- Department of Plant Pathology, North Dakota State University, Fargo, ND, United States
Worldwide, Ascochyta blight is caused by a complex of host-specific fungal pathogens, including Ascochyta pisi, Didymella pinodes, and Didymella pinodella. The application of foliar fungicides is often necessary for disease management, but a better understanding of pathogen prevalence, aggressiveness, and fungicide sensitivity is needed to optimize control. Leaf and stem samples were obtained from 56 field pea production fields in 14 counties in North Dakota from 2017 to 2020 and isolates were collected from lesions characteristic of Ascochyta blight. Based on fungal characteristics and sequencing the ITS1-5.8S-ITS2 region, 73% of isolates were confirmed to be D. pinodes (n = 177) and 27% were A. pisi (n = 65). Across pathogens, aggressiveness was similar among some isolates in greenhouse assays. The in vitro pyraclostrobin sensitivity of all D. pinodes isolates collected from 2017 to 2020 was lower than that of the three baseline isolates. Sensitivity of 91% of A. pisi isolates collected in 2019 and 2020 was lower than the sensitivity of two known sensitive isolates. Resistance factors (Rf) from mean EC50 values of pyraclostrobin baseline/known sensitive isolates to isolates collected from 2017 to 2020 ranged from 2 to 1,429 for D. pinodes and 1 to 209 for A. pisi. In vitro prothioconazole sensitivity of 91% of D. pinodes isolates collected from 2017 to 2020 was lower than the sensitivity of the baseline isolates and 98% of A. pisi isolates collected from 2019 to 2020 was lower than the sensitivity of the known sensitive isolates. Prothioconazole Rf ranged from 1 to 338 for D. pinodes and 1 to 127 for A. pisi. Based on in vitro results, 92% of D. pinodes and 98% of A. pisi isolates collected displayed reduced-sensitivity/resistance to both fungicides when compared to baseline/known sensitive isolates. Disease control under greenhouse conditions of both pathogens provided by both fungicides was significantly lower in isolates determined to be reduced-sensitive or resistant in in vitro assays when compared to sensitive. Results reported here reinforce growers desperate need of alternative fungicides and/or management tools to fight Ascochyta blight in North Dakota and neighboring regions.
1 Introduction
Field pea (Pisum sativum L.) production has increased substantially in North America over the last two decades. North Dakota ranks second in field pea production in the United States behind Montana, with 98,000 ha harvested in 2021 (United States Department of Agriculture National Agriculture Statistics Service USDA-NASS, 2022). Ascochyta blight is among the most important diseases of field pea in North America (Tivoli and Baninza, 2007) and disease progression is favored by cool, wet weather from bloom until mid-pod development. In peas, Ascochyta blight is caused by a complex of host-specific fungal pathogens (Tivoli and Baninza, 2007; Davidson et al., 2009; Davidson et al., 2021). Ascochyta pisi Lib, Didymella pinodes (Berk. & A. Bloxam) Petr., and Didymella pinodella (L.K. Jones) Qian Chen & L. Cai have been documented as infecting field pea in the northern Great Plains region of North America (Owati et al., 2019). These three fungal pathogens have been associated with the disease in many field pea producing countries including the USA (Peever et al., 2007), Australia (Ali et al., 1978), Canada (Beasse et al., 1999), China (Liu et al., 2016), France (Le May et al., 2018), and Spain (Barilli et al., 2016). Phoma koolunga (Davidson et al., 2009), Phoma herbarum (Li et al., 2011), Boerema exigua var. exigua (Li et al., 2012), and Phoma glomerata (Tran et al., 2014) are associated with the disease complex, also known as black spot of field pea in Australia. P. koolunga has been widely distributed in Australia and has become an important component of the Ascochyta blight complex of field pea (Davidson et al., 2012; Tran et al., 2014; Keirnan et al., 2020).
Ascochyta blight pathogens infect all above-ground parts of the pea plant and it is difficult to distinguish among symptoms caused by these fungi (Skoglund et al., 2011; Barbetti et al., 2021). Symptoms include purplish black to brown lesion on stems, leaves, and pods (Figure 1). D. pinodella most typically causes foot rot and has been the most frequently isolated pathogen from pea roots in Sweden and Denmark (Persson et al., 1997; Tivoli and Baninza, 2007). Seed quality and quantity are reduced by Ascochyta blight through seed discoloration and deceleration of seed development, respectively. Ascochyta blight can be misdiagnosed as bacterial blight (Pseudomonas syringae pv. pisi) or Septoria blight (Septoria pisi), both of which can be observed with Ascochyta blight (Barbetti et al., 2021). D. pinodes has been reported as the predominant and most aggressive pathogen, causing up to 70% yield loss; therefore, this species has been the focus among the Ascochyta blight pathogens (Tivoli and Baninza, 2007). A. pisi was the predominant pathogen recovered from seeds collected in Montana and has been reported as a pathogen of field peas in South Dakota (Mathew et al., 2010; Owati et al., 2020); however, causal agents of Ascochyta blight of field peas in North Dakota have not been reported.
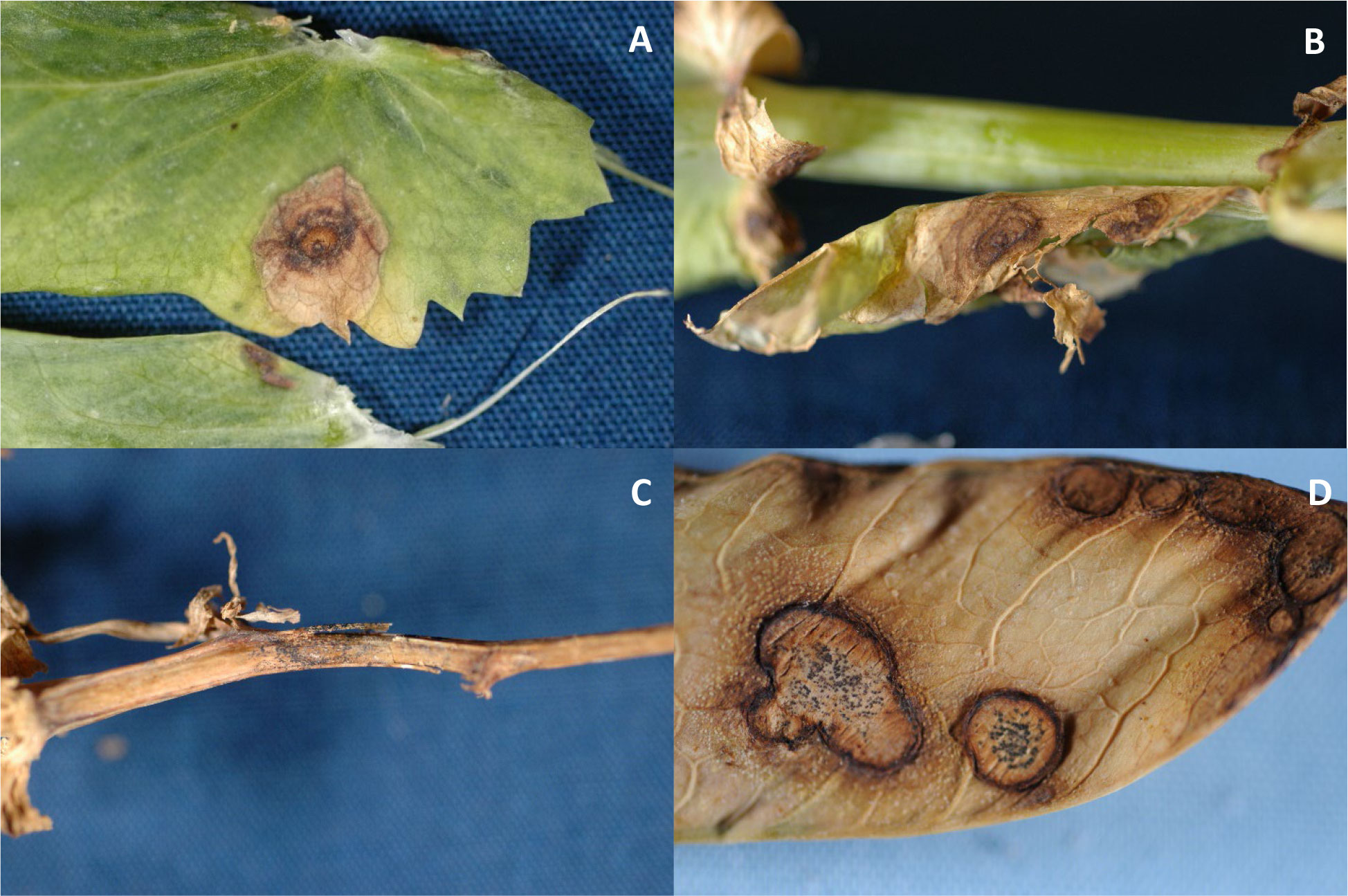
Figure 1 Symptoms of Ascochyta blight on leaves (A, B), a stem (C), and pod (D) (Photos: S. G. Markell).
The fungi that cause Ascochyta blight in North America overwinter in the soil, on debris, and can be seed-transmitted (Skoglund et al., 2011; Barilli et al., 2016). Air-borne ascospores of D. pinodes develop from pseudothecia on infected stubble, can travel long distances via wind, and can place even new fields at risk (Tivoli and Baninza, 2007; Schmidt, 2021). Pycnidiospores (conidia from pycnidia) of A. pisi and D. pinodella are spread to other plants via rain splash (Davidson et al., 2021). D. pinodes and D. pinodella produce chlamydospores while A. pisi does not. In the initial description, D. pisi was determined to have a longer latent period and caused less severe infections when compared to P. pinodes and A. pinodella in greenhouse evaluations (Chilvers et al., 2009). Subsequent research reported that isolates of P. pinodes were more aggressive in the greenhouse than isolates of D. pinodella, D. pisi, and Phoma spp. (Owati et al., 2020).
Effective disease management requires an integrated approach including crop rotation, use of pathogen-free certified seed, and fungicide seed treatments. Application of foliar fungicides should initiate at early flowering if the canopy is dense and cool, wet weather persists. Typically, a single fungicide application can provide adequate disease reductions if disease pressure is low (Schmidt, 2021). A second fungicide application 10- to 14-days after the first is warranted if symptoms “spread upward” in the crop canopy and favorable conditions persist. The use of locally systemic and translaminar fungicides has increased recently due to the importance of the crop in the northern Great Plains region and high disease pressure present (Schmidt, 2021). Five quinone outside inhibitor (QoI; Fungicide Resistance Action Committee [FRAC] code 11), succinate dehydrogenase inhibitor (SDHI; FRAC code 7), and demethylation inhibitor (DMI; FRAC code 3) fungicides are currently labeled as solo or pre-mixed products for the management of Ascochyta blight of field pea in North Dakota (Friskop et al., 2022).
QoI fungicides play an important role in the control of many fungi and fungal-like organisms. QoI fungicides have a single-site mode of action, interfering with electron transfer at the outer quinol oxidation site (Qo) of the cytochrome bc1 complex, also known as the complex III of the mitochondrial respiration chain (Bartlett et al., 2002). QoI fungicides have been classified as being high-risk for the development of resistance and field reduced-sensitivity/resistance has been reported in over 50 fungal pathogens (Pasche et al., 2004), Ishii, 2008; Bolton et al., 2013 Reimann and Deising, 2005), including. Ascochyta rabiei in commercial chickpea production systems in the prairie provinces of Canada and the northern Great Plains region of the US (Chang et al., 2007; Wise et al., 2009).
Resistance to QoI fungicides in D. pinodes has been reported in Alberta and Saskatchewan, likely due to increased selection pressure caused by sequential applications of the QoI pyraclostrobin (Headline ®, BASF Corporation, RTP, NC, USA) in intensive field pea production systems (Bowness et al., 2016). In that study, 6% of D. pinodes isolates collected in 2010 and 2011 demonstrated high levels of insensitivity to the fungicide: nine from central Alberta, five from northern Alberta, and five from south central Saskatchewan (Bowness, 2013). The 19 D. pinodes isolates characterized as insensitive exhibited from 667- to 1800-fold decreased in vitro sensitivity to pyraclostrobin when compared to the baseline isolates. Although the majority of isolates tested remained sensitive to pyraclostrobin, the development of QoI reduced-sensitive population in the prairie provinces in Canada was concerning for field pea producers in major field pea production regions of the US. From 2014 to 2016, 131 A. pisi isolates collected in Montana were determined to be sensitive to pyraclostrobin based on a discriminatory dose of 5 µg/ml (Owati et al., 2017). Reduced efficacy of QoI fungicides was first observed in D. pinodes in North Dakota in 2017; however, the distribution and frequency of insensitive isolates in the state has not been evaluated (Markell et al., 2018).
DMI fungicides are one of four classes of sterol biosynthesis inhibitors with broad-spectrum activity on many plant pathogens (Thomas et al., 2012). The mode of action of the DMI fungicides is the inhibition of fungal cell membrane development by interfering with ergosterol biosynthesis. Interference with this mechanism leads to disruption of membrane function, leakage of cytoplasmic contents, and hyphal death (Brent and Hollomon, 2007). Insensitivity to DMI fungicides has been reported in several phytopathogenic fungi since the 1980s (Fletcher and Wolfe, 1981; Schepers, 1983). Since that time, field resistance to DMI fungicides has developed in over 30 phytopathogenic fungi (Sheridan et al., 1985; Elad, 1992; Karaoglanidis et al., 2000; Reimann and Deising, 2005; Spolti et al., 2014). Despite their site-specific mode of action, DMI fungicides are considered to be at medium risk for fungicide resistance development FRAC Code List (2022). The development of insensitivity to DMI fungicides is a multi-step process, where decreases in disease control occur gradually (Brent and Hollomon, 2007). Prothioconazole (BayerCropScience, St. Louis, MO, USA) was first registered for use on field pea in 2007, and later, in combination with trifloxystrobin as Delaro® SC (Bayer CropScience). Registration labels for both fungicides include limits of no more than three applications per season (Friskop et al., 2022).
There have been few reports assessing in vitro fungicide sensitivity of Ascochyta blight pathogens of field pea to prothioconazole. In vitro prothioconazole EC50 values ranged from 0.70 to 9.34 µg/ml for 52 baseline D. pinodes isolates collected from the US and prairie provinces of Canada (Delgado et al., 2011). In vitro sensitivity of a 2012 D. pinodes population from Montana was generally lower (EC50 = 0.25 to 0.83 µg/ml) than was observed for the previously determined baseline population (Lonergan et al., 2015). A single D. pinodes isolate was reported with reduced-sensitivity in vitro to prothioconazole in a study conducted in China; however, only six isolates were evaluated (Liu et al., 2016). DMI fungicide sensitivity in D. pinodes and A. pisi pathogen populations from North Dakota have not been evaluated.
The objectives of this study were to determine: 1) the causal Ascochyta blight foliar pathogens of field pea present in North Dakota and their aggressiveness, 2) the in vitro sensitivity of D. pinodes and A. pisi populations from across field pea growing regions of North Dakota to pyraclostrobin and prothioconazole fungicides, and 3) the effect of any in vitro sensitivity shift on disease control.
2 Materials and methods
2.1 Pathogen collection, identification and maintenance
Isolates collected from 2017 to 2020 were obtained from infected leaf samples collected from field pea production fields in North Dakota and research plots located at North Dakota State University (NDSU) Research Extension Centers in Carrington, Langdon, Minot, and Williston (Supplementary Tables S1, S2). Symptomatic field pea leaves and stems containing lesions typical of Ascochyta blight were examined under a dissecting microscope for the presence of pycnidia. Tissue sections with lesions were surface sterilized in 1% NaOCl for 30 s, rinsed three times with sterile deionized water (SDW), and allowed to dry for 10 min in a laminar flow hood (Bowness et al., 2016). Tissue sections were aseptically transferred onto full-strength potato dextrose agar (PDA) (Difco™ Becton, Dickinson, and Company, Sparks, MD, USA) amended with streptomycin and neomycin (50 mg per milliliter). Cultures were incubated at 20 ± 2°C for 10 days under a cycle of alternating 12 h of white fluorescent light and 12 h of darkness (Onfroy et al., 1999). Individual isolates were differentiated morphologically by comparing conidial size, number of conidial cells, and macroscopic appearance of colony growth on media (Peever et al., 2007; Skoglund et al., 2011). Cultures generated from a single conidium transferred to fresh PDA and incubated for 14 days under the conditions previously described were considered distinct isolates. For working cultures (up to 6 months), 2.5-mm agar plugs bearing pycnidia were excised using a sterilized cork-borer, placed in a 1.5-ml sterile centrifuge tube with 1 ml of SDW, and stored at 4°C. The identity of the isolates collected was confirmed by PCR amplification and sequencing part of the rDNA internal transcribed spacer (ITS) region using primers ITS1 and ITS4 (Golani et al., 2016). Each isolates confirmed as acausal pathogen of Ascochyta blight was preserved for long-term cryogenic storage following previously described methods (Wise et al., 2008). Briefly, 2 µl of a conidial suspension was pipetted onto Whatman no. 1 filter paper that had been cut into small strips, autoclaved, and aseptically placed on the surface of PDA. After incubating for 14 days under conditions previously described, filter paper sections colonized by the fungus were aseptically removed, dried overnight in a laminar flow hood, placed in sterile 15-ml centrifuge tubes capped and sealed with Parafilm, and preserved at -80°C. Herbarium specimens were prepared for each plant sample from which D. pinodes and A. pisi isolates were obtained. Reference isolates were obtained for use in aggressiveness and fungicide sensitivity assays. Three D. pinodes isolates (Ap-1, Ap-8, and Mp-1) collected in 2001 were recovered from long-term cryogenic storage (Supplementary Table S1; Delgado et al., 2011). Two A. pisi isolates (D2 and Dp-3) previously tested to be sensitive to QoI fungicides were generously provided by Dr. Mary Burrows at Montana State University, Bozeman, MT, USA (Supplementary Table S2; Owati et al., 2017).
2.2 In vitro sensitivity of D. pinodes and A. pisi isolates to pyraclostrobin and prothioconazole
Pyraclostrobin and prothioconazole sensitivity was determined via mycelial growth assays conducted as described previously (Wise et al., 2011; Bowness et al., 2016). Working cultures were transferred onto PDA and were incubated under 24 h fluorescent light at 20 ± 2°C. After seven days, 5 mm agar plugs were excised from the leading edge of growth and inverted onto 100 × 15 mm Petri plates containing quarter-strength PDA (1/4 PDA) amended with technical grade formulations of pyraclostrobin (95% active ingredient [a.i.]) (BASF Corporation, Research Triangle Park, NC, USA). The fungicide was dissolved in acetone to reach final concentrations of 0, 0.01, 0.1, 1, 10, and 100 mg/ml (Bowness et al., 2016). Salicylhydroxamic acid (SHAM) (Sigma Chemical Company, St. Louis, MO), which has been previously determined to inhibit the alternative respiratory pathway, was dissolved in methanol at 100 µg/ml. The final concentration of both acetone and methanol in the media was 0.1% by volume. Technical grade prothioconazole (98.4% a.i.) (Bayer CropScience, Raleigh, NC, USA) was dissolved in acetone to reach final concentrations of 0, 0.01, 0.1, 1, 10, and 100 mg/ml. All amendments were added to the autoclaved media after it had cooled to 55°C.
Three baseline D. pinodes and two known sensitive A. pisi isolates were evaluated for in vitro sensitivity to both fungicides. The D. pinodes isolates were collected in 2001 and have no exposure to pyraclostrobin or prothioconazole. The A. pisi isolates were tested in previous research and were determined to be sensitive to pyraclostrobin (Owati et al., 2017). D. pinodes isolates collected from North Dakota in 2017 (11 isolates), 2018 (11 isolates), 2019 (69 isolates), and 2020 (44 isolates) were tested for in vitro sensitivity to both fungicides (Table 1; Supplementary Table S1). Additionally, A. pisi isolates collected in 2019 (44 isolates) and 2020 (six isolates) were tested for in vitro sensitivity to both fungicides (Table 1; Supplementary Table S2). D. pinodes control isolates Ap-1, Ap-8, and Mp-1 and A. pisi control isolates D-1 and DP-1 were included in in vitro assays as internal controls to determine assay reproducibility for each pathogen (Wong and Wilcox, 2002). Two perpendicular measurements of mycelial growth for each isolate at all fungicide concentrations were recorded, with the original plug diameter (5 mm) subtracted, after incubation at 20 ± 2°C in darkness for 14 days (Bowness et al., 2016).
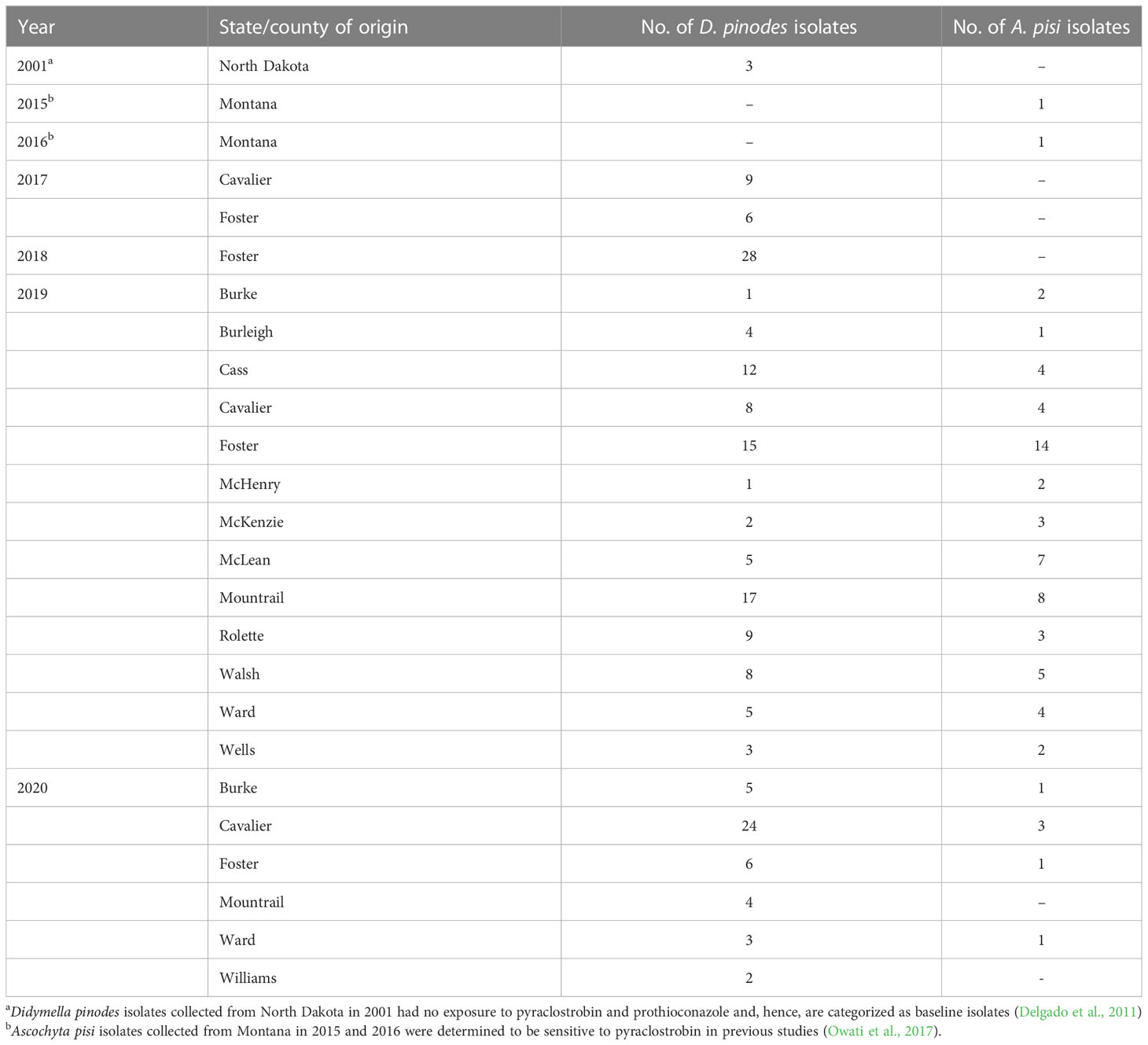
Table 1 Collection information for Didymella pinodes and Ascochyta pisi isolates collected in North Dakota and Montana from 2001 to 2020.
2.3 Aggressiveness of D. pinodes and A. pisi isolates
To determine if D. pinodes and A. pisi isolates were pathogenic to field peas and estimate aggressiveness, trials on a subset of isolates were conducted under greenhouse conditions in the NDSU Agriculture Experiment Station Jack Dalrymple Research Complex. Selected D. pinodes isolates included four collected in 2017, two collected in 2018 and two collected in 2001 known to be sensitive to both fungicides (used as baseline). Selected A. pisi isolates included four collected in 2019 and two known to be sensitive to both fungicides (collected in 2015 and 2016) (Table 2). Three seeds of an Ascochyta blight susceptible field pea cultivar ‘DS Admiral’ (Andersen et al., 2002) were sown in each 15.2 × 8.9 × 8.9 cm pot containing Sunshine Mix LC1 (Sun Gro Horticulture Inc., Bellevue, WA, USA). After emergence, plants were thinned to obtain one uniformly sized plant per pot and maintained at 25 ± 2°C with daily application of water. After three weeks, plants were inoculated with a conidial suspension produced from four- to seven-day-old-cultures of a single isolate of D. pinodes or A. pisi maintained on pea agar (Field pea cultivar ‘DS Admiral’, 50 g; agar, 15 g; and distilled water, 1000 ml) under 24 h fluorescent light at 22 ± 2°C. Conidia were harvested by adding 0.01% Tween 20 (100 µl Tween 20 in 1000 ml distilled water) and scraping the agar surface with a cotton swab. The suspension was filtered through a double layer of cheesecloth and adjusted to 1 × 105 conidia/ml using a hemocytometer. The conidial suspension was applied to the plants to run-off using a paint-spray gun (Anest Iwata-Medea Inc, Portland, OR, USA). To avoid cross-infection among isolates, inoculated plants were incubated in individual humidity chambers (Phytotronic Inc., Earth City, MO, USA) for 48 h at >95% relativity humidity and 22 ± 2°C and transferred to confinement chambers (plastic chambers with open ceiling) on greenhouse benches. Ascochyta blight severity was rated visually at 14 days post inoculation (DPI) on the four lowest leaves (four sub-samples) based on a visual scale of 0 to 5: 0 = no disease; 1 = a few dispersed lesions; 2 = several lesions; 3 = 10 to 15% of leaf area necrotic; 4 = 50% of the leaf areas dehydrated and/or covered by lesions; 5 = 75 to 100% of the leaf area dehydrated and/or necrotic (Figure 2; adapted from Onfroy et al., 1999).
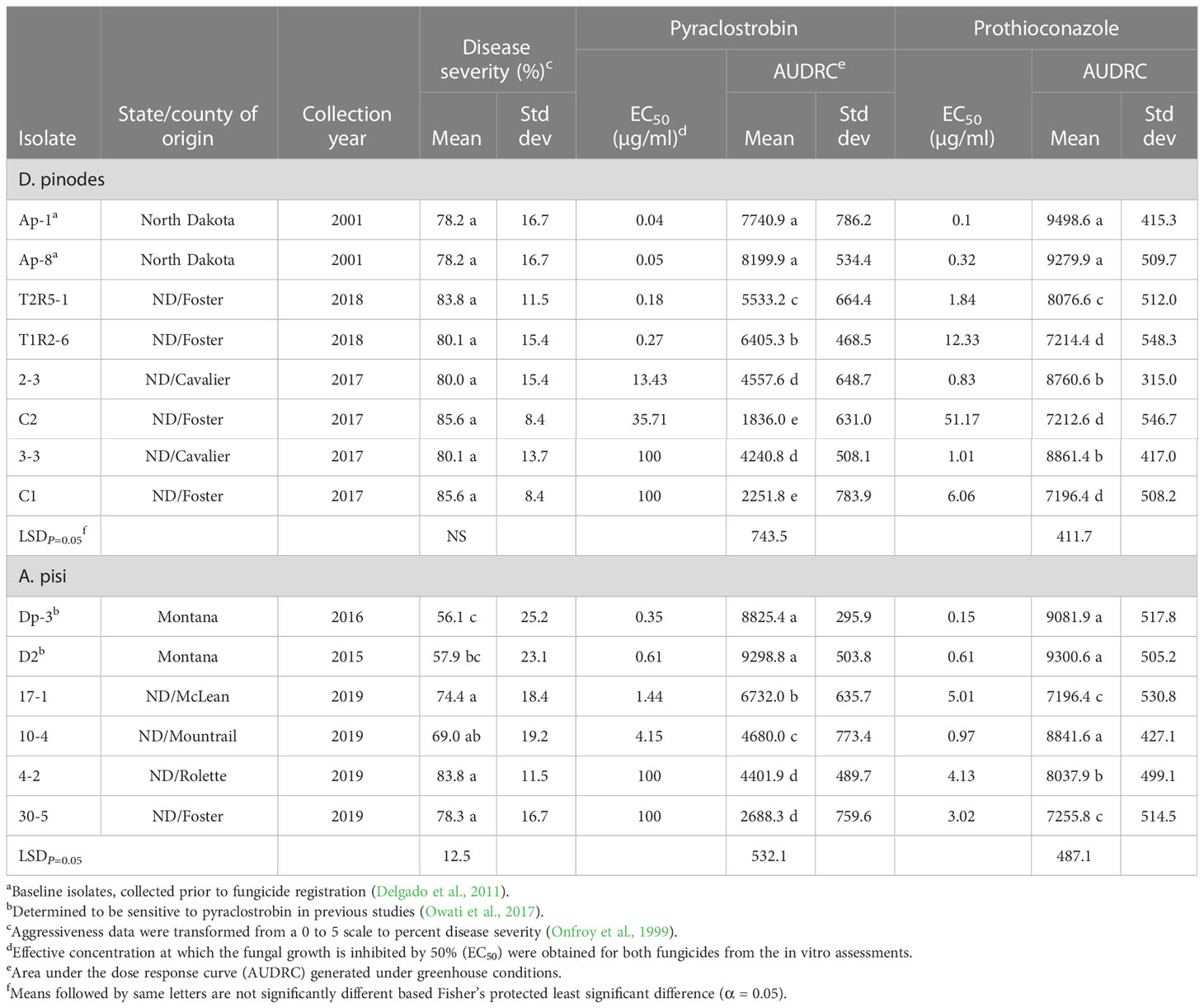
Table 2 Aggressiveness, in vitro and in vivo fungicide sensitivities for Didymella pinodes and Ascochyta pisi isolates.
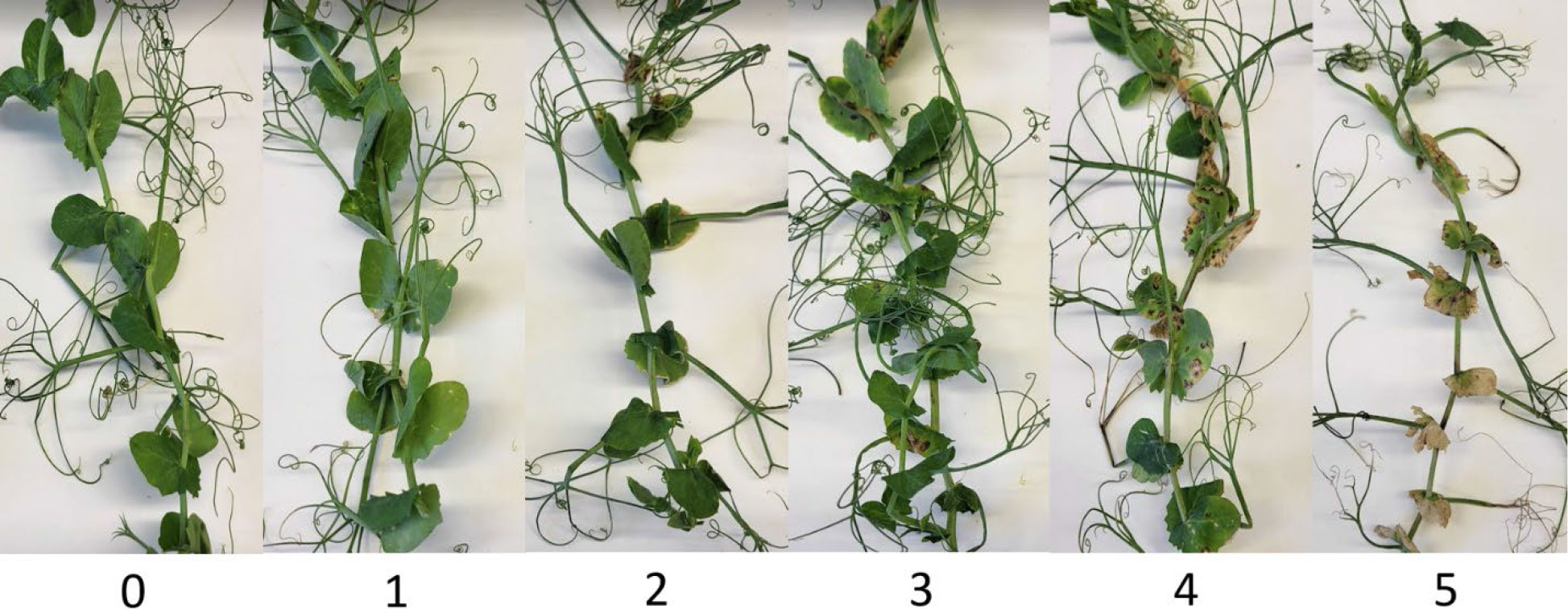
Figure 2 Ascochyta blight severity rating scale used to evaluate disease in the greenhouse; 0 = no disease; 1 = a few dispersed lesions; 2 = several lesions; 3 = 10 to 15% of leaf area necrotic; 4 = 50% of the leaf area dehydrated and/or covered by lesions; 5 = 75 to 100% of the leaf area dehydrated and/or necrotic (Adapted from Onfroy et al., 1999).
2.4 In vivo efficacy of pyraclostrobin and prothioconazole on D. pinodes and A. pisi
The impact of an in vitro shift in sensitivity of D. pinodes and A. pisi to pyraclostrobin and prothioconazole on disease control was determined under greenhouse conditions using the same subset of D. pinodes and A. pisi isolates evaluated for pathogenicity and aggressiveness. The in vivo fungicide efficacy assays were conducted as a 24-h preventive test (Fonseka and Gudmestad, 2016). Pea plants, reared as described above for pathogenicity and aggressiveness trials, were treated with a commercial formulation of pyraclostrobin (23.3% a.i.) (Headline ® SC, BASF Corporation) or prothioconazole (41% a.i.) (Proline ® SC, Bayer CropScience). Ten-fold fungicide dilutions (0, 0.1, 1, 10, and 100 µg active ingredient/ml) were applied to plants to obtain a dose response curve. Fungicide was applied using a Generation II Research Sprayer (Devries Manufacturing, Hollandale, MN, USA) at approximately 400 kPa and plants were returned to the greenhouse room. Twenty-four hours after fungicide application, plants were inoculated with a D. pinodes or A. pisi conidial suspension and disease severity was assessed as described above for aggressiveness assays.
2.5 Statistical analyses
All in vitro experiments for each pathogen × fungicide combination were performed twice in a completely random design with two replications (plates) at each fungicide concentration. EC50 values were calculated for each isolate using the percentage reduction in mycelial growth relative to the non-fungicide amended control (Fonseka and Gudmestad, 2016). Data were regressed against the log10 fungicide concentration and the EC50 value was determined by interpolation of 50% intercept using the Statistical Analysis System (SAS© Institute Inc., Cary, NC, USA). EC50 values of <0.01 and >100 were considered as 0.01 and 100 µg/ml, respectively, for final analysis (Budde-Rodriguez et al., 2021). The assay reproducibility calculations generated approximate limits for 95% confidence intervals for the internal controls in every trial (Wong and Wilcox, 2002). Within pathogens and fungicides, the F-test was used to determine homogeneity of variance across in vitro experiments and Fisher’s protected least significant difference (LSD) test was used for mean separation of EC50 values across years isolates were collected (α = 0.05). A resistance factor (Rf) was calculated by dividing the EC50 value of each isolate collected from 2017 to 2020 by the mean EC50 value of the baseline/known sensitive isolates for each pathogen and fungicide (Brent and Hollomon, 2007).
All greenhouse experiments were conducted twice in split-plot randomized complete block designs with D. pinodes or A. pisi isolates as the main plot and fungicide concentration as split-plots. Five replications (five pots) were tested for each isolate × fungicide concentration. Ordinal rating data were converted to percent disease control by each pathogen isolate × fungicide combination using the formula [1 – (Disease rating/disease rating in non-treated plants) × 100]. Homogeneity of variance among two independent trials was determined via Levene’s test (Milliken and Johnson, 1992). Data from both trials were combined, and “trial” was considered as a random effect. Data from aggressiveness assays were treated as randomized complete blocks nested within fungicide where both “block” and “fungicide” were considered random effects in the model. Analysis of variance was implemented with the Glimmix procedure in SAS version 9.4. The area under the dose-response curve (AUDRC) was calculated to determine significant differences in disease control provided by pyraclostrobin or prothioconazole (Fonseka and Gudmestad, 2016). Fisher’s protected least significant difference (LSD; α = 0.05) test was used for the mean separation of isolate aggressiveness within each pathogen and AUDRC within each pathogen and fungicide. Regression models were implemented within fungicides to elucidate the relationship between disease control and in vitro sensitivity of the isolates used in the greenhouse trials. AUDRC was modeled with a linear-log simple regression to EC50. Models were fitted with the “lm” function in R (R Core Team, 2022), and figures were produced with the package ggplot2 (Wickham, 2016).
3 Results
3.1 Pathogen identification
Leaf samples with characteristic Ascochyta blight lesions were obtained from 56 field pea production fields in 14 counties in North Dakota from 2017 to 2020 (Table 1; Figure 3). D. pinodes was recovered most frequently (73%; n = 177) and from all years and all counties surveyed. A. pisi (27%; n = 65) and was recovered in 2019 and 2020 from all counties surveyed with the exception of Williams county in northwestern North Dakota. Although D. pinodes was the predominant pathogen isolated from the counties surveyed in 2019 and 2020, A. pisi was the dominant species isolated from McHenry (67%), McKenzie (60%), and McLean (58%) counties.
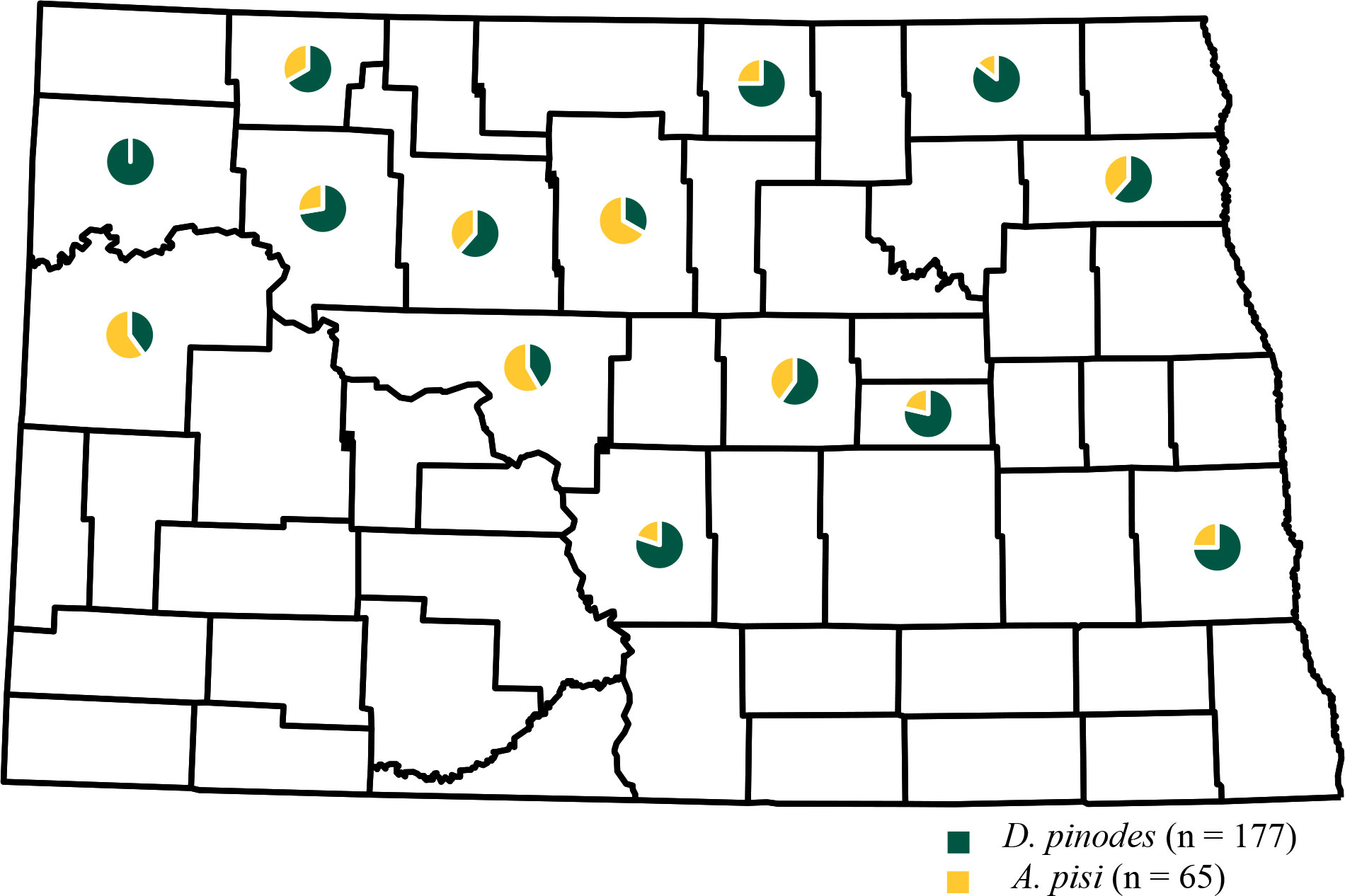
Figure 3 Geographic frequency distribution for Didymella pinodes collected from 2017 to 2020 and Ascochyta pisi isolates collected from 2019 to 2020 in North Dakota.
3.2 In vitro sensitivity of D. pinodes and A. pisi isolates to pyraclostrobin and prothioconazole
Radial growth assays uncovered an in vitro shift in fungicide sensitivity among isolates evaluated (Figure 4). Variances were homogenous for D. pinodes (P = 0.6095) and A. pisi (P = 0.2755); thus, trials were combined within pathogens. EC50 values of the three baseline D. pinodes isolates ranged from 0.04 to 0.12 µg/ml for pyraclostrobin (Figure 5A; Supplementary Table S1), within the previously established baseline of 0.03 to 0.29 µg/ml (Bowness et al., 2016). EC50 values of D. pinodes isolates collected from 2017 to 2020 ranged from 0.17 to >100 µg/ml. In vitro sensitivity of 100% (n = 135) of isolates collected from 2017 to 2020 was lower than the sensitivity of the three baseline isolates evaluated during the current study. When compared to the previously established baseline (0.03 to 0.29 µg/ml; Bowness et al., 2016), 96% (129) of D. pinodes isolates were less sensitive to pyraclostrobin. Thirty-six percent (n = 49) of isolates displayed EC50 values >100 µg/ml. Rf for D. pinodes in vitro pyraclostrobin sensitivity ranged from 2.4 to 1,429. EC50 values of the two known sensitive A. pisi isolates included in this study were 0.35 (Dp-3) and 0.61 µg/ml (D2) for pyraclostrobin (Figure 5B; Supplementary Table S2). EC50 values of A. pisi isolates collected from 2019 to 2020 ranged from 0.56 to 100 µg/ml. In vitro sensitivity of 98% (n = 49) of A. pisi isolates collected from 2019 to 2020 were lower than the sensitivity of the two known sensitive isolates tested during this evaluation. Eighteen percent of A. pisi isolates displayed EC50 values >100 µg/ml pyraclostrobin. Rf for A. pisi in vitro sensitivity ranged from 1.2 to 208.3.

Figure 4 In vitro assays comparing a baseline (left) and a 2017 to 2020 (right) Didymella pinodes isolate to pyraclostrobin. The fungicide concentration increases from 0.0 ppm (left) to 100 ppm (right).
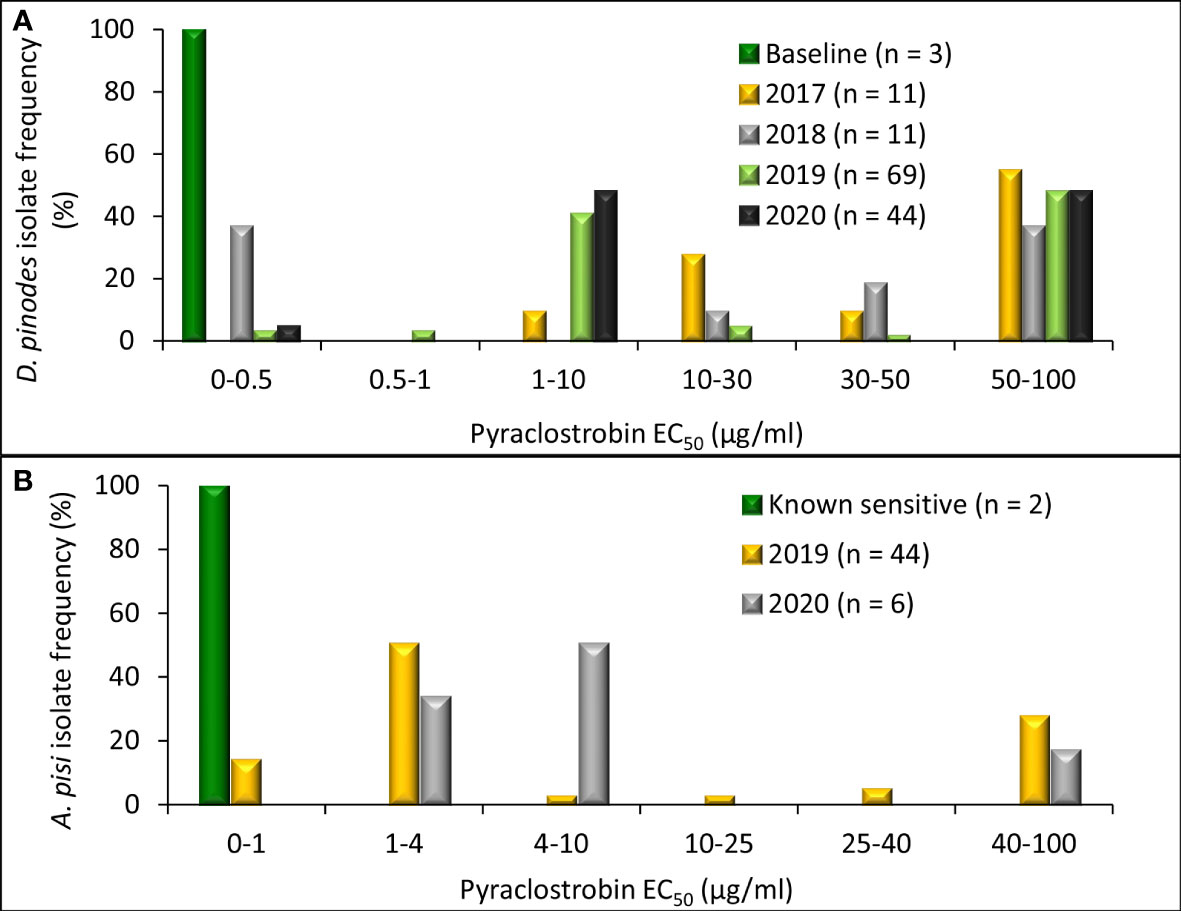
Figure 5 Frequency distribution of in vitro sensitivity to pyraclostrobin based on methods to determine the effective concentration that inhibits mycelial growth by 50% when compared with the non-fungicide-amended (EC50 µg/ml) for (A) Didymella pinodes (n = 138) isolates collected from 2001 (baseline; Delgado et al., 2011) and 2017 to 2020 and (B), Ascochyta pisi (n = 52) isolates collected from 2015 and 2016 (determined to be sensitive to QoI fungicides; Owati et al., 2017), and isolates collected in 2019 and 2020).
Independent ANOVA of in vitro prothioconazole sensitivity assays determined that variances were homogenous for D. pinodes (P = 0.1690) and A. pisi (P = 0.8356); thus, trials were combined within pathogens. EC50 values of the three baseline D. pinodes isolates ranged from 0.10 to 0.32 µg/ml for prothioconazole (Figure 6A; Supplementary Table S1). This is below the range of the previously established baseline of 0.70 to 9.34 µg/ml (Delgado et al., 2011) and below or within the 2012 Montana population of 0.25 to 0.83 µg/ml (Lonergan et al., 2015). EC50 values of D. pinodes isolates collected from 2017 to 2020 ranged from 0.10 to 67.6 µg/ml for prothioconazole. In vitro prothioconazole sensitivities of 91% (n = 123) of isolates collected from 2017 to 2020 were lower than the sensitivities of the three baseline D. pinodes isolates evaluated during the current study. Nineteen percent (26 isolates) had lower sensitivity than the previously established prothioconazole baseline (0.70 to 9.34 µg/ml; Delgado et al., 2011) and 83% (112 isolates) displayed lower sensitivity than the 2012 Montana population (0.25 to 0.83 µg/ml; Lonergan et al., 2015). Rf for D. pinodes in vitro pyraclostrobin sensitivity ranged from 0.5 to 337.9. EC50 values of the two known sensitive A. pisi isolates included in this study were 0.15 (Dp-3) and 0.61 µg/ml (D2) for prothioconazole (Figure 6B; Supplementary Table S2). EC50 values of A. pisi isolates collected from 2019 to 2020 ranged from 0.34 to 48.3 µg/ml. In vitro prothioconazole sensitivity of 98% (n = 49) of A. pisi isolates collected in 2019 and 2020 were lower than the two known sensitive A. pisi isolates tested during this evaluation. Rf for A. pisi in vitro sensitivity ranged from 0.9 to 127.0 for prothioconazole.
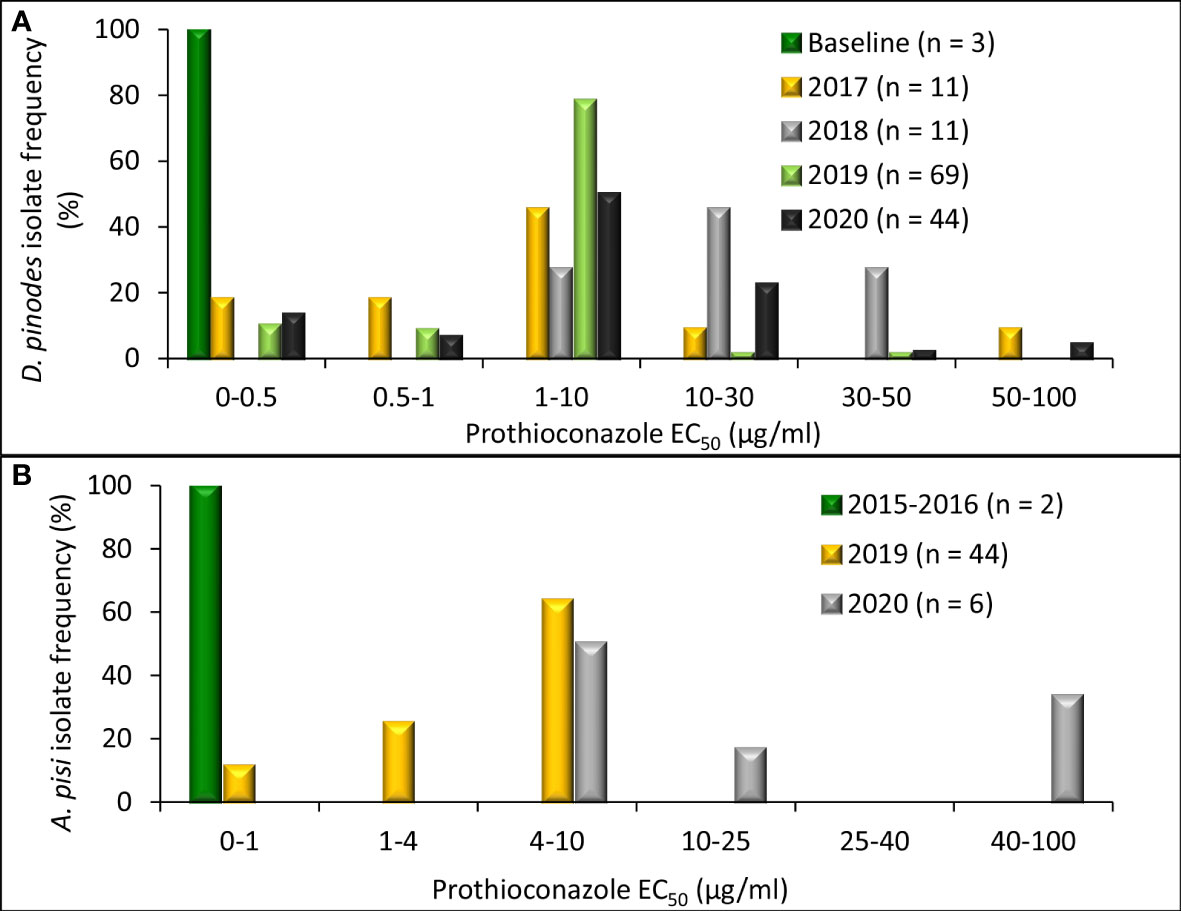
Figure 6 Frequency distribution of in vitro sensitivity to prothioconazole based on methods to determine the effective concentration that inhibits mycelial growth by 50% when compared with the non-fungicide-amended (EC50 µg/ml) for (A), Didymella pinodes (n = 138) isolates collected from 2001 (baseline; Delgado et al., 2011) and 2017 to 2020 and (B), Ascochyta pisi (n = 52) isolates collected from 2015 and 2016 (Owati et al., 2017), and isolates collected in 2019 and 2020).
Mean EC50 values of baseline D. pinodes isolates was 0.07 µg/ml for pyraclostrobin and was 0.20 µg/ml for prothioconazole (Figure 7A). Mean sensitivities of isolates collected from 2017 to 2020 were significantly lower than the mean of the baseline isolates for both fungicides. No significant difference in sensitivity was observed across isolates within years for non-baseline populations for pyraclostrobin. Mean prothioconazole sensitivity of D. pinodes isolates collected in 2018 was significantly lower when compared to isolates collected in 2017, 2019, and 2020. Mean EC50 values of known sensitive A. pisi isolates were 0.48 µg/ml for pyraclostrobin and 0.38 µg/ml for prothioconazole (Figure 7B). Mean sensitivities of isolates collected in 2019 to 2020 were significantly lower than the mean sensitivities of baseline isolates for both fungicides. A. pisi isolates collected in 2019 and 2020 did not differ in mean sensitivity to pyraclostrobin; however, isolates collected in 2020 displayed significantly lower mean sensitivity to prothioconazole when compared to isolates collected in 2019.
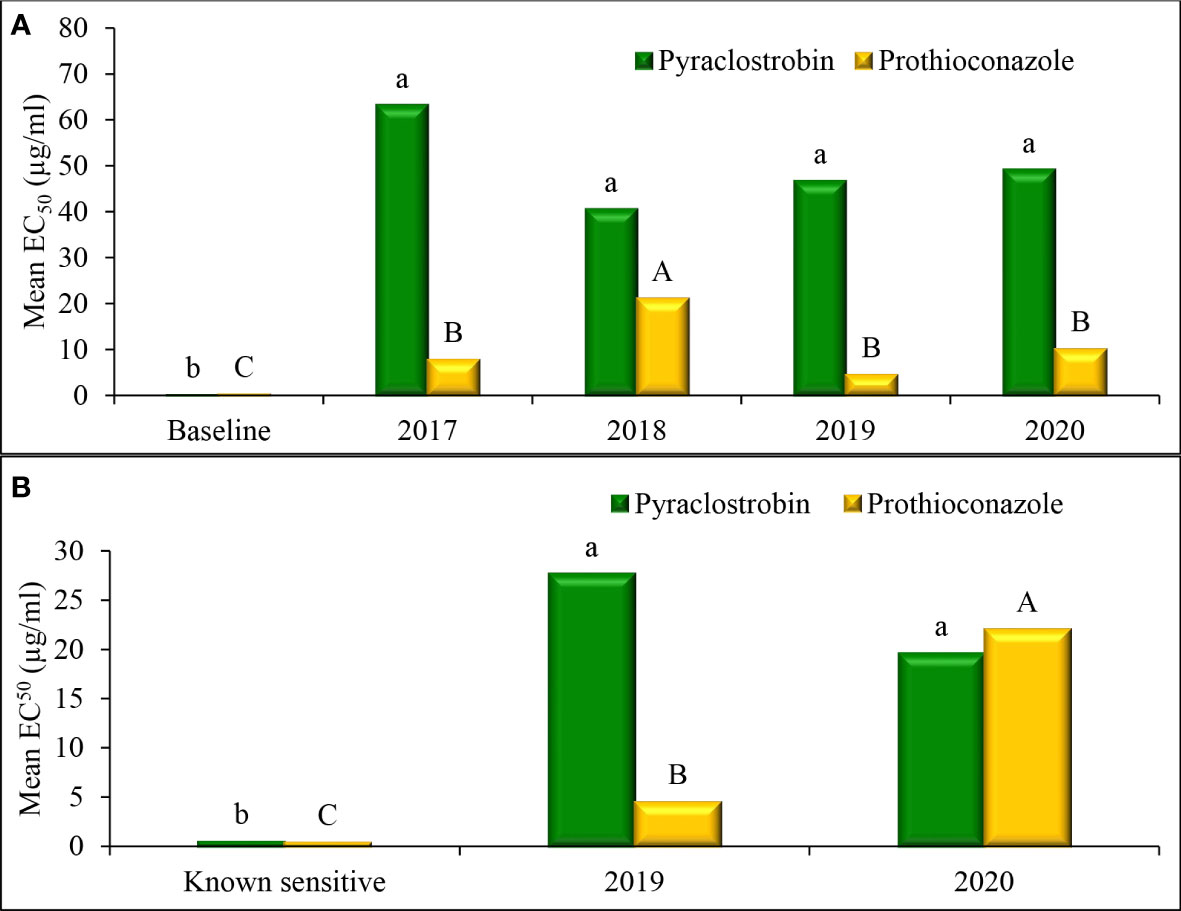
Figure 7 Mean effective concentration that inhibits mycelial growth by 50% compared with the non-fungicide-amended (EC50 µg/ml) for in vitro isolate sensitivity of (A) Didymella pinodes (n = 138) and (B) Ascochyta pisi (n = 52), to pyraclostrobin and prothioconazole across years. Within species, columns with the same letter are not significantly different based on Fisher’s protected least significant difference (α = 0.05).
Pearson correlation analysis comparing EC50 values for pyraclostrobin and prothioconazole sensitivities of D. pinodes isolates collected from 2017 to 2020 was not significant (r = -0.1128; P = 0.1927); however, sensitivities of a sub-set of isolates appear to be correlated (Figure 8A). Pearson correlation analysis comparing EC50 values for pyraclostrobin and prothioconazole sensitivities of individual A. pisi isolates collected from 2019 to 2020 was not significant (r = -0.2172; P = 0.1297) (Figure 8B). However, in vitro sensitivities for both pyraclostrobin and prothioconazole of 92% (n = 124) of D. pinodes and 98% (n = 49) of A. pisi isolates collected during the disease survey from 2017 to 2020 were lower than the sensitivities of the baseline/known sensitive isolates evaluated during the current study (Supplementary Table S1; Supplementary Table S2).
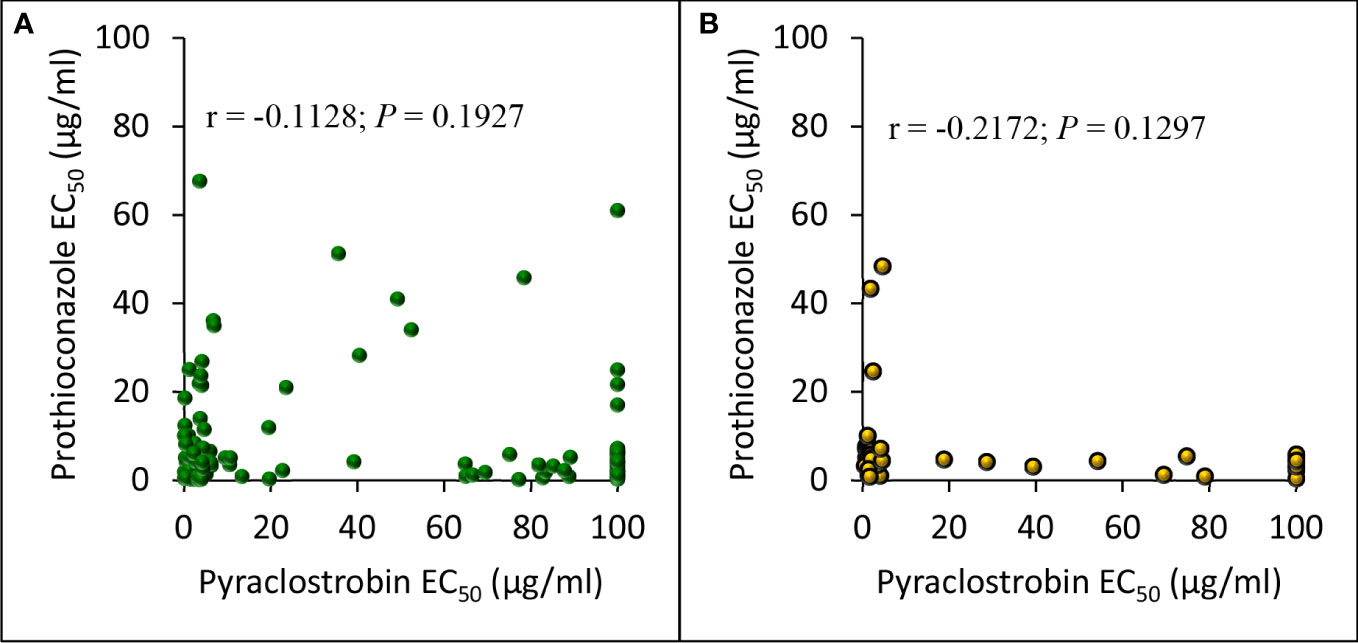
Figure 8 Pearson’s correlation between in vitro pyraclostrobin and prothioconazole sensitivity (EC50 µg/ml) of (A), Didymella pinodes (n = 135) and (B), Ascochyta pisi (n = 50) isolates collected from 2017 to 2020, and 2019 to 2020, respectively.
3.3 Aggressiveness of D. pinodes and A. pisi isolates
All eight D. pinodes and six A. pisi isolates evaluated in the greenhouse were determined to be pathogenic to cultivar ‘DS Admiral’. Disease severity caused by isolates of D. pinodes ranged from 78 to 86% and was not significantly different across the eight isolates evaluated (Table 2). Significant differences were observed across the six A. pisi isolates evaluated, where disease severity ranged from 56 to nearly 84%. Isolate Dp-3, collected in 2016, was significantly less aggressive than all other A. pisi isolates with the exception of isolate D2, collected in Montana in 2015. Aggressiveness of A. pisi isolate D2 was not different than isolate 10-4 collected from North Dakota in 2019. No significant difference in aggressiveness was observed among the four A. pisi isolates collected from North Dakota in 2019.
3.4 In vivo efficacy of pyraclostrobin and prothioconazole on D. pinodes and A. pisi
Independent analysis of pyraclostrobin in vivo disease control experiments for D. pinodes (P = 0.1086) and A. pisi (P = 0.6314) determined that variances were homogenous; thus, trials were combined within pathogen for further analysis. Significant differences in disease control provided by pyraclostrobin were observed among D. pinodes isolates (Table 2; Figure 9A). Statistical analyses divided isolates into five groups based on AUDRC, with the two baseline isolates controlled at a significantly higher level than the six non-baseline isolates evaluated. Non-baseline isolates were generally broken down by 10-fold decreases in in vitro sensitivity. As in vitro sensitivity decreased (increasing EC50 value), control provided by the fungicide decreased (decreasing AUDRC). Disease control provided by pyraclostrobin of the four isolates of A. pisi collected in 2019 was significantly lower than that of known sensitive isolates D2 and Dp-3 (Table 2; Figure 9B). A. pisi isolates collected in 2019 were divided into three statistical groups following trends in in vitro sensitivity.
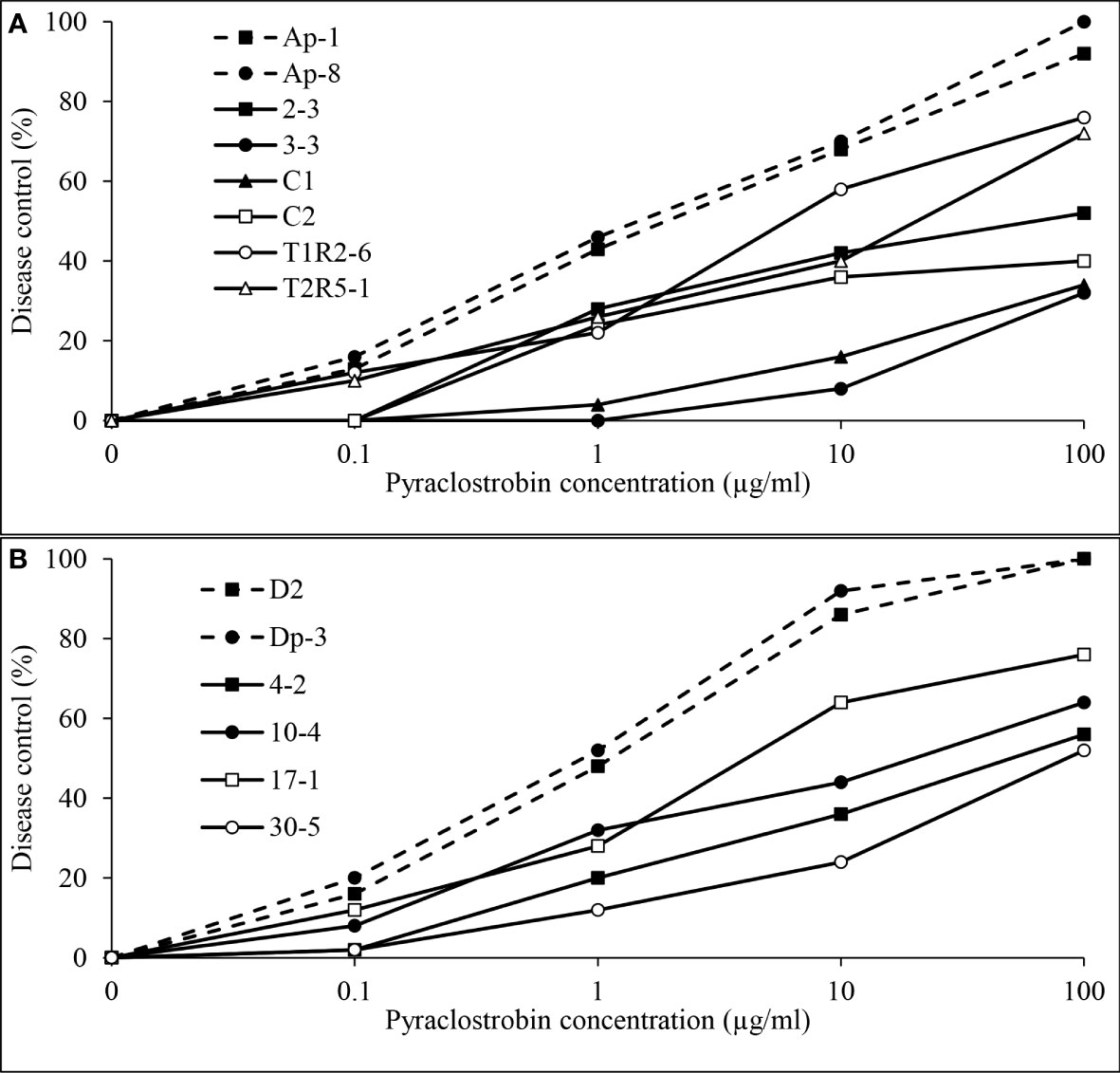
Figure 9 Mean in vivo percentage disease control by pyraclostrobin of (A), two baseline (Ap-1 and Ap-8; Delgado et al., 2011) and six Didymella pinodes isolates collected in 2017 and 2018 and (B), two Ascochyta pisi isolates collected from 2015 and 2016 (D2 and Dp-3 determined to be sensitive to pyraclostrobin; Owati et al., 2017) and four isolates collected in 2019 as determined in greenhouse assays.
Independent analysis of prothioconazole in vivo disease control experiments for D. pinodes (P = 0.7465) and A. pisi (P = 0.5217) determined that variances were homogenous and repetitions were combined within pathogen for further analysis. Disease control provided by prothioconazole of non-baseline isolates of D. pinodes was significantly lower than control of baseline isolates (Table 2; Figure 10A). Control of non-baseline isolates was statistically classified into three groups. Similar to the observations with pyraclostrobin, with decreasing in vitro sensitivity, disease control provided by prothioconazole also decreased (Table 2). Disease control of three isolates of A. pisi collected in 2019 was significantly less than the disease control provided by prothioconazole on known sensitive isolates (Table 2; Figure 10B). One isolate, 10-4, displayed similar prothioconazole in vitro sensitivity to known sensitive A. pisi isolates and was controlled similarly to those isolates under greenhouse conditions (Table 2). Linear-log simple regression analysis disclosed a significant relationship (r2 from 0.63 to 0.88) between in vitro EC50 values and in vivo AUDRC for all four fungicide:pathogen combinations (Figures 11A-D).
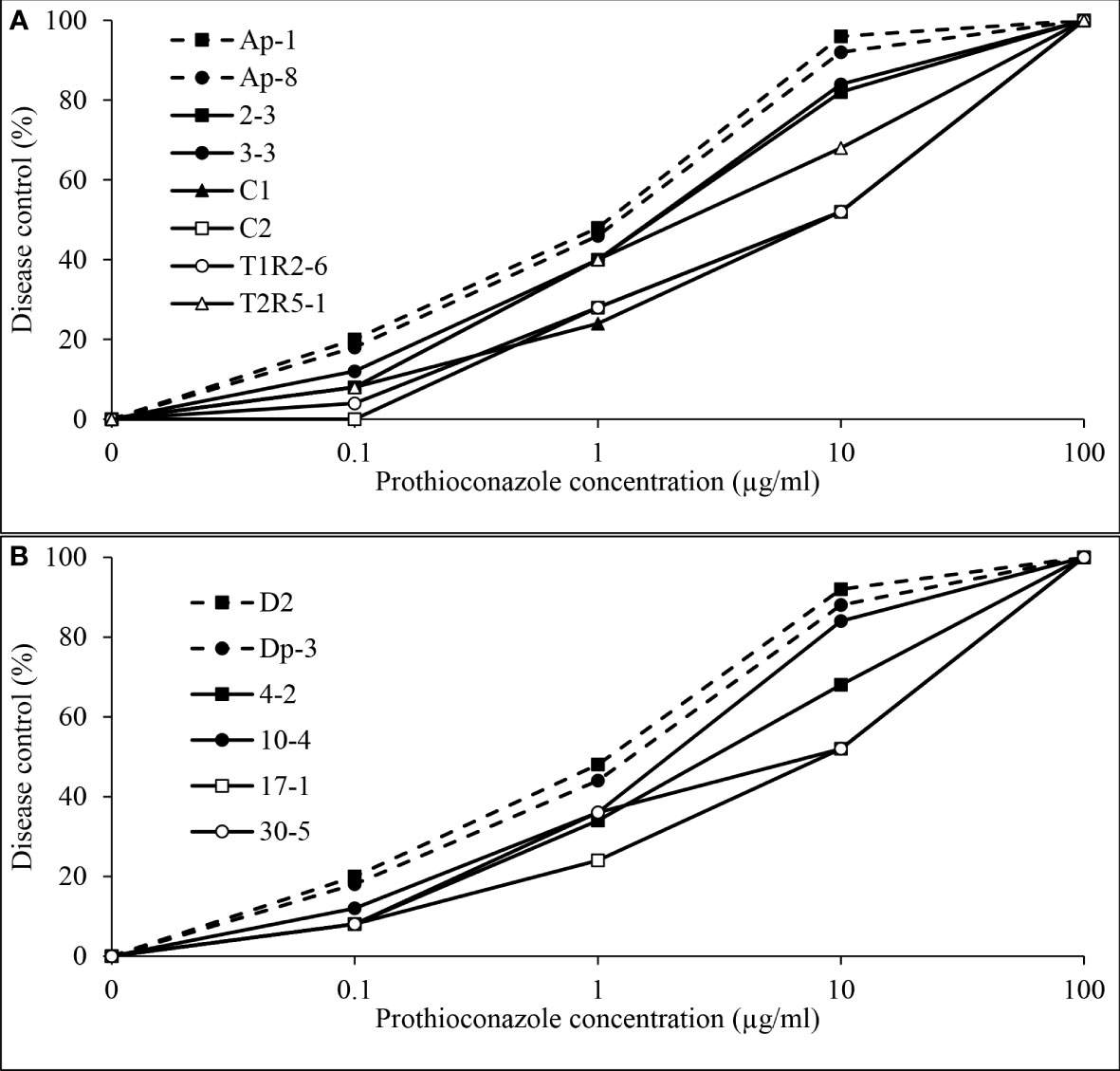
Figure 10 Mean in vivo percentage disease control by prothioconazole of (A), two baseline (Ap-1 and Ap-8; Delgado et al., 2011) and six Didymella pinodes isolates collected in 2017 and 2018 and (B), two Ascochyta pisi isolates collected from 2015 and 2016 (D2 and Dp-3; Owati et al., 2017) and four isolates collected in 2019 as determined in greenhouse assays.
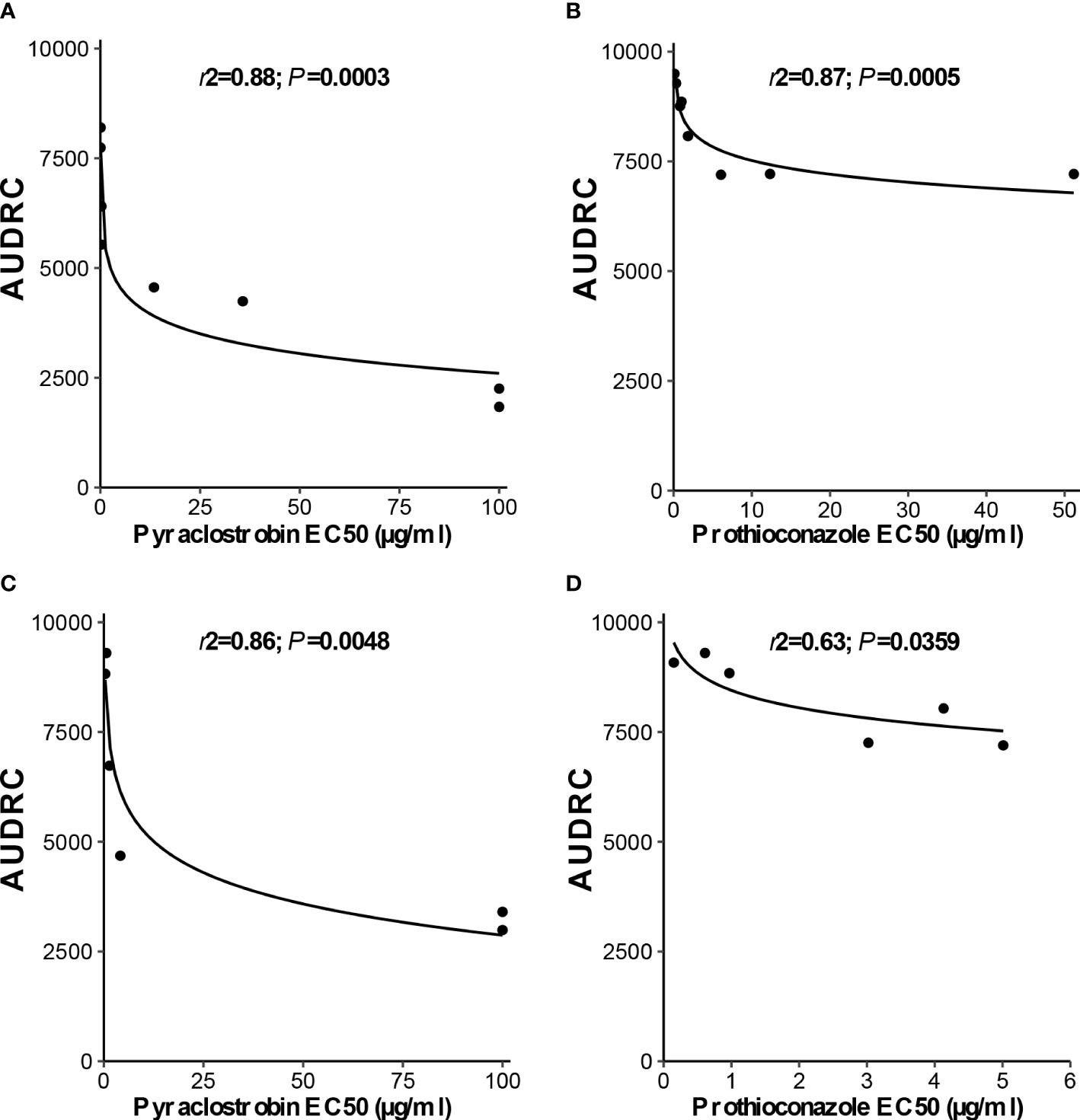
Figure 11 Linear-log simple regression comparing in vitro (A, C), pyraclostrobin and (B, D), prothioconazole sensitivity (EC50 µg/ml) and area under dose response curve values for (A, B), Didymella pinodes and (C, D), Ascochyta pisi isolates.
4 Discussion
Results from this research demonstrate that D. pinodes is the dominant foliar Ascochyta blight pathogen in North Dakota, but that A. pisi coexists and plays an important role in the state, particularly in some regions. This study also established a robust survey and monitoring approach to examine pathogen sensitivity to fungicides, and reports a shift of sensitivity of D. pinodes and A. pisi to pyraclostrobin and prothioconazole in in vitro and greenhouse assays. Pathogen prevalence, aggressiveness and fungicide sensitivity presented in this study contribute crucial knowledge which helps determine research priorities and integrated pest management recommendations to growers in North Dakota and the region.
Three fungal pathogens are associated with the Ascochyta blight disease complex in North America (Aveskamp et al., 2010). D. pinodella has been associated with disease complex in field pea seed lots tested in Montana and Saskatchewan (Sivachandra Kumar and Banniza, 2017; Owati et al., 2020), and became the prevalent species in Canada in the mid-1960s after the introduction of host resistance to A. pisi (Tivoli and Baninza, 2007). However, this pathogen was not detected during the North Dakota survey. This is likely because we isolated only from foliar and stem lesions with symptoms characteristic of Ascochyta blight; D. pinodella has been frequently isolated from pea roots, which were not evaluated during the current study (Persson et al., 1997). Differences in environmental conditions, location, and cropping systems also have been reported to impact the ecological balance among species involved in the Ascochyta blight complex of field pea (Barbetti et al., 2021).
The current research determined that all D. pinodes and A. pisi isolates evaluated were pathogenic on cultivar ‘DS Admiral’, and no difference was observed in aggressiveness among eight isolates of D. pinodes. Aggressiveness of two of six A. pisi isolates fell within the range of the D. pinodes isolates, while two A. pisi isolates from Montana were significantly less aggressive than A. pisi isolates recently collected from North Dakota. These results are only somewhat consistent with previous research indicating higher aggressiveness of D. pinodes when compared to A. pisi (Owati et al., 2020). Collection and storage conditions may have contributed to observed differences.
This is the first report we are aware of monitoring sensitivity of D. pinodes and A. pisi populations to pyraclostrobin and prothioconazole across multiple years. Evaluating a large number of isolates from a wider geographic area is critical in fungicide sensitivity monitoring, especially when loss of disease control has not been detected (Russell, 2008). In vitro inhibition of mycelial growth is less resource intensive than evaluating spore germination and has been demonstrated effective in detecting shifts in pyraclostrobin sensitivity in D. pinodes (Bowness et al., 2016). This previous research demonstrated that despite the EC50 values of the baseline D. pinodes isolates being considerably lower using spore germination when compared to mycelial growth, no differences were observed in the classification of isolates as sensitive or resistant when comparing methods. During our evaluations, in vitro sensitivity of the three baseline D. pinodes isolates collected in 2001 from North Dakota fell within the previously established baseline established using the mycelial growth assay. In vitro sensitivity of the two A. pisi isolates obtained from Montana were previously determined to be sensitive to pyraclostrobin using a discriminatory dose and a mismatch amplification mutation assay to detect the G143A mutation; therefore, direct comparisons could not be made to the in vitro EC50 results generated in our study (Owati et al., 2017).
Reductions in the efficacy of pyraclostrobin for the management of field pea Ascochyta blight were first observed in North Dakota in 2017 (Markell et al., 2018). At that time, D. pinodes isolates were obtained from typical Ascochyta blight foliar lesions from two field sites where fungicide efficacy appeared uncharacteristically low. All 14 isolates displayed reduced-sensitivity/resistance to pyraclostrobin with Rf ranging from 89.0 to 1,428.6 and reductions in efficacy were confirmed under greenhouse conditions. These observations prompted us to initiate a widescale monitoring effort to obtain a thorough understanding of the frequency and distribution of fungicide reduced-sensitivity/resistance in Ascochyta blight pathogens. Among D. pinodes and A. pisi isolates collected from 2017 to 2020, pyraclostrobin resistance and/or reduced-sensitivity were ubiquitous across sampling sites in North Dakota. All isolates collected from 2017 to 2020 were less sensitive to pyraclostrobin than the three D. pinodes baseline isolates. In addition, 96% of North Dakota isolates were less sensitive to the fungicide than the previously established baseline (Bowness et al., 2016). No baseline is available for A. pisi; therefore, our comparisons were made to two isolates previously determined to be sensitive to pyraclostrobin and again, nearly all isolates collected in 2019 and 2020 displayed lower sensitivities. The in vitro results were well-supported by greenhouse evaluations conducted across a series of 10-fold fungicide dilutions. These findings have prompted recommendations to growers that they discontinue the use of QoI fungicides for the management of Ascochyta blight in field peas.
The first field observations in North Dakota suggesting reduction in efficacy and control for pyraclostrobin occurred in 2017; however, it is unclear when resistance first developed. Results from a previous study indicated that 6% of the 304 D. pinodes isolates collected in 2010 and 2011 were highly insensitive to pyraclostrobin (Bowness et al., 2016). No isolates collected in the US displayed insensitivity, including isolates collected from North Dakota in 2010 and 2011. Insensitive D. pinodes isolates represented populations that potentially were exposed to the class of chemistry for up to eight years in Alberta and Saskatchewan. The shift in in vitro sensitivity was supported by in planta studies (Bowness et al., 2016). No reduced-sensitivity or resistance to pyraclostrobin was detected among 810 A. pisi isolates collected from 2014 to 2016 from a survey of grower fields and seed lots in Montana (Owati et al., 2017).
The Rf for D. pinodes and A. pisi isolates evaluated in this study to pyraclostrobin ranged from 2.4 to 1,428.6, and 1.2 to 208.3, respectively. A greater than 500-fold shift in sensitivity in some D. pinodes isolates and 36% of isolates displaying EC50 values >100 µg/ml lead to the hypothesis that these isolates contain the G143A mutation (Gisi et al., 2002; Wise et al., 2009; Owati et al., 2017). A similar situation was observed with A. pisi isolates where 18% of isolates displayed EC50 values >100 µg/ml pyraclostrobin. The large observed range in Rf suggests that the F129L mutation also may be present in some isolates evaluated (Pasche et al., 2004; Pasche and Gudmestad, 2008). Significant differences in control by pyraclostrobin observed among reduced-sensitive/resistant isolates of D. pinodes and A. pisi under greenhouse conditions support the hypothesis that both the F129L and G143A mutations may be present. The presence of both mutations has been reported previously in Pyricularia grisea and Pyrenophora tritici-repentis (Kim et al., 2003; Sierotzki et al., 2007). Therefore, further work is important to determine the mechanism of reduced sensitivity/resistance in these two Ascochyta blight pathogens.
Little research has been conducted evaluating sensitivity of Ascochyta blight pathogens to prothioconazole. A prothioconazole sensitivity monitoring program was established in North Dakota due to the detection of QoI reduced sensitivity/resistance. Baseline isolates of D. pinodes and known sensitive isolates of A. pisi evaluated in the current study displayed relatively low levels of variability in response to prothioconazole, with the difference between the most- and least-sensitive isolates 3.2- and 4.1-fold, respectively. High levels of variability in prothioconazole response were reported for 29 D. pinodes isolates collected from the Pacific Northwest region of the US and 23 isolates collected from Alberta and Saskatchewan (Delgado et al., 2011). A bimodal pattern of sensitivity, as was observed with these baseline isolates, can result from natural variability in isolate sensitivity, or represent distinct fungicide sensitive and insensitive populations (Brent and Hollomon, 2007). However, by definition, these baseline D. pinodes isolates had no previous exposure to any DMI fungicide (Delgado et al., 2011). Prothioconazole sensitivity of 30 D. pinodes isolates collected from northeastern Montana in 2012 was similar to that observed for the most sensitive isolates of the baseline population, despite potentially being to exposed to the fungicide (Delgado et al., 2011; Lonergan et al., 2015). Sensitivity of a majority (82%) of D. pinodes isolates collected in North Dakota from 2017 to 2020 fell within the previously established baseline for prothioconazole (Delgado et al., 2011). However, 83% were less sensitive than the 2012 D. pinodes isolates from Montana which displayed a much narrower range in sensitivity (Lonergan et al., 2015). More than 90% of D. pinodes and A. pisi isolates collected from 2017 to 2020 were less sensitive to prothioconazole than the baseline/known sensitive isolates tested within our study. This suggests either the presence of a naturally occurring prothioconazole reduced-sensitive and/or resistant D. pinodes and A. pisi pathogen population in North Dakota, or more likely, that isolates may have been exposed to prothioconazole (Damicone and Smith, 2009). Previous studies reported A. rabiei isolates exposed to prothioconazole for as little as one growing season exhibited a significant decrease in in vitro sensitivity when compared to the baseline isolates (Wise et al., 2011). We are not aware of previous research studies assessing in vitro fungicide sensitivity of A. pisi to prothioconazole.
Previous studies suggest that in vitro sensitivity assays are useful in predicting shifts in fungicide efficacy, but it is necessary to conduct in vivo studies on a subset of isolates with representative in vitro sensitivities to confirm that in vitro shifts accurately correspond to reductions in disease control (Wise et al., 2009). While sensitivity of one D. pinodes isolate from Zhejiang Province, China aligned with reduced-sensitive isolates evaluated in the current study, in vitro results were not supported by in vivo fungicide efficacy evaluations in that study (Liu et al., 2016). In the greenhouse studies performed here, disease control provided by pyraclostrobin and prothioconazole was significantly lower in isolates of D. pinodes and A. pisi displaying reduced-sensitivity/resistance in in vitro assays when compared to sensitive. A 5- to 10-fold shift in in vitro sensitivity resulted in a significant reduction in disease control provided by both fungicides under greenhouse conditions. When pyraclostrobin was applied at the highest concentration (100 µg/ml), reductions in disease severity on plants inoculated with D. pinodes isolates with in vitro EC50 values of >100 µg/ml were 32 and 34%, which are commercially unacceptable levels of disease control (Wise et al., 2009). The relationship between in vitro and in vivo results is supported by the regression analyses. However, these results do not confirm a significant shift in prothioconazole sensitivity is occurring in North Dakota. To date, no loss of disease control provided by that fungicide has been reported from field pea production fields in the state.
5 Conclusions
Ascochyta blight of field pea can be devastating when the disease is inadequately managed. The results reported here reveal the diversity of pathogen populations of the Ascochyta blight complex of field pea present in North Dakota along with a shift in sensitivity of D. pinodes and A. pisi to pyraclostrobin and prothioconazole. Despite the presence of A. pisi isolates displaying similar aggressiveness to D. pinodes isolates, D. pinodes is the most abundant species in the Ascochyta blight pathogen complex of field pea in the state and is likely the most important pathogen causing Ascochyta blight. D. pinodes is considered high-risk pathogen for fungicide resistance development due to sexual recombination that increases variation in morphology, physiology, and aggressiveness. A large majority of D. pinodes and A. pisi isolates collected during this survey displayed some level of reduced-sensitivity or resistance to both fungicides in in vitro and greenhouse assays. Reductions in control of Ascochyta blight in field peas by pyraclostrobin have been reported, but no such observations have been made for prothioconazole. Therefore, growers should discontinue the use of pyraclostrobin for the management of Ascochyta blight. Results of this study provide the foundation for the development of timely, relevant and economically-viable disease management recommendations that will limit yield loss, guide future research, and potentially delay resistance development to the limited number of fungicides available to field pea producers in North Dakota.
Data availability statement
The original contributions presented in the study are included in the article/Supplementary Material. Further inquiries can be directed to the corresponding author.
Author contributions
DF: methodology, investigation, data curation, writing - review & editing. SM: writing – review & editing. MZ: statistical analyses – review & editing. ME: writing – review & editing. JP: writing – review & editing, supervision, project administration, funding procurement. All authors contributed to the article and approved the submitted version.
Funding
This work was supported by Northern Pulse Growers Association.
Acknowledgments
We thank K. Zitnick-Anderson, M. Bolgrean, and R. Lamppa for technical assistance. A. Kalil, M. Wunsch, and V. Chapara are acknowledged for their contributions to the disease survey.
Conflict of interest
The authors declare that the research was conducted in the absence of any commercial or financial relationships that could be construed as a potential conflict of interest.
Publisher’s note
All claims expressed in this article are solely those of the authors and do not necessarily represent those of their affiliated organizations, or those of the publisher, the editors and the reviewers. Any product that may be evaluated in this article, or claim that may be made by its manufacturer, is not guaranteed or endorsed by the publisher.
Supplementary material
The Supplementary Material for this article can be found online at: https://www.frontiersin.org/articles/10.3389/fpls.2023.1165269/full#supplementary-material
References
Ali, S. M., Nitschke, L. F., Dube, A. J., Krause, M. R., Cameron, B. (1978). Selection of pea lines for resistance to pathotypes of Ascochyta pinodes, A. pisi and Phoma medicaginis var. pinodella. Aust. J. Agric. Res. 29, 841–849. doi: 10.1071/AR9780841
Andersen, L., Warkentin, T., Philipp, O., Xue, A., Sloan, A. (2002). DS Admiral field pea. Can. J. Plant Sci. 82, 751–752. doi: 10.4141/P02-023
Aveskamp, M. M., de Gruyter, J., Woudenberg, J. H. C., Verkley, G. J. M., Crous, P. W. (2010). Highlights of the Didymellaceae: a polyphasic approach to characterize Phoma and related pleosporalean genera. Stud. Mycol. 65, 1–60. doi: 10.3114/sim.2010.65.01
Barbetti, M. J., Khan, T. N., Pritchard, I., Lamichhane, J. R., Aubertot, J. N., Corrales, D. C. (2021). Challenges with managing disease complexes during application of different measures against foliar diseases of field pea. Plant Dis. 105, 616–627. doi: 10.1094/PDIS-07-20-1470-RE
Barilli, E., Cobos, M. J., Rubiales, D. (2016). Clarification on host range of Didymella pinodes the causal agent of pea ascochyta blight. Front. Plant Sci. 7. doi: 10.3389/fpls.2016.00592
Bartlett, D. W., Clough, J. M., Godwin, J. R., Hall, A. A., Hamer, M., Parr-Dobrzanski, B. (2002). The strobilurin fungicides. Pest Manage. Sci. 58, 649–662. doi: 10.1002/ps.520
Beasse, C., Ney, B., Tivoli, B. (1999). Effects of pod infection by Mycosphaerella pinodes on yield components of pea (Pisum sativum). Ann. Appl. Biol. 135, 359–367. doi: 10.1111/j.1744-7348.1999.tb00862.x
Bolton, M. D., Rivera, V., Secor, G. (2013). Identification of the G143A mutation associated with QoI resistance in Cercospora beticola field isolates from Michigan, united states. Pest Manage. Sci. 69, 35–39. doi: 10.1002/ps.3358
Bowness, R. (2013). Sensitivity of mycosphaerella pinodes to pyraclostrobin and optimizing fungicide application in field pea. (University of Alberta, Edmonton, Canada). Masters Thesis 203. doi: 10.7939/R3NT2S
Bowness, R., Gossen, B. D., Chang, K. F., Goswami, R., Willenborg, C. J., Holtz, M. (2016). Sensitivity of Mycosphaerella pinodes to pyraclostrobin fungicide. Plant Dis. 100, 192–199. doi: 10.1094/PDIS-03-15-0350-RE
Brent, K. J., Hollomon, D. W. (2007) Fungicide resistance in crop pathogens: how can it be managed? Available at: https://www.frac.info/docs/default-source/publications/monographs/monograph-1.
Budde-Rodriguez, S., Celoy, R. M., Mallik, I., Pasche, J. S., Gudmestad, N. C. (2021). Impact of SDH mutations in Alternaria solani on recently developed sdhi fungicides adepidyn and solatenol. Plant Dis. 105, 3015–3024. doi: 10.1094/PDIS-12-20-2718-RE
Chang, K. F., Ahmed, H. U., Hwang, S. F., Gossen, B. D., Strelkov, S. E., Blade, S. F. (2007). Sensitivity of field populations of Ascochyta rabiei to chlorothalonil, mancozeb and pyraclostrobin fungicides and effect of strobilurin fungicides on the progress of ascochyta blight of chickpea. Can. J. Plant Sci. 87, 937–944. doi: 10.4141/CJPS07019
Chilvers, M. I., Rogers, J. D., Dugan, F. M., Stewart, J. E., Chen, W., Peever, T. L. (2009). Didymella pisi sp. nov., the teleomorph of ascochyta pisi. Mycol. Res. 113, 391–400. doi: 10.1016/j.mycres.2008.11.017
Damicone, J., Smith, D. (2009). Fungicide resistance management (Stillwater, OK: Oklahoma State University Cooperative Extension Service).
Davidson, J. A., Gossen, B. D., Chen, W. (2021). Ascochyta complex in compendium of pea diseases and pests. 3rd edition (St. Paul, MN: APS Press). doi: 10.1094/9780890546567.fm
Davidson, J. A., Hartley, D., Priest, M., Krysinska-Kaczmarek Herdina, M., McKay, A. (2009). A new species of Phoma causes ascochyta blight symptoms on field peas (Pisum sativum) in south Australia. Mycologia 101, 120–128. doi: 10.3852/07-199
Davidson, J. A., Krysinska-Kaczmarek Herdina, M., McKay, A., Scott, E. S. (2012). Comparison of cultural growth and in planta quantification of Didymella pinodes, Phoma koolunga and Phoma medicaginis var. pinodella, causal agents of ascochyta blight on field pea (Pisum sativum). Mycologia 104, 93–101. doi: 10.3852/11-118
Delgado, J. A., Stoppler, T., Gossen, B. D., Chang, K. F., Dugan, F., Markell, S. G., et al. (2011). Sensitivity of Ascochyta pinodes populations to prothioconazole. Can. J. Plant Sci. 91, 388.
Elad, Y. (1992). Reduced sensitivity of Botrytis cinerea to two sterol biosynthesis-inhibiting fungicides: fenetrazole and fenethanil. Plant Pathol. 41, 47–54. doi: 10.1111/j.1365-3059.1992.tb02315.x
Fletcher, J. T., Wolfe, M. S. (1981). Insensitivity of Erysiphe graminis f. sp. hordei to triadimefon, triadimenol and other fungicides. proceedngs bright. Crop Prot. Conf. Pests Dis. (2), 633–640.
Fonseka, D. L., Gudmestad, N. C. (2016). Spatial and temporal sensitivity of Alternaria species associated with potato foliar diseases to demethylation inhibiting and anilino-pyrimidine fungicides. Plant Dis. 100, 1848–1857. doi: 10.1094/PDIS-01-16-0116-RE
FRAC Code List. (2022). Fungal control agents sorted by cross-resistance pattern and mode of action (including coding for FRAC Groups on product labels). Available at: frac.info/docs/default-source/publications/frac-code-list/frac-code-list-2022--final.pdf?sfvrsn=b6024e9a_2.
Friskop, A., Markell, S. G., Khan, M. (2022). North Dakota field crop plant disease management guide (Fargo, ND: North Dakota State University Cooperative Extension Service).
Gisi, U., Sierotzki, H., Cook, A., McCaffery, A. (2002). Mechanisms influencing the evolution of resistance to qo inhibitor fungicides. Pest Manage. Sci. 58, 859–867. doi: 10.1002/ps.565
Golani, M., Abbo, S., Sherman, A., Frenkel, O., Shtienberg, D. (2016). The temperature response and aggressiveness of Peyronellaea pinodes isolates originating from wild and domesticated Pisum sp. in Israel. Phytopathology 106, 824–832. doi: 10.1094/PHYTO-11-15-0306-R
Ishii, H. (2008). “Fungicide research in Japan, an overview. In: modern fungicides and antifungal compounds IV,” in Proceedings of the 15th International Reinhardsbrunn Symposium. Eds. Dehne H-W, Gisi U, Kuck K-h, Russell P E, Lyr H.
Karaoglanidis, G. S., Ioannidis, P. M., Thanassoulopoulos, C. C. (2000). Reduced sensitivity of cercospora beticola isolates to sterol-demethylation-inhibiting fungicides. Plant Pathol. 49, 567–572. doi: 10.1046/j.1365-3059.2000.00488.x
Keirnan, E. C., Davidson, J. A., Correll, R. L., Scott, E. S. (2020). Host range investigation of Phoma koolunga, a causal agent of ascochyta blight of field pea. australas. Plant Pathol. 49, 707–719. doi: 10.1007/s13313-020-00747-z
Kim, Y. S., Dixon, E. W., Vincelli, P., Farman, M. L. (2003). Field resistance to strobilurin (QoI) fungicides in Pyricularia grisea caused by mutations in the mitochondrial cytochrome b gene. Phytopathology 93, 891–900. doi: 10.1094/PHYTO.2003.93.7.891
Le May, C., Chilvers, M. I., Saucereau, A. L., Guibert, M., Peever, T. L. (2018). Spatiotemporal distribution of Ascochyta pinodes and Ascochyta pinodella during the winter growing season in France. Plant Pathol. 67, 1031–1045. doi: 10.1111/ppa.12823
Li, Y. P., You, M. P., Finnegan, P., Khan, T. N., Lanoiselet, V., Eyres, N. (2012). First report of black spot caused by Boeremia exigua var. exigua on field pea in Australia. Plant Dis. 96, 148–149. doi: 10.1094/PDIS-08-11-0637
Li, Y. P., You, M. P., Khan, T. N., Finnegan, P., Barbetti, M. J. (2011). First report of Phoma herbarum on field pea (Pisum sativum) in Australia. Plant Dis. 95, 1590–1590. doi: 10.1094/PDIS-07-11-0594
Liu, N., Xu, S., Yao, X., Zhang, G., Mao, W., Hu, Q. (2016). Studies on the control of ascochyta blight in field peas (Pisum sativum l.) caused by Ascochyta pinodes in zhejiang province, china. front. Microbiol 7. doi: 10.3389/fmicb.2016.00481
Lonergan, E., Pasche, J., Skoglund, L., Burrows, M. (2015). Sensitivity of Ascochyta species infecting pea, lentil, and chickpea to boscalid, fluxapyroxad, and prothioconazole. Plant Dis. 99, 1254–1260. doi: 10.1094/PDIS-06-14-0620-RE
Markell, S. G., Pasche, J. S., Wunsch, M. (2018). Confirmed report of fungicide resistance in the field pea Ascochyta/Mycosphaerella blight pathogen. North Dakota State Univ. Coop. Ext. Service 8, 9–10.
Mathew, F. M., Goswami, R. S., Markell, S. G., Osborne, L., Tande, C., Ruden, B. (2010). First report of ascochyta blight of field pea caused by ascochyta pisi in south Dakota. Plant Dis. 94, 789. doi: 10.1094/PDIS-94-6-0789A
Milliken, G. A., Johnson, D. E. (1992). “One-way treatment structure in a completely randomized design with heterogeneous errors,” in Analysis of messydata (London: Chapman and Hall), 16–28.
Onfroy, C., Tivoli, B., Corbiere, R., Bouznad, Z. (1999). Cultural, molecular and pathogenic variability of Mycosphaerella pinodes and Phoma medicaginis var. pinodella isolates from dried pea (Pisum sativum) in France. Plant Pathol. 48, 218–229. doi: 10.1046/j.1365-3059.1999.00323.x
Owati, A., Agindotan, B., Burrows, M. (2019). Development and application of real-time and conventional SSR-PCR assays for rapid and sensitive detection of Didymella pisi associated with ascochyta blight of dry pea. Plant Dis. 103, 2751–2758. doi: 10.1094/PDIS-02-19-0381-RE
Owati, A., Agindotan, B., Burrows, M. (2020). Characterization of fungal species associated with ascochyta blight of dry pea in Montana and north America and development of a differential medium for their detection. Plant Heal. Prog. 21, 262–271. doi: 10.1094/PHP-05-20-0037-RS
Owati, A. S., Agindotan, B., Pasche, J. S., Burrows, M. (2017). The detection and characterization of QoI-resistant Didymella rabiei causing ascochyta blight of chickpea in Montana. Front. Plant Sci. 8. doi: 10.3389/fpls.2017.01165
Pasche, J. S., Gudmestad, N. C. (2008). Prevalence, competitive fitness and impact of the F129L mutation in Alternaria solani from the united states. Crop Prot. 27, 427–435. doi: 10.1016/j.cropro.2007.07.011
Pasche, J. S., Wharam, C. M., Gudmestad, N. C. (2004). Shift in sensitivity of Alternaria solani in response to QoI fungicides. Plant Dis. 88, 181–187. doi: 10.1094/PDIS.2004.88.2.181
Peever, T. L., Barve, M. P., Stone, L. J., Kaiser, W. J. (2007). Evolutionary relationships among Ascochyta species infecting wild and cultivated hosts in the legume tribes cicereae and vicieae. Mycologia 99, 59–77. doi: 10.1080/15572536.2007.11832601
Persson, L., Bødker, L., Larsson-Wikström, M. (1997). Prevalence and pathogenicity of foot and root rot pathogens of pea in southern Scandinavia. Plant Dis. 81, 171–174. doi: 10.1094/PDIS.1997.81.2.171
R Core Team (2022). R: a language and environment for statistical computing (Vienna, Austria: R Foundation for Statistical Computing). Available at: https://www.R-project.org/.
Reimann, S., Deising, H. B. (2005). Inhibition of efflux transporter-mediated fungicide resistance in Pyrenophora tritici-repentis by a derivative of 4′-hydroxyflavone and enhancement of fungicide activity. Appl. Environ. Microbiol. 71, 3269–3275. doi: 10.1128/AEM.71.6.3269-3275.2005
Russell, P. E. (2008). Fungicide resistance action committee (FRAC). Outlooks Pest Manag 17, 119–121. doi: 10.1564/17jun07
Schepers, H. T. A. M. (1983). Decreased sensitivity of Sphaerotheca fuliginea to fungicides which inhibit ergosterol biosynthesis. Netherlands J. Plant Pathol. 89, 185–187. doi: 10.1007/BF01999846
Schmidt, L. (2021). The pea report: all things ascochyta (Carman, MB: Manitoba Pulse and Soybean Growers).
Sheridan, J. E., Grbavac, N., Sheridan, M. H. (1985). Triadimenol insensitivity in Pyrenophora teres. Trans. Br. Mycol. Soc 85, 338–341. doi: 10.1016/S0007-1536(85)80198-4
Sierotzki, H., Frey, R., Wullschleger, J., Palermo, S., Karlin, S., Godwin, J., et al. (2007). Cytochrome b gene sequence and structure of Pyrenophora teres and P. tritici-repentis and implications for QoI resistance. Pest Manage. Sci.: formerly Pesticide Sci. 63, 225–233. doi: 10.1002/ps.1330
Sivachandra Kumar, N. T., Banniza, S. (2017). Assessment of the effect of seed infection with Ascochyta pisi on pea in western Canada. Front. Plant Sci. 8. doi: 10.3389/fpls.2017.00933
Skoglund, L. G., Harveson, R. M., Chen, W., Dugan, F., Schwartz, H. F., Markell, S. G. (2011). Ascochyta blight of peas. Plant Heal. Prog. 12. doi: 10.1094/php-2011-0330-01-rs
Spolti, P., Del Ponte, E. M., Dong, Y., Cummings, J. A., Bergstrom, G. C. (2014). Triazole sensitivity in a contemporary population of Fusarium graminearum from new York wheat and competitiveness of a tebuconazole-resistant isolate. Plant Dis. 98, 607–613. doi: 10.1094/PDIS-10-13-1051-RE
Thomas, A., Langston, D. B., Sanders, H. F., Stevenson, K. L. (2012). Relationship between fungicide sensitivity and control of gummy stem blight of watermelon under field conditions. Plant Dis. 96, 1780–1784. doi: 10.1094/PDIS-02-12-0129-RE
Tivoli, B., Baninza, S. (2007). Comparison of the epidemiology of ascochyta blights on grain legumes. Can. J. Plant Pathol. 119, 59–76. doi: 10.1007/s10658-007-9117-9
Tran, H. S., Li, Y. P., You, M. P., Khan, T. N., Pritchard, I., Barbetti, M. J. (2014). Temporal and spatial changes in the pea black spot disease complex in Western Australia. Plant Dis. 98, 790–796. doi: 10.1094/PDIS-08-13-0806-RE
United States Department of Agriculture and National Agriculture Statistics Service (2022). Available at: https://usda.library.cornell.edu/concern/publications/tm70mv177.
Wickham, H. (2016). “ggplot2: elegant graphics for data analysis,”(Springer-Verlag New York), ISBNISBN 978-3-319-24277-4. 212. doi: 10.1007/978-0-387-98141-3
Wise, K. A., Bradley, C. A., Markell, S., Pasche, J., Delgado, J. A., Goswami, R. S. (2011). Sensitivity of Ascochyta rabiei populations to prothioconazole and thiabendazole. Crop Prot. 30, 1000–1005. doi: 10.1016/j.cropro.2011.01.009
Wise, K. A., Bradley, C. A., Pasche, J. S., Gudmestad, N. C. (2009). Resistance to QoI fungicides in Ascochyta rabiei from chickpea in the northern great plains. Plant Dis. 93, 528–536. doi: 10.1094/PDIS-93-5-0528
Wise, K. A., Bradley, C. A., Pasche, J. S., Gudmestad, N. C., Dugan, F. M., Chen, W. (2008). Baseline sensitivity of Ascochyta rabiei to azoxystrobin, pyraclostrobin, and boscalid. Plant Dis. 92, 295–300. doi: 10.1094/PDIS-92-2-0295
Keywords: Ascochyta blight, Didymella pinodes, Ascochyta pisi, pyraclostrobin, prothioconazole, fungicide resistance, Pisum sativum L.
Citation: Fonseka DL, Markell SG, Zaccaron ML, Ebert MK and Pasche JS (2023) Ascochyta blight in North Dakota field pea: the pathogen complex and its fungicide sensitivity. Front. Plant Sci. 14:1165269. doi: 10.3389/fpls.2023.1165269
Received: 13 February 2023; Accepted: 29 June 2023;
Published: 02 August 2023.
Edited by:
Weidong Chen, Agricultural Research Service, United StatesReviewed by:
Prabhakaran Sambasivam, Griffith University, AustraliaMalkhan Singh Gurjar, Indian Agricultural Research Institute (ICAR), India
Syama Chatterton, Agriculture and Agri-Food Canada (AAFC), Canada
Copyright © 2023 Fonseka, Markell, Zaccaron, Ebert and Pasche. This is an open-access article distributed under the terms of the Creative Commons Attribution License (CC BY). The use, distribution or reproduction in other forums is permitted, provided the original author(s) and the copyright owner(s) are credited and that the original publication in this journal is cited, in accordance with accepted academic practice. No use, distribution or reproduction is permitted which does not comply with these terms.
*Correspondence: Julie S. Pasche, Julie.Pasche@NDSU.edu