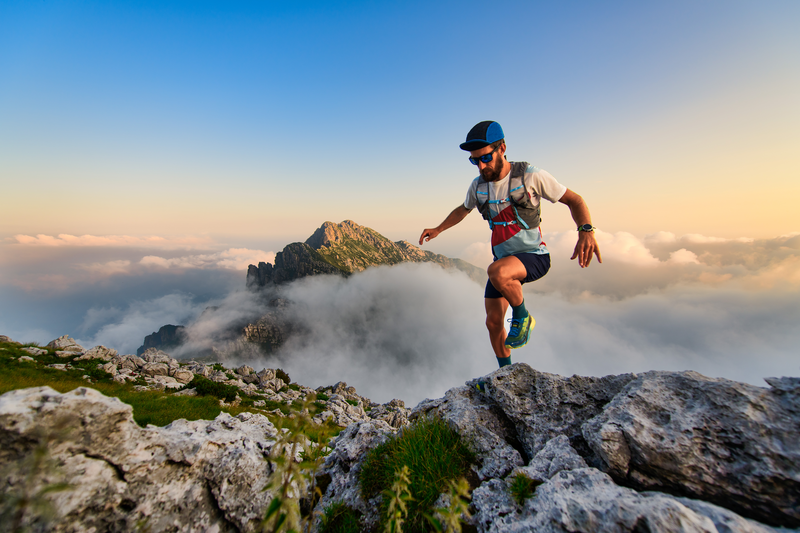
94% of researchers rate our articles as excellent or good
Learn more about the work of our research integrity team to safeguard the quality of each article we publish.
Find out more
REVIEW article
Front. Plant Sci. , 22 August 2023
Sec. Plant Breeding
Volume 14 - 2023 | https://doi.org/10.3389/fpls.2023.1160695
This article is part of the Research Topic Generating Useful Genetic Variation in Crops by Induced Mutation, Volume III View all 6 articles
Since its introduction in 2000, the TILLING strategy has been widely used in plant research to create novel genetic diversity. TILLING is based on chemical or physical mutagenesis followed by the rapid identification of mutations within genes of interest. TILLING mutants may be used for functional analysis of genes and being nontransgenic, they may be directly used in pre-breeding programs. Nevertheless, classical mutagenesis is a random process, giving rise to mutations all over the genome. Therefore TILLING mutants carry background mutations, some of which may affect the phenotype and should be eliminated, which is often time-consuming. Recently, new strategies of targeted genome editing, including CRISPR/Cas9-based methods, have been developed and optimized for many plant species. These methods precisely target only genes of interest and produce very few off-targets. Thus, the question arises: is it the end of TILLING era in plant studies? In this review, we recap the basics of the TILLING strategy, summarize the current status of plant TILLING research and present recent TILLING achievements. Based on these reports, we conclude that TILLING still plays an important role in plant research as a valuable tool for generating genetic variation for genomics and breeding projects.
Mutation induction through chemical or physical mutagenesis has long been used in plant breeding as it creates new alleles and can lead to the development of new, agronomically important traits (for further reading see Maluszynski et al., 2017). It is also used in genetic studies for functional analysis of mutated genes. There are two main approaches to such studies: “forward” and “reverse”. The classical forward approach starts with the identification of an interesting phenotype and then the mutated gene responsible for this phenotype can be searched for, usually through mapping and positional cloning. The “reverse” approach is the other way around – first, the mutation within the gene of interest is identified and afterward the phenotype caused by this mutation is analyzed (Griffiths et al., 2004).
TILLING (Targeting Induced Local Lesion IN Genomes) was first developed for Arabidopsis thaliana as an alternative to insertional mutagenesis. It was described as a reverse genetic strategy that combines traditional random chemical mutagenesis with rapid mutational screening for induced lesions in genes of interest (McCallum et al., 2000a; McCallum et al., 2000b). However, once established, a TILLING population may be used for forward genetic studies as well. Immediately after its invention, TILLING has been adapted and deployed for large-scale screening of induced mutations not only in Arabidopsis but also in other plants (reviewed in Colbert et al., 2001; Henikoff et al., 2004; Stemple, 2004). At the beginning of TILLING, one of the biggest limitations of this technology was the necessity of knowing the sequence of the gene of interest (or at least its fragment) and TILLING relied on the emergence of practical genome sequence tools. However, as more and more plant species have been fully sequenced in recent years and there is an enormous amount of sequence data and bioinformatic tools for their analysis, these issues are becoming less limiting. Additionally, throughout the years TILLING is being continuously modified to make it more robust, especially in terms of mutation identification (discussed in the next section). The general scheme of TILLING strategy is described in detail in Szarejko et al, 2017 and Szurman-Zubrzycka et al., 2017, and illustrated briefly in Figure 1.
Figure 1 The general flow of traditional TILLING strategy. The first step is the mutagenesis of seeds. The seeds are propagated into further generations and DNA is isolated from M2 plants individually. The next step is DNA pooling, followed by the PCR reactions for specific amplicons. There are many methods of mutation identification - either direct or based on heteroduplex analysis. After the identification of a particular plant carrying a mutation in a gene of interest, the phenotypic evaluation should be performed to assign the gene function. DHPLC, Denaturing High-Performance Liquid Chromatography; HRM, High-Resolution Melting; CEL I- endonuclease extracted from a Celery Juice Extract; NGS, Next Generation Sequencing.
The goal of mutagenesis for TILLING is to obtain a highly mutagenized population to increase the probability of finding a mutation in every gene. Thus, it is crucial to select a proper mutagen and its dose. The sensitivity for mutagen treatment varies between plant species and even between various genotypes of the same species. In general, the lower the mutation frequency, the larger population is needed, which leads to higher costs and labor efforts. On the other hand, too high mutation frequency may cause lethality or sterility of M1 plants. The most popular mutagen used for the creation of TILLING populations is EMS (Ethyl methanesulphonate) which is an alkylating agent causing mainly G/C to A/T transitions (Kurowska et al., 2011). The mutation density in different TILLING populations is very diverse and ranges from ~1/7 Mb to 1/20 kb (Table 1; Till et al., 2018). Once the dose of a mutagen is optimized, the TILLING platform may be created. For generatively propagated crops, the material to be mutagenized is usually the seeds (Jankowicz-Cieslak et al., 2011). This is the best choice because seed mutagenesis is technically easy, there are no temporal or developmental stage restrictions for treatment, relatively high mutagen doses can be applied to dormant seeds, and they are easy to store and transport. Only mutations induced in generative line cells in the seed embryo are inherited, hence all molecular analyses aimed at the identification of induced mutations should be performed starting with the M2 generation. In regard to vegetatively propagated crops, such as banana (Musa paradisica L.), peppermint (Mentha L.) or sugarcane (Saccharum officinarum), the generation of stable mutants is more problematic. The meristematic buds (e.g. tubers, bulbs, rhizomes) or cell or tissue cultures in vitro are usually target materials for mutagenesis (Jankowicz-Cieslak et al., 2012; Jankowicz-Cieslak and Till, 2016). The chimera dissociation usually has to be performed e.g. by adventitious regeneration from a single somatic cell or by somatic embryogenesis (Geier, 2012). For generatively propagated plants, only one seed from the M1 plant should be propagated into the next generation (M2) in order to avoid the repetitions of the same mutations during screening. A very important aspect is the maintenance of mutagenized generations – each M2 plant and its M3 progeny should be assigned a unique code and seeds from each plant should be collected separately.
The mutation screening is performed on the M2 generation, because of the chimerism of the M1 generation owing to the fact that seeds are multi-cellular and only a subset of the mutagenized cells are responsible for germline development. The DNA should be isolated from each M2 plant individually (one M2 plant per M1 parent) and is usually pooled prior to screening for mutations. There are many screening methods aimed at mutation identification - they differ in sensitivity, capacity, difficulty, and costs. These methods are briefly described in the following section. Depending on the methods of mutation screening used, there is a different schema of DNA sample pooling. The M2 plants (and further generation) may be also directly phenotypically characterized for a forward genetic approach. Importantly, the proper maintenance of seeds from all generations (in a seed bank) and DNA stocks isolated individually from M2 plants are crucial to make the TILLING platform long-lasting. In order to make a TILLING platform publicly available, it is indispensable to create a database where all data about phenotypes, mutations identified and seed availability is collected.
The first TILLING population was developed for Arabidopsis thaliana in 2000. Because TILLING is a method that can be easily applied to most organisms, shortly afterwards new TILLING populations for other plant species were produced. Examples of TILLING platforms created for various, diploid and polyploid, plant species are presented in Table 1.
The discovery that mutations can be induced revolutionized plant breeding. The first crop mutant variety (‘Chlorina’ – a variety of tobacco) was introduced in the 1930s (Tollenaar, 1934; Tollenaar, 1938) and methods of mutation induction with the use of physical or chemical mutagens have long been established for many plant species. In the TILLING approach, mutation induction is followed by mutation identification in the genes of interest. The detection of mutations at the DNA base level became possible with the development of sequencing methods beginning in the 1970s. In recent years DNA sequencing methodologies have experienced a renaissance, with methods continually evolving. This is resulting in higher throughput and lower costs for mutation discovery and other applications.
In the first TILLING experiments performed on Arabidopsis thaliana the method of point mutation identification was based on DHPLC (Denaturing High-Performance Liquid Chromatography) (McCallum et al., 2000a; McCallum et al., 2000b). Arabidopsis seeds were treated with EMS, DNA was isolated from M2 individuals and pooled, PCR reactions for selected gene regions were performed and after denaturation and annealing (allowing heteroduplex formation in the case of mutation appearance) the DHPLC method was used. This method allows the detection of heteroduplex in a pool as an additional peak in the chromatogram, because of differential melting kinetics of homo- and heteroduplexes of DNA. After the identification of the potential mutations in particular M2 plants, the mutant PCR products were sequenced to confirm the mutation. The DHPCL method is very sensitive, however, it is relatively low throughput. Another method used for direct analysis of heteroduplex appearance is HRM (High-Resolution Melting) which is very fast but restricted only to fragments of max. 400 bp length (Gundry et al., 2003; Szurman-Zubrzycka et al., 2017). In order to increase the efficiency of mutation discovery, single-strand-specific nucleases began to be used (Colbert et al., 2001). The most commonly used endonuclease is CEL I which recognizes heteroduplexes and cuts them in a position of mismatch (position of induced mutation). The observation of cleaved products was typically performed with the use of LI-COR sequencers, where up to 768 samples may be analyzed on one gel, in the case of 8-fold DNA pooling (Colbert et al., 2001). For the first two decades of TILLING research, this was the most common method of mutation discovery. To reduce the cost of TILLING screening it is possible to visualize the products of digestion on an agarose gel, but this method is not very sensitive and some mutations may go unnoticed. Recently, capillary sequencers (e.g. AdvanceCE 96 FS or Fragment Analyzer) are also becoming the instruments of choice for the identification of heteroduplex cleavage products (Jost et al., 2019).
Currently, the rapid identification of all mutations present in the mutant genome may be done through the NGS approach (‘TILLING by Sequencing’), either directly or using different strategies of sample pooling (one-, two- or three-dimensional) (e.g. Tsai et al., 2011; Fanelli et al., 2021). The huge restriction for broad usage of NGS for TILLING is its cost, however, NGS is becoming noticeably cheaper over time. Another problem is the production of very large datasets for bioinformatic analysis. Nevertheless, the collection of genomic data from individual plants from mutagenized populations opens up new possibilities for TILLING approach. One can simply screen collected data for plants carrying mutations in a gene (regions) of interest and order the seeds from a developer of TILLING population (Krasileva et al, 2017). To reduce the size of obtained data and to minimalize the acquisition of unnecessary data, ‘TILLING by Sequencing’ may be restricted only to coding fragments of DNA, when performing exome capture instead of whole genome sequencing. NGS methods may be also applied to screen a set of pooled PCR amplicons instead of sequencing of whole genome/exome. There are many different NGS technologies described up till now, with Illumina being the most popular in TILLING projects. However, we can suppose that in the future the long-read sequencing technologies, such as PacBio and Oxford Nanopore sequencing, or new technology like Linked-Read (10xGenomics), will also be used in TILLING strategy. This can contribute to increasing sequencing accuracy or improving the balance between costs and depth of sample sequencing. The methods of mutation identification used in different TILLING projects are shown in Table 1. Recently, Wang et al. (2023) showed a very exciting example of how to boost wheat functional genomics using a mutant population of common winter wheat variety KN9204 generated by EMS treatment, similar to the majority of TILLING population, however, authors did not describe their strategy as TILLING. Studies aimed to obtain gene-indexed mutants in every coding gene in the wheat genome, which is a huge effort from one side, but offers great opportunities from the other. The use of NGS technology, specifically exome capture sequencing of 2,090 mutant lines revealed that for almost all coding genes (99%) this goal was achieved. Potentially, it might be also the direction of progress in the analysis of TILLING population in other plant species.
Another new approach used to screen mutagenized populations is called FIND-IT (Fast Identification of Nucleotide variants by droplet DigITal PCR) (Knudsen et al., 2022). It provides ultrafast screening (10 days) for targeted genetic variants of interest (at single-nucleotide resolution). FIND-IT combines large-scale sample pooling with highly sensitive droplet digital PCR (ddPCR) for genotyping. It was efficiently validated for barley by fast screening of variant libraries from 500,000 individuals and isolating more than 125 targeted gene knockouts and other interesting variants (Knudsen et al., 2022).
Even though the first TILLING service was developed more than 20 years ago, this strategy is still broadly used in plant research studies, especially for agronomically important species. TILLING mutants are used to study the genetic bases of many important traits related to plant development and response to various biotic and abiotic stresses. TILLING is also used to create genotypes with improved important agronomic traits e.g. related to climate change, and hence it holds great promise for addressing global challenges in agriculture and food security and may be helpful in achieving some of the Sustainable Development Goals (SDGs) adopted by the United Nations General Assembly in 2015 as part of the 2030 Agenda for Sustainable Development (https://sdgs.un.org). The newest achievements generated through the TILLING approach are illustrated in Table 2, where we describe the goal of using TILLING in a particular study, a species and genotype used for TILLING screening, a gene and encoded protein analyzed, its biological function, the created mutants and their characteristics.
Table 2 The recent achievements of TILLING in basic studies of gene function and/or breeding of commercial cultivars.
There is a threat that in the future we will face even more severe than now problems in terms of climate change and global warming. Extreme weather events such as heatwaves, drought, wildfires on the one hand, and flooding on the other, salinization of the soil, or violent weather phenomena like hurricanes, will happen more often. We are all witnesses of global climate change. It has been noticed that in the years 2011-2022 the temperature increased by 1.09°C (IPCC, 2022). The newest prediction presented by the sixth Assessment Report of the Intergovernmental Panel on Climate Change (IPCC, 2022) showed that in the 21st century, we can expect the global mean temperature to increase between 1 to 5.7°C, depending on the scenarios, i.e. taking into account the level of greenhouse gas emission. Global warming already affects agriculture, and in the long term also food security. It has been estimated by a global-scale meta-analysis that one Celsius degree increase in global mean temperature will lead to a yield loss in four major crops: wheat (Triticum aestivum L., 6%), rice (Oryza sativa L, 3.2%), maize (Zea mays, 7.4%), and soybean (Glycine max L., 3.1%) (Zhao et al., 2017). The TILLING approach has been used to study response to abiotic stresses imposed by climate changes (drought, salinity, Al toxicity) in many important crops.
Drought belongs to abiotic stresses that affect crop productivity most strongly. Drought stress tolerance is a complex trait, therefore a great number and variety of genes can be selected for analysis by TILLING and both, drought-tolerant and drought-sensitive mutants have been created (Collin et al., 2020; Daszkowska-Golec et al., 2020; Marzec et al., 2020). In barley (Hordeum vulgare L.), a number of mutants in different genes encoding proteins engaged in response to drought e.g. bZIP transcription factor HvABI5 (ABA Insensitive 5), a protein involved in RNA metabolism - HvCBP20 (Cap-Binding Protein 20), or a subunit of farnesyltransferase HvERA1 (Enhanced Response to ABA1) have been developed. Some of induced alleles led to the increased tolerance to drought stress and influenced such physiological traits as stomata closure, relative water content (RWC), photosynthesis performance, membrane permeability, pigment content in leaves, and wax deposition (Daszkowska-Golec et al., 2018; Collin et al., 2020; Daszkowska-Golec et al., 2020). Tolerance to salinity, another severe abiotic stress in many agricultural regions, has also been studied using TILLING approach. The yellow mustard (Brassica rapa ssp. trilocularis) mutant carrying a substitution in a gene encoding Ca2+ cation exchanger transporter (CAX) showed a higher biomass, better photosynthetic performance, higher water use efficiency, and increased accumulation of a myo-inositol, which influenced osmotic protection under salt treatment and increased tolerance to salt stress (Navarro-León et al., 2021). Another example of employing the TILLING technique for obtaining a more salt tolerant crop comes from barrel clover (Medicago truncatula) (de Lorenzo et al., 2009). Two mutants carrying changes in SRLK (Surface Receptor-like Protein Kinase) gene showed insensitivity to the salt stress treatment in terms of root growth. This gene encodes a membrane-located receptor kinase, which plays an important role in stress signal transduction (de Lorenzo et al., 2009). In rice, nine genes associated with membrane transport – OsAKT1 (Similar to AKT1-like potassium channel), OsHKT6 (Oryza sativa high-affinity K+ transporter), OsNSCC2 (Translocation protein Sec62 family protein), OsCAX2 (Sodium/calcium exchanger protein), OsHAK11 (Potassium transporter), OsP5CS1 (Delta-1-pyrroline-5-carboxylate synthetase), OsNHX (Sodium/hydrogen Exchanger), OsNAC60 (Similar to NAM/CUC2-like protein), OsSOS1 (Similar to Na+/H+ Antiporter) were subjected for TILLING analysis (Hwang et al., 2017). In total nine mutants carrying changes in five (OsAKT1, OsHKT6, OsNSCC2, OsHAK11, OsSOS1) of nine investigated genes exhibited higher tolerance to salt treatment compared to the wild type, in regards to root and shoot growth.
TILLING mutants have been employed also in studies related to plant response to heavy metals or to aluminum (Al3+) toxicity in acid soils. Decreased heavy metal accumulation in tobacco (Nicotiana tabacum) was achieved by TILLING analysis of two genes – HMA2S and HMA4T, which encode heavy metal transporters: cadmium (Cd) and zinc (Zn). They play a role in root-to-shoot metal transport. In the identified mutants, compared to the wild type, the levels of Cd and Zn in leaves decreased by 39-97% and 35-48%, respectively (Gao et al., 2020). The homolog of one of these genes – HMA4a was selected for TILLING analysis in yellow mustard (B. rapa ssp. trilocularis). The mutant with higher tolerance to Cd and Zn was identified, which may be used in Cd phytoremediation programs (Blasco et al., 2019; Navarro-Leon et al., 2019).
Aluminum is the most common metal in the Earth’s crust. In acidic soils, it solubilizes to phytotoxic Al3+ ions that cause root growth inhibition and may lead to yield reduction (Mossor-Pietraszewska, 2001; Bhalerao and Prabhu, 2013). Crop sensitivity to toxic Al3+ ions has become an important agronomic issue because of the progressive acidification of arable lands due to industrialization, acid rain, and the overuse of ammonia- and amide-containing fertilizers. It is known that Al3+ ions induce DNA damage and activate the DDR (DNA Damage Response) pathway, which leads to cell cycle inhibition and activation of DNA repair mechanisms (Nezames et al., 2012; Jaskowiak et al., 2018). TILLING analysis has been performed in barley for the ATR gene, which encodes kinase activated upon DNA damage that is involved in DDR signaling. Two of identified mutants show increased Al tolerance, resulting from disturbances in the DDR pathway leading to normal cell cycle progression despite DNA damage caused by Al3+ (Szurman-Zubrzycka et al., 2019b). In general, increasing Al tolerance in barley is a very important task due to the fact that it is the most Al-sensitive species among cereal crops (Ishikawa et al., 2000; Wang et al., 2006; Taylor and Greene, 2003; Szurman-Zubrzycka et al., 2021).
Domestication and selection processes led to narrowing down the genetic variability for many crops. Nowadays, in breeding programs, it is necessary to reverse this process and TILLING-derived plant lines may possess desired genetic variability. Product quality is very important for consumers and, depending on the crop species, it may be related to various aspects including flavor and taste, health-promotion traits, sustainable production without the use of chemical inputs, quality, and nutritional content, or prolonged fruit shelf-life (Gascuel et al., 2017). There are many examples of using TILLING for improving product quality traits. In soybean (Glycine max L.), mutants in genes encoding four fatty acid desaturases that control saturated/unsaturated fatty acid ratio were isolated in a TILLING population that was used to decipher the oil biosynthesis pathway. Among these mutants, there were lines with improved seed oil composition – elevated oleic acid and lower polyunsaturated fatty acids contents (Lakhssassi et al., 2021a; Lakhssassi et al., 2021b). It should be noted that oils with increased levels of oleic acid are desired in the human diet. On the other hand, TILLING can be used for producing mutants with decreased content of undesired compounds or even free of them.
Low phytic acid mutants identification was a goal of Sashidhar’s and co-workers’ studies (2020) in oilseed rape (Brassica napus L.), a polyploid species. Phytic acid is the major phosphorous storage compound in seeds of this species (2-4%), but due to the fact that it cannot be metabolized, similarly to glucosinolates, tannins, and phenolic acids, it is considered to be an antinutritive compound (Thompson et al., 1990; Campbell et al., 2016). Researchers identified TILLING mutants carrying changes in two paralogs of Bn2-PGK2 (2-Phosphoglyceric Acid Kinase) genes. The double mutant for Bn2-PGK2 had significantly reduced phytic acid contents, which is potentially beneficial for the human diet (Sashidhar et al., 2020).
Wheat is one of the most important crops worldwide, being a major source of energy and nutrition, but because of the high content of gluten, it cannot be eaten by some people with health issues (celiac disease). The TILLING strategy has been used to identify wheat genotypes with reduced content of immunogenic gluten proteins (Wen et al., 2022). Double mutants in durum wheat and triple mutants in common wheat with complete activity suppression of DME (DEMETER, 5-methylcytosine DNA glycosylase/lyase) and DRE2 (an iron-sulfur cluster biogenesis enzyme) displayed reduced content of immunogenic gluten proteins while retaining essential baking properties. Composition of starch is another quality trait that has been modified through TILLING in both common and durum wheat. The ratio of amylose:amylopectin influences the properties of starch. Resistant starch with higher amylose content is associated with health benefits. TILLING analysis in common wheat for two genes encoding starch synthases (SSIIa and SSIIIa) led to the identification of mutants with higher amylose content, with triple mutants (in A, B, and D genomes) having the strongest phenotypes (Schoen et al., 2021; Fahy et al., 2022).
Enrichment of provitamin A content in durum wheat (Triticum durum) grain is another example of the use of TILLING strategy to modify genes responsible for nutritional value. Vitamin A deficiency (VAD) is a public health problem in more than half of all countries, especially those in Africa and South-East Asia. The most severe effects of this deficiency are seen in young children and pregnant women in low-income countries. The transgenic approach to address VAD problem has been undertaken in rice (Oryza sativa L.) and genetically engineered ‘Golden Rice’ with enhanced provitamin A content was finally approved for cultivation in 2021 in The Philippines, after almost 20 years of studies (www.isaaa.org). In durum wheat, the increase of β-carotene content by more than 70% was achieved by TILLING of two genes, HYD1 (β-carotene hydroxylase 1) and LcyE (Lycopene ϵ-cyclase) (Sestili et al., 2019; Garcia Molina et al., 2021). Owing to the polyploid nature of durum wheat (4X), it was necessary to identify mutations in target genes located in both subgenomes (A and B).
A spectacular example of breeding success with the use of TILLING strategy also comes from wheat (in this case common wheat). For this species, tolerance to herbicide glyphosate (N-phosphonomethyl-glycine) was introduced through a nontransgenic approach (Moehs et al., 2021). The double wheat mutant, which carries missense mutations in A and D subgenomes in the gene EPSPS (5-enolpyruvylshiki-mate-3-phosphate synthase) showed enhanced tolerance to this herbicide (Green, 2018). Taking into account that glyphosate is the world’s most widely employed herbicide and genetically modified (GM) glyphosate-tolerant cultivars of maize, soybean and cotton have been widely used, the new sources of glyphosate tolerance may be very important for breeding. It should also be underlined that GM wheat tolerant to glyphosate (Roundup Ready™ wheat), developed by Monsanto in 2004, has never been cultivated in any country, including the USA (www.isaaa.org).
In tomato (Solanum lycopersicum L.), the improvement of fruit shelf-life has been a frequently studied trait, modified by TILLING for breeding purposes (Okabe et al., 2011; Okabe et al., 2012; Mubarok et al., 2015; Minoia et al., 2016; Brisou et al., 2021). One example is a study of Brisou et al. (2021) who identified mutants carrying changes in the SlACO1 (ACC oxidase 1) gene. The mutants exhibited decreased ethylene production and conductivity, which led to enhanced shelf-life and firmness. Another study aimed at the development of TILLING mutants with changed ethylene metabolism, shelf-life duration and fruit softening was performed for the Ethylene Receptor gene (SlETR1) (Mubarok et al., 2015). TILLING mutants with prolonged shelf-life were also identified through TILLING of Expansin 1 (SlExp1) involved in cell wall expansions and loosening. The improvement of fruit shelf-life in these mutants was attributed to altered cell wall polysaccharide composition (Minoia et al., 2016).
The biggest advantage of TILLING, contrary to transgenic techniques, is that it is applicable to all species regardless of their genome size or transformation potential. There is no need to use sophisticated tissue cultures that for some species or genotypes are impossible to maintain. After traditional mutagenesis used for the creation of TILLING population, mutations can be found in every gene of interest. In general, the higher density of mutations the smaller population is needed to be analyzed to find mutations within each gene. With the use of TILLING one can identify the series of alleles (potentially giving “weak” and “strong” phenotypes), not only knock-outs. It is of special importance, especially for genes that are crucial for plants to survive, whose total inactivation may be lethal. For them, the sublethal alleles may be required for phenotypic analysis. Advantage from the reduction of protein activity obtained with TILLING-mutants vs. total turn off of the gene function using RNAi technique can rely also on avoiding some disruption during plant growth and development, which was indicated e.g. in research in tobacco, where RNAi mutants were characterized by necrotic lesions or increased water content in leaves (Liedschulte et al., 2017). Importantly, the analysis of multiple “weak” and “strong” alleles allows the deepening of knowledge on gene function. In barley analysis of the HvHox1 (homeodomain-leucine-zipper1) gene, which controls the row-type character of a spike, showed that three forms carrying missense mutation presented different phenotypes compared with the parent cultivar ‘Barke’. It reflects the different influences of substitutions on the protein function. Two mutants presented intermediate phenotypes, another one a six-row type spike, while cultivar ‘Barke’ was two-rowed (Gottwald et al., 2009). Another example of an increase in knowledge about gene function comes from Arabidopsis and mutant generated by TILLING in ABP (Auxin Binding Protein 1) gene - abp1-5 (Henikoff et al., 2004). This mutant carries a missense mutation leading to substitution in the auxin binding pocket of ABP1, which might alter the auxin binding process. Studies using the TILLING mutant led to the revealing of many auxin-related roles for ABP1, while null alleles, appeared to be embryo-lethal (Enders et al., 2015). TILLING could be also the technique of choice when complete loss of function of the investigated gene is undesired in breeding programs. An example of the necessity to obtain so-called “weak” alleles (that can be provided by TILLING) was shown e.g. in the study of Liedschulte et al. (2017) on the reduction of cadmium in leaves of tobacco (Nicotiana tabacum). The low level of Cd might be „beneficial” for smokers because it leads to lower Cd accumulation in their bodies. The study conducted on inhabitants of the Upper Silesia region in Poland indicated that smokers accumulated a twice higher level of Cd compared with non-smokers (Bem et al., 1993). First, two heavy metal transporter homeologous genes - HMA4.1 and HMA4.2 were silenced using an RNAi (RNA interference) approach. This was successful for Cd reduction in tobacco leaves, however negative effects on plant development, including retarded growth, necrotic lesions, altered leaf morphology, and increased water content have been observed. Application of the TILLING strategy led to removing this impact, and mutants with both, a lower content of Cd in leaves and no negative developmental effects have been identified. In order to minimize the phenotypic effects, the different mutation combinations in analyzed genes have been investigated. It has been proven, that complete functional loss obtained by induction of nonsense mutation in one homeologous HMA4 gene and the functional reduction in the other HMA4 gene obtained by induction of missense mutation led to the best results and these mutation combinations are the best choice for breeding programs in tobacco (Liedschulte et al., 2017).
The TILLING strategy has been used for more than 20 years, during which it was modified and improved. In general, as described in previous sections, the screening methods keep getting better, faster, and cheaper, which makes TILLING a robust tool for functional genomics. This can be seen especially in terms of sequencing technology, but currently, the use of TILLING-by-sequencing is limited to species for which reference genomes are available. The software for mutation analysis is also evolving together with TILLING. As an example, the PARSESNP tool (Taylor and Greene, 2003) was very useful at the beginning of TILLING research, however, it is not active anymore, whereas new tools are arising e.g. Mutation Finder Annotator (https://github.com/bjtill/Mutation-Finder-Annotator-CLI) that can be used for TILLING by sequencing. It is noteworthy that TILLING can also be conducted without any sophisticated apparatus, utilizing only basic laboratory equipment (e.g. with product visualization on agarose gel). Such a low-cost approach is suitable for implementation in developing countries with limited laboratory infrastructure, however, it is important to acknowledge that it may result in reduced sensitivity when it comes to identifying mutations.
A significant advantage of TILLING is that once established, a TILLING platform is long-lasting and may be used for both, forward and reverse screening. Finally, most important from an agronomic point of view, non-GMO mutants are created, as in TILLING strategy there is no need for transformation. Hence, new TILLING alleles may be used not only for functional analysis of genes but also serve as a valuable resource for crop improvement because they can be directly used in breeding programs without regulatory restrictions that exist in some countries for the material labeled as GMO.
TILLING, like any other approach, has also some limitations. Establishing TILLING platform may be time- and labor-consuming, but once established it may serve as a source of mutation for many years. However, the biggest disadvantage of TILLING is, without a doubt, the presence of background mutations that can affect the phenotype and, hence, impede gene function analysis. It was shown that in an abp1-5 mutant carrying change in the ABP (Auxin Binding Protein 1) gene of Arabidopsis, which was identified by TILLING approach, after whole-genome sequencing the additional 8,000 single nucleotide polymorphisms were identified (Enders et al., 2015). It was also calculated that for barley, in a TILLING population with mutational density of 1/500 kb, there are ca. 10,000 mutations in the genome of each plant (Szurman-Zubrzycka et al., 2018). However, it has to be noted that the coding part of a genome is usually very small (in the case of barley, it is 1.4%; Mascher et al., 2017), so most of these 10,000 mutations are in the noncoding sequences, with the lower probability of impacting the phenotype. What is more, some of those in coding regions may be silent or cause amino-acid substitutions that do not affect the protein activity. Taking it all into account, the total number of background mutations that was problematic in terms of functional analysis is low enough to be removed from the background by backcrosses with the parent variety. One backcross of a TILLING mutant with a parent variety reduces the number of background mutations by half, and performing a higher number of backcrosses can be recommended. However, it should be kept in mind that multiple backcrossing will prolong the time before phenotyping. The F1 generation is heterozygous in terms of analyzed mutation. The homozygous mutants should be selected from the BCF2 generation. Mutations are very often recessive, which means that only individuals carrying a mutation in a homozygous state should be phenotyped (Okabe et al., 2011). Such backcrossing is often time-consuming and the high density of background mutations is considered to be the main problem in the application of TILLING strategy as a reverse genetic tool. This problem is avoided in the modern targeted genome editing methods, such as CRISPR/Cas9 (reviewed in Gaj et al, 2013; Mei et al., 2016; Liu et al., 2021) and TALEN (reviewed in Becker and Boch, 2021), which are two powerful genome editing (GE) tools that have revolutionized plant biotechnology. In the case of these GE methods, called also New Genomic Techniques (NGTs), the mutation can be introduced specifically to the target position within the gene of interest, and the probability of off-target mutation appearance is relatively low. It is the main advantage of CRISPR/Cas9 and TALEN over TILLING. Both, CRISPR/Cas9 and TALENs have been successfully employed in plant research, and being the precise and efficient methods for modifying plant genomes, they have opened up new possibilities for plant breeding and biotechnology. It has to be kept in mind, that in some countries materials produced through these technologies are labeled as GMOs, even though the stable CRISPR/Cas9 or TALEN mutants do not carry any transgene and do not possess foreign DNA. However, the regulatory landscape in this regard is continuously evolving, and the legal status of CRISPR/Cas9 mutants is undergoing changes, reflecting the shifting perspectives and emerging legislative frameworks in various jurisdictions (e.g. the newest, dated July 5th 2023, proposal, for a REGULATION OF THE EUROPEAN PARLIAMENT AND OF THE COUNCIL on plants obtained by certain new genomic techniques and their food and feed, and amending Regulation (EU) 2017/625).
In general, TILLING and GE approaches should complement each other in functional genetic studies or in pre-breeding programs. There are several examples of joined usage of these approaches to study gene function. For example, Wang and co-workers by using CRISPR/Cas9 and TILLING strategies demonstrated that TaGW2 homoeologs are negative regulators of grain size and weight, that contribute additively to the phenotype. The obtained TILLING and CRISPR/Cas9 mutants provide the opportunity to combine mutant alleles in various configurations, enabling fine-tuning of the phenotypic outcomes (Wang et al., 2018). However, it is also needed to be highlighted that for many plant species, including those agronomically important, there is no efficient protocol for transformation. Hence, GE technologies may be applied only to a limited number of species or to a limited number of cultivars of particular species (e.g. a protocol of barley transformation is efficient only for one cultivar - ‘Golden Promise’), whereas TILLING may be applied to any plant species and genotype.
The problem of background mutation was also reduced in a FIND-IT approach, because of using a mutagenized population with very low mutation density (Knudsen et al., 2022). This is a powerful barley platform generated with the use of NaN3, possessing high capacity for the detection of premature stop codons, which was demonstrated by the identification of one hundred such changes in genes of interest. Mutations that cause the emergence of a premature stop codon have a high probability of disrupting the function of the encoded protein. However, these types of mutations are detected with low frequency as compared with other types of mutations (silent, missense) in previously reported plant TILLING populations (Kurowska et al., 2011; Szurman-Zubrzycka et al., 2018, Gao et al., 2020; Brisou et al., 2021).
We are currently in the so-called postgenomic era. Due to the high throughput methods of genome sequencing, there is more information about sequences than about gene function. Functional analysis of genes lags behind the acquisition of new sequences of whole genomes. It is well established that induced mutations have been a powerful tool in functional genomics and breeding for over 80 years of their use in plant research. Establishing a TILLING platform may be time-consuming, however for many species, these platforms have already been developed and the identification of TILLING mutants is really fast. Additionally, one can predict that as technologies of mutation detection are getting better and faster, so TILLING will too. As an example, FIND-IT, the new TILLING-based approach, provides ultrafast screening for variants of interest (within 10 days). The bottleneck is usually the characterization of identified mutants – determining the phenotypic consequence of the mutation, but this is a problem of each mutation technology, including gene editing by CRISPR/Cas9. In terms of functional genetics studies, it is always an added value to combine different approaches that complement each other, e. g. TILLING and CRISPR/Cas9 strategies that produce different types of mutants. It is also worth reminding that TILLING mutants are not GMOs so they can be directly used, without regulations, in pre-breeding programs around the world to improve crop performance.
MS-Z and IS conceive the manuscript. MS-Z and MK wrote the manuscript; MK generated tables; BT and IS revised and edited manuscript. All authors approved the submitted version.
This work was supported by the National Science Centre, Poland (grant Beethoven Life1 2018/31/F/NZ2/03952).
The authors declare that the research was conducted in the absence of any commercial or financial relationships that could be construed as a potential conflict of interest.
All claims expressed in this article are solely those of the authors and do not necessarily represent those of their affiliated organizations, or those of the publisher, the editors and the reviewers. Any product that may be evaluated in this article, or claim that may be made by its manufacturer, is not guaranteed or endorsed by the publisher.
Adeyanju, A. O., Sattler, S. E., Rich, P. J., Rivera-Burgos, L. A., Xu, X., Ejeta, G. (2021). Sorghum brown midrib19 (Bmr19) gene links lignin biosynthesis to folate metabolism. Genes 12 (5), 660. doi: 10.3390/genes12050660
Alcock, T. D., Thomas, C. L., Ó Lochlainn, S., Pongrac, P., Wilson, M., Moore, C., et al. (2021). Magnesium and calcium overaccumulate in the leaves of a schengen3 mutant of Brassica rapa. Plant Physiol. 186 (3), 1616–1631. doi: 10.1093/plphys/kiab150
Avni, R., Zhao, R., Pearce, S., Jun, Y., Uauy, C., Tabbita, F., et al. (2014). Functional characterization of GPC-1 genes in hexaploid wheat. Planta 239 (2), 313–324. doi: 10.1007/s00425-013-1977-y
Becker, S., Boch, J. (2021). TALE and TALEN genome editing technologies. Gene Genome Editing 2, 10007. doi: 10.1016/j.ggedit.2021.100007
Bem, E. M., Orlowski, C., Piotrowski, J. K., Januszewski, K., Pajak, J. (1993). Cadmium, zinc, copper, and metallothionein levels in the kidney and liver of inhabitants of upper Silesia (Poland). Int. Arch. Occup. Environ. Health 65 (1), 57–63. doi: 10.1007/BF00586060
Bhalerao, S., Prabhu, D. (2013). Aluminium toxicity in plants – a review. J. Appl. Chem. 2, 447–474.
Blasco, B., Navarro-León, E., Ruiz, J. M. (2019). Study of Zn accumulation and tolerance of HMA4 TILLING mutants of Brassica rapa grown under Zn deficiency and Zn toxicity. Plant Sci. 287, 110201. doi: 10.1016/j.plantsci.2019.110201
Braatz, J., Harloff, H.-J., Emrani, N., Elisha, C., Heepe, L., Gorb, S. N., et al. (2018). The effect of INDEHISCENT point mutations on silique shatter resistance in oilseed rape (Brassica napus). Theor. Appl. Genet. 131 (4), 959–971. doi: 10.1007/s00122-018-3051-4
Brisou, G., Piquerez, S. J. M., Minoia, S., Marcel, F., Cornille, A., Carriero, F., et al. (2021). Induced mutations in SlE8 and SlACO1 control tomato fruit maturation and shelf-life. J. Exp. Bot. 72 (20), 6920–6932. doi: 10.1093/jxb/erab330
Cain, A. B., Yu, S., Tian, L. (2022). Mutational analysis of a wheat O-methyltransferase involved in flavonoid metabolism. Plants 11 (2), 164. doi: 10.3390/plants11020164
Caldwell, D. G., McCallum, N., Shaw, P., Muehlbauer, G. J., Marshall, D. F., Waugh, R. (2004). A structured mutant population for forward and reverse genetics in Barley (Hordeum vulgare L.). Plant J. 40 (1), 143–150. doi: 10.1111/j.1365-313X.2004.02190.x
Campbell, L., Rempel, C. B., Wanasundara, J. P. (2016). Canola/rapeseed protein: future opportunities and directions–workshop proceedings of IRC. Plants 5, e17. doi: 10.3390/plants5020017
Chawade, A., Sikora, P., Bräutigam, M., Larsson, M., Vivekanand, V., Nakash, M. A., et al. (2010). Development and characterization of an oat TILLING-population and identification of mutations in lignin and beta-glucan biosynthesis genes. BMC Plant Biol. 10, 86. doi: 10.1186/1471-2229-10-86
Colbert, T., Till, B., Tompa, R., Reynolds, S., Steine, M., Yeung, A., et al. (2001). High-throughput screening for induced point mutations. Plant Physiology 126, 2, 480–484. doi: 10.1104/pp.126.2.480
Collin, A., Daszkowska-Golec, A., Kurowska, M., Szarejko, I. (2020). Barley ABI5 (Abscisic Acid INSENSITIVE 5) is involved in abscisic acid-dependent drought response. Front. Plant Sci. 11. doi: 10.3389/fpls.2020.01138
Cooper, J. L., Till, B. J., Laport, R. G., Darlow, M. C., Kleffner, J. M., Jamai, A., et al. (2008). TILLING to detect induced mutations in soybean. BMC Plant Biol. 8, 9. doi: 10.1186/1471-2229-8-9
Dahmani-Mardas, F., Troadec, C., Boualem, A., Lévêque, S., Alsadon, A. A., Aldoss, A. A., et al. (2010). Engineering melon plants with improved fruit shelf life using the TILLING approach. PloS One 5 (12), e15776. doi: 10.1371/journal.pone.0015776
Dalmais, M., Schmidt, J., Le Signor, C., Moussy, F., Burstin, J., Savois, V., et al. (2008). UTILLdb, a Pisum sativum in silico forward and reverse genetics tool. Genome Biol. 9 (2), R43. doi: 10.1186/gb-2008-9-2-r43
Daszkowska-Golec, A., Karcz, J., Plociniczak, T., Sitko, K., Szarejko, I. (2020). Cuticular waxes-A shield of barley mutant in CBP20 (Cap-Binding Protein 20) gene when struggling with drought stress. Plant Science: An. Int. J. Exp. Plant Biol. 300, 110593. doi: 10.1016/j.plantsci.2020.110593
Daszkowska-Golec, A., Skubacz, A., Marzec, M., Slota, M., Kurowska, M., Gajecka, M., et al. (2017). Mutation in hvCBP20 (Cap binding protein 20) adapts barley to drought stress at phenotypic and transcriptomic levels. Front. Plant Sci. 8. doi: 10.3389/fpls.2017.00942
Daszkowska-Golec, A., Skubacz, A., Sitko, K., Słota, M., Kurowska, M., Szarejko, I. (2018). Mutation in barley ERA1 (Enhanced Response to ABA1) gene confers better photosynthesis efficiency in response to drought as revealed by transcriptomic and physiological analysis. Environ. Exp. Bot. 148, 12–26. doi: 10.1016/j.envexpbot.2018.01.003
de Lorenzo, L., Merchan, F., Laporte, P., Thompson, R., Clarke, J., Sousa, C., et al. (2009). A novel plant leucine-rich repeat receptor kinase regulates the response of Medicago truncatula roots to salt stress. Plant Cell. 21 (2), 668–680. doi: 10.1105/tpc.108.059576
Elahi, N., Duncan, R. W., Stasolla, C. (2015). Decreased seed oil production in FUSCA3 Brassica napus mutant plants. Plant Physiol. Biochem. 96, 222–230. doi: 10.1016/j.plaphy.2015.08.002
Enders, T. A., Oh, S., Yang, Z., Montgomery, B. L., Strader, L. C. (2015). Genome sequencing of arabidopsis abp1-5 reveals second-site mutations that may affect phenotypes. Plant Cell. 27 (7), 1820–1826. doi: 10.1105/tpc.15.00214
Fahy, B., Gonzalez, O., Savva, G. M., Ahn-Jarvis, J. H., Warren, F. J., Dunn, J., et al. (2022). Loss of starch synthase IIIa changes starch molecular structure and granule morphology in grains of hexaploid bread wheat. Sci. Rep. 12 (1), 10806. doi: 10.1038/s41598-022-14995-0
Fanelli, V., Ngo, K. J., Thompson, V. L., Silva, B. R., Tsai, H., Sabetta, W., et al. (2021). A TILLING by sequencing approach to identify induced mutations in sunflower genes. Sci. Rep. 11 (1), 9885. doi: 10.1038/s41598-021-89237-w
Fusi, R., Rosignoli, S., Lou, H., Sangiorgi, G., Bovina, R., Pattem, J. K., et al. (2022). Root angle is controlled by EGT1 in cereal crops employing an antigravitropic mechanism. Proc. Natl. Acad. Sci. United States America 119 (31), e2201350119. doi: 10.1073/pnas.2201350119
Gady, A. L., Hermans, F. W., Van de Wal, M. H., van Loo, E. N., Visser, R. G., Bachem, C. W. (2009). Implementation of two high through-put techniques in a novel application: Detecting point mutations in large EMS mutated plant populations. Plant Methods 5, 13. doi: 10.1186/1746-4811-5-13
Gaj, T., Gersbach, C. A., Barbas, C. F. (2013). ZFN, TALEN, and CRISPR/Cas-based methods for genome engineering. Trends Biotechnol. 31, 397–405. doi: 10.1016/j.tibtech.2013.04.004
Gao, Y., Quan, S., Lyu, B., Tian, T., Liu, Z., Nie, Z., et al. (2022). Barley transcription factor HvNLP2 mediates nitrate signaling and affects nitrogen use efficiency. J. Exp. Bot. 73 (3), 770–783. doi: 10.1093/jxb/erab245
Gao, Y.-L., Yao, X.-F., Li, W.-Z., Song, Z.-B., Wang, B.-W., Wu, Y.-P., et al. (2020). An efficient TILLING platform for cultivated tobacco. J. Integr. Plant Biol. 62 (2), 165–180. doi: 10.1111/jipb.12784
Garcia Molina, M. D., Botticella, E., Beleggia, R., Palombieri, S., De Vita, P., Masci, S., et al. (2021). Enrichment of provitamin A content in durum wheat grain by suppressing β-carotene hydroxylase 1 genes with a TILLING approach. Theor. Appl. Genet. 134 (12), 4013–4024. doi: 10.1007/s00122-021-03944-6
Gascuel, Q., Diretto, G., Monforte, A. J., Fortes, A. M., Granell, A. (2017). Use of natural diversity and biotechnology to increase the quality and nutritional content of tomato and grape. Front. Plant Sci. 12. doi: 10.3389/fpls.2017.00652
Geier, T. (2012). “Chimeras: Properties and Dissociation in Vegetatively Propagated Plants,” in Plant Mutation Breeding and Biotechnology. Eds. Shu, Forester, Nakagawa (Wallingford, UK and Rome, Italy: CAB International and FAO), 191–201.
Gottwald, S., Bauer, P., Komatsuda, T., Lundqvist, U., Stein, N. (2009). TILLING in the two-rowed barley cultivar „Barke” reveals preferred sites of functional diversity in the gene HvHox1. BMC Res. Notes 2, 258. doi: 10.1186/1756-0500-2-258
Green, J. M. (2018). The rise and future of glyphosate and glyphosate-resistant crops. Pest Manag Sci. 74 (5), 1035–1039. doi: 10.1002/ps.4462
Griffiths, A., Wessler, S., Lewontin, R., Gelbart, W., Suzuki, D., Miller, J. (2004). “Dissection of Gene Function,” in An Introduction to Genetic Analysis, 8th Edition. Ed. Freeman, W. H. (New York, NY).
Gruszka, D., Gorniak, M., Glodowska, E., Wierus, E., Oklestkova, J., Janeczko, A., et al. (2016). A reverse-genetics mutational analysis of the barley hvDWARF gene results in identification of a series of alleles and mutants with short stature of various degree and disturbance in BR biosynthesis allowing a new insight into the process. Int. J. Mol. Sci. 17 (4), E600. doi: 10.3390/ijms17040600
Gundry, C. N., Vandersteen, J. G., Reed, G. H., Pryor, R. J., Chen, J., Wittwer, C. T. (2003). Amplicon melting analysis with labeled primers: a closed-tube method for differentiating homozygotes and heterozygotes. Clin. Chem. 49 (3), 396–406. doi: 10.1373/49.3.396
Guo, Y., Hans, H., Christian, J., Molina, C. (2014). Mutations in single FT- and TFL1-paralogs of rapeseed (Brassica napus L.) and their impact on flowering time and yield components. Front. Plant Sci. 5. doi: 10.3389/fpls.2014.00282
Harloff, H.-J., Lemcke, S., Mittasch, J., Frolov, A., Wu, J. G., Dreyer, F., et al. (2012). A mutation screening platform for rapeseed (Brassica napus L.) and the detection of sinapine biosynthesis mutants. Theor. Appl. Genet. 124 (5), 957–969. doi: 10.1007/s00122-011-1760-z
Hawkins, E., Chen, J., Watson-Lazowski, A., Ahn-Jarvis, J., Barclay, J. E., Fahy, B., et al. (2021). STARCH SYNTHASE 4 is required for normal starch granule initiation in amyloplasts of wheat endosperm. New Phytol. 230 (6), 2371–2386. doi: 10.1111/nph.17342
Henikoff, S., Till, B. J., Comai, L. (2004). TILLING. Traditional mutagenesis meets functional genomics. Plant Physiol. 135 (2), 630–636. doi: 10.1104/pp.104.041061
Hwang, J. E., Jang, D.-S., Lee, K. J., Ahn, J.-W., Kim, S. H., Kang, S.-Y., et al. (2017). Identification of gamma ray irradiation-induced mutations in membrane transport genes in a rice population by TILLING. Genes Genet. Syst. 91 (5), 245–256. doi: 10.1266/ggs.15-00052
IPCC (2022). Climate change 2022: Impacts, adaptation and vulnerability. Available at: https://reliefweb.int/report/world/climate-change-2022-impacts-adaptation-and-vulnerability?gclid=Cj0KCQjwz8emBhDrARIsANNJjS4W_7ZgjlM972WT6SZbMMCSkwWD23yIezs9DmVRDvVFdw2rD2DrQ0EaAiacEALw_wcB.
Ishikawa, S., Wagatsuma, T., Sasaki, R., Ofei-Manu, P. (2000). Comparison of the amount of citric and malic acids in Al media of seven plant species and two cultivars each in five plant species. Soil Sci. Plant Nutr. 46, 751–758. doi: 10.1080/00380768.2000.10409141
Janeczko, A., Gruszka, D., Pociecha, E., Dziurka, M., Filek, M., Jurczyk, B., et al. (2016). Physiological and biochemical characterisation of watered and drought-stressed barley mutants in the HvDWARF gene encoding C6-oxidase involved in brassinosteroid biosynthesis. Plant Physiol. Biochem. 99, 126–141. doi: 10.1016/j.plaphy.2015.12.003
Jankowicz-Cieslak, J., Huynh, O. A., Bado, S., Matijevic, M., Till, B. J. (2011). Reverse-genetics by TILLING expands through the plant kingdom. Emir. J. Food Agric. 23, 290–300.
Jankowicz-Cieslak, J., Huynh, O. A., Brozynska, M., Nakitandwe, J., Till, B. J. (2012). Induction, rapid fixation and retention of mutations in vegetatively propagated banana. Plant Biotechnol. J. 10 (9), 1056–1066. doi: 10.1111/j.1467-7652.2012.00733.x
Jankowicz-Cieslak, J., Till, B. J. (2016). Chemical mutagenesis of seed and vegetatively propagated plants using EMS. Curr. Protoc. Plant Biol. 1 (4), 617–635. doi: 10.1002/cppb.20040
Jaskowiak, J., Kwasniewska, J., Szurman-Zubrzycka, M., Rojek-Jelonek, M., Larsen, P. B., Szarejko, I. (2020). Al-tolerant barley mutant hvatr.g shows the ATR-regulated DNA damage response to maleic acid hydrazide. Int. J. Mol. Sci. 21 (22), E8500. doi: 10.3390/ijms21228500
Jaskowiak, J., Tkaczyk, O., Slota, M., Kwasniewksa, J., Szarejko, I. (2018). Analysis of aluminium toxicity in Hordeum vulgare roots with an emphasis on DNA integrity and cell cycle. PloS One 13, e0193156. doi: 10.1371/journal.pone.0193156
Jiang, C., Lei, M., Guo, Y., Gao, G., Shi, L., Jin, Y., et al. (2022). A reference-guided TILLING by amplicon-sequencing platform supports forward and reverse genetics in barley. Plant Commun. 3 (4), 100317. doi: 10.1016/j.xplc.2022.100317
Jost, M., Szurman-Zubrzycka, M., Gajek, K., Szarejko, I., Stein, N. (2019). TILLING in barley. Methods Mol. Biol. 1900, 73–94. doi: 10.1007/978-1-4939-8944-7_6
Karaman, K., Kizil, S., Başak, M., Uzun, B., Yol, E. (2021). Development of EMS-induced Mutagenized Groundnut Population and Discovery of Point Mutations in the ahFAD2 and Ara h 1 Genes by TILLING. J. Oleo Sci. 70 (11), 1631–1640. doi: 10.5650/jos.ess21075
Kirschner, G. K., Rosignoli, S., Guo, L., Vardanega, I., Imani, J., Altmüller, J., et al. (2021). ENHANCED GRAVITROPISM 2 encodes a STERILE ALPHA MOTIF-containing protein that controls root growth angle in barley and wheat. Proc. Natl. Acad. Sci. U.S.A. 118, e2101526118. doi: 10.1073/pnas.2101526118
Knoll, J. E., Ramos, M. L., Zeng, Y., Holbrook, C. C., Chow, M., Chen, S., et al. (2011). TILLING for allergen reduction and improvement of quality traits in peanut (Arachis hypogaea L.). BMC Plant Biol. 11, 81. doi: 10.1186/1471-2229-11-81
Knudsen, S., Wendt, T., Dockter, C., Thomsen, H. C., Rasmussen, M., Egevang Jørgensen, M., et al. (2022). FIND-IT: Accelerated trait development for a green evolution. Sci. Adv. 8 (34), eabq2266. doi: 10.1126/sciadv.abq2266
Krasileva, K. V., Vasquez-Gross, H. A., Howell, T., Bailey, P., Paraiso, F., Clissold, L., et al. (2017). Uncovering hidden variation in polyploid wheat. Proc. Natl. Acad. Sci. U.S.A. 114 (6), E913–E921. doi: 10.1073/pnas.1619268114
Kurowska, M., Daszkowska-Golec, A., Gruszka, D., Marzec, M., Szurman, M., Szarejko, I., et al. (2011). TILLING – a shortcut in functional genomics. J. Appl. Genet. 52, 371–390. doi: 10.1007/s13353-011-0061-1
Kurowska, M., Labocha-Pawłowska, A., Gnizda, D., Maluszynski, M., Szarejko, I. (2012). ). Molecular analysis of point mutations in a barley genome exposed to MNU and gamma rays. Mutat. Res. 738–739, 52–70. doi: 10.1016/j.mrfmmm.2012.08.008
Lababidi, S., Mejlhede, N., Rasmussen, S. K., Backes, G., Al-Said, W., Baum, M., et al. (2009). Identification of barley mutants in the cultivar ‘Lux’ at the Dhn loci through TILLING. Plant Breed. 128, 332–336. doi: 10.1111/j.1439-0523.2009.01640.x
Lakhssassi, N., Lopes-Caitar, V. S., Knizia, D., Cullen, M. A., Badad, O., El Baze, A., et al. (2021a). TILLING-by-sequencing+ Reveals the role of novel fatty acid desaturases (GmFAD2-2s) in increasing soybean seed oleic acid content. Cells 10 (5), 1245. doi: 10.3390/cells10051245
Lakhssassi, N., Zhou, Z., Cullen, M. A., Badad, O., El Baze, A., Chetto, O., et al. (2021b). TILLING-by-sequencing+ to decipher oil biosynthesis pathway in soybeans: A new and effective platform for high-throughput gene functional analysis. Int. J. Mol. Sci. 22 (8), 4219. doi: 10.3390/ijms22084219
Li, M., Hensel, G., Melzer, M., Junker, A., Tschiersch, H., Ruwe, H., et al. (2021). Mutation of the ALBOSTRIANS ohnologous gene hvCMF3 impairs chloroplast development and thylakoid architecture in barley. Front. Plant Sci. 12. doi: 10.3389/fpls.2021.732608
Li, S., Liu, S.-M., Fu, H.-W., Huang, J.-Z., Shu, Q.-Y. (2018). High-resolution melting-based TILLING of γ ray-induced mutations in rice. J. Zhejiang University- Sci. B 19 (8), 620–629. doi: 10.1631/jzus.B1700414
Liedschulte, V., Laparra, H., Battey, J. N., Schwaar, J. D., Broye, H., Mark, R., et al. (2017). Impairing both HMA4 homeologs is required for cadmium reduction in tobacco. Plant Cell Environ. 40 (3), 364–377. doi: 10.1111/pce.12870
Liu, T., Jiang, G.-Q., Yao, X.-F., Liu, C.-M. (2021). The leucine-rich repeat receptor-like kinase OsERL plays a critical role in anther lobe formation in rice. Biochem. Biophys. Res. Commun. 563, 85–91. doi: 10.1016/j.bbrc.2021.05.059
Liu, Q., Yang, F., Zhang, J., Liu, H., Rahman, S., Islam, S., et al. (2021). Application of CRISPR/cas9 in crop quality improvement. Int. J. Mol. Sci. 22 (8), 4206. doi: 10.3390/ijms22084206
Lochlainn, S.Ó., Amoah, S., Graham, N. S., Alamer, K., Rios, J. J., Kurup, S., et al. (2011). High Resolution Melt (HRM) analysis is an efficient tool to genotype EMS mutants in complex crop genomes. Plant Methods 7, 43. doi: 10.1186/1746-4811-7-43
Maluszynski, M., Szarejko, I., Maluszynska, J., Szurman-Zubrzycka, M. (2017). “Mutation Techniques,” in Encyclopedia of Applied Sciences, vol. 2 . Eds. Thomas, B., Murray, B. G., Murphy, D. J. (Waltham, MA: Academic Press), 215–228.
Marko, D., El-Shershaby, A., Carriero, F., Summerer, S., Petrozza, A., Iannacone, R., et al. (2019). Identification and characterization of a thermotolerant TILLING allele of heat shock binding protein 1 in tomato. Genes 10 (7), E516. doi: 10.3390/genes10070516
Marzec, M., Daszkowska-Golec, A., Collin, A., Melzer, M., Eggert, K., Szarejko, I. (2020). Barley strigolactone signalling mutant hvd14.d reveals the role of strigolactones in abscisic acid-dependent response to drought. Plant Cell Environ. 43 (9), 2239–2253. doi: 10.1111/pce.13815
Marzec, M., Gruszka, D., Tylec, P., Szarejko, I. (2016). Identification and functional analysis of the HvD14 gene involved in strigolactone signaling in Hordeum vulgare. Physiologia Plantarum 158 (3), 341–355. doi: 10.1111/ppl.12460
Mascher, M., Gundlach, H., Himmelbach, A., Beier, A., Twardziok, S., Wicker, T., et al. (2017). A chromosome conformation capture ordered sequence of the barley genome. Nature 544, 427–433. doi: 10.1038/nature22043
McCallum, C. M., Comai, L., Greene, E. A., Henikoff, S. (2000a). Targeting Induced Local Lesions IN Genomes (TILLING) for plant functional genomics. Plant Physiol. 123, 439–442. doi: 10.1104/pp.123.2.439
McCallum, C. M., Comai, L., Greene, E. A., Henikoff, S. (2000b). Targeted screening for induced mutations. Nat. Biotechnol. 18, 455–457. doi: 10.1038/74542
Mei, Y., Wang, Y., Chen, H., Sun, Z. S., Ju, X. D. (2016). Recent progress in CRISPR/Cas9 technology. J. Genet. Genomics 43, 63–75. doi: 10.1016/j.jgg.2016.01.001
Mendiondo, G. M., Gibbs, D. J., Szurman-Zubrzycka, M., Korn, A., Marquez, J., Szarejko, I., et al. (2016). Enhanced waterlogging tolerance in barley by manipulation of expression of the N-end rule pathway E3 ligase PROTEOLYSIS6. Plant Biotechnol. J. 14 (1), 40–50. doi: 10.1111/pbi.12334
Minoia, S., Boualem, A., Marcel, F., Troadec, C., Quemener, B., Cellini, F., et al. (2016). Induced mutations in tomato SlExp1 alter cell wall metabolism and delay fruit softening. Plant Sci. 242, 195–202. doi: 10.1016/j.plantsci.2015.07.001
Minoia, S., Petrozza, A., D’Onofrio, O., Piron, F., Mosca, G., Sozio, G., et al. (2010). A new mutant genetic resource for tomato crop improvement by TILLING technology. BMC Res. Notes 3, 69. doi: 10.1186/1756-0500-3-69
Moehs, C. P., Austill, W. J., Facciotti, D., Holm, A., Loeffler, D., Lu, Z., et al. (2021). Development of non-transgenic glyphosate tolerant wheat by TILLING. PloS One 16 (9), e0245802. doi: 10.1371/journal.pone.0245802
Mossor-Pietraszewska, T. (2001). Effect of aluminium on plant growth and metabolism. Acta Biochim. Pol. 48, 673–686. doi: 10.18388/abp.2001_3902
Mubarok, S., Hoshikawa, K., Okabe, Y., Yano, R., Tri, M. D., Ariizumi, T., et al. (2019). Evidence of the functional role of the ethylene receptor genes SlETR4 and SlETR5 in ethylene signal transduction in tomato. Mol. Genet. Genomics 294 (2), 301–313. doi: 10.1007/s00438-018-1505-7
Mubarok, S., Okabe, Y., Fukuda, N., Ariizumi, T., Ezura, H. (2015). Potential use of a weak ethylene receptor mutant, sletr1-2, as breeding material to extend fruit shelf life of tomato. J. Agric. Food Chem. 63 (36), 7995–8007. doi: 10.1021/acs.jafc.5b02742
Navarro-León, E., Oviedo-Silva, J., Ruiz, J. M., Blasco, B. (2019a). Possible role of HMA4a TILLING mutants of Brassica rapa in cadmium phytoremediation programs. Ecotoxicology Environ. Saf. 180, 88–94. doi: 10.1016/j.ecoenv.2019.04.081
Navarro-León, E., Paradisone, V., López-Moreno, F. J., Rios, J. J., Esposito, S., Blasco, B. (2021). Effect of CAX1a TILLING mutations on photosynthesis performance in salt-stressed Brassica rapa plants. Plant Sci. 311, 111013. doi: 10.1016/j.plantsci.2021.111013
Navarro-León, E., Ruiz, J. M., Albacete, A., Blasco, B. (2019b). Effect of CAX1a TILLING mutations and calcium concentration on some primary metabolism processes in Brassica rapa plants. J. Plant Physiol. 237, 51–60. doi: 10.1016/j.jplph.2019.04.002
Nezames, C., Sjogren, C., Barajas, J., Larsen, P. (2012). The Arabidopsis cell cycle checkpoint regulators TANMEI/ALT2 and ATR mediate the active process of aluminum-dependent root growth inhibition. Plant Cell 24, 608–621. doi: 10.1105/tpc.112.095596
Okabe, Y., Ariizumi, T., Ezura, H. (2013). Updating the micro-tom TILLING platform. Breed. Sci. 63 (1), 42–48. doi: 10.1270/jsbbs.63.42
Okabe, Y., Asamizu, E., Ariizumi, T., Shirasawa, K., Tabata, S., Ezura, H. (2012). Availability of Micro-Tom mutant library combined with TILLING in molecular breeding of tomato fruit shelf-life. Breed. Sci. 62 (2), 202–208. doi: 10.1270/jsbbs.62.202
Okabe, Y., Asamizu, E., Saito, T., Matsukura, C., Ariizumi, T., Brès, C., et al. (2011). Tomato TILLING technology: Development of a reverse genetics tool for the efficient isolation of mutants from Micro-Tom mutant libraries. Plant Cell Physiol. 52 (11), 1994–2005. doi: 10.1093/pcp/pcr134
Perry, J., Brachmann, A., Welham, T., Binder, A., Charpentier, M., Groth, M., et al. (2009). TILLING in Lotus japonicus identified large allelic series for symbiosis genes and revealed a bias in functionally defective ethyl methanesulfonate alleles toward glycine replacements. Plant Physiol. 151 (3), 1281–1291. doi: 10.1104/pp.109.142190
Perry, J. A., Wang, T. L., Welham, T. J., Gardner, S., Pike, J. M., Yoshida, S., et al. (2003). A TILLING reverse genetics tool and a web-accessible collection of mutants of the legume Lotus japonicus. Plant Physiol. 131 (3), 866–871. doi: 10.1104/pp.102.017384
Piron, F., Nicolaï, M., Minoïa, S., Piednoir, E., Moretti, A., Salgues, A., et al. (2010). An induced mutation in tomato eIF4E leads to immunity to two potyviruses. PloS One 5 (6), e11313. doi: 10.1371/journal.pone.0011313
Sabetta, W., Alba, V., Blanco, A., Montemurro, C. (2011). sunTILL: A TILLING resource for gene function analysis in sunflower. Plant Methods 7 (1), 20. doi: 10.1186/1746-4811-7-20
Sashidhar, N., Harloff, H.-J., Jung, C. (2020). Identification of phytic acid mutants in oilseed rape (Brassica napus) by large-scale screening of mutant populations through amplicon sequencing. New Phytol. 225 (5), 2022–2034. doi: 10.1111/nph.16281
Schoen, A., Joshi, A., Tiwari, V., Gill, B. S., Rawat, N. (2021). Triple null mutations in starch synthase SSIIa gene homoeologs lead to high amylose and resistant starch in hexaploid wheat. BMC Plant Biol. 21 (1), 74. doi: 10.1186/s12870-020-02822-5
Schreiber, M., Barakate, A., Uzrek, N., Macaulay, M., Sourdille, A., Morris, J., et al. (2019). A highly mutagenised barley (cv. Golden Promise) TILLING population coupled with strategies for screening-by-sequencing. Plant Methods 15, 99. doi: 10.1186/s13007-019-0486-9
Sestili, F., Garcia-Molina, M. D., Gambacorta, G., Beleggia, R., Botticella, E., De Vita, P., et al. (2019). Provitamin A biofortification of durum wheat through a TILLING approach. Int. J. Mol. Sci. 20 (22), E5703. doi: 10.3390/ijms20225703
Slade, A. J., Fuerstenberg, S. I., Loeffler, D., Steine, M. N., Facciotti, D. (2005). A reverse genetic, nontransgenic approach to wheat crop improvement by TILLING. Nat. Biotechnol. 23 (1), 75–81. doi: 10.1038/nbt1043
Slade, A. J., McGuire, C., Loeffler, D., Mullenberg, J., Skinner, W., Fazio, G., et al. (2012). Development of high amylose wheat through TILLING. BMC Plant Biol. 12, 69. doi: 10.1186/1471-2229-12-69
Slade, A. J., Fuerstenberg, S. I., Loeffler, D., Steine, M. N., Facciotti, D. (2005). A reverse genetic, nontransgenic approach to wheat crop improvement by TILLING. Nat. Biotechnol. 23 (1), 75–81. doi: 10.1038/nbt1043
Song, Z., Sui, X., Li, M., Gao, Y., Li, W., Zhao, L., et al. (2020). Development of a nornicotine-reduced flue-cured tobacco line via EMS mutagenesis of nicotine N-demethylase genes. Plant Signal Behav. 15 (2), 1710053. doi: 10.1080/15592324.2019.1710053
Stemple, D. (2004). TILLING – a high-throughput harvest for functional genomics. Nat. Rev. Genet. 5, 145–150. doi: 10.1038/nrg1273
Stephenson, P., Baker, D., Girin, T., Perez, A., Amoah, S., King, G. J., et al. (2010). A rich TILLING resource for studying gene function in Brassica rapa. BMC Plant Biol. 10, 62. doi: 10.1186/1471-2229-10-62
Stolarek, M., Gruszka, D., Braszewska-Zalewska, A., Maluszynski, M. (2015a). Alleles of newly identified barley gene HvPARP3 exhibit changes in efficiency of DNA repair. DNA Repair 28, 116–130. doi: 10.1016/j.dnarep.2015.02.018
Stolarek, M., Gruszka, D., Braszewska-Zalewska, A., Maluszynski, M. (2015b). Functional analysis of the new barley gene HvKu80 indicates that it plays a key role in double-strand DNA break repair and telomere length regulation. Mutagenesis 30 (6), 785–797. doi: 10.1093/mutage/gev033
Szarejko, I., Szurman-Zubrzycka, M., Nawrot, M., Marzec, M., Gruszka, D., Kurowska, M., et al. (2017). “Creation of a TILLING population in barley after chemical mutagenesis with sodium azide and MNU,” in Biotechnologies for Plant Mutation Breeding. Protocols. Eds. Jankowicz-Cieślak, J., Tai, T. H., Kumlehn, J., Till, B. J. (Switzerland: Springer Open, Springer International Publishing), 91–111. doi: 10.1007/978-3-319-45021-6_6
Szurman-Zubrzycka, M., Baran, B., Stolarek-Januszkiewicz, M., Kwaśniewska, J., Szarejko, I., Gruszka, D. (2019a). The dmc1 Mutant Allows an Insight Into the DNA Double-Strand Break Repair During Meiosis in Barley (Hordeum vulgare L.). Front. Plant Sci. 10. doi: 10.3389/fpls.2019.00761
Szurman-Zubrzycka, M., Chmielewska, B., Gajewska, P., Szarejko, I. (2017). “Mutation detection by analysis of DNA heteroduplexes in TILLING populations of diploid species,” in Biotechnologies for Plant Mutation Breeding. Protocols. Eds. Jankowicz-Cieślak, J., Tai, T. H., Kumlehn, J., Till, B. J. (Switzerland: Springer Open, Springer International Publishing), 281–304. doi: 10.1007/978-3-319-45021-6_18
Szurman-Zubrzycka, M., Nawrot, M., Jelonek, J., Dziekanowski, M., Kwasniewska, J., Szarejko, I. (2019b). ATR, a DNA damage signaling kinase, is involved in aluminum response in barley. Front. Plant Sci. 10. doi: 10.3389/fpls.2019.01299
Szurman-Zubrzycka, M., Chwiałkowska, K., Niemira, M., Kwaśniewski, M., Nawrot, M., Gajecka, M., et al. (2021). Aluminium or low pH - which is the bigger enemy of barley? Transcriptome analysis of barley root meristem under Al and low pH stress. Front. Genet. 12. doi: 10.3389/fgene.2021.675260
Szurman-Zubrzycka, M. E., Zbieszczyk, J., Marzec, M., Jelonek, J., Chmielewska, B., Kurowska, M. M., et al. (2018). HorTILLUS-A rich and renewable source of induced mutations for forward/reverse genetics and pre-breeding programs in barley (Hordeum vulgare L.). Front. Plant Sci. 9. doi: 10.3389/fpls.2018.00216
Takei, H., Shinozaki, Y., Yano, R., Kashojiya, S., Hernould, M., Chevalier, C., et al. (2019). Loss-of-function of a tomato receptor-like kinase impairs male fertility and induces parthenocarpic fruit set. Front. Plant Sci. 10. doi: 10.3389/fpls.2019.00403
Talamè, V., Bovina, R., Sanguineti, M. C., Tuberosa, R., Lundqvist, U., Salvi, S. (2008). TILLMore, a resource for the discovery of chemically induced mutants in barley. Plant Biotechnol. J. 6 (5), 477–485. doi: 10.1111/j.1467-7652.2008.00341.x
Taylor, N., Greene, E. (2003). PARSESNP: a tool for the analysis of nucleotide polymorphisms. Nucleic Acids Research 31, 3808–3811. doi: 10.1093/nar/gkg574
Thompson, L. U. (1990). “Phytates in canola/rapeseed,” in Canola andrapeseed: production, chemistry, nutrition and processing technology. Ed. Shahidi, F. (Boston, MA,USA: Springer), 173–192.
Till, B. J., Cooper, J., Tai, T. H., Colowit, P., Greene, E. A., Henikoff, S., et al. (2007). Discovery of chemically induced mutations in rice by TILLING. BMC Plant Biol. 7, 19. doi: 10.1186/1471-2229-7-19
Till, B. J., Datta, S., Jankowicz-Cieslak, J. (2018). “TILLING: The Next Generation,” in Plant Genetics and Molecular Biology. Advances in Biochemical Engineering/Biotechnology, vol. 164 . Eds. Varshney, R., Pandey, M., Chitikineni, A. (Cham: Springer). doi: 10.1007/10_2017_54
Till, B. J., Reynolds, S. H., Greene, E. A., Codomo, C. A., Enns, L. C., Johnson, J. E., et al. (2003). Large-scale discovery of induced point mutations with high-throughput TILLING. Genome Res. 13 (3), 524–530. doi: 10.1101/gr.977903
Till, B. J., Reynolds, S. H., Weil, C., Springer, N., Burtner, C., Young, K., et al. (2004). Discovery of induced point mutations in maize genes by TILLING. BMC Plant Biol. 4, 12. doi: 10.1186/1471-2229-4-12
Tollenaar, D. (1934). Untersuchungen ueber Mutation bei Tabak. I. Entstehungsweise une Wesen kuenstlich erzeugter Gen-Mutanten. Genetica 16, 111–152. doi: 10.1007/BF01837860
Tollenaar, D. (1938). Untersuchungen euber Mutation bei Tabak. II. Einige kuenstlich erzeugte Chromosom-Mutanten. Genetica 20, 285–294. doi: 10.1007/BF01508112
Tramontano, A., Jarc, L., Jankowicz-Cieslak, J., Hofinger, B. J., Gajek, K., Szurman-Zubrzycka, M., et al. (2019). Fragmentation of pooled PCR products for highly multiplexed TILLING. G3 9 (8), 2657–2666. doi: 10.1534/g3.119.400301
Tsai, H., Howell, T., Nitcher, R., Missirian, V., Watson, B., Ngo, K. J., et al. (2011). Discovery of rare mutations in populations: TILLING by sequencing. Plant Physiol. 156, 1257–1268. doi: 10.1104/pp.110.169748
Uauy, C., Paraiso, F., Colasuonno, P., Tran, R. K., Tsai, H., Berardi, S., et al. (2009). A modified TILLING approach to detect induced mutations in tetraploid and hexaploid wheat. BMC Plant Biol. 9, 115. doi: 10.1186/1471-2229-9-115
Vernoud, V., Lebeigle, L., Munier, J., Marais, J., Sanchez, M., Pertuit, D., et al. (2021). β-amyrin synthase1 controls the accumulation of the major saponins present in pea (Pisum sativum). Plant Cell Physiol. 62 (5), 784–797. doi: 10.1093/pcp/pcab049
Wang, D., Li, Y., Wang, H., Xu, Y., Yang, Y., Zhou, Y., et al. (2023). Boosting wheat functional genomics via an indexed EMS mutant library of KN9204. Plant Commun. 21, 100593. doi: 10.1016/j.xplc.2023.100593
Wang, J., Raman, H., Zhang, G., Mendham, N., Zhou, M. (2006). Aluminum tolerance in barley (Hordeum vulgare L.): physiological mechanisms, genetics and screening methods. J. Zhejiang Univ. Sci. B 7, 769–787. doi: 10.1111/j.1469-8137.2008.02619.x
Wang, W., Simmonds, J., Oan, Q., Davidson, D., He, F., Battal, A., et al. (2018). Gene editing and mutagenesis reveal inter-cultivar differences and additivity in the contribution of TaGW2 homoeologues to grain size and weight in wheat. Theor. Appl. Genet. 121, 2463–2475. doi: 10.1007/s00122-018-3166-7
Wen, N., Osorio, C. E., Brew-Appiah, R. A. T., Mejías, J. H., Alam, T., Kashyap, S., et al. (2022). Targeting induced local lesions in the wheat DEMETER and DRE2 genes, responsible for transcriptional derepression of wheat gluten proteins in the developing endosperm. Front. Nutr. 9. doi: 10.3389/fnut.2022.847635
Xin, Z., Wang, M. L., Barkley, N. A., Burow, G., Franks, C., Pederson, G., et al. (2008). Applying genotyping (TILLING) and phenotyping analyses to elucidate gene function in a chemically induced sorghum mutant population. BMC Plant Biol. 8, 103. doi: 10.1186/1471-2229-8-103
Yan, Z., Appiano, M., van Tuinen, A., Meijer-Dekens, F., Schipper, D., Gao, D., et al. (2021). Discovery and characterization of a novel tomato mlo mutant from an EMS mutagenized micro-tom population. Genes 12 (5), 719. doi: 10.3390/genes12050719
Yano, R., Hoshikawa, K., Okabe, Y., Wang, N., Dung, P. T., Imriani, P. S., et al. (2019). Multiplex exome sequencing reveals genome-wide frequency and distribution of mutations in the „Micro-Tom” Targeting Induced Local Lesions in Genomes (TILLING) mutant library. Plant Biotechnol. 36 (4), 223–231. doi: 10.5511/plantbiotechnology.19.0830a
Zhang, D., Guo, X., Xu, Y., Li, H., Ma, L., Yao, X., et al. (2019). OsCIPK7 point-mutation leads to conformation and kinase-activity change for sensing cold response. J. Integr. Plant Biol. 61 (12), 1194–1200. doi: 10.1111/jipb.12800
Keywords: TILLING, mutagenesis, crops, reverse genetics, functional genomics, mutations, plants, NGTs
Citation: Szurman-Zubrzycka M, Kurowska M, Till BJ and Szarejko I (2023) Is it the end of TILLING era in plant science? Front. Plant Sci. 14:1160695. doi: 10.3389/fpls.2023.1160695
Received: 07 February 2023; Accepted: 19 July 2023;
Published: 22 August 2023.
Edited by:
Apichart Vanavichit, Kasetsart University, ThailandReviewed by:
Aamir Raina, Aligarh Muslim University, IndiaCopyright © 2023 Szurman-Zubrzycka, Kurowska, Till and Szarejko. This is an open-access article distributed under the terms of the Creative Commons Attribution License (CC BY). The use, distribution or reproduction in other forums is permitted, provided the original author(s) and the copyright owner(s) are credited and that the original publication in this journal is cited, in accordance with accepted academic practice. No use, distribution or reproduction is permitted which does not comply with these terms.
*Correspondence: Miriam Szurman-Zubrzycka, bWlyaWFtLnN6dXJtYW5AdXMuZWR1LnBs
Disclaimer: All claims expressed in this article are solely those of the authors and do not necessarily represent those of their affiliated organizations, or those of the publisher, the editors and the reviewers. Any product that may be evaluated in this article or claim that may be made by its manufacturer is not guaranteed or endorsed by the publisher.
Research integrity at Frontiers
Learn more about the work of our research integrity team to safeguard the quality of each article we publish.