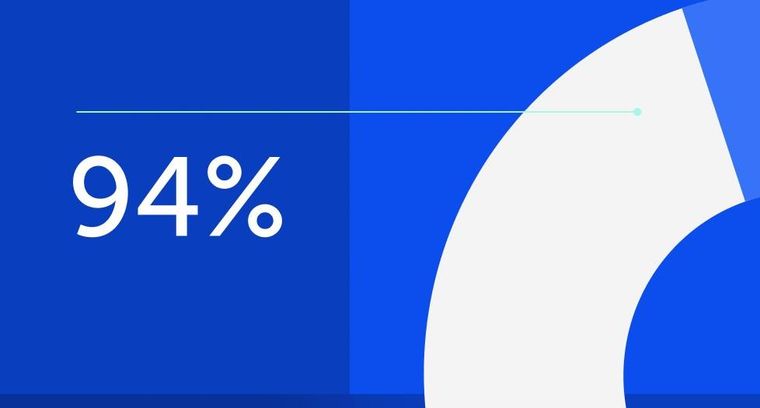
94% of researchers rate our articles as excellent or good
Learn more about the work of our research integrity team to safeguard the quality of each article we publish.
Find out more
ORIGINAL RESEARCH article
Front. Plant Sci., 09 March 2023
Sec. Plant Metabolism and Chemodiversity
Volume 14 - 2023 | https://doi.org/10.3389/fpls.2023.1155297
This article is part of the Research TopicSecondary Metabolites as Multifunctional Molecules in the Changing Environment of Plant GrowthView all 6 articles
The aim of this study was to determine intra- and interspecies variation in the qualitative and quantitative composition of methanol-soluble metabolites in the leaves of three Digitalis species (D. lanata, D. ferruginea, and D. grandiflora) from the central Balkans. Despite the steady use of foxglove constituents for human health as valuable medicinal products, populations of the genus Digitalis (Plantaginaceae) have been poorly investigated to describe their genetic and phenetic variation. Following untargeted profiling using UHPLC-LTQ Orbitrap MS, by which we identified a total of 115 compounds, 16 compounds were quantified using the UHPLC(–)HESI–QqQ-MS/MS approach. In total, 55 steroid compounds, 15 phenylethanoid glycosides, 27 flavonoids, and 14 phenolic acid derivatives were identified across the samples with D. lanata and D. ferruginea showing a great similarity, while 15 compounds were characteristic only for D. grandiflora. The phytochemical composition of methanol extracts, considered here as complex phenotypes, are further examined along multiple levels of biological organization (intra- and interpopulation) and subsequently subjected to chemometric data analysis. The quantitative composition of the selected set of 16 chemomarkers belonging to the classes of cardenolides (3 compounds) and phenolics (13 compounds) pointed to considerable differences between the taxa studied. D. grandiflora and D. ferruginea were found to be richer in phenolics as compared to cardenolides, which otherwise predominate in D. lanata over other compounds. PCA revealed lanatoside C, deslanoside, hispidulin, and p-coumaric acid to be the main compounds contributing to the differences between D. lanata on one side and D. grandiflora and D. ferruginea on the other, while p-coumaric acid, hispidulin, and digoxin contribute to the diversification between D. grandiflora and D. ferruginea. However, quantitative variation in the metabolite content within species was faint with mild population diversification visible in D. grandiflora and particularly in D. ferruginea. This pointed to the highly conserved content and ratio of targeted compounds within the analyzed species, which was not severely influenced by the geographic origin or environmental conditions. The presented metabolomics approach might have, along with morphometrics and molecular genetics studies, a high information value for further elucidation of the relationships among taxa within the genus Digitalis.
Both genotyping and phenotyping of a robust sample set of a species under study are essential to properly understand the variation in its populations. The variation itself is the main prerequisite for a species to “outwit” environmental pressures and to prolong its survival on the planet. In medicinal plants, assessing the genetic and phenetic variation of wild plant populations enables appropriate planning of selective breeding to ensure favorable yield. Besides crop and timber improvement programs, medicinal plants, being a great part of the modern world health care system (Fabricant and Farnsworth, 2001), are in the focus of plant breeders. In fact, around 25% of drugs commonly used worldwide is derived from plants (Mishra et al., 2013).
During the Middle Ages, foxglove (Digitalis spp., family Plantaginaceae) has been considered to have multifarious healing properties (Aronson and Aronson, 1986) and the first reports of its role as an agent in the congestive heart failure treatment dated from the year 1250 (Williams, 1861). Today, D. purpurea L. and, more notably, D. lanata Ehrh. are both widely collected from nature and field-cultivated to extract cardenolides, being among the best producers of these compounds in the plant world (Clemente et al., 2011). One of them, digoxin, is mainly produced in Europe from dried leaves of D. lanata, reaching up to 1.5% dry weight (Kennedy, 1978; Bown, 1995; Clemente et al., 2011). There is a vast diversity of cardenolides within the genus Digitalis with more than one hundred different compounds isolated, the most commercially attractive, besides digoxin, being lanatoside C, digitoxin, and acetyldigitoxin (Clemente et al., 2011; Kreis, 2017). Other compounds, such as digitanols, steroidal saponins, anthranoids, phenols, sterols, and polysaccharides, have been identified in various Digitalis species (see Clemente et al., 2011 and Kreis, 2017 for reviews). Today, with the development of synthetic substitute drugs for cardiac diseases, foxgloves are given “a second chance” (Kreis, 2017) as potent agents against various viruses as well as for the treatment of cystic fibrosis and several cancer types (Bertol et al., 2011).
Out of the 27 recognized species of the genus Digitalis (Plants of the World Online | Kew Science, 2023), 5 are found in the Balkan Peninsula: D. lanata, D. laevigata Waldst. & Kit., D. ferruginea L., D. viridiflora Lindl., and D. grandiflora Mill. (syn. D. ambigua Murray), the first three belonging to the section Globiflorae and the last two to expanded “Maranthae” (Bräuchler et al., 2004). Two more species, namely D. ikarica (P.H.Davis) Strid and D. fuscescens Waldst. & Kit., can be found in the Balkans, the former inhabiting several North Aegean and Dodecanese islands, while the latter being reported to represent a hybrid between D. grandiflora and D. purpurea (Bræmer et al., 1927). Among these, D. lanata is somewhat better studied in terms of genetic and phenetic diversity and by its potential to accumulate various specialized metabolites (Braga et al., 1997; Yücesan et al., 2018). Few data are available for the other species listed (Kennedy, 1978; Katanić et al., 2017; Kaska et al., 2020). Surprisingly, variation in metabolite fingerprints both between and within wild populations is pretty much neglected, having in mind that it can render starting material for selective breeding or at least for the selection of specific genotypes that would accumulate metabolite amounts appropriate for the pharmaceutical industry. Nevertheless, according to EURISCO (Kotni et al., 2023), many landraces are stored in seed collections. Therefore, phytochemical characterization of an appropriate sample set consisting of as many populations as possible and represented by a sufficient number of individuals is an evident prerequisite to perceive the extent of variation of specialized metabolites in Digitalis species. Since the Balkan Peninsula represents one of the two centers of the genus diversity and considering that D. lanata, D. ferruginea, and D. grandiflora are most commonly found throughout it, the three species were selected for the study. In a greater perception, D. lanata grows across the southeast Europe and Turkey (and has been introduced into North America and several central European and Asian countries), D. ferruginea, besides the Balkan Peninsula, inhabits the Apennine Peninsula, Asia Minor, and the Caucasian region, while D. grandiflora is spread throughout Europe (except for several westernmost and northernmost countries) and northwest Asia, but has been introduced into North America. Central Balkan populations of these three species contain few individuals (several dozen at maximum, pers. obs.), which implicates that they might be at risk of extinction due to the bottleneck effect. Therefore, the protection of degraded populations should be considered and the level of their genetic variation must be assessed (Nebauer et al., 2000). However, only D. grandiflora has been studied in this sense (Boronnikova et al., 2007). The detection of population differentiation by studying either genetic or phenetic variation (or preferably both) provides valuable information for planning conservation measures and conducting monitoring as well as for sampling germplasm for ex situ conservation (Nazir et al., 2008; Clemente et al., 2011).
The present study is aimed at determining, disentangling, and interpreting the chemical diversity of three Digitalis species growing in the Balkan Peninsula, to setup the footprint for meaningful biodiversity conservation strategies and to propose the means for sustainable utilization of bioresources. Furthermore, this study will establish the background for future elucidation of molecular aspects of chemical diversity by adopting state-of-the art omics technologies and will enable the development of alternative strategies for the production of bioactive compounds through biotechnology approaches. The specific objective of this study was to select high-resolution chemical markers to estimate both inter- and intrapopulation variability of Digitalis species by applying a targeted metabolomic approach.
All reagents and solvents used were of analytical grade. Acetonitrile, formic acid (both MS grade), and methanol (HPLC grade) were purchased from Merck (Darmstadt, Germany). Ultra-pure water (Water Purification System, New Human Power I Integrate, Human Corporation, Republic of Korea) was used to prepare standard solutions and blanks. Analytical standards of protocatechuic acid, syringic acid, p-hydroxybenzoic acid, 5-O-caffeoylquinic acid, caffeic acid, aesculetin, isoorientin, p-coumaric acid, quercetin 3-O-glucoside, naringin, luteolin, hispidulin, and isorhamnetin were purchased from Sigma Aldrich (Steinheim, Germany). Standards of deslanoside, lanatoside C, and digoxin were provided by Professor Yang Ye and Dr. Chunping Tang (Shanghai Institute of Materia Medica- SIMM, Chinese Academy of Sciences — CAS, China).
Leaves from the flowering stems were collected from individual plants during June, July, and August of 2020 and 2021 from wild populations in the central part of the Balkan Peninsula. Localities and the list of 28 populations of three Digitalis species (Digitalis grandiflora, D. lanata, and D. ferruginea) are listed in Table 1 and their spatial distribution is presented in Figure 1. Plants were identified in the field by the authors and classified by referring to The World Flora Online (WFO) database (The World Flora Online, 2023) and Plants of the World Online | Kew Garden (Plants of the World Online | Kew Science, 2023). The corresponding voucher specimens are deposited in the herbarium of the University of Belgrade, Serbia (BEOU, acronym follows Thiers, 2023) with the voucher numbers listed in Table 1.
Table 1 The list of Digitalis grandiflora, D. lanata and D. ferruginea accessions used in the study.
Figure 1 Map presenting the populations of Digitalis grandifloraa, D. lanata, and D. ferruginea originating from the central Balkan Peninsula analyzed within the present study. For the population labels, please refer to Table 1.
After harvesting, leaves were immediately transferred into plastic zip-bags containing silica gel. Prior to methanol extraction, leaves were ground to a fine powder using liquid nitrogen. Approximately 50 mg of dry plant material was extracted with 1 ml of 80% methanol overnight at room temperature. The next day, samples were sonicated (Sonorex Bandelin Electronic, Berlin, Germany) for 1 h and subsequently centrifuged at 10,000g for 10 min. The supernatants were filtered through 0.2-mm cellulose filters (Agilent Technologies, Santa Clara USA) and stored at 4°C until use.
Identification of metabolites in methanol extracts of the three foxglove species was done by an untargeted approach using an Accela UHPLC system connected to a linear ion trap–Orbitrap hybrid mass spectrometer (LTQ OrbiTrap XL, Thermo Fisher Scientific, Bremen, Germany) with heated electrospray ionization (HESI). Methanol extracts of D. lanata, D. ferruginea, and D. grandiflora were prepared from leaves collected in 2020 in Zavojsko jezero (population accession code No. DLZAV), Zaovine (population accession code No. DFZAO), and Debelo brdo (population accession code No. DADB), respectively (Table 1).
Separations of compounds were performed on a Hypersil Gold C18 column (50 × 2.1 mm, 1.9 µm; Thermo Fisher Scientific) at 40°C. The mobile phase consisted of (A) water + 0.1% formic acid and (B) acetonitrile + 0.1% formic acid. A linear gradient program at a flow rate of 0.300 mL/min was used: 0.0–1.0 min 5% (B), 1.0–14.0 min from 5% to 95% (B), 14.0–14.2 min from 95% to 5% (B), and 5% (B) for 6 min. The injection volume was 5 µL.
The mass spectrometer was operated in either negative or positive ionization mode, depending on the compound class. The HESI-source parameters were described by Koprivica et al. (2018). MS spectra were acquired by full range acquisition covering 100–1500 m/z. The data-dependent MS/MS events were always performed on the most intense ions detected in the full scan MS. The ions of interest were isolated in the ion trap with an isolation width of 5 ppm and activated with 35% collision energy levels.
Metabolites were identified according to the corresponding spectral characteristics: mass spectra, accurate mass, characteristic fragmentation patterns, and corresponding retention time. Tentative identification of various metabolites was achieved by studying their MSn spectra and comparing them with the available literature on spectroscopic and mass data for compounds detected previously in the genus Digitalis and other related species (Supplementary Table 1). Xcalibur software (version 2.1) was used for the instrument control, data acquisition, and data analysis.
Quantification of the targeted compounds was performed using a Dionex Ultimate 3000 UHPLC system connected to a triple-quadrupole (QqQ) mass spectrometer (TSQ Quantum Access Max, Thermo Fisher Scientific, Bremen, Germany). Metabolite quantification was performed across 259 individual plants belonging to the three study species (Table 1).
A Syncronis C18 analytical column (100 × 2.1 mm) with 1.7 µm particle size (Thermo Fisher Scientific, Bremen, Germany) was used for the chromatographic separation. The flow rate and the composition of the mobile phases as well as the gradient elution program are described in the previous section (2.4.1.). The mass detector was equipped with a HESI source operated in the negative ionization mode. The parameters of the HESI source and the other mass detector settings were previously described by Banjanac et al. (2017).
The selected reaction monitoring (SRM) mode of the instrument was used for the quantification of the targeted compounds in the samples. The compounds were quantified by direct comparison with commercial standards. Calibration curves revealed good linearity, with r2 values exceeding 0.99 (peak areas vs. concentration). The total amount of each compound was evaluated by calculation of the peak area and is expressed as mg/kg. The concentration of digoxin is expressed via the calibration curve of deslanoside, because the available amount of digoxin standard was insufficient to obtain the calibration curve with appropriate calibration levels.
The quantitative data were analyzed by two unsupervised methods, principal component analyses (PCA) and hierarchical cluster analyses (HCA), as well as one supervised learning method, linear discriminant analysis (LDA). For HCA the input variables were scaled to the [0, 1] range. HCA was performed based on Euclidean distances with cluster agglomeration using Ward’s (Ward, 1963) minimum variance method. LDA was performed with a presumption of the equal prior probability of classes. The correlation matrix for the quantitative data was constructed using Pearson’s correlation coefficients. All statistical analyses were performed in the Past 4 software (version 4.12; Hammer et al., 2001).
Although some data on the phytochemical content of D. grandiflora, D. ferruginea, and especially D. lanata are available in the literature, these species are only scarcely investigated for the phytochemical interpopulation variability (Yücesan et al., 2018). Several previous studies have reported carbohydrates, iridoids, and caffeoyl phenylethanoid glycosides as suitable chemomarkers for the Plantaginaceae family, including the genus Digitalis (Taskova et al., 2005). Cardenolides and phenolics were not considered in this regard. On the other hand, tracing the amounts of commercially attractive compounds can provide valuable information to study the diversity of Digitalis species in the phylogenetic context. This may also highlight their characteristic populations’ metabolite profiles allowing to pick out the genotypes that produce pharmaceutically interesting compounds. More than 100 different cardenolides have been isolated from the genus Digitalis, and previous studies have been focused mainly on several well-known and exploited Digitalis species (mostly D. purpurea and D. lanata). However, to search for novel compounds, there is a necessity for in-depth screening of various Digitalis species for their metabolite composition, which may render bioactive agents for the treatment of various diseases. On the other hand, cardenolides occur in multi-compound mixtures whose composition represents complex phenotypes that may vary along multiple dimensions and levels of biological organization. Digitalis species are characterized by the presence of a vast array of phenolic compounds, among which flavonoids, mainly belonging to the flavone and 3-methoxyflavone groups, predominate. These two large groups of bioactive compounds and their diversity both within and among Digitalis species deserve a considerable attention. Simultaneous tracing of their composition in samples can ensure characterization of phenotypes in an ecologically and evolutionary meaningful way and enable the elucidation of the relations among and within group of compounds pointing out to the links between their biosynthetic pathways.
According to Kreis (2017), HPLC-MS procedures for assessing the metabolic footprints of cardenolides found in Digitalis spp. are highly recommended. Identification of metabolites present in the methanol extracts of three foxglove species (D. lanata, D. ferruginea, and D. grandiflora) was achieved by high-resolution mass spectrometry (HRMS) in combination with MSn fragmentation. The representative base peak chromatograms of the three species are shown in Supplementary Figure 1. Using this technique, the molecular formula of an unknown compound can be determined through the exact mass, and its structure can be proposed and solved by studying its fragmentation pathway. Using the UHPLC-LTQ OrbiTrap MS technique in both positive and negative ionization modes, totally 115 compounds were identified based on their monoisotopic masses, MSn fragmentation, and previously reported MS data (Table 2; Supplementary Table 1). The consulted literature that contained NMR and MS data used to confirm the identification of the given compounds is listed in Supplementary Table 1. All identified compounds were classified into seven groups: steroidal glycosides (43 compounds), steroid aglycones (12 compounds), phenylethanoid glycosides (15 compounds), flavonoid glycosides (18 compounds), flavonoid aglycones (9 compounds), phenolic acid derivatives (14 compounds), and 6 compounds belonging to other classes. In addition to the expected cardiac glycosides, steroidal saponins as well as pregnane and furostanol glycosides were also present in the analyzed extracts. A detailed LC/MS qualitative analysis revealed very similar profiles of steroidal glycosides in the extracts of D. lanata and D. ferruginea leaves, in particular of digoxin, deslanoside, and lanatosides A, B, and C, which is consistent to earlier reports (Kennedy, 1978; Braga et al., 1997; Verma et al., 2014; Yücesan et al., 2018). On the other hand, these compounds were not detected in leaves of D. grandiflora although digoxin has been reported in small amounts in an earlier study (Kennedy, 1978). Table 2 specifies which of the identified compounds were previously detected in any Digitalis species, while for a certain number of compounds it can be concluded that they were identified for the first time in Digitalis species.
Table 2 High resolution MS data on the metabolites identified in the three studied Digitalis species.
The largest number of identified compounds (55 in total) belongs to the group of steroids (cardenolides, pregnane glycosides, furostane-type and spirostane-type steroidal saponins), 43 of which are steroidal glycosides and 12 are steroidal aglycones. Different sugar derivatives can be found as sugar components of these cardenolides and other steroid glycosides. The most common is digitoxose, which gives 130 Da as a neutral loss in the mass spectrum, followed by different hexoses (162 Da), acetyldigitoxose (172 Da), digitalose (160 Da), and less often deoxyhexose (146 Da) (Ravi et al., 2020). As can be seen from Table 2, some of these steroid derivatives are named after a certain Digitalis species (e.g., digitalin, purpureagitoside, lanatoside, purpurea glycoside), while some compounds are named trivially as furostanol or spirostanol glycosides. All derivatives of steroidal aglycones are, due to their polarity, identified only in the positive ionization mode. For all listed compounds (except compound 23) from the group of steroids, Supplementary Table 1 lists the literature sources in which the given compounds were previously isolated or identified. Compound 23 was identified as digoxigenin 3-O-dideoxyhexoside and its proposed structure and fragmentation pathway are shown in Supplementary Figure 2. The MS2 base peak of this compound at 519 m/z resulted from the loss of one deoxyhexose residue (146 Da), while the loss of one more residue of deoxyhexose (146 Da) yields the mass of the deprotonated aglycone digoxigenin at 373 m/z (MS3 base peak). Further, neutral loss of H2O (18 Da) and CO2 (44 Da) results in MS4 base peak at 311 m/z. Interestingly, a compound with this structure has not yet been identified but has been synthesized as a digitoxin-related compound and used to test its anticytomegalovirus activity (Cai et al., 2014).
A total of 15 compounds was identified from the group of phenylethanoid glycosides (PG), all of which except decaffeoyl acteoside isomers (compounds 56 and 57) were previously identified in various Digitalis species (Table 2). All the three examined Digitalis species proved to be rich in PG, as previously reported (Brieger et al., 1995), except for compound 69 (forsythiaside), which was identified only in the extract of D. grandiflora. Compounds from the group of phenylethanoid glycosides specific for Digitalis have been shown to be potential tumor inhibitors - cytotoxic activity of the PG isolated from D. davisiana showed a strong impact on HEp-2 cells (Kutluay et al., 2019), while PG extracts from D. purpurea showed PKCα-inhibitory bioactivity (Zhou et al., 1998).
All 18 identified flavonoid glycosides belong to the flavone subgroup and are represented by hydroxyl, methyl, and methoxy derivatives of apigenin and luteolin (Table 2). Compound 71 (apigenin 6,8-di-C-hexoside) is a C-glycoside, while all others (compounds 72-88) are identified as 7-O-glycosides. Flavonoid C-glycosides were not found to be common for Digitalis species, but compound 71 was previously identified in the related genus Plantago, belonging to the same family Plantaginaceae (Kawashty et al., 1994). Among the derivatives of flavonoid O-glycosides, hexosides (neutral loss of 162 Da), hexuronides (neutral loss of 176 Da), and two acylhexosides (compound 83 and 88) were found. The presence of three glycosides (compounds 73, 76, and 77) with two hexuronic acid units was confirmed by the specific MS2 fragmentation, in which the fragment 351 m/z (dihexuronyl–H) was formed as a base peak. Usually, the MS2 base peak actually represents the mass of the deprotonated aglycone, which was not the case here, but the disaccharide residue had the highest abundance. In the next fragmentation stage, the MS3 base peak at 193 m/z was formed by the loss of 158 Da (dihexuronyl–H2O). A similar fragmentation pathway was noticed for compound 73 (luteolin 7-O-hexosyl-hexuronide), with the difference that its MS2 base peak corresponds to the mass of the deprotonated aglycone – luteolin (285 m/z). It was previously reported that leaves of D. purpurea contained luteolin 7-O-hexosyl-hexuronide (Harborne, 1963). Compound 86 (jaceosidin 7-O-hexuronide) has not been detected in Digitalis species so far, but its aglycone jaceosidin (Imre et al., 1984) and 7-O-glucoside of jaceosidin (Hiermann et al., 1977) have been reported. Using the exact mass of compound 88 with molecular ion at 563.14063 m/z in the positive ionization mode, its molecular formula C26H26O14 was generated. By studying its fragmentation pattern, it was concluded that dihydroxy-dimethoxyflavone, malonic acid and hexose had to be present in its structure. In the first stage of fragmentation, the malonylhexose unit was lost and the MS2 base peak at 315 m/z was formed, which corresponds to the mass of protonated dihydroxy-dimethoxyflavone. Further, in MS3 fragmentation, the loss of the CH3 group (15 Da) resulted in only one fragment at 300 m/z, while in MS4 fragmentation, a base peak at 168 m/z was observed. The fragment ion at 168 m/z was formed by the specific RDA fragmentation of flavonoids and it can be designated as a 1,3A+–CH3 fragment (Tsimogiannis et al., 2007), which further leads to the conclusion that this flavonoid has two hydroxyl groups and one methoxy group on the A ring. In view of all the above-mentioned facts, 6-methoxy-4’-methylapigenin or pectolinaringenin was proposed as the aglycone of this compound, which was otherwise common for Digitalis species (Imre et al., 1984). A proposed structure and a fragmentation pathway of this compound, trivially assigned as pectolinaringenin 7-O-malonylhexoside, is depicted in Supplementary Figure 3.
Among the nine identified flavonoid aglycones (Table 2), eight were flavone derivatives and only one (compound 96) was identified as flavonol – santin. Santin belongs to the subgroup of flavonoids termed O-methylated flavonols and it was earlier isolated from D. orientalis (Imre et al., 1984). The presence of compounds 92–94 was confirmed by comparing their mass spectra with the analytical standards, while the other compounds from this group were proven by determination of the exact masses and fragmentation pathways (ApSimon et al., 1963; Hiermann et al., 1977; Imre et al., 1984).
Derivatives of phenolic acids found in this study (Table 2) were previously reported to be specific for Digitalis species and were actually derivatives of hydroxycinnamic acid (Kırmızıbekmez et al., 2009; Katanić et al., 2017; Kaska et al., 2020). In this study, a certain number of hydroxybenzoic acid derivatives (compounds 98, 100, and 102) were also found. Three compounds (102, 104, and 105) were identified by direct comparison with the corresponding standards. A significant number of hexosyl derivatives of phenolic acids were detected, giving a specific fragmentation with the neutral loss of 162 Da.
Six compounds that structurally do not belong to any of the above-mentioned groups are included in the “other compounds” category (Table 2). The presence of two derivatives of coumarins, aesculetin (compound 110), and its 6-O-glucoside – aesculin (compound 112) was confirmed by comparison with the analytical standards. Compound 111, hebitol II (O-acyl carbohydrate), was found only in the D. lanata extract in this study, and its fragmentation was consistent with previously published MS data (Friščić et al., 2016). Compound 113, lignan syringaresinol, has not been previously detected in Digitalis species, but its fragmentation is in agreement with the literature data (Jiang et al., 2019). The last two compounds (114 and 115), digitoemodin and ω-hydroxyziganein-1-methyl ether, are anthraquinones previously isolated from D. cariensis (Imre et al., 1994).
Targeted metabolic profiling was conducted with the aim of quantifying 16 bioactive compounds in methanol extracts of the three analyzed Digitalis species. We were able to quantify 3 compounds from the class of cardenolides: digoxin, lanatoside C, and deslanoside (syn. desacetyllanatoside C). In addition, we quantified 7 phenolic compounds, derivatives of either hydroxycinnamic (5-O-caffeoylquinic acid, caffeic acid, p-coumaric acid, and aesculetin) or hydroxybenzoic acid (p-hydroxybenzoic acid, syringic acid, and protocatechuic acid). Three Digitalis species have also been investigated with respect to their flavonoid content by targeting totally 6 compounds belonging to flavones (luteolin, hispidulin, isoorientin), flavonols (quercetin, isorhamnetin), and flavanones (naringin). The results indicate that the most abundant phenolic acids of D. grandiflora belong to the group of hydroxybenzoic acids (protocatechuic, syringic, and p-hydroxybenzoic acid), while p-coumaric acid predominates among hydrohycinnamic acids (Gašić et al., 2023). Dominant phenolic acids in D. lanata and D. ferruginea are p-coumaric acid and p-hydroxybenzoic acid (Gašić et al., 2023), which slightly coincides with previous findings, reporting chlorogenic and caffeic acids (Katanić et al., 2017) or ferulic acid (Kaska et al., 2020) as the most abundant in D. ferruginea. Hispidulin and other flavonoids belonging to the flavone and 3-methoxyflavone groups are especially abundant in Digitalis species (Kaska et al., 2020), which is also confirmed in the present study. Naringin, luteolin, and hispidulin are the most abundant flavonoids in D. grandiflora (Gašić et al., 2023). In D. lanata hispidulin predominates, while D. ferruginea leaves are characterized by the prevalence of hispidulin and naringin.
The content of the main cardenolides in D. lanata varies with the growth stages (Freier, 1977; Braga et al., 1997) and is further influenced by the leaves’ maturity (Vogel and Luckner, 1981), growth conditions, vegetation stage, collection time, drying method, and storage conditions and duration (Balakina et al., 2005). Within the present study, the profiling of specialized metabolites has been performed using silica gel-dried leaf samples collected from blooming plants in their second year of growth, as recommended by Clemente et al. (2011) at the stage when the content of pharmaceutically interesting compounds is at the optimal level. Leaves at the same developmental stage were collected to further reduce the effect of developmental stage on the content of compounds of interest. All the three targeted cardenolides were present in D. lanata, with lanatoside C predominating (Gašić et al., 2023). According to the literature, D. lanata is known to contain primary cardenolide glycosides including lanatoside A, B, and C series (Braga et al., 1997; Pérez-Alonso et al., 2012; Yücesan et al., 2018). On the other hand, D. grandiflora has been reported to contain lanatoside A and B, but lanatoside C was absent (Wichtl et al., 1987). This is in accordance with the present study, since we were able to quantify digoxin in D. grandiflora leaves, while lanatoside C and deslanoside were not detected or were present in trace amounts (Gašić et al., 2023). Major cardenolides in leaves of D. ferruginea were digoxin and deslanoside, which coincides to a previous study (Verma et al., 2014) that reported digitoxigenin and digoxigenin as the dominant cardenolides in this species.
The content and the ratio of cardenolides in Digitalis species are regulated by the activity of enzymes responsible for the conversion of primary glycosides such as lanatoside A, lanatoside B, and lanatoside C into corresponding secondary glycosides digitoxin, gitoxin, and digoxin, respectively (Pellati et al., 2009). The hydrolysis of lanatoside C to form digoxin proceeds in two stages (Figure 2A) and involves the enzyme digilanidase and one deacetylation step (Cobb, 1976). Deslanoside (desacetyllanatoside C) is a product of the lanatoside C metabolism and a precursor of digoxin (Figure 2A). The hydrolysis of lanatoside C usually occurs after the damage of leaf tissue or plant harvesting. It has been suggested that the absence or low enzymatic activity in the plant material favors the preservation of primary glycosides in D. lanata (Balakina et al., 2005). On the contrary, if the enzymes are sufficiently active, a spontaneous enzymatic degradation of primary to secondary glycosides occurs, resulting in an extremely complex profile in which both primary and secondary glycosides are present in different ratios, depending on both genetic background and post-harvest processing methodologies (Balakina et al., 2005). Within the present study, harvested leaves were immediately stored on silica gel, which enabled their fast dehydration and prevented degradation of primary into secondary glycosides. Thus, we were able to trace the species- and genotype-specific content and ratio of the targeted cardenolides in D. lanata, D. ferruginea, and D. grandiflora leaves. Accordingly, we may conclude that D. lanata is characterized by the predominance of lanatoside C, which is moderately converted to digoxin, most probably via deslanoside (Figure 2A), as this intermediate is present in significant amounts in leaves of this species. The alternative digoxin precursor, acetyldigoxin, was not recorded in any analyzed taxon (Table 2). On the other hand, D. grandiflora leaves most likely display prominent activity of the enzymes responsible for the conversion of primary into secondary glycosides, as digoxin is found to be the major cardenolide in this species (Gašić et al., 2023). Lanatoside C and its metabolite deslanoside were absent in the majority of the analyzed D. grandiflora accessions or were present in trace amounts in several samples. In D. ferruginea, lanatoside C is, most likely, efficiently converted into digoxin, the dominant cardenolide compound. The conversion probably takes place via deslanoside intermediate, as this compound is the second most abundant compound in this species.
Figure 2 A simplified scheme of the lanatoside C hydrolysis that results in the formation of digoxin in two stages and involves the enzyme digilanidase and one deacetylation step (A). The conversion goes via either deslanoside (desacetyllanatoside C) or acetyldigoxin. D. lanata (a) is a rich source of lanatoside C, D. ferruginea (b) contains predominantly deslanoside and digoxin, while D. grandiflora (c) is abundant in digoxin. Correlation analysis of the compounds’ quantitative data was performed (B), and the correlation matrix was constructed using Pearson’s correlation algorithm. Color scale indicates a positive (blue) or a negative (red) correlation.
We further performed the pairwise correlation analysis by calculating the Pearson’s correlation coefficient (Figure 2B) to provide further evidence for the stated presumptions. Significant positive correlations were observed between the contents of lanatoside C and deslanoside. Digitoxin was negatively correlated with the other two cardenolides analyzed here, which is not surprising taking into account that this secondary glycoside emerges by the deacetylation of lanatoside C to deslanoside, which then undergoes hydrolysis. Cardenolides were further positively correlated with hydroxybenzoic acids and flavones hispidulin and isorhamnetin. Positive correlations were mainly observed within the group of phenolics, with the exception of syringic acid, which was negatively correlated with the majority of analyzed compounds.
To better understand the relationship between the analyzed taxa (D. grandiflora, D. lanata, and D. ferruginea), we performed PCA analysis on phytochemical dataset acquired for 16 targeted compounds (Figure 3A). PC1 and PC2 cumulatively explained 83.56% of the total variance. D. lanata is clearly separated from the other two Digitalis species along the PC1, explaining 61.63% of the total variation. On the other hand, D. grandiflora and D. ferruginea segregate along the PC2, which explains 21.93% of the variability. The major contributors to PC1 are lanatoside C, deslanoside, hispidulin, and p-coumaric acid (Figure 3B). Along the PC2, samples are distinguished mainly by p-coumaric acid, hispidulin, and digoxin (Figure 3C). This unsupervised multivariate method offered a glimpse of possible usefulness of the selected combination of metabolites for phytochemical determination of the three Digitalis species. Although we presumed that D. lanata and D. ferruginea, phylogenetically closely related taxa (Bräuchler et al., 2004) would show greater similarity in phytochemical profiles, this was not the case.
Figure 3 Principal component analysis (PCA) biplot with the first two PCs explaining 83.56% of total variance (A). For each species 95% confidence ellipses are presented: black − Digitalis grandiflora, red − D. lanata, green − D. ferruginea. Different symbols indicate affiliation of populations. Participation of the variables in the first two PCs is indicated by the corresponding vectors and by loading plots presented separately for PC1 (B) and PC2 (C). Variables protocatechuic acid , A; syringic acid, B; p-hydroxybenzoic acid, C; 5-O-caffeoylquinic acid, D; caffeic acid, E; p-coumaric acid, F; aesculetin, G; isoorientin, H; quercetin-3-O-glucoside, I; naringin, J; luteolin, K; hispidulin, L; isorhamnetin, M; digoxin, N; deslanoside, O; lanatoside C, P. For the interpretation of population abbreviations in the figure legend, please refer to Table 1.
Based on the HCA analysis (Supplementary Figure S4), a similar conclusion to that derived from PCA can be drawn regarding the phytochemical relationships among the analyzed Digitalis taxa. However, HCA more clearly depictured the populations’ linkages and indicated clustering of populations based on their geographical distribution.
Having in mind that distinct metabolic pathways of the 16 compounds may be reflected on different accumulation patterns across the studied populations within the three Digitalis species, one would expect huge quantitative variation in their profiles, since interpopulation variation in metabolite accumulation has been previously reported for other Digitalis species (Nebauer et al., 1999; Usai et al., 2007). However, our results imply poor population differentiation in this regard for all the three studied species. As shown in Figures 4–6, a divergence of two populations from the central sample cloud in the PCA can be observed for D. ferruginea (Figure 6A) and only mild diversification of several populations of D. grandiflora can be noticed in the LDA (Figure 4B). In particular, all individuals representing the population DABARE and 5 individuals each from DAZ and DADZ cluster separately from the main sample cloud in the HCA matrix formed by the remaining samples belonging to 12 other populations of D. grandiflora (Figure 4C). The three populations mentioned are from Dinaric karst areas with limestone as the basal rock, located at the high altitudes (Table 1), which could be the reason for their segregation from the common cluster, since soil characteristics are reportedly related to cardenolide production (Roca-Pérez et al., 2004). The most abundant compound in the majority of D. grandiflora populations, p-hydroxybenzoic acid, is in the same time the compound that varies at the most, followed by two flavonoid aglycones, hispidulin and luteolin (Figure 4A). Although present in greater amounts than in D. lanata, digoxin is evenly quantified across the populations of D. grandiflora (Gašić et al., 2023). As the species with the broadest areal among the three studied species, D. grandiflora shows rather surprisingly low variation in the metabolite profiles, despite being represented by the largest sample set. D. grandiflora is a biennial plant, occurring as a basal rosette of leaves during the first year, while the second-year rosette leaves wither rapidly during flowering stem elongation. To uniform the sampling procedure, leaves of the same developmental stage originating from flowering stems were collected for the analyses. Delayed flowering of D. grandiflora in higher altitudes enabled us to perform sampling throughout summer seasons and, presented results indicate that sampling period had no significant influence on the content of metabolites. An earlier study (Boronnikova et al., 2007) that estimated the partitioning of molecular variation showed that only a small fraction of the species’ genetic variation was present between populations, while most of it resided within populations (about 90%, depending on the type of molecular marker used). Therefore, the weak population differentiation seen in the metabolite profiles would most certainly have its ground in their low genetic differentiation. Further studies on the genetic background of these populations currently planned by the same team of authors will undoubtedly clarify this hypothesis.
Figure 4 Principal component analysis (PCA) biplot with the first two PCs explaining 84.38% of the total variance (A) among D. grandiflora accessions. For fifteen D. grandiflora populations (each labeled by a different symbol) 95% confidence ellipses are presented. Participation of the variables (compounds) in the first two PCs is indicated by the corresponding loading plots. Linear discriminant analysis (LDA) (B) represents the same fifteen populations of D. grandiflora with 95% confidence ellipses. Heatmap of the scaled quantitative data (C) with the samples arranged according to the hierarchical cluster analysis (Ward’s method of cluster agglomeration). Compounds’ labels are the same as in Figure 3. For the interpretation of population abbreviations in the figure legend, please refer to Table 1.
Figure 5 Principal component analysis (PCA) biplot with the first two PCs explaining 83.90% of the total variance (A) among D. lanata accessions. For six D. lanata populations (each labeled by a different symbol) 95% confidence ellipses are presented. Participation of the variables (compounds) in the first two PCs is indicated by the corresponding loading plots. Linear discriminant analysis (LDA) (B) represents the same six populations of D. lanata with 95% confidence ellipses. Heatmap of the scaled quantitative data (C) with the samples arranged according to the hierarchical cluster analysis (Ward’s method of cluster agglomeration). Compounds’ labels are the same as in Figure 3. For the interpretation of population abbreviations in the figure legend, please refer to Table 1.
The sample matrix made of 6 populations of D. lanata presented in Figure 5A is much more homogeneous, while clustering fails to offer the resolution to distinguish potential groups. Hispidulin and p-coumaric acid are the major factors of the summary metabolite variation among the studied populations of this species (Figure 5A). They are followed by the two most abundant cardenolide compounds in D. lanata, lanatoside C and deslanoside. The four compounds are also leading in the quantified amounts throughout the studied populations (Gašić et al., 2023). Nearly 50 years ago, D. lanata genotypes were actually quite often assessed but only with respect to cardenolide content (Weiler and Zenk, 1976; Weiler and Westekemper, 1979; Wichtl et al., 1987) and no comprehensive study based on a broader metabolite profiling has been performed in this species. However, these articles report on a remarkable variation in the accumulation of cardenolides in plants originating in different geographic regions. In our study, we were able to collect samples from 6 populations having the maximum distance of 200 km and growing in similar habitats, and only mild differences in metabolic profiles were anticipated. Sampling across a broader species’ range would most probably bring about better resolution to differentiate populations based on their metabolite footprints.
In D. ferruginea, the whole population DFPR along with 5 individuals from other 4 populations form a separate cluster (Figure 6C), while LDA differentiates the population DFZAO from the main cloud formed by the majority of the remaining individuals that belong to 5 other populations. The reason for this differentiation could be the same as for D. grandiflora, namely the limestone soil the plants have been grown on. The most accountable compounds for this differentiation are digoxin, hispidulin, and p-coumaric acid (Figure 6A) but for the different reasons. Hispidulin in a higher amount is quantified in the population DFPR only, while the other two compounds are the dominant specialized metabolites across the studied D. ferruginea populations, but their quantities vary among these (Gašić et al., 2023).
Figure 6 Principal component analysis (PCA) biplot with the first two PCs explaining 83.84% of the total variance (A) among D. ferruginea accessions. For seven D. ferruginea populations (each labeled by a different symbol) 95% confidence ellipses are presented. Participation of the variables (compounds) in the first two PCs is indicated by the corresponding loading plots. Linear discriminant analysis (LDA) (B) represents the same seven populations of D. ferruginea with 95% confidence ellipses. Heatmap of the scaled quantitative data (C) with the samples arranged according to the hierarchical cluster analysis (Ward’s method of cluster agglomeration). Compounds’ labels are the same as in Figure 3. For the interpretation of population abbreviations in the figure legend, please refer to Table 1.
The results point to the fact that the content and ratio of targeted compounds is highly conserved within the analyzed Digitalis species, and is only moderately modified in response to environmental conditions, such as the soil type and altitude above sea level. To increase the resolution of discrimination at the inter- and intrapopulation level, it is possible to include the additional subset of chemomarkers, belonging to the same or other classes of metabolites, which is the course of our further work. Together with morphometrics and molecular markers, chemomarkers can provide a deeper insight into phylogenetic relationships and overall genetic variation among and within Digitalis taxa. Another aspect of this study provides data relevant for the conservation biology studies of the genus Digitalis.
The conservation of individual species, populations, and biodiversity in general is increasingly becoming one of the leading premises of applicative science. The data generated in the present study, which refer to the diversity of bioactive compounds in natural populations of three Digitalis species, thus representing their variation at the phenotypic level, are the starting point for the implementation of conservation strategies. As stated above, digoxin and other commercially interesting cardenolides are mainly isolated from natural sources, which might severely reduce the natural diversity in wild populations. The logical extension of this research is the study of diversity at the genotype level using various molecular markers, which is also the course of our further work.
The original contributions presented in the study are publicly available. This data can be found here: http://radar.ibiss.bg.ac.rs/handle/123456789/5448.
DMi, TB, BŠ, and UG conceived and designed the experiments. TB, JB, MM, NA, SD, MS, JN, LP, MT, SŽ, DMa, BF, and TL performed the experiments. UG and DMi performed the phytochemical characterization of samples. DMi was responsible for the statistical data analysis. UG, TB, BŠ, JB, and DMi organized and wrote the manuscript with editing from all the authors. All authors contributed to the article and approved the submitted version.
This work is financed by the Ministry of Education, Science and Technological Development of the Republic of Serbia (Contract numbers 451-03-68/2022-14/200007 and 451-03-47/2023-01/200007).
The authors would like to acknowledge Professor Yang Ye and Dr. Chunping Tang from Shanghai Institute of Materia Medica (SIMM, Chinese Academy of Sciences - CAS, China) for kindly providing the standards of lanatoside C, deslanoside, and digoxin for the analytical purposes.
The authors declare that the research was conducted in the absence of any commercial or financial relationships that could be construed as a potential conflict of interest.
All claims expressed in this article are solely those of the authors and do not necessarily represent those of their affiliated organizations, or those of the publisher, the editors and the reviewers. Any product that may be evaluated in this article, or claim that may be made by its manufacturer, is not guaranteed or endorsed by the publisher.
The Supplementary Material for this article can be found online at: https://www.frontiersin.org/articles/10.3389/fpls.2023.1155297/full#supplementary-material
ApSimon, J. W., Haynes, N. B., Sim, K. Y., Whalley, W. B. (1963). 705. 5, 7, 4′-Trihydroxy-3′, 6-dimethoxyflavone, a pigment from Digitalis lanata l. J. Chem. Soc, 3780–3782. doi: 10.1039/JR9630003780
Aronson, J. K., Aronson, J. K. (1986). An account of the foxglove and its medical uses 1785-1985: Incorporating a facsimile of William withering’s `An account of the foxglove and some of its medical uses’ (1785) (Oxford, New York: Oxford University Press).
Balakina, M. V., Zvonkova, E. N., Dyumaev, K. M., Bykov, V. A. (2005). HPLC control of the quality of Digitalis lanata raw material. Pharm. Chem. J. 39, 587–592. doi: 10.1007/s11094-006-0025-7
Banjanac, T., Dragićević, M., Šiler, B., Gašić, U., Bohanec, B., Nestorović Živković, J., et al. (2017). Chemodiversity of two closely related tetraploid Centaurium species and their hexaploid hybrid: Metabolomic search for high-resolution taxonomic classifiers. Phytochemistry 140, 27–44. doi: 10.1016/j.phytochem.2017.04.005
Bertol, J. W., Rigotto, C., de Pádua, R. M., Kreis, W., Barardi, C. R. M., Braga, F. C., et al. (2011). Antiherpes activity of glucoevatromonoside, a cardenolide isolated from a Brazilian cultivar of Digitalis lanata. Antivir. Res. 92, 73–80. doi: 10.1016/j.antiviral.2011.06.015
Boronnikova, S. V., Kokaeva, Z. G., Gostimsky, S. A., Dribnokhodova, O. P., Tikhomirova, N. N. (2007). Analysis of DNA polymorphism in a relict uralian species, large-flowered foxglove (Digitalis grandiflora mill.), using RAPD and ISSR markers. Russ. J. Genet. 43, 530–535. doi: 10.1134/S1022795407050080
Bræmer, L., Welté, J., Lavialle, P. (1927). L’Hybridation dans le genre Digitalis l., Digitalis purpurascens Roth. B. Soc Bot. Fr. 74, 351–355. doi: 10.1080/00378941.1927.10831476
Braga, F. C., Kreis, W., Récio, R. A., de Oliveira, A. B. (1997). Variation of cardenolides with growth in a Digitalis lanata Brazilian cultivar. Phytochemistry 45, 473–476. doi: 10.1016/S0031-9422(96)00809-6
Bräuchler, C., Meimberg, H., Heubl, G. (2004). Molecular phylogeny of the genera Digitalis l. and Isoplexis (Lindley) loudon (Veronicaceae) based on ITS-and trnL-f sequences. Plant Syst. Evol. 248, 111–128. doi: 10.1007/s00606-004-0145-z
Brieger, D., Liedtke, S., Weber, R., Kirschke, M., Lichius, J. J. (1995). Ein neues, verbascosidähnliches esterglucosid aus den blättern von Digitalis lanata ehrh. - maxosid. Pharmazie 50, 707–708.
Cai, H., Wang, H.-Y. L., Venkatadri, R., Fu, D.-X., Forman, M., Bajaj, S. O., et al. (2014). Digitoxin analogues with improved anticytomegalovirus activity. ACS Med. Chem. Lett. 5, 395–399. doi: 10.1021/ml400529q
Clemente, E. S., Müller-Uri, F., Nebauer, S. G., Segura, J., Kreis, W., Arrillaga, I. (2011). “Digitalis,” in Wild crop relatives: Genomic and breeding resources: Plantation and ornamental crops. Ed. Kole, C. (Berlin, Heidelberg: Springer), 73–112. doi: 10.1007/978-3-642-21201-7_5
Cobb, P. H. (1976). Application of high-performance liquid chromatography to the separation of cardenolides and the assay of digoxin in Digitalis lanata leaf. Analyst 101, 768–776. doi: 10.1039/AN9760100768
Fabricant, D. S., Farnsworth, N. R. (2001). The value of plants used in traditional medicine for drug discovery. Environ. Health Persp. 109, 69–75. doi: 10.1289/ehp.01109s169
Freier, R. (1977). Untersuchungen zur biogenese der herzwirksamen glykoside in digitalis lanata EHRH. Dissertation, Philipps-Universität Marburg.
Friščić, M., Bucar, F., Hazler Pilepić, K. (2016). LC-PDA-ESI-MSn analysis of phenolic and iridoid compounds from Globularia spp. J. Mass Spectrom. 51, 1211–1236. doi: 10.1002/jms.3844
Gašić, U., Mišić, D., Šiler, B., Banjanac, T. (2023). Data from: Metabolomics quantitative data of three foxglove species (genus Digitalis, fam. Plantaginaceae) from Central Balkan Peninsula. IBISS RADaR - Digital Repository of the Institute for Biological Research "Siniša Stanković"-National Institute of the Republic of Serbia, University of Belgrade. Version:draft. http://radar.ibiss.bg.ac.rs/handle/123456789/5448.
Hammer, Ø., Harper, D. A., Ryan, P. D. (2001). PAST: paleontological statistics software package for education and data analysis. Palaeontol. Electron. 4, 1–9.
Harborne, J. B. (1963). Plant polyphenols. x. flavone and aurone glycosides of Antirrhinum. Phytochemistry 2, 327–334. doi: 10.1016/S0031-9422(00)84856-6
Hiermann, A., Kartnig, T., Seligmann, O., Wagner, H. (1977). Flavonoids in the leaves of Digitalis lanata (Ehrhart). Planta Med. 32, 24–26. doi: 10.1055/s-0028-1097553
Imre, S., Ertürk, S., Imre, Z. (1994). Two new anthraquinones from Digitalis cariensis. Z. Naturforsch. C 49, 684–686. doi: 10.1515/znc-1994-9-1020
Imre, S., Islimyeli, S., Öztunc, A., Büyüktimkin, N. (1984). Flavonoid aglycones in some Digitalis species. Planta Med. 50, 360–360. doi: 10.1055/s-2007-969735
Jiang, Y., Liu, R., Chen, J., Liu, M., Liu, M., Liu, B., et al. (2019). Application of multifold characteristic ion filtering combined with statistical analysis for comprehensive profiling of chemical constituents in anti-renal interstitial fibrosis I decoction by ultra-high performance liquid chromatography coupled with hybrid quadrupole-orbitrap high resolution mass spectrometry. J. Chromatogr. A 1600, 197–208. doi: 10.1016/j.chroma.2019.04.051
Kaska, A., Deniz, N., Çiçek, M., Mammadov, R. (2020). The screening of Digitalis ferruginea l. subsp. ferruginea for toxic capacities, phenolic constituents, antioxidant properties, mineral elements and proximate analysis. Food Sci. Technol. 41, 505–512. doi: 10.1590/fst.08620
Katanić, J., Ceylan, R., Matić, S., Boroja, T., Zengin, G., Aktumsek, A., et al. (2017). Novel perspectives on two Digitalis species: Phenolic profile, bioactivity, enzyme inhibition, and toxicological evaluation. S. Afr. J. Bot. 109, 50–57. doi: 10.1016/j.sajb.2016.12.004
Kawashty, S. A., Abdalla, M. F., Saleh, N. A. M. (1994). Flavonoids of Plantago species in Egypt. Biochem. Syst. Ecol. 22, 729–733. doi: 10.1016/0305-1978(94)90058-2
Kennedy, A. J. (1978). Cytology and digoxin production in hybrids between Digitalis lanata and D. grandiflora. Euphytica 27, 267–272. doi: 10.1007/BF00039142
Kırmızıbekmez, H., Celep, E., Masullo, M., Bassarello, C., Yeşilada, E., Piacente, S. (2009). Phenylethyl glycosides from Digitalis lanata. Helv. Chim. Acta 92, 1845–1852. doi: 10.1002/hlca.200900049
Koprivica, M. R., Trifković, J.Đ., Dramićanin, A. M., Gašić, U. M., Akšić, M. M. F., Milojković-opsenica, D. M. (2018). Determination of the phenolic profile of peach (Prunus persica l.) kernels using UHPLC–LTQ OrbiTrap MS/MS technique. Eur. Food Res. Technol. 244, 2051–2064. doi: 10.1007/s00217-018-3116-2
Kotni, P., van Hintum, T., Maggioni, L., Oppermann, M., Weise, S. (2023). EURISCO update 2023: the European search catalogue for plant genetic resources, a pillar for documentation of genebank material. Nucleic Acids Res. 51, D1465–D1469. doi: 10.1093/nar/gkac852
Kreis, W. (2017). The foxgloves (Digitalis) revisited. Planta Med. 83, 962–976. doi: 10.1055/s-0043-111240
Kutluay, V. M., Ishiuchi, K., Makino, T., Saracoglu, I. (2019). Cytotoxic phenylethanoid glycosides from Digitalis davisiana Heywood: Evaluation of structure activity relationships and chemotaxonomical significance of isolated compounds. Fitoterapia 135, 90–98. doi: 10.1016/j.fitote.2019.04.009
Mishra, K. P., Sharma, N., Diwaker, D., Ganju, L., Singh, S. B. (2013). Plant derived antivirals: a potential source of drug development. J. Virol. Antivir. Res. 2, 2. doi: 10.4172/2324-8955.1000109
Nazir, R., Reshi, Z., Wafai, B. A. (2008). Reproductive ecology of medicinally important Kashmir Himalayan species of Digitalis l. Plant Spec. Biol. 23, 59–70. doi: 10.1111/j.1442-1984.2008.00214.x
Nebauer, S. G., Del Castillo-Agudo, L., Segura, J. (1999). Cardenolide variation within and among natural populations of Digitalis obscura. J. Plant Physiol. 154, 426–430. doi: 10.1016/S0176-1617(99)80278-8
Nebauer, S. G., del Castillo-Agudo, L., Segura, J. (2000). An assessment of genetic relationships within the genus Digitalis based on PCR-generated RAPD markers. Theor. Appl. Genet. 100, 1209–1216. doi: 10.1007/s001220051426
Pellati, F., Bruni, R., Bellardi, M. G., Bertaccini, A., Benvenuti, S. (2009). Optimization and validation of a high-performance liquid chromatography method for the analysis of cardiac glycosides in Digitalis lanata. J. Chromatogr. A 1216, 3260–3269. doi: 10.1016/j.chroma.2009.02.042
Pérez-Alonso, N., Capote, A., Gerth, A., Jiménez, E. (2012). Increased cardenolides production by elicitation of Digitalis lanata shoots cultured in temporary immersion systems. Plant Cell Tiss. Organ Cult. 110, 153–162. doi: 10.1007/s11240-012-0139-4
Plants of the World Online | Kew Science (2023) Plants of the world online. Available at: https://powo.science.kew.org/ (Accessed January 27, 2023).
Ravi, B. G., Guardian, M. G. E., Dickman, R., Wang, Z. Q. (2020). Profiling and structural analysis of cardenolides in two species of Digitalis using liquid chromatography coupled with high-resolution mass spectrometry. J. Chromatogr. A 1618, 460903. doi: 10.1016/j.chroma.2020.460903
Roca-Pérez, L., Boluda, R., Pérez-Bermúdez, P. (2004). Soil-plant relationships, micronutrient contents, and cardenolide production in natural populations of Digitalis obscura. J. Plant Nutr. Soil. Sci. 167, 79–84. doi: 10.1002/jpln.200320336
Taskova, R. M., Gotfredsen, C. H., Jensen, S. R. (2005). Chemotaxonomic markers in digitalideae (Plantaginaceae). Phytochemistry 66, 1440–1447. doi: 10.1016/j.phytochem.2005.04.020
The World Flora Online (2023). Available at: http://www.worldfloraonline.org/ (Accessed January 27, 2023).
Thiers, B. (2023) Index herbariorum: A global directory of public herbaria and associated staff. new York botanical garden’s virtual herbarium. Available at: http://sweetgum.nybg.org/ih (Accessed January 24, 2023).
Tsimogiannis, D., Samiotaki, M., Panayotou, G., Oreopoulou, V. (2007). Characterization of flavonoid subgroups and hydroxy substitution by HPLC-MS/MS. Molecules 12, 593–606. doi: 10.3390/12030593
Usai, M., Atzei, A. D., Marchetti, M. (2007). Cardenolides content in wild sardinian Digitalis purpurea l. populations. Nat. Prod. Res. 21, 798–804. doi: 10.1080/14786410701218291
Verma, S. K., Yucesan, B., Sahin, G., Gurel, E. (2014). Embryogenesis, plant regeneration and cardiac glycoside determination in Digitalis ferruginea subsp. ferruginea l. Plant Cell Tiss. Organ Cult. 119, 625–634. doi: 10.1007/s11240-014-0562-9
Vogel, E., Luckner, M. (1981). Distribution of cardenolides in Digitalis lanata. Planta Med. 41, 161–165. doi: 10.1055/s-2007-971693
Ward, J. H. (1963). Hierarchical grouping to optimize an objective function. J. Am. Stat. Assoc. 58, 236–244. doi: 10.1080/01621459.1963.10500845
Weiler, E. W., Westekemper, P. (1979). Rapid selection of strains of Digitalis lanata EHRH. with high digoxin Content1. Planta Med. 35, 316–322. doi: 10.1055/s-0028-1097223
Weiler, E. W., Zenk, M. H. (1976). Radioimmunoassay for the determination of digoxin and related compounds in Digitalis lanata. Phytochemistry 15, 1537–1545. doi: 10.1016/S0031-9422(00)88933-5
Wichtl, M., Buhl, W., Huesmann, G. (1987). Digitalis l. bekannte und weing bekannte vertreter einer wichtigen arzneipflanzengattung. Deutsche Apotheker Zeitung 127, 2391–2400.
Williams, J. (Ed.) (1861). “The physicians of myddvai: Meddygon myddvai, or the medical practice of the celebrated rhiwallon and his sons, of myddvai,” in Caermarthenshire, physicians to Rhys gryg, lord of dynevor and ystrad towy, about the middle of the thirteenth century (Felinfach: Llanerch).
Yücesan, B. B., Eker, İ., Lazzarini, L. E. S., Aslam, N., Mohammed, A., Gürel, E. (2018). Shoot-tip cultivation and cardenolide content analysis of natural populations of Digitalis lanata ehrh. subsp. lanata (Wooly foxglove) in Thrace region. Uluslararası Tarım ve Yaban Hayatı Bilimleri Dergisi 4, 55–62. doi: 10.24180/ijaws.390582
Keywords: UHPLC-LTQ OrbiTrap MS, cardiac glycosides, steroids, phenylethanoids, flavonoids, Digitalis grandiflora, D. lanata, D. ferruginea
Citation: Gašić U, Banjanac T, Šiler B, Božunović J, Milutinović M, Aničić N, Dmitrović S, Skorić M, Nestorović Živković J, Petrović L, Todorović M, Živković S, Matekalo D, Filipović B, Lukić T and Mišić D (2023) Variation in the chemical profiles of three foxglove species in the central Balkans. Front. Plant Sci. 14:1155297. doi: 10.3389/fpls.2023.1155297
Received: 31 January 2023; Accepted: 23 February 2023;
Published: 09 March 2023.
Edited by:
Maciej Strzemski, Medical University of Lublin, PolandReviewed by:
Sylwia Zielińska, Wroclaw Medical University, PolandCopyright © 2023 Gašić, Banjanac, Šiler, Božunović, Milutinović, Aničić, Dmitrović, Skorić, Nestorović Živković, Petrović, Todorović, Živković, Matekalo, Filipović, Lukić and Mišić. This is an open-access article distributed under the terms of the Creative Commons Attribution License (CC BY). The use, distribution or reproduction in other forums is permitted, provided the original author(s) and the copyright owner(s) are credited and that the original publication in this journal is cited, in accordance with accepted academic practice. No use, distribution or reproduction is permitted which does not comply with these terms.
*Correspondence: Uroš Gašić, dXJvcy5nYXNpY0BpYmlzcy5iZy5hYy5ycw==; Danijela Mišić, ZG1pc2ljQGliaXNzLmJnLmFjLnJz
Disclaimer: All claims expressed in this article are solely those of the authors and do not necessarily represent those of their affiliated organizations, or those of the publisher, the editors and the reviewers. Any product that may be evaluated in this article or claim that may be made by its manufacturer is not guaranteed or endorsed by the publisher.
Research integrity at Frontiers
Learn more about the work of our research integrity team to safeguard the quality of each article we publish.