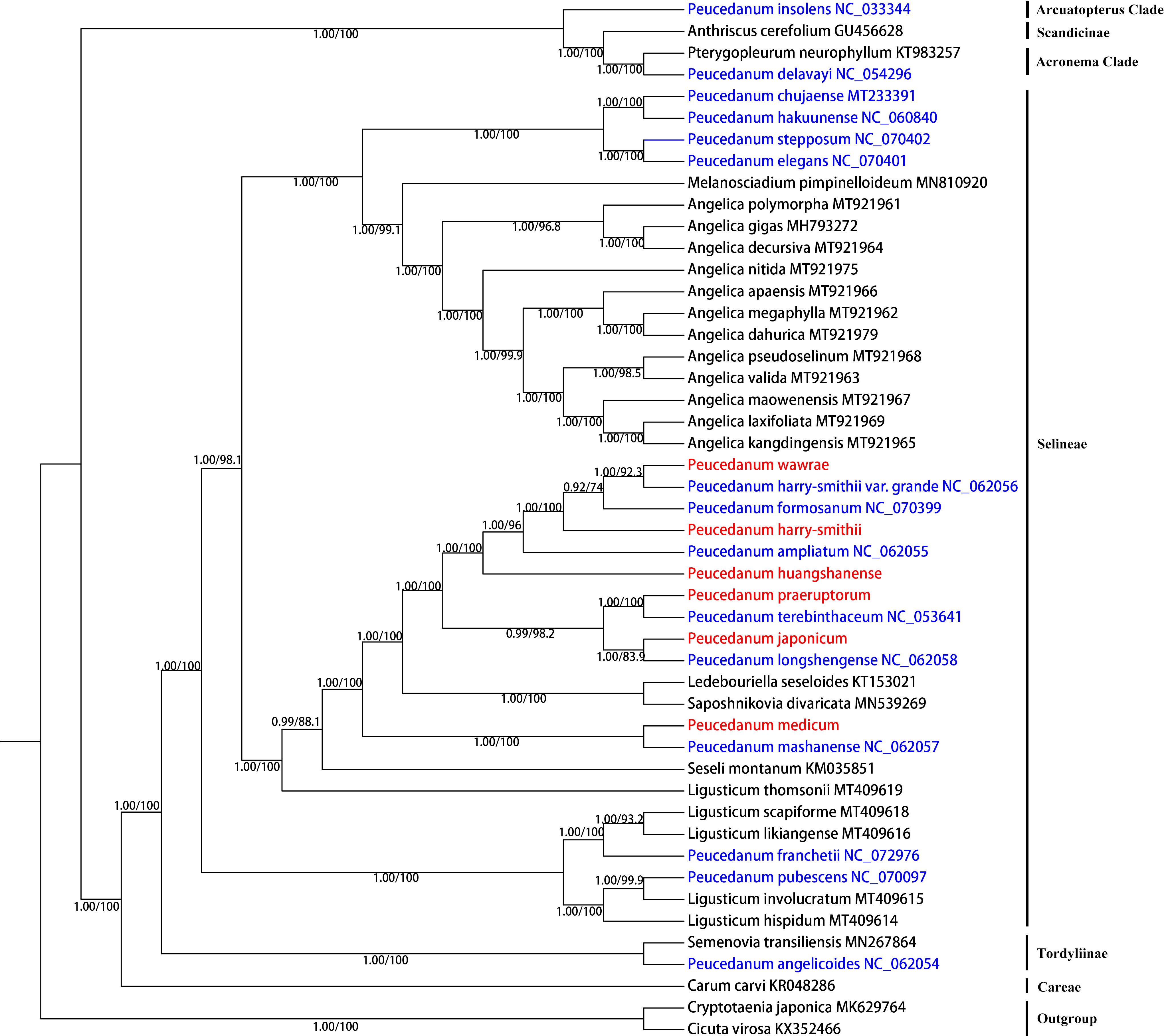
Figure 5 Phylogenetic relationships inferred from maximum likelihood (ML) and Bayesian inference (BI) analyses based on the complete chloroplast genomes. Numbers represent Bayesian posterior probabilities (PP) and maximum likelihood bootstrap values (BS).
3.6 Quantitative determination of ten coumarins in the Peucedanum species
A further quantitative analysis was performed to explore the differences in the components between the underground parts of the plants of the Peucedanum genus. We simultaneously determined the ten components of the samples using HPLC, and the results are shown in Figure 6 and Supplementary Table 4. The contents of the ten compounds were different in these samples. Ten compounds were found in P. praeruptorum, P. wawrae, and P. harry-smithii, but the first three compounds were not detected in some samples. Nodakenin was not detected in a P. praeruptorum, whereas xanthotoxin was not detected in another P. praeruptorum. Umbelliferone, psoralen, and xanthotoxin were not detected in few P. wawrae, and praeruptorin B was not detected in some P. wawrae. Three samples of P. harry-smithii—nodakenin, psoralen, and xanthotoxin—were not detected separately. Praeruptorin E was not detected in the samples of P. japonicum. Xanthotoxin was not detected in one P. japonicum, but praeruptorin A was detected in this sample. None of the P. medicum isolates contained praeruptorin B or E, and praeruptorin A was detected only in few P. medicum, which lacked umbelliferone and Psoralen.
The praeruptorin A content in most of P. praeruptorum was the highest. The praeruptorin E content in a small amount of P. praeruptorum was the highest, followed by praeruptorin A. The content of praeruptorin B was the second highest in some P. praeruptorum, but the content of praeruptorin E was the second highest in other P. praeruptorum. In individual P. japonicum the peucedanol content was the highest, followed by praeruptorin B. The contents of these two components were the opposite in other P. japonicum. Nodakenin content was highest in most P. wawrae. The content of bergapten or imperatorin was the second highest and praeruptorin B was the highest in half of P. harry-smithii, while the content of praeruptorin E was the highest in the other half of P. harry-smithii. The umbelliferone content in P. huangshanense was the highest, followed by psoralen and praeruptorin B. xanthotoxin, bergapten, and xanthotoxin were present only in small amounts in P. huangshanense. According to the cluster results, among the six species of the Peucedanum genus, the contents of various compounds in P. japonicum and P. harry-smithii were relatively close, P. huangshanense was similar to them, P. wawrae and P. praeruptorum were relatively close to each other, and P. medicum was a single branch.
4 Discussion
4.1 Speculation of the medicinal value of P huangshanense
Qianhu has a long history as a traditional Chinese medicine. Therefore, illustrations of the medicinal plants of Qianhu were found in ancient herbal books. According to the results of textual research on illustrations in Bencao literature, it can be inferred that at least five species of Peucedanum have been used as the original plants of Qianhu. Therefore, we collected these species of Peucedanum for our research.
The phylogenetic tree based on ITS sequences showed that the genetic relationship between P. huangshanense and the sister group of P. longshengense and P. formosanum was closest (Figure 3). However, the result of the phylogenetic analysis based on the whole chloroplast genome revealed that the genetic relationship between P. huangshanense and P. ampliatum was closest, followed by the relationship between P. huangshanense and P. harry-smithii (Figure 5). From the results of the cluster analysis of the compound content, the components of P. huangshanense were the most similar to those of P. japonicum and P. harry-smithii. Pharmacologists believe that medicinal plants containing similar chemical components often have similar clinical efficacies (Hao and Xiao, 2020; Gong et al., 2022). Chinese Herbal Medicine records that P. japonicum has the effects of clearing heat and relieving cough, diuresis, and detoxification. Yingqianhu has the effects of reducing Qi, resolving phlegm, dispersing wind, and clearing heat. It is often used to treat wind-heat cough, thick sputum, chest tightness, and asthma (Zhang, 2020). The Standard of Traditional Chinese Medicine in Shaanxi Province (2015 edition) states the plant sources of Yingqianhu include P. harry-smithii, P. harry-smithii var. subglabrum, and P. harry-smithii var. grande (Qiu et al., 2019). Therefore, we think that P. harry-smithii has the same effect as Yingqianhu. Based on the efficacy of P. japonicum and P. harry-smithii, we speculated that P. huangshanense could evacuate wind heat, relieve cough, and resolve phlegm.
According to the analysis of 10 coumarins in the six Peucedanum species, the content of umbelliferone in P. huangshanense was highest, followed by psoralen and praeruptorin B. Umbelliferolide (7-hydroxycoumarin) has various pharmacological properties, such as antioxidation, anti-hyperglycemia, antitumor, anti-inflammatory, anti-hyperlipidemia, immunomodulatory, hemostatic, and antiulcerogenic properties (Li et al., 2018; Cruz et al., 2020). Psoralen has been reported to be used to inhibit tumors, for example central nervous system malignant tumors, breast cancer, erythroleukemia, and oral carcinoma, as well as having anti-inflammatory, anti-diabetic, anti-microbial, and osteogenesis effects (Thakur et al., 2020). Praeruptorin B can inhibit osteoclast formation, thus treating osteoporosis and exerting anti-cancer effects (Lin et al., 2020; Xu et al., 2022). Pyranocoumarin can relieve neuropathic pain and neuroinflammatory reactions and exert anti-inflammatory effects (Onder and Trendafilova, 2022). Based on the main coumarin components of P. huangshanense, it can be speculated that P. huangshanense may has effects of anti-cancer, anti-inflammatory, anti-diabetic, bacteriostatic, and osteoporosis. Furthermore, these effects could be transformed into resolving phlegm to stop cough and dispersing wind heat. However, these specific effects need to be verified through further pharmacological experiments.
4.2 Discovery of molecular markers for phylogenetic classification of Peucedanum
DNA barcoding is a method used to identify species based on short and standardized DNA fragments (Gao et al., 2019). In most angiosperms, the chloroplast genome is maternally inherited, with a low recombination rate and abundant mutation sites that can accurately identify medicinal plants (Teske et al., 2020). Plastid markers, such as rbcL, matK, psbA, and trnH, have been widely used in medicinal species identification (Mahima et al., 2022). At present, 12 chloroplast genomes of the genus Peucedanum have been compared to screen hotspot mutation regions, providing potential molecular markers for species division and population genetic research of Peucedanum (Liu et al., 2022).
In this study, six complete chloroplast genomes of the genus Peucedanum were assembled, and the complete chloroplast genomes of P. huangshanense and P. harry-smithii were published for the first time. Comparison of 13 chloroplast genomes of the genus Peucedanum showed relatively conservative genome structures, and all had typical tetrad structures. In terms of gene number—except for one gene deletion in P. delavayi, P. praeruptorum, and P. harry-smithii var. grande—the gene number of other species were consistent. The distribution of Simple Sequences Repeat (SSR) was also consistent, with the LSC region being the most distributed, and the IR and SSC regions being less distributed. In addition, we observed obvious diversity in the 13 chloroplast genomes of Peucedanum. First, the chloroplast genome size varied greatly from 142,494 bp (P. angelicoides) to 155,552 bp (P. delavayi). Second, it can be seen from the map of Peucedanum chloroplast genomes that there are two ycf2 genes in P. medicum, and there is an inversion of the trnD-trnY-trnE gene in P. huangshanense, which can be used as a specific molecular marker for species identification. Third, SSR analysis showed that only a small number of hexanucleotide repeats were found in P. japonicum, P. praeruptorum, P. longshengense, and P. mashanense. The number of trinucleotide repeats in P. angelicoides was 10 and only 2–4 in the other species. In addition, we found 14 hotspot mutation regions in the sliding window analysis, which has the potential to develop DNA barcoding for the identification of Peucedanum species.
During the evolution of the chloroplast genome, the differences of SC/IR boundaries between different species are often observed, which further leads to change in the size of the chloroplast genomes. In this study, the IR regions of P. delavayi, P. mashanense, and P. medicum expanded the most, while the complete chloroplast genomes became larger, and the LSC region also changed greatly. The regions of IR are the most conserved parts of chloroplast genomes, but their contraction and expansion can account for the size differences between them. Therefore, IR regions can be used to explain variations in chloroplast genome size (Liu et al., 2019; Xue et al., 2019). In this study, 13 species of Peucedanum were roughly divided into four types according to the IR boundary analysis results, which also provided a basis for the species classification of Peucedanum.
In addition, based on both ITS and the chloroplast genomes, the phylogenetic trees showed that the genus Peucedanum is not monophyletic but divided into four clades. Most species of Peucedanum are distributed in the Selenae Clade, while the samples of Selineae were also not clustered into a clade. This conclusion supports the results of previous research (Liu et al., 2022). The phylogenetic tree based on ITS and chloroplast genome was slightly different. And the result of the phylogenetic analysis is also different from the morphological classification of Peucedanum, which indicates that the systematic classification of Peucedanum requires further study.
5 Conclusion
In this study, on the basis of textual research on the illustration of Qianhu in Bencao literature, we chose five medicinal of Peucedanum and P. huangshanense together for subsequent research. Six complete chloroplast genomes were successfully assembled, and chloroplast genomes of P. huangshanense and P. harry-smithii were reported for the first time. Phylogenetic analysis showed that the phylogenetic tree based on the complete chloroplast genome had high support and resolution, and the genetic relationship between P. huangshanense and P. ampliatum was closest, followed by the relationship between P. huangshanense and P. harry-smithii. In addition, ten coumarins from the samples were analyzed using HPLC. The results of chemical composition clustering showed that the contents and types of compounds in P. huangshanense, P. japonicum, and P. harry-smithii were the most similar. Overall, our research has laid the foundation for future studies on the utilization of P. huangshanense in traditional medicine. This can also be used as a reference for the research of new medicinal resources.
Data availability statement
The datasets presented in this study can be found in online repositories. The names of the repository/repositories and accession number(s) can be found in the article/Supplementary Material.
Author contributions
HS, LH, and HP conceived and designed the experiments. HS, SC, and LJ performed the experiments and writing—original draft. HS and ZT validated and analyzed the data. HP investigated and collected the samples. M’eC guided the literature research. All authors contributed to the article and approved the submitted version.
Funding
This work was supported by the National Key Research and Development Program of China (2022YFC3500903), Innovation Team and Talents Cultivation Program of National Administration of Traditional Chinese Medicine (ZYYCXTD-D-202005), and CAMS Innovation Fund for Medical Sciences (2019-I2M-5-065). Anhui Provincial Natural Science. Foundation (2208085QH269) and Talent Supporting Program of Anhui University of Chinese Medicine (2022rcyb020).
Conflict of interest
The authors declare that the research was conducted in the absence of any commercial or financial relationships that could be construed as a potential conflict of interest.
Publisher’s note
All claims expressed in this article are solely those of the authors and do not necessarily represent those of their affiliated organizations, or those of the publisher, the editors and the reviewers. Any product that may be evaluated in this article, or claim that may be made by its manufacturer, is not guaranteed or endorsed by the publisher.
Supplementary material
The Supplementary Material for this article can be found online at: https://www.frontiersin.org/articles/10.3389/fpls.2023.1179915/full#supplementary-material
References
Amiryousefi, A., Hyvönen, J., Poczai, P. (2018). IRscope: an online program to visualize the junction sites of chloroplast genomes. Bioinf. (Oxford England) 34 (17), 3030–3031. doi: 10.1093/bioinformatics/bty220
Chen, C. J., Chen, H., Zhang, Y., Thomas, H. R., Frank, M. H., He, Y. H., et al. (2020). TBtools: an integrative toolkit developed for interactive analyses of big biological data. Mol. Plant 13, 1194–1202. doi: 10.1016/j.molp.2020.06.009
Chen, L. L., Chu, S. S., Zhang, L., Xie, J., Dai, M., Wu, X., et al. (2019). Tissue-specific metabolite profiling on the different parts of bolting and unbolting Peucedanum praeruptorum Dunn (Qianhu) by laser microdissection combined with UPLC-Q/TOF-MS and HPLC-DAD. Molecules (Basel Switzerland) 24 (7), 1439. doi: 10.3390/molecules24071439
Chen, S. F., Zhou, Y. Q., Chen, Y. R., Gu, J. (2018). Fastp: an ultra-fast all-in-one FASTQ preprocessor. Bioinf. (Oxford England) 34 (17), 1884–1890. doi: 10.1093/bioinformatics/bty560
Chu, S. S., Chen, L. L., Xie, H. Q., Xie, J., Zhao, Y. J., Tong, Z. Z., et al. (2020). Comparative analysis and chemical profiling of different forms of peucedani radix. J. Pharm. Biomed. Anal. 189, 113410. doi: 10.1016/j.jpba.2020.113410
Chu, S. S., Wang, D. Q., Peng, H. S., Huang, L. Q. (2020). Peucedanum huangshanense (Apiaceae), a new species from anhui, China. Phytotaxa 430 (1), 17–24. doi: 10.11646/phytotaxa.430.1.3
Committee for the Pharmacopoeia of PR China. (2020). Pharmacopoeia of PR China, part I (Beijing: China Medical Science and Technology Press).
Cruz, L. F., Figueiredo, G. F., Pedro, L. P., Amorin, Y. M., Andrade, J. T., Passos, T. F., et al. (2020). Umbelliferone (7-hydroxycoumarin): a non-toxic antidiarrheal and antiulcerogenic coumarin. Biomed. Pharmacother. 129, 110432. doi: 10.1016/j.biopha.2020.110432
Danna, C., Bazzicalupo, M., Ingegneri, M., Smeriglio, A., Trombetta, D., Burlando, B., et al. (2022). Anti-inflammatory and wound healing properties of leaf and rhizome extracts from the medicinal plant Peucedanum ostruthium (L.) w. d. j. Koch. Molecules (Basel Switzerland) 27 (13), 4271. doi: 10.3390/molecules27134271
Darling, A. C., Mau, B., Blattner, F. R., Perna, N. T. (2004). Mauve: multiple alignment of conserved genomic sequence with rearrangements. Genome Res. 14 (7), 1394–1403. doi: 10.1101/gr.2289704
Elkins, K. M. (2013). Analysis of deoxyribonucleic acid (DNA) sequence data using BioEdit. Forensic DNA Biol. 15, 129–132. doi: 10.1016/B978-0-12-394585-3.00015-8
Frazer, K. A., Pachter, L., Poliakov, A., Rubin, E. M., Dubchak, I. (2004). VISTA: computational tools for comparative genomics. Nucleic Acids Res. 32 (Web Server issue), W273–W279. doi: 10.1093/nar/gkh458
Gao, Z. T., Liu, Y., Wang, X. Y., Wei, X. M., Han, J. P. (2019). DNA Mini-barcoding: a derived barcoding method for herbal molecular identification. Front. Plant Sci. 10. doi: 10.3389/fpls.2019.00987
Gong, X., Yang, M., He, C. N., Bi, Y. Q., Zhang, C. H., Li, M. H., et al. (2022). Plant pharmacophylogeny: review and future directions. Chin. J. Integr. Med. 28 (6), 567–574. doi: 10.1007/s11655-020-3270-9
Greiner, S., Lehwark, P., Bock, R. (2019). Organellar genome DRAW (OGDRAW) version 1.3.1: expanded toolkit for the graphical visualization of organellar genomes. Nucleic Acids Res. 47 (W1), W59–W64. doi: 10.1093/nar/gkz238
Hao, D. C., Xiao, P. G. (2020). Pharmaceutical resource discovery from traditional medicinal plants: pharmacophylogeny and pharmacophylogenomics. Chin. Herbal Medicines 12 (2), 104–117. doi: 10.1016/j.chmed.2020.03.002
Jin, J. J., Yu, W. B., Yang, J. B., Song, Y., dePamphilis, C. W., Yi, T. S., et al. (2020). GetOrganelle: a fast and versatile toolkit for accurate de novo assembly of organelle genomes. Genome Biol. 21 (1), 241. doi: 10.1186/s13059-020-02154-5
Kalyaanamoorthy, S., Minh, B. Q., Wong, T. K. F., von Haeseler, A., Jermiin, L. S. (2017). ModelFinder: fast model selection for accurate phylogenetic estimates. Nat. Methods 14 (6), 587–589. doi: 10.1038/nmeth.4285
Kanazawa, R., Morimoto, R., Horio, Y., Sumitani, H., Isegawa, Y. (2022). Inhibition of influenza virus replication by apiaceae plants, with special reference to Peucedanum japonicum (Sacna) constituents. J. Ethnopharmacology 292, 115243. doi: 10.1016/j.jep.2022.115243
Katoh, K., Standley, D. M. (2013). MAFFT multiple sequence alignment software version 7: improvements in performance and usability. Mol. Biol. Evol. 30 (4), 772–780. doi: 10.1093/molbev/mst010
Khruengsai, S., Sripahco, T., Rujanapun, N., Charoensup, R., Pripdeevech, P. (2021). Chemical composition and biological activity of peucedanum dhana a. ham essential oil. Sci. Rep. 11 (1), 19079. doi: 10.1038/s41598-021-98717-y
Kimura, M. (1980). A simple method for estimating evolutionary rate of base substitutions through comparative studies of nucleotide sequences. J. Mol. Evol. 16, 111–120. doi: 10.1007/BF01731581
Lee, J., Lee, Y. J., Kim, J., Bang, O. S. (2015). Pyranocoumarins from root extracts of Peucedanum praeruptorum Dunn with multidrug resistance reversal and anti-inflammatory activities. Molecules (Basel Switzerland) 20 (12), 20967–20978. doi: 10.3390/molecules201219738
Li, K., Fan, H., Yin, P. P., Yang, L. G., Xue, Q., Li, X., et al. (2018). Structure-activity relationship of eight high content flavonoids analyzed with a preliminary assignscore method and their contribution to antioxidant ability of flavonoids-rich extract from scutellaria baicalensis shoots. Arab. J. Chem. 11, 159–170. doi: 10.1016/j.arabjc.2017.08.002
Lin, C. L., Hung, T. W., Ying, T. H., Lin, C. J., Hsieh, Y. H., Chen, C. M. (2020). Praeruptorin b mitigates the metastatic ability of human renal carcinoma cells through targeting CTSC and CTSV expression. Int. J. Mol. Sci. 21 (8), 2919. doi: 10.3390/ijms21082919
Liu, X., Chang, E. M., Liu, J. F., Huang, Y. N., Wang, Y., Yao, N. (2019). Complete chloroplast genome sequence and phylogenetic analysis of Quercus bawanglingensis Huang, Li et xing, a vulnerable oak tree in China. Forests 10, 587. doi: 10.3390/F10070587
Liu, C. K., Lei, J. Q., Jiang, Q. P., Zhou, S. D., He, X. J. (2022). The complete plastomes of seven Peucedanum plants: comparative and phylogenetic analyses for the Peucedanum genus. BMC Plant Biol. 22 (1), 101. doi: 10.1186/s12870-022-03488-x
Mahima, K., Sunil Kumar, K. N., Rakhesh, K. V., Rajeswaran, P. S., Sharma, A., Sathishkumar, R. (2022). Advancements and future prospective of DNA barcodes in the herbal drug industry. Front. Pharmacol. 13. doi: 10.3389/fphar.2022.947512
Mudunuri, S. B., Nagarajaram, H. A. (2007). IMEx: imperfect microsatellite extractor. Bioinformatics 23, 1181–1187. doi: 10.1093/bioinformatics/btm097
Nguyen, L. T., Schmidt, H. A., von Haeseler, A., Minh, B. Q. (2015). IQ-TREE: a fast and effective stochastic algorithm for estimating maximum likelihood phylogenies. Mol. Biol. Evol. 32, 268–274. doi: 10.1093/molbev/msu300
Onder, A., Trendafilova, A. (2022). A review on anomalin: a natural bioactive pyranocoumarin from the past to the future. Chem. biodiversity 19 (9), e202200167. doi: 10.1002/cbdv.202200167
Palmioli, A., Bertuzzi, S., De Luigi, A., Colombo, L., La Ferla, B., Salmona, M., et al. (2019). bioNMR-based identification of natural anti-aβ compounds in Peucedanum ostruthium. Bioorganic Chem. 83, 76–86. doi: 10.1016/j.bioorg.2018.10.016
Qiu, G. Y., Li, Y., Li, X. C., Cheng, X. L., Wei, F., Kang, S., et al. (2019). Study on the chemical constituents and quality control methods of the medicinal materials of Peucedanum. China Pharm. 33 (4), 446–459. doi: 10.16153/j.1002-77777.2019.04.015
Ronquist, F., Teslenko, M., van der Mark, P., Ayres, D. L., Darling, A., Höhna, S., et al. (2012). MrBayes 3.2: efficient Bayesian phylogenetic inference and model choice across a large model space. Systematic Biol. 61 (3), 539–542. doi: 10.1093/sysbio/sys029
Rozas, J., Ferrer-Mata, A., Sanchez-DelBarrio, J. C., Guirao-Rico, S., Librado, P., Ramos-Onsins, S. E., et al. (2017). DnaSP 6: DNA sequence polymorphism analysis of large data sets. Mol. Biol. Evol. 34, 3299–3302. doi: 10.1093/molbev/msx248
Sarkhail, P. (2014). Traditional uses, phytochemistry and pharmacological properties of the genus Peucedanum: a review. J. Ethnopharmacology 156, 235–270. doi: 10.1016/j.jep.2014.08.034
Shi, L. C., Chen, H. M., Jiang, M., Wang, L. Q., Wu, X., Huang, L. F., et al. (2019). CPGAVAS2, an integrated plastome sequence annotator and analyzer. Nucleic Acids Res. 47 (W1), W65–W73. doi: 10.1093/nar/gkz345
Song, Y. L, Jing, W. H., Yan, R., Wang, Y. T. (2015). Research progress of the studies on the roots of Peucedanum praeruptorum Dunn (Peucedani radix). Pakistan J. Pharm. Sci. 28 (1), 71–81. doi: 10.1254/jphs.13237FP
Swindell, S. R., Plasterer, T. N. (1997). Sequence data analysis guidebook || SEQMAN. Methods Mol. Biol. 70 (6), 75–89. doi: 10.1385/0-89603-358-9:75
Teske, D., Peters, A., Möllers, A., Fischer, M. (2020). Genomic profiling: the strengths and limitations of chloroplast genome-based plant variety authentication. J. Agric. Food Chem. 68 (49), 14323–14333. doi: 10.1021/acs.jafc.0c03001
Thakur, A., Sharma, R., Jaswal, V. S., Nepovimova, E., Chaudhary, A., Kuca, K. (2020). Psoralen: a biologically important coumarin with emerging applications. Mini Rev. Medicinal Chem. 20 (18), 1838–1845. doi: 10.2174/1389557520666200429101053
Wen, J., Xie, D. F., Price, M., Ren, T., Deng, Y. Q., Gui, L. J., et al. (2021). Backbone phylogeny and evolution of apioideae (Apiaceae): new insights from phylogenomic analyses of plastome data. Mol. Phylogenet. Evol. 161, 107183. doi: 10.1016/j.ympev.2021.107183
Xu, K. B., Chen, Z. Y., Hou, J. L., Dong, C. L., Shi, C. G., Gao, L. L., et al. (2022). Praeruptorin b inhibits osteoclastogenesis by targeting GSTP1 and impacting on the s-glutathionylation of IKKβ. Biomed. Pharmacother. 154, 113529. doi: 10.1016/j.biopha.2022.113529
Xue, S., Shi, T., Luo, W., Ni, X., Iqbal, S., Ni, Z., et al. (2019). Comparative analysis of the complete chloroplast genome among Prunus mume, P. armeniaca, and P. salicina. Hortic. Res. 6, 89. doi: 10.1038/s41438-019-0171-1
Yadegari, S., Saidijam, M., Moradi, M., Dastan, D., Mahdavinezhad, A. (2020). Aerial parts of Peucedanum chenur have anti-cancer properties through the induction of apoptosis and inhibition of invasion in human colorectal cancer cells. Iranian Biomed. J. 24 (5), 314–323. doi: 10.29252/ibj.24.5.309
Zhang, J. (2020). Study on the quality standard of 6 local medicinal materials in Gansu Province. master’s thesis. (Lanzhou: Lanzhou University)
Zhang, D., Gao, F. L., Jakovlic, I., Zou, H., Zhang, J., Li, W. X., et al. (2020). PhyloSuite: an integrated and scalable desktop platform for streamlined molecular sequence data management and evolutionary phylogenetics studies. Mol. Ecol. Resour. 20 (1), 348–355. doi: 10.1111/1755-0998.13096
Keywords: Peucedanum huangshanense, chloroplast genome, Chinese medicine, herbal textual research, medicinal value, Qianhu
Citation: Sun H, Chu S, Jiang L, Tong Z, Cheng M, Peng H and Huang L (2023) Integrative analysis of chloroplast genome, chemicals, and illustrations in Bencao literature provides insights into the medicinal value of Peucedanum huangshanense. Front. Plant Sci. 14:1179915. doi: 10.3389/fpls.2023.1179915
Received: 06 March 2023; Accepted: 03 July 2023;
Published: 04 August 2023.
Edited by:
Michelle Waycott, University of Adelaide, AustraliaReviewed by:
Hanghui Kong, Chinese Academy of Sciences (CAS), ChinaKhurram Shahzad, Chinese Academy of Sciences (CAS), China
Copyright © 2023 Sun, Chu, Jiang, Tong, Cheng, Peng and Huang. This is an open-access article distributed under the terms of the Creative Commons Attribution License (CC BY). The use, distribution or reproduction in other forums is permitted, provided the original author(s) and the copyright owner(s) are credited and that the original publication in this journal is cited, in accordance with accepted academic practice. No use, distribution or reproduction is permitted which does not comply with these terms.
*Correspondence: Huasheng Peng, aHNwZW5nQDEyNi5jb20=; Luqi Huang, aHVhbmdsdXFpMDFAMTI2LmNvbQ==
†These authors have contributed equally to this work