- 1State Key Laboratory of Subtropical Silviculture, Zhejiang A&F University, Lin’an, Zhejiang, China
- 2Key Laboratory of Carbon Cycling in Forest Ecosystems and Carbon Sequestration of Zhejiang Province, Zhejiang A&F University, Lin’an, Zhejiang, China
- 3College of Environmental and Resource Sciences, Zhejiang A&F University, Lin’an, Zhejiang, China
- 4Jiyang College, Zhejiang A&F University, Zhuji, Zhejiang, China
- 5Tropical Silviculture and Forest Ecology, University of Göttingen, Göttingen, Germany
- 6Department of Environmental and Biological Sciences, University of Eastern Finland, Joensuu, Finland
Stem respiration (Rs) plays a vital role in ecosystem carbon cycling. However, the measured efflux on the stem surface (Es) is not always in situ Rs but only part of it. A previously proposed mass balance framework (MBF) attempted to explore the multiple partitioning pathways of Rs, including sap-flow-transported and internal storage of Rs, in addition to Es. This study proposed stem photosynthesis as an additional partitioning pathway to the MBF. Correspondingly, a double-chamber apparatus was designed and applied on newly sprouted Moso bamboo (Phyllostachys edulis) in leafless and leaved stages. Rs of newly sprouted bamboo were twice as high in the leafless stage (7.41 ± 2.66 μmol m−2 s−1) than in the leaved stage (3.47 ± 2.43 μmol m−2 s−1). Es accounted for ~80% of Rs, while sap flow may take away ~2% of Rs in both leafless and leaved stages. Culm photosynthesis accounted for ~9% and 13% of Rs, respectively. Carbon sequestration from culm photosynthesis accounted for approximately 2% of the aboveground bamboo biomass in the leafless stage. High culm photosynthesis but low sap flow during the leafless stage and vice versa during the leaved stage make bamboo an outstanding choice for exploring the MBF.
1 Introduction
Stem respiration (Rs) is a complex physiological process involving enzyme-catalyzed reactions, which is thought to be mainly influenced by temperature (Amthor, 2000; Atkin et al., 2005). We synthesized and analyzed measured stem-surface CO2 efflux (Es) of 191 woody plant species from 66 published papers, and the results confirmed the significant positive effects of temperature on Es (P < 0.01; Figure S1). Still, we found the temperature can only explain 20–42% variation of Rs, which corroborated the varying explaining power of temperature (17–75%) to the variance of stem respiration in other studies (Zhu et al., 2012). Furtherly, several studies observed the decoupling between respiration and temperature, including the time lag between respiration and temperature (Saveyn et al., 2008), the “midday depression” of stem respiration (Saveyn et al., 2008). One of the hypotheses explaining this decoupling is that the measured Es was not in situ but apparent Rs (Ceschia et al., 2002; McGuire and Teskey, 2002; McGuire and Teskey, 2004), i.e., Es is only one part of Rs. The gap between Rs and Es may suggest some missing efflux (Emiss) being ignored by the conventional approach. Therefore, a mass balance framework (MBF) on Rs was proposed by McGuire and Teskey (2004) based on previous ideas and observations (McGuire and Teskey, 2002).
According to the MBF, CO2 produced by Rs at a given position of a stem is allocated into three pathways: 1) CO2 efflux released from stem surface, i.e., Es, 2) CO2 efflux transported in sap flow (ET), and 3) CO2 efflux stored internally (EI). Therefore, Emiss includes both ET and EI. Compared with Es measured outside a stem, the other two parts are more challenging to detect and quantify. Based on the MBF, CO2 released by respiring cells in woody tissues could be dissolved in xylem sap and transported upward by sap rather than diffusing into the atmosphere directly (McGuire and Teskey, 2004; Teskey et al., 2007; Aubrey and Teskey, 2009). The isotopic tracing method (14C or 13C) proved the existence of ET on some trees (Bloemen et al., 2013; Salomón et al., 2019; Salomón et al., 2020). Moreover, up to 17% of the upward transported ET could be refixed by leaf photosynthesis (Bloemen et al., 2013). In contrast, EI was limited by CO2 saturation in sap according to equilibrium reactions, which were pH-dependent and more challenging to measure in situ (Teskey et al., 2007).
Besides, in some species with abundant stem chloroplasts, a fourth partitioning pathway was proposed, i.e., the CO2 reused by the culm/stem photosynthesis (Ep) (Pfanz et al., 2002; Wittmann et al., 2006; Berveiller et al., 2007; Teskey et al., 2007; Cernusak and Cheesman, 2015; Wittmann and Pfanz, 2018). Ep was confirmed with measured chlorophyll fluorescence on six coniferous and two broad-leaved tree species (Berveiller et al., 2007) and with an isotope tracing method on several C3 and CAM species (Kocurek et al., 2015). Furthermore, Ep was estimated continuously by comparing the theoretical Rs derived from stem temperature and Es on boreal Scots pines (Pinus sylvestris L.; Tarvainen et al., 2018). However, such a method of calculating Ep might still overestimate Ep when ignoring ET and EI. To resolve this problem in this study, we proposed an experimental apparatus including a pair of simultaneously monitoring chambers (one transparent and another light-proof; Figure 1). By applying one pair of chambers on the same stem and comparing the difference of Rs and Es between the chambers, we could derive Ep (see Section 2.3). In this way, we could avoid measuring ET and EI in situ, which is difficult to accurately measure due to the current limited techniques.
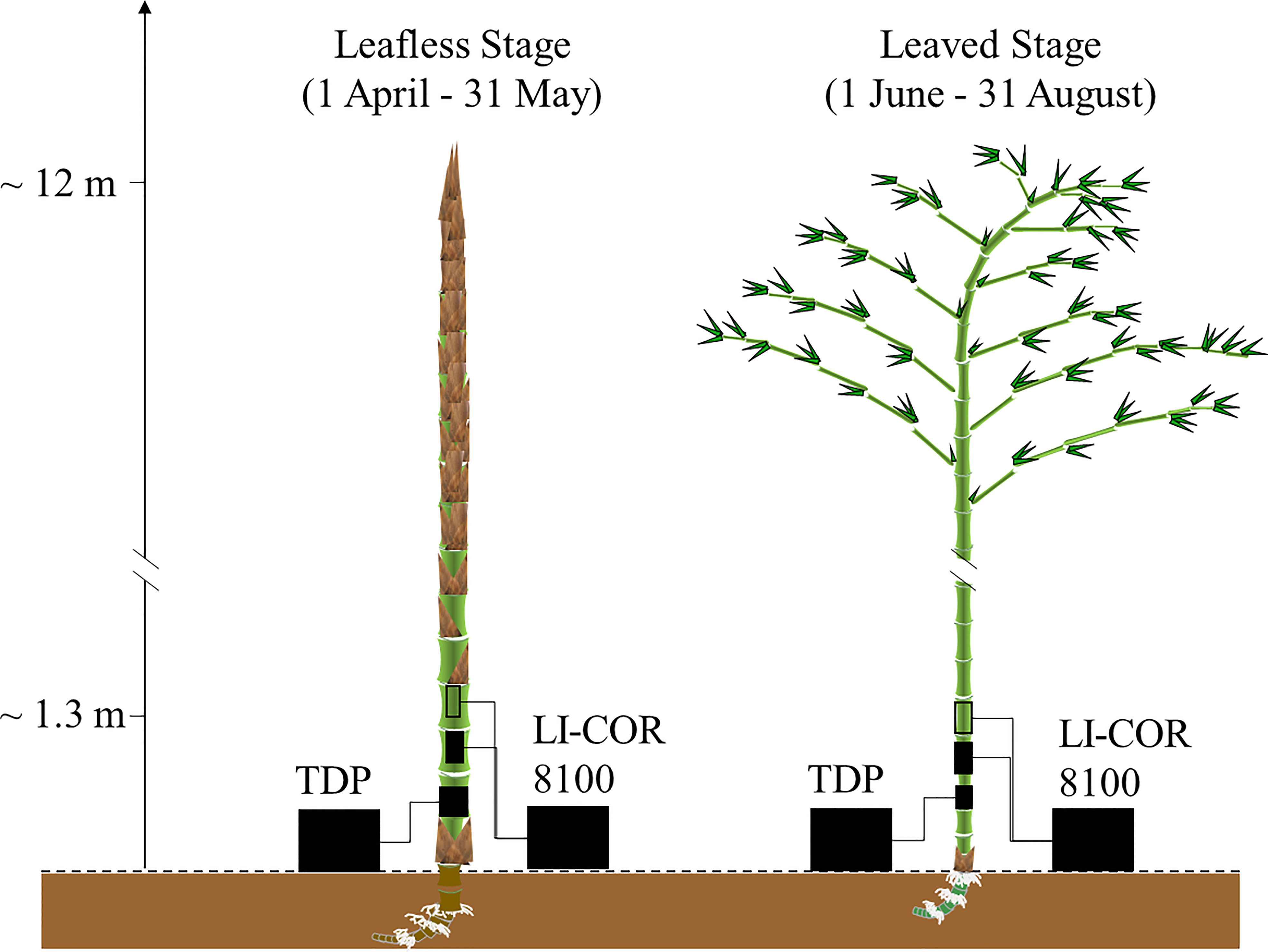
Figure 1 Bamboo phenology and experimental design. This study monitored sap flow with a self-built thermal dissipation probe based on the classical Granier type (TDP). In addition, CO2 efflux in the field was measured with LI-COR 8100 (Li-cor Inc., USA) using transparent and light-proof chambers.
To test the designed method, we chose Moso bamboo (Phyllostachys edulis (Carriere) J. Houzeau) as the experimental object. Unlike tree stems, bamboo culm has a smooth circular surface and a hollow cavity of each internode. Such characteristics make monitoring and measuring efflux pathways much easier and more accessible. Firstly, the traditional chambers measuring Es (Zhu et al., 2012; Darenova et al., 2018; Katayama et al., 2019; Helm et al., 2021) could be easily attached to the smooth circular bamboo culm surface with better sealing. Secondly, as the hollow cavity occupies the most volume of the internode, the thin culm wall (~1 cm thickness in an internode with 10 cm diameter at breast height) may have limited space for EI, which may reduce the disturbance for estimating the other allocated CO2 efflux (e.g., ET and Ep). At last, the observed large amount of chlorophyll in bamboo culms (Wang et al., 2013) implied the existence of Ep, thus making a possible examination of the four pathways of the MBF, which suggested Moso bamboo could be a fantastic model plant for exploring MBF.
As the most distributed bamboo species in China (National Forestry and Grassland Administration, 2019; FAO, 2020), Moso bamboo grows very fast, taking only 6–8 weeks for a newly sprouted culm to finish its ~12 m height growth; such a process was named “explosive growth” (Wang et al., 2019; Mei et al., 2020; Li et al., 2022). Especially the newly sprouted culms finish their height growth without leaves, which means they may primarily rely on an external supply of water (Fang et al., 2019; Gu et al., 2019; Wu et al., 2019; Mei et al., 2020) and carbon (Wei et al., 2019; Li et al., 2022) from other established elder culms in the leafless stage. The previous studies found that the water use patterns in the leafless and leaved stages were opposite (Fang et al., 2019; Tong et al., 2021), characterizing peak values of the sap flux density at midnight and midday, respectively. As the newly expanded leaves will lead to increasing transpiration and sap flow in the culms, we hypothesized our first assumption that sap flow might take away more CO2 efflux and influence more negatively to Es in the leaved stage than in the leafless stage. Additionally, the increasing ET may decrease the effect of culm photosynthesis. Thus, our second assumption was that Ep played a more significant role in the leafless stage than in the leaved stage. In summary, MBF theory was tested with a two-chambers experimental apparatus on Moso bamboo in this study, examining the role of sap flow and culm photosynthesis to the culm CO2 efflux in leafless and leaved stages.
2 Materials and methods
2.1 Study site and bamboo culms
The experiment was conducted in the experimental garden (30°15’55” N, 119°42’47” E, 13 m asl) of Zhejiang A&F University in Hangzhou, located in southeast China. The climate of the study site belongs to the subtropical monsoon climate zone. The annual mean temperature was 17.6 ± 0.4°C, and the annual rainfall was 1579 ± 263 mm averaged from 2008 to 2017 (mean ± std; Mei et al., 2020). The studied bamboo stand produces a similar amount of bamboo shoots each year, different from the on-and-off-year bamboo forests. The upper canopy of bamboo culm that grew before 2015 was cut to avoid crushing by snow in winter, while the culms that developed after 2015 have whole canopies.
This study selected ten newly sprouted bamboo culms developed in the spring of 2018 and 2019, respectively. The selected culms had no visible damage from pests and diseases. Furthermore, they were in good condition, e.g., with an evenly rounded form, green shoot tips, brown hair on the cover of the culm sheaths, and no apparent indications of degradation. In April, the culms started falling off the sheaths at the breast height, developing leaves from the end of May, and finished expanding most of their new leaves by August (Figure 1). Therefore, the experiment was conducted from April to August, when newly sprouted culms were easily installed and monitored. More detailed bamboo phenology refers to another study conducted on the same site (Mei et al., 2020).
2.2 CO2 efflux measurement
Es (μmol m−2 s−1) from the bamboo culm was measured with a self-sealing chamber designed following (Zhu et al., 2012). The chamber contains a cuvette covered with a 5*10 cm transparent PVC board and surrounded by 8 mm thick silicone foam to seal the gap between the chamber and the culm surface. Two plastic tubes with a row of holes were placed inside the cuvette on its left and right side. Two ends of each tube were connected to a T jointer that settled out of the cuvette, allowing airflow in and out. In application, the chamber was attached to the culm surface and fixed tightly with two belts to the bamboo culm (Figure 2).
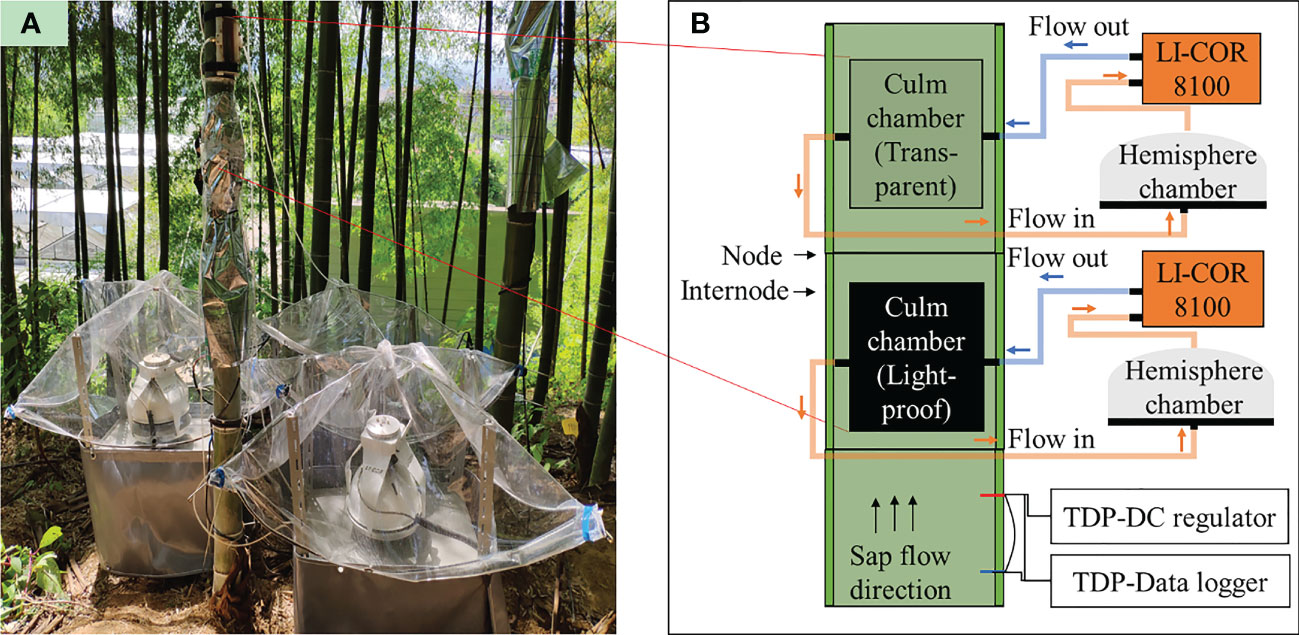
Figure 2 (A) Field installation and (B) schematic presentation of the equipment for monitoring CO2 efflux (Es, μmol m−2 s−1) in the field. The black and grey areas on the culm refer to light-proof and transparent culm chambers (double-chamber method). Each culm chamber was connected to an LI-Cor 8100 and a sealed hemisphere chamber. A pair of TDP was placed on an internode below the light-proof culm chamber. The sap flow direction was almost upward in the daytime.
The culm chamber was connected to a modified detector (LI-8100, Li-cor Inc., USA) to measure CO2 flux continuously. The LI-8100 contained an infrared CO2 analyzer and a hemisphere chamber designed. The hemisphere chamber was connected to the culm chamber with a tube in this study. Gas flowed into the hemisphere chamber from the culm chamber, mixed eventually, and sucked into the infrared CO2 analyzer by a build-in pump of the LI-8100. After measurement in the analyzer, the gas was pumped back into the culm chamber (Figure 2). When the measurement was running, the hemisphere chambers were closed, and the gas path of measurement became a closed-loop path. The chamber system was temporally closed for 5 min during measurements, and the air from the chamber was circulated using a pump from Li-cor 8100. The CO2 analyzer measured the CO2 concentration of the flow (ppm) and its temperature (°C). For each measurement, CO2 concentration started from an ambient value to a maximum accumulated concentration with a one-minute stepwise, given 30 s of a dead band, which excludes data from the beginning measurements and 50 cm2 of covered culm surface by the chamber, CO2 efflux (µmol m−2 s−1) can be derived with a linear model and recorded by the analyzer. After each measurement, the hemisphere chamber lifted, and air in both chambers was exchanged with the atmosphere for another 5 minutes.
Measurements were conducted from the beginning of April to the end of August in 2018 and 2019. A transparent and a light-proof chamber (double-chamber method) were installed separately on neighboring internodes for all studied culms (Figure 2). Two LI-8100s were equipped to both culm chambers and measured Es simultaneously for three to five sunny days on a culm and then moved to another. Due to the rapid growth of freshly sprouted culms and limited days in each phenological stage, four culms in each year were measured in both 2018 and 2019.
2.3 Culm photosynthetic rate
In-situ respired CO2 (Rs) was supposed to be released through four pathways (eq.1), including efflux from the culm surface (Es), stored at the position (EI), dissolved through sap flow (ET), and refixed by culm photosynthesis (Ep), respectively.
Respiration is an enzyme-driven metabolism that is strongly influenced by temperature. Therefore, theoretical Rs (Rs_theory, µmol m−2 s−1) can be derived with the following model:
where T is culm temperature (°C), which is assumed to be the same as the temperature of airflow measured by the infrared CO2 analyzer; a and b are parameters derived from nighttime Es and T, during when ET and Ep are assumed to be zero due to limited sap flow and photosynthetic photon flux density (PPFD). EI is also supposed to be zero under balanced conditions during the night. Therefore, Rs_theory equals to Es and parameters a and b could be derived from night-time observations (i.e., Es and T).
Es was always unequal to in situ respiration due to multiple partitioning pathways of respired CO2. The amount of the carbon loss of Rs_theory that was not released through the culm surface (Es) but through other pathways was named “missing efflux” in this study. A missing efflux rate (Emiss) can be derived from Rs_theory − Es for each observation step during a day.
To calculate culm photosynthesis, a transparent and a light-proof chamber were installed separately on neighboring internodes (Figure 2). Missing efflux rates for transparent (Emiss) and light-proof (Emiss) chambers were derived. Two equations can be derived from eq.1 for the transparent and light-proof chamber, respectively:
As the two chambers were installed on the neighboring segments of a culm, we assumed that sap flow was similar and CO2 exchange to the storage of the hollow part was almost the same. Therefore, ET−tp equals ET−lp, and EI−tp equals EI−lp. Therefore, culm photosynthesis can be derived from eq.3 and eq.4.
2.4 Sap flow measurement
Sap flux density on bamboo culms, where CO2 efflux was measured, was simultaneously monitored with a self-constructed thermal dissipation probe (TDP, Granier, 1987). A pair of TDP include one heating and one reference probes which are able to detect temperatures at the installation positions. The principle of TDP to measure sap flux is that the temperature will decrease as sap flux increases and bring heat around the heating probe away. The probes (1 cm in length) were modified from the 2-cm-length Granier-type probe (Granier, 1987) specifically for giant bamboo. The application and other specifications of self-build TDP refer to former studies on bamboo (Fang et al., 2019; Mei et al., 2020; Tong et al., 2021). When installing TDP on the culms, the heating and the reference probes were installed with a 10-cm vertical space at upper and lower positions. The upper heating probe was heated with 0.1 W power, and the lower reference probe was left unheated. The temperature difference between the two probes was recorded as voltage difference by a datalogger and multiplexers (CR1000, AM16/32, Campbell Inc., USA). Sap flux density (g m−2 s−1) was derived using the Moso bamboo-specific formula (Tong et al., 2021).
Where Js is sap flux density (g m−2 s−1), Vmax is the maximum output voltage in a day, which usually appears at night when sap flow is almost zero. v is a mean output voltage every 10 minutes. Finally, μ is the age-specific parameter for Moso bamboo (Tong et al., 2021).
2.5 Micrometeorological observations
Two micrometeorological stations were set up above and below the bamboo canopy. The above-canopy station was located about 10 m from the stand in an open field. Photosynthetic Photon flux density (PPFD, LI190R, Campbell Scientific, USA), air temperature, and humidity (HMP155A, Campbell Inc., USA) were measured in the above canopy station. The below-canopy station was placed in the stand to monitor radiation below the canopy (LI190R, Campbell Scientific, USA) and soil moisture (CS616, Campbell Inc., USA). Three soil moisture probes were placed at the study site. Meteorological data were collected by dataloggers (CR1000, Campbell Inc., USA).
2.6 Data analysis and statistics
Daily patterns of hourly Es, Emiss were plotted for culms in transparent and light-proof chambers, and so was hourly Ep for culms in transparent chambers in the leafless and leaved stage of the newly sprouted culms.
The difference in stem surface temperature, measured efflux, and theoretical respired efflux between transparent and light-proof chambers were examined with Student’s t-test (parametric method) and Signed Rank methods (nonparametric method) if data was normal and non-normal distribution, respectively.
Daily accumulated Emiss and Ep were examined to determine if they had linear relationships with daily accumulated sap flux density and environmental variables (radiation above and below canopy, air temperature, air humidity, soil moisture). And significant regressions were plotted.
Furtherly, stepwise linear multiple regression models predicting Es, Emiss, and Ep with environmental factors were conducted to explore the comparative implications of varying factors. Only variables entered in the models were kept for analysis.
Percentages of daily Es, Emiss to Rs_theory were calculated for culms in transparent and light-proof chambers. Further, the daily Ep to Rs_theory ratio was calculated for transparent chamber culms. As Emiss and sap flow had significant positive relationships, as examined above, we supposed the regression R2 between them could be a proxy of ET to Emiss, which was furtherly multiplied by the Percentage of Emiss to Rs_theory to obtain the Percentage of ET to Rs_theory. At last, the Percentage of EI to Rs_theory was calculated by subtracting the above three parts from 1.
Except for Figures 1, 2, all the other figures and analyses were performed with SAS 9.4 (SAS Institute Inc., Cary, NC, USA).
3 Results
3.1 CO2 efflux (Es) of freshly sprouted Moso bamboo
On freshly sprouted Moso bamboo culms at both phenological stages (leafless and leaved), Es showed a similar daily pattern, i.e., higher value in the daytime and lower value in the nighttime (Figure 3). Es increased rapidly at around 6:00 in the morning, reached its maximum value at 14:00–15:00, and decreased afterward.
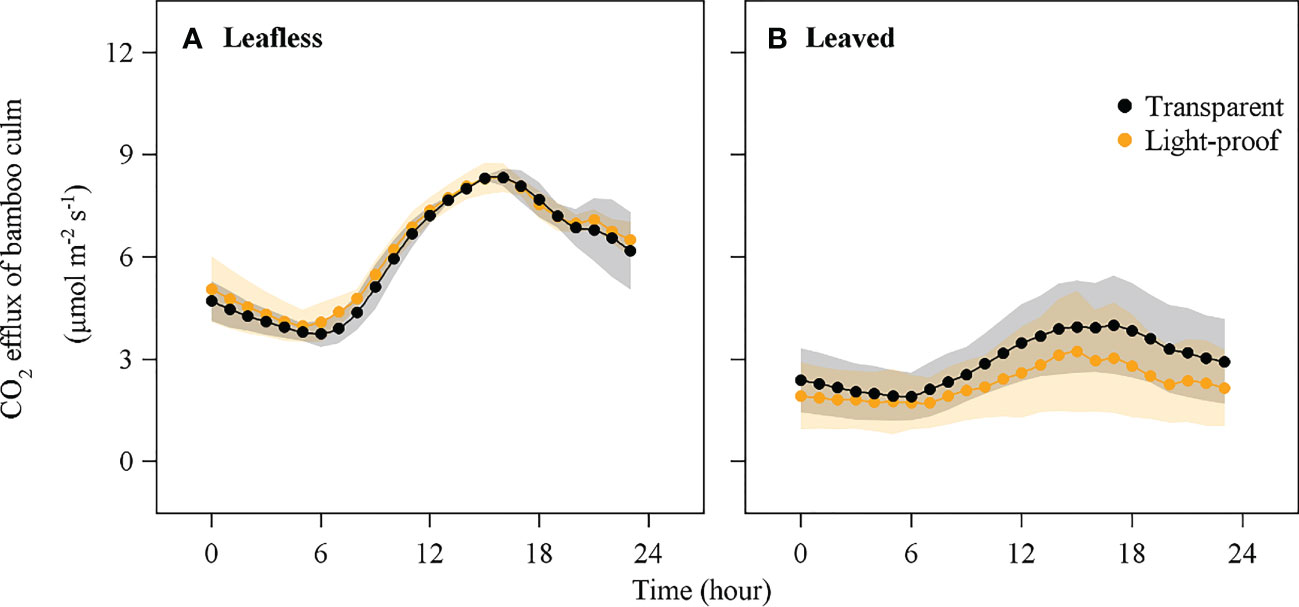
Figure 3 CO2 efflux of bamboo culm (Es) in the transparent and light-proof chambers in leafless (A) and leaved (B) stages (mean ± std, n = 8).
There was no significant difference in Es between transparent and light-proof chambers in the leafless stage (P < 0.05), while Es in transparent chambers were significantly higher than that in light-proof chambers in the leaved stage (P < 0.05; Table 1). In contrast, in both types of chambers, Es was significantly higher in the leafless stage than in the leaved stage (P < 0.05). The maximum value of Es in a day was 8.5 ± 0.9 μmol m−2 s−1 in the leafless stage and 3.6 ± 1.5 μmol m−2 s−1 in the leaved stage. Mean Es was 4.7 ± 1.1 μmol m−2 s−1 in the leafless stage and 2.3 ± 0.9 μmol m−2 s−1 in the leaved stage (Figure 3).
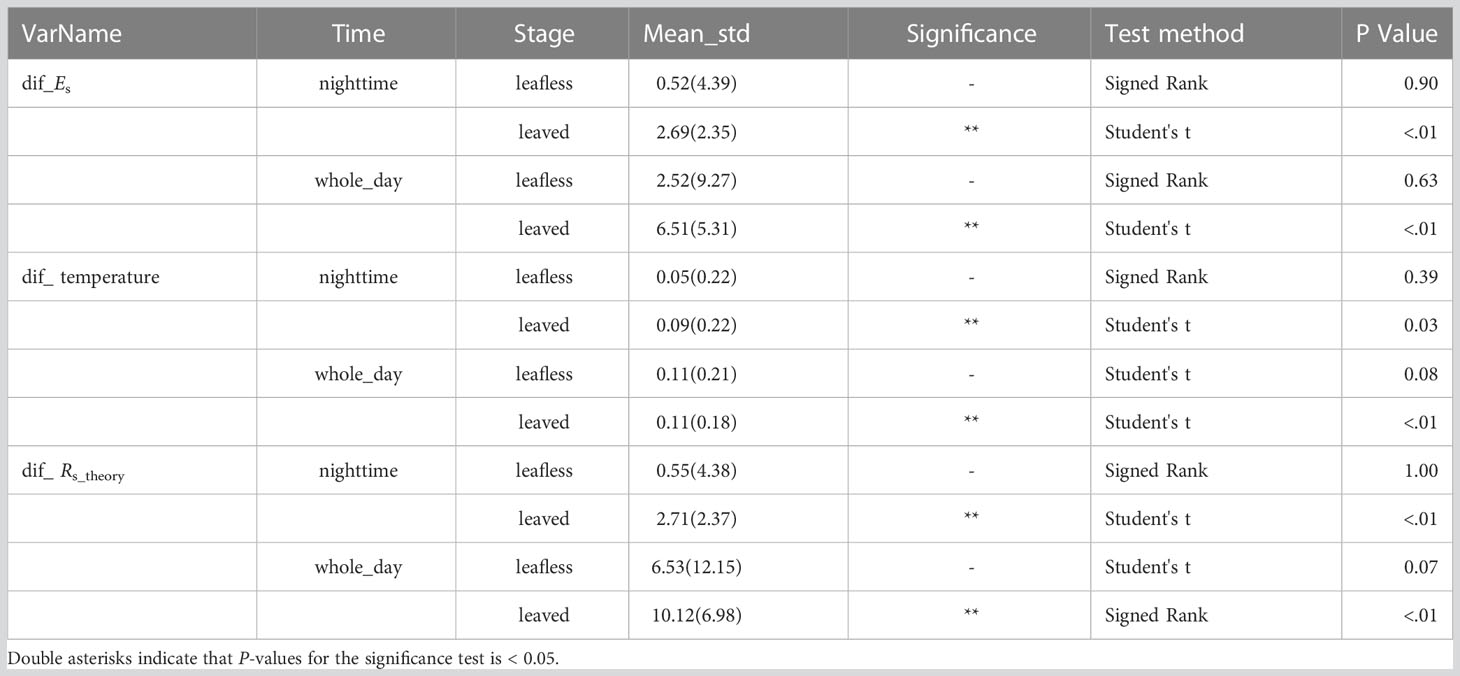
Table 1 The difference in measured efflux (dif_Es), stem surface temperature (dif_ temperature), and theoretical respired efflux (dif_Rs_theory) between transparent and light-proof chambers.
3.2 Impact factors of Es
This study conducted a stepwise multiple regression to explore the comprehensive impacts of the varying environmental factors on Es in transparent chambers (Table 2). For both leafless and leaved stages, three variables (sap flux density, below-canopy radiation, and temperature) entered the models (P < 0.01 and R2 = 0.7). However, the leading impact factor differed in the two stages, i.e., sap flux (model variation explained = 67.6%) for the leafless stage and below-canopy radiation (model variation explained = 78.6%) for the leaved stage. In both stages, sap flux density and below-canopy radiation exerted significant negative and positive effects on Es. In contrast, below-canopy temperature shifted its impact on Es from positive to negative when the newly sprouted culms changed their status from leafless to leaved. To find more relationships between sap flux density and Es, we calculated the residuals of the model of log-transformed Es and air temperature in the leafless and leaved stages to eliminate the effect of temperature. Then sap flux density significantly negatively affected Es (Figure 4), respectively.
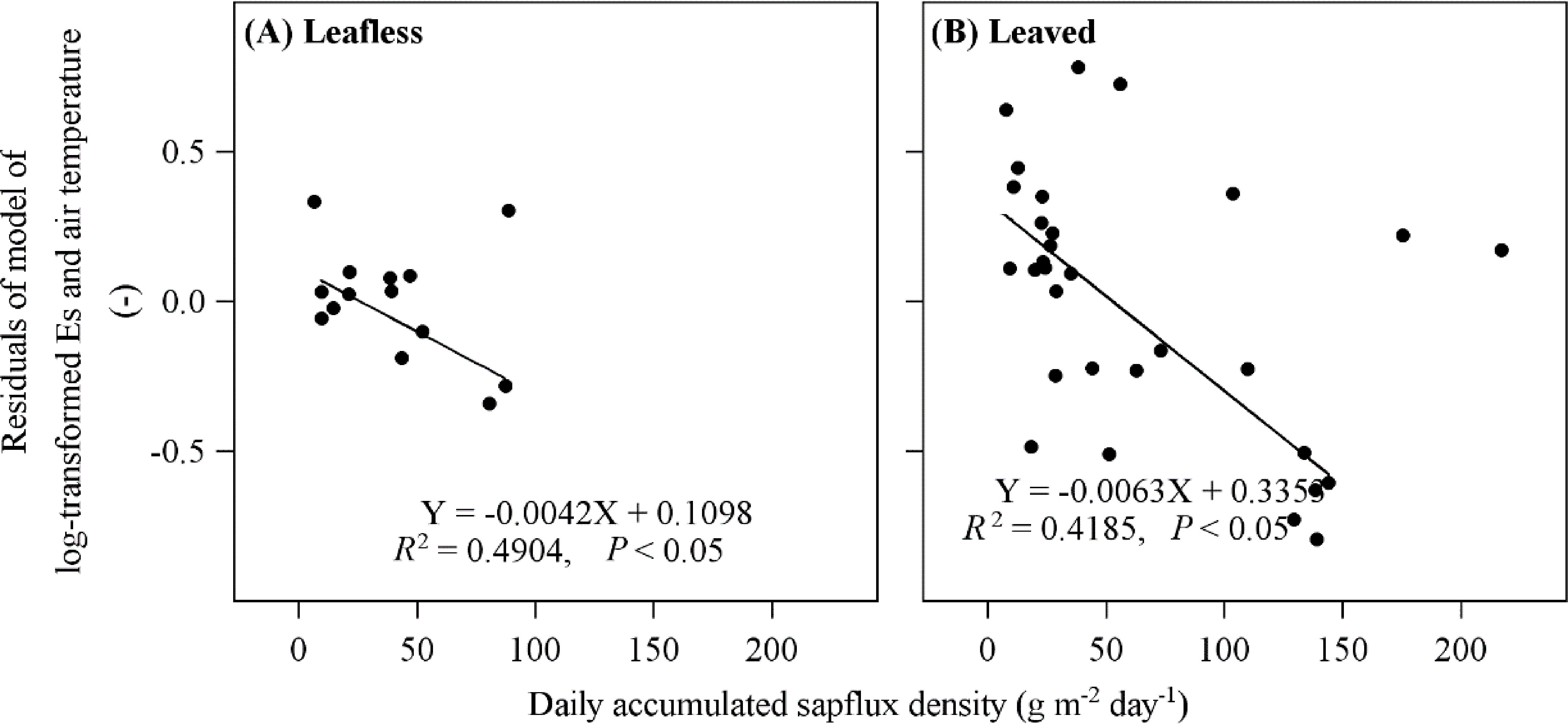
Figure 4 Relationship between daily accumulated sap flux density and residuals of the log-transformed stem-surface CO2 efflux (Es) model and air temperature in leafless (A) and leaved (B) stages of the newly sprouted culms. One and two abnormal points were excluded from the regressions in leafless (A) and leaved (B) stages, respectively.
3.3 Culm photosynthesis
In transparent chambers, EP presented a typical single-peak pattern with a maximum value around mid-day and a lowest at night (Figure 5). Daily accumulated Ep was 81.74 ± 42.40 mmol m−2 day−1 and 39.05 ± 6.65 mmol m−2 day−1 in leafless and leaved stages, respectively, which meant ~9.0% (with a maximum value of 33.4%) and 13% (with a maximum value of 40.4%) of Rs, respectively. Ep contributed half of the daily accumulated Emiss (Table 3). In addition, daily accumulated Ep has a significant correlation (P < 0.05) with daily accumulated below-canopy radiation in both stages (Figure 6).
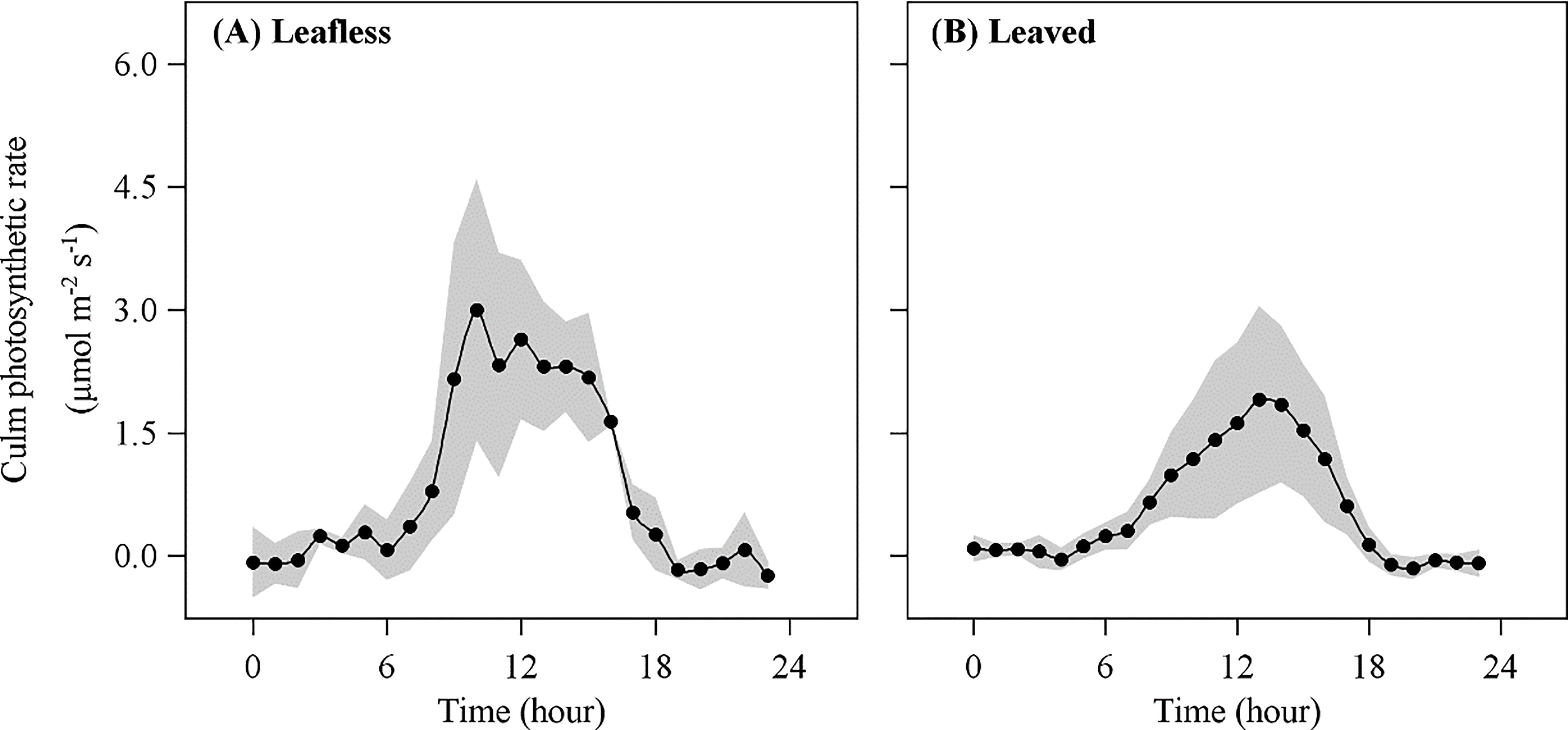
Figure 5 Culm photosynthetic rate (Ep) in leafless (A) and leaved (B) stages of the newly sprouted culms.

Table 3 Partitioning percentages of culm-respired CO2 efflux flowing to the four pathways, i.e., surface (Es), photosynthesis (Ep), sap flow (ET), and internal storage (EI).
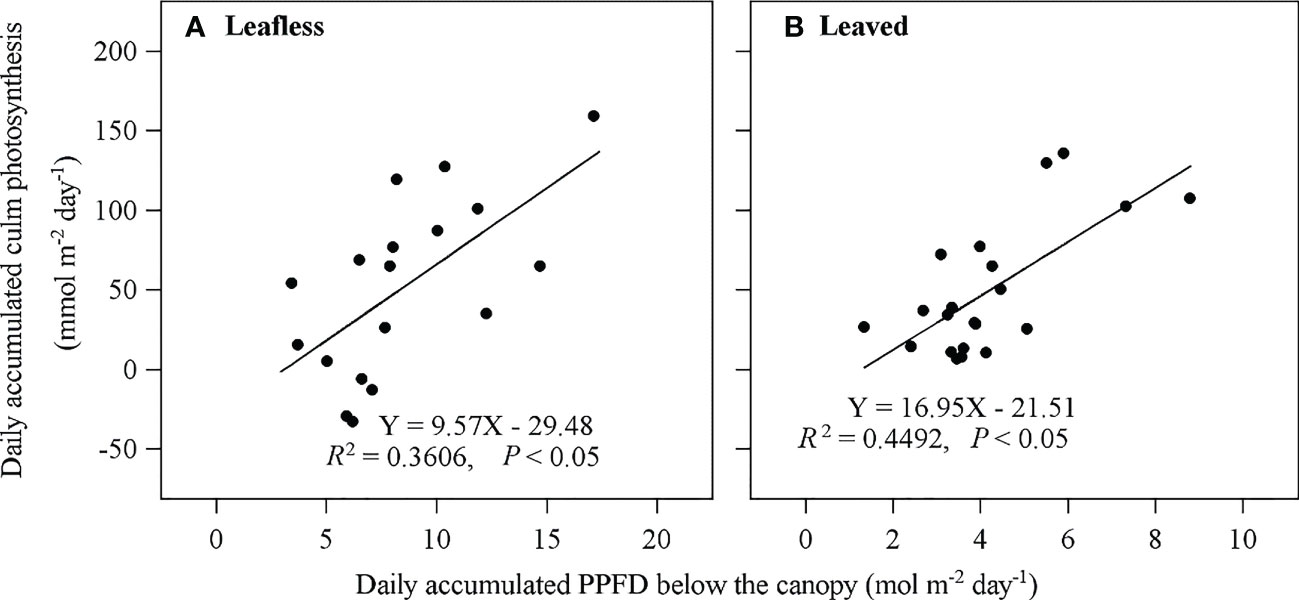
Figure 6 Relationship between daily accumulated culm photosynthesis (Ep) and daily accumulated radiation (PPFD) below the canopy in leafless (A) and leaved (B) stages the newly sprouted culms.
3.4 Missing efflux of in situ respired CO2 and its impact factors
An apparent Emiss was observed during daytime in the leafless and leaved stages for both the transparent and light-proof chambers (Figures 7, 8). There was no significant difference in daily accumulated Emiss between leafless and leaved stages for each type of chamber (P > 0.05). In contrast, a significant difference was observed between the transparent and light-proof chambers for both stages (P < 0.05). In both stages, daily accumulated Emiss accounts for ~10% and 20% of Rs in transparent and light-proof chambers, respectively (Table 3).
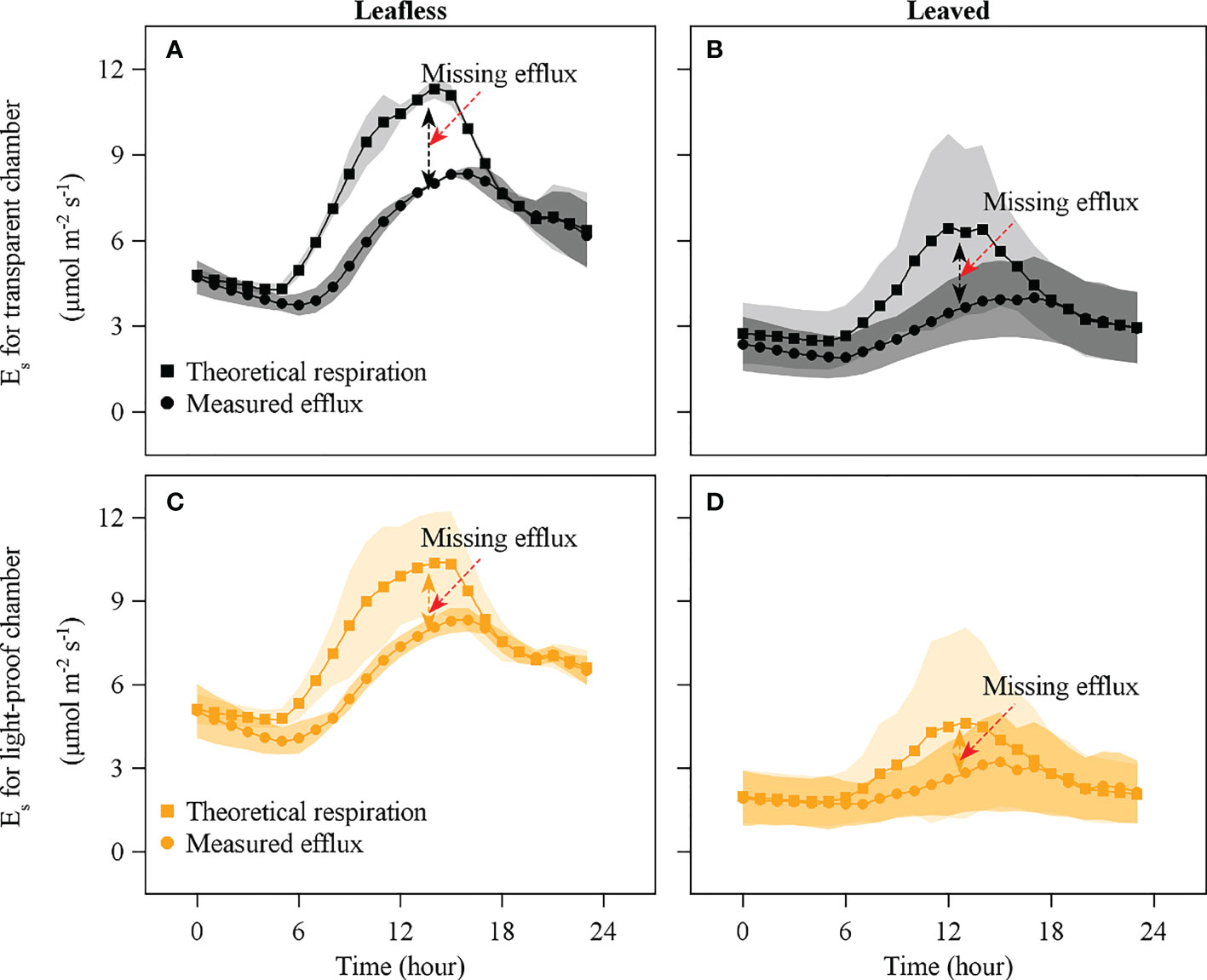
Figure 7 Comparison of theoretical respiration (squares) and measured efflux (dots) with transparent (black) and light-proof chambers (orange) in leafless (A), (C) and leaved (B), (D) stages. Note that Es is the CO2 efflux released from the culm surface, and missing efflux is the difference between the theoretical respiration and Es.
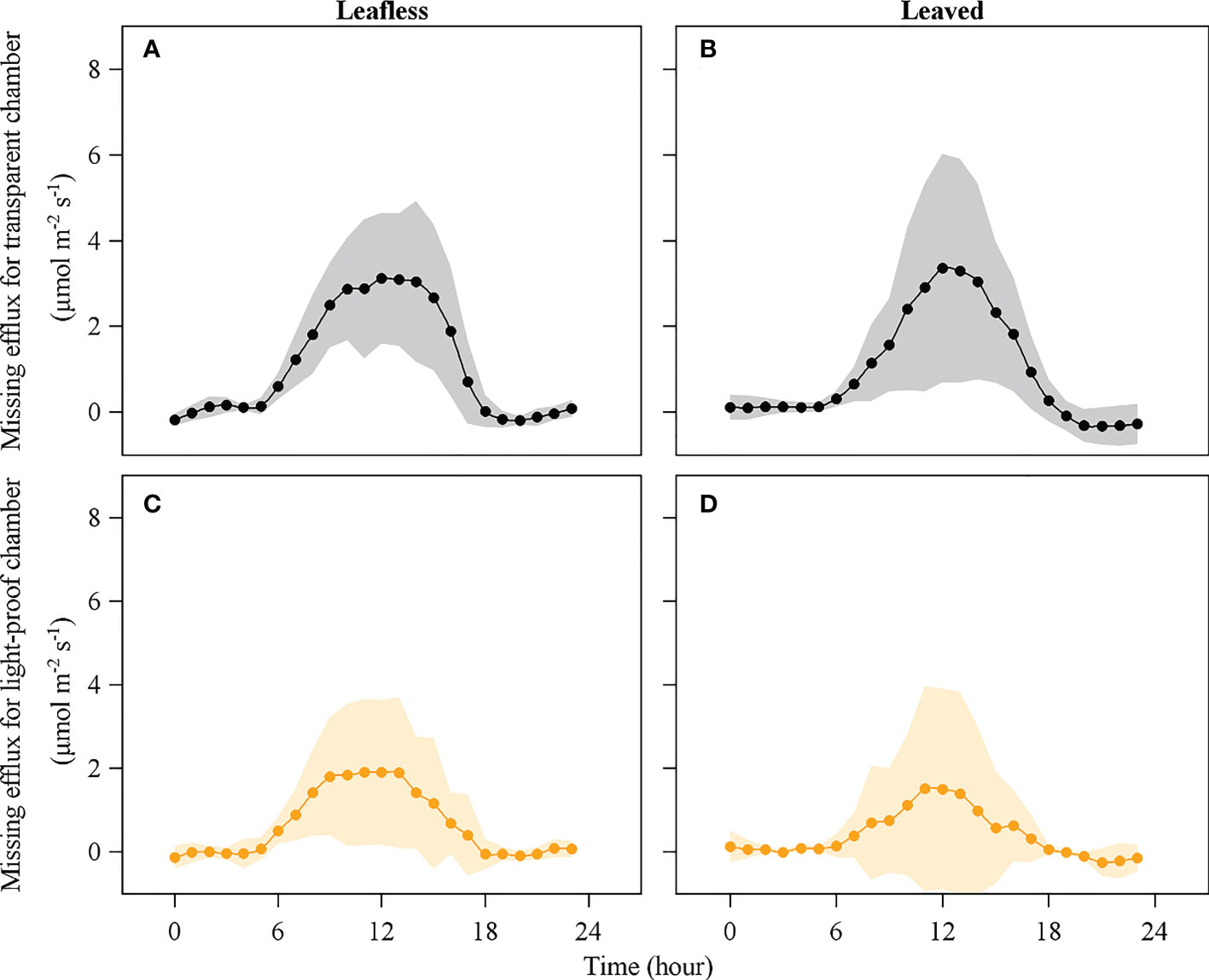
Figure 8 Missing efflux of culm respired CO2 in leafless (A), (C) and leaved (B), (D) stages for transparent and light-proof chambers. Note that missing efflux is the difference between the theoretical culm respiration and the surface-released CO2 efflux.
Daily accumulated Emiss had significantly negative (R2 = 0.27) and positive (R2 = 0.16) relationships with daily accumulated sap flux in leafless and leaved stages, respectively (P < 0.05; Figure 9), which may indirectly indicate a similar contribution (~27 and 16%) of ET to Emiss. In this case, the results may furtherly mean that ET could merely account for ~2% of Rs. As Emiss consisted of ET and EI, the contribution of EI to Rs could be ~7–9% by subtracting ET from Emiss (Table 3).
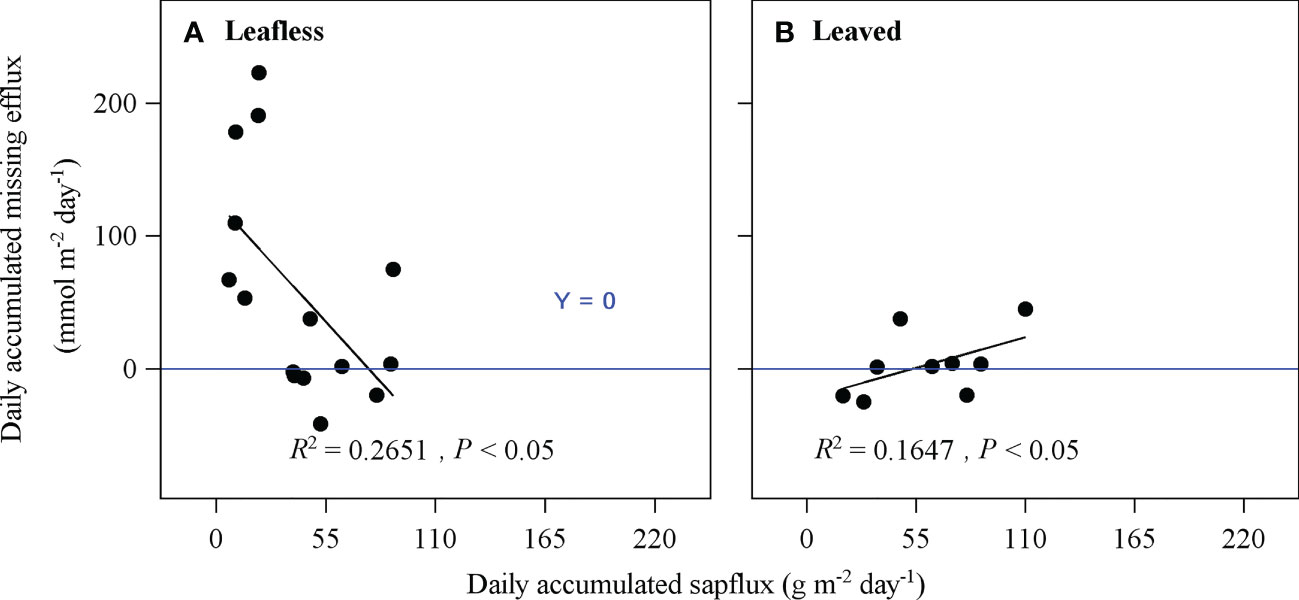
Figure 9 Relationships of daily accumulated sap flux and daily accumulated missing efflux of the light-proof chamber (i.e., exclude culm photosynthesis) in leafless (A) and leaved (B) stages. Note that missing efflux is the difference between the theoretical culm respiration and the surface-released CO2 efflux.
In stepwise multiple regression predicting Emiss, two variables entered the models for both leafless (P < 0.01; R2 = 0.58) and leaved stages (P < 0.01; R2 = 0.32; Table 4). Below-canopy radiation was the most influential variable for both stages, explaining 61% and 58% of the model variation in leafless and leaved stages, respectively. In contrast, the second most significant variable was different, i.e., below-canopy temperature and sap flux for leafless and leaved stages, respectively.
4 Discussion
4.1 CO2 efflux of newly sprouted Moso bamboo culm between leafless and leaved stages
Compared to the averaged Es (2.04 ± 2.03 μmol m−2 s−1) of all the studied tree/bamboo species in our synthesized data pool (Figure S1), the newly sprouted Moso bamboo culms released a much higher and similar Es in leafless and leaved stages, respectively. Furtherly, compared with Es in the previous studies on bamboo (Table 5), Es in the leaved stage in this study was similar to the value reported on 1–2-year-old Moso bamboo in China (2.3 μmol m−2 s−1; Xiao et al., 2010) and 4-month-old ones in Japan (1.9 ± 0.46 μmol m−2 s−1; Uchida et al., 2022) but much lower than Es of 6-month-old Bambusa vulgaris (6.9 μmol m−2 s−1; Zachariah et al., 2016). Es of Moso bamboo measured in situ showed a decreasing trend with increasing ages (Table 5, and Xiao et al., 2010; Uchida et al., 2022), almost coinciding with findings based on harvested culm segments from another previous study (Isagi et al., 1997).
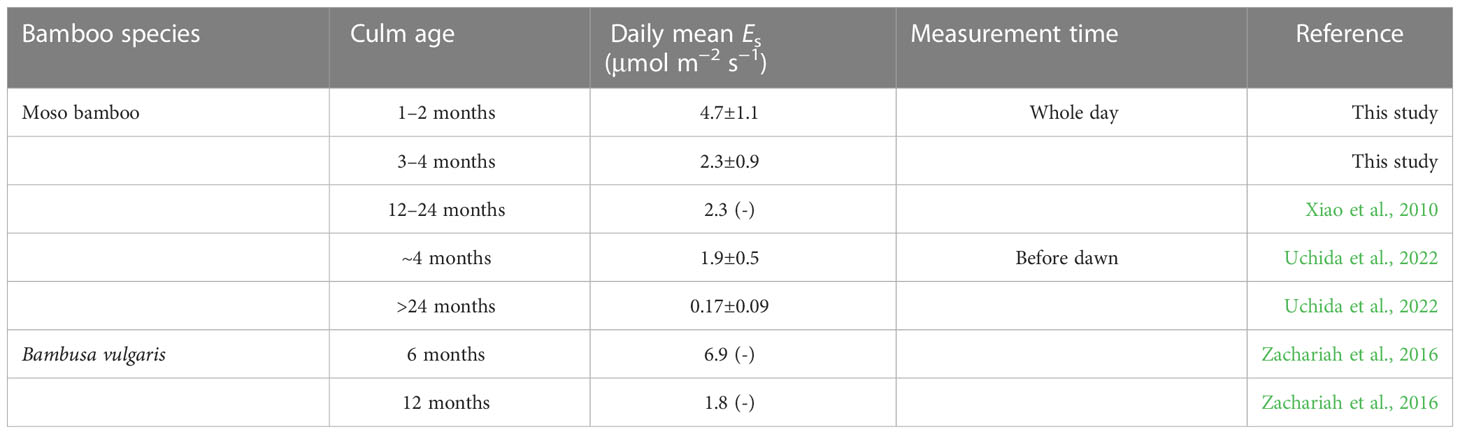
Table 5 Comparison of bamboo culm efflux of this study and bamboos in former studies, and contribution of culm photosynthesis to theoretical in situ respiration.
The decreasing trend of Es by aging could be attributed to potentially reduced maintenance respiration in the cytoplasm, which could be squeezed by the thicking cell wall of parenchyma (Uchida et al., 2022) and fiber (Huang et al., 2015). In the leafless stage, the current-year newly sprouted culms elongated their internodes with increasing cell length and the number of parenchymas and high lignin and cellulose content (Chen et al., 2022), which meant active growth respiration consuming a mass of carbohydrates, e.g., starch (Uchida et al., 2022). The rule that growth stimulated higher Es was also supported in trees in several studies (Ryan, 1990; Maier, 2001; Vose and Ryan, 2002; Lavigne et al., 2004). In this study, the newly sprouted Moso bamboo culms have about twice as high Es in leafless stages than in leaved stages (Figure 3). If taking the after-leaved Es as a reference for maintenance respiration, growth respiration during the leafless stage accounted for more than 50% of the total respiration. Therefore, growth could contribute mainly to the higher Es of newly sprouted bamboo culm in the leafless stage.
For both leafless and leaved stages, three variables (sap flux density, below-canopy radiation, and temperature) entered the models for predicting Es (P < 0.01 and R2 = 0.7; Table 2). However, the leading impact factor differed in the two stages, i.e., sap flow for the leafless stage and below-canopy radiation for the leaved stage (Table 2). In both stages, sap flux density had significantly negative correlations with Es, which is consistent with some former studies (McGuire and Teskey, 2004; Bowman et al., 2005). Bowman et al. (2005) found that sap flow could interpret variables of Es among different heights, directions, and individuals. Direct monitoring of dissolved CO2 concentration in sap flow for Fagus grandifolia, Liquidambar styraciflua, and Platanus occidentalis showed 13–71% of the respired CO2 was transported through sap flow and hence positively correlated with sap flow (McGuire and Teskey, 2004). A considerable increase in sap flow for freshly sprouted Moso bamboo in the same site was observed (Mei et al., 2020), which may take away the in situ respired CO2 and reduce Es after leaved. However, sap flow can only explain 5.4% of the variation of Es in the leaved stage (Table 2). Such a result does not fit our first assumption that sap might take away more CO2 efflux and negatively influence Es in the leaved stage more than in the leafless stage.
4.2 Roles of culm photosynthesis on carbon partitioning of in situ respired CO2
In this study, culm photosynthesis was assumed to equal the difference value of Emiss between transparent and light-proof chambers. The two-chamber design attempted to avoid errors in calculating culm photosynthesis that was introduced by CO2 partitioning through other pathways, e.g., sap flow, compared with the single-chamber design reported by Tarvainen et al. (Tarvainen et al., 2018). The single-chamber design works on one assumption that carbon partitioning through sap flow was ignorable (Tarvainen et al., 2018). However, some former studies did not support this assumption (McGuire and Teskey, 2002; Saveyn et al., 2008). Therefore, the two-chamber design applied in this study was expected to improve the estimation accuracy in culm photosynthesis.
As one of the partitioning pathways for in situ respired CO2, culm photosynthesis was supposed to reduce Es (Pfanz et al., 2002; Wittmann et al., 2006). The above assumption was indicated by observing a smaller Es with culm photosynthesis in this study (Table S2). Due to the high radiation dependence of photosynthesis and reduced radiation below the canopy with expanded leaves for a specific freshly sprouted culm, culm photosynthesis was assumed to reduce in the leaved stage. The above assumption was also confirmed in this study, i.e., a significantly reduced PPFD below the canopy after June (7.05 ± 4.72 vs. 4.37 ± 1.97 mol m−2 day−1 in the leafless and leaved stages, respectively), along with a significantly reduced culm photosynthesis in the leaved stage (Figures 5, 6). Higher culm photosynthesis in leafless stages helps recycle more carbon loss due to respiration for freshly sprouted culms. However, Ep has a relative contribution (~10%) to the partitioning of Rs in both stages, which does not fit our hypothesis that its effect may be reduced in the leaved stage due to the increased sap flow.
To explore whether Es of this study falls into a reasonable range, we collected Es of 195 tree species in different climate zones from articles published before February 13, 2020 (Figure S1). As a result, Es of Moso bamboo in this study falls in a promising position close to the respiration-temperature regression line of subtropical tree species (Figure S1). It was predicted to have a lower Es on species with culm photosynthesis. The green bamboo culm was proved to have photosynthesis (Liu et al., 2013; Wang et al., 2013) and hence lower Es. Almost all the studied bamboo Es falls close to/below the respiration-temperature regression line based on the collected worldwide dataset (Figure S1), which was in line with the above assumption, except for one case study on Bambusa vulgaris conducted in the tropical zone.
In this study, the daily accumulated Ep was significantly positively correlated with daily accumulated PPFD below the canopy (Figure 6), agreeing with previous expectations. As one of the partitioning pathways of respired CO2, culm photosynthesis could recycle about 9–13% of the in situ respired CO2 (Table 3). Culm photosynthesis could be essential to trees originally located in high-latitude areas worldwide. By synthesizing Es data from articles published before February 13, 2020, we found a significant relationship between Es and temperature in each climate zone (Figure S1). From the dataset, we observed a lower Es on tree species with non-culm photosynthesis (temperate zone 2.64 μmol m−2 s−1; subtropical zone 2.24 μmol m−2 s−1) compared to tree species with culm photosynthesis (temperate zone 3.18 μmol m−2 s−1; subtropical zone 4.15 μmol m−2 s−1). As a photo-induced physiological process, culm photosynthesis could be more likely to appear in forests with fewer-layer canopies than multi-layer canopies. With increasing latitude, forest composition and structure tend to be simpler, allowing more light to penetrate the stand canopy. Although Moso bamboo is considered an evergreen species, it renews leaves in early spring when explosive growth occurs every two years (Mei et al., 2020), leading to higher radiation below the canopy during this stage (Figure 6B). The spring leafing phenology seems to favor culm photosynthesis and the growth of young bamboo culms (Mei et al., 2020).
4.3 Missing efflux and partitioning of in situ respired CO2
An apparent Emiss was observed during daytime in the leafless and leaved stages for both the transparent and light-proof chambers (Figures 7, 8, and Table 3). Ep accounted for 50% of Emiss, equaling a partitioning of 10% of Rs. Therefore, the left ~10% of Rs might be partitioned to the pathways of sap flow and internal storage, i.e., ET and EI. However, the experimental design in this study can not precisely distinguish ET and EI with direct measurement, which meant either ET or EI might account for 10% of Rs at most in extreme cases. Alternatively, we roughly estimated the ET could merely account for ~2% of Rs via the correlations between Emiss and sap flow (Figure 9), thus deriving the percentage of EI (~8%).
In stepwise multiple regression predicting Emiss, two variables entered the models for both leafless and leaved stages (Table 4). Below-canopy radiation was the most influential variable for both stages. The result indirectly confirmed the partitioning pathway of culm photosynthesis, considering the positive relations between radiation and photosynthesis.
For a newly sprouted culm with a DBH of 10.77 cm and a height of 13.41 m, its estimated aboveground biomass was about 12.105 kg calculated with an allometric equation (, Zhang et al., 2016). Based on daily accumulated EP and growth rates (48.99 cm d–1) in leafless stages (Chen et al., 2022), we found the total fixed carbon from culm photosynthesis accounted for 2.40% of the aboveground biomass of bamboo in leafless stages.
Consistent with some previous studies (McGuire and Teskey, 2004; Teskey et al., 2007; Aubrey and Teskey, 2009), daily accumulated sap flux density was proved to have a significant negative effect on Emiss (Figure 9). The above correlation may confirm the carbon partitioning of in situ respired CO2 taken by sap flow. In addition, a significant positive relationship between sap flux density and Emiss was found in the leaved stage in this study (Figure 9B), which also implied a possible carbon partitioning of in situ respired CO2 through sap flow. However, as mentioned above, sap flux density can only explain 5.4% of the variation of Es in the leaved stage (Table 2).
5 Conclusion
The applied double-chamber method was used to estimate culm photosynthesis in newly sprouted Moso bamboo, which could provide a powerful way to test the mass balance framework (MBF) on stem respiration (Rs). By measuring and analyzing culm photosynthesis, sap flux, and micrometeorological factors above and below the canopy, we found 80% of in situ Rs were released through the culm surface of Moso bamboo. In comparison, culm photosynthesis and sap flux reallocated approximately 10% and 2% of in situ Rs. Despite higher culm photosynthesis in the leafless stage and a higher sap flow in the leaved stage, the carbon partitioning pathways indicated by MBF have no significant difference between the two phenological stages. Considering the dynamic change of the partitioning components of in situ Rs and the handleability of the developed method on bamboo culm, we think bamboo is a good choice to explore the MBF.
Data availability statement
The original contributions presented in the study are included in the article/Supplementary Material. Further inquiries can be directed to the corresponding authors.
Author contributions
TM, GZ and CY planned and designed the research; CY, KH, YZ and TM performed experiments, and conducted fieldwork; CY, QZ and DF analyzed data; and CY, QZ, KH, DF, DH, HD, YS, FB, TM, GZ wrote or revised the manuscript. CY and QZ contributed equally. All authors contributed to the article and approved the submitted version.
Funding
The research was supported by the National Nature Science Foundation of China (grant number: 32001102), Zhejiang Provincial Natural Science Foundation (grant number: Q19C160022), the Key Research and Development Program of Zhejiang Province (2021C02005), Scientific Research Foundation of Zhejiang A&F University (grant number: 2020FR050), Scientific Research Foundation of Jiyang College of Zhejiang A&F University (grant number: 05251700038), Overseas Expertise Introduction Project for Discipline Innovation (111 Project D18008).
Conflict of interest
The authors declare that the research was conducted in the absence of any commercial or financial relationships that could be construed as a potential conflict of interest.
Publisher’s note
All claims expressed in this article are solely those of the authors and do not necessarily represent those of their affiliated organizations, or those of the publisher, the editors and the reviewers. Any product that may be evaluated in this article, or claim that may be made by its manufacturer, is not guaranteed or endorsed by the publisher.
Supplementary material
The Supplementary Material for this article can be found online at: https://www.frontiersin.org/articles/10.3389/fpls.2023.1154232/full#supplementary-material
References
Amthor, J. (2000). The McCree–de Wit–Penning de Vries–Thornley respiration paradigms: 30 years later. Ann. Bot. 86, 1–20. doi: 10.1006/anbo.2000.1175
Atkin, O. K., Bruhn, D., Tjoelker, M. G. (2005). “Response of plant respiration to changes in temperature: mechanisms and consequences of variations in Q10 values and acclimation,” in Plant respiration: From cell to ecosystem. Eds. Lambers, H., Ribas-Carbo, M. (Dordrecht: Springer Netherlands), 95–135. doi: 10.1007/1-4020-3589-6_7
Aubrey, D. P., Teskey, R. O. (2009). Root-derived CO2 efflux via xylem stream rivals soil CO2 efflux. New Phytol. 184, 35–40. doi: 10.1111/j.1469-8137.2009.02971.x
Berveiller, D., Kierzkowski, D., Damesin, C. (2007). Interspecific variability of stem photosynthesis among tree species. Tree Physiol. 27, 53–61. doi: 10.1093/treephys/27.1.53
Bloemen, J., McGuire, M. A., Aubrey, D. P., Teskey, R. O., Steppe, K. (2013). Transport of root-respired CO2via the transpiration stream affects aboveground carbon assimilation and CO2 efflux in trees. New Phytol. 197, 555–565. doi: 10.1111/j.1469-8137.2012.04366.x
Bloemen, J., McGuire, M. A., Aubrey, D. P., Teskey, R. O., Steppe, K. (2013). Assimilation of xylem-transported CO2 is dependent on transpiration rate but is small relative to atmospheric fixation. J. Exp. Bot. 64, 2129–2138. doi: 10.1093/jxb/ert071
Bowman, W. P., Barbour, M. M., Turnbull, M. H., Tissue, D. T., Whitehead, D., Griffin, K. L. (2005). Sap flow rates and sapwood density are critical factors in within-and between-tree variation in CO2 efflux from stems of mature Dacrydium cupressinum trees. New Phytol. 167, 815–828. doi: 10.1111/j.1469-8137.2005.01478.x
Cernusak, L. A., Cheesman, A. W. (2015). The benefits of recycling: how photosynthetic bark can increase drought tolerance. New Phytol. 208, 995–997. doi: 10.1111/nph.13723
Ceschia, R., Damesin, C., Lebaube, S., Pontailler, J., Dufrêne, R. (2002). Spatial and seasonal variations in stem respiration of beech trees (Fagus sylvatica). Ann. For. Sci. 59, 801–812. doi: 10.1051/forest:2002078
Chen, M., Guo, L., Ramakrishnan, M., Fei, Z., Vinod, K. K., Ding, Y., et al. (2022). Rapid growth of Moso bamboo (Phyllostachys edulis): Cellular roadmaps, transcriptome dynamics, and environmental factors. Plant Cell 34, 3577–3610. doi: 10.1093/plcell/koac193
Darenova, E., Acosta, M., Pokorny, R., Pavelka, M. (2018). Variability in temperature dependence of stem CO2 efflux from Norway spruce trees. Tree Physiol. 38, 1333–1344. doi: 10.1093/treephys/tpy006
Fang, D., Mei, T., Röll, A., Hölscher, D. (2019). Water transfer between bamboo culms in the period of sprouting. Front. Plant Sci. 10. doi: 10.3389/fpls.2019.00786
FAO (2020). Global forest resources assessment 2020: Main report (Rome: Food and Agriculture Organization of the United Nations). doi: 10.4060/ca9825en
Granier, A. (1987). Evaluation of transpiration in a Douglas-fir stand by means of sap flow measurements. Tree Physiol. 3, 309–320. doi: 10.1093/treephys/3.4.309
Gu, D., He, W., Huang, K., Otieno, D., Zhou, C., He, C., et al. (2019). Transpiration of Moso bamboo in southern China is influenced by ramet age, phenology, and drought. For. Ecol. Manage. 450, 117–126. doi: 10.1016/j.foreco.2019.117526
Helm, J., Hartmann, H., Göbel, M., Hilman, B., Herrera Ramírez, D., Muhr, J. (2021). Low-cost chamber design for simultaneous CO2 and O2 flux measurements between tree stems and the atmosphere. Tree Physiol. 41, 1767–1780. doi: 10.1093/treephys/tpab022
Huang, X., Jin, Q., Jiu, L., Jian, F., Bai, D., Si, M. (2015). Variation in anatomical characteristics of bamboo, Bambusa rigida. JSM 44, 17–23. doi: 10.17576/jsm-2015-4401-03
Isagi, Y., Kawahara, T., Kamo, K., Ito, H. (1997). Net production and carbon cycling in a bamboo Phyllostachys pubescens stand. Plant Ecol. 130, 41–52. doi: 10.1023/A:1009711814070
Katayama, A., Kume, T., Ichihashi, R., Nakagawa, M. (2019). Vertical variation in wood CO2 efflux is not uniformly related to height: measurement across various species and sizes of bornean tropical rainforest trees. Tree Physiol. 39, 1000–1008. doi: 10.1093/treephys/tpz022
Kocurek, M., Kornas, A., Pilarski, J., Tokarz, K., Lüttge, U., Miszalski, Z. (2015). Photosynthetic activity of stems in two clusia species. Trees 29, 1029–1040. doi: 10.1007/s00468-015-1182-7
Lavigne, M., Little, C., Riding, R. (2004). Changes in stem respiration rate during cambial reactivation can be used to refine estimates of growth and maintenance respiration. New Phytol. 162, 81–93. doi: 10.1111/j.1469-8137.2004.01004.x
Li, X., Ye, C., Fang, D., Zeng, Q. F., Cai, Y. J., Du, H., et al. (2022). Non-structural carbohydrate and water dynamics of Moso bamboo during its explosive growth period. Front. Forests Global Change 5, 938–941. doi: 10.3389/ffgc.2022.938941
Liu, L., Wang, Y., Wang, X. (2013). Correlation between pigment content and reflectance spectrum of Phyllostachys pubescens stems during its rapid growth stage. Acta Ecologica Sin. 33, 2703–2711. doi: 10.5846/stxb201201200118
Maier, C. A. (2001). Stem growth and respiration in loblolly pine plantations differing in soil resource availability. Tree Physiol. 21, 1183–1193. doi: 10.1093/treephys/21.16.1183
McGuire, M. A., Teskey, R. O. (2002). Microelectrode technique for in situ measurement of carbon dioxide concentrations in xylem sap of trees. Tree Physiol. 22, 807–811. doi: 10.1093/treephys/22.11.807
McGuire, M. A., Teskey, R. O. (2004). Estimating stem respiration in trees by a mass balance approach that accounts for internal and external fluxes of CO2. Tree Physiol. 24, 571–578. doi: 10.1093/treephys/24.5.571
Mei, T., Liu, X., Fang, D., Zhou, G., Ye, C., Li, P., et al. (2020). Spring leafing phenology favors younger culms of Moso bamboo: aspects from water use relations. Front. Plant Sci. 11. doi: 10.3389/fpls.2020.00550
National Forestry and Grassland Administration (2019). Chinese Forest resources report (2014-2018) (Beijing: China Forestry Publishing House).
Pfanz, H., Aschan, G., Langenfeld-Heyser, R., Wittmann, C., Loose, M. (2002). Ecology and ecophysiology of tree stems: corticular and wood photosynthesis. Naturwissenschaften 89, 147–162. doi: 10.1007/s00114-002-0309-z
Ryan, M. G. (1990). Growth and maintenance respiration in stems of Pinus contorta and Picea engelmannii. Can. J. For. Res. 20, 48–57. doi: 10.1139/x90-008
Salomón, R. L., De Roo, L., Bodé, S., Boeckx, P., Steppe, K. (2019). Isotope ratio laser spectroscopy to disentangle xylem-transported from locally respired CO2 in stem CO2 efflux. Tree Physiol. 39, 819–830. doi: 10.1093/treephys/tpy152
Salomón, R. L., De Roo, L., Oleksyn, J., De Pauw, D. J. W., Steppe, K. (2020). TReSpire – a biophysical TRee stem respiration model. New Phytol. 225, 2214–2230. doi: 10.1111/nph.16174
Saveyn, A., Steppe, K., McGuire, M. A., Lemeur, R., Teskey, R. O. (2008). Stem respiration and carbon dioxide efflux of young Populus deltoides trees in relation to temperature and xylem carbon dioxide concentration. Oecologia 154, 637–649. doi: 10.1007/s00442-007-0868-y
Tarvainen, L., Wallin, G., Lim, H., Linder, S., Oren, R., Ottosson Löfvenius, M., et al. (2018). Photosynthetic refixation varies along the stem and reduces CO2 efflux in mature boreal Pinus sylvestris trees. Tree Physiol. 38, 558–569. doi: 10.1093/treephys/tpx130
Teskey, R. O., Saveyn, A., Steppe, K., McGuire, M. A. (2007). Origin, fate and significance of CO2 in tree stems. New Phytol. 177, 17–32. doi: 10.1111/j.1469-8137.2007.02286.x
Tong, C., Zhang, X., Xie, J., Mei, T., Fang, D., Li, Y. (2021). Water use strategies of different aged Moso bamboo culms under summer drought. For. Ecol. Manage. 498, 119567. doi: 10.1016/j.foreco.2021.119567
Uchida, E. M., Katayama, A., Yasuda, Y., Enoki, T., Otsuki, K., Koga, S., et al. (2022). Age-related changes in culm respiration of Phyllostachys pubescens culms with their anatomical and morphological traits. Front. Forests Global Change 5. doi: 10.3389/ffgc.2022.868732
Vose, J. M., Ryan, M. G. (2002). Seasonal respiration of foliage, fine roots, and woody tissues in relation to growth, tissue N, and photosynthesis. Global Change Biol. 8, 182–193. doi: 10.1046/j.1365-2486.2002.00464.x
Wang, T., Li, Q., Lou, S., Yang, Y., Peng, L., Lin, Z., et al. (2019). GSK3/shaggy-like kinase 1 ubiquitously regulates cell growth from arabidopsis to Moso bamboo (Phyllostachys edulis). Plant Sci. 283, 290–300. doi: 10.1016/j.plantsci.2019.03.015
Wang, X., Liu, L., Zhang, J., Wang, Y., Wen, G., Gao, R., et al. (2013). Changes of photosynthetic pigment and photosynthetic enzyme activity in stems of Phyllostachys pubescens during rapid growth stage after shooting. Chin. J. Plant Ecol. 36, 456–462. doi: 10.3724/SP.J.1258.2012.00456
Wei, Q., Guo, L., Jiao, C., Fei, Z., Chen, M., Cao, J., et al. (2019). Characterization of the developmental dynamics of the elongation of a bamboo internode during the fast growth stage. Tree Physiol. 39, 1201–1214. doi: 10.1093/treephys/tpz063
Wittmann, C., Pfanz, H. (2018). More than just CO2-recycling: corticular photosynthesis as a mechanism to reduce the risk of an energy crisis induced by low oxygen. New Phytol. 219, 551–564. doi: 10.1111/nph.15198
Wittmann, C., Pfanz, H., Loreto, F., Centritto, M., Pietrini, F., Alessio, G. (2006). Stem CO2 release under illumination: corticular photosynthesis, photorespiration or inhibition of mitochondrial respiration? Plant Cell Environ. 29, 1149–1158. doi: 10.1111/j.1365-3040.2006.01495.x
Wu, X.-P., Liu, S., Luan, J., Wang, Y., Cai, C. (2019). Responses of water use in Moso bamboo (Phyllostachys heterocycla) culms of different developmental stages to manipulative drought. For. Ecosyst. 6, 31. doi: 10.1186/s40663-019-0189-8
Xiao, F. M., Xiong, C., Zhang, X., Qi, L., Xu, H., Zhang, T. (2010). “A measurement of Moso bamboo plantation community respiration,” in Proceedings of the 9th China Forestry Youth Academic Conference, Cheng du. 1–7.
Zachariah, E. J., Sabulal, B., Nair, D. N. K., Johnson, A. J., Kumar, C. S. P. (2016). Carbon dioxide emission from bamboo culms. Plant Biol. 18, 400–405. doi: 10.1111/plb.12435
Zhang, Y., Yue, X., Qi, L., Jiang, Z., Shi, L. (2016). Estimation of Phyllostachys edulis forest biomass in southern Wuyishan Mountain using allometric equation and geostatistical technique. Chin. J. Ecol. 35, 1957–1962. doi: 10.13292/j.1000-4890.201607.036
Keywords: explosive growth, stem respiration, sap flow, leaf phenology, stem photosynthesis
Citation: Ye C, Zeng Q, Hu K, Fang D, Hölscher D, Du H, Shi Y, Zhou Y, Berninger F, Mei T and Zhou G (2023) Partitioning of respired CO2 in newly sprouted Moso bamboo culms. Front. Plant Sci. 14:1154232. doi: 10.3389/fpls.2023.1154232
Received: 30 January 2023; Accepted: 30 March 2023;
Published: 20 April 2023.
Edited by:
Attila Ördög, University of Szeged, HungaryReviewed by:
Mattia Terzaghi, University of Bari Aldo Moro, ItalyXimeng Li, Minzu University of China, China
Copyright © 2023 Ye, Zeng, Hu, Fang, Hölscher, Du, Shi, Zhou, Berninger, Mei and Zhou. This is an open-access article distributed under the terms of the Creative Commons Attribution License (CC BY). The use, distribution or reproduction in other forums is permitted, provided the original author(s) and the copyright owner(s) are credited and that the original publication in this journal is cited, in accordance with accepted academic practice. No use, distribution or reproduction is permitted which does not comply with these terms.
*Correspondence: Tingting Mei, ttmei@zafu.edu.cn; Guomo Zhou, zhougm@zafu.edu.cn
†These authors have contributed equally to this work